Gurumuda Networks

Mass and weight – problems and solutions
Solved problems in Newton’s laws of motion – Mass, and weight
1. The weight of a 1 kg mass at the surface of the Earth is… g = 9.8 m/s 2
Mass (m) = 1 kg
The acceleration due to gravity at the surface of the Earth (g) = 9.8 m/s 2
Wanted: weight (w)
m = mass (The SI unit of mass is the kilogram, kg)
g = acceleration due to gravity (The SI unit of g is m/s 2 )
w = weight (The SI unit of w is kg m/s 2 or Newton)
w = (1 kg)(9.8 m/s 2 ) = 9.8 kg m/s 2 = 9.8 Newton
(a) Draw the force of gravity (weight) that act on the object when the object is at rest on a table, as shown in figure (a).
(b) Draw the force of gravity (weight) and it’s components that act on an object sliding down an inclined plane , as shown in figure (b)

The direction of the weight is downward toward the center of the Earth.
w x = the horizontal component of the weight and w y = the vertical component of the weight
3. The mass of a box is 1 kg and acceleration due to gravity is 9.8 m/s 2 . Find (a) weight (b) the horizontal component and the vertical component of the weight.

Weight : w = m g = (1 kg)(9.8 m/s 2 ) = 9.8 kg m/s 2 = 9.8 Newton
The horizontal component of the weight :
w x = w sin 30 o = (9,8 N)(0,5) = 4.9 Newton
The vertical component of the weight :
w y = w cos 30 o = (9.8 N)(0.5√3) = 4.9√3 Newton
[wpdm_package id=’458′]
- Mass and weight
- Normal force
- Newton’s second law of motion
- Friction force
- Motion on the horizontal surface without friction force
- The motion of two bodies with the same acceleration on the rough horizontal surface with the friction force
- Motion on the inclined plane without friction force
- Motion on the rough inclined plane with the friction force
- Motion in an elevator
- The motion of bodies connected by cord and pulley
- Two bodies with the same magnitude of accelerations
- Rounding a flat curve – dynamics of circular motion
- Rounding a banked curve – dynamics of circular motion
- Uniform motion in a horizontal circle
- Centripetal force in uniform circular motion

Share this:
Leave a comment cancel reply.
You must be logged in to post a comment.
This site uses Akismet to reduce spam. Learn how your comment data is processed .
Discover more from Physics
Subscribe now to keep reading and get access to the full archive.
Type your email…
Continue reading
5 Newton’s Laws of Motion
5.4 mass and weight, learning objectives.
By the end of the section, you will be able to:
- Explain the difference between mass and weight
- Explain why falling objects on Earth are never truly in free fall
- Describe the concept of weightlessness
Mass and weight are often used interchangeably in everyday conversation. For example, our medical records often show our weight in kilograms but never in the correct units of newtons. In physics, however, there is an important distinction. Weight is the pull of Earth on an object. It depends on the distance from the center of Earth. Unlike weight, mass does not vary with location. The mass of an object is the same on Earth, in orbit, or on the surface of the Moon.
Units of Force
The equation [latex] {F}_{\text{net}}=ma [/latex] is used to define net force in terms of mass, length, and time. As explained earlier, the SI unit of force is the newton. Since [latex] {F}_{\text{net}}=ma, [/latex]
Although almost the entire world uses the newton for the unit of force, in the United States, the most familiar unit of force is the pound (lb), where 1 N = 0.225 lb. Thus, a 225-lb person weighs 1000 N.
Weight and Gravitational Force
When an object is dropped, it accelerates toward the center of Earth. Newton’s second law says that a net force on an object is responsible for its acceleration. If air resistance is negligible, the net force on a falling object is the gravitational force, commonly called its weight [latex] \overset{\to }{w} [/latex], or its force due to gravity acting on an object of mass m . Weight can be denoted as a vector because it has a direction; down is, by definition, the direction of gravity, and hence, weight is a downward force. The magnitude of weight is denoted as w . Galileo was instrumental in showing that, in the absence of air resistance, all objects fall with the same acceleration g . Using Galileo’s result and Newton’s second law, we can derive an equation for weight.
Consider an object with mass m falling toward Earth. It experiences only the downward force of gravity, which is the weight [latex] \overset{\to }{w} [/latex]. Newton’s second law says that the magnitude of the net external force on an object is [latex] {\overset{\to }{F}}_{\text{net}}=m\overset{\to }{a}. [/latex] We know that the acceleration of an object due to gravity is [latex] \overset{\to }{g}, [/latex] or [latex] \overset{\to }{a}=\overset{\to }{g} [/latex]. Substituting these into Newton’s second law gives us the following equations.
The gravitational force on a mass is its weight. We can write this in vector form, where [latex] \overset{\to }{w} [/latex] is weight and m is mass, as
In scalar form, we can write
Since [latex] g=9.80\,{\text{m/s}}^{2} [/latex] on Earth, the weight of a 1.00-kg object on Earth is 9.80 N:
When the net external force on an object is its weight, we say that it is in free fall , that is, the only force acting on the object is gravity. However, when objects on Earth fall downward, they are never truly in free fall because there is always some upward resistance force from the air acting on the object.
Acceleration due to gravity g varies slightly over the surface of Earth, so the weight of an object depends on its location and is not an intrinsic property of the object. Weight varies dramatically if we leave Earth’s surface. On the Moon, for example, acceleration due to gravity is only [latex] {1.67\,\text{m/s}}^{2} [/latex]. A 1.0-kg mass thus has a weight of 9.8 N on Earth and only about 1.7 N on the Moon.
The broadest definition of weight in this sense is that the weight of an object is the gravitational force on it from the nearest large body, such as Earth, the Moon, or the Sun. This is the most common and useful definition of weight in physics. It differs dramatically, however, from the definition of weight used by NASA and the popular media in relation to space travel and exploration. When they speak of “weightlessness” and “microgravity,” they are referring to the phenomenon we call “free fall” in physics. We use the preceding definition of weight, force [latex] \overset{\to }{w} [/latex] due to gravity acting on an object of mass m , and we make careful distinctions between free fall and actual weightlessness.
Be aware that weight and mass are different physical quantities, although they are closely related. Mass is an intrinsic property of an object: It is a quantity of matter. The quantity or amount of matter of an object is determined by the numbers of atoms and molecules of various types it contains. Because these numbers do not vary, in Newtonian physics, mass does not vary; therefore, its response to an applied force does not vary. In contrast, weight is the gravitational force acting on an object, so it does vary depending on gravity. For example, a person closer to the center of Earth, at a low elevation such as New Orleans, weighs slightly more than a person who is located in the higher elevation of Denver, even though they may have the same mass.
It is tempting to equate mass to weight, because most of our examples take place on Earth, where the weight of an object varies only a little with the location of the object. In addition, it is difficult to count and identify all of the atoms and molecules in an object, so mass is rarely determined in this manner. If we consider situations in which [latex] \overset{\to }{g} [/latex] is a constant on Earth, we see that weight [latex] \overset{\to }{w} [/latex] is directly proportional to mass m , since [latex] \overset{\to }{w}=m\overset{\to }{g}, [/latex] that is, the more massive an object is, the more it weighs. Operationally, the masses of objects are determined by comparison with the standard kilogram, as we discussed in Units and Measurement . But by comparing an object on Earth with one on the Moon, we can easily see a variation in weight but not in mass. For instance, on Earth, a 5.0-kg object weighs 49 N; on the Moon, where g is [latex] {1.67\,\text{m/s}}^{2} [/latex], the object weighs 8.4 N. However, the mass of the object is still 5.0 kg on the Moon.
Clearing a Field
A farmer is lifting some moderately heavy rocks from a field to plant crops. He lifts a stone that weighs 40.0 lb. (about 180 N). What force does he apply if the stone accelerates at a rate of [latex] 1.5\,{\text{m/s}}^{2}? [/latex]
We were given the weight of the stone, which we use in finding the net force on the stone. However, we also need to know its mass to apply Newton’s second law, so we must apply the equation for weight, [latex] w=mg [/latex], to determine the mass.
No forces act in the horizontal direction, so we can concentrate on vertical forces, as shown in the following free-body diagram. We label the acceleration to the side; technically, it is not part of the free-body diagram, but it helps to remind us that the object accelerates upward (so the net force is upward).
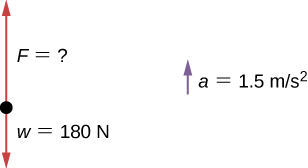
[latex] \begin{array}{ccc}\hfill w& =\hfill & mg\hfill \\ \hfill m& =\hfill & \frac{w}{g}=\frac{180\,\text{N}}{9.8\,{\text{m/s}}^{2}}=18\,\text{kg}\hfill \\ \hfill \sum F& =\hfill & ma\hfill \\ \hfill F-w& =\hfill & ma\hfill \\ \hfill F-180\,\text{N}& =\hfill & (18\,\text{kg})(1.5\,{\text{m/s}}^{2})\hfill \\ \hfill F-180\,\text{N}& =\hfill & 27\,\text{N}\hfill \\ \hfill F& =\hfill & 207\,\text{N}=210\,\text{N to two significant figures}\hfill \end{array} [/latex]
Significance
To apply Newton’s second law as the primary equation in solving a problem, we sometimes have to rely on other equations, such as the one for weight or one of the kinematic equations, to complete the solution.
Check Your Understanding
For (Example) , find the acceleration when the farmer’s applied force is 230.0 N.
[latex] a=2.78\,{\text{m/s}}^{2} [/latex]
Can you avoid the boulder field and land safely just before your fuel runs out, as Neil Armstrong did in 1969? This version of the classic video game accurately simulates the real motion of the lunar lander, with the correct mass, thrust, fuel consumption rate, and lunar gravity. The real lunar lander is hard to control.
Use this interactive simulation to move the Sun, Earth, Moon, and space station to see the effects on their gravitational forces and orbital paths. Visualize the sizes and distances between different heavenly bodies, and turn off gravity to see what would happen without it.
- Mass is the quantity of matter in a substance.
- The weight of an object is the net force on a falling object, or its gravitational force. The object experiences acceleration due to gravity.
- Some upward resistance force from the air acts on all falling objects on Earth, so they can never truly be in free fall.
- Careful distinctions must be made between free fall and weightlessness using the definition of weight as force due to gravity acting on an object of a certain mass.
Conceptual Questions
What is the relationship between weight and mass? Which is an intrinsic, unchanging property of a body?
How much does a 70-kg astronaut weight in space, far from any celestial body? What is her mass at this location?
The astronaut is truly weightless in the location described, because there is no large body (planet or star) nearby to exert a gravitational force. Her mass is 70 kg regardless of where she is located.
Which of the following statements is accurate?
(a) Mass and weight are the same thing expressed in different units.
(b) If an object has no weight, it must have no mass.
(c) If the weight of an object varies, so must the mass.
(d) Mass and inertia are different concepts.
(e) Weight is always proportional to mass.
When you stand on Earth, your feet push against it with a force equal to your weight. Why doesn’t Earth accelerate away from you?
The force you exert (a contact force equal in magnitude to your weight) is small. Earth is extremely massive by comparison. Thus, the acceleration of Earth would be incredibly small. To see this, use Newton’s second law to calculate the acceleration you would cause if your weight is 600.0 N and the mass of Earth is [latex] 6.00\,×\,{10}^{24}\,\text{kg} [/latex].
How would you give the value of [latex] \overset{\to }{g} [/latex] in vector form?
The weight of an astronaut plus his space suit on the Moon is only 250 N. (a) How much does the suited astronaut weigh on Earth? (b) What is the mass on the Moon? On Earth?
a. [latex] \begin{array}{ccc}\hfill {w}_{\text{Moon}}& =\hfill & m{g}_{\text{Moon}}\hfill \\ \hfill m& =\hfill & 150\,\text{kg}\hfill \\ \hfill {w}_{\text{Earth}}& =\hfill & 1.5\,×\,{10}^{3}\,\text{N}\hfill \end{array} [/latex]; b. Mass does not change, so the suited astronaut’s mass on both Earth and the Moon is [latex] 150\,\text{kg.} [/latex]
Suppose the mass of a fully loaded module in which astronauts take off from the Moon is [latex] 1.00\,×\,{10}^{4} [/latex] kg. The thrust of its engines is [latex] 3.00\,×\,{10}^{4} [/latex] N. (a) Calculate the module’s magnitude of acceleration in a vertical takeoff from the Moon. (b) Could it lift off from Earth? If not, why not? If it could, calculate the magnitude of its acceleration.
A rocket sled accelerates at a rate of [latex] {49.0\,\text{m/s}}^{2} [/latex]. Its passenger has a mass of 75.0 kg. (a) Calculate the horizontal component of the force the seat exerts against his body. Compare this with his weight using a ratio. (b) Calculate the direction and magnitude of the total force the seat exerts against his body.
a. [latex] \begin{array}{ccc}\hfill {F}_{\text{h}}& =\hfill & 3.68\,×\,{10}^{3}\,\text{N and}\hfill \\ \hfill w& =\hfill & 7.35\,×\,{10}^{2}\,\text{N}\hfill \\ \hfill \frac{{F}_{\text{h}}}{w}& =\hfill & 5.00\,\text{times greater than weight}\hfill \end{array} [/latex];
b. [latex] \begin{array}{ccc}\hfill {F}_{\text{net}}& =\hfill & 3750\,\text{N}\hfill \\ \hfill \theta & =\hfill & 11.3\text{°}\,\text{from horizontal}\hfill \end{array} [/latex]
Repeat the previous problem for a situation in which the rocket sled decelerates at a rate of [latex] {201\,\text{m/s}}^{2} [/latex]. In this problem, the forces are exerted by the seat and the seat belt.
A body of mass 2.00 kg is pushed straight upward by a 25.0 N vertical force. What is its acceleration?
[latex] \begin{array}{ccc}\hfill w& =\hfill & 19.6\,\text{N}\hfill \\ \hfill {F}_{\text{net}}& =\hfill & 5.40\,\text{N}\hfill \\ \hfill {F}_{\text{net}}& =\hfill & ma⇒a=2.70\,{\text{m/s}}^{2}\hfill \end{array} [/latex]
A car weighing 12,500 N starts from rest and accelerates to 83.0 km/h in 5.00 s. The friction force is 1350 N. Find the applied force produced by the engine.
A body with a mass of 10.0 kg is assumed to be in Earth’s gravitational field with [latex] g=9.80\,{\text{m/s}}^{2} [/latex]. What is its acceleration?
[latex] 0.60\hat{i}-8.4\hat{j}\,{\text{m/s}}^{2} [/latex]
A fireman has mass m ; he hears the fire alarm and slides down the pole with acceleration a (which is less than g in magnitude). (a) Write an equation giving the vertical force he must apply to the pole. (b) If his mass is 90.0 kg and he accelerates at [latex] 5.00\,{\text{m/s}}^{2}, [/latex] what is the magnitude of his applied force?
A baseball catcher is performing a stunt for a television commercial. He will catch a baseball (mass 145 g) dropped from a height of 60.0 m above his glove. His glove stops the ball in 0.0100 s. What is the force exerted by his glove on the ball?
When the Moon is directly overhead at sunset, the force by Earth on the Moon, [latex] {F}_{\text{EM}} [/latex], is essentially at [latex] 90\text{°} [/latex] to the force by the Sun on the Moon, [latex] {F}_{\text{SM}} [/latex], as shown below. Given that [latex] {F}_{\text{EM}}=1.98\,×\,{10}^{20}\,\text{N} [/latex] and [latex] {F}_{\text{SM}}=4.36\,×\,{10}^{20}\,\text{N}, [/latex] all other forces on the Moon are negligible, and the mass of the Moon is [latex] 7.35\,×\,{10}^{22}\,\text{kg}, [/latex] determine the magnitude of the Moon’s acceleration.
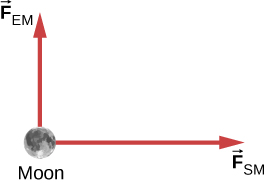
- OpenStax University Physics. Authored by : OpenStax CNX. Located at : https://cnx.org/contents/[email protected]:Gofkr9Oy@15 . License : CC BY: Attribution . License Terms : Download for free at http://cnx.org/contents/[email protected]
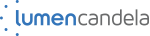
Privacy Policy
5.4 Mass and Weight
Learning objectives.
By the end of this section, you will be able to:
- Explain the difference between mass and weight
- Explain why objects falling through the air are never truly in free fall
- Describe the concept of weightlessness
Mass and weight are often used interchangeably in everyday conversation. For example, our medical records often show our weight in kilograms but never in the correct units of newtons. In physics, however, there is an important distinction. Weight is the pull of Earth on an object. It depends on the distance from the center of Earth. Unlike weight, mass does not vary with location. The mass of an object is the same on Earth, in orbit, or on the surface of the Moon.
Units of Force
The equation F net = m a F net = m a is used to define net force in terms of mass, length, and time. As explained earlier, the SI unit of force is the newton. Since F net = m a , F net = m a ,
Although almost the entire world uses the newton for the unit of force, in the United States, the most familiar unit of force is the pound (lb), where 1 N = 0.225 lb. Thus, a 225-lb person weighs 1000 N.
Weight and Gravitational Force
When an object is dropped, it accelerates toward the center of Earth. Newton’s second law says that a net force on an object is responsible for its acceleration. If air resistance is negligible, the net force on a falling object is the gravitational force, commonly called its weight w → w → , or its force due to gravity acting on an object of mass m . Weight can be denoted as a vector because it has a direction; down is, by definition, the direction of gravity, and hence, weight is a downward force. The magnitude of weight is denoted as w . Galileo was instrumental in showing that, in the absence of air resistance, all objects fall with the same acceleration g . Using Galileo’s result and Newton’s second law, we can derive an equation for weight.
Consider an object with mass m falling toward Earth. It experiences only the downward force of gravity, which is the weight w → w → . Newton’s second law says that the magnitude of the net external force on an object is F → net = m a → . F → net = m a → . We know that the acceleration of an object due to gravity is g → , g → , or a → = g → a → = g → . Substituting these into Newton’s second law gives us the following equations.
The gravitational force on a mass is its weight. We can write this in vector form, where w → w → is weight and m is mass, as
In scalar form, we can write
Since g = 9.80 m/s 2 g = 9.80 m/s 2 on Earth, the weight of a 1.00-kg object on Earth is 9.80 N:
When the net external force on an object is its weight, we say that it is in free fall , that is, the only force acting on the object is gravity. However, when objects on Earth fall downward through the air, they are never truly in free fall because there is always some upward resistance force from the air acting on the object.
Acceleration due to gravity g varies slightly over the surface of Earth, so the weight of an object depends on its location and is not an intrinsic property of the object. Weight varies dramatically if we leave Earth’s surface. On the Moon, for example, acceleration due to gravity is only 1.62 m/s 2 1.62 m/s 2 . A 1.0-kg mass thus has a weight of 9.8 N on Earth and only about 1.6 N on the Moon.
The broadest definition of weight in this sense is that the weight of an object is the gravitational force on it from the nearest large body, such as Earth, the Moon, or the Sun. This is the most common and useful definition of weight in physics. It differs dramatically, however, from the definition of weight used by NASA and the popular media in relation to space travel and exploration. When they speak of “weightlessness” and “microgravity,” they are referring to the phenomenon we call “free fall” in physics. We use the preceding definition of weight, force w → w → due to gravity acting on an object of mass m , and we make careful distinctions between free fall and actual weightlessness.
Be aware that weight and mass are different physical quantities, although they are closely related. Mass is an intrinsic property of an object: It is a quantity of matter. The quantity or amount of matter of an object is determined by the numbers of atoms and molecules of various types it contains. Because these numbers do not vary, in Newtonian physics, mass does not vary; therefore, its response to an applied force does not vary. In contrast, weight is the gravitational force acting on an object, so it does vary depending on gravity. For example, a person closer to the center of Earth, at a low elevation such as New Orleans, weighs slightly more than a person who is located in the higher elevation of Denver, even though they may have the same mass.
It is tempting to equate mass to weight, because most of our examples take place on Earth, where the weight of an object varies only a little with the location of the object. In addition, it is difficult to count and identify all of the atoms and molecules in an object, so mass is rarely determined in this manner. If we consider situations in which g → g → is a constant on Earth, we see that weight w → w → is directly proportional to mass m , since w → = m g → , w → = m g → , that is, the more massive an object is, the more it weighs. Operationally, the masses of objects are determined by comparison with the standard kilogram, as we discussed in Units and Measurement . But by comparing an object on Earth with one on the Moon, we can easily see a variation in weight but not in mass. For instance, on Earth, a 5.0-kg object weighs 49 N; on the Moon, where g is 1.67 m/s 2 1.67 m/s 2 , the object weighs 8.4 N. However, the mass of the object is still 5.0 kg on the Moon.
Example 5.8
Clearing a field, significance, check your understanding 5.6.
For Example 5.8 , find the acceleration when the farmer’s applied force is 230.0 N.
As an Amazon Associate we earn from qualifying purchases.
This book may not be used in the training of large language models or otherwise be ingested into large language models or generative AI offerings without OpenStax's permission.
Want to cite, share, or modify this book? This book uses the Creative Commons Attribution License and you must attribute OpenStax.
Access for free at https://openstax.org/books/university-physics-volume-1/pages/1-introduction
- Authors: William Moebs, Samuel J. Ling, Jeff Sanny
- Publisher/website: OpenStax
- Book title: University Physics Volume 1
- Publication date: Sep 19, 2016
- Location: Houston, Texas
- Book URL: https://openstax.org/books/university-physics-volume-1/pages/1-introduction
- Section URL: https://openstax.org/books/university-physics-volume-1/pages/5-4-mass-and-weight
© Jan 19, 2024 OpenStax. Textbook content produced by OpenStax is licensed under a Creative Commons Attribution License . The OpenStax name, OpenStax logo, OpenStax book covers, OpenStax CNX name, and OpenStax CNX logo are not subject to the Creative Commons license and may not be reproduced without the prior and express written consent of Rice University.
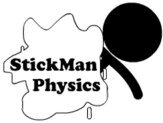
StickMan Physics
Animated Physics Lessons
Mass and Weight
Learn the difference between mass (m) and weight (F w ). Find out how to convert to mass from weight and from weight to mass.
Watch the video as we go through the content and problems set or go through the same material below
Learning Targets
- I know the difference between mass and weight
- I can calculate mass from weight and weight from mass using F w = mg
- I can draw a Force Diagram of an object on a horizontal surface of any planet
Mass is a measurement of matter (molecular composition) of an object. Since an objects molecular composition remains when location changes, mass never changes.
Weight is a measure of the force of gravity on an objects mass. This is directly related to mass but will change with location as the force and acceleration due to gravity changes.
Weight equals mass times gravity
- On the Earth's surface, the accepted value for acceleration due to gravity (g) is 9.81 m/s 2
- In some lessons we had you use a less specific rounded number of 10 m/s 2
Weight Changes When (g) Changes
On Earth, your weight is caused by the earths pull on you. In a future lesson we will see that weight is the result of the your mass, the earths mass, and the distance between you and Earth.
We will solve problems on this page using an average surface acceleration due to gravity
- More massive interstellar objects have a higher surface acceleration due to gravity ( g ).
- The higher the acceleration due to gravity ( g ) the higher the weight ( F w )
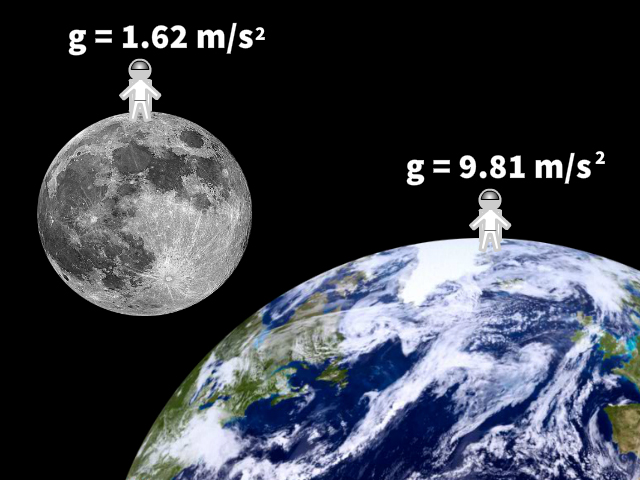
Mass, Radius, and Acceleration due to Gravity of Planets
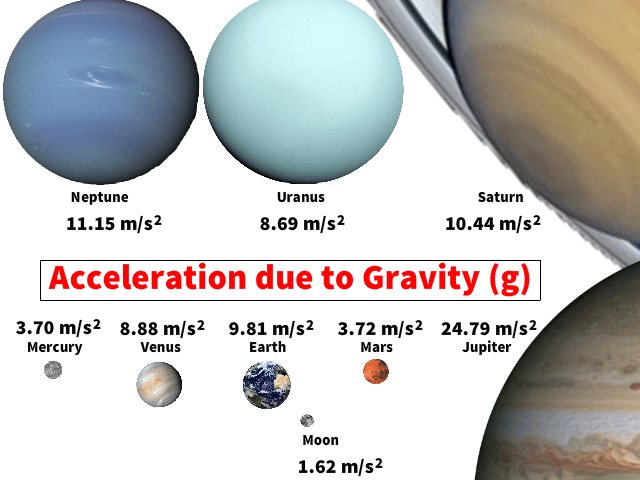
Mass, Radius, and Acceleration due to Gravity of Other Interstellar Objects
Example questions (mass and weight).
1. What is 76 kg Natalia's weight on earth where the acceleration due to gravity is 9.81 m/s 2 ?
See Solution
F w = (76)(9.81)= 745.56 N
2. What is Natalia's weight on the moon where the acceleration due to gravity is 1.62 m/s 2 ?
F w = (76)(1.62)= 123.12 N
3. If 76 kg Natalia went to Neptune where the surface acceleration due to gravity is 11.15 m/s 2 , would her mass or weight change and what would each be?
Mass would still be 76 kg wither location and weight would change
F w = (76)(11.15) = 847.4 N
Normal Force Equals Weight on a Horizontal Surface
Notice in the force diagrams :
- Mass ( m ) of 10 kg does not change
- Weight ( F w ) changes with the acceleration due to gravity
- The normal ( F N )force changes with weight ( F w )
Normal force is the force created by weight on a surface pushing back upwards perpendicular to the surface. Perpendicular to any horizontal surface will be up.
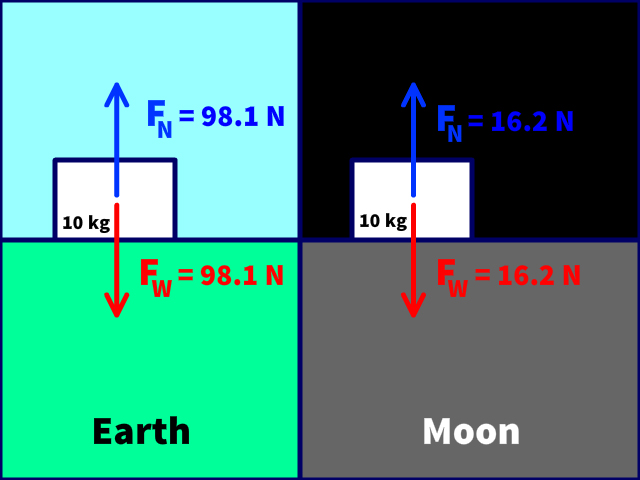
Problem Set
1. What is the weight of a 25 kg object on Pluto where the acceleration due to gravity is 0.62 m/s 2 ?
F w = 15.5 N
2. Draw a force diagram including the normal force of this 25 kg object on a horizontal surface of Pluto where the acceleration due to gravity is 0.62 m/s 2 ?
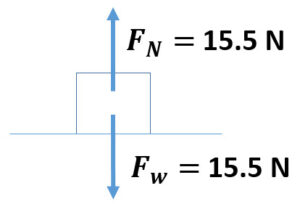
3. What is the mass of a 25 kg object on Pluto where the acceleration due to gravity is 0.62 m/s 2 ?
m = 25 kg (does not change)
4. What is the weight of a 25 kg object on Mars where the acceleration due to gravity is 3.72 m/s 2 ?
5. What is the mass of a 100 N object on the Moon where the acceleration due to gravity is 1.62 m/s 2 ?
6. Draw a force diagram including the normal force of a 100 N object on the surface of the Moon where the acceleration due to gravity is 1.62 m/s 2 ?
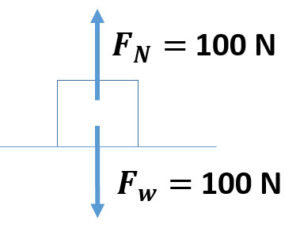
7. If you weigh 1177.2 N on Earth where the acceleration due to gravity is 9.81 m/s 2 what is your mass on Uranus where the acceleration due to gravity is 8.69 m/s 2 ?
8. If you weigh 1177.2 N on Earth where the acceleration due to gravity is 9.81 m/s 2 what is your weight on Uranus where the acceleration due to gravity is 8.69 m/s 2 ?
F w = 1042.8 N
Mass and Weight Quiz
Joe has a mass of 54 kg. What is his mass on the moon where the acceleration due to gravity is 1.62 m/s 2 ?
Mass does not change. Weight does depending on the acceleration due to gravity.
Joe has a mass of 54 kg. What is his weight on the moon where the acceleration due to gravity is 1.62 m/s 2 ?
g = 1.62 m/s 2
F w = (54)(1.62) = 87.48 N
Joe has a mass of 54 kg. What is his weight on the earth?
g = 9.8 m/s 2
F w = (54)(9.8) = 529.2 N
Which changes depending on your location?
Mass (kg) never changes because it is a measure of the matter that you are composed of. You are the same matter on earth or on the moon.
Weight (F w ) changes when g changes. g changes depending on your altitude on earth, in space, or on another planet (it is not always 9.8 m/s 2 which is why many classes use a more rounded 10 m/s 2 instead.
Which is the term for the molecules that make up an object.
Matter is composed of the atoms/molecules that make up an object
What would happen to your weight if you were on a planet with twice the acceleration due to gravity?
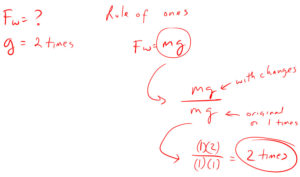
Your score is
- Continue to Newton's Third Law
- Back to the Main Forces Page
- Back to the Stickman Physics Home Page
- Equation Sheet
Terms and Conditions - Privacy Policy
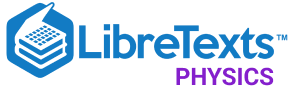
- school Campus Bookshelves
- menu_book Bookshelves
- perm_media Learning Objects
- login Login
- how_to_reg Request Instructor Account
- hub Instructor Commons
- Download Page (PDF)
- Download Full Book (PDF)
- Periodic Table
- Physics Constants
- Scientific Calculator
- Reference & Cite
- Tools expand_more
- Readability
selected template will load here
This action is not available.
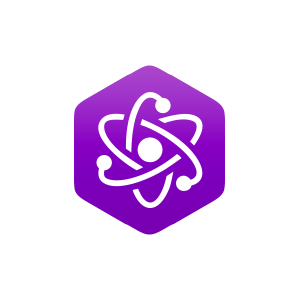
6.12: Mass and Weight
- Last updated
- Save as PDF
- Page ID 18159
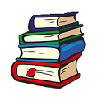
Learning Objectives
- Explain the difference between mass and weight
- Explain why falling objects on Earth are never truly in free fall
- Describe the concept of weightlessness
Mass and weight are often used interchangeably in everyday conversation. For example, our medical records often show our weight in kilograms but never in the correct units of newtons. In physics, however, there is an important distinction. Weight is the pull of Earth on an object. It depends on the distance from the center of Earth. Unlike weight, mass does not vary with location. The mass of an object is the same on Earth, in orbit, or on the surface of the Moon.
Units of Force
The equation F net = ma is used to define net force in terms of mass, length, and time. As explained earlier, the SI unit of force is the newton. Since F net = ma,
\[1\; N = 1\; kg \cdotp m/s^{2} \ldotp \nonumber\]
Although almost the entire world uses the newton for the unit of force, in the United States, the most familiar unit of force is the pound (lb), where 1 N = 0.225 lb. Thus, a 225-lb person weighs 1000 N.
Weight and Gravitational Force
When an object is dropped, it accelerates toward the center of Earth. Newton’s second law says that a net force on an object is responsible for its acceleration. If air resistance is negligible, the net force on a falling object is the gravitational force, commonly called its weight \(\vec{w}\), or its force due to gravity acting on an object of mass m. Weight can be denoted as a vector because it has a direction; down is, by definition, the direction of gravity, and hence, weight is a downward force. The magnitude of weight is denoted as w. Galileo was instrumental in showing that, in the absence of air resistance, all objects fall with the same acceleration g. Using Galileo’s result and Newton’s second law, we can derive an equation for weight.
Consider an object with mass m falling toward Earth. It experiences only the downward force of gravity, which is the weight \(\vec{w}\). Newton’s second law says that the magnitude of the net external force on an object is \(\vec{F}_{net} = m \vec{a}\). We know that the acceleration of an object due to gravity is \(\vec{g}\), or \(\vec{a} = \vec{g}\). Substituting these into Newton’s second law gives us the following equations.
Defintion: Weight
The gravitational force on a mass is its weight. We can write this in vector form, where \(\vec{w}\) is weight and m is mass, as
\[\vec{w} = m \vec{g} \ldotp \label{5.8}\]
In scalar form, we can write
\[w = mg \ldotp \label{5.9}\]
Since g = 9.80 m/s 2 on Earth, the weight of a 1.00-kg object on Earth is 9.80 N:
\[w = mg = (1.00\; kg)(9.80 m/s^{2}) = 9.80\; N \ldotp\]
When the net external force on an object is its weight, we say that it is in free fall , that is, the only force acting on the object is gravity. However, when objects on Earth fall downward, they are never truly in free fall because there is always some upward resistance force from the air acting on the object.
Acceleration due to gravity g varies slightly over the surface of Earth, so the weight of an object depends on its location and is not an intrinsic property of the object. Weight varies dramatically if we leave Earth’s surface. On the Moon, for example, acceleration due to gravity is only 1.67 m/s 2 . A 1.0-kg mass thus has a weight of 9.8 N on Earth and only about 1.7 N on the Moon.
The broadest definition of weight in this sense is that the weight of an object is the gravitational force on it from the nearest large body, such as Earth, the Moon, or the Sun. This is the most common and useful definition of weight in physics. It differs dramatically, however, from the definition of weight used by NASA and the popular media in relation to space travel and exploration. When they speak of “weightlessness” and “microgravity,” they are referring to the phenomenon we call “free fall” in physics. We use the preceding definition of weight, force \(\vec{w}\) due to gravity acting on an object of mass m, and we make careful distinctions between free fall and actual weightlessness.
Be aware that weight and mass are different physical quantities, although they are closely related. Mass is an intrinsic property of an object: It is a quantity of matter. The quantity or amount of matter of an object is determined by the numbers of atoms and molecules of various types it contains. Because these numbers do not vary, in Newtonian physics, mass does not vary; therefore, its response to an applied force does not vary. In contrast, weight is the gravitational force acting on an object, so it does vary depending on gravity. For example, a person closer to the center of Earth, at a low elevation such as New Orleans, weighs slightly more than a person who is located in the higher elevation of Denver, even though they may have the same mass.
It is tempting to equate mass to weight, because most of our examples take place on Earth, where the weight of an object varies only a little with the location of the object. In addition, it is difficult to count and identify all of the atoms and molecules in an object, so mass is rarely determined in this manner. If we consider situations in which \(\vec{g}\) is a constant on Earth, we see that weight \(\vec{w}\) is directly proportional to mass m, since \(\vec{w} = m \vec{g}\), that is, the more massive an object is, the more it weighs. Operationally, the masses of objects are determined by comparison with the standard kilogram, as we discussed in Units and Measurement . But by comparing an object on Earth with one on the Moon, we can easily see a variation in weight but not in mass. For instance, on Earth, a 5.0-kg object weighs 49 N; on the Moon, where g is 1.67 m/s 2 , the object weighs 8.4 N. However, the mass of the object is still 5.0 kg on the Moon.
Example \(\PageIndex{1}\): Clearing a Field
A farmer is lifting some moderately heavy rocks from a field to plant crops. He lifts a stone that weighs 40.0 lb. (about 180 N). What force does he apply if the stone accelerates at a rate of 1.5 m/s 2 ?
We were given the weight of the stone, which we use in finding the net force on the stone. However, we also need to know its mass to apply Newton’s second law, so we must apply the equation for weight, w = mg, to determine the mass.
No forces act in the horizontal direction, so we can concentrate on vertical forces, as shown in the following free-body diagram. We label the acceleration to the side; technically, it is not part of the free-body diagram, but it helps to remind us that the object accelerates upward (so the net force is upward).
\[w = mg \nonumber \]
\[m = \frac{w}{g} = \frac{180\; N}{9.8\; m/s^{2}} = 18\; kg \nonumber\]
\[\sum F = ma \nonumber\]
\[F - w = ma \nonumber\]
\[F - 180\; N = (18\; kg)(1.5\; m/s^{2}) \nonumber\]
\[F - 180\; N = 27\; N \nonumber\]
\[F = 207\; N = 210\; N\; \text{ to two significant figures} \nonumber\]
Significance
To apply Newton’s second law as the primary equation in solving a problem, we sometimes have to rely on other equations, such as the one for weight or one of the kinematic equations, to complete the solution.
Exercise \(\PageIndex{1}\)
For \(\PageIndex{1}\), find the acceleration when the farmer’s applied force is 230.0 N
Can you avoid the boulder field and land safely just before your fuel runs out, as Neil Armstrong did in 1969? This version of the classic video game accurately simulates the real motion of the lunar lander, with the correct mass, thrust, fuel consumption rate, and lunar gravity. The real lunar lander is hard to control.
practice problem 1
Use the weight formula.
W = mg
Solve for mass. Substitute one newton for weight and one standard earth gravity for gravity.
The 96.7 gram tangerine comes closest to this value. Not all tangerines weigh 98.7 grams, however, so this is only a rule of thumb. There are certainly apples, bananas, oranges, tomatoes, and other fruits out there with a mass of approximately 102 grams and thus a weight of approximately one newton.
Those of you familiar with multiple choice tests should have eliminated the chicken egg as a possible answer. A chicken egg is only metaphorically the "fruit of the chicken".
practice problem 2
Here's the way I usually do it — using values I've memorized from years of use.
Here's a more accurate way to do it — using values that are exact by definition.
Not quite a quarter pound, but you get the idea.
The fraction 9 40 gives a decimal expansion of 0.225, which is accurate to three significant figures. Not my favorite fraction, but it gets the job done. With sixteen avoirdupois ounces in a pound, one newton is also about 3½ ounces.
practice problem 3
Practice problem 4.
- PRO Courses Guides New Tech Help Pro Expert Videos About wikiHow Pro Upgrade Sign In
- EDIT Edit this Article
- EXPLORE Tech Help Pro About Us Random Article Quizzes Request a New Article Community Dashboard This Or That Game Popular Categories Arts and Entertainment Artwork Books Movies Computers and Electronics Computers Phone Skills Technology Hacks Health Men's Health Mental Health Women's Health Relationships Dating Love Relationship Issues Hobbies and Crafts Crafts Drawing Games Education & Communication Communication Skills Personal Development Studying Personal Care and Style Fashion Hair Care Personal Hygiene Youth Personal Care School Stuff Dating All Categories Arts and Entertainment Finance and Business Home and Garden Relationship Quizzes Cars & Other Vehicles Food and Entertaining Personal Care and Style Sports and Fitness Computers and Electronics Health Pets and Animals Travel Education & Communication Hobbies and Crafts Philosophy and Religion Work World Family Life Holidays and Traditions Relationships Youth
- Browse Articles
- Learn Something New
- Quizzes Hot
- This Or That Game New
- Train Your Brain
- Explore More
- Support wikiHow
- About wikiHow
- Log in / Sign up
- Education and Communications
- Classical Mechanics
How Find the Weight of an Object Using Mass and Gravity
Last Updated: March 19, 2024 Fact Checked
This article was co-authored by Sean Alexander, MS and by wikiHow staff writer, Johnathan Fuentes . Sean Alexander is an Academic Tutor specializing in teaching mathematics and physics. Sean is the Owner of Alexander Tutoring, an academic tutoring business that provides personalized studying sessions focused on mathematics and physics. With over 15 years of experience, Sean has worked as a physics and math instructor and tutor for Stanford University, San Francisco State University, and Stanbridge Academy. He holds a BS in Physics from the University of California, Santa Barbara and an MS in Theoretical Physics from San Francisco State University. This article has been fact-checked, ensuring the accuracy of any cited facts and confirming the authority of its sources. This article has been viewed 1,352,106 times.
If you’re taking a physics class, you’ll probably be asked to calculate weight from mass. But how do you do this, exactly? We’ve got you covered. While it sounds tricky, calculating weight from mass is very straightforward if you know which formula to use. This article will teach you that formula, plus how to use it when you encounter physics problems in class. As a bonus, we’ve included some practice problems to help these concepts sink in. Keep reading to learn how to calculate weight from mass on your next physics quiz, test, or homework assignment.
Things You Should Know
- The weight of an object equals the force of gravity exerted on that object. The mass of an object is always the same, but its weight changes depending on gravity.
Weight from Mass Calculation Help

Calculating Weight

- If you're using meters , the gravitational acceleration at the Earth's surface is 9.8 m/s 2 . Always use m/s 2 for acceleration, unless you’re instructed to do otherwise.
- If you're asked to use feet , instead of meters , the gravitational acceleration on Earth is 32.2 ft/s 2 . This is the same unit, it's just converted from meters to feet . Luckily, you’re very unlikely to encounter a problem with acceleration written in ft/s 2 .

- The gravitational acceleration on the moon is different from the gravitational acceleration on the Earth. Acceleration due to gravity on the moon is about 1.622 m/s 2 , or about 1/6 of the acceleration that it is here on Earth. That's why you weigh 1/6 of your Earth-weight on the moon. [4] X Research source
- The gravitational acceleration on the sun is different from the gravitational acceleration on the Earth and moon. Acceleration due to gravity on the sun is about 274.0 m/s 2 , or about 28 times the acceleration that it is here on Earth. That's why you would weigh 28 times your Earth-weight on the sun (if you could survive!). [5] X Research source

Sample Problems

Catching Mistakes

- You only have weight while you're " wait "ing on Earth, but even " mass "tronauts have mass.

- 1 pound-force = ~4.448 newtons
- 1 foot = ~0.3048 meters
Community Q&A

Addendum: Weights Expressed in kgf
- A Newton is a SI-unit. Quite often the weight is expressed in kilogramforce or kgf. This is not a SI-unit, therefore less impeccable. But it is very convenient for comparing weights anywhere with weights on Earth.
- 1 kgf = 9.8166 N.
- Divide the calculated number of Newtons by 9.80665, or use the last column when available.
- The weight of the 101 kg astronaut is 101.3 kgf on the North Pole, and 16.5 kgf on the moon.
- What is an SI-unit? It stands for Systeme International d'Unites, a complete metric system of units of measurement for scientists.
- The most difficult part is understanding the difference between weight and mass as people tend to use the words 'weight' and 'mass' interchangeably. They use kilograms for weight, when they should use Newton, or at least kilogramforce. Even your doctor may discuss your weight, when he meant to discuss your mass. Thanks Helpful 0 Not Helpful 0
- The gravitational acceleration g can also be expressed in N/kg. 1 N/kg = 1 m/s 2 exactly. So the numbers remain the same. Thanks Helpful 0 Not Helpful 0
- An astronaut with a mass of 100 kg will weigh 983.2 N on the North Pole, and 162.0 N on the moon. On a neutron star, he'll weigh even more, but he probably won't notice. Thanks Helpful 0 Not Helpful 0

You Might Also Like

- ↑ https://www.grc.nasa.gov/www/k-12/VirtualAero/BottleRocket/airplane/wteq.html
- ↑ https://web.mst.edu/~reflori/be150/Dyn%20Lecture%20Videos/F=ma%20Particle%20Straight%20Line%201/F=ma%20Particle%20Straight%20Line%201.pdf
- ↑ https://www.physicsclassroom.com/class/1DKin/Lesson-5/Acceleration-of-Gravity
- ↑ https://hypertextbook.com/facts/2004/MichaelRobbins.shtml
- ↑ https://www.smartconversion.com/otherInfo/gravity_of_planets_and_the_sun.aspx
About This Article

To find weight when you already know the mass, use the formula weight = mass times gravitational acceleration. Remember that on the surface of the earth, gravitational acceleration is always 9.8 m/s^2, so simply plug in the mass and multiply it by 9.8 to get the weight in newtons. For sample problems and tips for checking your answer and catching mistakes, read on! Did this summary help you? Yes No
- Send fan mail to authors
Reader Success Stories

Angelina Ross
May 29, 2017
Did this article help you?

Jun 6, 2018

Modupe Ikusebiala
Apr 27, 2016

Featured Articles

Trending Articles

Watch Articles

- Terms of Use
- Privacy Policy
- Do Not Sell or Share My Info
- Not Selling Info
Don’t miss out! Sign up for
wikiHow’s newsletter
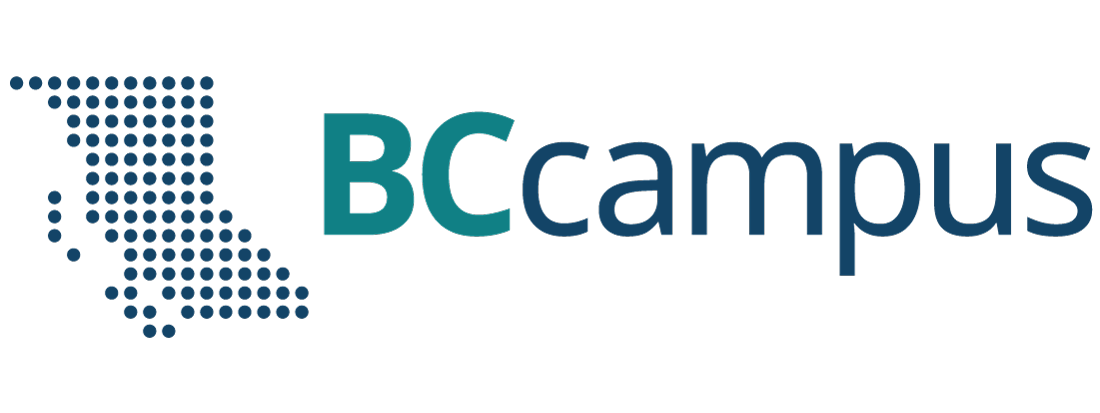
Want to create or adapt books like this? Learn more about how Pressbooks supports open publishing practices.
Newton’s Laws of Motion
Mass and Weight
Learning objectives.
By the end of the section, you will be able to:
- Explain the difference between mass and weight
- Explain why falling objects on Earth are never truly in free fall
- Describe the concept of weightlessness
Mass and weight are often used interchangeably in everyday conversation. For example, our medical records often show our weight in kilograms but never in the correct units of newtons. In physics, however, there is an important distinction. Weight is the pull of Earth on an object. It depends on the distance from the center of Earth. Unlike weight, mass does not vary with location. The mass of an object is the same on Earth, in orbit, or on the surface of the Moon.
Units of Force

Although almost the entire world uses the newton for the unit of force, in the United States, the most familiar unit of force is the pound (lb), where 1 N = 0.225 lb. Thus, a 225-lb person weighs 1000 N.
Weight and Gravitational Force

In scalar form, we can write

When the net external force on an object is its weight, we say that it is in free fall , that is, the only force acting on the object is gravity. However, when objects on Earth fall downward, they are never truly in free fall because there is always some upward resistance force from the air acting on the object.

Be aware that weight and mass are different physical quantities, although they are closely related. Mass is an intrinsic property of an object: It is a quantity of matter. The quantity or amount of matter of an object is determined by the numbers of atoms and molecules of various types it contains. Because these numbers do not vary, in Newtonian physics, mass does not vary; therefore, its response to an applied force does not vary. In contrast, weight is the gravitational force acting on an object, so it does vary depending on gravity. For example, a person closer to the center of Earth, at a low elevation such as New Orleans, weighs slightly more than a person who is located in the higher elevation of Denver, even though they may have the same mass.

Solution No forces act in the horizontal direction, so we can concentrate on vertical forces, as shown in the following free-body diagram. We label the acceleration to the side; technically, it is not part of the free-body diagram, but it helps to remind us that the object accelerates upward (so the net force is upward).
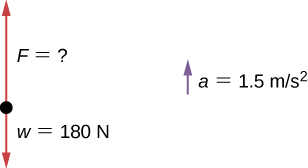
Significance To apply Newton’s second law as the primary equation in solving a problem, we sometimes have to rely on other equations, such as the one for weight or one of the kinematic equations, to complete the solution.
Check Your Understanding For (Figure) , find the acceleration when the farmer’s applied force is 230.0 N.

Can you avoid the boulder field and land safely just before your fuel runs out, as Neil Armstrong did in 1969? This version of the classic video game accurately simulates the real motion of the lunar lander, with the correct mass, thrust, fuel consumption rate, and lunar gravity. The real lunar lander is hard to control.
Use this interactive simulation to move the Sun, Earth, Moon, and space station to see the effects on their gravitational forces and orbital paths. Visualize the sizes and distances between different heavenly bodies, and turn off gravity to see what would happen without it.
- Mass is the quantity of matter in a substance.
- The weight of an object is the net force on a falling object, or its gravitational force. The object experiences acceleration due to gravity.
- Some upward resistance force from the air acts on all falling objects on Earth, so they can never truly be in free fall.
- Careful distinctions must be made between free fall and weightlessness using the definition of weight as force due to gravity acting on an object of a certain mass.
Conceptual Questions
What is the relationship between weight and mass? Which is an intrinsic, unchanging property of a body?
How much does a 70-kg astronaut weight in space, far from any celestial body? What is her mass at this location?
The astronaut is truly weightless in the location described, because there is no large body (planet or star) nearby to exert a gravitational force. Her mass is 70 kg regardless of where she is located.
Which of the following statements is accurate?
(a) Mass and weight are the same thing expressed in different units.
(b) If an object has no weight, it must have no mass.
(c) If the weight of an object varies, so must the mass.
(d) Mass and inertia are different concepts.
(e) Weight is always proportional to mass.
When you stand on Earth, your feet push against it with a force equal to your weight. Why doesn’t Earth accelerate away from you?

The weight of an astronaut plus his space suit on the Moon is only 250 N. (a) How much does the suited astronaut weigh on Earth? (b) What is the mass on the Moon? On Earth?
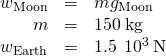
A body of mass 2.00 kg is pushed straight upward by a 25.0 N vertical force. What is its acceleration?
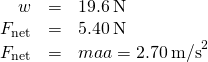
A car weighing 12,500 N starts from rest and accelerates to 83.0 km/h in 5.00 s. The friction force is 1350 N. Find the applied force produced by the engine.

A baseball catcher is performing a stunt for a television commercial. He will catch a baseball (mass 145 g) dropped from a height of 60.0 m above his glove. His glove stops the ball in 0.0100 s. What is the force exerted by his glove on the ball?

Mass and Weight Copyright © 2016 by cnxuniphysics. All Rights Reserved.
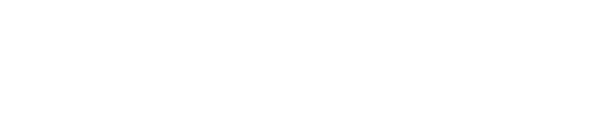
Or search by topic
Number and algebra
- The Number System and Place Value
- Calculations and Numerical Methods
- Fractions, Decimals, Percentages, Ratio and Proportion
- Properties of Numbers
- Patterns, Sequences and Structure
- Algebraic expressions, equations and formulae
- Coordinates, Functions and Graphs
Geometry and measure
- Angles, Polygons, and Geometrical Proof
- 3D Geometry, Shape and Space
- Measuring and calculating with units
- Transformations and constructions
- Pythagoras and Trigonometry
- Vectors and Matrices
Probability and statistics
- Handling, Processing and Representing Data
- Probability
Working mathematically
- Thinking mathematically
- Mathematical mindsets
- Cross-curricular contexts
- Physical and digital manipulatives
For younger learners
- Early Years Foundation Stage
Advanced mathematics
- Decision Mathematics and Combinatorics
- Advanced Probability and Statistics

Resources tagged with: Mass and weight
There are 20 NRICH Mathematical resources connected to Mass and weight , you may find related items under Measuring and calculating with units .
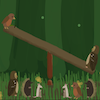
Seesaw Shenanigans
A group of animals has made a seesaw in the woods. How can you make the seesaw balance?
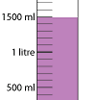
Place Your Orders
Can you rank these sets of quantities in order, from smallest to largest? Can you provide convincing evidence for your rankings?
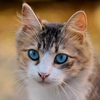
Discuss and Choose
This activity challenges you to decide on the 'best' number to use in each statement. You may need to do some estimating, some calculating and some research.
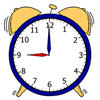
Order, Order!
Can you place these quantities in order from smallest to largest?
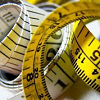
All in a Jumble
My measurements have got all jumbled up! Swap them around and see if you can find a combination where every measurement is valid.
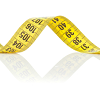
Being Resilient - Primary Measures
Measure problems at primary level that may require resilience.
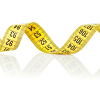
Being Resourceful - Primary Measures
Measure problems at primary level that require careful consideration.
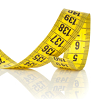
Being Collaborative - Primary Measures
Measure problems for primary learners to work on with others.
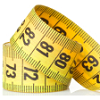
Being Curious - Primary Measures
Measure problems for inquiring primary learners.
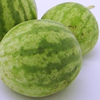
Watermelons
These watermelons have been entered into a competition. Use the information to work out the number of points each one was awarded.
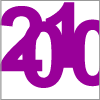
2010: A Year of Investigations
This article for teachers suggests ideas for activities built around 10 and 2010.
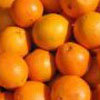
Weighing Fruit
Can you use this information to estimate how much the different fruit selections weigh in kilos and pounds?
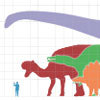
Working with Dinosaurs
This article for teachers suggests ways in which dinosaurs can be a great context for discussing measurement.
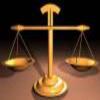
Measure for Measure
This article, written for students, looks at how some measuring units and devices were developed.
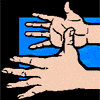
Weigh to Go
This article for teachers recounts the history of measurement, encouraging it to be used as a spring board for cross-curricular activity.
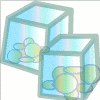
Money Measure
How can you use just one weighing to find out which box contains the lighter ten coins out of the ten boxes?
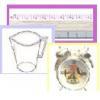
Do You Measure Up?
A game for two or more players that uses a knowledge of measuring tools. Spin the spinner and identify which jobs can be done with the measuring tool shown.
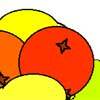
Oranges and Lemons
On the table there is a pile of oranges and lemons that weighs exactly one kilogram. Using the information, can you work out how many lemons there are?
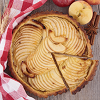
Grandma found her pie balanced on the scale with two weights and a quarter of a pie. So how heavy was each pie?
What's My Weight?
There are four equal weights on one side of the scale and an apple on the other side. What can you say that is true about the apple and the weights from the picture?
WORD PROBLEMS INVOLVING MASS AND WEIGHT
The mass of an object is the amount of matter it contains.
Example 1 :
If one egg has a mass of 55 g, find the total mass of eggs in 50 cartons, each containing 12 eggs.
Mass of 1 egg = 55 g
Number of eggs in each carton = 12
Total number of cartons = 50
Total mass = 55 x 12 x 50
= 33000 g
1000g = 1 kg
= 33 kg
Example 2 :
Find the total mass of 32 chocolates, each of mass 28 grams.
Number of chocolates = 32
Mass of each chocolate = 28 grams
Total mass = 32 x 28
= 896 grams
Example 3 :
If a clothes peg has a mass of 6.5 g, how many pegs are there in a 13 kg box?
Mass of 1 cloth peg = 6.5 g
Mass of box = 13 kg
1000 grams = 1 kg
Converting kg to grams, we get
= 13000 grams
Number of pegs = 13000/6.5
= 2000 pegs
Example 4 :
If a roof tile has mass 1.25 kilograms, how many tiles could a van with a load limit of 8 tonnes carry?
1000 kg = 1 tonne
8 tonnes = 8(1000)
= 8000 kg
Mass of 1 roof tile = 1.25 kg
Number of roof tiles carried by the van = 8000/1.25
= 6400 tiles
Example 5 :
Find the total mass in tonnes of 3500 books, each with mass 800 grams.
Mass of each book = 800 grams
Total number of books = 3500
Mass of 3500 books = 800 x 3500
= 2800000 grams
= 2800000/1000
= 2800 kg
1000 kg = 1 tonne
= 2800/1000
= 2.8 tonnes
Example 6 :
If the mass of 8000 oranges is 1.04 tonnes, what is the average mass of one orange?
Total number of oranges = 8000
Weight of oranges = 1.04 tonnes
= 1.04 (1000)
= 1040 kg
= 1040(1000)
= 1040000 grams
Average weight of 1 orange = 1040000 / 8000
= 130 grams
So, mass of 1 orange is 130 grams.

Apart from the stuff given above, if you need any other stuff in math, please use our google custom search here.
Kindly mail your feedback to [email protected]
We always appreciate your feedback.
© All rights reserved. onlinemath4all.com
- Sat Math Practice
- SAT Math Worksheets
- PEMDAS Rule
- BODMAS rule
- GEMDAS Order of Operations
- Math Calculators
- Transformations of Functions
- Order of rotational symmetry
- Lines of symmetry
- Compound Angles
- Quantitative Aptitude Tricks
- Trigonometric ratio table
- Word Problems
- Times Table Shortcuts
- 10th CBSE solution
- PSAT Math Preparation
- Privacy Policy
- Laws of Exponents
Recent Articles

Honors Algebra 2 Problems on Solving Logarithmic Equations
Apr 03, 24 11:00 PM
Honors Algebra 2 Solving Exponential Equations with Logarithms
Apr 03, 24 10:57 PM
A Problem Solving Plan in Mathematics
Apr 03, 24 08:37 PM
The home of mathematics education in New Zealand.
- Forgot password ?
- Resource Finder
Weighty Problems
The Ministry is migrating nzmaths content to Tāhurangi. Relevant and up-to-date teaching resources are being moved to Tāhūrangi (tahurangi.education.govt.nz). When all identified resources have been successfully moved, this website will close. We expect this to be in June 2024. e-ako maths, e-ako Pāngarau, and e-ako PLD 360 will continue to be available.
For more information visit https://tahurangi.education.govt.nz/updates-to-nzmaths
This unit comprises six problems for students to apply and interpret measurement of mass. Students are also introduced to the concepts of net and gross mass.
- Select the appropriate standard unit of measurement for a specific application.
- Measure masses with appropriate measuring devices.
- Measure net and gross mass.
Mass is the force created by gravity acting of on an object. Mass is felt as weight, a force that pulls the object towards the centre of the Earth. Mass is measured in units based on grams, and tonnes. Larger or smaller units are created by combining or equally partitioning these units. One kilogram is a combination of 1000 grams (kilo means 1000). One milligram is 1/1000 of a gram and one microgram is 1/ 1 000 000 of a gram. The units for mass come from the mass of water. One cubic metre of water has a mass of 1 tonne, or 1000 kilograms. One millilitre of water has a mass of one gram.
Note that in the New Zealand Curriculum document, “weight” and “mass” are used interchangeably. In a science context, the definition of “force created by gravity acting on an object” would often be equated with weight, not mass. Consider the scientific knowledge of your students (e.g. are they studying forces in science). It may be more appropriate to define mass as the amount of matter in an object (measured in kilograms) and weight using the adorementioned definition (measured in Newtons, N).
The learning opportunities in this unit can be differentiated by providing or removing support to students and by varying the task requirements. Ways to support students include:
- letting students attempt problems using physical materials as much as possible, so they develop a ‘feel’ for the benchmark units
- directly modelling measurement with tools, like digital scales for mass
- providing opportunities for students to copy the correct use of tools
- clarifying the language of measurement units, such as “kilogram” as a mass that is made up of 1000 grams
- clarifying the meaning of symbols, e.g. 45g as 45 grams, and 45kg as 45 kilograms; 45t as 45 tonnes
- encouraging students to work collaboratively (mahi tahi) to share and justify their ideas
- easing the calculation demands by providing calculators where appropriate.
Tasks can be varied in many ways including:
- reducing the complexity of the numbers involved in the station tasks, e.g. whole number versus fraction dimensions. The choice of measurement units influences the difficulty of calculation as well as the level of precision
- allowing physical solutions with manipulatives before requiring abstract (in the head) anticipation of measures
- creating or using models of standard units, e.g. 1 litre of water for the mass of 1 kilogram
- reducing the demands for a product, e.g. less calculations and words, and more diagrams and models.
The context for this unit can be adapted to suit the interests and cultural backgrounds of your students. Use the interests of your students to create contexts that will engage them. Students may be interested in the mass of rugby players. Students from large whānau, or who prepare food for large numbers of people, may relate to measuring quantities to scale up recipes. Carrying heavy objects was a major problem for pre-European Māori. How did they carry heavy loads, or move waka? Counting on Frank by Rod Clement may inspire some students to look for eccentric ways to apply measurement to their daily lives. For example, the human body is 60% water, by mass. How much water is in their body?
Te reo Māori vocabulary terms such as maihea (weight / mass), karamu (gram), manokaramu (kilogram), and tana (tonne) could be introduced in this unit and used throughout other mathematical learning.
- Station 1: Copymaster 1 , $1, $2 and 50c coins, scales (preferably digital and able to weigh in grams), metre ruler
- Station 2: Copymaster 2 , calculator
- Station 3: Copymaster 3 , calculator
- Station 4: Copymaster 4 , access to the internet.
- Station 5: Copymaster 5 , 1L measuring jug, five different sized plastic containers, scales, eyedropper
- Station 6: Copymaster 6 , can of dog food, supermarket bags, calculator
The following six stations provide a range of problems for students to apply and interpret measurement of mass. Consider what would be the most effective method for introducing these to your class. You could work on them as a whole class and provide support to groups of students. Alternatively, you could use another relevant igniting activity to introduce the context for learning, before directing students to work on one or more of these stations independently, or in small groups. These stations could serve as the basis for learning in different sessions, or could be used as one session. At the conclusion of these stations, students draw on the problems presented and create their own stations to be used in other lessons.
Station 1: A kilo of coins
You have won a prize which can be just one of the following:
- 1 kilogram of $1 coins
- A 1.5 metre long trail of $2 coins (lying flat and touching)
- A 0.5 metre high stack of 50c coins
What is your choice?
1 kg of $1 coins (1000 ÷ 8 = 125 coins, so $125)
1.5 metre of $2 coins (1500 ÷ 26.5 = 57 coins, so $114)
0.5 metre stack of 50c coins (500 ÷ 1.7 = 294 coins, so $147)
Station 2: Largest Lasagne
This problem could be adapted to reflect food that is meaningful to your students (e.g. the largest tray of pani popo).
The world’s largest lasagne was made in 2012 at a restaurant in Wieliczka, Poland. It weighed 4865 kg and measured 25 m x 2.5 m.
The ingredients were:
2500kg of pasta, 800kg of mince, 400kg of mozzarella cheese, 100kg of peas, 100kg of carrots, and equal amounts of white sauce and tomato sauce.
- How much did the white sauce and tomato sauce weigh?
- What would be the size of a 500g piece from the lasagne?
- How many people could be fed with the whole lasagne? Show how you arrived at your estimate.
- The other ingredients total 3900kg so the sauces must weigh 4865 – 3900 = 965 kg. 500L of each sauce was used. Does that sound right?
- A 500g piece would be about 1/10 000 of the whole lasagne. One way is to cut both the length and width into 100 parts, since 100 x 100 = 10 000. A single piece would measure 25cm x 2.5cm. That’s a bit skinny so 12.5cm x 5cm might work better.
- The lasagne was actually cut into 10 000 pieces so that’s how many people were fed. Each piece had a mass of 0.486 kg or 486 grams. That is a good serving of lasagne.
Station 3: Weighing Tonnes
Konsihiki was the largest active sumo wrestler in the world with a mass of 287 kg. Now he is retired.
How many Konishikis weigh as much as 1 tonne?
Make a table of tonne weights using objects in the classroom. Remember that 1000 kg is a tonne.
The number of Konshihikis in 1 tonne equals 3.48, about 3 and ½ of him.
To find how many of any object make 1 tonne, divided 1 000 by the weight of the object in kilograms. For example, if a schoolbag weighs 5kg then 1 000 ÷ 5 = 200 make 1 tonne.
Station 4: Jumbo facts
Find out facts about the mass of very large animals and make a report about these animals for the class. To get you started here are some facts about the Blue Whale, which can be seen in New Zealand waters.
The blue whale is the largest animal living on Earth. It can reach up to nearly 30 metres in length and weigh up to 180 tonnes (t). Their tongues alone can weigh as much as some elephants and their hearts are huge, weighing a whopping 180kg. They have the largest babies on Earth. When they are first born they can be 8 metres (m) in length and weigh 4000kg. Imagine a jet engine that registers at 140 decibels. A blue whale, when it calls, registers at 188 decibels. Compare the facts about the Blue Whale with the large African elephant
The African elephant is the biggest animal on land. Fully grown the male can be 7 metres long, 3.2 metres tall at the shoulder and have a mass of 6500kg. Its tusks can weigh as much as 100kg each. The largest pair of tusks on record are in the British Museum and weigh 133kg each. What combination of animals could be equal to the elephant's weight?
For example, it takes 6500 ÷ 5 = 1300 big domestic cats to weigh 1 elephant or 130 big dogs.
How many rhinoceroses, lions, giraffes, or hippopotamuses weigh the same as an elephant?
Answers will vary depending on what other animals your students research.
Station 5: Mass of water
Measure out one litre (l) of water.
- What is the mass of one litre of water? If 1L = 1000ml, what is the mass of 1mL of water?
For each container, estimate the capacity of the container, measure it to check, estimate the mass of water when the container is full, and find the mass of the water using scales.
Record your results like this:
- How many drops of water are needed to fill each container?
- What is the mass of a single drop?
- 1 litre (l) of water has a mass of 1 kilogram (1000 grams). 1 millilitre (mL) of water has a mass of 1 gram.
Answers depend on the size of the containers. Here is an example:
- About 20 drops make 1 ml of water. Find the capacity of the container in mL then multiply by 20 to get the number of drops.
- A single drop has a mass of 1/20 of 1g, that’s 0.05g.
Station 6: Frank’s arms
Counting on Frank by Rod Clement (1990; Harper Collins Publishers: Sydney) has some great ideas for measurement investigations. You can view readings of the book on YouTube if you cannot source a copy of the book. One of the ideas introduced in the story is about Frank carrying a trolley load of cans to the supermarket.
- Trolleys measure 60 litres or 80 litres. What do those measures mean?
- How heavy do you think Frank’s load of cans is?
- How many cans are you able to carry in a reusable supermarket bag?
How did you work that out?
Here is another task based on Counting on Frank that you may choose to use.
- 1 litre is a unit of capacity, but it is also used as a unit of volume. One litre measures 10cm x 10cm x 10cm. 60 litres is 60 times that size.
- Frank has 47 cans. They could be 420g cans so they would weigh 47 x 420g = 19 740g or 19.740 kg (about 20 kg). If the cans are bigger, say 820g, then the mass equals 47 times the mass of one can.
- You could get 24 x 420g cans in a supermarket bag, or 36 cans if you add another layer. The mass of the bag would be 24 x 420g = 10.080 kg or 36 x 420g = 15.12 kg.
Dear family and whānau,
In class we have talked a lot about weight this week. In particular, we discovered that Konshiki, the largest Sumo wrestler, weighs 226kg, a Blue Whale weighs 180 tonnes and an elephant weighs 6500kg. What do you think the Blue Whale weighs in kg?
Your child has been asked to look for other facts associated with weight by reading the newspaper or doing some reading on the internet. They should record what they find out. Please encourage them to discuss their findings with you.
You could support them further by weighing items at home or cooking with them.
Figure It Out
Some links from the Figure It Out series which you may find useful are:
- Measurement, Level 3-4: Can You, page 5; Breaking Bags, page 9; Egging you on, page 21
- Sport, Level 3-4, Scrum Power, page 20
- Measurement, Level 4: Taking Off, pages 6 & 7; Weighty Water, pages 12 & 13
Log in or register to create plans from your planning space that include this resource.
- Printer-friendly version
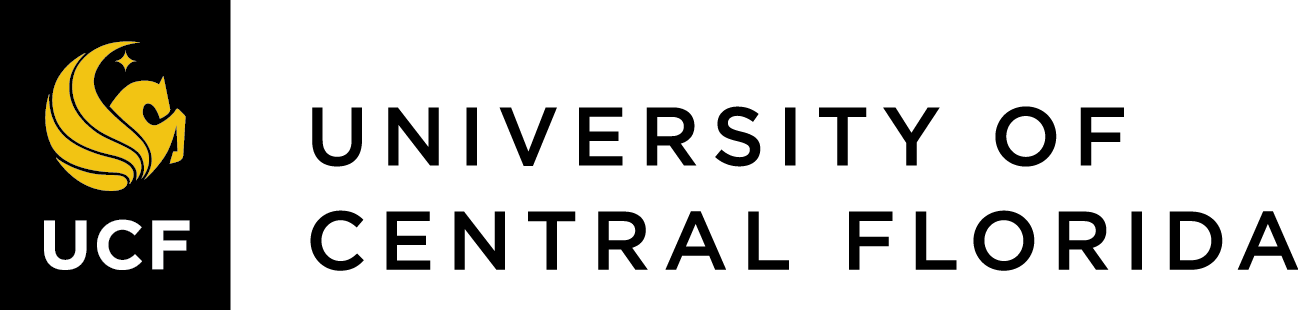
5 Newton’s Laws of Motion
5.4 Mass and Weight
Learning objectives.
By the end of the section, you will be able to:
- Explain the difference between mass and weight
- Explain why falling objects on Earth are never truly in free fall
- Describe the concept of weightlessness
Mass and weight are often used interchangeably in everyday conversation. For example, our medical records often show our weight in kilograms but never in the correct units of newtons. In physics, however, there is an important distinction. Weight is the pull of Earth on an object. It depends on the distance from the center of Earth. Unlike weight, mass does not vary with location. The mass of an object is the same on Earth, in orbit, or on the surface of the Moon.
Units of Force
The equation [latex]{F}_{\text{net}}=ma[/latex] is used to define net force in terms of mass, length, and time. As explained earlier, the SI unit of force is the newton. Since [latex]{F}_{\text{net}}=ma,[/latex]
Although almost the entire world uses the newton for the unit of force, in the United States, the most familiar unit of force is the pound (lb), where 1 N = 0.225 lb. Thus, a 225-lb person weighs 1000 N.
Weight and Gravitational Force
When an object is dropped, it accelerates toward the center of Earth. Newton’s second law says that a net force on an object is responsible for its acceleration. If air resistance is negligible, the net force on a falling object is the gravitational force, commonly called its weight [latex]\mathbf{\overset{\to }{w}}[/latex], or its force due to gravity acting on an object of mass m . Weight can be denoted as a vector because it has a direction; down is, by definition, the direction of gravity, and hence, weight is a downward force. The magnitude of weight is denoted as w . Galileo was instrumental in showing that, in the absence of air resistance, all objects fall with the same acceleration g . Using Galileo’s result and Newton’s second law, we can derive an equation for weight.
Consider an object with mass m falling toward Earth. It experiences only the downward force of gravity, which is the weight [latex]\mathbf{\overset{\to }{w}}[/latex]. Newton’s second law says that the magnitude of the net external force on an object is [latex]{\mathbf{\overset{\to }{F}}}_{\text{net}}=m\mathbf{\overset{\to }{a}}.[/latex] We know that the acceleration of an object due to gravity is [latex]\mathbf{\overset{\to }{g}},[/latex] or [latex]\mathbf{\overset{\to }{a}}=\mathbf{\overset{\to }{g}}[/latex]. Substituting these into Newton’s second law gives us the following equations.
The gravitational force on a mass is its weight. We can write this in vector form, where [latex]\mathbf{\overset{\to }{w}}[/latex] is weight and m is mass, as
In scalar form, we can write
Since [latex]g=9.80\,{\text{m/s}}^{2}[/latex] on Earth, the weight of a 1.00-kg object on Earth is 9.80 N:
When the net external force on an object is its weight, we say that it is in free fall , that is, the only force acting on the object is gravity. However, when objects on Earth fall downward, they are never truly in free fall because there is always some upward resistance force from the air acting on the object.
Acceleration due to gravity g varies slightly over the surface of Earth, so the weight of an object depends on its location and is not an intrinsic property of the object. Weight varies dramatically if we leave Earth’s surface. On the Moon, for example, acceleration due to gravity is only [latex]{1.67\,\text{m/s}}^{2}[/latex]. A 1.0-kg mass thus has a weight of 9.8 N on Earth and only about 1.7 N on the Moon.
The broadest definition of weight in this sense is that the weight of an object is the gravitational force on it from the nearest large body, such as Earth, the Moon, or the Sun. This is the most common and useful definition of weight in physics. It differs dramatically, however, from the definition of weight used by NASA and the popular media in relation to space travel and exploration. When they speak of “weightlessness” and “microgravity,” they are referring to the phenomenon we call “free fall” in physics. We use the preceding definition of weight, force [latex]\mathbf{\overset{\to }{w}}[/latex] due to gravity acting on an object of mass m , and we make careful distinctions between free fall and actual weightlessness.
Be aware that weight and mass are different physical quantities, although they are closely related. Mass is an intrinsic property of an object: It is a quantity of matter. The quantity or amount of matter of an object is determined by the numbers of atoms and molecules of various types it contains. Because these numbers do not vary, in Newtonian physics, mass does not vary; therefore, its response to an applied force does not vary. In contrast, weight is the gravitational force acting on an object, so it does vary depending on gravity. For example, a person closer to the center of Earth, at a low elevation such as New Orleans, weighs slightly more than a person who is located in the higher elevation of Denver, even though they may have the same mass.
It is tempting to equate mass to weight, because most of our examples take place on Earth, where the weight of an object varies only a little with the location of the object. In addition, it is difficult to count and identify all of the atoms and molecules in an object, so mass is rarely determined in this manner. If we consider situations in which [latex]\mathbf{\overset{\to }{g}}[/latex] is a constant on Earth, we see that weight [latex]\mathbf{\overset{\to }{w}}[/latex] is directly proportional to mass m , since [latex]\mathbf{\overset{\to }{w}}=m\mathbf{\overset{\to }{g}},[/latex] that is, the more massive an object is, the more it weighs. Operationally, the masses of objects are determined by comparison with the standard kilogram, as we discussed in Units and Measurement . But by comparing an object on Earth with one on the Moon, we can easily see a variation in weight but not in mass. For instance, on Earth, a 5.0-kg object weighs 49 N; on the Moon, where g is [latex]{1.67\,\text{m/s}}^{2}[/latex], the object weighs 8.4 N. However, the mass of the object is still 5.0 kg on the Moon.
Clearing a Field
A farmer is lifting some moderately heavy rocks from a field to plant crops. He lifts a stone that weighs 40.0 lb. (about 180 N). What force does he apply if the stone accelerates at a rate of [latex]1.5\,{\text{m/s}}^{2}?[/latex]
We were given the weight of the stone, which we use in finding the net force on the stone. However, we also need to know its mass to apply Newton’s second law, so we must apply the equation for weight, [latex]w=mg[/latex], to determine the mass.
No forces act in the horizontal direction, so we can concentrate on vertical forces, as shown in the following free-body diagram. We label the acceleration to the side; technically, it is not part of the free-body diagram, but it helps to remind us that the object accelerates upward (so the net force is upward).
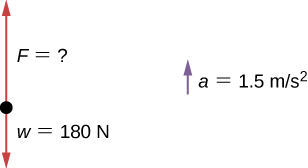
Significance
To apply Newton’s second law as the primary equation in solving a problem, we sometimes have to rely on other equations, such as the one for weight or one of the kinematic equations, to complete the solution.
Check Your Understanding
For (Example) , find the acceleration when the farmer’s applied force is 230.0 N.
[latex]a=2.78\,{\text{m/s}}^{2}[/latex]
Can you avoid the boulder field and land safely just before your fuel runs out, as Neil Armstrong did in 1969? This version of the classic video game accurately simulates the real motion of the lunar lander, with the correct mass, thrust, fuel consumption rate, and lunar gravity. The real lunar lander is hard to control.
Use this interactive simulation to move the Sun, Earth, Moon, and space station to see the effects on their gravitational forces and orbital paths. Visualize the sizes and distances between different heavenly bodies, and turn off gravity to see what would happen without it.
- Mass is the quantity of matter in a substance.
- The weight of an object is the net force on a falling object, or its gravitational force. The object experiences acceleration due to gravity.
- Some upward resistance force from the air acts on all falling objects on Earth, so they can never truly be in free fall.
- Careful distinctions must be made between free fall and weightlessness using the definition of weight as force due to gravity acting on an object of a certain mass.
Conceptual Questions
What is the relationship between weight and mass? Which is an intrinsic, unchanging property of a body?
How much does a 70-kg astronaut weight in space, far from any celestial body? What is her mass at this location?
The astronaut is truly weightless in the location described, because there is no large body (planet or star) nearby to exert a gravitational force. Her mass is 70 kg regardless of where she is located.
Which of the following statements is accurate?
(a) Mass and weight are the same thing expressed in different units.
(b) If an object has no weight, it must have no mass.
(c) If the weight of an object varies, so must the mass.
(d) Mass and inertia are different concepts.
(e) Weight is always proportional to mass.
When you stand on Earth, your feet push against it with a force equal to your weight. Why doesn’t Earth accelerate away from you?
The force you exert (a contact force equal in magnitude to your weight) is small. Earth is extremely massive by comparison. Thus, the acceleration of Earth would be incredibly small. To see this, use Newton’s second law to calculate the acceleration you would cause if your weight is 600.0 N and the mass of Earth is [latex]6.00\times {10}^{24}\,\text{kg}[/latex].
How would you give the value of [latex]\mathbf{\overset{\to }{g}}[/latex] in vector form?
The weight of an astronaut plus his space suit on the Moon is only 250 N. (a) How much does the suited astronaut weigh on Earth? (b) What is the mass on the Moon? On Earth?
a. [latex]\begin{array}{ccc}\hfill {w}_{\text{Moon}}& =\hfill & m{g}_{\text{Moon}}\hfill \\ \hfill m& =\hfill & 150\,\text{kg}\hfill \\ \hfill {w}_{\text{Earth}}& =\hfill & 1.5\times {10}^{3}\,\text{N}\hfill \end{array}[/latex]; b. Mass does not change, so the suited astronaut’s mass on both Earth and the Moon is [latex]150\,\text{kg.}[/latex]
Suppose the mass of a fully loaded module in which astronauts take off from the Moon is [latex]1.00\times {10}^{4}[/latex] kg. The thrust of its engines is [latex]3.00\times {10}^{4}[/latex] N. (a) Calculate the module’s magnitude of acceleration in a vertical takeoff from the Moon. (b) Could it lift off from Earth? If not, why not? If it could, calculate the magnitude of its acceleration.
A rocket sled accelerates at a rate of [latex]{49.0\,\text{m/s}}^{2}[/latex]. Its passenger has a mass of 75.0 kg. (a) Calculate the horizontal component of the force the seat exerts against his body. Compare this with his weight using a ratio. (b) Calculate the direction and magnitude of the total force the seat exerts against his body.
a. [latex]\begin{array}{ccc}\hfill {F}_{\text{h}}& =\hfill & 3.68\times {10}^{3}\,\text{N and}\hfill \\ \hfill w& =\hfill & 7.35\times {10}^{2}\,\text{N}\hfill \\ \hfill \frac{{F}_{\text{h}}}{w}& =\hfill & 5.00\,\text{times greater than weight}\hfill \end{array}[/latex];
b. [latex]\begin{array}{ccc}\hfill {F}_{\text{net}}& =\hfill & 3750\,\text{N}\hfill \\ \hfill \theta & =\hfill & 11.3^\circ\,\text{from horizontal}\hfill \end{array}[/latex]
Repeat the previous problem for a situation in which the rocket sled decelerates at a rate of [latex]{201\,\text{m/s}}^{2}[/latex]. In this problem, the forces are exerted by the seat and the seat belt.
A body of mass 2.00 kg is pushed straight upward by a 25.0 N vertical force. What is its acceleration?
[latex]\begin{array}{ccc}\hfill w& =\hfill & 19.6\,\text{N}\hfill \\ \hfill {F}_{\text{net}}& =\hfill & 5.40\,\text{N}\hfill \\ \hfill {F}_{\text{net}}& =\hfill & ma\Rightarrow a=2.70\,{\text{m/s}}^{2}\hfill \end{array}[/latex]
A car weighing 12,500 N starts from rest and accelerates to 83.0 km/h in 5.00 s. The friction force is 1350 N. Find the applied force produced by the engine.
A body with a mass of 10.0 kg is assumed to be in Earth’s gravitational field with [latex]g=9.80\,{\text{m/s}}^{2}[/latex]. What is its acceleration?
[latex]0.60\mathbf{\hat{i}}-8.4\mathbf{\hat{j}}\,{\text{m/s}}^{2}[/latex]
A fireman has mass m ; he hears the fire alarm and slides down the pole with acceleration a (which is less than g in magnitude). (a) Write an equation giving the vertical force he must apply to the pole. (b) If his mass is 90.0 kg and he accelerates at [latex]5.00\,{\text{m/s}}^{2},[/latex] what is the magnitude of his applied force?
A baseball catcher is performing a stunt for a television commercial. He will catch a baseball (mass 145 g) dropped from a height of 60.0 m above his glove. His glove stops the ball in 0.0100 s. What is the force exerted by his glove on the ball?
When the Moon is directly overhead at sunset, the force by Earth on the Moon, [latex]{F}_{\text{EM}}[/latex], is essentially at [latex]90^\circ[/latex] to the force by the Sun on the Moon, [latex]{F}_{\text{SM}}[/latex], as shown below. Given that [latex]{F}_{\text{EM}}=1.98\times {10}^{20}\,\text{N}[/latex] and [latex]{F}_{\text{SM}}=4.36\times {10}^{20}\,\text{N},[/latex] all other forces on the Moon are negligible, and the mass of the Moon is [latex]7.35\times {10}^{22}\,\text{kg},[/latex] determine the magnitude of the Moon’s acceleration.
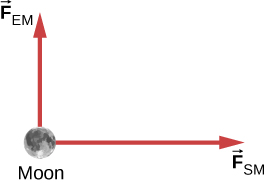
5.4 Mass and Weight Copyright © 2016 by OpenStax. All Rights Reserved.
Share This Book
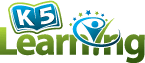
Reading & Math for K-5
- Kindergarten
- Learning numbers
- Comparing numbers
- Place Value
- Roman numerals
- Subtraction
- Multiplication
- Order of operations
- Drills & practice
- Measurement
- Factoring & prime factors
- Proportions
- Shape & geometry
- Data & graphing
- Word problems
- Children's stories
- Leveled Stories
- Context clues
- Cause & effect
- Compare & contrast
- Fact vs. fiction
- Fact vs. opinion
- Main idea & details
- Story elements
- Conclusions & inferences
- Sounds & phonics
- Words & vocabulary
- Reading comprehension
- Early writing
- Numbers & counting
- Simple math
- Social skills
- Other activities
- Dolch sight words
- Fry sight words
- Multiple meaning words
- Prefixes & suffixes
- Vocabulary cards
- Other parts of speech
- Punctuation
- Capitalization
- Narrative writing
- Opinion writing
- Informative writing
- Cursive alphabet
- Cursive letters
- Cursive letter joins
- Cursive words
- Cursive sentences
- Cursive passages
- Grammar & Writing
Breadcrumbs
- Word Problems
- Mass & weight
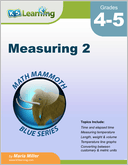
Download & Print Only $7.70
Mass word problems
Word problem worksheets: mass and weight.
These word problems involve mass or weight. Problems involve the addition, subtraction, multiplication or division of amounts with units of mass . There are worksheets on both customary units (ounces & pounds) and metric units (gm, kg). Students are not asked to convert between the two systems.

Ounces, pounds:
Grams, kilograms:
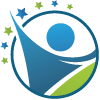
These worksheets are available to members only.
Join K5 to save time, skip ads and access more content. Learn More
More word problem worksheets
Explore all of our math word problem worksheets , from kindergarten through grade 5.
What is K5?
K5 Learning offers free worksheets , flashcards and inexpensive workbooks for kids in kindergarten to grade 5. Become a member to access additional content and skip ads.
Our members helped us give away millions of worksheets last year.
We provide free educational materials to parents and teachers in over 100 countries. If you can, please consider purchasing a membership ($24/year) to support our efforts.
Members skip ads and access exclusive features.
Learn about member benefits
This content is available to members only.
- Forgot Password?
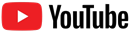
- TPC and eLearning
- Read Watch Interact
- What's NEW at TPC?
- Practice Review Test
- Teacher-Tools
- Subscription Selection
- Seat Calculator
- Ad Free Account
- Edit Profile Settings
- Classes (Version 2)
- Student Progress Edit
- Task Properties
- Export Student Progress
- Task, Activities, and Scores
- Metric Conversions Questions
- Metric System Questions
- Metric Estimation Questions
- Significant Digits Questions
- Proportional Reasoning
- Acceleration
- Distance-Displacement
- Dots and Graphs
- Graph That Motion
- Match That Graph
- Name That Motion
- Motion Diagrams
- Pos'n Time Graphs Numerical
- Pos'n Time Graphs Conceptual
- Up And Down - Questions
- Balanced vs. Unbalanced Forces
- Change of State
- Force and Motion
- Mass and Weight
- Match That Free-Body Diagram
- Net Force (and Acceleration) Ranking Tasks
- Newton's Second Law
- Normal Force Card Sort
- Recognizing Forces
- Air Resistance and Skydiving
- Solve It! with Newton's Second Law
- Which One Doesn't Belong?
- Component Addition Questions
- Head-to-Tail Vector Addition
- Projectile Mathematics
- Trajectory - Angle Launched Projectiles
- Trajectory - Horizontally Launched Projectiles
- Vector Addition
- Vector Direction
- Which One Doesn't Belong? Projectile Motion
- Forces in 2-Dimensions
- Being Impulsive About Momentum
- Explosions - Law Breakers
- Hit and Stick Collisions - Law Breakers
- Case Studies: Impulse and Force
- Impulse-Momentum Change Table
- Keeping Track of Momentum - Hit and Stick
- Keeping Track of Momentum - Hit and Bounce
- What's Up (and Down) with KE and PE?
- Energy Conservation Questions
- Energy Dissipation Questions
- Energy Ranking Tasks
- LOL Charts (a.k.a., Energy Bar Charts)
- Match That Bar Chart
- Words and Charts Questions
- Name That Energy
- Stepping Up with PE and KE Questions
- Case Studies - Circular Motion
- Circular Logic
- Forces and Free-Body Diagrams in Circular Motion
- Gravitational Field Strength
- Universal Gravitation
- Angular Position and Displacement
- Linear and Angular Velocity
- Angular Acceleration
- Rotational Inertia
- Balanced vs. Unbalanced Torques
- Getting a Handle on Torque
- Torque-ing About Rotation
- Properties of Matter
- Fluid Pressure
- Buoyant Force
- Sinking, Floating, and Hanging
- Pascal's Principle
- Flow Velocity
- Bernoulli's Principle
- Balloon Interactions
- Charge and Charging
- Charge Interactions
- Charging by Induction
- Conductors and Insulators
- Coulombs Law
- Electric Field
- Electric Field Intensity
- Polarization
- Case Studies: Electric Power
- Know Your Potential
- Light Bulb Anatomy
- I = ∆V/R Equations as a Guide to Thinking
- Parallel Circuits - ∆V = I•R Calculations
- Resistance Ranking Tasks
- Series Circuits - ∆V = I•R Calculations
- Series vs. Parallel Circuits
- Equivalent Resistance
- Period and Frequency of a Pendulum
- Pendulum Motion: Velocity and Force
- Energy of a Pendulum
- Period and Frequency of a Mass on a Spring
- Horizontal Springs: Velocity and Force
- Vertical Springs: Velocity and Force
- Energy of a Mass on a Spring
- Decibel Scale
- Frequency and Period
- Closed-End Air Columns
- Name That Harmonic: Strings
- Rocking the Boat
- Wave Basics
- Matching Pairs: Wave Characteristics
- Wave Interference
- Waves - Case Studies
- Color Addition and Subtraction
- Color Filters
- If This, Then That: Color Subtraction
- Light Intensity
- Color Pigments
- Converging Lenses
- Curved Mirror Images
- Law of Reflection
- Refraction and Lenses
- Total Internal Reflection
- Who Can See Who?
- Formulas and Atom Counting
- Atomic Models
- Bond Polarity
- Entropy Questions
- Cell Voltage Questions
- Heat of Formation Questions
- Reduction Potential Questions
- Oxidation States Questions
- Measuring the Quantity of Heat
- Hess's Law
- Oxidation-Reduction Questions
- Galvanic Cells Questions
- Thermal Stoichiometry
- Molecular Polarity
- Quantum Mechanics
- Balancing Chemical Equations
- Bronsted-Lowry Model of Acids and Bases
- Classification of Matter
- Collision Model of Reaction Rates
- Density Ranking Tasks
- Dissociation Reactions
- Complete Electron Configurations
- Elemental Measures
- Enthalpy Change Questions
- Equilibrium Concept
- Equilibrium Constant Expression
- Equilibrium Calculations - Questions
- Equilibrium ICE Table
- Ionic Bonding
- Lewis Electron Dot Structures
- Limiting Reactants
- Line Spectra Questions
- Mass Stoichiometry
- Measurement and Numbers
- Metals, Nonmetals, and Metalloids
- Metric Estimations
- Metric System
- Molarity Ranking Tasks
- Mole Conversions
- Name That Element
- Names to Formulas
- Names to Formulas 2
- Nuclear Decay
- Particles, Words, and Formulas
- Periodic Trends
- Precipitation Reactions and Net Ionic Equations
- Pressure Concepts
- Pressure-Temperature Gas Law
- Pressure-Volume Gas Law
- Chemical Reaction Types
- Significant Digits and Measurement
- States Of Matter Exercise
- Stoichiometry Law Breakers
- Stoichiometry - Math Relationships
- Subatomic Particles
- Spontaneity and Driving Forces
- Gibbs Free Energy
- Volume-Temperature Gas Law
- Acid-Base Properties
- Energy and Chemical Reactions
- Chemical and Physical Properties
- Valence Shell Electron Pair Repulsion Theory
- Writing Balanced Chemical Equations
- Mission CG1
- Mission CG10
- Mission CG2
- Mission CG3
- Mission CG4
- Mission CG5
- Mission CG6
- Mission CG7
- Mission CG8
- Mission CG9
- Mission EC1
- Mission EC10
- Mission EC11
- Mission EC12
- Mission EC2
- Mission EC3
- Mission EC4
- Mission EC5
- Mission EC6
- Mission EC7
- Mission EC8
- Mission EC9
- Mission RL1
- Mission RL2
- Mission RL3
- Mission RL4
- Mission RL5
- Mission RL6
- Mission KG7
- Mission RL8
- Mission KG9
- Mission RL10
- Mission RL11
- Mission RM1
- Mission RM2
- Mission RM3
- Mission RM4
- Mission RM5
- Mission RM6
- Mission RM8
- Mission RM10
- Mission LC1
- Mission RM11
- Mission LC2
- Mission LC3
- Mission LC4
- Mission LC5
- Mission LC6
- Mission LC8
- Mission SM1
- Mission SM2
- Mission SM3
- Mission SM4
- Mission SM5
- Mission SM6
- Mission SM8
- Mission SM10
- Mission KG10
- Mission SM11
- Mission KG2
- Mission KG3
- Mission KG4
- Mission KG5
- Mission KG6
- Mission KG8
- Mission KG11
- Mission F2D1
- Mission F2D2
- Mission F2D3
- Mission F2D4
- Mission F2D5
- Mission F2D6
- Mission KC1
- Mission KC2
- Mission KC3
- Mission KC4
- Mission KC5
- Mission KC6
- Mission KC7
- Mission KC8
- Mission AAA
- Mission SM9
- Mission LC7
- Mission LC9
- Mission NL1
- Mission NL2
- Mission NL3
- Mission NL4
- Mission NL5
- Mission NL6
- Mission NL7
- Mission NL8
- Mission NL9
- Mission NL10
- Mission NL11
- Mission NL12
- Mission MC1
- Mission MC10
- Mission MC2
- Mission MC3
- Mission MC4
- Mission MC5
- Mission MC6
- Mission MC7
- Mission MC8
- Mission MC9
- Mission RM7
- Mission RM9
- Mission RL7
- Mission RL9
- Mission SM7
- Mission SE1
- Mission SE10
- Mission SE11
- Mission SE12
- Mission SE2
- Mission SE3
- Mission SE4
- Mission SE5
- Mission SE6
- Mission SE7
- Mission SE8
- Mission SE9
- Mission VP1
- Mission VP10
- Mission VP2
- Mission VP3
- Mission VP4
- Mission VP5
- Mission VP6
- Mission VP7
- Mission VP8
- Mission VP9
- Mission WM1
- Mission WM2
- Mission WM3
- Mission WM4
- Mission WM5
- Mission WM6
- Mission WM7
- Mission WM8
- Mission WE1
- Mission WE10
- Mission WE2
- Mission WE3
- Mission WE4
- Mission WE5
- Mission WE6
- Mission WE7
- Mission WE8
- Mission WE9
- Vector Walk Interactive
- Name That Motion Interactive
- Kinematic Graphing 1 Concept Checker
- Kinematic Graphing 2 Concept Checker
- Graph That Motion Interactive
- Two Stage Rocket Interactive
- Rocket Sled Concept Checker
- Force Concept Checker
- Free-Body Diagrams Concept Checker
- Free-Body Diagrams The Sequel Concept Checker
- Skydiving Concept Checker
- Elevator Ride Concept Checker
- Vector Addition Concept Checker
- Vector Walk in Two Dimensions Interactive
- Name That Vector Interactive
- River Boat Simulator Concept Checker
- Projectile Simulator 2 Concept Checker
- Projectile Simulator 3 Concept Checker
- Hit the Target Interactive
- Turd the Target 1 Interactive
- Turd the Target 2 Interactive
- Balance It Interactive
- Go For The Gold Interactive
- Egg Drop Concept Checker
- Fish Catch Concept Checker
- Exploding Carts Concept Checker
- Collision Carts - Inelastic Collisions Concept Checker
- Its All Uphill Concept Checker
- Stopping Distance Concept Checker
- Chart That Motion Interactive
- Roller Coaster Model Concept Checker
- Uniform Circular Motion Concept Checker
- Horizontal Circle Simulation Concept Checker
- Vertical Circle Simulation Concept Checker
- Race Track Concept Checker
- Gravitational Fields Concept Checker
- Orbital Motion Concept Checker
- Angular Acceleration Concept Checker
- Balance Beam Concept Checker
- Torque Balancer Concept Checker
- Aluminum Can Polarization Concept Checker
- Charging Concept Checker
- Name That Charge Simulation
- Coulomb's Law Concept Checker
- Electric Field Lines Concept Checker
- Put the Charge in the Goal Concept Checker
- Circuit Builder Concept Checker (Series Circuits)
- Circuit Builder Concept Checker (Parallel Circuits)
- Circuit Builder Concept Checker (∆V-I-R)
- Circuit Builder Concept Checker (Voltage Drop)
- Equivalent Resistance Interactive
- Pendulum Motion Simulation Concept Checker
- Mass on a Spring Simulation Concept Checker
- Particle Wave Simulation Concept Checker
- Boundary Behavior Simulation Concept Checker
- Slinky Wave Simulator Concept Checker
- Simple Wave Simulator Concept Checker
- Wave Addition Simulation Concept Checker
- Standing Wave Maker Simulation Concept Checker
- Color Addition Concept Checker
- Painting With CMY Concept Checker
- Stage Lighting Concept Checker
- Filtering Away Concept Checker
- InterferencePatterns Concept Checker
- Young's Experiment Interactive
- Plane Mirror Images Interactive
- Who Can See Who Concept Checker
- Optics Bench (Mirrors) Concept Checker
- Name That Image (Mirrors) Interactive
- Refraction Concept Checker
- Total Internal Reflection Concept Checker
- Optics Bench (Lenses) Concept Checker
- Kinematics Preview
- Velocity Time Graphs Preview
- Moving Cart on an Inclined Plane Preview
- Stopping Distance Preview
- Cart, Bricks, and Bands Preview
- Fan Cart Study Preview
- Friction Preview
- Coffee Filter Lab Preview
- Friction, Speed, and Stopping Distance Preview
- Up and Down Preview
- Projectile Range Preview
- Ballistics Preview
- Juggling Preview
- Marshmallow Launcher Preview
- Air Bag Safety Preview
- Colliding Carts Preview
- Collisions Preview
- Engineering Safer Helmets Preview
- Push the Plow Preview
- Its All Uphill Preview
- Energy on an Incline Preview
- Modeling Roller Coasters Preview
- Hot Wheels Stopping Distance Preview
- Ball Bat Collision Preview
- Energy in Fields Preview
- Weightlessness Training Preview
- Roller Coaster Loops Preview
- Universal Gravitation Preview
- Keplers Laws Preview
- Kepler's Third Law Preview
- Charge Interactions Preview
- Sticky Tape Experiments Preview
- Wire Gauge Preview
- Voltage, Current, and Resistance Preview
- Light Bulb Resistance Preview
- Series and Parallel Circuits Preview
- Thermal Equilibrium Preview
- Linear Expansion Preview
- Heating Curves Preview
- Electricity and Magnetism - Part 1 Preview
- Electricity and Magnetism - Part 2 Preview
- Vibrating Mass on a Spring Preview
- Period of a Pendulum Preview
- Wave Speed Preview
- Slinky-Experiments Preview
- Standing Waves in a Rope Preview
- Sound as a Pressure Wave Preview
- DeciBel Scale Preview
- DeciBels, Phons, and Sones Preview
- Sound of Music Preview
- Shedding Light on Light Bulbs Preview
- Models of Light Preview
- Electromagnetic Radiation Preview
- Electromagnetic Spectrum Preview
- EM Wave Communication Preview
- Digitized Data Preview
- Light Intensity Preview
- Concave Mirrors Preview
- Object Image Relations Preview
- Snells Law Preview
- Reflection vs. Transmission Preview
- Magnification Lab Preview
- Reactivity Preview
- Ions and the Periodic Table Preview
- Periodic Trends Preview
- Reaction Rates Preview
- Ammonia Factory Preview
- Stoichiometry Preview
- Gaining Teacher Access
- Tasks and Classes
- Tasks - Classic
- Subscription
- Subscription Locator
- 1-D Kinematics
- Newton's Laws
- Vectors - Motion and Forces in Two Dimensions
- Momentum and Its Conservation
- Work and Energy
- Circular Motion and Satellite Motion
- Thermal Physics
- Static Electricity
- Electric Circuits
- Vibrations and Waves
- Sound Waves and Music
- Light and Color
- Reflection and Mirrors
- About the Physics Interactives
- Task Tracker
- Usage Policy
- Newtons Laws
- Vectors and Projectiles
- Forces in 2D
- Momentum and Collisions
- Circular and Satellite Motion
- Balance and Rotation
- Electromagnetism
- Waves and Sound
- Forces in Two Dimensions
- Work, Energy, and Power
- Circular Motion and Gravitation
- Sound Waves
- 1-Dimensional Kinematics
- Circular, Satellite, and Rotational Motion
- Einstein's Theory of Special Relativity
- Waves, Sound and Light
- QuickTime Movies
- About the Concept Builders
- Pricing For Schools
- Directions for Version 2
- Measurement and Units
- Relationships and Graphs
- Rotation and Balance
- Vibrational Motion
- Reflection and Refraction
- Teacher Accounts
- Task Tracker Directions
- Kinematic Concepts
- Kinematic Graphing
- Wave Motion
- Sound and Music
- About CalcPad
- 1D Kinematics
- Vectors and Forces in 2D
- Simple Harmonic Motion
- Rotational Kinematics
- Rotation and Torque
- Rotational Dynamics
- Electric Fields, Potential, and Capacitance
- Transient RC Circuits
- Light Waves
- Units and Measurement
- Stoichiometry
- Molarity and Solutions
- Thermal Chemistry
- Acids and Bases
- Kinetics and Equilibrium
- Solution Equilibria
- Oxidation-Reduction
- Nuclear Chemistry
- NGSS Alignments
- 1D-Kinematics
- Projectiles
- Circular Motion
- Magnetism and Electromagnetism
- Graphing Practice
- About the ACT
- ACT Preparation
- For Teachers
- Other Resources
- Newton's Laws of Motion
- Work and Energy Packet
- Static Electricity Review
- Solutions Guide
- Solutions Guide Digital Download
- Motion in One Dimension
- Work, Energy and Power
- Frequently Asked Questions
- Purchasing the Download
- Purchasing the CD
- Purchasing the Digital Download
- About the NGSS Corner
- NGSS Search
- Force and Motion DCIs - High School
- Energy DCIs - High School
- Wave Applications DCIs - High School
- Force and Motion PEs - High School
- Energy PEs - High School
- Wave Applications PEs - High School
- Crosscutting Concepts
- The Practices
- Physics Topics
- NGSS Corner: Activity List
- NGSS Corner: Infographics
- About the Toolkits
- Position-Velocity-Acceleration
- Position-Time Graphs
- Velocity-Time Graphs
- Newton's First Law
- Newton's Second Law
- Newton's Third Law
- Terminal Velocity
- Projectile Motion
- Forces in 2 Dimensions
- Impulse and Momentum Change
- Momentum Conservation
- Work-Energy Fundamentals
- Work-Energy Relationship
- Roller Coaster Physics
- Satellite Motion
- Electric Fields
- Circuit Concepts
- Series Circuits
- Parallel Circuits
- Describing-Waves
- Wave Behavior Toolkit
- Standing Wave Patterns
- Resonating Air Columns
- Wave Model of Light
- Plane Mirrors
- Curved Mirrors
- Teacher Guide
- Using Lab Notebooks
- Current Electricity
- Light Waves and Color
- Reflection and Ray Model of Light
- Refraction and Ray Model of Light
- Classes (Legacy Version)
- Teacher Resources
- Subscriptions

- Newton's Laws
- Einstein's Theory of Special Relativity
- About Concept Checkers
- School Pricing
- Newton's Laws of Motion
- Newton's First Law
- Newton's Third Law
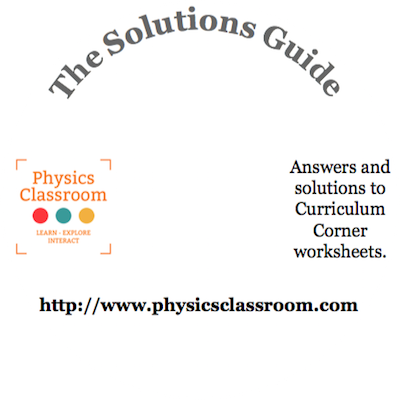
Worksheet on Word Problem on Measuring Mass
Practice the questions given in the worksheet on word problem on measuring mass (i.e. addition and subtraction). Addition and subtraction in grams and kilograms is done in the similar way as in the case of ordinary numbers.
1. Jason purchased 7 kg 200 g of sugar, 9 kg 395 g of rice. What is the total weight which Jason carried?
2. Rachel bought 8 kg 400 g tomatoes, 2 kg 130 g brinjal and 7 kg 300 g watermelon from the green grocer. How many kilograms of fruits and vegetables did she buy?
3. A rickshaw-puller is carrying two persons weighting 52 kg 250 g and 37 kg 700 g. What is the total weight of the two persons carried by the rickshaw-puller?
4. The total weight of Tania’s bag is 45 kg 750 g and Diana’s bag is 43 kg 950 g. Whose bag is heavier and by how much?
5. A truck was loaded with 352 kg 100 g of pumpkins and 207 kg 432 g of watermelons. Find the total weight carried by the truck.
6. Weight of a pile of English newspaper is 16 kg 270 g and that of French newspaper is 18 kg 227 g. If both the piles are tied together, what will be the total weight of the bundle?
7. A shopkeeper sold 67 kg 626 g of wheat on Saturday and 125 kg 200 g of wheat on Sunday. Find the total weight of wheat sold on both the days.
8. A grocer puts apples and plums together on the weighing machine. The total weight shown on the machine is 8 kg. If the weight of the plums is 2 kg 700 g, then what is the weight of the apples?
9. Jasmine purchased 7 kg 302 g of rice and Melissa purchased 3 kg 598 g more. What quantity of rice did Jasmine and Melissa purchase?
10. The weight of a chocolate carton is 20 kg. Each carton has 10 packets. Each packet has 25 chocolates. How many chocolates are there? Find the weight of each chocolate presuming that weight of carton and packets is negligible.
11. Brian sold 123 kg 231 g of newspapers and 200 kg of magazines. Find the total quantity of articles sold.
12. Ron takes care of deer and wild bulls in the zoo. The deer weighs 182 kg 290 g and a wild bull weighs 753 kg 380 g. Answer the given questions.
(i) If the both the animals stand on a weighing machine together what will be the total weight shown on the machine?
(ii) By how much is a bull heavier than deer?
(iii) If a deer eats 15 kg 250 g grass in a week. How much grass will be eaten by a deer in 42 days?
13. Aaron weight 49 kg 357 g and Ron weights 32 kg 458 g. Who weighs less and by how much?
14. If the total weight of 24 bulls having same weight is 487200 kg. What is the weight of each bull?
15. Father bought 10 kg 750 g of fruits (mangoes and apples). He had 6 kg 860 g of mangoes. What is the weight of apples?
16. Nina bought 5 kg of fruits. On the way home, she ate 750 g of fruits. How much fruit did she take back home?
17. Nancy brought a cake weighing 5 kg 675 g. Approximately 2 kg 395 g of cake is distributed among the children. What quantity of cake is left?
18. Rita weighs 13 kg 250 g. Her elder brother is three times heavier than Rita. What is her brother’s weight?
19. Mother purchased 9 kg 357 g of sweets and snacks for the occasion. Out of which 6 kg 458 g were consumed. What quantity of sweets and snacks were left?
20. A box can carry a total weight of 27 kg. Candies have to be packed inside the box. If the weight of each candy is 30 g, how many candies can be packed inside the box?
21. A shopkeeper purchased 287 kg 500 g of orange. Later on, he found that 98 kg 300 g of oranges were rotten. Find the quantity of oranges in good condition.
22. A lift can withstand a weight of 500 kg. There are 7 people standing to go in the lift. Given below are their weights. Find out if all of them can go inside the lift altogether. If no, then why?

23. Mary weighs 63 kg 59 g and Alex weighs 59 kg 36 g. Who weighs less and by how much?
24. Kellie’s weight is 55 kg 330 g. The doctor says that according to her height, her weight should be 62 kg. How much weight should she gain?
25. How much heavier is the toffee packet which has mass 1 kg 845 g in comparison to 500 g of chocolates?
Answers for the worksheet on word problem on measuring mass (i.e. addition and subtraction) are given below.
1. 16 kg 595 g
2. 17 kg 830 g
3. 89 kg 950 g
4. Tania’s bag is 1 kg 800 g
5. 559 kg 532 g
6. 34 kg 497 g
7. 192 kg 826 g
8. 5 kg 300 g
9. 10 kg 900 g
10. 250, 80 g
11. 323 kg 231 g
12. (i) 935 kg 670 g
(ii) 571 kg 90 g
(iii) 91 kg 500 g
13. Ron weighs less by 16 kg 899 g
14. 20,300 kg
15. 3 kg 890 g
16. 4 kg 250 g
17. 3 kg 280 g
18. 39 kg 750 g
19. 2 kg 899 g
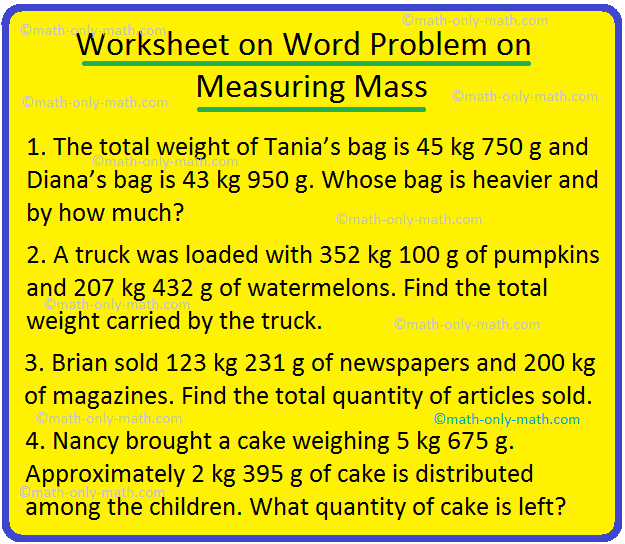
21. 189 kg 200 g
22. All can go, 425 kg 350 g
23. Alex weighs less by 4 kg 23 g
24. 6 kg 670 g
25. 1 kg 345 g
Measurement of Mass:
What is Mass?
Conversion of Standard Unit of Mass
Conversion of Measuring Mass
Addition of Mass
Subtraction of Mass
Addition and Subtraction of Measuring Mass
Worksheet on Conversion of Mass
Worksheet on Addition of Mass
Worksheet on Third Grade Measurement of Mass
Worksheet on Subtraction of Mass
3rd Grade Math Worksheets
3rd Grade Math Lessons
From Worksheet on Word Problem on Measuring Mass to HOME PAGE
New! Comments
Didn't find what you were looking for? Or want to know more information about Math Only Math . Use this Google Search to find what you need.
- Preschool Activities
- Kindergarten Math
- 1st Grade Math
- 2nd Grade Math
- 3rd Grade Math
- 4th Grade Math
- 5th Grade Math
- 6th Grade Math
- 7th Grade Math
- 8th Grade Math
- 9th Grade Math
- 10th Grade Math
- 11 & 12 Grade Math
- Concepts of Sets
- Probability
- Boolean Algebra
- Math Coloring Pages
- Multiplication Table
- Cool Maths Games
- Math Flash Cards
- Online Math Quiz
- Math Puzzles
- Binary System
- Math Dictionary
- Conversion Chart
- Homework Sheets
- Math Problem Ans
- Free Math Answers
- Printable Math Sheet
- Funny Math Answers
- Employment Test
- Math Patterns
- Link Partners
- Privacy Policy

Recent Articles

Months of the Year | List of 12 Months of the Year |Jan, Feb, Mar, Apr
Apr 02, 24 02:08 PM
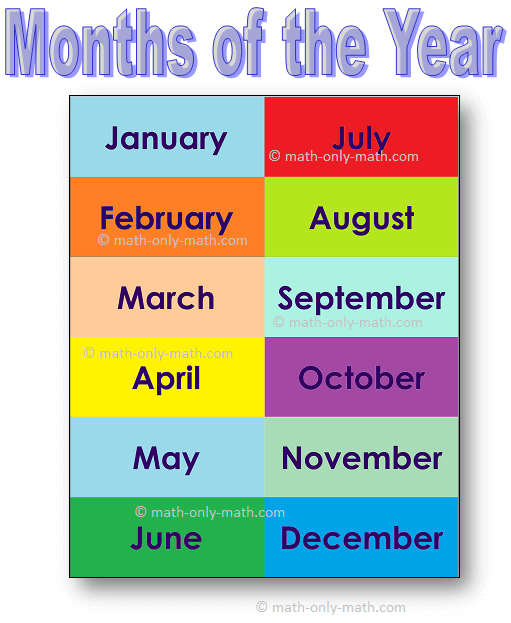
Division of Two-Digit by a One-Digit Numbers | Dividing Larger Numbers
Apr 02, 24 11:58 AM
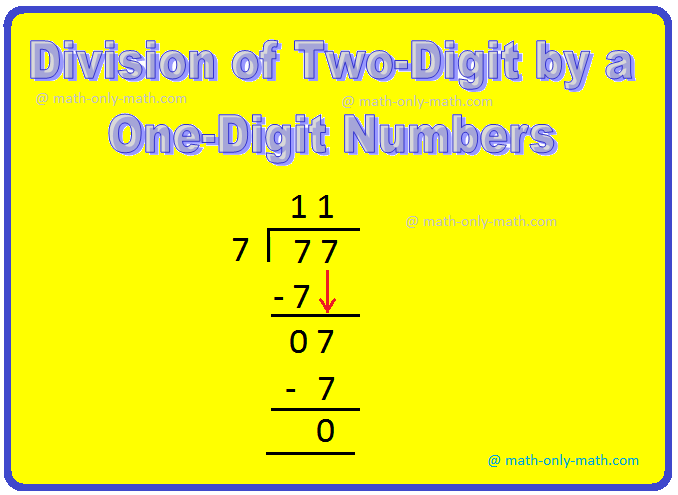
Terms Used in Division | Dividend | Divisor | Quotient | Remainder
Apr 01, 24 05:38 PM
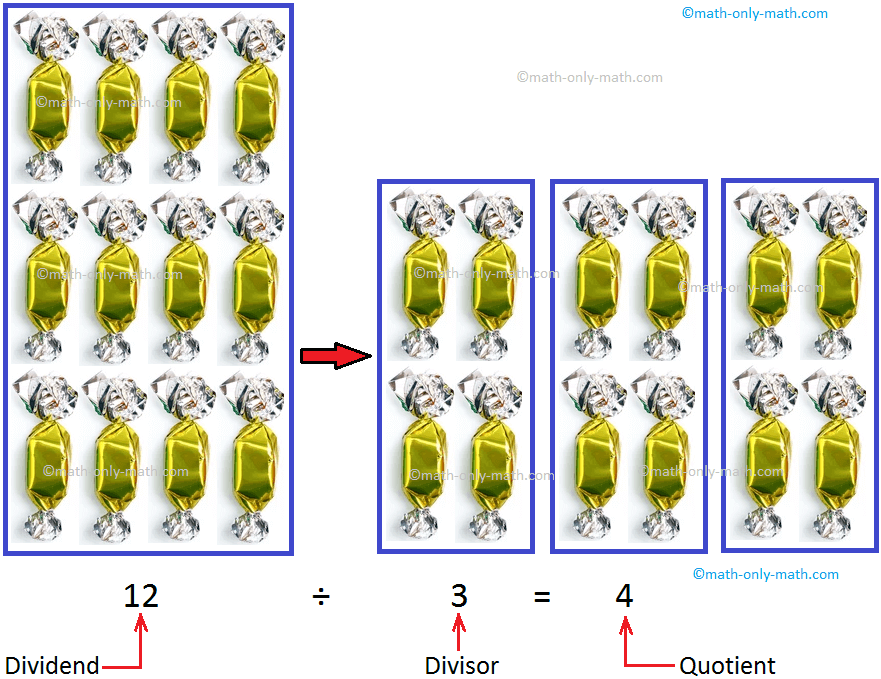
Multiply a Number by a 2-Digit Number | Multiplying 2-Digit by 2-Digit
Apr 01, 24 04:52 PM
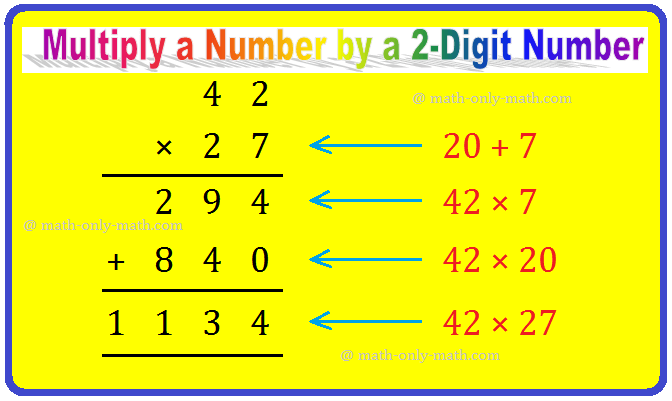
Mental Math on Multiplication Worksheet | Multiplication Facts|Answers
Apr 01, 24 04:04 PM
© and ™ math-only-math.com. All Rights Reserved. 2010 - 2024.
- Physics Formulas
Weight Formula
Weight is not anything but the force gravity experiences. It is represented by W and Newton is its SI unit. It is articulated as the product of mass and acceleration due to gravity. So the weight of a given object will show variation according to the gravity in that particular space. So, objects with similar mass appear in different weights across different planets.
Formula of Weight
The formula for weight is articulated as,
W=mg
- Weight of the object is W
- Mass of the object is m
- Acceleration due to gravity is g
Solved Examples
Numerical associated to weight calculations are provided underneath:
Problem 1: Compute the weight of a body on the moon if the mass is 60Kg? g is given as 1.625 m/s 2 . Answer :
It is known that, m = 60 kg and
g = 1.625 m/s 2
Formula for weight is, W = mg W = 60×1.625 W = 97.5 N
Problem 2: Compute the weight of a body on earth whose mass is 25 kg? Answer :
It is known that, m = 25 kg and
g = 9.8 m/s 2
Formula for weight is, W = mg W = 25×9.8 W = 245 N
Leave a Comment Cancel reply
Your Mobile number and Email id will not be published. Required fields are marked *
Request OTP on Voice Call
Post My Comment

- Share Share
Register with BYJU'S & Download Free PDFs
Register with byju's & watch live videos.
These are our mass and weight Word Problems worksheets for 2nd grade math school. Click on the previews to go to download page.
Worksheet by math grade levels:
Our grade 2 math worksheets are free and printable in PDF format. Based on the Singaporean math curriculum for second graders, these math worksheets are made for students in grade level 2. However, also students in other grade levels can benefit from doing these math worksheets. Feel free to print them. Our math worksheets cover important math topics such as: whole numbers, spelling, place value, skip counting, addition and subtraction, multiplication tables, basic division facts, fractions, mixed operations, geometry, graphing, picture graphs, measurement of time, mass, length and volume.
Our first grade math worksheets are free and printable in PDF format. Based on the Singaporean math school curriculum for grade 1 students, these 1st level math worksheets are made for students in school, tutoring or online math education. Our grade 1 math worksheets cover topics such as: whole numbers, spelling of basic numbers up to 10 or 100 and first grade math operations, grade 1addition and subtraction, place value, skip counting, introduction to division and multiplication, first grade geometry and basic shapes, easy picture graphs, length, volume and mass measurement and beginners number patterns.

IMAGES
VIDEO
COMMENTS
3. The mass of a box is 1 kg and acceleration due to gravity is 9.8 m/s 2. Find (a) weight (b) the horizontal component and the vertical component of the weight. Solution. Weight : w = m g = (1 kg)(9.8 m/s 2) = 9.8 kg m/s 2 = 9.8 Newton. The horizontal component of the weight :
Explain the difference between mass and weight; ... To apply Newton's second law as the primary equation in solving a problem, we sometimes have to rely on other equations, such as the one for weight or one of the kinematic equations, to complete the solution. ... [latex] {201\,\text{m/s}}^{2} [/latex]. In this problem, the forces are exerted ...
The equation Fnet = ma F net = m a is used to define net force in terms of mass, length, and time. As explained earlier, the SI unit of force is the newton. Since Fnet = ma, F net = m a, 1N = 1kg ⋅m/s2. 1 N = 1 kg · m/s 2. Although almost the entire world uses the newton for the unit of force, in the United States, the most familiar unit of ...
Mass and Weight. The Mass and Weight Concept Builder is a tool that challenges the learner to distinguish between the concepts of mass and weight. In addition to the conceptual aspect of these two concepts, students will also perform calculations of mass and weight. There are a total of 28 questions organized into nine different Question Groups ...
Weight Changes When (g) Changes. On Earth, your weight is caused by the earths pull on you. In a future lesson we will see that weight is the result of the your mass, the earths mass, and the distance between you and Earth. We will solve problems on this page using an average surface acceleration due to gravity
Weight and mass are independent of any physical state of a matter, such as solid, gas, liquid, or plasma. Weight and mass have definite units and dimensions. Both mass and weight are measurable quantities. Weight and mass depend on the amount of matter in a body. 4. The mass of an object can be zero. True. False.
Be aware that weight and mass are different physical quantities, although they are closely related. Mass is an intrinsic property of an object: It is a quantity of matter. ... To apply Newton's second law as the primary equation in solving a problem, we sometimes have to rely on other equations, such as the one for weight or one of the ...
1 N. g. 9.8 m/s 2. m =. 0.102 kg = 102 g. The 96.7 gram tangerine comes closest to this value. Not all tangerines weigh 98.7 grams, however, so this is only a rule of thumb. There are certainly apples, bananas, oranges, tomatoes, and other fruits out there with a mass of approximately 102 grams and thus a weight of approximately one newton.
The weight of an object equals the force of gravity exerted on that object. The mass of an object is always the same, but its weight changes depending on gravity. Use the formula. w = m ∗ g {\displaystyle w=m*g} to calculate weight from mass. In this formula, w {\displaystyle w} = weight (in N),
Word problems with mass. Emily needs 4 eggs for baking her cake. All of the eggs have the same mass. Emily knows the mass of 1 egg (shown below). What is the total mass of 4 eggs? Stuck? Review related articles/videos or use a hint. Learn for free about math, art, computer programming, economics, physics, chemistry, biology, medicine, finance ...
Course: UP Class 9th Science > Unit 9. Lesson 2: Week 2. Mass & weight. Mass and Weight. What is Pressure? Pressure and Buoyancy. Archimedes principle. Archimedes principle.
Are mass and weight the same thing? This video explains the difference between mass and weight. This video also has four different example problems for how t...
Weight is the pull of Earth on an object. It depends on the distance from the center of Earth. Unlike weight, mass does not vary with location. The mass of an object is the same on Earth, in orbit, or on the surface of the Moon. Units of Force. The equation is used to define net force in terms of mass, length, and time.
Resources tagged with: Mass and weight Types All types Problems Articles Games Age range All ages 5 to 11 7 to 14 11 to 16 14 to 18 Challenge level There are 20 NRICH Mathematical resources connected to Mass and weight , you may find related items under Measuring and calculating with units .
Notice on the scale: in order to change from grams to kilograms you have to move up. Therefore, we have to divide by 1,000: 1 kg = 1,000 g … then 600 g = 600 / 1000 kg … then 1,000 kg = 0.6 kg. Now we add: 2.25 kg + 0.6 kg = 2.85 kg. Thus, the answer to this problem is: At birth, Sara weighed 2.85 kg.
Total mass = 55 x 12 x 50 = 33000 g. 1000g = 1 kg = 33 kg. Example 2 : Find the total mass of 32 chocolates, each of mass 28 grams. Solution : Number of chocolates = 32. Mass of each chocolate = 28 grams. Total mass = 32 x 28 = 896 grams. Example 3 : If a clothes peg has a mass of 6.5 g, how many pegs are there in a 13 kg box? Solution :
Mass is the force created by gravity acting of on an object. Mass is felt as weight, a force that pulls the object towards the centre of the Earth. Mass is measured in units based on grams, and tonnes. Larger or smaller units are created by combining or equally partitioning these units. One kilogram is a combination of 1000 grams (kilo means 1000).
1.7 Solving Problems in Physics. 1 Chapter Review. 2 Vectors. Introduction. 2.1 Scalars and Vectors. 2.2 Coordinate Systems and Components of a Vector. 2.3 Algebra of Vectors. ... Explain the difference between mass and weight; Explain why falling objects on Earth are never truly in free fall;
These word problems involve mass or weight. Problems involve the addition, subtraction, multiplication or division of amounts with units of mass. There are worksheets on both customary units (ounces & pounds) and metric units (gm, kg). Students are not asked to convert between the two systems. Ounces, pounds:
If the PDF does not show in the window above, then you can access it directly here: Mass and Weight (PDF) The Curriculum Corner contains a complete ready-to-use curriculum for the high school physics classroom. This collection of pages comprise worksheets in PDF format that developmentally target key concepts and mathematics commonly covered in ...
Answers for the worksheet on word problem on measuring mass (i.e. addition and subtraction) are given below. Answers: 1. 16 kg 595 g. 2. 17 kg 830 g. 3. 89 kg 950 g. 4.
Problem 1: Compute the weight of a body on the moon if the mass is 60Kg? g is given as 1.625 m/s 2. g = 1.625 m/s 2. Problem 2: Compute the weight of a body on earth whose mass is 25 kg? g = 9.8 m/s 2. The formula for weight is given as the product of mass and acceleration due to gravity. The solved numericals helps in understanding the formula ...
These are our mass and weight Word Problems worksheets for 2nd grade math school. Click on the previews to go to download page. Mass Problems (level 2) Mass Problems (level 2) Mass Problems (level 2) Worksheet by math grade levels: Grade 1 sheets. Grade 2 sheets. Grade 3 sheets. Grade 4 sheets.