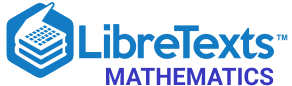
- school Campus Bookshelves
- menu_book Bookshelves
- perm_media Learning Objects
- login Login
- how_to_reg Request Instructor Account
- hub Instructor Commons
- Download Page (PDF)
- Download Full Book (PDF)
- Periodic Table
- Physics Constants
- Scientific Calculator
- Reference & Cite
- Tools expand_more
- Readability
selected template will load here
This action is not available.
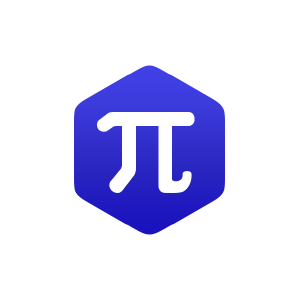

4.5: Linear Approximations and Differentials
- Last updated
- Save as PDF
- Page ID 93389
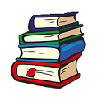
Learning Objectives
- Describe the linear approximation to a function at a point.
- Write the linearization of a given function.
- Draw a graph that illustrates the use of differentials to approximate the change in a quantity.
- Calculate the relative error and percentage error in using a differential approximation.
We have just seen how derivatives allow us to compare related quantities that are changing over time. In this section, we examine another application of derivatives: the ability to approximate functions locally by linear functions. Linear functions are the easiest functions with which to work, so they provide a useful tool for approximating function values. In addition, the ideas presented in this section are generalized later in the text when we study how to approximate functions by higher-degree polynomials Introduction to Power Series and Functions.
Linear Approximation of a Function at a Point
Consider a function \(f\) that is differentiable at a point \(x=a\). Recall that the tangent line to the graph of \(f\) at \(a\) is given by the equation
\[y=f(a)+f'(a)(x−a). \nonumber \]
For example, consider the function \(f(x)=\frac{1}{x}\) at \(a=2\). Since \(f\) is differentiable at \(x=2\) and \(f'(x)=−\frac{1}{x^2}\), we see that \(f'(2)=−\frac{1}{4}\). Therefore, the tangent line to the graph of \(f\) at \(a=2\) is given by the equation
\[y=\frac{1}{2}−\frac{1}{4}(x−2). \nonumber \]
Figure \(\PageIndex{1a}\) shows a graph of \(f(x)=\frac{1}{x}\) along with the tangent line to \(f\) at \(x=2\). Note that for \(x\) near \(2\), the graph of the tangent line is close to the graph of \(f\). As a result, we can use the equation of the tangent line to approximate \(f(x)\) for \(x\) near \(2\). For example, if \(x=2.1\), the \(y\) value of the corresponding point on the tangent line is
\[y=\frac{1}{2}−\frac{1}{4}(2.1−2)=0.475. \nonumber \]
The actual value of \(f(2.1)\) is given by
\[f(2.1)=\frac{1}{2.1}≈0.47619. \nonumber \]
Therefore, the tangent line gives us a fairly good approximation of \(f(2.1)\) (Figure \(\PageIndex{1b}\)). However, note that for values of \(x\) far from \(2\), the equation of the tangent line does not give us a good approximation. For example, if \(x=10\), the \(y\)-value of the corresponding point on the tangent line is
\[y=\frac{1}{2}−\frac{1}{4}(10−2)=\frac{1}{2}−2=−1.5, \nonumber \]
whereas the value of the function at \(x=10\) is \(f(10)=0.1.\)

In general, for a differentiable function \(f\), the equation of the tangent line to \(f\) at \(x=a\) can be used to approximate \(f(x)\) for \(x\) near \(a\). Therefore, we can write
\(f(x)≈f(a)+f'(a)(x−a)\) for \(x\) near \(a\).
We call the linear function
\[L(x)=f(a)+f'(a)(x−a) \label{linearapprox} \]
the linear approximation, or tangent line approximation , of \(f\) at \(x=a\). This function \(L\) is also known as the linearization of \(f\) at \(x=a.\)
To show how useful the linear approximation can be, we look at how to find the linear approximation for \(f(x)=\sqrt{x}\) at \(x=9.\)
Example \(\PageIndex{1}\): Linear Approximation of \(\sqrt{x}\)
Find the linear approximation of \(f(x)=\sqrt{x}\) at \(x=9\) and use the approximation to estimate \(\sqrt{9.1}\).
Since we are looking for the linear approximation at \(x=9,\) using Equation \ref{linearapprox} we know the linear approximation is given by
\[L(x)=f(9)+f'(9)(x−9). \nonumber \]
We need to find \(f(9)\) and \(f'(9).\)
\(f(x)=\sqrt{x}⇒f(9)=\sqrt{9}=3\)
\(f'(x)=\frac{1}{2\sqrt{x}}⇒f'(9)=\frac{1}{2\sqrt{9}}=\frac{1}{6}\)
Therefore, the linear approximation is given by Figure \(\PageIndex{2}\).
\[L(x)=3+\frac{1}{6}(x−9) \nonumber \]
Using the linear approximation, we can estimate \(\sqrt{9.1}\) by writing
\[\sqrt{9.1}=f(9.1)≈L(9.1)=3+\frac{1}{6}(9.1−9)≈3.0167. \nonumber \]
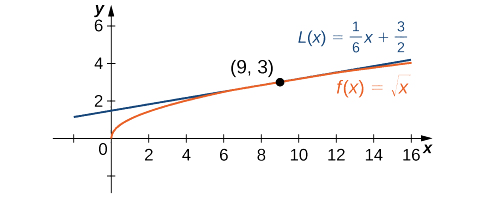
Using a calculator, the value of \(\sqrt{9.1}\) to four decimal places is \(3.0166\). The value given by the linear approximation, \(3.0167\), is very close to the value obtained with a calculator, so it appears that using this linear approximation is a good way to estimate \(\sqrt{x}\), at least for x near \(9\). At the same time, it may seem odd to use a linear approximation when we can just push a few buttons on a calculator to evaluate \(\sqrt{9.1}\). However, how does the calculator evaluate \(\sqrt{9.1}\)? The calculator uses an approximation! In fact, calculators and computers use approximations all the time to evaluate mathematical expressions; they just use higher-degree approximations.
Exercise \(\PageIndex{1}\)
Find the local linear approximation to \(f(x)=\sqrt[3]{x}\) at \(x=8\). Use it to approximate \(\sqrt[3]{8.1}\) to five decimal places.
\(L(x)=f(a)+f'(a)(x−a)\)
\(L(x)=2+\frac{1}{12}(x−8);\) \(2.00833\)
Example \(\PageIndex{2}\): Linear Approximation of \(\sin x \)
Find the linear approximation of \(f(x)=\sin x \) at \(x=\frac{π}{3}\) and use it to approximate \(\sin(62°).\)
First we note that since \(\frac{π}{3}\) rad is equivalent to \(60°\), using the linear approximation at \(x=π/3\) seems reasonable. The linear approximation is given by
\(L(x)=f(\frac{π}{3})+f'(\frac{π}{3})(x−\frac{π}{3}).\)
We see that
\(f(x)=\sin x ⇒f(\frac{π}{3})=\sin(\frac{π}{3})=\frac{\sqrt{3}}{2}\)
\(f'(x)=\cos x ⇒f'(\frac{π}{3})=\cos(\frac{π}{3})=\frac{1}{2}\)
Therefore, the linear approximation of \(f\) at \(x=π/3\) is given by Figure \(\PageIndex{3}\).
\(L(x)=\frac{\sqrt{3}}{2}+\frac{1}{2}(x−\frac{π}{3})\)
To estimate \(\sin(62°)\) using \(L\), we must first convert \(62°\) to radians. We have \(62°=\frac{62π}{180}\) radians, so the estimate for \(\sin(62°)\) is given by
\(\sin(62°)=f(\frac{62π}{180})≈L(\frac{62π}{180})=\frac{\sqrt{3}}{2}+\frac{1}{2}(\frac{62π}{180}−\frac{π}{3})=\frac{\sqrt{3}}{2}+\frac{1}{2}(\frac{2π}{180})=\frac{\sqrt{3}}{2}+\frac{π}{180}≈0.88348.\)
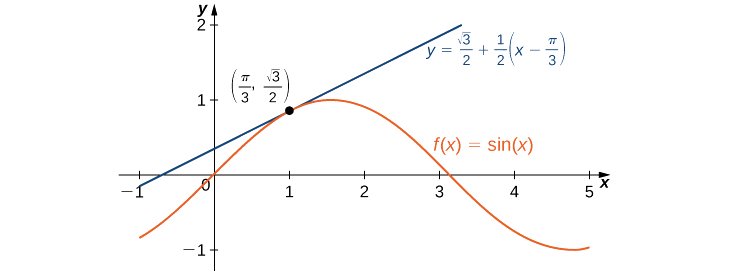
Exercise \(\PageIndex{2}\)
Find the linear approximation for \(f(x)=\cos x \) at \(x=\frac{π}{2}.\)
\(L(x)=−x+\frac{π}{2}\)
Linear approximations may be used in estimating roots and powers. In the next example, we find the linear approximation for \(f(x)=(1+x)^n\) at \(x=0\), which can be used to estimate roots and powers for real numbers near \(1\). The same idea can be extended to a function of the form \(f(x)=(m+x)^n\) to estimate roots and powers near a different number \(m\).
Example \(\PageIndex{3}\): Approximating Roots and Powers
Find the linear approximation of \(f(x)=(1+x)^n\) at \(x=0\). Use this approximation to estimate \((1.01)^3.\)
The linear approximation at \(x=0\) is given by
\(L(x)=f(0)+f'(0)(x−0).\)
\(f(x)=(1+x)^n⇒f(0)=1\)
\(f'(x)=n(1+x)^{n−1}⇒f'(0)=n,\)
the linear approximation is given by Figure \(\PageIndex{4a}\).
\(L(x)=1+n(x−0)=1+nx\)
We can approximate \((1.01)^3\) by evaluating \(L(0.01)\) when \(n=3\). We conclude that
\((1.01)^3=f(1.01)≈L(1.01)=1+3(0.01)=1.03.\)

Exercise \(\PageIndex{3}\)
Find the linear approximation of \(f(x)=(1+x)^4\) at \(x=0\) without using the result from the preceding example.
\(f'(x)=4(1+x)^3\)
\(L(x)=1+4x\)
Differentials
We have seen that linear approximations can be used to estimate function values. They can also be used to estimate the amount a function value changes as a result of a small change in the input. To discuss this more formally, we define a related concept: differentials . Differentials provide us with a way of estimating the amount a function changes as a result of a small change in input values.
When we first looked at derivatives, we used the Leibniz notation \(dy/dx\) to represent the derivative of \(y\) with respect to \(x\). Although we used the expressions \(dy\) and \(dx\) in this notation, they did not have meaning on their own. Here we see a meaning to the expressions \(dy\) and \(dx\). Suppose \(y=f(x)\) is a differentiable function. Let \(dx\) be an independent variable that can be assigned any nonzero real number, and define the dependent variable \(dy\) by
\[dy=f'(x)\,dx. \label{diffeq} \]
It is important to notice that \(dy\) is a function of both \(x\) and \(dx\). The expressions \(dy\) and \(dx\) are called differentials. We can divide both sides of Equation \ref{diffeq} by \(dx,\) which yields
\[\frac{dy}{dx}=f'(x). \label{inteq} \]
This is the familiar expression we have used to denote a derivative. Equation \ref{inteq} is known as the differential form of Equation \ref{diffeq}.

Example \(\PageIndex{4}\): Computing Differentials
For each of the following functions, find \(dy\) and evaluate when \(x=3\) and \(dx=0.1.\)
- \(y=x^2+2x\)
- \(y=\cos x \)
The key step is calculating the derivative. When we have that, we can obtain \(dy\) directly.
a. Since \(f(x)=x^2+2x,\) we know \(f'(x)=2x+2\), and therefore
\(dy=(2x+2)\,dx.\)
When \(x=3\) and \(dx=0.1,\)
\(dy=(2⋅3+2)(0.1)=0.8.\)
b. Since \(f(x)=\cos x , f'(x)=−\sin(x).\) This gives us
\(dy=−\sin x \,dx.\)
\(dy=−\sin(3)(0.1)=−0.1\sin(3).\)
Exercise \(\PageIndex{4}\)
For \(y=e^{x^2}\), find \(dy\).
\(dy=f'(x)\,dx\)
\(dy=2xe^{x^2}dx\)
We now connect differentials to linear approximations. Differentials can be used to estimate the change in the value of a function resulting from a small change in input values. Consider a function \(f\) that is differentiable at point \(a\). Suppose the input \(x\) changes by a small amount. We are interested in how much the output \(y\) changes. If \(x\) changes from \(a\) to \(a+dx\), then the change in \(x\) is \(dx\) (also denoted \(Δx\)), and the change in \(y\) is given by
\[Δy=f(a+dx)−f(a). \nonumber \]
Instead of calculating the exact change in \(y\), however, it is often easier to approximate the change in \(y\) by using a linear approximation. For \(x\) near \(a, f(x)\) can be approximated by the linear approximation (Equation \ref{linearapprox})
\[L(x)=f(a)+f'(a)(x−a). \nonumber \]
Therefore, if \(dx\) is small,
\[f(a+dx)≈L(a+dx)=f(a)+f'(a)(a+dx−a). \nonumber \]
\[f(a+dx)−f(a)≈L(a+dx)−f(a)=f'(a)\,dx. \nonumber \]
In other words, the actual change in the function \(f\) if \(x\) increases from \(a\) to \(a+dx\) is approximately the difference between \(L(a+dx)\) and \(f(a)\), where \(L(x)\) is the linear approximation of \(f\) at \(a\). By definition of \(L(x)\), this difference is equal to \(f'(a)\,dx\). In summary,
\[Δy=f(a+dx)−f(a)≈L(a+dx)−f(a)=f'(a)\,dx=dy. \nonumber \]
Therefore, we can use the differential \(dy=f'(a)\,dx\) to approximate the change in \(y\) if \(x\) increases from \(x=a\) to \(x=a+dx\). We can see this in the following graph.

We now take a look at how to use differentials to approximate the change in the value of the function that results from a small change in the value of the input. Note the calculation with differentials is much simpler than calculating actual values of functions and the result is very close to what we would obtain with the more exact calculation.
Example \(\PageIndex{5}\): Approximating Change with Differentials
Let \(y=x^2+2x.\) Compute \(Δy\) and \(dy\) at \(x=3\) if \(dx=0.1.\)
The actual change in \(y\) if \(x\) changes from \(x=3\) to \(x=3.1\) is given by
\(Δy=f(3.1)−f(3)=[(3.1)^2+2(3.1)]−[3^2+2(3)]=0.81.\)
The approximate change in \(y\) is given by \(dy=f'(3)\,dx\). Since \(f'(x)=2x+2,\) we have
\(dy=f'(3)\,dx=(2(3)+2)(0.1)=0.8.\)
Exercise \(\PageIndex{5}\)
For \(y=x^2+2x,\) find \(Δy\) and \(dy\) at \(x=3\) if \(dx=0.2.\)
\(dy=f'(3)\,dx, \;Δy=f(3.2)−f(3)\)
\(dy=1.6, \; Δy=1.64\)
Calculating the Amount of Error
Any type of measurement is prone to a certain amount of error. In many applications, certain quantities are calculated based on measurements. For example, the area of a circle is calculated by measuring the radius of the circle. An error in the measurement of the radius leads to an error in the computed value of the area. Here we examine this type of error and study how differentials can be used to estimate the error.
Consider a function \(f\) with an input that is a measured quantity. Suppose the exact value of the measured quantity is \(a\), but the measured value is \(a+dx\). We say the measurement error is \(dx\) (or \(Δx\)). As a result, an error occurs in the calculated quantity \(f(x)\). This type of error is known as a propagated error and is given by
Since all measurements are prone to some degree of error, we do not know the exact value of a measured quantity, so we cannot calculate the propagated error exactly. However, given an estimate of the accuracy of a measurement, we can use differentials to approximate the propagated error \(Δy.\) Specifically, if \(f\) is a differentiable function at \(a\),the propagated error is
\[Δy≈dy=f'(a)\,dx. \nonumber \]
Unfortunately, we do not know the exact value \(a.\) However, we can use the measured value \(a+dx,\) and estimate
\[Δy≈dy≈f'(a+dx)\,dx. \nonumber \]
In the next example, we look at how differentials can be used to estimate the error in calculating the volume of a box if we assume the measurement of the side length is made with a certain amount of accuracy.
Example \(\PageIndex{6}\): Volume of a Cube
Suppose the side length of a cube is measured to be \(5\) cm with an accuracy of \(0.1\) cm.
- Use differentials to estimate the error in the computed volume of the cube.
- Compute the volume of the cube if the side length is (i) \(4.9\) cm and (ii) \(5.1\) cm to compare the estimated error with the actual potential error.
a. The measurement of the side length is accurate to within \(±0.1\) cm. Therefore,
\(−0.1≤dx≤0.1.\)
The volume of a cube is given by \(V=x^3\), which leads to
\(dV=3x^2dx.\)
Using the measured side length of \(5\) cm, we can estimate that
\(−3(5)^2(0.1)≤dV≤3(5)^2(0.1).\)
\(−7.5≤dV≤7.5.\)
b. If the side length is actually \(4.9\) cm, then the volume of the cube is
\(V(4.9)=(4.9)^3=117.649\text{cm}^3.\)
If the side length is actually \(5.1\) cm, then the volume of the cube is
\(V(5.1)=(5.1)^3=132.651\text{cm}^3.\)
Therefore, the actual volume of the cube is between \(117.649\) and \(132.651\). Since the side length is measured to be 5 cm, the computed volume is \(V(5)=5^3=125.\) Therefore, the error in the computed volume is
\(117.649−125≤ΔV≤132.651−125.\)
\(−7.351≤ΔV≤7.651.\)
We see the estimated error \(dV\) is relatively close to the actual potential error in the computed volume.
Exercise \(\PageIndex{6}\)
Estimate the error in the computed volume of a cube if the side length is measured to be \(6\) cm with an accuracy of \(0.2\) cm.
\(dV=3x^2dx\)
The volume measurement is accurate to within \(21.6\,\text{cm}^3\).
The measurement error \(dx\ (=Δx)\) and the propagated error \(Δy\) are absolute errors. We are typically interested in the size of an error relative to the size of the quantity being measured or calculated. Given an absolute error \(Δq\) for a particular quantity, we define the relative error as \(\frac{Δq}{q}\), where \(q\) is the actual value of the quantity. The percentage error is the relative error expressed as a percentage. For example, if we measure the height of a ladder to be \(63\) in. when the actual height is \(62\) in., the absolute error is 1 in. but the relative error is \(\frac{1}{62}=0.016\), or \(1.6\%\). By comparison, if we measure the width of a piece of cardboard to be \(8.25\) in. when the actual width is \(8\) in., our absolute error is \(\frac{1}{4}\) in., whereas the relative error is \(\frac{0.25}{8}=\frac{1}{32}\), or \(3.1\%.\) Therefore, the percentage error in the measurement of the cardboard is larger, even though \(0.25\) in. is less than \(1\) in.
Example \(\PageIndex{7}\): Relative and Percentage Error
An astronaut using a camera measures the radius of Earth as \(4000\) mi with an error of \(±80\) mi. Let’s use differentials to estimate the relative and percentage error of using this radius measurement to calculate the volume of Earth, assuming the planet is a perfect sphere.
Solution: If the measurement of the radius is accurate to within \(±80,\) we have
\(−80≤dr≤80.\)
Since the volume of a sphere is given by \(V=(\frac{4}{3})πr^3,\) we have
\(dV=4πr^2dr.\)
Using the measured radius of \(4000\) mi, we can estimate
\(−4π(4000)^2(80)≤dV≤4π(4000)^2(80).\)
To estimate the relative error, consider \(\dfrac{dV}{V}\). Since we do not know the exact value of the volume \(V\), use the measured radius \(r=4000\) mi to estimate \(V\). We obtain \(V≈(\frac{4}{3})π(4000)^3\). Therefore the relative error satisfies
\(\frac{−4π(4000)^2(80)}{4π(4000)^3/3}≤\dfrac{dV}{V}≤\frac{4π(4000)^2(80)}{4π(4000)^3/3},\)
which simplifies to
\(−0.06≤\dfrac{dV}{V}≤0.06.\)
The relative error is \(0.06\) and the percentage error is \(6\%\).
Exercise \(\PageIndex{7}\)
Determine the percentage error if the radius of Earth is measured to be \(3950\) mi with an error of \(±100\) mi.
Use the fact that \(dV=4πr^2dr\) to find \(dV/V\).
Key Concepts
- A differentiable function \(y=f(x)\) can be approximated at \(a\) by the linear function
\(L(x)=f(a)+f'(a)(x−a).\)
- For a function \(y=f(x)\), if \(x\) changes from \(a\) to \(a+dx\), then
is an approximation for the change in \(y\). The actual change in \(y\) is
\(Δy=f(a+dx)−f(a).\)
- A measurement error \(dx\) can lead to an error in a calculated quantity \(f(x)\). The error in the calculated quantity is known as the propagated error. The propagated error can be estimated by
\(dy≈f'(x)\,dx.\)
- To estimate the relative error of a particular quantity \(q\), we estimate \(\frac{Δq}{q}\).
Key Equations
- Linear approximation
- A differential
Please ensure that your password is at least 8 characters and contains each of the following:
- a special character: @$#!%*?&

IMAGES
VIDEO
COMMENTS
2) Determine the necessary conditions such that the linear approximation function is constant. Use a graph to prove your result. Answer. \ (f' (a) = 0\) 3) Explain why the linear approximation becomes less accurate as you increase the distance between \ (x\) and \ (a\). Use a graph to prove your argument.
Math. Calculus. Calculus questions and answers. Homework: 4.5) Linear Approximation HW ⓧ 4.5.38 of the by.
Example 4.5.1: Linear Approximation of √x. Find the linear approximation of f(x) = √x at x = 9 and use the approximation to estimate √9.1. Solution. Since we are looking for the linear approximation at x = 9, using Equation 4.5.1 we know the linear approximation is given by. L(x) = f(9) + f ′ (9)(x − 9).
View 4_5_Homework.pdf from MATH MISC at University of Nevada, Reno. 4.5 Linear Approximation Homework Problems 1 - 4, Find the tangent line approximation of each function at the given value = . ... Examine Figure 4 on the right and answer the following questions about elevation. Remember, contours can run off the edge of the map and return back ...
Click the card to flip 👆. a. Write the equation of the line that represents the linear approximation to the following function at the given point a. 16 - 5x² at a = 4; f(4.1) b. Use the linear approximation to estimate the given function value.
How can we use the tangent line to approximate the y-value of function f? 1.First of all, we find the tangent line of the point that close to the approximate point. 2. Use Formula: L (x)=f' (a) (x-a)+f (a), where a is the point x you picked. 3. Plug in the approximate x ( one you want to find the y-value from) to the L (x). underestimate is ...
View 4.5 Linear Approximation HW.pdf from MATH 405 at University of Phoenix. 4.5 Linear Approximation Homework Problems 1 - 4, Find the tangent line approximation of each function at the given value ... 4-5_Homework-key.pdf. Crestwood High School. MATH GEOMETRY. 4.6_hw.pdf. Solutions Available. ... Show work that leads to your answer. B ...
4.5 Linear Approximation Homework Answers Flamingo Math | added by users. 4644 kb/s. 3527. Search results. ... 4.5 linear approximation homework answers mathswatch vle answers gcse guia para examen ipn 2024 edexcel a level exam timetable january 2024 ccna exam fees in rupees
View Homework Help - Worksheet 4.5 Solutions.pdf from MATH 1172 at Ohio State University. 4.5: Linear Approximation and Differentials Problem 1 (a) Find the linearization, L(x), of the function f (x)
Michelle C. AP Calculus AB Big Bundle Curriculum. Jean Adams produces high-quality instructional materials. The curriculums she has created are second to none. The guided notes are thorough and provide the students with examples that promote critical thinking skills and prepare the student for the homework section.
(iv) Find the linear approximation, L(x). (v) Compute the approximate value of the expression using the linear approximation. (vi) Compare the approximated value to the value given by your calculator. (vii) Compare dyand yusing the value given by your calculator. Solution: (a)cos 31ˇ 180 (i) f(x) = cosx (ii) a= 30ˇ 180 = ˇ 6 (iii) x= 31ˇ ...
Calculus. Calculus questions and answers. Homework: 4.5 - Linear Approximations Save Score: 1.5 of 2 pts 5 of 21 (21 complete) HW Score: 97.83%, 22.5 of 23 pts 4.5.19 Question Help a. Write the equation of the line that represents the linear approximation to the following function at the given point a b.
calc_4.4_packet.pdf. File Size: 251 kb. File Type: pdf. Download File. Want to save money on printing? Support us and buy the Calculus workbook with all the packets in one nice spiral bound book. Solution manuals are also available.
View 4-5_Homework-key.pdf from MATH GEOMETRY at Crestwood High School. 4.5 Key Linear Approximation Homework Problems 1 - 4, Find the tangent line approximation of each function at the given ... Examine Figure 4 on the right and answer the following questions about elevation. Remember, contours can run off the edge of the map and return back ...
test to help you meet your assessment needs. ***** NOTE: Each lesson in this product has been revised and realigned to match the new Topics and pacing of the Fall 2019 CED Binder. Your AP Calculus AB students will have a set of Guided Notes and a comprehensive homework assignment, plus a daily content quiz with
View 4.5 Linear Approximation HW KEY.pdf from MATH AB at Macarthur High School. 17 7. Find the linearization of the function f (x) = x + 3 at x = 1 and use it to approximate v4.05 . Determine
Free math problem solver answers your algebra homework questions with step-by-step explanations.
See Answer See Answer See Answer done loading Question: Homework: HW15 (4.5) Linear Approximation & Differentials Score: 0 of 5 pts 2 of 9 (8 complete) 4.6.29 a. Write the equation of the line that represents the linear approximation to the following function at the given point a. b. Use the linear approximation to estimate the given quantity c ...
What is the value found using the linear approximation? Convert any degree measures to radians. cos 24 (Type an exact answer, using as needed.) help! please write LEGIBALLY
Question: Use linear approximation to find a decimal approximation of 4.5 : Answer: 4.5≈ This is Section 3.1 Problem 42: For y=f (x)=xex−5, when x=5 and dx=0.1 : dy= Hence the linear approximation using dy is f (5.1)≈f (5)+dy)=. Show transcribed image text. There are 2 steps to solve this one.