

Climate Change: Evidence and Causes: Update 2020 (2020)
Chapter: conclusion, c onclusion.
This document explains that there are well-understood physical mechanisms by which changes in the amounts of greenhouse gases cause climate changes. It discusses the evidence that the concentrations of these gases in the atmosphere have increased and are still increasing rapidly, that climate change is occurring, and that most of the recent change is almost certainly due to emissions of greenhouse gases caused by human activities. Further climate change is inevitable; if emissions of greenhouse gases continue unabated, future changes will substantially exceed those that have occurred so far. There remains a range of estimates of the magnitude and regional expression of future change, but increases in the extremes of climate that can adversely affect natural ecosystems and human activities and infrastructure are expected.
Citizens and governments can choose among several options (or a mixture of those options) in response to this information: they can change their pattern of energy production and usage in order to limit emissions of greenhouse gases and hence the magnitude of climate changes; they can wait for changes to occur and accept the losses, damage, and suffering that arise; they can adapt to actual and expected changes as much as possible; or they can seek as yet unproven “geoengineering” solutions to counteract some of the climate changes that would otherwise occur. Each of these options has risks, attractions and costs, and what is actually done may be a mixture of these different options. Different nations and communities will vary in their vulnerability and their capacity to adapt. There is an important debate to be had about choices among these options, to decide what is best for each group or nation, and most importantly for the global population as a whole. The options have to be discussed at a global scale because in many cases those communities that are most vulnerable control few of the emissions, either past or future. Our description of the science of climate change, with both its facts and its uncertainties, is offered as a basis to inform that policy debate.
A CKNOWLEDGEMENTS
The following individuals served as the primary writing team for the 2014 and 2020 editions of this document:
- Eric Wolff FRS, (UK lead), University of Cambridge
- Inez Fung (NAS, US lead), University of California, Berkeley
- Brian Hoskins FRS, Grantham Institute for Climate Change
- John F.B. Mitchell FRS, UK Met Office
- Tim Palmer FRS, University of Oxford
- Benjamin Santer (NAS), Lawrence Livermore National Laboratory
- John Shepherd FRS, University of Southampton
- Keith Shine FRS, University of Reading.
- Susan Solomon (NAS), Massachusetts Institute of Technology
- Kevin Trenberth, National Center for Atmospheric Research
- John Walsh, University of Alaska, Fairbanks
- Don Wuebbles, University of Illinois
Staff support for the 2020 revision was provided by Richard Walker, Amanda Purcell, Nancy Huddleston, and Michael Hudson. We offer special thanks to Rebecca Lindsey and NOAA Climate.gov for providing data and figure updates.
The following individuals served as reviewers of the 2014 document in accordance with procedures approved by the Royal Society and the National Academy of Sciences:
- Richard Alley (NAS), Department of Geosciences, Pennsylvania State University
- Alec Broers FRS, Former President of the Royal Academy of Engineering
- Harry Elderfield FRS, Department of Earth Sciences, University of Cambridge
- Joanna Haigh FRS, Professor of Atmospheric Physics, Imperial College London
- Isaac Held (NAS), NOAA Geophysical Fluid Dynamics Laboratory
- John Kutzbach (NAS), Center for Climatic Research, University of Wisconsin
- Jerry Meehl, Senior Scientist, National Center for Atmospheric Research
- John Pendry FRS, Imperial College London
- John Pyle FRS, Department of Chemistry, University of Cambridge
- Gavin Schmidt, NASA Goddard Space Flight Center
- Emily Shuckburgh, British Antarctic Survey
- Gabrielle Walker, Journalist
- Andrew Watson FRS, University of East Anglia
The Support for the 2014 Edition was provided by NAS Endowment Funds. We offer sincere thanks to the Ralph J. and Carol M. Cicerone Endowment for NAS Missions for supporting the production of this 2020 Edition.
F OR FURTHER READING
For more detailed discussion of the topics addressed in this document (including references to the underlying original research), see:
- Intergovernmental Panel on Climate Change (IPCC), 2019: Special Report on the Ocean and Cryosphere in a Changing Climate [ https://www.ipcc.ch/srocc ]
- National Academies of Sciences, Engineering, and Medicine (NASEM), 2019: Negative Emissions Technologies and Reliable Sequestration: A Research Agenda [ https://www.nap.edu/catalog/25259 ]
- Royal Society, 2018: Greenhouse gas removal [ https://raeng.org.uk/greenhousegasremoval ]
- U.S. Global Change Research Program (USGCRP), 2018: Fourth National Climate Assessment Volume II: Impacts, Risks, and Adaptation in the United States [ https://nca2018.globalchange.gov ]
- IPCC, 2018: Global Warming of 1.5°C [ https://www.ipcc.ch/sr15 ]
- USGCRP, 2017: Fourth National Climate Assessment Volume I: Climate Science Special Reports [ https://science2017.globalchange.gov ]
- NASEM, 2016: Attribution of Extreme Weather Events in the Context of Climate Change [ https://www.nap.edu/catalog/21852 ]
- IPCC, 2013: Fifth Assessment Report (AR5) Working Group 1. Climate Change 2013: The Physical Science Basis [ https://www.ipcc.ch/report/ar5/wg1 ]
- NRC, 2013: Abrupt Impacts of Climate Change: Anticipating Surprises [ https://www.nap.edu/catalog/18373 ]
- NRC, 2011: Climate Stabilization Targets: Emissions, Concentrations, and Impacts Over Decades to Millennia [ https://www.nap.edu/catalog/12877 ]
- Royal Society 2010: Climate Change: A Summary of the Science [ https://royalsociety.org/topics-policy/publications/2010/climate-change-summary-science ]
- NRC, 2010: America’s Climate Choices: Advancing the Science of Climate Change [ https://www.nap.edu/catalog/12782 ]
Much of the original data underlying the scientific findings discussed here are available at:
- https://data.ucar.edu/
- https://climatedataguide.ucar.edu
- https://iridl.ldeo.columbia.edu
- https://ess-dive.lbl.gov/
- https://www.ncdc.noaa.gov/
- https://www.esrl.noaa.gov/gmd/ccgg/trends/
- http://scrippsco2.ucsd.edu
- http://hahana.soest.hawaii.edu/hot/

Climate change is one of the defining issues of our time. It is now more certain than ever, based on many lines of evidence, that humans are changing Earth's climate. The Royal Society and the US National Academy of Sciences, with their similar missions to promote the use of science to benefit society and to inform critical policy debates, produced the original Climate Change: Evidence and Causes in 2014. It was written and reviewed by a UK-US team of leading climate scientists. This new edition, prepared by the same author team, has been updated with the most recent climate data and scientific analyses, all of which reinforce our understanding of human-caused climate change.
Scientific information is a vital component for society to make informed decisions about how to reduce the magnitude of climate change and how to adapt to its impacts. This booklet serves as a key reference document for decision makers, policy makers, educators, and others seeking authoritative answers about the current state of climate-change science.
READ FREE ONLINE
Welcome to OpenBook!
You're looking at OpenBook, NAP.edu's online reading room since 1999. Based on feedback from you, our users, we've made some improvements that make it easier than ever to read thousands of publications on our website.
Do you want to take a quick tour of the OpenBook's features?
Show this book's table of contents , where you can jump to any chapter by name.
...or use these buttons to go back to the previous chapter or skip to the next one.
Jump up to the previous page or down to the next one. Also, you can type in a page number and press Enter to go directly to that page in the book.
Switch between the Original Pages , where you can read the report as it appeared in print, and Text Pages for the web version, where you can highlight and search the text.
To search the entire text of this book, type in your search term here and press Enter .
Share a link to this book page on your preferred social network or via email.
View our suggested citation for this chapter.
Ready to take your reading offline? Click here to buy this book in print or download it as a free PDF, if available.
Get Email Updates
Do you enjoy reading reports from the Academies online for free ? Sign up for email notifications and we'll let you know about new publications in your areas of interest when they're released.
- Open access
- Published: 04 January 2021
Climate change and health in North America: literature review protocol
- Sherilee L. Harper ORCID: orcid.org/0000-0001-7298-8765 1 ,
- Ashlee Cunsolo 2 ,
- Amreen Babujee 1 ,
- Shaugn Coggins 1 ,
- Mauricio Domínguez Aguilar 3 &
- Carlee J. Wright 1
Systematic Reviews volume 10 , Article number: 3 ( 2021 ) Cite this article
27k Accesses
11 Citations
9 Altmetric
Metrics details
Climate change is a defining issue and grand challenge for the health sector in North America. Synthesizing evidence on climate change impacts, climate-health adaptation, and climate-health mitigation is crucial for health practitioners and decision-makers to effectively understand, prepare for, and respond to climate change impacts on human health. This protocol paper outlines our process to systematically conduct a literature review to investigate the climate-health evidence base in North America.
A search string will be used to search CINAHL®, Web of Science™, Scopus®, Embase® via Ovid, and MEDLINE® via Ovid aggregator databases. Articles will be screened using inclusion/exclusion criteria by two independent reviewers. First, the inclusion/exclusion criteria will be applied to article titles and abstracts, and then to the full articles. Included articles will be analyzed using quantitative and qualitative methods.
This protocol describes review methods that will be used to systematically and transparently create a database of articles published in academic journals that examine climate-health in North America.
Peer Review reports
The direct and indirect impacts of climate change on human health continue to be observed globally, and these wide-ranging impacts are projected to continue to increase and intensify this century [ 1 , 2 ]. The direct climate change effects on health include rising temperatures, which increase heat-related mortality and morbidity [ 3 , 4 , 5 ], and increased frequency and intensity of storms, resulting in increased injury, death, and psychological stressors [ 2 , 6 , 7 , 8 ]. Indirect climate change impacts on health occur via altered environmental conditions, such as climate change impacts on water quality and quantity, which increase waterborne disease [ 9 , 10 , 11 , 12 , 13 ]; shifting ecosystems, which increase the risk of foodborne disease [ 14 , 15 , 16 ], exacerbate food and nutritional security [ 17 , 18 ], and change the range and distribution of vectors that cause vectorborne disease [ 19 , 20 ]; and place-based connections and identities, leading to psycho-social stressors and potential increases in negative mental health outcomes and suicide [ 6 , 8 ]. These wide-ranging impacts are not uniformly or equitably distributed: children, the elderly, those with pre-existing health conditions, those experiencing lower socio-economic conditions, women, and those with close connections to and reliance upon the local environment (e.g. Indigenous Peoples, farmers, fishers) often experience higher burdens of climate-health impacts [ 1 , 2 , 21 ]. Indeed, climate change impacts on human health not only are dependent on exposure to climatic and environmental changes, but also depend on climate change sensitivity and adaptive capacity—both of which are underpinned by the social determinants of health [ 1 , 22 , 23 ].
The inherent complexity, great magnitude, and widespread, inequitable, and intersectional distribution of climate change impacts on health present an urgent and grand challenge for the health sector this century [ 2 , 24 , 25 ]. Climate-health research and evidence is critical for informing effective, equitable, and timely adaptation responses and strategies. For instance, research continues to inform local to international climate change and health vulnerability and adaptation assessments [ 26 ]. However, to create evidence-based climate-health adaptation strategies, health practitioners, researchers, and policy makers must sift and sort through vast and often unmanageable amounts of information. Indeed, the global climate-health evidence base has seen exponential growth in recent years, with tens of thousands of articles published globally this century [ 22 , 25 , 27 , 28 ]. Even when resources are available to parse through the evidence base, the available research evidence may not be locally pertinent to decision-makers, may provide poor quality of evidence, may exclude factors important to decision-makers, may overlook temporal and geographical scales over which decision-makers have impact, and/or may not produce information in a timely manner [ 29 , 30 , 31 , 32 , 33 , 34 , 35 , 36 , 37 ].
Literature reviews that utilize systematic methods present a tool to efficiently and effectively integrate climate-health information and provide data to support evidence-based decision-making. Furthermore, literature reviews that use systematic methods are replicable and transparent, reduce bias, and are ultimately intended to improve reliability and accuracy of conclusions. As such, systematic approaches to identify, explore, evaluate, and synthesize literature separates insignificant, less rigorous, or redundant literature from the critical and noteworthy studies that are worthy of exploration and consideration [ 38 ]. As such, a systematic approach to synthesizing the climate-health literature provides invaluable information and adds value to the climate-health evidence base from which decision-makers can draw from. Therefore, we aim to systematically and transparently create a database of articles published in academic journals that examine climate-health in North America. As such, we outline our protocol that will be used to systematically identify and characterize literature at the climate-health nexus in North America.
This protocol was designed in accordance with the Preferred Reporting Items for Systematic Review and Meta-Analyses (PRISMA) Guidelines [ 39 , 40 ] and presented in accordance with the PRISMA-P checklist.
Research questions
Research on climate change and human health encompasses a diverse range of health outcomes, climate change exposures, populations, and study designs. Given the breadth and depth of information needed by health practitioners and decision-makers, a variety of research questions will be examined (Table 1 ).
Search strategy
The search strategy, including the search string development and selection of databases, was developed in consultation with a research librarian and members of the research team (SLH, AC, and MDA). The search string contains terms related to climate change [ 41 , 42 ], human health outcomes [ 1 , 25 , 43 , 44 ], and study location (Table 2 ). Given the interdisciplinary nature of the climate-health nexus and to ensure that our search is comprehensive, the search string will be used to search five academic databases:
CINAHL® will be searched to capture unique literature not found in other databases on common disease and injury conditions, as well as other health topics;
Web of Science™ will be searched to capture a wide range of multi-disciplinary literature;
Scopus® will be searched to capture literature related to medicine, technology, science, and social sciences;
Embase® via Ovid will be searched to capture a vast range of biomedical sciences journals; and
MEDLINE® via Ovid will be searched to capture literature on biomedical and health sciences.
No language restrictions will be placed on the search. Date restrictions will be applied to capture literature published on or after 01 January 2013, in order to capture literature published after the Intergovernmental Panel on Climate Change (IPCC) Fifth Assessment Report (which assessed literature accepted for publication prior to 31 August 2013). An initial test search was conducted on June 10, 2019, and updated on February 14, 2020; however, the search will be updated to include literature published within the most recent full calendar year prior to publication.
To explore the sensitivity of our search and capture any missed articles, (1) a snowball search will be conducted on the reference lists of all the literature that meet the inclusion criteria and (2) a hand search of three relevant disciplinary journals will be conducted:
Environmental Health Perspectives , an open access peer-reviewed journal that is a leading disciplinary journal within environmental health sciences;
The Lancet , a peer-reviewed journal that is the leading disciplinary journal within public health sciences; and
Climatic Change , a peer-reviewed journal covering cross-disciplinary literature that is a leading disciplinary journal for climate change research.
Citations will be downloaded from the databases and uploaded into Mendeley™ reference management software to facilitate reference management, article retrieval, and removal of duplicate citations. Then, de-duplicated citations will be uploaded into DistillerSR® to facilitate screening.
Article selection
Inclusion and exclusion criteria.
To be included, articles must evaluate or examine the intersection of climate change and human health in North America (Fig. 1 ). Health is defined to include physical, mental, emotional, and social health and wellness [ 1 , 25 , 43 , 44 ] (Fig. 1 ). This broad definition will be used to examine the nuanced and complex direct and indirect impacts of climate change on human health. To examine the depth and breadth of climate change impacts on health, climate change contexts are defined to include seasonality, weather parameters, extreme weather events, climate, climate change, climate variability, and climate hazards [ 41 , 42 ] (Fig. 1 ). However, articles that discuss climate in terms of indoor work environments, non-climate hazards due to geologic events (e.g. earthquakes), and non-anthropogenic climate change (e.g. due to volcanic eruptions) will be excluded. This broad definition of climate change contexts will be used in order to examine the wide range and complexity of climate change impacts on human health. To be included, articles need to explicitly link health outcomes to climate change in the goal statement, methods section, and/or results section of the article. Therefore, articles that discuss both human health and climate change—but do not link the two together—will be excluded. The climate-health research has to take place in North America to be included. North America is defined to include Canada, the USA, and Mexico in order to be consistent with the IPCC geographical classifications; that is, in the Fifth Assessment Report, the IPCC began confining North America to include Canada, Mexico, and the USA [ 45 ] (Fig. 1 ). Articles published in any language will be eligible for inclusion. Articles need to be published online on or after 01 January 2013 to be included. No restrictions will be placed on population type (i.e. all human studies will be eligible for inclusion).
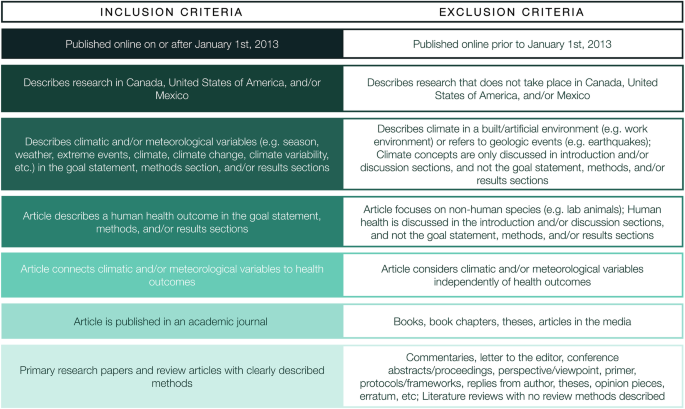
Inclusion and exclusion criteria to review climate change and health literature in North America
Level 1 screening
The title and abstract of each citation will be examined for relevance. A stacked questionnaire will be used to screen the titles and abstracts; that is, when a criterion is not met, the subsequent criteria will not be assessed. When all inclusion criteria are met and/or it is unclear whether or not an inclusion criterion is met (e.g. “unsure”), the article will proceed to Level 2 screening. If the article meets any exclusion criteria, it will not proceed to Level 2 screening. Level 1 screening will be completed by two independent reviewers, who will meet to resolve any conflicts via discussion. The level of agreement between reviewers will be evaluated by dividing the total number of conflicts by the total number of articles screened for Level 1.
Level 2 screening
The full text of all potentially relevant articles will be screened for relevance. A stacked questionnaire will also be used to screen the full texts. In Level 2 screening, only articles that meet all the inclusion criteria will be included in the review (i.e. “unsure” will not be an option). Level 2 screening will be completed by two independent reviewers, who will meet to resolve any conflicts via discussion. The level of agreement between reviewers will be evaluated by dividing the total number of conflicts by the total number of articles screened for Level 2 (Fig. 2 ).
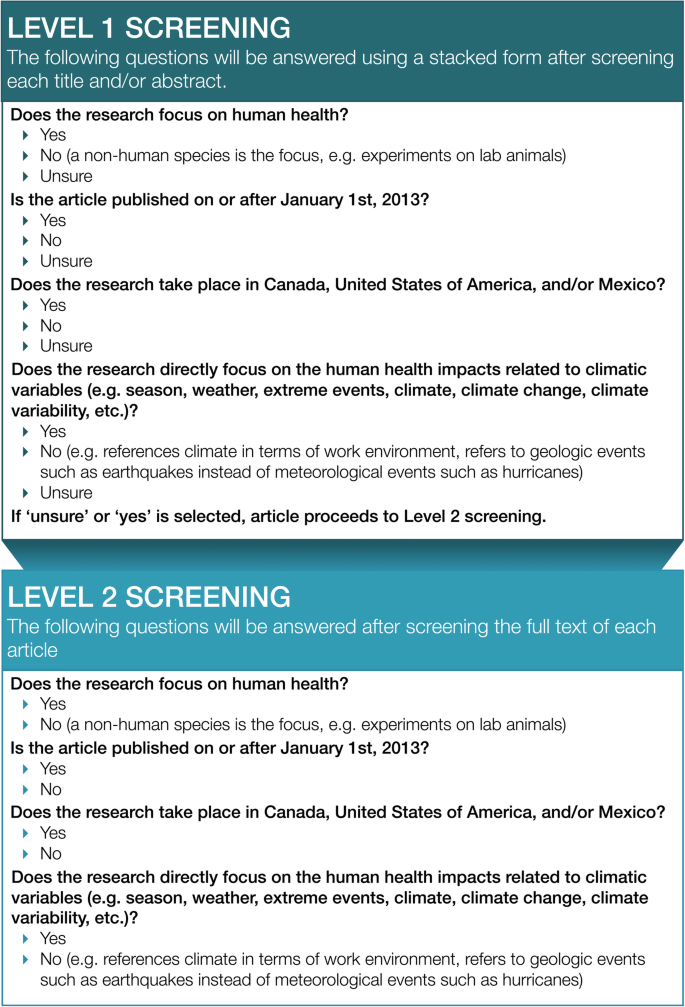
Flow chart of screening questions for the literature review on climate change and health in North America
Data extraction and analysis
A data extraction form will be created in DistillerSR® ( Appendix 2 ) and will be tested by three data extractors on a sample of articles to allow for calibration on the extraction process (i.e. 5% of articles if greater than 50 articles, 10% of articles if less than or equal to 50 articles). After completing the calibration process, the form will be adapted based on feedback from the extractors to improve usability and accuracy. The data extractors will then use the data extraction form to complete data extraction. Reviewers will meet regularly to discuss and resolve any further issues in data extraction, in order to ensure the data extraction process remains consistent across reviewers.
Data will be extracted from original research papers (i.e. articles containing data collection and analysis) and review articles that reported a systematic methodology. This data extraction will focus on study characteristics, including the country that the data were collected in, focus of the study (i.e. climate change impact, adaptation, and/or mitigation), weather variables, climatic hazards, health outcomes, social characteristics, and future projections. The categories within each study characteristic will not be mutually exclusive, allowing more than one response/category to be selected under each study characteristic. For the country of study, Canada, the USA, and/or Mexico will be selected if the article describes data collection in each country respectively. Non-North American regions will be selected if the article not only collects data external to North America, but also includes data collection within Canada, the USA, and/or Mexico. For the study focus, data will be extracted on whether the article focuses on climate change impacts, adaptation, and/or mitigation within the goals, methods, and/or results sections of the article. Temperature, precipitation, and/or UV radiation will be selected for weather variables if the article utilizes these data in the goal, methods, and/or results sections. Data will be extracted on the following climatic hazards if the article addresses them in the goal, methods, and/or results sections: heat events (e.g. extreme heat, heat waves), cold events (e.g. extreme cold, winter storms), air quality (e.g. pollution, parts per million (PPM) data, greenhouse gas emissions), droughts, flooding, wildfires, hurricanes, wildlife changes (including changes in disease vectors such as ticks or mosquitos), vegetation changes (including changes in pollen), freshwater (including drinking water), ocean conditions (including sea level rise and ocean acidity/salinity/temperature changes), ice extent/stability/duration (including sea ice and freshwater ice), coastal erosion, permafrost changes, and/or environmental hazards (e.g. exposure to sewage, reduced crop productivity).
Data will be extracted on the following health outcomes if the article focuses on them within the goal, methods, and/or results sections: heat-related morbidity and/or mortality, respiratory outcomes (including asthma, chronic obstructive pulmonary disease), cardiovascular outcomes (including heart attacks or stroke), urinary outcomes (e.g. urinary tract infections, renal failure), dermatologic concerns, mental health and wellness (e.g. suicide, emotional health), fetal health/birth outcomes and/or maternal health, cold exposure, allergies, nutrition (including nutrient deficiency), waterborne disease, foodborne disease, vectorborne disease, injuries (including accidents), and general morbidity and/or mortality. Data on the following social characteristics will also be extracted from the articles if they are included in the goal, methods, and/or results sections of the article: access to healthcare, sex and/or gender, age, income, livelihood (including data on employment, occupation), ethnicity, culture, Indigenous Peoples, rural/remote communities (“rural”, “remote”, or similar terminology must be explicitly mentioned), urban communities (“urban”, “city”, “metropolitan”, or similar terminology must be explicitly used), coastal communities (use of “coastal”, or similar terms must be explicitly mentioned), residence location (zipcode/postal code, neighbourhood, etc.), level of education, and housing (e.g. data on size, age, number of windows, air conditioning). Finally, data will be collected on future projections, including projections that employ qualitative and/or quantitative methods that are included in the goal, methods, and/or results sections of the article.
Descriptive statistics and regression modelling will be used to examine publication trends. Data will be visualized through the use of maps, graphs, and other visualization techniques as appropriate. To enable replicability and transparency, a PRISMA flowchart will be created to illustrate the article selection process and reasons for exclusion. Additionally, qualitative thematic analyses will be conducted. These analyses will utilize constant-comparative approaches to identify patterns across articles through the identification, development, and refinement of codes and themes. Article excerpts will be grouped under thematic categories in order to explore connections in article characteristics, methodologies, and findings.
Quality appraisal of studies included in the systematic scoping review will be performed using a framework based on the Mixed Methods Appraisal Tool (MMAT) [ 46 ] and the Confidence in the Evidence from Reviews of Qualitative Research (CERQual) tool [ 47 ]. This will enable appraisal of evidence in reviews that contain qualitative, quantitative, and mixed methods studies, as well as appraisal of methodological limitations in included qualitative studies. These tools may be adapted to include additional questions as required in order to fit the scope and objectives of the review. A minimum of two reviewers will independently appraise the included articles and discuss judgements as needed. The findings will be made available as supplementary material for the review.
Climate-health literature reviews using systematic methods will be increasingly critical in the health sector, given the depth and breadth of the growing body of climate change and health literature, as well as the urgent need for evidence to inform climate-health adaptation and mitigation strategies. To support and encourage the systematic and transparent identification and synthesis of climate-health information, this protocol describes our approach to systematically and transparently create a database of articles published in academic journals that examine climate-health in North America.
Availability of data and materials
Not applicable.
Abbreviations
Confidence in the Evidence from Reviews of Qualitative Research
Intergovernmental Panel on Climate Change
Mixed Methods Appraisal Tool
Parts per million
Preferred Reporting Items for Systematic review and Meta-Analyses
Preferred Reporting Items for Systematic review and Meta-Analyses, Protocol Extension
- United States of America
Ultraviolet
Smith KR, Woodward A, Campbell-Lendrum D, Chadee DD, Honda Y, Liu Q, et al. Human health: impacts, adaptation, and co-benefits. In: Field CB, Barros VR, Dokken DJ, Mach KJ, Mastrandrea MD, Bilir TE, et al., editors. Climate Change 2014: impacts, adaptation, and vulnerability part A: global and sectoral aspects contribution of Working Group II to the Fifth Assessment Report of the Intergovernmental Panel on Climate Change. Cambridge, UK and New York, USA: Cambridge University Press; 2014. p. 709–54.
Google Scholar
Watts N, Amann M, Ayeb-Karlsson S, Belesova K, Bouley T, Boykoff M, et al. The Lancet Countdown on health and climate change: from 25 years of inaction to a global transformation for public health. Lancet. 2018;391(10120):581–630.
Article Google Scholar
Son JY, Liu JC, Bell ML. Temperature-related mortality: a systematic review and investigation of effect modifiers. Environ Res Lett. 2019;14:073004.
Campbell S, Remenyi TA, White CJ, Johnston FH. Heatwave and health impact research: a global review. Heal Place. 2018;53:210–8.
Sanderson M, Arbuthnott K, Kovats S, Hajat S, Falloon P. The use of climate information to estimate future mortality from high ambient temperature: a systematic literature review. PLoS One. 2017: e0180369.
Rataj E, Kunzweiler K, Garthus-Niegel S. Extreme weather events in developing countries and related injuries and mental health disorders - a systematic review. BMC Public Health. 2016;16:1020.
Saulnier DD, Brolin Ribacke K, von Schreeb J. No Calm after the storm: a systematic review of human health following flood and storm disasters. Prehosp Disaster Med. 2017;32(5):568–79.
Cunsolo A, Neville E. Ecological grief as a mental health response to climate change-related loss. Nat Clim Chang. 2018;8:275–81.
Levy K, Woster AP, Goldstein RS, Carlton EJ. Untangling the impacts of climate change on waterborne diseases: a systematic review of relationships between diarrheal diseases and temperature, rainfall, flooding, and drought. Environ Sci Technol. 2016;50:4905–22.
Article CAS Google Scholar
Semenza JC, Herbst S, Rechenburg A, Suk JE, Höser C, Schreiber C, et al. Climate change impact assessment of food- and waterborne diseases. Crit Rev Environ Sci Technol. 2012;42(8):857–90.
Cann K, Thomas D, Salmon R, W-J AP, Kay D. Extreme water-related weather events and waterborne disease. Epidemiol Infect. 2013;141:671–86.
Andrade L, O’Dwyer J, O’Neill E, Hynds P. Surface water flooding, groundwater contamination, and enteric disease in developed countries: a scoping review of connections and consequences. Environ Pollut. 2018;236:540–9.
Harper SL, Wright C, Masina S, Coggins S. Climate change, water, and human health research in the Arctic. Water Secur. 2020;10:100062.
Park MS, Park KH, Bahk GJ. Interrelationships between multiple climatic factors and incidence of foodborne diseases. Int J Environ Res Public Health. 2018;15:2482.
Lake IR, Barker GC. Climate change, foodborne pathogens and illness in higher-income countries. Curr Environ Heal Rep. 2018;5(1):187–96.
Lake IR, Gillespie IA, Bentham G, Nichols GL, Lane C, Adak GK, et al. A re-evaluation of the impact of temperature and climate change on foodborne illness. Epidemiol Infect. 2009;137(11):1538–47.
Lake IR, Hooper L, Abdelhamid A, Bentham G, Boxall AB. a. A, Draper A, et al. Climate change and food security: health impacts in developed countries. Environ Health Perspect. 2012;120(11):1520–6.
Springmann M, Mason-D’Croz D, Robinson S, Garnett T, Godfray HCJ, Gollin D, et al. Global and regional health effects of future food production under climate change: a modelling study. Lancet. 2016;387(10031):1937–46.
Campbell-Lendrum D, Manga L, Bagayoko M, Sommerfeld J. Climate change and vector-borne diseases: what are the implications for public health research and policy? Philos Trans R Soc London. 2015;370:20130552.
Sweileh WM. Bibliometric analysis of peer-reviewed literature on climate change and human health with an emphasis on infectious diseases. Glob Health. 2020;16(1):1–17.
Ford J. Indigenous health and climate change. Am J Public Health. 2012;102(7):1260–6.
Butler CD. Climate change, health and existential risks to civilization: a comprehensive review (1989–2013). Int J Environ Res Public Health. 2018;15(10):2266.
Tong S, Ebi K. Preventing and mitigating health risks of climate change. Environ Res. 2019;174:9–13.
Ebi KL, Hess JJ. The past and future in understanding the health risks of and responses to climate variability and change. Int J Biometeorol. 2017;61(S1):71–80.
Hosking J, Campbell-Lendrum D. How well does climate change and human health research match the demands of policymakers? A scoping review. Environ Health Perspect. 2012;120(8):1076–82.
Berry P, Enright PM, Shumake-Guillemot J, Villalobos Prats E, Campbell-Lendrum D. Assessing health vulnerabilities and adaptation to climate change: a review of international progress. Int J Environ Res Public Health. 2018;15(12):2626.
Verner G, Schütte S, Knop J, Sankoh O, Sauerborn R. Health in climate change research from 1990 to 2014: positive trend, but still underperforming. Glob Health Action. 2016;9:30723.
Ebi KL, Hasegawa T, Hayes K, Monaghan A, Paz S, Berry P. Health risks of warming of 1.5 °C, 2 °C, and higher, above pre-industrial temperatures. Environ Res Lett. 2018;13(6):063007.
Bäckstrand K. Civic science for sustainability: reframing the role of experts, policy-makers and citizens in environmental governance. Glob Environ Polit. 2003;3(4):24–41.
Susskind L, Jain R, Martyniuk A. Better environmental policy studies: how to design and conduct more effective analyses. Washington, DC: Island Press; 2001. p. 256.
Holmes J, Clark R. Enhancing the use of science in environmental policy-making and regulation. Environ Sci Policy. 2008;11(8):702–11.
Pearce T, Ford J, Duerden F, Smit B, Andrachuk M, Berrang-Ford L, et al. Advancing adaptation planning for climate change in the Inuvialuit Settlement Region (ISR): a review and critique. Reg Environ Chang. 2011;11(1):1–17.
Gearheard S, Shirley J. Challenges in community-research relationships: learning from natural science in Nunavut. Arctic. 2007;60(1):62–74.
Brownson R, Royer C, Ewing R, McBride T. Researchers and policymakers: travelers in parallel universes. Am J Prev Med. 2006;30(2):164–72.
Feldman P. Improving communication between researchers and policy makers in long-term care or, researchers are from Mars; policy makers are from Venus. Gerontologist. 2001;41(3):312–21.
Pearce TD, Ford JD, Laidler GJ, Smit B, Duerden F, Allarut M, et al. Community collaboration and climate change research in the Canadian Arctic. Polar Res. 2009;28(1):10–27.
Duerden F, Beasley E, Riewe R, Oakes J. Assessing community vulnerabilities to environmental change in the Inuvialuit region. Climate Change: Linking Traditional and Scientific Knowledge. Winnipeg, MB: Aboriginal Issues Press; 2006.
Mulrow CD. Rationale for systematic reviews. BMJ. 1994;309(6954):597–9.
Tricco A, Lillie E, Zarin W, O’Brien KK, Colquhoun H, Levac D. PRISMA extension for scoping reviews (PRISMA-ScR): checklist and explanation. Ann Intern Med. 2018;169:467–73.
Moher D, Liberati A, Tetzlaff J, Altman D. The Prisma Group. Preferred reporting items for systematic reviews and meta-analyses: the PRISMA statement. Ann Intern Med. 2009;151(4):264–9.
IPCC. In: Stocker TF, Qin D, Plattner G-K, Tignor M, Allen SK, Boschung J, et al., editors. Climate Change 2013: The Physical Science Basis. Contribution of Working Group I to the Fifth Assessment Report of the Intergovernmental Panel on Climate Change. Cambridge: Cambridge University Press; 2013. 1535 pp.
IPCC. Managing the risks of extreme events and disasters to advance climate change adaptation. A special report of Working Groups I and II of the Intergovernmental Panel on Climate Change. In: Field CB, Barros V, Stocker TF, Qin D, Dokken DJ, Ebi KL, et al., editors. Cambridge: Cambridge University Press; 2012. p. 582.
Woodward A, Smith KR, Campbell-Lendrum D, Chadee DD, Honda Y, Liu Q, et al. Climate change and health: on the latest IPCC report. Lancet. 2014;383(9924):1185–9.
Confalonieri U, Menne B, Akhtar R, Ebi KL, Hauengue M, Kovats RS, et al. Human Health. In: Parry ML, Canziani OF, Palutikof JP, van der Linden PJ, Hanson CE, editors. Climate change 2007: impacts, adaptation and vulnerability contribution of Working Group II to the Fourth Assessment Report of the Intergovernmental Panel on Climate Change. Cambridge: Cambridge University Press; 2007. p. 391–431.
IPCC. Climate Change 2014: Impacts, adaptation, and vulnerability. Part B: regional aspects. Contribution of Working Group II to the Fifth Assessment Report of the Intergovernmental Panel on Climate Change. In: Barros VR, Field CB, Dokken DJ, Mastrandrea MD, Mach KJ, Bilir TE, et al., editors. Climate change 2014 impacts, adaptation and vulnerability: part A: global and sectoral aspects. Cambridge, UK and New York, USA: Cambridge University Press; 2014. p. 688.
Hong Q, Pluye P, Fàbregues S, Bartlett G, Boardman F, Cargo M, et al. Mixed Methods Appraisal Tool (MMAT), Version 2018. Registration of copyright (#1148552), Canadian Intellectual Property Office, Industry Canada; 2018. p. 1–11.
Lewin S, Glenton C, Munthe-Kaas H, Carlsen B, Colvin CJ, Gülmezoglu M, et al. Using qualitative evidence in decision making for health and social interventions: an approach to assess confidence in findings from qualitative evidence syntheses (GRADE-CERQual). PLoS Med. 2015;12(10):1001895.
Download references
Acknowledgements
We would like to thank Maria Tan at the University of Alberta Library for the advice, expertise and guidance provided in developing the search strategy for this protocol. Special thanks to those who assisted with methodology refinement, including Etienne de Jongh, Katharine Neale, and Tianna Rusnak.
Funding was provided by the Canadian Institutes for Health Research (to SLH and AC). The funding body had no role in the design of the study and collection, analysis, and interpretation of data and in writing the manuscript.
Author information
Authors and affiliations.
School of Public Health, University of Alberta, ECHA, 11405 87 Ave NW, Edmonton, AB, T6G 1C9, Canada
Sherilee L. Harper, Amreen Babujee, Shaugn Coggins & Carlee J. Wright
School of Arctic & Subarctic Studies, Labrador Institute of Memorial University, 219 Hamilton River Road, Stn B, PO Box 490, Happy Valley-Goose Bay, NL, A0P 1E0, Canada
Ashlee Cunsolo
Unidad de Ciencias Sociales, Universidad Autónoma de Yucatán, Calle 61 x 66 # 525. Col. Centro, Mérida, Yucatán, México
Mauricio Domínguez Aguilar
You can also search for this author in PubMed Google Scholar
Contributions
SLH, AC, and MDA contributed to the conceptualization, methodology, writing, and editing of the manuscript. AB contributed to the methodology, writing, and editing of the manuscript. SC contributed to the writing and editing of the manuscript. CJW contributed to visualization, writing, and editing of the manuscript. The authors have read and approved the final manuscript.
Corresponding author
Correspondence to Sherilee L. Harper .
Ethics declarations
Ethics approval and consent to participate, consent for publication, competing interests.
The authors declare that they have no competing interests.
Additional information
Publisher’s note.
Springer Nature remains neutral with regard to jurisdictional claims in published maps and institutional affiliations.
Supplementary Information
Additional file 1..
Search strategy for CINAHL®, Web of Science™, Scopus®, Embase® via Ovid, and MEDLINE® via Ovid.
Data extraction form
- *Categories were not mutually exclusive; that is, more than one category could be selected
Rights and permissions
Open Access This article is licensed under a Creative Commons Attribution 4.0 International License, which permits use, sharing, adaptation, distribution and reproduction in any medium or format, as long as you give appropriate credit to the original author(s) and the source, provide a link to the Creative Commons licence, and indicate if changes were made. The images or other third party material in this article are included in the article's Creative Commons licence, unless indicated otherwise in a credit line to the material. If material is not included in the article's Creative Commons licence and your intended use is not permitted by statutory regulation or exceeds the permitted use, you will need to obtain permission directly from the copyright holder. To view a copy of this licence, visit http://creativecommons.org/licenses/by/4.0/ . The Creative Commons Public Domain Dedication waiver ( http://creativecommons.org/publicdomain/zero/1.0/ ) applies to the data made available in this article, unless otherwise stated in a credit line to the data.
Reprints and permissions
About this article
Cite this article.
Harper, S.L., Cunsolo, A., Babujee, A. et al. Climate change and health in North America: literature review protocol. Syst Rev 10 , 3 (2021). https://doi.org/10.1186/s13643-020-01543-y
Download citation
Received : 30 October 2020
Accepted : 23 November 2020
Published : 04 January 2021
DOI : https://doi.org/10.1186/s13643-020-01543-y
Share this article
Anyone you share the following link with will be able to read this content:
Sorry, a shareable link is not currently available for this article.
Provided by the Springer Nature SharedIt content-sharing initiative
- Climate Change
- Human Health
- Mental Health
- North America
Systematic Reviews
ISSN: 2046-4053
- Submission enquiries: Access here and click Contact Us
- General enquiries: [email protected]
- AI Content Shield
- AI KW Research
- AI Assistant
- SEO Optimizer
- AI KW Clustering
- Customer reviews
- The NLO Revolution
- Press Center
- Help Center
- Content Resources
- Facebook Group
Outline for Climate Change Essay: Writing an Essay
Table of Contents
Changes in weather patterns and conditions are a result of climate change.
A number of factors are causing the climate to change quickly right now. It goes without saying that the temperature of the entire planet is rising. Do you consider it to be a severe issue? Yes, melting glaciers are causing sea levels to rise, as you may have heard.
Hazardous elements like pollution, coal burning, the release of industrial waste into the atmosphere, etc., have caused a significant change in the climate. Everything above will have an impact on the environment and its resources.
You need to raise public awareness of the problem of climate change in addition to taking strict environmental protection and preservation measures. What’s the best way to raise awareness about the issue?
Well, write an essay on the issue! But how are you going to write an essay on climate change without a structure or an outline? That’s what we’re going to show you today! Keep reading to find out more about the outline for climate change essay!
What’s Climate Change?
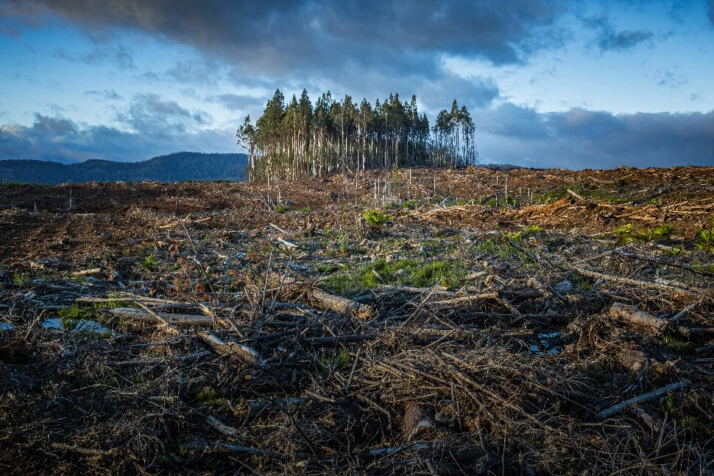
To begin with, if someone needs to write an essay about climate change, they should have an idea of what they will write about. A significant issue facing the entire world, climate change frequently forces millions of people to relocate and causes devastating natural disasters. Global warming is commonly linked to climate change. But this phenomenon has a lot more facets than just this one.
A whole region or settlement may become utterly intolerable to live in as a result of climate change. However, it might also have a detrimental impact on natural processes, which would have an unintended ripple effect throughout the entire planet.
Weather patterns as a whole are changing due to climate change. We can infer that changes to the typical climatic conditions are caused by climate change. As a result of these changes, there will be more severe storms, heat waves, floods, melting glaciers, etc.
The livelihood of people and other living things is being impacted by the numerous climate changes that our planet is undergoing. Among the features of climate change is global warming. These elements cause the atmosphere to release greenhouse gases like carbon dioxide. Examine the causes of climate change listed below.
All forms of life on Earth will be affected if the current climate change situation persists in the same way. Sea levels will rise, and the Earth’s temperature will increase. The monsoon patterns will change, and there will be more frequent storms, volcanic eruptions, and other natural disasters.
There will be disruptions to the Earth’s biological and ecological balance. As a result of environmental pollution, people won’t be able to breathe clean air or drink clean water. The end of earthly life is imminent.
Outline for Climate Change Essay
Here’s an example of an outline for climate change essay that you can use in your own. This outline covers the introduction, the main body paragraphs, and finally, the conclusion. We will also give some examples of topics that you can cover in your climate change (or global warming) essay.
Introduction
Here, you can give why climate change came to be and how the industrial revolution caused it to accelerate. You can mention why we called this phenomenon global warming at first and why we now refer to it as climate change. Remember, in the introduction part of your climate change essay. You have to include a thesis statement.
A thesis statement is a phrase that summarizes the subject and aim of your essay. A strong thesis statement will guide the format of your essay and help your audience comprehend the concepts you’ll be addressing in it.
Your paper’s introduction should contain your thesis statement somewhere, usually in the final phrase. Typically, a thesis will be one sentence long. But if your topic is complex, you might find that splitting the thesis statement into two sentences works better.
Main Body Paragraphs
This is the meat of your essay on climate change. There are a couple of topics that you can mention here to keep the essay going. We’ll look at a couple of interesting subjects or themes right now.
What Causes Climate Change?
- Construction of industries during the Industrial Revolution
- Human activities involving the use of fossil fuels are of concern.
- Greenhouse gas usage
- Increasing deforestation
- Gas emissions and chemical leaks
- Platonic movement
- Ozone layer degradation
- Inefficient transportation humans with car infrastructure
- Energy generation using fossil fuels in the 21st century
- Lack of government intervention in those who cause climate change
- Human activities that affect the environment in a negative turn
- The need to extract Earth’s resources
- The problem of human over consumption
- The constant need for new energy sources every year
- The decimation of plant life for human overpopulation on Earth
- Humans damaging the environment and the animal and plant life across the Earth
- The increased amount of pollutants that affect the atmosphere
What Are The Effects of Climate Change and Global Warming?
- Elevated global temperature
- Major catastrophes across different countries on Earth
- Melting Ice Caps
- Sea levels are rising.
- Polluted oceans
- The hottest years we have witnessed thanks to global warming
- Continuous cyclones, typhoons, hurricanes, etc.
- Heatwaves caused by global warming
- Substantial rains
- The process of decomposition and higher temperatures
- Seasonal growth
- Reduced Earth Fertility
- Water supplies reducing on a global scale, affecting all life in the world
- Cities around the world are underwater due to climate change and global warming.
- Extreme weather events due to increased carbon dioxide
How Can We Tackle Climate Change and Global Warming?
- Cutting back on fossil fuels
- Expanding the number of forests and trees
- Utilizing renewable energy sources
- The introduction of carbon-free technology
- Environmentally friendly transportation
- Utilizing more renewable sources that exist in nature, such as solar and geothermal power.
Concluding Remarks
We hope our guide on writing an essay on climate change and the environment had positive effects on your writing process. If you are still unsure about how to continue your climate change essay, there’s another solution you should know.
You can use an essay outline tool like the one we have at INK. This helps to create the structure of your climate change essay for you! This way, you can devote more time to researching instead of thinking about the structure.
Best of luck to you in writing essay of the effects of climate change on the environment. We hope our post was able to help you increase awareness on how human activity affects the Earth!
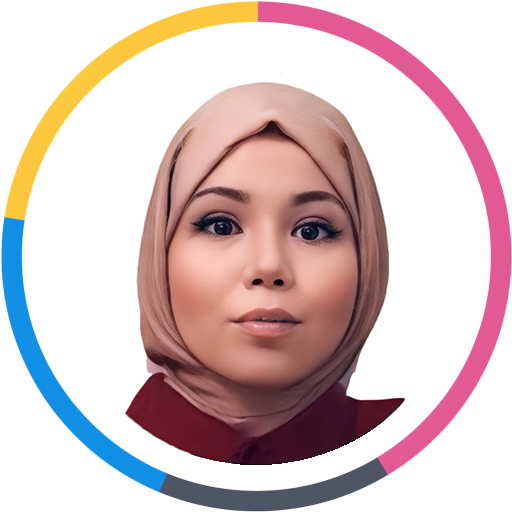
Abir Ghenaiet
Abir is a data analyst and researcher. Among her interests are artificial intelligence, machine learning, and natural language processing. As a humanitarian and educator, she actively supports women in tech and promotes diversity.
Explore All Essay Outline Tool Articles
How to write a synthesis essay outline.
One of the most interesting assignments you could have is writing a synthesis essay. For a college or university student,…
- Essay Outline Tool
Learning the Structure of an Informational Essay
Academic writing assignments, primarily essays, are required of all college and university students. That’s because they think it will aid…
The Correct Way to Structure an Article
Writing non-fiction has a set format that can be followed, which makes it not all that different from writing fiction.…
Exploring the Structure of a Response Essay
You will typically be expected to write in a formal and impersonal voice when you are given the assignment of…
Writing a Persuasive Essay? Use This Structure!
Writing essays is a requirement of your academic program as a college student. Whether you love them or loathe them,…
Writing a Proposal Essay? Read This!
Are you writing a proposal essay? To write it correctly, we have to know what a proposal essay actually is.…

Scientists outline a bold solution to climate change, biodiversity loss, social injustice
An international team of scientists led by Oregon State University researchers has used a novel 500-year dataset to frame a "restorative" pathway through which humanity can avoid the worst ecological and social outcomes of climate change.
In addition to charting a possible new course for society, the researchers say their "paradigm shifting" plan can support climate modeling and discussion by providing a set of actions that strongly emphasize social and economic justice as well as environmental sustainability.
Oregon State's William Ripple, former OSU postdoctoral researcher Christopher Wolf and collaborators argue their scenario should be included in climate models along with the five "shared socioeconomic pathways," or SSPs, that are used by the U.N.'s Intergovernmental Panel on Climate Change.
"We understand that our proposed scenario may be a major challenge to implement given current trends in emissions, a lack of political will and widespread social denial, but its merits can't even be honestly debated if it's not included in the suite of options," said Ripple, distinguished professor of ecology in the OSU College of Forestry. "We're arguing for radical incrementalism: achieving massive change through small, short-term steps. And we're offering a much-needed contrast to many other climate scenarios, which may be more aligned with the status quo, which isn't working."
Ripple and co-authors from the United States, the Netherlands and Australia present their restorative pathway in a paper published today in Environmental Research Letters . They say the pathway is inspired by a unique compilation of Earth system variables that vividly illustrate how humanity's resource demands have exploded since 1850, indicating ecological overshoot.
"The supporting data underscore the urgent need for action," said Wolf, now a scientist with Corvallis-based Terrestrial Ecosystems Research Associates. "The growth in human population, gross domestic product and energy consumption, primarily reliant on fossil fuels, has led to an extraordinary surge in greenhouse gas emissions, dramatically altering land use and triggering a massive biodiversity decline."
The authors note that current climate change modeling relies on multiple assumptions and factors related to policy options and societal developments. An international team of climate scientists, economists and energy systems modelers developed the SSPs, which are used to derive greenhouse gas emissions scenarios under different sets of policies that assume continued and significant GDP growth through 2100.
"The SSPs describe plausible developments that in the future would lead to different challenges for climate change mitigation and adaptation," Wolf said. "They're based on five narratives that describe alternative socioeconomic developments, some more sustainable than others. Our scenario focuses on reducing the consumption of primary resources to a level that keeps environmental pressures within planetary boundaries, with per capita GDP stabilizing over time."
Wolf, Ripple and collaborators took a long-term look back at a range of variables: fossil fuel emissions, human population, GDP, land use, greenhouse gas concentrations, global temperature, vertebrate wildlife species abundance, income inequality and meat production.
Collectively, the data paint a comprehensive picture of the profound changes Earth has undergone, say the authors, who include Jillian Gregg of Terrestrial Ecosystems Research Associates, Detlef P. van Vuuren with the Netherlands Environmental Assessment Agency and Manfred Lenzen of the University of Sydney.
"The income share variable extends back to 1820 and shows how the top 10% have consistently received at least 50% of all income, illustrating global economic inequality over the long term," Ripple said. "The restorative pathway would represent a more equitable and resilient world with a focus on nature preservation as a natural climate solution; societal well-being and quality of life; equality and high levels of education for girls and women, resulting in low fertility rates and higher standards of living; and a rapid transition toward renewable energy."
Unlike some of the current shared socioeconomic pathways, the restorative pathway does not rely on the development of carbon capture technologies, nor does it assume continued economic growth as the SSPs do.
"By prioritizing large-scale societal change, our proposed pathway could limit warming much more effectively than pathways that support rising resource consumption by wealthy nations," Ripple said. "We aim to bend the curves on a wide range of planetary vital signs with a holistic vision for addressing climate change, biodiversity loss and socioeconomic injustice. Our work presents a case for how humanity can embark on the journey of saving the world from these environmental and social crises."
In October 2023, Ripple, Wolf and 10 other U.S. and global scientists published research in BioScience that showed the Earth's vital signs have worsened beyond anything humans have yet seen, to the point that life on the planet is imperiled.
Ripple is also the co-author of another BioScience paper, published today, that examines climate change and the related biodiversity crisis from a cosmic perspective using "long-term planetary thinking," he said.
- Endangered Animals
- Endangered Plants
- Environmental Awareness
- Environmental Issues
- Environmental Policies
- Resource Shortage
- Ocean Policy
- Global warming controversy
- Paleoclimatology
- Climate change mitigation
- Kyoto Protocol
- Scientific opinion on climate change
- Temperature record of the past 1000 years
- Climate engineering
- Global climate model
Story Source:
Materials provided by Oregon State University . Original written by Steve Lundeberg. Note: Content may be edited for style and length.
Journal References :
- William J Ripple, Christopher Wolf, Detlef P van Vuuren, Jillian W Gregg, Manfred Lenzen. An environmental and socially just climate mitigation pathway for a planet in peril . Environmental Research Letters , 2024; 19 (2): 021001 DOI: 10.1088/1748-9326/ad059e
- Francisco Garcia-Gonzalez, William J Ripple, Aurelio F Malo. Scientists’ warning to humanity for long-term planetary thinking on biodiversity and humankind preservation, a cosmic perspective . BioSciences , 2024 DOI: 10.1093/biosci/biad108
Cite This Page :
Explore More
- Emergence of Animals: Magnetic Field Collapse
- Ice Shelves Crack from Weight of Meltwater Lakes
- Countries' Plans to Remove CO2 Not Enough
- Toward Robots With Human-Level Touch Sensitivity
- 'Doubling' in Origin of Cancer Cells
- New Catalyst for Using Captured Carbon
- Random Robots Are More Reliable
- Significant Discovery in Teleportation Research
- Orangutan Treats Wound With Pain-Relieving Plant
- 75,000-Year-Old Neanderthal from Burial Cave
Trending Topics
Strange & offbeat.
- Skip to Guides Search
- Skip to breadcrumb
- Skip to main content
- Skip to footer
- Skip to chat link
- Report accessibility issues and get help
- Go to Penn Libraries Home
- Go to Franklin catalog
Critical Writing: Waking Up to Climate Change: Researching the White Paper
- Getting started
- News and Opinion Sites
- Academic Sources
- Grey Literature
- Substantive News Sources
- What to Do When You Are Stuck
- Understanding a citation
- Examples of Quotation
- Examples of Paraphrase
- Chicago Manual of Style: Citing Images
- Researching the Op-Ed
- Researching Prospective Employers
- Resume Resources
- Cover Letter Resources
Research the White Paper
Researching the White Paper:
The process of researching and composing a white paper shares some similarities with the kind of research and writing one does for a high school or college research paper. What’s important for writers of white papers to grasp, however, is how much this genre differs from a research paper. First, the author of a white paper already recognizes that there is a problem to be solved, a decision to be made, and the job of the author is to provide readers with substantive information to help them make some kind of decision--which may include a decision to do more research because major gaps remain.
Thus, a white paper author would not “brainstorm” a topic. Instead, the white paper author would get busy figuring out how the problem is defined by those who are experiencing it as a problem. Typically that research begins in popular culture--social media, surveys, interviews, newspapers. Once the author has a handle on how the problem is being defined and experienced, its history and its impact, what people in the trenches believe might be the best or worst ways of addressing it, the author then will turn to academic scholarship as well as “grey” literature (more about that later). Unlike a school research paper, the author does not set out to argue for or against a particular position, and then devote the majority of effort to finding sources to support the selected position. Instead, the author sets out in good faith to do as much fact-finding as possible, and thus research is likely to present multiple, conflicting, and overlapping perspectives. When people research out of a genuine desire to understand and solve a problem, they listen to every source that may offer helpful information. They will thus have to do much more analysis, synthesis, and sorting of that information, which will often not fall neatly into a “pro” or “con” camp: Solution A may, for example, solve one part of the problem but exacerbate another part of the problem. Solution C may sound like what everyone wants, but what if it’s built on a set of data that have been criticized by another reliable source? And so it goes.
For example, if you are trying to write a white paper on the opioid crisis, you may focus on the value of providing free, sterilized needles--which do indeed reduce disease, and also provide an opportunity for the health care provider distributing them to offer addiction treatment to the user. However, the free needles are sometimes discarded on the ground, posing a danger to others; or they may be shared; or they may encourage more drug usage. All of those things can be true at once; a reader will want to know about all of these considerations in order to make an informed decision. That is the challenging job of the white paper author. The research you do for your white paper will require that you identify a specific problem, seek popular culture sources to help define the problem, its history, its significance and impact for people affected by it. You will then delve into academic and grey literature to learn about the way scholars and others with professional expertise answer these same questions. In this way, you will create creating a layered, complex portrait that provides readers with a substantive exploration useful for deliberating and decision-making. You will also likely need to find or create images, including tables, figures, illustrations or photographs, and you will document all of your sources.
Courtney Warren

Connect to a Librarian Live Chat or "Ask a Question"
- Librarians staff live chat from 9-5 Monday through Friday . You can also text to chat: 215-543-7674
- You can submit a question 24 hours a day and we aim to respond within 24 hours
- You can click the "Schedule Appointment" button above in librarian's profile box (to the left), to schedule a consultation with her in person or by video conference.
- You can also make an appointment with a Librarian by subject specialization .
- Connect by email with a subject librarian
Find more easy contacts at our Quick Start Guide
- Next: Getting started >>
- Last Updated: Sep 25, 2023 4:30 PM
- URL: https://guides.library.upenn.edu/wakinguptoclimatechangelibguide
- Share full article
Advertisement
Subscriber-only Newsletter
Climate Forward
Your most pressing climate questions.
Introducing Ask NYT Climate, where we’ll explore how climate intersects with your everyday life.

By Ryan McCarthy
I’m the new editor of the Climate Forward newsletter.
Are traffic circles better for the environment than four-way stops? Will the oceans be too hot for fish to survive? Is green hydrogen a thing?
Over the past few years, we here at the Climate desk have received hundreds of smart, often highly specific, questions from our readers about what they can do in their daily lives to affect climate change. To answer some of these questions, this week we’ve launched “ Ask NYT Climate ,” which is dedicated to exploring how climate intersects with your life.
Our first edition is about the perhaps counterintuitive idea that buying stuff online can actually be better for the planet than driving to a store. And if you’ve got a question you want us to answer, send it via the form at the bottom of this page .
To get a sense of how the biggest issues in the climate world intertwine with our lives, I also turned to our reporters and asked them two things: “What’s the most common question you hear from readers?” and “What are the biggest questions your sources are trying to answer right now?”
Our warming planet
“When I tell people I write about science and the natural world, the questions, I think, kind of stop,” said Raymond Zhong . He was only half-joking.
He writes about what climate scientists are thinking and researching. But on the core issues of climate change, he pointed out, the science is largely settled.
“A lot of the most basic questions people have about climate change were answered by scientists long ago,” Zhong said. We know what’s warming the earth: emissions of carbon dioxide, largely from human sources. And we know stopping global warming requires moving away from fossil fuels.
That said, the question he most often gets is some version of “What can I do?” (These are the kinds of tricky questions — which kinds of personal choices matter more than others — that will be a focus of Ask NYT Climate.)
Many of Zhong’s sources in the scientific community are investigating a somewhat different question: What role have we all played in this year’s record-breaking heat, and what that might tell us about the future? To answer that, some of them are looking back to the ancient world .
“The question, broadly, is: Has something deeper in the climate system changed?” Zhong said. We know many of the reasons the planet was so hot last year, but scientists are trying to discern the exact effect of other variables, including El Niño, or of aerosols emitted from ships’ smokestacks, which in a twist can have a cooling effect on the planet.
“Is 2023 the harbinger of something worse? That’s a deep question,” he said, “and it’s challenging the notion that we’ve already solved all the big questions.”
The scale of the problem
Coral Davenport , who covers climate change policy from Washington, also says she gets the “What can I do?” question a lot. In fact, it’s the most common thing our reporters hear from readers.
Individual actions can add up, of course. For example, food choices do have consequences. Earlier, we answered your questions about the climate implications of what we eat .
But Davenport said she often has to remind people of the sheer scope of climate change. Moving the global energy system away from fossil fuels is a gargantuan undertaking.
“As a problem, as a policy issue,” Davenport said, “it is arguably the most gigantic problem in the world.”
Climate change, she said, “absolutely cannot be solved unless it’s by gigantic entities working together. It has to be massive and global.” Davenport has recently covered things like the Biden administration’s pause on building a new natural gas export terminal , Republican attacks on electric vehicles and drilling regulations .
What do Davenport’s sources want to know? Many say they simply want to understand what the government’s rules will be. “I hear a lot of frustration,” Davenport said, “from companies caught in a regulatory whiplash” between Democratic and Republican administrations.
Automakers and electric utilities in particular, she said, tend to complain about climate rules that come and go as political winds change in Washington. But those changes, and those complaints, have become more extreme than in the past, she said.
How to protect nature
“One question I get is, ‘Is there any hope?’” said Catrin Einhorn , who covers biodiversity, wildlife ecosystems and nature. She’s covered things like ocean protection treaties , vanishing kestrels and the decline of California salmon .
The Climate desk has written about the hope vs. despair debate extensively over the past few years . We’re coming off the hottest year on record , but there are growing reasons to think the world can make significant progress, perhaps even quickly. “There are many pathways, spelled out by rigorous research. Each has tradeoffs,” wrote Somini Sengupta in our interactive Climate FAQ, which is built around the questions people ask of it.
Many of Einhorn’s sources, meanwhile, are focused on quantifying biodiversity loss and finding ways to slow it down. Globally, the rate of species extinction is currently at least tens to hundreds of times higher than the average over the past 10 million years. “Climate change is actually an easier problem to solve than biodiversity loss,” Einhorn said. “Biodiversity loss is even more sprawling and also harder to measure than greenhouse gas emissions.”
And, she says, her sources are obsessed with the big question of how we can reorient our economy — and a growing population that consumes more and more — in ways that don’t take as severe a toll on the natural world.
Three places where change is happening
Glaciers are shrinking , coral reefs are in crisis and last year was the hottest on record . Atmospheric concentrations of carbon dioxide , the main greenhouse gas, have passed a dangerous new threshold as people continue to burn fossil fuels. Is anyplace making progress on climate change?
The short answer is: It’s complicated, but yes.
Uruguay has pivoted in less than a decade to generating almost all its electricity from a diverse mix of renewables.
In China, an electric car that costs just $5,000 is suddenly one of the biggest sellers.
Paris is transforming itself into a city of bikes. The percentage of trips taken by bicycle within Paris more than doubled between 2020 and 2024, from 5 to 11 percent , thanks in part to new bike lanes set up during the coronavirus pandemic.
Steps like these, taken individually, aren’t enough to avoid the most serious consequences of climate change — worsening droughts, intensified storms and human suffering. Still, they show how some places are pulling off significant local changes very quickly. Read more here. — Delger Erdenesanaa
More climate news
The Cadillac Lyriq is the new darling of the E.V. industry, Bloomberg reports .
Ecuador is considering more debt-for-nature swaps after last year’s record-breaking $1.6 billion swap deal, Reuters reports .
Heatmap News examines why pollsters may be underestimating climate change as a political issue.
Rooftop solar generates so much electricity in California that on certain days gigawatts of power are “curtailed,” or essentially thrown away, per The Washington Post .
Thanks for being a subscriber.
Read past editions of the newsletter here .
If you’re enjoying what you’re reading, please consider recommending it to others. They can sign up here . Browse all of our subscriber-only newsletters here . And follow The New York Times on Instagram , Threads , Facebook and TikTok at @nytimes.
Reach us at [email protected] . We read every message, and reply to many!
Learn More About Climate Change
Have questions about climate change? Our F.A.Q. will tackle your climate questions, big and small .
Cattle ranches have ruled the Amazon for decades. Now, new companies are selling something else: the ability of trees to lock away planet-warming carbon.
Paris is becoming a city of bikes. Across China, people are snapping up $5,000 electric cars. Here’s a look at a few bright spots for emission reductions.
In theory, online shopping can be more efficient than driving to the store. But you may still want to think before you add to cart.
“Buying Time,” a new series from The New York Times, looks at the risky ways humans are starting to manipulate nature to fight climate change.
Did you know the ♻ symbol doesn’t mean something is actually recyclable ? Read on about how we got here, and what can be done.
ORIGINAL RESEARCH article
Correlates of climate change skepticism.
- 1 Department of Experimental Psychology, Division of Psychology and Language Sciences, University College London, London, United Kingdom
- 2 Department of Leadership and Organisational Behaviour, Norwegian Business School (BI), Oslo, Norway
While much research has examined the correlates of climate change beliefs from an alarmist perspective, less work has systematically measured climate change skepticism. This study aims to create a comprehensive tool capturing climate skeptics’ beliefs and test its association with individual difference variables. 502 European adults completed a 22-item questionnaire on climate change (CC) skepticism as well as measures of ambiguity tolerance, belief in a just world (BJW), dark-side personality traits, and self-esteem. Principal components analysis revealed a four dimension structure of CC. Political ideology was the most consistent and significant predictor across the climate change skepticism factors. Dark-side traits, also played a role. Future research should further validate this measure and explore how climate change information could be tailored to different audiences. Understanding the nuances and causes of climate skepticism can enable more effective communication to promote sustainability.
Introduction
Anthropogenic climate change is a momentous challenge, with grave consequences for human societies and the natural ecosystems. Despite overwhelming consensus from the scientific community and frequent alerts as to the dangers of current fossil-fuel based practices, a large number of people continue to resist policies intended to curb carbon emissions. A section of the public is unwilling to incur the costs of changing energy sources. However, the most alarming are those who oppose solutions to climate change because they do not believe the assertions made by scientists that anthropogenic climate change is real ( Bolsen et al., 2015 ; Douglas and Sutton, 2015 ; Carmichael and Brulle, 2017 ).
As a result, climate change remains a highly divisive issue. This has led to various labels attached to people like “alarmists” and “deniers”: the former insisting on immediate and dramatic changes around a wide range of behaviors, while the latter deny many of the central claims of the climate change scientists. One central question of interest is what personal characteristics are associated with these two extreme groups. Also, nearly all studies concerning beliefs about climate change use statements and concepts from an “alarmist” position while this study does the opposite using primarily “denial” concepts.
There are also other less extreme groups that could be labeled “skeptics” who challenge some of the scientific reports and recommendations and the “concerned” who are worried by, and eager to reverse, some aspects of climate change. Whether a government chooses to initiate mitigation and adaptation policy efforts highly depends on public beliefs and perception of climate change risks. Consequently, research efforts have primarily concentrated on the deniers who, it is suggested, are essentially conspiracy theorists ( Hornsey et al., 2018 ). Strong oppositions from this portion of the public have thwarted efforts to create a low-carbon economy and have caused controversy for a number a renewable energy development ( Devine-Wright et al., 2022 ).
Inevitably, the public debate on climate change has attracted researchers from many different disciplines ( Leiserowitz, 2006 ; Egan and Mullin, 2012 ; Goeminne, 2012 ; Leiserowitz et al., 2013 ; Bolsen et al., 2015 ; van der Linden, 2015 ; Dunlap et al., 2016 ; Pepermans and Maeseele, 2016 ; Carmichael and Brulle, 2017 ; Lahn and Sundqvist, 2017 ; Saunders, 2017 ; Uscinski and Olivella, 2017 ; Sellbom et al., 2020 ; Zummo et al., 2020 ; Tangney, 2021 ). This literature seems to have been dominated by philosophers, political scientists and sociologists who inevitably focus on the issue through their particular theoretical and research lenses. Although there is a great deal of work by psychologists on conspiracy theories and inoculation from climate change disinformation ( Uscinski and Olivella, 2017 ; Cook et al., 2018 ; Compton et al., 2021 ), less work has been dedicated to measuring climate change skepticism in a systematic way. This is an important gap as by some accounts’ skeptic beliefs may represent a softer side of the denialist claims ( Whitmarsh, 2011 ). Understanding these attitudes in turn may inform campaigns aimed at increasing compliance with mitigation policies ( Spence and Pidgeon, 2009 ). Thus, the aim of the current study is to create a comprehensive tool that encapsulates climate skeptics beliefs. More importantly relatively few studies have looked at individual difference variables like personality or belief systems. In this study we explore both, such as the Just World Belief construct which is concerned with concepts of fate and deservingness. Further we examine “dark-side” personality traits which have been linked to beliefs in conspiracy theories ( Furnham and Grover, 2021 ). We also examine self-esteem and whether this is related to climate change beliefs.
Before delving into the details of the study, a general description of climate change denial is warranted. There are five techniques associated with denialists arguments: conspiracy theories, fake experts, impossible expectations, misrepresentations and logical fallacies, and cherry- picking ( Diethelm and McKee, 2009 ). These techniques help people maintain their doubts about the occurrence of climate change, its causes and impacts. Where one falls on the climate change skepticism spectrum then depends on the form of denial employed. This in turn reflects the problematic way in which the public perceives climate change information. Poortinga et al. (2011) notes that this has created enormous heterogeneity in the construct. Rahmstorf (2004) distinguishes between trend skeptics who deny climate change; attribution skeptics , who do not deny that the climate may be changing, but deny human involvement; and impact skeptics who do not deny human-caused climate change, but do not believe it will have detrimental impacts. Leiserowitz et al. (2021) extended this categorization in the US to comprise six audience segments based on their responses to climate change information, which they have termed the six Americas: The Alarmed, Concerned, Cautious, Disengaged, Doubtful, and Dismissive. In 2020 the latter three made up 26% of the US population and typically represent climate deniers in line with Rahmstorf’s (2004) categorization. However, recent findings have shown climate change to be a more politically fueled issue in the US than in other countries, which renders this categorization difficult to apply to other populations ( Hornsey et al., 2018 ).
In recent years, the terms ‘deniers’, ‘contrarians’ ‘dissenters’ and ‘skeptics’ have been used interchangeably to describe the portion of the public that take scientific uncertainty as an absence of consensus on climate change, or who publicly misrepresent climate science and attack scientific claims ( McCright, 2007 ; Anderegg and Harold, 2009 ). This is in line with Tobler et al. (2012) and Malpass et al. (2007) who argue that climate skeptics tend to distrust scientific facts and harbor doubts about individual responsibility for climate change. However, Whitmarsh (2011) noted that climate change denial is a multidimensional construct comprised of both an active rejection of climate science, and more general attitudinal ambivalence and uncertainty about climate change. The portion of the public who is uncertain about the facts of climate change is typically larger ( Leiserowitz et al., 2010 , 2021 ). Having a multitude of constructs under pejorative terms such as ‘climate dissenter’ has led to a lack of clarity regarding the proportion of the public that endorses such claims. Moreover it hampers attempts to establish a synergistic relationship between climate science, policy and society ( O’Neill and Boykoff, 2010 ).
Therefore, we sought to construct a comprehensive tool that reliably measures climate change deniers beliefs, as well as test its association to individual constructs that typically predict skeptical beliefs.
Psychological correlates of climate denialism
Psychological research has shown that adopting denialist thinking is linked to absence of control ( Whitson and Galinsky, 2008 ), uncertainty ( Whitson et al., 2015 ) and perceived self-esteem threats ( Cichocka et al., 2015 ). This is especially true in the case of existential threats such as climate change, which put the desire for control in jeopardy and frame unsustainable actions as malevolent ( Uscinski and Olivella, 2017 ; Douglas et al., 2019 ) thus potentially threatening one’s identity. These reactions are further complicated by political ideologies, as seen in the relationship between support for Trump’s policies, aversion to wealth redistribution, and climate change skepticism, especially among those with high political interest ( Panno et al., 2019 ). Moreover, conservative political orientation, amplified by high political interest, has been shown to predict climate change denial through ideological pathways like right-wing authoritarianism and social dominance orientation ( Carrus and Leone, 2018 ). This suggests that identity-protecting cognition and neoliberal beliefs might interact, often leading to a lowering of risk perceptions and denial of climate change ( Haltinner and Sarathchandra, 2018 ). Thus, public beliefs about climate change are shaped by a complex interplay of individual differences, psychological orientations, political ideologies, and knowledge of climate science ( Whitmarsh, 2011 ). A question of interest then is how people’s identities as well as their way of coping with the inherent uncertainty of climate change impact climate change beliefs. To explore this, we included measures of Tolerance of Ambiguity and Self-esteem to explore their relation to climate change skepticism.
Another question concerns how diverse worldviews shape the interpretation of climate change information ( Hornsey, 2021 ). A worldview that is particularly relevant to climate change skepticism is Belief in a Just World: The BJW concept was identified over 50 years ago and concentrates on the tendency of people to blame victims of misfortunes for their own fate ( Lerner and Miller, 1978 ; Lerner, 1980 ). The central idea is that people have a fundamental need to believe that the (social) world is a just place and that this belief is functionally necessary for them to develop principles of deservingness. People are confronted with difficult social problems such as why some people get ill, are abused, descend into poverty etc. while others do not. They also have to make sense of their own misfortunes. The idea of the BJW is that it helps answer some of these very difficult moral, political and social questions. Previous studies have shown that people holding BJW tend to be more skeptical of climate change, especially when messages are expressed in terms of the detrimental consequences of climate change for human societies ( Feinberg and Willer, 2011 ). It is thought that such messages contradict the belief that the world is just, thus producing denial. Therefore, we were interested in exploring how this factor relates to climate change beliefs.
Finally, we were interested in examining the relationship between personality and climate skepticism. Many studies have tested the association between Personality measures and climate change beliefs. Several studies have shown that Big-5 traits such as Openness and Conscientiousness favorably influence climate beliefs and sustainable behavior ( Brick and Lewis, 2016 ). Here we were interested in the relatively underexplored dark-side personality measures (essentially sub-clinical personality disorders) and climate skepticism. These have been as measured by the new five-dimensional measure (PID-5-BF). This has five dimensions:
I. Negative Affectivity (Anxiousness, Emotional lability, Hostility, Perseveration, Lack of restricted affectivity, Separation insecurity, Submissiveness), II. Detachment (Anhedonia, Depressivity, Intimacy avoidance, Suspiciousness, Withdrawal), III. Antagonism (Attention seeking, Callousness, Deceitfulness, Grandiosity, Manipulativeness), IV. Disinhibition (Distractibility, Impulsivity, Irresponsibility, Lack of rigid perfectionism, Risk taking), and V . Psychoticism (Eccentricity, Perceptual dysregulation, Unusual beliefs and experiences). Several studies have shown that dark personality traits such as Antagonism are typically associated with need for control, insensitivity to untrustworthiness cues, and gullibility which renders people vulnerable to conspiracy beliefs ( Hart et al., 2021 ; Miller et al., 2021 ; Cichocka et al., 2022 ). Considering the vast literature on the link between climate change denial and conspiratorial ideation we sought to explore whether such traits could account for climate change skeptics’ beliefs.
Aims of the study
The first innovative aspect of the study is our measure of CC beliefs. While there are a number of measures in this area, there is no ‘gold standard’ for measuring climate change beliefs with psychometrically robust and proven tools. Various studies have employed a multiple-choice format in which respondents are asked to select the factors they believe cause climate change. A variation of this is asking people to indicate the degree to which they believe climate change is caused by human activities ( Poortinga et al., 2011 ; Goldberg et al., 2019 ). However, most researchers construct their own measurement tools and infer degree of belief in climate change from the level of agreement with various statements. These measures tend to be characterized by two factors. The first, is that tend to be short and often of unproven psychometric validity.
The second is that they often unashamedly have more items from the alarmist perspective. This in turn has resulted in conflicting reports of climate change beliefs and sometimes artificially low levels of denial. For instance, in 2010 beliefs in anthropogenic climate change ranged from 50.4 to 94% for people in Australia depending on the measurement tool used ( Leviston et al., 2011 ). It is known that subtle wording and response options can have a major impact on test results, thus rendering conclusions drawn from these studies often elusive ( Greenhill et al., 2014 ). One issue of considerable interest to questionnaire researchers is whether agreeing with a positive statement about any issue is much the same as disagreeing with a negative statement. Therefore, in this study we sought to craft a questionnaire from the denialist perspective in order to assess its validity in measuring climate change skepticism.
The first phase of the study involved the selection of several statements that are in line with the climate change denial. There have been a wealth of papers from many disciplines on the beliefs of deniers which are very clearly documented and set out in a comprehensive entry into Wikipedia. This was a source of climate skeptics’ statements.
The first aim of the study was to test the reliability of the CC instrument in measuring climate skeptics beliefs. The second aim was to test how these beliefs associate with various attitudinal, demographic, personality and worldview measures. We expected based on previous research that BJW would be positively associated with CC skepticism (H1). Based on the literature on identity protecting cognition we also expected that TOA would be negatively associated with CC skepticism (H2) and Self-esteem would be negatively associated with CC Skepticism (H3). As there is no extensive research on the dark side personality measures and CC skepticism we made a tentative prediction that dark side DSM factors would be positively associated with CC skepticism (H4) based on the general characteristics associated with each dimension of personality.
Participants
There were 502 participants, 254 males and 248 females. They ranged in age from 30–69 years old, with a modal age of 36. In all 70.9% were graduates. With regard to their religious beliefs (1 = Not At All to 9 = Very ), they scored a mean of 3.80 ( SD = 3.01). They rated their political views from (1) Very Conservative to (9) Very Liberal, with a mean of 5.83 ( SD = 1.81). They rated “I am an optimist” from (10) Agree to (1) Disagree, with a mean of 6.74 ( SD = 2.15). Asked 2% said they were vegan, 7% vegetarian and the remainder (91%) neither. They stated their religious views on (1) Not religious to (9) Very Religious scale with a mean score of 2.88 (SD = 2.54).
Questionnaires
Climate change.
This questionnaire was created for this study and based on many statements from the Wikipedia entry on Climate Change Denial. Twenty-two statements were mainly taken from this entry as well as other papers on the topic. These are shown in Table 1 . They were piloted for clarity. The instructions read “There is still a lot of debate about climate change. This short questionnaire asks you to rate how much you agree or disagree about a number of statements that var ious people have made about the topic .”
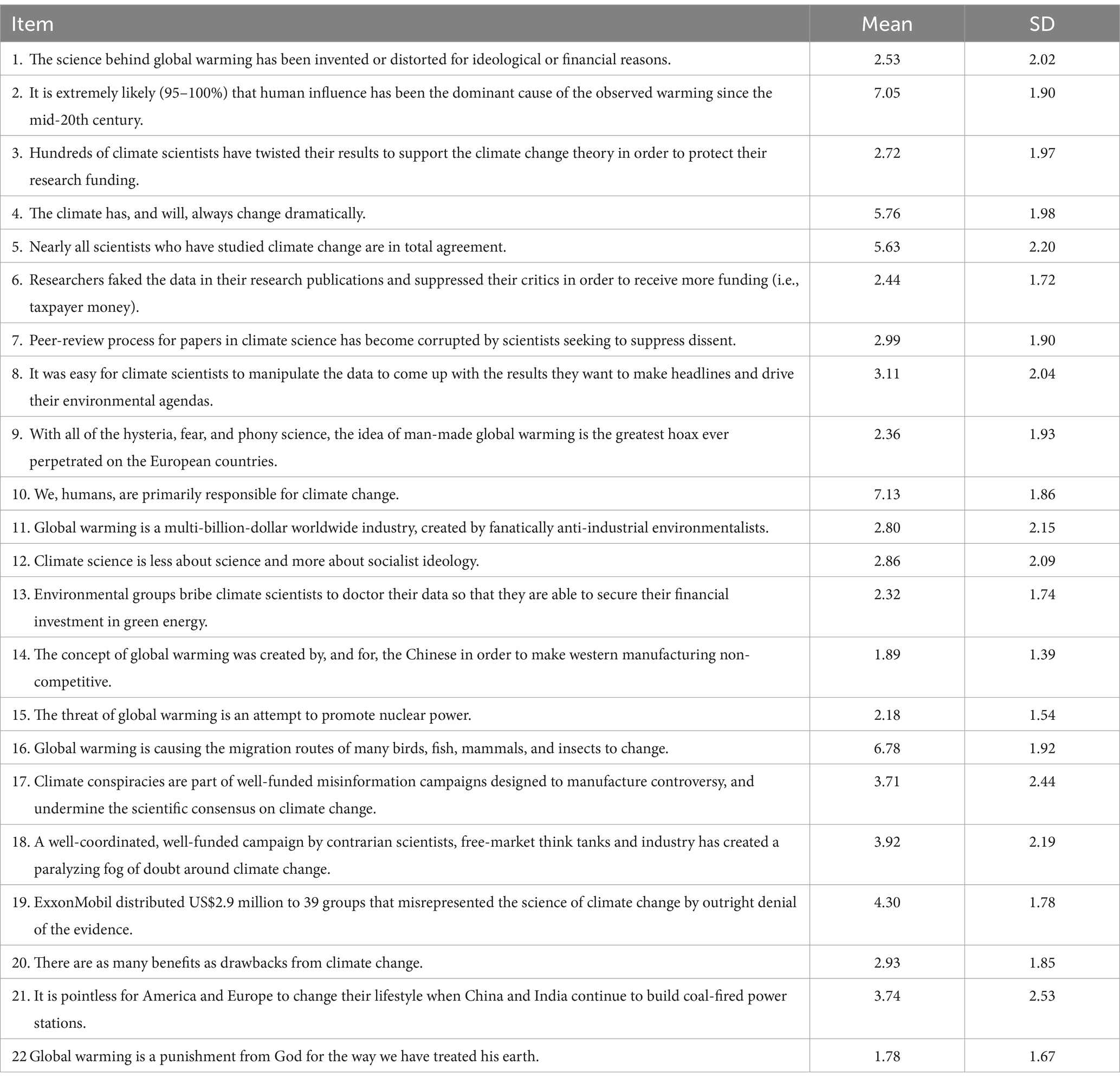
Table 1 . Means and SDs for each of the 22 items (9 = very strongly agree; 1 = very strongly disagree).
Self-esteem
The self-esteem measure ( Furnham et al., 2020 ) comprised four other factors on a scale from 1–100: Physical Attractiveness ( M = 52.58; SD = 17.58), Physical Health ( M = 61.76, SD = 19.93), Intelligence (IQ) ( M = 67.88, SD = 14.28) and Emotional Intelligence ( M = 67.35, SD = 16.68).
The Alpha for these four items was 0.69, and they were summed together forming a variable labeled Self-Esteem.
Global Belief in a Just World
This is a seven-item measure. Hellman et al. (2008) found the Global Belief in a Just World Scale ( Lipkus, 1991 ) produced the highest average reliability score ( α = 0.81) compared to the Just World Scale ( Rubin and Peplau, 1973 ; α = 0.64) and the Just World Scale Revised ( Rubin and Peplau, 1975 ; α = 0.68). In this study the alpha was 0.90.
Tolerance of ambiguity
This is a 12 item scale by Herman et al. (2010) which was devised to improve on the earlier scale of Budner (1962) with an alpha of 0.73. The authors demonstrate an improved factor structure and internal consistency for the TOA compared to the measure of Budner.

DSM-5—brief form (PID-5-BF)
The Personality Inventory for DSM ( Krueger et al., 2012 ; Díaz-Batanero et al., 2019 ) is a 25-item self-rated assessment scale which assesses five personality trait domains [Negative Affect (0.74), Detachment (0.60), Antagonism (0.68), Disinhibition (0.72) and Psychoticism (0.75)] with each trait domain consisting of 5 items. It has been validated by a number of psychometric studies in different countries ( Al-Dajani et al., 2016 ; Sellbom et al., 2020 ).
Departmental ethical approval was gained prior to data collection. Data was collected on Prolific and participants were compensated for their time at the set rate. Data cleansing took part before the formal analysis.
Table 1 shows the means and SDs for each of the statements. Scores, on the nine-point scale ranged from 1.78 (Global warming is a punishment from God for the way we have treated his earth) to 7.13 (We, humans, are primarily responsible for climate change). Standard Deviations varied from 1.39 (The concept of global warming was created by, and for, the Chinese in order to make western manufacturing non-competitive) to 2.53 (It is pointless for America and Europe to change their lifestyle when China and India continue to build coal-fired power stations.). In general, the level of CC denial was low-to-moderate. The highest mean agreement score was with the statements aligned with anthropogenic climate change views. This is in line with a general trend in recent years showing level of skepticism to be on the decline ( Leiserowitz et al., 2021 ) and climate change to be considered a serious problem by one in five Europeans. It is noteworthy that this trend appears to hold independently of whether questions are framed from the denialist perspective or the alarmist perspective.
These data were then treated to a Varimax and Promax rotated principal component factor analysis. The results of both analyses were very similar. We used results from the Varimax analysis which showed a four factor solution which in total accounted for around ¾ of the variance (see Table 2 ). The emergent factors have some conceptual similarity with Rahmstorf’s (2004) categorization. The alphas for the four factors were 0.77, 0.70, 0.71 and 0.45, respectively, suggesting the first three were internally reliable and coherent. In particular Factor 1 resembles both trend skepticism and attribution skepticism whereas Factor 4 is conceptually similar to impact skepticism. In general, these represent the uncertainty relating to the veracity of Climate science claims, thus forming the basis of the CC skepticism scale. It is noteworthy that items corresponding to climate conspiracies and misinformation loaded more strongly on Factor 3 suggesting these might be conceptually different from general misinformation and climate science skepticism.
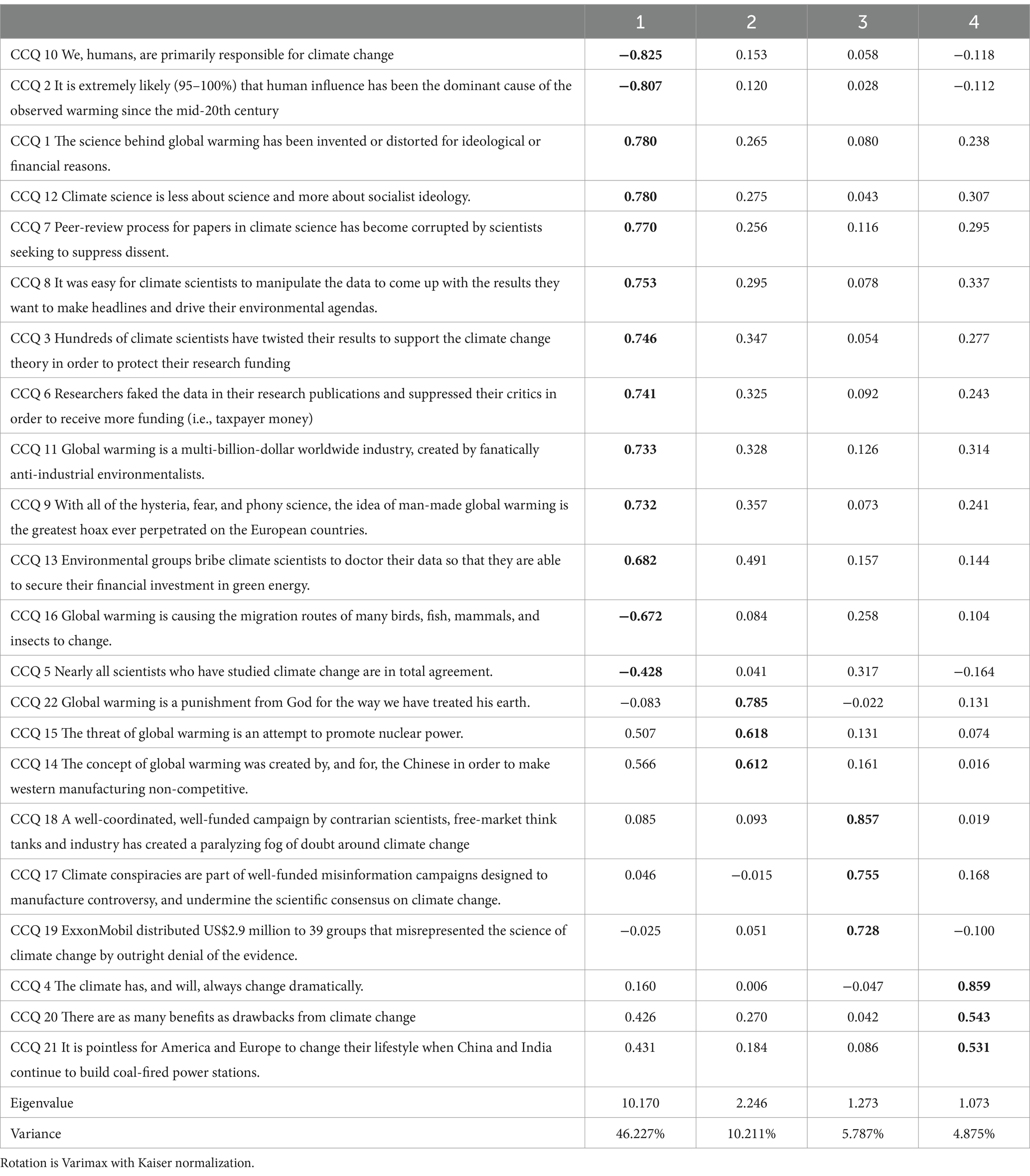
Table 2 . PCA analysis of the 22 items.
The overall scale showed good internal consistency with a Cronbach α = 0.83. There were no problems concerning sampling adequacy or sphericity based on KMO statistics (0.94) and Bartlett’s test ( p < 0.001). The rest of the scales: Self-esteem, Belief in a Just World, Tolerance of Ambiguity, and DSM factors were collapsed to form a singular score.
Correlational analysis
We performed correlational analysis for all variables of interest and the four factors of CC skepticism (see Table 3 ). The highest correlations were obtained between BJW and CC one ( r = 0.17) and CC four ( r = 0.21), TOA and CC two (−0.16) and CC four (−0.12). In terms of Personality, positive correlations emerged between Detachment and CCthree, Disinhibition and Psychotism and CC two ( r = 0.18 and r = 0.16 respectively) and CCthree ( r = 0.16 and r = 0.15 respectively), and Self- Esteem and CCthree ( r = −11). Table 3 shows some of the hypotheses were confirmed for certain factors of CC skepticism. The expected negative correlation between TOA, Self-esteem and CC skepticism is in line with previous studies ( Jessani and Harris, 2018 ) and predictions. And the predicted positive association between BJW and CC skepticism was obtained for three out of the four factors in line with previous findings ( Feinberg and Willer, 2011 ). Positive associations were obtained between dark side personality traits and all CC skepticism factors in line with predictions.
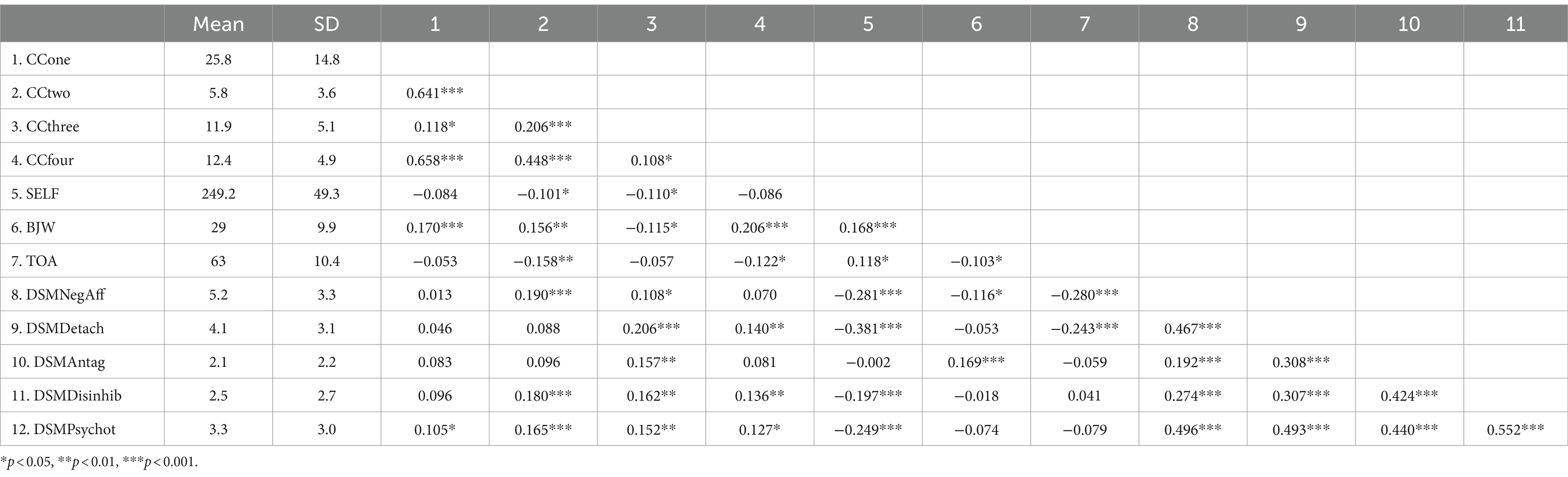
Table 3 . Correlation between CC factors and scales.
We were also interested in exploring the relationship between political ideology and climate change skepticism. We found a strong negative correlation between political ideology and most CC skepticism factors, such that the more right leaning tended to be more skeptical of climate change. This is a well-documented finding in the literature ( Hornsey et al., 2016 ). However, a significant positive relationship emerged between political ideology and CCthree ( r = 0.13) and BJW and CC three ( r = 0.12) contrary to predictions, which might be because this factor is measuring a unique dimension of CC skepticism.
Regression analysis
Linear regressions were performed between demographic variables, education, political ideology, BJW, Self-esteem, TOA, DSM and the CC skepticism factors. These are shown in Table 4 . The regression analyses revealed that DSM Disinhibition emerged as a significant predictor for CC Two and CC Four. Political orientation was found to be a notable predictor for the majority of the CC factors. Education level was a significant predictor for three out of the four factors. Religious beliefs were found to significantly influence CC Two. Age and gender also contributed to the prediction model, with age emerging as a significant predictor for the first CC factor and gender for the third. The whole model tests were significant with reasonable fit accounting for a good proportion of variance in skepticism R 2 = 0.16 for CCOne, R 2 = 0.16 for CCTwo, R 2 = 0.11 for CCThree, and R 2 = 0.19 for CCFour.
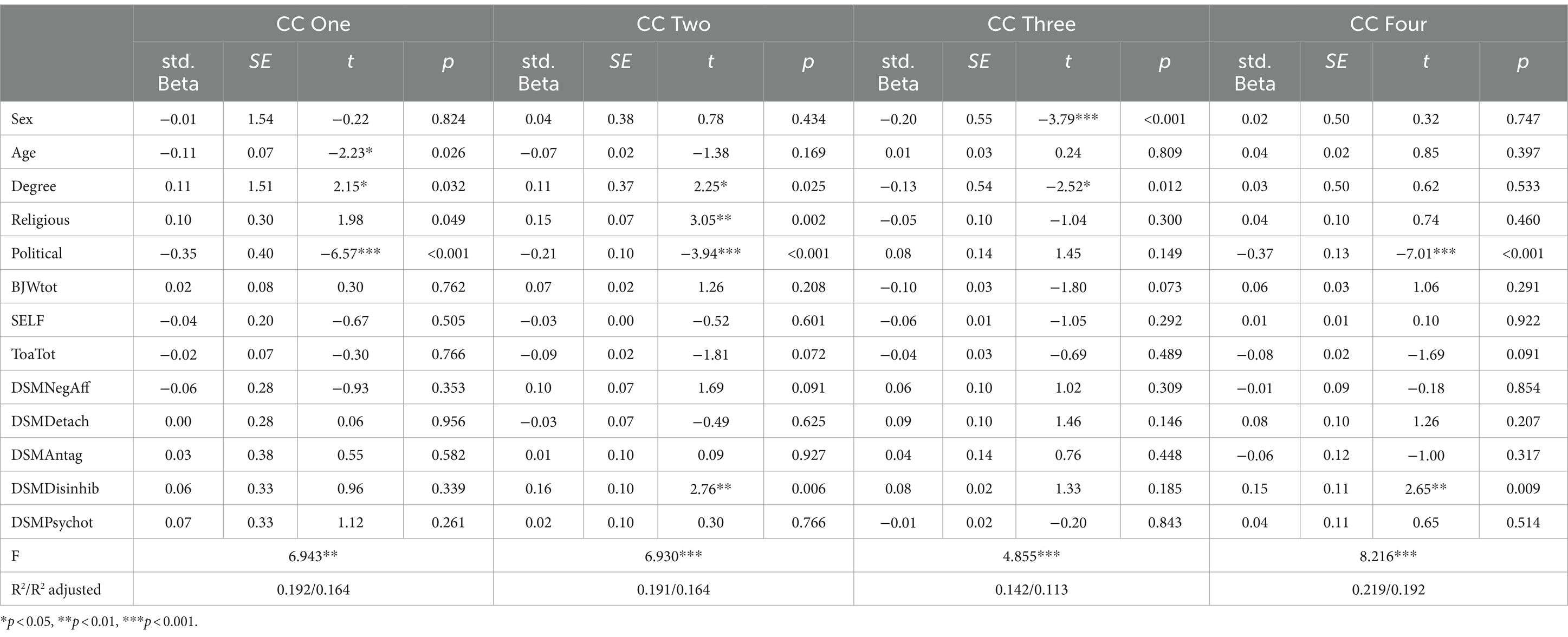
Table 4 . Regression onto the four factors.
In order to examine the relative importance of Personality factors above demographic variables, a hierarchical regression was performed as well onto all CC Skepticism factors. This is shown in Table 5 . For CCone, the first model including Age, Religious views, Sex and Political orientation was significant F (4,396) = 19.027, p < 0.001. This model accounts for R 2 = 0.16 of the variance in CC one. Looking at the individual predictors, Political views b = −0.39, 95% CI[−3.6,-2.2], t (396) = 8.08, p < 0.001 have an impact on CC One. The next two models are also significant but none of the added individual predictors reach significance.
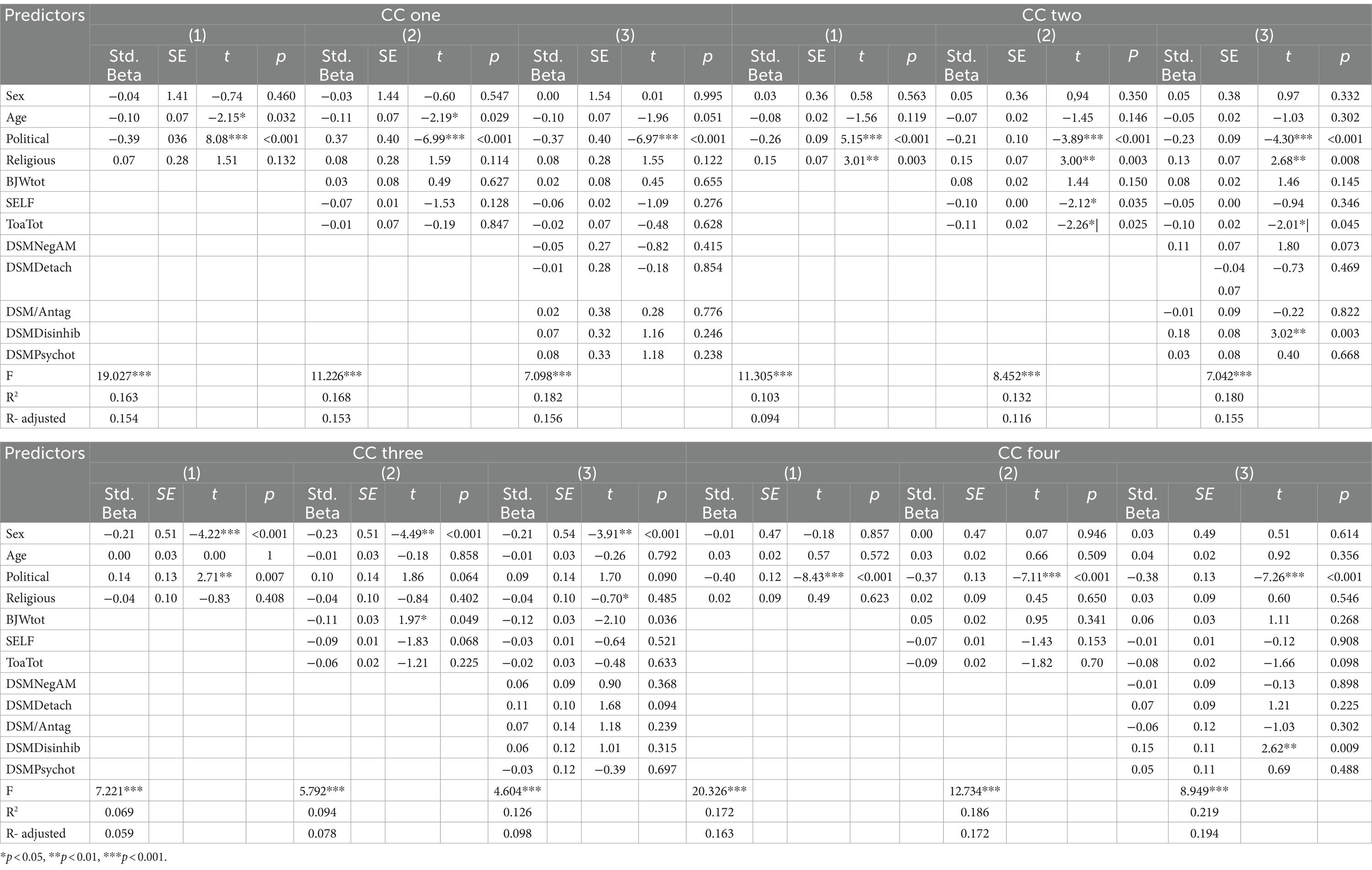
Table 5 . Hierarchical regression onto the 4 factors.
For CC Two the first model was significant F (4,397) = 11.305, p < 0.001 accounting for R 2 = 0.09 of the variance. Both Political views b = −0.26, 95% CI[−0.65,-0.29], t (397) = 5.15, p < 0.001 and Religious views b = 0.15, 95% CI[0.07,0.35], t (396) = 3.01, p < 0.05 were significant predictors of CC Two. The second model was significant F (7,396) = 8.452, p < 0.01 and accounts for R 2 = 0.11 of the variance adding 2% of explained variance. The third model including personality factors was significant F (12,396) = 7.04 and adds 4% of explained variance in CCtwo with Disinhibition b = 0.18, 95% CI[0.08,0.39], t (397) = 3.02, p < 0.01 emerging as a significant predictor of CC Two.
For CC Three the first model is significant F (4,396) = 7.221, p < 0.001 accounting for R 2 = 0.06 of the variance. Sex is a significant predictor of CC Three b = −0.21, 95% CI[−3.2,-1.15], t (396) = − 4.2, p < 0.001. The second model is significant F (7,396) = 5.792, p < 0.001 and accounts for R 2 = 0.08 of the variance. Looking at the individual predictors, BJW barely reaches significance. This model adds 2% of explained variance. The third model including personality factors was significant F (12,397) = 4.880 and adds 2% of explained variance in CC Three.
For CC Four the first model is significant F (4,396) = 4.517, p < 0.001 accounting for R 2 = 0.16 of the variance. Political views is a significant predictor of CC Four b = −0.40, 95% CI[−1.2,-0.77], t (397) = −8.43, p < 0.001. The second model is significant F (7,396) = 12.73, p < 0.001 and accounts for R 2 = 0.17 of the variance. The third model including personality factors was significant F (12,396) = 8.94 and adds 2% of explained variance in CC Four. Here Disinhibition emerged as a significant predictor of CC Four b = 0.15, 95% CI[0.07,0.48], t (396) = 2.6, p < 0.01.
A plethora of research explores the characteristics of environmental attitudes and correlates of climate change beliefs ( Hornsey et al., 2016 ). However, few scholars have attempted to examine climate change beliefs from the denialist perspective. Notable exceptions include work done by Whitmarsh (2011) and Poortinga et al. (2011) . The majority of psychometric tools employed to examine Climate Change beliefs tend to include items that may skew responses toward more ‘alarmist’ attitudes. In this study we carried out an investigation of Climate Change beliefs through a new tool constructed from the denialist perspective. The study explored how widespread skeptical beliefs are in Europe and to what extent they are associated worldviews such as Just World Beliefs and Political ideology and attitude aspects, such as Tolerance of ambiguity. We also set out to test the association between Dark Side personality facets and climate change skepticism, a relatively underexplored factor in the climate change attitudes literature.
The majority of participants tended to agree with human-caused climate change and the scientific consensus regarding anthropogenic climate change. These results are in line with a general trend showing certain types of skepticism surrounding climate change may be declining while level of concern is on the rise ( Saad, 2017a , b ). Other studies have reported alarmed segments to have risen by 9% since 2008 and the Dismissive and Disengaged segments to have dropped by 1 and 9%, respectively, (e.g., Leiserowitz et al., 2021 ). However, such trend observations should be taken with caution as previous reports mostly concern the US population and use different measurement tools. Most studies have primarily reported on levels of agreement with the causes of climate change as a human-made or naturally occurring phenomenon ( Poortinga et al., 2011 ; Leiserowitz et al., 2021 ). The current results extend this line of findings by showing that levels of agreement with conspiratorial rhetoric and disinformation surrounding climate change are among the lowest. This suggests that these types of beliefs are not prevalent in the public discourse. Another possibility might be that outright climate change denial might be pervasive only among those who are very right leaning ( Roser-Renouf et al., 2014 ) which made up only 5% of our sample.
The measure of Climate Change beliefs devised from the denialist perspective showed a clear multidimensional structure. Items concerning the trustworthiness of climate scientist claims tended to be quite different from conspiracy-related items and items relating to the impacts of climate change. These observations have been echoed in previous research and deserve further investigation ( Poortinga et al., 2011 ; Whitmarsh, 2011 ). It might be that a level of uncertainty surrounding climate science is a softer stance than outright denial of scientific data and conspiracy-laden rhetoric and each may be impacted by a different set of attitudinal variables. Exploring these dimensions further may aid intervention attempts aimed at clarifying climate science uncertainties and solidifying the knowledge required to take climate action. Although the instrument devised here showed high reliability, further studies are needed to ascertain its validity in capturing climate skeptics’ beliefs.
Similarly to previous studies ( Carrus and Leone, 2018 ; Panno et al., 2019 ), we find that certain ideologies tend to be more related to climate beliefs. For instance, conservatives tended to be more prone to climate skepticism than liberals. Recently, Hornsey et al. (2018) found that the ideological divide in climate change beliefs is more prevalent in the US, suggesting the issue of climate change has been more politicized there than in other nations. Considering that our sample was mostly European, this goes contrary to Hornsey’s findings and seems to be an international phenomenon. However, we had only a single item for Political beliefs. Further studies may attempt to replicate these findings with a more extensive instrument of political beliefs and behaviors, particularly those associated with conservatism.
Just World Beliefs correlated more strongly with CC factors than any other worldview measures. This suggests that perceptions of unpredictability and unfairness may be particularly pronounced in the case of climate change messages and that these belief violations may inspire denialist convictions. Previous findings support the notion that emphasizing the threat of climate change exacerbates climate denialism ( Feinberg and Willer, 2011 ). However, this worldview is hardly mentioned in the climate change literature. Yet several reports seem to suggest that climate skepticism may be a coping strategy employed by people confronted with uncomfortable truths about the devastating effects of climate change. Indeed, avoiding or refusing to assimilate negative information is such a pervasive coping mechanism that it has been termed the ‘ostrich effect’ ( Sullivan et al., 2010 ; Grzesiak-Feldman, 2013 ). Many scholars have noted that framing climate message in ways that align with people’s belief systems may avoid negative impacts such as climate denial ( Panno et al., 2019 ; Goldberg et al., 2020 ). As we did not test this experimentally, further studies may consider testing how variations in the way climate information is presented might interact with worldviews such as BJW to determine climate beliefs, and how these in turn may impact climate action.
An interesting finding from our data related to the dark side personality measures. DSM explained about 2% of variance in climate skepticism measures, which is a typical finding in personality research. This was the only factor that remained significant after controlling for demographic variables and political views. As other measures tended to intercorrelate, personality showed a unique contribution to climate change skepticism. Importantly, measures such as Disinhibition predicted responses to impact-related factors, and did not predict factors concerning trend and attribution skepticism. Whereas traits such as Antagonism were strongly correlated with conspiracy-related factors. This suggests that certain types of skepticism are diversly impacted by dark side personality types. Somewhat parallel to current findings, previous research has found dark side traits such as disinhibition and psychopathy to predict low endorsement of health-related behaviors and intent to knowingly expose others to risk during the COVID-19 pandemic. A similar argument could be made for the association between a reckless disregard of the risk of climate change (in the form of climate change conspiracy/impact beliefs and unsustainable behaviors) and dark side personality measures. This warrants further investigation.
Given the widespread agreement among the majority of participants on human-caused climate change, it becomes evident that policy efforts should focus on reinforcing this consensus and translating it into action. Policy recommendations could include the development of educational campaigns that address the identified gap between climate change acknowledgment and the misconceptions surrounding it. Such campaigns should be tailored to the nuanced spectrum of beliefs, particularly targeting groups with Just World Beliefs, and should aim to mitigate the ‘ostrich effect’ by presenting information in a manner that resonates with their worldviews. Furthermore, the association between dark side personality traits and climate change skepticism suggests that policy-making should consider psychological factors when designing communication strategies. For example, policies could support the creation of narrative-based messaging that effectively counters conspiracy theories and disinhibited views on the impacts of climate change ( Constantino and Weber, 2021 ). By recognizing the emotional and psychological components of climate change skepticism, policies can be more effective in promoting sustainable behaviors.
Like all studies this had limitations. It would be desirable to have other studies using our questionnaire to replicate the factor structure. We acknowledge that many of the items were taken from Wikipedia and other studies and it is possible that we missed out on some important themes which only future studies could correct. Moreover, those who work on attitude measurement may be concerned with some items being two long or too technical in use of jargon though we did pilot it. Clearly the measure, still unique in its perspective, requires more work. Next, we were restricted to self-report measures with common method variance problems. It would also have been interesting to know more about the participants’ political beliefs and attitudes given how important they appear to be on this issue. It would be most desirable to replicate the findings on a bigger and more representative population.
Whether a government or society takes steps to mitigate the threats associated with climate change is highly dependent on public beliefs. These latter in turn serve as an indicators of the likelihood of endorsement for various policies to curb carbon emissions. As such, belief in human causality is a pre-condition to acknowledge the problem of climate change in the first place. Yet discrepancies in belief systems are only part of the story when it comes to engaging different audience segments with solutions.
As with all correlational data, the current findings are merely suggestive of interesting constructs that may account for some of the observed variability in climate beliefs and do not offer insights into causal relations. However, many studies examining the impact of information on climate beliefs tend to conclude that the communication of facts and figures is insufficient in changing minds ( Moser, 2010 ; Goldberg et al., 2020 ; Brosch and Steg, 2021 ). Thus, recognizing the dimensions and causes of climate skepticism and the characteristics associated with those that espouse such beliefs may allow communicators to move away from a knowledge deficit model (i.e., providing more information based on the premise that the public is ignorant of the facts) toward a more inclusive framework that may advance targeted communications with a higher chance of success.
The pattern of results observed here is a further indicator that there is a high level of awareness of climate change and its causes. Future research should aim to expand upon the current study’s findings by employing a multifaceted approach to understanding climate change beliefs. This could involve longitudinal studies to assess how climate change beliefs evolve over time and in response to major environmental events. Additionally, experimental research that tests different methods of presenting climate change information could be invaluable in understanding how to counteract denialist attitudes. To enhance the public’s perception of climate-related phenomena, future studies could explore the use of personalized information and storytelling that align with individuals’ existing belief systems ( Hornsey and Fielding, 2017 ). This approach could potentially reduce defensive responses and increase the openness to scientific evidence.
Future studies would do well to assess a number of other participant variables like their endorsement of conspiracy theories, the participation in local and national politics as well as their personal behaviors associated with energy use and conservation. There has been a great deal of work on how to best address and combat conspiracy theories in general ( Lewandowsky et al., 2022 ; O’Mahony et al., 2023 ). By addressing these elements, the research community can play a pivotal role in aiding society’s transition toward a more informed and proactive stance on climate change. This measure may prove useful in attempting to try to change beliefs about CC.
Data availability statement
The original contributions presented in the study are included in the article/ Supplementary material , further inquiries can be directed to the corresponding author.
Ethics statement
The studies involving humans were approved by Norwegian Business School Ethics committee EP/2018/007. The studies were conducted in accordance with the local legislation and institutional requirements. The ethics committee/institutional review board waived the requirement of written informed consent for participation from the participants or the participants’ legal guardians/next of kin because data collected online anonymously.
Author contributions
JL: Formal analysis, Supervision, Writing – original draft, Writing – review & editing. AF: Conceptualization, Supervision, Writing – review & editing.
The author(s) declare that no financial support was received for the research, authorship, and/or publication of this article.
Conflict of interest
The authors declare that the research was conducted in the absence of any commercial or financial relationships that could be construed as a potential conflict of interest.
Publisher’s note
All claims expressed in this article are solely those of the authors and do not necessarily represent those of their affiliated organizations, or those of the publisher, the editors and the reviewers. Any product that may be evaluated in this article, or claim that may be made by its manufacturer, is not guaranteed or endorsed by the publisher.
Supplementary material
The Supplementary material for this article can be found online at: https://www.frontiersin.org/articles/10.3389/fpsyg.2024.1328307/full#supplementary-material
Al-Dajani, N., Gralnick, T. M., and Bagby, R. M. (2016). A Psychometric Review of the Personality Inventory for DSM-5 (PID-5): Current Status and Future Directions. J. Pers. Assess. 98, 62–81. doi: 10.1080/00223891.2015.1107572
Crossref Full Text | Google Scholar
Anderegg, W. R. L., and Harold, J. (2009) Climate science and the dynamics of expert consensus. Publication of the Center for Conservation Biology . Stanford, CA: Stanford University.
Google Scholar
Bolsen, T., Druckman, J. N., and Cook, F. L. (2015). Citizens’, scientists’, and policy advisors’ beliefs about global warming. Ann. Am. Acad. Polit. Soc. Sci. 658, 271–295. doi: 10.1177/0002716214558393
Brick, C., and Lewis, G. J. (2016). Unearthing the “green” personality: Core traits predict environmentally friendly behavior. Environ. Behav. 48, 635–658. doi: 10.1177/0013916514554695
Brosch, T., and Steg, L. (2021). Leveraging emotion for sustainable action. One Earth 4, 1693–1703. doi: 10.1016/j.oneear.2021.11.006
PubMed Abstract | Crossref Full Text | Google Scholar
Budner, S. (1962). Intolerance of ambiguity as a personality variable. J. Pers. 30, 29–50. doi: 10.1111/j.1467-6494.1962.tb02303.x
Carmichael, J. T., and Brulle, R. J. (2017). Elite cues, media coverage, and public concern: an integrated path analysis of public opinion on climate change, 2001–2013. Environ. Polit. 26, 1–21. doi: 10.1080/09644016.2016.1263433
Carrus, P., and Leone, L. (2018). The moderating role of interest in politics on the relations between conservative political orientation and denial of climate change. Soc. Nat. Resour. 31, 1103–1117. doi: 10.1080/08941920.2018.1463422
Cichocka, A., Marchlewska, M., and Biddlestone, M. (2022). Why do narcissists find conspiracy theories so appealing? Curr. Opin. Psychol. 47:101386. doi: 10.1016/j.copsyc.2022.101386
Cichocka, A., Marchlewska, M., Golec de Zavala, A., and Olechowski, M. (2015). “Grandiose delusions. Collective narcissism, secure in-group identification, and belief in conspiracies” in The Psychology of Conspiracy Theories . eds. M. Bilewicz, A. Cicochka, and W. Soral (London: Routledge)
Compton, J., van der Linden, S., Cook, J., and Basol, M. (2021). Inoculation theory in the post-truth era: Extant findings and new frontiers for contested science, misinformation, and conspiracy theories. Soc. Personal. Psychol. Compass 15:e12602. doi: 10.1111/spc3.12602
Constantino, S. M., and Weber, E. U. (2021). Decision-making under the deep uncertainty of climate change: The psychological and political agency of narratives. Curr. Opin. Psychol. 42, 151–159. doi: 10.1016/j.copsyc.2021.11.001
Cook, J., Ellerton, P., and Kinkead, D. (2018). Deconstructing climate misinformation to identify reasoning errors. Environ. Res. Lett. 13:24018. doi: 10.1088/1748-9326/aaa49f
Devine-Wright, P., Whitmarsh, L., Gatersleben, B., O’Neill, S., Hartley, S., Burningham, K., et al. (2022). Placing people at the heart of climate action. PLoS Clim. 1:e0000035. doi: 10.1371/journal.pclm.0000035
Díaz-Batanero, C., Ramírez-López, J., Domínguez-Salas, S., Fernández-Calderón, F., and Lozano, Ó. M. (2019). Personality Inventory for DSM-5-Short Form (PID-5-SF). Assessment 26, 853–866. doi: 10.1177/1073191117739980
Diethelm, P., and McKee, M. (2009). Denialism: what is it and how should scientists respond? Europ. J. Public Health 19, 2–4. doi: 10.1093/eurpub/ckn139
Douglas, K. M., and Sutton, R. M. (2015). Climate change: Why the conspiracy theories are dangerous. Bull. At. Sci. 71, 98–106. doi: 10.1177/0096340215571908
Douglas, K. M., Uscinski, J. E., Sutton, R. M., Cichocka, A., Nefes, T., Ang, C. S., et al. (2019). Understanding conspiracy theories. Polit. Psychol. 40, 3–35. doi: 10.1111/pops.12568
Dunlap, R. E., McCright, A. M., and Yarosh, J. H. (2016). The political divide on climate change: Partisan polarization widens in the U.S. Environ. Sci. Policy Sustain. Dev. 58, 4–23. doi: 10.1080/00139157.2016.1208995
Egan, P. J., and Mullin, M. (2012). Turning personal experience into political attitudes: The effect of local weather on Americans’ perceptions about global warming. J. Polit. 74, 796–809. doi: 10.1017/S0022381612000448
Feinberg, M., and Willer, R. (2011). Apocalypse soon? Dire messages reduce belief in global warming by contradicting just-world beliefs. Psychol. Sci. 22, 34–38. doi: 10.1177/0956797610391911
Furnham, A., and Grover, S. (2021). Do you have to be mad to believe in Conspiracy Theories? Personality Disorders and Conspiracy Theories. Int. J. Soc. Psychiatry 68:1614. doi: 10.1177/00207640211031614
Furnham, A., Horne, G., and Grover, S. (2020). Correlates of the militant extremist mindset. Front. Psychol. 11:558136. doi: 10.3389/fpsyg.2020.02250
Goeminne, G. (2012). Lost in Translation: Climate Denial and the Return of the Political. Global Environ. Polit. 12, 1–8. doi: 10.1162/GLEP_a_00104
Goldberg, M. H., Gustafson, A., and van der Linden, S. (2020). Leveraging Social Science to Generate Lasting Engagement with Climate Change Solutions. One Earth 3, 314–324. doi: 10.1016/j.oneear.2020.08.011
Goldberg, M. H., van der Linden, S., Ballew, M. T., Rosenthal, S. A., Gustafson, A., and Leiserowitz, A. (2019). The Experience of Consensus: Video as an Effective Medium to Communicate Scientific Agreement on Climate Change. Sci. Commun. 41, 659–673. doi: 10.1177/1075547019874361
Greenhill, M., Leviston, Z., Leonard, R., and Walker, I. (2014). Assessing climate change beliefs: Response effects of question wording and response alternatives. Public Underst. Sci. 23, 947–965. doi: 10.1177/0963662513480117
Grzesiak-Feldman, M. (2013). The effect of high-anxiety situations on conspiracy thinking. Curr. Psychol. 32, 100–118. doi: 10.1007/s12144-013-9165-6
Haltinner, K., and Sarathchandra, D. (2018). Climate change skepticism as a psychological coping strategy. Sociol. Compass 12, 1–10. doi: 10.1111/soc4.12586
Hart, W., Breeden, C. J., and Lambert, J. (2021). Exploring a vulnerable side to dark personality: People with some dark triad features are gullible and show dysfunctional trusting. Personal. Individ. Differ. 181:111030. doi: 10.1016/j.paid.2021.111030
Hellman, C. M., Muilenburg-Trevino, E. M., and Worley, J. A. (2008). The Belief in a Just World: An Examination of Reliability Estimates Across Three Measures. J. Pers. Assess. 90, 399–401. doi: 10.1080/00223890802108238
Herman, J. L., Stevens, M. J., Bird, A. W., Mendenhall, M. E., and Oddou, G. (2010). The Tolerance for Ambiguity Scale: Towards a more refined measure for international management research. Int. J. Intercult. Relat. 34, 58–65. doi: 10.1016/j.ijintrel.2009.09.004
Hornsey, M. J. (2021). The role of worldviews in shaping how people appraise climate change. Curr. Opin. Behav. Sci. 42, 36–41. doi: 10.1016/j.cobeha.2021.02.021
Hornsey, M. J., and Fielding, K. S. (2017). Attitude roots and Jiu Jitsu persuasion: Understanding and overcoming the motivated rejection of science. Am. Psychol. 72, 459–473. doi: 10.1037/a0040437
Hornsey, M. J., Harris, E. A., Bain, P. G., and Fielding, K. S. (2016). Meta-analyses of the determinants and outcomes of belief in climate change. Nat. Clim. Chang. 6, 622–626. doi: 10.1038/nclimate2943
Hornsey, M. J., Harris, E. A., and Fielding, K. S. (2018). Relationships among conspiratorial beliefs, conservatism and climate scepticism across nations. Nat. Clim. Chang. 8, 614–620. doi: 10.1038/s41558-018-0157-2
Jessani, Z., and Harris, P. B. (2018). Personality, politics, and denial: Tolerance of ambiguity, political orientation and disbelief in climate change. Personal. Individ. Differ. 131, 121–123. doi: 10.1016/j.paid.2018.04.033
Krueger, R. F., Derringer, J., Markon, K. E., Watson, D., and Skodol, A. E. (2012). Initial construction of a maladaptive personality trait model and inventory for DSM-5. Psychol. Med. 42, 1879–1890. doi: 10.1017/S0033291711002674
Lahn, B., and Sundqvist, G. (2017). Science as a “fixed point”? Quantification and boundary objects in international climate politics. Environ. Sci. Pol. 67, 8–15. doi: 10.1016/j.envsci.2016.11.001
Leiserowitz, A. (2006). Climate change risk perception and policy preferences: The role of affect, imagery, and values. Clim. Chang. 77, 45–72. doi: 10.1007/s10584-006-9059-9
Leiserowitz, A., Maibach, E. W., Roser-Renouf, C., Feinberg, G., and Howe, P.. (2013). Climate Change in the American Mind: Americans’ Global Warming Beliefs and Attitudes in April 2013. Available at: https://ssrn.com/abstract=2298705
Leiserowitz, A., Maibach, E., Roser-Renouf, C., and Smith, N.. (2010) Climate change in the American Mind: Americans’ global warming beliefs and attitudes in June 2010. Yale University and George Mason University . New Haven, CT: Yale Project on Climate Change Communication.
Leiserowitz, A., Roser-Renouf, C., Marlon, J., and Maibach, E. (2021). Global Warming’s Six Americas: A review and recommendations for climate change communication. Curr. Opin. Behav. Sci. 42, 97–103. doi: 10.1016/j.cobeha.2021.04.007
Lerner, M. J. (1980). The Belief in a Just World: A Fundamental Delusion . New York: Plenum Press.
Lerner, M. J., and Miller, D. T. (1978). Just world research and the attribution process: Looking back and ahead. Psychol. Bull. 85, 1030–1051. doi: 10.1037/0033-2909.85.5.1030
Leviston, Z., Leitch, A., Greenhill, M., Leonard, R., and Walker, I. (2011). Australians’ views of climate change . Canberra: CSIRO.
Lewandowsky, S., Armaos, K. D., Bruns, H., Schmid, P., Holford, D. L., Hahn, U., et al. (2022). When Science Becomes Embroiled in Conflict: Recognizing the Public’s Need for Debate while Combating Conspiracies and Misinformation. Ann. Am. Acad. Polit. Soc. Sci. 700, 26–40. doi: 10.1177/00027162221084663
Lipkus, I. M. (1991). The construction and preliminary validation of a global belief in a just world scale and the exploratory analysis of the multidimensional belief in a just world scale. Personal. Individ. Differ. 12, 1171–1178. doi: 10.1016/0191-8869(91)90081-L
Malpass, A., Barnett, C., Clarke, N., and Cloke, P. (2007). “Problematizing choice: responsible consumers and sceptical citizens” in Governance, consumers and citizens (London: Palgrave Macmillan), 231–256.
McCright, A. M. (2007). “Dealing with climate change contrarian” in Creating a Climate for Change: Communicating Climate Change and Facilitating Social Change . eds. S. C. Moser and L. Dilling (Cambridge: Cambridge University Press), 200–212.
Miller, J. D., Back, M. D., Lynam, D. R., and Wright, A. G. (2021). Narcissism today: What we know and what we need to learn. Curr. Dir. Psychol. Sci. 30, 519–525. doi: 10.1177/09637214211044109
Moser, S. C. (2010). Communicating climate change: History, challenges, process and future directions. Wiley Interdiscip. Rev. Clim. Chang. 1, 31–53. doi: 10.1002/wcc.11
O’Mahony, C., Brassil, M., Murphy, G., and Linehan, C. (2023). The efficacy of interventions in reducing belief in conspiracy theories: A systematic review. PLoS One 18:e0280902. doi: 10.1371/journal.pone.0280902
O’Neill, S. J., and Boykoff, M. (2010). Climate denier, skeptic, or contrarian? Proc. Natl. Acad. Sci. 107:E151. doi: 10.1073/pnas.101050710
Panno, A., Carrus, G., and Leone, L. (2019). Attitudes towards Trump policies and climate change: The key roles of aversion to wealth redistribution and political interest. J. Soc. Issues 75, 153–168. doi: 10.1111/josi.12318
Pepermans, Y., and Maeseele, P. (2016). The politicization of climate change: problem or solution? Wiley Interdiscip. Rev. Clim. Chang. 7, 478–485. doi: 10.1002/wcc.405
Poortinga, W., Spence, A., Whitmarsh, L., Capstick, S., and Pidgeon, N. F. (2011). Uncertain climate: An investigation into public scepticism about anthropogenic climate change. Global Linking Political Stance, Big 5 Personality Traits and Anthropogenic Climate Change Denial. environmental change 21, 1015–1024. doi: 10.1016/j.gloenvcha.2011.03.001
Rahmstorf, S. (2004). The climate sceptics . Potsdam: Potsdam Institute for Climate Impact Research.
Roser-Renouf, C., Stenhouse, N., Rolfe-Redding, J., Maibach, E. W., and Leiserowitz, A. (2014). Engaging Diverse Audiences with Climate Change: Message Strategies for Global Warming’s Six Americas. SSRN Electron. J. , 1–33. doi: 10.2139/ssrn.2410650
Rubin, Z., and Peplau, A. (1973). Belief in a just world and reactions to another’s lot: A study of participants in the national draft lottery. J. Soc. Issues 29, 73–93. doi: 10.1111/j.1540-4560.1973.tb00104.x
Rubin, Z., and Peplau, L. A. (1975). Who believes in a just world? J. Soc. Issues 31, 65–89. doi: 10.1111/j.1540-4560.1975.tb00997.x
Saad, L. (2017a). Global warming concern at three-decade high in U.S. Available at: http://news.gallup.com/poll/206030/global-warming-concern-three-decade-high.aspx
Saad, L. (2017b). Half in U.S. are now concerned global warming believers: Available at: http://news.gallup.com/poll/207119/half-concerned-global-warming-believers.aspx
Saunders, K. L. (2017). The impact of elite frames and motivated reasoning on beliefs in a global warming conspiracy: The promise and limits of trust. Res. Polit. doi: 10.1177/2053168017717602
Sellbom, M., Solomon-Krakus, S., Bach, B., and Bagby, R. M. (2020). Validation of Personality Inventory for DSM-5 (PID-5) Algorithms to Assess ICD-11 Personality Trait Domains in a Psychiatric Sample. Psychol. Assess. 32, 40–49. doi: 10.1037/pas0000746
Spence, A., and Pidgeon, N. (2009). Psychology, climate change & sustainable bahaviour. Environ. Sci. Policy Sustain. Dev. 51, 8–18. doi: 10.1080/00139150903337217
Sullivan, D., Landau, M. J., and Rothschild, Z. K. (2010). An existential function of enemyship: Evidence that people attribute influence to personal and political enemies to compensate for threats to control. J. Pers. Soc. Psychol. 98, 434–449. doi: 10.1037/a0017457
Tangney, P. (2021). Are “Climate Deniers” Rational Actors? Applying Weberian Rationalities to Advance Climate Policymaking. Environ. Commun. 15, 1077–1091. doi: 10.1080/17524032.2021.1942117
Tobler, C., Visschers, V., and Siegrist, M. (2012). Addressing climate change: determinants of consumers’ willingness to act and to support policy measures. J. Environ. Psychol. 32, 197–207. doi: 10.1016/j.jenvp.2012.02.001
Uscinski, J. E., and Olivella, S. (2017). The conditional effect of conspiracy thinking on attitudes toward climate change. Research & Politics , 4, 1–9. doi: 10.1177/2053168017743105
van der Linden, S. (2015). The conspiracy-effect: Exposure to conspiracy theories (about global warming) decreases pro-social behavior and science acceptance. Personal. Individ. Differ. 87, 171–173. doi: 10.1016/j.paid.2015.07.045
Whitmarsh, L. (2011). Scepticism and uncertainty about climate change: Dimensions, determinants and change over time. Glob. Environ. Chang. 21, 690–700. doi: 10.1016/j.gloenvcha.2011.01.016
Whitson, J. A., and Galinsky, A. D. (2008). Lacking control increases illusory pattern perception. Science 322, 115–117. doi: 10.1126/science.1159845
Whitson, J. A., Galinsky, A. D., and Kay, A. (2015). The emotional roots of conspiratorial perceptions, system justification, and belief in the paranormal. J. Exp. Soc. Psychol. 56, 89–95. doi: 10.1016/j.jesp.2014.09.002
Zummo, L., Gargroetzi, E., and Garcia, A. (2020). Youth voice on climate change: using factor analysis to understand the intersection of science, politics, and emotion. Environ. Educ. Res. 26, 1207–1226. doi: 10.1080/13504622.2020.1771288
Keywords: climate change, skepticism, climate change denial, Just World Beliefs, political beliefs
Citation: Leka J and Furnham A (2024) Correlates of climate change skepticism. Front. Psychol . 15:1328307. doi: 10.3389/fpsyg.2024.1328307
Received: 27 October 2023; Accepted: 12 March 2024; Published: 24 April 2024.
Reviewed by:
Copyright © 2024 Leka and Furnham. This is an open-access article distributed under the terms of the Creative Commons Attribution License (CC BY) . The use, distribution or reproduction in other forums is permitted, provided the original author(s) and the copyright owner(s) are credited and that the original publication in this journal is cited, in accordance with accepted academic practice. No use, distribution or reproduction is permitted which does not comply with these terms.
*Correspondence: Jona Leka, [email protected]
Disclaimer: All claims expressed in this article are solely those of the authors and do not necessarily represent those of their affiliated organizations, or those of the publisher, the editors and the reviewers. Any product that may be evaluated in this article or claim that may be made by its manufacturer is not guaranteed or endorsed by the publisher.
Outline History of Nuclear Energy
- The science of atomic radiation, atomic change and nuclear fission was developed from 1895 to 1945, much of it in the last six of those years.
- Over 1939-45, most development was focused on the atomic bomb.
- From 1945 attention was given to harnessing this energy in a controlled fashion for naval propulsion and for making electricity.
- Since 1956 the prime focus has been on the technological evolution of reliable nuclear power plants.
Exploring the nature of the atom
Uranium was discovered in 1789 by Martin Klaproth, a German chemist, and named after the planet Uranus.
Ionising radiation was discovered by Wilhelm Rontgen in 1895, by passing an electric current through an evacuated glass tube and producing continuous X-rays. Then in 1896 Henri Becquerel found that pitchblende (an ore containing radium and uranium) caused a photographic plate to darken. He went on to demonstrate that this was due to beta radiation (electrons) and alpha particles (helium nuclei) being emitted. Villard found a third type of radiation from pitchblende: gamma rays, which were much the same as X-rays. Then in 1896 Pierre and Marie Curie gave the name 'radioactivity' to this phenomenon, and in 1898 isolated polonium and radium from the pitchblende. Radium was later used in medical treatment. In 1898 Samuel Prescott showed that radiation destroyed bacteria in food.
In 1902 Ernest Rutherford showed that radioactivity, as a spontaneous event emitting an alpha or beta particle from the nucleus, created a different element. He went on to develop a fuller understanding of atoms and in 1919 he fired alpha particles from a radium source into nitrogen and found that nuclear rearrangement was occurring, with formation of oxygen. Niels Bohr was another scientist who advanced our understanding of the atom and the way electrons were arranged around its nucleus through to the 1940s.
By 1911 Frederick Soddy discovered that naturally-radioactive elements had a number of different isotopes (radionuclides), with the same chemistry. Also in 1911, George de Hevesy showed that such radionuclides were invaluable as tracers, because minute amounts could readily be detected with simple instruments.
In 1932 James Chadwick discovered the neutron. Also in 1932 Cockcroft and Walton produced nuclear transformations by bombarding atoms with accelerated protons, then in 1934 Irene Curie and Frederic Joliot found that some such transformations created artificial radionuclides. The next year Enrico Fermi found that a much greater variety of artificial radionuclides could be formed when neutrons were used instead of protons.
Fermi continued his experiments, mostly producing heavier elements from his targets, but also, with uranium, some much lighter ones. At the end of 1938 Otto Hahn and Fritz Strassmann in Berlin showed that the new lighter elements were barium and others which were about half the mass of uranium, thereby demonstrating that atomic fission had occurred. Lise Meitner and her nephew Otto Frisch, working under Niels Bohr, then explained this by suggesting that the neutron was captured by the nucleus, causing severe vibration leading to the nucleus splitting into two not quite equal parts. They calculated the energy release from this fission as about 200 million electron volts. Frisch then confirmed this figure experimentally in January 1939.
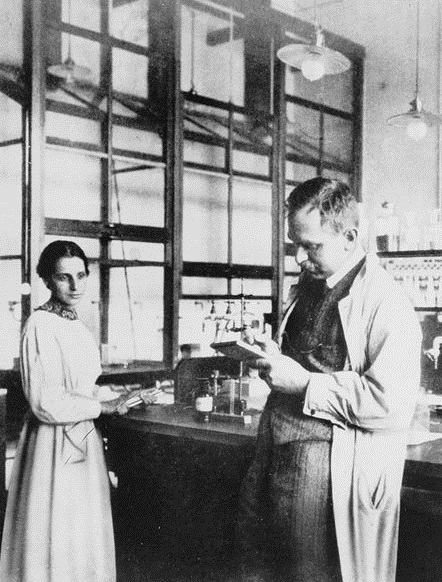
Lise Meitner and Otto Hahn, c. 1913
This was the first experimental confirmation of Albert Einstein's paper putting forward the equivalence between mass and energy, which had been published in 1905.
Harnessing nuclear fission
These 1939 developments sparked activity in many laboratories. Hahn and Strassmann showed that fission not only released a lot of energy, but that it also released additional neutrons which could cause fission in other uranium nuclei and possibly a self-sustaining chain reaction leading to an enormous release of energy. This suggestion was soon confirmed experimentally by Joliot and his co-workers in Paris, and Leo Szilard working with Fermi in New York.
Bohr soon proposed that fission was much more likely to occur in the uranium-235 isotope than in U-238 and that fission would occur more effectively with slow-moving neutrons than with fast neutrons. The latter point was confirmed by Szilard and Fermi, who proposed using a 'moderator' to slow down the emitted neutrons. Bohr and Wheeler extended these ideas into what became the classical analysis of the fission process, and their paper was published only two days before war broke out in 1939.
Another important factor was that U-235 was then known to comprise only 0.7% of natural uranium, with the other 99.3% being U-238, with similar chemical properties. Hence the separation of the two to obtain pure U-235 would be difficult and would require the use of their very slightly different physical properties. This increase in the proportion of the U-235 isotope became known as 'enrichment'.
The remaining piece of the fission/atomic bomb concept was provided in 1939 by Francis Perrin who introduced the concept of the critical mass of uranium required to produce a self-sustaining release of energy. His theories were extended by Rudolf Peierls at Birmingham University and the resulting calculations were of considerable importance in the development of the atomic bomb. Perrin's group in Paris continued their studies and demonstrated that a chain reaction could be sustained in a uranium-water mixture (the water being used to slow down the neutrons) provided external neutrons were injected into the system. They also demonstrated the idea of introducing neutron-absorbing material to limit the multiplication of neutrons and thus control the nuclear reaction (which is the basis for the operation of a nuclear power station).
Peierls had been a student of Werner Heisenberg, who from April 1939 presided over the German nuclear energy project under the German Ordnance Office. Initially this was directed towards military applications, and by the end of 1939 Heisenberg had calculated that nuclear fission chain reactions might be possible. When slowed down and controlled in a 'uranium machine' (nuclear reactor), these chain reactions could generate energy; when uncontrolled, they would lead to a nuclear explosion many times more powerful than a conventional explosion. It was suggested that natural uranium could be used in a uranium machine, with heavy water moderator (from Norway), but it appears that researchers were unaware of delayed neutrons which would enable a nuclear reactor to be controlled. Heisenberg noted that they could use pure uranium-235, a rare isotope, as an explosive, but he apparently believed that the critical mass required was higher than was practical.
In the summer of 1940, Carl Friedrich von Weizsäcker, a younger colleague and friend of Heisenberg's, drew upon publications by scholars working in Britain, Denmark, France, and the USA to conclude that if a uranium machine could sustain a chain reaction, then some of the more common uranium-238 would be transmuted into 'element 94', now called plutonium. Like uranium-235, element 94 would be an incredibly powerful explosive. In 1941, von Weizsäcker went so far as to submit a patent application for using a uranium machine to manufacture this new radioactive element.
By 1942 the military objective was wound down as impractical, requiring more resources than available. The priority became building rockets. However, the existence of the German Uranverein project provided the main incentive for wartime development of the atomic bomb by Britain and the USA.
Nuclear physics in Russia
Russian nuclear physics predates the Bolshevik Revolution by more than a decade. Work on radioactive minerals found in central Asia began in 1900 and the St Petersburg Academy of Sciences began a large-scale investigation in 1909. The 1917 Revolution gave a boost to scientific research and over 10 physics institutes were established in major Russian towns, particularly St Petersburg, in the years which followed. In the 1920s and early 1930s many prominent Russian physicists worked abroad, encouraged by the new regime initially as the best way to raise the level of expertise quickly. These included Kirill Sinelnikov, Pyotr Kapitsa and Vladimir Vernadsky.
By the early 1930s there were several research centres specialising in nuclear physics. Kirill Sinelnikov returned from Cambridge in 1931 to organise a department at the Ukrainian Institute of Physics and Technology (later renamed Kharkov Institute of Physics and Technology, KIPT) in Kharkov, which had been set up in 1928. Academician Abram Ioffe formed another group at the Leningrad Physics and Technical Institute (FTI), later becoming independent as the Ioffe Institute, including the young Igor Kurchatov. Ioffe was its first director, through to 1950.
By the end of the decade, there were cyclotrons installed at the Radium Institute and Leningrad FTI (the biggest in Europe). But by this time many scientists were beginning to fall victim to Stalin's purges – half the staff of Kharkov Institute, for instance, was arrested in 1939. Nevertheless, 1940 saw great advances being made in the understanding of nuclear fission including the possibility of a chain reaction. At the urging of Kurchatov and his colleagues, the Academy of Sciences set up a "Committee for the Problem of Uranium" in June 1940 chaired by Vitaly Khlopin, and a fund was established to investigate the central Asian uranium deposits. The Radium Institute had a factory in Tartarstan used by Khlopin to produce Russia’s first high-purity radium. Germany's invasion of Russia in 1941 turned much of this fundamental research to potential military applications.
Conceiving the atomic bomb
British scientists had kept pressure on their government. The refugee physicists Peierls and Frisch (who had stayed in England with Peierls after the outbreak of war), gave a major impetus to the concept of the atomic bomb in a three-page document known as the Frisch-Peierls Memorandum. In this they predicted that an amount of about 5kg of pure U-235 could make a very powerful atomic bomb equivalent to several thousand tonnes of dynamite. They also suggested how such a bomb could be detonated, how the U-235 could be produced, and what the radiation effects might be in addition to the explosive effects. They proposed thermal diffusion as a suitable method for separating the U-235 from the natural uranium. This memorandum stimulated a considerable response in Britain at a time when there was little interest in the USA.
A group of eminent scientists known as the MAUD Committee was set up in Britain and supervised research at the Universities of Birmingham, Bristol, Cambridge, Liverpool and Oxford. The chemical problems of producing gaseous compounds of uranium and pure uranium metal were studied at Birmingham University and Imperial Chemical Industries (ICI). Dr Philip Baxter at ICI made the first small batch of gaseous uranium hexafluoride for Professor James Chadwick in 1940. ICI received a formal contract later in 1940 to make 3kg of this vital material for the future work. Most of the other research was funded by the universities themselves.
Two important developments came from the work at Cambridge. The first was experimental proof that a chain reaction could be sustained with slow neutrons in a mixture of uranium oxide and heavy water, ie. the output of neutrons was greater than the input. The second was by Bretscher and Feather based on earlier work by Halban and Kowarski soon after they arrived in Britain from Paris. When U-235 and U-238 absorb slow neutrons, the probability of fission in U-235 is much greater than in U-238. The U-238 is more likely to form a new isotope U-239, and this isotope rapidly emits an electron to become a new element with a mass of 239 and an Atomic Number of 93. This element also emits an electron and becomes a new element of mass 239 and Atomic Number 94, which has a much greater half-life. Bretscher and Feather argued on theoretical grounds that element 94 would be readily fissionable by slow and fast neutrons, and had the added advantages that it was chemically different to uranium and therefore could easily be separated from it.
This new development was also confirmed in independent work by McMillan and Abelson in the USA in 1940. Dr Kemmer of the Cambridge team proposed the names neptunium for the new element # 93 and plutonium for # 94 by analogy with the outer planets Neptune and Pluto beyond Uranus (uranium, element # 92). The Americans fortuitously suggested the same names, and the identification of plutonium in 1941 is generally credited to Glenn Seaborg.
Developing the concepts
By the end of 1940 remarkable progress had been made by the several groups of scientists coordinated by the MAUD Committee and for the expenditure of a relatively small amount of money. All of this work was kept secret, whereas in the USA several publications continued to appear in 1940 and there was also little sense of urgency.
By March 1941 one of the most uncertain pieces of information was confirmed - the fission cross-section of U-235. Peierls and Frisch had initially predicted in 1940 that almost every collision of a neutron with a U-235 atom would result in fission, and that both slow and fast neutrons would be equally effective. It was later discerned that slow neutrons were very much more effective, which was of enormous significance for nuclear reactors but fairly academic in the bomb context. Peierls then stated that there was now no doubt that the whole scheme for a bomb was feasible provided highly enriched U-235 could be obtained. The predicted critical size for a sphere of U-235 metal was about 8kg, which might be reduced by use of an appropriate material for reflecting neutrons. However, direct measurements on U-235 were still necessary and the British pushed for urgent production of a few micrograms.
The final outcome of the MAUD Committee was two summary reports in July 1941. One was on 'Use of Uranium for a Bomb' and the other was on 'Use of Uranium as a Source of Power'. The first report concluded that a bomb was feasible and that one containing some 12 kg of active material would be equivalent to 1,800 tons of TNT and would release large quantities of radioactive substances which would make places near the explosion site dangerous to humans for a long period. It estimated that for a plant to produce 1kg of U-235 per day it would cost £5 million and would require a large skilled labour force that was also needed for other parts of the war effort. Suggesting that the Germans could also be working on the bomb, it recommended that the work should be continued with high priority in cooperation with the Americans, even though they seemed to be concentrating on the future use of uranium for power and naval propulsion.
The second MAUD Report concluded that the controlled fission of uranium could be used to provide energy in the form of heat for use in machines, as well as providing large quantities of radioisotopes which could be used as substitutes for radium. It referred to the use of heavy water and possibly graphite as moderators for the fast neutrons, and that even ordinary water could be used if the uranium was enriched in the U-235 isotope. It concluded that the 'uranium boiler' had considerable promise for future peaceful uses but that it was not worth considering during the present war. The Committee recommended that Halban and Kowarski should move to the USA where there were plans to make heavy water on a large scale. The possibility that the new element plutonium might be more suitable than U-235 was mentioned, so that the work in this area by Bretscher and Feather should be continued in Britain.
The two reports led to a complete reorganisation of work on the bomb and the 'boiler'. It was claimed that the work of the committee had put the British in the lead and that "in its fifteen months' existence it had proved itself one of the most effective scientific committees that ever existed". The basic decision that the bomb project would be pursued urgently was taken by the Prime Minister, Winston Churchill, with the agreement of the Chiefs of Staff.
The reports also led to high level reviews in the USA, particularly by a Committee of the National Academy of Sciences, initially concentrating on the nuclear power aspect. Little emphasis was given to the bomb concept until 7 December 1941, when the Japanese attacked Pearl Harbour and the Americans entered the war directly. The huge resources of the USA were then applied without reservation to developing atomic bombs.
The Manhattan Project
The Americans increased their effort rapidly and soon outstripped the British. Research continued in each country with some exchange of information. Several of the key British scientists visited the USA early in 1942 and were given full access to all of the information available. The Americans were pursuing three enrichment processes in parallel: Professor Lawrence was studying electromagnetic separation at Berkeley (University of California), E. V. Murphree of Standard Oil was studying the centrifuge method developed by Professor Beams, and Professor Urey was coordinating the gaseous diffusion work at Columbia University. Responsibility for building a reactor to produce fissile plutonium was given to Arthur Compton at the University of Chicago. The British were only examining gaseous diffusion.
In June 1942 the US Army took over process development, engineering design, procurement of materials and site selection for pilot plants for four methods of making fissionable material (because none of the four had been shown to be clearly superior at that point) as well as the production of heavy water. With this change, information flow to Britain dried up. This was a major setback to the British and the Canadians who had been collaborating on heavy water production and on several aspects of the research program. Thereafter, Churchill sought information on the cost of building a diffusion plant, a heavy water plant and an atomic reactor in Britain.
After many months of negotiations an agreement was finally signed by Mr Churchill and President Roosevelt in Quebec in August 1943, according to which the British handed over all of their reports to the Americans and in return received copies of General Groves' progress reports to the President. The latter showed that the entire US program would cost over $1,000 million, all for the bomb, as no work was being done on other applications of nuclear energy.
Construction of production plants for electromagnetic separation (in calutrons) and gaseous diffusion was well under way. An experimental graphite pile constructed by Fermi had operated at the University of Chicago in December 1942 – the first controlled nuclear chain reaction.
Enrico Fermi, c. 1943-1949 (National Archives and Records Administration)
A full-scale production reactor for plutonium was being constructed at Argonne, with further ones at Oak Ridge and then Hanford, plus a reprocessing plant to extract the plutonium. Four plants for heavy water production were being built, one in Canada and three in the USA. A team under Robert Oppenheimer at Los Alamos in New Mexico was working on the design and construction of both U-235 and Pu-239 bombs. The outcome of the huge effort, with assistance from the British teams, was that sufficient Pu-239 and highly enriched U-235 (from calutrons and diffusion at Oak Ridge) was produced by mid-1945. The uranium mostly originated from the Belgian Congo.
The first atomic device tested successfully at Alamagordo in New Mexico on 16 July 1945. It used plutonium made in a nuclear pile. The teams did not consider that it was necessary to test a simpler U-235 device. The first atomic bomb, which contained U-235, was dropped on Hiroshima on 6 August 1945. The second bomb, containing Pu-239, was dropped on Nagasaki on 9 August. That same day, the USSR declared war on Japan. On 10 August 1945 the Japanese Government surrendered.
The Soviet bomb
Initially Stalin was not enthusiastic about diverting resources to develop an atomic bomb, until intelligence reports suggested that such research was under way in Germany, Britain and the USA. Consultations with Academicians Ioffe, Kapitsa, Khlopin and Vernadsky convinced him that a bomb could be developed relatively quickly and he initiated a modest research program in 1942. Igor Kurchatov, then relatively young and unknown, was chosen to head it and in 1943 he became Director of Laboratory No.2 recently established on the outskirts of Moscow. This was later renamed LIPAN, then became the Kurchatov Institute of Atomic Energy. Overall responsibility for the bomb program rested with Security Chief Lavrenti Beria and its administration was undertaken by the First Main Directorate (later called the Ministry of Medium Machine Building).
Research had three main aims: to achieve a controlled chain reaction; to investigate methods of isotope separation; and to look at designs for both enriched uranium and plutonium bombs. Attempts were made to initiate a chain reaction using two different types of atomic pile: one with graphite as a moderator and the other with heavy water. Three possible methods of isotope separation were studied: counter-current thermal diffusion, gaseous diffusion and electromagnetic separation.
After the defeat of Nazi Germany in May 1945, German scientists were "recruited" to the bomb program to work in particular on isotope separation to produce enriched uranium. This included research into gas centrifuge technology in addition to the three other enrichment technologies.
The test of the first US atomic bomb in July 1945 had little impact on the Soviet effort, but by this time, Kurchatov was making good progress towards both a uranium and a plutonium bomb. He had begun to design an industrial scale reactor for the production of plutonium, while those scientists working on uranium isotope separation were making advances with the gaseous diffusion method.
It was the bombing of Hiroshima and Nagasaki the following month which gave the program a high profile and construction began in November 1945 of a new city in the Urals which would house the first plutonium production reactors -- Chelyabinsk-40 (Later known as Chelyabinsk-65 or the Mayak production association). This was the first of ten secret nuclear cities to be built in the Soviet Union. The first of five reactors at Chelyabinsk-65 came on line in 1948. This town also housed a processing plant for extracting plutonium from irradiated uranium.
As for uranium enrichment technology, it was decided in late 1945 to begin construction of the first gaseous diffusion plant at Verkh-Neyvinsk (later the closed city of Sverdlovsk-44), some 50 kilometres from Yekaterinburg (formerly Sverdlovsk) in the Urals. Special design bureaux were set up at the Leningrad Kirov Metallurgical and Machine-Building Plant and at the Gorky (Nizhny Novgorod) Machine Building Plant. Support was provided by a group of German scientists working at the Sukhumi Physical Technical Institute.
In April 1946 design work on the bomb was shifted to Design Bureau-11 – a new centre at Sarova some 400 kilometres from Moscow (subsequently the closed city of Arzamas-16). More specialists were brought in to the program including metallurgist Yefim Slavsky who was given the immediate task of producing the very pure graphite Kurchatov needed for his plutonium production pile constructed at Laboratory No. 2 known as F-1. The pile was operated for the first time in December 1946. Support was also given by Laboratory No.3 in Moscow – now the Institute of Theoretical and Experimental Physics – which had been working on nuclear reactors.
Work at Arzamas-16 was influenced by foreign intelligence gathering and the first device was based closely on the Nagasaki bomb (a plutonium device). In August 1947 a test site was established near Semipalatinsk in Kazakhstan and was ready for the detonation two years later of the first bomb, RSD-1. Even before this was tested in August 1949, another group of scientists led by Igor Tamm and including Andrei Sakharov had begun work on a hydrogen bomb.
Revival of the 'nuclear boiler'
By the end of World War II, the project predicted and described in detail only five and a half years before in the Frisch-Peierls Memorandum had been brought to partial fruition, and attention could turn to the peaceful and directly beneficial application of nuclear energy. Post-war, weapons development continued on both sides of the "iron curtain", but a new focus was on harnessing the great atomic power, now dramatically (if tragically) demonstrated, for making steam and electricity.
In the course of developing nuclear weapons the Soviet Union and the West had acquired a range of new technologies and scientists realised that the tremendous heat produced in the process could be tapped either for direct use or for generating electricity. It was also clear that this new form of energy would allow development of compact long-lasting power sources which could have various applications, not least for shipping, and especially in submarines.
The first nuclear reactor to produce electricity (albeit a trivial amount) was the small Experimental Breeder reactor (EBR-1) designed and operated by Argonne National Laboratory and sited in Idaho, USA. The reactor started up in December 1951.
In 1953 President Eisenhower proposed his "Atoms for Peace" program, which reoriented significant research effort towards electricity generation and set the course for civil nuclear energy development in the USA.
In the Soviet Union, work was under way at various centres to refine existing reactor designs and develop new ones. The Institute of Physics and Power Engineering (FEI) was set up in May 1946 at the then-closed city of Obninsk, 100 km southwest of Moscow, to develop nuclear power technology. The existing graphite-moderated channel-type plutonium production reactor was modified for heat and electricity generation and in June 1954 the world's first nuclear powered electricity generator began operation at the FEI in Obninsk. The AM-1 (Atom Mirny – peaceful atom) reactor was water-cooled and graphite-moderated, with a design capacity of 30 MWt or 5 MWe. It was similar in principle to the plutonium production reactors in the closed military cities and served as a prototype for other graphite channel reactor designs including the Chernobyl-type RBMK (reaktor bolshoi moshchnosty kanalny – high power channel reactor) reactors. AM-1 produced electricity until 1959 and was used until 2000 as a research facility and for the production of isotopes.
Also in the 1950s FEI at Obninsk was developing fast breeder reactors (FBRs) and lead-bismuth reactors for the navy. In April 1955 the BR-1 ( bystry reaktor – fast reactor) fast neutron reactor began operating. It produced no power but led directly to the BR-5, which started up in 1959 with a capacity of 5 MWt, and which was used to do the basic research necessary for designing sodium-cooled FBRs. It was upgraded and modernised in 1973 and then underwent major reconstruction in 1983 to become the BR-10 with a capacity of 8 MWt which is now used to investigate fuel endurance, to study materials and to produce isotopes.
The main US effort was under Admiral Hyman Rickover, which developed the pressurised water reactor (PWR) for naval (particularly submarine) use. The PWR used enriched uranium oxide fuel and was moderated and cooled by ordinary (light) water. The Mark 1 prototype naval reactor started up in March 1953 in Idaho, and the first nuclear-powered submarine, USS Nautilus , was launched in 1954. In 1959 both USA and USSR launched their first nuclear-powered surface vessels.
The Mark 1 reactor led to the US Atomic Energy Commission building the 60 MWe Shippingport demonstration PWR reactor in Pennsylvania, which started up in 1957 and operated until 1982.
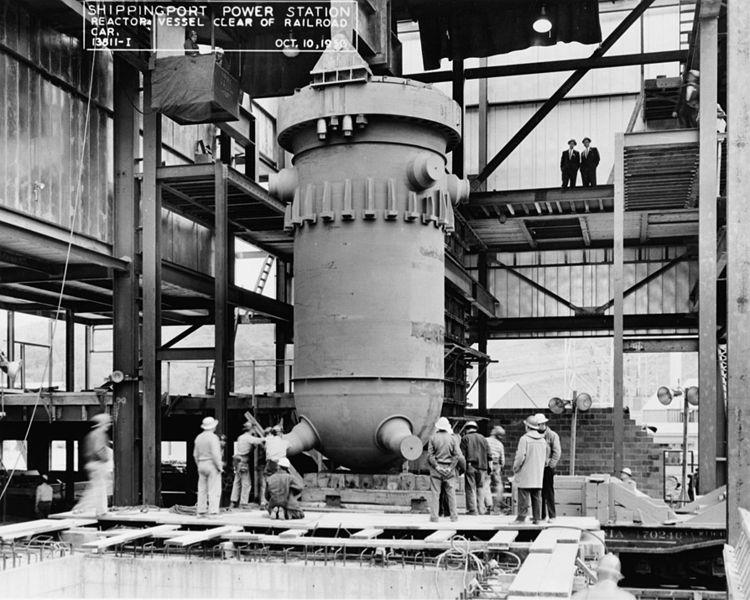
Installation of reactor vessel at Shippingport, the first commercial US nuclear power plant (US Library of Congress)
Since the USA had a virtual monopoly on uranium enrichment in the West, British development took a different tack and resulted in a series of reactors fuelled by natural uranium metal, moderated by graphite, and gas-cooled. The first of these 50 MWe Magnox types, Calder Hall 1, started up in 1956 and ran until 2003. However, after 1963 (and 26 units) no more were commenced. Britain next embraced the advanced gas-cooled reactor (using enriched oxide fuel) before conceding the pragmatic virtues of the PWR design.
Nuclear energy goes commercial
In the USA, Westinghouse designed the first fully commercial PWR of 250 MWe, Yankee Rowe, which started up in 1960 and operated to 1992. Meanwhile the boiling water reactor (BWR) was developed by the Argonne National Laboratory, and the first one, Dresden-1 of 250 MWe, designed by General Electric, was started up earlier in 1960. A prototype BWR, Vallecitos, ran from 1957 to 1963. By the end of the 1960s, orders were being placed for PWR and BWR reactor units of more than 1000 MWe.
Canadian reactor development headed down a quite different track, using natural uranium fuel and heavy water as a moderator and coolant. The first unit started up in 1962. This CANDU design continues to be refined.
France started out with a gas-graphite design similar to Magnox and the first reactor started up in 1956. Commercial models operated from 1959. It then settled on three successive generations of standardised PWRs, which was a very cost-effective strategy.
In 1964 the first two Soviet nuclear power plants were commissioned. A 100 MW boiling water graphite channel reactor began operating in Beloyarsk (Urals). In Novovoronezh (Volga region) a new design – a small (210 MW) pressurised water reactor (PWR) known as a VVER (veda-vodyanoi energetichesky reaktor– water cooled power reactor) – was built.
The first large RBMK (1,000 MW – high-power channel reactor) started up at Sosnovy Bor near Leningrad in 1973, and in the Arctic northwest a VVER with a rated capacity of 440 MW began operating. This was superseded by a 1000 MWe version which became a standard design.
In Kazakhstan the world's first commercial prototype fast neutron reactor (the BN-350) started up in 1972 with a design capacity of 135 MWe (net), producing electricity and heat to desalinate Caspian seawater. In the USA, UK, France and Russia a number of experimental fast neutron reactors produced electricity from 1959, the last of these closing in 2009. This left Russia's BN-600 as the only commercial fast reactor, until joined by a BN-800 in 2016.
Around the world, with few exceptions, other countries have chosen light-water designs for their nuclear power programmes, so that today 69% of the world capacity is PWR and 20% BWR.
The nuclear power brown-out and revival
From the late 1970s to about 2002 the nuclear power industry suffered some decline and stagnation. Few new reactors were ordered, the number coming on line from mid 1980s little more than matched retirements, though capacity increased by nearly one third and output increased 60% due to capacity plus improved load factors. The share of nuclear in world electricity from mid 1980s was fairly constant at 16-17%. Many reactor orders from the 1970s were cancelled. The uranium price dropped accordingly, and also because of an increase in secondary supplies. Oil companies which had entered the uranium field bailed out, and there was a consolidation of uranium producers.
However, by the late 1990s the first of the third-generation reactors was commissioned – Kashiwazaki-Kariwa 6 – a 1350 MWe Advanced BWR, in Japan. This was a sign of the recovery to come.
In the new century several factors combined to revive the prospects for nuclear power. First was the realisation of the scale of projected increased electricity demand worldwide, but particularly in rapidly-developing countries. Secondly was the awareness of the importance of energy security – the prime importance of each country having assured access to affordable energy, and particularly to dispatchable electricity able to meet demand at all times. Thirdly was the need to limit carbon emissions due to concerns about climate change.
These factors coincided with the availability of a new generation of nuclear power reactors, and in 2004 the first of the late third-generation units was ordered for Finland – a 1600 MWe European PWR (EPR). A similar unit is being built in France, and two new Westinghouse AP1000 units are under construction in the USA.
But plans in Europe and North America are overshadowed by those in Asia, particularly China and India. China alone plans and is building towards a huge increase in nuclear power capacity by 2030, and has more than one hundred further large units proposed and backed by credible political determination and popular support. Many of these are the latest Western design, or adaptations thereof. Others are substantially local designs.
The history of nuclear power thus starts with science in Europe, blossoms in the UK and USA with the latter's technological and economic might, languishes for a few decades, then has a new growth spurt in east Asia. In the process, over 17,000 reactor-years of operation have been accumulated in providing a significant proportion of the world’s electricity.
Notes & references
General sources.
Atomic Rise and Fall, the Australian Atomic Energy Commission 1953-1987 , by Clarence Hardy, Glen Haven, 1999. Chapter 1 provides the major source for 1939-45 Radiation in Perspective, OECD NEA, 1997 Nuclear Fear , by Spencer Weart, Harvard UP, 1988 Judith Perera (Russian material) Alexander Petrov, ITER Domestic Agency of the Russian Federation, A Short History of the Ioffe Institute Mark Walker, Nazis and the Bomb , NOVA (November 2005) Carl H. Meyer and Günter Schwarz, The Theory of Nuclear Explosives that Heisenberg did not Present to the German Military , Max Planck Institute for the History of Science, Preprint #467 (2015)
Related information
Thank you for visiting nature.com. You are using a browser version with limited support for CSS. To obtain the best experience, we recommend you use a more up to date browser (or turn off compatibility mode in Internet Explorer). In the meantime, to ensure continued support, we are displaying the site without styles and JavaScript.
- View all journals
- My Account Login
- Explore content
- About the journal
- Publish with us
- Sign up for alerts
- Open access
- Published: 17 April 2024
The economic commitment of climate change
- Maximilian Kotz ORCID: orcid.org/0000-0003-2564-5043 1 , 2 ,
- Anders Levermann ORCID: orcid.org/0000-0003-4432-4704 1 , 2 &
- Leonie Wenz ORCID: orcid.org/0000-0002-8500-1568 1 , 3
Nature volume 628 , pages 551–557 ( 2024 ) Cite this article
90k Accesses
3502 Altmetric
Metrics details
- Environmental economics
- Environmental health
- Interdisciplinary studies
- Projection and prediction
Global projections of macroeconomic climate-change damages typically consider impacts from average annual and national temperatures over long time horizons 1 , 2 , 3 , 4 , 5 , 6 . Here we use recent empirical findings from more than 1,600 regions worldwide over the past 40 years to project sub-national damages from temperature and precipitation, including daily variability and extremes 7 , 8 . Using an empirical approach that provides a robust lower bound on the persistence of impacts on economic growth, we find that the world economy is committed to an income reduction of 19% within the next 26 years independent of future emission choices (relative to a baseline without climate impacts, likely range of 11–29% accounting for physical climate and empirical uncertainty). These damages already outweigh the mitigation costs required to limit global warming to 2 °C by sixfold over this near-term time frame and thereafter diverge strongly dependent on emission choices. Committed damages arise predominantly through changes in average temperature, but accounting for further climatic components raises estimates by approximately 50% and leads to stronger regional heterogeneity. Committed losses are projected for all regions except those at very high latitudes, at which reductions in temperature variability bring benefits. The largest losses are committed at lower latitudes in regions with lower cumulative historical emissions and lower present-day income.
Similar content being viewed by others
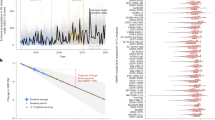
Climate damage projections beyond annual temperature
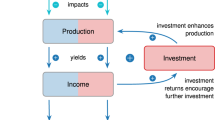
Investment incentive reduced by climate damages can be restored by optimal policy
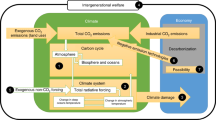
Climate economics support for the UN climate targets
Projections of the macroeconomic damage caused by future climate change are crucial to informing public and policy debates about adaptation, mitigation and climate justice. On the one hand, adaptation against climate impacts must be justified and planned on the basis of an understanding of their future magnitude and spatial distribution 9 . This is also of importance in the context of climate justice 10 , as well as to key societal actors, including governments, central banks and private businesses, which increasingly require the inclusion of climate risks in their macroeconomic forecasts to aid adaptive decision-making 11 , 12 . On the other hand, climate mitigation policy such as the Paris Climate Agreement is often evaluated by balancing the costs of its implementation against the benefits of avoiding projected physical damages. This evaluation occurs both formally through cost–benefit analyses 1 , 4 , 5 , 6 , as well as informally through public perception of mitigation and damage costs 13 .
Projections of future damages meet challenges when informing these debates, in particular the human biases relating to uncertainty and remoteness that are raised by long-term perspectives 14 . Here we aim to overcome such challenges by assessing the extent of economic damages from climate change to which the world is already committed by historical emissions and socio-economic inertia (the range of future emission scenarios that are considered socio-economically plausible 15 ). Such a focus on the near term limits the large uncertainties about diverging future emission trajectories, the resulting long-term climate response and the validity of applying historically observed climate–economic relations over long timescales during which socio-technical conditions may change considerably. As such, this focus aims to simplify the communication and maximize the credibility of projected economic damages from future climate change.
In projecting the future economic damages from climate change, we make use of recent advances in climate econometrics that provide evidence for impacts on sub-national economic growth from numerous components of the distribution of daily temperature and precipitation 3 , 7 , 8 . Using fixed-effects panel regression models to control for potential confounders, these studies exploit within-region variation in local temperature and precipitation in a panel of more than 1,600 regions worldwide, comprising climate and income data over the past 40 years, to identify the plausibly causal effects of changes in several climate variables on economic productivity 16 , 17 . Specifically, macroeconomic impacts have been identified from changing daily temperature variability, total annual precipitation, the annual number of wet days and extreme daily rainfall that occur in addition to those already identified from changing average temperature 2 , 3 , 18 . Moreover, regional heterogeneity in these effects based on the prevailing local climatic conditions has been found using interactions terms. The selection of these climate variables follows micro-level evidence for mechanisms related to the impacts of average temperatures on labour and agricultural productivity 2 , of temperature variability on agricultural productivity and health 7 , as well as of precipitation on agricultural productivity, labour outcomes and flood damages 8 (see Extended Data Table 1 for an overview, including more detailed references). References 7 , 8 contain a more detailed motivation for the use of these particular climate variables and provide extensive empirical tests about the robustness and nature of their effects on economic output, which are summarized in Methods . By accounting for these extra climatic variables at the sub-national level, we aim for a more comprehensive description of climate impacts with greater detail across both time and space.
Constraining the persistence of impacts
A key determinant and source of discrepancy in estimates of the magnitude of future climate damages is the extent to which the impact of a climate variable on economic growth rates persists. The two extreme cases in which these impacts persist indefinitely or only instantaneously are commonly referred to as growth or level effects 19 , 20 (see Methods section ‘Empirical model specification: fixed-effects distributed lag models’ for mathematical definitions). Recent work shows that future damages from climate change depend strongly on whether growth or level effects are assumed 20 . Following refs. 2 , 18 , we provide constraints on this persistence by using distributed lag models to test the significance of delayed effects separately for each climate variable. Notably, and in contrast to refs. 2 , 18 , we use climate variables in their first-differenced form following ref. 3 , implying a dependence of the growth rate on a change in climate variables. This choice means that a baseline specification without any lags constitutes a model prior of purely level effects, in which a permanent change in the climate has only an instantaneous effect on the growth rate 3 , 19 , 21 . By including lags, one can then test whether any effects may persist further. This is in contrast to the specification used by refs. 2 , 18 , in which climate variables are used without taking the first difference, implying a dependence of the growth rate on the level of climate variables. In this alternative case, the baseline specification without any lags constitutes a model prior of pure growth effects, in which a change in climate has an infinitely persistent effect on the growth rate. Consequently, including further lags in this alternative case tests whether the initial growth impact is recovered 18 , 19 , 21 . Both of these specifications suffer from the limiting possibility that, if too few lags are included, one might falsely accept the model prior. The limitations of including a very large number of lags, including loss of data and increasing statistical uncertainty with an increasing number of parameters, mean that such a possibility is likely. By choosing a specification in which the model prior is one of level effects, our approach is therefore conservative by design, avoiding assumptions of infinite persistence of climate impacts on growth and instead providing a lower bound on this persistence based on what is observable empirically (see Methods section ‘Empirical model specification: fixed-effects distributed lag models’ for further exposition of this framework). The conservative nature of such a choice is probably the reason that ref. 19 finds much greater consistency between the impacts projected by models that use the first difference of climate variables, as opposed to their levels.
We begin our empirical analysis of the persistence of climate impacts on growth using ten lags of the first-differenced climate variables in fixed-effects distributed lag models. We detect substantial effects on economic growth at time lags of up to approximately 8–10 years for the temperature terms and up to approximately 4 years for the precipitation terms (Extended Data Fig. 1 and Extended Data Table 2 ). Furthermore, evaluation by means of information criteria indicates that the inclusion of all five climate variables and the use of these numbers of lags provide a preferable trade-off between best-fitting the data and including further terms that could cause overfitting, in comparison with model specifications excluding climate variables or including more or fewer lags (Extended Data Fig. 3 , Supplementary Methods Section 1 and Supplementary Table 1 ). We therefore remove statistically insignificant terms at later lags (Supplementary Figs. 1 – 3 and Supplementary Tables 2 – 4 ). Further tests using Monte Carlo simulations demonstrate that the empirical models are robust to autocorrelation in the lagged climate variables (Supplementary Methods Section 2 and Supplementary Figs. 4 and 5 ), that information criteria provide an effective indicator for lag selection (Supplementary Methods Section 2 and Supplementary Fig. 6 ), that the results are robust to concerns of imperfect multicollinearity between climate variables and that including several climate variables is actually necessary to isolate their separate effects (Supplementary Methods Section 3 and Supplementary Fig. 7 ). We provide a further robustness check using a restricted distributed lag model to limit oscillations in the lagged parameter estimates that may result from autocorrelation, finding that it provides similar estimates of cumulative marginal effects to the unrestricted model (Supplementary Methods Section 4 and Supplementary Figs. 8 and 9 ). Finally, to explicitly account for any outstanding uncertainty arising from the precise choice of the number of lags, we include empirical models with marginally different numbers of lags in the error-sampling procedure of our projection of future damages. On the basis of the lag-selection procedure (the significance of lagged terms in Extended Data Fig. 1 and Extended Data Table 2 , as well as information criteria in Extended Data Fig. 3 ), we sample from models with eight to ten lags for temperature and four for precipitation (models shown in Supplementary Figs. 1 – 3 and Supplementary Tables 2 – 4 ). In summary, this empirical approach to constrain the persistence of climate impacts on economic growth rates is conservative by design in avoiding assumptions of infinite persistence, but nevertheless provides a lower bound on the extent of impact persistence that is robust to the numerous tests outlined above.
Committed damages until mid-century
We combine these empirical economic response functions (Supplementary Figs. 1 – 3 and Supplementary Tables 2 – 4 ) with an ensemble of 21 climate models (see Supplementary Table 5 ) from the Coupled Model Intercomparison Project Phase 6 (CMIP-6) 22 to project the macroeconomic damages from these components of physical climate change (see Methods for further details). Bias-adjusted climate models that provide a highly accurate reproduction of observed climatological patterns with limited uncertainty (Supplementary Table 6 ) are used to avoid introducing biases in the projections. Following a well-developed literature 2 , 3 , 19 , these projections do not aim to provide a prediction of future economic growth. Instead, they are a projection of the exogenous impact of future climate conditions on the economy relative to the baselines specified by socio-economic projections, based on the plausibly causal relationships inferred by the empirical models and assuming ceteris paribus. Other exogenous factors relevant for the prediction of economic output are purposefully assumed constant.
A Monte Carlo procedure that samples from climate model projections, empirical models with different numbers of lags and model parameter estimates (obtained by 1,000 block-bootstrap resamples of each of the regressions in Supplementary Figs. 1 – 3 and Supplementary Tables 2 – 4 ) is used to estimate the combined uncertainty from these sources. Given these uncertainty distributions, we find that projected global damages are statistically indistinguishable across the two most extreme emission scenarios until 2049 (at the 5% significance level; Fig. 1 ). As such, the climate damages occurring before this time constitute those to which the world is already committed owing to the combination of past emissions and the range of future emission scenarios that are considered socio-economically plausible 15 . These committed damages comprise a permanent income reduction of 19% on average globally (population-weighted average) in comparison with a baseline without climate-change impacts (with a likely range of 11–29%, following the likelihood classification adopted by the Intergovernmental Panel on Climate Change (IPCC); see caption of Fig. 1 ). Even though levels of income per capita generally still increase relative to those of today, this constitutes a permanent income reduction for most regions, including North America and Europe (each with median income reductions of approximately 11%) and with South Asia and Africa being the most strongly affected (each with median income reductions of approximately 22%; Fig. 1 ). Under a middle-of-the road scenario of future income development (SSP2, in which SSP stands for Shared Socio-economic Pathway), this corresponds to global annual damages in 2049 of 38 trillion in 2005 international dollars (likely range of 19–59 trillion 2005 international dollars). Compared with empirical specifications that assume pure growth or pure level effects, our preferred specification that provides a robust lower bound on the extent of climate impact persistence produces damages between these two extreme assumptions (Extended Data Fig. 3 ).
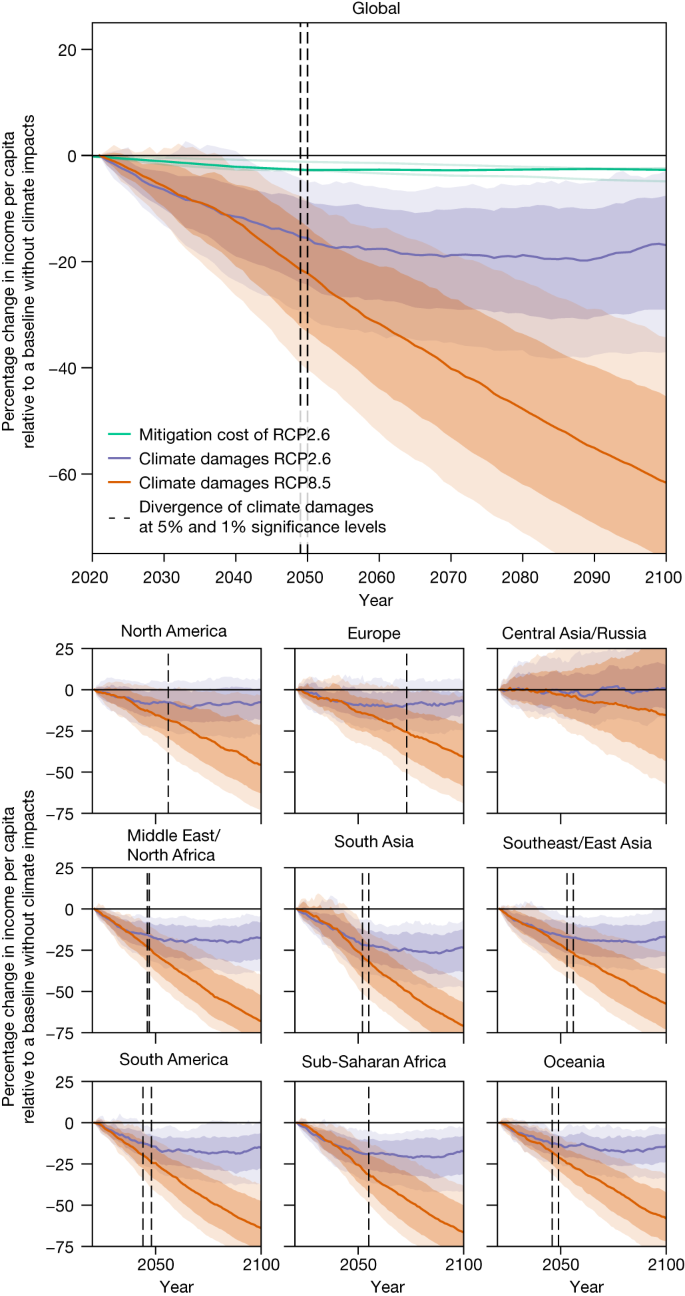
Estimates of the projected reduction in income per capita from changes in all climate variables based on empirical models of climate impacts on economic output with a robust lower bound on their persistence (Extended Data Fig. 1 ) under a low-emission scenario compatible with the 2 °C warming target and a high-emission scenario (SSP2-RCP2.6 and SSP5-RCP8.5, respectively) are shown in purple and orange, respectively. Shading represents the 34% and 10% confidence intervals reflecting the likely and very likely ranges, respectively (following the likelihood classification adopted by the IPCC), having estimated uncertainty from a Monte Carlo procedure, which samples the uncertainty from the choice of physical climate models, empirical models with different numbers of lags and bootstrapped estimates of the regression parameters shown in Supplementary Figs. 1 – 3 . Vertical dashed lines show the time at which the climate damages of the two emission scenarios diverge at the 5% and 1% significance levels based on the distribution of differences between emission scenarios arising from the uncertainty sampling discussed above. Note that uncertainty in the difference of the two scenarios is smaller than the combined uncertainty of the two respective scenarios because samples of the uncertainty (climate model and empirical model choice, as well as model parameter bootstrap) are consistent across the two emission scenarios, hence the divergence of damages occurs while the uncertainty bounds of the two separate damage scenarios still overlap. Estimates of global mitigation costs from the three IAMs that provide results for the SSP2 baseline and SSP2-RCP2.6 scenario are shown in light green in the top panel, with the median of these estimates shown in bold.
Damages already outweigh mitigation costs
We compare the damages to which the world is committed over the next 25 years to estimates of the mitigation costs required to achieve the Paris Climate Agreement. Taking estimates of mitigation costs from the three integrated assessment models (IAMs) in the IPCC AR6 database 23 that provide results under comparable scenarios (SSP2 baseline and SSP2-RCP2.6, in which RCP stands for Representative Concentration Pathway), we find that the median committed climate damages are larger than the median mitigation costs in 2050 (six trillion in 2005 international dollars) by a factor of approximately six (note that estimates of mitigation costs are only provided every 10 years by the IAMs and so a comparison in 2049 is not possible). This comparison simply aims to compare the magnitude of future damages against mitigation costs, rather than to conduct a formal cost–benefit analysis of transitioning from one emission path to another. Formal cost–benefit analyses typically find that the net benefits of mitigation only emerge after 2050 (ref. 5 ), which may lead some to conclude that physical damages from climate change are simply not large enough to outweigh mitigation costs until the second half of the century. Our simple comparison of their magnitudes makes clear that damages are actually already considerably larger than mitigation costs and the delayed emergence of net mitigation benefits results primarily from the fact that damages across different emission paths are indistinguishable until mid-century (Fig. 1 ).
Although these near-term damages constitute those to which the world is already committed, we note that damage estimates diverge strongly across emission scenarios after 2049, conveying the clear benefits of mitigation from a purely economic point of view that have been emphasized in previous studies 4 , 24 . As well as the uncertainties assessed in Fig. 1 , these conclusions are robust to structural choices, such as the timescale with which changes in the moderating variables of the empirical models are estimated (Supplementary Figs. 10 and 11 ), as well as the order in which one accounts for the intertemporal and international components of currency comparison (Supplementary Fig. 12 ; see Methods for further details).
Damages from variability and extremes
Committed damages primarily arise through changes in average temperature (Fig. 2 ). This reflects the fact that projected changes in average temperature are larger than those in other climate variables when expressed as a function of their historical interannual variability (Extended Data Fig. 4 ). Because the historical variability is that on which the empirical models are estimated, larger projected changes in comparison with this variability probably lead to larger future impacts in a purely statistical sense. From a mechanistic perspective, one may plausibly interpret this result as implying that future changes in average temperature are the most unprecedented from the perspective of the historical fluctuations to which the economy is accustomed and therefore will cause the most damage. This insight may prove useful in terms of guiding adaptation measures to the sources of greatest damage.
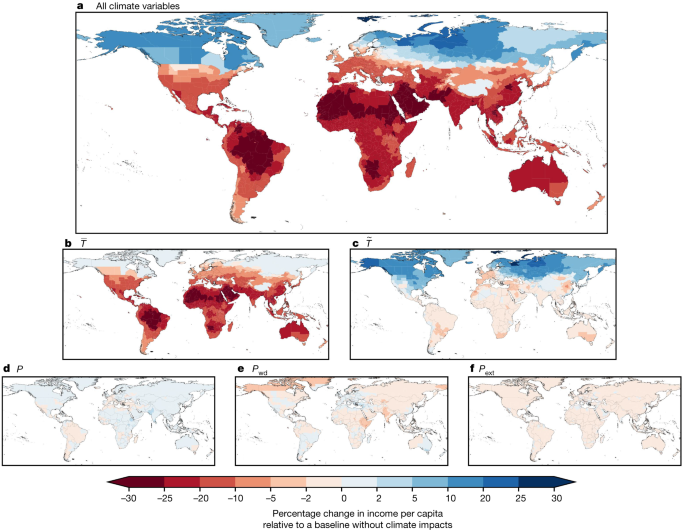
Estimates of the median projected reduction in sub-national income per capita across emission scenarios (SSP2-RCP2.6 and SSP2-RCP8.5) as well as climate model, empirical model and model parameter uncertainty in the year in which climate damages diverge at the 5% level (2049, as identified in Fig. 1 ). a , Impacts arising from all climate variables. b – f , Impacts arising separately from changes in annual mean temperature ( b ), daily temperature variability ( c ), total annual precipitation ( d ), the annual number of wet days (>1 mm) ( e ) and extreme daily rainfall ( f ) (see Methods for further definitions). Data on national administrative boundaries are obtained from the GADM database version 3.6 and are freely available for academic use ( https://gadm.org/ ).
Nevertheless, future damages based on empirical models that consider changes in annual average temperature only and exclude the other climate variables constitute income reductions of only 13% in 2049 (Extended Data Fig. 5a , likely range 5–21%). This suggests that accounting for the other components of the distribution of temperature and precipitation raises net damages by nearly 50%. This increase arises through the further damages that these climatic components cause, but also because their inclusion reveals a stronger negative economic response to average temperatures (Extended Data Fig. 5b ). The latter finding is consistent with our Monte Carlo simulations, which suggest that the magnitude of the effect of average temperature on economic growth is underestimated unless accounting for the impacts of other correlated climate variables (Supplementary Fig. 7 ).
In terms of the relative contributions of the different climatic components to overall damages, we find that accounting for daily temperature variability causes the largest increase in overall damages relative to empirical frameworks that only consider changes in annual average temperature (4.9 percentage points, likely range 2.4–8.7 percentage points, equivalent to approximately 10 trillion international dollars). Accounting for precipitation causes smaller increases in overall damages, which are—nevertheless—equivalent to approximately 1.2 trillion international dollars: 0.01 percentage points (−0.37–0.33 percentage points), 0.34 percentage points (0.07–0.90 percentage points) and 0.36 percentage points (0.13–0.65 percentage points) from total annual precipitation, the number of wet days and extreme daily precipitation, respectively. Moreover, climate models seem to underestimate future changes in temperature variability 25 and extreme precipitation 26 , 27 in response to anthropogenic forcing as compared with that observed historically, suggesting that the true impacts from these variables may be larger.
The distribution of committed damages
The spatial distribution of committed damages (Fig. 2a ) reflects a complex interplay between the patterns of future change in several climatic components and those of historical economic vulnerability to changes in those variables. Damages resulting from increasing annual mean temperature (Fig. 2b ) are negative almost everywhere globally, and larger at lower latitudes in regions in which temperatures are already higher and economic vulnerability to temperature increases is greatest (see the response heterogeneity to mean temperature embodied in Extended Data Fig. 1a ). This occurs despite the amplified warming projected at higher latitudes 28 , suggesting that regional heterogeneity in economic vulnerability to temperature changes outweighs heterogeneity in the magnitude of future warming (Supplementary Fig. 13a ). Economic damages owing to daily temperature variability (Fig. 2c ) exhibit a strong latitudinal polarisation, primarily reflecting the physical response of daily variability to greenhouse forcing in which increases in variability across lower latitudes (and Europe) contrast decreases at high latitudes 25 (Supplementary Fig. 13b ). These two temperature terms are the dominant determinants of the pattern of overall damages (Fig. 2a ), which exhibits a strong polarity with damages across most of the globe except at the highest northern latitudes. Future changes in total annual precipitation mainly bring economic benefits except in regions of drying, such as the Mediterranean and central South America (Fig. 2d and Supplementary Fig. 13c ), but these benefits are opposed by changes in the number of wet days, which produce damages with a similar pattern of opposite sign (Fig. 2e and Supplementary Fig. 13d ). By contrast, changes in extreme daily rainfall produce damages in all regions, reflecting the intensification of daily rainfall extremes over global land areas 29 , 30 (Fig. 2f and Supplementary Fig. 13e ).
The spatial distribution of committed damages implies considerable injustice along two dimensions: culpability for the historical emissions that have caused climate change and pre-existing levels of socio-economic welfare. Spearman’s rank correlations indicate that committed damages are significantly larger in countries with smaller historical cumulative emissions, as well as in regions with lower current income per capita (Fig. 3 ). This implies that those countries that will suffer the most from the damages already committed are those that are least responsible for climate change and which also have the least resources to adapt to it.
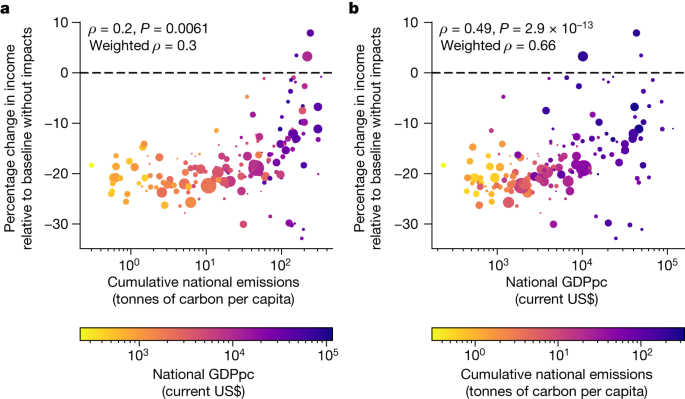
Estimates of the median projected change in national income per capita across emission scenarios (RCP2.6 and RCP8.5) as well as climate model, empirical model and model parameter uncertainty in the year in which climate damages diverge at the 5% level (2049, as identified in Fig. 1 ) are plotted against cumulative national emissions per capita in 2020 (from the Global Carbon Project) and coloured by national income per capita in 2020 (from the World Bank) in a and vice versa in b . In each panel, the size of each scatter point is weighted by the national population in 2020 (from the World Bank). Inset numbers indicate the Spearman’s rank correlation ρ and P -values for a hypothesis test whose null hypothesis is of no correlation, as well as the Spearman’s rank correlation weighted by national population.
To further quantify this heterogeneity, we assess the difference in committed damages between the upper and lower quartiles of regions when ranked by present income levels and historical cumulative emissions (using a population weighting to both define the quartiles and estimate the group averages). On average, the quartile of countries with lower income are committed to an income loss that is 8.9 percentage points (or 61%) greater than the upper quartile (Extended Data Fig. 6 ), with a likely range of 3.8–14.7 percentage points across the uncertainty sampling of our damage projections (following the likelihood classification adopted by the IPCC). Similarly, the quartile of countries with lower historical cumulative emissions are committed to an income loss that is 6.9 percentage points (or 40%) greater than the upper quartile, with a likely range of 0.27–12 percentage points. These patterns reemphasize the prevalence of injustice in climate impacts 31 , 32 , 33 in the context of the damages to which the world is already committed by historical emissions and socio-economic inertia.
Contextualizing the magnitude of damages
The magnitude of projected economic damages exceeds previous literature estimates 2 , 3 , arising from several developments made on previous approaches. Our estimates are larger than those of ref. 2 (see first row of Extended Data Table 3 ), primarily because of the facts that sub-national estimates typically show a steeper temperature response (see also refs. 3 , 34 ) and that accounting for other climatic components raises damage estimates (Extended Data Fig. 5 ). However, we note that our empirical approach using first-differenced climate variables is conservative compared with that of ref. 2 in regard to the persistence of climate impacts on growth (see introduction and Methods section ‘Empirical model specification: fixed-effects distributed lag models’), an important determinant of the magnitude of long-term damages 19 , 21 . Using a similar empirical specification to ref. 2 , which assumes infinite persistence while maintaining the rest of our approach (sub-national data and further climate variables), produces considerably larger damages (purple curve of Extended Data Fig. 3 ). Compared with studies that do take the first difference of climate variables 3 , 35 , our estimates are also larger (see second and third rows of Extended Data Table 3 ). The inclusion of further climate variables (Extended Data Fig. 5 ) and a sufficient number of lags to more adequately capture the extent of impact persistence (Extended Data Figs. 1 and 2 ) are the main sources of this difference, as is the use of specifications that capture nonlinearities in the temperature response when compared with ref. 35 . In summary, our estimates develop on previous studies by incorporating the latest data and empirical insights 7 , 8 , as well as in providing a robust empirical lower bound on the persistence of impacts on economic growth, which constitutes a middle ground between the extremes of the growth-versus-levels debate 19 , 21 (Extended Data Fig. 3 ).
Compared with the fraction of variance explained by the empirical models historically (<5%), the projection of reductions in income of 19% may seem large. This arises owing to the fact that projected changes in climatic conditions are much larger than those that were experienced historically, particularly for changes in average temperature (Extended Data Fig. 4 ). As such, any assessment of future climate-change impacts necessarily requires an extrapolation outside the range of the historical data on which the empirical impact models were evaluated. Nevertheless, these models constitute the most state-of-the-art methods for inference of plausibly causal climate impacts based on observed data. Moreover, we take explicit steps to limit out-of-sample extrapolation by capping the moderating variables of the interaction terms at the 95th percentile of the historical distribution (see Methods ). This avoids extrapolating the marginal effects outside what was observed historically. Given the nonlinear response of economic output to annual mean temperature (Extended Data Fig. 1 and Extended Data Table 2 ), this is a conservative choice that limits the magnitude of damages that we project. Furthermore, back-of-the-envelope calculations indicate that the projected damages are consistent with the magnitude and patterns of historical economic development (see Supplementary Discussion Section 5 ).
Missing impacts and spatial spillovers
Despite assessing several climatic components from which economic impacts have recently been identified 3 , 7 , 8 , this assessment of aggregate climate damages should not be considered comprehensive. Important channels such as impacts from heatwaves 31 , sea-level rise 36 , tropical cyclones 37 and tipping points 38 , 39 , as well as non-market damages such as those to ecosystems 40 and human health 41 , are not considered in these estimates. Sea-level rise is unlikely to be feasibly incorporated into empirical assessments such as this because historical sea-level variability is mostly small. Non-market damages are inherently intractable within our estimates of impacts on aggregate monetary output and estimates of these impacts could arguably be considered as extra to those identified here. Recent empirical work suggests that accounting for these channels would probably raise estimates of these committed damages, with larger damages continuing to arise in the global south 31 , 36 , 37 , 38 , 39 , 40 , 41 , 42 .
Moreover, our main empirical analysis does not explicitly evaluate the potential for impacts in local regions to produce effects that ‘spill over’ into other regions. Such effects may further mitigate or amplify the impacts we estimate, for example, if companies relocate production from one affected region to another or if impacts propagate along supply chains. The current literature indicates that trade plays a substantial role in propagating spillover effects 43 , 44 , making their assessment at the sub-national level challenging without available data on sub-national trade dependencies. Studies accounting for only spatially adjacent neighbours indicate that negative impacts in one region induce further negative impacts in neighbouring regions 45 , 46 , 47 , 48 , suggesting that our projected damages are probably conservative by excluding these effects. In Supplementary Fig. 14 , we assess spillovers from neighbouring regions using a spatial-lag model. For simplicity, this analysis excludes temporal lags, focusing only on contemporaneous effects. The results show that accounting for spatial spillovers can amplify the overall magnitude, and also the heterogeneity, of impacts. Consistent with previous literature, this indicates that the overall magnitude (Fig. 1 ) and heterogeneity (Fig. 3 ) of damages that we project in our main specification may be conservative without explicitly accounting for spillovers. We note that further analysis that addresses both spatially and trade-connected spillovers, while also accounting for delayed impacts using temporal lags, would be necessary to adequately address this question fully. These approaches offer fruitful avenues for further research but are beyond the scope of this manuscript, which primarily aims to explore the impacts of different climate conditions and their persistence.
Policy implications
We find that the economic damages resulting from climate change until 2049 are those to which the world economy is already committed and that these greatly outweigh the costs required to mitigate emissions in line with the 2 °C target of the Paris Climate Agreement (Fig. 1 ). This assessment is complementary to formal analyses of the net costs and benefits associated with moving from one emission path to another, which typically find that net benefits of mitigation only emerge in the second half of the century 5 . Our simple comparison of the magnitude of damages and mitigation costs makes clear that this is primarily because damages are indistinguishable across emissions scenarios—that is, committed—until mid-century (Fig. 1 ) and that they are actually already much larger than mitigation costs. For simplicity, and owing to the availability of data, we compare damages to mitigation costs at the global level. Regional estimates of mitigation costs may shed further light on the national incentives for mitigation to which our results already hint, of relevance for international climate policy. Although these damages are committed from a mitigation perspective, adaptation may provide an opportunity to reduce them. Moreover, the strong divergence of damages after mid-century reemphasizes the clear benefits of mitigation from a purely economic perspective, as highlighted in previous studies 1 , 4 , 6 , 24 .
Historical climate data
Historical daily 2-m temperature and precipitation totals (in mm) are obtained for the period 1979–2019 from the W5E5 database. The W5E5 dataset comes from ERA-5, a state-of-the-art reanalysis of historical observations, but has been bias-adjusted by applying version 2.0 of the WATCH Forcing Data to ERA-5 reanalysis data and precipitation data from version 2.3 of the Global Precipitation Climatology Project to better reflect ground-based measurements 49 , 50 , 51 . We obtain these data on a 0.5° × 0.5° grid from the Inter-Sectoral Impact Model Intercomparison Project (ISIMIP) database. Notably, these historical data have been used to bias-adjust future climate projections from CMIP-6 (see the following section), ensuring consistency between the distribution of historical daily weather on which our empirical models were estimated and the climate projections used to estimate future damages. These data are publicly available from the ISIMIP database. See refs. 7 , 8 for robustness tests of the empirical models to the choice of climate data reanalysis products.
Future climate data
Daily 2-m temperature and precipitation totals (in mm) are taken from 21 climate models participating in CMIP-6 under a high (RCP8.5) and a low (RCP2.6) greenhouse gas emission scenario from 2015 to 2100. The data have been bias-adjusted and statistically downscaled to a common half-degree grid to reflect the historical distribution of daily temperature and precipitation of the W5E5 dataset using the trend-preserving method developed by the ISIMIP 50 , 52 . As such, the climate model data reproduce observed climatological patterns exceptionally well (Supplementary Table 5 ). Gridded data are publicly available from the ISIMIP database.
Historical economic data
Historical economic data come from the DOSE database of sub-national economic output 53 . We use a recent revision to the DOSE dataset that provides data across 83 countries, 1,660 sub-national regions with varying temporal coverage from 1960 to 2019. Sub-national units constitute the first administrative division below national, for example, states for the USA and provinces for China. Data come from measures of gross regional product per capita (GRPpc) or income per capita in local currencies, reflecting the values reported in national statistical agencies, yearbooks and, in some cases, academic literature. We follow previous literature 3 , 7 , 8 , 54 and assess real sub-national output per capita by first converting values from local currencies to US dollars to account for diverging national inflationary tendencies and then account for US inflation using a US deflator. Alternatively, one might first account for national inflation and then convert between currencies. Supplementary Fig. 12 demonstrates that our conclusions are consistent when accounting for price changes in the reversed order, although the magnitude of estimated damages varies. See the documentation of the DOSE dataset for further discussion of these choices. Conversions between currencies are conducted using exchange rates from the FRED database of the Federal Reserve Bank of St. Louis 55 and the national deflators from the World Bank 56 .
Future socio-economic data
Baseline gridded gross domestic product (GDP) and population data for the period 2015–2100 are taken from the middle-of-the-road scenario SSP2 (ref. 15 ). Population data have been downscaled to a half-degree grid by the ISIMIP following the methodologies of refs. 57 , 58 , which we then aggregate to the sub-national level of our economic data using the spatial aggregation procedure described below. Because current methodologies for downscaling the GDP of the SSPs use downscaled population to do so, per-capita estimates of GDP with a realistic distribution at the sub-national level are not readily available for the SSPs. We therefore use national-level GDP per capita (GDPpc) projections for all sub-national regions of a given country, assuming homogeneity within countries in terms of baseline GDPpc. Here we use projections that have been updated to account for the impact of the COVID-19 pandemic on the trajectory of future income, while remaining consistent with the long-term development of the SSPs 59 . The choice of baseline SSP alters the magnitude of projected climate damages in monetary terms, but when assessed in terms of percentage change from the baseline, the choice of socio-economic scenario is inconsequential. Gridded SSP population data and national-level GDPpc data are publicly available from the ISIMIP database. Sub-national estimates as used in this study are available in the code and data replication files.
Climate variables
Following recent literature 3 , 7 , 8 , we calculate an array of climate variables for which substantial impacts on macroeconomic output have been identified empirically, supported by further evidence at the micro level for plausible underlying mechanisms. See refs. 7 , 8 for an extensive motivation for the use of these particular climate variables and for detailed empirical tests on the nature and robustness of their effects on economic output. To summarize, these studies have found evidence for independent impacts on economic growth rates from annual average temperature, daily temperature variability, total annual precipitation, the annual number of wet days and extreme daily rainfall. Assessments of daily temperature variability were motivated by evidence of impacts on agricultural output and human health, as well as macroeconomic literature on the impacts of volatility on growth when manifest in different dimensions, such as government spending, exchange rates and even output itself 7 . Assessments of precipitation impacts were motivated by evidence of impacts on agricultural productivity, metropolitan labour outcomes and conflict, as well as damages caused by flash flooding 8 . See Extended Data Table 1 for detailed references to empirical studies of these physical mechanisms. Marked impacts of daily temperature variability, total annual precipitation, the number of wet days and extreme daily rainfall on macroeconomic output were identified robustly across different climate datasets, spatial aggregation schemes, specifications of regional time trends and error-clustering approaches. They were also found to be robust to the consideration of temperature extremes 7 , 8 . Furthermore, these climate variables were identified as having independent effects on economic output 7 , 8 , which we further explain here using Monte Carlo simulations to demonstrate the robustness of the results to concerns of imperfect multicollinearity between climate variables (Supplementary Methods Section 2 ), as well as by using information criteria (Supplementary Table 1 ) to demonstrate that including several lagged climate variables provides a preferable trade-off between optimally describing the data and limiting the possibility of overfitting.
We calculate these variables from the distribution of daily, d , temperature, T x , d , and precipitation, P x , d , at the grid-cell, x , level for both the historical and future climate data. As well as annual mean temperature, \({\bar{T}}_{x,y}\) , and annual total precipitation, P x , y , we calculate annual, y , measures of daily temperature variability, \({\widetilde{T}}_{x,y}\) :
the number of wet days, Pwd x , y :
and extreme daily rainfall:
in which T x , d , m , y is the grid-cell-specific daily temperature in month m and year y , \({\bar{T}}_{x,m,{y}}\) is the year and grid-cell-specific monthly, m , mean temperature, D m and D y the number of days in a given month m or year y , respectively, H the Heaviside step function, 1 mm the threshold used to define wet days and P 99.9 x is the 99.9th percentile of historical (1979–2019) daily precipitation at the grid-cell level. Units of the climate measures are degrees Celsius for annual mean temperature and daily temperature variability, millimetres for total annual precipitation and extreme daily precipitation, and simply the number of days for the annual number of wet days.
We also calculated weighted standard deviations of monthly rainfall totals as also used in ref. 8 but do not include them in our projections as we find that, when accounting for delayed effects, their effect becomes statistically indistinct and is better captured by changes in total annual rainfall.
Spatial aggregation
We aggregate grid-cell-level historical and future climate measures, as well as grid-cell-level future GDPpc and population, to the level of the first administrative unit below national level of the GADM database, using an area-weighting algorithm that estimates the portion of each grid cell falling within an administrative boundary. We use this as our baseline specification following previous findings that the effect of area or population weighting at the sub-national level is negligible 7 , 8 .
Empirical model specification: fixed-effects distributed lag models
Following a wide range of climate econometric literature 16 , 60 , we use panel regression models with a selection of fixed effects and time trends to isolate plausibly exogenous variation with which to maximize confidence in a causal interpretation of the effects of climate on economic growth rates. The use of region fixed effects, μ r , accounts for unobserved time-invariant differences between regions, such as prevailing climatic norms and growth rates owing to historical and geopolitical factors. The use of yearly fixed effects, η y , accounts for regionally invariant annual shocks to the global climate or economy such as the El Niño–Southern Oscillation or global recessions. In our baseline specification, we also include region-specific linear time trends, k r y , to exclude the possibility of spurious correlations resulting from common slow-moving trends in climate and growth.
The persistence of climate impacts on economic growth rates is a key determinant of the long-term magnitude of damages. Methods for inferring the extent of persistence in impacts on growth rates have typically used lagged climate variables to evaluate the presence of delayed effects or catch-up dynamics 2 , 18 . For example, consider starting from a model in which a climate condition, C r , y , (for example, annual mean temperature) affects the growth rate, Δlgrp r , y (the first difference of the logarithm of gross regional product) of region r in year y :
which we refer to as a ‘pure growth effects’ model in the main text. Typically, further lags are included,
and the cumulative effect of all lagged terms is evaluated to assess the extent to which climate impacts on growth rates persist. Following ref. 18 , in the case that,
the implication is that impacts on the growth rate persist up to NL years after the initial shock (possibly to a weaker or a stronger extent), whereas if
then the initial impact on the growth rate is recovered after NL years and the effect is only one on the level of output. However, we note that such approaches are limited by the fact that, when including an insufficient number of lags to detect a recovery of the growth rates, one may find equation ( 6 ) to be satisfied and incorrectly assume that a change in climatic conditions affects the growth rate indefinitely. In practice, given a limited record of historical data, including too few lags to confidently conclude in an infinitely persistent impact on the growth rate is likely, particularly over the long timescales over which future climate damages are often projected 2 , 24 . To avoid this issue, we instead begin our analysis with a model for which the level of output, lgrp r , y , depends on the level of a climate variable, C r , y :
Given the non-stationarity of the level of output, we follow the literature 19 and estimate such an equation in first-differenced form as,
which we refer to as a model of ‘pure level effects’ in the main text. This model constitutes a baseline specification in which a permanent change in the climate variable produces an instantaneous impact on the growth rate and a permanent effect only on the level of output. By including lagged variables in this specification,
we are able to test whether the impacts on the growth rate persist any further than instantaneously by evaluating whether α L > 0 are statistically significantly different from zero. Even though this framework is also limited by the possibility of including too few lags, the choice of a baseline model specification in which impacts on the growth rate do not persist means that, in the case of including too few lags, the framework reverts to the baseline specification of level effects. As such, this framework is conservative with respect to the persistence of impacts and the magnitude of future damages. It naturally avoids assumptions of infinite persistence and we are able to interpret any persistence that we identify with equation ( 9 ) as a lower bound on the extent of climate impact persistence on growth rates. See the main text for further discussion of this specification choice, in particular about its conservative nature compared with previous literature estimates, such as refs. 2 , 18 .
We allow the response to climatic changes to vary across regions, using interactions of the climate variables with historical average (1979–2019) climatic conditions reflecting heterogenous effects identified in previous work 7 , 8 . Following this previous work, the moderating variables of these interaction terms constitute the historical average of either the variable itself or of the seasonal temperature difference, \({\hat{T}}_{r}\) , or annual mean temperature, \({\bar{T}}_{r}\) , in the case of daily temperature variability 7 and extreme daily rainfall, respectively 8 .
The resulting regression equation with N and M lagged variables, respectively, reads:
in which Δlgrp r , y is the annual, regional GRPpc growth rate, measured as the first difference of the logarithm of real GRPpc, following previous work 2 , 3 , 7 , 8 , 18 , 19 . Fixed-effects regressions were run using the fixest package in R (ref. 61 ).
Estimates of the coefficients of interest α i , L are shown in Extended Data Fig. 1 for N = M = 10 lags and for our preferred choice of the number of lags in Supplementary Figs. 1 – 3 . In Extended Data Fig. 1 , errors are shown clustered at the regional level, but for the construction of damage projections, we block-bootstrap the regressions by region 1,000 times to provide a range of parameter estimates with which to sample the projection uncertainty (following refs. 2 , 31 ).
Spatial-lag model
In Supplementary Fig. 14 , we present the results from a spatial-lag model that explores the potential for climate impacts to ‘spill over’ into spatially neighbouring regions. We measure the distance between centroids of each pair of sub-national regions and construct spatial lags that take the average of the first-differenced climate variables and their interaction terms over neighbouring regions that are at distances of 0–500, 500–1,000, 1,000–1,500 and 1,500–2000 km (spatial lags, ‘SL’, 1 to 4). For simplicity, we then assess a spatial-lag model without temporal lags to assess spatial spillovers of contemporaneous climate impacts. This model takes the form:
in which SL indicates the spatial lag of each climate variable and interaction term. In Supplementary Fig. 14 , we plot the cumulative marginal effect of each climate variable at different baseline climate conditions by summing the coefficients for each climate variable and interaction term, for example, for average temperature impacts as:
These cumulative marginal effects can be regarded as the overall spatially dependent impact to an individual region given a one-unit shock to a climate variable in that region and all neighbouring regions at a given value of the moderating variable of the interaction term.
Constructing projections of economic damage from future climate change
We construct projections of future climate damages by applying the coefficients estimated in equation ( 10 ) and shown in Supplementary Tables 2 – 4 (when including only lags with statistically significant effects in specifications that limit overfitting; see Supplementary Methods Section 1 ) to projections of future climate change from the CMIP-6 models. Year-on-year changes in each primary climate variable of interest are calculated to reflect the year-to-year variations used in the empirical models. 30-year moving averages of the moderating variables of the interaction terms are calculated to reflect the long-term average of climatic conditions that were used for the moderating variables in the empirical models. By using moving averages in the projections, we account for the changing vulnerability to climate shocks based on the evolving long-term conditions (Supplementary Figs. 10 and 11 show that the results are robust to the precise choice of the window of this moving average). Although these climate variables are not differenced, the fact that the bias-adjusted climate models reproduce observed climatological patterns across regions for these moderating variables very accurately (Supplementary Table 6 ) with limited spread across models (<3%) precludes the possibility that any considerable bias or uncertainty is introduced by this methodological choice. However, we impose caps on these moderating variables at the 95th percentile at which they were observed in the historical data to prevent extrapolation of the marginal effects outside the range in which the regressions were estimated. This is a conservative choice that limits the magnitude of our damage projections.
Time series of primary climate variables and moderating climate variables are then combined with estimates of the empirical model parameters to evaluate the regression coefficients in equation ( 10 ), producing a time series of annual GRPpc growth-rate reductions for a given emission scenario, climate model and set of empirical model parameters. The resulting time series of growth-rate impacts reflects those occurring owing to future climate change. By contrast, a future scenario with no climate change would be one in which climate variables do not change (other than with random year-to-year fluctuations) and hence the time-averaged evaluation of equation ( 10 ) would be zero. Our approach therefore implicitly compares the future climate-change scenario to this no-climate-change baseline scenario.
The time series of growth-rate impacts owing to future climate change in region r and year y , δ r , y , are then added to the future baseline growth rates, π r , y (in log-diff form), obtained from the SSP2 scenario to yield trajectories of damaged GRPpc growth rates, ρ r , y . These trajectories are aggregated over time to estimate the future trajectory of GRPpc with future climate impacts:
in which GRPpc r , y =2020 is the initial log level of GRPpc. We begin damage estimates in 2020 to reflect the damages occurring since the end of the period for which we estimate the empirical models (1979–2019) and to match the timing of mitigation-cost estimates from most IAMs (see below).
For each emission scenario, this procedure is repeated 1,000 times while randomly sampling from the selection of climate models, the selection of empirical models with different numbers of lags (shown in Supplementary Figs. 1 – 3 and Supplementary Tables 2 – 4 ) and bootstrapped estimates of the regression parameters. The result is an ensemble of future GRPpc trajectories that reflect uncertainty from both physical climate change and the structural and sampling uncertainty of the empirical models.
Estimates of mitigation costs
We obtain IPCC estimates of the aggregate costs of emission mitigation from the AR6 Scenario Explorer and Database hosted by IIASA 23 . Specifically, we search the AR6 Scenarios Database World v1.1 for IAMs that provided estimates of global GDP and population under both a SSP2 baseline and a SSP2-RCP2.6 scenario to maintain consistency with the socio-economic and emission scenarios of the climate damage projections. We find five IAMs that provide data for these scenarios, namely, MESSAGE-GLOBIOM 1.0, REMIND-MAgPIE 1.5, AIM/GCE 2.0, GCAM 4.2 and WITCH-GLOBIOM 3.1. Of these five IAMs, we use the results only from the first three that passed the IPCC vetting procedure for reproducing historical emission and climate trajectories. We then estimate global mitigation costs as the percentage difference in global per capita GDP between the SSP2 baseline and the SSP2-RCP2.6 emission scenario. In the case of one of these IAMs, estimates of mitigation costs begin in 2020, whereas in the case of two others, mitigation costs begin in 2010. The mitigation cost estimates before 2020 in these two IAMs are mostly negligible, and our choice to begin comparison with damage estimates in 2020 is conservative with respect to the relative weight of climate damages compared with mitigation costs for these two IAMs.
Data availability
Data on economic production and ERA-5 climate data are publicly available at https://doi.org/10.5281/zenodo.4681306 (ref. 62 ) and https://www.ecmwf.int/en/forecasts/datasets/reanalysis-datasets/era5 , respectively. Data on mitigation costs are publicly available at https://data.ene.iiasa.ac.at/ar6/#/downloads . Processed climate and economic data, as well as all other necessary data for reproduction of the results, are available at the public repository https://doi.org/10.5281/zenodo.10562951 (ref. 63 ).
Code availability
All code necessary for reproduction of the results is available at the public repository https://doi.org/10.5281/zenodo.10562951 (ref. 63 ).
Glanemann, N., Willner, S. N. & Levermann, A. Paris Climate Agreement passes the cost-benefit test. Nat. Commun. 11 , 110 (2020).
Article ADS CAS PubMed PubMed Central Google Scholar
Burke, M., Hsiang, S. M. & Miguel, E. Global non-linear effect of temperature on economic production. Nature 527 , 235–239 (2015).
Article ADS CAS PubMed Google Scholar
Kalkuhl, M. & Wenz, L. The impact of climate conditions on economic production. Evidence from a global panel of regions. J. Environ. Econ. Manag. 103 , 102360 (2020).
Article Google Scholar
Moore, F. C. & Diaz, D. B. Temperature impacts on economic growth warrant stringent mitigation policy. Nat. Clim. Change 5 , 127–131 (2015).
Article ADS Google Scholar
Drouet, L., Bosetti, V. & Tavoni, M. Net economic benefits of well-below 2°C scenarios and associated uncertainties. Oxf. Open Clim. Change 2 , kgac003 (2022).
Ueckerdt, F. et al. The economically optimal warming limit of the planet. Earth Syst. Dyn. 10 , 741–763 (2019).
Kotz, M., Wenz, L., Stechemesser, A., Kalkuhl, M. & Levermann, A. Day-to-day temperature variability reduces economic growth. Nat. Clim. Change 11 , 319–325 (2021).
Kotz, M., Levermann, A. & Wenz, L. The effect of rainfall changes on economic production. Nature 601 , 223–227 (2022).
Kousky, C. Informing climate adaptation: a review of the economic costs of natural disasters. Energy Econ. 46 , 576–592 (2014).
Harlan, S. L. et al. in Climate Change and Society: Sociological Perspectives (eds Dunlap, R. E. & Brulle, R. J.) 127–163 (Oxford Univ. Press, 2015).
Bolton, P. et al. The Green Swan (BIS Books, 2020).
Alogoskoufis, S. et al. ECB Economy-wide Climate Stress Test: Methodology and Results European Central Bank, 2021).
Weber, E. U. What shapes perceptions of climate change? Wiley Interdiscip. Rev. Clim. Change 1 , 332–342 (2010).
Markowitz, E. M. & Shariff, A. F. Climate change and moral judgement. Nat. Clim. Change 2 , 243–247 (2012).
Riahi, K. et al. The shared socioeconomic pathways and their energy, land use, and greenhouse gas emissions implications: an overview. Glob. Environ. Change 42 , 153–168 (2017).
Auffhammer, M., Hsiang, S. M., Schlenker, W. & Sobel, A. Using weather data and climate model output in economic analyses of climate change. Rev. Environ. Econ. Policy 7 , 181–198 (2013).
Kolstad, C. D. & Moore, F. C. Estimating the economic impacts of climate change using weather observations. Rev. Environ. Econ. Policy 14 , 1–24 (2020).
Dell, M., Jones, B. F. & Olken, B. A. Temperature shocks and economic growth: evidence from the last half century. Am. Econ. J. Macroecon. 4 , 66–95 (2012).
Newell, R. G., Prest, B. C. & Sexton, S. E. The GDP-temperature relationship: implications for climate change damages. J. Environ. Econ. Manag. 108 , 102445 (2021).
Kikstra, J. S. et al. The social cost of carbon dioxide under climate-economy feedbacks and temperature variability. Environ. Res. Lett. 16 , 094037 (2021).
Article ADS CAS Google Scholar
Bastien-Olvera, B. & Moore, F. Persistent effect of temperature on GDP identified from lower frequency temperature variability. Environ. Res. Lett. 17 , 084038 (2022).
Eyring, V. et al. Overview of the Coupled Model Intercomparison Project Phase 6 (CMIP6) experimental design and organization. Geosci. Model Dev. 9 , 1937–1958 (2016).
Byers, E. et al. AR6 scenarios database. Zenodo https://zenodo.org/records/7197970 (2022).
Burke, M., Davis, W. M. & Diffenbaugh, N. S. Large potential reduction in economic damages under UN mitigation targets. Nature 557 , 549–553 (2018).
Kotz, M., Wenz, L. & Levermann, A. Footprint of greenhouse forcing in daily temperature variability. Proc. Natl Acad. Sci. 118 , e2103294118 (2021).
Article CAS PubMed PubMed Central Google Scholar
Myhre, G. et al. Frequency of extreme precipitation increases extensively with event rareness under global warming. Sci. Rep. 9 , 16063 (2019).
Min, S.-K., Zhang, X., Zwiers, F. W. & Hegerl, G. C. Human contribution to more-intense precipitation extremes. Nature 470 , 378–381 (2011).
England, M. R., Eisenman, I., Lutsko, N. J. & Wagner, T. J. The recent emergence of Arctic Amplification. Geophys. Res. Lett. 48 , e2021GL094086 (2021).
Fischer, E. M. & Knutti, R. Anthropogenic contribution to global occurrence of heavy-precipitation and high-temperature extremes. Nat. Clim. Change 5 , 560–564 (2015).
Pfahl, S., O’Gorman, P. A. & Fischer, E. M. Understanding the regional pattern of projected future changes in extreme precipitation. Nat. Clim. Change 7 , 423–427 (2017).
Callahan, C. W. & Mankin, J. S. Globally unequal effect of extreme heat on economic growth. Sci. Adv. 8 , eadd3726 (2022).
Diffenbaugh, N. S. & Burke, M. Global warming has increased global economic inequality. Proc. Natl Acad. Sci. 116 , 9808–9813 (2019).
Callahan, C. W. & Mankin, J. S. National attribution of historical climate damages. Clim. Change 172 , 40 (2022).
Burke, M. & Tanutama, V. Climatic constraints on aggregate economic output. National Bureau of Economic Research, Working Paper 25779. https://doi.org/10.3386/w25779 (2019).
Kahn, M. E. et al. Long-term macroeconomic effects of climate change: a cross-country analysis. Energy Econ. 104 , 105624 (2021).
Desmet, K. et al. Evaluating the economic cost of coastal flooding. National Bureau of Economic Research, Working Paper 24918. https://doi.org/10.3386/w24918 (2018).
Hsiang, S. M. & Jina, A. S. The causal effect of environmental catastrophe on long-run economic growth: evidence from 6,700 cyclones. National Bureau of Economic Research, Working Paper 20352. https://doi.org/10.3386/w2035 (2014).
Ritchie, P. D. et al. Shifts in national land use and food production in Great Britain after a climate tipping point. Nat. Food 1 , 76–83 (2020).
Dietz, S., Rising, J., Stoerk, T. & Wagner, G. Economic impacts of tipping points in the climate system. Proc. Natl Acad. Sci. 118 , e2103081118 (2021).
Bastien-Olvera, B. A. & Moore, F. C. Use and non-use value of nature and the social cost of carbon. Nat. Sustain. 4 , 101–108 (2021).
Carleton, T. et al. Valuing the global mortality consequences of climate change accounting for adaptation costs and benefits. Q. J. Econ. 137 , 2037–2105 (2022).
Bastien-Olvera, B. A. et al. Unequal climate impacts on global values of natural capital. Nature 625 , 722–727 (2024).
Malik, A. et al. Impacts of climate change and extreme weather on food supply chains cascade across sectors and regions in Australia. Nat. Food 3 , 631–643 (2022).
Article ADS PubMed Google Scholar
Kuhla, K., Willner, S. N., Otto, C., Geiger, T. & Levermann, A. Ripple resonance amplifies economic welfare loss from weather extremes. Environ. Res. Lett. 16 , 114010 (2021).
Schleypen, J. R., Mistry, M. N., Saeed, F. & Dasgupta, S. Sharing the burden: quantifying climate change spillovers in the European Union under the Paris Agreement. Spat. Econ. Anal. 17 , 67–82 (2022).
Dasgupta, S., Bosello, F., De Cian, E. & Mistry, M. Global temperature effects on economic activity and equity: a spatial analysis. European Institute on Economics and the Environment, Working Paper 22-1 (2022).
Neal, T. The importance of external weather effects in projecting the macroeconomic impacts of climate change. UNSW Economics Working Paper 2023-09 (2023).
Deryugina, T. & Hsiang, S. M. Does the environment still matter? Daily temperature and income in the United States. National Bureau of Economic Research, Working Paper 20750. https://doi.org/10.3386/w20750 (2014).
Hersbach, H. et al. The ERA5 global reanalysis. Q. J. R. Meteorol. Soc. 146 , 1999–2049 (2020).
Cucchi, M. et al. WFDE5: bias-adjusted ERA5 reanalysis data for impact studies. Earth Syst. Sci. Data 12 , 2097–2120 (2020).
Adler, R. et al. The New Version 2.3 of the Global Precipitation Climatology Project (GPCP) Monthly Analysis Product 1072–1084 (University of Maryland, 2016).
Lange, S. Trend-preserving bias adjustment and statistical downscaling with ISIMIP3BASD (v1.0). Geosci. Model Dev. 12 , 3055–3070 (2019).
Wenz, L., Carr, R. D., Kögel, N., Kotz, M. & Kalkuhl, M. DOSE – global data set of reported sub-national economic output. Sci. Data 10 , 425 (2023).
Article PubMed PubMed Central Google Scholar
Gennaioli, N., La Porta, R., Lopez De Silanes, F. & Shleifer, A. Growth in regions. J. Econ. Growth 19 , 259–309 (2014).
Board of Governors of the Federal Reserve System (US). U.S. dollars to euro spot exchange rate. https://fred.stlouisfed.org/series/AEXUSEU (2022).
World Bank. GDP deflator. https://data.worldbank.org/indicator/NY.GDP.DEFL.ZS (2022).
Jones, B. & O’Neill, B. C. Spatially explicit global population scenarios consistent with the Shared Socioeconomic Pathways. Environ. Res. Lett. 11 , 084003 (2016).
Murakami, D. & Yamagata, Y. Estimation of gridded population and GDP scenarios with spatially explicit statistical downscaling. Sustainability 11 , 2106 (2019).
Koch, J. & Leimbach, M. Update of SSP GDP projections: capturing recent changes in national accounting, PPP conversion and Covid 19 impacts. Ecol. Econ. 206 (2023).
Carleton, T. A. & Hsiang, S. M. Social and economic impacts of climate. Science 353 , aad9837 (2016).
Article PubMed Google Scholar
Bergé, L. Efficient estimation of maximum likelihood models with multiple fixed-effects: the R package FENmlm. DEM Discussion Paper Series 18-13 (2018).
Kalkuhl, M., Kotz, M. & Wenz, L. DOSE - The MCC-PIK Database Of Subnational Economic output. Zenodo https://zenodo.org/doi/10.5281/zenodo.4681305 (2021).
Kotz, M., Wenz, L. & Levermann, A. Data and code for “The economic commitment of climate change”. Zenodo https://zenodo.org/doi/10.5281/zenodo.10562951 (2024).
Dasgupta, S. et al. Effects of climate change on combined labour productivity and supply: an empirical, multi-model study. Lancet Planet. Health 5 , e455–e465 (2021).
Lobell, D. B. et al. The critical role of extreme heat for maize production in the United States. Nat. Clim. Change 3 , 497–501 (2013).
Zhao, C. et al. Temperature increase reduces global yields of major crops in four independent estimates. Proc. Natl Acad. Sci. 114 , 9326–9331 (2017).
Wheeler, T. R., Craufurd, P. Q., Ellis, R. H., Porter, J. R. & Prasad, P. V. Temperature variability and the yield of annual crops. Agric. Ecosyst. Environ. 82 , 159–167 (2000).
Rowhani, P., Lobell, D. B., Linderman, M. & Ramankutty, N. Climate variability and crop production in Tanzania. Agric. For. Meteorol. 151 , 449–460 (2011).
Ceglar, A., Toreti, A., Lecerf, R., Van der Velde, M. & Dentener, F. Impact of meteorological drivers on regional inter-annual crop yield variability in France. Agric. For. Meteorol. 216 , 58–67 (2016).
Shi, L., Kloog, I., Zanobetti, A., Liu, P. & Schwartz, J. D. Impacts of temperature and its variability on mortality in New England. Nat. Clim. Change 5 , 988–991 (2015).
Xue, T., Zhu, T., Zheng, Y. & Zhang, Q. Declines in mental health associated with air pollution and temperature variability in China. Nat. Commun. 10 , 2165 (2019).
Article ADS PubMed PubMed Central Google Scholar
Liang, X.-Z. et al. Determining climate effects on US total agricultural productivity. Proc. Natl Acad. Sci. 114 , E2285–E2292 (2017).
Desbureaux, S. & Rodella, A.-S. Drought in the city: the economic impact of water scarcity in Latin American metropolitan areas. World Dev. 114 , 13–27 (2019).
Damania, R. The economics of water scarcity and variability. Oxf. Rev. Econ. Policy 36 , 24–44 (2020).
Davenport, F. V., Burke, M. & Diffenbaugh, N. S. Contribution of historical precipitation change to US flood damages. Proc. Natl Acad. Sci. 118 , e2017524118 (2021).
Dave, R., Subramanian, S. S. & Bhatia, U. Extreme precipitation induced concurrent events trigger prolonged disruptions in regional road networks. Environ. Res. Lett. 16 , 104050 (2021).
Download references
Acknowledgements
We gratefully acknowledge financing from the Volkswagen Foundation and the Deutsche Gesellschaft für Internationale Zusammenarbeit (GIZ) GmbH on behalf of the Government of the Federal Republic of Germany and Federal Ministry for Economic Cooperation and Development (BMZ).
Open access funding provided by Potsdam-Institut für Klimafolgenforschung (PIK) e.V.
Author information
Authors and affiliations.
Research Domain IV, Research Domain IV, Potsdam Institute for Climate Impact Research, Potsdam, Germany
Maximilian Kotz, Anders Levermann & Leonie Wenz
Institute of Physics, Potsdam University, Potsdam, Germany
Maximilian Kotz & Anders Levermann
Mercator Research Institute on Global Commons and Climate Change, Berlin, Germany
Leonie Wenz
You can also search for this author in PubMed Google Scholar
Contributions
All authors contributed to the design of the analysis. M.K. conducted the analysis and produced the figures. All authors contributed to the interpretation and presentation of the results. M.K. and L.W. wrote the manuscript.
Corresponding author
Correspondence to Leonie Wenz .
Ethics declarations
Competing interests.
The authors declare no competing interests.
Peer review
Peer review information.
Nature thanks Xin-Zhong Liang, Chad Thackeray and the other, anonymous, reviewer(s) for their contribution to the peer review of this work. Peer reviewer reports are available.
Additional information
Publisher’s note Springer Nature remains neutral with regard to jurisdictional claims in published maps and institutional affiliations.
Extended data figures and tables
Extended data fig. 1 constraining the persistence of historical climate impacts on economic growth rates..
The results of a panel-based fixed-effects distributed lag model for the effects of annual mean temperature ( a ), daily temperature variability ( b ), total annual precipitation ( c ), the number of wet days ( d ) and extreme daily precipitation ( e ) on sub-national economic growth rates. Point estimates show the effects of a 1 °C or one standard deviation increase (for temperature and precipitation variables, respectively) at the lower quartile, median and upper quartile of the relevant moderating variable (green, orange and purple, respectively) at different lagged periods after the initial shock (note that these are not cumulative effects). Climate variables are used in their first-differenced form (see main text for discussion) and the moderating climate variables are the annual mean temperature, seasonal temperature difference, total annual precipitation, number of wet days and annual mean temperature, respectively, in panels a – e (see Methods for further discussion). Error bars show the 95% confidence intervals having clustered standard errors by region. The within-region R 2 , Bayesian and Akaike information criteria for the model are shown at the top of the figure. This figure shows results with ten lags for each variable to demonstrate the observed levels of persistence, but our preferred specifications remove later lags based on the statistical significance of terms shown above and the information criteria shown in Extended Data Fig. 2 . The resulting models without later lags are shown in Supplementary Figs. 1 – 3 .
Extended Data Fig. 2 Incremental lag-selection procedure using information criteria and within-region R 2 .
Starting from a panel-based fixed-effects distributed lag model estimating the effects of climate on economic growth using the real historical data (as in equation ( 4 )) with ten lags for all climate variables (as shown in Extended Data Fig. 1 ), lags are incrementally removed for one climate variable at a time. The resulting Bayesian and Akaike information criteria are shown in a – e and f – j , respectively, and the within-region R 2 and number of observations in k – o and p – t , respectively. Different rows show the results when removing lags from different climate variables, ordered from top to bottom as annual mean temperature, daily temperature variability, total annual precipitation, the number of wet days and extreme annual precipitation. Information criteria show minima at approximately four lags for precipitation variables and ten to eight for temperature variables, indicating that including these numbers of lags does not lead to overfitting. See Supplementary Table 1 for an assessment using information criteria to determine whether including further climate variables causes overfitting.
Extended Data Fig. 3 Damages in our preferred specification that provides a robust lower bound on the persistence of climate impacts on economic growth versus damages in specifications of pure growth or pure level effects.
Estimates of future damages as shown in Fig. 1 but under the emission scenario RCP8.5 for three separate empirical specifications: in orange our preferred specification, which provides an empirical lower bound on the persistence of climate impacts on economic growth rates while avoiding assumptions of infinite persistence (see main text for further discussion); in purple a specification of ‘pure growth effects’ in which the first difference of climate variables is not taken and no lagged climate variables are included (the baseline specification of ref. 2 ); and in pink a specification of ‘pure level effects’ in which the first difference of climate variables is taken but no lagged terms are included.
Extended Data Fig. 4 Climate changes in different variables as a function of historical interannual variability.
Changes in each climate variable of interest from 1979–2019 to 2035–2065 under the high-emission scenario SSP5-RCP8.5, expressed as a percentage of the historical variability of each measure. Historical variability is estimated as the standard deviation of each detrended climate variable over the period 1979–2019 during which the empirical models were identified (detrending is appropriate because of the inclusion of region-specific linear time trends in the empirical models). See Supplementary Fig. 13 for changes expressed in standard units. Data on national administrative boundaries are obtained from the GADM database version 3.6 and are freely available for academic use ( https://gadm.org/ ).
Extended Data Fig. 5 Contribution of different climate variables to overall committed damages.
a , Climate damages in 2049 when using empirical models that account for all climate variables, changes in annual mean temperature only or changes in both annual mean temperature and one other climate variable (daily temperature variability, total annual precipitation, the number of wet days and extreme daily precipitation, respectively). b , The cumulative marginal effects of an increase in annual mean temperature of 1 °C, at different baseline temperatures, estimated from empirical models including all climate variables or annual mean temperature only. Estimates and uncertainty bars represent the median and 95% confidence intervals obtained from 1,000 block-bootstrap resamples from each of three different empirical models using eight, nine or ten lags of temperature terms.
Extended Data Fig. 6 The difference in committed damages between the upper and lower quartiles of countries when ranked by GDP and cumulative historical emissions.
Quartiles are defined using a population weighting, as are the average committed damages across each quartile group. The violin plots indicate the distribution of differences between quartiles across the two extreme emission scenarios (RCP2.6 and RCP8.5) and the uncertainty sampling procedure outlined in Methods , which accounts for uncertainty arising from the choice of lags in the empirical models, uncertainty in the empirical model parameter estimates, as well as the climate model projections. Bars indicate the median, as well as the 10th and 90th percentiles and upper and lower sixths of the distribution reflecting the very likely and likely ranges following the likelihood classification adopted by the IPCC.
Supplementary information
Supplementary information, peer review file, rights and permissions.
Open Access This article is licensed under a Creative Commons Attribution 4.0 International License, which permits use, sharing, adaptation, distribution and reproduction in any medium or format, as long as you give appropriate credit to the original author(s) and the source, provide a link to the Creative Commons licence, and indicate if changes were made. The images or other third party material in this article are included in the article’s Creative Commons licence, unless indicated otherwise in a credit line to the material. If material is not included in the article’s Creative Commons licence and your intended use is not permitted by statutory regulation or exceeds the permitted use, you will need to obtain permission directly from the copyright holder. To view a copy of this licence, visit http://creativecommons.org/licenses/by/4.0/ .
Reprints and permissions
About this article
Cite this article.
Kotz, M., Levermann, A. & Wenz, L. The economic commitment of climate change. Nature 628 , 551–557 (2024). https://doi.org/10.1038/s41586-024-07219-0
Download citation
Received : 25 January 2023
Accepted : 21 February 2024
Published : 17 April 2024
Issue Date : 18 April 2024
DOI : https://doi.org/10.1038/s41586-024-07219-0
Share this article
Anyone you share the following link with will be able to read this content:
Sorry, a shareable link is not currently available for this article.
Provided by the Springer Nature SharedIt content-sharing initiative
By submitting a comment you agree to abide by our Terms and Community Guidelines . If you find something abusive or that does not comply with our terms or guidelines please flag it as inappropriate.
Quick links
- Explore articles by subject
- Guide to authors
- Editorial policies
Sign up for the Nature Briefing newsletter — what matters in science, free to your inbox daily.


COMMENTS
Climate change has become a widespread topic in recent years. This a problem that resulted from the emission of greenhouse gases that affect our environment. ... This paper discusses and compares the factors that contribute to climate change by humans and nature, some effects of climate change, and some solutions that have been developed to ...
Climate change (CC) ... Secondly, to explore issues in this area, propose an outline of research for future work. The third aim of the study is to synthesize the previous literature on climate change, various sectors, and their mitigation measurement. ... Paper presented at the Healthcare [PMC free article]
This paper will discuss urban sustainability in the context of. climate change and address the following research objectives: (1) to examine how. climate change is affecting urban areas; (2) to assess how cities can enhance urban. sustainability by addressing climate change; (3) to discuss resources available for city.
The rapid anthropogenic climate change that is being experienced in the early twenty-first century is intimately entwined with the health and functioning of the biosphere. ... In our introduction we outline the themes, introduce the papers in the thematic issue, and conclude with a synthesis of the main findings of the Forum. ... Research Trend ...
To understand how urgent and durable climate action can be facilitated, we first need to unify the extensive corpus of research on climate action. This is what this. Correspondence: jale.tosun@ipw ...
C ONCLUSION. This document explains that there are well-understood physical mechanisms by which changes in the amounts of greenhouse gases cause climate changes. It discusses the evidence that the concentrations of these gases in the atmosphere have increased and are still increasing rapidly, that climate change is occurring, and that most of ...
Background Climate change is a defining issue and grand challenge for the health sector in North America. Synthesizing evidence on climate change impacts, climate-health adaptation, and climate-health mitigation is crucial for health practitioners and decision-makers to effectively understand, prepare for, and respond to climate change impacts on human health. This protocol paper outlines our ...
4 / x Climate Change 2021: Summary for All x Climate change today Global warming has already caused widespread, rapid and intensifying changes. Some changes are unprecedented in thousands or even millions of years Climate change is more than simply the world getting hotter; we are experiencing widespread changes across the atmosphere, land,
The rapid growth of climate change research presents challenges for IPCC assessments and their stated aim of being comprehensive, objective and transparent. Here the authors use topic modelling to ...
Nature Climate Change is dedicated to publishing the most significant research across the physical and social sciences on the impacts of global climate change and its implications for the economy ...
are affected by the stress caused by climate change (APA, 2020). People say they would do more to reduce their contribution to climate change, but they feel they don't have enough resources or skills to make necessary changes (APA, 2020). To learn to mitigate and adapt to climate change, people need to navigate through these challenges.
This book outlines the impact of climate change in four developing country regions: Africa, Asia, Latin America and small island developing States; the vulnerability of these regions to future climate change; current adaptation plans, strategies and actions; and future adaptation options and needs.
activities in a dedicated climate change module or as part of related topics. We suggest that you use our 'Introduction to Climate Change' presentation in a lesson or assembly before running these activities with your students. Teacher briefings on Considerations When Teaching Climate Change and Climate Justice can be found in the appendix. 3
Climate change could have a devastating effect on human and environmental health. Potential effects of climate change on human health include higher rates of respiratory and heat-related illness, increased prevalence of vector-borne and waterborne diseases, food and water insecurity, and malnutrition. Persons who are elderly, sick, or poor are especially vulnerable to these potential ...
Outline for Climate Change Essay. Here's an example of an outline for climate change essay that you can use in your own. This outline covers the introduction, the main body paragraphs, and finally, the conclusion. We will also give some examples of topics that you can cover in your climate change (or global warming) essay.
Scientists outline a bold solution to climate change, biodiversity loss, social injustice. ScienceDaily . Retrieved May 1, 2024 from www.sciencedaily.com / releases / 2024 / 01 / 240109121104.htm
We outline how neuroscience can be used to: (1) investigate the negative impact of climate change on the human brain; (2) identify ways to adapt; (3) understand the neural substrates of decisions ...
Unlike a school research paper, the author does not set out to argue for or against a particular position, and then devote the majority of effort to finding sources to support the selected position. Instead, the author sets out in good faith to do as much fact-finding as possible, and thus research is likely to present multiple, conflicting ...
Analysis of Warren et al. ( 2018) on a global scale on the effects of climate change on the distribution of insects, vertebrates and plants indicated that even with 2 °C temperature increase, approximately 18% of insects, 16% of plants and 8% of vertebrates species are projected to loose > 50% geographic range; this falls to 6% for insects, 8% ...
Climate change affects crop production by means of direct, indirect, and socio-economic effects as described in Figure 1. Furthermore, climate change (drought, flood, high temperature, storm etc.) events are increased dramatically as reported by Food and Agriculture Organization (FAO) and as shown in Figure 2. Figure 1.
2015. TLDR. Investigation of relationships between Iowa farmers' trust in environmental or agricultural interest groups as sources of climate information, climate change beliefs, perceived climate risks to agriculture, and support for adaptation and mitigation responses indicates that beliefs varied with trust, and beliefs had a significant ...
together to address the health impacts of climate change and health inequities. Through the . NIH Climate . Change and Health Initiative launched in 2022, NIH is taking action to expand research funding and support to address these urgent and complex problems. As the climate continues to change, and weather-related events such as floods,
In this episode, we're starting to think about climate change education, moreover, what is education's role in tackling the climate crisis? As well as the audio below, which you can play from the page, you can listen to this and other episodes by going to the website , Spotify , Apple Podcasts , or Google Podcasts .
Climate change will affect the adoption of residential rooftop solar photovoltaics by changing the patterns of both electricity generation and demand. This research projects that climate change ...
But on the core issues of climate change, he pointed out, the science is largely settled. "A lot of the most basic questions people have about climate change were answered by scientists long ago ...
1 Department of Experimental Psychology, Division of Psychology and Language Sciences, University College London, London, United Kingdom; 2 Department of Leadership and Organisational Behaviour, Norwegian Business School (BI), Oslo, Norway; While much research has examined the correlates of climate change beliefs from an alarmist perspective, less work has systematically measured climate ...
Your report is vital in helping our editors decide if the manuscript meets the journal's criteria for publication, and we ask you to keep the following factors in mind when you write your report ...
With this change, information flow to Britain dried up. This was a major setback to the British and the Canadians who had been collaborating on heavy water production and on several aspects of the research program. Thereafter, Churchill sought information on the cost of building a diffusion plant, a heavy water plant and an atomic reactor in ...
Global projections of macroeconomic climate-change damages typically consider impacts from average annual and national temperatures over long time horizons1-6. Here we use recent empirical ...