
An official website of the United States government
The .gov means it’s official. Federal government websites often end in .gov or .mil. Before sharing sensitive information, make sure you’re on a federal government site.
The site is secure. The https:// ensures that you are connecting to the official website and that any information you provide is encrypted and transmitted securely.
- Publications
- Account settings
Preview improvements coming to the PMC website in October 2024. Learn More or Try it out now .
- Advanced Search
- Journal List


Split-Brain: What We Know Now and Why This is Important for Understanding Consciousness
Edward h. f. de haan.
1 Department of Psychology, University of Amsterdam, Amsterdam, the Netherlands
Paul M. Corballis
2 School of Psychology, University of Auckland, Auckland, New Zealand
Steven A. Hillyard
3 School of Health Sciences, University of California Dan Diego, La Jolla, CA USA
Carlo A. Marzi
4 School of Medicine and Surgery, University of Verona, Verona, Italy
5 Sackler Centre for Consciousness Science, Sussex University, Brighton, UK
Victor A. F. Lamme
6 Klinik für Neurologie, Universitätsklinikum Köln, Kerpener Str, 62 Köln, Germany
7 Dipartimento di Medicina Sperimentale e Clinica, Via Tronto 10/A, 60020 Ancona, Italy
Elizabeth Schechter
8 Department of Philosophy, Washington University, St. Louis, MO USA
9 Department of Philosophy, Monash University, Melbourne, Australia
Michael Corballis
Recently, the discussion regarding the consequences of cutting the corpus callosum (“split-brain”) has regained momentum (Corballis, Corballis, Berlucchi, & Marzi, Brain , 141 (6), e46, 2018 ; Pinto et al., Brain, 140 (5), 1231–1237, 2017a ; Pinto, Lamme, & de Haan, Brain, 140 (11), e68, 2017 ; Volz & Gazzaniga, Brain , 140 (7), 2051–2060, 2017 ; Volz, Hillyard, Miller, & Gazzaniga, Brain , 141 (3), e15, 2018 ). This collective review paper aims to summarize the empirical common ground, to delineate the different interpretations, and to identify the remaining questions. In short, callosotomy leads to a broad breakdown of functional integration ranging from perception to attention. However, the breakdown is not absolute as several processes, such as action control, seem to remain unified. Disagreement exists about the responsible mechanisms for this remaining unity. The main issue concerns the first-person perspective of a split-brain patient. Does a split-brain harbor a split consciousness or is consciousness unified? The current consensus is that the body of evidence is insufficient to answer this question, and different suggestions are made with respect to how future studies might address this paucity. In addition, it is suggested that the answers might not be a simple yes or no but that intermediate conceptualizations need to be considered.
Introduction
The term “split-brain” refers to patients in whom the corpus callosum has been cut for the alleviation of medically intractable epilepsy. Since the earliest reports by van Wagenen and Herren ( 1940 ) and Akelaitis ( 1941 , 1943 ) on the repercussions of a split-brain, two narratives have emerged. First and foremost is the functional description, pioneered by Gazzaniga, Sperry and colleagues (Gazzaniga, Bogen, & Sperry, 1963 ; Gazzaniga, Bogen, & Sperry, 1962 ; Sperry, 1968 ), in which the intricacies, the exceptions, the effects of different testing conditions, and the experimental confounds have been delineated by decades of extensive research with a relatively small group of patients (Berlucchi, Aglioti, Marzi, & Tassinari, 1995 ; Corballis, 1994 ; Corballis et al., 2010 ; Corballis, 2003 ; Luck, Hillyard, Mangun, & Gazzaniga, 1989 ; Pinto, Lamme, & de Haan, 2017b ; Volz, Hillyard, Miller, & Gazzaniga, 2018 ). It is important to note that even in this small group there are differences. In some patients all commissures were severed (“commissurotomy”), in others only the corpus callosum was cut (“callosotomy”) and some patients fall somewhere in between these two boundaries. Now, the search term “split-brain” results in a total of 2848 publications in the database of the Web-of-Science and 29,300 hits on Google Scholar, indicating a wealth of detailed information. The other depiction of split-brain patients entails the first-person perspective of the split-brain. In other words, “what is it like” to be a split-brain patient? It is especially this perspective that has captured the attention of the general press, popular science books and basic textbooks. By its nature, this second narrative lacks the detail of the functional description of the phenomenon, but it captures the intriguing question of how unity of consciousness is related to brain processes. Dominant in this description is the idea that in a split-brain each hemisphere houses an independent conscious agent. This notion, and particularly the concept of an isolated but conscious right hemisphere that is unable to express its feelings, desires or thinking due a lack of language, has captured the imagination (Gazzaniga, 2014 ).
It is important to clarify what we mean by unified consciousness. Here, we use the term in the sense of “subject unity” as defined by Bayne (Bayne & Chalmers, 2003 ; Bayne, 2008 ; Bayne, 2010 ). Subject unity is present if all the experiences generated in a system belong to one subject. In other words, if a system contains a first person perspective, then subject unity is preserved if that system only contains one such perspective, but subject unity is absent if the system contains multiple first person perspectives. Thus, in our definition of conscious unity, consciousness in a split-brain is split if each cortical hemisphere houses an independent conscious agent.
The view that consciousness is split in a split-brain has significantly impacted cognitive neuroscience at large. For instance, currently dominant theories about conscious awareness - the Integrated Information Theory (Tononi, 2005 ; Tononi, 2004 ) and the Global Neuronal Workspace Theory (Dehaene & Naccache, 2001 ; Dehaene, Kerszberg, & Changeux, 1998 ) - may be critically dependent on the validity of this view. Both theories imply that without massive communication between different subsystems, for instance cortical hemispheres, independent conscious agents arise. Thus, if the split consciousness view is invalid, these theories may be critically challenged.
The idea of split consciousness in a split-brain had its origin in the early split-brain studies (Gazzaniga, 1967 ; Gazzaniga, 1975 ; Gazzaniga et al., 1962 ; Sperry, 1968 ). These studies tested patients primarily in the two perceptual domains where processing is largely restricted to the contralateral hemisphere, that is vision and touch. In these early studies, stimuli, for instance objects, that were presented to the left hemisphere either physically in the right hand or as an image in the right visual half-field, could be readily named (as the left hemisphere is dominant for language) or pointed out with the right hand (which is controlled by the left hemisphere). The patient’s behavior became intriguing when the stimuli were presented in the left visual field or in the left hand. Now the patient, or at least the verbal left hemisphere, appeared oblivious to the fact that there had been a stimulus at all but was nevertheless able to select the correct object from an array of alternatives presented to the left hand or the left visual half-field (see Fig. 1 ). In a particularly dramatic recorded demonstration, the famous patient “Joe” was able to draw a cowboy hat with his left hand in response to the word “Texas” presented in his left visual half field. His commentary (produced by the verbal left hemisphere) showed a complete absence of insight into why his left hand had drawn this cowboy hat. Another astonishing example involved the same patient. MacKay and MacKay ( 1982 ) flashed a digit in the left visual field and trained the patient to play a version of ‘20 questions’ across hemispheres. The left hemisphere guessed the answer vocally, and the right hemisphere provided responses by pointing ‘up’ (meaning ‘guess a higher number’) or ‘down’ with the left hand. In this way the patient managed to vocalize the right answer. This suggests two independent conscious agents communicating with each other (one steering the left hand, the other agent controlling vocal expressions). However, note that an alternative interpretation is possible. Perhaps the patient knows the answer but finds it hard to vocalize. The ‘20 questions’ then simply help him in finding the correct vocalization.

One of the most well-known split-brain findings is that the patient claims verbally not to have seen the stimulus in the left visual field, yet indicates the identity of it with their left hand. This suggests that the left hemisphere (controlling verbal output) is blind to the left visual field, while the right hemisphere (controlling the left hand) does perceive it
Thus, these early observations suggested that there is no meaningful communication between the two hemispheres in split-brain patients. This led to the hypothesis that there might be two separate conscious agents, a left hemisphere that is able to talk to us and can explain what it sees and feels, and a mute right hemisphere that cannot communicate in language but that can nevertheless show that it has perceived and recognized objects and words. However, over time this view has eroded somewhat due to several anomalies (even right from the start) that may challenge this view.
Common Ground
An early observation, suggesting some remaining unification concerned what Joe Bogen called the “social ordinariness” of split-brain patients. Apart from a number of anecdotal incidents in the subacute phase following the surgery, these patients seem to behave in a socially ordinary manner and they report feeling unchanged after the operation (Bogen, Fisher, & Vogel, 1965 ; Pinto et al., 2017a ; R. W. Sperry, 1968 ; R. Sperry, 1984 ), although their extra-experiment behavior has not been systematically observed in great detail (Schechter, 2018 ). While the right hemisphere appears to be better at recognizing familiarity from faces, self-face recognition, that is the ability to realise immediately that a presented photograph represents you, appears to be available equally to both hemispheres in a split-brain patient (Uddin et al., 2008 ; Uddin, 2011 ). Thus, it seems unlikely that a mute but conscious right hemisphere would not have made itself known one way or the other. Thus, right from the start a paradox arose. The controlled lab tests suggested that consciousness is split in split-brain patients. Yet, everyday experiences of the patient and their close ones suggests that only one person exists in a split-brain. Additionally, it has been suggested that the two separate consciousnesses-hypothesis presumes that in the intact brain (before surgery) both hemispheres were conscious but connected via the corpus callosum, and they only became dissociable due to the operation. This casts doubt on the viability of the two consciousness view.
Crucially, the lab tests themselves were not always supportive of the split-consciousness view. Multiple experimental results showed that capacity for communication between the hemispheres varies both across patients and across tasks. For instance, a central observation in split-brain patients concerns the inability to compare visual stimuli across the midline. In other words, when one stimulus is presented to the left visual hemifield and the other to the right hemifield, the patient cannot accurately indicate whether both stimuli are the same or not, although they can do so when both stimuli are presented within one visual field. This is consistent with the notion that each hemisphere independently perceives the contralateral visual field, and that an intact corpus callosum is necessary for integration. Although there are indeed many examples of split-brain patients who are incapable of comparing stimuli across the midline, prominent examples can also be found of patients who can compare stimuli across the midline (Johnson, 1984 ; but see Seymour, Reuter-Lorenz, & Gazzaniga, 1994 ). This points to an important problem in the field, namely, individual differences. One aspect that may be important for individual differences is handedness differences. Variations in handedness may lead to differences in language capabilities in the right hemisphere, and could even underly differences in inter-hemispheric integration.
Moreover, under certain circumstances nearly all tested split-brain patients seem able to compare, or integrate, particular types of stimuli across the two visual half-fields (see Fig. Fig.2). 2 ). An early demonstration of across hemifields integration is the study by Eviatar and Zaidel ( 1994 ). They showed that split-brain patients could accurately indicate the identity and shape of upper- and lower-case letters in either hemifield, regardless of with which hand they responded, for instance accurately identifying the letter A in the left visual field with the right hand. Yet these patients were mostly unable to compare these same stimuli across visual fields. In another experiment, two tilted lines were presented with a gap between them. The lines were positioned in such a way that extending them across the gap would either cause the lines to coincide or to run in parallel. When split-brain patients indicate whether the lines are parallel or coincident, they are highly accurate, even when both line segments are located in different half-fields (Corballis, 1995 ; Pinto, de Haan, Lamme, & Fabri, n.d. ; Sergent, 1987 ; Trevarthen & Sperry, 1973 ). Another example of visual integration across the midline involves apparent motion. When two dots are presented in succession at a short distance (2 to 14 visual degrees), split-brain patients are able to accurately indicate whether the dots create apparent motion, or that they were presented simultaneously or with delays too long to provoke apparent motion. Critically, they are able to do so even when one dot appears in the left visual field, and the other in the right visual field (Knapen et al., 2012 ; Naikar & Corballis, 1996 ; Ramachandran, Cronin-Golomb, & Myers, 1986 ). Clearly, under specific conditions, there is meaningful communication between the two hemispheres in the absence of the corpus callosum.

Although most split-brain patients cannot compare visual features such as shape and object identity across the midline, other features, such as good continuation of lines, and apparent motion, are integrated without a corpus callosum
Another observation that suggests some form of unity across the two visual half fields concerns detection and localization of stimulation, for instance, a brief flash (see, for example, an early study on the response times to light flashes with the ipsi- or contralateral hand: Clarke & Zaidel, 1989 ). Several investigations (Corballis, Corballis, Fabri, Paggi, & Manzoni, 2005 ; Pinto et al., 2017a ; Trevarthen & Sperry, 1973 ) have demonstrated convincingly that split-brain patients can accurately report the presence and location of stimuli for any position in the whole visual field, with either hand, and even verbally. Accurate detection and localization appears to be possible for all patients and all stimuli (different shapes, figures, equiluminant stimuli) tested so far. Thus, when patients in earlier studies said that they saw “nothing” when a stimulus was presented in the left visual half-field, they may have meant that they could see it but that they could not identify or retrieve the name of the object.
Other findings point to a crucial difference between the degree of lateralization of visual-perceptual processing and producing overt responses. Perception appears to be more split, while responding remains largely unified. Whether a stimulus appears in the left or the right visual hemifield strongly impacts performance of split-brain patients. However, response type (left hand, right hand or verbally) seems to have a much smaller, or no effect at all. For instance, Pinto et al., 2017a ) found that the split-brain patient was much better at matching pictures to sample stimuli in the left visual field. Yet, for the exact same stimuli matching pictures to verbal labels was vastly superior when the stimuli appeared in the right visual field. Crucially, response type did not play any role. The patient was better in matching pictures to sample for stimuli in the left visual field, even if they responded verbally or with the right hand. Similarly, Levy, Trevarthen, and Sperry ( 1972 ) presented split-brain patients with chimeric or composite faces, that is, one half-face in each visual field. Subsequently the patient either matched the chimeric face to sample, or attached a verbal label to it. Verbal matching was mostly based on the half-face in the right visual field, while matching to sample was mainly driven by the half-face in the left visual field. But crucially, the latter was the case, independent of whether the patient responded with the left or the right hand.
Thus, it seems that in split-brain patients perceptual processing is largely split, yet response selection and action control appear to be unified under certain conditions. This, by itself, does not prove whether a split-brain houses one or two conscious agents. One explanation could be that the split-brain houses two agents, each having their own experiences, who synchronize their behavioral output through various means. Another possible explanation is that a split-brain houses one agent who experiences an unintegrated stream of information who controls the entire body, comparable to watching a movie where sight and sound are out-of-sync. At any rate, these findings challenge the previously mentioned classic split-brain description, which is still found in reviews and text books (Gray, 2002 ; Wolman, 2012 ). In this classic characterization the patient indicates that they saw nothing when a stimulus appeared in the left visual field. Yet, to their own verbal surprise, the left hand correctly draws the stimulus. The aforementioned examples of unity in action control suggests that these effects may depend on the type and complexity of the response that is required.
Interpretations
There are three, not-mutually exclusive, hypotheses concerning the mechanisms involved in, seemingly, preserved unity in the split-brain. The first notion is that information is transferred subcortically. The second idea is that ipsilateral motor control underlies unity in action control. The third idea claims that information transfer is based on varies forms of inter-hemispheric collaboration, including subtle behavioral cues. The first proposal (Corballis Corballis, Berlucchi, & Marzi, 2018 ; de Haan et al., 2019 ; Pinto, Lamme, & de Haan, 2017b ; Pinto et al., 2017a ; Savazzi et al., 2007 ; Mancuso, Uddin, Nani, Costa, & Cauda, 2019 ) suggests that the multitude of subcortical connections that are spared during surgery are responsible for the transfer of information. As was initially pointed out by Trevarthen ( 1968 ) and Trevarthen and Sperry ( 1973 ) and recently stressed by Pinto, de Haan, and Lamme ( 2017a ) and Corballis et al. ( 2018 ), there are many commissures (white matter tracts that connect homologous structures on both sides of the central nervous system) and decussations (bundles that connect different structures on both sides) that link nuclei that are known to be involved in perceptual processing. The importance of these commisural connections for transferring visual information in split-brain patients has been highlighted by Trevarthen and Sperry ( 1973 ). Moreover, the role of these connections in a split-brain has recently been demonstrated by bilateral fMRI activations in the first somatosensory cortex, after unilateral stimulation of trunk midline touch receptors (Fabri et al., 2006 ) and in the second somatic sensory area after unilateral stimulation of hand pain receptors (Fabri, Polonara, Quattrini, & Salvolini, 2002 ). Uddin and colleagues used low-frequency BOLD fMRI resting state imaging to investigate functional connectivity between the two hemispheres in a patient in whom all major cerebral commissures had been cut (Uddin et al., 2008 ). Compared to control subjects, the patient’s interhemispheric correlation scores fell within the normal range for at least two symmetrical regions. In addition, Nomi and colleagues suggested that split-brain patients might rely particularly on dorsal and ventral pontine decussations of the cortico-cerebellar interhemispheric pathways as evidenced by increased fractional anisotropy (FA) on diffusion weighted imaging (Nomi, Marshall, Zaidel, Biswal, Castellanos, Dick, Uddin & Mooshagian, 2019). Interhemispheric exchange of information also seems to occur in the domain of taste sensitivity, activation of primary gustatory cortex in the fronto-parietal operculum was reported in both hemispheres after unilateral gustatory stimulation of the tongue receptors (Mascioli, Berlucchi, Pierpaoli, Salvolini, Barbaresi, Fabri, & Polonara, 2015 ). Note that patients may differ with respect to how many of these connections have been cut, and this might also explain some of the individual variance among patients. Moreover, in all patients subcortical structures remain intact. For instance, the superior colliculus is known to integrate visual information from both hemispheres and project information to both hemispheres (Meredith & Stein, 1986 ; Comoli et al., 2003 ). Such structures may support attentional networks, and may enable the right hemisphere to attend to the entire visual field. In turn, attentional unity could help in unifying cognitive and motor control, which may subserve ipsilateral motor control.
The second point concerns the ipsilateral innervation of the arms. Manual action is not strictly lateralized, and the proximal (but not the distal) parts of the arm are controlled bilaterally, although the ipsilateral contribution remains undetermined. This could explain why split-brain patients may respond equally well with both hands in certain experimental conditions (Corballis, 1995 ; Gazzaniga, Bogen, & Sperry, 1967 ; Pinto, de Haan, & Lamme, 2017a ). First, there is substantial evidence that bilateral cortical activations can be observed during unilateral limb movements in healthy subjects. In addition, ipsilesional motor problems in arm control have been observed in patients with unilateral cortical injuries, and finally there is evidence from electrocorticography with implanted electrodes for localization of epileptic foci showing similar spatial and spectral encoding of contralateral and ipsilateral limb kinematics (Bundy, Szrama, Pahwa, & Leuthardt, 2018 ). While these observations argue convincingly for a role in action control by the ipsilateral hemisphere, they do not prove that a hemisphere on it’s own can purposefully control the movements of the ipsilateral hand. Thus, the role of ipsilateral arm-hand control in explaining split-brain findings is currently not settled.
The third hypothesis argues that in addition to whatever direct neural communication may exist between the hemispheres, they may inform one another via strategic cross-cueing processes (Volz & Gazzaniga, 2017 ; Volz et al., 2018 ). The split-brain patients underwent surgery many years prior to testing, and the separated perceptual systems have had ample time to learn how to compensate for the lack of commissural connections. For example, subtle cues may be given by minimal movements of the eyes or facial muscles, which might not even be visible to an external observer but are capable of encoding, for example, the location of a stimulus for the hemisphere that did not “see” it. A cross-cueing mechanism might also allow one hemisphere to convey to the other which one of a limited set of known items had been shown (Gazzaniga & Hillyard, 1971 ; Gazzaniga, 2013 ).
Finally, it is possible to entertain combinations of the different explanations. For instance, it is conceivable that in the subacute phase following split-brain surgery the hemispheres are ineffective in communicating with each other. During this initial phase, phenomena such as an “alien hand” - that is a hand moving outside conscious control of the (verbal) person - may be present. In the ensuing period, the patients may have learned to utilize the information that is exchanged via subcortical connections, ipsilateral motor control or cross-cueing to coordinate the processing of the two hemispheres. In such a way, the patient may counteract some of the effects of losing the corpus callosum.
What do We Need to Know?
This paper aims to contribute to the agenda for the next decade of split-brain research. Full split-brain surgery is rare these days, and it is important that we try to answer the central questions while these patients are still available for study. In order to examine the variations between patients it would be useful to test as many of the available patients as possible with the same tests.
One important goal is to map out precisely how much functionality and information is still integrated across hemispheres in the split-brain, and what the underlying principles are. For instance, in some cases the two hemispheres seem to carry out sensory-motor tasks, such as visual search, independently from one another (Arguin et al., 2000 ; Franz, Eliassen, Ivry, & Gazzaniga, 1996 ; Hazeltine, Weinstein, & Ivry, 2008 ; Luck, Hillyard, Mangun, & Gazzaniga, 1994 ; Luck et al., 1989 ), while in other cases functions such as attentional blink, or attentional cueing, seem to be integrated across hemispheres (Giesbrecht & Kingstone, 2004 ; Holtzman, Volpe, & Gazzaniga, 1984 ; Holtzman, Sidtis, Volpe, Wilson, & Gazzaniga, 1981 ; Pashler et al., 1994 ; Ptito, Brisson, Dell’Acqua, Lassonde, & Jolicœur, 2009 ). An important challenge is to unveil why some cognitive functions can be carried out independently in the separated hemispheres while other functions engage both hemispheres. Furthermore, it is now clear that accurate detection and localization is possible across the whole visual field, and there is some evidence that even more information concerning visual images can be transferred between hemispheres. Although we have some understanding of what types of information can be transferred in the visual domain, our knowledge base in the somatosensory domain is much more limited. This is probably due to a bias throughout cognitive neuroscience and psychology, leading to a strong focus on vision in split-brain research. It is important to collect converging evidence by investigating the somatosensory system which is also strongly lateralized. Note that in somatosensory processing transfer between hemispheres (about 80% correct for the bimanual conditions) has been observed for basic same-different matching of real objects (Fabri, Del Pesce et al., 2005 ).
Another important goal is to obtain a more detailed description of the perceptual, cognitive and linguistic capabilities of the disconnected right hemisphere. For understanding unity of mind, two capabilities specifically are crucial. First, experiments investigating aspects of the conscious mind often go beyond simple visual processing, and future studies will thus critically depend on testing high-level cognitive abilities of both hemispheres. Specifically, language abilities, crucial for understanding questions and instructions, will likely play a pivotal role. Thus, the first question is to what extent the right hemisphere is capable of language processing. Note that complicated instructions (Gazzaniga, Smylie, Baynes, Hirst, & McCleary, 1984 ; Pinto et al., 2017a ; Zaidel, 1983 ), for instance relating to mental imagery (Johnson, Corballis, & Gazzaniga, 2001 ; Kosslyn, Holtzman, Farah, & Gazzaniga, 1985 ; Sergent & Corballis, 1990 ), seem to be well within the reach of the right hemisphere. Moreover, right hemisphere language capabilities seem to improve over time (Gazzaniga, Volpe, Smylie, Wilson, & LeDoux, 1979 ; Gazzaniga et al., 1996 ). Longitudinal language tests (for instance with a Token test: De Renzi & Vignolo, 1962 ) would further illuminate the extent of right hemisphere language processing.
Second, unveiling to what extent each hemisphere is capable of subserving consciousness at all seems relevant for unity of mind as well. If the disconnected right hemisphere can produce full-blown consciousness, then questions regarding unity of mind are clearly more pertinent then if the right hemisphere only produces minimal amounts of consciousness. Right hemisphere consciousness can be studied through novel neural paradigms (Bekinschtein et al., 2009 ; Casali et al., 2013 ; Pitts, Metzler, & Hillyard, 2014 ; Shafto & Pitts, 2015 ). For instance, Bekinschtein et al. employed EEG to measure if the brain detected irregularities (as indicated by an event-related potential [ERP] signal called the P3) in different states of consciousness. They found that when consciousness was reduced, local irregularities were still detected - for instance after three high auditory tones a low tone evoked a P3. However, global irregularities - several times a low tone followed three high tones, then on the critical trial three high tones were followed by another high tone - did not evoke a P3 when consciousness was reduced. Crucially, when consciousness was unimpaired both local and global irregularities evoked a P3 response. Right hemisphere consciousness may also be studied in other patient groups where interhemispheric communication is hampered. One particularly interesting group are post-hemispherotomy patients (Lew, 2014 ). These patients have been surgically treated to disconnect an entire hemisphere (usually for intractable epilepsy), but unlike hemispherectomy patients the disconnected hemisphere remains in place in the cranium and remains vascularized.
Clearly, the central question, whether each hemisphere supports an independent conscious agent, is not settled yet. Novel paradigms in this respect could lead to progress. For instance, a pivotal question is whether each hemisphere makes its own decisions independent of the other hemisphere. If each hemisphere produces its own autonomous conscious agent then this should be the case. That is, if two agents are asked to freely choose a random number, then the odds that they consistently pick the same number are small. And vice versa, if each hemisphere makes its own conscious decisions, independent of the other hemisphere, then this seems to rule out unity of mind. Note that each hemisphere making its own decisions is different from information processing occurring independently per hemisphere. Unconscious information processing is almost certainly split across hemispheres in a split-brain. However, this does not prove that consciousness is split or unified. Even in a healthy brain, where consciousness is unified, many unconscious processes run independently, and in parallel.
One way to tackle the central question is by having the hemispheres respond to questions in parallel. Overt behavior most likely does not allow for this, due to bilateral motor control processes sketched earlier. However, perhaps parallel responding is possible if the hemispheres produce covert responses. For instance, the patient could be asked to pick one of four options and indicate their choice by carrying out certain content-specific mental imagery tasks. This imagery can then be decoded in parallel from each hemisphere using neuroimaging techniques (see Owen et al., 2006 for a similar approach with vegetative state patients). If each hemisphere harbors an autonomous conscious agent, then it is highly unlikely that the two hemispheres will consistently make the same choices. Thus, if the choices are uncorrelated across hemispheres, then this may critically challenge the unified mind view.
Another way to tackle the question of unified consciousness in the split-brain is to employ ERPs as markers of concurrent conscious processing in the left and right hemispheres. For instance, in one study (Kutas, Hillyard, Volpe, & Gazzaniga, 1990 ) visual targets were presented either separately to the left or right visual field or to both visual fields simultaneously. It was found that the P300 - a signal possibly reflecting conscious processing of a visual target (Dehaene & Changeux, 2011 ; Dehaene, Charles, King, & Marti, 2014 ; Salti, Bar-Haim, & Lamy, 2012 ) - was reduced for bilateral targets. This suggests some type of integration of conscious processing. Studies employing ERPs may indicate whether conscious processing is unified, while unconscious processing is split, which would be suggestive of unified consciousness.
Conclusions
In summary, the pivotal issue in split-brain research is whether dividing the brain divides consciousness. That is, do we find evidence for the existence of one, or two conscious agents in a split-brain? Note that intermediate results may be found. Perhaps some measures indicate unified consciousness while others do not. This would then provoke further interesting questions on the unity of consciousness. What are the crucial measures for unity of consciousness? If intermediate results are found, more unconventional possibilities should be entertained as well. For instance, although difficult to fathom, some philosophers have suggested that a split-brain does not contain one or two observers, but a non-whole number of conscious agents (Nagel, 1971 ; Perry, 2009 ), for instance one and a half first-person perspectives. If evidence for this position is found, then its implications would stretch beyond split-brain patients. It would suggest that our intuitions on the indivisibility of the experiential self may be mistaken. One way to think of this is as with the difference between conscious and unconscious processing. Perhaps this is not a dichotomous distinction, but a continuum between more or less conscious. Similarly, perhaps the existence of a first-person perspective is not dichotomous, but gradual as well. Another possibility is that a split-brain does contain a whole number of conscious agents, but that consciousness across these agents is only partially unified. That is, the agents share some conscious experiences and decisions, but not all (Lockwood, 1989 ; Schechter, 2014 ; Schechter, 2018 ; Schechter, 2013 ). Finally, another way to look at this is in terms of ‘dissociation’, as in depersonalization (Phillips et al., 2001 ; Sierra et al., 2002 ). Perhaps the number of agents is not altered, but the agent feels depersonalized in some situations, and therefore no longer feels that they control the actions, or even experience the information, that has just occurred in their brain.
New findings on the unity of consciousness in a split-brain could fundamentally impact currently dominant consciousness theories. Global Neuronal Workspace Theory (Dehaene & Naccache, 2001 ; Dehaene et al., 1998 ) asserts that consciousness arises when information that is processed in unconscious (or preconscious) modules is broadcast to a central ‘workspace’, primarily residing in frontal regions of the brain. Although not very explicit on the unity of consciousness in split-brain patients, Global Neuronal Workspace Theory seems to endorse the split consciousness idea, given that each hemisphere has its own prefrontal hub, enabling broadcasting of whatever information is processed in that hemisphere.
Integrated Information Theory (Tononi, 2005 ; Tononi, 2004 ) has specifically addressed the issue of split brain (for instance in Tononi, 2004 ). Integrated Information Theory asserts that ‘phi’, a measure of how integrated information is, determines the level of consciousness. The higher phi, the more conscious a system is. Moreover, local maxima in phi correspond to conscious agents. If in a system all subsystems are highly interconnected, then phi is highest for the system as a whole, and local maxima are absent. Thus, such a system produces only one conscious agent. However, if subsystems only exchange minimal amounts of information, then phi per subsystem is higher than phi for the system as whole. In such a case each subsystem creates its own conscious agent. In a split-brain, connectedness, that is integration of information, is much higher within than across hemispheres. Therefore, according to Integrated Information Theory consciousness should be split in a split-brain.
Recurrent Processing theory (Lamme & Roelfsema, 2000 ; Lamme, 2004 ; Lamme, 2010 ) argues for the independence of consciousness from attention, access, or report. This theory has addressed the issue of report specifically, making the case that consciousness and reportability, whether verbal or manual, should be viewed as entirely independent (Lamme, 2006 ; Tsuchiya, Wilke, Frässle, & Lamme, 2015 ). Crucially, this theory states that even in the normal mind, ‘islands’ of unattended yet conscious information reside (Lamme, 2006 ). In these cases, all the information, although functionally unintegrated, is nonetheless experienced by the same mind. Support for this view comes from findings in multiple object tracking (Pinto, Howe, Cohen, & Horowitz, 2010 ; Pinto, Scholte, & Lamme, 2012 ; Pylyshyn & Storm, 1988 ). Here, evidence indicates that when moving objects in two visual hemifields are tracked, attention is split (Howe, Cohen, Pinto, & Horowitz, 2010 ) and each hemisphere processes the relevant information in the contralateral visual field independently of the other (Alvarez & Cavanagh, 2005 ; Cavanagh & Alvarez, 2005 ; Drew, Mance, Horowitz, Wolfe, & Vogel, 2014 ). That is, the left hemisphere only tracks the right visual field and vice versa. Yet, although the visual information is not integrated across hemispheres, from a first person perspective, it seems clear that the subject experiences all moving objects across the entire visual field. Another example of the dissociation between consciousness and reportability is the so-called partial report paradigm (Pinto et al., 2017b ; Pinto, Sligte, Shapiro, & Lamme, 2013 ; Sligte, Scholte, & Lamme, 2008 ; Sperling, 1960 ). In these paradigms subjects seem to remember more than they can report. Thus, reportability and consciousness are dissociated. Perhaps in split-brain patients this dissociation is simply more pronounced. That is, consciousness remains unified, but reportability has become more dissociated, thereby inducing the appearance of two independent agents. In sum, according to the Recurrent Processing theory, integration of information is not needed for a unified mind, implying that the mind may remain unified when the brain is split. Thus, different theories of consciousness have different predictions on the unity of mind in split-brain patients, and await the results of further investigation into this intriguing phenomenon.
Acknowledgements
This work was supported by an Advanced Investigator Grant by the European Research Council (ERC grant FAB4V (#339374) to EdH and a Templeton grant (ID# 61382, "Towards understanding a unified mind") to YP and VL.
Compliance with Ethical Standards
The authors report no competing interests.
Publisher’s Note
Springer Nature remains neutral with regard to jurisdictional claims in published maps and institutional affiliations.
- Akelaitis AJ. Studies on the corpus callosum: II. The higher visual functions in each homonymous field following complete section of the corpus callosum. Archives of Neurology and Psychiatry. 1941; 45 (5):788–796. [ Google Scholar ]
- Akelaitis AJ. Studies on the corpus callosum: VII. Study of language functions (tactile and visual lexia and graphia) unilaterally following section of the corpus callosum. Journal of Neuropathology & Experimental Neurology. 1943; 2 (3):226–262. [ Google Scholar ]
- Alvarez GA, Cavanagh P. Independent resources for attentional tracking in the left and right visual hemifields. Psychological Science. 2005; 16 (8):637–643. [ PubMed ] [ Google Scholar ]
- Arguin M, Lassonde M, Quattrini A, Del Pesce M, Foschi N, Papo I. Divided visuo-spatial attention systems with total and anterior callosotomy. Neuropsychologia. 2000; 38 (3):283–291. [ PubMed ] [ Google Scholar ]
- Bayne T. The unity of consciousness and the split-brain syndrome. The Journal of Philosophy. 2008; 105 (6):277–300. [ Google Scholar ]
- Bayne, T. (2010). The unity of consciousness. Oxford: Oxford University Press.
- Bayne T, Chalmers DJ. What is the unity of consciousness? In: Cleeremans A, editor. The unity of consciousness: Binding, integration and dissociation. Oxford, England: Oxford University press; 2003. pp. 23–58. [ Google Scholar ]
- Bekinschtein TA, Dehaene S, Rohaut B, Tadel F, Cohen L, Naccache L. Neural signature of the conscious processing of auditory regularities. Proceedings of the National Academy of Sciences of the United States of America. 2009; 106 (5):1672–1677. [ PMC free article ] [ PubMed ] [ Google Scholar ]
- Berlucchi G, Aglioti S, Marzi C, Tassinari G. Corpus callosum and simple visuomotor integration. Neuropsychologia. 1995; 33 (8):923–936. [ PubMed ] [ Google Scholar ]
- Bogen JE, Fisher E, Vogel P. Cerebral commissurotomy: A second case report. Jama. 1965; 194 (12):1328–1329. [ PubMed ] [ Google Scholar ]
- Bundy DT, Szrama N, Pahwa M, Leuthardt EC. Unilateral, 3D arm movement kinematics are encoded in ipsilateral human cortex. The Journal of neuroscience : the official journal of the Society for Neuroscience. 2018; 38 (47):10042–10056. [ PMC free article ] [ PubMed ] [ Google Scholar ]
- Casali AG, Gosseries O, Rosanova M, Boly M, Sarasso S, Casali KR, et al. A theoretically based index of consciousness independent of sensory processing and behavior. Science Translational Medicine. 2013; 5 (198):198ra105. [ PubMed ] [ Google Scholar ]
- Cavanagh P, Alvarez GA. Tracking multiple targets with multifocal attention. Trends in Cognitive Sciences. 2005; 9 (7):349–354. [ PubMed ] [ Google Scholar ]
- Clarke JM, Zaidel E. Simple reaction times to lateralized light flashes. Varieties of interhemispheric communication routes. Brain. 1989; 112 :849–870. [ PubMed ] [ Google Scholar ]
- Comoli E, Coizet V, Boyes J, Bolam JP, Canteras NS, Quirk RH, Overton PG, Redgrave P. A direct projection from superior colliculus to substantia nigra for detecting salient visual events. Nature Neuroscience. 2003; 6 (9):974–980. [ PubMed ] [ Google Scholar ]
- Corballis MC. Can commissurotomized subjects compare digits between the visual fields? Neuropsychologia. 1994; 32 (12):1475–1486. [ PubMed ] [ Google Scholar ]
- Corballis MC. Visual integration in the split brain. Neuropsychologia. 1995; 33 (8):937–959. [ PubMed ] [ Google Scholar ]
- Corballis MC, Birse K, Paggi A, Manzoni T, Pierpaoli C, Fabri M. Mirror-image discrimination and reversal in the disconnected hemispheres. Neuropsychologia. 2010; 48 (6):1664–1669. [ PubMed ] [ Google Scholar ]
- Corballis MC, Corballis PM, Berlucchi G, Marzi CA. Perceptual unity in the split brain: The role of subcortical connections. Brain. 2018; 141 (6):e46. [ PubMed ] [ Google Scholar ]
- Corballis MC, Corballis PM, Fabri M, Paggi A, Manzoni T. Now you see it, now you don't: Variable hemineglect in a commissurotomized man. Cognitive Brain Research. 2005; 25 (2):521–530. [ PubMed ] [ Google Scholar ]
- Corballis PM. Visuospatial processing and the right-hemisphere interpreter. Brain and Cognition. 2003; 53 (2):171–176. [ PubMed ] [ Google Scholar ]
- de Haan EH, Fabri M, Dijkerman HC, Foschi N, Lattanzi S, Pinto Y. Unified tactile detection and localisation in split-brain patients. Cortex. 2019; 124 :217–223. [ PMC free article ] [ PubMed ] [ Google Scholar ]
- De Renzi, A., & Vignolo, L. A. (1962). Token test: A sensitive test to detect receptive disturbances in aphasics. Brain: A Journal of Neurology, 85 , 665–678. 10.1093/brain/85.4.665 [ PubMed ]
- Dehaene S, Changeux J. Experimental and theoretical approaches to conscious processing. Neuron. 2011; 70 (2):200–227. [ PubMed ] [ Google Scholar ]
- Dehaene S, Charles L, King J, Marti S. Toward a computational theory of conscious processing. Current Opinion in Neurobiology. 2014; 25 :76–84. [ PMC free article ] [ PubMed ] [ Google Scholar ]
- Dehaene S, Kerszberg M, Changeux JP. A neuronal model of a global workspace in effortful cognitive tasks. Proceedings of the National Academy of Sciences of the United States of America. 1998; 95 (24):14529–14534. [ PMC free article ] [ PubMed ] [ Google Scholar ]
- Dehaene S, Naccache L. Towards a cognitive neuroscience of consciousness: Basic evidence and a workspace framework. Cognition. 2001; 79 (1):1–37. [ PubMed ] [ Google Scholar ]
- Drew T, Mance I, Horowitz TS, Wolfe JM, Vogel EK. A soft handoff of attention between cerebral hemispheres. Current Biology. 2014; 24 (10):1133–1137. [ PMC free article ] [ PubMed ] [ Google Scholar ]
- Eviatar Z, Zaidel E. Letter matching within and between the disconnected hemispheres. Brain and Cognition. 1994; 25 (1):128–137. [ PubMed ] [ Google Scholar ]
- Fabri M, Del Pesce M, Paggi A, Polonara G, Bartolini M, Salvolini U, et al. Contribution of posterior corpus callosum to the interhemispheric transfer of tactile information. Cognitive Brain Research. 2005; 24 (1):73–80. [ PubMed ] [ Google Scholar ]
- Fabri M, Polonara G, Mascioli G, Paggi A, Salvolini U, Manzoni T. Contribution of the corpus callosum to bilateral representation of the trunk midline in the human brain: An fMRI study of callosotomized patients. European Journal of Neuroscience. 2006; 23 (11):3139–3148. [ PubMed ] [ Google Scholar ]
- Mascioli, G., Berlucchi, G., Pierpaoli, C., Salvolini, U., Barbaresi, P., Fabri, M. and Polonara, G. (2015) Functional MRI cortical activations from unilateral tactile-taste stimulations of the tongue. Physiology and Behavior, 151 , 221–229. 10.1016/j.physbeh.2015.07.031. [ PubMed ]
- Fabri M, Polonara G, Quattrini A, Salvolini U. Mechanical noxious stimuli cause bilateral activation of parietal operculum in callosotomized subjects. Cerebral Cortex. 2002; 12 (4):446–451. [ PubMed ] [ Google Scholar ]
- Franz EA, Eliassen JC, Ivry RB, Gazzaniga MS. Dissociation of spatial and temporal coupling in the bimanual movements of callosotomy patients. Psychological Science. 1996; 7 (5):306–310. [ Google Scholar ]
- Gazzaniga M, Bogen J, Sperry R. Dyspraxia following division of the cerebral commissures. Archives of Neurology. 1967; 16 (6):606–612. [ PubMed ] [ Google Scholar ]
- Gazzaniga MS. The split brain in man. Scientific American. 1967; 217 (2):24–29. [ PubMed ] [ Google Scholar ]
- Gazzaniga MS. Review of the split brain. Journal of Neurology. 1975; 209 (2):75–79. [ PubMed ] [ Google Scholar ]
- Gazzaniga MS. Shifting gears: Seeking new approaches for mind/brain mechanisms. Annual Review of Psychology. 2013; 64 (1):1–20. [ PubMed ] [ Google Scholar ]
- Gazzaniga MS. The split-brain: Rooting consciousness in biology. Proceedings of the National Academy of Sciences of the United States of America. 2014; 111 (51):18093–18094. [ PMC free article ] [ PubMed ] [ Google Scholar ]
- Gazzaniga MS, Bogen JE, Sperry RW. Some functional effects of sectioning the cerebral commissures in man. Proceedings of the National Academy of Sciences of the United States of America. 1962; 48 :1765–1769. [ PMC free article ] [ PubMed ] [ Google Scholar ]
- Gazzaniga MS, Bogen JE, Sperry RW. Laterality effects in somesthesis following cerebral commissurotomy in man. Neuropsychologia. 1963; 1 (3):209–215. [ Google Scholar ]
- Gazzaniga MS, Eliassen JC, Nisenson L, Wessinger CM, Fendrich R, Baynes K. Collaboration between the hemispheres of a callosotomy patient: Emerging right hemisphere speech and the left hemisphere interpreter. Brain. 1996; 119 (4):1255–1262. [ PubMed ] [ Google Scholar ]
- Gazzaniga MS, Hillyard SA. Language and speech capacity of the right hemisphere. Neuropsychologia. 1971; 9 (3):273–280. [ PubMed ] [ Google Scholar ]
- Gazzaniga MS, Smylie CS, Baynes K, Hirst W, McCleary C. Profiles of right hemisphere language and speech following brain bisection. Brain and Language. 1984; 22 (2):206–220. [ PubMed ] [ Google Scholar ]
- Gazzaniga MS, Volpe B, Smylie CS, Wilson DH, LeDoux JE. Plasticity in speech organization following commissurotomy. Brain. 1979; 102 (4):805–815. [ PubMed ] [ Google Scholar ]
- Giesbrecht B, Kingstone A. Right hemisphere involvement in the attentional blink: Evidence from a split-brain patient. Brain and Cognition. 2004; 55 (2):303–306. [ PubMed ] [ Google Scholar ]
- Gray, P. (2002). The nervous system. Psychology (pp. 160-161) worth publishers, New York, NY, USA.
- Hazeltine E, Weinstein A, Ivry RB. Parallel response selection after callosotomy. Journal of Cognitive Neuroscience. 2008; 20 (3):526–540. [ PubMed ] [ Google Scholar ]
- Holtzman JD, Sidtis JJ, Volpe BT, Wilson DH, Gazzaniga MS. Dissociation of spatial information for stimulus localization and the control of attention. Brain : A Journal of Neurology. 1981; 104 (Pt 4):861–872. [ PubMed ] [ Google Scholar ]
- Holtzman JD, Volpe BT, Gazzaniga MS. Spatial orientation following commisural section. In: Parasuram R, Davies DR, editors. Varieties of attention. Orlando (FL): Academic press; 1984. pp. 375–394. [ Google Scholar ]
- Howe PD, Cohen MA, Pinto Y, Horowitz TS. Distinguishing between parallel and serial accounts of multiple object tracking. Journal of Vision. 2010; 10 (8):11–11. [ PMC free article ] [ PubMed ] [ Google Scholar ]
- Johnson LE. Bilateral visual cross-integration by human forebrain commissurotomy subjects. Neuropsychologia. 1984; 22 (2):167–175. [ PubMed ] [ Google Scholar ]
- Johnson SH, Corballis PM, Gazzaniga MS. Within grasp but out of reach: Evidence for a double dissociation between imagined hand and arm movements in the left cerebral hemisphere. Neuropsychologia. 2001; 39 (1):36–50. [ PubMed ] [ Google Scholar ]
- Knapen T, Pinto Y, Scholte HS, Lamme V, Foschi N, Fabri M. Perception of apparent motion in a split-brain observer. Journal of Vision. 2012; 12 (9):548–548. [ Google Scholar ]
- Kosslyn SM, Holtzman JD, Farah MJ, Gazzaniga MS. A computational analysis of mental image generation: Evidence from functional dissociations in split-brain patients. Journal of Experimental Psychology: General. 1985; 114 (3):311–341. [ PubMed ] [ Google Scholar ]
- Kutas M, Hillyard SA, Volpe BT, Gazzaniga MS. Late positive event-related potentials after commissural section in humans. Journal of Cognitive Neuroscience. 1990; 2 (3):258–271. [ PubMed ] [ Google Scholar ]
- Lamme VA. Separate neural definitions of visual consciousness and visual attention; a case for phenomenal awareness. Neural Networks. 2004; 17 (5):861–872. [ PubMed ] [ Google Scholar ]
- Lamme VA. Towards a true neural stance on consciousness. Trends in Cognitive Sciences. 2006; 10 (11):494–501. [ PubMed ] [ Google Scholar ]
- Lamme VA. How neuroscience will change our view on consciousness. Cognitive Neuroscience. 2010; 1 (3):204–220. [ PubMed ] [ Google Scholar ]
- Lamme VA, Roelfsema PR. The distinct modes of vision offered by feedforward and recurrent processing. Trends in Neurosciences. 2000; 23 (11):571–579. [ PubMed ] [ Google Scholar ]
- Levy J, Trevarthen C, Sperry RW. Perception of bilateral chimeric figures following hemispheric deconnexion. Brain : A Journal of Neurology. 1972; 95 (1):61–78. [ PubMed ] [ Google Scholar ]
- Lew SM. Hemispherectomy in the treatment of seizures: A review. Translational Pediatrics. 2014; 3 (3):208–217. [ PMC free article ] [ PubMed ] [ Google Scholar ]
- Lockwood M. Mind, brain and the quantum: The compound 'I' . Oxford: Basil Blackwell; 1989. [ Google Scholar ]
- Luck SJ, Hillyard SA, Mangun GR, Gazzaniga MS. Independent hemispheric attentional systems mediate visual-search in split-brain patients. Nature. 1989; 342 (6249):543–545. [ PubMed ] [ Google Scholar ]
- Luck SJ, Hillyard SA, Mangun GR, Gazzaniga MS. Independent attentional scanning in the separated hemispheres of split-brain patients. Journal of Cognitive Neuroscience. 1994; 6 (1):84–91. [ PubMed ] [ Google Scholar ]
- MacKay DM, MacKay V. Explicit dialogue between left and right half-systems of split brains. Nature. 1982; 295 (5851):690–691. [ PubMed ] [ Google Scholar ]
- Mancuso L, Uddin LQ, Nani A, Costa T, Cauda F. Brain functional connectivity in individuals with callosotomy and agenesis of the corpus callosum: A systematic review. Neuroscience & Biobehavioral Reviews. 2019; 105 :231–248. [ PubMed ] [ Google Scholar ]
- Meredith MA, Stein BE. Visual, auditory, and somatosensory convergence on cells in superior colliculus results in multisensory integration. Journal of Neurophysiology. 1986; 56 (3):640–662. [ PubMed ] [ Google Scholar ]
- Nagel T. Brain bisection and the unity of consciousness. Synthese. 1971; 22 (3–4):396–413. [ Google Scholar ]
- Naikar N, Corballis MC. Perception of apparent motion across the retinal midline following commissurotomy. Neuropsychologia. 1996; 34 (4):297–309. [ PubMed ] [ Google Scholar ]
- Owen AM, Coleman MR, Boly M, Davis MH, Laureys S, Pickard JD. Detecting awareness in the vegetative state. Science (New York, N.Y.) 2006; 313 (5792):1402. [ PubMed ] [ Google Scholar ]
- Pashler, H., Luck, S. J., Hillyard, S. A., Mangun, G. R., OʼBrien, S., & Gazzaniga, M. S. (1994). Sequential operation of disconnected cerebral hemispheres in split-brain patients. Neuroreport, 5 , 2381–2384. [ PubMed ]
- Perry, J. (2009). Diminished and fractured selves. Personal Identity and Fractured Selves , 129–162.
- Phillips ML, Medford N, Senior C, Bullmore ET, Suckling J, Brammer MJ, Andrew C, Sierra M, Williams SCR, David AS. Depersonalization disorder: Thinking without feeling. Psychiatry Research: Neuroimaging. 2001; 108 (3):145–160. [ PubMed ] [ Google Scholar ]
- Pinto Y, de Haan EHF, Lamme VAF. The split-brain phenomenon revisited: A single conscious agent with split perception. Trends in Cognitive Sciences. 2017; 21 (11):835–851. [ PubMed ] [ Google Scholar ]
- Pinto, Y., de Haan, E. H. F., Lamme, V. A. F., & Fabri, M. (n.d.). Intact gestalt and gist processing in split-brain patients.
- Pinto Y, Howe PD, Cohen MA, Horowitz TS. The more often you see an object, the easier it becomes to track it. Journal of Vision. 2010; 10 (10):4–4. [ PMC free article ] [ PubMed ] [ Google Scholar ]
- Pinto Y, Scholte HS, Lamme V. Tracking moving identities: After attending the right location, the identity does not come for free. PLoS One. 2012; 7 (8):e42929. [ PMC free article ] [ PubMed ] [ Google Scholar ]
- Pinto Y, Sligte IG, Shapiro KL, Lamme VAF. Fragile visual short-term memory is an object-based and location-specific store. Psychonomic Bulletin & Review. 2013; 20 (4):732–739. [ PubMed ] [ Google Scholar ]
- Pinto, Y., Lamme, V. A., & de Haan, E. H. (2017). Cross-cueing cannot explain unified control in split-brain patients. Brain , 140 (11), e68. [ PubMed ]
- Pinto, Y., Neville, D. A., Otten, M., Corballis, P. M., Lamme, V. A., de Haan, E. H., et al. (2017a). Split brain: Divided perception but undivided consciousness. Brain , 140 (5), 1231–1237. [ PubMed ]
- Pinto, Y., Vandenbroucke, A. R., Otten, M., Sligte, I. G., Seth, A. K., & Lamme, V. A. F. (2017b). Conscious visual memory with minimal attention. Journal of Experimental Psychology: General , 146 (2), 214–226. [ PubMed ]
- Pitts MA, Metzler S, Hillyard SA. Isolating neural correlates of conscious perception from neural correlates of reporting one's perception. Frontiers in Psychology. 2014; 5 :1078. [ PMC free article ] [ PubMed ] [ Google Scholar ]
- Ptito A, Brisson B, Dell’Acqua R, Lassonde M, Jolicœur P. The attentional blink within and across the hemispheres: Evidence from a patient with a complete section of the corpus callosum. Biological Psychology. 2009; 82 (1):64–69. [ PubMed ] [ Google Scholar ]
- Pylyshyn ZW, Storm RW. Tracking multiple independent targets: Evidence for a parallel tracking mechanism. Spatial Vision. 1988; 3 (3):179–197. [ PubMed ] [ Google Scholar ]
- Ramachandran V, Cronin-Golomb A, Myers J. Perception of apparent motion by commissurotomy patients. Nature. 1986; 320 (6060):358–359. [ PubMed ] [ Google Scholar ]
- Salti M, Bar-Haim Y, Lamy D. The P3 component of the ERP reflects conscious perception, not confidence. Consciousness and Cognition. 2012; 21 (2):961–968. [ PubMed ] [ Google Scholar ]
- Savazzi S, Fabri M, Rubboli G, Paggi A, Tassinari CA, Marzi CA. Interhemispheric transfer following callosotomy in humans: Role of the superior colliculus. Neuropsychologia. 2007; 45 (11):2417–2427. [ PubMed ] [ Google Scholar ]
- Schechter E. The unity of consciousness: Subjects and objectivity. Philosophical Studies. 2013; 165 (2):671–692. [ Google Scholar ]
- Schechter E. Partial unity of consciousness. In: Bennett DJ, Hill CS, editors. Sensory integration and the unity of consciousness. Cambridge, MA: MIT press; 2014. pp. 347–374. [ Google Scholar ]
- Schechter E. Self-consciousness and "split" brains: The minds' I. Oxford, UK: Oxford University Press; 2018. [ Google Scholar ]
- Sergent J. A new look at the human split brain. Brain. 1987; 110 (5):1375–1392. [ PubMed ] [ Google Scholar ]
- Sergent J, Corballis MC. Generation of multipart images in the disconnected cerebral hemispheres. Bulletin of the Psychonomic Society. 1990; 28 (4):309–311. [ Google Scholar ]
- Seymour SE, Reuter-Lorenz PA, Gazzaniga MS. The disconnection syndrome - basic findings reaffirmed. Brain. 1994; 117 (1):105–115. [ PubMed ] [ Google Scholar ]
- Shafto JP, Pitts MA. Neural signatures of conscious face perception in an inattentional blindness paradigm. Journal of Neuroscience. 2015; 35 (31):10940–10948. [ PMC free article ] [ PubMed ] [ Google Scholar ]
- Sierra M, Senior C, Dalton J, McDonough M, Bond A, Phillips ML, O'Dwyer AM, David AS. Autonomic response in depersonalization disorder. Archives of General Psychiatry. 2002; 59 (9):833–838. [ PubMed ] [ Google Scholar ]
- Sligte IG, Scholte HS, Lamme VAF. Are there multiple visual short-term memory stores? PLoS One. 2008; 3 (2):e1699. [ PMC free article ] [ PubMed ] [ Google Scholar ]
- Sperling G. The information available in brief visual presentations. Psychological Monographs: General and Applied. 1960; 74 (11):1–29. [ Google Scholar ]
- Sperry R. Consciousness, personal identity and the divided brain. Neuropsychologia. 1984; 22 (6):661–673. [ PubMed ] [ Google Scholar ]
- Sperry RW. Hemisphere deconnection and unity in conscious awareness. American Psychologist. 1968; 23 (10):723–733. [ PubMed ] [ Google Scholar ]
- Tononi G. An information integration theory of consciousness. BMC Neuroscience. 2004; 5 (1):42. [ PMC free article ] [ PubMed ] [ Google Scholar ]
- Tononi G. Consciousness, information integration, and the brain. Progress in Brain Research. 2005; 150 :109–126. [ PubMed ] [ Google Scholar ]
- Trevarthen C, Sperry RW. Perceptual unity of the ambient visual system in human commissurotomy patients. Brain. 1973; 96 (3):547–570. [ PubMed ] [ Google Scholar ]
- Trevarthen CB. Two mechanisms of vision in primates. Psychologische Forschung. 1968; 31 (4):299–337. [ PubMed ] [ Google Scholar ]
- Tsuchiya N, Wilke M, Frässle S, Lamme VA. No-report paradigms: Extracting the true neural correlates of consciousness. Trends in Cognitive Sciences. 2015; 19 (12):757–770. [ PubMed ] [ Google Scholar ]
- Uddin LQ. Brain connectivity and the self: The case of cerebral disconnection. Consciousness and Cognition. 2011; 20 (1):94–98. [ PMC free article ] [ PubMed ] [ Google Scholar ]
- Uddin LQ, Mooshagian E, Zaidel E, Scheres A, Margulies DS, Kelly AMC, Shehzad Z, Adelstein JS, Castellanos FX, Biswal BB, Milham MP. Residual functional connectivity in the split-brain revealed with resting-state fMRI. Neuroreport. 2008; 19 (7):703–709. [ PMC free article ] [ PubMed ] [ Google Scholar ]
- Van Wagenen WP, Herren RY. Surgical division of commissural pathways in the corpus callosum: Relation to spread of an epileptic attack. Archives of Neurology and Psychiatry. 1940; 44 (4):740. [ Google Scholar ]
- Volz LJ, Gazzaniga MS. Interaction in isolation: 50 years of insights from split-brain research. Brain. 2017; 140 (7):2051–2060. [ PubMed ] [ Google Scholar ]
- Volz LJ, Hillyard SA, Miller MB, Gazzaniga MS. Unifying control over the body: Consciousness and cross-cueing in split-brain patients. Brain. 2018; 141 (3):e15. [ PubMed ] [ Google Scholar ]
- Wolman D. A tale of two halves. Nature. 2012; 483 (7389):260–263. [ PubMed ] [ Google Scholar ]
- Zaidel, E. (1983). A response to Gazzaniga: Language in the right hemisphere, convergent perspectives. American Psychologist, 38 (5), 542–546. 10.1037/0003-066X.38.5.542 [ PubMed ]
Split-Brain: What We Know Now and Why This is Important for Understanding Consciousness
Affiliations.
- 1 Department of Psychology, University of Amsterdam, Amsterdam, the Netherlands. [email protected].
- 2 School of Psychology, University of Auckland, Auckland, New Zealand.
- 3 School of Health Sciences, University of California Dan Diego, La Jolla, CA, USA.
- 4 School of Medicine and Surgery, University of Verona, Verona, Italy.
- 5 Sackler Centre for Consciousness Science, Sussex University, Brighton, UK.
- 6 Department of Psychology, University of Amsterdam, Amsterdam, the Netherlands.
- 7 Klinik für Neurologie, Universitätsklinikum Köln, Kerpener Str, 62, Köln, Germany.
- 8 Dipartimento di Medicina Sperimentale e Clinica, Via Tronto 10/A, 60020, Ancona, Italy.
- 9 Department of Philosophy, Washington University, St. Louis, MO, USA.
- 10 Department of Philosophy, Monash University, Melbourne, Australia.
- PMID: 32399946
- PMCID: PMC7305066
- DOI: 10.1007/s11065-020-09439-3
Recently, the discussion regarding the consequences of cutting the corpus callosum ("split-brain") has regained momentum (Corballis, Corballis, Berlucchi, & Marzi, Brain, 141(6), e46, 2018; Pinto et al., Brain, 140(5), 1231-1237, 2017a; Pinto, Lamme, & de Haan, Brain, 140(11), e68, 2017; Volz & Gazzaniga, Brain, 140(7), 2051-2060, 2017; Volz, Hillyard, Miller, & Gazzaniga, Brain, 141(3), e15, 2018). This collective review paper aims to summarize the empirical common ground, to delineate the different interpretations, and to identify the remaining questions. In short, callosotomy leads to a broad breakdown of functional integration ranging from perception to attention. However, the breakdown is not absolute as several processes, such as action control, seem to remain unified. Disagreement exists about the responsible mechanisms for this remaining unity. The main issue concerns the first-person perspective of a split-brain patient. Does a split-brain harbor a split consciousness or is consciousness unified? The current consensus is that the body of evidence is insufficient to answer this question, and different suggestions are made with respect to how future studies might address this paucity. In addition, it is suggested that the answers might not be a simple yes or no but that intermediate conceptualizations need to be considered.
Keywords: Consciousness agents; Epilepsy; Lateralization; Split-brain; Visual perception.
Publication types
- Research Support, Non-U.S. Gov't
- Consciousness / physiology*
- Corpus Callosum / physiopathology
- Split-Brain Procedure*
Thank you for visiting nature.com. You are using a browser version with limited support for CSS. To obtain the best experience, we recommend you use a more up to date browser (or turn off compatibility mode in Internet Explorer). In the meantime, to ensure continued support, we are displaying the site without styles and JavaScript.
- View all journals
- Explore content
- About the journal
- Publish with us
- Sign up for alerts
- Published: 01 August 2005
Forty-five years of split-brain research and still going strong
- Michael S. Gazzaniga 1
Nature Reviews Neuroscience volume 6 , pages 653–659 ( 2005 ) Cite this article
10k Accesses
277 Citations
32 Altmetric
Metrics details
Forty-five years ago, Roger Sperry, Joseph Bogen and I embarked on what are now known as the modern split-brain studies. These experiments opened up new frontiers in brain research and gave rise to much of what we know about hemispheric specialization and integration. The latest developments in split-brain research build on the groundwork laid by those early studies. Split-brain methodology, on its own and in conjunction with neuroimaging, has yielded insights into the remarkable regional specificity of the corpus callosum as well as into the integrative role of the callosum in the perception of causality and in our perception of an integrated sense of self.
This is a preview of subscription content, access via your institution
Relevant articles
Open Access articles citing this article.
A review of combined functional neuroimaging and motion capture for motor rehabilitation
- Emanuel A. Lorenz
- , Xiaomeng Su
- & Nina Skjæret-Maroni
Journal of NeuroEngineering and Rehabilitation Open Access 03 January 2024
A frontal transcallosal inhibition loop mediates interhemispheric balance in visuospatial processing
- Yanjie Wang
- , Zhaonan Chen
- … Siyu Zhang
Nature Communications Open Access 25 August 2023
Mapping lesion, structural disconnection, and functional disconnection to symptoms in semantic aphasia
- Nicholas E. Souter
- , Xiuyi Wang
- … Elizabeth Jefferies
Brain Structure and Function Open Access 04 July 2022
Access options
Subscribe to this journal
Receive 12 print issues and online access
176,64 € per year
only 14,72 € per issue
Buy this article
- Purchase on Springer Link
- Instant access to full article PDF
Prices may be subject to local taxes which are calculated during checkout
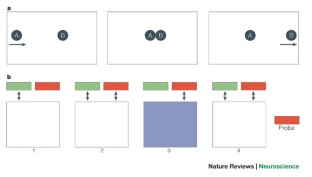
Lashley, K. S. In search of the engram. Symp. Soc. Exp. Biol. 4 , 454–482 (1950).
Google Scholar
Hebb, D. O. The Organization of Behavior: a Neuropsychological Theory (Wiley, New York, USA, 1949).
Sperry, R. W. Chemoaffinity in the orderly growth of nerve fiber patterns and connections. Proc. Natl Acad. Sci. USA 50 , 703–710 (1963).
CAS PubMed Google Scholar
Weiss, P. A. In vitro experiments on the factors determining the course of the outgrowing nerve fiber. J. Exp. Zool. 68 , 393–448 (1934).
Van Wagenen, W. P & Herren, R. Y. Surgical division of commissural pathways in the corpus callosum: relation to spread of an epileptic attack. Arch. Neurol. Psychiatry 44 , 740–759 (1940).
Bogen, J. E. & Vogel, P. J. Cerebral commissurotomy in man. Bull. Los Angel. Neuro. Soc. 27 , 169–172 (1962).
Akelaitis, A. J. A study of gnosis, praxis and language following section of the corpus callosum and anterior commissure. J. Neurosurg. 1 , 94–102 (1944).
Myers, R. E. Function of the corpus callosum in interocular transfer. Brain 79 , 358–363 (1956).
Myers, R. E. & Sperry, R. W. Interhemispheric communication through the corpus callosum: mnemonic carry-over between the hemispheres. Arch. Neurol. Psychiatry 80 , 298–303 (1958).
CAS Google Scholar
Gazzaniga, M. S. Split brain research: a personal history. Cornell Univ. Alumni Q. 45 , 2–12 (1982).
Lettvin, J. Y. 1981 Nobel prize for physiology or medicine. Science 214 , 517–520 (1981).
Gazzaniga, M. S. Cerebral specialization and interhemispheric communication: does the corpus callosum enable the human condition? Brain 123 , 1293–1326 (2000).
PubMed Google Scholar
Zaidel, E. in Handbook of Neuropsychology Vol. 4 (eds Boller, F. & Grafman, J.) 115–150 (Elsevier, Amsterdam, 1991).
Funnell, M. G., Corballis, P. M. & Gazzaniga, M. S. Handbook of Neuropsychology 2nd Edn Vol. 1 (eds Boller, F. & Grafman, J.) 103–120 (Elsevier, Amsterdam, 2000).
Milner, B. in Interhemispheric Relations and Cerebral Dominance (ed. Mountcastle, V. B.) 177–198 (Johns Hopkins Press, Baltimore, Maryland, 1962).
Zaidel, E. & Peters, A. M. Phonological encoding and ideographic reading by the disconnected right hemisphere: two case studies. Brain and Language 14 , 205–234 (1981).
Zaidel, E. in The Dual Brain (eds Benson, D. F. & Zaidel, E.) 205–231 (Guildford, New York, 1985).
Baynes, K., Eliassen, J. C., Lutsep, H. L & Gazzaniga, M. S. Modular organization of cognitive systems masked by interhemispheric integration. Science 280 , 902–905 (1998).
Nebes, R. Superiority of the minor hemisphere in commissurotomized man on a test of figural unification. Brain 95 , 633–638 (1972).
Nebes, R. Perception of spatial relationships by the right and left hemispheres of a commissurotomized man. Neuropsychologia 11 , 285–289 (1973).
Forster, B. A., Corballis, P. M. & Corballis, M. C. Effect of luminance on successiveness discrimination in the absence of the corpus callosum. Neuropsychologia 38 , 441–450 (2000).
Corballis, M. C. & Sergent, J. Imagery in a commissurotomized patient. Neuropsychologia 26 , 13–26 (1988).
Corballis, P. M., Funnell, M. G. & Gazzaniga, M. S. A dissociation between spatial and identity matching in callosotomy patients. Neuroreport 10 , 2183–2187 (1999).
Funnell, M. G., Corballis, P. M. & Gazzaniga, M. S. A deficit in perceptual matching in the left hemisphere of a callosotomy patient. Neuropsychologia 37 , 1143–1154 (1999).
Corballis, P. M., Fendrich, R., Shapley, R. & Gazzaniga, M. S. Illusory contours and amodal completion: evidence for a functional dissociation in callosotomy patients. J. Cogn. Neurosci. 11 , 459–466 (1999).
Luck, S. J., Hillyard, S. A., Mangun, G. R. & Gazzaniga, M. S. Independent hemispheric attentional systems mediate visual search in split-brain patients. Nature 342 , 543–545 (1989).
Lambert, A. J. Interhemispheric interaction in the split-brain. Neuropsychologia 29 , 941–948 (1991).
Corballis, M. C. Split decisions: problems in the interpretation of results from commissurotomized subjects. Behav. Brain Res. 64 , 163–172 (1994).
Levy, J. & Trevarthen, C. Metacontrol of hemispheric function in human split-brain patients. J. Exp. Psychol. Hum. Percept. Perform. 2 , 299–312 (1976).
Holtzman, J. D. & Gazzaniga, M. S. Dual task interactions due exclusively to limits in processing resources. Science 218 , 1325–1327 (1982).
Weissman, D. H. & Banich, M. T. The cerebral hemispheres cooperate to perform complex but not simple tasks. Neuropsychology 14 , 41–59 (2000).
Belger, A. & Banich, M. T. Costs and benefits of integrating information between the cerebral hemispheres: a computational perspective. Neuropsychology 12 , 380–398 (1998).
Banich, M. T. & Belger, A. Interhemispheric interaction: how do the hemispheres divide and conquer a task? Cortex 26 , 77–94 (1990).
Gordon, H. W., Bogen, J. E. & Sperry, R. W. Absence of deconnexion syndrome in two patients with partial section of the neocommissures. Brain 94 , 327–336 (1971).
Gazzaniga, M. S. & Freedman, H. Observations on visual processes after posterior callosal section. Neurology 23 , 1126–1130 (1973).
Risse, G. L., Gates, J., Lund, G., Maxwell, R. & Rubens, A. Interhemispheric transfer in patients with incomplete section of the corpus-callosum. Anatomic verification with magnetic resonance imaging. Arch. Neurol. 46 , 437–443 (1989).
Gazzaniga, M. S. The split brain in man. Sci. Am. 217 , 24–29 (1967).
Corballis, M. C. Visual integration in the split brain. Neuropsychologia 33 , 937–959 (1995).
Baynes, K. Language and reading in the right hemisphere: highways or byways of the brain? J. Cogn. Neurosci. 2 , 159–179 (1990).
Gazzaniga, M. S. Interhemispheric communication of visual learning. Neuropsychologia 4 , 183–189 (1966).
Seymour, S. A., Reuter-Lorenz, P. A. & Gazzaniga, M. S. The disconnection syndrome: basic findings reaffirmed. Brain 117 , 105–115 (1994).
Gazzaniga, M. S., Bogen, J. E. & Sperry, R. W. Observations on visual perception after disconnexion of the cerebral hemispheres in man. Brain 88 , 221–236 (1965).
Funnell, M. G., Corballis, P. M. & Gazzaniga, M. S. Cortical and subcortical interhemispheric interactions following partial and complete callosotomy. Arch. Neurol. 57 , 185–189 (2000).
Funnell, M. G., Corballis, P. M. & Gazzaniga, M. S. Insights into functional specificity of the human corpus callosum. Brain 123 , 920–926 (2000).
Fabri, M. et al. Posterior corpus callosum and interhemispheric transfer of somatosensory information: an fMRI and neuropsychological study of a partially callosotomized patient. J. Cogn. Neurosci. 13 , 1071–1079 (2001).
Ihori, N., Kawamura, M., Fukuzawa, K. & Kamaki, M. Somesthetic disconnection syndromes in patients with callosal lesions. Eur. Neurol. 44 , 65–71 (2000).
Arguin, M. et al. Divided visuo-spatial attention systems with total and anterior callosotomy. Neuropsychologia 15 , 295–302 (2000).
Basser, P. J. & Jones, D. K. Diffusion-tensor MRI: theory, experimental design and data analysis - a technical review. NMR Biomed. 15 , 456–467 (2002).
Basser, P. J., Mattiello, J. & LeBihan, D. Estimation of the effective self-diffusion tensor from the NMR spin echo. J. Magn. Reson. B 103 , 247–254 (1994).
Basser, P. J., Mattiello, J. & LeBihan, D. MR diffusion tensor spectroscopy and imaging. Biophys. J. 66 , 259–267 (1994).
CAS PubMed PubMed Central Google Scholar
Le Bihan, D. Looking into the functional architecture of the brain with diffusion MRI. Nature Rev. Neurosci. 4 , 469–480 (2003).
Sundgren, P. C. et al. Diffusion tensor imaging of the brain: review of clinical applications. Neuroradiology 46 , 339–350 (2004).
Chepuri, N. B. et al. Diffusion anisotropy in the corpus callosum. Am. J. Neuroradiol. 23 , 803–808 (2002).
Baird, A. A., Colvin, M. K., Van Horn, J. D., Inati, S. & Gazzaniga, M. S. Functional connectivity: integrating behavioral, DTI and fMRI data sets. J. Cogn. Neurosci. 17 , 687–693 (2005).
Warrington, E. K. & Taylor, A. M. The contribution of the right parietal lobe to object recognition. Cortex 9 , 152–164 (1973).
Humphreys, G. W., Price, C. J. & Riddoch, M. J. From objects to names: a cognitive neuroscience approach. Psychol. Res. 62 , 118–130 (1999).
Colvin, M. K., Funnell, M. G., Hahn, B. & Gazzaniga, M. S. Identifying functional channels in the corpus callosum: correlating interhemispheric transfer time with white matter organization. Poster presented at the annual meeting of the Society for Neuroscience, San Diego, California, 2004. J. Cogn. Neurosci. 139 (suppl. 5), (2005).
Aboitiz, F. & Montiel, J. One hundred million years of interhemispheric communication: the history of the corpus callosum. Braz. J. Med. Biol. Res. 36 , 409–420 (2003).
LaMantia, A. S. & Rakic, P. Cytological and quantitative characteristics of four cerebral commissures in the rhesus monkey. J. Comp. Neurol. 291 , 520–537 (1990).
Banich, M. T. The missing link: the role of interhemispheric interaction in attentional processing. Brain Cogn. 36 , 128–157 (1998).
Cabeza, R. Hemispheric asymmetry reduction in older adults: the HAROLD model. Psychol. Aging 17 , 85–100 (2002).
Colvin, M. K., Wig, G. S., Kelley, W. M., Grafton, S. T. & Gazzaniga, M. S. Callosal organization predicts the level and effect of right frontal activity during verbal encoding on subsequent memory in healthy young adults. Soc. Neurosci. Abstr. 204.4 (2005).
Colvin, M. K. Individual differences in callosal organization: relationship to interhemispheric communication and hemispheric asymmetries. Diss. Abstr. (in the press).
Leslie, A. M. & Keeble, S. Do six-month-old infants perceive causality? Cognition 25 , 265–288 (1987).
Michotte, A. The Perception of Causality (Basic Books, New York, USA, 1963) (Translated from original, published 1946).
Roser, M. E., Fugelsang, J. A., Dunbar, K. N., Corballis, P. M. & Gazzaniga, M. S. Dissociating causal perception and causal inference in the brain. Neuropsychology (in the press).
Fugelsang, J. A., Roser, M. E., Corballis, P. M., Gazzaniga, M. S. & Dunbar, K. N. Brain mechanisms underlying perceptual causality. Cogn. Brain Res. (in the press).
Turk, D. J., Heatherton, T. F., Macrae, C. N., Kelley, W. M. & Gazzaniga, M. S. Out of contact, out of mind: the distributed nature of self. Ann. NY Acad. Sci. 1001 , 65–78 (2003).
Gazzaniga, M. S. One brain — two minds? Am. Sci. 60 , 311–317 (1972).
Gazzaniga, M. S. & Smylie, C. S. Facial recognition and brain asymmetries: clues to underlying mechanisms. Ann. Neurol. 13 , 536–540 (1983).
DeRenzi, E. Prosopagnosia in two patients with CT scan evidence of damage confined to the right-hemisphere. Neuropsychologia 24 , 385–389 (1986).
Landis, T., Cummings, J. L., Christen, L., Bogen, J. E. & Imhof, H. G. Are unilateral right posterior cerebral lesions sufficient to cause prosopagnosia? Clinical and radiological findings in six additional patients. Cortex 22 , 243–252 (1986).
Michel, F., Poncet, M. & Signoret, J. L. Les lesions responsables de la prosopagnosie sont-elles toujours bilateral. Rev. Neurol. (Paris) 145 , 764–770 (1989) (in French).
Wada, Y. & Yamamoto, T. Selective impairment of facial recognition due to a haematoma restricted to the right fusiform and lateral occipital region. J. Neurol. Neurosurg. Psychiatry 71 , 254–257 (2001).
Whiteley, A. M. & Warrington, E. K. Prosopagnosia: a clinical, psychological, and anatomical study of three patients. J. Neurol. Neurosurg. Psychiatry 40 , 395–403 (1977).
Keenan, J. P., Nelson, A., O'Connor, M. & Pascual-Leone, A. Neurology: self-recognition and the right hemisphere. Nature 409 , 305 (2001).
Keenan, J. P. et al. Left hand advantage in a self-face recognition task. Neuropsychologia 37 , 1421–1425 (1999).
Keenan, J. P., Ganis, G, Freund, S. & Pascual-Leone, A. Self-face identification is increased with left hand responses. Laterality 5 , 259–268 (2000).
Conway, M. A. et al. A positron emission tomography (PET) study of autobiographical memory retrieval. Memory 7 , 679–702 (1999).
Conway, M. A. & Pleydell-Pearch, C. W. The construction of autobiographical memories in the self-memory system. Psychol. Rev. 107 , 261–288 (2000).
Kircher, T. T. et al. The neural correlates of intentional and incidental self processing. Neuropsychologia 40 , 683–692 (2002).
Maguire, E. A. & Mummery, C. J. Differential modulation of a common memory retrieval network revealed by positron emission tomography. Hippocampus 9 , 54–61 (1999).
Turk, D. J. Mike or me? Self-recognition in a split-brain patient. Nature Neurosci. 5 , 841–842 (2002).
Cooney, J. W. & Gazzaniga, M. S. Neurologic disorders and the structure of human consciousness. Trends Cogn. Sci. 7 , 161–164 (2003).
Erikson, T. C. Spread of epileptic discharge. Arch. Neurol. Psychiatry 43 , 429–452 (1940).
Gazzaniga, M. S., Bogen, J. E. & Sperry, R. W. Some functional effects of sectioning the cerebral commissures in man. Proc. Natl Acad. Sci. USA 48 , 1765–1769 (1962).
Gazzaniga, M. S. Effects of commissurotomy on a preoperatively learned visual discrimination. Exp. Neurol. 8 , 14–19 (1963).
Gazzaniga, M. S., Bogen, J. E. & Sperry, R. W. Dyspraxia following division of cerebral commissures. Arch. Neurol. 16 , 606–612 (1967).
Bogen, J. E. & Gazzaniga, M. S. Cerebral commissurotomy in man — minor hemisphere dominance for certain visuospatial functions. J. Neurosurg. 23 , 394–399 (1965).
Gazzaniga, M. S. & LeDoux, J. The Integrated Mind (Plenum, New York, USA, 1978).
Corballis, P. M. Visuospatial processing and the right-hemisphere interpreter. Brain Cogn. 53 , 171–176 (2003).
Download references
Acknowledgements
This research was supported by National Institutes of Health grants to the author. It was also supported by a graduate reseach fellowship from the National Science Foundation to M. Colvin. I would like to thank my collaborators, M. Colvin, M. Funnell, M. Roser and D. Turk, for their scientific input as well as their assistance in reviewing this paper. I would also like to thank R. Townsend for her editorial assistance.
Author information
Authors and affiliations.
the Center for Cognitive Neuroscience, 6162 Moore Hall, Dartmouth College, Hanover, 03755-3547, New Hampshire, USA
Michael S. Gazzaniga
You can also search for this author in PubMed Google Scholar
Ethics declarations
Competing interests.
The author declares no competing financial interests.
Related links
Further information.
Center for Cognitive Neuroscience
Rights and permissions
Reprints and permissions
About this article
Cite this article.
Gazzaniga, M. Forty-five years of split-brain research and still going strong. Nat Rev Neurosci 6 , 653–659 (2005). https://doi.org/10.1038/nrn1723
Download citation
Issue Date : 01 August 2005
DOI : https://doi.org/10.1038/nrn1723
Share this article
Anyone you share the following link with will be able to read this content:
Sorry, a shareable link is not currently available for this article.
Provided by the Springer Nature SharedIt content-sharing initiative
This article is cited by
- Xiaomeng Su
- Nina Skjæret-Maroni
Journal of NeuroEngineering and Rehabilitation (2024)
- Zhaonan Chen
Nature Communications (2023)
Impact of corpus callosum integrity on functional interhemispheric connectivity and cognition in healthy subjects
- Michele Porcu
- Luigi Cocco
Brain Imaging and Behavior (2023)
Impaired neurovascular coupling and cognitive deficits in anti-N-methyl-D-aspartate receptor encephalitis
- Yuanyuan Guo
- Yanghua Tian
Brain Imaging and Behavior (2022)
- Elizabeth Jefferies
Brain Structure and Function (2022)
Quick links
- Explore articles by subject
- Guide to authors
- Editorial policies
Sign up for the Nature Briefing newsletter — what matters in science, free to your inbox daily.

- Search Menu
- CNS Injury and Stroke
- Epilepsy and Sleep
- Movement Disorders
- Multiple Sclerosis/Neuroinflammation
- Neuro-oncology
- Neurodegeneration - Cellular & Molecular
- Neuromuscular Disease
- Neuropsychiatry
- Pain and Headache
- Advance articles
- Editor's Choice
- Author Guidelines
- Submission Site
- Why publish with this journal?
- Open Access
- About Brain
- Editorial Board
- Advertising and Corporate Services
- Journals Career Network
- Self-Archiving Policy
- Dispatch Dates
- Terms and Conditions
- Journals on Oxford Academic
- Books on Oxford Academic

Article Contents
Introduction, separated information processing in both hemispheres, lateralization of function, non-neural interhemispheric integration the concept of cross-cueing, the split-brain and concepts of neurological lesions, implications for understanding consciousness.
- < Previous
Interaction in isolation: 50 years of insights from split-brain research
- Article contents
- Figures & tables
- Supplementary Data
Lukas J. Volz, Michael S. Gazzaniga, Interaction in isolation: 50 years of insights from split-brain research, Brain , Volume 140, Issue 7, July 2017, Pages 2051–2060, https://doi.org/10.1093/brain/awx139
- Permissions Icon Permissions
Fifty years ago, one of the first studies that showed the neuropsychological consequences of sectioning the corpus callosum, that great bundle of fibres that connects the two cerebral hemispheres, was published in Brain ( Gazzaniga and Sperry, 1967 ). With the help of several patients who have undergone this procedure and generously given of their time as willing participants in research, a gold mine of information about the way brains function has been ferreted out. Research studies in the ensuing years have both confirmed and extended the findings, not only in the original patient group, but other groups as well. The insights gained from testing these so called ‘split-brain’ patients have contributed to the evolving field of cognitive neuroscience and have helped establish information processing models for how the brain governs behaviour and cognition.
The original ‘split-brain’ patients tested in California had undergone a complete transection of the corpus callosum and the anterior and hippocampal commissures (with some minor variance occurring between subjects) to alleviate intractable, severe epilepsy, which it did. Twenty years before, testing of another group of similar split-brain patients in Rochester, New York ( cf. Akelaitis, 1941 ) had not revealed any discernible differences between pre- and post-surgical behaviour, suggesting that not much would be learned from this new group. Using a behavioural testing device (which had not been used in New York) that allowed information to be fed to either hemisphere independent of the other, however, revealed that these patients were to provide a unique opportunity to investigate the separate functions of the two cerebral hemispheres ( Fig. 1 ).

Tachistoscope. Presenting visual stimuli with a tachistoscope allows selective presentation of visual information to one hemisphere at a time. Patients were asked to fix their gaze on the centre of the translucent screen, upon which the examiner projects visual stimuli for 0.1 s. Information projected onto the left half of the screen is subsequently processed by the right hemisphere, whereas stimuli presented in the right visual field are processed by the left hemisphere. The short presentation interval prevents visual information on one side of the screen from being processed by both hemispheres due to eye movements. Modified from Gazzaniga (2000) , with permission.
‘In general the post-surgical studies indicate a striking functional independence of the gnostic activities of the two hemispheres. Perceptual, cognitive, mnemonic, learned and volitional activities persist in each hemisphere, but can proceed separately in each case outside the realm of awareness of the other hemisphere.’
The goal of this article is to outline some of the challenges in interpreting the experience of interacting with split-brain patients. After briefly summarizing some elementary and uncontroversial findings derived from split-brain patients, we will focus on more controversial points that remain the topic of ongoing debate. In particular, we will review the concept of cross-cueing, which is a crucial and tangible reality when interpreting split-brain results. This may resonate with any reader who has had the experience of working with neurological patients.
The starting point for many split-brain experiments is to provide information to one hemisphere at a time ( Fig. 1 ). This is most easily accomplished through the visual system, thanks to its tidy anatomy ( Fig. 2 ). If you stare straight ahead at a spot, information on the right side of space perceived by both eyes will end up in the left hemisphere and information on the left side of space will end up in the right hemisphere. This is true for all of us, including our split-brain patients. Since our hemispheres are connected, it is natural for our brains to stitch the two sides together and create a unified visual world ( Gazzaniga et al. , 1965 ). Yet, for the split-brain patient with no such connection, each hemisphere sees only the opposite half of the space.

Neuroanatomical basis for processing of visual information. When fixating the centre of the screen (cross), visual information presented on the left half of the screen (blue square) is processed by neurons located in the nasal half of the retina in the left eye and lateral half of the retina in the right eye. While the latter directly project into the right hemisphere, axons of retinal neurons in the nasal half of the left eye (blue) cross from the left to the right hemisphere in the optic chiasm. As a result, visual stimuli presented to the left visual field are processed by the right hemisphere, while stimuli presented to the right visual field (red circle) are processed by the left hemisphere.
This neat separation of visual input makes it possible to provide visual information to one hemisphere of split-brain patients without the knowledge of the other hemisphere. For example, when an object is shown in the right visual field, the visual information travels to the left hemisphere and the patient is effortlessly able to name it ( Fig. 3 A). When shown to the left visual field, however, the information travels to the right hemisphere, and when asked, the patient will typically answer that no object was seen ( Fig. 3 B). This phenomenon is easily explained by the fact that most people’s speech centre is located in their left hemisphere. When the hemispheres are separated, the left will be capable of naming an object, while the right hemisphere stays mute. Moreover, the left hemisphere will also eagerly answer the question intended for the right hemisphere. When it hears the question directed to the right hemisphere asking what the object was, the left hemisphere correctly and honestly reports that it did not see anything at all.

Separated information processing. ( A ) When two different letters are presented in each visual field, the patient will report the letter projected onto the right half of the screen (‘R’, processed by the verbally dominant left hemisphere). The letter presented on the left half of the screen (‘B’, processed by the right hemisphere) is not verbally reported, but can be identified via tactile information using the left hand (controlled by the right hemisphere). ( B ) If visual stimuli are exclusively presented in the left visual field (processed by the right hemisphere), they can again be identified by the patient via tactile information from the left hand (also processed by the right hemisphere). Intriguingly, the patient will verbally report that he did not see any stimulus, due to the lack of information in the verbal left hemisphere. Modified from Sperry et al. (1969) , with permission.
Now picture yourself listening to the completely normal looking person sitting in front of you saying that he did not see the object. He sounds absolutely sure about this. One might jump to the conclusion that the right hemisphere did not perceive the stimulus. Yet this interpretation drastically changes when the right hemisphere is asked to communicate non-verbally. For example, when instructed to point out the object from a group of objects with the left hand, patients reliably identify the object that had been presented to the right hemisphere. Not just better than chance. Every time.
From an anatomical perspective, this hardly seems surprising: the right hemisphere perceives and processes the visual input and then uses its loyal henchman, the left hand, to point it out. The left hand does this because it receives its neuronal input from corticospinal fibres that originate from the right hemisphere. Phenomenologically for the onlooker, however, the observation is far more challenging: the left hand is now confidently pointing out the object that the person just categorically and confidently denied seeing. This is where things get really interesting. Ask the person why he is pointing to that object. Since the left hemisphere and its speech centre do not know what the right hemisphere saw and do not know why the left hand is pointing to a particular object, one might think that the person would once again answer correctly and honestly by admitting ignorance with a simple ‘I don’t know’. This never happens. The left hemisphere always comes up with a story about why the left hand is doing what it is doing, ‘It is pointing to the apple because I like red’. The results of this very simple experiment led to numerous questions and more testing of the split-brain patients, resulting in more intriguing answers and inferences which are well summarized by the notion of the ‘left hemisphere interpreter’ ( Fig. 4 ; for a detailed account see Gazzaniga and LeDoux, 1978 ; Gazzaniga, 2000 ).

Example of the left hemisphere interpreter. In a classic test, a chicken claw was shown to the (speaking) left hemisphere and a snow scene was shown to the (silent) right hemisphere. Patient P.S. easily picked out related pictures from a set of eight options. His left hand chose a snow shovel and his right hand chose a chicken. When asked why he had picked those particular pictures, P.S. said, ‘Oh, that’s simple. The chicken claw goes with the chicken, and you need a shovel to clean out the chicken shed’. Modified from Gazzaniga (2000) , with permission.
On the one hand, the strict separation of information processing seems to be a logical consequence of well understood basic neuroanatomy. At the same time, however, interpreting the consequences of two independent information processing systems housed in the same body challenges our intuitive understanding of fundamental aspects of psychology, such as conscious awareness of perception (when one hemisphere reports, ‘I didn’t see anything’) or agency (yet the other chooses the correct object) and causation (‘because I like red’), which ultimately led to the question how these independent systems can coexist and coordinate a single physical body despite the lack of direct, neural interaction. And there was the other nagging notion: can a flick of a knife really produce two separate-consciousness autonomous brains? If so, what exactly does that mean for, say, personal identity?
The fact that the left hemisphere jumps in to offer an explanation whenever asked, even if it does not know what its counterpart to the right is up to, may suggest that the right hemisphere is unable to process language at all. While, indeed, the right hemisphere is typically, at first, not capable of speech production, it does, however, understand both spoken and written language. Since auditory stimuli are typically processed bilaterally, the experimental design had to be adjusted to test lateralization of phoneme processing. For example, after verbally presenting a target word (perceived by both hemispheres) such as ‘chair’, a series of words was visually presented to the right hemisphere only. The left hand then successfully indicated that it recognized the target word by pointing to it ( Gazzaniga and Sperry, 1967 ). To accomplish this, the spoken word had to be interpreted by the right hemisphere in order to produce the correct response from the left hand, since only the right hemisphere could see the list of words from which to choose. In a similar fashion, the right hemisphere can also process the semantic meaning of short sentences. For example, changing the initial verbal target from a single word to a description (‘Used to tell the time’), also leads to a correct response with the left hand pointing to ‘clock’ from a list of words.
Despite the obvious dominance of the left hemisphere, various follow-up experiments have established and further characterized that both hemispheres possess the ability to process language independently. In a complementary fashion, the right hemisphere shows superior specialization for visuospatial processing, as observed in tasks involving part-whole relations, spatial relationships, apparent motion detection, mental rotation, spatial matching and mirror image discrimination (for further details see Gazzaniga, 2005 ).
More recent findings suggest that in the split-brain, the right hemisphere may be specialized to infer causality from physical interactions, whereas the left hemisphere may be involved in more abstract inference of causality ( Roser et al. , 2005 ). The right hemisphere is also better at recognizing familiar faces and human faces. The clinical observation that prosopagnosia typically occurs after lesions to the right hemisphere converges with results from split-brain research ( Turk et al. , 2002 ), as well as neuroimaging findings in both healthy subjects and neurological patients alike ( Rossion et al. , 2011 ). It also appears that the right hemisphere plays a major role in our ability to determine what the intentions of another person might be ( Young and Saxe, 2009 ). Even more startling the right hemisphere can develop speech following callosal section ( Gazzaniga et al. , 1979 , 1984 ; Baynes et al. , 1995 ).
The fact that the split-brain separately processes information in each hemisphere has been replicated numerous times for various domains and, by itself, constitutes an uncontroversial and accepted concept. The degree of hemispheric separation, however, is a topic of ongoing debate. Does surgically disconnecting (most) cortical interhemispheric fibres result in two distinct conscious systems? Are the two hemispheres each perceiving the world and processing information in a slightly different fashion, leading to two independent minds constructing and following their own respective goals?
A first objection might be that two completely separated neural systems should have trouble coordinating one body, given that each of these systems governs the motor function of half of the body. Indeed, some split-brain patients transiently experienced symptoms of an alien hand syndrome, where typically the left hand is perceived to be moving as if following its own goals with a reduced experience of agency over those movements ( Gazzaniga, 2015 ). Moreover, for some patients an intermanual conflict was observed. For example, when trying to arrange a set of blocks with both hands, one hand often undoes what the other has just arranged rather than cooperating to optimize task performance ( Gazzaniga, 2015 ). It is no surprise that the right hemisphere, with its specialized skills for visuospatial reasoning, runs circles around the left hemisphere outperforming it ‘hands down’ in this task. Yet very quickly after surgery, patients are able to walk and run while avoiding obstacles ( Holtzman et al. , 1981 ), even swim ( Gazzaniga, 2015 ), dance and play the piano ( Akelaitis, 1941 ).
Such behaviours critically rely on the coordinated interactions between the hemispheres and the movements they control. It seems almost impossible that two separated hemispheres should be able to swim or play piano, naturally leading to the question of whether the split-brain uses some alternative mysterious non-callosal pathway to transfer information. Could visual information from both hemi-fields be transferred via non-callosal fibres and used to adjust motor controls to avoid bumping into objects while walking or running? While in monkeys, visual information can indeed be exchanged between hemispheres via the anterior commissure, a similar mechanism has been ruled out in humans ( Gazzaniga, 2005 ).
A more likely explanation lies in behavioural ‘cross-cueing’ between hemispheres. A popular analogy illustrating the concept of cross-cueing lies in the coordinated behaviour displayed by conjoined twins. If two unquestionably independent brains control one body, as is the case if the conjunction is sufficiently high, we see a wonderful example of two distinct neural systems integrating information without direct pathways linking the two. Abby and Brittany Hensel are such a pair, each with different desires, likes and dislikes, and personalities. They are conjoined at the chest and torso with a single pair of arms and legs. Even though Abby controls one arm and leg and Brittany the other, they are athletically coordinated. By picking up on behavioural cues, for example when Brittany perceives a movement initiated by Abby (and vice versa), they are able to unconsciously and effortlessly coordinate their movements to a degree that allows them to do such things as play softball.
Split-brain patients might be in a related situation—in some instances only one hemisphere may have access to crucial information needed to perform a certain task. With the abundant amount of constant practice starting right after the surgery, it seems logical that split-brain patients quickly develop nuanced ways to integrate such crucial pieces of information, even in the absence of fibre bundles carrying it from one hemispheres to the other. Since patients are used to constantly relying on cross-cueing, these subtle behavioural cues, which allow them to accomplish complex behaviour, can turn into a profound problem for an experimenter who is trying to test the hemispheres in isolation.
In a manner similar to a patient with early dementia, who creatively dodges questions that would reveal his inability to recall recent events, a split-brain patient will use cueing mechanisms when faced with a task that requires integration of information between hemispheres. Neither of these patients, however, intend to trick the examiner. Their intent, like anyone’s, is simply to perform as well as they can when faced with a challenge. Over the decades, various findings seemed to support the notion of information integration across hemispheres in split-brain patients at first glance. Yet this support dissolved when meticulous re-examination prevented any possibility of cross-cueing ( Gazzaniga and Hillyard, 1971 ). Depending on the experimental design, this can be highly challenging or even impossible ( Seymour et al. , 1994 ).
Recently, Pinto et al. (2017) investigated the degree to which processing of visual information is segregated between hemispheres in two split-brain patients. In line with the canonical interpretation of independent visual processing, they observed that visual stimuli could not be compared across visual half-fields. The authors, however, also observed that some features, such as the presence or location of visual stimuli, were correctly reported throughout the entire visual field for responses obtained verbally or with either hand ( Pinto et al. , 2017 ). This seems at odds with two separated perceptual streams of information. For example, how can the patients verbally report or indicate with their right hand (both controlled by the left hemisphere) whether a visual stimulus was presented to the left visual half-field (i.e. the right hemisphere)? The authors conclude that a certain degree of information exchange has to occur between hemispheres through non-callosal fibres. They suggest that although the information is not sufficient to inform the other hemisphere about its details, there is enough to let it know if and where a stimulus was presented.
These findings can easily be explained by cross-cueing, even though the authors quickly discarded this explanation in their discussion. By characterizing cross-cueing as ‘behavioural tricks, such as touching the left hand with the right hand’ the authors reveal that they underestimate the potential range and subtlety of cueing behaviour, which has been flushed out over decades. In fact, their data and observations fall nicely in line with previous observations of non-neural communication occurring via cross-cueing.
As noted by the authors, the amount of information transferred from one hemisphere to the other by cross-cueing is limited. Accordingly, the patients answered the simple question of whether a visual stimulus was presented or not (almost) perfectly. With the more difficult question of the stimulus’s localization, the answers were not so perfect: though reported above chance level, there was a higher error rate (see Figure 2 in Pinto et al. , 2017 ). Thus, cueing binary information (stimulus/no stimulus) is easy for two separated hemispheres, even without a highly obvious manoeuvre such as touching hands. Informing the other hemisphere about the location of the stimulus is more difficult, however, as readily reflected in the increased error rates. The fact that patients localized stimuli above chance level, even in the crossed case (e.g. stimulus presented to the left hemisphere and response with left hand), can be explained by the experimental design: while an eye-tracking device made sure that a patient fixated on the centre of the screen during the presentation of the visual stimulus, the patients did not have to focus their gaze on the centre of the screen while consecutively indicating the stimulus location. Because split-brain patients have the capacity to cross-cue the location of visual stimuli by eye movements (a glance to the upper-left or right would be cue enough), this allowed them to cue the opposite hemisphere ( Gazzaniga, 1969 ).
Even without the cue of eye movements, intriguing previous data suggest that attentional capacities can be controlled by either hemisphere in split-brain patients, hence giving yet another alternative explanation for the above chance localization of visual stimuli ( Fig. 5 ; Holtzman et al. , 1981 ). For example, after a visual stimulus was exclusively perceived by the right hemisphere, it can direct the attention of the left hemisphere to the given spot in the consecutive relocation condition, by using eye movements or neural connections via collicular-cortical projections or the intact anterior commissure ( Holtzman et al. , 1981 ). In summary, cross-cueing directing hemispheric attention may well explain the findings, rendering the concluded direct inter-hemispheric transfer of visual information unnecessary. This explanation is also in perfect agreement with the observation that two stimuli simultaneously presented in different visual half-fields, could not be compared by the patients (in line with the canonical view of two independent processing systems).

Interhemispheric transfer of spatial location. In this experiment, patients were instructed to locate target stimuli by fixating them with their right eye, while the left eye was occluded. In the first condition, the target stimulus location was highlighted ( A and B ). Unsurprisingly, subjects correctly moved their right eye to the target location when the target was presented in the left visual field, processed by the right hemisphere (within-field trial). In the second between-field condition ( B ), the subject was required to move the eyes to the relative point in the right visual field (not processed by the right hemisphere). Split-brain subjects were able to do this, suggesting cross-integration of spatial information between hemispheres. In the second part of the experiment, information on the identity of the target was presented, either within the left visual field (processed by the right hemisphere, C ) or in the right visual field (not processed by the right hemisphere, D ). While patients had no problems correctly identifying the indicated target stimulus in within-field trials ( C ), they had to guess the target-identity in between-field trials ( D ), as reflected by chance-level accuracy. Hence, while crude information on the spatial localization of a stimulus can be cross-integrated between hemispheres ( B ), more complex information such as stimulus identity ( D ) is not integrated in split-brain patients. Modified from Gazzaniga (1995) , with permission.

Cross-cueing mechanism and mirror neurons
If cross-cueing indeed plays a prominent role in integrating information between hemispheres lacking direct neural connections, how does one hemisphere express content in a way that allows the other hemisphere to understand it? As mentioned above, an obvious possibility lies in initiating a motor action that is perceived by the other hemisphere, for example touching the right hand with the left or tapping a finger. But many more subtle possibilities exist. For example, some of the facial musculature is innervated bilaterally. Thus, a contraction instigated by one hemisphere can attract the other hemisphere’s attention. As discussed above, eye movements and direction of attention via subcortical pathways may be particularly suitable ways to convey the location of a stimulus.
The success of cross-cueing critically relies on the capacity of the recipient hemisphere to decipher the meaning of a given cue. This leads to the question of whether specific mechanisms are involved in the perception and interpretation of cues. Does each hemisphere possess neural circuitry that specializes in picking up, deciphering and potentially even anticipating actions initiated by the other hemisphere? A suitable candidate for this job may be mirror neurons, a set of neurons in the cortical motor system that are active each time an individual performs an action or observes another individual performing the same action ( Rizzolatti et al. , 1996 ). While the initial studies described the mirror mechanism for hand movements with neuronal representations in the ventral premotor cortex, similar neurons have been reported throughout a parieto-frontal network, reacting to a range of different actions, including movements of the mouth and face ( Rizzolatti and Sinigaglia, 2010 ). Could these specialized neurons also be activated in one hemisphere of a split-brain when it detects an action initiated by the other hemisphere? Indeed, when healthy subjects imitate actions, mirror neurons in the hemisphere not controlling the motor output show stronger activation than in the contralateral hemisphere’s network that performs the actual movement ( Aziz-zadeh et al. , 2006 ). Moreover, mirror neurons in the parietal cortex have been characterized as encoding the goal of a perceived action ( Rizzolatti and Sinigaglia, 2010 ), thus making them prominent candidates to decode action cues.
The sports’ world illuminates just how specialized the prediction of movements can be. For example, standing at bat, a skilled baseball player, unconsciously predicting a fastball’s trajectory from the pitcher’s movement, initiates his swing before the ball even leaves the pitcher’s hands. Similarly, the split-brain may rely on the mirror neuron network to become more and more efficient at interpreting and, in the case of sequences of cues, even anticipating such cues thrown to it by the other hemisphere. While this hypothesis remains pure speculation, it may explain how split-brain patients become more adept at using cross-cueing over time and some have even gained the capacity to produce simple speech, such as one-word utterances, from the formerly mute right hemisphere ( cf. Gazzaniga, 2000 ).
How could that formerly mute right hemisphere possibly learn to speak? This skill can emerge years after surgery in some patients and may partially rely on neural plasticity in the right hemisphere. As discussed above, the right hemisphere understands words and hence readily represents their semantic meaning. What could be holding back the right hemisphere’s verbal floodgates may be that it lacks the capacity to coordinate muscle activation in order to produce intelligible speech. Over those intervening years, every time a split-brain patient uses the left hemisphere to speak, the right hemisphere will perceive both intonation-related movements in the thorax, neck and face, and the auditory result. Using the capacity of the mirror neuron system, the right hemisphere might be able to emulate movements to produce speech-related motor output itself. Support for this hypothesis stems from the observation that some ‘audiovisual’ mirror neurons discharge both when seeing or hearing an action, such as when ripping paper or snapping a stick in two ( Kohler et al. , 2002 ). Such neurons may help to evolve the skill to generate motor commands that result in production of simple speech. How difficult it must be to accomplish this complex task is clear to anyone who has tried to speak a foreign language with a perfect accent, a major challenge even with both hemispheres on the job.
Beyond the insights into the functional specialization of the hemispheres and how much hemispheric integration is necessary to produce behaviour, the split-brain also offers a unique perspective on our understanding of brain lesions. In 1965, Norman Geschwind published his seminal paper entitled ‘ Disconnexion syndromes in animals and man ’ ( Geschwind, 1965 ), which reinvigorated the much older idea that the disconnection of communication pathways may lead to specific patterns of functional impairment, introduced by Karl Wernicke (1874). The prototypical example for a disconnection syndrome is conduction aphasia, where a person understands what they hear, can speak fluently, but may use the wrong words or parts of words and has difficulty or is unable to repeat spoken phrases. This condition is produced by lesions to the bundle of neural fibres connecting Broca’s area, which is responsible for the motor component of language and Wernicke’s area, responsible for the sensory component of language. Thus, the clinical observation linking lesions in communication pathways to specific deficits presented neuroscience a path worth pursuing, paving the way for the concept of distributed functional networks, a hot topic in contemporary neuroscience (for a review see Catani and ffytche, 2005 ).
While the split-brain is clearly an example of a disconnexion syndrome, it provides an opportunity that other examples of disconnection syndromes do not. This is the opportunity to study the presence of mental capacities, not the absence of mental capacity caused by lesions ( Gazzaniga, 2015 ). For example, in some patients, the corpus callosum was surgically sectioned in stages over a period of months, in the hope that the patient’s seizures could be controlled without sectioning the entire structure. Testing patients throughout this process revealed the functional organization of the corpus callosum: the more posterior regions transfer basic sensory information that relates to vision, audition and somatosensory information, while anterior regions are involved in the transfer of attentional resources and higher cognitive information ( cf. Gazzaniga, 2005 ). Moreover, split-brain research led to the development of several methodological advances that derived from questions specifically occurring in split-brain patients. One such question lies in accurately assessing the surgical result of the sectioning, that is, the actual extent of the corpus callosum sectioning. This led to the development of a specific neuroimaging approach that allows one to assess the extent of callosal disconnection in split-brain patients ( Gazzaniga et al. , 1985 ; Corballis et al. , 2001 ) and callosal lesions due to all kinds of pathologies ( Fig. 6 ).
![research paper on split brain Imaging the corpus callosum. The necessity to determine the extent of the callosotomy in split-brain patients motivated the advancement of neuroimaging methodology to investigate if the corpus callosum was entirely resected or if residual fibres allow information transfer between hemispheres. The first assessment of a split-brain patient via MRI in 1985 suggested two remaining interhemispheric connections in the anterior and posterior end of the corpus callosum (bright spots in white boxes). Reassessment of the same patient with advanced imaging technology (higher spatial resolution and 3D acquisition) in 2001 confirmed the remaining anterior connection, while showing that the posterior fibres were clearly severed. Modern imaging techniques allow reconstruction of callosal fibres from diffusion imaging data [diffusion spectrum imaging (DSI)] and hence a more direct assessment of corpus callosum integrity. Modified from Corballis et al. (2001), with permission.](https://oup.silverchair-cdn.com/oup/backfile/Content_public/Journal/brain/140/7/10.1093_brain_awx139/2/m_awx139f6.jpeg?Expires=1716321704&Signature=InGvJ3E2G50MwJ3WHpZj-E57Q2L9bScup5ttTcR19YJfBfmZUqhw80bnx9pHnDyBEJLjUZcwtalInct22tIanhfQXdSgnRbYUfLIYb6455YMa9-92zcsaNau0KA8~dFqtlKOJzJuTFn-KxS2lT1O3bdwk-5iCV5TR3Yh41qGpL7gj6J2-CtkK46kcsJsz6yGj5IL77xFl661-hhsbFuFXAzydoVdcaR5WPLhQl9s-xdJThvp4HkJUIU-Nva2PLDIVQ5JfyDnwtKeVDPCP6yuLYWiJBuY3RLmTlVVs4XQufuSz7CujgKUdp1TtcZ8D1AiPEwnUZmOB2tD0hu1VMcS7w__&Key-Pair-Id=APKAIE5G5CRDK6RD3PGA)
Imaging the corpus callosum. The necessity to determine the extent of the callosotomy in split-brain patients motivated the advancement of neuroimaging methodology to investigate if the corpus callosum was entirely resected or if residual fibres allow information transfer between hemispheres. The first assessment of a split-brain patient via MRI in 1985 suggested two remaining interhemispheric connections in the anterior and posterior end of the corpus callosum (bright spots in white boxes). Reassessment of the same patient with advanced imaging technology (higher spatial resolution and 3D acquisition) in 2001 confirmed the remaining anterior connection, while showing that the posterior fibres were clearly severed. Modern imaging techniques allow reconstruction of callosal fibres from diffusion imaging data [diffusion spectrum imaging (DSI)] and hence a more direct assessment of corpus callosum integrity. Modified from Corballis et al. (2001) , with permission.
Besides the various insights on aspects of functional specialization of the hemispheres or the functional anatomy of the corpus callosum that were obtained from split-brain work, these extraordinary cases of separated hemispheres raise an even more general question: how much integration of information between specialized brain modules is necessary to give rise to our skilled behaviour and to create our unique experience of the world around us? It seems puzzling that the verbal IQ and problem solving capacities of split-brain patients are typically unaffected by the surgery. Moreover, patients do not report any difference in the nature of their personal experience—despite the fact that their hemispheres are separated, they report that they experience a single consciousness ( cf. Gazzaniga, 2000 ). Not surprisingly, theoretical frameworks of consciousness often include the split-brain as a test-case for their respective theory. Yet claims of support are made regardless of whether conscious experience is interpreted to result from the integration of regional resources, as in the Global Workspace Theory ( cf. Baars, 1997 ) or the Information Integration Theory ( cf. Tononi and Koch, 2015 ) or, in contrast, is hypothesized to stem from focal activity, as suggested by the local recurrent processing theory of consciousness for example ( cf. Lamme, 2006 ).
A set of observations from split-brain experiments may be particularly suitable to inform such theoretical frameworks of consciousness. In several domains of problem-solving, the left hemisphere shows fundamentally different strategic tendencies compared to the right hemisphere. For example, the right hemisphere adheres to factual knowledge when asked to identify previously presented stimuli and thus outperforms the left hemisphere, which falsely recognizes similar yet unseen objects ( Phelps and Gazzaniga, 1992 ). This observation is in line with the notion that the left hemisphere ‘gets the gist’ and tends to integrate information into theories, which can help to predict future events and offer a coherent interpretative framework. Interpretive qualities unique to the left hemisphere were also observed in a probability-guessing paradigm ( Wolford et al. , 2000 ) where it tries to find patterns, i.e. a ‘theory’ in random events. The left hemisphere is not shy to interpret the behaviour of or physiological responses evoked by emotional stimuli presented to the right hemisphere, even when it is bound to fail to come up with a veridical story due to the lack of critical information exclusively present in the right hemisphere. Why would the left hemisphere interpreter bother to do so? By constantly offering explanations for what it perceives, the left hemisphere interpreter may generate a feeling in all of us that we are integrated and unified ( Gazzaniga, 2000 ). Hence, the interpretive function that strings events together to form our seemingly coherent autobiographies is hosted by the left hemisphere.
Of course, the distinct interpretive capacities of both hemispheres are but a small piece in the puzzle of deciphering the neurobiological foundations that give rise to our conscious experience of the world. These findings also intriguingly illustrate the vast scope of impactful insights that can be gained from the persistent study of a unique group of neurological patients.
L.J.V. and M.S.G. thankfully acknowledge funding by the SAGE Center for the Study of the Mind, University of California.
Akelaitis AJ . Psychobiological studies following section of the corpus callosum. A preliminary report . Am J Psychiatry 1941 ; 97 : 1147 – 57 .
Google Scholar
Aziz-zadeh L , Koski L , Zaidel E , Mazziotta J , Iacoboni M . Lateralization of the human mirror neuron system . J Neurosci 2006 ; 26 : 2964 – 70 .
Baars BJ . In the Theater of Consciousness: Global Workspace Theory, A Rigorous Scientific Theory of Consciousness . Journal of Consciousness Studies 1997 ; 4 : 292 – 309 .
Baynes K , Wessinger CM , Fendrich R , Gazzaniga MS . The emergence of the capacity to name left visual field stimuli in a callosotomy patient: implications for functional plasticity . Neuropsychologia 1995 ; 33 : 1225 – 42 .
Catani M , ffytche DH . The rises and falls of disconnection syndromes . Brain 2005 ; 128 : 2224 – 39 .
Corballis PM , Inati S , Funnell MG , Grafton ST , Gazzaniga MS . MRI assessment of spared fibers following callosotomy: a second look . Neurology 2001 ; 57 : 1345 – 6 .
Gazzaniga MS . Cross-cuing mechanisms control and ipsilateral monkeys eye-hand in split-brain monkeys . Exp Neurol 1969 ; 23 : 11 – 17 .
Gazzaniga MS . Principles of human brain organization derived from split-brain studies . Neuron 1995 ; 14 : 217 – 28 .
Gazzaniga MS . Cerebral specialization and interhemispheric communication: does the corpus callosum enable the human condition? Brain 2000 ; 123 : 1293 – 326 .
Gazzaniga MS . Forty-five years of split-brain research and still going strong . Nat Rev Neurosci 2005 ; 6 : 653 – 9 .
Gazzaniga MS . Tales from both sides of the brain: a life in neuroscience . New York : Ecco/HarperCollins ; 2015 .
Google Preview
Gazzaniga MS , Bogen JE , Sperry RW . Observations on visual perception after disconnexion of the cerebral hemispheres in man . Brain 1965 ; 88 : 221 – 36 .
Gazzaniga MS , Hillyard SA . Language and speech capacity of the right hemisphere . Neuropsychologia 1971 ; 9 : 273 – 80 .
Gazzaniga MS , Holtzman JD , Deck MD , Lee BC . MRI assessment of human callosal surgery with neuropsychological correlates . Neurology 1985 ; 35 : 1763 – 6 .
Gazzaniga MS , LeDoux JE . The integrated mind . New York : Plenum Press ; 1978 .
Gazzaniga MS , Smylie CS , Baynes K , Hirst W , McCleary C . Profiles of right hemisphere language and speech following brain bisection . Brain Lang 1984 ; 22 : 206 – 20 .
Gazzaniga MS , Sperry RW . Language after section of the cortical commissures . Brain 1967 ; 90 : 131 – 48 .
Gazzaniga MS , Volpe BT , Smylie CS , Wilson DH , Le Doux JE . Plasticity in speech organization following commissurotomy . Brain 1979 ; 102 : 805 – 15 .
Geschwind N . Disconnection syndromes in animals and man . Brain 1965 ; 88 : 237 – 644 .
Holtzman JD , Sidtis JJ , Volpe BT , Wilson DH , Gazzaniga MS . Dissociation of spatial information for stimulus localization and the control of attention . Brain 1981 ; 104 : 861 – 72 .
Kohler E , Keysers C , Umiltà MA , Fogassi L , Gallese V , Rizzolatti G . Hearing sounds, understanding actions: action representation in mirror neurons . Science 2002 ; 297 : 846 – 8 .
Lamme VAF . Towards a true neural stance on consciousness . Trends Cogn Sci 2006 ; 10 : 494 – 501 .
Phelps EA , Gazzaniga MS . Hemispheric differences in mnemonic processing: the effects of left hemisphere interpretation . Neuropsychologia 1992 ; 30 : 293 – 7 .
Pinto Y , Neville DA , Otten M , Corballis PM , Lamme VAF , de Haan EHF et al. Split brain: divided perception but undivided consciousness . Brain 2017 : pii : aww358 .
Rizzolatti G , Fadiga L , Gallese V , Fogassi L . Premotor cortex and the recognition of motor actions . Cogn Brain Res 1996 ; 3 : 131 – 41 .
Rizzolatti G , Sinigaglia C . The functional role of the parieto-frontal mirror circuit: interpretations and misinterpretations . Nat Rev Neurosci 2010 ; 11 : 264 – 74 .
Roser ME , Fugelsang JA , Dunbar KN , Corballis PM , Gazzaniga MS . Dissociating processes supporting causal perception and causal inference in the brain . Neuropsychology 2005 ; 19 : 591 – 602 .
Rossion B , Dricot L , Goebel R , Busigny T . Holistic face categorization in higher order visual areas of the normal and prosopagnosic brain: toward a non-hierarchical view of face perception . Front Hum Neurosci 2011 ; 4 : 225 .
Seymour SE , Reuter-lorenz PA , Gazzaniga MS . The disconnection syndrome: Basic findings reaffirmed . Brain 1994 ; 117 : 105 – 15 .
Sperry RW , Gazzaniga MS , Bogen JE . Interhemispheric relationships: the neocortical commissures; syndromes of hemisphere disconnection . Handb Clin Neurol 1969 ; 4 : 273 – 90 .
Tononi G , Koch C . Consciousness: here, there and everywhere? Phil Trans R Soc B 2015 ; 370 : 20140167 .
Turk DJ , Heatherton TF , Kelley WM , Funnell MG , Gazzaniga MS , Macrae CN . Mike or me? Self-recognition in a split-brain patient . Nat Neurosci 2002 ; 5 : 841 – 2 .
Wernicke C . The aphasia symptom-complex. 1874. Breslau, Cohn and Weigert . Translated in: Eling P, editor. Reader in the history of aphasia. Vol. 4. Amsterdam: John Benjamins; 1994. p. 69–89 .
Wolford G , Miller MB , Gazzaniga MS . The left hemisphere’s role in hypothesis formation . J Neurosci 2000 ; 20 : RC64 .
Young L , Saxe R . Innocent intentions: a correlation between forgiveness for accidental harm and neural activity . Neuropsychologia 2009 ; 47 : 2065 – 72 .
Email alerts
Citing articles via, looking for your next opportunity.
- Contact the editorial office
- Guarantors of Brain
- Recommend to your Library
Affiliations
- Online ISSN 1460-2156
- Print ISSN 0006-8950
- Copyright © 2024 Guarantors of Brain
- About Oxford Academic
- Publish journals with us
- University press partners
- What we publish
- New features
- Open access
- Institutional account management
- Rights and permissions
- Get help with access
- Accessibility
- Advertising
- Media enquiries
- Oxford University Press
- Oxford Languages
- University of Oxford
Oxford University Press is a department of the University of Oxford. It furthers the University's objective of excellence in research, scholarship, and education by publishing worldwide
- Copyright © 2024 Oxford University Press
- Cookie settings
- Cookie policy
- Privacy policy
- Legal notice
This Feature Is Available To Subscribers Only
Sign In or Create an Account
This PDF is available to Subscribers Only
For full access to this pdf, sign in to an existing account, or purchase an annual subscription.
One Head, Two Brains
How a radical epilepsy treatment in the early 20th century paved the way for modern-day understandings of perception, consciousness, and the self
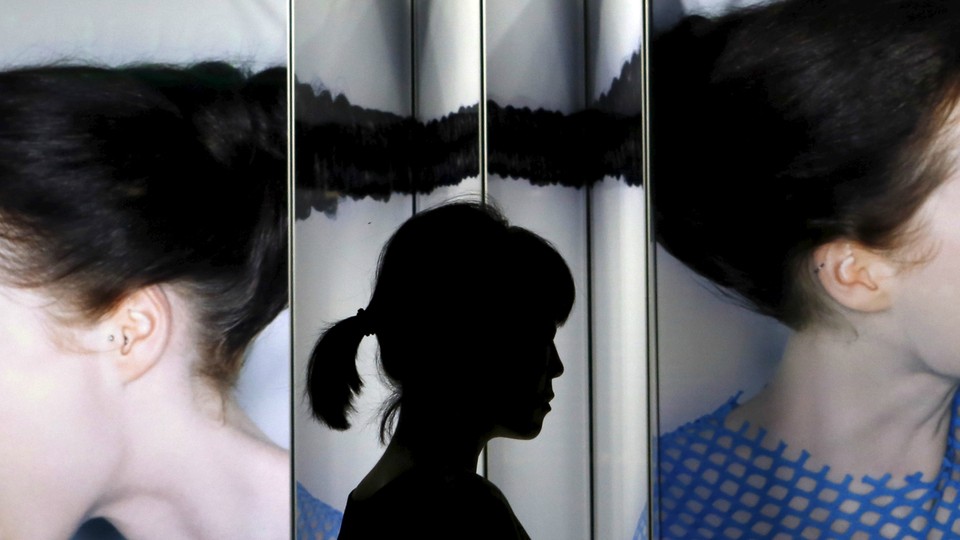
In 1939, a group of 10 people between the ages of 10 and 43, all with epilepsy, traveled to the University of Rochester Medical Center, where they would become the first people to undergo a radical new surgery.
The patients were there because they all struggled with violent and uncontrollable seizures. The procedure they were about to have was untested on humans, but they were desperate—none of the standard drug therapies for seizures had worked.
Between February and May of 1939, their surgeon William Van Wagenen, Rochester’s chief of neurosurgery, opened up each patient’s skull and cut through the corpus callosum, the part of the brain that connects the left hemisphere to the right and is responsible for the transfer of information between them. It was a dramatic move: By slicing through the bundle of neurons connecting the two hemispheres, Van Wagenen was cutting the left half of the brain away from the right, halting all communication between the two.
In a paper he and a colleague published in the Journal of the American Medical Association in 1940, Van Wagenen explained his reasoning: He had developed the idea for the surgery after observing two epilepsy patients with brain tumors located in the corpus callosum. The patients had experienced frequent convulsive seizures in the early stages of their cancer, when the tumors were still relatively small masses in the brain—but as the tumors grew, they destroyed the corpus callosum, and the seizures eased up.
“In other words, as the corpus callosum was destroyed, generalized convulsive seizures became less frequent,” Van Wagenen wrote in the 1940 paper, noting that “as a rule, consciousness is not lost when the spread of the epileptic wave is not great or when it is limited to one cerebral cortex.” Based on the cases of the cancer patients—and some other clinical observations —Van Wagenen believed that destroying the corpus callosum of his patients would block the spread of the electrical impulses that lead to seizures, so that a seizure that began in the left hemisphere, for example, stayed in the left hemisphere.
The surgery worked for most of the patients : In his paper, Van Wagenen reported that seven of the 10 experienced seizures that were less frequent or less severe.
Between 1941 and 1945, Van Wagenen’s colleague, the University of Rochester psychiatrist A. J. Akelaitis, tested the patients to see if they had experienced any cognitive or behavioral changes as a result of the invasive procedure. After giving the patients a series of assessments—an I.Q. test, a memory test, motor-skills assessments, and interviews—he reported that most of the patients had the same levels of cognitive functioning after the surgery as before, and displayed no behavioral or personality changes. Though the brain hemispheres of split-brain patients had been disconnected, he wrote in a 1944 paper in the Journal of Neurosurgery , they were otherwise normal.
Or so it seemed.
When Michael Gazzaniga first learned about the Rochester patients as an undergraduate research intern in 1960, he was curious—and skeptical.
Gazzaniga’s timing was fortuitous: Roger Sperry, who headed the neuroscience lab where Gazzaniga worked at the California Institute of Technology, had begun split-brain research on cats and monkeys just a few years earlier. Sperry found that severing the corpus callosum of those animals had affected their behavior and cognitive functioning.
In one experiment with split-brain cats, for example, Sperry would cover one of the animal’s eyes and then teach it to differentiate between a triangle and a square. Once the cats learned to do that, Sperry switched the covering from one eye to the other and tested the them to see if they recalled their new knowledge. They didn’t. “The split-brain cat,” as one neurosurgeon wrote in an overview of Sperry’s work, “has to learn all over again.” As Sperry noted, this suggested that the two hemispheres were not communicating with each other, and that each was learning the task on its own.
If the Rochester patients’ left and right brains were also no longer communicating, Sperry and his colleagues believed, then they must be experiencing some sort of change, too.
The question was still bothering Gazzaniga by the time he returned to Sperry’s lab as a graduate student in 1961: What kind of change was it? Would human brains react the same way as those of the animals in Sperry’s lab?
“In monkeys,” Gazzaniga told me, “sectioning the corpus callosum led to the right hand not knowing what the left hand was doing. I wanted to know if we would see a similar result in humans.”
The researchers didn’t have to wait long to begin looking for the answer. In the summer of 1961, as Gazzaniga was preparing to return to Sperry’s lab as a graduate student, a young neurosurgeon at Caltech named Joseph Bogen approached Sperry about the opportunity to study a split-brain patient—and Sperry, who had been working exclusively with animals, seized the chance to work on his first human case.
The patient Bogen had in mind was a man in his late forties named William Jenkins, a World War II veteran who had been hit in the head with the butt of a German officer’s rifle after parachuting behind enemy lines. Jenkins’ doctors believed that this was the likely origin of the uncontrollable seizures he later developed; when he returned to the U.S. after the war and sought treatment, he discovered that no drugs worked to contain the seizures.
In 1961, as a last-ditch effort, Bogen suggested that he have split-brain surgery. Sperry assigned Gazzaniga to conduct some standard pre-operative neurological tests, and Bogen and a colleague performed the procedure in February of 1962. After a few months of post-surgery monitoring, Bogen found that the severity and frequency of Jenkins’ seizures had abated, but he still did not know if the surgery had produced other unintended consequences. So about a month after the surgery, Bogen sent Jenkins to Sperry and Gazzaniga for cognitive testing. In doing so, he kicked off a line of work that would turn the two men into pioneers of split-brain research, eventually earning Sperry a share of the Nobel Prize in 1981—and causing scientists to reconsider long-held ideas about the brain and the self.
The cognitive tests performed on the 10 original Rochester patients hadn’t tested each brain hemisphere separately; believing that this was one reason why the patients hadn’t shown any changes after surgery, Sperry and Gazzaniga decided to run tests for both the left and right sides of Jenkins’s brain.
Recommended Reading

The Personality Trait That Makes People Feel Comfortable Around You

Loving Your Job Is a Capitalist Trap

The Seven Habits That Lead to Happiness in Old Age
In one of the first split-brain studies that the pair designed, published in August 1962 in the Proceedings of the National Academy of Sciences , Gazzaniga invited Jenkins into the lab and had him stare straight ahead at a dot. As he was staring ahead, Gazzaniga flashed a picture of a square on a screen to the right of where his eyes were staring, meaning the image would be processed by Jenkins’ left brain. ( Because of the way the brain is wired , if a patient looks straight ahead, something quickly flashed to the left of his gaze will be processed by the right side of the brain, and vice versa. The brain’s hemispheres control activity mainly on the opposite side of the body—the left hemisphere controls the action of the right hand, for example, while the right hemisphere moves the left hand.)
When Gazzaniga asked Jenkins what he saw, Jenkins was able to describe the square. Then Gazzaniga tried the same thing on the other side, flashing the same image to the left of Jenkins’ gaze. When he asked Jenkins again what he saw, though, Jenkins said he saw nothing.
Intrigued, Gazzaniga pulled another image, this time of a circle, to flash on Jenkins’s right and left sides separately, as he had done with the square.
Instead of asking Jenkins to describe the object, though, he asked him to point to it. When the image was on Jenkins’ right side (left brain), he lifted his right hand (controlled by the left brain) to point to it. When the circle flashed on his left side (right brain), he lifted his left hand (controlled by the right brain) to point to it.
The fact that Jenkins was able to point to the circle with both hands told Gazzaniga that each of Jenkins’ hemispheres had processed the sight of the circle. It also meant that in the previous trial, both of Jenkins’s hemispheres had processed the square—even though Jenkins said, when his right brain processed the sight, that he saw nothing. At that point, scientists had known for about a century that language arises from the left hemisphere; given that, the researchers later reasoned, Jenkins could only talk about the square when its picture was flashed to his right eye (left brain). On the other side, even though Jenkins had seen the square, he could not speak about it.
Between 1962 and 1967, Sperry and Gazzaniga worked together to perform dozens of additional experiments with Jenkins and other split-brain patients. In one set of studies conducted in 1962 and 1963, Gazzaniga presented Jenkins with four multicolored blocks. Then, he showed Jenkins a picture of the blocks arranged in a certain order, and asked him to make the same arrangement with the blocks in front of him.
Because the right brain handles visual-motor capacity, Gazzaniga was unsurprised to see that Jenkins’ right hemisphere excelled at this task: Using his left hand, Jenkins was immediately able to arrange the blocks correctly. But when he tried to do the very same task with his right hand, he couldn’t. He failed, badly.
“It couldn’t even get the overall organization of how the blocks should be positioned, in a 2x2 square,” Gazzaniga later wrote of Jenkins’ left hemisphere in his memoir, Tales from Both Sides of the Brain . “It just as often would arrange them in a 3+1 shape.”
But more surprising was this: As the right hand kept trying to get the blocks to match up to the picture, the more capable left hand would creep over to the right hand to intervene, as if it realized how incompetent the right hand was. This occurred so frequently that Gazzaniga eventually asked Jenkins to sit on his left hand so it wouldn’t butt in.
When Gazzaniga let Jenkins use both hands to solve the problem in another trial, he again saw the two brain hemispheres at odds with one another. “One hand tried to undo the accomplishments of the other,” he wrote. “The left hand would make a move to get things correct and the right hand would undo the gain. It looked like two separate mental systems were struggling for their view of the world.”
The more information the split-brain researchers discovered, the more they wondered: If the two sides of the brain functioned so independently of each other, how do people—ordinary people and split-brain patients alike—experience a single, cohesive reality?
In a 1977 study with a 15-year-old split-brain patient from Vermont identified as P. S., Gazzaniga (then a professor at Dartmouth) and his graduate assistant Joseph LeDoux performed a visual test similar to the one Jenkins had undergone years earlier. The researchers asked P. S. to stare straight ahead at a dot, and then flashed a picture of a chicken foot to the brain’s left hemisphere and a picture of a snowy scene to the brain’s right hemisphere. Directly in front of the patient—so that he could process the sight with both hemispheres—was a series of eight other pictures. When the researchers asked him to point to the ones that went with the images he saw, P. S. pointed to the picture of a chicken head and a picture of a snow shovel.
So far, the results were as expected: Each hemisphere had led P. S. to choose an image that went along with the one that he had seen from that side moments earlier. The surprise came when the researchers asked him why he chose these two totally unrelated images.
Because the left hemisphere, which controls language, had not processed the snowy scene, they believed P. S. wouldn’t be able to verbally articulate why he chose the snow shovel. “The left brain doesn’t know why,” Gazzaniga told me. “That information is in the right hemisphere.” Neither hemisphere knew what the other had seen, and because the two sides of his brain were unable to communicate, P.S. should have been confused when Gazzaniga asked him why he had picked the two images he did.
But as Gazzaniga recalled in his memoir, P. S. didn’t skip a beat: “Oh, that’s simple,” the patient told them. “The chicken claw goes with the chicken, and you need a shovel to clean out the chicken shed.”
Here’s what happened, as the researchers later deduced: Rather leading him to simply say, “I don’t know” to Gazzaniga’s question, P.S.’s left brain concocted an answer as to why he had picked those two images. In a brief instant, the left brain took two unconnected pieces of information it had received from the environment—the two images—and told a story that drew a connection between them.
Gazzaniga went on to replicate the findings of this study many times with various co-authors: When faced with incomplete information, the left brain can fill in the blanks. Based on these findings Gazzaniga developed the theory that the left hemisphere is responsible for our sense of psychological unity—the fact that we are aware of and reflect upon what is happening at any given moment.
“It’s the part of the brain,” Gazzaniga told me, “that takes disparate points of information in and weaves them into a storyline and meaning. That it’s central gravity.”
In addition to answering questions of brain specialization, split-brain research also examined some of the ways in which the left and right hemispheres are autonomous agents. Jenkins’ left and right hands started fighting over how to arrange the blocks, for example, because the two hemispheres are—as Gazzaniga told me—“two separate minds, all in one head.”
As he further explained in Tales from Both Sides of the Brain : “The notion that there is an ‘I’ or command center in the brain was an illusion.”
Among psychologists, the idea wasn’t exactly new; figures like Sigmund Freud and William James had previously theorized about a “divided self,” with Freud arguing that the mind is divided into the ego, the superego, and the id. But split-brain research was arguably one of the first scientific demonstrations that the divided self has a real, physical basis—a demonstration that, in turn, raised new questions about the relationship between the mind and the brain.
“The demonstration that you could in effect split consciousness by splitting anatomy—by just making a tiny change in anatomy … It was one of the most remarkable results in neuroscience, with huge implications,” said Patricia Churchland, a philosopher at the University of California, San Diego, whose work focuses on the relationship between philosophy and neuroscience. “If you thought that consciousness and mental states were independent of the brain, then this should have been a real wake-up call.”
Helping to illuminate the relationship between the mind and the brain, according to the cognitive psychologist Steven Pinker, is one of split-brain research’s most important contributions to modern psychology and neuroscience. “The fact that each hemisphere supports its own coherent, conscious stream of thought highlights that consciousness is a product of brain activity,” he told me. “The notion that there is a single entity called consciousness , without components or parts, is false.”
Today’s therapies for seizures are more advanced than those of the mid-20th century, and split-brain surgery is now exceedingly rare —Michael Miller, a neuroscientist at the University of California at Santa Barbara who did graduate work with Gazzaniga, told me the last one he heard of was performed around 10 years ago. Many of the split-brain patients that Gazzaniga, Sperry, and their colleagues studied have passed away.
Though the research on split-brain patients has slowed dramatically, Miller believes that the field still has something left to offer. He’s currently working on a study currently working with a patient to answer the question: Does each hemisphere of the brain reflect on and evaluate itself in a unique way?
“We know that the two hemispheres have different strategies for thinking,” Miller told me, “and we’re curious about how that might change their reflection of themselves. Does the left hemisphere think of itself as a sad person while the right one think of itself as a happy person? We are having each hemisphere evaluate itself to find out.”
Miller’s study uses a test called the “trait-judgment task”: A trait like happy or sad flashes on a screen, and research subjects indicate whether the trait describes them. Miller has slightly modified this task for his split-brain patients—in his experiments, he flashes the trait on a screen straight in front of the subject’s gaze, so that both the left and right hemispheres process the information. Then, he quickly flashes the words “me” and “not me” to one side of the subject’s gaze—so that they’re processed only by one hemisphere—and the subject is instructed to point at the trait on the screen when Miller flashes the appropriate descriptor. (For example, if the screen reads “happy,” an unhappy left hemisphere would lead a subject to point when Miller flashes “not me” to the right side of the subject’s gaze, and to stay still when he flashes “me.”) If the subject reacts differently on each side—in this example, if the subject points to the screen when “me” is flashed to the right hemisphere—then Miller believes there must be a disconnect between the self-concept contained in each side of the brain.
Miller’s research is ongoing. But, he said, if the study finds that each hemisphere evaluates itself differently from the other, it could add a new layer of understanding to how divided the mind really is.
“Split-brain patients give you a unique glimpse into a state of consciousness you wouldn’t see otherwise,” Miller told me.
“There is something quite unique in interacting with a split-brain patient,” he added. “All the interactions you are engaging in are with left hemisphere, and you can suddenly manipulate things to interact with right hemisphere and it’s a completely different experience. A completely different consciousness.”
Final dates! Join the tutor2u subject teams in London for a day of exam technique and revision at the cinema. Learn more →
Reference Library
Collections
- See what's new
- All Resources
- Student Resources
- Assessment Resources
- Teaching Resources
- CPD Courses
- Livestreams
Study notes, videos, interactive activities and more!
Psychology news, insights and enrichment
Currated collections of free resources
Browse resources by topic
- All Psychology Resources
Resource Selections
Currated lists of resources
Study Notes
Biopsychology: Hemispheric Lateralisation & Split Brain Research
Last updated 10 Apr 2017
- Share on Facebook
- Share on Twitter
- Share by Email
Lateralisation is the idea that the two halves of the brain are functionally different and that each hemisphere has functional specialisations, e.g. the left is dominant for language, and the right excels at visual motor tasks.
The two hemispheres are connected through nerve fibres called the corpus callosum, which facilitate interhemispheric communication: allowing the left and right hemispheres to ‘talk to’ one another.
Split-Brain Research
Sperry and Gazzaniga (1967) were the first to investigate hemispheric lateralisation with the use of split-brain patients.
Background: Split-brain patients are individuals who have undergone a surgical procedure where the corpus callosum, which connects the two hemispheres, is cut. This procedure, which separates the two hemispheres, was used as a treatment for severe epilepsy.
Aim: The aim of their research was to examine the extent to which the two hemispheres are specialised for certain functions.
Method: An image/word is projected to the patient’s left visual field (which is processed by the right hemisphere) or the right visual field (which is processed by the left hemisphere). When information is presented to one hemisphere in a split-brain patient, the information is not transferred to the other hemisphere (as the corpus callosum is cut).

Sperry and Gazzaniga conducted many different experiments, including describe what you see tasks, tactile tests, and drawing tasks.
In the describe what you see task, a picture was presented to either the left or right visual field and the participant had to simply describe what they saw.
In the tactile test , an object was placed in the patient’s left or right hand and they had to either describe what they felt, or select a similar object from a series of alternate objects.
Finally, in the drawing task , participants were presented with a picture in either their left or right visual field, and they had to simply draw what they saw.
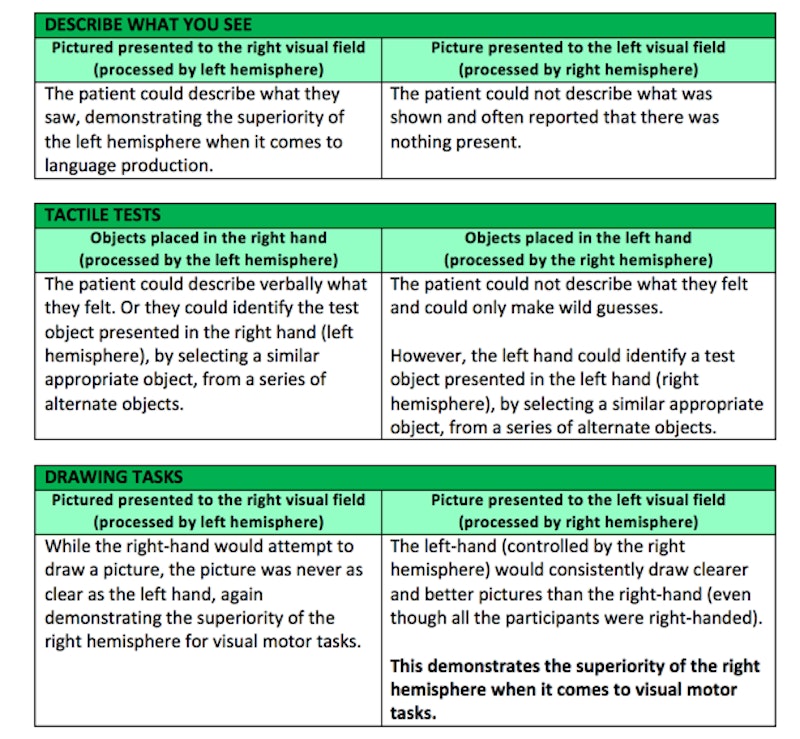
Conclusion: The findings of Sperry and Gazzaniga’s research highlights a number of key differences between the two hemispheres. Firstly, the left hemisphere is dominant in terms of speech and language. Secondly, the right hemisphere is dominant in terms of visual-motor tasks.
- Biopsychology
- Hemispheric Lateralisation
- Split Brain Research
You might also like
The biological approach, reflecting on… mirror neurons.
1st August 2016
Students as Co-planners and Teachers - Free Resource Download
5th August 2016
Could you kill for your food?
14th August 2016
An Interactive Atlas of The Brain
9th January 2017
Using Play-Doh In Biopsychology Lessons
14th January 2017
Q&A from AQA: Evaluation in Biopsychology
13th January 2017
Psychology Weekly Update & Newsletter
16th January 2017
Our subjects
- › Criminology
- › Economics
- › Geography
- › Health & Social Care
- › Psychology
- › Sociology
- › Teaching & learning resources
- › Student revision workshops
- › Online student courses
- › CPD for teachers
- › Livestreams
- › Teaching jobs
Boston House, 214 High Street, Boston Spa, West Yorkshire, LS23 6AD Tel: 01937 848885
- › Contact us
- › Terms of use
- › Privacy & cookies
© 2002-2024 Tutor2u Limited. Company Reg no: 04489574. VAT reg no 816865400.
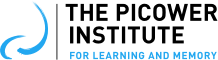
Paper: To understand cognition—and its dysfunction—neuroscientists must learn its rhythms
Thought emerges and is controlled in the brain via the rhythmically and spatially coordinated activity of millions of neurons, scientists argue in a new article. Understanding cognition and its disorders requires studying it at that level.
It could be very informative to observe the pixels on your phone under a microscope, but not if your goal is to understand what a whole video on the screen shows. Cognition is much the same kind of emergent property in the brain . It can only be understood by observing how millions of cells act in coordination, argues a trio of MIT neuroscientists. In a new article , they lay out a framework for understanding how thought arises from the coordination of neural activity driven by oscillating electric fields—also known as brain “waves” or “rhythms.”
Historically dismissed solely as byproducts of neural activity, brain rhythms are actually critical for organizing it, write Picower Professor Earl Miller and research scientists Scott Brincat and Jefferson Roy in Current Opinion in Behavioral Science . And while neuroscientists have gained tremendous knowledge from studying how individual brain cells connect and how and when they emit “spikes” to send impulses through specific circuits, there is also a need to appreciate and apply new concepts at the brain rhythm scale, which can span individual, or even multiple, brain regions.
“Spiking and anatomy are important but there is more going on in the brain above and beyond that,” said senior author Miller, a faculty member in The Picower Institute for Learning and Memory and the Department of Brain and Cognitive Sciences at MIT. “There’s a whole lot of functionality taking place at a higher level, especially cognition.”
The stakes of studying the brain at that scale, the authors write, might not only include understanding healthy higher-level function but also how those functions become disrupted in disease.
“Many neurological and psychiatric disorders, such as schizophrenia, epilepsy and Parkinson’s involve disruption of emergent properties like neural synchrony,” they write. “We anticipate that understanding how to interpret and interface with these emergent properties will be critical for developing effective treatments as well as understanding cognition.”
The emergence of thoughts
The bridge between the scale of individual neurons and the broader-scale coordination of many cells is founded on electric fields, the researchers write. Via a phenomenon called “ephaptic coupling,” the electrical field generated by the activity of a neuron can influence the voltage of neighboring neurons, creating an alignment among them. In this way, electric fields both reflect neural activity but also influence it. In a paper in 2022 , Miller and colleagues showed via experiments and computational modeling that the information encoded in the electric fields generated by ensembles of neurons can be read out more reliably than the information encoded by the spikes of individual cells. In 2023 Miller’s lab provided evidence that rhythmic electrical fields may coordinate memories between regions.
At this larger scale, in which rhythmic electric fields carry information between brain regions, Miller’s lab has published numerous studies showing that lower-frequency rhythms in the so-called “beta” band originate in deeper layers of the brain’s cortex and appear to regulate the power of faster-frequency “gamma” rhythms in more superficial layers. By recording neural activity in the brains of animals engaged in working memory games the lab has shown that beta rhythms carry “top down” signals to control when and where gamma rhythms can encode sensory information, such as the images that the animals need to remember in the game.
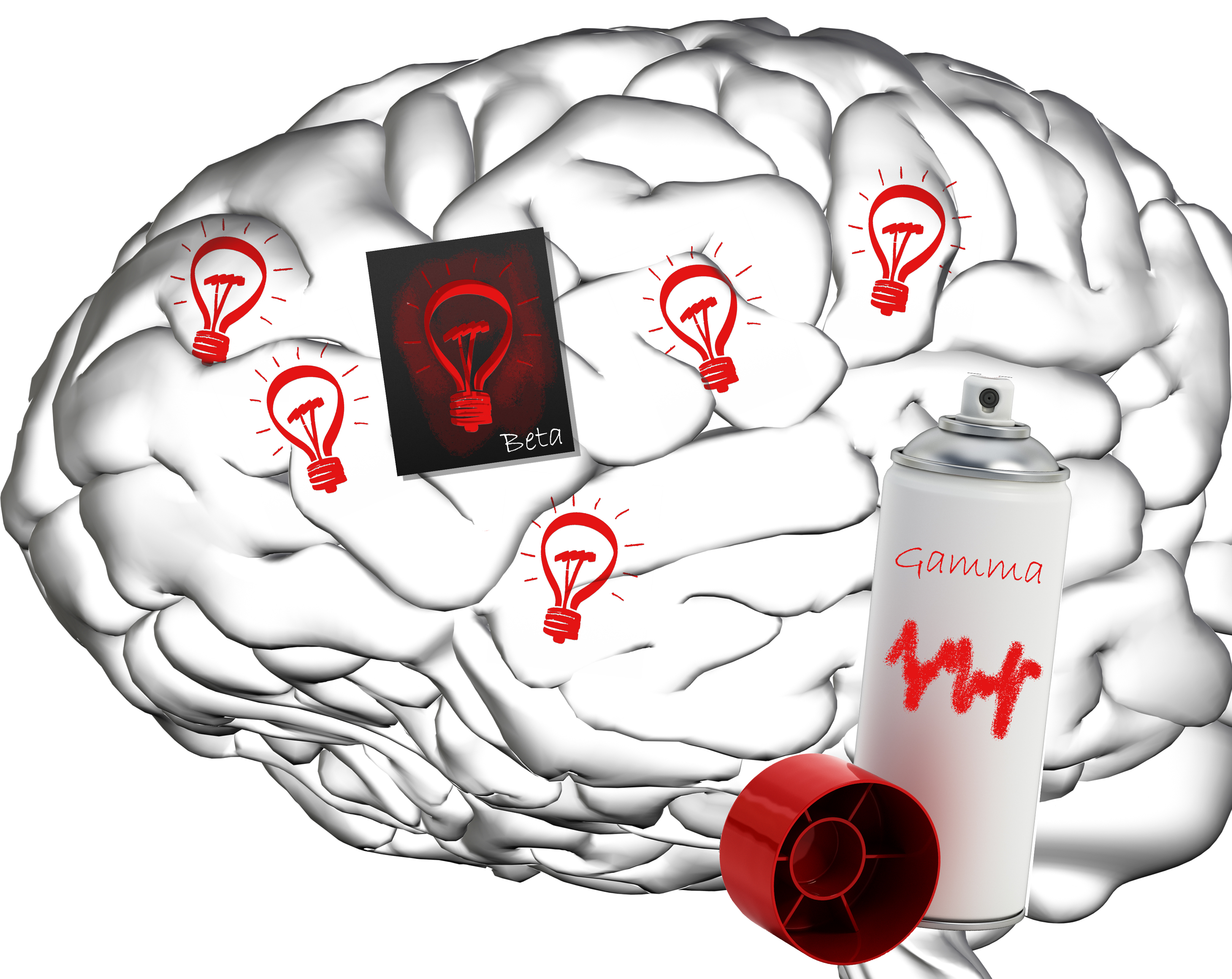
Some of the lab’s latest evidence suggests that beta rhythms apply this control of cognitive processes to physical patches of the cortex, essentially acting like stencils that pattern where and when gamma can encode sensory information into memory, or retrieve it. According to this theory, which Miller calls “ Spatial Computing ,” beta can thereby establish the general rules of a task (for instance, the back and forth turns required to open a combination lock), even as the specific information content may change (for instance, new numbers when the combination changes). More generally, this structure also enables neurons to flexibly encode more than one kind of information at a time, the authors write, a widely observed neural property called “mixed selectivity.” For instance, a neuron encoding a number of the lock combination can also be assigned, based on which beta-stenciled patch it is in, the particular step of the unlocking process that the number matters for.
In the new study Miller, Brincat and Roy suggest another advantage consistent with cognitive control being based on an interplay of large-scale coordinated rhythmic activity: “Subspace coding.” This idea postulates that brain rhythms organize the otherwise massive number of possible outcomes that could result from, say, 1,000 neurons engaging in independent spiking activity. Instead of all the many combinatorial possibilities, many fewer “subspaces” of activity actually arise, because neurons are coordinated, rather than independent. It is as if the spiking of neurons is like a flock of birds coordinating their movements. Different phases and frequencies of brain rhythms provide this coordination, aligned to amplify each other, or offset to prevent interference. For instance, if a piece of sensory information needs to be remembered, neural activity representing it can be protected from interference when new sensory information is perceived.
“Thus the organization of neural responses into subspaces can both segregate and integrate information,” the authors write.
The power of brain rhythms to coordinate and organize information processing in the brain is what enables functional cognition to emerge at that scale, the authors write. Understanding cognition in the brain, therefore, requires studying rhythms.
“Studying individual neural components in isolation—individual neurons and synapses—has made enormous contributions to our understanding of the brain and remains important,” the authors conclude. “However, it’s becoming increasingly clear that, to fully capture the brain’s complexity, those components must be analyzed in concert to identify, study, and relate their emergent properties.”
Related Articles
Study reveals a universal pattern of brain wave frequencies.
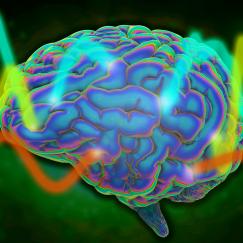
Anesthesia blocks sensation by cutting off communication within the cortex
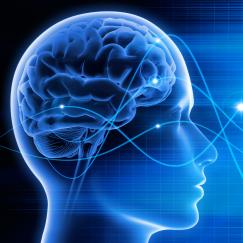
Anesthesia technology precisely controls unconsciousness in animal tests
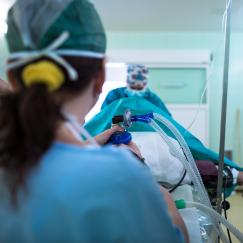
A multifunctional tool for cognitive neuroscience
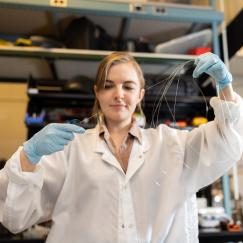
Help | Advanced Search
Computer Science > Computer Vision and Pattern Recognition
Title: umbrae: unified multimodal decoding of brain signals.
Abstract: We address prevailing challenges of the brain-powered research, departing from the observation that the literature hardly recover accurate spatial information and require subject-specific models. To address these challenges, we propose UMBRAE, a unified multimodal decoding of brain signals. First, to extract instance-level conceptual and spatial details from neural signals, we introduce an efficient universal brain encoder for multimodal-brain alignment and recover object descriptions at multiple levels of granularity from subsequent multimodal large language model (MLLM). Second, we introduce a cross-subject training strategy mapping subject-specific features to a common feature space. This allows a model to be trained on multiple subjects without extra resources, even yielding superior results compared to subject-specific models. Further, we demonstrate this supports weakly-supervised adaptation to new subjects, with only a fraction of the total training data. Experiments demonstrate that UMBRAE not only achieves superior results in the newly introduced tasks but also outperforms methods in well established tasks. To assess our method, we construct and share with the community a comprehensive brain understanding benchmark BrainHub. Our code and benchmark are available at this https URL .
Submission history
Access paper:.
- HTML (experimental)
- Other Formats
References & Citations
- Google Scholar
- Semantic Scholar
BibTeX formatted citation

Bibliographic and Citation Tools
Code, data and media associated with this article, recommenders and search tools.
- Institution
arXivLabs: experimental projects with community collaborators
arXivLabs is a framework that allows collaborators to develop and share new arXiv features directly on our website.
Both individuals and organizations that work with arXivLabs have embraced and accepted our values of openness, community, excellence, and user data privacy. arXiv is committed to these values and only works with partners that adhere to them.
Have an idea for a project that will add value for arXiv's community? Learn more about arXivLabs .
- Download PDF
- Share X Facebook Email LinkedIn
- Permissions
Fatal Traffic Risks With a Total Solar Eclipse in the US
- 1 Department of Medicine, University of Toronto, Toronto, Ontario, Canada
- 2 Evaluative Clinical Science Platform, Sunnybrook Research Institute, Toronto, Ontario, Canada
- 3 Institute for Clinical Evaluative Sciences, Toronto, Ontario, Canada
- 4 Division of General Internal Medicine, Sunnybrook Health Sciences Centre, Toronto, Ontario, Canada
- 5 Center for Leading Injury Prevention Practice Education & Research, Toronto, Ontario, Canada
- 6 Department of Medicine, University of British Columbia, Vancouver, British Columbia, Canada
- 7 Centre for Clinical Epidemiology & Evaluation, University of British Columbia, Vancouver, British Columbia, Canada
A total solar eclipse occurs when the moon temporarily obscures the sun and casts a dark shadow across the earth. This astronomical spectacle has been described for more than 3 millennia and can be predicted with high precision. Eclipse-related solar retinopathy (vision loss from staring at the sun) is an established medical complication; however, other medical outcomes have received little attention. 1
Read More About
Redelmeier DA , Staples JA. Fatal Traffic Risks With a Total Solar Eclipse in the US. JAMA Intern Med. Published online March 25, 2024. doi:10.1001/jamainternmed.2023.5234
Manage citations:
© 2024
Artificial Intelligence Resource Center
Best of JAMA Network 2022
Browse and subscribe to JAMA Network podcasts!
Others Also Liked
Select your interests.
Customize your JAMA Network experience by selecting one or more topics from the list below.
- Academic Medicine
- Acid Base, Electrolytes, Fluids
- Allergy and Clinical Immunology
- American Indian or Alaska Natives
- Anesthesiology
- Anticoagulation
- Art and Images in Psychiatry
- Artificial Intelligence
- Assisted Reproduction
- Bleeding and Transfusion
- Caring for the Critically Ill Patient
- Challenges in Clinical Electrocardiography
- Climate and Health
- Climate Change
- Clinical Challenge
- Clinical Decision Support
- Clinical Implications of Basic Neuroscience
- Clinical Pharmacy and Pharmacology
- Complementary and Alternative Medicine
- Consensus Statements
- Coronavirus (COVID-19)
- Critical Care Medicine
- Cultural Competency
- Dental Medicine
- Dermatology
- Diabetes and Endocrinology
- Diagnostic Test Interpretation
- Drug Development
- Electronic Health Records
- Emergency Medicine
- End of Life, Hospice, Palliative Care
- Environmental Health
- Equity, Diversity, and Inclusion
- Facial Plastic Surgery
- Gastroenterology and Hepatology
- Genetics and Genomics
- Genomics and Precision Health
- Global Health
- Guide to Statistics and Methods
- Hair Disorders
- Health Care Delivery Models
- Health Care Economics, Insurance, Payment
- Health Care Quality
- Health Care Reform
- Health Care Safety
- Health Care Workforce
- Health Disparities
- Health Inequities
- Health Policy
- Health Systems Science
- History of Medicine
- Hypertension
- Images in Neurology
- Implementation Science
- Infectious Diseases
- Innovations in Health Care Delivery
- JAMA Infographic
- Law and Medicine
- Leading Change
- Less is More
- LGBTQIA Medicine
- Lifestyle Behaviors
- Medical Coding
- Medical Devices and Equipment
- Medical Education
- Medical Education and Training
- Medical Journals and Publishing
- Mobile Health and Telemedicine
- Narrative Medicine
- Neuroscience and Psychiatry
- Notable Notes
- Nutrition, Obesity, Exercise
- Obstetrics and Gynecology
- Occupational Health
- Ophthalmology
- Orthopedics
- Otolaryngology
- Pain Medicine
- Palliative Care
- Pathology and Laboratory Medicine
- Patient Care
- Patient Information
- Performance Improvement
- Performance Measures
- Perioperative Care and Consultation
- Pharmacoeconomics
- Pharmacoepidemiology
- Pharmacogenetics
- Pharmacy and Clinical Pharmacology
- Physical Medicine and Rehabilitation
- Physical Therapy
- Physician Leadership
- Population Health
- Primary Care
- Professional Well-being
- Professionalism
- Psychiatry and Behavioral Health
- Public Health
- Pulmonary Medicine
- Regulatory Agencies
- Reproductive Health
- Research, Methods, Statistics
- Resuscitation
- Rheumatology
- Risk Management
- Scientific Discovery and the Future of Medicine
- Shared Decision Making and Communication
- Sleep Medicine
- Sports Medicine
- Stem Cell Transplantation
- Substance Use and Addiction Medicine
- Surgical Innovation
- Surgical Pearls
- Teachable Moment
- Technology and Finance
- The Art of JAMA
- The Arts and Medicine
- The Rational Clinical Examination
- Tobacco and e-Cigarettes
- Translational Medicine
- Trauma and Injury
- Treatment Adherence
- Ultrasonography
- Users' Guide to the Medical Literature
- Vaccination
- Venous Thromboembolism
- Veterans Health
- Women's Health
- Workflow and Process
- Wound Care, Infection, Healing
- Register for email alerts with links to free full-text articles
- Access PDFs of free articles
- Manage your interests
- Save searches and receive search alerts

An official website of the United States government
Here’s how you know
Official websites use .gov A .gov website belongs to an official government organization in the United States.
Secure .gov websites use HTTPS A lock ( Lock A locked padlock ) or https:// means you’ve safely connected to the .gov website. Share sensitive information only on official, secure websites.
JavaScript appears to be disabled on this computer. Please click here to see any active alerts .
EPA Scientists Contribute to Paper in Nature that Shows Common Household Chemicals May Disrupt Brain Development
Published April 16, 2024
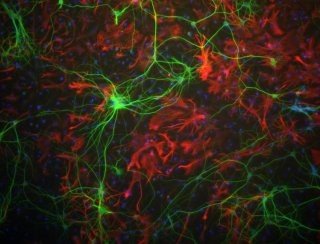
There are many existing chemicals that have incomplete data about their potential toxicity and effects on human health. Children are a particularly susceptible population and chemical exposure can disrupt critical periods in their development, especially brain development.
EPA scientists contributed to the development of data on over 1,800 compounds using a high-throughput developmental screen. Their research determined that quaternary compounds, a class of chemicals that are common in personal care products and disinfectants, may disrupt brain development in children. The findings were published in Nature .
High-throughput screening is a process that allows scientists to quickly test chemicals to see how they interact with a specific biological target or cell type. After screening over 1,800 compounds, researchers identified quaternary compounds as a class that may affect oligodendrocytes by impacting cell health. Oligodendrocytes are a type of cell in the central nervous system. Oligodendrocytes play an important role in neuron function and longevity and their disruption can lead to cognitive and motor disability. Additionally, some organophosphate flame retardants disrupted oligodendrocyte growth in a cell-based, in vitro model. Organophosphate flame retardants are commonly found in household items such as furniture and electronics.
While oligodendrocytes are crucial for brain development, their susceptibility to various chemicals has been studied less than other brain cells, such as neurons. This high-throughput screen is a first step in identifying chemicals that may affect oligodendrocyte development and shows how these methods can be used to identify potentially toxic chemicals quickly and efficiently.
The study suggests quaternary compounds and organophosphate flame retardants impact oligodendrocyte health in in vitro models, but further research is needed to confirm effects and determine the full impact of exposure. High-throughput screening is a powerful tool but it is only one part of EPA’s tiered toxicity testing strategy that prioritizes chemicals based on bioactivity so they can be tested with more traditional methods.
EPA scientists are leading and contributing to chemical safety research as part of their mission to protect human health and the environment.
Learn more about the science:
- Evaluating the Effects of Chemicals on Nervous System Development
- High-Throughput Toxicology
- Understanding Exposures in Children’s Environments
- Assessing and Managing Chemicals under TSCA
- Science Matters Home
- Researchers at Work Profiles
- All Stories

IMAGES
VIDEO
COMMENTS
This paper aims to contribute to the agenda for the next decade of split-brain research. Full split-brain surgery is rare these days, and it is important that we try to answer the central questions while these patients are still available for study. In order to examine the variations between patients it would be useful to test as many of the ...
A depiction of the traditional view of the split brain syndrome (top) versus what we actually found in two split-brain patients across a wide variety of tasks (bottom).The canonical idea of split-brain patients is that they cannot compare stimuli across visual half-fields (left), because visual processing is not integrated across hemispheres.This is what we found as well.
This paper aims to contribute to the agenda for the next decade of split-brain research. Full split-brain surgery is rare these days, and it is important that we try to answer the central questions while these patients are still available for study. ... M. S. (2017). Interaction in isolation: 50 years of insights from split-brain research ...
As the opportunities for split-brain research dwindle, Gazzaniga is busy trying to digitize the archive of recordings of tests with cohort members, some of which date back more than 50 years.
Introduction. The term split-brain refers to patients in whom the corpus. " ". callosum has been cut for the alleviation of medically intrac-table epilepsy. Since the earliest reports by van Wagenen and Herren (1940) and Akelaitis (1941, 1943) on the repercus-sions of a split-brain, two narratives have emerged.
The split-brain: Rooting consciousness in biology ARTICLES. Current Issue Latest Articles Special Features List ... It was the paper that launched 50 y of intense research on the brain mechanisms that underlie the human conscious experience. All of the subsequent knowledge that has been revealed has been the product of the idea to "just try ...
The main issue concerns the first-person perspective of a split-brain patient. Does a split-brain harbor a split consciousness or is consciousness unified? The current consensus is that the body of evidence is insufficient to answer this question, and different suggestions are made with respect to how future studies might address this paucity.
The evolution of split-brain testing has led us to new frontiers (for reviews of split-brain research, see Refs 12-14, 37-39). In the early days of split-brain testing, research with split ...
human split brain—the theory that the right and left hemispheres of the brain can act independently, have diff erent strengths, and separate agendas. Gazzaniga tells the story of how the split-brain theory was developed and talks about the friends, patients, and family who stood by him on his journey through the research and discoveries.
Although the 1967 paper's title puts the focus on language processing, its abstract addresses implications far beyond language processing: ... Moreover, split-brain research led to the development of several methodological advances that derived from questions specifically occurring in split-brain patients. One such question lies in accurately ...
A tale of split-brain research. In his new book— Tales from both sides of the brain: a life in neuroscience —Michael Gazzaniga describes a heartfelt story of his life in neuroscience in the form of a memoir. The book presents a review of Gazzaniga's work on the human split brain—the theory that the right and left hemispheres of the brain ...
This collective review paper aims to summarize the empirical common ground, to delineate the different interpretations, and to identify the remaining questions about the split-brain patient's first-person perspective. Recently, the discussion regarding the consequences of cutting the corpus callosum ("split-brain") has regained momentum (Corballis, Corballis, Berlucchi, & Marzi, Brain, 141 ...
The term split brain is used to denote a patient. in whom the cortical commissures have been severed, for the relief of epilepsy that cannot be controlled by. medication, in an operation known as ...
The author displays one of the tachistoscopes he built for the first tests of split-brain subjects. ... It was the paper that launched 50 y of intense research on the brain mechanisms that underlie the human conscious experience. All of the subsequent knowledge that has been revealed has been the product of the idea to "just try it ...
Split-brain methodology has yielded insights into the remarkable regional specificity of the corpus callosum as well as into the integrative role of thecallosum in the perception of causality and in the authors' perception of an integrated sense of self. Forty-five years ago, Roger Sperry, Joseph Bogen and I embarked on what are now known as the modern split-brain studies.
If percent difference was not listed, the percent was calculated from the data in the paper using 100*[(Observed-Expected)/Expected] where Observed was the DID patient result and Expected result was the healthy control. ... Progress in Brain Research, 167 (2007), pp. 307-310, 10.1016/S0079-6123(07)67029-. View in Scopus Google Scholar. Elzinga ...
In a paper he and a colleague published in the Journal of the American Medical ... But split-brain research was arguably one of the first scientific demonstrations that the divided self has a real ...
In the 1970s, when the modern era of split-brain research began, the idea of mapping the cortical circuits of perception, memory and cognition was revolutionary. While Karl Lashley was heavily committed to the idea that neurons had little specificity1, Donald Hebb was strenuously arguing the opposite2. Roger Sperry's ongoing work on neural ...
Split-Brain Research Sperry and Gazzaniga (1967) were the first to investigate hemispheric lateralisation with the use of split-brain patients. Background: Split-brain patients are individuals who have undergone a surgical procedure where the corpus callosum, which connects the two hemispheres, is cut. This procedure, which separates the two ...
Historically dismissed solely as byproducts of neural activity, brain rhythms are actually critical for organizing it, write Picower Professor Earl Miller and research scientists Scott Brincat and Jefferson Roy in Current Opinion in Behavioral Science. And while neuroscientists have gained tremendous knowledge from studying how individual brain ...
We address prevailing challenges of the brain-powered research, departing from the observation that the literature hardly recover accurate spatial information and require subject-specific models. To address these challenges, we propose UMBRAE, a unified multimodal decoding of brain signals. First, to extract instance-level conceptual and spatial details from neural signals, we introduce an ...
Antiretroviral Drugs for HIV Treatment and Prevention in Adults - 2022 IAS-USA Recommendations CONSERVE 2021 Guidelines for Reporting Trials Modified for the COVID-19 Pandemic Creation and Adoption of Large Language Models in Medicine Global Burden of Cancer, 2010-2019 Global Burden of Long COVID Global Burden of Melanoma Global Burden of Skin ...
EPA scientists contributed to the development of data on over 1,800 compounds using a high-throughput developmental screen. Their research determined that quaternary compounds, a class of chemicals that are common in personal care products and disinfectants, may disrupt brain development in children. The findings were published in Nature.