Ion thrusters for electric propulsion: Scientific issues developing a niche technology into a game changer
Affiliations.
- 1 Institute of Experimental Physics I, Justus Liebig University, Heinrich-Buff-Ring 16, 35392 Giessen, Germany.
- 2 Institute of Theoretical Physics, Justus Liebig University, Heinrich-Buff-Ring 16, 35392 Giessen, Germany.
- 3 Department of Electrical Engineering, University of Applied Sciences, Wiesenstr. 14, 35390 Giessen, Germany.
- 4 ONERA, Palaiseau 91120, France.
- 5 Institute of Experimental Physics II, Justus Liebig University, Heinrich-Buff-Ring 16, 35392 Giessen, Germany.
- PMID: 32611046
- DOI: 10.1063/5.0010134
The transition from old space to new space along with increasing commercialization has a major impact on space flight, in general, and on electric propulsion (EP) by ion thrusters, in particular. Ion thrusters are nowadays used as primary propulsion systems in space. This article describes how these changes related to new space affect various aspects that are important for the development of EP systems. Starting with a historical overview of the development of space flight and of the technology of EP systems, a number of important missions with EP and the underlying technologies are presented. The focus of our discussion is the technology of the radio frequency ion thruster as a prominent member of the gridded ion engine family. Based on this discussion, we give an overview of important research topics such as the search for alternative propellants, the development of reliable neutralizer concepts based on novel insert materials, as well as promising neutralizer-free propulsion concepts. In addition, aspects of thruster modeling and requirements for test facilities are discussed. Furthermore, we address aspects of space electronics with regard to the development of highly efficient electronic components as well as aspects of electromagnetic compatibility and radiation hardness. This article concludes with a presentation of the interaction of EP systems with the spacecraft.
Help | Advanced Search

Physics > Plasma Physics
Title: three-dimensional kinetic simulation of an ion thruster plume with carbon backsputtering in a vacuum chamber.
Abstract: Gridded ion thrusters are tested in ground vacuum chambers to verify their performance when deployed in space. However, the presence of high background pressure and conductive walls in the chamber leads to facility effects that increase uncertainty in the performance of the thruster in space. To address this issue, this study utilizes a fully kinetic simulation to investigate the facility effects on the thruster plume. The in-chamber condition shows a downstream neutral particle density 100 times larger than the in-space case due to ion neutralization at the wall and limited vacuum pump capability, resulting in a significant difference in the density and distribution of charge-exchange ions. The flux, energy, and angle of charge-exchange ions incident on the chamber wall are found to be altered by the electron sheath, which can only be simulated by the fully kinetic approach, as opposed to the conventionally used quasi-neutral Boltzmann approach. We also examine the effect of backsputtering, another important facility effect, and find that it does not necessarily require a fully kinetic simulation as the incident flux and energy of the sampled charge-exchange ion are negligibly small. Finally, we demonstrate that the carbon deposition rate on the thruster is significantly influenced by the angular dependence of the sputtered carbon, with a nearly 50% effect.
Submission history
Access paper:.
- Other Formats

References & Citations
- Google Scholar
- Semantic Scholar
BibTeX formatted citation

Bibliographic and Citation Tools
Code, data and media associated with this article, recommenders and search tools.
- Institution
arXivLabs: experimental projects with community collaborators
arXivLabs is a framework that allows collaborators to develop and share new arXiv features directly on our website.
Both individuals and organizations that work with arXivLabs have embraced and accepted our values of openness, community, excellence, and user data privacy. arXiv is committed to these values and only works with partners that adhere to them.
Have an idea for a project that will add value for arXiv's community? Learn more about arXivLabs .
Thank you for visiting nature.com. You are using a browser version with limited support for CSS. To obtain the best experience, we recommend you use a more up to date browser (or turn off compatibility mode in Internet Explorer). In the meantime, to ensure continued support, we are displaying the site without styles and JavaScript.
- View all journals
- Explore content
- About the journal
- Publish with us
- Sign up for alerts
- Published: 21 November 2018
Flight of an aeroplane with solid-state propulsion
- Haofeng Xu 1 ,
- Yiou He 2 ,
- Kieran L. Strobel 1 ,
- Christopher K. Gilmore 1 ,
- Sean P. Kelley 1 ,
- Cooper C. Hennick 1 ,
- Thomas Sebastian 3 ,
- Mark R. Woolston 3 ,
- David J. Perreault 2 &
- Steven R. H. Barrett 1
Nature volume 563 , pages 532–535 ( 2018 ) Cite this article
58k Accesses
157 Citations
1508 Altmetric
Metrics details
- Aerospace engineering
- Electrical and electronic engineering
- Fluid dynamics
- Plasma physics
Since the first aeroplane flight more than 100 years ago, aeroplanes have been propelled using moving surfaces such as propellers and turbines. Most have been powered by fossil-fuel combustion. Electroaerodynamics, in which electrical forces accelerate ions in a fluid 1 , 2 , has been proposed as an alternative method of propelling aeroplanes—without moving parts, nearly silently and without combustion emissions 3 , 4 , 5 , 6 . However, no aeroplane with such a solid-state propulsion system has yet flown. Here we demonstrate that a solid-state propulsion system can sustain powered flight, by designing and flying an electroaerodynamically propelled heavier-than-air aeroplane. We flew a fixed-wing aeroplane with a five-metre wingspan ten times and showed that it achieved steady-level flight. All batteries and power systems, including a specifically developed ultralight high-voltage (40-kilovolt) power converter, were carried on-board. We show that conventionally accepted limitations in thrust-to-power ratio and thrust density 4 , 6 , 7 , which were previously thought to make electroaerodynamics unfeasible as a method of aeroplane propulsion, are surmountable. We provide a proof of concept for electroaerodynamic aeroplane propulsion, opening up possibilities for aircraft and aerodynamic devices that are quieter, mechanically simpler and do not emit combustion emissions.
This is a preview of subscription content, access via your institution
Access options
Access Nature and 54 other Nature Portfolio journals
Get Nature+, our best-value online-access subscription
24,99 € / 30 days
cancel any time
Subscribe to this journal
Receive 51 print issues and online access
185,98 € per year
only 3,65 € per issue
Buy this article
- Purchase on Springer Link
- Instant access to full article PDF
Prices may be subject to local taxes which are calculated during checkout
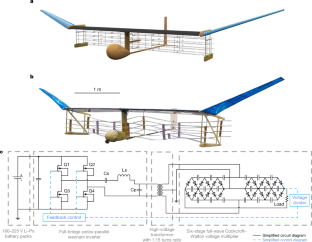
Similar content being viewed by others
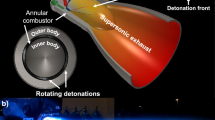
Experimental validation of rotating detonation for rocket propulsion
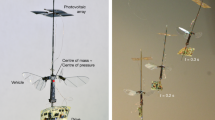
Untethered flight of an insect-sized flapping-wing microscale aerial vehicle
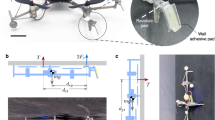
Energy efficient perching and takeoff of a miniature rotorcraft
Data availability.
The data that support the findings of this study are available from the corresponding author upon reasonable request.
Chattock, A. P., Walker, W. E. & Dixon, E. H. IV. On the specific velocities of ions in the discharge from points. Phil. Mag . 1 , 79–98 (1901).
Article CAS Google Scholar
Stuetzer, O. M. Ion-drag pumps. J. Appl. Phys . 31 , 136–146 (1960).
Article ADS Google Scholar
Christenson, E. A. & Moller, P. S. Ion-neutral propulsion in atmospheric media. AIAA J . 5 , 1768–1773 (1967).
Wilson, J., Perkins, H. D. & Thompson, W. K. An Investigation Of Ionic Wind Propulsion. Report No. NASA/TM 2009–215822 (NASA, 2009).
Masuyama, K. & Barrett, S. R. H. On the performance of electrohydrodynamic propulsion. Proc. R. Soc. A 469 , 20120623 (2013).
Monrolin, N., Ploouraboué, F. & Praud, O. Electrohydrodynamic thrust for in-atmosphere propulsion. AIAA J . 55 , 4296–4305 (2017).
Article CAS ADS Google Scholar
Gilmore, C. K. & Barrett, S. R. H. Electrohydrodynamic thrust density using positive corona-induced ionic winds for in-atmosphere propulsion. Proc. R. Soc. A 471 , 20140912 (2015).
Loeb, L. B. Electrical Coronas: Their Basic Physical Mechanisms (Univ. California Press, Berkeley, 1965).
Google Scholar
Melcher, J. R. Traveling-wave induced electroconvection. Phys. Fluids 9 , 1548 (1966).
Allen, P. H. G. & Karayiannis, T. G. Electrohydrodynamic enhancement of heat transfer and fluid flow. Heat Recovery Syst . 15 , 389–423 (1995).
Drew, D. S., Lambert, N. O., Schindler, C. B. & Pister, K. S. J. Toward controlled flight of the ionocraft: a flying microrobot using electrohydrodynamic thrust with onboard sensing and no moving parts. IEEE Robotics Automation Lett . 3 , 2807–2813 (2018).
Article Google Scholar
Cumpsty, N. & Heyes, A. Jet Propulsion (Cambridge Univ. Press, Cambridge, 1998).
Leishman, J. G. Principles of Helicopter Aerodynamics (Cambridge Univ. Press, Cambridge, 2000).
Hoburg, W. & Abbeel, P. Geometric programming for aircraft design optimization. AIAA J . 52 , 2414–2426 (2014).
Gu, W. J. & Liu, R. A study of volume and weight vs. frequency for high-frequency transformers. In Power Electronics Specialists Conf . 1123–1129 (IEEE, 1993).
He, Y., Woolston, M. R. & Perreault, D. J. Design and implementation of a lightweight high-voltage power converter for electro-aerodynamic propulsion. In IEEE Workshop on Control and Modeling for Power Electronics http://doi.org/10.1109/COMPEL.2017.8013315 (IEEE, 2017).
Hsu, W. C., Chen, J. F., Hsieh, Y. P. & Wu, Y. M. Design and steady-state analysis of parallel resonant DC–DC converter for high-voltage power generator. IEEE Trans. Power Electronics 32 , 957–966 (2017).
McFarland, M. W. (ed.) The Papers of Wilbur and Orville Wright (McGraw-Hill, New York, 1953).
Download references
Acknowledgements
The work was also contributed to by many undergraduate students from 2010–2018 as part of MIT’s Undergraduate Research Opportunities Program (UROP), as part of MIT’s Minority Students Research Program (MSRP), or as part of MIT’s summer research exchange program with Imperial College London (IROP). These students include Y. K. Tey, P. Kandangwa, W. B. Rideout, J. Epps, S. O’Neill, M. Adams, J. M. Salinas, N. H. Rodman, I. L. LaJoie, W. A. Rutter, A. J. Sanders, N. J. Martorell, I. Vallina Garcia, J. P. Liguori, K. Dasadhikari, B. J. Scalzo Dees, M. H. Knowles, D. W. Fellows and D. P. Aaradhya. In addition, we thank A. Brown, T. Tao, C. Tan, P. Lozano, J. Peraire and C. Guerra-Garcia for technical discussions and advice, in some cases as part of student thesis committees. K. Masuyama, A. Dexter and J. Payton contributed to the project in its earlier phases. J. Leith and J. L. Freeman contributed to the financial and procurement administration for the project. F. Allroggen contributed to the resource management for the project. We also thank the laboratory staff at MIT AeroAstro for their help with the design, fabrication and flight testing of the EAD aircraft, in particular D. Robertson, T. Billings, A. Zolnik and T. Numan. Finally, we thank the MIT Department of Athletics, Physical Education, and Recreation for access to space for indoor flight testing, in particular S. Lett. This work was funded through MIT Lincoln Laboratory Autonomous Systems Line, the Professor Amar G. Bose Research Grant, and through the Singapore-MIT Alliance for Research and Technology (SMART). The work was also funded through the Charles Stark Draper and Leonardo career development chairs at MIT. This material is based on work supported by the Assistant Secretary of Defense for Research and Engineering under Air Force Contract No. FA8721-05-C-0002 and/or FA8702-15-D-0001. Any opinions, findings, conclusions or recommendations expressed in this material are those of the author(s) and do not necessarily reflect the views of the Assistant Secretary of Defense for Research and Engineering.
Reviewer information
Nature thanks D. Drew, K. Pister, F. Plouraboué and H. Smith for their contribution to the peer review of this work.
Author information
Authors and affiliations.
Department of Aeronautics and Astronautics, Massachusetts Institute of Technology, Cambridge, MA, USA
Haofeng Xu, Kieran L. Strobel, Christopher K. Gilmore, Sean P. Kelley, Cooper C. Hennick & Steven R. H. Barrett
Department of Electrical Engineering and Computer Science, Massachusetts Institute of Technology, Cambridge, MA, USA
Yiou He & David J. Perreault
Massachusetts Institute of Technology Lincoln Laboratory, Cambridge, MA, USA
Thomas Sebastian & Mark R. Woolston
You can also search for this author in PubMed Google Scholar
Contributions
S.R.H.B. conceived the aeroplane. H.X. and C.K.G. designed the aeroplane. Y.H., D.J.P. and M.R.W. developed the electrical power systems. H.X., Y.H., K.L.S., C.K.G., S.P.K. and C.C.H. built and tested the aeroplane. H.X. piloted the aeroplane. K.L.S. and S.P.K. performed wind tunnel tests. S.R.H.B., D.J.P. and T.S. coordinated the project.
Corresponding author
Correspondence to Steven R. H. Barrett .
Ethics declarations
Competing interests.
The authors declare no competing interests.
Additional information
Publisher’s note: Springer Nature remains neutral with regard to jurisdictional claims in published maps and institutional affiliations.
Extended data figures and tables
Extended data fig. 1 schematic of propulsion system electrodes..
Not to scale. The emitting electrode is a 32 American Wire Gauge (32 AWG; 0.2 mm diameter) stainless steel wire, held at 60 mm spacing from the collecting electrode by 3D-printed spacers. The collecting electrode is a National Advisory Committee for Aeronautics (NACA) 0010 airfoiled foam section covered in a thin layer of aluminium foil. The electrodes are 3 m in span (into the page).
Extended Data Fig. 2 HVPC output voltage and input power for a single flight (number 9).
The HVPC is designed to ramp up to the final voltage over 20 s while the aeroplane is on the launcher. The aircraft was in flight for 10–12 s. During flight, the HVPC regulates the output voltage to maintain 40.3 kV.
Supplementary information
Supplementary video 1.
Undistorted camera footage from flight 9, with position and energy from camera tracking annotated.
Supplementary Video 2
Undistorted camera footage from unpowered glide 2, with position and energy from camera tracking annotated.
Rights and permissions
Reprints and permissions
About this article
Cite this article.
Xu, H., He, Y., Strobel, K.L. et al. Flight of an aeroplane with solid-state propulsion. Nature 563 , 532–535 (2018). https://doi.org/10.1038/s41586-018-0707-9
Download citation
Received : 22 June 2018
Accepted : 09 October 2018
Published : 21 November 2018
Issue Date : 22 November 2018
DOI : https://doi.org/10.1038/s41586-018-0707-9
Share this article
Anyone you share the following link with will be able to read this content:
Sorry, a shareable link is not currently available for this article.
Provided by the Springer Nature SharedIt content-sharing initiative
- Solid-state Actuators
- Thrust Density
- Steady Level Flight
- High Voltage Power Converter (HVPC)
- Geometric Programming
This article is cited by
Effect of the high voltage waveform on the ionic wind produced by a needle-to-plate dielectric barrier discharge.
- Eric Moreau
- Etienne Defoort
Scientific Reports (2022)
Toroidal counter electrode for ionic propulsion
- Marius Chirita
- Adrian Ieta
Enhancement of Ion Acceleration Efficiency by Multi-stage and Accelerating Electrodes Coupling
- Sang-Jun Kim
- Ung-Hui Yun
- Jin-Gyu Kim
Journal of Electrical Engineering & Technology (2022)
Ionic wind review-2020: advancement and application in thermal management
- Saravanan Venkatesh
- Saurav Pramanik
Sādhanā (2021)
High-voltage transformer
- Jorge A. Gordillo
Electrical Engineering (2020)
By submitting a comment you agree to abide by our Terms and Community Guidelines . If you find something abusive or that does not comply with our terms or guidelines please flag it as inappropriate.
Quick links
- Explore articles by subject
- Guide to authors
- Editorial policies
Sign up for the Nature Briefing newsletter — what matters in science, free to your inbox daily.

Gridded Ion Thrusters (NEXT-C)
NEXT Ion Engine Test Firing
NASA’s Evolutionary Xenon Thruster(NEXT) is a gridded-ion thruster. In an ion thruster, ions are accelerated by electrostatic forces. The electric fields used for acceleration are generated by electrodes positioned at the downstream end of the thruster. Each set of electrodes, called ion optics or grids, contains thousands of coaxial apertures. Each set of apertures acts as a lens that electrically focuses ions through the optics. NASA’s ion thrusters use a two-electrode system, where the upstream electrode (called the screen grid) is charged highly positive, and the downstream electrode (called the accelerator grid) is charged highly negative. Since the ions are generated in a region of high positive and the accelerator grid’s potential is negative, the ions are attracted toward the accelerator grid and are focused out of the discharge chamber through the apertures, creating thousands of ion jets. The stream of all the ion jets together is called the ion beam. The thrust force is the force that exists between the upstream ions and the accelerator grid. The exhaust velocity of the ions in the beam is based on the voltage applied to the optics. While a chemical rocket’s top speed is limited by the thermal capability of the rocket nozzle, the ion thruster’s top speed is limited by the voltage that is applied to the ion optics (which is theoretically unlimited).
NEXT is projected to be about three times as powerful as the NSTAR used on Dawn and Deep Space 1 spacecraft. NEXT affords larger delivered payloads, smaller launch vehicle size, and other mission enhancements compared to chemical and other electric propulsion technologies for Discovery, New Frontiers, Mars Exploration, and Flagship outer-planet exploration missions. Glenn Research Center manufactured the test engine’s core ionization chamber, and Aerojet Rocketdyne designed and built the ion acceleration assembly. The first two flight units will be available in early 2019.
Performance
The NEXT engine is a type of electric propulsion in which thruster systems use electricity to accelerate the xenon propellant to speeds of up to 90,000mph (145,000km/h or 40 km/s). NEXT can produce 6.9 kW thruster power and 236 mN thrust . It can be throttled down to 0.5kW power, and has a specific impulse of 4,190 seconds (compared to 3,120 for NSTAR ).The NEXT thruster has demonstrated a total impulse of 17 MN·s; which is the highest total impulse ever demonstrated by an ion thruster. [2] A beam extraction area 1.6 times that of NSTAR allows higher thruster input power while maintaining low voltages and ion current densities, thus maintaining thruster longevity.
In December 2009, the prototype had completed an 48,000 hour (5.5 years) test. Thruster performance characteristics, measured over the entire throttle range of the thruster, were within predictions and the engine showed little signs of degradation and is ready for mission opportunities.
The first two flight units will be available in early 2019, in time for possible use on a New Frontiers-4 mission. After that, the NEXT-C engine will be made commercially available for purchase by both NASA and Industry through Aerojet Rocketdyne.
Power Processing Unit
The Power Processing Unit on the NEXT-C Ion Thruster controls the power output to the thrusters. Two flight power processing units (PPUs) are being developed by the Aerojet Rocketdyne Corporation in Redmond, Washington and ZIN Technologies in Cleveland, Ohio, in support of the NEXT-C Project.
The power processing unit operates from two sources: a wide input 80 to 160 V high-power bus and a nominal 28 V low-power bus. The unit includes six power supplies. Four power supplies (beam, accelerator, discharge, and neutralizer keeper) are needed for steady state operation, while two cathode heater power supplies (neutralizer and discharge) are utilized during thruster startup. The unit in total delivers up to 7 kW of regulated power to a single gridded-ion thruster.
Asteroid Impact and Deflection Assessment (AIDA) Mission
Comet astrobiology exploration sample return (caesar).
Ion propulsion has been studied since the early 1960’s. NASA Glenn Research Center has had a long history of leadership in the area of electric propulsion, and is currently NASA’s lead center for ion propulsion.
NASA Solar Electric Propulsion Technology Application Readiness (NSTAR) Ion Thruster
The NASA Solar Technology Application Readiness (NSTAR) program provided a single string, primary IPS to the Deep Space 1 spacecraft. The 30-cm ion thruster operates over a 0.5 kW to 2.3 kW input power range providing thrust from 19 mN to 92 mN. The specific impulse ranges from 1900 s at 0.5 kW to 3100 s at 2.3 kW. The flight thruster and PPU design requirements were derived with the aid of about 50 development tests and a series of wear-tests at NASA GRC and JPL of 2000 hours, 1000 hours, and 8193 hours using engineering model thrusters. The flight-set masses for the thruster, PPU, and DCIU were 8.2 kg, 14.77 kg, and 2.51 kg, respectively. About 1.7 kg mass was added to the PPU top plate to satisfy the DS1 micrometeoroid requirements. The power cable between the thruster and PPU was comprised of two segments which were connected at a field junction. The thruster cable mass was 0.95 kg, and the PPU cable mass was 0.77 kg. The xenon storage and feed system dry mass was about 20.5 kg. A total of 82 kg of xenon was loaded for the flight. Thrusters and PPUs were manufactured for NASA GRC by Hughes, and the DCIU was built by Spectrum Astro, Inc. The feed system development was a collaborative effort between JPL and Moog, Inc.
The DS1 spacecraft was launched on October 24, 1998. In-space testing and the IPS technology demonstrations were completed within the next three months. By April 27, 1999, the primary thrusting of the NSTAR engine system, required to encounter the asteroid Braille, was completed. The thrusting time at the end of April was 1764 hours. Thruster input power levels were varied from 0.48 kW to 1.94 kW. On July 26, 1999 DS1 obtained spectrometer data and images of Braille fifteen minutes after the flyby.
Info on Contract
Link to contractors, publications, press releases.
Thanks for contacting us! We will get in touch with you shortly.
Ion Electric Propulsion System Electric Breakdown Problems: Causes, Impacts and Protection Strategies
Ieee account.
- Change Username/Password
- Update Address
Purchase Details
- Payment Options
- Order History
- View Purchased Documents
Profile Information
- Communications Preferences
- Profession and Education
- Technical Interests
- US & Canada: +1 800 678 4333
- Worldwide: +1 732 981 0060
- Contact & Support
- About IEEE Xplore
- Accessibility
- Terms of Use
- Nondiscrimination Policy
- Privacy & Opting Out of Cookies
A not-for-profit organization, IEEE is the world's largest technical professional organization dedicated to advancing technology for the benefit of humanity. © Copyright 2024 IEEE - All rights reserved. Use of this web site signifies your agreement to the terms and conditions.
Suggestions or feedback?
MIT News | Massachusetts Institute of Technology
- Machine learning
- Social justice
- Black holes
- Classes and programs
Departments
- Aeronautics and Astronautics
- Brain and Cognitive Sciences
- Architecture
- Political Science
- Mechanical Engineering
Centers, Labs, & Programs
- Abdul Latif Jameel Poverty Action Lab (J-PAL)
- Picower Institute for Learning and Memory
- Lincoln Laboratory
- School of Architecture + Planning
- School of Engineering
- School of Humanities, Arts, and Social Sciences
- Sloan School of Management
- School of Science
- MIT Schwarzman College of Computing
MIT engineers fly first-ever plane with no moving parts
Press contact :, media download.
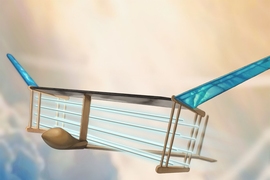
*Terms of Use:
Images for download on the MIT News office website are made available to non-commercial entities, press and the general public under a Creative Commons Attribution Non-Commercial No Derivatives license . You may not alter the images provided, other than to crop them to size. A credit line must be used when reproducing images; if one is not provided below, credit the images to "MIT."
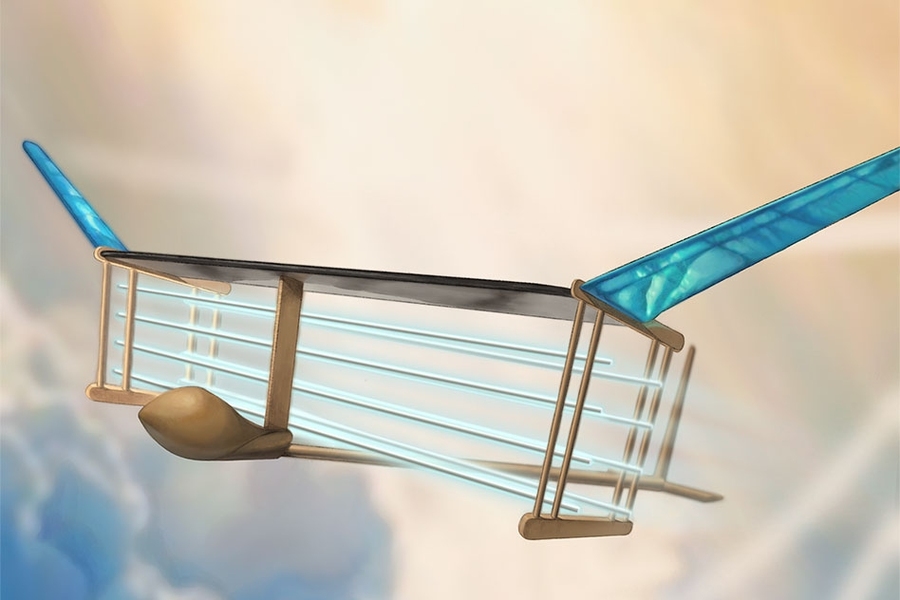
Previous image Next image
Since the first airplane took flight over 100 years ago, virtually every aircraft in the sky has flown with the help of moving parts such as propellers, turbine blades, or fans that produce a persistent, whining buzz.
Now MIT engineers have built and flown the first-ever plane with no moving parts. Instead of propellers or turbines, the light aircraft is powered by an “ionic wind” — a silent but mighty flow of ions that is produced aboard the plane, and that generates enough thrust to propel the plane over a sustained, steady flight.
Unlike turbine-powered planes, the aircraft does not depend on fossil fuels to fly. And unlike propeller-driven drones, the new design is completely silent.
“This is the first-ever sustained flight of a plane with no moving parts in the propulsion system,” says Steven Barrett, associate professor of aeronautics and astronautics at MIT. “This has potentially opened new and unexplored possibilities for aircraft which are quieter, mechanically simpler, and do not emit combustion emissions.”
He expects that in the near-term, such ion wind propulsion systems could be used to fly less noisy drones. Further out, he envisions ion propulsion paired with more conventional combustion systems to create more fuel-efficient, hybrid passenger planes and other large aircraft.
Barrett and his team at MIT have published their results today in the journal Nature .
Hobby crafts
Barrett says the inspiration for the team’s ion plane comes partly from the movie and television series, “Star Trek,” which he watched avidly as a kid. He was particularly drawn to the futuristic shuttlecrafts that effortlessly skimmed through the air, with seemingly no moving parts and hardly any noise or exhaust.
“This made me think, in the long-term future, planes shouldn’t have propellers and turbines,” Barrett says. “They should be more like the shuttles in ‘Star Trek , ’ that have just a blue glow and silently glide.”
About nine years ago, Barrett started looking for ways to design a propulsion system for planes with no moving parts. He eventually came upon “ionic wind,” also known as electroaerodynamic thrust — a physical principle that was first identified in the 1920s and describes a wind, or thrust, that can be produced when a current is passed between a thin and a thick electrode. If enough voltage is applied, the air in between the electrodes can produce enough thrust to propel a small aircraft.
For years, electroaerodynamic thrust has mostly been a hobbyist’s project, and designs have for the most part been limited to small, desktop “lifters” tethered to large voltage supplies that create just enough wind for a small craft to hover briefly in the air. It was largely assumed that it would be impossible to produce enough ionic wind to propel a larger aircraft over a sustained flight.
“It was a sleepless night in a hotel when I was jet-lagged, and I was thinking about this and started searching for ways it could be done,” he recalls. “I did some back-of-the-envelope calculations and found that, yes, it might become a viable propulsion system,” Barrett says. “And it turned out it needed many years of work to get from that to a first test flight.”
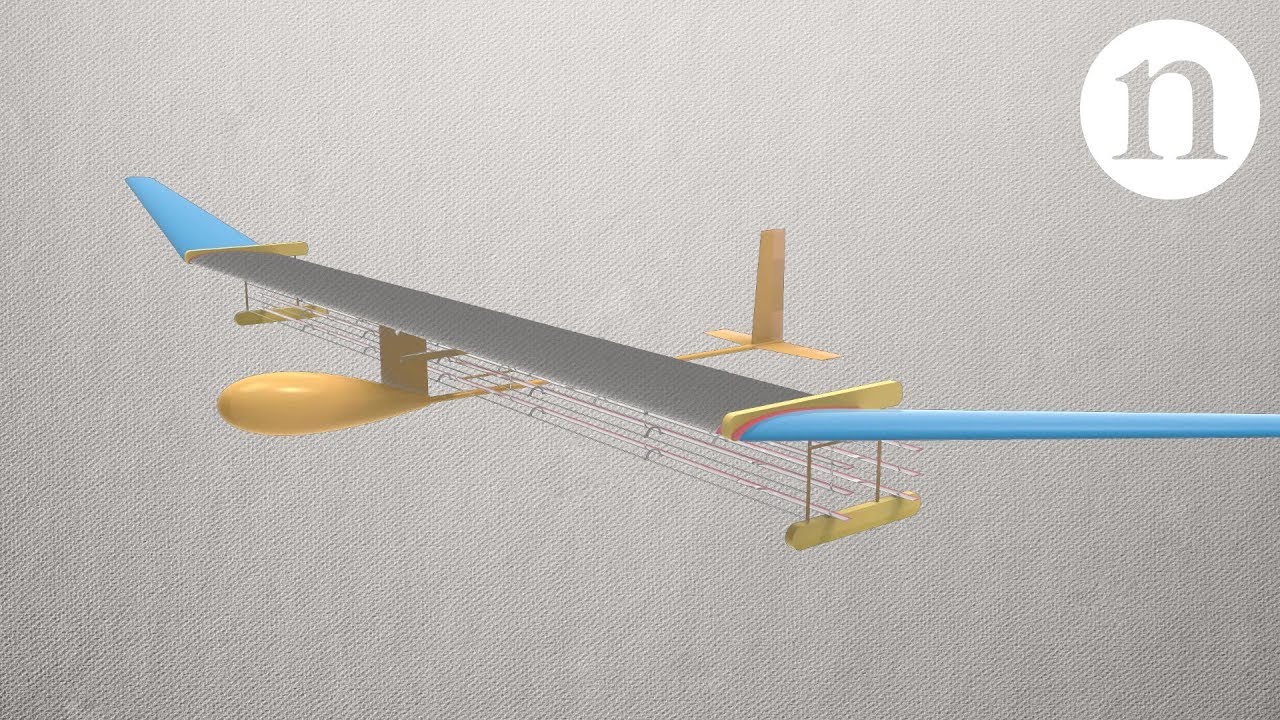
Ions take flight
The team’s final design resembles a large, lightweight glider. The aircraft, which weighs about 5 pounds and has a 5-meter wingspan, carries an array of thin wires, which are strung like horizontal fencing along and beneath the front end of the plane’s wing. The wires act as positively charged electrodes, while similarly arranged thicker wires, running along the back end of the plane’s wing, serve as negative electrodes.
The fuselage of the plane holds a stack of lithium-polymer batteries. Barrett's ion plane team included members of Professor David Perreault’s Power Electronics Research Group in the Research Laboratory of Electronics, who designed a power supply that would convert the batteries’ output to a sufficiently high voltage to propel the plane. In this way, the batteries supply electricity at 40,000 volts to positively charge the wires via a lightweight power converter.
Once the wires are energized, they act to attract and strip away negatively charged electrons from the surrounding air molecules, like a giant magnet attracting iron filings. The air molecules that are left behind are newly ionized, and are in turn attracted to the negatively charged electrodes at the back of the plane.
As the newly formed cloud of ions flows toward the negatively charged wires, each ion collides millions of times with other air molecules, creating a thrust that propels the aircraft forward.
Undistorted camera footage from unpowered glide 2, with position and energy from camera tracking annotated. Credit: Steven Barrett
The team, which also included Lincoln Laboratory staff Thomas Sebastian and Mark Woolston, flew the plane in multiple test flights across the gymnasium in MIT’s duPont Athletic Center — the largest indoor space they could find to perform their experiments. The team flew the plane a distance of 60 meters (the maximum distance within the gym) and found the plane produced enough ionic thrust to sustain flight the entire time. They repeated the flight 10 times, with similar performance.
Undistorted camera footage from flight 9, with position and energy from camera tracking annotated. Sped up 2x. Credit: Steven Barrett
“This was the simplest possible plane we could design that could prove the concept that an ion plane could fly,” Barrett says. “It’s still some way away from an aircraft that could perform a useful mission. It needs to be more efficient, fly for longer, and fly outside.”
The new design is a “big step” toward demonstrating the feasibility of ion wind propulsion, according to Franck Plouraboue, senior researcher at the Institute of Fluid Mechanics in Toulouse, France, who notes that researchers previously weren’t able to fly anything heavier than a few grams.
“The strength of the results are a direct proof that steady flight of a drone with ionic wind is sustainable,” says Plouraboue, who was not involved in the research. “[Outside of drone applications], it is difficult to infer how much it could influence aircraft propulsion in the future. Nevertheless, this is not really a weakness but rather an opening for future progress, in a field which is now going to burst.”
Barrett’s team is working on increasing the efficiency of their design, to produce more ionic wind with less voltage. The researchers are also hoping to increase the design’s thrust density — the amount of thrust generated per unit area. Currently, flying the team’s lightweight plane requires a large area of electrodes, which essentially makes up the plane’s propulsion system. Ideally, Barrett would like to design an aircraft with no visible propulsion system or separate controls surfaces such as rudders and elevators.
“It took a long time to get here,” Barrett says. “Going from the basic principle to something that actually flies was a long journey of characterizing the physics, then coming up with the design and making it work. Now the possibilities for this kind of propulsion system are viable.”
This research was supported, in part, by MIT Lincoln Laboratory Autonomous Systems Line, the Professor Amar G. Bose Research Grant, and the Singapore-MIT Alliance for Research and Technology (SMART). The work was also funded through the Charles Stark Draper and Leonardo career development chairs at MIT.
Share this news article on:
Press mentions.
Prof. Steven Barrett speaks with Forbes reporter Jeremy Bogaisky about the new plane he developed that is propelled by an ion drive, noting that he is working to embed a prolusion system within the skin of the aircraft. “There’s no reason to think long-term that airplane designs with electroaerodynamic propulsion need look at all like an airplane today,” explains Barrett.
The Economist highlights how MIT researchers have developed the first plane that is powered by an ion drive and has no moving parts. “The use of an ion drive means the MIT craft contains no moving propulsion parts in the form of propellers or jet engines,” The Economist explains. “It can fly silently and without direct emissions from burning fossil fuels.”
CNN reporter Helen Regan highlights a new solid-state plane developed by MIT researchers that has no moving parts and does not require fossil fuels. “The flight is a milestone in ‘ionic wind’ technology,” explains Regan, “and could pave the way for quieter and environmentally cleaner aircraft in the future.”
Scientific American
Scientific American reporter Angus Chen writes about how Prof. Steven Barrett has created the first-ever airplane that is powered by ionic wind thrusters and has no moving parts. “[Barrett] has demonstrated something truly unique,” says Prof. Mitchell Walker of the Georgia Institute of Technology.
A Nature editorial highlights the historic breakthrough achieved by MIT researchers who developed the first plane that is propelled by ionic wind and has no moving parts. Nature writes that the plane is a “remarkable machine,” adding that “anyone who watches the machine fly can surely see glimpses of a future with cleaner and quieter aircraft.”
The Conversation
In an article for The Conversation , Prof. Steven Barrett details how he was inspired by science fiction movies to create an airplane that makes no noise, has no moving parts and does not require fossil fuels to operate. Barrett explains that he hopes the new technology “could be used in larger aircraft to reduce noise and even allow an aircraft’s exterior skin to help produce thrust.”
Popular Science
Writing for Popular Science , Rob Verger highlights how MIT researchers have built and flown “a radically different type of plane that is thrust through the air using just electricity and the movement of ions, a type of silent drive without moving parts out of science fiction.”

The Washington Post
MIT researchers have built a new electric plane that has no moving parts and is propelled by “ionic wind,” reports Joel Achenbach for The Washington Post . Franck Plouraboué of Toulouse University, explains that the new plane creates “an opening for future progress, in a field which is now going to burst.”
Reuters reporter Will Dunham writes that a new plane without moving parts developed by MIT researchers is a “radical new approach toward flying.” The plane could one day lead to “ultra-efficient and nearly silent airplanes that have no moving control surfaces like rudders or elevators, no moving propulsion system like propellers or turbines, and no direct combustion emissions like you get with burning jet fuel,” explains Prof. Steven Barrett.
Associated Press
Inspired by “Star Trek,” Prof. Steven Barrett has developed a new silent airplane that does not require fossil fuels to operate and is powered by ionic wind thrusters, reports Malcom Ritter for the AP. Ritter explains that the technology that powers the plane could eventually be used “in airplane-like drones that perform tasks like environmental monitoring and surveillance.”
Previous item Next item
Related Links
- Flight of an aeroplane with solid-state propulsion
- Steven Barrett
- MIT Electric Aircraft Initiative
- Power Electronics Research Group
- Research Laboratory of Electronics
- MIT Lincoln Laboratory
- More Videos From the MIT Electric Aircraft Initiative
- Department of Aeronautics and Astronautics
Related Topics
- Aeronautical and astronautical engineering
Related Articles
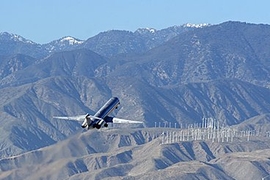
A mighty wind
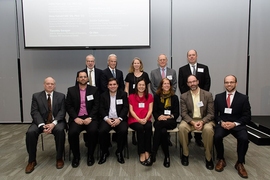
Bose Grants for 2017 reward bold and unconventional research visions
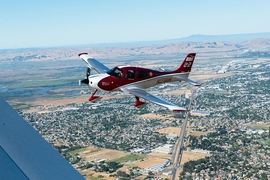
Unfriendly skies: Piston engine aircraft pose a significant health threat
More mit news.
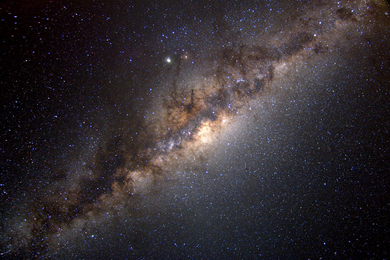
MIT researchers discover the universe’s oldest stars in our own galactic backyard
Read full story →
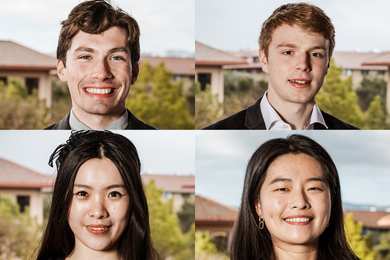
Four from MIT named 2024 Knight-Hennessy Scholars
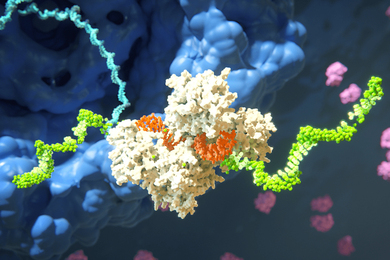
Taking RNAi from interesting science to impactful new treatments
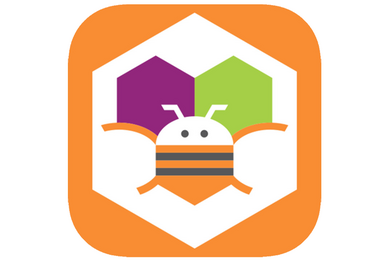
The power of App Inventor: Democratizing possibilities for mobile applications
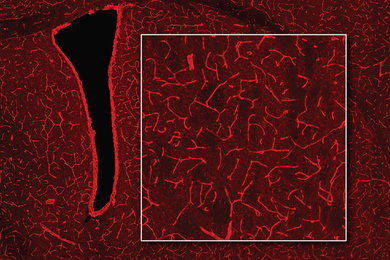
Using MRI, engineers have found a way to detect light deep in the brain
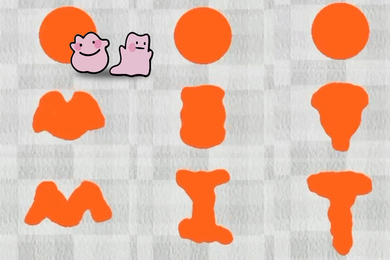
A better way to control shape-shifting soft robots
- More news on MIT News homepage →
Massachusetts Institute of Technology 77 Massachusetts Avenue, Cambridge, MA, USA
- Map (opens in new window)
- Events (opens in new window)
- People (opens in new window)
- Careers (opens in new window)
- Accessibility
- Social Media Hub
- MIT on Facebook
- MIT on YouTube
- MIT on Instagram
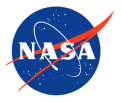
Suggested Searches
- Climate Change
- Expedition 64
- Mars perseverance
- SpaceX Crew-2
- International Space Station
- View All Topics A-Z
Humans in Space
Earth & climate, the solar system, the universe, aeronautics, learning resources, news & events.
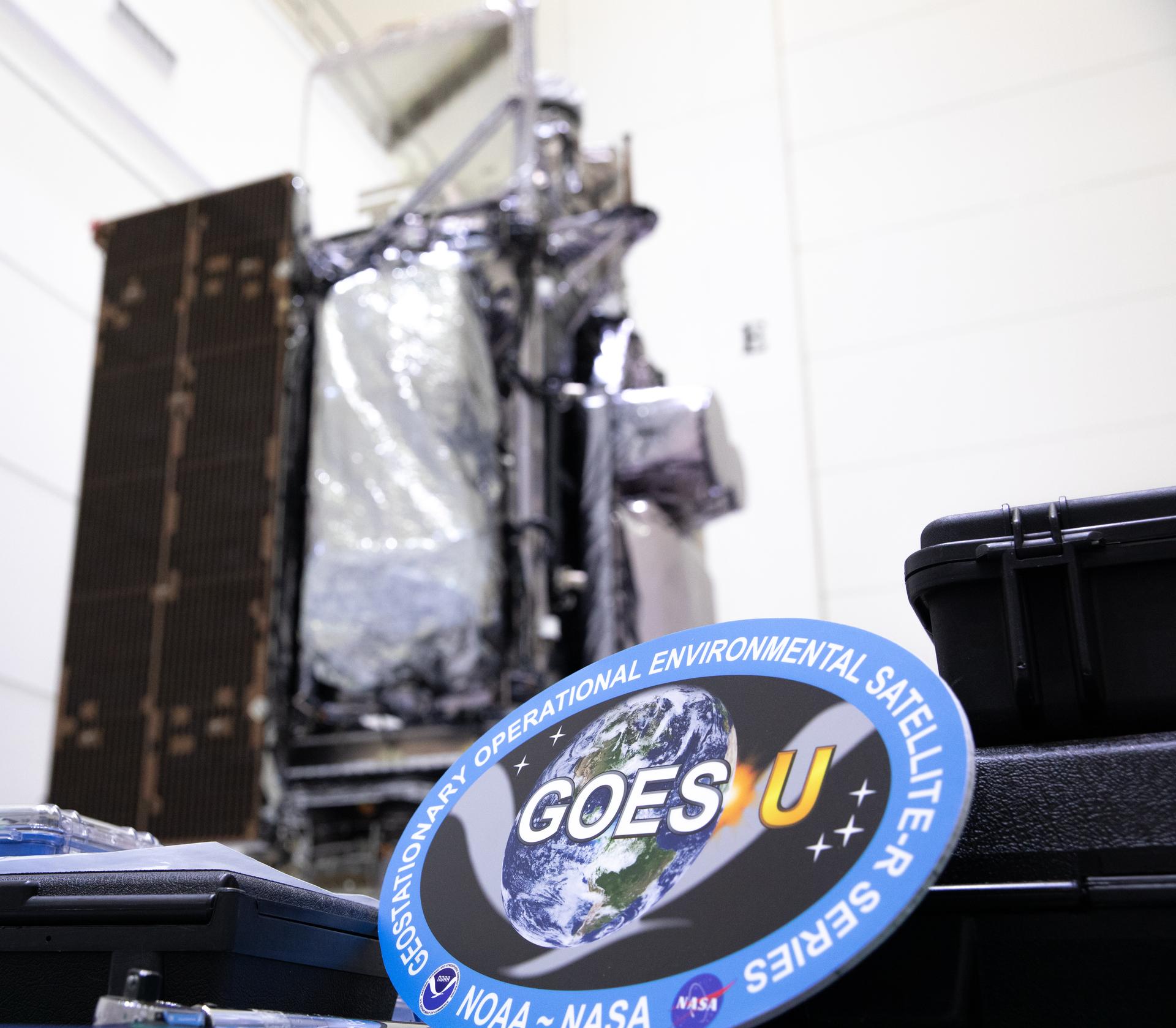
NASA Invites Social Creators for Launch of NOAA Weather Satellite
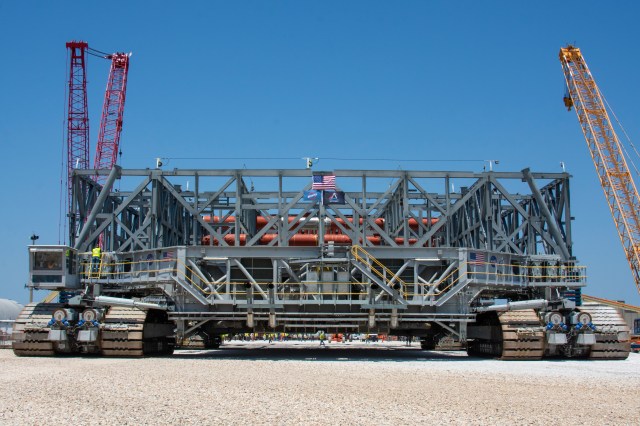
NASA’s New Mobile Launcher Stacks Up for Future Artemis Missions
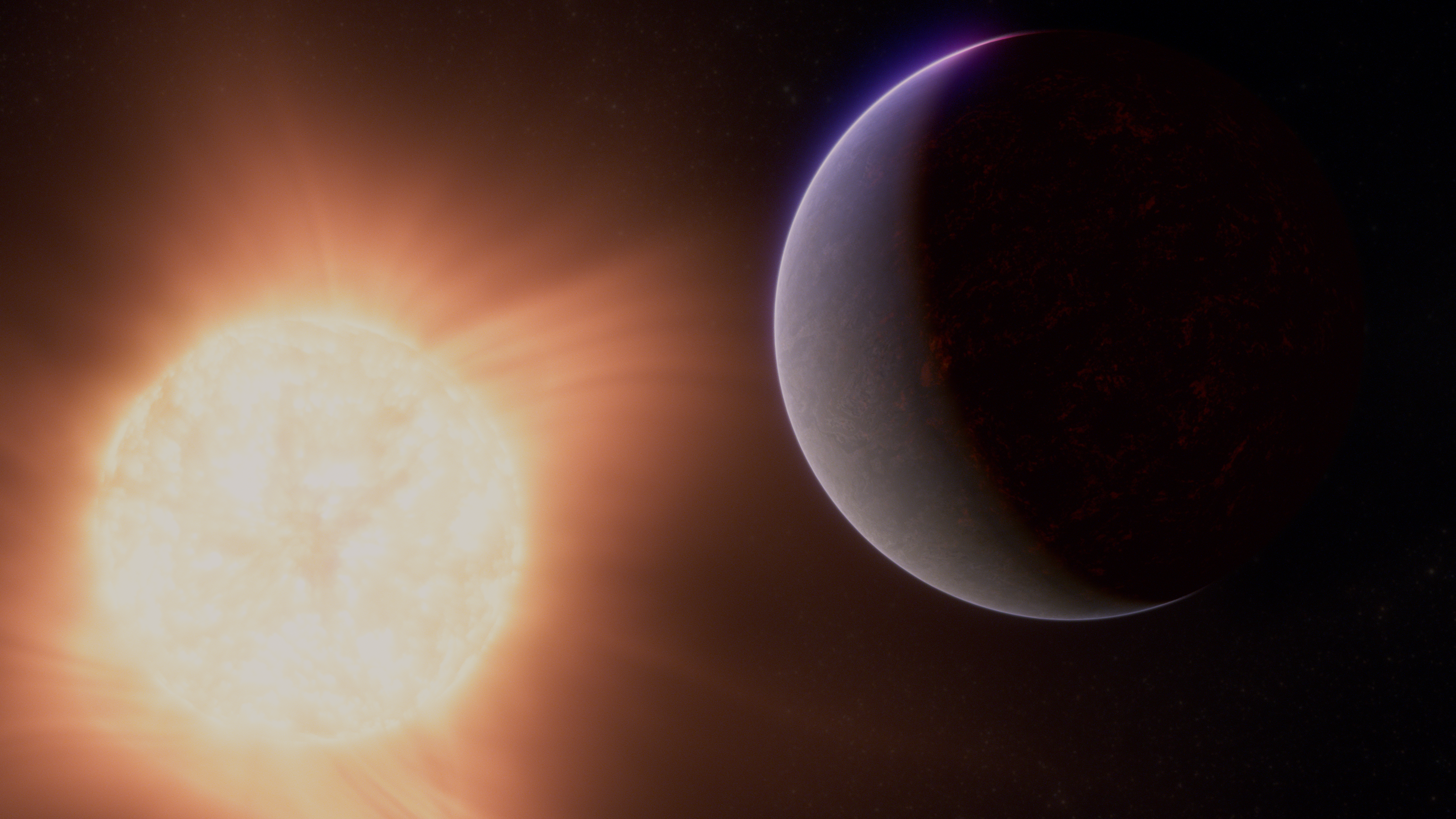
NASA’s Webb Hints at Possible Atmosphere Surrounding Rocky Exoplanet
- Search All NASA Missions
- A to Z List of Missions
- Upcoming Launches and Landings
- Spaceships and Rockets
- Communicating with Missions
- James Webb Space Telescope
- Hubble Space Telescope
- Why Go to Space
- Astronauts Home
- Commercial Space
- Destinations
- Living in Space
- Explore Earth Science
- Earth, Our Planet
- Earth Science in Action
- Earth Multimedia
- Earth Science Researchers
- Pluto & Dwarf Planets
- Asteroids, Comets & Meteors
- The Kuiper Belt
- The Oort Cloud
- Skywatching
- The Search for Life in the Universe
- Black Holes
- The Big Bang
- Dark Energy & Dark Matter
- Earth Science
- Planetary Science
- Astrophysics & Space Science
- The Sun & Heliophysics
- Biological & Physical Sciences
- Lunar Science
- Citizen Science
- Astromaterials
- Aeronautics Research
- Human Space Travel Research
- Science in the Air
- NASA Aircraft
- Flight Innovation
- Supersonic Flight
- Air Traffic Solutions
- Green Aviation Tech
- Drones & You
Technology Transfer & Spinoffs
- Space Travel Technology
- Technology Living in Space
- Manufacturing and Materials
- Science Instruments
- For Kids and Students
- For Educators
- For Colleges and Universities
- For Professionals
- Science for Everyone
- Requests for Exhibits, Artifacts, or Speakers
- STEM Engagement at NASA
- NASA's Impacts
- Centers and Facilities
- Directorates
- Organizations
- People of NASA
- Internships
- Our History
- Doing Business with NASA
- Get Involved
- Aeronáutica
- Ciencias Terrestres
- Sistema Solar
- All NASA News
- Video Series on NASA+
- Newsletters
- Social Media
- Media Resources
- Upcoming Launches & Landings
- Virtual Events
- Sounds and Ringtones
- Interactives
- STEM Multimedia
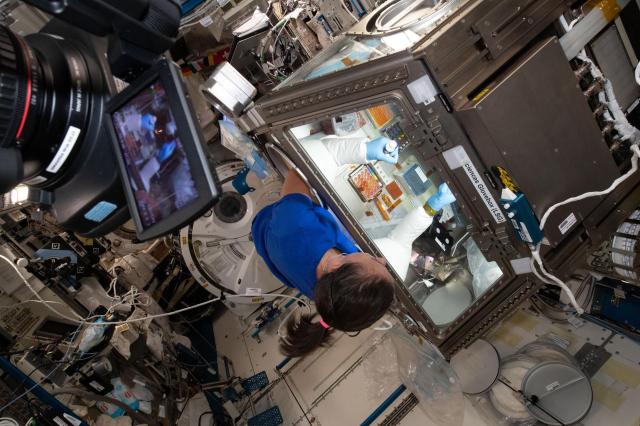
Station Science 101 | Research in Microgravity: Higher, Faster, Longer
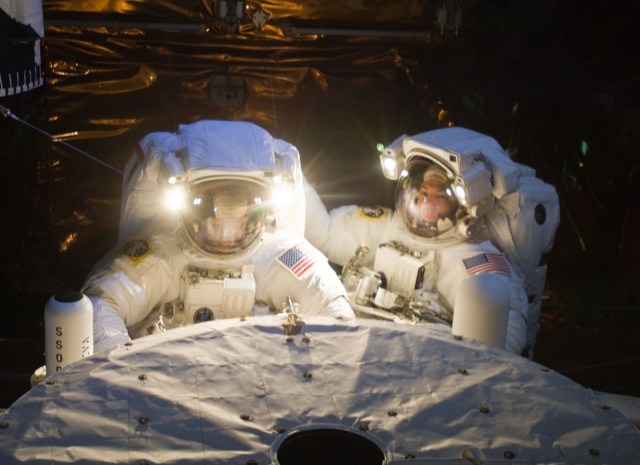
15 Years Ago: STS-125, the Final Hubble Servicing Mission
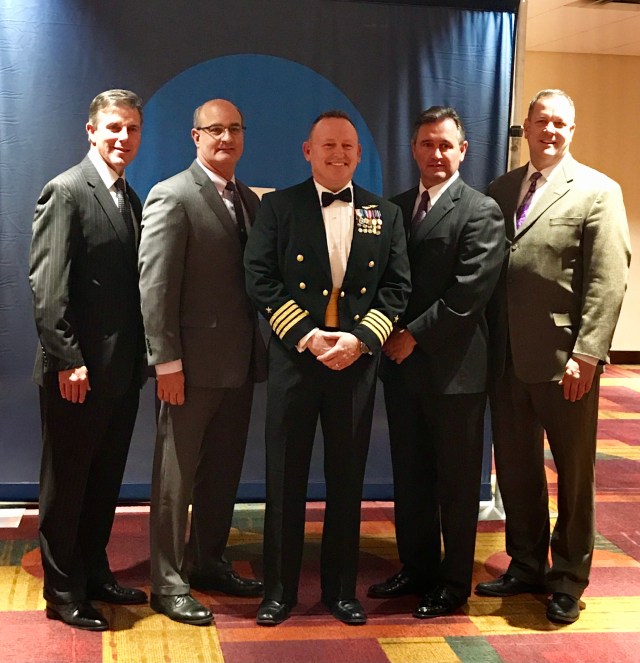
NASA Mission Strengthens 40-Year Friendship
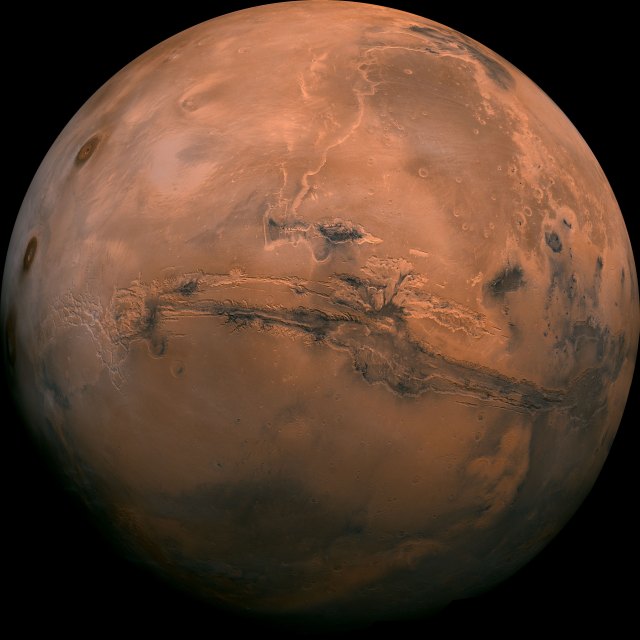
NASA Selects Commercial Service Studies to Enable Mars Robotic Science
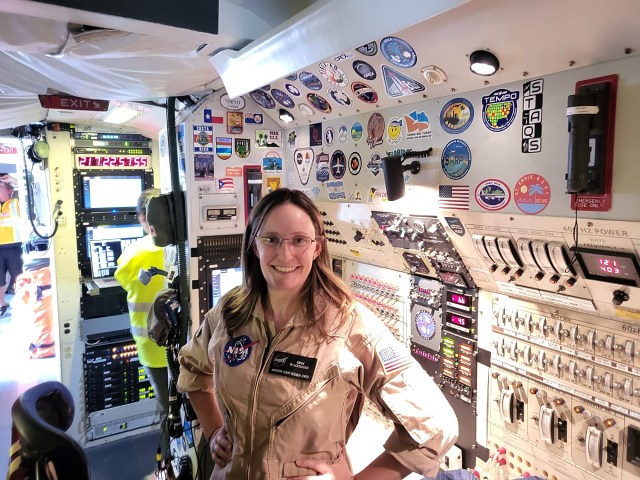
Meet NASA Women Behind World’s Largest Flying Laboratory
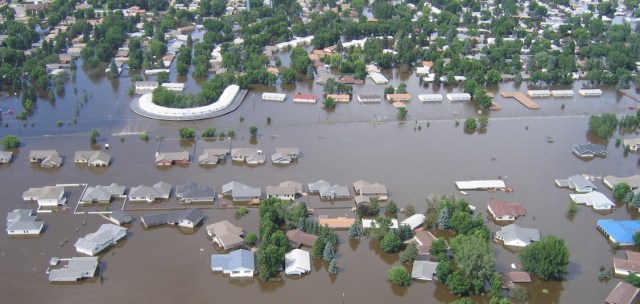
International SWOT Mission Can Improve Flood Prediction
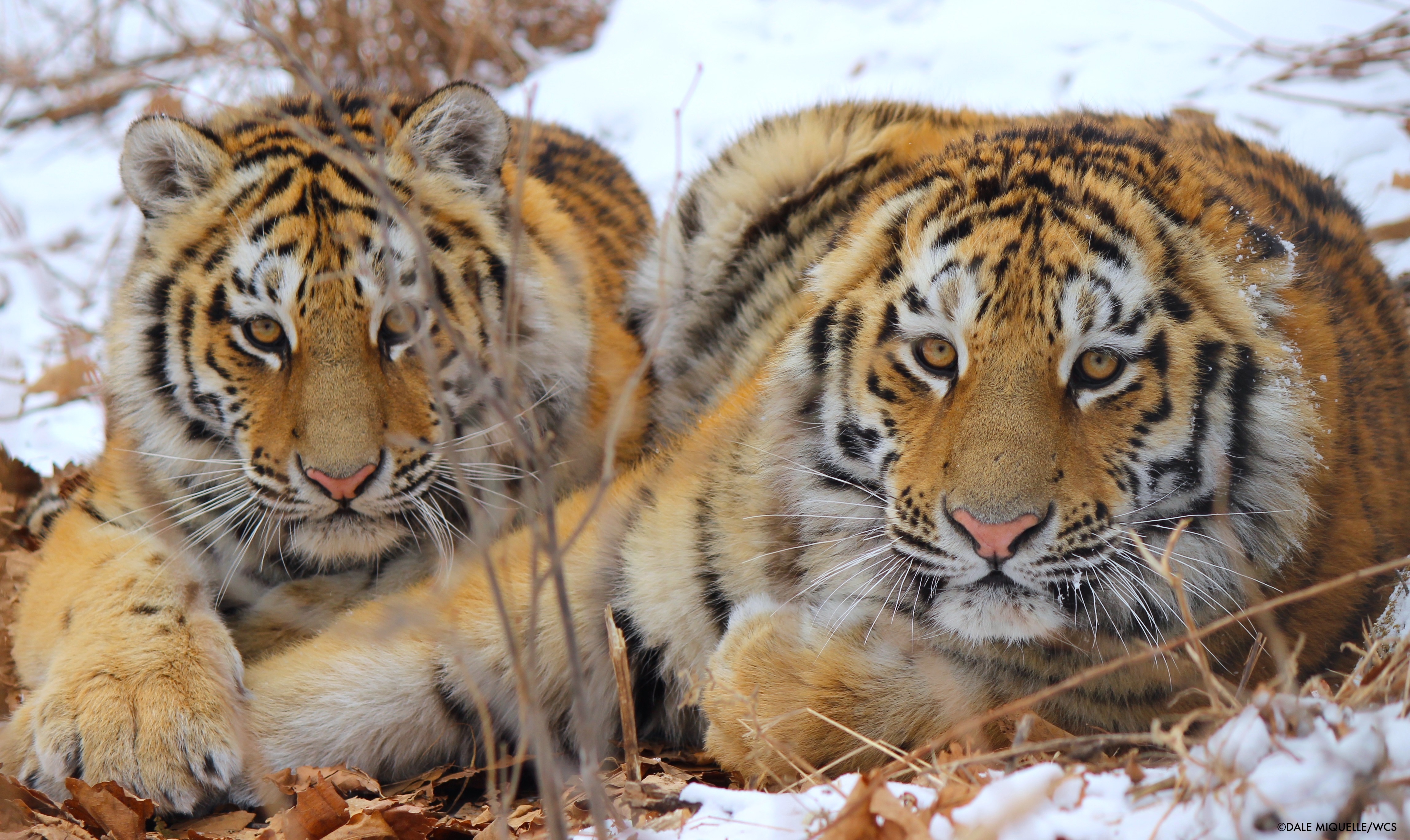
NASA Is Helping Protect Tigers, Jaguars, and Elephants. Here’s How.
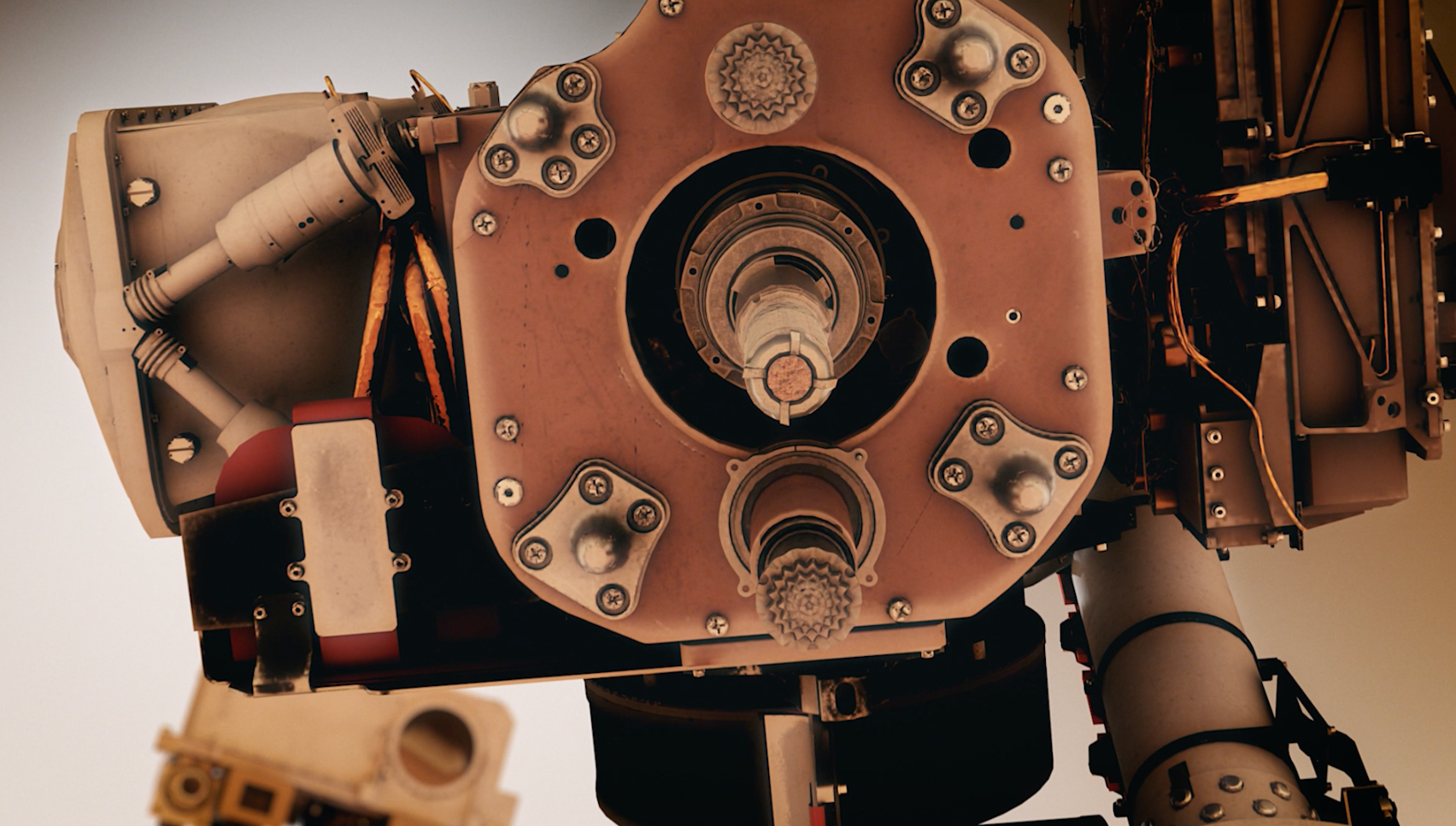
C.26 Rapid Mission Design Studies for Mars Sample Return Correction and Other Documents Posted
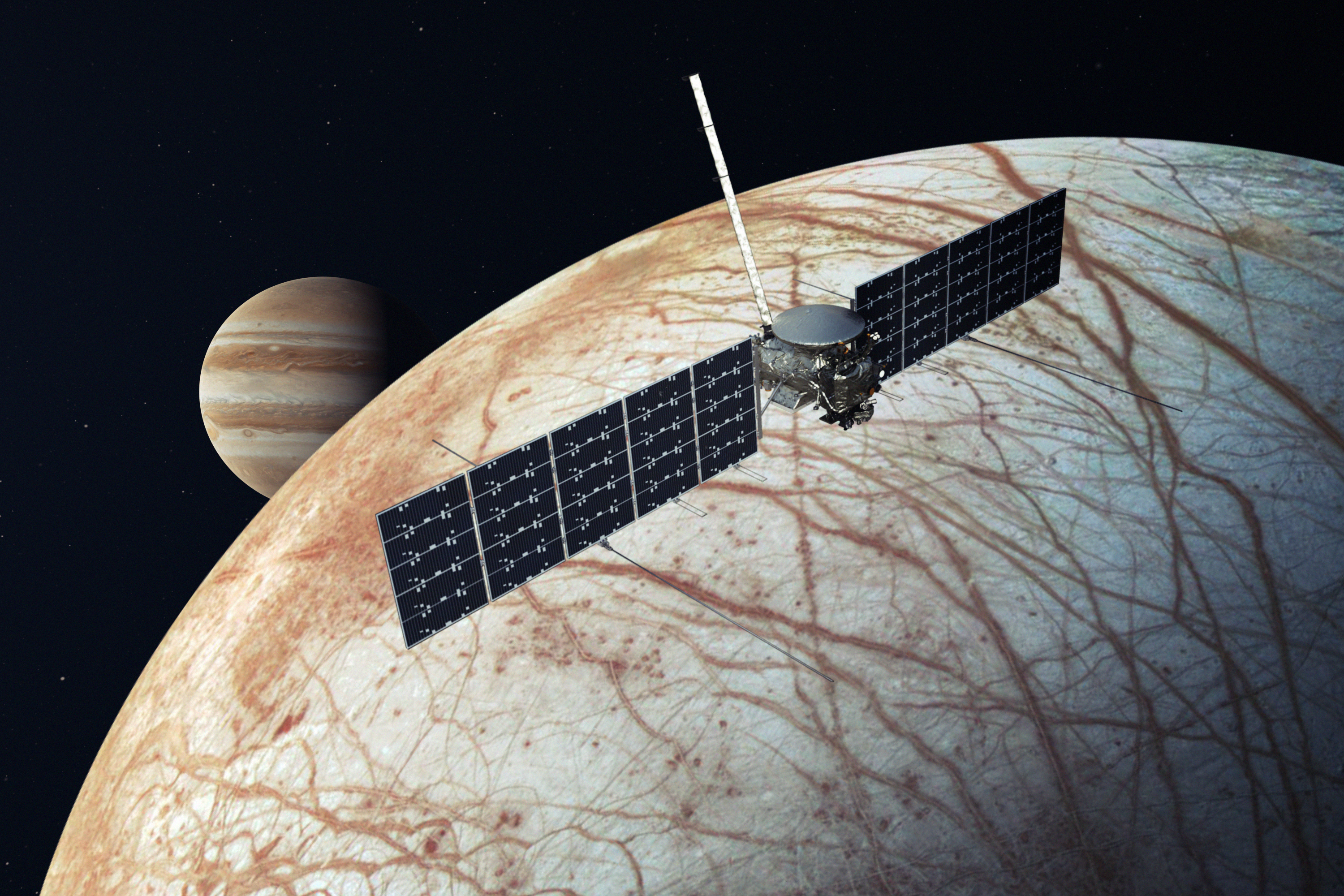
NASA Selects Students for Europa Clipper Intern Program
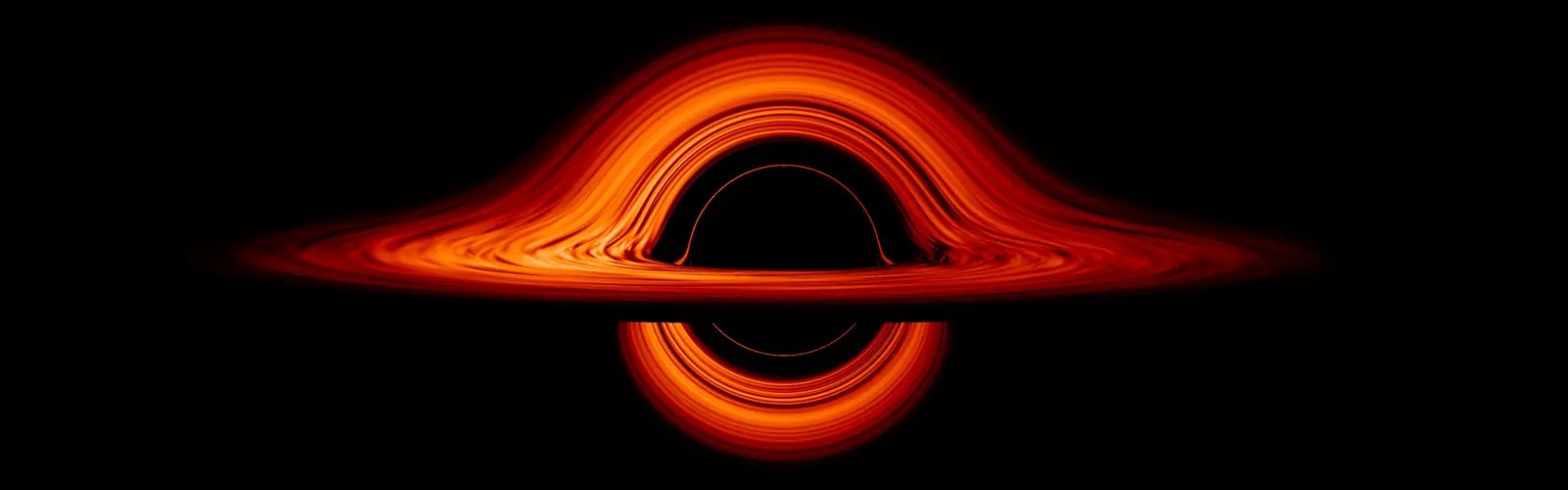
The Big Event, 2024
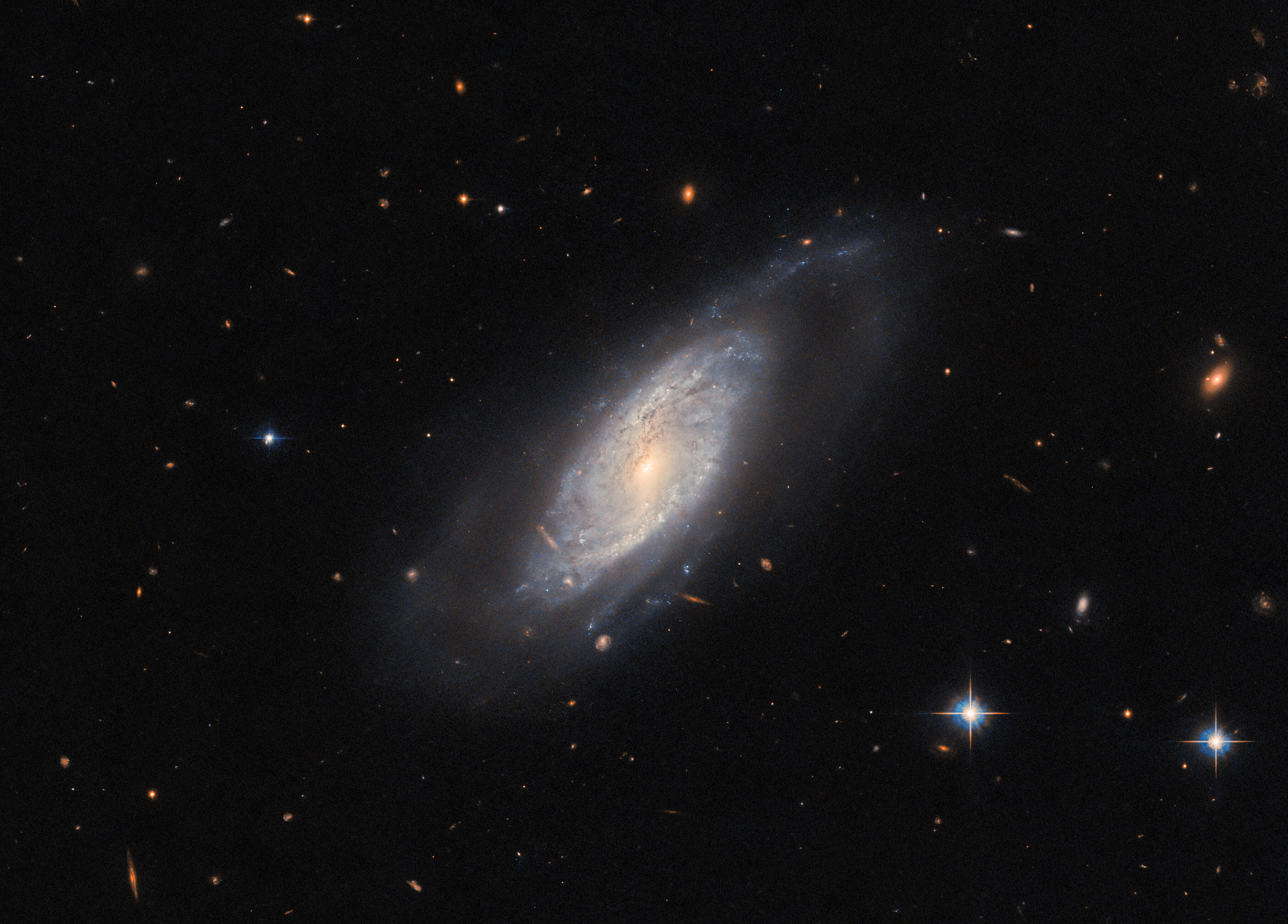
Hubble Glimpses a Star-Forming Factory
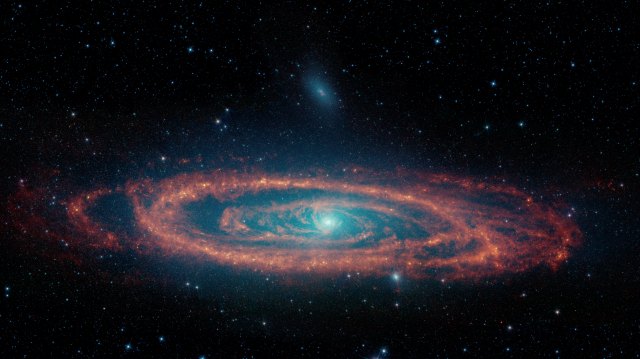
NASA Images Help Explain Eating Habits of Massive Black Hole
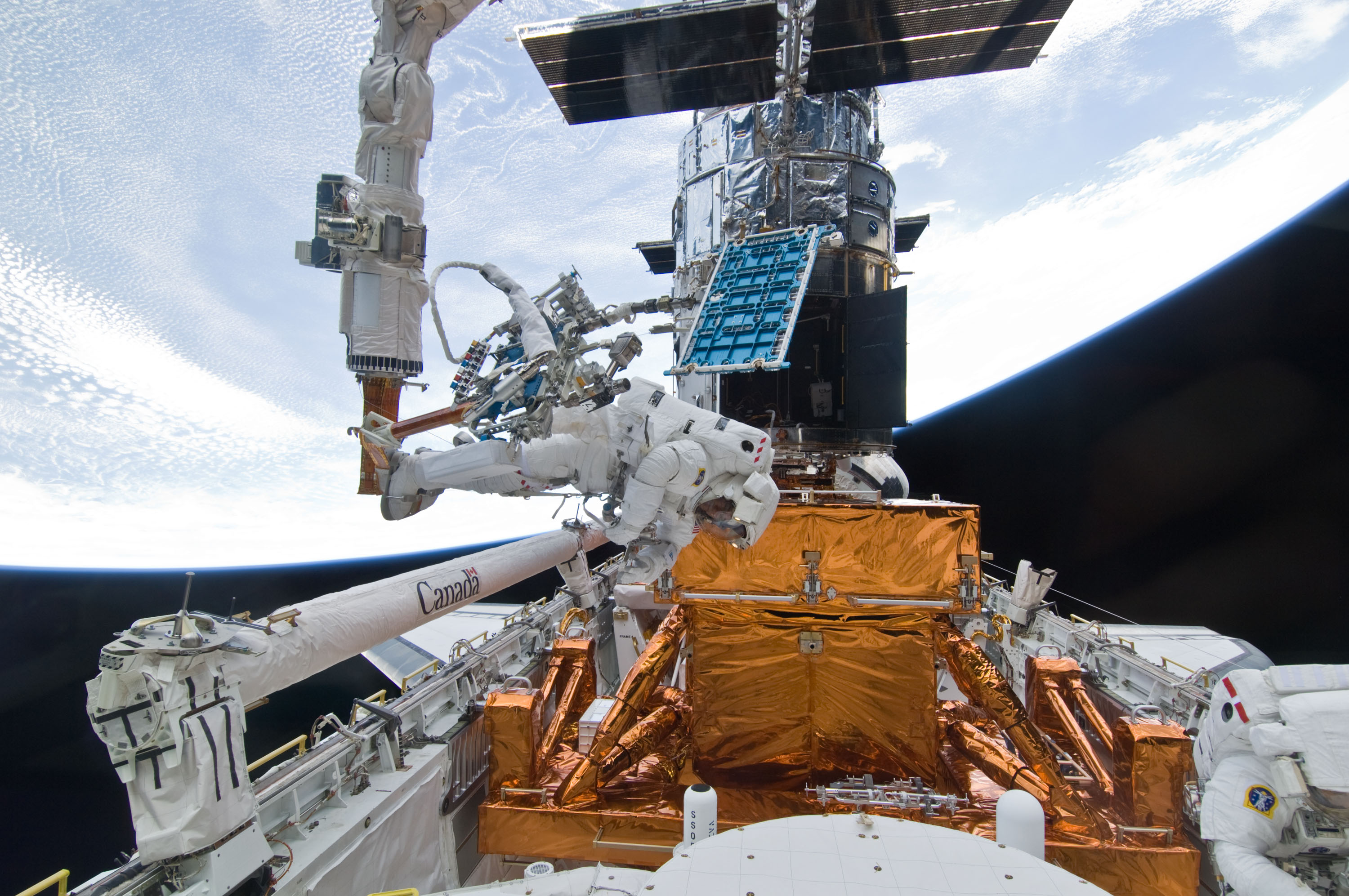
Hubble Celebrates the 15th Anniversary of Servicing Mission 4
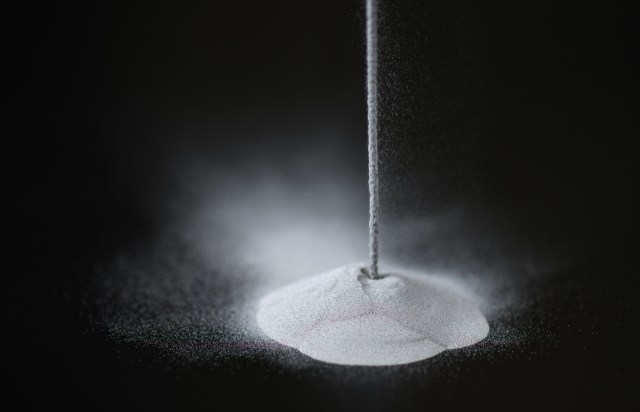
NASA Licenses 3D-Printable Superalloy to Benefit US Economy
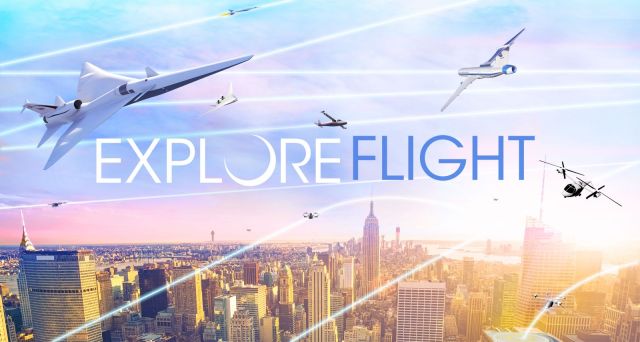
ARMD Solicitations
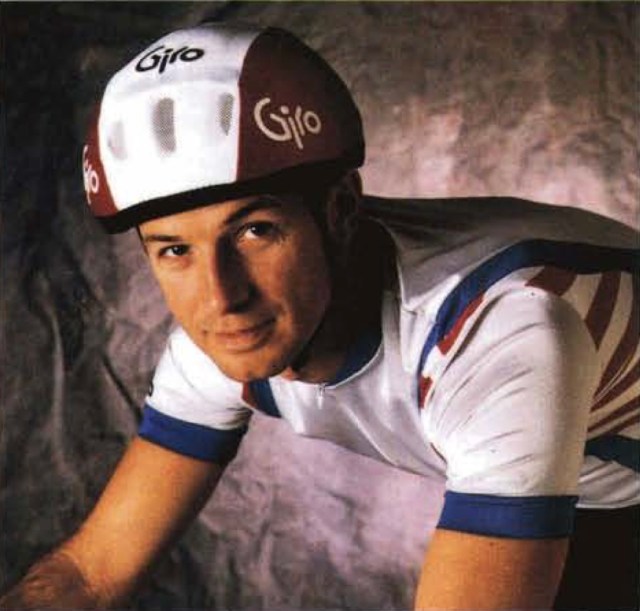
Tech Today: A NASA-Inspired Bike Helmet with Aerodynamics of a Jet
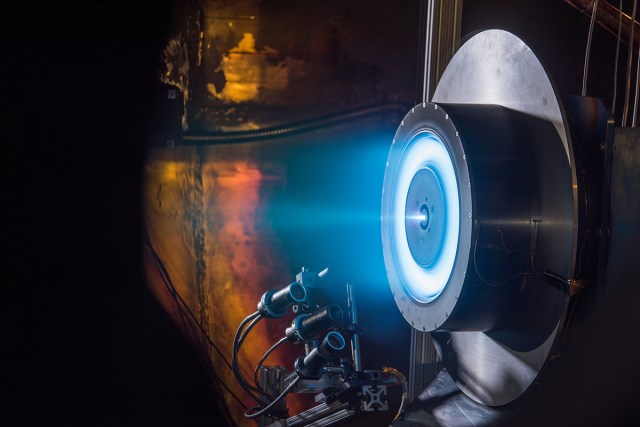
Tech Today: NASA’s Ion Thruster Knowhow Keeps Satellites Flying

Latest Join Artemis News and Features
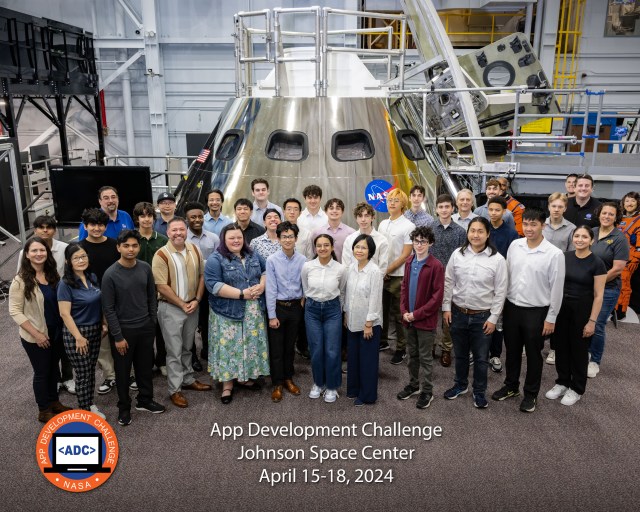
NASA Challenge Gives Artemis Generation Coders a Chance to Shine
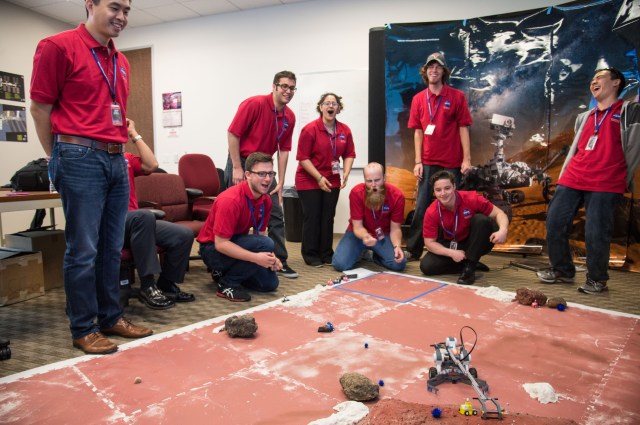
NASA Community College Aerospace Scholars
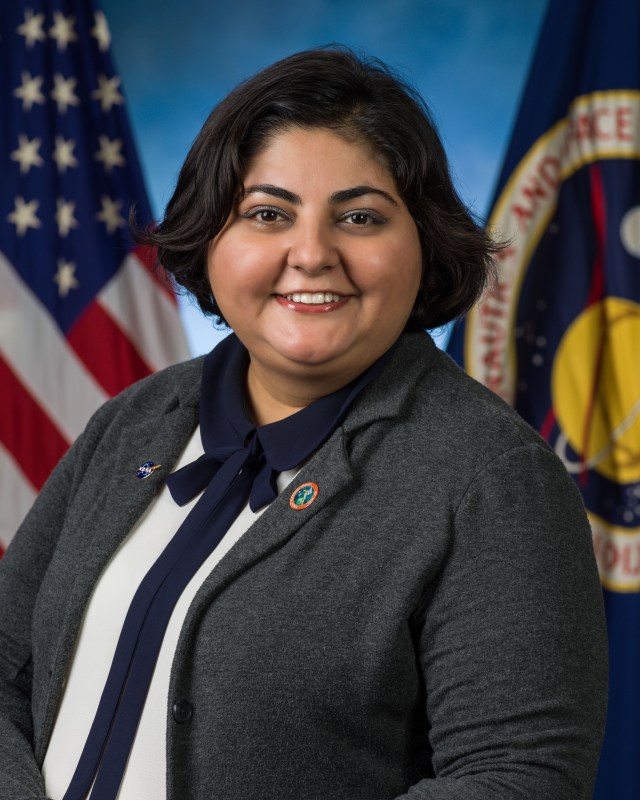
Johnson Celebrates AA and NHPI Heritage Month: Kimia Seyedmadani
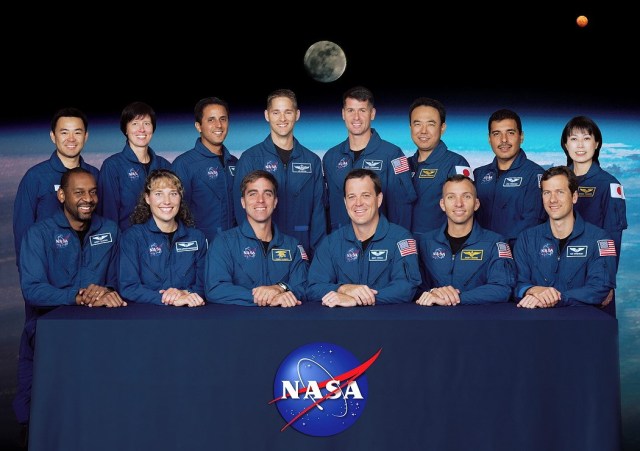
20 Years Ago: NASA Selects its 19th Group of Astronauts
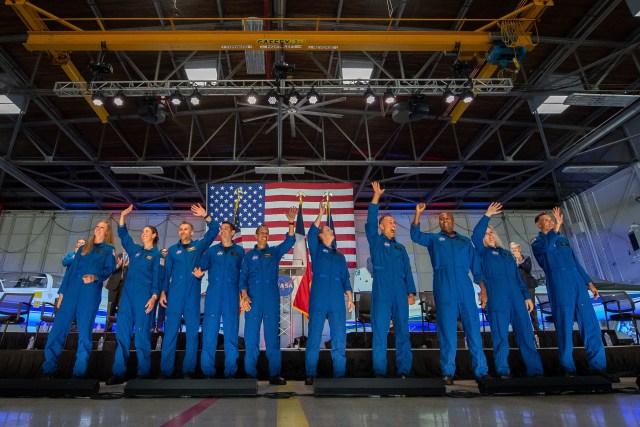
Diez maneras en que los estudiantes pueden prepararse para ser astronautas
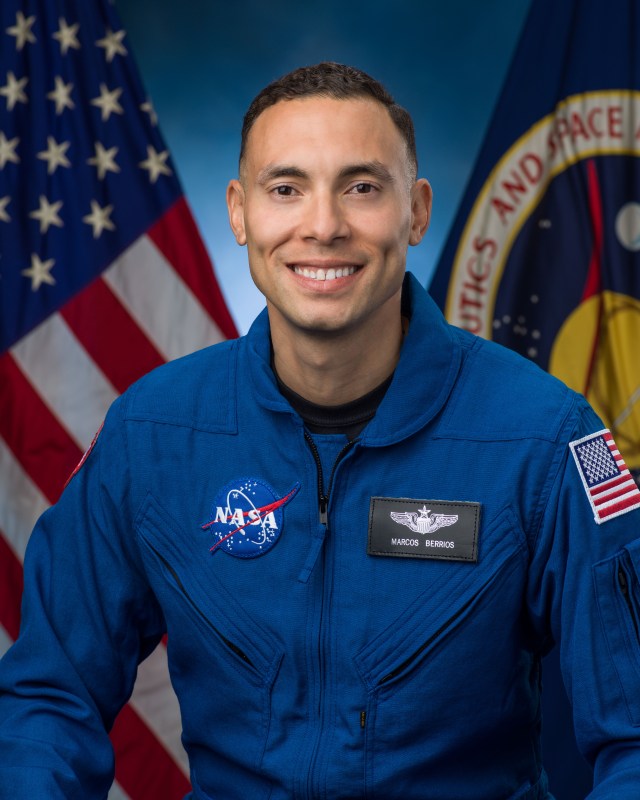
Astronauta de la NASA Marcos Berríos
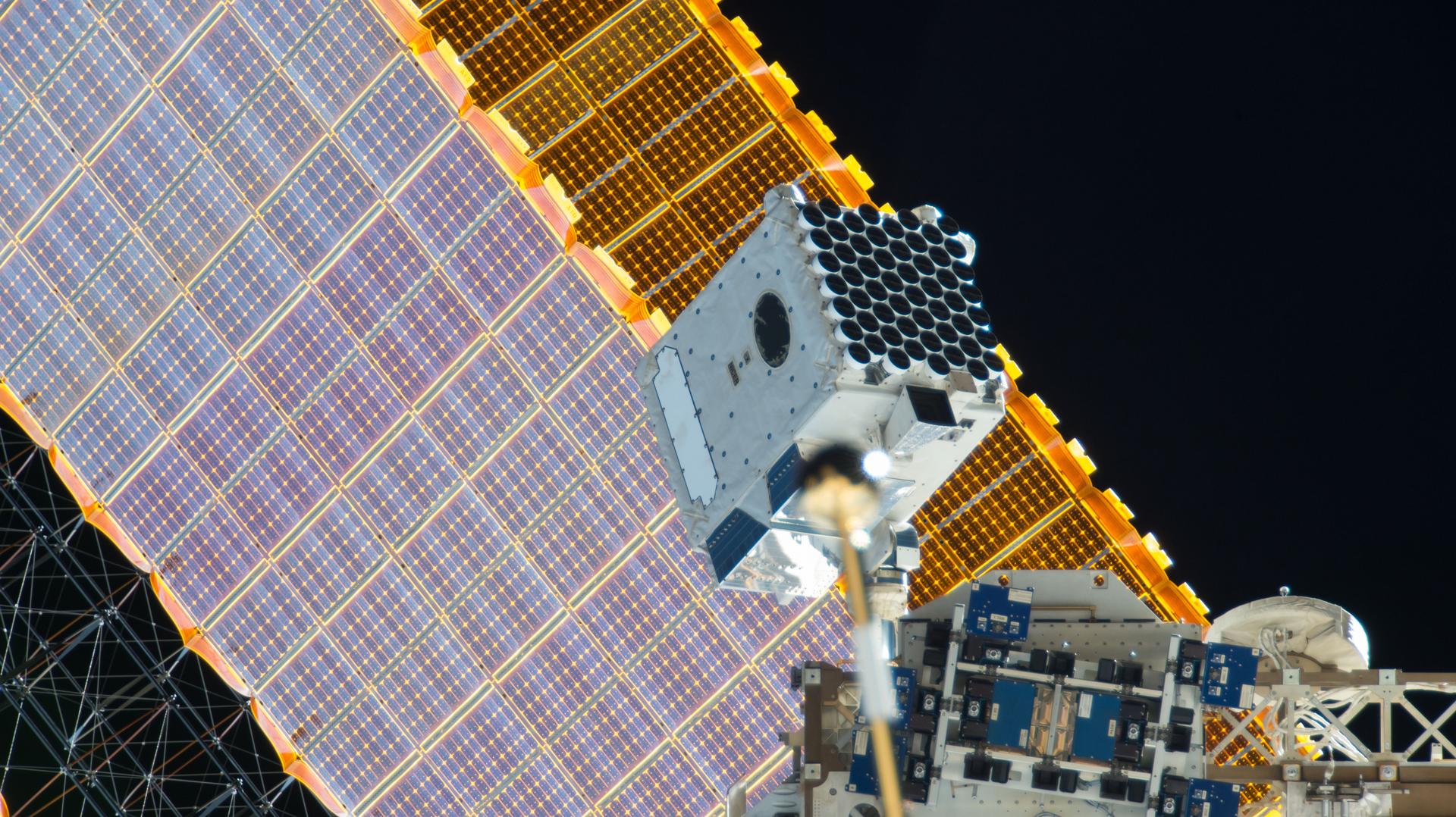
Resultados científicos revolucionarios en la estación espacial de 2023
Andrew Wagner
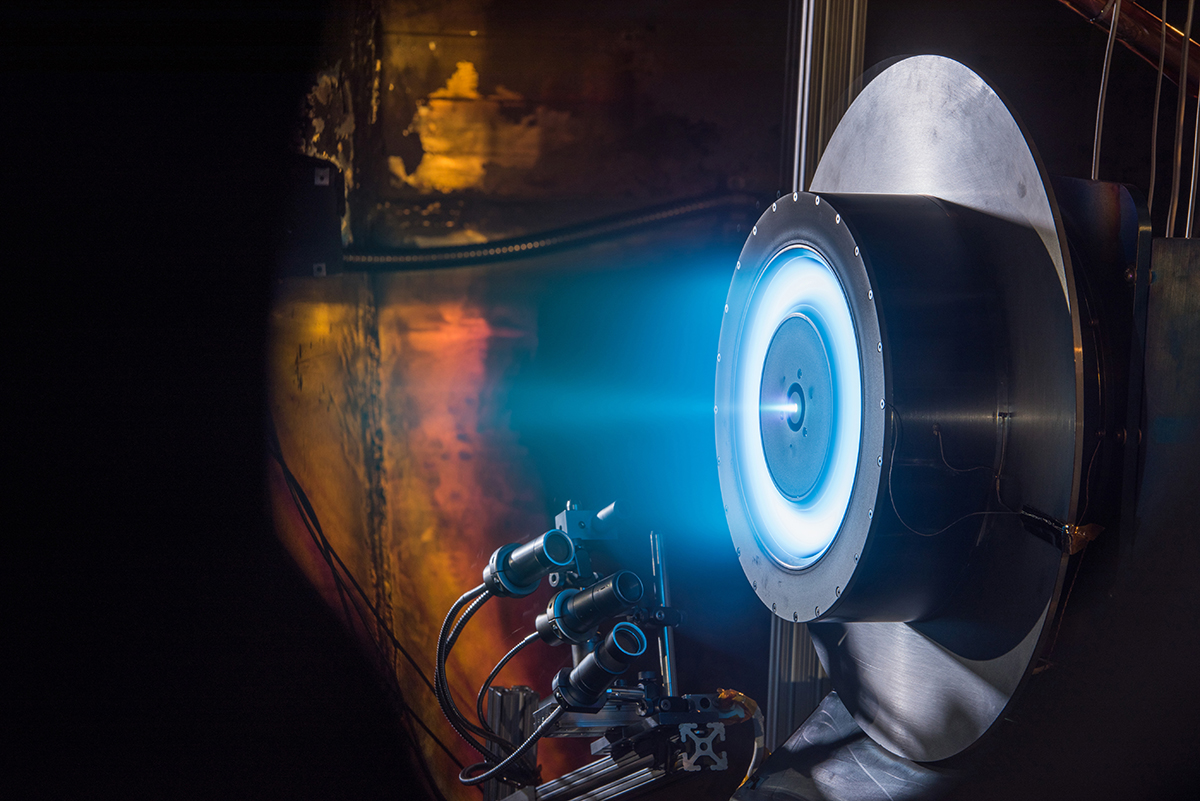
In low Earth orbit, satellites face a constant challenge – a tiny amount of atmospheric drag that, over time, causes them to slow down and decay their orbit. To combat this, spacecraft rely on in-space thrusters to adjust positioning and boost orbits. However, most of these thrusters use heavy, expensive chemical propellants. This is where the game-changing ion thrusters come in, offering a more efficient and cost-effective solution for satellite operations.
Orbion Space Technology, based in Houghton, Michigan, was established in 2016. Recognizing a market need, the company set out to find innovative ways to either extend the lifespan of satellites in orbit or increase their payload capacity. This ambitious goal necessitated the development of a thruster that could operate efficiently with minimal fuel consumption, leading to the creation of the company’s Aurora thruster.
Hall-effect thrusters, an advanced ion propulsion technology , use electricity rather than chemical reactions to propel spacecraft. Orbion’s founders saw the technology grow from an experimental concept to being regularly used on missions across the solar system. Still, the company had to turn to the experts to make these thrusters viable for satellite operators.
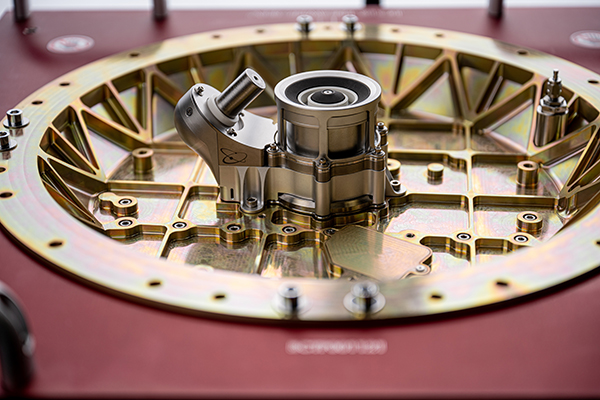
NASA’s Glenn Research Center in Cleveland leads the development of ion thrusters for the agency, designing and evaluating thrusters for missions like Dawn and DART and the agency’s Gateway lunar space station. Orbion entered into a Data Usage Agreement with NASA Glenn to receive detailed information from the development of these engines and a non-exclusive evaluation license. One of the reasons Orbion turned to NASA was its advancements in materials research for ion thrusters and the Glenn-developed cathode heater, which improves electrical efficiency and operating life.
This work resulted in Orbion’s Aurora thrusters being just as capable as those that NASA builds for its deep space science and exploration missions. Orbion has since sold several Aurora thrusters to government and private sector companies, including a recent contract with a large commercial satellite operator for its new constellation of Earth-observing spacecraft.
Related Terms
Glenn Research Center
- Technology Transfer
Explore More
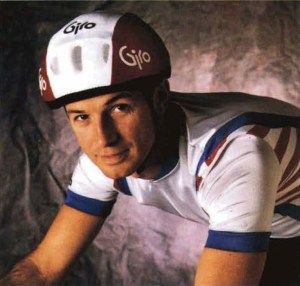
1942: Engine Roars to Life in First Test at Future NASA Glenn
Discover related topics.
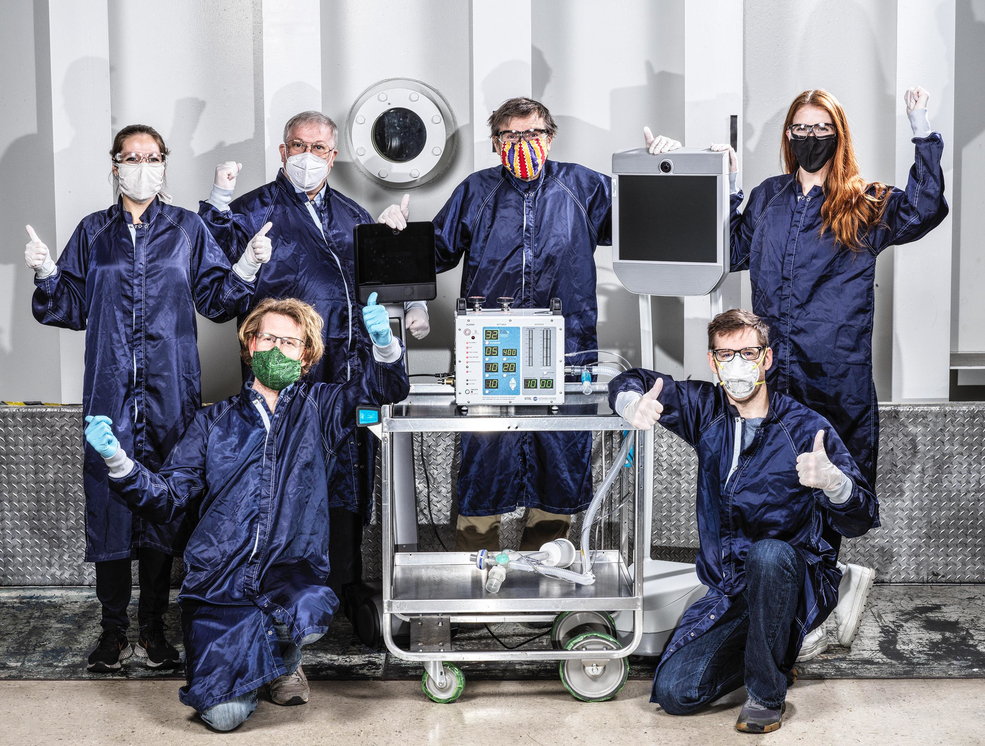
Solar Electric Propulsion
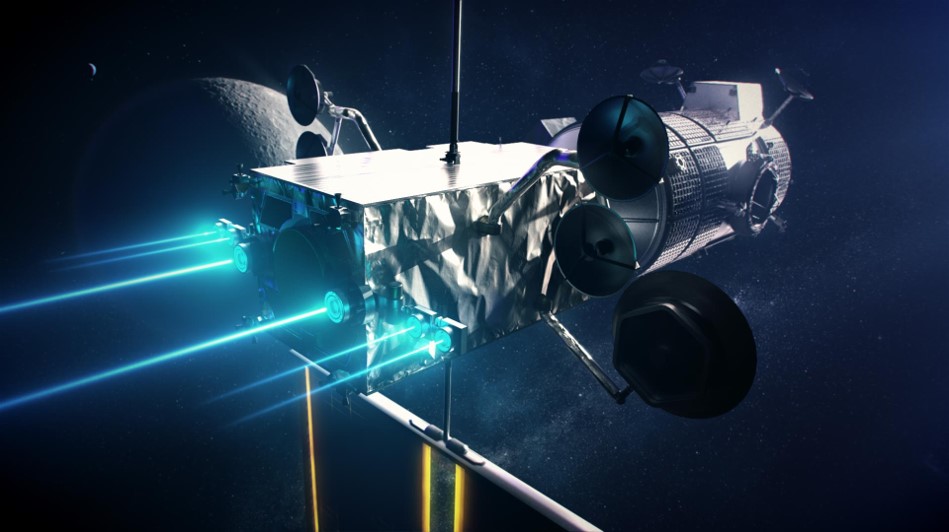
Innovative Ion Propulsion: Sustaining Satellite Orbits with NASA’s Ion Thruster Expertise
P ositioning and maintaining satellites in their designated orbits is a persistent challenge. Factors such as atmospheric friction, although very faint in low Earth orbit, can gradually diminish a satellite’s speed and potentially reduce its orbital integrity. Traditional solutions involve using thrusters powered by chemical propellants, which are burdensome in terms of weight and cost. A modern and efficient alternative to this issue is found in ion thrusters, a technology that promises to revolutionize the maintenance of satellite orbits.
Orbion Space Technology, operating out of Houghton, Michigan since 2016, aimed to tackle the problem. The company has been dedicated to the dual objectives of lengthening satellite lifespans and maximizing their payload capabilities. To achieve these aims, Orbion focused on developing a thruster named Aurora enthused by minimal fuel usage yet high efficiency.
These thrusters are modeled after the Hall-effect technology, which leverages electric power rather than chemical interactions for thrust, transitioning from experimental stages to frequent application in various space ventures. However, to ensure their product met the industry’s requirements, Orbion needed to collaborate with those more experienced in the field.
At the forefront of ion thruster progression is NASA’s Glenn Research Center, which conducts research to augment these technologies for high-profile missions, including Dawn, DART, and the Gateway lunar space station. By entering agreements with NASA Glenn, Orbion gained access to extensive data and expertise, particularly benefiting from NASA’s advanced material studies for ion thrusters and an innovative cathode heater that heightens the electrical efficiency and operational lifespan.
The partnership with NASA culminated in Orbion’s Aurora thruster achieving a level of performance parallel to that of NASA’s thrusters crafted for exploratory and scientific deep-space ventures. The result has seen Orbion successfully market its thrusters, earning contracts with numerous clients, including a recent agreement to equip a new fleet of commercial satellites focused on Earth observation.
FAQ Section
Q1: What are ion thrusters and how do they work?
A1: Ion thrusters utilize electric power to accelerate ions and create thrust, allowing spacecraft adjustments and orbit maintenance with higher efficiency compared to traditional chemical propellants.
Q2: What is the significance of NASA’s ion thruster technology?
A2: NASA’s ion thruster technology, researched and developed at Glenn Research Center, has been pivotal in enabling long-duration deep-space missions and is crucial for the sustainability of satellites in low Earth orbit.
Q3: Who is Orbion Space Technology?
A3: Orbion Space Technology is a company that specializes in the development of efficient and reliable space thrusters, such as the Aurora thruster, in a bid to extend satellite lifetimes and improve payload capacities.
Q4: How did Orbion Space Technology benefit from working with NASA?
A4: Orbion gained access to advanced data, material research, and expert knowledge through agreements with NASA, enabling them to enhance their thruster technology and commercial viability.
Q5: What makes Orbion’s Aurora thrusters comparable to NASA’s thrusters?
A5: Aurora thrusters incorporate NASA’s advancements in material science and cathode heater technology, making them comparable in performance and longevity to thrusters that NASA uses for its own deep-space missions.
As satellites continue to populate our lower Earth orbit, the demand for smart, efficient, and economical technologies to sustain their operations is undeniably crucial. Ion thrusters represent a quantum leap in satellite propulsion, providing a lighter, more cost-effective, and potent alternative to traditional methods. NASA’s expertise within this realm has not only propelled its own missions but also bolstered the capabilities of industry players such as Orbion Space Technology. As a result, satellite technology remains robustly on course, ensuring the steady flow of data and services upon which our modern world so heavily relies.

COMMENTS
Ion thrusters are nowadays used as primary propulsion systems in space. The article describes how these changes related to NEW SPACE affect various aspects that are important for the development ...
Ion thrusters are characterized by the electrostatic acceleration of ions extracted from a plasma source. 24 The ion accelerator consists of electrically biased multi-aperture grids, typically two or three, which are called ion optics. The grid in contact with the plasma in the discharge chamber is called screen grid, the second one is called accelerator (accel) grid, and the third one, used ...
Propellant cost comparison. High-purity iodine is not needed in our propulsion system, and the total propellant cost for a purity of 99.5% was approximately US$60, with an additional cost below US ...
a, Thrust to power for different types of real electrical engine.Key observations: electrostatic thrusters have the highest efficiencies, with Hall thrusters typically <55% and ion engines <75% ...
This paper investigates whether biased electrodes can improve the performance of a microwave-discharge water ion thruster, which has been proposed as a propulsion system for CubeSats. ... However, they concluded that research into water ion thrusters was at the initial stage and that the performance had not reached the level needed for actual ...
The focus of our discussion is the technology of the radio frequency ion thruster as a prominent member of the gridded ion engine family. Based on this discussion, we give an overview of important research topics such as the search for alternative propellants, the development of reliable neutralizer concepts based on novel insert materials, as ...
The focus of our discussion is the technology of the radio frequency ion thruster as a prominent member of the gridded ion engine family. Based on this discussion, we give an overview of important research topics such as the search for alternative propellants, the development of reliable neutralizer concepts based on novel insert materials, as ...
Gridded ion thrusters are tested in ground vacuum chambers to verify their performance when deployed in space. However, the presence of high background pressure and conductive walls in the chamber leads to facility effects that increase uncertainty in the performance of the thruster in space. To address this issue, this study utilizes a fully kinetic simulation to investigate the facility ...
The flight distances of 55 m and durations of 12 s for the heavier-than-air aircraft with solid-state propulsion described here compare well with the powered flight of the first heavier-than-air ...
Therefore, this paper developed a torsional pendulum thrust measuring device for an existing 8 cm ion thruster, carried out research on the calibration method of the thruster under different working conditions, carried out a micro thrust measurement experiment under a wide working range of the thruster based on a reasonable calibration method ...
The T6 ion thruster is providing in-space propulsion for the BepiColombo mission, currently en route to Mercury. ... Electric Rocket Propulsion Soc. Paper IEPC-2017-060, Atlanta, GA, Oct. 2017. Google Scholar ... This research was carried out at the Jet Propulsion Laboratory, California Institute of Technology, under a contract with the ...
The NEXT engine is a type of electric propulsion in which thruster systems use electricity to accelerate the xenon propellant to speeds of up to 90,000mph (145,000km/h or 40 km/s). NEXT can produce 6.9 kW thruster power and 236 mN thrust. It can be throttled down to 0.5kW power, and has a specific impulse of 4,190 seconds (compared to 3,120 for ...
Study of an Ion Thruster Final Degree Project - Report Bachelor's Degree in Aerospace Technology Engineering Author: Carlos Sánchez Lara Director: Josep Oriol Lizandra Dalmases Terrassa, 22nd June, 2016 . ii. Abstract iii Polytechnic University of Catalonia (UPC) Abstract
Past Ion Propulsion. The NASA Glenn Research Center has . been a leader in ion propulsion tech-nology development since the late 1950s, with its first test in space— the Space Electric Rocket Test 1— flying on July 20, 1964. From 1998 to 2001, the NASA Solar Technology Application Readiness (NSTAR) ion propulsion system enabled the Deep
The research challenges for electric propulsion technologies are examined in the context of s-curve development cycles. It is shown that the need for research is driven both by the application as well as relative maturity of the technology. For flight qualified systems such as moderately-powered Hall thrusters and gridded ion thrusters, there are open questions related to testing fidelity and ...
This paper reviews recent iodine electric propulsion research and development activities at the NASA Glenn Research Center (GRC). Activities included (i) investigation of the iodine ... in-space propulsion is xenon-fed Hall-effect and gridded-ion thrusters. Such systems have not only been demonstrated by NASA on the Dawn and Deep Space 1 ...
Ion electric propulsion systems have been widely used in satellite station keeping, attitude control, and orbit transfer due to their advantages of high specific impulse, good flexibility, and high reliability. The electric breakdown might happen at grids or across isolators due to the disturbing effects of the power source or environment. The electric breakdown can induce spurious effects in ...
capability. This lack of small satellite maneuverability is where the ion Electrospray Propulsion Systems really begins to shine. 1.2 Enter electrospray propulsion In simple terms, ion electrospray propulsion operates via application of a high voltage between an "extractor" electrode and an ionic liquid propellant, from which, ions are
However, this paper mainly focuses on the performance of the ion wind thruster when the air pressure is .87-20 kPa and the altitude is 10-32 km in near space. It is the first time to systematically test the ion-wind thruster under the low pressure corresponding to the near space and obtain the thrust characteristics of the near space thruster.
The Economist highlights how MIT researchers have developed the first plane that is powered by an ion drive and has no moving parts. "The use of an ion drive means the MIT craft contains no moving propulsion parts in the form of propellers or jet engines," The Economist explains. "It can fly silently and without direct emissions from burning fossil fuels."
Tech Today: NASA's Ion Thruster Knowhow Keeps Satellites Flying. This Hall-effect thruster, shown being tested at Glenn Research Center, turns electricity and inert gas into force that could propel a spacecraft. Orbion Space Technology was founded to bring the high efficiency of these thrusters to small commercial satellites, and the company ...
A2: NASA's ion thruster technology, researched and developed at Glenn Research Center, has been pivotal in enabling long-duration deep-space missions and is crucial for the sustainability of ...