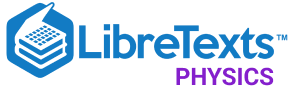
- school Campus Bookshelves
- menu_book Bookshelves
- perm_media Learning Objects
- login Login
- how_to_reg Request Instructor Account
- hub Instructor Commons
- Download Page (PDF)
- Download Full Book (PDF)
- Periodic Table
- Physics Constants
- Scientific Calculator
- Reference & Cite
- Tools expand_more
- Readability
selected template will load here
This action is not available.
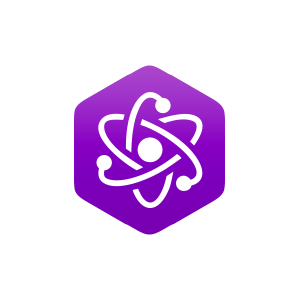

6.5: Potential Energy and Conservation of Energy
- Last updated
- Save as PDF
- Page ID 14470
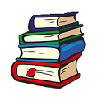
Conservative and Nonconservative Forces
Conservative force—a force with the property that the work done in moving a particle between two points is independent of the path it takes.
learning objectives
- Describe properties of conservative and nonconservative forces
A conservative force is a force with the property that the work done in moving a particle between two points is independent of the path taken. Equivalently, if a particle travels in a closed loop, the net work done (the sum of the force acting along the path multiplied by the distance travelled) by a conservative force is zero.
A conservative force is dependent only on the position of the object. If a force is conservative, it is possible to assign a numerical value for the potential at any point. When an object moves from one location to another, the force changes the potential energy of the object by an amount that does not depend on the path taken. Gravity and spring forces are examples of conservative forces.
If a force is not conservative , then defining a scalar potential is not possible, because taking different paths would lead to conflicting potential differences between the start and end points. Nonconservative forces transfer energy from the object in motion (just like conservative force), but they do not transfer this energy back to the potential energy of the system to regain it during reverse motion. Instead, they transfer the energy from the system in an energy form which can not be used by the force to transfer it back to the object in motion. Friction is one such nonconservative force.
Path Independence of Conservative Force
Work done by the gravity in a closed path motion is zero. We can extend this observation to other conservative force systems as well. We imagine a closed path motion. We imagine this closed path motion be divided in two motions between points A and B as diagramed in Fig 1. Starting from point A to point B and then ending at point A via two work paths named 1 and 2 in the figure. The total work by the conservative force for the round trip is zero:
Motion Along Different Paths : Motion along different paths. For a conservative force, work done via different path is the same.
\[\mathrm{W = W_{AB1}+W_{BA2} = 0.}\]
Let us now change the path for motion from A to B by another path, shown as path 3. Again, the total work by the conservative force for the round trip via new route is zero: \(\mathrm{W = W_{AB3}+W_{BA2} = 0.}\).
Comparing two equations, \(\mathrm{W_{AB1} = W_{AB3}}\). This is true for an arbitrary path. Therefore, work done for motion from A to B by conservative force along any paths are equal.
What is Potential Energy?
Potential energy is the energy difference between the energy of an object in a given position and its energy at a reference position.
- Relate the potential energy and the work
Potential energy is often associated with restoring forces such as a spring or the force of gravity. The action of stretching the spring or lifting the mass of an object is performed by an external force that works against the force field of the potential. This work is stored in the force field as potential energy. If the external force is removed the force field acts on the body to perform the work as it moves the body back to its initial position, reducing the stretch of the spring or causing the body to fall. The more formal definition is that potential energy is the energy difference between the energy of an object in a given position and its energy at a reference position.
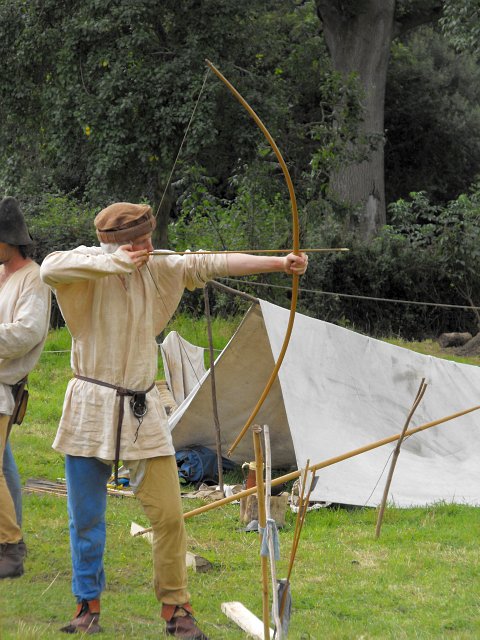
Potential Energy in a Bow and Arrow : In the case of a bow and arrow, the energy is converted from the potential energy in the archer’s arm to the potential energy in the bent limbs of the bow when the string is drawn back. When the string is released, the potential energy in the bow limbs is transferred back through the string to become kinetic energy in the arrow as it takes flight.
If the work for an applied force is independent of the path, then the work done by the force is evaluated at the start and end of the trajectory of the point of application. This means that there is a function U ( x ), called a “potential,” that can be evaluated at the two points x ( t = t 1 ) and x ( t 2 ) to obtain the work over any trajectory between these two points. It is tradition to define this function with a negative sign so that positive work is represented as a reduction in the potential:
\[\begin{align}\mathrm{W} & \mathrm{= \int_{C}F⋅dx= \int_{x(t_1)}^{x(t_2)}F⋅dx} \\ & \mathrm{=U(x(t_1))−U(x(t_2))=−ΔU.} \end{align}\]
Examples of Potential Energy
There are various types of potential energy, each associated with a particular type of force. More specifically, every conservative force gives rise to potential energy. For example, the work of an elastic force is called elastic potential energy; work done by the gravitational force is called gravitational potential energy; and work done by the Coulomb force is called electric potential energy.
Gravitational energy is the potential energy associated with gravitational force, as work is required to move objects against gravity.
- Generate an equation that can be used to express the gravitational potential energy near the earth
Gravitational energy is the potential energy associated with gravitational force (a conservative force), as work is required to elevate objects against Earth’s gravity. The potential energy due to elevated positions is called gravitational potential energy, evidenced, for example, by water held in an elevated reservoir or behind a dam (as an example, shows Hoover Dam). If an object falls from one point to another point inside a gravitational field, the force of gravity will do positive work on the object, and the gravitational potential energy will decrease by the same amount.
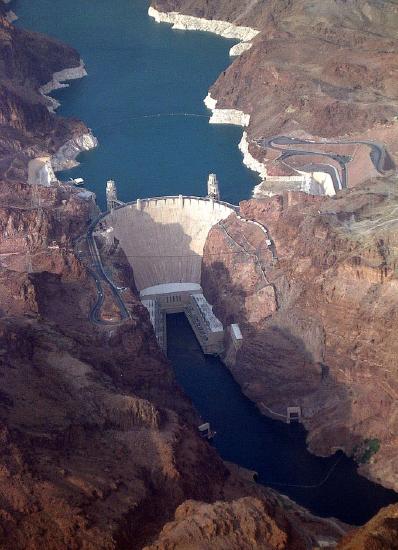
Hoover Dam : Hoover dam uses the stored gravitational potential energy to generate electricity.
Potential Near Earth
Gravitational potential energy near the Earth can be expressed with respect to the height from the surface of the Earth. (The surface will be the zero point of the potential energy. ) We can express the potential energy (gravitational potential energy) as:
\[\mathrm{PE=mgh,}\]
where PE = potential energy measured in joules (J), m = mass of the object (measured in kg), and h = perpendicular height from the reference point (measured in m); g = gravitational acceleration (9.8m/s 2 ). Near the surface of the Earth, g can be considered constant.
General Formula
However, over large variations in distance, the approximation that g is constant is no longer valid. Instead, we must use calculus and the general mathematical definition of work to determine gravitational potential energy. For the computation of the potential energy we can integrate the gravitational force, whose magnitude is given by Newton’s law of gravitation (with respect to the distance r between the two bodies). Using that definition, the gravitational potential energy of a system of masses m and M at a distance r using gravitational constant G is:
\[\mathrm{U(r) = \int_r (G \dfrac{mM}{r'^{2}} ) dr' = -G \dfrac{mM}{r} + K, } \]
where K is the constant of integration. Choosing the convention that K =0 makes calculations simpler, albeit at the cost of making U negative. For this choice, the potential at infinity is defined as 0.
When a spring is stretched/compressed from its equilibrium position by x, its potential energy is give as \(\mathrm{U=\frac{1}{2}kx^2}\).
- Explain how potential energy is stored in springs
Spring force is conservative force, given by the Hooke’s law: \(\mathrm{F = -kx}\), where k is spring constant, measured experimentally for a particular spring and x is the displacement. We would like to obtain an expression for the work done to the spring. From the conservation of mechanical energy (Check our Atom on “Conservation of Mechanical Energy), the work should be equal to the potential energy stored in spring. The displacement x is usually measured from the position of “neutral length” or “relaxed length” – the length of spring corresponding to situation when spring is neither stretched nor compressed. We shall identify this position as the origin of coordinate reference (x=0).
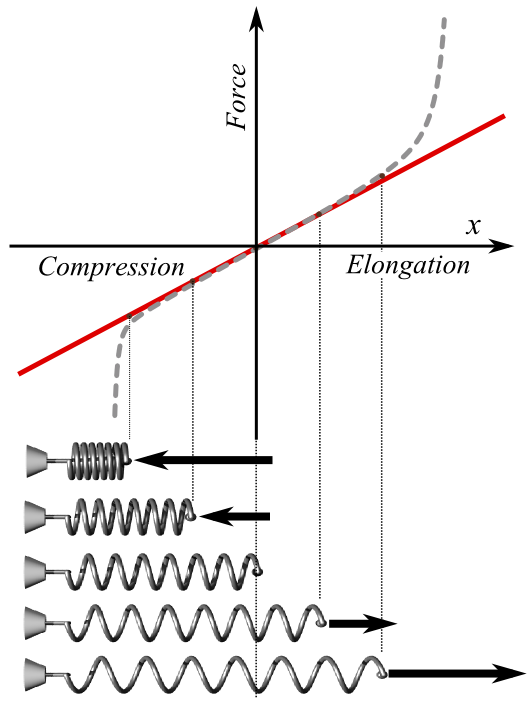
Hooke’s Law : Plot of applied force F vs. elongation X for a helical spring according to Hooke’s law (solid line) and what the actual plot might look like (dashed line). Red is used extension, blue for compression. At bottom, schematic pictures of spring states corresponding to some points of the plot; the middle one is in the relaxed state (no force applied).
Let \(\mathrm{x = 0}\) and \(\mathrm{x = x_f (>0)}\) be the initial and final positions of the block attached to the string. As the block slowly moves, we do work W on the spring: \(\mathrm{W=\int_{0}^{x_f}(kx)dx=\frac{1}{2}kx_f^2}\). When we stretch the spring. We have to apply force in the same direction as the displacement. (Technically, work is given as the inner product of the two vectors: force and displacement. \(\mathrm{W=F⋅Δx}\)). Therefore, the overall sign in the integral is +, not -.
If the block is gently released from the stretched position (\mathrm{x = x_f}\), the stored potential energy in the spring will start to be converted to the kinetic energy of the block, and vice versa. Neglecting frictional forces, Mechanical energy conservation demands that, at any point during its motion,
\[\begin{align} \mathrm{Total \; Energy} & \mathrm{= \dfrac{1}{2}mv^2+\dfrac{1}{2}kx^2} \\ & \mathrm{=\dfrac{1}{2}kx_f^2=constant.} \end{align}\]
From the energy conservation, we can estimate that, by the time the block reaches x=0 position, its speed will be \(\mathrm{v(x=0)=\sqrt{\frac{k}{m}}x_f}\). The block will keep oscillating between x = -x f and x f .
Conservation of Mechanical Energy
Conservation of mechanical energy states that the mechanical energy of an isolated system remains constant without friction.
- Formulate the principle of the conservation of the mechanical energy
Conservation of mechanical energy states that the mechanical energy of an isolated system remains constant in time, as long as the system is free of all frictional forces. In any real situation, frictional forces and other non-conservative forces are always present, but in many cases their effects on the system are so small that the principle of conservation of mechanical energy can be used as a fair approximation. An example of a such a system is shown in. Though energy cannot be created nor destroyed in an isolated system, it can be internally converted to any other form of energy.
A Mechanical System : An example of a mechanical system: A satellite is orbiting the Earth only influenced by the conservative gravitational force and the mechanical energy is therefore conserved. This acceleration is represented by a green acceleration vector and the velocity is represented by a red velocity vector.
Let us consider what form the work -energy theorem takes when only conservative forces are involved (leading us to the conservation of energy principle). The work-energy theorem states that the net work done by all forces acting on a system equals its change in kinetic energy (KE). In equation form, this is:
\[\mathrm{W_{net}=\dfrac{1}{2}mv^2−\dfrac{1}{2}mv_0^2=ΔKE.}\]
If only conservative forces act, then \(\mathrm{W_{net} = W_c}\), where W c is the total work done by all conservative forces. Thus, \(\mathrm{W_c = \Delta KE}\).
Now, if the conservative force, such as the gravitational force or a spring force, does work, the system loses potential energy (PE). That is, \(\mathrm{W_c = -PE}\). Therefore,
\[\mathrm{−ΔPE=ΔKE}\]
This equation means that the total kinetic and potential energy is constant for any process involving only conservative forces. That is,
\[\mathrm{KE+PE=const \; or \; KE_i+PE_i=KE_f+PE_f,}\]
where i and f denote initial and final values. This equation is a form of the work-energy theorem for conservative forces; it is known as the conservation of mechanical energy principle.
Remember that the law applies to the extent that all the forces are conservative, so that friction is negligible. The total kinetic plus potential energy of a system is defined to be its mechanical energy (\(\mathrm{KE+PE}\)). In a system that experiences only conservative forces, there is a potential energy associated with each force, and the energy only changes form between KE and various types of PE (with the total energy remaining constant).
Conservation of Mechanical Energy : Worked example.
Problem Solving With the Conservation of Energy
To solve a conservation of energy problem determine the system of interest, apply law of conservation of energy, and solve for the unknown.
- Identify steps necessary to solve a conservation of energy problem
Problem-solving Strategy
You should follow a series of steps whenever you are problem solving:
Determine the system of interest and identify what information is given and what quantity is to be calculated. For example, let’s assume you have the problem with car on a roller coaster. You know that the cars of a roller coaster reach their maximum kinetic energy (KEKE) when at the bottom of their path. When they start rising, the kinetic energy begins to be converted to gravitational potential energy (PEgPEg). The sum of kinetic and potential energy in the system should remain constant, if losses to friction are ignored.
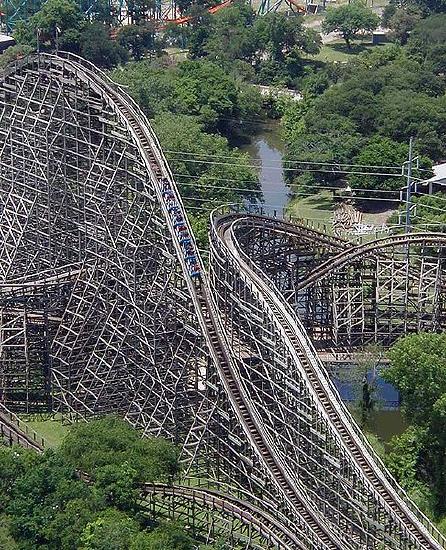
Determining Energy : The cars of a roller coaster reach their maximum kinetic energy when at the bottom of their path. When they start rising, the kinetic energy begins to be converted to gravitational potential energy. The sum of kinetic and potential energy in the system remains constant, ignoring losses to friction.
Examine all the forces involved and determine whether you know or are given the potential energy from the work done by the forces. Then use step three or step four.
If you know the potential energies (\(\mathrm{PE}\)) for the forces that enter into the problem, then forces are all conservative, and you can apply conservation of mechanical energy simply in terms of potential and kinetic energy. The equation expressing conservation of energy is:
\[\mathrm{KE_i+PE_i=KE_f+PE_f.}\]
If you know the potential energy for only some of the forces, then the conservation of energy law in its most general form must be used:
\[\mathrm{KE_i+PE_i+W_{nc}+OE_i=KE_f+PE_f+OE_f}\]
where \(\mathrm{O_E}\) stand for all other energies, and \(\mathrm{W_{nc}}\) stands for work done by non-conservative forces. In most problems, one or more of the terms is zero, simplifying its solution. Do not calculate \(\mathrm{W_c}\), the work done by conservative forces; it is already incorporated in the \(\mathrm{PE}\) terms.
You have already identified the types of work and energy involved (in step two). Before solving for the unknown, eliminate terms wherever possible to simplify the algebra. For example, choose height \(\mathrm{h=0}\) at either the initial or final point—this will allow to set \(\mathrm{PEg}\) at zero. Then solve for the unknown in the customary manner.
Check the answer to see if it is reasonable. Once you have solved a problem, reexamine the forms of work and energy to see if you have set up the conservation of energy equation correctly. For example, work done against friction should be negative, potential energy at the bottom of a hill should be less than that at the top, and so on.
Energy conservation : Part of a series of videos on physics problem-solving. The problems are taken from “The Joy of Physics. ” This one deals with energy conservation. The viewer is urged to pause the video at the problem statement and work the problem before watching the rest of the video.
Problem Solving with Dissipative Forces
In the presence of dissipative forces, total mechanical energy changes by exactly the amount of work done by nonconservative forces (W c ).
- Express the energy conservation relationship that can be applied to solve problems with dissipative forces
INTRODUCTION
We have seen a problem-solving strategy with the conservation of energy in the previous section. Here we will adopt the strategy for problems with dissipative forces. Since the work done by nonconservative (or dissipative) forces will irreversibly alter the energy of the system, the total mechanical energy (KE + PE) changes by exactly the amount of work done by nonconservative forces (W c ). Therefore, we obtain \(\mathrm{KE_i+PE_i+W_{nc}=KE_f+PE_f}\), where KE and PE represent kinetic and potential energies respectively. Therefore, using the new energy conservation relationship, we can apply the same problem-solving strategy as with the case of conservative forces.
Consider the situation shown in, where a baseball player slides to a stop on level ground. Using energy considerations, calculate the distance the 65.0-kg baseball player slides, given that his initial speed is 6.00 m/s and the force of friction against him is a constant 450 N.
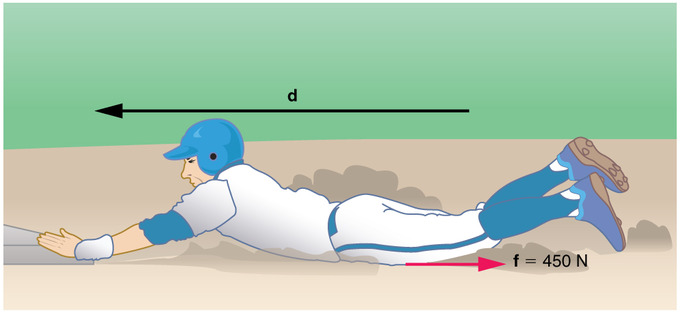
Fig 1 : The baseball player slides to a stop in a distance d. In the process, friction removes the player’s kinetic energy by doing an amount of work fd equal to the initial kinetic energy.
Strategy: Friction stops the player by converting his kinetic energy into other forms, including thermal energy. In terms of the work-energy theorem, the work done by friction (f), which is negative, is added to the initial kinetic energy to reduce it to zero. The work done by friction is negative, because f is in the opposite direction of the motion (that is, \(\mathrm{θ=180º}\), and so \(\mathrm{ \cos θ=−1}\)). Thus \(\mathrm{W_{nc} = -fd}\). The equation simplifies to \(\mathrm{\frac{1}{2}mv_i^2−fd=0}\).
Solution: Solving the previous equation for d and substituting known values yields, we get d = 2.60 m. The most important point of this example is that the amount of nonconservative work equals the change in mechanical energy.
- If a particle travels in a closed loop, the net work done (the sum of the force acting along the path multiplied by the distance travelled) by a conservative force is zero.
- Conservative force is dependent only on the position of the object. If a force is conservative, it is possible to assign a numerical value for the potential at any point.
- Nonconservative force transfer the energy from the system in an energy form which can not be used by the force to transfer back to the object in motion.
- If the work for an applied force is independent of the path, then the work done by the force is evaluated at the start and end of the trajectory of the point of application. This means that there is a function U(x), called a ” potential “.
- It is tradition to define the potential function with a negative sign so that positive work is represented as a reduction in the potential.
- Every conservative force gives rise to potential energy. Examples are elastic potential energy, gravitational potential energy, and electric potential energy.
- Gravitational potential energy near the earth can be expressed with respect to the height from the surface of the Earth as PE = mgh. g = gravitational acceleration (9.8m/s 2 ). Near the surface of the Earth, g can be considered constant.
- Over large variations in distance, the approximation that g is constant is no longer valid and a general formula should be used for the potential. It is given as: \(\mathrm{U(r)= \int_r (G\frac{mM}{r′^2})dr′=−G\frac{mM}{r} +K}\).
- Choosing the convention that the constant of integration K=0 assumes that the potential at infinity is defined to be 0.
- The displacement of spring x is usually measured from the position of “neutral length ” or “relaxed length”. Often, it is most convenient to identify this position as the origin of coordinate reference (x=0).
- If the block is gently released from the stretched position (\(\mathrm{x = x_f}\)), energy conservation tells us that \(\mathrm{\frac{1}{2}mv^2+\frac{1}{2}kx^2=\frac{1}{2}kx_f^2=constant}\).
- If the block is released from the stretched position (\(\mathrm{x = x_f}\)), by the time the block reaches x=0 position, its speed will be \(\mathrm{v(x=0)=\sqrt{\frac{k}{m}}x_f}\). The block will keep oscillating between \(\mathrm{x = -x_f}\) and \(\mathrm{x_f}\).
- The conservation of mechanical energy can be written as “\(\mathrm{KE + PE = const}\)”.
- Though energy cannot be created nor destroyed in an isolated system, it can be internally converted to any other form of energy.
- In a system that experiences only conservative forces, there is a potential energy associated with each force, and the energy only changes form between KE and various types of PE, with the total energy remaining constant.
- If you know the potential energies for the forces that enter into the problem, then forces are all conservative, and you can apply conservation of mechanical energy simply in terms of potential and kinetic energy. The equation expressing conservation of energy is: \(\mathrm{KE_i+PE_i=KE_f+PE_f.}\) .
- If you know the potential energy for only some of the forces, then the conservation of energy law in its most general form must be used: \(\mathrm{KE_i+PE_i+W_{nc}+OE_i=KE_f+PE_f+OE_f}\) , where OE stands for all other energies.
- Once you have solved a problem, always check the answer to see if it is reasonable.
- Using the new energy conservation relationship \[\mathrm{KE_i+PE_i+W_{nc}=KE_f+PE_f}\], we can apply the same problem-solving strategy as with the case of conservative forces.
- The most important point is that the amount of nonconservative work equals the change in mechanical energy.
- The work done by nonconservative (or dissipative) forces will irreversibly dissipated in the system.
- potential : A curve describing the situation where the difference in the potential energies of an object in two different positions depends only on those positions.
- Coulomb force : the electrostatic force between two charges, as described by Coulomb’s law
- conservative force : A force with the property that the work done in moving a particle between two points is independent of the path taken.
- Hooke’s law : the principle that the stress applied to a solid is directly proportional to the strain produced. This law describes the behavior of springs and solids stressed within their elastic limit.
- conservation : A particular measurable property of an isolated physical system does not change as the system evolves.
- isolated system : A system that does not interact with its surroundings, that is, its total energy and mass stay constant.
- frictional force : Frictional force is the force resisting the relative motion of solid surfaces, fluid layers, and material elements sliding against each other.
- kinetic energy : The energy possessed by an object because of its motion, equal to one half the mass of the body times the square of its velocity.
- potential energy : The energy an object has because of its position (in a gravitational or electric field) or its condition (as a stretched or compressed spring, as a chemical reactant, or by having rest mass)
- dissipative force : A force resulting in dissipation, a process in which energy (internal, bulk flow kinetic, or system potential) is transformed from some initial form to some irreversible final form.
LICENSES AND ATTRIBUTIONS
CC LICENSED CONTENT, SHARED PREVIOUSLY
- Curation and Revision. Provided by : Boundless.com. License : CC BY-SA: Attribution-ShareAlike
CC LICENSED CONTENT, SPECIFIC ATTRIBUTION
- Conservative force. Provided by : Wikipedia. Located at : en.Wikipedia.org/wiki/Conservative_force . License : CC BY-SA: Attribution-ShareAlike
- Sunil Kumar Singh, Conservative Force. September 17, 2013. Provided by : OpenStax CNX. Located at : http://cnx.org/content/m14104/latest/ . License : CC BY: Attribution
- curl. Provided by : Wiktionary. Located at : en.wiktionary.org/wiki/curl . License : CC BY-SA: Attribution-ShareAlike
- potential. Provided by : Wikipedia. Located at : en.Wikipedia.org/wiki/potential . License : CC BY-SA: Attribution-ShareAlike
- gradient. Provided by : Wiktionary. Located at : en.wiktionary.org/wiki/gradient . License : CC BY-SA: Attribution-ShareAlike
- Sunil Kumar Singh, Conservative Force. February 3, 2013. Provided by : OpenStax CNX. Located at : http://cnx.org/content/m14104/latest/ . License : CC BY: Attribution
- Potential energy. Provided by : Wikipedia. Located at : en.Wikipedia.org/wiki/Potential_energy . License : CC BY-SA: Attribution-ShareAlike
- Coulomb force. Provided by : Wiktionary. Located at : en.wiktionary.org/wiki/Coulomb_force . License : CC BY-SA: Attribution-ShareAlike
- Potential energy. Provided by : Wikipedia. Located at : en.Wikipedia.org/wiki/Potential_energy . License : CC BY: Attribution
- Free High School Science Texts Project, Gravity and Mechanical Energy: Potential Energy. September 17, 2013. Provided by : OpenStax CNX. Located at : http://cnx.org/content/m40047/latest/ . License : CC BY: Attribution
- conservative force. Provided by : Wikipedia. Located at : en.Wikipedia.org/wiki/conservative%20force . License : CC BY-SA: Attribution-ShareAlike
- Dam. Provided by : Wikipedia. Located at : en.Wikipedia.org/wiki/Dam . License : CC BY: Attribution
- Sunil Kumar Singh, Work by Spring Force. September 17, 2013. Provided by : OpenStax CNX. Located at : http://cnx.org/content/m14102/latest/ . License : CC BY: Attribution
- Hooke's law. Provided by : Wiktionary. Located at : en.wiktionary.org/wiki/Hooke's_law . License : CC BY-SA: Attribution-ShareAlike
- HookesLawForSpring-English. Provided by : Wikipedia. Located at : en.Wikipedia.org/wiki/File:HookesLawForSpring-English.png . License : CC BY-SA: Attribution-ShareAlike
- OpenStax College, Conservative Forces and Potential Energy. September 17, 2013. Provided by : OpenStax CNX. Located at : http://cnx.org/content/m42149/latest/ . License : CC BY: Attribution
- Mechanical energy. Provided by : Wikipedia. Located at : en.Wikipedia.org/wiki/Mechanical_energy%23Conservation_and_interconversion_of_energy . License : CC BY-SA: Attribution-ShareAlike
- conservation. Provided by : Wikipedia. Located at : en.Wikipedia.org/wiki/conservation . License : CC BY-SA: Attribution-ShareAlike
- frictional force. Provided by : Wikipedia. Located at : en.Wikipedia.org/wiki/frictional%20force . License : CC BY-SA: Attribution-ShareAlike
- isolated system. Provided by : Wiktionary. Located at : en.wiktionary.org/wiki/isolated_system . License : CC BY-SA: Attribution-ShareAlike
- Mechanical energy. Provided by : Wikipedia. Located at : en.Wikipedia.org/wiki/Mechanical_energy%23Conservation_and_interconversion_of_energy . License : CC BY: Attribution
- Conservation of Mechanical Energy. Located at : http://www.youtube.com/watch?v=n637fBmj9Ko . License : Public Domain: No Known Copyright . License Terms : Standard YouTube license
- OpenStax College, Conservation of Energy. September 17, 2013. Provided by : OpenStax CNX. Located at : http://cnx.org/content/m42151/latest/ . License : CC BY: Attribution
- Kinetic energy. Provided by : Wikipedia. Located at : en.Wikipedia.org/wiki/Kinetic_energy . License : CC BY-SA: Attribution-ShareAlike
- kinetic energy. Provided by : Wikipedia. Located at : en.Wikipedia.org/wiki/kinetic%20energy . License : CC BY-SA: Attribution-ShareAlike
- potential energy. Provided by : Wikipedia. Located at : en.Wikipedia.org/wiki/potential%20energy . License : CC BY-SA: Attribution-ShareAlike
- Energy conservation. Located at : http://www.youtube.com/watch?v=TIzC5TBIJNM . License : Public Domain: No Known Copyright . License Terms : Standard YouTube license
- Provided by : Wikimedia. Located at : http://upload.wikimedia.org/Wikipedia/commons/thumb/3/30/Wooden_roller_coaster_txgi.jpg/486px-Wooden_roller_coaster_txgi.jpg . License : CC BY-SA: Attribution-ShareAlike
- OpenStax College, Nonconservative Forces. September 17, 2013. Provided by : OpenStax CNX. Located at : http://cnx.org/content/m42150/latest/ . License : CC BY: Attribution
- dissipative force. Provided by : Wikipedia. Located at : en.Wikipedia.org/wiki/dissipative%20force . License : CC BY-SA: Attribution-ShareAlike
- OpenStax College, Nonconservative Forces. February 3, 2013. Provided by : OpenStax CNX. Located at : http://cnx.org/content/m42150/latest/ . License : CC BY: Attribution
If you're seeing this message, it means we're having trouble loading external resources on our website.
If you're behind a web filter, please make sure that the domains *.kastatic.org and *.kasandbox.org are unblocked.
To log in and use all the features of Khan Academy, please enable JavaScript in your browser.
Physics library
Course: physics library > unit 5.
- Introduction to work and energy
- Work and energy (part 2)
- Conservation of energy
- What are energy and work?
- What is kinetic energy?
- What is gravitational potential energy?
- What is conservation of energy?
- Work and the work-energy principle
- Work as the transfer of energy
- Work example problems
- Work as area under curve
- Thermal energy from friction
- What is thermal energy?
Work/energy problem with friction
- Conservative forces
- What is power?
Want to join the conversation?
- Upvote Button navigates to signup page
- Downvote Button navigates to signup page
- Flag Button navigates to signup page

Video transcript
About me and why I created this physics website.
Energy Problems
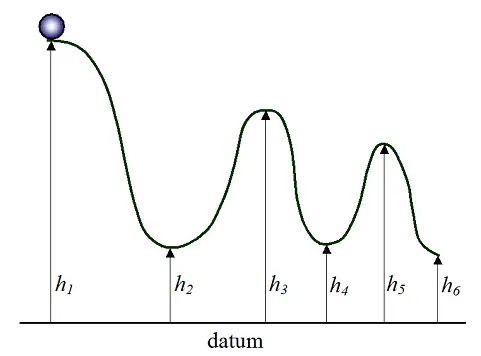
- Search Website
Real World Applications — for high school level and above
- Amusement Parks
- Battle & Weapons
- Engineering
- Miscellaneous
Education & Theory — for high school level and above
- Useful Formulas
- Physics Questions
- Example Mechanics Problems
- Learn Physics Compendium

Kids Section
- Physics For Kids
- Science Experiments
- Science Fair Ideas
- Science Quiz
- Science Toys
- Teacher Resources
- Commercial Disclosure
- Privacy Policy
© Copyright 2009-2024 real-world-physics-problems.com


- Science Notes Posts
- Contact Science Notes
- Todd Helmenstine Biography
- Anne Helmenstine Biography
- Free Printable Periodic Tables (PDF and PNG)
- Periodic Table Wallpapers
- Interactive Periodic Table
- Periodic Table Posters
- How to Grow Crystals
- Chemistry Projects
- Fire and Flames Projects
- Holiday Science
- Chemistry Problems With Answers
- Physics Problems
- Unit Conversion Example Problems
- Chemistry Worksheets
- Biology Worksheets
- Periodic Table Worksheets
- Physical Science Worksheets
- Science Lab Worksheets
- My Amazon Books
Potential And Kinetic Energy Example Problem – Work and Energy Examples
Potential energy is energy attributed to an object by virtue of its position. When the position is changed, the total energy remains unchanged but some potential energy gets converted into kinetic energy . The frictionless roller coaster is a classic potential and kinetic energy example problem.
The roller coaster problem shows how to use the conservation of energy to find the velocity or position or a cart on a frictionless track with different heights. The total energy of the cart is expressed as a sum of its gravitational potential energy and kinetic energy. This total energy remains constant across the length of the track.
Potential And Kinetic Energy Example Problem

A cart travels along a frictionless roller coaster track. At point A, the cart is 10 m above the ground and traveling at 2 m/s. A) What is the velocity at point B when the cart reaches the ground? B) What is the velocity of the cart at point C when the cart reaches a height of 3 m? C) What is the maximum height the cart can reach before the cart stops?
The total energy of the cart is expressed by the sum of its potential energy and its kinetic energy.
Potential energy of an object in a gravitational field is expressed by the formula
where PE is the potential energy m is the mass of the object g is the acceleration due to gravity = 9.8 m/s 2 h is the height above the measured surface.
Kinetic energy is the energy of the object in motion. It is expressed by the formula
KE = ½mv 2
where KE is the kinetic energy m is the mass of the object v is the velocity of the object.
The total energy of the system is conserved at any point of the system. The total energy is the sum of the potential energy and the kinetic energy.
Total E = KE + PE
To find the velocity or position, we need to find this total energy. At point A, we know both the velocity and the position of the cart.
Total E = KE + PE Total E = ½mv 2 + mgh Total E = ½m(2 m/s) 2 + m(9.8 m/s 2 )(10 m) Total E = ½m(4 m 2 /s 2 ) + m(98 m 2 /s 2 ) Total E = m(2 m 2 /s 2 ) + m(98 m 2 /s 2 ) Total E = m(100 m 2 /s 2 )
We can leave the mass value as it appears for now. As we complete each part, you will see what happens to this variable.
The cart is at ground level at point B, so h = 0 m.
Total E = ½mv 2 + mgh Total E = ½mv 2 + mg(0 m) Total E = ½mv 2
All of the energy at this point is kinetic energy. Since total energy is conserved, the total energy at point B is the same as the total energy at point A.
Total E at A = Total Energy at B m(100 m 2 /s 2 ) = ½mv 2
Divide both sides by m 100 m 2 /s 2 = ½v 2
Multiply both sides by 2 200 m 2 /s 2 = v 2
v = 14.1 m/s
The velocity at point B is 14.1 m/s.
At point C, we know only a value for h (h = 3 m).
Total E = ½mv 2 + mgh Total E = ½mv 2 + mg(3 m)
As before, the total energy is conserved. Total energy at A = total energy at C.
m(100 m 2 /s 2 ) = ½mv 2 + m(9.8 m/s 2 )(3 m) m(100 m 2 /s 2 ) = ½mv 2 + m(29.4 m 2 /s 2 )
Divide both sides by m
100 m 2 /s 2 = ½v 2 + 29.4 m 2 /s 2 ½v 2 = (100 – 29.4) m 2 /s 2 ½v 2 = 70.6 m 2 /s 2 v 2 = 141.2 m 2 /s 2 v = 11.9 m/s
The velocity at point C is 11.9 m/s.
The cart will reach its maximum height when the cart stops or v = 0 m/s.
Total E = ½mv 2 + mgh Total E = ½m(0 m/s) 2 + mgh Total E = mgh
Since total energy is conserved, the total energy at point A is the same as the total energy at point D.
m(100 m 2 /s 2 ) = mgh
100 m 2 /s 2 = gh
100 m 2 /s 2 = (9.8 m/s 2 ) h
The maximum height of the cart is 10.2 m.
A) The velocity of the cart at ground level is 14.1 m/s. B) The velocity of the cart at a height of 3 m is 11.9 m/s. C) The maximum height of the cart is 10.2 m.
This type of problem has one main key point: total energy is conserved at all points of the system. If you know the total energy at one point, you know the total energy at all points.
Related Posts
- energy-transformations.pdf Identify a device, event, phenomenon, or process whereby one form of energy is transformed into another.
- Write something else.
- Write something different.
- Write something completely different.
7.6 Conservation of Energy
Learning objectives.
By the end of this section, you will be able to:
- Explain the law of the conservation of energy.
- Describe some of the many forms of energy.
- Define efficiency of an energy conversion process as the fraction left as useful energy or work, rather than being transformed, for example, into thermal energy.
Law of Conservation of Energy
Energy, as we have noted, is conserved, making it one of the most important physical quantities in nature. The law of conservation of energy can be stated as follows:
Total energy is constant in any process. It may change in form or be transferred from one system to another, but the total remains the same.
We have explored some forms of energy and some ways it can be transferred from one system to another. This exploration led to the definition of two major types of energy—mechanical energy KE + PE KE + PE and energy transferred via work done by nonconservative forces ( W nc ) ( W nc ) . But energy takes many other forms, manifesting itself in many different ways, and we need to be able to deal with all of these before we can write an equation for the above general statement of the conservation of energy.
Other Forms of Energy than Mechanical Energy
At this point, we deal with all other forms of energy by lumping them into a single group called other energy ( OE OE ). Then we can state the conservation of energy in equation form as
All types of energy and work can be included in this very general statement of conservation of energy. Kinetic energy is KE KE , work done by a conservative force is represented by PE PE , work done by nonconservative forces is W nc W nc , and all other energies are included as OE OE . This equation applies to all previous examples; in those situations OE OE was constant, and so it subtracted out and was not directly considered.
Making Connections: Usefulness of the Energy Conservation Principle
The fact that energy is conserved and has many forms makes it very important. You will find that energy is discussed in many contexts, because it is involved in all processes. It will also become apparent that many situations are best understood in terms of energy and that problems are often most easily conceptualized and solved by considering energy.
When does OE OE play a role? One example occurs when a person eats. Food is oxidized with the release of carbon dioxide, water, and energy. Some of this chemical energy is converted to kinetic energy when the person moves, to potential energy when the person changes altitude, and to thermal energy (another form of OE OE ).
Some of the Many Forms of Energy
What are some other forms of energy? You can probably name a number of forms of energy not yet discussed. Many of these will be covered in later chapters, but let us detail a few here. Electrical energy is a common form that is converted to many other forms and does work in a wide range of practical situations. Fuels, such as gasoline and food, carry chemical energy that can be transferred to a system through oxidation. Chemical fuel can also produce electrical energy, such as in batteries. Batteries can in turn produce light, which is a very pure form of energy. Most energy sources on Earth are in fact stored energy from the energy we receive from the Sun. We sometimes refer to this as radiant energy , or electromagnetic radiation, which includes visible light, infrared, and ultraviolet radiation. Nuclear energy comes from processes that convert measurable amounts of mass into energy. Nuclear energy is transformed into the energy of sunlight, into electrical energy in power plants, and into the energy of the heat transfer and blast in weapons. Atoms and molecules inside all objects are in random motion. This internal mechanical energy from the random motions is called thermal energy , because it is related to the temperature of the object. These and all other forms of energy can be converted into one another and can do work.
Table 7.1 gives the amount of energy stored, used, or released from various objects and in various phenomena. The range of energies and the variety of types and situations is impressive.
Problem-Solving Strategies for Energy
You will find the following problem-solving strategies useful whenever you deal with energy. The strategies help in organizing and reinforcing energy concepts. In fact, they are used in the examples presented in this chapter. The familiar general problem-solving strategies presented earlier—involving identifying physical principles, knowns, and unknowns, checking units, and so on—continue to be relevant here.
Step 1. Determine the system of interest and identify what information is given and what quantity is to be calculated. A sketch will help.
Step 2. Examine all the forces involved and determine whether you know or are given the potential energy from the work done by the forces. Then use step 3 or step 4.
Step 3. If you know the potential energies for the forces that enter into the problem, then forces are all conservative, and you can apply conservation of mechanical energy simply in terms of potential and kinetic energy. The equation expressing conservation of energy is
Step 4. If you know the potential energy for only some of the forces, possibly because some of them are nonconservative and do not have a potential energy, or if there are other energies that are not easily treated in terms of force and work, then the conservation of energy law in its most general form must be used.
In most problems, one or more of the terms is zero, simplifying its solution. Do not calculate W c W c , the work done by conservative forces; it is already incorporated in the PE PE terms.
Step 5. You have already identified the types of work and energy involved (in step 2). Before solving for the unknown, eliminate terms wherever possible to simplify the algebra. For example, choose h = 0 h = 0 at either the initial or final point, so that PE g PE g is zero there. Then solve for the unknown in the customary manner.
Step 6. Check the answer to see if it is reasonable . Once you have solved a problem, reexamine the forms of work and energy to see if you have set up the conservation of energy equation correctly. For example, work done against friction should be negative, potential energy at the bottom of a hill should be less than that at the top, and so on. Also check to see that the numerical value obtained is reasonable. For example, the final speed of a skateboarder who coasts down a 3-m-high ramp could reasonably be 20 km/h, but not 80 km/h.
Transformation of Energy
The transformation of energy from one form into others is happening all the time. The chemical energy in food is converted into thermal energy through metabolism; light energy is converted into chemical energy through photosynthesis. In a larger example, the chemical energy contained in coal is converted into thermal energy as it burns to turn water into steam in a boiler. This thermal energy in the steam in turn is converted to mechanical energy as it spins a turbine, which is connected to a generator to produce electrical energy. (In all of these examples, not all of the initial energy is converted into the forms mentioned. This important point is discussed later in this section.)
Another example of energy conversion occurs in a solar cell. Sunlight impinging on a solar cell (see Figure 7.19 ) produces electricity, which in turn can be used to run an electric motor. Energy is converted from the primary source of solar energy into electrical energy and then into mechanical energy.
Even though energy is conserved in an energy conversion process, the output of useful energy or work will be less than the energy input. The efficiency Eff Eff of an energy conversion process is defined as
Table 7.2 lists some efficiencies of mechanical devices and human activities. In a coal-fired power plant, for example, about 40% of the chemical energy in the coal becomes useful electrical energy. The other 60% transforms into other (perhaps less useful) energy forms, such as thermal energy, which is then released to the environment through combustion gases and cooling towers.
PhET Explorations
Masses and springs.
A realistic mass and spring laboratory. Hang masses from springs and adjust the spring stiffness and damping. You can even slow time. Transport the lab to different planets. A chart shows the kinetic, potential, and thermal energies for each spring.
- 1 Representative values
As an Amazon Associate we earn from qualifying purchases.
This book may not be used in the training of large language models or otherwise be ingested into large language models or generative AI offerings without OpenStax's permission.
Want to cite, share, or modify this book? This book uses the Creative Commons Attribution License and you must attribute OpenStax.
Access for free at https://openstax.org/books/college-physics-2e/pages/1-introduction-to-science-and-the-realm-of-physics-physical-quantities-and-units
- Authors: Paul Peter Urone, Roger Hinrichs
- Publisher/website: OpenStax
- Book title: College Physics 2e
- Publication date: Jul 13, 2022
- Location: Houston, Texas
- Book URL: https://openstax.org/books/college-physics-2e/pages/1-introduction-to-science-and-the-realm-of-physics-physical-quantities-and-units
- Section URL: https://openstax.org/books/college-physics-2e/pages/7-6-conservation-of-energy
© Jan 19, 2024 OpenStax. Textbook content produced by OpenStax is licensed under a Creative Commons Attribution License . The OpenStax name, OpenStax logo, OpenStax book covers, OpenStax CNX name, and OpenStax CNX logo are not subject to the Creative Commons license and may not be reproduced without the prior and express written consent of Rice University.
- The Magazine
- Stay Curious
- The Sciences
- Environment
- Planet Earth
And The Biggest Unsolved Problems In Physics Are...
When a researcher asked nobel prize winning physicists and other scientists whether there was any new physics left to discover, this is what they said..
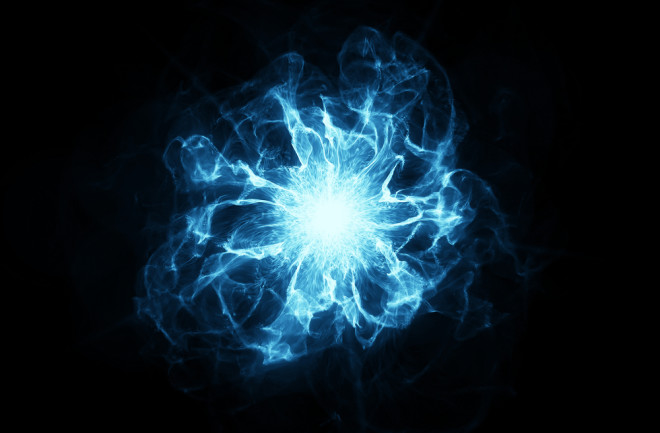
The story has been told many times. At the beginning of the 20th century, physics seemed essentially solved. One or two puzzling phenomena like the photoelectric effect and concerns over black body radiation had scientists scratching their heads but a general feeling prevailed that solving these issues would be dotting the i’s and crossing the t’s.
In the event, these seemingly minor problems required an entire revolution for the laws of physics. And the result — quantum theory and relativity — have become cornerstones of modern science and provided the intellectual bedrock for our 21st century world.
That raises an interesting question for young researchers looking for potential revolutions of the future. For them, the question is: what are the most interesting unsolved problems in science today?
Now they have an answer thanks to the work of Suzy Lidstrom at Texas A&M University at College Station who has compiled a set of answers to this question given by some of the planet’s leading thinkers. By asking these researchers — Nobel prize winners and aspiring young researchers among them — whether there will be new physics, she has created a fascinating insight into the “big problems” of physics and into the opportunities, challenges and potential missteps ahead.
Cosmic Mysteries
The paper begins with William Philips, of laser cooling Nobel Prize fame, arguing that the universe promises new physics galore because only about 5 percent of it is visible to us. The properties of the rest, in the form of dark matter and dark energy, are entirely unknown, and so will offer a rich vein of discovery for science.
Gerrard t’Hooft, with an electroweak interaction Nobel Prize under his belt, takes up the baton with the knotty problem of topological phenomenon in physics and its potential for future discovery.
The composition of the galaxy, Solar System, the Earth plus everything that exists on it are determined by the elements produced in some of the most violent processes in the universe — supernovas, the collisions between neutron stars and between black holes. But scientists know little about the way matter behaves in these events so predicting the abundance of elements is hard.
That will begin to change when an experiment called FAIR — the Facility for Antiproton and Ion Research — comes online in 2027, as explained by Karlheinz Langanke, a theoretical nuclear physicist at the Technical University of Darmstadt, in Germany, and one of the driving forces behind its construction.
One refreshing perspective is the iconoclastic view of Jim Baggot who decries an over-obsession in modern physics with topics such as string theory and the multiverse. He points out that discussion on these topics is largely metaphysical because there is little or no experimental evidence to inform thinking. Science would be better served, he suggests, by focusing on solid state physics or quantum information “where new physics is happening all the time”.
Others point to the nature of entanglement and the problem of how the measurement of a quantum state in one part of the universe can instantaneously influence the state of another in a distant region of the universe. Nobody knows how this happens.
And, of course, no discussion of unsolved problems would be complete without asking how the brain works and what approach will help unravel the mysteries of consciousness.
These are truly profound problems that will require partnerships with biologists, linguists, computer scientists, neuroscientists and others. Whether the physics community is well prepared for these kinds of collaborations is left unanswered.
Nature of Information
A fascinating corollary is the discussion of the role of information in the nature of life. One way of thinking about reproduction is as the transmission of biological information from one generation to the next. Lazaro Castanedo and colleagues point out that in the 20th century, engineers led by Claude Shannon developed powerful models for describing the transmission of information from one location to another. They ask whether the specific problems of information transmission in biology might help develop this classical theory of communication even further.
And so on. This is a fascinating paper that sets out many of the biggest problems in physics in a way that largely avoids verbosity and so is easily accessible.
There is another problem that this paper perfectly demonstrates but fails to mention explicitly — the gender bias in physics.
A quick look at the list of authors makes this bias all too clear and Figure 1, showing many of the authors at a meeting in Prague, is an embarrassment of elderly, white men. Shamefully, that’s probably a fair reflection of the state of modern physics.
If the community really has the ambition to take on the mysteries of the universe in all their glory, it will need all the expertise it can get. That means regardless of gender, race and ethnicity.
Ref: The sounds of science a symphony for many instruments and voices part II : arxiv.org/abs/2404.11724
Already a subscriber?
Register or Log In
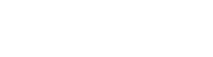
Keep reading for as low as $1.99!
Sign up for our weekly science updates.
Save up to 40% off the cover price when you subscribe to Discover magazine.

My Research and Related Info
How to solve physics problems.
This is a quick two page guide I made back when I was at Penn State tutoring first and second year physics.
Downloadable PDF .

- © University of Delaware
- Legal Notices
- Accessibility Notice
- International edition
- Australia edition
- Europe edition
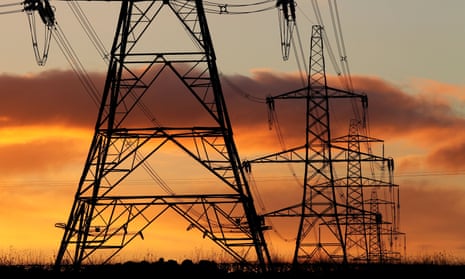
Capacity crunch: why the UK doesn’t have the power to solve the housing crisis
Our inadequate electricity network is stopping the building of thousands of new homes. And the necessary move to low-carbon heating and cars is only increasing demand
O xford has a severe housing problem. With house prices 12 times the average salary, it has become one of the least affordable cities in the country . Its council house waiting list has grown to more than 3,000 households, with many having to live in temporary accommodation.
An obvious solution is to build more homes, but those trying to do this face a big barrier: electricity.
“When I talk to developers or potential developers, one of the first questions they now ask me is about grid capacity,” says Susan Brown, leader of Oxford city council.
With housing developments competing for power against energy-hungry tech companies and the city’s increasingly electrified transport network, connection prospects are a matter of concern for housebuilders.
“The problem [for developers] is securing sufficient energy, and the time it takes to connect to the grid,” Brown says.
The council estimates that 26,000 new homes will be needed in and around the city by 2040 , but it fears any building plans could be delayed by capacity constraints. In the nearby market town of Bicester, this has already happened.
“The latest expansion in Bicester was supposed to see an additional 7,000 homes and a large commercial zone built, but they’ve been put on pause because grid reinforcements are needed to get them further,” says Brown, who is also a vice-chair of the District Councils’ Network, a cross-party group of 169 district and unitary councils in England. “I don’t think Oxfordshire is unique at all. I know it is becoming quite a big issue across the country.”
This inability to build enough homes is not a new problem. For the past decade, sluggish building rates have meant the government’s target of 300,000 homes a year has been repeatedly missed , while the housing crisis has worsened.
Funding and planning issues are routinely blamed for the country’s supply issues; a lack of electricity supply has rarely been given as a reason why homes are not built – until recently.
“Securing capacity has always been a priority, but over the past 18 months, it has become a significant roadblock and a real challenge for developers across the country,” says Vicki Spiers, chair of the Independent Networks Association, whose members are responsible for connecting 80% of new UK homes to the grid.
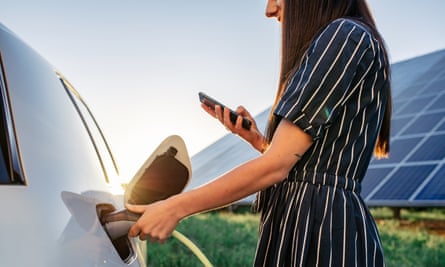
And as households move away from using fossil fuels to heat homes and power cars, the challenge will only grow.
“We can see examples of developments being knocked back because of grid capacity,” says energy expert Adam Bell of Stonehaven Consulting. “That problem will only get worse in the future without expansion of the distribution network.”
Just like the capacity issues facing housing developments, the country’s electricity system is complex, and growing more so.
National Grid is responsible for the transmission network, which acts as a motorway transporting electricity from power sources to other parts of the country along high-voltage lines.
After this come 14 distribution network operators (DNOs), the equivalent of A-roads, all responsible for distribution within a region. These connect either directly to housing developments or, more often, through independent network operators.
In a country where electricity use is expected to increase by 50% by 2035, as millions of electric cars and domestic heat pumps are plugged in, the transmission network will require significant upgrades, at a cost of tens of billions of pounds. But it is at the distribution level, the A-roads, that the biggest roadblocks come for housing developments.
The operators are often left waiting for major National Grid transmission upgrades to provide extra capacity to their network, but the five-year funding cycles set by regulator Ofgem mean local upgrade plans can become outdated as demand changes.
Bell says: “These [cycles] made sense in a slow-paced world where demand grew through economic growth and population growth, but now that we are electrifying stuff, more data centres are coming online and the view taken five years ago is going to be wrong.”
Even when upgrades or new connections are agreed on, completion can take years. Spiers says this is forcing more and more developers to mothball building projects.
The Observer has spoken to several developers who have had to scale down, change or scrap plans because of electricity capacity issues.
One developer was forced to shrink a development of more than 80 homes to fewer than 20 because it could not secure enough electricity. Another said capacity limitations meant it had had to cut the number of electric car chargers on a site.
In an extreme example, one building firm scrapped a planned development of almost 100 homes after a £570,000 charge to secure a new connection made the scheme unviable.
The most high-profile example of electricity shortages causing housing delays is in the west London boroughs of Hounslow, Hillingdon and Ealing. The area has become popular over recent years with data centre developers, thanks to the fibre optic cables that run along the M4 corridor to the Atlantic. Crucially, for an area with a large housing shortage, they have sucked up capacity that could have been used for vital housing schemes.
In 2022, the Greater London Authority sounded the alarm over the power shortages, warning that all developments in the area could be paused until 2035, when new transmission connections would be constructed.
Building of new infrastructure has now restarted and the National Grid and Scottish and Southern Electricity Network (SSEN) have come up with solutions to flatten out demand. These have included accelerating smaller housing developments, and striking deals with developers to build developments in phases to better match the extra capacity added to the network.
But while progress has been made and 7,800 homes are now connected, 3,900 planned units, including 1,855 affordable homes, are still waiting to secure a power supply.
Situations like the one in west London will become more common as the government’s Future Homes Standard legislation turbocharges the electrification of homes. The new law, which will come into force next year, will reduce the maximum permitted carbon emissions of all new homes by 75%-80%.
David Adams, strategic adviser at the Future Homes Hub (which was set up to develop a long-term delivery plan for the sector) says this will effectively ban the use of fossil fuels in homes, and make electric heat pumps the only option.
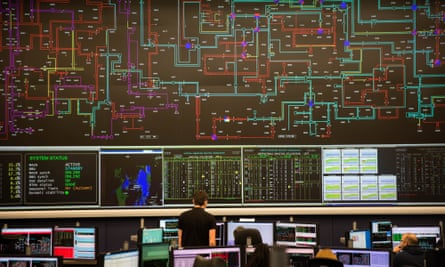
According to the National Grid electricity system operator (ESO), the body that ensures supply meets demand on the network, heat pumps will require 80 terawatt hours a year by 2050, if the country is to hit its decarbonisation goal. This is four times the current usage, but achieving this could be hampered by capacity constraints.
Last year, Reading borough council raised concerns that its net zero housing ambitions could be curtailed because of SSEN restrictions on new connections. On a planned development of more than 220 homes with heat pumps, nearly two-thirds converted back to gas after capacity concerns were raised.
“We had a developer that was willing to fit air source heat pumps, fit electric car chargers, but couldn’t get the capacity, and had to come back to planning so it could fit gas boilers,” says Micky Leng, the council’s planning lead. “This is a massive issue that has the potential to put the country’s race to net zero at risk.”
The Energy Network Association, the body that represents district network operators, says connection issues are rare for housing projects, but where they do occur operators are working to find solutions. It adds that in most cases connections usually take a little over a year from a request being made, and that the past year has seen its members deliver enough additional capacity to power a million homes.
But the Home Builders Federation says this is becoming a bigger issue for its members, and will only get worse. A spokesperson says: “Moving away from gas for heating will increase electricity usage and we have seen elsewhere that if there isn’t the grid capacity, the answer is to not allow any new housing.”
Upgrading infrastructure at both National Grid and the distribution network will be key to this.
Adams at the Future Home Hub suggests that smart energy technologies could provide a solution by using existing capacity more efficiently, and that installing batteries in housing schemes could also help.
DNOs are also increasing adopting “flexibility models”, in which they buy excess energy from local generators of power, for example homes or businesses with solar panels, to increase grid supply.
For Brown, the solutions for Oxfordshire can’t come soon enough. She says: “What concerns me is that it is delaying housing, making it more expensive, and it is affecting our ability to make housing zero carbon, which is so important.”
- Energy industry
- The Observer
- Construction industry
- Climate crisis
- Electric, hybrid and low-emission cars
Most viewed
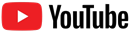
- TPC and eLearning
- What's NEW at TPC?
- Read Watch Interact
- Practice Review Test
- Teacher-Tools
- Subscription Selection
- Seat Calculator
- Ad Free Account
- Edit Profile Settings
- Classes (Version 2)
- Student Progress Edit
- Task Properties
- Export Student Progress
- Task, Activities, and Scores
- Metric Conversions Questions
- Metric System Questions
- Metric Estimation Questions
- Significant Digits Questions
- Proportional Reasoning
- Acceleration
- Distance-Displacement
- Dots and Graphs
- Graph That Motion
- Match That Graph
- Name That Motion
- Motion Diagrams
- Pos'n Time Graphs Numerical
- Pos'n Time Graphs Conceptual
- Up And Down - Questions
- Balanced vs. Unbalanced Forces
- Change of State
- Force and Motion
- Mass and Weight
- Match That Free-Body Diagram
- Net Force (and Acceleration) Ranking Tasks
- Newton's Second Law
- Normal Force Card Sort
- Recognizing Forces
- Air Resistance and Skydiving
- Solve It! with Newton's Second Law
- Which One Doesn't Belong?
- Component Addition Questions
- Head-to-Tail Vector Addition
- Projectile Mathematics
- Trajectory - Angle Launched Projectiles
- Trajectory - Horizontally Launched Projectiles
- Vector Addition
- Vector Direction
- Which One Doesn't Belong? Projectile Motion
- Forces in 2-Dimensions
- Being Impulsive About Momentum
- Explosions - Law Breakers
- Hit and Stick Collisions - Law Breakers
- Case Studies: Impulse and Force
- Impulse-Momentum Change Table
- Keeping Track of Momentum - Hit and Stick
- Keeping Track of Momentum - Hit and Bounce
- What's Up (and Down) with KE and PE?
- Energy Conservation Questions
- Energy Dissipation Questions
- Energy Ranking Tasks
- LOL Charts (a.k.a., Energy Bar Charts)
- Match That Bar Chart
- Words and Charts Questions
- Name That Energy
- Stepping Up with PE and KE Questions
- Case Studies - Circular Motion
- Circular Logic
- Forces and Free-Body Diagrams in Circular Motion
- Gravitational Field Strength
- Universal Gravitation
- Angular Position and Displacement
- Linear and Angular Velocity
- Angular Acceleration
- Rotational Inertia
- Balanced vs. Unbalanced Torques
- Getting a Handle on Torque
- Torque-ing About Rotation
- Properties of Matter
- Fluid Pressure
- Buoyant Force
- Sinking, Floating, and Hanging
- Pascal's Principle
- Flow Velocity
- Bernoulli's Principle
- Balloon Interactions
- Charge and Charging
- Charge Interactions
- Charging by Induction
- Conductors and Insulators
- Coulombs Law
- Electric Field
- Electric Field Intensity
- Polarization
- Case Studies: Electric Power
- Know Your Potential
- Light Bulb Anatomy
- I = ∆V/R Equations as a Guide to Thinking
- Parallel Circuits - ∆V = I•R Calculations
- Resistance Ranking Tasks
- Series Circuits - ∆V = I•R Calculations
- Series vs. Parallel Circuits
- Equivalent Resistance
- Period and Frequency of a Pendulum
- Pendulum Motion: Velocity and Force
- Energy of a Pendulum
- Period and Frequency of a Mass on a Spring
- Horizontal Springs: Velocity and Force
- Vertical Springs: Velocity and Force
- Energy of a Mass on a Spring
- Decibel Scale
- Frequency and Period
- Closed-End Air Columns
- Name That Harmonic: Strings
- Rocking the Boat
- Wave Basics
- Matching Pairs: Wave Characteristics
- Wave Interference
- Waves - Case Studies
- Color Addition and Subtraction
- Color Filters
- If This, Then That: Color Subtraction
- Light Intensity
- Color Pigments
- Converging Lenses
- Curved Mirror Images
- Law of Reflection
- Refraction and Lenses
- Total Internal Reflection
- Who Can See Who?
- Formulas and Atom Counting
- Atomic Models
- Bond Polarity
- Entropy Questions
- Cell Voltage Questions
- Heat of Formation Questions
- Reduction Potential Questions
- Oxidation States Questions
- Measuring the Quantity of Heat
- Hess's Law
- Oxidation-Reduction Questions
- Galvanic Cells Questions
- Thermal Stoichiometry
- Molecular Polarity
- Quantum Mechanics
- Balancing Chemical Equations
- Bronsted-Lowry Model of Acids and Bases
- Classification of Matter
- Collision Model of Reaction Rates
- Density Ranking Tasks
- Dissociation Reactions
- Complete Electron Configurations
- Elemental Measures
- Enthalpy Change Questions
- Equilibrium Concept
- Equilibrium Constant Expression
- Equilibrium Calculations - Questions
- Equilibrium ICE Table
- Intermolecular Forces Questions
- Ionic Bonding
- Lewis Electron Dot Structures
- Limiting Reactants
- Line Spectra Questions
- Mass Stoichiometry
- Measurement and Numbers
- Metals, Nonmetals, and Metalloids
- Metric Estimations
- Metric System
- Molarity Ranking Tasks
- Mole Conversions
- Name That Element
- Names to Formulas
- Names to Formulas 2
- Nuclear Decay
- Particles, Words, and Formulas
- Periodic Trends
- Precipitation Reactions and Net Ionic Equations
- Pressure Concepts
- Pressure-Temperature Gas Law
- Pressure-Volume Gas Law
- Chemical Reaction Types
- Significant Digits and Measurement
- States Of Matter Exercise
- Stoichiometry Law Breakers
- Stoichiometry - Math Relationships
- Subatomic Particles
- Spontaneity and Driving Forces
- Gibbs Free Energy
- Volume-Temperature Gas Law
- Acid-Base Properties
- Energy and Chemical Reactions
- Chemical and Physical Properties
- Valence Shell Electron Pair Repulsion Theory
- Writing Balanced Chemical Equations
- Mission CG1
- Mission CG10
- Mission CG2
- Mission CG3
- Mission CG4
- Mission CG5
- Mission CG6
- Mission CG7
- Mission CG8
- Mission CG9
- Mission EC1
- Mission EC10
- Mission EC11
- Mission EC12
- Mission EC2
- Mission EC3
- Mission EC4
- Mission EC5
- Mission EC6
- Mission EC7
- Mission EC8
- Mission EC9
- Mission RL1
- Mission RL2
- Mission RL3
- Mission RL4
- Mission RL5
- Mission RL6
- Mission KG7
- Mission RL8
- Mission KG9
- Mission RL10
- Mission RL11
- Mission RM1
- Mission RM2
- Mission RM3
- Mission RM4
- Mission RM5
- Mission RM6
- Mission RM8
- Mission RM10
- Mission LC1
- Mission RM11
- Mission LC2
- Mission LC3
- Mission LC4
- Mission LC5
- Mission LC6
- Mission LC8
- Mission SM1
- Mission SM2
- Mission SM3
- Mission SM4
- Mission SM5
- Mission SM6
- Mission SM8
- Mission SM10
- Mission KG10
- Mission SM11
- Mission KG2
- Mission KG3
- Mission KG4
- Mission KG5
- Mission KG6
- Mission KG8
- Mission KG11
- Mission F2D1
- Mission F2D2
- Mission F2D3
- Mission F2D4
- Mission F2D5
- Mission F2D6
- Mission KC1
- Mission KC2
- Mission KC3
- Mission KC4
- Mission KC5
- Mission KC6
- Mission KC7
- Mission KC8
- Mission AAA
- Mission SM9
- Mission LC7
- Mission LC9
- Mission NL1
- Mission NL2
- Mission NL3
- Mission NL4
- Mission NL5
- Mission NL6
- Mission NL7
- Mission NL8
- Mission NL9
- Mission NL10
- Mission NL11
- Mission NL12
- Mission MC1
- Mission MC10
- Mission MC2
- Mission MC3
- Mission MC4
- Mission MC5
- Mission MC6
- Mission MC7
- Mission MC8
- Mission MC9
- Mission RM7
- Mission RM9
- Mission RL7
- Mission RL9
- Mission SM7
- Mission SE1
- Mission SE10
- Mission SE11
- Mission SE12
- Mission SE2
- Mission SE3
- Mission SE4
- Mission SE5
- Mission SE6
- Mission SE7
- Mission SE8
- Mission SE9
- Mission VP1
- Mission VP10
- Mission VP2
- Mission VP3
- Mission VP4
- Mission VP5
- Mission VP6
- Mission VP7
- Mission VP8
- Mission VP9
- Mission WM1
- Mission WM2
- Mission WM3
- Mission WM4
- Mission WM5
- Mission WM6
- Mission WM7
- Mission WM8
- Mission WE1
- Mission WE10
- Mission WE2
- Mission WE3
- Mission WE4
- Mission WE5
- Mission WE6
- Mission WE7
- Mission WE8
- Mission WE9
- Vector Walk Interactive
- Name That Motion Interactive
- Kinematic Graphing 1 Concept Checker
- Kinematic Graphing 2 Concept Checker
- Graph That Motion Interactive
- Two Stage Rocket Interactive
- Rocket Sled Concept Checker
- Force Concept Checker
- Free-Body Diagrams Concept Checker
- Free-Body Diagrams The Sequel Concept Checker
- Skydiving Concept Checker
- Elevator Ride Concept Checker
- Vector Addition Concept Checker
- Vector Walk in Two Dimensions Interactive
- Name That Vector Interactive
- River Boat Simulator Concept Checker
- Projectile Simulator 2 Concept Checker
- Projectile Simulator 3 Concept Checker
- Hit the Target Interactive
- Turd the Target 1 Interactive
- Turd the Target 2 Interactive
- Balance It Interactive
- Go For The Gold Interactive
- Egg Drop Concept Checker
- Fish Catch Concept Checker
- Exploding Carts Concept Checker
- Collision Carts - Inelastic Collisions Concept Checker
- Its All Uphill Concept Checker
- Stopping Distance Concept Checker
- Chart That Motion Interactive
- Roller Coaster Model Concept Checker
- Uniform Circular Motion Concept Checker
- Horizontal Circle Simulation Concept Checker
- Vertical Circle Simulation Concept Checker
- Race Track Concept Checker
- Gravitational Fields Concept Checker
- Orbital Motion Concept Checker
- Angular Acceleration Concept Checker
- Balance Beam Concept Checker
- Torque Balancer Concept Checker
- Aluminum Can Polarization Concept Checker
- Charging Concept Checker
- Name That Charge Simulation
- Coulomb's Law Concept Checker
- Electric Field Lines Concept Checker
- Put the Charge in the Goal Concept Checker
- Circuit Builder Concept Checker (Series Circuits)
- Circuit Builder Concept Checker (Parallel Circuits)
- Circuit Builder Concept Checker (∆V-I-R)
- Circuit Builder Concept Checker (Voltage Drop)
- Equivalent Resistance Interactive
- Pendulum Motion Simulation Concept Checker
- Mass on a Spring Simulation Concept Checker
- Particle Wave Simulation Concept Checker
- Boundary Behavior Simulation Concept Checker
- Slinky Wave Simulator Concept Checker
- Simple Wave Simulator Concept Checker
- Wave Addition Simulation Concept Checker
- Standing Wave Maker Simulation Concept Checker
- Color Addition Concept Checker
- Painting With CMY Concept Checker
- Stage Lighting Concept Checker
- Filtering Away Concept Checker
- InterferencePatterns Concept Checker
- Young's Experiment Interactive
- Plane Mirror Images Interactive
- Who Can See Who Concept Checker
- Optics Bench (Mirrors) Concept Checker
- Name That Image (Mirrors) Interactive
- Refraction Concept Checker
- Total Internal Reflection Concept Checker
- Optics Bench (Lenses) Concept Checker
- Kinematics Preview
- Velocity Time Graphs Preview
- Moving Cart on an Inclined Plane Preview
- Stopping Distance Preview
- Cart, Bricks, and Bands Preview
- Fan Cart Study Preview
- Friction Preview
- Coffee Filter Lab Preview
- Friction, Speed, and Stopping Distance Preview
- Up and Down Preview
- Projectile Range Preview
- Ballistics Preview
- Juggling Preview
- Marshmallow Launcher Preview
- Air Bag Safety Preview
- Colliding Carts Preview
- Collisions Preview
- Engineering Safer Helmets Preview
- Push the Plow Preview
- Its All Uphill Preview
- Energy on an Incline Preview
- Modeling Roller Coasters Preview
- Hot Wheels Stopping Distance Preview
- Ball Bat Collision Preview
- Energy in Fields Preview
- Weightlessness Training Preview
- Roller Coaster Loops Preview
- Universal Gravitation Preview
- Keplers Laws Preview
- Kepler's Third Law Preview
- Charge Interactions Preview
- Sticky Tape Experiments Preview
- Wire Gauge Preview
- Voltage, Current, and Resistance Preview
- Light Bulb Resistance Preview
- Series and Parallel Circuits Preview
- Thermal Equilibrium Preview
- Linear Expansion Preview
- Heating Curves Preview
- Electricity and Magnetism - Part 1 Preview
- Electricity and Magnetism - Part 2 Preview
- Vibrating Mass on a Spring Preview
- Period of a Pendulum Preview
- Wave Speed Preview
- Slinky-Experiments Preview
- Standing Waves in a Rope Preview
- Sound as a Pressure Wave Preview
- DeciBel Scale Preview
- DeciBels, Phons, and Sones Preview
- Sound of Music Preview
- Shedding Light on Light Bulbs Preview
- Models of Light Preview
- Electromagnetic Radiation Preview
- Electromagnetic Spectrum Preview
- EM Wave Communication Preview
- Digitized Data Preview
- Light Intensity Preview
- Concave Mirrors Preview
- Object Image Relations Preview
- Snells Law Preview
- Reflection vs. Transmission Preview
- Magnification Lab Preview
- Reactivity Preview
- Ions and the Periodic Table Preview
- Periodic Trends Preview
- Intermolecular Forces Preview
- Melting Points and Boiling Points Preview
- Reaction Rates Preview
- Ammonia Factory Preview
- Stoichiometry Preview
- Nuclear Chemistry Preview
- Gaining Teacher Access
- Tasks and Classes
- Tasks - Classic
- Subscription
- Subscription Locator
- 1-D Kinematics
- Newton's Laws
- Vectors - Motion and Forces in Two Dimensions
- Momentum and Its Conservation
- Work and Energy
- Circular Motion and Satellite Motion
- Thermal Physics
- Static Electricity
- Electric Circuits
- Vibrations and Waves
- Sound Waves and Music
- Light and Color
- Reflection and Mirrors
- About the Physics Interactives
- Task Tracker
- Usage Policy
- Newtons Laws
- Vectors and Projectiles
- Forces in 2D
- Momentum and Collisions
- Circular and Satellite Motion
- Balance and Rotation
- Electromagnetism
- Waves and Sound
- Atomic Physics
- Forces in Two Dimensions
- Work, Energy, and Power
- Circular Motion and Gravitation
- Sound Waves
- 1-Dimensional Kinematics
- Circular, Satellite, and Rotational Motion
- Einstein's Theory of Special Relativity
- Waves, Sound and Light
- QuickTime Movies
- About the Concept Builders
- Pricing For Schools
- Directions for Version 2
- Measurement and Units
- Relationships and Graphs
- Rotation and Balance
- Vibrational Motion
- Reflection and Refraction
- Teacher Accounts
- Task Tracker Directions
- Kinematic Concepts
- Kinematic Graphing
- Wave Motion
- Sound and Music
- About CalcPad
- 1D Kinematics
- Vectors and Forces in 2D
- Simple Harmonic Motion
- Rotational Kinematics
- Rotation and Torque
- Rotational Dynamics
- Electric Fields, Potential, and Capacitance
- Transient RC Circuits
- Light Waves
- Units and Measurement
- Stoichiometry
- Molarity and Solutions
- Thermal Chemistry
- Acids and Bases
- Kinetics and Equilibrium
- Solution Equilibria
- Oxidation-Reduction
- Nuclear Chemistry
- NGSS Alignments
- 1D-Kinematics
- Projectiles
- Circular Motion
- Magnetism and Electromagnetism
- Graphing Practice
- About the ACT
- ACT Preparation
- For Teachers
- Other Resources
- Newton's Laws of Motion
- Work and Energy Packet
- Static Electricity Review
- Solutions Guide
- Solutions Guide Digital Download
- Motion in One Dimension
- Work, Energy and Power
- TaskTracker
- Other Tools
- Algebra Based Physics
- Frequently Asked Questions
- Purchasing the Download
- Purchasing the CD
- Purchasing the Digital Download
- About the NGSS Corner
- NGSS Search
- Force and Motion DCIs - High School
- Energy DCIs - High School
- Wave Applications DCIs - High School
- Force and Motion PEs - High School
- Energy PEs - High School
- Wave Applications PEs - High School
- Crosscutting Concepts
- The Practices
- Physics Topics
- NGSS Corner: Activity List
- NGSS Corner: Infographics
- About the Toolkits
- Position-Velocity-Acceleration
- Position-Time Graphs
- Velocity-Time Graphs
- Newton's First Law
- Newton's Second Law
- Newton's Third Law
- Terminal Velocity
- Projectile Motion
- Forces in 2 Dimensions
- Impulse and Momentum Change
- Momentum Conservation
- Work-Energy Fundamentals
- Work-Energy Relationship
- Roller Coaster Physics
- Satellite Motion
- Electric Fields
- Circuit Concepts
- Series Circuits
- Parallel Circuits
- Describing-Waves
- Wave Behavior Toolkit
- Standing Wave Patterns
- Resonating Air Columns
- Wave Model of Light
- Plane Mirrors
- Curved Mirrors
- Teacher Guide
- Using Lab Notebooks
- Current Electricity
- Light Waves and Color
- Reflection and Ray Model of Light
- Refraction and Ray Model of Light
- Classes (Legacy Version)
Teacher Resources
- Subscriptions

- Newton's Laws
- Einstein's Theory of Special Relativity
- About Concept Checkers
- School Pricing
- Newton's Laws of Motion
- Newton's First Law
- Newton's Third Law
The Physics Classroom has been devoted to helping students, teachers, and classrooms since the 1990s. We are as passionate about that mission now as we have ever been. If you are a teacher of Physics or Physical Science, we encourage you to use our Video Tutorial with your students. And we also encourage you to consider the use of other resources on our website that coordinate with the video. We have listed a few below to help you get started.
Curriculum Corner, Work, Energy, and Power Section: Energy
Teacher Toolkits, Work-Energy Relationships
The Calculator Pad, Work and Energy Problem Set, Problems #20-28
Minds On Physics, Work and Energy Module. Missions WE8
Minds On Physics, Work and Energy Section: Missions WE10
Concept Builders, Work and Energy section: Energy Analysis 1
Concept Builder, Work and Energy Section: Energy Analsysis 2
Physics Interactives, Work and Energy section, Roller Coaster Simulation
The Physics Classroom Tutorial, Work, Energy, and Power Chapter, Applications and Practice Questions

COMMENTS
Problem 2: Hans Full is pulling on a rope to drag his backpack to school across the ice. He pulls upwards and rightwards with a force of 22.9 Newtons at an angle of 35 degrees above the horizontal to drag his backpack a horizontal distance of 129 meters to the right. Determine the work (in Joules) done upon the backpack.
Problem Set WE1: Work 1. Use the work equation to calculate the work done, a force value, or a displacement value. Includes 8 problems. Problem Set WE2: Work 2. Use the work equation to calculate the work done, a force value, or a displacement value. Includes 6 problems. Problem Set WE3: Work and Power 1.
The first thing you need to identify when solving an energy problem is whether the mechanical energy (kinetic + potential) is conserved or not in the situation described by the problem. The basic equation that you will have to learn to solve this type of problems is the following: Where E C is the kinetic energy of the particle and W the work ...
7.3: Gravitational Potential Energy. 7. In Example, we calculated the final speed of a roller coaster that descended 20 m in height and had an initial speed of 5 m/s downhill. Suppose the roller coaster had had an initial speed of 5 m/s uphill instead, and it coasted uphill, stopped, and then rolled back down to a final point 20 m below the start.
Introduction to work and energy. Work and energy (part 2) Conservation of energy. What are energy and work? What is kinetic energy? What is gravitational potential energy? What is conservation of energy? Work and the work-energy principle. Work as the transfer of energy.
Energy conservation: Part of a series of videos on physics problem-solving. The problems are taken from "The Joy of Physics. " This one deals with energy conservation. The viewer is urged to pause the video at the problem statement and work the problem before watching the rest of the video.
This is a statement of the work-energy theorem, which is expressed mathematically as. W = ΔKE = 1 2mv22 − 1 2mv21. W = Δ K E = 1 2 m v 2 2 − 1 2 m v 1 2. The subscripts 2 and 1 indicate the final and initial velocity, respectively. This theorem was proposed and successfully tested by James Joule, shown in Figure 9.2.
Unit test. Level up on all the skills in this unit and collect up to 1,100 Mastery points! In physics, the work goes beyond 9-5. Let's see how physicists define the term "work", and how work relates to the concepts of kinetic energy, potential energy, conservation of energy, and mechanical advantage.
Since friction is always an opposing force you subtract this from the 38.5KJ and get the 8455J mentioned. This is the kinetic energy so 1/2mv^2 and you then multiply both sides by 2 and get 16910 = mv^2. The mass is 90kg so divide both sides by 90 and get v^2=187.8889. Square root this and you end up with 13.7m/s.
Combining these three equations we can solve for VG . Answer: VG = [ (10 g /7) ( h1 − h3 )] 1/2. Hint and answer for Problem # 4. The friction force produces an additional energy term in the energy equation. The energy equation is (1/2) MV2 = (1/2) ks2 + Mgμks, where Mg is the normal force, and s is the sliding distance, which is equal to ...
Solution: The total energy of the cart is expressed by the sum of its potential energy and its kinetic energy. Potential energy of an object in a gravitational field is expressed by the formula. PE = mgh. where. PE is the potential energy. m is the mass of the object. g is the acceleration due to gravity = 9.8 m/s 2.
Our mission is to improve educational access and learning for everyone. OpenStax is part of Rice University, which is a 501 (c) (3) nonprofit. Give today and help us reach more students. Help. OpenStax. This free textbook is an OpenStax resource written to increase student access to high-quality, peer-reviewed learning materials.
Using our problem-solving strategy, the first step is to define that we are interested in the particle-Earth system. Second, only the gravitational force is acting on the particle, which is conservative (step 3). We neglect air resistance in the problem, and no work is done by the string tension, which is perpendicular to the arc of the motion.
numerical. A 55 kg human cannonball is shot out the mouth of a 4.5 m cannon with a speed of 18 m/s at an angle of 60°. (Friction and air resistance are negligible in this problem. You may not use Newton's laws or the equations of motion to solve these problems. Think conservation of energy.)
Work, Energy and Power: Problem Set Overview There are 20 ready-to-use problem sets on the topic of Work, Energy, and Power. The problems target your ability to use equations related to work and power, to calculate the kinetic, potential and total mechanical energy, and to use the work-energy relationship in order to determine the final speed, stopping distance or final height of an object.
Problems practice. energy-transformations.pdf Identify a device, event, phenomenon, or process whereby one form of energy is transformed into another. Write something else. Write something different. Write something completely different. numerical. problem
Work-Energy Practice Problems Video Tutorial. The Work-Energy Practice Problems Video Tutorial focuses on the use of work-energy concepts to solve problems. After providing a background and a short strategy, Mr. H steps through detailed solutions to six example problems involving work and energy. Learn how to use the concepts to solve for speed ...
How to Solve Energy Problems. 1.Identify the Problem. Energy is never created or destroyed, although under action of a force it can change in form. Therefore, the idea of conservation of energy always applies. It is only useful for solving a problem, however, when you have enough information to track the changes in energy. You can track changes ...
Energy Problem Solving The main thing to always keep prescient in your mind is that the total energy before must equal the total energy after. If some energy has transferred out of or into the system via work, you calculate that work done and include it in the energy sum equation.
Energy Problem Solving. The main thing to always keep prescient in your mind is that the total energy before must equal the total energy after. If some energy has transferred out of or into the system via work, you calculate that work done and include it in the energy sum equation. Generally work done by friction is listed on the 'after' side ...
You have already identified the types of work and energy involved (in step 2). Before solving for the unknown, eliminate terms wherever possible to simplify the algebra. For example, choose h = 0 h = 0 at either the initial or final point, so that PE g PE g is zero there. Then solve for the unknown in the customary manner. Step 6.
The story has been told many times. At the beginning of the 20th century, physics seemed essentially solved. One or two puzzling phenomena like the photoelectric effect and concerns over black body radiation had scientists scratching their heads but a general feeling prevailed that solving these issues would be dotting the i's and crossing the t's.
How To Solve Physics Problems. This is a quick two page guide I made back when I was at Penn State tutoring first and second year physics. Downloadable PDF. This entry was posted in Uncategorized on March 9, 2024 . ← Externalities, Subsidies, and Taxpayer Cost of Fossil Fuels.
The resources below provide an opportunity to do this. We encourage learners to solidify their learning through the use of one or more of the following resources. Calculator Pad, Work and Energy Problem Set, Problems #20 - #28. Our Calculator Pad section consists of a collection of problems on varied topics, Each problem comes with an answer ...
O xford has a severe housing problem. With house prices 12 times the average salary, it has become one of the least affordable cities in the country. Its council house waiting list has grown to ...
Each toolkit includes annotated links to vetted resources from across the web that we feel reliably support the specific topic. Give this one on work and energy a try. The Calculator Pad, Work and Energy Problem Set, Problems #20-28. The Calculator Pad consists of problems with answers and audio-guided solutions.