Advertisement
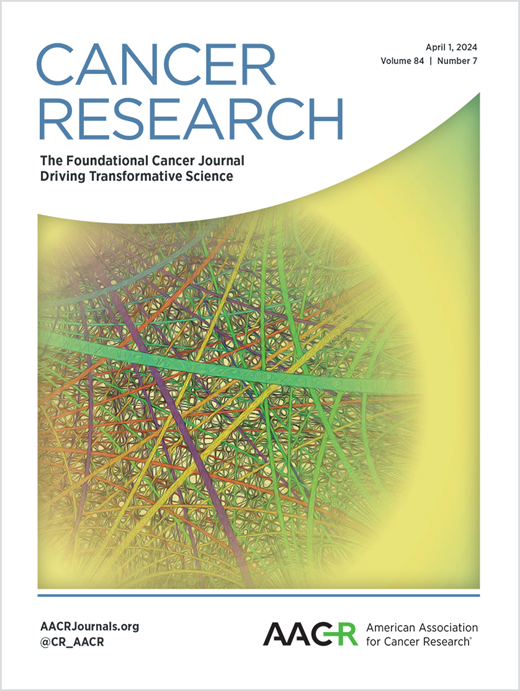

About the Journal
Cancer Research publishes impactful original studies, reviews, and opinion pieces of high significance to the broad cancer research community. Cancer Research seeks manuscripts that offer conceptual or technological advances leading to basic and translational insights into cancer biology. Read More About the Journal
Editor-in-Chief
Christine A. Iacobuzio-Donahue
Impact Factor
Featured articles.

Exploring Ferroptosis-Inducing Therapies for Cancer Treatment: Challenges and Opportunities
Guang Lei; Boyi Gan

Chromatin Remodelers Are Regulators of the Tumor Immune Microenvironment
Apoorvi Chaudhri; Gregory Lizee; Patrick Hwu; Kunal Rai

Personalized Cancer Vaccines Directed against Tumor Mutations: Building Evidence from Mice to Humans
Edward F. Fritsch; Patrick A. Ott
Trending - Altmetric
The Twitter live feed is not currently active.
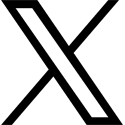
Keep up to date with Cancer Research
Follow @CR_AACR
- Online First
- Collections
- Online ISSN 1538-7445
- Print ISSN 0008-5472
AACR Journals
- Blood Cancer Discovery
- Cancer Discovery
- Cancer Epidemiology, Biomarkers & Prevention
- Cancer Immunology Research
- Cancer Prevention Research
- Cancer Research
- Cancer Research Communications
- Clinical Cancer Research
- Molecular Cancer Research
- Molecular Cancer Therapeutics
- Info for Advertisers
- Information for Institutions/Librarians

- Privacy Policy
- Copyright © 2023 by the American Association for Cancer Research.
This Feature Is Available To Subscribers Only
Sign In or Create an Account
Journal of Cancer Research and Clinical Oncology
- The official journal of the German Cancer Society (Deutsche Krebsgesellschaft)
- Fully open access (OA) as of January 1, 2024
- Alwin Krämer
Latest articles
Identification and validation of tryptophan metabolism-related lncrnas in lung adenocarcinoma prognosis and immune response.
- Mingjun Gao
- Mengmeng Wang
- Xiaolin Wang
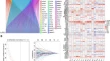
Prospects of molecular hydrogen in cancer prevention and treatment
- Wenchang Zhou
- Changhong Miao
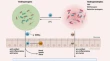
Enzyme-labeled liquid-based cytology (ELLBC): a new noninvasive diagnostic method for bladder cancers
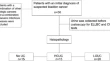
Resveratrol ameliorates glioblastoma inflammatory response by reducing NLRP3 inflammasome activation through inhibition of the JAK2/STAT3 pathway
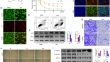
Decision regret of cancer patients after radiotherapy: results from a cross-sectional observational study at a large tertiary cancer center in Germany
- Alexander Rühle
- Leonie Wieland
- Clemens Seidel
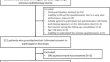
Journal updates
Journal of cancer research and clinical oncology is now fully open access.
We are excited to announce that the Journal of Cancer Research and Clinical Oncology has now become a fully open-access (OA) journal as of January 2024. This means that we will only be publishing articles as Open Access meaning content will be freely available to readers worldwide, enabling the widest possible dissemination and reuse.
Journal information
- Biological Abstracts
- CAB Abstracts
- Chemical Abstracts Service (CAS)
- Current Contents/Life Sciences
- Google Scholar
- INIS Atomindex
- Japanese Science and Technology Agency (JST)
- OCLC WorldCat Discovery Service
- Pathway Studio
- Science Citation Index Expanded (SCIE)
- Semantic Scholar
- TD Net Discovery Service
- UGC-CARE List (India)
Rights and permissions
Springer policies
© Springer-Verlag GmbH Germany, part of Springer Nature
- Find a journal
- Publish with us
- Track your research
Treatment Research
In a new study in mice, researchers showed they could enhance radiation therapy by boosting levels of the BAMBI protein in MDSC immune cells in the tumor microenvironment. After radiation, T cells flooded into the tumor and killed tumors elsewhere in the body.
In a clinical trial, people being treated for cancer who participated in virtual mind–body fitness classes were less likely to be hospitalized, and had shorter stays when they were hospitalized, than people who did not take the classes.
NCI’s James H. Doroshow, M.D., reflects on the accomplishments of NCI-MATCH, a first-of-its-kind precision medicine cancer trial, and gives an overview of three new successor trials: ComboMATCH, MyeloMATCH, and iMATCH.
A new study, conducted largely in mice, may help explain why a currently used molecular marker—called mismatch repair deficiency—doesn’t always work to predict which patients will respond to immunotherapies called immune checkpoint inhibitors.
New approach may increase the effectiveness of T-cell-based immunotherapy treatments against solid tumors.
A cancer-infecting virus engineered to tamp down a tumor’s ability to suppress the immune system shrank tumors in mice, a new study shows. The modified oncolytic virus worked even better when used along with an immune checkpoint inhibitor.
Despite recommendations, a new analysis shows few people with cancer undergo germline testing to learn if their cancer may have been caused by gene changes inherited from a parent. Germline testing can help doctors determine the best treatments for a patient and help identify people whose family members may be at higher risk of cancer.
ComboMATCH will consist of numerous phase 2 cancer treatment trials that aim to identify promising drug combinations that can advance to larger, more definitive clinical trials.
A new study has compared three formulations of an mRNA vaccine designed to treat cancers caused by human papillomavirus (HPV) infections. All three vaccines showed promise in mice.
Researchers have identified a mechanism by which cancer cells develop specific genetic changes needed to become resistant to targeted therapies. They also showed that this process, called non-homologous end-joining (NHEJ), can potentially be disrupted.
For some people with cancer, is 6 months of immunotherapy the only treatment they might ever need? Or 4 weeks of immunotherapy followed by minor surgery? Results from several small clinical trials suggest these scenarios may be bona fide possibilities.
Two research teams have developed ways of overcoming barriers that have limited the effectiveness of CAR T-cell therapies, including engineering ways to potentially make them effective against solid tumors like pancreatic cancer and melanoma.
In people with cancer treated with immune checkpoint inhibitors, a rare, but often fatal, side effect is inflammation in the heart, called myocarditis. Researchers have now identified a potential chief cause of this problem: T cells attacking a protein in heart cells called α-myosin.
Researchers have modified a chemo drug, once abandoned because it caused serious gut side effects, so that it is only triggered in tumors but not normal tissues. After promising results in mice, the drug, DRP-104, is now being tested in a clinical trial.
Two research teams have developed a treatment approach that could potentially enable KRAS-targeted drugs—and perhaps other targeted cancer drugs—flag cancer cells for the immune system. In lab studies, the teams paired these targeted drugs with experimental antibody drugs that helped the immune system mount an attack.
Inflammation is considered a hallmark of cancer. Researchers hope to learn more about whether people with cancer might benefit from treatments that target inflammation around tumors. Some early studies have yielded promising results and more are on the horizon.
NCI researchers are developing an immunotherapy that involves injecting protein bits from cytomegalovirus (CMV) into tumors. The proteins coat the tumor, causing immune cells to attack. In mice, the treatment shrank tumors and kept them from returning.
FDA has approved the combination of the targeted drugs dabrafenib (Tafinlar) and trametinib (Mekinist) for nearly any type of advanced solid tumor with a specific mutation in the BRAF gene. Data from the NCI-MATCH trial informed the approval.
People with cancer who take immunotherapy drugs often develop skin side effects, including itching and painful rashes. New research in mice suggests these side effects may be caused by the immune system attacking new bacterial colonies on the skin.
Researchers have developed tiny “drug factories” that produce an immune-boosting molecule and can be implanted near tumors. The pinhead-sized beads eliminated tumors in mice with ovarian and colorectal cancer and will soon be tested in human studies.
Women are more likely than men to experience severe side effects from cancer treatments such as chemotherapy, targeted therapy, and immunotherapy, a new study finds. Researchers hope the findings will increase awareness of the problem and help guide patient care.
Research to improve CAR T-cell therapy is progressing rapidly. Researchers are working to expand its use to treat more types of cancer and better understand and manage its side effects. Learn how CAR T-cell therapy works, which cancers it’s used to treat, and current research efforts.
Experts say studies are needed on how to best transition telehealth from a temporary solution during the pandemic to a permanent part of cancer care that’s accessible to all who need it.
Removing immune cells called naive T cells from donated stem cells before they are transplanted may prevent chronic graft-versus-host disease (GVHD) in people with leukemia, a new study reports. The procedure did not appear to increase the likelihood of patients’ cancer returning.
A specific form of the HLA gene, HLA-A*03, may make immune checkpoint inhibitors less effective for some people with cancer, according to an NCI-led study. If additional studies confirm the finding, it could help guide the use of these commonly used drugs.
The success of mRNA vaccines for COVID-19 could help accelerate research on using mRNA vaccine technology to treat cancer, including the development of personalized cancer vaccines.
Aneuploidy—when cells have too many or too few chromosomes—is common in cancer cells, but scientists didn’t know why. Two new studies suggest that aneuploidy helps the cells survive treatments like chemotherapy and targeted therapies.
New research suggests that fungi in the gut may affect how tumors respond to cancer treatments. In mice, when bacteria were eliminated with antibiotics, fungi filled the void and impaired the immune response after radiation therapy, the study found.
FDA has approved belumosudil (Rezurock) for the treatment of chronic graft-versus-host disease (GVHD). The approval covers the use of belumosudil for people 12 years and older who have already tried at least two other therapies.
In lab studies, the antibiotic novobiocin showed promise as a treatment for cancers that have become resistant to PARP inhibitors. The drug, which inhibits a protein called DNA polymerase theta, will be tested in NCI-supported clinical trials.
A drug called avasopasem manganese, which has been found to protect normal tissues from radiation therapy, can also make cancer cells more vulnerable to radiation treatment, a new study in mice suggests.
While doctors are familiar with the short-term side effects of immune checkpoint inhibitors, less is known about potential long-term side effects. A new study details the chronic side effects of these drugs in people who received them as part of treatment for melanoma.
Cholesterol-lowering drugs known as PCSK9 inhibitors may improve the effectiveness of cancer immune checkpoint inhibitors, according to studies in mice. The drugs appear to improve the immunotherapy drugs’ ability to find tumors and slow their growth.
Researchers have developed a nanoparticle that trains immune cells to attack cancer. According to the NCI-funded study, the nanoparticle slowed the growth of melanoma in mice and was more effective when combined with an immune checkpoint inhibitor.
A comprehensive analysis of patients with cancer who had exceptional responses to therapy has revealed molecular changes in the patients’ tumors that may explain some of the exceptional responses.
Researchers are developing a new class of cancer drugs called radiopharmaceuticals, which deliver radiation therapy directly and specifically to cancer cells. This Cancer Currents story explores the research on these emerging therapies.
FDA has recently approved two blood tests, known as liquid biopsies, that gather genetic information to help inform treatment decisions for people with cancer. This Cancer Currents story explores how the tests are used and who can get the tests.
Cancer cells with a genetic feature called microsatellite instability-high (MSI-high) depend on the enzyme WRN to survive. A new NCI study explains why and reinforces the idea of targeting WRN as a treatment approach for MSI-high cancer.
Efforts to contain the opioid epidemic may be preventing people with cancer from receiving appropriate prescriptions for opioids to manage their cancer pain, according to a new study of oncologists’ opioid prescribing patterns.
The gene-editing tool CRISPR is changing the way scientists study cancer, and may change how cancer is treated. This in-depth blog post describes how this revolutionary technology is being used to better understand cancer and create new treatments.
FDA’s approval of pembrolizumab (Keytruda) to treat people whose cancer is tumor mutational burden-high highlights the importance of genomic testing to guide treatment, including for children with cancer, according to NCI Director Dr. Ned Sharpless.
Patients with acute graft-versus-host disease (GVHD) that does not respond to steroid therapy are more likely to respond to the drug ruxolitinib (Jakafi) than other available treatments, results from a large clinical trial show.
NCI is developing the capability to produce cellular therapies, like CAR T cells, to be tested in cancer clinical trials at multiple hospital sites. Few laboratories and centers have the capability to make CAR T cells, which has limited the ability to test them more broadly.
An experimental drug may help prevent the chemotherapy drug doxorubicin from harming the heart and does so without interfering with doxorubicin’s ability to kill cancer cells, according to a study in mice.
In people with blood cancers, the health of their gut microbiome appears to affect the risk of dying after receiving an allogeneic hematopoietic stem cell transplant, according to an NCI-funded study conducted at four hospitals across the globe.
A novel approach to analyzing tumors may bring precision cancer medicine to more patients. A study showed the approach, which analyzes gene expression using tumor RNA, could accurately predict whether patients had responded to treatment with targeted therapy or immunotherapy.
Bone loss associated with chemotherapy appears to be induced by cells that stop dividing but do not die, a recent study in mice suggests. The researchers tested drugs that could block signals from these senescent cells and reverse bone loss in mice.
Some experts believe that proton therapy is safer than traditional radiation, but research has been limited. A new observational study compared the safety and effectiveness of proton therapy and traditional radiation in adults with advanced cancer.
In people with cancer, the abscopal effect occurs when radiation—or another type of localized therapy—shrinks a targeted tumor but also causes untreated tumors in the body to shrink. Researchers are trying to better understand this phenomenon and take advantage of it to improve cancer therapy.
An experimental drug, AMG 510, that targets mutated forms of the KRAS protein completely shrank tumors in cancer mouse models and data from a small clinical trial show that it appears to be active against different cancer types with a KRAS mutation.
Researchers have engineered an oncolytic virus to kill cancer cells and boost the immune response against tumors. In a new study, the virus provided T cells around tumors with a hormone they need for their own cell-killing functions.
FDA has approved entrectinib (Rozlytrek) for the treatment of children and adults with tumors bearing an NTRK gene fusion. The approval also covers adults with non-small cell lung cancer harboring a ROS1 gene fusion.
A new NCI-supported study showed that altering cancer cell metabolism by feeding mice a diet very low in the nutrient methionine improved the ability of chemotherapy and radiation therapy to shrink tumors.
An NCI-funded clinical trial is testing the immunotherapy drug nivolumab (Opdivo) in people who have advanced cancer and an autoimmune disease, such as rheumatoid arthritis, lupus, or multiple sclerosis, who are often excluded from such trials.
Researchers have identified a protein called CD24 that may be a new target for cancer immunotherapy. The protein is a ‘don’t eat me’ signal that prevents immune cells called macrophages from engulfing and eating cells.
Injecting cells undergoing necroptosis, a form of cell death, into tumors in mice kickstarted an immune response against the tumors, researchers have found. When combined with immunotherapy, the treatment was effective at eliminating tumors in mice.
Researchers have identified proteins that may play a central role in transforming T cells from powerful destroyers to depleted bystanders that can no longer harm cancer cells. The findings could lead to strategies for boosting cancer immunotherapies.
Did you know that NCI supports clinical trials of new treatments for pet dogs with cancer? Learn more about NCI’s comparative oncology studies and how they may also help people with cancer.
Researchers have discovered a potential way to turn on one of the most commonly silenced tumor-suppressor proteins in cancer, called PTEN. They also found a natural compound, I3C, that in lab studies could flip the on switch.
New findings from a clinical trial suggest that a single dose of radiation therapy may control painful bone metastases as effectively as multiple lower doses of radiation therapy.
The expanding use of cancer immunotherapy has revealed a variety of side effects associated with this treatment approach. Researchers are now trying to better understand how and why these side effects occur and develop strategies for better managing them.
The investigational immunotherapy drug bintrafusp alfa (also called M7824), a bifunctional fusion protein, shrank the tumors of some patients with advanced HPV-related cancers, according to results from a phase 1 clinical trial.
A new study provides insight into how cancer immunotherapy works and suggests ways to enhance the treatment’s effectiveness. The NCI-led study, published in Science, examined the effect of high potassium levels on T cells.
Pain is a common and much-feared symptom among people with cancer and long-term survivors. As more people survive cancer for longer periods, there is a renewed interest in developing new, nonaddictive approaches for managing their chronic pain.
- Editorial board
- About this journal
- Author info
- Submit manuscript
- Manuscript login
- Current issue
- Volume 15; 2024
- Volume 14; 2023
- Volume 13; 2022
- Volume 12; 2021
- Volume 11; 2020
- Cover images
- Index & coverage
- Special issues
International Journal of Biological Sciences
International Journal of Medical Sciences
Theranostics
Journal of Genomics
Nanotheranostics
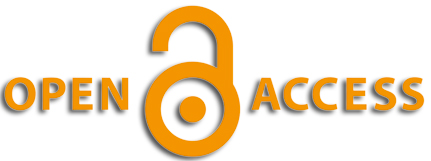
Research Paper Mendelian randomization combined with multi-omics explores the relationship between heart failure and cancer Tian Sun, Na Mei, Yanting Su, Shigang Shan, Wenbin Qian, Mengxi Li, Zhenwang Zhang J. Cancer 2024; 15(10): 2928-2939. doi:10.7150/jca.94142 Abstract Full text PDF
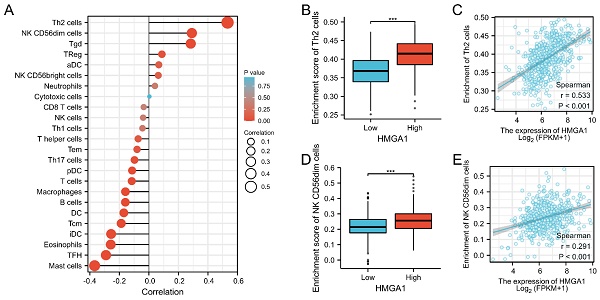
Research Paper HMGA1 is a Prognostic Biomarker and Correlated with Glycolysis in Lung Adenocarcinoma Yu Ma, XiaoYu Ma, BuLin Du, XueNa Li, YaMing Li J. Cancer 2024; 15(10): 2913-2927. doi:10.7150/jca.89056 Abstract Full text PDF
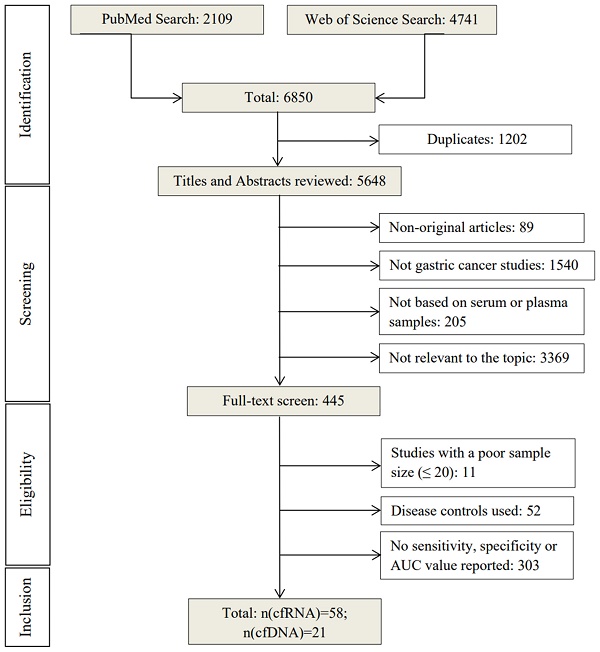
Research Paper Cell-free Nucleic Acid as Promising Diagnostic Biomarkers for Gastric Cancer: a Systematic Review Qun Zhang, Zhouyuan Du, Xiaoxiao Wang, Fen Li, Yi Liu, Jingjing Sun, Lin Zhang, Yong Xiao, Xiaoming Lu, Haixin Yu, Tao Liu J. Cancer 2024; 15(10): 2900-2912. doi:10.7150/jca.92704 Abstract Full text PDF
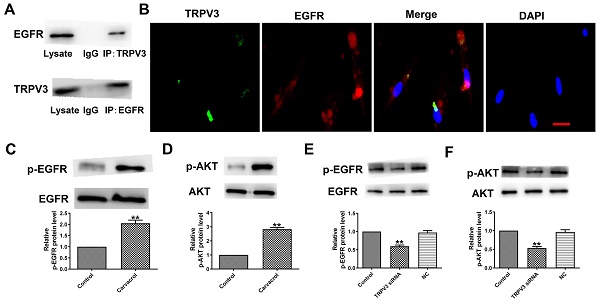
Research Paper TRPV3 regulates Breast Cancer Cell Proliferation and Apoptosis by EGFR/AKT pathway Yan Xie, Hyo In Kim, Qianzhi Yang, Jinghao Wang, Wei Huang J. Cancer 2024; 15(10): 2891-2899. doi:10.7150/jca.93940 Abstract Full text PDF
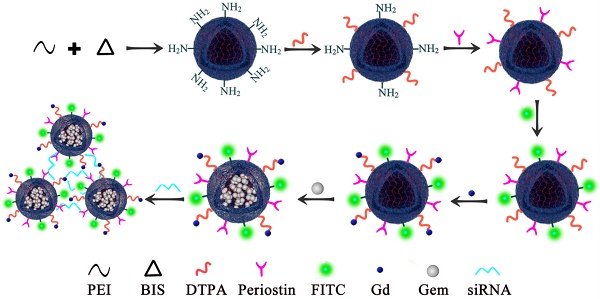
Research Paper Ultrasound-targeted microbubble destruction improves the suppression and magnetic resonance imaging of pancreatic cancer with polyethyleneimine nanogels Yang Liu, Yuanqiong Deng, Paul E Constanthin, Fan Li J. Cancer 2024; 15(10): 2880-2890. doi:10.7150/jca.93802 Abstract Full text PDF
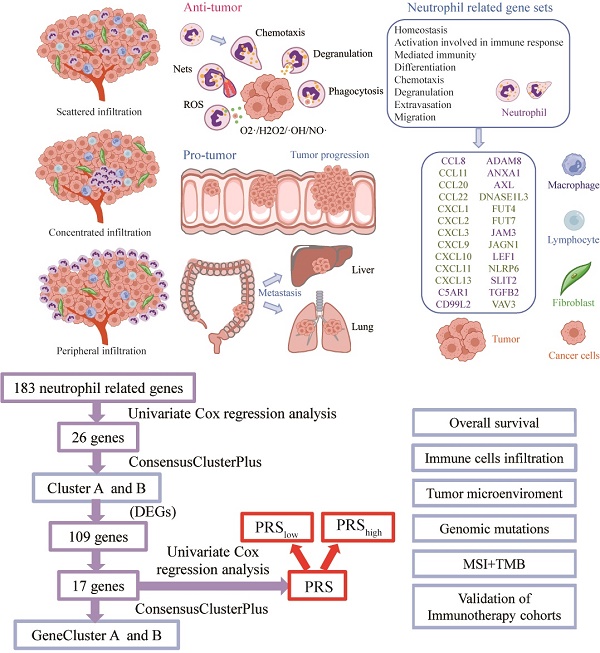
Research Paper Construction and multicohort validation of a colon cancer prognostic risk score system based on big data of neutrophil-associated differentially expressed genes Yunxi Yang, Cheng Lu, Linbin Li, Chunfang Zheng, Yifan Wang, Jiahui Chen, Bingwei Sun J. Cancer 2024; 15(9): 2866-2879. doi:10.7150/jca.94560 Abstract Full text PDF
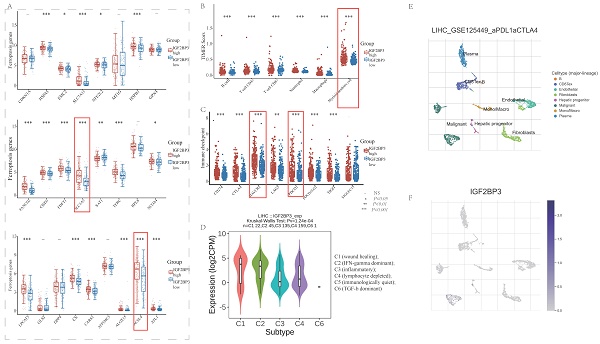
Research Paper Comprehensive analysis of IGF2BP3 with expression features, prognosis, immune modulation and stemness in hepatocellular carcinoma and pan-cancer Sha Qin, Haoer Jin, Yan Li, Xue Chen, Jiang He, Juxiong Xiao, Yan Qin, Chuyi Liu, Yitao Mao, Luqing Zhao J. Cancer 2024; 15(9): 2845-2865. doi:10.7150/jca.92768 Abstract Full text PDF
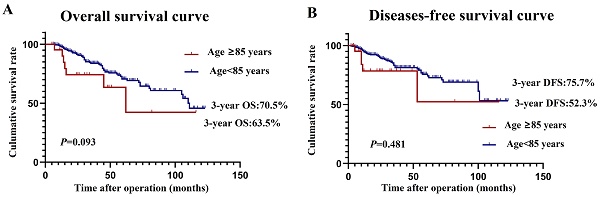
Research Paper Safety and Efficacy Analysis of Radical Surgery for 403 Patients with Colon Cancer over 80 Years Old Ruilong Niu, Mandula Bao, Yujuan Jiang, Jinjie Zhang, Xiaowei Qin, Kangkang Zhang, Jianjun Bi, Wei Xing, Wei Guo, Jianwei Liang J. Cancer 2024; 15(9): 2837-2844. doi:10.7150/jca.94016 Abstract Full text PDF
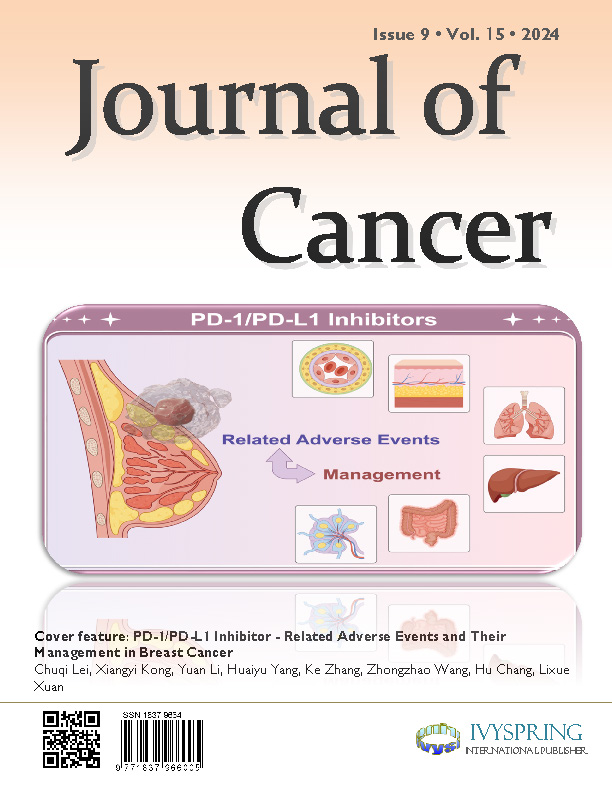
Indexed in PubMed and SCI expanded; full texts in PubMed Central and Europe PMC . ...
A note on plagiarism (please read before submission) : There is a zero-tolerance policy towards plagiarism in this journal. Manuscripts are screened for plagiarism at any stages. If a manuscript is already published and plagiarism is still detected or later reported by readers, it will be retracted and the authors' institutions and department heads will be notified to take actions. This includes data fabrication and image manipulation.
Research Paper Microbial Diversity in Children with Gastroenteritis in the Amazon Region of Brazil: Development and Validation of a Molecular Method for Complete Sequencing of Viral Genomes Juliana Merces Hernandez, Giovanna Brunetta SantAna Almeida, Ana Caroline Rodrigues Portela, Jedson Ferreira Cardoso, Edivaldo Costa Sousa Junior, Maria Silvia Sousa Lucena, Márcio Roberto Teixeira Nunes, Yvone Benchimol Gabbay, Luciana Damascena Silva J. Genomics 2024; 12: 47-54. doi:10.7150/jgen.94116 [ Abstract ] [ Full text ] [ PDF ]
JCA Top cited papers:
- Anahid Jewett, et al. Dual Functions of Natural Killer Cells in Selection and Differentiation of Stem Cells; Role in Regulation of Inflammation and Regeneration of Tissues. Cited by 62
- Jan Trøst Jørgensen, Maria Hersom. HER2 as a Prognostic Marker in Gastric Cancer - A Systematic Analysis of Data from the Literature. Cited by 204
- Chikezie O. Madu, Yi Lu . Novel diagnostic biomarkers for prostate cancer. Cited by 223
- Ohtani H, et al. A meta-analysis of the short-and long-term results of randomized controlled trials that compared laparoscopy-assisted and conventional open surgery for colorectal cancer. Cited by 167
- Dario Sangiolo . Cytokine Induced Killer Cells as Promising Immunotherapy for Solid Tumors. Cited by 193
- Sara A. Lari, Henry M. Kuerer . Biological Markers in DCIS and Risk of Breast Recurrence: A Systematic Review. Cited by 131
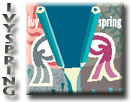
©2024 Ivyspring International Publisher . Terms of use
- Open access
- Published: 29 March 2024
Exploring the dynamic interplay between exosomes and the immune tumor microenvironment: implications for breast cancer progression and therapeutic strategies
- Sahar Safaei 1 ,
- Manouchehr Fadaee 1 ,
- Omid Rahbar Farzam 1 ,
- Amirhossein Yari 1 , 2 ,
- Elham Poursaei 3 ,
- Cynthia Aslan 4 ,
- Sahar Samemaleki 5 ,
- Dariush Shanehbandi 1 ,
- Behzad Baradaran 1 &
- Tohid Kazemi 1
Breast Cancer Research volume 26 , Article number: 57 ( 2024 ) Cite this article
98 Accesses
Metrics details
Breast cancer continues to pose a substantial worldwide health concern, demanding a thorough comprehension of the complex interaction between cancerous cells and the immune system. Recent studies have shown the significant function of exosomes in facilitating intercellular communication and their participation in the advancement of cancer. Tumor-derived exosomes have been identified as significant regulators in the context of breast cancer, playing a crucial role in modulating immune cell activity and contributing to the advancement of the illness. This study aims to investigate the many effects of tumor-derived exosomes on immune cells in the setting of breast cancer. Specifically, we will examine their role in influencing immune cell polarization, facilitating immunological evasion, and modifying the tumor microenvironment. Furthermore, we explore the nascent domain of exosomes produced from immune cells and their prospective involvement in the prevention of breast cancer. This paper focuses on new research that emphasizes the immunomodulatory characteristics of exosomes produced from immune cells. It also explores the possibility of these exosomes as therapeutic agents or biomarkers for the early identification and prevention of breast cancer. The exploration of the reciprocal connections between exosomes formed from tumors and immune cells, together with the rising significance of exosomes derived from immune cells, presents a potential avenue for the advancement of novel approaches in the field of breast cancer therapy and prevention.
Introduction
Breast cancer (BC) is the most common cancer among women in most countries, with the highest mortality and morbidity rates [ 1 ]. Despite the earlier detection and improved clinical management, there is still a high death rate among women because of treatment failure and metastasis [ 2 ]. BC can be divided based on its molecular characteristics into luminal A, luminal B, HER2, estrogen, and progesterone-positive receptors, and triple-negative BC (TNBC). Further subcategories of TNBC include basal-like 1 and 2, immunomodulatory, luminal androgen receptor, mesenchymal, and mesenchymal stem cell-like [ 3 , 4 ]. TNBC and HER2-positive breast cancers are more likely to metastasize to other areas of the body, such as the brain, bone, lung, liver, and abdominal cavity [ 5 , 6 ].
In recent decades, there has been a dramatic increase in the literature associated with the involvement of the immune system in BC [ 7 ]. It is well known that the immune system plays an important role in the development, progression, and control of BC [ 8 ]. The complex relationship between the immune system and cancer cells is characterized by the theory of “cancer immunoediting” (CI) [ 9 ], which was initially developed by Dunn et al. [ 10 ] CI postulates a dual role of the immune system in cancer: host-protective and tumor-promoting actions [ 11 ]. CI consists of “three Es”: elimination, equilibrium, and escape [ 12 , 13 ]. In the ‘elimination’ phase, all tumor cells may be destroyed by the immune system [ 13 ]. During the second phase, the opposing forces remain balanced and cancer growth is still under control. Escape is the terminal stage of immunoediting, in which cancer cell variants effectively evade immune pressure, replicate progressively, and become clinically apparent [ 13 ]. In light of this, manipulating the immune system and boosting its defenses against cancer may be one of the keys to controlling cancer [ 14 ]. This idea is known as cancer immunotherapy [ 14 ]. Exosomes (Exo) have emerged as potential sources of biomarkers for early detection and prognosis in breast cancer. Several studies have identified specific molecules, such as proteins, nucleic acids, and microRNAs, within exosomes that show promise as diagnostic and prognostic markers. MiRNAs are small non-coding RNA molecules that regulate gene expression. For example, miRNA-21, miRNA-155, and miRNA-210 have been found to be upregulated in breast cancer-derived exosomes and are associated with tumor growth, metastasis, and poor prognosis [ 15 , 16 , 17 ]. In addition, Proteins such as human epidermal growth factor receptor 2 (HER2), and mucin 1 (MUC1) have been detected in exosomes isolated from breast cancer patients. Changes in the levels of these proteins in exosomes have been associated with tumor progression and can potentially serve as diagnostic and prognostic markers [ 18 , 19 ]. The field of exosome research is rapidly evolving, and ongoing studies continue to identify new potential markers. The most common immunotherapy approaches in BC are non-specific immune stimulation, immune checkpoint blockade, adoptive cell transfer, and vaccination [ 20 , 21 ]. Immune checkpoint inhibitors targeting programmed cell death 1 (PD-1), programmed cell death 1 ligand 1 (PD-L1), and cytotoxic T-lymphocyte-associated protein 4 (CTLA-4) have revolutionized cancer treatment by eliciting durable objective responses in multiple cancer types, often leading to improved overall survival [ 22 , 23 ]. According to accumulating evidence, PD-1/PD-L1 inhibitors have the potential to induce durable clinical responses in some patients with metastatic TNBC and are likely to have clinically meaningful results in rare ER + HER2-BC patients as well [ 11 ]. Recent evidence has confirmed exosome-based immunotherapy as a potential new approach in the treatment of BC [ 24 , 25 , 26 ]. Exosomes are nano-sized small extracellular vesicles with endogenous origin and capacity to transport various types of proteins, lipids, RNA, microRNA (miR), and DNAs [ 27 , 28 ]. Exosomes protect their cargo contents from degradation (especially RNA molecules) in systemic circulation [ 29 ]. In addition, their small size enables them to overcome the blood–brain barrier and penetrate deep into the tissues [ 29 , 30 ].
Exosomes may serve as a robust and feasible vehicle for delivering immune-modulatory agents into specific target cells to either stimulate antitumor immunity or exert immunosuppressive effects [ 31 ]. In addition, Exosomes can deliver factors involved in DNA repair pathways, enhancing the repair of DNA double-strand breaks and promoting cell survival and radioresistance [ 32 ]. They can also transfer anti-apoptotic proteins that protect cancer cells from radiation-induced cell death [ 33 ]. Exosomes secreted from HER2-positive cell lines, SKBR3 and BT-474, are reportedly enriched in antigen processing/presentation-related proteins, including HSPA5 (heat shock 70 kDa protein 5), CALR (calreticulin), and PSME2 (proteasomeactivator complex subunit 2), and glycolysis/gluconeogenesis-related proteins, such as TPI1 (triosephosphate isomerase 1) and PGAM1(phosphoglycerate mutase 1) [ 34 ]. Interestingly, it has also been shown that SKBR3 and BT-474 derived-exosomes contain a full-length HER2 active molecule which can bound to trastuzumab and inhibit drug’s activity [ 35 ].
In this review, we aim to highlight the potential of using exosomes in the field of BC immunotherapy. We describe the known functions of BC-derived exosomes and immune cell-derived exosomes in immune modulation. Next, we point out how exosome-based immunotherapy can have significant therapeutic effects on BC progression. And finally, we will discuss the current knowledge on the possible applications of exosome-based immunotherapy in BC and the future direction of these fascinating vesicles in cancer therapy.
Biogenesis of exosomes and their biological importance
Extracellular vesicles (EVs) are membrane-bound vesicles that are commonly classified into three main subtypes: exosomes, microvesicles (MVs), and apoptotic bodies [ 36 ]. For the first time, Johnstone et al. used the word “exosome” to describe small membrane vesicles formed in multivesicular bodies (MVBs) in the late 1980s [ 37 ]. Exosomes are generated by various cell types and loaded with a myriad of bioactive molecules, including nucleic acids, proteins, lipids, and other metabolites, which mediate cell–cell communication via their cargo contents [ 38 ]. They have a diameter of 30–150 nm, a typical “cup dish” shape, and a density of 1.13–1.19 g/mL [ 39 ].
The complex process that leads to the biogenesis of exosomes may be divided into three main steps: first, the early and late endosomes are formed; then, intraluminal vesicles (ILVs) are formed inside the late endosomes, which leads to the formation of MVBs; and finally, upon the fusion of MVBs with the plasma membrane, ILVs are secreted throughout body fluids (blood, saliva, urine, etc.) by exocytosis and are called exosomes [ 40 , 41 ]. Exosomes can be released during normal and pathological states from a majority of cell types [ 42 ].
The process of cargo sorting into the ILVs is mediated via two opposing sorting systems namely, endosomal sorting complexes required for the transport (ESCRT) dependent and ESCRT-independent pathways [ 41 ]. Four ESCRT proteins (ESCRT-0, ESCRT-I, ESCRT-II, and ESCRT-III), tumor susceptibility gene 101 (TSG101), and ALIX are mainly involved in the transition of endosomes to exosomes [ 43 , 44 ]. The ESCRT-I protein Tsg101, together with the ubiquitinated proteins, triggers activation of the ESCRT-II complex and mediates the oligomerization of the ESCRT-III complex [ 45 ]. ESCRT-III machinery contributes to vesicle release into the endosome and scission [ 40 ]. Vacuolar protein sorting associate protein 4 (VPS4) and ATPase degrade the ESCRT-III complex as the final step of exosome formation [ 40 , 46 ]. However, under certain conditions, the biogenesis of exosomes can occur via an ESCRT-independent pathway. This process can be mediated by lipids such as ceramides and cholesterol [ 47 ].
Exosomes participate in several normal and pathological conditions, such as pregnancy, immune responses, cardiovascular disorders, viral pathogenesis, neurological disorders, and cancer [ 48 ]. According to the origin of the donor cell, exosomes play a variety of roles, including immune response, antigen presentation, programmed cell death, angiogenesis, inflammation, coagulation, etc. [ 27 , 49 ] (Figure 1 ).
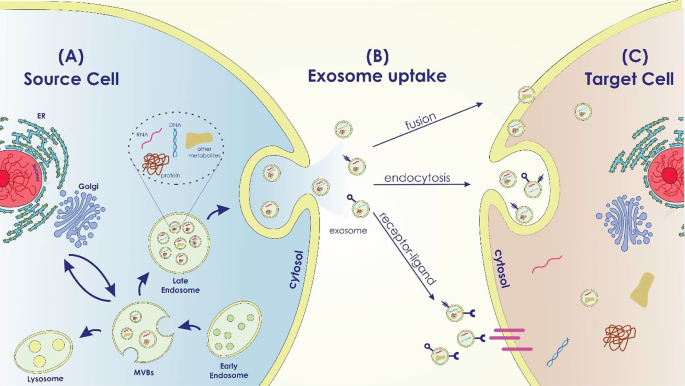
Exosome Biogenesis in the Source Cell, Uptake, and Cargo Transfer ( a ) Exosome Biogenesis in the Source Cell: The intracellular mechanisms involved in exosome biogenesis within the source cell are depicted in this area of the image. It displays the early endosome formation process, the sorting of different cargo molecules into the developing exosomes, and the inward budding of the endosomal membrane to generate multivesicular bodies (MVBs). Labeled cargo molecules consist of proteins, RNAs (miRNAs and mRNAs), lipids, and other bioactive compounds. ( b ) Exosome Uptake Routes by Target Cells: This portion of the diagram shows the various ways that target cells can absorb exosomes. It demonstrates a variety of exosome uptake processes, including as receptor-mediated interactions, membrane fusion, and endocytosis. ( c ) Cargo Transfer and Impacts in the Target Cell: The cargo transfer that occurs when exosomes enter the target cell and the ensuing impacts are the main topics of this portion of the picture. It shows how certain cellular processes are activated and how exosome cargo is released into the target cell’s cytoplasm
TDEs effect on immune cells
Over the past 10 years, the study of exosomes has observed increasing attention, representing a high-potential area for their application in cancer as biomarkers and therapeutic tools [ 50 ]. Tumor-derived exosomes (TDEs) are important immune adjusters in the tumor milieu. TDEs reduce immune cell cytotoxicity in the microenvironment of tumor cells by transporting suppressive cargo to these cells, to facilitate tumorigenesis [ 51 ]. TDEs have been implicated in all stages of BC progression (tumor initiation, growth, dissemination, and metastatic spread) [ 2 ]. As part of their immunological functions, exosomes modulate antigen presentation, immune activation, immune surveillance, intercellular communication, and immune suppression [ 52 , 53 ]. On the other hand, the expression of PD-1 on the surface of BC-derived exosomes can blunt T-cell activation, effectively enabling tumor cells to escape immune surveillance [ 54 ]. The functions of exosomes secreted from cancer cells are discussed in more detail in the following sections (Fig. 2 ).
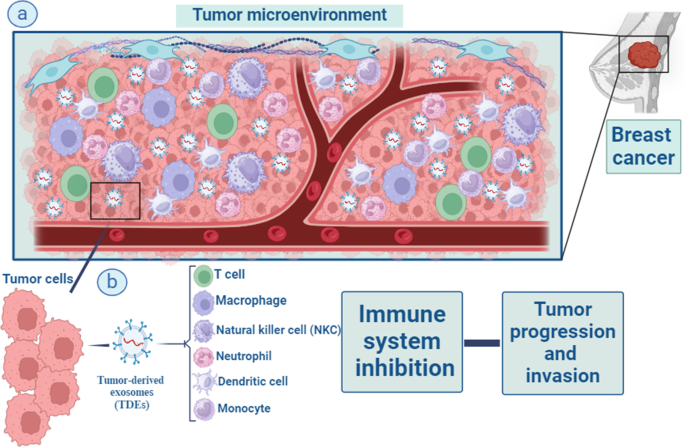
Macrophages, T lymphocytes, natural killer (NK) cells, dendritic cells, neutrophils, and monocytes are all part of the intricate web of immune cells that surrounds tumour and impacts cancer development and outcome via their interactions with tumour cells. Important immune modifiers in the tumor environment are something called tumor-derived exosomes (TDEs) ( a ). TDEs enhance the development of tumors by lowering and suppressing the level of immune cells cytotoxicity and activities in the microenvironment around tumor cells. They do this by transferring suppressive cargo to these cells ( b )
NK cells, a subset of innate effector lymphocytes, need constant renewal through bone marrow cells. It is well known that Natural killer (NK) cells are highly effective in attacking tumor cells. NK cells directly eradicate tumors using death receptors and cytolytic particles. There is evidence that Fas ligand (FasL) expressed on the surface of NK cell-derived exosomes is involved in killing Fas + tumor cells [ 55 ]. They defend against metastatic and initial tumor cells, and enhance adaptive immunity by releasing cytokines to inhibit target cell growth [ 56 ].
TDEs can integrate with the NK cell membrane, obstructing NK cells’ antitumor activity by carrying their cargo to NK cells [ 57 , 58 ]. In addition, the interplay among receptors on NK cells and their TDE ligands excites multiple downstream inhibitory signaling pathways that suppress NK cells’ cytotoxic function [ 51 ]. Examples of NK cells activating receptors that TDEs downregulate include NKp46, NKG2C, NKp30, and NKG2D [ 59 ]. The exosomes-derived breast carcinoma cell line T47d, even in the presence of IL-15 stimulation, inhibits immunological functions via reducing NKG2D receptor expression [ 60 ]. The ratio of CD56 dim and CD56 bright NK cells in patients with breast cancer in relation to negative control was remarkably increased and decreased, respectively [ 61 ].
Stimulating NK cells by releasing (IFN-γ) and (TNF-α) promote the antitumor immune reaction. However, purified exosomes from mouse breast cancer cells debilitated the production of IFN-γ from IL-2– activated NK cells and, so impaired NK cell proliferative capacities. TDEs also significantly inhibited human NK cell proliferation in response to IL-2 through inhibiting Janus kinase 3(Jak3)-mediated signal transducers and activators of transcription 5(STAT 5) stimulation. Moreover, TDEs intensely decreased perforin release in IL-2 motivated NK cells [ 62 ]. Wen et al. demonstrated that breast cancer–derived exosomes reduced NK cells’ number and anticancer abilities [ 63 ] (Fig. 3 ).
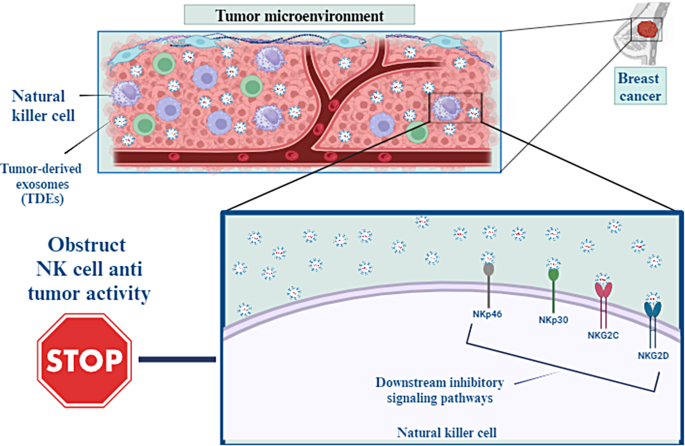
TDEs can integrate with the membrane of NK cells, which may inhibit the antitumor activity of NK cells by transporting their payload to these cells. Furthermore, the interaction between receptors on NK cells and the TDE ligands that bind to them activates many downstream inhibitory signaling pathways, which in turn reduce the cytotoxic activity of NK cells
T-CD8 + cells
A subpopulation of T cells known as T-CD8 + cells play a crucial role in the immunological tumor microenvironment of cancer patients because of their capacity for cytotoxicity. Through the use of major histocompatibility complex class I (MHC-I) molecules and the T cell receptor (TCR) found on T-CD8 + cells, they are able to identify tumor-associated antigens expressed on the surface of cancer cells. Tumor progression, invasion, and resistance to therapies are closely related to tumor microenvironment (TME) interactions. Cancer cells can evade antitumor immunity through packaging immunosuppressive mediators, such as FasL, TNF-associated apoptosis inducing ligand (TRAIL), transforming growth factor-β (TGF-β), and PD-L1 into their exosomes and affect T-CD8 + cell activity [ 64 ].
A high level of PD-L1 expression is associated with a poor prognosis in various types of tumors. PD-L1 binds to the PD-1 receptor which is expressed on almost all immune cells and inactivates their function. So, immune checkpoint inhibitors target the PD1/PD-L1 axis in cancer immunotherapy. However, anti-PD-L1 antibodies are inefficient at blocking exosomal PD-L1, so this phenomenon can promote immunotherapy resistance [ 65 ]. In head and neck squamous cell cancers (HNSCC) patients, the level of PD-L1 on exosomes, but not the level of soluble PD-L1, correlates with the progression of the disease and poor prognosis [ 66 ]. In a study, TNBC exosomes overexpressing PD-L1 reduced T-CD8 + numbers and inhibited their activities, whereas the differentiation of pro-tumorigenic cells including myeloid-derived suppressor cells (MDSCs) and M2 macrophages increased in TME [ 67 ]. T-CD8 + granzyme B secretion is also inhibited by exosomal PD-L1 in vivo, thereby protecting and promoting tumor growth [ 54 ]. Chatterjee et al. showed that high level of transforming growth factor beta (TGF-β) is correlated with secretion of PD-L1 carrying exosomes from BC cells [ 68 ]. Synergistic effects of TGF-β and TDEs expressing high PD-L1 impair T-CD8 + function by suppressing T cell receptor (TCR) signaling mediated phosphorylation (Fig. 4 ).
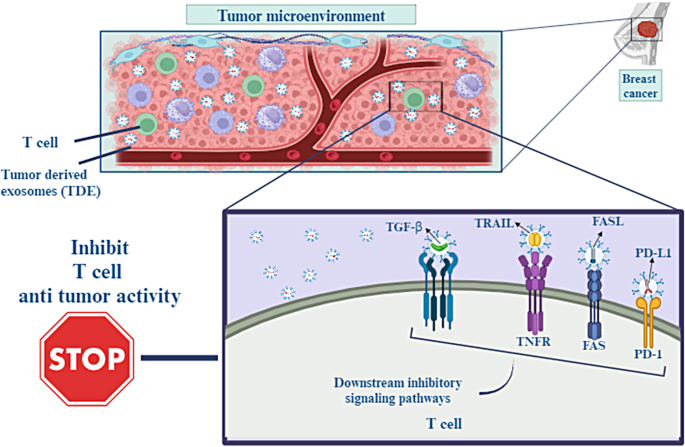
Interactions within the tumor microenvironment (TME) are directly connected to the growth of cancer, its ability to invade surrounding tissue, and resistance to treatment. Cancer cells can resist antitumor immunity by packing immunosuppressive mediators including FasL, TNF-associated apoptosis-inducing ligand (TRAIL), transforming growth factor- β (TGF- β), and PD-L1 into their exosomes. This allows the cancer cells to circumvent the immune system
Regulatory T cells (Tregs) assist tumor cells in evading immune system responses by secreting immunosuppressive cytokines including IL-10 and TGF-β1. The presence of Tregs within tumors is closely linked to cancer prognosis [ 69 ]. Studies have shown that exosomes obtained from tumor cells promote the production and proliferation of Tregs. CD25 − CD4 + T cells can be transformed into FoxP3 + CD4 + CD25 high T cells via coculture with exosomes derived from tumors. Exosomal microRNA-214, in terms of its mechanism, suppresses the expression of phosphatase and tensin homolog (PTEN) in T cells and induces regulatory T cells (Tregs) to secrete IL-10, which ultimately leads to the promotion of tumor growth [ 70 ]. Exosomes of mutant KRAS lung tumor cells promote the conversion of naïve T cells into Tregs, a phenomenon also seen following the cDNA transfection of mutant KRAS. DNA of exosomal mutant KRAS causes this transformation, as evidenced by the increased presence of FoxP3 + Tregs in cancer tissues of mutant KRAS patients compared to those with wild type (WT) KRAS [ 71 ]. Moreover, in breast cancer, exosomal long noncoding RNA (lncRNA) SNHG16 has been shown to promote the development of CD73 + γδ1 Treg cells in breast cancer by sequestering miR-16-4p, leading to the suppression of SMAD5, activation of the TGF-b1/SMAD5 pathway, and ultimately increasing CD73 expression [ 72 ].
Myeloid-derived suppressor cells (MDSCs) are immature myeloid-derived cells with various morphologies present in the tumor microenvironment, possessing potent immune suppressive capabilities [ 73 ]. The aggregation of MDSCs inhibits antigen processing and it’s presentation and releases several immunosuppressive substances that disrupt immune responses [ 74 ]. Several studies have indicated that the suppressive function of MDSCs on T cells can be potentiated by cancer exosomes. Exosomal HSP70 in renal carcinoma promotes cell growth and boosts the activity of MDSCs by activating TLR2 signaling [ 75 ]. Meanwile, miR-181a and miR-9 and from exosomes produced from breast cancer promote tumor development and immune escape by boosting the activity of MDSCs through activating the JAK/STAT signaling pathway. This is achieved by individually suppressing SOCS3 and PIAS3 [ 76 ]. In addition, Exosomes derived from breast cancer contain TGF-β1 and PGE2, which promote the aggregation of MDSCs and facilitate tumor progression [ 77 ].
Non-immune cells
Exosomes play a significant role in modulating the tumor microenvironment by interacting with various stromal cell types, including cancer-associated fibroblasts (CAFs), endothelial cells, and adipocytes [ 78 ]. BC-Exos can educate and activate CAFs, leading to the secretion of growth factors, cytokines, and extracellular matrix components that promote tumor growth and invasion. Moreover, exosomes can transfer oncogenic signals, such as transforming growth factor-beta (TGF-β), to CAFs, further promoting tumor progression and epithelial-mesenchymal transition (EMT) [ 79 ]. In addition, BC-Exos stimulate angiogenesis by promoting endothelial cell migration, proliferation, and tube formation. These exosomes carry pro-angiogenic factors, including vascular endothelial growth factor (VEGF), fibroblast growth factor (FGF), and matrix metalloproteinases (MMPs), which enhance the formation of new blood vessels to support tumor growth and metastasis [ 80 , 81 ].
In summary, exosomes have the ability to influence the composition and functions of the tumor microenvironment. By inducing phenotypic changes in cancer-associated fibroblasts and immune cells, they promote the secretion of factors that contribute to radioresistance or immunosuppression. This altered microenvironment provides a supportive niche for surviving cancer cells and contributes to treatment resistance [ 82 ].
Exosomes-based immunotherapy approaches for BC
Surgical resection, chemotherapy, radiotherapy, hormone therapy, and radiation therapy are currently available to breast cancer patients for treatment. However, drug resistance and toxicity to healthy tissues are two issues that plague all current treatments [ 83 ]. So, developing targeted, non-toxic, and non-immunogenic delivery technologies is crucial. Exosomes and the tumor microenvironment are becoming increasingly attractive targets for clinical applications. This is mainly because of their adaptable function in carcinogenesis in terms of cancer diagnosis and response to treatment [ 84 ].
Precision BC treatment has been significantly improved by nanoparticle-based technology. Exosomes are natural nanoparticles carrying chemotherapeutic drugs, photosensitizers or antitumor drugs. For cancer therapy, exosomes are particularly appealing drug delivery system due to their excellent biosafety, low immunogenicity, carrier properties, and nanoscale penetration effect [ 85 ] (Table 1 ). The exosomes primary advantage over other nanoparticles is inherent capacity to transport molecules of biological significance. This feature has prompted research in treatment choices drugs including the utilization of nucleic acid or enactment of the immune system.
Dendritic-based exosomes
Dendritic cells (DCs) play key role in the immune response by capturing, processing and presenting antigens (Ag) to immature T lymphocytes and activating them. This creates a crucial link between innate and adaptive immune responses. However, tumor-associated DC with immunosuppressive activity can induce T cell tolerance and progressive tumor growth. In order to take advantage of DC’s capacity to initiate and enhance anti-tumor immunity, novel DC-targeted therapies must be developed [ 93 ].
In recent years, DC-derived exosomes (Dex) have been gaining interest as an alternative delivery method for tumor antigens. Dex carry antigen presenting particles such as MHC-I and MHC-II, CD80, CD86 and Hsp 70 and 90 [ 94 ]. T cells can be stimulated both directly and indirectly by Dex. In a direct manner, T cells are induced by MHC and co-stimulatory molecules on the Dex membrane [ 95 ]. However, this mechanism is not effective in activation of naïve T cells due to insufficiency of TCR-cross linking and co-stimulation [ 96 ]. As opposed to direct mechanisms, indirect mechanisms produce more potent T-cell responses against antigens since bystander DCs efficiently activate antigen-specific T cells in response to antigen substances transferred by Dex. Dex can induce T cell responses against the tumor by transferring MHC-peptide complexes to the tumor cell surface. One study showed that Dex treated human breast adenocarcinoma cells, SK-BR-3, increased IFN-γ secretion by CD3 + primed T cells [ 97 ] (Fig. 5 a).
NK derived exosome
In cancer immunotherapy, natural killer (NK) cells are being investigated for adoptive cell therapy as intrinsic lymphocytes that facilitate tumor immune surveillance [ 98 ]. It has also been demonstrated that NK cells control the immune response in other, non-cell activation-dependent ways, one of which is through exosomes [ 55 ]. NK derived exosomes (NK-Exos) containing NK cell’s prototype markers, e.g. CD56 and cytotoxic proteins such as FasL and perforin, enables them to direct their cytolytic activity into tumor tissue sites [ 99 ]. Zhu et al. developed exosome mimetics (NK-EM) by extruding NK cells through filters with increasingly smaller pores. NK-EM showed powerful tumor-killing activity against cancers such as glioblastoma and BC in mice as compared to NK-Exo at low and high doses [ 100 ]. Kaban and colleagues, transduced lentivirally NK cell line NK92MI to express and load BCL-2 siRNA (siBCL-2) into NK-Exos. siBCL-2 NK-Exos prompted intrinsic pathway of apoptosis in BC cells via targeting BCL-2, without influencing non-dangerous cells [ 101 ] (Fig. 5 b).
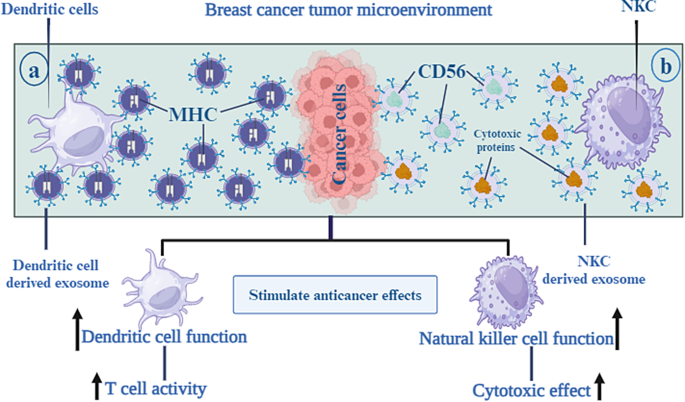
As an alternate delivery mechanism for tumor antigens, DC-derived exosomes, also known as Dex, have been attracting a lot of attention recently. Antigen-presenting particles like MHC-I and MHC-II, CD80 and CD86, and Hsp 70 and 90 are carried by dex in the body. This enables the DC to increase Tcell activity towards tumor tissue areas ( a ). NK-derived exosomes, also known as NK-Exos, are vesicles that include NK cells’ prototype markers, such as CD56, together with cytotoxic proteins like FasL and perforin. This enables the NK cells to guide their cytolytic activity towards tumor tissue areas ( b )
Exosome based vaccines
Cancer immunotherapy mainly relies on CD8 + T cells to promote Cytotoxic T-lymphocyte (CTL) activity, initiate tumor-specific CTLs in lymphoid organs, and establish lasting anti-cancer immunity [ 102 ]. Utilizing an EXO-based vaccine by activating the immune response is a promising cancer treatment option because the immune system avoids tumors. Wang et al. used HER2/neu-specific Neu-T cell derived exosome (TEXO) and HER2-TEXO vaccines by transfecting DCs to stimulate HER2/neu-specific CTLs and study anti-tumor activity. They demonstrated that novel T cell-based exosome vaccine was effective in inducing protective immunity against tumor cells and may be an alternative therapeutic method for HER2 + breast cancer, especially for women with trastuzumab-resistant HER2 + breast cancer [ 103 ]. Additionally, vaccination with exosome-loaded T cells (exosome-T) has the capacity to reverse CD4 + CD25 + Treg cell-mediated immunosuppression and initiate long-term CTL memory, making it an appealing method for generating immune responses against human cancers [ 104 , 105 ]. Also, the HER2-specific exosome-T vaccine was just recently industrialized to effectively boost the immune system of the patient against HER2-positive breast cancer [ 106 ]. By utilizing the transmembrane domain of the human platelet-derived growth factor receptor to demonstration two distinct kinds of monoclonal antibodies on the external of an exosome, the brand-new synthetic poly valent antibody redirected exosome (SMART-EXO) was created. SMART-EXOs with the breast cancer-related HER2 and EGFR receptors can activate CTLs by targeting the CD3 receptor on the surface of T cells. CTLs then display highly effective and specific anti-tumor activity in vitro and in vivo [ 107 , 108 ]. On the other hand, CAR-T exosomes have high safety and efficiency, which could enhance BC immunotherapy [ 109 ]. MSLN-positive TNBC cells and xenograft tumors growth were effectively inhibited by CAR-T cell exosomes secreting granzyme B and perforin without any overt side effects [ 110 ].
Macrophage-based exosomes
Macrophages are one of the innate immune cells that play a critical role in TME. Macrophages have to major subtypes: M1 and M2. M1 macrophages have pro-inflammatory function and their polarization is due to several factors including Th1 cytokines, lipopolysaccharide (LPS), and Toll-like receptor (TLR) agonists. However, Th2 cytokines including IL-4 and IL-13, polarize macrophages to M2 phenotype that exert anti-inflammatory function and has involved in tissue repair and hemostasis [ 111 ]. Tumor-associated macrophages (TAMs) are one of the M2 subtypes that polarize from macrophages in the TME and facilitate tumor growth and angiogenesis. IL-6 signaling through signal transducer and activator of transcription 3 (STAT3) transcription factor phosphorylation, induce TAMs development and tumorigenesis. Ham et al. revealed that BC-derived exosomes render IL-6Rβ (gp130) to macrophages and stimulate STAT3 signaling, thereby enhancing pro-tumorigenic microenvironment [ 112 ].
Suppressing the M2 phenotype or promoting the M1 phenotype in macrophages may be a therapeutic approach for the treatment of cancer. A number of strategies are available to inhibit M2 macrophage attraction and differentiation, including blocking the prostaglandin E2, IL-6, and STAT3 activation loops and preventing tumors from releasing growth factors [ 111 ]. Interferon-gamma (IFN-γ) administration or Notch signaling activation are two other approaches for polarizing M2 macrophages to M1 phenotype. Additionally, it has been established that miRNAs play a crucial role in controlling macrophage polarization. For instance, mir-155 inhibited M2/Th2 responses by suppressing CCAAT/enhancer-binding protein beta (C/EBPβ) and interleukin 13 receptor (IL-13R) [ 113 ]. MiR-125b by downregulating of IFN regulatory factor 4 proved the potential to activate M1 polarization [ 114 ]. Moreover, by transfecting exosomes of MDA-MB-231 cells with miR-130 and miR-33, macrophages were polarized to the M1 phenotype that inhibited tumor growth [ 115 ]. MiR-130 was transported via tumor-derived exosomes to M2 macrophages, suppressed tumor cells growth, migration, or invasion [ 116 ] (Fig. 6 ).
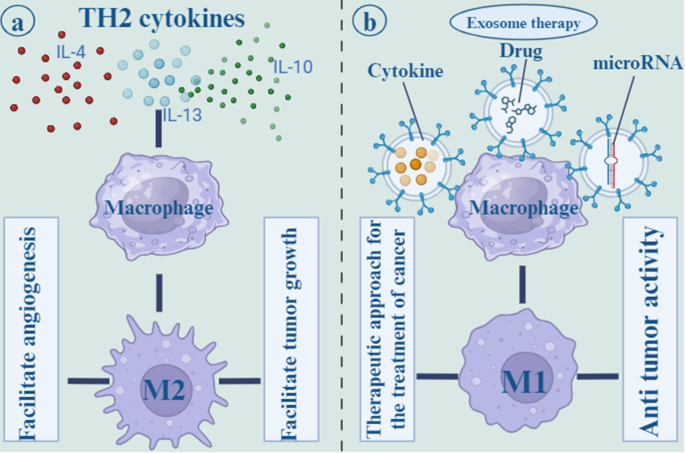
Th2 cytokines, such as IL-4, IL-13, and IL-10 polarize macrophages to the M2 phenotype, which is known to have an anti-inflammatory effect and to play a role in tissue repair and hemostasis. the M2 subtypes, which originate from macrophages in the tumor microenvironment and promote tumor development as well as angiogenesis ( a ). Other methods for polarizing M2 macrophages to the M1 phenotype include the infusion of cytokines and drugs. Additionally, it has been shown that microRNAs are an essential component in the regulation of the polarization of macrophages. For example, mir-155 was able to suppress M2/Th2 responses ( b )
Exosome-coated targeting substance
A new class of tumor-targeting drugs and vector models that target tumor cells within breast cells involves mesenchymal stem cell (MSC) exosomes expressing suicide or tumor-killing genes [ 117 ]. In the field of cancer treatment, mesenchymal stem cells are increasingly being sought after because of their ability to differentiate into a wide range of cell types and their powerful immunomodulatory and regenerative potentials [ 118 ]. Due to the lack of MHC-II and co-stimulatory ligands, MSCs exhibit poor immunogenicity. They also have good biosafety and are capable of homing to several organs thanks to the production of unique surface molecules [ 119 ]. Gene edited MSC-derived exosomes can overexpress a therapeutic RNA or protein to suppress tumors. The treatment of breast cancer can be achieved using MSC exosomes that express suicide or tumor-killing genes [ 117 ]. Generally modified MSC exosomes can be effective targeting delivery systems for killing HER2 + tumor cells [ 120 ]. O’Brien et al. demonstrated that EVs containing miR-379 suppressed breast tumor through regulation of cyclooxygenase-2 (COX-2) expression [ 121 ]. Vakhshiteh et al. harnessed the migration and invasion of MDA-MB-231 cells through exosome secreted by genetically modified dental pulp MSCs (DPSCs) that overexpress miR-34a [ 122 ]. Furthermore, LNA-antimir 142-3p was transported effectively by MSCs-Exo and reduced miR-142-3p and miR-150 expression in breast CSC-like cells [ 123 ].
Wang et al. targeted HER2 + ve human breast cancer cells via EVs loaded with HChrR6-encoding mRNA and expressing anti-HER2 scFv antibody batch on their surface called “EXO-DEPT”. HChrR6 is a bacterial enzyme that converts 6-chloro-9-nitro-5-oxo-5 H-benzo-(a)-phenoxazine (CNOB) into the cytotoxic drug 9-p amino-6-chloro-5 H-benzo[a]phenoxazine-5-one (MCHB). After treatment, EXO-DEPTs, but not undirected EVs, plus CNOB inhibited almost complete growth of orthotopic BT474 xenografts in vivo [ 124 ]. Similarly, Forterre et al. successfully delivered functional HChrR6 mRNA via EVs to HER2 + ve human breast cancer cells. When combined with the prodrug CB1954, they stopped the growth of HER2 + human breast cancer xenografts in athymic mice by activating the prodrug [ 125 ]. Overall, these systems are developing, and the successful implementation of them will also contribute to breast cancer treatment.
Drug delivery carriers
Exosomes can be utilized to transfer conventional clinical chemotherapy drugs to targeted sites, thus reducing their toxicity and improving their enrichment effect [ 126 ]. Tran et al. loaded aspirin, as an anti-cancer agent, into exosomes that enhanced drug dissolution and homing targeting effect. Aspirin-loaded exosomes exerts cytotoxicity effects on breast and colorectal cancers [ 127 ]. Yu et al. showed that erastin-loaded exosomes labeled with folic acid suppressed the proliferation and migration of MDA-MB-231 cells and induced ferroptosis through the depletion of intracellular glutathione and activation of ROS [ 88 ]. In another study, exosomes loaded with paclitaxel (PTX) inhibited tumor progression of MDA-MB-231 cells and were considered an effective drug delivery carrier for BC [ 128 ].
Doxorubicin (DOX), a common chemotherapeutic remedy for breast cancer, has been shown to decrease the risk of relapse by up to 8% and death by 6.5%. However, the side effects of congestive heart failure and medicine resistance necessitate switching patients taking doxorubicin to fewer effective therapies. Tian et al. engineered immature DCs (imDCs) expressing Lamp2b, an exosomal membrane protein, fused to αv integrin-specific iRGD peptide. Exosome derived from imDCs loaded with DOX and targeted to αv integrin- positive BC cells. iRGD exosomes efficiently inhibited tumor growth without noticeable toxicity [ 129 ]. Li et al. introduced milk exosomes containing DOX to the BC cells with specifically CD44-targeting hyaluronic acid (HA). The HA-mExo-Dox effectively targeted CD44-expressed BC cells and induced apoptosis in vitro [ 130 ]. Additionally, Hadla and colleagues confirmed that, in comparison to the free drug, DOX-encapsulated exosomes had less of an impact on cardiac toxicity and other tissues. As a result, the dose of DOX can be enhanced, resulting in a targeted cytotoxic effect on breast cancer cells [ 126 ].
Clinical implications
Achieving an optimal balance between maximal effectiveness and minimum side effects for conventional drugs used to combat tumors is often difficult due to tumor heterogeneity and biological barriers. Many anti-cancer agents in clinical practice have poor bioavailability and little in vivo stability, causing adverse damage to normal cells. Technologies based on exosomes offer exciting approaches to diagnosing and treating BC. Therapeutic agents can be delivered more efficiently using nanoplatforms, which are innovative dosage forms. Besides uploading drugs, nanoplatforms have the ability to target active molecules as well [ 131 ].
Nanoplatforms are mainly used to target tumors by enhancing the permeability and retention (EPR) of tumor internals or by interacting with antigens overexpressed on tumor surfaces. A unique carrier property and biosafety make exosomes ideal for developing precisely targeted approaches. In this review, current methods of exosomes in BC treatment are discussed specifically, but there are several challenges to overcome. The production and isolation methods need optimization to obtain sufficient quantities of exosomes with consistent quality and purity. Standardization of isolation, characterization, and quality control methods is crucial for safety and reproducibility. Determining the best administration route is challenging, and different routes need evaluation for effectiveness and safety. Achieving targeted delivery to specific tissues or cells is difficult, but strategies like engineering exosomes with specific ligands are being explored. Loading therapeutic cargo into exosomes is challenging due to limited capacity, and efficient methods are needed for stability and preservation. Exosomes from different cell sources may have varying immunogenicity profiles, so minimizing immune responses is important. Lastly, long-term safety and potential off-target effects or accumulation concerns require careful evaluation [ 132 , 133 ].
However, through genetic editing, researchers can precisely manipulate specific genes to increase or decrease their expression, thus enhancing anti-tumor exosome production. Exosome surface modification during nanocarrier production can improve exosome recruitment and abundance at tumor locations as well as the recognition of a specific target. Additionally, chemotherapeutics and other therapeutic chemicals like phototherapy and photothermal therapy can be delivered using exosomes as a delivery vehicle. On the other hand, the majority of the current studies involve cell and animal research, and are now in the preclinical stage. It is required to do extensive research on the creation of exosome carriers, how they actually work in the human body, and the management of side effects to establish the combination of both the efficacy and safety of exosome delivery as a way to finally achieve clinical use.
Data availability
Not applicable.
Bray F, Ferlay J, Soerjomataram I, Siegel RL, Torre LA, Jemal A. Global cancer statistics 2018: GLOBOCAN estimates of incidence and mortality worldwide for 36 cancers in 185 countries. Cancer J Clin. 2018;68(6):394–424.
Article Google Scholar
Giordano C, La Camera G, Gelsomino L, Barone I, Bonofiglio D, Andò S, Catalano S. The biology of exosomes in breast cancer progression: dissemination, immune evasion and metastatic colonization. Cancers. 2020;12(8):2179.
Article CAS PubMed PubMed Central Google Scholar
Gupta P, Neupane YR, Parvez S, Kohli K. Recent advances in targeted nanotherapeutic approaches for breast cancer management. Nanomedicine. 2021;16(29):2605–31.
Article CAS PubMed Google Scholar
Harbeck N, Penault-Llorca F, Cortes J, Gnant M, Houssami N, Poortmans P et al. Breast cancer (primer) Nat. Rev Dis Primers. 2019;66.
Cheang MC, Martin M, Nielsen TO, Prat A, Voduc D, Rodriguez-Lescure A, et al. Defining breast cancer intrinsic subtypes by quantitative receptor expression. Oncologist. 2015;20(5):474–82.
Wong GL, Abu Jalboush S, Lo H-W. Exosomal MicroRNAs and organotropism in breast cancer metastasis. Cancers. 2020;12(7):1827.
Amens JN, Bahçecioglu G, Zorlutuna P. Immune System effects on breast Cancer. Cell Mol Bioeng. 2021;14(4):279–92.
Article PubMed PubMed Central Google Scholar
Emens LA. Breast cancer immunobiology driving immunotherapy: vaccines and immune checkpoint blockade. Expert Rev Anticancer Ther. 2012;12(12):1597–611.
Smyth MJ, Dunn GP, Schreiber RD. Cancer immunosurveillance and immunoediting: the roles of immunity in suppressing tumor development and shaping tumor immunogenicity. Adv Immunol. 2006;90:1–50.
CAS PubMed Google Scholar
Dunn GP, Bruce AT, Ikeda H, Old LJ, Schreiber RD. Cancer immunoediting: from immunosurveillance to tumor escape. Nat Immunol. 2002;3(11):991–8.
Emens LA. Breast Cancer Immunotherapy: facts and HopesBreast Cancer Immunotherapy. Clin Cancer Res. 2018;24(3):511–20.
Edechi CA, Ikeogu N, Uzonna JE, Myal Y. Regulation of immunity in breast cancer. Cancers. 2019;11(8):1080.
Dunn GP, Old LJ, Schreiber RD. The three Es of cancer immunoediting. Annu Rev Immunol. 2004;22:329–60.
Nam GH, Choi Y, Kim GB, Kim S, Kim SA, Kim IS. Emerging prospects of exosomes for cancer treatment: from conventional therapy to immunotherapy. Adv Mater. 2020;32(51):2002440.
Article CAS Google Scholar
Hussen BM, Abdullah KH, Abdullah SR, Majeed NM, Mohamadtahr S, Rasul MF, et al. New insights of miRNA molecular mechanisms in breast cancer brain metastasis and therapeutic targets. Noncoding RNA Res. 2023;8(4):645–60.
Wang H, He D, Wan K, Sheng X, Cheng H, Huang J, et al. In situ multiplex detection of serum exosomal microRNAs using an all-in-one biosensor for breast cancer diagnosis. Analyst. 2020;145(9):3289–96.
Yi Y, Wu M, Zeng H, Hu W, Zhao C, Xiong M, et al. Tumor-derived exosomal non-coding RNAs: the emerging mechanisms and potential clinical applications in breast Cancer. Front Oncol. 2021;11:738945.
Zhou Q, Wang J, Zhang H, Sun L, Liu J, Meng L, Li J. Tumor-derived exosomes RNA expression profiling identifies the prognosis, immune characteristics, and treatment in HR+/HER2-breast cancer. Aging. 2023;15(16):8471–86.
Zhang J, Shi J, Liu W, Zhang K, Zhao H, Zhang H, Zhang Z. A simple, specific and on-off type MUC1 fluorescence aptasensor based on exosomes for detection of breast cancer. Sens Actuators B. 2018;276:552–9.
Kennedy LB, Salama AK. A review of cancer immunotherapy toxicity. Cancer J Clin. 2020;70(2):86–104.
Xu Z, Zeng S, Gong Z, Yan Y. Exosome-based immunotherapy: a promising approach for cancer treatment. Mol Cancer. 2020;19(1):1–16.
Lipson EJ, Forde PM, Hammers H-J, Emens LA, Taube JM, Topalian SL, editors. Antagonists of PD-1 and PD-L1 in cancer treatment. Seminars in oncology. Elsevier; 2015.
Emens LA, Ascierto PA, Darcy PK, Demaria S, Eggermont AM, Redmond WL, et al. Cancer immunotherapy: opportunities and challenges in the rapidly evolving clinical landscape. Eur J Cancer. 2017;81:116–29.
Kamerkar S, LeBleu VS, Sugimoto H, Yang S, Ruivo CF, Melo SA, et al. Exosomes facilitate therapeutic targeting of oncogenic KRAS in pancreatic cancer. Nature. 2017;546(7659):498–503.
Théry C, Ostrowski M, Segura E. Membrane vesicles as conveyors of immune responses. Nat Rev Immunol. 2009;9(8):581–93.
Article PubMed Google Scholar
Pitt JM, Charrier M, Viaud S, André F, Besse B, Chaput N, Zitvogel L. Dendritic cell–derived exosomes as immunotherapies in the fight against cancer. J Immunol. 2014;193(3):1006–11.
Théry C, Zitvogel L, Amigorena S. Exosomes: composition, biogenesis and function. Nat Rev Immunol. 2002;2(8):569–79.
Tkach M, Théry C. Communication by extracellular vesicles: where we are and where we need to go. Cell. 2016;164(6):1226–32.
Kalluri R. The biology and function of exosomes in cancer. J Clin Investig. 2016;126(4):1208–15.
Aslan C, Maralbashi S, Salari F, Kahroba H, Sigaroodi F, Kazemi T, Kharaziha P. Tumor-derived exosomes: implication in angiogenesis and antiangiogenesis cancer therapy. J Cell Physiol. 2019;234(10):16885–903.
Tran T-H, Mattheolabakis G, Aldawsari H, Amiji M. Exosomes as nanocarriers for immunotherapy of cancer and inflammatory diseases. Clin Immunol. 2015;160(1):46–58.
Steinbichler TB, Dudás J, Skvortsov S, Ganswindt U, Riechelmann H. Skvortsova I-I. therapy resistance mediated by exosomes. Mol Cancer. 2019;18(1):1–11.
Pu X, Ma S, Gao Y, Xu T, Chang P, Dong L. Mesenchymal stem cell-derived exosomes: biological function and their therapeutic potential in radiation damage. Cells. 2020;10(1):42.
Klinke DJ, Kulkarni YM, Wu Y, Byrne-Hoffman C. Inferring alterations in cell‐to‐cell communication in HER2 + breast cancer using secretome profiling of three cell models. Biotechnol Bioeng. 2014;111(9):1853–63.
Ciravolo V, Huber V, Ghedini GC, Venturelli E, Bianchi F, Campiglio M, et al. Potential role of HER2-overexpressing exosomes in countering trastuzumab‐based therapy. J Cell Physiol. 2012;227(2):658–67.
Maas SL, Breakefield XO, Weaver AM. Extracellular vesicles: unique intercellular delivery vehicles. Trends Cell Biol. 2017;27(3):172–88.
Johnstone RM, Adam M, Hammond J, Orr L, Turbide C. Vesicle formation during reticulocyte maturation. Association of plasma membrane activities with released vesicles (exosomes). J Biol Chem. 1987;262(19):9412–20.
Ni Z, Zhou S, Li S, Kuang L, Chen H, Luo X, et al. Exosomes: roles and therapeutic potential in osteoarthritis. Bone Res. 2020;8(1):1–18.
Théry C, Amigorena S, Raposo G, Clayton A. Isolation and characterization of exosomes from cell culture supernatants and biological fluids. Curr Protocols cell Biology. 2006;30(1):3. 1–3. 9.
Kumar DN, Chaudhuri A, Aqil F, Dehari D, Munagala R, Singh S, et al. Exosomes as Emerging Drug Delivery and Diagnostic modality for breast Cancer: recent advances in isolation and application. Cancers. 2022;14(6):1435.
Chen H, Wang L, Zeng X, Schwarz H, Nanda HS, Peng X, Zhou Y. Exosomes, a new star for targeted delivery. Front Cell Dev Biology. 2021:2827.
Beach A, Zhang H-G, Ratajczak MZ, Kakar SS. Exosomes: an overview of biogenesis, composition and role in ovarian cancer. J Ovarian Res. 2014;7(1):1–11.
Schmidt O, Teis D. The ESCRT machinery. Curr Biol. 2012;22(4):R116–20.
Colombo M, Moita C, Van Niel G, Kowal J, Vigneron J, Benaroch P, et al. Analysis of ESCRT functions in exosome biogenesis, composition and secretion highlights the heterogeneity of extracellular vesicles. J Cell Sci. 2013;126(24):5553–65.
Mercier V, Laporte MH, Destaing O, Blot B, Blouin CM, Pernet-Gallay K, et al. ALG-2 interacting protein-X (Alix) is essential for clathrin-independent endocytosis and signaling. Sci Rep. 2016;6(1):1–15.
Février B, Raposo G. Exosomes: endosomal-derived vesicles shipping extracellular messages. Curr Opin Cell Biol. 2004;16(4):415–21.
Trajkovic K, Hsu C, Chiantia S, Rajendran L, Wenzel D, Wieland F, et al. Ceramide triggers budding of exosome vesicles into multivesicular endosomes. Science. 2008;319(5867):1244–7.
Kalluri R, LeBleu VS. The biology, function, and biomedical applications of exosomes. Science. 2020;367(6478):eaau6977.
Gurunathan S, Kang M-H, Kim J-H. A comprehensive review on factors influences biogenesis, functions, therapeutic and clinical implications of exosomes. Int J Nanomed. 2021;16:1281.
Panigrahi AR, Srinivas L, Panda J. Exosomes: insights and therapeutic applications in cancer. Translational Oncol. 2022;21:101439.
Hong C-S, Sharma P, Yerneni SS, Simms P, Jackson EK, Whiteside TL, Boyiadzis M. Circulating exosomes carrying an immunosuppressive cargo interfere with cellular immunotherapy in acute myeloid leukemia. Sci Rep. 2017;7(1):1–10.
Greening DW, Gopal SK, Xu R, Simpson RJ, Chen W, editors. Exosomes and their roles in immune regulation and cancer. Seminars in cell & developmental biology. Elsevier; 2015.
Barros FM, Carneiro F, Machado JC, Melo SA. Exosomes and immune response in cancer: friends or foes? Front Immunol. 2018;9:730.
Yang Y, Li C-W, Chan L-C, Wei Y, Hsu J-M, Xia W, et al. Exosomal PD-L1 harbors active defense function to suppress T cell killing of breast cancer cells and promote tumor growth. Cell Res. 2018;28(8):862–4.
Lugini L, Cecchetti S, Huber V, Luciani F, Macchia G, Spadaro F, et al. Immune surveillance properties of human NK cell-derived exosomes. J Immunol. 2012;189(6):2833–42.
Huntington ND, Cursons J, Rautela J. The cancer–natural killer cell immunity cycle. Nat Rev Cancer. 2020;20(8):437–54.
Liu J, Ren L, Li S, Li W, Zheng X, Yang Y, et al. The biology, function, and applications of exosomes in cancer. Acta Pharm Sinica B. 2021;11(9):2783–97.
Hegmans JP, Gerber PJ, Lambrecht BN, Exosomes. Functional Proteomics: Springer; 2008. p. 97–109.
Whiteside TL. Immune modulation of T-cell and NK (natural killer) cell activities by TEXs (tumour-derived exosomes). Biochem Soc Trans. 2013;41(1):245–51.
Clayton A, Tabi Z. Exosomes and the MICA-NKG2D system in cancer. Blood Cells Molecules Dis. 2005;34(3):206–13.
Bauernhofer T, Kuss I, Henderson B, Baum AS, Whiteside TL. Preferential apoptosis of CD56dim natural killer cell subset in patients with cancer. Eur J Immunol. 2003;33(1):119–24.
Liu C, Yu S, Zinn K, Wang J, Zhang L, Jia Y, et al. Murine mammary carcinoma exosomes promote tumor growth by suppression of NK cell function. J Immunol. 2006;176(3):1375–85.
Wen SW, Sceneay J, Lima LG, Wong CS, Becker M, Krumeich S, et al. The Biodistribution and Immune Suppressive effects of breast Cancer–derived ExosomesExosomes regulate Immune Composition in Metastatic organs. Cancer Res. 2016;76(23):6816–27.
Xu HY, Li N, Yao N, Xu XF, Wang HX, Liu XY, Zhang Y. CD8 + T cells stimulated by exosomes derived from RenCa cells mediate specific immune responses through the FasL/Fas signaling pathway and, combined with GM–CSF and IL–12, enhance the anti–renal cortical adenocarcinoma effect. Oncol Rep. 2019;42(2):866–79.
Poggio M, Hu T, Pai C-C, Chu B, Belair CD, Chang A, et al. Suppression of exosomal PD-L1 induces systemic anti-tumor immunity and memory. Cell. 2019;177(2):414–27. e13.
Theodoraki M-N, Yerneni SS, Hoffmann TK, Gooding WE, Whiteside TL. Clinical significance of PD-L1 + exosomes in plasma of Head and Neck Cancer PatientsPD-L1 + exosomes in plasma of HNC patients. Clin Cancer Res. 2018;24(4):896–905.
Wang Y, Goliwas KF, Severino PE, Hough KP, Van Vessem D, Wang H, et al. Mechanical strain induces phenotypic changes in breast cancer cells and promotes immunosuppression in the tumor microenvironment. Lab Invest. 2020;100(12):1503–16.
Chatterjee S, Chatterjee A, Jana S, Dey S, Roy H, Das MK, et al. Transforming growth factor beta orchestrates PD-L1 enrichment in tumor-derived exosomes and mediates CD8 T-cell dysfunction regulating early phosphorylation of TCR signalome in breast cancer. Carcinogenesis. 2021;42(1):38–47.
Wada J, Onishi H, Suzuki H, Yamasaki A, Nagai S, Morisaki T, Katano M. Surface-bound TGF-β1 on effusion-derived exosomes participates in maintenance of number and suppressive function of regulatory T-cells in malignant effusions. Anticancer Res. 2010;30(9):3747–57.
Yin Y, Cai X, Chen X, Liang H, Zhang Y, Li J, et al. Tumor-secreted miR-214 induces regulatory T cells: a major link between immune evasion and tumor growth. Cell Res. 2014;24(10):1164–80.
Kalvala A, Wallet P, Yang L, Wang C, Li H, Nam A, et al. Phenotypic switching of naïve T cells to immune-suppressive Treg-like cells by mutant KRAS. J Clin Med. 2019;8(10):1726.
Ni C, Fang Q-Q, Chen W-Z, Jiang J-X, Jiang Z, Ye J, et al. Breast cancer-derived exosomes transmit lncRNA SNHG16 to induce CD73 + γδ1 Treg cells. Signal Transduct Target Therapy. 2020;5(1):41.
Kumar V, Patel S, Tcyganov E, Gabrilovich DI. The nature of myeloid-derived suppressor cells in the tumor microenvironment. Trends Immunol. 2016;37(3):208–20.
Filipazzi P, Bürdek M, Villa A, Rivoltini L, Huber V, editors. Recent advances on the role of tumor exosomes in immunosuppression and disease progression. Seminars in cancer biology. Elsevier; 2012.
Gao Y, Xu H, Li N, Wang H, Ma L, Chen S, et al. Renal cancer-derived exosomes induce tumor immune tolerance by MDSCs-mediated antigen-specific immunosuppression. Cell Communication Signal. 2020;18(1):1–14.
Jiang M, Zhang W, Zhang R, Liu P, Ye Y, Yu W, et al. Cancer exosome-derived miR-9 and miR-181a promote the development of early-stage MDSCs via interfering with SOCS3 and PIAS3 respectively in breast cancer. Oncogene. 2020;39(24):4681–94.
Xiang X, Poliakov A, Liu C, Liu Y, Deng Zb, Wang J, et al. Induction of myeloid-derived suppressor cells by tumor exosomes. Int J Cancer. 2009;124(11):2621–33.
Fu H, Yang H, Zhang X, Xu W. The emerging roles of exosomes in tumor–stroma interaction. J Cancer Res Clin Oncol. 2016;142:1897–907.
Yan Z, Sheng Z, Zheng Y, Feng R, Xiao Q, Shi L, et al. Cancer-associated fibroblast-derived exosomal miR-18b promotes breast cancer invasion and metastasis by regulating TCEAL7. Cell Death Dis. 2021;12(12):1120.
Lee J-K, Park S-R, Jung B-K, Jeon Y-K, Lee Y-S, Kim M-K, et al. Exosomes derived from mesenchymal stem cells suppress angiogenesis by down-regulating VEGF expression in breast cancer cells. PLoS ONE. 2013;8(12):e84256.
Zhu Y, Tao Z, Chen Y, Lin S, Zhu M, Ji W, et al. Exosomal MMP-1 transfers metastasis potential in triple-negative breast cancer through PAR1-mediated EMT. Breast Cancer Res Treat. 2022;193(1):65–81.
Tung KH, Ernstoff MS, Allen C, Shu S. A review of exosomes and their role in the Tumor Microenvironment and Host-Tumor Macroenvironment. J Immunol Sci. 2019;3(1):4–8.
Yu Dd, Wu Y, Shen Hy L, Mm C, Wx Z, Xh, et al. Exosomes in development, metastasis and drug resistance of breast cancer. Cancer Sci. 2015;106(8):959–64.
György B, Hung ME, Breakefield XO, Leonard JN. Therapeutic applications of extracellular vesicles: clinical promise and open questions. Annu Rev Pharmacol Toxicol. 2015;55:439.
Liang Y, Duan L, Lu J, Xia J. Engineering exosomes for targeted drug delivery. Theranostics. 2021;11(7):3183.
Huang L, Rong Y, Tang X, Yi K, Qi P, Hou J, et al. Engineered exosomes as an in situ DC-primed vaccine to boost antitumor immunity in breast cancer. Mol Cancer. 2022;21(1):1–19.
Gong C, Tian J, Wang Z, Gao Y, Wu X, Ding X, et al. Functional exosome-mediated co-delivery of doxorubicin and hydrophobically modified microRNA 159 for triple-negative breast cancer therapy. J Nanobiotechnol. 2019;17(1):1–18.
Yu M, Gai C, Li Z, Ding D, Zheng J, Zhang W, et al. Targeted exosome-encapsulated erastin induced ferroptosis in triple negative breast cancer cells. Cancer Sci. 2019;110(10):3173–82.
Zhao L, Gu C, Gan Y, Shao L, Chen H, Zhu H. Exosome-mediated siRNA delivery to suppress postoperative breast cancer metastasis. J Controlled Release. 2020;318:1–15.
Ohno S-i, Takanashi M, Sudo K, Ueda S, Ishikawa A, Matsuyama N, et al. Systemically injected exosomes targeted to EGFR deliver antitumor microRNA to breast cancer cells. Mol Ther. 2013;21(1):185–91.
Han D, Wang K, Zhang T, Gao G-C, Xu H. Natural killer cell-derived exosome-entrapped paclitaxel can enhance its anti-tumor effect. Eur Rev Med Pharmacol Sci. 2020;24(10).
Hashemi ZS, Ghavami M, Kiaie SH, Mohammadi F, Barough MS, Khalili S, et al. Novel delivery of sorafenib by natural killer cell-derived exosomes-enhanced apoptosis in triple-negative breast cancer. Nanomedicine. 2023;18(5):437–53.
Wylie B, Macri C, Mintern JD, Waithman JJC. Dendritic cells and cancer: from biology to therapeutic intervention. 2019;11(4):521.
Boer MC, Joosten SA, Ottenhoff THJF. Regulatory T-cells at the interface between human host and pathogens in infectious diseases and vaccination. 2015;6:217.
Admyre C, Johansson SM, Paulie S, Gabrielsson S. Direct exosome stimulation of peripheral humanT cells detected by ELISPOT. Eur J Immunol. 2006;36(7):1772–81.
Vincent-Schneider H, Stumptner‐Cuvelette P, Lankar D, Pain S, Raposo G, Benaroch P, Bonnerot C. Exosomes bearing HLA‐DR1 molecules need dendritic cells to efficiently stimulate specific T cells. Int Immunol. 2002;14(7):713–22.
Romagnoli GG, Zelante BB, Toniolo PA, Migliori IK, Barbuto JAM. Dendritic cell-derived exosomes may be a tool for cancer immunotherapy by converting tumor cells into immunogenic targets. Front Immunol. 2015;5:692.
O’Sullivan TE, Sun JC, Lanier LL. Natural killer cell memory. Immunity. 2015;43(4):634–45.
Di Pace AL, Tumino N, Besi F, Alicata C, Conti LA, Munari E, et al. Characterization of human NK cell-derived exosomes: role of DNAM1 receptor in exosome-mediated cytotoxicity against tumor. Cancers. 2020;12(3):661.
Zhu L, Gangadaran P, Kalimuthu S, Oh JM, Baek SH, Jeong SY, et al. Novel alternatives to extracellular vesicle-based immunotherapy–exosome mimetics derived from natural killer cells. Artif Cells Nanomed Biotechnol. 2018;46(sup3):166–79.
Kaban K, Hinterleitner C, Zhou Y, Salva E, Kantarci AG, Salih HR, Märklin M. Therapeutic silencing of Bcl-2 using Nk cell-derived exosomes as a novel therapeutic approach in breast cancer. Cancers. 2021;13(10):2397.
Borst J, Ahrends T, Bąbała N, Melief CJ, Kastenmüller W. CD4 + T cell help in cancer immunology and immunotherapy. Nat Rev Immunol. 2018;18(10):635–47.
Wang L, Xie Y, Ahmed KA, Ahmed S, Sami A, Chibbar R, et al. Exosomal pMHC-I complex targets T cell-based vaccine to directly stimulate CTL responses leading to antitumor immunity in transgenic FVBneuN and HLA-A2/HER2 mice and eradicating trastuzumab-resistant tumor in athymic nude mice. Breast Cancer Res Treat. 2013;140(2):273–84.
Hao S, Liu Y, Yuan J, Zhang X, He T, Wu X, et al. Novel exosome-targeted CD4 + T cell vaccine counteracting CD4 + 25 + regulatory T cell-mediated immune suppression and stimulating efficient central memory CD8 + CTL responses. J Immunol. 2007;179(5):2731–40.
Xie Y, Wang L, Freywald A, Qureshi M, Chen Y, Xiang J. A novel T cell-based vaccine capable of stimulating long-term functional CTL memory against B16 melanoma via CD40L signaling. Cell Mol Immunol. 2013;10(1):72–7.
Li R, Chibbar R, Xiang J. Novel EXO-T vaccine using polyclonal CD4 + T cells armed with HER2-specific exosomes for HER2-positive breast cancer. OncoTargets Therapy. 2018;11:7089.
Shi X, Cheng Q, Hou T, Han M, Smbatyan G, Lang JE, et al. Genetically engineered cell-derived nanoparticles for targeted breast cancer immunotherapy. Mol Ther. 2020;28(2):536–47.
Cheng Q, Shi X, Zhang Y. Reprogramming exosomes for Immunotherapy. Cell Reprogramming for Immunotherapy: Springer; 2020. pp. 197–209.
Book Google Scholar
Cao Y, Rodgers DT, Du J, Ahmad I, Hampton EN, Ma JS, et al. Design of switchable chimeric antigen receptor T cells targeting breast cancer. Angew Chem Int Ed. 2016;55(26):7520–4.
Yang P, Cao X, Cai H, Feng P, Chen X, Zhu Y, et al. The exosomes derived from CAR-T cell efficiently target mesothelin and reduce triple-negative breast cancer growth. Cell Immunol. 2021;360:104262.
Heusinkveld M, van Der Burg SH. Identification and manipulation of tumor associated macrophages in human cancers. J Translational Med. 2011;9:1–14.
Ham S, Lima LG, Chai EPZ, Muller A, Lobb RJ, Krumeich S, et al. Breast cancer-derived exosomes alter macrophage polarization via gp130/STAT3 signaling. Front Immunol. 2018;9:871.
O’Connell RM, Taganov KD, Boldin MP, Cheng G, Baltimore D. MicroRNA-155 is induced during the macrophage inflammatory response. Proceedings of the National Academy of Sciences. 2007;104(5):1604-9.
Chaudhuri AA, So AY-L, Sinha N, Gibson WS, Taganov KD, O’Connell RM, Baltimore D. MicroRNA-125b potentiates macrophage activation. J Immunol. 2011;187(10):5062–8.
Moradi-Chaleshtori M, Bandehpour M, Soudi S, Mohammadi-Yeganeh S, Hashemi SM. In vitro and in vivo evaluation of anti-tumoral effect of M1 phenotype induction in macrophages by miR-130 and miR-33 containing exosomes. Cancer Immunol Immunother. 2021;70:1323–39.
Moradi-Chaleshtori M, Shojaei S, Mohammadi-Yeganeh S, Hashemi SM. Transfer of miRNA in tumor-derived exosomes suppresses breast tumor cell invasion and migration by inducing M1 polarization in macrophages. Life Sci. 2021;282:119800.
Altanerova U, Jakubechova J, Benejova K, Priscakova P, Pesta M, Pitule P, et al. Prodrug suicide gene therapy for cancer targeted intracellular by mesenchymal stem cell exosomes. Int J Cancer. 2019;144(4):897–908.
Lee J, Henderson K, Massidda MW, Armenta-Ochoa M, Im BG, Veith A, et al. Mechanobiological conditioning of mesenchymal stem cells for enhanced vascular regeneration. Nat Biomedical Eng. 2021;5(1):89–102.
Li H, Jin Y, Zhao Y, Li W, He Z, Zhang Q, et al. Targeted cell therapy for partial-thickness cartilage defects using membrane modified mesenchymal stem cells by transglutaminase 2. Biomaterials. 2021;275:120994.
Gomari H, Forouzandeh Moghadam M, Soleimani M. Targeted cancer therapy using engineered exosome as a natural drug delivery vehicle. OncoTargets Therapy. 2018:5753–62.
O’brien K, Khan S, Gilligan K, Zafar H, Lalor P, Glynn C, et al. Employing mesenchymal stem cells to support tumor-targeted delivery of extracellular vesicle (EV)-encapsulated microRNA-379. Oncogene. 2018;37(16):2137–49.
Vakhshiteh F, Rahmani S, Ostad SN, Madjd Z, Dinarvand R, Atyabi F. Exosomes derived from miR-34a-overexpressing mesenchymal stem cells inhibit in vitro tumor growth: a new approach for drug delivery. Life Sci. 2021;266:118871.
Naseri Z, Oskuee RK, Forouzandeh-Moghadam M, Jaafari MR. Delivery of LNA-antimiR-142-3p by mesenchymal stem cells-derived exosomes to breast cancer stem cells reduces tumorigenicity. Stem cell Reviews Rep. 2020;16:541–56.
Wang J-H, Forterre AV, Zhao J, Frimannsson DO, Delcayre A, Antes TJ, et al. Anti-HER2 scfv-directed extracellular vesicle-mediated mRNA-based gene delivery inhibits growth of HER2-positive human breast tumor xenografts by prodrug activation. Mol Cancer Ther. 2018;17(5):1133–42.
Forterre AV, Wang J-H, Delcayre A, Kim K, Green C, Pegram MD, et al. Extracellular vesicle–mediated in Vitro transcribed mRNA delivery for treatment of HER2 + breast Cancer xenografts in mice by Prodrug CB1954 without General ToxicitySide Effect–Free GDEPT in mice by CB1954 (Tretazicar). Mol Cancer Ther. 2020;19(3):858–67.
Hadla M, Palazzolo S, Corona G, Caligiuri I, Canzonieri V, Toffoli G, Rizzolio F. Exosomes increase the therapeutic index of doxorubicin in breast and ovarian cancer mouse models. Nanomedicine. 2016;11(18):2431–41.
Tran PH, Wang T, Yin W, Tran TT, Barua HT, Zhang Y, et al. Development of a nanoamorphous exosomal delivery system as an effective biological platform for improved encapsulation of hydrophobic drugs. Int J Pharm. 2019;566:697–707.
Kalimuthu S, Gangadaran P, Rajendran RL, Zhu L, Oh JM, Lee HW, et al. A new approach for loading anticancer drugs into mesenchymal stem cell-derived exosome mimetics for cancer therapy. Front Pharmacol. 2018;9:1116.
Tian Y, Li S, Song J, Ji T, Zhu M, Anderson GJ, et al. A doxorubicin delivery platform using engineered natural membrane vesicle exosomes for targeted tumor therapy. Biomaterials. 2014;35(7):2383–90.
Li D, Yao S, Zhou Z, Shi J, Huang Z, Wu Z. Hyaluronan decoration of milk exosomes directs tumor-specific delivery of doxorubicin. Carbohydr Res. 2020;493:108032.
Jung I, Shin S, Baek M-C, Yea K. Modification of immune cell-derived exosomes for enhanced cancer immunotherapy: current advances and therapeutic applications. Exp Mol Med. 2024:1–13.
Yamashita T, Takahashi Y, Takakura Y. Possibility of exosome-based therapeutics and challenges in production of exosomes eligible for therapeutic application. Biol Pharm Bull. 2018;41(6):835–42.
Willis GR, Kourembanas S, Mitsialis SA. Toward exosome-based therapeutics: isolation, heterogeneity, and fit-for-purpose potency. Front Cardiovasc Med. 2017;4:63.
Download references
Acknowledgements
The authors would like to thank all the staff of the Immunology Research Center of Tabriz University of Medical Sciences for the sincere collaboration and support during the period of conducting this study.
No funding.
Author information
Authors and affiliations.
Immunology Research Center, Tabriz University of Medical Sciences, Gholghasht Ave, Tabriz, Iran
Sahar Safaei, Manouchehr Fadaee, Omid Rahbar Farzam, Amirhossein Yari, Dariush Shanehbandi, Behzad Baradaran & Tohid Kazemi
Department of Biology, Tabriz Branch, Islamic Azad University, Tabriz, Iran
Amirhossein Yari
Biotechnology Research Center, Tabriz University of Medical Sciences, Tabriz, Iran
Elham Poursaei
Research Center for Integrative Medicine in Aging, Tabriz University of Medical Sciences, Tabriz, Iran
Cynthia Aslan
Department of Immunology, Tabriz University of Medical Sciences, Tabriz, Iran
Sahar Samemaleki
You can also search for this author in PubMed Google Scholar
Contributions
T.K., B.B. and D.Sh. designed the manuscript. S.S., E.P., C.A. and S.S. wrote the main manuscript textM.F. and O.R.F. revised the manuscript.A.Y. prepared Figs. 1 , 2 , 3 , 4 , 5 and 6 . All authors reviewed the manuscript.
Corresponding author
Correspondence to Tohid Kazemi .
Ethics declarations
Ethical approval, competing interests.
The authors declare no competing interests.
Additional information
Publisher’s note.
Springer Nature remains neutral with regard to jurisdictional claims in published maps and institutional affiliations.
Rights and permissions
Open Access This article is licensed under a Creative Commons Attribution 4.0 International License, which permits use, sharing, adaptation, distribution and reproduction in any medium or format, as long as you give appropriate credit to the original author(s) and the source, provide a link to the Creative Commons licence, and indicate if changes were made. The images or other third party material in this article are included in the article’s Creative Commons licence, unless indicated otherwise in a credit line to the material. If material is not included in the article’s Creative Commons licence and your intended use is not permitted by statutory regulation or exceeds the permitted use, you will need to obtain permission directly from the copyright holder. To view a copy of this licence, visit http://creativecommons.org/licenses/by/4.0/ . The Creative Commons Public Domain Dedication waiver ( http://creativecommons.org/publicdomain/zero/1.0/ ) applies to the data made available in this article, unless otherwise stated in a credit line to the data.
Reprints and permissions
About this article
Cite this article.
Safaei, S., Fadaee, M., Farzam, O.R. et al. Exploring the dynamic interplay between exosomes and the immune tumor microenvironment: implications for breast cancer progression and therapeutic strategies. Breast Cancer Res 26 , 57 (2024). https://doi.org/10.1186/s13058-024-01810-z
Download citation
Received : 31 October 2023
Accepted : 18 March 2024
Published : 29 March 2024
DOI : https://doi.org/10.1186/s13058-024-01810-z
Share this article
Anyone you share the following link with will be able to read this content:
Sorry, a shareable link is not currently available for this article.
Provided by the Springer Nature SharedIt content-sharing initiative
- Breast cancer
- Tumor-derived exosomes
- Immune cell
- Tumor microenvironment
- Immune cell-derived exosomes
- Immunotherapy
Breast Cancer Research
ISSN: 1465-542X
- Submission enquiries: [email protected]

An official website of the United States government
The .gov means it’s official. Federal government websites often end in .gov or .mil. Before sharing sensitive information, make sure you’re on a federal government site.
The site is secure. The https:// ensures that you are connecting to the official website and that any information you provide is encrypted and transmitted securely.
- Publications
- Account settings
Preview improvements coming to the PMC website in October 2024. Learn More or Try it out now .
- Advanced Search
- Journal List
- J Oral Maxillofac Pathol
- v.25(2); May-Aug 2021
Immunotherapy: The future of cancer treatment
Manisha sahu.
Department of Oral Pathology and Microbiology, Chhattisgarh Dental College and Research Institute, Rajnandgaon, Chhattisgarh, India
Hemakumari Suryawanshi
Head and neck squamous cell carcinomas (HNSCCs) are one of the most common cancers worldwide. A large number of patients are diagnosed with locally advanced disease and require multimodal treatment approaches. Standard treatment modalities ranging from surgery to chemotherapy and radiation are yielding mixed results. To overcome this hurdle, newer innovative approaches are required to reduce the morbidity and mortality of the patients. In the last few decades, immunotherapy has become an important part of treating some types of cancer. The immune system plays a key role in the development, establishment and progression of HNSCC. A greater understanding of the dysregulation and evasion of the immune system in the evolution and progression of HNSCC provides the basis for improved therapies and outcomes for patients. Newer types of immune treatments are now being studied, and they will impact how we treat cancer in the future. This article provides a brief overview of the current immunotherapeutic strategies for cancer with emphasis on HNSCC.
INTRODUCTION
Head-and-neck squamous cell cancers (HNSCCs) are one of the most common cancers worldwide.[ 1 ] The treatment of HNSCC varies by tumor site and stage, however the standard treatment include surgery, radiation and cytotoxic chemotherapy.[ 2 ] Despite advances in treatment, more than half of all cancers recur locoregionally or distantly.[ 3 ] Cancer immunotherapy is emerging as a beneficial tool for cancer treatment by activating the immune system to produce antitumor effects. In 1891, Dr. William Coley, the father of immunotherapy, made the first attempt to stimulate the immune system for improving a cancer patient's condition by intratumoral injections of inactivated bacterial toxin.[ 4 ]
All cancers arise as a result of somatic genomic alterations. These alterations arise sequentially and give rise to the progressively more aggressive and invasive phenotypes during tumorigenesis. Such genomic variations could give rise to tumor antigens, which could be recognized by the immune system as nonself and elicit cellular immunwponses.[ 5 ] However, avoiding immune destruction is one of the hallmarks of cancer. HNSCCs are highly immunosuppressive malignancy with high mutational burden. Cancer cells have evolved multiple mechanisms, such as defects in antigen presentation machinery, the upregulation of negative regulatory pathways and the recruitment of immunosuppressive cell populations to escape immune surveillance. There has been extensive research on the complex and dynamic interaction between tumor cells and host immune cells which has led to the development of currently approved immunotherapies. Immunotherapy is designed to either actively target a specific antigen on the tumor or enhance the host's immune system.[ 1 , 5 ]
Cancer immunotherapy was voted “breakthrough of the year” by Science in 2013 and has revolutionized the field of oncology.[ 6 ] The cancer immunotherapy aims at harnessing the specificity and killing mechanisms of the immune system to target and eradicate malignant cells. The Society for Immunotherapy of Cancer established the Cancer Immunotherapy Guideline-Head and Neck Cancer subcommittee to provide evidence-based recommendations on how best to incorporate immunotherapies into practice for the treatment of patients with HNSCC.[ 7 ] The present article provides a brief overview of the current immunotherapeutic strategies for cancer with emphasis on HNSCC.
TUMOR IMMUNOLOGY
Immune system.
The immune system is comprised of the innate and adaptive immune system. The innate immune system includes dendritic cells (DCs), natural killer cells (NK), macrophages, neutrophils, eosinophils, basophils and mast cells. Innate immune cells do not require prior stimulation by antigens and act as a first line of defense against foreign antigens. The adaptive immune system includes B lymphocytes, CD4+ helper T lymphocytes and CD8+ cytotoxic T lymphocytes, and requires formal presentation by antigen-presenting cells (APCs) for its activation. The adaptive immune system generates antigen-specific T- and B-cell lymphocytes.[ 8 ]
Immunoediting
Each cell is estimated to experience over 20,000 DNA damaging events each day which are normally repaired. Cells which are not repaired and which acquire malignant or potentially malignant changes are then usually recognized and killed by the tumor immunosurveillance system. However, tumor cells develop mechanisms to thwart immune recognition and response, a dynamic process termed immunoediting that leads to immune escape.[ 8 ]
There is now an improved understanding of the complex interaction between immune system and tumor cells. The theory of Immune Editing was put forth by Schreiber et al . where they hypothesize that the body's immune system interacts with the tumor in three distinct phases namely elimination, equilibrium and escape.[ 9 , 10 ] The cancer immunosurveillance hypothesis developed by Burnet and Thomas is now considered a component of cancer immunoediting.
The elimination phase refers to the initial damage and possible destruction of tumor cells by the innate immune system, followed by presentation of the tumor antigens in the cellular debris to DCs which then present them to T-cells and thereby create tumor-specific CD4+ and CD8+ T-cells. These help destroy the remaining tumor cells if elimination is complete. The equilibrium phase occurs when any tumor cells survive the initial elimination attempt but are not able to progress, being maintained in a state of equilibrium with the immune cells. In the escape phase, cancer cells grow and metastasize due to loss of control by the immune system.[ 8 ]
Escape mechanisms of HNSCC
HNSCC is one of the most immunosuppressive human tumors.[ 11 ] Tumor is able to evade immune destruction not only by modulating its own cellular characteristics but also by creating its own “tumor microenvironment (TME).” Many signaling molecules and cell types play a role in tumor-driven immune tolerance, from cytokines to both the innate and adaptive arms of the cellular immune system.[ 12 ]

MOLECULAR ESCAPE MECHANISMS
Tumor-derived factors.
The production of immunosuppressive cytokines, including transforming growth factor (TGF)-b, interleukin (IL)-6 and IL-10 inhibit T cell proliferation and effector functions. Tumor cells also deplete local micronutrients and overexpress indoleamine 2,3-dioxygenase, an enzyme responsible for depletion of tryptophan, which hinders T cell proliferation and activation. It has also been shown that exosomes secreted by HNSCC are enriched for suppressive compounds (including cyclooxygenase-2, TGF-b, programmed death 1 [Programmed cell death receptor 1] and cytotoxic T lymphocyte antigen 4 [Cytotoxic T lymphocyte associated molecule 4]) that promote CD8+ T cell apoptosis, inhibit CD4+ T cell proliferation, upregulate (regulatory T cells) Tregs and impair NK cell function.[ 12 ]
Beyond secreted cytokines and metabolites, HNSCCs have developed mechanisms of human leukocyte antigen (HLA) modulation for immune escape. HNSCC provoke genetic alterations in key genes associated with processing and presentation of neoantigens, including signal transducer and activator of signal 1 deficiency and downregulated transporter for antigen processing, without significantly affecting HLA expression itself.[ 12 ]
Suppressive cellular tumor infiltrate
HNSCC regulate and recruit immune populations capable of modulating T and NK cell responses, including Tregs, myeloid-derived suppressor cells, tumor-associated macrophages and cancer-associated fibroblasts. Immunomodulation enacted by these various cell populations contributes to a tumor-promoted microenvironment.[ 12 ]
IMMUNE CHECKPOINTS
In the healthy state, effector functions of the immune system must be held in check to prevent damage to self (autoimmunity) or prolonged activation. Checkpoint molecules are generally thought of as primarily immunosuppressive and the key inhibitory checkpoint receptors are PD-1, CTLA4, lymphocyte activating gene 3 and T cell immunoglobulin (IgG) and mucin domain-containing 3.[ 12 ]
Immunotherapy can be broadly divided into active and passive.[ 4 ]
Active immunotherapy
The active approach involves directing the host immune system to tumor-associated antigens on the surface of tumors. These antigens can be specific proteins or carbohydrates that are exclusively expressed or overly expressed in tumor cells.
Passive immunotherapy
Passive immunotherapy involves enhancing the standard anticancer response by the immune system using monoclonal antibodies (MoAbs), lymphocytes and cytokines.
TYPES OF IMMUNOTHERAPY
Adoptive cell therapy (act).
The central premise in ACT is that T cells are crucial for eliminating cancer cells and hence transfer of T cells in expanded numbers can augment anti-tumor immunity.[ 13 ] Adoptive cell therapy (ACT) utilizing either tumor-infiltrating lymphocyte (TIL)-derived T cells or T cells genetically engineered to express tumor recognizing receptors has emerged as a powerful and potentially curative therapy for several cancers.[ 14 ]
TYPES OF ADOPTIVE CELL THERAPY
Tumor-infiltrating lymphocyte therapy (unmodified cells).
ACT involves isolating TILs from cancers, growing them in culture and then reintroducing them to the patient who has undergone a lymphocyte depleting preparatory regimen. There is now a greater number of activated cells available, enhancing the body's anti-tumor immune response.
Engineered T-cell receptor therapy (modified cells)
With modern genetic engineering technology, specific antigen receptors can be introduced into T cells allowing them to recognize tumor specific antigens. These lymphocytes can then be produced on a large scale and used in patients. T-cell receptor (TCR)-modified T cells exert antigen recognition in a major histocompatibility complex-dependent manner.
The first evidence of the feasibility and clinical potency of TCR gene therapy targeting the melanoma differentiation antigen MART-1, present in approximately 80%–95% of melanomas was demonstrated in 17 patients with progressive metastatic melanoma.[ 15 ]
Chimeric antigen receptor T-cell therapy (modified cells)
Chimeric antigen receptor (CAR) is introduced to the T lymphocyte surface with help of a viral vector [ Figure 1 ]. CARs are specialized structures with both antigen binding as well as intracellular signaling apparatus such that they can recognize antigens independent of APCs and can also drive cellular activation.[ 13 ]

Chimeric antigen receptor T-cell therapy is a type of treatment in which a patient's T cells (a type of immune cell) are changed in the laboratory so they will bind to cancer cells and kill them. Credit: National Cancer Institute US
IMMUNE CHECKPOINT INHIBITORS
Immune checkpoints are the normal components of immune system. T-cell activation is functionally determined by antigen presentation along with many co-stimulatory and co-inhibitory signals. In the presence of these co-inhibitory signals, the stimulatory signal will fail, leading to the induction of T-cell anergy or apoptosis, weakening the immune response due to failure to induce cytotoxicity.[ 16 , 17 ] Co-inhibitory molecules called checkpoints prevent exaggerated immune response and maintains immune tolerance in normal physiological conditions. These inhibitory checkpoints are overexpressed in the TME, contributing to tumor-promoting immunosuppression. These checkpoints are blocked by checkpoint inhibitors which are MoAbs.[ 18 ]
The most widely used targets for immune checkpoint inhibitors are
- CTLA4 – Cytotoxic T lymphocyte-associated molecule 4
- PD1 – Programmed cell death receptor 1
- Programmed cell death ligand 1.
The two major classes of checkpoint inhibitors currently used are anti CTLA-4 antibodies (e.g., Ipilimumab) and anti PD-1 [ Figure 2 ] (Nivolumab and Pembrolizumab) antibodies.[ 18 ]

Checkpoint proteins, such as PD-L1 on tumor cells and PD-1 on T cells, help keep immune responses in check. The binding of PD-L1 to PD-1 keeps T cells from killing tumor cells in the body (left panel). Blocking the binding of PD-L1 to PD-1 with an immune checkpoint inhibitor (anti-PD-L1 or anti-PD-1) allows the T cells to kill tumor cells (right panel). Credit: National Cancer Institute US
TARGETED MONOCLONAL ANTIBODIES
It is a form of active immunity was MoAbs target specific antigen present on cancer cells. MoAbs can either be unconjugated or be conjugated with therapeutic drugs that would produce a cytotoxic effect on cancer cells. Overexpression of epidermal growth factor receptor (EGFR) has been noted in up to 90% of HNSCC and upon binding of EGF promotes tumor cell proliferation, angiogenesis and metastasis. MoAbs such as cetuximab and panitumumab are EGFR targeted therapies; they are proven to be effective against HNSCC either alone or in combination with radiotherapy. Cetuximab is a mouse–human chimeric IgG1 Ab targeting EGFR whereas Panitumumab is a fully human IgG2 antibody.[ 19 , 20 , 21 ]
Other promising antigens of HNSCC which can be targeted.
Vascular endothelial growth factor (VEGF) AND VEGFR, insulin-like growth factor receptor which are overexpressed in HNSCC.
ONCOLYTIC VIRUS THERAPY
Oncolytic virus therapy originated from the finding that virus-infected tumor cells are destroyed by the cytopathic effects of viruses.
Viruses are infectious agents that are capable of infecting living cells, hijacking their genetic machinery, which allows the viruses to replicate inside them.In this therapy genetically modified viruses are used to infect tumor cells. The virus-infected tumor cells are destroyed by the cytopathic effects of viruses which stimulate a pro inflammatory environment to augment systemic antitumor immunity. Cell death induced by viral infections in tumor tissues also promotes inherent tumor immunity in cancer patients. Several DNA and RNA viruses have been proposed as candidates for this treatment through in vitro and in vivo studies as well as clinical trials. They are termed “oncolytic viruses” as they are designed to target tumor cell specifically.[ 5 , 22 ]
The first oncolytic virus therapy was approved by the US Food and Drug Administration (FDA) in 2015 – talimogene laherparepvec (T-VEC) for the treatment of melanoma. T-Vec, also known as Imlygic, a genetically modified herpes simplex virus, demonstrates impressive clinical benefits for patients with advanced melanoma and has been approved for the treatment of unresectable metastatic melanoma.
CANCER VACCINES
Cancer vaccines are sub classified as
- Prophylactic vaccines
- Therapeutic cancer vaccines.
Prophylactic vaccines are used for the prevention of primary and secondary cancer which is aimed at reducing cancer incidence, morbidity and mortality.
They are designed to alert the immune system to a specific virus so that it can recognize and attack the virus before it is able to cause an infection. This type of vaccine is administered to healthy individuals.[ 23 ]
The U. S. FDA have approved two prophylactic vaccines, including one for hepatitis B virus that can cause hepatocellular carcinoma and another for human papillomavirus (Gardasil) accounting for about 70% of cervical cancer.[ 24 ]
Therapeutic cancer vaccines are administrated to cancer patients and designed to eradicate cancer cells through strengthening patient's own immune responses. Based on their content, they may be classified into several major categories, which include cell vaccines (tumor or immune cell), protein/peptide vaccines and genetic (DNA, RNA) vaccines.
Bacillus Calmette–Guérin (BCG) and sipuleucel-T (Provenge ® ) are two examples of therapeutic cancer vaccines. The BCG vaccine is approved for patients with early-stage bladder cancer and sipuleucel-T (Provenge ® ) are approved for asymptomatic metastatic castrate-resistant prostate cancer. Sipuleucel-T works by inducing an immune response, targeting the prostatic acid phosphatase antigen, which is overexpressed in the majority prostate cancers.[ 23 , 24 ]
Cytokines are molecular messengers that allow the cells of the immune system to communicate with one another to generate a coordinated, robust, but self-limited response to a target antigen. Cytokines are secreted or membrane-bound proteins that act as mediators of intercellular signaling to regulate homeostasis of the immune system. They are produced by cells of innate and adaptive immunity in response to microbes and tumor antigens.[ 25 , 26 ]
Two cytokines currently approved by the FDA for clinical purposes are interferon α (IFN α) and IL-2.
Interferon α
These cytokines when injected subcutaneously in renal cell carcinoma have shown tumor regression.[ 27 ] These have shown excellent results in stage 3 melanoma. The combination of IFN α and IL-2 showed partial response and higher toxicity. IFN α plays multifaceted roles in tumor control, including directly eradicating tumor cells through inducing senescence and apoptosis and boosting effective antitumor immune responses through the stimulation of DC maturation and the enhancement of T-cell cytotoxicity.
Interleukin-2
It is an US FDA-approved cytokine for metastatic melanoma.[ 27 ] These cytokines increase level of NK cells and TILs in the lesion. Perilymphatic IL-2 administration has increased the survival rate of patients with HNSCC; increased tumor reactive T cells were found in patients who underwent MoAb therapy after surgery.
Cancer immunology is a rapidly evolving field. Understanding the behavior of cancer cells, identifying target antigens and detailing immune system pathways have allowed scientists to explore new approaches in immunotherapies. Despite impressive advances in immunotherapies there are few challenges including limited response rates, the inability to predict clinical efficacy and potential side effects such as autoimmune reactions or cytokine storm. In order to augment responses, rational combinations of immunotherapeutic agents and new immunotherapy technologies are being vigorously investigated.
Financial support and sponsorship
Conflicts of interest.
There are no conflicts of interest.
- Share full article
Advertisement
Supported by
More Studies by Columbia Cancer Researchers Are Retracted
The studies, pulled because of copied data, illustrate the sluggishness of scientific publishers to address serious errors, experts said.

By Benjamin Mueller
Scientists in a prominent cancer lab at Columbia University have now had four studies retracted and a stern note added to a fifth accusing it of “severe abuse of the scientific publishing system,” the latest fallout from research misconduct allegations recently leveled against several leading cancer scientists.
A scientific sleuth in Britain last year uncovered discrepancies in data published by the Columbia lab, including the reuse of photos and other images across different papers. The New York Times reported last month that a medical journal in 2022 had quietly taken down a stomach cancer study by the researchers after an internal inquiry by the journal found ethics violations.
Despite that study’s removal, the researchers — Dr. Sam Yoon, chief of a cancer surgery division at Columbia University’s medical center, and Changhwan Yoon, a more junior biologist there — continued publishing studies with suspicious data. Since 2008, the two scientists have collaborated with other researchers on 26 articles that the sleuth, Sholto David, publicly flagged for misrepresenting experiments’ results.
One of those articles was retracted last month after The Times asked publishers about the allegations. In recent weeks, medical journals have retracted three additional studies, which described new strategies for treating cancers of the stomach, head and neck. Other labs had cited the articles in roughly 90 papers.
A major scientific publisher also appended a blunt note to the article that it had originally taken down without explanation in 2022. “This reuse (and in part, misrepresentation) of data without appropriate attribution represents a severe abuse of the scientific publishing system,” it said .
Still, those measures addressed only a small fraction of the lab’s suspect papers. Experts said the episode illustrated not only the extent of unreliable research by top labs, but also the tendency of scientific publishers to respond slowly, if at all, to significant problems once they are detected. As a result, other labs keep relying on questionable work as they pour federal research money into studies, allowing errors to accumulate in the scientific record.
“For every one paper that is retracted, there are probably 10 that should be,” said Dr. Ivan Oransky, co-founder of Retraction Watch, which keeps a database of 47,000-plus retracted studies. “Journals are not particularly interested in correcting the record.”
Columbia’s medical center declined to comment on allegations facing Dr. Yoon’s lab. It said the two scientists remained at Columbia and the hospital “is fully committed to upholding the highest standards of ethics and to rigorously maintaining the integrity of our research.”
The lab’s web page was recently taken offline. Columbia declined to say why. Neither Dr. Yoon nor Changhwan Yoon could be reached for comment. (They are not related.)
Memorial Sloan Kettering Cancer Center, where the scientists worked when much of the research was done, is investigating their work.
The Columbia scientists’ retractions come amid growing attention to the suspicious data that undergirds some medical research. Since late February, medical journals have retracted seven papers by scientists at Harvard’s Dana-Farber Cancer Institute . That followed investigations into data problems publicized by Dr. David , an independent molecular biologist who looks for irregularities in published images of cells, tumors and mice, sometimes with help from A.I. software.
The spate of misconduct allegations has drawn attention to the pressures on academic scientists — even those, like Dr. Yoon, who also work as doctors — to produce heaps of research.
Strong images of experiments’ results are often needed for those studies. Publishing them helps scientists win prestigious academic appointments and attract federal research grants that can pay dividends for themselves and their universities.
Dr. Yoon, a robotic surgery specialist noted for his treatment of stomach cancers, has helped bring in nearly $5 million in federal research money over his career.
The latest retractions from his lab included articles from 2020 and 2021 that Dr. David said contained glaring irregularities . Their results appeared to include identical images of tumor-stricken mice, despite those mice supposedly having been subjected to different experiments involving separate treatments and types of cancer cells.
The medical journal Cell Death & Disease retracted two of the latest studies, and Oncogene retracted the third. The journals found that the studies had also reused other images, like identical pictures of constellations of cancer cells.
The studies Dr. David flagged as containing image problems were largely overseen by the more senior Dr. Yoon. Changhwan Yoon, an associate research scientist who has worked alongside Dr. Yoon for a decade, was often a first author, which generally designates the scientist who ran the bulk of the experiments.
Kun Huang, a scientist in China who oversaw one of the recently retracted studies, a 2020 paper that did not include the more senior Dr. Yoon, attributed that study’s problematic sections to Changhwan Yoon. Dr. Huang, who made those comments this month on PubPeer, a website where scientists post about studies, did not respond to an email seeking comment.
But the more senior Dr. Yoon has long been made aware of problems in research he published alongside Changhwan Yoon: The two scientists were notified of the removal in January 2022 of their stomach cancer study that was found to have violated ethics guidelines.
Research misconduct is often pinned on the more junior researchers who conduct experiments. Other scientists, though, assign greater responsibility to the senior researchers who run labs and oversee studies, even as they juggle jobs as doctors or administrators.
“The research world’s coming to realize that with great power comes great responsibility and, in fact, you are responsible not just for what one of your direct reports in the lab has done, but for the environment you create,” Dr. Oransky said.
In their latest public retraction notices, medical journals said that they had lost faith in the results and conclusions. Imaging experts said some irregularities identified by Dr. David bore signs of deliberate manipulation, like flipped or rotated images, while others could have been sloppy copy-and-paste errors.
The little-noticed removal by a journal of the stomach cancer study in January 2022 highlighted some scientific publishers’ policy of not disclosing the reasons for withdrawing papers as long as they have not yet formally appeared in print. That study had appeared only online.
Roland Herzog, the editor of the journal Molecular Therapy, said that editors had drafted an explanation that they intended to publish at the time of the article’s removal. But Elsevier, the journal’s parent publisher, advised them that such a note was unnecessary, he said.
Only after the Times article last month did Elsevier agree to explain the article’s removal publicly with the stern note. In an editorial this week , the Molecular Therapy editors said that in the future, they would explain the removal of any articles that had been published only online.
But Elsevier said in a statement that it did not consider online articles “to be the final published articles of record.” As a result, company policy continues to advise that such articles be removed without an explanation when they are found to contain problems. The company said it allowed editors to provide additional information where needed.
Elsevier, which publishes nearly 3,000 journals and generates billions of dollars in annual revenue , has long been criticized for its opaque removals of online articles.
Articles by the Columbia scientists with data discrepancies that remain unaddressed were largely distributed by three major publishers: Elsevier, Springer Nature and the American Association for Cancer Research. Dr. David alerted many journals to the data discrepancies in October.
Each publisher said it was investigating the concerns. Springer Nature said investigations take time because they can involve consulting experts, waiting for author responses and analyzing raw data.
Dr. David has also raised concerns about studies published independently by scientists who collaborated with the Columbia researchers on some of their recently retracted papers. For example, Sandra Ryeom, an associate professor of surgical sciences at Columbia, published an article in 2003 while at Harvard that Dr. David said contained a duplicated image . As of 2021, she was married to the more senior Dr. Yoon, according to a mortgage document from that year.
A medical journal appended a formal notice to the article last week saying “appropriate editorial action will be taken” once data concerns had been resolved. Dr. Ryeom said in a statement that she was working with the paper’s senior author on “correcting the error.”
Columbia has sought to reinforce the importance of sound research practices. Hours after the Times article appeared last month, Dr. Michael Shelanski, the medical school’s senior vice dean for research, sent an email to faculty members titled “Research Fraud Accusations — How to Protect Yourself.” It warned that such allegations, whatever their merits, could take a toll on the university.
“In the months that it can take to investigate an allegation,” Dr. Shelanski wrote, “funding can be suspended, and donors can feel that their trust has been betrayed.”
Benjamin Mueller reports on health and medicine. He was previously a U.K. correspondent in London and a police reporter in New York. More about Benjamin Mueller
- Alzheimer's disease & dementia
- Arthritis & Rheumatism
- Attention deficit disorders
- Autism spectrum disorders
- Biomedical technology
- Diseases, Conditions, Syndromes
- Endocrinology & Metabolism
- Gastroenterology
- Gerontology & Geriatrics
- Health informatics
- Inflammatory disorders
- Medical economics
- Medical research
- Medications
- Neuroscience
- Obstetrics & gynaecology
- Oncology & Cancer
- Ophthalmology
- Overweight & Obesity
- Parkinson's & Movement disorders
- Psychology & Psychiatry
- Radiology & Imaging
- Sleep disorders
- Sports medicine & Kinesiology
- Vaccination
- Breast cancer
- Cardiovascular disease
- Chronic obstructive pulmonary disease
- Colon cancer
- Coronary artery disease
- Heart attack
- Heart disease
- High blood pressure
- Kidney disease
- Lung cancer
- Multiple sclerosis
- Myocardial infarction
- Ovarian cancer
- Post traumatic stress disorder
- Rheumatoid arthritis
- Schizophrenia
- Skin cancer
- Type 2 diabetes
- Full List »
share this!
April 2, 2024
This article has been reviewed according to Science X's editorial process and policies . Editors have highlighted the following attributes while ensuring the content's credibility:
fact-checked
peer-reviewed publication
trusted source
One in five people with cancer participate in medical research studies
by Fred Hutchinson Cancer Center
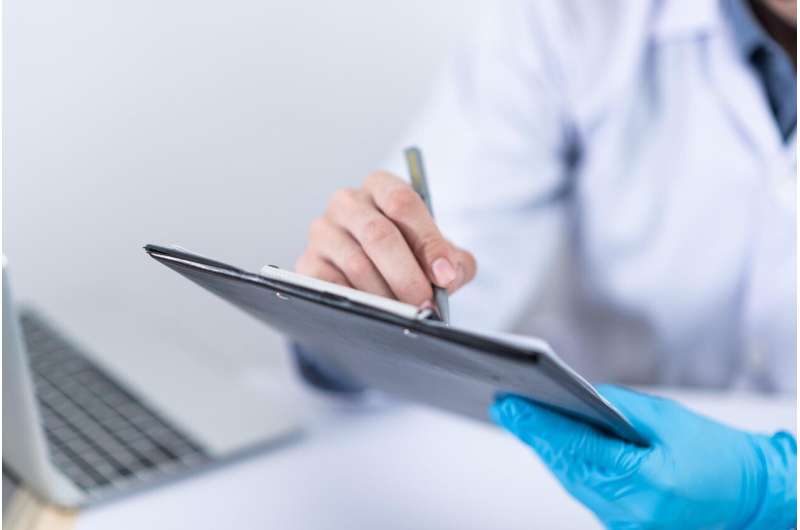
Researchers from Fred Hutchinson Cancer Center, the American Cancer Society Cancer Action Network and peer institutions released new findings in the Journal of Clinical Oncology showing that when all types of cancer research studies are considered, at least one in five people with cancer, or 21.9%, participate in some form of clinical research.
The study evaluated all categories of cancer studies, such as treatment trials, biorepository studies and quality of life studies—the first time an estimate of participation in all types of cancer studies has been reported. Moreover, enrollment in cancer treatment trials was 7.1%, a notably higher participation rate than previous estimates of 2%–3%.
The study also found that enrollment in treatment trials was over five-fold higher at National Cancer Institute-designated cancer centers than at community sites (21.6% versus 4.1%), reflecting the impact that NCI funding for staff and infrastructure has on an institution's ability to offer trials and recruit patients.
Using deidentified accreditation data provided by the Commission on Cancer, the study updated decades-old estimates for participation in cancer research.
This expanded analysis includes more than 70% of people diagnosed with cancer in the U.S. each year who received care at a variety of clinical settings, from community hospitals and academic medical centers to NCI-designated comprehensive cancer centers. Additionally, the study reflects the broad spectrum of cancer research including different study types and those sponsored by industry, government and other sources.
"As we work to increase participation in cancer research studies and make them more accessible to patients, we need an inclusive, accurate assessment of current participation to inform these policies," said Joseph Unger, Ph.D., MS, a health services researcher and biostatistician at Fred Hutch and lead author of the study.
"While we knew that patients play a significant role in advancing all types of cancer research, now we better understand just how commonly people are participating in all types of cancer studies today."
While previous estimates of participation in cancer research studies were derived solely from government-sponsored trials, the study authors used patient data from a diverse range of trial sponsors and care settings for this analysis. Importantly, the data included settings such as community hospitals, where a majority of U.S. cancer patients receive care.
"We know that most patients with cancer will participate in a clinical trial if given the chance, and the level of enrollment we see at NCI-designated cancer centers shows what participation can be when patients are offered trials," stated Mark Fleury, Ph.D., a policy principle at ACS CAN and senior author of the study.
"These findings emphasize the need to offer more patients in community settings the chance to participate and that will require an investment in these sites that currently isn't there."
People with cancer enrolled in many different types of clinical studies. The study found the following participation rates in each type of clinical study: biorepository (12.9%), treatment (7.1%), registry (7.3%), genetic (3.6%), quality-of-life (2.8%), diagnostic (2.5%) and economic studies (2.4%).
Expanding the types of cancer clinical studies in this analysis demonstrates that there are a variety of ways people choose to participate in cancer research beyond the previous assessments, which were based only on participation in treatment studies.
"Cancer clinical research, in all of its forms, simply cannot be conducted without the contributions of people with cancer," emphasized Dr. Unger.
"These contributions are much more extensive than was previously recognized. Cancer clinical research is a true partnership between those with cancer and those who study and treat cancer."
Explore further
Feedback to editors

New report presents a global plan to combat prostate cancer
2 hours ago

Dogs may provide new insights into human aging and cognition
3 hours ago

AI's ability to detect tumor cells could be key to more accurate bone cancer prognoses
4 hours ago

Novel pre-clinical models help advance therapeutic development for antibiotic-resistant bacterial infections

Far-UVC light can virtually eliminate airborne virus in an occupied room, study shows
5 hours ago

Las Vegas mass shooting survivors continue to struggle with major depression, PTSD

Combining food taxes and subsidies can lead to healthier grocery purchases for low-income households

New insights into muscle health: How innervation influences the recovery process
6 hours ago

Simulations reveal mechanism behind protein buildup in Parkinson's disease

Increasing positive affect in adolescence could lead to improved health and well-being in adulthood
7 hours ago
Related Stories

More than half of cancer patients willing to enroll in clinical trials
Oct 13, 2020

Study paves way for solutions to boost pediatric clinical trial enrollment
Feb 12, 2024

Better cancer trials could be around the corner
Mar 21, 2024

Study finds people of color more likely to participate in cancer clinical trials
Apr 28, 2021
Research shows structural barriers are the biggest reason for low participation in clinical trials
Feb 19, 2019

Expanded Medicaid coverage linked to higher participation in cancer clinical trials
Aug 17, 2023
Recommended for you

New insights into aggressive breast cancer and potential treatment options
8 hours ago

Investigators develop novel treatment for T-cell leukemias and lymphomas
9 hours ago
Let us know if there is a problem with our content
Use this form if you have come across a typo, inaccuracy or would like to send an edit request for the content on this page. For general inquiries, please use our contact form . For general feedback, use the public comments section below (please adhere to guidelines ).
Please select the most appropriate category to facilitate processing of your request
Thank you for taking time to provide your feedback to the editors.
Your feedback is important to us. However, we do not guarantee individual replies due to the high volume of messages.
E-mail the story
Your email address is used only to let the recipient know who sent the email. Neither your address nor the recipient's address will be used for any other purpose. The information you enter will appear in your e-mail message and is not retained by Medical Xpress in any form.
Newsletter sign up
Get weekly and/or daily updates delivered to your inbox. You can unsubscribe at any time and we'll never share your details to third parties.
More information Privacy policy
Donate and enjoy an ad-free experience
We keep our content available to everyone. Consider supporting Science X's mission by getting a premium account.
E-mail newsletter
Thank you for visiting nature.com. You are using a browser version with limited support for CSS. To obtain the best experience, we recommend you use a more up to date browser (or turn off compatibility mode in Internet Explorer). In the meantime, to ensure continued support, we are displaying the site without styles and JavaScript.
- View all journals
- Explore content
- About the journal
- Publish with us
- Sign up for alerts
- 27 March 2024
Cancer-vaccine trials give reasons for optimism
- Liam Drew 0
Liam Drew is a freelance writer based near London, UK.
You can also search for this author in PubMed Google Scholar
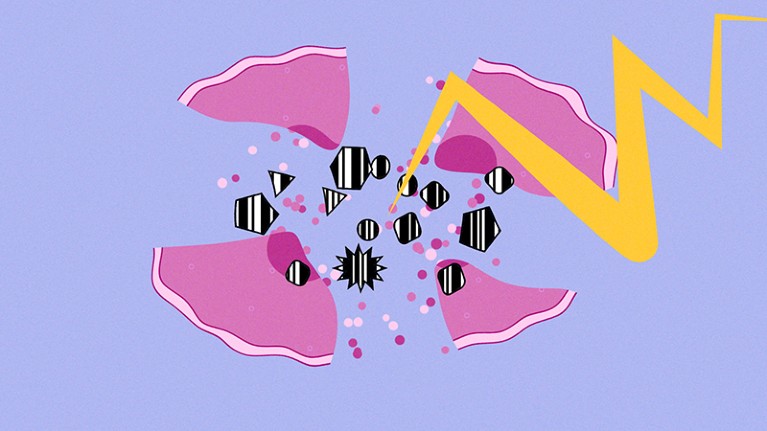
Illustration: Andrew Khosravani
Most people think of immunization as a way to prevent infectious disease. Vaccines contain proteins that the immune system can use to identify a pathogen, such as a virus, enabling the body to respond forcefully to it in future. But the immune system doesn’t only defend against foreign invaders — it also responds to threats from within, such as cancer.
Just as pathogens carry distinguishing proteins, known as antigens, so too do cancer cells. The immune system constantly detects and destroys mutating cells, and usually prevents tumours from developing. But sometimes cancer cells acquire mechanisms to evade the immune system. To tackle these cells, some researchers are turning to vaccines — not to prevent cancer, but to treat it.
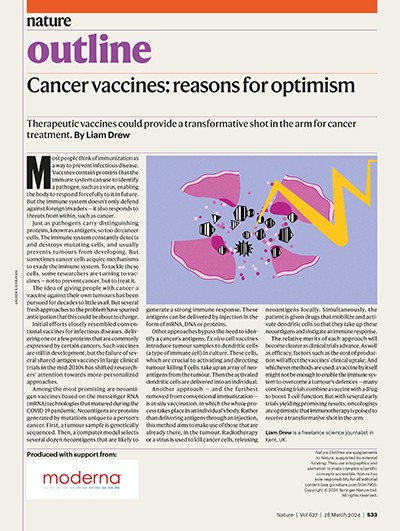
Nature Outline: Cancer vaccines
The idea of giving people with cancer a vaccine against their own tumours has been pursued for decades to little avail. But several fresh approaches to the problem have spurred anticipation that this could be about to change.
Initial efforts closely resembled conventional vaccines for infectious diseases, delivering one or a few proteins that are commonly expressed by certain cancers. Such vaccines are still in development, but the failure of several shared-antigen vaccines in large clinical trials in the mid-2010s has shifted researchers’ attention towards more-personalized approaches.
Among the most promising are neoantigen vaccines based on the messenger RNA (mRNA) technologies that matured during the COVID-19 pandemic. Neoantigens are proteins generated by mutations unique to a person’s cancer. First, a tumour sample is genetically sequenced. Then, a computer model selects several dozen neoantigens that are likely to generate a strong immune response. These antigens can be delivered by injection in the form of mRNA, DNA or proteins.
Other approaches bypass the need to identify a cancer’s antigens. Ex vivo cell vaccines introduce tumour samples to dendritic cells (a type of immune cell) in culture. These cells, which are crucial to activating and directing tumour-killing T cells, take up an array of neoantigens from the tumour. Then the activated dendritic cells are delivered into an individual.
Another approach — and the furthest removed from conventional immunization — is in situ vaccination, in which the whole process takes place in an individual’s body. Rather than delivering antigens through an injection, this method aims to make use of those that are already there, in the tumour. Radiotherapy or a virus is used to kill cancer cells, releasing neoantigens locally. Simultaneously, the patient is given drugs that mobilize and activate dendritic cells so that they take up these neoantigens and instigate an immune response.
The relative merits of each approach will become clearer as clinical trials advance. As well as efficacy, factors such as the cost of production will affect the vaccines’ clinical uptake. And whichever methods are used, a vaccine by itself might not be enough to enable the immune system to overcome a tumour’s defences — many continuing trials combine a vaccine with a drug to boost T-cell function. But with several early trials yielding promising results, oncologists are optimistic that immunotherapy is poised to receive a transformative shot in the arm.
Nature 627 , S33 (2024)
doi: https://doi.org/10.1038/d41586-024-00840-z
This article is part of Nature Outline: Cancer vaccines , a supplement produced with the financial support from Moderna . Nature maintains full independence in all editorial decisions related to the content. About this content .
Related Articles
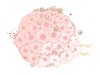
Sponsor feature: Revolutionizing cancer research with the power of mRNA
- Personalized medicine
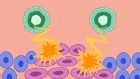
Video: Cancer-busting vaccines
Outline 27 MAR 24
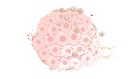
How does a cancer vaccine work?
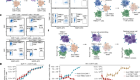
TRBC1-targeting antibody–drug conjugates for the treatment of T cell cancers
Article 27 MAR 24
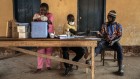
Mobile delivery of COVID-19 vaccines improved uptake in rural Sierra Leone
News & Views 13 MAR 24
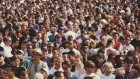
Ambitious survey of human diversity yields millions of undiscovered genetic variants
News 19 FEB 24
Postdoctoral Associate- Cell Biology
Houston, Texas (US)
Baylor College of Medicine (BCM)
Head of ClinicalTrials.gov
National Institutes of Health (NIH) National Library of Medicine (NLM) National Center for Biotechnology Information (NCBI) Information Engineering...
Washington D.C. (US)
National Library of Medicine, National Center for Biotechnology Information
POSTDOCTORAL FELLOWSHIP IN SYSTEMS BIOLOGY: PRECISION VACCINE PROGRAM (PVP) - BOSTON CHILDREN'
The Data Management and Analysis Core (DMAC) within the Precision Vaccine Program (PVP) at Boston Children’s Hospital and Harvard Medical School (H...
Boston, Massachusetts
Boston Children's Hospital - Department of Pediatrics
2024 Recruitment notice Shenzhen Institute of Synthetic Biology: Shenzhen, China
The wide-ranging expertise drawing from technical, engineering or science professions...
Shenzhen,China
Shenzhen Institute of Synthetic Biology
Global Talent Recruitment (Scientist Positions)
Global Talent Gathering for Innovation, Changping Laboratory Recruiting Overseas High-Level Talents.
Beijing, China
Changping Laboratory
Sign up for the Nature Briefing newsletter — what matters in science, free to your inbox daily.
Quick links
- Explore articles by subject
- Guide to authors
- Editorial policies

IMAGES
COMMENTS
According to the International Agency for Research on Cancer (IARC), in 2020 there were approximately 19.3 million new cases of cancer, and 10 million deaths by this disease, 6 while 23.8 million cases and 13.0 million deaths are projected to occur by 2030. 73 In this regard, it is clear the increasing role that environmental factors ...
Cancer Research publishes original studies, reviews, and opinion pieces on cancer biology and translational research. Browse the current issue, impact factor, editorial board, and featured articles on various topics of cancer.
Nature Cancer aims to publish the most significant advances across the full spectrum of cancer research in the life, physical, applied and social sciences, ...
Cancer, an international interdisciplinary journal of the American Cancer Society, publishes high-impact, peer-reviewed original articles and solicited content on the latest clinical research findings.Spanning the breadth of oncology disciplines, Cancer delivers something for everyone involved in cancer research, risk reduction, treatment, and patient care.
The growing global burden of cancer is rapidly exceeding the current cancer control capacity. More than 19 million new cancer cases were diagnosed in 2020 worldwide, and 10 million people died of cancer.1 By 2040, that burden is expected to increase to around 30 million new cancer cases annually and 16 million deaths from cancer according to the Global Cancer Observatory.
Targeted therapies have swiftly taken a prominent position in cancer research and clinical oncology in recent decades, thanks to the molecular insights into oncogenic processes and mechanisms ...
Read the latest Research articles from Nature Cancer. ... De Blank and colleagues examine data from childhood cancer survivors diagnosed between 1970 and 1999 and find that exposure to radiation ...
The Journal of Cancer Research and Clinical Oncology publishes content within the fields of experimental and clinical oncology. Topics covered include, but are not limited to: carcinogenesis, molecular biology, developments in tumor therapy, general and laboratory diagnoses, diagnostic and experimental pathology, oncologic surgery, and epidemiology.
Journal of Cancer Research and Therapeutics. 19(5):1075-1078, Jul-Sep 2023. Abstract. Favorite; PDF; Permissions Open. Original Article The adherence to the American Association for the Study of Liver Diseases 2018 guidelines in the management of hepatocellular carcinoma and its impact on survival ...
Keywords. 1.1. Introduction. Cancer is the uninhibited growth and development of abnormal cells in the body, and is one of the foremost reasons of deaths throughout the world ( Paul and Jindal, 2017 ). These abnormal cells are commonly designated as cancerous cells, tumorous cells, or malignant cells. In 2018, cancer accounted for an estimated ...
Top 100 in Cancer - 2022. This collection highlights our most downloaded* cancer papers published in 2022. Featuring authors from aroud the world, these papers showcase valuable research from an ...
The clinical validation cohort included 10,258 persons, 7861 of whom met eligibility criteria and were evaluable. A total of 83.1% of the participants with colorectal cancer detected by ...
The impact of cancer is increasing significantly day by day. Tobacco is 22% responsible for causing cancer, 15% cancer is caused due some infections like HIV, hepatitis b, Epstein-Barretc, and 10% ...
New research suggests that fungi in the gut may affect how tumors respond to cancer treatments. In mice, when bacteria were eliminated with antibiotics, fungi filled the void and impaired the immune response after radiation therapy, the study found. FDA Approves Belumosudil to Treat Chronic Graft-Versus-Host Disease.
Research Paper Construction and multicohort validation of a colon cancer prognostic risk score system based on big data of neutrophil-associated differentially expressed genes Yunxi Yang, Cheng Lu, Linbin Li, Chunfang Zheng, Yifan Wang, Jiahui Chen, Bingwei Sun J. Cancer 2024; 15(9): 2866-2879. doi:10.7150/jca.94560 Abstract Full text PDF
New research published in JAMA Oncology shows that an estimated 1 in 9 cancer diagnoses were missed in the USA during the first part of the COVID-19 pandemic (March-December, 2020), translating to 134 395 undiagnosed cancers during that time . The authors, led by Todd Burus (Markey Cancer Center, University of Kentucky, Lexington, KY, USA), calculated the missing diagnoses by comparing ...
Tel: +91-7834871362, +91-7055064227. 1. Cancer: An Overview. Garima Mathur, Sumitra Nain and Pramod Kumar Sha rma. 12 1. School of Medical and Allied Scien ces, Galgotia university, Greater Noida ...
Top 100 in Cancer. This collection highlights our most downloaded* cancer papers published in 2021. Featuring authors from aroud the world, these papers showcase valuable research from an ...
This paper focuses on new research that emphasizes the immunomodulatory characteristics of exosomes produced from immune cells. It also explores the possibility of these exosomes as therapeutic agents or biomarkers for the early identification and prevention of breast cancer.
Cancer immunotherapy is emerging as a beneficial tool for cancer treatment by activating the immune system to produce antitumor effects. ... There has been extensive research on the complex and dynamic interaction between tumor cells and host immune cells which has led to the development of currently approved immunotherapies.
"For every one paper that is retracted, there are probably 10 that should be," said Dr. Ivan Oransky, co-founder of Retraction Watch, which keeps a database of 47,000-plus retracted studies.
Read the latest Research articles in Cancer from Scientific Reports. ... Cancer incidence trends in New York State and associations with common population-level exposures 2010-2018: an ...
Cancer clinical research is a true partnership between those with cancer and those who study and treat cancer." More information: Joseph Unger et al, Journal of Clinical Oncology (2024).
A new research paper was published on the cover of Aging (listed by MEDLINE/PubMed as "Aging (Albany NY)" and "Aging-US" by Web of Science) Volume 16, Issue 6, entitled, "Altered brain morphology ...
Illustration: Andrew Khosravani. Most people think of immunization as a way to prevent infectious disease. Vaccines contain proteins that the immune system can use to identify a pathogen, such as ...