- Search Menu
- Advanced Articles
- Editor's Choice
- Author Guidelines
- Publish with us
- Submission Site
- Open Access
- Self-Archiving Policy
- About Clean Energy
- About the National Institute of Clean and Low-Carbon Energy
- Editorial Board
- Instructions for Reviewers
- Advertising & Corporate Services
- Journals Career Network
- Journals on Oxford Academic
- Books on Oxford Academic


Article Contents
Introduction, 1 installed capacity and application of solar energy worldwide, 2 the role of solar energy in sustainable development, 3 the perspective of solar energy, 4 conclusions, conflict of interest statement.
- < Previous
Solar energy technology and its roles in sustainable development

- Article contents
- Figures & tables
- Supplementary Data
Ali O M Maka, Jamal M Alabid, Solar energy technology and its roles in sustainable development, Clean Energy , Volume 6, Issue 3, June 2022, Pages 476–483, https://doi.org/10.1093/ce/zkac023
- Permissions Icon Permissions
Solar energy is environmentally friendly technology, a great energy supply and one of the most significant renewable and green energy sources. It plays a substantial role in achieving sustainable development energy solutions. Therefore, the massive amount of solar energy attainable daily makes it a very attractive resource for generating electricity. Both technologies, applications of concentrated solar power or solar photovoltaics, are always under continuous development to fulfil our energy needs. Hence, a large installed capacity of solar energy applications worldwide, in the same context, supports the energy sector and meets the employment market to gain sufficient development. This paper highlights solar energy applications and their role in sustainable development and considers renewable energy’s overall employment potential. Thus, it provides insights and analysis on solar energy sustainability, including environmental and economic development. Furthermore, it has identified the contributions of solar energy applications in sustainable development by providing energy needs, creating jobs opportunities and enhancing environmental protection. Finally, the perspective of solar energy technology is drawn up in the application of the energy sector and affords a vision of future development in this domain.

With reference to the recommendations of the UN, the Climate Change Conference, COP26, was held in Glasgow , UK, in 2021. They reached an agreement through the representatives of the 197 countries, where they concurred to move towards reducing dependency on coal and fossil-fuel sources. Furthermore, the conference stated ‘the various opportunities for governments to prioritize health and equity in the international climate movement and sustainable development agenda’. Also, one of the testaments is the necessity to ‘create energy systems that protect and improve climate and health’ [ 1 , 2 ].
The Paris Climate Accords is a worldwide agreement on climate change signed in 2015, which addressed the mitigation of climate change, adaptation and finance. Consequently, the representatives of 196 countries concurred to decrease their greenhouse gas emissions [ 3 ]. The Paris Agreement is essential for present and future generations to attain a more secure and stable environment. In essence, the Paris Agreement has been about safeguarding people from such an uncertain and progressively dangerous environment and ensuring everyone can have the right to live in a healthy, pollutant-free environment without the negative impacts of climate change [ 3 , 4 ].
In recent decades, there has been an increase in demand for cleaner energy resources. Based on that, decision-makers of all countries have drawn up plans that depend on renewable sources through a long-term strategy. Thus, such plans reduce the reliance of dependence on traditional energy sources and substitute traditional energy sources with alternative energy technology. As a result, the global community is starting to shift towards utilizing sustainable energy sources and reducing dependence on traditional fossil fuels as a source of energy [ 5 , 6 ].
In 2015, the UN adopted the sustainable development goals (SDGs) and recognized them as international legislation, which demands a global effort to end poverty, safeguard the environment and guarantee that by 2030, humanity lives in prosperity and peace. Consequently, progress needs to be balanced among economic, social and environmental sustainability models [ 7 ].
Many national and international regulations have been established to control the gas emissions and pollutants that impact the environment [ 8 ]. However, the negative effects of increased carbon in the atmosphere have grown in the last 10 years. Production and use of fossil fuels emit methane (CH 4 ), carbon dioxide (CO 2 ) and carbon monoxide (CO), which are the most significant contributors to environmental emissions on our planet. Additionally, coal and oil, including gasoline, coal, oil and methane, are commonly used in energy for transport or for generating electricity. Therefore, burning these fossil fuel s is deemed the largest emitter when used for electricity generation, transport, etc. However, these energy resources are considered depleted energy sources being consumed to an unsustainable degree [ 9–11 ].
Energy is an essential need for the existence and growth of human communities. Consequently, the need for energy has increased gradually as human civilization has progressed. Additionally, in the past few decades, the rapid rise of the world’s population and its reliance on technological developments have increased energy demands. Furthermore, green technology sources play an important role in sustainably providing energy supplies, especially in mitigating climate change [ 5 , 6 , 8 ].
Currently, fossil fuels remain dominant and will continue to be the primary source of large-scale energy for the foreseeable future; however, renewable energy should play a vital role in the future of global energy. The global energy system is undergoing a movement towards more sustainable sources of energy [ 12 , 13 ].
Power generation by fossil-fuel resources has peaked, whilst solar energy is predicted to be at the vanguard of energy generation in the near future. Moreover, it is predicted that by 2050, the generation of solar energy will have increased to 48% due to economic and industrial growth [ 13 , 14 ].
In recent years, it has become increasingly obvious that the globe must decrease greenhouse gas emissions by 2050, ideally towards net zero, if we are to fulfil the Paris Agreement’s goal to reduce global temperature increases [ 3 , 4 ]. The net-zero emissions complement the scenario of sustainable development assessment by 2050. According to the agreed scenario of sustainable development, many industrialized economies must achieve net-zero emissions by 2050. However, the net-zero emissions 2050 brought the first detailed International Energy Agency (IEA) modelling of what strategy will be required over the next 10 years to achieve net-zero carbon emissions worldwide by 2050 [ 15–17 ].
The global statistics of greenhouse gas emissions have been identified; in 2019, there was a 1% decrease in CO 2 emissions from the power industry; that figure dropped by 7% in 2020 due to the COVID-19 crisis, thus indicating a drop in coal-fired energy generation that is being squeezed by decreasing energy needs, growth of renewables and the shift away from fossil fuels. As a result, in 2020, the energy industry was expected to generate ~13 Gt CO 2 , representing ~40% of total world energy sector emissions related to CO 2 . The annual electricity generation stepped back to pre-crisis levels by 2021, although due to a changing ‘fuel mix’, the CO 2 emissions in the power sector will grow just a little before remaining roughly steady until 2030 [ 15 ].
Therefore, based on the information mentioned above, the advantages of solar energy technology are a renewable and clean energy source that is plentiful, cheaper costs, less maintenance and environmentally friendly, to name but a few. The significance of this paper is to highlight solar energy applications to ensure sustainable development; thus, it is vital to researchers, engineers and customers alike. The article’s primary aim is to raise public awareness and disseminate the culture of solar energy usage in daily life, since moving forward, it is the best. The scope of this paper is as follows. Section 1 represents a summary of the introduction. Section 2 represents a summary of installed capacity and the application of solar energy worldwide. Section 3 presents the role of solar energy in the sustainable development and employment of renewable energy. Section 4 represents the perspective of solar energy. Finally, Section 5 outlines the conclusions and recommendations for future work.
1.1 Installed capacity of solar energy
The history of solar energy can be traced back to the seventh century when mirrors with solar power were used. In 1893, the photovoltaic (PV) effect was discovered; after many decades, scientists developed this technology for electricity generation [ 18 ]. Based on that, after many years of research and development from scientists worldwide, solar energy technology is classified into two key applications: solar thermal and solar PV.
PV systems convert the Sun’s energy into electricity by utilizing solar panels. These PV devices have quickly become the cheapest option for new electricity generation in numerous world locations due to their ubiquitous deployment. For example, during the period from 2010 to 2018, the cost of generating electricity by solar PV plants decreased by 77%. However, solar PV installed capacity progress expanded 100-fold between 2005 and 2018. Consequently, solar PV has emerged as a key component in the low-carbon sustainable energy system required to provide access to affordable and dependable electricity, assisting in fulfilling the Paris climate agreement and in achieving the 2030 SDG targets [ 19 ].
The installed capacity of solar energy worldwide has been rapidly increased to meet energy demands. The installed capacity of PV technology from 2010 to 2020 increased from 40 334 to 709 674 MW, whereas the installed capacity of concentrated solar power (CSP) applications, which was 1266 MW in 2010, after 10 years had increased to 6479 MW. Therefore, solar PV technology has more deployed installations than CSP applications. So, the stand-alone solar PV and large-scale grid-connected PV plants are widely used worldwide and used in space applications. Fig. 1 represents the installation of solar energy worldwide.
![research articles on solar energy Installation capacity of solar energy worldwide [20].](https://oup.silverchair-cdn.com/oup/backfile/Content_public/Journal/ce/6/3/10.1093_ce_zkac023/2/m_zkac023_fig1.jpeg?Expires=1717067481&Signature=1Gw03YnyM52~~-twsZWPYiYuu~YvoGRG~VBFGDOaXRU6HhxHDB-Tk1eQ9G~tmKSd-Y~23xlKR7vOoxu4uGWQgs1NzLE0ovAuGyPzTyt2mxYtZg4LATtB6VOC1DIsgpa2UBiWW9kDFZBJFdGPBTbY1XLzj9o25-Xz~cJ6DugW8CdZTbl50sgfANpGKCWHVXxYTCD-o1Loo3-~HVGp7tpGa4EMGpe1oNwXjec5DNIcCd2E60XKqnnTUKBwuQyvG9H3oftpounbAqkup~MnZ~EtqOUTVL5ttFcSd7s2Of2u7BI6IRcPwVsPmolA~ASEx~hIVk0E0AkC6HqMHfKzfRmGMQ__&Key-Pair-Id=APKAIE5G5CRDK6RD3PGA)
Installation capacity of solar energy worldwide [ 20 ].
1.2 Application of solar energy
Energy can be obtained directly from the Sun—so-called solar energy. Globally, there has been growth in solar energy applications, as it can be used to generate electricity, desalinate water and generate heat, etc. The taxonomy of applications of solar energy is as follows: (i) PVs and (ii) CSP. Fig. 2 details the taxonomy of solar energy applications.

The taxonomy of solar energy applications.
Solar cells are devices that convert sunlight directly into electricity; typical semiconductor materials are utilized to form a PV solar cell device. These materials’ characteristics are based on atoms with four electrons in their outer orbit or shell. Semiconductor materials are from the periodic table’s group ‘IV’ or a mixture of groups ‘IV’ and ‘II’, the latter known as ‘II–VI’ semiconductors [ 21 ]. Additionally, a periodic table mixture of elements from groups ‘III’ and ‘V’ can create ‘III–V’ materials [ 22 ].
PV devices, sometimes called solar cells, are electronic devices that convert sunlight into electrical power. PVs are also one of the rapidly growing renewable-energy technologies of today. It is therefore anticipated to play a significant role in the long-term world electricity-generating mixture moving forward.
Solar PV systems can be incorporated to supply electricity on a commercial level or installed in smaller clusters for mini-grids or individual usage. Utilizing PV modules to power mini-grids is a great way to offer electricity to those who do not live close to power-transmission lines, especially in developing countries with abundant solar energy resources. In the most recent decade, the cost of producing PV modules has dropped drastically, giving them not only accessibility but sometimes making them the least expensive energy form. PV arrays have a 30-year lifetime and come in various shades based on the type of material utilized in their production.
The most typical method for solar PV desalination technology that is used for desalinating sea or salty water is electrodialysis (ED). Therefore, solar PV modules are directly connected to the desalination process. This technique employs the direct-current electricity to remove salt from the sea or salty water.
The technology of PV–thermal (PV–T) comprises conventional solar PV modules coupled with a thermal collector mounted on the rear side of the PV module to pre-heat domestic hot water. Accordingly, this enables a larger portion of the incident solar energy on the collector to be converted into beneficial electrical and thermal energy.
A zero-energy building is a building that is designed for zero net energy emissions and emits no carbon dioxide. Building-integrated PV (BIPV) technology is coupled with solar energy sources and devices in buildings that are utilized to supply energy needs. Thus, building-integrated PVs utilizing thermal energy (BIPV/T) incorporate creative technologies such as solar cooling [ 23 ].
A PV water-pumping system is typically used to pump water in rural, isolated and desert areas. The system consists of PV modules to power a water pump to the location of water need. The water-pumping rate depends on many factors such as pumping head, solar intensity, etc.
A PV-powered cathodic protection (CP) system is designed to supply a CP system to control the corrosion of a metal surface. This technique is based on the impressive current acquired from PV solar energy systems and is utilized for burying pipelines, tanks, concrete structures, etc.
Concentrated PV (CPV) technology uses either the refractive or the reflective concentrators to increase sunlight to PV cells [ 24 , 25 ]. High-efficiency solar cells are usually used, consisting of many layers of semiconductor materials that stack on top of each other. This technology has an efficiency of >47%. In addition, the devices produce electricity and the heat can be used for other purposes [ 26 , 27 ].
For CSP systems, the solar rays are concentrated using mirrors in this application. These rays will heat a fluid, resulting in steam used to power a turbine and generate electricity. Large-scale power stations employ CSP to generate electricity. A field of mirrors typically redirect rays to a tall thin tower in a CSP power station. Thus, numerous large flat heliostats (mirrors) are used to track the Sun and concentrate its light onto a receiver in power tower systems, sometimes known as central receivers. The hot fluid could be utilized right away to produce steam or stored for later usage. Another of the great benefits of a CSP power station is that it may be built with molten salts to store heat and generate electricity outside of daylight hours.
Mirrored dishes are used in dish engine systems to focus and concentrate sunlight onto a receiver. The dish assembly tracks the Sun’s movement to capture as much solar energy as possible. The engine includes thin tubes that work outside the four-piston cylinders and it opens into the cylinders containing hydrogen or helium gas. The pistons are driven by the expanding gas. Finally, the pistons drive an electric generator by turning a crankshaft.
A further water-treatment technique, using reverse osmosis, depends on the solar-thermal and using solar concentrated power through the parabolic trough technique. The desalination employs CSP technology that utilizes hybrid integration and thermal storage allows continuous operation and is a cost-effective solution. Solar thermal can be used for domestic purposes such as a dryer. In some countries or societies, the so-called food dehydration is traditionally used to preserve some food materials such as meats, fruits and vegetables.
Sustainable energy development is defined as the development of the energy sector in terms of energy generating, distributing and utilizing that are based on sustainability rules [ 28 ]. Energy systems will significantly impact the environment in both developed and developing countries. Consequently, the global sustainable energy system must optimize efficiency and reduce emissions [ 29 ].
The sustainable development scenario is built based on the economic perspective. It also examines what activities will be required to meet shared long-term climate benefits, clean air and energy access targets. The short-term details are based on the IEA’s sustainable recovery strategy, which aims to promote economies and employment through developing a cleaner and more reliable energy infrastructure [ 15 ]. In addition, sustainable development includes utilizing renewable-energy applications, smart-grid technologies, energy security, and energy pricing, and having a sound energy policy [ 29 ].
The demand-side response can help meet the flexibility requirements in electricity systems by moving demand over time. As a result, the integration of renewable technologies for helping facilitate the peak demand is reduced, system stability is maintained, and total costs and CO 2 emissions are reduced. The demand-side response is currently used mostly in Europe and North America, where it is primarily aimed at huge commercial and industrial electricity customers [ 15 ].
International standards are an essential component of high-quality infrastructure. Establishing legislative convergence, increasing competition and supporting innovation will allow participants to take part in a global world PV market [ 30 ]. Numerous additional countries might benefit from more actively engaging in developing global solar PV standards. The leading countries in solar PV manufacturing and deployment have embraced global standards for PV systems and highly contributed to clean-energy development. Additional assistance and capacity-building to enhance quality infrastructure in developing economies might also help support wider implementation and compliance with international solar PV standards. Thus, support can bring legal requirements and frameworks into consistency and give additional impetus for the trade of secure and high-quality solar PV products [ 19 ].
Continuous trade-led dissemination of solar PV and other renewable technologies will strengthen the national infrastructure. For instance, off-grid solar energy alternatives, such as stand-alone systems and mini-grids, could be easily deployed to assist healthcare facilities in improving their degree of services and powering portable testing sites and vaccination coolers. In addition to helping in the immediate medical crisis, trade-led solar PV adoption could aid in the improving economy from the COVID-19 outbreak, not least by providing jobs in the renewable-energy sector, which are estimated to reach >40 million by 2050 [ 19 ].
The framework for energy sustainability development, by the application of solar energy, is one way to achieve that goal. With the large availability of solar energy resources for PV and CSP energy applications, we can move towards energy sustainability. Fig. 3 illustrates plans for solar energy sustainability.

Framework for solar energy applications in energy sustainability.
The environmental consideration of such applications, including an aspect of the environmental conditions, operating conditions, etc., have been assessed. It is clean, friendly to the environment and also energy-saving. Moreover, this technology has no removable parts, low maintenance procedures and longevity.
Economic and social development are considered by offering job opportunities to the community and providing cheaper energy options. It can also improve people’s income; in turn, living standards will be enhanced. Therefore, energy is paramount, considered to be the most vital element of human life, society’s progress and economic development.
As efforts are made to increase the energy transition towards sustainable energy systems, it is anticipated that the next decade will see a continued booming of solar energy and all clean-energy technology. Scholars worldwide consider research and innovation to be substantial drivers to enhance the potency of such solar application technology.
2.1 Employment from renewable energy
The employment market has also boomed with the deployment of renewable-energy technology. Renewable-energy technology applications have created >12 million jobs worldwide. The solar PV application came as the pioneer, which created >3 million jobs. At the same time, while the solar thermal applications (solar heating and cooling) created >819 000 jobs, the CSP attained >31 000 jobs [ 20 ].
According to the reports, although top markets such as the USA, the EU and China had the highest investment in renewables jobs, other Asian countries have emerged as players in the solar PV panel manufacturers’ industry [ 31 ].
Solar energy employment has offered more employment than other renewable sources. For example, in the developing countries, there was a growth in employment chances in solar applications that powered ‘micro-enterprises’. Hence, it has been significant in eliminating poverty, which is considered the key goal of sustainable energy development. Therefore, solar energy plays a critical part in fulfilling the sustainability targets for a better plant and environment [ 31 , 32 ]. Fig. 4 illustrates distributions of world renewable-energy employment.
![research articles on solar energy World renewable-energy employment [20].](https://oup.silverchair-cdn.com/oup/backfile/Content_public/Journal/ce/6/3/10.1093_ce_zkac023/2/m_zkac023_fig4.jpeg?Expires=1717067481&Signature=bPO154VJCGdyXGfjX52uwbqRmk0PgIhx3TtDL43euIaxRFZ6iRNSj9OQJlphZVmcC2dPkGWCmtkZJazYNwmVmIlkLALcTzUnK7pv81XZJdMnbWyAPw~wvcL0vKOCQTa1Y63b6EZFPwTtvoTDNwQ7Eu2NssBuWUCWpDs6wu9yPXxkiCw77-2slwAOv4cMZJzOejMy9kA2AN1K6~IyJRxL10FAbc6WH8PbCIflWG5xenfgKsoxJtNnysoD24K2hmr8ybfButsILixw-N295OAq-RrcGBzoKgLcJ9aep87F80I6sXGoFY0d727g~aeLJpP5I1UjQ3I0Y72eThbp8Y4AeA__&Key-Pair-Id=APKAIE5G5CRDK6RD3PGA)
World renewable-energy employment [ 20 ].
The world distribution of PV jobs is disseminated across the continents as follows. There was 70% employment in PV applications available in Asia, while 10% is available in North America, 10% available in South America and 10% availability in Europe. Table 1 details the top 10 countries that have relevant jobs in Asia, North America, South America and Europe.
List of the top 10 countries that created jobs in solar PV applications [ 19 , 33 ]
Solar energy investments can meet energy targets and environmental protection by reducing carbon emissions while having no detrimental influence on the country’s development [ 32 , 34 ]. In countries located in the ‘Sunbelt’, there is huge potential for solar energy, where there is a year-round abundance of solar global horizontal irradiation. Consequently, these countries, including the Middle East, Australia, North Africa, China, the USA and Southern Africa, to name a few, have a lot of potential for solar energy technology. The average yearly solar intensity is >2800 kWh/m 2 and the average daily solar intensity is >7.5 kWh/m 2 . Fig. 5 illustrates the optimum areas for global solar irradiation.
![research articles on solar energy World global solar irradiation map [35].](https://oup.silverchair-cdn.com/oup/backfile/Content_public/Journal/ce/6/3/10.1093_ce_zkac023/2/m_zkac023_fig5.jpeg?Expires=1717067481&Signature=pmAzuQgCJhNyWPaQouLIUOhRL7xCfGGlcB8Mco7KB4isH5wEkQmzWvr6IC2oPcKXUip~RVbik0y5RH3UVxRCYbhFMEv1a2XAUh5B2MmElmvLM6Rek7qpBSBD-nXmM83azj~IiuJ0Oe4lgljxNOJrh58SIV7OQEIsXAqIMybEpJiCL5SwTSaHEHSNSKzLjQqIb7g7fFeOXpINO72fI49NYycKi3gfVqn8B97OAUBMCuSywsnweNLOquCltoy5A8KsoRIUKMsgMHoldFpXxmqKwB7HIMVfd25naQs3m18XyLUq8hAgt2iv1HHkNrxDwSiWvS7jwmUrZSfmyLdxMQUXwg__&Key-Pair-Id=APKAIE5G5CRDK6RD3PGA)
World global solar irradiation map [ 35 ].
The distribution of solar radiation and its intensity are two important factors that influence the efficiency of solar PV technology and these two parameters vary among different countries. Therefore, it is essential to realize that some solar energy is wasted since it is not utilized. On the other hand, solar radiation is abundant in several countries, especially in developing ones, which makes it invaluable [ 36 , 37 ].
Worldwide, the PV industry has benefited recently from globalization, which has allowed huge improvements in economies of scale, while vertical integration has created strong value chains: as manufacturers source materials from an increasing number of suppliers, prices have dropped while quality has been maintained. Furthermore, the worldwide incorporated PV solar device market is growing fast, creating opportunities enabling solar energy firms to benefit from significant government help with underwriting, subsides, beneficial trading licences and training of a competent workforce, while the increased rivalry has reinforced the motivation to continue investing in research and development, both public and private [ 19 , 33 ].
The global outbreak of COVID-19 has impacted ‘cross-border supply chains’ and those investors working in the renewable-energy sector. As a result, more diversity of solar PV supply-chain processes may be required in the future to enhance long-term flexibility versus exogenous shocks [ 19 , 33 ].
It is vital to establish a well-functioning quality infrastructure to expand the distribution of solar PV technologies beyond borders and make it easier for new enterprises to enter solar PV value chains. In addition, a strong quality infrastructure system is a significant instrument for assisting local firms in meeting the demands of trade markets. Furthermore, high-quality infrastructure can help reduce associated risks with the worldwide PV project value chain, such as underperforming, inefficient and failing goods, limiting the development, improvement and export of these technologies. Governments worldwide are, at various levels, creating quality infrastructure, including the usage of metrology i.e. the science of measurement and its application, regulations, testing procedures, accreditation, certification and market monitoring [ 33 , 38 ].
The perspective is based on a continuous process of technological advancement and learning. Its speed is determined by its deployment, which varies depending on the scenario [ 39 , 40 ]. The expense trends support policy preferences for low-carbon energy sources, particularly in increased energy-alteration scenarios. Emerging technologies are introduced and implemented as quickly as they ever have been before in energy history [ 15 , 33 ].
The CSP stations have been in use since the early 1980s and are currently found all over the world. The CSP power stations in the USA currently produce >800 MW of electricity yearly, which is sufficient to power ~500 000 houses. New CSP heat-transfer fluids being developed can function at ~1288 o C, which is greater than existing fluids, to improve the efficiency of CSP systems and, as a result, to lower the cost of energy generated using this technology. Thus, as a result, CSP is considered to have a bright future, with the ability to offer large-scale renewable energy that can supplement and soon replace traditional electricity-production technologies [ 41 ]. The DESERTEC project has drawn out the possibility of CSP in the Sahara Desert regions. When completed, this investment project will have the world’s biggest energy-generation capacity through the CSP plant, which aims to transport energy from North Africa to Europe [ 42 , 43 ].
The costs of manufacturing materials for PV devices have recently decreased, which is predicted to compensate for the requirements and increase the globe’s electricity demand [ 44 ]. Solar energy is a renewable, clean and environmentally friendly source of energy. Therefore, solar PV application techniques should be widely utilized. Although PV technology has always been under development for a variety of purposes, the fact that PV solar cells convert the radiant energy from the Sun directly into electrical power means it can be applied in space and in terrestrial applications [ 38 , 45 ].
In one way or another, the whole renewable-energy sector has a benefit over other energy industries. A long-term energy development plan needs an energy source that is inexhaustible, virtually accessible and simple to gather. The Sun rises over the horizon every day around the globe and leaves behind ~108–1018 kWh of energy; consequently, it is more than humanity will ever require to fulfil its desire for electricity [ 46 ].
The technology that converts solar radiation into electricity is well known and utilizes PV cells, which are already in use worldwide. In addition, various solar PV technologies are available today, including hybrid solar cells, inorganic solar cells and organic solar cells. So far, solar PV devices made from silicon have led the solar market; however, these PVs have certain drawbacks, such as expenditure of material, time-consuming production, etc. It is important to mention here the operational challenges of solar energy in that it does not work at night, has less output in cloudy weather and does not work in sandstorm conditions. PV battery storage is widely used to reduce the challenges to gain high reliability. Therefore, attempts have been made to find alternative materials to address these constraints. Currently, this domination is challenged by the evolution of the emerging generation of solar PV devices based on perovskite, organic and organic/inorganic hybrid materials.
This paper highlights the significance of sustainable energy development. Solar energy would help steady energy prices and give numerous social, environmental and economic benefits. This has been indicated by solar energy’s contribution to achieving sustainable development through meeting energy demands, creating jobs and protecting the environment. Hence, a paramount critical component of long-term sustainability should be investigated. Based on the current condition of fossil-fuel resources, which are deemed to be depleting energy sources, finding an innovative technique to deploy clean-energy technology is both essential and expected. Notwithstanding, solar energy has yet to reach maturity in development, especially CSP technology. Also, with growing developments in PV systems, there has been a huge rise in demand for PV technology applications all over the globe. Further work needs to be undertaken to develop energy sustainably and consider other clean energy resources. Moreover, a comprehensive experimental and validation process for such applications is required to develop cleaner energy sources to decarbonize our planet.
The authors declare that they have no known competing financial interests or personal relationships that could have appeared to influence the work reported in this paper.
World Health Organization . COP26 Special Report on Climate Change and Health: The Health Argument for Climate Action. Geneva : World Health Organization , 2021 .
Google Scholar
Google Preview
Hunter DB , Salzman JE , Zaelke D . Glasgow Climate Summit: COP26. UCLA School of Law, Public Law Research Paper No. 22-02. 2021 . doi: org/10.2139/ssrn.4005648 30 March 2022 , date last accessed).
UNFCCC . Paris Agreement-Status of Ratification, United Nations Framework Convention on Climate , 2016 . https://unfccc.int/process/the-paris-agreement/status-of-ratification ( 25 January 2022 , date last accessed).
UNFCCC . The Paris Agreement. Archived from the original on 19 March 2021 . Retrieved 18 September 2021 . https://unfccc.int/process-and-meetings/the-paris-agreement/the-paris-agreement ( 2 February 2022 , date last accessed).
Watts RG. Engineering Response to Climate Change. 2nd edn. Boca Raton, FL : CRC Press , 2013 .
Sorensen B. Renewable Energy: Physics, Engineering, Environmental Impacts, Economics and Planning . 4th edn. London : Academic Press , 2010 .
IEA, IRENA, WMO, WBG, WHO . Tracking SDG7: The Energy Progress Report 2021. Washington, DC : The World Bank , 2021 .
Edenhofer O , Pichs-Madruga R , Sokona Y , et al. Renewable Energy Sources and Climate Change Mitigation: Special Report of the Intergovernmental Panel on Climate Change. Cambridge : Cambridge University Press , 2011 .
Roaf S , Roaf S , Crichton D , et al. Adapting buildings and Cities for Climate Change: A 21st Century Survival Guide . 2nd edn. Oxford : Architectural Press , 2009 .
Sims RE . Renewable energy: a response to climate change . Solar Energy , 2004 , 76 : 9 – 17 .
Muneer T. Solar Radiation and Daylight Models. 2nd edn, London : Routledge , 2004 .
Martin J . ‘Green growth’: from a growing eco-industry to economic sustainability . Energy Policy , 2012 , 48 : 13 – 21 .
IRENA. A Roadmap to 2050: International Renewable Energy Agency: Global energy Transformation. Abu Dhabi : IRENA , 2018 .
Kost C , Mayer JN , Thomsen J , et al. Levelized Cost of Electricity Renewable Energy Technologies. Freiburg : Fraunhofer Institute for Solar Energy Systems (ISE), 2013 , 144 .
Cozzi L , Gould T , Bouckart S , et al. World Energy Outlook 2020. Paris : International Energy Agency , 2020 .
Ku AY , de Souza A , McRobie J , et al. Zero-emission public transit could be a catalyst for decarbonization of the transportation and power sectors . Clean Energy , 2021 , 5 : 492 – 504 .
Bouckaert S , Pales AF , McGlade C , et al. Net Zero by 2050: A Roadmap for the Global Energy Sector. Paris : International Energy Agency , 2021 .
Fraas LM . History of solar cell development . Low-cost Solar Electric Power. 2014 : 1 – 12 . doi: 10.1007/978-3-319-07530-31 .
Gahrens S , Alessandra S , Steinfatt K. Trading Into a Bright Energy Future. The Case for Open, High-Quality Solar Photovoltaic Markets . Abu Dhabi : IRENA , 2021 , 1 – 44 . https://irena.org/-/media/Files/IRENA/Agency/Publication/2021/Jul/IRENA_WTO_Trading_Energy_Future_2021.pdf ( 21 April 2022 , date last accessed).
IRENA . Solar Energy—International Renewable Energy Agency . 2021 . www.irena.org/solar ( 2 February 2022 , date last accessed).
Honsberg C , Bowden S . Sun Position Calculator . 2014 . http://pveducation org/pvcdrom/properties-of-sunlight/sun-position-calculator ( 25 January 2022 , date last accessed).
Green MA , Hishikawa Y , Dunlop ED , et al. Solar cell efficiency tables (version 52) . Progress in Photovoltaics , 2018 , 26 : 427 – 436 .
Kylili A , Fokaides PA . Investigation of building integrated photovoltaics potential in achieving the zero energy building target . Indoor Built Environment , 2014 , 23 : 92 – 106 .
Maka AO , O’Donovan TS . A review of thermal load and performance characterisation of a high concentrating photovoltaic (HCPV) solar receiver assembly . Solar Energy , 2020 , 206 : 35 – 51 .
Mohamed ET , Maka AO , Mehmood M , et al. Performance simulation of single and dual-junction GaInP/GaAs tandem solar cells using AMPS-1D . Sustainable Energy Technologies Assessments , 2021 , 44 : 101067 .
Maka AO , O’Donovan TS . Dynamic performance analysis of solar concentrating photovoltaic receiver by coupling of weather data with the thermal-electrical model . Thermal Science Engineering Progress , 2021 , 24 : 100923 .
Maka AO , O’Donovan TS . Transient thermal-electrical performance modelling of solar concentrating photovoltaic (CPV) receiver . Solar Energy , 2020 , 211 : 897 – 907 .
Radovanovic M , Popov S , Dodic S. Sustainable Energy Management. Cambridge, MA : Academic Press , 2012 .
Salvarli MS , Salvarli H . For sustainable development: future trends in renewable energy and enabling technologies . In: Al Al Qubeissi M, El-kharouf A, Soyhan HS (eds). Qubeissi M , El-kharouf A , Soyhan HS (eds). Renewable Energy-Resources, Challenges and Applications . London : IntechOpen , 2020 .
Maka AO , Salem S , Mehmood M . Solar photovoltaic (PV) applications in Libya: challenges, potential, opportunities and future perspectives . Cleaner Engineering Technology , 2021 , 51 : 100267 .
IRENA . Renewable Energy and Jobs—Annual Review 2021, (REJ) . 2021 . https://www.irena.org/publications/2021/Oct/Renewable-Energy-and-Jobs-Annual-Review-2021 ( 2 January 2022 , date last accessed).
Obaideen K , AlMallahi MN , Alami AH , et al. On the contribution of solar energy to sustainable developments goals: case study on Mohammed bin Rashid Al Maktoum Solar Park . International Journal of Thermofluids , 2021 , 12 : 100123 .
IRENA . International Renewable Energy Agency, Renewable Energy and Jobs—Annual Review 2020. Abu Dhabi : IRENA , 2020 .
Strielkowski W , Civín L , Tarkhanova E , et al. Renewable energy in the sustainable development of electrical power sector: a review . Energies , 2021 , 14 : 8240 .
Grid-Arendal . Natural Resources—Solar Power (Potential) . 2008 . https://www.grida.no/resources/7308 ( 9 February 2022 , date last accessed).
Kannan N , Vakeesan D . Solar energy for future world: a review . Renewable Sustainable Energy Reviews , 2016 , 62 : 1092 – 1105 .
Löf GO , Duffie JA , Smith CO . World distribution of solar radiation . Solar Energy , 1966 , 10 : 27 – 37 .
Kabir E , Kumar P , Kumar S , et al. Solar energy: potential and future prospects . Renewable Sustainable Energy Reviews , 2018 , 82 : 894 – 900 .
Johansson TB , Goldemberg J. Energy for Sustainable Development: A Policy Agenda. New York : United Nations Development Programme (UNDP) , 2002 .
Lowe R , Drummond P . Solar, wind and logistic substitution in global energy supply to 2050—barriers and implications . Renewable Sustainable Energy Reviews , 2022 , 153 : 111720 .
Asmelash E , Prakash G. Future of Solar Photovoltaic: Deployment, Investment, Technology, Grid Integration and Socio-economic Aspects . Abu Dhabi : IRENA , 2019 .
Griffiths S . Strategic considerations for deployment of solar photovoltaics in the Middle East and North Africa . Energy Strategy Reviews , 2013 , 2 : 125 – 131 .
Hafner M , Tagliapietra S , El Andaloussi EH . Outlook for Electricity and Renewable Energy in Southern and Eastern Mediterranean Countries. WP4b, Energy and Climate Change Mitigations, MEDPROTechnical Report No. 16/October 2012 . www.medpro-foresight.eu ( 25 January 2022 , date last accessed).
Martí A , Luque A. Next Generation Photovoltaics: High Efficiency Through Full Spectrum Utilization . 1st edn. Boca Raton, FL : CRC Press , 2003 .
Dimroth F , Kurtz S . High-efficiency multijunction solar cells . MRS Bulletin , 2007 , 32 : 230 – 235 .
Kashmir J . Solar Energy for Sustainable Development . 2018 . https://www.dailyexcelsior.com/solar-energy-sustainable-development/ ( 15 January 2022 , date last accessed).
Email alerts
Citing articles via.
- Advertising and Corporate Services
Affiliations
- Online ISSN 2515-396X
- Print ISSN 2515-4230
- Copyright © 2024 National Institute of Clean-and-Low-Carbon Energy
- About Oxford Academic
- Publish journals with us
- University press partners
- What we publish
- New features
- Open access
- Institutional account management
- Rights and permissions
- Get help with access
- Accessibility
- Advertising
- Media enquiries
- Oxford University Press
- Oxford Languages
- University of Oxford
Oxford University Press is a department of the University of Oxford. It furthers the University's objective of excellence in research, scholarship, and education by publishing worldwide
- Copyright © 2024 Oxford University Press
- Cookie settings
- Cookie policy
- Privacy policy
- Legal notice
This Feature Is Available To Subscribers Only
Sign In or Create an Account
This PDF is available to Subscribers Only
For full access to this pdf, sign in to an existing account, or purchase an annual subscription.
- Future Energy Systems Center
- Studies and reports
- Funding opportunities
- Basic energy science
- Built environment and infrastructure
- Climate and environment
- Conventional energy
- Developing world
- Energy efficiency
- Nuclear energy
- Policy and economics
- Power distribution and energy storage
- Renewable energy
- Transportation
- Undergraduate education
- Graduate & postdoctoral
- Online education
- Education research
- Current members
- Energy Futures
- In the media
- Affiliations
The Future of Solar Energy
Read the report.
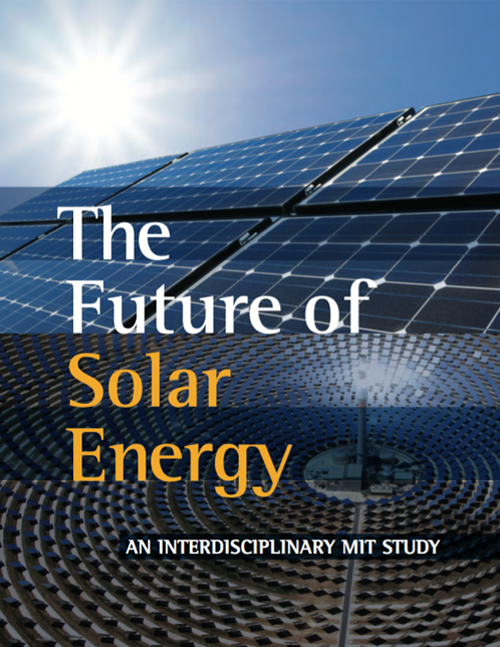
Executive summary (PDF) Full report (PDF)
The Future of Solar Energy considers only the two widely recognized classes of technologies for converting solar energy into electricity — photovoltaics (PV) and concentrated solar power (CSP), sometimes called solar thermal) — in their current and plausible future forms. Because energy supply facilities typically last several decades, technologies in these classes will dominate solar-powered generation between now and 2050, and we do not attempt to look beyond that date. In contrast to some earlier Future of studies, we also present no forecasts — for two reasons. First, expanding the solar industry dramatically from its relatively tiny current scale may produce changes we do not pretend to be able to foresee today. Second, we recognize that future solar deployment will depend heavily on uncertain future market conditions and public policies — including but not limited to policies aimed at mitigating global climate change.
As in other studies in this series, our primary aim is to inform decision-makers in the developed world, particularly the United States. We concentrate on the use of grid-connected solar-powered generators to replace conventional sources of electricity. For the more than one billion people in the developing world who lack access to a reliable electric grid, the cost of small-scale PV generation is often outweighed by the very high value of access to electricity for lighting and charging mobile telephone and radio batteries. In addition, in some developing nations it may be economic to use solar generation to reduce reliance on imported oil, particularly if that oil must be moved by truck to remote generator sites. A companion working paper discusses both these valuable roles for solar energy in the developing world.
Related publications
Shaping photovoltaic array output to align with changing wholesale electricity price profiles
December 2019
Spatial and temporal variation in the value of solar power across United States electricity markets
Solar heating for residential and industrial processes
Related news
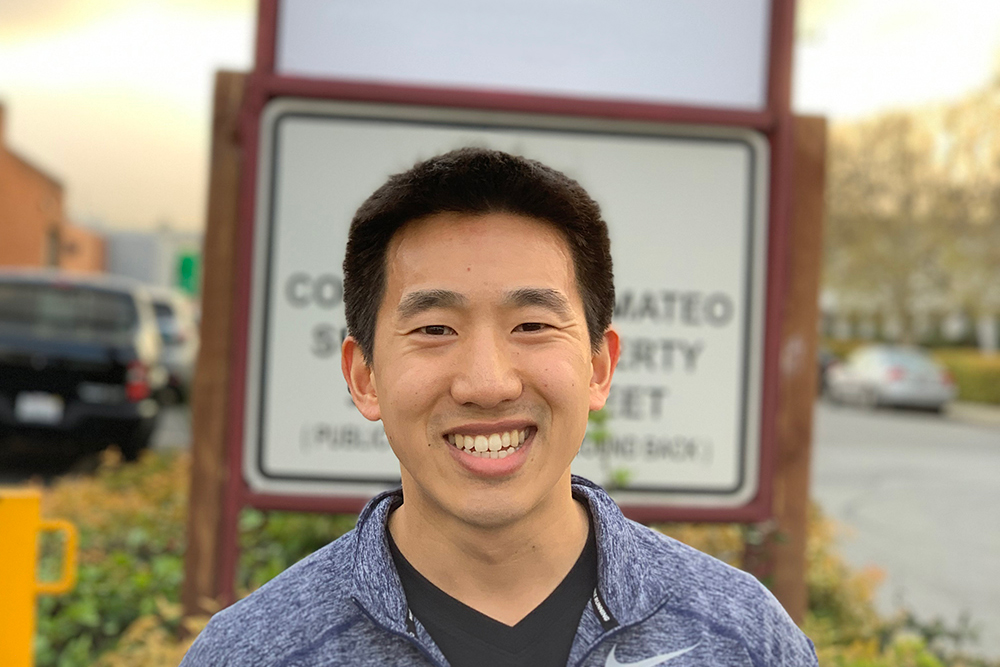
MIT Energy Initiative Director Robert Armstrong shares perspectives on past successes and ongoing and future energy projects at the Institute.
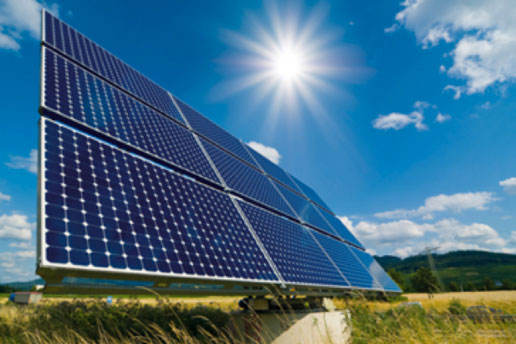
Suggestions or feedback?
MIT News | Massachusetts Institute of Technology
- Machine learning
- Social justice
- Black holes
- Classes and programs
Departments
- Aeronautics and Astronautics
- Brain and Cognitive Sciences
- Architecture
- Political Science
- Mechanical Engineering
Centers, Labs, & Programs
- Abdul Latif Jameel Poverty Action Lab (J-PAL)
- Picower Institute for Learning and Memory
- Lincoln Laboratory
- School of Architecture + Planning
- School of Engineering
- School of Humanities, Arts, and Social Sciences
- Sloan School of Management
- School of Science
- MIT Schwarzman College of Computing
Researchers find benefits of solar photovoltaics outweigh costs
Press contact :.
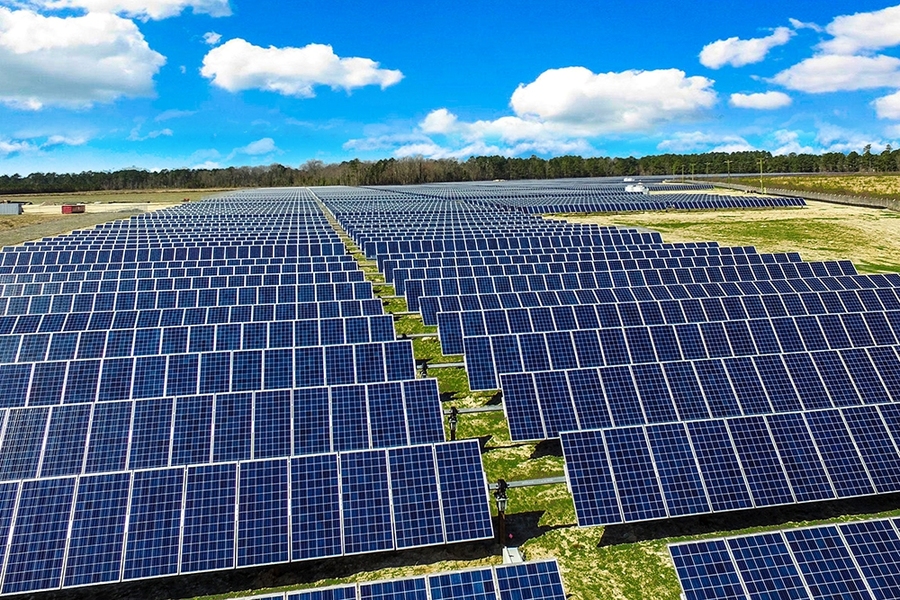
Previous image Next image
Over the past decade, the cost of solar photovoltaic (PV) arrays has fallen rapidly. But at the same time, the value of PV power has declined in areas that have installed significant PV generating capacity. Operators of utility-scale PV systems have seen electricity prices drop as more PV generators come online. Over the same time period, many coal-fired power plants were required to install emissions-control systems, resulting in declines in air pollution nationally and regionally. The result has been improved public health — but also a decrease in the potential health benefits from offsetting coal generation with PV generation.
Given those competing trends, do the benefits of PV generation outweigh the costs? Answering that question requires balancing the up-front capital costs against the lifetime benefits of a PV system. Determining the former is fairly straightforward. But assessing the latter is challenging because the benefits differ across time and place. “The differences aren’t just due to variation in the amount of sunlight a given location receives throughout the year,” says Patrick R. Brown PhD ’16, a postdoc at the MIT Energy Initiative. “They’re also due to variability in electricity prices and pollutant emissions.”
The drop in the price paid for utility-scale PV power stems in part from how electricity is bought and sold on wholesale electricity markets. On the “day-ahead” market, generators and customers submit bids specifying how much they’ll sell or buy at various price levels at a given hour on the following day. The lowest-cost generators are chosen first. Since the variable operating cost of PV systems is near zero, they’re almost always chosen, taking the place of the most expensive generator then in the lineup. The price paid to every selected generator is set by the highest-cost operator on the system, so as more PV power comes on, more high-cost generators come off, and the price drops for everyone. As a result, in the middle of the day, when solar is generating the most, prices paid to electricity generators are at their lowest.
Brown notes that some generators may even bid negative prices. “They’re effectively paying consumers to take their power to ensure that they are dispatched,” he explains. For example, inflexible coal and nuclear plants may bid negative prices to avoid frequent shutdown and startup events that would result in extra fuel and maintenance costs. Renewable generators may also bid negative prices to obtain larger subsidies that are rewarded based on production.
Health benefits also differ over time and place. The health effects of deploying PV power are greater in a heavily populated area that relies on coal power than in a less-populated region that has access to plenty of clean hydropower or wind. And the local health benefits of PV power can be higher when there’s congestion on transmission lines that leaves a region stuck with whatever high-polluting sources are available nearby. The social costs of air pollution are largely “externalized,” that is, they are mostly unaccounted for in electricity markets. But they can be quantified using statistical methods, so health benefits resulting from reduced emissions can be incorporated when assessing the cost-competitiveness of PV generation.
The contribution of fossil-fueled generators to climate change is another externality not accounted for by most electricity markets. Some U.S. markets, particularly in California and the Northeast, have implemented cap-and-trade programs, but the carbon dioxide (CO 2 ) prices in those markets are much lower than estimates of the social cost of CO 2 , and other markets don’t price carbon at all. A full accounting of the benefits of PV power thus requires determining the CO 2 emissions displaced by PV generation and then multiplying that value by a uniform carbon price representing the damage that those emissions would have caused.
Calculating PV costs and benefits
To examine the changing value of solar power, Brown and his colleague Francis M. O’Sullivan, the senior vice president of strategy at Ørsted Onshore North America and a senior lecturer at the MIT Sloan School of Management, developed a methodology to assess the costs and benefits of PV power across the U.S. power grid annually from 2010 to 2017.
The researchers focused on six “independent system operators” (ISOs) in California, Texas, the Midwest, the Mid-Atlantic, New York, and New England. Each ISO sets electricity prices at hundreds of “pricing nodes” along the transmission network in their region. The researchers performed analyses at more than 10,000 of those pricing nodes.
For each node, they simulated the operation of a utility-scale PV array that tilts to follow the sun throughout the day. They calculated how much electricity it would generate and the benefits that each kilowatt would provide, factoring in energy and “capacity” revenues as well as avoided health and climate change costs associated with the displacement of fossil fuel emissions. (Capacity revenues are paid to generators for being available to deliver electricity at times of peak demand.) They focused on emissions of CO 2 , which contributes to climate change, and of nitrogen oxides (NO x ), sulfur dioxide (SO 2 ), and particulate matter called PM 2.5 — fine particles that can cause serious health problems and can be emitted or formed in the atmosphere from NO x and SO 2 .
The results of the analysis showed that the wholesale energy value of PV generation varied significantly from place to place, even within the region of a given ISO. For example, in New York City and Long Island, where population density is high and adding transmission lines is difficult, the market value of solar was at times 50 percent higher than across the state as a whole.
The public health benefits associated with SO 2 , NO x , and PM 2.5 emissions reductions declined over the study period but were still substantial in 2017. Monetizing the health benefits of PV generation in 2017 would add almost 75 percent to energy revenues in the Midwest and New York and fully 100 percent in the Mid-Atlantic, thanks to the large amount of coal generation in the Midwest and Mid-Atlantic and the high population density on the Eastern Seaboard.
Based on the calculated energy and capacity revenues and health and climate benefits for 2017, the researchers asked: Given that combination of private and public benefits, what upfront PV system cost would be needed to make the PV installation “break even” over its lifetime, assuming that grid conditions in that year persist for the life of the installation? In other words, says Brown, “At what capital cost would an investment in a PV system be paid back in benefits over the lifetime of the array?”
Assuming 2017 values for energy and capacity market revenues alone, an unsubsidized PV investment at 2017 costs doesn’t break even. Add in the health benefit, and PV breaks even at 30 percent of the pricing nodes modeled. Assuming a carbon price of $50 per ton, the investment breaks even at about 70 percent of the nodes, and with a carbon price of $100 per ton (which is still less than the price estimated to be needed to limit global temperature rise to under 2 degrees Celsius), PV breaks even at all of the modeled nodes.
That wasn’t the case just two years earlier: At 2015 PV costs, PV would only have broken even in 2017 at about 65 percent of the nodes counting market revenues, health benefits, and a $100 per ton carbon price. “Since 2010, solar has gone from one of the most expensive sources of electricity to one of the cheapest, and it now breaks even across the majority of the U.S. when considering the full slate of values that it provides,” says Brown.
Based on their findings, the researchers conclude that the decline in PV costs over the studied period outpaced the decline in value, such that in 2017 the market, health, and climate benefits outweighed the cost of PV systems at the majority of locations modeled. “So the amount of solar that’s competitive is still increasing year by year,” says Brown.
The findings underscore the importance of considering health and climate benefits as well as market revenues. “If you’re going to add another megawatt of PV power, it’s best to put it where it’ll make the most difference, not only in terms of revenues but also health and CO 2 ,” says Brown.
Unfortunately, today’s policies don’t reward that behavior. Some states do provide renewable energy subsidies for solar investments, but they reward generation equally everywhere. Yet in states such as New York, the public health benefits would have been far higher at some nodes than at others. State-level or regional reward mechanisms could be tailored to reflect such variation in node-to-node benefits of PV generation, providing incentives for installing PV systems where they’ll be most valuable. Providing time-varying price signals (including the cost of emissions) not only to utility-scale generators, but also to residential and commercial electricity generators and customers, would similarly guide PV investment to areas where it provides the most benefit.
Time-shifting PV output to maximize revenues
The analysis provides some guidance that might help would-be PV installers maximize their revenues. For example, it identifies certain “hot spots” where PV generation is especially valuable. At some high-electricity-demand nodes along the East Coast, for instance, persistent grid congestion has meant that the projected revenue of a PV generator has been high for more than a decade. The analysis also shows that the sunniest site may not always be the most profitable choice. A PV system in Texas would generate about 20 percent more power than one in the Northeast, yet energy revenues were greater at nodes in the Northeast than in Texas in some of the years analyzed.
To help potential PV owners maximize their future revenues, Brown and O’Sullivan performed a follow-on study focusing on ways to shift the output of PV arrays to align with times of higher prices on the wholesale market. For this analysis, they considered the value of solar on the day-ahead market and also on the “real-time market,” which dispatches generators to correct for discrepancies between supply and demand. They explored three options for shaping the output of PV generators, with a focus on the California real-time market in 2017, when high PV penetration led to a large reduction in midday prices compared to morning and evening prices.
- Curtailing output when prices are negative: During negative-price hours, a PV operator can simply turn off generation. In California in 2017, curtailment would have increased revenues by 9 percent on the real-time market compared to “must-run” operation.
- Changing the orientation of “fixed-tilt” (stationary) solar panels: The general rule of thumb in the Northern Hemisphere is to orient solar panels toward the south, maximizing production over the year. But peak production then occurs at about noon, when electricity prices in markets with high solar penetration are at their lowest. Pointing panels toward the west moves generation further into the afternoon. On the California real-time market in 2017, optimizing the orientation would have increased revenues by 13 percent, or 20 percent in conjunction with curtailment.
- Using 1-axis tracking: For larger utility-scale installations, solar panels are frequently installed on automatic solar trackers, rotating throughout the day from east in the morning to west in the evening. Using such 1-axis tracking on the California system in 2017 would have increased revenues by 32 percent over a fixed-tilt installation, and using tracking plus curtailment would have increased revenues by 42 percent.
The researchers were surprised to see how much the optimal orientation changed in California over the period of their study. “In 2010, the best orientation for a fixed array was about 10 degrees west of south,” says Brown. “In 2017, it’s about 55 degrees west of south.” That adjustment is due to changes in market prices that accompany significant growth in PV generation — changes that will occur in other regions as they start to ramp up their solar generation.
The researchers stress that conditions are constantly changing on power grids and electricity markets. With that in mind, they made their database and computer code openly available so that others can readily use them to calculate updated estimates of the net benefits of PV power and other distributed energy resources.
They also emphasize the importance of getting time-varying prices to all market participants and of adapting installation and dispatch strategies to changing power system conditions. A law set to take effect in California in 2020 will require all new homes to have solar panels. Installing the usual south-facing panels with uncurtailable output could further saturate the electricity market at times when other PV installations are already generating.
“If new rooftop arrays instead use west-facing panels that can be switched off during negative price times, it’s better for the whole system,” says Brown. “Rather than just adding more solar at times when the price is already low and the electricity mix is already clean, the new PV installations would displace expensive and dirty gas generators in the evening. Enabling that outcome is a win all around.”
Patrick Brown and this research were supported by a U.S. Department of Energy Office of Energy Efficiency and Renewable Energy (EERE) Postdoctoral Research Award through the EERE Solar Energy Technologies Office. The computer code and data repositories are available here and here .
This article appears in the Spring 2020 issue of Energy Futures, the magazine of the MIT Energy Initiative.
Share this news article on:
Related links.
- Paper: “Shaping photovoltaic array output to align with changing wholesale electricity price profiles.”
- Paper: “Spatial and temporal variation in the value of solar power across United States electricity markets.”
- Report: “The Future of Solar Energy”
- Patrick Brown
- MIT Energy Initiative
- Energy Futures magazine
Related Topics
- MIT Sloan School of Management
- Climate change
- Photovoltaics
- Environment
- Sustainability
- Renewable energy
Related Articles
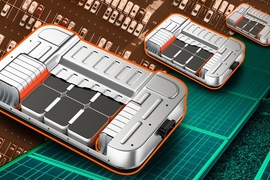
Solar energy farms could offer second life for electric vehicle batteries
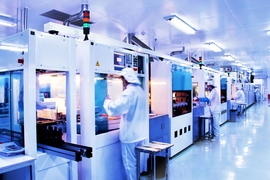
For cheaper solar cells, thinner really is better
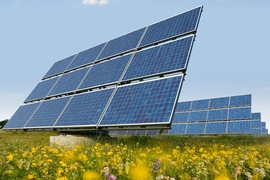
MITEI releases report on the future of solar energy
Previous item Next item
More MIT News
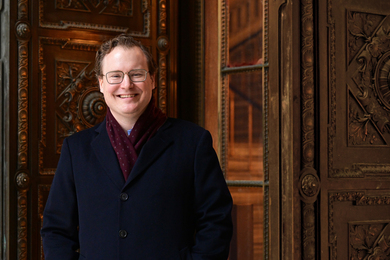
Exploring the history of data-driven arguments in public life
Read full story →
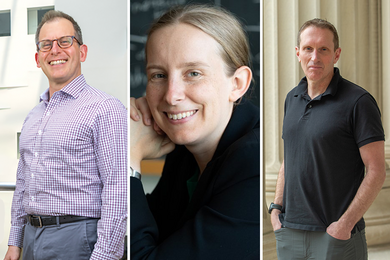
Three from MIT awarded 2024 Guggenheim Fellowships
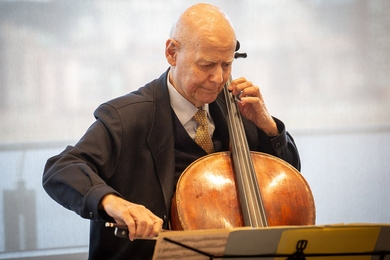
A musical life: Carlos Prieto ’59 in conversation and concert
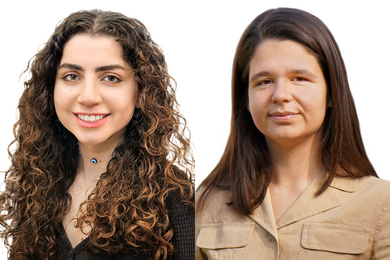
Two from MIT awarded 2024 Paul and Daisy Soros Fellowships for New Americans
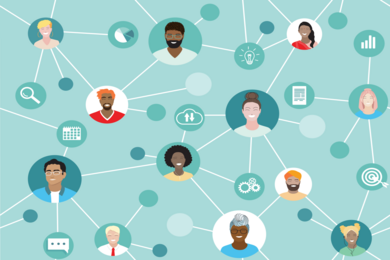
MIT Emerging Talent opens pathways for underserved global learners
The MIT Edgerton Center’s third annual showcase dazzles onlookers
- More news on MIT News homepage →
Massachusetts Institute of Technology 77 Massachusetts Avenue, Cambridge, MA, USA
- Map (opens in new window)
- Events (opens in new window)
- People (opens in new window)
- Careers (opens in new window)
- Accessibility
- Social Media Hub
- MIT on Facebook
- MIT on YouTube
- MIT on Instagram

An official website of the United States government
The .gov means it’s official. Federal government websites often end in .gov or .mil. Before sharing sensitive information, make sure you’re on a federal government site.
The site is secure. The https:// ensures that you are connecting to the official website and that any information you provide is encrypted and transmitted securely.
- Publications
- Account settings
Preview improvements coming to the PMC website in October 2024. Learn More or Try it out now .
- Advanced Search
- Journal List
- v.45(Suppl 1); 2016 Jan

Solar energy for electricity and fuels
Olle inganäs.
Biomolecular and Organic Electronics, IFM, Linköpings Universitet, 58183 Linköping, Sweden
Villy Sundström
Chemical Physics, Lund University, P.O. Box 124, 22100 Lund, Sweden
Solar energy conversion into electricity by photovoltaic modules is now a mature technology. We discuss the need for materials and device developments using conventional silicon and other materials, pointing to the need to use scalable materials and to reduce the energy payback time. Storage of solar energy can be achieved using the energy of light to produce a fuel. We discuss how this can be achieved in a direct process mimicking the photosynthetic processes, using synthetic organic, inorganic, or hybrid materials for light collection and catalysis. We also briefly discuss challenges and needs for large-scale implementation of direct solar fuel technologies.
Introduction
Renewable energies are still dominated by bio-energy and hydro-energy. This technology is well developed and more energy can be produced but are inherently limited (Fig. 1 ). Vegetation exists on some 80 million km 2 with an average net bio-energy production of around 0.1 W/m 2 . Higher production would require active nitrogen. Hydro-energy is even more limited.

A comparison of finite and renewable planetary energy reserves measured in TW/years. Total recoverable reserves are shown in TWy for the finite resources, and yearly potential for the renewables. OTEC = Ocean thermal energy conversion. One TWy is 8760 TWh. This figure does not include shale gas or shale oil nor the energy available in methane hydrate. Source: Perez and Perez ( 2009 )
Photovoltaic electricity from solar cells has undergone a rapid development and is rapidly being used both in minor private systems as well as in large-scale installations connected to the national grids. Wind energy has also reach maturity and undergoes presently a rapid worldwide implementation. The main challenge with the renewable energy is the intermittency requiring major storage or large-scale integration.
This article focuses on solar energy, identifying the need for breakthroughs in more efficient ways to produce (i) electricity from more powerful and cost efficient solar cells (ii) the possibility of direct conversion of solar energy into fluid (such as ethanol or methanol) or gas forms (methane of hydrogen), and (iii) the need for producing significantly higher bioconversion including breeding of special plants and genetic engineering of cyanobacteria.
The annual flow of energy from the sun dwarfs all other non-renewable energy flows and stocks, and is several orders of magnitude above what humankind needs (Fig. 1 ). Very major secondary flows of solar energy are concentrated by the thermal engine of the atmosphere, supplying flow of water for hydropower, and flow of air for wind power. From inside the Earth, geothermal heat is delivered from nuclear processes. All these are very small by comparison to the solar influx. The solar influx over land on a horizontal surface varies considerably between different regions and with the diurnal and annual cycle. The most favorable areas are in the subtropics where values can reach almost 300 W/m 2 in annual average, while in parts of northern Europe values can be as low as around 50 W/m 2 with very low values during winter. The diurnal variation of the solar energy influx matches somewhat but not perfectly the activity patterns of societies. There is therefore a great need to store solar derived energy as electrical or chemical energy, to be used at some later time.
Photovoltaic
Current scientific status.
Photovoltaic devices generate electrical power upon illumination (Fig. 2 ). The electrical current is generated as photons from sunlight are absorbed and charges are generated in a semiconductor material. Semiconductors such as silicon absorb a large fraction of sunlight, but below the absorption edge (band gap) of the semiconductor, no absorption occurs. This controls the photocurrent and thus the electrical power of the devices, in combination with the voltage which is influenced by very basic physics and materials science parameters.

a The solar radiation arriving at the surface of the earth is distributed over different wavelengths of light, with tails extending far out in the infrared and invisible region. With semiconductor materials, light with energy higher/wavelengths shorter than the band gap of the material can be absorbed (shadow in graph), but none below the band gap. b The semiconductor is contacted with electrodes and exposed to solar light. Photocurrent J sc from the devices depends on absorption and charge generation in the active material; the photo-voltage V oc basically depends on the materials. c The current–voltage curve shows both these parameters, and the device is used to deliver electricity at the maximum power delivery point, indicated with a star
The science of photovoltaic is a mature field. Novel photovoltaic materials arrive, and scientists later learn how to accommodate these novel phenomena to the scientific terminology of photovoltaics. The thermodynamic limitations analyzed by Shockley and Queisser (S–Q) in ( 1961 ) resulted in a maximum efficiency of slightly over 33 % in a single-junction device using a band gap of 1.1–1.4 eV. These limitations are due to heat losses from high energy photons above the single-band gap, and from loss of photons not absorbed below the band gap. It is also based on the equilibrium flux of photons to the photovoltaic device from the sun, and the flow of photons to the universe from the photovoltaic device. This is a description that is valid for well established materials, like crystalline and poly-crystalline silicon-based photovoltaic, but also for amorphous inorganic semiconductors in thin film format, as well as newer organics in wet or dry state conditions, and new hybrid organic–inorganic materials. Though the science is mature, the implications of this science have not always been fully acknowledged. The recent improvement, to 28.8 % power conversion efficiency (PCE), of high performance gallium arsenide (GaAs) single-junction solar cells, has been accomplished by photonic engineering, to manipulate the balance between the incoming and outgoing photon flux, with the reward coming as voltage from the cell (Miller et al. 2012 ).
Improved energy conversion is found in multi-junction solar cells, or tandem solar cells, where the heat and transmission losses are minimized by use of two, three, or more different materials of different band gaps, and connecting these devices in electrical series. These are very high performance solar cells, with power conversion efficiencies up to 44.7 % under the direct sun spectrum at very high concentrations. These solar cells are mostly used in space, or under concentrated solar light on earth. The delicate growth of several compound semiconductors on top of each other to create these structures results in a higher material and processing cost. Therefore, these solar cells are mostly relevant together with concentrating optical elements, and thus require high performance, low-cost concentrators, which only work well in direct sunlight. Such concentrator systems are today becoming more widely available and applications in the multi MW range have been realized.
Attempts to use the part of the solar spectrum found in the infrared and far infrared, carrying 50 % of the solar energy, for photovoltaic energy conversion is of relevance, in particular in combination with tandems. For single-band gap devices, the optimum band gap is 1.1–1.4 eV, and the lower energy part of the solar spectrum is sacrificed.
Current technological developments
Developments of silicon photovoltaic markets and technology.
The goal of 0.4 €/W p for photovoltaic (PV) modules is close to appear in the silicon photovoltaic market, where the wholesale price is now 0.5 €/W p , and where the financial difficulties of most companies indicates that variable costs in their production are c. 0.4 €/W p . The dramatic reductions of silicon PV sales prices (to 20 % during the last 5 years) are mainly due to market pressure resulting from oversupply, increased production volumes leading to cost reduction by scale, and to some degree also due to technological advancement. Extrapolating that pace of technological improvement in the near future, short-term market and technology analysis indicate that by year 2017 the production cost of 0.3 €/W p will be reached. The learning curve of silicon PV may, however, decelerate as sales prices go so low that new technology cannot be acquired and financed, or by reaching the technology limits given by the requirement for crystalline layers with – due to the indirect band gap of silicon—large thickness.
However, already today photovoltaic electricity generation cost can be as low as 12 €cents/KWh in countries like France or Germany and 8 €cents/KWh in the south of Europe. Within the next decade solar electricity from photovoltaics is likely to become the cheapest form of renewable energy in most parts of the world. Silicon is an established photovoltaic material, it is widely available, non-toxic and it is likely to dominate the market for the foreseeable future. However, production cost of electricity is not the only element, as the electricity system cost represents typically 40–60 % of the final cost.
This major cost reduction of silicon PV puts significant pressure on all alternative technologies like thin film inorganic photovoltaics (e.g., a-Si, CdTe, CIGS) which lost in market share during recent years, but improved somewhat in record performance numbers. All PV technologies in the future will have to approach higher conversion efficiencies to reduce the contribution of all other module components. Whether there is a place for low efficiency, low-cost solar cells to compete with silicon photovoltaic is now more doubtful than 5 years back, as the cost of silicon has dropped.
The combination of silicon PV with compound semiconductors (thin film III-V compounds or nanowires) in hybrid tandem photovoltaic promises big improvements of power conversion efficiencies, and may be one of the most attractive pathways for rapid improvement in PCE. Also these approaches can benefit from the advances in silicon photovoltaic like lower material costs and enhanced manufacturing technologies.
Hybrid organic/inorganic solar cells
High performance dye sensitized solar cells, where an organic compound in contact with a high band gap inorganic semiconductor (e.g., TiO 2 , ZnO, ZrO 2 ) generates charge upon photon absorption, still require a redox liquid electrolyte for best performance. Alternative redox design using cobalt compounds has improved efficiencies to 13 %. Solid state versions of the dye sensitized solar cells have lower PCE. A new inorganic absorber, a perovskite based on lead halogen compound first used for dye sensitized solar cells, has rapidly demonstrated that this compound can be used at its best (18 % power conversion efficiency) in thin film geometries (Jeon Jeon et al. 2015 ), as optical absorption and electronic transport is sufficient in this material; the advantages of the dye sensitized cells are thereby removed for this absorber.
Solid state organic solar cells
Development of organic donor/acceptor-based photovoltaic (OPV), where donor and acceptor are typically organic molecules or polymers, is accelerating, with best efficiencies now at 12 % (Heliatek 2012 ; Mitsubishi 2013 ). The photo-voltage from these materials is controlled by a charge transfer state generated at the interface between the donor/acceptor (Vandewal et al. 2009 ). Though the basic physics of these photovoltaic materials is now somewhat mature, there is still a large room for improvement by chemical design. With improvement of 1 % unit per year, and limits to PCE still above 20 %, there is reason to expect 15–20 % PCE as an outcome of novel materials.
As the active materials in OPVs are carbon based, these are generally very scalable materials. The production methods for OPV include printing from solvents or evaporation of molecules through vacuum. Both are amenable for large area deposition, with somewhat different potential speed of production and capital costs of equipment. Printing is the faster and cheaper, and rapidly scalable to produce large areas of photovoltaic modules on the global scale.
For both OPV and dye sensitized cells, they operate well under low lights, high temperatures and considerably improve the light harvesting compared to silicon devices. The extra yield of almost a 1/3 from an organic-based module will give the same amount of kWh from a 15 % organic module as compared to a 20 % silicon module.
In the comparison of early organic photovoltaic systems, which have no market share yet, issues of lifetime and module efficiency are largely unresolved. Whether they will be sufficient to compete with mature silicon photovoltaic is not clear, and they will most probably not enter into power plants but rather be used in building integration.
Needs for breakthroughs
Scalability of semiconductor materials.
Silicon- or carbon-based materials, which can be scaled up, deliver 25 % (Si) and 12–13 % (carbon based) power conversion efficiency in best lab devices. Several of the competing thin film technologies suffer from a dependence on rare or toxic materials which reduces their potential to play a significant role when photovoltaics will be used in large-scale systems. Therefore, the use of earth abundant materials or efficient recycling concepts will be important for large-scale deployment of the technologies. Removing materials limitations due to less abundant elements (for active materials e.g., indium, gallium, tellurium, for current collectors silver) by substitution with more abundant elements (e.g., zinc, sulfur or copper, aluminum) will be a general and critical target.
Decreasing the energy payback time of solar cells
Measures and means needed
- Reducing the energy input in photovoltaic modules production. Low-temperature processes, substrates with low embodied energy, repeated use of substrates for growth of high quality materials/devices, closure of the materials cycle during the life cycle of devices;
- Extending the technical lifetime of high performance photovoltaic by improved encapsulation and protection.
Materials design
- Designing the electronic properties of carbon-based materials by understanding and controlling the path from single molecular structures via nano-morphology to film growth. A prime example is bulk heterojunctions in organic solid state solar cells which need a nanometer control of morphology in the key active layer.
Areas where scientific and materials breakthroughs may be possible and of potential importance
- Multiple-band gap tandem solar cells incorporating scalable materials;
- Light concentration through far field optics for multiple-band gap materials;
- Nanophotonics in dielectrics and semiconductors for light-matter coupling, light trapping/ light management;
- Plasmonics for light-matter coupling, to direct energy to the semiconductor structure in photovoltaic devices;
- Combination with solar fuel generation, e.g., hydrogen generation by photovoltaic layers with catalyst electrodes.
Identification of research needs of the coming decades
Nanophotonic strategies for light trapping in thin structures.
The well established Yablonovitch limit for light trapping in the limit of geometrical optics is not relevant when arriving at thin film structures that must be analyzed with the wave optics approach. This goes for thin film devices with extremely thin absorbers, and new strategies for optical compression of solar light into such thin absorbers have been proposed. Considerable experimental developments are necessary to exploit such strategies, shaping, and integrating thin film absorbers in a benign optical environment.
Transparent electrode materials, not relying on elements of low abundance
- Carbon based or abundant metal oxide transparent conductors for electrodes;
- New combinations of device/module assembly to generate lower currents and higher voltages for decrease of losses in photovoltaic modules.
Beyond S–Q limits?
The perennial quest for ways of moving beyond the Shockley–Queisser limits for single- and multiple-band gap absorbers in photovoltaic devices may require new approaches for generating electricity. When developing hybrid approaches based on high performance semiconductors, the added energy conversion with novel materials and structures may include up-conversion. Without a band gap alternative physical mechanisms must be found. Current developments in thermoelectrics points to new combinations of photovoltaics and thermoelectrics; optical antennas and rectennas show interesting avenues for creating absorbing structures somewhere between geometric antennas and molecular/elemental semiconductors with defined band gaps.
Solar fuels: Artificial photosynthesis
Direct conversion of solar energy into a fuel mimicking the catalytic processes of photosynthesis would be a way to solve the problem of storing solar energy. A direct process would minimize the number of steps needed from light to fuel, and therefore potentially allow for high conversion efficiencies. The raw material for the (storage) fuel is an important key aspect for the solar fuels. The desirable raw material must be essentially inexhaustible, cheap, and widely available. Most scientists target water as the raw material. Processes in which water is split (oxidized) into its constituents by solar energy can become large contributors to shift away from fossil fuels on a global scale.
The target fuel is another important issue. Many scientists target hydrogen as the solar fuel. When water is used as starting material this is natural for scientific reasons. However, the transition to a hydrogen-based economy is not easy. The hydrogen technologies have their own hurdles to overcome and technological issues to tackle before hydrogen can become a worldwide spread fuel. An alternative is to use CO 2 itself as a second raw material (together with water) to create a carbon-based solar fuel. This might have lower technological problems on a shorter term but will not remove CO 2 from the energy cycle (see Fig. 1 ). In addition, some of the science involved in developing methods for fuel production is most likely more difficult than for hydrogen production.
The envisaged key components of an assembly for production of a solar fuel (H 2 ) using water as raw material are illustrated in Fig. 3 (Hammarström and Hammes-Schiffer 2009 ; Cogdell et al. 2013 ). A photo-sensitizer (PS) absorbs the light from the sun and delivers energized electrons to the proton reducing catalysts (PRC) that generates the fuel, H 2 . The electrons delivered by the sensitizer are replenished by oxidizing (splitting) water with the help of a water splitting catalyst (WSC). In an operating device the WSC and PRC functions would most likely be driven by different sensitizers and physically separated and located on different photo-electrodes in contact with water.

Schematic picture of an artificial photosynthesis assembly consisting of a light collecting photosensitizer (PS), a proton reducing catalyst (PRC), and a water splitting catalyst (WSC)
Predicted power conversion efficiencies: Direct processes for solar fuel
Today, no complete device for (direct) solar fuel generation exists, but sensitizers and catalysts that perform the individual reactions have been developed. Nevertheless, a maximum theoretical Shockley–Queisser (S–Q) efficiency of ~30 % for (direct) solar fuel generation (hydrogen and oxygen from water) can be estimated for a single-band gap sensitized process and assuming a realistic value (~0.15 V) for the over-potential (Hanna and Nozik 2006 ; Blankenship et al. 2011 ). It is foreseeable that the time curve for the development of solar fuel generation devices, with respect both to efficiency and cost, will be faster than for the different PV technologies, because the development to some extent will benefit from progress already made in neighboring fields. The most important being the scientific level in light management, electron and proton transfer, material and nano-science, biochemical, and natural photosynthesis. Technological advances in characterization methods, such as time resolved spectroscopy of many kinds, synchrotrons and new laser techniques, are also vital. Sensitizers and catalysts available today frequently contain elements that are scarce, expensive, or toxic. For large-scale commercial implementation of solar fuels produced by artificial photosynthesis technologies materials based on abundant and environmentally benign elements have to be developed, which most likely will take an additional 10 years or more. This restriction is analogous to PV technology limitations.
In comparison, for solar fuel generation relying on a combination of PV and electrolysis, the thermodynamic limits are given by the S–Q treatment in combination with conditions for electrolysis. For the case of photoelectrolysis, with production of H 2 and O 2 , the over potentials for oxidation of water to oxygen and reduction of protons to hydrogen must be added to the thermodynamic potential of 1.23 V for dissociation. Driven by solar cells, this requires more than one cell, added in series, to generate sufficient voltage for electrolysis. With electrolysis having a quantum efficiency of 80–90 %, and a single-band gap cell of 33 % power conversion efficiency, the maximum efficiency from photon to hydrogen may be limited to ≈30 %. The “artificial leaf” construction of Nocera ( 2012 ), comprising a triple junction amorphous silicon photovoltaic cell interfaced with hydrogen- and oxygen-evolving catalysts made from a ternary alloy (NiMoZn) and a cobalt_phosphate cluster (Co-OEC), respectively, is an example of where this technology stands today. The reported overall efficiency of this device is 2.5 %.
Methods to produce direct solar fuels
At present there is no direct process that works on any technological scale. The large potential in the direct processes make them nevertheless attractive future technologies and their development is expected to be require heavy research initiatives in several fields.
As mentioned above and illustrated in Fig. 3 , the key steps for direct solar fuel production mimicking photosynthesis are two catalytic reactions, oxidation of water and reduction of a substrate to produce the fuel. If the substrate is protons a relatively well established catalytic process yields the hydrogen fuel. A carbon-based fuel, for example, an alcohol, might be easier to use, but is probably more difficult to make since it would involve less established catalytic processes. A highly critical aspect is that the catalysts should be made from earth abundant metals like cobalt, manganese, iron and nickel, while scarce elements like iridium, indium, palladium, platinum, rhenium, and ruthenium are not available in amounts large enough to allow development of processes and materials of a scale sufficient to replace fossil fuels.
Molecular and semiconductor nanostructure processes. The light-driven catalyst can be molecular (Hammarström and Hammes-Schiffer 2009 ; Sundström 2009 ; Nocera and Guldi 2009 ; Kamat and Bisquert 2013 ) or non-molecular (Cook et al. 2010 ; Poddutoori et al. 2011 ; Vagnini et al. 2013 ; Joya et al. 2013 ). The physical limitations are equal and the scientific problems are of equal magnitude. The development of catalysts that can assist the light-driven oxidation of water is the main research problem. The capture of solar energy and the formation of hydrogen are easier to achieve. Systems combining the two reactions (light-driven water oxidation and hydrogen formation) have the highest potential with respect to solar energy to fuel conversion of all systems envisioned today (Fig. 3 ).
The catalysts and maybe the entire system for artificial photosynthesis can be entirely molecular in nature. They can thereby be varied through small, deliberate synthetic modifications to improve and fine-tune their properties. They are also amenable to studies with high-level molecular or kinetic spectroscopy. Thereby, the catalytic process can be followed and understood to a very detailed level. A special angle to this research involves biomimetic approaches. Here, knowledge and chemical principles from extremely efficient enzymes are applied in entirely synthetic systems for artificial photosynthesis. A successful scientific example involves the development of di-iron catalysts for the reduction of protons to hydrogen. These are designed from deep knowledge about the structure and function of so called hydrogenase enzymes and there are recent examples of very efficient catalysts in the category (Tamagnini et al. 2007 ). Another example involves mimics of the natural photosynthetic reaction centres, with both catalysis and light harvesting in future artificial photosynthesis devices (Magnuson et al. 2009 ).
In non-molecular systems the light-driven catalysis occurs on metal surfaces, semiconductors or nanostructured carbon-based materials while the catalysts involved for water splitting are often cores of metal oxides, sometimes doped with other metals. A severe limitation is that systems many times are based on catalysts made from scarce and expensive metals. Another disadvantage when compared to the molecular systems is that it is much more difficult to study the mechanism for the reactions involved. An important advantage is that many non-molecular systems are seemingly sturdier against degradation and inactivation while most molecular systems studied to date are unstable and easily break during illumination. It is not obvious that this situation will always prevail when more functional systems have been better characterized. An analogy is the Grätzel cell where the individual parts are fairly unstable but the integrated system is very stable.
A rapid development involves ideas where molecular and non-molecular systems are mixed. They are sometimes known as hybrid systems and the science is very broad and collaborative, involving several scientific fields. Here, the solar energy capture system is semiconductor based or made from some other nanotechnology while the chemistry is carried out by linked molecular catalysts. These catalysts are then made from abundant materials like cobalt, iron, manganese, or nickel similar to the “purely” molecular systems described above. Often catalysis is driven by light via incorporation of a photo-sensitizer between the semiconductor and the catalyst. It is not unlikely that these mixed hybrid systems will become dominant in research and maybe technology since they combine advantages from both fields.
Thermochemical cycles. A totally different technology is the employment of thermal processes (Pagliaro et al. 2010 ) for solar fuels production, which involve generation of very high temperatures in closed environments to split water into its constituents directly. This results in a solar fuel when the high temperature is achieved in a reaction vessel in a solar tower by concentration of solar energy in a heliostat. The technology represents interesting engineering and physical science and is very demanding technically involving very high temperatures and huge systems like heliostats. They are mainly suitable to very sunny locations.
Direct solar fuel needs for breakthroughs
Efficient photo-sensitizers and photocatalysts.
For large-scale implementation of direct solar fuel technologies, water will necessarily be the ultimate electron source for reducing the fuel-producing substrate, protons, CO 2 , etc. New and highly efficient catalysts for water oxidation and fuel generation are urgently needed, as well as sensitizers based on abundant elements:
- Photo-sensitizers frequently contain noble metals (ruthenium), which have to be replaced with cheap and abundant elements. Iron is one example but dyes based on Fe and similar transition metals have unfavorable properties for solar energy conversion, necessitating scientific breakthroughs. At the same time fully organic dyes are appearing. Thus, it can be expected that sensitizers suitable for most applications and for large-scale implementation are likely to appear in not too distant future.
- To achieve highly efficient catalysts for water oxidation and hydrogen (liquid fuel) generation is a considerably more difficult and complex problem. Catalysts may be either molecular complex or solid state (e.g., metal oxide) based. Understanding the mechanisms of O-O and H-H bond formation is key to the development of efficient catalysts. Most of today’s catalysts have low efficiencies and are frequently based on scarce or noble metals, making them unsuitable for large-scale commercial implementation. Here, scientific breakthroughs are needed, both concerning efficiency and developing new catalysts based on abundant and cheap elements. Proof-of-concept catalysts with high efficiencies, based on metals like ruthenium, are likely to appear within the next 10 years, but catalysts suitable for commercial applications most likely will take considerably more time.
Liquid fuels
Liquid solar fuels would facilitate the use of existing infrastructure for the utilization of the fuel. By combining the protons and electrons released as a result of water oxidation with CO 2 in a catalytic process, alcohols or other liquid fuels could be synthesized. This is a scientifically challenging task and there are today no good catalysts for CO 2 reduction. Another problem is that the concentration of CO 2 in the atmosphere is low, necessitating an energy-consuming concentration step, or coupling of the solar fuel production to CO 2 -emissions from e.g., a fossil-fuel power plant or a CCS plant (see MacElroy 2016).
Functional devices for direct solar fuel production
No complete device for (direct) solar fuel production exists. New concepts to assemble sensitizers and catalysts into a functional device producing a fuel through light-driven water splitting have to be developed. One possibility that is considered within the field is to attach sensitizer-catalyst assemblies to a nanostructured electrode surface and placing these electrodes in separate compartments, one for fuel (hydrogen) generation and the other for oxygen evolution. Here, suitable electrode systems have to be developed—methods for sensitizer-catalyst assembly attachment to the electrode must be developed, and electrochemical properties of catalysts and electrode materials matched. Considering that this development is still in its scientific infancy, it will probably take more than 10 years until efficient proof-of-concept systems have been developed and considerably more time until commercially suitable devices are available.
Scalability of photo-sensitizers and catalysts
Like for PV, large-scale implementation of solar fuel technology requires cheap and earth abundant materials. Today’s molecular or semiconductor photo-sensitizers and photocatalysts often contain scarce, noble (and expensive), or toxic metals, which obviously need to be replaced by more abundant and benign elements. This may take a considerable amount of time and effort, since the photophysical, photochemical, and catalytic properties of materials based on the abundant elements are frequently very different from those of the metals used today. It will require extensive development work to achieve requested properties and efficiencies of the abundant materials.
Acknowledgments
VS would like to thank S. Styring for useful discussions and for providing parts of the text on solar fuels.
Biographies
is a Professor of Biomolecular and Organic Electronic at Linköping University. His research interests are in polymer optoelectronics and bioelectronics, conjugated polymer physics, and polymer electrochemistry.
is a Professor of Chemical Physics, Lund University. His research interests are in light-induced processes in photosynthesis and materials for solar energy conversion. Various forms of time resolved laser spectroscopy are used in this research.
Contributor Information
Olle Inganäs, Email: es.uil.mfi@gnilo .
Villy Sundström, Email: [email protected] .
- Blankenship RE, Tiede DM, Barber J, Brudvig GW, Fleming G, Ghirardi M, Gunner MR, Junge W, et al. Comparing photosynthetic and photovoltaic efficiencies and recognizing the potential for improvement. Science. 2011; 332 :805–809. doi: 10.1126/science.1200165. [ PubMed ] [ CrossRef ] [ Google Scholar ]
- Cogdell RJ, Gardiner AT, Molina PI, Cronin L. The use and misuse of photosynthesis in the quest for novel methods to harness solar energy to make fuel. Philosophical Transactions of the Royal Society A. 2013; 371 :20110603. doi: 10.1098/rsta.2011.0603. [ PubMed ] [ CrossRef ] [ Google Scholar ]
- Cook TR, Dogutan DK, Reece SY, Surendranath Y, Teets TS, Nocera DG. Solar energy supply and storage for the legacy and nonlegacy worlds. Chemical Reviews. 2010; 110 :6474–6502. doi: 10.1021/cr100246c. [ PubMed ] [ CrossRef ] [ Google Scholar ]
- Hammarström L, Hammes-Schiffer S. Artificial photosynthesis and solar fuels. Accounts of Chemical Research. 2009; 42 :1859–1860. doi: 10.1021/ar900267k. [ PubMed ] [ CrossRef ] [ Google Scholar ]
- Hanna MC, Nozik AJ. Solar conversion efficiency of photovoltaic and photoelectrolysis cells with carrier multiplication absorbers. Journal of Applied Physics. 2006; 100 :074510. doi: 10.1063/1.2356795. [ CrossRef ] [ Google Scholar ]
- Heliatek. 2012. Retrieved January 10, 2015 from www.heliatek.com .
- Jeon NJ, Noh JH, Yang WS, Kim YC, Ryu S, Seo J, Seok SI. Compositional engineering of perovskite materials for high-performance solar cells. Nature. 2015; 517 :476–480. doi: 10.1038/nature14133. [ PubMed ] [ CrossRef ] [ Google Scholar ]
- Joya KS, Joya YF, Ocakoglu K, van de Krol R. Water-splitting catalysis and solar fuel devices: Artificial leaves on the move. Angewandte Chemie International Edition. 2013; 52 :10426–10437. doi: 10.1002/anie.201300136. [ PubMed ] [ CrossRef ] [ Google Scholar ]
- Kamat P, Bisquert J. Solar fuels. Photocatalytic hydrogen generation. Journal of Physical Chemistry C. 2013; 117 :14873–14875. doi: 10.1021/jp406523w. [ CrossRef ] [ Google Scholar ]
- Magnuson A, Johansson O, Polivka T, Anderlund M, Lindblad P, Styring S, Ott S, Lomoth R, et al. Biomimetic and microbial approaches to solar fuel generation. Accounts of Chemical Research. 2009; 42 :1899–1909. doi: 10.1021/ar900127h. [ PubMed ] [ CrossRef ] [ Google Scholar ]
- Miller OD, Yablonovitch E, Kurtz SR. Strong internal and external luminescence as solar cells approach the Shockley–Queisser limit. IEEE Journal of Photovoltaics. 2012; 2 :303–311. doi: 10.1109/JPHOTOV.2012.2198434. [ CrossRef ] [ Google Scholar ]
- Mitsubishi. 2013. Retrieved January 10, 2015 from http://www.m-kagaku.co.jp/english/r_td/strategy/technology/topics/opv/ .
- Nocera DG. The artificial leaf. Accounts of Chemical Research. 2012; 45 :767–776. doi: 10.1021/ar2003013. [ PubMed ] [ CrossRef ] [ Google Scholar ]
- Nocera, D., and D. Guldi. (ed.). 2009. Themed issue: Renewable energy, Chemical Society Reviews . 38: 1–300.
- Pagliaro M, Konstandopoulos AG, Ciriminna R, Palmisano G. Solar hydrogen: Fuel of the near future. Energy & Environmental Science. 2010; 3 :279–287. doi: 10.1039/b923793n. [ CrossRef ] [ Google Scholar ]
- Perez, R., and M. Perez. 2009. A fundamental look at energy reserves for the planet. IEA SHC Solar Update. Retrieved from: http://www.asrc.cestm.albany.edu/perez/Kit/pdf/a-fundamental-look-at%20the-planetary-energy-reserves.pdf .
- Poddutoori P, Co DT, Samuel APS, Kim CH, Vagnini MT, Wasielewski MR. Photoinitiated multistep charge separation in ferrocene–zinc porphyrin–diiron hydrogenase model complex triads. Energy & Environmental Science. 2011; 4 :244–2450. doi: 10.1039/c1ee01334c. [ CrossRef ] [ Google Scholar ]
- Shockley W, Queisser HJ. Detailed balance limit of efficiency of p–n junction solar cells. Journal of Applied Physics. 1961; 32 :510. doi: 10.1063/1.1736034. [ CrossRef ] [ Google Scholar ]
- Sundström, V. (ed.). 2009. Solar energy conversion Dalton Transactions. Themed Issue volume 45. [ PubMed ]
- Tamagnini P, Leita E, Oliveira P, Ferreira D, Pinto F, Harris D, Heidorn T, Lindblad P. Cyanobacterial hydrogenases: Diversity, regulation and applications. FEMS Microbiology Reviews. 2007; 31 :692–720. doi: 10.1111/j.1574-6976.2007.00085.x. [ PubMed ] [ CrossRef ] [ Google Scholar ]
- Vagnini MT, Mara MW, Harpham MR, Huang J, Shelby ML, Chen LX, Wasielewski MR. Interrogating the photogenerated Ir(IV) state of a water oxidation catalyst using ultrafast optical and X-ray absorption spectroscopy. Chemical Science. 2013; 4 :3863–3873. doi: 10.1039/c3sc51511g. [ CrossRef ] [ Google Scholar ]
- Vandewal K, Tvingstedt K, Gadisa A, Inganäs O, Manca JV. On the origin of the open-circuit voltage of polymer–fullerene solar cells. Nature Materials. 2009; 8 :904–909. doi: 10.1038/nmat2548. [ PubMed ] [ CrossRef ] [ Google Scholar ]
Numbers, Facts and Trends Shaping Your World
Read our research on:
Full Topic List
Regions & Countries
- Publications
- Our Methods
- Short Reads
- Tools & Resources
Read Our Research On:

Home solar panel adoption continues to rise in the U.S.
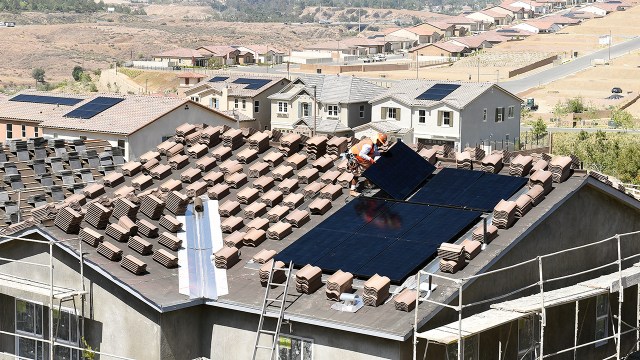
President Joe Biden recently signed into law new legislation that includes larger investments in renewable energy and measures to address climate change. Among its provisions is a 30% solar tax credit that could spur more Americans to “go solar” over the next decade.
While residential solar power currently generates just a fraction of the country’s overall electricity, it has continued to grow rapidly in recent years, despite COVID-19-related supply chain issues , import restrictions and other obstacles .
Residential solar power installations rose by 34% from 2.9 gigawatts in 2020 to 3.9 gigawatts in 2021, according to data from the U.S. Energy Information Administration (EIA), a government agency that collects and analyzes information about the energy industry. And in the second quarter of 2022, residential solar set its fifth consecutive quarterly growth record, according to the Solar Energy Industries Association .
Pew Research Center conducted this analysis to understand Americans’ adoption of home solar panels. It relies on data from the U.S. Energy Information Administration and the Solar Energy Industries Association , among other sources.
The analysis also draws from a Center survey of 10,237 U.S. adults conducted from Jan. 24-30, 2022. Everyone who took part in the survey is a member of the Center’s American Trends Panel (ATP), an online survey panel that is recruited through national, random sampling of residential addresses. This way, nearly all U.S. adults have a chance of selection. The survey is weighted to be representative of the U.S. adult population by gender, race, ethnicity, partisan affiliation, education and other categories. Read more about the ATP’s methodology .
Here are the questions used for this analysis, along with responses, and its methodology .
Even with the new federal tax credit – and other available incentives, including state tax incentives – home solar panels are expensive. The average installation cost of a residential solar panel system so far this year can range from $16,870 to $23,170 after applying the federal solar tax credit, according to EnergySage , though other factors, such as the type of solar panel , can affect the price. Supply chain constraints have also increased the cost of solar panels since the start of the COVID-19 pandemic.
Residential solar power still generates less electricity than large utility-scale solar, such as solar panel farms. And all solar power together generates only a small amount of the electricity used in the United States. In 2021, solar generated just 3% of all utility-scale electricity , a far smaller share than natural gas (38%) or coal (22%).
A January Pew Research Center survey found that 8% of U.S. homeowners said they have already installed solar panels and an additional 39% have given serious thought to it in the past year. The survey was conducted before the 30% federal tax credit became law in August.
The share of homeowners who say they have installed solar panels is up from 6% in a 2019 survey and 4% in 2016. But the share who said in January that they have given serious thought to installing solar panels is down 7 percentage points from 2019.
Residential solar by region
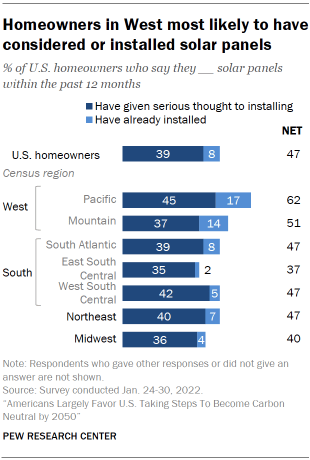
In the January survey, homeowners in Western states were especially likely to have considered or already installed solar panels at their homes, as past Center surveys have also found. Among homeowners in the Pacific region, 45% say they’ve given serious thought to installing solar panels to generate electricity for their home, while another 17% say they’ve already done this. About half of homeowners in the Mountain region (51%) also say they have either given serious thought to installing or already installed solar panels.
The survey is consistent with EIA data, which shows that California has by far the greatest electricity-generating capacity from small-scale residential solar power . Arizona and Texas are next in electricity-generating capacity from residential solar power.
Interest in home solar panels differs by party
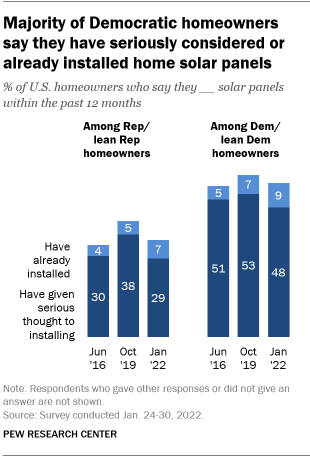
Democratic and Democratic-leaning homeowners are more likely than Republican and GOP-leaning homeowners to say they’ve installed or given serious thought in the past year to installing solar panels at their home to generate electricity (57% vs. 36%), according to the January survey. Past Center surveys have also found that Democrats are more likely than Republicans to say they have already installed or given serious thought to installing solar panels.
When asked about possible reasons for installing solar panels, almost all homeowners who have installed them or considered doing so (92%) see saving money on bills as a reason. Democrats and Republicans who have installed or considered installing home solar panels are about equally likely to say this.
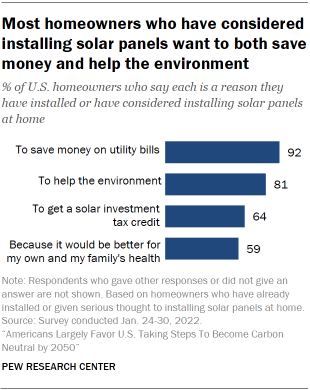
Most homeowners who said in January that they’ve installed or seriously considered installing solar panels at home said helping the environment was a motivation for doing so (81%). About six-in-ten (59%) said they did so because it would be better for their health and their family’s health. Another 64% cited solar investment tax credits as a reason.
The federal tax credit for home solar panels was 26% of the installation costs prior to the recent law, which increased that percentage to 30% through 2032. Many states give additional tax credits for installing solar panels.
Note: Here are the questions used for this analysis, along with responses, and its methodology .
- Climate, Energy & Environment
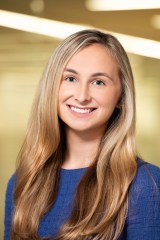
Rebecca Leppert is a copy editor at Pew Research Center
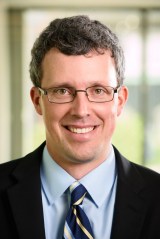
Brian Kennedy is a senior researcher focusing on science and society research at Pew Research Center
How Republicans view climate change and energy issues
How americans view future harms from climate change in their community and around the u.s., americans continue to have doubts about climate scientists’ understanding of climate change, growing share of americans favor more nuclear power, why some americans do not see urgency on climate change, most popular.
1615 L St. NW, Suite 800 Washington, DC 20036 USA (+1) 202-419-4300 | Main (+1) 202-857-8562 | Fax (+1) 202-419-4372 | Media Inquiries
Research Topics
- Age & Generations
- Coronavirus (COVID-19)
- Economy & Work
- Family & Relationships
- Gender & LGBTQ
- Immigration & Migration
- International Affairs
- Internet & Technology
- Methodological Research
- News Habits & Media
- Non-U.S. Governments
- Other Topics
- Politics & Policy
- Race & Ethnicity
- Email Newsletters
ABOUT PEW RESEARCH CENTER Pew Research Center is a nonpartisan fact tank that informs the public about the issues, attitudes and trends shaping the world. It conducts public opinion polling, demographic research, media content analysis and other empirical social science research. Pew Research Center does not take policy positions. It is a subsidiary of The Pew Charitable Trusts .
Copyright 2024 Pew Research Center
Terms & Conditions
Privacy Policy
Cookie Settings
Reprints, Permissions & Use Policy
Notification: View the latest site access restrictions, updates, and resources related to the coronavirus (COVID-19) »
NREL's solar research strives to enable reliable, low-cost solar energy at scale—on the grid and beyond the grid.
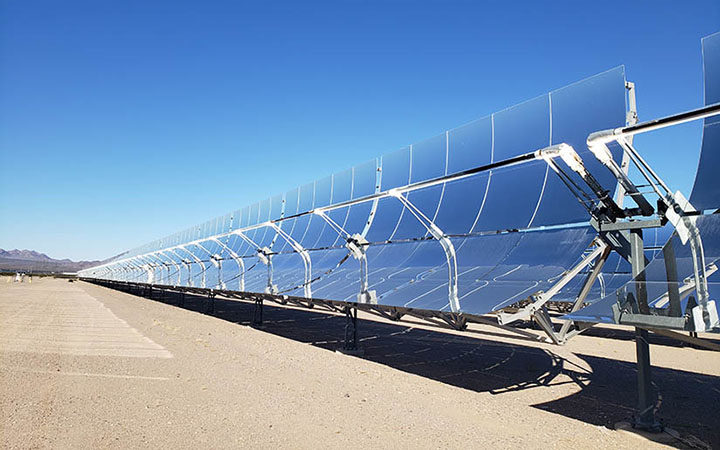
Postdocs Study Impact of Turbulent Winds on Concentrating Solar Power
The study will help predict the impact of wind conditions on concentrating solar power performance and more
Photovoltaic Research
Concentrating solar power research, solar market research and analysis, solar grid and systems integration, solar tech to market.
News Release: NREL Proof of Concept Shows Path to Easier Recycling of Solar Modules
April 25, 2024
NREL Researchers Outline Path Forward for Tandem Solar Cells
NREL Shows Live Grid Impacts From Total Solar Eclipse
April 8, 2024
Solar Energy Basics

Solar Newsletters
Read the latest edition and subscribe to the solar newsletter .
For a focus on NREL's solar analysis work, subscribe to the solar market research and analysis newsletter .
- News & Events
- Contact & Visit
- Faculty & Staff
- McCormick Advisory Council
- Departments & Institutes
- Diversity Data
- Faculty Journal Covers
- Areas of Study
- Bachelor's Degrees
- Music & Engineering
- Combined BS / MS Program Collapse Combined BS / MS Program Submenu
- Murphy Scholars Program Projects
- Undergraduate Honors
- Certificates & Minors Collapse Certificates & Minors Submenu
- Integrated Engineering Studies
- Engineering First® Program
- Theme Requirement
- Research Opportunities
- Personal & Career Development
- Global Opportunities
- Existing Groups
- McCormick Community
- Transfer AP/IB Credits
- ABET Course Partitioning
- Enrollment and Graduation Data
- Full-time Master's
- Part-time Master's
- MS with Interdepartmental Minors
- Application Checklist
- Application FAQs
- Financial Aid
- International Students
- Student Groups
- Career & Professional Development
- All Areas of Study
- Departments & Programs
- Apply to Northwestern Engineering
- Faculty Fellows
- Office of the Dean
- Administration, Finance, Facilities, & Planning
- Alumni Relations & Development
- Career Development
- Corporate Engagement
- Customer Service Center
- Faculty Affairs
- Global Initiatives
- Graduate Studies
- Information Technology
- Marketing & Communications
- McCormick Advising System
- Personal Development StudioLab
- Professional Education
- Research Offices
- Undergraduate Engineering
- Newsletter Signup
- Information for the Media
- Tech Room Finder
Researchers Take a Step Closer to Better, More Affordable Solar Cells
Innovative technique leads to perovskite-based solar cells with record-breaking efficiency, the problem:.
Scaling single-junction perovskite solar cells (PSCs) has been challenging.
A new technique applied during crystal formation that allows PSCs with an ‘inverted’ or ‘pin’ structure – known for their stability – to exhibit high efficiency.
Why it Matters:
The breakthrough means PSCs are closer to scaling, bringing them nearer their potential to contribute to the decarbonization of the electricity supply.
Professor Ted Sargent, Research Assistant Professor Bin Chen, Postdoctoral Researcher Hao Chen, Postdoctoral Fellow Cheng Liu
An international team of researchers, including a group from Northwestern Engineering and Northwestern Chemistry , has set a new world record for power conversion efficiency (PCE) of single-junction perovskite solar cells (PSCs).
These solar cells – created from an emerging solar material – have the potential to generate greater solar energy at a lower cost than today’s industry-standard silicon solar cells, but scaling the technology has its challenges. Until now, PSCs have shown either high stability and lower efficiency or vice versa, depending on their structure.
Yet this team’s work has resulted in a highly stable, highly efficient 0.05cm 2 perovskite solar cell with a PCE of 26.15 percent certified by a National Renewable Energy Laboratory -accredited facility. The prior certified world record published in a scientific journal was 25.73 percent.
A 1.04 cm 2 device had a certified power conversion efficiency of 24.74 percent, also a record for its size. The best devices retained 95 percent of their initial PCE following 1,200 hours of continuous solar illumination at a temperature of 65 degrees.
“Perovskite-based solar cells have the potential to contribute to the decarbonization of the electricity supply once we finalize their design, achieve the union of performance and durability, and scale the devices,” said Ted Sargent , Lynn Hopton Davis and Greg Davis Professor of Chemistry and Electrical and Computer Engineering at Northwestern University, co-executive director of the Paula M. Trienens Institute for Sustainability and Energy , and co-corresponding author of the paper. “Our team has discovered a new technique applied during crystal formation that allows PSCs with an ‘inverted’ or ‘pin’ structure – known for their stability – to exhibit high efficiency. It’s the best of both worlds.”
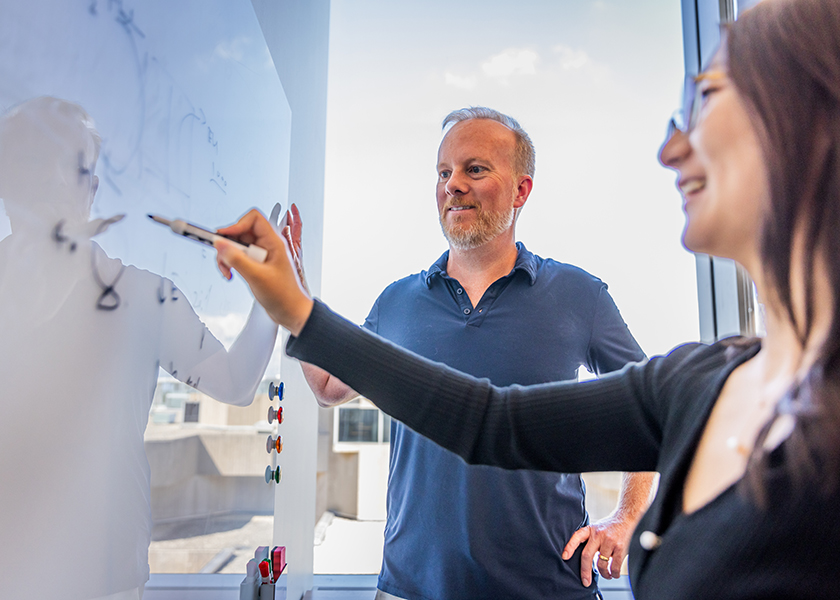
Our team has discovered a new technique applied during crystal formation that allows perovskite solar cells with an ‘inverted’ or ‘pin’ structure – known for their stability – to exhibit high efficiency. It’s the best of both worlds.
Ted Sargent Lynn Hopton Davis and Greg Davis Professor of Chemistry and Electrical and Computer Engineering
"Until today, a promising and more stable perovskite solar cell - inverted perovskite solar cells - have suffered lower energy efficiencies than those achieved in their non-inverted counterparts. This work represents an important milestone by crossing the efficiency-parity threshold," said Zhijun Ning, co-corresponding author and assistant professor at ShanghaiTech University.
Findings were reported April 11 in the journal Science.
A new approach to treating defects
The basic structure of “inverted” PSCs consists of an outer electron-transporting layer (ETL), a hole transporting layer (HTL), an anode, and a cathode. The energetic losses for the cells occur primarily at the interfaces between the perovskites and the ETL and HTL layers in places where there are tiny defects in the crystals.
Prior attempts at reducing energy loss have included the use of additive or surface treatments to passivate the defects. Sargent’s team noted that the molecules in these treatments bonded at a single site on the defects in a perpendicular orientation, forcing the electrons to travel a long distance up through the material, causing resistance and lowering efficiency.
The team set out to find a molecule that would bond on two neighboring sites on the defects in a horizontal orientation, reducing the distance the electrons needed to travel and improving efficiency. They identified one molecule – 4- chlorobenzenesulfonate – that could lay down at the surface of the perovskites by forming strong Cl-Pb and SO 3 -Pb bonds with the undercoordinated Pb 2+ and led to improved performance of the devices.
“By carefully selecting molecules that lie flat on the perovskite surface, binding to two sites simultaneously, our new strategy reduced the interface resistance: the result is much higher fill factor in solar cells, reaching 95 percent of the theoretical limit," said Jian Xu, co-first author and postdoctoral fellow at the University of Toronto.
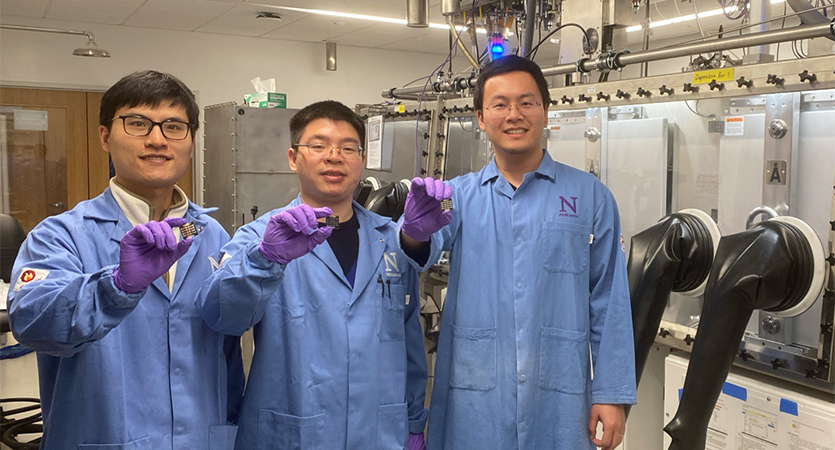
“Not only did the addition of these molecules improve efficiency, they also simplified the manufacturing process,” noted Hao Chen , a postdoctoral researcher at Northwestern Engineering and co-first author of the paper. “When added to the perovskites precursor, these molecules automatically go to the surface of the perovskite layer to patch defects during the crystallization process. This removes the need to treat the surface defects, an extra step that often results in uneven coverage of passivators and poor stability of the devices.”
This discovery builds on prior research conducted by the Sargent Group , which has explored various strategies to improve PSC performance and stability to make them a viable alternative to silicon solar cells. Next, the team will look toward scaling the devices.
“Northwestern is really at the forefront of renewable energy technology research,” said Bin Chen , co-corresponding author and research assistant professor at Northwestern Engineering. “By focusing on stable inverted perovskites and making breakthroughs in their performance, we are developing a solar technology that can be a gamechanger in the field.”
"With the efficiency discrepancy solved, the large and growing perovskite community will focus even more of its firepower on the inverted perovskite solar cell architecture in light of its stability advantages," said Aidan Maxwell, co-first author of the paper and a graduate student at the University of Toronto.
“We were thrilled when we achieved an independently certified efficiency of 26.1 percent for inverted perovskite solar cells: this was the first to surpass the record for the conventional structure,” added Cheng Liu , postdoctoral fellow at Northwestern Chemistry and co-first author of the paper. “The accomplishment motivates not only our own team but will also inspires further collective efforts across the wide and productive global perovskite community."
Additional authors on the paper include Yi Yang, Abdulaziz S. R. Bati, Yuan Liu, and Mercouri G. Kanatzidis of Northwestern Chemistry; Haoyue Wan, Zaiwei Wang, Lewei Zeng, Junke Wang, Sam Teale, Yanjiang Liu, Sjoerd Hoogland, Peter Serles, and Tobin Filleter of the University of Toronto; Wei Zhou and Qilin Zhou of ShanghaiTech University; Makhsud I. Saidaminov of the University of Victoria; and Muzhi Li and Nicholas Rolston of Arizona State University.
Get our news in your inbox.
Sign up for our newsletter.
Check out our magazine.
Find more in depth stories and get to know Northwestern Engineering.
Solar Energy
Though costly to implement, solar energy offers a clean, renewable source of power.
Solar energy is the technology used to harness the sun's energy and make it useable. As of 2011 , the technology produced less than one tenth of one percent of global energy demand.
Many are familiar with so-called photovoltaic cells, or solar panels, found on things like spacecraft, rooftops, and handheld calculators. The cells are made of semiconductor materials like those found in computer chips. When sunlight hits the cells, it knocks electrons loose from their atoms. As the electrons flow through the cell, they generate electricity.
On a much larger scale, solar-thermal power plants employ various techniques to concentrate the sun's energy as a heat source. The heat is then used to boil water to drive a steam turbine that generates electricity in much the same fashion as coal and nuclear power plants, supplying electricity for thousands of people.
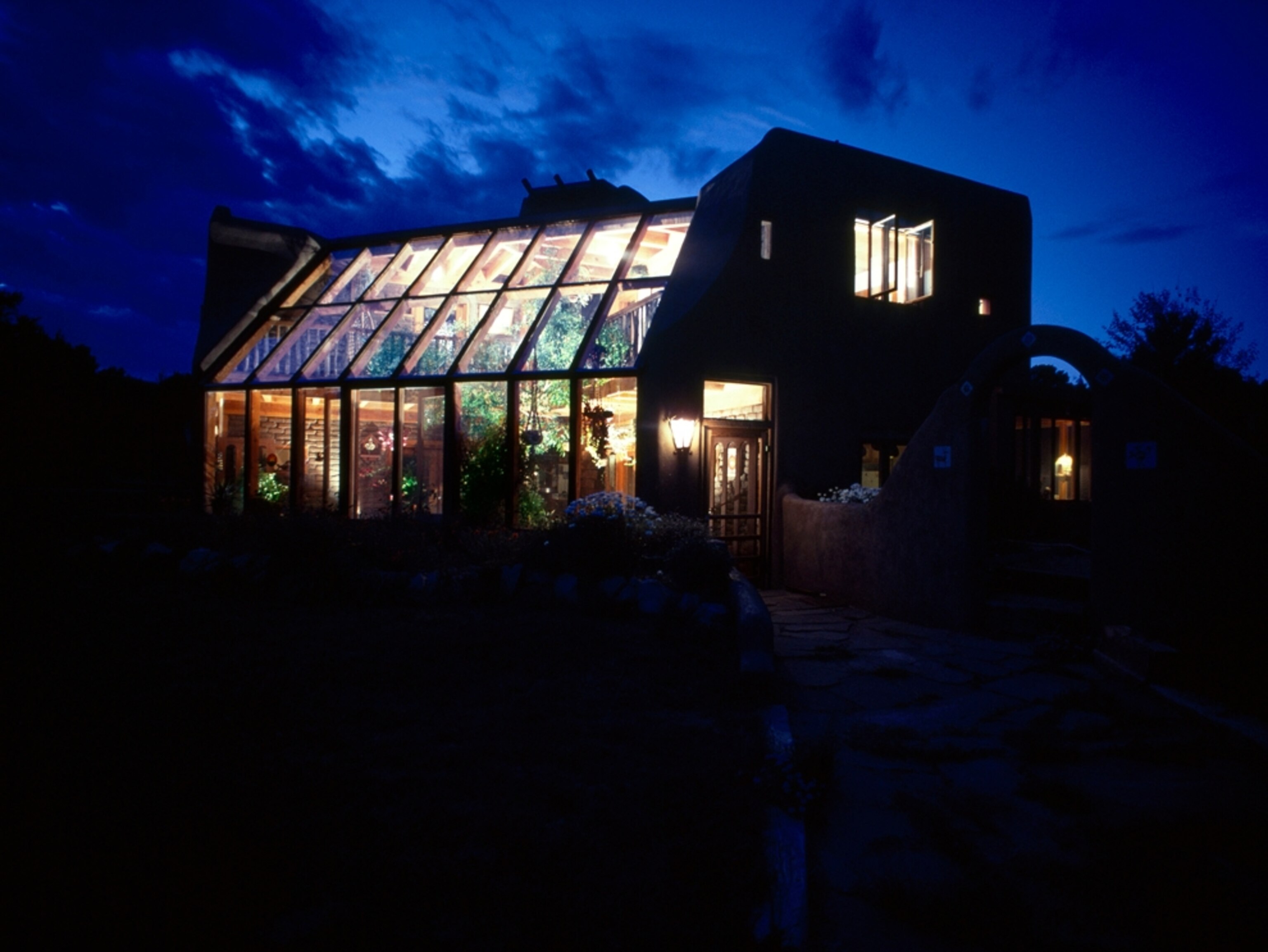
The sun has produced energy for billions of years. Every hour the sun beams more energy onto Earth than it needs to satisfy global energy needs for an entire year.
How to Harness Solar Power
In one technique, long troughs of U-shaped mirrors focus sunlight on a pipe of oil that runs through the middle. The hot oil then boils water for electricity generation. Another technique uses moveable mirrors to focus the sun's rays on a collector tower, where a receiver sits. Molten salt flowing through the receiver is heated to run a generator.
Other solar technologies are passive. For example, big windows placed on the sunny side of a building allow sunlight to heat-absorbent materials on the floor and walls. These surfaces then release the heat at night to keep the building warm. Similarly, absorbent plates on a roof can heat liquid in tubes that supply a house with hot water.
Solar energy is lauded as an inexhaustible fuel source that is pollution- and often noise-free. The technology is also versatile. For example, solar cells generate energy for far-out places like satellites in Earth orbit and cabins deep in the Rocky Mountains as easily as they can power downtown buildings and futuristic cars.
Solar energy doesn't work at night without a storage device such as a battery, and cloudy weather can make the technology unreliable during the day. Solar technologies are also very expensive and require a lot of land area to collect the sun's energy at rates useful to lots of people.
Despite the drawbacks, solar energy use has surged at about 20 percent a year over the past 15 years, thanks to rapidly falling prices and gains in efficiency. Japan, Germany, and the United States are major markets for solar cells. With tax incentives, and efficient coordination with energy companies , solar electricity can often pay for itself in five to ten years.
FREE BONUS ISSUE
Related topics.
- SOLAR POWER
- ENVIRONMENT AND CONSERVATION
You May Also Like
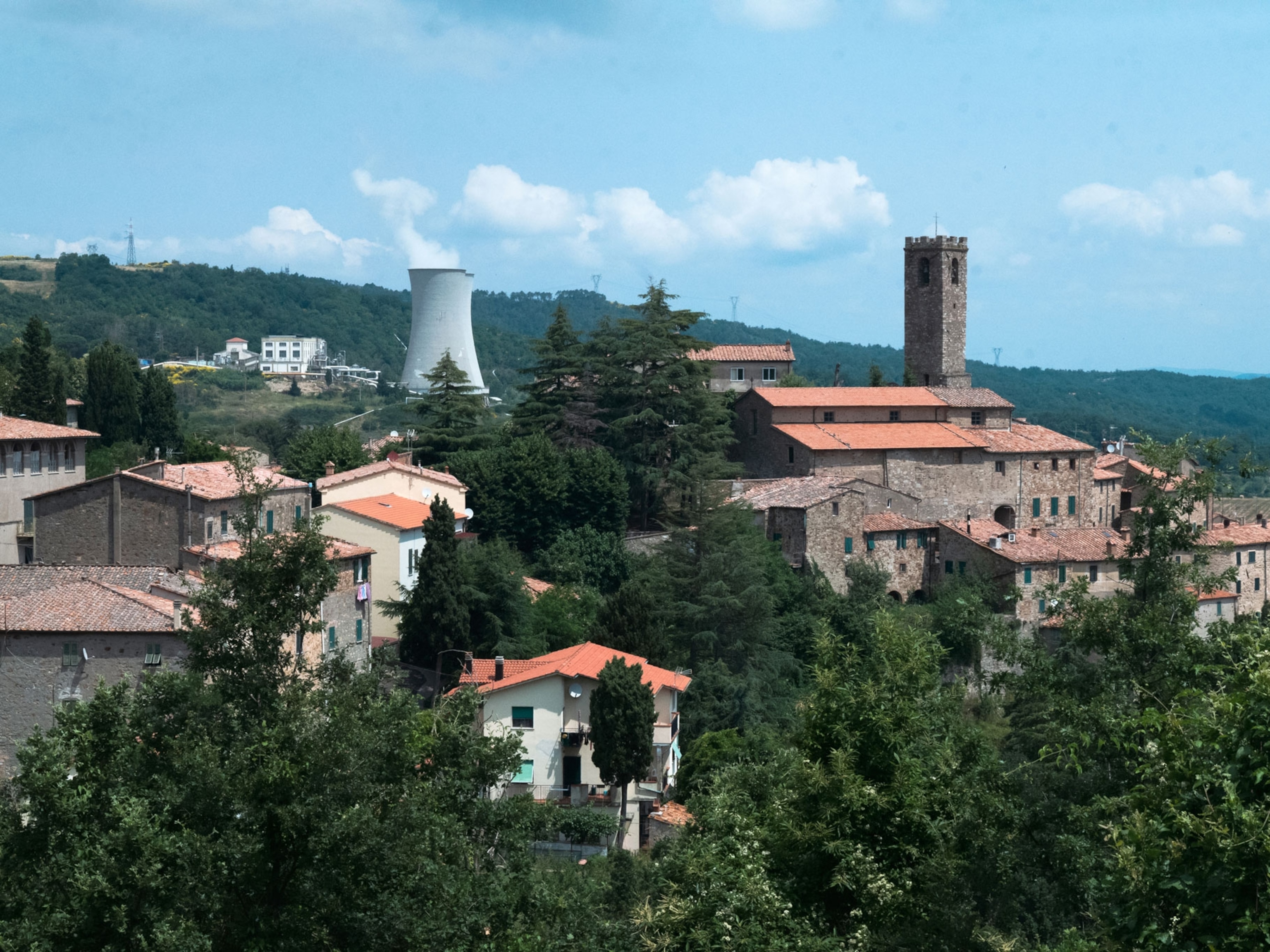
Can energy harnessed from Earth’s interior help power the world?
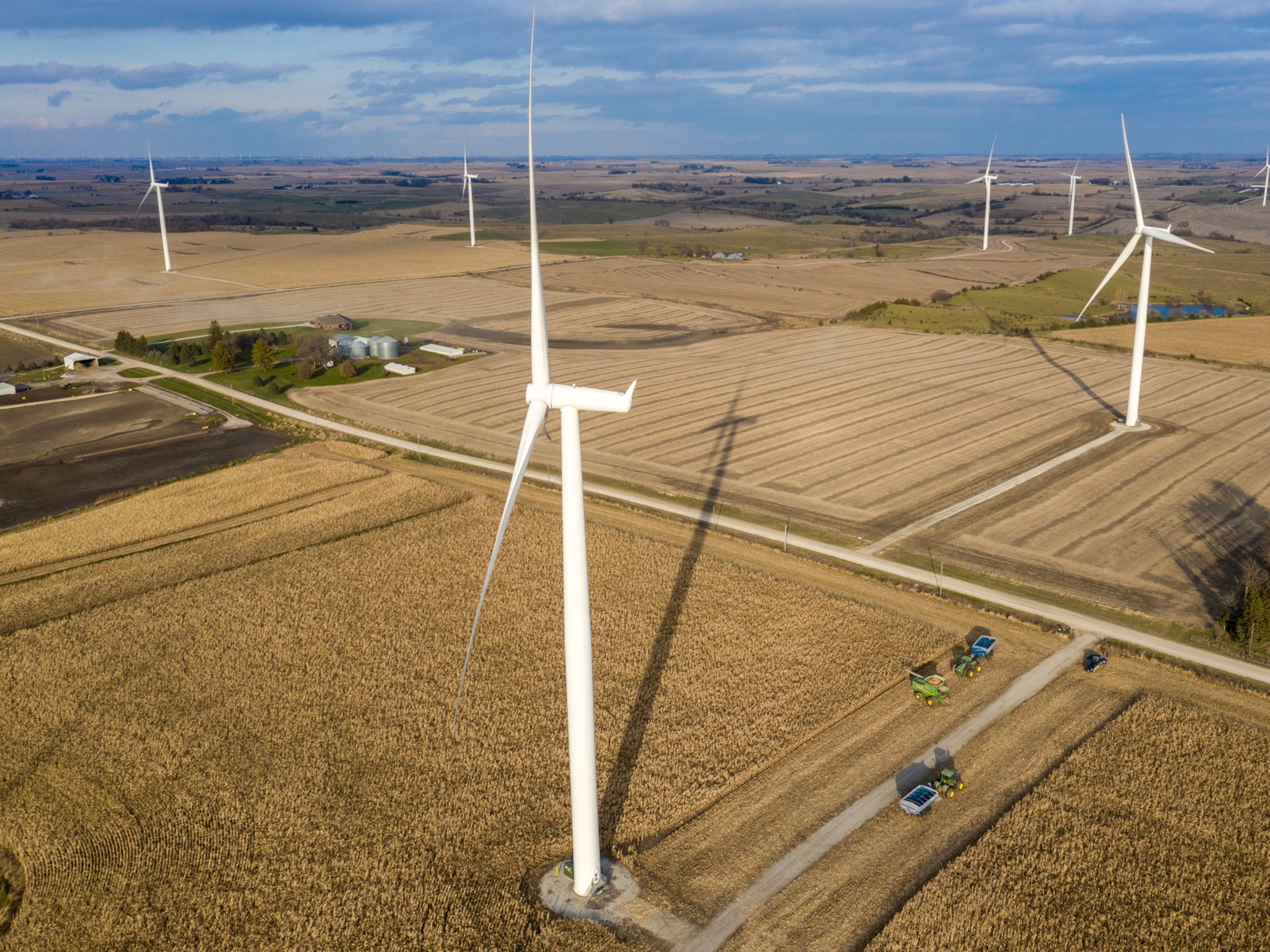
How the historic climate bill will dramatically reduce U.S. emissions
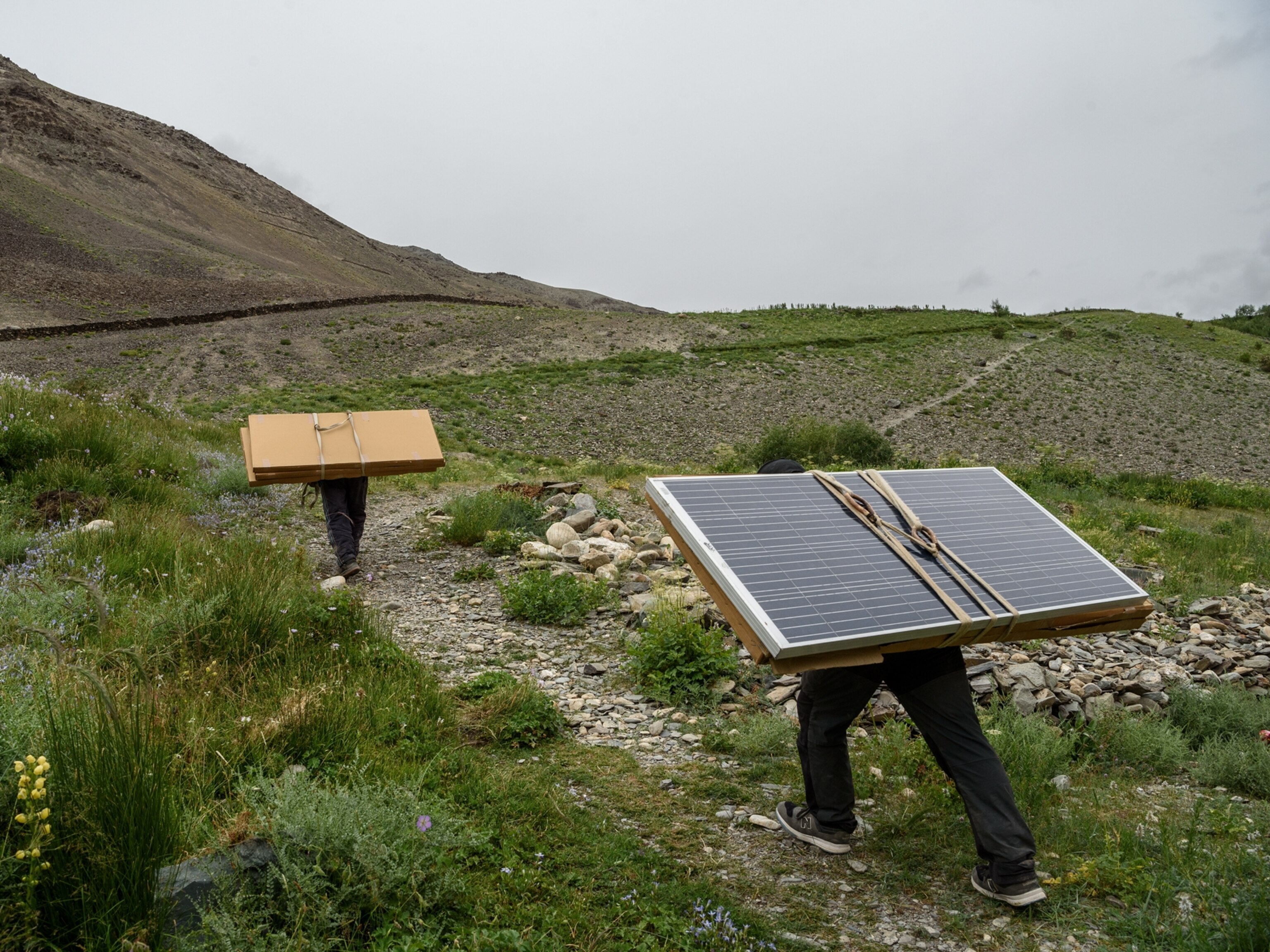
India bets its energy future on solar—in ways both small and big
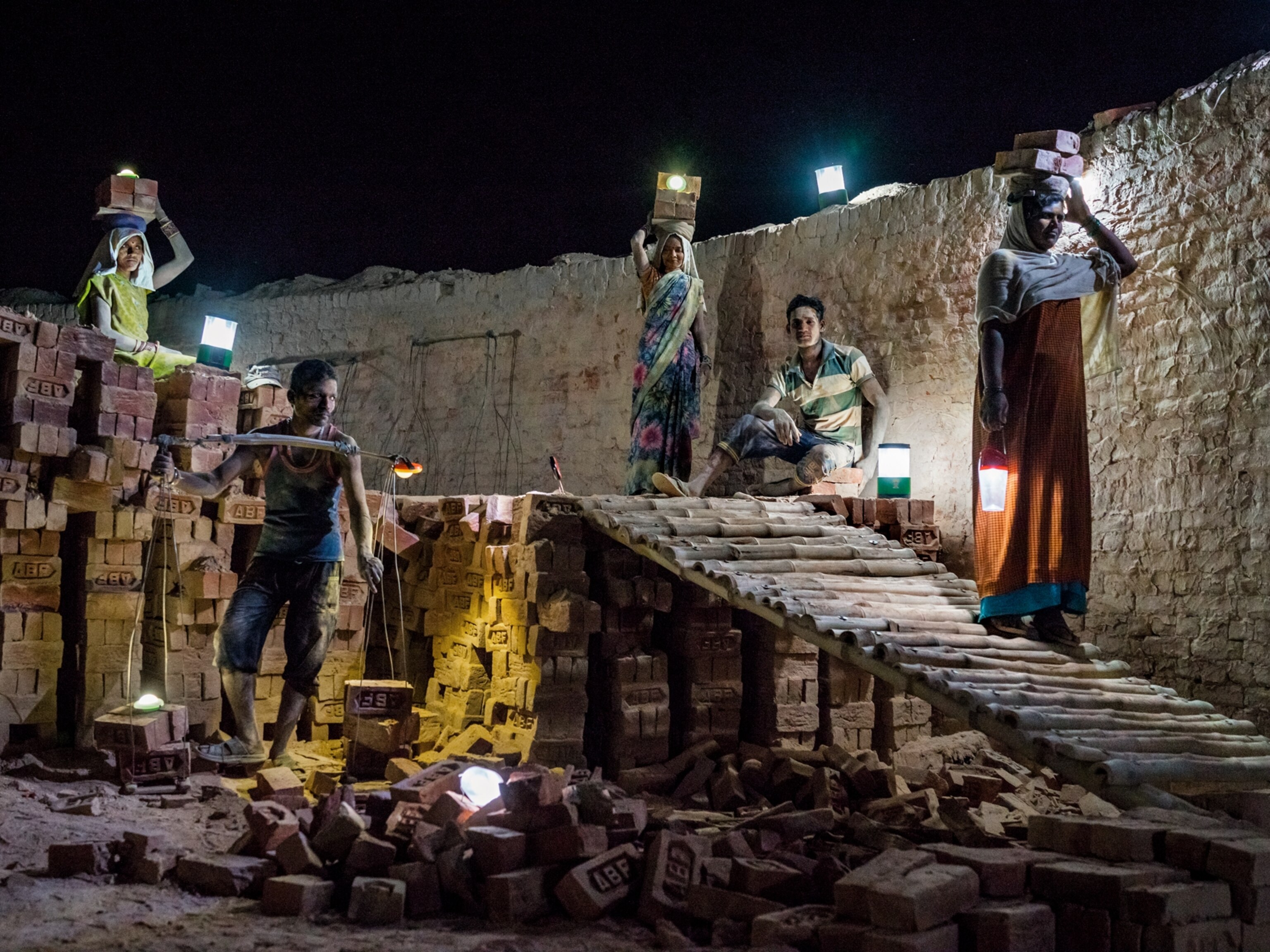
How solar lanterns are giving power to the people
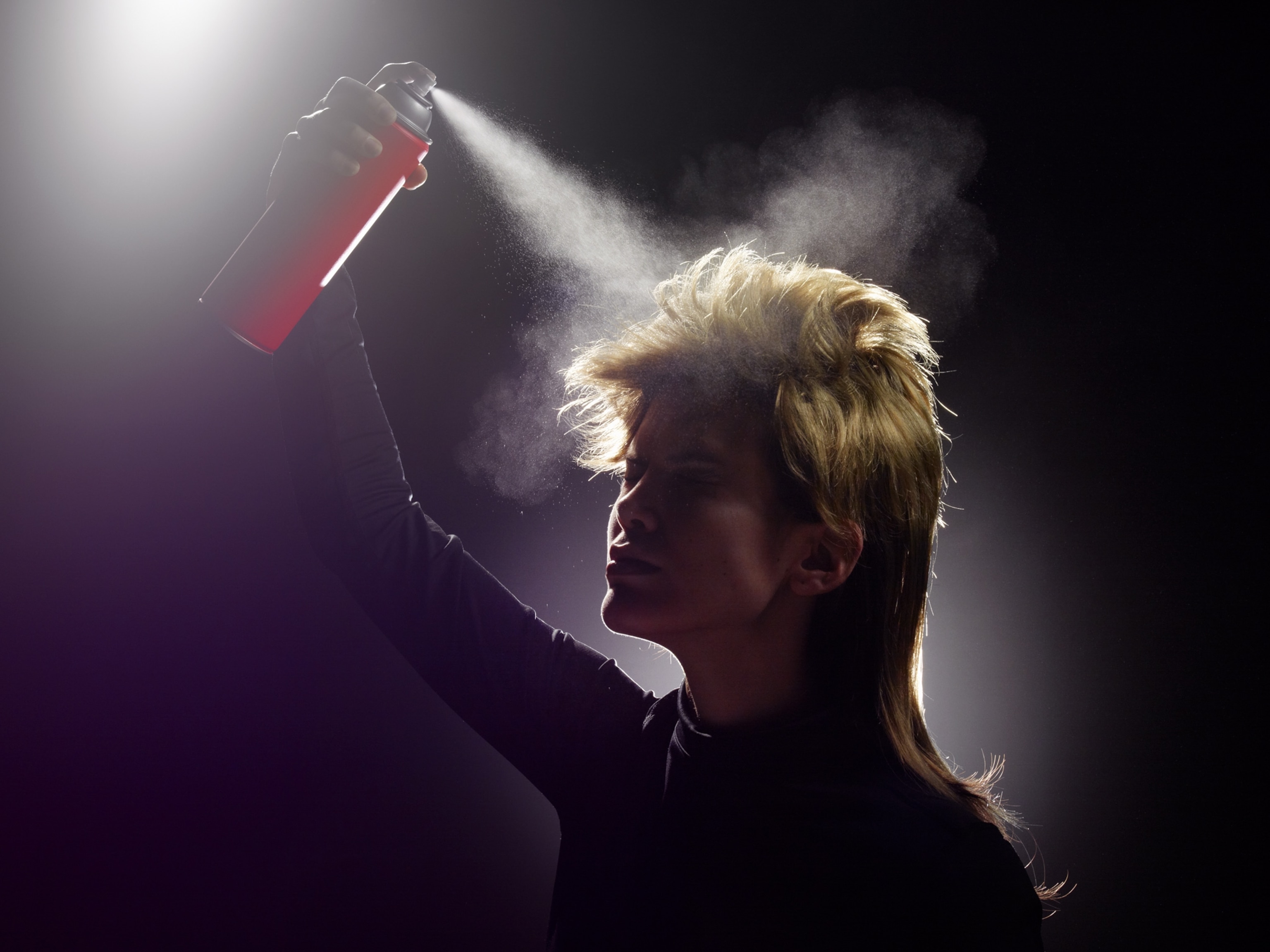
What is the ozone layer, and why does it matter?
- Environment
- Perpetual Planet
History & Culture
- History & Culture
- History Magazine
- Mind, Body, Wonder
- Paid Content
- Terms of Use
- Privacy Policy
- Your US State Privacy Rights
- Children's Online Privacy Policy
- Interest-Based Ads
- About Nielsen Measurement
- Do Not Sell or Share My Personal Information
- Nat Geo Home
- Attend a Live Event
- Book a Trip
- Inspire Your Kids
- Shop Nat Geo
- Visit the D.C. Museum
- Learn About Our Impact
- Support Our Mission
- Advertise With Us
- Customer Service
- Renew Subscription
- Manage Your Subscription
- Work at Nat Geo
- Sign Up for Our Newsletters
- Contribute to Protect the Planet
Copyright © 1996-2015 National Geographic Society Copyright © 2015-2024 National Geographic Partners, LLC. All rights reserved

Maintenance work is planned for Wednesday 1st May 2024 from 9:00am to 11:00am (BST).
During this time, the performance of our website may be affected - searches may run slowly and some pages may be temporarily unavailable. If this happens, please try refreshing your web browser or try waiting two to three minutes before trying again.
We apologise for any inconvenience this might cause and thank you for your patience.
Sustainable Energy & Fuels
Design and development of nanostructured photocatalysts for large-scale solar green hydrogen generation.

* Corresponding authors
a Department of Chemistry, Siksha ‘O’ Anusandhan Deemed to be University Bhubaneswar, Odisha, India E-mail: [email protected] , [email protected]
The production of clean hydrogen through artificial photosynthesis is the most intriguing research topic that offers hope for meeting the world's energy demands. The evolution of green hydrogen via visible light-driven photocatalysis is challenging but feasible. Photocatalytic solar power systems primarily rely on utilizing the complete range of solar spectrum. The synthesis of an optimal photocatalyst should address all the influencing parameters with an efficient scaling method, which remains yet to be elucidated despite several advancements in photocatalytic water-splitting applications. Real-time solutions are necessary to overcome the lack of photocatalytic efficacy of semiconducting nanomaterials in solar-powered systems. In addition to the proposal of designing solar-powered systems for hydrogen generation, this review paves the way for highlighting the difficulties associated with water reduction methods. It also offers some strategies to improve charge separation and migration in a semiconducting photocatalyst by enhancing light absorption and altering their band positions. Moreover, a cost-effective, eco-friendly, and photostable heterogeneous nanocatalyst must be designed for visible light-harvesting water-splitting processes. This article reports various nanomaterial-based photocatalysts, which act as the base surface for photocatalytic solar water splitting. These include oxides, chalcogenides, and nitrides of metals, noble metals, plasmonic metals, ultrathin 2D covalent–organic frameworks (COFs), metal–organic frameworks (MOFs), and metal-free polymeric graphitic carbon nitrides. The integration of multi-component nano-materials can be more appropriate than single-component photocatalysts to maximize their catalytic activity. Thin-film photocatalysis is considered the most effective method for increasing hydrogen production rates compared to powder suspension-based photocatalysis. This article presents the latest advancements in thin film-based photocatalytic technology, outlining all the critical factors, prerequisites, and techniques for thin film preparation. Future research on advanced photocatalysis focuses on harvesting green hydrogen for in situ carbon dioxide reduction, fine chemical synthesis, nitrogen fixation, and hydrogen peroxide synthesis. Experimentally, photocatalytic solar-powered systems utilize natural sun light. However, the synthesis of ideal photocatalysts via effective scaling approaches remains a challenge. This paper paves the way for finding solutions and designing a practical solar-powered system for green hydrogen production.
Article information
Download citation, permissions.

P. K. Sahu, A. Champati, A. Pradhan and B. Naik, Sustainable Energy Fuels , 2024, Advance Article , DOI: 10.1039/D4SE00056K
To request permission to reproduce material from this article, please go to the Copyright Clearance Center request page .
If you are an author contributing to an RSC publication, you do not need to request permission provided correct acknowledgement is given.
If you are the author of this article, you do not need to request permission to reproduce figures and diagrams provided correct acknowledgement is given. If you want to reproduce the whole article in a third-party publication (excluding your thesis/dissertation for which permission is not required) please go to the Copyright Clearance Center request page .
Read more about how to correctly acknowledge RSC content .
Social activity
Search articles by author.
This article has not yet been cited.
Advertisements
Is Geothermal Power Heating Up as an Energy Source?
Long confined to regions with volcanic activity, the method of harnessing energy from the Earth promises to become much more versatile thanks to new technologies
Katarina Zimmer, Knowable Magazine
:focal(750x432:751x433)/https://tf-cmsv2-smithsonianmag-media.s3.amazonaws.com/filer_public/d8/de/d8de1cef-91c5-41f3-af36-3c58935e5337/geothermal-update-500x288.jpg)
Glistening in the dry expanses of the Nevada desert is an unusual kind of power plant that harnesses energy not from the sun or wind, but from the Earth itself.
The site, known as Project Red, pumps water thousands of feet into the ground, down where rocks are hot enough to roast a turkey. Around the clock, the structure sucks the heated water back up; it is then used to power generators. Since last November, this carbon-free, Earth-borne power has been flowing onto a local grid in Nevada.
Geothermal energy, though it’s continuously radiating from Earth’s super-hot core, has long been a relatively niche source of electricity, largely limited to volcanic regions like Iceland where hot springs bubble from the ground. But geothermal enthusiasts have dreamed of sourcing Earth power in places without such specific geological conditions—like Project Red’s Nevada site, developed by energy startup Fervo Energy .
Such next-generation geothermal systems have been in the works for decades, but they’ve proved expensive and technologically difficult, and have sometimes even triggered earthquakes. Some experts hope that newer efforts like Project Red may now, finally, signal a turning point, by leveraging techniques that were developed in oil and gas extraction to improve reliability and cost efficiency.
The advances have garnered hopes that with enough time and money, geothermal power—which currently generates less than 1 percent of the world’s electricity , and 0.4 percent of electricity in the United States —could become a mainstream energy source. Some posit that geothermal could be a valuable tool in transitioning the energy system off of fossil fuels, because it can provide a continuous backup to intermittent energy sources like solar and wind . “It’s been, to me, the most promising energy source for a long time,” says energy engineer Roland Horne of Stanford University. “But now that we’re moving toward a carbon-free grid, geothermal is very important.”
A rocky start
Geothermal energy works best with two things: heat, plus rock that is permeable enough to carry water. In places where molten rock sizzles close to the surface, water will seep through porous volcanic rock, warm up and bubble upward as hot water, steam or both.
If the water or steam is hot enough—ideally at least around 300 degrees Fahrenheit—it can be extracted from the ground and used to power generators for electricity. In Kenya, nearly 50 percent of electricity generated comes from geothermal. Iceland produces 25 percent of its electricity from this source, while New Zealand makes about 18 percent and the state of California generates 6 percent.
Some natural geothermal resources are still untapped, such as in the western U.S., says geologist Ann Robertson-Tait , president of GeothermEx, a geothermal energy consulting division at the oilfield services company SLB. But by and large, we’re running out of natural, high-quality geothermal resources, pushing experts to consider ways of extracting geothermal energy from areas where the energy is much harder to access. “There’s so much heat in the Earth,” Robertson-Tait says. But, she adds, “much of it is locked inside rock that isn’t permeable.”
/https://tf-cmsv2-smithsonianmag-media.s3.amazonaws.com/filer_public/a6/39/a63915a1-f058-46d9-bcc7-3613b78f72bf/gettyimages-1177027231.jpg)
Tapping that heat requires deep drilling and creating cracks in these non-volcanic, dense rocks to allow water to flow through them. Since 1970, engineers have been developing “enhanced geothermal systems” (EGS) that do just that, applying methods similar to the hydraulic fracturing—or fracking—used to suck oil and gas out of deep rocks. Water is pumped at high pressure into wells, up to several miles deep, to blast cracks into the rocks. The cracked rock and water create an underground radiator where water heats before rising to the surface through a second well. Dozens of such EGS installations have been built in the U.S., Europe, Australia and Japan—most of them experimental and government-funded—with mixed success.
Famously, one EGS plant in South Korea was abruptly shuttered in 2017 after having probably caused a 5.5 magnitude earthquake ; fracking of any kind can add pressure to nearby tectonic faults. Other issues were technological—some plants didn’t create enough fractures for good heat exchange, or fractures traveled in the wrong direction and failed to connect the two wells.
Some efforts, however, turned into viable power plants, including several German and French systems built between 1987 and 2012 in the Rhine Valley. There, engineers made use of existing fractures in the rock.
But overall, there just hasn’t been enough interest to develop EGS into a more reliable and lucrative technology, says geophysicist Dimitra Teza of the energy research institute Fraunhofer IEG in Karlsruhe, Germany, who helped develop some of the Rhine Valley EGS systems. “It has been quite tough for the industry.”
/https://tf-cmsv2-smithsonianmag-media.s3.amazonaws.com/filer_public/83/91/8391fa25-3a25-4789-8b98-0992b1e40cec/g-geothermal-energy-types.png)
New momentum
Solutions exist for both safety and technological problems. There are, in fact, robust protocols for avoiding earthquakes , such as by not drilling near active faults. Long-term monitoring of the operating EGS plants in France and Germany has documented only minor tremors, building confidence in the safety of the technology. Importantly, drilling and fracking methodology has improved by leaps and bounds, thanks to the boom in oil and gas extraction from shale rocks that began in the 2010s. “Since then, we’ve seen a renewed interest in EGS as a concept, because the techniques that are central to EGS were perfected and brought down significantly in cost during that time,” says Wilson Ricks , an energy systems researcher at Princeton University.
In 2015, for instance, the U.S. Department of Energy launched a research site in Utah dedicated to advancing EGS technologies . Several new North American startups, including Sage Geosystems and E2E Energy Solutions , are developing new EGS systems in Texas and Canada, respectively. The most advanced is Fervo Energy, which has applied several techniques from the shale industry at its Nevada site; the electricity now supplies a local grid that includes energy-sucking data storage centers owned by Google. (Google partnered with Fervo to develop the plant.)
Engineers drilled almost 8,000 feet downward into the Nevada rock, reaching temperatures of nearly 380 degrees Fahrenheit, and then, at the bottom, drilled another 3,250-foot horizontal well to expand the area of hot rock that the system touches—a technique used in oil and gas extraction in order to maximize yield. The company also fractured the surrounding rock at several sites along the horizontal well to create a more extensive web of cracks for water to trickle through. Technologically speaking, compared to earlier EGS efforts, “they are, in fact, a big step forward,” says Horne, who is on Fervo’s scientific advisory board.
/https://tf-cmsv2-smithsonianmag-media.s3.amazonaws.com/filer_public/bf/ac/bfac74d4-82f2-453b-b3ed-0cb85d6586c6/fervo_energy_project_red_2022.jpg)
It remains to be seen how these new EGS systems perform in the long term. One advantage of systems like Fervo’s is that they can be made more profitable by taking advantage of energy price fluctuations , according to recent research by Ricks, a Princeton colleague and several experts at Fervo Energy. Operators could plug the exit wells, causing water to accumulate inside the system, building up pressure and heat. Then the energy could be extracted during times when it is most valuable—such as during cloudy or windless periods when solar or wind aren’t working.
Still, such systems would have to be significantly scaled up to be commercially viable, Ricks says. Although Project Red provided enough steam to generate 3.5 megawatts, enough to power more than 2,500 homes and more than any other EGS plant , it’s still relatively small; a nuclear or coal plant can easily have an output of 1,000 megawatts, while large solar or traditional geothermal plants often produce several hundred megawatts.
What the EGS field needs right now, Ricks says, is the funding to build and test more such systems to inspire investor confidence. “This all needs to be very well proven, out to the point where the perceived risk is low,” he says.
A turning point for geothermal?
To that end, the U.S. Department of Energy recently awarded $60 million in funding to three demonstration projects for EGS and related technologies as part of a broader initiative to speed up EGS development . One 2019 report from the agency estimated that, with advances in EGS, geothermal power could represent around 60 gigawatts (60,000 megawatts) of installed capacity in the U.S. by 2050 , generating 8.5 percent of the country’s electricity—a more than 20-fold increase from today.
Even an increase of a few percentage points could aid in a global energy transition that’s aiming to get to net zero carbon emissions by 2050. “If in 15, 20 years, EGS is viable, I think it could play a huge part,” says Nils Angliviel de La Beaumelle , who recently co-wrote an article on the global outlook for renewable energy in the Annual Review of Environment and Resources .
Other geothermal technologies may also help. Some companies are exploring the feasibility of “super hot rock” geothermal —essentially, a young, extreme variant of EGS that involves drilling down even deeper into Earth’s crust, to a depth where water reaches a “supercritical” vapor-like state that allows it to carry much more energy than either steam or liquid. In southern Germany, the energy company Eavor is building the world’s first “closed-loop” geothermal system : Once pipes funnel water into the deep rock, the system fans out into a network of parallel boreholes, without water ever penetrating the rock. That’s a more predictable—albeit less efficient—way of warming water, as it doesn’t involve uncertainties around fracturing the rock in the right way, Teza says. “I’m really excited to see that there’s investment into these technologies,” she says. “I think it can only help.”
On the whole, it’s an important moment for geothermal energy—and not just for providing carbon-free electricity, Robertson-Tait says. Geothermal brines hauled out of the Earth are rich in lithium and other critical minerals that can be used to build green technologies like solar panels and EV batteries. There’s a growing push to use direct geothermal heat to warm buildings, either through shallow heat pumps for residential buildings or larger systems designed for entire districts —as Paris and Munich already have.
Some oil and gas companies, recognizing that a change is coming, are increasingly interested in building geothermal systems of various kinds, says Robertson-Tait. “Our Earth is geothermal,” she says, “and so I think we owe it to ourselves to do everything we can to use it.”
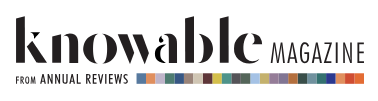
Get the latest stories in your inbox every weekday.
Thank you for visiting nature.com. You are using a browser version with limited support for CSS. To obtain the best experience, we recommend you use a more up to date browser (or turn off compatibility mode in Internet Explorer). In the meantime, to ensure continued support, we are displaying the site without styles and JavaScript.
- View all journals
- Explore content
- About the journal
- Publish with us
- Sign up for alerts
- Published: 19 April 2024
Climate change will impact the value and optimal adoption of residential rooftop solar
- Mai Shi ORCID: orcid.org/0000-0002-6430-0315 1 , 2 ,
- Xi Lu ORCID: orcid.org/0000-0002-5063-3776 1 , 3 &
- Michael T. Craig ORCID: orcid.org/0000-0002-3031-5041 2 , 4
Nature Climate Change ( 2024 ) Cite this article
745 Accesses
96 Altmetric
Metrics details
- Energy and behaviour
- Energy economics
- Energy supply and demand
Rooftop solar adoption is critical for residential decarbonization and hinges on its value to households. Climate change will probably affect the value of rooftop solar through impacts on rooftop solar generation and cooling demand, but no studies have quantified this effect. In this study, we quantified household-level effects of climate change on rooftop solar value and techno-economically optimal capacity by integrating empirical demand data for over 2,000 US households across 17 cities, household-level simulation and optimization models, and downscaled weather data for historic and future climates. We found that climate change will increase the value of rooftop solar to households by up to 19% and increase techno-economically optimal household capacity by up to 25% by the end of the century under a Representative Concentration Pathway 4.5 scenario. This increased value is robust across cities, households, future warming scenarios and retail tariff structures. Researchers, installers and policymakers should capture this increasing value to maximize household and system value of rooftop solar.
This is a preview of subscription content, access via your institution
Access options
Access Nature and 54 other Nature Portfolio journals
Get Nature+, our best-value online-access subscription
24,99 € / 30 days
cancel any time
Subscribe to this journal
Receive 12 print issues and online access
195,33 € per year
only 16,28 € per issue
Buy this article
- Purchase on Springer Link
- Instant access to full article PDF
Prices may be subject to local taxes which are calculated during checkout
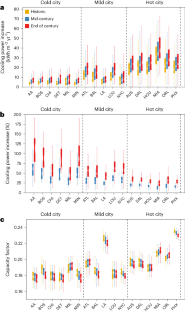
Similar content being viewed by others
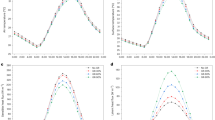
Green roofs save energy in cities and fight regional climate change
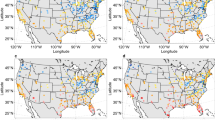
Impacts of climate change, population growth, and power sector decarbonization on urban building energy use
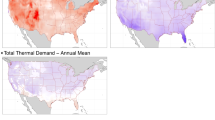
Differential effects of climate change on average and peak demand for heating and cooling across the contiguous USA
Data availability.
The individual hourly cooling behaviour data can be obtained from Ecobee upon request 38 . The future TGW climate data are from the US Department of Energy Office of Scientific and Technical Information 39 and are publicly available. Other data sources are provided in Methods and Supplementary Information . The household-level VOS and optimal solar capacity data are available via Figshare at https://doi.org/10.6084/m9.figshare.25296250.v1 (ref. 64 ).
Code availability
The codes (python scripts) are available via Figshare at https://doi.org/10.6084/m9.figshare.25296250.v1 (ref. 64 ), including model codes (HHAC model, solar potential mapping and VOS optimization) and figure production codes (Figs. 1 – 5 ).
IPCC Special Report on Global Warming of 1.5 °C (eds Masson-Delmotte, V. et. al.) (WMO, 2018).
IPCC Climate Change 2022: Impacts, Adaptation and Vulnerability (eds Pörtner, H.-O. et al.) (Cambridge Univ. Press, 2022).
Bouckaert, S., McGlade, C., Remme, U. & Wanner, B. Net Zero by 2050: A Roadmap for the Global Energy Sector (IEA, 2021).
Larson, E. et. al. Net-Zero America: Potential Pathways, Infrastructure, and Impacts (Princeton Univ., 2021).
Zhang, S. & Chen, W. Assessing the energy transition in China towards carbon neutrality with a probabilistic framework. Nat. Commun. 13 , 87 (2022).
Article Google Scholar
Jones-Albertus, B. et. al. Solar Futures Study (Office of Energy Efficiency and Renewable Energy, US Department of Energy, 2021).
Schulte, E., Scheller, F., Sloot, D. & Bruckner, T. A meta-analysis of residential PV adoption: the important role of perceived benefits, intentions and antecedents in solar energy acceptance. Energy Res. Soc. Sci. 84 , 102339 (2022).
Alipour, M., Salim, H., Stewart, R. A. & Sahin, O. Residential solar photovoltaic adoption behaviour: end-to-end review of theories, methods and approaches. Renew. Energy 170 , 471–486 (2021).
Ashraf Fauzi, M., Hanis Zainal Abidin, N., Mohd Suki, N. & Mokhtar Albshir Budiea, A. Residential rooftop solar panel adoption behavior: bibliometric analysis of the past and future trends. Renew. Energy Focus 45 , 1–9 (2023).
Shimada, H. & Honda, T. What drives households’ choices of residential solar photovoltaic capacity? Energy Policy 168 , 113120 (2022).
Jacksohn, A., Grösche, P., Rehdanz, K. & Schröder, C. Drivers of renewable technology adoption in the household sector. Energy Econ. 81 , 216–226 (2019).
Hansen, L. et. al. A Review of Solar PV Benefit and Cost studies (Rocky Mountain Institute, 2013).
Sexton, S., Kirkpatrick, A. J., Harris, R. I. & Muller, N. Z. Heterogeneous solar capacity benefits, appropriability, and the costs of suboptimal siting. J. Assoc. Environ. Resour. Econ. 8 , 1209–1244 (2021).
Google Scholar
Meriläinen, A., Puranen, P., Kosonen, A. & Ahola, J. Optimization of rooftop photovoltaic installations to maximize revenue in Finland based on customer class load profiles and simulated generation. Sol. Energy 240 , 422–434 (2022).
Beuse, M., Dirksmeier, M., Steffen, B. & Schmidt, T. S. Profitability of commercial and industrial photovoltaics and battery projects in South-East Asia. Appl. Energy 271 , 115218 (2020).
Shi, M. et al. Opportunity of rooftop solar photovoltaic as a cost-effective and environment-friendly power source in megacities. iScience 25 , 104890 (2022).
Article CAS Google Scholar
Tongsopit, S. et al. The economics of solar PV self-consumption in Thailand. Renew. Energy 138 , 395–408 (2019).
Taylor, M. et al. Value of Solar: Program Design and Implementation Considerations (National Renewable Energy Laboratory, 2015).
Ossenbrink, J. How feed-in remuneration design shapes residential PV prosumer paradigms. Energy Policy 108 , 239–255 (2017).
O’Shaughnessy, E., Barbose, G., Wiser, R., Forrester, S. & Darghouth, N. The impact of policies and business models on income equity in rooftop solar adoption. Nat. Energy 6 , 84–91 (2021).
Lu, X. et al. Combined solar power and storage as cost-competitive and grid-compatible supply for China’s future carbon-neutral electricity system. Proc. Natl Acad. Sci. USA 118 , e2103471118 (2021).
Adeyemi-Kayode, T. M., Misra, S., Maskeliunas, R. & Damasevicius, R. A bibliometric review of grid parity, energy transition and electricity cost research for sustainable development. Heliyon 9 , e15532 (2023).
Lang, T., Ammann, D. & Girod, B. Profitability in absence of subsidies: a techno-economic analysis of rooftop photovoltaic self-consumption in residential and commercial buildings. Renew. Energy 87 , 77–87 (2016).
Waibel, C., Hsieh, S. & Schlueter, A. Impact of demand response on BIPV and district multi-energy systems design in Singapore and Switzerland. In Carbon Neutral Cities—Energy Efficiency and Renewables in the Digital Era (CISBAT 2021) Vol. 2042 (The Electrochemical Society, 2021).
Ramasamy, V. et al. U.S. Solar Photovoltaic System and Energy Storage Cost Benchmarks, With Minimum Sustainable Price Analysis: Q1 2022 (National Renewable Energy Laboratory, 2022).
Feron, S., Cordero, R. R., Damiani, A. & Jackson, R. B. Climate change extremes and photovoltaic power output. Nat. Sustain. 4 , 270–276 (2021).
Crook, J. A., Jones, L. A., Forster, P. M. & Crook, R. Climate change impacts on future photovoltaic and concentrated solar power energy output. Energy Environ. Sci. 4 , 3101–3109 (2011).
Jerez, S. et al. The impact of climate change on photovoltaic power generation in Europe. Nat. Commun. 6 , 10014 (2015).
Wild, M., Folini, D., Henschel, F., Fischer, N. & Müller, B. Projections of long-term changes in solar radiation based on CMIP5 climate models and their influence on energy yields of photovoltaic systems. Sol. Energy 116 , 12–24 (2015).
Gernaat, D. E. H. J. et al. Climate change impacts on renewable energy supply. Nat. Clim. Change 11 , 119–125 (2021).
Deroubaix, A. et al. Large uncertainties in trends of energy demand for heating and cooling under climate change. Nat. Commun. 12 , 5197 (2021).
Ortiz, L., González, J. E. & Lin, W. Climate change impacts on peak building cooling energy demand in a coastal megacity. Environ. Res. Lett. 13 , 094008 (2018).
Laine, H. S. et al. Meeting global cooling demand with photovoltaics during the 21st century. Energy Environ. Sci. 12 , 2706–2716 (2019).
Isaac, M. & van Vuuren, D. P. Modeling global residential sector energy demand for heating and air conditioning in the context of climate change. Energy Policy 37 , 507–521 (2009).
Kan, X., Hedenus, F., Reichenberg, L. & Hohmeyer, O. Into a cooler future with electricity generated from solar photovoltaic. iScience 25 , 104208 (2022).
Wang, H. et al. Optimization of clustering analysis of residential electricity consumption behavior. In Proc . 14th IEEE Conference on Industrial Electronics and Applications (ICIEA) 418–423 (IEEE, 2019).
Zhu, K., Victoria, M., Andresen, G. B. & Greiner, M. Impact of climatic, technical and economic uncertainties on the optimal design of a coupled fossil-free electricity, heating and cooling system in Europe. Appl. Energy 262 , 114500 (2020).
Donate Your Data Researcher Handbook (Ecobee, 2019).
Jones, A. D. et al. IM3/HyperFACETS Thermodynamic Global Warming (TGW) Simulation Datasets (v1.0.0) (US Department of Energy Office of Scientific and Technical Information, 2022).
Dessler, A. E. A determination of the cloud feedback from climate variations over the past decade. Science 330 , 1523–1527 (2010).
Power Sector Carbon Index (Scott Institute for Energy Innovation, Carnegie Mellon University, 2017); https://www.emissionsindex.org
Narvaez, G., Bressan, M., Pantoja, A. & Giraldo, L. F. Climate change impact on photovoltaic power potential in South America. Environ. Res. Commun. 5 , 081004 (2023).
Zhang, J., You, Q. & Ullah, S. Changes in photovoltaic potential over China in a warmer future. Environ. Res. Lett. 17 , 114032 (2022).
Lei, Y. et al. Co-benefits of carbon neutrality in enhancing and stabilizing solar and wind energy. Nat. Clim. Change https://doi.org/10.1038/s41558-023-01692-7 (2023).
Naderi, S., Pignatta, G., Heslop, S., MacGill, I. & Chen, D. Demand response via pre-cooling and solar pre-cooling: a review. Energy Build. 272 , 112340 (2022).
Hunter, C. A. et al. Techno-economic analysis of long-duration energy storage and flexible power generation technologies to support high-variable renewable energy grids. Joule 5 , 2077–2101 (2021).
Craig, M. T. et al. A review of the potential impacts of climate change on bulk power system planning and operations in the United States. Renew. Sustain. Energy Rev. 98 , 255–267 (2018).
Hayhoe, K. et al. in Fourth National Climate Assessment. Volume II: Impacts, Risks, and Adaptation in the United States 72–144 (U.S. Global Change Research Program, 2018).
Craig, M. T. et al. Overcoming the disconnect between energy system and climate modeling. Joule 6 , 1405–1417 (2022).
Local Climatological Data (LCD) (National Oceanic and Atmospheric Administration, 2023); https://www.ncei.noaa.gov/data/local-climatological-data/access/
Reyna, J. et al. U.S. Building Stock Characterization Study: A National Typology for Decarbonizing U.S. Buildings (National Renewable Energy Laboratory, 2022).
Literature library. Goodman Air Conditioning and Heating https://www.goodmanmfg.com/support/literature-library (2023).
Wildstein, P. J., Craig, M. T. & Vaishnav, P. Participant overrides can halve the reliability value of direct load control programs. Energy Build. 299 , 113606 (2023).
Gagnon, P., Margolis, R., Melius, J., Phillips, C. & Elmore, R. Rooftop Solar Photovoltaic Technical Potential in the United States: A Detailed Assessment 70 (National Renewable Energy Laboratory, 2016).
Holmgren, W., Hansen, C. & Mikofski, M. pvlib python: a python package for modeling solar energy systems. J. Open Source Softw. 3 , 884 (2018).
Erbs, D. G., Klein, S. A. & Duffie, J. A. Estimation of the diffuse radiation fraction for hourly, daily and monthly-average global radiation. Sol. Energy 28 , 293–302 (1982).
2021 Annual Technology Baseline (NREL, 2021); https://atb.nrel.gov/
Burleyson, C., Thurber, T. & Vernon, C. Projections of Hourly Meteorology by County Based on the IM3/HyperFACETS Thermodynamic Global Warming (TGW) Simulations (v1.0.0) (MSD-LIVE Data Repository, 2023); https://doi.org/10.57931/1960548
Jones, A. D. et al. Continental United States climate projections based on thermodynamic modification of historical weather. Sci. Data 10 , 664 (2023).
Kumar P. Bias-correction 0.4 (Python, 2022); https://pypi.org/project/bias-correction/
Sengupta, M. et al. The National Solar Radiation Data Base (NSRDB). Renew. Sustain. Energy Rev. 89 , 51–60 (2018).
Gillett, M. P., Betkoski, J. W. III & Caron, M. A. Annual Residential Renewable Energy Tariff Program Review and Rate Setting (Public Utilities Regulatory Authority, State of Connecticut, 2021).
Time-of-use (TOU) rate plans. South California Edison https://www.sce.com/residential/rates/Time-Of-Use-Residential-Rate-Plans (2023).
Shi, M. S. et. al. Climate change will impact the value and optimal adoption of residential rooftop solar. Figshare https://doi.org/10.6084/m9.figshare.25296250.v1 (2024).
Download references
Acknowledgements
This work was supported by the National Natural Science Foundation of China (grant nos. 72025401, 72243007 and 72140003), the National Key Research and Development Program of China (Grant Nos. 2022YFC3702902, 2022YFC3702900 and 2023YFE0204600), the Carbon Neutrality and Energy System Transformation (CNEST) Project and the Ordos-Tsinghua Innovative & Collaborative Research Program in Carbon Neutrality. M.T.C. thanks the U.S. National Science Foundation under grant no. 2142421 for funding. We thank P. Wildstein for building modelling and grid tariff analysis and R. Jain for building simulations.
Author information
Authors and affiliations.
State Key Joint Laboratory of Environment Simulation and Pollution Control, School of Environment, Tsinghua University, Beijing, China
Mai Shi & Xi Lu
School for Environment and Sustainability, University of Michigan, Ann Arbor, MI, USA
Mai Shi & Michael T. Craig
Institute for Carbon Neutrality, Tsinghua University, Beijing, China
Department of Industrial and Operations Engineering, University of Michigan, Ann Arbor, MI, USA
Michael T. Craig
You can also search for this author in PubMed Google Scholar
Contributions
M.S. developed the research concept, designed and performed the analysis, collected data, wrote the code and drafted the paper. M.T.C contributed to the development of the concept, reviewed the codes and revised the narrative structure and language of the paper. X.L. contributed to the draft paper and its revision.
Corresponding authors
Correspondence to Xi Lu or Michael T. Craig .
Ethics declarations
Competing interests.
The authors declare no competing interests.
Peer review
Peer review information.
Nature Climate Change thanks Amarasinghage T. D. Perera, Zhili Wang and the other, anonymous, reviewer(s) for their contribution to the peer review of this work.
Additional information
Publisher’s note Springer Nature remains neutral with regard to jurisdictional claims in published maps and institutional affiliations.
Supplementary information
Supplementary information.
Supplementary Methods 1–7, Results 1–8, Figs. 1–43 and Tables 1–4.
Rights and permissions
Springer Nature or its licensor (e.g. a society or other partner) holds exclusive rights to this article under a publishing agreement with the author(s) or other rightsholder(s); author self-archiving of the accepted manuscript version of this article is solely governed by the terms of such publishing agreement and applicable law.
Reprints and permissions
About this article
Cite this article.
Shi, M., Lu, X. & Craig, M.T. Climate change will impact the value and optimal adoption of residential rooftop solar. Nat. Clim. Chang. (2024). https://doi.org/10.1038/s41558-024-01978-4
Download citation
Received : 20 July 2023
Accepted : 08 March 2024
Published : 19 April 2024
DOI : https://doi.org/10.1038/s41558-024-01978-4
Share this article
Anyone you share the following link with will be able to read this content:
Sorry, a shareable link is not currently available for this article.
Provided by the Springer Nature SharedIt content-sharing initiative
Quick links
- Explore articles by subject
- Guide to authors
- Editorial policies
Sign up for the Nature Briefing newsletter — what matters in science, free to your inbox daily.


IMAGES
VIDEO
COMMENTS
3 The perspective of solar energy. Solar energy investments can meet energy targets and environmental protection by reducing carbon emissions while having no detrimental influence on the country's development [32, 34].In countries located in the 'Sunbelt', there is huge potential for solar energy, where there is a year-round abundance of solar global horizontal irradiation.
Research 18 Apr 2024 Nature Energy P: 1-10 Flexible quasi-2D perovskite solar cells with high specific power and improved stability for energy-autonomous drones
Photocatalytic solar hydrogen production from water on a 100-m 2 scale. Carbon-neutral hydrogen can be produced through photocatalytic water splitting, as demonstrated here with a 100-m 2 array of ...
Read the latest Research articles in Solar energy from Scientific Reports ... Ultra-thin Ag/Si heterojunction hot-carrier photovoltaic conversion Schottky devices for harvesting solar energy at ...
The journal covers research on integrated solar energy systems and their applications, optimised solar energy solutions and energy storage, hybrid energy systems including mini- and micro-power systems. Moreover, the Journal welcomes articles related to research and development in direct and indirect solar energy utilization, with special focus ...
The Official Journal of the International Solar Energy Society® Solar Energy, the official journal of the International Solar Energy Society®, is devoted exclusively to the science and technology of solar energy applications. ISES is an UN-accredited membership-based NGO founded in 1954. For over 60 years, ISES members from more than 100 countries have undertaken the product research and ...
Full report (PDF) The Future of Solar Energy considers only the two widely recognized classes of technologies for converting solar energy into electricity — photovoltaics (PV) and concentrated solar power (CSP), sometimes called solar thermal) — in their current and plausible future forms. Because energy supply facilities typically last ...
Benefits of solar photovoltaic energy generation outweigh the costs, according to new research from the MIT Energy Initiative. Over a seven-year period, decline in PV costs outpaced decline in value; by 2017, market, health, and climate benefits outweighed the cost of PV systems.
The building is the best carrier of solar energy utilization in the city, under the dual pressure of energy crisis and environmental pollution. ... We invite researchers and scientists to submit high-quality original research articles that report fundamental research activities, field and laboratory experiments, innovative operational ...
See all (44) Learn more about Research Topics. Part of an innovative journal, this section covers direct energy conversion technologies, materials and device science necessary for large-scale deployment of cost-effective solar technologies.
Solar Energy Materials & Solar Cells is intended as a vehicle for the dissemination of research results on materials science and technology related to photovoltaic, photothermal and photoelectr…. View full aims & scope. $4430. Article publishing charge.
Solar energy conversion into electricity by photovoltaic modules is now a mature technology. We discuss the need for materials and device developments using conventional silicon and other materials, pointing to the need to use scalable materials and to reduce the energy payback time. Storage of solar energy can be achieved using the energy of ...
The Future of Solar is Bright. by Emily Kerr. figures by Abagail Burrus. The Sun emits enough power onto Earth each second to satisfy the entire human energy demand for over two hours. Given that it is readily available and renewable, solar power is an attractive source of energy. However, as of 2018, less than two percent of the world's ...
Solar energy systems are being installed in more diverse settings. New cell designs, materials, packaging and racking technologies are advancing to market within months. ... Research articles News ...
Submission. Solar Energy welcomes submissions of the following article types: Brief Research Report, Correction, Data Report, Editorial, General Commentary, Hypothesis & Theory, Methods, Mini Review, Opinion, Original Research, Perspective, Policy and Practice Reviews, Review, Technology and Code. All manuscripts must be submitted directly to the section Solar Energy, where they are peer ...
Abstract — Solar Energy is the prime important. source of energy, and it has continued to gain. popularity globally. As of 2018, about 486 GW of solar. PV was installed worldwide. One of the key ...
The sun, widely regarded as the most potent resource for renewable energy, presents an unceasing and omnipresent solution to combat the global energy crisis spurred by fossil fuel exhaustion. ... Research Article. An experimental study of the effect of solar tracking technology on the performance of a single phase open thermosyphon: a case study.
Pew Research Center conducted this analysis to understand Americans' adoption of home solar panels. It relies on data from the U.S. Energy Information Administration and the Solar Energy Industries Association, among other sources.. The analysis also draws from a Center survey of 10,237 U.S. adults conducted from Jan. 24-30, 2022.
67. JRTE-2020. Solar Energy Technology. Sumedha R.G. Weliwaththage, Udara S.P.R. Arach chige. Faculty of Technology, University of Sri Jayewardenepura, Sri Lanka. Abstract Energy resources can ...
NREL's solar research strives to enable reliable, low-cost solar energy at scale—on the grid and beyond the grid. Postdocs Study Impact of Turbulent Winds on Concentrating Solar Power The study will help predict the impact of wind conditions on concentrating solar power performance and more
An international team of researchers, including a group from Northwestern Engineering and Northwestern Chemistry, has set a new world record for power conversion efficiency (PCE) of single-junction perovskite solar cells (PSCs).. These solar cells - created from an emerging solar material - have the potential to generate greater solar energy at a lower cost than today's industry-standard ...
Solar energy is the most widely available energy resource on Earth, and its economic attractiveness is improving fast in a cycle of increasing investments. ... Research articles Reviews & Analysis ...
Solar Energy. Though costly to implement, solar energy offers a clean, renewable source of power. Solar energy is the technology used to harness the sun's energy and make it useable. As of 2011 ...
Nov. 2, 2020 — Solar power has shown immense potential as a futuristic, 'clean' source of energy. No wonder environmentalists worldwide have been looking for ways to advance the current solar ...
Yet adding a perovskite cell produces a theoretical maximum efficiency of roughly 45%. "It's offering the potential to get 25-50% more power out of the panels. I think that's an exciting ...
The production of clean hydrogen through artificial photosynthesis is the most intriguing research topic that offers hope for meeting the world's energy demands. The evolution of green hydrogen via visible light-driven photocatalysis is challenging but feasible. Photocatalytic solar power systems primarily rely on
Table 1, which includes four bibliometric articles found in the scientific literature that somehow approach solar PV energy, was developed aiming to explain the inexistence of publications with bibliometric features related to the solar PV energy management term.It presents the author's name, the journal where it was published, as well as the article's title and goal.
One advantage of systems like Fervo's is that they can be made more profitable by taking advantage of energy price fluctuations, according to recent research by Ricks, a Princeton colleague and ...
Climate change will affect the adoption of residential rooftop solar photovoltaics by changing the patterns of both electricity generation and demand. This research projects that climate change ...
An electrolyzer sits on a truck bed at the Advanced Clean Energy Storage project being constructed in Delta, Utah. Photo: Chevron