

Materials Advances
Nanomaterials: a review of synthesis methods, properties, recent progress, and challenges.

* Corresponding authors
a Center of Research Excellence in Desalination & Water Treatment, King Fahd University of Petroleum and Minerals, Dhahran 31261, Saudi Arabia E-mail: [email protected] , [email protected]
b Center for Environment and Water, King Fahd University of Petroleum and Minerals, Dhahran 31261, Saudi Arabia
c Interdisciplinary Research Center for Membranes and Water Security, King Fahd University of Petroleum and Minerals, Dhahran 31261, Saudi Arabia
d Department of Chemical & Biological Engineering, University of Alabama, Tuscaloosa, Alabama 35487-0203, USA E-mail: [email protected] , [email protected]
e Department of Mechanical Engineering, King Fahd University of Petroleum and Minerals, Dhahran 31261, Saudi Arabia
Nanomaterials have emerged as an amazing class of materials that consists of a broad spectrum of examples with at least one dimension in the range of 1 to 100 nm. Exceptionally high surface areas can be achieved through the rational design of nanomaterials. Nanomaterials can be produced with outstanding magnetic, electrical, optical, mechanical, and catalytic properties that are substantially different from their bulk counterparts. The nanomaterial properties can be tuned as desired via precisely controlling the size, shape, synthesis conditions, and appropriate functionalization. This review discusses a brief history of nanomaterials and their use throughout history to trigger advances in nanotechnology development. In particular, we describe and define various terms relating to nanomaterials. Various nanomaterial synthesis methods, including top-down and bottom-up approaches, are discussed. The unique features of nanomaterials are highlighted throughout the review. This review describes advances in nanomaterials, specifically fullerenes, carbon nanotubes, graphene, carbon quantum dots, nanodiamonds, carbon nanohorns, nanoporous materials, core–shell nanoparticles, silicene, antimonene, MXenes, 2D MOF nanosheets, boron nitride nanosheets, layered double hydroxides, and metal-based nanomaterials. Finally, we conclude by discussing challenges and future perspectives relating to nanomaterials.
Article information

Download Citation
Permissions.

N. Baig, I. Kammakakam and W. Falath, Mater. Adv. , 2021, 2 , 1821 DOI: 10.1039/D0MA00807A
This article is licensed under a Creative Commons Attribution-NonCommercial 3.0 Unported Licence . You can use material from this article in other publications, without requesting further permission from the RSC, provided that the correct acknowledgement is given and it is not used for commercial purposes.
To request permission to reproduce material from this article in a commercial publication , please go to the Copyright Clearance Center request page .
If you are an author contributing to an RSC publication, you do not need to request permission provided correct acknowledgement is given.
If you are the author of this article, you do not need to request permission to reproduce figures and diagrams provided correct acknowledgement is given. If you want to reproduce the whole article in a third-party commercial publication (excluding your thesis/dissertation for which permission is not required) please go to the Copyright Clearance Center request page .
Read more about how to correctly acknowledge RSC content .
Social activity
Search articles by author, advertisements.
- Search Menu
- Advance Articles
- Author Guidelines
- Open Access Options
- Self-Archiving Policy
- About International Journal of Low-Carbon Technologies
- Editorial Board
- Advertising and Corporate Services
- Journals Career Network
- Dispatch Dates
- Journals on Oxford Academic
- Books on Oxford Academic

Article Contents
1. introduction, 2. systematic literature review: methods and materials, 3. results of the bibliographic analysis of data, 4. applications of nanotechnology in ev, 5. summary of key research findings, 6. conclusions, declaration of competing interest, acknowledgements, author contributions.
- < Previous
A systematic review of nanotechnology for electric vehicles battery

- Article contents
- Figures & tables
- Supplementary Data
Pulkit Kumar, Harpreet Kaur Channi, Atul Babbar, Raman Kumar, Javed Khan Bhutto, T M Yunus Khan, Abhijit Bhowmik, Abdul Razak, Anteneh Wogasso Wodajo, A systematic review of nanotechnology for electric vehicles battery, International Journal of Low-Carbon Technologies , Volume 19, 2024, Pages 747–765, https://doi.org/10.1093/ijlct/ctae029
- Permissions Icon Permissions
Nanotechnology has increased electric vehicle (EV) battery production, efficiency and use. Nanotechnology is explored in this electric car battery illustration. Nanoscale materials and topologies research has increased battery energy density, charge time and cycle life. Nanotubes, graphene and metal oxides improve energy storage, flow and charging/discharge. Solid-state and lithium-air high-energy batteries are safer, more energy dense and more stable using nanoscale catalysts. Nanotechnology improves battery parts. Nanostructured fluids reduce lithium dendrite, improving batteries. Nanocoating electrodes may reduce damage and extend battery life. Nanotechnology benefits the planet. Nanomaterials allow battery parts to employ ordinary, safe materials instead of rare, harmful ones. Nanotechnology promotes battery recycling, reducing waste. Change does not influence stable, cost-effective or scalable items. Business opportunities for nanotechnology-based EV batteries need more research. High-performance, robust and environmentally friendly batteries might make electric cars more popular and transportation more sustainable with research and development. An outline of EV battery nanotechnology researchexamines the publication patterns, notable articles, collaborators and contributions. This issue was researched extensively, indicating interest. Research focuses on anode materials, energy storage and battery performance. A research landscape assessment demonstrates EV battery nanotechnology’s growth and future. A comprehensive literature review examined nanosensors in EVs. Our study provides a solid foundation for understanding the current state of research, identifying major trends and discovering nanotechnology breakthroughs in EV sensors by carefully reviewing, characterizing and rating important papers.
The future of nanotechnology with electric vehicles (EVs) is uncertain. Researchers and engineers use nano-manipulating materials to boost EVs’ speed, efficiency and longevity [ 1 ]. Nanotechnology makes coatings for EVs, battery technology, energy harvesting, sensors, catalysis and lightweight materials possible [ 2 ]. By enhancing energy storage, charging speed, component weight and durability, nanotechnology makes electric automobiles more efficient and practical, which could help circumvent transportation system limitations. A cleaner, greener and more sustainable transportation future will be ushered in as nanotechnology improves the capabilities of electric automobiles and increases their widespread acceptability. Nanotechnology’s quick growth has generated exciting potential for the EV revolution [ 3 ]. The use of nanotechnology can improve the performance, efficiency and longevity of EVs. Battery technology, lightweight materials, energy harvesting, sensors, catalysis and coatings are all examples of applications where scientists and engineers have taken advantage of nanoparticles’ unique capabilities [ 4 ]. The batteries used in EVs are improving because of nanotechnology [ 5 ].
Graphene and carbon nanotubes are two examples of nanoscale materials that improve energy storage, electrode surface area and electrode conductivity. The lightweight materials made possible by nanotechnology may also impact EV design [ 6 ]. Nanomaterial composites, including carbon nanotubes and nanofibers, have superior strength-to-weight ratios. Lightening the load improves the vehicle’s mileage, range and handling. EVs use nanotechnology for energy collection. Nanogenerators convert the kinetic energy produced by the car’s motion and vibrations into electrical power. Nanostructured solar panels on the vehicle’s surface capture sun energy for EV charging [ 7 ].
Nanotechnology allows for the development sophisticated sensors that keep tabs on and control the operation of EVs. The susceptible and accurate nanosensors can measure temperature, pressure and gas concentrations, increase safety, give better battery management and enhance vehicle performance. Catalysis and fuel cell technologies, which provide alternatives to EV batteries, rely heavily on nanotechnology [ 8 ]. Using nanocatalysts, fuel cells can convert energy more cleanly. Fuel cells are an option for EVs because of their longer ranges and shorter recharging times [ 9 ]. Intelligent coatings and self-repairing materials can be produced via nanotechnology for EVs. Scratches, corrosion and weathering are all thwarted by nanocoating, and the coating’s self-healing properties repair minor damage [ 10 ]. These coatings’ durability, hydrophobicity and UV resistance increase EVs’ aesthetics, longevity and sustainability. As progress in nanotechnology advances, the outlook for EVs improves. Nanotechnology can make transportation systems more effective, practical and environmentally friendly [ 11 ]. Figure 1 shows the few primary ways nanotechnology can be applied to EVs. Cleaner, greener transportation may lie in the hands of EVs that utilize nanoparticles and nanoscale engineering.

Nanotechnology in EVs.
To further explore how EV batteries might be improved, it is vital to provide a more comprehensive explanation of the specific roles played by nanotubes, graphene, metal oxides and nanoscale catalysts. Nanomaterials are crucial in enhancing different performance characteristics of EV batteries [ 12 ]. The remarkable conductivity features of nanotubes and graphene enable efficient electron transit, hence increasing the overall conductivity of the battery. Metal oxides enhance batteries’ energy storage capacity, improving their efficiency in storing and releasing energy during charge and discharge processes [ 13 ]. In addition, catalysts at the nanoscale operate as promoters to enhance the speed of reaction kinetics, optimizing the charge and discharge efficiency. Furthermore, integrating these nanomaterials improves EV batteries’ security and durability by addressing concerns regarding excessive heat generation [ 14 ] and establishing a more substantial structural foundation [ 15 ]. An in-depth investigation into the unique effects of each nanomaterial on energy storage [ 16 ], conductivity, charge/discharge processes, safety and stability issues offers a detailed comprehension of their combined influence on the advancement of EV battery technology [ 17 ].
Nanotechnology has become a major force in improving the performance and economy of batteries for EVs. Materials like nanotubes, graphene, metal oxides and nanoscale catalysts play key roles in this. Nanotubes and graphene are great for storing energy because they are highly conductive and have a lot of surface area [ 15 ]. This makes them better electrode materials for lithium-ion batteries, which are widely used in EVs. These materials make it easier for electrons and ions to move faster, which leads to more energy and faster charging and discharging. On the other hand, metal oxides are great alternatives to standard cathode materials because they have higher capacity and better cycling stability. Their nanoscale size makes charge transfer even more efficient, which lowers the energy lost during cycles [ 18 ]. Graphene is a single layer of carbon atoms grouped in a hexagonal lattice. It is very good at conducting electricity and is also very strong. It makes the conductivity better when added to battery electrodes, which speeds up the charging and discharging processes. Nanotubes, like carbon nanotubes, have a lot of surface area and can carry electricity well. This has helped make electrode materials that are light and work well. Metal oxides, such as manganese oxide and titanium oxide, have better electrochemical qualities [ 19 ]. This meets the need for materials in EV batteries that have a higher energy density and last longer between cycles.
Moreover, tiny catalysts have completely changed the electrochemical processes that happen inside batteries. These helpers, which are usually made of precious metals like platinum and palladium, make the processes that reduce and release oxygen easier in fuel cells and metal–air batteries. This makes them work better overall. Nanostructuring these catalysts also makes them more active, which means they can convert and store energy more efficiently. When it comes to safety, nanomaterials help make improved battery systems possible [ 20 ]. Nanoscale coatings and additives can make lithium-ion batteries safer by making them more stable at high temperatures, lowering the risk of burning, and stopping dendrites from forming. Nanomaterials also make things more stable, which means batteries last longer. This means they don’t need to be replaced as often, and throwing away batteries has less of an effect on the world [ 21 ]. According to the researchers, adding nanotubes, graphene, metal oxides and tiny catalysts to the design and make-up of EV batteries is a big step forward for making them safer, more stable and better at storing energy and conducting electricity. As scientists continue to study and improve these nanotechnology uses, the chances of making electric transportation more efficient and environmentally friendly grow [ 22 ].
1.1. Importance of nanosensors
Sanguesa et al. (2021) reviewed EV battery technological trends, charging techniques and future research challenges and prospects. They discussed the pros and cons of lead-acid, nickel-metal hydride, lithium-ion and lithium-sulfur EV batteries. They also discussed EV charging standards and modes such as AC and DC, slow and rapid, wireless and bidirectional. They discussed nanosensors’ high sensitivity, low power consumption, miniaturization, fabrication, integration and calibration concerns for battery management [ 23 ]. A literature overview on EV technology and its various uses, including smart grid, vehicle to grid, and vehicle to home, was presented. Energy efficiency, emissions reduction, grid stability, battery cost, range anxiety and charging infrastructure were only some of the topics covered. Several global EV programs and projects were highlighted, including Tesla, Nissan Leaf and Dubai Smart City [ 24 ]. A systematic literature review (SLR) on EV consumer acceptance was published by Han et al. [ 25 ]. They synthesized the methodology, ideas and variables from 57 peer-reviewed publications published between 2015 and 2022 on EV purchase, behavior and usage intentions. They found that attitudes, norms, perceived behavioral control, awareness, knowledge, personal values, emotions and social influence affect EV adoption. They also highlighted literature gaps and suggested additional research [ 25 ]. The authors of Basu et al. (2018) provided a synopsis of EVs and EV sensors. The advantages and disadvantages of the various varieties of EVs, including fuel cell EVs (FCEVs), battery EVs (BEVs) and hybrid EVs (HEVs), were discussed. Additionally, they deliberated on the evolving landscape of micro-electro-mechanical system- (MEMS) based miniaturization for sensors and devices utilized in diverse EV applications and the various types of sensors associated with battery and position monitoring [ 26 ].
1.2. Literature review
The low-carbon mobility transition relies on plug-in EVs, including battery and hybrid EVs. Daramy-Williams et al. (2019) found many user experience topics, including driving and travel behaviors, vehicle interactions and subjective factors. EVs have increased the utilization of electric batteries. Many studies have developed and improved battery cell voltage equalization methods by adding noteworthy features [ 27 ]. These cell equalization techniques were the subject of a thorough and methodical review (Das et al., 2020). This SLR focuses on recent efforts that have built energy management storage system (EMSs) for HEVs [ 28 ]. The study conducted by Torreglosa et al. (2020) aimed to provide a quantitative analysis of the chosen works. Notwithstanding advancements in driving range and recharge alternatives, these and additional market impediments persist, rendering the present market share of BEVs inconsequential [ 29 ]. In light of the advancement of hydrogen fuel cell stacks, an emerging powertrain architecture concept for N1 class-type cars was described (Castillo et al., 2020). The fuel cell extended range electric vehicles (FC-EREV)concept combines a battery-electric arrangement with a hydrogen-powered fuel cell stack that acts as a range extender. The lithium-ion battery in EVs can sustain an operational temperature range of 15°C to 35°C employing a battery thermal management system (BTMS) [ 30 ].
Tete et al. (2021) conducted a comprehensive review of experimental and numerical analyses of BTMS utilized in electric and hybrid vehicles. This review encompassed approaches such as air, liquid, phase change material, heat pipe and refrigeration cooling for battery cooling systems. EVs need high-energy batteries. The maximum capacity of lithium-air battery theory using graphene under optimal electron conduction conditions and the experimental maximum obtained by optimizing the structure geometry, examples of structural engineering using carbon fiber and carbon nanotubes in cathode fabrication to perform the reaction properly while providing space for lithium oxide placement, are examined [ 31 ]. Suryatna et al. (2022) described the battery’s mechanism and analyze its constituent parts. As a result of the growth of green logistics and the support of new energy car development policies both domestically and globally, logistics and distribution have embraced EVs as an alternative to traditional fuel vehicles [ 32 ]. Ye et al. (2022) examined the most recent breakthroughs in EV routing models and solution algorithms in logistics and distribution. Passenger vehicles contribute significantly to glasshouse gas (GHG) emissions; thus, precise and current estimates of the comparative emissions of the key types of alternative power trains are essential to support evidence-based policy recommendations [ 33 ]. A systematic review and harmonization of the most recent scientific literature on this subject was presented in Raugei (2022). The findings show that battery BEVs are the most promising option for decarbonizing the passenger vehicle fleet across all global regions studied, with the potential for −70% reductions in GHG emissions compared to conventional gasoline-powered internal combustion engine vehicles. EVs are becoming increasingly prevalent as many nations set net-zero carbon targets for the foreseeable future [ 34 ].
The status, characteristics and application scope of global lithium ion battery (LIB) safety standards and regulations were examined by Lai et al. (2022). The rational test standard upgrade is reviewed in light of recent EV and energy storage power plant fires. Direct drive offers improved systematic economy, more flexible wheel control and better passenger comfort by eliminating the gearbox and transmission [ 35 ]. Cai et al. (2022) reviewed vehicle direct-drive methods and contemporary electrical machine improvements for direct-drive propulsion systems. FCEVs for long-haul applications can reduce road freight CO 2 emissions until long-distance battery-electric mobility matures, depending on the hydrogen fuel source [ 36 ]. Pardhi et al. (2022) examined FCEV powertrain topologies for long-distance HD applications, as well as their operating constraints, cooling requirements, waste heat recovery methods, cutting-edge powertrain control, energy and thermal management strategies, as well as over-the-air route data-based predictive powertrain management with V2X connectivity. Batteries powering EVs provide a promising way to reduce pollution and uncertainty.
In contrast to battery degeneration’s scalability and temporal constraints, machine learning (ML) approaches offer a non-invasive, accurate and low-processing solution [ 37 ]. Sharma and Bora (2023) evaluated these problems objectively and comprehensively. EVs are becoming mainstream as more governments set near-term net-zero carbon ambitions [ 38 ]. Senol et al. (2023) conducted a comprehensive literature analysis in their study, which focused on integrating power networks and Li-ion battery technologies, particularly emphasizing their performance under unfavorable weather circumstances [ 39 ]. Increasing multiscale modeling and design for battery efficiency and safety management was reviewed by Kiran MD et al. (2024). This article shows how machine learning-based data analysis in battery research has advanced, setting the groundwork for cloud and digital battery management to produce trustworthy onboard applications [ 40 ]. Electron and ion transport affect the battery’s energy production under application conditions and how much energy can be used [ 41 ]. The transport mechanisms of ions and electrons for active materials, in addition to positive and negative composite electrodes, were examined by Quilty et al. (2023). Simultaneously, contemporary EVs exhibit a confident capacity to reduce fossil fuel consumption [ 42 ]. Gevorkov et al. (2023) reviewed and analyzed multiport converters’ key characteristics, topologies, pros and cons and applications. Operando characterization is not new, but techniques that can track commercial battery properties under realistic conditions have unlocked a trove of chemical, thermal and mechanical data that could revolutionize lithium-ion device development and use [ 43 ]. The innovative dual-ion battery that is built on aluminum and has a cathode made of three-dimensional graphene possesses high-energy density, low cost and the ability to charge and discharge faster than other batteries, making it an ideal choice for grid storage and personal gadgets by Zhang et al. (2016) [ 44 ]. A novel calcium-ion battery with a high discharge voltage and 95% capacity retention can function consistently at room temperature. This battery has the potential to serve as an alternative to lithium-ion batteries by Wang et al. (2018) [ 45 ]. For sodium-based energy storage applications, this study conducted by Mu et al. (2020) successfully synthesizes high-performance anodes with high-fraction active materials. These anodes achieve good rate capability and cycling stability [ 46 ]. Zhang et al. (2023) examined the difficulties encountered by power systems that heavily rely on inverter-based resources (IBR), specifically emphasizing the inadequate capacity to support voltage and frequency. The authors suggest reorganizing the virtual synchronous generator (VSG’s) control blocks by aligning the control blocks of a VSG with the control perspective of conventional synchronous generators. The reorganization above streamlines and clarifies the control pathway governing the system’s active and reactive power output, enabling easier virtual inertia and attenuation parameters to be adjusted. The article presents a compact signal model for IBR controlled by VSG, including voltage and current loops modules, instantaneous power calculation and an LC (inductors (L) and capacitors (C)) filter [ 47 ]. A current discrepancy that occurs during charge and discharging in high-temperature superconducting (HTS) non-insulation closed-loop coils may result in novel phenomena, such as a rapid decrease or increase in magnetic field at particular positions, and may influence operational current judgment by Lu et al. (2022) [ 48 ]. Wu et al. (2023) centers on the advancements in rare earth-barium-copper oxides superconducting tapes, with a specific emphasis on the nanocomposite EuBa2Cu3O7-δ superconducting films. These films hold significant importance in the context of high-field magnet applications. The scientists utilize a pulsed laser deposition methodology with an exceedingly rapid growth rate of up to 100 nm/s—two orders of magnitude higher than traditional approaches. This enables them to accomplish both rapid growth and a substantial capacity to transport field current [ 49 ]. Shen et al. (2024) have presented an innovative energy management approach that seeks to optimize the functionality of energy storage systems in EVs, specifically focusing on reducing the aging of lithium-ion batteries caused by high-frequency power requirements. Fuzzy logic control and ensemble empirical mode decomposition are incorporated into the proposed method. At the outset, the power demand of EVs is decomposed into intrinsic mode function components. Subsequently, permutation entropy is utilized to reconstruct each component into low- or high-frequency components [ 50 ]. A proposal was made by Zhao et al. (2023) for the preview-based human-like trajectory planning model (PHTPM), which is subsequently evaluated and assessed through comparative and generalizability tests. The findings indicate that the implementation of the driver preview feature empowers PHTPM to precisely emulate the attributes of proficient drivers during left turns while surpassing them during right turns [ 51 ]. A critical scenario search technique for intelligent vehicle testing that is based on the social cognitive optimization algorithm has been suggested, and the findings show that the proposed method has the potential to increase both the search efficiency, and the coverage of important scenarios was figured out by Zhu et al. (2023) [ 52 ]. This research presents a novel model that has the potential to improve our understanding of the behavior of lithium deposition and pave the way for the development of stable and secure lithium metal anodes through the utilization of bimetallic metal organic framework- (MOF) derived materials in the construction of three-dimensional frameworks studied by Wei et al. (2023) [ 53 ]. Based on the findings of the analysis conducted by Yue et al. (2023), it can be concluded that the stability of the road system in the presence of incident effects is directly connected to the severity of the event, the signal control strategy, the penetration rate and the spatial distribution of autonomous vehicles. In conclusion, simulation results are carried out to demonstrate the efficacy of our suggested event management policy in enhancing the rate of recovery and the stability of road networks [ 54 ]. In order to solve the issue in which the response quality is decreased as a result of factors such as parameter mismatch and disturbance, an adaptive disturbance observer-based improved super-twisting sliding mode control (ISTSMC-ADOB) has been developed. With the purpose of achieving adaptive compensation, avoiding the usage of high-gain feedback, and expanding the applicability of the conventional disturbance observer, an adaptive disturbance observer, also known as an ADOB, was intentionally constructed by Lu et al. (2022) [ 55 ]. Yu et al. (2023) has discussed the difficulties that arise when attempting to implement aqueous zinc-ion batteries in practical applications. The paper focuses on the unstable electrode/electrolyte interface that is related to inhomogeneous zinc deposition and side reactions. The solution that has been offered involves the utilization of L-carnitine (L-CN) as an effective addition for the purpose of stabilizing electrodes and extending the lifespan of batteries. When L-CN is present in minute quantities, it exhibits a remarkable synergy between quaternary ammonium cations, COO− anions and hydroxyl groups. This synergy can influence the electrochemical deposition/insertion of Zn 2+ and the activity of water molecules [ 56 ]. Hou et al. (2023) have centered on developing a self-powered, lightweight biomimetic mouse whisker sensor (BMWS) that draws inspiration from the extraordinarily perceptive whisker detection exhibited by mice. The BMWS, unlike its predecessors that attempted to imitate animal whiskers, surmounts drawbacks, including a cumbersome design, dependence on external power and restricted application scenarios. By utilizing the triboelectric effect and a meticulously engineered framework, the BMWS exhibits exceptional capabilities in detecting collisions and maintaining signal stability. Intelligent early warning, direction identification, hole width discrimination and real-time distance sensing are among the many duties in which it excels [ 57 ]. A novel mechanism and technology for directed energy deposition-arc in the production of large parts, referred to as alternating-arc-based additive manufacturing and enabled by a polarity-switching self-adaptive shunt, are presented Yan et al. (2023). The experimental outcomes demonstrate that the proposed system facilitates the alternating passage of current through the welding wire and the substrate, generating electronegative arcs at the anode with the wire and electropositive arcs at the cathode with the substrate. By modulating the arcs, decoupling control between thermal force and mass transfer is accomplished. The electropositive arc is responsible for cathode cleaning and molten pool temperature, whereas the electronegative arc regulates wire melting, particle size and temperature [ 58 ]. A battery–supercapacitor hybrid energy storage system and an accompanying energy management strategy are proposed in the article by Wang et al. (2024) in a response to the growing need for compact motors with high output torque, specifically for use in mobile robotics. This method draws inspiration from automobiles’ high-capacity hybrid energy systems. In conditions of minimal torque, the motor operates on battery power, with any excess power being directed toward charging the supercapacitor. When faced with torque overload conditions, the motor is supplied with high current by swiftly discharging the supercapacitor, which guarantees an instantaneous increase in output power. The objective of the energy management strategy is to regulate the supercapacitor’s charging and discharging processes to prevent interference with the motor’s power supply from the battery and maintain current stability and control during discharge [ 59 ].

Process of SLR.

Flow chart of selection of data for bibliometric analysis.
The resources utilized in this SLR comprise bibliometric data extracted from various sources, such as journal articles, conference papers, scholarly surveys, books, essays and review articles. These sources are analyzed to determine author affiliations, country collaboration maps, citation patterns, keywords plus and the frequency of author keywords. The present study employs a systematic procedure to select research publications about nanomaterials in EVs. The literature search involved comprehensive exploration using databases like Scopus. Key search terms included ‘nanotechnology in EV batteries,’ ‘electrode nanocoating’ and related variations. Potential biases in article selection were mitigated by employing rigorous inclusion criteria, prioritizing peer-reviewed studies and avoiding undue influence from commercial interests. Figure 2 shows the process of SLR.
The Scopus article research selections were restricted in the first step, as shown in Figure 3 and Table 1 . From 1991 to July 2023, this analysis uncovered 2361 publications concerning EVs, nanotechnology, nanomaterials and sustainable development. We have 2322 articles remaining after eliminating concise surveys, notes, erratum editorials and letters. After publication status-based exclusions of research papers, 2309 remained. The final subjects that were excluded from consideration were the following: astronomy and physics, pharmacology, toxicology, pharmaceutics, earth and planetary science, health profession, agriculture and biological science, neuroscience, immunology, microbiology, business and management, accounting, economics, econometrics and finance, arts and humanities and dentistry. One thousand five hundred forty products were remaining.
Bibliometric analysis is a method that quantifies scientific publications by examining citation patterns. Citations, according to a bibliometric study, reveal the impact, influence and connections of academic journals [ 60 ]. The bibliometric analysis theory posits that scientific knowledge is transmitted through scholarly publications, wherein the number and caliber of citations gauge the scientific significance of an article or publication it garners [ 61 ]. Bibliometric researchers utilize citation data to quantify author output, journal influence, collaboration patterns and research trends. According to this theory, more people cite influential articles [ 62 ].
Additionally, intellectual networks between authors, institutions and fields may be reflected in citations [ 63 , 64 ]. By quantifying these citation patterns, bibliometric analysis evaluates research output, influence and collaboration [ 63 ]. Bibliometric research is regulated by mathematical and statistical models, including co-citation analysis, citation counts, h-index and bibliographic coupling. Academics can quantify and contrast scholastic output, discern notable works and authors and monitor the evolution of specific publications or research domains through these techniques. Citation patterns can disclose the structure and dynamics of scientific knowledge, aiding policymakers, institutions and researchers in making informed decisions regarding funding allocation, research evaluation and scholarly communication, according to bibliometric analysis [ 65 ].
By examining the bibliography, the most cited journals are determined. Energy and environmental science, nanoenergy, ACS Applied Materials and Interfaces and the Journal of Materials Chemistry , all of which have contributed to the study of nanomaterials in EVs, are utilized in the proposed research. Numerous scholarly periodicals indicate that nanomaterials are an emerging trend for energy storage and lightweight materials to reduce the weight of EVs, as demonstrated by this review [ 66 ]. Bibliometric analysis is an expert methodology that assists researchers in assessing the advancement of various approaches through the statistical distribution of data about citation analysis, keywords, author affiliation and country of contribution. The top journals are shown in Table 2 .
Detailed analysis of nanomaterials in EV data is presented in this section. In recent decades, the quantity of publications and literary sources is evaluated in Sections 3.1 and 3.2. Additionally, the country production analysis is presented in Section 3.3. The thematic evaluation of the keywords in the publications is presented in Section 3.4. Additionally, the collaboration map of the top 15 nations, most cited countries, country production over time and source dynamics is provided in Section 3.5. Section 3.6 provides a comprehensive analysis of the findings regarding author keywords, most common terms, a tree map of keywords and word clouds. Section 3.7 presents three field plots depicting the relationships between author keywords, author countries and keywords plus.
3.1. Literature analysis of publications
Figure 4 illustrates the annual distribution of publications from 1991 to 2023. However, upon examining the graph, we have identified a substantial fluctuation in the number of publications on nanomaterials in EVs. The number of publications in this field was ‘0’ before 2003 but rose after 2005. However, between 2012 and 2021, there has been a significant increase in the number of publications devoted to investigations. The calculated annual growth rate was approximately 12.79%.
3.2. Institutions
A visual representation of the collaborations between the five most prestigious institutions worldwide and their publications over the last 30 years is presented in Figure 5 . As stated, five major institutions contribute to the Scopus Journal, with Nanyang Technological University ranking first with 945 publications and Beijing University ranking second with 586 publications. Simultaneously, the University of Science and Technology of China, the School of Material Science and Engineering and Tsinghua University ranked third, fourth and fifth in the Scopus database from 1991 to 2023, with net publications of 528, 475 and 409, respectively.
Main information of data taken from Scopus.
Top 15 journals in the research area under the study, 3.3. analysis of countries.
Diverse nations have contributed to academic journals concerning the application of nanotechnology to EVs. The cumulative number of publications originates from the 10 countries in Figure 6 . Based on the analysis, China holds the highest position regarding the total number of articles. Following that, India and the United States occupied the second and third positions, respectively, with 1584 and 480 publications, while China produced 2370 articles within the specified period.
3.4. Thematic evaluation of keywords
Based on the evaluation of keywords from 1991 to 2023, as shown in Figure 7 , several authors have repeatedly utilized a small number of keywords. The following terms were examined following the analysis: self-powered sensors, batteries, triboelectric nanogenerator, nanotechnology, renewable energy, lithium-ion batteries, lithium-ion batteries, electrode material and electrochemical performance.

Annual distribution of the publications.

Top 5 productive institutions.

Top 15 countries with the maximum number of publications.

Thematic evaluation of keywords.
3.5. Country collaboration
The collaborative efforts of numerous nations are illustrated in Figure 8 , and Figure 9 shows the cloud-based structure of the various country collaborations. Based on the bibliometric survey’s analysis, the following countries have been identified as having the greatest number of collaborations in the field of nanotechnology for EVs: China, India, the United States, South Korea, Germany, Japan, the United Kingdom, the Philippines, Singapore, France, Australia, Canada, Italy, Spain and Malaysia.

Country collaboration map.

Cloud-based structure of the various country collaborations.
3.6. Keywords
Each node in Figure 10a corresponds to a distinct keyword. We opted to include only the top 50 out of 1000 keywords. The prevailing research topics identified were ‘nanotechnology,’ ‘lithium,’ ‘electrodes,’ ‘electric batteries,’ ‘nanostructured materials’ and ‘energy efficiency.’ A cloud structure of keywords is depicted in Figure 10b , which resembles the analysis in Figure 10a ; however, the distinction between the two figures lies in the quantity of keywords. The authors spent the most time gathering the following 50 keywords for the cloud of keywords: ‘nanotechnology,’ ‘lithium-ion batteries,’ ‘lithium,’ ‘electrodes,’ ‘electric batteries’ and lithium compounds. In contrast, Figure 10c illustrates the tree map structure of keywords as they appear in the publications of various authors.

a. Keyword co-occurrence network. b Keywords cloud of top 50 keywords. c. Tree map of keywords.
3.7. Three-field plot
The three field plots between author keywords, author countries and keywords plus were depicted in Figure 11 . The preceding 20-author keywords, utilized by most authors in their publications, are displayed on the left side of the plot. The author countries to which they belong are then indicated in the middle. The top 20 countries were analyzed, with China, the United States, Korea and India ranking the highest. The concluding segment of the narrative presents keywords plus, which includes the top 20 keywords and those used by the author. Concerning the keywords, this plot is structured to examine the research field and the authors’ collaborative network.

Three field plots between author keywords, author countries and keywords plus.
3.8. Bibliometric analysis challenges
Researchers may encounter several problems and restrictions when using R-Studio Biblioshiny for bibliometric analysis. One big problem is that bibliographic records are very different from one another. This is because different sources may use different formats and terminologies, which makes it hard to combine and standardize data. It might also be hard to ensure the bibliometric study’s accuracy if the data’s quality and completeness vary from database to database [ 67 ]. Another problem is that scientific literature is always changing; new papers are always being added, and databases might not be updated very often, which could mean the results are incomplete or outdated. Also, full-text articles may not always be available or easily accessible, which could affect the study’s completion. Researchers may also have trouble developing relevant search queries because the buzzwords and the criteria they use to include or leave out information can greatly affect the results [ 68 ]. Even with these problems, R-Studio Biblioshiny for bibliometric analysis is still a useful tool that helps researchers get around many of these issues and learn important things about the scholarly world.
Additionally, picking the right bibliometric indicators and measures presents different problems. To measure the effect, output and teamwork, researchers must be meticulous in picking the right factors. If you misinterpret metrics or only use a small group of indicators, you might come to the wrong conclusions about the importance of certain study topics or authors [ 69 ]. Also, because some fields are changing and combining different areas of study, it might be hard to correctly group papers into the right research domains. When doing bibliometric analysis, it is also very important to think about ethics, especially when there are disagreements about who wrote a paper or when someone cites themselves in a paper. To ensure the results are accurate, it is important to balance automated data extraction and human verification [ 70 ]. There may also be problems with how bibliometric analyses can be repeated since different researchers may get different results depending on how they read parameters or how the data sources are changed. Even with these problems and restrictions, R-Studio Biblioshiny has an easy-to-use design and many tools that can help with many of them. As software is constantly updated and made better, some problems may be solved. This makes bibliometric analysis a useful and ever-evolving way to understand the scholarly scene. As the scientific literature changes quickly, researchers should stay alert and deal with these problems to ensure their results are strong and correct [ 71 ].
Nanotechnology is currently leading the way in transforming numerous aspects of EV technology. Nanoscale materials, including carbon nanotubes and graphene, are important in battery technology because they improve electrode conductivity, surface area and energy storage. This leads to enhanced energy density, prolonged battery life and accelerated charging, effectively mitigating apprehensions regarding charging durations and range anxiety [ 72 ]. In addition to batteries, nanomaterials can generate lightweight and robust materials for EV construction. This is demonstrated through the integration of carbon nanotubes and nanofibers into composites. This phenomenon fortifies the structure and positively impacts the vehicle’s energy efficiency, range and overall performance [ 73 ]. Energy harvesting in EVs is further facilitated by nanotechnology, as nanostructured solar panels capture solar radiation, and nanogenerators convert mechanical energy into electrical power, thereby contributing to sustainable electricity generation [ 74 ]. Incorporating nanomaterials into supercapacitors and energy storage improves regenerative braking and power delivery, increasing energy storage and charge–discharge rates [ 75 ]. Nanosensors, renowned for their accuracy and sensitivity, provide advantages to EV control and monitoring systems by facilitating the observation of gas, pressure, temperature and concentrations for enhanced performance and safety.
Furthermore, progress in catalysis and fuel cell technologies is facilitated by nanotechnology, which has the potential to supplant conventional EV batteries and enhance the efficiency of energy conversion [ 76 ]. Intelligent coatings and self-healing materials, enabled by nanotechnology, safeguard EV surfaces against corrosion, weathering and abrasion by forming hydrophobic, UV-resistant and durable coatings that increase longevity. Fundamentally, nanotechnology manifests as a paradigm-shifting influence, initiating an era of enhanced EV performance, sustainability and innovation [ 77 ]. Figure 12 shows nanotechnology’s applications, challenges and future perspectives in EVs.

Nanotechnology for EVs.
4.1. Challenges of nanotechnology in EVs
Although nanotechnology can greatly enhance EVs, it presents considerable obstacles that must be thoughtfully assessed and resolved inventively. The significant challenge of scalability arises from the difficulties associated with mass-producing nanotechnology processes, which hinder the consistent and superior integration of nanomaterials into EV components. The complexities inherent in nanotechnology further complicate issues about scalability, thereby demanding progress in manufacturing methodologies to guarantee extensive implementation [ 78 , 79 ]. Nanotechnology materials and processes are more expensive to manufacture; thus, cost-effectiveness is essential. Nanomaterials in EV batteries and lightweight components may raise production costs, affecting EV affordability and consumer acceptability. Another major challenge is EV nanoparticle and nanostructure stability. Nanomaterials must be durable and reliable to maintain EV performance and safety, especially under harsh operational conditions [ 80 ].
These stability difficulties require continual research and development to improve nanomaterial robustness and compatibility with EV dynamics. In EV nanotechnology integration, environmental concerns are essential [ 81 ]. Nanomaterial manufacturing, use and disposal must meet environmental goals to address health and ecological problems throughout the life cycle of nanotechnology in EVs. Research and collaboration between the scientific and manufacturing sectors aim to overcome these limitations and advance nanotechnology’s use in EVs. Scalability, cost-effectiveness, long-term stability and environmental impact can be addressed to realize nanotechnology’s transformative promise in EVs, enabling sustainable, efficient and widely accessible electric mobility [ 82–84 ].
4.2. Commercial potential of nanotechnology in EV batteries
The discourse on the commercial viability of nanotechnology-enabled EV batteries involves a comprehensive examination of the promising breakthroughs and the obstacles and limitations that hinder their widespread use. Nanotechnology has become a robust and influential factor in energy storage, specifically EV batteries [ 85 ]. These technological developments are crucial for achieving higher performance metrics, including increased energy storage capacity, enhanced conductivity, efficient charge and discharge cycles and enhanced safety and stability. When considering the economic potential of nanotechnology in EV batteries, it is crucial to carefully examine the obstacles that could impede its smooth absorption into the market [ 86 ].
An essential component of this topic revolves around comprehending the market’s preparedness for EV batteries that incorporate nanotechnology. Although the potential advantages are significant, the market must be sufficiently prepared to adopt these technical advancements. Market preparation covers various elements, including establishing infrastructure for manufacturing and distribution, creating regulatory frameworks that can handle emerging technology and promoting consumer awareness and acceptance [ 87 ]. Evaluating the present level of preparedness offers valuable information regarding the timeframe and viability of implementing nanotechnology-enhanced EV batteries on a broader scope. Simultaneously, it is crucial to tackle the obstacles posed by incorporating nanotechnology in EV batteries. The issues encompass a wide spectrum, from intricate technological obstacles to financial constraints. Nanomaterials, including nanotubes, graphene and metal oxides, provide remarkable advancements at the molecular scale. However, their ability to be scaled up and their cost-effectiveness in industrial applications must be thoroughly evaluated. The complexities of production processes, potential environmental consequences and the requirement for specialized knowledge provide problems that require careful and thorough consideration [ 88 ].
Moreover, the conversation should include the possible obstacles to the adoption that could hinder the universal approval of nanotechnology-enabled EV batteries. Barriers can appear in different ways, such as economic, technological and societal issues. Economic factors encompass the aggregate expenses of production, prospective price increases for consumers and the economic viability for producers [ 89 ]. Technological impediments encompass challenges such as the requirement for standardized testing protocols, concerns regarding reliability and compatibility issues with current infrastructure. The success of nanotechnology-enabled EV batteries is heavily influenced by societal variables, including how the public perceives and accepts new technologies. The economic feasibility of EV batteries enhanced by nanotechnology also depends on effectively addressing safety issues and environmental consequences [ 90 ]. Although nanomaterials provide advancements in battery safety and stability, it is crucial to conduct a comprehensive assessment of the potential hazards related to the manufacturing and disposal of nanomaterials. Establishing or modifying regulatory frameworks is necessary to guarantee the secure and conscientious incorporation of nanotechnology into the electric car industry [ 91 ].
Strong competition in the EV sector complicates commercializing nanotechnology-enabled batteries. Given the ongoing advancements in traditional battery technologies and other energy storage solutions, it is crucial to consider the placement of nanotech-enabled batteries in this changing landscape [ 92 ]. An essential aspect of evaluating the commercial success of nanotechnology-enabled EV batteries is comprehending the competitive dynamics, developing distinctive selling factors and distinguishing them from existing technologies. Moreover, the influence of government regulations and incentives in defining the commercial path of nanotechnology-enabled EV batteries should not be underestimated [ 93 ]. Implementing supportive regulations, financial incentives and research funding can greatly expedite the implementation and acceptance of these advanced energy storage options. On the other hand, if there are no well-defined norms or obstacles in regulations, it could impede innovation and discourage investment in the emerging market of nanotechnology-enabled EV batteries [ 94 ].
4.3. Nanoscale breakthroughs boost battery performance
One of the most important factors in improving the overall performance of EV batteries is the adoption of nanoscale enhancements, which are advances on a smaller scale. The essential components of the battery, such as electrodes and catalysts, are subjected to transformations due to using materials such as nanotubes, graphene, metal oxides and nanoscale catalysts [ 95 ]. The nanoscale dimensions of these materials contribute to an increase in surface area, an improvement in conductivity and an enhancement in electrochemical characteristics. Due to these enhancements, the battery’s cycle life is extended, its charge and discharge rates are accelerated and its energy density is increased [ 96 ]. Furthermore, the improvements in nanotechnology also address safety concerns by reducing the dangers of overheating and limiting the creation of dendrites. As a result, the cumulative effect of these small-scale advancements dramatically raises the overall efficiency, reliability and sustainability of EV batteries, paving the way for a more optimistic future in electric transportation [ 86 ].
4.4. Future perspectives of nanotechnology
A comprehensive evaluation of the environmental consequences of incorporating nanomaterials and nanotechnology processes into EVs is imperative. This underscores the criticality of assessing health and environmental risks to ascertain nanotechnology’s feasibility and ecological sustainability in this domain. When considering the future, nanotechnology can fundamentally transform numerous facets of EV technology [ 97 ]. Nanomaterials possessing enhanced energy storage properties such as higher energy densities, faster charging capabilities and longer cycle lifetimes hold promise for substantially augmenting EV batteries’ range, efficiency and overall performance. Furthermore, continuous investigations into nanomaterials have the potential to yield sophisticated lightweight materials that integrate composites and nanoscale structures. Such materials would augment EV construction materials’ strength while decreasing their weight. Consequently, this could enhance maneuverability, range and energy efficiency [ 98 , 99 ].
Nanotechnology can create smart, functional surfaces with self-cleaning, anti-fogging and anti-icing qualities that improve EV visibility and efficiency while reducing maintenance. Nanosensors in EVs enable real-time battery health, temperature and performance monitoring, improving charging, safety and maintenance [ 100 ]. As research advances, sustainable nanomaterials that use abundant, non-toxic resources to make the EV sector more environmentally friendly become a priority. As EV nanomaterial recycling reduces resource depletion and waste throughout the lifecycle of EVs, it supports a circular economy. These advances demonstrate the continuous commitment to leverage nanotechnology’s transformative potential in EVs, balancing innovation with environmental responsibility for a sustainable electric mobility future [ 101 , 102 ].
Readers interested in delving more into nanotechnology in batteries will find that internet resources that clarify complicated topics are an excellent place to begin their exploration [ 103 ]. Websites like Nano.gov provide explanations of nanotechnology and its uses that are suitable for beginners. These explanations include the role that nanotechnology plays in battery technology. In addition, educational sites such as Khan Academy offer illuminating video lessons on nanoscience and its applications in the real world, which makes the process of learning more interesting and accessible [ 104 ]. Exploring articles on respected science websites such as Scientific American or National Geographic can provide in-depth yet understandable insights into how nanomaterials contribute to breakthroughs in battery efficiency. This is especially helpful for individuals who prefer a more hands-on approach [ 105 ]. The last point is that books such as Nanotechnology for Dummies by Earl Boysen and Nancy Boysen offer a straightforward guide to comprehending nanotechnology. This makes it an invaluable resource for readers anxious to dispel the mystery surrounding this fascinating field [ 106 ].
This study conducted a systematic literature analysis to analyze bibliometric data from various sources to gain insights into the changing research landscape of nanomaterials in EVs. The study primarily examined author affiliations, nation collaboration maps, citation patterns, keywords and the frequency of author keywords. The research publication selection process is depicted in Figure 2 , highlighting the meticulousness and thoroughness of the literature evaluation. Between 1991 and July 2023, the research yielded 2361 articles about EVs, nanotechnology, nanomaterials and sustainable development. By applying the exclusion criteria, the selection was narrowed down to 1540 articles that were deemed relevant, resulting in a strong dataset for bibliometric analysis. Prominent journals such as Energy and Environmental Science , Nano Energy , ACS Applied Materials and Interfaces and the Journal of Materials Chemistry emphasized the importance of nanoparticles in the context of EVs. The significance of bibliometric analysis in comprehending the impact, influence and interconnections among academic journals was underscored. Citation patterns were employed to assess the scientific value of articles, monitor research trends and evaluate collaboration networks. The study demonstrated the ever-changing nature of how scientific knowledge is spread and highlighted the importance of bibliometric research in guiding politicians, institutions and researchers when making decisions. Figure 3 depicts how the Scopus article research selections were reduced in the first step.
Section 4 included an in-depth examination of nanomaterials in EVs, including a study of publishing trends, country production, thematic evaluation of keywords, collaboration maps and field plots. Figure 4 depicts the yearly dissemination of publications from 1991 to 2023. It shows a notable increase in research efforts beginning in 2005, with an estimated annual growth rate of around 12.79%. The collaboration map depicted in Figure 5 prominently showcased the significant contributions made by prominent universities, with Nanyang Technological University and Beijing University taking the lead in research endeavors. Figure 6 provides an overview of the worldwide contributions to nanotechnology in EVs, with China, India and the United States as the primary countries leading in this field. The analysis of keywords ( Figure 7 ) revealed the consistent presence of terms such as self-powered sensors, batteries, triboelectric nanogenerator, nanotechnology, renewable energy, lithium-ion batteries, electrode material and electrochemical performance. These terms indicate the main themes of the research. Figures 8 and 9 depict the cooperative endeavors of several nations, with particular emphasis on China, India, the United States, South Korea, Germany, Japan, the United Kingdom, the Philippines, Singapore, France, Australia, Canada, Italy, Spain and Malaysia, which are recognized as significant contributors in this sector. Figures 10 and 11 visually depict keyword analysis, revealing the most common study topics and the network of writers who collaborated. A list of the 50 most significant keywords was compiled, which included terms such as ‘nanotechnology,’ ‘lithium,’ ‘electrodes,’ ‘electric batteries,’ ‘nanostructured materials,’ and ‘energy efficiency.’ The treemap structure ( Figure 10c ) displayed the distribution of keywords in publications by different authors, providing a detailed comprehension of the research landscape. To summarize, this SLR thoroughly examines nanomaterials in EVs, utilizing bibliometric methodologies to uncover patterns in research, collaborations and important theme areas. The results emphasize the increasing significance of nanotechnology in the progression of EV technologies. Research gaps may exist in comprehending the precise applications of nanomaterials, tackling issues related to scalability and cost-efficiency, and investigating potential environmental consequences. Potential areas for future research could prioritize interdisciplinary investigations, technological advancements and sustainable methodologies to facilitate the incorporation of nanomaterials into EV technologies. The findings obtained from this work provide valuable contributions to the wider discussion on the convergence of nanotechnology and electric cars, offering guidance for future research efforts in this rapidly changing and developing subject.
In conclusion, the bibliometric analysis conducted on nanotechnology in EVs provides valuable insights into this field’s research landscape and trends. The study highlights the growing interest and research output dedicated to nanotechnology’s applications in electric cars, showcasing its significance and potential impact. By examining publication trends, influential articles, collaborating institutions and key contributors, the analysis helps identify the key areas of research focus and the leading contributors in the field. This information can guide future research directions, collaboration opportunities and resource allocation. The analysis also highlights the challenges and opportunities associated with nanotechnology in EVs. It reveals the need to address scalability, cost-effectiveness, long-term stability and environmental impact to realize the potential of nanotechnology in this domain fully.
Moreover, the bibliometric analysis is a foundation for further exploration and understanding of nanotechnology in EVs. It provides a basis for researchers, policymakers and industry stakeholders to assess the progress made, identify knowledge gaps and develop strategies to accelerate the development and adoption of nanotechnology in EVs. Overall, the bibliometric analysis of nanotechnology in EVs is a valuable tool that enhances our understanding of the research landscape, highlights emerging trends and offers insights into the future perspectives of this exciting field. It paves the way for further research and collaboration, ultimately contributing to advancing and implementing nanotechnology in EVs for a greener and more sustainable transportation future.
The authors declare that there is no conflict of interest in this work.
The authors extend their appreciation to the Deanship of Scientific Research at King Khalid University, Saudi Arabia, for funding this work through the Research Group Program under grant no. RGP 2/88/44.
Pulkit Kumar: Conceptualization, Methodology, Software, Writing – Original Draft, Data Curation, Writing – Review & Editing; Harpreet Kaur Channi: Conceptualization, Methodology, Supervision, Writing – Review & Editing, Formal analysis; Atul Babbar: Investigation, Supervision, Resources, Formal analysis; Raman Kumar: Investigation, Supervision, Conceptualization, Methodology, Formal analysis, Writing – Review & Editing; Javed Khan Bhutto: Formal analysis, Investigation, Supervision T.M. Yunus Khan: Investigation, Visualization; Abhijit Bhowmik: Formal analysis, Investigation, Supervision; Abdul Razak: Formal analysis, Investigation, Supervision; Anteneh Wogasso Wodajo: Formal analysis, Visualization, Supervision
Thomas J , Patil RS , John J . et al. A comprehensive outlook of scope within exterior automotive plastic substrates and its coatings . Coatings (Basel) 2023 ; 13 :1569. https://doi.org/10.3390/coatings13091569 .
Google Scholar
Channi HK , Kumar R . The role of smart sensors in smart city . Smart Sensor Networks: Analytics, Sharing and Control 2022 ; 92 : 27 – 48 . https://doi.org/10.1007/978-3-030-77214-7_2 .
Parameswaran AK . et al. Recent progress of nanotechnology in the research framework of all-solid-state batteries . Nano Energy 2023 ; 105 :107994. https://doi.org/10.1016/j.nanoen.2022.107994 .
Kaur H , Kumar R , Kumar P . et al. 2024 . Efficient and cost-effective renewable energy integration of photovoltaic and hydro in rural India using homer pro: a case study of Chupki, Punjab. In Talpa Sai PHVS, Potnuru S, Avcar M, Ranjan Kar V (eds). Intelligent Manufacturing and Energy Sustainability: Proceedings of ICIMES 2023 . Springer . 281 – 91 .
Google Preview
Saraogi AK , Ibrahim M , Sangeethkumar E . et al. Battery materials for electric vehicle—a comprehensive review . Mater Today: Proc 2023 ; 72 : 2206 – 11 .
Naik N , Suresh P , Yadav S . et al. A review on composite materials for energy harvesting in electric vehicles . Energies 2023 ; 16 : 3348 . https://doi.org/10.3390/en16083348 .
Haghani M , Sprei F , Kazemzadeh K . et al. Trends in electric vehicles research . Transp Res Part D: Transp Environ 2023 ; 123 :103881. https://doi.org/10.1016/j.trd.2023.103881 .
Khan FNU , Rasul MG , Sayem A . et al. Design and optimization of lithium-ion battery as an efficient energy storage device for electric vehicles: a comprehensive review . J Energy Storage 2023 ; 71 :108033. https://doi.org/10.1016/j.est.2023.108033 .
Saha RK , Kumar R , Dev N . et al. Structural modeling and analysis of fuel cell: a graph-theoretic approach . Comput Sci 2023 ; 9 :e1510. https://doi.org/10.7717/peerj-cs.1510 .
Hameed MM , Mansor MB , Azau MAM . et al. Computational design and analysis of LiFePO4 battery thermal management system (BTMS) using thermoelectric cooling/thermoelectric generator (TEC–TEG) in electric vehicles (EVs) . J Energy Storage 2023 ; 72 :108394. https://doi.org/10.1016/j.est.2023.108394 .
Malik S , Muhammad K , Waheed Y . Nanotechnology: a revolution in modern industry . Molecules 2023 ; 28 : 661 . https://doi.org/10.3390/molecules28020661 .
Wali S . et al. Grid-connected lithium-ion battery energy storage system towards sustainable energy: a patent landscape analysis and technology updates . J Energy Storage 2024 ; 77 :109986. https://doi.org/10.1016/j.est.2023.109986 .
Shahzad K , Cheema II . Low-carbon technologies in automotive industry and decarbonizing transport . J Power Sources 2024 ; 591 :233888. https://doi.org/10.1016/j.jpowsour.2023.233888 .
Ahmad A , Prakash O , Sarangi SK . et al. Thermal and CFD analyses of sustainable heat storage-based passive greenhouse dryer operating in no-load condition . Sustain For 2023 ; 15 : 12067 . https://doi.org/10.3390/su151512067 .
Sharma YK , Kumar Y , Sharma S . et al. Nanotechnologies in the renewable energy sector . Renewable Energy Innovations: Biofuels, Solar, and Other Technologies 2024 ; 41 – 82 . https://doi.org/10.1002/9781119785712.ch2 .
Shu X . et al. Sustainability assessment of energy storage technologies based on commercialization viability: MCDM model . Sustain For 2023 ; 15 : 4707 . [Online]. Available: https://www.mdpi.com/2071–1050/15/6/4707 .
Nazir S , Zhang JM , Junaid M . et al. Metal-based nanoparticles: basics, types, fabrications and their electronic applications . Z Phys Chem 2024 ; 0 . https://doi.org/10.1515/zpch-2023-0375 .
Alemu MA , Worku AK , Getie MZ . Recent advances in electrically rechargeable transition metal-based-air batteries for electric mobility . Inorg Chem Commun 2024 ; 159 :111742. https://doi.org/10.1016/j.inoche.2023.111742 .
Cao J , Ji Y , Shao Z . Nanotechnologies in ceramic electrochemical cells . Chem Soc Rev 2024 ; 53 : 450 – 501 . https://doi.org/10.1039/D3CS00303E .
Aryanfar A , Elias F , Goddard WA . Enhancing the thermal dissipation in batteries via inclusion of central heat sink . J Electrochem Energy Convers Storage 2024 ; 21 . https://doi.org/10.1115/1.4062712 .
Mishra S , Mishra AK . 2024 . Supercapacitors: carbon-based nanostructures for supercapacitor application. In Sonkar P, Ganesan V (eds). Nanomaterials for Sustainable Energy Applications . CRC Press . 77 – 100 .
Zhao Y , Geng C , Wang L . et al. Design and modification of metal sulfide-based catalysts for lithium-sulfur batteries . Particuology 2024 ; 86 : 86 – 100 . https://doi.org/10.1016/j.partic.2023.04.010 .
Sanguesa JA , Torres-Sanz V , Garrido P . et al. A review on electric vehicles: technologies and challenges . Smart Cities 2021 ; 4 : 372 – 404 . [Online]. Available: https://doi.org/10.3390/smartcities4010022 . Accessed on (15 November, 2023) . https://www.mdpi.com/2624–6511/4/1/22 .
Ali MU , Zafar A , Nengroo SH . et al. Towards a smarter battery management system for electric vehicle applications: a critical review of lithium-ion battery state of charge estimation . Energies 2019 ; 12 : 446 . https://doi.org/10.3390/en12030446 .
Han D , Hosamo H , Ying C . et al. A comprehensive review and analysis of nanosensors for structural health monitoring in bridge maintenance: innovations, challenges, and future perspectives . Appl Sci 2023 ; 13 : 11149 . [Online]. Available: . https://www.mdpi.com/2076–3417/13/20/11149 . Accessed on (20 November, 2023) .
Basu AK , Tatiya S , Bhattacharya S . Overview of electric vehicles (EVs) and EV sensors . Sensors for Automotive and Aerospace Applications 2019 ; 107 – 22 . https://doi.org/10.1007/978-981-13-3290-6_7 .
Daramy-Williams E , Anable J , Grant-Muller S . A systematic review of the evidence on plug-in electric vehicle user experience . Transp Res Part D: Transp Environ 2019 ; 71 : 22 – 36 . https://doi.org/10.1016/j.trd.2019.01.008 .
Das UK . et al. Advancement of lithium-ion battery cells voltage equalization techniques: a review . Renew Sustain Energy Rev 2020 ; 134 :110227. https://doi.org/10.1016/j.rser.2020.110227 .
Torreglosa JP , Garcia-Triviño P , Vera D . et al. Analyzing the improvements of energy management systems for hybrid electric vehicles using a systematic literature review: how far are these controls from rule-based controls used in commercial vehicles? Appl Sci 2020 ; 10 : 8744 . https://doi.org/10.3390/app10238744 .
Castillo O , Álvarez R , Domingo R . Opportunities and barriers of hydrogen–electric hybrid powertrain vans: a systematic literature review . Processes 2020 ; 8 : 1261 . https://doi.org/10.3390/pr8101261 .
Tete PR , Gupta MM , Joshi SS . Developments in battery thermal management systems for electric vehicles: a technical review . J Energy Storage 2021 ; 35 :102255. https://doi.org/10.1016/j.est.2021.102255 .
Suryatna A , Raya I , Thangavelu L . et al. A review of high-energy density lithium-air battery technology: investigating the effect of oxides and nanocatalysts . J Chem 2022 ; 2022 : 1 – 32 . https://doi.org/10.1155/2022/2762647 .
Ye C , He W , Chen H . Electric vehicle routing models and solution algorithms in logistics distribution: a systematic review . Environ Sci Pollut Res 2022 ; 29 : 57067 – 90 . https://doi.org/10.1007/s11356-022-21559-2 .
Raugei M . Update on the life-cycle GHG emissions of passenger vehicles: literature review and harmonization . Energies 2022 ; 15 : 7163 . https://doi.org/10.3390/en15197163 .
Lai X , Yao J , Jin C . et al. A review of lithium-ion battery failure hazards: test standards, accident analysis, and safety suggestions . Batteries 2022 ; 8 : 248 . https://doi.org/10.3390/batteries8110248 .
Cai S , Kirtley JL , Lee CH . Critical review of direct-drive electrical machine systems for electric and hybrid electric vehicles . IEEE Trans Energy Convers 2022 ; 37 : 2657 – 68 . https://doi.org/10.1109/TEC.2022.3197351 .
Pardhi S , Chakraborty S , Tran D-D . et al. A review of fuel cell powertrains for long-haul heavy-duty vehicles: technology, hydrogen, energy and thermal management solutions . Energies 2022 ; 15 : 9557 . https://doi.org/10.3390/en15249557 .
Sharma P , Bora BJ . A review of modern machine learning techniques in the prediction of remaining useful life of lithium-ion batteries . Batteries 2023 ; 9 : 13 . https://doi.org/10.3390/batteries9010013 .
Senol M , Bayram IS , Naderi Y . et al. Electric vehicles under low temperatures: a review on battery performance, charging needs, and power grid impacts . IEEE Access 2023 ; 11 : 39879 – 912 . https://doi.org/10.1109/ACCESS.2023.3268615 .
Kiran MD , B R LY , Babbar A . et al. Tribological properties of CNT-filled epoxy-carbon fabric composites: optimization and modelling by machine learning . J Mater Res Technol 2024 ; 28 : 2582 – 601 . https://doi.org/10.1016/j.jmrt.2023.12.175 .
He R , Xie W , Wu B . et al. Towards interactional management for power batteries of electric vehicles . RSC Adv 2023 ; 13 : 2036 – 56 . https://doi.org/10.1039/D2RA06004C .
Quilty CD , Wu D , Li W . et al. Electron and ion transport in lithium and lithium-ion battery negative and positive composite electrodes . Chem Rev 2023 ; 123 : 1327 – 63 . https://doi.org/10.1021/acs.chemrev.2c00214 .
Gevorkov L , Domínguez-García JL , Romero LT . et al. Modern MultiPort converter technologies: a systematic review . Appl Sci 2023 ; 13 : 2579 . https://doi.org/10.3390/app13042579 .
Zhang X , Tang Y , Zhang F . et al. A novel aluminum–graphite dual-ion battery . Adv Energy Mater 2016 ; 6 : 1502588 . https://doi.org/10.1002/aenm.201502588 .
Wang M , Jiang C , Zhang S . et al. Reversible calcium alloying enables a practical room-temperature rechargeable calcium-ion battery with a high discharge voltage . Nat Chem 2018 ; 10 : 667 – 72 . https://doi.org/10.1038/s41557-018-0045-4 .
Mu S , Liu Q , Kidkhunthod P . et al. Molecular grafting towards high-fraction active nanodots implanted in N-doped carbon for sodium dual-ion batteries . Natl Sci Rev 2020 ; 8 : nwaa178 . https://doi.org/10.1093/nsr/nwaa178 .
Zhang X , Gong L , Zhao X . et al. Voltage and frequency stabilization control strategy of virtual synchronous generator based on small signal model . Energy Rep 2023 ; 9 : 583 – 90 . https://doi.org/10.1016/j.egyr.2023.03.071 .
Lu L , Wu W , Gao Y . et al. Study on current discrepancy and redistribution of HTS non-insulation closed-loop coils during charging/discharging and subsequent transient process toward steady-state operation . Supercond Sci Technol 2022 ; 35 :095001. https://doi.org/10.1088/1361-6668/ac7dfe .
Wu Y , Wu H , Zhao Y . et al. Metastable structures with composition fluctuation in cuprate superconducting films grown by transient liquid-phase assisted ultra-fast heteroepitaxy . Mater Today Nano 2023 ; 24 :100429. https://doi.org/10.1016/j.mtnano.2023.100429 .
Shen Y , Xie J , He T . et al. CEEMD-fuzzy control energy management of hybrid energy storage systems in electric vehicles . IEEE Trans Energy Convers 2024 ; 39 : 555 – 66 . https://doi.org/10.1109/TEC.2023.3306804 .
Zhao J , Song D , Zhu B . et al. A human-like trajectory planning method on a curve based on the driver preview mechanism . IEEE Trans Intell Transp Syst 2023 ; 24 : 11682 – 98 . https://doi.org/10.1109/TITS.2023.3285430 .
Zhu B , Sun Y , Zhao J . et al. A critical scenario search method for intelligent vehicle testing based on the social cognitive optimization algorithm . IEEE Trans Intell Transp Syst 2023 ; 24 : 7974 – 86 . https://doi.org/10.1109/TITS.2023.3268324 .
Wei T , Zhou Y , Sun C . et al. An intermittent lithium deposition model based on CuMn-bimetallic MOF derivatives for composite lithium anode with ultrahigh areal capacity and current densities . Nano Res 2023 ; 1 – 7 . https://doi.org/10.1007/s12274-023-6187-8 .
Yue W , Li C , Wang S . et al. Cooperative incident management in mixed traffic of CAVs and human-driven vehicles . IEEE Trans Intell Transp Syst 2023 ; 24 : 12462 – 76 . https://doi.org/10.1109/TITS.2023.3289983 .
Lu Y , Tan C , Ge W . et al. Adaptive disturbance observer-based improved super-twisting sliding mode control for electromagnetic direct-drive pump . Smart Mater Struct 2022 ; 32 :017001.
Yu H , Chen D , Ni X . et al. Reversible adsorption with oriented arrangement of a zwitterionic additive stabilizes electrodes for ultralong-life Zn-ion batteries . Energ Environ Sci 2023 ; 16 : 2684 – 95 . https://doi.org/10.1039/D3EE00982C .
Hou X , Xin L , Fu Y . et al. A self-powered biomimetic mouse whisker sensor (BMWS) aiming at terrestrial and space objects perception . Nano Energy 2023 ; 118 :109034. https://doi.org/10.1016/j.nanoen.2023.109034 .
Yan Z , Hu Q , Jiang F . et al. Mechanism and technology evaluation of a novel alternating-arc-based directed energy deposition method through polarity-switching self-adaptive shunt . Addit Manuf 2023 ; 67 :103504. https://doi.org/10.1016/j.addma.2023.103504 .
Wang Z , Li J , Hu C . et al. Hybrid energy storage system and management strategy for motor drive with high torque overload . J Energy Storage 2024 ; 75 :109432. https://doi.org/doi:10.1016/j.est.2023.109432 .
Sidhu AS , Singh S , Kumar R . Bibliometric analysis of entropy weights method for multi-objective optimization in machining operations . Mater Today: Proc 2022 ; 50 : 1248 – 55 . https://doi.org/10.1016/j.matpr.2021.08.132 .
Kumar R , Rani S , Awadh MA . Exploring the application sphere of the internet of things in industry 4.0: a review, bibliometric and content analysis . Sensor 2022 ; 22 : 4276 . https://doi.org/10.3390/s22114276 .
Kumar R , Goel P . Exploring the domain of interpretive structural modelling (ISM) for sustainable future panorama: a bibliometric and content analysis . Arch Comput Methods Eng 2022 ; 29 : 2781 – 810 . https://doi.org/10.1007/s11831-021-09675-7 .
Kaur S , Kumar R , Kaur R . et al. Piezoelectric materials in sensors: bibliometric and visualization analysis . Mater Today: Proc 2022 ; 65 : 3780 – 6 . https://doi.org/10.1016/j.matpr.2022.06.484 .
A. S. Sidhu , S. Singh , and R. Kumar , "Bibliometric analysis of entropy weights method for multi-objective optimization in machining operations," in 2nd International Conference on Functional Materials, Manufacturing and Performances, ICFMMP 2021 , Volume 50, Part 5 , 2021 , Elsevier Ltd , pp. 1248 – 1255 , [Online]. Available: https://www.scopus.com/inward/record.uri?eid=2-s2.0-85124946918&doi=10.1016%2fj.matpr.2021.08.132&partnerID=40&md5=06e988ed2dd8421efbcc139f893d1dcb . Accessed on (20 November, 2023) .
Kumar R , Singh S , Sidhu AS . et al. Bibliometric analysis of specific energy consumption (SEC) in machining operations: a sustainable response . Sustain For 2021 ; 13 :5617. [Online]. Available: https://doi.org/10.3390/su13105617 . https://www.mdpi.com/2071-1050/13/10/5617 .
Rani S , Kumar R . Bibliometric review of actuators: key automation technology in a smart city framework . Mater Today: Proc 2022 ; 60 : 1800 – 7 . https://doi.org/10.1016/j.matpr.2021.12.469 .
Paganin L , Borsato M . A critical review of design for reliability-a bibliometric analysis and identification of research opportunities . Procedia Manuf 2017 ; 11 : 1421 – 8 . https://doi.org/10.1016/j.promfg.2017.07.272 .
Donthu N , Kumar S , Mukherjee D . et al. How to conduct a bibliometric analysis: an overview and guidelines . J Bus Res 2021 ; 133 : 285 – 96 . https://doi.org/doi:10.1016/j.jbusres.2021.04.070 .
Agbodjan YS , Wang J , Cui Y . et al. Bibliometric analysis of zero energy building research, challenges and solutions . Sol Energy 2022 ; 244 : 414 – 33 . https://doi.org/10.1016/j.solener.2022.08.061 .
Xiong H , Wang Y , Guo X . et al. Current status and future challenges of groundwater vulnerability assessment: a bibliometric analysis . J Hydrol 2022 ; 615 :128694. https://doi.org/10.1016/j.jhydrol.2022.128694 .
Akhtar MN , Haleem A , Javaid M . Exploring the advent of Medical 4.0: a bibliometric analysis systematic review and technology adoption insights . Informatics Health 2024 ; 1 : 16 – 28 . https://doi.org/10.1016/j.infoh.2023.10.001 .
Wong K , Dia S . Nanotechnology in batteries . J Energy Resour Technol 2017 ; 139 :014001. https://doi.org/10.1115/1.4034860 .
Yang B , Ji J , Zhang X . et al. Passive cooling of lithium-ion batteries based on flexible phase change materials: molecular structure, interactions and mechanistic aspects . J Mol Liq 2023 ; 391 :123340. https://doi.org/10.1016/j.molliq.2023.123340 .
Kumar M , Farwaha HS , Kumar R . et al. Thermal performance evaluation of solar collector with rice husk graphene-PCM: bioengineering approach . Case Stud Therm Eng 2023 ; 52 :103773. https://doi.org/10.1016/j.csite.2023.103773 .
Chen Y , Fan Z , Liang L . et al. Nanotechnology in modern practical electric vehicles . Highl Sci Eng Technol 2023 ; 46 : 1 – 7 . https://doi.org/10.54097/hset.v46i.7656 .
Mahardhika SP , Putriani O . 2023 . A review of artificial intelligence-enabled electric vehicles in traffic congestion management. In ICSEDTI 2022: Proceedings of the 1st International Conference on Sustainable Engineering Development and Technological Innovation, ICSEDTI 2022, 11–13 October 2022 . Tanjungpinang, Indonesia : European Alliance for Innovation . 255 .
Sun L . Nanotechnology in the field of electric vehicles . Highl Sci Eng Technol 2023 ; 43 : 327 – 32 . https://doi.org/10.54097/hset.v43i.7436 .
Wang Y . Application of advanced nanotechnology in electric vehicles . Highl Sci Eng Technol 2023 ; 46 : 8 – 13 . https://doi.org/10.54097/hset.v46i.7657 .
Shukla S , Khan R , Saxena A . et al. Future of modern society: sustainability in green nanotechnology . Nanoremediation 2023 ; 393 – 410 . https://doi.org/10.1016/B978-0-12-823874-5.00001-2 .
Kurapati SK , Mahendar Reddy N , Sujithra R . et al. Nanomaterials and nanostructures in additive manufacturing: properties, applications, and technological challenges . Nanotechnology-Based Additive Manufacturing: Product Design, Properties and Applications 2023 ; 1 : 53 – 102 . https://doi.org/10.1002/9783527835478.ch3 .
Madheswaran DK , Vengatesan S , Varuvel EG . et al. Nanofluids as a coolant for polymer electrolyte membrane fuel cells: recent trends, challenges, and future perspectives . J Clean Prod 2023 ; 424 :138763. https://doi.org/10.1016/j.jclepro.2023.138763 .
Zero N . Nano-technology to achieve net zero goals . MBMST 2023 2023 ; 392 : 32 .
Bosu S , Rajamohan N . Recent advancements in hydrogen storage-comparative review on methods, operating conditions and challenges . Int J Hydrogen Energy 2024 ; 52 : 352 – 70 . https://doi.org/10.1016/j.ijhydene.2023.01.344 .
Shilkov VI , Anikin YV , Grishchenko YO . 2023 . Promising innovations for green economy and cleaner production in agriculture. In Trukhachev VI (ed). Unlocking Digital Transformation of Agricultural Enterprises: Technology Advances, Digital Ecosystems, and Innovative Firm Governance . Springer . 177 – 87 .
Soukupová G , Jindra M , Lapka T . et al. Novel silicon nanoparticles-based carbonized polypyrrole nanotube composites as anode materials for Li-ion batteries . J Power Sources 2024 ; 593 :233976. https://doi.org/10.1016/j.jpowsour.2023.233976 .
Poorshakoor E , Darab M . Advancements in the development of nanomaterials for lithium-ion batteries: a scientometric review . J Energy Storage 2024 ; 75 :109638. https://doi.org/10.1016/j.est.2023.109638 .
Hussain W , Sulaman M , Sandali Y . et al. An extensive exploration of non-conventional sources for preparing NiS and its potential applications in battery and photodegradation processes . Mater Sci Eng B 2024 ; 299 :116918. https://doi.org/10.1016/j.mseb.2023.116918 .
Gao S-L , Qin Z-X , Wang B-F . et al. Lithium recovery from the spent lithium-ion batteries by commercial acid-resistant nanofiltration membranes: a comparative study . Desalination 2024 ; 572 :117142. https://doi.org/10.1016/j.desal.2023.117142 .
Thomas F , Mahdi L , Lemaire J . et al. Technological advances and market developments of solid-state batteries: a review . Materials 2024 ; 17 : 239 . https://doi.org/10.3390/ma17010239 .
Schiavi PG , Marrani AG , Russina O . et al. Aqueous electrochemical delithiation of cathode materials as a strategy to selectively recover lithium from waste lithium-ion batteries . J Energy Chem 2024 ; 88 : 144 – 53 . https://doi.org/10.1016/j.jechem.2023.09.040 .
Kharabati S , Saedodin S . A systematic review of thermal management techniques for electric vehicle batteries . J Energy Storage 2024 ; 75 :109586. https://doi.org/10.1016/j.est.2023.109586 .
Divya N , Saju M , Roshna A . et al. 2024 . Smart nanomaterials in energy applications. In Tailored Functional Materials for Clean and Sustainable Development . Apple Academic Press . 263 – 80 .
Karjalainen P , Leinonen V , Olin M . et al. Real-world emissions of nanoparticles, particulate mass and black carbon from a plug-in hybrid vehicle compared to conventional gasoline vehicles . Environ Adv 2024 ; 15 :100454. https://doi.org/10.1016/j.envadv.2023.100454 .
Munonde TS , Raphulu MC . Review on titanium dioxide nanostructured electrode materials for high-performance lithium batteries . J Energy Storage 2024 ; 78 :110064. https://doi.org/10.1016/j.est.2023.110064 .
Lim JM , Jang YS , van T. Nguyen H . et al. Advances in high-voltage supercapacitors for energy storage systems: materials and electrolyte tailoring to implementation . Nanoscale Adv 2023 ; 5 : 615 – 26 . https://doi.org/10.1039/D2NA00863G .
Shi R , Shen Z , Yue Q . et al. Advances in functional organic material-based interfacial engineering on metal anodes for rechargeable secondary batteries . Nanoscale 2023 ; 15 : 9256 – 89 . https://doi.org/10.1039/D3NR01306E .
Edoziuno FO , Adediran AA , Nwaeju CC . et al. 2023 . Nanomaterials. In Verma C, Srivastava V, ... Ebenso EE (eds). Smart Anticorrosive Materials . Elsevier . 19 – 30 . https://doi.org/10.1016/B978-0-323-95158-6.00023-0 .
Huang Z , Li Y , Xu Y . Diverse nanotechnology applications for electric vehicles . Highl Sci Eng Technol 2023 ; 43 : 438 – 49 . https://doi.org/10.54097/hset.v43i.7462 .
Aravindan M . et al. Fuelling the future: a review of non-renewable hydrogen production and storage techniques . Renew Sustain Energy Rev 2023 ; 188 :113791.
Raj KS , Baskaran N , Nair PP . et al. Current scenario and future perspective of food waste into Li-ion based batteries—a critical review . J Hazard Mater Adv 2023 ; 10 :100317. https://doi.org/10.1016/j.hazadv.2023.100317 .
Yang Z . Nanomaterials as well their applications and effects in batteries . Highl Sci Eng Technol 2023 ; 32 : 73 – 82 . https://doi.org/10.54097/hset.v32i.4944 .
Mahdavi Dehkharghani F , Ghahremanlou M , Zandi Z . et al. Future energy and therapeutic perspectives of green nano-technology: recent advances and challenges . Nano Micro Biosyst 2023 ; 2 : 11 – 21 .
Shah SSA , Zafar HK , Javed MS . et al. Mxenes for Zn-based energy storage devices: nano-engineering and machine learning . Coord Chem Rev 2024 ; 501 :215565. https://doi.org/10.1016/j.ccr.2023.215565 .
Li Z , Zhang Y , Zhang S . et al. Phase change materials for lithium-ion battery thermal management systems: a review . J Energy Storage 2024 ; 80 :110259. https://doi.org/10.1016/j.est.2023.110259 .
Karthikeyan B , Velvizhi G . A state-of-the-art on the application of nanotechnology for enhanced biohydrogen production . Int J Hydrogen Energy 2024 ; 52 : 536 – 54 . https://doi.org/10.1016/j.ijhydene.2023.04.237 .
Xie H , Ahmad T , Zhang D . et al. Community-based virtual power plants’ technology and circular economy models in the energy sector: a techno-economy study . Renew Sustain Energy Rev 2024 ; 192 :114189. https://doi.org/10.1016/j.rser.2023.114189 .
Email alerts
Citing articles via, affiliations.
- Online ISSN 1748-1325
- Print ISSN 1748-1317
- Copyright © 2024 Oxford University Press
- About Oxford Academic
- Publish journals with us
- University press partners
- What we publish
- New features
- Open access
- Institutional account management
- Rights and permissions
- Get help with access
- Accessibility
- Advertising
- Media enquiries
- Oxford University Press
- Oxford Languages
- University of Oxford
Oxford University Press is a department of the University of Oxford. It furthers the University's objective of excellence in research, scholarship, and education by publishing worldwide
- Copyright © 2024 Oxford University Press
- Cookie settings
- Cookie policy
- Privacy policy
- Legal notice
This Feature Is Available To Subscribers Only
Sign In or Create an Account
This PDF is available to Subscribers Only
For full access to this pdf, sign in to an existing account, or purchase an annual subscription.
Thank you for visiting nature.com. You are using a browser version with limited support for CSS. To obtain the best experience, we recommend you use a more up to date browser (or turn off compatibility mode in Internet Explorer). In the meantime, to ensure continued support, we are displaying the site without styles and JavaScript.
- View all journals
- Explore content
- About the journal
- Publish with us
- Sign up for alerts
Review Articles
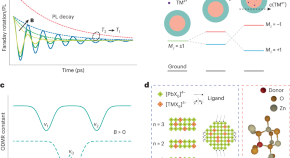
Engineering colloidal semiconductor nanocrystals for quantum information processing
This Review highlights the current potential for colloidal quantum dots for applications in quantum sensing and quantum circuits.
- Jawaher Almutlaq
- Edward H. Sargent
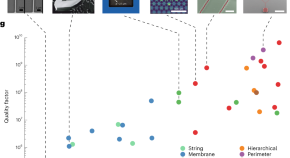
Ultrahigh-quality-factor micro- and nanomechanical resonators using dissipation dilution
This Review discusses advances in engineering high-quality-factor strained nanomechanical resonators with applications in precision measurements.
- Nils Johan Engelsen
- Alberto Beccari
- Tobias Jan Kippenberg
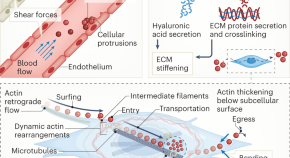
Evidence and therapeutic implications of biomechanically regulated immunosurveillance in cancer and other diseases
This Review highlights the current understanding of mechanisms underlying the mechanical changes occurring in diseased and immune cells and discusses new approaches to leverage and target biomechanical cues for immune engineering at various length scales for therapeutic interventions.
- Vincent Mittelheisser
- Valentin Gensbittel
- Jacky G. Goetz
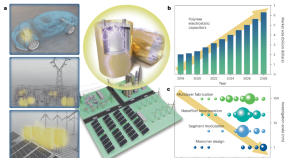
Polymer nanocomposite dielectrics for capacitive energy storage
The Review discusses the state-of-the-art polymer nanocomposites from three key aspects: dipole activity, breakdown resistance and heat tolerance for capacitive energy storage applications.
- Minzheng Yang
- Mengfan Guo
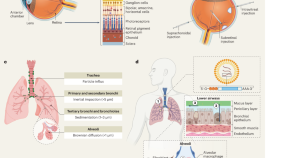
Strategies for non-viral vectors targeting organs beyond the liver
Nanoparticles naturally accumulate in the liver; this can be a major limitation to any therapy needing delivery to other organs or tissues. Here the authors review the reason for predominant liver uptake and explore different strategies used to target non-viral gene delivery nanoparticles to other organs and tissues.
- Jeonghwan Kim
- Yulia Eygeris
- Gaurav Sahay
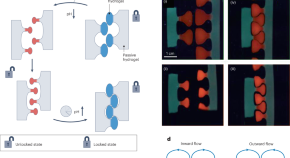
Interlinking spatial dimensions and kinetic processes in dissipative materials to create synthetic systems with lifelike functionality
This Review aims to spur developments in next-generation functional materials by highlighting design rules to interconnect length and timescales.
- Oleg E. Shklyaev
- Anna C. Balazs
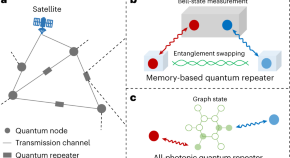
Telecom-band quantum dot technologies for long-distance quantum networks
The Review discusses epitaxial quantum dot devices emitting in the telecom bands for quantum network devices.
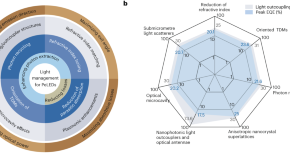
Light management for perovskite light-emitting diodes
This Review analyses the mechanisms of light extraction from perovskite light-emitting diodes and suggests new approaches towards ultrahigh electroluminescence efficiencies.
- Baodan Zhao
- Maria Vasilopoulou
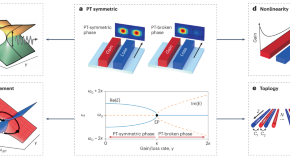
Exceptional points and non-Hermitian photonics at the nanoscale
This Review discusses the latest theoretical progress related to exceptional points in non-Hermitian physics and the associated implications for emerging technologies in nanophotonics.
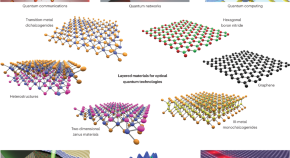
Layered materials as a platform for quantum technologies
This Review highlights the role of transition metal dichalcogenides, hexagonal boron nitride and stacked heterostructures in applications in quantum communication, computation, sensing and single-photon detection.
- Alejandro R.-P. Montblanch
- Matteo Barbone
- Andrea C. Ferrari
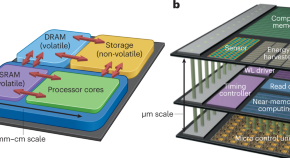
Wurtzite and fluorite ferroelectric materials for electronic memory
This Review presents the most recent ferroelectric materials with wurtzite structure and emphasizes applications in memory and storage-based microelectronic hardware.
- Kwan-Ho Kim
- Ilya Karpov
- Deep Jariwala
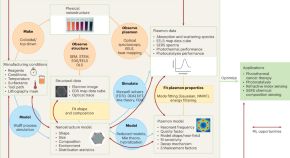
Machine learning for nanoplasmonics
This Review illustrates opportunities for the nanophotonics community when adopting machine learning approaches.
- Jean-Francois Masson
- John S. Biggins
- Emilie Ringe
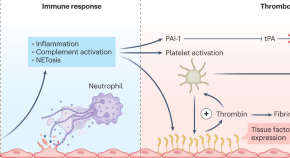
The potential impact of nanomedicine on COVID-19-induced thrombosis
This Review analyses the possibilities that a nanomedicine approach offers to tackle COVID-19-induced thrombosis and the associated challenges.
- Peije Russell
- Nicolas H. Voelcker
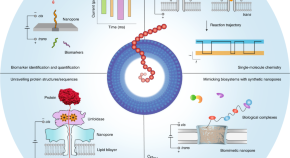
Nanopore-based technologies beyond DNA sequencing
This Review discusses the latest advances in nanopore technologies beyond DNA sequencing.
- Yi-Lun Ying
- Zheng-Li Hu
- Yi-Tao Long
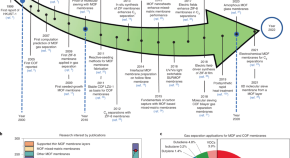
Metal–organic frameworks and covalent organic frameworks as disruptive membrane materials for energy-efficient gas separation
This critical Review discusses the molecular sieving behaviour of metal–organic framework and covalent organic framework membranes as thin supported layers and mixed-matrix membranes. This behaviour is different from that of classical zeolite membranes.
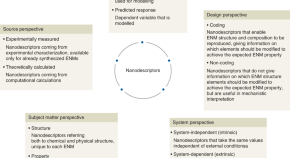
Representing and describing nanomaterials in predictive nanoinformatics
This Review discusses how a comprehensive system for defining nanomaterial descriptors can enable a safe-and-sustainable-by-design concept for engineered nanomaterials.
- Ewelina Wyrzykowska
- Alicja Mikolajczyk
- Tomasz Puzyn
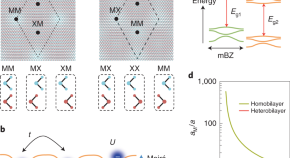
Semiconductor moiré materials
This Review elaborates on the recent developments and the future opportunities and challenges of fundamental research on semiconductor moiré materials, with a particular focus on transition metal dichalcogenides.
- Kin Fai Mak
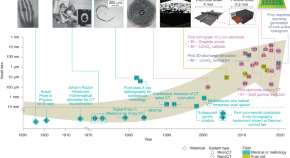
Bridging nano- and microscale X-ray tomography for battery research by leveraging artificial intelligence
This Review discusses how artificial intelligence and machine learning algorithms can be used in combination with X-ray computed tomography to study the composition and the dynamics of microstructures in battery materials with nanoscale resolution.
- Jonathan Scharf
- Mehdi Chouchane
- Ying Shirley Meng
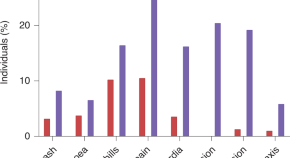
Applying lessons learned from nanomedicines to understand rare hypersensitivity reactions to mRNA-based SARS-CoV-2 vaccines
This perspective analyses the adverse reactions reported for mRNA-based SARS-CoV-2 vaccines in the light of infusion reactions to nanomedicines, which display similar outcomes, suggests possible mechanisms and offers a safety roadmap for vaccine developers.
- Janos Szebeni
- Marina A. Dobrovolskaia
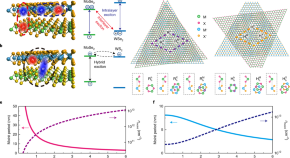
Excitons in semiconductor moiré superlattices
This Review discusses the recent progress in the emerging field of exciton phenomena in semiconductor moiré superlattices.
Quick links
- Explore articles by subject
- Guide to authors
- Editorial policies

- Architecture and Design
- Asian and Pacific Studies
- Business and Economics
- Classical and Ancient Near Eastern Studies
- Computer Sciences
- Cultural Studies
- Engineering
- General Interest
- Geosciences
- Industrial Chemistry
- Islamic and Middle Eastern Studies
- Jewish Studies
- Library and Information Science, Book Studies
- Life Sciences
- Linguistics and Semiotics
- Literary Studies
- Materials Sciences
- Mathematics
- Social Sciences
- Sports and Recreation
- Theology and Religion
- Publish your article
- The role of authors
- Promoting your article
- Abstracting & indexing
- Publishing Ethics
- Why publish with De Gruyter
- How to publish with De Gruyter
- Our book series
- Our subject areas
- Your digital product at De Gruyter
- Contribute to our reference works
- Product information
- Tools & resources
- Product Information
- Promotional Materials
- Orders and Inquiries
- FAQ for Library Suppliers and Book Sellers
- Repository Policy
- Free access policy
- Open Access agreements
- Database portals
- For Authors
- Customer service
- People + Culture
- Journal Management
- How to join us
- Working at De Gruyter
- Mission & Vision
- De Gruyter Foundation
- De Gruyter Ebound
- Our Responsibility
- Partner publishers

Your purchase has been completed. Your documents are now available to view.
Nanotechnology Reviews
- Type: Journal
- Language: English
- Publisher: De Gruyter
- First published: January 20, 2012
- Publication Frequency: 1 Issue per Year
- Audience: Scientists and engineers in nanotechnology and all involved disciplines

An official website of the United States government
The .gov means it’s official. Federal government websites often end in .gov or .mil. Before sharing sensitive information, make sure you’re on a federal government site.
The site is secure. The https:// ensures that you are connecting to the official website and that any information you provide is encrypted and transmitted securely.
- Publications
- Account settings
Preview improvements coming to the PMC website in October 2024. Learn More or Try it out now .
- Advanced Search
- Journal List
- Front Microbiol
- PMC10168541
A review on nanoparticles: characteristics, synthesis, applications, and challenges
The significance of nanoparticles (NPs) in technological advancements is due to their adaptable characteristics and enhanced performance over their parent material. They are frequently synthesized by reducing metal ions into uncharged nanoparticles using hazardous reducing agents. However, there have been several initiatives in recent years to create green technology that uses natural resources instead of dangerous chemicals to produce nanoparticles. In green synthesis, biological methods are used for the synthesis of NPs because biological methods are eco-friendly, clean, safe, cost-effective, uncomplicated, and highly productive. Numerous biological organisms, such as bacteria, actinomycetes, fungi, algae, yeast, and plants, are used for the green synthesis of NPs. Additionally, this paper will discuss nanoparticles, including their types, traits, synthesis methods, applications, and prospects.
1. Introduction
Nanotechnology evolved as the achievement of science in the 21st century. The synthesis, management, and application of those materials with a size smaller than 100 nm fall under the interdisciplinary umbrella of this field. Nanoparticles have significant applications in different sectors such as the environment, agriculture, food, biotechnology, biomedical, medicines, etc. like; for treatment of waste water ( Zahra et al., 2020 ), environment monitoring ( Rassaei et al., 2011 ), as a functional food additives ( Chen et al., 2023 ), and as a antimicrobial agents ( Islam et al., 2022 ). Cutting-edge properties of NPs such as; nature, biocompatibility, anti-inflammatory and antibacterial activity, effective drug delivery, bioactivity, bioavailability, tumor targeting, and bio-absorption have led to a growth in the biotechnological, and applied microbiological applications of NPs.
A particle of matter with a diameter of one to one hundred nanometers (nm) is commonly referred to as a nanoparticle or ultrafine particle. Nanoparticles frequently exhibit distinctive size-dependent features, mostly due to their tiny size and colossal surface area. The periodic boundary conditions of the crystalline particle are destroyed when the size of a particle approaches the nano-scale with the characteristic length scale close to or smaller than the de Broglie wavelength or the wavelength of light ( Guo et al., 2013 ). Because of this, many of the physical characteristics of nanoparticles differ significantly from those of bulk materials, leading to a wide range of their novel uses ( Hasan, 2015 ).
2. Emergence of nanotechnology
Nanotechnology emerged in the 1980s due to the convergence of experimental advances such as the invention of the scanning tunneling microscope in 1981 and the discovery of fullerenes in 1985 ( Bayda et al., 2019 ), with the elucidation. The popularization of a conceptual framework for nanotechnology goals began with the publication of the book Engines of Creation in 1986 ( Bayda et al., 2019 ).
2.1. Early stage of NPs
Carbon nanotubes have been discovered in pottery from Keeladi, India, dating from around 600–300 BC ( Bayda et al., 2019 ; Kokarneswaran et al., 2020 ). Cementite nanowires have been discovered in Damascus steel, a material that dates back to around 900 AD; nevertheless, its origin and creation method are unclear ( Kokarneswaran et al., 2020 ). However, it is unknown how they developed or whether the material containing them was used on purpose.
2.2. Discovery of C, Ag, Zn, Cu, and Au nanoparticles
Carbon NPs were found in 1991, and Iijima and Ichihashi announced the single-wall carbon nanotube synthesis with a diameter of 1 nanometer in 1993 ( Chen et al., 2021 ). Carbon nanotubes (CNTs), also known as Bucky tubes, are a kind of nanomaterial made up of a two-dimensional hexagonal lattice of carbon atoms. They are bent one way and joined to produce a hollow cylindrical cylinder. Carbon nanotubes are carbon allotropes that fall between Fullerene (0 dimensional) and Grapheme (2 dimensional) ( Chen et al., 2021 ).
In addition, M. C. Lea reported that the synthesis of citrate-stabilized silver colloid almost 120 years ago ( Nowack et al., 2011 ). This process produces particles with an average diameter of 7 to 9 nm. Nanoscale size and citrate stabilization are analogous to recent findings on nanosilver production employing silver nitrate and citrate ( Majeed Khan et al., 2011 ). The use of proteins to stabilize nanosilver has also been documented as early as 1902 ( Nowack et al., 2011 ; Beyene et al., 2017 ). Since 1897, a nanosilver known as “Collargol” has been made commercially and used for medicinal purposes ( Nowack et al., 2011 ). Collargol, a type of silver nanoparticle, has a particle size of about 10 nanometers (nm). This was determined as early as 1907, and it was found that the diameter of Collargol falls within the nanoscale range. In 1953, Moudry developed a different type of silver nanoparticle called gelatin-stabilized silver nanoparticles, with a diameter ranging from 2–20 nm. These nanoparticles were produced using another method than Collargol. The necessity of nanoscale silver was recognized by the creators of nanosilver formulations decades ago, as seen by the following remark from a patent: “for optimal efficiency, the silver must be disseminated as particles of colloidal size less than 25 nm in crystallite size”( Nowack et al., 2011 ).
Gold NPs (AuNPs) have a long history in chemistry, going back to the Roman era when they were used to decorate glassware by staining them. With the work of Michael Faraday, who may have been the first to notice that colloidal gold solutions have characteristics different from bulk gold, the contemporary age of AuNP synthesis began more than 170 years ago. Michael Faraday investigated the making and factors of colloidal suspensions of “Ruby” gold in 1857. They are among the magnetic nanoparticles due to their distinctive optical and electrical characteristics. Under specific illumination circumstances, Faraday showed how gold nanoparticles might create solutions of various colors ( Bayda et al., 2019 ; Giljohann et al., 2020 ).
3. Classification of NPs
Nanoparticles (NPs) are categorized into the following classes based on their shape, size, and chemical characteristics;
3.1. Carbon-based NPs
Fullerenes and carbon nanotubes (CNTs) are the two essential sub-categories of carbon-based NPs. NPs of globular hollow cages, like allotropic forms of carbon, are found in fullerenes. Due to their electrical conductivity, high strength, structure, electron affinity, and adaptability, they have sparked significant economic interest. These materials have organized pentagonal and hexagonal carbon units, each of which is sp2 hybridized. While CNTs are elongated and form 1–2 nm diameter tubular structures. These fundamentally resemble graphite sheets rolling on top of one another. Accordingly, they are referred to as single-walled (SWNTs), double-walled (DWNTs), or multi-walled carbon nanotubes (MWNTs) depending on how many walls are present in the rolled sheets ( Elliott et al., 2013 ; Astefanei et al., 2015 ).
3.2. Metal NPs
Metal NPs are purely made of metals. These NPs have distinctive electrical properties due to well-known localized surface Plasmon resonance (LSPR) features. Cu, Ag, and Au nanoparticles exhibit a broad absorption band in the visible region of the solar electromagnetic spectrum. Metal NPs are used in several scientific fields because of their enhanced features like facet, size, and shape-controlled synthesis of metal NPs ( Khan et al., 2019 ).
3.3. Ceramics NPs
Ceramic NPs are tiny particles made up of inorganic, non-metallic materials that are heat-treated and cooled in a specific way to give particular properties. They can come in various shapes, including amorphous, polycrystalline, dense, porous, and hollow, and they are known for heat resistance and durable properties. Ceramic NPs are used in various applications, including coating, catalysts, and batteries ( Sigmund et al., 2006 ).
3.4. Lipid-based NPs
These NPs are helpful in several biological applications because they include lipid moieties. Lipid NPs typically have a diameter of 10–1,000 nm and are spherical. Lipid NPs, i.e., polymeric NPs, have a solid lipid core and a matrix consisting of soluble lipophilic molecules ( Khan et al., 2019 ).
3.5. Semiconductor NPs
Semiconductor NPs have qualities similar to metals and non-metals. That is why Semiconductor NPs have unique physical and chemical properties that make them useful for various applications. For example, semiconductor NPs can absorb and emit light and can be used to make more efficient solar cells or brighter light-emitting diodes (LEDs). They can make smaller and faster electronic devices, such as transistors, and can be used in bio imaging and cancer therapy ( Biju et al., 2008 ).
3.6. Polymeric NPs
Polymeric NPs with a size between 1 and 1,000 nm can have active substances surface-adsorbed onto the polymeric core or entrapped inside the polymeric body. These NPs are often organic, and the term polymer nanoparticle (PNP) is commonly used in the literature to refer to them. They resemble Nano spheres or Nano capsules for the most part ( Khan et al., 2019 ; Zielińska et al., 2020 ).
4. Types of different metal-based NPs
Metal NPs are purely made of metal precursors. Due to well-known localized surface plasmon resonance (LSPR) characteristics, these NPs possess unique optoelectrical properties. NPs of the alkali and noble metals, i.e., Cu, Ag, and Au, have a broad absorption band in the visible zone of the solar electromagnetic spectrum. The facet, size, and shape-controlled synthesis of metal NPs are essential in present-day cutting-edge materials ( Dreaden et al., 2012 ; Khan et al., 2019 ).
4.1. Silver nanoparticles (AgNPs)
AgNPs are particles with a size range of 1–100 nanometers made of silver. They have unique physical and chemical properties due to their small size, high surface area-to-volume ratio, and ability to absorb and scatter light in the visible and near-infrared range. Because of their relatively small size and high surface-to-volume ratios, which cause chemical and physical differences in their properties compared to their bulk counterparts, silver nanoparticles may exhibit additional antimicrobial capabilities not exerted by ionic silver ( Shenashen et al., 2014 ).
Besides, AgNPs can be created in various sizes and forms depending on the manufacturing process, the most common of which is chemical reduction. The AgNPs were created by chemically reducing a 12 mM AgNO3 aqueous solution. The reaction was carried out in an argon environment using 70 mL of this solution containing PVP (keeping the molar ratio of the repeating unit of PVP and Ag equal to 34) and 21 mL of Aloe Vera. The mixture was agitated in ultrasonic for 45 min at ambient temperature, then heated 2°C/min to 80°C and left for 2 h to generate a transparent solution with tiny suspended particles that must be removed by simple filtering ( Shenashen et al., 2014 ; Gloria et al., 2017 ).
4.2. Zinc nanoparticles (ZnONPs)
Zinc nanoparticles (ZnONPs) are particles with a size range of 1–100 nm made of zinc. Zinc oxide (ZnO) NPs are a wide band gap semiconductor with a room temperature energy gap of 3.37 eV. Its catalytic, electrical, optoelectronic, and photochemical capabilities have made it widely worthwhile ( Kumar S.S. et al., 2013 ). ZnO nanostructures are ideal for catalytic reaction processes ( Chen and Tang, 2007 ). Laser ablation, hydrothermal methods, electrochemical depositions, sol-gel method, chemical vapor deposition, thermal decomposition, combustion methods, ultrasound, microwave-assisted combustion method, two-step mechanochemical-thermal synthesis, anodization, co-precipitation, electrophoretic deposition, and precipitation processes are some methods for producing ZnO nanoparticles ( Madathil et al., 2007 ; Moghaddam et al., 2009 ; Ghorbani et al., 2015 ).
4.3. Copper nanoparticles (CuNPs)
Copper nanoparticles (CuNPs) comprise a size range of 1–100 nm of copper-based particles ( Khan et al., 2019 ). Cu and Au metal fluorescence have long been known to exist. For excitation at 488 nm, a fluorescence peak centering on the metals’ interband absorption edge has been noted. Additionally, it was noted that the fluorescence peaked at the same energy at two distinct excitation wavelengths (457.9–514.5 and 300–400 nm), and the high-energy tail somewhat grows with increased photon energy pumping. A unique, physical, top-down EEW approach has been used to create Cu nanoparticles. The EEW method involves sending a current of *1,010 A/m2 (1,010 A/m2) across a thin Cu wire, which explodes on a Cu plate for a duration of 10–6 s ( Siwach and Sen, 2008 ).
4.4. Gold nanoparticles (AuNPs)
Gold nanoparticles(AuNPs) are nanometers made of gold. They have unique physical and chemical properties and can absorb and scatter light in the visible and near-infrared range ( Rad et al., 2011 ; Compostella et al., 2017 ).
Scientists around the turn of the 20th century discovered anisotropic AuNPs. Zsigmond ( Li et al., 2014 ) said that gold particles “are not always spherical when their size is 40 nm or lower” in his book, released in 1909. Additionally, he found anisotropic gold particles of various colors. Zsigmondy won the Nobel Prize in 1925 for “his demonstration of the heterogeneous character of colloidal solutions and the methods he utilized” and for developing the ultramicroscope, which allowed him to see the forms of Au particles. He noticed that gold frequently crystallized into a six-sided leaf shape ( Li et al., 2014 ).
AuNPs are the topic of extensive investigation due to their optical, electrical, and molecular-recognition capabilities, with numerous prospective or promised uses in a wide range of fields, including electron microscopy, electronics, nanotechnology, materials science, and biomedicine ( Rad et al., 2011 ).
4.5. Aluminum nanoparticles (AlNPs)
Aluminum nanoparticles (AlNPs) are nanoparticles made of aluminum. Aluminum nanoparticles’ strong reactivity makes them promising for application in high-energy compositions, hydrogen generation in water processes, and the synthesis of alumina 2D and 3D structures ( Lerner et al., 2016 ).
4.6. Iron nanoparticles (FeNPs)
Iron nanoparticles(FeNPs) are particles with a size range of 1−100 nanometers ( Khan et al., 2019 ) made of iron. FeNPs have several potential applications, including their use as catalysts, drug delivery systems, sensors, and energy storage and conversion. They have also been investigated for use in photovoltaic and solar cells and water purification and environmental remediation. FeNPs can also be used in magnetic resonance imaging (MRI) as contrast agents to improve the visibility of tissues and organs. They can also be used in magnetic recording media, such as hard disk drives ( Zhuang and Gentry, 2011 ; Jamkhande et al., 2019 ).
As with any NPs, there are potential health and safety concerns associated with using FeNPs, e.g., FeNPs are used to deliver drugs to specific locations within the body, such as cancer cells and used in MRI, and used to remove contaminants from water ( Farrell et al., 2003 ; Zhuang and Gentry, 2011 ). Tables 1 , ,2 2 show the characteristics of metal-based nanoparticles and the techniques to study their characteristics, respectively.
Characteristics of metal based nanoparticles.
Different analytical techniques and their purposes in studying nanoparticles.
5. Approaches for the synthesis of metal NPs
There are mainly three types of approaches for the synthesis of NPs: the physical, chemical, and biological approaches. The physical approach is also called the top-down approach, while chemical and biological approaches are collectively called the bottom-up approach. The biological approach is also named green systems of NPs. All these approaches are further sub-categorized into various types based upon their method adopted. Figure 1 illustrates each approach’s reported methods for synthesizing NPs.

Approaches of NPs synthesis.
5.1. Top down/physical approach
Bulk materials are fragmented in top-down methods to create nano-structured materials ( Figure 2 ). They are additionally known as physical approaches ( Baig et al., 2021 ). The following techniques can achieve a top-down approach;

Difference between top-down and bottom-up approaches.
5.1.1. Mechanical milling
The mechanical milling process uses balls inside containers and may be carried out in various mills, typically planetary and shaker mills, which is an impact process with high energy ( Gorrasi and Sorrentino, 2015 ). Mechanical milling is a practical approach for creating materials at the nanoscale from bulk materials. Aluminum alloys that have been strengthened by oxide and carbide, spray coatings that are resistant to wear, nanoalloys based on aluminum, nickel, magnesium, and copper, and a variety of other nanocomposite materials may all be created mechanically. A unique class of nanoparticles known as ball-milled carbon nanomaterials has the potential to meet the needs for energy storage, energy conversion, and environmental remediation ( Yadav et al., 2012 ; Lyu et al., 2017 ).
5.1.2. Electrospinning
Typically, it is used to create nanofibers from various materials, most often polymers ( Ostermann et al., 2011 ). A technique for creating fibers called electrospinning draws charged threads from polymer melts or solutions up to fiber sizes of a few hundred nanometers ( Chronakis, 2010 ). Coaxial electrospinning was a significant advancement in the field of electrospinning. The spinneret in coaxial electrospinning is made up of two coaxial capillaries. Core-shell nanoarchitectures may be created in these capillaries using two viscous liquids, a viscous liquid as the shell and a non-viscous liquid as the core ( Du et al., 2012 ). Core-shell and hollow polymer, inorganic, organic, and hybrid materials have all been developed using this technique ( Kumar R. et al., 2013 ).
5.1.3. Laser ablation
A microfeature can be made by employing a laser beam to vaporize a single material ( Tran and Wen, 2014 ). Laser ablation synthesis produces nanoparticles by striking the target material with an intense laser beam. Due to the high intensity of the laser irradiation used in the laser ablation process, the source material or precursor vaporizes, causing the production of nanoparticles ( Amendola and Meneghetti, 2009 ). Laser ablation is an environmentally friendly for producing noble metal nanoparticles ( Baig et al., 2021 ). This method may be used to create a wide variety of nanomaterials, including metal nanoparticles, carbon nanomaterials, oxide composites, and ceramics ( Su and Chang, 2018 ; Baig et al., 2021 ).
5.1.4. Sputtering
Microparticles of a solid material are expelled off its surface during the phenomenon known as sputtering, which occurs when the solid substance is assaulted by intense plasma or gas particles ( Behrisch, 1981 ). According to the incident gaseous ion energy, energetic gaseous ions used in the sputtering deposition process physically expel tiny atom clusters off the target surface ( Muñoz-García et al., 2009 ). The sputtering method is intriguing because it is more affordable than electron-beam lithography, and the composition of the sputtered nanomaterials is similar to the target material with fewer contaminants ( Baig et al., 2021 ).

5.1.5. Electron explosion
In this technique, a thin metal wire is subjected to a high current pulse that causes an explosion, evaporation, and ionization. The metal becomes vaporized and ionized, expands, and cools by reacting with the nearby gas or liquid medium. The condensed vapor finally forms the nanoparticles ( Joh et al., 2013 ). Electron explosion method because it produces plasma from the electrical explosion of a metallic wire, which may produce nanoparticles from a Pt solution without using a reducing agent ( Joh et al., 2013 ).
5.1.6. Sonication
The most crucial step in the creation of nanofluids is sonication. After the mixture has been magnetically stirred in a magnetic stirrer, sonication is performed in an ultrasonication path, ultrasonic vibrator, and mechanical homogenizer. Sonicators have become the industry standard for Probe sonication and are noticeably more powerful and effective when compared to ultrasonic cleaner baths for nanoparticle applications. Probe sonication is highly effective for processing nanomaterials (carbon nanotubes, graphene, inks, metal oxides, etc.) ( Zheng et al., 2010 ).
5.1.7. Pulsed wire discharge method
This is the most used method for creating metal nanoparticles. A pulsating current causes a metal wire to evaporate, producing a vapor that is subsequently cooled by an ambient gas to form nanoparticles. This plan may quickly produce large amounts of energy ( Patil et al., 2021 ).
5.1.8. Arc discharge method
Two graphite rods are adjusted in a chamber with a constant helium pressure during the Arc Discharge procedure. It is crucial to fill the chamber with helium because oxygen or moisture prevents the synthesis of fullerenes. Arc discharge between the ends of the graphite rods drives the vaporization of carbon rods. Achieving new types of nanoparticles depends significantly on the circumstances in which arc discharge occurs. The creation of several nanostructured materials may be accomplished with this technique ( Berkmans et al., 2014 ). It is well-recognized for creating carbon-based materials such as fullerenes, carbon nanohorns (CNHs), carbon nanotubes ( Shi et al., 2000 ), few-layer graphene, and amorphous spherical carbon nanoparticles ( Kumar R. et al., 2013 ).
5.1.9. Lithography
Lithography typically uses a concentrated beam of light or electrons to create nanoparticles, a helpful technique ( Pimpin and Srituravanich, 2012 ). Masked and maskless lithography are the two primary categories of lithography. Without a mask, arbitrary nano-pattern printing is accomplished in maskless lithography. Additionally, it is affordable and easy to apply ( Brady et al., 2019 ).
5.2. Bottom-up approach
Tiny atoms and molecules are combined in bottom-up methods to create nano-structured particles ( Figure 2 ; Baig et al., 2021 ). These include chemical and biological approaches:
5.2.1. Chemical vapor deposition (CVD)
Through a chemical process involving vapor-phase precursors, a thin coating is created on the substrate surface during CVD ( Dikusar et al., 2009 ). Precursors are deemed appropriate for CVD if they exhibit sufficient volatility, high chemical purity, strong evaporation stability, cheap cost, a non-hazardous nature, and long shelf life. Additionally, its breakdown should not leave behind any contaminants. Vapor phase epitaxy, metal-organic CVD, atomic layer epitaxy, and plasma-enhanced CVD are only a few CVD variations. This method’s benefits include producing very pure nanoparticles that are stiff, homogeneous, and strong ( Ago, 2015 ). CVD is an excellent approach to creating high-quality nanomaterials ( Machac et al., 2020 ). It is also well-known for creating two-dimensional nanoparticles ( Baig et al., 2021 ).
5.2.2. Sol-gel process
A wet-chemical approach, called the sol-gel method, is widely utilized to create nanomaterials ( Das and Srivasatava, 2016 ; Baig et al., 2021 ). Metal alkoxides or metal precursors in solution are condensed, hydrolyzed, and thermally decomposed. The result is a stable solution or sol. The gel gains greater viscosity as a result of hydrolysis or condensation. The particle size may be seen by adjusting the precursor concentration, temperature, and pH levels. It may take a few days for the solvent to be removed, for Ostwald ripening to occur, and for the phase to change during the mature stage, which is necessary to enable the growth of solid mass. To create nanoparticles, the unstable chemical ingredients are separated. The generated material is environmentally friendly and has many additional benefits thanks to the sol-gel technique ( Patil et al., 2021 ). The uniform quality of the material generated, the low processing temperature, and the method’s ease in producing composites and complicated nanostructures are just a few of the sol-gel technique’s many advantages ( Parashar et al., 2020 ).
5.2.3. Co-precipitation
It is a solvent displacement technique and is a wet chemical procedure. Ethanol, acetone, hexane, and non-solvent polymers are examples of solvents. Polymer phases can be either synthetic or natural. By mixing the polymer solution, fast diffusion of the polymer-solvent into the non-solvent phase of the polymer results. Interfacial stress at two phases results in the formation of nanoparticles ( Das and Srivasatava, 2016 ). This method’s natural ability to produce high quantities of water-soluble nanoparticles through a straightforward process is one of its key benefits. This process is used to create many commercial iron oxide NP-based MRI contrast agents, including Feridex, Reservist, and Combidex ( Baig et al., 2021 ; Patil et al., 2021 ).
5.2.4. Inert gas condensation/molecular condensation
Metal NPs are produced using this method in large quantities. Making fine NPs using the inactive gas compression approach has been widespread, which creates NPs by causing a metallic source to disappear in an inert gas. At an attainable temperature, metals evaporate at a tolerable pace. Copper metal nanoparticles are created by vaporizing copper metal inside a container containing argon, helium, or neon. The atom quickly loses its energy by cooling the vaporized atom with an inert gas after it boils out. Liquid nitrogen is used to cool the gases, forming nanoparticles in the range of 2–100 nm ( Pérez-Tijerina et al., 2008 ; Patil et al., 2021 ).
5.2.5. Hydrothermal
In this method, for the production of nanoparticles, hydrothermal synthesis uses a wide temperature range from ambient temperature to extremely high temperatures. Comparing this strategy to physical and biological ones offers several benefits. At higher temperature ranges, the nanomaterials produced by hydrothermal synthesis could become unstable ( Banerjee et al., 2008 ; Patil et al., 2021 ).
5.2.6. Green/biological synthesis
The synthesis of diverse metal nanoparticles utilizing bioactive agents, including plant materials, microbes, and various biowastes like vegetable waste, fruit peel waste, eggshell, agricultural waste, algae, and so on, is known as “green” or “biological” nanoparticle synthesis ( Kumari et al., 2021 ). Developing dependable, sustainable green synthesis technologies is necessary to prevent the formation of undesirable or dangerous byproducts ( Figure 3 ). The green synthesis of nanoparticles also has several advantages, including being straightforward, affordable, producing NPs with high stability, requiring little time, producing non-toxic byproducts, and being readily scaled up for large-scale synthesis ( Malhotra and Alghuthaymi, 2022 ).

Schematic diagram for biosynthesis of NPs.
5.2.6.1. Biological synthesis using microorganisms
Microbes use metal capture, enzymatic reduction, and capping to create nanoparticles. Before being converted to nanoparticles by enzymes, metal ions are initially trapped on the surface or interior of microbial cells ( Ghosh et al., 2021 ). Use of microorganisms (especially marine microbes) for synthesis of metalic NPs is environmental friendly, fast and economical ( Patil and Kim, 2018 ). Several microorganisms are used in the synthesis of metal NPs, including:
Biosynthesis of NPs by bacteria: A possible biofactory for producing gold, silver, and cadmium sulfide nanoparticles is thought to be bacterial cells. It is known that bacteria may produce inorganic compounds either inside or outside of their cells ( Hulkoti and Taranath, 2014 ). Desulforibrio caledoiensis ( Qi et al., 2013 ), Enterococcu s sp. ( Rajeshkumar et al., 2014 ), Escherichia coli VM1 ( Maharani et al., 2016 ), and Ochrobactrum anhtropi ( Thomas et al., 2014 ) based metal NPs are reported previously for their potential photocatalytic properties ( Qi et al., 2013 ), antimicrobial activity ( Rajeshkumar et al., 2014 ), and anticancer activity ( Maharani et al., 2016 ).
Extracellular synthesis of NPs by bacteria: The microorganisms’ extracellular reductase enzymes shrink the silver ions to the nanoscale range. According to protein analysis of microorganisms, the NADH-dependent reductase enzyme carries out the bio-reduction of silver ions to AgNPs. The electrons for the reductase enzyme come from NADH, which is subsequently converted to NAD+. The enzyme is also oxidized simultaneously when silver ions are reduced to nanosilver. It has been noted that bio-reduction can occasionally be caused by nitrate-dependent reductase. The decline occurs within a few minutes in the quick extracellular creation of nanoparticles ( Mathew et al., 2010 ). At pH 7, the bacterium R. capsulata produced gold nanoparticles with sizes ranging from 10−20 nm. Numerous nanoplates and spherical gold nanoparticles were produced when the pH was changed to four ( Sriram et al., 2012 ). By adjusting the pH, the gold nanoparticles’ form may be changed. Gold nanoparticle shape was controlled by regulating the proton content at various pH levels. The bacteria R. capsulata ’s release cofactor NADH and NADH-dependent enzymes may cause the bioreduction of Au (3+) to Au (0) and the generation of gold nanoparticles. By using NADH-dependent reductase as an electron carrier, it is possible to start the reduction of gold ions ( Sriram et al., 2012 ).
Intracellular synthesis of NPs by bacteria: Three processes are involved in the intracellular creation of NPs: trapping, bioreduction, and capping. The cell walls of microorganisms and ions charge contribute significantly to creating NPs in the intracellular route. This entails specific ion transit in the presence of enzymes, coenzymes, and other molecules in the microbial cell. Microbes have a range of polysaccharides and proteins in their cell walls, which function as active sites for the binding of metal ions ( Slavin et al., 2017 ). Not all bacteria can produce metal and metal oxide nanoparticles. The only ions that pose a significant hazard to microorganisms are heavy metal ions, which, in response to a threat, cause the germs to react by grabbing or trapping the ions on the cell wall via electrostatic interactions. This occurs because a metal ion is drawn to the cell wall’s carboxylate groups, including cysteine and polypeptides, and certain enzymes with a negative charge ( Zhang et al., 2011 ).
Additionally, the electron transfers from NADH via NADH-dependent educates, which serves as an electron carrier and is located inside the plasma membrane, causing the trapped ions to be reduced into the elemental atom. The nuclei eventually develop into NPs and build up in the cytoplasm or the pre-plasmic space. On the other hand, the stability of NPs is provided by proteins, peptides, and amino acids found inside cells, including cysteine, tyrosine, and tryptophan ( Mohd Yusof et al., 2019 ).
Biosynthesis of NPs by fungi: Because monodisperse nanoparticles with distinct dimensions, various chemical compositions, and sizes may be produced, the biosynthesis of nanoparticles utilizing fungus is frequently employed. Due to the existence of several enzymes in their cells and the ease of handling, fungi are thought to be great candidates for producing metal and metal sulfide nanoparticles ( Mohanpuria et al., 2008 ).
The nanoparticles were created on the surface of the mycelia. After analyzing the results and noting the solution, it was determined that the Ag + ions are initially trapped on the surface of the fungal cells by an electrostatic interaction between gold ions and negatively charged carboxylate groups, which is facilitated by enzymes that are present in the mycelia’s cell wall. Later, the enzymes in the cell wall reduce the silver ions, causing the development of silver nuclei. These nuclei then increase as more Ag ions are reduced and accumulate on them.
The TEM data demonstrate the presence of some silver nanoparticles both on and inside the cytoplasmic membrane. The findings concluded that the Ag ions that permeate through the cell wall were decreased by enzymes found inside the cytoplasm and on the cytoplasmic membrane. Also possible is the diffusion of some silver nanoparticles over the cell wall and eventual cytoplasmic entrapment ( Mukherjee et al., 2001 ; Hulkoti and Taranath, 2014 ).
It was observed that the culture’s age does not affect the shape of the synthesized gold nanoparticles. However, the number of particles decreased when older cells were used. The different pH levels produce a variety of shapes of gold nanoparticles, indicating that pH plays a vital role in determining the shape. The incubation temperature also played an essential role in the accumulation of the gold nanoparticles. It was observed that the particle growth rate was faster at increased temperature levels ( Mukherjee et al., 2001 ; Ahmad et al., 2003 ). The form of the produced gold nanoparticles was shown to be unaffected by the age of the culture. However, when older cells were utilized, the particle count fell. The fact that gold nanoparticles take on various forms at different pH levels suggests that the pH is crucial in determining the shape. The incubation temperature significantly influenced the accumulation of the gold nanoparticles. It was found that higher temperatures caused the particle development rate to accelerate ( Mukherjee et al., 2001 ; Ahmad et al., 2003 ). Verticillium luteoalbum is reported to synthesize gold nanoparticles of 20–40 nm in size ( Erasmus et al., 2014 ). Aspergillus terreus and Penicillium brevicompactum KCCM 60390 based metal NPs are reported for their antimicrobial ( Li G. et al., 2011 ) and cytotoxic activities ( Mishra et al., 2011 ), respectively.
Biosynthesis of NPs using actinomycetes: Actinomycetes have been categorized as prokaryotes since they share significant traits with fungi. They are sometimes referred to as ray fungi ( Mathew et al., 2010 ). Making NPs from actinomycetes is the same as that of fungi ( Sowani et al., 2016 ). Thermomonospora sp., a new species of extremophilic actinomycete, was discovered to produce extracellular, monodispersed, spherical gold nanoparticles with an average size of 8 nm ( Narayanan and Sakthivel, 2010 ). Metal NPs synthesized by Rhodococcus sp. ( Ahmad et al., 2003 ) and Streptomyces sp. Al-Dhabi-87 ( Al-Dhabi et al., 2018 ) are reported for their antimicrobial activities.
Biosynthesis of NPs using algae: Algae have a high concentration of polymeric molecules, and by reducing them, they may hyper-accumulate heavy metal ions and transform them into malleable forms. Algal extracts typically contain pigments, carbohydrates, proteins, minerals, polyunsaturated fatty acids, and other bioactive compounds like antioxidants that are used as stabilizing/capping and reducing agents ( Khanna et al., 2019 ). NPs also have a faster rate of photosynthesis than their biosynthetic counterparts. Live or dead algae are used as model organisms for the environmentally friendly manufacturing process of bio-nanomaterials, such as metallic NPs ( Hasan, 2015 ). Ag and Au are the most extensively researched noble metals to synthesized NPs by algae either intracellularly or extracellularly ( Dahoumane et al., 2017 ). Chlorella vulgaris ( Luangpipat et al., 2011 ), Chlorella pyrenoidosa ( Eroglu et al., 2013 ), Nanochloropsis oculata ( Xia et al., 2013 ), Scenedesmus sp. IMMTCC-25 ( Jena et al., 2014 ) based metal NPs are reported for their potential catalytic ( Luangpipat et al., 2011 ; Eroglu et al., 2013 ) and, antimicrobial ( Eroglu et al., 2013 ; Jena et al., 2014 ) activities along with their use in Li-Ion batteries ( Xia et al., 2013 ).
Intracellular synthesis of NPs using algae: In order to create intracellular NPs, algal biomass must first be gathered and thoroughly cleaned with distilled water. After that, the biomass (living algae) is treated with metallic solutions like AgNO3. The combination is then incubated at a specified pH and a specific temperature for a predetermined time. Finally, it is centrifuged and sonicated to produce the extracted stable NPs ( Uzair et al., 2020 ).
Extracellular synthesis of NPs using algae: Algal biomass is first collected and cleaned with distilled water before being used to synthesize NPs extracellularly ( Uzair et al., 2020 ). The following three techniques are frequently utilized for the subsequent procedure:
(i) A particular amount of time is spent drying the algal biomass (dead algae), after which the dried powder is treated with distilled water and filtered.
(ii) The algal biomass is sonicated with distilled water to get a cell-free extract.
(iii) The resultant product is filtered after the algal biomass has been rinsed with distilled water and incubated for a few hours (8–16 h).
5.2.6.2. Biological synthesis using plant extracts
The substance or active ingredient of the desired quality extracted from plant tissue by treatment for a particular purpose is a plant extract ( Jadoun et al., 2021 ). Plant extracts are combined with a metal salt solution at room temperature to create nanoparticles. Within minutes, the response is finished. This method has been used to create nanoparticles of silver, gold, and many other metals ( Li X. et al., 2011 ). Nanoparticles are biosynthesized using a variety of plants. It is known that the kind of plant extract, its concentration, the concentration of the metal salt, the pH, temperature, and the length of contact time all have an impact on how quickly nanoparticles are produced as well as their number and other properties ( Mittal and Chisti, 2013 ). A leaf extract from Polyalthia longifolia was used to create silver nanoparticles, the average particle size was around 58 nm ( Kumar and Yadav, 2009 ; Kumar et al., 2016 ).
Acacia auriculiformis ( Saini et al., 2016 ), Anisomeles indica ( Govindarajan et al., 2016 ), Azadirachta indica ( Velusamy et al., 2015 ), Bergenia ciliate ( Phull et al., 2016 ), Clitoria ternatea , Solanum nigrum ( Krithiga et al., 2013 ), Coffea arabica ( Dhand et al., 2016 ), Coleus forskohlii ( Naraginti et al., 2016 ), Curculigo orchioides ( Kayalvizhi et al., 2016 ), Digitaria radicosa ( Kalaiyarasu et al., 2016 ), Dioscorea alata ( Pugazhendhi et al., 2016 ), Diospyros paniculata ( Rao et al., 2016 ), Elephantopus scaber ( Kharat and Mendhulkar, 2016 ), Emblica officinalis ( Ramesh et al., 2015 ), Euphorbia antiquorum L. ( Rajkuberan et al., 2017 ), Ficus benghalensis ( Nayak et al., 2016 ), Lantana camara ( Ajitha et al., 2015 ), Cinnamomum zeylanicum ( Soni and Sonam, 2014 ), and Parkia roxburghii ( Paul et al., 2016 ) are the few examples of plants which are reported for the green synthesis of metal NPs (i.e., AgNPs). These were evaluated for their antifilaria activity ( Saini et al., 2016 ), mosquitocidal activity ( Govindarajan et al., 2016 ), antibacterial activity ( Velusamy et al., 2015 ), catalytic activity ( Edison et al., 2016 ), antioxidant activity ( Phull et al., 2016 ), and Cytotoxicity ( Patil et al., 2017 ).
5.2.6.3. Biological synthesis using biomimetic
“Biomimetic synthesis” typically refers to chemical processes that resemble biological synthesis carried out by living things ( Dahoumane et al., 2017 ). In the biomimetic approach, proteins, enzymes, cells, viruses, pollen, and waste biomass are used to synthesize NPs. Two categories are used to classify biomimetic synthesis:
Functional biomimetic synthesis uses various materials and approaches to emulate particular characteristics of natural materials, structures, and systems ( Zan and Wu, 2016 ).
Process biomimetic synthesis is a technique that aims to create different desirable nanomaterials/structures by imitating the synthesis pathways, processes, or procedures of natural chemicals and materials/structures. For instance, several distinctive nano-superstructures (such as satellite structures, dendrimer-like structures, pyramids, cubes, 2D nanoparticle arrays, 3D AuNP tubes, etc.) have been put together in vitro by simulating the protein manufacturing process ( Zan and Wu, 2016 ).
6. Applications of NPs
6.1. applications of nps in environment industry.
Due to their tiny size and distinctive physical and chemical characteristics, NPs appeal to various environmental applications. The properties of nanoparticals and their advantages are illustrated in Figure 4 . The following are some possible NP uses in the environment.

Properties of nanoparticals and their advantages.
6.1.1. Bioremediation
Nanoparticles (NPs) can remove environmental pollutants, such as heavy metals from water or organic contaminants from soil ( Zhuang and Gentry, 2011 ). For example, silver nanoparticles (AgNPs) effectively degrade certain pollutants, such as organic dyes and compounds found in wastewater. Several nanomaterials have been considered for remediation purposes, such as nanoscale zeolites, metal oxides, and carbon nanotubes and fibers ( Zhuang and Gentry, 2011 ). Nanoscale particles used in remediation can access areas that larger particles cannot. They can be coated to facilitate transport and prevent reaction with surrounding soil matrices before reacting with contaminants. One widely used nanomaterial for remediation is Nanoscale zerovalent iron (nZVI). It has been used at several hazardous waste sites to clean up chlorinated solvents that have contaminated groundwater ( Elliott et al., 2013 ). Removing heavy metals such as mercury, lead, thallium, cadmium, and arsenic from natural water has attracted considerable attention because of their adverse effects on environmental and human health. Superparamagnetic iron oxide NPs are an effective sorbent material for this toxic soft material. So, no measurements of engineered NPs in the environment have been available due to the absence of analytical methods able to quantify the trace concentration of NPs ( Elliott et al., 2013 ).
6.1.2. Sensors in environment
Nanotechnology/NPs are already being used to improve water quality and assist in environmental clean-up activities ( Pradeep, 2009 ). Their potential use as environmental sensors to monitor pollutants is also becoming viable NPs can be used as sensors to detect the presence of certain compounds in the environment, such as heavy metals or pollutants. The nano-sensors small size and wide detection range provide great flexibility in practical applications. It has been reported that nanoscale sensors can be used to detect microbial pathogens and biological compounds, such as toxins, in aqueous environments ( Yadav et al., 2010 ). NPS can be designed to selectively bind to specific types of pollutants, allowing them to be detected at low concentrations. For example, gold nanoparticles (AuNPs) have been used as sensors for the detection of mercury in water ( Theron et al., 2010 ).
6.1.3. Catalysts in environment
Nanoparticles (NPs) are used as catalysts in chemical reactions, such as in the production of biofuels or environmental remediation processes, and to catalyze biomass conversion into fuels, such as ethanol or biodiesel. For example, platinum nanoparticles (PtNPs) have been explored for use in the production of biofuels due to their ability to catalyze the conversion of biomass into fuels ( Lam and Luong, 2014 ). PtNPs also showed promising sensing properties; for example, Using Pt NPs, the Hg ions were quantified in the range of 50–500 nM in MilliQ, tap, and groundwater samples, and the limit of quantifications for Hg ions were 16.9, 26, and 47.3 nM. The biogenic PtNPs-based probe proved to be applicable for detecting and quantifying Hg ions ( Kora and Rastogi, 2018 ).
Overall, NPs have significant potential for use in the environment and are being actively researched for a variety of applications.
6.2. Applications of NPs in medicine industry
Nanoparticles (NPs) have unique physical and chemical properties due to their small size, making them attractive for use in various applications, including the medicine industry. Some potential applications of NPs in medicine include:
6.2.1. Drug delivery
Technological interest has been given to AuNPs due to their unique optical properties, ease of synthesis, and chemical stability. The particles can be used in biomedical applications such as cancer treatment ( Sun et al., 2014 ), biological imaging ( Abdulle and Chow, 2019 ), chemical sensing, and drug delivery. Sun et al. (2014) mentioned in detail about two different methods of controlled release of drugs associated with NPs, which were (1) sustained (i.e., diffusion-controlled and erosion-controlled) and (2) stimuli-responsive (i.e., pH-sensitive, enzyme-sensitive, thermoresponsive, and photosensitive). Figure 5 illustrates that how NPs acts as targeted delivery of medicines to treat cancer cells ( Figure 5A ) and therapeutic gene delivery to synthesis proteins of interests in targeted cells ( Figure 5B ). NPs can deliver drugs to specific body areas, allowing for more targeted and effective treatment ( Siddique and Chow, 2020 ). For example AgNPs have been explored for use in drug delivery due to their stability and ability to accumulate in certain types of cancerous tumors ( Siddique and Chow, 2020 ). ZnONPs have also been explored for drug delivery due to their ability to selectively target cancer cells ( Anjum et al., 2021 ). CuNPs have been shown to have antimicrobial properties and are being explored for drug delivery to treat bacterial infections ( Yuan et al., 2018 ). AuNPs have unique optical, electrical, and catalytic properties and are being explored for drug delivery due to their ability to accumulate in certain cancerous tumors. Silver NPs (AgNPs) have been incorporated into wound dressings, bone cement, and implants ( Schröfel et al., 2014 ).

Application of nanoparticles as; targated drug delivery (A) , and therapeutic protein generation in targated cells (B) .
6.2.2. Diagnostics
Nanoparticles (NPs) can be used as imaging agents to help visualize specific body areas. For example, iron oxide nanoparticles (Fe 3 O 4 NPs) have been used as magnetic resonance imaging (MRI) contrast agents to help visualize tissues and organs ( Nguyen et al., 2013 ). AuNPs have unique optical, electrical, and catalytic properties and are being explored for diagnostics due to their ability to accumulate in certain cancerous tumors ( Siddique and Chow, 2020 ).
6.2.3. Tissue engineering
Nanoparticles (NPs) can help stimulate the growth and repair of tissues and organs. For example, titanium dioxide nanoparticles (TiO2 NPs) have been explored for tissue engineering due to their ability to stimulate the growth of bone cells ( Kim et al., 2014 ).
6.2.4. Antimicrobials
Some NPs, such as silver nanoparticles (AgNPs) and copper nanoparticles (CuNPs), have strong antimicrobial properties and are being explored for use in a variety of medical products, such as wound dressings and medical devices ( Hoseinzadeh et al., 2017 ).
Overall, NPs have significant potential for use in the medical industry and are being actively researched for various applications. However, it is essential to carefully consider the potential risks and benefits of using NPs in medicine and ensure their safe and responsible use.
6.3. Applications of NPs in agriculture industry
There are several ways in which nanoparticles (NPs) have the potential to alter the agricultural sector. NPs may be used in agriculture for a variety of reasons, including:
6.3.1. Pesticides and herbicides
Nanoparticles (NPs) can be used to deliver pesticides and herbicides in a targeted manner, reducing the number of chemicals needed and minimizing the potential for environmental contamination ( Khan et al., 2019 ). AgNPs and CuNPs have antimicrobial properties, making them potentially useful for controlling pests and diseases in crops. They can also be used as delivery systems for active ingredients, allowing for more targeted application and reducing the potential for environmental contamination ( Hoseinzadeh et al., 2017 ; Dangi and Verma, 2021 ).
It is important to note that using metal NPs in pesticides and herbicides is still in the early stages of development. More research is needed to understand their potential impacts on human health and the environment ( Dangi and Verma, 2021 ).
6.3.2. Fertilizers and plant growth
Nano fertilizers offer an opportunity for efficiently improving plant mineral nutrition. Some studies have shown that nanomaterials can be more effective than conventional fertilizers, with a controlled release of nutrients increasing the efficiency of plant uptake and potentially reducing adverse environmental outcomes associated with the loss of nutrients in the broader environment. However, other studies have found that nanomaterial has the same or even less effective effectiveness than conventional fertilizers. NPs used to deliver fertilizers to plants more efficiently, reducing the amount of fertilizer needed, and reducing the risk of nutrient runoff ( Kopittke et al., 2019 ).
Ag ( Jaskulski et al., 2022 ), Zn ( Song and Kim, 2020 ), Cu, Au, Al, and Fe ( Kopittke et al., 2019 ) based NPs have been shown to have fertilizing properties and plant growth-promoting properties, and may help provide essential nutrients to plants and improve plant growth and yield. It is important to note that the use of NPs in fertilizers is still in the early stages of development. More research is needed to understand their potential impacts on human health and the environment.
6.3.3. Food safety
Nanoparticles (NPs) can detect and eliminate pathogens in food products, improving food safety, and reducing the risk of foodborne illness ( Zhuang and Gentry, 2011 ).
6.3.4. Water purification
Nanoparticles (NPs) can purify irrigation water, reducing the risk of crop contamination and improving crop yield ( Zhuang and Gentry, 2011 ). Using NPs in agriculture can improve crop yields, reduce agriculture’s environmental impact, and improve food products’ safety and quality.
6.4. Applications of NPs in food industry
Numerous applications for nanoparticles (NPs) in the food sector are possible, including:
6.4.1. Food processing and food preservation/food packaging
Nanoparticles (NPs) can be used to improve the efficiency and performance of food processing operations, such as grinding, mixing, and drying, e.g., AgNPs have been used as a natural antimicrobial agent in food processing operations, helping to prevent the growth of bacteria and other microorganisms ( Dangi and Verma, 2021 ) and also NPs are used to enhance the performance of materials used in food packaging, making them more resistant to pollutants like moisture and gases.
6.4.2. Food fortification
Nanoparticles (NPs) can deliver essential nutrients to food products, such as vitamins and minerals, more efficiently and effectively. e.g., Fe 2 O 3 , and CuNPs have been used to fortify food products with iron, and Cu is an essential nutrient necessary for the metabolism of iron and other nutrients. Iron is an essential nutrient often lacking in many people’s diets, particularly in developing countries ( Kopittke et al., 2019 ).
6.4.3. Sensors
Nanoparticles (NPs) used to improve the sensitivity and specificity of food sensors, allowing them to detect a broader range of substances or signals ( Yadav et al., 2010 ).
Overall, using NPs in the food industry can improve the performance, safety, and nutritional value of a wide range of food products and processes.
6.5. Applications of NPs in electronics industry and automotive industry
In many aspects, nanoparticles (NPs) can transform the electronics sector. NPs may be used in a variety of electrical applications, such as:
6.5.1. Display technologies/storage devices
Nanoparticles (NPs) can be used to improve the performance of displays ( Park and Choi, 2019 ; Bahadur et al., 2021 ; Triana et al., 2022 ), such as LCD and OLED displays, by enhancing the brightness, color, and contrast of the image, such as silver NPs and gold NPs, have been explored for use in LCD and OLED displays as a means of improving the conductivity of the display ( Gwynne, 2020 ). NPs improve the performance and durability of energy storage devices, such as batteries and supercapacitors, by increasing energy density and charging speed. Zinc oxide nanoparticles (ZnO NPs) have the potential to be used in energy storage devices, such as batteries and supercapacitors, due to their ability to store and release energy ( Singh et al., 2011 ).
6.5.2. Data storage
Nanoparticles (NPs) can improve the capacity and speed of data storage devices, such as hard drives and flash drives. Magnetic NPs, such as iron oxide NPs, have been explored for use in data storage devices, such as hard drives, due to their ability to store, and retrieve data using magnetism. These NPs are often composed of a magnetic metal, such as iron, cobalt, or nickel. They can be magnetized and demagnetized, allowing them to store and retrieve data ( Ahmad et al., 2021 ).
Overall, the use of NPs in electronics has the potential to improve the performance and efficiency of a wide range of electronic devices and systems.
Applications of NPs in chemical industry: The chemical industry might be entirely transformed by nanoparticles (NPs) in various ways. The following are potential uses for NPs in the chemical industry ( Salem and Fouda, 2021 ).
6.5.3. Chemical processing/catalysis
Nanoparticles (NPs) can be used as catalysts in chemical reactions, allowing them to be carried out more efficiently and at lower temperatures. Some examples of metal NPs that have been used as catalysts in the chemical industry include: PtNPs have been used as catalysts in a variety of chemical reactions, including fuel cell reactions ( Bhavani et al., 2021 ), hydrogenation reactions, and oxidation reactions ( Lara and Philippot, 2014 ), PdNPs have been used as catalysts in a variety of chemical reactions, including hydrogenation reactions and cross-coupling reactions ( Pérez-Lorenzo, 2012 ), FeNPs have been used as catalysts in a variety of chemical reactions, including hydrolysis reactions ( Jiang and Xu, 2011 ), and oxygen reduction reactions, NiNPs have been used as catalysts in a variety of chemical reactions, including hydrogenation reactions, and hydrolysis reactions ( Salem and Fouda, 2021 ).
6.5.4. Separation and purification
NPs are used to separate and purify chemicals and other substances, such as gases and liquids, by exploiting their size-based properties ( Hollamby et al., 2010 ). Several types of metal nanoparticles (NPs) have been explored for use in separation and purification processes in the chemical industry, including Fe 2 O 3 NPs have been used to separate and purify gases, liquids, and chemicals. They have also been used to remove contaminants from water ( Pradeep, 2009 ; Siddique and Chow, 2020 ). AgNPs have been used to purify water and remove contaminants ( Pradeep, 2009 ), such as bacteria and viruses. They have also been used to remove heavy metals from water and other substances ( Zhuang and Gentry, 2011 ). AuNPs have been used to purify water and remove contaminants, such as bacteria and viruses ( Siddique and Chow, 2020 ). They have also been used to separate and purify gases and liquids ( Zhuang and Gentry, 2011 ). AlNPs have been used to remove contaminants from water and other substances, such as oils and fuels. They have also been used to purify gases ( Zhuang and Gentry, 2011 ).
6.6. Applications of NPs in defense industry
Nanoparticles (NPs) can be used to improve the efficiency and performance of chemical processing operations, such as refining and synthesizing chemicals ( Schröfel et al., 2014 ). Nanoparticles (NPs) have the potential to be used in the defense industry in several ways, including:
6.6.1. Sensors
Nanoparticles (NPs) can improve the sensitivity and specificity of sensors used in defense systems, such as sensors for detecting chemical, biological, or radiological threats ( Zheng et al., 2010 ).
6.6.2. Protective coatings
Nanoparticles (NPs) can improve the performance and durability of protective coatings applied to defense equipment, such as coatings resistant to chemical or biological agents. For example, metal NPs can improve the mechanical properties and durability of the coating, making it more resistant to wear and corrosion. For example, adding Al or Zn based NPs to a polymer coating can improve its corrosion resistance. In contrast, adding Ni or Cr-based NPs can improve their wear resistance ( Rangel-Olivares et al., 2021 ).
6.6.3. Weapons
Nanoparticles (NPs) are used as weapons against viruses, bacteria, etc, ( Ye et al., 2020 ) and as well as in the development of armor and protective materials. There have been some reports of the potential use of NPs in military and defense applications, such as in the development of armor and protective materials. For example, adding nanoparticles, such as ceramic or metal NPs, to polymers or other materials can improve their mechanical properties and make them more resistant to damage. In addition, there have been reports of the use of NPs in developing sensors and detection systems for defense purposes.
6.6.4. Manufacturing
Nanoparticles (NPs) can improve the performance and durability of materials used in defense equipment, such as armor or structural materials. Metal NPs can be used in materials by adding them as a filler or reinforcement in polymers. For example, the addition of metal NPs such as aluminum (Al), copper (Cu), or nickel (Ni) to polymers can improve the mechanical properties, thermal stability, and electrical conductivity of the resulting composite material ( Khan et al., 2019 ).
Metal NPs can also make functional materials, such as catalysts and sensors. For example, metal NPs, such as gold (Au), and platinum (Pt), can be used as catalysts in various chemical reactions due to their high surface area and ability to adsorb reactants ( Zheng et al., 2010 ).
6.6.5. Energy storage
Nanoparticles (NPs) can improve the performance and efficiency of energy storage systems used in defense systems, such as batteries or fuel cells ( Morsi et al., 2022 ). In batteries, nanoparticles can be used as a cathode material to increase the battery’s energy density, rate capability, and cycling stability. For example, lithium cobalt oxide (LiCoO 2 ) nanoparticles have been used as cathode materials in lithium-ion batteries due to their high capacity and good rate performance. In addition, nanoparticles of transition metal oxides, such as iron oxide (Fe 2 O 3 ), and manganese oxide (MnO 2 ), have been used as cathode materials in rechargeable lithium batteries due to their high capacity and good rate performance. In supercapacitors, nanoparticles can be used as the active material in the electrodes to increase the specific surface area, leading to an increase in the device’s capacitance ( Morsi et al., 2022 ). Using NPs in the defense industry can improve defense systems’ performance, efficiency, and safety.
7. Future perspectives
Metal nanoparticles (NPs) have many potential applications in various fields, including electronics, energy storage, catalysis, and medicine. However, there are also several challenges and potential future directions for developing and using metal NPs.
One major challenge is synthesizing and processing metal NPs with precise size and shape control. Many methods for synthesizing metal NPs involve high temperatures and harsh chemical conditions, which can be challenging to scale up for large-scale production. In addition, the size and shape of metal NPs can significantly impact their properties and potential applications, so it is essential to synthesize NPs with precise size and shape control.
Another challenge is the environmental impact of metal NPs. Some metal NPs, such as silver NPs, can be toxic to aquatic life and may have other environmental impacts. There is a need for more research on the environmental effects of metal NPs and the development of more environmentally friendly (Green) synthesis and processing methods.
In terms of future directions, one promising area is the use of metal NPs for energy storage, conversion, and protection of the environment. For example, metal NPs could be used to improve batteries’ performance or develop more efficient solar cells. In addition, metal NPs could be used in catalysis to improve the efficiency of chemical reactions. There is also ongoing research on metal NPs in medicine, including drug delivery and cancer therapy.
Author contributions
KAA: conceptualization, methodology, validation, formal analysis, investigation, writing – original draft, writing – review and editing, and visualization.
Acknowledgments
The author thanks Prof. Dr. Mona M. Sobhy, Department of Reproductive Diseases, Animal Reproduction Research Institute, ARC, Giza, Egypt, and Dr. Omar Hewedy, University of Guelph, Canada, for the critical reading of the manuscript.
Conflict of interest
The author declares that the research was conducted in the absence of any commercial or financial relationships that could be construed as a potential conflict of interest.
Publisher’s note
All claims expressed in this article are solely those of the authors and do not necessarily represent those of their affiliated organizations, or those of the publisher, the editors and the reviewers. Any product that may be evaluated in this article, or claim that may be made by its manufacturer, is not guaranteed or endorsed by the publisher.
- Abdulle A., Chow J. C. (2019). Contrast enhancement for portal imaging in nanoparticle-enhanced radiotherapy: A Monte Carlo phantom evaluation using flattening-filter-free photon beams. Nanomaterials 9 : 920 . 10.3390/nano9070920 [ PMC free article ] [ PubMed ] [ CrossRef ] [ Google Scholar ]
- Ago H. (2015). “ CVD growth of high-quality single-layer graphene ,” in Frontiers of Graphene and Carbon Nanotubes , Ed. Matsumoto K. (Berlin: Springer; ), 3–20. 10.1007/978-4-431-55372-4_1 [ CrossRef ] [ Google Scholar ]
- Ahmad A., Alsaad A., Al-Bataineh Q. M., Al-Akhras M.-A. H., Albataineh Z., Alizzy K. A., et al. (2021). Synthesis and characterization of ZnO NPs-doped PMMA-BDK-MR polymer-coated thin films with UV curing for optical data storage applications. Polymer Bull. 78 1189–1211. 10.1007/s00289-020-03155-x [ CrossRef ] [ Google Scholar ]
- Ahmad A., Senapati S., Khan M. I., Kumar R., Ramani R., Srinivas V., et al. (2003). Intracellular synthesis of gold nanoparticles by a novel alkalotolerant actinomycete. Rhodococcus species. Nanotechnology 14 : 824 . 10.1088/0957-4484/14/7/323 [ CrossRef ] [ Google Scholar ]
- Ahmad T., Wani I. A., Ahmed J., Al-Hartomy O. A. (2014). Effect of gold ion concentration on size and properties of gold nanoparticles in TritonX-100 based inverse microemulsions. Appl. Nanosci. 4 491–498. 10.1007/s13204-013-0224-y [ CrossRef ] [ Google Scholar ]
- Ajitha B., Reddy Y. A. K., Reddy P. S. (2015). Green synthesis and characterization of silver nanoparticles using Lantana camara leaf extract. Mater. Sci. Eng. C 49 373–381. 10.1016/j.msec.2015.01.035 [ PubMed ] [ CrossRef ] [ Google Scholar ]
- Al-Dhabi N. A., Mohammed Ghilan A.-K., Arasu M. V. (2018). Characterization of silver nanomaterials derived from marine Streptomyces sp. al-dhabi-87 and its in vitro application against multidrug resistant and extended-spectrum beta-lactamase clinical pathogens. Nanomaterials 8 : 279 . 10.3390/nano8050279 [ PMC free article ] [ PubMed ] [ CrossRef ] [ Google Scholar ]
- Amendola V., Meneghetti M. (2009). Laser ablation synthesis in solution and size manipulation of noble metal nanoparticles. Phys. Chem. Chem. Phys. 11 3805–3821. 10.1039/b900654k [ PubMed ] [ CrossRef ] [ Google Scholar ]
- Anjum S., Hashim M., Malik S. A., Khan M., Lorenzo J. M., Abbasi B. H., et al. (2021). Recent advances in zinc oxide nanoparticles (Zno nps) for cancer diagnosis, target drug delivery, and treatment. Cancers 13 : 4570 . 10.3390/cancers13184570 [ PMC free article ] [ PubMed ] [ CrossRef ] [ Google Scholar ]
- Astefanei A., Núñez O., Galceran M. T. (2015). Characterisation and determination of fullerenes: a critical review. Anal. Chim. Acta 882 1–21. [ PubMed ] [ Google Scholar ]
- Bahadur P. S., Jaiswal S., Srivastava R., Kumar A. (2021). “ Advanced application of nanotechnology in engineering ,” in Proceedings of the 2021 International Conference on Technological Advancements and Innovations (ICTAI) , (Piscataway, NJ: IEEE; ), 92–95. [ Google Scholar ]
- Baig N., Kammakakam I., Falath W. (2021). Nanomaterials: A review of synthesis methods, properties, recent progress, and challenges. Mater. Adv. 2 1821–1871. [ Google Scholar ]
- Banerjee A., Krishna R., Das B. (2008). Size controlled deposition of Cu and Si nano-clusters by an ultra-high vacuum sputtering gas aggregation technique. Appl. Phys. A 90 299–303. [ Google Scholar ]
- Bayda S., Adeel M., Tuccinardi T., Cordani M., Rizzolio F. (2019). The history of nanoscience and nanotechnology: from chemical–physical applications to nanomedicine. Molecules 25 : 112 . 10.3390/molecules25010112 [ PMC free article ] [ PubMed ] [ CrossRef ] [ Google Scholar ]
- Behrisch R. (1981). Sputtering by Particle Bombardment Springer Verlag. Berlin-Heidelberg: Springer. [ Google Scholar ]
- Berkmans A. J., Jagannatham M., Priyanka S., Haridoss P. (2014). Synthesis of branched, nano channeled, ultrafine and nano carbon tubes from PET wastes using the arc discharge method. Waste Manag. 34 2139–2145. 10.1016/j.wasman.2014.07.004 [ PubMed ] [ CrossRef ] [ Google Scholar ]
- Beyene H. D., Werkneh A. A., Bezabh H. K., Ambaye T. G. (2017). Synthesis paradigm and applications of silver nanoparticles (AgNPs), a review. Sustain. Mater. Technol. 13 18–23. [ Google Scholar ]
- Bhattacharjee S. (2016). DLS and zeta potential–what they are and what they are not? J. Control. Release 235 337–351. [ PubMed ] [ Google Scholar ]
- Bhavani K. S., Anusha T., Brahman P. K. (2021). Platinum nanoparticles decorated on graphitic carbon nitride-ZIF-67 composite support: An electrocatalyst for the oxidation of butanol in fuel cell applications. Int. J. Hydr. Energy 46 9199–9214. [ Google Scholar ]
- Biju V., Itoh T., Anas A., Sujith A., Ishikawa M. (2008). Semiconductor quantum dots and metal nanoparticles: syntheses, optical properties, and biological applications. Anal. Bioanal. Chem. 391 2469–2495. [ PubMed ] [ Google Scholar ]
- Brady B., Wang P. H., Steenhoff V., Brolo A. G. (2019). “ Nanostructuring solar cells using metallic nanoparticles ,” in Metal Nanostructures for Photonics , eds Kassab L. R. P., De Araujo C. B. (Amsterdam: Elsevier; ), 197–221. [ Google Scholar ]
- Cadene A., Durand-Vidal S., Turq P., Brendle J. (2005). Study of individual Na-montmorillonite particles size, morphology, and apparent charge. J. Colloid Interf. Sci. 285 719–730. 10.1016/j.jcis.2004.12.016 [ PubMed ] [ CrossRef ] [ Google Scholar ]
- Chen J., Zhu X. (2016). Magnetic solid phase extraction using ionic liquid-coated core–shell magnetic nanoparticles followed by high-performance liquid chromatography for determination of Rhodamine B in food samples. Food Chem. 200 10–15. 10.1016/j.foodchem.2016.01.002 [ PubMed ] [ CrossRef ] [ Google Scholar ]
- Chen J., Guo Y., Zhang X., Liu J., Gong P., Su Z., et al. (2023). Emerging nanoparticles in food: sources, application, and safety. J. Agricult. Food Chem. 71 3564–3582. [ PubMed ] [ Google Scholar ]
- Chen J., Wei S., Xie H. (2021). “ A brief introduction of carbon nanotubes: history, synthesis, and properties ,” in Proceedings of the Journal of Physics: Conference Series , (United Kingdom: IOP Publishing; ), 012184. 10.1088/1742-6596/1948/1/012184 [ CrossRef ] [ Google Scholar ]
- Chen J.-C., Tang C.-T. (2007). Preparation and application of granular ZnO/Al2O3 catalyst for the removal of hazardous trichloroethylene. J. Hazardous Mater. 142 88–96. 10.1016/j.jhazmat.2006.07.061 [ PubMed ] [ CrossRef ] [ Google Scholar ]
- Chronakis I. S. (2010). Micro-/nano-fibers by electrospinning technology: processing, properties and applications. Micromanufact. Eng. Technol. 2010 264–286. 10.1016/B978-0-8155-1545-6.00016-8 [ CrossRef ] [ Google Scholar ]
- Compostella F., Pitirollo O., Silvestri A., Polito L. (2017). Glyco-gold nanoparticles: synthesis and applications. Beilstein J. Org. Chem. 13 1008–1021. 10.3762/bjoc.13.100 [ PMC free article ] [ PubMed ] [ CrossRef ] [ Google Scholar ]
- Dahoumane S. A., Mechouet M., Wijesekera K., Filipe C. D., Sicard C., Bazylinski D. A., et al. (2017). Algae-mediated biosynthesis of inorganic nanomaterials as a promising route in nanobiotechnology–a review. Green Chem. 19 552–587. 10.1039/C6GC02346K [ CrossRef ] [ Google Scholar ]
- Dangi K., Verma A. K. (2021). Efficient & eco-friendly smart nano-pesticides: Emerging prospects for agriculture. Mater. Today Proc. 45 3819–3824. [ Google Scholar ]
- Das S., Srivasatava V. C. (2016). Synthesis and characterization of ZnO–MgO nanocomposite by co-precipitation method. Smart Sci. 4 190–195. [ Google Scholar ]
- De La Calle I., Menta M., Klein M., Séby F. (2018). Study of the presence of micro-and nanoparticles in drinks and foods by multiple analytical techniques. Food Chem. 266 133–145. 10.1016/j.foodchem.2018.05.107 [ PubMed ] [ CrossRef ] [ Google Scholar ]
- Delvallée A., Feltin N., Ducourtieux S., Trabelsi M., Hochepied J. (2015). Direct comparison of AFM and SEM measurements on the same set of nanoparticles. Measur. Sci. Technol. 26 : 085601 . [ Google Scholar ]
- Dhand V., Soumya L., Bharadwaj S., Chakra S., Bhatt D., Sreedhar B. (2016). Green synthesis of silver nanoparticles using Coffea arabica seed extract and its antibacterial activity. Mater. Sci. Eng. C 58 36–43. 10.1016/j.msec.2015.08.018 [ PubMed ] [ CrossRef ] [ Google Scholar ]
- Dikusar A., Globa P., Belevskii S., Sidel’nikova S. (2009). On limiting rate of dimensional electrodeposition at meso-and nanomaterial manufacturing by template synthesis. Surf. Eng. Appl. Electrochem. 45 171–179. [ Google Scholar ]
- Dragovic R. A., Gardiner C., Brooks A. S., Tannetta D. S., Ferguson D. J., Hole P., et al. (2011). Sizing and phenotyping of cellular vesicles using nanoparticle tracking analysis. Nanomedicine 7 780–788. [ PMC free article ] [ PubMed ] [ Google Scholar ]
- Dreaden E. C., Alkilany A. M., Huang X., Murphy C. J., El-Sayed M. A. (2012). The golden age: gold nanoparticles for biomedicine. Chem. Soc. Rev. 41 2740–2779. 10.1039/c1cs15237h [ PMC free article ] [ PubMed ] [ CrossRef ] [ Google Scholar ]
- Du P., Song L., Xiong J., Li N., Xi Z., Wang L., et al. (2012). Coaxial electrospun TiO2/ZnO core–sheath nanofibers film: Novel structure for photoanode of dye-sensitized solar cells. Electrochim. Acta 78 392–397. [ Google Scholar ]
- Edison T. N. J. I., Lee Y. R., Sethuraman M. G. (2016). Green synthesis of silver nanoparticles using Terminalia cuneata and its catalytic action in reduction of direct yellow-12 dye. Pectrochimica Acta Part A 161 122–129. 10.1016/j.saa.2016.02.044 [ PubMed ] [ CrossRef ] [ Google Scholar ]
- Elliott J. A., Shibuta Y., Amara H., Bichara C., Neyts E. C. (2013). Atomistic modelling of CVD synthesis of carbon nanotubes and graphene. Nanoscale 5 6662–6676. 10.1039/c3nr01925j [ PubMed ] [ CrossRef ] [ Google Scholar ]
- Erasmus M., Cason E. D., Van Marwijk J., Botes E., Gericke M., Van Heerden E. (2014). Gold nanoparticle synthesis using the thermophilic bacterium Thermus scotoductus SA-01 and the purification and characterization of its unusual gold reducing protein. Gold Bull. 47 245–253. [ Google Scholar ]
- Eroglu E., Chen X., Bradshaw M., Agarwal V., Zou J., Stewart S. G., et al. (2013). Biogenic production of palladium nanocrystals using microalgae and their immobilization on chitosan nanofibers for catalytic applications. RSC Adv. 3 1009–1012. [ Google Scholar ]
- Essajai R., Benhouria Y., Rachadi A., Qjani M., Mzerd A., Hassanain N. (2019). Shape-dependent structural and magnetic properties of Fe nanoparticles studied through simulation methods. RSC Adv. 9 22057–22063. 10.1039/c9ra03047f [ PMC free article ] [ PubMed ] [ CrossRef ] [ Google Scholar ]
- Falke S., Betzel C. (2019). “ Dynamic light scattering (DLS) ,” in Radiation in Bioanalysis , eds Pereira A. S., Tavares P., Limão-Vieira P. (Berlin: Springer; ), 173–193. [ Google Scholar ]
- Farrell D., Majetich S. A., Wilcoxon J. P. (2003). Preparation and characterization of monodisperse Fe nanoparticles. J. Phys. Chem. B 107 11022–11030. [ Google Scholar ]
- Feng L., Xuan Z., Ma J., Chen J., Cui D., Su C., et al. (2015). Preparation of gold nanorods with different aspect ratio and the optical response to solution refractive index. J. Exp. Nanosci. 10 258–267. [ Google Scholar ]
- Ghorbani H. R., Mehr F. P., Pazoki H., Rahmani B. M. (2015). Synthesis of ZnO nanoparticles by precipitation method. Orient. J. Chem. 31 1219–1221. [ Google Scholar ]
- Ghosh S., Ahmad R., Zeyaullah M., Khare S. K. (2021). Microbial nano-factories: synthesis and biomedical applications. Front. Chem. 9 : 194 . 10.3389/fchem.2021.626834 [ PMC free article ] [ PubMed ] [ CrossRef ] [ Google Scholar ]
- Giljohann D. A., Seferos D. S., Daniel W. L., Massich M. D., Patel P. C., Mirkin C. A. (2020). Gold nanoparticles for biology and medicine. Spherical Nucleic Acids 49 3280–3294. [ PMC free article ] [ PubMed ] [ Google Scholar ]
- Giurlani W., Innocenti M., Lavacchi A. (2018). X-ray microanalysis of precious metal thin films: thickness and composition determination. Coatings 8 : 84 . [ Google Scholar ]
- Gloria E. C., Ederley V., Gladis M., César H., Jaime O., Oscar A., et al. (2017). “ Synthesis of silver nanoparticles (AgNPs) with antibacterial activity ,” in Proceedings of the Journal of Physics: Conference Series , (United Kingdom: IOP Publishing; ), 012023. [ Google Scholar ]
- Gorrasi G., Sorrentino A. (2015). Mechanical milling as a technology to produce structural and functional bio-nanocomposites. Green Chem. 17 2610–2625. [ Google Scholar ]
- Govindarajan M., Rajeswary M., Veerakumar K., Muthukumaran U., Hoti S., Benelli G. (2016). Green synthesis and characterization of silver nanoparticles fabricated using Anisomeles indica: mosquitocidal potential against malaria, dengue and Japanese encephalitis vectors. Exp. Parasitol. 161 40–47. 10.1016/j.exppara.2015.12.011 [ PubMed ] [ CrossRef ] [ Google Scholar ]
- Graf C., Vossen D. L., Imhof A., Van Blaaderen A. (2003). A general method to coat colloidal particles with silica. Langmuir 19 6693–6700. [ PubMed ] [ Google Scholar ]
- Greczynski G., Hultman L. (2020). X-ray photoelectron spectroscopy: towards reliable binding energy referencing. Progr. Mater. Sci. 107 : 100591 . [ Google Scholar ]
- Guo D., Xie G., Luo J. (2013). Mechanical properties of nanoparticles: basics and applications. J. Phys. D 47 : 013001 . [ Google Scholar ]
- Guo W., Pleixats R., Shafir A. (2015). Water-soluble gold nanoparticles: from catalytic selective Nitroarene reduction in water to refractive index sensing. Chem. An Asian J. 10 2437–2443. 10.1002/asia.201500290 [ PubMed ] [ CrossRef ] [ Google Scholar ]
- Gwynne K. (2020). Enhancement of the Photostability of Blue Phosphorescence Using Plasmonic Surfaces. New Brunswick, NJ: Rutgers University-School of Graduate Studies. [ Google Scholar ]
- Haasch R. T. (2014). “ X-ray photoelectron spectroscopy (XPS) and auger electron spectroscopy (AES) ,” in Practical Materials Characterization , Ed. Sardela M. (Berlin: Springer; ), 93–132. [ Google Scholar ]
- Hasan S. (2015). A review on nanoparticles: their synthesis and types. Res. J. Recent Sci. 2277 : 2502 . [ Google Scholar ]
- Holder C. F., Schaak R. E. (2019). Tutorial on Powder X-ray Diffraction for Characterizing Nanoscale Materials. Washington, DC: ACS Publications. [ PubMed ] [ Google Scholar ]
- Hollamby M. J., Eastoe J., Chemelli A., Glatter O., Rogers S., Heenan R. K., et al. (2010). Separation and purification of nanoparticles in a single step. Langmuir 26 6989–6994. 10.1021/la904225k [ PubMed ] [ CrossRef ] [ Google Scholar ]
- Hoo C. M., Starostin N., West P., Mecartney M. L. (2008). A comparison of atomic force microscopy (AFM) and dynamic light scattering (DLS) methods to characterize nanoparticle size distributions. J. Nanopart. Res. 10 89–96. [ Google Scholar ]
- Hortin J., Anderson A., Britt D., Jacobson A., Mclean J. (2020). Copper oxide nanoparticle dissolution at alkaline pH is controlled by dissolved organic matter: influence of soil-derived organic matter, wheat, bacteria, and nanoparticle coating. Environ. Sci. 7 2618–2631. [ Google Scholar ]
- Hoseinzadeh E., Makhdoumi P., Taha P., Hossini H., Stelling J., Amjad Kamal M. (2017). A review on nano-antimicrobials: metal nanoparticles, methods and mechanisms. Curr. Drug Metab. 18 120–128. [ PubMed ] [ Google Scholar ]
- Hulkoti N. I., Taranath T. (2014). Biosynthesis of nanoparticles using microbes—a review. Colloids Surf. B Biointerf. 121 474–483. 10.1016/j.colsurfb.2014.05.027 [ PubMed ] [ CrossRef ] [ Google Scholar ]
- Islam F., Shohag S., Uddin M. J., Islam M. R., Nafady M. H., Akter A., et al. (2022). Exploring the journey of zinc oxide nanoparticles (ZnO-NPs) toward biomedical applications. Materials 15 : 2160 . 10.3390/ma15062160 [ PMC free article ] [ PubMed ] [ CrossRef ] [ Google Scholar ]
- Jadoun S., Arif R., Jangid N. K., Meena R. K. (2021). Green synthesis of nanoparticles using plant extracts: A review. Environ. Chem. Lett. 19 355–374. [ Google Scholar ]
- Jamkhande P. G., Ghule N. W., Bamer A. H., Kalaskar M. G. (2019). Metal nanoparticles synthesis: An overview on methods of preparation, advantages and disadvantages, and applications. J. Drug Deliv. Sci. Technol. 53 101174 . [ Google Scholar ]
- Jana N. R., Earhart C., Ying J. Y. (2007). Synthesis of water-soluble and functionalized nanoparticles by silica coating. Chem. Mater. 19 5074–5082. [ Google Scholar ]
- Jaskulski D., Jaskulska I., Majewska J., Radziemska M., Bilgin A., Brtnicky M. (2022). Silver Nanoparticles (AgNPs) in urea solution in laboratory tests and field experiments with crops and vegetables. Materials 15 : 870 . 10.3390/ma15030870 [ PMC free article ] [ PubMed ] [ CrossRef ] [ Google Scholar ]
- Jayaraman V., Ghosh S., Sengupta A., Srivastava S., Sonawat H., Narayan P. K. (2014). Identification of biochemical differences between different forms of male infertility by nuclear magnetic resonance (NMR) spectroscopy. J. Assist. Reproduct. Genet. 31 1195–1204. 10.1007/s10815-014-0282-4 [ PMC free article ] [ PubMed ] [ CrossRef ] [ Google Scholar ]
- Jena J., Pradhan N., Nayak R. R., Dash B. P., Sukla L. B., Panda P. K., et al. (2014). Microalga Scenedesmus sp.: a potential low-cost green machine for silver nanoparticle synthesis. J. Microbiol. Biotechnol. Adv. 24 522–533. 10.4014/jmb.1306.06014 [ PubMed ] [ CrossRef ] [ Google Scholar ]
- Jiang H.-L., Xu Q. (2011). Catalytic hydrolysis of ammonia borane for chemical hydrogen storage. Catal. Today 170 56–63. [ Google Scholar ]
- Joh D.-W., Jung T.-K., Lee H.-S., Kim D.-H. (2013). Synthesis of nanoparticles using electrical explosion of Ni wire in Pt solution. J. Nanosci. Nanotechnol. 13 6092–6094. 10.1166/jnn.2013.7677 [ PubMed ] [ CrossRef ] [ Google Scholar ]
- Kahle M., Kleber M., Jahn R. (2002). Review of XRD-based quantitative analyses of clay minerals in soils: the suitability of mineral intensity factors. Geoderma 109 191–205. [ Google Scholar ]
- Kalaiyarasu T., Karthi N., Sharmila G. V., Manju V. (2016). In vitro assessment of antioxidant and antibacterial activity of green synthesized silver nanoparticles from Digitaria radicosa leaves. Asian J. Pharm. Clin. Res. 9 297–302. [ Google Scholar ]
- Kayalvizhi T., Ravikumar S., Venkatachalam P. (2016). Green synthesis of metallic silver nanoparticles using Curculigo orchioides rhizome extracts and evaluation of its antibacterial, larvicidal, and anticancer activity. J. Environ. Eng. 142 : C4016002 . [ Google Scholar ]
- Khan A., Rashid R., Murtaza G., Zahra A. (2014). Gold nanoparticles: synthesis and applications in drug delivery. Trop. J. Pharm. Res. 13 1169–1177. [ Google Scholar ]
- Khan I., Saeed K., Khan I. (2019). Nanoparticles: Properties, applications and toxicities. Arab. J. Chem. 12 908–931. [ Google Scholar ]
- Khanna P., Kaur A., Goyal D. (2019). Algae-based metallic nanoparticles: Synthesis, characterization and applications. J. Microbiol Methods 163 : 105656 . 10.1016/j.mimet.2019.105656 [ PubMed ] [ CrossRef ] [ Google Scholar ]
- Kharat S. N., Mendhulkar V. D. (2016). Synthesis, characterization and studies on antioxidant activity of silver nanoparticles using Elephantopus scaber leaf extract. Mater. Sci. Eng. C 62 719–724. 10.1016/j.msec.2016.02.024 [ PubMed ] [ CrossRef ] [ Google Scholar ]
- Kim J.-H., Sheikh F. A., Ju H. W., Park H. J., Moon B. M., Lee O. J., et al. (2014). 3D silk fibroin scaffold incorporating titanium dioxide (TiO2) nanoparticle (NPs) for tissue engineering. Int. J. Biol. Macromol. 68 158–168. 10.1016/j.ijbiomac.2014.04.045 [ PubMed ] [ CrossRef ] [ Google Scholar ]
- Kohl H., Reimer L. (2008). Transmission Electron Microscopy. Berlin: Springer Series in Optical Sciences, 36. [ Google Scholar ]
- Kokarneswaran M., Selvaraj P., Ashokan T., Perumal S., Sellappan P., Murugan K. D., et al. (2020). Discovery of carbon nanotubes in sixth century BC potteries from Keeladi, India. Sci. Rep. 10 1–6. 10.1038/s41598-020-76720-z [ PMC free article ] [ PubMed ] [ CrossRef ] [ Google Scholar ]
- Kopittke P. M., Lombi E., Wang P., Schjoerring J. K., Husted S. (2019). Nanomaterials as fertilizers for improving plant mineral nutrition and environmental outcomes. Environ. Sci. 6 3513–3524. 10.3390/biology10111123 [ PMC free article ] [ PubMed ] [ CrossRef ] [ Google Scholar ]
- Kora A. J., Rastogi L. (2018). Peroxidase activity of biogenic platinum nanoparticles: A colorimetric probe towards selective detection of mercuric ions in water samples. Sens. Actuators B Chem. 254 690–700. [ Google Scholar ]
- Kreizer M., Ratner D., Liberzon A. (2010). Real-time image processing for particle tracking velocimetry. Exp. Fluids 48 105–110. [ Google Scholar ]
- Krithiga N., Jayachitra A., Rajalakshmi A. (2013). Synthesis, characterization and analysis of the effect of copper oxide nanoparticles in biological systems. Ind. J. Ns 1 6–15. [ Google Scholar ]
- Kumar R., Singh R. K., Dubey P. K., Kumar P., Tiwari R. S., Oh I.-K. (2013). Pressure-dependent synthesis of high-quality few-layer graphene by plasma-enhanced arc discharge and their thermal stability. J. Nanopart. Res. 15 1–10. [ Google Scholar ]
- Kumar S. S., Venkateswarlu P., Rao V. R., Rao G. N. (2013). Synthesis, characterization and optical properties of zinc oxide nanoparticles. Int. Nano Lett. 3 1–6. [ Google Scholar ]
- Kumar V., Yadav S. K. (2009). Plant-mediated synthesis of silver and gold nanoparticles and their applications. J. Chem. Technol. Biotechnol. 84 151–157. [ Google Scholar ]
- Kumar V., Bano D., Mohan S., Singh D. K., Hasan S. H. (2016). Sunlight-induced green synthesis of silver nanoparticles using aqueous leaf extract of Polyalthia longifolia and its antioxidant activity. Mater. Lett. 181 371–377. [ Google Scholar ]
- Kumari S. C., Dhand V., Padma P. N. (2021). Green synthesis of metallic nanoparticles: a review. Nanomaterials 2021 259–281. [ Google Scholar ]
- Lam E., Luong J. H. (2014). Carbon materials as catalyst supports and catalysts in the transformation of biomass to fuels and chemicals. ACS Catal. 4 3393–3410. [ Google Scholar ]
- Lara P., Philippot K. (2014). The hydrogenation of nitroarenes mediated by platinum nanoparticles: an overview. Catal. Sci. Technol. 4 2445–2465. [ Google Scholar ]
- Lerner M. I., Glazkova E. A., Lozhkomoev A. S., Svarovskaya N. V., Bakina O. V., Pervikov A. V., et al. (2016). Synthesis of Al nanoparticles and Al/AlN composite nanoparticles by electrical explosion of aluminum wires in argon and nitrogen. Powder Technol. 295 307–314. [ Google Scholar ]
- Lewczuk B., Szyryńska N. (2021). Field-emission scanning electron microscope as a tool for large-area and large-volume ultrastructural studies. Animals 11 : 3390 . 10.3390/ani11123390 [ PMC free article ] [ PubMed ] [ CrossRef ] [ Google Scholar ]
- Li G., He D., Qian Y., Guan B., Gao S., Cui Y., et al. (2011). Fungus-mediated green synthesis of silver nanoparticles using Aspergillus terreus. Int. J. Mol. Sci. 13 466–476. 10.3390/ijms13010466 [ PMC free article ] [ PubMed ] [ CrossRef ] [ Google Scholar ]
- Li N., Zhao P., Astruc D. (2014). Anisotropic gold nanoparticles: synthesis, properties, applications, and toxicity. Angewand. Chem. Int. Edn. 53 1756–1789. [ PubMed ] [ Google Scholar ]
- Li T., Senesi A. J., Lee B. (2016). Small angle X-ray scattering for nanoparticle research. Chem. Rev. 116 11128–11180. 10.1021/acs.chemrev.5b00690 [ PubMed ] [ CrossRef ] [ Google Scholar ]
- Li X., Xu H., Chen Z.-S., Chen G. (2011). Biosynthesis of nanoparticles by microorganisms and their applications. J. Nanomater. 2011 : 270974 . [ Google Scholar ]
- Luangpipat T., Beattie I. R., Chisti Y., Haverkamp R. G. (2011). Gold nanoparticles produced in a microalga. J. Nanopart. Res. 13 6439–6445. [ Google Scholar ]
- Lyon L. A., Keating C. D., Fox A. P., Baker B. E., He L., Nicewarner S. R., et al. (1998). Raman spectroscopy. Anal. Chem. 70 341–362. [ PubMed ] [ Google Scholar ]
- Lyu H., Gao B., He F., Ding C., Tang J., Crittenden J. C. (2017). Ball-milled carbon nanomaterials for energy and environmental applications. ACS Sust. Chem. Eng. 5 9568–9585. 10.1016/j.biortech.2020.123613 [ PubMed ] [ CrossRef ] [ Google Scholar ]
- Machac P., Cichon S., Lapcak L., Fekete L. (2020). Graphene prepared by chemical vapour deposition process. Graph. Technol. 5 9–17. [ Google Scholar ]
- Madathil A. N. P., Vanaja K., Jayaraj M. (2007). “ Synthesis of ZnO nanoparticles by hydrothermal method ,” in Nanophotonic materials IV , eds Gaburro Z., Cabrini S. (Bellingham, WA: SPIE; ), 47–55. [ Google Scholar ]
- Maharani V., Sundaramanickam A., Balasubramanian T. J. E. (2016). In vitro anticancer activity of silver nanoparticle synthesized by Escherichia coli VM1 isolated from marine sediments of Ennore southeast coast of India. Enzyme Microb. Technol. 95 146–154. 10.1016/j.enzmictec.2016.09.008 [ PubMed ] [ CrossRef ] [ Google Scholar ]
- Majeed Khan M. A., Kumar S., Ahamed M., Alrokayan S. A., Alsalhi M. S. (2011). Structural and thermal studies of silver nanoparticles and electrical transport study of their thin films. Nanosc. Res. Lett. 6 1–8. [ PMC free article ] [ PubMed ] [ Google Scholar ]
- Malhotra S. P. K., Alghuthaymi M. A. (2022). Biomolecule-assisted biogenic synthesis of metallic nanoparticles. Agri-Waste Microb. Product. Sust. Nanomater. 2022 139–163. [ Google Scholar ]
- Mathew L., Chandrasekaran N., Mukherjee A. (2010). “ Biomimetic synthesis of nanoparticles: science, technology & applicability ,” Biomimetics learning from nature , Ed. Mukherjee A. (Norderstedt: Books on Demand; ). [ Google Scholar ]
- Mishra A., Tripathy S. K., Wahab R., Jeong S.-H., Hwang I., Yang Y.-B., et al. (2011). Microbial synthesis of gold nanoparticles using the fungus Penicillium brevicompactum and their cytotoxic effects against mouse mayo blast cancer C 2 C 12 cells. Appl. Microbiol. Biotechnol. Adv. 92 617–630. 10.1007/s00253-011-3556-0 [ PubMed ] [ CrossRef ] [ Google Scholar ]
- Mittal A., Chisti Y. (2013). Synthesis of metallic nanoparticles using plant extracts. Biotechnol. Adv. 31 346–356. [ PubMed ] [ Google Scholar ]
- Moghaddam A. B., Nazari T., Badraghi J., Kazemzad M. (2009). Synthesis of ZnO nanoparticles and electrodeposition of polypyrrole/ZnO nanocomposite film. Int. J. Electrochem. Sci. 4 247–257. [ Google Scholar ]
- Mohanpuria P., Rana N. K., Yadav S. K. (2008). Biosynthesis of nanoparticles: technological concepts and future applications. J. Nanopart. Res. 10 507–517. [ Google Scholar ]
- Mohd Yusof H., Mohamad R., Zaidan U. H., Rahman A. (2019). Microbial synthesis of zinc oxide nanoparticles and their potential application as an antimicrobial agent and a feed supplement in animal industry: a review. J. Anim. Sci. Biotechnol. 10 1–22. 10.1186/s40104-019-0368-z [ PMC free article ] [ PubMed ] [ CrossRef ] [ Google Scholar ]
- Morsi M., Abdelrazek E., Ramadan R., Elashmawi I., Rajeh A. (2022). Structural, optical, mechanical, and dielectric properties studies of carboxymethyl cellulose/polyacrylamide/lithium titanate nanocomposites films as an application in energy storage devices. Polymer Test. 114 107705 . [ Google Scholar ]
- Mott D., Galkowski J., Wang L., Luo J., Zhong C.-J. (2007). Synthesis of size-controlled and shaped copper nanoparticles. Langmuir 23 5740–5745. [ PubMed ] [ Google Scholar ]
- Mukherjee P., Ahmad A., Mandal D., Senapati S., Sainkar S. R., Khan M. I., et al. (2001). Fungus-mediated synthesis of silver nanoparticles and their immobilization in the mycelial matrix: a novel biological approach to nanoparticle synthesis. Nano Lett. 1 515–519. [ Google Scholar ]
- Muñoz-García J., Vázquez L., Cuerno R., Sánchez-García J. A., Castro M., Gago R. (2009). “ Self-organized surface nanopatterning by ion beam sputtering ,” in Toward Functional Nanomaterials , Ed. Wang Z. M. (Berlin: Springer; ), 323–398. [ Google Scholar ]
- Naraginti S., Kumari P. L., Das R. K., Sivakumar A., Patil S. H., Andhalkar V. V. (2016). Amelioration of excision wounds by topical application of green synthesized, formulated silver and gold nanoparticles in albino Wistar rats. Mater. Sci. Eng. C 62 293–300. 10.1016/j.msec.2016.01.069 [ PubMed ] [ CrossRef ] [ Google Scholar ]
- Narayanan K. B., Sakthivel N. (2010). Biological synthesis of metal nanoparticles by microbes. Adv. Colloid Interf. Sci. 156 1–13. [ PubMed ] [ Google Scholar ]
- Nayak D., Ashe S., Rauta P. R., Kumari M., Nayak B. (2016). Bark extract mediated green synthesis of silver nanoparticles: evaluation of antimicrobial activity and antiproliferative response against osteosarcoma. Mater. Sci. Eng. C 58 44–52. 10.1016/j.msec.2015.08.022 [ PubMed ] [ CrossRef ] [ Google Scholar ]
- Ndolomingo M. J., Meijboom R. (2016). Determination of the surface area and sizes of supported copper nanoparticles through organothiol adsorption—Chemisorption. Appl. Surf. Sci. 390 224–235. [ Google Scholar ]
- Newbury D. E., Ritchie N. W. (2013). Is scanning electron microscopy/energy dispersive X-ray spectrometry (SEM/EDS) quantitative? Scanning 35 141–168. 10.1002/sca.21041 [ PubMed ] [ CrossRef ] [ Google Scholar ]
- Nguyen K. T., Menon J. U., Jadeja P. V., Tambe P. P., Vu K., Yuan B. (2013). Nanomaterials for photo-based diagnostic and therapeutic applications. Theranostics 3 152–166. [ PMC free article ] [ PubMed ] [ Google Scholar ]
- Nowack B., Krug H. F., Height M. (2011). 120 Years of Nanosilver History: Implications for Policy Makers. Washington, DC: ACS Publications. [ PubMed ] [ Google Scholar ]
- Önal E. S., Yatkın T., Aslanov T., Ergüt M., Özer A. (2019). Biosynthesis and characterization of iron nanoparticles for effective adsorption of Cr (VI). Int. J. Chem. Eng. 2019 : 2716423 . [ Google Scholar ]
- Ostermann R., Cravillon J., Weidmann C., Wiebcke M., Smarsly B. M. (2011). Metal–organic framework nanofibers via electrospinning. Chem. Commun. 47 442–444. [ PubMed ] [ Google Scholar ]
- Parashar M., Shukla V. K., Singh R. (2020). Metal oxides nanoparticles via sol–gel method: a review on synthesis, characterization and applications. J. Mater. Sci. 31 3729–3749. [ Google Scholar ]
- Park C. Y., Choi B. (2019). Enhanced light extraction from bottom emission oleds by high refractive index nanoparticle scattering layer. Nanomaterials 9 : 1241 . 10.3390/nano9091241 [ PMC free article ] [ PubMed ] [ CrossRef ] [ Google Scholar ]
- Patil M. P., Kim G.-D. (2018). Marine microorganisms for synthesis of metallic nanoparticles and their biomedical applications. Colloids Surf. B Biointerf. 172 487–495. [ PubMed ] [ Google Scholar ]
- Patil M. P., Ngabire D., Thi H. H. P., Kim M.-D., Kim G.-D. (2017). Eco-friendly synthesis of gold nanoparticles and evaluation of their cytotoxic activity on cancer cells. J. Clust. Sci. 28 119–132. [ Google Scholar ]
- Patil N., Bhaskar R., Vyavhare V., Dhadge R., Khaire V., Patil Y. (2021). Overview on methods of synthesis of nanoparticles. Int. J. Curr. Pharm. Res. 13 11–16. [ Google Scholar ]
- Patois E., Capelle M., Palais C., Gurny R., Arvinte T. (2012). Evaluation of nanoparticle tracking analysis (NTA) in the characterization of therapeutic antibodies and seasonal influenza vaccines: pros and cons. J. Drug Deliv. Sci. Technol. 22 427–433. [ Google Scholar ]
- Paul B., Bhuyan B., Purkayastha D. D., Dhar S. S. (2016). Photocatalytic and antibacterial activities of gold and silver nanoparticles synthesized using biomass of Parkia roxburghii leaf. J. Photochem. Photobiol. B Biol. 154 1–7. 10.1016/j.jphotobiol.2015.11.004 [ PubMed ] [ CrossRef ] [ Google Scholar ]
- Pérez-Lorenzo M. (2012). Palladium nanoparticles as efficient catalysts for Suzuki cross-coupling reactions. J. Phys. Chem. Lett. 3 167–174. [ Google Scholar ]
- Pérez-Tijerina E., Pinilla M. G., Mejía-Rosales S., Ortiz-Méndez U., Torres A., José-Yacamán M. (2008). Highly size-controlled synthesis of Au/Pd nanoparticles by inert-gas condensation. Faraday Discuss. 138 353–362. 10.1039/b705913m [ PubMed ] [ CrossRef ] [ Google Scholar ]
- Phull A.-R., Abbas Q., Ali A., Raza H., Zia M., Haq I.-U. (2016). Antioxidant, cytotoxic and antimicrobial activities of green synthesized silver nanoparticles from crude extract of Bergenia ciliata. Fut. J. Pharm. Sci. 2 31–36. [ Google Scholar ]
- Pimpin A., Srituravanich W. (2012). Review on micro-and nanolithography techniques and their applications. Eng. J. 16 37–56. [ Google Scholar ]
- Pradeep T. (2009). Noble metal nanoparticles for water purification: a critical review. Thin Solid Films 517 6441–6478. [ Google Scholar ]
- Praseptiangga D., Zahara H. L., Widjanarko P. I., Joni I. M., Panatarani C. (2020). Preparation and FTIR spectroscopic studies of SiO2-ZnO nanoparticles suspension for the development of carrageenan-based bio-nanocomposite film. 100005 . [ Google Scholar ]
- Pugazhendhi S., Sathya P., Palanisamy P., Gopalakrishnan R. (2016). Synthesis of silver nanoparticles through green approach using Dioscorea alata and their characterization on antibacterial activities and optical limiting behavior. J. Photochem. Photobiol. B Biol. 159 155–160. 10.1016/j.jphotobiol.2016.03.043 [ PubMed ] [ CrossRef ] [ Google Scholar ]
- Qi P., Zhang D., Wan Y. (2013). Sulfate-reducing bacteria detection based on the photocatalytic property of microbial synthesized ZnS nanoparticles. Anal. Chim. Acta 800 65–70. 10.1016/j.aca.2013.09.015 [ PubMed ] [ CrossRef ] [ Google Scholar ]
- Rad A. G., Abbasi H., Afzali M. H. (2011). Gold nanoparticles: synthesising, characterizing and reviewing novel application in recent years. Phys. Proc. 22 203–208. [ Google Scholar ]
- Rahmati-Abkenar M., Manteghian M. (2020). Effect of silver nanoparticles on the solubility of methane and ethane in water. J. Nat. Gas Sci. Eng. 82 : 103505 . [ Google Scholar ]
- Rajeshkumar S., Ponnanikajamideen M., Malarkodi C., Malini M., Annadurai G. (2014). Microbe-mediated synthesis of antimicrobial semiconductor nanoparticles by marine bacteria. J. Nanostruct. Chem. 4 1–7. [ Google Scholar ]
- Rajkuberan C., Prabukumar S., Sathishkumar G., Wilson A., Ravindran K., Sivaramakrishnan S. (2017). Facile synthesis of silver nanoparticles using Euphorbia antiquorum L. latex extract and evaluation of their biomedical perspectives as anticancer agents. J. Saudi Chem. Soc. 21 911–919. [ Google Scholar ]
- Ramesh P., Kokila T., Geetha D. (2015). Plant mediated green synthesis and antibacterial activity of silver nanoparticles using Emblica officinalis fruit extract. Spectrochim. Acta Part A 142 339–343. 10.1016/j.saa.2015.01.062 [ PubMed ] [ CrossRef ] [ Google Scholar ]
- Rangel-Olivares F. R., Arce-Estrada E. M., Cabrera-Sierra R. (2021). Synthesis and characterization of polyaniline-based polymer nanocomposites as anti-corrosion coatings. Coatings 11 : 653 . [ Google Scholar ]
- Rao N. H., Lakshmidevi N., Pammi S., Kollu P., Ganapaty S., Lakshmi P. (2016). Green synthesis of silver nanoparticles using methanolic root extracts of Diospyros paniculata and their antimicrobial activities. Mater. Sci. Eng. C 62 553–557. 10.1016/j.msec.2016.01.072 [ PubMed ] [ CrossRef ] [ Google Scholar ]
- Rassaei L., Marken F., Sillanpää M., Amiri M., Cirtiu C. M., Sillanpää M. (2011). Nanoparticles in electrochemical sensors for environmental monitoring. TrAC Trends Anal. Chem. 30 1704–1715. [ Google Scholar ]
- Rocha F. S., Gomes A. J., Lunardi C. N., Kaliaguine S., Patience G. S. (2018). Experimental methods in chemical engineering: Ultraviolet visible spectroscopy—UV-Vis. Can. J. Chem. Eng. 96 2512–2517. [ Google Scholar ]
- Saini P., Saha S. K., Roy P., Chowdhury P., Babu S. P. S. (2016). Evidence of reactive oxygen species (ROS) mediated apoptosis in Setaria cervi induced by green silver nanoparticles from Acacia auriculiformis at a very low dose. Exp. Parasitol. 160 39–48. 10.1016/j.exppara.2015.11.004 [ PubMed ] [ CrossRef ] [ Google Scholar ]
- Saldarriaga J. F., Aguado R., Pablos A., Amutio M., Olazar M., Bilbao J. (2015). Fast characterization of biomass fuels by thermogravimetric analysis (TGA). Fuel 140 744–751. [ Google Scholar ]
- Salem S. S., Fouda A. (2021). Green synthesis of metallic nanoparticles and their prospective biotechnological applications: an overview. Biol. Trace Element Res. 199 344–370. 10.1007/s12011-020-02138-3 [ PubMed ] [ CrossRef ] [ Google Scholar ]
- Salopek B., Krasic D., Filipovic S. (1992). Measurement and application of zeta-potential. Rudarsko-Geolosko-Naftni Zbornik 4 : 147 . [ Google Scholar ]
- Saw M. J., Ghosh B., Nguyen M. T., Jirasattayaporn K., Kheawhom S., Shirahata N., et al. (2019). High aspect ratio and post-processing free silver nanowires as top electrodes for inverted-structured photodiodes. ACS Omega 4 13303–13308. 10.1021/acsomega.9b01479 [ PMC free article ] [ PubMed ] [ CrossRef ] [ Google Scholar ]
- Schröfel A., Kratošová G., Šafa r ̄ ík I., Šafa r ̄ íková M., Raška I., Shor L. M. (2014). Applications of biosynthesized metallic nanoparticles–a review. Acta Biomater. 10 4023–4042. [ PubMed ] [ Google Scholar ]
- Shenashen M. A., El-Safty S. A., Elshehy E. A. (2014). Synthesis, morphological control, and properties of silver nanoparticles in potential applications. Part. Part. Syst. Char. 31 293–316. [ Google Scholar ]
- Shi Z., Lian Y., Liao F. H., Zhou X., Gu Z., Zhang Y., et al. (2000). Large scale synthesis of single-wall carbon nanotubes by arc-discharge method. J. Phys. Chem. Solids 61 1031–1036. 10.1166/jnn.2001.012 [ PubMed ] [ CrossRef ] [ Google Scholar ]
- Siddiqi K. S., Husen A. (2016). Green synthesis, characterization and uses of palladium/platinum nanoparticles. Nanosc. Res. Lett. 11 1–13. 10.1186/s11671-016-1695-z [ PMC free article ] [ PubMed ] [ CrossRef ] [ Google Scholar ]
- Siddique S., Chow J. C. (2020). Gold nanoparticles for drug delivery and cancer therapy. Appl. Sci. 10 : 3824 . [ Google Scholar ]
- Sigmund W., Yuh J., Park H., Maneeratana V., Pyrgiotakis G., Daga A. (2006). Processing and structure relationships in electrospinning of ceramic fiber systems. J. Am. Ceramic Soc. 89 395–407. [ Google Scholar ]
- Singh R. P., Shukla V. K., Yadav R. S., Sharma P. K., Singh P. K., Pandey A. C. (2011). Biological approach of zinc oxide nanoparticles formation and its characterization. Adv. Mater. Lett. 2 313–317. [ Google Scholar ]
- Siwach O. P., Sen P. (2008). Synthesis and study of fluorescence properties of Cu nanoparticles. J. Nanopart. Res. 10 107–114. [ Google Scholar ]
- Slavin Y. N., Asnis J., Häfeli U. O., Bach H. (2017). Metal nanoparticles: understanding the mechanisms behind antibacterial activity. J. Nanobiotechnol. 15 1–20. [ PMC free article ] [ PubMed ] [ Google Scholar ]
- Song U., Kim J. (2020). Zinc oxide nanoparticles: a potential micronutrient fertilizer for horticultural crops with little toxicity. Horticult. Environ. Biotechnol. 61 625–631. [ Google Scholar ]
- Soni N., Sonam P. (2014). Green nanoparticles for mosquito control. Sci. World J. 2014 1–6. [ PMC free article ] [ PubMed ] [ Google Scholar ]
- Sowani H., Mohite P., Munot H., Shouche Y., Bapat T., Kumar A. R., et al. (2016). Green synthesis of gold and silver nanoparticles by an actinomycete Gordonia amicalis HS-11: mechanistic aspects and biological application. Process Biochem. 51 374–383. [ Google Scholar ]
- Sriram M. I., Kalishwaralal K., Barathmanikanth S., Gurunathani S. (2012). Size-based cytotoxicity of silver nanoparticles in bovine retinal endothelial cells. Nanosci. Methods 1 56–77. [ Google Scholar ]
- Stepanov A. L., Nuzhdin V. I., Valeev V. F., Kreibig U. (2011). “ Optical properties of metal nanoparticles ,” in Proceedings of the ICONO 2010: International Conference on Coherent and Nonlinear Optics , (Bellingham, WA: SPIE; ), 543–552. [ Google Scholar ]
- Su S. S., Chang I. (2018). “ Review of production routes of nanomaterials ,” in Commercialization of nanotechnologies–a case study approach , eds Brabazon D., Pellicer E., Zivic F., Sort J., Baró M. D., Grujovic N., Choy K.-L. (Berlin: Springer; ), 15–29. [ Google Scholar ]
- Sugihartono I., Dianisya D., Isnaeni I. (2018). “ Crystal structure analyses of ZnO nanoparticles growth by simple wet chemical method ,” in Proceedings of the IOP Conference Series: Materials Science and Engineering , (Bristol: IOP Publishing; ), 012077. [ Google Scholar ]
- Sun T., Zhang Y. S., Pang B., Hyun D. C., Yang M., Xia Y. J. A. C. I. E. (2014). Engineered nanoparticles for drug delivery in cancer therapy. Angew. Chem. Int. Ed. Engl. 53 12320–12364. [ PubMed ] [ Google Scholar ]
- Tavakoli A. H., Maram P. S., Widgeon S. J., Rufner J., Van Benthem K., Ushakov S., et al. (2013). Amorphous alumina nanoparticles: structure, surface energy, and thermodynamic phase stability. J. Phys. Chem. C 117 17123–17130. 10.1021/jp405820g [ CrossRef ] [ Google Scholar ]
- Theron J., Eugene Cloete T., De Kwaadsteniet M. (2010). Current molecular and emerging nanobiotechnology approaches for the detection of microbial pathogens. Crit. Rev. Microbiol. 36 318–339. 10.3109/1040841X.2010.489892 [ PubMed ] [ CrossRef ] [ Google Scholar ]
- Thomas R., Janardhanan A., Varghese R. T., Soniya E., Mathew J., Radhakrishnan E. (2014). Antibacterial properties of silver nanoparticles synthesized by marine Ochrobactrum sp. Braz. J. Microbiol. 45 1221–1227. 10.1590/s1517-83822014000400012 [ PMC free article ] [ PubMed ] [ CrossRef ] [ Google Scholar ]
- Titus D., Samuel E. J. J., Roopan S. M. (2019). “ Nanoparticle characterization techniques ,” in Green synthesis, characterization and applications of nanoparticles , eds Shukla A., Iravani S. (Amsterdam: Elsevier; ), 303–319. 10.1016/B978-0-08-102579-6.00012-5 [ CrossRef ] [ Google Scholar ]
- Tran V., Wen X. (2014). “ Rapid prototyping technologies for tissue regeneration ,” in Rapid prototyping of biomaterials , Ed. Narayan R. (Sawston: Woodhead Publishing; ), 97–155. 10.1533/9780857097217.97 [ CrossRef ] [ Google Scholar ]
- Triana M. A., Hsiang E.-L., Zhang C., Dong Y., Wu S.-T. (2022). Luminescent nanomaterials for energy-efficient display and healthcare. ACS Energy Lett. 7 1001–1020. [ Google Scholar ]
- Uzair B., Liaqat A., Iqbal H., Menaa B., Razzaq A., Thiripuranathar G., et al. (2020). Green and cost-effective synthesis of metallic nanoparticles by algae: Safe methods for translational medicine. Bioengineering 7 : 129 . 10.3390/bioengineering7040129 [ PMC free article ] [ PubMed ] [ CrossRef ] [ Google Scholar ]
- Van Thai P., Abe S., Kosugi K., Saito N., Takahashi K., Sasaki T., et al. (2019). Size/shape control of gold nanoparticles synthesized by alternating current glow discharge over liquid: The role of pH. Mater. Res. Expr. 6 : 095074 . [ Google Scholar ]
- Velusamy P., Das J., Pachaiappan R., Vaseeharan B., Pandian K. (2015). Greener approach for synthesis of antibacterial silver nanoparticles using aqueous solution of neem gum ( Azadirachta indica L.). Indus. Crops Products 66 103–109. [ Google Scholar ]
- Wang P., Menzies N. W., Lombi E., Sekine R., Blamey F. P. C., Hernandez-Soriano M. C., et al. (2015). Silver sulfide nanoparticles (Ag2S-NPs) are taken up by plants and are phytotoxic. Nanotoxicology 9 1041–1049. 10.3109/17435390.2014.999139 [ PubMed ] [ CrossRef ] [ Google Scholar ]
- Wang Z., Li H., Tang F., Ma J., Zhou X. (2018). A facile approach for the preparation of nano-size zinc oxide in water/glycerol with extremely concentrated zinc sources. Nanosc. Res. Lett. 13 1–9. 10.1186/s11671-018-2616-0 [ PMC free article ] [ PubMed ] [ CrossRef ] [ Google Scholar ]
- Wiesendanger R., Güntherodt H.-J. (2013). Scanning tunneling microscopy III: theory of STM and related scanning probe methods. Berlin: Springer Science & Business Media. [ Google Scholar ]
- Xia Y., Xiao Z., Dou X., Huang H., Lu X., Yan R., et al. (2013). Green and facile fabrication of hollow porous MnO/C microspheres from microalgaes for lithium-ion batteries. ACS Nano 7 7083–7092. 10.1021/nn4023894 [ PubMed ] [ CrossRef ] [ Google Scholar ]
- Yadav R., Dwivedi S., Kumar S., Chaudhury A. (2010). Trends and perspectives of biosensors for food and environmental virology. Food Environ. Virol. 2 53–63. [ Google Scholar ]
- Yadav T. P., Yadav R. M., Singh D. P. (2012). Mechanical milling: a top down approach for the synthesis of nanomaterials and nanocomposites. Nanosci. Nanotechnol. 2 22–48. [ Google Scholar ]
- Yang W., Wang L., Mettenbrink E. M., Deangelis P. L., Wilhelm S. (2021). Nanoparticle toxicology. Annu. Rev. Pharmacol. Toxicol. 61 269–289. [ PubMed ] [ Google Scholar ]
- Ye Q., Chen W., Huang H., Tang Y., Wang W., Meng F., et al. (2020). Iron and zinc ions, potent weapons against multidrug-resistant bacteria. Appl. Microbiol. Biotechnol. 104 5213–5227. 10.1007/s00253-020-10600-4 [ PubMed ] [ CrossRef ] [ Google Scholar ]
- Yuan P., Ding X., Yang Y. Y., Xu Q. H. (2018). Metal nanoparticles for diagnosis and therapy of bacterial infection. Adv. Healthc. Mater. 7 : 1701392 . [ PubMed ] [ Google Scholar ]
- Zahra Z., Habib Z., Chung S., Badshah M. A. (2020). Exposure route of TiO2 NPs from industrial applications to wastewater treatment and their impacts on the agro-environment. Nanomaterials 10 : 1469 . 10.3390/nano10081469 [ PMC free article ] [ PubMed ] [ CrossRef ] [ Google Scholar ]
- Zan G., Wu Q. (2016). Biomimetic and bioinspired synthesis of nanomaterials/nanostructures. Adv. Mater. 28 2099–2147. [ PubMed ] [ Google Scholar ]
- Zhang X., Yan S., Tyagi R., Surampalli R. (2011). Synthesis of nanoparticles by microorganisms and their application in enhancing microbiological reaction rates. Chemosphere 82 489–494. [ PubMed ] [ Google Scholar ]
- Zheng Z., Zhang X., Carbo D., Clark C., Nathan C.-A., Lvov Y. (2010). Sonication-assisted synthesis of polyelectrolyte-coated curcumin nanoparticles. Langmuir 26 7679–7681. 10.1021/la101246a [ PMC free article ] [ PubMed ] [ CrossRef ] [ Google Scholar ]
- Zhou C., Wang Y., Du L., Yao H., Wang J., Luo G. (2016). Precipitation preparation of high surface area and porous nanosized ZnO by continuous gas-based impinging streams in unconfined space. Indus. Eng. Chem. Res. 55 11943–11949. [ Google Scholar ]
- Zhou M., Wei Z., Qiao H., Zhu L., Yang H., Xia T. (2009). Particle size and pore structure characterization of silver nanoparticles prepared by confined arc plasma. J. Nanomater. 2009 : 968058 . [ Google Scholar ]
- Zhuang J., Gentry R. W. (2011). “ Environmental application and risks of nanotechnology: a balanced view ,” in Biotechnology and Nanotechnology Risk Assessment: Minding and Managing the Potential Threats around Us , eds Ripp S., Henry T. (Washington, DC: ACS Publications; ), 41–67. 10.3390/ijerph16234848 [ CrossRef ] [ Google Scholar ]
- Zielińska A., Carreiró F., Oliveira A. M., Neves A., Pires B., Venkatesh D. N., et al. (2020). Polymeric nanoparticles: production, characterization, toxicology and ecotoxicology. Molecules 25 : 3731 . 10.3390/molecules25163731 [ PMC free article ] [ PubMed ] [ CrossRef ] [ Google Scholar ]
Repurposing simvastatin in cancer treatment: an updated review on pharmacological and nanotechnological aspects
- Published: 15 May 2024
Cite this article
- Nargis Ara 1 ,
- Abdul Hafeez 1 &
- Shom Prakash Kushwaha 2
57 Accesses
Explore all metrics
Management of cancer is challenging due to non-targeting and high side effect issues. Drug repurposing is an innovative method for employing medications for other disease therapy in addition to their original use. Simvastatin, a 3-hydroxy-3-methylglutaryl coenzyme-A reductase inhibitor, is a lipid-lowering drug that is being studied for the treatment of cancer in various in vitro and in vivo models. Nanotechnology offers a potential platform for incorporation of drugs with enhanced pharmaceutical (solubility, release characteristics, stability, etc.) and biological characteristics (targeting, pharmacokinetic, pharmacodynamic). Utilizing a variety of resources such as Scopus, Springer, Web of Science, Elsevier, Bentham Science, Taylor & Francis, and PubMed, a thorough literature search was carried out by looking through electronic records published between 2003 and 2024. The keywords used were simvastatin, drug repurposing, anti-cancer simvastatin, pharmaceutical properties of simvastatin, simvastatin nanoformulations, simvastatin patents, clinical trials, etc. Numerous articles were looked for, filtered, checked out, and incorporated. Pure simvastatin has been researched as a repurposed medication for the treatment of cancer in several in vitro and in vivo models, such as carcinoma of the lung, colon, liver, prostate, breast, and skin. Simvastatin also incorporated into different nanocarriers (nanosuspensions, microparticles/nanoparticles, liposomes, and nanostructured lipid carriers) and showed improvement in solubility, bioavailability, drug loading, release kinetics, and targeting. Clinical trial and patent reports suggest potential of simvastatin in cancer therapy. The preclinical studies of pure simvastatin in in vitro and in vivo models showed the potential for its ability to inhibit cancer cell growth and further incorporation into nanoformulations strengthened its preclinical and pharmaceutical characteristics.
This is a preview of subscription content, log in via an institution to check access.
Access this article
Price includes VAT (Russian Federation)
Instant access to the full article PDF.
Rent this article via DeepDyve
Institutional subscriptions
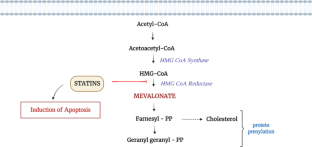
Similar content being viewed by others
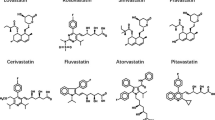
Statins: a repurposed drug to fight cancer

Looking at NSAIDs from a historical perspective and their current status in drug repurposing for cancer treatment and prevention
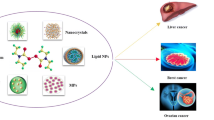
Recent advances in the delivery of disulfiram: a critical analysis of promising approaches to improve its pharmacokinetic profile and anticancer efficacy
Data availability.
No datasets were generated or analysed during the current study.
Abdoul-Azize S, Buquet C, Li H, Picquenot JM, Vannier JP (2018) Integration of Ca 2+ signaling regulates the breast tumor cell response to simvastatin and doxorubicin. Oncogene. 37:4979–93
Article CAS PubMed Google Scholar
Alhakamy NA, Fahmy UA, Ahmed OA, Caruso G, Caraci F (2020) Chitosan coated microparticles enhance simvastatin colon targeting and pro-apoptotic activity. Mar Drugs 18:226
Article CAS PubMed PubMed Central Google Scholar
Amin F, Fathi F, Reiner Ž, Banach M, Sahebkar A (2022) The role of statins in lung cancer. Arch Med Sci 18:141
CAS PubMed Google Scholar
Anzar N, Mirza MA, Anwer K, Khuroo T, Alshetaili AS et al (2018) Preparation, evaluation and pharmacokinetic studies of spray dried PLGA polymeric submicron particles of simvastatin for the effective treatment of breast cancer. J Mol Liq 249:609–16
Article CAS Google Scholar
Bjørnstad R, Reiten IN, Knudsen KS, Schjøtt J, Herfindal L (2022) A liposomal formulation of simvastatin and doxorubicin for improved cardioprotective and anti-cancer effect. Int J Pharm 629:122379
Article PubMed Google Scholar
Bruinsmann FA, Alves AD, de Fraga Dias A, Silva LF, Visioli F, Pohlmann AR, Figueiró F, Sonvico F, Guterres SS (2022) Nose-to-brain delivery of simvastatin mediated by chitosan-coated lipid-core nanocapsules allows for the treatment of glioblastoma in vivo. Int J Pharm 616:121563
Chan KK, Oza AM, Siu LL (2003) The statins as anticancer agents. Clin Cancer Res 9:10–9
Chen Y, Li LB, Zhang J, Tang DP, Wei JJ (2018) Simvastatin, but not pravastatin, inhibits the proliferation of esophageal adenocarcinoma and squamous cell carcinoma cells: a cell-molecular study. Lipids Health Dis 17:290
Cho KJ, Cho BC, Yun MR, Pyo KH, Kang HN (2019). Use of statin-based drug for treatment of EML4-ALK-positive non-small cell lung cancer progressing on ALK inhibitor. United States of America Patent 20190046514
Clendening J, Penn L (2012) Targeting tumor cell metabolism with statins. Oncogene 31:4967–4978
Dehghankelishadi P, Maritz MF, Dmochowska N, Badiee P, Cheah E (2022) Formulation of simvastatin within high density lipoprotein enables potent tumour radiosensitisation. J Control Release 346:98–109
Desai SA, Manjappa AS, Khulbe P (2022). Combination drug therapy for treatment of osteosarcoma. Indian Patent 202221039544
Dinić J, Efferth T, García-Sosa AT, Grahovac J, Padrón JM (2021) Repurposing old drugs to fight multidrug resistant cancers. Drug Resist Updat 52:100713
Article Google Scholar
Duarte JA, de Barros AL, Leite EA (2021) The potential use of simvastatin for cancer treatment: a review. Biomed Pharmacother 141:111858
Duleba AJ, Banaszewska B, Spaczynski RZ, Pawelczyk L (2006) Simvastatin improves biochemical parameters in women with polycystic ovary syndrome: results of a prospective, randomized trial. Fertil Steril 85:996–1001. https://doi.org/10.1016/j.fertnstert.2005.09.030
Edis Z, Wang J, Waqas MK, Ijaz M, Ijaz M (2021) Nanocarriers-mediated drug delivery systems for anticancer agents: an overview and perspectives. Int J Nanomedicine 16:1313–1330
Article PubMed PubMed Central Google Scholar
Elakkad YE, Mohamed SN, Abuelezz NZ (2021) Potentiating the cytotoxic activity of a novel simvastatin-loaded cubosome against breast cancer cells: insights on dual cell death via ferroptosis and apoptosis. Breast cancer: targets and therapy 13:675–89. https://doi.org/10.2147/BCTT.S336712
Endo A (2004) The origin of the statins. Atheroscler Suppl 5:125–130. https://doi.org/10.1016/j.atherosclerosissup.2004.08.033
Etemad L, Salmasi Z, Kalat SA, Moshiri M, Zamanian J (2023) An overview on nanoplatforms for statins delivery: perspective study for safe and effective therapy methods. Environ Res 8:116572
Fahmy UA, Aljaeid BM (2016) Combined strategy for suppressing breast carcinoma MCF-7 cell lines by loading simvastatin on alpha lipoic acid nanoparticles. Expert Opin Drug Deliv 13:1653–60
Famta P, Shah S, Fernandes V, Kumar KC, Bagasariya D (2022) Quality by design (QbD) assisted fabrication & evaluation of simvastatin loaded nano-enabled thermogel for melanoma therapy. Intl J Pharm 628:122270
Fan Y, Cui Y, Hao W, Chen M, Liu Q (2021) Carrier-free highly drug-loaded biomimetic nanosuspensions encapsulated by cancer cell membrane based on homology and active targeting for the treatment of glioma. Bioact Mater 6:4402–14
CAS PubMed PubMed Central Google Scholar
Fatehi HA (2019) Current perspectives on statins as potential anti-cancer therapeutics: clinical outcomes and underlying molecular mechanisms. Transl Lung Cancer Res. 8:692–699. https://doi.org/10.21037/tlcr.2019.09.08
Francis CB, Robbi LS (2021). Triple drug combination (metformin, simvastatin, digoxin) for targeted treatment of pancreatic cancer. US Patent 20210100770
Han JY, Lee SH, Yoo NJ, Hyung LS, Moon YJ, Yun T, Kim HT, Lee JS (2011) A randomized phase II study of gefitinib plus simvastatin versus gefitinib alone in previously treated patients with advanced non-small cell lung cancer. Clin Cancer Res 17:1553–1560. https://doi.org/10.1158/1078-0432.CCR-10-2525
Higgins MJ, Prowell TM, Blackford AL, Byrne C, Khouri NF et al (2012) A short-term biomarker modulation study of simvastatin in women at increased risk of a new breast cancer. Breast Cancer Res Treat 131:915–924. https://doi.org/10.1007/s10549-011-1858-7
Huynh NT, Passirani C, Saulnier P, Benoit JP (2009) Lipid nanocapsules: a new platform for nanomedicine. Int J Pharm 379:201–9
Hwang KE, Na KS, Park DS, Choi KH, Kim BR (2011) Apoptotic induction by simvastatin in human lung cancer A549 cells via Akt signaling dependent down-regulation of survivin. Investigational new drugs. 29:945–52
Istvan E (2003) Statin inhibition of HMG-CoA reductase: a 3-dimensional view. Atheroscler Suppl 4:3–8. https://doi.org/10.1016/s1567-5688(03)00003-5
Jiang W, Hu JW, He XR (2021) Statins: a repurposed drug to fight cancer. J Exp Clin Cancer Res 40:241
Joharatnam-Hogan N, Alexandre L, Yarmolinsky J, Lake B, Capps N (2021) Statins as potential chemoprevention or therapeutic agents in cancer: a model for evaluating repurposed drugs. Curr Oncol Rep 23:1–3
Karimi B, Ashrafi M, Shomali T, Yektaseresht A (2019) Therapeutic effect of simvastatin on DMBA-induced breast cancer in mice. Fundam Clin Pharmacol 33:84–93
Kaushik I, Ramachandran S, Prasad S, Srivastava SK (2021) Drug rechanneling: a novel paradigm for cancer treatment. Seminars in cancer biology, 68th, vol Academic. Press, New York, pp 279–290
Google Scholar
Kumbhar PS, Manjappa AS, Shah RR, Nadaf SJ, Disouza JI (2023) Nanostructured lipid carrier–based gel for repurposing simvastatin in localized treatment of breast cancer: formulation design, development, and in vitro and in vivo characterization. AAPS Pharm Sci Tech. 24:106
La Rosa JC (2001) Pleiotropic effects of statins and their clinical significance. Am J Card 88:291–3
Lee Y, Lee SH, Lee GK, Lim EJ, Han JY (2023) A randomized phase II study of irinotecan plus cisplatin with or without simvastatin in ever-smokers with extended disease small cell lung cancer. Cancer Res Treat 55:885–893. https://doi.org/10.4143/crt.2023.283
Li Y, Fu J, Yuan X, Hu C (2014) Simvastatin inhibits the proliferation of A549 lung cancer cells through oxidative stress and up-regulation of SOD2. Die Pharmazie-An Int J Pharm Sci 69:610–4
CAS Google Scholar
Liqin Z, Dayong L, Fei H, Jinkai Z, Yu ZH (2021). Application of simvastatin in preparation of medicine for preventing or treating liver cancer. China Patent 114177168
Liyuan Y, Yi Z, Stefan M, Gina CYC, Ruoxiang W (2019). Compounds and methods to sensitize cancer cells to tyrosine kinase inhibitors. European Patent 3529315
Lourenço T, Vale N (2023) Pharmacological efficacy of repurposing drugs in the treatment of prostate cancer. Int J Mol Sci 24:4154
Lu C, Li X, Ren Y, Zhang X (2021) Disulfiram: a novel repurposed drug for cancer therapy. Cancer Chemoth Pharm 87:159–72
Mahmoud K, Teaima M, Attia Y, El-Nabarawi M, Swidan S (2023) Size-optimized simvastatin TPGS modified lipid nanocapsules for targeting epithelial-to-mesenchymal transition in hepatocellular carcinoma: role of PTEN/AKT signaling. Expert Opin Drug Deliv 20:703–719
Malik JA, Ahmed S, Jan B, Bender O, Al Hagbani T (2022) Drugs repurposed: an advanced step towards the treatment of breast cancer and associated challenges. Biomed Pharmacother 145:112375
Manu KA, Shanmugam MK, Li F (2014) Simvastatin sensitizes human gastric cancer xenograft in nude mice to capecitabine by suppressing nuclear factor-kappa B-regulated gene products. J Mol Med. 92:267–276
Manuel GCJ (2016). Combinations of methotrexate and simvastatin for use in the treatment of osteosarcoma. European Patent 3028704
Matusewicz L, Filip-Psurska B, Psurski M, Tabaczar S, Podkalicka J et al (2019) EGFR-targeted immunoliposomes as a selective delivery system of simvastatin, with potential use in treatment of triple-negative breast cancers. Int J Pharm 569:118605
Matusewicz L, Czogalla A, Sikorski AF (2020) Attempts to use statins in cancer therapy: An update. Tumor Biol 42:1010428320941760
Moon AR, Kang SO (2008). Pharmaceutical composition containing simvastatin as an effective ingredient for treatment of breast cancer, especially inhibiting breast cancer metastasis. Korean Patent 1020080042333
Mussi SV, Torchilin VP (2013) Recent trends in the use of lipidic nanoparticles as pharmaceutical carriers for cancer therapy and diagnostics. J Mater Chem B 1:5201–9
Nabi R, Alvi SS, Saeed M, Ahmad S, Khan MS (2019) Glycation and HMG-CoA reductase inhibitors: implication in diabetes and associated complications. Curr Diabetes Rev. 15:213–23
Neuvonen PJ, Backman JT, Niemi M (2008) Pharmacokinetic comparison of the potential over-the-counter statins simvastatin, lovastatin, fluvastatin and pravastatin. Clin Pharmacokinet 47:463–74
Patel KK, Sehgal VS, Kashfi K (2022) Molecular targets of statins and their potential side effects: not all the glitter is gold. Eur J Pharmacol 922:174906
Piplani H, Marek-Iannucci S, Sin J, Hou J, Takahashi T (2019). Simvastatin induces autophagic flux to restore cerulein-impaired phagosome-lysosome fusion in acute pancreatitis. Biochimica et Biophysica Acta (BBA)-Molecular Basis of Disease. 1865:165530
Rodrigues R, Duarte D, Vale N (2022) Drug repurposing in cancer therapy: influence of patient’s genetic background in breast cancer treatment. Int J Mol Sci 23:4280
Safwat S, Hathout RM, Ishak RA, Mortada ND (2017) Augmented simvastatin cytotoxicity using optimized lipid nanocapsules: a potential for breast cancer treatment. J Liposome Res 27:1–10
Sahoo NG, Abbas A, Li CM (2008) Micro/nanoparticle design and fabrication for pharmaceutical drug preparation and delivery applications. Curr Drug Ther 3:78–97
Sally S et al (2017) Statins anticancer targeted delivery systems: re-purposing an old molecule. J Pharm Pharmacol 69:613–624
Sarkar S, Osama K, Mohammad Sajid Jamal Q, Amjad Kamal M, Sayeed U (2017) Advances and implications in nanotechnology for lung cancer management. Curr Drug Metab 18:30–8
Saxena A, Becker D, Preeshagul I, Lee K, Katz EB (2015) Therapeutic effects of repurposed therapies in non-small cell lung cancer: what is old is new again. The Oncologist. 20:934–45
Schein CH (2021) Repurposing approved drugs for cancer therapy. Br Med Bull 137:13–27
Schmidmaier R, Baumann P, Bumeder I, Meinhardt G, Straka C, Emmerich B (2007) First clinical experience with simvastatin to overcome drug resistance in refractory multiple myeloma. Eur J Haematol 79:240–3
Shahid A, Khan MM, Ahmad U, Haider MF, Ali A (2022) Exploring liposomes for lung cancer therapy. Crit Rev Ther Drug Carr Syst. https://doi.org/10.1615/CritRevTherDrugCarrierSyst.2021037912
Shahrukh S, Jain N, Shah S, Famta P, Vambhurkar G (2024) Quality by design enabled tumor microenvironment-responsive simvastatin-loaded liposomes for prostate cancer management. J Drug Deliv Sci Technol 13:105474
Sheikh SY, Ansari WA, Hassan F, Khan MF, Faiyaz SSM, Akhtar Y, Khan AR, Nasibullah M (2023) Drug repurposing and high-throughput screening against phosphomannomutase for the treatment of cutaneous leishmaniasis. Orient J Chem 39:1–10
Sheikh SY, Ansari WA, Hassan F, Faruqui T, Khan MF, Akhter Y (2023) Drug repositioning to discover novel ornithine decarboxylase inhibitors against visceral leishmaniasis. J Mol Recognit 24:3021
Shen YY, Yuan Y, Du YY, Pan YY (2015) Molecular mechanism underlying the anticancer effect of simvastatin on MDA-MB-231 human breast cancer cells. Mol Med Rep 2:623–630
Sherif AY, Harisa GI, Shahba AA, Nasr FA, Taha EI, Alqahtani AS (2023) Assembly of nanostructured lipid carriers loaded gefitinib and simvastatin as hybrid therapy for metastatic breast cancer: codelivery and repurposing approach. Drug Dev Res. https://doi.org/10.1002/ddr.22097
Shid RL, Dhole SN, Kulkarni N, Shid SL (2015) Formulation and evaluation of nanosuspension of simvastatin. Int J Pharm Sci Nanotechnol 8:2858–73. https://doi.org/10.37285/ijpsn.2015.8.2.9
Shinde ME, Sonawane MP, Maru AD (2021) Formulation and evaluation of nanosuspension as an alternative approach for solubility enhancement of simvastatin. Int J Pharm Sci Rev Res 71:97–101
Shuhaili MF, Samsudin IN, Stanslas J, Hasan S, Thambiah SC (2017) Effects of different types of statins on lipid profile: a perspective on Asians. Int J Endocrinol Metab 15:e43319
Singh PP, Lemanu DP, Soop M, Bissett IP, Harrison J, Hill AG (2016) Perioperative simvastatin therapy in major colorectal surgery: a prospective, double-blind randomized controlled trial. J Am Coll Surg 223:308-320.e1. https://doi.org/10.1016/j.jamcollsurg.2016.04.004
Spampanato C, De Maria S, Sarnataro M, Giordano E, Zanfardino M (2012) Simvastatin inhibits cancer cell growth by inducing apoptosis correlated to activation of Bax and down-regulation of BCL-2 gene expression. Int J Oncol 40:935–41
Subramaniam B, Siddik ZH, Nagoor NH (2020) Optimization of nanostructured lipid carriers: understanding the types, designs, and parameters in the process of formulations. J Nanoparticle Res 22:1–29
Taymouri S, Ahmadi Z, Mirian M, Tavakoli N (2021) Simvastatin nanosuspensions prepared using a combination of pH-sensitive and timed-release approaches for potential treatment of colorectal cancer. Pharm Dev Technol 26:335–48
Thomas O, Lagarce F (2013) Lipid nanocapsules: a nanocarrier suitable for scale-up process. J Drug Deliv Technol 23:555–9
Tilija Pun N, Jeong CH (2021) Statin as a potential chemotherapeutic agent: current updates as a monotherapy, combination therapy, and treatment for anti-cancer drug Resistance. Pharmaceuticals 14:470
Towers CG, Wodetzki D, Thorburn A (2019) Autophagy and cancer: modulation of cell death pathways and cancer cell adaptations. J Cell Biol 219:201909033
Varshosaz J, Hajhashemi V, Soltanzadeh S (2011) Lipid nanocapsule-based gels for enhancement of transdermal delivery of ketorolac tromethamine. J Drug Deliv. https://doi.org/10.1155/2011/571272
Vered S (2013). Simvastatin and anastrozole in treating postmenopausal women with ductal carcinoma in situ or invasive breast cancer. National Library of Medicine. https://www.clinicaltrials.gov/study/NCT00354640 . Accessed 28 March 2024
Wang G, Cao R, Wang Y, Qian G, Dan HC (2016) Simvastatin induces cell cycle arrest and inhibits proliferation of bladder cancer cells via PPARγ signalling pathway. Sci Rep. 6:35783
Wang F, Liu W, Ning J, Wang J, Lang Y (2018) Simvastatin suppresses proliferation and migration in non-small cell lung cancer via pyroptosis. Int J Biol Sci 14:406–417
Wang Y, Wei Z, Pan K, Li J, Chen Q (2020) The function and mechanism of ferroptosis in cancer. Apoptosis. 2511:786–98
Yang B, Shi J (2020) Developing new cancer nanomedicines by repurposing old drugs. Angew Chem, Int Ed Engl 59:21829–38
Yang F, Bettadapura SN, Smeltzer MS, Zhu H, Wang S (2022) Pyroptosis and pyroptosis-inducing cancer drugs. Acta Pharmacologica Sinica. 43:2462–73
Yao X, Xie R, Cao Y (2021) Simvastatin induced ferroptosis for triple-negative breast cancer therapy. J Nanobiotechnol 19:311. https://doi.org/10.1186/s12951-021-01058-1
Yonghua Y, Xiaoxiao J, Xinhui K, Zhaohu L, Zhuqing Z (2017). Pharmaceutical composition for treating triple-negative breast cancer. China Patent106310268
Yongzhuo H, Weimin Y (2020). Medical composition, liposome containing medical composition and use. China Patent 110898066
Young SP (2017). Trial of simvastatin and gemcitabine in pancreatic cancer patients. National Library of Medicine. https://www.clinicaltrials.gov/study/NCT00944463
Yu X, Pan Y, Ma H, Li W (2013) Simvastatin inhibits proliferation and induces apoptosis in human lung cancer cells. Oncol Res. 20:351–7
Zhiyuan Z, Congcong L, Jun L (2020). Application of combination of avermectin and simvastatin and pharmaceutical composition of avermectin and simvastatin in preparation of medicine for treating pancreatic cancer. China Patent 110898055
Zhou Y, Tashiro J, Kamatani S, Irie N, Suzuki A, Ishikawa T, Warita K, Oltvai ZN, Warita T (2023) HMG-CoA reductase degrader, SR-12813, counteracts statin-induced upregulation of HMG-CoA reductase and augments the anticancer effect of atorvastatin. Biochem Biophys Res Commun 677:13–9
Zhou D, Yan X, Xiao L, Wang J, Wei J (2024) Gold capped mesoporous bioactive glass guides bone regeneration via BMSCs recruitment and drug adaptive release. Chem Eng J 20:150546
Download references
Acknowledgements
The authors would like to acknowledge the Faculty of Pharmacy, Integral University for continuous support and guidance during the writing process of this work.
Author information
Authors and affiliations.
Department of Pharmaceutics, Faculty of Pharmacy, Integral University, Lucknow, 226026, India
Nargis Ara & Abdul Hafeez
Department of Pharmaceutical Chemistry, Faculty of Pharmacy, Integral University, Lucknow, 226026, India
Shom Prakash Kushwaha
You can also search for this author in PubMed Google Scholar
Contributions
All the authors contributed to the study conception and design. Data collection and main manuscript text writing were performed by Nargis Ara and Abdul Hafeez. Data analysis was performed by Shom Prakash Kushwaha. The first draft of the manuscript was written by Nargis Ara, and Abdul Hafeez reviewed and commented on previous versions of the manuscript. All the authors reviewed and approved the final manuscript. The authors confirm that no paper mill and artificial intelligence was used.
Corresponding author
Correspondence to Abdul Hafeez .
Ethics declarations
Ethics approval.
Not applicable.
Competing interests
The authors declare no competing interests.
Additional information
Publisher's note.
Springer Nature remains neutral with regard to jurisdictional claims in published maps and institutional affiliations.
Rights and permissions
Springer Nature or its licensor (e.g. a society or other partner) holds exclusive rights to this article under a publishing agreement with the author(s) or other rightsholder(s); author self-archiving of the accepted manuscript version of this article is solely governed by the terms of such publishing agreement and applicable law.
Reprints and permissions
About this article
Ara, N., Hafeez, A. & Kushwaha, S.P. Repurposing simvastatin in cancer treatment: an updated review on pharmacological and nanotechnological aspects. Naunyn-Schmiedeberg's Arch Pharmacol (2024). https://doi.org/10.1007/s00210-024-03151-2
Download citation
Received : 28 January 2024
Accepted : 06 May 2024
Published : 15 May 2024
DOI : https://doi.org/10.1007/s00210-024-03151-2
Share this article
Anyone you share the following link with will be able to read this content:
Sorry, a shareable link is not currently available for this article.
Provided by the Springer Nature SharedIt content-sharing initiative
- Drug repurposing
- Simvastatin
- Pharmacological aspect
- Nanotechnology
- 3-Hydroxy-3-methylglutaryl coenzyme-A reductase inhibitor
- Anticancer activity
- Find a journal
- Publish with us
- Track your research

European Observatory for Nanomaterials
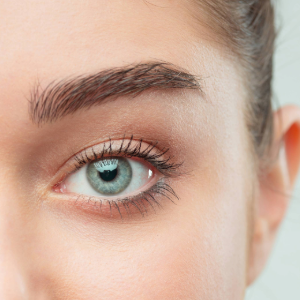
Discover the insights from Prof. Barbara Pierscionek in the latest Nanopinion, where she highlights groundbreaking advancements in nanotechnology aimed at treating eye diseases, especially those related to aging such as cataracts and retinal diseases. These innovations promise more effective and less invasive treatment options, marking significant progress in ocular health research.
News More news RSS
The Joint Research Centre (JRC) has launched a call for project proposals to access the JRC Nanobiotechnology Laboratory for researchers and scientists from EU Member States and a call for Training and Capacity Building (T&CB). The deadline for both calls is 21 May 2024.
Read the latest Nanopinion by Dr. Ester Carregal-Romero, Prof. Jesús-Cabello, and Dr. Susana Carregal-Romero where they introduce the benefits of Safe and Sustainable by Design (SSbD) approach for nanomaterials and present applications of Calcium Carbonate in new biomedical systems.
The European Union Observatory for Nanomaterials (EUON) invites proposals for upcoming studies aimed at addressing knowledge gaps to nanomaterials within the EU market.
Nanomaterials and health

Are substances in nanoforms more dangerous than they are in their normal size? Some are and some are not. Just like any other chemical substance, nanomaterials have to be assessed separately.

Nanomaterials are not only used in consumer products, but also in new and innovative medical treatments. Research has shown that nanoparticles can be used to damage and even destroy cancer cells from within.
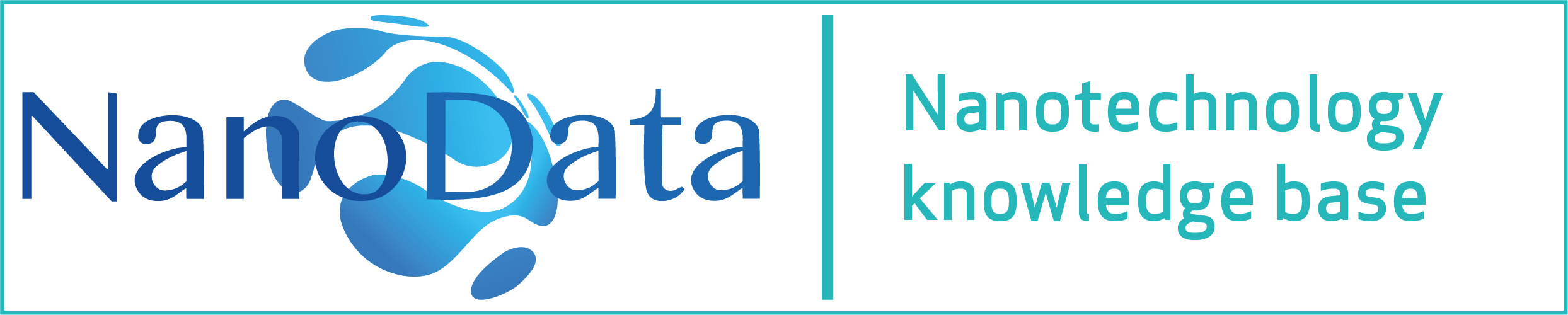
Infographics
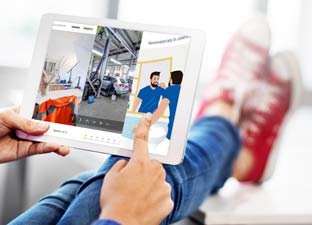
Visit the interactive Infographics to learn more about nanomaterials around us and in the most popular products.
More on the web
Electron vortices in graphene detected 13 May | Source: ETH Zurich
New Method Streamlines Development of Poly(2-oxazoline) Nanomedicines 09 May | Source: AZO Nano
Oregon State researchers develop device for better delivery of gene therapy for lung disease 07 May | Source: Oregon State University
Oxford Instruments awarded King’s Award for Enterprise in Innovation 06 May | Source: Oxford Instruments
Sylvester Researchers Develop Nanoparticles to Tackle Brain Metastases 06 May | Source: Miller School of Medicine
Welcome to the ECHA website. This site is not fully supported in Internet Explorer 7 (and earlier versions). Please upgrade your Internet Explorer to a newer version.
Close Do not show this message again
This website uses cookies to ensure you get the best experience on our websites.
Close Find out more on how we use cookies.

IMAGES
VIDEO
COMMENTS
Nanotechnology is a relatively new field of science and technology that studies tiny objects (0.1-100 nm). ... in this review, we present a thorough overview of NPs in general on their properties and therapeutic uses in different fields of science and technology, with specific emphasis on their therapeutic applications. ... The literature ...
Nanotechnology is the study of the controlling the matter on an atom and molecular scale. Generally nanotechnology deals with structures sized between 1-100 nanometers in at least one. dimension ...
Nanotechnology is a relatively new field of science and technology that studies tiny objects (0.1-100 nm). Due to various positive attributes displayed by the biogenic synthesis of nanoparticles (NPs) such as cost-effectiveness, none to negligible environmental hazards, and biological reduction served as an attractive alternative to its counterpart chemical methods.
This review discusses a brief history of nanomaterials and their use throughout history to trigger advances in nanotechnology development. In particular, we describe and define various terms relating to nanomaterials. Various nanomaterial synthesis methods, including top-down and bottom-up approaches, are discussed.
The application of nanotechnology in medicine and healthcare is referred to as nanomedicine, and it has been used to combat some of the most common diseases, including cardiovascular diseases and cancer. The present review provides an overview of the recent advances of nanotechnology in the aspects of imaging and drug delivery.
Novel nano-organisms, called nanobes, are gaining interest among nanotechnology researchers as they are found during off-shore petroleum exploration on Triassic and Jurassic sandstones in Western Australia . These nanobes contains 20-150 nm diameter individual cells that are composed of a carbon, oxygen, nitrogen, DNA, membrane-bound ...
A Literature Review of Nanotechnology. Divesh Garg. Published 2021. Materials Science, Environmental Science, Engineering. Many sectors, including information technology, electricity, environmental science, pharmacy, home security, food safety and transport allow nanotechnology to significantly improve and even revolutionise, among others.
This review article will cover the recent advanced applications of nanotechnology in different industries, mainly agriculture, food, cosmetics, medicine, healthcare, automotive, oil and gas industries, chemical, and mechanical industries [2,3]. Moreover, a brief glimpse of the drawbacks of nanotechnology will be highlighted for each industry to ...
History of nanotechnology. Human dreams and imagination often give rise to new science and technology. Nanotechnology, a 21st-century frontier, was born out of such dreams. Nanotechnology is defined as the understanding and control of matter at dimensions between 1 and 100 nm where unique phenomena enable novel applications. 1 Although human ...
Nature Nanotechnology - This analysis leverages a large-scale literature review, text mining, statistics and machine learning to identify trends, shortcomings and future opportunities in developing...
Nanotechnology: A Brief Literature Review M. Ellin Doyle, Ph.D. Food Research Institute, University of Wisconsin-Madison, Madison, WI 53706 [email protected] Contents
A comprehensive literature review examined nanosensors in EVs. Our study provides a solid foundation for understanding the current state of research, identifying major trends and discovering nanotechnology breakthroughs in EV sensors by carefully reviewing, characterizing and rating important papers.
The Review discusses the state-of-the-art polymer nanocomposites from three key aspects: dipole activity, breakdown resistance and heat tolerance for capacitive energy storage applications ...
2006. TLDR. This paper seeks to review a limited subset of products that contain nanoscale materials, assess the available data for evaluating the consumer exposures and potential hazards associated with these products, and discuss the capacity of U.S. regulatory agencies to address the potential risks associated withThese products. Expand.
The literature review is not claimed to be exhaustive, but the sample presents a broad range of published research papers giving ample insight into what scholars discuss when addressing the communication of nanotechnology to the public. The analysis was inspired by Carol Bacchi's (1999, 2012) approach to policy analysis. Her analytical and ...
Jeon, Nanotechnology, big things from a tiny world: a review, International Journal of u- and e-Service, Science and Technology 2(3) (2009), 29-37. [3] M. Ellin Doyle, Nanotechnology: A Brief Literature Review, FRI Briefings, 2006. [4] Mark T. Lusk and L. D. Carr, Nanoengineering defect structures on grapheme, Physical
REVIEW OF LITERATURE 2.1. NANOTECHNOLOGY Nanotechnology is a multidisciplinary field, as it combines the knowledge from different disciplines: chemistry, physics, and biology amongst others ...
The term "nanotechnology" is composed of the unit prefix ... At the first level, a literature review was conducted to describe and illuminate the existing status of nanoethics considerations. To this purpose, scientific databases, including PubMed, Scopus, and Web of Science, were searched using nanoethics, nanotechnology, nanomedicine ...
The Nanotechnology Reviews is a respected journal on nanoscience and nanotechnology, where almost all the papers are co-authored by Engineers. Among many top notch Review Papers, the 60% of the published papers in the Nanotechnology Reviews (NTREV) are Research Articles and Regular Papers. Ranked among 22 out of 160 journals in the category ...
Nanotechnology is a developing new technology. It offers many benefits in numerous fields including food, 1,2 cosmetics, 3 medicine, 4 and agriculture. 2,5 The US National Nanotechnology Initiative (NNI) defines nanotechnology as "the understanding and control of matter at dimensions between approximately 1 and 100 nm, where unique phenomena enable novel applications". 6-8 In addition to ...
The development of nanotechnology and nanomaterials offers promising signs for a change in the way of construction and geotechnical projects. ... Walubita LF (2014) Application of nano-technology in pavement engineering: a literature review. In: Application of nanotechnology in pavements, geological disasters, and foundation settlement control ...
Nanotechnology evolved as the achievement of science in the 21st century. ... These NPs are often organic, and the term polymer nanoparticle (PNP) is commonly used in the literature to refer to them. They resemble Nano spheres or Nano capsules for the most part (Khan ... A review on nano-antimicrobials: metal nanoparticles, methods and ...
The nano-carrier has the ability to co-wrap a range of medications, provide drug co-delivery, and produce more consistent in vivo behaviour. Based on this, a study was conducted to develop a liposome nano drug delivery system that would mix gefitinib (1.5 mg) with SMV (1 mg) in order to treat non-small-cell lung cancer and provide an anti ...
The European Union Observatory for Nanomaterials (EUON) invites proposals for upcoming studies aimed at addressing knowledge gaps to nanomaterials within the EU market. Read the latest Nanopinion by Dr Eleonora Cara and Dr Federico Ferrarese Lupi. They introduce sequential infiltration synthesis (SIS) to produce innovative nanomaterials and ...