Advertisement

Introduction
Vitamin b 12 deficiency: the hematological perspective, past and present, pathobiology of b 12 deficiency, variations on the theme and the b 12 -folate nexus, causes of b 12 deficiency, diagnosis of b 12 deficiency, serum b 12 levels, serum holotc, serum or plasma mma and hcy, assays of b 12 absorption and intrinsic factor antibodies, prevention and treatment of b 12 deficiency, vitamin b 12 deficiency from the perspective of a practicing hematologist.

- Split-Screen
- Request Permissions
- Cite Icon Cite
- Search Site
- Open the PDF for in another window
Ralph Green; Vitamin B 12 deficiency from the perspective of a practicing hematologist. Blood 2017; 129 (19): 2603–2611. doi: https://doi.org/10.1182/blood-2016-10-569186
Download citation file:
- Ris (Zotero)
- Reference Manager
B 12 deficiency is the leading cause of megaloblastic anemia, and although more common in the elderly, can occur at any age. Clinical disease caused by B 12 deficiency usually connotes severe deficiency, resulting from a failure of the gastric or ileal phase of physiological B 12 absorption, best exemplified by the autoimmune disease pernicious anemia. There are many other causes of B 12 deficiency, which range from severe to mild. Mild deficiency usually results from failure to render food B 12 bioavailable or from dietary inadequacy. Although rarely resulting in megaloblastic anemia, mild deficiency may be associated with neurocognitive and other consequences. B 12 deficiency is best diagnosed using a combination of tests because none alone is completely reliable. The features of B 12 deficiency are variable and may be atypical. Timely diagnosis is important, and treatment is gratifying. Failure to diagnose B 12 deficiency can have dire consequences, usually neurological. This review is written from the perspective of a practicing hematologist.
Traditionally, vitamin B 12 deficiency has been considered to lie within the scope and expertise of hematologists. This assignation has deep historical roots, going back to the earliest recognition of the disease that acquired the eponymic title of Addisonian pernicious anemia following the somewhat vague description by the Guy’s Hospital physician, Thomas Addison, of “a very remarkable form of general anemia occurring without any discoverable cause whatsoever.” It was the astute clinical observations of Richard Cabot, William Osler, and others that brought the picture of the syndromic disease with its classical triad of associated jaundice, glossitis, and myeloneuropathy into sharper focus, as nicely recorded in William Castle’s historical review of the disease. 1 Coller, 2 in his commentary to mark the 70th anniversary of Blood , wrote: “The most dramatic and far reaching event in hematology in the United States in the pre- Blood period was Minot and Murphy’s 1926 report that feeding liver to patients with pernicious anemia could cure this otherwise fatal disorder. This dramatic breakthrough was an enormous stimulus to hematologic investigation.” A quest for the active principle in liver that made it possible to “cure” pernicious anemia ushered in the era of Big Pharma in a race to identify and produce the compound that ultimately became known as vitamin B 12 . Elucidation of the physiology of B 12 and its intricate mechanism of assimilation made it clear that there was a myriad of causes of B 12 deficiency. 3
Because of the often conspicuous hematological manifestations of B 12 deficiency, it remained largely within the domain of hematology. However, as ever more sensitive methods were developed to assess subtle degrees of deficiency of the vitamin, 4 , 5 it became clear that the effects of B 12 deficiency were not restricted to the hematopoietic system but were often overshadowed by neurological complications and were sometimes entirely absent. 6 Just as folate deficiency is associated with effects beyond anemia, 7 B 12 deficiency also can be associated with nonhematological complications, including increased risk of neural tube defect pregnancy, cognitive impairment, osteopenia, and vascular occlusive disease, perhaps attributable to the accumulation of homocysteine (Hcy) that occurs in B 12 deficiency. 3 Even so, because the most conspicuous manifestations of established B 12 deficiency affect the blood and bone marrow and are a leading cause of macrocytic and megaloblastic anemia, it is ultimately the practicing hematologist who remains front and center of the clinical diagnosis and management of patients with suspected or confirmed B 12 deficiency.
This review is written from the perspective of a practicing hematologist who might suspect B 12 deficiency during a routine patient encounter or who might see a patient in consultation for anemia as part of a complex medical problem. An understanding of the normal physiology and its perturbations in disease is a key factor to the understanding of the causes and manifestations of B 12 deficiency. The clinical features in a given case of B 12 deficiency may range from the typical “textbook” picture through any 1 of a kaleidoscopic variety of atypical presentations that can befuddle the unwary.
In an adult, the total body B 12 store is 3 to 5 mg, and the recommended daily intake (RDI) is 2.4 μg. 8 Natural food sources of B 12 are restricted to food of animal origin. As a consequence, it is a micronutrient that is often in critically short supply, particularly among vegetarian or vegan populations who, through culture, poverty, or conviction, subsist on diets that lack or are poor in B 12 . Were it not for efficient conservation of biliary B 12 through enterohepatic reabsorption, 9-11 symptomatic B 12 deficiency would occur more frequently among vegans.
Complex mechanisms are in place to render B 12 bioavailable, protect it during intestinal transit, and then absorb and retain the precious vitamin for cellular uptake 3 , 12 ( Figure 1 ). It is remarkable that B 12 is the required cofactor for only 2 biochemical reactions in humans, 3 yet the effects of B 12 deficiency are not only profound but protean. The several possible reasons for the broad spectrum of manifestations fall into the broad categories of genetic variation and acquired comorbidities.
![case study megaloblastic anemia Figure 1. Normal pathway of B12 absorption and cellular uptake. Food B12 is released in the stomach and binds to salivary HC. In the small intestine, food B12 and biliary B12 are released from HC by pancreatic proteases and bind to intrinsic factor (IF). The IF-B12 complex then binds to the cubam (Cubilin [Cub]–amnionless [Amn]) receptor in the terminal ileum for internalization and release to plasma where it is bound by TC. TC delivers B12 to the TC receptor (CD320) on cells, and following release in the cell, B12 is reduced and converted to adenosylcobalamin in the mitochondria and methylcobalamin in the cytosol, where they serve as cofactors for the 2 B12-dependent reactions. CoA, coenzyme A; THF, tetrahydrofolate. Professional illustration by Patrick Lane, ScEYEnce Studios.](https://ash.silverchair-cdn.com/ash/content_public/journal/blood/129/19/10.1182_blood-2016-10-569186/4/m_blood569186f1.jpeg?Expires=1719080892&Signature=YaakP9Da4yET8z9ysoVjhqjPfVbYtduJM9DBwwvQ662tx2-gBhRn2eAXclDwo-weFkmCnrJ5bPmZdpMxWFKjeXJpa1nKH0XPYOc-OKGOvvBkfUgGIYEl~76K8EyE-YqIYI2XEaOE7OYpz3quBImUhRzw3UJqj25PkIQMm9HQulLdVgicfv71wM22jVzjsez7P~zuuZzjX4fjJprciXKOOeJ8nRCDCLLCw208JZoMza6kjYhtraXUph0IBsQH9X7oDAitJpRUrWxXdeCPnS~~dqsN6Llpwa0st7ajrJVYWze~QHjkauCROqE11uxGr9XHPuak-wWOGkX5K6KmsFxcKw__&Key-Pair-Id=APKAIE5G5CRDK6RD3PGA)
Normal pathway of B 12 absorption and cellular uptake. Food B 12 is released in the stomach and binds to salivary HC. In the small intestine, food B 12 and biliary B 12 are released from HC by pancreatic proteases and bind to intrinsic factor (IF). The IF-B 12 complex then binds to the cubam (Cubilin [Cub]–amnionless [Amn]) receptor in the terminal ileum for internalization and release to plasma where it is bound by TC. TC delivers B 12 to the TC receptor (CD320) on cells, and following release in the cell, B 12 is reduced and converted to adenosylcobalamin in the mitochondria and methylcobalamin in the cytosol, where they serve as cofactors for the 2 B 12 -dependent reactions. CoA, coenzyme A; THF, tetrahydrofolate. Professional illustration by Patrick Lane, ScEYEnce Studios.
Depletion of body B 12 stores resulting from insufficient capture of the vitamin from dietary sources because of either inadequate intake or malabsorption eventually leads to a state of deficiency. When a certain threshold of deficiency is reached, the supply of B 12 becomes inadequate to support biochemical pathways requiring the vitamin, leading to disruption of the functional and ultimately the structural integrity of cells. Absent of any underlying perturbation of B 12 -dependent pathways that occur in individuals who harbor inborn errors involving intracellular B 12 assimilation and processing, 13 , 14 the major determinant of the severity of B 12 deficiency and whether it leads to either megaloblastic anemia, demyelinating neurological disease, or both appears to be whether there is abrogation of the normal physiological axis of B 12 absorption. Normal B 12 absorption requires intact gastric production of intrinsic factor as well as a functioning cubam receptor for the B 12 -intrinsic factor complex in the terminal ileum. 3 , 12 , 15
B 12 and folate are intimately connected through their cooperative roles in one-carbon metabolism, and the hematological complications seen in deficiency of either vitamin are indistinguishable. Both are caused by impaired DNA synthesis that results in a prolongation of the S phase of the cell cycle 16 and causes maturation arrest. 17 Prolongation of the cell cycle is associated with delayed migration of the DNA replication fork and the annealing of DNA fragments synthesized from the lagging strand. 18 The retardation of DNA replication in megaloblasts arises from failure of the folate-dependent conversion of deoxyuridine to deoxythymidine. The deoxyuridine triphosphate that accumulates is incorporated into DNA in place of thymidine 5′-triphosphate by the somewhat promiscuous DNA polymerase enzyme. 19 The normal process of excision-repair of U-A mismatches in DNA fail for persistent lack of thymidine 5′-triphosphate. Repetitive iterations of defective DNA repair ultimately lead to DNA strand breaks, fragmentation, and apoptotic cell death. 20 , 21
The morphological appearances of these biochemical lesions are seen as megaloblastic changes in the marrow, which consist of red cell precursors that are larger than normal with a lack of synchronous maturation of the nucleus and cytoplasm ( Figure 2 ). There is a preponderance of basophilic erythroblasts over more mature hemoglobinized forms, creating the appearance of a maturation arrest. The myeloid-to-erythroid ratio falls and may even show reversal (<1:1), due to varying degrees of both apparent erythroid hyperplasia caused by maturation arrest and granulocytic hypoplasia. Megaloblastic features in the granulocyte precursors consist of giant metamyelocyte and band forms containing large horseshoe-shaped nuclei ( Figure 2 ). Megaloblastic megakaryocytes may be seen with abnormally large and polylobated nuclei, sometimes with detached lobes and absent cytoplasmic granulation.

Photomicrograph of bone marrow in a patient with pernicious anemia. (A) Megaloblastic change in the nucleus of an erythroid precursors consisting of variegated finely granular chromatin (“salt-and-pepper” appearance) in contrast to the ground-glass texture of normal proerythroblasts. With progressive maturation, chromatin condensation occurs at a slower pace than normal, giving rise to darker aggregates that fuse nonhomogeneously and impart to the nucleus a characteristic latticelike appearance. Undisturbed maturation of the cytoplasm as hemoglobin forms in a cell with an immature-appearing nucleus results in cells that are conspicuous for their lack of synchrony between nuclear and cytoplasmic development. (B) A megaloblastic (“giant”) granulocyte precursor. Original magnification ×100; Wright-Giemsa stain.
All megaloblastic anemias display similar clinical features. Absent of any sudden acceleration in the rate of B 12 depletion, such as occurs following exposure to nitrous oxide, 22 , 23 anemia develops slowly, and symptoms including weakness, palpitations, fatigue, light-headedness, and shortness of breath may not occur until anemia is fairly profound, because compensatory cardiopulmonary changes mitigate tissue hypoxia. The melding of severe pallor with jaundice caused by hemolysis produces a peculiar lemon-yellow skin color.
All formed blood elements are affected by the ineffective megaloblastic hematopoiesis, but erythrocytes show the most marked changes, both in size and in shape, with large oval macrocytes and prominent anisopoikilocytosis. In general, the degree of anemia corresponds with the severity of the red cell morphologic changes. When the hematocrit falls <20%, megaloblasts may appear in the blood. The morphologic features of megaloblastic anemia may be grossly exaggerated in splenectomized patients or in whom there is functional asplenism as occurs in celiac disease or sickle cell anemia when circulating megaloblasts and bizarre red cell morphology may be present. 24
The anemia is macrocytic (mean corpuscular volume 100-150 fl or more); mild macrocytosis may be the earliest evidence of a megaloblastic process, but because of longevity of red cells, there is a gradual shift in mean corpuscular volume as comingling occurs with older normocytic red cells. Anisocytosis becomes more marked, and the earliest measurable change in red cell indices is an increase in the red cell distribution width.
Neutrophils typically show hypersegmentation of their nuclei, beyond the usual 3 to 5 lobes, and may contain 6 or more lobes. 25 Hypersegmented neutrophils are an early sign of megaloblastosis and may persist for many days after treatment. 25 However, neutrophil hypersegmentation does not appear to be a sensitive indicator of mild B 12 deficiency. 26 Leukopenia and thrombocytopenia may be present but only rarely cause clinical problems. Thrombocytopenia may be severe, when it may be confused with thrombotic thrombocytopenic purpura. 27 , 28 Platelet production is reduced to 10% of what would be expected from the megakaryocyte mass, 29 reflecting ineffective thrombopoiesis, and platelets may be functionally abnormal. 30
Cytogenetic changes, when they occur, are nonspecific and show elongated and broken chromosomes, changes that are usually corrected within 2 days of treatment, although some abnormalities may remain for months. 21 , 31
What determines the particular manifestations of B 12 deficiency in a given individual depends on several factors, some of which are understood, others not. Two clear examples of what influences the clinical presentation in a given patient are the rate of development and the degree of severity of deficiency. The extent to which absorption of B 12 is compromised, whether partial or complete and whether absorption is totally abrogated or whether it relates only to poor bioavailability of food B 12 is also important. Polymorphic differences in genes involved in the complex repertoire that comprises the pathways of B 12 absorption, assimilation, cellular metabolism, and plasma transport of the vitamin ( Figure 1 ) are known to affect the susceptibility to develop B 12 deficiency. 32 , 33 Whether these genetic factors can also influence the disease phenotype in B 12 deficiency is not well understood at this time. Another factor that may play a role in the susceptibility of an individual to B 12 deficiency is the composition of their gastrointestinal microbiome. Some microbiota are capable of degrading B 12 , which may affect bioavailability of the vitamin and also lead to the generation of B 12 analogs. 34 B 12 analogs have been identified in plasma and tissues 35 and have been invoked as a possible cause of some of the manifestations of B 12 deficiency. 36 Host-microbial interactions have also been implicated as a possible initiating factor in autoimmune gastritis leading to pernicious anemia. In this proposed mechanism, chronic Helicobacter pylori infection may, through molecular mimicry of H + K + ATPase, evoke a host immune response that involves CD4 + T cells through a Fas-dependent mechanism 37 and leads to destruction of the gastric mucosa. 38 , 39
Nutrient-nutrient interactions are known to play a role in the manifestations of B 12 deficiency. The best known of these is concomitant iron deficiency, which can mask the macrocytosis typically seen in B 12 deficiency. The same is true for other microcytic disorders like α- or β-thalassemia trait. 40 , 41
An important B 12 nutrient interaction is with folate. In B 12 deficiency, there is disruption of normal folate cycling for regeneration of methylene-tetrahydrofolate, the form required to sustain synthesis of thymidine for DNA replication. Folate becomes effectively “trapped” as methylfolate, 42 because B 12 is required for its conversion to tetrahydrofolate in the methionine synthase reaction ( Figure 3 ). Trapping of methylfolate creates a state of functional folate deficiency. Supply of folic acid to a B 12 -deficient patient can intermittently bypass this block through reduction of folic acid to dihydrofolate and then tetrahydrofolate, thereby partially or temporarily alleviating the anemia. Alleviation of the anemia masks the underlying B 12 deficiency and allows the neurological damage from B 12 deprivation to continue unabated. There is well-described evidence in the early literature that amounts of folic acid exceeding 1 mg daily given to patients with pernicious anemia were fraught with deleterious outcome. 3 , 8 Although at first ameliorating hematological features of the disease, even at times with temporary improvement in neurological symptoms, continued administration of folic acid would precipitate or aggravate neurological complications, usually with subsequent recurrence of the anemia. 8 , 43 Linked to these observations are reports of dissociation between neurological and hematological manifestations in B 12 -deficient patients 6 as well as an inverse correlation between the degree of anemia and the severity of neurological involvement. 44 , 45 There is some evidence that this relationship might be related to higher serum folate concentrations in patients with exclusively or predominantly neurological manifestations. 44 More recently, and occurring in the wake of national folic acid fortification programs designed to reduce neural tube defect pregnancies, there have been several reports from longitudinal population studies that individuals with low serum B 12 levels, who had associated high serum folate levels, had significantly higher levels of methylmalonic acid (MMA) and Hcy and were more likely to show cognitive decline and have lower hemoglobin concentrations than those with low B 12 but without high serum folate. 46-48 Moreover, individuals with low serum B 12 and high serum folate had depressed levels of holotranscobalamin (holoTC), indicating an apparent depletion of circulating active B 12 when serum folate was high. 48 A report that the prevalence of anemia in patients with low B 12 levels before and after the introduction of folic acid fortification was unchanged argues against the proposition that food fortification may have caused an increase in masking the hematological complications of B12 deficiency. 49 However, it is possible that if there is any deleterious effect of folate in B 12 -deficient persons, this occurs only in individuals consuming amounts of folate well in excess of the recommended safe upper limit. 8

Intersections of B 12 and folate metabolism, the methionine cycle, folate cycle, and DNA synthesis showing the methyl folate “trap.” The key intersection of B 12 and folate occurs at the methionine synthase (MS) reaction in which the one-carbon methyl group of methyltetrahydrofolate (MethylTHF) is transferred to Hcy to form methionine. The cofactor for this reaction is B 12 in the form of methylcobalamin. The folate product tetrahydrofolate regains a one-carbon methylene group through the serine hydroxymethyltransferase (SHMT) reaction, and the resulting methylenetetrahydrofolate is essential for conversion of deoxyuridine to thymidine in the thymidylate synthase (TS) reaction. This reaction is rate limiting for DNA synthesis. In B 12 deficiency, folate becomes trapped as methylTHF. Administration of folic acid can temporarily overcome this block through dihydrofolate reductase (DHFR) reduction to tetrahydrofolate. The other product of the MS reaction, the essential amino acid methionine, after adenosylation to S - adenosyl-methionine (SAM), serves as a universal methyl donor in numerous methyltransferase reactions. The product, S - adenosyl-homocysteine (SAH), undergoes reversible hydrolysis by the enzyme adenosyl-homocysteine hydrolase (AHCY hydrolase), yielding Hcy and thus completing the methionine or remethylation cycle. Not shown in this figure is the alternative transsulfuration pathway for Hcy disposal, which requires vitamin B 6 . 8 ATP, adenosine triphosphate; DHFR, dihydrofolate reductase; H + , proton; MTHFR, methylene tetrahydrofolate reductase; NADP + , NAD phosphate; NADPH + , reduced NAD phosphate. Professional illustration by Patrick Lane, ScEYEnce Studios.
There are several causes and varying degrees of severity of B 12 depletion leading to deficiency ( Table 1 ). From the hematological standpoint, it is convenient to divide the causes of B 12 deficiency into those that frequently lead to megaloblastic anemia or overt neurological complications and those that usually do not. 3 , 50 The features that distinguish the severe from the mild category of B 12 deficiency are summarized in Table 2 . The separation is based on pathophysiologic considerations and the degree of severity of the deficiency that occurs. The causes that are listed as severe usually involve disease processes that disrupt some aspect of the physiological pathway for B 12 absorption comprising intrinsic factor and the cubam receptor in the terminal ileum. Undiagnosed or untreated, these conditions ultimately advance to a level of depletion of B 12 that manifests the clinical features of B 12 deficiency, either hematological or neurological or both. The exemplar of this category of B 12 deficiency is pernicious anemia. The slow evolution of this disease reflects the rate at which the autoimmune process disables the manufacture of intrinsic factor in gastric parietal cells leading to the inexorable depletion of the body B 12 store. Gastrectomy emulates abrogation of intrinsic factor production but with surgical suddenness. Similar temporal considerations apply in the case of ileal disease vs surgical resection. In the case of chemical inactivation of B 12 by nitrous oxide, depending on the frequency and duration of its use and the state of B 12 reserves, deficiency can develop either suddenly or insidiously. 22 , 23
Causes of vitamin B 12 deficiency
Severe and mild categories of B 12 deficiency
The causes of mild B 12 deficiency, on the other hand, involve either a disruption of the ability to render natural dietary B 12 bioavailable or a primary dietary lack of B 12 that is obtained among unsupplemented vegans or, to a lesser extent, among vegetarians. 51 There are several conditions that disrupt the normal processes, as discussed in the review by Nielsen et al 12 and depicted in Figure 1 , whereby food B 12 is rendered bioavailable for absorption through the physiological intrinsic factor-cubam receptor pathway ( Table 1 ). Disruption of the mechanisms to render dietary B 12 bioavailable all involve a failure of adequate gastric acid production, disrupting the proteolytic activity of peptic digestion. 52 A similar failure of the digestive process applies in the case of chronic pancreatic disease, 53 in which the release of B 12 from salivary haptocorrin (HC) in the upper small intestine is disrupted through lack of bicarbonate and trypsin production. 54 There are some less common causes of B 12 deficiency that do not fit nicely into either category, such as infestation with the fish tapeworm, Diphyllobothrium latum , in which the degree of deficiency and hence its clinical severity can vary considerably. 55
Two pathophysiologic processes contribute to the anemia resulting from B 12 deficiency. In addition to the ineffective erythropoiesis caused by intramedullary apoptosis of megaloblastic erythroid precursors, 20 the erythrocytes that are produced have increased rigidity associated with abnormal red cell membrane proteins leading to shortened red cell survival. 56 , 57 The resulting hemolysis is associated with a 30% to 50% reduction in red cell lifespan. Plasma bilirubin is increased, 58 as is serum lactic dehydrogenase (LDH), 59 with LDH-1 predominating over LDH-2. 60 Serum AST levels are, however, often normal. 61 There is an increase in serum erythropoietin levels, but the increase is relatively modest, compared with other anemias of similar severity. 62 Another feature arising from the ineffective erythropoiesis is a block in iron utilization, resulting in increased serum iron and ferritin levels, 63 but with increased levels of soluble serum transferrin receptor, presumably related to hemolysis. 64 Corresponding to the increase in LDH, there may be an increase in serum muramidase caused by increased granulocyte turnover. 65
Although often used as the first-line screening test for B 12 deficiency, serum B 12 measurement used in isolation has a generally poor sensitivity and specificity for reliable detection of B 12 deficiency. 4 , 5 A low serum B 12 level does not always indicate B 12 deficiency, and a serum B 12 within the reference range does not always connote normalcy. There are several reasons serum B 12 is not low in all patients with B 12 deficiency. In part, this relates to the distribution of B 12 on the 2 major plasma B 12 binding proteins. Normally, the major fraction (70% to 90%) of circulating B 12 is bound to HC, which is unavailable for immediate delivery to cells. The other 10% to 30% is bound to transcobalamin (TC), the functional B 12 transport protein. Consequently, if levels of the HC-bound fraction are conserved, the total serum B 12 level may lie within the normal reference range, despite lowered levels of the important TC-bound fraction. An extreme example of this is seen in a B 12 -deficient patient with normal serum B 12 levels who has an underlying myeloproliferative disease with high HC levels. 66 In almost 50% of patients with low vitamin B 12 levels, levels of the biochemical markers, MMA and Hcy, were found to be normal, and these patients had no hematologic or neurologic response to B 12 replacement therapy, suggesting that the low B 12 values were false positive results. 67 Serum B 12 levels are usually normal in functional B 12 deficiency, resulting from exposure to nitrous oxide, which chemically inactivates the methylcobalamin at the active site of the methionine synthase during its catalytic cycle. 68 Serum B 12 levels are also usually normal in TC deficiency, and inborn errors of cobalamin metabolism. 69 Conversely, serum B 12 levels may be low in the presence of normal tissue B 12 in vegetarians, 70 in subjects taking megadoses of ascorbic acid, 71 in inherited “benign” HC deficiency, 72 , 73 and in a substantial proportion of patients with megaloblastic anemia resulting from folate deficiency (30%). 4
The B 12 bound to HC comprises 70% to 90% of the total plasma B 12 , yet is unavailable for cellular delivery. That TC is the critical plasma B 12 binding protein is underscored by the fact that inherited TC deficiency is associated with serious hematological and neurological sequelae and, if untreated, fatal outcome, 74 whereas HC deficiency has no morbid consequence. 73 Theoretically, measurement of the TC-bound fraction of the plasma B 12 (holoTC), also termed “active B 12 ,” 75 should be more relevant for assessing functional B 12 status, even though it constitutes only 10% to 30% of the total plasma B 12 . Increasingly, holoTC measurement is being used for clinical assessment of B 12 status, either singly 3 , 76 , 77 or in combination with the total serum B 12 with or without measurement of the metabolites MMA and Hcy. 78-80 In addition, holoTC levels also reflect B 12 absorptive capacity. 81-83
Because they are the substrates of the 2 B 12 -dependent reactions, elevated levels of MMA and Hcy are sensitive indicators of tissue B 12 deficiency. Their levels are high in >90% of B 12 -deficient patients and increase before serum B 12 falls to subnormal levels. 4 , 5 Even when there is no manifest evidence of clinical B 12 deficiency, and serum B 12 levels are not low, elevated levels of MMA and Hcy can be considered as sensitive biomarkers of a subclinical underlying state of B 12 deficiency, which may potentially progress to a state of manifest B 12 deficiency with its attendant clinical complications that may remain subtle, often being only neurological 84 or may become more exuberant. 3 , 85 , 86 MMA measurements can be carried out on either plasma or serum, whereas Hcy is best measured in plasma, because cellular release of Hcy in a clotted blood sample can alter Hcy levels. 87 , 88 Elevated plasma MMA and/or elevated Hcy are both indicators of B 12 deficiency in patients without impaired renal function or an inherited defect in cobalamin processing enzymes. 4 , 13 , 14 , 89 Of the 2, MMA measurement is both more sensitive and more specific, and elevated MMA will persist for several days, even after B 12 treatment. MMA elevation is seen only in B 12 deficiency, unlike Hcy levels that also increase in folate and pyridoxine deficiencies, as well as in hypothyroidism. 4 However, certain intestinal microbes synthesize propionate, a precursor of MMA, and when there is bacterial overgrowth in the small intestine, as occurs in blind loops following gastrointestinal surgery, microbial-derived MMA may contribute to elevations in plasma MMA. 5 , 90 Although measurement of these metabolites may be used in population screening for B 12 deficiency, the finding of an isolated elevation of plasma MMA should not be taken as proof of clinically attributable B 12 deficiency, absent of any ancillary measurements to support that diagnosis or any demonstration of a therapeutic response to the administration of B 12 . 90 , 91
There is currently no approved test in routine clinical use to measure B 12 absorption since the Schilling test became obsolete. Lack of a validated B 12 absorption test hampers accurate diagnosis of pernicious anemia as the cause of B 12 deficiency and clinical investigations related to all causes of B 12 malabsorption. 92 One possible test that shows promise, the Cobasorb test, is based on the measurement of the change in holoTC following oral administration of nonradiolabeled cobalamin. 82 , 93 , 94 An alternative approach has been described using accelerator mass spectrometry to quantify 14 C in the blood following an orally administered dose of [ 14 C]-cyanocobalamin. 95
In absence of any test for B 12 absorption, definitive diagnosis of pernicious anemia is problematic and depends on the demonstration of circulating antibodies to intrinsic factor and gastric parietal cells. Antibodies to intrinsic factor can be of 2 types, varying according to the epitope on the intrinsic factor molecule to which they are directed. For diagnostic purposes, the so-called “blocking” type, directed against the B 12 binding site, is measured, as this type not only is highly specific for pernicious anemia but also is the species present in 70% of patients. 96 Antibodies to parietal cells, although present in 90% of patients with pernicious anemia, are less specific, as they can occur in simple atrophic gastritis and in autoimmune thyroid disease. 97
Regarding prevention of B 12 deficiency, the Institute of Medicine Food and Nutrition Board has defined the RDI for adults at 2.4 μg daily but with the caveat that individuals 51 years and older obtain most of this amount through consuming foods fortified with B 12 or in a B 12 -containing supplement. 8 This rider is added in consideration of the high prevalence of food B 12 malabsorption caused by gastric dysfunction among the elderly. Assuming that the lowest possible MMA level is consistent with optimal well-being, a large segment of the population may exist in a state of precarious B 12 balance, as evidenced by the fact that concentrations of serum MMA leveled off to a nadir in healthy individuals consuming 4 to 7 μg B 12 daily. 98 One of the possible implications of this finding is that individuals consuming less B 12 may have a narrow margin of safety in the event that they were to develop any condition that further compromised their state of B 12 repletion. Provided the physiologic intrinsic factor–cubam pathway for physiologic B 12 absorption is intact, a daily supplement of B 12 of 10 μg or more would suffice to prevent B 12 deficiency or to maintain adequate B 12 status in individuals with food B 12 malabsorption caused by gastric dysfunction, including atrophic gastritis or the chronic use of drugs that impair acid production, such as proton pump inhibitors. 12 , 50 The defined RDI notwithstanding, it is important to recognize that individuals with pernicious anemia or any other condition that interdicts the physiological intrinsic factor cubam absorption pathway would not benefit from the additional Institute of Medicine recommendation.
It is worth noting that prospective interventional trials using Hcy-lowering vitamin supplements containing B 12 in subjects at high risk through suboptimal baseline B vitamin status show a slowing of cognitive decline and of cerebral atrophy. 99 Considering that vitamin B 12 deficiency is the dominant modifiable cause of hyperhomocysteinemia in the post–folic acid fortification era, 100 it is reasonable to conclude that B 12 adequacy is important to maintain, and this becomes progressively more relevant with advancing age.
Concerning treatment of confirmed B 12 deficiency, well-defined guidelines have been enunciated, 50 , 101 the details of which still apply. Some important principles need emphasizing. Where the cause of the deficiency is not known or irreversible, treatment must be lifelong. In general, the form and dosage of treatment depend first on whether the intrinsic factor-dependent pathway is intact or not. If not intact, then the choices lie between intramuscular injection of 1000 μg B 12 (cyanocobalamin in the United States; hydroxocobalamin in Europe) given every other day for 1 to 2 weeks followed by weekly injections for a month and then tapered to once a month indefinitely. Only ∼10% of each B 12 dose is retained. The alternative to injected B 12 is high-dose oral B 12 . Between 1% and 4% of an oral dose of B 12 is absorbed passively, even when the intrinsic factor-dependent pathway is abrogated. 102 Consequently, oral replacement therapy with B 12 , which was used successfully in the past, 103 has again come into vogue, 104 because of convenience and cost. In most instances, however, it would be prudent to “top up” a B 12 -deficient patient through parenteral injection before switching to the oral route for maintenance, with due vigilance concerning compliance, particularly in the elderly. Because the passive route of absorption of B 12 applies to all mucosal surfaces, approved sublingual and intranasal formulations of B 12 are also available. It should be noted that patients with pernicious anemia at times report that the recommended treatment schedule is not adequate to relieve all their neurological symptoms and therefore often request, or may even treat themselves with, B 12 injections more frequently than the guidelines suggest. No biological basis for this apparent increased requirement for B 12 replacement is known, but because there are no reports of adverse effects associated with excess B 12 intake, there is no reason to advise against this practice. 8
Although considered an “old” disease, new information is constantly accruing about B 12 deficiency, the broad array of its effects, and methods for its diagnosis. B 12 deficiency primarily affects the hematopoietic system, but its effects extend to other tissues and organs, most notably the nervous system. The spectrum of clinical presentations is broad so that diagnosis depends first on a high index of suspicion and then on the judicious application of appropriate testing. Because B 12 deficiency is amenable to simple replacement therapy, diagnosis is critical. Several questions still remain unanswered concerning B 12 deficiency, including the possible harmful effects of high folate levels in subjects with low B 12 status, particularly with respect to neurological damage. Other newer areas of investigation that may provide better insights into the variability of expression of B 12 deficiency include genetic analysis and the effects of the microbiome.
Contribution: R.G. wrote the manuscript.
Conflict-of-interest disclosure: The author declares no competing financial interests.
Correspondence: Ralph Green, Department of Pathology and Laboratory Medicine, University of California, Davis, 4400 V St, PATH Building, Sacramento, CA 95817; e-mail: [email protected] .
This feature is available to Subscribers Only
- Previous Article
- Next Article
Email alerts
Affiliations.
- Current Issue
- First edition
- Collections
- Submit to Blood
- About Blood
- Subscriptions
- Public Access
- Permissions
- Blood Classifieds
- Advertising in Blood
- Terms and Conditions
American Society of Hematology
- 2021 L Street NW, Suite 900
- Washington, DC 20036
- TEL +1 202-776-0544
- FAX +1 202-776-0545
ASH Publications
- Blood Advances
- Blood Neoplasia
- Blood Vessels, Thrombosis & Hemostasis
- Hematology, ASH Education Program
- ASH Clinical News
- The Hematologist
- Publications
- Privacy Policy
- Cookie Policy
- Terms of Use
This Feature Is Available To Subscribers Only
Sign In or Create an Account

ROBERT C. LANGAN, MD, FAAFP, AND ANDREW J. GOODBRED, MD
Am Fam Physician. 2017;96(6):384-389
Patient information : A handout on this topic is available at https://familydoctor.org/vitamin-b-12 .
Author disclosure: No relevant financial affiliations.
Vitamin B 12 deficiency is a common cause of megaloblastic anemia, various neuropsychiatric symptoms, and other clinical manifestations. Screening average-risk adults for vitamin B 12 deficiency is not recommended. Screening may be warranted in patients with one or more risk factors, such as gastric or small intestine resections, inflammatory bowel disease, use of metformin for more than four months, use of proton pump inhibitors or histamine H 2 blockers for more than 12 months, vegans or strict vegetarians, and adults older than 75 years. Initial laboratory assessment should include a complete blood count and serum vitamin B 12 level. Measurement of serum methylmalonic acid should be used to confirm deficiency in asymptomatic high-risk patients with low-normal levels of vitamin B 12 . Oral administration of high-dose vitamin B 12 (1 to 2 mg daily) is as effective as intramuscular administration for correcting anemia and neurologic symptoms. Intramuscular therapy leads to more rapid improvement and should be considered in patients with severe deficiency or severe neurologic symptoms. Absorption rates improve with supplementation; therefore, patients older than 50 years and vegans or strict vegetarians should consume foods fortified with vitamin B 12 or take vitamin B 12 supplements. Patients who have had bariatric surgery should receive 1 mg of oral vitamin B 12 per day indefinitely. Use of vitamin B 12 in patients with elevated serum homocysteine levels and cardiovascular disease does not reduce the risk of myocardial infarction or stroke, or alter cognitive decline.
Vitamin B 12 (cobalamin) is a water-soluble vitamin obtained through the ingestion of fish, meat, and dairy products, as well as fortified cereals and supplements. 1 , 2 It is coabsorbed with intrinsic factor, a product of the stomach's parietal cells, in the terminal ileum after being extracted by gastric acid 1 , 2 ( Figure 1 3 ) . Vitamin B 12 is crucial for neurologic function, red blood cell production, and DNA synthesis, and is a cofactor for three major reactions: the conversion of methylmalonic acid to succinyl coenzyme A; the conversion of homocysteine to methionine; and the conversion of 5-methyltetrahydrofolate to tetrahydrofolate. 1 , 2
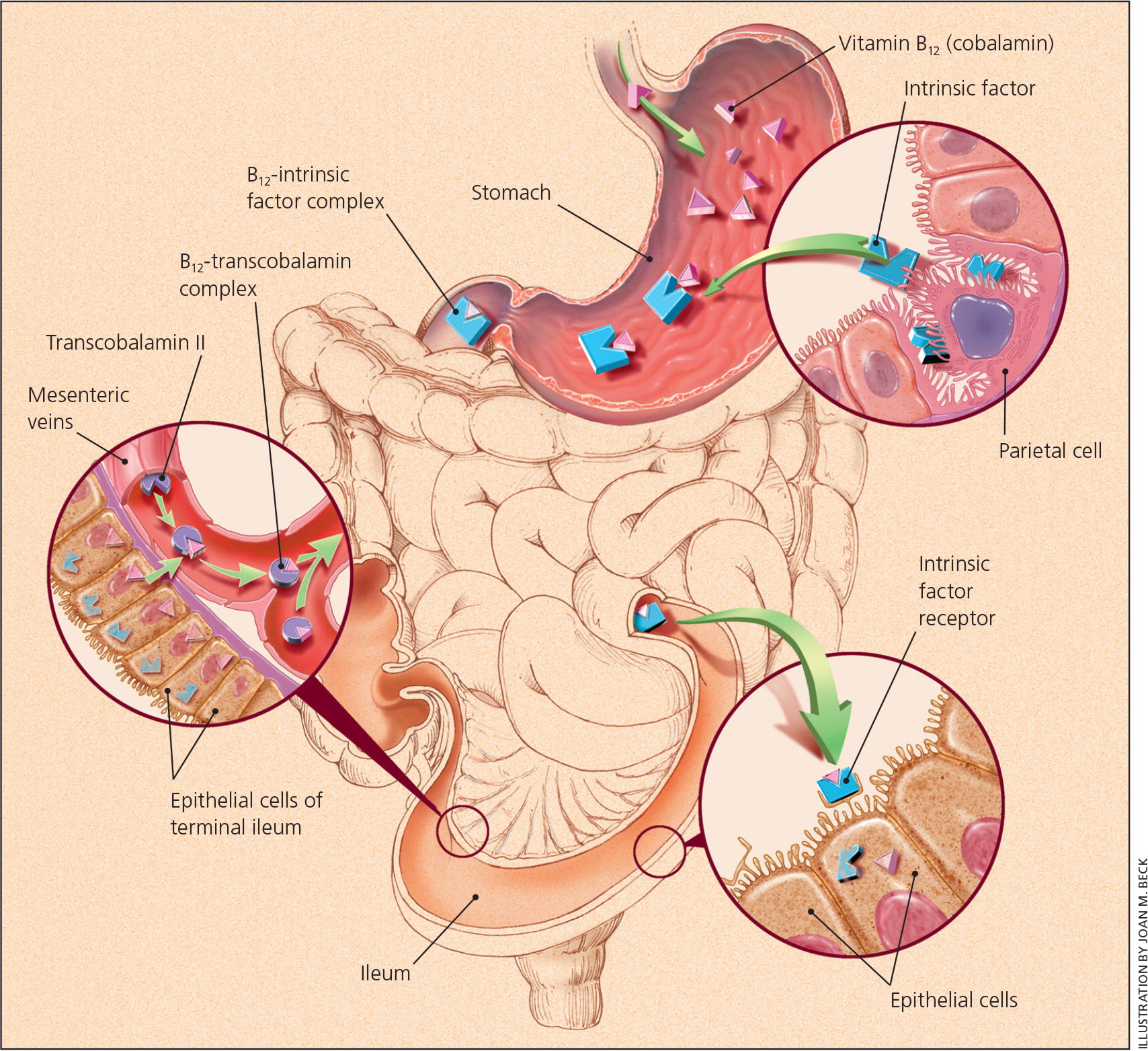
In the United States and the United Kingdom, the prevalence of vitamin B 12 deficiency is approximately 6% in persons younger than 60 years, and nearly 20% in those older than 60 years. 1 Latin American countries have a clinical or subclinical deficiency rate of approximately 40%. 1 The prevalence is 70% in Kenyan school children, 80% in East Indian preschool-aged children, and 70% in East Indian adults. 1 Certain risk factors increase the prevalence of vitamin B 12 deficiency ( Table 1 ) . 4 , 5 Dietary insufficiency, pernicious anemia (i.e., an autoimmune process that reduces available intrinsic factor and subsequent absorption of vitamin B 12 1 , 2 , 6 , 7 ), and long-term use of metformin or acid-suppressing medications have been implicated in B 12 deficiency. 8 , 9
A multicenter randomized controlled trial of 390 patients with diabetes mellitus showed that those taking 850 mg of metformin three times per day had an increased risk of vitamin B 12 deficiency (number needed to harm = 14 per 4.3 years) and low vitamin B 12 levels (number needed to harm = 9 per 4.3 years) vs. placebo. 8 This effect increased with duration of therapy, and patients had an unclear prophylactic supplementation response. 8 A case-control study that compared 25,956 patients who had vitamin B 12 deficiency with 184,199 control patients found a significantly increased risk of vitamin B 12 deficiency in patients who had taken proton pump inhibitors (odds ratio = 1.65) or histamine H 2 blockers (odds ratio = 1.25) for at least two years. 9 In light of these findings, long-term use of these medications should be periodically reassessed, particularly in patients with other risk factors for vitamin B 12 deficiency. 8 , 9
Manifestations
Vitamin B 12 deficiency affects multiple systems, and sequelae vary in severity from mild fatigue to severe neurologic impairment 1 , 2 , 6 , 10 ( Table 2 4 , 10 ) . The substantial hepatic storage of vitamin B 12 can delay clinical manifestations for up to 10 years after the onset of deficiency. 11 Bone marrow suppression is common and potentially affects all cell lines, with megaloblastic anemia being most common. 1 , 2 , 6 The resultant abnormal erythropoiesis can trigger other notable abnormal laboratory findings, such as decreased haptoglobin levels, high lactate dehydrogenase levels, and elevated reticulocyte count. 1 , 2 , 6 Symptoms typically include being easily fatigued with exertion, palpitations, and skin pallor. 1 , 2 , 6 Skin hyperpigmentation, glossitis, and infertility have also been reported. 1 , 2 , 6 Neurologic manifestations are caused by progressive demyelination and can include peripheral neuropathy, areflexia, and the loss of proprioception and vibratory sense. Areflexia can be permanent if neuronal death occurs in the posterior and lateral spinal cord tracts. 1 , 2 , 6 , 12 Dementia-like disease, including episodes of psychosis, is possible with more severe and chronic deficiency. 1 , 12 Clinical evaluation seems to show an inverse relationship between the severity of megaloblastic anemia and the degree of neurologic impairment. 2
Maternal vitamin B 12 deficiency during pregnancy or while breastfeeding may lead to neural tube defects, developmental delay, failure to thrive, hypotonia, ataxia, and anemia. 4 , 13 – 16 Women at high risk or with known deficiency should supplement with vitamin B 12 during pregnancy or while breastfeeding. 4 , 14 – 16
Screening and Diagnosis
Screening persons at average risk of vitamin B 12 deficiency is not recommended. 17 Screening should be considered in patients with risk factors, and diagnostic testing should be considered in those with suspected clinical manifestations. 1 , 2 , 6 , 18
The recommended laboratory evaluation for patients with suspected vitamin B 12 deficiency includes a complete blood count and serum vitamin B 12 level. 2 , 19 – 21 A level of less than 150 pg per mL (111 pmol per L) is diagnostic for deficiency. 1 , 2 Serum vitamin B 12 levels may be artificially elevated in patients with alcoholism, liver disease, or cancer because of decreased hepatic clearance of transport proteins and resultant higher circulating levels of vitamin B 12 ; physicians should use caution when interpreting laboratory results in these patients. 22 , 23 In patients with a normal or low-normal serum vitamin B 12 level, complete blood count results demonstrating macrocytosis, or suspected clinical manifestations, a serum methylmalonic acid level is an appropriate next step 1 , 2 , 6 , 18 and is a more direct measure of vitamin B 12 's physiologic activity. 1 , 2 Although not clinically validated or available for widespread use, measurement of holotranscobalamin, the metabolically active form of vitamin B 12 , is an emerging method of detecting deficiency. 1 , 2 , 18 Table 3 lists the relative sensitivities and specificities of various laboratory tests. 24
Pernicious anemia refers to one of the hematologic manifestations of chronic auto-immune gastritis, in which the immune system targets the parietal cells of the stomach or intrinsic factor itself, leading to decreased absorption of vitamin B 12 . 1 Asymptomatic autoimmune gastritis likely precedes gastric atrophy by 10 to 20 years, followed by the onset of iron-deficiency anemia that occurs as early as 20 years before vitamin B 12 deficiency pernicious anemia. 25
Patients diagnosed with vitamin B 12 deficiency whose history and physical examination do not suggest an obvious dietary or malabsorptive etiology should be tested for pernicious anemia with anti-intrinsic factor antibodies (positive predictive value = 95%), particularly if other autoimmune disorders are present. 1 , 2 , 6 , 18 Patients with pernicious anemia may have hematologic findings consistent w ith normocytic anemia. 1 If anti-intrinsic factor results are negative but suspicion for pernicious anemia remains, an elevated serum gastrin level is consistent with the diagnosis. 2 The Schilling test, which was once the diagnostic standard for pernicious anemia, is no longer available in the United States. Figure 2 presents an approach to diagnosing vitamin B 12 deficiency and pernicious anemia. 18 , 26
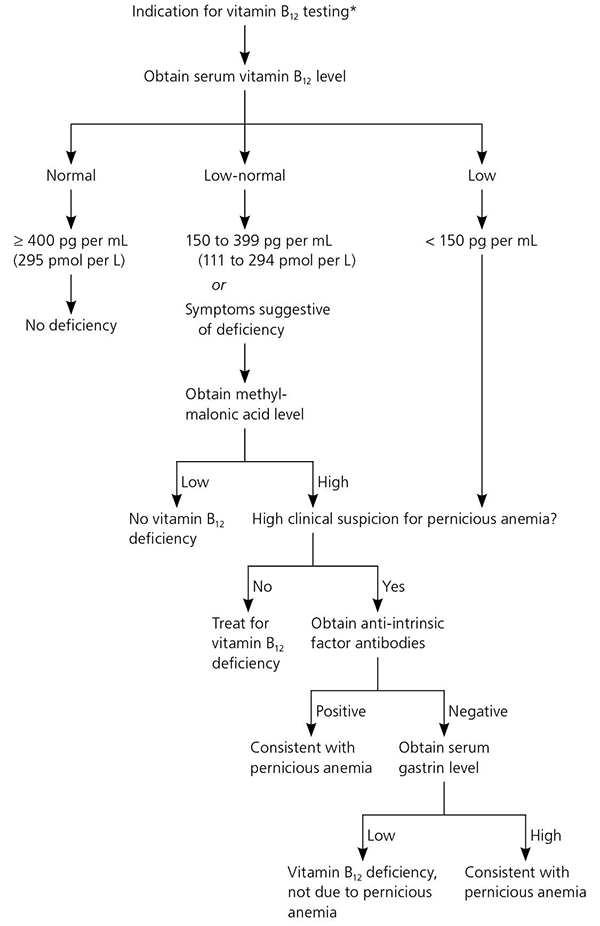
Vitamin B 12 deficiency can be treated with intramuscular injections of cyanocobalamin or oral vitamin B 12 therapy. Approximately 10% of the standard injectable dose of 1 mg is absorbed, which allows for rapid replacement in patients with severe deficiency or severe neurologic sy mptoms. 2 Guidelines from the British Society for Haematology recommend injections three times per week for two weeks in patients without neurologic deficits. 18 If neurologic deficits are present, injections should be given every other day for up to three weeks or until no further improvement is noted. Table 4 lists the usual times until improvement for abnormalities associated with vitamin B 12 deficiency. 27 In general, patients with an irreversible cause should be treated indefinitely, whereas those with a reversible cause should be treated until the deficiency is corrected and symptoms resolve. 1 If vitamin B 12 deficiency coexists with folate deficiency, vitamin B 12 should be replaced first to prevent subacute combined degeneration of the spinal cord. 1 The British Society for Haematology does not recommend retesting vitamin B 12 levels after treatment has been initiated, and no guidelines address the optimal interval for screening high-risk patients. 18
A 2005 Cochrane review involving 108 patients with vitamin B 12 deficiency found that high-dose oral replacement (1 mg to 2 mg per day) was as effective as parenteral administration for correcting anemia and neurologic symptoms. 28 However, oral therapy does not improve serum methylmalonic acid levels as well as intramuscular therapy, although the clinical relevance is unclear. 29 There is also a lack of data on the long-term benefit of oral therapy when patients do not take daily doses. 2 There is insufficient data to recommend other formulations of vitamin B 12 replacement (e.g., nasal, sublingual, subcutaneous). 2 The British Society for Haematology recommends intramuscular vitamin B 12 for severe deficiency and malabsorption syndromes, whereas oral replacement may be considered for patients with asymptomatic, mild disease with no absorption or compliance concerns. 18
Because of potential interactions from prolonged medication use, physicians should consider screening patients for vitamin B 12 deficiency if they have been taking proton pump inhibitors or H 2 blockers for more than 12 months, or metformin for more than four months. 5 The average intake of vitamin B 12 in the United States is 3.4 mcg per day, and the recommended dietary allowance is 2.4 mcg per day for adult men and nonpregnant women, and 2.6 mcg per day for pregnant women. 30 Patients older than 50 years may not be able to adequately absorb dietary vitamin B 12 and should consume food fortified with vitamin B 12 . 30 Vegans and strict vegetarians should be counseled to consume fortified cereals or supplements to prevent deficiency. The American Society for Metabolic and Bariatric Surgery recommends that patients who have had bariatric surgery take 1 mg of oral vitamin B 12 per day indefinitely. 31
Vitamin B12 and Hyperhomocysteinemia
Vitamin B 12 deficiency is a much more common cause of hyperhomocysteinemia in developed countries than folate deficiency because of widespread fortification of food with folate. Although epidemiologic studies have shown an association between vascular disease and hyperhomocysteinemia, large randomized controlled trials have shown that lowering homocysteine levels in these patients does not reduce the number of myocardial infarctions or strokes, or improve mortality rates. 32 Similarly, an association between elevated homocysteine levels and cognitive impairment has been noted, but subsequent vitamin B 12 replacement does not have preventive or therapeutic benefit. 33
This article updates previous articles on this topic by Langan and Zawistoski , 4 and by Oh and Brown . 3
Data Sources : A PubMed search was completed in Clinical Queries using the key terms vitamin B 12 , cobalamin, deficiency, and treatment. The search included meta-analyses, randomized controlled trials, clinical trials, and reviews. Also searched were the Agency for Healthcare Research and Quality evidence reports, Clinical Evidence, the Cochrane database, Essential Evidence, the Institute for Clinical Systems Improvement, the National Guideline Clearinghouse database, and the U.S. Preventive Services Task Force. Search dates: March 1, 2016; October 20, 2016; and June 9, 2017.
Hunt A, Harrington D, Robinson S. Vitamin B 12 deficiency. BMJ. 2014;349:g5226.
Stabler SP. Clinical practice. Vitamin B 12 deficiency. N Engl J Med. 2013;368(2):149-160.
Brown DL, Oh R. Vitamin B 12 deficiency. Am Fam Physician. 2003;67(5):979-986.
Langan RC, Zawistoski KJ. Update on vitamin B 12 deficiency. Am Fam Physician. 2011;83(12):1425-1430.
Agency for Healthcare Research and Quality. Guideline summary: cobalamin (vitamin B 12 ) deficiency—investigation and management. January 1, 2012. https://www.guideline.gov/summaries/summary/38881 . Accessed October 13, 2016.
Dali-Youcef N, Andrès E. An update on cobalamin deficiency in adults. QJM. 2009;102(1):17-28.
Toh BH, van Driel IR, Gleeson PA. Pernicious anemia. N Engl J Med. 1997;337(20):1441-1448.
de Jager J, Kooy A, Lehert P, et al. Long term treatment with metformin in patients with type 2 diabetes and risk of vitamin B 12 deficiency: randomised placebo controlled trial. BMJ. 2010;340:c2181.
Lam JR, Schneider JL, Zhao W, Corley DA. Proton pump inhibitor and histamine 2 receptor antagonist use and vitamin B 12 deficiency. JAMA. 2013;310(22):2435-2442.
Derin S, Koseoglu S, Sahin C, Sahan M. Effect of vitamin B 12 deficiency on olfactory function. Int Forum Allergy Rhinol. 2016;6(10):1051-1055.
Carmel R. Current concepts in cobalamin deficiency. Annu Rev Med. 2000;51:357-375.
Reynolds E. Vitamin B 12 , folic acid, and the nervous system. Lancet Neurol. 2006;5(11):949-960.
Molloy AM, Kirke PN, Troendle JF, et al. Maternal vitamin B 12 status and risk of neural tube defects in a population with high neural tube defect prevalence and no folic acid fortification. Pediatrics. 2009;123(3):917-923.
Dror DK, Allen LH. Effect of vitamin B 12 deficiency on neurodevelopment in infants: current knowledge and possible mechanisms. Nutr Rev. 2008;66(5):250-255.
Centers for Disease Control and Prevention (CDC). Neurologic impairment in children associated with maternal dietary deficiency of cobalamin—Georgia, 2001. MMWR Morb Mortal Wkly Rep. 2003;52(4):61-64.
Hay G, Johnston C, Whitelaw A, Trygg K, Refsum H. Folate and cobalamin status in relation to breastfeeding and weaning in healthy infants. Am J Clin Nutr. 2008;88(1):105-114.
U.S. Preventive Services Task Force. A-Z topic guide. http://www.uspreventiveservicestaskforce.org/uspstopics.htm#AZ . Accessed May 24, 2016.
Devalia V, Hamilton MS, Molloy AM British Committee for Standards in Haematology. Guidelines for the diagnosis and treatment of cobalamin and folate disorders. Br J Haematol. 2014;166(4):496-513.
Carmel R, Green R, Rosenblatt DS, Watkins D. Update on cobalamin, folate, and homocysteine. Hematology Am Soc Hematol Educ Program. 2003:62-81.
Kaferle J, Strzoda CE. Evaluation of macrocytosis. Am Fam Physician. 2009;79(3):203-208.
Stabler SP, Allen RH. Megaloblastic anemias. In: Cecil RL, Goldman L, Ausiello DA, eds. Cecil Textbook of Medicine . 22nd ed. Philadelphia, Pa.: Saunders; 2004:1050–1057.
Arendt JF, Nexo E. Cobalamin related parameters and disease patterns in patients with increased serum cobalamin levels. PLoS One. 2012;7(9):e45979.
Andrès E, Serraj K, Zhu J, Vermorken AJ. The pathophysiology of elevated vitamin B 12 in clinical practice. QJM. 2013;106(6):505-515.
Oberley MJ, Yang DT. Laboratory testing for cobalamin deficiency in megaloblastic anemia. Am J Hematol. 2013;88(6):522-526.
Toh BH. Pathophysiology and laboratory diagnosis of pernicious anemia. Immunol Res. 2017;65(1):326-330.
Moridani M, Ben-Poorat S. Laboratory investigation of vitamin B 12 deficiency. Lab Med. 2006;37(3):166-174.
Carmel R. How I treat cobalamin (vitamin B 12 ) deficiency. Blood. 2008;112(16):2214-2221.
Vidal-Alaball J, Butler CC, Cannings-John R, et al. Oral vitamin B 12 versus intramuscular vitamin B 12 for vitamin B 12 deficiency. Cochrane Database Syst Rev. 2005(3):CD004655.
Kuzminski AM, Del Giacco EJ, Allen RH, Stabler SP, Lindenbaum J. Effective treatment of cobalamin deficiency with oral cobalamin. Blood. 1998;92(4):1191-1198.
Institute of Medicine. Dietary Reference Intakes for Thiamin, Riboflavin, Niacin, Vitamin B 6 , Folate, Vitamin B 12 , Pantothenic Acid, Biotin, and Choline . Washington, DC: National Academy Press; 1998.
Mechanick JI, Youdim A, Jones DB, et al. Clinical practice guidelines for the perioperative nutritional, metabolic, and nonsurgical support of the bariatric surgery patient—2013 update: cosponsored by American Association of Clinical Endocrinologists, the Obesity Society, and American Society for Metabolic & Bariatric Surgery. Surg Obes Relat Dis. 2013;9(2):159-191.
Yang HT, Lee M, Hong KS, Ovbiagele B, Saver JL. Efficacy of folic acid supplementation in cardiovascular disease prevention: an updated meta-analysis of randomized controlled trials. Eur J Intern Med. 2012;23(8):745-754.
Nachum-Biala Y, Troen AM. B-vitamins for neuroprotection: narrowing the evidence gap. Biofactors. 2012;38(2):145-150.
Continue Reading
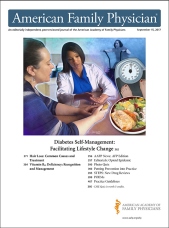
More in AFP
More in pubmed.
Copyright © 2017 by the American Academy of Family Physicians.
This content is owned by the AAFP. A person viewing it online may make one printout of the material and may use that printout only for his or her personal, non-commercial reference. This material may not otherwise be downloaded, copied, printed, stored, transmitted or reproduced in any medium, whether now known or later invented, except as authorized in writing by the AAFP. See permissions for copyright questions and/or permission requests.
Copyright © 2024 American Academy of Family Physicians. All Rights Reserved.
- HEMATOLOGY CASE STUDY
- HEMATOLOGY LECTURES
- PRACTICAL HEMATOLOGY
- Privacy Policy
- Site Policy
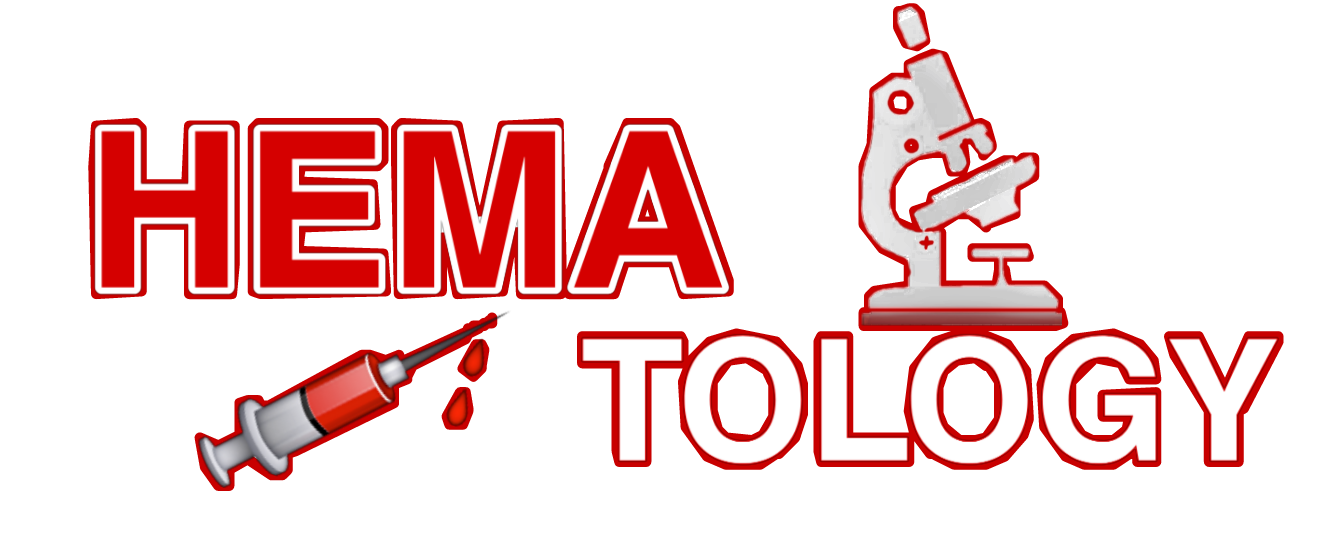
www.hematology-lessons.com
Case study (6)- megaloblastic anemia.

Examination:
Investigations:, peripheral blood smear.
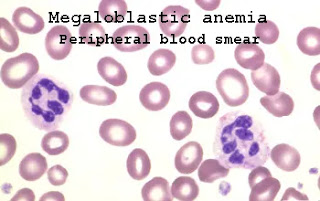
Further Laboratory Studies
Latest articles from : hematology case study, post a comment.
- Search Menu
- Sign in through your institution
- Advance Articles
- Supplements
- Author Guidelines
- Submission Site
- Open Access
- Self-Archiving Policy
- Advertising & Corporate Services
- Advertising
- Reprints and ePrints
- Sponsored Supplements
- Branded Books
- Journals Career Network
- About Paediatrics & Child Health
- About The Canadian Paediatric Society
- Editorial Board
- Journals on Oxford Academic
- Books on Oxford Academic
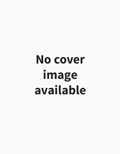
Article Contents
Case 2 diagnosis: megaloblastic anemia due to dietary cobalamin deficiency, clinical pearls, recommended reading.
- < Previous
Case 2: A pale infant – not a typical case of iron deficiency
- Article contents
- Figures & tables
- Supplementary Data
Michelle P Wong, Louis Wadsworth, John K Wu, David Dix, Case 2: A pale infant – not a typical case of iron deficiency, Paediatrics & Child Health , Volume 13, Issue 6, July/August 2008, Pages 507–511, https://doi.org/10.1093/pch/13.6.507a
- Permissions Icon Permissions
A 15-month-old boy presented to his doctor when his mother became concerned about his diet. Despite the introduction of solids at six months of age, he was almost exclusively breastfed and a ‘picky eater’. His parents were partial vegetarians, with little meat intake. His mother had noticed that he was pale with gait disturbance and occasional falls. His developmental history was otherwise unremarkable. His past medical history included a term delivery with no complications and an uneventful postnatal period. After initial bloodwork, he was referred to a paediatric hematologist.
On examination, he was pale. His weight and height were between the 5th to 10th and 15th percentiles, respectively. A systolic murmur grade 2/6 was present. The patient's bloodwork included a complete blood count with white blood cell count 5.2×10 9 /L (normal 5.3×10 9 /L to 16×10 9 /L), neutrophils 1×10 9 /L (normal 1×10 9 /L to 6.5×10 9 /L), hemoglobin 83 g/L (normal 103 g/L to 135 g/L), mean corpuscular volume 107 fL (normal 70 fL to 86 fL) and platelet count 311×10 9 /L (normal 200×10 9 /L to 550×10 9 /L). His reticulocyte count was 31×10 9 /L (normal 40×10 9 /L to 120×10 9 /L), and his peripheral blood smear showed occasional oval macrocytes and hypersegmented neutrophils. A bone marrow aspirate was performed, and the images demonstrated classic findings of a particular diagnosis.
Additional investigations for macrocytic anemia included serum folate 33.3 nmol/L (normal greater than 6.8 nmol/L), and vitamin B 12 (cobalamin) 44 pmol/L (deficient range lower than 107 pmol/L). Based on the test results and the bone marrow aspirate findings of hypersegmented neutrophils, megaloblasts and giant metamyelocytes, a diagnosis of megaloblastic anemia (MA) was made.
Bloodwork was also performed on the mother. Serum folate was normal at 30.9 nmol/L (greater than 6.81 nmol/L) and serum vitamin B 12 was low-normal at 209 pmol/L (normal 133 pmol/L to 675 pmol/L). Maternal hemoglobin level and mean corpuscular volume (MCV) were normal.
Given the history, the likely cause of MA and cobalamin deficiency was reduced dietary intake. The toddler was treated with intramuscular cyanocobalamin injections (20 μg daily for seven days, then 100 μg weekly for four weeks) and a dietician was consulted. Oral cobalamin therapy was also an option and would have been effective, but for convenience and to ensure compliance, the parenteral route was chosen.
Two months later, he had improved significantly with a normal hemoglobin level (107 g/L) and MCV (83 fl). He was more energetic and interactive. His gait had returned to normal and his falls had decreased. His diet had improved with increased intake of meats, eggs, milk, fruits and vegetables, supplemented with PediaSure (Abbott Nutrition, Canada). Eight months after presentation, his development was appropriate for age and he was not anemic, despite being off therapy for several months. Maintenance of normal counts after discontinuation of therapy and adherence to a balanced diet are consistent with a dietary etiology for MA.
MA describes a group of disorders characterized by defective DNA synthesis. Morphological hallmarks in the marrow are megaloblasts and giant metamyelocytes displaying nuclear to cytoplasmic asynchrony. A megaloblast has a nucleus that is immature relative to the cytoplasm because the nucleus has impaired DNA synthesis, but hemoglobinization, which is dependant on ribosomal function, continues normally in the cytoplasm. There are many causes of MA, some of which are listed in Table 1 .
Causes of megaloblastic anemia
The case described demonstrates MA in a paediatric patient who was a ‘picky eater’ and was found to have macrocytic anemia. The insidious onset can delay diagnosis. It is important to diagnose this condition early to avoid the symptoms of anemia, as well as the neurological sequelae, including loss of vibration sensation and potential progression to spastic ataxia due to demyelination of the dorsal and lateral columns of the spinal cord. An approach to the workup of suspected MA in paediatric patients is proposed.
History and physical examination
Many patients are asymptomatic, and a diagnosis of MA is made incidentally when macrocytosis is found on routine bloodwork. There may be a history of poor food intake, prolonged breastfeeding, and a maternal history of vegetarian and vegan diets or autoimmune disorders. Nonspecific symptoms include irritability, weight loss, diarrhea or constipation. The clinical features are primarily those of a classic ‘lemon yellow’ pallor because of the combination of anemia and jaundice. Severe cases may have marked anorexia, weight loss, glossitis and angular cheilosis. Neurological effects may be manifested by failure to reach developmental milestones and may include paresthesias, muscle weakness and impaired intellectual development.
Screening bloodwork
The complete blood count shows macrocytic anemia. The MCV can be normal when there is concomitant microcytosis (thalassemia trait or iron deficiency). The peripheral blood smear shows oval macrocytes and hypersegmented neutrophils (five or more lobes) ( Figure 1 ). If a child is being breastfed, maternal bloodwork must be performed to exclude maternal vitamin deficiencies.

Peripheral blood smear demonstrating a hypersegmented neutrophil
Diagnostic tests
Folate and cobalamin levels are critical diagnostic blood tests. Red blood cell folate levels may be a better indicator of body folate because recent changes in dietary intake and hemolysis of the specimen will interfere with serum folate levels. Patients deficient in folate have low assay results, but a significant proportion of cobalamin-deficient patients will also have low red cell folate assays because cobalamin is a cofactor in folate metabolism. Cobalamin assays have limitations when correlating clinical deficiency with low-normal assay levels in some patients. Concentrations of the cobalamin carrier protein transcobalamin 1 can influence serum levels of vitamin B 12 . The assays for folate and vitamin B 12 levels are generally robust and convenient for diagnosing deficient states.
A bone marrow biopsy is an invasive procedure and less frequently performed with the availability of diagnostic blood tests, but it can be warranted to expedite diagnosis. The marrow can provide a morphological diagnosis within a matter of minutes; however, it requires skilled physician resources. A bone marrow aspirate in MA shows hypercel-lularity with ineffective hematopoiesis and megaloblastic erythropoiesis ( Figure 2 ), giant myeloid precursors (giant metamyelocytes) ( Figures 2 and 3 ), increased iron stores and, less commonly, hyperpolyploid megakaryocytes. When no explanation for cytopenias is found, bone marrow studies must be performed to exclude bone marrow failure, hematological malignancy or metastatic tumour.

Bone marrow aspirate demonstrating megaloblastic hematopoiesis with a megaloblast (white arrow) and a giant metamye-locyte (black arrow)

Bone marrow aspirate demonstrating megaloblastic hematopoiesis with a giant metamyelocyte (black arrow)
Additional tests and special tests
Schilling test:.
When Addisonian pernicious anemia (PA) is suspected, a Schilling test may be performed to assess cobalamin absorption. The test measures urinary excretion of orally administered radioactive cobalamin, with and without added intrinsic factor. PA is rare in children. The Schilling test would be helpful, and should be performed when there is a need to distinguish PA from the rarer malabsorptive errors of cobalamin absorption that are listed in Table 1 .
Total plasma homocysteine, serum methylmalonate and urinary excretion of methylmalonate:
Vitamin B 12 , but not folate, is required in methylmalonate (MMA) metabolism. Increased total plasma homocysteine and MMA levels are associated with cobalamin deficiency. Total plasma homocysteine level is elevated in both folate and vitamin B 12 deficiency, and is less specific than MMA. These tests, however, may be useful in suspected presymptomatic deficiency when the patient is not anemic and cobalamin levels are in the low-normal range. Elevated total plasma homocysteine and MMA levels may signal functional vitamin B 12 deficiency ( 1 ). Availability of these tests may limit their diagnostic application.
The etiology of folate or cobalamin deficiency must be determined because most causes are preventable or treatable. In developed countries, MA is more commonly caused by cobalamin rather than folate deficiency because many foods are folate-supplemented. Cobalamin deficiency must be ruled out before administering folic acid because treatment with folic acid alone, in the presence of cobalamin deficiency, can cause or exacerbate irreversible neurological damage.
MA can occur in children when there is a history of ‘picky eating’, poverty, chronic hemolysis such as hereditary spherocytosis, diets low in animal products or prolonged breastfeeding. Human milk cobalamin concentrations have been found to be lower in vegetarian mothers compared with omnivorous mothers ( 2 ). Breastfeeding mothers can have clinical ( 3 ) or subclinical cobalamin deficiency, the latter having low-normal cobalamin levels ( 1 ). As such, infants of cobalamin-deficient mothers are at risk of developing MA. Cobalamin deficiency may also occur in infants born to mothers with PA; however, this risk is largely theoretical because women with PA are usually infertile ( 4 ).
Early diagnosis of MA in childhood is imperative to prevent neurological consequences in infants who remain untreated. Increased awareness leading to early diagnosis and appropriate, timely therapy can prevent irreversible neurological effects for the child with MA. This is especially important during the critical period of neurodevelopment in early childhood.
Prolonged breastfed infants may be at risk of developing MA, especially if the mother consumes a vegetarian or vegan diet.
Cobalamin deficiency must be ruled out before instituting folate therapy.
Cobalamin deficiency may have neuropsychiatric as well as hematological manifestations, and early diagnosis is imperative to prevent irreversible neurological damage.
Measurement of serum MMA is important in the detection of presymptomatic cobalamin deficiency.
Google Scholar
Google Preview
Email alerts
Citing articles via, looking for your next opportunity.
- About Paediatrics & Child Health
- Recommend to Your Librarian
- Advertising and Corporate Services
Affiliations
- Online ISSN 1918-1485
- Print ISSN 1205-7088
- Copyright © 2024 Oxford University Press
- About Oxford Academic
- Publish journals with us
- University press partners
- What we publish
- New features
- Open access
- Institutional account management
- Rights and permissions
- Get help with access
- Accessibility
- Media enquiries
- Oxford University Press
- Oxford Languages
- University of Oxford
Oxford University Press is a department of the University of Oxford. It furthers the University's objective of excellence in research, scholarship, and education by publishing worldwide
- Copyright © 2024 Oxford University Press
- Cookie settings
- Cookie policy
- Privacy policy
- Legal notice
This Feature Is Available To Subscribers Only
Sign In or Create an Account
This PDF is available to Subscribers Only
For full access to this pdf, sign in to an existing account, or purchase an annual subscription.
- CONNECT COMMUNITY
- CE ORGANIZER
- LABUCATE VIRTUAL LEARNING
- MEMBER CENTRAL
- Constituent Societies
- Membership Packages
- Registration
- Accommodations
- Poster Abstract Submissions
- Poster Information
- Partner Engagement
- eCLECtic Blog
- Laboratory Educators Institute
- CE Organizer Data Transfer
- Labucate Virtual Learning
- Online P.A.C.E. Courses
- Labvocate Symposium
- Social Media
- Society News Now
- ASCLS Today
- ASCLS Connect Community
- Clinical Laboratory Science Journal
- The Bench Connection
- Ascending Professionals Forum
- Developing Professionals Forum
- House of Delegates
- Governance Resources
- Volunteer Opportunities
- Scientific Assemblies
- Lab Week-MLPW
- Mentorship Program
- Legislative Symposium
- ASCLS Political Action Committee
- Patient Safety
- Labvocate Action Center
- Position Papers
- Career Center – Job Search
- Career Center – Employers
- How do I become a laboratory professional?
- Online Academic Programs
- Certification Information
- DCLS Body of Knowledge
- Career Recruitment Tool Kit
- Laboratory Science Careers Website
- PACE Providers List
- Mission Vision Statement
- Code of Ethics
- Board of Directors
- Leadership Directories
- Leadership Resources
- Past Presidents
- Professional Staff
- Awards and Scholarships
- Education & Research Fund
- Industry Support
- ASCLS Voices Under 40
- Case Study: Vitamin B12 Deficiency
Volume 35 Number 4 | August 2021
Minh kosfeld, phd, mlt(ascp) cm, patient physical examination on admission.
Our patient is a previously healthy 56-year-old white man who presents with progressive neuropathy and declining mental status over several months. The patient initially developed numbness of his fingertips and the balls of his feet and began to lose motor control of his hands, which manifested as dropping objects or flinging them as he tried to pick them up. As symptoms progressed, he had visual tracking problems that were severe enough to interfere with driving a car, and he developed short-term memory loss and slowing of cognitive function.
Laboratory Results
Diagnosis and treatment.
Based on the clinical presentation and laboratory findings, a diagnosis of psychomotor regression due to B12 deficiency was made. The patient was then treated with a series of B12 intramuscular injections, which resulted in rapid remission of associated neurological symptoms. In follow-up laboratory examinations, his B12 level was normalized in two months (606 pg/mL), and MMA in eight months (197 nmol/L).
B12 is the largest and most complex of the water-soluble B vitamins. Since it contains cobalt, compounds with B12 activity are collectively called “cobalamins.” For humans, the only natural dietary sources of B12 are animal products (meat, dairy), in which it is bound to protein. The B12 absorption mechanism is complex, requiring several transporter proteins. First, it must be freed from the food matrix by gastric HCl and pepsin. Once liberated, it is transported to the duodenum by haptocorrin (transcobalamin I), a cobalamin-binding protein produced in the saliva. In the duodenum, pancreatic digestive enzymes free B12 from haptocorrin, allowing it to bind intrinsic factor (IF), a transporter protein synthesized by the parietal cells of the gastric mucosa. This B12-IF complex travels to the terminal ileum where it is absorbed and the B12 is separated from IF. B12 then is delivered to peripheral tissues and the liver by transcobalamin II and haptocorrin, respectively. 1,2
B12 is essential for DNA synthesis, hematopoiesis, and myelination. In different metabolically active forms, it functions as a cofactor for two different enzymes. As methylcobalamin, B12 activates cytoplasmic methionine synthase to convert homocysteine to the essential amino acid methionine. Methionine is required for the formation of S-adenosylmethionine, a universal methyl donor for almost 100 different substrates, including DNA, RNA, proteins, and lipids. As adenosylcobalamin, B12 activates the mitochondrial L-methylmalonyl-CoA mutase to convert L-methylmalonyl-CoA to succinyl-CoA in the metabolism of propionate, a short-chain fatty acid. 3,4 In B12 deficiency, these cofactors are unavailable, causing homocysteine and MMA to accumulate.
Despite our understanding of the metabolic disturbances resulting from B12 deficiency, its pathogenesis is not as well understood. Deficiency of B12 can lead to two major clinical syndromes, megaloblastic anemia and neuropathy. 1,3,4 It is thought that severe B12 deficiency interferes with the synthesis of DNA needed for hematopoiesis, leading to megaloblastic anemia, the appearance of hypersegmented neutrophils, and possible pancytopenia. 5 Regarding neuropathy, it is thought that long-term B12 deficiency may lead to impaired synthesis of ethanolamine, phospholipids, and sphingomyelin, resulting in altered myelin integrity. 6 An enigmatic feature of B12 deficiency is that its clinical presentations vary and may entail only hematologic or neurological abnormalities, or both. 1
Common diagnostic lab tests for B12 deficiency typically begin with serum B12 measurement and a complete blood count (CBC). Low B12 levels and evidence of megaloblastic anemia (decreased RBC, Hgb, Hct, WBC, platelet count, increased MCV, large oval RBCs and hypersegmented neutrophils) indicate B12 deficiency. For ambiguous results, MMA and homocysteine levels should also be measured to confirm. 2,4 Serum MMA is the most sensitive marker of B12 status where its increase indicates decreased tissue B12. However, it also rises with renal insufficiency and tends to be higher in older adults. Plasma homocysteine is a sensitive indicator for early B12 deficiency where it rises quickly as B12 status declines. However, it also rises in folate or B6 deficiency and especially with renal insufficiency, reducing its specificity. 3,4 Additional tests include serum or RBC folate to differentiate causes of macrocytic anemia, and Anti-IF or Anti-parietal cell antibodies to confirm pernicious anemia. 1,2
Caregivers should be aware of the different clinical presentations of B12 deficiency and screen for it in high-risk populations. Risk factors include insufficient dietary B12 intake (vegetarians), lack of intrinsic factor (autoimmune pernicious anemia), food-bound malabsorption (atrophic gastritis of aging or chronic H. pylori infection), gastrointestinal surgery (post gastrectomy or ileal resection), pancreatic or intestinal disorders (chronic pancreatitis, Crohn’s, or Celiac disease), genetic disorders (Transcobalamin II deficiency), and use of certain drugs (long-term use of metformin, H2 receptor antagonists or Proton-pump inhibitors). 1,2,3
Diagnosis of B12 deficiency can be complicated since symptoms may be vague and lab test results can be equivocal. Despite the low B12, high MMA, and significant neurologic symptoms, this patient’s homocysteine level was normal, and he was not anemic. With no known risk factor for B12 deficiency, assays were done for anti-IF antibodies to evaluate for pernicious anemia and AChR binding antibodies for Myasthenia Gravis. However, this patient’s positive symptomatic and serologic responses to B12 supplementation suggest that simple vitamin B12 deficiency was the etiology. Fortunately, B12 therapy reversed his most severe neurological symptoms, although that may not always be the case. Since the reason for the deficiency is unknown, he will continue to receive B12 supplementation indefinitely.
- Michael J Shipton and Jecko Thachil, Vitamin B12 deficiency–A 21st century perspective. Clin Med (Lond). 2015 Apr; 15(2): 145–150. https://www.ncbi.nlm.nih.gov/pmc/articles/PMC4953733/
- https://labtestsonline.org/conditions/vitamin-b12-and-folate-deficiencies
- Vitamin B12. https://ods.od.nih.gov/factsheets/VitaminB12-HealthProfessional/
- Bishnu Prasad Devkota. Methylmalonic Acid. https://emedicine.medscape.com/article/2108967-overview#showall
- Mark J Koury, Prem Ponka. New insights into erythropoiesis: the roles of folate, vitamin B12, and iron. Annu Rev Nutr. 2004;24:105-31. https://pubmed.ncbi.nlm.nih.gov/15189115/
- Brahim El Hasbaoui, Nadia Mebrouk, Salahiddine Saghir, Abdelhkim El Yajouri, Rachid Abilkassem, and Aomar Agadr. Vitamin B12 deficiency: case report and review of literature. https://www.ncbi.nlm.nih.gov/pmc/articles/PMC8140678/
Minh Kosfeld is Director/Assistant Professor, Investigative and Medical Sciences Program, in the Department of Clinical Health Sciences at Saint Louis University in Missouri.
- Case report
- Open access
- Published: 20 September 2021
Megaloblastic anemia-related iron overload and erythroid regulators: a case report
- Nicolas Vallet 1 ,
- Jean-Baptiste Delaye 2 ,
- Martine Ropert 3 ,
- Amélie Foucault 4 , 5 ,
- Noémie Ravalet 4 , 5 ,
- Sophie Deriaz 6 ,
- Thomas Chalopin 1 ,
- Hélène Blasco 2 ,
- François Maillot 6 ,
- Olivier Hérault 4 , 5 &
- Emmanuel Gyan 1 , 5
Journal of Medical Case Reports volume 15 , Article number: 463 ( 2021 ) Cite this article
7246 Accesses
2 Citations
2 Altmetric
Metrics details
In ineffective erythropoiesis, hepcidin synthesis is suppressed by erythroid regulators, namely erythroferrone and growth differentiation factor-15. For the first time, the hypothesis that iron overload in megaloblastic anemia may be related to ineffective erythropoiesis is explored by describing the kinetics of hepcidin, erythroferrone, and growth differentiation factor-15 levels in a patient diagnosed with megaloblastic anemia associated with iron overload.
Case presentation
An 81-year-old Caucasian male was admitted for fatigue. He had type-2 diabetes previously treated with metformin, ischemic cardiac insufficiency, and stage-3 chronic kidney disease. Vitiligo was observed on both hands. Biological tests revealed normocytic non-regenerative anemia associated with hemolysis, thrombocytopenia, and elevated sideremia, ferritin, and transferrin saturation levels. Megaloblastic anemia was confirmed with undetectable blood vitamin B12 and typical cytological findings like hyper-segmented neutrophils in blood and megaloblasts in bone marrow. The patient received vitamin B12 supplementation. At 3 months, biological parameters reached normal values. Hepcidin kinetics from diagnosis to 3 months inversely correlated with those of erythroferrone and growth differentiation factor-15.
Conclusions
This case suggests that iron-overload mechanisms of dyserythropoietic anemias may apply to megaloblastic anemias.
Peer Review reports
Iron overload related to hemolysis and ineffective erythropoiesis is a feature of megaloblastic anemia. The latter mechanism is yet to be explored [ 1 , 2 ]. In ineffective erythropoiesis, hepcidin synthesis is suppressed by erythroid regulators, namely erythroferrone (ERFE) [ 3 ] and growth differentiation factor-15 (GDF15) [ 4 ]. Hepcidin is essential for iron homeostasis, as it induces the endocytosis of ferroportin, lowering iron absorption and circulating iron levels through iron sequestration by hepatocytes, macrophages, and duodenal enterocytes [ 5 ]. Herein, we describe the kinetics of hepcidin, ERFE, and GDF15 levels in a patient diagnosed with megaloblastic anemia associated with iron overload. We hypothesized that ineffective erythropoiesis was associated with high levels of ERFE and GDF15 at the time of diagnosis, possibly associated with suppressed hepcidin, thus explaining the iron overload.
An 81-year-old Caucasian male was referred to emergency department for fatigue associated with profound anemia. He had type-2 diabetes previously treated with metformin, ischemic cardiac insufficiency, and stage-3 chronic kidney disease (CKD, CKD-Epidemiology Collaboration [CKD-EPI] estimated glomerular filtration rate: 32 mL/minute/1.73 m 2 ). There were no clinical signs of infectious or tumoral disease. Vitiligo was observed on both hands. First biological tests revealed normocytic non-regenerative anemia associated with hemolysis, thrombocytopenia, and elevated sideremia, ferritin, and transferrin saturation (TSAT) levels. Vitamin B12 was undetectable, whereas vitamin B9 and C-reactive protein levels were normal (Table 1 ). He received one pack of red blood cells (RBC), then was admitted to internal medicine department. A peripheral blood smear showed hypersegmented neutrophils, and a bone-marrow smear showed hypercellularity and clear signs of dyserythropoiesis and blocked maturation of erythroid cells (Fig. 1 a, b). Stomach endoscopy and biopsies showed chronic gastritis and fundic atrophy. Though metformin may have participated in this condition [ 1 ], a diagnosis of megaloblastic anemia caused by pernicious anemia was preferred considering the profound anemia and vitiligo, even if blood antiparietal cells or anti-intrinsic-factor antibodies were not detected. After two other packs of RBC and five daily intramuscular vitamin B12 injections, he was discharged and continued oral vitamin B12 supplementation. Three-month (M3) biological control revealed that platelet count and hemoglobin, sideremia, and TSAT levels normalized (Table 1 ). Ferritin returned to normal values at 7 months (361 µg/L).
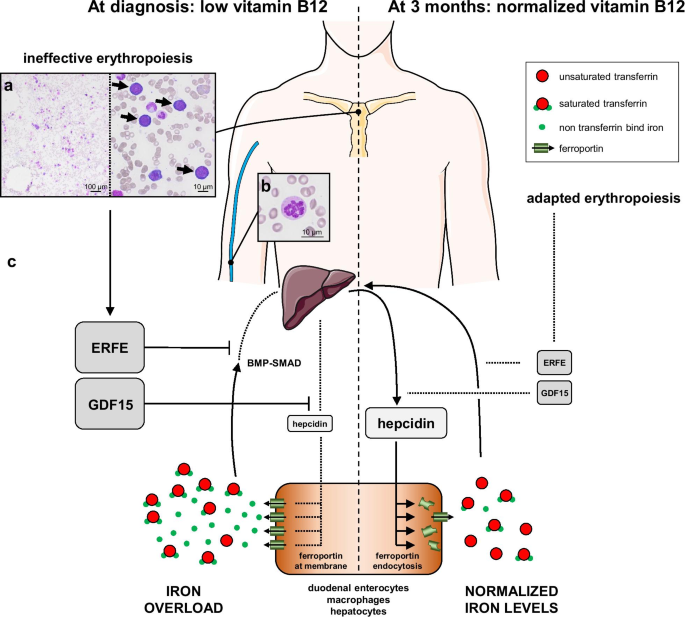
Proposed integrative interpretation of the results obtained from this case report. a May–Grünwald Giemsa staining of a bone-marrow smear showing hypercellularity (left) and intense basophilic megaloblasts (right, arrows). b Peripheral blood smear revealing increased neutrophil cell volume with a hypersegmented nucleus. c Hypothetical mechanism of the pathogenesis of iron overload in megaloblastic anemia. Relative changes in the size of the protein names represent their down- or upregulation. ERFE erythroferrone, GDF15 growth differentiation factor-15
We tested our hypothesis by analyzing the samples at the time of diagnosis and at M3 after receiving informed consent of the patient. Serum hepcidin, ERFE, and GDF15 levels were assessed by ELISA (hepcidin-25: S-1337, Peninsula; ERFE: ERF-001, Intrinsic LifeSciences; GDF15: DGD150, R&D Systems). Serum erythropoietin (EPO) was measured by chemiluminescence (UniCel DxL, Beckman Coulter).
ERFE and GDF15 levels were higher than those of age-matched healthy controls (HC) at diagnosis, dropping to HC levels at M3, supporting our hypothesis. The level of hepcidin was lower at the time of diagnosis than M3 but remained within reference levels, and its kinetics from diagnosis to M3 inversely correlated with those of EPO, ERFE, and GDF15 (Table 1 ).
Discussion and conclusions
Given the high level of TSAT, the level of hepcidin at diagnosis was inappropriately low, suggesting suppression by an erythroid regulator. The influence of CKD [ 6 , 7 ] and metformin [ 8 ] may explain why the hepcidin levels were comparable to those of HC, but its range is consistent with that found in CKD patients [ 7 ]. A metabolic syndrome may also explain high ferritin levels. But these potential biases were internally controlled by comparing two time points before and after vitamin B12 and hemoglobin normalization, when comorbidities and treatments were comparable. ERFE and GDF15 levels were higher than in HC from our institution and previous studies [ 9 , 10 ]. ERFE levels at diagnosis were comparable to those of patients with pyruvate-kinase deficiency but lower than those with β-thalassemia [ 9 ]. The inverse correlation of EPO and ERFE kinetics is consistent with that observed in EPO-treated healthy humans [ 3 ] and CKD mouse [ 6 ] models. The GDF15 level at diagnosis was comparable to that of congenital dyserythropoietic anemia type-1 [ 11 ]. Despite the undetectable vitamin B12 levels and typical cytological abnormalities that define megaloblastic anemia, the mean corpuscular volume (MCV) was unexpectedly not macrocytic [ 1 ]. The patient had no history of iron deficiency, inflammation, or red-blood-cell transfusion. GDF15 was recently shown to stimulate erythroid precursor growth in mice [ 12 ]. Cell-cycle acceleration in erythroid precursors may have hidden macrocytosis, but whether high levels of GDF15 may have affected the MCV remains to be demonstrated. Intramedullary hemolysis, suggested by bilirubin, lactate dehydrogenase, and haptoglobin levels, can also explain iron overload [ 2 ]. However, hemolysis alone cannot explain the higher ERFE and GDF15 levels. In sickle-cell diseases (SCD), in which hemolysis is dominant compared with dyserythropoiesis, the ERFE and GDF15 levels and their correlation with hepcidin levels were not comparable to those observed in β-thalassemia [ 13 ]. Additionally, the hepcidin level was inappropriately low relative to that at M3 when TSAT was normal, reinforcing the hypothesis that an erythroid regulator suppressed hepcidin synthesis.
ERFE suppresses hepcidin synthesis by sequestering bone morphogenetic protein (BMP) receptor ligands [ 5 ]. Its synthesis starts upon EPO stimulation through the JAK2-STAT5 pathway in erythroblasts [ 14 ]. ERFE levels are elevated in human congenital or acquired dyserythropoietic diseases associated with iron overload [ 3 , 15 ]. GDF15 was shown to suppress hepcidin synthesis in the context of β-thalassemia [ 4 ] and congenital dyserythropoietic anemia [ 11 ]. GDF15 is produced by erythroblasts, but its mechanisms of synthesis and hepcidin suppression are not fully understood [ 4 , 10 ].
In conclusion, we found the kinetics of hepcidin, ERFE, and GDF15 to be comparable to those described in iron overload associated with ineffective erythropoiesis (Fig. 1 c). This case broadens the spectrum of iron-overload mechanisms in dyserythropoietic anemias to vitamin B12 deficiency, in which hepcidin levels inversely correlate with those of erythroid regulators, suggesting suppression by ERFE and GDF15.
Availability of data and materials
The dataset analyzed during the current study is available from the corresponding author on reasonable request.

Abbreviations
Bone morphogenetic protein
Chronic kidney disease
Erythropoietin
- Erythroferrone
Growth differentiation factor-15
Three months
Mean corpuscular volume
Red blood cells
Sickle cell disease
Transferrin saturation
Green R. Vitamin B12 deficiency from the perspective of a practicing hematologist. Blood. 2017;129(19):2603–11.
Article CAS Google Scholar
Ho C-H, You J-Y, Chau W-K, et al . Diagnostic value of serum transferrin receptor and glycosylated hemoglobin on hemolytic anemia. Ann Hematol. 2003;82(4):228–30.
Ganz T, Jung G, Naeim A, et al . Immunoassay for human serum erythroferrone. Blood. 2017;130(10):1243–6.
Tanno T, Bhanu NV, Oneal PA, et al . High levels of GDF15 in thalassemia suppress expression of the iron regulatory protein hepcidin. Nat Med. 2007;13(9):1096–101.
Camaschella C, Nai A, Silvestri L. Iron metabolism and iron disorders revisited in the hepcidin era. Haematologica. 2020;105(2):260–72.
Article Google Scholar
Hanudel MR, Rappaport M, Chua K, et al . Levels of the erythropoietin-responsive hormone erythroferrone in mice and humans with chronic kidney disease. Haematologica. 2018;103(4):e141–2.
Coll AP, Chen M, Taskar P, et al . GDF15 mediates the effects of metformin on body weight and energy balance. Nature. 2020;578(7795):444–8.
Ashby DR, Gale DP, Busbridge M, et al . Plasma hepcidin levels are elevated but responsive to erythropoietin therapy in renal disease. Kidney Int. 2009;75(9):976–81.
van Vuren AJ, Eisenga MF, van Straaten S, et al . Interplay of erythropoietin, fibroblast growth factor 23, and erythroferrone in patients with hereditary hemolytic anemia. Blood Adv. 2020;4(8):1678–82.
Theurl I, Finkenstedt A, Schroll A, et al . Growth differentiation factor 15 in anaemia of chronic disease, iron deficiency anaemia and mixed type anaemia. Br J Haematol. 2010;148(3):449–55.
Casanovas G, Swinkels DW, Altamura S, et al . Growth differentiation factor 15 in patients with congenital dyserythropoietic anaemia (CDA) type II. J Mol Med. 2011;89(8):811–6.
Hao S, Xiang J, Wu D-C, et al . Gdf15 regulates murine stress erythroid progenitor proliferation and the development of the stress erythropoiesis niche. Blood Adv. 2019;3(14):2205–17.
Mangaonkar AA, Thawer F, Son J, et al . Regulation of iron homeostasis through the erythroferrone-hepcidin axis in sickle cell disease. Br J Haematol. 2020;189(6):1204–9.
Kautz L, Jung G, Valore EV, Rivella S, Nemeth E, Ganz T. Identification of erythroferrone as an erythroid regulator of iron metabolism. Nat Genet. 2014;46(7):678–84.
Bondu S, Alary A-S, Lefèvre C, et al . A variant erythroferrone disrupts iron homeostasis in SF3B1-mutated myelodysplastic syndrome. Sci Transl Med. 2019. https://doi.org/10.1126/scitranslmed.aav5467 .
Article PubMed PubMed Central Google Scholar
Download references
Acknowledgements
Fig. 1 was created using illustrations from Servier Medical Art by Servier, licensed under a Creative Commons Attribution 3.0 Unported License. http://smart.servier.com .
Not applicable
Author information
Authors and affiliations.
Department of Hematology and Cell Therapy, Tours University Hospital, 2 Boulevard Tonnellé, 37044, Tours Cedex, France
Nicolas Vallet, Thomas Chalopin & Emmanuel Gyan
Department of Biochemistry and Molecular Biology, Tours University Hospital, Tours, France
Jean-Baptiste Delaye & Hélène Blasco
Department of Biochemistry, CHU Rennes, Rennes, France
Martine Ropert
Department of Biological Hematology, Tours University Hospital, Tours, France
Amélie Foucault, Noémie Ravalet & Olivier Hérault
CNRS ERL7001 LNOX, EA 3549, Tours University, Tours, France
Amélie Foucault, Noémie Ravalet, Olivier Hérault & Emmanuel Gyan
Department of Internal Medicine, Tours University Hospital, Tours, France
Sophie Deriaz & François Maillot
You can also search for this author in PubMed Google Scholar
Contributions
EG and NV designed the study and wrote the paper. JBD designed the study and performed the ERFE and GDF15 assays. MR performed the hepcidin assays, and AF performed the cytological analysis. All authors critically reviewed the paper, and read and approved the final version of the manuscript.
Corresponding author
Correspondence to Emmanuel Gyan .
Ethics declarations
Ethics approval and consent to participate.
Patient and controls gave their consent to participate. Analyses were conducted according to Helsinki declaration.
Consent for publication
Written informed consent was obtained from the patient for publication of this case report and any accompanying images. A copy of the written consent is available for review by the Editor-in-Chief of this journal.
Competing interests
The authors declare that they have no competing interests.
Additional information
Publisher’s note.
Springer Nature remains neutral with regard to jurisdictional claims in published maps and institutional affiliations.
Rights and permissions
Open Access This article is licensed under a Creative Commons Attribution 4.0 International License, which permits use, sharing, adaptation, distribution and reproduction in any medium or format, as long as you give appropriate credit to the original author(s) and the source, provide a link to the Creative Commons licence, and indicate if changes were made. The images or other third party material in this article are included in the article's Creative Commons licence, unless indicated otherwise in a credit line to the material. If material is not included in the article's Creative Commons licence and your intended use is not permitted by statutory regulation or exceeds the permitted use, you will need to obtain permission directly from the copyright holder. To view a copy of this licence, visit http://creativecommons.org/licenses/by/4.0/ . The Creative Commons Public Domain Dedication waiver ( http://creativecommons.org/publicdomain/zero/1.0/ ) applies to the data made available in this article, unless otherwise stated in a credit line to the data.
Reprints and permissions
About this article
Cite this article.
Vallet, N., Delaye, JB., Ropert, M. et al. Megaloblastic anemia-related iron overload and erythroid regulators: a case report. J Med Case Reports 15 , 463 (2021). https://doi.org/10.1186/s13256-021-03065-0
Download citation
Received : 16 December 2020
Accepted : 16 August 2021
Published : 20 September 2021
DOI : https://doi.org/10.1186/s13256-021-03065-0
Share this article
Anyone you share the following link with will be able to read this content:
Sorry, a shareable link is not currently available for this article.
Provided by the Springer Nature SharedIt content-sharing initiative
- Vitamin B12
- Iron overload
- Erythropoiesis
Journal of Medical Case Reports
ISSN: 1752-1947
- Submission enquiries: Access here and click Contact Us
- General enquiries: [email protected]

Case Report
Korean J Clin Pathol 1982; 2(1): 27-32
Published online February 1, 1982
Copyright © Korean Society for Laboratory Medicine.
A Case Report of Megaloblastic Anemia
Chun Hee Lee, M.D., Sook-Ja Park, M.D.
Department of Cinical Pathology of Chun Ju Presbyterian Medical Center
Background : A case of megaloblastic anemia of 30 year-old married female is presented and the literatures of the case are briefly reviewed. This patient was second adimitted to the department of General Sugery of Presbyterian Medical Center on March 30th, 1982, because of repair of colostomy and anemia . debridement was done. On 86 th hospital day she was discharged with T-loop colostomy state. This patient was second adimitted to .the department of General Sugery of Presbyterian Medical Center on Match 30 th, 1982, because of repair of colostomy and anemia. On physical examination at second admission time, her gross appearance was chronic ill looking state Pale face and anemic conjunctiva were also noted. T-loop colostomy state was remained. On the laboratory examination peripheral blood smears disclosed macrocytic anemia with moderate poikilocytosis. Moderate leukopenia with shift to left change and many hypersegmented neutrophils were found. The first bone marrow showed moderate erythroid hyperplasia, most of which were megaloblastic appearance and giant metamyelocytes and myelocytes with hypersegmented neutrophils were also noted. Megakaryocytes are adequate in number and maturation. Serum folic acid level is mild decreased, but serum vitamin B12 level within normal limit. Follow up bone marrow showed same a. first bone marrow except for relative myeloid hyperplasia and moderate toxic granulation of myeloid series. And last bone marrow study disclosed more myeloid hyperplasia and severe toxic granulation. On 77th hospital day she was expired because of septicemia. Method : Result : Conclusion :
Aritcle Tools

Stats or Metrics
- Download 615
Share this article on :


An official website of the United States government
The .gov means it’s official. Federal government websites often end in .gov or .mil. Before sharing sensitive information, make sure you’re on a federal government site.
The site is secure. The https:// ensures that you are connecting to the official website and that any information you provide is encrypted and transmitted securely.
- Publications
- Account settings
Preview improvements coming to the PMC website in October 2024. Learn More or Try it out now .
- Advanced Search
- Journal List
- Med J Armed Forces India
- v.74(4); 2018 Oct
A descriptive study of clinico-hematological profile of megaloblastic anemia in a tertiary care hospital
Navjyot kaur.
a Assistant Professor (Medicine), Command Hospital (Southern Command), Pune 411040, India
b Former Director General Medical Services (Army), Integrated Headquarters, Ministry of Defence, New Delhi, India
Sanjeevan Sharma
c Associate Professor (Medicine), Command Hospital (Southern Command), Pune 411040, India
Puja Dudeja
d Associate Professor, Department of Community Medicine, Armed Forces Medical College, Pune 411040, India
Pankaj Puri
e Brig Med, HQ 12 Corps, C/o 56 APO, India
Megaloblastic Anemia (MA) is a relatively common disease, yet the data on prevalence of MA remains scarce. This study was conducted to study the prevalence and clinico-hematological profile of MA.
This was a cross-sectional study done on 1150 adult anemic patients. All patients diagnosed to have MA were studied for clinico-hematological and etiological profile. Nerve conduction studies (NCS) were done in all.
MA was present in 3.6% cases of anemia. Severe anemia was seen in 9.7% of anemic patients and 75% of MA cases ( p < 0.05). Forty five percent of MA patients presented with pancytopenia. Vitamin B12 and folic acid deficiency were documented in 40% and 25% cases respectively while combined deficiency was noted in 35% of all MA cases. There was no co-relation between severity of anemia and deficiency of either of the vitamins (Fischer exact test: 0.530). Among MA patients, 35% were vegetarians while 65% consumed mixed diet. There was no association between vegetarian diet and Vit B12 deficiency ( p = 0.3137). An additional etiology was more commonly found in patients on mixed diet [92%; 24/26] as compared to those on vegetarian diet [50%; 7/14] ( p = 0.04). NCS was abnormal in 14 patients (35%). Overt clinical neuropathy was present in 12 cases of MA, while subclinical neuropathy was seen in 2 cases.
MA is one of common causes of pancytopenia and severe anemia. Diet plays an important role in causation of MA in vegetarians. An alternative etiology is however, more likely to be found in patients on mixed diet. While overt neurological abnormalities are common in MA, subclinical neuropathy is uncommon.
Introduction
The prevalence of megaloblastic anemia (MA) reported by various Indian studies ranges from 02% to 40%. 1 , 2 , 3 , 4 , 5 , 6 Most of these studies were carried out in children and in hospitalized patients. MA remains the commonest cause of macrocytic anemia 7 and pancytopenia. 8 , 9 , 10 , 11 , 12 It can have a varied clinical and hematological presentation. 13 , 14 , 15 While overt neurological syndromes associated with MA are well documented, there is paucity of data on the existence of subclinical neurological manifestations in MA with one study published in British Journal in 1980 having shown a prevalence of 25%. 16 In the current study, we calculated the prevalence of MA in adult patients of anemia, studied its clinico-hematological profile and the existence of overt and subclinical neuropathy in patients of MA.
Material and methods
We did a cross sectional study over a period of 18 months (Nov 2011–Apr 2013) at a tertiary care hospital of Maharashtra. All patients of anemia managed over a period of 18 months were evaluated. Pregnant and lactating women, children less than 12 years and the patients already on Vit B12/folic acid replacement or who have already been transfused were excluded. A written informed consent was obtained from all patients included in the study.
Hemogram was measured using 2 ml of ethylene diamine tetraacetic acid anticoagulated blood in automated Beckman Coulter 500 FC series analyzer. Anemia was defined as hemoglobin (Hb) < 13 g/dL in males and Hb < 12 g/dL in females, as per WHO definition of anemia. Severe anemia was defined as Hb < 8 g/dL. All patients of anemia with mean corpuscular volume (MCV) > 100 fL were evaluated with peripheral blood smear (PBS), bone marrow studies, Vit B12 and folic acid levels. Peripheral blood smear suggestive of MA was defined by presence of macro-ovalocytosis, anisocytosis, poikilocytosis, hypersegmented neutrophils and pancytopenia. The diagnosis of MA was confirmed with bone marrow aspiration studies where presence of hypercellular marrow with increased erythroid/myeloid ratio, megaloblasts, giant bands and metamyelocytes and decreased megakariocytes favor the diagnosis of MA. Vit B12 and folic acid levels were measured using chemiluminescence method. Normal levels of Vit B12 and folic acid were defined as 211–911 pg/ml and >5.38 ng/ml respectively. A detailed dietary history was taken. Non vegetarian diet was defined as diet comprising predominately of animal food, mixed diet as one containing food of both plant and animal origin, vegetarian diet comprised of food of plant origin and milk, whereas pure vegan diet was defined as vegetarian diet less the milk. History of intake of drugs including proton pump inhibitor (PPIs) was taken along with history of previous surgery if any. All patients were asked about history of alcohol intake. Significant alcohol consumption was defined as more than 30 g of alcohol intake per day. History of neurological symptoms if any was noted. All patients were subjected to a detailed clinical examination.
Further evaluation of patients with MA included Liver Function Tests (LFTs), Lactate Dehydrogenase (LDH), Upper Gastrointestinal Endoscopy (UGIE) and biopsy from stomach and duodenum for Histopathological Examination (HPE) besides the biopsies for Helicobacter Pylori (H. Pylori) detection by Rapid Urease Test (RUT). Additional tests like anti tissue transglutaminase antibodies (anti TTG antibodies), stool for cryptosporidiosis (for 03 days), Human Immunodeficiency Virus 1 and 2 (HIV 1 and 2) antibodies and colonoscopy were done if indicated. Parietal cell antibodies were looked for when there was evidence of atrophic gastritis or when there was suspicion of an auto-immune disease. Nerve Conduction Studies (NCS) were done in all patients of MA to document any subclinical neuropathy.
Statistical analysis: The continuous variables were analyzed using unpaired t test, while proportions and qualitative variables were analyzed using chi-square and Fisher's exact test. A ‘ p ’ value of less than or equal to 0.05 was considered statistically significant.
A total of 1150 patients were diagnosed to have anemia, of which 59 patients were excluded as they have already been transfused or were on hematinics. Remaining 1091 (404 males and 687 females) patients were screened for macrocytosis (MCV > 100 fL). Macrocytosis was seen in 57/1091 patients. Three patients refused to give consent; the rest 54 patients were evaluated for MA with PBS, bone marrow studies, Vit B12 and folic acid levels. Forty patients (30 males and 10 females) were diagnosed to have MA ( Fig. 1 ) which constituted 3.6% (95% CI: 2.5–4.7%) of all cases of anemia.

Consort diagram.
Mean age of MA patients was 36.15 ± 17.6 years which was significantly lower than mean age of all anemia cases (41.3 ± 15.3 years) ( p = 0.03). Highest incidence of MA was noted in the age group between 16 and 20 years. Thirty-five percent (14/40) of MA patients and thirty six percent (397/1091) of total patients of anemia consumed vegetarian diet and there was no statistical difference between two cohorts ( p = 0.89) as depicted in Table 1 . Similarly, there was no statistical difference between significant alcohol consumption and regular PPI consumption between two groups ( p = 0.62 and p = 0.41 respectively).
Baseline characteristics.
Symptoms and signs
Easy fatigability was the commonest symptom. One patient came with Congestive Cardiac Failure (CCF) due to anemia while two patients reported with acute Deep Vein Thrombosis (DVT) due to hyperhomocysteinemia secondary to Vit B12 deficiency. Thirty percent patients (12/40) presented with overt neurological symptoms and signs. While all twelve patients had evidence of peripheral neuropathy; two patients in addition presented with ataxia and had posterior column signs including positive Romberg's sign. Table 2 summarizes the symptoms and signs in our cohort of MA patients.
Symptoms and signs in megaloblastic anemia.
DVT, deep vein thrombosis.
Investigations
Megaloblastic anemia (MA) constituted 3.4% (95% CI: 2.5–4.7%) of all cases of anemia. Severe anemia (Hb < 8 g/dL) was seen in 9.7% (106/1091) of all anemia cases and 75% (30/40) of MA cases ( p < 0.05). Pancytopenia was noted in 45% (18/40) of MA patients. Hyper segmented neutrophils were noted in 57.5% (23/40) patients while ovalo-macrocytosis was documented in 45% (18/40) patients. Mean Hb noted in MA patients was 6.39 g/dL (± 1.95) and MCV recorded was 117.5 fL (± 5.082) with a mean red cell distribution width (RDW) of 21.53 (± 4.63). MCV > 110 fL and RDW ≥ 18 each correlated with a diagnosis of MA ( p = 0.001). LDH activity was increased in all patients with MA. Mean LDH recorded in patient with MA was 3208 IU/L (range 589–9110 IU/L). Lower Hb level was associated with higher values of LDH. Indirect hyperbilirubinemia was seen in 50% (20/40) patients of MA; mean bilirubin recorded was 2.18 mg% (± 1.3).
Out of total 40 patients of MA, Vit B12 deficiency was noted in 40% (16/40) patients, folic acid deficiency in 25% (10/40) patients and 35% (14/40) patients had combined deficiency of Vit B12 and folic acid. There was no co-relation between the severity of anemia and vitamin B12 and/or folic acid levels, neither was there any co-relation between deficiency of a particular vitamin or combined deficiency and severity of anemia ( Table 3 ).
Co-relation between deficiency of a particular vitamin or combined deficiency and severity of anemia.
Fischer's exact test value: 0.530.
Gastrointestinal investigations
Fourteen (14/40) patients tested positive for Helicobacter Pylori by RUT. Atrophic gastritis was reported in nine biopsies and parietal cell antibodies were done in all these patients. One out of these nine patients, had positive parietal cell and intrinsic factor antibody and was diagnosed to have pernicious anemia. Villous atrophy in duodeneal biopsies was seen in 6/40 patients.TTG antibodies were detected in two patients with villous atrophy while four patients were diagnosed to have tropical sprue. Colonoscopy was done in three patients which was essentially normal.
Homocysteine levels were raised in two patients who presented with DVT; one of them had only Vit B12 deficiency while other had combined deficiency of Vit B12 and folic acid.
Diet and Megaloblastic Anemia (MA)
For analyzing the possible causative factors of MA, we divided the patients into two groups: Group 1 who consumed mixed diet (26/40) and Group 2 who consumed vegetarian diet (14/40). There were no vegans in our patients with MA. Combined Vit B12 and folic acid deficiency was found in 10 patients who consumed mixed diet and in 05 patients who consumed vegetarian food. Exclusive Vit B12 deficiency was found in 08 patients on vegetarian diet and in 09 patients on mixed diet while exclusive folic acid deficiency was noted in one vegetarian patient and in 07 patients on mixed diet. The possible etiological factors associated with MA seen in our study were H. Pylori infection (14/40;35%), chronic use of PPI (12/40;30%), atrophic gastritis (9/40;22.5%), significant alcohol consumption (8/40;20%), tropical sprue (4/40;10%), celiac sprue (2/40;5%), Acquired Immune Deficiency Syndrome (2/40;5%), pernicious anemia (1/40; 2.5%) and intestinal resection (1/40;2.5%). Out of 14 patients who were vegetarians, only 7 (50%) had one or more additional etiology whereas 24/26 (92.30%) patients who consumed mixed diet had one or more than one etiology. The etiological work up was more likely to be negative in vegetarians (fischer's exact test value: 0.04). This implies that diet plays an important role in causation of MA in vegetarians. There was no statistical association between type of diet and deficiency of particular vitamin as depicted in Table 4 .
Association between diet and type of vitamin deficiency.
Chisquare test: 0.3137 (no significant association between diet and type of vitamin deficiency).
Nerve Conduction Studies (NCS)
Twelve patients (12/40) had clinical evidence of large sensory fiber involvement which was confirmed by NCS. Apart from these, two patients had evidence of subclinical neuropathy (demyelinating type) as demonstrated by delayed latencies on NCS. There was no co-relation between Hb levels and neurological findings clinically or on NCS. Out of 14 patients who had clinical and/or electrophysiological neurological abnormalities, 50% (7/14) had deficiency of Vit B12 alone; 28.57%(4/14) had deficiency of folic acid alone and 21.43% (3/14) had deficiency of both vitamin B12 and folic acid.
Most of the studies on prevalence of MA have been done in children and the prevalence of MA in these studies have been documented anywhere between 2% and 42%. 1 , 2 , 3 , 4 , 5 , 17 The prevalence of MA in our adult patients of anemia was 3.6%. Most of our patients of MA were males (30/40). This observation may be due to the fact that females tend to be more iron deficient and in presence of iron deficiency, the MCV won’t rise above 100 fL even with co-existent Vit B12 or folic acid deficiency. We could not do the iron profile of our whole anemia cohort because of financial constraints and also as it was beyond the scope of our study. The male preponderance for MA has been noted in another study by Maktouf et al. 18 While studies have shown an increased prevalence of MA in vegetarians, 19 , 20 majority of our patients consumed mixed diet (65%) and none of them was a pure vegan. Though the limitation of our finding was that the number of MA patients was small; but still we would like to point out an important observation that diet does seem to play a role, as the etiological work up was more likely to be negative in vegetarian group (Fischer's exact test value: 0.04). There was no significant association between diet and type of vitamin deficiency (Chisquare test: 0.3137). RUT for Helicobacter Pylori was positive in 12/26 patients (35%). This organism has been postulated to play a role in causing MA in many studies 21 , 22 , 23 , 24 ; though causal role could not be established. History of chronic intake of PPI was given by 12/40 (30%) patients. These drugs may play a role in malabsorption of cobalamin. 25 , 26 Whether patients take PPI to ameliorate the mucosal effects of megaloblastic anemia or the use of PPI lead to megaloblastosis is not clear. 17
Vitamin B12 deficiency was found in 40% of our patients, folic acid deficiency was seen in 25% while combined deficiency was recorded in 35% patients. The Indian series from 1960s documented folate deficiency to be more common cause of MA. 27 , 28 Subsequent studies done in 1980s and 1990s highlighted that Vit B12 deficiency is far more common than folate deficiency. 5 , 29 , 30 , 31 , 32 Increased prevalence of Vit B12 deficiency as compared to folic acid deficiency has been reported from countries outside India also. 33 , 34 , 35 , 36 Indirect hyperbilirubinemia and raised LDH are commonly found in MA which occur due to ineffective erythropoeisis. Lower Hb level was associated with higher values of LDH as has also been noticed by Emerson et al. 37
In our study, MA accounted for 28.30% of all cases of severe anemia. This has also been shown in another study where MA accounted for 42.5% of all cases of severe anemia. 38 In our study, 01 patient presented with CCF, 02 patients had DVT on presentation, 02 patients presented with ataxia, while 05 patients were admitted as case of fever with hepatomegaly and/or hepatosplenomegaly. As many as 18 patients presented with pancytopenia. Such varied presentations of MA have been documented in earlier studies also. 8 , 9 , 10 , 11 , 12 , 15 , 39 , 40 , 41 , 42 , 43 , 44 MA can mimic aplastic anemia, leukemia and myelodysplastic syndrome. Even an expert hemato-pathologist may find it difficult to differentiate between myelodysplastic syndrome and megaloblastosis on bone marrow without the support of cytogenetics. Overt neurological manifestations were found in 30% patients as has been documented in the literature earlier. 16 The sub-clinical neurological manifestations were documented only in 02 patients unlike the previous report where a prevalence of 25% was reported. There was no relation between the neurological manifestations and the Hb levels as was demonstrated in other studies also. 45
Conclusions
Prevalence of MA in adult patients with anemia was found to be 3.6%. MA is one of the common causes of severe anemia and constituted 28.30% of all causes of severe anemia. MA is not restricted to vegetarians only and is also seen quite commonly in those who consume non vegetarian food. However, the people who take vegetarian diet are more likely to have negative etiology thus emphasizing the role of diet in MA patients. The presentation of MA can be varied. While overt neurological abnormalities are common in MA, subclinical neuropathy is uncommon.
Limitations
The prevalence of MA in cohort of anemia may be a little under-estimation as we did not evaluate the patients whose MCV was less than 100 fL, so we could have missed MA when it co-existed with iron deficiency or with other causes of microcytic anemia. It was a cross sectional study so we could not establish the causal relationship to various etiological factors studied.
Conflicts of interest
The authors have none to declare.

IMAGES
VIDEO
COMMENTS
In a study including 201 adults with well-documented cobalamin deficiency, approximately 10% of the patients were found to have life-threatening haematological manifestations. 3 Among these were pancytopenia (5%), severe anaemia (defined as a haemoglobin level <6.0 g/dL; 2.5%) and haemolytic anaemia (1.5%). Our patient had severe macrocytic ...
B 12 deficiency is the leading cause of megaloblastic anemia, and although more common in the elderly, can occur at any age. Clinical disease caused by B 12 deficiency usually connotes severe deficiency, resulting from a failure of the gastric or ileal phase of physiological B 12 absorption, best exemplified by the autoimmune disease pernicious anemia. . There are many other causes of B 12 ...
Megaloblastic anemia (MA) encompasses a heterogeneous group of macrocytic anemias characterized by the presence of large red blood cell precursors called megaloblasts in the bone marrow.[1] This condition is due to impaired DNA synthesis, which inhibits nuclear division. Cytoplasmic maturation, mainly dependent on RNA and protein synthesis, is less impaired. This leads to an asynchronous ...
In a study by Tahlan et al. 3 the incidence of low-grade fever in nutritional megaloblastic anemia varied from 28% to 60% ... The present report described a case of megaloblastic anemia in a middle-aged female patient, who presented with low-grade pyrexia, pancytopenia, macrocytosis (114.3 fL), very high LDH ...
Vitamin B12 deficiency is a common cause of megaloblastic anemia, various neuropsychiatric symptoms, and other clinical manifestations. ... 8 A case-control study that compared 25,956 patients who ...
Megaloblastic anemia causes macrocytic anemia from ineffective red blood cell production and intramedullary hemolysis. The most common causes are folate (vitamin B9) deficiency and cobalamin (vitamin B12) deficiency. Megaloblastic anemia can be diagnosed based on characteristic morphologic and laboratory findings. However, other benign and neoplastic diseases need to be considered ...
Vitamin B 12 deficiency causes reversible megaloblastic anemia, demyelinating neurologic disease, or both. •. Autoimmune gastritis (pernicious anemia) is the most common cause of severe ...
1 INTRODUCTION. Megaloblastic anemia (MA) in the form of autoimmune pernicious anemia (PA) was described by physicians as early as the 1800s. A case report described a patient with numbness of the upper extremities, large red blood cells, atrophic gastric mucosa, and hypercellular bone marrow with increased erythroblasts. 1 Whipple, Minot, and Murphy earned a Nobel Prize in 1934 for the ...
for which vitamin B12 levels were evaluated, and it was found to be decreased. Megaloblastic anemia is an important cause of cytopenias, but to best of our knowledge, there are not many studies quoting its incidence. Keywords: Anemia, Megaloblastic, Pancytopenia, Vitamin B12 Sciences, Karimnagar which is a teritiary care centre that
We report a case of severe pancytopenia in a 15-year-old patient due to a severe deficiency in vitamin B12 and folic acid, probably of nutritional origin. The clinical and biological course was favorable after vitamin supplementation. With this case, we discuss the diagnostic approach of pancytopenia with megaloblastic anemia in children and adolescents, as well as the mechanisms involved in ...
Case Study (6)- Megaloblastic anemia History: A 63-year-old woman checked by her general practitioner (GP) complaining of extreme tiredness. She has been increasingly fatigued over the past year but in recent weeks she has become breathless on exertion and complained of headaches.
CASE 2 DIAGNOSIS: MEGALOBLASTIC ANEMIA DUE TO DIETARY COBALAMIN DEFICIENCY. ... bone marrow studies must be performed to exclude bone marrow failure, hematological malignancy or metastatic tumour. Figure 2. Open in new tab Download slide. Bone marrow aspirate demonstrating megaloblastic hematopoiesis with a megaloblast (white arrow) and a giant ...
Vitamin B 12 is essential for normal blood synthesis and neurological function. Vitamin B 12 deficiency may cause megaloblastic anemia, subacute combined degeneration of the cord and psychiatric illness ("megaloblastic madness"). Notably, the degree of bone marrow suppression is typically inversely related to both the presence and severity of neurological involvement.
The present study describes a case of an elderly patient that was hospitalized secondary to hypotension and delirium. Physical examination at admission revealed bilateral positive Babinski's sign. Laboratory examination revealed severe anemia. ... Megaloblastic anemia is a nutritional anemia induced by deficiencies of folic acid and/or ...
Deficiency of B12 can lead to two major clinical syndromes, megaloblastic anemia and neuropathy. 1,3,4 It is thought that severe B12 deficiency interferes with the synthesis of DNA needed for hematopoiesis, leading to megaloblastic anemia, the appearance of hypersegmented neutrophils, and possible pancytopenia. 5 Regarding neuropathy, it is ...
Iron overload related to hemolysis and ineffective erythropoiesis is a feature of megaloblastic anemia. The latter mechanism is yet to be explored [1, 2].In ineffective erythropoiesis, hepcidin synthesis is suppressed by erythroid regulators, namely erythroferrone (ERFE) [] and growth differentiation factor-15 (GDF15) [].Hepcidin is essential for iron homeostasis, as it induces the endocytosis ...
vere megaloblastic anemia and its 2 most com-mon causes: folate (vitamin B 9) and cobalamin (vitamin B 12) defi ciency, with 2 representative case studies. MEGALOBLASTIC ANEMIA OVERVIEW Megaloblastic anemia is caused by defec-tive DNA synthesis involving hematopoi-etic precursors, resulting in ineffective red
Macrocytic anemia may be classified as megaloblastic, due to impaired DNA synthesis in erythrocyte precursors, or nonmegaloblastic, due to various other etiologies (Table 1) [1]. Table 1. In the Western world, about 90% of megaloblastic anemias result from either alcohol-induced folate deficiency or B12 deficiency [1].
Megaloblastic Anemia. Megaloblastic anemia is a form of macrocytic anemia, a blood disorder that happens when your bone marrow produces stem cells that make abnormally large red blood cells. Megaloblastic anemia is a type of vitamin deficiency anemia that happens when you don't get enough vitamin B12 and/or vitamin B9 (folate).
Background :A case of megaloblastic anemia of 30 year-old married female is presented and the literatures of the case are briefly reviewed.This patient was second adimitted to the department of General Sugery of Presbyterian Medical Center on March 30th, 1982, because of repair of colostomy and anemia . debridement was done.
In this case study, we have discussed the history, presentation, and laboratory workup of a patient with refractory anemia and elevated mean corpuscular volume and mean corpuscular hemoglobin on long-term zidovudine therapy coupled with vitamin B12 deficiency, thus supporting a diagnosis of megaloblastic anemia.
Study C09-001r (N=30) revealed a safety profile that was similar to that which was observed in the two prospective studies. Study C09-001r included 19 pediatric patients less than 18 years of age. Overall, the safety of eculizumab in pediatric patients with aHUS enrolled in Study C09-001r appeared similar to that observed in adult patients.
Megaloblastic Anemia (MA) is a relatively common disease, yet the data on prevalence of MA remains scarce. This study was conducted to study the prevalence and clinico-hematological profile of MA.This was a cross-sectional study done on 1150 adult anemic ...