
Research plan
The research plan is a document in which the PhD student develops a plan for his/her thesis. It should include an introduction, a brief state of the art to put in context the plan, the motivation and objectives of the work, the work done so far, a plan, detailing tasks and time planning, and a list of conferences and indexed journals were the work can be presented or submitted to. A typical table of content is
- Cover page including the text “Research plan”, tentative title of the thesis, PhD program, name of the PhD student, name of the advisor(s) and date.
- Brief summary
- Introduction (state of the art, motivation, objectives)
- Work done so far
- Work plan (work to be done, tasks and time planning, tentative list of conferences and indexed journals, stays, etc). This must include a 1--page schedule of the forthcoming work until the end of the Thesis .
A short document of 10-30 pages is recommended, with annexes for details.
The panel is composed by 3 doctors with relevant expertise in the field, as president, secretary (from UPC) and vocal. As a general rule, the panel is composed by 2 researchers associated to the DMA program and an external one. In any case, the panel must include at least 1 researcher associated to the DMA program, and three of them cannot belong to the same research group. Finally any of the members of the panel can be a coauthor of the PhD student.
[These rules have been approved by the Board of Studies of DMA at a meeting 05/07/2018, and included the condition of coauthor at the CADMAMAMME meeting 08/04/2021]
Presentation
The presentation should last no more than 30 min, followed by comments and questions from the panel.
- the pdf document of the research plan
- the form detailing the panel proposal (president, secretary and vocal), the date and time for the presentation, and the information for each one of the members of the panel.
- A scan of the registration form , previously signed by the advisors and the student.
- An updated version of the Document d'activitats del Doctorand (DAD)
Please, contact the PhD program admin or the coordinator for further information.

251+ Math Research Topics [2024 Updated]
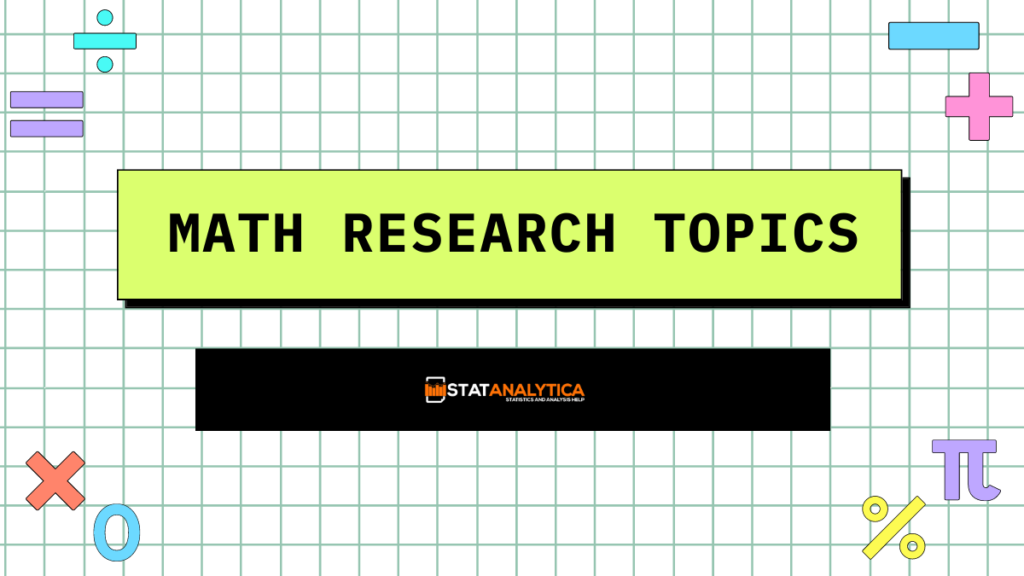
Mathematics, often dubbed as the language of the universe, holds immense significance in shaping our understanding of the world around us. It’s not just about crunching numbers or solving equations; it’s about unraveling mysteries, making predictions, and creating innovative solutions to complex problems. In this blog, we embark on a journey into the realm of math research topics, exploring various branches of mathematics and their real-world applications.
How Do You Write A Math Research Topic?
Writing a math research topic involves several steps to ensure clarity, relevance, and feasibility. Here’s a guide to help you craft a compelling math research topic:
- Identify Your Interests: Start by exploring areas of mathematics that interest you. Whether it’s pure mathematics, applied mathematics, or interdisciplinary topics, choose a field that aligns with your passion and expertise.
- Narrow Down Your Focus: Mathematics is a broad field, so it’s essential to narrow down your focus to a specific area or problem. Consider the scope of your research and choose a topic that is manageable within your resources and time frame.
- Review Existing Literature: Conduct a thorough literature review to understand the current state of research in your chosen area. Identify gaps, controversies, or unanswered questions that could form the basis of your research topic.
- Formulate a Research Question: Based on your exploration and literature review, formulate a clear and concise research question. Your research question should be specific, measurable, achievable, relevant, and time-bound (SMART).
- Consider Feasibility: Assess the feasibility of your research topic in terms of available resources, data availability, and research methodologies. Ensure that your topic is realistic and achievable within the constraints of your project.
- Consult with Experts: Seek feedback from mentors, advisors, or experts in the field to validate your research topic and refine your ideas. Their insights can help you identify potential challenges and opportunities for improvement.
- Refine and Iterate: Refine your research topic based on feedback and further reflection. Iterate on your ideas to ensure clarity, coherence, and relevance to the broader context of mathematics research.
- Craft a Title: Once you have finalized your research topic, craft a compelling title that succinctly summarizes the essence of your research. Your title should be descriptive, engaging, and reflective of the key themes of your study.
- Write a Research Proposal: Develop a comprehensive research proposal outlining the background, objectives, methodology, and expected outcomes of your research. Your research proposal should provide a clear roadmap for your study and justify the significance of your research topic.
By following these steps, you can effectively write a math research topic that is well-defined, relevant, and poised to make a meaningful contribution to the field of mathematics.
251+ Math Research Topics: Beginners To Advanced
- Prime Number Distribution in Arithmetic Progressions
- Diophantine Equations and their Solutions
- Applications of Modular Arithmetic in Cryptography
- The Riemann Hypothesis and its Implications
- Graph Theory: Exploring Connectivity and Coloring Problems
- Knot Theory: Unraveling the Mathematics of Knots and Links
- Fractal Geometry: Understanding Self-Similarity and Dimensionality
- Differential Equations: Modeling Physical Phenomena and Dynamical Systems
- Chaos Theory: Investigating Deterministic Chaos and Strange Attractors
- Combinatorial Optimization: Algorithms for Solving Optimization Problems
- Computational Complexity: Analyzing the Complexity of Algorithms
- Game Theory: Mathematical Models of Strategic Interactions
- Number Theory: Exploring Properties of Integers and Primes
- Algebraic Topology: Studying Topological Invariants and Homotopy Theory
- Analytic Number Theory: Investigating Properties of Prime Numbers
- Algebraic Geometry: Geometry Arising from Algebraic Equations
- Galois Theory: Understanding Field Extensions and Solvability of Equations
- Representation Theory: Studying Symmetry in Linear Spaces
- Harmonic Analysis: Analyzing Functions on Groups and Manifolds
- Mathematical Logic: Foundations of Mathematics and Formal Systems
- Set Theory: Exploring Infinite Sets and Cardinal Numbers
- Real Analysis: Rigorous Study of Real Numbers and Functions
- Complex Analysis: Analytic Functions and Complex Integration
- Measure Theory: Foundations of Lebesgue Integration and Probability
- Topological Groups: Investigating Topological Structures on Groups
- Lie Groups and Lie Algebras: Geometry of Continuous Symmetry
- Differential Geometry: Curvature and Topology of Smooth Manifolds
- Algebraic Combinatorics: Enumerative and Algebraic Aspects of Combinatorics
- Ramsey Theory: Investigating Structure in Large Discrete Structures
- Analytic Geometry: Studying Geometry Using Analytic Methods
- Hyperbolic Geometry: Non-Euclidean Geometry of Curved Spaces
- Nonlinear Dynamics: Chaos, Bifurcations, and Strange Attractors
- Homological Algebra: Studying Homology and Cohomology of Algebraic Structures
- Topological Vector Spaces: Vector Spaces with Topological Structure
- Representation Theory of Finite Groups: Decomposition of Group Representations
- Category Theory: Abstract Structures and Universal Properties
- Operator Theory: Spectral Theory and Functional Analysis of Operators
- Algebraic Number Theory: Study of Algebraic Structures in Number Fields
- Cryptanalysis: Breaking Cryptographic Systems Using Mathematical Methods
- Discrete Mathematics: Combinatorics, Graph Theory, and Number Theory
- Mathematical Biology: Modeling Biological Systems Using Mathematical Tools
- Population Dynamics: Mathematical Models of Population Growth and Interaction
- Epidemiology: Mathematical Modeling of Disease Spread and Control
- Mathematical Ecology: Dynamics of Ecological Systems and Food Webs
- Evolutionary Game Theory: Evolutionary Dynamics and Strategic Behavior
- Mathematical Neuroscience: Modeling Brain Dynamics and Neural Networks
- Mathematical Physics: Mathematical Models in Physical Sciences
- Quantum Mechanics: Foundations and Applications of Quantum Theory
- Statistical Mechanics: Statistical Methods in Physics and Thermodynamics
- Fluid Dynamics: Modeling Flow of Fluids Using Partial Differential Equations
- Mathematical Finance: Stochastic Models in Finance and Risk Management
- Option Pricing Models: Black-Scholes Model and Beyond
- Portfolio Optimization: Maximizing Returns and Minimizing Risk
- Stochastic Calculus: Calculus of Stochastic Processes and Itô Calculus
- Financial Time Series Analysis: Modeling and Forecasting Financial Data
- Operations Research: Optimization of Decision-Making Processes
- Linear Programming: Optimization Problems with Linear Constraints
- Integer Programming: Optimization Problems with Integer Solutions
- Network Flow Optimization: Modeling and Solving Flow Network Problems
- Combinatorial Game Theory: Analysis of Games with Perfect Information
- Algorithmic Game Theory: Computational Aspects of Game-Theoretic Problems
- Fair Division: Methods for Fairly Allocating Resources Among Parties
- Auction Theory: Modeling Auction Mechanisms and Bidding Strategies
- Voting Theory: Mathematical Models of Voting Systems and Social Choice
- Social Network Analysis: Mathematical Analysis of Social Networks
- Algorithm Analysis: Complexity Analysis of Algorithms and Data Structures
- Machine Learning: Statistical Learning Algorithms and Data Mining
- Deep Learning: Neural Network Models with Multiple Layers
- Reinforcement Learning: Learning by Interaction and Feedback
- Natural Language Processing: Statistical and Computational Analysis of Language
- Computer Vision: Mathematical Models for Image Analysis and Recognition
- Computational Geometry: Algorithms for Geometric Problems
- Symbolic Computation: Manipulation of Mathematical Expressions
- Numerical Analysis: Algorithms for Solving Numerical Problems
- Finite Element Method: Numerical Solution of Partial Differential Equations
- Monte Carlo Methods: Statistical Simulation Techniques
- High-Performance Computing: Parallel and Distributed Computing Techniques
- Quantum Computing: Quantum Algorithms and Quantum Information Theory
- Quantum Information Theory: Study of Quantum Communication and Computation
- Quantum Error Correction: Methods for Protecting Quantum Information from Errors
- Topological Quantum Computing: Using Topological Properties for Quantum Computation
- Quantum Algorithms: Efficient Algorithms for Quantum Computers
- Quantum Cryptography: Secure Communication Using Quantum Key Distribution
- Topological Data Analysis: Analyzing Shape and Structure of Data Sets
- Persistent Homology: Topological Invariants for Data Analysis
- Mapper Algorithm: Method for Visualization and Analysis of High-Dimensional Data
- Algebraic Statistics: Statistical Methods Based on Algebraic Geometry
- Tropical Geometry: Geometric Methods for Studying Polynomial Equations
- Model Theory: Study of Mathematical Structures and Their Interpretations
- Descriptive Set Theory: Study of Borel and Analytic Sets
- Ergodic Theory: Study of Measure-Preserving Transformations
- Combinatorial Number Theory: Intersection of Combinatorics and Number Theory
- Additive Combinatorics: Study of Additive Properties of Sets
- Arithmetic Geometry: Interplay Between Number Theory and Algebraic Geometry
- Proof Theory: Study of Formal Proofs and Logical Inference
- Reverse Mathematics: Study of Logical Strength of Mathematical Theorems
- Nonstandard Analysis: Alternative Approach to Analysis Using Infinitesimals
- Computable Analysis: Study of Computable Functions and Real Numbers
- Graph Theory: Study of Graphs and Networks
- Random Graphs: Probabilistic Models of Graphs and Connectivity
- Spectral Graph Theory: Analysis of Graphs Using Eigenvalues and Eigenvectors
- Algebraic Graph Theory: Study of Algebraic Structures in Graphs
- Metric Geometry: Study of Geometric Structures Using Metrics
- Geometric Measure Theory: Study of Measures on Geometric Spaces
- Discrete Differential Geometry: Study of Differential Geometry on Discrete Spaces
- Algebraic Coding Theory: Study of Error-Correcting Codes
- Information Theory: Study of Information and Communication
- Coding Theory: Study of Error-Correcting Codes
- Cryptography: Study of Secure Communication and Encryption
- Finite Fields: Study of Fields with Finite Number of Elements
- Elliptic Curves: Study of Curves Defined by Cubic Equations
- Hyperelliptic Curves: Study of Curves Defined by Higher-Degree Equations
- Modular Forms: Analytic Functions with Certain Transformation Properties
- L-functions: Analytic Functions Associated with Number Theory
- Zeta Functions: Analytic Functions with Special Properties
- Analytic Number Theory: Study of Number Theoretic Functions Using Analysis
- Dirichlet Series: Analytic Functions Represented by Infinite Series
- Euler Products: Product Representations of Analytic Functions
- Arithmetic Dynamics: Study of Iterative Processes on Algebraic Structures
- Dynamics of Rational Maps: Study of Dynamical Systems Defined by Rational Functions
- Julia Sets: Fractal Sets Associated with Dynamical Systems
- Mandelbrot Set: Fractal Set Associated with Iterations of Complex Quadratic Polynomials
- Arithmetic Geometry: Study of Algebraic Geometry Over Number Fields
- Diophantine Geometry: Study of Solutions of Diophantine Equations Using Geometry
- Arithmetic of Elliptic Curves: Study of Elliptic Curves Over Number Fields
- Rational Points on Curves: Study of Rational Solutions of Algebraic Equations
- Galois Representations: Study of Representations of Galois Groups
- Automorphic Forms: Analytic Functions with Certain Transformation Properties
- L-functions: Analytic Functions Associated with Automorphic Forms
- Selberg Trace Formula: Tool for Studying Spectral Theory and Automorphic Forms
- Langlands Program: Program to Unify Number Theory and Representation Theory
- Hodge Theory: Study of Harmonic Forms on Complex Manifolds
- Riemann Surfaces: One-dimensional Complex Manifolds
- Shimura Varieties: Algebraic Varieties Associated with Automorphic Forms
- Modular Curves: Algebraic Curves Associated with Modular Forms
- Hyperbolic Manifolds: Manifolds with Constant Negative Curvature
- Teichmüller Theory: Study of Moduli Spaces of Riemann Surfaces
- Mirror Symmetry: Duality Between Calabi-Yau Manifolds
- Kähler Geometry: Study of Hermitian Manifolds with Special Symmetries
- Algebraic Groups: Linear Algebraic Groups and Their Representations
- Lie Algebras: Study of Algebraic Structures Arising from Lie Groups
- Representation Theory of Lie Algebras: Study of Representations of Lie Algebras
- Quantum Groups: Deformation of Lie Groups and Lie Algebras
- Algebraic Topology: Study of Topological Spaces Using Algebraic Methods
- Homotopy Theory: Study of Continuous Deformations of Spaces
- Homology Theory: Study of Algebraic Invariants of Topological Spaces
- Cohomology Theory: Study of Dual Concepts to Homology Theory
- Singular Homology: Homology Theory Defined Using Simplicial Complexes
- Sheaf Theory: Study of Sheaves and Their Cohomology
- Differential Forms: Study of Multilinear Differential Forms
- De Rham Cohomology: Cohomology Theory Defined Using Differential Forms
- Morse Theory: Study of Critical Points of Smooth Functions
- Symplectic Geometry: Study of Symplectic Manifolds and Their Geometry
- Floer Homology: Study of Symplectic Manifolds Using Pseudoholomorphic Curves
- Gromov-Witten Invariants: Invariants of Symplectic Manifolds Associated with Pseudoholomorphic Curves
- Mirror Symmetry: Duality Between Symplectic and Complex Geometry
- Calabi-Yau Manifolds: Ricci-Flat Complex Manifolds
- Moduli Spaces: Spaces Parameterizing Geometric Objects
- Donaldson-Thomas Invariants: Invariants Counting Sheaves on Calabi-Yau Manifolds
- Algebraic K-Theory: Study of Algebraic Invariants of Rings and Modules
- Homological Algebra: Study of Homology and Cohomology of Algebraic Structures
- Derived Categories: Categories Arising from Homological Algebra
- Stable Homotopy Theory: Homotopy Theory with Stable Homotopy Groups
- Model Categories: Categories with Certain Homotopical Properties
- Higher Category Theory: Study of Higher Categories and Homotopy Theory
- Higher Topos Theory: Study of Higher Categorical Structures
- Higher Algebra: Study of Higher Categorical Structures in Algebra
- Higher Algebraic Geometry: Study of Higher Categorical Structures in Algebraic Geometry
- Higher Representation Theory: Study of Higher Categorical Structures in Representation Theory
- Higher Category Theory: Study of Higher Categorical Structures
- Homotopical Algebra: Study of Algebraic Structures in Homotopy Theory
- Homotopical Groups: Study of Groups with Homotopical Structure
- Homotopical Categories: Study of Categories with Homotopical Structure
- Homotopy Groups: Algebraic Invariants of Topological Spaces
- Homotopy Type Theory: Study of Foundations of Mathematics Using Homotopy Theory
In conclusion, the world of mathematics is vast and multifaceted, offering endless opportunities for exploration and discovery. Whether delving into the abstract realms of pure mathematics or applying mathematical principles to solve real-world problems, mathematicians play a vital role in advancing human knowledge and shaping the future of our world.
By embracing diverse math research topics and interdisciplinary collaborations, we can unlock new possibilities and harness the power of mathematics to address the challenges of today and tomorrow. So, let’s embark on this journey together as we unravel the mysteries of numbers and explore the boundless horizons of mathematical inquiry.
Related Posts
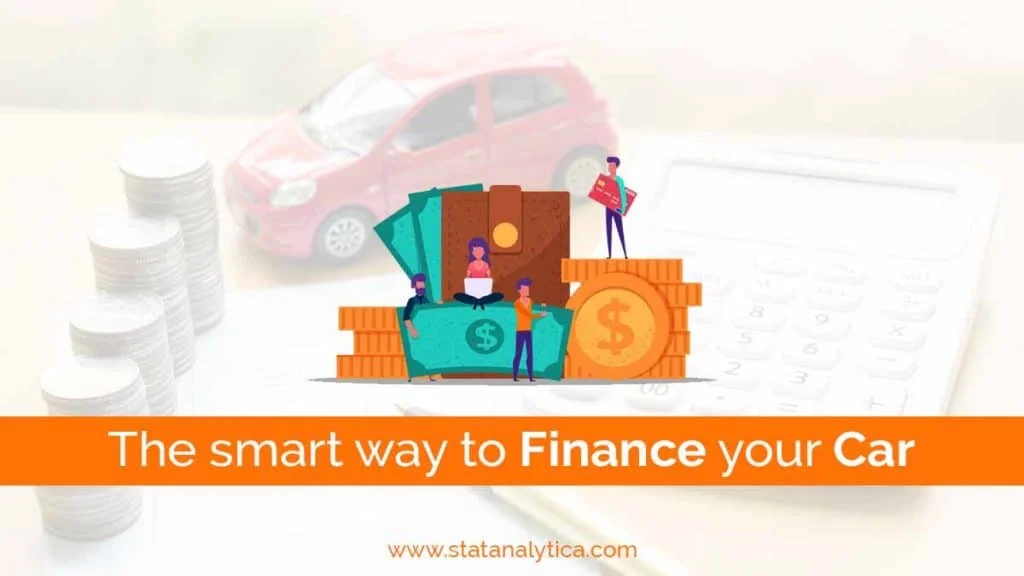
Step by Step Guide on The Best Way to Finance Car
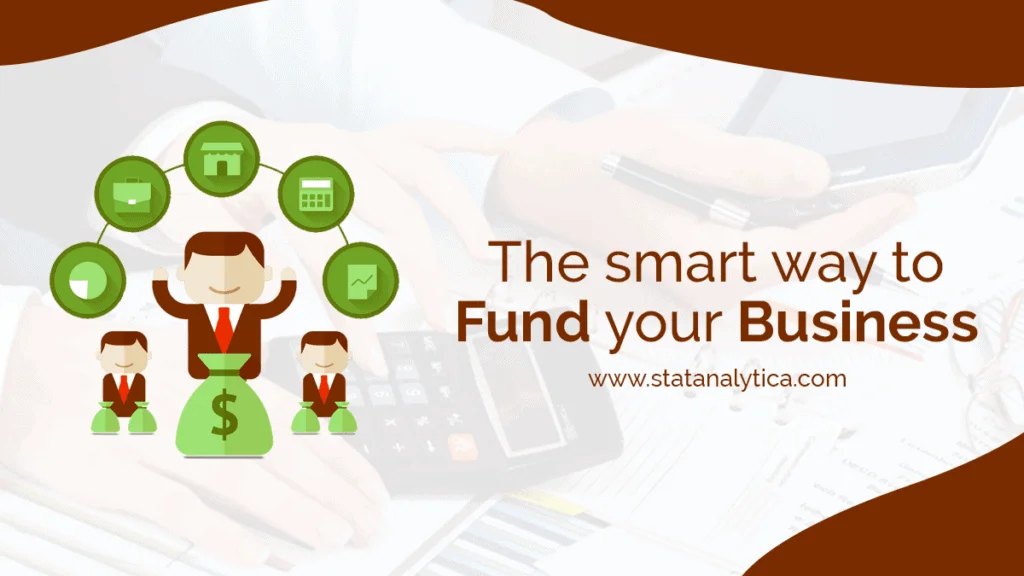
The Best Way on How to Get Fund For Business to Grow it Efficiently
Applied Mathematics Research
In applied mathematics, we look for important connections with other disciplines that may inspire interesting and useful mathematics, and where innovative mathematical reasoning may lead to new insights and applications.
Applied Mathematics Fields
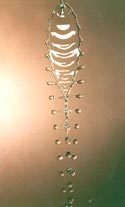
- Combinatorics
- Computational Biology
- Physical Applied Mathematics
- Computational Science & Numerical Analysis
- Theoretical Computer Science
- Mathematics of Data
Applied Math Committee
Mathematical Analysis
In a rough division of mathematics, mathematical analysis deals with inequalities and limits. in some of its branches, such as asymptotic analysis, these aspects of the subject matter are readily apparent. in others, such as operator algebras, they are concealed in the topology of an algebra or its structure as an infinite-dimensional vector space. our department offers a wealth of different perspectives on mathematical analysis..
Chairs: Sung Jin Oh and Daniel Tataru
Mathematical Analysis Faculty, Courses, Dissertations
Senate faculty, graduate students, visiting faculty, meet our faculty, michael christ, l. craig evans, steven n. evans, f. alberto grünbaum, ole h. hald, jenny harrison, svetlana jitomirskaya, michael j. lindsey, c. keith miller, john c. neu, sung-jin oh, james pitman, fraydoun rezakhanlou, marc a. rieffel, james a. sethian, nikhil srivastava, john strain, daniel tataru, dan-virgil voiculescu, franziska weber, alan d. weinstein, jon wilkening, mariusz wodzicki.
- 1 of 2 Grid: People (Current page)
- 2 of 2 Grid: People
- next › Grid: People
- last » Grid: People

- How we work

How to Compose a Quality Research Proposal for Mathematics
Best tips on what will make your research proposal for mathematics stand out.
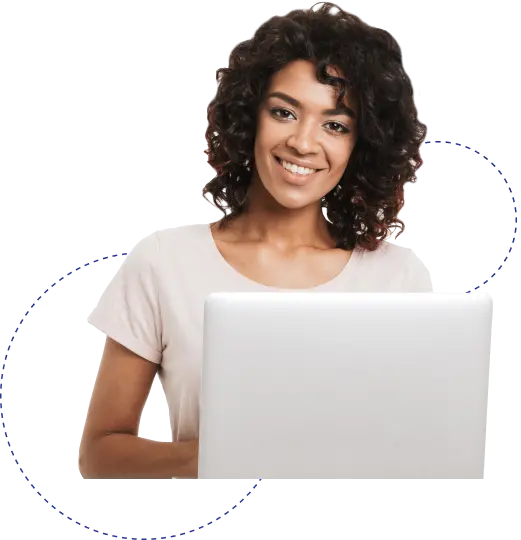
What is a Research Proposal in Mathematics Education?
This one is an official document representing the significance of the study. It’s a kind of plan you submit to the supervisors or the funding committee to share information about your research goals, as well as investigation issues, objectives, and methods related to teaching, learning, program development, or assessment in mathematics education.
Crafting an impactful research proposal for mathematics is extremely hard, although utterly exciting. Most students’ problems begin when choosing mathematics research areas . Other issues are often related to a lack of fundamental knowledge and complexity of particular topics & concepts, predicting the potential study influence, or suggesting effective issue solutions. Ordinary time management issues may also create a lot of hassle.
Except for the difficulty factor, there’s the need to follow strict structural and formatting guidelines. Obviously, writing professionals can easily compose a good text. Learners, on the other hand, are often doing this for the first time. Despite all the different approaches to crafting proposals, almost always, mathematics research encompasses the following parts:
- Problem overview.
- (Potential) solutions.
- Costs (both in human efforts and budgetary ones).
- Possible benefits.
Of course, the action research proposal in mathematics content will change according to the specific mathematics research topics and corresponding college guidelines. We believe it is completely obvious that writing such a complex task requires extraneous amounts of effort and time. As a result, many learners decide to delegate these assignments to seasoned experts, freeing up their time for more important responsibilities.
How to Write Best Research Proposal in Mathematics Easily
Despite all the different approaches, almost always, a mathematical survey encompasses the following parts:
- Choosing a Specific Topic. Formulate clearly the problem you are going to solve, explain its relevance, and articulate a concise, well-structured question or hypothesis your study aims to answer. If you have several research proposal in mathematics topics, try to choose a specific one that interests you and have enough materials to study.
- Highlighting Importance: Formulate the significance of your investigation and its possible implications on the mathematics field. Justify why it is significant and how it enhances knowledge.
- Tailoring to Audience: Adapt your engineering proposal to your target audience, whether it’s an academic committee, potential advisors, or funding agencies. Take into account their interests, worries, and expectations.
- Conducting a Literature Review: Thoroughly examine existing literature related to your chosen topic and prepare the theoretical background for your action research proposal in mathematics.
- Outlining Your Methodology: Describe the methods and techniques you plan to use to investigate the chosen mathematics issue. Explain why these methods are appropriate and how you will apply them.
- Proposing a Timeline: Create a realistic timeline that outlines your mathematics project’s key milestones and stages.
- Budget and Resources: Estimate the necessary research resources, including materials, software, equipment, and any potential travel. Justify the budget allocation based on the requirements of your work.
- Writing a Clear and Compelling Paper: Craft a well-structured and engaging research proposal in mathematics education following the guidelines provided by your institution or funding agency.
- Citations: Properly cite all sources you reference in your paper using the appropriate citation style (e.g., APA, MLA, Chicago).
- Proofreading and Formatting: Check the text for grammatical errors, typos, and formatting issues. Ensure consistency in style and formatting throughout the document.
Review Our Sample of Action Research Proposal in Mathematics
We recommend you check the following sample of action research proposal in mathematics to see for yourself the quality of the work. It includes such parts as:
- Introduction
- Background of the Study
- Statement of the Research
- Limitation of the Study
- References.
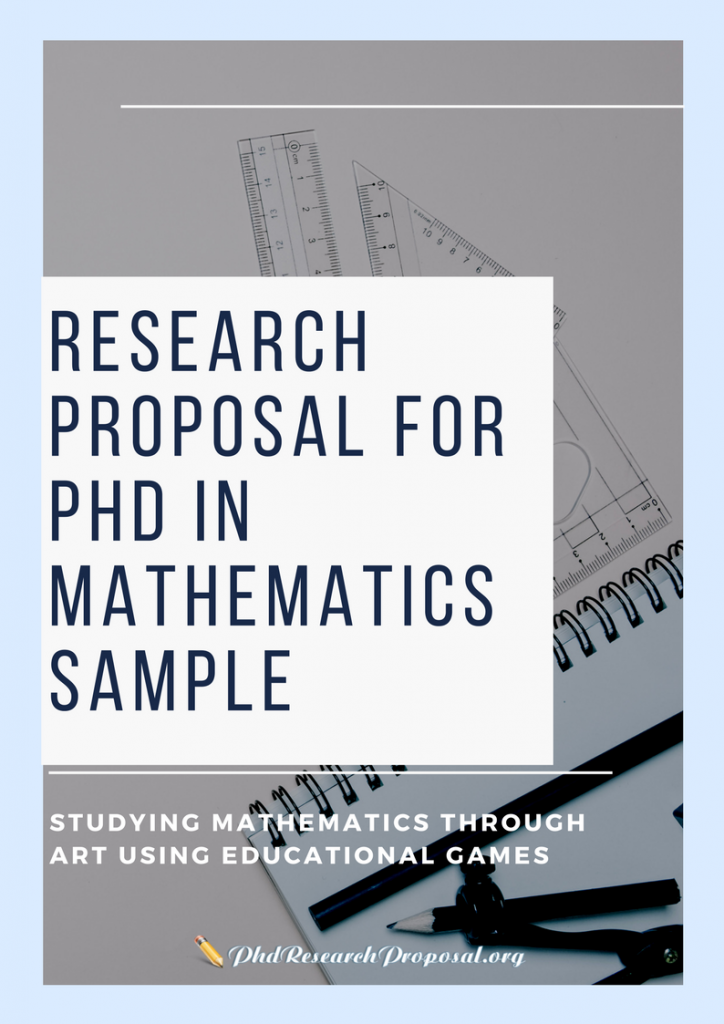
Click and DOWNLOAD FULL Research Proposal for PhD in Mathematics
Some topics for action research proposal in mathematics.
In case you lack inspiration, we’ve listed a few mathematics topics to give you some inspiration.
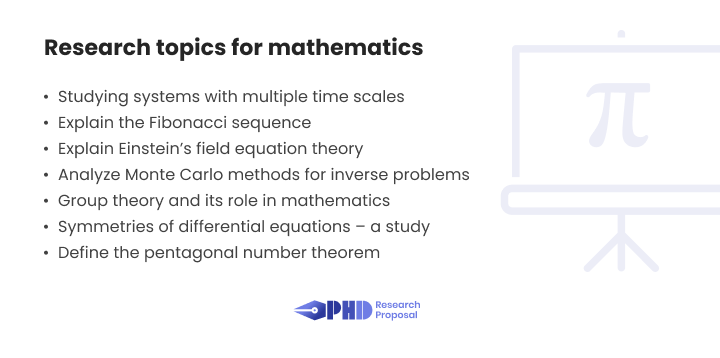
In addition, many experts recommend moving away from traditional research proposal topics in mathematics and looking more broadly. Mathematical theoretical concepts are at the core of various fields, covering many engineering, architectural, and business industries. You can also find good ideas by checking out studies in information and computer technology.
Quite a few finance and accounting fields also involve mathematics concepts. The same can be said about logistics, social and political sciences, business, and management. Perhaps some ideas are not on the surface, and you will have to dig deeper. However, as a result, you will be able to identify several worthy solutions that can not only take your research proposal to the next level but also make it more relevant, valuable, and effective.
How to Start Writing Mathematics Research Proposal?
Sometimes, it may be difficult to get started because of the abovementioned factors. Nevertheless, we recommend a few techniques to help you get a grip on your writing.
- Set goals you are sure to accomplish and move step by step, sticking to a certain schedule ( e.g., use the Pomodoro technique).
- Take some ready-made examples of proposals dedicated to working with theoretical frameworks, as a mathematics research proposal may have different approaches to presenting your research than any others related to applied solutions.
- Acquire the support of your supervisor – any writing prompts, guidance, one-to-one conversations, etc. Getting help isn’t a weakness but a testament to your desire to develop a better project proposal.
You can also try to start a mathematics research proposal by preparing a theoretical base and additional materials, visuals, graphs, diagrams, etc. First, it will allow you to focus and choose a direction for your work. Secondly, having a variety of ready-made materials is ideal for combating the fear of a blank sheet. In addition, a well-designed research proposal involving many mathematics visuals has greater value and, in all likelihood, will be better received by the target audience.
Get Research Proposal for Mathematics Worthy of Your Tutor’s Approval
Do you need writing professionals to complete your mathematics paper quickly and without problems? We are one of the top services on the market – picking us over others is an obvious decision.
The service benefits are plentiful, covering almost all client experience areas, but that is not the end of it all! We also offer extra features:
- “Top” and “First-Rate” writer options.
- Continuous chat with writers and email updates.
- Revisions during the writing process and free edits after getting the final result.
- Plagiarism report and list of sources.
- Help with revision and final proofreading.
Even more importantly, our service heavily prioritizes customer safety. Whenever students are purchasing mathematics research proposal – their banking information is secured. No third party will access confidential client data. The website integrates exclusively safe buying options: Visa & Mastercard. Further enhancing security, our platform encompasses modern web security protocols, protecting customer connection to our servers.
With the help of our specialists, you no longer have to puzzle over how to write best research proposal in mathematics. Finally, just take a breath from your overwhelming academic loads by entrusting our experts.
Contact us and fill out the order form to make your life easier!

Upload Files
Thank you for your request!
We will get in touch with you shortly!
Please, try one more time.
We use cookies to enhance our website for you. Proceed if you agree to this policy or learn more about it.
- Essay Database >
- Essays Samples >
- Essay Types >
- Research Proposal Example
Mathematics Research Proposals Samples For Students
24 samples of this type
Regardless of how high you rate your writing abilities, it's always an appropriate idea to check out an expertly written Research Proposal example, especially when you're dealing with a sophisticated Mathematics topic. This is exactly the case when WowEssays.com database of sample Research Proposals on Mathematics will prove useful. Whether you need to brainstorm a fresh and meaningful Mathematics Research Proposal topic or survey the paper's structure or formatting peculiarities, our samples will provide you with the required data.
Another activity area of our write my paper company is providing practical writing assistance to students working on Mathematics Research Proposals. Research help, editing, proofreading, formatting, plagiarism check, or even crafting fully original model Mathematics papers upon your demand – we can do that all! Place an order and buy a research paper now.
Research Proposal On Whiteboard on Mathematics Classroom
Virtual architecture model, smashing research proposal about research questions, impacts of interactive boards in mathematics classrooms..
- What are the effects of white board technology on mathematics student’s performance and attention? - Do mathematics students engage better while using interactive white boards than without the white board?
Research description
Purpose statement research proposals example, impact of interactive board in mathematics classroom.
Don't waste your time searching for a sample.
Get your research proposal done by professional writers!
Just from $10/page
Example Of Research Proposal On The Correlation Between Student Anxiety And Academic Performance In Low-Level College
This paper examines the correlative effects of stress levels on examination results, particularly in areas of academic study where students are already struggling. The research examines the test scores and stress levels of remedial and basic-level mathematics students in the undergraduate level, tracking their changes in stress level over time and the effects that changes in stress level has on a student’s academic performance in in-class mathematics examinations.
Introduction
Research proposal on research hypotheses:, education research proposal samples, free math group studying discourse community research proposal sample, common core standards in education: research proposal research proposal sample, introduction, free research proposal on the relationship between preschool programs and kindergarten readiness, the research aim research proposal template for faster writing, research outline proposal: development of geometry by the hippocrates of chios.
Introduction Hippocrates made mathematical advancement during the Golden Age in which fundamental concepts of geometry began thriving in the social dynamics (Huffman, and Filolaos 197). Some of the two core mathematic techniques that occurred during this era include axiomatic techniques to geometry and introduction of paradoxes by the Zeno of Elea (Bell 138). Principally, the paradoxes were concerned with the determination of infinite and infinitesimal mathematic concepts (Klette, and Azriel 455).
Good Research Proposal About Project-Based Learning
This research proposal will employ a longitudinal experimental design to investigate student learning using Project-based learning for middle school literature classrooms within the same school. The results will be measured by IOWA testing every term over a 3 year time period and compared to the results of the students from traditional classroom setting. Assessment scores will be the dependent variables. The literature review of six peer-reviewed articles is included. The purpose of the study, hypotheses, methodology, participants, procedure, instruments and data analysis will be described.
Radiation Detector Failures Research Proposals Examples
Proper research proposal example about the impact of computers on individual learning, unknown cumulative distribution function research proposal, problem statement research proposal example.
Dropping Out or Pushed Out: The Impact of High School Dropout Rate Relative To High Stakes Testing Policy In The State Of Michigan
The Research Project Research Proposal Examples
Doctor candidate:.
Philosophy of Transformation: Differentiation or Subtraction Summary in Key Words Philosophy of transformation; Deleuze; Badiou; differentiation, subtraction, (re-, de-) territorialization; folding; plane of consistency; events (interventions); truth procedure; subject; the capitalism; the democratic materialism
Summary of the Theme and the Aim of the Project
Research questions: a sample research proposal for inspiration & mimicking, exemplar research proposal on winston educational foundation to write after, improvements in public education.
1598 Camarillo Street
Anaheim, California
818.432.2465
Dear City Councilman Roberts:
Example of components of a computer research proposal.
Introduction The application of computers is widespread in the current generation. Computers form a fundamental part of our daily lives as well as activities. The application of computers ranges from simple mathematical computations in devices like calculators to complex mathematical computations in industries. Virtually everything depends on computers for operation and completion of various tasks and processes in equal measure. The use of computer presents a variety of advantages over other alternative methods of accomplishing tasks (Krishnamoorthy, et al, 2009).
Background Research
Good recidivism among paroled inmates: discussion research proposal example.
(Institute)
Returning to prison for paroled inmates: Introduction
Good research proposal on p(g,s,r)= p(g/s,r)p(s/r)p(r), good research proposal about gcu: res 880.
Dropping Out or Pushed Out: The Impact of High School Dropout Rate Relative to High Stakes Testing Policy in Wayne County, State of Michigan
Dissertation Prospectus Dropping Out or Pushed Out: The Impact of High School Dropout Rate Relative to High Stakes Testing Policy in Wayne County, State of Michigan <Insert Chair Name>
Dissertation Prospectus
Preparing design and technology students for the future research proposal, research proposal on nursing homes, statement of the problem.
Old age is associated with several mental illnesses, which culminate into other psychosocial issues. For example, dementia and other related conditions such as the Creutzfeudz Jacob’s disease – as caused by advanced senility – have become common in the modern world. Usually, these diseases affect old people, which become more and more problematic as the age advances. The role of caring for the old people has therefore become very vital, usually requiring increased care and special treatment to these people.
Password recovery email has been sent to [email protected]
Use your new password to log in
You are not register!
By clicking Register, you agree to our Terms of Service and that you have read our Privacy Policy .
Now you can download documents directly to your device!
Check your email! An email with your password has already been sent to you! Now you can download documents directly to your device.
or Use the QR code to Save this Paper to Your Phone
The sample is NOT original!
Short on a deadline?
Don't waste time. Get help with 11% off using code - GETWOWED
No, thanks! I'm fine with missing my deadline
Advertisement
Implementation-related research in mathematics education: the search for identity
- Survey Paper
- Open access
- Published: 01 September 2021
- Volume 53 , pages 975–989, ( 2021 )
Cite this article
You have full access to this open access article
- Boris Koichu ORCID: orcid.org/0000-0001-7298-8175 1 ,
- Mario Sánchez Aguilar ORCID: orcid.org/0000-0002-1391-9388 2 &
- Morten Misfeldt ORCID: orcid.org/0000-0002-6481-4121 3
4493 Accesses
11 Citations
4 Altmetric
Explore all metrics
Implementation has always been a paramount concern of mathematics education, but only recently has the conceptualizing and theorizing work on implementation as a phenomenon begun in our field. In this survey paper, we conduct a hermeneutic review of mathematics education research identified as related to the implementation problematics. The first cycle of the review is based on examples of studies published in mathematics education journals during the last 40 years. It is organized according to five reasons for developing implementation research. The second cycle concerns 15 papers included in this special issue and is organized by four themes, as follows: objects of implementation, stakeholders in implementation, implementation vs. scaling up, and implementability of mathematics education research. The paper is concluded with a refined glossary of implementation-related terms and suggestions for future research.
Similar content being viewed by others
The use of cronbach’s alpha when developing and reporting research instruments in science education.
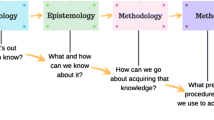
A Medical Science Educator’s Guide to Selecting a Research Paradigm: Building a Basis for Better Research
Ethical Considerations of Conducting Systematic Reviews in Educational Research
Avoid common mistakes on your manuscript.
1 Introduction
As long as research and practice co-exist in the field of mathematics education, implementation and implementability have always been of paramount concern. Already in 1979, Bruckheimer pointed out the difficulty of implementing innovative curriculum materials in the mathematics classroom, and indicated the existence of “inhibitors of curriculum implementation” (Bruckheimer, 1979 , p. 44). He argued that these inhibitors can be properties of the specific curriculum design, but they can also result from an interplay with the overall structure of the educational system. In 1996, Niss identified the implementation problem as one of the main problems of mathematics education research that has to do with establishing the structural and organizational framework for conducting mathematics education, providing the necessary resources for conducting mathematics teaching, and addressing issues related to the philosophy and modes of assessment (Niss, 1996 ). In his characterization of mathematics education as an academic discipline, Niss ( 1999 ) suggested that the implementation problem calls for theoretical scrutiny:
[i]t is fair to claim that the overarching, ultimate end of the whole enterprise is to promote/improve students’ learning of mathematics and acquisition of mathematical competencies. It is worth pointing out that the very specification of the terms just used (‘promote’, ‘improve’ ‘students’ (what students are being considered?) ‘learning’, ‘mathematics’, ‘acquisition’, ‘mathematical competencies’) is in itself a genuine didactic task. (p. 5)
Niss identified the need to theorize such terms as ‘promote’ and ‘improve’ that to a large extent express the raison d'être for implementation of mathematics education research in mathematics education practice. However, it is only recently that conceptualizing and theorizing work on implementation has begun in our field, though it has been pursued for a while in some other fields, most notably in health care (e.g., Eccles & Mittman, 2006 ).
Let us recall several landmarks. Confrey et al. ( 2000 ) introduced the idea of ‘implementation research’ as a means to link applied psychology and systemic reform in mathematics education. Burkhardt and Schoenfeld ( 2003 ) reflected on implementation in mathematics education from the perspective of bridging the gap between research and practice by developing appropriate tools and structures. Remillard ( 2005 ) suggested that a particular case of implementation—‘curriculum use’—can be usefully explored as a participatory relationship between teachers and curriculum materials (this idea was further developed in research on curriculum ergonomics, Choppin et al., 2018 ). Maaß and Artigue ( 2013 ) interpreted implementation as setting a planned intervention or innovation in motion so that new research results lead to the development of new interventions and these are further disseminated. Cai et al. ( 2017 ) considered ‘classroom implementation’, that is, implementation of research-based learning opportunities in the classroom. All these approaches are related, but put forwards different aspects of the implementation problem and imply complementary agendas for action.
The diversity of approaches to implementation was particularly salient in two collections of papers written by participants in Thematic Working Group 23 of the 10th and 11th Congress of the European Society for Research in Mathematics Education (CERME). This group was established in 2017 at CERME10 with a special focus on implementation and replication research (Jankvist et al., 2017 ). Participants of CERME11 collaboratively produced a tentative conceptualization of implementation in mathematics education in an attempt to accommodate the diversity (see Aguilar et al., 2019 , p. 8). A slight modification of that definition was used in the call for papers for this special issue. In this call, implementation was referred to as a change-oriented process of endorsing an action plan based on a relatively well-defined resource (such as a research finding, a digital tool, a curriculum, a textbook, or an institutional policy) that occurs in interaction of a community of the resource proponents and a community of the resource adapters, leading to a gradual shift in agency over the resource and the action plan, from the proponents to the adapters, and also leading to changes in communication and practice of both communities. (This definition is further refined towards the end of this paper).
Even more recently, Artigue ( 2021 ) analyzed theoretical resources that are likely to support implementation studies, and argued that resources either internal or external to the field of mathematics education can be useful, though in different ways. In addition, Cobb and Jackson ( 2021 ) presented elements of theory of action as an empirically-grounded theoretical resource for guiding implementation initiatives at scale. Of special note is that Artigue’s and Cobb and Jackson’s ( 2021 ) papers were published in an inauguration issue of a new journal entitled Implementation and Replication Studies in Mathematics Education (IRME). The appearance of IRME is another indication of how vibrant and timely the implementation and implementability problematique in mathematics education is today.
This special issue (hereafter SI) aims at further foregrounding the implementation problem, by showing different ways to conceptualize and work with the implementation aspects of mathematics education research. The motivation for this focus is the observation that implementation still more often than not remains in the background, and not in the forefront of mathematics education research. Fifteen papers of this SI essentially represent the specificity of implementation and implementation-related research in mathematics education. In line with Artigue ( 2021 ), we presume that though implementation in mathematics education can be informed by theoretical developments in other fields of study (e.g., Century & Cassata, 2016 ; Nilsen, 2015 ), the specificity of the mathematics education ecology (Blomhøj, 2021 ) requires considering implementation in connection with epistemology and ontology of mathematics as a discipline/subject, as well as in relation to the specific ways in which mathematics education is organized nationally and internationally.
In order to better understand the nature of implementation in mathematics education as it has been developing so far, we first survey past empirical research concerning aspects of implementation in mathematics education without theorizing implementation as a phenomenon (Sect. 3 ). We then provide a detailed introduction to all the papers of this SI (Sect. 4 ). A characteristic feature of this SI is that the authors—an international group of scholars who were invited to share their work designed a-priori as ‘implementation research’ or present their ‘regular’ research via implementation-related lenses—are very explicit about what implementation and implementation research means for them. Since we are entering a research realm that is not yet fully institutionalized and agreed upon, we choose to conduct the survey in accordance with a hermeneutic approach to reviewing the literature; the details of this approach are explained in Sect. 2 . We conclude, in Sect. 5 , with a refined glossary of implementation-related terms and suggestions for future research.
2 Hermeneutic approach to the literature on implementation in mathematics education
Although hermeneutic literature reviews are used in such fields as health science and well-being (Lawler et al., 2019 ; Valentine et al., 2021 ), they are not common in educational research. According to Boell and Cecez-Kecmanovic ( 2014 ), a hermeneutic literature review presumes continuous engagement with a growing body of literature, during which increased understanding and insights are sought. This approach allows engaging in a dialogue with the literature in search of new meanings (Smythe & Spence, 2012 ). Practically, a hermeneutic literature review is guided by two circles, namely, search and acquisition, and analysis and interpretation. In the first circle, publications relevant to the topic of interest are identified. Here one often prioritizes working in depth with a relatively small set of highly relevant publications, and allows the reading to influence the search and inclusion criteria in an iterative fashion (Boell & Cecez-Kecmanovic, 2014 ). The analysis and interpretation circle is developed through the reading of the selected publications, and leads to classifications, critical assessment, and development of arguments about the studied topic.
Accordingly, the aim of the hermeneutic review is not an exhaustive characterization of the chosen topic, but rather to reach a saturation point, whereby insights from an in-depth reading of part of the body of the literature are sufficiently comprehensive. This point is evident from the diminished feeling of novelty when reading additional literature. However, this saturation is where one of the limitations of a hermeneutic literature review lies: it has the goal of obtaining insights without considering all evidence surrounding a particular issue as in a systematic literature review. In the case of the hermeneutical review that we report here, it is very likely that certain literature that might be considered under the umbrella of ‘implementation research’ has not been considered, due to the design of the review itself, for example, by the choice of the initial inclusion criteria.
In our case, we began the search by exploring the research journals in the field of mathematics education, according to Williams and Leatham’s ( 2017 ) classifications. Footnote 1 By means of the review management software Covidence ( http://www.covidence.org ), we identified 137 papers published in these journals during the last 40 years, which contain the key term ‘implement’ (or derived terms such as ‘implementation’ or ‘implementing’) in the title, in the abstract, or in the keywords. Browsing through the located papers showed a huge variety of ways in which the word ‘implementation’ was used. This led us to introduce an additional inclusion criterion: we decided that the papers of interest should not only concern possible implementation of the presented findings, but conform to the working definition of implementation research proposed in Century and Cassata’s ( 2016 ) overview of the landscape of implementation research in education. In particular, they provided a conceptual ground made up of notions and distinctions, including their definitions of innovation, implementation and implementation research. These definitions were often cited by the participants in the TSG23 of CERME11. According to Century and Cassata ( 2016 ), implementation research is “a systematic inquiry regarding innovations enacted in controlled settings or in ordinary practice, the factors that influence innovation enactment, and the relationships between innovations, influential factors, and outcomes.” (p. 170).
Thus, among the papers identified, we looked for those papers that empirically addressed a research question on the implementation of an innovation, probably among other research questions. These papers were then grouped according to five reasons for developing implementation research suggested by Century and Cassata ( 2016 ). The reasons are as follows: (i) inform innovation design and development; (ii) understand whether (and to what extent) the innovation achieves desired outcomes for the target population; (iii) understand relationships between influential factors, innovation enactment, and outcomes; (iv) improve innovation design, use, and support in practice settings; (v) develop theory (ibid, p. 174). The interpretation of selected papers according to the five reasons is presented in Sect. 3 . Within each reason-category, the interpretation went beyond the motivation for conducting the research, and attended to how it was conducted and what was discovered or concluded. This extended focus eventually led us to the development of four new themes, as follows: (i) objects of implementation, (ii) configurations of stakeholders in implementation; (iii) implementation vs. scaling up; (iv) the question of implementability of mathematics education research. These themes emerge in Sect. 3 with regard to past research, and are then systematically put forth in Sect. 4 in relation to the papers included in this SI. That is, whereas Century and Cassata’s ( 2016 ) five reasons were chosen at the early stage of our reading as a tool to frame and demarcate the past literature, the four themes emerged from this reading and were further used as a frame for discussing papers included in this SI. Eventually, these four themes served as a springboard for a discussion of what implementation-related research in mathematics education currently is, and of how this SI contributes to the field.
3 Implementation research in the mathematics education literature
As explained, this survey embraces studies that are not explicitly identified by their authors as ‘implementation research’, but are interpreted as such by us, in accordance with the working definition offered by Century and Cassata ( 2016 ). We use Century and Cassata’s ( 2016 ) five reasons as an organizational structure, and illustrate each reason through a small number of studies. Each study was selected for relating in a particularly clear way to one reason in question. In addition, an effort was made to diversify the selected studies with respect to where and when they were conducted.
3.1 Reason 1. Inform innovation design and development
This reason is germane to those studies that “examine questions about what the innovation could and/or should be, the extent to which an innovation is feasible in particular settings, and its utility from the perspective of the end users” (Century & Cassata, 2016 , p. 174). It includes studies that “examine the creation of an innovation, its qualities and characteristics; understand place feasibility and usability of the innovation; or create an innovation customized for a time, and context” (p. 174).
A study by Confrey et al. ( 2017 ) can serve as a characteristic example. The study describes “the creation of a tool to meet the design challenge of scaffolding improved curricular coherence when practitioners use a variety of resources to build curriculum.” (p. 732). This tool—a digital learning system called Math-Mapper (M-M) that includes visual maps of mathematical content called Relational Learning Clusters (RLC)—is the innovation in question. The paper first provides a detailed description of the innovation and its theoretical underpinnings. It then reports on three pilot studies where the innovation was enacted by the end-users—mathematics teachers and students from two school districts in the US—in order to test its feasibility and usability in practice. The first study explored how the M-M supported a process of curriculum revisions. A testimony of one of the teachers who “called her experience of the previous curriculum as ‘chaotic’ and celebrated the new one as ‘calm’” (Confrey et al., 2017 , p. 726) is presented as an illustration of feasibility and usability of the system. The second pilot study explored sixth grade students’ performance on one of the RLCs, based on quantitative data collected from several hundred students. The third study explored the students’ feedback after experiencing assessment that was organized in accordance with the M-M principles. Here the authors briefly summarized more than 1000 students’ reflective reports and exemplified them through selected quotes. They cautiously concluded that the data “supports the validity of emphasis on learner-centeredness of curriculum coherence” (ibid., p. 732). Overall, in spite of the relatively broad scope of the research (two districts, tens of teachers, hundreds of students), the authors’ final remark is that “the reported studies only offer an initial glimpse on M-M’s potential effects on curriculum and instruction” (p. 732) and that further studies are needed to explore outcomes of implementation, especially when conducted without ongoing support of the R&D team, and also to refine the design of the innovation.
Studies having a similar research focus—that is, studies that report on initial enactment of an innovation when its proponents are heavily involved—are not rare in the reviewed literature, though the nature of the innovations varies. Such studies concern, for example, innovative assessment systems (Ernest, 1984 ), electronic textbooks (Hoch et al., 2018 ), instructional guidelines (Colonnese, 2018 ), classroom pedagogies (Sullivan et al., 2013 ), instructional sequences (Stylianides & Stylianides, 2009 ), and professional development courses (Kuzle & Biehler, 2015 ).
3.2 Reason 2. If and how the innovation achieves desired outcomes for the target population
Studies in this group focus on examining the efficacy and effectiveness of innovations, and explore their emerging outcomes (Century & Cassata, 2016 ). This kind of research tries to determine whether the implementation of an innovation produces the expected results in the target population (students, teachers, schools, etc.)
Research of this kind was reported by Prediger et al. ( 2019 ). The study explored effects of combining three implementation strategies for upscaling professional development, namely, the community-based strategy, the material-based strategy and the systemic strategy. This combination, used in a professional development program, Mastering Math, in Germany, was the innovation at stake. The authors not only provided an existence proof of the viability of combining these strategies, but explored the effects of the innovation enactment on the participating teachers and students. The first two research questions of this study were as follows: (i) What are the effects of the research-based implementation and professional development program on teachers’ perception of materials and of their cooperation in professional learning communities? (ii) What are the effects of the research-based implementation and professional development program on students’ learning gains compared to a control group of students supported by the teachers outside the program? (p. 364). The data for the first question were collected via a questionnaire filled out by 63 teachers in the Mastering Math program. The data for the second question were collected using pre- and post-tests offered to about 600 students of the intervention group and to about 400 students of the control group. The results were encouraging at both teacher and student levels. However, the authors acknowledge methodological limitations of the study, and conclude by saying that “a combination of strategies can be effective” (p. 361, italics added).
Identification of ‘effects’ produced by innovations is an important and recurring theme in implementation research in mathematics education. Effects are reported with qualifiers (e.g. time—sometimes, often; quantity—some, most) and with different degrees of confidence, and are subjects of many qualifications, but we suggest that Reason-2 studies can be grouped into the following four categories:
Studies that focus on identifying effects of innovative teacher professional development programs and interventions (e.g., Beswick & Jones, 2011 ; Ferrini-Mundy et al., 2007 ); the aforementioned study by Prediger et al. ( 2019 ) belongs to this type.
Studies related to effects of curriculum reforms and innovative curricula. For instance, there are studies that analyze effects of curriculum reform on students’ knowledge and achievements depending on the level of fidelity of the implementation (e.g., Balfanz et al., 2006 ). There are also studies that investigate whether the implementation of curriculum innovations changes teachers’ instructional practice (e.g., Obara & Sloan, 2010 ).
Studies that analyze effects of implementing particular teaching approaches. Examples are as follows: (i) a study by Polotskaia and Savard ( 2018 ), who investigated effects of the relational paradigm on Canadian students' competencies in solving additive word problems; (ii) a study by Adam ( 2004 ) that explored outcomes of the implementation of an ethnomathematics curriculum unit for Maldivian students. This study relied on data collected from seven teachers and about 200 students, and reported an increase in measures related to motivation and interest, awareness of mathematics in society, and the understanding of mathematical concepts.
Studies that analyze outcomes of the implementation of technological innovations. These studies are less common in the literature reviewed. An example is the work of Hoch et al. ( 2018 ) on the design and implementation of an electronic mathematics textbook, where researchers tried to identify an overall effect of ‘time on task’ on students’ task success during initial instruction of fractions.
Reason-2 studies are highly diverse methodologically, ranging from relatively large-scope controlled experiments (as in Prediger et al., 2019 ), to relatively small-scope (mainly) qualitative studies (as in Adam, 2004 ). However, they all have a comparative component. For example, the study described by Adam ( 2004 ) does not include a control group, but the students were asked to indicate if they would prefer to study mathematics the way they learned in an ethnographic unit as implemented, or in a regular way. Generally speaking, the scope of the studies in this category varies, but they seem to conform to the maxim formulated by Adam ( 2004 ): “[t]he practical intervention was moderated by what was practically possible” (p. 57).
3.3 Reason 3. Understand relationships between influential factors, enactment, and outcomes
Reason 3 embraces studies that explore relationships between factors that can influence the innovation enactment and sometimes also attend to relationships between the contextual factors and outcomes of the implementation. These are studies that explore how, why, when, where, and with whom an innovation is effective (Century & Cassata, 2016 , pp. 174–175). Identifying and understanding these factors are fundamental to implementation research, which makes Reason 3 one of the most frequently observed in the literature. Also, it is often the case that studies considered within Reason 3 are also included in some of the other reasons. In fact, the four categories used to classify the studies under Reason 2 can be used to organize the studies included in Reason 3 as well.
The work by Manouchehri and Goodman ( 2000 ) is a particularly salient illustration of Reason 3. It addresses the implementation of curriculum reforms and curricular innovations. In this work, the researchers used a qualitative case study to investigate the process of evaluation and implementation of an NCTM Standards-based textbook by two mathematics teachers over a period of two years. Their findings suggest that teachers’ mathematical knowledge is one of the factors that most influences the way teachers evaluate and implement the textbook. Another illustration can be found in the study by Wright ( 2014 ) that analyzed the effectiveness of a teaching model as an instructional tool for the topic of percentages, ratio and proportions. As part of the results of this study, the researcher reported that the successful implementation of the teaching model “is dependent on the teacher noticing and responding to the layers of understanding demonstrated by students and the careful selection of materials, problems and situations.” (p. 101).
In spite of the indicated overlap between Reason-2 and Reason-3 studies, there is also a characteristic difference: Reason-3 studies attend to individual differences among the participants and to contextual specificities of the implementation settings, whereas Reason-2 studies tend to report general effects. However, both Reason-2 and Reason-3 studies present implemented innovations as stable entities (e.g., a textbook or a curriculum unit), and are less explicit about iterative processes of the innovation design and re-design, as in studies driven by Reason 4.
3.4 Reason 4. Improve innovation design, use, and support in practice settings
Century and Cassata ( 2016 ) considered in this category studies that focus on improving the innovation and its implementation in order to improve outcomes as intended. Some of these studies identify or develop supports needed for improving the use of innovations in practice. Other studies may instead be more focused on design iterations in order to improve the innovation itself.
The Reason-4 studies can be exemplified by the work of Clark-Wilson and Hoyles ( 2019 ). This study is part of a long-term research project in the UK that initially focused on the design of curriculum units embedding digital technology for learning mathematics. Later the project entered a phase of upscaling and dissemination. The researchers acknowledged the barriers and obstacles that may emerge when new end-users of the innovation join the project. As an action plan for overcoming the obstacles, they proposed to design a web-based ‘professional development toolkit’ that can support mathematics teachers’ who implement the units in their classrooms beyond the timeline of the funded project. They also proposed the research basis for designing such a toolkit, including design principles.
Reason 4 manifests itself also in those studies that adopt a design research methodology with a focus on curriculum innovations. A characteristic example is the work by Kwon et al. ( 2015 ), where the researchers designed and implemented an inquiry-based multivariable calculus course containing various opportunities for students to discuss and argue. One of the goals of the study was to derive design principles, that is, to discover the characteristics of the instructional design that would supports students’ argumentation. To achieve this goal, the authors adopted a design research methodology with iterative cycles comprising design, implementation, and reflection stages.
3.5 Reason 5. Develop theory
According to Century and Cassata ( 2016 ), this reason is characteristic of studies that aim to enhance our understanding of educational change by devising theories and frameworks. This type of study is not common in the reviewed mathematics education literature.
Reason-5 studies can be illustrated by the work of Maaß and Doorman ( 2013 ), in which a theoretical model for a widespread implementation of inquiry-based learning (IBL) is proposed. The authors acknowledge that it is not easy to change day-to-day teaching on a large scale, and therefore it is necessary to consider in depth the question of how to promote a widespread uptake of IBL in day-to-day teaching. To this end, Maaß and Doorman ( 2013 ) introduced a model for the dissemination and implementation of IBL. The authors explained the complexity of the model, including its theoretical basis, its iterative approach to evaluation and refinement, and its intended contributions to theory and practice.
The work by Jankvist and Niss ( 2015 ) is another example of Reason-5 research. These authors offer a framework for designing and implementing an in-service teacher education program in which findings from mathematics education research are put into practice. In particular, this program had the aim of helping teachers identify students with genuine learning difficulties in mathematics, investigate the nature of these difficulties, and carry out research-based interventions to assist the students in overcoming them.
One more example, already mentioned in Sect. 1 , by Cobb and Jackson ( 2021 ), presented elements of theory of action and supported the claim of its usability in the context of a large-scale program in the US. Additional examples of this type are overviewed as part of this SI.
3.6 Intermediate remarks
In conclusion of this section, we would like to make three remarks. First , it should not be surprising that mathematics education implementation research, identified as such in accordance with Century and Cassata’s ( 2016 ) working definition, embraces studies that could be, and actually are, characterized by their authors as design research, intervention studies, controlled experiments, teaching experiments, etc. However, not every design research or controlled experiment would qualify. It is the authors’ decision to study enactment of a resource/innovation/approach not only in the context in which it was originally developed but in a new context, that makes the study fit Century and Cassata’s definition. Second , some authors self-identify their studies as ‘background research’ or as ‘research accompanying an implementation project’, but rarely can we find ‘implementation research’ as an explicitly stated type of research. This is in contrast to some fields of study other than mathematics education (cf., Century & Cassata, 2016 ; Eccles & Mittman, 2006 ). In light of this observation, we choose to use the term ‘implementation-related research’ rather than ‘implementation research’ henceforth. The third remark is that studies on long-term consequences of implementation of innovations—that is, what happens when the innovation proposers step out and the innovation remains essentially in the hands of its end-users—are extremely rare in the reviewed mathematics education literature.
4 Implementation-related research in this special issue
4.1 a general overview of the si.
Classifying the SI papers according to Century and Cassata’s ( 2016 ) five reasons for conducting implementation research turned out to be not especially informative. This is because most of these papers simultaneously encompass several reasons. For example, Jaworski and Potari ( 2021 ) mentioned all five reasons as the motivation for their study, and many authors developed arguments that concern four reasons (e.g., Burkhardt & Schoenfeld, 2021 ; Krainer, 2021 ; Prytz, 2021 ) or three (e.g., Devlin, 2021 ; Jankvist et al., 2021a , 2021b ; Valoyes-Chávez & Felmer, 2021 ; Wang et al., 2021 ). In other words, most of the papers simultaneously elaborate on design and/or re-design issues, on the interplay of factors that influence the enactment and on aspects of implementation-related theory development. Of course, the multitude of the reasons for conducting research does not mean that the SI papers are unfocused. It is rather indicative of the specific setting of this SI, which was conceived as a platform for the collective search for identity of implementation as a sub-domain in mathematics education research (cf. a search for the identity of mathematics education as a research domain described in the book edited by Sierpinska & Kilpatrick, 1998 ). It can also be an indication of the mathematics education research tradition that treats design, enactment and transfer across contexts as interwoven and theory-laden (Adler et al., 2005 ; Niss, 1996 , 1999 ).
As could be expected in a rapidly developing but not yet institutionalized field of study, the SI papers contain diverse suggestions about what ‘implementation’ and ‘implementation research’ are or should be in mathematics education. Some authors situate their work in one of the existing conceptualizations of ‘implementation’ and ‘implementation research’ (see Sect. 1 ) whereas others challenge some of the basic assumptions on which the existing conceptualizations rely. The unifying feature is that all the papers describe and explore efforts made in pursuit of some educational change in natural (or fairly natural) mathematics education habitats. In this way, the SI papers come close to ecological perspectives, which consider innovations as “ecological disruptions for the didactic system in which they are implemented” (Artigue, 2021 , p. 31).
With respect to the types of argument the authors construct in order to make their main points, six paper can be tagged as theoretical , i.e., papers in which a theoretical argument representing the authors’ position is construed and then supported by illustrative examples taken from the authors’ past studies, and nine papers that can be tagged as empirical , in which the original data sets are analyzed within a particular implementation-related theoretical perspective.
As explained in Sect. 2 , the reading of the past literature and the SI papers led us to identification of four themes that we deem particularly important for further discussion in our community. Each theme represents diversity and even controversy in the authors’ approaches, which allows us to seize a valuable opportunity to map the implementation-related mathematics education research landscape (as represented by this particular collection of papers), by comparing and contrasting different approaches. These themes are developed in Sects. 4.2 – 4.6 .
4.2 Objects of implementation
For Century and Cassata ( 2016 ), the object of implementation is innovation , which is broadly defined as “programs, interventions, technologies, processes, approaches, methods, strategies, or policies that involve a change (e.g., in behavior or practice) for the individuals (end users) enacting them” (p. 170). This notion is succinctly denoted as “the it” or “the focus of change” (ibid). In the context of this SI, characteristics of objects of implementation are important as they seem to relate strongly to preferences in research. Two characterizations are in order. The first is related to the medium of an innovation, that is, the form in which an innovation ‘exists’ or is proposed so that it could be implemented by someone. The second is related to the extent to which ‘the focus of change’ is predefined (by the proponents) or co-constructed (by the proposers and the adapters).
As for the first characterization, we see (i) innovations, in which know-how is encapsulated in a relatively stable material artefact around which the human activity is developed (e.g., a collection of published instructional materials), and (ii) innovations which mainly exist in the form of human interactions driven by ideas or principles (e.g., a community of inquiry). Footnote 2 Innovations of the second type can be supported by material artefacts but do not fully depend on them; they rather depend on a communicational infrastructure and rules of interaction between the involved individuals and communities. We refer to these two types of objects of implementation as material-centered and interaction-centered , respectively.
Examples of material-centered objects of implementation are present in the papers by Burkhardt and Schoenfeld (e.g., a collection of instructional materials on the website of the Mathematics Assessment Program) and Karsenty (a collection of recorded mathematics lessons on the website of the VIDEO-LM project). Interaction-centered objects of implementation are described in the papers by Jaworski and Potari (the developmental model for enhancing an inquiry stance), Pinto and Koichu (practices and processes of disciplined inquiry), Roesken-Winter et al. (insights from past research with teachers and facilitators conducted by the authors), among others.
Of course, there are also implementation programs, in which nearly symmetrical attention is given to both material-centered and interaction-center innovations that complement each other (e.g., Diego-Mantecón et al., 2021 ; Jankvist et al., 2021a , 2021b ; Karsenty, 2021 ; Roesken-Winter et al., 2021 ; Wang et al., 2021 ). Such programs concern innovations that can be seen as mixed or material-interactive . Existence of such programs implies that the above dichotomy is blurred. However, we deem it useful because it can partially account for certain differences in the authors’ foci of research attention. Namely, studies on implementation of material-centered innovations more readily discuss implementation outcomes, whereas studies focusing on interaction-centered innovations are more deliberate about processes of implementation. In addition, it seems that material objects of implementation favor the ‘improvement of practice at scale’ problematique, while interactional objects of implementation favor ‘bridging research and practice’ agendas.
And what about mathematics education research as an object of implementation? Overall, this seems to belong to the interaction-centered type of object, or to the mixed material-interactive type, but never to the material-centered type only. Actually, research as an object of implementation is alluded to in many studies as a conjunction of approaches, resources, organizational models or theories developed in the past within an organizational frame called ‘research’ (e.g., Burkhardt & Schoenfeld, 2021 ; Wang et al., 2021 ). Some authors avoid the use of the collocation ‘implementation of research’ and talk about ‘implementation of research-based innovations’ (e.g., Jaworski & Potari, 2021 ; Roesken-Winter et al., 2021 ). The other authors, however, adhere to the ‘implementation of research’ language. For example, Pinto and Koichu ( 2021 ) talk about implementation of research as teachers’ engagement with procedures and constructs of disciplined educational inquiry. The engagement occurs in the context of a community of inquiry and is mediated by a set of boundary objects. Some of these objects are material (e.g., a written guide for data-collection and analysis), and others are purely interactive (e.g., practices developed in the community for making decisions about further action). Kontorovich and Bartlett ( 2021 ) and Cai and Hwang ( 2021 ) describe implementation of research as incorporating in teaching tasks of particular types that have been extensively explored in the past, namely, scriptwriting tasks and problem-posing tasks, respectively. In these studies, teacher-researcher interactions towards developing a predisposition for the use of the chosen types of tasks are analyzed in depth, and material artefacts supporting implementation (e.g., specific tasks) are co-constructed in due course of the interactions.
The second characterization of objects of implementation—by whether an innovation as ‘the object of change’ is predefined and stable or gradually co-constructed and flexible—results in quite a different classification. For example, the TBM project presented by Jaworski and Potari ( 2021 ) had a clear predefined goal, namely, to promote inquiry as a way of being, by means of implementing at scale the developmental PD model developed in prior research. Likewise, a project described by Tamborg ( 2021 ) aimed to achieve a pre-defined goal, namely, to promote an objective-oriented approach to teaching (i.e., an approach in which teaching is driven by well-defined learning objectives) by means of making a particular digital platform mandatory for all teachers of the country. Both projects have pre-defined goals but differ in the types of their objects of implementation, which were interaction-centered and material-interactive respectively. Interestingly, both studies focus on tensions and pitfalls of implementation. In contrast, the studies, in which ‘the object of change’ is not pre-defined but co-constructed under a flexible theoretical umbrella (e.g., Cai & Hwang, 2021 ; Valoyes-Chávez & Felmer, 2021 ) are essentially described as ‘success stories’, in the following meaning: they extensively report on learning gains in the process of implementation (and sometimes also in its outcomes; see Wang et al., 2021 ), and focus less on tensions and pitfalls of the implementation processes. This leads us to a paramount question, addressed in the next section: who decides on what ‘the object of change’ should or can be, and on what is this decision based?
4.3 Configurations of stakeholders in implementation
Krainer ( 2021 ) presents the following four idealized implementation scenarios representing four different configurations of stakeholders: (i) teachers identify a practical problem and search for the solution—policy makers and researchers are not involved, local implementation is successful but scaling up is hardly possible; (ii) researchers propose an innovation—a group of teachers successfully implement it, policy makers are informed of the success but scaling up is uncertain; (iii) policy makers identify a systemic problem—researchers advise, there are disagreements about focus, timescale and resources, action is uncertain; (iv) a policy maker and a practitioner consider an innovative program while trying to address different problems—there are issues related to scope, expectations, timescale and operation, action is initially impossible, more thinking, possibly including researchers, is needed.
Three types of stakeholders—practitioners, researchers, policy makers—interact in these scenarios, as they do in the papers of this SI. Nevertheless, some scenarios are more commonly addressed in research than others. The first scenario, describing a situation where practitioners initiate educational change and pursue it by themselves, is absent in this SI and in the reviewed literature. This is not to say that such situations cannot exist in practice: perhaps they exist but are not documented. The same holds for scenario (iv). However, two studies of the SI are quite close to scenario (i). Cai and Hwang ( 2021 ) describe a teacher who is interested in improving her lessons and seeks collaboration with a researcher, who proposes that the teacher adapt a research-based innovation. In the second case, Kontorovich and Bartlett ( 2021 ) describe a researcher who approaches a practitioner with an idea for an innovation while pursuing his research agenda, and collaboration becomes possible only when the researcher adjusts his research agenda to the agenda of the practitioner, who eventually implements the innovation for the sake of addressing a teaching need. In both cases, scaling up of the implementation is not considered as realistic. Scenario (ii) is present in the study by Pinto and Koichu ( 2021 ): researchers offer an innovative PD program, in which experienced teacher-participants enter the shoes of education researchers and implement the innovation—teaching aligned with the educational research cycle—in order to improve aspects of their teaching. Scaling up is uncertain. Implementation initiatives described by Burkhardt and Schoenfeld ( 2021 ), Devlin ( 2021 ), Diego-Mantecón et al. ( 2021 ), Jaworski and Potari ( 2021 ), Karsenty ( 2021 ) and Roesken-Winter et al. ( 2021 ) partially conform to scenario (ii), in that the change is initiated by researchers but not necessarily in collaboration with policy makers, who enter the picture later on. Scaling up is achieved to different extents. Finally, scenario (iii), in which policy makers initiate the change, on their own or in collaboration with researchers, appears in papers by Krainer ( 2021 ), Tamborg ( 2021 ), Valoyes-Chávez and Felmer ( 2021 ) and Wang et al., ( 2021 ). Not surprisingly, projects in which policy makers are involved from the beginning always aim to implement at scale, projects in which researchers are proponents of innovations vary in this respect, and projects initiated by teachers are neutral with regard to upscaling.
Furthermore, in three SI papers, by Jankvist et al., ( 2021a , 2021b ), Krainer ( 2021 ), and Prytz ( 2021 ), the complexity of relationships between the stakeholders is the topic of investigation. Here we would like to briefly dwell on the study by Prytz ( 2021 ), which reveals not only the complexity, but also dynamics of the various roles of stakeholders in the long run. While exploring the history of mathematics education reforms in Sweden, Prytz describes how the role of researches has evolved from proponents of innovations to be adapted by teachers in a highly centralized educational system in the 1960s, to supporters and explorers of teacher-initiated innovations in a highly decentralized educational system in the 2010s.
Last but not least, innovations are conceived and enacted not out of the blue but in order to resolve what is seen by particular stakeholders as an acute problem (Bryk et al., 2015 ; Krainer, 2021 ). We tentatively observe that implementation projects initiated by researchers, practitioners or policy makers tend to have different justifications. Researcher-initiated projects tend to put forward theoretical justifications of proposed innovations (e.g., we know that Problem Based Learning in transdisciplinary contexts is important, so let’s implement it, as in the work of Diego-Mantecón et al., 2021 ). Practitioner-initiated projects stem from the description of a problem of practice (e.g., a teacher is unsatisfied with her students’ achievements and motivation, as in Cai & Hwang, 2021 ). Policy-maker initiatives usually stem from a systemic problem (e.g., poor alignment of teaching with the objectives identified as important by officials, as in Tamborg, 2021 ). This SI contains only one example where all three stakeholders are involved and seem to co-exist in harmony: it is the study of the Just Do Math program in Taiwan (Wang et al., 2021 ), which during several years reached the national level and was considered successful by various measures. In this case, communication channels among different stakeholders were carefully designed as part of the overarching research-driven model of implementation from the very beginning.

4.4 Implementation vs. scaling up
Implementation is often interpreted as the application in practice of an innovation generated by a group of specialists, with the aspiration of disseminating the innovation and scaling it up (Maaß & Doorman, 2013 ). A conceptualization of implementation reflecting these perspectives was provided by Maaß and Artigue ( 2013 ): “Implementation is what happens when a planned intervention, an innovation, is set in motion. When an intervention is designed, its designers often do not aim merely for a small-scale implementation, but also wish to disseminate their ideas, materials, etc.” (p. 779).
Judging from scenarios and examples discussed in Sect. 4.3 , implementation and scaling up can be seen as related but not synonymous notions. They both presume that an innovation designed by a group of specialists in a particular context is then enacted in new contexts, with or without direct involvement of the original specialists. The difference is subtle: scaling up presumes that the new contexts are larger (in terms of numbers of students, teachers, schools or districts) than the initial context, whereas implementation is concerned with how an innovation works across contexts , as Cai and Hwang ( 2021 ) suggest. Footnote 3 The former approach always values dissemination, and the latter approach can value dissemination, but also some other important goals, such as resolving teaching problems in particular classrooms. Let us refer to the first approach as implementation at scale , and to the second one as local implementation (this is to follow ‘classroom implementation’ introduced by Cai et al., 2017 ).
Implementation at scale is at the center of most of the papers in this SI. However, what counts as a worthy scale of implementation is very idiosyncratic. For example, Jaworski and Potari ( 2021 ) describe an innovation (the developmental model) conceived and designed in the context of a learning community comprising 12–15 didacticians and about 30 teachers, which was then enacted in five districts in Norway. Devlin ( 2021 ) describe gamified mathematical activities designed by a small R&D team that are eventually used by hundreds of thousands of students over the world. Roesken-Winter et al. ( 2021 ) describe an 18-year-long Mastering Math project, in which the innovation (research-based curriculum materials) was developed in-house over six years, was enacted in first three schools, then in 40 schools, and eventually in more than 200 schools. Prytz ( 2021 ) describes a developmental project involving 400 teachers followed by the large-scale Boost project involving the majority of mathematics teachers in Sweden. He concludes: “[w]hat is possible with 400 teachers in one municipality is perhaps not possible with 76 percent of all Swedish mathematics teachers” (this issue). Karsenty ( 2021 ) reports dissemination of the project SHLAV as follows: it began from 2 schools (6 teachers, 100 students) in the pilot phase and extended to 32 schools (191 teachers and 4759 students) in the implementation phase. Burkhardt and Schoenfeld ( 2021 ) describe the impact of the Connected Mathematics project in terms of its dissemination among practitioners and researchers: apparently (the exact numbers are not available), they talk about thousands of teacher-users and hundreds of researcher-users.
Not surprisingly—a similar observation was made by Venkat and Graven ( 2017 ) regarding material vs. human PD resources—material-centered objects of implementation can be scaled up more readily than interaction-centered objects of implementation. However, what is striking in the diversity of scope attained in the abovementioned projects is that different scholars seem to assign very different meaning to implementation as ‘enactment’ or ‘setting in motion’. We have already observed that types of implementation differ depending on the type of the implementation objects (Sect. 4.2 ). To this we now add an observation informed by the distinction made by Karsenty ( 2021 ): there are two kinds of scaling up—of the setting when the program is implemented in new contexts as a package to be used essentially ‘as is’, and of values, where practitioners, stimulated by the program’s ideas, attain full agency over how to use these ideas in practice. Thus, ‘enactment’ as a phenomenon is tied to the degree of professional autonomy of its users, as well as to the degree to which proponents of an innovation feel that credit belongs to them even when the innovation is essentially modified or used in unexpected ways, or is used in conjunction with other innovations developed in other projects. Footnote 4 Further theorizing of such notions as ‘enactment’ and ‘setting in motion’ is needed as part of theorizing ‘implementation at scale’ in mathematics education.
We now turn to examples of local implementation in this SI. Four studies, namely those by Cai and Hwang ( 2021 ), Diego-Mantecón et al. ( 2021 ), Kontorovich and Bartlett ( 2021 ) and Pinto and Koichu ( 2021 ), belong to this category. The unifying feature of these studies is that they operate with interaction-based objects of implementation (see Sect. 4.2 ), which are deeply rooted in specific insights from focused sub-domains of mathematics education research. (To recall, the sub-domains are problem posing in Cai and Hwang’s case, Problem Based Learning in transdisciplinary contexts in Diego-Mantecón et al.’s case, scriptwriting tasks in Kontorovich and Bartlett’s case, and teacher inquiry with a special focus on empowering school students to ask good questions in mathematics lessons in Pinto and Koichu’s case.)
A natural question is, what added value can there be in considering these small-scale studies as instances of implementation-related research? Moreover, would it not be more natural to consider them as design studies or teaching experiments, given that the methodologies applied in these studies bear clear signs of these types of research? Our response is two-fold. First, we would like to reiterate (see Sect. 3 ) that it is not a particular methodology, but rather specific research questions focusing on ‘implementation’ that matter. Second, we would like to rely on Burkhardt and Schoenfeld’s ( 2021 ) assertion: “In education, it is rare for a single research result to form the basis for a change in practice” (this issue). We agree that it is rare, and value that this is the case in the four studies in question. We deem that dealing with the challenge of changing practice based on specific research results is what gives merit to these studies within the implementation problematique. Speaking more generally, some changes in practice are so desirable and simultaneously so difficult to achieve, that even a small-scope implementation is of academic value, especially if it is documented in detail and can inform the field in ways germane to in-depth qualitative research. For example, the study by Kontorovich and Bartlett ( 2021 ) contains a fine-grained analysis of interactions between the mathematics education researcher and the mathematics lecturer that result in the inclusion of an unorthodox assignment in a lecture-based mathematics course for undergraduate students. This is a rare achievement. The analysis cannot be generalized directly, but it is theorized in the paper and therefore can be useful for constructing researcher-practitioner interactions in future projects, including large-scale ones.
Speaking on the benefits of small-scale qualitative studies, it is of note that most of the large-scale implementation projects mentioned above are accompanied by this type of research. For example, the Mastering Math project (Roesken-Winter et al., 2021 ) in Germany was informed and supported by a series of small-scale design and intervention studies. A national-level implementation project in Denmark presented by Tamborg ( 2021 ) was analyzed in light of the data collected from a group of seven teachers. An aspect of a large-scale APRA project in Chile was explored by Valoyes‑Chávez and Felmer ( 2021 ) by means of a case study of one participant. Furthermore, the data corpus on the Teaching Better Mathematics project in Norway that Jaworski and Potari ( 2021 ) present consists of reflections of the leading didacticians of the project. Jankvist et al., ( 2021a , 2021b ) and Prytz ( 2021 ) explored the long-term reform of mathematics education in Sweden as an educational case study that relies on the analysis of relevant documents and insights from past studies. As a matter of fact, in this SI only the national-level To-Do-Math project in Taiwan (Wang et al., 2021 ) is accompanied by systematic analysis of quantitative data collected at a scale comparable with the scale of the project.
The following trend seems to emerge: there is often a gap between the scope of the implementation projects and the scope of the related research. The gap is fully understandable: as a rule, mathematics education research is conducted by relatively small research teams having limited capacity for collecting and processing large-scale data, and especially large-scale qualitative data. Paraphrasing Adam’s ( 2004 ) maxim quoted in Sect. 3.2 , even in large-scale projects research is restricted by what is practically possible. Simultaneously, we recognize that establishing normative relationships between the project-scope and the research-scope requires more thinking. On the one hand, not everything in large-scale projects should and can be documented and explored. On the other hand, the role of the large scope of a project in small-scale studies accompanying the project should remain visible.
4.5 Implementability of mathematics education research
Research-based innovations differ in their implementability . Drawing on Levine and Cooper ( 1991 ), Jankvist et al., ( 2021a , 2021b ) refer to implementability of a research-based innovation in an educational context as an indicator of “how realistic and feasible it is for practitioners to implement an innovation arising from interpreted research results” (this issue, italics added). In turn, interpretation of research results is necessary in order to make them accessible for the intended end users. Therefore, implementability of research-based innovations depends on who interprets and mediates them, and how.
We have already discussed mathematics education research as an object of implementation in Sect. 4.2 . We now unfold that discussion by considering implementability of mathematics education research products, including research findings, theoretical frameworks and experiences (Aguilar et al., 2019 ).
As for specific research findings , we have discussed that their implementation is possible but rare (Sect. 4.4 ). In three cases of local implementation included in this SI (Cai & Hwang, 2021 ; Kontorovich & Bartlett, 2021 ; Pinto & Koichu, 2021 ), special attention was given to artefacts supporting implementation. Cai and Hwang ( 2021 ) give special merit to tangible artefacts that emerge from teaching. These artefacts, they argue, can mediate between research and practice. In the two other studies, the boundary object notion (e.g., Star, 2010 ) is put forward. Studies in the implementation-at-scale category, which usually do not operate with specific findings but with clusters of findings developed in realms of mathematics education research (e.g., research on conceptual learning), are also attuned to mediation of research findings through artefacts. For example, Roesken-Winter et al. ( 2021 ) discuss how material implementation strategy (i.e., the use of research-based instructional materials with students, teachers and facilitators) can support the scaling up processes. In sum, implementability of research findings seems to be closely related to ways by which these findings are interpreted and transposed into artefacts accessible to end users. Last but not least, mediation of research findings in the form of advice to policy makers is also considered, for example, by Krainer ( 2021 ), but implementability of this sort of mediation is uncertain.
Unfolding implementability of theoretical frameworks seems to be even trickier than the implementability of research findings. Though all projects and studies in this SI are driven by theories and theoretical frameworks, no claims are made for their (direct) implementation. While not surprising, it is a phenomenon that requires interpretation. Ours stems from a seemingly paradoxical idea of Mason about mathematics education research as an enterprise. Mason ( 1998 , 2002 ) suggested that the main enterprise of mathematics education researchers is to learn about themselves through interactions with others (cf. Sect. 1 on the improvement mathematics education as the raison d'être for the existence of mathematics education research). To extend Mason’s idea, one can argue that theories and conceptual frameworks deeply affect their creators and sometimes additional researchers who are willing to study them and are trained to put them into action. However, the influence of mathematics education theory on other stakeholders of implementation (i.e., practitioners and policy makers) is hindered for many reasons (see, e.g., Jankvist et al., 2021a , 2021b ). Practically, in light of the current view of implementation as a complex endeavor based on communication of many parties, and also in light of the call to make implementation an integral part of research (Cai & Hwang, 2021 ; Cai et al., 2017 ), creators of theories might consider how to coin their concepts and constructs so that at least some of these would be communicable beyond the research community. Otherwise, the evident complexity of conceptual apparatuses used by mathematics education researchers may pose yet another obstacle for implementability of research.
Finally, implementability of research experiences is considered in two studies of the SI. It is the main topic of the study by Pinto and Koichu ( 2021 ), and an aspect of the developmental model of Jaworski and Potari ( 2021 ). In particular, Pinto and Koichu’s ( 2021 ) study shows how the border between practices of disciplined inquiry (i.e., research practices) and of teacher inquiry can be blurred when both parties productively collaborate over specially designed boundary objects without an expectation of reaching full consensus. Implementability of research experiences, is, however, a peripheral topic in this SI, though it is extensively discussed elsewhere (e.g., Cobb, 2000 ; Kieran et al., 2012 ).
5 Concluding remarks
We have engaged with a growing body of mathematics education studies that relates to the implementation of innovations in a more or less direct manner. To make sense of this body of studies, we followed a hermeneutical approach in which five reasons for developing implementation research (Century & Cassata, 2016 ) were instrumental for the initial organization of the literature. The hermeneutic approach, applied to past research and to the studies included in this SI, allowed our understanding to evolve towards the identification of four themes that we deem important for further research and practice, namely, objects of implementation (two categorizations are offered), stakeholders in implementation (possible configurations are illustrated), emerging differences between implementation and scaling up (a distinction between dissemination and implementation across contexts is substantiated), and implementability of mathematics education research (implementability of different research products is discussed).
In what follows, we propose a refined conceptualization of the notion of implementation in mathematics education , which may serve as an organizational framework for future research, by signaling the key actors and processes within this enterprise. In addition, a conceptualization of implementation-related research is also offered in conjunction with suggestions for future research.
We conceptualize implementation in mathematics education as an ecological disruption to a particular mathematics education system, through the gradual endorsement of innovation in conjunction with an action plan aimed at resolving what is perceived as a problem by (at least some of) the stakeholders involved. The defining feature of implementation is that it occurs in interaction between the innovation and plan proponents and the innovation adapters . At the beginning of the implementation, the innovation proponents have the ultimate agency over the innovation and the associated action plan. During the implementation process, the innovation adapters experience some or all of the following sub-processes: (1) constructing agency over the innovation, (2) gradually changing within-community communication or across-community communication, (3) gradually changing practice so that it accommodates the innovation, (4) adapting the innovation to their needs and aspirations. These sub-processes reflect back on the proponents, including evolution of the innovation, of the associated action plan and of the theories underlying their development. The implementation process is iterative and ends when the innovation stops being perceived by the stakeholders as an ecological disruption. To this end, implementation can succeed (e.g., the adapted innovation is eventually integrated in the system) or fail (i.e., the innovation is rejected explicitly or tacitly so that it stops influencing the system).
While recognizing the specificity of mathematics education as a field of study, we prefer to talk about implementation-related research (IRR) rather than on implementation research. We refer to IRR as a disciplined inquiry of implementation (as specified above) that aims at creating new theoretical and practical knowledge on the use of innovations beyond contexts in which they have been created.
Given the apparent inclusiveness of the above conceptualization and the broadly recognized fact that ‘good research’ presumes that its results are to be used by someone or for something, one may wonder what is not implementation and what is not IRR. Well, quite a few types of examples can be constructed and illustrated, by negation of one or more of the above-listed characteristic features of implementation. However, it might be more productive to ask what the core and future direction of IRR may be. The papers in this SI suggest a focus on understanding and creating alignment between various stakeholders’ different goals in relation to implementation. Researchers in IRR should be aware of what types of goals are in play in relation to the implementation problem (Niss, 1996 ), by whom these goals are articulated and what rationality they are based on (Krainer, 2021 ). For now, we see developing epistemic tools for better understanding the alignment between implementation stakeholders as one of the key concerns for IRR as it moves forward.
In addition, we see the pursuit of another type of alignment—between theoretical resources used in mathematics education IRR studies and theoretical resources developed in other fields of study or in other sub-domains of mathematics education research (e.g., curriculum use)—as another important developmental direction for IRR. This type of alignment work has been visible in CERME10 and CERME11 (e.g., Jankvist et al., 2019 ), is continued in this SI, and, hopefully, will be continued on pages of the aforementioned newborn journal IRME, Implementation and Replication Studies in Mathematics Education ( Artigue, 2021 ; Cobb & Jackson, 2021 ; Jankvist et al., 2021a ), among other platforms.
Two recent initiatives, this SI and IRME, arose from the discussions and collective reflections that took place at CERME11. As mentioned, the goal of this SI was to foreground further the implementation problem in our community. The goal of IRME is to provide a stable space for academic interaction that could further support research-based improvement of mathematics education. Since we, the authors of this paper, are involved in both initiatives, we would like to finish this paper with an open invitation to mathematics education practitioners, researchers, didacticians and policymakers to use IRME as a platform for continuing the discussion of the implementation problem in mathematics education.
We refer to Educational Studies in Mathematics, Journal for Research in Mathematics Education, The Journal of Mathematical Behavior, For the Learning of Mathematics, Mathematical Thinking and Learning, Journal of Mathematics Teacher Education, ZDM—Mathematics Education, Mathematics Education Research Journal, International Journal of Mathematical Education in Science and Technology, School Science and Mathematics, International Journal of Science and Mathematics Education, Recherches en Didactique des Mathématiques, and Research in Mathematics Education.
Admittedly, this categorization resonates with Adler’s ( 2000 ) categorization of resources in school mathematics. Adler distinguishes between human resources (such as teacher–pupil ratios, class size, teacher qualifications), material resources (such as technologies, textbooks, mathematical objects and everyday objects) and social and cultural resources (such as language and time).
A related discussion can be found in the editorial by Cai et al. ( 2020 ), in which they introduce the replimentation notion. Replimentation refers to the duality of goals that researchers may pursue: to implement an innovation in new contexts to scale it up, and to treat the new implementations as replications with variations to explore the innovation.
Admittedly, the second part of this observation (about the innovation proposers) is inspired by Rogers’ ( 2003 ) discussion of phenomena pertinent to diffusion of innovations and by Remillard’s ( 2005 ) observation that ‘fidelity’ is a problematic concept when applied to curriculum use.
Adam, S. (2004). Ethnomathematical ideas in the curriculum. Educational Studies in Mathematics, 16 (2), 49–68. https://doi.org/10.1007/BF03217395
Article Google Scholar
Adler, J. (2000). Conceptualising resources as a theme for teacher education. Journal of Mathematics Teacher Education , 3 (3), 205–224. https://doi.org/10.1023/A:1009903206236
Adler, J., Ball, D., Krainer, K., Lin, F.-L., & Novotna, J. (2005). Reflections on an emerging field: Researching mathematics teacher education. Educational Studies in Mathematics, 60 (3), 359–381. https://doi.org/10.1007/s10649-005-5072-6
Aguilar, M. S., Kuzle, A., Wæge, K., & Misfeldt, M. (2019). Introduction to the papers of TWG23: Implementation of research findings in mathematics education. In U. T. Jankvist, M. van der Heuvel-Panhuizen, & M. Veldhuis (Eds.), Proceedings of the Eleventh Congress of the European Society for Research in Mathematics Education (pp. 4355–4362). Freudenthal Group & Freudenthal Institute; Utrecht University; ERME.
Artigue, M. (2021). Implementation studies in mathematics education: What theoretical resources? Implementation and Replication Studies in Mathematics Education, 1 (1), 21–52. https://doi.org/10.1163/26670127-01010002
Balfanz, R., Mac Iver, D. J., & Byrnes, V. (2006). The implementation and impact of evidence-based mathematics reforms in high-poverty middle schools: A multi-site, multi-year study. Journal for Research in Mathematics Education, 37 (1), 33–64. https://doi.org/10.2307/30035051
Beswick, K., & Jones, T. (2011). Taking professional learning to isolated schools: Perceptions of providers and principals, and lessons for effective professional learning. Mathematics Education Research Journal, 23 (2), 83–105. https://doi.org/10.1007/s13394-011-0006-3
Blomhøj, M. (2021). Samspil mellem fagdidaktisk forskning og udvikling af matematikundervisning—belyst gennem erfaringer fra et udviklingsprojekt i undersøgende matematikundervisning [Interaction between didactic research and development of mathematics teaching—Viewed through experiences in a development project in exploratory mathematics teaching]. Sammenlignende Fagdidaktik, 6 , 29–50. https://doi.org/10.7146/sammenlignendefagdidaktik.vi6.127569
Boell, S. K., & Cecez-Kecmanovic, D. (2014). A hermeneutic approach for conducting literature reviews and literature searches. Communications of the Association for Information Systems, 34 , 257–286. https://doi.org/10.17705/1CAIS.03412 Article number 12.
Bruckheimer, M. (1979). Creative implementation. In P. Tamir, A. Blum, A. Hofstein, & N. Sabar (Eds.), Proceedings of the Bat-Sheva Seminar on curriculum implementation and its relationships to curriculum development in science (pp. 43–49). The Weizmann Institute of Science.
Bryk, A. S., Gomez, L. M., Grunow, A., & LeMahieu, P. G. (2015). Learning to improve: How America’s schools can get better at getting better . Harvard Education Press.
Google Scholar
Burkhardt, H., & Schoenfeld, A. (2021). Not just “implementation”: The synergy of research and practice in an engineering research approach to educational design and development. ZDM - Mathematics Education . https://doi.org/10.1007/s11858-020-01208-z
Burkhardt, H., & Schoenfeld, A. H. (2003). Improving educational research: Toward a more useful, more influential, and better-funded enterprise. Educational Researcher, 32 (9), 3–14. https://doi.org/10.3102/0013189X032009003
Cai, J., & Hwang, S. (2021). What does it mean to make implementation integral to research? ZDM - Mathematics Education , 53 (5). https://doi.org/10.1007/s11858-021-01301-x
Cai, J., Morris, A., Hohensee, C., Hwang, S., Robison, V., Cirillo, M., Kramer, S., & Hiebert, J. (2020). Working across contexts: Scaling up or replicating with variations? Journal for Research in Mathematics Education, 51 (3), 258–267. https://doi.org/10.5951/jresemtheduc-2020-0007
Cai, J., Morris, A., Hohensee, C., Hwang, S., Robison, V., & Hiebert, J. (2017). Making classroom implementation an integral part of research. Journal for Research in Mathematics Education, 48 (4), 342–347. https://doi.org/10.5951/jresematheduc.48.4.0342
Century, J., & Cassata, A. (2016). Implementation research: Finding common ground on what, how, why, where, and who. Review of Research in Education, 40 (1), 169–215. https://doi.org/10.3102/0091732X16665332
Choppin, J., McDuffie, A. R., Drake, C., & Davis, J. (2018). Curriculum ergonomics: Conceptualizing the interactions between curriculum design and use. International Journal of Educational Research, 92 , 75–85. https://doi.org/10.1016/j.ijer.2018.09.015
Clark-Wilson, A., & Hoyles, C. (2019). A research-informed web-based professional development toolkit to support technology-enhanced mathematics teaching at scale. Educational Studies in Mathematics, 102 (3), 343–359. https://doi.org/10.1007/s10649-018-9836-1
Cobb, P. (2000). Conducting teaching experiments in collaboration with teachers. In A. Kelly & R. Lesh (Eds.), Handbook of research design in mathematics and science education (pp. 307–333). Lawrence Erlbaum.
Cobb, P., & Jackson, K. (2021). An empirically grounded system of supports for improving the quality of mathematics teaching on a large scale. Implementation and Replication Studies in Mathematics Education, 1 (1), 77–110. https://doi.org/10.1163/26670127-01010004
Colonnese, M. W. (2018). The development of instructional guidelines for elementary mathematical writing. School Science and Mathematics, 120 (3), 129–143. https://doi.org/10.1111/ssm.12391
Confrey, J., Castro-Filho, J., & Wilhelm, J. (2000). Implementation research as a means to link systemic reform and applied psychology in mathematics education. Educational Psychologist, 35 (3), 179–191. https://doi.org/10.1207/S15326985EP3503_4
Confrey, J., Gianopulos, G., McGowan, W., Shah, M., & Belcher, M. (2017). Scaffolding learner-centered curricular coherence using learning maps and diagnostic assessments designed around mathematics learning trajectories. ZDM - Mathematics Education, 49 (5), 717–734. https://doi.org/10.1007/s11858-017-0869-1
Devlin, K. (2021). Creating an educational tool that uses interactive representations to provide a new approach to mathematics learning. ZDM - Mathematics Education . https://doi.org/10.1007/s11858-021-01235-4
Diego-Mantecón, J.-M., Prodromou, T., Lavicza, Z., Blanco, T., & Oritz-Laso, Z. (2021). Are STEAM project-based learning implementations suitable to mathematically prepare our teenagers for the current society? ZDM - Mathematics Education , 53(5).
Eccles, M. P., & Mittman, B. S. (2006). Welcome to Implementation Science . Implementation Science . https://doi.org/10.1186/1748-5908-1-1
Ernest, P. (1984). The CXC mathematics examination: A Caribbean innovation in assessment. Educational Studies in Mathematics, 15 (4), 397–412. https://doi.org/10.1007/BF00311114
Ferrini-Mundy, J., Burrill, G., & Schmidt, W. H. (2007). Building teacher capacity for implementing curricular coherence: Mathematics teacher professional development tasks. Journal of Mathematics Teacher Education, 10 (4–6), 311–324. https://doi.org/10.1007/s10857-007-9053-9
Hoch, S., Reinhold, F., Werner, B., Richter-Gebert, J., & Reiss, K. (2018). Design and research potential of interactive textbooks: The case of fractions. ZDM - Mathematics Education, 50 (5), 839–848. https://doi.org/10.1007/s11858-018-0971-z
Jankvist, U. T., Aguilar, M. S., Ärlebäck, J. B., & Wæge, K. (2017). Introduction to the papers of TWG23: Implementation of research findings in mathematics education. In T. Dooley & G. Gueudet (Eds.), Proceedings of the Tenth Congress of the European Society for Research in Mathematics Education (pp. 3769–3775). DCU Institute of Education; ERME.
Jankvist, U., Aguilar, M. S., Dreyøe, J., & Misfeldt, M. (2019). Adapting implementation research frameworks for mathematics education. In U. Jankvist, M. van den Heuvel-Panhuizen, & M. Veldhuis (Eds), Proceedings of the Eleventh Congress of the European Society for Research in Mathematics Education (pp. 4405–4412). Freudenthal Group & Freudenthal Institute; ERME.
Jankvist, U. F., Aguilar, M. S., Misfeldt, M., & Koichu, B. (2021a). Launching Implementation and Replication Studies in Mathematics Education (IRME). Implementation and Replication Studies in Mathematics Education, 1 (1), 1–19. https://doi.org/10.1163/26670127-01010001
Jankvist, U. T., Gregersen, R. M., & Lauridsen, S. D. (2021b). Illustrating the need for a ‘Theory of Change’ in implementation processes. ZDM - Mathematics Education . https://doi.org/10.1007/s11858-021-01238-1
Jankvist, U. T., & Niss, M. (2015). A framework for designing a research-based “maths counsellor” teacher programme. Educational Studies in Mathematics, 90 (3), 259–284. https://doi.org/10.1007/s10649-015-9629-8
Jaworski, B., & Potari, D. (2021). Implementation of a developmental model of teachers’ and didacticians’ learning through inquiry: Design, operationalisation and outcomes. ZDM - Mathematics Education . https://doi.org/10.1007/s11858-021-01290-x
Karsenty, R. (2021). Implementing professional development programs for mathematics teachers at scale: What counts as success? ZDM - Mathematics Education . https://doi.org/10.1007/s11858-021-01250-5
Kieran, C., Krainer, K., & Shaughnessy, J. M. (2012). Linking research to practice: Teachers as key stakeholders in mathematics education research. In M. A. Clements, A. J. Bishop, C. Keitel, J. Kilpatrick, & F. K. S. Leung (Eds.), Third international handbook of mathematics education. Springer. https://doi.org/10.1007/978-1-4614-4684-2_12
Chapter Google Scholar
Kontorovich, I., & Bartlett, P. (2021). Implementation of research on scriptwriting in an undergraduate mathematics course: A study of teacher-researcher collaboration. ZDM - Mathematics Education . https://doi.org/10.1007/s11858-021-01281-y
Krainer, K. (2021). Implementation as interaction of research, practice and policy. Considerations from the Austrian initiative IMST. ZDM - Mathematics Education , 53 (5). https://doi.org/10.1007/s11858-021-01300-y
Kuzle, A., & Biehler, R. (2015). Examining mathematics mentor teachers’ practices in professional development courses on teaching data analysis: Implications for mentor teachers’ programs. ZDM - Mathematics Education, 47 (1), 39–51. https://doi.org/10.1007/s11858-014-0663-2
Kwon, O. N., Bae, Y., & Oh, K. H. (2015). Design research on inquiry-based multivariable calculus: Focusing on students’ argumentation and instructional design. ZDM - Mathematics Education, 47 (6), 997–1011. https://doi.org/10.1007/s11858-015-0726-z
Lawler, J., Trevatt, P., Elliot, C., & Leary, A. (2019). Does the diabetes specialist nursing workforce impact the experiences and outcomes of people with diabetes? A hermeneutic review of the evidence. Human Resources for Health, 17 , 65. https://doi.org/10.1186/s12960-019-0401-5
Levine, D. U., & Cooper, E. J. (1991). The change process and its implications in teaching thinking. In L. Idol & B. Jones (Eds.), Educational values and cognitive instruction (pp. 387–408). Routledge.
Maaß, K., & Artigue, M. (2013). Implementation of inquiry-based learning in day-to-day teaching: A synthesis. ZDM - Mathematics Education, 45 (6), 779–795. https://doi.org/10.1007/s11858-013-0528-0
Maaß, K., & Doorman, M. (2013). A model for a widespread implementation of inquiry-based learning. ZDM - Mathematics Education, 45 (6), 887–899. https://doi.org/10.1007/s11858-013-0505-7
Manouchehri, A., & Goodman, T. (2000). Implementing mathematics reform: The challenge within. Educational Studies in Mathematics, 42 (1), 1–34. https://doi.org/10.1023/A:1004011522216
Mason, J. (1998). Researching from the inside in mathematics education. In A. Sierpinska & J. Kilpatrick (Eds.), Mathematics education as a research domain: A search for identity (pp. 357–377). Kluwer.
Mason, J. (2002). Researching your own practice: The discipline of noticing . Routledge.
Book Google Scholar
Nilsen, P. (2015). Making sense of implementation theories, models and frameworks. Implementation Science, 10 , 53. https://doi.org/10.1186/s13012-015-0242-0
Niss, M. (1996). Mathematics in society. In R. Biehler, R. W. Scholz, R. Sträßer, & B. Winkelmann (Eds.), Didactics of mathematics as a scientific discipline (pp. 367–378). Kluwer.
Niss, M. (1999). Aspects of the nature and state of research in mathematics education. Educational Studies in Mathematics, 40 (1), 1–24. https://doi.org/10.1023/A:1003715913784
Obara, S., & Sloan, M. (2010). Classroom experiences with new curriculum materials during the implementation of performance standards in mathematics: A case study of teachers coping with change. International Journal of Science and Mathematics Education, 8 (2), 349–372. https://doi.org/10.1007/s10763-009-9176-9
Pinto, A., & Koichu, B. (2021). Implementation of mathematics education research as crossing the boundary between disciplined inquiry and teacher inquiry. ZDM - Mathematics Education . https://doi.org/10.1007/s11858-021-01286-7
Polotskaia, E., & Savard, A. (2018). Using the relational paradigm: Effects on pupils’ reasoning in solving additive word problems. Research in Mathematics Education, 20 (1), 70–90. https://doi.org/10.1080/14794802.2018.1442740
Prediger, S., Fischer, C., Selter, C., & Schöber, C. (2019). Combining material- and community-based implementation strategies for scaling up: The case of supporting low-achieving middle school students. Educational Studies in Mathematics, 102 (3), 361–378. https://doi.org/10.1007/s10649-018-9835-2
Prytz, J. (2021). When research met policy: A history of innovation and a complicated relationship in three Swedish development projects in mathematics education, 1960–2018. ZDM - Mathematics Education . https://doi.org/10.1007/s11858-021-01247-0
Remillard, J. T. (2005). Examining key concepts in research on teachers’ use of mathematics curricula. Review of Educational Research, 75 (2), 211–246. https://doi.org/10.3102/00346543075002211
Roesken-Winter, B., Stahnke, R., Prediger, S., & Gasteiger, H. (2021). Towards a research base for implementation strategies addressing mathematics teachers and facilitators. ZDM - Mathematics Education . https://doi.org/10.1007/s11858-021-01220-x
Rogers, E. M. (2003). Diffusion of innovations (5th ed.). Free Press.
Sierpinska, A., & Kilpatrick, J. (Eds.). (1998). Mathematics education as a research domain: A search for identity . Kluwer.
Smythe, E., & Spence, D. (2012). Re-viewing literature in hermeneutic research. International Journal of Qualitative Methods, 11 (1), 12–25. https://doi.org/10.1177/160940691201100102
Star, S. L. (2010). This is not a boundary object: Reflections on the origin of a concept. Science, Technology, and Human Values, 35 (5), 601–617. https://doi.org/10.1177/0162243910377624
Stylianides, G. J., & Stylianides, A. J. (2009). Facilitating the transition from empirical arguments to proof. Journal for Research in Mathematics Education, 40 (3), 314–352. https://doi.org/10.5951/jresematheduc.40.3.0314
Sullivan, P., Jorgensen, R., Boaler, J., & Lerman, S. (2013). Transposing reform pedagogy into new contexts: Complex instruction in remote Australia. Mathematics Education Research Journal, 25 (1), 173–184. https://doi.org/10.1007/s13394-013-0069-4
Tamborg, A. L. (2021). Improving mathematics teaching via digital platforms? Implementation processes seen through the lens of instrumental genesis. ZDM - Mathematics Education . https://doi.org/10.1007/s11858-021-01282-x
Valentine, N., Durning, S., Shanahan, E. M., & Schuwirth, L. (2021). Fairness in human judgement in assessment: A hermeneutic literature review and conceptual framework. Advances in Health Sciences Education, 26 (2), 713–738. https://doi.org/10.1007/s10459-020-10002-1
Valoyes-Chávez, L., & Felmer, P. (2021). “She was probing me to see if I knew”: Becoming a credible and confident PD facilitator. ZDM - Mathematics Education . https://doi.org/10.1007/s11858-021-01283-w
Venkat, H., & Graven, M. (2017). Changing teaching through a resources approach. In H. Venkat & M. Graven (Eds.), Improving primary mathematics education, teaching and learning (pp. 163–178). Palgrave Macmillan.
Wang, T. Y., Lin, F.L. & Yang, K.L. (2021). Success factors for a national problem-driven program aimed at enhancing affective performance in mathematics learning. ZDM - Mathematics Education , 53 (5). https://doi.org/10.1007/s11858-021-01285-8
Williams, S. R., & Leatham, K. R. (2017). Journal quality in mathematics education. Journal for Research in Mathematics Education, 48 (4), 369–396. https://doi.org/10.5951/jresematheduc.48.4.0369
Wright, V. (2014). Frequencies as proportions: Using a teaching model based on Pirie and Kieren’s model of mathematical understanding. Mathematics Education Research Journal, 26 (1), 101–128. https://doi.org/10.1007/s13394-014-0118-7
Download references
Acknowledgements
The authors of this paper gratefully acknowledge the collaboration of Linda Marie Ahl who assisted us with the identification of papers included in the literature review. We also sincerely thank to Jason Cooper and three reviewers whose valuable comments helped us to finalize the paper.
Author information
Authors and affiliations.
Weizmann Institute of Science, Rehovot, Israel
Boris Koichu
CICATA Legaria, Instituto Politécnico Nacional, Mexico City, Mexico
Mario Sánchez Aguilar
University of Copenhagen, Copenhagen, Denmark
Morten Misfeldt
You can also search for this author in PubMed Google Scholar
Corresponding author
Correspondence to Boris Koichu .
Additional information
Publisher's note.
Springer Nature remains neutral with regard to jurisdictional claims in published maps and institutional affiliations.
Rights and permissions
Open Access This article is licensed under a Creative Commons Attribution 4.0 International License, which permits use, sharing, adaptation, distribution and reproduction in any medium or format, as long as you give appropriate credit to the original author(s) and the source, provide a link to the Creative Commons licence, and indicate if changes were made. The images or other third party material in this article are included in the article's Creative Commons licence, unless indicated otherwise in a credit line to the material. If material is not included in the article's Creative Commons licence and your intended use is not permitted by statutory regulation or exceeds the permitted use, you will need to obtain permission directly from the copyright holder. To view a copy of this licence, visit http://creativecommons.org/licenses/by/4.0/ .
Reprints and permissions
About this article
Koichu, B., Aguilar, M.S. & Misfeldt, M. Implementation-related research in mathematics education: the search for identity. ZDM Mathematics Education 53 , 975–989 (2021). https://doi.org/10.1007/s11858-021-01302-w
Download citation
Accepted : 23 August 2021
Published : 01 September 2021
Issue Date : October 2021
DOI : https://doi.org/10.1007/s11858-021-01302-w
Share this article
Anyone you share the following link with will be able to read this content:
Sorry, a shareable link is not currently available for this article.
Provided by the Springer Nature SharedIt content-sharing initiative
- Implementation
- Implementation research
- Implementability of mathematics education research
- Stakeholders in implementation
- Find a journal
- Publish with us
- Track your research
May 31, 2024
10 min read
Math Can Help Solve Social Justice Problems
Mathematicians are working on ways to use their field to tackle major social issues, such as social inequality and the need for gender equity
By Rachel Crowell & Nature magazine
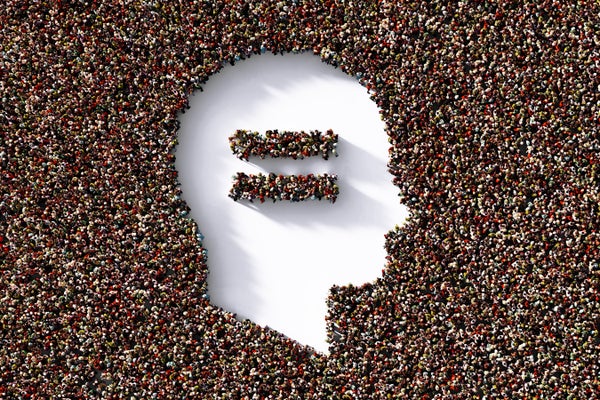
MicroStockHub/Getty Images
When Carrie Diaz Eaton trained as a mathematician, they didn’t expect their career to involve social-justice research. Growing up in Providence, Rhode Island, Diaz Eaton first saw social justice in action when their father, who’s from Peru, helped other Spanish-speaking immigrants to settle in the United States.
But it would be decades before Diaz Eaton would forge a professional path to use their mathematical expertise to study social-justice issues. Eventually, after years of moving around for education and training, that journey brought them back to Providence, where they collaborated with the Woonasquatucket River Watershed Council on projects focused on preserving the local environment of the river’s drainage basin, and bolstering resources for the surrounding, often underserved communities.
By “thinking like a mathematician” and leaning on data analysis, data science and visualization skills, they found that their expertise was needed in surprising ways, says Diaz Eaton, who is now executive director of the Institute for a Racially Just, Inclusive, and Open STEM Education at Bates College in Lewiston, Maine.
On supporting science journalism
If you're enjoying this article, consider supporting our award-winning journalism by subscribing . By purchasing a subscription you are helping to ensure the future of impactful stories about the discoveries and ideas shaping our world today.
For example, the council identified a need to help local people to better connect with community resources. “Even though health care and education don’t seem to fall under the purview of a watershed council, these are all interrelated issues,” Diaz Eaton says. Air pollution can contribute to asthma attacks, for example. In one project, Diaz Eaton and their collaborators built a quiz to help community members to choose the right health-care option, depending on the nature of their illness or injury, immigration status and health-insurance coverage.
“One of the things that makes us mathematicians, is our skills in logic and the questioning of assumptions”, and creating that quiz “was an example of logic at play”, requiring a logic map of cases and all of the possible branches of decision-making to make an effective quiz, they say.
Maths might seem an unlikely bedfellow for social-justice research. But applying the rigour of the field is turning out to be a promising approach for identifying, and sometimes even implementing, fruitful solutions for social problems.
Mathematicians can experience first-hand the messiness and complexity — and satisfaction — of applying maths to problems that affect people and their communities. Trying to work out how to help people access much-needed resources, reduce violence in communities or boost gender equity requires different technical skills, ways of thinking and professional collaborations compared with breaking new ground in pure maths. Even for an applied mathematician like Diaz Eaton, transitioning to working on social-justice applications brings fresh challenges.
Mathematicians say that social-justice research is difficult yet fulfilling — these projects are worth taking on because of their tremendous potential for creating real-world solutions for people and the planet.
Data-driven research
Mathematicians are digging into issues that range from social inequality and health-care access to racial profiling and predictive policing. However, the scope of their research is limited by their access to the data, says Omayra Ortega, an applied mathematician and mathematical epidemiologist at Sonoma State University in Rohnert Park, California. “There has to be that measured information,” Ortega says.
Fortunately, data for social issues abound. “Our society is collecting data at a ridiculous pace,” Ortega notes. Her mathematical epidemiology work has examined which factors affect vaccine uptake in different communities. Her work has found, for example, that, in five years, a national rotavirus-vaccine programme in Egypt would reduce disease burden enough that the cost saving would offset 76% of the costs of the vaccine. “Whenever we’re talking about the distribution of resources, there’s that question of social justice: who gets the resources?” she says.
Lily Khadjavi’s journey with social-justice research began with an intriguing data set.
About 15 years ago, Khadjavi, a mathematician at Loyola Marymount University in Los Angeles, California, was “on the hunt for real-world data” for an undergraduate statistics class she was teaching. She wanted data that the students could crunch to “look at new information and pose their own questions”. She realized that Los Angeles Police Department (LAPD) traffic-stop data fit that description.
At that time, every time that LAPD officers stopped pedestrians or pulled over drivers, they were required to report stop data. Those data included “the perceived race or ethnicity of the person they had stopped”, Khadjavi notes.
When the students analysed the data, the results were memorable. “That was the first time I heard students do a computation absolutely correctly and then audibly gasp at their results,” she says. The data showed that one in every 5 or 6 police stops of Black male drivers resulted in a vehicle search — a rate that was more than triple the national average, which was about one out of every 20 stops for drivers of any race or ethnicity, says Khadjavi.
Her decision to incorporate that policing data into her class was a pivotal moment in Khadjavi’s career — it led to a key publication and years of building expertise in using maths to study racial profiling and police practice. She sits on California’s Racial Identity and Profiling Advisory Board , which makes policy recommendations to state and local agencies on how to eliminate racial profiling in law enforcement.
In 2023, she was awarded the Association for Women in Mathematics’ inaugural Mary & Alfie Gray Award for Social Justice, named after a mathematician couple who championed human rights and equity in maths and government.
Sometimes, gaining access to data is a matter of networking. One of Khadjavi’s colleagues shared Khadjavi’s pivotal article with specialists at the American Civil Liberties Union. In turn, these specialists shared key data obtained through public-records requests with Khadjavi and her colleague. “Getting access to that data really changed what we could analyse,” Khadjavi says. “[It] allowed us to shine a light on the experiences of civilians and police in hundreds of thousands of stops made every year in Los Angeles.”
The data-intensive nature of this research can be an adjustment for some mathematicians, requiring them to develop new skills and approach problems differently. Such was the case for Tian An Wong, a mathematician at the University of Michigan-Dearborn who trained in number theory and representation theory.
In 2020, Wong wanted to know more about the controversial issue of mathematicians collaborating with the police, which involves, in many cases, using mathematical modelling and data analysis to support policing activities. Some mathematicians were protesting about the practice as part of a larger wave of protests around systemic racism , following the killing of George Floyd by police in Minneapolis, Minnesota. Wong’s research led them to a technique called predictive policing, which Wong describes as “the use of historical crime and other data to predict where future crime will occur, and [to] allocate policing resources based on those predictions”.
Wong wanted to know whether the tactics that mathematicians use to support police work could instead be used to critique it. But first, they needed to gain some additional statistics and data analysis skills. To do so, Wong took an online introductory statistics course, re-familiarized themself with the Python programming language, and connected with colleagues trained in statistical methods. They also got used to reading research papers across several disciplines.
Currently, Wong applies those skills to investigating the policing effectiveness of a technology that automatically locates gunshots by sound. That technology has been deployed in parts of Detroit, Michigan, where community members and organizations have raised concerns about its multimillion-dollar cost and about whether such police surveillance makes a difference to public safety.
Getting the lay of the land
For some mathematicians, social-justice work is a natural extension of their career trajectories. “My choice of mathematical epidemiology was also partially born out of out of my love for social justice,” Ortega says. Mathematical epidemiologists apply maths to study disease occurrence in specific populations and how to mitigate disease spread. When Ortega’s PhD adviser mentioned that she could study the uptake of a then-new rotovirus vaccine in the mid-2000s, she was hooked.
Mathematicians, who decide to jump into studying social-justice issues anew, must do their homework and dedicate time to consider how best to collaborate with colleagues of diverse backgrounds.
Jonathan Dawes, an applied mathematician at the University of Bath, UK, investigates links between the United Nations’ Sustainable Development Goals (SDGs) and their associated target actions. Adopted in 2015, the SDGs are “a universal call to action to end poverty, protect the planet, and ensure that by 2030 all people enjoy peace and prosperity,” according to the United Nations , and each one has a number of targets.
“As a global agenda, it’s an invitation to everybody to get involved,” says Dawes. From a mathematical perspective, analysing connections in the complex system of SDGs “is a nice level of problem,” Dawes says. “You’ve got 17 Sustainable Development Goals. Between them, they have 169 targets. [That’s] an amount of data that isn’t very large in big-data terms, but just big enough that it’s quite hard to hold all of it in your head.”
Dawes’ interest in the SDGs was piqued when he read a 2015 review that focused on how making progress on individual goals could affect progress on the entire set. For instance, if progress is made on the goal to end poverty how does that affect progress on the goal to achieve quality education for all, as well as the other 15 SDGs?
“If there’s a network and you can put some numbers on the strengths and signs of the edges, then you’ve got a mathematized version of the problem,” Dawes says. Some of his results describe how the properties of the network change if one or more of the links is perturbed, much like an ecological food web. His work aims to identify hierarchies in the SDG networks, pinpointing which SDGs should be prioritized for the health of the entire system.
As Dawes dug into the SDGs, he realized that he needed to expand what he was reading to include different journals, including publications that were “written in very different ways”. That involved “trying to learn a new language”, he explains. He also kept up to date with the output of researchers and organizations doing important SDG-related work, such as the International Institute for Applied Systems Analysis in Laxenburg, Austria, and the Stockholm Environment Institute.
Dawes’ research showed that interactions between the SDGs mean that “there are lots of positive reinforcing effects between poverty, hunger, health care, education, gender equity and so on.” So, “it’s possible to lift all of those up” when progress is made on even one of the goals. With one exception: managing and protecting the oceans. Making progress on some of the other SDGs could, in some cases, stall progress for, or even harm, life below water.
Collaboration care
Because social-justice projects are often inherently cross-disciplinary, mathematicians studying social justice say it’s key in those cases to work with community leaders, activists or community members affected by the issues.
Getting acquainted with these stakeholders might not always feel comfortable or natural. For instance, when Dawes started his SDG research, he realized that he was entering a field in which researchers already knew each other, followed each other’s work and had decades of experience. “There’s a sense of being like an uninvited guest at a party,” Dawes says. He became more comfortable after talking with other researchers, who showed a genuine interest in what he brought to the discussion, and when his work was accepted by the field’s journals. Over time, he realized “the interdisciplinary space was big enough for all of us to contribute to”.
Even when mathematicians have been invited to join a team of social-justice researchers, they still must take care, because first impressions can set the tone.
Michael Small is an applied mathematician and director of the Data Institute at the University of Western Australia in Perth. For much of his career, Small focused on the behaviour of complex systems, or those with many simple interacting parts, and dynamical systems theory, which addresses physical and mechanical problems.
But when a former vice-chancellor at the university asked him whether he would meet with a group of psychiatrists and psychologists to discuss their research on mental health and suicide in young people, it transformed his research. After considering the potential social impact of better understanding the causes and risks of suicide in teenagers and younger children, and thinking about how the problem meshed well with his research in complex systems and ‘non-linear dynamics’, Small agreed to collaborate with the group.
The project has required Small to see beyond the numbers. For the children’s families, the young people are much more than a single data point. “If I go into the room [of mental-health professionals] just talking about mathematics, mathematics, mathematics, and how this is good because we can prove this really cool theorem, then I’m sure I will get push back,” he says. Instead, he notes, it’s important to be open to insights and potential solutions from other fields. Listening before talking can go a long way.
Small’s collaborative mindset has led him to other mental-health projects, such as the Transforming Indigenous Mental Health and Wellbeing project to establish culturally sensitive mental-health support for Indigenous Australians.
Career considerations
Mathematicians who engage in social-justice projects say that helping to create real-world change can be tremendously gratifying. Small wants “to work on problems that I think can do good” in the world. Spending time pursuing them “makes sense both as a technical challenge [and] as a social choice”, he says.
However, pursuing this line of maths research is not without career hurdles. “It can be very difficult to get [these kinds of] results published,” Small says. Although his university supports, and encourages, his mental-health research, most of his publications are related to his standard mathematics research. As such, he sees “a need for balance” between the two lines of research, because a paucity of publications can be a career deal breaker.
Diaz Eaton says that mathematicians pursuing social-justice research could experience varying degrees of support from their universities. “I’ve seen places where the work is supported, but it doesn’t count for tenure [or] it won’t help you on the job market,” they say.
Finding out whether social-justice research will be supported “is about having some really open and transparent conversations. Are the people who are going to write your recommendation letters going to see that work as scholarship?” Diaz Eaton notes.
All things considered, mathematicians should not feel daunted by wading into solving the world’s messy problems, Khadjavi says: “I would like people to follow their passions. It’s okay to start small.”
This article is reproduced with permission and was first published on May 22, 2024 .
- Current Students
Journal Highlights Math Research by Professor and Student
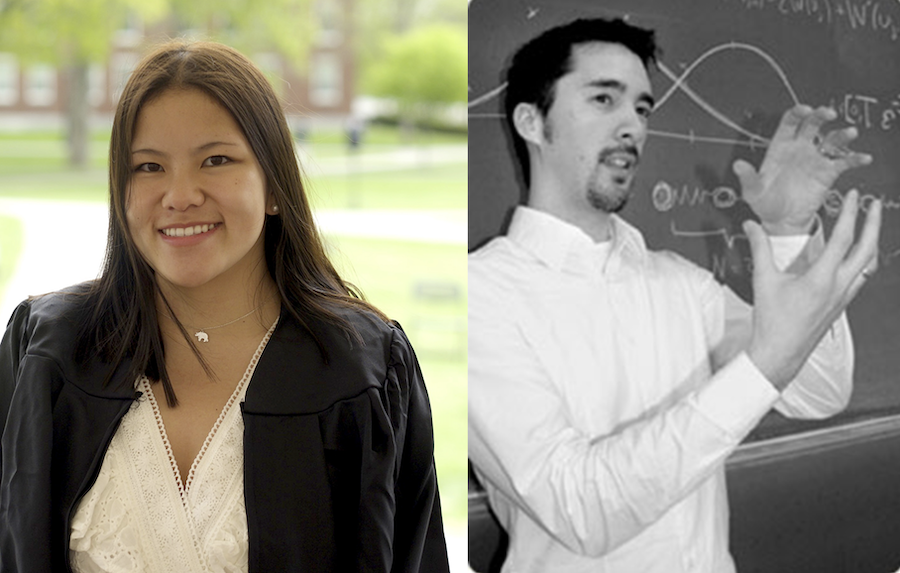
The paper , "Modulation instability and wavenumber bandgap breathers in a time layered phononic lattice," is the result of a yearslong collaboration of Chong, Wallace, and two researchers at CalTech.
Their project focused on how pressure waves can be manipulated by materials. It began in 2021 with a theoretical component—which Wallace worked on for her honors theses, " Instability in a Time-Modulated Lattice .” She is now wrapping up a master's degree in applied mathematics at Columbia University.
Wallace said she reached out to Chong in 2021 to inquire whether she could assist him with a research project. She had excelled in his ordinary differential equations and partial differential equations classes, Chong said, and he was just launching a new research program. So he invited her to work as his research assistant in the summer, a partnership that extended through her senior year.
The work Wallace completed in the first stage of the research program was followed by numerical and experimental simulations, conducted by Chong and his Caltech teammates, which was then backed up with rigorous mathematical proofs in a follow-up paper. “It is a nice spectrum that goes from Evy's analytical computations to computer simulations, the laboratory, and to mathematical proof,” Chong said.
New Insights
Their April 11 paper is garnering attention because it opens new ground in the field of physical energy. Their project specifically focused on understanding how pressure waves are affected by media that varies over time. (Pressure waves, of which the most commonly known is a sound wave, are set off when a mass or object is compressed or decompressed by an external force, such as an explosion.)
Traditionally, researchers have experimented with pressure waves using media that vary in space while they remain constant in time.
For those who are not familiar with his field, Chong came up with a helpful analogy to explain his research, comparing the time-varying media he and Wallace studied to a floor, and the waves passing over the media to a person walking across that floor. “A traditional floor might have a pattern of alternating spots of rough and smooth areas,” he began. “As you’re walking across the floor, how your feet touch the ground will affect how you walk along it.”
In contrast, a medium that changes over time resembles a wacky floor in an amusement park fun house. Rather than having both smooth and rough patches, it is entirely smooth one moment before shifting to a rough surface, switching back and forth between these two patterns. “How you walk through that medium will be different than walking across a traditional floor,” Chong said.
Wallace's original calculations showed exactly where this system would be unstable, which is desirable. When a system is stable, “it means the amplitude of the wave doesn't grow,” Chong said, whereas an unstable system results in a wave that amplifies quite a bit.
“What Evy did was to tell us exactly what material parameters would be needed for that to happen, an exact formula for when and under what circumstances you get interesting instability,” he said. “Having a formula that exact is quite rare. Usually you have to approximate it or do some numerical simulations.”
By the way, the materials they studied aren't linoleum or wood panels or anything most of us would recognize as typical flooring materials. “Our experiment consists of magnets,” Chong said. They looked at variables such as the distance and strength of magnets, as well as the effect of dampening from air friction. “Each of those properties has a parameter that you can tune, so having a formula saying this combination of parameters leads to amplification is very helpful.”
What is of particular interest to researchers working in this area is how to maintain the force of a wave or signal, because over time, all waves degrade. Each electrical circuit, for example, needs an amplifier to work, Chong said.
“So when talking about the applications of our work, our system would act as an amplifier,” he added. “Based on what Evy did, you would know exactly how to make the material so that the wave would amplify.”
Possible applications
Though Chong and his team are working on a basic research level, in the long term, understanding the systems they are studying could lead to innovations in “acoustic logic,” Chong explained, which is like the logic underlying electrical circuits, but which don't use electricity but rather pressure waves. They could even help create a situation where “acoustic circuits” could operate with the use of ambient vibrations only, and thus avoid detection from sensors.
“We’re still doing work that is more fundamental,” Chong said. “But understanding how these systems behave could contribute to that the much-longer project of having a goal like that.”
Wallace's undergraduate work with Chong led to a research collaboration with a Columbia faculty member. With him, Wallace expanded Chong's one-dimensional study to two dimensions.
Starting this type of high-level research at Bowdoin, Wallace said, “synthesized everything I had learned at Bowdoin and learned about math in general.” She continued, “Doing research allowed me to take what I learned out of the classroom and apply it to something I could see in the the real world, and see how it would be very important and relevant.”
The journal, which is open access, and Bowdoin College provided support to cover the publication fees via the Fletcher Family Research Grant. Wallace's research was supported by a National Science Foundation grant and the Bowdoin Kaufman Family Fellowship .
Related Stories
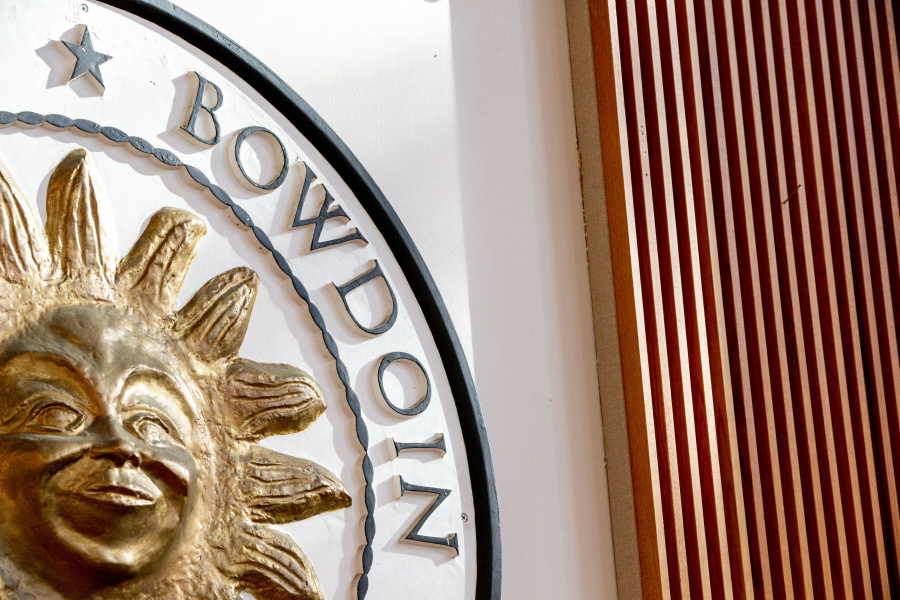
- Make a Gift
- Request Info
- Offices & Services A-Z
- Accessibility
Cookie Acceptance Needed
This website would like to use cookies to collect information to improve your browsing experience. Please review our Privacy Statement for more information. Do you accept?
accept deny
College of Sciences and Mathematics Homepage
- Toggle Search
- Find People
COSAM News Articles 2024 05 Virtual lab meetings improve undergraduate research experience and foster diversity in academia
Virtual lab meetings improve undergraduate research experience and foster diversity in academia
Published: 05/29/2024
By: Maria Gebhardt
Moisés A. Bernal , assistant professor in the Department of Biological Sciences at Auburn University, is collaborating with researchers Kathleen Lotterhos (Northeast University), Megan Phifer-Rixey (Drexel University), and Torrance Hanley , (Sacred Heart University) to evaluate the effectiveness of virtual lab meetings in demystifying the hidden curriculum in academia, incentivize primary research among students without previous experience, and foster participation of underrepresented groups in STEM.
The “Biological Practices” article entitled A virtual lab meeting training program mutually benefits mentees and host labs was recently published in Proceedings B, dives into the specific details that are needed to develop a virtual meetings program from scratch, and reports on the experiences by both mentors and mentees. The first step on the NSF-funded Virtual Lab Meeting Program (LaMP) was to match research mentors (i.e. faculty in research intensive institutions) with students in STEM fields (i.e. at institutions with limited research support), Bernal indicated.
“The program matched mentors and mentees based on affinity of research topics, and mentees were provided with a $500 stipend if they participated in at least 10 meetings,” Bernal said. “This is important because the students had an incentive to participate at the meetings and actively exchange ideas with a research lab.”
The team collected data that shared insight of the value of the LaMP experience.
“When we analyzed the data, we saw a gap that exists between the current mentors and the mentees,” he said. “The mentees were a much more diverse group then the mentors.”
The data included racial and sexual diversity among mentees participating. However, the faculty did not exhibit as much diversity, which highlights the need for this type of program among undergraduate and graduate students.
"Our results clearly show that this program worked to extend the professional networks of traditionally under-represented groups in the sciences, with little extra time commitment on the part of the participating labs,” said Lotterhos. “We hope these results will stimulate similar efforts across STEM disciplines."
And those efforts can be easily integrated into a lab.
“For faculty, this is a small-time investment, because we are already conducting regular lab meetings and we can simply bring on a new mentee into this experience” Bernal added. The results from the study show that the mentees learned new information on specific techniques and study systems, while developing a broader network of personal connections in academia. Further, the mentees learned how to discuss and present research as well as how to discuss a manuscript and the overall writing process. Meanwhile, mentors indicated it was beneficial to gain novel perspectives on how to discuss peer-reviewed manuscripts, and gain knowledge on other study systems presented by the mentees. Overall, the survey results indicate that the program was useful for staff and students in the mentor’s lab as well as the mentees, and participants strongly recommend this program to future participants.
Bernal spoke about his time with a virtual student in his lab.
“It was a positive experience because I was able to have someone in my lab with a novel perspective on how to discuss manuscripts and to look at research problems from a new angle” he explained. “This program helps to develop and expand professional networks for participants. It also helps them prepare to apply to graduate school and learn how to build their CVs over time.”
Overall, the LaMP initiative represents an effective way of connecting researchers with students in STEM, while fostering the participation of underrepresented groups in academia.
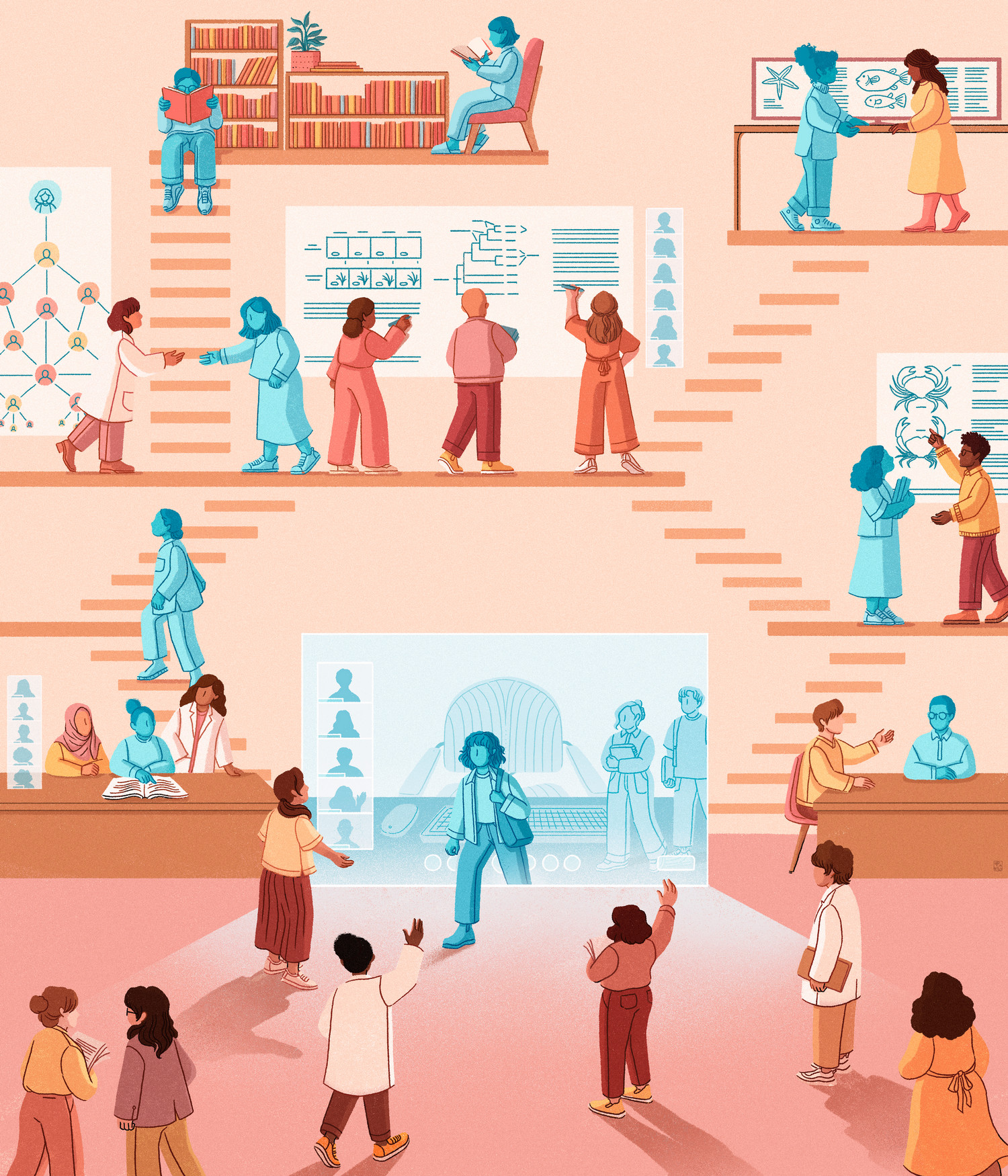
Latest Headlines
- Auburn alumnus bridges Auburn University and the National Renewable Energy Laboratory in DOE's Energy Earthshots Initiative 05/28/2024
- Auburn hosts CBMS Conference: Mathematical Methods for Novel Metamaterials 05/28/2024
- Auburn biologists publish first work on avian migration conducted in the AU MitoMobile 05/21/2024
- 2024 Department of Chemistry and Biochemistry awards honor faculty, staff and students 04/27/2024
- Department of Geosciences celebrates Earth Day 04/23/2024
Stay Connected
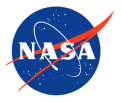
Suggested Searches
- Climate Change
- Expedition 64
- Mars perseverance
- SpaceX Crew-2
- International Space Station
- View All Topics A-Z
- Humans in Space
Earth & Climate
The solar system, the universe, aeronautics, learning resources, news & events.
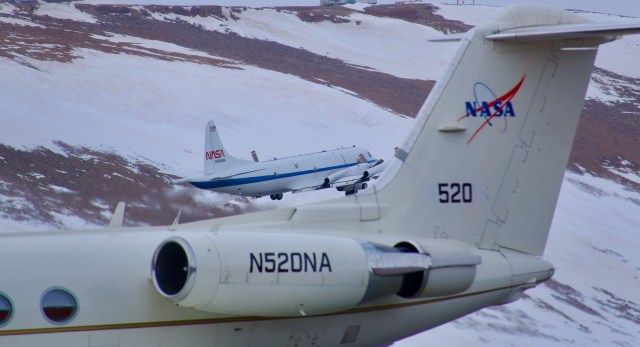
NASA Mission Flies Over Arctic to Study Sea Ice Melt Causes
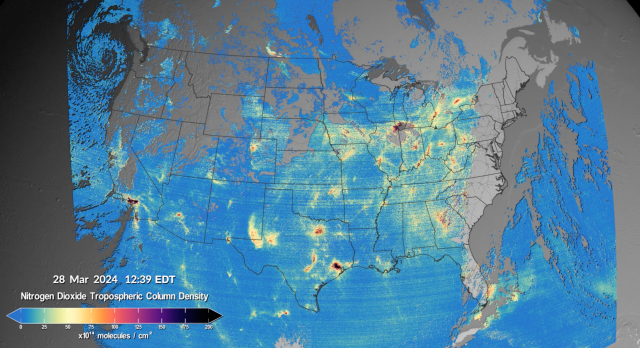
NASA Releases New High-Quality, Near Real-Time Air Quality Data
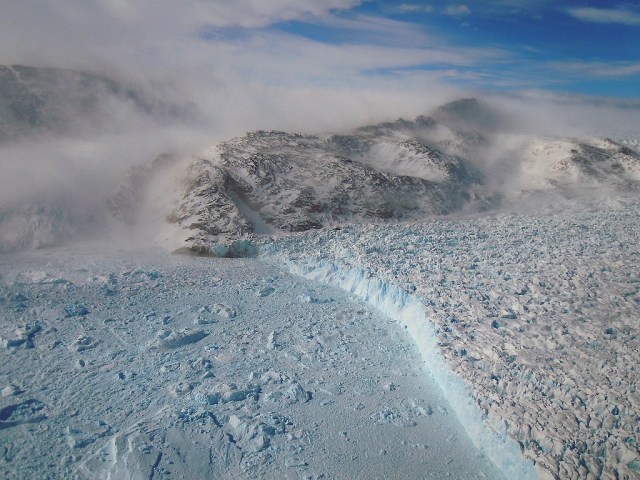
Twin NASA Satellites Ready to Help Gauge Earth’s Energy Balance
- Search All NASA Missions
- A to Z List of Missions
- Upcoming Launches and Landings
- Spaceships and Rockets
- Communicating with Missions
- James Webb Space Telescope
- Hubble Space Telescope
- Why Go to Space
- Astronauts Home
- Commercial Space
- Destinations
Living in Space
- Explore Earth Science
- Earth, Our Planet
- Earth Science in Action
- Earth Multimedia
- Earth Science Researchers
- Pluto & Dwarf Planets
- Asteroids, Comets & Meteors
- The Kuiper Belt
- The Oort Cloud
- Skywatching
- The Search for Life in the Universe
- Black Holes
- The Big Bang
- Dark Energy & Dark Matter
- Earth Science
- Planetary Science
- Astrophysics & Space Science
- The Sun & Heliophysics
- Biological & Physical Sciences
- Lunar Science
- Citizen Science
- Astromaterials
- Aeronautics Research
- Human Space Travel Research
- Science in the Air
- NASA Aircraft
- Flight Innovation
- Supersonic Flight
- Air Traffic Solutions
- Green Aviation Tech
- Drones & You
- Technology Transfer & Spinoffs
- Space Travel Technology
- Technology Living in Space
- Manufacturing and Materials
- Science Instruments
- For Kids and Students
- For Educators
- For Colleges and Universities
- For Professionals
- Science for Everyone
- Requests for Exhibits, Artifacts, or Speakers
- STEM Engagement at NASA
- NASA's Impacts
- Centers and Facilities
- Directorates
- Organizations
- People of NASA
- Internships
- Our History
- Doing Business with NASA
- Get Involved
- Aeronáutica
- Ciencias Terrestres
- Sistema Solar
- All NASA News
- Video Series on NASA+
- Newsletters
- Social Media
- Media Resources
- Upcoming Launches & Landings
- Virtual Events
- Sounds and Ringtones
- Interactives
- STEM Multimedia
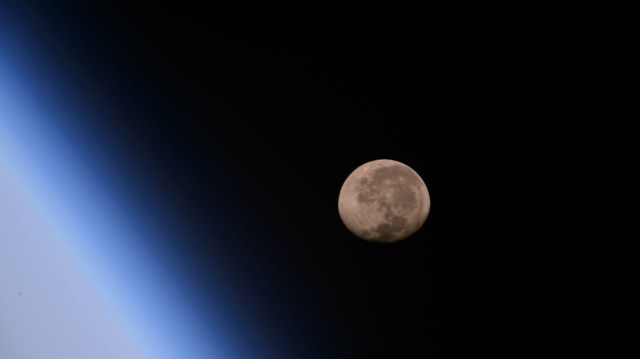
Space Station Research Advances NASA’s Plans to Explore the Moon, Mars
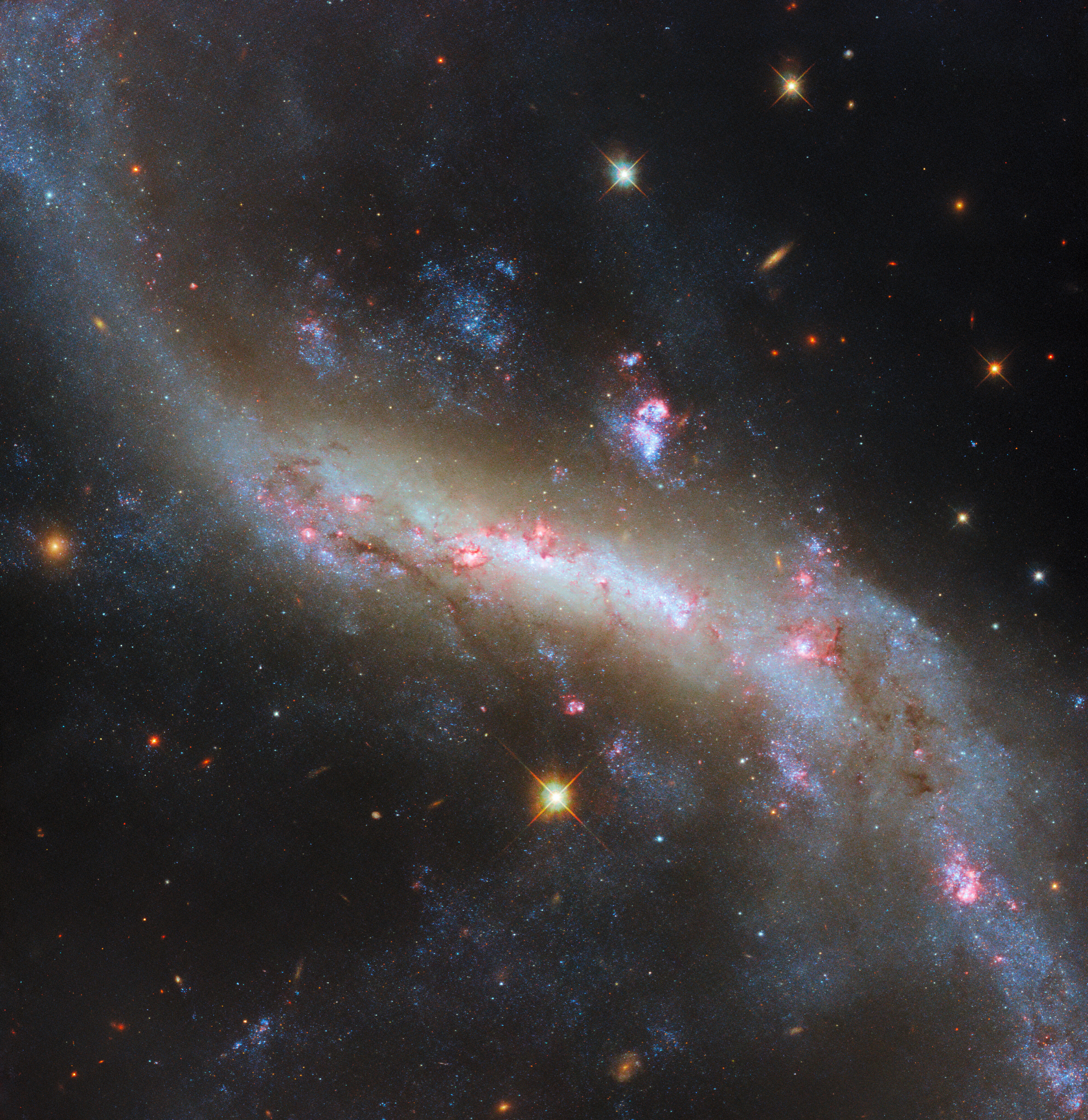
Hubble Views the Lights of a Galactic Bar
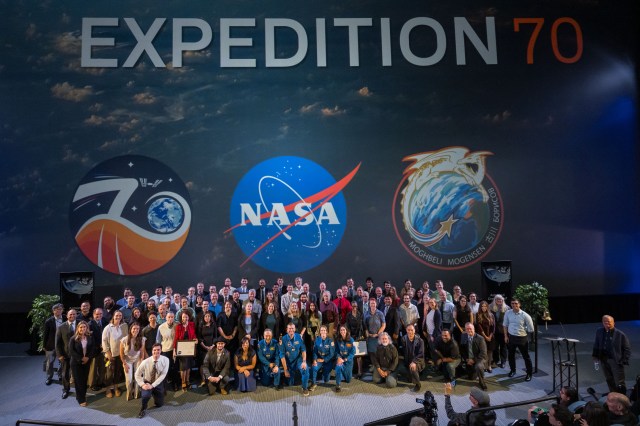
Welcome Back to Planet Earth, Expedition 70 Crew!
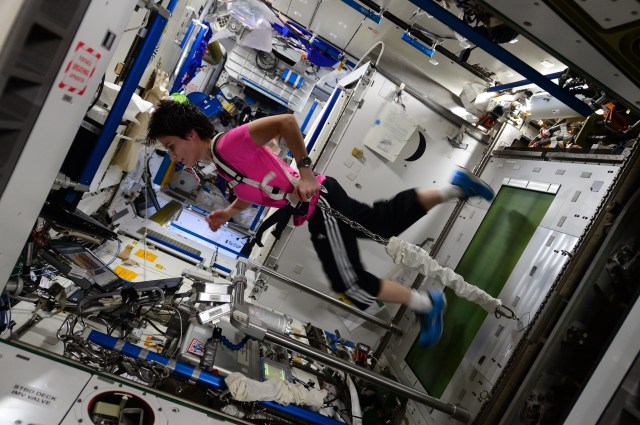
Astronaut Exercise
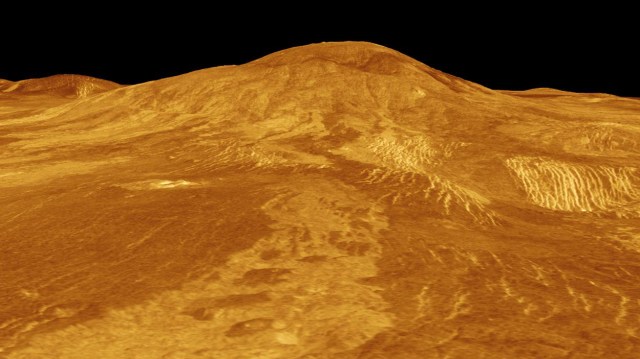
Ongoing Venus Volcanic Activity Discovered With NASA’s Magellan Data
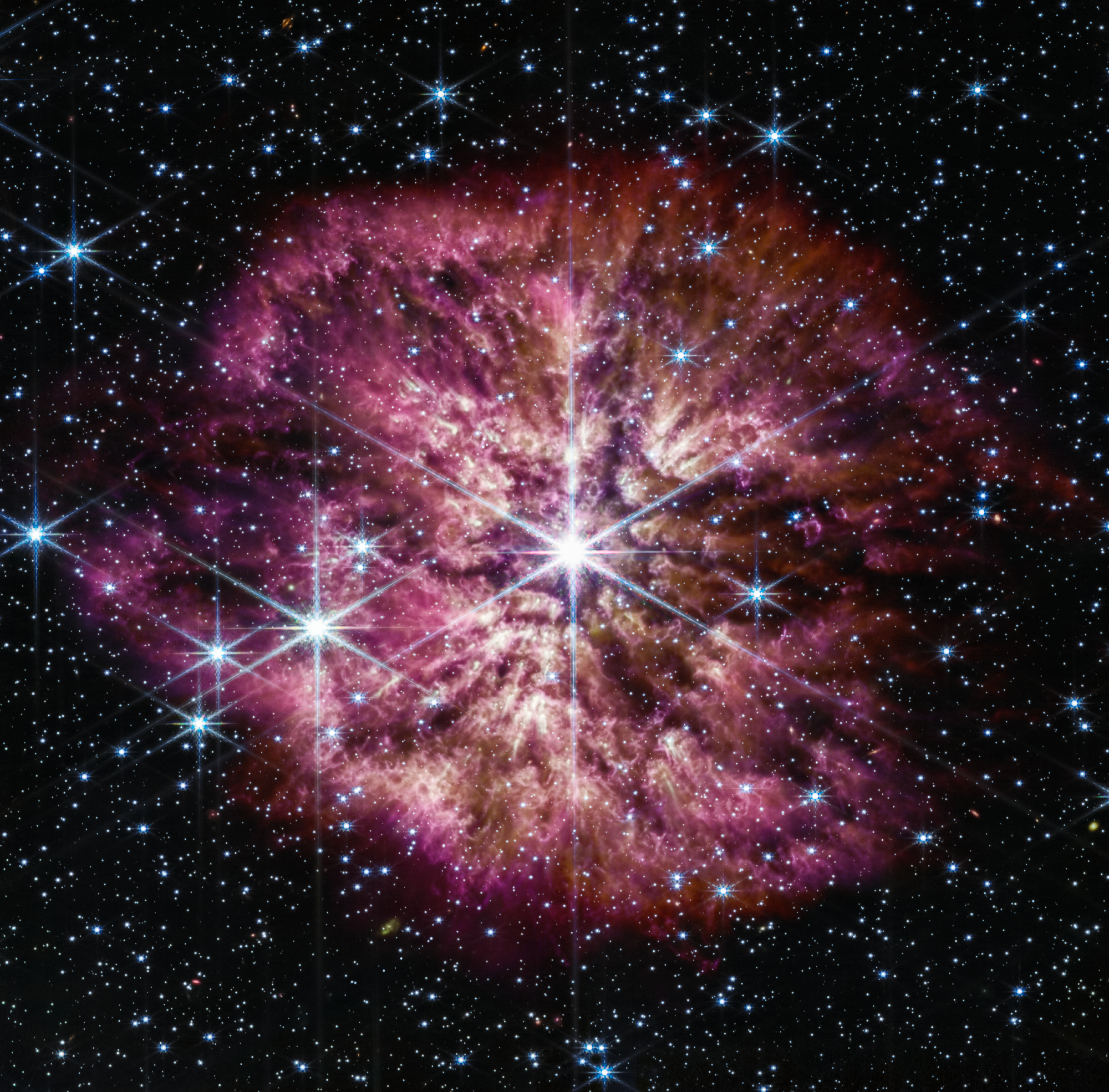
C.12 Planetary Instrument Concepts for the Advancement of Solar System Observations POC Change
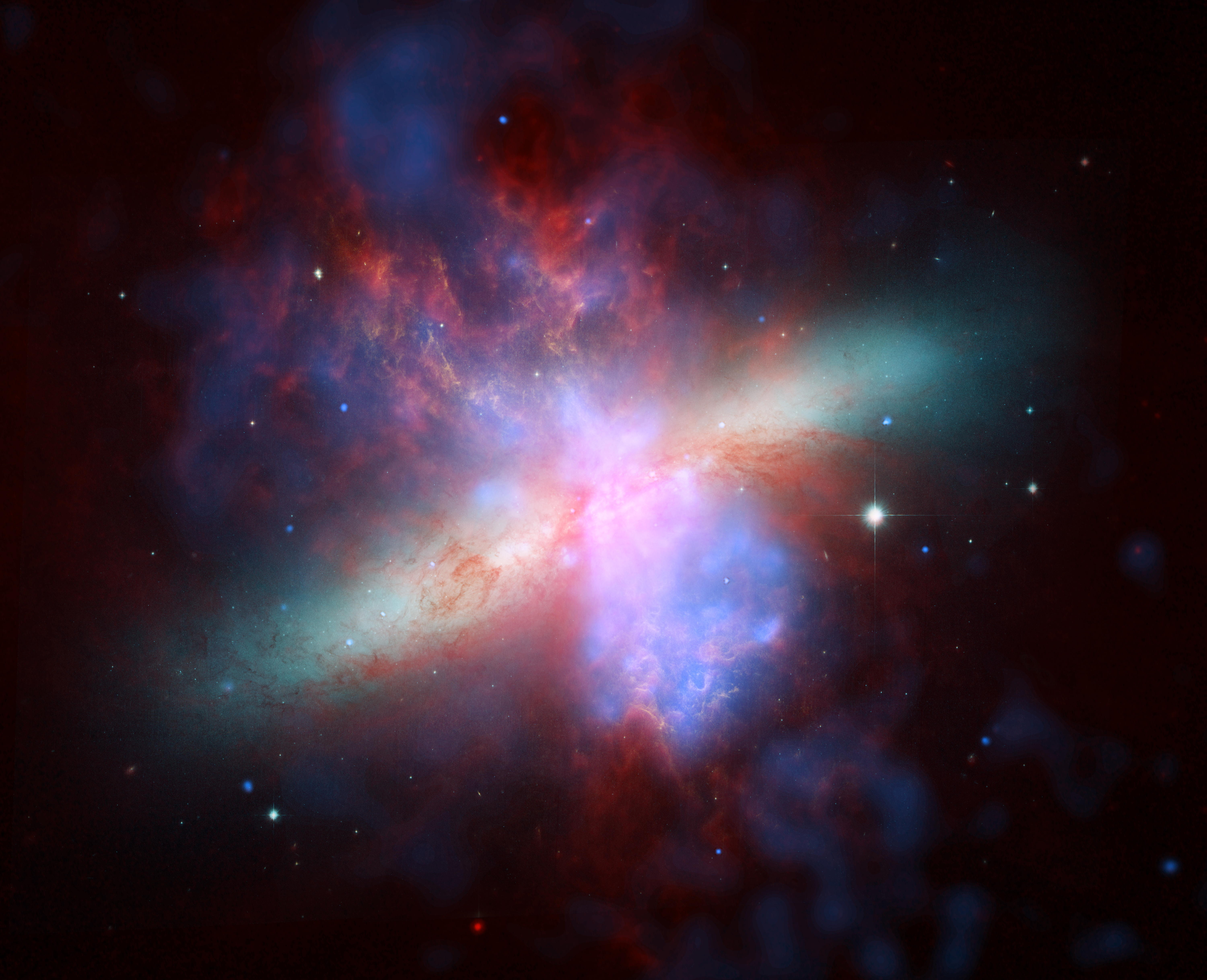
June’s Night Sky Notes: Constant Companions: Circumpolar Constellations, Part III
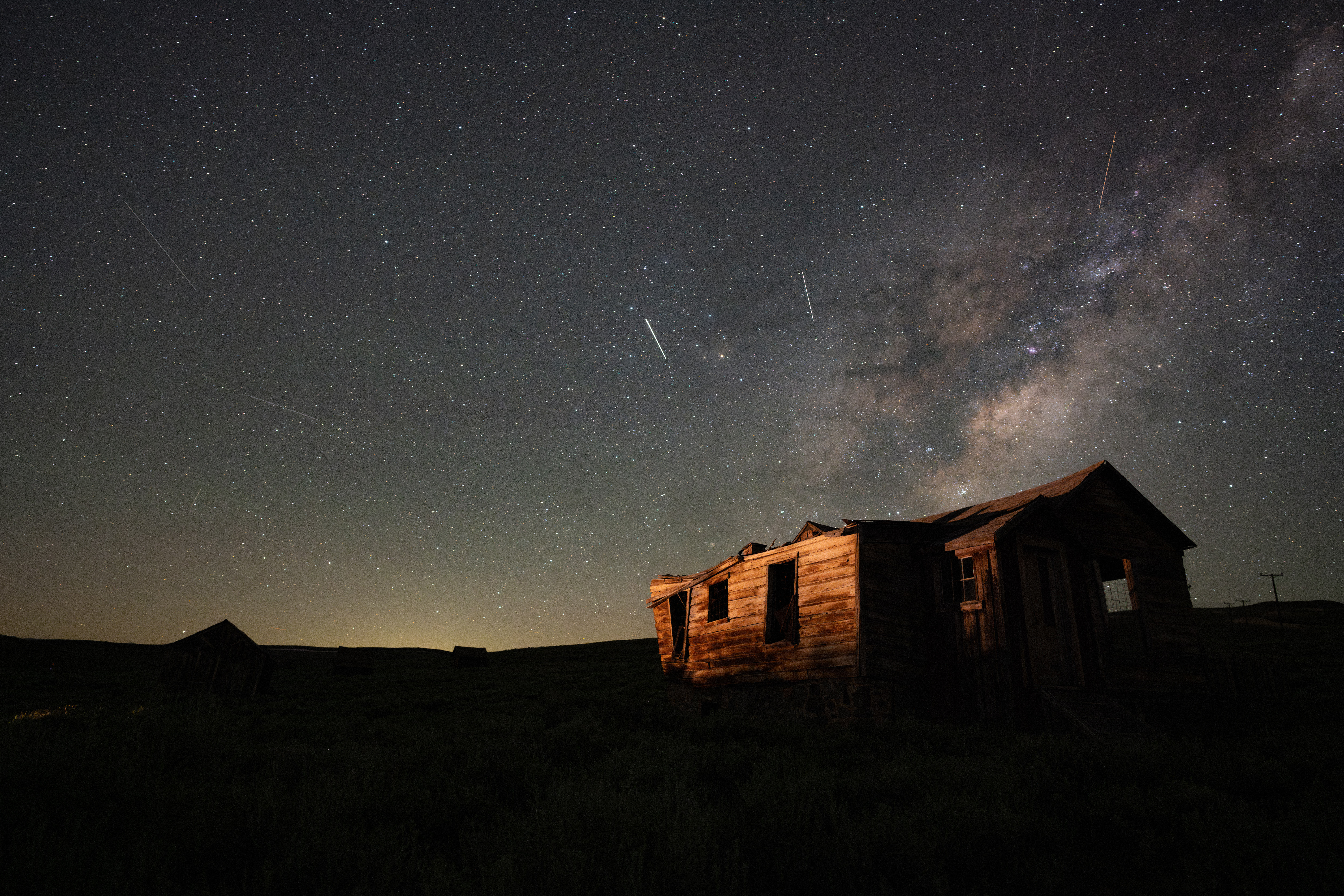
What’s Up: June 2024 Skywatching Tips from NASA
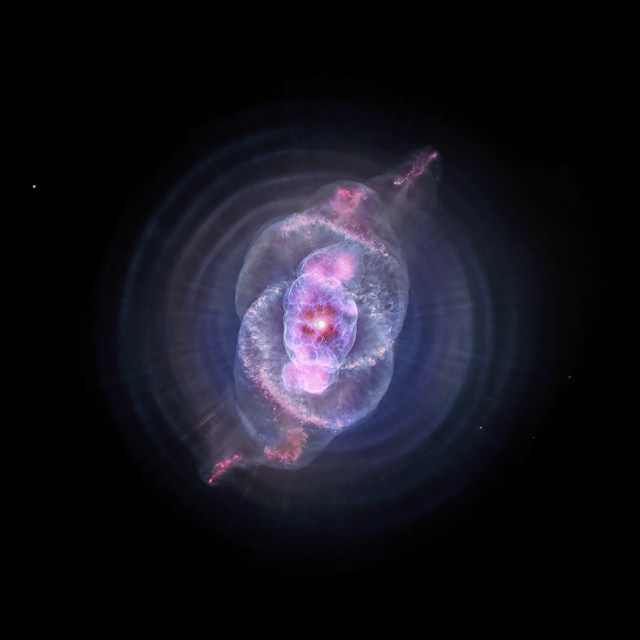
Travel Through Data From Space in New 3D Instagram Experiences
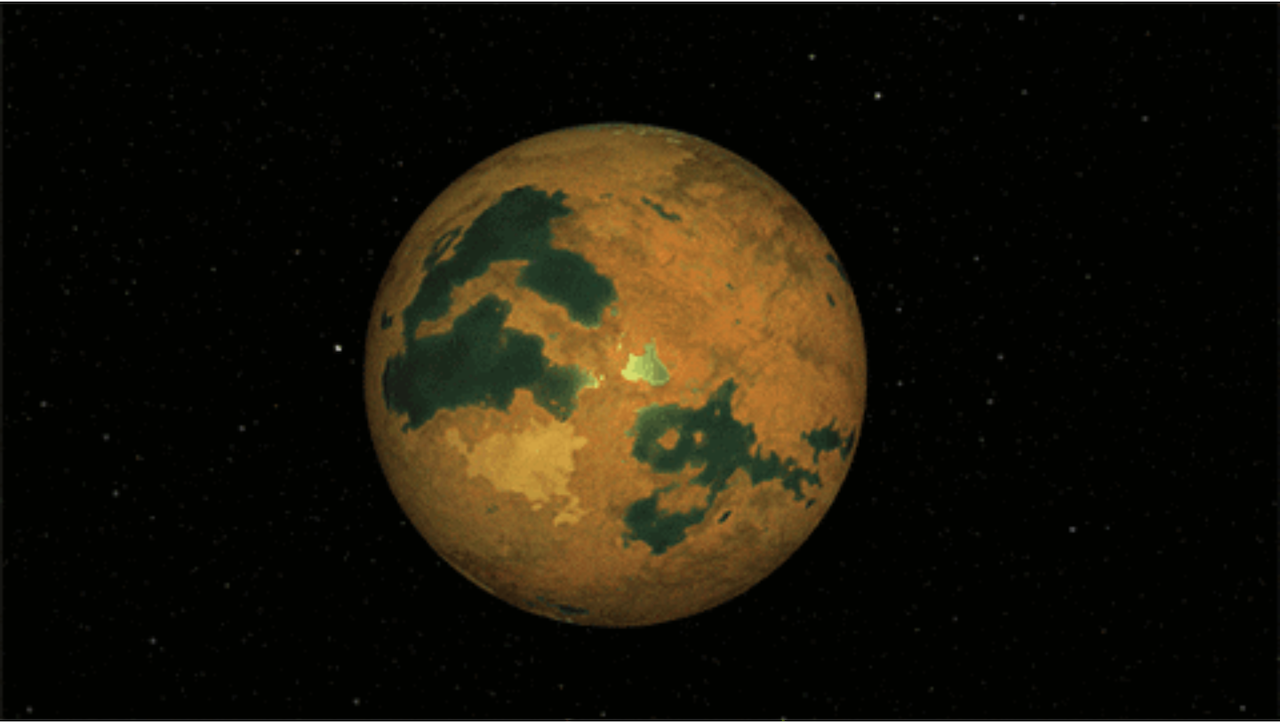
Discovery Alert: Spock’s Home Planet Goes ‘Poof’
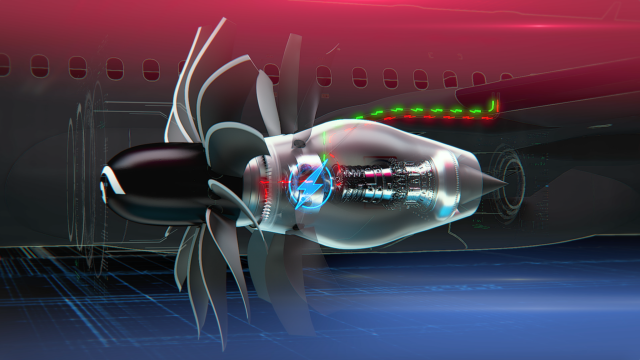
NASA, Industry to Start Designing More Sustainable Jet Engine Core
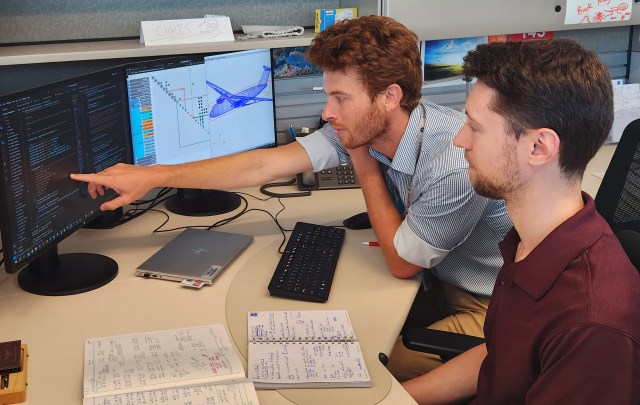
Aviary: A New NASA Software Platform for Aircraft Modelling

NASA’s X-59 Passes Milestone Toward Safe First Flight
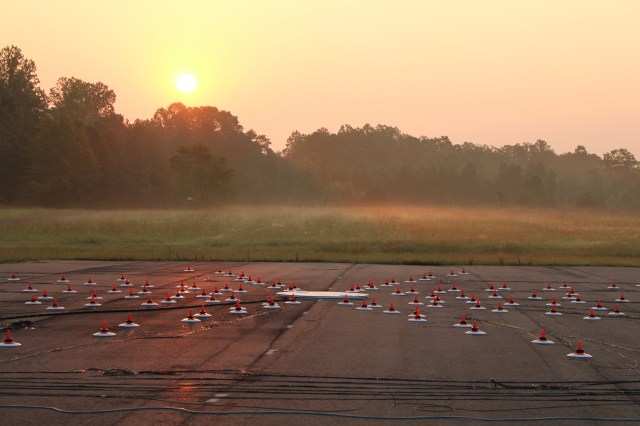
Tech Today: Measuring the Buzz, Hum, and Rattle
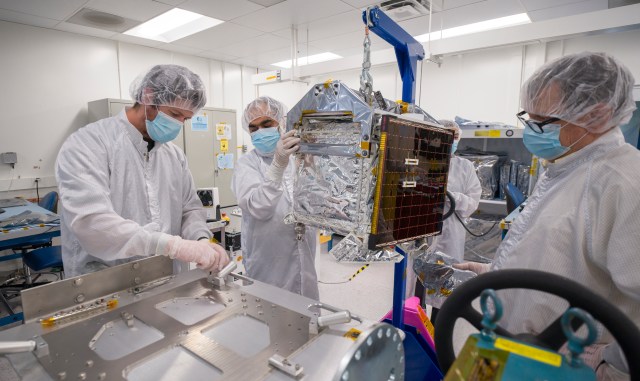
NASA to Measure Moonquakes With Help From InSight Mars Mission
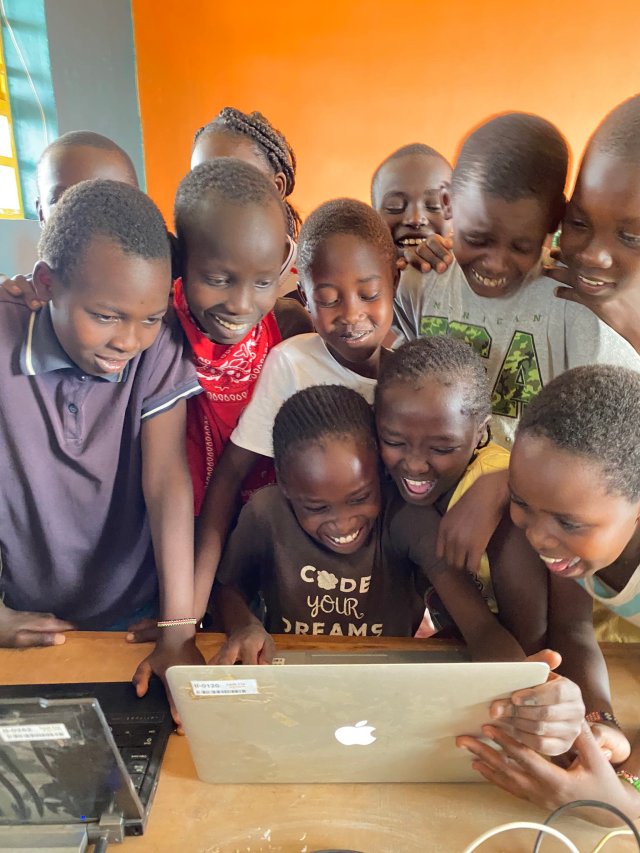
NASA Around the World: Interns Teach Virtual Lessons in Kenya
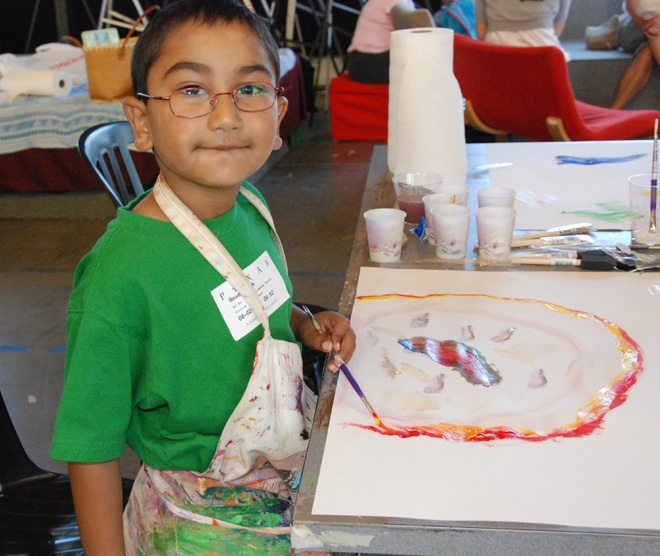
The Moon and Amaey Shah
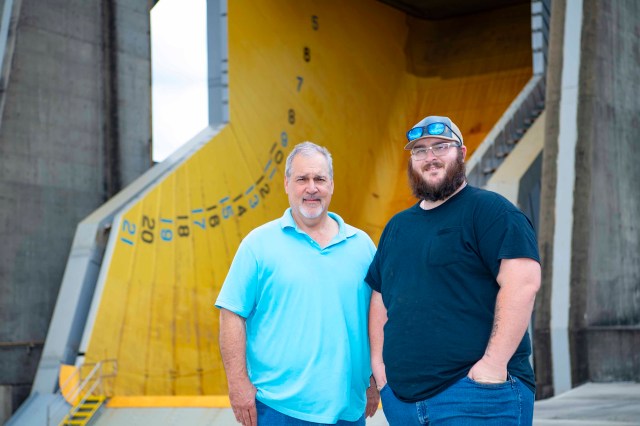
NASA Stennis Helps Family Build a Generational Legacy
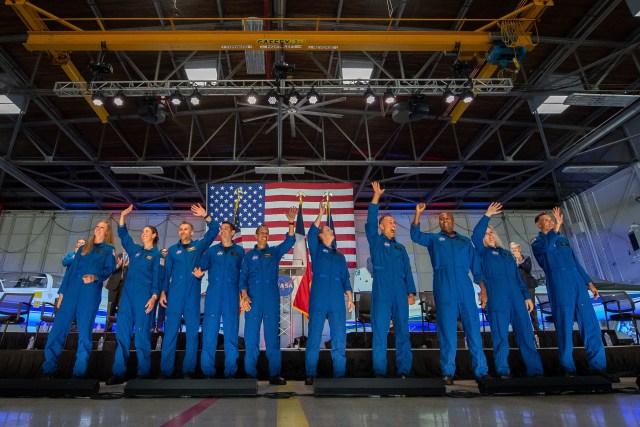
Diez maneras en que los estudiantes pueden prepararse para ser astronautas
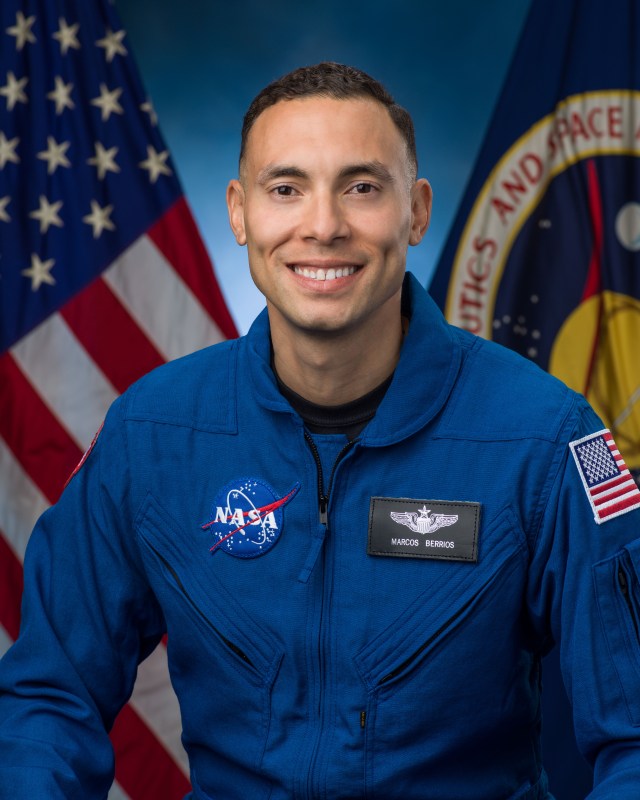
Astronauta de la NASA Marcos Berríos
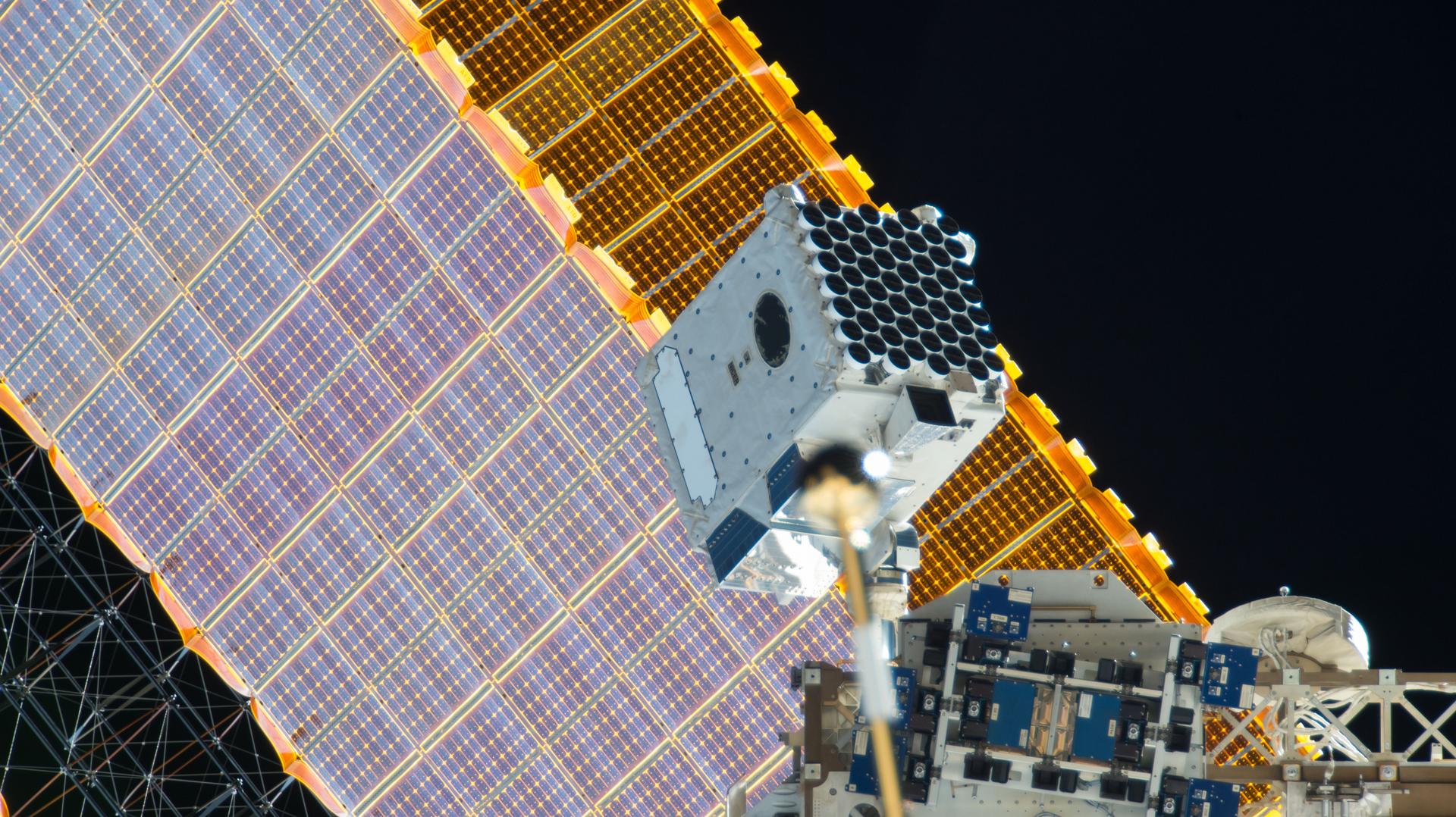
Resultados científicos revolucionarios en la estación espacial de 2023
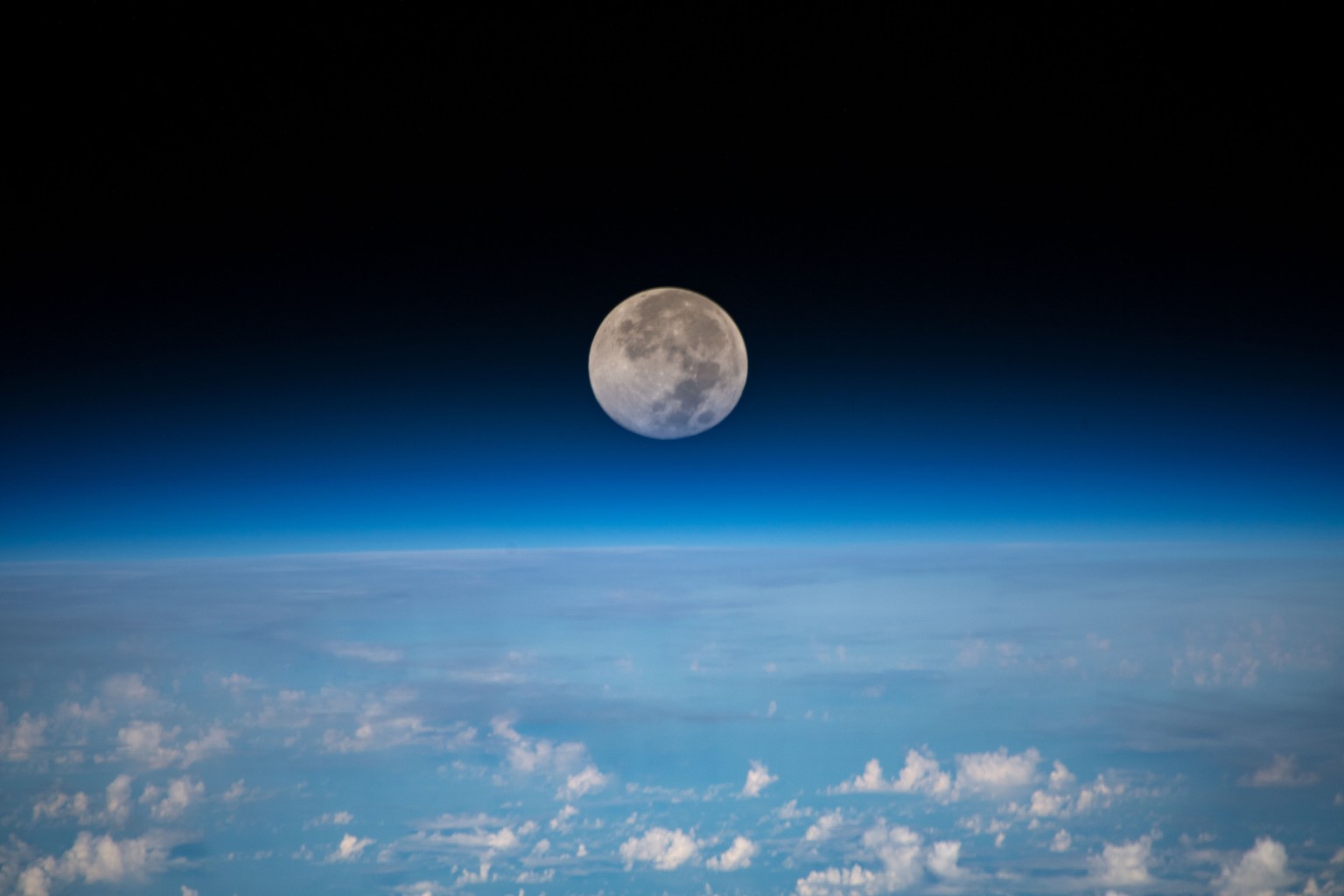
Melissa L. Gaskill
Tracking tiny stowaways, getting there and back, refining radiation detection, robot helpers.
Space, the saying goes, is hard. And the farther humans go, the harder it can get.
Some of the challenges on missions to explore the Moon and Mars include preventing microbial contamination of these destinations, navigating there safely, protecting crew members and hardware from radiation, and maintaining and repairing equipment.
Research on the International Space Station is helping NASA scientists develop tools and processes to ensure success on these important missions. Here are highlights from some of the investigations making space a little easier.
Bacteria and fungi live in and on all humans and all around us on Earth. Most of these microorganisms are beneficial or harmless but introducing them to other celestial bodies could adversely affect our ability to study ecosystems on those other worlds.
Crew members will conduct a spacewalk to collect samples near space station life support system vents for ISS External Microorganisms , an investigation to assess whether the orbiting laboratory releases microorganisms into space. Results could provide insight into the potential for organisms to survive and reproduce in space and help researchers determine which microbes would most likely contaminate other planetary bodies visited by crewed missions.
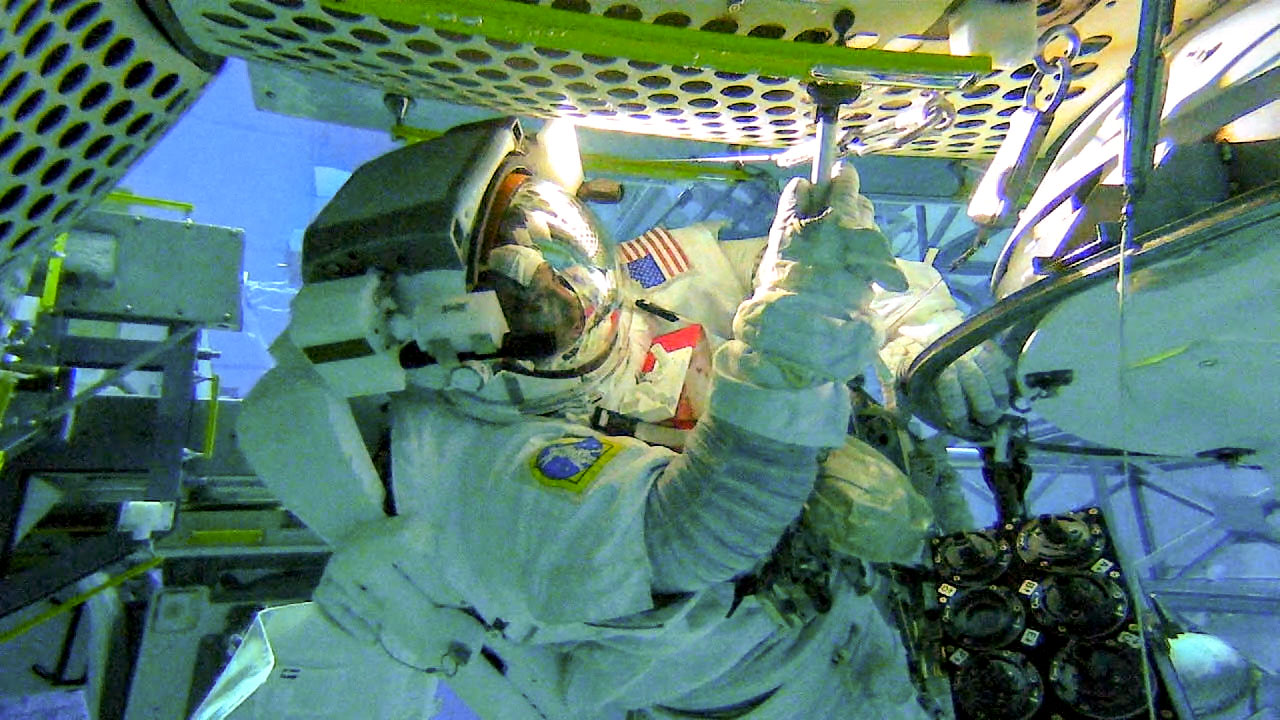
A miniature, hand-held digital microscope designed to make in-flight medical diagnoses, the Moon Microscope , also can test water, food, and surfaces for contamination. The device images samples at high resolution and processes data on web-enabled devices such as phones or tablets. Multiple users can access the microscope simultaneously, and some applications run autonomously.
Spacecraft must have sophisticated high-tech systems for navigating. Sextant Navigation tests the function of sextants in microgravity as an emergency backup navigation technique for Artemis and other future exploration missions. These mechanical devices have guided navigators for centuries, and Gemini and Apollo missions demonstrated they were useful for astronauts.

Missions beyond low Earth orbit increase exposure to radiation, which can pose a hazard to human health and interfere with equipment operation. As NASA prepares for future missions, providing adequate protection is vital.
The Hybrid Electronic Radiation Assessor, or HERA, was built to serve as a primary radiation detection system for the Orion spacecraft, which will carry crews into orbit around the Moon. The International Space Station Hybrid Electronic Radiation Assessor investigation modified the system to operate on the space station to provide researchers input for use on future exploration missions.
Artemis HERA on Space Station further modified the radiation detection system so researchers could continue to evaluate the hardware in the space radiation environment prior to Artemis II.
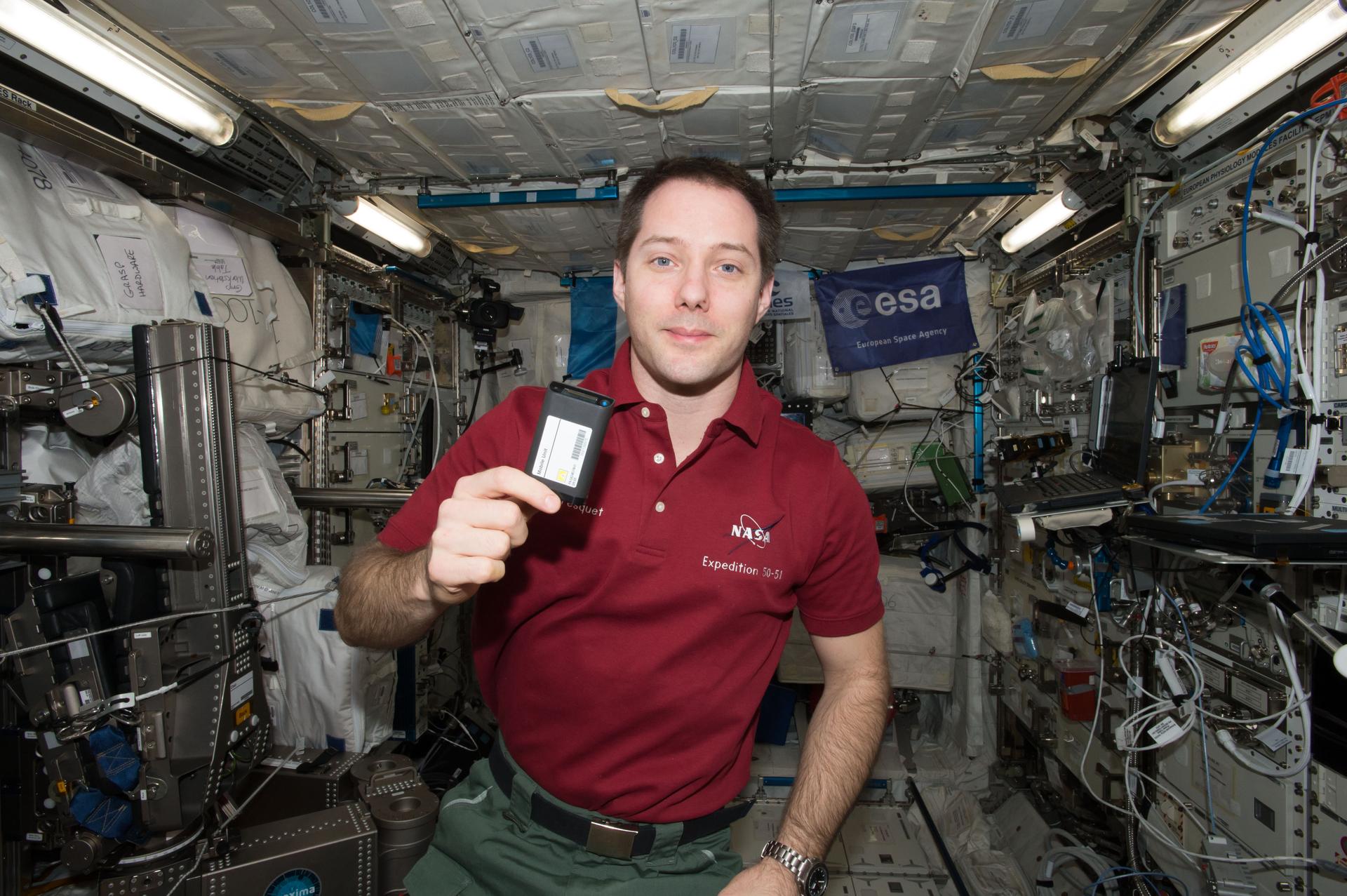
Active-Dosimeters , an investigation led by ESA (European Space Agency), tested a wearable system to measure radiation exposure to crew members on the space station and how it changes with the station’s orbit and altitude. Data from the wearable dosimeter improved radiation risk assessments and could lead to better protection for astronauts, including the ability to quickly respond to changes in exposure throughout future exploration missions.
On future exploration missions, robotic technology can help crew members with basic tasks, monitor and maintain equipment, and conduct operations such as sample collection, reducing the need to expose astronauts to harsh environments. Integrated System for Autonomous and Adaptive Caretaking demonstrates using autonomous robots to transfer and unpack cargo and to track and respond to maintenance issues such as leaks and fires, which could protect valuable equipment and reduce costly repairs on future missions. The investigation uses the space station’s Astrobee and Robonaut robots.
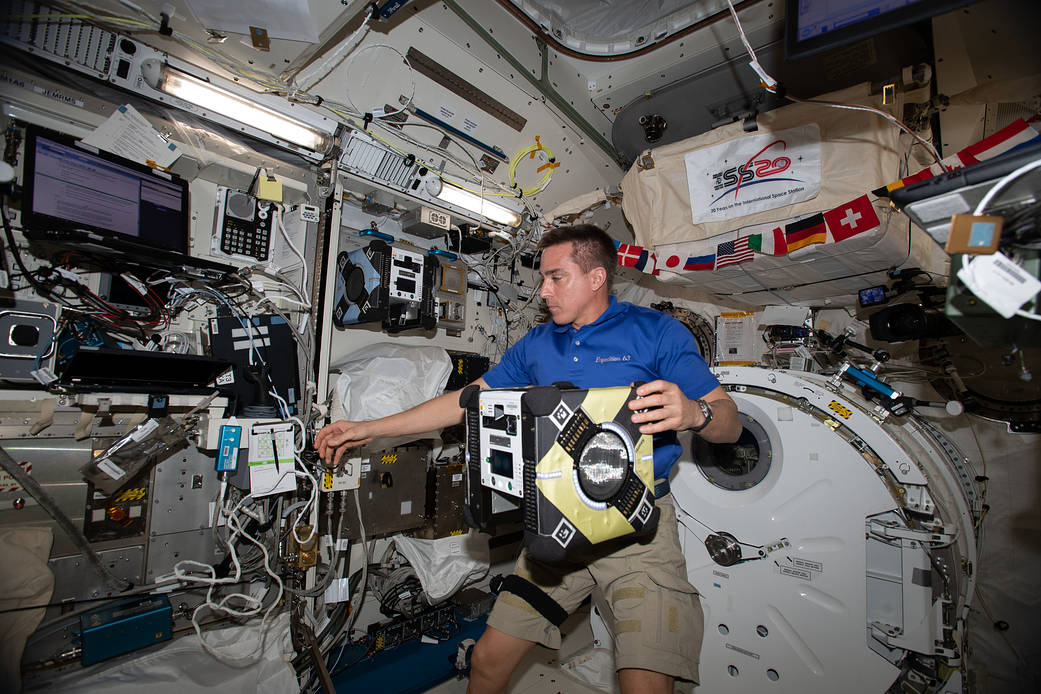
Multi-Resolution Scanning uses the station’s Astrobees to test sensors and robotics to support automated 3D sensing, mapping, and situational awareness functions. On future Gateway and lunar surface missions, such systems could automatically detect defects and conduct remote maintenance and autonomous operation of vehicles such as rovers.
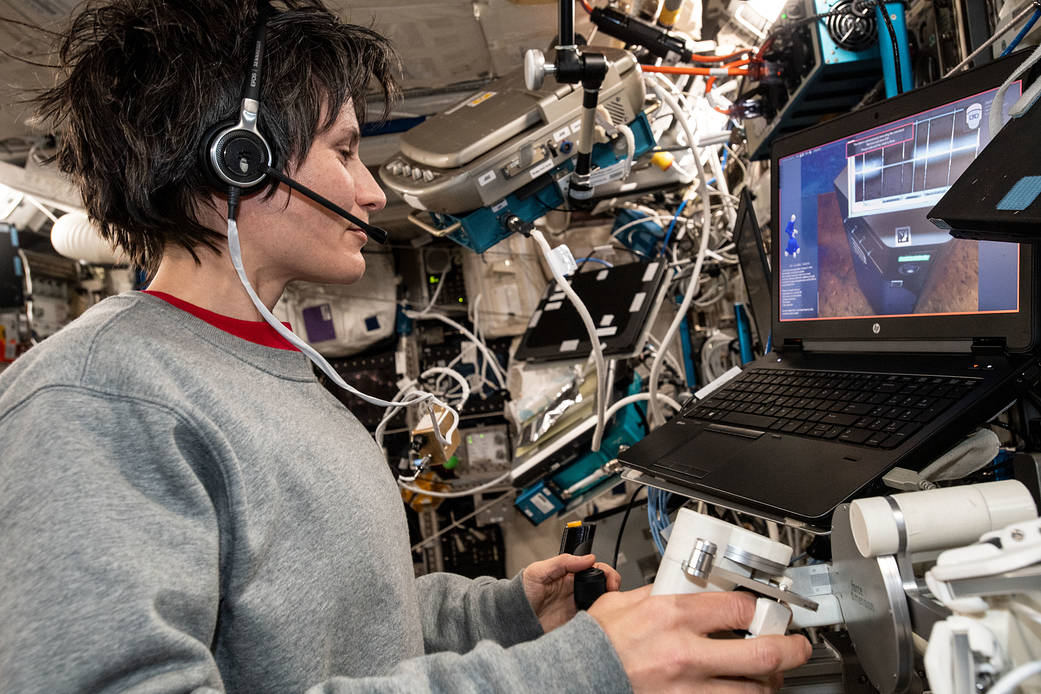
Surface Avatar evaluates crew operation of multiple autonomous robots in space. The investigation also assesses crew member responsiveness to feedback on the consoles used to operate robots remotely, which supports design of effective setups for operating robots on the ground from a spacecraft orbiting above. Results contribute to the development of other uses of robotic assistance such as returning samples from Mars and asteroids.
Melissa Gaskill International Space Station Research Communications Team NASA’s Johnson Space Center
Search this database of scientific experiments to learn more about those mentioned above.
Discover More Topics
Space Station Research and Technology
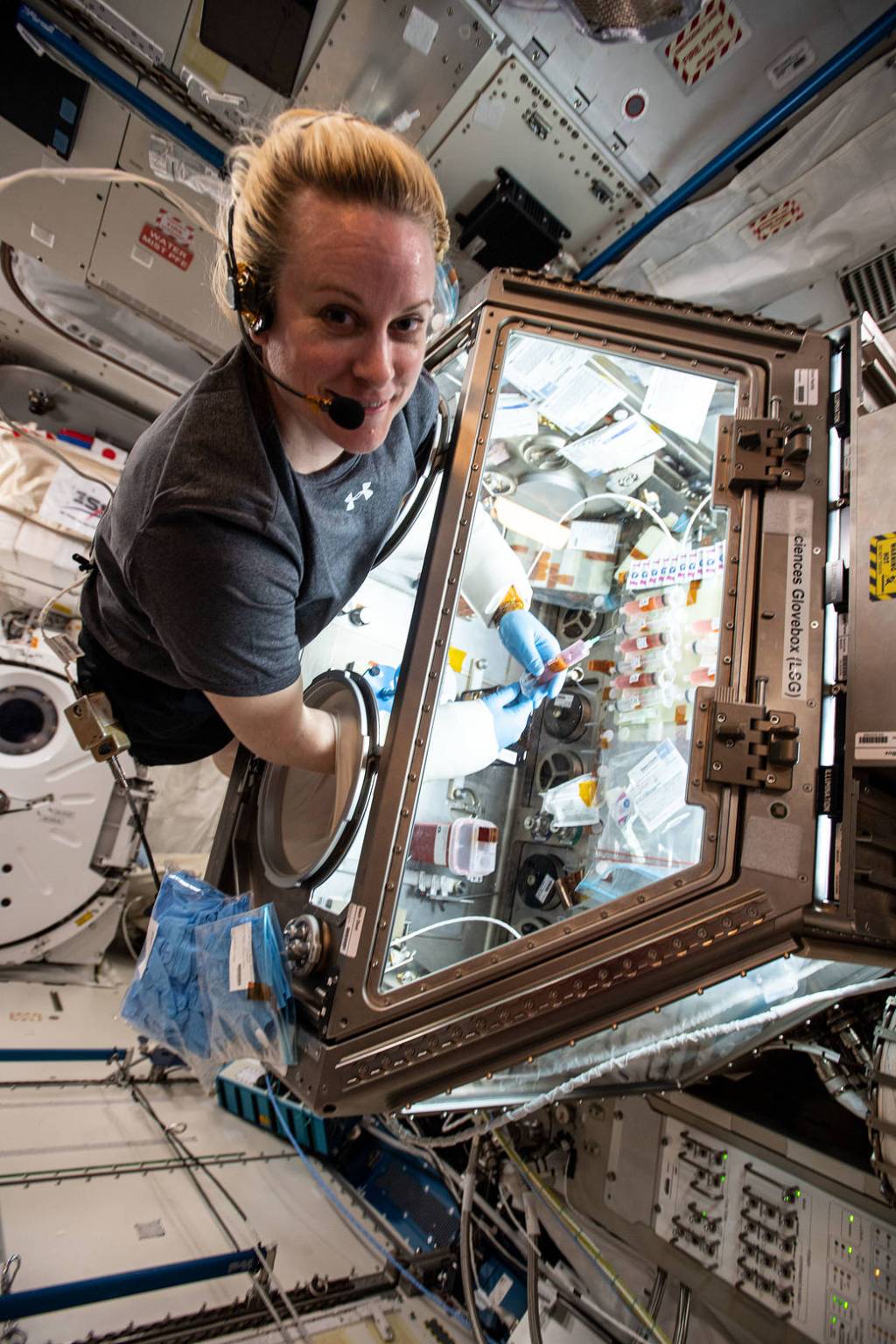
Station Science 101: Biology and Biotechnology
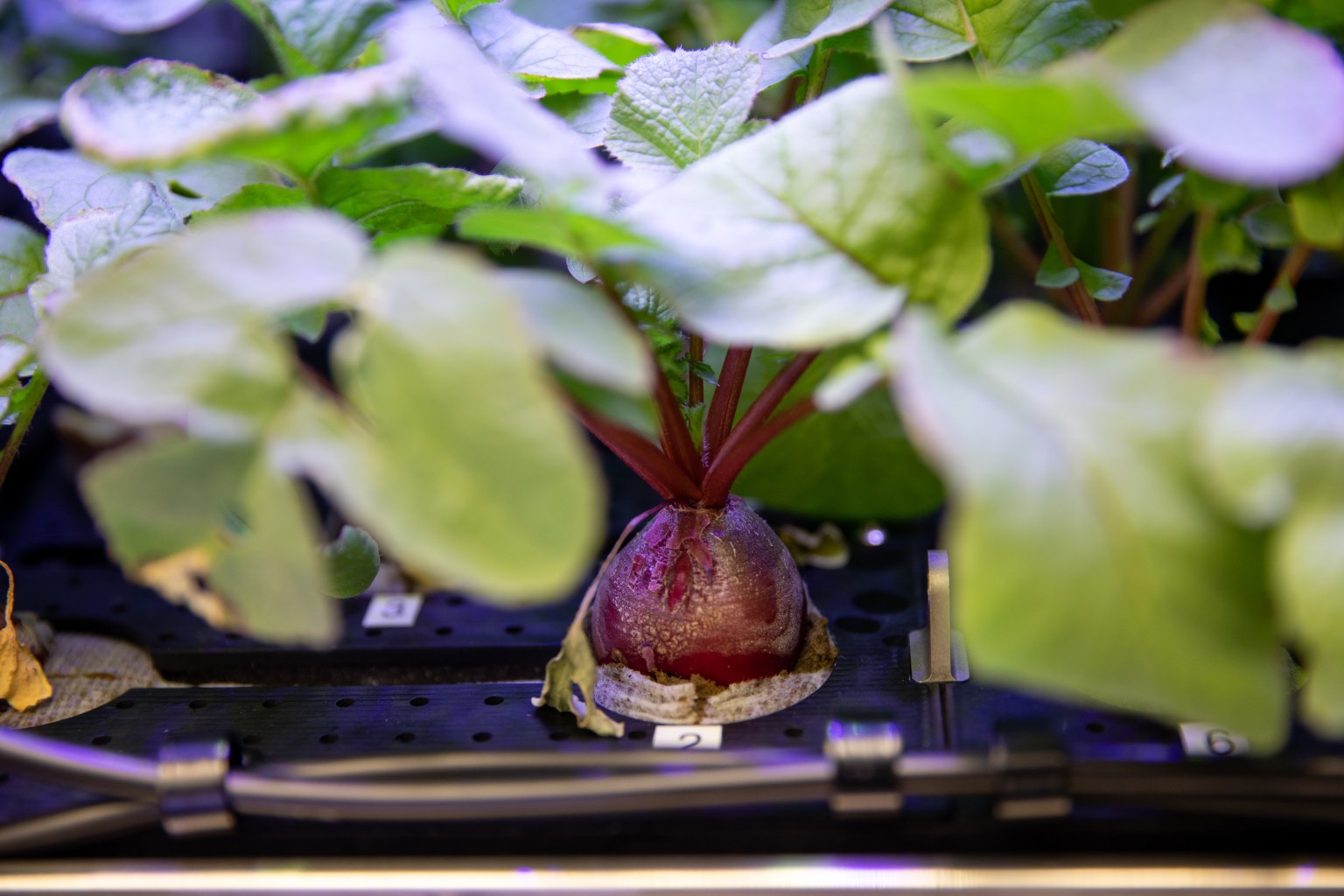
Related Terms
- ISS Research
- Cell and Molecular Biology
- Human Research Program
- International Space Station (ISS)
- Johnson Space Center
- Microbiology
- Science & Research

IMAGES
VIDEO
COMMENTS
1. When applying for PhD programs outside your home university, the university you apply to usually requires a research statement from you. However, this isn't the case when you reach out for a Bachelor's or Master's thesis topic to a professor you know from your home university (in mathematics). You would just approach a supervisor and express ...
The research plan is a document in which the PhD student develops a plan for his/her thesis. It should include an introduction, a brief state of the art to put in context the plan, the motivation and objectives of the work, the work done so far, a plan, detailing tasks and time planning, and a list of conferences and indexed journals were the work can be presented or submitted to.
Since you're applying for a fellowship, it's competitive. So you have to make a few points in your proposal: [ WHY] that the problem you're studying is interesting and worthwhile. [ HOW] that your line of attack (briefly sketched) is plausible. [ WHO] and that you have the right skills to execute this plan.
Mathematics in a Research Proposal Emily Clader Good proposal writing is, in many ways, just good math-ematical writing, which is just good writing, period. Nei-ther of these equivalences is entirely true, however, and examining their nuances is a helpful way to probe the question of what exactly makes a strong research proposal in mathematics.
The research plan template must be filled out and signed by all Applied Mathematics Ph.D. students, their advisor and mentor, and emailed to the Graduate Chair and Graduate Program Assistant by no later than the end of the third year of study. Complete details can be found in the graduate supplement. Research Plan Template. Example Research Plan.
In applied mathematics, we look for important connections with other disciplines that may inspire interesting and useful mathematics, and where innovative mathematical reasoning may lead to new insights and applications. Combinatorics. Computational Biology. Physical Applied Mathematics. Computational Science & Numerical Analysis.
This volume provides accessible and self-contained research problems designed for undergraduate student projects, and simultaneously promotes the development of sustainable undergraduate research programs. The chapters in this work span a variety of topical areas of pure and applied mathematics and mathematics education.
251+ Math Research Topics: Beginners To Advanced. Prime Number Distribution in Arithmetic Progressions. Diophantine Equations and their Solutions. Applications of Modular Arithmetic in Cryptography. The Riemann Hypothesis and its Implications. Graph Theory: Exploring Connectivity and Coloring Problems.
Even if it is standard in other fields, it's possible that most mathematics proposals would not include one. @AnonymousMathematician From my experience this past application season, required research proposals were standard in graduate applications in Europe (for math). I never saw one that required a methodology section, though.
Math 598 - Non-Thesis Research or Math 698 - Non-Dissertation Research; Fourth/Fifth/Sixth Years. Electives and MATH 699 - Dissertation Research. Preferred electives: MATH 684 Complex Analysis II; MATH 686 Functional Analysis. MATH 677 Topics in Algebra may be taken more than once. ... Sample Study Plan for Mathematics Education.
Honor's Research Proposal for Sciences and Mathematics (HO 401 Research Proposal, etc.) Structure and Format Overview. The following is a general overview of how most students in the Department of Sciences and Mathematics should structure their HO 401 Honors Project Proposals.
Microsoft Word - ED504828.doc. INCREASING STUDENT LEARNING IN MATHEMATICS WITH THE USE OF. COLLABORATIVE TEACHING STRATEGIES. Jenna Di Fatta, B.A. Sarah Garcia, B. S. Stephanie Gorman, B. S. An Action Research Proposal Submitted to the Graduate Faculty of the School of.
Applied Mathematics Research Advisor and Dissertation Plan. Instructions: Fill out this form with your doctoral dissertation advisor and return to the Graduate Program Chair and Graduate Program Coordinator no later than the end of Spring Semester of your 3rd year. Your faculty mentor must be an APPM faculty member with a graduate faculty ...
E-mail: [email protected] Overview: I am dedicated to research in applied mathematics. I received my Ph.D. in 2013 under guidance of Sorin Mitran at UNC. While at UNC I also worked with Greg Forest and Laura Miller. After my Ph.D. I took a postdoctoral fellowship at Duke University as part of the mathematical-biology research group;
A PhD research proposal is a document that outlines your proposed research topic for your PhD in mathematics.It is an important part of your application, and it is your opportunity to demonstrate ...
Abstract. PhD in Applied Mathematics - Research Proposal Scope of the research project: development of a game theory-based mathematical model for an autonomous machine decision-making system, with ...
Applied Mathematics Research. In applied mathematics, we look for important connections with other disciplines that may inspire interesting and useful mathematics, and where innovative mathematical reasoning may lead to new insights and applications. Applied Mathematics Fields The mathematics of surface tension. Combinatorics; Computational Biology
Mathematical Analysis. In a rough division of mathematics, mathematical analysis deals with inequalities and limits. In some of its branches, such as asymptotic analysis, these aspects of the subject matter are readily apparent. in others, such as operator algebras, they are concealed in the topology of an algebra or its structure as an ...
This research will focus on the technologies that are used in the Mathematics academe, especially focusing on learning Mathematics via art, through the use of video game. As of the moment, traditional approaches in education have resulted in a mismatch between the lessons taught to students, and what the industry currently needs. (Nelman, 2002).
Proposing a Timeline: Create a realistic timeline that outlines your mathematics project's key milestones and stages. Budget and Resources: Estimate the necessary research resources, including materials, software, equipment, and any potential travel. Justify the budget allocation based on the requirements of your work.
Before the pandemic (2019), we asked: On what themes should research in mathematics education focus in the coming decade? The 229 responses from 44 countries led to eight themes plus considerations about mathematics education research itself. The themes can be summarized as teaching approaches, goals, relations to practices outside mathematics education, teacher professional development ...
Journal metrics Editorial board. Research in Mathematics is a broad open access journal publishing all aspects of mathematics including pure, applied, and interdisciplinary mathematics, and mathematical education and other fields. The journal primarily publishes research articles, but also welcomes review and survey articles, and case studies.
The Problem. Problem-solving in mathematics and reading comprehension go hand in hand. Solving math problems entails the students applying two skills at the same time: reading and computing. It is a double-edged sword. As a public school teacher of sixth-grade mathematics for five years, I have encountered many pupils who are poor in both ...
24 samples of this type. Regardless of how high you rate your writing abilities, it's always an appropriate idea to check out an expertly written Research Proposal example, especially when you're dealing with a sophisticated Mathematics topic. This is exactly the case when WowEssays.com database of sample Research Proposals on Mathematics will ...
Implementation has always been a paramount concern of mathematics education, but only recently has the conceptualizing and theorizing work on implementation as a phenomenon begun in our field. In this survey paper, we conduct a hermeneutic review of mathematics education research identified as related to the implementation problematics. The first cycle of the review is based on examples of ...
Research proposal Name: Sally-Ann Robertson Student No: 70H2867 Degree: PhD (full thesis) Field of research: Mathematics education Provisional title: The role of language in supporting children's numeracy development: Exploring Grade 4 teachers' use of classroom talk in mathematics lessons Supervisor: Professor Mellony Graven
Maths might seem an unlikely bedfellow for social-justice research. But applying the rigour of the field is turning out to be a promising approach for identifying, and sometimes even implementing ...
Journal Highlights Math Research by Professor and Student. The journal Physical Review Research in its April issue tagged a paper by Associate Professor of Mathematics Chris Chong and Evelyn Wallace '22 as an "editor's suggestion" and featured it on its homepage. The paper, "Modulation instability and wavenumber bandgap breathers in a time ...
"The program matched mentors and mentees based on affinity of research topics, and mentees were provided with a $500 stipend if they participated in at least 10 meetings," Bernal said. "This is important because the students had an incentive to participate at the meetings and actively exchange ideas with a research lab."
Space Station Research Advances NASA's Plans to Explore the Moon, Mars. Space, the saying goes, is hard. And the farther humans go, the harder it can get. Some of the challenges on missions to explore the Moon and Mars include preventing microbial contamination of these destinations, navigating there safely, protecting crew members and ...