- Geographical skills and enquiry
- Human geography
- Physical geography
- Careers Spotlights
- Skills Boosts
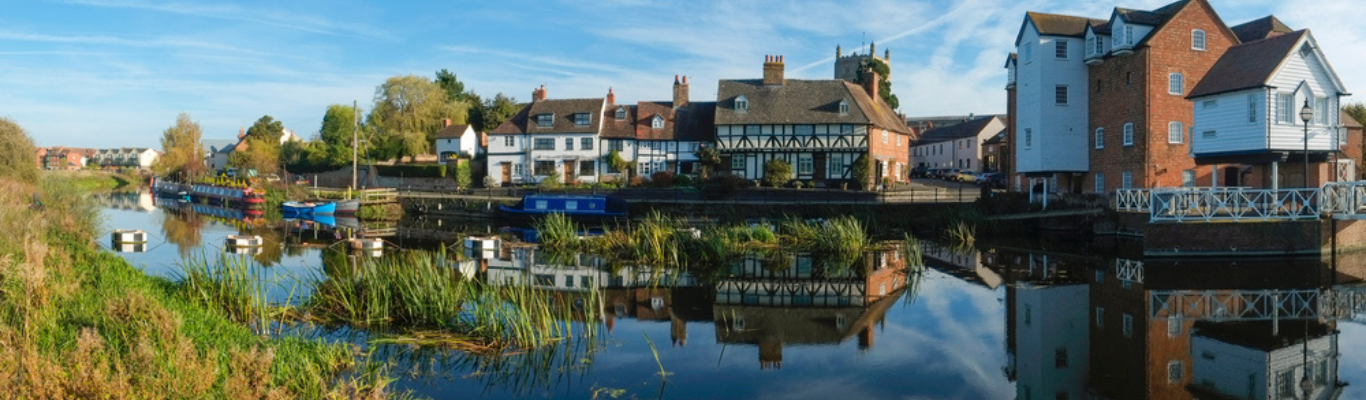

River management: a case study of the River Severn
In this unit you’ll compare hard and soft engineering methods for managing the flood hazard on the River Severn.
Then try the quiz to see how much you know about river management and measures put in place to help manage the risk of floods.
BACK TO GCSE MENU
Open PowerPoint
Take the quiz
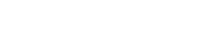
Get in Touch
The Geographical Association 160 Solly Street, Sheffield, S1 4BF
0114 296 0088 [email protected]
Stay Social
Cookies Privacy Terms Sitemap
© 2020 The Geographical Association. Charity no. 1135148. Company no. 07139068. Website by QWeb Ltd
AQA GCSE Geography

Case Study: River Tees
The River Tees, located in Northern England, flows from its source at Cross Fell in the Pennines travelling 130 km until it reaches its mouth at Teesside, where it meets the North Sea.
With a drainage basin spanning 1,800 km², the River Tees undergoes various processes that shape its course, influenced by both natural and human factors.
Upper Section: Steep-Sided Valleys and High Force Waterfall
In the upper course of the River Tees (near its source), the landscape is characterised by mountains, hills and steep slopes.
There are V-shaped valleys in this region that form as the river descends rapidly through the moorlands. The force of the water erodes the rock, resulting in the formation of steep-sided valleys with a distinctive V-shape. Large rocks within the riverbed contribute to abrasion, which vertically erodes the riverbed.
A notable feature in the upper course is the High Force waterfall. This waterfall is formed by the interaction of a resistant igneous rock called dolerite, locally known as Whin Sill, with a softer rock called limestone.

The hydraulic action erodes the softer rock more rapidly, causing undercutting and leaving the harder rock to overhang. Over time, the unsupported hard rock collapses, causing the waterfall to move upstream and gradually leaving behind a gorge.
Middle Section: Meanders and Flood Plains
As the River Tees enters its middle section, adding tributaries increases its water volume, making the river wider and deeper.
Attrition in the upper section reduces large rocks to smaller pebbles. This reduces friction in the river, allowing it to flow faster. The increased energy from faster flow leads to more hydraulic action.
This process contributes to the formation of meanders, characterised by lateral erosion and the development of a floodplain. These features can be observed around Barnard Castle along the river’s course.
Lower Section: Enlarged Meanders and Levees
In the lower course of the River Tees, the meanders continue to grow, and the floodplain expands further.

This transformation is evident in the areas of Darlington and Yarm. In some locations, meanders may evolve into oxbow lakes.
Another feature present in the lower section is the formation of levees. Levees form when the river overflows during floods and deposits sediment along its banks. Over time, this repeated deposition builds up the levees, which help contain the river within its channel during future flood events.
Estuary: River Tees’ Meeting with the North Sea
Finally, the River Tees reaches its estuary, the point where it flows into the North Sea.
The estuary serves as a transition zone between the freshwater river and the sea’s saltwater, displaying unique ecological characteristics.
You’ve used 0 of your 10 free revision notes for the month
Sign up to get unlimited access to revision notes, quizzes, audio lessons and more.
Last updated: April 27th, 2024
Please read these terms and conditions carefully before using our services.
Definitions
For these Terms and Conditions:
- Affiliate means an entity that controls, is controlled by or is under common control with a party, where “control” means ownership of 50% or more of the shares, equity interest or other securities entitled to vote for the election of directors or other managing authority.
- Account means a unique account created for you to access our services or some of our services.
- Country refers to the United Kingdom
- Company refers to Shalom Education Ltd, 86 London Road, (Kingsland Church), Colchester, Essex, CO3 9DW, and may be referred to as ‘we’, ‘us’, ‘our’, or ‘Shalom Education’ in this agreement.
- Device means any device that can access the Service, such as a computer, a mobile phone or a digital tablet.
- Feedback means feedback, innovations or suggestions sent by You regarding the attributes, performance or features of our service.
- Free Trial refers to a limited period of time that may be free when purchasing a subscription.
- Orders mean a request by you to purchase services or resources from us.
- Promotions refer to contests, sweepstakes or other promotions offered by us through our website or official social media platforms.
- Services refer to our website, resources and tutoring service.
- Subscriptions refer to the services or access to the service offered on a subscription basis by the company to you.
- Terms and Conditions (also referred to as “Terms”) mean these Terms and Conditions that form the entire agreement between you and Shalom Education Ltd regarding the use of the services we offer.
- Third-party Social Media Service means any services or content (including data, information, products or services) provided by a third party that may be displayed, included or made available on the website.
- Website refers to Shalom-education.com, accessible from https://www.shalom-education.com
- You means the individual accessing or using our services, or the company, or other legal entity on behalf of which such individual is accessing or using our services.
- Tutor refers to an individual that teaches a single pupil or a small group of students which have registered with Shalom Education Ltd.
- Tutee refers to a student or a pupil that has registered for tutoring with Shalom Education Ltd, which is administered through our tutoring platform.
Acknowledgement
Thank you for choosing Shalom Education Tuition for your educational needs. These terms and conditions outline the rules and regulations for the use of our services, and the agreement that will govern your relationship with us.
By accessing or using our services, you accept and agree to be bound by these terms and conditions, and our privacy policy , which describes our policies and procedures for the collection, use, and disclosure of your personal information when you use our website.
It is important that you read both documents carefully before using our services, as they outline your rights and obligations as a user of our services. If you do not agree to these terms and conditions or our privacy policy, please do not use our services. We hope you have a positive and educational experience with Shalom Education Tuition.
Signing up for Tutoring or Membership Accounts
By signing up for tutoring or membership accounts through the website, you confirm that you have the legal ability to enter into a binding contract.
Your information
When you place an order, we may ask you to provide certain information, such as your name, email, phone number, credit card details and billing address.
You confirm that you have the right to use the payment method you choose, and that the information you provide is accurate and complete. By submitting your information, you give us permission to share it with payment processing third parties to complete your order.
Order cancellation
We reserve the right to cancel your order at any time for various reasons, including but not limited to:
- Unavailability of services (e.g. no tutors available)
- Errors in the description or prices of services
- Errors in your order
- Suspected fraud or illegal activity
Cancelling your order
Any services that you purchase can only be returned in accordance with these terms and conditions. Our Returns Policy forms a part of these Terms and Conditions.
In general, you have the right to cancel your order and receive a full refund within 14 days of placing it. However, you cannot cancel an order for services that are made to your specifications or are clearly personalised, or for services that you have already received in part.
Money-Back Guarantee: If you are not satisfied with the quality of your tutoring session, you may be eligible for a full or partial refund or credit. To request a refund or credit, please contact us within 24 hours after the end of the session and provide a detailed explanation of your dissatisfaction. We will review your request and, if approved, will issue a refund or credit to your account.
- Please note that refunds or credits may not be available for all types of tutoring services and may be subject to fees or other charges. For more information, please contact us.
Errors and inaccuracies
We strive to provide accurate and up-to-date information about the service we offer, but we cannot guarantee that everything will be completely accurate and up-to-date at all times. Prices, product images, descriptions, availability, and services may be inaccurate, incomplete, or out of date.
We reserve the right to change or update any information, and to correct errors, inaccuracies, or omissions at any time without prior notice.
Prices policy
We reserve the right to change our prices at any time before accepting your order.
All tutoring services and membership accounts purchased on our website must be paid for in full at the time of purchase, for the required time of use. We accept a variety of payment methods, including credit cards, debit cards, and online payment services like PayPal.
Your payment card may be subject to validation checks and authorisation by your card issuer. If we do not receive the necessary authorisation, we cannot be held responsible for any delays or failure to deliver your order.
Subscriptions
Subscription period.
Our tutoring services are available with a pay-as-you-go option or a subscription option that is billed on a monthly or annual basis. The tutoring account subscription will end at the end of the period. You can choose the subscription option that best suits your needs and cancel at any time.
Our membership accounts are billed monthly or annually and do not automatically renew after the period. You can choose to renew your membership account at the end of the period if you wish to continue your membership.
Subscription cancellations (tutoring services)
You can cancel your tutoring subscription renewal by contacting us at [email protected].
You can cancel your learning resources subscription renewal through your account settings or by contacting us at [email protected].
Please note that you will not receive a refund for fees you have already paid for your current subscription period, and you will be able to access the service until the end of your current subscription period.
We need accurate and complete billing information from you, including your full name, address, postal code, telephone number, and a valid payment method. If automatic billing fails, you will not receive tutoring services until a payment is made. If payment is not made within a reasonable time period, your account may be terminated.
Fee changes
We reserve the right to modify the subscription fees at any time. Any change in fees will take effect at the end of your current subscription period.
We will give you reasonable notice of any fee changes so you have the opportunity to cancel your subscription before the changes take effect. If you continue to use the service after the fee change, you agree to pay the modified amount.
In general, paid subscription fees are non-refundable. However, we may consider certain refund requests on a case-by-case basis and grant them at our discretion.
We may offer free trials of our subscriptions at our discretion. You may be asked to provide billing information to sign up for a free trial. If you do provide billing information, you will not be charged until the free trial period ends.
On the last day of the free trial, unless you have cancelled your subscription, you will be automatically charged the applicable subscription fees for the plan you have chosen. We reserve the right to modify or cancel free trial offers at any time without notice.
From time to time, we may offer promotions through the Service, such as discounts, special offers, or contests. These promotions may be governed by separate rules and regulations.
If you choose to participate in a promotion, please review the applicable rules and our privacy policy carefully. In the event of a conflict between the promotion rules and these terms and conditions, the promotion rules will take precedence.
Please note that any promotion may be modified or discontinued at any time, and we reserve the right to disqualify any participant who violates the rules or engages in fraudulent or dishonest behaviour. By participating in a promotion, you agree to be bound by the applicable rules and our decisions, which are final and binding in all matters related to the promotion.
User Accounts
In order to access certain features of our services, you may be required to create an account. When you create an account, you agree to provide accurate, complete, and current information about yourself as prompted by the account registration process. If you provide any false, inaccurate, outdated, or incomplete information, or if we have reasonable grounds to suspect that you have done so, we reserve the right to suspend or terminate your account.
You are solely responsible for maintaining the confidentiality of your account and password, and you agree to accept responsibility for all activities that occur under your account. If you believe that your account has been compromised or that there has been any unauthorised access to it, you must notify us immediately.
You may not use a username that is already in use by another user, that belongs to another person or entity without appropriate authorisation, or that is offensive, vulgar, or obscene. We reserve the right to remove or reclaim any username that we determine, in our sole discretion, to be inappropriate, infringing, or otherwise in violation of these terms and conditions.
Tutee Agreements
As a tutee using our service, you agree to the following terms and conditions:
- If you are under 18 years of age, you must have consent from a parent or guardian to register and that parent/guardian must enter into an agreement with Shalom Education to provide tuition services.
- You must contact a tutor through the Shalom Education tutoring platform. Any other means of communication is prohibited.
- You agree not to publish any abusive comments about a tutor or another tutee on the Shalom Education website or any other place. This includes defamatory or derogatory comments.
- You must not request a tutor to complete your coursework, essays, or other assignments given to you in your various schools but can request for support towards that.
- You agree not to make any agreement or arrangement with a tutor which is intended to circumvent the relationship between Shalom Education and the Tutor.
Expectations
- Lessons will be held at the frequency and duration agreed upon with us.
- Shalom Education will provide any necessary materials or resources for the lessons.
- You are expected to be punctual for lessons and to provide adequate notice for cancellations or rescheduling.
- You are expected to provide any necessary materials or resources for the lessons (e.g. pens, pencils or a protractor).
Responsibilities
- If you cancel a lesson less than 12 hours before the lesson is due to commence, you may be charged a cancellation fee of 50% of the lesson fee. If you cancel a lesson less than 2 hours before the scheduled start time of a lesson, you will be charged the full lesson fee.
Dispute Resolution
- If you have any concerns or issues with your tutoring experience, please contact us at [email protected] as soon as possible. We will work with you and your tutor to try to resolve any disputes or issues in a fair and reasonable manner.
Termination
- You may terminate your lessons with a tutor at any time by providing reasonable notice, as specified in your contract with us.
By using our service, you acknowledge that you have read and understand this agreement and agree to be bound by its terms. If you have any questions or concerns, please do not hesitate to contact us.
- Revision Notes
- Unlimited Quizzes
- Audio Content
- Progress Tracking
- No Advertisements

Hi there! Want to study 2x more effectively?
Sign up now for exclusive access to interactive quizzes, audio lessons and more educational tools..
Sign me up!
Last updated: December 27, 2022
- Orders mean a request by you to purchase services from us.
- Promotions refer to contests, sweepstakes or other promotions offered by us through the website.
- Tutor refers to an individual who teaches a single pupil or a small group of students who have registered with Shalom Education Ltd.
- Tutee refers to a student or a pupil who has registered for tutoring with Shalom Education Ltd, which is administered through our tutoring platform.
When you place an order, we may ask you to provide certain information, such as your name, email, phone number, credit card details, and billing address.
Subscription cancellations
You can cancel your subscription renewal through your account settings or by contacting us. Please note that you will not receive a refund for fees you have already paid for your current subscription period, and you will be able to access the service until the end of your current subscription period.
We need accurate and complete billing information from you, including your full name, address, postal code, telephone number, and valid payment method. If automatic billing fails, you will not receive tutoring services until a payment is made. If payment is not made within a reasonable time period, your account may be terminated.
Tutor Agreements
As a tutor working with Shalom Education, you agree to the following terms and conditions:
Tutor relationships
- You are at least eighteen years of age and have the necessary qualifications and/or experience to provide tuition in the subjects specified on your CV.
- You will not make any agreements with a tutee, any member of the tutee’s family, or the tutee’s guardian that are intended to circumvent the relationship between our service and the tutee and to benefit you at our expense. This includes the exchange of private information (e.g. phone numbers, emails or social media accounts etc).
- You will not, during the period of any tutoring session with a tutee and for a period of six months from the conclusion of the last tutoring session, give any tuition services to that tutee.
- You will use our platform as your only means of communication with tutees, and any other methods are strictly prohibited.
- You will not be abusive towards a tutee or their nominee on our website or any other place.
- You are expected to maintain a professional and respectful relationship with tutees at all times.
- You will not engage in any inappropriate or illegal behaviour while working with tutees, including but not limited to harassment or discrimination.
- You are expected to follow all applicable laws and regulations while providing tutoring services.
Tutor responsibilities
- You will not complete any coursework, essays, or other assignments on behalf of the tutee. However, you can offer support.
- You will be punctual and communicate with us if you are running late or need to reschedule a lesson.
- You are expected to provide high-quality tutoring services to tutees, including preparing appropriate lesson plans.
- You will be responsible for ensuring that you have the necessary resources and equipment to provide effective tutoring services, such as a reliable internet connection and computer if tutoring online.
- You will be expected to keep confidential any personal or sensitive information that you may learn about a tutee while working with them.
Tutor compensation
- You will not request or accept any payments from a tutee, or their nominee.
- The company reserves the right to withhold payment to tutors if it believes lessons are being booked outside our platform.
- The company will pay you a fee for your tutoring services according to the rates agreed between you and the company.
- The company will be responsible for collecting payment from the tutee.
- If you are self-employed, we expect you to provide us with your UTR number and you are expected to pay your own tax to HMRC
Tutor termination
- As a tutor working with the company, you may terminate your relationship with a tutee at any time by providing reasonable notice.
- The company may also terminate your relationship with a tutee at any time, for any reason, such as if the tutee is no longer in need of tutoring services or if the tutee expresses dissatisfaction with your services.
- The company may terminate your use of its service if you breach any of the terms and conditions outlined in this agreement.
- The company may also terminate your use of its service if it determines that you are no longer fit to provide tutoring services or if it receives multiple complaints about your performance.
Copyright Policy
We respect the intellectual property rights of others and expect our users to do the same. It is our policy to respond to any claim that content posted on our service infringes the copyright or other intellectual property rights of any person.
If you are a copyright owner or authorised on behalf of one and you believe that your copyrighted work has been copied in a way that constitutes copyright infringement, please provide our copyright agent with the following information:
- An electronic or physical signature of the person authorised to act on behalf of the owner of the copyright’s interests.
- A description of the copyrighted work that you claim has been infringed, including the URL (web page address) of the location where the copyrighted work exists or a copy of the copyrighted work.
- Identification of the URL or other specific location on our service where the material that you claim is infringing is located.
- Your address, telephone number, and email address.
- A statement by you that you have a good faith belief that the disputed use is not authorized by the copyright owner, its agent, or the law.
- A statement by you, made under penalty of perjury, that the information in your notice is accurate and that you are the copyright owner or are authorised to act on the copyright owner’s behalf.
You may be held accountable for damages (including costs and attorneys’ fees) for misrepresenting that any content is infringing your copyright.
Upon receipt of a notification, we will take whatever action, in our sole discretion, we deem appropriate, including removal of the challenged content from our service. If you believe that your content has been removed in error, please contact us at [email protected] .
Intellectual Property
The original content on our services (excluding content provided by you or other users), features, and functionality are and will remain the exclusive property of Shalom Education Ltd and its licensors. This includes, but is not limited to, text, graphics, images, logos, software, and other materials on our website and any proprietary technology used in the operation of our services.
Our service is protected by copyright, trademark, and other laws of both the United Kingdom and foreign countries. Our trademarks and trade dress may not be used in connection with any product or service without the prior written consent of Shalom Education Ltd. All other trademarks not owned by Shalom Education Ltd that appear on our service are the property of their respective owners.
By using our service, you acknowledge and agree that any intellectual property rights, including copyrights, trademarks, patents, and trade secrets, in the content and materials provided by Shalom Education Ltd or accessed through our service are the sole property of Shalom Education Ltd or the respective owners of such rights. You agree not to use, reproduce, modify, distribute, or create derivative works of such content and materials without the express written permission of Shalom Education Ltd or the respective owners of such rights.
You are responsible for protecting your own intellectual property rights and for obtaining any necessary licenses or permissions from the owners of any third-party intellectual property that you may use in connection with your use of our service. Shalom Education Ltd will not be liable for any claims or damages arising from your use of intellectual property that infringes the rights of others.
If you believe that your intellectual property rights have been violated on our service, please contact us at [email protected] .
Your Feedback to Us
By submitting any feedback or suggestions to the company, you agree to assign to the company all rights, titles, and interests in such feedback or suggestions. If for any reason such assignment is ineffective, you agree to grant the company a non-exclusive, perpetual, irrevocable, royalty-free, worldwide right and license to use, reproduce, disclose, sublicense, distribute, modify, and exploit such feedback or suggestions without restriction.
You acknowledge that the company may use your feedback or suggestions for any purpose, including to improve the company’s products or services, and that the company is under no obligation to compensate you for your feedback or suggestions.
Links to Other Websites
Our Service may contain links to third-party websites or services that are not owned or controlled by Shalom Education Ltd. These links are provided for your convenience only and do not imply endorsement by our business of the linked website or service. We have no control over and assume no responsibility for the content, privacy policies, or practices of any third-party websites or services.
By using our Service, you acknowledge and agree that the use of any third-party websites or services is at your own risk. Shalom Education Ltd does not endorse or guarantee the accuracy or reliability of any content or materials on third-party websites or services, and we are not responsible for any errors or omissions.
In no event will Shalom Education Ltd be liable for any damage or loss caused or alleged to be caused by or in connection with the use of or reliance on any content, goods, or services available on or through any third-party websites or services. This limitation of liability applies to all claims.
We recommend that you carefully read the terms and conditions and privacy policies of any third-party websites or services that you visit, as they may differ from the terms and policies of our own services. If you have any concerns or questions about a third-party website or service linked from our service, we encourage you to contact the site or service directly for more information.
Shalom Education Ltd reserves the right to terminate or suspend your account at any time, without prior notice or liability, for any reason whatsoever, including but not limited to breach of these terms and conditions, fraudulent or illegal activity, or any other conduct that we deem inappropriate or harmful to our business or other users.
Upon termination, your right to use our services will immediately cease, and any outstanding balances or fees owed to Shalom Education Ltd must be paid in full. If you wish to terminate your account, you may simply stop using our services and contact us to request the closure of your account.
Any personal information or user-generated content associated with your account will be retained in accordance with our privacy policy, unless otherwise required by law.
You acknowledge and agree that Shalom Education Ltd will not be liable to you or any third party for any termination of your access to our services. You further agree that any rights or obligations that survive the termination of your account, such as indemnification or confidentiality obligations, will remain in effect.
“AS IS” and “AS AVAILABLE” Disclaimer
The service is provided to you “As is” and “As available” and with all faults and defects without warranty of any kind. While we make every effort to ensure the accuracy and reliability of our services, we cannot guarantee that they will be error-free or uninterrupted.
To the maximum extent permitted by applicable law, our business and its affiliates and licensors and service providers disclaim all warranties, expressed or implied, including but not limited to warranties of merchantability, fitness for a particular purpose, title and non-infringement. We do not make any representations or warranties that our services will meet your requirements, achieve any intended results, be compatible with any other software or services, operate without interruption, or be error-free.
We do not guarantee the accuracy, completeness, reliability, or timeliness of the information, content, or materials provided through our services.
We do not guarantee that our services or any content or materials provided through our services will be free from viruses, malware, or other harmful components. It is your responsibility to protect your device and system from such threats, and we recommend that you use appropriate security measures and virus protection software.
Some jurisdictions do not allow the exclusion of certain types of warranties or limitations on the applicable statutory rights of a consumer, so some or all of the above exclusions and limitations may not apply to you. In such cases, the exclusions and limitations set forth in this section shall be applied to the greatest extent enforceable under applicable law.
By using our services, you acknowledge and agree that your use is at your own risk, and that you are solely responsible for any damage or loss that may result from your use of our services.
Governing Law and Jurisdiction
These terms and your use of the service shall be governed by and construed in accordance with the laws of the country, excluding its conflicts of law rules. Any disputes arising out of or in connection with these terms or the use of the service shall be resolved through the courts of the country and you hereby consent to the exclusive jurisdiction of such courts.
Disputes Resolution
If you have any concerns or disputes related to the service, you agree to try to resolve the issue informally by contacting us first.
You can try contacting the company through email ([email protected]) or by phone (01206657616) to see if we can come to an agreement or find a solution to your issue. This can be a quick and effective way to address any concerns or issues you may have, without the need for formal legal proceedings.
We will make every effort to address your concerns and reach a mutually satisfactory resolution. If we are unable to resolve the dispute informally, you may choose to bring the matter to alternative dispute resolution through a mediator or arbitrator. Any such alternative dispute resolution proceedings will be conducted in accordance with the laws of the country and will be confidential. You agree that any such dispute will be resolved on an individual basis and that class or collective actions are not permitted.
Severability and Waiver
Severability.
If any provision of these terms and conditions is found to be invalid or unenforceable, that provision will be enforced to the maximum extent possible, and the remaining provisions will remain in full force and effect.
The failure to exercise a right or to require the performance of an obligation under these terms and conditions shall not affect a party’s ability to exercise such right or require such performance at any time in the future. Similarly, the waiver of a breach of these terms and conditions shall not constitute a waiver of any subsequent breach.
Changes to These Terms and Conditions
We may update or change these terms and conditions at any time, at our sole discretion. If we make a material change to these terms, we will provide reasonable notice, such as by posting a notification on our website or through email, at least 30 days before the change takes effect. It is your responsibility to regularly check these terms and conditions for any updates or changes.
By continuing to access or use our Service after any updates or changes to these terms, you agree to be bound by the revised terms. If you do not agree to the updated or changed terms, in whole or in part, please stop using the website and our services.
If you have any questions about these terms and conditions, You can contact us:
- By email: [email protected]
- By visiting this page on our website: https://www.shalom-education.com/contact-us
- By phone number: 01206657616
Username or Email Address
Remember Me
Registration confirmation will be emailed to you.
Thank you for visiting nature.com. You are using a browser version with limited support for CSS. To obtain the best experience, we recommend you use a more up to date browser (or turn off compatibility mode in Internet Explorer). In the meantime, to ensure continued support, we are displaying the site without styles and JavaScript.
- View all journals
- My Account Login
- Explore content
- About the journal
- Publish with us
- Sign up for alerts
- Open access
- Published: 22 April 2024
Global patterns in river water storage dependent on residence time
- Elyssa L. Collins ORCID: orcid.org/0000-0002-8054-8468 1 , 2 , 3 ,
- Cédric H. David ORCID: orcid.org/0000-0002-0924-5907 1 ,
- Ryan Riggs ORCID: orcid.org/0000-0001-6834-9469 4 ,
- George H. Allen ORCID: orcid.org/0000-0001-8301-5301 5 ,
- Tamlin M. Pavelsky 3 ,
- Peirong Lin 6 ,
- Ming Pan 7 ,
- Dai Yamazaki ORCID: orcid.org/0000-0002-6478-1841 8 ,
- Ross K. Meentemeyer ORCID: orcid.org/0000-0002-1247-6212 2 , 9 &
- Georgina M. Sanchez ORCID: orcid.org/0000-0002-2365-6200 2
Nature Geoscience ( 2024 ) Cite this article
3234 Accesses
163 Altmetric
Metrics details
- Climate sciences
Accurate assessment of global river flows and stores is critical for informing water management practices, but current estimates of global river flows exhibit substantial spread and estimates of river stores remain sparse. Estimates of river flows and stores are hampered by uncertainties in land runoff, an unobserved quantity that provides water input to rivers. Here we leverage global river flow observations and an ensemble of land surface models to generate a globally gauge-corrected monthly river flow and storage dataset. We estimate a global river storage mean (± monthly variability) of 2,246 ± 505 km 3 and a global continental flow of 37,411 ± 7,816 km 3 yr −1 . Our global river water storage time series demonstrates that flow wave residence time is a fundamental driver that can double or halve river water stores and their variability. We also reconcile the wide range in previous estimates of monthly variability in global river flows. We identify previously underappreciated freshwater sources to the ocean from the Maritime Continent (Indonesia, Malaysia and Papua New Guinea) amounting to 1.6 times the Congo River and illustrate our capability of detecting severe anthropogenic water withdrawals.
Similar content being viewed by others
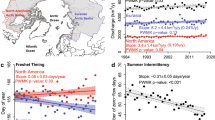
Recent changes to Arctic river discharge
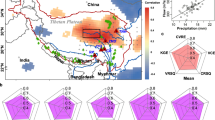
Reconstructed eight-century streamflow in the Tibetan Plateau reveals contrasting regional variability and strong nonstationarity
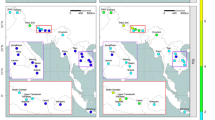
Future hydrology and hydrological extremes under climate change in Asian river basins
Rivers are considered the most renewable, most accessible and, hence, most sustainable source of freshwater. Accordingly, several studies have sought to quantify the water in our world’s rivers 1 , 2 , 3 , 4 , 5 , 6 , 7 , 8 , 9 , 10 , 11 , 12 , 13 , 14 , 15 , 16 , 17 , 18 , 19 , 20 . Yet, surprisingly little is known about the average and temporal variability of global river water storage, as well as the temporal variability of global river flows. Nearly all estimates of global river water storage 1 , 2 , 3 , 5 , 6 , 7 , 13 , 20 trace back to a report published as part of the UNESCO International Hydrological Decade from 1965 to 1974 (ref. 2 ), and only one study quantifies temporal variability 20 . Notably, to our knowledge, there are no previously published time series of global river storage. A recent study mentioned such computations, but it focused on their participation in total terrestrial water storage variability 20 . Meanwhile, estimates of average global river flows are ubiquitous 1 , 2 , 3 , 4 , 5 , 6 , 7 , 8 , 9 , 10 , 11 , 12 , 13 , 14 , 15 , 16 , 17 , 18 , 19 ; however, the temporal variability of flows has received much less attention and reported values have considerable spread 14 , 15 , 18 , 19 . Consequently, our understanding of global river water storage and of temporal variability of global river flows has so far been limited. A more complete characterization of global historical river discharge and water storage is therefore critical to advancing our understanding of the world’s waters.
In situ stream gauges around the world provide key information on the spatial and temporal distribution of river discharge. However, the spatial and temporal coverage of in situ measurements are severely limited. Gauges are sparsely distributed globally, with placement bias towards specific environmental conditions (for example, large rivers 21 ). Moreover, data sharing constraints across political boundaries, in combination with a worldwide decrease in stream gauge reporting, have further constrained the amount of river discharge observations available for scientific research 22 , 23 , 24 , 25 , 26 . Modelling approaches such as river network routing, which at global scales commonly uses gridded runoff from a land surface model (LSM) as input, can be used to seamlessly estimate river discharge and water storage around the world, including in ungauged basins 27 . Yet, the quality of river water estimates produced from these models is greatly influenced by the resolution of the underlying hydrography 28 and by uncertainties present in the input runoff data 29 . For global river discharge and water storage estimates to be most useful, the uncertainties in these simulations must be constrained by observations 28 , 29 . Substantial progress has been made to correct for uncertainties and biases from runoff inputs that influence river discharge outputs in regional, continental and global river network routing 28 , 30 , 31 , 32 , 33 . However, the majority of past correction approaches are either computationally expensive 31 —limiting the geographic extent over which they can be applied—or rely on modelled reference data that contain errors 28 , 30 , 32 . Given that surface and subsurface runoff are not directly measurable at the global scale, discharge observations from in situ stream gauge networks provide the closest proxy. Seeking to combine the strengths of models and in situ observations, one study leveraged runoff fields and observations at river outlets (that is, a ‘hybrid’ approach) to generate global discharge estimates 16 . However, surprisingly, a global hybrid methodology has yet to be developed that leverages gauges beyond river outlets to produce high-resolution estimates of river discharge that are spatially seamless and match average monthly observations where available. In this Article, we derive such a method and apply it to generate the first globally corrected monthly river flow and storage dataset, which we name Mean Discharge Runoff and Storage (MeanDRS). Using MeanDRS, we quantify total discharge to the ocean, reconciling the wide range in previous estimates of monthly variability in continental flow. Our study also identifies previously underappreciated freshwater sources to the ocean, and produces the first time series of global river water storage, demonstrating residence time as a fundamental driver of river water stores.
Global discharge aided by observations
We used a global database of monthly discharge observations at 998 locations along with monthly runoff outputs from an ensemble of LSMs for 1980–2009 to bias-correct simulated runoff and route it through a recent high-resolution river network containing ~3 million reaches 28 , 30 . Our novel bias correction approach, called long-term inverse routing (LTIR; Methods ), compares average discharge simulations and average observations to calculate temporally constant multiplicative correction factors for runoff in all river reaches located upstream of available discharge observations. These corrections are then applied at each time step to generate global 30-year (1980–2009) corrected monthly estimates of mean river discharge extrapolated from and consistent with in situ observations. We evaluated the geographic distribution of common discharge metrics (for example, normalized bias, Nash–Sutcliffe efficiency (NSE)) at the monthly time scale (Extended Data Figs. 1 – 3 ) and found that the bias-corrected estimates provided a better statistical match to observed discharge at gauges than uncorrected estimates; 99% of the 998 gauges showed no normalized bias (the difference between simulated and observed temporal average, relative to the observed temporal average), as expected from bias correction (see ‘Model validation’ section in Methods for an explanation of the remaining 1%). Our corrections also led to improvements in other metrics: 55% of gauges showed improvements in normalized standard deviation of error (NSTDERR), 75% in normalized root mean square error (NRMSE) and 75% in NSE (a measure of how well the simulated time series matches the observed). The improvements in simulation metrics are further visually confirmed by discharge time series (see Extended Data Fig. 4 for example hydrographs before and after correction). More details are provided in Methods and Supplementary Information , including independent validation results.
Evaluating the sign and magnitude of our corrections along with the spatial distribution of river discharge estimates globally enables us to understand and visualize the impact of our gauge-based corrections (Fig. 1 ). Global estimates of river discharge averaged across 30 years of monthly simulations ranged from 0 to 192,683 m 3 s −1 across all river reaches for uncorrected simulations (Extended Data Fig. 5 ). Using our LTIR approach ( Methods ), we developed multiplicative runoff correction factors for 29% of river reaches globally. The remaining 71% of river reaches were not located upstream of a gauge used in this analysis. Correction factors can be positive or negative. Positive factors between 0 and 1 lead to a decrease in discharge, and those greater than 1 lead to an increase in discharge. Negative correction factors are indicative of hydrological inconsistencies in the gauge network where upstream discharge is greater than downstream discharge, that is, a distinct fingerprint of anthropogenic water withdrawals. Note that the magnitude of hydrological inconsistencies, and therefore withdrawals, is a direct result of gauge observations and can therefore be trusted. Of the correction factors developed, 95% were positive (51% of which led to a decrease in discharge and the remaining 49% to an increase) and 5% were negative. After applying LTIR and then propagating corrections downstream via routing through the river network, 31% of river reaches were impacted globally, which is 918 times more river reaches than gauges (the 998 gauges used in this analysis cover 0.03% of global river reaches). Our average corrected discharge estimates ranged from −12,064 to 195,849 m 3 s −1 (Fig. 1a ), with negative discharges due to the aforementioned hydrological inconsistencies. These seemingly erroneous negative discharge values are not only justifiable from the mass conservation (that is, water balance) perspective, they also specifically highlight regions of the world characterized by intense water management 34 , 35 , 36 , 37 , including water withdrawals: the south-western United States, south-eastern Australia and countries in South America (for example, Brazil, Peru and Colombia) and Africa (South Africa, Botswana and Namibia; Fig. 1a ). Our methodology can therefore be used to detect severe anthropogenic water withdrawals.
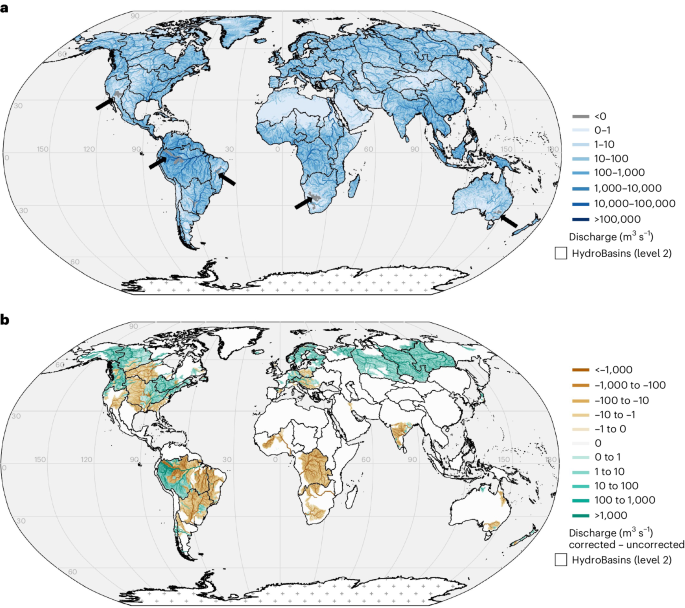
a , Corrected global river discharge estimates. Black arrows point to locations that result in negative river discharges, which are indicative of the human footprint on the water cycle. b , The difference between corrected and uncorrected estimates. Green areas indicate positive differences and, therefore, locations where the corrected simulations resulted in greater discharge estimates than uncorrected. Brown areas indicate negative differences and, therefore, locations where the corrected simulations resulted in smaller discharge estimates than uncorrected. Crosshairs in Antarctica indicate no data. Maps created in QGIS, using graticules from Natural Earth.
Differences in 30-year average river discharge between corrected and uncorrected simulations ranged from −20,432 to 63,694 m 3 s −1 (Fig. 1b ). Positive differences indicate that the average corrected estimates are higher than the average uncorrected estimates, while negative differences indicate that corrected estimates are lower than uncorrected. Most of the world’s river reaches (69%) display no difference between average corrected and uncorrected simulations, largely due to the lack of available gauge data in those locations. Positive differences (15% of river reaches) are located in northern Europe, north-eastern and north-western United States (including Alaska), Canada, Russia, Peru, Bolivia and portions of western Brazil, Argentina, Chile, India, Australia, Japan and New Zealand. Negative differences (16% of river reaches) are located across the southern and central United States, central Canada, eastern Brazil, Argentina, Paraguay, various countries in Africa (for example, South Africa and Democratic Republic of the Congo) and Europe (for example, Spain and Poland), Iran, Australia and India. Note that using even just a few gauges (for example, Africa and Australia; Extended Data Fig. 1 ) can lead to large differences in our estimates of basin-level discharge (Fig. 1b ). Further visualization of our corrections as a percentage of average river discharge confirms spatial consistency in our correction factors for each gauge subbasin, while also highlighting inland subbasins that underwent large positive and negative corrections (for example, south-western United States and Russia; Supplementary Fig. 1 ).
We expect that the spatial information gained from our corrections will be important for the land surface modelling community to evaluate and calibrate simulated runoff outputs, an otherwise unobservable quantity. The corrected discharge can also provide an estimate of the state-of-the-art in global river discharge, offering future opportunities for comparison with the Surface Water and Ocean Topography (SWOT) mission that has begun such retrievals from space 22 . SWOT may also show similar evidence of severe water withdrawals—the spatial coverage of space-based estimates from SWOT and sparser ground observations used with LTIR can together be leveraged to document and quantify the human footprint on the water cycle.
Total discharge to the global ocean
Given that continental river discharge into oceans is a key feature of Earth’s water cycle, we summed all discharge values at coastal river termini globally (with the exception of Antarctica) and plotted the monthly time series for 1980–2009 (Fig. 2 ). After confirming that the ensemble of LSMs outperforms simulations from any single LSM, we found that the temporal average and monthly variability (that is, standard deviation) of water discharged into the ocean from rivers is 37,808 ± 6,704 km 3 yr −1 (average ± variability) for uncorrected and 37,411 ± 7,816 km 3 yr −1 for corrected simulations. While the LTIR approach results in large differences in discharge at the river reach scale (Fig. 1b ), uncorrected and corrected estimates produce similar results when globally summed at the coast (positive and negative differences cancel each other out on spatial aggregate), hence building confidence in the global sum even if some regions did not benefit from corrections due to lacking observations. Both uncorrected and corrected averages of total ocean discharge in our study are consistent with previous estimates in the literature, which range from 29,485 to 45,900 km 3 yr −1 (Extended Data Table 1 ) 1 , 2 , 3 , 4 , 5 , 6 , 7 , 8 , 9 , 10 , 11 , 12 , 13 , 14 , 15 , 16 , 17 , 18 , 19 . These values are also encompassed within our simulated monthly variability (Fig. 2 ). The topic of monthly variability in total discharge to the ocean has received much less attention, and reported values range from 4,800 to 16,164 km 3 yr −1 (refs. 15 , 18 ). Other studies did not report variability but include graphical time series that allow making an inference (1,116 km 3 yr −1 (ref. 19 ) and 3,606 km 3 yr −1 (ref. 14 )). The previously reported and inferred estimates are equivalent to a monthly variability that is between 3% and 45% of the corresponding reported temporal average (Extended Data Table 1 ). Our own estimates of monthly standard deviations for uncorrected (6,704 km 3 yr −1 ) and corrected (7,816 km 3 yr −1 ) are equivalent to 18% (uncorrected) and 21% (corrected) of the temporal average and therefore are in the middle of the limited number of prior studies. Yet, our comparison of coefficients of variations between our simulations and observations (Extended Data Fig. 3 and Methods ) suggests that the magnitude of our discharge variability generally matches observations (where available) and can hence help reconcile the sizable range in temporal variability among prior values.
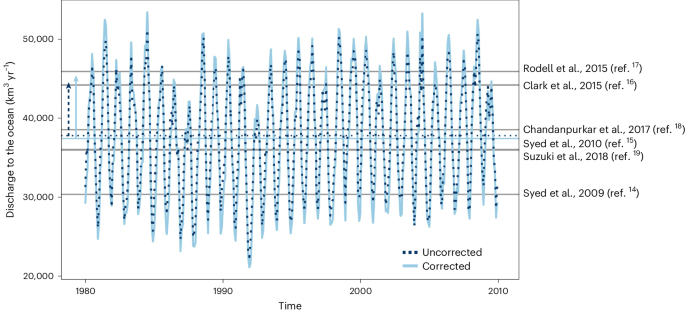
Uncorrected and corrected global monthly discharge accumulated into the ocean for 1980–2009. The estimated average is indicated as horizontal coloured dotted (uncorrected) and solid (corrected) lines. An arrow in proportion to the plot units on the left interior of the plot indicates temporal variability (that is, one standard deviation). Previous long-term estimates in the literature (2009 and after) are indicated with a grey line and associated reference on the right exterior. Additional previous estimates in the literature before 2009 are included in Extended Data Table 1 .
We also estimated which hydrologic regions contribute most to global discharge and variability (Fig. 3 ). Our findings are mostly consistent with previous knowledge ( Methods ); however, we found that the Maritime Continent (Indonesia, Malaysia and Papua New Guinea) discharges 8% of the global total, that is, the equivalent of 1.6 times the Congo River (5%). Little attention has been given to Maritime Continent basins 11 , 16 , 19 in previous global discharge studies; however, one study found that the islands of Oceania and Southeast Asia are important contributors of ocean discharge, thus supporting our finding 16 . We suspect the region might have escaped prior scrutiny in part because its largest rivers and streams (including Mahakam, Kapuas, Sepik and Fly) are poorly observed (for example, Fly River is one of the largest rivers in the world, yet it is ungauged). Accounting for these large aggregate water fluxes from the Maritime Continent could impact ocean circulation models and change our understanding of carbon/sediment/solute delivery to the ocean. Note that, in our analysis, the Maritime Continent did not benefit from corrections, and therefore the simulations were not constrained by observations, which may influence the accuracy of estimates. However, additional analysis further supports our finding (Supplementary Text 5 ) 38 , 39 .
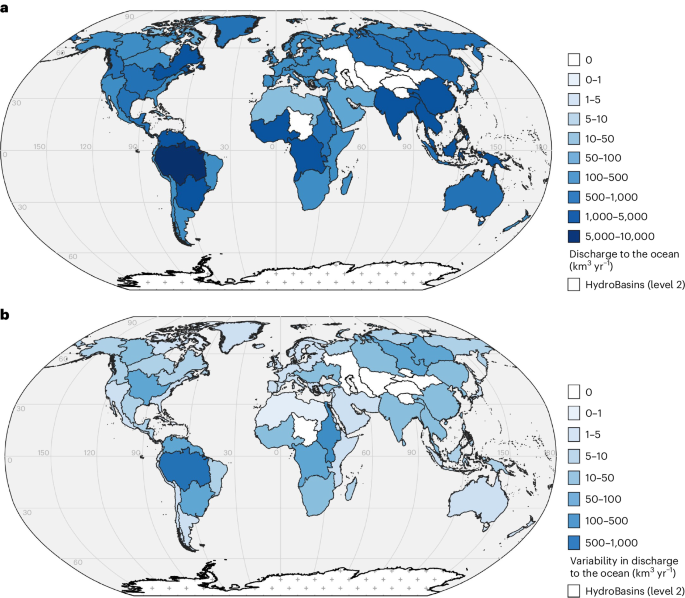
a , b , Corrected estimates of global hydrologic contributors to average ( a ) and variability ( b ) of discharge accumulated into the ocean for 1980–2009. Crosshairs in Antarctica indicate no data. Maps created in QGIS, using graticules from Natural Earth.
Implications for river water storage
To provide a global assessment of the spatial and temporal variations of river water storage, we produced monthly estimates for each river reach (including those in endorheic basins), which we summed spatially (Fig. 4 ) and averaged temporally (Fig. 5 ). Our estimates of storage depend on river flow wave residence time and on the monthly discharge at each river reach (see equation ( 15 ) in Methods ). Residence time is computed as river length divided by the speed (that is, celerity) of river flow waves. Wave celerity—despite being unobserved—is perhaps the most fundamental parameter of global river routing models, and a range of values tends to be used at such scale 40 . We used three characteristic values for propagation speed (that is, celerity) for each river reach with resulting residence times (short, medium and long) to calculate a range of possible storage estimates. Note that our analysis assumes spatial consistency in short, medium and long residence times, while the world’s rivers and streams are likely to include a distribution thereof. This conscious assumption allows drawing lower and upper bounds for potential storage and its temporal variability and, hence, constrain expected values. We found that the global average and monthly variability (standard deviation) of river water storage is 1,246 ± 225 km 3 (short residence time), 2,181 ± 394 km 3 (medium) and 3,116 ± 564 km 3 (long) for uncorrected simulations (Fig. 4 ). For corrected simulations (also in Fig. 4 ), storage average and variability are 1,283 ± 288 km 3 (short), 2,246 ± 505 km 3 (medium) and 3,208 ± 721 km 3 (long). Both uncorrected and corrected estimates of average water storage are in the same order of magnitude relative to previous estimates in the literature, which range from 1,200 to 2,858 km 3 (refs. 1 , 2 , 3 , 5 , 6 , 7 , 13 , 20 ). Our storage estimates by basin (Fig. 5 ) are also commensurate with the limited number of prior studies. For further evaluation of our estimates, see Supplementary Text 7 (refs. 20 , 41 ).
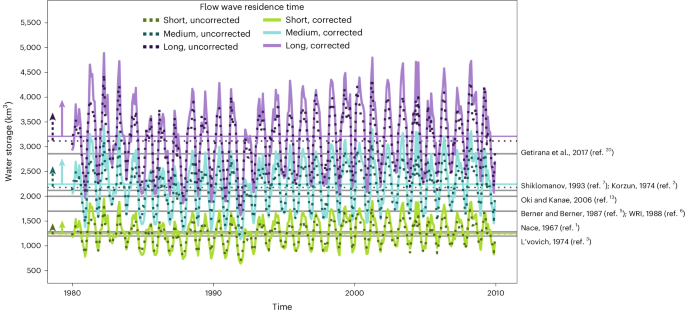
Uncorrected and corrected global monthly water storage for each flow wave residence time for 1980–2009. The estimated average is indicated as horizontal coloured dotted (uncorrected) and solid (corrected) lines. An arrow in proportion to the plot units on the left interior indicates temporal variability (that is, one standard deviation). Previous long-term estimates in the literature are indicated on each plot with a grey line and associated reference on the right exterior.
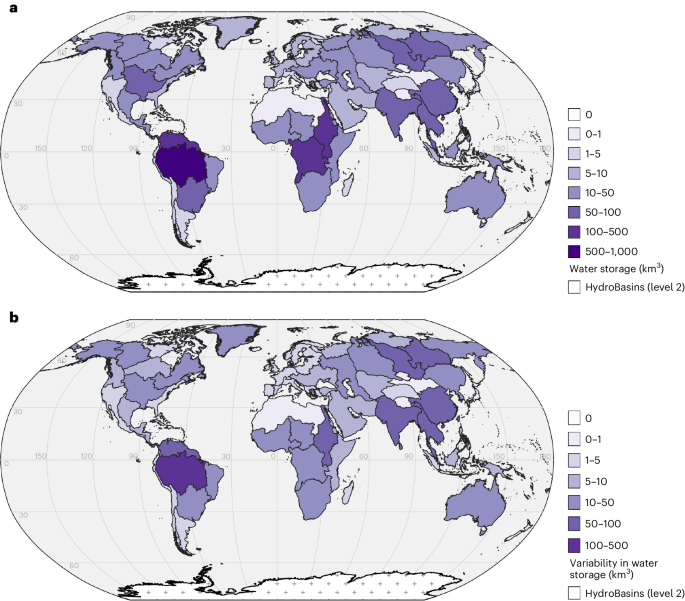
a , b , Corrected estimates of global hydrologic contributors to average ( a ) and variability ( b ) of river water storage for 1980–2009. Values are displayed for the medium flow wave residence time. Crosshairs in Antarctica indicate no data. Maps created in QGIS, using graticules from Natural Earth.
The impact of wave celerity on discharge computations has long been known 40 . However, given the considerable range we found in mean water storage across residence times (Fig. 4 ), we suggest that knowledge of flow wave propagation celerity is as critical as river discharge to accurately estimating global river water storage, which has so far not been appreciated. Longer residence times (that is, slower flow wave celerity) lead to larger estimates of mean global river water storage, but also to greater temporal variability (Fig. 4 ). One of the future challenges in global river water science is therefore bound to be the accurate estimation of residence times, a globally unobserved quantity that is currently determined from empirical equations 42 . Ongoing satellite measurements of changes in surface water storage 22 can be expected to narrow the likely range of variability, thus helping refine understanding of wave propagation in Earth’s rivers, an unanticipated benefit beyond SWOT mission requirements 43 . In turn, accurate estimates of wave celerity may help support flood warning systems 42 .
Hydrography
Several hydrography datasets are available as the river network for global routing. Here, we used the vector-based river network called Multi-Error-Removed Improved Terrain (MERIT) Hydro (v0.7) Basins (v1.0) due to the high spatial resolution of its underlying digital elevation model (~90 m) and to the geographic coverage above 60° N (refs. 28 , 30 , 44 ). MERIT Hydro Basins was derived from the MERIT Hydro digital elevation model by using a 25 km 2 channelization threshold, which resulted in ~3 million river reaches and catchments globally, as well as 61 hydrologic regions. The MERIT Hydro Basins dataset also contains derived attributes for each individual reach polyline and each associated catchment polygon (for example, reach length, downstream reach and catchment contributing area).
Runoff estimates
The primary dynamic (that is, time variable) input file for our monthly routing was the lateral inflow into each river reach. To partially alleviate uncertainty in the runoff outputs from different LSMs, we used an ensemble of three LSMs from version 2.0 of the Global Land Data Assimilation System (GLDAS; Supplementary Text 1 ) 45 . Specifically, we averaged the sum of monthly surface and subsurface runoff outputs from Variable Infiltration Capacity 46 , 47 , Catchment Land Surface Model 48 and Noah 49 , 50 , 51 , all of which have a spatial resolution of 1°. The gridded runoff was converted into lateral inflow to each river reach using a centroid-based approach—the centroid of each catchment is used to identify the corresponding LSM grid cell 52 before multiplying the runoff by the area of the catchment. The three-model ensemble average inflow was calculated on a monthly time step across the 30-year period of interest for this study, which is January 1980 to December 2009.
Discharge observations
We compiled an extensive global database of in situ gauges (for bias correction and model evaluation) by collecting daily gauge data from a combination of international and national organizations (Supplementary Table 1 ) 53 , 54 , 55 , 56 , 57 , 58 , 59 , 60 , 61 , 62 , 63 , 64 , 65 . We removed gauges located within ~100 m of each other, on the basis that the same exact gauge from the same organization can be included in multiple independent databases, hence filtering for duplicates 66 . In total, 45,837 daily gauge records were collected. We subset the database to only those gauges that had 95% daily availability for the 1980–2009 study period and to those with an average discharge greater than or equal to 100 m 3 s −1 (based on the 1980–2009 average; n = 1,148). To identify the locations of river gauges along the MERIT Hydro river network, we mapped the gauges using a distance buffer, as well as order of magnitude and duplicate gauge checks (Supplementary Text 2 ). The mapping process resulted in a final dataset of 1,001 gauges, three of which did not benefit our corrections due to limitations in our method (see ‘Discharge corrections’ section). Since we are modelling at a monthly temporal resolution, we calculated monthly average discharge for each gauge across the 1980–2009 time period (360 time steps).
Given that potential temporal trends in discharge observations cannot be explicitly accounted for in our long-term correction method (see ‘Discharge corrections’ section), we performed a trend analysis and identified that 39% of the gauges had a statistically significant trend, although of minimal magnitude (Supplementary Text 3 ). We note that this is a limitation of the methodology.

Discharge estimates
Lateral inflow can be used with the monthly mass conservation equation to determine monthly average flows throughout a river network with r river reaches with limited negative impact of neglecting horizontal transfer times 29 , as traditionally done through lumped river models as
where I is the r × r identity matrix, N is the r × r river network matrix (for example, ref. 27 ), Q e is an r -sized vector of external lateral inflows (e) entering each river reach, and Q is an r -sized vector of river discharge outflows exiting each reach. Given that lumped river models accumulate runoff from upstream to downstream without accounting for horizontal travel time from land to rivers or within the river system, we applied the model on a monthly time step. Lumped routing at this time scale can produce a fair approximation of discharge except for the largest basins of the world; however, it is fair even at the scale of the Colorado and Columbia river basins 29 . The monthly ensemble average inflow was used as input to the lumped routing model to generate uncorrected ensemble river discharge estimates across the 30 year period.
Discharge corrections
To generate corrected estimates of river discharge, we developed a novel inverse routing algorithm called LTIR that is capable of correcting bias in long-term mean lateral inflow and long-term mean discharge together. Our approach allows for corrections of lateral inflows upstream of gauges while matching observed discharge values, with impacts on discharge estimates both upstream and downstream of gauges.
Extended Data Fig. 6 shows a schematic that summarizes much of our notation for an example river network containing five reaches and two gauges and illustrates the mathematical derivation that follows. We use a river network with r river reaches and g gauges (with g < r ), S the g × r observation selector matrix (for example, ref. 45 ), t for time, \({{\mathbf{Q}}}^{{\mathrm{e}}}\left(t\right)\) an r -sized vector of simulated monthly external lateral water inflows (e) entering upstream of each river reach, Q ( t ) an r -sized vector of simulated monthly water outflows exiting each reach and q ( t ) a g -sized vector of monthly discharge observations. Vinculum symbols are used to indicate long-term means; for example, \(\overline{{{\mathbf{Q}}}^{{\mathrm{e}}}}\) is the long-term mean of \({{\mathbf{Q}}}^{{\mathrm{e}}}\left(t\right)\) . Double-struck symbols are used to indicated corrected quantities; for example, \({\mathbb{Q}}\) is the corrected equivalent to discharge Q .
The long-term continuity equation enforces equality between the downstream outflows \(\overline{{\mathbf{Q}}}\) and the upstream inflows \(\overline{{{\mathbf{Q}}}^{{\mathrm{e}}}}+{{N}}\times \overline{{\mathbf{Q}}}\) (that is, the sum of lateral inflows \(\overline{{{\mathbf{Q}}}^{{\mathrm{e}}}}\) and inflows from upstream reaches \({{N}}\times \overline{{\mathbf{Q}}}\) ). This is traditionally done through lumped river models, and can be described in matrix–vector form (for example, ref. 29 ) for both simulated and corrected states as
Enforcing that long-term corrected discharge equals observations at gauges leads to
Equations ( 2 ) and ( 3 ) together give rise to an ‘inverse routing’ problem for which an r -sized vector of corrected lateral inflow \(\overline{{{\mathbb{Q}}}^{{\mathrm{e}}}}\) is the unknown as
Equation ( 4 ) is a g × r linear system with r unknowns, that is, an underdetermined problem with an infinite number of solutions, and one must therefore narrow the mathematical problem down. Because the number of equations is the same as the number of gauges ( g ), one might first focus on the individual subbasins associated with each one of the gauges. Let \(\overline{{{\mathbf{q}}}^{{\mathrm{e}}}}\) be a g -sized array with long-term means of total lateral inflows for these individual subbasins. As a preliminary step, let \(\overline{{{\mathbb{Q}}}^{{\mathrm{e}}{{\upalpha }}}}\) be one of the infinite number of solutions to equation ( 4 ) that crudely applies the total lateral inflow of each subbasin solely at river reaches that are home to a gauge such that
where S t is the transpose of S and the Greek letter superscript is used for incremental versions of corrected estimates for \(\overline{{{\mathbb{Q}}}^{{\mathrm{e}}}}\) , with α being the first such estimate. Combining equations ( 4 ) and ( 5 ) leads to
Equation ( 6 ) is now a g × g linear system with the g unknowns of \(\overline{{{\mathbf{q}}}^{{\mathrm{e}}}}\) , and can therefore be solved. Using n , the g × g matrix describing connectivity among gauges, equation ( 6 ) can also be seen as a continuity equation that relates the total lateral inflow within each subbasin to the outflow of each subbasin as
As a result, and while the shape of \(\overline{{{\mathbb{Q}}}^{{\mathrm{e}}{{\upalpha }}}}\) was based on a crude assumption, it reveals that the inverse routing problem of equation ( 4 ) can be reduced to solve for \(\overline{{{\mathbf{q}}}^{{\mathrm{e}}}}\) , the total lateral inflows of each subbasin. This in turn offers multiple avenues for constructing valid options for \(\overline{{{\mathbb{Q}}}^{{\mathrm{e}}}}\) from \(\overline{{{\mathbf{q}}}^{{\mathrm{e}}}}\) at the subbasin level. To do so, one must first understand how the various elements of \(\overline{{{\mathbf{Q}}}^{{\mathrm{e}}}}\) get accumulated downstream of a river network for which the connection between subbasins were removed, creating the ‘disconnected’ discharge \(\overline{{{\mathbf{Q}}}_{{\mathrm{D}}}}\) , valid for both simulated and corrected states as
\(\overline{{{\mathbb{Q}}}_{{\mathrm{D}}}}\) can be seen as the long-term mean of discharge at every reach of a river network where the connectivity downstream of each gauge was removed and with long-term mean inflow \(\overline{{{\mathbb{Q}}}^{{\mathrm{e}}}}\) . Provided adequate corrections are made, \({{S}}\times \overline{{{\mathbb{Q}}}_{{\mathrm{D}}}}\) (that is, the values of \(\overline{{{\mathbb{Q}}}_{{\mathrm{D}}}}\) at river reaches that have a gauge) should be equal to \(\overline{{{\mathbf{q}}}^{{\mathrm{e}}}}\) (that is, the total lateral inflows for these individual subbasins), thus
We can now look for multiplicative scalars, one per subbasin, stored in a g -sized vector λ and allowing to correct \({S}\times \overline{{{\mathbf{Q}}}_{{\mathrm{D}}}}\) into \({{S}}\times {\overline{{{\mathbb{Q}}}_{{\mathrm{D}}}}}\) as
where ⊗ is elementwise multiplication. Equations ( 9 ) and ( 10 ) together allow the computation of λ as
where \(\oslash\) is elementwise division. The multiplicative scalars stored for each subbasin in λ can then be applied for each river reach of the relevant subbasin and stored in an r -sized vector Λ by applying the following transformation:
where ⊝ is elementwise subtraction and used here to ensure that places with no gauge retain their initial value. We can then build a corrected lateral inflow vector \(\overline{{{\mathbb{Q}}}^{{\mathrm{e}}{\mathbf{\upbeta }}}}\) , with β being the second version of the corrected estimates, such that the lateral inflow values of each subbasin are proportional to the initial values of \(\overline{{{\mathbf{Q}}}^{{\mathrm{e}}}}\) thus
Equation ( 13 ) is a linear transformation that can equally be applied at the monthly time step as
Overall, our inverse routing methodology can hence be summarized in six implementation steps (Extended Data Fig. 7 ): (1) determine \(\overline{{{\mathbf{q}}}^{{\mathrm{e}}}}\) from \(\overline{{\mathbf{q}}}\) using equation ( 6 ), (2) determine \({{S}}\times \overline{{{\mathbf{Q}}}_{{\mathrm{D}}}}\) from \(\overline{{{\mathbf{Q}}}^{{\mathrm{e}}}}\) using equation ( 8 ), (3) determine λ from \(\overline{{{\mathbf{q}}}^{{\mathrm{e}}}}\) and \(\overline{{{\mathbf{Q}}}_{{\mathrm{D}}}}\) using equation ( 11 ), (4) determine Λ from λ using equation ( 12 ), (5) computing \({{\mathbb{Q}}}^{{\mathrm{e}}{\mathbf{\upbeta }}}(t)\) from Λ and \({{\mathbf{Q}}}^{{\mathrm{e}}}(t)\) for all monthly time steps using equation ( 14 ) and (6) determine \({\mathbb{Q}}(t)\) from \({{\mathbb{Q}}}^{{\mathrm{e}}{\mathbf{\upbeta }}}(t)\) using equation ( 2 ).
Note that the design of this methodology is flawed in the cases in which occasional elements of \({{S}}\times \overline{{{\mathbb{Q}}}_{{\mathrm{D}}}}\) are null, that is, when the total lateral inflow within given subbasins is zero, in which case a multiplicative scaling correction is bound to fail. Such a challenge was encountered for three subbasins when using the full 1,001 gauge dataset for correction; three gauges were dropped from the analysis, resulting in a final correction gauge dataset of size 998.
Discharge evaluation metrics
To evaluate the performance of bias correction, we calculated test statistics to compare uncorrected and corrected simulations with observations where in situ observations exist. Before applying the correction to our full gauge dataset, we first ensured independent evaluation by splitting the gauge observation dataset into 70% for calibration (that is, correction; n = 702) and 30% for validation ( n = 299; Supplementary Text 4 ). For each of the calibration, validation and full gauge datasets, we used monthly observations and simulations to calculate normalized absolute bias (NBIAS; absolute value of observed minus simulated; normalized using the mean of the observations), NSTDERR, NRMSE and NSE (a measure of how well the simulated time series matches the observed) 67 . For the final correction with the full gauge dataset, we also calculate coefficient of variation (CV) for monthly observations, uncorrected simulations and corrected simulations.
Model validation
After validating our correction algorithm with the independent dataset (Supplementary Text 4 ), we performed the correction with the full gauge dataset ( n = 998; see ‘Discharge corrections’ section), and evaluated the model performance at each of the 998 gauges (Extended Data Table 2 ). The mean/median NBIAS decreased from 0.45/0.28 for uncorrected simulations to 0.00/0.00 for corrected simulations, and 99% of the 998 gauges showed an improvement in NBIAS (Extended Data Fig. 1a ). Note that four gauges worsened minimally in NBIAS after correction, which is due to using all simulated monthly discharge values and only available monthly observed discharge to calculate our correction factors but then calculating NBIAS only at time steps in which observations were available. We confirmed that bias went to 0.00 at these gauges when using all time steps to calculate NBIAS rather than only time steps in which observations were available. Reduction in normalized bias led to improvements in the other model test statistics. The mean/median NSTDERR changed from 0.84/0.61 for uncorrected simulations to 0.68/0.63 for corrected simulations (Extended Data Fig. 1b ), hence showing very limited impacts of our bias correction on the temporal variability of flow errors. The mean/median NRMSE decreased from 0.99/0.73 for uncorrected simulations to 0.68/0.63 for corrected simulations (Extended Data Fig. 2a ), indicating that all of NRMSE was composed of NSTDERR. The mean/median NSE increased from −3.74/0.07 for uncorrected simulations to −0.79/0.38 for corrected simulations (Extended Data Fig. 2b ), which indicates that corrected simulations better matched observations than uncorrected simulations; 55% of gauges showed improvements in NSTDERR, 75% in NRMSE and 75% in NSE. Improvements in test statistics can be seen across most of the world, except for portions of north-western and north-eastern United States, western and south-eastern Canada, western Brazil, western Argentina, northern Australia and northern Europe. Deteriorations in NSTDERR were also present in central Europe and New Zealand.
For each gauge location, we calculated the CV and found that the mean/median was 0.76/0.73 for observations, 0.86/0.79 for uncorrected simulations and 0.87/0.79 for corrected simulations. Based on mean/median values, simulations showed slightly greater variability than observations, with minimally greater variability in corrected simulations. After fitting a linear regression enforcing a zero-intercept between simulated CV and observed CV, we found slopes of 0.98 (uncorrected) and 0.99 (corrected) and R 2 of 0.84 (uncorrected) and 0.83 (corrected; Extended Data Fig. 3 ), showing that observed and simulated CV were close to the line of unity (that is, observed CV = simulated CV) and that ~83% of the variance was explained. However, the regression residuals failed the Shapiro–Wilks normality test (uncorrected P value of 1.43 × 10 −12 ; corrected P value <2.2 × 10 −16 ), hence limiting valid inference.
Future studies might consider correcting for NSTDERR in addition to bias, which can be expected to have a positive impact on NRMSE, and probably also on NSE. The broad improvement in bias, NSE and NRMSE reported here—with limited impacts on NSTDERR and on CV—are therefore sufficient for the stated purpose of our study, which is correcting bias and evaluating global discharge and storage in rivers.
Estimates of discharge into the ocean
The uncorrected and corrected river discharge estimates at coastal river termini were used to calculate the global total discharge into the ocean, along with its variability. Coastal river termini were identified by extracting all river reaches with no downstream river reach and then selecting all river reaches within 200 m of the coastline. Using a buffer from the coastline was necessary to remove river termini located in the middle of continents. Global discharge into the ocean was calculated on a monthly time step by summing the discharge for all coastal river termini ( n = 48,200). Variability was calculated as the standard deviation of total discharge into the ocean across the time series.
Across the globe, average discharge into the ocean is highest for the Amazon (18% of global discharge for uncorrected and 18% for corrected simulations), South America north of the Amazon (for example, Orinoco, Catatumbo; 6%, 6%), the Congo (6%, 5%) and Ganges–Brahmaputra (5%, 5%) basins (Fig. 3a ). Variability in discharge to the ocean is highest in the Amazon, Nile, La Plata and Congo basins (Fig. 3b ).
Water storage estimates
Each of Earth’s river reaches can fundamentally be reduced to an individual control volume. At steady state, assuming that water is incompressible and neglecting friction by viscous forces, this control volume follows Bernoulli’s principle. In turn, the river reach becomes ruled by a linear relationship between water storage and water flow, and involving residence time. Assuming that residence times are much shorter than 1 month, such a relationship can be applied at the monthly time scale. Under the same steady-state assumption, the Muskingum method 68 for river routing also reduces to a linear storage–discharge relationship:
where V is the storage volume and k is the Muskingum time parameter that is known to be related to the celerity—or speed—of flow wave propagation 69 (Supplementary Text 6 ). To calculate Muskingum k , we divided the length of each river reach by a reference celerity for the flow wave of 1 km h −1 , and then multiplied that quantity by a scaling factor λ specific to the Muskingum k (λ k )—generating a unique value for each reach and for each residence time characterization. Based on our experience with automated parameter estimation for the Muskingum method (Extended Data Table 3 ) and that all used the same reference value 27 , 29 , 52 , 70 , 71 , 72 , we used a low (0.20), medium (0.35) and high (0.50) value of λ k to calculate three different possible sets of Muskingum k values (for short, medium and long flow wave residence times) associated to each river reach. After confirming that our residence times are indeed much shorter than 1 month (mean/median values are 1.87 h/1.37 h, 3.27 h/2.39 h and 4.67 h/3.41 h for short, medium and long experiments, respectively), we calculated global water storage using each Muskingum k on a monthly time step by summing the storage for all river reaches. Variability was calculated as the standard deviation of river water storage across the monthly time series.
On average, the majority of river water is stored in the Amazon (34% of global river water storage for uncorrected and 38% for corrected simulations), Congo (8%, 6%), Nile (5%, 5%) and South America north of the Amazon (for example, Orinoco, Catatumbo; 5%, 5%) basins (Fig. 5a ). Water storage variability is the highest in the Amazon, Nile, Ganges–Brahmaputra, La Plata and South America north of the Amazon basins (Fig. 5b ).
Methodological limitations
We believe that there are several avenues for future research and refinement. First, in this study, we focus on the impacts of observations and parameters (that is, flow wave propagation celerity) on estimates of global water storage; however, future work could explore the impacts of multi-model runoff uncertainty by using more LSMs and atmospheric forcings. Second, the correction algorithm creates a multiplicative factor based on the average discharge across the 30-year study period and therefore does not incorporate a constraint on river discharge extremes (high and low flows) or on variability. Future work could focus on correcting the amplitude in addition to the average. Third, an assumption of our approach is that errors in river discharge are attributed solely to errors in runoff. Such errors could also be due to lack of representation of other components of the water cycle (for example, lakes and wetlands) in our routing model; future work could further explore potential errors, particularly with the release of SWOT data. Lastly, human influences on the water cycle (for example, water withdrawals, dams and reservoirs) are not directly incorporated into the routing model, as the only aspect of anthropogenic influence we are looking at is observed water balance. While the gauge observations used for correction and the range of celerity that we use to calculate global river water storage (which were optimized from real case studies) indirectly inform the model of some anthropogenic activities, explicit incorporation of such activities may improve river discharge and storage estimates 73 .
Data availability
Global river discharge and water storage data (‘MeanDRS’) are made publicly available via Zenodo at https://doi.org/10.5281/zenodo.8248069 (ref. 74 ). We follow the guidelines ( https://doi.org/10.5281/zenodo.10161527 ) of NASA’s Transform to OPen Science (TOPS) mission for our open science practices.
Code availability
Our software is publicly available via Zenodo at https://doi.org/10.5281/zenodo.3236649 (ref. 75 ) and via GitHub at https://github.com/c-h-david/rrr (ref. 76 ). We follow the guidelines ( https://doi.org/10.5281/zenodo.10161527 ) of NASA’s Transform to OPen Science (TOPS) mission for our open science practices.
Nace, R. L. Are We Running Out of Water? (United States Department of the Interior, Geological Survey, 1967).
Korzun, V. I. World Water Balance and Water Resources of the Earth (USSR, National Committee for the IHD, Chief Administration of Hydrometeorological Service, 1974).
L’vovich, M. I. World Water Resources and their Future (American Geophysical Union, 1974).
Baumgartner, A. & Reichel, E. The World Water Balance: Mean Annual Global, Continental and Maritime Precipitation, Evaporation and Run-off (Elsevier, 1975).
Berner, E. K. & Berner, R. A. The Global Water Cycle: Geochemistry and Environment (Prentice-Hall, 1987).
World Resources Institute. World Resources 1988-89: An Assessment of the Resource Base that Supports the Global Economy 374 (Basic Books, Inc., 1988).
Shiklomanov, I. in Water in Crisis: A Guide to the World’s Freshwater Resources 13–24 (Oxford Univ. Press, 1993).
Fekete, B., Vörösmarty, C. & Grabs, W. Global Composite Runoff Fields on Observed River Discharge and Simulated Water Balances (Global Runoff Data Centre, 2000).
Vörösmarty, C. J., Green, P., Salisbury, J. & Lammers, R. B. Global water resources: vulnerability from climate change and population growth. Science 289 , 284–288 (2000).
Article Google Scholar
Oki, T. et al. Global assessment of current water resources using total runoff integrating pathways. Hydrol. Sci. J. 46 , 983–995 (2001).
Dai, A. & Trenberth, K. E. Estimates of freshwater discharge from continents: latitudinal and seasonal variations. J. Hydrometeorol. 3 , 660–687 (2002).
Syvitski, J. P. M., Vörösmarty, C. J., Kettner, A. J. & Green, P. Impact of humans on the flux of terrestrial sediment to the global coastal ocean. Science 308 , 376–380 (2005).
Article CAS Google Scholar
Oki, T. & Kanae, S. Global hydrological cycles and world water resources. Science 313 , 1068–1072 (2006).
Syed, T. H., Famiglietti, J. S. & Chambers, D. P. GRACE-based estimates of terrestrial freshwater discharge from basin to continental scales. J. Hydrometeorol. 10 , 22–40 (2009).
Syed, T. H., Famiglietti, J. S., Chambers, D. P., Willis, J. K. & Hilburn, K. Satellite-based global-ocean mass balance estimates of interannual variability and emerging trends in continental freshwater discharge. Proc. Natl Acad. Sci. USA 107 , 17916–17921 (2010).
Clark, E. A., Sheffield, J., van Vliet, M. T. H., Nijssen, B. & Lettenmaier, D. P. Continental runoff into the oceans (1950–2008). J. Hydrometeorol. 16 , 1502–1520 (2015).
Rodell, M. et al. The observed state of the water cycle in the early twenty-first century. J. Clim. 28 , 8289–8318 (2015).
Chandanpurkar, H. A., Reager, J. T., Famiglietti, J. S. & Syed, T. H. Satellite- and reanalysis-based mass balance estimates of global continental discharge (1993–2015). J. Clim. 30 , 8481–8495 (2017).
Suzuki, T. et al. A dataset of continental river discharge based on JRA-55 for use in a global ocean circulation model. J. Oceanogr. 74 , 421–429 (2018).
Getirana, A., Kumar, S., Girotto, M. & Rodell, M. Rivers and floodplains as key components of global terrestrial water storage variability. Geophys. Res. Lett. 44 , 10,359–10,368 (2017).
Krabbenhoft, C. A. et al. Assessing placement bias of the global river gauge network. Nat. Sustain. 5 , 586–592 (2022).
Alsdorf, D. E., Rodríguez, E. & Lettenmaier, D. P. Measuring surface water from space. Rev. Geophys . 45 , RG2002 (2007).
Hannah, D. M. et al. Large-scale river flow archives: importance, current status and future needs. Hydrol. Process. 25 , 1191–1200 (2011).
Famiglietti, J. S. et al. Satellites provide the big picture. Science 349 , 684–685 (2015).
Fekete, B. M. et al. Time for in situ renaissance. Science 349 , 685–686 (2015).
Ruhi, A., Messager, M. L. & Olden, J. D. Tracking the pulse of the Earth’s fresh waters. Nat. Sustain. 1 , 198–203 (2018).
David, C. H. et al. River network routing on the NHDPlus dataset. J. Hydrometeorol. 12 , 913–934 (2011).
Lin, P. et al. Global reconstruction of naturalized river flows at 2.94 million reaches. Water Resour. Res. 55 , 6499–6516 (2019).
David, C. H. et al. Analytical propagation of runoff uncertainty into discharge uncertainty through a large river network. Geophys. Res. Lett. 46 , 8102–8113 (2019).
Yang, Y. et al. Global reach-level 3-hourly river flood reanalysis (1980–2019). Bull. Am. Meteorol. Soc. 102 , E2086–E2105 (2021).
Emery, C. M. et al. Large-scale hydrological model river storage and discharge correction using a satellite altimetry-based discharge product. Hydrol. Earth Syst. Sci. 22 , 2135–2162 (2018).
Beck, H. E., de Roo, A. & van Dijk, A. I. J. M. Global maps of streamflow characteristics based on observations from several thousand catchments. J. Hydrometeorol. 16 , 1478–1501 (2015).
Pan, M. & Wood, E. F. Inverse streamflow routing. Hydrol. Earth Syst. Sci. 17 , 4577–4588 (2013).
Gleick, P. H. Roadmap for sustainable water resources in southwestern North America. Proc. Natl Acad. Sci. USA 107 , 21300–21305 (2010).
Huang, Z. et al. Reconstruction of global gridded monthly sectoral water withdrawals for 1971–2010 and analysis of their spatiotemporal patterns. Hydrol. Earth Syst. Sci. 22 , 2117–2133 (2018).
Hou, J., van Dijk, A. I. J. M., Beck, H. E., Renzullo, L. J. & Wada, Y. Remotely sensed reservoir water storage dynamics (1984–2015) and the influence of climate variability and management at a global scale. Hydrol. Earth Syst. Sci. 26 , 3785–3803 (2022).
Döll, P., Fiedler, K. & Zhang, J. Global-scale analysis of river flow alterations due to water withdrawals and reservoirs. Hydrol. Earth Syst. Sci. 13 , 2413–2432 (2009).
Lan, C.-W., Lo, M.-H., Chou, C. & Kumar, S. Terrestrial water flux responses to global warming in tropical rainforest areas. Earths Future 4 , 210–224 (2016).
Kabuya, P. M., Hughes, D. A., Tshimanga, R. M., Trigg, M. A. & Bates, P. Establishing uncertainty ranges of hydrologic indices across climate and physiographic regions of the Congo River Basin. J. Hydrol. Reg. Stud. 30 , 100710 (2020).
Oki, T., Nishimura, T. & Dirmeyer, P. Assessment of annual runoff from land surface models using Total Runoff Integrating Pathways (TRIP). J. Meteorol. Soc. Jpn 77 , 235–255 (1999).
Coss, S. et al. Channel water storage anomaly: a new remotely sensed quantity for global river analysis. Geophys. Res. Lett. 50 , e2022GL100185 (2023).
Allen, G. H., David, C. H., Andreadis, K. M., Hossain, F. & Famiglietti, J. S. Global estimates of river flow wave travel times and implications for low-latency satellite data. Geophys. Res. Lett. 45 , 7551–7560 (2018).
Desai, S. Surface Water and Ocean Topography Mission (SWOT). Project Science Requirements Document . Rev B . (NASA JPL, 2018).
Yamazaki, D. et al. MERIT Hydro: a high-resolution global hydrography map based on latest topography dataset. Water Resour. Res. 55 , 5053–5073 (2019).
Rodell, M. et al. The global land data assimilation system. Bull. Am. Meteorol. Soc. 85 , 381–394 (2004).
Wood, E. F., Lettenmaier, D., Liang, X., Nijssen, B. & Wetzel, S. W. Hydrological modeling of continental-scale basins. Annu. Rev. Earth Planet. Sci. 25 , 279–300 (1997).
Liang, X., Lettenmaier, D. P., Wood, E. F. & Burges, S. J. A simple hydrologically based model of land surface water and energy fluxes for general circulation models. J. Geophys. Res. Atmos. 99 , 14415–14428 (1994).
Koster, R. D., Suarez, M. J., Ducharne, A., Stieglitz, M. & Kumar, P. A catchment-based approach to modeling land surface processes in a general circulation model: 1. Model structure. J. Geophys. Res. Atmos. 105 , 24809–24822 (2000).
Betts, A. K., Chen, F., Mitchell, K. E. & Janjić, Z. I. Assessment of the land surface and boundary layer models in two operational versions of the NCEP Eta model using FIFE data. Mon. Weather Rev. 125 , 2896–2916 (1997).
Chen, F., Janjić, Z. & Mitchell, K. Impact of atmospheric surface-layer parameterizations in the new land-surface scheme of the NCEP Mesoscale Eta model. Bound. Layer. Meteorol. 85 , 391–421 (1997).
Ek, M. B. et al. Implementation of Noah land surface model advances in the National Centers for Environmental Prediction operational mesoscale Eta model. J. Geophys. Res. Atmos. 108 , 8851 (2003).
David, C. H., Yang, Z.-L. & Hong, S. Regional-scale river flow modeling using off-the-shelf runoff products, thousands of mapped rivers and hundreds of stream flow gauges. Environ. Model. Softw. 42 , 116–132 (2013).
ArcticNET v1.0. ArcticNET https://russia-arcticnet.sr.unh.edu (2022)
Australian Bureau of Meteorology Hydrologic Reference Stations . Australian Bureau of Meteorology http://www.bom.gov.au/ (2022).
National Water and Sanitation Agency. Brazil National Water Agency https://www.gov.br/ana/en/national_water_agency (2022).
National Water Data Archive: HYDAT. Canada National Water Data Archive https://www.canada.ca/en/environment-climate-change/services/water-overview/quantity/monitoring/survey/data-products-services/national-archive-hydat.html (2022).
Chile Center for Climate and Resilience Research CR2. Chilean research center on climate, climate change and resilience https://www.cr2.cl/eng/ (2022).
Henck, A. C., Montgomery, D. R., Huntington, K. W. & Liang, C. Monsoon control of effective discharge, Yunnan and Tibet. Geology 38 , 975–978 (2010).
Schmidt, A. H., Montgomery, D. R., Huntington, K. W. & Liang, C. The question of communist land degradation: new evidence from local erosion and basin-wide sediment yield in Southwest China and Southeast Tibet. Ann. Assoc. Am. Geogr. 101 , 477–496 (2011).
GRDC Data Portal. The Global Runoff Data Centre https://portal.grdc.bafg.de/applications/public.html?publicuser=PublicUser (2023).
India-WRIS. India Water Resources Information System https://indiawris.gov.in/wris/#/ (2022).
Japanese Water Information System. Ministry of Land, Infrastructure, Transport and Tourism https://www.mlit.go.jp/en/ (2022).
Anuario de Aforos Digital. Government of Spain http://datos.gob.es/es/catalogo/e00125801-anuario-de-aforos/resource/4836b826-e7fd-4a41-950c-89b4eaea0279 (2022).
RID River Discharge Data. Thailand Royal Irrigation Department http://hydro.iis.u-tokyo.ac.jp/GAME-T/GAIN-T/routine/rid-river/disc_d.html (2022).
USGS Current Water Data for the Nation. US Geological Survey https://waterdata.usgs.gov/nwis/rt (2022).
Crochemore, L. et al. Lessons learnt from checking the quality of openly accessible river flow data worldwide. Hydrol. Sci. J. 65 , 699–711 (2020).
Nash, J. E. & Sutcliffe, J. V. River flow forecasting through conceptual models part I—a discussion of principles. J. Hydrol. 10 , 282–290 (1970).
McCarthy, G. T. The Unit Hydrograph and Flood Routing (Army Engineer District, Providence, 1938).
Cunge, J. A. On the subject of a flood propagation computation method (Muskingum method). J. Hydraul. Res. 7 , 205–230 (1969).
David, C. H., Habets, F., Maidment, D. R. & Yang, Z.-L. RAPID applied to the SIM-France model. Hydrol. Process. 25 , 3412–3425 (2011).
David, C. H., Yang, Z.-L. & Famiglietti, J. S. Quantification of the upstream-to-downstream influence in the Muskingum method and implications for speedup in parallel computations of river flow. Water Resour. Res. 49 , 1–18 (2013).
David, C. H., Famiglietti, J. S., Yang, Z.-L. & Eijkhout, V. Enhanced fixed-size parallel speedup with the Muskingum method using a trans-boundary approach and a large subbasins approximation. Water Resour. Res. 51 , 1–25 (2015).
Tavakoly, A. A. et al. Continental-scale river flow modeling of the mississippi river basin using high-resolution NHDPlus dataset. J. Am. Water Resour. Assoc. 53 , 258–279 (2017).
Collins, E. et al. RRR input and output files corresponding to “Global patterns in river water storage dependent on residence time”. Zenodo https://doi.org/10.5281/zenodo.8248069 (2024).
David, C. H. c-h-david/rrr: rrr. Zenodo https://doi.org/10.5281/zenodo.3236649 (2024).
David, C. H. Reproducible Routing Rituals (RRR). GitHub https://github.com/c-h-david/rrr (2024).
Download references
Acknowledgements
E.L.C. and C.H.D. were supported by the Jet Propulsion Laboratory, California Institute of Technology, under a contract with the National Aeronautics and Space Administration, including grants from the Terrestrial Hydrology (NNH17ZDA001N-THP) and Earth Science U.S. Participating Investigator (NNH20ZDA001N-EUSPI) programmes. We thank M. Skrip at the Center for Geospatial Analytics for comments on an earlier draft of the paper.
Author information
Authors and affiliations.
Jet Propulsion Laboratory, California Institute of Technology, Pasadena, CA, USA
Elyssa L. Collins & Cédric H. David
Center for Geospatial Analytics, North Carolina State University, Raleigh, NC, USA
Elyssa L. Collins, Ross K. Meentemeyer & Georgina M. Sanchez
Department of Earth, Marine and Environmental Sciences, University of North Carolina, Chapel Hill, NC, USA
Elyssa L. Collins & Tamlin M. Pavelsky
Department of Geography, Texas A&M University, College Station, TX, USA
Department of Geosciences, Virginia Polytechnic Institute and State University, Blacksburg, VA, USA
George H. Allen
Institute of Remote Sensing and GIS, School of Earth and Space Sciences, Peking University, Beijing, China
Peirong Lin
Center for Western Weather and Water Extremes, Scripps Institution of Oceanography, University of California, San Diego, La Jolla, CA, USA
Global Hydrological Prediction Center, Institute of Industrial Science, The University of Tokyo, Komaba, Tokyo, Japan
Dai Yamazaki
Department of Forestry and Environmental Resources, North Carolina State University, Raleigh, NC, USA
Ross K. Meentemeyer
You can also search for this author in PubMed Google Scholar
Contributions
E.L.C. assisted with developing the method, performed the analysis and drafted the paper. C.H.D. conceptualized the study, developed the method, assisted with the analysis, reviewed and edited the paper, and provided funding. R.R. and G.H.A. provided the gauge database and reviewed and edited the paper. T.M.P., R.K.M. and G.M.S. provided funding and reviewed and edited the paper. P.L., M.P. and D.Y. reviewed and edited the paper.
Corresponding authors
Correspondence to Elyssa L. Collins or Cédric H. David .
Ethics declarations
Competing interests.
The authors declare no competing interests.
Peer review
Peer review information.
Nature Geoscience thanks Doug Alsdorf, Aaron Anthony Boone, Steve Coss and Ali Nazemi for their contribution to the peer review of this work. Primary handling editor: Tom Richardson, in collaboration with the Nature Geoscience team.
Additional information
Publisher’s note Springer Nature remains neutral with regard to jurisdictional claims in published maps and institutional affiliations.
Extended data
Extended data fig. 1 difference between corrected and uncorrected river discharge test statistics..
( a ) normalized bias (NBIAS) and ( b ) normalized root mean square error (NRMSE). Blue indicates an improvement in the metric, and red a deterioration in the metric. Crosshairs in Antarctica indicate no data. Maps created in QGIS, using graticules from Natural Earth.
Extended Data Fig. 2 Difference between corrected and uncorrected river discharge test statistics.
( a ) normalized standard error (NSTDERR) and ( b ) Nash-Sutcliffe efficiency (NSE). Blue indicates an improvement in the metric, and red a deterioration in the metric. Crosshairs in Antarctica indicate no data. Maps created in QGIS, using graticules from Natural Earth.
Extended Data Fig. 3 Coefficient of variation (CV).
CV is shown for observations vs. simulations for both uncorrected and corrected simulations. We fit a linear regression to both simulation types—the equations and R 2 are displayed on the figure.
Extended Data Fig. 4 Example hydrographs for six rivers.
Hydrographs show observations, uncorrected simulations, and corrected simulations for six rivers: ( a ) Amazon, ( b ) Congo, ( c ) Paraná, ( d ) Yenesei, ( e ) Mississippi, and ( f ) Lena. Each plot is labeled with the river ID (top left), and uncorrected and corrected Nash-Sutcliffe efficiency (NSE; top middle and right).
Extended Data Fig. 5 Global 30-year mean river discharge, uncorrected.
Ensemble 30-yr average uncorrected global river discharge estimates. Crosshairs in Antarctica indicate no data. Map created in QGIS, using graticules from Natural Earth.
Extended Data Fig. 6 Schematic of the correction algorithm.
Schematic summarizing and illustrating our mathematical notation and derivation for our correction algorithm for an example river network containing five reaches and two gauges.
Extended Data Fig. 7 Illustration of the implementation of the correction algorithm.
Illustration of the six implementation steps of our correction algorithm for an example river network containing five reaches and two gauges.
Supplementary information
Supplementary information.
Supplementary Texts 1–7, Tables 1–4 and Figs. 1 and 2.
Rights and permissions
Open Access This article is licensed under a Creative Commons Attribution 4.0 International License, which permits use, sharing, adaptation, distribution and reproduction in any medium or format, as long as you give appropriate credit to the original author(s) and the source, provide a link to the Creative Commons licence, and indicate if changes were made. The images or other third party material in this article are included in the article’s Creative Commons licence, unless indicated otherwise in a credit line to the material. If material is not included in the article’s Creative Commons licence and your intended use is not permitted by statutory regulation or exceeds the permitted use, you will need to obtain permission directly from the copyright holder. To view a copy of this licence, visit http://creativecommons.org/licenses/by/4.0/ .
Reprints and permissions
About this article
Cite this article.
Collins, E.L., David, C.H., Riggs, R. et al. Global patterns in river water storage dependent on residence time. Nat. Geosci. (2024). https://doi.org/10.1038/s41561-024-01421-5
Download citation
Received : 18 May 2023
Accepted : 15 March 2024
Published : 22 April 2024
DOI : https://doi.org/10.1038/s41561-024-01421-5
Share this article
Anyone you share the following link with will be able to read this content:
Sorry, a shareable link is not currently available for this article.
Provided by the Springer Nature SharedIt content-sharing initiative
Quick links
- Explore articles by subject
- Guide to authors
- Editorial policies
Sign up for the Nature Briefing: Anthropocene newsletter — what matters in anthropocene research, free to your inbox weekly.

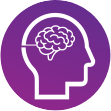
- Revision notes >
- IGCSE Geography Revision Notes >
- Theme 2: Natural Environment
River Case Study: The Mississippi
- – Length – 3,800 km
- – Width – Flood Plain is 200 km wide at its widest point
- – Releases 600 million tonnes of sediment of load each year (into the sea and along its course)
- – Flows through 10 states
- – Carries 13% of all freight traffic in the USA
1. What Economic Opportunities are Offered by The River Mississippi?
– 11 million tourists each year in the upper reaches of river
– tourism contributes $1.2 Billion to the economy each year
– Sports Fishing – $100 milling, Waterfowl hunting – $58 million
– 29 power plants provide energy to vast array of factories and homes
– Water from river used for cooling in 80% of Energy Production Facilities
– Silt deposits formed on huge flood plains made area around the river among the most fertile
regions of the USA (sugar canes, rice, tobacco, and cotton plantations)
– River provides main transport routes for exports around the world
– Today over 100 million of commodities transported down the river every year (including 56%
of the nation’s corn, and 41% of soybean exports)
2. What problems/hazards are Presented by the Mississippi?
– Despite efforts there has been major floods over the last 50 years
– Particularly severe flood in 1993 (see below)
– Many people live along the river’s flood plains
– Many scientists have argued that overflowing in a natural part of the river, and should be left to
happen (prevention measures should be abandoned)
– One of the worst areas of flooding is the point when the rivers enters its delta at the Gulf of
Mexico and spreads through distributaries e.g. Hurricane Katrina storm surge at the mouth of the Mississippi in New Orleans
– Careful management is needed to stop the build-up of sediments causing even more floods
– The river’s length of 3,800 km means that it’s very difficult to prevent flooding
– needs constant dredging to allow for ship traffic
Great flood of 1993”
- Between April and October 1993
- 30,000 square miles flooded
- Affected both the Missouri river (tributary of Mississippi) and Mississippi basins
- Caused by a series of storms with heavy rainfall (less time to absorb into the soil or infiltrate) throughout period, in some places 750% more rainfall than usual with rainfall amounts of (125 to 175 mm) in 24 hours
- Lower than average temperatures (less evaporation)
- By the beginning of June the ground was already saturated meaning that continued rainfall went straight into river systems
- St. Louis, river levels were nearly 20 feet (6 m) above flood stage.
- 700 privately built agricultural levees were overtopped or destroyed along the Missouri River.
- Navigation on the Mississippi and Missouri River had been closed since early July resulting in a loss of $2 million (1993) per day in commerce.
- Approximately 100,000 homes were destroyed as a result of the flooding, 15 million acres (60,000 km²) of farmland inundated
- 32 people died
- Approximately $15–20 billion in damages
- River levels breached levees and floodwalls (levees)
3. What Managements/Solutions Have Been Tried/Planned for The River?
https://www.bbc.co.uk/education/clips/z3bqxnb
Dams, spillways, artificially strengthened levees, river straightening, flood warning systems, afforestation
– 6 dams along the Missouri, 1600 km chain of 105 reservoirs: these hold back water until river levels have lowered and can manage more water
– 9 dams have been built along the Tennessee river (also a tributary)
– Afforestation in the upper Mississippi drainage basin system: planting of trees increases interception and increases lag time
– The Bonnet Carré floodway (9 km long spillway) diverts excess water from the Mississippi, 50km north of New Orleans
– River straightening e.g. one 530 km stretch of river has been shortened by almost 300 km by cutting through meanders. This increases the gradient and speed with which flood waters can be carried away.
– Reinforced levees using concrete blocks: success in St Louis in 1993 as river levels almost reached the full height of levee but it just managed to hold them back.
Still got a question? Leave a comment
Leave a comment, cancel reply.
Save my name, email, and website in this browser for the next time I comment.
Let's get acquainted ? What is your name?
Nice to meet you, {{name}} what is your preferred e-mail address, nice to meet you, {{name}} what is your preferred phone number, what is your preferred phone number, just to check, what are you interested in, when should we call you.
It would be great to have a 15m chat to discuss a personalised plan and answer any questions
What time works best for you? (UK Time)
Pick a time-slot that works best for you ?
How many hours of 1-1 tutoring are you looking for?
My whatsapp number is..., for our safeguarding policy, please confirm....
Please provide the mobile number of a guardian/parent
Which online course are you interested in?
What is your query, you can apply for a bursary by clicking this link, sure, what is your query, thank you for your response. we will aim to get back to you within 12-24 hours., lock in a 2 hour 1-1 tutoring lesson now.
If you're ready and keen to get started click the button below to book your first 2 hour 1-1 tutoring lesson with us. Connect with a tutor from a university of your choice in minutes. (Use FAST5 to get 5% Off!)
River Tees Example

It also provides an example of how river basins can be managed against flooding.

River Tees Catchment factors
Physical (natural Factors) The River Tees catchment is located in the north east of England. It has three main rivers, the River Tees, the River Skerne and the River Leven. The River Tees drains the eastern slopes of Cross Fell in the Pennines and flows eastward to the North Sea. The length of the channel from source to sea is approximately 160 kilometres. The River Tees rises on the slopes of Cross Fell at a height of 893metres. The area receives over 2,000 millimetres of rain each year. The rainfall reaches the river quickly because the slopes are steep and very little water can infiltrate due to the impermeable rocks and saturated peat. After heavy rainfall or when the snow melts in the spring the river level can rise quickly and the water also flows downstream quickly. This can cause the ‘Tees roll’ or ‘Tees wave’, when the river level may rise as much as a metre in 15 minutes. There is a long history of flooding along the River Tees, especially in its lower course.
The catchment has areas with distinctly different characteristics. The rivers in the Upper Tees have steep channel gradients and valley sides. In the mid-catchment, the valley widens out and channel slopes become much gentler. The lower catchment is close to sea level and predominantly tidal in nature.
Human Factors
The Tees Barrage forms an artificial barrier between the Tees Estuary and the upstream catchment. This helps maintain water levels for amenity purposes and eliminates tidal effects further upstream. Land use in the west of the area is mainly moorland and pasture. On the lower slopes and middle catchment the land use changes to a greater amount of pasture and woodland.
To the east land use is mainly arable farmland interspersed with large built up areas, including Middlesbrough and Stockton-on-Tees. Approximately 687,000 people live within the catchment area concentrated in the main towns of Darlington, Hartlepool, Middlesbrough, Redcar and Cleveland, and Stockton-on-Tees. There are also large industrial areas such as the ICI chemical works. Flood damage can run into hundreds of thousands of pounds. There is also a huge demand for water for use in homes, industry and agriculture.
Flood events along the River Tees “ The Tees catchment has a long history of flooding with reported flooding dating back over 400 years. Over the years, a number of engineering schemes have been implemented to reduce the risk of flooding in the catchment. At present over 9,600 properties including some key infrastructure buildings are at risk of flooding (not taking into account defences) during the one per cent flood event. In the future due to climate change this number is expected to increase to 11,230 .” David Dangerfield, Director – Yorkshire and North East Environment Agency Tees Catchment Flood Management Plan
Historic events On the night of 16/17th November 1771 the North East of England suffered some of the worst and most destructive flooding on record. The river Tees burst its banks in a number of places with Yarm being one of the areas worst affected. At the height of the flood some stretches of Yarm High Street were submerged in 20 feet of water. The floods were caused by a sudden thaw of the ice in upper Teesdale, and a cloud burst over the Pennines. The rain began in the early hours of Saturday morning and continued heavily throughout the day and night without a break. On the main street alone, six houses were completely destroyed and many more left uninhabitable. The Shambles was washed away and the town’s main church was also damaged. ‘The pews were upturned and tossed about, the pulpit was overturned and several windows were shattered’. Nine of the townspeople lost their lives in the floods. In one home alone where three people were staying, two drowned, and one survived by hanging from the top of a window for nineteen hours with floodwater up to his chin. Source The river flooded again in 1995 and 2015. Within the Tees catchment the main consequences of flooding occur in the urban areas of the catchment. In total there are almost 8,500 residential properties and over 1,200 commercial properties at risk of flooding. This means almost three per cent of the catchment population is at risk from the one per cent flood event.This means that flood management is definitely needed in this catchment

Above - Properties at risk of flooding on River Tees
Flood Management along the Tees
The Flood defence scheme in Yarm Since the 1995 flood event a new flood defence scheme costing £2.1 million has been built. They have also used improved flood warning systems which liaise with the Meteorological Office, police and other emergency services. New development has discouraged building on low-lying and flood-prone land (land is used for activities not damaged by inundation e.g. playing fields, parks, urban forests/walks etc.) In the Yarm area the Environment Agency have;
Put in Reinforced concrete walls with metal flood gates for access by people and vehicles Used Earth Embankments Put in Gabions to protect walls and embankments from erosion Included Fishing platforms, street lighting and replanting to improve the environment Kept building materials in keeping with existing architecture
Cow Green Reservoir

NEXT TOPIC - Hydrographs
©2015 Cool Geography
- Copyright Policy
- Privacy & Cookies
- Testimonials
- Feedback & support


welcome to the geography portal!
- 0 Shopping Cart
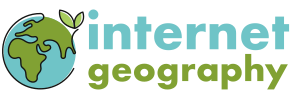
Managing the Mekong River – A transboundary water issue
Educas GCSE Geography > Case Studies > Managing the Mekong River – A transboundary water issue
The Mekong River, located in southeast Asia, is the twelfth longest river in the world. Six countries share the river and its tributaries. The source of the river is in China. The river flows southeast to its mouth in the South China Sea in Vietnam. The boundary of the river basin is shown in the map below.
The Mekong River has traditionally experienced seasonal changes in discharge. During the monsoon season, extensive flooding has occurred along the course of the river. Outside of the monsoon season, the region has experienced significant droughts.
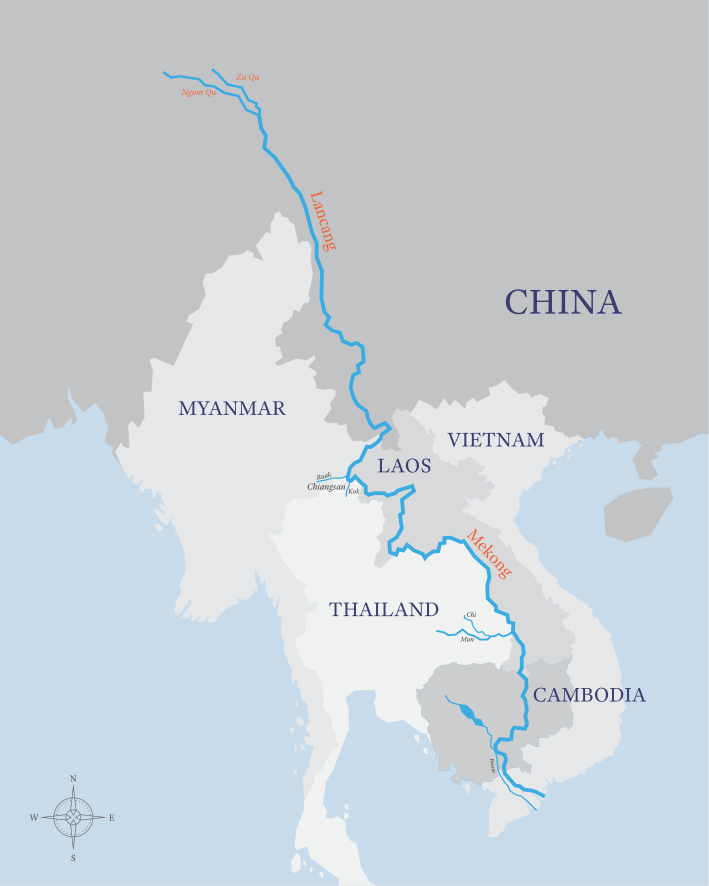
The Mekong River
What are the impacts of building dams on the Mekon River in China?
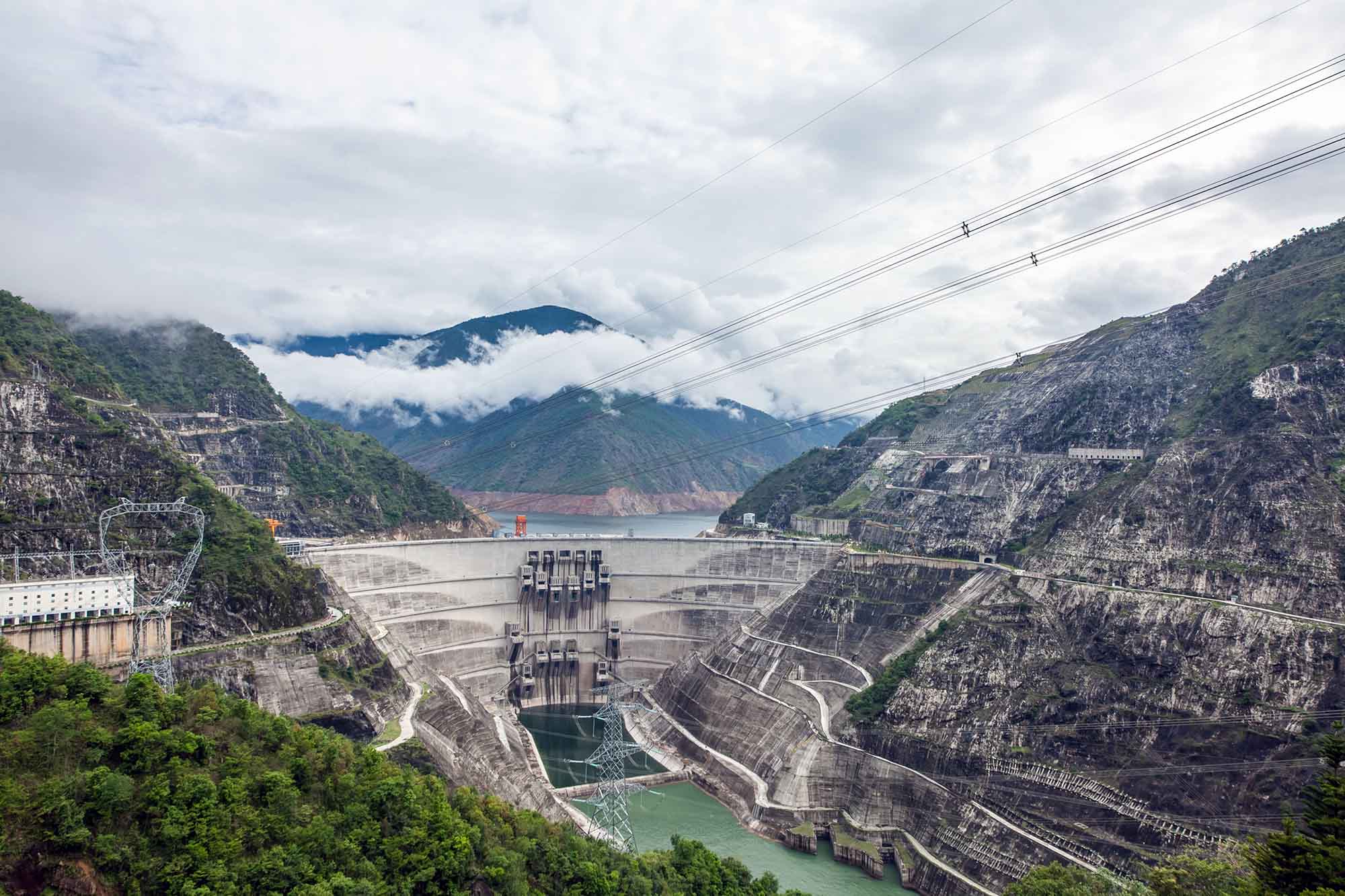
The Xiaowan Dam on The Mekong River in China
Along the course of the River Mekong, each country relies on the river for water supply and food. For example, in Cambodia, fishermen catch around 2 million tonnes of fish a year. Also, flooding during the monsoon season deposits fertile sediment onto the flood plain , which supports 80% of rice production, which depends on these floods. However, constructing dams along the river in China has interfered with the river’s flow, leading to reduced flooding and lower fish stocks. Additionally, the reduced flow of the river has led to sediment banks forming downstream, which has led to larger boats, such as ferries, running aground.
The effects of dam-building projects in China include:
- Land has been flooded to form reservoirs behind dams, leading to the loss of land farmland and the displacement of people (over 200,000 had to be moved when the Xayaburi Dam in Laos was being built between 2010-2019);
- In September 2015, heavy monsoon rain led to dangerously high water levels behind a Chinese-built HEP Nuozhadu Damdam. Water was released to avoid the collapse of the dam, leading to homes being flooded, affecting 1,571 families;
- Cheap hydro-electric power has supported the growth of China’s economy;
- More people and industry have a safe and reliable water supply;
- Water supply is available throughout the year in areas that previously suffered from seasonal drought;
- Jobs in construction and hydroelectric power have provided opportunities for people in China.
The effects of dam-building projects in the lower river basin (Cambodia) include:
- Reduced water levels and river velocity cause increased deposition , which has led to the formation of sandbanks that impede navigation;
- Fish stocks have been reduced, leading to a drop in income for fishermen;
- Monsoon floods are much smaller, meaning less sediment is deposited, reducing the fertility of valuable agricultural land used for rice farming;
- In 2019, fishermen in the Lower Mekon Basin blamed the combination of low monsoon rainfall and the opening of the Xayburi dam on its most severe drought in 100 years;
- Dams are evening the river’s flow, reducing the flood’s size. This harms fishing as the seasonal rise and fall in the river flow is required to spread out fish into lakes and ponds on the flood plain where they are caught.
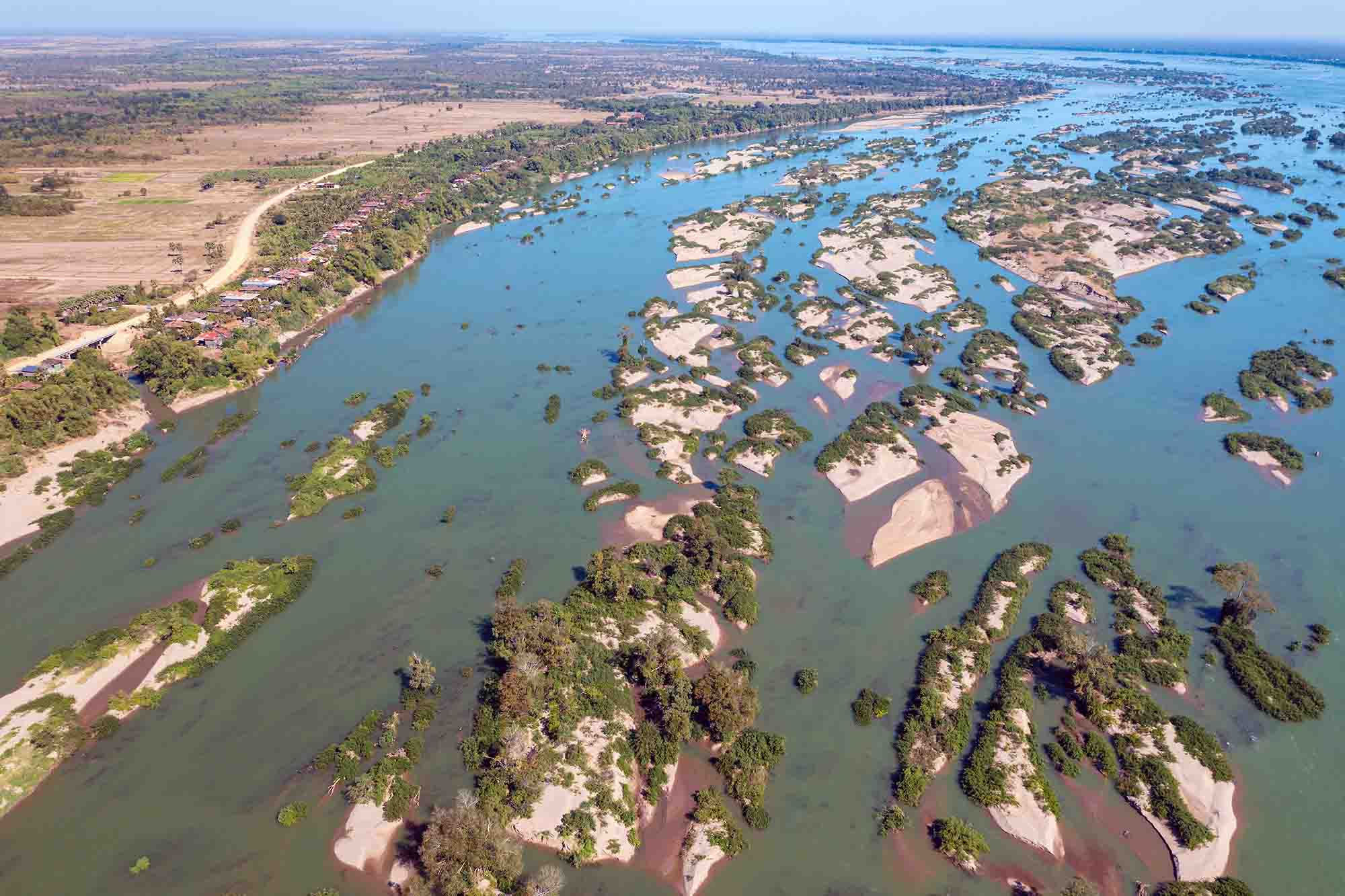
Sand bars and islands on the Mekong River in Cambodia formed by deposition due to reduced discharge.
Dams on international rivers, like the Mekong, can create conflict between the countries dependent on the water. Constructing a dam upstream alters the flow downstream. As more water is used in China, less arrives in Cambodia, risking the rice harvest.
What strategies are being used in Cambodia to reduce flood and drought impact?
The Cambodian government is considering a range of options to reduce the risk of flooding and drought:
- Collecting flood data and issuing forecasts through a flood control centre
- Risk assessing flooding in each community
- Providing advice to communities on how to protect households from flooding
- Constructing defences such as flood walls and embankments
- Building dams to control the flow of water
- Improving planning so homes are not constructed on floodplains
- Coordinating with neighbouring countries about the management of the river
- Run an annual flood conference with neighbouring countries
- Support neighbouring countries with aid during extreme events
Geography and Seasonal Patterns
The Mekong River, the twelfth longest in the world, flows through six Southeast Asian countries, from China to the South China Sea in Vietnam. It experiences seasonal changes, with monsoon-induced flooding and significant droughts outside the monsoon season.
Impact of Dams in China
Dam construction on the Mekong in China has reduced flooding and lowered fish stocks in downstream countries. Over 200,000 people were displaced due to the Xayaburi Dam in Laos, and water released from a Chinese dam in 2015 flooded homes, affecting 1,571 families.
Economic and Environmental Consequences
The dams have supported China’s economic growth and provided reliable water supply and hydroelectric jobs. However, they have caused land loss, sediment buildup, impeded navigation, and decreased fish stocks, affecting agriculture and fishing in Cambodia.
Reduced Fertility and Navigation Issues
In the lower Mekong basin, reduced water levels have led to sediment accumulation and the formation of sandbanks, hampering navigation. The decrease in monsoon floods has also reduced agricultural land fertility, which is crucial for rice farming.
Transboundary Conflicts
Dam construction alters the river flow, leading to conflicts between countries. As China uses more water, downstream countries like Cambodia face risks to their rice harvests due to reduced water flow.
Flood and Drought Management in Cambodia
Cambodia is adopting strategies to mitigate flood and drought impacts, including flood data collection, community risk assessments, construction of defences, improved planning, and international cooperation with neighbouring countries.
Test Yourself
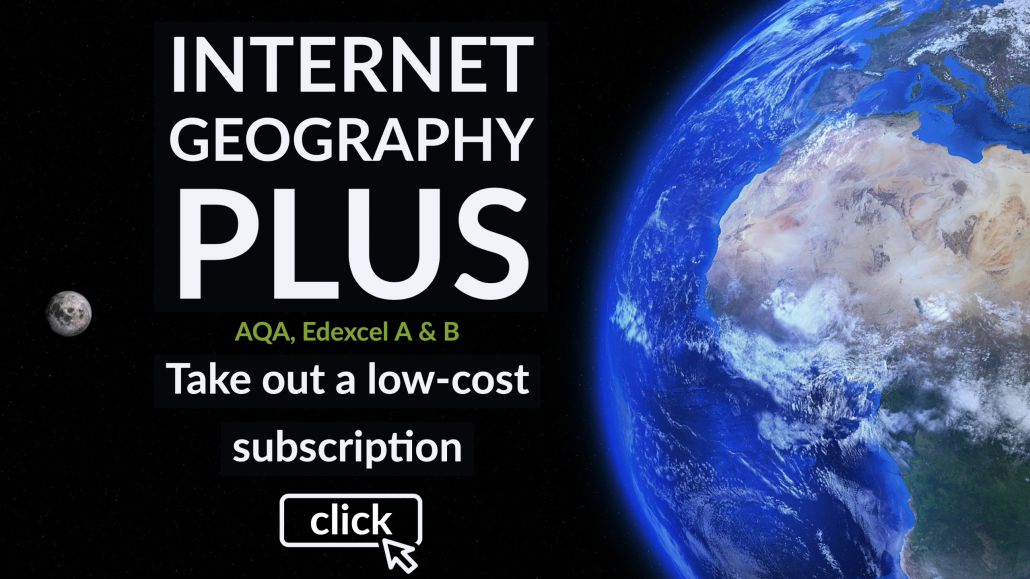
Premium Resources
Please support internet geography.
If you've found the resources on this page useful please consider making a secure donation via PayPal to support the development of the site. The site is self-funded and your support is really appreciated.
Share this:
- Click to share on Twitter (Opens in new window)
- Click to share on Facebook (Opens in new window)
- Click to share on Pinterest (Opens in new window)
- Click to email a link to a friend (Opens in new window)
- Click to share on WhatsApp (Opens in new window)
- Click to print (Opens in new window)
If you've found the resources on this site useful please consider making a secure donation via PayPal to support the development of the site. The site is self-funded and your support is really appreciated.
Search Internet Geography
Top posts and pages.

Latest Blog Entries

Pin It on Pinterest
- Click to share
- Print Friendly

IMAGES
VIDEO
COMMENTS
The River Severn Case Study - landforms of erosion and deposition. The River Severn (Afon Hafren) is the UK's longest river at 354 km (220 miles) long. The upper, middle and lower course of the River Severn. The source of the River Severn is on the slopes of Plynlimon (the highest point of the Cambrian Mountains) in mid-Wales at around 600 ...
Case study - River Tees. The River Tees is located in the north of England. The. source. of the River Tees is located in the Pennines and it flows east to its. mouth. where the river joins the ...
River landforms: a case study of the River Severn. The Severn is the UK's longest river: in this unit you'll use online map tools and images to investigate the landforms from the Severn's source to its mouth and understand the physical processes that created them. Try the quiz to see how much you know about the river features created by ...
River Case Study: The River Wye. The Wye River is over 150 miles (120 km) long, it is the 5th longest river in the UK and descends over 700m along its course. Both the Wye and Severn rise, within a few miles of each other, in the foothills of the Cambrian Mountains in Plynlimon, Wales. The Wye is a Site of Special Scientific Interest, a Special ...
GCSE; OCR; River landforms - OCR Case study - river basin - River Tees. Erosional landforms include V-shaped valleys, interlocking spurs, waterfalls and gorges. Meanders and oxbow lakes use ...
River management: a case study of the River Severn. In this unit you'll compare hard and soft engineering methods for managing the flood hazard on the River Severn. Then try the quiz to see how much you know about river management and measures put in place to help manage the risk of floods.
This work by PMT Education is licensed under CC BY-NC-ND 4.0. In other parts of the river - closer to Yarm near the mouth of the river - these large meanders have formed ox-bow lakes. Yarm is important to the River Tees because it tends to be the location of any flooding. Over time, this has helped to build the river's levees here.
Learn about and revise river landforms, whether created through erosion or deposition, with GCSE Bitesize Geography (Edexcel). ... Edexcel Case study - river landforms: River Tees.
Case study of river landforms on the River TeesThis is the forty-sixth video for the AQA GCSE 9-1 Geography course, and the thirteenth video of the Physical...
AQA GCSE Geography Physical Landscapes in the UK Case Study: River Tees. The River Tees, located in Northern England, flows from its source at Cross Fell in the Pennines travelling 130 km until it reaches its mouth at Teesside, where it meets the North Sea. With a drainage basin spanning 1,800 km², the River Tees undergoes various processes ...
We estimate a global river storage mean (± monthly variability) of 2,246 ± 505 km3 and a global continental flow of 37,411 ± 7,816 km3 yr−1. Our global river water storage time series ...
River Case Study: The Mississippi. - Length - 3,800 km. - Width - Flood Plain is 200 km wide at its widest point. - Releases 600 million tonnes of sediment of load each year (into the sea and along its course) - Flows through 10 states. - Carries 13% of all freight traffic in the USA.
Physical (natural Factors) The River Tees catchment is located in the north east of England. It has three main rivers, the River Tees, the River Skerne and the River Leven. The River Tees drains the eastern slopes of Cross Fell in the Pennines and flows eastward to the North Sea. The length of the channel from source to sea is approximately 160 ...
Trans evaporation process/albedo (Snow cover has reduced in the Elk Mountains, Colorado) Dust from industry has led to greater levels of trans-evaporation from glaciers. 22 Transbasin diversion projects (Pipe-works) have led to the Colorado flowing at 50% of its traditional capacity. Study with Quizlet and memorize flashcards containing terms ...
welcome to the geography portal! On this page you will find an interactive map with some of the best case studies and examples for A-Level - with fact files, links, further thinking, analysis and much more!
The Nile River Basin extends through ten countries and has two major tributaries, the White Nile and the Blue Nile. The White Nile begins at Lake Victoria and in the mountains of Burundi, Rwanda, and the Democratic Republic of Congo ("DRC"). The Blue Nile is composed of waters from Ethiopia, Eritrea, and Sudan. The Blue and White Nile meet at.
A-Level Geography: River Exe Case Study (Water and Carbon) 12 terms. Alfie_Rumsey4. Preview. A Level Geography- River Exe. 15 terms. abbieks. ... Amazon - Case Study for AQA A-Level Geography. 10 terms. Gubfree. Preview. The River Exe case Study, Water and Carbon. 11 terms. tomb283. Preview. biosphere ( soil ) 14 terms. izzy19286. Preview ...
indus climate. > Snowmelt occurs in the spring. > Monsoon season in summer brings precipitation. > Dry season from October to March. indus geology/soil. > River basin is mostly igneous and metamorphic rocks - tends to be impermeable. > River can swell in volume during monsoon season. Study with Quizlet and memorize flashcards containing terms ...
From 1950 to 2012, the Indus River basin in Pakistan witnessed 22 significant floods. These calamities resulted in the unfortunate loss of over 9,300 lives, impacted upwards of 10,000 villages, and led to direct economic setbacks of roughly $20 billion. The 2010 floods stand out as the most detrimental in Pakistan's history.
Recreational, such as - canoeing, kayaking, rock climbing. agriculture - with water used from river for irrigation. limestone quarrying. fishing. Study with Quizlet and memorize flashcards containing terms like Where is the source of the river wye located?, What is the length of the river wye?, Where is the mouth of the river located? and more.
The Mekong River, located in southeast Asia, is the twelfth longest river in the world. Six countries share the river and its tributaries. The source of the river is in China. The river flows southeast to its mouth in the South China Sea in Vietnam. The boundary of the river basin is shown in the map below.