Reimagining Design with Nature: ecological urbanism in Moscow
- Reflective Essay
- Published: 10 September 2019
- Volume 1 , pages 233–247, ( 2019 )

Cite this article
- Brian Mark Evans ORCID: orcid.org/0000-0003-1420-1682 1
978 Accesses
2 Citations
Explore all metrics
The twenty-first century is the era when populations of cities will exceed rural communities for the first time in human history. The population growth of cities in many countries, including those in transition from planned to market economies, is putting considerable strain on ecological and natural resources. This paper examines four central issues: (a) the challenges and opportunities presented through working in jurisdictions where there are no official or established methods in place to guide regional, ecological and landscape planning and design; (b) the experience of the author’s practice—Gillespies LLP—in addressing these challenges using techniques and methods inspired by McHarg in Design with Nature in the Russian Federation in the first decade of the twenty-first century; (c) the augmentation of methods derived from Design with Nature in reference to innovations in technology since its publication and the contribution that the art of landscape painters can make to landscape analysis and interpretation; and (d) the application of this experience to the international competition and colloquium for the expansion of Moscow. The text concludes with a comment on how the application of this learning and methodological development to landscape and ecological planning and design was judged to be a central tenant of the winning design. Finally, a concluding section reflects on lessons learned and conclusions drawn.
This is a preview of subscription content, log in via an institution to check access.
Access this article
Price includes VAT (Russian Federation)
Instant access to the full article PDF.
Rent this article via DeepDyve
Institutional subscriptions
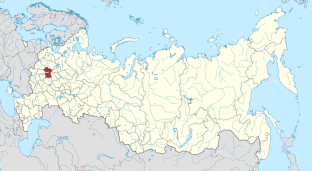
Similar content being viewed by others
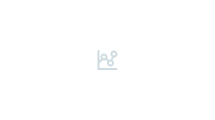
The politics of designing with nature: reflections from New Orleans and Dhaka
Acknowledgements
The landscape team from Gillespies Glasgow Studio (Steve Nelson, Graeme Pert, Joanne Walker, Rory Wilson and Chris Swan) led by the author and all our collaborators in the Capital Cities Planning Group.
Author information
Authors and affiliations.
Mackintosh School of Architecture, The Glasgow School of Art, 167 Renfrew Street, Glasgow, G3 6BY, UK
Brian Mark Evans
You can also search for this author in PubMed Google Scholar
Corresponding author
Correspondence to Brian Mark Evans .
Rights and permissions
Reprints and permissions
About this article
Evans, B.M. Reimagining Design with Nature: ecological urbanism in Moscow. Socio Ecol Pract Res 1 , 233–247 (2019). https://doi.org/10.1007/s42532-019-00031-5
Download citation
Received : 17 March 2019
Accepted : 13 August 2019
Published : 10 September 2019
Issue Date : October 2019
DOI : https://doi.org/10.1007/s42532-019-00031-5
Share this article
Anyone you share the following link with will be able to read this content:
Sorry, a shareable link is not currently available for this article.
Provided by the Springer Nature SharedIt content-sharing initiative
- Design With Nature
- Find a journal
- Publish with us
- Track your research
Thank you for visiting nature.com. You are using a browser version with limited support for CSS. To obtain the best experience, we recommend you use a more up to date browser (or turn off compatibility mode in Internet Explorer). In the meantime, to ensure continued support, we are displaying the site without styles and JavaScript.
- View all journals
- Explore content
- About the journal
- Publish with us
- Sign up for alerts
Latest science news, discoveries and analysis
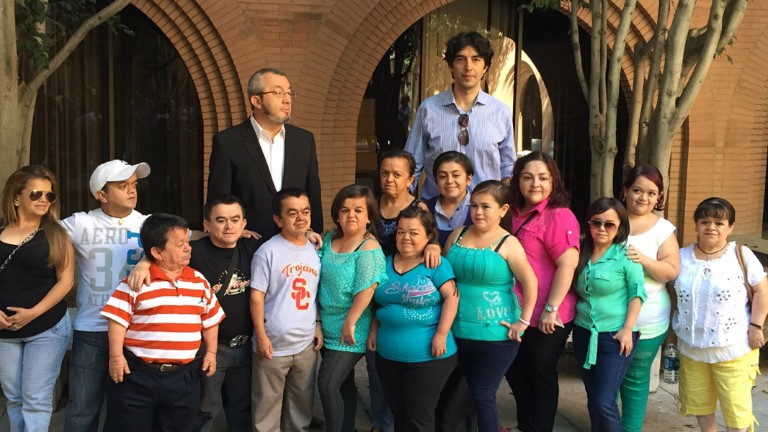
Could a rare mutation that causes dwarfism also slow ageing?
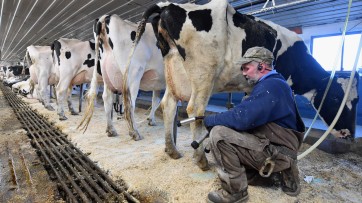
Bird flu in US cows: is the milk supply safe?
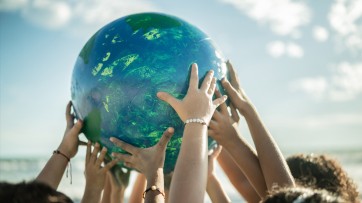
Future of Humanity Institute shuts: what's next for ‘deep future’ research?
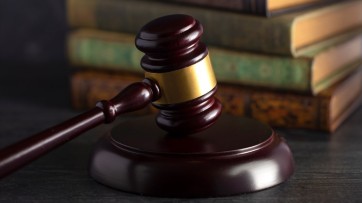
Judge dismisses superconductivity physicist’s lawsuit against university
Nih pay raise for postdocs and phd students could have us ripple effect, hello puffins, goodbye belugas: changing arctic fjord hints at our climate future, china's moon atlas is the most detailed ever made, ‘shut up and calculate’: how einstein lost the battle to explain quantum reality, ecologists: don’t lose touch with the joy of fieldwork chris mantegna.
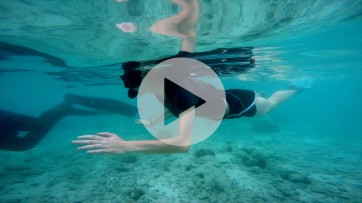
Should the Maldives be creating new land?
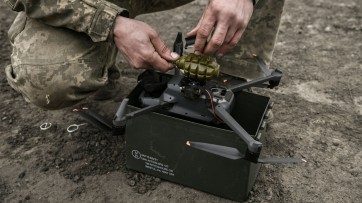
Lethal AI weapons are here: how can we control them?
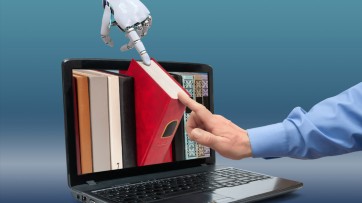
Algorithm ranks peer reviewers by reputation — but critics warn of bias
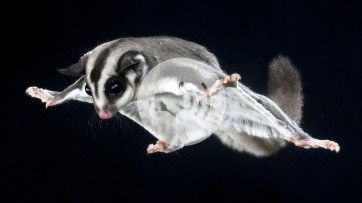
How gliding marsupials got their ‘wings’
Bird flu virus has been spreading in us cows for months, rna reveals, audio long read: why loneliness is bad for your health, nato is boosting ai and climate research as scientific diplomacy remains on ice, rat neurons repair mouse brains — and restore sense of smell.
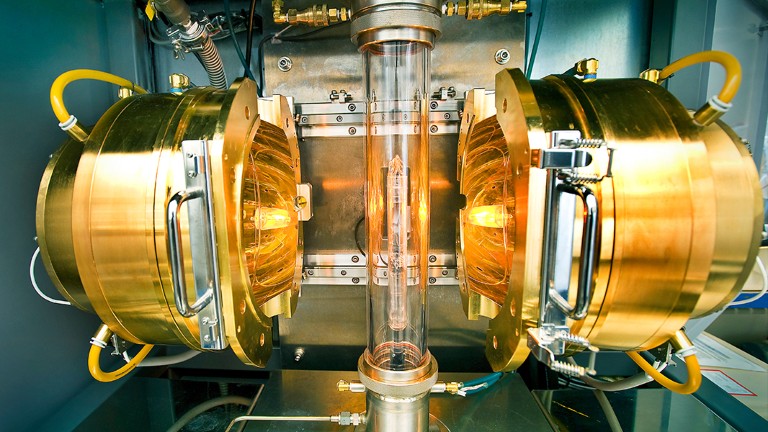
Retractions are part of science, but misconduct isn’t — lessons from a superconductivity lab
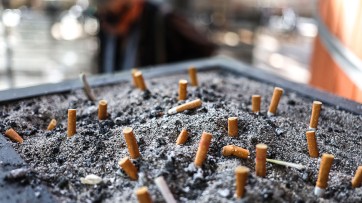
Any plan to make smoking obsolete is the right step
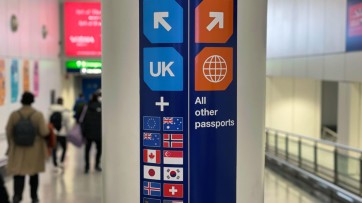
Citizenship privilege harms science
European ruling linking climate change to human rights could be a game changer — here’s how charlotte e. blattner, will ai accelerate or delay the race to net-zero emissions, current issue.
The Maldives is racing to create new land. Why are so many people concerned?
Surprise hybrid origins of a butterfly species, stripped-envelope supernova light curves argue for central engine activity, optical clocks at sea, research analysis.
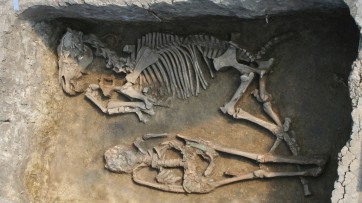
Ancient DNA traces family lines and political shifts in the Avar empire
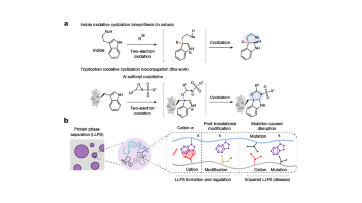
A chemical method for selective labelling of the key amino acid tryptophan
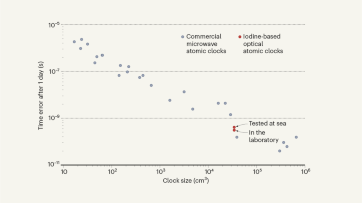
Robust optical clocks promise stable timing in a portable package
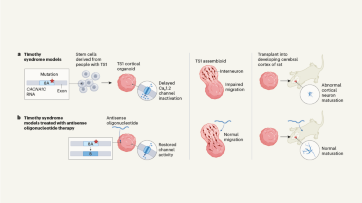
Targeting RNA opens therapeutic avenues for Timothy syndrome
Bioengineered ‘mini-colons’ shed light on cancer progression, galaxy found napping in the primordial universe, tumours form without genetic mutations, marsupial genomes reveal how a skin membrane for gliding evolved.
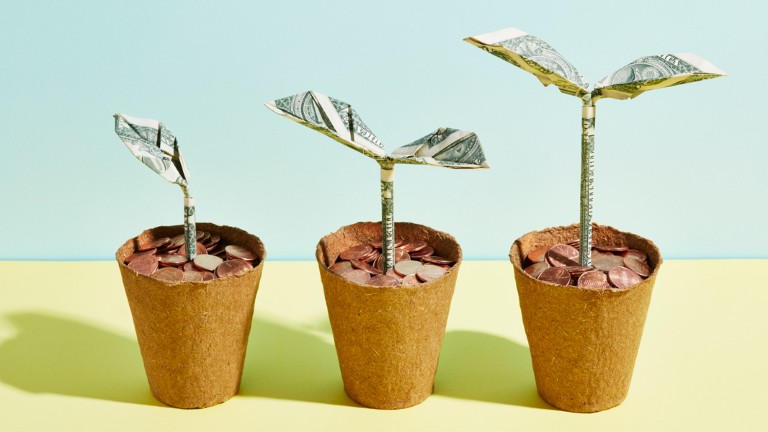
Scientists urged to collect royalties from the ‘magic money tree’
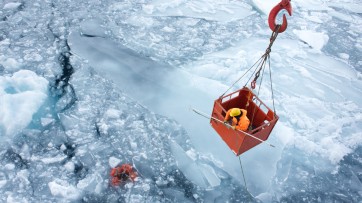
Breaking ice, and helicopter drops: winning photos of working scientists
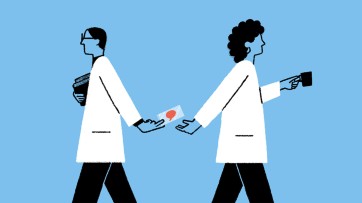
Shrouded in secrecy: how science is harmed by the bullying and harassment rumour mill
Want to make a difference try working at an environmental non-profit organization, how ground glass might save crops from drought on a caribbean island, books & culture.
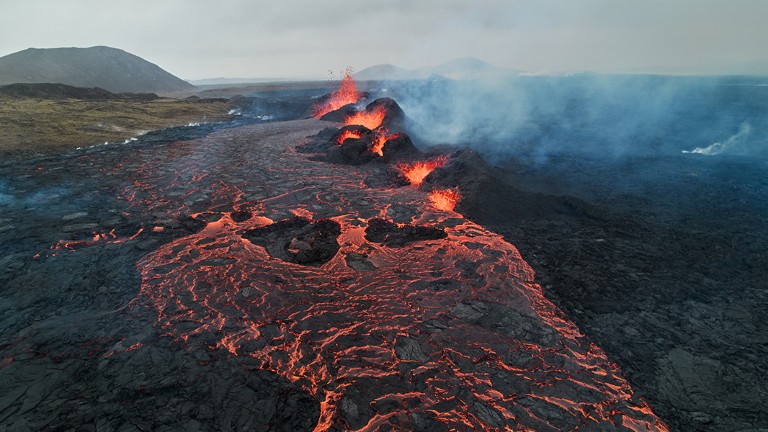
How volcanoes shaped our planet — and why we need to be ready for the next big eruption
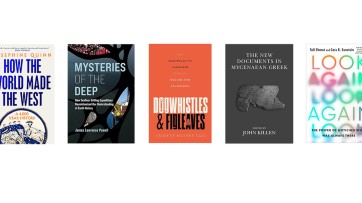
Dogwhistles, drilling and the roots of Western civilization: Books in brief
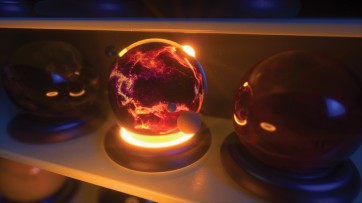
Cosmic rentals
Las borinqueñas remembers the forgotten puerto rican women who tested the first pill, dad always mows on summer saturday mornings, nature podcast.
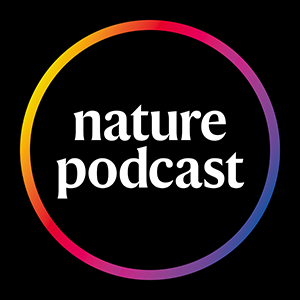
Latest videos
Nature briefing.
An essential round-up of science news, opinion and analysis, delivered to your inbox every weekday.

Quick links
- Explore articles by subject
- Guide to authors
- Editorial policies

Discover CALS
See how our current work and research is bringing new thinking and new solutions to some of today's biggest challenges.
- Agriculture
- Applied Economics
- Climate Change
- Communication
- Environment
- Global Development
- Health + Nutrition
Tracking down dairy’s greenhouse gas footprint

- Animal Science
The Nutrient Management Spear Program (NMSP) has been working hard to evaluate greenhouse gas (GHG) emissions on New York dairy farms. The dairy sustainability team, led by Research Associate Olivia Godber , helps farmers identify opportunities for improvement and discover which management practices have already successfully reduced their GHG emissions. Read on to find out more about the project and its importance, and to learn how to join.
What does it take to figure out the GHG emissions of dairy farms?
The NMSP team focuses on annual emissions of three primary greenhouse gasses (carbon dioxide, methane, and nitrous oxide). They collect data directly from farmers and analyze it with Cool Farm Tool – an online tool adopted by companies in many countries around the world– to estimate the farm’s overall greenhouse gas emissions, potential soil carbon sequestration, above ground biodiversity and water use for crop production. NMSP uses this tool to assess the emissions from different sources on dairy farms, including cows, cow manure, crops grown or purchased for feed, and the energy and fuel used.

"Completing the NMSP survey requires you to take a closer look at all aspects of your operation,” said Beth White, of Elkendale Farm. “Like a financial benchmark project, the final report allows you to assess your environmental footprint, find areas to improve and track your progress."
“ Cool Farm Tool has an active international development team which is continuously improving its software based on feedback from farmers, corporations and other users,” said Godber. “They try not to ask for too much data or effort from farmers, but they still ask for enough to provide meaningful results.”
What has the NMSP team discovered?
"We have Cool Farm Tool results from 48 New York dairy farms accounting for just under 15% of the annual milk production in the state – around 2 billion pounds of milk,” Godber reported.

Currently, the NMSP team is working with dairies in New York. Findings so far show that most of the dairies in the program are producing milk with emissions under 1.0 kilogram of carbon dioxide equivalents per kilogram of fat- and protein-corrected milk, though there is quite a range.
As more researchers in different regions work on similar GHG projects, there will be more opportunities for national and international comparisons of progress in dairy GHG emissions.
Why is it important to track on-farm GHG emissions?
“It is important for individual dairy farms to be able to know their farm footprint,” said Karl Czymmek , dairy climate leadership specialist for PRO-DAIRY . “With this information, they can more easily identify practices best suited to their farm’s resources which could help reduce greenhouse gas emissions.”
“In many ways, things farmers have always been trying to do – such as increase crop yield and milk production with fewer inputs – will continue to help reduce greenhouse gas emissions per gallon of milk,” said Czymmek.
“Tracking results over time through a greenhouse gas footprint tool helps farmers meet stewardship goals set by the dairy industry, food company targets and public policy objectives,” Czymmek continued.
We asked Beth White of Elkendale Farm – one of many farms in upstate New York that has participated in this program – for her perspective. "Completing the NMSP survey requires you to take a closer look at all aspects of your operation,” she said. “Like a financial benchmark project, the final report allows you to assess your environmental footprint, find areas to improve, and track your progress."
Where do we go from here?
“This project is key to helping us understand how New York dairy farms are performing in terms of their greenhouse gas emissions,” said Godber. “As the program continues to grow and farms with different characteristics and management practices contribute to the project, we can start to identify strengths and opportunities for reducing greenhouse gasses at the individual farm level and within the New York dairy industry as a whole.”
There are a few options that can be easier to integrate into annual practices than others. For example, by adding and retaining organic matter, cover cropping and reduced tillage can increase carbon sequestration and organic matter in the soil, lowering some GHG emissions.

“Manure treatment and storage is a significant source of GHG emissions on dairy farms that store manure to protect water quality,” Czymmek noted. He explained that there are a number of practices that can reduce manure emissions, including anaerobic digestion– a process that enhances the production of methane and captures it for use as renewable energy while conserving nutrients to grow crops that are used to feed the cows.
“Our goal is to grow the percentage of milk that completes a greenhouse gas footprint assessment each year to 50% or more,” said Quirine Ketterings , professor of nutrient management in agricultural ecosystems in the Department of Animal Science and lead for NMSP. “By doing that, we can learn from farms what is feasible and identify key performance indicators for improvements in future years.”
If you’re interested in learning more about the GHG emissions project, or other projects related to dairy sustainability or on-farm research, take a look at the NMSP website . If you are a farmer interested in estimating your greenhouse gas footprint, contact Olivia Godber with questions or a request to join the project.
Madeline Hanscom is a writer for the Nutrient Management Spear Program.
Keep Exploring

A team of mostly first-year students created Brewing CommuniTea, an Earl Grey tea ice cream with caramel swirls and buttery shortbread pieces inspired by Cornell’s theme year.
- Food Science

We openly share valuable knowledge.
Sign up for more insights, discoveries and solutions.
Main Navigation
- Contact NeurIPS
- Code of Ethics
- Code of Conduct
- Create Profile
- Journal To Conference Track
- Diversity & Inclusion
- Proceedings
- Future Meetings
- Exhibitor Information
- Privacy Policy
NeurIPS 2024
Conference Dates: (In person) 9 December - 15 December, 2024
Homepage: https://neurips.cc/Conferences/2024/
Call For Papers
Author notification: Sep 25, 2024
Camera-ready, poster, and video submission: Oct 30, 2024 AOE
Submit at: https://openreview.net/group?id=NeurIPS.cc/2024/Conference
The site will start accepting submissions on Apr 22, 2024
Subscribe to these and other dates on the 2024 dates page .
The Thirty-Eighth Annual Conference on Neural Information Processing Systems (NeurIPS 2024) is an interdisciplinary conference that brings together researchers in machine learning, neuroscience, statistics, optimization, computer vision, natural language processing, life sciences, natural sciences, social sciences, and other adjacent fields. We invite submissions presenting new and original research on topics including but not limited to the following:
- Applications (e.g., vision, language, speech and audio, Creative AI)
- Deep learning (e.g., architectures, generative models, optimization for deep networks, foundation models, LLMs)
- Evaluation (e.g., methodology, meta studies, replicability and validity, human-in-the-loop)
- General machine learning (supervised, unsupervised, online, active, etc.)
- Infrastructure (e.g., libraries, improved implementation and scalability, distributed solutions)
- Machine learning for sciences (e.g. climate, health, life sciences, physics, social sciences)
- Neuroscience and cognitive science (e.g., neural coding, brain-computer interfaces)
- Optimization (e.g., convex and non-convex, stochastic, robust)
- Probabilistic methods (e.g., variational inference, causal inference, Gaussian processes)
- Reinforcement learning (e.g., decision and control, planning, hierarchical RL, robotics)
- Social and economic aspects of machine learning (e.g., fairness, interpretability, human-AI interaction, privacy, safety, strategic behavior)
- Theory (e.g., control theory, learning theory, algorithmic game theory)
Machine learning is a rapidly evolving field, and so we welcome interdisciplinary submissions that do not fit neatly into existing categories.
Authors are asked to confirm that their submissions accord with the NeurIPS code of conduct .
Formatting instructions: All submissions must be in PDF format, and in a single PDF file include, in this order:
- The submitted paper
- Technical appendices that support the paper with additional proofs, derivations, or results
- The NeurIPS paper checklist
Other supplementary materials such as data and code can be uploaded as a ZIP file
The main text of a submitted paper is limited to nine content pages , including all figures and tables. Additional pages containing references don’t count as content pages. If your submission is accepted, you will be allowed an additional content page for the camera-ready version.
The main text and references may be followed by technical appendices, for which there is no page limit.
The maximum file size for a full submission, which includes technical appendices, is 50MB.
Authors are encouraged to submit a separate ZIP file that contains further supplementary material like data or source code, when applicable.
You must format your submission using the NeurIPS 2024 LaTeX style file which includes a “preprint” option for non-anonymous preprints posted online. Submissions that violate the NeurIPS style (e.g., by decreasing margins or font sizes) or page limits may be rejected without further review. Papers may be rejected without consideration of their merits if they fail to meet the submission requiremhttps://www.overleaf.com/read/kcffhyrygkqc#85f742ents, as described in this document.
Paper checklist: In order to improve the rigor and transparency of research submitted to and published at NeurIPS, authors are required to complete a paper checklist . The paper checklist is intended to help authors reflect on a wide variety of issues relating to responsible machine learning research, including reproducibility, transparency, research ethics, and societal impact. The checklist forms part of the paper submission, but does not count towards the page limit.
Supplementary material: While all technical appendices should be included as part of the main paper submission PDF, authors may submit up to 100MB of supplementary material, such as data, or source code in a ZIP format. Supplementary material should be material created by the authors that directly supports the submission content. Like submissions, supplementary material must be anonymized. Looking at supplementary material is at the discretion of the reviewers.
We encourage authors to upload their code and data as part of their supplementary material in order to help reviewers assess the quality of the work. Check the policy as well as code submission guidelines and templates for further details.
Use of Large Language Models (LLMs): We welcome authors to use any tool that is suitable for preparing high-quality papers and research. However, we ask authors to keep in mind two important criteria. First, we expect papers to fully describe their methodology, and any tool that is important to that methodology, including the use of LLMs, should be described also. For example, authors should mention tools (including LLMs) that were used for data processing or filtering, visualization, facilitating or running experiments, and proving theorems. It may also be advisable to describe the use of LLMs in implementing the method (if this corresponds to an important, original, or non-standard component of the approach). Second, authors are responsible for the entire content of the paper, including all text and figures, so while authors are welcome to use any tool they wish for writing the paper, they must ensure that all text is correct and original.
Double-blind reviewing: All submissions must be anonymized and may not contain any identifying information that may violate the double-blind reviewing policy. This policy applies to any supplementary or linked material as well, including code. If you are including links to any external material, it is your responsibility to guarantee anonymous browsing. Please do not include acknowledgements at submission time. If you need to cite one of your own papers, you should do so with adequate anonymization to preserve double-blind reviewing. For instance, write “In the previous work of Smith et al. [1]…” rather than “In our previous work [1]...”). If you need to cite one of your own papers that is in submission to NeurIPS and not available as a non-anonymous preprint, then include a copy of the cited anonymized submission in the supplementary material and write “Anonymous et al. [1] concurrently show...”). Any papers found to be violating this policy will be rejected.
OpenReview: We are using OpenReview to manage submissions. The reviews and author responses will not be public initially (but may be made public later, see below). As in previous years, submissions under review will be visible only to their assigned program committee. We will not be soliciting comments from the general public during the reviewing process. Anyone who plans to submit a paper as an author or a co-author will need to create (or update) their OpenReview profile by the full paper submission deadline. Your OpenReview profile can be edited by logging in and clicking on your name in https://openreview.net/ . This takes you to a URL "https://openreview.net/profile?id=~[Firstname]_[Lastname][n]" where the last part is your profile name, e.g., ~Wei_Zhang1. The OpenReview profiles must be up to date, with all publications by the authors, and their current affiliations. The easiest way to import publications is through DBLP but it is not required, see FAQ . Submissions without updated OpenReview profiles will be desk rejected. The information entered in the profile is critical for ensuring that conflicts of interest and reviewer matching are handled properly. Because of the rapid growth of NeurIPS, we request that all authors help with reviewing papers, if asked to do so. We need everyone’s help in maintaining the high scientific quality of NeurIPS.
Please be aware that OpenReview has a moderation policy for newly created profiles: New profiles created without an institutional email will go through a moderation process that can take up to two weeks. New profiles created with an institutional email will be activated automatically.
Venue home page: https://openreview.net/group?id=NeurIPS.cc/2024/Conference
If you have any questions, please refer to the FAQ: https://openreview.net/faq
Ethics review: Reviewers and ACs may flag submissions for ethics review . Flagged submissions will be sent to an ethics review committee for comments. Comments from ethics reviewers will be considered by the primary reviewers and AC as part of their deliberation. They will also be visible to authors, who will have an opportunity to respond. Ethics reviewers do not have the authority to reject papers, but in extreme cases papers may be rejected by the program chairs on ethical grounds, regardless of scientific quality or contribution.
Preprints: The existence of non-anonymous preprints (on arXiv or other online repositories, personal websites, social media) will not result in rejection. If you choose to use the NeurIPS style for the preprint version, you must use the “preprint” option rather than the “final” option. Reviewers will be instructed not to actively look for such preprints, but encountering them will not constitute a conflict of interest. Authors may submit anonymized work to NeurIPS that is already available as a preprint (e.g., on arXiv) without citing it. Note that public versions of the submission should not say "Under review at NeurIPS" or similar.
Dual submissions: Submissions that are substantially similar to papers that the authors have previously published or submitted in parallel to other peer-reviewed venues with proceedings or journals may not be submitted to NeurIPS. Papers previously presented at workshops are permitted, so long as they did not appear in a conference proceedings (e.g., CVPRW proceedings), a journal or a book. NeurIPS coordinates with other conferences to identify dual submissions. The NeurIPS policy on dual submissions applies for the entire duration of the reviewing process. Slicing contributions too thinly is discouraged. The reviewing process will treat any other submission by an overlapping set of authors as prior work. If publishing one would render the other too incremental, both may be rejected.
Anti-collusion: NeurIPS does not tolerate any collusion whereby authors secretly cooperate with reviewers, ACs or SACs to obtain favorable reviews.
Author responses: Authors will have one week to view and respond to initial reviews. Author responses may not contain any identifying information that may violate the double-blind reviewing policy. Authors may not submit revisions of their paper or supplemental material, but may post their responses as a discussion in OpenReview. This is to reduce the burden on authors to have to revise their paper in a rush during the short rebuttal period.
After the initial response period, authors will be able to respond to any further reviewer/AC questions and comments by posting on the submission’s forum page. The program chairs reserve the right to solicit additional reviews after the initial author response period. These reviews will become visible to the authors as they are added to OpenReview, and authors will have a chance to respond to them.
After the notification deadline, accepted and opted-in rejected papers will be made public and open for non-anonymous public commenting. Their anonymous reviews, meta-reviews, author responses and reviewer responses will also be made public. Authors of rejected papers will have two weeks after the notification deadline to opt in to make their deanonymized rejected papers public in OpenReview. These papers are not counted as NeurIPS publications and will be shown as rejected in OpenReview.
Publication of accepted submissions: Reviews, meta-reviews, and any discussion with the authors will be made public for accepted papers (but reviewer, area chair, and senior area chair identities will remain anonymous). Camera-ready papers will be due in advance of the conference. All camera-ready papers must include a funding disclosure . We strongly encourage accompanying code and data to be submitted with accepted papers when appropriate, as per the code submission policy . Authors will be allowed to make minor changes for a short period of time after the conference.
Contemporaneous Work: For the purpose of the reviewing process, papers that appeared online within two months of a submission will generally be considered "contemporaneous" in the sense that the submission will not be rejected on the basis of the comparison to contemporaneous work. Authors are still expected to cite and discuss contemporaneous work and perform empirical comparisons to the degree feasible. Any paper that influenced the submission is considered prior work and must be cited and discussed as such. Submissions that are very similar to contemporaneous work will undergo additional scrutiny to prevent cases of plagiarism and missing credit to prior work.
Plagiarism is prohibited by the NeurIPS Code of Conduct .
Other Tracks: Similarly to earlier years, we will host multiple tracks, such as datasets, competitions, tutorials as well as workshops, in addition to the main track for which this call for papers is intended. See the conference homepage for updates and calls for participation in these tracks.
Experiments: As in past years, the program chairs will be measuring the quality and effectiveness of the review process via randomized controlled experiments. All experiments are independently reviewed and approved by an Institutional Review Board (IRB).
Financial Aid: Each paper may designate up to one (1) NeurIPS.cc account email address of a corresponding student author who confirms that they would need the support to attend the conference, and agrees to volunteer if they get selected. To be considered for Financial the student will also need to fill out the Financial Aid application when it becomes available.

An official website of the United States government
The .gov means it’s official. Federal government websites often end in .gov or .mil. Before sharing sensitive information, make sure you’re on a federal government site.
The site is secure. The https:// ensures that you are connecting to the official website and that any information you provide is encrypted and transmitted securely.
- Publications
- Account settings
Preview improvements coming to the PMC website in October 2024. Learn More or Try it out now .
- Advanced Search
- Journal List

Observations of greenhouse gases as climate indicators
Lori bruhwiler.
1 NOAA Global Monitoring Laboratory, Boulder, CO USA
Sourish Basu
2 NASA Goddard Space Flight Center, Greenbelt, MD USA
3 Universities Space Research Association, Columbia, MD USA
James H. Butler
Abhishek chatterjee, ed dlugokencky, melissa a. kenney.
4 University of Minnesota Institute on the Environment, Saint Paul, MN USA
Allison McComiskey
5 Brookhaven National Laboratory, Environmental & Climate Sciences Department, Upton, NY USA

Stephen A. Montzka
Diane stanitski, associated data.
All data used in this study are freely available for download from https://www.esrl.noaa.gov/gmd/ .
Humans have significantly altered the energy balance of the Earth’s climate system mainly not only by extracting and burning fossil fuels but also by altering the biosphere and using halocarbons. The 3rd US National Climate Assessment pointed to a need for a system of indicators of climate and global change based on long-term data that could be used to support assessments and this led to the development of the National Climate Indicators System (NCIS). Here we identify a representative set of key atmospheric indicators of changes in atmospheric radiative forcing due to greenhouse gases (GHGs), and we evaluate atmospheric composition measurements, including non-CO 2 GHGs for use as climate change indicators in support of the US National Climate Assessment. GHG abundances and their changes over time can provide valuable information on the success of climate mitigation policies, as well as insights into possible carbon-climate feedback processes that may ultimately affect the success of those policies. To ensure that reliable information for assessing GHG emission changes can be provided on policy-relevant scales, expanded observational efforts are needed. Furthermore, the ability to detect trends resulting from changing emissions requires a commitment to supporting long-term observations. Long-term measurements of greenhouse gases, aerosols, and clouds and related climate indicators used with a dimming/brightening index could provide a foundation for quantifying forcing and its attribution and reducing error in existing indicators that do not account for complicated cloud processes.
Introduction
Humans have significantly perturbed the energy balance of the climate system mainly not only by extracting and burning fossil fuels but also through land use changes and halocarbon usage. Agriculture and waste treatment also release nitrous oxide and methane to the atmosphere, and both are strong greenhouse gases (GHGs). In response to anthropogenic GHG emissions, the global atmosphere has warmed over the last half century. By 2017, global mean surface temperature (GMST) was found to be about 1 °C above preindustrial values and increasing at 0.2 °C/decade (Allen et al. 2018 ). This trend is slightly higher (4–8%) if global mean surface air temperature (GSAT) rather than blended air and sea surface temperature is considered (Cowtan et al. 2015 ; Richardson et al. 2018 ).
Understanding changes in atmospheric composition is essential for estimating changes in the energy balance of the atmosphere. Quantifying exchanges of GHGs among the biosphere, oceans, and atmosphere as well as anthropogenic emissions can also be useful indicators of global change but are more difficult to quantify. Time-dependent emission estimates are crucial for evaluating success of emission reduction strategies, and identifying trends in natural exchange processes can further understanding of how natural processes respond to climate change.
The 3rd US National Climate Assessment pointed to a need for a system of indicators of climate and global change based on long-term data that could be used to support assessments (Corell et al. 2014 ). Kenney et al. ( 2014 , 2016 ) described development and prototypes of such a system, the National Climate Indicators System (NCIS), to establish a “system of physical, natural, and societal indicators that communicate and inform decisions about key aspects of the physical climate, climate impacts, vulnerabilities, and preparedness.” Its primary purpose is to support the sustained US National Climate Assessment (Buizer et al. 2013 ) by providing long-term information that is regularly updated and comparative to a baseline of change. Indicators are reference tools that can be constructed from measured data, modeled data, or an index and they can facilitate advancement and communication of scientific understanding, inform decision-making, and demonstrate progress in achieving management objectives. Indicators relevant to key US systems and sectors, such as indicators for the atmosphere including greenhouse gases, are required by the 1990 Global Change Research Act and are of broad concern to the US public (see Kenney et al. 2018 , this issue). A proof-of-concept indicator system was released by the US Global Change Research Program in 2015 ( http://www.globalchange.gov/explore/indicators ) and included Annual Greenhouse Gas Index (AGGI) and Atmospheric Carbon Dioxide indicators. This paper revisits and expands on the conceptual model for these indicators.
Observations of atmospheric greenhouse gases are useful climate indicators because they can be used to show the influence of human activities (e.g., emissions) on the climate system. Carbon dioxide (CO 2 ) is included in USGCRP Climate Indicators, while atmospheric burdens of methane (CH 4 ), nitrous oxide (N 2 O), synthetic ozone depleting gases, and other long-lived halogenated gases and their changes over time (including over the past 800,000 years) are included in the EPA’s set of key climate indicators. Both the EPA and the USGCRP emphasize the utility of climate indicators for communication about changing climate, environmental assessment, and informed decision-making. A related effort is the collection of Essential Climate Variables (ECVs) identified by the World Meteorological Organization (WMO, https://public.wmo.int/en/programmes/global-climate-observing-system/essential-climate-variables ). The criteria for ECVs include how critical a variable is for characterizing the changing climate system, and whether it is feasible and cost effective to observe that particular variable. The WMO also defines a set of climate monitoring principles that lay out needs for continuity and compatibility of data sets, data management, maintenance of long-term measurements, and focus on under-observed regions.
The objective of this paper is to identify a representative set of key atmospheric indicators of changes in atmospheric radiative forcing driven by greenhouse gases. In particular, we evaluate the use of atmospheric composition measurements as climate change indicators. We present a conceptual model of climate forcing used to identify potential indicators (Section 2 ), the proposed key indicators (Sections 3 , 4 , and 5 ), and research and indicator development opportunities (Section 6). Such indicators provide an overview of the climate forcing that causes the climate impacts and vulnerabilities, and, when tracked over time, can be a measure of large-scale mitigation response effectiveness.
Global surface temperature change is an essential indicator of changing climate, and climate change policies have targeted limits in global temperature increase relative to the pre-industrial era. The recognition of an approximate linear relationship between cumulative CO 2 emissions and temperature increase has allowed for estimation of remaining CO 2 emissions consistent with temperature change targets (Allen et al. 2009 ; Gregory et al. 2009 ; Matthews et al. 2009 ). Clearly, observational records from which global atmospheric burdens of CO 2 can be deduced provide an important link between cumulative emissions, which could be considered climate indicators, and global temperature change. Such observations allow confirmation of the linear relationship (Gillett et al. 2013 ) and could be useful for detecting departures from the linear relationship between cumulative emissions and temperature change if feedback processes become significant.
Non-CO 2 climate forcers or short-lived climate pollutants (SLCPs), such as CH 4 , N 2 O, and manufactured halogenated gases, complicate the simple connection between cumulative CO 2 emissions and temperature. Some SLCPs have lifetimes as short as a decade, implying that the effects of emissions at a particular time will not significantly influence temperatures decades later. On the other hand, some short-lived climate pollutants (SLCPs) are much more effective at trapping heat in the climate system than CO 2 so that reducing their emissions could provide short term benefit. It may also be economically advantageous to reduce SCLP emissions. For example, fugitive emissions of CH 4 from oil and gas production have economic value. For policy considerations, it is useful to have metrics that allow tradeoffs between CO 2 and SCLP emissions to be evaluated; however, incorporating SLCPs into cumulative carbon budgets is difficult due to the range and uncertainties of atmospheric lifetimes. Metrics designed to provide simple quantifications of the joint effects of SLCPs and CO 2 emissions on atmospheric radiative forcing range from looking at GWPs and GTPs over short- and long-time horizons, to using simple or medium complexity models to estimate climate-relevant equivalence between SLCP and CO 2 emissions (Tanaka et al. 2010 , 2019 ; Jenkins et al. 2018 ; Cain et al. 2019 ; Collins et al. 2020 ). Observational records of the global abundances of SLCPs are essential for computing and evaluating these metrics.
A conceptual model of climate forcing indicators
Kenney et al. ( 2018 , this issue) point to the need for a conceptual model to justify climate indicators. A conceptual model is communicated by a simple diagram that shows how a complex system functions, and measurable quantities that can be used to characterize important components of the system. Figure Figure1 1 shows a conceptual model of atmospheric composition climate indicators. Radiative forcing is the quantity that needs to be quantified over time, and in Section 4 , we describe how it can be estimated using observations of major GHGs and used as a climate indicator. We also propose that atmospheric concentrations of major non-CO 2 GHGs are indicators of changes in anthropogenic and natural sources and sinks. Atmospheric concentrations of GHGs underlay radiative forcing estimates and change over time due to anthropogenic emissions and natural processes that regulate exchanges of these gases among atmosphere, terrestrial biosphere, and oceans (Section 3 ). Many SLCPs are also removed in the atmosphere by chemical or photolytic loss.

Conceptual model for greenhouse gas (GHG) climate indicators. Indicators proposed in this study are atmospheric concentrations of non-CO 2 GHGs (CO 2 is already included in the National Climate Indicator System (NCIS)). Atmospheric GHG concentrations are directly observable and can be monitored over time. They can also be used to estimate radiative forcing. The Annual Greenhouse Gas Index (AGGI) is also included in the NCIS and quantifies the change in radiative forcing due to GHGs relative to 1990. Solid arrows indicate sources and sinks of atmospheric GHGs. Observations constraining these processes could be useful future climate indicators, but fluxes of GHGs are at present difficult to quantify at large spatial and temporal scales
Information about fluxes, if constrained by observable quantities and updated over time, could also be useful indicators (Section 5 ). Additional indicators could be identified to quantify observed changes in radiative fluxes at the Earth’s surface as a foundation for understanding the role of GHGs versus the impacts of changing aerosol and cloud distributions due to changes in both emissions (aerosol) and changes in atmospheric circulations (clouds) from natural and anthropogenic processes. These future climate indicators would be more difficult to quantify and would involve the use of models, process level measurements, and inventories of emissions. As such, use of these quantities as possible future climate indicators is still an active area of research.
Atmospheric measurements of radiatively active atmospheric constituents as climate indicators
In this section, we review the role of major GHGs in climate forcing. We discuss the role of emission inventories for anthropogenic sources and point out their value as climate indicators. Long-term observational records of major greenhouse gases are essential for evaluating bottom-up understanding of emissions and for evaluating the effectiveness of mitigation policies (Canadell et al. 2010 ). Figure Figure2 2 shows observations of major greenhouse gases and how they have changed over time.

Global average abundances of the major, well-mixed, long-lived greenhouse gases—carbon dioxide, methane, nitrous oxide, CFC-12, and CFC-11—from the NOAA global air sampling network are plotted since the beginning of 1979
The strong greenhouse gas sulfur hexafluoride (SF 6 ), a manufactured gas that is used as an electrical insulator, provides an instructive example of the importance of atmospheric measurements. Emissions of SF 6 reported to the UNFCCC (which includes only Annex 1 Countries) were found by Rigby et al. ( 2010 ) to be much lower than those derived using global atmospheric observations of SF 6 . Monitoring atmospheric concentrations of greenhouse gases is essential as an evidence-based, independent method for assessing the effects of strategies to reduce emissions.
Emission inventories: the “bottom-up” approach
The framework of using indicators to measure progress towards emission and sustainable development goals has long been employed by the emission inventory community. For example, the UNEP Economics and Trade Unit developed a standardized methodology for estimating GHG emissions from companies and other organizations (Thomas et al. 2000 ). The California Air Resources Board produces ongoing report of GHG emissions and economic indicators related to emissions (CARB 2020 ). Indicators in support of the UN’s sustainable development goals were described by Schmidt-Traub et al. ( 2015 ) and include indicators designed to measure progress towards achieving climate goals, such as energy efficiency and decarbonization strategies as well as quantifying emissions from industry, transportation, and agriculture.
Emission inventories are constructed by use of economic activity factors (e.g., number of gas wells, power plants, cows) combined with emission factors (emissions per unit emitter). This kind of approach is known as a “bottom-up” approach, and an updated discussion of how inventories are developed is given by Birdsey et al. ( 2018 ). Emission estimates are often produced on a country-wide basis and reported to the UNFCCC for use in policy decisions, although some emissions datasets are spatially disaggregated (e.g., EDGAR—Emissions Database for Global Atmospheric Research, Joint Research Center) (Olivier and Janssens-Maenhout 2012 ; Crippa et al. 2020 ). Bottom-up emission datasets can have considerable uncertainties, for example, they may not capture all processes leading to emissions. Emissions of CO 2 occur during combustion of fossil fuels and statistics for fossil fuel consumption are considered relatively, though not perfectly, well known. On the other hand, CH 4 emissions from fossil fuels result from unintentional leakage or venting during production, storage, and transport and are much more difficult to quantify. New extraction technologies have led to increased natural gas production, focusing attention on leakage from drilling, storage, and transport of fossil fuel (e.g., Alvarez et al. 2012 ; Peischl et al. 2015 ; Pétron et al. 2014 ). In particular, new sources of potential leakage were identified to explain higher emissions inferred from atmospheric observations compared to those estimated using bottom-up methods. Multi-decadal time series of greenhouse gas observations are essential for evaluating emission inventories; however, it is often difficult to use atmospheric concentration to attribute emissions to particular sources. For source attribution, bottom- up inventories are needed and therefore could be considered climate indicators as well. Ideally, bottom-up inventories should be spatially disaggregated so that they can be evaluated for consistency with top-down atmospheric concentration data. Atmospheric observations and emission inventories are complementary for our understanding of the processes and activities affecting climate forcing, and for devising effective and efficient ways to mitigate them (NAS 2018 ).
Carbon dioxide
Measurements of air trapped in ice cores and ongoing analysis of modern air samples show that global atmospheric CO 2 has increased from 280 ppm (parts per million of dry air, MacFarling Meure et al. 2006 ) in the pre-industrial atmosphere to over 413 ppm as of July 2020 ( http://www.esrl.noaa.gov/gmd/ccgg/trends/global.html ). This increase has been shown to be due to human activities, especially the burning of fossil fuels with small contributions from cement production (Tans 2009 ) and land use change. By burning fossil fuels, humans are accelerating the part of the natural geologic carbon cycle that transfers carbon in rocks and sediments to the atmosphere, processes with timescales of ten to hundreds of millennia. Since 1870, cumulative emissions of CO 2 have totaled 440 ± 20 PgC (Friedlingstein et al. 2019 ), while cumulative emissions of carbon from land use change since 1750 have been estimated at 205 ± 60 PgC ( Friedlingstein et al. 2019 ). Roughly half of anthropogenic emissions remain in the atmosphere with the remainder taken up by the oceans and natural terrestrial biosphere (Ciais et al. 2013 ). As of 2019, CO 2 accounts for 2/3 (2.076 Wm −2 out of 3.140 Wm −2 ) of the total anthropogenic climate forcing from long-lived gases (not including ozone, aerosols, and clouds) (Hofmann et al. 2006 updated at http://www.esrl.noaa.gov/gmd/aggi/ ).
Current CH 4 abundance in the atmosphere is unprecedented over at least the last 800,000 years (Loulergue et al. 2008 ), increasing by 160% since preindustrial times. Atmospheric network observations (mainly NOAA and the AGAGE network) show that global CH 4 increased rapidly until the late 1990s, leveled off during the early 2000s, and has begun to increase rapidly again since 2006 (Rigby et al. 2008 ; Dlugokencky et al. 2009 ). The cause of the renewed atmospheric increase is currently not well understood.
Estimates reported by Saunois et al. ( 2020 ) and Jackson et al. ( 2020 ) for anthropogenic emissions are 357 TgCH 4 year −1 (range 334–392 TgCH 4 year −1 ) for 2008–2017. Production of fossil fuels account for 30–35% of all anthropogenic emissions. Livestock, agriculture, and landfills and sewage account for another ~ 60%, with the remainder due to biomass and biofuel burning. A recent study suggested that emissions from fossil fuels and geologic emissions may be 20–60% higher than previously thought, requiring compensating reduction in microbial anthropogenic emissions (Schwietzke et al. 2016 ) in order to match observed global average CH 4 abundance and its growth. Natural emissions, mainly from wetlands and terrestrial aquatic systems, are thought to make up ~ 40% of global emissions. CH 4 has an atmospheric chemical sink that is in approximate balance with its sources, reaction with the hydroxyl radical (OH). All sinks, including small contributions from other reactions and a microbial soil sink, result in about a 10-year atmospheric lifetime of CH 4 . OH has an extremely short atmospheric residence time and is difficult to directly measure. Its global abundance is usually inferred using observations of methyl chloroform (CH 3 CCl 3 ), an anthropogenic gas for which emissions are thought to be well known and for which reaction with OH is the main atmospheric loss (e.g., Krol and Lelieveld 2003 ; Montzka et al. 2011 ).
CH 4 contributed 0.52 Wm −2 in 2019 to global total anthropogenic radiative forcing, about a quarter of that due to CO 2 (Hofmann et al. 2006 updated at http://www.esrl.noaa.gov/gmd/aggi/ ). Including carbon cycle-climate feedbacks, the global warming potential (GWP100) of CH 4 is 34; on a per mass basis, its emissions are 34 times more effective at trapping heat in the climate system than an equivalent emission of CO 2 over a 100-year time horizon (Myhre et al. 2013 ). Due to its atmospheric lifetime of about a decade, CH 4 has a significantly higher GWP20 (GWP over a 20-year time horizon) of 70. Global temperature change potential (GTP; Shine et al. 2005 ) is metric comparing the change in surface temperature due to emission pulses of non-CO 2 GHGs and CO 2 . This metric captures the relative impact of a non-CO 2 GHG at a particular time usually chosen for policy relevance. The GTP of CH 4 for 20- and 100-year horizons (including carbon cycle climate feedbacks) is 70 and 11 (Myhre et al. 2013 ).
Nitrous oxide
The atmospheric budget of nitrous oxide (N 2 O) is not well understood, and its abundance in the atmosphere is growing at a rate of 0.94 ppb year −1 (Dlugokencky, NOAA/GML, www.esrl.noaa.gov/gmd/ccgg/trends_n2o ). With an atmospheric lifetime of ~120 years (Davidson and Kanter 2014 ), it is destroyed mainly in the stratosphere by reaction with excited state atomic oxygen and photolysis. Global natural emissions (ocean upwelling and soil emissions) are thought to be 10–12 Tg N 2 O-N year −1 (Davidson and Kanter 2014 ).
Anthropogenic emissions are estimated at 5.2–5.5 TgN 2 O-N year −1 . About 66% of this is likely due to agriculture, mostly not only from use of industrially produced fertilizer but also from cultivation of nitrogen-fixing crops (Röckmann and Levin 2005 ; Park et al. 2012 ). Another 15% of total emissions come from fossil fuel combustion. Biomass burning contributes 11% of global emissions, with remaining emissions coming from other sources such as waste waters. Population growth nearly guarantees that N 2 O will continue to increase in the atmosphere since producing enough food to feed the growing human population will rely on fertilizer use (Hansen et al. 2017 ).
N 2 O has a GWP100 of 298 and an atmospheric lifetime of ~120 years (Davidson and Kanter 2014 ). In 2019, N 2 O contributed 0.2 Wm −2 to global anthropogenic radiative forcing, about a tenth of that due to CO 2 (Hofmann et al. 2006 , updated at http://www.esrl.noaa.gov/gmd/aggi/ ). The GWP20 of N 2 O is relatively close to its GWP100, 268. In terms of global temperature change potential, for N 2 O, GTP100 is 297 and GTP20 is 284. Note that in comparison to CH 4 , the variation among these metrics is small, a reflection of the long atmospheric N 2 O lifetime.
Halocarbons
Halogenated gases have been produced by industry in significant quantities since the mid-twentieth century to meet societal needs for refrigeration, air conditioning, insulation, solvents and fire protection. Measurements of air extracted from snow in Antarctica confirm that most of these halogenated chemicals (chlorofluorocarbons, hydrochlorofluorocarbons, hydrofluorocarbons, halons, and some chlorinated gases) were not present in the atmosphere in appreciable quantities before the mid-twentieth century.
Many industrially produced halogenated gases are resistant to destruction by natural processes. Once these chemicals escape from refrigerators or foams to the atmosphere, they persist for decades to centuries. They are powerful GHGs, with GWP100s that are up to 14,000 times larger than CO 2 . Atmospheric concentrations of halogenated gases are much lower (by 10 6 ) than that of CO 2 , so their contribution to climate forcing today is only about 17% as large as that from CO 2 .
The main means by which halocarbons are destroyed and removed from the atmosphere is by photolysis in the stratosphere leading to reactive forms of chlorine and bromine that deplete stratospheric ozone. This realization led to the 1987 Montreal Protocol on Substances that Deplete the Ozone Layer, and phase-out in the global production of these chemicals. Because halocarbons are also strong GHGs, the Montreal Protocol has been the most successful global climate treaty to date (Velders et al. 2007 ). Future warming from these compounds will depend on the effectiveness of controls on the next generation of substitutes for ozone-depleting compounds, namely the HFCs. The Kigali Amendment to the Montreal Protocol was agreed to in late 2016 to phase down and stabilize HFC use and associated climate warming but has not yet been ratified by all parties.
The annual greenhouse gas index
The effect of anthropogenic GHGs on the energy budget of the climate system is quantified using the concept of radiative forcing. The change in radiative forcing over time is a useful climate indicator because it is directly related to the surface temperature change.
Radiative forcing is defined as the change in net radiative flux (downward minus upward in Wm −2 ) at the tropopause due to a perturbation from an external driver, after allowing the stratosphere to come to radiative-dynamical equilibrium (Myhre et al. 2013 ). Radiative forcing is usually defined relative to 1750, the start of the industrial era. A net positive change results in warming of the climate system. External perturbations include atmospheric concentration changes due to anthropogenic emissions of greenhouse gases, changes in aerosols, and changes in albedo due to land use change. Clouds and water vapor are considered internal to the climate system, but changes in atmospheric temperature and relative humidity, possibly driven by changes in atmospheric composition, can affect cloudiness.
Changes in long-lived, well mixed greenhouse gases, such as CO 2 , CH 4 , N 2 O, and halogenated compounds such as CFCs make up the largest and most certain component of radiative forcing, because their concentrations and atmospheric growth rates can be accurately measured in the atmosphere. The effects of anthropogenic emissions on trace gas atmospheric concentrations and radiative forcing is also relatively well understood in comparison to, for example, the radiative forcing contribution from changing cloudiness and associated cloud-climate feedbacks.
An example of a radiative forcing climate indicator is the NOAA Annual Greenhouse Gas Index (AGGI), which uses long-term global observations of important greenhouse gases to calculate changes in radiative forcing relative to 1990 (the baseline year for the Kyoto Protocol). Total radiative forcing from these main greenhouse gases is calculated using IPCC-recommended expressions derived from atmospheric radiative transfer models to convert greenhouse gas concentration increases relative to 1750 to instantaneous radiative forcing (Ramaswamy et al. 2001 ). Feedbacks due to water vapor and ozone depletion are not considered in this calculation because they are less well quantified. Other spatially heterogeneous, short-lived, climate forcing agents, such as aerosols and tropospheric ozone, have uncertain global magnitudes and also are not considered in the calculation of the AGGI. Changes in radiative forcing before 1978 are derived from atmospheric measurements of CO 2 started in the late 1950s (Keeling 1958 ) and from measurements of CO 2 and other greenhouse gases in air trapped in snow and ice in Antarctica and Greenland (Etheridge et al. 1996 ; Butler et al. 1999 ).
The AGGI was introduced in 2006 based on measurements through 2004 (Hofmann et al. 2006 ) and has been updated annually. For 2019, the AGGI was 1.45, indicating that total direct radiative forcing of anthropogenic changes in long-lived greenhouse gases since 1750 has increased 45% since 1990.
Figure Figure3 3 shows radiative forcing for major GHGs and 15 minor long-lived halogenated gases. Many of these minor gases are also ozone-depleting gases and are regulated by the Montreal Protocol. CO 2 dominates the total forcing with CH 4 making up half of the remaining forcing and N 2 O and CFCs accounting for most of the remainder. Five major greenhouse gases (CO 2 , CH 4 , N 2 O, CFC-11, and CFC-12) account for about 96% of the direct radiative forcing by long-lived greenhouse gas increases since 1750. Note that increasing atmospheric CO 2 accounts for most of the growth in radiative forcing since 1990, about 80%.

Radiative forcing, relative to 1750, for long-lived greenhouse gases. The NOAA Annual Greenhouse Gas Index (AGGI), which is indexed to 1 for 1990, is shown on the right axis
Had the ozone-depleting gases not been regulated by the Montreal Protocol and its amendments, it is estimated that climate forcing in 2010 would have been as much as 0.3 W m −2 greater in 2010 (Velders et al. 2007 ), which is more than half of the increase in radiative forcing due to CO 2 alone since 1990. Of the ozone-depleting gases and their substitutes, the largest contributors to direct warming in 2019 were CFC-12, followed by CFC-11, HCFC-22, CFC-113, and HCFC-134a.
Potential climate indicators
Cloud climate feedbacks are difficult to quantify, and model predictions are highly uncertain, but understanding cloud-climate feedbacks and effects on the surface radiation budget is critical for quantifying the ultimate effect of greenhouse gases on climate. A potential climate indicator is global dimming/brightening (e.g., Long et al. 2009 ; Wild 2012 , 2009 ) which quantifies the change in downwelling solar radiation at the Earth’s surface through time, and is an integrated function of all climate forcers and feedbacks, thus providing the highest-level constraint on radiative forcing. Long-term measurements of greenhouse gases, aerosols, and clouds and related climate indicators used with a dimming/brightening index could provide a foundation for quantifying forcing and reducing error in existing indicators that do not account for complicated cloud processes.
Earth system models (ESMs) suggest that there is a near-linear relationship between cumulative emissions of CO 2 and changes in global surface air temperature (e.g., Allen et al. 2009 ; Gregory et al. 2009 ; Matthews et al. 2009 ). Gillet et al. ( 2013 ) showed that this emergent property from the ESMs, referred to as the transient climate response (TCRE), is consistent with both global temperature observations and estimates of cumulative emissions. The TCRE is frequently used to estimate cumulative emission limits for targeted global temperature increases. However, the slopes of the TCRE linear relationships vary among models due to differences in how carbon cycle feedbacks are represented in models (MacDougall et al. 2016 ; Friedlingstein et al. 2014 ). Observational constraints on carbon cycle feedbacks could help to constrain TCRE and lead to improvements in ESMs.
About half of all anthropogenic CO 2 emissions to date have been taken up by the oceans and terrestrial biosphere (Ciais et al. 2013 ). The size of this “carbon emission discount” will likely change in the future as more carbon accumulates in the oceans, as humans alter landscapes, as climate changes, and atmospheric composition continues to evolve. Efforts to limit global average temperature increases and hopefully avoid the most catastrophic consequences of climate change could be affected by how natural fluxes of carbon and nitrogen change in response to human activities and changing climate.
Coupled carbon cycle–climate models forced with scenarios for future emissions suggest that carbon cycle changes in response to anthropogenic climate change will likely be a positive (additive) feedback on climate, enhancing warming. The magnitude of the projected feedback is uncertain, and a wide range of future atmospheric carbon abundances is predicted by models (Friedlingstein et al. 2014 ). There are many possible processes that can result in positive carbon cycle feedbacks. A warmer climate can lead to increased fires and droughts, resulting in less uptake and storage of carbon by the terrestrial biosphere. Warming is expected to decrease carbon uptake in the tropics and mid-latitudes (a positive feedback), while in the high latitudes, a warmer climate may lead to increased productivity of the terrestrial biosphere and carbon uptake (a negative feedback), although this may be offset by increases in respiration and release of some of the vast amounts of carbon stored in soils and lakes. Increased atmospheric CO 2 may cause a fertilization effect on global vegetation leading to increased carbon storage, but the importance of this effect and the factors (e.g., nutrients) which could limit it are highly uncertain.
Land use change can directly affect climate since deforestation and agriculture alter carbon storage in soils and biomass. Fertilizer use also has impacts on the global nitrogen budget and may increase carbon storage while also affecting productivity in marine and freshwater environments as it is washed into marine environments. On the other hand, increased fertilizer use can not only result in increased food production but also increased emissions of the powerful greenhouse gas, N 2 O. Creation or drainage of wetlands can alter CH 4 emissions (Kolka et al. 2018 ). For example, drainage of wetlands can lead to lower CH 4 emissions but higher CO 2 emissions as a result of lower anerobic respiration and higher aerobic respiration.
Increasing atmospheric CO 2 also has implications for ocean waters (Cooley et al. 2018 ). As the partial pressure of CO 2 in the atmosphere increases, ocean carbon increases and waters become more acidic. Eventually, ocean waters may acidify enough to dissolve calcium carbonate (CaCO 3 ) shells of ocean organisms, possibly significantly perturbing ocean ecosystems and reducing productivity of ocean waters.
Frozen Arctic soils are another potentially important carbon cycle-climate feedback (Schuur et al. 2018 ). An estimated 1700 PgC is frozen in Arctic soils, and warming has proceeded in the Arctic faster than any other region. Current understanding is that ~130–160 PgC, primarily as CO 2 , could be released over the next century (Schuur et al. 2013 ). On an annual basis, Schuur et al. ( 2015 ) estimate that annual carbon releases, if at a constant rate, could be lower than annual fossil fuel emissions (~9 PgCyear −1 ), but comparable to land use change (0.9 PgCyear −1 ). A small percentage of Arctic soil carbon could be released as CH 4 , a more powerful greenhouse gas.
Understanding and identifying feedbacks between GHGs and climate, such as those mentioned above, at a variety of spatial and temporal scales is of first-order importance (Huntzinger et al. 2018 ). The ability to supply food to a growing global population could be threatened by changes in productivity of marine and land ecosystems. Efforts to reduce GHG emissions could also be made significantly less effective by carbon cycle feedbacks.
Disentangling changes in atmospheric composition that are due to human activities from those due to natural processes is a fundamental scientific challenge. Many GHGs are such as the CFCs, SF 6 , and other halocarbons, are manufactured and do not occur in nature, unlike CO 2 , CH 4 , and N 2 O which have complex sources and sinks that overlap in space and time. GHGs can have removal processes that vary over time, and accounting for these changes is also important when quantitatively estimating the change in radiative forcing due to emissions, especially for short-lived species.
A potential climate indicator that could be useful for tracking anthropogenic CO 2 emissions was introduced by Hofmann et al. ( 2006 ), who estimated the anthropogenic contribution to observed global average CO 2 mole fraction by subtracting the pre-industrial value of 280 ppm. They noted that the resulting anthropogenic CO 2 mole fraction could be fit to an exponential function with a doubling time of approximately 30 years, and suggested that this doubling time is linked with global population growth. Figure Figure4 4 shows an extension of the Hofmann et al. ( 2009 ) analysis for recent years using identical fit parameters. A clear trend away from the exponential growth curve can be seen after 2008 implying that growth in anthropogenic CO 2 is decelerating, possibly due to increased energy efficiency and slower economic growth for these years. Note that in 2015 and 2016, there was an uptick in CO 2 that is likely related to a strong El Niño that caused a net release of carbon from the terrestrial biosphere as well as economic growth. While this event illustrates the difficulty in separating anthropogenic and natural signals over short time periods as well as further disentangling the response of the land and the ocean components (Chatterjee et al. 2017 ), longer-term changes are driven almost exclusively by human activities (Tans 2009 ). It would clearly be ideal to be able to subtract a natural signal from the curves shown in Fig. 4 ; however, doing so requires either conclusions drawn from uncertain terrestrial and ocean carbon exchange models, or additional observational information.

(Top) De-seasonalized global average anthropogenic CO 2 (red), estimated by subtracting the pre- industrial value of 280 ppm. The black curve shows an exponential fit to observations using the fit parameters of Hofmann et al. ( 2009 ) that results in an emission doubling time of about 30 years. ( Bottom ) The difference between the exponential fit of Hofmann et al. ( 2009 ) and observed global average CO 2
Observations of trace species with budgets that are related to major GHGs can be helpful for discriminating between anthropogenic and natural contributions. For example, measurements of δ 13 C(CH 4 ) can be useful for partitioning emissions between thermogenic (fossil fuels and natural geologic sources) and natural and anthropogenic microbial sources (Schaefer et al. 2016 ; Nisbet et al. 2016 ). Radiocarbon ( 14 CO 2 and 14 CH 4 ) can be useful for identifying fossil fuel emissions since all of the 14 C in fossils fuels has decayed away over the millions of years it took to transform organic to fossil carbon (Graven 2015 ; Basu et al. 2020 ). Ethane (C 2 H 6 ) and other hydrocarbons can in theory be used to constrain CH 4 emissions from oil and gas production; however, the emission ratios must be well characterized over space and time (Peischl et al. 2015 ; Peischl et al. 2016 ). In addition, COS has been used to constrain uptake of CO 2 as a result of photosynthesis (Montzka et al. 2007 ). The use of measurements of related species to constrain GHG budgets is currently an active area of research that may lead to additional robust climate indicators.
Using remote sensing to monitor atmospheric composition
In situ observational approaches allow for high-quality measurements of atmospheric composition; however, they are limited in spatial and temporal coverage. Over the past decade, remote sensing of atmospheric constituents from satellites has emerged as a potential new technique to quantify the time evolution of atmospheric GHGs. Retrievals of column average abundances from remote sensors use emission or absorption spectra at the top of the atmosphere (TOA). Early GHG satellite instruments such as the Atmospheric Infrared Sounder (AIRS) and the Thermal Emission Sounder (TES) were primarily built for estimating air temperature and water vapor content, and GHG retrievals were incidental benefits from having TOA thermal infrared spectra. The resulting GHG estimates were primarily of the upper troposphere and stratosphere, which are inadequate for estimating surface fluxes.
Satellites such as SCanning Imaging Absorption SpectroMeter for Atmospheric CHartographY (SCIAMACHY), Greenhouse Gases Observing Satellite (GOSAT), TROPOspheric Monitoring Instrument (TROPOMI), and Orbiting Carbon Observatory (OCO) have been built specifically for estimating GHG mole fractions from space. These satellites are sensitive to short wave infrared (SWIR) radiation of sunlight reflected from the Earth’s surface, and provide estimates of the vertical column average CO 2 and CH 4 mole fraction including significant sensitivity to the lower troposphere. More such observing systems are planned for the near future, including the Geostationary Carbon Observatory (GeoCARB), MicroCarb, GOSAT-2, and CarbonSat. In theory, GHG estimates from these satellites could be used to track near-surface GHG mole fractions and thereby estimate surface processes behind their fluxes, including their response to climate variability. In practice, the accuracy requirements on satellite sensors of GHGs are daunting, since the signal in the total column that they need to detect is an order of magnitude smaller than the near-surface signal. As a result, small biases (~0.5 ppm for CO 2 and 1–2 ppb for CH 4 ) in total column estimates can significantly alter the inferred surface fluxes of the respective species and skew conclusions about their climate response. Current estimates of CO 2 and CH 4 from existing satellite instruments have spatially coherent biases which make it challenging to use them to track carbon climate feedbacks. However, significant progress has been made in this area over the past decade, and it is possible that over the next decade these biases will be further reduced to acceptable levels. Commercial and non-governmental organizations are also developing space-based observing systems with very high spatial resolution (1 km or less) for detecting methane leaks, especially from oil and gas operations (Crisp et al. 2018 , App. 5). Examples include GHGSat, MethaneSAT, and microsatellites planned by Bluefield Technologies. Observing systems such as these could provide essential improvements to our ability to quantify fugitive emissions of CH 4 from anthropogenic sources.
Conclusions
We propose that long-term observations of atmospheric greenhouse gases be considered as climate indicators, and we have presented a conceptual model showing the relationship between emissions, fluxes, concentration, and radiative forcing. Knowledge of the GHG atmospheric abundances and their change over time allows us to compute and track the associated radiative forcing, as is being done with the Annual Greenhouse Gas Index, for example. GHG abundances and their changes over time also provide valuable information on the success of climate mitigation policies and insights into possible carbon-climate feedback processes that may change the effectiveness of those policies. To ensure that reliable information for assessing GHG emission changes can be provided at need spatial and temporal scales, expanded observational efforts are needed. Furthermore, the ability to detect trends resulting from changing emissions requires a commitment to supporting long-term observations. Efforts to improve and continue bottom-up techniques are also be needed since the interplay of bottom-up and top-down approaches is vital to the success of understanding and mitigating climate change.
Acknowledgements
Data used in this study were collected by the NOAA Global Monitoring Laboratory which supported the work of Bruhwiler, Dlugokencky, Montzka, Butler, and Stanitski.
Author contribution
All authors contributed to the development of this paper by contributing text. The paper concept was developed by Kenney, Butler, and Stanitski. The original draft was prepared by Bruhwiler.
Bruhwiler, Butler, Montzka, Dlugencky, and Stanitski are employed by the NOAA Global Monitoring Laboratory. The work of Chatterjee was supported by funding from the NASA Grant/Cooperative Agreement NNX17AD69A and 80NSSC20K0006. Kenney was supported by the National Oceanic and Atmospheric Administration grants NA09NES4400006 and NA14NES4320003 (Cooperative Climate and Satellites-CICS) at the University of Maryland/ESSIC.
Data availability
Declarations.
The authors declare no competing interests.
This article is part of a topical collection on “National Indicators of Climate Changes, Impacts, and Vulnerability” edited by Melissa A. Kenney and Anthony C. Janetos.
Publisher’s note
Springer Nature remains neutral with regard to jurisdictional claims in published maps and institutional affiliations.
- Allen MR, Frame DJ, Huntingford C, Jones CD, Lowe JA, Meinshausen M, Meinshausen N (2009) Warming caused by cumulative carbon emissions towards the trillionth tonne. Nature, 458(7242):1163–6. 10.1038/nature08019.PMID:19407800 [ PubMed ]
- Allen M. R. et al. (2018) Framing and context. in “Global warming of 1.5 °C. An IPCC Special Report on the impacts of global warming of 1.5 °C above pre-industrial levels and related global greenhouse gas emission pathways, in the context of strengthening the global response to the threat of climate change, sustainable development, and efforts to eradicate poverty.” (IPCC).
- Alvarez AR, Pacala SW, Winebrake JJ, Chameides WL, Hamburg SP. Greater focus needed on methane leakage from natural gas infrastructure. PNAS. 2012; 109 (17):6435–6440. doi: 10.1073/pnas.1202407109. [ PMC free article ] [ PubMed ] [ CrossRef ] [ Google Scholar ]
- Basu S, Lehman SJ, Miller JB, Andrews AE, Sweeney C, Gurney KR, Xu X, Southon J, Tans PP. Estimating US fossil fuel CO 2 emissions from measurements of 14 CO 2 in atmospheric CO 2 . PNAS. 2020; 117 (24):13300–13,307. doi: 10.1073/pnas.1919032117. [ PMC free article ] [ PubMed ] [ CrossRef ] [ Google Scholar ]
- Birdsey R, Gurwick NP, Gurney KR, Shrestha G, Mayes MA, Najjar RG, Reed SC, Romero-Lankao P. Appendix D. Carbon measurement approaches and accounting frameworks. In: Cavallaro N, Shrestha G, Birdsey R, Mayes MA, Najjar RG, Reed SC, Romero-Lankao P, Zhu Z, editors. Second State of the Carbon Cycle Report (SOCCR2): a sustained assessment report. Washington, DC: U.S. Global Change Research Program; 2018. pp. 834–838. [ Google Scholar ]
- Buizer, J. L., P. Fleming, S. L. Hays, K. Dow, C. B. Field, D. Gustafson, A. Luers, and R. H. Moss (2013) Preparing the nation for change: building a sustained national climate assessment process. National Climate Assessment and Development Advisory Committee (NCADAC) Rep., 73 pp., https://sncaadvisorycom-mittee.noaa.gov/Portals/0/Meeting-Documents/NCA-SASRWG_Report_Print.pdf .
- Butler J, Battle M, Bender M et al. (1999) A record of atmospheric halocarbons during the twentieth century from polar firn air. Nature 399:749–755. 10.1038/21586
- California Air Resources Board (CARB) (2020) California greenhouse gas emissions for 2000 to 2018, trends of emissions and other indicators, https://ww3.arb.ca.gov/cc/inventory/pubs/reports/2000_2018/ghg_inventory_trends_00–18.pdf
- Canadell JG, Ciais P, Dhakal S, et al. Interactions of the carbon cycle, human activity and the climate system: a research portfolio. Curr Opin Environ Sustain. 2010; 2 :301–311. doi: 10.1016/j.cosust.2010.08.003. [ CrossRef ] [ Google Scholar ]
- Cain M, Lynch J, Allen MR, et al. Improved calculation of warming-equivalent emissions for short-lived climate pollutants. npj Clim Atmos Sci. 2019; 2 :29. doi: 10.1038/s41612-019-0086-4. [ PMC free article ] [ PubMed ] [ CrossRef ] [ Google Scholar ]
- Chatterjee A, Gierach MM, Sutton AJ, Feely RA, Crisp D, Eldering A, Gunson MR, O’Dell CW, Stephens BB, Schimel DS. Influence of El Niño on atmospheric CO 2 over the tropical Pacific Ocean: findings from NASA’s OCO-2 mission. Science. 2017; 358 :6360. doi: 10.1126/science.aam5776. [ PMC free article ] [ PubMed ] [ CrossRef ] [ Google Scholar ]
- Ciais P, Sabine C, Bala G, et al. Carbon and other biogeochemical cycles. In: Stocker TF, Qin D, Plattner G-K, Tignor M, Allen SK, Boschung J, Nauels A, Xia Y, Bex V, Midgley PM, et al., editors. Climate change 2013: the physical science basis. Contribution of Working Group I to the Fifth Assessment Report of the Intergovernmental Panel on Climate Change. Cambridge, United Kingdom and New York, NY, USA: Cambridge University Press; 2013. [ Google Scholar ]
- Collins WJ, Frame DJ, Fuglestvedt JS, Shine KP. Environ. Res Lett. 2020; 15 :024018. doi: 10.1088/1748-9326/ab6039. [ CrossRef ] [ Google Scholar ]
- Cooley SR, Moore DJP, Alin SR, et al. In: Chapter 17: Biogeochemical effects of rising atmospheric carbon dioxide. In Second State of the Carbon Cycle Report (SOCCR2): a sustained assessment report. Cavallaro N, Shrestha G, Birdsey R, Mayes MA, Najjar RG, Reed SC, Romero-Lankao P, Zhu Z, et al., editors. Washington, DC: U.S. Global Change Research Program; 2018. pp. 690–727. [ Google Scholar ]
- Corell RW, Liverman D, Dow K, Ebi KL, Kunkel K, Mearns LO, Melillo J (2014) Ch. 29: Research needs for climate and global change assessments. In: Melillo JM, Richmond T, Yohe GW (eds) Climate Change Impacts in the United States: The Third National Climate Assessment . U.S. Global Change Research Program, pp 707–718. 10.7930/J03R0QR3
- Cowtan K, Hausfather Z, Hawkins E, Jacobs P, Mann ME, Miller SK, et al. Robust comparison of climate models with observations using blended land air and ocean sea surface temperatures. Geophys Res Lett. 2015; 42 :6526–6534. doi: 10.1002/2015GL064888. [ CrossRef ] [ Google Scholar ]
- Crippa M, Solazzo E, Huang G, et al. High resolution temporal profiles in the Emissions Database for Global Atmospheric Research. Sci Data. 2020; 7 :121. doi: 10.1038/s41597-020-0462-2. [ PMC free article ] [ PubMed ] [ CrossRef ] [ Google Scholar ]
- Crisp, D., Y. Meijer, R. Munro et al. (2018) A constellation architecture for monitoring carbon dioxide and methane from space, Committee on Earth Observation Satellites. http://ceos.org/document_management/Virtual_Constellations/ACC/Documents/CEOS_AC-VC_GHG_White_Paper_Publication_Draft2_20181111.pdf
- Davidson EA, Kanter D. Inventories and scenarios of nitrous oxide emissions. Environ Res Lett. 2014; 9 :105,012. doi: 10.1088/1748-9326/9/10/105012. [ CrossRef ] [ Google Scholar ]
- Dlugokencky EJ, Bruhwiler L, White, et al. Observational constraints on recent increases in the atmospheric CH 4 burden. Geophys Res Lett. 2009; 36 :L18803. doi: 10.1029/2009GL039780. [ CrossRef ] [ Google Scholar ]
- Etheridge DM, Steele LP, Langenfelds RL, Francey RJ. Natural and anthropogenic changes in atmospheric CO 2 over the last 1000 years from air in Antarctic ice and firn. J Geophys Res. 1996; 101 :4115–4128. doi: 10.1029/95JD03410. [ CrossRef ] [ Google Scholar ]
- Friedlingstein P, Jones MW, O’Sullivan, et al. Global carbon budget 2019. Earth Syst Sci Data. 2019; 11 :1783–1838. doi: 10.5194/essd-11-1783-2019. [ CrossRef ] [ Google Scholar ]
- Friedlingstein P, Meinshausen M, Arora VK, Jones CD, Anav A, Liddicoat SK, Knutti R. Uncertainties in CMIP5 climate projections due to carbon cycle feedbacks. J Clim. 2014; 27 (2):511–526. doi: 10.1175/JCLI-D-12-00579.1. [ CrossRef ] [ Google Scholar ]
- Gillett NP, Arora VK, Matthews D, Allen MR (2013) Constraining the ratio of global warming to cumulative CO emissions using CMIP5 simulations. J Clim 26(18):6844–6858. 10.1175/JCLI-D-12-00476.1
- Graven HD. Impact of fossil fuel emissions on radiocarbon. PNAS. 2015; 112 (31):9542–9545. doi: 10.1073/pnas.1504467112. [ PMC free article ] [ PubMed ] [ CrossRef ] [ Google Scholar ]
- Gregory JM, Jones CD, Cadule P, Friedlingstein P (2009) Quantifying carbon cycle feedbacks. J Clim 22(19):5232–5250. 10.1175/2009JCLI2949.1
- Hansen J, Sato M, Kharecha P, et al. Young people’s burden: requirement of negative CO 2 emissions. Earth Syst Dyn. 2017; 8 :577–616. doi: 10.5194/esd-8-577-2017. [ CrossRef ] [ Google Scholar ]
- Hofmann DJ, Butler JH, Conway TJ, Dlugokencky EJ, Elkins JW, Masarie K, Montzka SA, Schnell RC, Tans PP. Tracking climate forcing: the Annual Greenhouse Gas Index. EOS Trans Am Geophys Union. 2006; 87 :46. doi: 10.1029/2006EO460002. [ CrossRef ] [ Google Scholar ]
- Hofmann, DJ, Butler JH, Tans PP (2009) A new look at atmospheric carbon dioxide, Atmos. Env. 43(12):2084–2086. 10.1016/jatmosenv.2008.12.028
- Huntzinger DN, Chatterjee A, Moore DJP, et al. Chapter 19: Future of the North American carbon cycle. In: Cavallaro N, Shrestha G, Birdsey R, Mayes MA, Najjar RG, Reed SC, Romero-Lankao P, Zhu Z, et al., editors. Second State of the Carbon Cycle Report (SOCCR2): a sustained assessment report. Washington, DC: U.S. Global Change Research Program; 2018. pp. 760–809. [ Google Scholar ]
- Jackson RB, Saunois M, Bousquet P, Canadell JG, Poulter B, Stavert AR, Bergamaschi P, Niwa Y, Segers A, Tsuruta A. Environ Res Lett. 2020; 15 :071,002. doi: 10.1088/1748-9326/ab9ed2. [ CrossRef ] [ Google Scholar ]
- Jenkins S, Millar RJ, Leach N, Allen MR. Framing climate goals in terms of cumulative CO 2 -forcing-equivalent emissions. Geophys Res Lett. 2018; 45 :2795–2804. doi: 10.1002/2017GL076173. [ CrossRef ] [ Google Scholar ]
- Keeling CD. The concentration and isotopic abundances of atmospheric carbon dioxide in rural areas. Geochim Cosmochim Acta. 1958; 13 :322–334. doi: 10.1016/0016-7037(58)90033-4. [ CrossRef ] [ Google Scholar ]
- Kenney, M.A., Janetos, A.C. & Gerst, M.D. (2018) A framework for national climate indicators. Clim Chang. 10.1007/s10584-018-2307-y
- Kenney MA, Janetos AC, Lough GC. Building an integrated U.S. National Climate Indicators System. In: Jacobs K, Moser S, Buizer J, editors. The US National Climate Assessment. Cham: Springer Climate. Springer; 2016. [ Google Scholar ]
- Kenney MA, Janetos AC et al. (2014) National climate indicators system report. National Climate Assessment Development and Advisory Committee.
- Kolka R, Trettin C, Tang W, et al. Chapter 13: Terrestrial wetlands. In: Cavallaro N, Shrestha G, Birdsey R, Mayes MA, Najjar RG, Reed SC, Romero-Lankao P, Zhu Z, et al., editors. Second State of the Carbon Cycle Report (SOCCR2): a sustained assessment report. Washington, DC: U.S. Global Change Research Program; 2018. pp. 507–567. [ Google Scholar ]
- Krol M, Lelieveld J. Can the variability in tropospheric OH be deduced from measurements of 1,1,1- trichloroethane (methyl chloroform)? J Geophys Res. 2003; 108 :D3. doi: 10.1029/2002JD002423. [ CrossRef ] [ Google Scholar ]
- Long CN, Dutton EG, Augustine JA, Wiscombe W, Wild M, McFarlane SA, Flynn CJ. Significant decadal brightening of downwelling shortwave in the continental United States. J Geophys Res. 2009; 114 :D00D06. doi: 10.1029/2008JD011263. [ CrossRef ] [ Google Scholar ]
- Loulergue L, Schilt A, Spahni R, Masson-Delmotte V, Blunier T, Lemieux B, Barnola J-M, Raynaud D, Stocker TF, Chappellaz J. Orbital and millennial-scale features of atmospheric CH 4 over the past 800,000 years. Nature. 2008; 453 :383–386. doi: 10.1038/nature06950. [ PubMed ] [ CrossRef ] [ Google Scholar ]
- MacDougall AH (2016) The transient reponse to cumulative CO emissions: a review. Curr Clim Change Rep 2:39–47. 10.1007/s40641-015-0030-6
- MacFarling Meure C, Etheridge D, Trudinger C, Steele P, Langenfelds R, van Ommen T, Smith A, Elkins J. Law dome CO 2 , CH 4 and N 2 O ice core records extended to 2000 years BP. Geophys Res Lett. 2006; 33 :L14810. doi: 10.1029/2006GL026152. [ CrossRef ] [ Google Scholar ]
- Matthews HD, Gillett NP, Stott PA, Zickfeld K (2009) The proportionality of global warming to cumulative carbon emissions. Nature 459(7248):829–832. 10.1038/nature08047 [ PubMed ]
- Montzka SA, Krol M, Dlugokencky E, Hall B, Jockel P, Lelieveld J. Small interannual variability of global atmospheric hydroxyl. Science. 2011; 331 (6013):67–69. doi: 10.1126/science.1197640. [ PubMed ] [ CrossRef ] [ Google Scholar ]
- Montzka SA, Calvert P, Hall BD, Elkins JW, Conway TJ, Tans PP, Sweeney C. On the global distribution, seasonality, and budget of atmospheric carbonyl sulfide (COS) and some similarities to CO 2 . J Geophys Res Atmos. 2007; 112 :D09302. doi: 10.1029/2006JD007665. [ CrossRef ] [ Google Scholar ]
- Myhre G, Shindell D, Bréon F-M, et al.et al. Anthropogenic and natural radiative forcing. In: Stocker TF, Qin D, Plattner G-K, et al.et al., editors. Climate change 2013: the physical science basis. Contribution of Working Group I to the Fifth Assessment Report of the Intergovernmental Panel on Climate Change. Cambridge, United Kingdom and New York, NY, USA: Cambridge University Press; 2013. [ Google Scholar ]
- National Academies of Sciences, Engineering, and Medicine (NAS) Improving the characterization of anthropogenic methane emissions in the United States. Washington, D.C.: The National Academies Press; 2018. [ PubMed ] [ Google Scholar ]
- Nisbet EG, Dlugokencky EJ, Manning, et al. Rising atmospheric methane: 2007–2014 growth and isotopic shift. Glob Biogeochem Cycles. 2016; 30 (9):1356–1370. doi: 10.1002/2016GB005406. [ CrossRef ] [ Google Scholar ]
- Olivier, J.G.J. and G. Janssens-Maenhout (2012) http://edgar.jrc.ec.europa.eu/docs/IEA_PARTIII.pdf .
- Park S, Croteau P, Boering K, et al. (2012) Trends and seasonal cycles in the isotopic composition of nitrous oxide since 1940. Nature Geosci 5:261–265. 10.1038//ngeo1421
- Peischl J, Karion A, Sweeney C, et al. Quantifying atmospheric methane emissions from oil and natural gas production in the Bakken shale region of North Dakota. J Geophys Res Atmos. 2016; 121 :6101–6111. doi: 10.1002/2015JD024631. [ CrossRef ] [ Google Scholar ]
- Peischl, J., Ryerson, T.B., Aikin, et al. (2015) Quantifying atmospheric methane emissions from the Haynesville, Fayetteville, and northeastern Marcellus shale gas production regions. J Geophys Res Atmos 120: 2119–2139. 10.1002/2014JD022697
- Pétron G, et al. A new look at methane and nonmethane hydrocarbon emissions from oil and natural gas operations in the Colorado Denver-Julesburg Basin. J Geophys Res Atmos. 2014; 119 :6836–6852. doi: 10.1002/2013JD021272. [ CrossRef ] [ Google Scholar ]
- Ramaswamy, et al. Radiative forcing of climate change, Chapter 1 in climate change 2001: the scientific basis. Cambridge UK and New York, NY USA: Cambridge Univ. Press; 2001. [ Google Scholar ]
- Richardson M, Cowtan K, Millar RJ. Global temperature definition affects achievement of long-term climate goals. Environ Res Lett. 2018; 13 :54,004. doi: 10.1088/1748-9326/aab305. [ CrossRef ] [ Google Scholar ]
- Rigby M, Mühle J, Miller BR, et al. History of atmospheric SF 6 from 1973 to 2008. Atmos Chem Phys. 2010; 10 :10,305–10,320. doi: 10.5194/acp-10-10,305-2010. [ CrossRef ] [ Google Scholar ]
- Rigby M, Prinn RG, Fraser, et al. Renewed growth of atmospheric methane. Geophys Res Lett. 2008; 35 :L22805. doi: 10.1029/2008gl036037. [ CrossRef ] [ Google Scholar ]
- Röckmann T, Levin I (2005) High-precision determination of the changing isotopic composition of atmospheric N 2 O from 1990 to 2002. J Geophys Res 110(D21304)
- Saunois M, Stavert AR, Poulter B, et al. The global methane budget 2000–2017. Earth Syst Sci Data. 2020; 12 :1561–1623. doi: 10.5194/essd-12-1561-2020. [ CrossRef ] [ Google Scholar ]
- Schaefer H, Mikaloff Fletcher SE, Veidt C et al (2016) A twenty-first century shift from fossil-fuel to biogenic methane emissions indicated by 13 CH 4 . Science. 10.1126/science.aad2705 [ PubMed ]
- Schmidt-Traub G, de la Mothe Karoubi E, Espey J (2015) Indicators and a monitoring framework for the sustainable development goals, launching a data revolution for the SDGs, a report to the Secretary General of the United Nations by the Leadership Council of the Sustainable Development Solutions Network. https://resources.unsdsn.org/indicators-and-a-monitoring-framework-for-sustainable-development-goals-launching-a-data-revolution-for-the-sdgs
- Schuur EAG, McGuire AD, Romanovsky V, Schädel C, Mack M. Chapter 11: Arctic and boreal carbon. In: Cavallaro N, Shrestha G, Birdsey R, Mayes MA, Najjar RG, Reed SC, Romero-Lankao P, Zhu Z, editors. Second State of the Carbon Cycle Report (SOCCR2): a sustained assessment report. Washington, DC, USA: U.S. Global Change Research Program; 2018. pp. 428–468. [ Google Scholar ]
- Schuur EAG, McGuire AD, Schädel C, et al. Climate change and the permafrost carbon feedback. Nature. 2015; 520 (7546):171–179. doi: 10.1038/nature14338. [ PubMed ] [ CrossRef ] [ Google Scholar ]
- Schuur EAG, Abbott BW, Bowden WB, Brovkin V, Camill P, et al. Expert assessment of vulnerability of permafrost carbon to climate change. Clim Chang. 2013; 119 :359–374. doi: 10.1007/s10584-013-0730-7. [ CrossRef ] [ Google Scholar ]
- Schwietzke S, Sherwood O, Bruhwiler L, et al. Upward revision of global fossil fuel methane emissions based on isotopic database. Nature. 2016; 538 :88–91. doi: 10.1038/nature19797. [ PubMed ] [ CrossRef ] [ Google Scholar ]
- Shine, K.P., Fuglestvedt, J.S., Hailemariam, K. et al. (2005) Alternatives to the global warming potential for comparing climate impacts of emissions of greenhouse gases. Clim Chang 68, 281–302 (2005). 10.1007/s10584-005-1146-9
- Tanaka K, Cavalett O, Collins WJ, et al. Asserting the climate benefits of the coal-to-gas shift across temporal and spatial scales. Nat Clim Chang. 2019; 9 :389–396. doi: 10.1038/s41558-019-0457-1. [ CrossRef ] [ Google Scholar ]
- Tanaka K, Peters GP, Fuglestvedt JS. Policy update: multicomponent climate policy: why do emission metrics matter? Carbon Manag. 2010; 1 (2):191–197. doi: 10.4155/cmt.10.28. [ CrossRef ] [ Google Scholar ]
- Tans P. An accounting of the observed increase in oceanic and atmospheric CO 2 and an outlook for the future. Oceanography. 2009; 22 (4):26–35. doi: 10.5670/oceanog.2009.94. [ CrossRef ] [ Google Scholar ]
- Thomas C, Tennant T, Rolls J (2000) The GHG indicator: UNEP guidelines for calculating greenhouse gas emissions for businesses and non-commercial organisations, UNEP Economics and Trade Unit, Geneva, Switzerland. https://www.unepfi.org/fileadmin/documents/ghg_indicator_2000.pdf
- Velders GJM, Andersen SO, Daniel JS, Fahey DW, McFarland M. The importance of the Montreal Protocol in protecting climate. PNAS. 2007; 104 :4814–4819. doi: 10.1073/pnas.0610328104. [ PMC free article ] [ PubMed ] [ CrossRef ] [ Google Scholar ]
- Wild M. Enlightening global dimming and brightening. Bull Am Meteorol Soc. 2012; 93 :27–37. doi: 10.1175/BAMS-D-11-00074.1. [ CrossRef ] [ Google Scholar ]
- Wild M (2009) Global dimming and brightening: a review. J Geophys Res. 10.1029/2008JD011470
Skip to Content
The U.S. labor market can affect ‘people who are not even here,’ research finds
- Share via Twitter
- Share via Facebook
- Share via LinkedIn
- Share via E-mail
A recently published paper co-authored by Brian Cadena finds deep connections between the U.S. and Mexican economies
That the job market in Phoenix can affect a child’s education in Mexico may strain credulity, but it’s nevertheless true, according to a recent paper co-authored by Brian Cadena , a University of Colorado Boulder associate professor of economics.
People from specific regions in Mexico tend to migrate to specific regions in the United States, and when U.S. work dries up in some areas, those migrants tend to return to Mexico, Cadena and his co-authors, María Esther Caballero of American University and Brian K. Kovak of Carnegie Mellon, found.
Their paper, published in the Journal of International Economics in November, explores the U.S. labor market’s influence on the lives of people in Mexico by comparing how neighboring Mexican counties, or “municipios,” fared during the Great Recession.
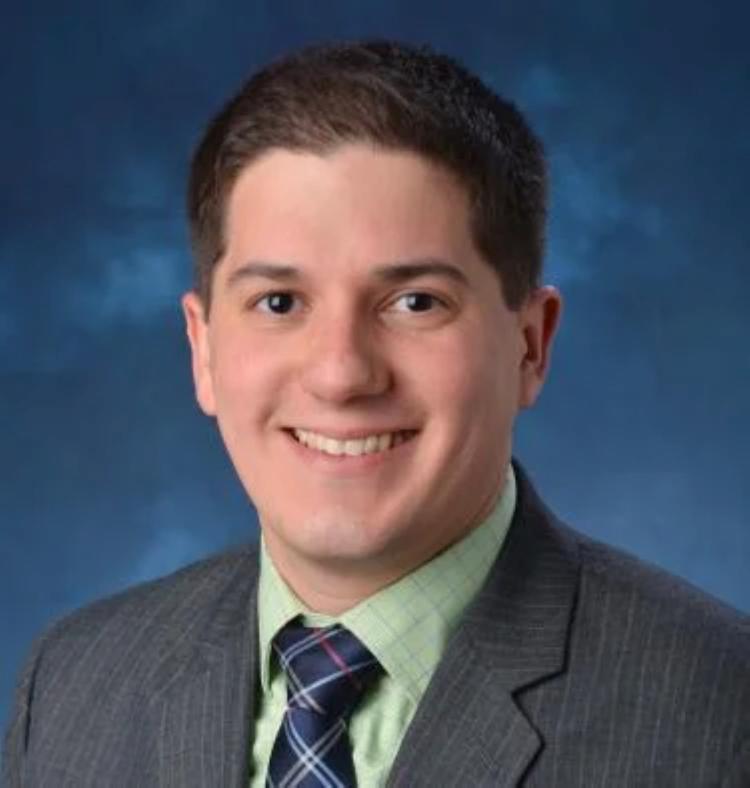
Brian Cadena, a CU Boulder associate professor of economics, and his research colleagues explore the U.S. labor market’s influence on the lives of people in Mexico by comparing how neighboring Mexican counties fared during the Great Recession.
To perform their analysis, Cadena, Caballero and Kovak drew upon data from the Matrícula Consular de Alta Seguridad (MCAS), a governmental organization that issues identity cards to Mexican migrants.
Unlike either the U.S. or Mexican census, MCAS provides in-depth, granular information on migrant workers, specifying the municipios they leave and where in the United States they settle.
MCAS is a treasure trove, says Cadena. But it wasn’t long ago that researchers didn’t know how to use it. Cadena, Caballero and Kovak changed that with another paper they published in 2018, which validated the MCAS data and thereby opened up a whole range of potential research.
“This identity-card data really allowed us to drill down and make tight comparisons between municipios,” says Cadena.
The strength of networks
A key finding that emerged from the MCAS data is that people from the same municipio often move to the same cities and states in the United States. “People follow their networks,” says Cadena. And these networks are so strong that migrants from nearby municipios often end up hundreds of miles apart in the States.
Migrants from the municipio of Dolores Hidalgo, for example, tend to move to Texas, while those from nearby Jaral del Progreso generally relocate to Chicago, California and the Southwest. Same region in Mexico, different time zones in the United States.
The close proximity of the municipios is important for the kind of research Cadena, Caballero and Kovak are doing, Cadena explains, because it cuts down on confounding variables. Neighboring municipios experience the same weather, suffer the same droughts, follow the same or similar laws, etc., which means differences in their economic outcomes are likely due to something they don’t share—the job market in the cities and states where their migrants moved.
To unearth these differences, Cadena, Caballero and Kovak measured the job-market losses in the U.S. regions linked to each municipio and then compared the economic outcomes in the municipios connected to harder-hit regions to those connected to softer-hit regions.
As it happens, labor demand in Texas survived the Great Recession relatively unscathed, so the municipios of the migrants who ventured there remained stable. The American Southwest, however, suffered some major blows, and so the municipios connected to that region exhibited several changes.
(Un)expected observations
Some of those changes were unsurprising, says Cadena.
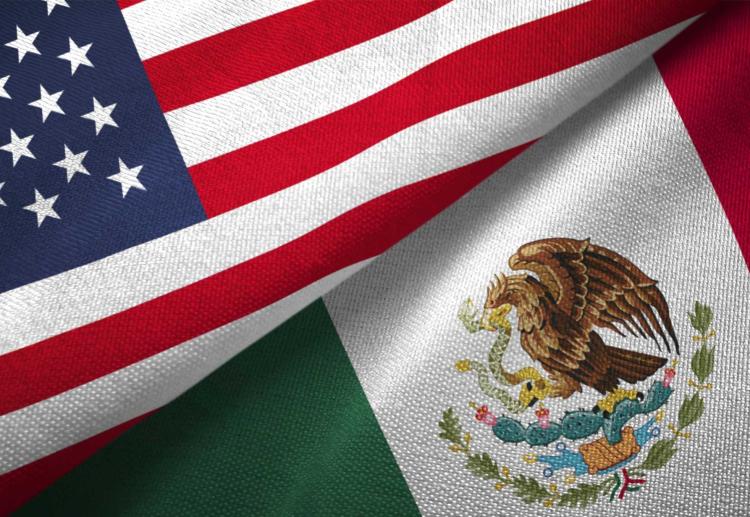
“One of the things we’re finding is how connected these two economies are," says CU Boulder researcher Brian Cadena of the United States and Mexico. On the one hand, the stark differences in what someone can earn and what the labor market looks like in one country as opposed to the other suggests that we have made the separation between those countries real and meaningful. On the other hand, we are certainly not islands.”
“When work dried up, more immigrants returned to Mexico, and fewer new immigrants came from that source community.” This then led to a fall in remittances, or money transfers from migrant workers to their families back in Mexico.
Yet Cadena, Caballero and Kovak also observed some changes they didn’t expect. One was that more women joined the Mexican workforce.
“This is called the added worker effect,” says Cadena. “When the primary earner of a household”—in this case, the migrant laborer—“loses their job, it’s a common reaction by the household to say, ‘Let’s send someone else to work.’”
Another unexpected change was a drop in school retention. “We found some suggestive evidence that a loss of jobs in the United States reduced investment in schooling in Mexico. We saw more schooling dropout, especially at transition ages, when kids move from one level of schooling to the next,” says Cadena.
Blurred lines and better choices
What do these findings suggest about the perceived separation between these two countries and their economies?
It makes that separation “a little fuzzier,” says Cadena.
“One of the things we’re finding is how connected these two economies are. On the one hand, the stark differences in what someone can earn and what the labor market looks like in one country as opposed to the other suggests that we have made the separation between those countries real and meaningful. On the other hand, we are certainly not islands.”
Realizing this, Cadena believes, could inform policymaking, specifically regarding immigration.
“When we’re thinking about immigration policy—when we’re thinking about all these things that affect the low-wage labor market—we are making policy that has a real and noticeable effect on the lives of people who are not even here,” he says.
“I’m not a politician, but I think that a more holistic sense of all the impacts of the choices we make as a country could help us make better choices.”
Did you enjoy this article? Subcribe to our newsletter. Passionate about economics? Show your support.
Related Articles
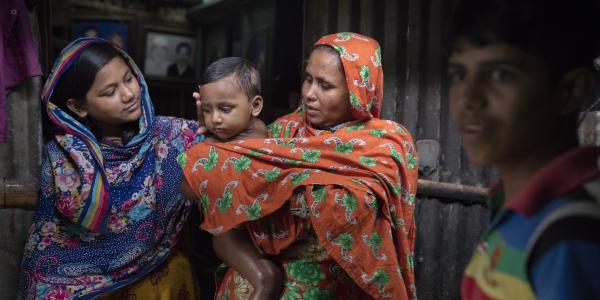
Early childhood health interventions have ‘big, multi-generation impacts,’ research finds

Understanding crime through both victims and offenders

Legal rights and legal reality diverge for single women in Nepal
- Division of Social Sciences
Help | Advanced Search
Computer Science > Robotics
Title: research on robot path planning based on reinforcement learning.
Abstract: This project has conducted research on robot path planning based on Visual SLAM. The main work of this project is as follows: (1) Construction of Visual SLAM system. Research has been conducted on the basic architecture of Visual SLAM. A Visual SLAM system is developed based on ORB-SLAM3 system, which can conduct dense point cloud mapping. (2) The map suitable for two-dimensional path planning is obtained through map conversion. This part converts the dense point cloud map obtained by Visual SLAM system into an octomap and then performs projection transformation to the grid map. The map conversion converts the dense point cloud map containing a large amount of redundant map information into an extremely lightweight grid map suitable for path planning. (3) Research on path planning algorithm based on reinforcement learning. This project has conducted experimental comparisons between the Q-learning algorithm, the DQN algorithm, and the SARSA algorithm, and found that DQN is the algorithm with the fastest convergence and best performance in high-dimensional complex environments. This project has conducted experimental verification of the Visual SLAM system in a simulation environment. The experimental results obtained based on open-source dataset and self-made dataset prove the feasibility and effectiveness of the designed Visual SLAM system. At the same time, this project has also conducted comparative experiments on the three reinforcement learning algorithms under the same experimental condition to obtain the optimal algorithm under the experimental condition.
Submission history
Access paper:.
- Other Formats

References & Citations
- Google Scholar
- Semantic Scholar
BibTeX formatted citation

Bibliographic and Citation Tools
Code, data and media associated with this article, recommenders and search tools.
- Institution
arXivLabs: experimental projects with community collaborators
arXivLabs is a framework that allows collaborators to develop and share new arXiv features directly on our website.
Both individuals and organizations that work with arXivLabs have embraced and accepted our values of openness, community, excellence, and user data privacy. arXiv is committed to these values and only works with partners that adhere to them.
Have an idea for a project that will add value for arXiv's community? Learn more about arXivLabs .
Suggestions or feedback?
MIT News | Massachusetts Institute of Technology
- Machine learning
- Social justice
- Black holes
- Classes and programs
Departments
- Aeronautics and Astronautics
- Brain and Cognitive Sciences
- Architecture
- Political Science
- Mechanical Engineering
Centers, Labs, & Programs
- Abdul Latif Jameel Poverty Action Lab (J-PAL)
- Picower Institute for Learning and Memory
- Lincoln Laboratory
- School of Architecture + Planning
- School of Engineering
- School of Humanities, Arts, and Social Sciences
- Sloan School of Management
- School of Science
- MIT Schwarzman College of Computing
Researchers detect a new molecule in space
Press contact :.
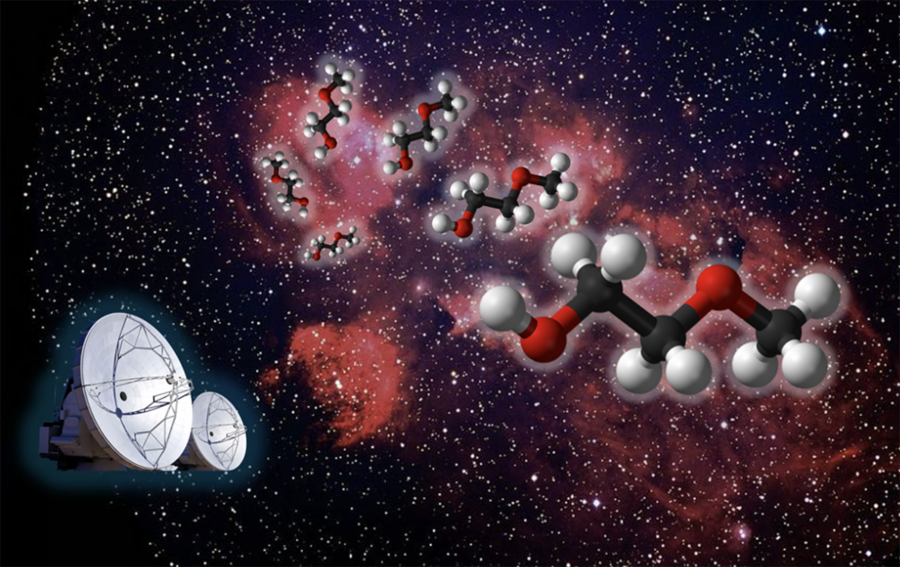
Previous image Next image
New research from the group of MIT Professor Brett McGuire has revealed the presence of a previously unknown molecule in space. The team's open-access paper, “ Rotational Spectrum and First Interstellar Detection of 2-Methoxyethanol Using ALMA Observations of NGC 6334I ,” appears in April 12 issue of The Astrophysical Journal Letters .
Zachary T.P. Fried , a graduate student in the McGuire group and the lead author of the publication, worked to assemble a puzzle comprised of pieces collected from across the globe, extending beyond MIT to France, Florida, Virginia, and Copenhagen, to achieve this exciting discovery.
“Our group tries to understand what molecules are present in regions of space where stars and solar systems will eventually take shape,” explains Fried. “This allows us to piece together how chemistry evolves alongside the process of star and planet formation. We do this by looking at the rotational spectra of molecules, the unique patterns of light they give off as they tumble end-over-end in space. These patterns are fingerprints (barcodes) for molecules. To detect new molecules in space, we first must have an idea of what molecule we want to look for, then we can record its spectrum in the lab here on Earth, and then finally we look for that spectrum in space using telescopes.”
Searching for molecules in space
The McGuire Group has recently begun to utilize machine learning to suggest good target molecules to search for. In 2023, one of these machine learning models suggested the researchers target a molecule known as 2-methoxyethanol.
“There are a number of 'methoxy' molecules in space, like dimethyl ether, methoxymethanol, ethyl methyl ether, and methyl formate, but 2-methoxyethanol would be the largest and most complex ever seen,” says Fried. To detect this molecule using radiotelescope observations, the group first needed to measure and analyze its rotational spectrum on Earth. The researchers combined experiments from the University of Lille (Lille, France), the New College of Florida (Sarasota, Florida), and the McGuire lab at MIT to measure this spectrum over a broadband region of frequencies ranging from the microwave to sub-millimeter wave regimes (approximately 8 to 500 gigahertz).
The data gleaned from these measurements permitted a search for the molecule using Atacama Large Millimeter/submillimeter Array (ALMA) observations toward two separate star-forming regions: NGC 6334I and IRAS 16293-2422B. Members of the McGuire group analyzed these telescope observations alongside researchers at the National Radio Astronomy Observatory (Charlottesville, Virginia) and the University of Copenhagen, Denmark.
“Ultimately, we observed 25 rotational lines of 2-methoxyethanol that lined up with the molecular signal observed toward NGC 6334I (the barcode matched!), thus resulting in a secure detection of 2-methoxyethanol in this source,” says Fried. “This allowed us to then derive physical parameters of the molecule toward NGC 6334I, such as its abundance and excitation temperature. It also enabled an investigation of the possible chemical formation pathways from known interstellar precursors.”
Looking forward
Molecular discoveries like this one help the researchers to better understand the development of molecular complexity in space during the star formation process. 2-methoxyethanol, which contains 13 atoms, is quite large for interstellar standards — as of 2021, only six species larger than 13 atoms were detected outside the solar system , many by McGuire’s group, and all of them existing as ringed structures.
“Continued observations of large molecules and subsequent derivations of their abundances allows us to advance our knowledge of how efficiently large molecules can form and by which specific reactions they may be produced,” says Fried. “Additionally, since we detected this molecule in NGC 6334I but not in IRAS 16293-2422B, we were presented with a unique opportunity to look into how the differing physical conditions of these two sources may be affecting the chemistry that can occur.”
Share this news article on:
Related links.
- McGuire Lab
- Department of Chemistry
Related Topics
- Space, astronomy and planetary science
- Astrophysics
Related Articles
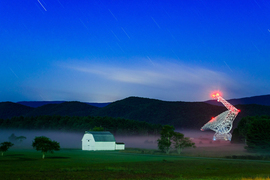
Found in space: Complex carbon-based molecules
Previous item Next item
More MIT News
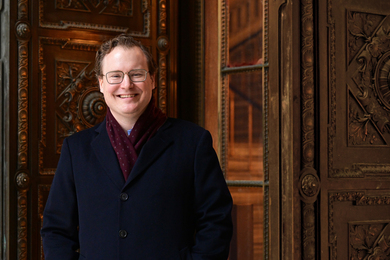
Exploring the history of data-driven arguments in public life
Read full story →
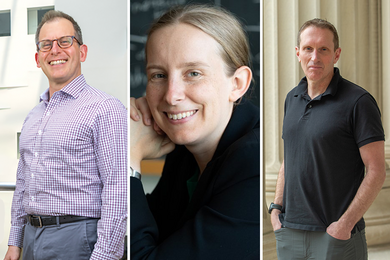
Three from MIT awarded 2024 Guggenheim Fellowships
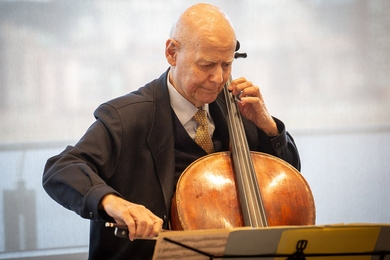
A musical life: Carlos Prieto ’59 in conversation and concert
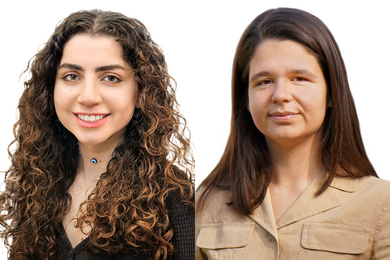
Two from MIT awarded 2024 Paul and Daisy Soros Fellowships for New Americans
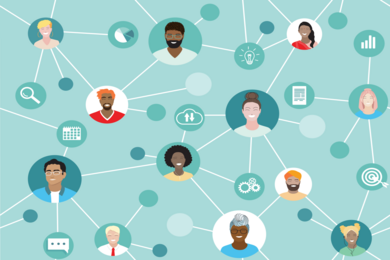
MIT Emerging Talent opens pathways for underserved global learners
The MIT Edgerton Center’s third annual showcase dazzles onlookers
- More news on MIT News homepage →
Massachusetts Institute of Technology 77 Massachusetts Avenue, Cambridge, MA, USA
- Map (opens in new window)
- Events (opens in new window)
- People (opens in new window)
- Careers (opens in new window)
- Accessibility
- Social Media Hub
- MIT on Facebook
- MIT on YouTube
- MIT on Instagram
Align Your Steps: Optimizing Sampling Schedules in Diffusion Models
Diffusion models (DMs) have established themselves as the state-of-the-art generative modeling approach in the visual domain and beyond. A crucial drawback of DMs is their slow sampling speed, relying on many sequential function evaluations through large neural networks. Sampling from DMs can be seen as solving a differential equation through a discretized set of noise levels known as the sampling schedule. While past works primarily focused on deriving efficient solvers, little attention has been given to finding optimal sampling schedules, and the entire literature relies on hand-crafted heuristics. In this work, for the first time, we propose Align Your Steps, a general and principled approach to optimizing the sampling schedules of DMs for high-quality outputs. We leverage methods from stochastic calculus and find optimal schedules specific to different solvers, trained DMs and datasets. We evaluate our novel approach on several image, video as well as 2D toy data synthesis benchmarks, using a variety of different solvers, and observe that our optimized schedules outperform previous handcrafted schedules in almost all experiments. Our method demonstrates the untapped potential of sampling schedule optimization, especially in the few-step synthesis regime.
Our optimized schedules can be used at inference time in a plug-and-play fashion. Please see our quickstart guide to get started with using our schedules in diffusers and the colab notebook for example code with Stable Diffusion 1.5 and SDXL.

The sampling schedule is iteratively optimized to reduce the discretization error. As the optimization proceeds, the generated images become sharper and more detailed.
Optimizing Sampling Schedules in Diffusion Models
Diffusion models (DMs) have proven themselves to be extremely reliable probabilistic generative models that can produce high-quality data. They have been successfully applied to applications such as image synthesis, image super-resolution, image-to-image translation, image editing, inpainting, video synthesis, text-to-3d generation, and even planning. However, sampling from DMs corresponds to solving a generative Stochastic or Ordinary Differential Equation (SDE/ODE) in reverse time and requires multiple sequential forward passes through a large neural network, limiting their real-time applicability.
Solving SDE/ODEs within the interval \([t_{min}, t_{max}]\) works by discretizing it into \(n\) smaller sub-intervals \(t_{min} = t_0 < t_1 < \dots < t_{n}=t_{max}\) called a sampling schedule, and numerically solving the differential equation between consecutive \(t_i\) values. Currently, most prior works adopt one of a handful of heuristic schedules, such as simple polynomials and cosine functions, and little effort has gone into optimizing this schedule. We attempt to fill this gap by introducing a principled approach for optimizing the schedule in a dataset and model specific manner, resulting in improved outputs given the same compute budget.
Assuming that \( P_{true} \) represents the distribution of running the reverse-time SDE (defined by the learnt model) exactly, and \( P_{disc} \) represents the distribution of solving it with Stochastic-DDIM and a sampling schedule, using the Girsanov theorem an upper bound can be derived for the Kullback-Leibler divergence between these two distributions (simplified; see paper for details) \[ D_{KL}(P_{true} || P_{disc}) \leq \underbrace{ \sum_{i=1}^{n} \int_{t_{i-1}}^{t_{i}} \frac{1}{t^3} \mathbb{E}_{x_t \sim p_t, x_{t_i} \sim p_{t_i | t}} || D_{\theta}(x_t, t) - D_{\theta}(x_{t_i}, t_i) ||_2^2 \ dt }_{= KLUB(t_0, t_1, \dots, t_n)} + constant \] A similar Kullback-Leibler Upper Bound (KLUB) can be found for other stochastic SDE solvers. Given this, we formulate the problem of optimizing the sampling schedule as minimizing the KLUB term with respect to its time discretization, i.e. the sampling scheduling. Monte-Carlo integration with importance sampling is used to estimate the expectation values and the schedule is optimized iteratively. We showcase the benefits of optimizing schedules on a 2D toy distribution (see visualization below).

Modeling a 2D toy distribution: Samples in (b), (c), and (d) are generated using 8 steps of SDE-DPM-Solver++(2M) with EDM, LogSNR, and AYS schedules, respectively. Each image consists of 100,000 sampled points.
Experimental Results
To evaluate the usefulness of optimized schedules, we performed rigorous quantitative experiments on standard image generation benchmarks (CIFAR10, FFHQ, ImageNet), and found that these schedules result in consistent improvements across the board in image quality (measured by FID) for a large variety of popular samplers. We also performed a user study for text-to-image models (specifically Stable Diffusion 1.5), and found that on average images generated with these schedules are preferred twice as much . Please see the paper for these results and evaluations.
Below, we showcase some text-to-image examples that illustrate how using an optimized schedule can generate images with more visual details and better text-alignment given the same number of forward evaluations (NFEs). We provide side-by-side comparisons between our optimized schedules against two of the most popular schedules used in practice (EDM and Time-Uniform). All images are generated with a stochastic ( casino ) or deterministic ( lock ) version of DPM-Solver++(2M) with 10 steps. Hover over the images for zoom-ins.
Stable Diffusion 1.5

casino Text prompt: "1girl, blue dress, blue hair, ponytail, studying at the library, focused" Model: Dreamshaper 8

casino Text prompt: "An enchanting forest path with sunlight filtering through the dense canopy, highlighting the vibrant greens and the soft, mossy floor"

casino Text prompt: "A digital Illustration of the Babel tower, 4k, detailed, trending in artstation, fantasy vivid colors"

casino Text prompt: "A glass-blown vase with a complex swirl of colors, illuminated by sunlight, casting a mosaic of shadows on a white table"

casino Text prompt: "A delicate glass pendant holding a single, luminous firefly, its glow casting warm, dancing shadows on the wearer's neck"

casino Text prompt: "A wise old owl wearing a velvet smoking jacket and spectacles, with a pipe in its beak, seated in a vintage leather armchair"

casino Text prompt: "A close-up portrait of a baby wearing a tiny spider-man costume, trending on artstation" Model: Dreamshaper 8
DeepFloyd-IF

casino Text prompt: "Capybara podcast neon sign"

casino Text prompt: "Long-exposure night photography of a starry sky over a mountain range, with light trails"

casino Text prompt: "A tranquil village nestled in a lush valley, with small, cozy houses dotting the landscape, surrounded by towering, snow-capped mountains under a clear blue sky. A gentle river meanders through the village, reflecting the warm glow of the sunrise"

casino Text prompt: "An ancient library buried beneath the earth, its halls lit by glowing crystals, with scrolls and tomes stacked in endless rows"

casino Text prompt: "A bustling spaceport on a distant planet, with ships of various designs taking off against a backdrop of twin moons"

casino Text prompt: "A set of ancient armor, standing as if worn by an invisible warrior, in front of a backdrop of medieval banners and weaponry."

casino Text prompt: "An elephant painting a colorful abstract masterpiece with its trunk, in a studio surrounded by amused onlookers."

casino Text prompt: "Tiger in construction gear, perched on aged wooden docks, formidable, curious, tiger on the waterfront, textured, vibrant, atmospheric, sharp focus, lifelike, professional lighting, cinematic, 8K"

casino Text prompt: "Cluttered house in the woods, anime, oil painting, high resolution, cottagecore, ghibli inspired, 4k"

casino Text prompt: "An old, creepy dollhouse in a dusty attic, with dolls posed in unsettling positions. Cobwebs, dim lighting, and the shadows of unseen presences create a chilling scene"

lock Text prompt: "A stunning, intricately detailed painting of a sunset in a forest valley, blending the rich, symmetrical styles of Dan Mumford and Marc Simonetti with astrophotography elements"

lock Text prompt: "Create a photorealistic scene of a powerful storm with swirling, dark clouds and fierce winds approaching a coastal village. Show villagers preparing for the storm, with detailed architecture reflecting a fantasy world"

lock Text prompt: "Cyberpunk cityscape with towering skyscrapers, neon signs, and flying cars"
Stable Video Diffusion
We also studied the effect of optimized schedules in video generation using the open-source image-to-video model Stable Video Diffusion. We find that using optimized schedules leads to more stable videos with less color distortions as the video progresses. Below we show side-by-side comparisons of videos generated with 10 DDIM steps using the two different schedules.

Amirmojtaba Sabour, Sanja Fidler, Karsten Kreis

IMAGES
VIDEO
COMMENTS
Solar greenhouses increase crop yield and quality, addressing global food security concerns. This paper presents an overview of current design trends in construction, current development technology for controlling and monitoring greenhouse microclimates, and the various systems available for managing greenhouse environments. ... Other research ...
This paper provides an in-depth examination of greenhouse design challenges as well as new greenhouse environment monitoring and wireless gateway technologies regarding the diversity of greenhouse climate management systems based on location and type of cultivation. Additionally, this work attempts to assist greenhouse engineers, researchers ...
In this paper, a comprehensive review of greenhouse design and cooling technologies in hot regions was presented, with a critical discussion and comparison between the various cooling systems. ... The review has explored research on design features of greenhouses, considered important for optimising greenhouse design and operation in hot ...
environmental effects. The term "greenhous e design" includes too many aspects to. be covered in one paper, therefore, this paper mainly addresses developments. related to possible effects of ...
Medium technology greenhouses are typically characterised by vertical walls. The. size of the medium technology greenhouses is more than 2m but less than 4 metres. tall and a total height usually ...
GREENHOUSE TECHNOLOGY: INTRODUCTION, TYPE AND PLANNING. January 2022. In book: Recent Advances in Agricultural Science and Technology for Sustainable India Part-I (pp.11-18) Publisher: Mahima ...
Several greenhouse energy saving technologies and management strategies have been developed in order to meet the needs for implementation of production systems with low and efficient energy use and low CO2 emissions. Towards this aim, a number of greenhouse concepts that make use of these technologies have been developed and tested, such as the closed greenhouse, the solar greenhouse, the ...
The research on the greenhouse sector has been making great advances as proved by the number of published research articles on agriculture, which has an increased average annual rate of 0.4% between 1999 and 2018, ... This paper aims to generate the knowledge, innovation, technologies, and practices needed to boost the local food production as ...
2.2. Data Processing. The Elsevier Scopus database has been selected to obtain the sample of studies to analyse, in the same way as other articles that have analysed the dynamics followed by a certain line of research [68,69,70,71].The search parameters selected include all the terms related to the principal topics of the research in greenhouse technology (GT), such as soil, energy, structure ...
Life on Earth is possible thanks to greenhouse effect. Without it, temperature on Earth's surface would be around -19°C, instead of the current average of 14°C. Greenhouse effect is produced by greenhouse gasses (GHG) like water vapor, carbon dioxide (CO 2), methane (CH 4), nitrous oxides (N x O) and ozone (O 3). GHG have natural and ...
Additionally, research into intelligent irrigation systems and smart greenhouses has expanded to fulfil the rising food demands brought on by population growth and to help farmers produce high-quality goods under challenging economic conditions (Jianjun et al., Citation 2013). Families require vegetables and other crops for daily use, but they ...
In this paper, we present the system architecture and design of a modern scientific greenhouse research facility for the purpose of Croatian Science Foundation's Project Urtica—BioFuture. Several sensor nodes are proposed in different locations: nutrient solution, environmental (inside and outside the greenhouse), and sensor nodes for ...
The greenhouse effect, despite all the controversy that surrounds the term, is actually one of the most well-established theories in atmospheric science. For example, with its dense CO2 atmosphere, Venus has temperatures near 700 K at its surface. Mars, with its very thin CO2 atmosphere, has temperatures of only 220 K.
The greenhouse effect is now commonly accepted by the scientific community, politicians and the general public. However, the misnomer 'greenhouse effect' has perpetuated, and there are a number of aspects of the effect which are poorly understood outside the atmospheric sciences. On such misconception is that greenhouse research is a recent ...
The twenty-first century is the era when populations of cities will exceed rural communities for the first time in human history. The population growth of cities in many countries, including those in transition from planned to market economies, is putting considerable strain on ecological and natural resources. This paper examines four central issues: (a) the challenges and opportunities ...
The clear effect of the greenhouse gases is the. stable heating of Earth's atmosphere and surface, thus, global warming. The abi lity of certain gases, greenhouse gases, to be transparent to ...
Significant challenges of the COVID-19 pandemic highlighted that features of a modern, sustainable and resilient city should not only relate to fulfilling economic and social urban strategies, but also to functional urban design, in particular, related to urban blue and green infrastructure (BGI). Using results from a web-based questionnaire survey conducted May-July 2020 in Moscow (Russia ...
Fig. 1 (a) and (b) show the year statistics and distribution of the selected papers' main index journals. It can be seen from Fig. 1 (a) that in the past five years, more and more attention has been paid to the research of intelligent greenhouse monitoring system. According to Fig. 1 (b), journal article accounts for the largest proportion of articles related to the above research, accounting ...
Political ecology, broadly, is a critical approach that interrogates nature-society relations from multiple post-positivist perspectives, unlike traditional ecology, where nature is presumed to be an objective reality independent of the society (Watts 2000). Moscow green spaces provide a good case study to use the political ecology approach ...
Find breaking science news and analysis from the world's leading research journal.
The Nutrient Management Spear Program (NMSP) has been working hard to evaluate greenhouse gas (GHG) emissions on New York dairy farms. The dairy sustainability team, led by Research Associate Olivia Godber, helps farmers identify opportunities for improvement and discover which management practices have already successfully reduced their GHG emissions.
Call For Papers Abstract submission deadline: May 15, 2024 01:00 PM PDT or Full paper submission deadline, including technical appendices and supplemental material (all authors must have an OpenReview profile when submitting): May 22, 2024 01:00 PM PDT or Author notification: Sep 25, 2024
Fig. 1. Conceptual model for greenhouse gas (GHG) climate indicators. Indicators proposed in this study are atmospheric concentrations of non-CO 2 GHGs (CO 2 is already included in the National Climate Indicator System (NCIS)). Atmospheric GHG concentrations are directly observable and can be monitored over time.
Theoretical propositions. The programme of housing renovation in the city of Moscow, Footnote 1 initiated by Mayor Sergey Sobyanin and approved by President Vladimir Putin in February 2017, has attracted much commentary among the domestic Russian audience and from international observers (see for example, Seddon Citation 2017).The programme promised to introduce significant improvements to the ...
Gene editing has the potential to solve fundamental challenges in agriculture, biotechnology, and human health. CRISPR-based gene editors derived from microbes, while powerful, often show significant functional tradeoffs when ported into non-native environments, such as human cells. Artificial intelligence (AI) enabled design provides a powerful alternative with potential to bypass ...
That the job market in Phoenix can affect a child's education in Mexico may strain credulity, but it's nevertheless true, according to a recent paper co-authored by Brian Cadena, a University of Colorado Boulder associate professor of economics. People from specific regions in Mexico tend to migrate to specific regions in the United States, and when U.S. work dries up in some areas, those ...
The natural. environment becomes unfavourable for crop production. Therefore, thermal cooling of greenhouse is required specially. during the peak per iod of summer. Cooling may be of. ventilation ...
This project has conducted research on robot path planning based on Visual SLAM. The main work of this project is as follows: (1) Construction of Visual SLAM system. Research has been conducted on the basic architecture of Visual SLAM. A Visual SLAM system is developed based on ORB-SLAM3 system, which can conduct dense point cloud mapping. (2) The map suitable for two-dimensional path planning ...
New research from the group of MIT Professor Brett McGuire has revealed the presence of a previously unknown molecule in space. The team's open-access paper, "Rotational Spectrum and First Interstellar Detection of 2-Methoxyethanol Using ALMA Observations of NGC 6334I," appears in April 12 issue of The Astrophysical Journal Letters. Zachary T.P. Fried, a graduate student in the McGuire ...
Please see the paper for these results and evaluations. Below, we showcase some text-to-image examples that illustrate how using an optimized schedule can generate images with more visual details and better text-alignment given the same number of forward evaluations (NFEs). We provide side-by-side comparisons between our optimized schedules ...