Thank you for visiting nature.com. You are using a browser version with limited support for CSS. To obtain the best experience, we recommend you use a more up to date browser (or turn off compatibility mode in Internet Explorer). In the meantime, to ensure continued support, we are displaying the site without styles and JavaScript.
- View all journals
- Explore content
- About the journal
- Publish with us
- Sign up for alerts
- 24 July 2019

The forgotten part of memory
- Lauren Gravitz 0
Lauren Gravitz is a science journalist in San Diego, California.
You can also search for this author in PubMed Google Scholar
Credit: Sam Falconer
Memories make us who we are. They shape our understanding of the world and help us to predict what’s coming. For more than a century, researchers have been working to understand how memories are formed and then fixed for recall in the days, weeks or even years that follow. But those scientists might have been looking at only half the picture. To understand how we remember, we must also understand how, and why, we forget.
Access options
Access Nature and 54 other Nature Portfolio journals
Get Nature+, our best-value online-access subscription
24,99 € / 30 days
cancel any time
Subscribe to this journal
Receive 51 print issues and online access
185,98 € per year
only 3,65 € per issue
Rent or buy this article
Prices vary by article type
Prices may be subject to local taxes which are calculated during checkout
Nature 571 , S12-S14 (2019)
doi: https://doi.org/10.1038/d41586-019-02211-5
This article is part of Nature Outlook: The brain , an editorially independent supplement produced with the financial support of third parties. About this content .
Berry, J. A., Cervantes-Sandoval, I., Nicholas, E. P. & Davis, R. L. Neuron 74 , 530–542 (2012).
Article PubMed Google Scholar
Migues, P. V. et al. J. Neurosci. 36 , 3481–3494 (2016).
Akers, K. G. et al. Science 344 , 598–602 (2014).
Schmitz, T. W., Correia, M. M., Ferreira, C. S., Prescot, A. P. & Anderson, M. C. Nature Commun. 8 , 1311 (2017).
Hulbert, J. C. & Anderson, M. C. J. Exp. Psychol. Gen. 147 , 1931–1949 (2018).
Download references
Related Articles
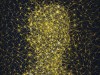
- Neuroscience
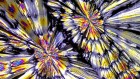
Ketamine is in the spotlight thanks to Elon Musk — but is it the right treatment for depression?
News Explainer 20 MAR 24
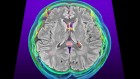
COVID’s toll on the brain: new clues emerge
News 20 MAR 24
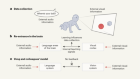
Can lessons from infants solve the problems of data-greedy AI?
News & Views 18 MAR 24
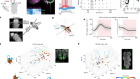
Motor neurons generate pose-targeted movements via proprioceptive sculpting
Article 20 MAR 24
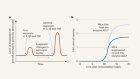
Astrocyte cells in the brain have immune memory
News & Views 20 MAR 24
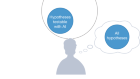
Artificial intelligence and illusions of understanding in scientific research
Perspective 06 MAR 24
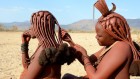
Why reciprocity is common in humans but rare in other animals
News & Views 21 FEB 24
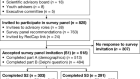
Making cities mental health friendly for adolescents and young adults
Analysis 21 FEB 24
Recruitment of Talent Positions at Shengjing Hospital of China Medical University
Call for top experts and scholars in the field of science and technology.
Shenyang, Liaoning, China
Shengjing Hospital of China Medical University
Assistant Professor in Plant Biology
The Plant Science Program in the Biological and Environmental Science and Engineering (BESE) Division at King Abdullah University of Science and Te...
Saudi Arabia (SA)
King Abdullah University of Science and Technology
Sir Run Run Shaw Hospital, School of Medicine, Zhejiang University, Warmly Welcomes Talents Abroad
“Qiushi” Distinguished Scholar, Zhejiang University, including Professor and Physician
No. 3, Qingchun East Road, Hangzhou, Zhejiang (CN)
Sir Run Run Shaw Hospital Affiliated with Zhejiang University School of Medicine
Postdoctoral Associate
Our laboratory at the Washington University in St. Louis is seeking a postdoctoral experimental biologist to study urogenital diseases and cancer.
Saint Louis, Missouri
Washington University School of Medicine Department of Medicine
Recruitment of Global Talent at the Institute of Zoology, Chinese Academy of Sciences (IOZ, CAS)
The Institute of Zoology (IOZ), Chinese Academy of Sciences (CAS), is seeking global talents around the world.
Beijing, China
Institute of Zoology, Chinese Academy of Sciences (IOZ, CAS)
Sign up for the Nature Briefing newsletter — what matters in science, free to your inbox daily.
Quick links
- Explore articles by subject
- Guide to authors
- Editorial policies
The effects of mnemonic variability and spacing on memory over multiple timescales
Affiliations.
- 1 Department of Psychology & Neuroscience, Temple University, Philadelphia PA 19122.
- 2 Department of Psychology, University of Pittsburgh, Pittsburgh, PA 15260.
- PMID: 38470923
- DOI: 10.1073/pnas.2311077121
The memory benefit that arises from distributing learning over time rather than in consecutive sessions is one of the most robust effects in cognitive psychology. While prior work has mainly focused on repeated exposures to the same information, in the real world, mnemonic content is dynamic, with some pieces of information staying stable while others vary. Thus, open questions remain about the efficacy of the spacing effect in the face of variability in the mnemonic content. Here, in two experiments, we investigated the contributions of mnemonic variability and the timescale of spacing intervals, ranging from seconds to days, to long-term memory. For item memory, both mnemonic variability and spacing intervals were beneficial for memory; however, mnemonic variability was greater at shorter spacing intervals. In contrast, for associative memory, repetition rather than mnemonic variability was beneficial for memory, and spacing benefits only emerged in the absence of mnemonic variability. These results highlight a critical role for mnemonic variability and the timescale of spacing intervals in the spacing effect, bringing this classic memory paradigm into more ecologically valid contexts.
Keywords: learning; long-term memory; spacing effect.
- Memory, Long-Term
- Mental Recall*
Grants and funding
- R21DA043568/HHS | NIH | National Institute on Drug Abuse (NIDA)
- R01DA055259/HHS | NIH | National Institute on Drug Abuse (NIDA)
- K01MH111991/HHS | NIH | National Institute of Mental Health (NIMH)
- 1651330/National Science Foundation (NSF)
- Search Menu
- Browse content in Arts and Humanities
- Browse content in Archaeology
- Anglo-Saxon and Medieval Archaeology
- Archaeological Methodology and Techniques
- Archaeology by Region
- Archaeology of Religion
- Archaeology of Trade and Exchange
- Biblical Archaeology
- Contemporary and Public Archaeology
- Environmental Archaeology
- Historical Archaeology
- History and Theory of Archaeology
- Industrial Archaeology
- Landscape Archaeology
- Mortuary Archaeology
- Prehistoric Archaeology
- Underwater Archaeology
- Urban Archaeology
- Zooarchaeology
- Browse content in Architecture
- Architectural Structure and Design
- History of Architecture
- Residential and Domestic Buildings
- Theory of Architecture
- Browse content in Art
- Art Subjects and Themes
- History of Art
- Industrial and Commercial Art
- Theory of Art
- Biographical Studies
- Byzantine Studies
- Browse content in Classical Studies
- Classical History
- Classical Philosophy
- Classical Mythology
- Classical Literature
- Classical Reception
- Classical Art and Architecture
- Classical Oratory and Rhetoric
- Greek and Roman Papyrology
- Greek and Roman Epigraphy
- Greek and Roman Law
- Greek and Roman Archaeology
- Late Antiquity
- Religion in the Ancient World
- Digital Humanities
- Browse content in History
- Colonialism and Imperialism
- Diplomatic History
- Environmental History
- Genealogy, Heraldry, Names, and Honours
- Genocide and Ethnic Cleansing
- Historical Geography
- History by Period
- History of Emotions
- History of Agriculture
- History of Education
- History of Gender and Sexuality
- Industrial History
- Intellectual History
- International History
- Labour History
- Legal and Constitutional History
- Local and Family History
- Maritime History
- Military History
- National Liberation and Post-Colonialism
- Oral History
- Political History
- Public History
- Regional and National History
- Revolutions and Rebellions
- Slavery and Abolition of Slavery
- Social and Cultural History
- Theory, Methods, and Historiography
- Urban History
- World History
- Browse content in Language Teaching and Learning
- Language Learning (Specific Skills)
- Language Teaching Theory and Methods
- Browse content in Linguistics
- Applied Linguistics
- Cognitive Linguistics
- Computational Linguistics
- Forensic Linguistics
- Grammar, Syntax and Morphology
- Historical and Diachronic Linguistics
- History of English
- Language Evolution
- Language Reference
- Language Acquisition
- Language Variation
- Language Families
- Lexicography
- Linguistic Anthropology
- Linguistic Theories
- Linguistic Typology
- Phonetics and Phonology
- Psycholinguistics
- Sociolinguistics
- Translation and Interpretation
- Writing Systems
- Browse content in Literature
- Bibliography
- Children's Literature Studies
- Literary Studies (Romanticism)
- Literary Studies (American)
- Literary Studies (Asian)
- Literary Studies (European)
- Literary Studies (Eco-criticism)
- Literary Studies (Modernism)
- Literary Studies - World
- Literary Studies (1500 to 1800)
- Literary Studies (19th Century)
- Literary Studies (20th Century onwards)
- Literary Studies (African American Literature)
- Literary Studies (British and Irish)
- Literary Studies (Early and Medieval)
- Literary Studies (Fiction, Novelists, and Prose Writers)
- Literary Studies (Gender Studies)
- Literary Studies (Graphic Novels)
- Literary Studies (History of the Book)
- Literary Studies (Plays and Playwrights)
- Literary Studies (Poetry and Poets)
- Literary Studies (Postcolonial Literature)
- Literary Studies (Queer Studies)
- Literary Studies (Science Fiction)
- Literary Studies (Travel Literature)
- Literary Studies (War Literature)
- Literary Studies (Women's Writing)
- Literary Theory and Cultural Studies
- Mythology and Folklore
- Shakespeare Studies and Criticism
- Browse content in Media Studies
- Browse content in Music
- Applied Music
- Dance and Music
- Ethics in Music
- Ethnomusicology
- Gender and Sexuality in Music
- Medicine and Music
- Music Cultures
- Music and Media
- Music and Religion
- Music and Culture
- Music Education and Pedagogy
- Music Theory and Analysis
- Musical Scores, Lyrics, and Libretti
- Musical Structures, Styles, and Techniques
- Musicology and Music History
- Performance Practice and Studies
- Race and Ethnicity in Music
- Sound Studies
- Browse content in Performing Arts
- Browse content in Philosophy
- Aesthetics and Philosophy of Art
- Epistemology
- Feminist Philosophy
- History of Western Philosophy
- Metaphysics
- Moral Philosophy
- Non-Western Philosophy
- Philosophy of Language
- Philosophy of Mind
- Philosophy of Perception
- Philosophy of Science
- Philosophy of Action
- Philosophy of Law
- Philosophy of Religion
- Philosophy of Mathematics and Logic
- Practical Ethics
- Social and Political Philosophy
- Browse content in Religion
- Biblical Studies
- Christianity
- East Asian Religions
- History of Religion
- Judaism and Jewish Studies
- Qumran Studies
- Religion and Education
- Religion and Health
- Religion and Politics
- Religion and Science
- Religion and Law
- Religion and Art, Literature, and Music
- Religious Studies
- Browse content in Society and Culture
- Cookery, Food, and Drink
- Cultural Studies
- Customs and Traditions
- Ethical Issues and Debates
- Hobbies, Games, Arts and Crafts
- Lifestyle, Home, and Garden
- Natural world, Country Life, and Pets
- Popular Beliefs and Controversial Knowledge
- Sports and Outdoor Recreation
- Technology and Society
- Travel and Holiday
- Visual Culture
- Browse content in Law
- Arbitration
- Browse content in Company and Commercial Law
- Commercial Law
- Company Law
- Browse content in Comparative Law
- Systems of Law
- Competition Law
- Browse content in Constitutional and Administrative Law
- Government Powers
- Judicial Review
- Local Government Law
- Military and Defence Law
- Parliamentary and Legislative Practice
- Construction Law
- Contract Law
- Browse content in Criminal Law
- Criminal Procedure
- Criminal Evidence Law
- Sentencing and Punishment
- Employment and Labour Law
- Environment and Energy Law
- Browse content in Financial Law
- Banking Law
- Insolvency Law
- History of Law
- Human Rights and Immigration
- Intellectual Property Law
- Browse content in International Law
- Private International Law and Conflict of Laws
- Public International Law
- IT and Communications Law
- Jurisprudence and Philosophy of Law
- Law and Politics
- Law and Society
- Browse content in Legal System and Practice
- Courts and Procedure
- Legal Skills and Practice
- Primary Sources of Law
- Regulation of Legal Profession
- Medical and Healthcare Law
- Browse content in Policing
- Criminal Investigation and Detection
- Police and Security Services
- Police Procedure and Law
- Police Regional Planning
- Browse content in Property Law
- Personal Property Law
- Study and Revision
- Terrorism and National Security Law
- Browse content in Trusts Law
- Wills and Probate or Succession
- Browse content in Medicine and Health
- Browse content in Allied Health Professions
- Arts Therapies
- Clinical Science
- Dietetics and Nutrition
- Occupational Therapy
- Operating Department Practice
- Physiotherapy
- Radiography
- Speech and Language Therapy
- Browse content in Anaesthetics
- General Anaesthesia
- Neuroanaesthesia
- Clinical Neuroscience
- Browse content in Clinical Medicine
- Acute Medicine
- Cardiovascular Medicine
- Clinical Genetics
- Clinical Pharmacology and Therapeutics
- Dermatology
- Endocrinology and Diabetes
- Gastroenterology
- Genito-urinary Medicine
- Geriatric Medicine
- Infectious Diseases
- Medical Toxicology
- Medical Oncology
- Pain Medicine
- Palliative Medicine
- Rehabilitation Medicine
- Respiratory Medicine and Pulmonology
- Rheumatology
- Sleep Medicine
- Sports and Exercise Medicine
- Community Medical Services
- Critical Care
- Emergency Medicine
- Forensic Medicine
- Haematology
- History of Medicine
- Browse content in Medical Skills
- Clinical Skills
- Communication Skills
- Nursing Skills
- Surgical Skills
- Browse content in Medical Dentistry
- Oral and Maxillofacial Surgery
- Paediatric Dentistry
- Restorative Dentistry and Orthodontics
- Surgical Dentistry
- Medical Ethics
- Medical Statistics and Methodology
- Browse content in Neurology
- Clinical Neurophysiology
- Neuropathology
- Nursing Studies
- Browse content in Obstetrics and Gynaecology
- Gynaecology
- Occupational Medicine
- Ophthalmology
- Otolaryngology (ENT)
- Browse content in Paediatrics
- Neonatology
- Browse content in Pathology
- Chemical Pathology
- Clinical Cytogenetics and Molecular Genetics
- Histopathology
- Medical Microbiology and Virology
- Patient Education and Information
- Browse content in Pharmacology
- Psychopharmacology
- Browse content in Popular Health
- Caring for Others
- Complementary and Alternative Medicine
- Self-help and Personal Development
- Browse content in Preclinical Medicine
- Cell Biology
- Molecular Biology and Genetics
- Reproduction, Growth and Development
- Primary Care
- Professional Development in Medicine
- Browse content in Psychiatry
- Addiction Medicine
- Child and Adolescent Psychiatry
- Forensic Psychiatry
- Learning Disabilities
- Old Age Psychiatry
- Psychotherapy
- Browse content in Public Health and Epidemiology
- Epidemiology
- Public Health
- Browse content in Radiology
- Clinical Radiology
- Interventional Radiology
- Nuclear Medicine
- Radiation Oncology
- Reproductive Medicine
- Browse content in Surgery
- Cardiothoracic Surgery
- Gastro-intestinal and Colorectal Surgery
- General Surgery
- Neurosurgery
- Paediatric Surgery
- Peri-operative Care
- Plastic and Reconstructive Surgery
- Surgical Oncology
- Transplant Surgery
- Trauma and Orthopaedic Surgery
- Vascular Surgery
- Browse content in Science and Mathematics
- Browse content in Biological Sciences
- Aquatic Biology
- Biochemistry
- Bioinformatics and Computational Biology
- Developmental Biology
- Ecology and Conservation
- Evolutionary Biology
- Genetics and Genomics
- Microbiology
- Molecular and Cell Biology
- Natural History
- Plant Sciences and Forestry
- Research Methods in Life Sciences
- Structural Biology
- Systems Biology
- Zoology and Animal Sciences
- Browse content in Chemistry
- Analytical Chemistry
- Computational Chemistry
- Crystallography
- Environmental Chemistry
- Industrial Chemistry
- Inorganic Chemistry
- Materials Chemistry
- Medicinal Chemistry
- Mineralogy and Gems
- Organic Chemistry
- Physical Chemistry
- Polymer Chemistry
- Study and Communication Skills in Chemistry
- Theoretical Chemistry
- Browse content in Computer Science
- Artificial Intelligence
- Computer Architecture and Logic Design
- Game Studies
- Human-Computer Interaction
- Mathematical Theory of Computation
- Programming Languages
- Software Engineering
- Systems Analysis and Design
- Virtual Reality
- Browse content in Computing
- Business Applications
- Computer Security
- Computer Games
- Computer Networking and Communications
- Digital Lifestyle
- Graphical and Digital Media Applications
- Operating Systems
- Browse content in Earth Sciences and Geography
- Atmospheric Sciences
- Environmental Geography
- Geology and the Lithosphere
- Maps and Map-making
- Meteorology and Climatology
- Oceanography and Hydrology
- Palaeontology
- Physical Geography and Topography
- Regional Geography
- Soil Science
- Urban Geography
- Browse content in Engineering and Technology
- Agriculture and Farming
- Biological Engineering
- Civil Engineering, Surveying, and Building
- Electronics and Communications Engineering
- Energy Technology
- Engineering (General)
- Environmental Science, Engineering, and Technology
- History of Engineering and Technology
- Mechanical Engineering and Materials
- Technology of Industrial Chemistry
- Transport Technology and Trades
- Browse content in Environmental Science
- Applied Ecology (Environmental Science)
- Conservation of the Environment (Environmental Science)
- Environmental Sustainability
- Environmentalist Thought and Ideology (Environmental Science)
- Management of Land and Natural Resources (Environmental Science)
- Natural Disasters (Environmental Science)
- Nuclear Issues (Environmental Science)
- Pollution and Threats to the Environment (Environmental Science)
- Social Impact of Environmental Issues (Environmental Science)
- History of Science and Technology
- Browse content in Materials Science
- Ceramics and Glasses
- Composite Materials
- Metals, Alloying, and Corrosion
- Nanotechnology
- Browse content in Mathematics
- Applied Mathematics
- Biomathematics and Statistics
- History of Mathematics
- Mathematical Education
- Mathematical Finance
- Mathematical Analysis
- Numerical and Computational Mathematics
- Probability and Statistics
- Pure Mathematics
- Browse content in Neuroscience
- Cognition and Behavioural Neuroscience
- Development of the Nervous System
- Disorders of the Nervous System
- History of Neuroscience
- Invertebrate Neurobiology
- Molecular and Cellular Systems
- Neuroendocrinology and Autonomic Nervous System
- Neuroscientific Techniques
- Sensory and Motor Systems
- Browse content in Physics
- Astronomy and Astrophysics
- Atomic, Molecular, and Optical Physics
- Biological and Medical Physics
- Classical Mechanics
- Computational Physics
- Condensed Matter Physics
- Electromagnetism, Optics, and Acoustics
- History of Physics
- Mathematical and Statistical Physics
- Measurement Science
- Nuclear Physics
- Particles and Fields
- Plasma Physics
- Quantum Physics
- Relativity and Gravitation
- Semiconductor and Mesoscopic Physics
- Browse content in Psychology
- Affective Sciences
- Clinical Psychology
- Cognitive Psychology
- Cognitive Neuroscience
- Criminal and Forensic Psychology
- Developmental Psychology
- Educational Psychology
- Evolutionary Psychology
- Health Psychology
- History and Systems in Psychology
- Music Psychology
- Neuropsychology
- Organizational Psychology
- Psychological Assessment and Testing
- Psychology of Human-Technology Interaction
- Psychology Professional Development and Training
- Research Methods in Psychology
- Social Psychology
- Browse content in Social Sciences
- Browse content in Anthropology
- Anthropology of Religion
- Human Evolution
- Medical Anthropology
- Physical Anthropology
- Regional Anthropology
- Social and Cultural Anthropology
- Theory and Practice of Anthropology
- Browse content in Business and Management
- Business Ethics
- Business Strategy
- Business History
- Business and Technology
- Business and Government
- Business and the Environment
- Comparative Management
- Corporate Governance
- Corporate Social Responsibility
- Entrepreneurship
- Health Management
- Human Resource Management
- Industrial and Employment Relations
- Industry Studies
- Information and Communication Technologies
- International Business
- Knowledge Management
- Management and Management Techniques
- Operations Management
- Organizational Theory and Behaviour
- Pensions and Pension Management
- Public and Nonprofit Management
- Strategic Management
- Supply Chain Management
- Browse content in Criminology and Criminal Justice
- Criminal Justice
- Criminology
- Forms of Crime
- International and Comparative Criminology
- Youth Violence and Juvenile Justice
- Development Studies
- Browse content in Economics
- Agricultural, Environmental, and Natural Resource Economics
- Asian Economics
- Behavioural Finance
- Behavioural Economics and Neuroeconomics
- Econometrics and Mathematical Economics
- Economic History
- Economic Systems
- Economic Methodology
- Economic Development and Growth
- Financial Markets
- Financial Institutions and Services
- General Economics and Teaching
- Health, Education, and Welfare
- History of Economic Thought
- International Economics
- Labour and Demographic Economics
- Law and Economics
- Macroeconomics and Monetary Economics
- Microeconomics
- Public Economics
- Urban, Rural, and Regional Economics
- Welfare Economics
- Browse content in Education
- Adult Education and Continuous Learning
- Care and Counselling of Students
- Early Childhood and Elementary Education
- Educational Equipment and Technology
- Educational Strategies and Policy
- Higher and Further Education
- Organization and Management of Education
- Philosophy and Theory of Education
- Schools Studies
- Secondary Education
- Teaching of a Specific Subject
- Teaching of Specific Groups and Special Educational Needs
- Teaching Skills and Techniques
- Browse content in Environment
- Applied Ecology (Social Science)
- Climate Change
- Conservation of the Environment (Social Science)
- Environmentalist Thought and Ideology (Social Science)
- Natural Disasters (Environment)
- Social Impact of Environmental Issues (Social Science)
- Browse content in Human Geography
- Cultural Geography
- Economic Geography
- Political Geography
- Browse content in Interdisciplinary Studies
- Communication Studies
- Museums, Libraries, and Information Sciences
- Browse content in Politics
- African Politics
- Asian Politics
- Chinese Politics
- Comparative Politics
- Conflict Politics
- Elections and Electoral Studies
- Environmental Politics
- European Union
- Foreign Policy
- Gender and Politics
- Human Rights and Politics
- Indian Politics
- International Relations
- International Organization (Politics)
- International Political Economy
- Irish Politics
- Latin American Politics
- Middle Eastern Politics
- Political Behaviour
- Political Economy
- Political Institutions
- Political Methodology
- Political Communication
- Political Philosophy
- Political Sociology
- Political Theory
- Politics and Law
- Public Policy
- Public Administration
- Quantitative Political Methodology
- Regional Political Studies
- Russian Politics
- Security Studies
- State and Local Government
- UK Politics
- US Politics
- Browse content in Regional and Area Studies
- African Studies
- Asian Studies
- East Asian Studies
- Japanese Studies
- Latin American Studies
- Middle Eastern Studies
- Native American Studies
- Scottish Studies
- Browse content in Research and Information
- Research Methods
- Browse content in Social Work
- Addictions and Substance Misuse
- Adoption and Fostering
- Care of the Elderly
- Child and Adolescent Social Work
- Couple and Family Social Work
- Developmental and Physical Disabilities Social Work
- Direct Practice and Clinical Social Work
- Emergency Services
- Human Behaviour and the Social Environment
- International and Global Issues in Social Work
- Mental and Behavioural Health
- Social Justice and Human Rights
- Social Policy and Advocacy
- Social Work and Crime and Justice
- Social Work Macro Practice
- Social Work Practice Settings
- Social Work Research and Evidence-based Practice
- Welfare and Benefit Systems
- Browse content in Sociology
- Childhood Studies
- Community Development
- Comparative and Historical Sociology
- Economic Sociology
- Gender and Sexuality
- Gerontology and Ageing
- Health, Illness, and Medicine
- Marriage and the Family
- Migration Studies
- Occupations, Professions, and Work
- Organizations
- Population and Demography
- Race and Ethnicity
- Social Theory
- Social Movements and Social Change
- Social Research and Statistics
- Social Stratification, Inequality, and Mobility
- Sociology of Religion
- Sociology of Education
- Sport and Leisure
- Urban and Rural Studies
- Browse content in Warfare and Defence
- Defence Strategy, Planning, and Research
- Land Forces and Warfare
- Military Administration
- Military Life and Institutions
- Naval Forces and Warfare
- Other Warfare and Defence Issues
- Peace Studies and Conflict Resolution
- Weapons and Equipment

- < Previous chapter
- Next chapter >

5 History of Memory
Hans J. Markowitsch, Professor of Physiological Psychology, University of Bielefeld
Angelica Staniloiu, Researcher and Senior Psychiatrist, University of Bielefeld
- Published: 02 November 2016
- Cite Icon Cite
- Permissions Icon Permissions
The historical roots of memory research—mainly from the period between 1870 and 1920—are described with emphasis on human memory. First, data from experimental psychology are reviewed; thereafter principal contributions from the old traditions of psychiatry and psychoanalysis are given and then relations between brain tissue damage and memory deficits are outlined. Experimental as well as clinical studies of that time already provided evidence for theoretical ideas on memory systems, which resemble those en vogue today. Furthermore, significant and still valid findings were reported on the contribution of bottleneck structures of the limbic system for mempry encoding. Among these, the mammillary bodies, thalamic nuclei, and the medial temporal lobe were most consistently mentioned. Furthermore, early findings from psychiatric patients revealed similar forms of dissociative states as found presently and indicated that these disease conditions occur much more in young than in older adults.
Memory connects innumerable single phenomena into a whole, and just as the body would be scattered like dust in countless atoms if the attraction of matter did not hold it together so consciousness—without the connecting power of memory—would fall apart in as many fragments as it contains moments. ( Hering, 1870 , p. 12; authors’ translation)
As is evident from the citation from Ewald Hering (who otherwise is known until today for his opponent-color theory), scientists from diverse backgrounds were attracted by the ability to memorize since long. And of course one could go much more backward in time than 1870 and still find numerous works on memory, especially from the old Greek who developed memory techniques, which during Middle Age were incorporated in the “ars memoriae” (see Yates, 1966 ). The complementary of memory, forgetting or the “ars oblivionis,” likewise was found already in writings of Homer and Ovid and had its blossoming during Middle Age (see Markowitsch & Brand, 2010 ).
As the present chapter attempts to integrate historical roots of neuroscientific with memory research, it will focus on the history of memory from the period of the detection of neurons onward, that is, principally from the time when Hering’s book appeared. Due to page limitations, it also will omit reference to any kind of animal research on memory (with the exception of mentioning Pavlov’s classical conditioning).
Of course, at the end of the second last century many investigations on the functions of memory and learning were done, frequently without much reference to the nervous system (Burnham, 1889a–d , 1903 ; Forel, 1885 ; Kennedy, 1898 ; Pick, 1886 ; Ribot, 1882 ; Titchener, 1895a , b ). Ebbinghaus’ (1885) division into short-term and long-term memory has to be cited here, as well as Ribot’s (1882) distinction of three meanings of memory (“the conservation of certain conditions, their reproduction, and their localization in the past”; p. 10). Ribot (1882) furthermore on page 71 distinguished between “temporary amnesia,” “periodical amnesia” and “progressive amnesia.” And Ribot is of course known for Ribot’s law or “law of regression,” stating that retrograde amnesia is usually unequally distributed in that way that the information closer to the present is more easily lost than information from the remote past (“last in, first out”).
Some years later the physiologist Pavlov (1928) published his results on the mechanisms of classical conditioning and the biologist Semon (1904) in an, at that time, very influential book introduced the state-dependency and fragility of episodic-autobiographical memory. He coined the term “ecphory” which decades later was reintroduced by Endel Tulving (1983) . Semon (1904) also stated that most forms of amnesia are not based on the loss of engrams but on the inability to ecphorize still fully intact engrams. He described ecphory as the process by which retrieval cues interact with stored information so that an image or a representation of the information in question appears. Retrieval cues may occur as other thought associations or as cues from the environment. If the retrieval cues are very different from those existent during encoding, distortions in remembrance may occur—a phenomenon taken up by Sigmund Freud in a number of variations ( Breuer & Freud, 1895 : Freud, 1901a , b , 1910 ). Hippokrates’ term “hysteria” became with Charcot (see Bogousslavsky, 2011 ), Janet ( 1894 , 1907 ), and Breuer and Freud (1895) a prominent concept and “hypnosis” a prominent tool for treatment of memory problems. Surprisingly, even Korbinian Brodmann (1897) , who otherwise is mainly known for his seminal work on cortical parcellations—the “Brodmann’s areas”—wrote about hypnosis. This research on hysteria brought the unconsciousness and implicit memories into the awareness of contemporary as well as subsequent generations. The French School of that time also introduced other terms such as anterograde and retrograde amnesia ( Charcot, 1892 ; Souques, 1892 ).
Remembrance and Forgetting
Remembrance and forgetting were in particular the topic of psychoanalysts (Freud 1898 , 1899 , 1901a , b ; Jung, 1905a–d ; Jung & Riklin, 1904 ). They emphasized that especially the most important things (the repressed, aversive complex) are forgotten. Jung (1905a) furthermore pointed to the role of “systematic” forgetting which might provoke the development of the Ganser syndrome (cf. the section on “ The Psychiatry of Memory ”; Staniloiu, Bender, Smolewska, Ellis, Abramowitz, & Markowitsch, 2009 ).
The ability to memorize was analyzed and discussed critically from psychological and neurological viewpoints as well (e.g., Pick, 1905 ). Boldt (1905) made three acoustic and four visual experiments on the ability to memorize: acoustic word memory (paired associate learning), picture memory (recognition of 5 out of 25 portraits), color memory, orientation, nonsense-words, memory for names (portraits with first and last names given), digit span. He used 35 patients as subjects, largely demented patients, but also one case each with Korsakoff’s psychosis and a hysterical psychosis. Boldt emphasized that especially patients with Korsakoff’s psychosis (he had data from additional Korsakoff subjects on record (Korsakoff patients, or at least the labeling of patients as being Korsakoff’s patients, were very common at the turn to the 20th century) manifested severe memory defects and retrograde amnesia. (However, one Korsakoff patient, a medical student, recovered completely.)
Boldt gave vivid descriptions of special abilities or disabilities of patients with particular professional backgrounds: A female wool-ware trader with senile dementia had kept an extraordinary memory for colors, because—as she explained herself—she had dealt with wool colors life-long. An architect had kept special abilities for orientation. On the other hand, one patient, belonging to “the species of head waiters” (“zur Spezies der Oberkellner gehörig,” p. 108), had severe problems in name, person, and digit memories; however, he still had a good remembrance of the regular guests (who were known to both the patient and the examiner “from his Berlin study period,” p. 109). Boldt’s investigation is of interest, as he used tasks that in part are still considered to be useful in examining the patient’s everyday memory and as at that early time he already emphasized the selectivity of mnestic disturbances, their interindividual variability, and the existence of relations to premorbid preferences and specially trained abilities.
Adalbert Gregor ( 1907 , 1909 ) explicitly stated that he intended to differentiate on the basis of applied tests between the stages of encoding and storage or retrieval. His subjects even had to learn poems and were retested between 1 and 120 days after initial learning. Gregor’s special interest lay in finding whether massed or distributed practice—or, as he formulated it, learning in toto and learning in parts—led to a better remembrance. He found that learning meaningless syllables profited better from more narrow spacing between learning sessions. For meaningless and meaningful words and stanzas spaced learning led to superior results compared to massed learning periods.
With respect to Korsakoff’s patients, Gregor found that they were able to acquire new associations, especially under conditions where they could carefully pay attention to all impressions and were given the possibility to repeat them frequently. Daily events of relevance to the patients that they could observe repeatedly on their own free will had the highest likeliness of being remembered. Gregor (1907) estimated that about 8 to 10% of the normal every-day events met these conditions.
Gregor (1907) was apparently among the first scientists who tested experimentally something that the earliest students of Korsakoff’s syndrome had observed repeatedly, namely that emotionally significant events have a higher probability of being remembered than more neutral ones (e.g., Bonhoeffer, 1901 ). Gregor argued that the strongly impaired time sense and ability to estimate time epochs hindered Korsakoff’s subjects in acquiring information in a way similar to normals.
Gregor furthermore pointed out that he quantified the remembrance of learned material on the basis of savings, and investigated the effects of temporal spacing on repeatedly presented material, of primacy and recency effects, and different error categories in the reproduction of learned material (e.g., semantic or phonemic errors). It was also common at that time to standardize the exposure time of material presented (words or pictures) by using apparatuses such as a “memory drum” (cf. Gregor, 1909 , p. 226; Ranschburg, 1901b , figures 1, 2) or by providing detailed instructions. The construction of learning curves was also common (Figure 5.1 ).
Another researcher of that time who devoted his career to the determination of memory functions was Ranschburg. He wanted to determine the extent of memory and the certainty of mnemonic knowledge (Ranschburg, 1901a , b ). He defined certainty as the percentage of incorrect remembrances; that is, of events when items were incorrectly recognized or reproduced but the appropriate correction was given immediately after presentation. He used 50 items, which were subdivided into 7 groups: memory for words (2 groups; acoustic memory), persons, colors, names, numbers, and orientation memory. Twelve pupils of about 12 years of age were involved, 12 so-called non-educated adults (ward keepers at the psychiatric clinic), 13 adults with higher education, and 12 so-called neurasthenic and 21 so-called paralytic patients. Ranschburg found that education enhanced the extent of memory but had an ambiguous influence on the certainty with which information was given. In his study, age had a positive influence on memory, especially for pair-associated and name memory. The patients were inferior throughout, but especially for words and word-pairs. Lastly, Ranschburg pointed out that the persons’ occupation was related to mnemonic abilities. Special memories were directly related to the job position: for example, waiters, teachers, or stockbrokers had retained special mnemonic abilities for numbers.
(a) Rate of word reproduction in 14 successive sessions in a patient with “progressive paralysis” (after Figure 1 of Gregor, 1909 ). (b) Learning-to-learn phenomenon in a “progressive paralytic” patient. Gregor (1909) had trained the patient to learn 4 blocks of 7 digits in each of 12 sessions and then constructed an averaged curve, stating that the patient had improved over time from repeated testing (after fig. 3 of Gregor, 1909 ).
In his book on “the diseased memory” Ranschburg (1911) first discussed the resemblance of memory research and what he called “pathological mnemology” with the head of Janus—there is anterograde and retrograde amnesia. He then described the kinds of memory loss that are most common in organic brain illnesses, including the memory for proper names. He explained this impairment with the frequently superfluous acquisition of names, which are then associated with a number of old, similar sounding names, with the consequence that they are often reproduced only approximately. Ranschburg considered factors of attention and inhibition as playing a major role in the memory for names. He then gave a number of examples of memory span and memory duration for visually presented words in various samples of normals, psychotics, and patients with brain damage. In the second part of his book, he described methods, techniques, and equipment for measuring memory abilities. An example is given in Figure 5.2 .
Early Methods for Memory Assessment and Memory Training
On the practical, applied site, partly sophisticated tests were invented. Examples, which were published in 1912 by the neurologist Schneider, are given in Figures 5.3 and 5.4 . Schneider wanted to measure functions of memory and combinatory abilities (“Ebbinghaus combinatory scheme”) with these tests. Several years later, in 1928, Schneider wrote a special review on memory disturbances.

The Ranschburg mnemometer connected to a Hipp-chronoscope and a Römer-sound measuring device (“Schallschlüssel”) for the exact measurement of reproduction times (reproduced from fig. 4 of Ranschburg, 1911 ).
Example of a test used by Schneider (1912) —so-called combinatory figures, which were used to investigate abilities of visual remembrance and combination in neurological patients (p. 571).

Examples of the so-called Ebbinghaus’ combinatory scheme in order to investigate neurological patients. Schneider (1912) wrote (p. 563): “For my purposes I used two rather short schemes, one of which is for people from the country-side, the other for those from the city.” The texts can be translated as follows:
Standard psychological tests were nonexistent and it was prevalent to rely on one’s own inventions for memory assessment (e.g., Brodmann, 1902 , 1904 ; Gregor, 1902 , 1907 , 1909 ). One example is Strümpell’s (1897) approach to test verbal memory. He just asked patients personal data about their job, all four-legged animals they knew, the names of trees and birds, or he instructed them to repeat sentences or names of cities (p. 407: “Stuttgart, Ulm, Friedrichshafen, Constanz”), or long words (p. 406: “Lotteriecommissionshausgesellschaft”). Bonhoeffer (1901 , p. 125) gave a short description on how to test memory in Korsakoff’s psychosis: “Mnemonic ability for the sensory modalities is tested, as is known, by presenting numbers, words, nonsense syllables, pictures, objects, and by repeatedly touching objects with the eyes closed. I have not investigated smell and taste. The older literature does not include anything on the ability of remembering within these sensory territories either.”
Francis Galton , a cousin of Charles Darwin, is considered to be the father of modern statistics. In 1879, he published an article, entitled “Psychometric Experiments,” in the journal Brain . Some of his experiments are still referred to in present-day evaluations of old (retrograde) memories (e.g., Crovitz & Schiffman, 1974 ).
In 1888 Emil Kraepelin gave a talk (published in 1889 ) at the Dorpat Medical Faculty in Estonia (nowadays named Tartu) in which he addressed effects of training on the duration of associations. He noted that even after 1 ¾ years many previously acquired associations were reproduced in spite of the subjects’ failure to realize that they had learned them—a phenomenon nowadays known as “priming” (or “perceptual memory”) ( Markowitsch & Staniloiu, 2012a ). Determining the duration of associations was also the main aim of the investigation that Trautscholdt carried out in Wilhelm Wundt’s “psychophysical laboratory” in 1880 and which was published in 1882 in the first volume of the Philosophische Studien , edited by Wundt.
Korbinian Brodmann, famous for his mapping of the cerebral cortex (e.g., Brodmann, 1909 , 1912 ) relied in his study from 1904 on procedures of Ebbinghaus, Müller, and Steffens (Ebbinghaus, 1885 , 1897 ; Müller & Schumann, 1894 ; Müller & Pilzecker, 1900 ; Steffens, 1900 ). These included principally the acquisition of nonsense syllables, measuring the influence of number of syllables and repetitions, and interval lengths. Comparable experiments were done by Ach (1901) , who, similar to Kraepelin in 1882 and 1892 and Kürz and Kraepelin in 1901 , intended to study the effects of drugs (alcohol, bromine, paraldehyde, caffeine) on the acquisition of verbal material. Ach found that alcohol reduced attention and speed of information processing, but concluded that alcohol did not impair memory performance—at least in the field he had investigated. For caffeine he noted improved comprehension and greater speed and accuracy in information processing; these effects were particularly evident under conditions of fatigue. In the early experiments in which alcohol was used, wine and champagne were apparently favorite test substances, as reviewed by Kraepelin (1882) .
Testing memory by associations was particular prominent at the turn to the 20th century. Aschaffenburg (1895) developed 15 categories of possible associations (see his Table XIV); he also designed standard recording sheets (p. 298 f). Four years later Aschaffenburg (1899) described changes in the formation of associations under the influence of exhaustion (testing after a night without sleep and eating). Lottie Steffens (1900) studied training of verses of poems in English in various ways in order to develop conditions for effective learning. The persons tested included her sister Laura, E. G. Müller, A. Pilzecker (still known today in experimental psychology for their work on retroactive and proactive interference; G. E. Müller & Pilzecker, 1900 ), and apparently a brother of Pilzecker.
At pretty much the same time, Pappenheim ( 1907 , 1908 ), working at the German Psychiatric Clinic in Prague, used quite a different way of assessing memory, namely what he termed “Assoziationsversuche.” These were of the sort, “What does the cat catch?—mice” or “bells—ring,” “pencil—writing.” Pappenheim also used meaningless syllables (after suggestions of Ebbinghaus, 1885 , and G. E. Müller & Schumann, 1894 , which were presented in the trochaic rhythm), normal words, poems, numbers and names, prose texts, tests of optic memorizing, recognition, and intelligence. In fact, from the citations given in Pappenheim (1908) alone, it becomes evident that after the turn of the century experimental psychology was quite engaged and active in inventing and applying mnemonic tests (e.g., Bleuler, 1905 ; Ebert & Meumann, 1905 ; Gregor, 1909 ; Lipmann, 1903 , 1904 ; Müller & Pilzecker, 1900 ; Müller & Schumann, 1894 ; Netschajeff, 1900 , 1902 ; Ranschburg, 1901a , 1911 ; Steffens, 1900 ; Stransky, 1905a–c) .
Comprehension, learning, and retention were investigated by Finzi (1901) . The selection of his 12 subjects is remarkable, the 3 females were described as “educated,” 7 of the 9 men had an M.D. degree, 1 a Ph.D., and 1 was a medical student. They were from Italy (1), Germany (4), the Netherlands (3), Belgium (1), North America (1), Norway (1), and Finland (1). Similarly Schneider (1901) tested comprehension and memory abilities in senile demented patients. His investigation was based on Finzi’s work.
Mention should also be made of the substantial contribution of Schneider (1912) on “clinical-psychological methods of investigation and their results.” Schneider first broadly reviewed the available techniques and stated that at that time puzzles and related games were already in use (p. 559). He then applied his test battery, consisting of pictorial material (which had to be interpreted or in which details had to be identified), puzzles, and verbal learning tests, to 25 healthy subjects (mainly hospital staff) and to a large number of psychiatric and neurological patients. He especially emphasized his repeated testing of three Korsakoff’s patients. Schneider emphasized the poor performance of Korsakoff’s patients, which was worse for reading of words and for the omission of learned material than in patients with depression, schizophrenia [“dementia praecox”], and paralysis. Schneider himself was not quite satisfied with his test battery. He concluded his article with the words: “we have to acknowledge that our methods do not allow an exact determination of psychic disturbances in a satisfactory manner” (p. 615).
Kurt Goldstein (1906) studied the ability to remember and the formation of associations. He used words, pictures, numbers, colors, and objects that had to be remembered, and tested memory for the alphabet, the months, days of the week, digits forward and backward, simple and compound multiplication, geographical and historical knowledge, the remembrance of prayers, and other items.
Breukink (1907) , a psychiatrist from Utrecht, described in detail his way of assessing memory (p. 115 ff). Tests of memory for earlier reminiscences in general were quite similar to present-day semi-structural autobiographical memory tests (e.g., Staniloiu, Borsutzky, Woermann, & Markowitsch, 2013 ).
The Psychiatry of Memory: Hysteria and Other Distortions of Memory
Simulating in general or feigning madness, has always been of special interest, reflected, for instance, in the dramatic literature (e.g., Shakespeare’s Hamlet or in the classical psychoanalytic Studien über Hysterie [Studies on hysteria] of Breuer & Freud, 1895 ; cf. also Freud, 1910 ).
Hysterical amnesia was diagnosed much more frequently 100 to 120 years ago than today (in part also related to war experiences: Bauer, 1917 ). Aside from soldiers, it was largely found in members of the female sex (cf., e.g., the case histories of Fräulein Anna O., Frau Emmy v. N., Miss Lucy R., Katharina, and Fräulein Elisabeth v. R., given in Breuer & Freud, 1895 ).
A detailed review on possible amnesic states—many of which were psychological in nature—was given by Heine (1911 , p. 55 f), who wrote, “The forensic significance of amnesia.” His list reflects the disease conditions related to memory disturbances, common 100 years ago:
Epileptic somnolence
Hysterical somnolence
States of unconsciousness and of mnestic activity after traumatic damage of the brain
Commotio cerebri
Attempt to hang oneself
Reanimation after hanging
States of somnolence with a relation to physiological sleep
Hypnotic states
Migraine-based somnolence
Affect-based somnolence
Toxic somnolence, or disturbance of mind
Complicated states after intoxication
Disease of the mind after CO-inhalation
Vasomotoric states of somnolence
Congestive (transitory mania)
Angiospastic (raptus melancholicus)
Transitory disturbances of mind after infectious diseases
Paralytic attacks
Retrograde amnesia without previous disturbances of consciousness
Korsakoff’s psychosis
There are numerous forms of psychogenic amnesic states and numerous attempts to test and treat them (cf. e.g., Markowitsch, 1999 ). Of special interest are old studies in which these twilight states were manipulated experimentally (e.g., Brodmann, 1897 ; Jahrreiss, 1928 ; Köhler, 1897 ; Leavitt, 1935 ; Lundholm, 1932 ; Prince, 1906 ). In Germany, Ganser ( 1898 , 1904 ) described “a peculiar hysterical state of somnolence” which up to today is characterized by vorbeireden (giving approximate answers such as “3” in response to the question “How many ears has a dog?) and disturbed consciousness, and which for many years was also subsumed under the category of psychogenic amnesic states (see Whitlock, 1967 ). (Nowadays it is classified differently in the DSM-5 , namely as a Dissociative Disorder Not Otherwise Specified). Frequently, it was and is diagnosed in the context of forensic situations ( C. G. Jung, 1902 ; Hey, 1904 ; Lücke, 1903 ; Matthies, 1908 ; Stertz, 1910 ; Markowitsch & Staniloiu, 2012b ; Staniloiu, Vitcu, & Markowitsch, 2011 ).
Similarly, so-called fugue-states (which are dissociative amnesias accompanied by leaving the usual place of living) were at the turn of the century frequently diagnosed (e.g., Burgl, 1900 ; Heilbronner, 1903 , 1905a , 1905b ; Woltär, 1906 ) and also considered to be sometimes accompanied by a forensic background ( Zingerle, 1912a ). Fugue states were frequently observed in children and young adults ( Bregman, 1899 ; Donath, 1899 ; Stier, 1912 ; cf. also Markowitsch, Fink, Thöne, Kessler, & Heiss, 1997 ) and were also named poriomania (Donath, 1899 , 1907 1908 ; Heilbronner, 1903 , 1905a , b ; Hey, 1904 ; Raecke, 1908 ; Schultze, 1903 ).
So-called multiple personality states in whom amnesia for the respective other personality state or states is a defining criterion were also frequently diagnosed in the time period between 1870 and 1920 ( Angell, 1906 ; Azam, 1876 ; Bregman, 1899 ; Burnett, 1925 ; Donath, 1908 ; Gordon, 1906 ; Pick, 1876 ; Prince, 1906 ; Read, 1923 ; Sidis & Goodhart, 1905 ; Viner, 1931 ; A. Wilson, 1903 ).
Interestingly, as in present times (e.g., Fujiwara, Brand, Kracht, Kessler, Diebel, Netz, & Markowitsch, 2008 ; Pommerenke, Staniloiu, Markowitsch, Eulitz, Gütler, & Dettmers, 2012 ), there were early reports of patients with a combination of amnesias with a combined organic and psychic background ( Bennett, 1878 ). Bennett, 1878 , p. 120) questioned the dichotomy between organic and psychic illnesses. He wrote: “symptoms of what is called hysteria may co-exist with organic disease of the brain—whether independent of it or the result, … Under any circumstances it serves to indicate what caution should be exercised in diagnosing, and more especially in treating, as hysteria, any nervous affection in women which may appear indefinite or mysterious.” (A case with related symptomatology after brain disease was reported in the same year in the same journal: Savage, 1878 .)
As an example, Dana described in 1894 “a case of amnesia or ‘double consciousness’.” The 24-year-old “active, intelligent, and healthy … man” (p. 571) had behavioral changes due to carbon monoxide poisoning by domestic gas. After having been rescued, he was quite disturbed and “did not know who he was or where he was, and … his conscious memory for everything connected with his past life was gone” (p. 572). His behavioral changes included the use and understanding of only the simplest words. After realizing where he was, he “pronounced many of the new words with a German accent” (p. 572), similar to the way his German attendant spoke. He did not know what marriage meant and did not remember (previously) familiar persons, although he seemed especially glad to see them. At first he was unable to read, but soon he learned to read and write simple sentences. Old musical memories existed and so did habits connected with courtesy.
Interestingly, “in argument he showed considerable dialectic skill and logical power, [but] he evidently could not understand any conceptions at all abstract” (p. 573). “The moon, the stars, the animals, his friends, all were mysteries he impatiently hastened to solve” (p. 574). While at the beginning he did not recognize his parents or sisters, from the beginning he said that he had always known his fiancée and subsequently his thoughts and feelings centered on her.
Exactly three months after his attack, his memory was completely restored. The recovery started during a visit in the evening at his fiancée’s; she thought he was in a condition worse than during the previous months, and said “he felt as though one half of his head was prickling and numb, found his memory restored…. He knew all his family at once and was plainly just the same man as before. But the three months was an entire blank to him” (p. 575). So he did not know Professor Dana whom he had not seen before the accident.
Although such an abrupt and nearly complete memory restoration, the total but time-limited loss of knowledge about his family and friends, and the age of the patient all favor psychogenic amnesia, the carbon monoxide poisoning provides a basis for an organic damage-based explanation. Possibly the poisoning but triggered the release or manifestation of an already existing tendency for psychogenic memory loss (cf. the review on similarities of organic and psychic amnesias of Markowitsch, 1996 ).
Many of the features present in Dana’s case closely resemble features and descriptions in psychogenic amnesic patients of the present. For instance, the patient of Pommerenke et al. (2012 , p. 107) postoperatively “did not recognize his friends, his grandmother and even himself in pictures from his youth…. He did not know which professional training or job he had had. He did not recall that fishing used to be his hobby. Furthermore, he did not know how to use a fishing rod. He encountered considerable difficulties with naming objects. He perceived his situation as if he stood in a white room, where the door behind him had been locked and he did not know where he had come from. Tests of anterograde memory were in the normal range or even above average.”
Depression and strong feelings of unreality were diagnosed in another most likely psychogenic amnesic case with additional somatic complaints (“an attack of severe pain in the head, face, and arms, lasting about 48 hours” ( Stevens, 1907 , p. 448). This patient had both severe anterograde and retrograde amnesia, similarly as a patient we had described more than a decade ago ( Markowitsch, Kessler, Van der Ven, Weber-Luxenburger, & Heiss, 1998 ; Markowitsch, Kessler, Weber-Luxenburger, Van der Ven, Albers, & Heiss, 2000 ).
Another example for retrograde amnesia with a psychic touch was given by Kohnstamm (1917) about a former soldier who had been buried alive but had no somatic complaints. The patient’s short-term memory was normal; however, he did not remember dates from history or geography, which he had learned at school. His musical repertoire, however, was still fully existent; while he was not aware of the musical titles, he was able to play them accurately. Similarly, he recognized his closest relatives, but not more distant friends, though he still was aware of the fact that he had known them. Kohnstamm (p. 375) concluded that the “quality of familiarity” had survived in the patient (cf. Markowitsch & Staniloiu, 2012a , fig. 1: the recent division of long-term memory into several systems of which both the “priming” and the “perceptual memory system” contain features preserved in this patient). The patient, however, had severe anterograde amnesia as well (but no trace of confabulation). Kohnstamm emphasized that the patient’s remembering was greatly facilitated by emotional stimuli and when he was interested and engaged in a topic (p. 378). He remarked that this observation might be used pedagogically and for memory training. When Kohnstamm stated that “knowledge of language, calculation, and requirements for daily living” (p. 380) was preserved, one is reminded of preserved semantic and procedural memory and of grossly impaired episodic-autobiographical memory. The same constellation is found in present-day case descriptions of patients with dissociative amnesia (e.g., Markowitsch & Staniloiu, 2013 ).
Neurology (Neuropathology) of Memory
Relations between brain damage and memory disturbances were the topic of a number of books from 1900 and before (e.g., Forel, 1885 ; Hering, 1870 ; Meynert, 1878 ; Ribot, 1882 ; Rittershain, 1871 ; Sollier, 1900 ). Some authors favored an anti-localizationist’s view ( Meynert, 1884 ; Monakow, 1904 , 1909 , 1914 ), while others propagated specific “responsible” loci for memory processing ( Bernhardt, 1874 ; Charcot, 1878 ; Exner, 1881 ; Ferrier, 1878 ; Guder, 1886 ; Hitzig, 1901 , 1903 ; Kleist, 1934 ; Liepmann, 1913 ; Solly, 1836 ; Ziehen, 1908 ). Usually, the cerebral cortex was considered as being the principal “memory structure”; subcortical structures were mentioned only later (e.g., Ewald, 1940 , who considered the mammillary bodies to be the center of a memory system). The preference given to the cortex was most likely related to the dominance of psychiatry over neurology at the time and the prevalence of dementia as a diagnosis. An example is Alzheimer’s (1904) paper on the anatomical bases of idiocy. “Dementia praecox, paralytic, epileptic, alcoholic dementia, states of weakness after certain other forms of mental illness” ( Alzheimer, 1904 , p. 497) were the dominant clinical diagnoses—so-called secondary dementias. Seven years earlier, in 1897, Alzheimer had published a manuscript that dealt principally with relations between amnesia and epilepsy ( Alzheimer, 1897 ). In this report he stated that he had no hint as to where in the cerebrum retrograde amnesia was localizable.
Aside from epilepsy, common causes for dementic processes were seen in infections (syphilis), various forms of encephalitis, and intoxications. Prevalent diagnoses were those of “progressive paralysis” (e.g., Gowers, 1878 ; Gregor, 1909 ) or senile dementia (e.g., Freund, 1889 ; Probst, 1903 ). Some of the best works on the localization of functions in the cerebral cortex stem from Goldstein (1927) , Kleist (1934) , and Monakow ( 1910 , 1914 ). Semon (1920) , who stated that his views generally were in close congruence with those of Monakow (1914) , was of the opinion that engrams certainly are not laid down in accordance with cyto- or myeloarchitectonic maps of the brain. Nevertheless, he assumed that the storage of engrams would occur in the chromatic substance of the neuronal nuclei (p. 157). Ziehen already concluded in 1908 that “only brain pathology has shown us that a general memory does not exist at all, but only subdivisions of memory or partial memories” (“Teilgedächtniss,” p. 16, “Partialgedächtnisse,” p. 17) and Paul stated in 1899 that “the degree or extent of amnesia is to a certain degree proportional to the duration of coma” (p. 264).
Boedeker (1905) described the interesting case of an “ordentlicher Universitätsprofessor,” 54 years of age, who developed Wernicke’s encephalopathy ( Wernicke, 1881 ). This case showed that remembrance frequently follows associations according to their sounds; the patient, for instance, answered that he lived in the “Fischborn” hospital, although in fact he lived in the “Fichtenhof.” After three weeks of hospitalization there was a nearly complete recovery, and after several further weeks he was giving lectures again. Boedeker’s second case was a “true chronic Korsakoff” (p. 314). When asked the same question as the previous patient, he answered “Fichtenhöhe”; he also concluded that he could not be ill: “When you have appetite, you can’t be ill, I say to myself, logically” (p. 316). However, some days later he repeatedly said that he had memory problems, especially with respect to the immediate past. Although he remembered specific dates from history correctly (“1648,” “1756–1763,” “about 800”), he thought that his two brothers had visited him in the hospital, though both had recently died. He attributed his memory problems to frequent masturbation in his youth (“… dass ich früher manustuprierte. Die Folgen bleiben ja nie aus.” [… that I masturbated in former times. The consequences are inevitable]). He was quick and accurate with calculations (“12 x 13,” “220–21”) and accurate with estimating numbers and in reporting four-figure numbers over time. But the discrepancy was striking between his ability to memorize “5679, 1235” over days and his inability to remember the deaths of several relatives and the fact that his daughter was 21, and not 7 years, as he estimated.
Burnham at the turn of the century seems to have been the American specialist for memory and memory problems (cf. Burnham, 1889a–d ); in 1903 he made an analysis of retrograde amnesia. His main thoughts were the following (p. 132):
In normal memory a process of organization is continually going on, a physical process of organization and a psychological process of repetition and association. In order for ideas to become a part of permanent memory, time must elapse for these processes of organization to be completed.
In cases of retrograde amnesia, the amnesia results from arrest of these processes of organization by shock or other causes. Memory is lost because it was never completely organized.
In normal memory these processes of organization are essential in order to fix impressions, and anything that interferes with them, fatigue, being in a hurry, distraction or excitement, hinders acquisition.
Hanging and Retrograde Amnesia
Unsuccessful attempts at hanging usually result in retrograde amnesia. Many articles of the old days dealt with this phenomenon as was described in the short review of Markowitsch (1992) .
Retrograde Amnesia after Cerebral Concussion
In 1894, Gussenbauer gave his introductory lecture as the new director of the Second Surgical Clinic of Vienna, centering on the consequences of cerebral concussion on behavior. He remarked that cerebral concussion might alter not only mnestic functions but intellectual behavior in general (p. 807). He wondered why some of the information lost might be recovered while other parts failed to do so. He described a patient whose concussion he could follow and investigate in detail from the very start, because he was an eyewitness: During mountain hike a friend of his lost his balance and fell on his head in the ice, which led to a short cardiac arrest, a cephalic hematomy, and wound. Gussenbauer noted the following (p. 808):
All memories for the last 24 hours, including imaginations, reflections, and affections were permanently lost. Even going over the same mountain route one year later failed to reinstate any remembrance.
Events from the previous two days were partially lost as well.
There was an anterograde amnesia for the next nine hours.
Some priming of emotionally significant memories from the last hours before the accident was possible.
Gussenbauer assumed that the effects of concussion were due to a partial ineffectivity of cortical neurons.
Alcohol-Related Brain Damage/Korsakoff’s Syndrome
Korsakoff’s psychosis can be characterized by focal intellectual deterioration that centers around problems in memory and by brain damage that is found most prominently and reliably in diencephalic structures. A vitamin B 1 deficiency—usually caused by high amounts of alcohol consumption—is the etiology. Another form of ethanol-based brain damage, alcoholic dementia, leads to more widespread deteriorations of intellect, personality, and brain damage than Korsakoff’s disease.
Though a variety of pathological conditions have been subsumed under the heading “Korsakoff’s disease” or “Korsakoff’s syndrome” (e.g., Brie, 1892 ; Lückerath, 1900 ; Meyer, 1904 ; Schulz, 1908 ; Weber, 1906 ), it might be worthwhile to call back to mind a differentiation made by Steinthal (1921) . He pointed out that a clear distinction should be made between the “Korsakoff’s symptom complex” and “Korsakoff’s psychosis.” Synonyms for the “Korsakoff’s symptom complex” would be “Korsakoff’s syndrome” and ‘the amnesic symptom complex.’ This symptom complex, according to Steinthal, could appear in “progressive paralysis, lues cerebri, brain tumors, after traumatic brain damage and strangulation, in the age-related illnesses of the brain, and –after various infections and toxic illnesses” (p. 287). He reserved the term “Korsakoff’s psychosis” for cases in which the symptom complex appeared after year-long alcohol abuse, that is, he based this term on an etiological moment.
Sergei S. Korsakoff (1854–1900), a psychiatrist in Moscow, published the first case descriptions in several Russian journals ( Archiv Psychiatrii [Charkow], Westnik Psychiatrii, Medizinskoje Oboszenije , and in the Jeschenedelnaja klinitscheskaja gaseta ), and as a book, On Alcoholic Paralysis , which appeared in 1887 in Russian (cited after Korsakoff, 1890 ; Korsakow, 1890 ). Later articles were published in French and German (Korsakoff, 1889 , 1890 ; Korsakow, 1890 , 1891 ; Korsakow & Serbski, 1892 ); an article published in Russian in 1887 was translated into English by Victor and Yakovlev (1955) . There were a number of articles and other contributions before Korsakoff’s publications that dealt with alcohol-related multiple neuritis (e.g., Annan, 1840 ; Huss, 1852 ; Canton, 1860 ; Dreschfeld, 1884 ; Moeli, 1883 ; Fischer, 1882 ; Lawson, 1878 ). Especially Wernicke’s (1881) acute, haemorrhagic, superior poliencephalitis, described in paragraph 47 of the second volume of his “Lehrbuch der Gehirnkrankheiten für Aerzte und Studirende,” was well-known. With his terminology Wernicke wanted to characterize a sudden, haemorrhagic infarct occurring at various loci of the gray matter dorsal to the medulla oblongata: “It is an independent, inflammatory, acute gray matter disease in the area of the eye muscle nerves, leading to death within 10–14 days” (p. 240). While Wernicke’s poliencephalitis is considered an acute form, Korsakoff’s disease is considered the chronic form of the two usually alcohol-related, diseases. The term “Korsakoff’s disease” had been suggested by P. Jolly at the International Medical Congress in Moscow in 1897 (cf. also Jolly, 1897 ).
At the beginning of the present century, Chotzen (1902) already described in detail which functions of the Korsakoff’s syndrome might recover in which sequence. First, orientation recovers, then the ability to memorize; thereafter, orientation for time recurs. However, some anterograde amnesia nearly always remains, together with a poor reaction toward the environment and a lack of interests. Retrograde amnesia, on the other hand, usually shrinks considerably according to Chotzen’s findings.
Korsakoff was of the opinion that in his cases, memory for recent events usually diminished, while it was spared for far remote events ( Korsakoff, 1890 , p. 479). His description of a typical Korsakoff’s patient can still be considered representative ( Korsakoff, 1890 , p. 479 f):
At the beginning of a talk the mental disturbance is hardly noticeable; he gives the impression of a person with full mental powers—talking with full reflection, drawing correct deductions on the basis of given premises, playing chess, or a game of cards, in short—he behaves like a mentally healthy subject; only after longer conversation may one notice that he mixes facts quite substantially, and that he is unable to keep in mind anything of what takes place in his environment: he does not remember having eaten, or whether he got up from bed. Sometimes he immediately forgets what has happened to him. Patients of this kind may read for hours one and the same page, because they are unable to keep the contents in mind. They can repeat 20 times the same conversation without realizing the continuous repetition of their stereotyped talk. He does not know persons he met only during the time of his illness, such as the doctor or the nurse, though he sees them consistently; he is always convinced of meeting them for the first time.
In his study “On a special form of a psychic disturbance, combined with multiple neuritis” ( Korsakow, 1890 ), he gave detailed case reports and a careful summary of the psychological changes (p. 700 ff), emphasizing the irritability of the patients, their disturbed association of ideas, and their memory problems. He also mentioned again that conscious and rational thinking may be preserved, while the amnesia is still profound (p. 701 f: “not knowing 5 min past lunch that he had just eaten”). “Pseudoreminiscences” or “false memories” were the specific topic of a study that he published in 1891. Korsakoff mentioned that these pseudoreminiscences appear in two forms—as déjà vu feeling and as the appearance of ideas or conceptions that had not occurred in reality. He had noted that most of these pseudoreminiscences had some relation to a true background, and so he hypothesized that they represent a rudimentary form of memory engrams (“The memory traces most likely correspond to the preservation of a function of neuronal elements, though in very minor intensity,” p. 410). He furthermore speculated that these rudimentary elements might then combine to “constant associative groups,” which are first unconscious but at a later stage may come to consciousness and thereby simulate real mem o ries.
A very influential book on Korsakoff’s disease was published in 1901 by Karl Bonhoeffer, a pupil of Wernicke (entitled The Acute Mental Diseases in Chronic Alcoholics .) The idea of four “cardinal elements” of the Korsakoff’s syndrome—a memory defect for current events ( Merkunfähigkeit ), retrograde amnesia ( Erinnerungsdefekt ), disorientation, and confabulation—was specified on p. 119 of his book. The memory defects are the most persistent and consistent of the four, as Korsakow (1890) had remarked earlier. Bonhoeffer, however, criticized that Korsakoff had failed to make a sharp distinction between anterograde and retrograde amnesia.
It is interesting that both Korsakow (1890) and Bonhoeffer (1901) emphasized that the amnesia is not complete, but that certain, usually emotionally significant, events can be memorized and that information that appeared to have been lost may be regained even after years (cf. the similar results of Markowitsch, Kessler, Bast-Kessler, & Riess, 1984 ; Markowitsch, Kessler, & Denzler, 1986 ).
Bonhoeffer further discussed the reduced apprehension of Korsakoff patients and referred to a peculiar and interesting case of Liepmann (1910) . Liepmann’s case was a 50-year-old journalist with an amnesic episode extending back about three decades, the border between preserved and lost remembrance dating to the year 1871. The patient, a philological, had a good remembrance of his former school friends, knew Latin syntax and could name his university professors. Asked about his occupation, he told the physician he was a student. Memory of the death of his parents (dating to the year 1874) was lost. All answers to political questions dated 30 years back in time. But he was well educated on questions of a political, historical, and moral nature.
Bonhoeffer criticized Liepmann’s description of the patient’s sharp border of memory loss; after all he had been able to calculate the age of Kaiser Wilhelm (I) correctly, though realizing that he would have to be 101 years old (in 1898). Bonhoeffer also argued that if the patient really only kept experiences up to the year 1871, he should have behaved like a 19-year-old adult (while in fact he behaved like a much older person, considering his available background of knowledge). Bonhoeffer concluded that there is no case with a total loss of that individuality which had accumulated over time; instead, the total character and degree of the mental maturity is preserved.
Again, these findings first point to the distinction between different long-term memory systems ( Markowitsch & Staniloiu, 2012a ) and, second, correspond to present-day descriptions of both psychogenic and brain-damaged patients with amnesia (e.g., Markowitsch, Cramon, & Schuri, 1993 ; Markowitsch & Staniloiu, 2013 ).
Serbsky (1907) , a pupil of Korsakoff, presented the case of an alcohol-based Korsakoff psychotic (aged 42 years), who had had severe memory problems, but was still able to think logically, could make jokes, and won games, and of another case, a female patient of 33 years, who drank large amounts of alcohol: every day, though usually only in the night, she had 1 to 1 ½ bottles of brandy, the same amount of schnapps, up to 10 bottles of beer, liqueurs, rowan-berry wine, and other alcoholic beverages. The woman survived but suffered feelings of jealousy for her husband. Serbsky ended his description by elaborating on a dilemma: The woman wanted frequent intercourse with her husband, but was not physically fit enough to become pregnant or have an abortion. Serbsky was of the opinion that coitus interruptus or reservatus might lead to epilepsy (in women), so he suggested as an alternative bringing the woman to a “special sanatorium.”
Neuroanatomy
Wernicke had already provided some hints in 1881 (hemorrhages surrounding the third and fourth ventricle). Probably the first article to give a clear statement on diencephalic damage in Korsakoff’s syndrome was from Gudden in 1896 ; however, his results were not generally acknowledged or referred to in the papers that appeared around the turn of the century. The same was true for Bonhoeffer’s (1901) findings on the neuropathology of Korsakoff’s disease. This is regrettable, as both authors had made very careful and detailed observations and furthermore had provided thorough reviews on previous cases.
A major contribution then came from Gamper ( 1928a , b ), an Austrian scientist who in 1930 became professor of psychiatry and neurology at the German University in Prague. Gamper had performed histological investigations on the brains of 16 chronic alcoholics who had died as Korsakoff’s psychotics. The average age of the nine men was 53.6 years and that of the seven women 47.7 years. Most patients died within 8–14 days after disease onset, while in three cases the illness lasted two months, and in one nine months. Gamper emphasized that he failed to find any characteristic, specific changes in the cortical mantle of any of the patients’ brains. On the other hand, marked changes were observed from the onset of the medulla oblongata up to the level of the anterior commissure. In all cases the mammillary bodies were regularly affected. Gamper stated that they apparently constituted the nodal points of the disease process and that they were also degenerated in cases in which no other areas were involved.
Furthermore, changes in the diencephalon were found in the nuclei along the medial wall of the third ventricle. The medial portions of the mediodorsal thalamic nuclei were affected, that is, areas that partly overlap with the nucleus reuniens. The nuclei parafascicularis, submedius, and reuniens were frequently damaged. Generally, however, destruction within the diencephalon was diminished in regions close to the dorsal and anterior thalamic boundaries, while lateral and anterior nuclei were not affected. In the subependymal area a glia proliferation was observed. This same, somewhat unexpected finding was documented in the cases reported on by Mair, Warrington, and Weiskrantz (1979 ; cf. their Figs. 8 and 15, or Figs. 15 and 17 of Markowitsch & Pritzel, 1985 ). In these cases also the mammillary nuclei and the most medial portions of the mediodorsal thalamic level were also degenerated (cf. their figs. 4 and 12, or figs. 14 and 16 of Markowitsch & Pritzel, 1985 ).
Gamper mentioned that Gudden first found atrophic mammillary bodies in the brains of Korsakoff’s patients with a longer disease process, and he furthermore remarked a strong proliferation of the vascular system and of glia cells and a restriction of the degenerative changes to nerve cells without invading the passing fiber systems.
It is important to note here that Gamper’s findings are frequently mentioned only in a curtailed form as if he had only mentioned the mammillary nuclei as the essential affected brain structures in Korsakoff’s psychosis, while in fact (with the exception of cortical areas) his minute descriptions include principally all the structures—if not more—that today have been found to be damaged in Korsakoff’s alcoholics. Furthermore, Gamper’s behavioral observations and the conclusions he drew from these remain valid today: He supposed that the principal deficit of Korsakoff’s patients lies in their inability to form a spatial-temporal background, “… which in normals comprehends in passive attentiveness the basis of the temporal-spatial integration of a distinct event complex in the mnestic continuum” ( Gamper, 1929 , p. 238). In referring to the finding that Korsakoff’s subjects frequently still possess the ability to perform the most complicated combinatory processes (calculating abilities), he argued that in experimental examination the ability to remember (short term) was hardly altered; what was altered in Gamper’s eyes is the ability to embed an event into its proper context, which therefore results in its loss.
Later studies principally confirmed the anatomical finding of dorsal (thalamic) and ventral (mammillary body) diencephalic degeneration in patients with Korsakoff’s syndrome, made by Gamper and his contemporaries ( Kant, 1932 ; Neubürger, 1929 ; see Markowitsch, 2010 ).
Diencephalic Amnesia of Other Backgrounds
About 1% of cerebral tumors are primarily thalamic. It is therefore not astonishing that already around 1900 the term “thalamic syndrome” became prominent (e.g., Dejerine & Roussy, 1906 ; Roussy, 1907 ; cf. also the review of Langworthy & Fox, 1937 ).
A larger series of thalamic and third ventricle tumors was included in the 368-page long book of Schuster (1902) . He began his introduction on thalamic tumors with the statement that these are “diversified” (p. 163). He referred to several cases that had been published in German- and non-German-language journals at the end of the 19th century and then gave a detailed description of one of his own cases. Schuster then listed in tabular form 16 further cases in which mental deterioration was a prominent symptom. In three of these cases pure memory disorder with intact intelligence was remarked. Ependymal cysts are rather frequently found in the third ventricle, as described by Bruns (1897 ; cf. Meyer, 1899 ; Piazza, 1909 ; Weisenburgh, 1910 ; S. A. K. Wilson, 1906 ).
Medial Temporal Lobe Amnesia
Schuster (1902) also described a number of patients with medial temporal lobe damage and pointed out that a number of them had memory problems. For example, his case number 9, a 52-year-old woman who shared a combination of amnesic and Klüver-Bucy-syndrome signs, had severe amnesic disturbances “and her mood suddenly changed to a happy, gay state, she felt completely healthy, … and laughed unmotivatedly” (p. 108). Schuster found a hemorrhagic focus in her left posterior temporal lobe which was surrounded by a tumor covering most of the temporal lobe. A number of the other cases reviewed by Schuster had “memory weaknesses,” “amnesic disturbances,” “Korsakoff’s psychosis,” and the like, as a consequence of temporal lobe damage; in two cases even “isolated memory weakness” was the diagnosis (p. 121). Well in time before Schuster, Harvey (1846) had a patient who had been kicked by a horse and died more than three months later. Autopsy showed that “the temporal region had entirely disappeared” (p. 506). The patient had defective memory, but no systematic attempt to measure his behavior was attempted.
Of special interest is the case description of Bechterew (1900) demonstrating “a brain with destruction of the anterior and medial parts of the cerebral cortex of both temporal lobes.” The bilateral destruction of the uncinate and hippocampal gyri was accompanied by an “extra-ordinary anterograde amnesia, and a partial retrograde amnesia.” As the patient during his lifetime had appeared in the sessions of the Russian Society of Psychiatrists and Neurologists in Moscow, as a Korsakoff’s case (“with polyneuritic psychoses”), Bechterew concluded that this symptomatology could occur after temporal lobe damage as well. If Scoville (1968) , who in 1954 had written about “The limbic lobe in man,” had known Bechterew’s report, he probably would have been more cautious with his surgery on patient H. M. ( Squire, 2009 ), as well as on other patients in whom he removed the medial temporal lobes bilaterally with the consequence of severe and lasting anterograde amnesia.
In 1954, less than one year after his surgery on patient H. M., Scoville wrote that he was really surprised from the outcome of a “bilateral resection of the uncus and amygdalum alone, or in conjunction with the entire pyriform amygdaloid hippocampal complex” (p. 64). He wrote that this surgery “has resulted in no marked physiologic or behavioral changes with the one exception of a very grave, recent memory loss , so severe as to prevent the patient from remembering the locations of the rooms in which he lives, the names of his close associates, or even the way to toilet and urinal” ( Scoville, 1954 , p. 64 f; his emphasis).

Schematic view of the extent of brain damage in the patient described by Zingerle (1912b) . The defects enclosed the whole ventral part of the right temporal lobe from lateral to medial. (After fig. 1 of Zingerle, 1912b .)
Another early case description pointing to the importance of the temporal lobe for memory was given by Zingerle (1912b) . Grossly viewed, the main portion of the right temporal lobe was absent along its total breadth and length, “as if it had been cut horizontally by a sharp knife” (Figure 5.5 ). Behaviorally, the 40-year-old patient was characterized by an inappropriate orientation with respect to space and time, and by marked memory disturbances with reduced intelligence. Zingerle’s case is remarkable as the tumor was unilateral, right-sided, and it isolated the amygdala and the hippocampal area from the rest of the brain, though marked damage was not detectable.
Otherwise, only around the time of the Second World War clear relations between memory and other intellectual deteriorations and bilateral hippocampal damage were given. Of special interest is Grünthal’s (1947) Case of a woman with mild diabetes who, after falling into a hypoglycemic coma quickly deteriorated in her intellectual level and manifested signs of a Klüver-Bucy syndrome. Both macroscopical and microscopical changes were confined to her hippocampi. Grünthal included altogether 11 photographs in his article, of which seven showed portions of the damaged hippocampal formation. The following is the English summary of his report (p. 15): “Dementia of high rate after acute circumscribed destruction of the cornu ammonis formation on both sides is described. Thence is to be concluded that the normal functioning of the cortex of the cornu ammonis is a necessary preliminary condition to the intellectual production of the cerebrum.”
Conclusions
The present survey on early research of memory functions in individuals with both normal and damaged brains revealed a surprisingly large amount of data which in part were later forgotten and rediscovered—in part probably due to the fact that English-speaking authors rarely read findings not published in their native language. The results from Bechterew (1900) on the functions of the hippocampal formation in memory, from Semon (1904) on state-dependency of memory or from Ziehen (1908) and Schneider (1912) on different memory systems, can be taken as examples. Also the interwovenness between neurology and psychiatry was denser around the turn of the second-last century than in the last decades. Only modern functional imaging methods brought a change by “narrowing the traditional gap between neurological and psychiatric approaches in defining a disease process” ( Markowitsch, 1999 , p. 331; cf. also Markowitsch, 1996 ; Pietrini, 2003 ).
Ach, N. ( 1901 ). Ueber die Beeinflussung der Auffassungsfähigkeit durch einige Arzneimittel [On the influence of some drugs on comprehension ability 2 ]. In E. Kraepelin (Ed.), Psychologische Arbeiten , Vol. 3 (pp. 203–288). Leipzig, Germany: Barth.
Google Scholar
Google Preview
Alzheimer, A. ( 1897 ). Ueber rückschreitende Amnesie bei der Epilepsie [On retrogressive amnesia in epilepsy]. Allgemeine Zeitschrift für Psychiatrie, 53, 483–499.
Alzheimer, A. ( 1904 ). Einiges über die anatomischen Grundlagen der Idiotie [Some remarks on the anatomical bases of idiocy]. Centralblatt für Nervenheilkunde und Psychiatrie, 27(15), 497–505.
Annan, S. ( 1840 ). Idiocy from alcoholism. Maryland Medical and Surgical Journal, 1, 333–334.
Angell, E. B. ( 1906 ). A case of double consciousness-amnesic type, with fabrication of memory. Journal of Abnormal Psychology, 1, 155–169.
Aschaffenburg, G. ( 1895 ). Experimentelle Studien über Associationen [Experimental studies on associations]. Psychologische Arbeiten, 1, 209–299.
Aschaffenburg, G. ( 1899 ). Experimentelle Studien über Associationen [Experimental studies on associations]. Psychologische Arbeiten, 2, 1–83.
Azam, M. ( 1876 ). Periodical amnesia; or, double consciousness. Journal of Nervous and Mental Disease, 3, 584–612.
Bauer, J. ( 1917 ). Hysterische Erkrankungen bei Kriegsteilnehmern [Hysteric diseases in combatants]. Archiv für Psychiatrie und Nervenkrankheiten, 57, 139–168.
Bechterew, W. von ( 1900 ). Demonstration eines Gehirns mit Zerstörung der vorderen und inneren Theile der Hirnrinde beider Schläfenlappen [Demonstration of a brain with destruction of the anterior and inner portions of the cerebral cortex of both temporal lobes]. Neurologisches Centralblatt, 19, 990–991.
Bennett, A. H. ( 1878 ). Case of cerebral tumour-symptoms simulating hysteria. Brain, 1, 114–120.
Bernhardt, M. ( 1874 ). Zur Frage von den Funktionen einzelner Theile der Hirnrinde des Menschen [On the question of the functions of individual parts of the human cerebral cortex]. Archiv für Psychiatrie und Nervenkrankheiten, 4, 480–481.
Bleuler, E. ( 1905 ). Diagnostische Assoziationsstudien V. Bewusstsein und Assoziation [Diagnostic association studies V. Consciousness and association]. Journal für Psychologie und Neurologie, 6, 126–154.
Boedeker, J. ( 1905 ). Ueber einen acuten (“Polioencephalitis superior haemorrhagica”) und einen chronischen Fall von Korsakow’scher Psychose [On an acute (“Polioencephalitis superior haemorrhagica”) and a chronic case of Korsakoff’s psychosis]. Archiv für Psychiatrie und Nervenkrankheiten, 40, 304–328.
Bogousslavsky, J. ( 2011 ). Hysteria after Charcot: Back to the future. Frontiers in Neurology and Neuroscience, 29, 137–161.
Boldt, K. ( 1905 ). Studien über Merkdefekte [Studies on defects of memorizing]. Monatsschrift für Psychiatrie und Neurologie, 17, 97–115.
Bonhoeffer, K. ( 1901 ). Die akuten Geisteskrankheiten der Gewohnheitstrinker [Acute mental illnesses of chronic drinkers] Jena: Fischer.
Bregman, L. E. ( 1899 ). Ueber den “Automatisme ambulatoire” (“Fugues,” “Dromomania”) [On the “automatisme ambulatoire” (“fugues,” “dromomania”)]. Neurologisches Centralblatt, 18, 776–781.
Breuer, J. , & Freud, S. ( 1895 ). Studien über Hysterie [Studies on hysteria]. Wien, Austria: Deuticke.
Breukink, H. ( 1907 ). Über Patienten mit Perseveration und symbolischen und aphasischen Erscheinungen [On patients with perseveration and symbolic and aphasic symptoms]. Journal für Psychologie und Neurologie, 9, 113–133 and 165–185.
Brie (no first name given). ( 1892 ). Ein Fall von Geistesstörung bei multipler Neuritis [A case of mental disturbance in multiple neuritis]. Allgemeine Zeitschrift für Psychiatrie und ihre Grenzgebiete, 48, 175–179.
Brodmann, K. ( 1897 ). Zur Methodik der hypnotischen Behandlung [On the methodology of hypnotic treatment]. Zeitschrift für Hypnotismus, Psychotherapie sowie andere Psychophysiologische und Psychopathologische Forschungen, 6, 1–10, 193–214.
Brodmann, K. ( 1902 ). Experimenteller und klinischer Beitrag zur Psychopathologie der polyneuritischen Psychose [Experimental and clinical contribution to the psychopathology of the polyneuritic psychoses]. Journal für Psychologie und Neurologie, 1, 225–246.
Brodmann, K. ( 1904 ). Experimenteller und klinischer Beitrag zur Psychopathologie der polyneuritischen Psychose. B. Experimenteller Teil. [Experimental and clinical contribution to the psychopathology of the polyneuritic psychoses. B. Experimental part.]. Journal für Psychologie und Neurologie, 3, 1–48.
Brodmann, K. ( 1909 ). Vergleichende Lokalisationslehre der Grosshirnrinde in ihren Prinzipien dargestellt auf Grund des Zellenbaues . [Comparative study of the localization in the cerebral cortex, demonstrated in its principles on the basis of cytoarchitecture]. Leipzig, Germany: Barth.
Brodmann, K. ( 1912 ). Ergebnisse über die vergleichende histologische Lokalisation der Grosshirnrinde mit besonderer Berücksichtigung des Stirnhirns [Results on the comparative histological study of the cerebral cortex with special emphasis on the frontal lobe]. Anatomischer Anzeiger, (Suppl.), 41 , 157–216.
Bruns, L. ( 1897 ). Die Geschwülste des Nervensystems [The tumors of the nervous system]. Berlin: Karger.
Burgl, G. ( 1900 ). Eine Reise in die Schweiz im epileptischen Dämmerzustande und die transitorischen Bewusstseinsstörungen der Epileptiker vor dem Strafrichter [A journey to Switzerland done in epileptic somnolence and the transitory disturbances of consciousness before the criminal judge]. Münchener medizinische Wochenschrift, No. 37, 1270–1273.
Burnett, C. T. ( 1925 ). Splitting the mind. Psychological Monographs, 34 (2), 1–132.
Burnham, W. H. ( 1889 a). Memory, historically and experimentally considered. I. An historical sketch of the older conceptions of memory. American Journal of Psychology, 2, 39–90.
Burnham, W. H. ( 1889 b). Memory, historically and experimentally considered. II. Modern conceptions of memory. American Journal of Psychology, 2, 225–270.
Burnham, W. H. ( 1889 c). Memory, historically and experimentally considered. III. Paramnesia. American Journal of Psychology, 2, 431–464.
Burnham, W. H. ( 1889 d). Memory, historically and experimentally considered. IV. Recent theories, experimental studies, conclusion. American Journal of Psychology, 2, 568–622.
Burnham, W. H. ( 1903 ). Retroactive amnesia: Illustrative cases and a tentative explanation. American Journal of Psychology, 14, 118–132.
Canton (n.n.g.) ( 1860 ). Chronic alcoholism with impending delirium tremens treated by suspension of the stimulus. Lancet, ii, 237–238.
Charcot, J. M. ( 1878 ). Ueber die Localisationen der Gehirn-Krankheiten [On the localization of brain diseases]. Stuttgart: Bonz & co.
Charcot, J. M. ( 1892 ). Sur un cas d’amnesie retro-anterograde. Revue de Medicine, 12, 81–96.
Chotzen, F. ( 1902 ). Zur Kenntnis der polyneuritischen Psychose [On our understanding of the polyneuritic psychosis]. Allgemeine Zeitschrift für Psychiatrie und ihre Grenzgebiete, 59, 498–534.
Crovitz, H. F. , & Schiffman, H. ( 1974 ). Frequency of episodic memories as a function of their age. Bulletin of the Psychonomic Society, 4, 517–518.
Dana, C.L. ( 1894 ). The study of a case of amnesia or “double consciousness. ” Psychological Review, 1, 570–580.
Dejerine, J. , & Roussy, G. ( 1906 ). Le syndrome thalamique [The thalamic syndrome]. Revue Neurologique, 14, 521–532.
Donath, J. ( 1899 ). Der epileptische Wandertrieb (Poriomanie) [The epileptic drive to wander (poriomania)]. Archiv für Psychiatrie und Nervenkrankheiten, 32, 335–355.
Donath, J. ( 1907 ). Weitere Beiträge zur Poriomanie [Further contributions on poriomania]. Archiv für Psychiatrie und Nervenkrankheiten, 42, 752–760.
Donath, J. ( 1908 ). Ueber hysterische Amnesie [On hysterical amnesia]. Archiv für Psychiatrie und Nervenkrankheiten, 44, 559–575.
Dreschfeld, J. ( 1884 ). On alcoholic paralysis. Brain, 7, 200–211.
DSM-5. ( 2013 ). Diagnostic and statistical manual of mental disorders (5th. ed.). Washington, DC: American Psychiatric Association.
Ebbinghaus, H. ( 1885 ). Über das Gedächtnis . Leipzig: Duncker & Humblot.
Ebbinghaus, H. ( 1897 ). Ueber eine neue Methode zur Prüfung geistiger Fähigkeiten und ihre Anwendung bei Schulkindern [On a new method for studying mental abilities, and its application on pupils]. Zeitschrift für Psychologie und Physiologie der Sinnesorgane, 13, 401–459.
Ebert, E. , & Meumann, E. ( 1905 ). Ueber einige Grundfragen der Psychologie der Uebungsphänomene im Bereiche des Gedächtnisses [On some basic questions of the psychology of practice phenomena in the field of memory]. Archiv für die gesamte Psychologie, 4, 1–232.
Ewald, G. ( 1940 ). Zur Frage der Lokalisation des amnestischen Symptomenkomplexes [On the question of the localization of the amnesic symptom complex]. Allgemeine Zeitschrift für Psychiatrie und ihre Grenzgebiete, 115, 220–237.
Exner, S. ( 1881 ). Untersuchungen über die Localisation der Funktionen in der Grosshirnrinde des Menschen [Investigations on the localization of functions in the human cerebral cortex]. Wien: Braumüller.
Ferrier, D. ( 1878 ). The localisation of cerebral disease . London: Elder & Co.
Finzi, J. ( 1901 ). Zur Untersuchung der Auffassungsfähigkeit und Merkfähigkeit [On the investigation of the abilities of comprehension and memory]. In E. Kraepelin (Ed.), Psychologische Arbeiten , Vol. 3 (pp. 289–384). Leipzig: Engelmann.
Fischer, G. ( 1882 ). Ueber eine eigenthümliche Spinalerkrankung bei Trinkern [On a peculiar spinal cord disease in alcoholics]. Archiv für Psychiatrie und Nervenkrankheiten, 13, 1–49.
Forel, A. ( 1885 ). Das Gedächtnis und seine Abnormitäten [Memory and its abnormal states]. Zürich: Orell Füssli & Co.
Freud, S. ( 1898 ). Zum psychischen Mechanismus der Vergesslichkeit [On the psychic mechanism of forgetfulness]. Monatsschrift für Psychiatrie und Neurologie, 1, 436–443.
Freud, S. ( 1899 ). Ueber Deckerinnerungen [On covered memories]. Monatsschrift für Psychiatrie und Neurologie, 2, 215–230.
Freud, S. ( 1901 a). Zum psychischen Mechanismus der Vergesslichkeit [On the psychic mechanism of forgetfulness]. Monatsschrift für Psychiatrie und Neurologie, 4/5, 436–443.
Freud, S. ( 1901 b). Zur Psychopathologie des Alltagslebens (Vergessen, Versprechen, Vergreifen) nebst Bemerkungen über eine Wurzel des Aberglaubens [On the psychopathology of daily life (forgetting, slips of the tongue, mistakes) together with remarks on the root of superstititon]. Monatsschrift für Psychiatrie und Neurologie, 10, 1–32, 95–143.
Freud, S. ( 1910 ). Über Psychoanalyse. Fünf Vorlesungen gehalten zur 20jährigen Gründungsfeier der Clark University in Worcester Mass. September 1909. [On psychoanalysis. Five lectures given on the occasion of the celebration of the 20th anniversary of the foundation of Clark University in Worcester Mass. September 1909]. Leipzig and Vienna: F. Deuticke.
Freund, C. S. ( 1889 ). Klinische Beiträge zur Kenntnis der generellen Gedächtnisschwäche [Clinical contributions to understanding general memory weakness]. Archiv für Psychiatrie und Nervenkrankheiten, 20, 441–457.
Fujiwara, E. , Brand, M. , Kracht, L. , Kessler, J. , Diebel, A. , Netz, J. , & Markowitsch, H.J. ( 2008 ). Functional retrograde amnesia: a multiple case study. Cortex, 44, 29–45.
Galton, F. ( 1879 ). Psychometric experiments. Brain, 2, 149–162.
Gamper, E. ( 1928 a).Zur Frage der Polioencephalitis haemorrhagica der chronischen Alkoholiker. Anatomische Befunde beim alkoholischen Korsakow und ihre Beziehungen zum klinischen Bild [On the question of polioencephalitis haemorrhagica of chronic alcoholics. Anatomical results in the chronic Korsakoff state and their relations to the clinical picture]. In: 17. Verhandlungen der Gesellschaft deutscher Nervenärzte, 1927 (pp. 122–129). Leipzig, Germany: Vogel.
Gamper, E. ( 1928 b). Zur Frage der Polioencephalitis haemorrhagica der chronischen Alkoholiker. Anatomische Befunde beim alkoholischen Korsakow und ihre Beziehungen zum klinischen Bild [On the question of polioencephalitis haemorrhagica of chronic alcoholics. Anatomical results in the chronic Korsakoff state and their relations to the clinical picture]. Deutsche Zeitschrift für Nervenheilkunde, 102, 352–359.
Gamper, E. ( 1929 ). Schlaf—Delirium tremens—Korsakowsches Syndrom [Sleep—delirium tremens—Korsakoff’s syndrome]. Zentralbaltt für Neurologie, 51, 236–239.
Ganser, S. J. ( 1898 ). Ueber einen eigenartigen hysterischen Dämmerzustand [On a peculiar hysterical state of somnolence]. Archiv für Psychiatrie und Nervenkrankheiten, 30, 633–640.
Ganser, S. J. ( 1904 ). Zur Lehre vom hysterischen Dämmerzustande [On the theory of the hysterical state of somnolence]. Archiv für Psychiatrie und Nervenkrankheiten, 38, 34–46.
Goldstein, K. ( 1906 ). Merkfähigkeit, Gedächtnis und Assoziation [Memory ability, memory, and association]. Zeitschrift für Psychologie, 41, 38–47, 117–144.
Goldstein, K. ( 1927 ). Die Lokalisation in der Grosshirnrinde nach den Erfahrungen am kranken Menschen [Localization in the cerebral cortex after experiences with sick persons]. In A. Bethe , G. von Bergmann , G. Embden , & A. Ellinger (Eds.), Handbuch der normalen und pathologischen Physiologie, Vol. 10. Spezielle Physiologie des Zentralnervensystems der Wirbeltiere (pp. 600–842). Berlin, Germany: Springer.
Gordon, A. ( 1906 ). On “double ego. ” American Journal of the Medical Sciences, 131, 480–486.
Gowers, W. R. ( 1878 ). On some symptoms of organic brain disease. Brain 1, 48–59.
Gregor, A. ( 1902 ). Beiträge zur Psychopathologie des Gedächtnisses [Contributions to the psychopathology of memory]. Monatsschrift für Psychiatrie und Neurologie, 15, 218–255, 339–386.
Gregor, A. ( 1907 ). Beiträge zur Kenntnis der Gedächtnisstörung bei der Korsakoffschen Psychose [Contributions to our understanding of memory disturbance in Korsakoff’s psychosis]. Monatsschrift für Psychiatrie und Neurologie, 21, 19–46, 148–167.
Gregor, A. ( 1909 ). Beiträge zur Psychopathologie des Gedächtnisses [Contributions to the psychopathology of memory]. Monatsschrift für Psychiatrie und Neurologie, 25, 218–255, 339–386.
Grünthal, E. ( 1947 ). Über das klinische Bild nach umschriebenem beiderseitigem Ausfall der Ammonshornrinde. Ein Beitrag zur Kenntnis der Funktion des Ammonshorns [On the clinical picture of circumscribed bilateral loss of Ammon’s horn’s cortex. A contribution to the function of the Ammon’s horn]. Monatsschrift für Psychiatrie und Neurologie, 113, 1–16.
Gudden, B. von ( 1896 ). Klinische und anatomische Beiträge zur Kenntnis der multiplen Alkoholneuritis nebst Bemerkungen über die Regenerationsvorgänge im peripheren Nervensystem [Clinical and anatomical contributions to an understanding of multiple alcohol neuritis together with remarks on the processes of regeneration in the peripheral nervous system]. Archiv für Psychiatrie und Nervenkrankheiten, 28, 643–741.
Guder, P. ( 1886 ). Die Geistesstörungen nach Kopfverletzungen unter besonderer Berücksichtigung ihrer gerichtsärztlichen Beurteilung [Mental disturbances after head injuries with special consideration of their evaluation by the forensic medical examiner]. Jena: Fischer.
Gussenbauer, K. ( 1894 ). Antrittsrede, anlässlich der Uebernahme der II. chirurgischen Klinik zu Wien [Inaugural lecture on the occasion of succeeding to director of the II. surgical clinic in Vienna]. Wiener klinische Wochenschrift, 7, 805–809.
Harvey, G. ( 1846 ). Instance of fracture of the cranium, with depression, and subsequent hernia cerebri. Lancet, ii, 503–506.
Heilbronner, K. ( 1903 ). Ueber Fugues und fugue-ähnliche Zustände [On fugues and fugue-related states]. Jahrbücher für Psychiatrie und Neurologie, 23, 107–206.
Heilbronner, K. ( 1905 a). Ueber Haftenbleiben und Stereotypie [On perseverating and stereotypy]. Allgemeine Zeitschrift für Psychiatrie und psychisch-gerichtliche Medizin, 62, 612–616.
Heilbronner, K. ( 1905 b). Ueber Haftenbleiben und Stereotypie [On persisting and stereotypy]. Monatsschrift für Psychiatrie und Neurologie, 18(Suppl.), 293–371.
Heine, R. ( 1911 ). Die forensische Bedeutung der Amnesie [The forensic significance of amnesia]. Vierteljahresschrift für gerichtliche Medicin, (3. Folge) , 42 , 51–93.
Hering, E. ( 1870 ). Ueber das Gedächtnis als eine allgemeine Funktion der organisierten Materie. Vortrag gehalten in der feierlichen Sitzung der Kaiserlichen Akademie der Wissenschaften in Wien am XXX. Mai MDCCCLXX . Leipzig, Germany: Akademische Verlagsgesellschaft. [transl.: Hering, E. (1895). Memory as a general function of organized matter . Chicago, IL: Open Court.]
Hey, J. ( 1904 ). Das Gansersche Symptom und seine klinische und forense Bedeutung [The Ganser symptom and its clinical and forensic importance]. Berlin: Hirschwald.
Hitzig, E. ( 1901 ). Aufklärung über einige Streitpunkte in der Localisationslehre [Clarifying some controversies inthe doctrine of localization]. Monatsschrift für Psychiatrie und Neurologie, 10, 457–458.
Hitzig, E. ( 1903 ). Einige Bemerkungen zu der Arbeit C. von Monakow’s “Ueber den gegenwärtigen Stand der Frage nach der Localisation im Grosshirn.” [Some remarks on the publication of C. von Monakow “On the present state of the question on the localization in the cerebrum]. Archiv für Psychiatrie und Nervenkrankheiten, 36, 907–913.
Huss, M. ( 1852 ). Alcoholismus chronicus . Stockholm, Sweden and Leipzig, Germany: Fritze.
Jahrreiss, W. ( 1928 ). Störungen des Denkens [Disturbances of thought]. In O. Bumke (Ed.), Handbuch der Geisteskrankheiten (Vol 1) (pp. 530–599). Berlin, Germany: Springer.
Janet, P. ( 1894 ). État mental des hystériques . Paris, France: Rueff.
Janet, P. ( 1907 ). The major symptoms of hysteria . New York, NY: Macmillan.
Jolly, P. ( 1897 ). Die psychischen Störungen bei Polyneuritis [Psychic disturbances in polyneuritis]. Neurologisches Centralblatt, 16, 916–917.
Jung, C. G. ( 1902 ). Ein Fall von hysterischem Stupor bei einer Untersuchungsgefangenen [A case of hysteric stupor in a prisoner on trial]. Journal für Psychologie und Neurologie, 1, 110–122.
Jung, C. G. ( 1905 a). Experimentelle Beobachtungen über das Erinnerungsvermögen [Experimental observations on remembrance]. Centralblatt für Nervenheilkunde und Psychiatrie, 28, 653–666.
Jung, C. G. ( 1905 b). Ueber das Verhalten der Reaktionszeit beim Assoziationsexperiment [On the behavior of reaction time in an association experiment]. Leipzig: Barth.
Jung, C. G. ( 1905 c). Diagnostische Assoziationsstudien. III. Beitrag: Analyse der Assoziationen eines Epileptikers [Diagnostic association studies. III. Contribution: Analysis of the associations of an epileptic]. Journal für Psychologie und Neurologie, 5, 73–90.
Jung, C. G. ( 1905 d). Diagnostische Assoziationsstudien. IV. Beitrag: Über das Verhalten der Reaktionszeit beim Assoziationsexperimente [Diagnostic association studies. IV. Contribution: On the behavior of reaction time in an association experiment] Journal für Psychologie und Neurologie, 6, 1–36.
Jung, C. G. , & Riklin, F. ( 1904 ). Diagnostische Assoziationsstudien. I. Beitrag: Experimentelle Untersuchungen über Assoziationen Gesunder [Diagnostic association studies. I. Contribution: Experimental investigations on associations of healthy people]. Journal für Psychologie und Neurologie, 4, 24–67.
Kant, F. ( 1932 ). Die Pseudoencephalitis Wernicke der Alkoholiker. (Polioencephalitis haemorrhagica superior acuta.) [Pseudoencephalitis of Wernicke in alcoholics. (Polioencephalitis haemorrhagica superior acuta.)]. Archiv für Psychiatrie und Nervenkrankheiten, 98, 702–768.
Kennedy, F. ( 1898 ). On the experimental investigation of memory. Psychological Review, 5, 477–499.
Kleist, K. ( 1934 ). Gehirnpathologie. Vornehmlich auf Grund der Kriegserfahrungen [Brain pathology. Based on war experiences]. Leipzig: Barth.
Köhler, F. ( 1897 ). Experimentelle Studien auf dem Gebiete des hypnotischen Somnambulismus [Experimental studies in hypnotic somnambulism]. Zeitschrift für Hypnotismus, Psychotherapie sowie andere psychophysiologische und psychopathologische Forschungen, 6, 357–374.
Kohnstamm, O. ( 1917 ). Über das Krankheitsbild der retro-anterograden Amnesie und die Unterscheidung des spontanen und lernenden Merkens [On case aspects of retro-anterograde amnesia and the differentiation of spontaneous and learned remembrance]. Monatsschrift für Psychiatrie und Neurologie, 41, 373–382.
Korsakoff, S. S. ( 1889 ). Etude mèdico-psychologique sur une forme des maladies de la memoire [Medico-psychological study on a form of memory disease]. Revue Philosophique, 5, 501–530.
Korsakoff, S. S. ( 1890 ). Eine psychische Störung combiniert mit multipler Neuritis (psychosis polyneuritica seu Cerebropathia psychia toxaemica) [A psychic disturbance combined with multiple neuritis (psychosis polyneuritica seu cerebropathia psychia toxaemica)]. Allgemeine Zeitschrift für Psychiatrie, 46, 475–485.
Korsakow, S. S. ( 1890 ). Ueber eine besondere Form psychischer Störung combiniert mit multipler Neuritis [On a special case of psychic disturbance combined with multiple neuritis]. Archiv für Psychiatrie und Nervenkrankheiten, 21, 669–704.
Korsakow, S. S. ( 1891 ). Erinnerungstäuschungen (Pseudoreminiscenzen) bei polyneuritischer Psychose [Delusions of memory (pseudoreminiscences) in polyneuritic psychosis]. Allgemeine Zeitschrift für Psychiatrie, 47, 390–410.
Korsakow, S. S. , & Serbski, W. ( 1892 ). Ein Fall von polyneuritischer Psychose mit Autopsie [A case of polyneuritic psychosis with autopsy]. Archiv für Psychiatrie und Nervenkrankheiten, 23, 112–134.
Kraepelin, E. ( 1882 ). Ueber die Einwirkung einiger medicamentöser Stoffe auf die Dauer einfacher psychischer Vorgänge [The influence of some medical drugs on the duration of simple psychic actions]. Philosophische Studien, 1, 573–605.
Kraepelin, E. ( 1889 ). Ueber den Einfluss der Uebung auf die Dauer von Associationen [The influence of practice on the duration of associations]. St. Petersburger Medicinische Wochenschrift, 14, 9–10.
Kraepelin, E. ( 1892 ). Ueber die Beeinflussung einfacher psychischer Vorgänge durch einige Arzneimittel [The influence of some drugs on simple psychic actions]. Jena: Gustav Fischer.
Kürz, E. , & Kraepelin, E. ( 1901 ). Ueber die Beeinflussung psychischer Vorgänge durch regelmässigen Alkoholgenuss [The influence of regular consumption of alcohol on psychic actions]. In E. Kraepelin (Ed.), Psychologische Arbeiten . Vol. 3 (pp. 417–457). Leipzig, Germany: Engelmann.
Langworthy, O. R. , & Fox, H. M. ( 1937 ). Thalamic syndrome. Syndrome of the posterior cerebral artery: A review. Archives of Internal Medicine, 60, 203–227.
Lawson, R. ( 1878 ). On the symptomatology of alcoholic brain disorders. Brain 1, 182–194.
Leavitt, F. H. ( 1935 ). The etiology of temporary amnesia. American Journal of Psychiatry, 91, 1079–1088.
Liepmann, H. ( 1910 ). Beitrag zur Kenntnis des amnestischen Symptomenkomplexes [Contribution to the knowledge of the amnesic symptom complex]. Neurologisches Zentralblatt, 29, 1147–1161.
Liepmann, H. ( 1913 ). Zur Lokalisation der Hirnfunktionen mit besonderer Berücksichtigung der Beteiligung der beiden Hemisphären an den Gedächtnisleistungen [On the localization of brain functions with special consideration of the contribution of both hemispheres in memory performance]. Zeitschrift für Psychologie, 63, 1–18.
Lipmann, O. ( 1903 ). Praktische Ergebnisse der experimentellen Untersuchung des Gedächtnisses [Practical results of the experimental investigation of memory]. Journal für Psychologie und Neurologie, Bd. II , 108–118.
Lipmann, O. ( 1904 ). Die Wirkung der einzelnen Wiederholungen auf verschieden starke und verschieden alte Assoziationen [The effect of single repetitions on differences in strong and differentially old associations]. Zeitschrift für Psychologie, 35, 195–233.
Lücke (no first name given) ( 1903 ). Ueber das Ganser’sche Symptom mit Berücksichtigung seiner forensischen Bedeutung [On the Ganser symptom with consideration of its forensic importance]. Allgemeine Zeitschrift für Psychiatrie, 60, 1–35.
Lückerath, M. ( 1900 ). Beitrag zu der Lehre von der Korsakowschen Psychose [Contribution to the theory of Korsakoff’s psychosis]. Neurologisches Zentralblatt, 19, 341–347.
Lundholm, H. ( 1932 ). The riddle of functional amnesia. Journal of Abnormal and Social Psychology, 26, 355–366.
Mair, W. G. P. , Warrington, E. K. , & Weiskrantz, L. ( 1979 ). Memory disorder in Korsakoff psychosis: A neuropathological and neuropsychological investigation of two cases. Brain, 102, 749–783.
Markowitsch, H. J. ( 1992 ). The neuropsychology of hanging: An historical perspective. Journal of Neurology, Neurosurgery and Psychiatry, 55, 507.
Markowitsch, H. J. ( 1996 ). Organic and psychogenic retrograde amnesia: Two sides of the same coin? Neurocase, 2, 357–371.
Markowitsch, H. J. ( 1999 ). Neuroimaging and mechanisms of brain function in psychiatric disorders. Current Opinion in Psychiatry, 12, 331–337.
Markowitsch, H. J. ( 2010 ). Korsakoff’s syndrome. In In G. F. Koob , M. Le Moal , & R. F. Thompson (Eds.), Encyclopedia of behavioral neuroscience , Vol. 2, R. Poldrack (Ed.) (pp. 131–136). Oxford, England: Academic Press.
Markowitsch, H. J. & Brand, M. ( 2010 ). Forgetting : A historical perspective. In S. Della Sala (Ed.), Forgetting (pp. 23–34). Hove, England: Psychology Press.
Markowitsch, H. J. , von Cramon, D. Y. , & Schuri, U. ( 1993 ). Mnestic performance profile of a bilateral diencephalic infarct patient with preserved intelligence and severe amnesic disturbances. Journal of Clinical and Experimental Neuropsychology, 15, 627–652.
Markowitsch, H. J. , Fink, G. R. , Thöne, A. I. M. , Kessler, J. , & Heiss, W.-D. ( 1997 ). Persistent psychogenic amnesia with a PET-proven organic basis. Cognitive Neuropsychiatry, 2, 135–158.
Markowitsch, H. J. , Kessler, J. , Bast-Kessler, C. , & Riess, R. ( 1984 ). Different emotional tones significantly affect recognition performance in patients with Korsakoff psychosis. International Journal of Neuroscience, 25, 145–159.
Markowitsch, H. J. , Kessler, J. , & Denzler, P. ( 1986 ). Recognition memory and psychophysiological responses towards stimuli with neutral and emotional content. A study with Korsakoff patients and recently detoxified and longterm abstinent alcoholics. International Journal of Neuroscience, 29, 1–35.
Markowitsch,H. J. , Kessler, J. , Van der Ven, C. , Weber-Luxenburger, G. , & Heiss, W.-D. ( 1998 ). Psychic trauma causing grossly reduced brain metabolism and cognitive deterioration. Neuropsychologia, 36, 77–82.
Markowitsch, H. J. , Kessler, J. , Weber-Luxenburger, G. , Van der Ven, C. , Albers, M. , & Heiss, W. D. ( 2000 ). Neuroimaging and behavioral correlates of recovery from mnestic block syndrome and other cognitive deteriorations. Neuropsychiatry Neuropsychology and Behavioral Neurology, 13, 60–66.
Markowitsch, H. J. , & Pritzel, M. ( 1985 ). The neuropathology of amnesia. Progress in Neurobiology, 25, 189–287.
Markowitsch, H. J. , & Staniloiu, S. ( 2012 a). Amnesic disorders. Lancet, 380(9851), 1229–1240.
Markowitsch, H. J. , & Staniloiu, A. ( 2012 b). Autonoetic consciousness and the self. In A. E. Cavanna & A. Nani (Eds.), Consciousness: States, mechanisms and disorders (pp. 85–110). New York: Nova Science.
Markowitsch, H. J. & Staniloiu, A. ( 2013 ). The impairment of recollection in functional amnesic states. Cortex, 49, 1494–1510.
Matthies (no first name given) ( 1908 ). Über einen Fall von hysterischem Dämmerzustand mit retrograder Amnesie [On a case of hysterical somnolence with retrograde amnesia]. Allgemeine Zeitschrift für Psychiatrie und ihre Grenzgebiete, 65, 188–206.
Meyer, E. ( 1899 ). Sarcom des III. Ventrikels mit Metastasen im IV. Ventrikel [Sarcoma of the III. ventricle with metastates in the IV. ventricle]. Archiv für Psychiatrie und Nervenkrankheiten, 32, 320–329.
Meyer, E. ( 1904 ). Korsakowscher Symptomenkomplex nach Gehirnerschütterung [Korsakoff’s symptom complex after cerebral concussion]. Neurologisches Zentralblatt, 23, 710–716.
Meynert, T. ( 1878 ). Ueber Fortschritte im Verständnis der krankhaften psychischen Gehirnzustände [On progress in understanding pathological psychic brain conditions]. Wien: Wilhelm Braumüller.
Meynert, T. ( 1884 ). Psychiatrie. Klinik der Erkrankungen des Vorderhirns, begründet auf dessen Bau, Leistungen und Ernährung [Psychiatry. Clinical description of diseases of the telencephalon, based on its construction, performance and nutrition]. Wien: Braumüller.
Moeli, C. ( 1883 ). Statistisches und Klinisches über Alcoholismus [Statistical and clinical facts on alcoholism]. Königliches Charité-Krankenhaus (Berlin): Charité-Annalen, 9, 524–548.
Monakow, C. von ( 1904 ). Ueber den gegenwärtigen Stand der Frage nach der Lokalisation im Grosshirn [On the current state of the question on the localization in the cerebrum]. Ergebnisse der Physiologie, II. Abt.: Biophysik und Psychophysik , 3–122.
Monakow, C. von ( 1909 ). Neue Gesichtspunkte in der Frage nach der Lokalisation im Grosshirn [New views on the question of localization in the cerebrum]. Zeitschrift für Psychologie, 54, 161–182.
Monakow, C. von ( 1910 ). Über Lokalisation der Hirnfunktionen [On localization of brain functions]. Wiesbaden: Bergmann.
Monakow, C. von ( 1914 ). Die Lokalisation im Grosshirn und der Abbau der Funktion durch kortikale Herde . Wiesbaden: Bergmann.
Müller, G.E. , & Pilzecker, A. ( 1900 ). Experimentelle Beiträge zur Lehre vom Gedächtnis [Experimental contribution on the concept of memory]. Zeitschrift für Psychologie, Ergänzungsband, 1.
Müller, G.E. , & Schumann, F. ( 1894 ). Experimentelle Beiträge zur Untersuchung des Gedächtnisses [Experimental contribution on the concept of memory]. Zeitschrift für Psychologie, 6, 81–190 and 257–339.
Netschajeff, A. ( 1900 ). Experimentelle Untersuchungen über die Gedächtnisentwicklung bei Schulkindern [Experimental investigations on the development of memory in school-age children]. Zeitschrift für Psychologie, 24, 321–351.
Netschajeff, A. ( 1902 ). Über Memorieren. Eine Skizze aus dem Gebiete der experimentellen pädagogischen Psychologie [On memorizing. A sketch from the area of experimental pedagogical psychology]. Berlin, Germany: Reuther & Reichard.
Neubürger, K. ( 1929 ). Demonstration von Hirnveränderungen bei Alkoholintoxikationen [Documentation of brain changes after alcoholic intoxication]. Allgemeine Zeitschrift für Psychiatrie und ihre Grenzgebiete, 91. (Cited after Kant, 1932, and after Neubürger, 1931.)
Pappenheim, M. ( 1907 ). Über die Kombination allgemeiner Gedächtnisschwäche und amnestischer Aphasie nach leichtem zerebralem Insult [On the combination of general memory weakness and amnesic aphasia after a minor cerebral insult]. Journal für Psychologie und Neurologie, 9, 201–214.
Pappenheim, M. ( 1908 ). Merkfähigkeit und Assoziationsversuch [Ability to memorize and an association experiment]. Zeitschrift für Psychologie, 46, 161–173.
Pavlov, I. P. ( 1928 ). Lectures on conditioned reflexes . New York, NY: International Publs.
Piazza, A. ( 1909 ). Ein Fall von Hirntumor [A case of brain tumor]. Berliner klinische Wochenschrift, 46, 1599–1604.
Pick, A. ( 1876 ). Zur Casuistik der Erinnerungstäuschungen [On the casuistry of illusions of remembrance]. Archiv für Psychiatrie und Nervenkrankheiten, 6, 568–574.
Pick, A. ( 1886 ). Zur Pathologie des Gedächtnisses [On the pathology of memory]. Archiv für Psychiatrie und Nervenkrankheiten, 17, 83–98.
Pick, A. ( 1905 ). Zur Psychologie des Vergessens bei Geistes- und Nervenkranken [The psychology of forgetting in psychiatric and neurological patients]. Archiv für kriminologische Anthropologie, 18, 251–261.
Pietrini, P. ( 2003 ). Toward a biochemistry of mind? American Journal of Psychiary, 160, 1907–1908.
Pommerenke, K. , Staniloiu, A. , Markowitsch, H.J. , Eulitz, H. , Gütler, R. , & Dettmers, C. ( 2012 ). Ein Fall von retrograder Amnesie nach Resektion eines Medullablastoms—psychogen/funktionell oder organisch? [A case of retrograde amnesia after resection of a medullablastoma—psychogenic, organic, or functional?] Neurologie & Rehabilitation, 18, 106–116.
Prince, M. ( 1906 ). The dissociation of a personality . London, England: Longmans, Green & Co.
Probst, M. ( 1903 ). Ueber durch eigenartigen Rindenschwund bedingten Blödsinn [On idiocy caused by a peculiar loss of the cerebral cortex]. Archiv für Psychiatrie und Nervenkrankheiten, 36, 762–792 (and 2 tables).
Raecke, J. ( 1908 ). Ueber epileptische Wanderzustände (Fugues, Poriomanie) [On epileptic states of wandering (fugues, poriomania)]. Archiv für Psychiatrie und Nervenkrankheiten, 43, 398–423.
Ranschburg, P. ( 1901 a). Studien über die Merkfähigkeit der Normalen, Nervenschwachen und Geisteskranken [Studies on the ability to memorize in normals, neurasthenics, and mental patients]. Monatsschrift für Psychiatrie und Neurologie, 9, 241–259.
Ranschburg, P. ( 1901 b). Apparat und Methode zur Untersuchung des (optischen) Gedächtnisses für medicinisch- und pädagogisch-psychologische Zwecke [Apparatus and method for the investigation of (optic) memory for medical and pedagogical-psychological purposes]. Monatsschrift für Psychiatrie und Neurologie, 10, 321–333.
Ranschburg, P. ( 1911 ). Das kranke Gedächtnis. Ergebnisse und Methoden der experimentellen Erforschung der alltäglichen Falschleistungen und der Pathologie des Gedächtnisses [The diseased memory. Results and methods of experimental research on common misperformance and the pathology of memory]. Leipzig: Barth.
Read, C. F. ( 1923 ). Hysterical amnesia following physical injury. A case study. Journal of Nervous and Mental Disease, 58, 513–524.
Ribot, T. ( 1882 ). Diseases of memory . New York, NY: D. Appleton and Co.
Rittershain, G. Ritter von ( 1871 ). Geistesleben [Life of the mind]. Wien, Austria: Wilhelm Braumüller.
Roussy, G. ( 1907 ). La couche optique. Le syndrome thalamique [The optic thalamus. The thalamic syndrome]. Paris, France: G. Steinheil.
Savage, G. H. ( 1878 ). Acute mania associated with abscess of the brain. Brain 1, 265–269.
Schneider, H. ( 1901 ). Ueber Auffassung und Merkfähigkeit beim Altersblödsinn [On comprehension and ability to memorize in senile dementia]. In E. Kraepelin (Ed.), Psychologische Arbeiten , Vol. 3 (pp. 458–481). Leipzig, Germany: Engelmann.
Schneider, K. ( 1912 ). Über einige klinisch-psychologische Untersuchungsmethoden und ihre Ergebnisse. Zugleich ein Beitrag zur Psychopathologie der Korsakowschen Psychose [On some clinical-pathological methods of investigation and their results. Simultaneously a contribution to the psychopathology of Korsakoff’s psychosis]. Zeitschrift für die gesamte Neurologie und Psychiatrie/Originalien, 8, 553–615.
Schneider, K. ( 1928 ). Die Störungen des Gedächtnisses [Disturbances of memory]. In O. Bumke (Ed.), Handbuch der Geisteskrankheiten , Vol. 1 (pp. 508–529). Berlin: Springer.
Schultze, E. ( 1903 ). Ueber krankhaften Wandertrieb [On poriomania]. Allgemeine Zeitschrift für Psychologie, 60, 795–814.
Schulz (no first name given) ( 1908 ). Korsakoff’sches Syndrom bei CO-Vergiftungen [Korsakoff’s syndrome after CO-intoxications]. Berliner Klinische Wochenschrift, 45, 1621–1623.
Schuster, P. ( 1902 ). Psychische Störungen bei Hirntumoren [Psychic disturbances in brain tumors]. Stuttgart, Germany: Enke.
Scoville, W. B. ( 1954 ). The limbic lobe in man. Journal of Neurosurgery, 11, 64–66.
Scoville, W. B. ( 1968 ). Amnesia after bilateral mesial temporal-lobe excision: Introduction to case H. M. Neuropsychologia, 6, 211–213.
Semon, R. ( 1904 ). Die Mneme als erhaltendes Prinzip im Wechsel des organischen Geschehens [Mneme as pertaining principle within the changes of organic occurrences]. Leipzig: Wilhelm Engelmann.
Semon, R. ( 1920 ). Bewusstseinsvorgang und Gehirnprozess [The processes of consciousness and of the brain]. Wiesbaden: J. F. Bergmann.
Serbsky, W. ( 1907 ). Die Korsakowsche Krankheit [Korsakoff’s disease]. Arbeiten aus dem neurologischen Institut der Wiener Universität, 15, 389–424.
Sidis, B. , & Goodhart, S. P. ( 1905 ). Multiple personality: An experimental investigation into the nature of human individuality . New York, NY: Appleton.
Sollier, P. ( 1900 ). Les probléme de la mémoire [Problems of memory]. Paris, France: Ancienne Librairie Germer Baillière.
Solly, S. ( 1836 ). The human brain . London, England: Longman, Rees, Orme, Brown, Green & Longman.
Souques, A. ( 1892 ). Essai sur l’amnesie retro-anterograde dans l’hysterie, les traumatismes cerebraux et l’alcoolisme chronique [Essay about retrograde-anterograde amnesia in hysteria, cerebral trauma and chronic alcoholism]. Revue de Medicine, 13, 367–401.
Squire, L. R. ( 2009 ). The legacy of patient H. M. for neuroscience. Neuron, 61, 6–9.
Staniloiu, A. , Bender, A. , Smolewska, K. , Ellis, J. , Abramowitz, C. , & Markowitsch, H. J. ( 2009 ). Ganser syndrome with work–related onset in a patient with a background of immigration. Cognitive Neuropsychiatry, 14, 180–198.
Staniloiu, A. , Borsutzky, S. , Woermann, F. & Markowitsch, H. J. ( 2013 ). Social cognition in a case of amnesia with neurodevelopmental mechanisms. Frontiers in Psychology, 24(4), Art. 342, 1–28. doi: 10.3389/fpsyg.2013.00342 10.3389/fpsyg.2013.00342
Staniloiu, A. , Vitcu, I. , & Markowitsch, H. J. ( 2011 ). Neuroimaging and dissociative disorders. In V. Chaudhary (Ed.), Advances in brain imaging (pp. 11–34). INTECH—Open Access Publ.
Steffens, L. ( 1900 ). Experimentelle Beiträge zur Lehre vom ökonomischen Lernen [Experimental contributions to the theory of economical learning]. Zeitschrift für Psychologie und Physiologie der Sinnesorgane, 22, 321–382.
Steinthal, E. ( 1921 ). Ein eigenartiger Fall Korsakowscher Psychose [A peculiar case of Korsakoff’s syndrome]. Zeitschrift für die gesamte Neurologie und Psychiatrie, 67, 287–310.
Stertz, G. ( 1910 ). Ueber psychogene Erkrankungen und Querulantenwahn nach Trauma nebst ihrer Bedeutung für die Begutachtungspraxis [On psychogenic diseases and delusions of querulous persons after trauma and their importance for the practice of expert reports. Zeitschrift für ärztliche Fortbildung, 7, 201–211.
Stevens, J. W. ( 1907 ). Korsakoff’s psychosis superimposed upon melancholia. Journal of Nervous and Mental Disease, 34, 447–458.
Stier, E. ( 1912 ). Wandertrieb und pathologisches Fortlaufen bei Kindern [Poriomania and pathological running away in children]. Jena: Gustav Fischer.
Stransky, E. ( 1905 a). Zur Lehre von der Amentia [On amentia]. Journal für Psychologie und Neurologie, 4, 158–171.
Stransky, E. ( 1905 b). Zur Lehre von der Amentia [On amentia]. Journal für Psychologie und Neurologie, 5, 18–36.
Stransky, E. ( 1905 c). Zur Lehre von der Amentia [On amentia]. Journal für Psychologie und Neurologie, 6, 37–83, and 155–191.
Strümpell, A. ( 1897 ). Ueber Störungen des Wortgedächtnisses und der Verknüpfung der Vorstellungen bei einem Kranken mit rechtsseitiger Hemiplegie [On disturbances of word memory and the combination of imaginations in a patient with right-sided hemiplegia]. Deutsche Zeitschrift für Nervenheilkunde , 397–415.
Titchener, E. B. ( 1895 a). The investigation of memory and association . New York: Macmillan.
Titchener, E. B. ( 1895 b). Affective memory. Philosophical Review, 4, 65–76.
Trautscholdt, M. ( 1882 ). Experimentelle Untersuchungen über die Association der Vorstellungen [Experimental investigations on the association of imaginations]. Philosophische Studien, 1, 213–250.
Tulving, E. ( 1983 ). Elements of episodic memory . Oxford: Clarendon Press.
Victor, M. , & Yakovlev, P. I. ( 1955 ). Translations of S. S. Korsakoff’s psychic disorder in conjunction with peripheral neuritis. Neurology, 5, 394–406.
Viner, N. ( 1931 ). Amnesia. Dual personality. With special reference to a case recalled by hypnotism. Canadian Medical Association Journal, 25, 147–152.
Weber (no first name given) ( 1906 ). Rudimentäre Formen der Korsakowschen Psychose [Rudimentary forms of Korsakoff’s psychosis]. Deutsche Medizinische Wochenschrift, 32, 485–486.
Weisenburgh, T. H. ( 1910 ). Tumours of the third ventricle. With the establishment of a symptom-complex. Brain, 33, 236–260.
Wernicke, C. ( 1881 ). Lehrbuch der Gehirnkrankheiten für Aerzte und Studirende , Vols. I and II [Textbook of brain diseases for physicians and students. Kassel, Germany: Fischer.
Whitlock, F. A. ( 1967 ). The Ganser syndrome. British Journal of Psychiatry, 113, 19–29.
Wilson, A. ( 1903 ). A case of double consciousness. Journal of Mental Science, 49, 640–658.
Wilson, S. A. K. ( 1906 ). Ectopia pupillae in certain mesencephalic lesions. Brain, 29, 524–536.
Woltär, O. ( 1906 ). Über den Bewusstseinszustand während der Fugue [On the state of consciousness during a fugue state]. Jahrbücher für Psychiatrie und Neurologie, 27, 125–143.
Yates, F. A. ( 1966 ). The art of memory . London: Routledge & Kegan Paul/PLC.
Ziehen, T. ( 1908 ). Das Gedächtnis [Memory]. Berlin: A. Hirschwald.
Zingerle, H. ( 1912 a). Ueber transitorische Geistesstörungen und deren forensische Beurteilung. Juristisch-psychiatrische Grenzfragen [On transitory mental disturbances and their forensic evaluation. Borderline or minor juridical-psychiatric cases]. Jena: Gustav Fischer.
Zingerle, H. ( 1912 b). Über einseitigen Schläfenlappendefekt beim Menschen [On a unilateral defect of the temporal lobe in man]. Journal für Psychologie und Neurologie, 18, 205–235.
- About Oxford Academic
- Publish journals with us
- University press partners
- What we publish
- New features
- Open access
- Institutional account management
- Rights and permissions
- Get help with access
- Accessibility
- Advertising
- Media enquiries
- Oxford University Press
- Oxford Languages
- University of Oxford
Oxford University Press is a department of the University of Oxford. It furthers the University's objective of excellence in research, scholarship, and education by publishing worldwide
- Copyright © 2024 Oxford University Press
- Cookie settings
- Cookie policy
- Privacy policy
- Legal notice
This Feature Is Available To Subscribers Only
Sign In or Create an Account
This PDF is available to Subscribers Only
For full access to this pdf, sign in to an existing account, or purchase an annual subscription.
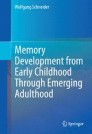
Memory Development from Early Childhood Through Emerging Adulthood pp 9–23 Cite as
A Brief History of Memory Development Research
- Wolfgang Schneider 2
- First Online: 01 January 2014
2637 Accesses
1 Citations
This chapter summarizes major historical trends in research on memory development. It shows that this research paradigm goes back to the beginning of the experimental study of memory near the end of the nineteenth century. Most of the early research was carried out in Western Europe and included both experimental studies on the development of memory functions and more applied research on children’s eyewitness testimony. Later on, Russian research on voluntary and involuntary memory and its development became more popular, dominating the field until the early 1960s. The transition to the modern era was observed from the mid-1960s on and stimulated by the “cognitive revolution” that influenced (developmental) experimental research in the USA and Europe.
- Behaviorism
- Explicit memory
- Eyewitness memory
- Implicit memory
- Infantile amnesia
- Involuntary memory
- Short-term memory
- Long-term memory and forgetting
- Verbal memory
- Voluntary memory
This is a preview of subscription content, log in via an institution .
Buying options
- Available as PDF
- Read on any device
- Instant download
- Own it forever
- Available as EPUB and PDF
- Compact, lightweight edition
- Dispatched in 3 to 5 business days
- Free shipping worldwide - see info
- Durable hardcover edition
Tax calculation will be finalised at checkout
Purchases are for personal use only
Author information
Authors and affiliations.
Department of Psychology, University of Würzburg, Würzburg, Germany
Wolfgang Schneider
You can also search for this author in PubMed Google Scholar
Corresponding author
Correspondence to Wolfgang Schneider .
Rights and permissions
Reprints and permissions
Copyright information
© 2015 Springer International Publishing Switzerland
About this chapter
Cite this chapter.
Schneider, W. (2015). A Brief History of Memory Development Research. In: Memory Development from Early Childhood Through Emerging Adulthood. Springer, Cham. https://doi.org/10.1007/978-3-319-09611-7_2
Download citation
DOI : https://doi.org/10.1007/978-3-319-09611-7_2
Published : 04 September 2014
Publisher Name : Springer, Cham
Print ISBN : 978-3-319-09610-0
Online ISBN : 978-3-319-09611-7
eBook Packages : Behavioral Science Behavioral Science and Psychology (R0)
Share this chapter
Anyone you share the following link with will be able to read this content:
Sorry, a shareable link is not currently available for this article.
Provided by the Springer Nature SharedIt content-sharing initiative
- Publish with us
Policies and ethics
- Find a journal
- Track your research
- Reference Manager
- Simple TEXT file
People also looked at
Perspective article, cognitive neuroscience perspective on memory: overview and summary.
- 1 Department of Psychology, Mount Allison University, Sackville, NB, Canada
- 2 Department of Industrial Engineering, College of Engineering, Jazan University, Jazan, Saudi Arabia
- 3 Department of Humanities and Social Sciences, Indian Institute of Technology Roorkee, Roorkee, India
- 4 Department of Design, Indian Institute of Technology Roorkee, Roorkee, India
This paper explores memory from a cognitive neuroscience perspective and examines associated neural mechanisms. It examines the different types of memory: working, declarative, and non-declarative, and the brain regions involved in each type. The paper highlights the role of different brain regions, such as the prefrontal cortex in working memory and the hippocampus in declarative memory. The paper also examines the mechanisms that underlie the formation and consolidation of memory, including the importance of sleep in the consolidation of memory and the role of the hippocampus in linking new memories to existing cognitive schemata. The paper highlights two types of memory consolidation processes: cellular consolidation and system consolidation. Cellular consolidation is the process of stabilizing information by strengthening synaptic connections. System consolidation models suggest that memories are initially stored in the hippocampus and are gradually consolidated into the neocortex over time. The consolidation process involves a hippocampal-neocortical binding process incorporating newly acquired information into existing cognitive schemata. The paper highlights the role of the medial temporal lobe and its involvement in autobiographical memory. Further, the paper discusses the relationship between episodic and semantic memory and the role of the hippocampus. Finally, the paper underscores the need for further research into the neurobiological mechanisms underlying non-declarative memory, particularly conditioning. Overall, the paper provides a comprehensive overview from a cognitive neuroscience perspective of the different processes involved in memory consolidation of different types of memory.
Introduction
Memory is an essential cognitive function that permits individuals to acquire, retain, and recover data that defines a person’s identity ( Zlotnik and Vansintjan, 2019 ). Memory is a multifaceted cognitive process that involves different stages: encoding, consolidation, recovery, and reconsolidation. Encoding involves acquiring and processing information that is transformed into a neuronal representation suitable for storage ( Liu et al., 2021 ; Panzeri et al., 2023 ). The information can be acquired through various channels, such as visual, auditory, olfactory, or tactile inputs. The acquired sensory stimuli are converted into a format the brain can process and retain. Different factors such as attention, emotional significance, and repetition can influence the encoding process and determine the strength and durability of the resulting memory ( Squire et al., 2004 ; Lee et al., 2016 ; Serences, 2016 ).
Consolidation includes the stabilization and integration of memory into long-term storage to increase resistance to interference and decay ( Goedert and Willingham, 2002 ). This process creates enduring structural modification in the brain and thereby has consequential effects on the function by reorganizing and strengthening neural connections. Diverse sources like sleep and stress and the release of neurotransmitters can influence memory consolidation. Many researchers have noted the importance of sleep due to its critical role in enabling a smooth transition of information from transient repositories into more stable engrams (memory traces) ( McGaugh, 2000 ; Clawson et al., 2021 ; Rakowska et al., 2022 ).
Retrieval involves accessing, selecting, and reactivating or reconstructing the stored memory to allow conscious access to previously encoded information ( Dudai, 2002 ). Retrieving memories depends on activating relevant neural pathways while reconstructing encoded information. Factors like contextual or retrieval cues and familiarity with the material can affect this process. Forgetting becomes a possibility if there are inadequate triggers for associated memory traces to activate upon recall. Luckily, mnemonic strategies and retrieval practice offer effective tools to enhance recovery rates and benefit overall memory performance ( Roediger and Butler, 2011 ).
Previous research implied that once a memory has been consolidated, it becomes permanent ( McGaugh, 2000 ; Robins, 2020 ). However, recent studies have found an additional phase called “reconsolidation,” during which stored memories, when reactivated, enter a fragile or liable state and become susceptible to modification or update ( Schiller et al., 2009 ; Asthana et al., 2015 ). The process highlights the notion that memory is not static but a dynamic system influenced by subsequent encounters. The concept of reconsolidation has much significance in memory modification therapies and interventions, as it offers a promising opportunity to target maladaptive or traumatic memories for modification specifically. However, more thorough investigations are needed to gain insight into the mechanisms and concrete implications of employing memory reconsolidation within therapeutic settings ( Bellfy and Kwapis, 2020 ).
The concept of memory is not reducible to a single unitary phenomenon; instead, evidence suggests that it can be subdivided into several distinct but interrelated constituent processes and systems ( Richter-Levin and Akirav, 2003 ). There are three major types of human memory: working memory, declarative memory (explicit), and non-declarative memory (implicit). All these types of memories involve different neural systems in the brain. Working memory is a unique transient active store capable of manipulating information essential for many complex cognitive operations, including language processing, reasoning, and judgment ( Atkinson and Shiffrin, 1968 ; Baddeley and Logie, 1999 ; Funahashi, 2017 ; Quentin et al., 2019 ). Previous models suggest the existence of three components that make up the working memory ( Baddeley and Hitch, 1974 ; Baddeley, 1986 ). One master component, the central executive, controls the two dependent components, the phonological loop (speech perception and language comprehension) and the visuospatial sketchpad (visual images and spatial impressions processing). Some models mention a third component known as the episodic buffer. It is theorized that the episodic buffer serves as an intermediary between perception, long-term memory, and two components of working memory (the phonological loop and visuospatial sketchpad) by storing integrated episodes or chunks from both sources ( Baddeley, 2000 ). Declarative memory (explicit memory) can be recalled consciously, including facts and events that took place in one’s life or information learned from books. It encompasses memories of both autobiographical experiences and memories associated with general knowledge. It is usually associated with the hippocampus–medial temporal lobe system ( Thompson and Kim, 1996 ; Ober, 2014 ). Non-declarative memory (implicit memory) refers to unconscious forms of learning such as skills, habits, and priming effects; this type of implicit learning does not involve conscious recollection but can include motor skill tasks that often require no thought prior to execution nor later recall upon completion. This type of memory usually involves the amygdala and other systems ( Thompson and Kim, 1996 ; Ober, 2014 ).
Working memory
Working memory is primarily associated with the prefrontal and posterior parietal cortex ( Sarnthein et al., 1998 ; Todd and Marois, 2005 ). Working memory is not localized to a single brain region, and research suggests that it is an emergent property arising from functional interactions between the prefrontal cortex (PFC) and the rest of the brain ( D’Esposito, 2007 ). Neuroimaging studies have explored the neural basis for the three components proposed by Baddeley and Hitch (1974) , the Central executive, the phonological loop, and the visuospatial sketch pad; there is evidence for the existence of a fourth component called the episodic buffer ( Baddeley, 2000 ).
The central executive plays a significant role in working memory by acting as the control center ( Shallice, 2002 ). It facilitates critical functions like attention allocation and coordination between the phonological loop and the visuospatial sketchpad ( Yu et al., 2023 ). Recent findings have illuminated the dual-functional network regulation, the cingulo-opercular network (CON) and the frontoparietal network (FPN), that underpins the central executive system ( Yu et al., 2023 ). The CON comprises the dorsal anterior cingulate cortex (dACC) and anterior insula (AI). In contrast, the FPN encompasses various regions, such as the dorsolateral prefrontal cortex (DLPFC) and frontal eye field (FEF), along with the intraparietal sulcus (IPS) ( Yu et al., 2023 ). Neuroimaging research has found evidence that elucidates the neural underpinnings of the executive attention control system to the dorsolateral prefrontal cortex (DLPFC) and the anterior cingulate cortex (ACC) ( Jung et al., 2022 ). The activation patterns indicate that the CON may have a broader top-down control function across the working memory process. At the same time, the FPN could be more heavily implicated in momentary control or processing at the trial level ( Yu et al., 2023 ). Evidence suggests that the central executive interacts with the phonological loop and visuospatial sketchpad to support working memory processes ( Baddeley, 2003 ; Buchsbaum, 2010 ; Menon and D’Esposito, 2021 ). The function, localization, and neural basis of this interaction are thought to involve the activation of specific brain regions associated with each component of working memory, as discussed in detail below.
The phonological loop is divided into two components: a storage system that maintains information (a few seconds) and a component involving subvocal rehearsal—which maintains and refreshes information in the working memory. Neuroanatomically, the phonological loop is represented in the Brodmann area (BA) 40 in the parietal cortex and the rehearsal components in BA 44 and 6, both situated in the frontal cortex ( Osaka et al., 2007 ). The left inferior frontal gyrus (Broca’s area) and the left posterior superior temporal gyrus (Wernicke’s area) has been proposed to play a critical role in supporting phonological and verbal working memory tasks, specifically the subvocal rehearsal system of the articulatory loop ( Paulesu et al., 1993 ; Buchsbaum et al., 2001 ; Perrachione et al., 2017 ). The phonological store in verbal short-term memory has been localized at the left supramarginal gyrus ( Graves et al., 2008 ; Perrachione et al., 2017 ).
Studies utilizing neuroimaging techniques have consistently yielded results indicating notable activation in these brain regions during phonological activities like recalling non-words and maintaining verbal information in memory ( Awh et al., 1996 ; Graves et al., 2008 ). During tasks that require phonological rehearsal, there was an increase in activation in the left inferior frontal gyrus ( Paulesu et al., 1993 ). Researchers have noted an increase in activity within the superior temporal gyrus-which plays a significant role in auditory processing-in individuals performing tasks that necessitate verbal information maintenance and manipulation ( Smith et al., 1998 ; Chein et al., 2003 ).
Additionally, lesion studies have provided further confirmation regarding the importance of these regions. These investigations have revealed that impairment in performing phonological working memory tasks can transpire following damage inflicted upon the left hemisphere, particularly on perisylvian language areas ( Koenigs et al., 2011 ). It is common for individuals with lesions affecting regions associated with the phonological loop, such as the left inferior frontal gyrus and superior temporal gyrus, to have difficulty performing verbal working memory tasks. Clinical cases involving patients diagnosed with aphasia and specific language impairments have highlighted challenges related to retaining and manipulating auditory information. For example, those who sustain damage specifically within their left inferior frontal gyrus often struggle with tasks involving phonological rehearsal and verbal working memory activities, and therefore, they tend to perform poorly in tasks that require manipulation or repetition of verbal stimuli ( Saffran, 1997 ; Caplan and Waters, 2005 ).
The visuospatial sketchpad is engaged in the temporary retention and manipulation of visuospatial facts, including mental pictures, spatial associations, and object placements ( Miyake et al., 2001 ). The visuospatial sketchpad is localized to the right hemisphere, including the occipital lobe, parietal and frontal areas ( Osaka et al., 2007 ). Ren et al. (2019) identified the localization of the visuospatial sketchpad, and these areas were the right infero-lateral prefrontal cortex, lateral pre-motor cortices, right inferior parietal cortex, and the dorsolateral occipital cortices ( Burbaud et al., 1999 ; Salvato et al., 2021 ). Moreover, the posterior parietal cortex and the intraparietal sulcus have been implicated in spatial working memory ( Xu and Chun, 2006 ). Additionally, some evidence is available for an increase in brain regions associated with the visuospatial sketchpad during tasks involving mental imagery and spatial processing. Neuroimaging studies have revealed increased neural activation in some regions of the parietal cortex, mainly the superior and posterior parietal cortex, while performing mental rotation tasks ( Cohen et al., 1996 ; Kosslyn et al., 1997 ). However, further research is needed to better understand the visuospatial working memory and its integration with other cognitive processes ( Baddeley, 2003 ). Lesions to the regions involving the visuospatial sketchpad can have detrimental effects on visuospatial working memory tasks. Individuals with lesions to the posterior parietal cortex may exhibit deficits in mental rotation tasks and may be unable to mentally manipulate the visuospatial representation ( Buiatti et al., 2011 ). Moreover, studies concerning lesions have shown that damage to the parietal cortex can result in short-term deficits in visuospatial memory ( Shafritz et al., 2002 ). Damage to the occipital cortex can lead to performance impairments in tasks that require the generation and manipulation of mental visual images ( Moro et al., 2008 ).
The fourth component of the working memory, termed episodic buffer, was proposed by Baddeley (2000) . The episodic buffer is a multidimensional but essentially passive store that can hold a limited number of chunks, store bound features, and make them available to conscious awareness ( Baddeley et al., 2010 ; Hitch et al., 2019 ). Although research has suggested that episodic buffer is localized to the hippocampus ( Berlingeri et al., 2008 ) or the inferior lateral parietal cortex, it is thought to be not dependent on a single anatomical structure but instead can be influenced by the subsystems of working memory, long term memory, and even through perception ( Vilberg and Rugg, 2008 ; Baddeley et al., 2010 ). The episodic buffer provides a crucial link between the attentional central executive and the multidimensional information necessary for the operation of working memory ( Baddeley et al., 2011 ; Gelastopoulos et al., 2019 ).
The interdependence of the working memory modules, namely the phonological loop and visuospatial sketchpad, co-relates with other cognitive processes, for instance, spatial cognition and attention allocation ( Repovs and Baddeley, 2006 ). It has been found that the prefrontal cortex (PFC) and posterior parietal cortex (PPC) have a crucial role in several aspects of spatial cognition, such as the maintenance of spatially oriented attention and motor intentions ( Jerde and Curtis, 2013 ). The study by Sellers et al. (2016) and the review by Ikkai and Curtis (2011) posits that other brain areas could use the activity in PFC and PPC as a guide and manifest outputs to guide attention allocation, spatial memory, and motor planning. Moreover, research indicates that verbal information elicits an activation response in the left ventrolateral prefrontal cortex (VLPFC) when retained in the phonological loop, while visuospatial information is represented by a corresponding level of activity within the right homolog region ( Narayanan et al., 2005 ; Wolf et al., 2006 ; Emch et al., 2019 ). Specifically, the study by Yang et al. (2022) investigated the roles of two regions in the brain, the right inferior frontal gyrus (rIFG) and the right supra-marginal gyrus (rSMG), as they relate to spatial congruency in visual working memory tasks. A change detection task with online repetitive transcranial magnetic stimulation applied concurrently at both locations during high visual WM load conditions determined that rIFG is involved in actively repositioning the location of objects. At the same time, rSMG is engaged in passive perception of the stability of the location of objects.
Recent academic studies have found evidence to support the development of a new working memory model known as the state-based model ( D’Esposito and Postle, 2015 ). This theoretical model proposes that the allocation of attention toward internal representations permits short-term retention within working memory ( Ghaleh et al., 2019 ). The state-based model consists of two main categories: activated LTM models and sensorimotor recruitment models; the former largely focuses upon symbolic stimuli categorized under semantic aspects, while the latter has typically been applied to more perceptual tasks in experiments. This framework posits that prioritization through regulating cognitive processes provides insight into various characteristics across different activity types, including capacity limitations, proactive interference, etcetera ( D’Esposito and Postle, 2015 ). For example, the paper by Ghaleh et al. (2019) provides evidence for two separate mechanisms involved in maintenance of auditory information in verbal working memory: an articulatory rehearsal mechanism that relies more heavily on left sensorimotor areas and a non-articulatory maintenance mechanism that critically relies on left superior temporal gyrus (STG). These findings support the state-based model’s proposal that attentional allocation is necessary for short-term retention in working memory.
State-based models were found to be consistent with the suggested storage mechanism as they do not require representation transfer from one dedicated buffer type; research has demonstrated that any population of neurons and synapses may serve as such buffers ( Maass and Markram, 2002 ; Postle, 2006 ; Avraham et al., 2017 ). The review by D’Esposito and Postle (2015) examined the evidence to determine whether a persistent neural activity, synaptic mechanisms, or a combination thereof support representations maintained during working memory. Numerous neural mechanisms have been hypothesized to support the short-term retention of information in working memory and likely operate in parallel ( Sreenivasan et al., 2014 ; Kamiński and Rutishauser, 2019 ).
Persistent neural activity is the neural mechanism by which information is temporarily maintained ( Ikkai and Curtis, 2011 ; Panzeri et al., 2023 ). Recent review by Curtis and Sprague (2021) has focused on the notion that persistent neural activity is a fundamental mechanism for memory storage and have provided two main arcs of explanation. The first arc, mainly underpinned by empirical evidence from prefrontal cortex (PFC) neurophysiology experiments and computational models, posits that PFC neurons exhibit sustained firing during working memory tasks, enabling them to store representations in their active state ( Thuault et al., 2013 ). Intrinsic persistent firing in layer V neurons in the medial PFC has been shown to be regulated by HCN1 channels, which contribute to the executive function of the PFC during working memory episodes ( Thuault et al., 2013 ). Additionally, research has also found that persistent neural firing could possibly interact with theta periodic activity to sustain each other in the medial temporal, prefrontal, and parietal regions ( Düzel et al., 2010 ; Boran et al., 2019 ). The second arc involves advanced neuroimaging approaches which have, more recently, enabled researchers to decode content stored within working memories across distributed regions of the brain, including parts of the early visual cortex–thus extending this framework beyond just isolated cortical areas such as the PFC. There is evidence that suggests simple, stable, persistent activity among neurons in stimulus-selective populations may be a crucial mechanism for sustaining WM representations ( Mackey et al., 2016 ; Kamiński et al., 2017 ; Curtis and Sprague, 2021 ).
Badre (2008) discussed the functional organization of the PFC. The paper hypothesized that the rostro-caudal gradient of a function in PFC supported a control hierarchy, whereas posterior to anterior PFC mediated progressively abstract, higher-order controls ( Badre, 2008 ). However, this outlook proposed by Badre (2008) became outdated; the paper by Badre and Nee (2018) presented an updated look at the literature on hierarchical control. This paper supports neither a unitary model of lateral frontal function nor a unidimensional abstraction gradient. Instead, separate frontal networks interact via local and global hierarchical structures to support diverse task demands. This updated perspective is supported by recent studies on the hierarchical organization of representations within the lateral prefrontal cortex (LPFC) and the progressively rostral areas of the LPFC that process/represent increasingly abstract information, facilitating efficient and flexible cognition ( Thomas Yeo et al., 2011 ; Nee and D’Esposito, 2016 ). This structure allows the brain to access increasingly abstract action representations as required ( Nee and D’Esposito, 2016 ). It is supported by fMRI studies showing an anterior-to-posterior activation movement when tasks become more complex. Anatomical connectivity between areas also supports this theory, such as Area 10, which has projections back down to Area 6 but not vice versa.
Finally, studies confirm that different regions serve different roles along a hierarchy leading toward goal-directed behavior ( Badre and Nee, 2018 ). The paper by Postle (2015) exhibits evidence of activity in the prefrontal cortex that reflects the maintenance of high-level representations, which act as top-down signals, and steer the circulation of neural pathways across brain networks. The PFC is a source of top-down signals that influence processing in the posterior and subcortical regions ( Braver et al., 2008 ; Friedman and Robbins, 2022 ). These signals either enhance task-relevant information or suppress irrelevant stimuli, allowing for efficient yet effective search ( D’Esposito, 2007 ; D’Esposito and Postle, 2015 ; Kerzel and Burra, 2020 ). The study by Ratcliffe et al. (2022) provides evidence of the dynamic interplay between executive control mechanisms in the frontal cortex and stimulus representations held in posterior regions for working memory tasks. Moreover, the review by Herry and Johansen (2014) discusses the neural mechanisms behind actively maintaining task-relevant information in order for a person to carry out tasks and goals effectively. This review of data and research suggests that working memory is a multi-component system allowing for both the storage and processing of temporarily active representations. Neural activity throughout the brain can be differentially enhanced or suppressed based on context through top-down signals emanating from integrative areas such as PFC, parietal cortex, or hippocampus to actively maintain task-relevant information when it is not present in the environment ( Herry and Johansen, 2014 ; Kerzel and Burra, 2020 ).
In addition, Yu et al. (2022) examined how brain regions from the ventral stream pathway to the prefrontal cortex were activated during working memory (WM) gate opening and closing. They defined gate opening as the switch from maintenance to updating and gate closing as the switch from updating to maintenance. The data suggested that cognitive branching increases during the WM gating process, thus correlating the gating process and an information approach to the PFC function. The temporal cortices, lingual gyrus (BA19), superior frontal gyri including frontopolar cortices, and middle and inferior parietal regions are involved in processes of estimating whether a response option available will be helpful for each case. During gate closing, on the other hand, medial and superior frontal regions, which have been associated with conflict monitoring, come into play, as well as orbitofrontal and dorsolateral prefrontal processing at later times when decreasing activity resembling stopping or downregulating cognitive branching has occurred, confirming earlier theories about these areas being essential for estimation of usefulness already stored within long-term memories ( Yu et al., 2022 ).
Declarative and non-declarative memory
The distinctions between declarative and non-declarative memory are often based on the anatomical features of medial temporal lobe regions, specifically those involving the hippocampus ( Squire and Zola, 1996 ; Squire and Wixted, 2011 ). In the investigation of systems implicated in the process of learning and memory formation, it has been posited that the participation of the hippocampus is essential for the acquisition of declarative memories ( Eichenbaum and Cohen, 2014 ). In contrast, a comparatively reduced level of hippocampal involvement may suffice for non-declarative memories ( Squire and Zola, 1996 ; Williams, 2020 ).
Declarative memory (explicit) pertains to knowledge about facts and events. This type of information can be consciously retrieved with effort or spontaneously recollected without conscious intention ( Dew and Cabeza, 2011 ). There are two types of declarative memory: Episodic and Semantic. Episodic memory is associated with the recollection of personal experiences. It involves detailed information about events that happened in one’s life. Semantic memory refers to knowledge stored in the brain as facts, concepts, ideas, and objects; this includes language-related information like meanings of words and mathematical symbol values along with general world knowledge (e.g., capitals of countries) ( Binder and Desai, 2011 ). The difference between episodic and semantic memory is that when one retrieves episodic memory, the experience is known as “remembering”; when one retrieves information from semantic memory, the experience is known as “knowing” ( Tulving, 1985 ; Dew and Cabeza, 2011 ). The hippocampus, medial temporal lobe, and the areas in the diencephalon are implicated in declarative memory ( Richter-Levin and Akirav, 2003 ; Derner et al., 2020 ). The ventral parietal cortex (VPC) is involved in declarative memory processes, specifically episodic memory retrieval ( Henson et al., 1999 ; Davis et al., 2018 ). The evidence suggests that VPC and hippocampus is involved in the retrieval of contextual details, such as the location and timing of the event, and the information is critical for the formation of episodic memory ( Daselaar, 2009 ; Hutchinson et al., 2009 ; Wiltgen et al., 2010 ). The prefrontal cortex (PFC) is involved in the encoding (medial PFC) and retrieval (lateral PFC) of declarative memories, specifically in the integration of information across different sensory modalities ( Blumenfeld and Ranganath, 2007 ; Li et al., 2010 ). Research also suggests that the amygdala may modulate other brain regions involved with memory processing, thus, contributing to an enhanced recall of negative or positive experiences ( Hamann, 2001 ; Ritchey et al., 2008 ; Sendi et al., 2020 ). Maintenance of the integrity of hippocampal circuitry is essential for ensuring that episodic memory, along with spatial and temporal context information, can be retained in short-term or long-term working memory beyond 15 min ( Ito et al., 2003 ; Rasch and Born, 2013 ). Moreover, studies have suggested that the amygdala plays a vital role in encoding and retrieving explicit memories, particularly those related to emotionally charged stimuli which are supported by evidence of correlations between hippocampal activity and amygdala modulation during memory formation ( Richter-Levin and Akirav, 2003 ; Qasim et al., 2023 ).
Current findings in neuroimaging studies assert that a vast array of interconnected brain regions support semantic memory ( Binder and Desai, 2011 ). This network merges information sourced from multiple senses alongside different cognitive faculties necessary for generating abstract supramodal views on various topics stored within our consciousness. Modality-specific sensory, motor, and emotional system within these brain regions serve specialized tasks like language comprehension, while larger areas of the brain, such as the inferior parietal lobe and most of the temporal lobe, participate in more generalized interpretation tasks ( Binder and Desai, 2011 ; Kuhnke et al., 2020 ). These regions lie at convergences of multiple perceptual processing streams, enabling increasingly abstract, supramodal representations of perceptual experience that support a variety of conceptual functions, including object recognition, social cognition, language, and the remarkable human capacity to remember the past and imagine the future ( Binder and Desai, 2011 ; Binney et al., 2016 ). The following section will discuss the processes underlying memory consolidation and storage within declarative memory.
Non-declarative (implicit) memories refer to unconscious learning through experience, such as habits and skills formed from practice rather than memorizing facts; these are typically acquired slowly and automatically in response to sensory input associated with reward structures or prior exposure within our daily lives ( Kesner, 2017 ). Non-declarative memory is a collection of different phenomena with different neural substrates rather than a single coherent system ( Camina and Güell, 2017 ). It operates by similar principles, depending on local changes to a circumscribed brain region, and the representation of these changes is unavailable to awareness ( Reber, 2008 ). Non-declarative memory encompasses a heterogenous collection of abilities, such as associative learning, skills, and habits (procedural memory), priming, and non-associative learning ( Squire and Zola, 1996 ; Camina and Güell, 2017 ). Studies have concluded that procedural memory for motor skills depends upon activity in diverse set areas such as the motor cortex, striatum, limbic system, and cerebellum; similarly, perceptual skill learning is thought to be associated with sensory cortical activation ( Karni et al., 1998 ; Mayes, 2002 ). Research suggests that mutual connections between brain regions that are active together recruit special cells called associative memory cells ( Wang et al., 2016 ; Wang and Cui, 2018 ). These cells help integrate, store, and remember related information. When activated, these cells trigger the recall of memories, leading to behaviors and emotional responses. This suggests that co-activated brain regions with these mutual connections are where associative memories are formed ( Wang et al., 2016 ; Wang and Cui, 2018 ). Additionally, observational data reveals that priming mechanisms within distinct networks, such as the “repetition suppression” effect observed in visual cortical areas associated with sensory processing and in the prefrontal cortex for semantic priming, are believed to be responsible for certain forms of conditioning and implicit knowledge transfer experiences exhibited by individuals throughout their daily lives ( Reber, 2008 ; Wig et al., 2009 ; Camina and Güell, 2017 ). However, further research is needed to better understand the mechanisms of consolidation in non-declarative memory ( Camina and Güell, 2017 ).
The process of transforming memory into stable, long-lasting from a temporary, labile memory is known as memory consolidation ( McGaugh, 2000 ). Memory formation is based on the change in synaptic connections of neurons representing the memory. Encoding causes synaptic Long-Term potentiation (LTP) or Long-Term depression (LTD) and induces two consolidation processes. The first is synaptic or cellular consolidation which involves remodeling synapses to produce enduring changes. Cellular consolidation is a short-term process that involves stabilizing the neural trace shortly after learning via structural brain changes in the hippocampus ( Lynch, 2004 ). The second is system consolidation, which builds on synaptic consolidation where reverberating activity leads to redistribution for long-term storage ( Mednick et al., 2011 ; Squire et al., 2015 ). System consolidation is a long-term process during which memories are gradually transferred to and integrated with cortical neurons, thus promoting their stability over time. In this way, memories are rendered less susceptible to forgetting. Hebb postulated that when two neurons are repeatedly activated simultaneously, they become more likely to exhibit a coordinated firing pattern of activity in the future ( Langille, 2019 ). This proposed enduring change in synchronized neuronal activation was consequently termed cellular consolidation ( Bermudez-Rattoni, 2010 ).
The following sections of this paper incorporate a more comprehensive investigation into various essential procedures connected with memory consolidation- namely: long-term potentiation (LTP), long-term depression (LTD), system consolidation, and cellular consolidation. Although these mechanisms have been presented briefly before this paragraph, the paper aims to offer greater insight into each process’s function within the individual capacity and their collective contribution toward memory consolidation.
Synaptic plasticity mechanisms implicated in memory stabilization
Long-Term Potentiation (LTP) and Long-Term Depression (LTP) are mechanisms that have been implicated in memory stabilization. LTP is an increase in synaptic strength, whereas LTD is a decrease in synaptic strength ( Ivanco, 2015 ; Abraham et al., 2019 ).
Long-Term Potentiation (LTP) is a phenomenon wherein synaptic strength increases persistently due to brief exposures to high-frequency stimulation ( Lynch, 2004 ). Studies of Long-Term Potentiation (LTP) have led to an understanding of the mechanisms behind synaptic strengthening phenomena and have provided a basis for explaining how and why strong connections between neurons form over time in response to stimuli.
The NMDA receptor-dependent LTP is the most commonly described LTP ( Bliss and Collingridge, 1993 ; Luscher and Malenka, 2012 ). In this type of LTP, when there is high-frequency stimulation, the presynaptic neuron releases glutamate, an excitatory neurotransmitter. Glutamate binds to the AMPA receptor on the postsynaptic neuron, which causes the neuron to fire while opening the NMDA receptor channel. The opening of an NMDA channel elicits a calcium ion influx into the postsynaptic neuron, thus initiating a series of phosphorylation events as part of the ensuing molecular cascade. Autonomously phosphorylated CaMKII and PKC, both actively functional through such a process, have been demonstrated to increase the conductance of pre-existing AMPA receptors in synaptic networks. Additionally, this has been shown to stimulate the introduction of additional AMPA receptors into synapses ( Malenka and Nicoll, 1999 ; Lynch, 2004 ; Luscher and Malenka, 2012 ; Bailey et al., 2015 ).
There are two phases of LTP: the early phase and the late phase. It has been established that the early phase LTP (E-LTP) does not require RNA or protein synthesis; therefore, its synaptic strength will dissipate in minutes if late LTP does not stabilize it. On the contrary, late-phase LTP (L-LTP) can sustain itself over a more extended period, from several hours to multiple days, with gene transcription and protein synthesis in the postsynaptic cell ( Frey and Morris, 1998 ; Orsini and Maren, 2012 ). The strength of presynaptic tetanic stimulation has been demonstrated to be a necessary condition for the activation of processes leading to late LTP ( Luscher and Malenka, 2012 ; Bailey et al., 2015 ). This finding is supported by research examining synaptic plasticity, notably Eric Kandel’s discovery that CREB–a transcription factor–among other cytoplasmic and nuclear molecules, are vital components in mediating molecular changes culminating in protein synthesis during this process ( Kaleem et al., 2011 ; Kandel et al., 2014 ). Further studies have shown how these shifts ultimately lead to AMPA receptor stabilization at post-synapses facilitating long-term potentiation within neurons ( Luscher and Malenka, 2012 ; Bailey et al., 2015 ).
The “synaptic tagging and capture hypothesis” explains how a weak event of tetanization at synapse A can transform to late-LTP if followed shortly by the strong tetanization of a different, nearby synapse on the same neuron ( Frey and Morris, 1998 ; Redondo and Morris, 2011 ; Okuda et al., 2020 ; Park et al., 2021 ). During this process, critical plasticity-related proteins (PRPs) are synthesized, which stabilize their own “tag” and that from the weaker synaptic activity ( Moncada et al., 2015 ). Recent evidence suggests that calcium-permeable AMPA receptors (CP-AMPARs) are involved in this form of heterosynaptic metaplasticity ( Park et al., 2018 ). The authors propose that the synaptic activation of CP-AMPARs triggers the synthesis of PRPs, which are then engaged by the weak induction protocol to facilitate LTP on the independent input. The paper also suggests that CP-AMPARs are required during the induction of LTP by the weak input for the full heterosynaptic metaplastic effect to be observed ( Park et al., 2021 ). Additionally, it has been further established that catecholamines such as dopamine plays an integral part in memory persistence by inducing PRP synthesis ( Redondo and Morris, 2011 ; Vishnoi et al., 2018 ). Studies have found that dopamine release in the hippocampus can enhance LTP and improve memory consolidation ( Lisman and Grace, 2005 ; Speranza et al., 2021 ).
Investigations into neuronal plasticity have indicated that synaptic strength alterations associated with certain forms of learning and memory may be analogous to those underlying Long-Term Potentiation (LTP). Research has corroborated this notion, demonstrating a correlation between these two phenomena ( Lynch, 2004 ). The three essential properties of Long-Term Potentiation (LTP) that have been identified are associativity, synapse specificity, and cooperativity ( Kandel and Mack, 2013 ). These characteristics provide empirical evidence for the potential role of LTP in memory formation processes. Specifically, associativity denotes the amplification of connections when weak stimulus input is paired with a powerful one; synapse specificity posits that this potentiating effect only manifests on synaptic locations exhibiting coincidental activity within postsynaptic neurons, while cooperativity suggests stimulated neuron needs to attain an adequate threshold of depolarization before LTP can be induced again ( Orsini and Maren, 2012 ).
There is support for the idea that memories are encoded by modification of synaptic strengths through cellular mechanisms such as LTP and LTD ( Nabavi et al., 2014 ). The paper by Nabavi et al. (2014) shows that fear conditioning, a type of associative memory, can be inactivated and reactivated by LTD and LTP, respectively. The findings of the paper support a causal link between these synaptic processes and memory. Moreover, the paper suggests that LTP is used to form neuronal assemblies that represent a memory, and LTD could be used to disassemble them and thereby inactivate a memory ( Nabavi et al., 2014 ). Hippocampal LTD has been found to play an essential function in regulating synaptic strength and forming memories, such as long-term spatial memory ( Ge et al., 2010 ). However, it is vital to bear in mind that studies carried out on LTP exceed those done on LTD; hence the literature on it needs to be more extensive ( Malenka and Bear, 2004 ; Nabavi et al., 2014 ).
Cellular consolidation and memory
For an event to be remembered, it must form physical connections between neurons in the brain, which creates a “memory trace.” This memory trace can then be stored as long-term memory ( Langille and Brown, 2018 ). The formation of a memory engram is an intricate process requiring neuronal depolarization and the influx of intracellular calcium ( Mank and Griesbeck, 2008 ; Josselyn et al., 2015 ; Xu et al., 2017 ). This initiation leads to a cascade involving protein transcription, structural and functional changes in neural networks, and stabilization during the quiescence period, followed by complete consolidation for its success. Interference from new learning events or disruption caused due to inhibition can abort this cycle leading to incomplete consolidation ( Josselyn et al., 2015 ).
Cyclic-AMP response element binding protein (CREB) has been identified as an essential transcription factor for memory formation ( Orsini and Maren, 2012 ). It regulates the expression of PRPs and enhances neuronal excitability and plasticity, resulting in changes to the structure of cells, including the growth of dendritic spines and new synaptic connections. Blockage or enhancement of CREB in certain areas can affect subsequent consolidation at a systems level–decreasing it prevents this from occurring, while aiding its presence allows even weak learning conditions to produce successful memory formation ( Orsini and Maren, 2012 ; Kandel et al., 2014 ).
Strengthening weakly encoded memories through the synaptic tagging and capture hypothesis may play an essential role in cellular consolidation. Retroactive memory enhancement has also been demonstrated in human studies, mainly when items are initially encoded with low strength but later paired with shock after consolidation ( Dunsmoor et al., 2015 ). The synaptic tagging and capture theory (STC) and its extension, the behavioral tagging hypothesis (BT), have both been used to explain synaptic specificity and the persistence of plasticity ( Moncada et al., 2015 ). STC proposed that electrophysiological activity can induce long-term changes in synapses, while BT postulates similar effects of behaviorally relevant neuronal events on learning and memory models. This hypothesis proposes that memory consolidation relies on combining two distinct processes: setting a “learning tag” and synthesizing plasticity-related proteins ( De novo protein synthesis, increased CREB levels, and substantial inputs to nearby synapses) at those tagged sites. BT explains how it is possible for event episodes with low-strength inputs or engagements can be converted into lasting memories ( Lynch, 2004 ; Moncada et al., 2015 ). Similarly, the emotional tagging hypothesis posits that the activation of the amygdala in emotionally arousing events helps to mark experiences as necessary, thus enhancing synaptic plasticity and facilitating transformation from transient into more permanent forms for encoding long-term memories ( Richter-Levin and Akirav, 2003 ; Zhu et al., 2022 ).
Cellular consolidation, the protein synthesis-dependent processes observed in rodents that may underlie memory formation and stabilization, has been challenging to characterize in humans due to the limited ability to study it directly ( Bermudez-Rattoni, 2010 ). Additionally, multi-trial learning protocols commonly used within human tests as opposed to single-trial experiments conducted with non-human subjects suggest there could be interference from subsequent information that impedes individual memories from being consolidated reliably. This raises important questions regarding how individuals can still form strong and long-lasting memories when exposed to frequent stimuli outside controlled laboratory conditions. Although this phenomenon remains undiscovered by science, it is of utmost significance for gaining a deeper understanding of our neural capacities ( Genzel and Wixted, 2017 ).
The establishment of distributed memory traces requires a narrow temporal window following the initial encoding process, during which cellular consolidation occurs ( Nader and Hardt, 2009 ). Once this period ends and consolidation has been completed, further protein synthesis inhibition or pharmacological disruption will be less effective at altering pre-existing memories and interfering with new learning due to the stabilization of the trace in its new neuronal network connections ( Nader and Hardt, 2009 ). Thus, systems consolidation appears critical for the long-term maintenance of memory within broader brain networks over extended periods after their formation ( Bermudez-Rattoni, 2010 ).
System consolidation and memory
Information is initially stored in both the hippocampus and neocortex ( Dudai et al., 2015 ). The hippocampus subsequently guides a gradual process of reorganization and stabilization whereby information present within the neocortex becomes autonomous from that in the hippocampal store. Scholars have termed this phenomenon “standard memory consolidation model” or “system consolidation” ( Squire et al., 2015 ).
The Standard Model suggests that information acquired during learning is simultaneously stored in both the hippocampus and multiple cortical modules. Subsequently, it posits that over a period of time which may range from weeks to months or longer, the hippocampal formation directs an integration process by which these various elements become enclosed into single unified structures within the cortex ( Gilboa and Moscovitch, 2021 ; Howard et al., 2022 ). These newly learned memories are then assimilated into existing networks without interference or compression when necessary ( Frankland and Bontempi, 2005 ). It is important to note that memory engrams already exist within cortical networks during encoding. They only need strengthening through links enabled by hippocampal assistance-overtime allowing remote memory storage without reliance on the latter structure. Data appears consistent across studies indicating that both AMPA-and NMDA receptor-dependent “tagging” processes occurring within the cortex are essential components of progressive rewiring, thus enabling longer-term retention ( Takeuchi et al., 2014 ; Takehara-Nishiuchi, 2020 ).
Recent studies have additionally demonstrated that the rate of system consolidation depends on an individual’s ability to relate new information to existing networks made up of connected neurons, popularly known as “schemas” ( Robin and Moscovitch, 2017 ). In situations where prior knowledge is present and cortical modules are already connected at the outset of learning, it has been observed that a hippocampal-neocortical binding process occurs similarly to when forming new memories ( Schlichting and Preston, 2015 ). The proposed framework involves the medial temporal lobe (MTL), which is involved in acquiring new information and binds different aspects of an experience into a single memory trace. In contrast, the medial prefrontal cortex (mPFC) integrates this information with the existing knowledge ( Zeithamova and Preston, 2010 ; van Kesteren et al., 2012 ). During consolidation and retrieval, MTL is involved in replaying memories to the neocortex, where they are gradually integrated with existing knowledge and schemas and help retrieve memory traces. During retrieval, the mPFC is thought to use existing knowledge and schemas to guide retrieval and interpretation of memory. This may involve the assimilation of newly acquired information into existing cognitive schemata as opposed to the comparatively slow progression of creating intercortical connections ( Zeithamova and Preston, 2010 ; van Kesteren et al., 2012 , 2016 ).
Medial temporal lobe structures are essential for acquiring new information and necessary for autobiographical (episodic) memory ( Brown et al., 2018 ). The consolidation of autobiographical memories depends on a distributed network of cortical regions. Brain areas such as entorhinal, perirhinal, and parahippocampal cortices are essential for learning new information; however, they have little impact on the recollection of the past ( Squire et al., 2015 ). The hippocampus is a region of the brain that forms episodic memories by linking multiple events to create meaningful experiences ( Cooper and Ritchey, 2019 ). It receives information from all areas of the association cortex and cingulate cortex, subcortical regions via the fornix, as well as signals originating within its entorhinal cortex (EC) and amygdala regarding emotionally laden or potentially hazardous stimuli ( Sorensen, 2009 ). Such widespread connectivity facilitates the construction of an accurate narrative underpinning each remembered episode, transforming short-term into long-term recollections ( Richter-Levin and Akirav, 2003 ).
Researchers have yet to establish a consensus regarding where semantic memory information is localized within the brain ( Roldan-Valadez et al., 2012 ). Some proponents contend that such knowledge is lodged within perceptual and motor systems, triggered when we initially associate with a given object. This point of view is supported by studies highlighting how neural activity occurs initially in the occipital cortex, followed by left temporal lobe involvement during processing and pertinent contributions to word selection/retrieval via activation of left inferior frontal cortices ( Patterson et al., 2007 ). Moreover, research indicates elevated levels of fusiform gyrus engagement (a ventral surface region encompassing both temporal lobes) occurring concomitantly with verbal comprehension initiatives, including reading and naming tasks ( Patterson et al., 2007 ).
Research suggests that the hippocampus is needed for a few years after learning to support semantic memory (factual information), yet, it is not needed for the long term ( Squire et al., 2015 ). However, some forms of memory remain dependent on the hippocampus, such as the retrieval of spatial memory ( Wiltgen et al., 2010 ). Similarly, the Multiple-trace theory ( Moscovitch et al., 2006 ), also known as the transformation hypothesis ( Winocur and Moscovitch, 2011 ), posits that hippocampal engagement is necessary for memories that retain contextual detail such as episodic memories. Consolidation of memories into the neocortex is theorized to involve a loss of specific finer details, such as temporal and spatial information, in addition to contextual elements. This transition ultimately results in an evolution from episodic memory toward semantic memory, which consists mainly of gist-based facts ( Moscovitch et al., 2006 ).
Sleep and memory consolidation
Sleep is an essential physiological process crucial to memory consolidation ( Siegel, 2001 ). Sleep is divided into two stages: Non-rapid Eye Movement (NREM) sleep and Rapid Eye Movement (REM) sleep. NREM sleep is divided into three stages: N1, N2, and N3 (AKA Slow Wave Sleep or SWS) ( Rasch and Born, 2013 ). Each stage displays unique oscillatory patterns and phenomena responsible for consolidating memories in distinct ways. The first stage, or N1 sleep, is when an individual transitions between wakefulness and sleep. This type of sleep is characterized by low-amplitude, mixed-frequency brain activity. N1 sleep is responsible for the initial encoding of memories ( Rasch and Born, 2013 ). The second stage, or N2 sleep, is characterized by the occurrence of distinct sleep spindles and K-complexes in EEG. N2 is responsible for the consolidation of declarative memories ( Marshall and Born, 2007 ). The third stage of sleep N3, also known as slow wave sleep (SWS), is characterized by low-frequency brain activity, slow oscillations, and high amplitude. The slow oscillations which define the deepest stage of sleep are trademark rhythms of NREM sleep. These slow oscillations are delta waves combined to indicate slow wave activity (SWA), which is implicated in memory consolidation ( Tononi and Cirelli, 2003 ; Stickgold, 2005 ; Kim et al., 2019 ). Sleep spindles are another trademark defining NREM sleep ( Stickgold, 2005 ). Ripples are high-frequency bursts, and when combined with irregularly occurring sharp waves (high amplitude), they form the sharp-wave ripple (SWR). These spindles and the SWRs coordinate the reactivation and redistribution of hippocampus-dependent memories to neocortical sites ( Ngo et al., 2020 ; Girardeau and Lopes-dos-Santos, 2021 ). The third stage is also responsible for the consolidation of procedural memories, such as habits and motor skills ( Diekelmann and Born, 2010 ). During SWS, there is minimal cholinergic activity and intermediate noradrenergic activity ( Datta and MacLean, 2007 ).
Finally, the fourth stage of sleep is REM sleep, characterized by phasic REMs and muscle atonia ( Reyes-Resina et al., 2021 ). During REM sleep, there is high cholinergic activity, serotonergic and noradrenergic activity are at a minimum, and high theta activity ( Datta and MacLean, 2007 ). REM sleep is also characterized by local increases in plasticity-related immediate-early gene activity, which might favor the subsequent synaptic consolidation of memories in the cortex ( Ribeiro, 2007 ; Diekelmann and Born, 2010 ; Reyes-Resina et al., 2021 ). The fourth stage of sleep is responsible for the consolidation of emotional memories and the integration of newly acquired memories into existing knowledge structures ( Rasch and Born, 2013 ). Studies indicate that the cholinergic system plays an imperative role in modifying these processes by toggling the entire thalamo-cortico-hippocampal network between distinct modes, namely high Ach encoding mode during active wakefulness and REM sleep and low Ach consolidation mode during quiet wakefulness and NREM sleep ( Bergmann and Staresina, 2017 ; Li et al., 2020 ). Consequently, improving neocortical hippocampal communication results in efficient memory encoding/synaptic plasticity, whereas hippocampo-neocortical interactions favor better systemic memory consolidation ( Diekelmann and Born, 2010 ).
The dual process hypothesis of memory consolidation posits that SWS facilitates declarative, hippocampus-dependent memory, whereas REM sleep facilitates non-declarative hippocampus-independent memory ( Maquet, 2001 ; Diekelmann and Born, 2010 ). On the other hand, the sequential hypothesis states that different sleep stages play a sequential role in memory consolidation. Memories are encoded during wakefulness, consolidated during NREM sleep, and further processed and integrated during REM sleep ( Rasch and Born, 2013 ). However, there is evidence present that contradicts the sequential hypothesis. A study by Goerke et al. (2013) found that declarative memories can be consolidated during REM sleep, suggesting that the relationship between sleep stages and memory consolidation is much more complex than a sequential model. Moreover, other studies indicate the importance of coordinating specific sleep phases with learning moments for optimal memory retention. This indicates that the timing of sleep has more influence than the specific sleep stages ( Gais et al., 2006 ). The active system consolidation theory suggests that an active consolidation process results from the selective reactivation of memories during sleep; the brain selectively reactivates newly encoded memories during sleep, which enhances and integrates them into the network of pre-existing long-term memories ( Born et al., 2006 ; Howard et al., 2022 ). Research has suggested that slow-wave sleep (SWS) and rapid eye movement (REM) sleep have complementary roles in memory consolidation. Declarative and non-declarative memories benefiting differently depending on which sleep stage they rely on ( Bergmann and Staresina, 2017 ). Specifically, during SWS, the brain actively reactivates and reorganizes hippocampo-neocortical memory traces as part of system consolidation. Following this, REM sleep is crucial for stabilizing these reactivated memory traces through synaptic consolidation. While SWS may initiate early plastic processes in hippocampo-neocortical memory traces by “tagging” relevant neocortico-neocortical synapses for later consolidation ( Frey and Morris, 1998 ), long-term plasticity requires subsequent REM sleep ( Rasch and Born, 2007 , 2013 ).
The active system consolidation hypothesis is not the only mechanism proposed for memory consolidation during sleep. The synaptic homeostasis hypothesis proposes that sleep is necessary for restoring synaptic homeostasis, which is challenged by synaptic strengthening triggered by learning during wake and synaptogenesis during development ( Tononi and Cirelli, 2014 ). The synaptic homeostasis hypothesis assumes consolidation is a by-product of the global synaptic downscaling during sleep ( Puentes-Mestril and Aton, 2017 ). The two models are not mutually exclusive, and the hypothesized processes probably act in concert to optimize the memory function of sleep ( Diekelmann and Born, 2010 ).
Non-rapid eye movement sleep plays an essential role in the systems consolidation of memories, with evidence showing that different oscillations are involved in this process ( Düzel et al., 2010 ). With an oscillatory sequence initiated by a slow frontal cortex oscillation (0.5–1 Hz) traveling to the medial temporal lobe and followed by a sharp-wave ripple (SWR) in the hippocampus (100–200 Hz). Replay activity of memories can be measured during this oscillatory sequence across various regions, including the motor cortex and visual cortex ( Ji and Wilson, 2006 ; Eichenlaub et al., 2020 ). Replay activity of memory refers to the phenomenon where the hippocampus replays previously experienced events during sharp wave ripples (SWRs) and theta oscillations ( Zielinski et al., 2018 ). During SWRs, short, transient bursts of high-frequency oscillations occur in the hippocampus. During theta oscillations, hippocampal spikes are ordered according to the locations of their place fields during behavior. These sequential activities are thought to play a role in memory consolidation and retrieval ( Zielinski et al., 2018 ). The paper by Zielinski et al. (2018) suggests that coordinated hippocampal-prefrontal representations during replay and theta sequences play complementary and overlapping roles at different stages in learning, supporting memory encoding and retrieval, deliberative decision-making, planning, and guiding future actions.
Additionally, the high-frequency oscillations of SWR reactivate groups of neurons attributed to spatial information encoding to align synchronized activity across an array of neural structures, which results in distributed memory creation ( Swanson et al., 2020 ; Girardeau and Lopes-dos-Santos, 2021 ). Parallel to this process is slow oscillation or slow-wave activity within cortical regions, which reflects synced neural firing and allows regulation of synaptic weights, which is in accordance with the synaptic homeostasis hypothesis (SHY). The SHY posits that downscaling synaptic strengths help incorporate new memories by avoiding saturation of resources during extended periods–features validated by discoveries where prolonged wakefulness boosts amplitude while it diminishes during stretches of enhanced sleep ( Girardeau and Lopes-dos-Santos, 2021 ).
During REM sleep, the brain experiences “paradoxical” sleep due to the similarity in activity to wakefulness. This stage plays a significant role in memory processing. Theta oscillations which are dominant during REM sleep, are primarily observed in the hippocampus, and these are involved in memory consolidation ( Landmann et al., 2014 ). There has been evidence of coherence between theta oscillations in the hippocampus, medial frontal cortex, and amygdala, which support their involvement in memory consolidation ( Popa et al., 2010 ). During REM sleep, phasic events such as ponto-geniculo-occipital waves originating from the brainstem coordinate activity across various brain structures and may contribute to memory consolidation processes ( Rasch and Born, 2013 ). Research has suggested that sleep-associated consolidation may be mediated by the degree of overlap between new and already known material whereby, if the acquired information is similar to the information one has learned, it is more easily consolidated during sleep ( Tamminen et al., 2010 ; Sobczak, 2017 ).
In conclusion, understanding more about how the brains cycle through different stages of sleep, including specific wave patterns, offers valuable insight into the ability to store memories effectively. While NREM sleep is associated with SWRs and slow oscillations, facilitating memory consolidation and synaptic downscaling, REM sleep, characterized by theta oscillations and phasic events, contributes to memory reconsolidation and the coordination of activity across brain regions. By exploring the interactions between sleep stages, oscillations, and memory processes, one may learn more about how sleep impacts brain function and cognition in greater detail.
Century has passed since we addressed memory, and several notable findings have moved from bench-to-bedside research. Several cross-talks between multidiscipline have been encouraged. Nevertheless, further research is needed into neurobiological mechanisms of non-declarative memory, such as conditioning ( Gallistel and Balsam, 2014 ). Modern research indicates that structural change that encodes information is likely at the level of the synapse, and the computational mechanisms are implemented at the level of neural circuitry. However, it also suggests that intracellular mechanisms realized at the molecular level, such as micro RNAs, should not be discounted as potential mechanisms. However, further research is needed to study the molecular and structural changes brought on by implicit memory ( Gallistel and Balsam, 2014 ).
The contribution of non-human animal studies toward our understanding of memory processes cannot be understated; hence recognizing their value is vital for moving forward. While this paper predominantly focused on cognitive neuroscience perspectives, some articles cited within this paper were sourced from non-human animal studies providing fundamental groundwork and identification of critical mechanisms relevant to human memories. A need persists for further investigation—primarily with humans—which can validate existing findings from non-human animals. Moving forward, it is prudent for researchers to bridge the gap between animal and human investigations done while exploring parallels and exploring unique aspects of human memory processes. By integrating findings from both domains, one can gain a more comprehensive understanding of the complexities of memory and its underlying neural mechanisms. Such investigations will broaden the horizon of our memory process and answer the complex nature of memory storage.
This paper attempted to provide an overview and summarize memory and its processes. The paper focused on bringing the cognitive neuroscience perspective on memory and its processes. This may provide the readers with the understanding, limitations, and research perspectives of memory mechanisms.
Data availability statement
The original contributions presented in this study are included in the article/supplementary material, further inquiries can be directed to the corresponding author.
Author contributions
SS and MKA: conceptualization, framework, and manuscript writing. AK: review and editing of the manuscript. All authors contributed to the article and approved the submitted version.
MKA was supported by the F.I.G. grant (IITR/SRIC/2741). The funding agency had no role in the preparation of the manuscript.
Acknowledgments
We gratefully thank students and Indian Institute of Technology Roorkee (IITR) office staff for their conditional and unconditional support. We also thank the Memory and Anxiety Research Group (MARG), IIT Roorkee for its constant support.
Conflict of interest
The authors declare that the research was conducted in the absence of any commercial or financial relationships that could be construed as a potential conflict of interest.
Publisher’s note
All claims expressed in this article are solely those of the authors and do not necessarily represent those of their affiliated organizations, or those of the publisher, the editors and the reviewers. Any product that may be evaluated in this article, or claim that may be made by its manufacturer, is not guaranteed or endorsed by the publisher.
Abraham, W. C., Jones, O. D., and Glanzman, D. L. (2019). Is plasticity of synapses the mechanism of long-term memory storage? NPJ Sci. Learn. 4:9. doi: 10.1038/s41539-019-0048-y
PubMed Abstract | CrossRef Full Text | Google Scholar
Asthana, M. K., Brunhuber, B., Mühlberger, A., Reif, A., Schneider, S., and Herrmann, M. J. (2015). Preventing the return of fear using reconsolidation update mechanisms depends on the met-allele of the brain derived neurotrophic factor val66met polymorphism. Int. J. Neuropsychopharmacol . 19:yv137. doi: 10.1093/ijnp/pyv137
Atkinson, R. C., and Shiffrin, R. M. (1968). “Human memory: a proposed system and its control processes,” in Psychology of learning and motivation , Vol. 2, eds K. W. Spence and J. T. Spence (Amsterdam: Elsevier), 89–195. doi: 10.1016/S0079-7421(08)60422-3
CrossRef Full Text | Google Scholar
Avraham, G., Leib, R., Pressman, A., Simo, L. S., Karniel, A., Shmuelof, L., et al. (2017). State-based delay representation and its transfer from a game of pong to reaching and tracking. Eneuro 4, ENEURO.179–ENEURO.117. doi: 10.1523/eneuro.0179-17.2017
Awh, E., Jonides, J., Smith, E. E., Schumacher, E. H., Koeppe, R. A., and Katz, S. (1996). Dissociation of storage and rehearsal in verbal working memory: evidence From Positron Emission Tomography. Psychol. Sci. 7, 25–31. doi: 10.1111/j.1467-9280.1996.tb00662.x
Baddeley, A. (2000). The episodic buffer: a new component of working memory? Trends Cogn. Sci. 4, 417–423. doi: 10.1016/S1364-6613(00)01538-2
Baddeley, A. (2003). Working memory: looking back and looking forward. Nat. Rev. Neurosci. 4, 829–839. doi: 10.1038/nrn1201
Baddeley, A. D. (1986). Working memory. Oxford: Oxford University Press.
Google Scholar
Baddeley, A. D., Allen, R. J., and Hitch, G. J. (2011). Binding in visual working memory: the role of the episodic buffer. Neuropsychologia 49, 1393–1400. doi: 10.1016/j.neuropsychologia.2010.12.042
Baddeley, A. D., and Hitch, G. (1974). “Working memory,” in Psychology of learning and motivation , Vol. 8, ed. G. A. Bower (Amsterdam: Elsevier), 47–89. doi: 10.1016/S0079-7421(08)60452-1
Baddeley, A. D., and Logie, R. H. (1999). “Working memory: the multiple-component model,” in Models of working memory , 1st Edn, eds A. Miyake and P. Shah (Cambridge: Cambridge University Press), 28–61. doi: 10.1017/CBO9781139174909.005
Baddeley, A., Allen, R. J., and Hitch, G. J. (2010). Investigating the episodic buffer. Psychol. Belgica 50, 223. doi: 10.5334/pb-50-3-4-223
Badre, D. (2008). Cognitive control, hierarchy, and the Rostro–caudal organization of the frontal lobes. Trends Cogn. Sci. 12, 193–200. doi: 10.1016/j.tics.2008.02.004
Badre, D., and Nee, D. E. (2018). Frontal cortex and the hierarchical control of behavior. Trends Cogn. Sci. 22, 170–188. doi: 10.1016/j.tics.2017.11.005
Bailey, C. H., Kandel, E. R., and Harris, K. M. (2015). Structural components of synaptic plasticity and memory consolidation. Cold Spring Harb. Perspect. Biol. 7:a021758. doi: 10.1101/cshperspect.a021758
Bellfy, L., and Kwapis, J. L. (2020). Molecular mechanisms of reconsolidation-dependent memory updating. Int. J. Mol. Sci. 21:6580. doi: 10.3390/ijms21186580
Bergmann, T. O., and Staresina, B. P. (2017). “Neuronal oscillations and reactivation subserving memory consolidation,” in Cognitive neuroscience of memory consolidation , eds N. Axmacher and B. Rasch (Cham: Springer), 185–207. doi: 10.1007/978-3-319-45066-7_12
Berlingeri, M., Bottini, G., Basilico, S., Silani, G., Zanardi, G., Sberna, M., et al. (2008). Anatomy of the episodic buffer: a Voxel-based morphometry study in patients with dementia. Behav. Neurol. 19, 29–34. doi: 10.1155/2008/828937
Bermudez-Rattoni, F. (2010). Is memory consolidation a multiple-circuit system? Proc. Natl. Acad. Sci. U.S.A. 107, 8051–8052. doi: 10.1073/pnas.1003434107
Binder, J. R., and Desai, R. H. (2011). The neurobiology of semantic memory. Trends Cogn. Sci. 15, 527–536. doi: 10.1016/j.tics.2011.10.001
Binney, R. J., Hoffman, P., and Lambon Ralph, M. A. (2016). Mapping the multiple graded contributions of the anterior temporal lobe representational hub to abstract and social concepts: evidence from distortion-corrected fmri. Cereb. Cortex 26, 4227–4241. doi: 10.1093/cercor/bhw260
Bliss, T. V., and Collingridge, G. L. (1993). A synaptic model of memory: long-term potentiation in the hippocampus. Nature 361, 31–39. doi: 10.1038/361031a0
Blumenfeld, R. S., and Ranganath, C. (2007). Prefrontal cortex and long-term memory encoding: an integrative review of findings from Neuropsychology and Neuroimaging. Neuroscientist 13, 280–291. doi: 10.1177/1073858407299290
Boran, E., Fedele, T., Klaver, P., Hilfiker, P., Stieglitz, L., Grunwald, T., et al. (2019). Persistent hippocampal neural firing and hippocampal-cortical coupling predict verbal working memory load. Sci. Adv. 5:eaav3687. doi: 10.1126/sciadv.aav3687
Born, J., Rasch, B., and Gais, S. (2006). Sleep to remember. Neuroscientist 12, 410–424. doi: 10.1177/1073858406292647
Braver, T. S., Gray, J. R., and Burgess, G. C. (2008). “Explaining the many varieties of working memory variation: dual mechanisms of cognitive control,” in Variation in working memory , 1st Edn, eds A. Conway, C. Jarrold, M. Kane, A. Miyake, and J. Towse (New York, NY: Oxford University Press), 76–106. doi: 10.1093/acprof:oso/9780195168648.003.0004
Brown, T. I., Rissman, J., Chow, T. E., Uncapher, M. R., and Wagner, A. D. (2018). Differential medial temporal lobe and parietal cortical contributions to real-world autobiographical episodic and autobiographical semantic memory. Sci. Rep. 8:6190. doi: 10.1038/s41598-018-24549-y
Buchsbaum, B. R. (2010). Neural basis of working memory. Encyclopedia of Behavioral Neuroscience 334–341. doi: 10.1016/b978-0-08-045396-5.00161-5
Buchsbaum, B. R., Hickok, G., and Humphries, C. (2001). Role of left posterior superior temporal gyrus in phonological processing for speech perception and production. Cogn. Sci. 25, 663–678. doi: 10.1207/s15516709cog2505_2
Buiatti, T., Mussoni, A., Toraldo, A., Skrap, M., and Shallice, T. (2011). Two qualitatively different impairments in making rotation operations. Cortex 47, 166–179. doi: 10.1016/j.cortex.2009.10.006
Burbaud, P., Camus, O., Caillé, J., Biolac, B., and Allard, M. (1999). Influence of individual strategies on brain activation patterns during cognitive tasks. J. Neuroradiol. 26, 59–65.
Camina, E., and Güell, F. (2017). The neuroanatomical, neurophysiological and psychological basis of memory: current models and their origins. Front. Pharmacol. 8:438. doi: 10.3389/fphar.2017.00438
Caplan, D., and Waters, G. (2005). The relationship between age, processing speed, working memory capacity, and language comprehension. Memory 13, 403–413. doi: 10.1080/09658210344000459
Chein, J. M., Ravizza, S. M., and Fiez, J. A. (2003). Using neuroimaging to evaluate models of working memory and their implications for language processing. J. Neurolinguist. 16, 315–339. doi: 10.1016/s0911-6044(03)00021-6
Clawson, B. C., Pickup, E. J., Ensing, A., Geneseo, L., Shaver, J., Gonzalez-Amoretti, J., et al. (2021). Causal role for sleep-dependent reactivation of learning-activated sensory ensembles for fear memory consolidation. Nat. Commun . 12, 1–13. doi: 10.1038/s41467-021-21471-2
Cohen, M. S., Kosslyn, S. M., Breiter, H. C., DiGirolamo, G. J., Thompson, W. L., Anderson, A. K., et al. (1996). Changes in cortical activity during mental rotation a mapping study using functional MRI. Brain 119, 89–100. doi: 10.1093/brain/119.1.89
Cooper, R. A., and Ritchey, M. (2019). Progression from feature-specific brain activity to hippocampal binding during episodic encoding. J. Neurosci. 40, 1701–1709. doi: 10.1523/jneurosci.1971-19.2019
Curtis, C. E., and Sprague, T. C. (2021). Persistent activity during working memory from front to back. Front. Neural Circ. 15:696060. doi: 10.3389/fncir.2021.696060
D’Esposito, M. (2007). From cognitive to neural models of working memory. Philos. Trans. R. Soc. B Biol. Sci. 362, 761–772. doi: 10.1098/rstb.2007.2086
D’Esposito, M., and Postle, B. R. (2015). The cognitive neuroscience of working memory. Annu. Rev. Psychol. 66, 115–142. doi: 10.1146/annurev-psych-010814-015031
Daselaar, S. M. (2009). Posterior midline and ventral parietal activity is associated with retrieval success and encoding failure. Front. Hum. Neurosci. 3:13. doi: 10.3389/neuro.09.013.2009
Datta, S., and MacLean, R. R. (2007). Neurobiological mechanisms for the regulation of mammalian sleep–wake behavior: reinterpretation of historical evidence and inclusion of contemporary cellular and molecular evidence. Neurosci. Biobehav. Rev. 31, 775–824. doi: 10.1016/j.neubiorev.2007.02.004
Davis, S. W., Wing, E. A., and Cabeza, R. (2018). Contributions of the ventral parietal cortex to declarative memory. Handb. Clin. Neurol. 151, 525–553. doi: 10.1016/b978-0-444-63622-5.00027-9
Derner, M., Dehnen, G., Chaieb, L., Reber, T. P., Borger, V., Surges, R., et al. (2020). Patterns of single-neuron activity during associative recognition memory in the human medial temporal lobe. Neuroimage 221:117214. doi: 10.1016/j.neuroimage.2020.117214
Dew, I. T. Z., and Cabeza, R. (2011). The porous boundaries between explicit and implicit memory: behavioral and neural evidence. Ann. N. Y. Acad. Sci. 1224, 174–190. doi: 10.1111/j.1749-6632.2010.05946.x
Diekelmann, S., and Born, J. (2010). The memory function of sleep. Nat. Rev. Neurosci. 11, 114–126. doi: 10.1038/nrn2762
Dudai, Y. (2002). Molecular bases of long-term memories: a question of persistence. Curr. Opin. Neurobiol. 12, 211–216. doi: 10.1016/s0959-4388(02)00305-7
Dudai, Y., Karni, A., and Born, J. (2015). The consolidation and transformation of memory. Neuron 88, 20–32. doi: 10.1016/j.neuron.2015.09.004
Dunsmoor, J. E., Murty, V. P., Davachi, L., and Phelps, E. A. (2015). Emotional learning selectively and retroactively strengthens memories for related events. Nature 520, 345–348. doi: 10.1038/nature14106
Düzel, E., Penny, W. D., and Burgess, N. (2010). Brain oscillations and memory. Curr. Opin. Neurobiol. 20, 143–149. doi: 10.1016/j.conb.2010.01.004
Eichenbaum, H., and Cohen, N. J. (2014). Can we reconcile the declarative memory and spatial navigation views on hippocampal function? Neuron 83, 764–770. doi: 10.1016/j.neuron.2014.07.032
Eichenlaub, J.-B., Jarosiewicz, B., Saab, J., Franco, B., Kelemen, J., Halgren, E., et al. (2020). Replay of learned neural firing sequences during rest in human motor cortex. Cell Rep. 31:107581. doi: 10.1016/j.celrep.2020.107581
Emch, M., von Bastian, C. C., and Koch, K. (2019). Neural correlates of verbal working memory: an fMRI meta-analysis. Front. Hum. Neurosci. 13:180. doi: 10.3389/fnhum.2019.00180
Frankland, P. W., and Bontempi, B. (2005). The organization of recent and remote memories. Nature Rev. Neurosci. 6, 119–130. doi: 10.1038/nrn1607
Frey, U., and Morris, R. G. M. (1998). Synaptic tagging: implications for late maintenance of hippocampal long-term potentiation. Trends Neurosci. 21, 181–188. doi: 10.1016/s0166-2236(97)01189-2
Friedman, N. P., and Robbins, T. W. (2022). The role of prefrontal cortex in cognitive control and executive function. Neuropsychopharmacology 47, 72–89. doi: 10.1038/s41386-021-01132-0
Funahashi, S. (2017). Working memory in the prefrontal cortex. Brain Sci. 7:49. doi: 10.3390/brainsci7050049
Gais, S., Lucas, B., and Born, J. (2006). Sleep after learning AIDS memory recall. Learn. Mem. 13, 259–262. doi: 10.1101/lm.132106
Gallistel, C. R., and Balsam, P. D. (2014). Time to rethink the neural mechanisms of learning and memory. Neurobiol. Learn. Mem. 108, 136–144. doi: 10.1016/j.nlm.2013.11.019
Ge, Y., Dong, Z., Bagot, R. C., Howland, J. G., Phillips, A. G., Wong, T. P., et al. (2010). Hippocampal long-term depression is required for the consolidation of Spatial Memory. Proc. Natl Acad. Sci. U.S.A. 107, 16697–16702. doi: 10.1073/pnas.1008200107
Gelastopoulos, A., Whittington, M. A., and Kopell, N. J. (2019). Parietal low beta rhythm provides a dynamical substrate for a working memory buffer. Proc. Natl Acad. Sci. U.S.A. 116, 16613–16620. doi: 10.1073/pnas.1902305116
Genzel, L., and Wixted, J. T. (2017). “Cellular and systems consolidation of declarative memory,” in Cognitive neuroscience of memory consolidation , eds N. Axmacher and B. Rasch (Berlin: Springer International Publishing), 3–16. doi: 10.1007/978-3-319-45066-7_1
Ghaleh, M., Lacey, E. H., Fama, M. E., Anbari, Z., DeMarco, A. T., and Turkeltaub, P. E. (2019). Dissociable mechanisms of verbal working memory revealed through multivariate lesion mapping. Cereb. Cortex 30, 2542–2554. doi: 10.1093/cercor/bhz259
Gilboa, A., and Moscovitch, M. (2021). No consolidation without representation: correspondence between neural and psychological representations in recent and remote memory. Neuron 109, 2239–2255. doi: 10.1016/j.neuron.2021.04.025
Girardeau, G., and Lopes-dos-Santos, V. (2021). Brain neural patterns and the memory function of sleep. Science 374, 560–564. doi: 10.1126/science.abi8370
Goedert, K. M., and Willingham, D. B. (2002). Patterns of interference in sequence learning and prism adaptation inconsistent with the consolidation hypothesis. Learn. Mem. 9, 279–292. doi: 10.1101/lm.50102
Goerke, M., Cohrs, S., Rodenbeck, A., Grittner, U., Sommer, W., and Kunz, D. (2013). Declarative memory consolidation during the first night in a sleep lab: the role of REM sleep and Cortisol. Psychoneuroendocrinology 38, 1102–1111. doi: 10.1016/j.psyneuen.2012.10.019
Graves, W. W., Grabowski, T. J., Mehta, S., and Gupta, P. (2008). The left posterior superior temporal gyrus participates specifically in accessing lexical phonology. J. Cogn. Neurosci. 20, 1698–1710. doi: 10.1162/jocn.2008.20113
Hamann, S. (2001). Cognitive and neural mechanisms of emotional memory. Trends Cogn. Sci. 5, 394–400. doi: 10.1016/S1364-6613(00)01707-1
Henson, R. N., Rugg, M. D., Shallice, T., Josephs, O., and Dolan, R. J. (1999). Recollection and familiarity in recognition memory: an event-related functional magnetic resonance imaging study. J. Neurosci. 19, 3962–3972. doi: 10.1523/jneurosci.19-10-03962.1999
Herry, C., and Johansen, J. P. (2014). Encoding of fear learning and memory in distributed neuronal circuits. Nat. Neurosci. 17, 1644–1654. doi: 10.1038/nn.3869
Hitch, G. J., Allen, R. J., and Baddeley, A. D. (2019). Attention and binding in visual working memory: two forms of attention and two kinds of buffer storage. Attent. Percept. Psychophys. 82, 280–293. doi: 10.3758/s13414-019-01837-x
Howard, M. D., Skorheim, S. W., and Pilly, P. K. (2022). A model of bi-directional interactions between complementary learning systems for memory consolidation of sequential experiences. Front. Syst. Neurosci. 16:972235. doi: 10.3389/fnsys.2022.972235
Hutchinson, J. B., Uncapher, M. R., and Wagner, A. D. (2009). Posterior parietal cortex and episodic retrieval: convergent and divergent effects of attention and memory. Learn. Mem. 16, 343–356. doi: 10.1101/lm.919109
Ikkai, A., and Curtis, C. E. (2011). Common neural mechanisms supporting spatial working memory, attention and motor intention. Neuropsychologia 49, 1428–1434. doi: 10.1016/j.neuropsychologia.2010.12.020
Ito, M., Kuroiwa, J., and Miyake, S. (2003). A neural network model of memory system using hippocampus. Electron. Commun. Japan 86, 86–97. doi: 10.1002/ecjc.1010
Ivanco, T. L. (2015). Long-term potentiation and long-term depression. International Encyclopedia of the Social & Behavioral Sciences 14, 358–365. doi: 10.1016/b978-0-08-097086-8.55034-x
Jerde, T. A., and Curtis, C. E. (2013). Maps of space in human frontoparietal cortex. J. Physiol. 107, 510–516. doi: 10.1016/j.jphysparis.2013.04.002
Ji, D., and Wilson, M. A. (2006). Coordinated memory replay in the visual cortex and hippocampus during sleep. Nat. Neurosci. 10, 100–107. doi: 10.1038/nn1825
Josselyn, S. A., Köhler, S., and Frankland, P. W. (2015). Finding the engram. Nat. Rev. Neurosci. 16, 521–534. doi: 10.1038/nrn4000
Jung, J., Lambon Ralph, M. A., and Jackson, R. L. (2022). Subregions of DLPFC display graded yet distinct structural and functional connectivity. J. Neurosci. 42, 3241–3252. doi: 10.1523/jneurosci.1216-21.2022
Kaleem, A., Hoessli, D. C., Haq, I., Walker-Nasir, E., Butt, A., Iqbal, Z., et al. (2011). CREB in long-term potentiation in hippocampus: role of post-translational modifications-studies in silico. J. Cell. Biochem. 112, 138–146. doi: 10.1002/jcb.22909
Kamiński, J., and Rutishauser, U. (2019). Between persistently active and activity-silent frameworks: novel vistas on the cellular basis of working memory. Ann. N. Y. Acad. Sci. 1464, 64–75. doi: 10.1111/nyas.14213
Kamiński, J., Sullivan, S., Chung, J. M., Ross, I. B., Mamelak, A. N., and Rutishauser, U. (2017). Persistently active neurons in human medial frontal and medial temporal lobe support working memory. Nat. Neurosci. 20, 590–601. doi: 10.1038/nn.4509
Kandel, E. R., and Mack, S. (2013). Principles of neural science. New York, NY: McGraw-Hill Medical.
Kandel, E. R., Dudai, Y., and Mayford, M. R. (2014). The molecular and systems biology of memory. Cell 157, 163–186. doi: 10.1016/j.cell.2014.03.001
Karni, A., Meyer, G., Rey-Hipolito, C., Jezzard, P., Adams, M. M., Turner, R., et al. (1998). The acquisition of skilled motor performance: fast and slow experience-driven changes in primary motor cortex. Proc. Natl. Acad. Sci. U.S.A. 95, 861–868. doi: 10.1073/pnas.95.3.861
Kerzel, D., and Burra, N. (2020). Capture by context elements, not attentional suppression of distractors, explains the PD with small search displays. J. Cogn. Neurosci. 32, 1170–1183. doi: 10.1162/jocn_a_01535
Kesner, R. P. (2017). Memory neurobiology?. Reference Module in Neuroscience and Biobehavioral Psychology 1–12. doi: 10.1016/b978-0-12-809324-5.03089-3
Kim, J., Gulati, T., and Ganguly, K. (2019). Competing roles of slow oscillations and delta waves in memory consolidation versus forgetting. Cell 179, 514–526.e13. doi: 10.1016/j.cell.2019.08.040
Koenigs, M., Acheson, D. J., Barbey, A. K., Solomon, J., Postle, B. R., and Grafman, J. (2011). Areas of left perisylvian cortex mediate auditory–verbal short-term memory. Neuropsychologia 49, 3612–3619. doi: 10.1016/j.neuropsychologia.2011.09.013
Kosslyn, S. M., Thompson, W. L., and Alpert, N. M. (1997). Neural Systems shared by visual imagery and visual perception: a positron emission tomography study. Neuroimage 6, 320–334. doi: 10.1006/nimg.1997.0295
Kuhnke, P., Kiefer, M., and Hartwigsen, G. (2020). Task-dependent recruitment of modality-specific and multimodal regions during conceptual processing. Cereb. Cortex 30, 3938–3959. doi: 10.1093/cercor/bhaa010
Landmann, N., Kuhn, M., Piosczyk, H., Feige, B., Baglioni, C., Spiegelhalder, K., et al. (2014). The reorganisation of memory during sleep. Sleep Med. Rev. 18, 531–541. doi: 10.1016/j.smrv.2014.03.005
Langille, J. J. (2019). Remembering to forget: a dual role for sleep oscillations in memory consolidation and forgetting. Front. Cell. Neurosci. 13:71. doi: 10.3389/fncel.2019.00071
Langille, J. J., and Brown, R. E. (2018). The synaptic theory of memory: a historical survey and reconciliation of recent opposition. Front. Syst. Neurosci. 12:52. doi: 10.3389/fnsys.2018.00052
Lee, H., Chun, M. M., and Kuhl, B. A. (2016). Lower parietal encoding activation is associated with sharper information and Better Memory. Cereb. Cortex 27, 2486–2499. doi: 10.1093/cercor/bhw097
Li, M., Lu, S., Wu, Y., and Zhong, N. (2010). “Functional segregation of memory encoding and retrieval in the prefrontal cortex,” in Proceedings of the IEEE/ICME International Conference on Complex Medical Engineering , (Gold Coast, QLD), 91–95. doi: 10.1109/iccme.2010.5558865
Li, Q., Song, J.-L., Li, S.-H., Westover, M. B., and Zhang, R. (2020). Effects of cholinergic neuromodulation on thalamocortical rhythms during NREM sleep: a model study. Front. Comput. Neurosci. 13:100. doi: 10.3389/fncom.2019.00100
Lisman, J. E., and Grace, A. A. (2005). The hippocampal-VTA loop: controlling the entry of information into long-term memory. Neuron 46, 703–713. doi: 10.1016/j.neuron.2005.05.002
Liu, J., Zhang, H., Yu, T., Ren, L., Ni, D., Yang, Q., et al. (2021). Transformative neural representations support long-term episodic memory. Sci. Adv. 7:eabg9715. doi: 10.1126/sciadv.abg9715
Luscher, C., and Malenka, R. C. (2012). NMDA receptor-dependent long-term potentiation and long-term depression (LTP/LTD). Cold Spring Harb. Perspect. Biol. 4, a005710–a005710. doi: 10.1101/cshperspect.a005710
Lynch, M. A. (2004). Long-term potentiation and memory. Physiol. Rev. 84, 87–136. doi: 10.1152/physrev.00014.2003
Maass, W., and Markram, H. (2002). Synapses as dynamic memory buffers. Neural Netw. 15, 155–161. doi: 10.1016/s0893-6080(01)00144-7
Mackey, W. E., Devinsky, O., Doyle, W. K., Golfinos, J. G., and Curtis, C. E. (2016). Human parietal cortex lesions impact the precision of spatial working memory. J. Neurophysiol. 116, 1049–1054. doi: 10.1152/jn.00380.2016
Malenka, R. C., and Bear, M. F. (2004). LTP and Ltd. Neuron 44, 5–21. doi: 10.1016/j.neuron.2004.09.012
Malenka, R. C., and Nicoll, R. (1999). Long-term potentiation–a decade of progress? Science 285, 1870–1874. doi: 10.1126/science.285.5435.1870
Mank, M., and Griesbeck, O. (2008). Genetically encoded calcium indicators. Chem. Rev. 108, 1550–1564. doi: 10.1021/cr078213v
Maquet, P. (2001). The role of sleep in learning and memory. Science 294, 1048–1052. doi: 10.1126/science.1062856
Marshall, L., and Born, J. (2007). The contribution of sleep to hippocampus-dependent memory consolidation. Trends Cogn. Sci. 11, 442–450. doi: 10.1016/j.tics.2007.09.001
Mayes, A. R. (2002). Memory disorders, organic. Encyclopedia of the Human Brain 2, 759–772. doi: 10.1016/b0-12-227210-2/00199-0
McGaugh, J. L. (2000). Memory–a century of consolidation. Science 287, 248–251. doi: 10.1126/science.287.5451.248
Mednick, S. C., Cai, D. J., Shuman, T., Anagnostaras, S., and Wixted, J. T. (2011). An opportunistic theory of cellular and systems consolidation. Trends Neurosci. 34, 504–514. doi: 10.1016/j.tins.2011.06.003
Menon, V., and D’Esposito, M. (2021). The role of PFC networks in cognitive control and executive function. Neuropsychopharmacology 47, 90–103. doi: 10.1038/s41386-021-01152-w
Miyake, A., Friedman, N. P., Rettinger, D. A., Shah, P., and Hegarty, M. (2001). How are visuospatial working memory, executive functioning, and spatial abilities related? A latent-variable analysis. J. Exp. Psychol. 130, 621–640. doi: 10.1037/0096-3445.130.4.621
Moncada, D., Ballarini, F., and Viola, H. (2015). Behavioral tagging: a translation of the synaptic tagging and capture hypothesis. Neural Plast. 2015, 1–21. doi: 10.1155/2015/650780
Moro, V., Berlucchi, G., Lerch, J., Tomaiuolo, F., and Aglioti, S. M. (2008). Selective deficit of mental visual imagery with intact primary visual cortex and visual perception. Cortex 44, 109–118. doi: 10.1016/j.cortex.2006.06.004
Moscovitch, M., Nadel, L., Winocur, G., Gilboa, A., and Rosenbaum, R. S. (2006). The cognitive neuroscience of remote episodic, semantic and spatial memory. Curr. Opin. Neurobiol. 16, 179–190. doi: 10.1016/j.conb.2006.03.013
Nabavi, S., Fox, R., Proulx, C. D., Lin, J. Y., Tsien, R. Y., and Malinow, R. (2014). Engineering A memory with ltd and LTP. Nature 511, 348–352. doi: 10.1038/nature13294
Nader, K., and Hardt, O. (2009). A single standard for memory: the case for reconsolidation. Nat. Rev. Neurosci. 10, 224–234. doi: 10.1038/nrn2590
Narayanan, N. S., Prabhakaran, V., Bunge, S. A., Christoff, K., Fine, E. M., and Gabrieli, J. D. E. (2005). The role of the prefrontal cortex in the maintenance of verbal working memory: an event-related fMRI analysis. Neuropsychology 19, 223–232. doi: 10.1037/0894-4105.19.2.223
Nee, D. E., and D’Esposito, M. (2016). The hierarchical organization of the lateral prefrontal cortex. eLife 5:e12112. doi: 10.7554/elife.12112
Ngo, H.-V., Fell, J., and Staresina, B. (2020). Sleep spindles mediate hippocampal-neocortical coupling during long-duration ripples. eLife 9:e57011. doi: 10.7554/elife.57011
Ober, B. A. (2014). Memory, explicit/implicit. Encyclopedia of the Neurological Sciences 2, 1042–1044. doi: 10.1016/b978-0-12-385157-4.00455-3
Okuda, K., Højgaard, K., Privitera, L., Bayraktar, G., and Takeuchi, T. (2020). Initial memory consolidation and the synaptic tagging and capture hypothesis. Eur. J. Neurosci. 54, 6826–6849. doi: 10.1111/ejn.14902
Orsini, C. A., and Maren, S. (2012). Neural and cellular mechanisms of fear and extinction memory formation. Neurosci. Biobehav. Rev. 36, 1773–1802. doi: 10.1016/j.neubiorev.2011.12.014
Osaka, N., Logie, R. H., and D’Esposito, M. (eds) (2007). The cognitive neuroscience of working memory. Oxford: Oxford University Press.
Panzeri, S., Janotte, E., Pequeńo-Zurro, A., Bonato, J., and Bartolozzi, C. (2023). Constraints on the design of neuromorphic circuits set by the properties of neural population codes. Neuromorphic Computing Eng. 3:012001. doi: 10.1088/2634-4386/acaf9c
Park, P., Kang, H., Georgiou, J., Zhuo, M., Kaang, B.-K., and Collingridge, G. L. (2021). Further evidence that CP-ampars are critically involved in synaptic tag and capture at hippocampal CA1 synapses. Mol. Brain 14:26. doi: 10.1186/s13041-021-00737-2
Park, P., Kang, H., Sanderson, T. M., Bortolotto, Z. A., Georgiou, J., Zhuo, M., et al. (2018). The role of calcium-permeable ampars in long-term potentiation at principal neurons in the rodent hippocampus. Front. Synaptic Neurosci. 10:42. doi: 10.3389/fnsyn.2018.00042
Patterson, K., Nestor, P. J., and Rogers, T. T. (2007). Where do you know what you know? The representation of semantic knowledge in the human brain. Nat. Rev. Neurosci. 8, 976–987. doi: 10.1038/nrn2277
Paulesu, E., Frith, C. D., and Frackowiak, R. S. (1993). The neural correlates of the verbal component of working memory. Nature 362, 342–345. doi: 10.1038/362342a0
Perrachione, T. K., Ghosh, S. S., Ostrovskaya, I., Gabrieli, J. D., and Kovelman, I. (2017). Phonological working memory for words and nonwords in cerebral cortex. J. Speech Lang. Hear. Res. 60, 1959–1979. doi: 10.1044/2017_jslhr-l-15-0446
Popa, D., Duvarci, S., Popescu, A. T., Léna, C., and Paré, D. (2010). Coherent amygdalocortical theta promotes fear memory consolidation during paradoxical sleep. Proc. Natl. Acad. Sci. U.S.A. 107, 6516–6519. doi: 10.1073/pnas.0913016107
Postle, B. R. (2006). Working memory as an emergent property of the mind and brain. Neuroscience 139, 23–38. doi: 10.1016/j.neuroscience.2005.06.005
Postle, B. R. (2015). The cognitive neuroscience of visual short-term memory. Curr. Opin. Behav. Sci. 1, 40–46. doi: 10.1016/j.cobeha.2014.08.004
Puentes-Mestril, C., and Aton, S. J. (2017). Linking network activity to synaptic plasticity during sleep: hypotheses and recent data. Front. Neural Circ. 11:61. doi: 10.3389/fncir.2017.00061
Qasim, S. E., Mohan, U. R., Stein, J. M., and Jacobs, J. (2023). Neuronal activity in the human amygdala and hippocampus enhances emotional memory encoding. Nat. Hum. Behav. 7, 754–764. doi: 10.1038/s41562-022-01502-8
Quentin, R., King, J.-R., Sallard, E., Fishman, N., Thompson, R., Buch, E. R., et al. (2019). Differential brain mechanisms of selection and maintenance of information during working memory. J. Neurosci. 39, 3728–3740. doi: 10.1523/jneurosci.2764-18.2019
Rakowska, M., Bagrowska, P., Lazari, A., Navarrete, M., Abdellahi, M. E., Johansen-Berg, H., et al. (2022). Cueing motor memory reactivation during NREM sleep engenders learning-related changes in precuneus and sensorimotor structures. bioRxiv 2022.01.-27.477838. doi: 10.1101/2022.01.27.477838
Rasch, B., and Born, J. (2007). Maintaining memories by reactivation. Curr. Opin. Neurobiol. 17, 698–703. doi: 10.1016/j.conb.2007.11.007
Rasch, B., and Born, J. (2013). About sleep’s role in memory. Physiol. Rev. 93, 681–766. doi: 10.1152/physrev.00032.2012
Ratcliffe, O., Shapiro, K., and Staresina, B. P. (2022). Fronto-medial theta coordinates posterior maintenance of working memory content. Curr. Biol. 32, 2121–2129.e3. doi: 10.1016/j.cub.2022.03.045
Reber, P. J. (2008). Cognitive neuroscience of declarative and nondeclarative memory. Hum. Learn. Biol. Brain Neurosci. 139, 113–123. doi: 10.1016/s0166-4115(08)10010-3
Redondo, R. L., and Morris, R. G. M. (2011). Making memories last: the synaptic tagging and capture hypothesis. Nat. Rev. Neurosci. 12, 17–30. doi: 10.1038/nrn2963
Ren, Z., Zhang, Y., He, H., Feng, Q., Bi, T., and Qiu, J. (2019). The different brain mechanisms of object and spatial working memory: voxel-based morphometry and resting-state functional connectivity. Front. Hum. Neurosci. 13:248. doi: 10.3389/fnhum.2019.00248
Repovs, G., and Baddeley, A. (2006). The multi-component model of working memory: explorations in experimental cognitive psychology. Neuroscience 139, 5–21. doi: 10.1016/j.neuroscience.2005.12.061
Reyes-Resina, I., Samer, S., Kreutz, M. R., and Oelschlegel, A. M. (2021). Molecular mechanisms of memory consolidation that operate during sleep. Front. Mol. Neurosci. 14:767384. doi: 10.3389/fnmol.2021.767384
Ribeiro, S. (2007). Novel experience induces persistent sleep-dependent plasticity in the cortex but not in the hippocampus. Front. Neurosci. 1:43–55. doi: 10.3389/neuro.01.1.1.003.2007
Richter-Levin, G., and Akirav, I. (2003). Emotional tagging of memory formation—In the search for neural mechanisms. Brain Res. Rev. 43, 247–256. doi: 10.1016/j.brainresrev.2003.08.005
Ritchey, M., Dolcos, F., and Cabeza, R. (2008). Role of amygdala connectivity in the persistence of emotional memories over time: an event-related fmri investigation. Cereb. Cortex 18, 2494–2504. doi: 10.1093/cercor/bhm262
Robin, J., and Moscovitch, M. (2017). Details, gist and schema: hippocampal–neocortical interactions underlying recent and remote episodic and spatial memory. Curr. Opin. Behav. Sci. 17, 114–123. doi: 10.1016/j.cobeha.2017.07.016
Robins, S. K. (2020). Stable engrams and neural dynamics. Philos. Sci. 87, 1130–1139. doi: 10.1086/710624
Roediger, H. L., and Butler, A. C. (2011). The critical role of retrieval practice in long-term retention. Trends Cogn. Sci. 15, 20–27. doi: 10.1016/j.tics.2010.09.003
Roldan-Valadez, E., García-Lázaro, H., Lara-Romero, R., and Ramirez-Carmona, R. (2012). Neuroanatomy of episodic and semantic memory in humans: a brief review of neuroimaging studies. Neurol. India 60:613. doi: 10.4103/0028-3886.105196
Saffran, N. M. (1997). Language and auditory-verbal short-term memory impairments: evidence for common underlying processes. Cogn. Neuropsychol. 14, 641–682. doi: 10.1080/026432997381402
Salvato, G., Peviani, V., Scarpa, P., Francione, S., Castana, L., Gallace, A., et al. (2021). Investigating visuo-spatial neglect and visual extinction during intracranial electrical stimulations: the role of the right inferior parietal cortex. Neuropsychologia 162:108049. doi: 10.1016/j.neuropsychologia.2021.108049
Sarnthein, J., Petsche, H., Rappelsberger, P., Shaw, G. L., and von Stein, A. (1998). Synchronization between prefrontal and posterior association cortex during human working memory. Proc. Natl. Acad. Sci. U.S.A. 95, 7092–7096. doi: 10.1073/pnas.95.12.7092
Schiller, D., Monfils, M.-H., Raio, C. M., Johnson, D. C., LeDoux, J. E., and Phelps, E. A. (2009). Preventing the return of fear in humans using reconsolidation update mechanisms. Nature 463, 49–53. doi: 10.1038/nature08637
Schlichting, M. L., and Preston, A. R. (2015). Memory integration: neural mechanisms and implications for behavior. Curr. Opin. Behav. Sci. 1, 1–8. doi: 10.1016/j.cobeha.2014
Sellers, K. K., Yu, C., Zhou, Z. C., Stitt, I., Li, Y., Radtke-Schuller, S., et al. (2016). Oscillatory dynamics in the frontoparietal attention network during sustained attention in the ferret. Cell Rep. 16, 2864–2874. doi: 10.1016/j.celrep.2016.08.055
Sendi, M. S., Kanta, V., Inman, C. S., Manns, J. R., Hamann, S., Gross, R. E., et al. (2020). “Amygdala stimulation leads to functional network connectivity state transitions in the hippocampus,” in Proceedings of the 2020 42nd Annual International Conference of the IEEE Engineering in Medicine & Biology Society (EMBC) , (Montreal, QC). doi: 10.1109/embc44109.2020.9176742
Serences, J. T. (2016). Neural mechanisms of information storage in visual short-term memory. Vis. Res. 128, 53–67. doi: 10.1016/j.visres.2016.09.010
Shafritz, K. M., Gore, J. C., and Marois, R. (2002). The role of the parietal cortex in visual feature binding. Proc. Natl. Acad. Sci. U.S.A. 99, 10917–10922. doi: 10.1073/pnas.152694799
Shallice, T. (2002). “Fractionation of the supervisory system,” in Principles of frontal lobe function , eds D. T. Stuss and R. T. Knight (Oxford: Oxford University Press), 261–277. doi: 10.1093/acprof:oso/9780195134971.003.0017
Siegel, J. M. (2001). The REM sleep-memory consolidation hypothesis. Science 294, 1058–1063. doi: 10.1126/science.1063049
Smith, E. E., Jonides, J., Marshuetz, C., and Koeppe, R. A. (1998). Components of verbal working memory: evidence from neuroimaging. Proc. Natl. Acad. Sci. U.S.A. 95, 876–882. doi: 10.1073/pnas.95.3.876
Sobczak, J. (2017). Mechanisms of memory consolidation (dissertation). PhD thesis. York: University of York.
Sorensen, K. E. (2009). The connections of the hippocampal region new observations on efferent connections in the guinea pig, and their functional implications*. Acta Neurol. Scand. 72, 550–560. doi: 10.1111/j.1600-0404.1985.tb00914.x
Speranza, L., di Porzio, U., Viggiano, D., de Donato, A., and Volpicelli, F. (2021). Dopamine: the neuromodulator of long-term synaptic plasticity, reward and movement control. Cells 10:735. doi: 10.3390/cells10040735
Squire, L. R., and Wixted, J. T. (2011). The cognitive neuroscience of human memory since H.M. Annu. Rev. Neurosci. 34, 259–288. doi: 10.1146/annurev-neuro-061010-113720
Squire, L. R., and Zola, S. M. (1996). Structure and function of declarative and nondeclarative memory systems. Proc. Natl. Acad. Sci. U.S.A. 93, 13515–13522. doi: 10.1073/pnas.93.24.13515
Squire, L. R., Genzel, L., Wixted, J. T., and Morris, R. G. (2015). Memory consolidation. Cold Spring Harb. Perspect. Biol. 7:a021766. doi: 10.1101/cshperspect.a021766
Squire, L. R., Stark, C. E. L., and Clark, R. E. (2004). The medial temporal lobe. Annu. Rev. Neurosci. 27, 279–306. doi: 10.1146/annurev.neuro.27.070203.144130
Sreenivasan, K. K., Curtis, C. E., and D’Esposito, M. (2014). Revisiting the role of persistent neural activity during working memory. Trends Cogn. Sci. 18, 82–89. doi: 10.1016/j.tics.2013.12.001
Stickgold, R. (2005). Sleep-dependent memory consolidation. Nature 437, 1272–1278. doi: 10.1038/nature04286
Swanson, R. A., Levenstein, D., McClain, K., Tingley, D., and Buzsáki, G. (2020). Variable specificity of memory trace reactivation during hippocampal sharp wave ripples. Curr. Opin. Behav. Sci. 32, 126–135. doi: 10.1016/j.cobeha.2020.02.008
Takehara-Nishiuchi, K. (2020). Prefrontal–hippocampal interaction during the encoding of New Memories. Brain Neurosci. Adv. 4, 239821282092558. doi: 10.1177/2398212820925580
Takeuchi, T., Duszkiewicz, A. J., and Morris, R. G. (2014). The synaptic plasticity and memory hypothesis: encoding, storage and persistence. Philos. Trans. R. Soc. B Biol. Sci. 369:20130288. doi: 10.1098/rstb.2013.0288
Tamminen, J., Payne, J. D., Stickgold, R., Wamsley, E. J., and Gaskell, M. G. (2010). Sleep spindle activity is associated with the integration of new memories and existing knowledge. The Journal of Neuroscience 30, 14356–14360. doi: 10.1523/jneurosci.3028-10.2010
Thomas Yeo, B. T., Krienen, F. M., Sepulcre, J., Sabuncu, M. R., Lashkari, D., Hollinshead, M., et al. (2011). The organization of the human cerebral cortex estimated by intrinsic functional connectivity. J. Neurophysiol. 106, 1125–1165. doi: 10.1152/jn.00338.2011
Thompson, R. F., and Kim, J. J. (1996). Memory systems in the brain and localization of a memory. Proc. Natl. Acad. Sci. U.S.A. 93, 13438–13444. doi: 10.1073/pnas.93.24.13438
Thuault, S. J., Malleret, G., Constantinople, C. M., Nicholls, R., Chen, I., Zhu, J., et al. (2013). Prefrontal cortex HCN1 channels enable intrinsic persistent neural firing and executive memory function. J. Neurosci. 33, 13583–13599. doi: 10.1523/jneurosci.2427-12.2013
Todd, J. J., and Marois, R. (2005). Posterior parietal cortex activity predicts individual differences in visual short-term memory capacity. Cogn. Affect. Behav. Neurosci. 5, 144–155. doi: 10.3758/cabn.5.2.144
Tononi, G., and Cirelli, C. (2003). Sleep and synaptic homeostasis: a hypothesis. Brain Res. Bull. 62, 143–150. doi: 10.1016/j.brainresbull.2003.09.004
Tononi, G., and Cirelli, C. (2014). Sleep and the price of plasticity: from synaptic and cellular homeostasis to memory consolidation and integration. Neuron 81, 12–34. doi: 10.1016/j.neuron.2013.12.025
Tulving, E. (1985). Memory and consciousness. Can. Psychol. 26, 1–12. doi: 10.1037/h0080017
van Kesteren, M. T. R., Ruiter, D. J., Fernández, G., and Henson, R. N. (2012). How schema and novelty augment memory formation. Trends Neurosci. 35, 211–219. doi: 10.1016/j.tins.2012.02.001
van Kesteren, M. T., Brown, T. I., and Wagner, A. D. (2016). Interactions between memory and new learning: insights from fMRI multivoxel pattern analysis. Front. Syst. Neurosci. 10:46. doi: 10.3389/fnsys.2016.00046
Vilberg, K. L., and Rugg, M. D. (2008). Memory retrieval and the PARIETAL CORTEX: a review of evidence from a dual-process perspective. Neuropsychologia 46, 1787–1799. doi: 10.1016/j.neuropsychologia.2008.01.004
Vishnoi, S., Naseem, M., Raisuddin, S., and Parvez, S. (2018). Behavioral tagging: plausible involvement of PKMZ, ARC and role of neurotransmitter receptor systems. Neurosci. Biobehav. Rev. 94, 210–218. doi: 10.1016/j.neubiorev.2018.07.009
Wang, J. H., Wang, D., Gao, Z., Chen, N., Lei, Z., Cui, S., et al. (2016). Both glutamatergic and Gabaergic neurons are recruited to be associative memory cells. Biophys. J. 110:481a. doi: 10.1016/j.bpj.2015.11.2571
Wang, J.-H., and Cui, S. (2018). Associative memory cells and their working principle in the brain. F1000Research 7:108. doi: 10.12688/f1000research.13665.1
Wig, G. S., Buckner, R. L., and Schacter, D. L. (2009). Repetition priming influences distinct brain systems: evidence from task-evoked data and resting-state correlations. J. Neurophysiol. 101, 2632–2648. doi: 10.1152/jn.91213.2008
Williams, J. N. (2020). The neuroscience of implicit learning. Language Learning 70, 255–307. doi: 10.1111/lang.12405
Wiltgen, B. J., Zhou, M., Cai, Y., Balaji, J., Karlsson, M. G., Parivash, S. N., et al. (2010). The hippocampus plays a selective role in the retrieval of detailed contextual memories. Curr. Biol. 20, 1336–1344. doi: 10.1016/j.cub.2010.06.068
Winocur, G., and Moscovitch, M. (2011). Memory transformation and systems consolidation. J. Int. Neuropsychol. Soc. 17, 766–780. doi: 10.1017/S1355617711000683
Wolf, R. C., Vasic, N., and Walter, H. (2006). Differential activation of ventrolateral prefrontal cortex during working memory retrieval. Neuropsychologia 44, 2558–2563. doi: 10.1016/j.neuropsychologia.2006.05.015
Xu, Y., and Chun, M. M. (2006). Dissociable neural mechanisms supporting visual short-term memory for objects. Nature 440, 91–95. doi: 10.1038/nature04262
Xu, Y., Zou, P., and Cohen, A. E. (2017). Voltage imaging with genetically encoded indicators. Curr. Opin. Chem. Biol. 39, 1–10. doi: 10.1016/j.cbpa.2017.04.005
Yang, P., Wang, M., Luo, C., Ni, X., and Li, L. (2022). Dissociable causal roles of the frontal and parietal cortices in the effect of object location on object identity detection: a TMS study. Exp. Brain Res. 240, 1445–1457. doi: 10.1007/s00221-022-06344-4
Yu, R., Han, B., Wu, X., Wei, G., Zhang, J., Ding, M., et al. (2023). Dual-functional network regulation underlies the Central Executive System in working memory. Neuroscience 524, 158–180. doi: 10.1016/j.neuroscience.2023.05.025
Yu, S., Rempel, S., Gholamipourbarogh, N., and Beste, C. (2022). A ventral stream-prefrontal cortex processing cascade enables working memory gating dynamics. Commun. Biol. 5:1086. doi: 10.1038/s42003-022-04048-7
Zeithamova, D., and Preston, A. R. (2010). Flexible memories: differential roles for medial temporal lobe and prefrontal cortex in cross-episode binding. J. Neurosci. 30, 14676–14684. doi: 10.1523/jneurosci.3250-10.2010
Zhu, Y., Zeng, Y., Ren, J., Zhang, L., Chen, C., Fernandez, G., et al. (2022). Emotional learning retroactively promotes memory integration through rapid neural reactivation and reorganization. eLife 11:e60190. doi: 10.7554/elife.60190
Zielinski, M. C., Tang, W., and Jadhav, S. P. (2018). The role of replay and Theta sequences in mediating hippocampal-prefrontal interactions for memory and cognition. Hippocampus 30, 60–72. doi: 10.1002/hipo.22821
Zlotnik, G., and Vansintjan, A. (2019). Memory: an extended definition. Front. Psychol. 10:2523. doi: 10.3389/fpsyg.2019.02523
Keywords : memory, cellular consolidation, cognitive neuroscience, hippocampus, sleep
Citation: Sridhar S, Khamaj A and Asthana MK (2023) Cognitive neuroscience perspective on memory: overview and summary. Front. Hum. Neurosci. 17:1217093. doi: 10.3389/fnhum.2023.1217093
Received: 04 May 2023; Accepted: 10 July 2023; Published: 26 July 2023.
Reviewed by:
Copyright © 2023 Sridhar, Khamaj and Asthana. This is an open-access article distributed under the terms of the Creative Commons Attribution License (CC BY) . The use, distribution or reproduction in other forums is permitted, provided the original author(s) and the copyright owner(s) are credited and that the original publication in this journal is cited, in accordance with accepted academic practice. No use, distribution or reproduction is permitted which does not comply with these terms.
*Correspondence: Manish Kumar Asthana, [email protected] , [email protected]
This article is part of the Research Topic
New Challenges and Future Perspectives in Cognitive Neuroscience
Logo Left Content
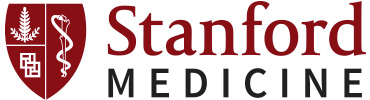
Logo Right Content
Stanford University School of Medicine blog
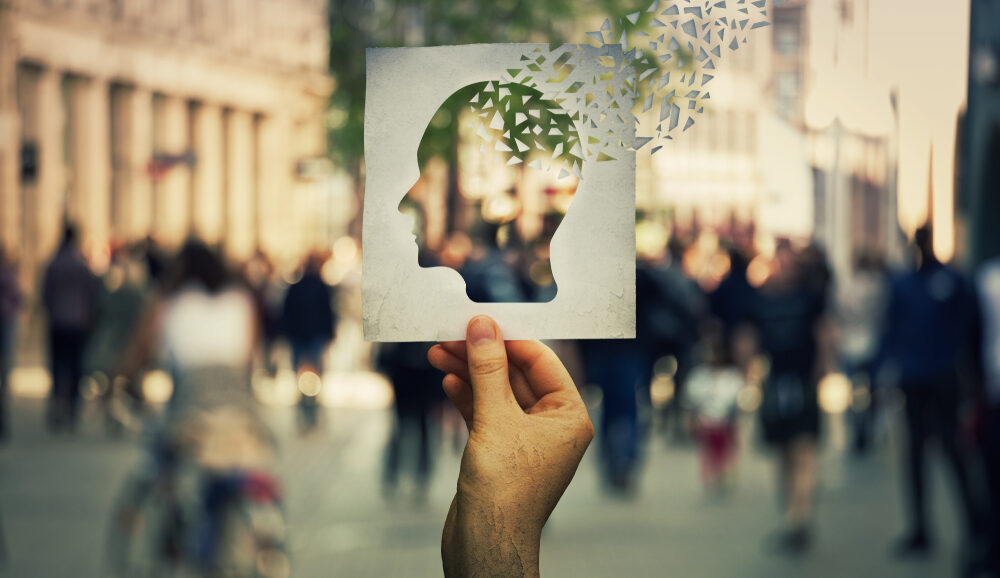
What really happens to our memory as we age?
For anyone over the age of 30 reading this article, here's some bad news for you: Your brain is already on the decline.
The good(ish) news? From the brain's peak performance in our mid-20s, that decline is gradual, said Stanford neurologist Sharon Sha , MD. Despite common lore about aging and major lapses in memory, the effects of healthy aging on cognitive functions are actually quite subtle.
For example, a young or middle-aged adult can remember a sequence of seven numbers, on average, while a person in their 60s without dementia can hold onto six digits. When asked to list as many animals as they can in a short time frame, a skill known as verbal fluency, adults over 55 can list about 4% fewer than those under 55 years old.
Around a generation ago, we assumed that when we get older, we dramatically lose our memory. That's really not the case. Sharon Sha
"Around a generation ago, we assumed that when we get older, we dramatically lose our memory," said Sha. "That's really not the case."
For all the talk about age, memory and cognitive ability overloading the news cycle in this presidential election year, it seems like a good time to consult memory experts. Sha leads Stanford Medicine's Memory Disorders Division and divides her time between clinical work with patients who have Alzheimer's disease and other forms of dementia and leading clinical trials in patients with these conditions.
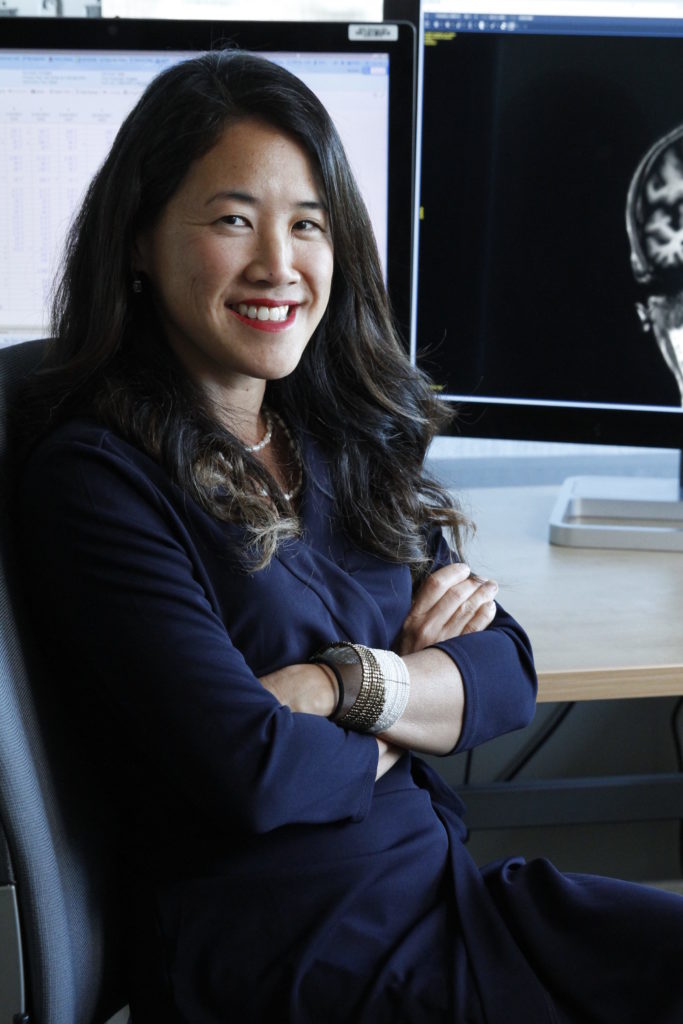
We asked her to discuss the links between aging and memory -- and what steps we can take to boost brain health. Her answers have been lightly edited for length and clarity.
What causes lapses in memory as we age?
Oh, that's a big bucket. Certain conditions like dementia, and specifically Alzheimer's disease, affect us more when we're older. The biggest risk factor for Alzheimer's disease is age. But beyond dementia, we think about a lot of other possible causes for memory lapses. When someone comes into the clinic and says they're having memory problems, we ask about medications, other psychiatric problems like anxiety and depression, and their sleep. There are a lot of factors that can affect memory and that are not necessarily expected in aging.
What's normal with memory and healthy aging, and what's not normal?
As we get older, we know we're going to get wrinkles and gray hair; similarly, there are normal age-related changes in our brains. Our processing speed -- how quickly we're thinking -- may slow down. The amount of content in our working memory may diminish. That short list of items you can keep in your head when going to the grocery story might get shorter, but it should not drop to zero. Although dementia is linked to age, it's not an inevitable part of getting older.
Although dementia is linked to age, it's not an inevitable part of getting older. Sharon Sha
How do you and other clinicians distinguish between normal aging and dementia?
There are screening tools that let us know if someone has a cognitive impairment that might indicate dementia. The definition of dementia also includes functional decline, meaning that someone is no longer able to live independently. If someone can no longer do their shopping or cooking, or remember to take their medications, that's concerning and beyond the expectation for normal aging.
There's also something we call mild cognitive impairment in which there is a cognitive decline from someone's baseline, but they are still functioning independently.
What happens to the brain when we lose memories?
We don't know exactly what happens biologically. But you can imagine that memory loss is part of the general atrophy and slowing down that happens to all parts of our bodies with age. We know there's a slight atrophy or shrinking of the brain with age, and that could include both a reduction in the volume and number of neurons as well as the insulation around neurons, called myelin. That loss of insulation also changes processing speed. And this is all normal -- just like you wouldn't expect to be as fast a runner at 80 as you were at 20.
When does the brain start that downward process?
It depends on the specific process, but generally speaking your brain is at its peak in terms of cognitive performance in your mid-20s. But if you're beyond your 20s, you probably recognize that you weren't making the best decisions at that point in your life. So, even if you're not at the peak of your brain function, what you have accrued now, if you're a couple decades beyond your mid-20s, is experience. It may take you longer to get to a decision, but that decision may be more likely to be right. I don't think any of us would trade our lived experience for a faster-working brain.
You probably recognize that you weren't making the best decisions (in your 20s). I don't think any of us would trade our lived experience for a faster-working brain. Sharon Sha
Are there things people can do to protect their memory and brain health?
This is the key question, because you can't fight aging, as much as you might want to. The benefit of aging is that you have all that experience, but how do we live and age healthfully? That's where research is supporting commonsense things like exercise. People often want to know what's the best kind of exercise. Any kind of exercise is better than sitting around. Aerobic exercise is the most studied in terms of brain health benefits, but smaller studies have also shown benefits for strength training and even being outside in nature. Just getting outside and moving your body is better than nothing.
We talk about cognitive stimulation, and anything that stimulates your brain in a positive way is great. If you hate crossword puzzles like I do, you're just going to get frustrated and that's not healthy, so pick something else. Learning a new sport, like pickleball, or a new kind of dance, is great for your brain because it's exercise, learning something new and giving you that social exposure.
We know from the pandemic that social isolation was bad for us, and part of it is that our brain needs that social interaction for fuel. In terms of actual fuel, the Mediterranean diet has been most well-studied in terms of brain health. However, if you are not of a Mediterranean background, culturally, you don't need to give up your food traditions. Just make sure you're getting those fruits and vegetables and lean proteins.
And finally, good sleep. If you have sleep apnea or other sleep problems, your brain is not getting what it needs to function at its best.
So, pretty much the stuff we know we should be doing, right?
Exactly. There's no easy pill, though everyone wants that magic prescription for brain health. It takes work. But it's never too late -- or too early -- to start taking care of your body and your brain.
Image: StunningArt
Related posts
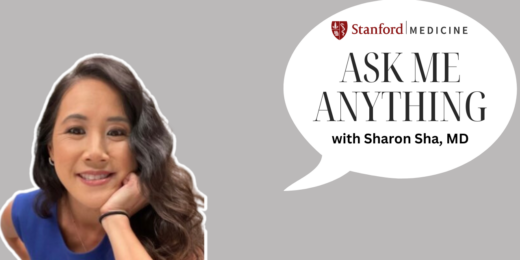
Ask Me Anything: Brain health and cognition
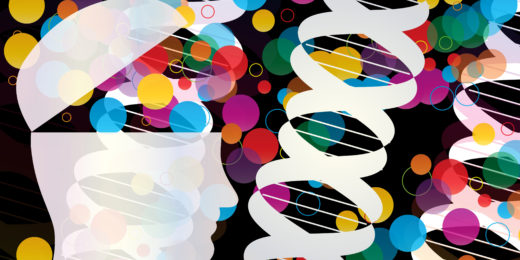
Clues from Down syndrome hint at new Alzheimer’s finding
Popular posts.
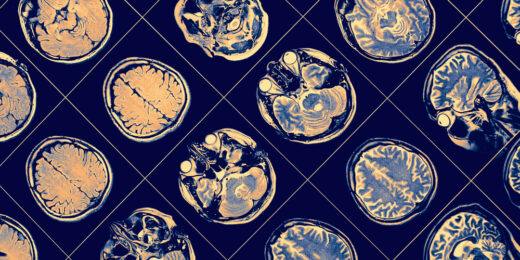

One step back: Why the new Alzheimer’s plaque-attack drugs don’t work
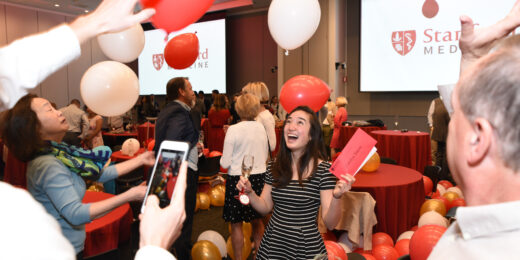
Match Day 101: How does the medical residency match work?
Advertisement
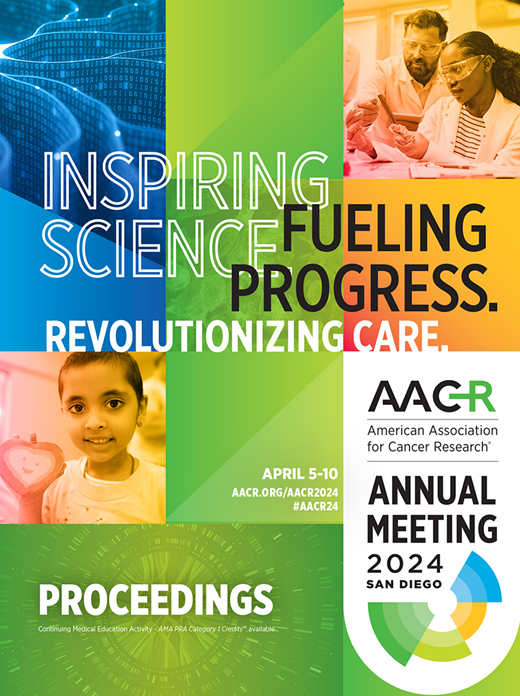
Abstract 5155: CD8 + T cells and CD4 + central memory T cells as biomarkers of perioperative anti-PD-1 therapy in combination with concurrent chemoradiotherapy for locally advanced G/GEJ adenocarcinoma
- Split-Screen
- Article contents
- Figures & tables
- Supplementary Data
- Peer Review
- Get Permissions
- Cite Icon Cite
- Search Site
- Version of Record March 22 2024
Yue Wang , Yawei Chen , Ju Yang , Xiaoyu Zhou , Yutao Wei , Qin Liu , Yang Yang , Wenxian Guan , Baorui Liu , Jia Wei; Abstract 5155: CD8 + T cells and CD4 + central memory T cells as biomarkers of perioperative anti-PD-1 therapy in combination with concurrent chemoradiotherapy for locally advanced G/GEJ adenocarcinoma. Cancer Res 15 March 2024; 84 (6_Supplement): 5155. https://doi.org/10.1158/1538-7445.AM2024-5155
Download citation file:
- Ris (Zotero)
- Reference Manager
Background: Gastric or gastroesophageal junction (G/GEJ) adenocarcinoma is one of the most common lethal malignancies in the world. Near half of the G/GEJ adenocarcinoma are locally advanced at diagnosis and with poor prognosis. Several clinical trials including SHARED (ChiCTR1900024428), which conducted in our center, demonstrated that perioperative anti-PD-1 therapy in combination with concurrent chemoradiotherapy for locally advanced G/GEJ cancers, could raise the pCR rate over 30%. However, the biomarkers for patients with pCR are nor clear.
Methods: Baseline tumor biopsies and post-treatment surgical tissues were collected for next-generation sequencing (NGS) and mass cytometry (CyTOF). The overall survival (OS) was measured from the date of surgery to the date of death or the last follow-up visit.
Results: 34 patients with locally advanced G/GEJ cancer received anti-PD-1 therapy (Sintilimab) in combination with concurrent chemoradiotherapy in our center. Our results met the pre-specified primary endpoint, with a pCR rate of 38.2% (13/34) and median DFS of 17.0 months. Tumor mutation burden (TMB) analyzed by NGS was higher in the pCR group than those not achieving pCR (non-pCR). Deletion of cytoband 7q35, where TPK1 locates, was significantly enriched in pCR groups. The RNA sequencing data revealed an enrichment of dendritic cells (DC), CD8 + T cells and cytotoxic cell signatures in the pCR group. Conversely, the signature for CD4 + central memory T cells (TCM) was observed to be lower in the pCR group. On the other hand, CyTOF data also demonstrated that the proportion of CD8 + T cells, CD8 + effector memory T cells (TEM) were both higher in pCR group at baseline, while CD4 + TCM was lower. Moreover, the proportion of specific subtype characterized by CD127 + CD27 + CD45RO + CXCR5 - CD4 + TCM was significantly lower in pCR patients and significantly reduced after treatment. As continuous variable, only CD8 + T cells correlated with OS.
Conclusions: It seemed that CD8 + T cells and CD4 + TCM cells could be predictive biomarkers of perioperative anti-PD-1 therapy in combination with cCRT for locally advanced G/GEJ. Furthermore, we found a new CD4 + TCM subtype defined with CD127 + CD27 + CD45RO + CXCR5 - could be novel biomarkers.
Citation Format: Yue Wang, Yawei Chen, Ju Yang, Xiaoyu Zhou, Yutao Wei, Qin Liu, Yang Yang, Wenxian Guan, Baorui Liu, Jia Wei. CD8 + T cells and CD4 + central memory T cells as biomarkers of perioperative anti-PD-1 therapy in combination with concurrent chemoradiotherapy for locally advanced G/GEJ adenocarcinoma [abstract]. In: Proceedings of the American Association for Cancer Research Annual Meeting 2024; Part 1 (Regular Abstracts); 2024 Apr 5-10; San Diego, CA. Philadelphia (PA): AACR; Cancer Res 2024;84(6_Suppl):Abstract nr 5155.
Citing articles via
Email alerts.
- Online First
- Collections
- Online ISSN 1538-7445
- Print ISSN 0008-5472
AACR Journals
- Blood Cancer Discovery
- Cancer Discovery
- Cancer Epidemiology, Biomarkers & Prevention
- Cancer Immunology Research
- Cancer Prevention Research
- Cancer Research
- Cancer Research Communications
- Clinical Cancer Research
- Molecular Cancer Research
- Molecular Cancer Therapeutics
- Info for Advertisers
- Information for Institutions/Librarians

- Privacy Policy
- Copyright © 2023 by the American Association for Cancer Research.
This Feature Is Available To Subscribers Only
Sign In or Create an Account
Microsoft Research Blog
Introducing garnet – an open-source, next-generation, faster cache-store for accelerating applications and services.
Published March 18, 2024
By Badrish Chandramouli , Partner Research Manager
Share this page
- Share on Facebook
- Share on Twitter
- Share on LinkedIn
- Share on Reddit
- Subscribe to our RSS feed
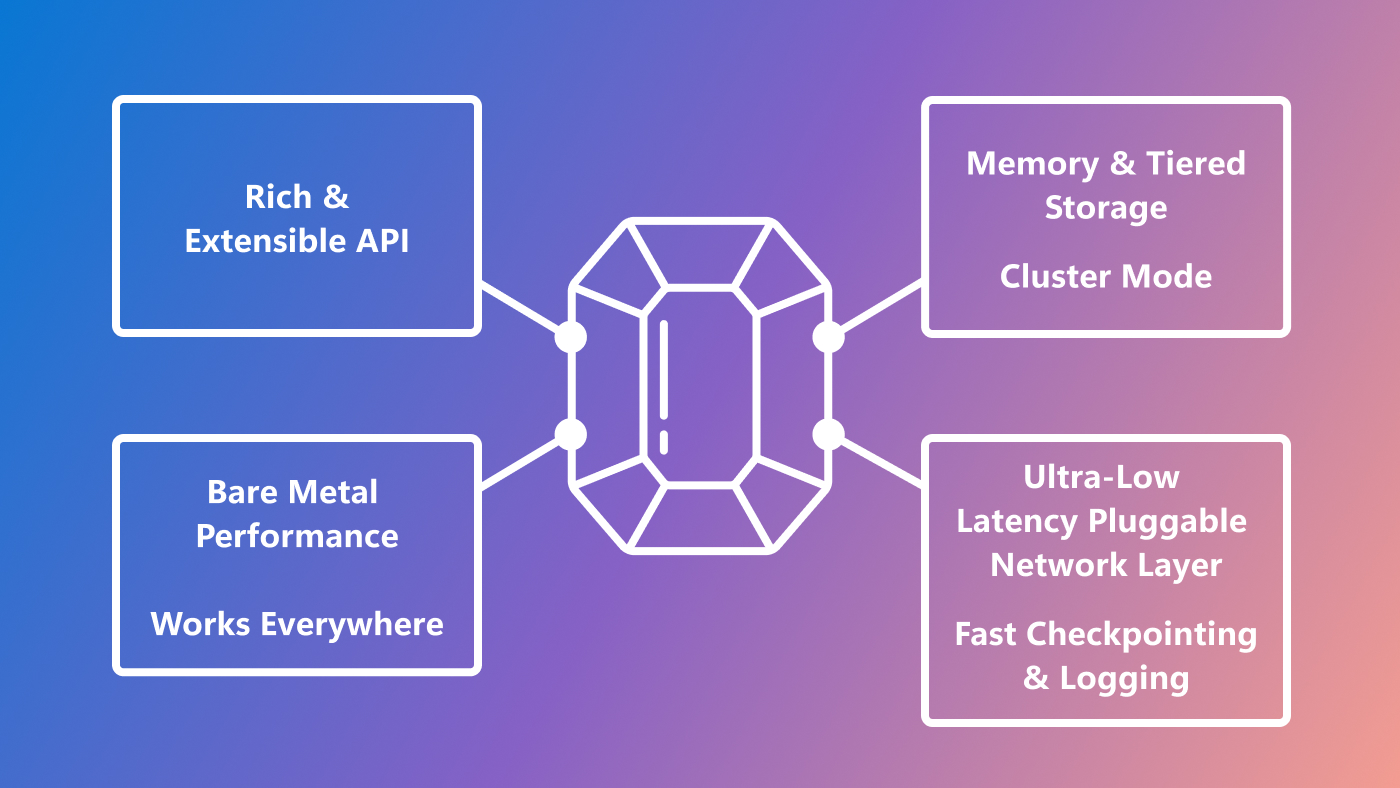
Researchers at Microsoft have been working for nearly a decade to address the increasing demand for data storage mechanisms to support the rapid advances in interactive web applications and services. Our new cache-store system called Garnet, which offers several advantages over legacy cache-stores, has been deployed in multiple use cases at Microsoft, such as those in the Windows & Web Experiences Platform, Azure Resource Manager, and Azure Resource Graph, and is now available as an open-source download at https://github.com/microsoft/garnet (opens in new tab) . In open sourcing Garnet, we hope to enable the developer community to benefit from its performance gains and capabilities, to build on our work, and to expand the Garnet ecosystem by adding new API calls and features. We also hope that the open sourcing will encourage follow-up academic research and open future collaboration opportunities in this important research area.
The cache-store problem
The growth of cloud and edge computing has brought an increasing number and range of applications and services that need to access, update, and transform data with higher efficiency, lower latencies, and lower costs than ever before. These applications and services often require significant operational spending on storage interactions, making this one of the most expensive and challenging platform areas today. A cache-store software layer, deployed as a separately scalable remote process, can ease these costs and improve application performance. This has fueled a growing cache-store industry, including many open-source systems, such as Redis, Memcached, KeyDB, and Dragonfly.
Unlike traditional remote cache-stores, which support a simple get/set interface, modern caches offer rich APIs and feature sets. They support raw strings, analytic data structures such as Hyperloglog, and complex data types such as sorted sets and hash. They allow users to checkpoint and recover the cache, create data shards, maintain replicated copies, and support transactions and custom extensions.
Spotlight: AI-POWERED EXPERIENCE
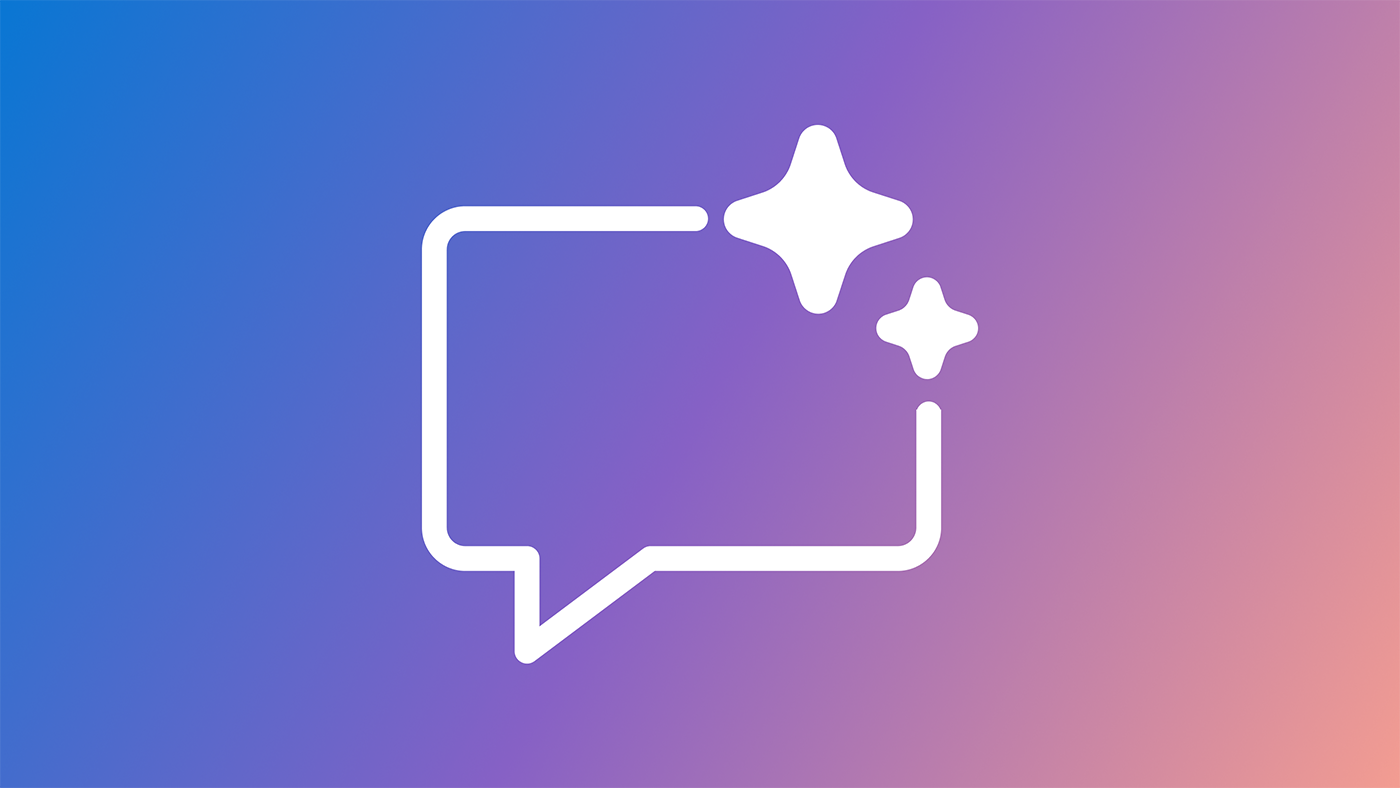
Microsoft research copilot experience
Discover more about research at Microsoft through our AI-powered experience
However, existing systems achieve this feature richness at a cost, by keeping the system design simple, which limits the ability to fully exploit the latest hardware capabilities (e.g., multiple cores, tiered storage, fast networks). Further, many of these systems are not explicitly designed to be easily extensible by app developers or to work well on diverse platforms and operating systems.
Introducing Garnet
At Microsoft Research, we have been investigating modern key-value database architectures since 2016. Our prior work, the FASTER (opens in new tab) embedded key-value library, which we open-sourced (opens in new tab) in 2018, demonstrated orders-of-magnitude better performance than existing systems, while focusing on the simple single-node in-process key-value model.
Starting in 2021, based on requirements from use-cases at Microsoft, we began building a new remote cache-store with all the necessary features to serve as a viable replacement to existing cache-stores. Our challenge was to maintain and enhance the performance benefits that we achieved in our earlier work, but in this more general and realistic network setting.
The result of this effort is Garnet – a new cache-store that offers several unique benefits:
- Garnet adopts the popular RESP wire protocol as a starting point, which makes it possible to use Garnet from unmodified Redis clients available in most programming languages today.
- Garnet offers much better scalability and throughput with many client connections and small batches, leading to cost savings for large apps and services.
- Garnet demonstrates better client latency at the 99 th and 99.9 th percentiles, which is critical to real-world scenarios.
- Based on the latest .NET technology, Garnet is cross-platform, extensible, and modern. It is designed to be easy to develop for and evolve, without sacrificing performance in the common case. We leveraged the rich library ecosystem of .NET for API breadth, with open opportunities for optimization. Thanks to our careful use of .NET, Garnet achieves state-of-the-art performance on both Linux and Windows.
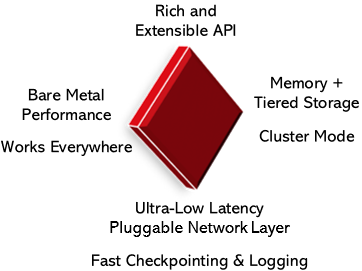
API features: Garnet supports a wide range of APIs including raw string, analytical, and object operations described earlier. It also implements a cluster mode with sharding, replication, and dynamic key migration. Garnet supports transactions in the form of client-side RESP transactions (opens in new tab) and our own server-side stored procedures in C# and allows users to define custom operations on both raw strings and new object types, all in the convenience of C#, leading to a lower bar for developing custom extensions.
Network, storage, cluster features: Garnet uses a fast and pluggable network layer, enabling future extensions such as leveraging kernel-bypass stacks. It supports secure transport layer security (TLS) communications as well as basic access control. Garnet’s storage layer, called Tsavorite, was forked from OSS FASTER, and includes strong database features such as thread scalability, tiered storage support (memory, SSD, and cloud storage), fast non-blocking checkpointing , recovery, operation logging for durability, multi-key transaction support, and better memory management and reuse. Finally, Garnet supports a cluster mode of operation – more on this later.
Performance preview
We illustrate a few key results comparing Garnet to leading open-source cache-stores. A more detailed performance comparison can be found on our website at https://microsoft.github.io/garnet/ (opens in new tab) .
We provision two Azure Standard F72s v2 virtual machines (72 vcpus, 144 GiB memory each) running Linux (Ubuntu 20.04), with accelerated TCP enabled. One machine runs different cache-store servers, and the other is dedicated to issuing workloads. We use our own benchmarking tool, called Resp.benchmark (opens in new tab) , to generate all results. We compare Garnet to the latest open-source versions of Redis (opens in new tab) (v7.2), KeyDB (opens in new tab) (v6.3.4), and Dragonfly (opens in new tab) (v6.2.11). We use a uniform random distribution of keys in these experiments (Garnet’s shared memory design benefits even more with skewed workloads). The data is pre-loaded onto each server, and fits in memory in these experiments.
Experiment 1: Throughput with varying number of client sessions
We start with large batches of GET operations (4096 requests per batch) and small payloads (8-byte keys and values) to minimize network overhead and compare the systems as we increase the number of client sessions. We see from Figure 1 that Garnet exhibits better scalability than Redis and KeyDB, while achieving higher throughput than all three baseline systems (the y-axis is log scale). Note that, while Dragonfly shows similar scaling behavior as Garnet, it is a pure in-memory system. Further, Garnet’s throughput relative to other systems remains strong when the database size (i.e., the number of distinct keys pre-loaded) is significantly larger, at 256 million keys, than what would fit in the processor caches.
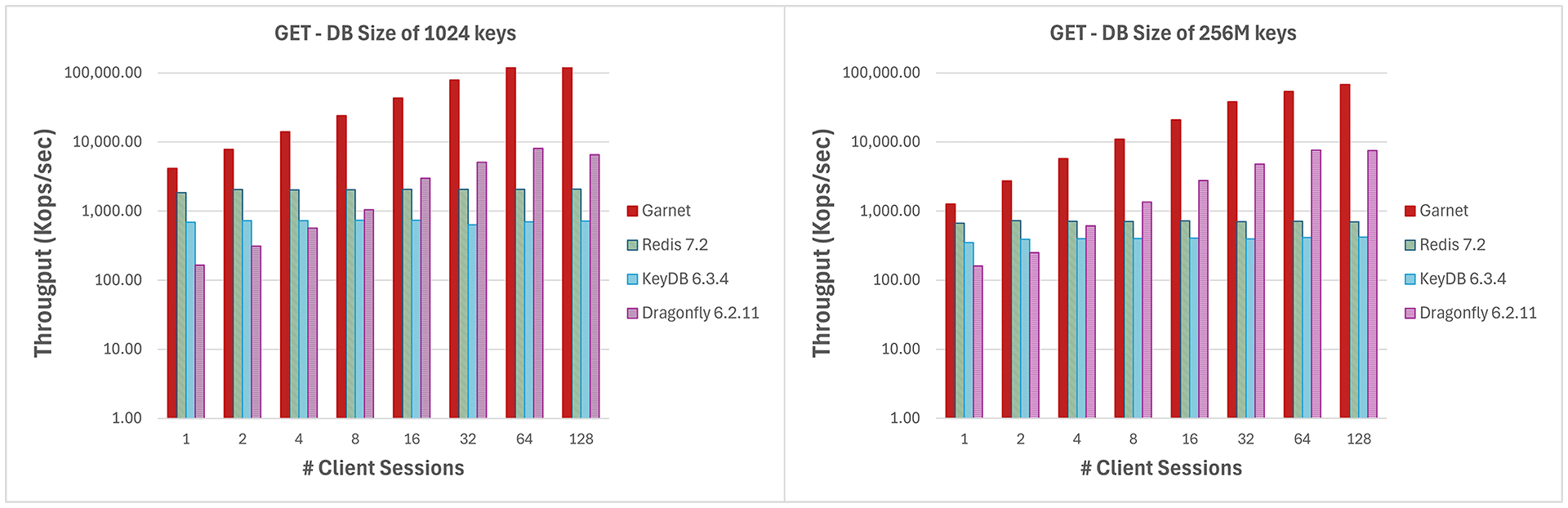
Experiment 2: Throughput with varying batch sizes
We next vary the batch size, with GET operations and a fixed number (64) of client sessions. We experiment with two different database sizes as before. Figure 2 shows that Garnet performs better even with no batching, and the gap increases even for very small batch sizes. Payload sizes are the same as before. Again, the y-axis is log scale.
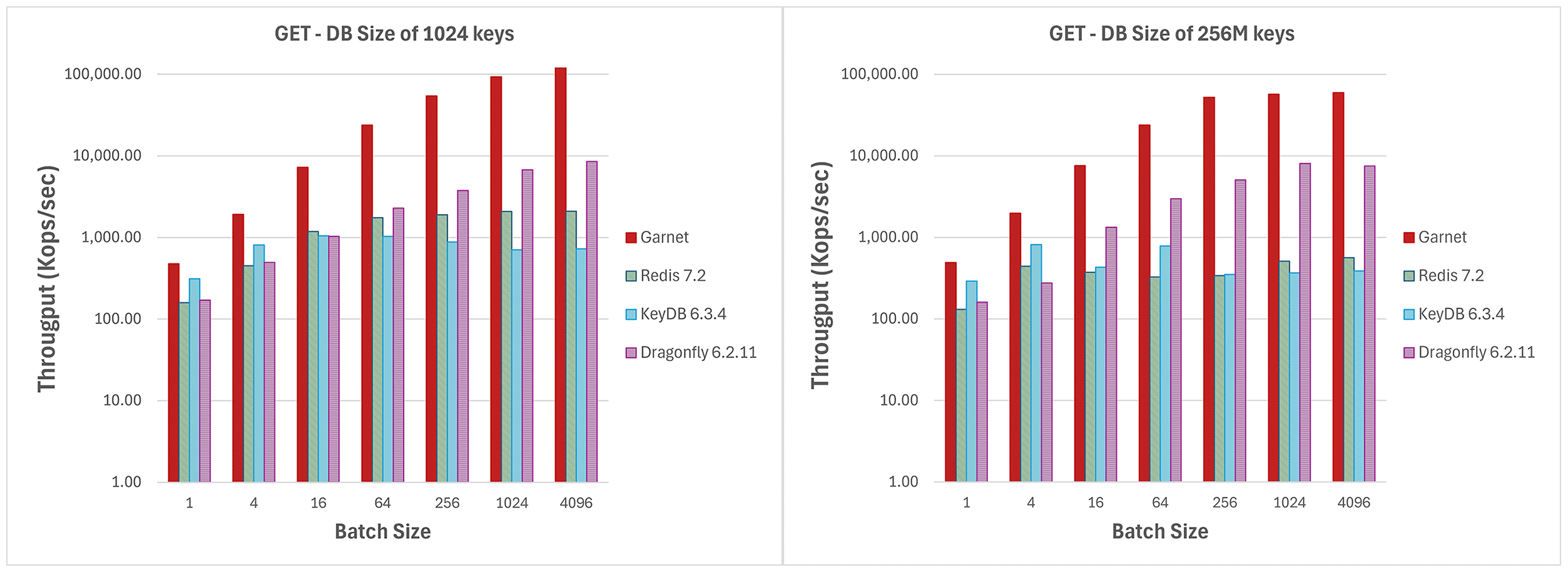
Experiment 3: Latency with varying number of client sessions
We next measure client-side latencies for the various systems. Figure 3 shows that, as we increase the number of client sessions, Garnet’s latency (measured in microseconds) at various percentiles stays much more stable and lower as compared to other systems. Here, we issue a mix of 80% GET and 20% SET operations, with no operation batching.
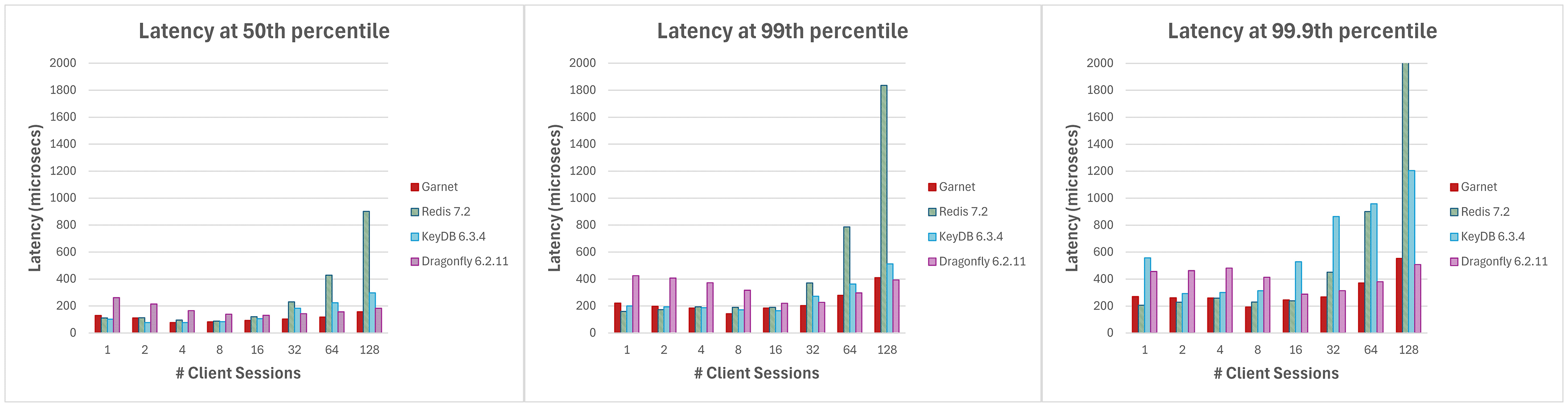
Experiment 4: Latency with varying batch sizes
Garnet’s latency is optimized for adaptive client-side batching and many sessions querying the system. We increase the batch sizes from 1 to 64 and plot latency at different percentiles below with 128 active client connections. We see in Figure 4 that Garnet’s latency is low across the board. As before, we issue a mix of 80% GET and 20% SET operations.
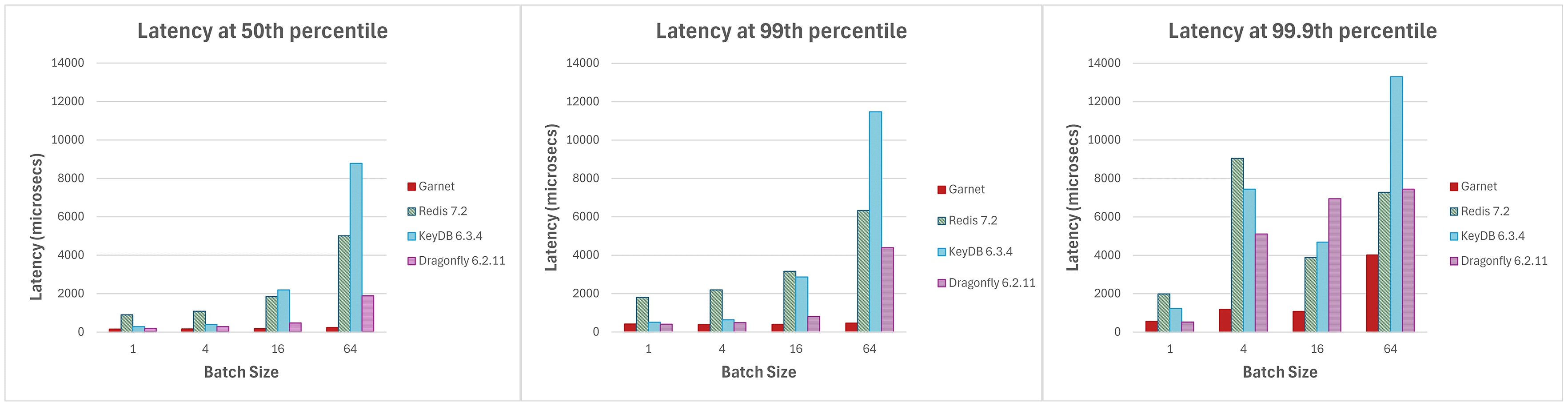
Other experiments
We have also experimented with other features and operation types and found Garnet to perform and scale well. Our documentation (opens in new tab) has more details, including how to run these experiments so that you can see the benefits for your own use cases.
Garnet’s design highlights
Garnet’s design re-thinks the entire cache-store stack – from receiving packets on the network, to parsing and processing database operations, to performing storage interactions. We build on top of years of research, with over 10 research papers published over the last decade. Figure 5 shows Garnet’s overall architecture. We highlight a few key ideas below.
Garnet’s network layer inherits a shared memory design inspired by our prior research on ShadowFax . TLS processing and storage interactions are performed on the IO completion thread, avoiding thread switching overheads in the common case. This approach allows CPU cache coherence to bring the data to the network, instead of traditional shuffle-based designs, which require data movement on the server.
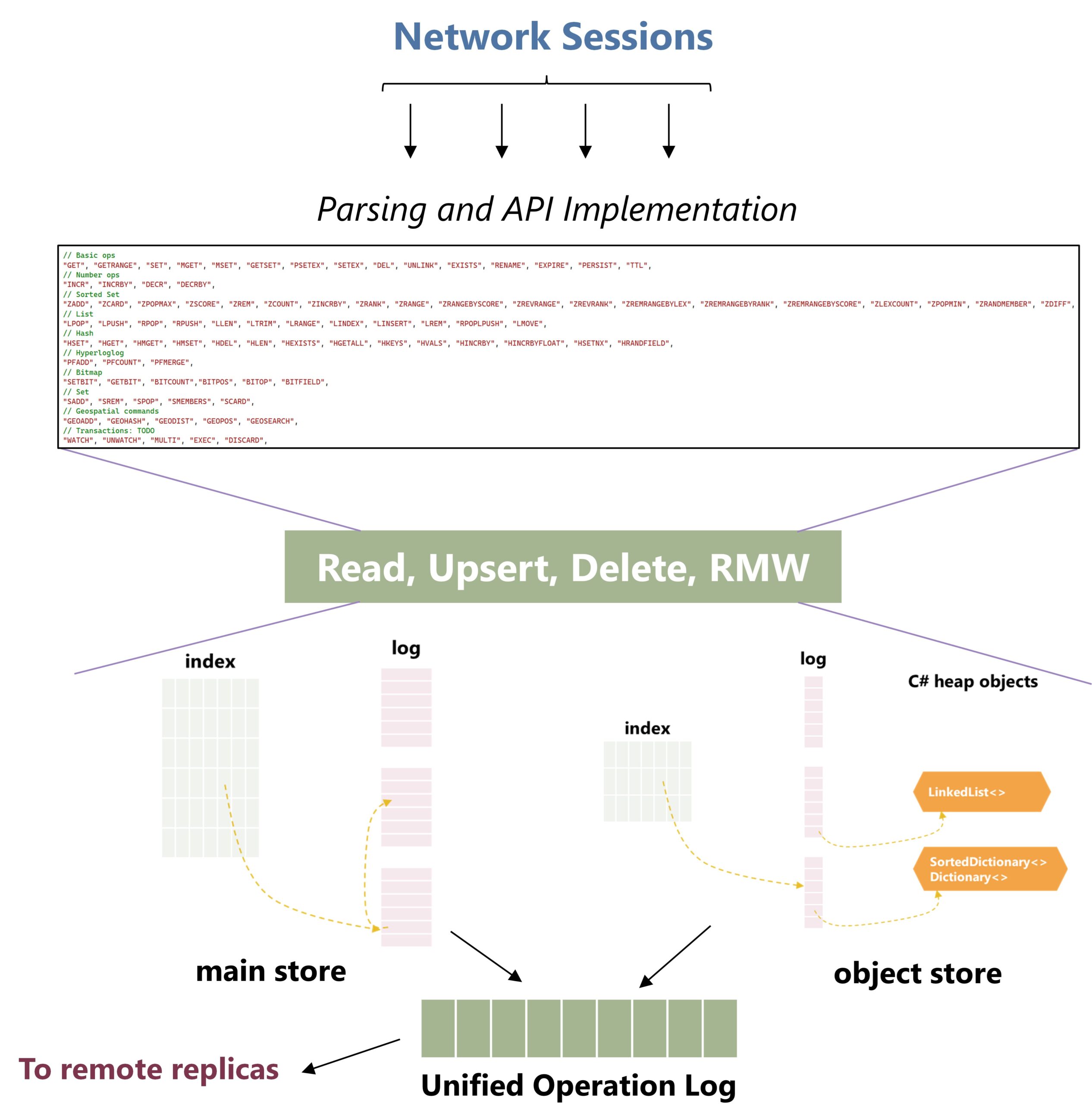
Garnet’s storage design consists of two Tsavorite key-value stores whose fates are bound by a unified operation log. The first store, called the “main store,” is optimized for raw string operations and manages memory carefully to avoid garbage collection. The second, and optional, “object store” is optimized for complex objects and custom data types, including popular types such as Sorted Set, Set, Hash, List, and Geo. Data types in the object store leverage the .NET library ecosystem for their current implementations. They are stored on the heap in memory (which makes updates very efficient) and in a serialized form on disk. In the future, we plan to investigate using a unified index and log to ease maintenance.
A distinguishing feature of Garnet’s design is its narrow-waist Tsavorite storage API, which is used to implement the large, rich, and extensible RESP API surface on top. This API consists of read, upsert, delete, and atomic read-modify-write operations, implemented with asynchronous callbacks for Garnet to interject logic at various points during each operation. Our storage API model allows us to cleanly separate Garnet’s parsing and query processing concerns from storage details such as concurrency, storage tiering, and checkpointing.
Garnet further adds support for multi-key transactions based on two-phase locking. One can either use RESP client-side transactions (MULTI/EXEC) or use our server-side transactional stored procedures in C#.
Cluster mode
In addition to single-node execution, Garnet supports a cluster mode, which allows users to create and manage a sharded and replicated deployment. Garnet also supports an efficient and dynamic key migration scheme to rebalance shards. Users can use standard Redis cluster commands to create and manage Garnet clusters, and nodes perform gossip to share and evolve cluster state. Overall, Garnet’s cluster mode is a large and evolving feature, and we will cover more details in subsequent posts.
Looking ahead
As Garnet is deployed in additional scenarios, we will continue to share those details in future articles. We also look forward to continuing to add new features and improvements to Garnet, as well as working with the open-source community.
Project contributors
Garnet Core: Badrish Chandramouli , Vasileios Zois , Lukas Maas , Ted Hart, Gabriela Martinez Sanchez, Yoganand Rajasekaran , Tal Zaccai , Darren Gehring , Irina Spiridonova .
Collaborators: Alan Yang, Pradeep Yadav, Alex Dubinkov, Venugopal Latchupatulla, Knut Magne Risvik , Sarah Williamson, Narayanan Subramanian, Saurabh Singh, Padmanabh Gupta, Sajjad Rahnama, Reuben Bond, Rafah Hosn , Surajit Chaudhuri , Johannes Gehrke , and many others.
Related publications
Faster: a concurrent key-value store with in-place updates, concurrent prefix recovery: performing cpr on a database, achieving high throughput and elasticity in a larger-than-memory store, meet the authors.

Badrish Chandramouli
Partner Research Manager
Continue reading
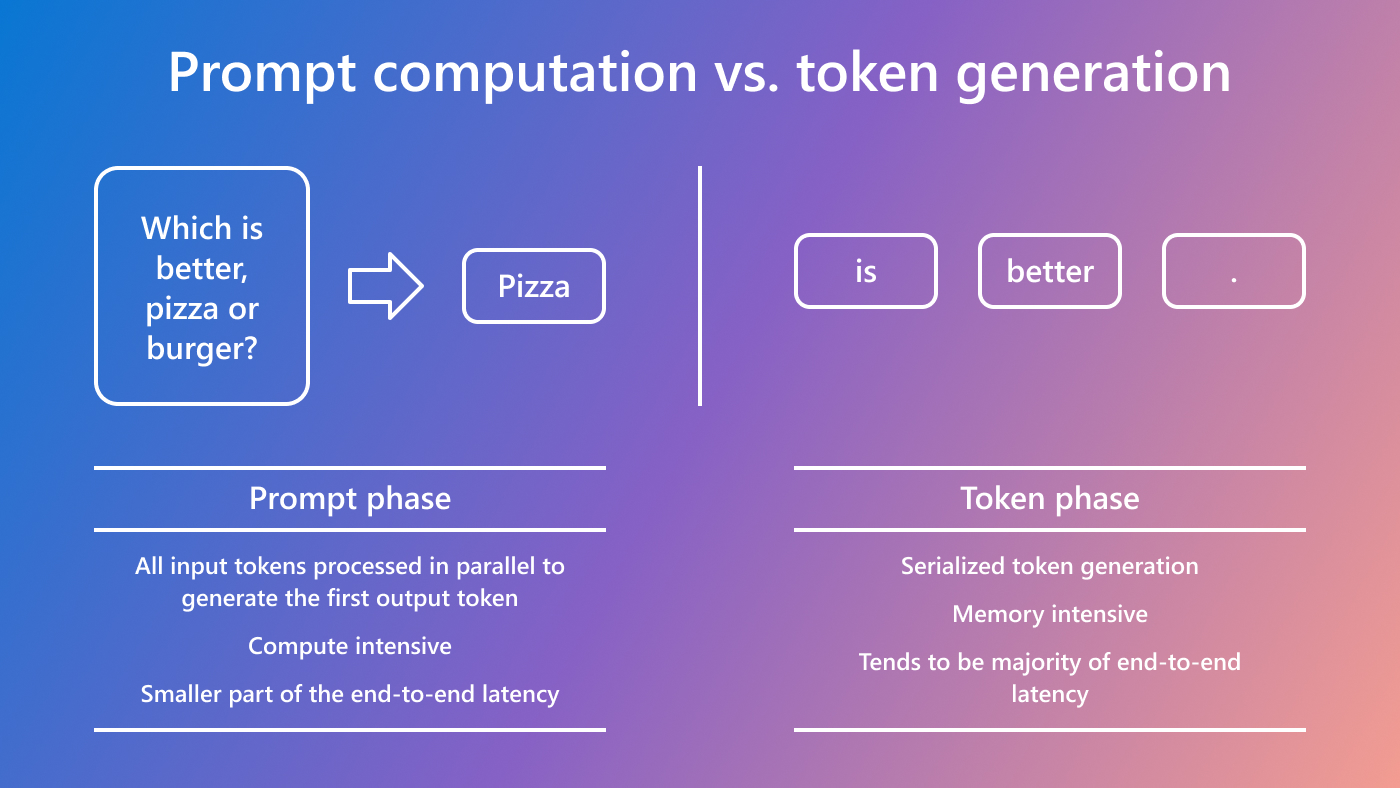
Splitwise improves GPU usage by splitting LLM inference phases

DeepSpeed ZeRO++: A leap in speed for LLM and chat model training with 4X less communication
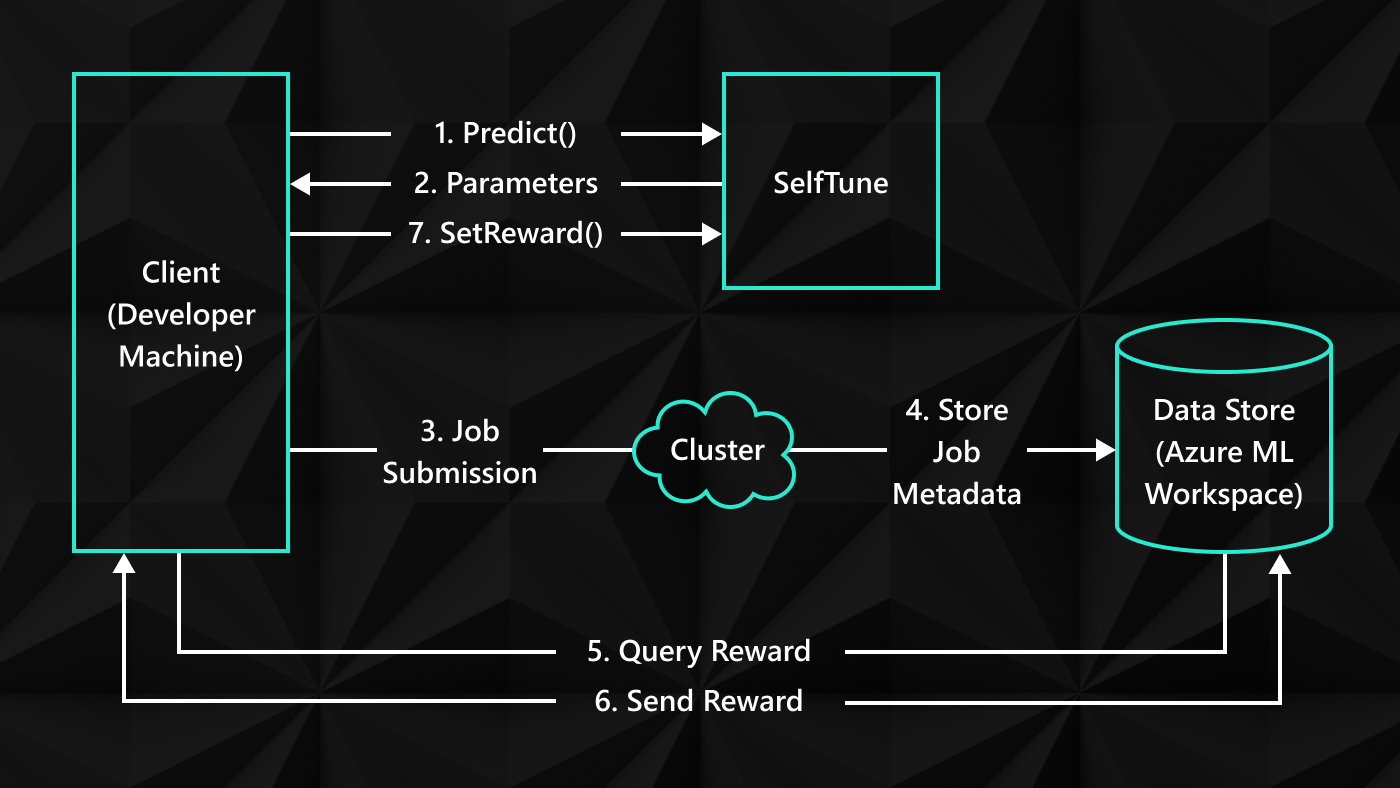
Automatic post-deployment management of cloud applications

DeepSpeed Compression: A composable library for extreme compression and zero-cost quantization
Research areas.

Research Groups
- Data Systems
Related projects
Related labs.
- Microsoft Research Lab - Redmond
- Follow on Twitter
- Like on Facebook
- Follow on LinkedIn
- Subscribe on Youtube
- Follow on Instagram
Share this page:

An official website of the United States government
The .gov means it’s official. Federal government websites often end in .gov or .mil. Before sharing sensitive information, make sure you’re on a federal government site.
The site is secure. The https:// ensures that you are connecting to the official website and that any information you provide is encrypted and transmitted securely.
- Publications
- Account settings
Preview improvements coming to the PMC website in October 2024. Learn More or Try it out now .
- Advanced Search
- Journal List
- APA Open Access
Sleep Deprivation and Memory: Meta-Analytic Reviews of Studies on Sleep Deprivation Before and After Learning
Chloe r. newbury.
1 Department of Psychology, Royal Holloway, University of London
Rebecca Crowley
Kathleen rastle, jakke tamminen.
This work was funded by Economic and Social Research Council grant ES/P001874/1 awarded to Kathleen Rastle and Jakke Tamminen.
Chloe R. Newbury and Rebecca Crowley contributed equally to this work.
The data and analysis scripts are available at osf.io/5gjvs/ .
Associated Data
Research suggests that sleep deprivation both before and after encoding has a detrimental effect on memory for newly learned material. However, there is as yet no quantitative analyses of the size of these effects. We conducted two meta-analyses of studies published between 1970 and 2020 that investigated effects of total, acute sleep deprivation on memory (i.e., at least one full night of sleep deprivation): one for deprivation occurring before learning and one for deprivation occurring after learning. The impact of sleep deprivation after learning on memory was associated with Hedges’ g = 0.277, 95% CI [0.177, 0.377]. Whether testing took place immediately after deprivation or after recovery sleep moderated the effect, with significantly larger effects observed in immediate tests. Procedural memory tasks also showed significantly larger effects than declarative memory tasks. The impact of sleep deprivation before learning was associated with Hedges’ g = 0.621, 95% CI [0.473, 0.769]. Egger’s tests for funnel plot asymmetry suggested significant publication bias in both meta-analyses. Statistical power was very low in most of the analyzed studies. Highly powered, preregistered replications are needed to estimate the underlying effect sizes more precisely.
Public Significance Statement
The health risks associated with lack of sleep are well known, but the consequences of missing one or more nights of sleep for learning and memory are less well appreciated. In two meta-analyses pooling studies across 5 decades of research, we found that total sleep deprivation before learning as well as after learning had a detrimental impact on memory for the newly learned materials. These data suggest sleep supports learning and memory in two ways: It prepares the brain for learning over the next day, and it helps strengthen new memories learned during the previous day.
There is a growing body of evidence suggesting a critical role of sleep in learning and memory ( Diekelmann & Born, 2010 ). On the one hand, offline memory consolidation during sleep benefits both declarative and procedural memories acquired during preceding wake ( Klinzing et al., 2019 ). On the other hand, memory encoding capacity has been argued to saturate gradually during wake, with sleep restoring this capacity ( Cirelli & Tononi, in press ; Tononi & Cirelli, 2012 ). These theoretical advances have been accompanied by practical societal concerns regarding the prevalence of poor sleep, especially for students ( Twenge et al., 2017 ) and shift workers ( Vidya et al., 2019 ). The proposed importance of sleep for memory processes has been supported by many studies showing detrimental effects of total sleep deprivation on the learning and retrieval of new information. Yet, the effect sizes associated with the total sleep deprivation impairment are variable, and some studies have failed to find significant effects altogether (e.g., Diekelmann et al., 2008 ). Therefore, we used a meta-analytic approach to estimate the effect size associated with the impact of sleep deprivation, both when the deprivation occurs before learning and when it occurs after learning.
Impact of Total Sleep Deprivation After Learning and Potential Moderators of the Effect
The active systems consolidation theory suggests that sleep after learning strengthens new memories (e.g., Klinzing et al., 2019 ; Kumaran et al., 2016 ; McClelland et al., 1995 ). Information learned during wakefulness is initially encoded rapidly in the hippocampus, where memories are stored separately from existing memory stores. Repeated reactivation of these new memories, primarily during slow-wave sleep (SWS), supports the strengthening of memory representations and leads to the integration of these memories in the neocortex. Such neocortical representations are less liable to disruption and form interrelated semantic networks with existing memories, yielding memory representations that allow abstraction, generalization, and discovery of statistical patterns across discrete memories ( Lewis & Durrant, 2011 ; Lewis et al., 2018 ; Stickgold & Walker, 2013 ). Notably, since the mechanisms outlined in this theory relate only to hippocampal-dependent memory consolidation ( Inostroza & Born, 2013 ), these mechanisms may primarily support the consolidation of declarative (or explicit) memory.
Effects of sleep on nondeclarative memory have also been observed; for example, sleep enhances motor skills such as finger-tapping sequence learning ( Korman et al., 2007 ; Walker et al., 2002 ; see King et al., 2017 for a review). However, this beneficial effect of sleep on procedural memories may be evident only when learning is intentional (explicit memory), rather than unintentional (implicit memory). Robertson et al. (2004) found that awareness of learning a finger-tapping task led to a sleep benefit, whereas improvements in implicit learning performance, when participants had little awareness of the task, were similar regardless of whether the retention interval contained sleep or wakefulness. Thus, there are suggestions that the mechanisms involved in the consolidation of hippocampal-dependent declarative memories may also facilitate the consolidation of some procedural tasks that rely on explicit learning and thus show some hippocampal-dependency ( Schönauer et al., 2014 ; Walker et al., 2005 ).
However, this latter theory does not take into account observations that some procedural tasks that do not rely on explicit learning or an intact hippocampus still show superior performance after sleep. Stickgold et al. (2000) found that a period of sleep after learning a visual discrimination task benefited later performance, and Schönauer et al. (2015) found that improvements in performance on a mirror-tracing task were only observed after a period of offline consolidation. Recent studies in both animals ( Sawangjit et al., 2018 ) and humans ( Schapiro et al., 2019 ) have suggested that the hippocampus may be involved in the sleep-dependent consolidation of memories that do not rely on the hippocampus during encoding. For example, Schapiro et al. (2019) trained amnesic patients with hippocampal damage on the motor sequence task, a classic procedural memory task typically considered to be non-hippocampus-dependent. The patients were able to learn the task equally well compared to matched controls, suggesting that the hippocampus is not required for learning of the task. However, while the controls showed the expected overnight consolidation benefit, the patients did not, leading the authors to conclude that the hippocampus may be involved in the consolidation of procedural tasks that do not require it for initial learning.
As reviewed above, theories of memory consolidation predict that depriving participants of sleep after learning should impair memory for the information encoded before sleep deprivation, relative to control conditions where participants are allowed to sleep normally after learning. In our first meta-analysis, we analyze the current research into both declarative and procedural memories to estimate the size of this sleep deprivation after learning effect. We focus on the literature using manipulations of total sleep deprivation, as this is the strongest and most direct manipulation to test theories of sleep-associated memory consolidation. In doing so, we exclude from our analyses studies of sleep restriction. In standard sleep restriction studies, participants are allowed to sleep for a shorter duration than they would do otherwise, and the manipulation typically continues for multiple nights. This chronic sleep restriction can have a detrimental impact on learning and memory (e.g., Cousins et al., 2018 ) although not always (e.g., Voderholzer et al., 2011 ). In selective sleep restriction studies, participants are only deprived of the first half of the night, rich in SWS, or the second half of the night, rich in rapid eye movement (REM) sleep (e.g., Plihal & Born, 1997 ). These studies can reveal important information about the precise brain mechanisms underlying benefits of sleep on memory. There are two theoretically motivated reasons for excluding both types of sleep restriction from our meta-analyses. Standard sleep restriction studies still allow participants to sleep for several hours each night, and it may be sufficient for sleep-associated memory consolidation to occur. Thus, these manipulations do not provide a strong test of the relevant theories, and their inclusion might lead us to underestimate the effect size associated with sleep deprivation. Selective sleep restriction studies on the other hand are designed to address the more fine-grained question of which specific sleep stages are the most beneficial for memory. Different theories make different predictions in this regard: For example, the active systems consolidation theory emphasizes the role of SWS ( Klinzing et al., 2019 ); the theory of Lewis et al. (2018) emphasizes REM (at least for learning involving creative thought); and the sequential theory of Giuditta (2014) proposes that an interaction between SWS and REM is key. However, all current theories make the common prediction that sleep should benefit memory more than wake. We do not attempt to adjudicate between the competing theories and therefore restrict our analysis to studies using total sleep deprivation where a clear prediction is made by all theories.
Where possible we use moderators to establish whether the effect size is modulated by variables that have been hypothesized to be important. For example, it is not clear whether sleep deprivation after learning impacts declarative and procedural memories similarly. If the neural and cognitive mechanisms associated with the consolidation of declarative and procedural memories differ, one may observe different effect sizes in studies targeting the two memory types. To test this hypothesis, we entered declarative versus procedural memory type as a moderator in our meta-analysis.
Some studies have tested the effects of sleep deprivation after one or more nights of recovery sleep while others have tested immediately after a night of sleep deprivation with no intervening recovery sleep. The primary reason for allowing recovery sleep before testing is that sleep deprivation has well-established impacts on attention (e.g., Lim & Dinges, 2008 ; Vargas et al., 2017 ) that may compromise performance in an immediate memory test and make it difficult to distinguish between effects of sleep deprivation due to fatigue and effects due to disruption to memory consolidation processes. Using recovery sleep to rule out potential effects of fatigue at test assumes that the first night of sleep following learning is of critical importance in memory consolidation and that consolidation may not occur in subsequent sleep periods or has a weaker impact after the first missed sleep opportunity. Although we are not aware of any studies that have explicitly tested this assumption, for example, by systematically manipulating the number of nights of sleep following learning, some support has been derived from studies such as that of Gais et al. (2007) who observed effects of one night of sleep deprivation after two recovery nights and even 6 months later.
Recent studies have cast doubt on the privileged status of the first night of sleep, however. Schönauer et al. (2015) found only a short-term cost of sleep deprivation after learning for hippocampal-dependent memories, with sleep deprivation after learning impairing retrieval of word pairs after one night of deprivation, but no difference in retrieval between the sleep and sleep deprivation conditions after two nights of recovery sleep. They suggested that for such hippocampal-dependent memories, the hippocampus may act as a temporary buffer, storing memories until the first sleep opportunity, even if that opportunity is delayed. Thus, sleep deprivation after learning would only have a detrimental effect on memory performance if there is no sleep opportunity before testing. In contrast, they found that procedural memories that do not rely on the hippocampus suffered a more long-term effect of sleep deprivation, supporting previous evidence that the first night of sleep is crucial for improving performance on procedural tasks ( Stickgold et al., 2000 ). Therefore, for procedural memories, the loss of the first night of sleep may be critical. Given the mixed evidence in the literature on the impact of recovery sleep, we entered the presence or absence of recovery sleep as a moderator in the meta-analysis. If the first night of sleep after learning is of critical importance, on the one hand, we should see a sleep deprivation impairment both in studies that test immediately after deprivation and in studies that test after recovery sleep. If, on the other hand, consolidation processes can be delayed, we should see a sleep deprivation impairment only in studies that test immediately (i.e., they provide no opportunity for delayed consolidation). The latter scenario is also consistent with the possibility that the sleep deprivation impairment is due entirely to fatigue at test (although see Schönauer et al., 2015 , for data suggesting fatigue does not impair memory recall).
Some studies of recognition memory have failed to find a beneficial effect of sleep on memory performance leading to a debate about whether sleep has no or only limited impact on recognition memory (e.g., Drosopoulos et al., 2005 ; Hu et al., 2006 ; Morgan et al., 2019 ; Rauchs et al., 2004 ; Stepan et al., 2017 ). The difference between recall and recognition tasks is particularly stark in the literature using the Deese–Roediger–McDermott (DRM) paradigm of false memory formation. Here, studies using recognition tasks have reported that sleep may reduce false memories ( Fenn et al., 2009 ; Lo et al., 2014 ), whereas studies using recall tasks have reported that sleep may increase false memories ( Diekelmann et al., 2010 ; McKeon et al., 2012 ; Pardilla-Delgado & Payne, 2017 ; Payne et al., 2009 ). Consequently, a recent meta-analysis of sleep studies using the DRM paradigm concluded that the impact of sleep on false memory is restricted to recall tasks ( Newbury & Monaghan, 2019 ). This meta-analysis also found that the sleep benefit for studied words (i.e., veridical memory) was dramatically larger in recall tasks than in recognition tasks ( g = 0.407 vs. g = 0.005). The discrepancy between recall and recognition tasks might be explained by dual-process accounts, which suggest that recognition memory has both an explicit recollection element as well as an implicit familiarity element (e.g., Jacoby, 1991 ; Yonelinas, 2002 ), and these two elements may rely on different neural structures, with only recollection depending on the hippocampus. Support for this account has come from findings that sleep only facilitates recognition memory based on recollection, not familiarity (e.g., Drosopoulos et al., 2005 ). On the other hand, studies that have directly compared memory for emotionally negative and neutral stimuli appear to suggest that sleep benefits recognition memory for emotional stimuli but not for neutral stimuli ( Alger et al., 2018 ; Hu et al., 2006 ; Payne et al., 2008 , 2015 ; see Kim & Payne, 2020 for a review) suggesting that sleep’s impact on recognition memory may be modulated by emotionality. Given the inconsistency in the existing literature on the extent to which sleep after learning benefits recognition memory tasks, it is important to quantify and compare the size of the sleep benefit across recognition and recall tasks. Thus, we entered memory type as a moderator in our meta-analysis.
Sleep has previously been found to improve emotional episodic memories more than neutral memories, with some studies suggesting a specific role of REM sleep in the consolidation of emotional memories (e.g., Groch et al., 2015 ; Payne & Kensinger, 2010 ; Wagner et al., 2001 ; see Kim & Payne, 2020 for a review). Two recent meta-analyses investigated the preferential role of sleep in emotional memory consolidation. Schäfer et al. (2020) found that sleep improved both emotional and neutral memory equally, with no evidence for preferential impact on emotional memory; in fact, the difference between emotional and neutral memory was larger after wake than sleep. However, Schäfer et al. (2020) did find that when their analysis was restricted to experiments that contrasted SWS and REM sleep, sleep that consisted primarily of REM did show a preferential consolidation effect for emotional memory, although the number of studies included in this analysis was small. Lipinska et al. (2019) also found no meta-analytic evidence in favor of sleep’s preferential impact on emotional memory. However, they did find that the preferential effect was larger in recall tasks compared to recognition tasks and in studies that controlled for initial learning. In the current meta-analysis, we shed new light on these issues by focussing on sleep deprivation manipulations. To investigate whether the effect of sleep deprivation on memory performance is modulated by emotionality of the to-be-remembered stimuli, we entered emotionality as a moderator in our meta-analysis.
Impact of Total Sleep Deprivation Before Learning and Potential Moderators of the Effect
Recent research has also proposed a role for sleep before learning. According to the synaptic homeostasis hypothesis ( Cirelli & Tononi, in press ; Tononi & Cirelli, 2003 , 2012 ), learning occurs during wake when a neuron detects a statistical regularity in its input and begins to fire in response to this regular input. In other words, successful learning requires neurons to be able to fire selectively in response to statistically regular patterns observed in the environment. To do so, strength of the synapses carrying these inputs must be increased. However, the neuron now faces the plasticity-selectivity dilemma. As an increasing number of input lines become strengthened, a larger range of input patterns can make the neuron fire, reducing the neuron’s ability to fire selectively. This loss of selectivity corresponds to reduced ability to encode new information. During sleep, the brain spontaneously activates both new information encoded during previous wake and information encoded in the past. Over the course of this activation, those synapses that are activated most strongly and consistently during wake survive, while at the same time, those synapses that were less activated are weakened. This weakening occurs primarily during the transitions between intracellular up and down states experienced during SWS. This competitive down-selection of weaker synapses restores memory encoding ability.
The restorative function of sleep is supported by evidence showing decreased episodic learning ability across a 6-hr retention interval in which participants remained awake, whereas encoding capacity was restored after a daytime nap ( Mander et al., 2011 ). Further, neuroimaging evidence suggests sleep deprivation prior to learning is associated with disrupted encoding-related functional activity in the bilateral posterior hippocampus ( Yoo et al., 2007 ; for similar findings, see Alberca-Reina et al., 2015 ; Drummond & Brown, 2001 ; Van Der Werf et al., 2009 ). Thus, sleep deprivation before learning may be detrimental specifically for the encoding of hippocampal-dependent declarative memories. Our second meta-analysis seeks to estimate the effect size associated with this impairment. As only two studies have looked at the impact of sleep deprivation on procedural learning when it occurs after deprivation, we were not able to assess the potential moderating effect of declarative versus procedural memory. We were also not able to use emotionality as a moderator here due to the low number of relevant studies. Yet, there are several studies that have used recall tasks and recognition memory tasks, so we were able to evaluate the moderating effects of recall versus recognition, as in the first meta-analysis.
The Present Meta-Analyses
Despite the breadth of evidence for an effect of total sleep deprivation both before and after learning on memory performance, there is no comprehensive review and analysis of the strength of the effect of sleep deprivation on long-term memory. Previous reviews and meta-analyses investigating a role of sleep deprivation have focused on tasks that are likely more susceptible to fatigue. Pilcher and Huffcutt (1996) conducted a meta-analytic review of the effect of sleep deprivation on cognitive and motor task performance in 19 primary studies and found that sleep deprivation had a significant impact on performance. Still, this meta-analysis does not address long-term memory performance. Similarly, Lim and Dinges (2010) found an effect of sleep deprivation on a range of cognitive tasks including attention, working memory, and short-term memory, though the size of the effect varied depending on the task (e.g., a nonsignificant effect on reasoning accuracy, but a large effect on attention). Finally, Harrison and Horne (2000b) in a review found that sleep deprivation impacted decision-making ability. The tasks studied in these reviews are often repetitive and monotonous (e.g., the Psychomotor Vigilance Task; Dinges & Powell, 1985 , the go/no go paradigm, and tests of serial addition), and they tend to probe lower-level functions that are particularly susceptible to fatigue, such as reaction times and processing speed. Therefore, the conditions of these studies are arguably better suited to finding adverse effects of sleep deprivation on performance than studies looking at higher-level learning and long-term memory. Thus, a review of the effects of sleep deprivation on such high-level, long-term memory is required.
Taking a meta-analytic approach will permit not only a quantitative assessment of the size of the main effect of sleep deprivation and its moderators but also an investigation of methodological quality within this literature including the statistical power of studies proposing to find a sleep deprivation effect. Variety in sample selection and methodological designs used within this literature raises the possibility of variations in methodological quality. Such variations could lead to biases in the meta-analysis by overestimating or underestimating the effect size ( Higgins et al., 2011 ). Thus, we developed a checklist to assess multiple aspects of methodological quality, including questions specifically relevant to the assessment of sleep effects (e.g., excluding participants with sleep disorders), questions on study design (e.g., within-subject vs. between-subjects design, and random allocation to conditions), and questions on data analysis (e.g., preregistration and a priori power analyses). We used similar questions to other meta-analyses in the sleep literature ( Lim & Dinges, 2010 ; Lo, Groeger, et al., 2016 ; Schäfer et al., 2020 ) and examined methodological quality as a continuous moderator in the analysis. The full methodological quality checklist is provided in Supplemental Appendix A .
It has been suggested that psychological science more broadly is currently suffering from a replication crisis due to low power, publication bias, selection biases, and analysis errors ( Nosek et al., 2015 ). Low power limits potential to detect genuine effects but also results in Type I errors and exaggerated effect sizes ( Ioannidis, 2005 ; Pollard & Richardson, 1987 ). Szucs and Ioannidis (2017) conducted an analysis of almost 4,000 cognitive neuroscience and psychology papers and found that the overall mean power to detect small, medium, and large effects was 17%, 49%, and 71%, respectively, with even lower power in the subfield of cognitive neuroscience. Given the convention that power to detect an effect size should be at least 80% ( Di Stefano, 2003 ), it is clear that a large number of studies within psychology are underpowered (see Button et al., 2013 ; for further evidence of low statistical power within neuroscience). In the sleep literature, sample sizes tend to be low, potentially due to the resource intensity of conducting these experiments. Thus, we investigated whether the low power seen more broadly in psychological science and neuroscience is also evident in the sleep deprivation literature. For each individual effect size entered into the meta-analysis, we calculated the study’s power (defined as power to detect our meta-analytic effect size) and investigated whether there is an association between a study’s power and the effect size observed in the study.
Search Strategy
For study selection, we generated the Boolean search term “Sleep AND (deprivation OR restriction OR loss) AND (learning OR memory OR conditioning)” and conducted a search in the electronic databases EBSCOhost (included PsycARTICLES, PsycEXTRA, PsycINFO, and PsycTESTS) and PubMED on July 29th, 2020. This search yielded 2,213 empirical articles published between January 01, 1970 and July 29, 2020 in peer-reviewed journals in English using human participants.
In line with best practice guidelines ( Rothstein et al., 2005 ; Siddaway et al., 2019 ), we ran several searches on July 13th, 2020, using the same search terms as above, to identify gray literature in an attempt to mitigate against publication bias. These searches yielded a total of 553 items. Specifically, we widened our search criteria in EBSCOhost and PubMED to include unpublished dissertations and theses, conference materials, and preprints; we searched the bioRxiv and PsyArXiv repositories for preprints; and we searched the ProQuest and OpenGrey (a European database in which national libraries submit unpublished studies) databases for unpublished dissertations and theses, conference materials, and for research grants and fellowship awards. Additionally, we contacted all authors who had published data included in our initial screening results to ask for unpublished data that fit our inclusion criteria, and this yielded one preprint article. We also had one in-press article during the search period that fits our inclusion criteria ( Tamminen et al., 2020 ) and was therefore included in our search results. In sum, we identified both published and unpublished data with search strategies spanning (a) peer-reviewed published articles, (b) in-press articles, (c) preprints uploaded to repositories, (d) unpublished dissertations and theses, (e) conference materials, and (f) research grants and fellowship awards.
We scanned the abstracts and full texts of all articles according to our inclusion and exclusion criteria, and separated them into articles that investigated the effect of sleep deprivation after learning, and those that investigated sleep deprivation before learning. Figure 1 displays a screening process flowchart showing that after exclusions were removed, 130 effect sizes (extracted from 45 reports) were included in the sleep deprivation after learning meta-analysis and 55 effect sizes (extracted from 31 reports) were included in the sleep deprivation before learning meta-analysis. The number of effect sizes included in each meta-analysis is greater than the number of full-text articles that fit our inclusion criteria. The reason for this is that several studies measured performance differences between a sleep deprivation and sleep control group using multiple tasks, multiple conditions (e.g., stimulus valence or procedural instructions), and across multiple time points. Effect sizes were calculated for each of these data sets within an article because each variation represents a different, yet correlated, measurement of the impact of sleep deprivation on memory. However, there were some studies that used multiple outcome measures to assess performance in a single task (e.g., accuracy and reaction time). Given that multiple outcome measures within the same task are different ways of assessing the same manipulation, we chose only one outcome measure for calculating an effect size in these instances, according to the following hierarchy from most to least preferred outcome measure: accuracy as measured by retention performance (i.e., performance change from training to test), accuracy at test only, reaction time measured by retention performance (i.e., performance change from training to test), and reaction time at test only. Further, in recognition tasks, if both signal detection analyses and analyses based on proportion correct were reported, we chose to include the signal detection measure only. For example, if a study reported both d -prime and reaction times in a recognition memory task (e.g., Tamminen et al., 2020 ), we only used the d -prime data. data.

A list of studies included in the two meta-analyses and their key properties can be found in Supplemental Appendix B (studies investigating sleep deprivation after learning) and Supplemental Appendix C (studies investigating sleep deprivation before learning), as well as at osf.io/5gjvs/ .
Inclusion/Exclusion Criteria
To select relevant studies, we applied the following inclusion/exclusion criteria.
- a Participants had to be healthy adults aged 18 years and older.
- b Studies must have included, as a primary independent variable, a manipulation of sleep deprivation that was a minimum of one night of total sleep deprivation with an appropriate sleep control condition consisting of one normal night of sleep. Residency studies (studies conducted in a medical setting) were excluded due to the lack of control over whether total sleep deprivation occurred (sleep deprivation is often reported despite naps having occurred on shift; e.g., Bartel et al., 2004 ; Guyette et al., 2013 ). Additionally, studies using sleep restriction protocols, which involve multiple nights of limited sleep duration rather than one or more nights of no sleep, were excluded because the neural and cognitive effects of sleep restriction may differ from those caused by total sleep deprivation ( Banks & Dinges, 2007 ; Lowe et al., 2017 ).
- c Studies must have included, as a primary dependent variable, at least one measure of learning or long-term memory where the task was described in sufficient detail to ascertain which cognitive function it assessed.
- d For the meta-analysis investigating sleep deprivation after learning, the cognitive task must have had a single encoding phase and a retrieval phase(s) that were temporally separated by either a period of sleep deprivation or an equivalent period of sleep. For the meta-analysis investigating sleep deprivation before learning, the single encoding phase and the retrieval phase(s) must have been temporally separated by a retention interval that had a minimum duration of at least 1 min, rather than being part of the same task session. The reason for this criterion is that our meta-analyses aimed to investigate effects of sleep deprivation on learning and long-term memory. The inclusion of studies with temporally indistinct encoding and retrieval phases would have included short-term and working memory tasks that form a separate body of literature ( Lim & Dinges, 2010 ), the analysis of which was beyond the scope of these meta-analyses.
- e In cases in which studies assessed the effects of other interventions (e.g., caffeine; Kilpeläinen et al., 2010 ) in ameliorating sleep deprivation effects, studies were included only if data could be obtained from the control sleep deprivation and control sleep groups. This criterion was included because the goal of the current meta-analyses was to assess effects of sleep deprivation in the absence of alertness-promoting strategies.
- f Studies must have reported sufficient statistical detail to calculate effect sizes (means, SD , F , and t ). When statistical details were not reported in the text, we either contacted corresponding authors to request relevant data or we extracted the data needed from published figures in the article using WebPlotDigitizer ( Rohatgi, 2019 ).
Methodological Quality
Through our survey of the literature, it became clear that sleep deprivation studies differ considerably in various aspects of methodological rigor (e.g., lack of control over adherence to sleep manipulations in the sleep deprivation and sleep control groups; Fischer et al., 2002 vs. complete control; Chatburn et al., 2017 ). For this reason, we assessed the methodological quality of each study entered into our meta-analyses and included this in our moderator analyses.
To assess methodological quality, we developed a 22-item checklist based on criteria for standard sleep deprivation experiments (e.g., preexperimental sleep monitored using actigraphy and exclusion of sleep disorders) and more general experimental psychology experiments (e.g., a priori power analysis and study design). For each item on the checklist, studies were scored with either a zero or a one according to whether they satisfied the criterion. To transform the total methodological quality score for each study into a risk of bias that reflects a rank of all the studies on a common scale, we normalized the total scores by dividing each study’s total methodological quality score by the maximum total methodological quality score that was achieved among all studies ( Stone et al., 2020 ). Lower values imply lower ranked studies (minimum score of 0) and higher values imply higher ranked studies (maximum score of 1) relative to the best study. The full methodological quality checklist can be found in Supplemental Appendix A .
Given that the checklist items form a multidimensional scale, the items were clustered according to the Downs and Black’s (1998) instrument for assessing methodological quality, which assesses five types of bias: “reporting,” “internal validity—bias,” “internal validity—confounding,” “power,” and “external validity.” The “reporting” cluster determines whether sufficient information is provided to make an unbiased assessment of study findings. In our methodological quality checklist, the items in this cluster referred to the reporting of exclusion criteria for participant characteristics (e.g., “Did the study exclude participants with a history of sleep disorders?”). The “internal validity—bias” cluster assesses whether biases were present in the intervention or outcome measure that would favor one experimental group [e.g., “Was interference for the sleep deprivation group low (nondemanding activities given and monitored in the lab)?”]. The “internal validity—confounding” cluster assesses whether biases were present in the selection and allocation of participants (e.g., “For within-group studies, was the order of deprivation and control conditions counterbalanced?”). The “power” cluster assesses whether a study used a priori power analyses to avoid Type II errors (e.g., “Did the study report an a priori power analysis with power set at 80% or higher and an α at .05 or lower?”). The Downs and Black’s (1998) “external validity” cluster determines the extent to which findings can be generalized to the population from which a sample was taken (e.g., “Were the staff, places, and facilities where the patients were treated, representative of the treatment the majority of patients receive?”; Downs & Black, 1998 , p. 383). Since the items in this cluster were designed for clinical intervention studies with nontypical populations, we dropped the external validity cluster from our checklist. In line with Cochrane Collaboration recommendations ( Higgins et al., 2011 ), the four clusters in our methodological quality checklist (hereon referred to as reporting, bias, confounding, and power) were then included in moderator analyses. The percentage of studies that passed on each item of the quality checklist for both Meta-Analysis 1 and Meta-Analysis 2 can be found in Supplemental Appendix D . Total methodological quality scores for each study, as well as the item-level ratings, can be found at osf.io/5gjvs/ .
Effect Size Calculation
Information on study means, standard deviation, and effect sizes for each item, as well as formulas used to calculate effect sizes, can be found at osf.io/5gjvs/ . We report the standardized mean difference in task performance between a sleep deprivation and sleep control group, with positive values indicating that sleep deprivation influenced learning and memory such that performance was significantly worsened compared to a sleep control group. For studies with independent samples (between-subjects designs), we computed Cohen’s d s based on the means and variance reported in each study for the sleep and sleep deprivation group. For within-subject designs, in which participants took part in both the sleep deprivation and sleep control conditions, we calculated Cohen’s d av , as recommended by Lakens (2013) .
Data Analysis
Overall meta-analytic effect size.
All analysis code can be found at osf.io/5gjvs/ . To analyze whether there was an overall meta-analytic effect of sleep deprivation versus overnight sleep on memory performance, we fitted a multilevel random-effects model using the R package metafor ( Viechtbauer, 2010 ). A random-effects model allows for inconsistencies between effect sizes from varying study designs, assuming systematic variability between effect sizes in addition to random sampling error. A random-effects model therefore provides more conservative effect size estimates than a fixed-effect model ( Borenstein et al., 2010 ). A multilevel model allows for the inclusion of both within-study effect sizes and between-study effect sizes ( Assink & Wibbelink, 2016 ). Many experiments included in the meta-analysis report multiple dependent effect sizes, such as results from multiple test sessions, multiple within-group experimental conditions (e.g., performance on emotional vs. neutral stimuli), or multiple outcomes (e.g., a procedural and declarative memory task). Including multiple dependent effect sizes from the same experiment violates the assumption of data independence assumed in a typical random-effects model. A multilevel meta-analysis accounts for such dependencies by modeling both within-study and between-study effects. Thus, we were able to model variance accounted for by (a) random error, (b) within-study differences among effect sizes within the same experiment, and (c) between-study differences across different experiments.
Heterogeneity
To investigate whether moderating variables may influence the size of the effect of sleep deprivation, we examined heterogeneity within the data set using the Q test ( Cochran, 1954 ). The Q test indicates whether there is heterogeneity within the data set and is calculated by the weighted sum of the squared deviations of individual study effect estimates and the overall effect across studies. Significant heterogeneity suggests that some of the variance within the data set may not be due to random sampling error, and thus moderating variables may influence the effect. Since we were interested in both the within-study and between-study variance, we ran two separate one-sided log-likelihood-ratio tests. As such, the fit of the overall multilevel model was compared to the fit of a model with only within-study variance and to a model with only between-study variance. This allowed us to determine whether it was necessary to account for both within- and between-study variances within our model.
Assink and Wibbelink (2016) suggest that such log-likelihood ratio tests may be subject to the issues of statistical power when the data set comprises a small number of effect sizes. Low statistical power may lead to nonsignificant effects of heterogeneity when in fact there is variance within or between studies. To account for this, it is recommended to also calculate the I 2 statistic, which indicates the percentage of variation across studies that is due to heterogeneity and that which is due to random sampling error ( Higgins & Thompson, 2002 ). Hunter and Schmidt (2004) suggest the 75% rule, such that if less than 75% of overall variance is attributed to sampling error, then moderating variables on the overall effect size should still be examined. Using the formula of Cheung (2014) , we calculated the percentage of variance that can be attributed to each level of our model.
However, although I 2 reports the proportion of variation in observed effect sizes, it does not provide us with absolute values that tell us the variance in true effects ( Borenstein et al., 2017 ). Thus, as recommended by Borenstein et al. (2011) , we report the τ 2 , which provide an estimate of the true effect size, and we report prediction intervals, which indicate that 95% of the time, effect sizes will fall within the range of those prediction intervals.
Publication Bias
To assess publication bias, we first examined a contour enhanced funnel plot. Funnel plots show each effect size plotted against its standard error, with contour lines corresponding to different levels of statistical significance. If studies are missing almost exclusively from the white area of nonsignificance, then there may be publication bias. If studies are missing from areas of statistical significance, the bias is likely due to other causes such as poor methodological quality, true heterogeneity, chance, or the bias may be artifactual ( Johnson, 2021 ; Sterne et al., 2011 ). We also conducted a variation of Egger’s regression test for funnel plot asymmetry ( Egger et al., 1997 ) that can be conducted with multilevel meta-analyses.
Meta-Analysis 1: Sleep Deprivation After Learning
This meta-analysis summarizes research from 45 reports investigating effects of sleep deprivation after learning (130 effect sizes) published in English between 1994 and 2020 across a total of 1,616 participants. All reports used healthy adult populations and deprived participants of one night of sleep postlearning. Notably, two reports from this meta-analysis also report data that are relevant to the sleep deprivation before learning meta-analysis ( Fischer et al., 2002 ; Tamminen et al., 2020 ). See Table 1 for central tendencies and frequency data for moderator and descriptive variables of studies included. The table shows that this literature is heavily biased toward young adults, severely limiting conclusions that can be drawn about older age groups. The literature predominantly uses between-groups designs rather than the statistically more powerful within-group designs, partly explaining and exacerbating the low power highlighted later in our analysis. Recognition memory and recall memory tasks are the most often employed measures of memory, and most of the literature probes declarative memory rather than procedural memory. We return to these memory type distinctions in our moderator analyses. Most studies used human observation to ensure participants in the sleep deprivation condition did not sleep during the night, but few ensured that they did not sleep during the day to the same standard. Low compliance during the day could possibly dilute the effect size. Finally, most but not all studies allowed recovery sleep after deprivation. We return to this in the moderator analyses. analyses.
The overall effect size for the mean difference in memory performance between the sleep deprivation and sleep control group, measured by Hedges’ g , was 0.277 ( SE = 0.050), indicating a small-to-medium effect according to Cohen’s categorization, and a significant difference from zero, 95% CI [0.177, 0.377], p < .001. Figure 2 displays a forest plot of the effect sizes. See Supplemental Appendix B for a summary of all studies included in the meta-analysis. meta-analysis.

Note . Effect sizes to the right indicate an effect of sleep deprivation after learning such that memory was significantly worse than in a sleep control group.
Some of the variance within the data set could not be explained by random error, highlighted by overall significant heterogeneity, Q (129) = 244.891, p < .001. An analysis of heterogeneity of between-study variance (Level 2) revealed a significant difference between a full and a reduced model ( p < .001), suggesting significant variability between studies. An analysis of heterogeneity of within-study variance (Level 3) also revealed a significant difference between a full model and a reduced model ( p < .001), suggesting significant variability between within-study effect sizes. We further calculated the I 2 statistic, which indicates the percentage of variance that could be attributed to each level of the model. Using the formula from Cheung (2014) , approximately 52% of variance can be attributed to sampling error, 14% to within-study variance, and 34% to between-study variance. Next, we calculated τ 2 , which provides a measure of the variance of the true effects; τ 2 = .026 for within-study variance, and τ 2 = .061 for between-study variance. Prediction intervals indicated that 95% of effect sizes would fall in the range of −0.316 and 0.870.
Based on the significant heterogeneity between studies, the large prediction intervals, as well as the 75% rule, such that moderators should be explored if less than 75% of the variance can be attributed to random sampling error ( Hunter & Schmidt, 2004 ), we therefore explored the effect of potential moderating variables on the direction of the effect.
Figure 3 shows a funnel plot of the effect sizes. Visual inspection of the funnel plot indicates that effect sizes are not evenly distributed across the funnel plot, raising the potential for publication bias in which studies reporting a positive effect are more likely to be published. Egger’s regression test reveals significant funnel plot asymmetry ( z = 2.297, p = .022), supporting this assessment of the funnel plot. Further visual inspection of the funnel plot reveals two potential outlier effect sizes in the area of high statistical significance; these potential outliers are characterized by large effect sizes and large standard error (therefore smaller sample sizes). These large effect sizes on the right-hand side of the plot suggest that there may be a bias in this literature toward publishing significant effects, regardless of the precision with which the study effect size can be estimated. However, the presence of multiple studies in the area of nonsignificance suggests other biases may also contribute to the asymmetry. asymmetry.

Because Egger’s test indicates the presence of publication bias, we sought to quantify the impact of this bias on the estimated effect size. We conducted a trim-and-fill analysis ( Duval & Tweedie, 2000 ), which calculates potential missing effect sizes to create a symmetric funnel plot and then provides an adjusted overall meta-analytic effect size based on this funnel plot symmetry. Although this is a well-used method to assess publication bias, it assumes that effect sizes are independent of each other. The current meta-analysis uses a multilevel approach, with dependencies between some effect sizes. The adjusted effect size should therefore be considered a preliminary estimate. The trim-and-fill method estimated 12 missing studies from the left-hand side of the funnel plot. With these effect sizes included, the adjusted overall meta-analytic effect size was smaller than the original effect size of g = 0.277, although it was still significantly greater than zero, Hedges’ g = 0.166, SE = 0.041, 95% CI [0.086, 0.247], p < .001.
Outlier Analysis
We explored whether outliers and influential cases may have significantly influenced the meta-analytic effect size. To identify the presence of outliers, we identified any effect sizes with studentized residuals greater than or smaller than three, which identified one effect size as an outlier ( Albouy, Sterpenich, et al., 2013 ). However, an outlier may not necessarily influence the size of the overall effect ( Viechtbauer & Cheung, 2010 ). Therefore, based on suggestions by Viechtbauer and Cheung, we conducted influential case analyses, to identify any effect sizes that exerted a significant influence on the size of the overall meta-analytic effect. We measured Cook’s distance to examine the influence of deleting each study on the overall size of the effect, and DFBETAs to examine the effect of deleting each study on individual parameter estimates. Cook’s analysis identified a further one effect size that was found to be an influential case ( Darsaud et al., 2011 , Know judgements). Removal of the outlier and influential case reduced the overall meta-analytic effect size to 0.271 (from 0.277). Since moderator analyses examine smaller subsets of effect sizes, we removed these two specific effect sizes from all moderator analyses conducted.
Moderator Analysis
We introduced four categorical moderating variables and analyzed the effect of each moderator separately on the size of the effect of sleep deprivation on learning and memory: (a) whether it was a declarative ( k = 108) or procedural ( k = 20) memory task, (b) for declarative tasks, whether task type was recall ( k = 42) or recognition ( k = 59), (c) whether participants received one or more recovery nights of sleep ( k = 83) or no recovery sleep ( k = 45), and (d) for those studies that investigated emotionality, whether the stimuli were emotional ( k = 31) or neutral ( k = 20). Supplemental Appendix B shows the classification of each study on these dimensions.
Whether participants received a recovery night of sleep before the test session as a moderator had a significant effect, Q (1) = 10.496, p < .001. Thus, we ran separate effect size analyses for those studies where participants had a night of recovery sleep and those that did not. For those studies that had one or more nights of recovery sleep, there was a small effect of sleep deprivation on learning and memory, Hedges’ g = 0.176 ( SE = 0.058), which is significantly different from zero, 95% CI [0.060, 0.292], p = .003; Q (82) = 133.766, p < .001. For those studies that did not have a night of recovery sleep, the effect size was larger, Hedges’ g = 0.410, SE = 0.044, 95% CI [0.320, 0.499], p < .001; Q (44) = 50.042, p = .246.
Whether the task probed declarative or procedural memory also had a significant moderating effect, Q (1) = 5.301, p = .021. We therefore ran separate effect size analyses for those studies that implemented a declarative memory task and those that implemented a procedural memory task. For those studies with a declarative memory task, there was a small effect of sleep deprivation on learning and memory, Hedges’ g = 0.218 ( SE = 0.055), which is significantly different from zero, 95% CI [0.109, 0.327], p < .001; Q (107) = 174.802, p < .001. For those studies with a procedural memory task, the effect size was larger, Hedges’ g = 0.449, SE = 0.083, 95% CI [0.276, 0.623], p < .001; Q (19) = 24.794, p = .167.
Since we found a significant moderating effect of both recovery sleep and task type (declarative or procedural), we ran a further analysis to investigate whether there was an interaction between the two significant moderators. The analysis revealed no significant interaction between recovery sleep and memory type, Q (1) = 0.804, p = .370, suggesting that whether participants received recovery sleep or not affected declarative and procedural memory task performance in a similar way. Whether studies used a recall or recognition task did not have a significant effect on the size of the effect of sleep deprivation on learning and memory. Studies using a recall task had a mean effect size of Hedges’ g = 0.209, SE = 0.082, 95% CI [0.045, 0.374], p = .014; Q (41) = 76.317, p < .001, and studies with a recognition task had an overall effect size of Hedges’ g = 0.175, SE = 0.077, 95% CI [0.021, 0.330], p = .027; Q (58) = 91.950, p = .003. Effect sizes associated with emotional and neutral stimuli were also not significantly different, with an overall effect size of Hedges’ g = 0.251, SE = 0.080, 95% CI [0.084, 0.417], p = .005; Q (19) = 25.789, p = .136, for studies using neutral stimuli, and an overall effect size of Hedges’ g = 0.207, SE = 0.085, 95% CI [0.033, 0.380], p = .021; Q (30) = 41.888, p = .073, for emotional stimuli.
We then introduced four continuous moderating variables and analyzed the effect of each moderator separately on the size of the effect of sleep deprivation on learning and memory: (a) methodological quality reporting cluster, (b) methodological quality bias cluster, (c) methodological quality confounding cluster, and (d) statistical power to find the mean effect size established in the meta-analysis ( g = 0.277). Since methodological quality was divided into clusters in our methodological quality checklist, we introduced these clusters (reporting, bias, and confounding) as moderators. We did not include the power cluster as a moderator, since the majority of studies did not calculate power and thus scored zero on this cluster, with only one study (contributing eight effect sizes) providing a power analysis. For each of the methodological quality clusters, we created a meta-analytic scatter plot using the metafor package ( Viechtbauer, 2010 ), showing the Hedges’ g of each individual study plotted against each moderator (see Figure 4 ). The figure shows that an effect size of zero falls within the 95% confidence interval in the reporting cluster for studies scoring 0.8 or higher, suggesting that these studies show no effect of sleep deprivation while the lower scoring studies do. Consistent with this observation, the reporting cluster was a significant moderator, Q (1) = 9.214, p = .002. Visual inspection of the plot for the confounding cluster suggests that studies with scores of 0.3 or lower on this cluster may not show an effect of sleep deprivation. However, this cluster did not show a statistically significant moderating effect, perhaps because there were no studies that scored below 0.3 on this cluster. Bias did not show a significant moderating effect either. either.

Note . The size of each point is proportional to the weight the study received in the analysis, with larger size indicating larger weight. The solid regression lines represent the effect size predicted by the meta-regression model as a function of each cluster score, with corresponding 95% confidence intervals.
To assess the achieved statistical power of each individual experiment to detect the mean meta-analytic effect size, we conducted a post hoc power analysis in G*Power ( Faul et al., 2007 ). For each study, we calculated the power to detect the mean meta-analytic effect size ( g = 0.277), as well as the power to detect the 95% upper and lower confidence intervals of the effect size. The distribution of the mean power and lower and upper confidence interval bounds of the power estimate are plotted in Figure 5 . We found the mean power to find the average meta-analytic effect to be 13.98% ( SD = 4.60%, range = 7.2%–30.2%). The moderator analysis revealed no significant effect of power on the size of the meta-analytic effect. All moderator analyses are reported in Table 2 . .

Note . See the online article for the color version of this figure.
Meta-Analysis 2: Sleep Deprivation Before Learning
This meta-analysis summarizes research from 31 reports investigating effects of sleep deprivation before learning (55 effect sizes) published in English between 1989 and 2020 across a total of 927 participants. All reports used healthy adult populations and deprived participants of one night of sleep prior to learning. Notably, two reports from this meta-analysis also include data that are relevant to the sleep deprivation after learning meta-analysis ( Fischer et al., 2002 ; Tamminen et al., 2020 ). See Table 3 for central tendencies and frequency data for moderator and descriptive variables of reports included. Again, the literature mostly involves young adults, leaving a gap in our understanding of how the effects of interest change with age. The discrepancy between use of between- and within-group designs is lower here than in the first meta-analysis, and recognition memory and recall memory tasks are roughly equally represented. However, nearly all studies look at declarative memory, suggesting that more work on procedural memory is needed. While most studies ensured compliance with the sleep deprivation manipulation with direct observation at night, few did so during the preceding day, potentially diluting the impact of sleep deprivation. deprivation.
Overall Sleep Deprivation Effect Size
The overall effect size for the mean difference in memory performance between the sleep deprivation and sleep control group, measured by Hedges’ g , was 0.621 ( SE = 0.074), indicating medium to large effect according to Cohen’s categorization, and a significant difference from zero, 95% CI [0.473, 0.769], p < .001. Figure 6 provides a forest plot of the effect sizes. See Supplemental Appendix C for a summary of all studies included in the meta-analysis. meta-analysis.

Note . Effect sizes to the right indicate an effect of sleep deprivation before learning such that memory was significantly worsened compared to a sleep control group.
Some of the variance within the data set could not be explained by random error, highlighted by overall significant heterogeneity, Q (54) = 118.166, p < .001. An analysis of heterogeneity of between-study variance (Level 2) also revealed a significant difference between a full and a reduced model ( p < .001), suggesting significant variability between studies. An analysis of heterogeneity of within-study variance (Level 3) revealed a significant difference between a full model and a reduced model ( p < .001), suggesting significant variability between within-study effect sizes. The I 2 statistic indicates that approximately 41% of variance can be attributed to sampling error, 9% to within-study variance, and 50% to between-study variance. τ 2 = .096 for between-study variance, and τ 2 = .017 for within-study variance, and prediction intervals indicated that 95% of effect sizes would fall in the range of −0.069 and 1.312. Based on this evidence for heterogeneity, we explored the effect of potential moderating variables on the direction of the effect.
Figure 7 shows a contour enhanced funnel plot of effect sizes. Similar to Meta-Analysis 1, visual inspection of the funnel plot indicates that effect sizes are not evenly distributed across the funnel plot, raising the potential for publication bias. Egger’s regression test supports this conclusion, indicating significant funnel plot asymmetry ( z = 3.363, p < .001). Further inspection of the funnel plot indicates that the majority of effect sizes are clustered toward the right side of the funnel plot. While many of these studies fall in the area of nonsignificance, there appear to be studies missing from the left-hand side of the plot. It is possible these missing studies are due to researchers being unable to publish findings that contradict their hypotheses, and that the bias indicated by Egger’s test may therefore be due to publication bias rather than other types of bias. bias.

Because Egger’s test indicates the presence of publication bias, we conducted a trim-and-fill analysis ( Duval & Tweedie, 2000 ), in the same way as in the first meta-analysis. The trim-and-fill method estimated seven missing studies from the left-hand side of the funnel plot. With these effect sizes included, the adjusted overall meta-analytic effect size was smaller than the original effect size of g = 0.621, although it was still significantly greater than zero, Hedges’ g = 0.463, SE = 0.070, 95% CI [0.326, 0.601], p < .001.
In the same way as the first meta-analysis, we explored whether any outliers and influential cases significantly influenced the size of the effect. We identified any effect sizes with studentized residuals greater than or smaller than three ( k = 1; Tempesta et al., 2016 , Recognition Task). Following recommendations by Viechtbauer and Cheung (2010) , influential case analysis (Cook’s distance and DFBETAs) identified two further effect sizes that may have had a significant influence on the results ( Poh & Chee, 2017 ; Yoo et al., 2007 ). Removal of the one outlier and two influential cases reduced the overall meta-analytic effect size to 0.525 (from 0.621). We removed these three specific outliers and influential cases from all moderator analyses.
We introduced the categorical moderating variable task type, recall ( k = 26) versus recognition ( k = 20). We excluded studies that used a different task type, including a recency discrimination task ( k = 3), a prototype learning task ( k = 2), and a finger-tapping task ( k = 1). Analysis of the moderator recall versus recognition revealed that the type of task used did not moderate the size of the effect, Q (1) = 0.028, p = .868. There were only two studies of procedural memory ( Fischer et al., 2002 ; McWhirter et al., 2015 ) and only five entries where the participants were given a night of recovery sleep (two further entries did not report whether recovery sleep was given). Likewise, only two studies investigated the effects of sleep deprivation on emotional memory ( Kaida et al., 2015 ; Tempesta et al., 2016 ). Thus, there was insufficient variability within the data set to assess whether one or more nights of recovery sleep, the type of memory (declarative, procedural), and emotionality moderated the size of the sleep deprivation effect.
We tested the influence of the three continuous moderating variables assessing methodological quality (reporting, bias, and confounding), as well as statistical power to detect the meta-analytic effect size, on the size of the sleep deprivation effect. No methodological quality cluster had a significant moderating effect. For each of the methodological quality clusters, we created a meta-analytic scatter plot (metafor package; Viechtbauer, 2010 ; see Figure 8 ). We did not include the power cluster as a moderator, since the majority of studies did not calculate power and thus scored zero on this cluster, with only two studies (contributing a total of 10 effect sizes) providing a power analysis. analysis.

We then investigated whether statistical power to find the meta-analytic effect moderated the size of the effect of sleep deprivation on memory. In the same way as in the first meta-analysis, for each study, we calculated the power to find the mean meta-analytic effect size, as well as the power to detect the upper and lower confidence interval bounds around the mean. The distribution of power to detect the three estimates is plotted in Figure 9 . We found the mean power to find the meta-analytic effect to be larger than in the first meta-analysis ( M = 54.77%, SD = 20.81%, range = 21.22%–98.06%). The moderator analysis revealed that power to find the mean meta-analytic effect size did not moderate the effect of sleep deprivation on memory, Q (1) = 3.179, p = .075. All moderator analyses are reported in Table 4 . .

The two meta-analyses presented here aimed to quantify the size of the effect of sleep deprivation after learning and before learning on memory performance. Based on previous evidence for an effect of sleep on both declarative and procedural memories ( Klinzing et al., 2019 ), we predicted that sleep deprivation would have a detrimental effect on learning and memory. We found that sleep deprivation after learning was associated with a mean effect size of g = 0.277. The effect size is positive, indicating that sleep deprivation has a detrimental rather than facilitatory impact on memory, as predicted by theory. Furthermore, the 95% confidence intervals around the mean do not cross zero, indicating that the effect size is statistically significantly higher than zero. For sleep deprivation before learning, we found an average effect size of g = 0.621. The effect size is positive, indicating that sleep deprivation before learning impairs rather than facilitates memory, as predicted by theory (e.g., Cirelli & Tononi, in press ; Tononi & Cirelli, 2012 ). The 95% confidence intervals around the mean do not cross zero, again indicating that the effect size is statistically significantly higher than zero. Following Cohen’s guidelines for categorizing effect sizes a small (0.20), medium (0.50), and large (0.8), the effect sizes above would correspond to small-to-medium and medium. However, given that these cutoff points are wholly arbitrary and were only ever intended to be used as a last resort, many now argue that effect sizes should be interpreted in the context of typical effect sizes observed in the relevant literature ( Correll et al., 2020 ; Funder & Ozer, 2019 ). According to Brysbaert (2019) , an effect size of d = 0.40 represents an average effect size in experimental psychology and has practical and theoretical relevance. Putting our meta-analytic effect sizes into this context, it appears that sleep deprivation before learning has an effect size somewhat larger than the average effect size in experimental psychology, while sleep deprivation after learning has a somewhat smaller than average effect size, although the latter varies as a function of both recovery sleep and memory type (declarative vs. procedural), as discussed in detail below.
Theory-Based Mediators
Despite the wide range of literature examining an effect of sleep deprivation on memory performance, this is, to our knowledge, the first time that the size of this effect has been formally quantified. A benefit of meta-analyses is that they allow for the investigation of potential moderating factors that may differentially influence the size of the meta-analytic effect. For deprivation after learning, we were able to investigate whether the first night of sleep is essential for the consolidation of newly acquired memories or whether a later sleep opportunity can compensate for the first night of sleep deprivation. We found that studies where memory was tested immediately after one night of sleep deprivation and before recovery sleep showed a significant sleep deprivation associated memory deficit ( g = 0.410). Critically, those studies that had one or more nights of recovery sleep prior to retrieval also showed a small but statistically significant memory deficit ( g = 0.176). Thus, memory impairments caused by sleep deprivation during the first postencoding night were still present but less severe when recovery sleep occurred before testing. On the one hand, this finding suggests that the first night of sleep after learning is important as its disruption is still felt even after recovery sleep. On the other hand, it also suggests that recovery sleep can to some extent mitigate the disruption of the first night of sleep by reducing the effect size by about 50%. While these data are consistent with theories arguing that, for hippocampal-dependent memories at least, the hippocampus may act as a buffer, retaining newly learned information until an offline consolidation opportunity is available ( Schönauer et al., 2015 ), the idea that consolidation processes can be spread over multiple nights of sleep is yet to be explicitly tested.
It is also difficult to establish the extent to which the smaller effect of sleep deprivation after recovery sleep on memory is due to the occurrence of a delayed consolidation opportunity or due to effects of fatigue being diminished. Recent work has suggested that fatigue at time of test might have little or no detrimental impact on tasks assessing long-term memory. Schönauer et al. (2015) found that sleep deprivation before a recall task did not impair memory for previously encoded and consolidated word pairs. Furthermore, despite a difference in the size of the effect of sleep deprivation, it is important to note that we still see a significant detrimental effect of sleep deprivation after learning even when a later sleep opportunity is permitted, albeit a smaller effect. An account based on fatigue alone is insufficient to explain this finding. Another potential alternative explanation for the decrease in effect size after recovery sleep could be based on interference. When tested after one night of sleep or sleep deprivation, participants in the sleep group will have experienced little interference from subsequent cognitive activity after learning. A large difference between the groups at this point could be due to sleep protecting new memories from interference rather than due to active consolidation processes. After one or more nights of recovery sleep, both groups will have experienced some degree of interference, and this could explain the reduction in the effect size. Further research is needed to adjudicate between these different accounts that could both contribute to the effect sizes we have observed.
For deprivation after learning, we also found that whether the task type was declarative or procedural had an effect on the size of the deprivation effect. Although both declarative and procedural tasks elicited a significant effect, a moderator analysis indicated that those studies implementing procedural memory tasks had significantly larger effect sizes on average ( g = .449) than declarative tasks ( g = .218). That both declarative and procedural memory tasks showed detrimental effects of sleep deprivation was unsurprising, given that a benefit of sleep has been observed for both declarative memories (e.g., Gais & Born, 2004 ; Talamini et al., 2008 ; Wagner et al., 2006 ) and procedural memories ( Korman et al., 2007 ; Schönauer et al., 2014 ; Walker et al., 2005 ). Our findings are also consistent with recent studies showing that sleep is beneficial even in tasks that do not require the hippocampus at learning (e.g., Schapiro et al., 2019 ). Although the current meta-analysis indicates a detrimental effect of sleep deprivation after learning on both declarative and procedural memories, the exact mechanisms that drive these effects are still debated, and thus it is unclear why procedural memories may show larger sleep deprivation effects. According to active systems consolidation theory, hippocampal-dependent declarative memories benefit from repeated reactivation of newly learned memories during sleep, supporting the strengthening of memory representations in the neocortex ( Born & Wilhelm, 2012 ; Walker & Stickgold, 2006 ). However, procedural memories that rely on implicit learning are unlikely to be dependent on such hippocampal–neocortical representations. It has been theorized that such implicit memories require more immediate offline consolidation to see a beneficial effect of sleep ( Schönauer et al., 2015 ; Stickgold et al., 2000 ). Thus, it may be that without an immediate sleep opportunity, the detrimental effects of sleep deprivation have a larger impact on procedural memory consolidation, whereas declarative memory consolidation is less impacted by the lack of an immediate sleep opportunity. However, we found no significant interaction between recovery sleep and task type, suggesting that for both declarative and procedural tasks, lack of an immediate sleep opportunity increased the size of the effect of sleep deprivation. Thus, recovery sleep had a similar impact on both procedural and declarative task performance, and procedural tasks elicited larger effect sizes than declarative tasks, regardless of whether recovery sleep occurred.
For deprivation after learning, we found no effect of emotional versus neutral memory on the size of the meta-analytic effect. Although some studies do suggest a preferential effect of sleep for emotional memories (e.g., Payne & Kensinger, 2010 ; Wagner et al., 2001 ), our findings join two recent meta-analyses that report no overall preferential effect of sleep on emotional memory consolidation ( Lipinska et al., 2019 ; Schäfer et al., 2020 ). These existing meta-analyses focussed on emotional memory and were able to uncover mediators that may reveal boundary conditions for the preferential effect; however, the number of studies in this domain is still low and more research is needed to establish the reliability of the effect.
For both deprivation after learning and deprivation before learning, we found no effect of the recall versus recognition moderator on the size of the meta-analytic effect. This is in contrast to some previous studies investigating the beneficial role of sleep on memory that have found a differential effect of recall versus recognition testing, and in contrast to the meta-analysis of Newbury and Monaghan (2019) , which looked at sleep studies using the DRM paradigm. Although performance on recall tasks repeatedly benefits from sleep, performance on recognition tasks has sometimes been found to show little or no offline consolidation benefit ( Ashton et al., 2018 ; Diekelmann et al., 2009 ; Drosopoulos et al., 2005 ; Gais et al., 2006 ; Hu et al., 2006 ). It is posited that, although recall tasks rely on explicit, hippocampal-dependent memory, recognition tasks could include both an explicit recollection and implicit familiarity element ( Jacoby, 1991 ), only the former of which benefits from sleep-associated consolidation. Thus, the mechanisms by which these two types of memories are consolidated may be different. Despite this, the present meta-analyses provide no evidence to suggest that performance on recall and recognition tasks are differentially affected by sleep deprivation either before or after sleep. Whether this finding extends to sleep paradigms other than total sleep deprivation remains to be established.
The null effects from our moderator analyses should be treated with caution, however, as we may not have adequate statistical power to detect smaller moderator effect sizes. Hempel et al. (2013) suggest that power to detect moderator effects is dependent on a combination of the amount of residual heterogeneity within the data set, the number of studies in the data set, the number of participants in the included studies, and the ratio of studies in the conditions compared against each other. Based on power simulations, Hempel et al. (2013) provide estimations of the approximate number of studies and participants required to detect categorical moderator effects of different effect sizes. We used data from these simulations to retrospectively assess the power of our moderator analyses to detect an effect. Since Hempel et al.’s simulations are not based on multilevel meta-analyses, the below estimates should be treated with caution when applied to our analyses and only considered as indicative.
For our deprivation after learning analysis, residual heterogeneity was τ 2 = .026 for within-study variance, and τ 2 = .061 for between-study variance. Therefore, based on a τ 2 of between 0 and 0.1, the simulations suggest that the moderator recall versus recognition was powered to detect an effect of around 0.2–0.3 (based on 100 trials, 20 participants per study, at 80% power). The emotionality moderator was powered to detect only large moderator effects of 0.3–0.4 (based on 50 trials, 20 participants per study, 80% power). For the deprivation before learning analysis, residual heterogeneity was τ 2 = .096 for between-study variance, and τ 2 = .017 for within-study variance. The simulations suggest that the moderator analysis of recall versus recognition was powered to detect only a large effect size of between 0.3 and 0.4 (based on 50 trials, 20 participants per study, at 80% power). Therefore, it appears that our moderator analyses were not sufficiently powered to detect small moderator effects and the null findings in these analyses should be considered preliminary. These analyses need to be repeated as more evidence accumulates over time.
There are other moderators that would be valuable to account for to increase the precision of our meta-analytic effect size, but that we could not include in our analyses due to the small number of studies available. For example, some studies included in the meta-analysis involved manipulations that the authors expected to reverse or eradicate the detrimental effect of sleep deprivation. Kolibius et al. (2021) predicted that large amounts of encoded information (640 word pairs) would increase forgetting in the sleep group compared to a sleep-deprived group. Similarly, Feld et al. (2016) hypothesized that those in a high memory load condition (360 word pairs) should no longer show a sleep benefit compared to a sleep-deprived condition. Vargas et al. (2019) examined memory for emotionally negative and neutral objects and backgrounds, but only predicted an impact of sleep deprivation on neutral objects. It is possible that the inclusion of studies such as these (or conditions within those studies where no sleep deprivation effect is predicted) may have artificially reduced our meta-analytic effect size. A mediator analysis would be the appropriate solution to establish whether this was the case, but the small number of relevant studies prevents this for now.
Quality-Based Moderators
The meta-analyses in this article suggest that there is a detrimental effect of sleep deprivation on learning and memory, and it is observed across a range of methodologies. However, our meta-analyses identified a number of potential limitations of the available data sets in this domain. Methodological quality scores ranged from 4 to 19 out of 22 in the studies investigating deprivation after learning; and they ranged from 7 to 19 in the studies investigating deprivation before learning.
We must be cautious in the way that we interpret the effects of methodological quality on the size of the effect of sleep deprivation. Valentine (2009) argues that methodological scales of this nature frequently lack operational specificity (e.g., that each item deserves equal weight) and include questions that are unclear. In an attempt to increase the validity of our methodological quality checklist, we designed our checklist based on the Downs and Black checklist ( 1998 ), with modified questions relevant to sleep studies. For the first meta-analysis, we found a significant mediating effect of the reporting cluster of our methodological quality checklist on the size of the sleep deprivation effect, Figure 4 shows that studies scoring highest on this cluster show no effect of sleep deprivation while the lower scoring studies do. The items in the reporting cluster are predominantly concerned with the number and nature of exclusion and inclusion criteria used in the study. It therefore appears that the studies showing higher effect sizes may have employed fewer such criteria. However, some of the scores on this cluster may be underestimated due to incomplete reporting. For example, studies stating that they only recruited healthy participants may have used other sleep-related inclusion and exclusion criteria, such as excluding participants who were taking medication that affects sleep or those who had recently traveled between time zones, without reporting these and may have scored higher on this cluster had these criteria been reported. We found no mediating effect of any cluster of methodological quality on the size of the effect of sleep deprivation for the second meta-analysis. Given that only one cluster of the quality score influenced the size of the effect in the first meta-analysis, and no clusters had a significant effect in the second meta-analysis, our effect size estimates are unlikely to be substantially biased by variation in methodological quality.
Taking a broader qualitative view of our quality checklist, we note that only one of the analyzed studies was preregistered, and only three justified their sample size with an a priori power analysis. Given that preregistration has become a mainstream practice only in the past few years ( Nosek & Lindsay, 2018 ), and that an a priori power analysis is part of the preregistration process, the low numbers here are unsurprising and are likely in line with the current broader field of experimental psychology. The key quality measures on study design were met by the clear majority of studies (e.g., equal group sizes, random allocation to groups or counterbalancing of conditions).
Power-Based Moderators
In the current meta-analyses, we calculated statistical power to find the meta-analytic effect size for each experiment and assessed whether statistical power significantly influenced the size of the effect of sleep deprivation. For sleep deprivation after learning, mean statistical power to find the meta-analytic effect size was just 14%; for sleep deprivation before learning, it was higher though still far less than optimal at 55%. Given that power is a function of the effect size, sample size, and the statistical test being employed, the difference in obtained power across the two research questions is understandable: As the effect size decreases, power to detect it decreases if sample size is held constant. Overall, these figures are closely in line with the broader field: For example, Szucs and Ioannidis (2017) found that within psychology and cognitive neuroscience, mean power to detect small, medium, and large effects (in Cohen’s terms) was 17%, 49%, and 71%, respectively.
Given the current convention that statistical power to find an effect is at 80% or higher ( Di Stefano, 2003 ), it is evident that the majority of the studies in these meta-analyses are underpowered. This is problematic as it increases the uncertainty around our meta-analytical effect sizes. To better understand the consequences of the uncertainty introduced by low power in the studies included in our meta-analyses, we investigated whether statistical power to find the mean meta-analytic effect size influenced the size of the sleep deprivation effect by entering obtained power as a moderator. For example, it might be the case that it is only low-powered studies that show an impact of sleep deprivation, while high-powered studies might show no impact. Such a pattern would suggest that our meta-analytic effect size might be overestimated as a consequence of low power. The opposite pattern would suggest that our effect size has been underestimated due to low power. For deprivation after learning, we found no moderating impact of statistical power on the size of the effect. In other words, both low- and high-powered studies yielded similar effect sizes. However, the validity of this analysis is reduced by the fact that there were no studies in this meta-analysis where power exceeded 33%, and therefore we have no way of knowing what effect sizes could be expected when power is higher. For deprivation before learning, we found a broader range of power extending from about 20% to over 90%. However, once again we found no statistically significant moderating impact of power on the size of the effect.
To gain a more precise estimate of the true effect size, future studies should use the current meta-analytic effect size as a guide in determining sample sizes that will yield high power. Studies planning to look at sleep deprivation after learning and running a two-tailed t -test for a between-subjects design with a sleep versus sleep deprivation manipulation would require a sample size of approximately 410 to have 80% power to detect the meta-analytic effect size. For a within-subjects design, the sample size required would be 105. For studies examining deprivation before learning, a two-tailed t -test with a between-subjects design would require a sample size of 82, whereas a within-subjects design would require a much smaller sample size of 23, to have 80% power to detect the meta-analytic effect size. The above numbers are rough indications only, and lower or higher sample sizes may be appropriate depending on the specific design of the experiment and the statistical analysis approach ( Brysbaert, 2019 ; Lakens & Caldwell, 2021 ).
It is clear that there is a significant discrepancy between the high-power sample sizes we have estimated above and the sample sizes found in the majority of the studies included in the current meta-analyses. This discrepancy is important as there are severe limitations to the strength of conclusions that can be drawn from underpowered individual studies (see, e.g., Brysbaert, 2019 , for a detailed discussion). Fraley and Vazire (2014) described three limitations: (a) underpowered studies are less likely than properly powered studies to detect a true effect; (b) underpowered studies are more likely to yield false-positive findings than properly powered studies; and (c) underpowered studies are less likely than properly powered studies to produce replicable findings. Wilson et al. (2020) further demonstrate that underpowered studies are likely to yield inflated effect sizes. Therefore, the results of any single underpowered study should be treated with caution, and a meta-analytic approach such as ours may be the more useful approach for extracting information from these studies. Yet, small-scale studies are not always completely uninformative; we return to this debate in the Conclusions section.
Conducting a meta-analysis allows for an estimation of publication bias within the literature. Publication bias is evident when there are a large number of published studies in the direction of the hypothesis, with few nonsignificant published studies ( Rosenthal, 1979 ). This can lead to overestimation of the size of the effect. We found statistically significant evidence of publication bias in both meta-analyses. Adjusting the deprivation after learning effect size for publication bias using the trim-and-fill method changed the effect size from 0.277 to 0.166, and changed the deprivation before learning effect size from 0.621 to 0.463, although these adjusted effect size should be treated with caution given that the trim-and-fill method was not designed for a multilevel approach. Nonetheless, both estimates remained significantly different from zero after the adjustment. To allow for more accurate effect size estimates in future meta-analyses, we suggest researchers in this field should adopt registered reports as an effective way of ensuring all results find their way into published literature.
Limitations
We focused specifically on the effects of total (overnight) sleep deprivation, and thus future meta-analyses are needed to establish whether the effect size is similar in studies using sleep restriction. We chose to concentrate on studies using total sleep deprivation because depriving a participant of all sleep is a stronger test of the hypothesis that sleep benefits memory than depriving them of a single stage of sleep or restricting their sleep for some hours over a period of time, as discussed in the Introduction section. An alternative approach could have been to include restriction studies and to conduct a moderator analysis to establish whether they lead to similar effect sizes as total deprivation. However, many sleep restriction studies in the literature are field studies that lack the rigorous controls we include in our inclusion criteria (e.g., lack of control over hours slept, Deary & Tait, 1987 ; inappropriate sleep control condition, Piérard et al., 2004 ), and therefore the number of eligible restriction studies would have been smaller than the number of total deprivation studies. As discussed earlier, such imbalance in number of studies can make moderator analyses insensitive ( Hempel et al., 2013 ).
Our search focused solely on English language reports, thus risking a mono-language bias ( Johnson, 2021 ). This restricts our ability to generalize the results of our meta-analyses to non-English language literature. In particular, by using English language sources only, there is the possibility that our search missed much of the gray literature such as PhD theses and conference abstracts written in other languages. The use of solely English language sources limits our understanding of any possible cross-cultural differences in effects of sleep on memory. Indeed, there are many cross-cultural differences in sleep habits (e.g., Cheung et al., 2021 ), and although we are not aware of any studies that have systematically compared sleep-associated memory consolidation effects across cultures, our reliance on English language literature means that we would not have captured such differences if they do exist.
We acknowledge that our inclusion criteria restrict our ability to draw conclusions beyond healthy, typical populations. We excluded studies that included participants under the age of 18 and studies that involved participants suffering from sleep disorders or psychiatric disorders. There is growing interest in understanding how sleep-associated memory consolidation in these groups might differ from healthy adults, however (e.g., Hoedlmoser, 2020 ; Manoach & Stickgold, 2019 ), and future meta-analyses addressing these questions will be valuable both for theoretical development and practical reasons. Finally, we note that there were 17 studies that we were unable to include in the analyses as the required statistical information was not reported (see Figure 1 ); unfortunately, the authors of these papers were unable to provide with the necessary data when contacted. Nonetheless, these studies made up a small proportion of the studies we identified as eligible and would be unlikely to change the conclusions we have drawn.
Conclusions
To conclude, the two meta-analyses presented here provide a comprehensive analysis of the impact of sleep deprivation after learning and before learning. The results of the first meta-analysis suggest that depriving participants of the first night of sleep after encoding new information results in lower performance at test, supporting the theories of sleep-associated memory consolidation (e.g., Diekelmann & Born, 2010 ; McClelland et al., 1995 ). This effect was larger before than after recovery sleep and larger in procedural memory tasks compared to declarative memory tasks. The results of the second meta-analysis suggest that sleep-deprived participants are able to encode less information than rested controls, supporting the theories that propose that sleep restores memory encoding capacity (e.g., Saletin & Walker, 2012 ; Tononi & Cirelli, 2012 ).
We found that levels of statistical power tended to be low, particularly in those studies looking at sleep deprivation after learning in which there was a small estimated effect size. Given that underpowered studies are ubiquitous across disciplines that use human participants ( Dumas-Mallet et al., 2017 ), new ways of interpreting low-powered studies are emerging. One particularly insightful interpretation has recently been offered by Wilson et al. (2020) . In short, Wilson and colleagues draw a distinction between “original science” and “replication science.” Original science is roughly science as practiced today, in that it uses Null Hypothesis Significance Testing combined with study designs whose power falls far short of the gold standard of high-N studies. Original science in this formulation serves an important and inexpensive screening function to identify effects that may be true and would therefore benefit from further, more costly examination of replication science. Replication science consists of high-N, highly powered, and costly direct replications of the key studies from original science, vital for verifying the preliminary results of original science. Applying this framework to the literature targeted in our meta-analyses, we propose that there is now sufficient evidence from original science to warrant a move to replication science in this field. No highly powered, preregistered direct replications looking at the role of sleep deprivation in learning and memory have been conducted thus far. The meta-analytic estimates of the relevant effect sizes provided here will facilitate the design of such urgently needed studies, while also allowing better informed sample size choice for continuing original science efforts.
Supplementary Material
References marked with * 1 indicate studies included in the first meta-analysis (sleep deprivation after learning) and references marked with * 2 indicate studies included in the second meta-analysis (sleep deprivation before learning).
- Alberca-Reina E., Cantero J. L., & Atienza M. (2015). Impact of sleep loss before learning on cortical dynamics during memory retrieval . NeuroImage , 123 , 51–62. 10.1016/j.neuroimage.2015.08.033 [ PubMed ] [ CrossRef ] [ Google Scholar ]
- * 1 Albouy G., Sterpenich V., Vandewalle G., Darsaud A., Gais S., Rauchs G., Desseilles M., Boly M., Dang-Vu T., Balteau E., Degueldre C., Phillips C., & Degueldre C. (2013). Interaction between hippocampal and striatal systems predicts subsequent consolidation of motor sequence memory . PLOS ONE , 8 ( 3 ). Article e59490. 10.1371/journal.pone.0059490 [ PMC free article ] [ PubMed ] [ CrossRef ] [ Google Scholar ]
- * 1 Albouy G., Vandewalle G., Sterpenich V., Rauchs G., Desseilles M., Balteau E., Degueldre C., Phillips C., Luxen A., & Maquet P. (2013). Sleep stabilizes visuomotor adaptation memory: A functional magnetic resonance imaging study . Journal of Sleep Research , 22 ( 2 ), 144–154. 10.1111/j.1365-2869.2012.01059.x [ PubMed ] [ CrossRef ] [ Google Scholar ]
- Alger S. E., Kensinger E. A., & Payne J. D. (2018). Preferential consolidation of emotionally salient information during a nap is preserved in middle age . Neurobiology of Aging , 68 , 34–47. 10.1016/j.neurobiolaging.2018.03.030 [ PMC free article ] [ PubMed ] [ CrossRef ] [ Google Scholar ]
- * 1 Ashton J., Harrington M. O., Langthorne D., Ngo H. V., & Cairney S. A. (2020). Sleep deprivation induces fragmented memory loss . Learning and Memory . 27 ( 4 ), 130–135. 10.1101/lm.050757.119 [ PMC free article ] [ PubMed ] [ CrossRef ] [ Google Scholar ]
- Ashton J. E., Cairney S. A., & Gaskell M. G. (2018). No effect of targeted memory reactivation during slow-wave sleep on emotional recognition memory . Journal of Sleep Research , 27 ( 1 ), 129–137. 10.1111/jsr.12542 [ PubMed ] [ CrossRef ] [ Google Scholar ]
- Assink M., & Wibbelink C. J. (2016). Fitting three-level meta-analytic models in R: A step-by-step tutorial . The Quantitative Methods for Psychology , 12 ( 3 ), 154–174. 10.20982/tqmp.12.3.p154 [ CrossRef ] [ Google Scholar ]
- * 1 Atienza M., & Cantero J. L. (2008). Modulatory effects of emotion and sleep on recollection and familiarity . Journal of Sleep Research , 17 ( 3 ), 285–294. 10.1111/j.1365-2869.2008.00661.x [ PubMed ] [ CrossRef ] [ Google Scholar ]
- Banks S., & Dinges D. F. (2007). Behavioral and physiological consequences of sleep restriction . Journal of Clinical Sleep Medicine , 3 ( 5 ), 519–528. 10.5664/jcsm.26918 [ PMC free article ] [ PubMed ] [ CrossRef ] [ Google Scholar ]
- Bartel P., Offermeier W., Smith F., & Becker P. (2004). Attention and working memory in resident anaesthetists after night duty: Group and individual effects . Occupational and Environmental Medicine , 61 ( 2 ), 167–170. 10.1136/oem.2002.006197 [ PMC free article ] [ PubMed ] [ CrossRef ] [ Google Scholar ]
- * 2 Basner M., Savitt A., Moore T. M., Port A. M., McGuire S., Ecker A. J., Nasrini J., Mollicone D. J., Mott C. M., McCann T., Dinges D. F., & Gur R. V. (2015). Development and validation of the cognition test battery for spaceflight . Aerospace Medicine and Human Performance , 86 ( 11 ), 942–952. 10.3357/AMHP.4343.2015 [ PMC free article ] [ PubMed ] [ CrossRef ] [ Google Scholar ]
- * 2 Bensimon G., Benoit D., Lacomblez L., Weiller E., Warot D., Weil J. S., & Puech A. J. (1991). Antagonism by modafinil of the psychomotor and cognitive impairment induced by sleep-deprivation in 12 healthy volunteers . European Psychiatry , 6 ( 2 ), 93–97. 10.1017/S0924933800000201 [ CrossRef ] [ Google Scholar ]
- * 2 Blagrove M., Cole-Morgan D., & Lambe H. (1994). Interrogative suggestibility: The effects of sleep deprivation and relationship with field dependence . Applied Cognitive Psychology , 8 ( 2 ), 169–179. 10.1002/acp.2350080207 [ CrossRef ] [ Google Scholar ]
- Borenstein M., Hedges L. V., Higgins J. P., & Rothstein H. R. (2010). A basic introduction to fixed-effect and random-effects models for meta-analysis . Research Synthesis Methods , 1 ( 2 ), 97–111. 10.1002/jrsm.12 [ PubMed ] [ CrossRef ] [ Google Scholar ]
- Borenstein M., Hedges L. V., Higgins J. P., & Rothstein H. R. (2011). Introduction to meta-analysis . Wiley. [ Google Scholar ]
- Borenstein M., Higgins J. P., Hedges L. V., & Rothstein H. R. (2017). Basics of meta-analysis: I2 is not an absolute measure of heterogeneity . Research Synthesis Methods , 8 ( 1 ), 5–18. 10.1002/jrsm.1230 [ PubMed ] [ CrossRef ] [ Google Scholar ]
- Born J., & Wilhelm I. (2012). System consolidation of memory during sleep . Psychological Research , 76 ( 2 ), 192–203. 10.1007/s00426-011-0335-6 [ PMC free article ] [ PubMed ] [ CrossRef ] [ Google Scholar ]
- * 1 Borragán G., Urbain C., Schmitz R., Mary A., & Peigneux P. (2015). Sleep and memory consolidation: Motor performance and proactive interference effects in sequence learning . Brain and Cognition , 95 , 54–61. 10.1016/j.bandc.2015.01.011 [ PubMed ] [ CrossRef ] [ Google Scholar ]
- * 2 Boyle J., Stanley N., James L. M., Wright N., Johnsen S., Arbon E. L., & Dijk D. J. (2012). Acute sleep deprivation: The effects of the AMPAKINE compound CX717 on human cognitive performance, alertness and recovery sleep . Journal of Psychopharmacology , 26 ( 8 ), 1047–1057. 10.1177/0269881111405353 [ PubMed ] [ CrossRef ] [ Google Scholar ]
- Brysbaert M. (2019). How many participants do we have to include in properly powered experiments? A tutorial of power analysis with reference tables . Journal of Cognition , 2 ( 1 ), Article 187. 10.5334/joc.72 [ PMC free article ] [ PubMed ] [ CrossRef ] [ Google Scholar ]
- Button K. S., Ioannidis J. P., Mokrysz C., Nosek B. A., Flint J., Robinson E. S., & Munafò M. R. (2013). Power failure: Why small sample size undermines the reliability of neuroscience . Nature Reviews Neuroscience , 14 ( 5 ), 365–376. 10.1038/nrn3475 [ PubMed ] [ CrossRef ] [ Google Scholar ]
- * 2 Chatburn A., Kohler M. J., Payne J. D., & Drummond S. P. (2017). The effects of sleep restriction and sleep deprivation in producing false memories . Neurobiology of Learning and Memory , 137 , 107–113. 10.1016/j.nlm.2016.11.017 [ PubMed ] [ CrossRef ] [ Google Scholar ]
- * 2 Chee M. W., Tan J. C., Parimal S., & Zagorodnov V. (2010). Sleep deprivation and its effects on object-selective attention . Neuroimage , 49 ( 2 ), 1903–1910. 10.1016/j.neuroimage.2009.08.067 [ PubMed ] [ CrossRef ] [ Google Scholar ]
- Cheung B. Y., Takemura K., Ou C., Gale A., & Heine S. J. (2021). Considering cross-cultural differences in sleep duration between Japanese and Canadian university students . PLOS ONE , 16 ( 4 ), Article e0250671. 10.1371/journal.pone.0250671 [ PMC free article ] [ PubMed ] [ CrossRef ] [ Google Scholar ]
- Cheung M. W. L. (2014). Modeling dependent effect sizes with three-level meta-analyses: A structural equation modeling approach . Psychological Methods , 19 ( 2 ), 211–229. 10.1037/a0032968 [ PubMed ] [ CrossRef ] [ Google Scholar ]
- * 2 Chuah L. Y., Chong D. L., Chen A. K., Rekshan W. R. III, Tan J. C., Zheng H., & Chee M. W. (2009). Donepezil improves episodic memory in young individuals vulnerable to the effects of sleep deprivation . Sleep , 32 ( 8 ), 999–1010. 10.1093/sleep/32.8.999 [ PMC free article ] [ PubMed ] [ CrossRef ] [ Google Scholar ]
- Cirelli C., & Tononi G. (in press). The why and how of sleep-dependent synaptic down-selection . Seminars in Cell and Developmental Biology . 10.1016/j.semcdb.2021.02.007 [ PMC free article ] [ PubMed ] [ CrossRef ] [ Google Scholar ]
- Cochran W. G. (1954). The combination of estimates from different experiments . Biometrics , 10 ( 1 ), 101–129. 10.2307/3001666 [ CrossRef ] [ Google Scholar ]
- Correll J., Mellinger C., McClelland G. H., & Judd C. M. (2020). Avoid Cohen’s “small”,“medium”, and “large” for power analysis . Trends in Cognitive Sciences , 24 ( 3 ), 200–207. 10.1016/j.tics.2019.12.009 [ PubMed ] [ CrossRef ] [ Google Scholar ]
- Cousins J. N., Sasmita K., & Chee M. W. (2018). Memory encoding is impaired after multiple nights of partial sleep restriction . Journal of Sleep Research , 27 ( 1 ), 138–145. 10.1111/jsr.12578 [ PubMed ] [ CrossRef ] [ Google Scholar ]
- * 1 Darsaud A., Dehon H., Lahl O., Sterpenich V., Boly M., Dang-Vu T., Desseilles M.,Gais S., Matarazzo L., Peters F., Schabus M., Schmidt C., Tinguely G., Vandewalle G., Luxen A., Maquet P., & Collette F. (2011). Does sleep promote false memories? . Journal of Cognitive Neuroscience , 23 ( 1 ), 26–40. 10.1162/jocn.2010.21448 [ PubMed ] [ CrossRef ] [ Google Scholar ]
- Deary I. J., & Tait R. (1987). Effects of sleep disruption on cognitive performance and mood in medical house officers . British Medical Journal (Clinical Research Ed.) , 295 ( 6612 ), 1513–1516. 10.1136/bmj.295.6612.1513 [ PMC free article ] [ PubMed ] [ CrossRef ] [ Google Scholar ]
- * 1 Deliens G., Gilson M., Schmitz R., & Peigneux P. (2013). Sleep unbinds memories from their emotional context . Cortex , 49 ( 8 ), 2221–2228. 10.1016/j.cortex.2012.11.014 [ PubMed ] [ CrossRef ] [ Google Scholar ]
- * 1 Deliens G., Schmitz R., Caudron I., Mary A., Leproult R., & Peigneux P. (2013b). Does recall after sleep-dependent memory consolidation reinstate sensitivity to retroactive interference? PLOS ONE , 8 ( 7 ), Article e68727. 10.1371/journal.pone.0068727 [ PMC free article ] [ PubMed ] [ CrossRef ] [ Google Scholar ]
- Di Stefano J. (2003). How much power is enough? Against the development of an arbitrary convention for statistical power calculations . Functional Ecology , 17 ( 5 ), 707–709. 10.1046/j.1365-2435.2003.00782.x [ CrossRef ] [ Google Scholar ]
- Diekelmann S., & Born J. (2010). The memory function of sleep . Nature Reviews Neuroscience , 11 ( 2 ), 114–126. 10.1038/nrn2762 [ PubMed ] [ CrossRef ] [ Google Scholar ]
- * 1 Diekelmann S., Born J., & Wagner U. (2010). Sleep enhances false memories depending on general memory performance . Behavioural Brain Research , 208 ( 2 ), 425–429. 10.1016/j.bbr.2009.12.021 [ PubMed ] [ CrossRef ] [ Google Scholar ]
- * 1 Diekelmann S., Landolt H. P., Lahl O., Born J., & Wagner U. (2008). Sleep loss produces false memories . PLOS ONE , 3 ( 10 ). Article e0250671. 10.1371/journal.pone.0003512 [ PMC free article ] [ PubMed ] [ CrossRef ] [ Google Scholar ]
- Diekelmann S., Wilhelm I., & Born J. (2009). The whats and whens of sleep-dependent memory consolidation . Sleep Medicine Reviews , 13 ( 5 ), 309–321. 10.1016/j.smrv.2008.08.002 [ PubMed ] [ CrossRef ] [ Google Scholar ]
- * 1 Diekelmann S., Wilhelm I., Wagner U., & Born J. (2013). Sleep to implement an intention . Sleep , 36 ( 1 ), 149–153. 10.5665/sleep.2322 [ PMC free article ] [ PubMed ] [ CrossRef ] [ Google Scholar ]
- Dinges D. F., & Powell J. W. (1985). Microcomputer analyses of performance on a portable, simple visual RT task during sustained operations . Behavior Research Methods, Instruments, and Computers , 17 ( 6 ), 652–655. 10.3758/BF03200977 [ CrossRef ] [ Google Scholar ]
- Downs S. H., & Black N. (1998). The feasibility of creating a checklist for the assessment of the methodological quality both of randomised and non-randomised studies of health care interventions . Journal of Epidemiology & Community Health , 52 ( 6 ), 377–384. 10.1136/jech.52.6.377 [ PMC free article ] [ PubMed ] [ CrossRef ] [ Google Scholar ]
- * 2 Drake C. L., Roehrs T. A., Burduvali E., Bonahoom A., Rosekind M., & Roth T. (2001). Effects of rapid versus slow accumulation of eight hours of sleep loss . Psychophysiology , 38 ( 6 ), 979–987. 10.1111/1469-8986.3860979 [ PubMed ] [ CrossRef ] [ Google Scholar ]
- Drosopoulos S., Wagner U., & Born J. (2005). Sleep enhances explicit recollection in recognition memory . Learning and Memory , 12 ( 1 ), 44–51. 10.1101/lm.83805 [ PMC free article ] [ PubMed ] [ CrossRef ] [ Google Scholar ]
- Drummond S. P., & Brown G. G. (2001). The effects of total sleep deprivation on cerebral responses to cognitive performance . Neuropsychopharmacology , 25 ( 5 ), S68–S73. 10.1016/S0893-133X(01)00325-6 [ PubMed ] [ CrossRef ] [ Google Scholar ]
- * 2 Drummond S. P., Brown G. G., Gillin J. C., Stricker J. L., Wong E. C., & Buxton R. B. (2000). Altered brain response to verbal learning following sleep deprivation . Nature , 403 ( 6770 ), 655–657. 10.1038/35001068 [ PubMed ] [ CrossRef ] [ Google Scholar ]
- * 2 Drummond S. P., Meloy M. J., Yanagi M. A., Orff H. J., & Brown G. G. (2005). Compensatory recruitment after sleep deprivation and the relationship with performance . Psychiatry Research: Neuroimaging , 140 ( 3 ), 211–223. 10.1016/j.pscychresns.2005.06.007 [ PubMed ] [ CrossRef ] [ Google Scholar ]
- Dumas-Mallet E., Button K. S., Boraud T., Gonon F., & Munafò M. R. (2017). Low statistical power in biomedical science: A review of three human research domains . Royal Society Open Science , 4 ( 2 ), Article 160254. 10.1098/rsos.160254 [ PMC free article ] [ PubMed ] [ CrossRef ] [ Google Scholar ]
- Duval S., & Tweedie R. (2000). Trim and fill: A simple funnel-plot–based method of testing and adjusting for publication bias in meta-analysis . Biometrics , 56 ( 2 ), 455–463. 10.1111/j.0006-341X.2000.00455.x [ PubMed ] [ CrossRef ] [ Google Scholar ]
- Egger M., Smith G. D., Schneider M., & Minder C. (1997). Bias in meta-analysis detected by a simple, graphical test . British Medical Journal , 315 ( 7109 ), 629–634. 10.1136/bmj.315.7109.629 [ PMC free article ] [ PubMed ] [ CrossRef ] [ Google Scholar ]
- * 1 Ertelt D., Witt K., Reetz K., Frank W., Junghanns K., Backhaus J., Tadic V., Pellicano A., Born J., Binkofski F., & Binkofski F. (2012). Skill memory escaping from distraction by sleep—Evidence from dual-task performance . PLOS ONE , 7 ( 12 ), Article e50983. 10.1371/journal.pone.0050983 [ PMC free article ] [ PubMed ] [ CrossRef ] [ Google Scholar ]
- * 2 Esposito M. J., Occhionero M., & Cicogna P. (2015). Sleep deprivation and time-based prospective memory . Sleep , 38 ( 11 ), 1823–1826. 10.5665/sleep.5172 [ PMC free article ] [ PubMed ] [ CrossRef ] [ Google Scholar ]
- * 2 Falleti M. G., Maruff P., Collie A., Darby D. G., & McStephen M. (2003). Qualitative similarities in cognitive impairment associated with 24 h of sustained wakefulness and a blood alcohol concentration of 0.05% . Journal of Sleep Research , 12 ( 4 ), 265–274. 10.1111/j.1365-2869.2003.00363.x [ PubMed ] [ CrossRef ] [ Google Scholar ]
- Faul F., Erdfelder E., Lang A. G., & Buchner A. (2007). G*Power 3: A flexible statistical power analysis program for the social, behavioral, and biomedical sciences . Behavior Research Methods , 39 ( 2 ), 175–191. 10.3758/BF03193146 [ PubMed ] [ CrossRef ] [ Google Scholar ]
- * 1 Feld G. B., Weis P. P., & Born J. (2016). The limited capacity of sleep-dependent memory consolidation . Frontiers in Psychology , 7 , Article 1368. 10.3389/fpsyg.2016.01368 [ PMC free article ] [ PubMed ] [ CrossRef ] [ Google Scholar ]
- Fenn K. M., Gallo D. A., Margoliash D., Roediger H. L. III, & Nusbaum H. C. (2009). Reduced false memory after sleep . Learning & Memory , 16 , 509–513. 10.1101/lm.1500808 [ PubMed ] [ CrossRef ] [ Google Scholar ]
- * 1 Ferrara M., Iaria G., De Gennaro L., Guariglia C., Curcio G., Tempesta D., & Bertini M. (2006). The role of sleep in the consolidation of route learning in humans: A behavioural study . Brain Research Bulletin , 71 ( 1–3 ), 4–9. 10.1177/1745691612459059 [ PubMed ] [ CrossRef ] [ Google Scholar ]
- * 1 Ferrara M., Iaria G., Tempesta D., Curcio G., Moroni F., Marzano C., De Gennaro L., & Pacitti C. (2008). Sleep to find your way: The role of sleep in the consolidation of memory for navigation in humans . Hippocampus , 18 ( 8 ), 844–851. 10.1002/hipo.20444 [ PubMed ] [ CrossRef ] [ Google Scholar ]
- * 1 * 2 Fischer S., Hallschmid M., Elsner A. L., & Born J. (2002). Sleep forms memory for finger skills . Proceedings of the National Academy of Sciences , 99 ( 18 ), 11987–11991. 10.1073/pnas.182178199 [ PMC free article ] [ PubMed ] [ CrossRef ] [ Google Scholar ]
- * 1 Fischer S., Nitschke M. F., Melchert U. H., Erdmann C., & Born J. (2005). Motor memory consolidation in sleep shapes more effective neuronal representations . Journal of Neuroscience , 25 ( 49 ), 11248–11255. 10.1523/JNEUROSCI.1743-05.2005 [ PMC free article ] [ PubMed ] [ CrossRef ] [ Google Scholar ]
- * 2 Forest G., & Godbout R. (2000). Effects of sleep deprivation on performance and EEG spectral analysis in young adults . Brain and Cognition , 43 ( 1–3 ), 195–200. [ PubMed ] [ Google Scholar ]
- Fraley R. C., & Vazire S. (2014). The N-pact factor: Evaluating the quality of empirical journals with respect to sample size and statistical power . PLOS ONE , 9 ( 10 ), Article e109019. 10.1371/journal.pone.0109019 [ PMC free article ] [ PubMed ] [ CrossRef ] [ Google Scholar ]
- Funder D. C., & Ozer D. J. (2019). Evaluating effect size in psychological research: Sense and nonsense . Advances in Methods and Practices in Psychological Science , 2 ( 2 ), 156–168. 10.1177/2515245919847202 [ CrossRef ] [ Google Scholar ]
- Gais S., Albouy G., Boly M., Dang-Vu T. T., Darsaud A., Desseilles M., Rauchs G., Schabus M., Sterpenich V., Vandewalle G., Maquet P., & Peigneux P. (2007). Sleep transforms the cerebral trace of declarative memories . Proceedings of the National Academy of Sciences , 104 ( 47 ), 18778–18783. 10.1073/pnas.0705454104 [ PMC free article ] [ PubMed ] [ CrossRef ] [ Google Scholar ]
- Gais S., & Born J. (2004). Declarative memory consolidation: Mechanisms acting during human sleep . Learning and Memory , 11 ( 6 ), 679–685. 10.1101/lm.80504. [ PMC free article ] [ PubMed ] [ CrossRef ] [ Google Scholar ]
- * 1 Gais S., Lucas B., & Born J. (2006). Sleep after learning aids memory recall . Learning and Memory , 13 ( 3 ), 259–262. 10.1101/lm.132106 [ PMC free article ] [ PubMed ] [ CrossRef ] [ Google Scholar ]
- * 2 Giacobbo B. L., Corrêa M. S., Vedovelli K., de Souza C. E. B., Spitza L. M., Gonçalves L., Gonçalves L., Paludo N., Molina R. D., da Rosa E. D., Argimon I. L., & Bromberg E. (2016). Could BDNF be involved in compensatory mechanisms to maintain cognitive performance despite acute sleep deprivation? An exploratory study . International Journal of Psychophysiology , 99 , 96–102. 10.1016/j.ijpsycho.2015.11.008 [ PubMed ] [ CrossRef ] [ Google Scholar ]
- Giuditta A. (2014). Sleep memory processing: The sequential hypothesis . Frontiers in Systems Neuroscience , 8 , Article 219. 10.3389/fnsys.2014.00219 [ PMC free article ] [ PubMed ] [ CrossRef ] [ Google Scholar ]
- * 2 Gorissen M., Tielemans M., & Coenen A. (1997). Alertness and memory after sleep deprivation and diazepam intake . Journal of Psychopharmacology , 11 ( 3 ), 233–239. 10.1177/026988119701100306 [ PubMed ] [ CrossRef ] [ Google Scholar ]
- * 1 Griessenberger H., Hödlmoser K., Heib D. P. J., Lechinger J., Klimesch W., & Schabus M. (2012). Consolidation of temporal order in episodic memories . Biological Psychology , 91 ( 1 ), 150–155. 10.1016/j.biopsycho.2012.05.012 [ PMC free article ] [ PubMed ] [ CrossRef ] [ Google Scholar ]
- Groch S., Zinke K., Wilhelm I., & Born J. (2015). Dissociating the contributions of slow-wave sleep and rapid eye movement sleep to emotional item and source memory . Neurobiology of Learning and Memory , 122 , 122–130. 10.1016/j.nlm.2014.08.013 [ PubMed ] [ CrossRef ] [ Google Scholar ]
- * 2 Grundgeiger T., Bayen U. J., & Horn S. S. (2014). Effects of sleep deprivation on prospective memory . Memory , 22 ( 6 ), 679–686. 10.1016/j.biopsycho.2012.05.012 [ PubMed ] [ CrossRef ] [ Google Scholar ]
- Guyette F. X., Morley J. L., Weaver M. D., Patterson P. D., & Hostler D. (2013). The effect of shift length on fatigue and cognitive performance in air medical providers . Prehospital Emergency Care , 17 ( 1 ), 23–28. 10.3109/10903127.2012.710719 [ PubMed ] [ CrossRef ] [ Google Scholar ]
- * 1 Harrington M. O., Nedberge K. M., & Durrant S. J. (2018). The effect of sleep deprivation on emotional memory consolidation in participants reporting depressive symptoms . Neurobiology of Learning and Memory , 152 , 10–19. 10.1016/j.nlm.2018.04.013 [ PubMed ] [ CrossRef ] [ Google Scholar ]
- * 2 Harrison Y., & Horne J. A. (2000a). Sleep loss and temporal memory . The Quarterly Journal of Experimental Psychology: Section A , 53 ( 1 ), 271–279. 10.1080/713755870 [ PubMed ] [ CrossRef ] [ Google Scholar ]
- Harrison Y., & Horne J. A. (2000b). The impact of sleep deprivation on decision making: A review . Journal of Experimental Psychology: Applied , 6 ( 3 ), 236–249. 10.1037/1076-898X.6.3.236 [ PubMed ] [ CrossRef ] [ Google Scholar ]
- * 2 Harrison Y., Horne J. A., & Rothwell A. (2000). Prefrontal neuropsychological effects of sleep deprivation in young adults—A model for healthy aging? Sleep , 23 ( 8 ), 1067–1073. 10.1093/sleep/23.8.1f [ PubMed ] [ CrossRef ] [ Google Scholar ]
- Hempel S., Miles J. N., Booth M. J., Wang Z., Morton S. C., & Shekelle P. G. (2013). Risk of bias: A simulation study of power to detect study-level moderator effects in meta-analysis . Systematic Reviews , 2 ( 1 ), 1–10. 10.1186/2046-4053-2-107 [ PMC free article ] [ PubMed ] [ CrossRef ] [ Google Scholar ]
- * 1 Herzog N., Friedrich A., Fujita N., Gais S., Jauch-Chara K., Oltmanns K. M., & Benedict C. (2012). Effects of daytime food intake on memory consolidation during sleep or sleep deprivation . PLOS ONE , 7 ( 6 ), Article e40298. 10.1371/journal.pone.0040298 [ PMC free article ] [ PubMed ] [ CrossRef ] [ Google Scholar ]
- Higgins J. P., Altman D. G., Gøtzsche P. C., Jüni P., Moher D., Oxman A. D., Savović J., Schulz K. F., Weeks L., & Sterne J. A. C., (2011). The cochrane collaboration’s tool for assessing risk of bias in randomised trials . British Medical Journal , 343 , Article d5928. 10.1136/bmj.d5928 [ PMC free article ] [ PubMed ] [ CrossRef ] [ Google Scholar ]
- Higgins J. P., & Thompson S. G. (2002). Quantifying heterogeneity in a meta-analysis . Statistics in Medicine , 21 ( 11 ), 1539–1558. 10.1002/sim.1186 [ PubMed ] [ CrossRef ] [ Google Scholar ]
- Hoedlmoser K. (2020). Sleep and memory in children . Current Sleep Medicine Reports , 6 ( 4 ), 280–289. 10.1007/s40675-020-00194-8 [ CrossRef ] [ Google Scholar ]
- Hu P., Stylos-Allan M., & Walker M. P. (2006). Sleep facilitates consolidation of emotional declarative memory . Psychological Science , 17 ( 10 ), 891–898. 10.1111/j.1467-9280.2006.01799.x [ PubMed ] [ CrossRef ] [ Google Scholar ]
- Hunter J. E., & Schmidt F. L. (2004). Methods of meta-analysis: Correcting error and bias in research findings . Sage Publications. [ Google Scholar ]
- * 1 Idzikowski C. (1984). Sleep and memory . British Journal of Psychology , 75 ( 4 ), 439–449. 10.1111/j.2044-8295.1984.tb01914.x [ PubMed ] [ CrossRef ] [ Google Scholar ]
- Inostroza M., & Born J. (2013). Sleep for preserving and transforming episodic memory . Annual Review of Neuroscience , 36 , 79–102. 10.1146/annurev-neuro-062012-170429 [ PubMed ] [ CrossRef ] [ Google Scholar ]
- Ioannidis J. P. (2005). Why most published research findings are false . PLoS Medicine , 2 ( 8 ), Article e124. 10.1371/journal.pmed.0020124 [ PMC free article ] [ PubMed ] [ CrossRef ] [ Google Scholar ]
- Jacoby L. L. (1991). A process dissociation framework: Separating automatic from intentional uses of memory . Journal of Memory and Language , 30 ( 5 ), 513–541. 10.1016/0749-596X(91)90025-F [ CrossRef ] [ Google Scholar ]
- Johnson B. T. (2021). Toward a more transparent, rigorous, and generative psychology . Psychological Bulletin , 147 ( 1 ), 1–15. 10.1037/bul0000317 [ PubMed ] [ CrossRef ] [ Google Scholar ]
- * 2 Kaida K., Niki K., & Born J. (2015). Role of sleep for encoding of emotional memory . Neurobiology of Learning and Memory , 121 , 72–79. 10.1016/j.nlm.2015.04.002 [ PubMed ] [ CrossRef ] [ Google Scholar ]
- Kilpeläinen A. A., Huttunen K. H., Lohi J. J., & Lyytinen H. (2010). Effect of caffeine on vigilance and cognitive performance during extended wakefulness . The International Journal of Aviation Psychology , 20 ( 2 ), 144–159. 10.1080/10508411003617847 [ CrossRef ] [ Google Scholar ]
- Kim S. Y., & Payne J. D. (2020). Neural correlates of sleep, stress, and selective memory consolidation . Current Opinion in Behavioral Sciences , 33 , 57–64. 10.1016/j.cobeha.2019.12.009 [ CrossRef ] [ Google Scholar ]
- King B. R., Hoedlmoser K., Hirschauer F., Dolfen N., & Albouy G. (2017). Sleeping on the motor engram: The multifaceted nature of sleep-related motor memory consolidation . Neuroscience and Biobehavioral Reviews , 80 , 1–22. 10.1016/j.neubiorev.2017.04.026 [ PubMed ] [ CrossRef ] [ Google Scholar ]
- Klinzing J. G., Niethard N., & Born J. (2019). Mechanisms of systems memory consolidation during sleep . Nature Neuroscience , 22 , 1598–1610. 10.1038/s41593-019-0467-3 [ PubMed ] [ CrossRef ] [ Google Scholar ]
- * 1 Kolibius L. D., Born J., & Feld G. B. (2021). Vast amounts of encoded items nullify but do not reverse the effect of sleep on declarative memory . Frontiers in Psychology , 11 , Article 3685. 10.3389/fpsyg.2020.607070 [ PMC free article ] [ PubMed ] [ CrossRef ] [ Google Scholar ]
- Korman M., Doyon J., Doljansky J., Carrier J., Dagan Y., & Karni A. (2007). Daytime sleep condenses the time course of motor memory consolidation . Nature Neuroscience , 10 ( 9 ), 1206–1213. 10.1038/nn1959 [ PubMed ] [ CrossRef ] [ Google Scholar ]
- Kumaran D., Hassabis D., & McClelland J. L. (2016). What learning systems do intelligent agents need? Complementary learning systems theory updated . Trends in Cognitive Sciences , 20 , 512–534. 10.1016/j.tics.2016.05.004 [ PubMed ] [ CrossRef ] [ Google Scholar ]
- * 1 Kuriyama K., Honma M., Yoshiike T., & Kim Y. (2013). Memory suppression trades prolonged fear and sleep-dependent fear plasticity for the avoidance of current fear . Scientific Reports , 3 , Article 2227. 10.1038/srep02227 [ PMC free article ] [ PubMed ] [ CrossRef ] [ Google Scholar ]
- * 1 Kuriyama K., Soshi T., & Kim Y. (2010). Sleep deprivation facilitates extinction of implicit fear generalization and physiological response to fear . Biological Psychiatry , 68 ( 11 ), 991–998. 10.1016/j.biopsych.2010.08.015 [ PubMed ] [ CrossRef ] [ Google Scholar ]
- * 1 Lahl O., & Pietrowsky R. (2006). Does the “sleep effect” on memory depend on sleep or on night time? Sleep and Hypnosis , 8 ( 2 ), 61–70. [ Google Scholar ]
- Lakens D. (2013). Calculating and reporting effect sizes to facilitate cumulative science: A practical primer for t -tests and ANOVAs . Frontiers in Psychology , 4 , Article 863. 10.3389/fpsyg.2013.00863 [ PMC free article ] [ PubMed ] [ CrossRef ] [ Google Scholar ]
- Lakens D., & Caldwell A. R. (2021). Simulation-based power analysis for factorial analysis of variance designs . Advances in Methods and Practices in Psychological Science , 4 , 1–14. 10.1177/2515245920951503 [ CrossRef ] [ Google Scholar ]
- Lewis P. A., & Durrant S. J. (2011). Overlapping memory replay during sleep builds cognitive schemata . Trends in Cognitive Sciences , 15 , 343–351. 10.1016/j.tics.2011.06.004 [ PubMed ] [ CrossRef ] [ Google Scholar ]
- Lewis P. A., Knoblich G., & Poe G. (2018). How memory replay in sleep boosts creative problem-solving . Trends in Cognitive Sciences , 22 , 491–503. 10.1016/j.tics.2018.03.009 [ PMC free article ] [ PubMed ] [ CrossRef ] [ Google Scholar ]
- Lim J., & Dinges D. (2008). Sleep deprivation and vigilant attention . Annals of the New York Academy of Sciences , 1129 ( 1 ), Article 305. 10.1196/annals.1417.002 [ PubMed ] [ CrossRef ] [ Google Scholar ]
- Lim J., & Dinges D. F. (2010). A meta-analysis of the impact of short-term sleep deprivation on cognitive variables . Psychological Bulletin , 136 ( 3 ), 375–389. 10.1037/a0018883 [ PMC free article ] [ PubMed ] [ CrossRef ] [ Google Scholar ]
- * 1 Lin C. C., & Yang C. M. (2014). Evidence of sleep-facilitating effect on formation of novel semantic associations: An event-related potential (ERP) study . Neurobiology of Learning and Memory , 116 , 69–78. 10.1016/j.nlm.2014.08.011 [ PubMed ] [ CrossRef ] [ Google Scholar ]
- Lipinska G., Stuart B., Thomas K. G., Baldwin D. S., & Bolinger E. (2019). Preferential consolidation of emotional memory during sleep: a meta-analysis . Frontiers in Psychology , 10 , Article 1014. 10.3389/fpsyg.2019.01014 [ PMC free article ] [ PubMed ] [ CrossRef ] [ Google Scholar ]
- * 2 Lo J. C., Chong P. L., Ganesan S., Leong R. L., & Chee M. W. (2016). Sleep deprivation increases formation of false memory . Journal of Sleep Research , 25 ( 6 ), 673–682. 10.1111/jsr.12436 [ PMC free article ] [ PubMed ] [ CrossRef ] [ Google Scholar ]
- Lo J. C., Groeger J. A., Cheng G. H., Dijk D. J., & Chee M. W. (2016). Self-reported sleep duration and cognitive performance in older adults: A systematic review and meta-analysis . Sleep Medicine , 17 , 87–98. 10.1016/j.sleep.2015.08.021 [ PubMed ] [ CrossRef ] [ Google Scholar ]
- Lo J. C., Sim S. K. Y., Chee M. W. L. (2014). Sleep reduces false memory in healthy older adults . Sleep , 37 , 665–671. 10.5665/sleep.3564 [ PMC free article ] [ PubMed ] [ CrossRef ] [ Google Scholar ]
- Lowe C. J., Safati A., & Hall P. A. (2017). The neurocognitive consequences of sleep restriction: A meta-analytic review . Neuroscience & Biobehavioral Reviews , 80 , 586–604. 10.1016/j.neubiorev.2017.07.010 [ PubMed ] [ CrossRef ] [ Google Scholar ]
- * 1 Maddox W. T., Glass B. D., Wolosin S. M., Savarie Z. R., Bowen C., Matthews M. D., & Schnyer D. M. (2009). The effects of sleep deprivation on information-integration categorization performance . Sleep , 32 ( 11 ), 1439–1448. 10.1093/sleep/32.11.1439 [ PMC free article ] [ PubMed ] [ CrossRef ] [ Google Scholar ]
- * 2 Maddox W. T., Glass B. D., Zeithamova D., Savarie Z. R., Bowen C., Matthews M. D., & Schnyer D. M. (2011). The effects of sleep deprivation on dissociable prototype learning systems . Sleep , 34 ( 3 ), 253–260. 10.1093/sleep/34.3.253 [ PMC free article ] [ PubMed ] [ CrossRef ] [ Google Scholar ]
- Mander B. A., Santhanam S., Saletin J. M., & Walker M. P. (2011). Wake deterioration and sleep restoration of human learning . Current Biology , 21 ( 5 ), R183–R184. 10.1016/j.cub.2011.01.019 [ PMC free article ] [ PubMed ] [ CrossRef ] [ Google Scholar ]
- Manoach D. S., & Stickgold R. (2019). Abnormal sleep spindles, memory consolidation, and schizophrenia . Annual Review of Clinical Psychology , 15 , 451–479. 10.1146/annurev-clinpsy-050718-095754 [ PMC free article ] [ PubMed ] [ CrossRef ] [ Google Scholar ]
- McClelland J. L., McNaughton B. L., & O’Reilly R. C. (1995). Why there are complementary learning systems in the hippocampus and neocortex: Insights from the successes and failures of connectionist models of learning and memory . Psychological Review , 102 ( 3 ), 419–457. 10.1037/0033-295X.102.3.419 [ PubMed ] [ CrossRef ] [ Google Scholar ]
- McKeon S., Pace-Schott E. F., Spencer R. M. C. (2012). Interaction of sleep and emotional content on the production of false memories . PLOS ONE , 7 , Article e49353. 10.1371/journal.pone.0049353 [ PMC free article ] [ PubMed ] [ CrossRef ] [ Google Scholar ]
- * 2 McWhirter K. K., Morrow A. S., Lee B. A., Bishu S., Zametkin A. J., Balkin T. J., Smith C. B., & Picchioni D. (2015). A pilot study on the encoding of a perceptual learning task following sleep deprivation . Perceptual and Motor Skills , 121 ( 1 ), 80–93. 10.2466/23.PMS.121c11x9 [ PMC free article ] [ PubMed ] [ CrossRef ] [ Google Scholar ]
- * 1 Mograss M. A., Guillem F., Brazzini-Poisson V., & Godbout R. (2009). The effects of total sleep deprivation on recognition memory processes: A study of event-related potential . Neurobiology of Learning and Memory , 91 ( 4 ), 343–352. 10.1016/j.nlm.2009.01.008 [ PubMed ] [ CrossRef ] [ Google Scholar ]
- * 1 Montemayor B. A. (2014). Musical task: Sleep deprivation and its effects on motor learning [Masters dissertation]. University of Houston-Clear Lake. Proquest. https://www.proquest.com/docview/1532140044?pq-origsite=gscholar&fromopenview=true [ Google Scholar ]
- Morgan D. P., Tamminen J., Seale-Carlisle T. M., & Mickes L. (2019). The impact of sleep on eyewitness identifications . Royal Society Open Science , 6 ( 12 ), Article 170501. 10.1098/rsos.170501 [ PMC free article ] [ PubMed ] [ CrossRef ] [ Google Scholar ]
- * 1 Nesca M., & Koulack D. (1994). Recognition memory, sleep and circadian rhythms . Canadian Journal of Experimental Psychology , 48 ( 3 ), 359–379. 10.1037/1196-1961.48.3.359 [ PubMed ] [ CrossRef ] [ Google Scholar ]
- Newbury C. R., Monaghan P. (2019). When does sleep affect veridical and false memory consolidation? A meta-analysis . Psychonomic Bulletin and Review , 26 , 387–400. 10.3758/s13423-018-1528-4 [ PMC free article ] [ PubMed ] [ CrossRef ] [ Google Scholar ]
- * 2 Nilsson L. G., Bäckman L., & Karlsson T. (1989). Priming and cued recall in elderly, alcohol intoxicated and sleep deprived subjects: A case of functionally similar memory deficits . Psychological Medicine , 19 ( 2 ), 423–433. 10.1017/s0033291700012460 [ PubMed ] [ CrossRef ] [ Google Scholar ]
- Nosek B. A., Alter G., Banks G. C., Borsboom D., Bowman S. D., Breckler S. J., Buck S., Chambers C. D., Chin G., Christensen G., Contestabile M., Dafoe A., Eich E., Freese J., Glennerster R., Goroff D., Green D. P., Hesse B., Humphreys M., et al.Yarkoni T. (2015). Promoting an open research culture . Science , 348 ( 6242 ), 1422–1425. 10.1126/science.aab2374 [ PMC free article ] [ PubMed ] [ CrossRef ] [ Google Scholar ]
- Nosek B. A., & Lindsay D. S. (2018, February 28). Preregistration becoming the norm in psychological science . APS Observer . https://www.psychologicalscience.org/observer/preregistration-becoming-the-norm-in-psychological-science [ Google Scholar ]
- * 2 Occhionero M., Cicogna P., & Esposito M. J. (2017). The effect of sleep loss on dual time-based prospective memory tasks . American Journal of Psychology , 130 ( 1 ), 93–103. 10.5406/amerjpsyc.130.1.0093 [ PubMed ] [ CrossRef ] [ Google Scholar ]
- * 1 Orban P., Rauchs G., Balteau E., Degueldre C., Luxen A., Maquet P., & Peigneux P. (2006). Sleep after spatial learning promotes covert reorganization of brain activity . Proceedings of the National Academy of Sciences , 103 ( 18 ), 7124–7129. 10.1073/pnas.0510198103 [ PMC free article ] [ PubMed ] [ CrossRef ] [ Google Scholar ]
- * 2 Pace-Schott E. F., Hutcherson C. A., Bemporad B., Morgan A., Kumar A., Hobson J. A., & Stickgold R. (2009). Failure to find executive function deficits following one night’s total sleep deprivation in university students under naturalistic conditions . Behavioral Sleep Medicine , 7 ( 3 ), 136–163. 10.1080/15402000902976671 [ PMC free article ] [ PubMed ] [ CrossRef ] [ Google Scholar ]
- Pardilla-Delgado E., & Payne J. D. (2017). The impact of sleep on true and false memory across long delays . Neurobiology of Learning and Memory , 137 , 123–133. 10.1016/j.nlm.2016.11.016 [ PubMed ] [ CrossRef ] [ Google Scholar ]
- Payne J. D., & Kensinger E. A. (2010). Sleep’s role in the consolidation of emotional episodic memories . Current Directions in Psychological Science , 19 , 290–295. 10.1177/0963721410383978 [ CrossRef ] [ Google Scholar ]
- Payne J. D., Kensinger E. A., Wamsley E. J., Spreng R. N., Alger S. E., Gibler K., Schacter D. L., & Stickgold R. (2015). Napping and the selective consolidation of negative aspects of scenes . Emotion , 15 , 176–186. 10.1037/a0038683 [ PMC free article ] [ PubMed ] [ CrossRef ] [ Google Scholar ]
- Payne J. D., Schacter D. L., Propper R. E., Huang L. W., Wamsley E. J., Tucker M. A., Walker M. P., & Stickgold R. (2009). The role of sleep in false memory formation . Neurobiology of Learning and Memory , 92 , 327–334. 10.1016/j.nlm.2009.03.007 [ PMC free article ] [ PubMed ] [ CrossRef ] [ Google Scholar ]
- Payne J. D., Stickgold R., Swanberg K., & Kensinger E. A. (2008). Sleep preferentially enhances memory for emotional components of scenes . Psychological Science , 19 ( 8 ), 781–788. 10.1111/j.1467-9280.2008.02157.x [ PMC free article ] [ PubMed ] [ CrossRef ] [ Google Scholar ]
- * 1 Peigneux P., Schmitz R., & Willems S. (2007). Cerebral asymmetries in sleep-dependent processes of memory consolidation . Learning and Memory , 14 ( 6 ), 400–406. 10.1101/lm.551207 [ PMC free article ] [ PubMed ] [ CrossRef ] [ Google Scholar ]
- Piérard C., Béracochéa D., Pérès M., Jouanin J. C., Liscia P., Satabin P., Martin S., Testylier G., Guézennec C. Y., & Beaumont M. (2004). Declarative memory impairments following a military combat course: Parallel neuropsychological and biochemical investigations . Neuropsychobiology , 49 ( 4 ), 210–217. 10.1159/000077369 [ PubMed ] [ CrossRef ] [ Google Scholar ]
- Pilcher J. J., & Huffcutt A. I. (1996). Effects of sleep deprivation on performance: A meta-analysis . Sleep , 19 ( 4 ), 318–326. 10.1093/sleep/19.4.318 [ PubMed ] [ CrossRef ] [ Google Scholar ]
- Plihal W., & Born J. (1997). Effects of early and late nocturnal sleep on declarative and procedural memory . Journal of Cognitive Neuroscience , 9 ( 4 ), 534–547. 10.1162/jocn.1997.9.4.534 [ PubMed ] [ CrossRef ] [ Google Scholar ]
- * 2 Poh J. H., & Chee M. W. (2017). Degradation of cortical representations during encoding following sleep deprivation . Neuroimage , 153 , 131–138. 10.1016/j.neuroimage.2017.01.080 [ PubMed ] [ CrossRef ] [ Google Scholar ]
- Pollard P., & Richardson J. T. (1987). On the probability of making Type I errors . Psychological Bulletin , 102 ( 1 ), 159–163. 10.1037/0033-2909.102.1.159 [ CrossRef ] [ Google Scholar ]
- * 1 Porcheret K., Holmes E. A., Goodwin G. M., Foster R. G., & Wulff K. (2015). Psychological effect of an analogue traumatic event reduced by sleep deprivation . Sleep , 38 ( 7 ), 1017–1025. 10.5665/sleep.4802 [ PMC free article ] [ PubMed ] [ CrossRef ] [ Google Scholar ]
- Rauchs G., Bertran F., Guillery-Girard B., Desgranges B., Kerrouche N., Denise P., Foret J., & Eustache F. (2004). Consolidation of strictly episodic memories mainly requires rapid eye movement sleep . Sleep , 27 ( 3 ), 395–401. 10.1093/sleep/27.3.395 [ PubMed ] [ CrossRef ] [ Google Scholar ]
- * 1 Rauchs G., Orban P., Schmidt C., Albouy G., Balteau E., Degueldre C., Schnackers C., Sterpenich V., Tinguely G., Luxen A., Maquet P., & Peigneux P. (2008). Sleep modulates the neural substrates of both spatial and contextual memory consolidation . PLOS ONE , 3 ( 8 ), Article e2949. 10.1371/journal.pone.0002949 [ PMC free article ] [ PubMed ] [ CrossRef ] [ Google Scholar ]
- Robertson E. M., Pascual-Leone A., & Press D. Z. (2004). Awareness modifies the skill-learning benefits of sleep . Current Biology , 14 ( 3 ), 208–212. 10.1016/j.cub.2004.01.027 [ PubMed ] [ CrossRef ] [ Google Scholar ]
- * 2 Roehrs T., Burduvali E., Bonahoom A., Drake C., & Roth T. (2003). Ethanol and sleep loss: A “dose” comparison of impairing effects . Sleep , 26 ( 8 ), 981–985. 10.1093/sleep/26.8.981 [ PubMed ] [ CrossRef ] [ Google Scholar ]
- Rohatgi A. (2019). WebPlotDigitizer (Version 4.2) . https://automeris.io/WebPlotDigitizer
- Rosenthal R. (1979). The file drawer problem and tolerance for null results . Psychological Bulletin , 86 ( 3 ), 638–641. 10.1037/0033-2909.86.3.638 [ CrossRef ] [ Google Scholar ]
- Rothstein H. R., Sutton A. J., & Borenstein M. (Eds.). (2005). Publication bias in meta-analysis: Prevention, assessment and adjustments . Wiley. [ Google Scholar ]
- Saletin J. M., & Walker M. P. (2012). Nocturnal mnemonics: Sleep and hippocampal memory processing . Frontiers in Neurology , 3 , Article 59. 10.3389/fneur.2012.00059 [ PMC free article ] [ PubMed ] [ CrossRef ] [ Google Scholar ]
- Sawangjit A., Oyanedel C. N., Niethard N., Salazar C., Born J., & Inostroza M. (2018). The hippocampus is crucial for forming non-hippocampal long-term memory during sleep . Nature 564 , 109–113. 10.1038/s41586-018-0716-8 [ PubMed ] [ CrossRef ] [ Google Scholar ]
- Schäfer S. K., Wirth B. E., Staginnus M., Becker N., Michael T., & Sopp M. R. (2020). Sleep’s impact on emotional recognition memory: A meta-analysis of whole-night, nap, and REM sleep effects . Sleep Medicine Reviews , 51 , Article 101280. 10.1016/j.smrv.2020.101280 [ PubMed ] [ CrossRef ] [ Google Scholar ]
- Schapiro A. C., Reid A. G., Morgan A., Manoach D. S., Verfaellie M., & Stickgold R. (2019). The hippocampus is necessary for the consolidation of a task that does not require the hippocampus for initial learning . Hippocampus , 29 , 1091–1100. 10.1002/hipo.23101 [ PMC free article ] [ PubMed ] [ CrossRef ] [ Google Scholar ]
- Schönauer M., Geisler T., & Gais S. (2014). Strengthening procedural memories by reactivation in sleep . Journal of Cognitive Neuroscience , 26 ( 1 ), 143–153. 10.1162/jocn_a_00471 [ PubMed ] [ CrossRef ] [ Google Scholar ]
- * 1 Schönauer M., Grätsch M., & Gais S. (2015). Evidence for two distinct sleep-related long-term memory consolidation processes . Cortex , 63 , 68–78. 10.1016/j.cortex.2014.08.005 [ PubMed ] [ CrossRef ] [ Google Scholar ]
- Siddaway A. P., Wood A. M., & Hedges L. V. (2019). How to do a systematic review: A best practice guide for conducting and reporting narrative reviews, meta-analyses, and meta-syntheses . Annual Review of Psychology , 70 , 747–770. 10.1146/annurev-psych-010418-102803 [ PubMed ] [ CrossRef ] [ Google Scholar ]
- Stepan M. E., Dehnke T. M., & Fenn K. M. (2017). Sleep and eyewitness memory: Fewer false identifications after sleep when the target is absent from the lineup . PLOS ONE , 12 , Article e0182907. 10.1371/journal.pone.0182907 [ PMC free article ] [ PubMed ] [ CrossRef ] [ Google Scholar ]
- Sterne J. A., Sutton A. J., Ioannidis J. P., Terrin N., Jones D. R., Lau J., Carpenter J., Rücker G., Harbord R. M., Schmid C. H., Tetzlaff J., Deeks J. J., Peters J., Macaskill P., Schwarzer G., Duval S., Altman D. G., Moher D., & Higgins J. P. T. (2011). Recommendations for examining and interpreting funnel plot asymmetry in meta-analyses of randomised controlled trials . British Medical Journal , 343 , Article d4002. 10.1136/bmj.d4002 [ PubMed ] [ CrossRef ] [ Google Scholar ]
- * 1 Sterpenich V., Albouy G., Boly M., Vandewalle G., Darsaud A., Balteau E., Dang-Vu T. T., Desseilles M., D’Argembeau A., Gais S., Rauchs G., Schabus M., Degueldre C., Luxen A., Collette F., & Maquet P. (2007). Sleep-related hippocampo-cortical interplay during emotional memory recollection . PLoS Biology , 5 ( 11 ), Article e282. 10.1371/journal.pbio.0050282 [ PMC free article ] [ PubMed ] [ CrossRef ] [ Google Scholar ]
- * 1 Sterpenich V., Albouy G., Darsaud A., Schmidt C., Vandewalle G., Vu T. T. D., Desseilles M., Phillips C., Degueldre C., Balteau E., Collette F., Luxen A., & Maquet P. (2009). Sleep promotes the neural reorganization of remote emotional memory . Journal of Neuroscience , 29 ( 16 ), 5143–5152. 10.1523/JNEUROSCI.0561-09.2009 [ PMC free article ] [ PubMed ] [ CrossRef ] [ Google Scholar ]
- * 1 Sterpenich V., Ceravolo L., & Schwartz S. (2017). Sleep deprivation disrupts the contribution of the hippocampus to the formation of novel lexical associations . Brain and Language , 167 , 61–71. 10.1016/j.bandl.2016.12.007 [ PubMed ] [ CrossRef ] [ Google Scholar ]
- * 1 Stickgold R., James L., & Hobson J. A. (2000). Visual discrimination learning requires sleep after training . Nature Neuroscience , 3 ( 12 ), 1237–1238. 10.1038/81756. [ PubMed ] [ CrossRef ] [ Google Scholar ]
- Stickgold R., & Walker M. P. (2013). Sleep-dependent memory triage: Evolving generalization through selective processing . Nature Neuroscience , 16 , 139–145. 10.1038/nn.3303 [ PMC free article ] [ PubMed ] [ CrossRef ] [ Google Scholar ]
- Stone J. C., Glass K., Munn Z., Tugwell P., & Doi S. A. (2020). Comparison of bias adjustment methods in meta-analysis suggests that quality effects modeling may have less limitations than other approaches . Journal of Clinical Epidemiology , 117 , 36–45. 10.1016/j.jclinepi.2019.09.010 [ PubMed ] [ CrossRef ] [ Google Scholar ]
- * 2 Stricker J. L., Brown G. G., Wetherell L. A., & Drummond S. P. (2006). The impact of sleep deprivation and task difficulty on networks of fMRI brain response . Journal of the International Neuropsychological Society , 12 ( 5 ), 591–597. 10.1017/S1355617706060851 [ PubMed ] [ CrossRef ] [ Google Scholar ]
- Szucs D., & Ioannidis J. P. (2017). Empirical assessment of published effect sizes and power in the recent cognitive neuroscience and psychology literature . PLoS Biology , 15 ( 3 ), Article e2000797. 10.1371/journal.pbio.2000797 [ PMC free article ] [ PubMed ] [ CrossRef ] [ Google Scholar ]
- Talamini L. M., Nieuwenhuis I. L., Takashima A., & Jensen O. (2008). Sleep directly following learning benefits consolidation of spatial associative memory . Learning and Memory , 15 ( 4 ), 233–237. 10.1101/lm.771608 [ PubMed ] [ CrossRef ] [ Google Scholar ]
- * 1 * 2 Tamminen J., Newbury C. R., Crowley R., Vinals L., Cevoli B., & Rastle K. (2020). Generalisation in language learning can withstand total sleep deprivation . Neurobiology of Learning and Memory , 173 , Article 107274. 10.1016/j.nlm.2020.107274 [ PubMed ] [ CrossRef ] [ Google Scholar ]
- * 1 Tempesta D., De Gennaro L., Natale V., & Ferrara M. (2015). Emotional memory processing is influenced by sleep quality . Sleep Medicine , 16 ( 7 ), 862–870. 10.1016/j.sleep.2015.01.024 [ PubMed ] [ CrossRef ] [ Google Scholar ]
- * 2 Tempesta D., Socci V., Coppo M., Ioio G. D., Nepa V., De Gennaro L., & Ferrara M. (2016). The effect of sleep deprivation on the encoding of contextual and non-contextual aspects of emotional memory . Neurobiology of Learning and Memory , 131 , 9–17. 10.1016/j.nlm.2016.03.007 [ PubMed ] [ CrossRef ] [ Google Scholar ]
- * 1 Tempesta D., Socci V., Ioio G. D., De Gennaro L., & Ferrara M. (2017). The effect of sleep deprivation on retrieval of emotional memory: A behavioural study using film stimuli . Experimental Brain Research , 235 ( 10 ), 3059–3067. 10.1007/s00221-01705043-z [ PubMed ] [ CrossRef ] [ Google Scholar ]
- Tononi G., & Cirelli C. (2003). Sleep and synaptic homeostasis: A hypothesis . Brain Research Bulletin , 62 ( 2 ), 143–150. 10.1016/j.brainresbull.2003.09.004 [ PubMed ] [ CrossRef ] [ Google Scholar ]
- Tononi G., & Cirelli C. (2012). Time to be SHY? Some comments on sleep and synaptic homeostasis . Neural Plasticity , 12 , 1–12. 10.1155/2012/415250 [ PMC free article ] [ PubMed ] [ CrossRef ] [ Google Scholar ]
- Twenge J. M., Krizan Z., & Hisler G. (2017). Decreases in self-reported sleep duration among US adolescents 2009–2015 and association with new media screen time . Sleep Medicine , 39 , 47–53. 10.1155/2012/415250 [ PubMed ] [ CrossRef ] [ Google Scholar ]
- Valentine J. C. (2009). Judging the quality of primary research. In The handbook of research synthesis and meta-analysis (2nd ed., pp. 129–146). Sage Publications. [ Google Scholar ]
- Van Der Werf Y. D., Altena E., Schoonheim M. M., Sanz-Arigita E. J., Vis J. C., De Rijke W., & Van Someren E. J. (2009). Sleep benefits subsequent hippocampal functioning . Nature Neuroscience , 12 ( 2 ), 122–123. 10.1038/nn.2253 [ PubMed ] [ CrossRef ] [ Google Scholar ]
- * 1 van Heugten–van der Kloet D., Giesbrecht T., & Merckelbach H. (2015). Sleep loss increases dissociation and affects memory for emotional stimuli . Journal of Behavior Therapy and Experimental Psychiatry , 47 , 9–17. 10.1016/j.jbtep.2014.11.002 [ PubMed ] [ CrossRef ] [ Google Scholar ]
- * 1 Vargas I., Payne J. D., Muench A., Kuhlman K. R., & Lopez-Duran N. L. (2019). Acute sleep deprivation and the selective consolidation of emotional memories . Learning and Memory , 26 ( 6 ), 176–181. 10.1101/lm.049312.119 [ PMC free article ] [ PubMed ] [ CrossRef ] [ Google Scholar ]
- Vargas I. P., Aguiar S. A., & Barela J. A. (2017). Effects of sleep deprivation on sustained attention in young adults . Brazilian Journal of Motor Behavior , 11 ( 1 ), 1–9. 10.20338/bjmb.v11i1.96 [ CrossRef ] [ Google Scholar ]
- Vidya S., Patlolla V. R. P., Kamuju N. R., Ampalam P., & Kalyan V. K. S. N. (2019). Impact of shift work on sleep and quality of life in industrial workers: A cross sectional study . Archives of Mental Health , 20 ( 2 ), Article 45. 10.4103/AMH.AMH_3_19 [ CrossRef ] [ Google Scholar ]
- Viechtbauer W. (2010). Conducting meta-analyses in R with the metafor package . Journal of Statistical Software , 36 ( 3 ), 1–48. 10.18637/jss.v036.i03 [ CrossRef ] [ Google Scholar ]
- Viechtbauer W., & Cheung M. W. L. (2010). Outlier and influence diagnostics for meta-analysis . Research Synthesis Methods , 1 ( 2 ), 112–125. 10.1002/jrsm.11 [ PubMed ] [ CrossRef ] [ Google Scholar ]
- Voderholzer U., Piosczyk H., Holz J., Landmann N., Feige B., Loessl B., Kpasz M., Doerr J. P., Riemann D., & Nissen C. (2011). Sleep restriction over several days does not affect long–term recall of declarative and procedural memories in adolescents . Sleep Medicine , 12 ( 2 ), 170–178. 10.1016/j.sleep.2010.07.017 [ PubMed ] [ CrossRef ] [ Google Scholar ]
- Wagner U., Gais S., & Born J. (2001). Emotional memory formation is enhanced across sleep intervals with high amounts of rapid eye movement sleep . Learning & Memory , 8 ( 2 ), 112–119. 10.1101/lm.36801 [ PMC free article ] [ PubMed ] [ CrossRef ] [ Google Scholar ]
- Wagner U., Hallschmid M., Rasch B., & Born J. (2006). Brief sleep after learning keeps emotional memories alive for years . Biological Psychiatry , 60 ( 7 ), 788–790. 10.1016/j.biopsych.2006.03.061 [ PubMed ] [ CrossRef ] [ Google Scholar ]
- Walker M. P., Brakefield T., Morgan A., Hobson J. A., & Stickgold R. (2002). Practice with sleep makes perfect: Sleep-dependent motor skill learning . Neuron , 35 ( 1 ), 205–211. 10.1016/S0896-6273(02)00746-8 [ PubMed ] [ CrossRef ] [ Google Scholar ]
- Walker M. P., & Stickgold R. (2006). Sleep, memory, and plasticity . Annual Review of Psychology , 57 , 139–166. 10.1146/annurev.psych.56.091103.070307 [ PubMed ] [ CrossRef ] [ Google Scholar ]
- Walker M. P., Stickgold R., Alsop D., Gaab N., & Schlaug G. (2005). Sleep-dependent motor memory plasticity in the human brain . Neuroscience , 133 ( 4 ), 911–917. 10.1016/j.neuroscience.2005.04.007 [ PubMed ] [ CrossRef ] [ Google Scholar ]
- Wilson B. M., Harris C. R., & Wixted J. T. (2020). Science is not a signal detection problem . Proceedings of the National Academy of Sciences , 117 ( 11 ), 5559–5567. 10.1073/pnas.1914237117 [ PMC free article ] [ PubMed ] [ CrossRef ] [ Google Scholar ]
- Yonelinas A. P. (2002). The nature of recollection and familiarity: A review of 30 years of research . Journal of Memory and Language , 46 ( 3 ), 441–517. 10.1006/jmla.2002.2864 [ CrossRef ] [ Google Scholar ]
- * 2 Yoo S. S., Hu P. T., Gujar N., Jolesz F. A., & Walker M. P. (2007). A deficit in the ability to form new human memories without sleep . Nature Neuroscience , 10 ( 3 ), 385–392. 10.1038/nn1851 [ PubMed ] [ CrossRef ] [ Google Scholar ]

IMAGES
COMMENTS
Working memory. Working memory is primarily associated with the prefrontal and posterior parietal cortex (Sarnthein et al., 1998; Todd and Marois, 2005).Working memory is not localized to a single brain region, and research suggests that it is an emergent property arising from functional interactions between the prefrontal cortex (PFC) and the rest of the brain (D'Esposito, 2007).
Abstract. Memory is the process of retaining of knowledge over a period for the function of affecting future actions. It can be divided into declarative and procedural types. The process of memory consolidation is done in the hippocampus. ... From a historical standpoint, the area of memory research from 1870 to 1920 was focused mainly on human ...
Abstract. Research in the last five years has made great strides toward mechanistic explanations of how the brain enables memory. This progress builds upon decades of research from two complementary strands: a Levels of Analysis approach and a Levels of Organization approach. We review how research in cognitive psychology and cognitive ...
Abstract. Learning and memorizing are two of the most remarkable abilities of our brain. Learning refers to a change in behavior and adaptation that results from acquiring knowledge about the ...
The Journal of Applied Research in Memory and Cognition (JARMAC) publishes the highest-quality applied research in memory and cognition, in the format of empirical reports, review articles, and target papers with invited peer commentary. The goal of this unique journal is to reach psychological scientists and other researchers working in this field and related areas, as well as professionals ...
The Journal of Experimental Psychology: Learning, Memory, and Cognition ® publishes original experimental and theoretical research on human cognition, with a special emphasis on learning, memory, language, and higher cognition.. The journal publishes impactful articles of any length, including literature reviews, meta-analyses, replications, theoretical notes, and commentaries on previously ...
Forgetting enables us as individuals, and as a species, to move forwards. "Evolution has achieved a graceful balance between the virtues of remembering and the virtues of forgetting," Anderson ...
Memory Studies affords recognition, form and direction to work in this nascent field, and provides a peer-reviewed, critical forum for dialogue and debate on the theoretical, empirical, and methodological issues central to a collaborative understanding of memory today.Memory Studies examines the social, cultural, cognitive, political and technological shifts affecting how, what and why ...
Journal metrics Editorial board. Memory publishes high quality papers in all areas of memory research, including experimental studies of memory (including laboratory-based research, everyday memory studies, and applied memory research), developmental, educational, neuropsychological, clinical and social research on memory.
Abstract The memory benefit that arises from distributing learning over time rather than in consecutive sessions is one of the most robust effects in cognitive psychology. While prior work has mainly focused on repeated exposures to the same information, in the real world, mnemonic content is dynamic, with some pieces of information staying ...
A cohort of 199 first-year Psychology undergraduates in Middlesex university took part in an experimental study on Memory as part of their module requirement. Their memory was tested using the ...
Abstract. This article presents a survey of research on memory development conducted within the last 120 years. It begins with an examination of historical trends and then focuses on developmental trends over the last three decades. The article concludes with some predictions of future research activities and trends in this classic domain of ...
Abstract. The present work reviewed the research results related to the impact of memory on academic achievement. ... The research in the relationships between memory and school learnings does not ...
Abstract. The historical roots of memory research—mainly from the period between 1870 and 1920—are described with emphasis on human memory. First, data from experimental psychology are reviewed; thereafter principal contributions from the old traditions of psychiatry and psychoanalysis are given and then relations between brain tissue damage and memory deficits are outlined.
In contrast, "memory" now is used to refer to storage of information in general, including in DNA, digital information storage, and neuro-chemical processes. Today, science has moved far beyond a popular understanding of memory as fixed, subjective, and personal. In the extended definition, it is simply the capacity to store and retrieve ...
The majority of research on visual memory has taken a compartmentalized approach, focusing exclusively on memory over shorter or longer durations, that is, visual working memory (VWM) or visual episodic long-term memory (VLTM), respectively. This tutorial provides a review spanning the two areas, with readers in mind who may only be familiar with one or the other. The review is divided into ...
Subsequent research (e.g., Lobsien 1902; Netschajeff 1900) confirmed this result in that memory span performance increased with age for all types of items, and memory was better for objects and labels than for sounds and abstract concepts.
This paper explores memory from a cognitive neuroscience perspective and examines associated neural mechanisms. It examines the different types of memory: working, declarative, and non-declarative, and the brain regions involved in each type. The paper highlights the role of different brain regions, such as the prefrontal cortex in working memory and the hippocampus in declarative memory.
Previous research has frequently shown the association between age, Neuroticism, and Openness with memory. However, few studies have investigated the interaction of these factors so far. The present study examined whether the relationship between age and memory is moderated by the Neuroticism and Openness using cross-sectional data from a sample of 550 Iranian participants.
ABSTRACT. What does science tell us about memory phenomena such as false and repressed memories? This issue is highly pressing as incorrect knowledge about these memory phenomena might contribute to egregious effects in the courtroom such as false accusations of abuse. ... Collectively, what research on false memory implantation has shown is ...
For anyone over the age of 30 reading this article, here's some bad news for you: Your brain is already on the decline. The good(ish) news? From the brain's peak performance in our mid-20s, that decline is gradual, said Stanford neurologist Sharon Sha, MD.Despite common lore about aging and major lapses in memory, the effects of healthy aging on cognitive functions are actually quite subtle.
Abstract. Human memory entails an active transaction between the mind and the reality that takes place in the larger cultural context. There has been an increasing awareness among memory ...
The reviewed studies indicate that stress does impair retrieval, particularly when induced with the TSST, in the afternoon, up to 45 minutes before the onset of the final memory test, in healthy young men. These results may inform future research on the impact of stress-induced cortisol surges on memory retrieval.
Abstract. Background: While Chimeric Antigen Receptor (CAR)T cell therapy has seen great success in pediatric and young adult acute lymphoblastic leukemia, up to 50% of patients will relapse following treatment. Increased memory formation and mitochondrial capacity, particularly in the CD4+ subset, have been linked to improved CART cell function and enhanced in vivo persistence, leading to ...
The research authors have shared their full poster presentation for updated details about their research abstract. Please see the digital file attached, under additional resources below, for these details. ... which may be affected by participant's memory or recall and may not accurately assess typical eating patterns. Factors that may also ...
Abstract. Cytotoxic CD8+ T cells play a key role in response to anti-PD-1/PD-L1 immune checkpoint inhibitors (ICI) therapy. However, the exact effector molecules that are regulated by PD-1 signals in cytotoxic CD8+ T cells have not been completely defined. Lack of this knowledge is a crucial problem in addressing how to overcome the low clinical response rate to ICI therapy. When exploring why ...
Abstract. Background: Gastric or gastroesophageal junction (G/GEJ) adenocarcinoma is one of the most common lethal malignancies in the world. Near half of the G/GEJ adenocarcinoma are locally advanced at diagnosis and with poor prognosis. Several clinical trials including SHARED (ChiCTR1900024428), which conducted in our center, demonstrated that perioperative anti-PD-1 therapy in combination ...
We build on top of years of research, with over 10 research papers published over the last decade. Figure 5 shows Garnet's overall architecture. We highlight a few key ideas below. Garnet's network layer inherits a shared memory design inspired by our prior research on ShadowFax. TLS processing and storage interactions are performed on the ...
The memory retention test utilized was patterned after Ebbinghaus's research on memory, a list of 50 3-letter nonsense syllables with a consonant-vowel-consonant pattern. ... [Show full abstract ...
There is a growing body of evidence suggesting a critical role of sleep in learning and memory (Diekelmann & Born, 2010).On the one hand, offline memory consolidation during sleep benefits both declarative and procedural memories acquired during preceding wake (Klinzing et al., 2019).On the other hand, memory encoding capacity has been argued to saturate gradually during wake, with sleep ...