Chemistry Learner
It's all about chemistry.
- Chemical Bonds
- Chemical Reactions
- Materials Chemistry
- Organic Chemistry
- Periodic Trends
- Periodic Table Groups
- How to Read Periodic Table
- Naming Covalent Compounds Worksheets
- Net Ionic Equation Worksheets
- Types of Chemical Reactions Worksheets
- Word Equations Worksheets
- Valence Electrons Worksheets
- Graphing Periodic Trends Worksheets
- Periodic Trends Ionization Energy Worksheets
- Atomic Structure And Isotopes Worksheets

Synthesis Reaction (Combination Reaction)
What is a synthesis reaction, how to identify a synthesis reaction, example of synthesis reaction, different types of synthesis reaction, examples of synthesis reactions in everyday life.
A synthesis reaction is a reaction in which two or more reactants chemically bond and combine to form a product. A synthesis reaction is also known as a combination reaction. Most synthesis reactions are exothermic reactions, i.e., heat is released during the reaction.
General Equation
The general chemical equation for a synthesis reaction is given by the following equation.
When writing an actual reaction, the reaction must be balanced.
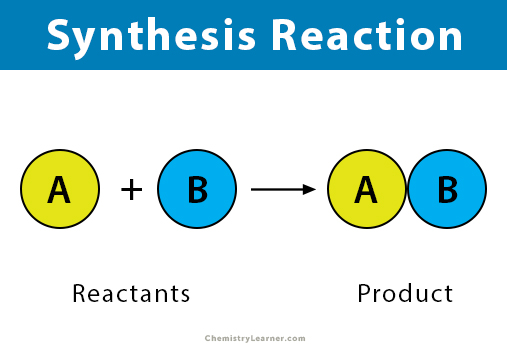
A synthesis reaction combines all the reactants of the reaction to form a product. To recognize a synthesis reaction, look for a product that contains all the reactant atoms.
An example of a combination reaction is the combination of aluminum (Al) and oxygen (O 2 ) to aluminum oxide (Al 2 O 3 ).
4 Al (s) + 3 O 2 (g) → 2 Al 2 O 3 (s)
There are three types of synthesis reaction.
1. Reaction between two elements
- The reaction between hydrogen (H 2 ) and nitrogen (N 2 ) to form ammonia (NH 3 )
N 2 (g) + 3 H 2 (g) → 2 NH 3 (g)
- The reaction between carbon (C) and oxygen (O 2 ) to form carbon dioxide (CO 2 )
C (s) + O 2 (g) → CO 2 (g)
- When sodium (Na) metal reacts with chlorine (Cl 2 ) gas, the reaction results in sodium chloride (NaCl), also known as common salt
2 Na (s) + Cl 2 (g) → 2 NaCl (s)
- The reaction between iron (Fe) and oxygen (O 2 ) results in iron (III) oxide (Fe 2 O 3 ), commonly known as rust. Rusting is a naturally occurring phenomenon.
4 Fe (s) + 3 O 2 (g) → 2 Fe 2 O 3 (s)
2. Reaction between two compounds
- When magnesium oxide (MgO) and carbon dioxide (CO 2 ) combine, the resulting product is magnesium carbonate (MgCO 3 )
MgO (s) + CO 2 (s) → MgCO 3 (s)
3. Reaction between an element and a compound
- The reaction between carbon monoxide (CO) and oxygen (O 2 ) yields carbon dioxide (CO 2 ).
2 CO (g) + O 2 (g) → 2 CO 2 (g)
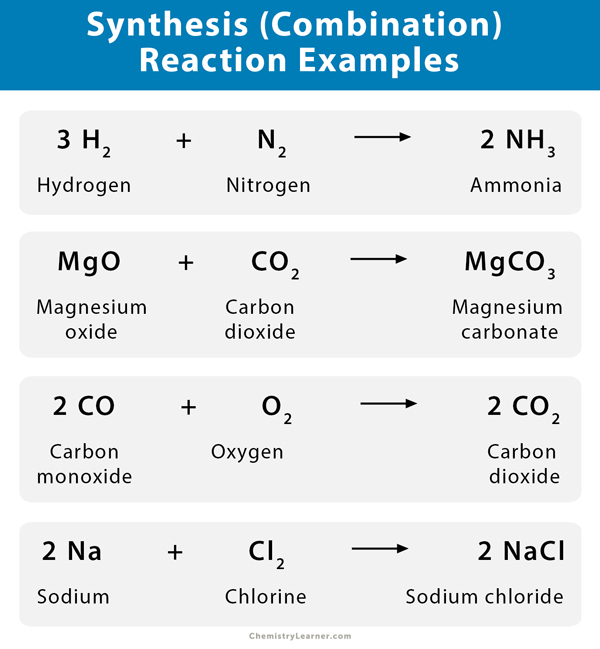
There are a few examples of the synthesis reaction in real and daily life. Almost all real-life examples are seen in the industry. During industrial production, the synthesis reaction plays a significant part in synthesizing new compounds.
- Synthesis of ammonia
- Commercial production of slaked lime (calcium hydroxide)
- Production of sodium chloride or common salt
- Preparation of hydrochloric acid and ammonium chloride
Other examples include
- Photosynthesis
Ans. A decomposition reaction is one when a substance decomposes into two or more products. This reaction is the opposite of what a combination does.
Ans. Yes. In fact, most common oxidation-reduction (redox) reactions are combination reactions.
Ans. Yes, a combination reaction can be an oxidation reaction if one of the reactants is oxygen.
Ans. While all combination reactions are exothermic, there can be an exception. The production of nitric oxide (NO) from nitrogen and oxygen is an endothermic reaction.
Ans. Dehydration synthesis is the formation of larger molecules from smaller reactants, followed by the loss of a water molecule.
Ans. Dehydration synthesis reactions build up the molecules and generally require energy, while hydrolysis reactions break down the molecules and generally release energy. The two are opposite to one another.
- Opentextbc.ca
- Chem.wisc.edu
- Cpanhd.sitehost.iu.edu
- Amrita.olabs.edu.in
- Chem.libretexts.org
Trending Topics
© 2024 ( Chemistry Learner )
Synthesis Reaction Description Plus Examples
Two or more simple substances combine to form more complex products
- Chemical Laws
- Periodic Table
- Projects & Experiments
- Scientific Method
- Biochemistry
- Physical Chemistry
- Medical Chemistry
- Chemistry In Everyday Life
- Famous Chemists
- Activities for Kids
- Abbreviations & Acronyms
- Weather & Climate
- Ph.D., Biomedical Sciences, University of Tennessee at Knoxville
- B.A., Physics and Mathematics, Hastings College
While there are many types of chemical reactions , they all fall into at least one of four broad categories: synthesis reactions, decomposition reactions , single displacement reactions, and double displacement reactions.
A synthesis reaction or direct combination reaction is a type of chemical reaction in which two or more simple substances combine to form a more complex product. The reactants may be elements or compounds, while the product is always a compound.
General Form of Synthesis Reactions
The general form of a synthesis reaction is:
A + B → AB
Examples of Synthesis Reactions
Here are some examples of synthesis reactions:
- Water: 2 H 2 (g) + O 2 (g) → 2 H 2 O(g)
- Carbon dioxide: 2 CO(g) + O 2 (g) → 2CO 2 (g)
- Ammonia: 3 H 2 (g) + N 2 (g) → 2 NH 3 (g)
- Aluminum oxide: 4 Al(s) + 3 O 2 (g) → 2 Al 2 O 3 (s)
- Iron sulfide: 8 Fe + S 8 → 8 FeS
- Potassium chloride: 2 K(s) + Cl 2 (g) → 2 KCl(s)
Recognizing Synthesis Reactions
The hallmark of a synthesis reaction is that a more complex product is formed from the reactants. One easy-to-recognize type of synthesis reaction occurs when two or more elements combine to form a compound. The other type of synthesis reaction happens when an element and a compound combine to form a new compound.
Basically, to identify this reaction, look for a product that contains all the reactant atoms. Be sure to count the number of atoms in both the reactants and the products. Sometimes when a chemical equation is written, "extra" information is given that might make it hard to recognize what is going on in a reaction. Counting numbers and types of atoms makes it easier to identify reaction types.
- Chemical Reaction Definition and Examples
- Synthesis Reaction Definition and Examples
- Simple Chemical Reactions
- Types of Chemical Reactions
- How Many Types of Chemical Reactions Are There?
- Single-Displacement Reaction Definition and Examples
- What Is a Chemical Reaction?
- Chemical Reaction Classification Practice Test
- Chemical Reaction vs. Chemical Equation
- Reactant Definition and Examples
- Decomposition Reaction Definition
- 4 Types of Inorganic Chemical Reactions
- What Is a Word Equation in Chemistry?
- Combustion Reactions in Chemistry
- Reaction Definition in Chemistry
- How to Calculate Theoretical Yield of a Reaction
A + B ---> AB
Mg + O 2 ---> MgO H 2 + O 2 ---> H 2 O K + Cl 2 ---> KCl Fe + O 2 ---> Fe 2 O 3
CaO + CO 2 ---> CaCO 3 Na 2 O + CO 2 ---> Na 2 CO 3 KCl + O 2 ---> KClO 3 Ba(ClO 3 ) 2 ---> BaCl 2 + O 2
CO 2 + H 2 O ---> C 6 H 12 O 6 + O 2
H 2 + O 2 ---> H 2 O 2
1) Direct union of two elements will produce a binary compound. 2) Metallic oxides and carbon dioxide react to produce carbonates. 3. Binary salts and oxygen react to produce a chlorate.
CaO + H 2 O ---> Ca(OH) 2 Na 2 O + H 2 O ---> NaOH N 2 O 5 + H 2 O ---> HNO 3 P 2 O 5 + H 2 O ---> H 3 PO 4
1) LiCl + O 2 ---> 2) Na 2 O + CO 2 ---> 3) SO 3 + H 2 O ---> 4) N 2 + H 2 --->
LiCl + O 2 are the products of a chlorate decomposing.
Chlorate is always ClO 3 ¯ Li is plus one
LiCl + O 2 ---> LiClO 3
Na 2 O + CO 2 are the products of a carbonate decomposing.
Carbonate is always CO 3 2 ¯ Na is plus one
Na 2 O + CO 2 ---> Na 2 CO 3
SO 3 + H 2 O are the products of an acid decomposing.
In SO 3 the S has an oxidation number of +6 H has its usual value of +1 and O has its usual value of -2
SO 3 + H 2 O ---> H 2 SO 4
N 2 + H 2 are the products of a binary compound decomposing.
N has a charge of -3 H has its usual value of +1
N 2 + H 2 ---> NH 3
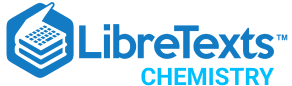
- school Campus Bookshelves
- menu_book Bookshelves
- perm_media Learning Objects
- login Login
- how_to_reg Request Instructor Account
- hub Instructor Commons
Margin Size
- Download Page (PDF)
- Download Full Book (PDF)
- Periodic Table
- Physics Constants
- Scientific Calculator
- Reference & Cite
- Tools expand_more
- Readability
selected template will load here
This action is not available.
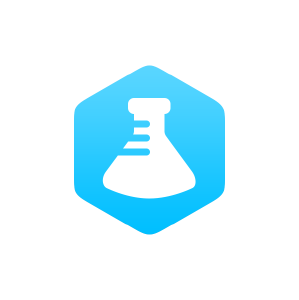
5.3: Types of Chemical Reactions
- Last updated
- Save as PDF
- Page ID 79224
\( \newcommand{\vecs}[1]{\overset { \scriptstyle \rightharpoonup} {\mathbf{#1}} } \)
\( \newcommand{\vecd}[1]{\overset{-\!-\!\rightharpoonup}{\vphantom{a}\smash {#1}}} \)
\( \newcommand{\id}{\mathrm{id}}\) \( \newcommand{\Span}{\mathrm{span}}\)
( \newcommand{\kernel}{\mathrm{null}\,}\) \( \newcommand{\range}{\mathrm{range}\,}\)
\( \newcommand{\RealPart}{\mathrm{Re}}\) \( \newcommand{\ImaginaryPart}{\mathrm{Im}}\)
\( \newcommand{\Argument}{\mathrm{Arg}}\) \( \newcommand{\norm}[1]{\| #1 \|}\)
\( \newcommand{\inner}[2]{\langle #1, #2 \rangle}\)
\( \newcommand{\Span}{\mathrm{span}}\)
\( \newcommand{\id}{\mathrm{id}}\)
\( \newcommand{\kernel}{\mathrm{null}\,}\)
\( \newcommand{\range}{\mathrm{range}\,}\)
\( \newcommand{\RealPart}{\mathrm{Re}}\)
\( \newcommand{\ImaginaryPart}{\mathrm{Im}}\)
\( \newcommand{\Argument}{\mathrm{Arg}}\)
\( \newcommand{\norm}[1]{\| #1 \|}\)
\( \newcommand{\Span}{\mathrm{span}}\) \( \newcommand{\AA}{\unicode[.8,0]{x212B}}\)
\( \newcommand{\vectorA}[1]{\vec{#1}} % arrow\)
\( \newcommand{\vectorAt}[1]{\vec{\text{#1}}} % arrow\)
\( \newcommand{\vectorB}[1]{\overset { \scriptstyle \rightharpoonup} {\mathbf{#1}} } \)
\( \newcommand{\vectorC}[1]{\textbf{#1}} \)
\( \newcommand{\vectorD}[1]{\overrightarrow{#1}} \)
\( \newcommand{\vectorDt}[1]{\overrightarrow{\text{#1}}} \)
\( \newcommand{\vectE}[1]{\overset{-\!-\!\rightharpoonup}{\vphantom{a}\smash{\mathbf {#1}}}} \)
Learning Outcomes
- Classify a reaction as combination, decomposition, single-replacement, double-replacement, or combustion.
- Predict the products and balance a combustion reaction.
Many chemical reactions can be classified as one of five basic types. Having a thorough understanding of these types of reactions will be useful for predicting the products of an unknown reaction. The five basic types of chemical reactions are combination, decomposition, single-replacement, double-replacement, and combustion. Analyzing the reactants and products of a given reaction will allow you to place it into one of these categories. Some reactions will fit into more than one category.
Combination Reactions
A combination reaction , also known as a synthesis reaction , is a reaction in which two or more substances combine to form a single new substance. Combination reactions can also be called synthesis reactions .The general form of a combination reaction is:
\[\ce{A} + \ce{B} \rightarrow \ce{AB}\]
One combination reaction is two elements combining to form a compound. Solid sodium metal reacts with chlorine gas to product solid sodium chloride.
\[2 \ce{Na} \left( s \right) + \ce{Cl_2} \left( g \right) \rightarrow 2 \ce{NaCl} \left( s \right)\]
Notice that in order to write and balance the equation correctly, it is important to remember the seven elements that exist in nature as diatomic molecules (\(\ce{H_2}\), \(\ce{N_2}\), \(\ce{O_2}\), \(\ce{F_2}\), \(\ce{Cl_2}\), \(\ce{Br_2}\), and \(\ce{I_2}\)).
One sort of combination reaction that occurs frequently is the reaction of an element with oxygen to form an oxide. Metals and nonmetals both react readily with oxygen under most conditions. Magnesium reacts rapidly and dramatically when ignited, combining with oxygen from the air to produce a fine powder of magnesium oxide.
\[2 \ce{Mg} \left( s \right) + \ce{O_2} \left( g \right) \rightarrow 2 \ce{MgO} \left( s \right)\]
Decomposition Reactions
A decomposition reaction is a reaction in which a compound breaks down into two or more simpler substances. The general form of a decomposition reaction is:
\[\ce{AB} \rightarrow \ce{A} + \ce{B}\]
Most decomposition reactions require an input of energy in the form of heat, light, or electricity.
Binary compounds are compounds composed of just two elements. The simplest kind of decomposition reaction is when a binary compound decomposes into its elements. Mercury (II) oxide, a red solid, decomposes when heated to produce mercury and oxygen gas.
\[2 \ce{HgO} \left( s \right) \rightarrow 2 \ce{Hg} \left( l \right) + \ce{O_2} \left( g \right)\]
A reaction is also considered to be a decomposition reaction even when one or more of the products is still a compound. A metal carbonate decomposes into a metal oxide and carbon dioxide gas. For example, calcium carbonate decomposes into calcium oxide and carbon dioxide.
\[\ce{CaCO_3} \left( s \right) \rightarrow \ce{CaO} \left( s \right) + \ce{CO_2} \left( g \right)\]
Metal hydroxides decompose on heating to yield metal oxides and water. Sodium hydroxide decomposes to produce sodium oxide and water.
\[2 \ce{NaOH} \left( s \right) \rightarrow \ce{Na_2O} \left( s \right) + \ce{H_2O} \left( g \right)\]
Single-Replacement Reactions
A single-replacement reaction is a reaction in which one element replaces a similar element in a compound . The general form of a single-replacement (also called single-displacement) reaction is:
\[\ce{A} + \ce{BC} \rightarrow \ce{AC} + \ce{B}\]
In this general reaction, element \(\ce{A}\) is a metal and replaces element \(\ce{B}\), also a metal, in the compound. When the element that is doing the replacing is a nonmetal, it must replace another nonmetal in a compound, and the general equation becomes:
\[\ce{Y} + \ce{XZ} \rightarrow \ce{XY} + \ce{Z}\]
\(\ce{Y}\) is a nonmetal and replaces the nonmetal \(\ce{Z}\) in the compound with \(\ce{X}\).
Magnesium is a more reactive metal than copper. When a strip of magnesium metal is placed in an aqueous solution of copper (II) nitrate, it replaces the copper. The products of the reaction are aqueous magnesium nitrate and solid copper metal.
\[\ce{Mg} \left( s \right) + \ce{Cu(NO_3)_2} \left( aq \right) \rightarrow \ce{Mg(NO_3)_2} \left( aq \right) + \ce{Cu} \left( s \right)\]
Many metals react easily with acids, and, when they do so, one of the products of the reaction is hydrogen gas. Zinc reacts with hydrochloric acid to produce aqueous zinc chloride and hydrogen (see figure below).
\[\ce{Zn} \left( s \right) + 2 \ce{HCl} \left( aq \right) \rightarrow \ce{ZnCl_2} \left( aq \right) + \ce{H_2} \left( g \right)\]
Figure 10.6.1: Zinc metal reacts with hydrochloric acid to give off hydrogen gas in a single-replacement reaction.
Figure 10.6.2: Pictured here is about 3 pounds of sodium metal reacting with water. Sodium metal reacts vigorously when dropped into a container of water, giving off hydrogen gas. A large piece of sodium will often generate so much heat that the hydrogen will ignite.
Double-Replacement Reactions
A double-replacement reaction is a reaction in which the positive and negative ions of two ionic compounds exchange places to form two new compounds . The general form of a double-replacement (also called double-displacement) reaction is:
\[\ce{AB} + \ce{CD} \rightarrow \ce{AD} + \ce{CB}\]
In this reaction, \(\ce{A}\) and \(\ce{C}\) are positively-charged cations, while \(\ce{B}\) and \(\ce{D}\) are negatively-charged anions. Double-replacement reactions generally occur between substances in aqueous solution. In order for a reaction to occur, one of the products is usually a solid precipitate, a gas, or a molecular compound such as water.
A precipitate forms in a double-replacement reaction when the cations from one of the reactants combine with the anions from the other reactant to form an insoluble ionic compound. When aqueous solutions of potassium iodide and lead (II) nitrate are mixed, the following reaction occurs.
\[2 \ce{KI} \left( aq \right) + \ce{Pb(NO_3)_2} \left( aq \right) \rightarrow 2 \ce{KNO_3} \left( aq \right) + \ce{PbI_2} \left( s \right)\]
Figure 10.6.3: When a few drops of lead (II) nitrate are added to a solution of potassium iodide, a yellow precipitate of lead (II) iodide immediately forms in a double-replacement reaction.
Combustion Reactions
A combustion reaction is a reaction in which a substance reacts with oxygen gas, releasing energy in the form of light and heat. Combustion reactions must involve \(\ce{O_2}\) as one reactant. The combustion of hydrogen gas produces water vapor (see figure below).
\[2 \ce{H_2} \left( g \right) + \ce{O_2} \left( g \right) + 2 \ce{H_2O} \left( g \right)\]
Notice that this reaction also qualifies as a combination reaction.
Figure 10.6.4: The Hindenburg was a hydrogen-filled airship that suffered an accident upon its attempted landing in New Jersey in 1937. The hydrogen immediately combusted in a huge fireball, destroying the airship and killing 36 people. The chemical reaction was a simple one: hydrogen combining with oxygen to produce water.
Many combustion reactions occur with a hydrocarbon, a compound made up solely of carbon and hydrogen. The products of the combustion of hydrocarbons are always carbon dioxide and water. Many hydrocarbons are used as fuel because their combustion releases very large amount of heat energy. Propane \(\left( \ce{C_3H_8} \right)\) is a gaseous hydrocarbon that is commonly used as the fuel source in gas grills.
\[\ce{C_3H_8} \left( g \right) + 5 \ce{O_2} \left( g \right) \rightarrow 3 \ce{CO_2} \left( g \right) + 4 \ce{H_2O} \left( g \right)\]
Example 10.6.1
Ethanol can be used as a fuel source in an alcohol lamp. The formula for ethanol is \(\ce{C_2H_5OH}\). Write the balanced equation for the combustion of ethanol.
Step 1: Plan the problem.
Ethanol and oxygen are the reactants. As with a hydrocarbon, the products of the combustion of an alcohol are carbon dioxide and water.
Step 2: Solve.
Write the skeleton equations: \(\ce{C_2H_5OH} \left( l \right) + \ce{O_2} \left( g \right) \rightarrow \ce{CO_2} \left( g \right) + \ce{H_2O} \left( g \right)\)
Balance the equation.
\[\ce{C_2H_5OH} \left( l \right) + 3 \ce{O_2} \left( g \right) \rightarrow 2 \ce{CO_2} \left( g \right) + 3 \ce{H_2O} \left( g \right)\]
Step 3: Think about your result.
Combustion reactions must have oxygen as a reactant. Note that the water that is produced is in the gas state rather that the liquid state because of the high temperatures that accompany a combustion reaction.
Supplemental Resources
- Simulation of the synthesis of water: http://www.dit.ncssm/edu/core/Chapte...Synthesis.html
- View the synthesis of calcium oxide at http://www.youtube.com/watch?v-dszSKIM5rqk
- View the reaction between copper and chlorine gas at http://www.youtube.com/watch?v-edLpxdERQZc
- Watch the decomposition of hydrogen peroxide at http://www.youtube.com/watch?v=oX5FyaqNx54
- Watch the decomposition of potassium chlorate at http://www.youtube.com/watch?v=svRIg_kzE68
- A video experiment of hydrogen replacement by calcium can be seen at http://www.youtube.com/watch?v=hjB96do_fRw
- A video experiment of magnesium metal reacting with hydrochloric acid can be viewed at http://www.youtube.com/watch?v=OBdgeJFzSec
- Watch an animation of a double-replacement reaction at http://www.dlt.ncssm.edu/core/Chapte...cidToBase.html
- A video experiment in which a precipitate is formed by reacting sodium chloride with silver nitrate can be seen at http://www.youtube.com/watch?v=eFF3El4mwok
- A video experiment of the double-replacement reaction between copper (II) sulfate and sodium sulfide can be viewed at http://www.youtube.com/watch?v=KkKBDcFfZWo
- A video of the double-replacement reaction between sodium sulfate and barium chloride can be viewed at http://www.youtube.com/watch?v=XaMyfjYLhxU
- View an in-depth explanation of the combustion reaction that occurs when you strike a match at http://www.pbs.org/wgbh/nova/cigarette/onfire.html
- View an exciting video demonstration that confirms the importance of oxygen in a combustion reaction at http://education.jlab.org/frost/life_candle.html
- View another video demonstration that confirms the importance of oxygen in a combustion reaction at http://education.jlab.org/frost/combustion.html
- Reaction Identification Practice: http://www.sciencegeek.net/Chemistry...tification.htm
Contributors
Allison Soult , Ph.D. (Department of Chemistry, University of Kentucky)
9.9 An Introduction to Organic Synthesis
9.9 • An Introduction to Organic Synthesis
As mentioned in the introduction, one of the purposes of this chapter is to use alkyne chemistry as a vehicle to begin looking at some of the general strategies used in organic synthesis—the construction of complex molecules in the laboratory. There are many reasons for carrying out the laboratory synthesis of an organic compound. In the pharmaceutical industry, new molecules are designed and synthesized in the hope that some might be useful new drugs. In the chemical industry, syntheses are done to devise more economical routes to known compounds. In academic laboratories, the synthesis of extremely complex molecules is sometimes done just for the intellectual challenge involved in mastering so difficult a subject. The successful synthesis route is a highly creative work that is sometimes described by such subjective terms as elegant or beautiful .
In this book, too, we will often devise syntheses of molecules from simpler precursors, but the purpose here is to learn. The ability to plan a successful multistep synthetic sequence requires a working knowledge of the uses and limitations of many different organic reactions. Furthermore, it requires the practical ability to piece together the steps in a sequence such that each reaction does only what is desired without causing changes elsewhere in the molecule. Planning a synthesis makes you approach a chemical problem in a logical way, draw on your knowledge of chemical reactions, and organize that knowledge into a workable plan—it helps you learn organic chemistry.
There’s no secret to planning an organic synthesis: all it takes is a knowledge of the different reactions and some practice. The only real trick is to work backward in what is often called a retrosynthetic direction. Don’t look at a potential starting material and ask yourself what reactions it might undergo. Instead, look at the final product and ask, “What was the immediate precursor of that product?” For example, if the final product is an alkyl halide, the immediate precursor might be an alkene, to which you could add HX. If the final product is a cis alkene, the immediate precursor might be an alkyne, which you could hydrogenate using the Lindlar catalyst. Having found an immediate precursor, work backward again, one step at a time, until you get back to the starting material. You have to keep the starting material in mind, of course, so that you can work back to it, but you don’t want that starting material to be your main focus.
Let’s work several examples of increasing complexity.
Worked Example 9.1
Devising a synthesis route.
How would you synthesize cis -2-hexene from 1-pentyne and an alkyl halide? More than one step is needed.
The product in this case is a cis-disubstituted alkene, so the first question is, “What is an immediate precursor of a cis-disubstituted alkene?” We know that an alkene can be prepared from an alkyne by reduction and that the right choice of experimental conditions will allow us to prepare either a trans-disubstituted alkene (using lithium in liquid ammonia) or a cis-disubstituted alkene (using catalytic hydrogenation over the Lindlar catalyst). Thus, reduction of 2-hexyne by catalytic hydrogenation using the Lindlar catalyst should yield cis -2-hexene.
Next ask, “What is an immediate precursor of 2-hexyne?” We’ve seen that an internal alkyne can be prepared by alkylation of a terminal alkyne anion. In the present instance, we’re told to start with 1-pentyne and an alkyl halide. Thus, alkylation of the anion of 1-pentyne with iodomethane should yield 2-hexyne.
Worked Example 9.2
How would you synthesize 2-bromopentane from acetylene and an alkyl halide? More than one step is needed.
What is an immediate precursor of an alkene? Perhaps an alkyne, which could be reduced.
What is an immediate precursor of a terminal alkyne? Perhaps sodium acetylide and an alkyl halide.
The desired product can be synthesized in four steps from acetylene and 1-bromopropane.
Worked Example 9.3
How would you synthesize 5-methyl-1-hexanol (5-methyl-1-hydroxyhexane) from acetylene and an alkyl halide?
What is an immediate precursor of a terminal alkene? Perhaps a terminal alkyne, which could be reduced.
What is an immediate precursor of 5-methyl-1-hexyne? Perhaps acetylene and 1-bromo-3-methylbutane.
As an Amazon Associate we earn from qualifying purchases.
This book may not be used in the training of large language models or otherwise be ingested into large language models or generative AI offerings without OpenStax's permission.
Want to cite, share, or modify this book? This book uses the Creative Commons Attribution-NonCommercial-ShareAlike License and you must attribute OpenStax.
Access for free at https://openstax.org/books/organic-chemistry/pages/1-why-this-chapter
- Authors: John McMurry, Professor Emeritus
- Publisher/website: OpenStax
- Book title: Organic Chemistry
- Publication date: Sep 20, 2023
- Location: Houston, Texas
- Book URL: https://openstax.org/books/organic-chemistry/pages/1-why-this-chapter
- Section URL: https://openstax.org/books/organic-chemistry/pages/9-9-an-introduction-to-organic-synthesis
© Jan 9, 2024 OpenStax. Textbook content produced by OpenStax is licensed under a Creative Commons Attribution-NonCommercial-ShareAlike License . The OpenStax name, OpenStax logo, OpenStax book covers, OpenStax CNX name, and OpenStax CNX logo are not subject to the Creative Commons license and may not be reproduced without the prior and express written consent of Rice University.
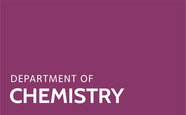
Synthesis is the production of chemical compounds by reaction from simpler materials. The construction of complex and defined new molecules is a challenging and complicated undertaking, and one that requires the constant development of new reactions, catalysts and techniques.
Synthesis projects underpin developments in a very wide range of areas. This makes chemical synthesis a unique and enabling science; it means that the design of new molecules can be put into practice so that the target compounds can be made and tested for interesting properties or activity.
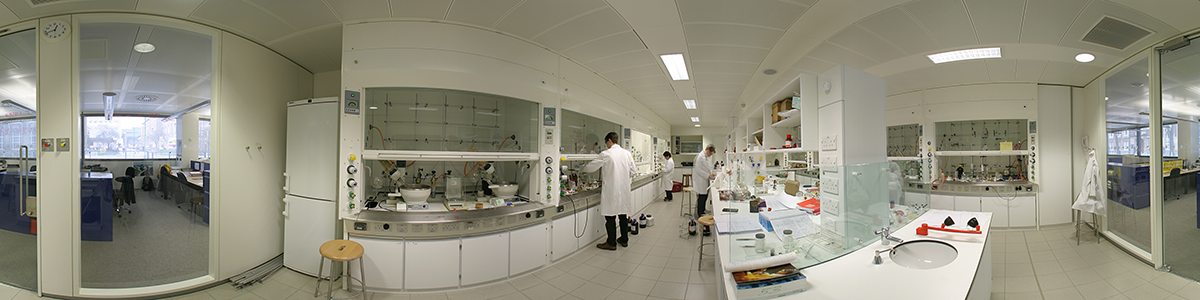
Areas in which synthesis is essential
Catalysts Catalysis is critical to a very wide range of industrial processes, encompassing both bulk and fine chemical manufacture. The rational design, synthesis and optimization of catalyst systems is therefore crucial to the development of more efficient, selective and environmentally tolerant processes. Research in this area is focussed on both metal-containing and metal-free systems, and targets not only better catalysts for existing processes but also entirely new catalytic transformations.
Medicine and drug discovery The development of new pharmaceutical products is an extremely important aspect of organic synthesis. This undertaking enables the discovery and optimisation of complex molecules with potent and selective biological activity. An understanding of synthetic chemistry allows balancing of chemical properties so that the molecules behave as desired in cells and patients. New reaction development is another essential facet of this work, because it opens up previously inaccessible routes to new compounds.
New materials The preparation of functional materials with custom-designed properties (e.g. electronic, optical, magnetic) is fundamental to breakthroughs in areas such as batteries, solar cell development, superconductors, smart materials etc., which hold much promise for future technologies. Oxford has a long-established track record in this area, with the fundamental synthetic work underpinning lithium ion battery technology having been carried out in the Department.
Chemical biology The synthesis of molecules that are designed to interact with and probe biological systems is very useful for investigating and understanding the processes involved in living systems. Such compounds allow us to understand fundamental biological processes more clearly, and to aid drug discovery through effective target validation.
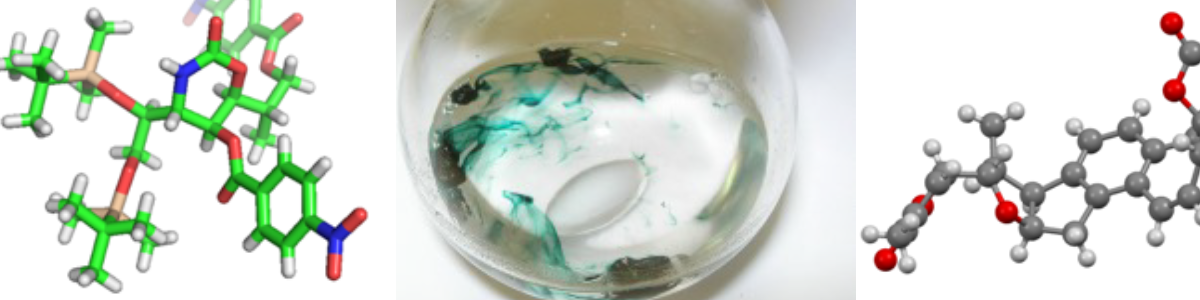
Natural products The history of medicines, flavourings and agrochemicals illustrates the central importance of natural products. Synthetic chemistry is very useful in mimicking Nature and allowing us to prepare complex molecules that are produced naturally but without disrupting the source itself. Such natural products, and analogues thereof, have myriad uses as drugs, flavourings and agrochemicals.
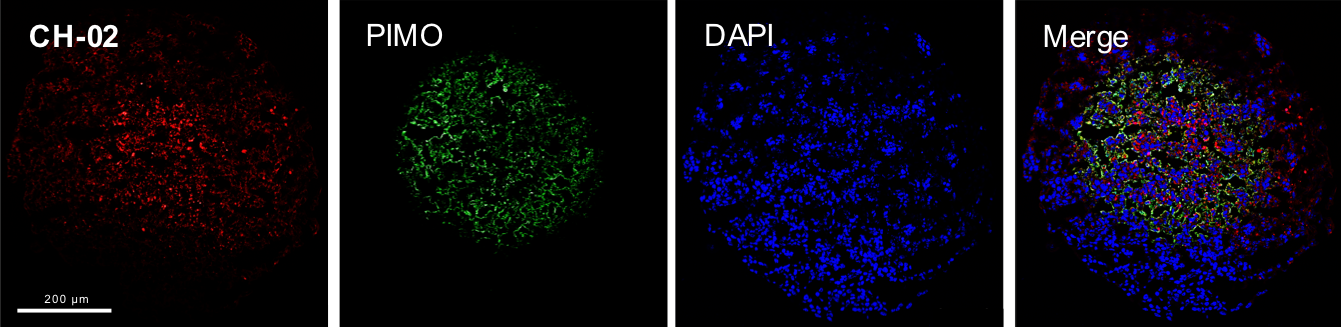
Imaging Synthetic dyes and probes have been extremely important in recent developments in imaging, which means that more powerful and less intrusive techniques can be used in the search for diseased or damaged tissue.
Theme co-ordinators
News and articles related to this theme, researchers associated with this theme.
Chemical Reactions and Equations
Chemical reactions, what is a chemical reaction.
A chemical reaction is in which the bonds are broken within reactant molecules, and new bonds are formed within product molecules in order to form a new substance.
Chemical reactions are all around us, from the metabolism of food in our body to how the light we get from the sun is the result of chemical reactions. Before beginning with chemical reactions, it is important to know about physical and chemical changes.
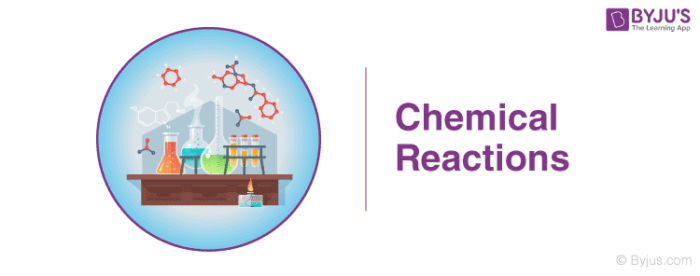
A burning candle is the best example of physical and chemical change. Take a candle and light it. As time passes, we can observe that the candle changes to wax. If you cover the candle with a jar, it will extinguish.
In the demonstration, burning of the candle is a chemical change while conversion of the candle to wax is a physical change. In a physical change, there is basically a change of state of the substance but in the case of a chemical change mostly a new substance is formed in which either energy is given off or absorbed. Thus, we can conclude that chemical changes are accompanied by certain physical changes.
Table of Content
Basic concepts of chemical reactions.
- Recommended Videos on Chemical Reactions
- Chemical equations
- Types of Chemical equations
Important Points to Remember
- Frequently Asked Questions – FAQs
- A Chemical Reaction is a process that occurs when two or more molecules interact to form a new product(s).
- Compounds that interact to produce new compounds are called reactants whereas the newly formed compounds are called products.
- Chemical reactions play an integral role in different industries, customs and even in our daily life. They are continuously happening in our general surroundings; for example, rusting of iron, pottery, fermentation of wine and so on.
- In a chemical reaction, a chemical change must occur which is generally observed with physical changes like precipitation, heat production, colour change etc.
- A reaction can take place between two atoms or ions or molecules, and they form a new bond and no atom is destroyed or created but a new product is formed from reactants.
- The rate of reaction depends on and is affected by factors like pressure, temperature, the concentration of reactants.
Recommended Videos
Chemical reactions and equations – all activities in one go.
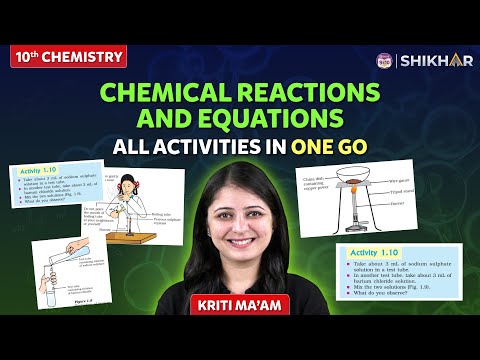
Chemical Equations
Due to the vast amounts of chemical reactions happening around us, a nomenclature was developed to simplify how we express a chemical reaction in the form of a chemical equation. A chemical equation is nothing but a mathematical statement which symbolizes the product formation from reactants while stating certain condition for which how the reaction has been conducted.
The reactants are on the left-hand side whereas the products formed are on the right-hand side. The reactants and products are connected by a one-headed or two-headed arrows. For example, a reaction
A + B → C + D
Here, A and B are the reactants, which react to form the products C and D. In an actual chemical equation, reactants are denoted by their chemical formula . In order to assure the law of conservation of mass, a chemical equation must be balanced i.e. the number of atoms on both sides must be equal. This is the balancing of the equation.
Let us consider an actual chemical reaction between Methane(CH₄) and Oxygen (O 2 ),
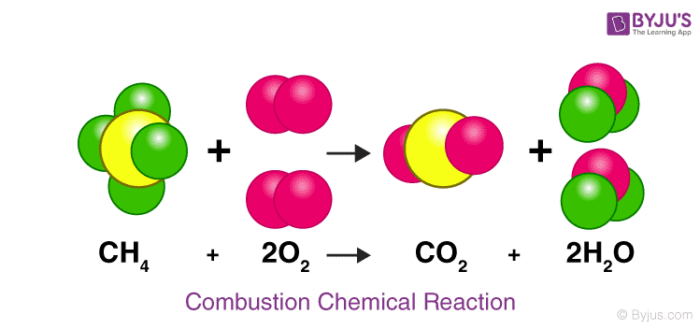
Here we can see how the number of each atom on the left side is balanced on the right side, as stated by the law of conservation of mass.
Types of Chemical Reactions
The basis for different types of reactions is the product formed, the changes that occur, the reactants involved and so on. Different types of reactions are
- Combustion reaction
- Decomposition reaction
- Neutralization reaction
- Redox Reaction
- Precipitation or Double-Displacement Reaction
- Synthesis reaction
1. Combustion Reaction
A combustion reaction is a reaction with a combustible material with an oxidizer to give an oxidized product. An oxidizer is a chemical a fuel requires to burn, generally oxygen. Consider the example of combustion of magnesium metal.
\(\begin{array}{l}2 Mg + O_2 \rightarrow 2 MgO + Heat\end{array} \)
Here, 2 magnesium atoms react with a molecule of oxygen producing 2 molecules of the compound magnesium oxide releasing some heat in the process.
2. Decomposition Reaction
A Decomposition reaction is a reaction in which a single component breaks down into multiple products. Certain changes in energy in the environment have to be made like heat, light or electricity breaking bonds of the compound. Consider the example of the decomposition of calcium carbonate giving out CaO (Quick Lime) which is a major component of cement.
\(\begin{array}{l}Ca C O_3 ( s ) \overset{Heat}{\rightarrow} Ca O ( s ) + CO_2 ( g ) \end{array} \)
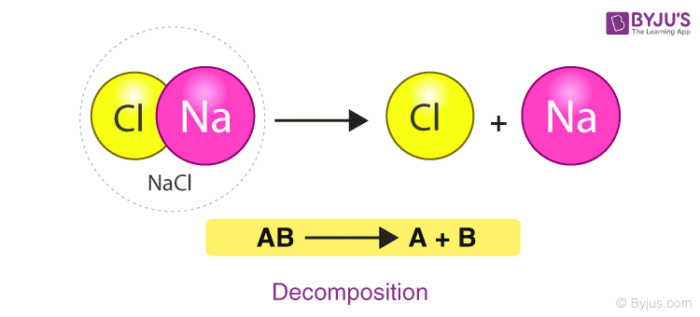
Here, the compound Calcium carbonate when heated breaks down into Calcium Oxide and Carbon Dioxide.
3. Neutralization Reaction
A Neutralization reaction is basically the reaction between an acid and a base giving salt and water as the products. The water molecule formed is by the combination of OH – ions and H + ions. The overall pH of the products when a strong acid and a strong base undergo a neutralization reaction will be 7. Consider the example of the neutralization reaction between Hydrochloric acid and Sodium Hydroxide giving out sodium chloride(Common Salt) and water.
\(\begin{array}{l}H Cl + NaOH \rightarrow NaCl +H_2O\end{array} \)
Here, an acid and a base, Hydrochloric acid and Sodium Hydroxide react in a neutralization reaction to produce Sodium Chloride(Common Salt) and water as the products.
4. Redox Reaction
A RED uction- OX idation reaction is a reaction in which there is a transfer of electrons between chemical species. Let us consider the example of an electrochemical cell-like redox reaction between Zinc and Hydrogen.
\(\begin{array}{l}Zn+2H^{+}\rightarrow Zn^{2+}+H_2\end{array} \)
Here, A Zinc atom reacts with 2 ions of positively charged hydrogen to which electrons get transferred from the zinc atom and hydrogen becomes a stable molecule and Zinc ion is the product.
5. Precipitation or Double-Displacement Reaction
It is a type of displacement reaction in which two compounds react and consequently, their anions and cations switch places forming two new products. Consider the example of the reaction between silver nitrate and sodium chloride. The products will be silver chloride and sodium nitrate after the double-displacement reaction.
\(\begin{array}{l}Ag N O_3 + Na Cl \rightarrow Ag Cl + Na N O_3\end{array} \)
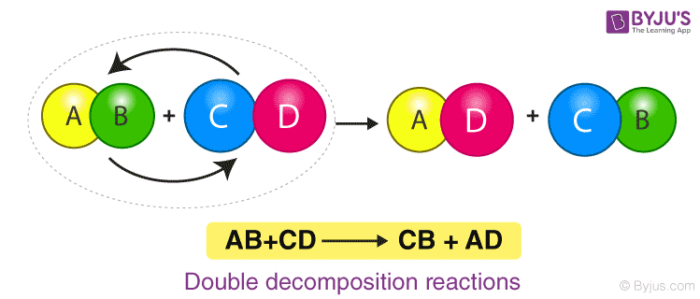
Here, Silver Nitrate and Sodium Chloride undergo a double displacement reaction. Wherein Silver replaces Sodium in Sodium Chloride and Sodium joins with Nitrate becoming Sodium Nitrate along with the Silver Chloride as the product.
6. Synthesis Reaction
A Synthesis reaction is one of the most basic types of reaction wherein multiple simple compounds combine under certain physical conditions giving out a complex product. The product will always be a compound. Let us consider the Synthesis reaction of sodium chloride with reactants solid sodium and chloride gas.
\(\begin{array}{l}2 Na ( s ) + Cl_{2} (g) \rightarrow 2 Na Cl ( s )\end{array} \)
Here, we have 2 Atoms of solid Sodium reacting with Chlorine gas giving out Sodium Chloride viz. Common Salt as the product.
- In a chemical change, a new compound is formed but in a physical change, the substance changes its state of existence.
- Atoms or ions or molecules which react to form a new substance are called reactants; the new atoms or molecules formed are products.
- A chemical reaction follows the law of conservation of mass. That is no atom is destroyed or created but only a new product is formed from reactants.
BYJU’S helps students by delivering chapter wise and detailed solutions to the questions of NCERT books. They can compare their answers with the sample answers given here – NCERT Solutions for class 10 Science Chapter 1 Chemical reactions and equations .
Related Videos
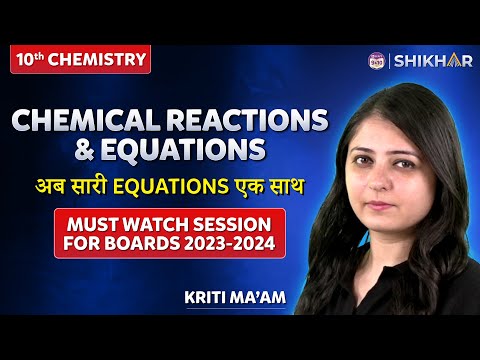
How Will Chemistry Help You Survive Alone on an Island?
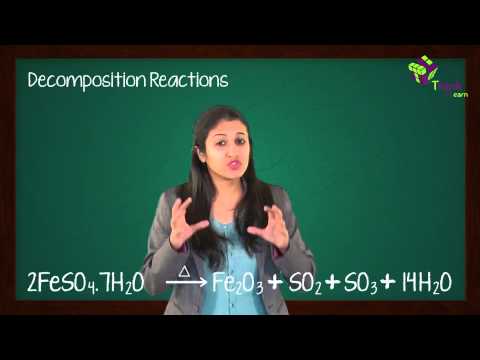
Frequently Asked Questions – FAQs
What is meant by a chemical reaction.
A Chemical Reaction is a process that occurs when two or more molecules collide with the right orientation and sufficient force to form a new product. In this process breaking and forming bonds between atoms takes place. Compounds that interact to produce new compounds are called reactants whereas the newly formed compounds are called products.
What is chemical reaction and equation?
A chemical reaction is in which the bonds are broken within reactant molecules, and new bonds are formed within product molecules in order to form a new substance. A chemical equation is nothing but a mathematical statement which symbolizes the product formation from reactants.
What are the chemical reaction types?
On the basis of the product formed, different types of reactions are Combustion reaction, Decomposition reaction, Neutralization reaction, Redox Reaction, Precipitation or Double-Displacement Reaction, Synthesis reaction.
What is a combustion chemical reaction?
A combustion reaction is a reaction in which a substance reacts with oxygen gas, releasing energy in the form of light and heat.

What is a chemical equation?
What is a decomposition reaction, what is a neutralization reaction, what is a redox reaction, what is precipitation or a double displacement reaction, what is a synthesis reaction.
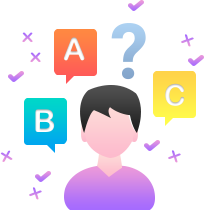
Put your understanding of this concept to test by answering a few MCQs. Click ‘Start Quiz’ to begin!
Select the correct answer and click on the “Finish” button Check your score and answers at the end of the quiz
Visit BYJU’S for all Chemistry related queries and study materials
Your result is as below
Request OTP on Voice Call
Leave a Comment Cancel reply
Your Mobile number and Email id will not be published. Required fields are marked *
Post My Comment

so easy to understand thank you for giving these resources for free
It nice😊😊😊😊😊 I was satisfied with this information 😊😊😊😊
So interesting easy way of learning
literally it really helped me a lot it is awsm
It is a very good learning platform
It is a very good learning platform for us

- Share Share
Register with BYJU'S & Download Free PDFs
Register with byju's & watch live videos.

Sciencing_Icons_Science SCIENCE
Sciencing_icons_biology biology, sciencing_icons_cells cells, sciencing_icons_molecular molecular, sciencing_icons_microorganisms microorganisms, sciencing_icons_genetics genetics, sciencing_icons_human body human body, sciencing_icons_ecology ecology, sciencing_icons_chemistry chemistry, sciencing_icons_atomic & molecular structure atomic & molecular structure, sciencing_icons_bonds bonds, sciencing_icons_reactions reactions, sciencing_icons_stoichiometry stoichiometry, sciencing_icons_solutions solutions, sciencing_icons_acids & bases acids & bases, sciencing_icons_thermodynamics thermodynamics, sciencing_icons_organic chemistry organic chemistry, sciencing_icons_physics physics, sciencing_icons_fundamentals-physics fundamentals, sciencing_icons_electronics electronics, sciencing_icons_waves waves, sciencing_icons_energy energy, sciencing_icons_fluid fluid, sciencing_icons_astronomy astronomy, sciencing_icons_geology geology, sciencing_icons_fundamentals-geology fundamentals, sciencing_icons_minerals & rocks minerals & rocks, sciencing_icons_earth scructure earth structure, sciencing_icons_fossils fossils, sciencing_icons_natural disasters natural disasters, sciencing_icons_nature nature, sciencing_icons_ecosystems ecosystems, sciencing_icons_environment environment, sciencing_icons_insects insects, sciencing_icons_plants & mushrooms plants & mushrooms, sciencing_icons_animals animals, sciencing_icons_math math, sciencing_icons_arithmetic arithmetic, sciencing_icons_addition & subtraction addition & subtraction, sciencing_icons_multiplication & division multiplication & division, sciencing_icons_decimals decimals, sciencing_icons_fractions fractions, sciencing_icons_conversions conversions, sciencing_icons_algebra algebra, sciencing_icons_working with units working with units, sciencing_icons_equations & expressions equations & expressions, sciencing_icons_ratios & proportions ratios & proportions, sciencing_icons_inequalities inequalities, sciencing_icons_exponents & logarithms exponents & logarithms, sciencing_icons_factorization factorization, sciencing_icons_functions functions, sciencing_icons_linear equations linear equations, sciencing_icons_graphs graphs, sciencing_icons_quadratics quadratics, sciencing_icons_polynomials polynomials, sciencing_icons_geometry geometry, sciencing_icons_fundamentals-geometry fundamentals, sciencing_icons_cartesian cartesian, sciencing_icons_circles circles, sciencing_icons_solids solids, sciencing_icons_trigonometry trigonometry, sciencing_icons_probability-statistics probability & statistics, sciencing_icons_mean-median-mode mean/median/mode, sciencing_icons_independent-dependent variables independent/dependent variables, sciencing_icons_deviation deviation, sciencing_icons_correlation correlation, sciencing_icons_sampling sampling, sciencing_icons_distributions distributions, sciencing_icons_probability probability, sciencing_icons_calculus calculus, sciencing_icons_differentiation-integration differentiation/integration, sciencing_icons_application application, sciencing_icons_projects projects, sciencing_icons_news news.
- Share Tweet Email Print
- Home ⋅
- Science ⋅
- Chemistry ⋅
How to Identify the 6 Types of Chemical Reactions
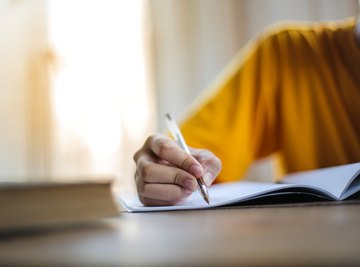
Examples of Chemical Synthesis
Chemical reactions are an essential part of technology, contributing to various human activities that are a part of our daily lives. Examples of chemical reactions that we encounter everyday include the burning of fuels and the making of wine and beer. Chemical reactions are also widely present in nature, from the chemical weathering of rocks, photosynthesis in plants and the respiration process in animals.
In the broader aspect, there are three types of reactions : physical, chemical and nuclear. Chemical reactions can be further divided into many categories. Six common types of chemical reactions are: synthesis, decomposition, single-displacement, double-displacement, combustion and acid-base reactions. Scientists classify them based on what happens when going from reactants to products. This is helpful in predicting the reactivity of reagents and the products formed from the reactions.
Types of Reactions
A chemical reaction is a process in which one or more substances, the reactants, undergo chemical transformation to form one or more different substances, the products. It is a process that involves rearrangement of the constituent atoms of the reactants to form products, without changing the nuclei of the atoms.
For instance, in a process used to manufacture soda and seltzer, carbon dioxide is bubbled into water under pressurized conditions and forms a new compound known as carbonic acid (H 2 CO 3 ). By this equation, you know a chemical reaction has occurred.
CO 2 (g) + H 2 O(l) —> H 2 CO 3 (aq)
A physical reaction is different from a chemical reaction. Physical changes only involve the change of state, for example, the freezing of water to ice and the sublimation of dry ice to carbon dioxide. In both scenarios, the chemical identity of reactants, H 2 O and CO 2 , did not change. The products are still made up of the same compounds as the reactants.
H 2 O(l) —> H 2 O(s)
CO 2 (s) —> CO 2 (g)
A nuclear reaction is also distinguished from a chemical reaction. It involves the collision of two nuclei to form one or more nuclides that are different from parent nuclei. For example, Ernest Rutherford performed the first artificial transmutation by exposing nitrogen gas to alpha particles, forming the isotope 17 O and ejecting a proton in this process. The element in the reactant changed, thus a reaction had taken place.
14 N + α —> 17 O + p
Types of Chemical Reactions
The most common types of chemical reactions are synthesis, decomposition, single displacement, double displacement, combustion and acid-base. However, such categorization is not exclusive. For example, an acid-base reaction can also be classified as a double displacement reaction.
Synthesis Reaction
A synthesis reaction is one in which two or more substances are combined to form a more complex one. The chemical equation for a general form of synthesis reaction is as follows:
A + B —> AB
One example of a synthesis reaction is the combination of iron (Fe) and sulfur (S) to form iron sulfide.
Fe(s) + S(s) —> FeS(s)
Another example is when sodium and chlorine gas are combined to produce a more complex molecule, the sodium chloride.
2Na(s) + Cl 2 (g) —> 2NaCl(s)
Decomposition Reaction
A decomposition reaction works quite the opposite to a synthesis reaction. It is a reaction where a more complex substance breaks apart into simpler ones. A general form of a decomposition reaction can be written as:
AB —> A + B
An example of a decomposition reaction is the electrolysis of water to form hydrogen and oxygen gas.
H 2 O(l) —> H 2 (g) + O 2 (g)
Decomposition can also be thermal, such as the conversion of carbonic acid to water and carbon dioxide under heating conditions. It is commonly seen in carbonated beverages.
H 2 CO 3 (aq) —> H 2 O(l) + CO 2 (g)
Single Displacement Reaction
Also known as the single replacement reaction, the single displacement reaction is when a pure element switches places with another element in a compound. It is in the general form:
A + BC —> AC + B
Many metals can react with a strong acid. For example, magnesium reacts with hydrochloric acid to form hydrogen gas and magnesium chloride. In this reaction, magnesium switches places with the hydrogen in hydrochloric acid.
Mg(s) + 2HCl(aq) —> H 2 (g) + MgCl 2 (aq)
Magnesium can also react with water to generate magnesium hydroxide and hydrogen gas.
Mg(s) + 2H 2 O(l) —> H 2 (g) + Mg(OH) 2 (aq)
Double Displacement
Another type of chemical reactions is double displacement, in which the cations of the two reactants switch places to form two completely different products. A general form of this reaction is:
AB + CD —> AD + CB
One example of a double displacement reaction is when barium chloride reacts with magnesium sulfate to form barium sulfate and magnesium chloride. In this reaction, barium and magnesium cations in the reactants switch places to new barium and magnesium compounds.
BaCl 2 + MgSO 4 —> BaSO 4 + MgCl 2
Another example is the reaction of lead nitrate with potassium iodide to form lead iodide and potassium nitrate.
Pb(NO 3 ) 2 + 2KI —> PbI 2 + 2KNO 3
In both cases, the reaction generates a precipitate (BaSO 4 and PbI 2 ) from two soluble reactants, so they are also grouped under precipitation reactions.
Combustion Reaction
A combustion reaction is an exothermic redox chemical reaction where a fuel reacts with oxygen to produce gaseous products. Although it is usually initiated by a form of energy, such as using a lit match to light a fire, the released heat provide energy for sustaining the reaction.
A complete combustion reaction occurs when excess oxygen is present and yields primarily common oxides such as carbon dioxide and sulfur dioxide. In order to ensure full combustion, the oxygen present has to be twice or three times the theoretical amount calculated by stoichiometry. A complete combustion of a hydrocarbon can be expressed in the form:
4C x H y + (4x+y)O 2 —> 4xCO 2 + 2yH 2 O + heat
Combustion of methane, which is a saturated hydrocarbon, releases substantial heat (891 kJ/mol) and can be summarized by the equation as follows:
CH 4 + 2O 2 —> CO 2 + 2H 2 O + heat
Naphthalene is another example of hydrocarbon and its complete combustion also generates carbon dioxide, water and heat.
C 10 H 8 + 12O 2 —> 10CO 2 + 4H 2 O + heat
Alcohols can also serve as a source of fuel for combustion, such as methanol.
CH 3 OH+ O 2 —> CO 2 + 2H 2 O + heat
An incomplete combustion occurs when there is not enough oxygen to fully react with the fuel to produce carbon dioxide and water. Such an example is when methane is burnt in a limited supply of oxygen to produce a combination of carbon monoxide, carbon dioxide, carbon ash and water. It can be expressed by the equations below, arranged by the amount of oxygen present.
Little oxygen:
CH 4 + O 2 —> C + 2H 2 O
Some oxygen:
2CH 4 + 3O 2 —> 2CO + 4H 2 O
More but not enough oxygen:
4CH 4 + 7O 2 —> 2CO + 2CO 2 + 8H 2 O
Too much carbon monoxide can result in air poisoning because it combines with hemoglobin to form carboxyhemoglobin and reduces its capacity for delivering oxygen. Therefore it is important to ensure complete combustion of fuel for household and industrial uses.
Acid-Base Reaction
The acid-base reaction is a reaction between an acid and a base, and water is one of the products. It is a special type of double displacement reaction (A and B switch places) and these chemical reaction examples are written as:
HA + BOH —> BA + H 2 O
A simple example of an acid-base reaction is when an antacid (calcium hydroxide) neutralizes stomach acid (hydrochloric acid).
Ca(OH) 2 + 2HCl —> CaCl 2 + 2H 2 O
Another example is the reaction of vinegar (acetic acid) with baking soda (sodium bicarbonate). In this process, water and carbon dioxide are formed but no heat is released, so it is not a combustion reaction.
CH 3 COOH + NaHCO 3 —> CH 3 COONa + H 2 O + CO 2
Related Articles
What is a synthesis reaction, three types of aqueous reactions, the differences between anionic and cationic single..., how to find heat reaction when zn reacts with hcl, safe combustion reaction experiments, what is being oxidized and what is being reduced in..., what is a double replacement reaction, four ways to speed up a chemical reaction, what are the reactants & products in a combustion reaction, what is anhydrous diethyl ether, what is a combustion reaction, what are the reactants in fermentation, what is the difference between reactants & products..., are combustion reactions exothermic, what is an endothermic reaction, difference between hydrogen peroxide & benzoyl peroxide, how to predict products in chemical reactions, examples of single replacement reactions.
- Encyclopedia Britannica: Chemical Reaction
- The Cavalcade o' Chemistry: The Six Types of Reactions
- Owlcation: Types of Chemical Reactions
- Chemistry LibreTexts: Classifying Chemical Reactions
- Combustion processes are rarely perfect. Realistically, you would see secondary combustion reactions. Secondary reactions often give out products such as carbon monoxide (CO). Carbon monoxide produced by this process indicates incomplete combustion. Though less prominent than the primary CO2-generating reaction, incomplete combustion matters. Running a car engine in a closed garage can be fatal—the small percentage of gas burned “incompletely” into CO adds up to toxic levels.
About the Author
Lan Luo has a PhD in Organic Chemistry from University of Chicago and a BS in Chemistry from Worcester Polytechnic Institute. She has years of research experience in asymmetric catalysis, natural product synthesis, drug discovery and drug delivery. She has served as a contributor for Synfacts and a reviewer for journal articles.
Find Your Next Great Science Fair Project! GO
Thank you for visiting nature.com. You are using a browser version with limited support for CSS. To obtain the best experience, we recommend you use a more up to date browser (or turn off compatibility mode in Internet Explorer). In the meantime, to ensure continued support, we are displaying the site without styles and JavaScript.
- View all journals
- My Account Login
- Explore content
- About the journal
- Publish with us
- Sign up for alerts
- Open access
- Published: 10 May 2024
Growing strings in a chemical reaction space for searching retrosynthesis pathways
- Federico Zipoli ORCID: orcid.org/0000-0001-8345-9965 1 , 2 ,
- Carlo Baldassari ORCID: orcid.org/0009-0008-1199-7113 1 ,
- Matteo Manica ORCID: orcid.org/0000-0002-8872-0269 1 ,
- Jannis Born ORCID: orcid.org/0000-0001-8307-5670 1 &
- Teodoro Laino 1 , 2
npj Computational Materials volume 10 , Article number: 101 ( 2024 ) Cite this article
Metrics details
- Organic chemistry
- Chemical synthesis
Machine learning algorithms have shown great accuracy in predicting chemical reaction outcomes and retrosyntheses. However, designing synthesis pathways remains challenging for existing machine learning models which are trained for single-step prediction. In this manuscript, we propose to recast the retrosynthesis problem as a string optimization problem in a data-driven fingerprint space, leveraging the similarity between chemical reactions and embedding vectors. Based on this premise, multi-step complex synthesis can be conceptualized as sequences that link multidimensional vectors (fingerprints) representing individual chemical reaction steps. We extracted an extensive corpus of chemical synthesis from patents and converted them into multidimensional strings. While optimizing the retrosynthetic path, we use the Euclidean metric to minimize the distance between the expanded trajectory of the growing retrosynthesis string and the corpus of extracted strings. By doing so, we promote the assembly of synthetic pathways that, in the chemical reaction space, will be more similar to existing retrosyntheses, thereby inheriting the strategic guidelines designed by human experts. We integrated this approach into the RXN platform ( https://rxn.res.ibm.com/ ) and present the method’s application to complex synthesis as well as its ability to produce better synthetic strategies than current methodologies.
Introduction
The retrosynthesis is the process of designing a synthetic route for a desired target molecule and requires the identification of optimal strategies to combine simpler molecules into a target product 1 . Frequently, retrosynthesis entails a series of reaction steps to synthesize those molecules from simpler precursor molecules. One of the main challenges in this process is exploring the large retrosynthesis hypergraph, which represents all possible synthetic routes for a given target molecule 2 , 3 , 4 , 5 , 6 , 7 , 8 , 9 , 10 , 11 , 12 , 13 , 14 , 15 , 16 , 17 , 18 , 19 , 20 , 21 , 22 , 23 , 24 , 25 , 26 , 27 , 28 , 29 , 30 . The pathways within the tree link the target product (i.e., the root) with all commercially available compounds (i.e., the leaves) which are identified by the algorithm through single-step disconnections.
The retrosynthesis tree is exponentially large because each retrosynthetic step can potentially branch into multiple alternatives, and the number of possible routes increases exponentially with the depth of the tree. Consequently, the sheer volume of potential synthetic routes can be overwhelming to explore using classical mathematical kernels, even for relatively small molecules.
The exploration of such hypergraphs requires the implementation of specific criteria to effectively filter the extensive array of disconnection options. One strategy relies on the evaluation and scoring on the pathways based on the confidence of each single-step retrosynthesis prediction, which can be individually evaluated. The idea is to consider the low confidence as a metric for an highly risky and most likely failing synthetic route. Therefore, steps with low confidence are not further propagated, giving higher priority to those with higher confidence 14 . Apart from filtering based on confidence values, single-step predictions that fail the round-trip check can also serve as additional targets to avoid further expansion. This check consists of applying a forward prediction model to the output of the single retro-step prediction and of verifying whether or not the result of this operation returns the desired product 14 . In other schemes, one could score and rank the different options, for example depending on the molecule availability, the corresponding cost, or by some metric related to green chemistry 31 .
Notwithstanding, most of the existing approaches exclusively use local information derived from single-step retrosynthesis and do not keep into account strategical decisions typical of a multi-step synthesis conceived by a human expert. In fact, when undertaking a multi-step synthesis, various strategic decisions can be made to streamline the process and optimize efficiency. One example is the strategic protection of functional groups. By selectively safeguarding specific groups early on, chemists can prevent unwanted reactions and ensure the desired transformations occur smoothly. In ideal cases, chemists follow the idea of introducing different protecting groups, targeting the removal of all protections in a single step at the final stage, saving time and minimizing the risk of side reactions. Another strategy involves utilizing robust and high-yielding reactions for key transformations, which can significantly impact the overall yield and simplify the synthesis route. Additionally, strategic retrosynthetic disconnections play a crucial role in planning the sequence of reactions. By identifying strategic bond disconnections, chemists can design efficient synthetic pathways and target specific intermediates or building blocks to assemble the final product. Lastly, the choice of reagents, catalysts, and reaction conditions is another strategic consideration. Selecting appropriate reaction parameters can enhance selectivity, improve yields, and expedite the overall synthesis process. These strategic decisions collectively contribute to the successful execution of complex multi-step syntheses 32 . Due to their dependence on single-step predictions, existing models lack a comprehensive understanding of the key strategies employed in multi-step retrosynthesis.
Recently, Thakkar et al. 29 described an approach aiming to improve retrosynthetic prediction systems by allowing chemists to have more control over the disconnections made during the exploration of the retrosynthesis tree. The method enables user-defined disconnections, creating a “human-in-the-loop” component that combines expert knowledge with deep learning. Their approach results in an increased diversity of predicted disconnections. With their method they improved decision-making strategies thus enhancing the chemist’s experience and facilitating user engagement that statistical and machine learning algorithms alone cannot encode due to insufficient training data and resulting model biases. Another recent approach to improve the search policy builds on reinforcement learning to introduce a notion of goal-driven synthesis planning that optimizes multistep synthesis routes toward specific building blocks 33 .
Furthermore, Chen et al. 21 introduced a method called “Retro*”, using an innovative neural-guided tree search approach for chemical retrosynthesis planning. Their method uses an A-like planning algorithm guided by a neural network trained on past retrosynthesis planning experiences. Their neural network learns synthesis costs for each molecule, assisting the search algorithm in choosing the most promising molecule node for expansion. In addition, the work by Ishida et al. introduces “ReTReK”, a data-driven computer-aided synthesis planning (CASP) application that integrates retrosynthesis knowledge into the evaluation of search directions. By incorporating adjustable parameters based on retrosynthesis knowledge, ReTReK successfully explores promising synthetic routes, demonstrating its preference for routes designed with the knowledge. The study addresses limitations in existing data-driven CASP applications by introducing rule-based techniques and evaluating performance using drug-like molecules, showcasing ReTReK’s potential to enhance both current and future data-driven CASP applications.
Recently, Pasquini and Stenta 30 showcased LinChemIn, a toolkit that simplifies the manipulation of reaction networks and enhances functionality for working with synthetic routes, promoting interaction between AI and human expertise in chemical analysis.
For a comprehensive review, we refer the reader to the evaluations provided by Zhong et al. 34 and Jiang et al. 35 .
However, the problem of designing chemical retrosynthesis is far more complex than removing potential biases from single-step retrosynthesis model or to steer routes toward specific precursors. It involves knowledge, experience and also a certain degree of creativity and intuition which goes beyond the state of the art of existing retrosynthesis algorithms. Similar to a strategy game, the evaluation of multi-step solutions requires holistic planning and thus may be more effectively conducted by considering a sequence of steps rather than solely focusing on individual steps. Hence, relying exclusively on the confidence of a single-step model to devise a synthetic route can potentially overlook crucial pathways and lead to sub-optimal or even erroneous predictions.
Our algorithm not only addresses strategic decisions but also extends its impact to enhance the efficiency of separation steps in multi-step synthesis planning. Unlike traditional approaches that rely solely on single-step retrosynthesis models, our method introduces a strategy for assembling single-step predictions into coherent retrosynthetic pathways.
By considering the entire sequence of steps, our approach provides a broader view of the synthesis process, including the crucial final stages where separation efficiency is crucial.
In this study, we present an algorithm that emulates human strategic decision-making when constructing an AI-driven retrosynthesis approach. The computational method facilitates the exploration of the retrosynthesis tree, which is constructed using conventional single-step machine learning predictions, leveraging the chemical knowledge derived from existing collections of multi-step retrosynthesis. By doing so, the algorithm effectively harnesses the expertise and available knowledge of human chemists readily accessible through retrosyntheses published in literature. The proposed method targets the task of assembling efficiently the single-step retro predictions and does not require any retraining of retrosynthesis models, since it makes used of existing pre-trained models. The algorithm focuses on using an embedding to represent sequences of chemical steps. It then compares the sequences of predictions with the sequences of steps in pre-existing datasets to prioritize retrosynthesis strategies. For the representation of the single-step chemical reaction, we leverage the work of Schwaller et al. 36 , who utilized embeddings of language models to build a chemical reaction fingerprint ( rxnfp ). Such reaction fingerprints capture structural and chemical properties, such as reactants, products, reaction context, and stereochemistry. These embeddings proved to be very successful to relate chemical reactions to specific reaction classes 37 , to predict reaction yield 38 or even to discovery novel Heck reactions 39 .
Here, we extend the concept of chemical reaction fingerprints to retrosynthesis routes, representing the sequences of steps contained in published retrosynthesis with a set of multidimensional strings in the fingerprint space. The core idea of the proposed algorithm is to construct the retrosynthesis tree by growing strings minimizing the distance between the predicted and each section of any pre-existing multidimensional string in the embedding space. The comparison can be extended to more intricate scenarios, where retrosyntheses are not linear trajectories but rather trees depicted by corresponding branched structures in the fingerprint space.
This method demonstrates better performance in terms of producing retrosynthesis with a smaller number of steps, protects/deprotects functional groups making decision across the entire length of the synthesis. The proposed approach makes better use of the different available reactions leading to steps which can occur in milder reaction conditions, avoiding for example the need for strong chemicals like organometals and powerful oxidants. We provide a few applications in the results section, which are illustrative of the potential of the methodology.
Data preprocessing and preparation
Our method relies on representing retrosynthesis routes as trajectories in the fingerprint space as described in the “Method” section. We assembled the corpus of retrosynthesis routes by processing the Pistachio dataset 40 , which is a large-scale dataset of chemical reactions extracted from US patents. The dataset contains over 4 million reactions. We assembled 1,202,092 linear and 187,478 branched pathways. Figure 1 a illustrates the distribution of number of steps for the linear and branched pathways consisting of at least two steps.
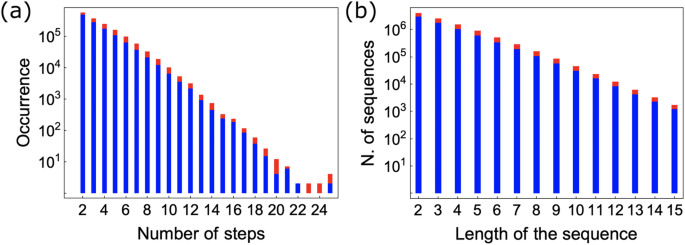
a Distribution of the tree depths of retrosynthesis routes in the database, linear reactions are shown in blue, while the count including the tree routes is shown in red. b The distribution of sequence lengths is shown in blue for linear routes and in red for both linear and tree routes.
To maximize the extraction of the human expert knowledge contained in all sequences, we extracted from each retrosynthesis route additional sub-routes of varying lengths, from a minimum of two steps up to the maximum length of each pathway. For example to build the datasets of sequences N-step long, we utilize all the retrosynthesis with length greater or equal to N. A retrosynthesis of length M, with M greater then or equal to N, produces M + 1 − N sequences of length N. However, if the number of steps exceeds 15, we truncate the sequence at 15 steps because, we explained in the “Method” section, the use of longer sequences does not add any performance improvements. Figure 1 b shows the final distribution of lengths of all sequences in our dataset. Figure 2 shows the Euclidean distance distribution of the fingerprints of the reaction SMILES in the Pistachio dataset. The histogram is the distribution of distances between randomly selected pairs of chemical reaction fingerprints. The number of pairs is large enough to give a converged distribution, which provides a numerical information on the range-distance values in the fingerprint space. We also observe a peak at shorter distances, centered at about 6, which relates almost entirely to distances among reactions from the same class, see Fig. S 1 a in the Supplementary Material. We verify this finding by computing the distance distributions of groups of chemical reaction SMILES belonging to the same reaction class, which we report in Supplementary Fig. 1 b. In addition, we computed the distance distribution of chemical reaction SMILES in the same class and in different classes using the curated dataset by Schneider et al. 41 . Supplementary Fig. 1 c shows a two-peaks distribution with a clear separation between the distribution of distances of SMILES in the same class, first peak, compared to the distribution among the remaining SMILES. Compared to Supplementary Fig. 1 a, Supplementary Fig. 1 c shows a more distinct separation between the reaction records compared to a more complex dataset. This clearer delineation can be attributed to the reduced number of reaction types in the simplified dataset of Schneider et al. 41 . We repeated the same analysis using cosine distance instead of Euclidean, which showed the same two-peak distribution. In the rest of the work, we will use the Euclidean distance because it better resolves the distribution of fingerprints in the same class compared to other metrics. To accelerate the computation and memory consumption, we employed PCA reduction, reducing the fingerprint embedding from 256 to 16 dimensions without significant loss of descriptive capacity, see “Methods” section for details. Figure 2 shows the distribution of distances in the reduced space and compared it with the original space. Our observations reveal that the PCA dimensionality reduction improves the separation between short-range and long-range distance peaks in both Pistachio and Schneider datasets.
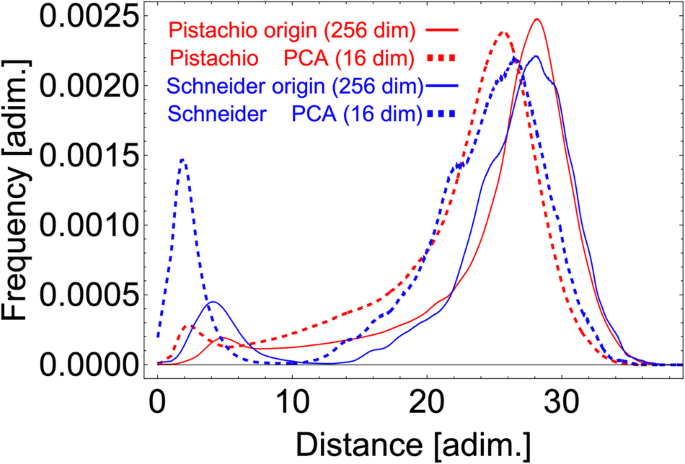
The Euclidean distances have been computed in the original fingerprint space of 256-dimensions (solid lines) and compared to the PCA 16-dim reduced space (dashed lines). The frequency of chemical reaction SMILES within the same class were centered around values of 6 in the original fingerprint space. Both plots have been normalized by the same total number of pair counts. The Pistachio distribution has been computed on subset of Pistachio data. In this case, the number of data points is large enough to give a converged distributions.
Method evaluation and comparative analysis
To evaluate the performance of our approach, we performed a comparison of this method based on multidimensional strings (see “Method” section) with an hypergraph search strategy 14 on a series of case studies.
We analyzed the routes obtained with both approaches on two molecules that are challenging for a search strategy based on hypergraph exploration ( Product A and B in Fig. 3 ).
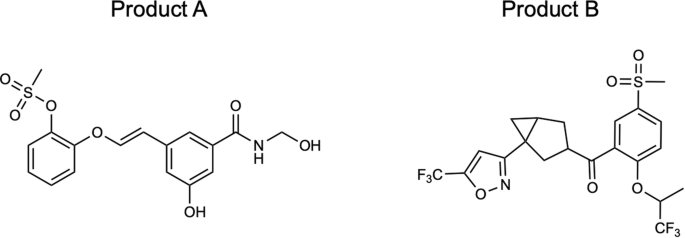
Two molecules, Product A and B, used as target input products that results challenging for a search relying on an hypergraph exploration strategy.
Our results demonstrate several advantages of our string-growth method compared to alternative approaches that rely on single-step confidences. Figures 4 and 5 show for both molecules the predictions obtained with the two approaches. In Fig. 4 , the proposed method finds solutions with less steps. With the standard approach the retrosynthetic tree counts 8 reaction steps, compared with 5 steps of the proposed method. Using the same settings and the same molecule, the proposed algorithm reaches commercial precursors more easily. Analyzing more carefully each step, it is possible to observe that the first two reactions are mostly the same: Amide formation at the first step and protection of the phenol at the second step, although the protecting group is different. From the third step instead the disconnections are different: with our algorithm, the intermediate is obtained through Heck coupling, without any possible interference, and the relative precursors are obtained in a single step from commercially available compounds. On the contrary, using the older algorithm the double bond is made through Horner-Wittig reaction, reaction that involves the use of strong bases, after the mesylation of the phenol, that due to his acidity can interfere. Even the two precursors of the Horner-Wittig reaction are not commercial and have to be made through complex reactions. Given these observations it seems like the hypergraph approach leads to worse intermediates during the retrosynthesis and it struggles to reach commercially available compounds, so that it has to force it through low confidence chemistry. Instead, in the proposed algorithm, the retrosynthesis is well designed and that is confirmed by the its shortness and higher reliability.
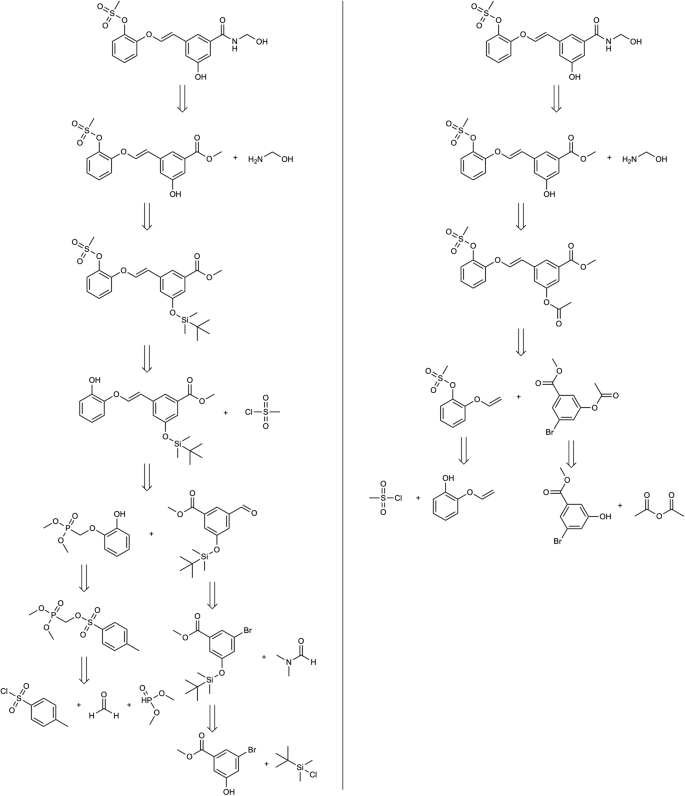
Retrosynthesis paths obtained with the proposed method (right) and with the standard confidence-based approach (left) for Product A of Fig. 3 .
Figure 5 illustrates an analogous comparison for Product B . The retrosynthesis depicted in Fig. 5 showcases two comparable routes in terms of length, but they differ in the choice of reactions employed. Both retrosynthetic pathways share the initial step of amide formation via the Steglich reaction, leading to the same set of intermediates. In the string-growth approach, the carboxylic acid is obtained through saponification of a commercially available ester, while the standard route involves the predicted formation of an ether through nucleophilic aromatic substitution. Although both routes are reliable, the saponification process is considerably easier to execute and thus preferable for chemists. In terms of the amine, both routes involve deprotection of a Boc protecting group. With the hypergraph exploration method, the bicyclic structure is made using a Simmons-Smith reaction from the alkene and the last disconnection is a Suzuki-Miyaura coupling. In the proposed approach the aromatic ring is obtained through [3 + 2] cycloaddition, favored by the aromaticity of the product, preceded by the formation of the oxime. The two path are built through different choices: in one case it is chosen to form the bicyclic structure and on the other side the formation of the isoxazole. Also in this case it has to be highlighted the preference of our approach for a more direct chemistry rather than reactions conducted under harsh conditions, despite the more direct path chosen.
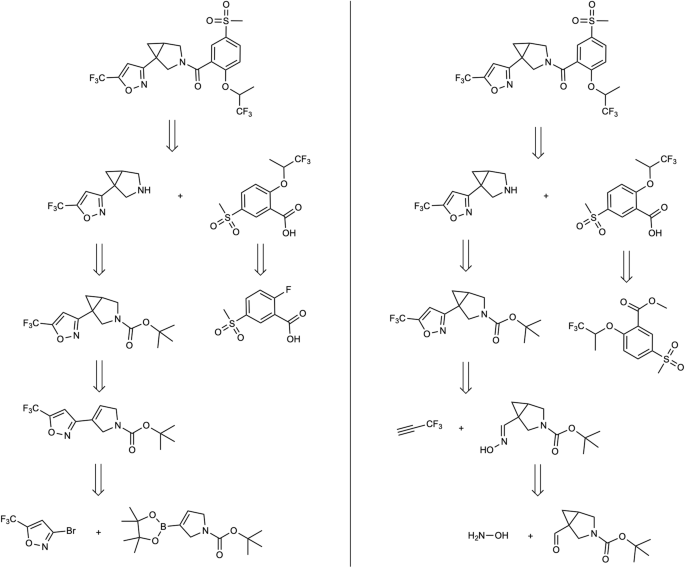
Retrosynthesis paths obtained with the standard confidence-based approach (left) and with the proposed method (right) for the Product B of Fig. 3 .
In the Supplementary Material, we show the retrosynthesis paths of Product A and B obtained via the method proposed by Chen et al. 21 , see Supplementary Figs. 5 and 6 , respectively. In these examples, we observe that the reaction pathways generated via the Retro* method are sub-optimal due to non-regioselective reactions.
To further analyze the impact of the proposed search strategy compared to a single-step confidence-based, we considered four additional examples where the latter is performing well. We employ the same settings as in the previous case studies with a focus on delineating the distinctions between the an hypergraph exploration approach and the proposed method. First, the comparison reported in Fig. 6 shows how predicted reaction pathways can exhibit a lot of similarities. The key distinction lies in the formation of the heteroaromatic chloride. The standard confidence-based approach relies on it being present in the building block, whereas the proposed method involves its creation under harsh conditions with POCl3. Nevertheless, this step streamlines the retrosynthetic process, allowing to initiate synthesis from a renewable building block.
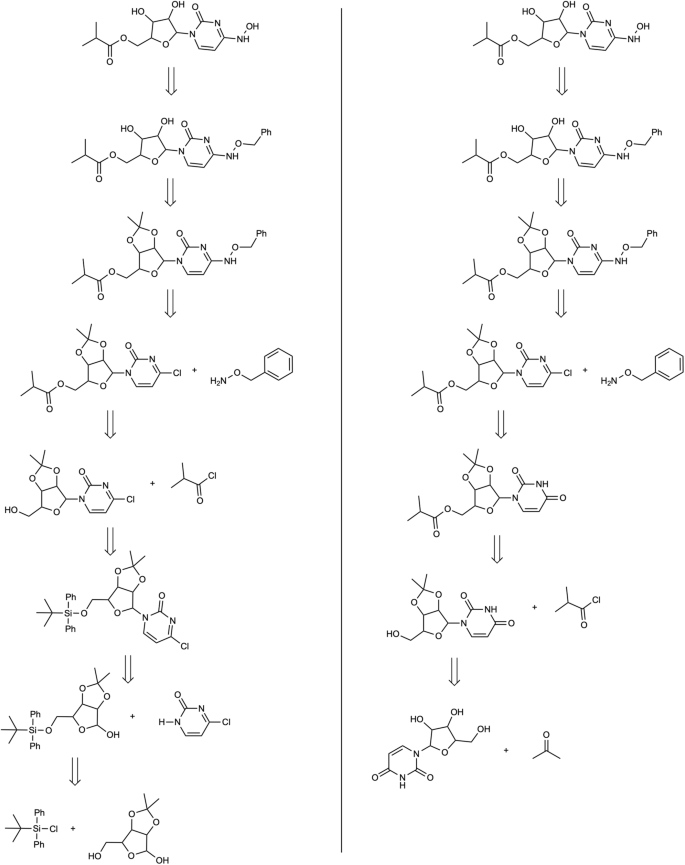
Retrosynthesis paths obtained with the standard confidence-based approach (left) and with the proposed method (right) with a key distinction in the formation of the heteroaromatic chloride.
Second, in Fig. 7 we can observe how two predicted routes can diverge in the construction of the core of the target molecule. The proposed method initiates with a cycloaddition to shape the three-membered cycle, and safeguarding the hydroxylic group enables manipulation of the opposing segment. In contrast, the standard confidence-based approach uses a Wittig reaction, followed by a hydroboration-oxidation sequence to achieve a comparable outcome. Third, in the molecule considered in Fig. 8 , we observe how the initial two disconnections remain identical. Subsequently, the proposed method capitalizes on the selectivity arising from two distinct halogen atoms. Ultimately, it tries to obtain the bicyclic aromatic structure with a nucleophilic substitution-carbonylamine condensation. Lastly, in Fig. 9 , the solution derived from the proposed method is more concise as it directly commences from the spyro-compound. In contrast, the hypergraph exploration method expends considerable effort in its construction. This underscores the string-growth approach’s proficiency in identifying a more direct route from a commercially available precursor. Additionally, the retrosyntheses are equivalent, differing only in the choice of protecting groups for the aminic group and the methodologies employed for its formation.
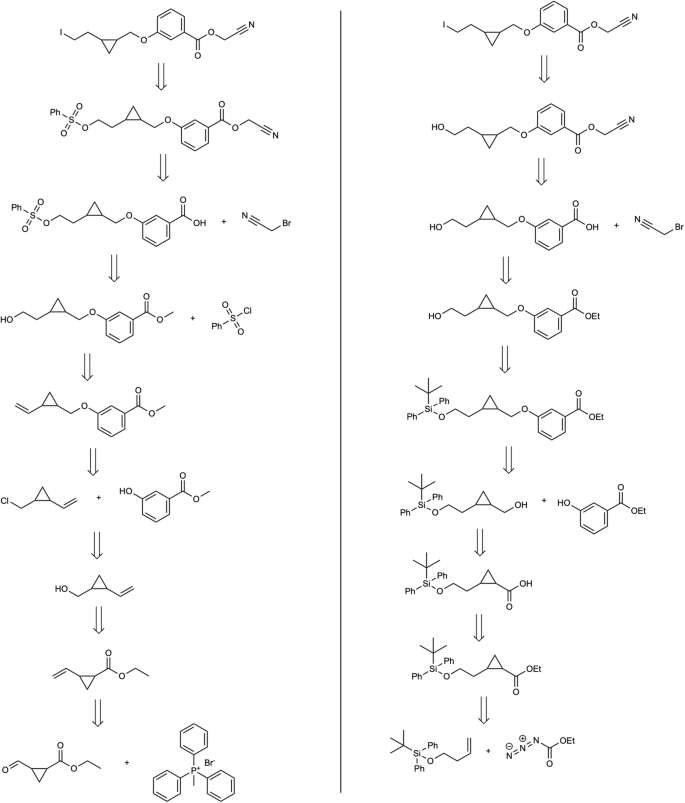
Retrosynthesis paths obtained with the standard confidence-based approach (left) and with the proposed method (right) where the formation of the core of the product highlights the different strategies.
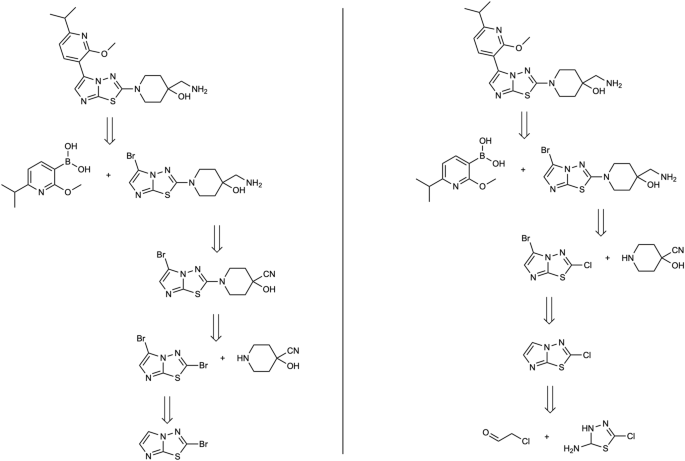
Retrosynthesis paths obtained with the standard confidence-based approach (left) and with the proposed method (right) where the proposed method exploit more the selectivity of the halogen atoms.
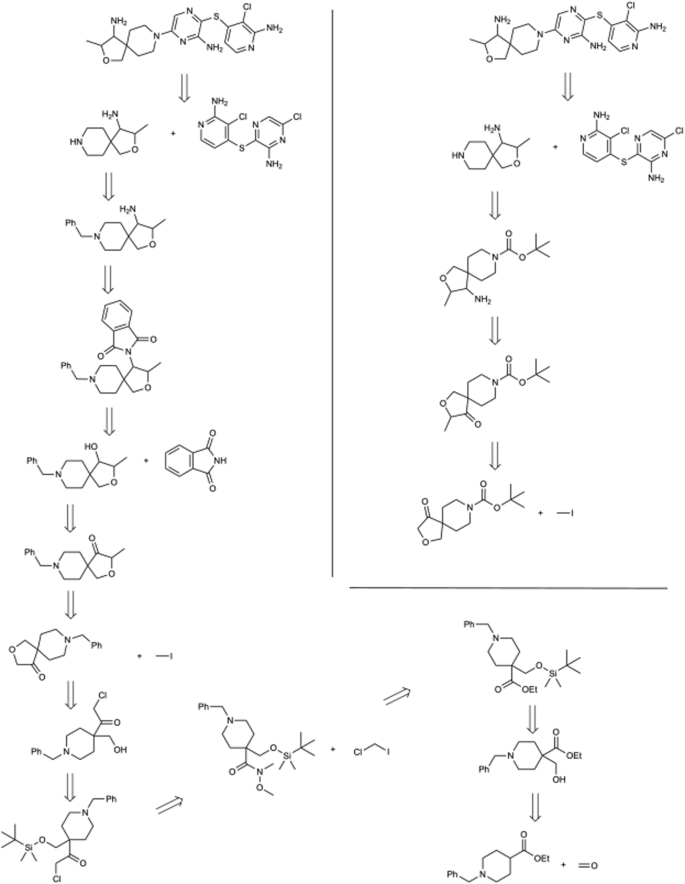
Retrosynthesis paths obtained with the standard confidence-based approach (left) and with the proposed method (right) with a more concise predicted route for the latter.
The overall effect of the proposed search approach is that the steps along the retrosynthetic tree are more interconnected, less focused on a single reaction and more on the quality of the resulting sequence, leading to shorter retrosynthesis with an higher quality and reliability of each step.
Figure 10 and Fig. S 4 in the Supplementary Material compare the length of the retrosynthesis obtained with the hypergraph search and our proposed method, which produces on average less deep retrosynthesis trees. The retrosyntheses planned by our proposed method overall consist of fewer reactions, see Fig. S 7 in the Supplementary Material, which compares the total number of reactions of retrosynthesis predicted via hypergraph search, our proposed method, and the “Retro * ” method 21 .
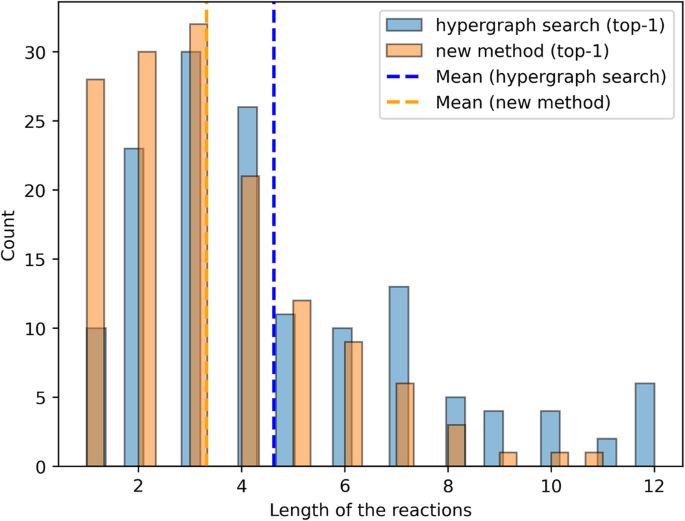
The histogram focuses on the frequency of tree depth for each product based on the top-1 results. Vertical blue and orange dashed lines indicate the means of the two distributions: hypergraph search and the proposed method, respectively. In the Supplementary Material Fig. S4 provides additional comparisons of the length of predicted retrosynthesis.
For reproducibility, in the Supplementary Material, we report the parameters configuration used in the RXN for Chemistry platform to produce the reported results, see Supplementary Fig. 2 .
We present a novel method for generating complete retrosynthesis pathways that incorporates human expert synthesis strategies, which are not captured by existing single-step prediction models. Our method utilizes chemical reaction fingerprints, commonly used for reaction classification, to capture multi-step strategies. Retrosynthetic routes are represented as strings in the fingerprint space. The fingerprints of published chemical routes are used to populate a database, which is then used to guide the expansion of the retrosynthesis tree by ranking branches using a score that favors pathways closer to those compiled by human experts. This approach prioritizes pathways that use similar retrosynthetic strategies, without requiring additional training of existing single-step prediction models.
Because at the time of this publication there are no benchmarks available for multi-steps synthesis, the testing a scale poses significant challenges. Therefore, we have engaged expert chemists among our authors to evaluate specific examples.
We demonstrate the effectiveness of our method by applying it to the synthesis of several products, which serve as prototypical examples to highlight the differences between our approach and the older statistical approach. In general we observe that the proposed algorithm shows improved results by creating shorter retrosynthesis paths. It efficiently handles the protection and deprotection of functional groups throughout the entire synthesis process. The method maximizes the utilization of various reactions, allowing for gentler reaction conditions for example eliminating the requirement for harsh substances such as organometals and potent oxidants.
Our proposed algorithm, which currently rewards synthesis routes closely resembling those documented in the Pistachio dataset, is designed to be adaptable to various preferences and constraints within the fingerprint space. By default, our scoring metric considers all the retrosyntheses in Pistachio equally important. Nothing prevents to customize our score function by assigning different weights to routes based on specific preferences or constraints, or, alternatively, combining with other metrics linked to trajectories in the fingerprint space, for instance, accounting for precise and unambiguous comparisons for improved resource management proposed by Andraos 42 or including concepts such as the one of strategic molecules proposed by Weber et al. 43 .
Our method relies on representing single-step chemical reactions with reaction fingerprints 36 . We build sequences of reaction steps of different lengths from the protocol synthesis contained in the Pistachio dataset 40 belonging to the same document ID. Each sequence of steps will be referenced as a string or trajectory in the fingerprints space. We compute the fingerprint of a synthetic pathway by concatenating the fingerprints of each step of the sequence and thus construct a database for all the synthetic procedures extracted from Pistachio. To promote the exploration and continuation of branches in the retrosynthesis tree that closely align with pre-existing trajectories derived from publications, we compare distances between the expanded pathway and any pre-existing string. The pathway with shorter distances to existing pathways will be prioritized for further exploration.
Chemical reaction fingerprints
Each step is identified by a chemical reaction in SMILES notation, which consists of a text string containing the precursors and the corresponding product separated by the “>>” token. The precursors are separated by a “.” token. In this work we used the fingerprints of Schwaller et al. 36 , which is an array of real numbers of length 256. The fingerprints of chemical reaction SMILES of the same reaction class are closer in space compared to the SMILES of different reaction classes.
Dimensionality reduction of the fingerprints of a chemical reaction
Expanding a retrosynthetic route close to humanly compiled pathways requires the operation on fingerprints of thousands of sequences during a single retrosynthesis prediction task. Each operation involved the calculation of the Euclidean distance between pairs of fingerprints. To speed up the computation, we performed a PCA reduction on the set of fingerprints of the chemical reactions, reducing the dimensionality from 256 to 64, 32, 16, and 8. We then computed the correlation between the distances in the original space and the reduced space and we identified a value of 16 being big enough to preserve the relative distances among chemical reaction SMILES.
Retrosynthesis route fingerprints
Given that each single reaction step is a point in the fingerprint space, a sequence of steps is can be thought of a string connecting the individual steps part of the synthetic pathway. Figure 11 shows two examples: for a linear and branched synthesis, see Fig. 11 a, b, respectively. The string contains the information both on the order of the steps in the sequence, but also on their nature. Branched synthesis, similar to linear synthesis, can be described in the same space, as it is shown in the second example illustrated in Fig. 11 b.
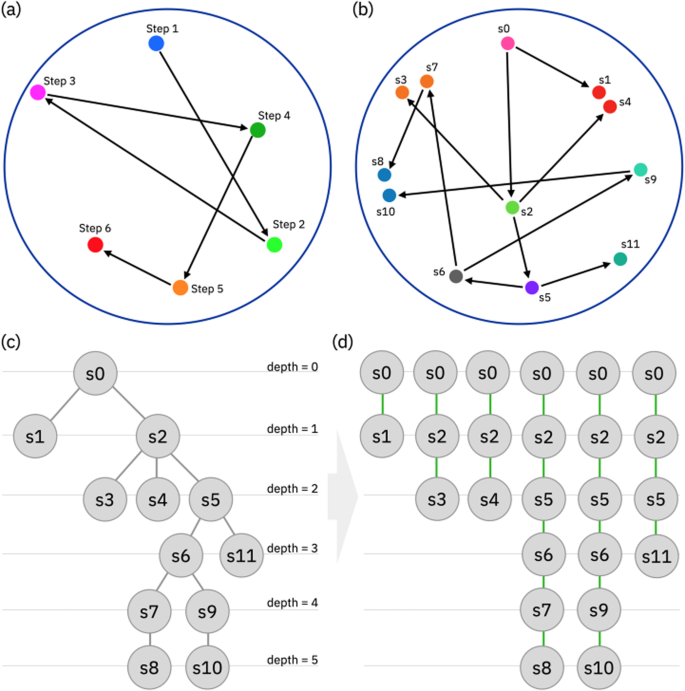
a Illustrative representation projected in two dimensions of sequence of chemical steps in the fingerprint space. This sequence could represent an entire linear synthesis or a smaller part consisting of six consecutive steps. Each of the six chemical steps maps to a specific point in the embedding space. The sequence is represented by a trajectory connecting all the points one after the other with a specific order. For visualization purposes, a two dimensions fingerprint space is used. b Same illustrative example as in ( a ) but for a non-linear synthesis, described via tree structure. Different trajectories merge in common nodes. c Graph representation of the retrosynthesis tree illustrated in ( b ). d Linear sequences derived from retrosynthesis tree. Each sequence connects one leaf of the tree to the common root. The root of the tree represents the last (time ordered) reaction step giving the target product. The root in at depth equal to zero. The leaves are at depth greater than zero. We use the convention to count the steps from the root to the leaves (backward).
The fingerprint of a retrosynthesis route (or string) is represented via an array built concatenating the fingerprint of each chemical reaction step, in the same order they appear in the sequence. A sequence of length N would be described by an array of N × 256 dimensions, if we use the original embedding space. The same sequence could be analogously described by an array N × 16 long using the representation in the PCA reduced space. This definition makes every string’s fingerprint depend on its particular length.
For example, considering the case of a linear pathway consisting of M = 6 steps as reported in Fig. 11 a, we can build M + 1 − N fingerprints of length N ( N ≤ M ). It is immediate to see how each retrosynthesis route will contribute only to sets shorter or at most of length equal to the maximum length of the extracted route. Similarly, we can extract linear sequences from branched pathways to augment the sequence dataset. An example is described in Fig. 11 .
To expedite the search of closest sequences, we employed the cKDTree class from the scipy library, which allows us to efficiently index each list of vectors and quickly retrieve the nearest neighbors of any given point. This indexing process was performed directly in the PCA reduced space, offering the advantage of a smaller indexed object size that needs to be stored and loaded into memory during runtime.
Algorithm of the fingerprint-driven retrosynthesis
The proposed method requires as input a target product, a single-step prediction model to preform single-step retrosynthesis predictions, and a model to convert chemical reaction SMILES into fingerprints. We use the fingerprint model of Schwaller et al. 36 and for the retro-predictions we use the models available on the RXN platform 44 , with the results presented in the next section relying on the use of the model with ID “2020-07-01”.
In retrosynthesis planning, a route is considered complete when all leaves of the retrosynthesis tree contain reaction SMILES with the precursors that are available. To this end, we use the database provided by eMolecules 45 to determine the availability of each molecule. The method involves a series of steps, which are exemplified in Fig. 12 for better understanding. The figure presents a simplified example of a reaction SMILES tree, along with the intermediate levels, highlighting the role of the precursor compounds.
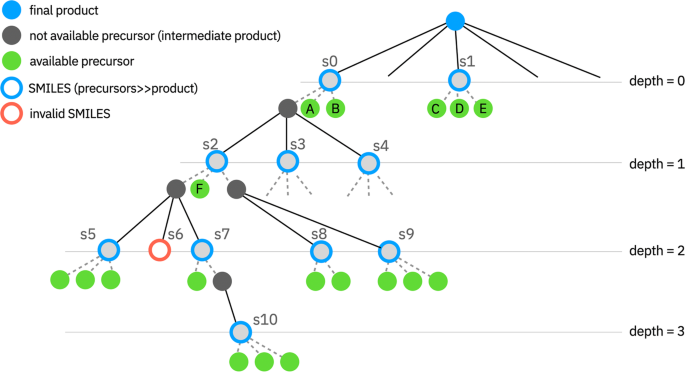
The color code is indicated in the legend of the figure. This representation not only indicates the reaction SMILES, it also shows the composition of the precursors of each SMILES. Green filled dots indicate available compounds, while black filled product are not available precursors that need further steps of synthesis.
Given a target product, the retrosynthesis route is expanded using the following steps:
The first step in our approach is to conduct a top-N single-step retro prediction for the desired product. For each prediction, we generate a reaction SMILES that follows the format of “precursors >> product”, where the precursors are separated by a dot. Subsequently, we calculate the fingerprint of each reaction SMILES.
We filter out all predictions that fail the round-trip check, in which a forward prediction model (in our case the Molecular Transformer 46 ) is used to confirm the correct prediction of the product from the precursors. This check is carried out by using the precursors predicted from the retro model as input for a forward model. If the resulting product from the forward prediction is the same as the original product, we consider the round prediction to be successful.
Our model predicts not only the reactants but also the reagents, solvents, and catalysts, leading to several single-step retro-predictions that may be quite similar. Although they have the same disconnection point, they use slightly different precursors. To ensure better exploration of the retrosynthesis tree and promote diversity, we use the chemical reaction fingerprints to remove similar predictions, that are too close in the fingerprint space. We use a fixed threshold of 2.0, which we determined by examining the distance distribution of these top-N predictions and testing thresholds between 1.0 and 2.5. The top-N predictions, which passed the round-trip check, are sorted by length with its maximum value being N. For each i in [1:N] and each j in [i + 1:N], if d i j < 2.0, we remove j . This filter action eliminates reactions that are too similar, expanding further only one of them, while saving computational time as the tree grows deeper. This step will retrain K single-step predictions, with K ∈ [1, N ].
Different to our previous approach 14 , here we do not filter based on the confidence of the forward model, thus avoiding biases on reaction classes that are under-represented and not sufficiently learned. In fact, sometimes there are effective retrosynthesis pathways that involve a single retro-step with low confidence, which would be penalized by confidence-based methods and possibly never explored.
For each of the K results obtained from the previous step, we examine the precursors to identify those that are not readily available. These precursors are deemed intermediate products that require synthesis. We maintain a list of completed reaction SMILES and those that require further prediction steps. Reaction SMILES with all available precursors are marked as completed. Completed pathways including the final product are considered successfully finished retrosynthesis, as the product can be directly obtained from the available precursors. In the example shown in Fig. 12 , the reaction labeled s1 is completed in a single step, and the corresponding chemical SMILES (s1) is located at depth zero.
When possible, our approach will return multiple routes, which are compatible with the time and execution constraints provided by the user. In the example of Fig. 12 , the prediction s0 contains two available precursors, A and B, and a third precursor that is not available. If compatible with the user settings, the method will continue to search for more routes expanding the leaves of the tree which correspond to reaction SMILES containing one or more non-available precursors. Precursors that are not available are considered intermediate products that need to be synthesized. During the execution we keep updating the lists of chemical reaction SMILES, which contain available precursors, and those that require further prediction steps. For example, in Fig. 12 , the reaction labeled s1 is completed in a single step, and the corresponding chemical SMILES is located at depth zero. If the maximum number of prediction is reached, the algorithm returns the results and terminates the execution, otherwise it continues by expanding the tree.
The tree expansions involve single-step retro predictions using as input all the non-available precursors of the non-completed reaction SMILES. In the example shown in Fig. 12 , these new predictions are located at depth one and are labeled as s2, s3, and s4.
If the precursors of each of the predictions s2, s3, and s4 were available, the chemical smiles s2, s3, and s4 would be leaves of the tree, and the solution would have three alternatives. In this case, we would return four routes: a single-step route [s1] and three 2-steps routes [s0, s2], [s0, s3], and [s0, s4]. For each route, we would compute the score and rank the solutions accordingly.
However, following the example of Fig. 12 , if two precursors of s2 were not available, we would continue the search for more routes involving s2. This process is repeated for each non-completed reaction SMILES until all routes have been explored or the maximum number of solutions requested by the user has been reached.
During the expansion of the retrosynthesis tree, the number of unfinished routes may increase, depending on the number of unavailable precursors. As this number grows, the algorithm computes a score to select which routes have to be further expanded and which should not. The selection is necessary to limit the exploration in favor of the computational time. Continuing with the example of Fig. 12 , we now compute the score for pathways consisting of two steps, for example s0 and s2. We compute the fingerprints of s0 and s2, and then we apply the PCA model: each fingerprint after PCA is a point in a 16-dimensional space. After concatenation of the two 16-dimensional arrays into a single array of length 32, we search for the closest five trajectories in the database using the cKDTree library. We store the average distance of the top five closest trajectories as a measure of how close or far the predicted [s0, s2] pathway is from the reported reactions. The same protocol will be applied for computing the score for all other alternative routes, [s0, s3] and [s0, s4] in the example. Although the sequences considered in this description consist of a length of two steps, as the tree depth grows, the score will compare longer sequences.
Among the unfinished routes, we select to expand further only a fraction, typically the top-M having the smallest distance from the database. The number M is usually between 10 and 20. The user can set the value of M to balance between the duration of the prediction and accuracy. Increasing M allows for the continuation of more routes, which results in more exploration but also a larger number of single-step retro predictions and a longer overall prediction time. On the contrary, a smaller value of M reduces exploration for a faster prediction.
After identifying the top M pathways, we search for intermediate compounds that are not available and require further synthesis. These intermediate products are stored in a list, and we perform single-step retro predictions to determine their precursors. In the simplified representation shown in Fig. 12 , these predictions are labeled as s5, s6, s7, s8, and s9. However, some predictions may result in invalid SMILES, such as s6, which are discarded.
If the precursors of s5, s8, and s9 are all available compounds, they do not require additional synthesis steps. At depth equal to two, there are three complete routes. One route is given by the single-step reaction s1. The other two routes are given by [s0, s2, s5, s7] and [s0, s2, s4, s8], both of which share the first two steps, [s0, s2].
The algorithm loops over the precursors and labels reactions as finished or to be continued based on the availability of compounds. The system also verifies that all branches of the tree have leaves containing only available compounds to compute the number of completed routes. Once the tree’s status is updated, we verify a set of stopping criteria that the user can set via the user interface.
The algorithm stops when at least one of the following criteria is met. The first termination is effective if the number of completed routes is greater than a user-defined threshold. At each prediction step, which occurs every time the tree increases in depth, there is a check to update the number of the new finished routes. The second termination criteria checks if the maximum depth of the tree is reached. Typically, the maximum depth is set to be between 6 and 15. Finally, if the runtime is exceeding a user provided value, the algorithm will terminate its execution. Whenever a stop condition is met, the execution proceeds directly to the final evaluation of the score and returns the solutions. If none of the stopping criteria are met, the algorithm proceeds to the next step.
As the sequences of steps become longer, further single-step retrosynthesis predictions are required. In this step, we describe how we compute the score for sequences of length greater than two, which was already been presented in the previous step.
To compute the score for a sequence of length N, we consider all sub-sequences of lengths ranging from two to N-1, with a maximum length of 15. We set a threshold of 40 for the distance between two steps in a sequence. This distance is computed in the 16-dimension embedding space after PCA. Any distance greater than the threshold is assumed to be equal to the threshold. This decision is based on the observation that if two steps belong to different classes, their distance is likely to be larger than the first minimum in the distance distribution. The threshold of 40 is already a much larger value than the location of the first minimum. Sub-sequences longer than 15 steps are excluded since it is very improbable to find such long sequences of steps close to each other. For each of these sequences, we search the corresponding database for the closest fingerprints and take the average distance of the closest five as the partial score. We compute the overall score of a sequence as the sum of partial scores from all sequences of different lengths within a specified range, thus accounting for different alignments between predicted sequences and those in the database.
The different pathways are ranked based on their scores, and those with the lowest scores are further expanded in the next single-step prediction iteration. For each expanded pathway, we search for all the nodes in the tree that are necessary to complete the retrosynthesis, which in turn requires all the leaves to represent available compounds. These additional nodes are expanded independently of their score.
After the stopping criteria is met, we compute the score of each route. The route can be either linear or a tree. In the case of a linear route, the score is calculated as described in the previous step. However, for a branched route, the score is computed summing the scores of all the pathways connecting the route to the leaves. Each of these pathways is linear and is scored in the same way as described before.
The final step of the algorithm occurs after the search has been completed, and it can unfold in two different scenarios. In the first scenario, no solution has been found, and the system returns a failed status. In this case, no solution can be displayed to the user. In the second scenario, at least one solution has been found, and the algorithm proceeds with the finalization steps.
In this finalization step, an additional score value y is computed and returned to the user interface using the formula y = 1/(1 + log( x + 1)), where x is the tree-route score computed in the previous step. While arbitrary, the scoring function was chosen to return a number between 0 and 1, with an higher value connected to a greater confidence in the designed retrosynthesis.
Data availability
We provide sample data to run the method ( https://github.com/rxn4chemistry/rxn-neb ). The Pistachio and emolecules data are available in 40 and 45 respectively.
Code availability
The experiments presented in the paper can be reproduced using the RXN platform via UI ( https://rxn.res.ibm.com ) or REST API (Python client: https://github.com/rxn4chemistry/rxn4chemistry ). Additionally, we provide and open source implementation of our algorithm that allows to run the method customizing: single-step forward/backward models, fingerprints model ( https://github.com/rxn4chemistry/rxn-neb ).
Corey, E. J. & Wipke, W. T. Computer-assisted design of complex organic syntheses. Science 166 , 178–192 (1969).
Article CAS PubMed Google Scholar
Szymkuć, S. et al. Computer-assisted synthetic planning: the end of the beginning. Angew. Chem. Int. Ed. 55 , 904–5937 (2016).
Article Google Scholar
Liu, B. et al. Retrosynthetic reaction prediction using neural sequence-to-sequence models. ACS Cent. Sci. 3 , 1103–1113 (2017).
Article CAS PubMed PubMed Central Google Scholar
Segler, M. H. S. & Waller, M. P. Neural-symbolic machine learning for retrosynthesis and reaction prediction. Chem. Eur. J. 23 , 5966–5971 (2017).
Dai, H., Li, C., Coley, C. W., Dai, B. & Song, L. Retrosynthesis prediction with conditional graph logic network. arXiv https://doi.org/10.48550/arXiv.2001.01408 (2020).
Segler, M. H. S., Preuss, M. & Waller, M. P. Planning chemical syntheses with deep neural networks and symbolic AI. Nature 555 , 604–610 (2018).
Chen, B., Shen, T., Jaakkola, T. S. & Barzilay, R. Learning to make generalizable and diverse predictions for retrosynthesis. arXiv https://doi.org/10.48550/arXiv.1910.09688 (2019).
Zheng, S., Rao, J., Zhang, Z., Xu, J. & Yang, Y. Predicting retrosynthetic reactions using self-corrected transformer neural networks. J. Chem. Inf. Model 60 , 47–55 (2020).
Coley, C. W. A robotic platform for flow synthesis of organic compounds informed by AI planning. Science 365 , eaax1566 (2019).
Schreck, J. S., Coley, C. W. & Bishop, K. J. M. Learning retrosynthetic planning through simulated experience. ACS Cent. Sci. 5 , 970–981 (2019).
Baylon, J. L., Cilfone, N. A., Gulcher, J. R. & Chittenden, T. W. Enhancing retrosynthetic reaction prediction with deep learning using multiscale reaction classification. J. Chem. Inf. Model 59 , 673–688 (2019).
Molga, K., Dittwald, P. & Grzybowski, B. A. Navigating around patented routes by preserving specific motifs along computer-planned retrosynthetic pathways. Chem 5 , 460–473 (2019).
Article CAS Google Scholar
Lee, A. A. et al. Molecular transformer unifies reaction prediction and retrosynthesis across pharma chemical space. Chem. Commun. 55 , 12152–12155 (2019).
Schwaller, P. et al. Predicting retrosynthetic pathways using transformer-based models and a hyper-graph exploration strategy. Chem. Sci. 11 , 3316–3325 (2020).
Karpov, P., Godin, G. & Tetko, I. V. A transformer model for retrosynthesis. In International Conference on Artificial Neural Networks 817–830 (2019).
Ishida, S., Terayama, K., Kojima, R., Takasu, K. & Okuno, Y. Prediction and interpretable visualization of retrosynthetic reactions using graph convolutional networks. J. Chem. Inf. Model 59 , 5026–5033 (2019).
Lin, K., Xu, Y., Pei, J. & Lai, L. Automatic retrosynthetic route planning using template-free models. Chem. Sci. 11 , 3355–3364 (2020).
Shi, C., Xu, M., Guo, H., Zhang, M. & Tang, J. A graph to graphs framework for retrosynthesis prediction. In Proceedings of the 37th International Conference on Machine Learning 8818–8827 (2020).
Genheden, S. Aizynthfinder: a fast, robust and flexible open-source software for retrosynthetic planning. J. Cheminform. 12 , 70 (2020).
Article PubMed PubMed Central Google Scholar
Tetko, I. V., Karpov, P., Van Deursen, R. & Godin, G. State-of-the-art augmented NLP transformer models for direct and single-step retrosynthesis. Nat. Commun. 11 , 5575 (2020).
Chen, B., Li, C., Dai, H. & Song, L. Retro*: learning retrosynthetic planning with neural guided A* search. In International Conference on Machine Learning , 1608–1616 (PMLR, 2020).
Mikulak-Klucznik, B. et al. Computational planning of the synthesis of complex natural products. Nature 588 , 83–88 (2020).
Badowski, T., Gajewska, E. P., Molga, K. & Grzybowski, B. A. Synergy between expert and machine-learning approaches allows for improved retrosynthetic planning. Angew. Chem. Int. Ed. Engl. 59 , 725–730 (2020).
Hasic, H. & Ishida, T. Single-step retrosynthesis prediction based on the identification of potential disconnection sites using molecular substructure fingerprints. J. Chem. Inf. Model 61 , 641–652 (2021).
Amol Thakkar, A. et al. Artificial intelligence and automation in computer aided synthesis planning. React. Chem. Eng. 6 , 27–51 (2021).
Mao, K. et al. Molecular graph enhanced transformer for retrosynthesis prediction. Neurocomputing 457 , 193–202 (2021).
Wang, X. R. et al. Retroprime: a diverse, plausible and transformer-based method for single-step retrosynthesis predictions. Chem. Eng. J. 420 , 129845 (2021).
Ishida, S., Terayama, K., Kojima, R., Takasu, K. & Okuno, Y. AI-driven synthetic route design incorporated with retrosynthesisknowledge. J. Chem. Inf. Model 62 , 1357–1367 (2022).
Thakkar, A. et al. Unbiasing retrosynthesis language models with disconnection prompts. ACS Cent. Sci. 9 , 1488 (2023).
Pasquini, M. & Stenta, M. LinChemIn: route arithmetic-operations on digital synthetic routes. J. Chem. Inf. Model. 64 , 1765–1771 (2024).
Lin, M. H., Tu, Z. & Coley, C. W. Improving the performance of models for one-step retrosynthesis through re-ranking. J. Cheminform. 14 , 15 (2022).
Warren, S. & Wyatt, P. Organic Synthesis: The Disconnection Approach 2nd ed. Wiley (2011).
Yu, Y. et al. GRASP: navigating retrosynthetic planning with goal-driven policy. Adv. Neural Inf. Process. Syst. 35 , 10257–10268 (2022).
Google Scholar
Zhong, Z. et al. Recent advances in deep learning for retrosynthesis. WIREs Comput. Mol. Sci. 14 , e1694 (2023).
Jiang, Y. et al. Artificial intelligence for retrosynthesis prediction. Engineering 25 , 32–50 (2023).
Schwaller, P. et al. Mapping the space of chemical reactions using attention-based neural networks. Nat. Mach. Intell. 3 , 144–152 (2021).
Janet, J. P., Tomberg, A. & Bostrőm, J. Reusability report: Learning the language of synthetic methods used in medicinal chemistry. Nat. Mach. Intell. 3 , 572–575 (2021).
Schwaller, P., Vaucher, A. C., Laino, T. & Reymond, J.-L. Prediction of chemical reaction yields using deep learning. Mach. Learn. Sci. Technol. 2 , 015016 (2021).
Wang, X. et al. From theory to experiment: transformer-based generation enables rapid discovery of novel reactions. J. Cheminform. 14 , 1–14 (2022).
Nextmove Software, Pistachio. https://www.nextmovesoftware.com/pistachio.html . Accessed 2021.
Schneider, N., Lowe, D. M., Sayle, R. A. & Landrum, G. A. Development of a novel fingerprint for chemical reactions and its application to large-scale reaction classification and similarity. J. Chem. Inf. Model. 55 , 39–53 (2015).
Andraos, J. On using tree analysis to quantify the material, input energy, and cost throughput efficiencies of simple and complex synthesis plans and networks: towards a blueprint for quantitative total synthesis and green chemistry. Org. Process Res. Dev. 10 , 212–240 (2006).
Weber, J. M., Lió, P. & Lapkin, A. A. Identification of strategic molecules for future circular supply chains using large reaction networks. React. Chem. Eng. 4 , 1969–1981 (2019).
IBM RXN for chemistry. https://rxn.res.ibm.com . Accessed August 2023.
eMolecules Database. https://www.emolecules.com . Accessed August 29, 2019.
Schwaller, P. et al. Molecular transformer: a model for uncertainty-calibrated chemical reaction prediction. ACS Cent. Sci. 5 , 1572–1583 (2019).
Download references
Acknowledgements
This publication was created as part of NCCR Catalysis (grant number 180544), a National Centre of Competence in Research funded by the Swiss National Science Foundation.
Author information
Authors and affiliations.
IBM Research Europe, Saümerstrasse 4, 8803, Rüschlikon, Switzerland
Federico Zipoli, Carlo Baldassari, Matteo Manica, Jannis Born & Teodoro Laino
National Center for Competence in Research-Catalysis (NCCR-Catalysis), Zurich, Switzerland
Federico Zipoli & Teodoro Laino
You can also search for this author in PubMed Google Scholar
Contributions
T.L. laid the foundational groundwork for the project through the initial design and conceptualization of the idea. F.Z. initiated the conceptual development of the project, expanded and adapted the original idea, and was primarily responsible for implementing the string approach. C.B. provided expert analysis and critical evaluation of the retrosynthesis strategy, ensuring the robustness and reliability of the proposed chemical synthesis. M.M. gave insights technical execution of the project, directly contributing to the implementation and integration of the project with the RXN platform. M.M. oversaw the process of open-sourcing of the project code and the assembling the scripts for file processing for easy accessibility and applicability of the work. J.B. was responsible for processing the data from the Pistachio and USPTO databases. T.L. also evaluated the retrosynthesis strategy, contributing to the strategic direction and methodological rigor of the research. All authors contributed significantly to the manuscript, collaborating on the drafting and critical revision of the text to ensure the clarity, accuracy, and coherence of the final publication.
Corresponding author
Correspondence to Federico Zipoli .
Ethics declarations
Competing interests.
The authors declare no competing interests.
Additional information
Publisher’s note Springer Nature remains neutral with regard to jurisdictional claims in published maps and institutional affiliations.
Supplementary information
Supplementary material, rights and permissions.
Open Access This article is licensed under a Creative Commons Attribution 4.0 International License, which permits use, sharing, adaptation, distribution and reproduction in any medium or format, as long as you give appropriate credit to the original author(s) and the source, provide a link to the Creative Commons licence, and indicate if changes were made. The images or other third party material in this article are included in the article’s Creative Commons licence, unless indicated otherwise in a credit line to the material. If material is not included in the article’s Creative Commons licence and your intended use is not permitted by statutory regulation or exceeds the permitted use, you will need to obtain permission directly from the copyright holder. To view a copy of this licence, visit http://creativecommons.org/licenses/by/4.0/ .
Reprints and permissions
About this article
Cite this article.
Zipoli, F., Baldassari, C., Manica, M. et al. Growing strings in a chemical reaction space for searching retrosynthesis pathways. npj Comput Mater 10 , 101 (2024). https://doi.org/10.1038/s41524-024-01290-x
Download citation
Received : 12 October 2023
Accepted : 26 April 2024
Published : 10 May 2024
DOI : https://doi.org/10.1038/s41524-024-01290-x
Share this article
Anyone you share the following link with will be able to read this content:
Sorry, a shareable link is not currently available for this article.
Provided by the Springer Nature SharedIt content-sharing initiative
Quick links
- Explore articles by subject
- Guide to authors
- Editorial policies
Sign up for the Nature Briefing newsletter — what matters in science, free to your inbox daily.


Chemical Communications
Synthesis of benzooxepane-fused cyclobutene derivatives via pd-catalyzed cascade reactions of haloarenes and diynylic ethers.
A tandem palladium-catalyzed Sonogashira coupling, propargyl-allenyl isomerization, and [2+2] cycloaddition sequence between electron-deficient haloarenes and 1,8-diynylic ethers is developed. The reaction shows good functional tolerance and proceeds under mild conditions to provide a new profile of benzooxepane-fused cyclobutene derivatives in moderate to high yields with high selectivity. The reaction mechanism is validated both by experimental studies and DFT calculations.
Supplementary files
- Supplementary information PDF (9948K)
- Crystal structure data CIF (830K)
Article information
Download citation, permissions.

S. Zhu, W. Huang, S. Liu, R. Yu, Y. Ma, H. Wang, R. Zhang, B. Liu, Y. Lan and R. Shen, Chem. Commun. , 2024, Accepted Manuscript , DOI: 10.1039/D4CC00999A
To request permission to reproduce material from this article, please go to the Copyright Clearance Center request page .
If you are an author contributing to an RSC publication, you do not need to request permission provided correct acknowledgement is given.
If you are the author of this article, you do not need to request permission to reproduce figures and diagrams provided correct acknowledgement is given. If you want to reproduce the whole article in a third-party publication (excluding your thesis/dissertation for which permission is not required) please go to the Copyright Clearance Center request page .
Read more about how to correctly acknowledge RSC content .
Social activity
Search articles by author.
This article has not yet been cited.
Advertisements

IMAGES
VIDEO
COMMENTS
A synthesis reaction or direct combination reaction reacts two or more simple elements or compounds to form a more complex product. A synthesis reaction is one of the four main types of chemical reactions, along with decomposition, single replacement, and double replacement reactions. Here is the synthesis reaction definition, examples of the reaction using elements and compounds, a look at ...
Synthesis Reaction Examples. In the simplest synthesis reactions, two elements combine to form a binary compound (a compound made of two elements). The combination of iron and sulfur to form iron (II) sulfide is an example of a synthesis reaction : 8 Fe + S 8 → 8 FeS. Another example of a synthesis reaction is the formation of potassium ...
A synthesis reaction is a reaction in which two or more reactants chemically bond and combine to form a product. A synthesis reaction is also known as a combination reaction. Most synthesis reactions are exothermic reactions, i.e., heat is released during the reaction. General Equation. The general chemical equation for a synthesis reaction is ...
A synthesis reaction or direct combination reaction is a type of chemical reaction in which two or more simple substances combine to form a more complex product. The reactants may be elements or compounds, while the product is always a compound.
Chemical synthesis. In chemistry, chemical synthesis ( chemical combination) is the artificial execution of chemical reactions to obtain one or several products. [1] This occurs by physical and chemical manipulations usually involving one or more reactions. In modern laboratory uses, the process is reproducible and reliable.
chemical synthesis, the construction of complex chemical compounds from simpler ones. It is the process by which many substances important to daily life are obtained. It is applied to all types of chemical compounds, but most syntheses are of organic molecules. Chemists synthesize chemical compounds that occur in nature in order to gain a ...
A synthesis reaction is a type of chemical reaction in which two or more simple substances combine to form a more complex product. The reactants may be elements or compounds, while the product is always a compound. The general equation for a synthesis reaction is: A + B → AB.
Synthesis Reactions. A synthesis reaction occurs when one or more compounds combines to form a complex compound. The simplest equation of synthesis reaction is illustrated below. An example of such a reaction is the reaction of silver with oxygen gas to form silver oxide: \[2Ag (s) +O_2 (g) \rightarrow 2AgO (s) \nonumber \]
A synthesis reaction occurs when two or more reactants combine to form a single product. This type of reaction is represented by the general equation: A + B → AB. An example of a synthesis reaction is the combination of sodium (Na) and chlorine (Cl) to produce sodium chloride (NaCl). This reaction is represented by the chemical equation: 2Na ...
Chemical reactions. Chemical reactions occur when chemical bonds between atoms are formed or broken. The substances that go into a chemical reaction are called the reactants, and the substances produced at the end of the reaction are known as the products. An arrow is drawn between the reactants and products to indicate the direction of the ...
Describes the basics of synthesis reactions, how to identify them, predict the product and balance the chemical equation. Two examples are also shown, synth...
In a synthesis reaction, two or more chemical species combine, forming a more complex product in the reaction. It is also called a direct reaction and is one of the most common chemical reactions. When the two or more reactants combine they make a larger compound. A synthesis reaction is the opposite of a decomposition reaction, which is when ...
Synthesis are, at this introductory level, almost always the reverse of a decomposition reaction. That means that two pieces join together to produce one, more complex compound. These pieces can be elements or simpler compounds. Complex simply means that the product compound has more atoms than the reactant molecules.
Chemical reaction, a process in which one or more substances, the reactants, are converted to one or more different substances, the products. ... chemists say either that they carry out a synthesis or that they synthesize the new material. Reactants are converted to products, and the process is symbolized by a chemical equation. For example ...
A synthesis reaction is a chemical reaction that results in the synthesis (joining) of components that were formerly separate ( Figure 2.12 a ). Again, nitrogen and hydrogen are reactants in a synthesis reaction that yields ammonia as the product. The general equation for a synthesis reaction is A + B → AB.
Combination reactions can also be called synthesis reactions .The general form of a combination reaction is: \[\ce{A} + \ce{B} \rightarrow \ce{AB}\] ... The chemical reaction was a simple one: hydrogen combining with oxygen to produce water. Many combustion reactions occur with a hydrocarbon, a compound made up solely of carbon and hydrogen ...
It is easy to find synthesis reaction examples in everyday life, such as the formation of table salt, NaCl, from its constituent elements Na (sodium) and Cl (chlorine). The general form of these reactions is A + B → AB. These reactions may fall into other chemical reaction types.
Planning a synthesis makes you approach a chemical problem in a logical way, draw on your knowledge of chemical reactions, and organize that knowledge into a workable plan—it helps you learn organic chemistry. There's no secret to planning an organic synthesis: all it takes is a knowledge of the different reactions and some practice. ...
Synthesis. Synthesis is the production of chemical compounds by reaction from simpler materials. The construction of complex and defined new molecules is a challenging and complicated undertaking, and one that requires the constant development of new reactions, catalysts and techniques. Synthesis projects underpin developments in a very wide ...
Chemical Reactions -A chemical reaction is in which the bonds are broken within reactant molecules, and new bonds are formed within product molecules in order to form a new substance. ... Synthesis is a chemical reaction in which multiple simple compounds combine under specific physical conditions to form a complex product. Example: 2 Na + Cl 2 ...
A reaction can be classified as physical, chemical or nuclear based on the changes from reactants to products. Common types of chemical reactions are synthesis, decomposition, single displacement, double displacement, combustion (burning of methane) and acid-base (antacid with HCl) reactions.
In chemical terms, photosynthesis is a light-energized oxidation-reduction process. (Oxidation refers to the removal of electrons from a molecule; reduction refers to the gain of electrons by a molecule.) In plant photosynthesis, the energy of light is used to drive the oxidation of water (H 2 O), producing oxygen gas (O 2 ), hydrogen ions (H ...
• A chemical change is the result of a chemical reaction. • In a chemical reaction, the atoms and molecules that interact with each other are called reactants. • In a chemical reaction, the atoms and molecules produced by the reaction are called products. • In a chemical reaction, only the atoms present in the reactants can end up in the
Based on this premise, multi-step complex synthesis can be conceptualized as sequences that link multidimensional vectors (fingerprints) representing individual chemical reaction steps.
Various pathways for synthesizing 1-butanol from renewable raw materials have been explored, with a particular focus on the Guerbet reactions. This study aimed to investigate the synthesis of 1-butanol from ethanol using Mg/Al mixed oxides derived from layered double hydroxides with Mg/Al molar ratios of 3:1, 5:1, 8:1, and 10:1.
Chemical Communications. Synthesis of Benzooxepane-Fused Cyclobutene Derivatives via Pd-Catalyzed Cascade Reactions of Haloarenes and Diynylic Ethers ... The reaction shows good functional tolerance and proceeds under mild conditions to provide a new profile of benzooxepane-fused cyclobutene derivatives in moderate to high yields with high ...