- NLN Certification
- Assessment Services
- CNEA Accreditation
- NLN Foundation
- Mission & Core Values
- History & Archives
- Board of Governors
- Senior Management
- Consultants & Speakers Bureau
- NLN Nominations
- Career Center
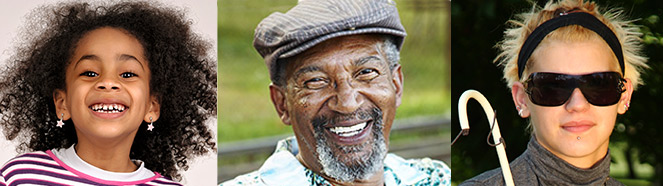

How to Use an Unfolding Case
ACE unfolding cases were written so that they can be modified to meet the needs of diverse curricula. Since preparation is key to a successful simulation experience, faculty should plan to read through each unfolding case before using it.
These cases were designed so that students can use the ACE Knowledge Domains and Essential Nursing Actions as a framework for thinking about the care and planning of their nursing interventions. Students should be introduced to these concepts before implementing the cases. They will be more successful in the simulation scenarios if they review the introductory monologues and the recommended resources and tools prior to the simulation.
We have included best practices but realize that treatments vary by region. Faculty may wish to include medications, treatments, and standards of care that are current practice in their own geographic areas.
No intentional errors were included in these cases, such as incorrect treatments or medication doses. However, in the Alzheimer's cases, the patient may have multiple providers and medication orders. An objective for some of the simulations is to use the Beers criteria to identify potentially inappropriate medications or combinations of medications for older adults. Faculty may wish to increase or decrease the complexity of the scenario depending on the level of students participating. Faculty may also wish to modify scenarios to provide an interprofessional educational experience for students. When redesigning for this purpose, we urge you to include the other health care professional(s) in the process to insure that the simulation accurately reflects their scope of practice.

Quality and Safety Education for Nurses
Strategy submission, using unfolding case studies to develop clinical forethought in novice nursing students.
Brenda Moench
RN, MS, CNE
Nursing Instructor
Institution:
University of Wisconsin Milwaukee
Competency Categories:
Patient-Centered Care, Safety
Learner Level(s):
Pre-Licensure ADN/Diploma, Pre-Licensure BSN
Learner Setting(s):
Strategy Type:
Case Studies
Learning Objectives:
Strategy Overview:
Submitted Materials:
Teaching-Strategy-QSEN-TS-241-1.docx - https://drive.google.com/open?id=1AECA5dGZdE6YPXxF0DmMMC8M9maipFe5&usp=drive_copy
Additional Materials:
Benner, P., Hughes, R.G., & Sutphen, M., (2008). Patient safety and quality: An evidence-based handbook for nurses. Rockville, MD: Agency for Healthcare Research and Quality. Tanner, C.A. (2006). Thinking like a nurse: A research-based model of clinical judgment in nursing. Journal of Nursing Education, 45, 204-211.
Evaluation Description:
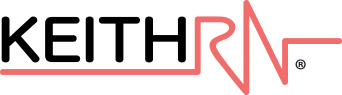
Why KeithRN Unfolding Case Studies Can Transform the Virtual (and Traditional) Clinical (1/2)
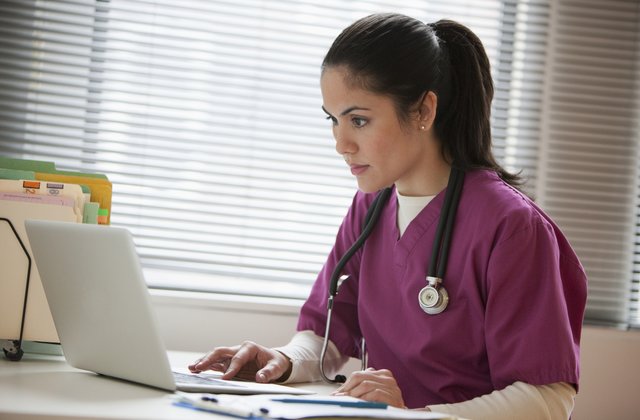
Keith Rischer – Ph.D., RN, CCRN, CEN
As a nurse with over 35 years of experience who remained in practice as an educator, I’ve witnessed the gap between how nursing is taught and how it is practiced, and I decided to do something about it! Read more…
The Ultimate Solution to Develop Clinical Judgment Skills
KeithRN’s Think Like a Nurse Membership
Access exclusive active learning resources for faculty and students, including KeithRN Case Studies, making it your go-to resource.
Sign up to receive a free KeithRN NextGen Case Study!
Develop clinical judgment skills with this innovative new case study on asthma to prepare your students for nextgen nclex and practice.
" * " indicates required fields
Keith Rischer
Helping graduate nurses transition to practice, recommended for you.
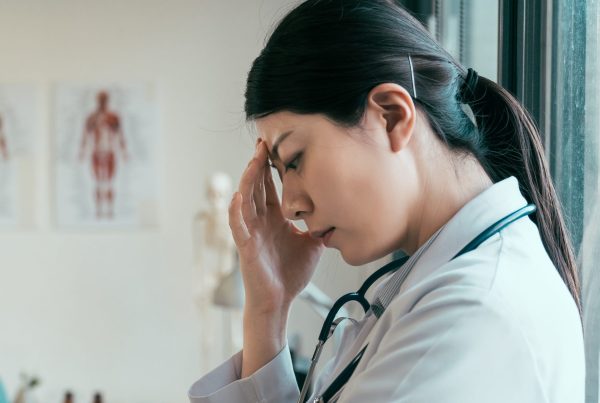
Writing Next Generation NCLEX-Style Case Study Questions
By Susan Sportsman, PhD, RN, ANEF, FAAN
Recently, our blog featured tips for developing Next Generation NCLEX (NGN)-style questions, particularly the revision of test questions faculty already include on their examinations. Revising these questions provides a strategy to adapt current test items to reflect the NGN process, since these knowledge questions represent many questions on the NGN exam.
Now let’s turn our attention to writing NGN Case Study questions . Although only three case studies will be on the NGN examination, all test-takers must respond to the six questions embedded in these case studies, regardless of how many total questions they receive. Case Studies are complex and require some thinking to create. However, I think they are fun to write—the exercise is very much like writing (a truly short) short story! Here are some suggestions for getting started.
- Begin with the objective(s) that emphasize the content you want students to apply. Keep in mind that the point of case studies (and NGN questions in general) is to evaluate how well students can apply content to a specific clinical situation.
- Develop a clinical problem that students must address that represents the objective(s) in your course. Ideas for this this problem might come from:
- Clinical situations highlighted in past tests (or in test banks to which you have access).
- Your own clinical experiences-or those of your colleagues.
- Content outlined in the NCLEX Test Design.
- Case Studies you use for class discussion or assignments for students.
- NCSBN’s Top Priority Knowledge Statements or Skills
- The introduction to the case study scenario should include:
- Client demographics (age, gender, brief and relevant social history)
- Client history
- Relevant environmental factors, such as where the client lives (own home, LTC facility, etc.)
- Normal/abnormal findings (e.g., Physical assessment findings, V/S, Lab values, psychological behaviors)
- Time considerations for the nurse/client
- Client/Nurse Interaction
- Recognize Cues: What matters most?
- Analyze Cues: What could it mean?
- Prioritize Hypothesis: Where do I go to start
- Generate Solution: What can I do?
- Take Action: What will I do?
- Evaluate Outcomes: Did it help?
Remember that the case study should represent situations that are appropriate for the level of student you are testing. Later in the curriculum the questions should represent clinical judgment that a novice nurse is expected to use in actual practice.
Starting the Process of Writing a Case Study
As you begin to write your own case studies, an example might be helpful in integrating the NGN components into an actual case study. The case study below was written for students in an early nursing clinical course, such as Fundamental in Nursing. In addition, the NGN Resources website also provides examples of case studies written at the level of a novice nurse.
Note: The templates we developed for this post can be found on our Resources page . Feel free to use them.

#1. Recognize Cues (Drag and Drop)
Drag & Drop the 4 cues the RN observed the initial assessment which require following up.

#2. Analyze Cues (Select All that Apply-N)
The RN is concerned about the client’s confusion. Identify factors that may be causes for this confusion. a. Hospital Environment * b. Early indication of Alzheimer’s Disease c. Use of Morphine for control of Pain * d. Anesthesia * e. Reduction in O2 f. Limited Sleep

#3. Prioritize Hypothesis (CLOZE)
Complete the following sentence by choosing from the following lists of options:

#4. Generate Solutions (Matrix)
Confusion continues to be a problem for the client on day three post-surgery.
Which of the possible interventions to the problem are indicated and which are contraindicated?

The RN notifies the surgeon of elevated TPR, drainage at surgical site, and pain and received orders.
#5. Take Action (Highlighting)
Upon receiving the following physician orders, highlight those which the nurse should implement right away.

#6. Evaluate Outcomes (Matrix)
The nurse has performed the interventions as ordered by the physician for the client. For each assessment finding, click to specify if the finding indicates that the client’s condition has improved, has not changed, or become worse.

Case study questions are an important part of the NGN emphasis on measuring test-takers’ clinical judgment competence. Hopefully, the example above will give you some ideas for developing your own case study questions. You may notice that these questions are not exactly formatted like those on the NGN website. Currently, most of us do not have the necessary software to completely mimic the NGN questions. But simple templates can come close. Please don’t let the differences in the format of these questions stop you from using case study questions in your testing and classroom teaching. Remember, the point of the NGN testing process is to assess new graduates’ ability to use good clinical judgment in a variety of clinical situations. Giving students the opportunity to practice clinical judgment is key to their success on NCLEX and in clinical practice.
Silvestri, L. (2020) Higher-Cognitive Level Test Question: A Starting Point for Creating Next Generation NCLEX Test Questions. White Paper. Elsevier.
Share this:
Leave a reply cancel reply, discover more from collaborative momentum consulting.
Subscribe now to keep reading and get access to the full archive.
Type your email…
Continue reading

An official website of the United States government
The .gov means it’s official. Federal government websites often end in .gov or .mil. Before sharing sensitive information, make sure you’re on a federal government site.
The site is secure. The https:// ensures that you are connecting to the official website and that any information you provide is encrypted and transmitted securely.
- Publications
- Account settings
Preview improvements coming to the PMC website in October 2024. Learn More or Try it out now .
- Advanced Search
- Journal List
- Biophys Rev (Melville)
- v.4(2); 2023 Jun
- PMC10903397

Thermal unfolding methods in drug discovery
Associated data.
Data sharing is not applicable to this article as no new data were created or analyzed in this study.
Thermal unfolding methods, applied in both isolated protein and cell-based settings, are increasingly used to identify and characterize hits during early drug discovery. Technical developments over recent years have facilitated their application in high-throughput approaches, and they now are used more frequently for primary screening. Widespread access to instrumentation and automation, the ability to miniaturize, as well as the capability and capacity to generate the appropriate scale and quality of protein and cell reagents have all played a part in these advances. As the nature of drug targets and approaches to their modulation have evolved, these methods have broadened our ability to provide useful chemical start points. Target proteins without catalytic function, or those that may be difficult to express and purify, are amenable to these methods. Here, we provide a review of the applications of thermal unfolding methods applied in hit finding during early drug discovery.
INTRODUCTION TO PROTEIN THERMAL UNFOLDING
Thermal unfolding methods have been employed increasingly as primary screening approaches during early drug discovery. These methods detect compounds that stabilize target proteins either in isolated protein assays or in the more physiological setting of the cell. As these broadly different settings suggest, the nature of the different detection methods is also broad with fluorescence, aggregation, antibody binding, mass spectrometry, and luminescence methods, all being employed to monitor the thermal unfolding process. However, the principle of the method is simple and common to all these detection approaches, the ability to discriminate between folded and unfolded states of a protein, in this case brought about by heating. The principles underlying protein thermal unfolding and denaturation have been studied since the late 19th century when Hardy and Whetham first described the processes of denaturation and agglutination of albumen. 1 Since then, we have recognized that temperature plays a central role in the balance between the different conformational states of proteins and thermal unfolding measurements of proteins began to be studied more widely following the introduction of the first adiabatic differential scanning calorimeter, applicable for studying protein unfolding by Peter Privalov in 1964. 2 Differential scanning calorimetry (DSC) follows protein unfolding by measuring the heat capacity of protein states and the excess heat associated with transitions that are induced by a change in temperature. This breakthrough allowed us to design experiments focused on understanding the nature of the forces that control the formation and stabilization of the three-dimensional structure of proteins and their functions. It also allowed the effects of ligand binding equilibria on macromolecular unfolding equilibria to be considered and studied. 3–5 Subsequently, DSC was demonstrated to be valuable in determining binding constants for very tightly binding ligands, which could not be accessed using traditional methods. 6 Whilst DSC was revolutionary in delivering thermodynamic studies on protein thermal unfolding, and measurements of ligand binding could be accessed using the technique, the throughput of the instrument limited wide application of thermal unfolding in hit identification. This situation changed in 2001 with the description of a fluorescence-based thermal shift assay applicable to screening in 384-well plates. 7 The Thermofluor™ approach, now also known as thermal shift assay (TSA) or differential scanning fluorimetry (DSF), provided a route by which high-throughput protein stabilization experiments could be undertaken without the need for a calorimeter and with reduced protein consumption per interaction. The method involves monitoring the unfolding process utilizing the change in fluorescence as an environmentally sensitive dye partitions itself into the unfolded protein. The decrease in the dielectric constant of the unfolded protein environment, compared to aqueous solution, results in a large increase in fluorescence of the dye as a function of protein unfolding with temperature. This method soon became widely adopted with the implementation of real-time polymerase chain reaction (PCR) systems coupled with the use of Sypro ® Orange dye to monitor the unfolding event. A modification to the method, whereby the requirement for the dye was removed, with detection relying on tryptophan or tyrosine fluorescence to monitor protein unfolding, was introduced and termed nanoDSF. Addressing two common issues facing DSF and other isolated protein assays in early drug discovery: the requirement for purified protein and the question of the physiological relevance of the target protein outside of its native cellular environment, a further development in thermal unfolding studies, the cellular thermal shift assay (CETSA), was presented in 2013. 8 For the first time, this allowed the use of thermal unfolding to detect compound-target engagement in cell lysates. The method has also been applied to intact cells, and the Western blot methodology described in the original publication has been extended to incorporate bead-based, 9 mass spectrometry-based, 10 and luminescence-based detection methods. 11 Thermal unfolding methods clearly represent powerful approaches to screen compounds for potential target engagement, and there is a wide choice of approaches dependent upon the nature of the target protein and the available reagents. Their common feature is that they exploit the thermodynamic stabilization of the protein occurring upon ligand binding, 12 allowing binding events to be indirectly detected. This review presents the principles of the different thermal unfolding methods that are employed in high-throughput screening, provides examples of screening outcomes, and highlights opportunities for future development.
Two potential issues that need consideration with thermal unfolding methods are (1) the potential change in pH of buffers used during thermal unfolding studies due to the enthalpy of ionization of the buffers used, and (2) the choice of scan rate. For buffers, such as Tris, with a reasonably large enthalpy of ionization (for Tris dpH/dT = −0.031), the pH can change by around 1.5 units over a 50 °C temperature range. Thus, the use of buffers with lower enthalpy of ionization (such as phosphate, dpH/dT = −0.0022, where the change over a 50 °C temperature range is only around 0.1 pH unit) is recommended, to avoid complications in interpreting the unfolding temperature and its stabilization by ligand binding. Scan rate dependence, which is the change in observed T m when the rate of temperature increase is varied, can indicate the presence of non-equilibrium processes, such as irreversible unfolding. Typical scan rates are often around 1 °C/min, although modern instruments, such as nanoDSF, can have much faster rates. These fast scan rates can be problematic as the temperature change may be faster than the equilibration of the system, leading to a correlation between the scan rate and the melting temperature. It is also possible that ligand binding may alter the kinetics of the relaxation process complicating interpretation. The observation of scan rate dependence precludes the interpretation of ligand binding and stabilization in terms of equilibrium thermodynamics.
DIFFERENTIAL SCANNING CALORIMETRY
Differential scanning calorimetry (DSC) 13,14 is a powerful method able to study thermally induced transitions between conformational states of biological macromolecules and is the only method to directly measure the enthalpy of this transition. Usually, this involves monitoring the folded to unfolded transition of proteins or nucleic acids. The differential scanning calorimeter measures the excess heat capacity (C p ) of a solution of the macromolecule of interest as a function of temperature. The transition is signified by an endothermic peak, where the value of C p is a maximum, which is centered on the melting temperature (T m ). The melting temperature is defined as the temperature at which 50% of the protein molecules are unfolded or, under ideal dynamic reversible two-state equilibrium conditions, the temperature at which any one molecule spends 50% of its time in the folded and 50% of its time in the unfolded state. Calculating the area under the thermogram yields the transition enthalpy (ΔH°), and the difference between the pre- and post-transition baseline yields the ΔC p . Analyzing the shape of the peak, by fitting a two-state model to enable determination of the van't Hoff enthalpy (ΔH° vH ) gives an indication of the size of the cooperative unit of the transition from native to denatured state or may indicate the presence of intermediates in the unfolding pathway. For a two-state reversible equilibrium, we expect ΔH° vH = ΔH° cal . Where ΔH° vH > ΔH° cal , this may indicate that the size of the cooperative unit may be larger than the monomeric form and could indicate that an oligomeric state is the cooperative unit. Observation of ΔH° vH < ΔH° cal may indicate that the transition involves unfolding intermediates or independent domains. Thus, DSC provides a low throughput, but thorough, thermodynamic characterization of macromolecular thermal unfolding. The method may be extended to evaluate the effect of ligands on macromolecular unfolding, by including, usually saturating concentrations of ligand in the thermal unfolding experiment. A shift of the transition peak to higher temperatures in the presence of ligand provides an indication of macromolecule-ligand binding, although there is not a simple relationship between the magnitude of the shift in T m and the ligand binding dissociation constant, K d (the relationship depends upon the contribution of the entropic and enthalpic contributions to the K d value and the concentrations of the protein and added ligand and the unfolding enthalpy). 6,15 The ability to fully characterize macromolecular thermal unfolding and the resulting effect of ligands, directly (without the need for additional equilibria associated with measuring the degree of unfolding) thus positions DSC as the benchmark method for comparison to other methods employed to study the thermal unfolding of isolated, purified macromolecules, such as differential scanning fluorimetry or nanoDSF ( Fig. 1 ).

Technologies that can be utilized to monitor the thermal unfolding of isolated protein samples. Several technologies can be utilized to monitor the thermal unfolding of isolated protein samples. Differential scanning calorimetry (DSC) detects the change in enthalpy of the transition between folded and unfolded states and is the most direct method; however, it is only capable of low throughput analysis. nanoDSF can analyze up to 48 samples in parallel and measures the intrinsic fluorescence of tryptophan residues within the protein structure as their exposure to the environment changes during protein unfolding. Differential scanning fluorimetry (DSF), while less direct, is capable of higher sample throughput and can be used to analyze samples prepared in a multi-well plate format. This dye-based method can be used to simultaneously analyze up to 384 or 1536 samples using multi-well plate-compatible thermocycler/PCR instruments. This form of DSF measures the extrinsic fluorescence generated by the inclusion of environmentally sensitive dyes such as Sypro ® Orange, which bind to exposed hydrophobic regions of the protein structure during unfolding.
Several technologies can be utilized to monitor the thermal unfolding of isolated protein samples. Differential scanning calorimetry detects the change in enthalpy of the transition between folded and unfolded states and is the most direct method; however, it is only capable of low throughput analysis. nanoDSF can analyze up to 48 samples in parallel and measures the intrinsic fluorescence of tryptophan residues within the protein structure as their exposure to the environment changes during protein unfolding. Differential scanning fluorimetry, while less direct, is capable of higher sample throughput and can be used to analyze samples prepared in a multi-well plate format. This dye-based method can be used to simultaneously analyze up to 384 or 1536 samples using multi-well plate-compatible thermocycler/PCR instruments. This form of DSF measures the extrinsic fluorescence generated by the inclusion of environmentally sensitive dyes such as Sypro ® Orange, which bind to exposed hydrophobic regions of the protein structure during unfolding.
PRINCIPLES OF DSF
Differential scanning fluorimetry is a convenient, cost-effective, and robust method used to measure the thermal stability of isolated proteins under a range of different conditions. 7 As with DSC, the assay is founded upon principles of protein thermodynamics, whereby proteins exist in equilibrium between their native (folded) state, favored under ambient conditions, and their denatured (unfolded) state. 16 Gradually increasing the energy of the environment through heating in a controlled manner acts to shift this equilibrium toward the unfolded state, generating a thermal denaturation profile that can be used to derive a melting temperature (T m ) [ Fig. 2(a) ]. The T m value is susceptible to changes in environment; changes in pH, buffer composition, or ligand binding can result in the formation of new molecular interactions which thermally stabilize a protein, leading to an increased T m . The ability to monitor changes in T m (ΔT m ) under different conditions has enabled DSF to be applied for multiple purposes within early drug discovery, from buffer screening to determine optimal conditions for protein stability 17–19 and crystallization 20 to ascertaining relative stabilities of isolated protein mutants or batch purifications. 21,22 Commonly, DSF is utilized for small molecule screening to indicate complex formation between a protein of interest and a compound, the occurrence of which usually manifests as a positive ΔT m value compared to screening of protein alone [ Fig. 2(c) ]. 23–26 It is possible for DSF traces to display greater than one unfolding transition, and therefore, more than one T m value. This can be due to the presence of multiple domains within a protein structure [ Fig. 2(b) ]. While one domain may exhibit stabilization upon ligand binding, the other may remain the same as in the native protein, offering information about potential binding site location. Knowledge of the oligomeric state of the protein can be advantageous before performing a DSF experiment as the presence of multiple states can also result in greater than one thermal transition.

(a) Typical dye-based DSF thermal denaturation curve for protein sample. At the starting temperature, the protein is present in its folded, native state. Fluorescence emission increases with temperature as the protein unfolds and SYPRO ® Orange co-operatively binds to exposed hydrophobic residues. The melting temperature (Tm) (dotted line) can be defined as the midpoint of the transition, or the temperature at which 50% of the protein is unfolded. Fluorescence decreases after the peak is largely attributed to protein aggregation causing shielding and exclusion of dye from hydrophobic residues. (b) Thermal denaturation profile of protein showing two distinct transitions (T m1 and T m2 ) indicating the presence of multiple structural domains. While T m1 remains the same as in the native protein, T m2 shifts to a higher temperature in the presence of a ligand, offering information about potential binding site location. (c) Thermal denaturation profiles of a protein in the absence and presence of a ligand. Ligand presence causes the Tm to shift positively (positive ΔT m ) indicative of thermal stabilization due to binding. Raw fluorescence emission is plotted as a function of temperature. (d) First derivative of thermal denaturation curve plotted as a function of temperature. The T m (dotted line) can also be defined as the inflection point, or peak, of the first derivative trace of the thermal shift curve, the temperature at which the steepest increase in fluorescence signal occurs.
Rather than observing the heat capacity of the protein (as in DSC), DSF monitors fluorescent signal as a function of temperature. Utilizing a fluorescence end point provides a precise, sensitive readout that is amenable to miniaturization within a multi-well plate format. The assay also benefits from much lower protein requirements than DSC. 27 These factors, coupled with the increased availability of lab instrumentation and software capable of monitoring unfolding across a temperature gradient (for example, instruments such as the LightCycler ® 480 from Roche), have driven increased application of DSF as an accessible method for high throughput screening within early drug discovery. 28–31 The source of fluorescence monitored can either be extrinsic, as in dye-based DSF methods, or intrinsic to protein structure, as in the label-free DSF approach.
DYE-BASED DSF
Dye-based DSF involves monitoring the extrinsic fluorescence of environmentally sensitive dyes which are incubated with the protein sample. Under aqueous conditions, dye fluorescence is quenched. However, during thermal unfolding, hydrophobic residues within the protein’s core are exposed and can interact non-covalently with dye molecules, increasing the quantum yield of the dye and producing a fluorescent signal. This typically occurs in a cooperative manner resulting in a sigmoidal thermal denaturation profile, or “melt transition,” across a defined temperature range. Most commonly, a temperature gradient ranging from room temperature to ∼95 °C is applied, with a ramp rate of approximately ∼1 °C/min. However, parallelisable and sensitive isothermal screening approaches have been described whereby the stability of a protein is compared at a constant temperature mimicking physiological conditions. 32 Although there are many commercially available extrinsic dyes, Sypro ® Orange (Thermo Fisher Scientific) is widely favored for DSF applications due to its high signal to background fluorescence in the presence of native protein. One caveat is the sensitivity of Sypro ® Orange dye to detergents; hydrophobic buffers and detergent presence cause high background fluorescence which can mask the assay signal and any resultant T m shift in the presence of ligand. Likewise, the presence of EDTA aggregates at basic pH has also been shown to induce interference. 33 Fluorescent molecular rotor dyes, such as probe 4-(dicyanovinyl)julolidine (DCVJ) and the commercially available dye Proteostat ® (Enzo Life Sciences), however, have been applied for monitoring unfolding under high-detergent conditions. 34,35 A comprehensive summary of other extrinsic dyes is given by Hawe et al. 36 and more recently Gao et al. 22 Sypro ® Orange dye has an excitation maximum of λ = ∼470–490 nm and an emission maximum of λ = ∼570–590 nm and is typically utilized at 1:1000–1:5000 of stock concentration diluted in DMSO. 37,38 However, extrinsic dye concentration is often optimized prior to each specific DSF assay undertaken.
As well as protein studies, dye-based DSF has been successfully applied for investigation into RNA stability. While intercalating dyes, such as Sybr ® Green, can affect RNA stability, sensitive analysis of structured RNA in the presence of stabilizing additives can be carried out through use of single-strand specific fluorescent dyes, such as Quant-iT™ RiboGreen ® (Thermo Fisher Scientific). 39

LABEL-FREE DSF
The label-free DSF approach removes the requirement for extrinsic dye molecules to be equilibrated with a protein sample. The technique instead relies upon monitoring the intrinsic fluorescence of tryptophan and/or tyrosine residues present within the protein structure, which alter as a result of changes in microenvironment polarity during protein unfolding. 40,41 Observing the ratio of fluorescence emission at λ = 330 and 350 nm as a function of temperature allows determination of a T m value for the protein sample of interest. Development of instrumentation such as the Prometheus NT.48 instrument (NanoTemper Technologies) has facilitated miniaturization of the label-free DSF assay for higher throughput application; nanoDSF allows for monitoring of up to 48 samples in parallel, each requiring only 10 μ l total volume. 42 While lower throughput and less sensitive than dye-based DSF, nanoDSF is applicable to a wider range of protein samples, such as those containing detergents which cause interference in the former technique. nanoDSF is thus suitable for use in monitoring the thermal stability of membrane proteins for optimal detergent selection. 43 Use of nanoDSF for the reliable determination of protein T m directly from crude cell lysates has also been described. 44 The label-free nature of nanoDSF also can be advantageous given that inclusion of extrinsic dyes such as Sypro ® Orange introduce a second binding equilibrium to the analysis. Dye binding can cause slight destabilization of the protein being monitored, and therefore underestimation of T m value. This effect is thought to be due to dye molecules selectively associating with aromatic residues, biasing the thermodynamic equilibrium toward the unfolded state; the extent of destabilization has been shown to be more pronounced for protein sequences containing a high proportion of charged or hydrophobic aromatic residues (e.g., Arg, Glu, Trp, and Phe). 42 Inclusion of excess extrinsic dye may also lead to competition with small molecule ligands for binding to the protein of interest, a factor that can be avoided through use of label-free DSF. Utilizing intrinsic fluorescence also offers the potential to follow refolding of a protein (i.e., transition from an unfolded to folded state), something that cannot be monitored using extrinsic dye. 45,46
ASSUMPTIONS AND LIMITATIONS OF DSF
While dye-based and label-free DSF techniques can be used to obtain valuable information regarding the thermal stability of isolated proteins, it is important to consider that successful implementation of both relies upon certain assumptions and that both are susceptible to their own limitations. The recent review by Gao et al. 22 provides an extensive summary of the key assumptions relating to both DSF techniques, some of which are discussed below.
First, dye-based DSF experiments rely upon the assumption that proteins being studied possess minimal exposed hydrophobic residues in their native state and that such proteins are stable and non-aggregated prior to unfolding. Proteins that are unstable, aggregated, or those that possess significant hydrophobic surface area can cause elevated background fluorescence at low temperature. Likewise, specific binding interactions between the protein and the extrinsic dye could prevent dye exposure to the aqueous environment, resulting in high background prior to unfolding. For small molecule screening, experiments are performed under the assumption that there is minimal interaction between compounds or other assay components and the extrinsic dye. Furthermore, screening collections can be specifically designed to minimize inclusion of compounds that interact with or sequester the dye, as presence of these can lead to either high background or low fluorescence, respectively.
A key assumption for nanoDSF small-molecule screening experiments is that compounds included are not intrinsically fluorescent with emission spectra overlapping that of the protein. This assumption also applies to dye-based DSF methods; however, such interference is less likely in Sypro ® Orange assays, due to a greater assay signal and high emission maximum of the dye. In nanoDSF, the assay signal correlates with the number of tryptophan residues within the protein sequence, and thus, this should be considered prior to setup. Equally, as signal sensitivity is lower than dye-based assays, it should be considered that the presence of multiple protein domains with different unfolding transitions may be difficult to characterize using a nanoDSF approach.
Despite the availability of automated DSF analysis software, Simeonov 47 discusses the fact that there is typically a requirement to conduct manual analysis of thermal melt transitions. Inspection of individual traces within DSF screening data is often required as noise, signal interferences, or secondary transitions can complicate T m determination leading to false positives and negatives. While greater ΔT m shifts are often associated with higher affinity binding, this relationship does not always apply. The extent of the thermal shift can be influenced by multiple factors, including the net interplay between both entropic and enthalpic contributions during binding. T m shift values are also influenced by additional equilibria present within the assay, for example, binding of a small molecule ligand to both the folded and unfolded protein states or dye itself binding to the protein. In this way, ligands with equal affinity can produce varying ΔT m values. 47 Although large-scale DSF screening can be conducted at a single ligand concentration to increase throughput, follow-up of suspected binders in concentration-response format is often applied in order to determine a “true ΔT m ,” for which the presence of the ligand under saturating conditions is a prerequisite, 47 although it should be recognized that the T m value increases with ligand concentration even beyond saturation, due to a positive entropy of mixing effect.
The observation of negative thermal shifts (i.e., decrease in T m ) in the presence of small molecules has also been shown by DSF. While ligand binding to a protein form is always associated with stabilization of that form, due to the lower free energy of the protein-ligand complex, negative thermal shifts or observed destabilization can occur in several scenarios. For example, ligand binding preferentially to the unfolded form of a protein over the native form can cause an observed decrease in T m relative to that of the protein in the absence of ligand. 48 Equally, if analysis is conducted on a heterogenous sample containing multiple protein populations, binding of a ligand preferentially to a minority population with a lower T m value could perturb the equilibrium leading to an observed decrease in T m . Other factors that have been associated with negative thermal shifts include covalent modification of the protein due to ligand binding, and altered ionic strength, depleting ions that would otherwise promote protein stabilization. 47 Inclusion of binding partners within a DSF assay, or their physiological presence in the case of cellular thermal shift assays, can complicate analysis further due to potential for additional interactions to occur. Due to difficulty in interpretation of negative thermal shift data, along with observations of nonspecific binding leading to decrease in T m , to date focus has largely been placed on ligands producing typical positive shifts. Comprehensive analysis into negative shift data and larger scale validation of negative shifting ligands would be beneficial to understanding the true value of this observation for future studies.
PRINCIPLES OF CETSA
The Cellular Thermal Shift Assay (CETSA) was developed following a collaboration between the Karolinska Institutet, Sweden and the University of Nanyang, Singapore and is the most recent of the thermal unfolding methods described here to be adapted to drug discovery. 8 CETSA, like other thermal shift assays, works on the principle that compound binding to a target protein alters the thermal stability of the target protein. 12,49 Unlike thermal unfolding methods such as DSC and DSF, CETSA is advantaged by its sensitivity to factors such as cell permeability of a compound, intracellular protein complex formation, and the impact of the presence/absence of cellular cofactors. 50 The general method for CETSA varies significantly depending on the application and the detection technology. Broadly, cells are co-incubated with compounds of interest for a short period. The cells are then heat-treated, usually using a thermal cycler or a heat block, and are subsequently lysed. Following cell lysis, the samples must usually be centrifuged, to separate the folded, soluble target of interest in the supernatant from the aggregated, melted target in the pellet. Compounds that alter the quantity of the folded soluble target of interest are posited to bind to the target of interest. 8
DETECTION METHODS LEVERAGED FOR CETSA
In the first CETSA experiments, Molina Martinez et al. used Western blot to detect the amount of soluble folded protein that remained following thermal destabilization of whole cells, lysed cells, or lysed tissues, in the presence and absence of compounds. 8 To generate a CETSA melt curve for a particular target protein, CETSA must be run across a range of temperatures, which means that target detection by Western blot is relatively low in terms of compound throughput. Therefore, the use of Western blot was rapidly followed by the development of other methods ( Fig. 3 ). Multiplexing of samples allows for higher throughput protein detection by mass spectrometry, 50 whilst AlphaScreen antibody/bead or NanoLuciferase protein detection reagents have enabled CETSA to be miniaturized into 384-well plate-based formats termed “AlphaCETSA” by PerkinElmer 51,52 and “BiTSA,” 11 “SplitLuc CETSA,” 53 or “NaLTSA.” 54 Though mass spectrometry remains lower throughput than AlphaScreen or NanoLuciferase-based detection technologies, mass spectrometry has the advantage of being totally label-free, requiring neither specific antibodies nor the introduction of a protein tag to the target of interest.

A range of technologies can be used to detect unfolding of the protein target(s) of interest for CETSA. Detection of the endogenous protein is enabled by mass spectrometry or antibody-based methods, whilst detection of genetically tagged target is most often based on NanoLuciferase technologies. Left to right images represent the target detection by (1) Western blot, 8 (2) AlphaScreen anti-target antibody pairs, 51 (3) immunofluorescence microscopy, 56,57 (4) mass spectrometry, 50 (5) acoustically dispensed reverse phase protein array, 55 (6) TIRF of anti-target antibody, bound to immobilized target, released following single cell lysis within a microfluidic device, 61 (7) monitoring of NanoLuciferase formed following the binding of LgBiT to the HiBiT-tagged target, 53 and (8) monitoring of NanoLuciferase engineered to be thermostable using a Gly-Ser linker, and thus suitable for real time CETSA. 58 (b) When applying CETSA to drug discovery, the concentration of the compound, the temperature of the melt event, or the treatment of the cells may all be varied to elucidate further information about the compound's mechanism of action. 8,49,62–64
The detection method for the target of interest continues to be developed to drive higher throughput compound screening in drug discovery. Acoustic droplet ejection technology has been used to enable 384-well plate-based reverse phase protein array (RPPA)-CETSA experiments, effectively providing a miniaturization of the Western blot approach with an imaging end point ( Fig. 3 ). 55 However, this method requires that the CETSA is performed on cell lysates; thus, information about compound membrane permeability is lost, along with all cellular protein that is not in the soluble fraction. Other groups have recently developed single-cell high content imaging (HCI)-CETSA where antibody detection is achieved by single-cell immunofluorescence ( Fig. 3 ). 56,57 Conversely to RPPA-CETSA, HCI-CETSA requires the CETSA experiment to be undertaken using intact cells. HCI-CETSA has been miniaturized to a 384-well plate-based format, and indeed Axelsson et al. 56 screened a library of >1000 compounds as proof-of-principle.
Most recently, the Henderson group, which originally implemented split NanoLuciferase HiBiT-CETSA, developed a 384-well plate thermal cycler capable of capturing luminescence ( Fig. 3 ). 53,58 They used this to detect their target of interest, which had been labeled with a NanoLuciferase engineered to be thermostable (termed “ThermLuc”). This allowed for the first instance of live cell real-time CETSA experiments and thus enabled the detection of melt curves for 384 samples within a 4-minute total melt/read cycle. Further miniaturization to 1536-well plates for high-throughput compound screening has been attempted, though remains challenging due to the requirement to separate the supernatant of the cell lysate from the pellet in all but real-time ThermLuc CETSA and a few target-dependent exceptions. 53,58 Research into CETSA method development over the last 10 years has enabled the first truly high-throughput drug discovery effort driven by CETSA, in which Rowlands et al. have undertaken a library screen of >300 000 compounds using the AlphaCETSA method. 59
Conversely, in drug discovery applications where compound throughput is not a priority, CETSA can be used to help to elucidate our understanding of the compound/target interaction. CETSA is particularly useful in proving cellular target engagement soon after high-throughput biochemical screens and alongside low-throughput phenotypic cellular screens. The use of mass spectrometry enables the detection of the wider proteome, and therefore also provides information about how a compound might affect the melting temperature (T m ) of thousands of different proteins. This method, known as thermal proteome profiling (TPP), mass spectrometry (MS)-CETSA or CETSA ® Explore can provide information about direct binding partners that appear to be co-stabilized or destabilized by the compound/target complex. 50,60 Another method that also aims to facilitate cellular target engagement studies, but does not improve compound throughput, is single-cell total internal reflection fluorescence microscopy (TIRF)-CETSA ( Fig. 3 ). 61 Relative to other CETSA methods that often require many cells per sample, this method requires only hundreds of cells per conditions, and thus can be used on rare patient-derived cells. The authors captured and lysed one cell at a time using a microfluidic chip and laser-based optical trapping, and then used TIRF microscopy to enable single-molecule antibody-based target detection.
There are very few publications that directly compare the findings of CETSA using different detection methods. Martinez et al. 53 have shown that 18 different HiBiT-tagged targets melt at similar T m values to the endogenous protein; meanwhile, Sanchez et al. 58 have benchmarked ThermLuc CETSA against SplitLuc CETSA and have shown strong agreement between the two methods across a panel of 29 previously identified binders to LDHA. On a smaller scale, Herledan et al. 55 have demonstrated that Western blot CETSA and RPPA CETSA produced concordant thermal shifts for three inhibitors of insulin-degrading enzyme (IDE), whilst Massey et al. 57 have shown that AlphaCETSA and HCI CETSA produced concordant thermal shifts for five inhibitors of p38a. Whilst these studies are encouraging, there are few studies to date that compare many binders or targets across different CETSA detection methods. It seems likely that different CETSA detection methods have different liabilities that might result in different putative binders being identified as false positives or false negatives. We therefore recommend that this be a focus of future drug discovery efforts to maximize the sensitivity and specificity of CETSA assays.
VARIATIONS TO THE CETSA FORMAT
CETSA is an extremely flexible method in its application to drug discovery which can be run in many different experimental formats to provide different information ( Fig. 3 ). To generate a classical CETSA melt curve, the temperature of the heat shock is varied whilst the concentration of the compound is fixed in excess. 8 As is the case for DSF, compounds that bind and stabilize the target will cause the T m of the target to increase, though the degree to which a compound (de-)stabilizes a target of interest does not necessarily correlate with the potency with which it binds to the target. 47 To obtain potency, the compound concentration is instead varied at a fixed temperature usually close to the target T m. 8 The concentration at which a particular compound stabilizes the target by 50% of the maximal possible stabilization effect that the compound can cause is used to determine the EC 50 and thereby provides an estimate of compound potency. This method variation is known as isothermal dose-response CETSA (ITDR-CETSA). If compound throughput is a particular priority, then isothermal CETSA, where the temperature of the heat shock and the compound concentration are both fixed, has been used to screen larger compound libraries of up to 1120 compounds. 56 We have since screened libraries of >300 000 compounds using this approach. 59
In 2D-CETSA, ITDR-CETSA and melt curves are combined such that the temperature of the heat shock and the compound concentration are varied in tandem, to access even more information. 64,65 For instance, some proteins might alter in abundance in response to increasing concentration of a particular compound, across all temperatures. These proteins are likely to be changing in expression or extractability due to compound treatment, rather than be direct binders that cause thermal stabilization, and might instead be involved in signaling pathways downstream of compound binding. Meanwhile, proteins that are thermally stabilized by the compound of interest even at very low compound concentrations might represent previously unknown off-target binding at high potency. 62 A variant of 2D-CETSA, termed compressed CETSA, has also been developed. 66 Samples melted at a range of different temperatures are pooled to increase the throughput relative to 2D-CETSA, whilst the output remains more robust than a truly isothermal approach.
The final variable that may be altered in a CETSA experiment is the cellular state. There are studies that have applied TPP/MS-CETSA across cells synchronized to different stages of the cell cycle, cells with different genetic knock out backgrounds, or to a cellular time series post-treatment. 49,62–64 Although to date these studies have not been undertaken with a direct drug discovery application, they highlight that unlike the other biophysical methods described here, CETSA enables compound potency to be interrogated in a label-free manner across a range of cellular states/conditions; therefore, CETSA holds great potential for impact in drug discovery.
Often, melting temperatures with and without ligand agree between DSF and CETSA experiments, for example. 67,68 However, it is possible that the additional complexity of the cellular system may not lead to correlations between CETSA experiments and those conducted on isolated proteins. In these cases, understanding of the protein system and its potential for interactions in a cellular context should be attempted to rationalize any differences in T m and ΔT m measurements.
The different methods used for monitoring thermal unfolding discussed above are applied at different stages and employ different reagents during the early part of drug discovery. They have a range of strengths and weaknesses, and the choice may depend upon the access to reagents and the different types of information required. Often it may be useful to combine methods as this may provide increased confidence in the engagement of the compound with the target. Table I provides a brief description of the pros and cons of the methods described.
Overview of the advantages and disadvantages of the different thermal unfolding methods applied in drug discovery.
Within AstraZeneca, thermal unfolding approaches are often utilized as primary screening approaches, either employing DSF at the 100k compound scale or CETSA at around 500k compound scale. Typically, following confirmation in the primary assay, hit compounds with the largest degree of stabilization are followed up with concentration response curves (in DSF) and concentration response curves and/or full melt curves in CETSA. Recognizing that apparent affinities measured in this way provide values at the melting temperature, applying biophysical approaches such as SPR provides additional confidence in the hits identified, before chemistry is applied. The degree of agreement between the thermal methods and the downstream biophysical methods is often target dependent.
ANALYSIS OF THERMAL UNFOLDING DATA
Most high-throughput thermal shift assays (DSC, DSF, and CETSA) focus on determination of the melting temperature of proteins (T m ) and often more specifically the changes in T m induced upon compound binding. 7 All three methods in their original form quantify protein stability either as protein in solution (CETSA), dye fluorescence (DSF), or the excess heat capacity (DSC) as a function of increasing temperature. Figure 2(c) illustrates the melting curve for a protein in an example of a DSF experiment in the absence and presence of a ligand. The ligand stabilizes the protein and increases its melting temperate as shown by the dashed lines.
For DSF, there are three general data analysis approaches to determining the T m and ΔT m upon binding. First, the derivative method [ Fig. 2(d) ] uses the smoothed first derivative of the melting curve signal. The T m is defined as the temperature at the inflection point, with the steepest increase in signal with temperature. Usually, a smoothing function such as a Savitzky–Golay filter is required to reduce experimental noise and produce a reliable T m estimate. The main advantages of the derivative method are that it is simple, quick, robust, and has fewer assumptions compared to the “thermodynamic” methods below. It can also help uncover proteins with multiple transitions manifesting as multiple peaks in the derivative signal. However, if those transitions are very close to each other, the T m estimates of the derivative may become more variable. In those cases, an even simpler model using the middle point between the bottom and the top of the curve may be more robust. The midpoint model approximates the T m as the intersection of the arithmetic mean of the top and bottom fluorescence plateaus with the melting curve. However, it is based on a linear relationship in the region between the bottom and the top, which is rarely seen in experimental data. Therefore, the midpoint method is generally recommended only when all other methods fail. The third group of methods relies on equilibrium and thermodynamic models of protein unfolding. It encompasses a wide continuum of methods with increasing levels of complexity and number of parameters.
Generally, when a protein is heated in thermal stability experiments, the relationship of folded (F) and unfolded (U) protein during heating can be represented as a reversible reaction which reaches equilibrium faster than the heat ramp rate.
The equilibrium constant between the folded and unfolded states is defined as
where [ U ] + [ F ] = [ P tot ] are the concentrations of the folded and unfolded protein, and P tot is the total concentration of all protein species. With that in mind, the fraction folded can be expressed as
K u is related to the difference in Gibbs free energy such that
where R is the universal gas constant, and T is the absolute temperature. Substitution leads to the Boltzmann equation:
In thermal stability experiments for CETSA and DSF, this is often expressed as
This equation is a convenient simplification used to determine the melting point, T m of the protein unfolding transition, i.e., the inflection point of the curve. It can incorporate a further constant or linear dependency for the start (S 0 ) and the end (S inf ) of the curve:
This sigmoidal fit, known as the Boltzmann fit, is in good agreement with the behavior of proteins in CETSA experiments where the melt-curves have a sigmoidal shape. That is why it is the most used model fitted to data from cellular thermal shift assays.
In contrast, biophysical techniques, such as DSC and DSF, applied to isolated protein systems can determine the thermodynamics of thermal unfolding. For example, the expression above for ΔG can be recast as
where ΔH u and ΔS u are the differences in entropy and enthalpy at the temperature for ΔG u . This relationship and the deviation from the simplistic sigmoid fit is readily observed in differential scanning fluorimetry and calorimetry studies (DSF and DSC).
As described by Robertson and Murphy in 1997, 69 the temperature dependence of ΔH u and ΔS u is governed by the change in heat capacity ΔC pu between the folded and unfolded states. In DSC experiments, ΔC pu is in principle simply the difference in heat capacities (C p ) (baselines) before and after unfolding. Due to solvent restructuring, it takes more heat to raise the temperature of a solution of unfolded protein compared to a folded protein with the same concentration. If the, usually small, temperature dependence of ΔC pu is ignored, the relationship of ΔG u on temperature ΔG u (T) can be expressed as
When the reference temperature T R = T m , the melting temperature of the protein, then ΔG u = 0 and Δ S u = Δ H u T m , and the relationship simplifies to the modified Gibbs−Helmholtz equation:
The experimentally observed equilibrium constant can then be expressed as
In the case of DSF data, the observed change in fluorescence can then be expressed as
In practice, the S 0 and S inf in DSF experiments often change with temperature, and the relationship can be modeled as linear: 70
where Y f , T m , Y u , T m are fluorescence intensities of the dye in the presence of folded and unfolded protein at the melting temperature T m , and m f and m u are the slopes describing the linear temperature dependency of dye fluorescence for the folded and unfolded states. This fit with linear dependency for the top and the bottom is the expression that is most commonly referred to as a “thermodynamic” fit or more confusingly “Boltzmann” fit.
This model has seven parameters: Y f , T m , Y u , T m , m f , m u , Δ H u , Δ C p u , and T m , which can result in overfitting to experimental data and poses problems with identifiability of individual parameters. For example, ΔC pu can often be poorly defined, and approaches have been suggested to measure it using separate DSC data 70 or to calculate it based on the number of amino acid residues (N res ) and area of the protein. 69,71
Robertson and Murphy 69 and Rees and Robertson 71 also report estimates for ΔH u and ΔS u which can be used as starting points to aid convergence during nonlinear regression analysis.
All the above considerations have focused on an equilibrium model of protein stability with a reversible transition between folded and unfolded protein and no intermediate states. The models above require the assumptions that ΔC pu does not change with temperature, that Δ S u = Δ H u T m along the whole temperature range, when that is only valid at the T m and that there are linear relationships for the temperature-dependent fluorescence signals. 70 Often, one or more of these assumptions may not hold, requiring more complex models be fitted to the experimentally observed data.
For example, it has been suggested, where Sypro ® Orange dye is used that a nonlinear model may better describe the post-transition behavior. 72 A common issue may be irreversible aggregation of the protein, following the initial reversible unfolding transition. Lumry–Eyring models have been proposed by Sanchez-Ruiz 73 to account for this irreversible aggregation process, and useful tools have recently become readily available in open-source code ( https://github.com/taiawu/dsfworld_package ) and applications ( https://gestwickilab.shinyapps.io/dsfworld/ ) to analyze these more complex situations. 74
In addition, it is important to bear in mind when fitting these more complex models that the magnitude and steepness of the pre- and post-unfolding baselines affect the entire fit and the values of the thermodynamic parameters both in simple linear models 69 and in the exponential decay models. 74 Therefore, melting points determined using the thermodynamic set of methods would often differ from those using the derivative or the midpoint methods.
High throughput thermal shift experiments also can provide information on the affinity of ligands for the target. The equation for the ligand binding equilibrium association constant at the T m is given in the original 7 publication for high-throughput DSF
where K L T m is the ligand association constant at T m , T m is the midpoint of the unfolding transition in the presence of ligand, T 0 is the midpoint of the unfolding transition in the absence of ligand, Δ H u T 0 is the enthalpy of protein unfolding in the absence of ligand at T 0 , Δ C p u T 0 is the change in heat capacity upon protein unfolding in the absence of ligand, [ L T m ] is the free ligand concentration at T m which is approximately L total when L total ≫ [ Protein ] total , R is the gas constant.
More recent work employing an isothermal approach 75 close to the T m has described a further simplified method for determination of the ligand association constant at temperatures close to T m
where [P T ] is the total protein concentration, and EC 50 is the ligand concentration where the fraction of unfolded protein is half of that observed in the absence of ligand. Note that the EC 50 in this case is not defined by the ligand concentration at which half of the protein is unfolded. f u 0 T is the fraction of protein that is unfolded at the T ≈ T m in the absence of ligand, and it can be determined directly from the thermal unfolding curve for the protein in the absence of ligand. The authors provide open-source code ( https://sourceforge.net/projects/dsf-fitting/ ) to perform the suggested isothermal analysis.
Of course, to be physiologically relevant, association constants need to be transposed to values at physiological temperatures. This may be accomplished using the expression
The binding constants at physiological temperatures can then be recalculated provided that Δ C p L T can be measured, for example, using isothermal titration calorimetry, or assuming a low value, so that the second term can be ignored. These assumptions simplify the estimate for K L T to
While Pantoliano et al. 7 suggest informed estimates of −10 to −15 kcal/moL for Δ H L T , if real enthalpies of binding differ substantially between ligands the ranking based on the association constants and their corrected estimates at physiological temperature would be unreliable. 76 Therefore, the binding enthalpy of the ligand (ΔH L T ) must be measured using calorimetric or van't Hoff methods.
Recent attempts have been made to quantify binding affinities using DSF data based on the isothermal method in nanoDSF experiments 77 and the authors provide readily available open tools for others to model and analyze their data.
Overall, with a plethora of commercial packages (such as GeneData Screener ) and open-source tools like DSF World, 74 IsothermalFitting, 75 FoldAffinity, 77 MeltDown, 78 and SimpleDSFViewer, 79 scientists can understand, model, and fit DSF data in a high-throughput manner to find, rank, and characterize compounds which interact with the target of interest.
CETSA MELT CURVE ANALYSIS
As discussed above, the CETSA melt curve data fits the Boltzmann equation well, and thus allows for an accurate determination of T m. 50,80 However, whilst for DSC and DSF experiments the ΔT m is a sufficient metric for identifying binding events, it has been suggested that for CETSA experiments, the ΔT m method may be too reductionist. This is because in DSF and DSC, the melt curves are determined from a definite amount of uncomplexed protein, but in CETSA experiments, the cellular environment could be driving changes in factors including target expression, localization, or complex formation. The result is that the melt curves report more than a simple thermal unfolding event, and therefore may vary in T m , slope and plateau position. 81 It is also possible that the T m of a compound might lie beyond the assayed temperature range. 82 As with DSC and DSF, there is a desire to be able to determine the degree of thermal shift at scale, i.e., for many compounds. Thus, there are several analysis approaches that have been developed to assess the degree of stabilization in an automated manner, for example, see recent developments in software packages such as TPP ( https://www.bioconductor.org/packages/release/bioc/html/TPP.html ).
FUTURE PERSPECTIVE
Thermal unfolding methods have had an important impact in early drug discovery, providing an understanding of protein stability, the thermodynamic detail around changes in stability upon ligand binding and by providing rapid and high-throughput approaches for identifying potential ligands. This impact is set to continue. However, we may expect to observe a shift from isolated protein primary screening approaches to those that make use of cell-based methods. The combination with CETSA, which does not rely on producing large quantities of purified protein, often for targets that are difficult to work with, opens the possibility for new screening cascades. For example, HiBIT CETSA methods will be used to identify potential hit molecules with follow-up using additional thermal unfolding methods but applied to far fewer compounds. This reduces the need for screening quantities of purified protein but ensures that rigorous characterization of actives from the primary screen is undertaken. We are already using thermal unfolding methods in this way, employing DSF to verify CETSA actives, using both single concentration and concentration response analysis, and bringing in additional biophysical methods once the confidence in those actives has been sufficiently demonstrated. The ability to undertake isothermal studies as well as thermal melting profiles and to combine different CETSA methods (SplitLuc and Alpha) alongside the isolated protein work in DSF and DSC is very powerful and forms the basis of a robust and rigorous downstream cascade. We expect that the MS-based methods will also feature strongly as thermal unfolding continues to have an important impact in hit identification and characterization.
AUTHOR DECLARATIONS
Conflict of interest.
The authors have no conflicts to disclose.
Author Contributions
Poppy Llowarch and Laura Usselmann contributed equally to this work.
Poppy Llowarch: Writing – original draft (equal); Writing – review & editing (equal). Laura Usselmann: Writing – original draft (equal); Writing – review & editing (equal). Delyan Ivanov: Writing – original draft (equal); Writing – review & editing (equal). Geoffrey Allan Holdgate: Conceptualization (lead); Supervision (lead); Writing – original draft (equal); Writing – review & editing (equal).
DATA AVAILABILITY
Thank you for visiting nature.com. You are using a browser version with limited support for CSS. To obtain the best experience, we recommend you use a more up to date browser (or turn off compatibility mode in Internet Explorer). In the meantime, to ensure continued support, we are displaying the site without styles and JavaScript.
- View all journals
- Explore content
- About the journal
- Publish with us
- Sign up for alerts
- Published: 14 May 2024
Top-down mass spectrometry of native proteoforms and their complexes: a community study
- Tanja Habeck ORCID: orcid.org/0000-0002-4325-6335 1 ,
- Kyle A. Brown ORCID: orcid.org/0000-0003-1255-9146 2 ,
- Benjamin Des Soye 3 ,
- Carter Lantz 4 ,
- Mowei Zhou ORCID: orcid.org/0000-0003-3575-3224 5 nAff11 ,
- Novera Alam 6 ,
- Md Amin Hossain 6 ,
- Wonhyeuk Jung 4 ,
- James E. Keener 7 ,
- Michael Volny ORCID: orcid.org/0000-0001-7456-5408 8 ,
- Jesse W. Wilson 5 ,
- Yujia Ying 9 ,
- Jeffrey N. Agar ORCID: orcid.org/0000-0003-2645-1873 6 , 10 ,
- Paul O. Danis 10 ,
- Ying Ge ORCID: orcid.org/0000-0001-5211-6812 2 , 10 ,
- Neil L. Kelleher ORCID: orcid.org/0000-0002-8815-3372 3 , 10 ,
- Huilin Li 9 ,
- Joseph A. Loo ORCID: orcid.org/0000-0001-9989-1437 4 , 10 ,
- Michael T. Marty ORCID: orcid.org/0000-0001-8115-1772 7 ,
- Ljiljana Paša-Tolić ORCID: orcid.org/0000-0001-9853-5457 5 , 10 ,
- Wendy Sandoval 8 &
- Frederik Lermyte ORCID: orcid.org/0000-0001-7371-4475 1
Nature Methods ( 2024 ) Cite this article
315 Accesses
11 Altmetric
Metrics details
- Mass spectrometry
The combination of native electrospray ionization with top-down fragmentation in mass spectrometry (MS) allows simultaneous determination of the stoichiometry of noncovalent complexes and identification of their component proteoforms and cofactors. Although this approach is powerful, both native MS and top-down MS are not yet well standardized, and only a limited number of laboratories regularly carry out this type of research. To address this challenge, the Consortium for Top-Down Proteomics initiated a study to develop and test protocols for native MS combined with top-down fragmentation of proteins and protein complexes across 11 instruments in nine laboratories. Here we report the summary of the outcomes to provide robust benchmarks and a valuable entry point for the scientific community.
This is a preview of subscription content, access via your institution
Access options
Access Nature and 54 other Nature Portfolio journals
Get Nature+, our best-value online-access subscription
24,99 € / 30 days
cancel any time
Subscribe to this journal
Receive 12 print issues and online access
251,40 € per year
only 20,95 € per issue
Buy this article
- Purchase on Springer Link
- Instant access to full article PDF
Prices may be subject to local taxes which are calculated during checkout

Similar content being viewed by others
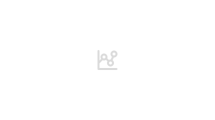
Accurate structure prediction of biomolecular interactions with AlphaFold 3
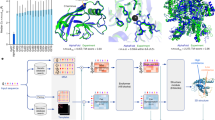
Highly accurate protein structure prediction with AlphaFold
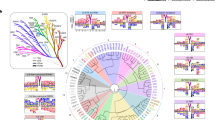
The intrinsic substrate specificity of the human tyrosine kinome
Data availability.
The raw MS data have been deposited to the ProteomeXchange Consortium via the PRIDE partner repository with the dataset identifier PXD047341 (refs. 72 , 73 ). The protein sequences can be accessed by the respective identifier (Supplementary Table 4 ) via https://www.uniprot.org/ .
Smith, L. M., Kelleher, N. L. & The Consortium for Top Down Proteomics Proteoform: a single term describing protein complexity. Nat. Methods 10 , 186–187 (2013).
Article CAS PubMed PubMed Central Google Scholar
Aebersold, R. et al. How many human proteoforms are there? Nat. Chem. Biol. 14 , 206–214 (2018).
Smith, L. M. & Kelleher, N. L. Proteoforms as the next proteomics currency. Science 359 , 1106–1107 (2018).
Dang, X. et al. The first pilot project of the consortium for top-down proteomics: a status report. Proteomics 14 , 1130–1140 (2014).
Chen, B., Brown, K. A., Lin, Z. & Ge, Y. Top-down proteomics: ready for prime time? Anal. Chem. 90 , 110–127 (2018).
Article CAS PubMed Google Scholar
Donnelly, D. P. et al. Best practices and benchmarks for intact protein analysis for top-down mass spectrometry. Nat. Methods 16 , 587–594 (2019).
Srzentic, K. et al. Interlaboratory study for characterizing monoclonal antibodies by top-down and middle-down mass spectrometry. J. Am. Soc. Mass Spectrom. 31 , 1783–1802 (2020).
Habeck, T. & Lermyte, F. Seeing the complete picture: proteins in top-down mass spectrometry. Essays Biochem. 67 , 283–300 (2023).
Brown, K. A., Melby, J. A., Roberts, D. S. & Ge, Y. Top-down proteomics: challenges, innovations, and applications in basic and clinical research. Expert Rev. Proteom. 17 , 719–733 (2020).
Article CAS Google Scholar
Smith, L. M. et al. The Human Proteoform Project: defining the human proteome. Sci. Adv. 7 , eabk0734 (2021).
Leney, A. C. & Heck, A. J. Native mass spectrometry: what is in the name? J. Am. Soc. Mass Spectrom. 28 , 5–13 (2017).
Robinson, C. V. Mass spectrometry: from plasma proteins to mitochondrial membranes. Proc. Natl Acad. Sci. USA 116 , 2814–2820 (2019).
Tamara, S., den Boer, M. A. & Heck, A. J. R. High-resolution native mass spectrometry. Chem. Rev. 122 , 7269–7326 (2022).
Bennett, J. L., Nguyen, G. T. H. & Donald, W. A. Protein-small molecule interactions in native mass spectrometry. Chem. Rev. 122 , 7327–7385 (2022).
Rogawski, R. & Sharon, M. Characterizing endogenous protein complexes with biological mass spectrometry. Chem. Rev. 122 , 7386–7414 (2022).
Skinner, O. S. et al. Top-down characterization of endogenous protein complexes with native proteomics. Nat. Chem. Biol. 14 , 36–41 (2018).
Ro, S. Y. et al. Native top-down mass spectrometry provides insights into the copper centers of membrane-bound methane monooxygenase. Nat. Commun. 10 , 2675 (2019).
Article PubMed PubMed Central Google Scholar
Gault, J. et al. Combining native and ‘omics’ mass spectrometry to identify endogenous ligands bound to membrane proteins. Nat. Methods 17 , 505–508 (2020).
Vimer, S. et al. Comparative structural analysis of 20S proteasome ortholog protein complexes by native mass spectrometry. ACS Cent. Sci. 6 , 573–588 (2020).
Schachner, L. F. et al. Decoding the protein composition of whole nucleosomes with Nuc-MS. Nat. Methods 18 , 303–308 (2021).
Melani, R. D. et al. Mapping proteoforms and protein complexes from king cobra venom using both denaturing and native top-down proteomics. Mol. Cell Proteom. 15 , 2423–2434 (2016).
Lermyte, F., Tsybin, Y. O., O’Connor, P. B. & Loo, J. A. Top or middle? Up or down? Toward a standard lexicon for protein top-down and allied mass spectrometry approaches. J. Am. Soc. Mass Spectrom. 30 , 1149–1157 (2019).
Zhou, M. et al. Higher-order structural characterisation of native proteins and complexes by top-down mass spectrometry. Chem. Sci. 11 , 12918–12936 (2020).
VanAernum, Z. L. et al. Rapid online buffer exchange for screening of proteins, protein complexes and cell lysates by native mass spectrometry. Nat. Protoc. 15 , 1132–1157 (2020).
McCabe, J. W. et al. Implementing digital-waveform technology for extended m / z range operation on a native dual-quadrupole FT-IM-orbitrap mass spectrometer. J. Am. Soc. Mass Spectrom. 32 , 2812–2820 (2021).
LeDuc, R. D. et al. ProForma: a standard proteoform notation. J. Proteome Res 17 , 1321–1325 (2018).
Schachner, L. F. et al. Standard proteoforms and their complexes for native mass spectrometry. J. Am. Soc. Mass Spectrom. 30 , 1190–1198 (2019).
Smith, L. M. et al. A five-level classification system for proteoform identifications. Nat. Methods 16 , 939–940 (2019).
Iacobucci, C. et al. First community-wide, comparative cross-linking mass spectrometry study. Anal. Chem. 91 , 6953–6961 (2019).
Masson, G. R. et al. Recommendations for performing, interpreting and reporting hydrogen deuterium exchange mass spectrometry (HDX-MS) experiments. Nat. Methods 16 , 595–602 (2019).
Allison, T. M. et al. Software requirements for the analysis and interpretation of native ion mobility mass spectrometry data. Anal. Chem. 92 , 10881–10890 (2020).
Allison, T. M. et al. Computational strategies and challenges for using native ion mobility mass spectrometry in biophysics and structural biology. Anal. Chem. 92 , 10872–10880 (2020).
Gabelica, V. et al. Recommendations for reporting ion mobility mass spectrometry measurements. Mass Spectrom. Rev. 38 , 291–320 (2019).
Konijnenberg, A., Butterer, A. & Sobott, F. Native ion mobility-mass spectrometry and related methods in structural biology. Biochim. Biophys. Acta 1834 , 1239–1256 (2013).
Konermann, L., Ahadi, E., Rodriguez, A. D. & Vahidi, S. Unraveling the mechanism of electrospray ionization. Anal. Chem. 85 , 2–9 (2013).
Hall, Z., Politis, A., Bush, M. F., Smith, L. J. & Robinson, C. V. Charge-state dependent compaction and dissociation of protein complexes: insights from ion mobility and molecular dynamics. J. Am. Chem. Soc. 134 , 3429–3438 (2012).
Rolland, A. D., Biberic, L. S. & Prell, J. S. Investigation of charge-state-dependent compaction of protein ions with native ion mobility-mass spectrometry and theory. J. Am. Soc. Mass Spectrom. 33 , 369–381 (2022).
Sobott, F., Hernandez, H., McCammon, M. G., Tito, M. A. & Robinson, C. V. A tandem mass spectrometer for improved transmission and analysis of large macromolecular assemblies. Anal. Chem. 74 , 1402–1407 (2002).
Sobott, F., McCammon, M. G., Hernandez, H. & Robinson, C. V. The flight of macromolecular complexes in a mass spectrometer. Philos. Trans. A Math. Phys. Eng. Sci. 363 , 379–389 (2005).
CAS PubMed Google Scholar
Rose, R. J., Damoc, E., Denisov, E., Makarov, A. & Heck, A. J. High-sensitivity Orbitrap mass analysis of intact macromolecular assemblies. Nat. Methods 9 , 1084–1086 (2012).
van de Waterbeemd, M. et al. High-fidelity mass analysis unveils heterogeneity in intact ribosomal particles. Nat. Methods 14 , 283–286 (2017).
Article PubMed Google Scholar
Fort, K. L. et al. Expanding the structural analysis capabilities on an Orbitrap-based mass spectrometer for large macromolecular complexes. Analyst 143 , 100–105 (2017).
McGee, J. P. et al. Voltage rollercoaster filtering of low-mass contaminants during native protein analysis. J. Am. Soc. Mass Spectrom. 31 , 763–767 (2020).
Snijder, J., Rose, R. J., Veesler, D., Johnson, J. E. & Heck, A. J. Studying 18 MDa virus assemblies with native mass spectrometry. Angew. Chem. Int. Ed. Engl. 52 , 4020–4023 (2013).
Behnke, J. S. & Urner, L. H. Emergence of mass spectrometry detergents for membrane proteomics. Anal. Bioanal. Chem. 415 , 3897–3909 (2023).
Borysik, A. J., Hewitt, D. J. & Robinson, C. V. Detergent release prolongs the lifetime of native-like membrane protein conformations in the gas-phase. J. Am. Chem. Soc. 135 , 6078–6083 (2013).
Reading, E. et al. The role of the detergent micelle in preserving the structure of membrane proteins in the gas phase. Angew. Chem. Int. Ed. Engl. 54 , 4577–4581 (2015).
Ives, A. N. et al. Using 10,000 fragment ions to inform scoring in native top-down proteomics. J. Am. Soc. Mass Spectrom. 31 , 1398–1409 (2020).
Lantz, C. et al. Native top-down mass spectrometry with collisionally activated dissociation yields higher-order structure information for protein complexes. J. Am. Chem. Soc. 144 , 21826–21830 (2022).
Paizs, B. & Suhai, S. Fragmentation pathways of protonated peptides. Mass Spectrom. Rev. 24 , 508–548 (2005).
Skinner, O. S. et al. Fragmentation of integral membrane proteins in the gas phase. Anal. Chem. 86 , 4627–4634 (2014).
Horn, D. M., Zubarev, R. A. & McLafferty, F. W. Automated reduction and interpretation of high resolution electrospray mass spectra of large molecules. J. Am. Soc. Mass Spectrom. 11 , 320–332 (2000).
Zamdborg, L. et al. ProSight PTM 2.0: improved protein identification and characterization for top down mass spectrometry. Nucleic Acids Res. 35 , W701–W706 (2007).
Mayampurath, A. M. et al. DeconMSn: a software tool for accurate parent ion monoisotopic mass determination for tandem mass spectra. Bioinformatics 24 , 1021–1023 (2008).
Li, L. & Tian, Z. Interpreting raw biological mass spectra using isotopic mass-to-charge ratio and envelope fingerprinting. Rapid Commun. Mass Spectrom. 27 , 1267–1277 (2013).
Liu, X. et al. Deconvolution and database search of complex tandem mass spectra of intact proteins: a combinatorial approach. Mol. Cell Proteom. 9 , 2772–2782 (2010).
Compton, P. D., Zamdborg, L., Thomas, P. M. & Kelleher, N. L. On the scalability and requirements of whole protein mass spectrometry. Anal. Chem. 83 , 6868–6874 (2011).
Guner, H. et al. MASH Suite: a user-friendly and versatile software interface for high-resolution mass spectrometry data interpretation and visualization. J. Am. Soc. Mass Spectrom. 25 , 464–470 (2014).
Cai, W. et al. MASH Suite Pro: a comprehensive software tool for top-down proteomics. Mol. Cell Proteom. 15 , 703–714 (2016).
Wu, Z. et al. MASH Explorer: a universal software environment for top-down proteomics. J. Proteome Res 19 , 3867–3876 (2020).
McIlwain, S. J. et al. Enhancing top-down proteomics data analysis by combining deconvolution results through a machine learning strategy. J. Am. Soc. Mass Spectrom. 31 , 1104–1113 (2020).
Larson, E. J. et al. MASH Native: a unified solution for native top-down proteomics data processing. Bioinformatics 39 , btad359 (2023).
Kafader, J. O. et al. Multiplexed mass spectrometry of individual ions improves measurement of proteoforms and their complexes. Nat. Methods 17 , 391–394 (2020).
Kafader, J. O. et al. Individual ion mass spectrometry enhances the sensitivity and sequence coverage of top-down mass spectrometry. J. Proteome Res. 19 , 1346–1350 (2020).
Worner, T. P. et al. Resolving heterogeneous macromolecular assemblies by Orbitrap-based single-particle charge detection mass spectrometry. Nat. Methods 17 , 395–398 (2020).
McGee, J. P. et al. Isotopic resolution of protein complexes up to 466 kDa using individual ion mass spectrometry. Anal. Chem. 93 , 2723–2727 (2021).
Marty, M. T. et al. Bayesian deconvolution of mass and ion mobility spectra: from binary interactions to polydisperse ensembles. Anal. Chem. 87 , 4370–4376 (2015).
Reid, D. J. et al. MetaUniDec: high-throughput deconvolution of native mass spectra. J. Am. Soc. Mass Spectrom. 30 , 118–127 (2019).
Marty, M. T. A universal score for deconvolution of intact protein and native electrospray mass spectra. Anal. Chem. 92 , 4395–4401 (2020).
Park, J. et al. Informed-proteomics: open-source software package for top-down proteomics. Nat. Methods 14 , 909–914 (2017).
Zhou, M., Pasa-Tolic, L. & Stenoien, D. L. Profiling of histone post-translational modifications in mouse brain with high-resolution top-down mass spectrometry. J. Proteome Res 16 , 599–608 (2017).
Deutsch, E. W. et al. The ProteomeXchange consortium in 2020: enabling ‘big data’ approaches in proteomics. Nucleic Acids Res. 48 , D1145–D1152 (2020).
Perez-Riverol, Y. et al. The PRIDE database resources in 2022: a hub for mass spectrometry-based proteomics evidences. Nucleic Acids Res. 50 , D543–D552 (2022).
Download references
Acknowledgements
We are grateful to all members of the Board of Directors of the CTDP for valuable discussions. We also thank B. Krichel and S. McIlwain (University of Wisconsin-Madison) and C. Hake (TU Darmstadt) for assistance with database searching. The CTDP, a 501c3 nonprofit corporation, is grateful for the generous support from Seer, ThermoFisher Scientific, Bruker, SCIEX, Lilly, Pfizer, Newomics and Agilent. M.T.M. thanks A. Makarov and K. Fort at ThermoFisher Scientific for support on the UHMR Q Exactive HF instrument. This work was supported by National Institutes of Health (NIH)/National Institute of General Medical Sciences (NIGMS) grant R35 GM128624 to M.T.M, NIH grant R01 GM125085 and S10 OD018475 to Y.G. and NIH/NIGMS grant P41 GM108569 to N.L.K., as well as NIH grant R01GM103479, R35GM145286, S10RR028893 and US Department of Energy grant DEFC02-02ER63421 to J.A.L. We acknowledge project award (10.46936/intm.proj.2019.51141/60006696) from the Environmental Molecular Sciences Laboratory, a Department of Energy Office of Science User Facility sponsored by the Biological and Environmental Research program under Contract No. DE-AC05-76RL01830 to M.Z. and J.W.W. and the Ruth L. Kirschstein National Research Service Award Program at NIH (GM007185) to C.L. We are grateful for funding by the Hessian Ministry for Science and Arts via the LOEWE project ‘TRABITA’ and by the Deutsche Forschungsgemeinschaft (grant nos. 461372424 and 524226614) to F.L. The funders had no role in the study design, data collection and analysis, decision to publish or preparation of the manuscript.
Author information
Present address: Zhejiang University, Zhejiang, China
Authors and Affiliations
Technische Universität Darmstadt, Darmstadt, Germany
Tanja Habeck & Frederik Lermyte
University of Wisconsin-Madison, Madison, WI, USA
Kyle A. Brown & Ying Ge
Northwestern University, Evanston, IL, USA
Benjamin Des Soye & Neil L. Kelleher
University of California, Los Angeles, CA, USA
Carter Lantz, Wonhyeuk Jung & Joseph A. Loo
Pacific Northwest National Laboratory, Richland, WA, USA
Mowei Zhou, Jesse W. Wilson & Ljiljana Paša-Tolić
Northeastern University, Boston, MA, USA
Novera Alam, Md Amin Hossain & Jeffrey N. Agar
University of Arizona, Tuscon, AZ, USA
James E. Keener & Michael T. Marty
Genentech Inc., San Francisco, CA, USA
Michael Volny & Wendy Sandoval
Sun Yat‐sen University, Guangzhou, China
Yujia Ying & Huilin Li
Consortium for Top-Down Proteomics, Cambridge, MA, USA
Jeffrey N. Agar, Paul O. Danis, Ying Ge, Neil L. Kelleher, Joseph A. Loo & Ljiljana Paša-Tolić
You can also search for this author in PubMed Google Scholar
Contributions
F.L., M.Z., B.D.S., J.A.L, C.L. and K.A.B. conceptualized the study. F.L., M.Z., B.D.S., C.L., K.A.B., Y.G., L.P.-T., W.S., J.A.L., J.N.A., N.L.K., M.T.M., H.L. and P.O.D. developed the methodology. T.H., K.A.B., B.D.S., C.L., M.Z., M.d.A.H., N.A., W.J., J.E.K., M.V., J.W.W. and Y.Y. performed the investigations. F.L. and T.H. performed formal analysis. F.L. and T.H. wrote the original draft of the manuscript. F.L., T.H., Y.G., L.P.-T., W.S., J.A.L., J.N.A., N.L.K., M.T.M., H.L. and P.O.D. contributed to the writing, review and editing of the final manuscript.
Corresponding author
Correspondence to Frederik Lermyte .
Ethics declarations
Competing interests.
N.L.K. is involved in entrepreneurial activities in top-down proteomics and consults for ThermoFisher Scientific. P.O.D. is the founder and principal of Eastwoods Consulting, providing business advisory services to life science companies. The remaining authors declare no competing interests.
Peer review
Peer review information.
Nature Methods thanks Alexander Leitner and the other, anonymous, reviewer(s) for their contribution to the peer review of this work. Peer reviewer reports are available. Primary Handling Editor: Arunima Singh, in collaboration with the Nature Methods team.
Additional information
Publisher’s note Springer Nature remains neutral with regard to jurisdictional claims in published maps and institutional affiliations.
Extended data
Supplementary information, supplementary information.
Supplementary Figs. 1–11, Tables 1–7, Protocol 1 and Survey 1.
Reporting Summary
Peer review file, rights and permissions.
Springer Nature or its licensor (e.g. a society or other partner) holds exclusive rights to this article under a publishing agreement with the author(s) or other rightsholder(s); author self-archiving of the accepted manuscript version of this article is solely governed by the terms of such publishing agreement and applicable law.
Reprints and permissions
About this article
Cite this article.
Habeck, T., Brown, K.A., Des Soye, B. et al. Top-down mass spectrometry of native proteoforms and their complexes: a community study. Nat Methods (2024). https://doi.org/10.1038/s41592-024-02279-6
Download citation
Received : 02 August 2023
Accepted : 10 April 2024
Published : 14 May 2024
DOI : https://doi.org/10.1038/s41592-024-02279-6
Share this article
Anyone you share the following link with will be able to read this content:
Sorry, a shareable link is not currently available for this article.
Provided by the Springer Nature SharedIt content-sharing initiative
Quick links
- Explore articles by subject
- Guide to authors
- Editorial policies
Sign up for the Nature Briefing: Translational Research newsletter — top stories in biotechnology, drug discovery and pharma.


IMAGES
COMMENTS
By using unfolding case studies that integrate all three paradigm shifts, you will accomplish two essential objectives: You are providing a meaningful learning activity that practices the essence of clinical reasoning that leads to correct clinical judgments in practice. Just like high fidelity simulation provides a replication of the clinical ...
the case study is presented in a classroom or clinical setting. The questions guide students through the application of the nursing process to the case study scenario. This case study can be completed synchronously or asynchronously, by an individual student or by a group. This case study can be completed in the classroom, in a clinical group, or
An unfolding case is one that evolves over time in a manner that is unpredictable to the learner. New situations develop and are revealed with each encounter. Every ACE unfolding case uses the highly regarded unfolding case model developed for Advancing Care Excellence for Seniors (ACE.S). Each case includes the following:
Unfolding Case Study that focuses on the concepts of Mobility, Pain, Perfusion, and Mood and Affect. Example of an Unfolding Case Study: Care of the Patient Experiencing Trauma Phase 1: Critical Care A 24-year old woman, Cheryl, was admitted to the trauma unit following a motor vehicle accident as a front-seat passenger.
ACE unfolding cases were written so that they can be modified to meet the needs of diverse curricula. Since preparation is key to a successful simulation experience, faculty should plan to read through each unfolding case before using it. These cases were designed so that students can use the ACE Knowledge Domains and Essential Nursing Actions ...
This article describes how an unfolding case study can be used to promote the development of clinical reasoning through students' self-reported perceptions, although at the same time facilitating collaboration among providers from various specialties. An unfolding case (evolving case) provides sequential information about a patient's illness trajectory as they experience the illness and ...
Clinical forethought can be introduced using unfolding case studies that encourage students to make a patient's values, preferences and beliefs an important factor in their care. Nurse Educators are challenged with writing case studies that require students to see how clinical judgment could facilitate safety and patient-centered care.
Clinical realities can also be replicated using realistic unfolding patient care scenarios in a simple unfolding case study that mirrors how a nurse encounters clinical realities in practice. As a nurse educator who has current experience and who has practiced in the critical-care float pool of a large metropolitan hospital, I have written most ...
The unfolding case study technique aligns well with Kolb's Theory of Experiential Learning and can be used to help students improve clinical reasoning and critical thinking skills. Description: An unfolding case study was used to simulate. clinical experience for students when clinical sites became unavailable to students due to COVID-19.
This capstone project evaluated if the use of an unfolding case study would result. in an increase in self-efficacy and therefore greater academic performance and the ability. to integrate essential knowledge domains for second year associate degree nursing. students.
Nurse practitioner students enrolled in their last adult-gerontology primary care didactic course were invited to participate in a study assessing their self-ef cacy after fi completing a mandatory unfolding case study assignment. The participants demonstrated increased self-ef cacy in several domains. fi 2022 Elsevier Inc.
Objective: The aim of this study was to determine how the use of unfolding case studies as a learning modality affected baccalaureate students' critical thinking skills in their Adult Health Theory course. The researcher compared course examination scores earned by nursing students who were taught using traditional case studies to scores ...
Unfolding case studies develop over time and are unpredictable to the learner. If well done, promotes experiential education and imagination. Students picture themselves as part of the scenario. Enables students to practice making decisions and evaluating the effects of those decisions in a non-threatening environment. When this is done in ...
How can you bring clinical realities to your online class and clinical replacement activity?Learn practical strategies to successfully use unfolding case stu...
Englund H (2020) Using unfolding case studies to develop critical thinking skills in baccalaureate nursing students: A pilot study, Nurse Education Today, 10.1016/j.nedt.2020.104542, 93, (104542), Online publication date: 1-Oct-2020.
Faculty will also receive tips on how to write unfolding case studies, an in-depth look at the new NGN item types, and test-taking strategies to share with students. White paper highlights. Research shows the decreased ability of new nursing graduates to make safe clinical decisions ; Clinical judgment is critically important for nursing practice
an evaluation of the case study. To develop this scripted unfolding case on delirium, the education consultant (V.K.) provided a template from her previous experience with case development. Step 1: Select Topic and Review the Literature The first step in developing a scripted unfolding case study is to select the topic, review the relevant lit-
This article describes a technique for using unfolding case studies to include such initiatives in the teaching of novice nursing students, as opposed to summative evaluation of their knowledge. Modeled after Kolb's experiential learning theory, a framework for unfolding case studies is presented, which proposes that instead of faculty ...
Before you attempt writing a case study, you need to understand how to design it. To do that, you need to be familiar with Layer 3 of the NCSBN's Clinical Judgment Measurement Model. Layer 3 illustrates the 6-step process that a nurse goes through in each client encounter. (See the numbered graphic below that depicts the 6 steps.)
Client/Nurse Interaction. The six questions in the case study should focus on the application of one of the cognitive skills highlighted in the Clinical Judgment Measurement Model (CJMM). Silvestri (2020) identifies questions that test-writers (and test-takers) ask themselves to understand each of the six cognitive skills.
In this insightful white paper, nurse education expert Donna Ignatavicius offers practical advice on how to effectively use single-episode case studies, unfolding case studies, and debriefing to promote student learning and critical thinking. Find out how you can put her valuable tips and strategies to work in your program to help strengthen ...
Write the case. The unfolding case study was reviewed and revised multiple times to ensure that the characters and story were authentic and that the objectives were met. SDOH were embedded into the story as the characters encountered life events. Write guidelines for facilitators. This step was the most difficult and time-consuming part of the ...
Cookies are used by this site. To decline or learn more, visit our Cookie Settings page.Cookie Settings page.
Addressing two common issues facing DSF and other isolated protein assays in early drug discovery: the requirement for purified protein and the question of the physiological relevance of the target protein outside of its native cellular environment, a further development in thermal unfolding studies, the cellular thermal shift assay (CETSA ...
The proteins used for this study were either bought from Sigma or (in the case of AqpZ) supplied by one of the participants. More information and catalog nos. can be found in Supplementary Table 4 .