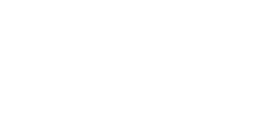

High Blood Pressure
Patient case study: elevated uric acid and high blood pressure.
by Peter Attia
Read Time 2 minutes
This audio clip is from “Ask Me Anything” (AMA) episode #15 — Real-world case studies—metabolic dysregulation, low testosterone, menopause, and more .
If you’re a member, you can now listen to this full episode on your private RSS feed or on our website . If you are not a member, learn more about the how to become a member and the benefits here .
Case study—Elevated uric acid and hypertension [1:10:55]
“Patient H”
- Body type: Normal BMI
- He was treated with lisinopril (ACEi) bringing him into the 130/90 range (still higher than ideal for 46 year old, especially if he’s interested in longevity)
- Uloric 40 mg QD
- Lipitor 20 mg
- 6/2020: Added Repatha.
- HTN: Father and Uncle
- CVD: Maternal and Paternal Grandfather.
- Essential HTN
- Mixed hyperlipidemia
- Atherosclerotic heart disease with evidence of calcification on CAC
- Low carb. No signs of metabolic syndrome or insulin resistance.
- Weight training 3x/week
- Zone2 training 3 hr a week.
- CAC of 88 – Approximately 85th percentile for age. (even more reason to be aggressive in treating blood pressure)
*To reiterate: This is a guy that on paper looked really good. He’s also very muscular and lean. He runs and exercises six days/week. He also eats well .
Looking at his initial labs—
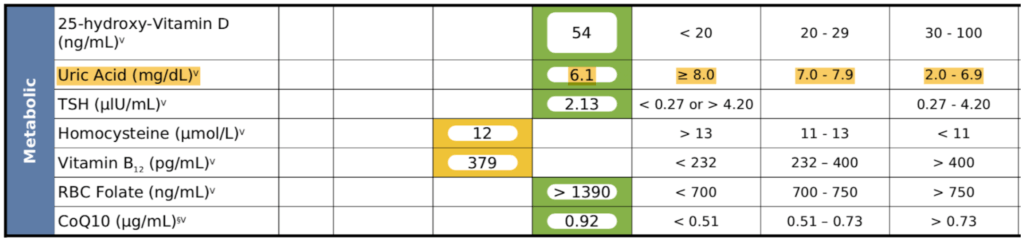
Figure 21. Patient H – Initial Labs when presenting with essential hypertension.
Observations :
- His uric acid was 6.1
- It was also quite stubborn—never went down with a dietary change (tweaking protein levels, reducing fructose, etc.)
- Peter could not get his uric acid below 6, and the target was below 5
- See Rick Johnson podcast to understand the importance of lowering uric acid
Labs after ~18 months on Uloric—
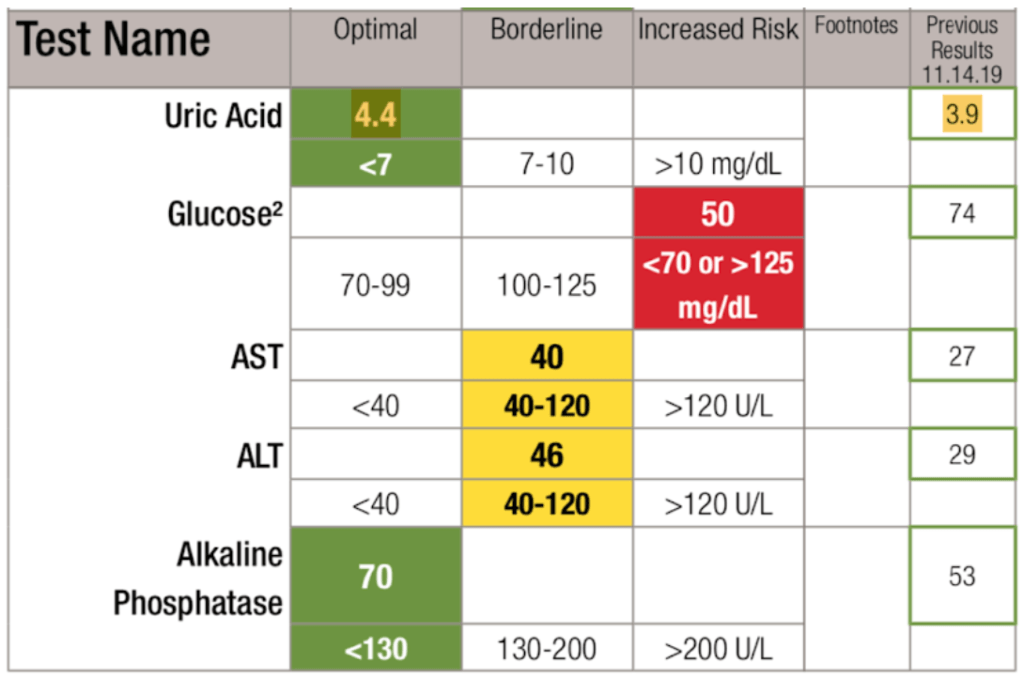
Figure 22. Labs after ~18 months on Uloric.
Observations :
- Uloric “fixed” uric acid
- His HTN resolved even with a persistently high homocysteine
- He no longer required ACE inhibitor (for blood pressure)
Concluding thoughts :
- This is a pretty unusual response to just lowering uric acid
- Do NOT go after blood pressure until you’ve got the uric acid below 5
- Only then start playing with the blood pressure medication
- *The broader point : Try to fix the insulin resistance and metabolic syndrome first (which in this case he didn’t have, but he did have the elevated uric acid) before you introduce drugs to treat blood pressure.
What determines uric acid levels?
- People who eat foods with lots of DNA and RNA (i.e., protein sources)
- Yeast in the beer
- But the biggest part may be genetics , says Peter ⇒ “ I don’t know why it is that in some patients you can remove all of the normal impediments to normal uric acid metabolism and it’s still elevated. ”
- Despite eating and exercising well, he can’t get his uric acid below 5.5 without using allopurinol
- He has a family history of hypertension
⇒ Make sure to check out Rick Johnson’s episode for more on the importance of managing uric acid levels
Peter’s internal standard for blood pressure : Below 120/80 without producing any symptoms of lightheadedness
Selected Links / Related Material
See episode of The Drive with Rick Johnson for the importance of managing uric acid : #87 – Rick Johnson, M.D.: Fructose—The common link in high blood pressure, insulin resistance, T2D, & obesity? | Peter Attia (peterattiamd.com) [40:00, 1:12:30]]
Other Content You May Like
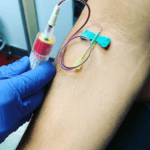
#124 – AMA #15: Real-world case studies—metabolic dysregulation, low testosterone, menopause, and more
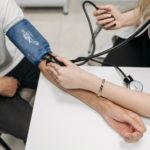
How Peter thinks about managing GFR & high blood pressure in his patients
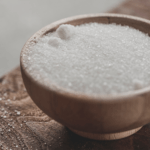
Rick Johnson, M.D. on the relationship between salt & blood pressure
Comment policy.
- Comments deemed to be spam or solely promotional in nature will be deleted. Including a link to relevant content is permitted, but comments should be relevant to the post topic.
- Comments including unnecessary profanity will be deleted.
- Comments containing language or concepts that could be deemed offensive will be deleted. Note this may include abusive, threatening, pornographic, offensive, misleading or libelous language.
- Comments that attack an individual directly will be deleted.
- Comments that harass other posters will be deleted. Please be respectful toward other contributors.
- Anonymous comments will be deleted. We only accept comment from posters who identify themselves.
- Comments requesting medical advice will not be responded to, as I am not legally permitted to practice medicine over the internet.
- The owner of this blog reserves the right to edit or delete any comments submitted to the blog without notice. This comment policy is subject to change at any time.
Introduction to My Longevity Tactics
Sign-up (free) to receive the 5 Tactics in my Longevity Toolkit delivered by email as a 5-day course.
You’ll also receive:
Non-lame, weekly emails on the latest strategies and tactics for increasing your lifespan, healthspan, and well-being (plus new podcast announcements).
- Hidden H-o-n-e-y-p-o-t
- Phone This field is for validation purposes and should be left unchanged.
- Reference Manager
- Simple TEXT file
People also looked at
Review article, physiology of hyperuricemia and urate-lowering treatments.
- 1 Pfizer Ltd., Cambridge, United Kingdom
- 2 DMPK, Oncology, IMED Biotech Unit, AstraZeneca, Cambridge, United Kingdom
- 3 IMED Biotech Unit, Medicinal Chemistry, Discovery Sciences, AstraZeneca, Cambridge, United Kingdom
Gout is the most common form of inflammatory arthritis and is a multifactorial disease typically characterized by hyperuricemia and monosodium urate crystal deposition predominantly in, but not limited to, the joints and the urinary tract. The prevalence of gout and hyperuricemia has increased in developed countries over the past two decades and research into the area has become progressively more active. We review the current field of knowledge with emphasis on active areas of hyperuricemia research including the underlying physiology, genetics and epidemiology, with a focus on studies which suggest association of hyperuricemia with common comorbidities including cardiovascular disease, renal insufficiency, metabolic syndrome and diabetes. Finally, we discuss current therapies and emerging drug discovery efforts aimed at delivering an optimized clinical treatment strategy.
Introduction
Hyperuricemia is a condition characterized by abnormally elevated levels of serum urate (sUA), while gout, the most common form of inflammatory arthritis, arises from the subsequent deposition of urate crystals when concentrations become saturated. Gout has been defined as “a progressive metabolic disease characterized by symptomatic hyperuricemia and deposition of monosodium urate (MSU) crystals in joints and soft tissues due to an imbalance in uric acid uptake, synthesis or excretion” (cited in ( 1 )). The initial clinical sign of an acute gout attack is severe disabling pain, usually involving a single joint, which typically spontaneously resolves over a period of a few days to weeks without intervention, although treatment with anti-inflammatory drugs such as colchicine, NSAIDs (non-steroidal anti-inflammatory drugs) and corticoids will generally improve symptoms more rapidly. Upon resolution of an acute attack the patient will enter a symptom-free interval, however flares can recur with increased frequency and duration if the underlying pathology is not addressed. If sUA values remain high, MSU crystal deposits can grow and expand to other sites leading to further inflammation and associated tissue/joint injury. Ultimately a subset of individuals will transition to chronic tophaceous gout which is characterized by nodular urate crystal deposits, recurrent flares and concurrent arthritis, which takes 11.6 years on average to occur from the initial flare (reviewed in ( 2 )).
It is often stated that the prevalence of hyperuricemia and gout has increased in recent years, although there are relatively few longitudinal studies in geographically diverse populations and increasing diagnosis rates may play a significant role. It has been highlighted that the distribution of gout varies significantly across the world which may reflect factors such as ethnicity, diet and socioeconomic factors ( 3 ). In the US the National Health and Nutrition Examination Survey [NHANES] 2007–2008 suggested estimating prevalence of gout hyperuricemia and gout at approximately 21 and 4%, respectively, an increase of 3.2 and 1.2% respectively when compared to the prior NHANES-III study conducted from 1988 to 1994 ( 4 , 5 ). Likewise, a review of data collected in the Australian population suggested an increase in the prevalence of gout from 0.5 to 1.7% from 1968 to 1995/6 ( 6 ). However, this trend is not universal and data collected in Taiwan over a similar time period, 1993–1996 and 2005–2008, to the US study showed a fall in the prevalence of hyperuricemia from 25.3 to 22.0% in men and from 16.7 to 9.7% in women ( 7 ). Some authors have argued that this is related to the epidemic of obesity and associated dietary shift toward foods rich in purines, alcohol consumption and fructose-sweetened drinks ( 8 , 9 ); however, this is still disputed and indeed, the impact of dietary intervention may be limited with respect to management of sUA ( 10 ).
Many epidemiological studies have shown that hyperuricemia and gout are associated with the development of hypertension, cardiovascular disease, chronic kidney disease and diabetes, potentially through crystal-independent modes of action ( 11 – 16 ). Interpretation of these studies is confounded by the specific definition of hyperuricemia that is applied, which in turn contributes to the controversy around the notion of urate having a causal role in these conditions ( 17 ). However, it is notable that EULAR (European League Against Rheumatism) now recommends that gout could be seen as a red flag for associated cardiovascular risk factors and co-morbidity, and that blood pressure, lipids and glucose be checked and treated if abnormal ( 18 ).
In this article, we review the current knowledge of the physiological and genetic factors involved of uric acid handling in humans. Furthermore, we discuss literature data which examine if hyperuricemia may be a factor in certain comorbidities such as hypertension, cardiovascular disease and chronic kidney disease. Finally, we highlight several current and future therapeutic options for the treatment of hyperuricemia and gout.
Uric acid is a weak diprotic acid with an aqueous pK a1 of 5.4 and pK a2 of 9.8 ( 19 ) (Figure 1 ). Consequently, at physiological pH, uric acid is predominantly (98–99%) found as the deprotonated urate anion. The solubility of uric acid at normal physiological pH is generally given as 6.8 mg/dL while the reference ranges for sUA are 3.5 to 7.2 mg/dL (210–430 μmol/L) and 2.6–6.0 mg/dL (155–360 μmol/L) in males and premenopausal females respectively ( 20 ). It is notable that the upper limit of the normal male reference range includes concentrations that exceed the concentration at which uric acid precipitates.
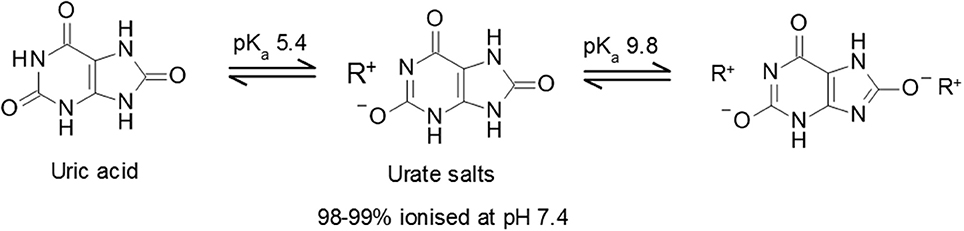
Figure 1 . Uric acid pKa and formation of urate salts. Structures of uric acid and urate salts including acid dissociation constants.
One interesting aspect of urate biology is the observation that the normal range for serum urate concentrations in humans, and some other primates, are significantly above the typical mammalian range of 0.5–2.0 mg/dL (30–120 μmol/L). The evolutionary drive for acquisition of comparatively high levels of circulating urate remains unclear, although it has been proposed to confer evolutionary advantages as an anti-oxidant, particularly in the context of neurodegenerative diseases such as Parkinson's ( 21 , 22 ). However, clinical data are conflicting and approaches seeking to increase urate levels have not shown a positive impact on disease outcomes ( 23 , 24 ). In contrast, while any potential benefits of high sUA levels remain to be elucidated it has been clearly demonstrated that hyperuricemia, in conjunction with genetic and/or environmental factors, can lead to significant health problems associated with urate crystal deposition.
Sources of Uric Acid
Uric acid is produced during the metabolism of both endogenous (daily synthesis rates approximate 300–400 mg) and exogenous (dietary contribution, approximately 300 mg) purines within a total pool size of 1,200 mg in healthy males (600 mg in females) on a purine-free diet ( 25 , 26 ). The relationship between diet and sUA is likely to be more complex than simple purine intake, for example beer and sweetened soft drinks (with high fructose corn syrup as a particularly maligned source) have both been shown to have impact on sUA levels independently of their purine content ( 9 , 27 , 28 ). Indeed, consistent with the notion of a direct relationship between fructose intake and high sUA levels, it has been shown that increased fructokinase activation leads to the rapid generation of uric acid which in turn upregulates fructokinase expression ( 29 , 30 ).
The biosynthesis of uric acid is catalyzed by the enzyme xanthine oxidase (XO, also known as xanthine oxidoreductase or XOR), coded for by the xanthine dehydrogenase gene XDH ( 31 ). The enzyme is normally present as an inactive NAD-dependent cytosolic dehydrogenase precursor, which is subsequently subjected to further processing by oxidation or proteolytic modification to form active enzyme. Xanthine oxidase is widely distributed throughout various organs including the liver, gut, lung, kidney, heart, and brain as well as the plasma and is involved in two stages of uric acid generation; conversion of hypoxanthine to xanthine and subsequently xanthine to uric acid (Figure 2 ).
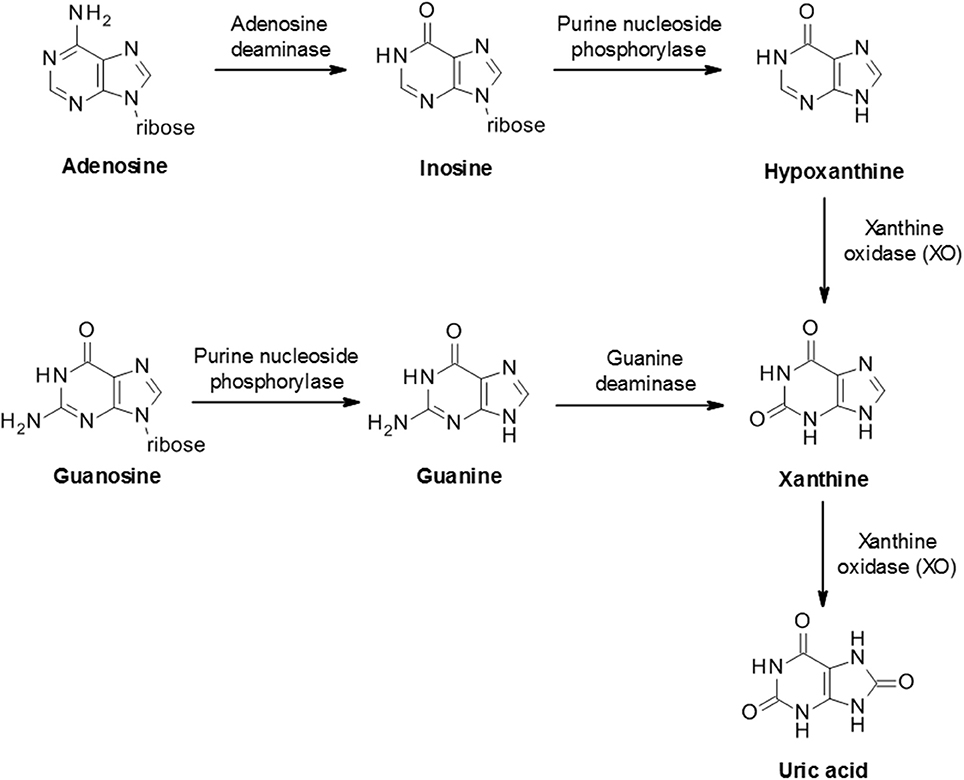
Figure 2 . Biosynthesis of uric acid from purines. Purine mononucleotides are catabolized to produce uric acid although the underlying pathway can vary in different tissues and cells. A schematic example pathway is shown.
Uric Acid Clearance
In most mammalian species uric acid is further metabolized by the enzyme uricase to the more soluble allantoin (Figure 3 ) which is subsequently excreted in the urine. However, humans and some higher order primates lack a functional uricase enzyme and therefore uric acid is the final breakdown product of the pathway ( 21 ). This discrepancy in uric acid handling between species can represent a significant challenge in the preclinical evaluation of urate lowering drugs during drug discovery.

Figure 3 . Uric acid metabolism via uricase. In humans and some primates, uric acid is the final product of the purine catabolism pathway. However, most animals further degrade uric acid to allantoic acid via the sequential actions of uricase, 5-hydroxyisourate hydrolase and allantoinase.
Urate elimination from humans occurs via two main routes; approximately two-thirds being excreted in urine with normal uricosuria levels of 620 ± 75 mg/day in adult, while the remainder is thought to be largely excreted via the gastrointestinal tract ( 25 , 26 , 32 , 33 ). Hyperuricemia may also be associated with hyperuricosuria (defined as urinary excretion of urate >800 mg/day in men and >750 mg/day in women). Urate elimination can be quantified as clearance (normal males: 8.7 ± 2.5 mL/min) or as fractional excretion of urate (FEUA) which indicates the net urate excretion by the kidney (normal males: 7.25 ± 2.98%). Healthy subjects have an average FEUA in the range of 6–8%, whereas gout patients generally have average FEUA of 3–5% ( 34 – 36 ). These observations are consistent with the notion that decreased renal excretion or low FEUA represents a major contributor to hyperuricemia as opposed to increased generation of uric acid.
Despite the high fraction of renally excreted uric acid, the process is more complex than simple glomerular filtration, with approximately 91–95% of filtered urate being reabsorbed in the proximal tubule. Reabsorption is a key factor underpinning the comparatively high levels of circulating urate and is primarily mediated by transporters that exchange intracellular anions for urate ( 37 , 38 ). Reabsorption and secretion of urate predominates in the S1 and S2 regions of the proximal tubule although it is not clear whether the secretion happens concomitantly with reabsorption and/or if there is post-reabsorptive secretion within the tubule. Ultimately, around 3–10% of the filtered urate emerges in the urine. Several transporters playing a role in reabsorption and secretion have been identified (Figure 4 ).
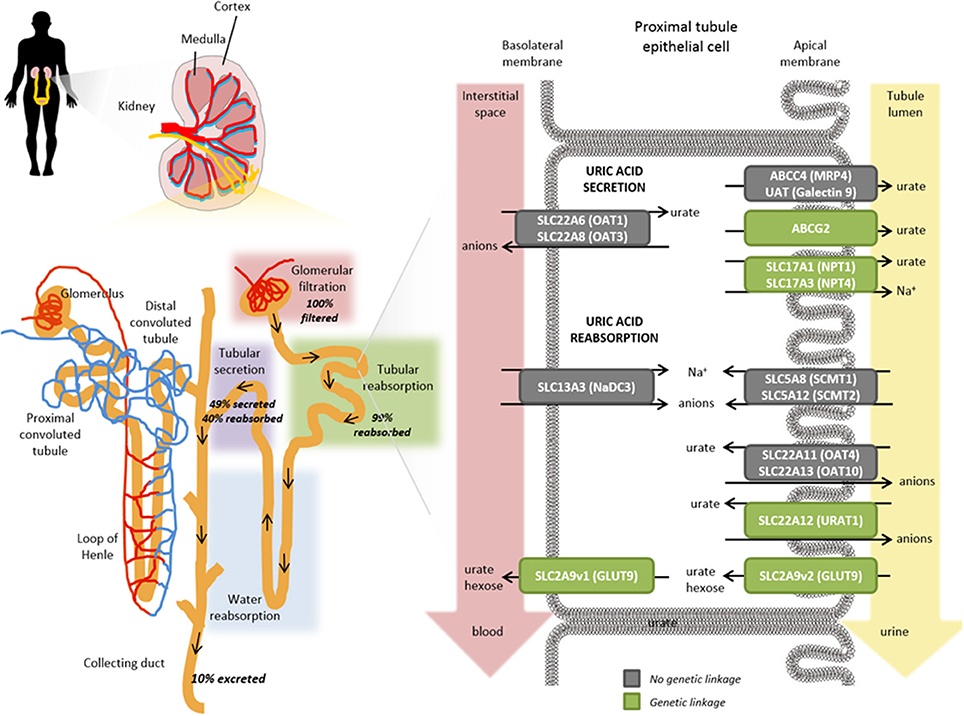
Figure 4 . Role of transporters in the renal proximal tubule on urate handling. Within an individual nephron in the kidney (yellow), filtration of water and solutes occurs in the glomerular capsule from the afferent arteriole into the renal tubule (pink shading). Tubular reabsorption (green shading) is predominantly mediated by the proximal convoluted tubule whereas tubular secretion extracts uric acid (and other substances) from peritubular capillaries (purple shading) and secretes them into the tubular fluid for urinary excretion. Urate transporters in renal proximal tubule epithelial cells actively mediate the secretion and reabsorption of urate. The balance between these processes determines the net excretion levels from the kidney. The anion transporters SLC22A6 (OAT1) and SLC22A8 (OAT3) localized on the basolateral membranes transport urate from the interstitial space in the blood depending on the gradients for exchanged anions but have not been shown to exhibit a genetic linkage with hyperuricemia or gout risk (gray box). On the apical membrane, ABCG2, SLC17A1 (NPT1), SLC17A3 (NPT4), ABCC4 (MRP4), UAT (Galectin 9) have all been shown to contribute to the secretory transport of urate into the tubule lumen and leading to urinary excretion; a number of these have been genetically associated with hyperuricemia and gout risk (green boxes). Exchange gradients upstream of urate anion exchange are enabled through the actions of SLC13A3 (NaD3), SLC5A8 (SCMT1), and SLC5A12 (SCMT2). In renal reabsorption, the apical urate-anion exchanger SLC22A12 (URAT1) has been shown to play a predominant role in urate homeostasis and indeed several variants have been identified to be associated with gout and hyperuricemia risk (green box). Additional contributions to urate reabsorption are mediated by SLC22A11 (OAT4) and SLC22A11 (OAT10) (gray boxes, not genetically associated with gout/hyperuricemia risk) and the short isoform of SLC2A9v2 (GLUT9, green box) on the apical membrane. The long isoform of SLC2A9v1 (GLUT9, green box) is the only known transporter to mediate basolateral efflux of urate back into circulation; which is in accordance with its genetic association for gout and hyperuricemia risk in addition to rare mutations associated with hypouricemia.
Urate Reabsorption Transporters
Urat1 (slc22a12).
The identification of URAT1 ( SLC22A12) as the dominant apical (luminal) urate exchanger in the human proximal tubule was a landmark event in the understanding of urate homeostasis ( 39 ). URAT1 is a 12-transmembrane domain protein, predominantly expressed on the apical brush border membrane of proximal tubule epithelial cells in the kidneys. URAT1 has been shown to transport urate with a K m of 371 ± 28 μM as well as other organic anions such as orotate, salicylate, lactate and nicotinate ( 39 – 41 ). URAT1-mediated urate transport is a tertiary active process dependent on sodium gradients which are initially established via basolateral Na + K + ATPases which actuate a number of apical Na + coupled organic anion transporters, in turn providing the driving force for urate reabsorption ( 42 , 43 ).
Clinical genetic studies have confirmed that loss-of-function mutations of URAT1 are associated with FEUA of 40–100% and extremely low serum urate levels (average levels of 0.93 mg/dL) ( 44 ) (Table 1 ). Interestingly, it has been shown that testosterone increases and estradiol decreases URAT1 protein levels in mice and it is interesting to speculate whether this would contribute to the increased hyperuricemia susceptibility in males and post-menopausal women ( 89 , 90 ). Additional genetic susceptibility toward hyperuricemia and gout via PDZK1 association is potentially through its known function of modulating the apical membrane localization of URAT1 ( 57 , 91 ) (Table 1 ). While URAT1 appears to be the predominant apical reabsorption transporter, other pathways are inferred given that FEUA is <100% even in patients with complete URAT1 loss of function mutations.
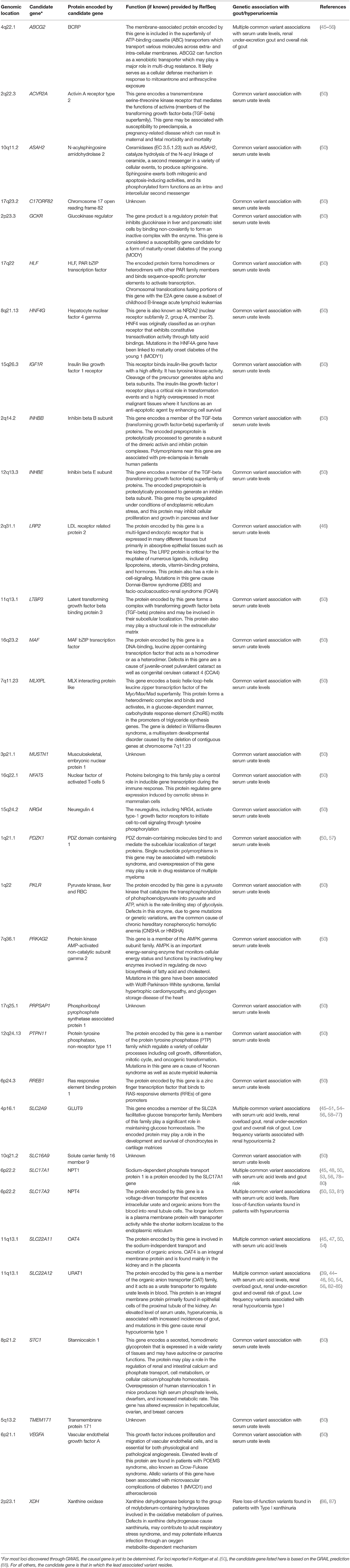
Table 1 . Genetic associations with urate levels.
URAT1 is now a well-established drug target with a number of primary and secondary uricosurics (drugs capable of increasing FEUA) such as benzbromarone, probenecid and lesinurad, known to derive at least part of their efficacy through this mechanism albeit, in earlier cases, this was not understood when they were developed ( 92 – 97 ). Interestingly, compounds such as pyrazinamide have been shown to trans-stimulate URAT1 activity to impact on vectorial transport of urate ( 72 , 98 ).
GLUT9 (SLC2A9)
Variants in glucose transporter 9 (GLUT9, also referred to as URATv1), coded for by the SLC2A9 gene, are strongly associated with both hyperuricemia and gout, a finding that has been successfully replicated in multiple studies ( 59 , 99 ) (Table 1 ). In addition, individuals with homozygous mutations in GLUT9 present with pronounced hypouricemia and hyperuricosuria with FEUA's of 100% or greater (indicative of net active urate secretion), response to fructose load, and a propensity for nephrolithiasis and exercise induced renal failure ( 62 , 84 , 100 – 102 ).
GLUT9 appears to function predominantly as a facilitative urate uniporter with at least some additional capacity for hexose transport ( 59 , 103 , 104 ). GLUT9-mediated urate transport is voltage dependent with currents recorded at physiological pH, but independent of sodium, chloride and other ions. Consequently, GLUT9 is distinct from other members of the glucose transporter (SLC2) family due to its substrate specificity and sequence identity although it shares common structural features such as 12 transmembrane helices, cytoplasmic termini, and an N-linked glycosylation site. Two isoforms of GLUT9 have been described that differ only by the first 29 residues of the N-terminal domains ( 104 ). The short isoform (GLUT9a) appears to be expressed at both apical and basolateral membranes in proximal tubule epithelium cells (and indeed may contribute to the import of urate from the peritubular interstitium and thus facilitate renal urate secretion). The long isoform (GLUT9b) is predominantly expressed on the basolateral membrane and is the only known basolateral efflux transporter for urate.
Interestingly, a positive relationship has been described between glycosuria and uricosuria suggesting that there could be interference between the tubular reabsorption of glucose and the tubular capacity to reabsorb urate ( 105 ). Indeed, it has been proposed that SGLT2 inhibitor treatment lowers serum uric acid through alteration of uric acid transport activity in renal tubules. This is consistent with clinical observations with canagliflozin treatment decreasing serum urate in patients with type 2 diabetes (a known co-morbidity of hyperuricemia), including those with baseline hyperuricemia ( 106 – 108 ). Therefore, studies which assess urate lowering therapy (ULT) efficacy should also consider ambient glycemia which could contribute to the uricosuric effect ( 109 ).
OAT4 ( SLC22A11 ) and OAT10 ( SLC22A13 )
Organic anion transporters 4 (OAT4) and 10 (OAT10) have a range of organic anion substrates and are expressed on the apical membrane of proximal tubule epithelium cells together with URAT1 ( SLC22A12 ) ( 99 ). While both transporters have been demonstrated to exhibit low levels of urate transport capabilities, only OAT4 has been associated with hyperuricemia and gout together with inefficient renal secretion ( 78 , 80 ).
Urate Excretion Transporters
Abcg2/bcrp ( abcg2 ).
Genetic variation in human ABCG2, an ATP-driven efflux pump on the apical membrane proximal tubule epithelial cells, has emerged as a major factor in human hyperuricemia and gout risk ( 78 , 110 , 111 ). Of note, the landmark paper from Ichida and colleagues shows that decreased extra-renal urate excretion caused by ABCG2 dysfunction contributes to clinical hyperuricemia; although paradoxically, urinary urate excretion is also increased by ABCG2 dysfunction implying further roles for regulation of urate levels by this transporter ( 110 ). However, ABCG2 is also expressed in the intestine and thus a contribution of gastrointestinal transport cannot be ruled out ( 110 , 112 ).
NPT1 ( SLC17A1 ) and NPT4 ( SLC17A3 )
GWAS (Genome Wide Association Study) studies have shown that NPT1 and NPT4 are associated with hyperuricemia and gout ( 38 , 55 , 79 , 99 ). Both NPT1 and NPT4 can transport urate in vitro and are localized on the apical membrane supporting the notion of a role in renal secretion of urate from the apical membrane ( 81 , 113 , 114 ). However, their relative importance to urate transport in proximal tubule epithelial cells remains incompletely defined.
OAT1 ( SLC22A6 ) and OAT3 ( SLC22A8 )
The basolateral entry of urate into renal proximal tubule cells is driven, at least partially, by the outwardly directed gradient for dicarboxylates, which in turn is generated by Na + -dependent uptake. Thus, urate exchange is significantly trans-stimulated by dicarboxylates ( 115 ). This is further complicated by the observation that OAT1 and OAT3 appear to exchange urate with divalent anions including dicarboxylates, suggesting that they are suited to basolateral entry of urate ( 38 , 116 ). However, there is no supporting genetic data for a role for these transporters in hyperuricemia.
Genetic Variation Influencing Uric Acid Handling
As indicated in the former sections on urate resorption and secretion transporters, much is now known about genetic variants contributing to sUA levels and gout through the discovery of rare monogenic disorders affecting uric acid homeostasis, from GWAS, and from candidate gene studies of common variants at the population level. Indeed, there is a strong overlap across genes implicated in monogenic uricemic traits and gout, in a range of genes in addition to those coding for uric acid transporters (Table 1 ). The largest GWAS to date, by the Global Urate Genetics Consortium (GUGC), studied >140,000 subjects of European ancestry and found 28 genetic loci associated with sUA levels and gout ( 50 ). The per-allele effect sizes on sUA ranged from 0.035 to 0.379 mg/dL. For gout, each allele contributed small effects on disease risk ranging from a 3% decrease to a 73% increase ( 50 ). The effects of common genetic variants found to be associated with hyperuricemia and gout in Europeans were of a similar magnitude when tested for association with subjects of other ancestries including African, Indian and Japanese ( 50 ). While GWAS have been pivotal in the identification of loci associated with sUA and gout risk, such studies do not provide a direct link to causal genes. For some loci there are obvious and highly likely causal genes, while at other loci the causal gene is far from clear and so GWAS findings should be interpreted with this in mind ( 117 ). In addition, genetics is a likely contributor to the well-documented observation that certain ethnic groups have a higher risk than others for hyperuricemia and gout ( 118 , 119 ). Consistent with this notion, GWAS of distinct ethnic groups has revealed some novel loci for gout and uric acid levels ( 46 , 48 , 49 ). However, in this context, it is worth emphasizing that while hyperuricemia is the central risk factor for gout development, there are other variables in play including age, dietary factors and medications which could be impactful across ethnicities. The heritability of sUA concentrations has been estimated at 42–73% ( 120 , 121 ), while early twin studies have led to estimates for the heritability of renal urate clearance at 60% and of fractional excretion of urate to be 87% ( 122 ). Further family studies have found a significant sUA correlation between siblings, parents and offspring though segregation analysis suggests this is likely due to multiple genetic factors rather than a major Mendelian gene ( 123 ).
Hypouricemia is defined when a serum urate concentration is less than or equal 2.0 mg/dL and is reported to occur in 0.8% of hospitalized patients and 0.2% of the general population ( 124 ). However, it is possible that the prevalence of hypouricemia is actually higher but undiagnosed ( 125 ). Hypouricemia can be associated with decreased fractional excretion of uric acid and increased xanthine excretion (e.g., hereditary xanthuria caused by an autosomal recessive deficiency of XDH ; ( 126 ). More typically, hypouricemia is associated with high fractional excretion of uric acid due to genetic causes; including mutations in genes such as SLC22A12 (URAT1) ( 39 , 127 ) and SLC2A9 (GLUT9) ( 62 , 84 ) or factors such as uricosuric usage, renal tubulopathy, neoplasias, and other conditions ( 124 , 127 ). The majority of individuals with hypouricemia are asymptomatic which suggests that it is potentially safe to lower sUA levels to <1 mg/dL although clinical management aims at maintaining sUA levels of 4–6 mg/dL. However, nephrolithiasis and exercise induced renal failure (EIRF) have been reported in individuals with mutations in either the SLC22A12 (URAT1) or SLC2A9 (GLUT9) but not XDH (XO) genes ( 82 , 128 , 129 ). The underlying mechanism is unclear and hypotheses include: (1) acute urate nephropathy caused by increased urate production during exercise, culminating in its intratubular precipitation; (2) ischaemic renal hypoperfusion secondary to vasoconstriction of the renal vessels, mediated by the production of oxygen free radicals during exercise or; (3) the accumulation of anions not eliminated in patients with URAT1 or GLUT9 gene mutations exerts a toxic tubular effect leading to acute tubular necrosis.
Hyperuricemia and Gout
Hyperuricemia is commonly defined as a serum urate concentration >6.8 mg/dL, based on the in vivo solubility of urate above which crystal deposition may occur leading to gout. However, it should be noted that alternative definitions of hyperuricemia are sometimes applied, a factor which needs to be considered when attempting comparative analysis of published data ( 17 ). The aqueous solubility of uric acid (6.8 mg/dL) is relatively low when compared to normal range of serum concentrations, and therefore a modest increase can elevate the risk of monosodium urate (MSU) crystal formation and precipitation, notably in the joints and urine reviewed in Chhana et al. ( 130 ).
Not all individuals with hyperuricemia go on to develop gout and therefore gout represents a subset of individuals with symptomatic hyperuricemia ( 131 ). Although sustained hyperuricemia is a prerequisite of crystal formation, it is not possible to accurately predict which individuals will go on to develop gout, even for those with very high sUA (reviewed in ( 132 )). There is no global consensus on approaches for asymptomatic hyperuricemia nor in response to acute gout attacks, with different strategies advocated by national or international guidelines ( 133 ), which range from reactive approaches to active sUA management such as that employed by the Japanese ( 18 , 134 – 136 ).
The process of crystal deposition leading to gout is reversible by means of reducing sUA levels below its saturation point and it can be surmised that the rate of crystal reduction will be modulated by both the total crystal load and reduction in sUA (reviewed in ( 137 )). While rapid dissolution of crystal deposits may be desirable in therapeutic sense, urate lowering therapy (ULT) initiation generally leads to increased flare rate and associated pain, potentially as a direct consequence of urate crystal dissolution leading to the removal of a protein deposit protecting the underlying surface from attack by inflammatory cells (reviewed in ( 138 )). Indeed, the increased flare rate when initiating ULT has been hypothesized to underpin the reported low levels of patient compliance ( 139 , 140 ) highlighting the need for appropriate prophylaxis in the first few months of the initiation phase ( 141 , 142 ); a consideration reflected in EULAR guidelines which recommend prophylaxis for the first 6 months of therapy ( 18 ). It is worth noting that treatment with anti-inflammatories alone may modulate the acute inflammatory response to crystals but it is unlikely to alter crystal deposition and ongoing joint damage. As a result, the patient may be unaware of progressive tophi formation and destruction of cartilage and bone. Better understanding of the extent of crystal deposition is being obtained with the advent of advanced imaging modalities including MRI, ultrasound and CT ( 143 ). MSU crystals are found in the synovial fluid in 12.5–90% of gout patients during otherwise asymptomatic phases, suggestive of ongoing inflammation and damage ( 144 ). Clinical observations confirm that dissolution of crystals by appropriate sUA lowering treatment results in reduction and ultimately elimination of chronic inflammation ( 145 , 146 ). Indeed, recently updated EULAR recommendations ( 18 ) now advocate active management of urate levels from the first presentation of acute gouty flares at <6 mg/dL (or <5 mg/dL in those with chronic, tophaceous gout) together with improved patient education, including the importance of compliance with long-term treatment, and appropriate pain prophylaxis.
Uric acid urolithiasis refers to the development of a stone or calculus composed of significant amounts of urate in the renal pelvis, ureter, or bladder and are reported to account for 5.0–16.5% of all kidney stones (reviewed in ( 147 – 149 )). Uric acid crystals may initiate calcium oxalate precipitation by the induction of heterogeneous nucleation. The glomerular filtrate of blood is usually acidified by the kidneys from a pH of approximately 7.4 to approximately 6.0 in the urine although this may vary from 4.5 to 8.0 depending on the individual. Accordingly, uric acid solubility is modulated as a function of its weakly acidic pK a and hence, individuals with more acidic urine (pH 5.5) are more likely to have increased uric acid stone formation than individuals with a normal pH range (pH 6.0–6.5) (reviewed in ( 147 )). Urinary alkalinisztion should therefore reduce stone growth/recurrence, and promote stone dissolution (reviewed in ( 2 )). Up to 20% of patients with gout develop kidney stones although stone formation may also occur in patients with normal urinary and serum levels of urate (reviewed in ( 147 )). MSU crystal deposits have been detected in the renal medulla of patients with gout and furthermore renal function has been shown to be improved after successful urate-lowering treatment in gout patients (reviewed in ( 150 )). This implies that the presence of MSU crystals in the kidneys and the associated inflammation may contribute to renal insufficiency. Hence, urate crystal elimination may contribute to improved renal function and reduce kidney injury or disease.
Epidemiology of Hyperuricemia and Associated Comorbidities
In addition to the previously mentioned increase in the prevalence of hyperuricemia and gout, cross-sectional case-control studies have found hyperuricemia to be comorbid with multiple common conditions, including cardiovascular events such as coronary artery disease and hypertension ( 151 – 153 ), chronic kidney disease ( 152 , 154 ) or type 2 diabetes (reviewed in ( 155 , 156 )). However, it is unknown whether hyperuricemia is a causal factor in the development of these conditions, is driven by the same risk factors, or if it is a consequence of the manifestation of these disorders. Unless it is directly causal, uric acid lowering is unlikely to be a successful strategy for the treatment of these co-morbid diseases.
If the genetic determinants of hyperuricemia are also associated with the risk of a disease, this provides evidence for causality for hyperuricemia for that disease. This reasoning underpins Mendelian randomization whereby naturally occurring genetic variants are used as instrumental variables to estimate the causal effect on a trait. As many of the common genetic determinants of uric acid levels have been revealed through GWAS, these instrumental variables have been used in several studies to test for the causality of uric acid in multiple disorders including sudden cardiac death ( 157 ), blood pressure ( 158 ), coronary heart disease ( 159 ), chronic kidney disease progression ( 102 ), type 2 diabetes ( 160 , 161 ), triglyceride levels ( 162 ) or adiposity ( 163 ). However, it remains a challenge to identify the underlying causal mechanisms behind any such associations given the large number of these instruments, with each contributing just a small effect on uric acid levels, across multiple genes. An additional consideration is that many patients with hyperuricemia and gout who develop major cardiovascular and renal events also possess several other known traditional risk factors which may be potentially confounding.
A recent review of the evidence for causality of hyperuricemia in disease concluded that the only robust evidence to date, based on both randomized clinical trials and/or Mendelian randomization, is that of a causal role for hyperuricemia in gout and nephrolithiasis ( 164 ). A limitation of the Mendelian randomization approach however is that the genetic instruments for serum urate levels may not adequately capture any effect of intracellular urate levels on disease so this should be considered when interpreting any negative findings ( 165 ). Trials seeking to determine whether urate-lowering therapy may influence outcomes in other diseases have been performed in small cohorts for short durations ( 166 , 167 ); we would like to see replication in larger cohorts with longitudinal assessment.
Cardiovascular Disease
Over the last decade, we have seen an accumulating body of evidence which implicates gout and/or uric acid elevation as an independent predictor for hypertension, atrial fibrillation and cardiovascular disease ( 150 , 155 , 168 – 171 ). However, clearly not all patients with hyperuricemia go on to develop cardiovascular disease and many of those who do also exhibit one or more other established risk factors.
Clinical observations showing an association between high uric acid levels and hypertension are further corroborated in the pediatric and adolescent populations where blood pressure values are significantly elevated (>95th percentile) in the presence of sUA levels of >5.5 mg/dL ( 172 – 174 ). Furthermore, independently of the pharmacological mechanism (both XO inhibitor and uricosuric), reduction of sUA has been shown to lower blood pressure in early primary hypertension in adolescents ( 173 , 175 ). This finding is supported by the observation that the nonsynonymous variant in SLC2A9 , rs16890979 (Val253Ile) is significantly associated both with a reduction in both uric acid and in blood pressure in an Amish cohort exposed to sodium-controlled diets ( 158 ). Finally, a number of studies have confirmed that people with elevated sUA are at risk of having high blood pressure, even if they otherwise appear to be perfectly healthy ( 152 , 176 ) and reviewed in Richette et al. ( 150 ).
Both experimental and clinical evidence suggests that deleterious effects of high uric acid levels on cardiovascular disease may occur at the vascular level. For example, it has been shown that high levels of uric acid are associated with low-grade inflammatory state and vascular activation of the renin-angiotensin system (reviewed in ( 155 )). Clinical data further supports the notion of endothelial dysfunction associated with sUA elevation and chronic inflammation ( 177 ) and reviewed in Richette et al. ( 150 ). An increasing number of researchers have suggested that XO plays an important role in various forms of ischemic and other types of tissue and vascular injuries, inflammatory diseases, and chronic heart failure (reviewed in ( 178 )). Interestingly, common variants in the XDH gene are associated with blood pressure and hypertension although these findings need to be further substantiated ( 179 ). Accordingly, XO inhibitors may have more profound effects through restoration of endothelial function as opposed to a lowering of sUA levels per se . However the studies were limited in size and careful meta-analyses may be warranted (reviewed in ( 170 )). This question of whether XO inhibitors have potential therapeutic benefit in cardiovascular disease, has been the subject of a number of clinical investigations ( 175 , 180 – 183 ). However, the impact of early intervention in patients with asymptomatic hyperuricemia (with or without crystal deposition) on vascular outcomes has not been definitively demonstrated ( 184 ). Given the long history of this class of drugs, in particular allopurinol, it is interesting to speculate on the potential value of mining medical record databases to understand the implications of long term urate lowering, although interpretation of such data may be undermined by lack of insight into patient adherence to therapy and by suboptimal dose management ( 185 – 187 ). Mendelian randomization studies have produced mixed results—in subjects from the Ludwigshafen Risk and Cardiovascular Health Study, a higher burden of variants associated with uric acid levels was associated both with an increased risk of cardiovascular death and sudden cardiac death ( 157 ). Meanwhile, White et al demonstrated that the evidence for causality of urate levels in coronary artery disease is dependent on the model used. Using the Egger method for Mendelian randomization, which accounts for unmeasured pleiotropy of the instrumental variables, they find that the evidence for causality using traditional Mendelian randomization approaches may be inflated ( 159 ). Finally, by excluding instrumental variants shown to be pleiotropic, Keenan et al found a lack of significant association between 14 urate-specific variants and risk of coronary heart disease, ischemic stroke and heart failure ( 188 ).
Kidney Disease
It is well accepted that hyperuricemia is associated with crystal-related pathologies such as nephrolithiasis. High plasma urate levels are associated with an increased risk of acute kidney injury (AKI) ( 154 , 189 , 190 ). Studies further suggest potential for renal injury in a manner analogous to cardiovascular outcomes due to renal vasoconstriction via inflammation, endothelial dysfunction and renin-antiotensin system activation (reviewed in ( 191 , 192 )). Interestingly, sUA reduction has been found to improve renal function in patients irrespective of gout presentation and a more comprehensive investigation of reno-protective potential for urate lowering therapies may be warranted ( 193 – 199 ). A small Mendelian randomization study supports the hypothesis that hyperuricemia, driven by an instrumental variant in SLC2A9 , is causal in chronic kidney disease progression ( 102 ). Contrary to expectations, a study using a genetic risk score composed of five instrumental variants in uric acid transporters was significantly associated with better (rather than worse) renal function ( 200 ). The authors speculate however that it is the activity of these uric acid transporters, rather than the serum urate levels, that are having the protective effect on renal function and that using fractional excretion of uric acid as the exposure in a Mendelian randomization study, instead of serum urate, would help to determine if this is the case.
Clinical data suggests that most gout sufferers under-excrete uric acid leading to a corresponding increase in sUA levels ( 78 , 110 , 201 ). The kidneys play a major role in the regulation of serum uric acid levels given the extensive handling of urate by the renal proximal tubules (as discussed in the physiology section); which adds to the debate whether hyperuricemia merely acts as an indicator of renal dysfunction or has a causative role. Accordingly, there is a wealth of clinical data which supports the hypothesis that hyperuricemia is both a predictor of onset and a modulator of progression for both acute kidney injury and for chronic kidney disease (CKD), the latter of which in particular is increasingly recognized as a global health problem ( 13 , 16 , 202 – 207 ). In contrast, other epidemiological studies have reported no significant relationship between hyperuricemia and CKD progression ( 208 ) and therefore the debate continues.
Type 2 Diabetes
In population-based studies, hyperuricemia was shown to be an independent risk factor for developing Type 2 diabetes (T2D) ( 60 , 209 ). In cardiovascular disease, there is a complex interplay of factors with obesity, insulin resistance and diuretic use all being associated with increased urate reabsorption confounding efforts to determine the relationship between sUA levels, kidney function and diabetes, in addition to other common co-morbidities or complications such as peripheral neuropathy ( 210 – 212 ). Consistent with the notion of addressing hyperuricemia to impact on symptoms, there are studies suggesting ULT beneficially modulates diabetic-associated phenotypes ( 194 , 213 – 216 ). Despite this, Mendelian randomization studies conclude that uric acid is not causal in type 2 diabetes ( 161 , 188 ), though the accompanying commentary for the former again highlights some of the limitations of Mendelian randomization using instruments that are context specific which may not represent what is happening at the physiological level ( 109 ).
The development of sodium glucose co-transporter 2 (SGLT2) inhibitors for the treatment of diabetes has rekindled interest and debate into the role of sUA in diabetes and CKD. SGLT2 inhibitors act via the kidneys in an insulin-independent manner to improve glycemic control but also reduce sUA levels without obvious uric acid nephrolithiasis ( 106 , 216 , 217 ). In one small study, it was shown that SGLT2 inhibitor treatment contributed to decreased plasma UA levels together with increased FEUA levels ( 109 ). It is not clear how SGLT2 inhibition impacts on uricosuria although one possibility could be through effects on GLUT9 via induction of glycosuria which in turn would contribute to higher levels of urate exchange across the apical membrane of tubular cells into the urine ( 105 , 106 , 218 ).
Taken together, these studies indicate that hyperuricemia is commonly observed in patients together with co-morbidities such as hypertension, cardiovascular disease, CKD and T2D. To date there are no conclusive data that modulation of uric acid levels reduces that risk ( 17 ). Therefore, there is no consensus on therapeutic approaches for these conditions in the context of hyperuricemia and indeed ULT are not indicated for these conditions. Should further studies support the notion that chronic hyperuricemia is associated with increased risk of these debilitating co-morbidities, and furthermore if active reduction of sUA levels proves to be protective, we wonder if this will enable a shift in clinical practice toward proactively monitoring of uric acid levels at least in the first instance.
Launched (Marketed and Withdrawn) Drugs for Hyperuricemia
There are several approved ULT drugs which fall into three main classes: reduction of uric acid synthesis (xanthine oxidase inhibitors); increasing uric acid excretion (uricosurics, e.g., URAT1 inhibitors); and enabling systemic metabolic hydrolysis of uric acid (urolytics, e.g., recombinant uricases) (Figure 5 , Table 2 ).
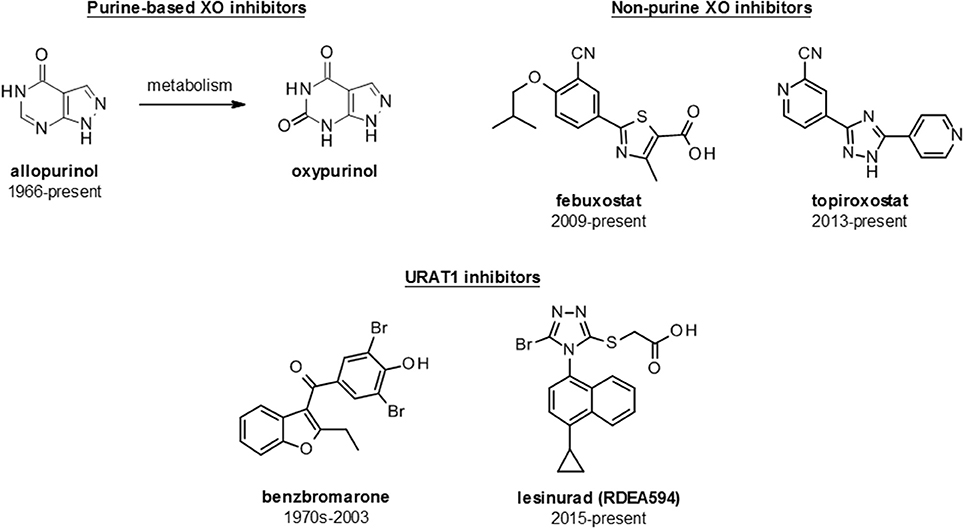
Figure 5 . Structures of launched (marketed and withdrawn) drugs for the management of hyperuricemia. Purine-based (allopurinol and oxypurinol) and non-purine based (febuxostat and topiroxostat) XO inhibitors are shown with dates of approval for clinical use. URAT1 inhibitors are represented by benzbromarone (withdrawn in 2003) and lesinurad (RDEA594).
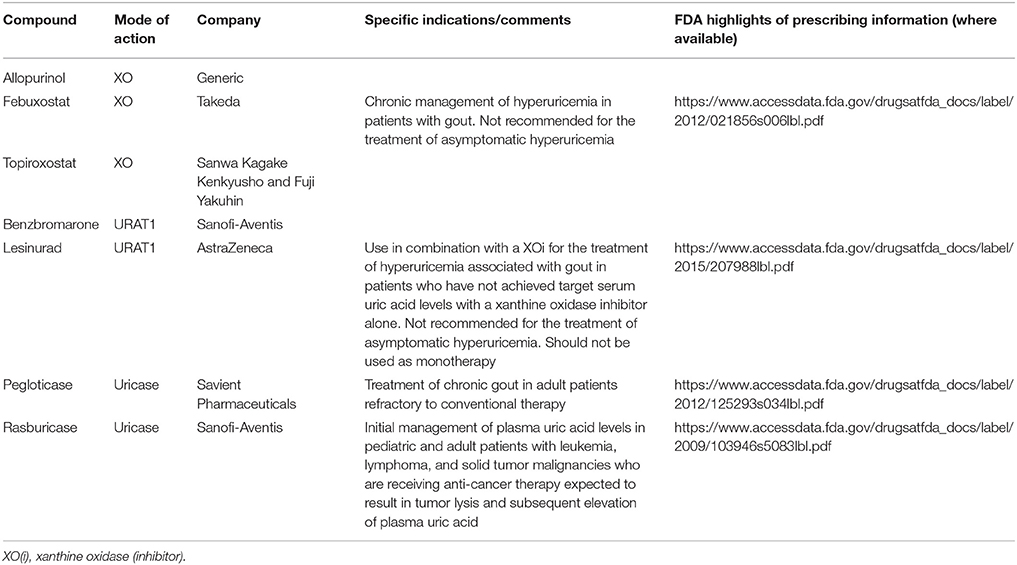
Table 2 . Launched (marketed and withdrawn) therapies for hyperuricemia/gout.
Xanthine Oxidase Inhibitors (XOi)
The inhibition of xanthine oxidase reduces endogenous production of uric acid and thus lowers sUA levels. Xanthine oxidase inhibitors, in the form of allopurinol, were the first class of urate lowering therapy to reach the market and remain the first line therapy for hyperuricemia and gout. Inhibitors fall into two main classes: the classical purine analogs (including allopurinol) and more recently developed non-purine analog compounds such as febuxostat and topiroxostat.
Allopurinol
Allopurinol, a structural isomer of hypoxanthine, has been the cornerstone of hyperuricemia and gout clinical management since its introduction in 1966; and indeed remains the current standard of care despite some tolerability issues and reportedly low patient compliance (reviewed in ( 219 )). It should be noted that patient adherence issues may simply reflect the high prevalence of allopurinol use over other urate lowering therapies, rather than reflecting any specific property of allopurinol.
Allopurinol itself is a relatively weak competitive XO inhibitor and is rapidly metabolized to the more potent oxypurinol, an isostere of xanthine, which is then renally cleared. Clinically, allopurinol is used for reducing uric acid levels particularly in the context of gouty arthritis and kidney stones/lithiasis. Additional indications include genetically linked enzyme disorders associated with uric acid overproduction, such as Lesch-Nyhan syndrome (HGPT deficiency), and myeloproliferative disease (tumor lysis syndrome). Allopurinol has been proposed to possess additional pharmacology, such as decreasing blood pressure and creatinine levels, which supports the notion that XO inhibition may have effects independent of urate lowering ( 220 ) and reviewed further in Richette et al. ( 150 ).
Although allopurinol remains the most frequently prescribed ULT, studies have suggested that less than 50% of patients taking the drug achieve a sUA level <6 mg/dL at an allopurinol dose of 300 mg/day ( 221 , 222 ) and reviewed further in ( 138 ). A more recent study by Jennings suggests that of 400 patients, 36% required allopurinol up-titration to achieve sUA levels of <6 mg/dL ( 223 ). The recommended starting dose of allopurinol in the USA is 100 mg/day with incremental dose increases each 2–4 weeks, up to 800 mg/day 1 (900 mg/day in Europe 224 )), until the target of sUA <6 mg/dL is achieved ( 134 , 135 ). However, in routine clinical practice, patients are often started at 100 mg daily and titrated up to 300 mg daily only, with the result that studies report between 36 and 50% of patients fail to achieve target sUA levels of less than 6 mg/dL ( 223 , 225 ) and discussed further in Shahid and Singh ( 226 ).
Allopurinol has been associated with several adverse effects including gastrointestinal effects, rash and Stevens-Johnson's syndrome ( 227 ). In addition, allopurinol hypersensitivity syndrome (AHS) is a rare but potentially lethal risk for 2–8% of patients ( 228 ). Complications can also arise when patients have renal impairments which may require dose reductions ( 229 ). Some drug interactions with ampicillin or amoxicillin are known to increase the incidence of skin rash when used in combination ( 230 ). More recently, structural understanding of XO together with rational drug development has enabled the discovery of novel, chemically diverse and more potent XO inhibitors such as febuxostat.
Febuxostat is a selective, non-purine, inhibitor of XO approved by the FDA in 2009, for management of patients with hyperuricemia in patients with gout but not for asymptomatic hyperuricemia 2 Febuxostat is approved for use at doses of 40 and 80 mg/day in the USA; and up to 120 mg/day in Europe and 10–60 mg/day in Japan ( 18 ). Febuxostat has a reported IC 50 of 1.8 nM, which is significantly more potent than allopurinol (IC 50 7.8 μM), and consequently has been shown to be more efficacious at doses of 80 or 120 mg for achieving target urate levels of <6 mg/dL vs. allopurinol at 100–300 mg daily ( 231 ). However, at the doses licensed for use in the USA, only 48–67% of those receiving febuxostat reach sUA levels <6 mg/dL ( 232 ).
Febuxostat clearance is predominantly via hepatic metabolism which suggests the potential at least for broader prescribing with respect to impaired renal function though this has not been fully assessed in the clinic. In addition, febuxostat has been reported to have fewer drug-drug interactions than allopurinol and is better tolerated in patients with AHS ( 233 ). It also has been reported to be a strong ABCG2 inhibitor, though the potential impact of this is not yet clear ( 234 ).
Interestingly, gout flares on commencement of treatment have been reported to be more frequent with febuxostat than with allopurinol, likely due to the more rapid and pronounced sUA reduction, an observation which has interesting implications with respect to considering the rate of sUA decrease for ULT strategies ( 235 ). While febuxostat is an option for patients with prior rash or hypersensitivity reaction to allopurinol, it is worth noting that a hypersensitivity reaction has also been reported with febuxostat ( 236 ). More recently, febuxostat was found to be noninferior to allopurinol with respect to rates of adverse cardiovascular events while all-cause mortality and cardiovascular mortality were reported to be higher with febuxostat than with allopurinol ( 237 ).
Clinical uptake of febuxostat has been relatively poor, likely in most part due to the cost compared to the generic allopurinol, with cost per tablet of $7 for febuxostat vs. $0.20–0.60 for allopurinol in the USA ( 226 , 238 , 239 ). In line with this, febuxostat has been suggested as second line to allopurinol in updated care guidelines ( 18 ).
Topiroxostat
Topiroxostat (FYX-051) is a structurally distinct, non-purine, selective XO inhibitor which was approved for use only in Japan in 2013 and was co-developed and marketed as Uriadec/Topiloric by Sanwa Kagake Kenkyusho and Fuji Yakuhin. It is available in oral tablets of 20, 40, and 60 mg doses and the general recommendation is to start with an initial 20 mg dose twice daily with the maximum approved dose being 80 mg twice daily with clinical efficacy reported at 120 mg/day ( 240 , 241 ). Topiroxostat has been shown to inhibit xanthine oxidase via formation of a hydroxylated 2-pyridine metabolite that forms a covalent linkage to the molybdenum via oxygen and also interacts with amino acid residues in the solvent channel ( 242 , 243 ).
Uricosurics
Studies have suggested that, despite the standard of care for hyperuricemia and gout being focussed on reduction of synthesis by XO inhibitors, the underlying cause of hyperuricemia in a notable subset of patients is due to renal underexcretion of uric acid ( 110 , 244 ). This suggests the application of uricosuric agents to increase renal urate excretion to be a rational approach for the treatment of hyperuricemia. However, by enhancing the renal clearance of uric acid, uricosurics may increase the risk of renal adverse events (e.g., nephrolithiasis) (reviewed in ( 245 )).
Historically, several drugs, including probenecid, sulfinpyrazone, fenofibrate and losartan, were serendipitously discovered to have uricosuric properties although the underlying pharmacology was not initially understood ( 35 , 139 , 246 ). Likewise, benzbromarone, the first drug specifically developed for its uricosuric properties, was discovered and developed without any understanding of its pharmacology at a molecular level (reviewed in ( 247 )). More recently, the identification of the URAT1 transporter and its role in urate re-uptake from the proximal tubule has shed light on the pharmacology of these compounds and enabled the development of a new generation of specifically targeted uricosuric compounds ( 39 ). Interestingly other transporters (e.g., GLUT9/ SLC2A9 ) have also been genetically linked with urate homeostasis and may provide further targets for novel uricosuric drug development in the future ( 39 , 59 , 218 ).
Currently uricosurics are recommended as a second line therapy when target sUA levels are not reached, particularly as add-on therapies in combination with XO inhibitors ( 134 , 135 ). Combination therapy with allopurinol and benzbromarone has been suggested to provide enhanced urate lowering and potentially more rapid resolution of tophi ( 145 ); a concept which has been applied to other XOi and uricosuric combinations.
Probenencid
Probenecid was originally introduced to prolong the action of antibiotics by reducing their renal clearance and functions as a non-selective inhibitor of organic anion transporters (reviewed in ( 248 )). URAT1 has now been demonstrated to be one of the molecular targets of probenecid and, while its lack of selectivity and subsequent potential for drug-drug interactions limit its clinical use as a uricosuric, it perhaps represents the prototypical URAT1 inhibitor and uricosuric mechanism for ULT ( 92 , 249 ).
Benzbromarone
Benzbromarone, first marketed in the 1970s, was the first compound specifically introduced as a uricosuric agent. The molecular basis of its pharmacology was not understood at the time of its introduction but it has subsequently been shown to be a potent URAT1 inhibitor (IC 50 22 nM) ( 96 ). Benzbromarone is effective as a single agent ULT with studies showing that 92% (22/24 patients) of gout patients reached target levels of serum urate from a 200 mg/day dose ( 250 ). However, it should be noted that in a subsequent study, where dose was escalated for both benzbromarone and allopurinol in patients failing to achieve adequate control on the starting dose, both compounds performed equally ( 251 ).
Like allopurinol, benzbromarone required dose titration from 50 to 200 mg once daily to achieve maximum efficacy but unlike allopurinol, benzbromarone could be used in patients with renal impairment. Given that uricosuric drug usage increases urate renal excretion, caution was advocated by clinicians where there was history of renal calculi so strategies such as urinary alkalinization to solubilize the uric acid were employed ( 245 , 252 ).
Benzbromarone was not approved in the USA and was withdrawn from the market in many other countries in 2003 following reported incidents of idiosyncratic hepatotoxicity (reviewed in ( 247 )).
Lesinurad (RDEA594)
Lesinurad (Zurampic ® ) is the first novel uricosuric to reach the market since benzbromarone ( 94 ). The primary mechanism of action of lesinurad derives from inhibition of URAT1, although it is also reported to be an inhibitor of OAT4 which could also potentially contribute to its efficacy; however, it is reported to be selective over related transporters such as OAT1 and OAT3 which may avoid some of the drug-drug interaction liabilities of non-selective compounds such as probenecid ( 94 , 253 – 255 , 273 ).
Following its acquisition by AstraZeneca in 2012, Ardea continued to progress the clinical programme. Phase 2 clinical studies have shown lesinurad to provide additional efficacy as an add-on therapy in individuals with inadequate urate reduction based on XOi's alone ( 256 – 258 ). Therefore, lesinurad was subsequently evaluated in three pivotal phase 3 trials in combination with XOi's; in combination with allopurinol in adults with gout showing inadequate response to allopurinol alone (CLEAR 1 and 2) and in combination with febuxostat in adults with tophaceous gout (CRYSTAL) ( 258 – 260 ).
The CLEAR 1 study demonstrated an increase in the proportion of patients achieving a serum UA level of <6.0 mg/dl after 6 months (the primary end point) with 54.2 and 59.2% in the lesinurad 200 mg plus allopurinol and lesinurad 400 mg plus allopurinol groups respectively, compared to 27.9% in those taking allopurinol alone. This was more broadly reflected across the duration of the trial with serum UA levels being lower at all time points assessed in both groups taking lesinurad plus allopurinol as compared with allopurinol alone. Lesinurad was reported to be generally well tolerated with the AE profile of the 200 mg lesinurad plus allopurinol dose comparable to that of allopurinol alone (73.1 and 68.7% respectively), while that of 400 mg lesinurad plus allopurinol was slightly higher (77.6%). Given the uricosuric mechanism of action, renal safety was highlighted as a potential risk and higher incidence of renal-related treatment-emergent adverse events (TEAEs) were observed with lesinurad 400 mg plus allopurinol (10.0%) compared with the lesinurad 200 mg plus allopurinol and allopurinol-alone groups (3.5 and 4.0%, respectively). In the lesinurad 400 mg plus allopurinol group a serious renal-related TEAE (renal failure) was reported in 1 patient (0.5%). The most common renal-related TEAE was elevations in serum creatinine ≥1.5 × baseline which occurred at higher rates in the lesinurad plus allopurinol groups (6.0 and 15.9% in the 200 mg and 400-mg lesinurad doses respectively) vs. allopurinol alone (1%). The CLEAR 2 trial showed similar findings with 55.4 and 66.5% in the lesinurad 200 mg plus allopurinol and lesinurad 400 mg plus allopurinol groups respectively, compared to 23.3% in those taking allopurinol alone achieving the target serum UA level of <6.0 mg/dl after 6 months. Likewise, the profile of renal-related TEAEs was comparable with lesinurad 400 mg plus allopurinol showing a higher rate (15.0%) compared with the lesinurad 200 mg plus allopurinol and allopurinol-alone groups (4.9 and 5.9%, respectively), again was mainly related to elevations in blood creatinine. Similar renal effects were also noted in a monotherapy trial with a 400 mg dose of lesinurad ( 261 ).
The CRYSTAL study investigated the safety and efficacy of lesinurad, in combination with febuxostat, in a 12-month trial in patients with tophaceous gout. The primary endpoint serum UA target, of <5.0 mg/dl after 6 months, was achieved by significantly more patients with febuxostat plus the addition of lesinurad 400 mg (76.1%; P < 0.0001), compared with febuxostat alone (46.8%). The 200 mg dose of lesinurad did not show a significant effect on the primary endpoint (56.6%; P = 0.13), however, at all other time points assessed, significantly more patients in this group achieved the serum UA target. There was no significant difference in the proportion of patients with complete tophus resolution between groups. However, the lesinurad (200 and 400 mg) plus febuxostat groups showed a greater reduction in the total target tophi area (50.1 and 52.9%, respectively) compared to febuxostat alone (28.3%). Overall rates of TEAEs were comparable across the group, while renal-related TEAEs were slightly increased in the lesinurad 200 mg plus febuxostat group, and in the lesinurad 400 mg plus febuxostat group (8.5 and 10.1%, respectively) compared with febuxostat (5.5%), again largely reflecting an increased incidence of elevated serum creatinine levels with lesinurad. No patients in the lesinurad 200 mg plus febuxostat group had a renal-related serious adverse event, while two patients in the lesinurad 400 mg plus febuxostat group (renal failure acute; renal failure chronic) and one patient in the febuxostat group (renal failure acute) had renal-related serious TEAEs.
Ultimately, the 200 mg dose of lesinurad received FDA approval at the end of 2015 for use in combination with a xanthine oxidase inhibitor for the treatment of hyperuricemia associated with gout in patients who have not achieved target serum uric acid levels with a xanthine oxidase inhibitor alone. Its use is contraindicated in patients with severe renal impairment, kidney transplant recipients, patients on dialysis, in tumor lysis syndrome (TLS) or Lesch-Nyhan syndrome) 3 For more information a number of focused reviews of this compound have recently been published providing a more in-depth description of its profile ( 262 – 264 ).
Urate Hydrolysis by Uricases (Pegloticase and Rasburicase)
Humans, and some primate species, differ from other mammals by the lack of a functional uricase enzyme. Uricase degrades uric acid into the more water soluble allantoin which is then eliminated readily through the kidney. Therefore, therapies based on the intravenous administration of a functional recombinant uricase enzyme have been developed which enable an immediate and significant reduction of sUA levels. Pegloticase, a PEGylated recombinant uricase was approved in 2010 for limited-patient use in the treatment of chronic refractory gout in patients with tophaceous deformities and complications ( 265 , 266 ). The National Institute of Health and Clinical Excellence supports the use of pegloticase as a third line treatment for tophaceous gout in patients where XO inhibitors and uricosurics are contraindicated 4 ; echoed by EULAR and ACR (American College of Rheumatology) guidelines ( 18 , 134 , 135 ). It is administered in 8 mg biweekly intravenous doses for at least 6 months. Clinical trials demonstrated a 47% response rate over placebo ( 266 ). However, likely due to the very rapid urate reduction caused by administration of pegloticase, its application has been associated with significant prevalence of acute flares. In addition, although pegloticase has been used effectively in the clinic it is also prone to immunogenic infusion reactions with a significant portion of the patients receiving pegloticase developing antibodies within the first few months of treatment ( 266 ). In clinical studies, infusion reaction adverse events occurred in 26–41% of patients including dyspnea, chest discomfort and flushing. These reactions were more common in patients in which significant levels of antibodies to pegloticase had developed. In order to reduce these infusion reactions, pre-treatment of patients with antihistamines or corticosteroids is carried out with the risk of complications that can lead to issues in patients with congestive heart failure ( 266 ).
A non-PEGylated uricase, rasburicase has also been approved for specifically treating hyperuricemia associated with tumor lysis syndrome as its quick mode of action and short half-life relative to pegloticase (8 h vs. 12 days) aligns well with this particular application ( 267 ). As a result, it was approved for the initial management of plasma uric acid levels in pediatric and adult patients with leukemia, lymphoma, and solid tumor malignancies who are receiving anti-cancer therapy expected to result in tumor lysis and subsequent elevation of plasma uric acid. Rasburicase also causes high rates of immunogenic infusion reactions, methemoglobinemia, hemolysis and anaphylaxis in patients which limit its more general use.
Emerging Clinical Treatment Approaches
Several factors may have influenced what appears to be a renewed interest in the development of treatments for hyperuricemia and gout in recent years, including: (1) emerging clinical perspective that gout and hyperuricemia are eminently treatable but under-treated conditions; (2) a growing understanding of relevant urate transporters in the kidney as potential drug targets enabling drug design; (3) observations that support the notion that hyperuricemia might contribute to a range of co-morbidities including hypertension, cardiovascular disease, stroke, type 2 diabetes, obesity, metabolic syndrome hyperlipidemia and chronic kidney disease; (4) adoption in Japan of an aggressive approach to the management of asymptomatic hyperuricemia creating a specific market opportunity.
In this section, we have endeaveoured to identify and discuss candidates which are actively undergoing clinical assessment as either urate lowering therapies or for the control of gout flares (Figure 6 , Table 3 ). Compounds which have not appeared to have advanced in clinical studies over the past 2 years, based on reported activity on Clintrials.gov, have been excluded as these are presumed to have been paused or stopped in clinical development although the rationale for this may not have been disclosed. A few notable examples of such compounds are briefly discussed at the end of the section based on scientific interest e.g., novel chemotypes for existing mechanisms, or a novel mechanism of action.
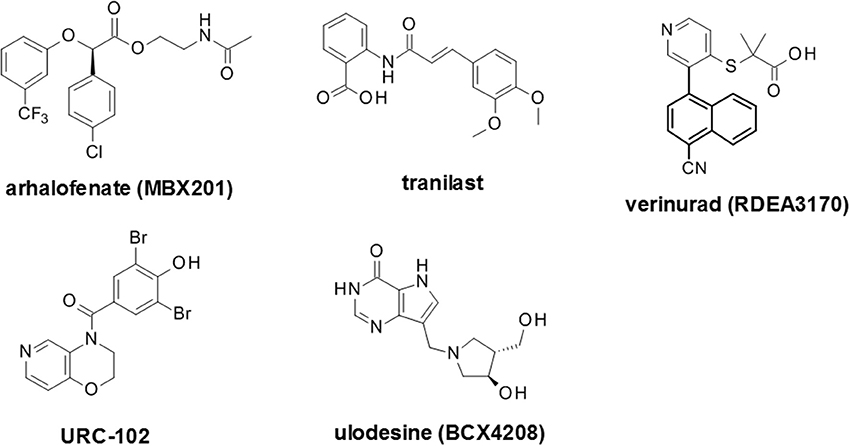
Figure 6 . Selected recent emerging clinical compounds (where structure disclosed). The structures for arhalofenate (MBX201), tranilast, verinurad (RDEA3170), URC-102 and ulodesine (BCX4208) are shown here.
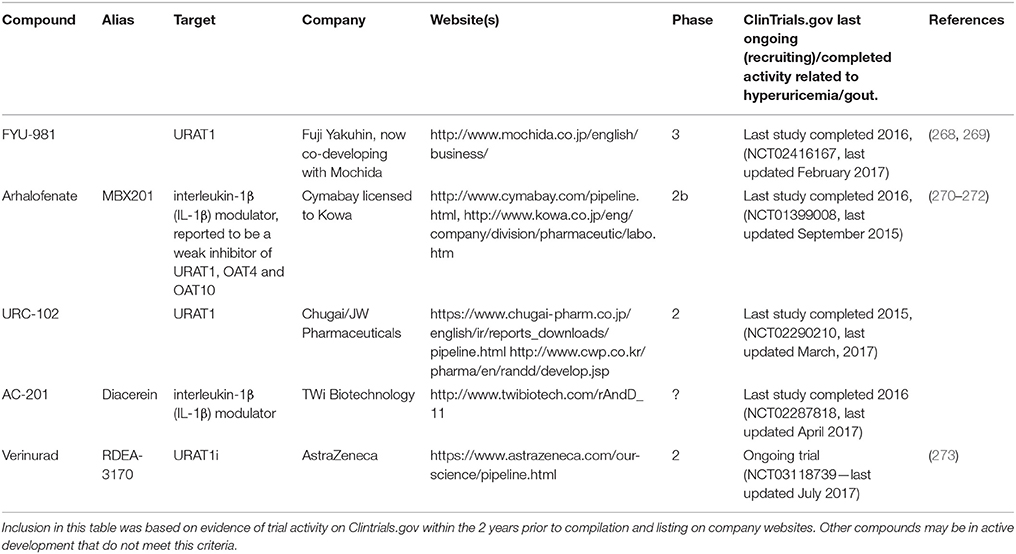
Table 3 . compounds currently in development for hyperuricemia/gout.
Uricosuric Compounds
Arhalofenate (mbx201).
Arhalofenate (Cymabay Therapeutics/Metabolex) is the ( R ) single enantiomer of the racemic drug halofenate that had been originally developed as a PPARγ inhibitor which was assessed in hyperlipidemia and diabetes but has since been repurposed as a gout drug based on urate lowering observations ( 274 ). Arhalofenate has been reported to act as a pro-drug which is cleaved in vivo to the active metabolite arhalofenic acid which has uricosuric activity attributable to the weak inhibition of a panel of renal uric acid transporters including URAT1, OAT4 and OAT10 5 Interestingly, arhalofenate appears to have additional effects with respect to reducing the IL-1β signaling cascade in response to monosodium urate crystals, in addition to decreasing triglycerides and enabling insulin sensitization. This has been reported to lead to reduced incidence of flares in patients with gout in a similar manner to colchicine, which also inhibits the release of IL-1β ( 272 , 275 , 276 ).
The potential for arhalofenate (600 and 800 mg) to reduce flare rate was tested against allopurinol (300 mg) and allopurinol (300 mg) + colchicine (0.6 mg); the efficacy of the compound, as measured by percentage reduction in serum urate and the proportion of patients with a serum UA level of <6 mg/dL was included as a secondary outcome ( 277 ). Treatment with 800 mg arhalofenate led to a decreased number of gout flares with a 46 and 41% vs. allopurinol and placebo groups respectively. While allopurinol (300 mg) + colchicine (0.6 mg) showed a numerically greater reduction to arhalofenate 800 mg it was not statistically different. At week 12 the mean reduction in sUA level from baseline was −12.5% in the 600 mg arhalofenate group and −16.5% in the 800 mg arhalofenate group, compared with −0.9% in the placebo group. This compared to −28.8% in the 300 mg allopurinol group and −24.9% in the 300 mg allopurinol + 0.6 mg colchicine group.
The efficacy and tolerability of arhalofenate have been evaluated both as a monotherapy at doses of 600 and 800 mg once daily and in combination with febuxostat 40 and 80 mg ( 276 ). The primary objectives of the study were to assess reduction in sUA with arhalofenate as a monotherapy and when combined with febuxostat as both absolute and percentage reductions from baseline, the percentage of patients achieving the target sUA of <6, <5, and <4 mg/dL, and the potential for interaction between the two compounds. Arhalofenate as a monotherapy showed a modest reduction in sUA of approximately 19–24%. However, in combination with 40 or 80 mg febuxostat all patients were reported to achieve the target sUA of <6 mg/dL. Additionally, more subjects (0 vs. 20% and 29 vs. 79% for 40 and 80 mg of febuxostat vs. combination with 800 mg arhalofenate) achieved the more stringent goal of <4 mg/dL. Combination with febuxostat did not markedly affect the pharmacokinetics of arhalofenate. Overall these results were an improvement over either arhalofenate or febuxostat monotherapies which were monitored as comparators.
Arhalofenate was recently licensed to Kowa Pharmaceuticals 6 suggesting the compound may progress to Phase 3 trials and, if successful, could become the first compound to combine urate-lowering and flare reduction in a single agent.
AC-201 (Diacerein/Diacetylrhein)
AC-201 (TWi biotechnology) is a small molecule IL-1β modulator that was originally developed for use in osteoarthritis. However, it has since been evaluated more broadly for metabolic indications including treatment of type 2 diabetes and gout flare prophylaxis. Indeed, AC-201 has been approved as diacerein for chronic rheumatic disease and is already in clinical use. However, clinical data suggested potential dual effects in reducing sUA levels through inhibition of reabsorption transporters in the kidney. This molecule is currently listed as being actively assessed in Phase 2 clinical development at 50 mg dosage (including a controlled release formulation). A trial was carried out to determine the capability to reduce flares and time of onset to flares for patients who started on urate lowering therapies 7 with the proportion of patients meeting the goal of <6.0 or <5.0 mg/dL assessed over 16 weeks. Overall the possible dual action of reduction in flares combined with increases in urate excretion could prove beneficial relative to other emerging drugs in this area and represents a potential competitor to arhalofenate with a dual anti-inflammatory/sUA lowering profile. However, currently there are only limited data available for this compound in the public domain ( 226 ).
RDEA3170 (verinurad)
Further optimization of the RDEA594 (lesinurad) template culminated in the development of RDEA3170 (verinurad) which reportedly has a URAT1 IC 50 of 25 nM and is currently in Phase 2 clinical trials ( 97 , 255 , 273 ). This is more potent than lesinurad which has been reported to have a URAT1 IC 50 of 3.4 μM. Verinurad completed a Phase I clinical trial, in Japanese patients with gout or with asymptomatic hyperuricemia, which was designed to demonstrate its urate lowering effects ( 94 , 278 ). Doses ranging from 5 to 12.5 mg per day were given and resulted in >60% decrease in the mean sUA levels in patients. Phase 2 trials of verinurad in combination with either febuxostat or allopurinol were then initiated ( 279 , 280 ).
FYU-981 (Fuji Yakuhin Co.) is in Phase 3 clinical trials for patients with hyperuricemia both with and without gout 8 However, despite being in advanced clinical trials, very little appears to have been published about this compound to date.
URC-102 (UR-1102)
URC-102 (JW Pharmaceutical) has recently been through Phase 2 clinical trials to assess efficacy and safety in gout patients at 0.25 mg to 2.0 mg doses 9 Published studies on this compound showed selective inhibition of the URAT1 transporter ( 281 ). However, no clinical data for this compound has been reported.
Selected Compounds Without Recent Clinical Progress
In addition to the aforementioned compounds which appear to be still actively progressing in clinical trials, there are a number of recent compounds for which no recent clinical activity has been reported. These include alternative novel XO inhibitors such as LC350189 by LG Life Sciences for the management of hyperuricemia in gout patients ( 282 ). Also, alternative uricosuric compounds were progressed into clinical trials, such as the proposed re-purposing of tranilast, an anti-inflammatory drug which has been used for many decades in Japan for bronchial asthma that had a secondary uricosuric effect. This uricosuric effect was later attributed to weak inhibition of GLUT9 in addition to URAT1, OAT4 and OAT10 ( 283 ). In addition, tranilast appears to inhibit a number of secretory urate transporters including NPT1, OAT1 and OAT3 which may also contribute to this effect to some extent. A novel dual XO and URAT1 inhibitor, PF-06743649, combined both inhibition of uric acid biosynthesis and increase urate excretion and was evaluated in partnership by Kissei Pharmaceuticals and Pfizer up to Phase 2 clinical trials for hyperuricemia and gout treatment, but was terminated due to emergent renal safety risks ( 284 ). Ulodesine (BCX4208) is also noteworthy as it represented a novel mode of action, outside of XO inhibitors, uricosuric and uricolytic agents. This is a potent purine nucleoside phosphorylase (PNP) inhibitor acting upstream of xanthine oxidase inhibitors ( 285 , 286 ).
Conclusions
Asymptomatic hyperuricemia appears to be increasing in prevalence. High levels of circulating uric acid have been associated with the increased risk for developing gout and kidney stones. Indeed, gout is the most common form of inflammatory arthritis with well-studied epidemiology. The natural history of gout can be summarized as asymptomatic hyperuricemia, acute gouty arthritis, intercritical period and chronic tophaceous gout; with diagnosis based on both laboratory and radiological features. The role of genetic predisposition is becoming more evident and new insights into the pathophysiology of hyperuricemia and gouty arthritis (both acute and chronic) allow for an even better understanding of the disease.
Gout is an eminently curable condition with approaches that include management of the inflammatory pain associated with flares, urate lowering therapies that address the underlying cause, as well as other approaches such as improved patient education. It has been suggested that clear visualization of treatment outcome could, in combination with improved patient education, help address the issue of poor patient compliance ( 287 ). If the treatment paradigm shifts further toward active management of hyperuricemia, we anticipate that patients will benefit from more facile methods for determining uric acid concentrations in biofluids which, together with increased availability of mobile applications (such as smart-device applications), has the potential to allow non-invasive self-assessment and subsequent disease management.
The associations between hyperuricemia and many co-morbidities such as hypertension, cardiovascular disease, diabetes, metabolic syndrome, dyslipidemia, acute and chronic kidney disease are well recognized. However, the causal relationship between hyperuricemia and these co-morbidities is still a subject of debate. Recent years have witnessed many experimental, epidemiological (including Mendelian randomization studies) and clinical therapeutic interventional studies seeking to test the hypothesis that hyperuricemia plays a pathophysiological role in these disease entities, many of which are important public health challenges. These investigations have been limited by the lack of a standardized approach for definition of hyperuricemia, and by duration and/or size. Nonetheless, the results are interesting enough to warrant further investigation with larger randomized controlled trials to establish the role of uric acid as a promising target for novel therapeutic interventions in the management of kidney and cardio-metabolic disease. Indeed, there have been notable efforts to standardize medical approaches regarding outcome measures, staging and management but there are still some notable gaps.
For example, despite ongoing efforts to educate practitioners on the appropriate titration of allopurinol (which is still often used as the therapy of choice), prescribed doses are typically around 300 mg in clinical practice which in turn leads to suboptimal outcomes. However, the increasing number of therapeutic options may help address this issue by removing requirements for dose titration, as is the case with newer XO inhibitors, or using combinations of XO inhibitors and uricosurics in patients who do not achieve sUA goals with XO inhibitors alone. Finally, further agents in development will likely benefit from our better understanding of pathophysiology. Taken together, there is genuine potential for further drug development to lead to better management in patients with hyperuricemia, gout and associated conditions in the coming years.
Author Contributions
CB conceived the manuscript. CB, RS, AP, CV, PL and RG collected and reviewed the literature and wrote the main body of the manuscript. PL and PD critically reviewed the manuscript. All authors approved the final version of the manuscript.
Conflict of Interest Statement
CB is a paid employee of Astex Pharmaceuticals. PD, RG, and PL are paid employees of Pfizer Ltd. RS and AP are paid employees of AstraZeneca and inventors on patents for URAT1 inhibitors (not in active clinical development at the time of submission). CV is a paid employee of Genomics plc.
Acknowledgments
We acknowledge the support of former colleagues who were members of the Pfizer Neusentis URAT1 team for relevant helpful discussions.
1. ^ https://www.accessdata.fda.gov/drugsatfda_docs/label/2017/209203s000lbl.pdf
2. ^ https://www.accessdata.fda.gov/drugsatfda_docs/label/2012/021856s006lbl.pdf
3. ^ https://www.accessdata.fda.gov/drugsatfda_docs/label/2015/207988lbl.pdf
4. ^ https://www.nice.org.uk/guidance/ta291
5. ^ http://ard.bmj.com/content/annrheumdis/71/Suppl_3/450.3.full.pdf
6. ^ http://www.kowapharma.com/newsroom/pr-2017-01-03/
7. ^ https://clinicaltrials.gov/ct2/show/NCT02287818
8. ^ https://clinicaltrials.gov/ct2/show/NCT03006445
9. ^ https://clinicaltrials.gov/ct2/show/NCT02290210
1. Brook RA, Forsythe A, Smeeding JE, Lawrence Edwards N. Chronic gout: epidemiology, disease progression, treatment and disease burden. Curr Med Res Opin . (2010) 26 :2813–21. doi: 10.1185/03007995.2010.533647
PubMed Abstract | CrossRef Full Text | Google Scholar
2. Burns CM, Wortmann RL. Latest evidence on gout management: what the clinician needs to know. Ther Adv Chronic Dis . (2012) 3 :271–286. doi: 10.1177/2040622312462056
3. Kuo CF, Grainge MJ, Zhang W, Doherty M. Global epidemiology of gout: prevalence, incidence and risk factors. Nat Rev Rheumatol . (2015) 11 :649–62. doi: 10.1038/nrrheum.2015.91
4. Zhu Y, Pandya BJ, Choi H.K. Prevalence of gout and hyperuricemia in the US general population: the National Health and Nutrition ExaminationSurvey 2007-2008. Arthritis Rheum . (2011) 63 :3136–41. doi: 10.1002/art.30520
CrossRef Full Text
5. Zhu Y, Pandya BJ, Choi H.K. Comorbidities of gout and hyperuricemia in the US general population:NHANES 2007-2008. Am J Med . (2012) 125 :679–87 e671. doi: 10.1016/j.amjmed.2011.09.033
6. Robinson PC, Taylor WJ, Merriman T.R. Systematic review of the prevalence of gout and hyperuricaemia in Australia. Intern Med J . (2012) 42 :997–1007. doi: 10.1111/j.1445-5994.2012.02794.x
7. Chuang SY, Lee SC, Hsieh YT, Pan W.H. Trends in hyperuricemia and gout prevalence: Nutrition and Health Survey in Taiwanfrom 1993-1996 to 2005-2008. Asia Pac J Clin Nutr . (2011) 20 :301–8.
8. Juraschek SP, Miller ER III, Gelber AC. Body mass index, obesity, and prevalent gout in the United Statesin 1988-1994 and 2007-2010. Arthritis Care Res . (2013) 65 :127–32. doi: 10.1002/acr.21791
9. Merriman TR, Dalbeth N, Johnson R.J. Sugar-sweetened beverages, urate, gout and genetic interaction. Pac Health Dialog . (2014) 20 :31–8.
PubMed Abstract | Google Scholar
10. Holland R, McGill NW. Comprehensive dietary education in treated gout patients does not further improve serum urate. Intern Med J . (2015) 45 :189–94. doi: 10.1111/imj.12661
CrossRef Full Text | Google Scholar
11. Kim SY, Guevara JP, Kim KM, Choi HK, Heitjan DF, Albert DA. Hyperuricemia and risk of stroke: a systematic review and meta-analysis. Arthritis Rheum . (2009) 61 :885–92. doi: 10.1002/art.24612
12. Kim SY, Guevara JP, Kim KM, Choi HK, Heitjan DF, Albert DA. Hyperuricemia and coronary heart disease: a systematic review and meta-analysis. Arthritis Care Res . (2010) 62 :170–80. doi: 10.1002/acr.20065
13. Li L, Yang C, Zhao Y, Zeng X, Liu F, Fu P. Is hyperuricemia an independent risk factor for new-onset chronic kidney disease?: A systematic review and meta-analysis based on observational cohort studies. BMC Nephrol . (2014) 15 :122. doi: 10.1186/1471-2369-15-122
14. Li M, Hou W, Zhang X, Hu L, Tang Z. Hyperuricemia and risk of stroke: a systematic review and meta-analysis of prospective studies. Atherosclerosis (2014) 232 :265–70. doi: 10.1016/j.atherosclerosis.2013.11.051
15. Wang J, Qin T, Chen J, Li Y, Wang L, Huang H, et al. Hyperuricemia and risk of incident hypertension: a systematic review and meta-analysis of observational studies. PLoS ONE (2014) 9 :e114259. doi: 10.1371/journal.pone.0114259
16. Kim S, Chang Y, Yun KE, Jung HS, Lee SJ, Shin H, et al. Development of nephrolithiasis in asymptomatic hyperuricemia: a cohort study. Am J Kidney Dis . (2017) 70 :173–81. doi: 10.1053/j.ajkd.2017.01.053
17. Stamp L, Dalbeth N. Urate-lowering therapy for asymptomatic hyperuricaemia: a need for caution. Semin Arthritis Rheum . (2017) 46 :457–64. doi: 10.1016/j.semarthrit.2016.07.015
PubMed Abstract | CrossRef Full Text
18. Richette P, Doherty M, Pascual E, Barskova V, Becce F, Castaneda-Sanabria J, et al. 2016 updated EULAR evidence-based recommendations for the management of gout. Ann Rheum Dis . (2017) 76 :29–42. doi: 10.1136/annrheumdis-2016-209707
19. Chong DP. Theoretical study of uric acid and its ions in aqueous solution. J Theor Comput Sci . (2013) 1 :104. doi: 10.4172/jtco.1000104
20. Desideri G, Castaldo G, Lombardi A, Mussap M, Testa A, Pontremoli R, et al. Is it time to revise the normal range of serum uric acid levels? Eur Rev Med Pharmacol Sci . (2014) 18 :1295–306.
21. Kratzer JT, Lanaspa MA, Murphy MN, Cicerchi C, Graves CL, Tipton PA, et al. Evolutionary history and metabolic insights of ancient mammalian uricases. Proc Natl Acad Sci USA . (2014) 111 :3763–8. doi: 10.1073/pnas.1320393111
22. Gao X, O'Reilly EJ, Schwarzschild MA, Ascherio A. Prospective study of plasma urate and risk of Parkinson disease in men and women. Neurology (2016) 86 :520–6. doi: 10.1212/WNL.0000000000002351
23. Paganoni S, Schwarzschild M.A. Urate as a Marker of Risk and Progression of Neurodegenerative Disease. Neurotherapeutics (2017) 14 :148–53. doi: 10.1007/s13311-016-0497-4
24. Wen M, Zhou B, Chen YH, Ma ZL, Gou Y, Zhang CL, et al. Serum uric acid levels in patients with Parkinson's disease: a meta-analysis. PLoS ONE (2017) 12 :e0173731. doi: 10.1371/journal.pone.0173731
25. Loffler W, Grobner W, Medina R, Zollner N. Influence of dietary purines on pool size, turnover, and excretion of uric acid during balance conditions. Isotope studiesusing 15N-uric acid. Res Exp Med . (1982) 181 :113–23. doi: 10.1007/BF01852188
26. Maiuolo J, Oppedisano F, Gratteri S, Muscoli C, Mollace V. Regulation of uric acid metabolism and excretion. Int J Cardiol . (2016) 213 :8–14. doi: 10.1016/j.ijcard.2015.08.109
27. Johnson RJ, Nakagawa T, Sanchez-Lozada LG, Shafiu M, Sundaram S, Le M, et al. Sugar, uric acid, and the etiology of diabetes and obesity. Diabetes (2013) 62 :3307–15. doi: 10.2337/db12-1814
28. Thottam GE, Krasnokutsky S, Pillinger M.H. Gout and metabolic syndrome: a tangled web. Curr Rheumatol Rep . (2017) 19 :60. doi: 10.1007/s11926-017-0688-y
29. Lanaspa MA, Sanchez-Lozada LG, Cicerchi C, Li N, Roncal-Jimenez CA, Ishimoto T, et al. Uric acid stimulates fructokinase and accelerates fructose metabolism in the development of fatty liver. PLoS ONE (2012) 7 :e47948. doi: 10.1371/journal.pone.0047948
30. Chaudhary K, Malhotra K, Sowers J, Aroor A. Uric Acid - key ingredient in the recipe for cardiorenal metabolic syndrome. Cardiorenal Med . (2013) 3 :208–20. doi: 10.1159/000355405
31. Pacher P, Nivorozhkin A, Szabo C. Therapeutic effects of xanthine oxidase inhibitors: renaissance half a century after the discovery of allopurinol. Pharmacol Rev . (2006) 58 :87–114. doi: 10.1124/pr.58.1.6
32. Sorensen LB. Role of the intestinal tract in the elimination of uric acid. Arthritis Rheum . (1965) 8 :694–706. doi: 10.1002/art.1780080429
33. Hyndman D, Liu S, Miner J.N. Urate handling in the human body. Curr Rheumatol Rep . (2016) 18 :34. doi: 10.1007/s11926-016-0587-7
34. Rieselbach RE, Sorensen LB, Shelp WD, Steele TH. Diminished renal urate secretion per nephron as a basis for primary gout. Ann Intern Med . (1970) 73 :359–66. doi: 10.7326/0003-4819-73-3-359
35. Garcia Puig J, Mateos Anton F, Lopez Jimenez M, Conthe Gutierrez P. Renal handling of uric acid in gout: impaired tubular transport of urate not dependent on serum urate levels. Metabolism (1986) 35 :1147–53. doi: 10.1016/0026-0495(86)90028-4
36. Mandal AK, Mount DB. The molecular physiology of uric acid homeostasis. Annu Rev Physiol . (2015) 77 :323–45. doi: 10.1146/annurev-physiol-021113-170343
37. Hediger MA, Johnson RJ, Miyazaki H, Endou H. Molecular physiology of urate transport. Physiology (2005) 20 :125–33. doi: 10.1152/physiol.00039.2004
38. Wright AF, Rudan I, Hastie ND, Campbell H. A ‘complexity' of urate transporters. Kidney Int . (2010) 78 :446–52. doi: 10.1038/ki.2010.206
39. Enomoto A, Kimura H, Chairoungdua A, Shigeta Y, Jutabha P, Cha SH, et al. Molecular identification of a renal urate anion exchanger that regulates blood urate levels. Nature (2002) 417 :447–52. doi: 10.1038/nature742
40. Ohtsu N, Anzai N, Fukutomi T, Kimura T, Sakurai H, Endou H. [Human renal urate transpoter URAT1 mediates the transport of salicylate]. Nihon Jinzo Gakkai Shi (2010) 52 :499–504.
41. Miura D, Anzai N, Jutabha P, Chanluang S, He X, Fukutomi T, et al. Human urate transporter 1 (hURAT1) mediates the transport of orotate. J Physiol Sci . (2011) 61 :253–7. doi: 10.1007/s12576-011-0136-0
42. Lu Y, Nakanishi T, Tamai I. Functional cooperation of SMCTs and URAT1 for renal reabsorption transport of urate. Drug Metab Pharmacokinet . (2013) 28 :153–8. doi: 10.2133/dmpk.DMPK-12-RG-070
43. Otani N, Ouchi M, Hayashi K, Jutabha P, Anzai N. Roles of organic anion transporters (OATs) in renal proximal tubules and their localization. Anat Sci Int . (2017) 92 :200–6. doi: 10.1007/s12565-016-0369-3
44. Ichida K, Hosoyamada M, Kamatani N, Kamitsuji S, Hisatome I, Shibasaki T, et al. Age and origin of the G774A mutation in SLC22A12 causing renal hypouricemia in Japanese. Clin Genet . (2008) 74 :243–51. doi: 10.1111/j.1399-0004.2008.01021.x
45. Li C, Li Z, Liu S, Wang C, Han L, Cui L, et al. Genome-wide association analysis identifies three new risk loci for gout arthritis in Han Chinese. Nat Commun . (2015) 6 :7041. doi: 10.1038/ncomms8041
46. Kolz M, Johnson T, Sanna S, Teumer A, Vitart V, Perola M, et al. Meta-analysis of 28,141 individuals identifies common variants within five new loci that influence uric acid concentrations. PLoS Genet . (2009) 5 :e1000504. doi: 10.1371/journal.pgen.1000504
47. Kamatani Y, Matsuda K, Okada Y, Kubo M, Hosono N, Daigo Y, et al. Genome-wide association study of hematological and biochemical traits in a Japanese population. Nat Genet . (2010) 42 :210–5. doi: 10.1038/ng.531
48. Yang Q, Kottgen A, Dehghan A, Smith AV, Glazer NL, Chen MH, et al. Multiple genetic loci influence serum urate levels and their relationship with gout and cardiovascular disease risk factors. Circ Cardiovasc Genet . (2010) 3 :523–30. doi: 10.1161/CIRCGENETICS.109.934455
49. Sulem P, Gudbjartsson DF, Walters GB, Helgadottir HT, Helgason A, Gudjonsson SA, et al. Identification of low-frequency variants associated with gout and serum uric acid levels. Nat Genet . (2011) 43 :1127–30. doi: 10.1038/ng.972
50. Karns R, Zhang G, Sun G, Rao Indugula S, Cheng H, Havas-Augustin D, et al. Genome-wide association of serum uric acid concentration: replication of sequence variants in an island population of the Adriatic coast of Croatia. Ann Hum Genet . (2012) 76 :121–7. doi: 10.1111/j.1469-1809.2011.00698.x
51. Kottgen A, Albrecht E, Teumer A, Vitart V, Krumsiek J, Hundertmark C, et al. Genome-wide association analyses identify 18 new loci associated with serum urate concentrations. Nat Genet . (2013) 45 :145–54. doi: 10.1038/ng.2500
52. Yang B, Mo Z, Wu C, Yang H, Yang X, He Y, et al. A genome-wide association study identifies common variants influencing serum uric acid concentrations in a Chinese population. BMC Med Genomics (2014) 7 :10. doi: 10.1186/1755-8794-7-10
53. Dong Z, Guo S, Yang Y, Wu J, Guan M, Zou H, et al. Association between ABCG2 Q141K polymorphism and gout risk affected by ethnicity and gender: a systematic review and meta-analysis. Int J Rheum Dis . (2015) 18 :382–91. doi: 10.1111/1756-185X.12519
54. Huffman JE, Albrecht E, Teumer A, Mangino M, Kapur K, Johnson T, et al. Modulation of genetic associations with serum urate levels by body-mass-index in humans. PLoS ONE (2015) 10 :e0119752. doi: 10.1371/journal.pone.0119752
55. Giri AK, Banerjee P, Chakraborty S, Kauser Y, Undru A, Roy S, et al. Genome wide association study of uric acid in Indian population and interaction of identified variants with Type 2 diabetes. Sci Rep . (2016) 6 :21440. doi: 10.1038/srep21440
56. Matsuo H, Yamamoto K, Nakaoka H, Nakayama A, Sakiyama M, Chiba T, et al. Genome-wide association study of clinically defined gout identifies multiple risk loci and its association with clinical subtypes. Ann Rheum Dis . (2016) 75 :652–9. doi: 10.1136/annrheumdis-2014-206191
57. Li M, Li Q, Li CG, Guo M, Xu JM, Tang YY, et al. Genetic polymorphisms in the PDZK1 gene and susceptibility to gout in male Han Chinese: a case-control study. Int J Clin Exp Med . (2015) 8 :13911–8.
58. Nakayama A, Nakaoka H, Yamamoto K, Sakiyama M, Shaukat A, Toyoda Y, et al. GWAS of clinically defined gout and subtypes identifies multiple susceptibility loci that include urate transporter genes. Ann Rheum Dis . (2017) 76 :869–77. doi: 10.1136/annrheumdis-2016-209632
59. Li S, Sanna S, Maschio A, Busonero F, Usala G, Mulas A, et al. The GLUT9 gene is associated with serum uric acid levels in Sardinia and Chianti cohorts. PLoS Genet . (2007) 3 :e194. doi: 10.1371/journal.pgen.0030194
60. Anzai N, Ichida K, Jutabha P, Kimura T, Babu E, Jin CJ, et al. Plasma urate level is directly regulated by a voltage-driven urate efflux transporter URATv1 (SLC2A9) in humans. J Biol Chem . (2008) 283 :26834–8. doi: 10.1074/jbc.C800156200
61. Dehghan A, van Hoek M, Sijbrands EJ, Hofman A, Witteman JC. High serum uric acid as a novel risk factor fortype 2 diabetes. Diabetes Care (2008) 31 :361–2. doi: 10.2337/dc07-1276
62. Doring A, Gieger C, Mehta D, Gohlke H, Prokisch H, Coassin S, et al. SLC2A9 influences uric acid concentrations with pronounced sex-specific effects. Nat Genet . (2008) 40 :430–6. doi: 10.1038/ng.107
63. Matsuo H, Chiba T, Nagamori S, Nakayama A, Domoto H, Phetdee K, et al. Mutations in glucose transporter 9 gene SLC2A9 cause renal hypouricemia. Am J Hum Genet . (2008) 83 :744–51. doi: 10.1016/j.ajhg.2008.11.001
64. McArdle PF, Parsa A, Chang YP, Weir MR, O'Connell JR, Mitchell BD, et al. Association of a common nonsynonymous variant in GLUT9 with serum uric acid levels in old order amish. Arthritis Rheum . (2008) 58 :2874–81. doi: 10.1002/art.23752
65. Vitart V, Rudan I, Hayward C, Gray NK, Floyd J, Palmer CN, et al. SLC2A9 is a newly identified urate transporter influencing serum urate concentration, urate excretion and gout. Nat Genet . (2008) 40 :437–42. doi: 10.1038/ng.106
66. Wallace C, Newhouse SJ, Braund P, Zhang F, Tobin M, Falchi M, et al. Genome-wide association study identifies genes for biomarkers of cardiovascular disease: serum urate and dyslipidemia. Am J Hum Genet . (2008) 82 :139–49. doi: 10.1016/j.ajhg.2007.11.001
67. Bahat H, Dinour D, Ganon L, Feldman L, Holtzman EJ, Goldman M. Non-urate transporter 1-related renal hypouricemia and acute renal failure in an Israeli-Arab family. Pediatr Nephrol . (2009) 24 :999–1003. doi: 10.1007/s00467-008-1093-6
68. Dinour D, Gray NK, Campbell S, Shu X, Sawyer L, Richardson W, et al. Homozygous SLC2A9 mutations cause severe renal hypouricemia. J Am Soc Nephrol . (2010) 21 :64–72. doi: 10.1681/ASN.2009040406
69. Charles BA, Shriner D, Doumatey A, Chen G, Zhou J, Huang H, et al. A genome-wide association study of serum uric acid in African Americans. BMC Med Genomics (2011) 4 :17. doi: 10.1186/1755-8794-4-17
70. Hamajima N, Okada R, Kawai S, Hishida A, Morita E, Yin G, et al. Significant association of serum uric acid levels with SLC2A9 rs11722228 among a Japanese population. Mol Genet Metab . (2011) 103 :378–82. doi: 10.1016/j.ymgme.2011.04.001
71. Kawamura Y, Matsuo H, Chiba T, Nagamori S, Nakayama A, Inoue H, et al. Pathogenic GLUT9 mutations causing renal hypouricemia type 2 (RHUC2). Nucleosides Nucleotides Nucleic Acids (2011) 30 :1105–11. doi: 10.1080/15257770.2011.623685
72. Shima Y, Nozu K, Nozu Y, Togawa H, Kaito H, Matsuo M, et al. Recurrent EIARF and PRES with severe renal hypouricemia by compound heterozygous SLC2A9 mutation. Pediatrics (2011) 127 :e1621–5. doi: 10.1542/peds.2010-2592
73. Nakanishi T, Ohya K, Shimada S, Anzai N, Tamai I. Functional cooperation of URAT1 (SLC22A12) and URATv1 (SLC2A9) in renal reabsorption of urate. Nephrol Dial Transplant . (2013) 28 :603–11. doi: 10.1093/ndt/gfs574
74. Voruganti VS, Kent JW Jr, Debnath S, Cole SA, Haack K, Goring HH, et al. Genome-wide association analysis confirms and extends the association of SLC2A9 with serum uric acid levels to Mexican Americans. Front Genet . (2013) 4 :279. doi: 10.3389/fgene.2013.00279
75. Chiba T, Matsuo H, Nagamori S, Nakayama A, Kawamura Y, Shimizu S, et al. Identification of a hypouricemia patient with SLC2A9 R380W, a pathogenic mutation for renal hypouricemia type 2. Nucleosides Nucleotides Nucleic Acids (2014) 33 :261–5. doi: 10.1080/15257770.2013.857781
76. Voruganti VS, Franceschini N, Haack K, Laston S, MacCluer JW, Umans JG, et al. Replication of the effect of SLC2A9 genetic variation on serum uric acid levels in American Indians. Eur J Hum Genet . (2014) 22 :938–43. doi: 10.1038/ejhg.2013.264
77. Androvitsanea A, Stylianou K, Maragkaki E, Tzanakakis M, Stratakis S, Petrakis I, et al. Vanishing urate, acute kidney injury episodes and a homozygous SLC2A9 mutation. Int Urol Nephrol . (2015) 47 :1035–6. doi: 10.1007/s11255-015-1005-1
78. Nagy R, Boutin TS, Marten J, Huffman JE, Kerr SM, Campbell A, et al. Exploration of haplotype research consortium imputation for genome-wide association studies in 20,032 Generation Scotland participants. Genome Med . (2017) 9 :23. doi: 10.1186/s13073-017-0414-4
79. Torres RJ, de Miguel E, Bailen R, Banegas JR, Puig JG. Tubular urate transporter gene polymorphisms differentiate patients with gout who have normal and decreased urinary uric acid excretion. J Rheumatol . (2014) 41 :1863–70. doi: 10.3899/jrheum.140126
80. Lytvyn Y, Skrtic M, Yang GK, Yip PM, Perkins BA, Cherney DZ. Glycosuria-mediated urinary uric acid excretion in patients with uncomplicated type 1 diabetes mellitus. Am J Physiol Renal Physiol . (2015) 308 :F77–83. doi: 10.1152/ajprenal.00555.2014
81. Ma L, Wei L, Chen H, Zhang Z, Yu Q, Ji Z, et al. Influence of urate-lowering therapies on renal handling of uric acid. Clin Rheumatol . (2016) 35 :133–41. doi: 10.1007/s10067-014-2806-9
82. Jutabha P, Anzai N, Kitamura K, Taniguchi A, Kaneko S, Yan K, et al. Human sodium phosphate transporter 4 (hNPT4/SLC17A3) as a common renal secretory pathway for drugs and urate. J Biol Chem . (2010) 285 :35123–32. doi: 10.1074/jbc.M110.121301
83. Tanaka M, Itoh K, Matsushita K, Matsushita K, Wakita N, Adachi M, et al. Two male siblings with hereditary renal hypouricemia and exercise-induced ARF. Am J Kidney Dis . (2003) 42 :1287–92. doi: 10.1053/j.ajkd.2003.08.032
84. Wakida N, Tuyen DG, Adachi M, Miyoshi T, Nonoguchi H, Oka T, et al. Mutations in human urate transporter 1 gene in presecretory reabsorption defect type of familial renal hypouricemia. J Clin Endocrinol Metab . (2005) 90 :2169–74. doi: 10.1210/jc.2004-1111
85. Dinour D, Bahn A, Ganon L, Ron R, Geifman-Holtzman O, Knecht A, et al. URAT1 mutations cause renal hypouricemia type 1 in Iraqi Jews. Nephrol Dial Transplant . (2011) 26 :2175–81. doi: 10.1093/ndt/gfq722
86. Ichida K, Amaya Y, Kamatani N, Nishino T, Hosoya T, Sakai O. Identification of two mutations in human xanthine dehydrogenase gene responsible for classical type I xanthinuria. J Clin Invest . (1997) 99 :2391–7. doi: 10.1172/JCI119421
87. Sakamoto N, Yamamoto T, Moriwaki Y, Teranishi T, Toyoda M, Onishi Y, et al. Identification of a new point mutation in the human xanthine dehydrogenase gene responsible for a case of classical type I xanthinuria. Hum Mol Genet . (2001) 108 :279–83.
88. Tin A, Woodward OM, Kao WH, Liu CT, Lu X, Nalls MA, et al. Genome-wide association study for serum urate concentrations and gout among African Americans identifies genomic risk loci and a novel URAT1 loss-of-function allele. Hum Mol Genet . (2011) 20 :4056–68. doi: 10.1093/hmg/ddr307
89. Hosoyamada M, Takiue Y, Shibasaki T, Saito H. The effect of testosterone upon the urate reabsorptive transport system in mouse kidney. Nucleosides Nucleotides Nucleic Acids (2010) 29 :574–79. doi: 10.1080/15257770.2010.494651
90. Takiue Y, Hosoyamada M, Kimura M, Saito H. The effect of female hormones upon urate transport systems in the mouse kidney. Nucleosides Nucleotides Nucleic Acids (2011) 30 :113–9. doi: 10.1080/15257770.2010.551645
91. Anzai N, Miyazaki H, Noshiro R, Khamdang S, Chairoungdua A, Shin HJ, et al. The multivalent PDZ domain-containing protein PDZK1 regulates transport activity of renal urate-anion exchanger URAT1 via its C terminus. J Biol Chem . (2004) 279 :45942–50. doi: 10.1074/jbc.M406724200
92. Raychaudhuri S, Plenge RM, Rossin EJ, Ng AC, International Schizophrenia Consortium, Purcell SM, et al. Identifying relationships among genomic disease regions: predicting genes at pathogenic SNP associations and rare deletions. PLoS Genet . (2009) 5 :e1000534. doi: 10.1371/journal.pgen.1000534
93. Shin HJ, Takeda M, Enomoto A, Fujimura M, Miyazaki H, Anzai N, et al. Interactions of urate transporter URAT1 in human kidney with uricosuric drugs. Nephrology (2011) 16 :156–62. doi: 10.1111/j.1440-1797.2010.01368.x
94. Wempe MF, Lightner JW, Miller B, Iwen TJ, Rice PJ, Wakui S, et al. Potent human uric acid transporter 1 inhibitors: in vitro and in vivo metabolism and pharmacokinetic studies. Drug Des Devel Ther . (2012) 6 :323–39. doi: 10.2147/DDDT.S35805
95. Miner J, Tan PK, Hyndman D, Liu S, Iverson C, Nanavati P, et al. Lesinurad, a novel, oral compound for gout, acts to decrease serum uric acid through inhibition of urate transporters in the kidney. Arthritis Res Ther . (2016) 18 :214. doi: 10.1186/s13075-016-1107-x
96. Pike A, Storer RI, Owen RM, Armstrong E, Benn CL, Bictash M, et al. The design, synthesis and evaluation of low molecular weight acidic sulfonamides as URAT1 inhibitors for the treatment of gout. MedChemComm (2016) 7 :1572–9. doi: 10.1039/C6MD00191B
97. Storer RI, Owen RM, Pike A, Benn CL, Armstrong E, Blakemore DC, et al. The discovery and evaluation of diaryl ether heterocyclic sulfonamides as URAT1 inhibitors for the treatment of gout. MedChemComm (2016) 7 :1587–95. doi: 10.1039/C6MD00190D
98. Tan PK, Liu S, Gunic E, Miner JN. Discovery and characterization of verinurad, a potent and specific inhibitor of URAT1 for the treatment of hyperuricemia and gout. Sci Rep . (2017) 7 :665. doi: 10.1038/s41598-017-00706-7
99. Ichida K, Hosoyamada M, Hisatome I, Enomoto A, Hikita M, Endou H, et al. Clinical and molecular analysis of patients with renal hypouricemia in Japan-influence of URAT1 gene on urinary urate excretion. J Am Soc Nephrol . (2004) 15 :164–73. doi: 10.1097/01.ASN.0000105320.04395.D0
100. Anzai N, Jutabha P, Amonpatumrat-Takahashi S, Sakurai H. Recent advances in renal urate transport: characterization of candidate transporters indicated by genome-wide association studies. Clin Exp Nephrol . (2012) 16 :89–95. doi: 10.1007/s10157-011-0532-z
101. Preitner F, Bonny O, Laverriere A, Rotman S, Firsov D, Da Costa A, et al. Glut9 is a major regulator of urate homeostasis and its genetic inactivation induces hyperuricosuria and urate nephropathy. Proc Natl Acad Sci USA . (2009) 106 :15501–6. doi: 10.1073/pnas.0904411106
102. Dalbeth N, House ME, Gamble GD, Horne A, Pool B, Purvis L, et al. Population-specific influence of SLC2A9 genotype on the acute hyperuricaemic response to a fructose load. Ann Rheum Dis . (2013) 72 :1868–73. doi: 10.1136/annrheumdis-2012-202732
103. Testa A, Mallamaci F, Spoto B, Pisano A, Sanguedolce MC, Tripepi G, et al. Association of a polymorphism in a gene encoding a urate transporter with CKD progression. Clin J Am Soc Nephrol . (2014) 9 :1059–65. doi: 10.2215/CJN.11041013
104. Doblado M, Moley KH. Facilitative glucose transporter 9, a unique hexose and urate transporter. Am J Physiol Endocrinol Metab . (2009) 297 :E831–5. doi: 10.1152/ajpendo.00296.2009
105. Witkowska K, Smith KM, Yao SY, Ng AM, O'Neill D, Karpinski E, et al. Human SLC2A9a and SLC2A9b isoforms mediate electrogenic transport of urate with different characteristics in the presence of hexoses. Am J Physiol Renal Physiol . (2012) 303 :F527–39. doi: 10.1152/ajprenal.00134.2012
106. Chino Y, Samukawa Y, Sakai S, Nakai Y, Yamaguchi J, Nakanishi T, et al. SGLT2 inhibitor lowers serum uric acid through alteration of uric acid transport activity in renal tubule by increased glycosuria. Biopharm Drug Dispos . (2014) 35 :391–404. doi: 10.1002/bdd.1909
107. Davies MJ, Trujillo A, Vijapurkar U, Damaraju CV, Meininger G. Effect of canagliflozin on serum uric acid in patients with type 2 diabetes mellitus. Diabetes Obes Metab . (2015) 17 :426–9. doi: 10.1111/dom.12439
108. Wang Y, Hu X, Liu X, Wang Z. An overview of the effect of sodium glucose cotransporter 2 inhibitor monotherapy on glycemic and other clinical laboratory parameters in type 2 diabetes patients. Ther Clin Risk Manag . (2016) 12 :1113–31. doi: 10.2147/TCRM.S112236
109. Zhao Y, Xu L, Tian D, Xia P, Zheng H, Wang L, et al. Effects of sodium-glucose co-transporter 2 (SGLT2) inhibitors on serum uric acid level: a meta-analysis of randomized controlled trials. Diabetes Obes Metab . (2018) 20 :458–62. doi: 10.1111/dom.13101
110. Sakiyama M, Matsuo H, Shimizu S, Nakashima H, Nakayama A, Chiba T, et al. A common variant of organic anion transporter 4 (OAT4/SLC22A11) gene is associated with renal underexcretion type gout. Drug Metab Pharmacokinet . (2014) 29 :208–10. doi: 10.2133/dmpk.DMPK-13-NT-070
111. Ichida K, Matsuo H, Takada T, Nakayama A, Murakami K, Shimizu T, et al. Decreased extra-renal urate excretion is a common cause of hyperuricemia. Nat Commun . (2012) 3 :764. doi: 10.1038/ncomms1756
112. Cleophas MC, Joosten LA, Stamp LK, Dalbeth N, Woodward OM, Merriman TR. ABCG2 polymorphisms in gout: insights into disease susceptibility and treatment approaches. Pharmgenomics Pers Med . (2017) 10 :129–42. doi: 10.2147/PGPM.S105854
113. Takada T, Ichida K, Matsuo H, Nakayama A, Murakami K, Yamanashi Y, et al. ABCG2 dysfunction increases serum uric acid by decreased intestinal urate excretion. Nucleosides Nucleotides Nucleic Acids (2014) 33 :275–81. doi: 10.1080/15257770.2013.854902
114. Iharada M, Miyaji T, Fujimoto T, Hiasa M, Anzai N, Omote H, et al. Type 1 sodium-dependent phosphate transporter (SLC17A1 Protein) is a Cl(-)-dependent urate exporter. J Biol Chem . (2010) 285 :26107–13. doi: 10.1074/jbc.M110.122721
115. Jutabha P, Anzai N, Wempe MF, Wakui S, Endou H, Sakurai H. Apical voltage-driven urate efflux transporter NPT4 in renal proximal tubule. Nucleosides Nucleotides Nucleic Acids (2011) 30 :1302–11. doi: 10.1080/15257770.2011.616564
116. Rebane EN, Orlov Yu N, Kazbekov EN, Lyubimov Y, Bulat MN. The mechanism of coupling of the organic anion exchange to Na(+)-dicarboxylate symport in basolateral membrane vesicles. Membr Cell Biol . (1998) 12 :51–6.
117. Bakhiya A, Bahn A, Burckhardt G, Wolff N. Human organic anion transporter 3 (hOAT3) can operate as an exchanger and mediate secretory urate flux. Cell Physiol Biochem . (2003) 13 :249–56. doi: 10.1159/000074539
118. Merriman TR. An update on the genetic architecture of hyperuricemia and gout. Arthritis Res Ther . (2015) 17 :98. doi: 10.1186/s13075-015-0609-2
119. DeBoer MD, Gurka MJ. Low sensitivity for the metabolic syndrome to detect uric acid elevations in females and non-Hispanic-black male adolescents: an analysis of NHANES 1999-2006. Atherosclerosis (2012) 220 :575–80. doi: 10.1016/j.atherosclerosis.2011.11.033
120. McAdams-DeMarco MA, Maynard JW, Baer AN, Kao LW, Kottgen A, Coresh J. A urate gene-by-diuretic interaction and gout risk in participants with hypertension: results from the ARIC study. Ann Rheum Dis . (2013) 72 :701–6. doi: 10.1136/annrheumdis-2011-201186
121. Whitfield JB, Martin NG. Inheritance and alcohol as factors influencing plasma uric acid levels. Acta Genet Med Gemellol . (1983) 32 :117–26. doi: 10.1017/S0001566000006401
122. Nath SD, Voruganti VS, Arar NH, Thameem F, Lopez-Alvarenga JC, Bauer R, et al. Genome scan for determinants of serum uric acid variability. J Am Soc Nephrol . (2007) 18 :3156–63. doi: 10.1681/ASN.2007040426
123. Emmerson BT, Nagel SL, Duffy DL, Martin NG. Genetic control of the renal clearance of urate: a study of twins. Ann Rheum Dis . (1992) 51 :375–7. doi: 10.1136/ard.51.3.375
124. Wilk JB, Djousse L, Borecki I, Atwood LD, Hunt SC, Rich SS, et al. Segregation analysis of serum uric acid in the NHLBI Family Heart Study. Hum Genet . (2000) 106 :355–9. doi: 10.1007/s004390051050
125. Esparza Martin N, Garcia Nieto V. Hypouricemia and tubular transport of uric acid. Nefrologia (2011) 31 :44–50. doi: 10.3265/Nefrologia.pre2010.Oct.10588
126. Kuwabara M, Niwa K, Ohtahara A, Hamada T, Miyazaki S, Mizuta E, et al. Prevalence and complications of hypouricemia in a general population: A large-scale cross-sectional study in Japan. PLoS ONE (2017d) 12 :e0176055. doi: 10.1371/journal.pone.0176055
127. Levartovsky D, Lagziel A, Sperling O, Liberman U, Yaron M, Hosoya T, et al. XDH gene mutation is the underlying cause of classical xanthinuria: a second report. Kidney Int . (2000) 57 :2215–20. doi: 10.1046/j.1523-1755.2000.00082.x
128. Sebesta I. Genetic disorders resulting in hyper- or hypouricemia. Adv Chronic Kidney Dis . (2012) 19 :398–403. doi: 10.1053/j.ackd.2012.06.002
129. Kaito H, Ishimori S, Nozu K, Shima Y, Nakanishi K, Yoshikawa N, et al. Molecular background of urate transporter genes in patients with exercise-induced acute kidney injury. Am J Nephrol . (2013) 38 :316–20. doi: 10.1159/000355430
130. Wakasugi M, Kazama JJ, Narita I, Konta T, Fujimoto S, Iseki K, et al. Association between hypouricemia and reduced kidney function: a cross-sectional population-based study in Japan. Am J Nephrol . (2015) 41 :138–46. doi: 10.1159/000381106
131. Chhana A, Lee G, Dalbeth N. Factors influencing the crystallization of monosodium urate: a systematic literature review. BMC Musculoskelet Disord . (2015) 16 :296. doi: 10.1186/s12891-015-0762-4
132. Campion EW, Glynn RJ, DeLabry LO. Asymptomatic hyperuricemia. Risks and consequences in the Normative Aging Study. Am J Med . (1987) 82 :421–6. doi: 10.1016/0002-9343(87)90441-4
133. Keenan RT. Limitations of the current standards of care for treating gout and crystal deposition in the primary care setting: a review. Clin Ther (2017) 39 :430–41. doi: 10.1016/j.clinthera.2016.12.011
134. Li Q, Li X, Kwong JS, Chen H, Sun X, Tian H, et al. Diagnosis and treatment for hyperuricaemia and gout: a protocol for a systematic review of clinical practice guidelines and consensus statements. BMJ Open (2017) 7 :e014928. doi: 10.1136/bmjopen-2016-014928
135. Khanna D, Fitzgerald JD, Khanna PP, Bae S, Singh MK, Neogi T, et al. 2012 American College of Rheumatology guidelines for management of gout. Part 1: systematic nonpharmacologic and pharmacologic therapeutic approaches to hyperuricemia. Arthritis Care Res . (2012a) 64 :1431–46. doi: 10.1002/acr.21772
136. Khanna D, Khanna PP, Fitzgerald JD, Singh MK, Bae S, Neogi T, et al. 2012 American College of Rheumatology guidelines for management of gout. Part 2: therapy and antiinflammatory prophylaxis of acute gouty arthritis. Arthritis Care Res . (2012b) 64 :1447–61. doi: 10.1002/acr.21773
137. Yamanaka H. Essence of the revised guideline for the management of hyperuricemia and gout. Japan Med Assoc J . (2012) 55 :324–9.
138. Pascual E, Andres M, Vela P. Gout treatment: should we aim for rapid crystal dissolution? Ann Rheum Dis . (2013a) 72 :635–7. doi: 10.1136/annrheumdis-2012-202594
139. Schlesinger N. Difficult-to-treat gouty arthritis:a disease warranting better management. Drugs (2011) 71 :1413–39. doi: 10.2165/11592290-000000000-00000
140. Harrold LR, Andrade SE, Briesacher BA, Raebel MA, Fouayzi H, Yood RA, et al. Adherence with urate-lowering therapies for the treatment of gout. Arthritis Res Ther . (2009) 11 :R46. doi: 10.1186/ar2659
141. Silva L, Miguel ED, Peiteado D, Villalba A, Mola M, Pinto J, et al. Compliance in gout patients. Acta Reumatol Port . (2010) 35 :466–74.
142. Feng X, Li Y, Gao W. Prophylaxis on gout flares after the initiation of urate-lowering therapy: a retrospective research. Int J Clin Exp Med . (2015) 8 :21460–5.
143. Yu J, Qiu Q, Liang L, Yang X, Xu H. Prophylaxis of acute flares when initiating febuxostat for chronic gouty arthritis in a real-world clinical setting. Mod Rheumatol . (2017) 28 :339–44. doi: 10.1080/14397595.2017.1318467
144. McQueen FM, Chhana A, Dalbeth N. Mechanisms of joint damage in gout: evidence from cellular and imaging studies. Nat Rev Rheumatol . (2012) 8 :173–81. doi: 10.1038/nrrheum.2011.207
145. Pascual E, Batlle-Gualda E, Martinez A, Rosas J, Vela P. Synovial fluid analysis for diagnosis of intercritical gout. Ann Intern Med . (1999) 131 :756–9. doi: 10.7326/0003-4819-131-10-199911160-00007
146. Perez-Ruiz F, Calabozo M, Pijoan JI, Herrero-Beites AM, Ruibal A. Effect of urate-lowering therapy on the velocity of size reduction of tophi in chronic gout. Arthritis Rheum . (2002) 47 :356–60. doi: 10.1002/art.10511
147. Pascual E, Martinez A, Ordonez S. Gout: the mechanism of urate crystal nucleation and growth. A hypothesis based in facts. Joint Bone Spine (2013b) 80 :1–4. doi: 10.1016/j.jbspin.2012.08.012
148. Ngo TC, Assimos DG. Uric Acid nephrolithiasis: recent progress and future directions. Rev Urol . (2007) 9 :17–27.
149. Spivacow FR, Del Valle EE, Lores E, Rey PG. Kidney stones: composition, frequency and relation to metabolic diagnosis. Medicina (2016) 76 :343–8.
150. Mandel NS, Mandel IC, Kolbach-Mandel AM. Accurate stone analysis: the impact on disease diagnosis and treatment. Urolithiasis (2017) 45 :3–9. doi: 10.1007/s00240-016-0943-0
151. Richette P, Perez-Ruiz F, Doherty M, Jansen TL, Nuki G, Pascual E, et al. Improving cardiovascular and renal outcomes in gout: what should we target? Nat Rev Rheumatol . (2014) 10 :654–61. doi: 10.1038/nrrheum.2014.124
152. Essex MN, Hopps M, Bienen EJ, Udall M, Mardekian J, Makinson GT. Evaluation of the relationship between serum uric acid levels and cardiovascular events in patients with gout: a retrospective analysis using electronic medical record data. J Clin Rheumatol . (2017) 23 :160–6. doi: 10.1097/RHU.0000000000000496
153. Kuwabara M, Niwa K, Hisatome I, Nakagawa T, Roncal-Jimenez CA, Andres-Hernando A, et al. Asymptomatic hyperuricemia without comorbidities predicts cardiometabolic diseases: five-year japanese cohort study. Hypertension (2017b) 69 :1036–44. doi: 10.1161/HYPERTENSIONAHA.116.08998
154. Kuwabara M, Niwa K, Nishihara S, Nishi Y, Takahashi O, Kario K, et al. Hyperuricemia is an independent competing risk factor for atrial fibrillation. Int J Cardiol . (2017c) 231 :137–42. doi: 10.1016/j.ijcard.2016.11.268
155. Kuwabara M, Bjornstad P, Hisatome I, Niwa K, Roncal-Jimenez CA, Andres-Hernando A, et al. Elevated serum uric acid level predicts rapid decline in kidney function. Am J Nephrol . (2017a) 45 :330–7. doi: 10.1159/000464260
156. Bardin T, Richette P. Impact of comorbidities on gout and hyperuricaemia: an update on prevalence and treatment options. BMC Med . (2017) 15 :123. doi: 10.1186/s12916-017-0890-9
157. Bove M, Cicero AF, Veronesi M, Borghi C. An evidence-based review on urate-lowering treatments: implications for optimal treatment of chronic hyperuricemia. Vasc Health Risk Manag . (2017) 13 :23–8. doi: 10.2147/VHRM.S115080
158. Kleber ME, Delgado G, Grammer TB, Silbernagel G, Huang J, Kramer BK, et al. Uric acid and cardiovascular events: a mendelian randomization study. J Am Soc Nephrol . (2015) 26 :2831–8. doi: 10.1681/ASN.2014070660
159. Parsa A, Brown E, Weir MR, Fink JC, Shuldiner AR, Mitchell BD, et al. Genotype-based changes in serum uric acid affect blood pressure. Kidney Int . (2012) 81 :502–7. doi: 10.1038/ki.2011.414
160. White J, Sofat R, Hemani G, Shah T, Engmann J, Dale C, et al. Plasma urate concentration and risk of coronary heart disease: a Mendelian randomisation analysis. Lancet Diabetes Endocrinol . (2016) 4 :327–36. doi: 10.1016/S2213-8587(15)00386-1
161. Pfister R, Barnes D, Luben R, Forouhi NG, Bochud M, Khaw KT, et al. No evidence for a causal link between uric acid and type 2 diabetes: a Mendelian randomisation approach. Diabetologia (2011) 54 :2561–9. doi: 10.1007/s00125-011-2235-0
162. Sluijs I, Holmes MV, van der Schouw YT, Beulens JW, Asselbergs FW, Huerta JM, et al. A mendelian randomization study of circulating uric acid and type 2 diabetes. Diabetes (2015) 64 :3028–36. doi: 10.2337/db14-0742
163. Rasheed H, Hughes K, Flynn TJ, Merriman TR. Mendelian randomization provides no evidence for a causal role of serum urate in increasing serum triglyceride levels. Circ Cardiovasc Genet . (2014) 7 :830–7. doi: 10.1161/CIRCGENETICS.114.000556
164. Lyngdoh T, Vuistiner P, Marques-Vidal P, Rousson V, Waeber G, Vollenweider P, et al. Serum uric acid and adiposity: deciphering causality using a bidirectional Mendelian randomization approach. PLoS ONE (2012) 7 :e39321. doi: 10.1371/journal.pone.0039321
165. Li X, Meng X, Timofeeva M, Tzoulaki I, Tsilidis KK, Ioannidis JP, et al. Serum uric acid levels and multiple health outcomes: umbrella review of evidence from observational studies, randomised controlled trials, and Mendelian randomisation studies. BMJ (2017) 357 :j2376. doi: 10.1136/bmj.j2376
166. Robinson PC, Choi HK, Do R, Merriman TR. Insight into rheumatological cause and effect through the use of Mendelian randomization. Nat Rev Rheumatol . (2016) 12 :486–96. doi: 10.1038/nrrheum.2016.102
167. Bose B, Badve SV, Hiremath SS, Boudville N, Brown FG, Cass A, et al. Effects of uric acid-lowering therapy on renal outcomes: a systematic review and meta-analysis. Nephrol Dial Transplant . (2014) 29 :406–13. doi: 10.1093/ndt/gft378
168. Mallat SG, Al Kattar S, Tanios BY, Jurjus A. Hyperuricemia, hypertension, and chronic kidney disease: an emerging association. Curr Hypertens Rep . (2016) 18 :74. doi: 10.1007/s11906-016-0684-z
169. Borghi C. The role of uric acid in the development of cardiovascular disease. Curr Med Res Opin . (2015) 31 (Suppl. 2):1–2. doi: 10.1185/03007995.2015.1087985
170. Borghi C, Rosei EA, Bardin T, Dawson J, Dominiczak A, Kielstein JT, et al. Serum uric acid and the risk of cardiovascular and renal disease. J Hypertens . (2015) 33 :1729–41; discussion 1741. doi: 10.1097/HJH.0000000000000701
171. Mancia G, Grassi G, Borghi C. Hyperuricemia, urate deposition and the association with hypertension. Curr Med Res Opin . (2015) 31 (Suppl. 2):15–19. doi: 10.1185/03007995.2015.1087981
172. Kawasoe S, Kubozono T, Yoshifuku S, Ojima S, Oketani N, Miyata M, et al. Uric acid level and prevalence of atrial fibrillation in a Japanese general population of 285,882. Circ J . (2016) 80 :2453–9. doi: 10.1253/circj.CJ-16-0766
173. Alper AB Jr., Chen W, Yau L, Srinivasan SR, Berenson GS, Hamm LL. Childhood uric acid predicts adult blood pressure: the Bogalusa Heart Study. Hypertension (2005) 45 :34–8. doi: 10.1161/01.HYP.0000150783.79172.bb
174. Feig DI. The role of uric acid in the pathogenesis of hypertension in the young. J Clin Hypertens . (2012) 14 :346–52. doi: 10.1111/j.1751-7176.2012.00662.x
175. Loeffler LF, Navas-Acien A, Brady TM, Miller ER III, Fadrowski JJ. Uric acid level and elevated blood pressure in US adolescents: National Health and Nutrition Examination Survey, 1999-2006. Hypertension (2012) 59 :811–7. doi: 10.1161/HYPERTENSIONAHA.111.183244
176. Soletsky B, Feig D.I. Uric acid reduction rectifies prehypertension in obese adolescents. Hypertension (2012) 60 :1148–56. doi: 10.1161/HYPERTENSIONAHA.112.196980
177. Mazza A, Lenti S, Schiavon L, Monte AD, Townsend DM, Ramazzina E, et al. Asymptomatic hyperuricemia is a strong risk factor for resistant hypertension in elderly subjects from general population. Biomed Pharmacother . (2017) 86 :590–4. doi: 10.1016/j.biopha.2016.11.104
178. Puddu P, Puddu GM, Cravero E, Vizioli L, Muscari A. Relationships among hyperuricemia, endothelial dysfunction and cardiovascular disease: molecular mechanisms and clinical implications. J Cardiol . (2012) 59 :235–42. doi: 10.1016/j.jjcc.2012.01.013
179. Schuchardt M, Herrmann J, Tolle M, van der Giet M. Xanthine oxidase and its role as target in cardiovascular disease: cardiovascular protection by enzyme inhibition? Curr Pharm Des . (2017) 23 :3391–404. doi: 10.2174/1381612823666170417130115
180. Scheepers LE, Wei FF, Stolarz-Skrzypek K, Malyutina S, Tikhonoff V, Thijs L, et al. Xanthine oxidase gene variants and their association with blood pressure and incident hypertension: a population study. J Hypertens . (2016) 34 :2147–54. doi: 10.1097/HJH.0000000000001077
181. Tani S, Nagao K, Hirayama A. Effect of febuxostat, a xanthine oxidase inhibitor, on cardiovascular risk in hyperuricemic patients with hypertension: a prospective, open-label, pilot study. Clin Drug Investig . (2015) 35 :823–31. doi: 10.1007/s40261-015-0349-8
182. Kojima S, Kojima S, Hifumi A, Soejima H, Ogawa H. Therapeutic strategy for efficient reduction of serum uric acid levels with allopurinol versus benzbromarone in hyperuricemic patients with essential hypertension - A randomized crossover study (terao study). Int J Cardiol . (2016) 224 :437–9. doi: 10.1016/j.ijcard.2016.09.073
183. Mackenzie IS, Ford I, Walker A, Hawkey C, Begg A, Avery A, et al. Multicentre, prospective, randomised, open-label, blinded end point trial of the efficacy of allopurinol therapy in improving cardiovascular outcomes in patients with ischaemic heart disease: protocol of the ALL-HEART study. BMJ Open (2016) 6 :e013774. doi: 10.1136/bmjopen-2016-013774
184. Kojima S, Matsui K, Ogawa H, Jinnouchi H, Hiramitsu S, Hayashi T, et al. Rationale, design, baseline characteristics of a study to evaluate the effect of febuxostat in preventing cerebral, cardiovascular, and renal events in patients with hyperuricemia. J Cardiol . (2017) 69 :169–75. doi: 10.1016/j.jjcc.2016.02.015
185. Larsen KS, Pottegard A, Lindegaard H, Hallas J. Is urate crystal precipitation a predictor of cardiovascular risk in hyperuricemic patients? A Danish cohort study. Arthritis Res Ther . (2015) 17 :304. doi: 10.1186/s13075-015-0822-z
186. Wei L, Mackenzie IS, Chen Y, Struthers AD, MacDonald TM. Impact of allopurinol use on urate concentration and cardiovascular outcome. Br J Clin Pharmacol . (2011) 71 :600–7. doi: 10.1111/j.1365-2125.2010.03887.x
187. Cottrell E, Crabtree V, Edwards JJ, Roddy E. Improvement in the management of gout is vital and overdue: an audit from a UK primary care medical practice. BMC Fam Pract . (2013) 14 :170. doi: 10.1186/1471-2296-14-170
188. Hatoum H, Khanna D, Lin SJ, Akhras KS, Shiozawa A, Khanna P. Achieving serum urate goal: a comparative effectiveness study between allopurinol and febuxostat. Postgrad Med . (2014) 126 :65–75. doi: 10.3810/pgm.2014.03.2741
189. Keenan T, Zhao W, Rasheed A, Ho WK, Malik R, Felix JF, et al. Causal assessment of serum urate levels in cardiometabolic diseases through a mendelian randomization study. J Am Coll Cardiol . (2016) 67 :407–16. doi: 10.1016/j.jacc.2015.10.086
190. Greenberg KI, McAdams-DeMarco MA, Kottgen A, Appel LJ, Coresh J, Grams ME. Plasma urate and risk of a hospital stay with AKI: the atherosclerosis risk in communities study. Clin J Am Soc Nephrol . (2015) 10 :776–83. doi: 10.2215/CJN.05870614
191. Xu X, Hu J, Song N, Chen R, Zhang T, Ding X. Hyperuricemia increases the risk of acute kidney injury: a systematic review and meta-analysis. BMC Nephrol . (2017) 18 :27. doi: 10.1186/s12882-016-0433-1
192. Ejaz AA, Mu W, Kang DH, Roncal C, Sautin YY, Henderson G, et al. Could uric acid have a role in acute renal failure? Clin J Am Soc Nephrol . (2007) 2 :16–21. doi: 10.2215/CJN.00350106
193. Shimada M, Dass B, Ejaz AA. Paradigm shift in the role of uric acid in acute kidney injury. Semin Nephrol . (2011) 31 :453–8. doi: 10.1016/j.semnephrol.2011.08.010
194. Perez-Ruiz F, Calabozo M, Herrero-Beites AM, Garcia-Erauskin G, Pijoan JI. Improvement of renal function in patients with chronic gout after proper control of hyperuricemia and gouty bouts. Nephron (2000) 86 :287–91. doi: 10.1159/000045783
195. Siu YP, Leung KT, Tong MK, Kwan TH. Use of allopurinol in slowing the progression of renal disease through its ability to lower serum uric acid level. Am J Kidney Dis . (2006) 47 :51–9. doi: 10.1053/j.ajkd.2005.10.006
196. Khan M, Khan RA, Islam F, Laghari J, Jamali SN. To study the efficacy of Losartan on urinary uric acid excretion in Thiazide induced hyperuricemic and hypertensive patients. Pak J Pharm Sci . (2011) 24 :583–7.
197. Maahs DM, Caramori L, Cherney DZ, Galecki AT, Gao C, Jalal D, et al. Uric acid lowering to prevent kidney function loss in diabetes: the preventing early renal function loss (PERL) allopurinol study. Curr Diab Rep . (2013) 13 :550–9. doi: 10.1007/s11892-013-0381-0
198. Kanji T, Gandhi M, Clase CM, Yang R. Urate lowering therapy to improve renal outcomes in patients with chronic kidney disease: systematic review and meta-analysis. BMC Nephrol . (2015) 16 :58. doi: 10.1186/s12882-015-0047-z
199. Chiba T, Matsuo H, Kawamura Y, Nagamori S, Nishiyama T, Wei L, et al. NPT1/SLC17A1 is a renal urate exporter in humans and its common gain-of-function variant decreases the risk of renal underexcretion gout. Arthritis Rheumatol . (2015) 67 :281–7. doi: 10.1002/art.38884
200. Sircar D, Chatterjee S, Waikhom R, Golay V, Raychaudhury A, Chatterjee S, et al. Efficacy of Febuxostat for Slowing the GFR Decline in Patients With C. K. D., and Asymptomatic Hyperuricemia: A 6-Month, Double-Blind, Randomized, Placebo-Controlled Trial. Am J Kidney Dis . (2015) 66 :945–50. doi: 10.1053/j.ajkd.2015.05.017
201. Hughes K, Flynn T, de Zoysa J, Dalbeth N, Merriman TR. Mendelian randomization analysis associates increased serum urate, due to genetic variation in uric acid transporters, with improved renal function. Kidney Int . (2014) 85 :344–51. doi: 10.1038/ki.2013.353
202. Goldfarb DS, MacDonald PA, Hunt B, Gunawardhana L. Febuxostat in gout: serum urate response in uric acid overproducers and underexcretors. J Rheumatol . (2011) 38 :1385–9. doi: 10.3899/jrheum.101156
203. Gillen DL, Worcester EM, Coe FL. Decreased renal function among adults with a history of nephrolithiasis: a study of NHANES III. Kidney Int . (2005) 67 :685–90. doi: 10.1111/j.1523-1755.2005.67128.x
204. Madero M, Sarnak MJ, Wang X, Greene T, Beck GJ, Kusek JW, et al. Uric acid and long-term outcomes in CKD. Am J Kidney Dis . (2009) 53 :796–803. doi: 10.1053/j.ajkd.2008.12.021
205. Chou YC, Kuan JC, Yang T, Chou WY, Hsieh PC, Bai CH, et al. Elevated uric acid level as a significant predictor of chronic kidney disease: a cohort study with repeated measurements. J Nephrol . (2015) 28 :457–62. doi: 10.1007/s40620-014-0158-9
206. Rodenbach KE, Schneider MF, Furth SL, Moxey-Mims MM, Mitsnefes MM, Weaver DJ, et al. Hyperuricemia and Progression of CKD in Children and Adolescents: The Chronic Kidney Disease in Children (CKiD) Cohort Study. Am J Kidney Dis . (2015) 66 :984–92. doi: 10.1053/j.ajkd.2015.06.015
207. Murphy D, McCulloch CE, Lin F, Banerjee T, Bragg-Gresham JL, Eberhardt MS, et al. Trends in prevalence of chronic kidney disease in the United States. Ann Intern Med . (2016) 165 :473–81. doi: 10.7326/M16-0273
208. Sarathy H, Henriquez G, Abramowitz MK, Kramer H, Rosas SE, Johns T, et al. Abdominal obesity, race and chronic kidney disease in young adults: results from NHANES 1999-2010. PLoS ONE (2016) 11 :e0153588. doi: 10.1371/journal.pone.0153588
209. Nashar K, Fried LF. Hyperuricemia and the progression of chronic kidney disease: is uric acid a marker or an independent risk factor? Adv Chronic Kidney Dis . (2012) 19 :386–91. doi: 10.1053/j.ackd.2012.05.004
210. Bhole V, Choi JW, Kim SW, de Vera M, Choi H. Serum uric acid levels and the risk of type 2 diabetes: a prospective study. Am J Med . (2010) 123 :957–61. doi: 10.1016/j.amjmed.2010.03.027
211. Araki S, Nishio Y, Araki A, Umegaki H, Sakurai T, Iimuro S, et al. Factors associated with progression of diabetic nephropathy in Japanese elderly patients with type 2 diabetes: sub-analysis of the Japanese Elderly Diabetes Intervention Trial. Geriatr Gerontol Int . (2012) 12 (Suppl. 1):127–33. doi: 10.1111/j.1447-0594.2011.00820.x
212. Xu Y, Zhu J, Gao L, Liu Y, Shen J, Shen C, et al. Hyperuricemia as an independent predictor of vascular complications and mortality in type 2 diabetes patients: a meta-analysis. PLoS ONE (2013) 8 :e78206. doi: 10.1371/journal.pone.0078206
213. Yu S, Chen Y, Hou X, Xu D, Che K, Li C, et al. Serum uric acid levels and diabetic peripheral neuropathy in type 2 diabetes: a systematic review and meta-analysis. Mol Neurobiol . (2016) 53 :1045–51. doi: 10.1007/s12035-014-9075-0
214. Liu P, Chen Y, Wang B, Zhang F, Wang D, Wang Y. Allopurinol treatment improves renal function in patients with type 2 diabetes and asymptomatic hyperuricemia: 3-year randomized parallel-controlled study. Clin Endocrinol . (2015) 83 :475–82. doi: 10.1111/cen.12673
215. Takir M, Kostek O, Ozkok A, Elcioglu OC, Bakan A, Erek A, et al. Lowering uric acid with allopurinol improves insulin resistance and systemic inflammation in asymptomatic hyperuricemia. J Investig Med . (2015) 63 :924–9. doi: 10.1097/JIM.0000000000000242
216. Ito H, Antoku S, Abe M, Omoto T, Shinozaki M, Nishio S, et al. Comparison of the renoprotective effect of febuxostat for the treatment of hyperuricemia between patients with and without type 2 diabetes mellitus: a retrospective observational study. Intern Med . (2016) 55 :3247–56. doi: 10.2169/internalmedicine.55.6791
217. Johnson RJ, Merriman T, Lanaspa MA. Causal or noncausal relationship of uric acid with diabetes. Diabetes (2015) 64 :2720–2. doi: 10.2337/db15-0532
218. Musso G, Gambino R, Cassader M, Pagano G. A novel approach to control hyperglycemia in type 2 diabetes: sodium glucose co-transport (SGLT) inhibitors: systematic review and meta-analysis of randomized trials. Ann Med . (2012) 44 :375–93. doi: 10.3109/07853890.2011.560181
219. Caulfield MJ, Munroe PB, O'Neill D, Witkowska K, Charchar FJ, Doblado M, et al. SLC2A9 is a high-capacity urate transporter in humans. PLoS Med . (2008) 5 :e197. doi: 10.1371/journal.pmed.0050197
220. Aung T, Myung G, FitzGerald JD. Treatment approaches and adherence to urate-lowering therapy for patients with gout. Patient PreferAdherence (2017) 11 :795–800. doi: 10.2147/PPA.S97927
221. Qu LH, Jiang H, Chen JH. Effect of uric acid-lowering therapy on blood pressure: systematic review and meta-analysis. Ann Med . (2017) 49 :142–56. doi: 10.1080/07853890.2016.1243803
222. Watts RW. Uric acid production with particular reference to the role of xanthine oxidase and its inhibition. Proc R Soc Med . (1966) 59 :287–92.
223. Becker MA, Schumacher HR Jr, Wortmann RL, MacDonald PA, Eustace D, Palo WA, et al. Febuxostat compared with allopurinol in patients with hyperuricemia and gout. N Engl J Med . (2005) 353 :2450–61. doi: 10.1056/NEJMoa050373
224. Jennings C G., Mackenzie I S., Flynn R, Ford I, Nuki G, De Caterina R, et al. Up-titration of allopurinol in patients with gout. Semin Arthritis Rheum . (2014) 44 :25–30. doi: 10.1016/j.semarthrit.2014.01.004
225. Jordan KM, Cameron JS, Snaith M, Zhang W, Doherty M, Seckl J, et al. British Society for Rheumatology and British Health Professionals in Rheumatology guideline for the management of gout. Rheumatology (2007) 46 :1372–4. doi: 10.1093/rheumatology/kem056a
226. Mikuls TR, Farrar JT, Bilker WB, Fernandes S, Saag KG. Suboptimal physician adherence to quality indicators for the management of gout and asymptomatic hyperuricaemia: results from the UK General Practice Research Database (GPRD). Rheumatology (2005) 44 :1038–42. doi: 10.1093/rheumatology/keh679
227. Shahid H, Singh JA. Investigational drugs for hyperuricemia. Expert Opin Invest Drugs (2015) 24 :1013–30. doi: 10.1517/13543784.2015.1051617
228. Halevy S, Ghislain P-D., Mockenhaupt M, Fagot J-P, Bouwes Bavinck Jan N, Sidoroff A, et al. Allopurinol is the most common cause of Stevens-Johnson syndrome and toxic epidermal necrolysis in Europe and Israel. J Am Acad Dermatol . (2008) 58 :25–32. doi: 10.1016/j.jaad.2007.08.036
229. Stamp LK, Day RO, Yun J. Allopurinol hypersensitivity: investigating the cause and minimizing the risk. Nat Rev Rheumatol . (2016) 12 :235–42. doi: 10.1038/nrrheum.2015.132
230. Chao J, Terkeltaub R. A critical reappraisal of allopurinol dosing, safety, and efficacy for hyperuricemia in gout. Curr Rheumatol Rep . (2009) 11 :135–40. doi: 10.1007/s11926-009-0019-z
231. Jick H, Porter JB. Potentiation of ampicillin skin reactions by allopurinol or hyperuricemia. J Clin Pharmacol . (1981) 21 :456–8. doi: 10.1002/j.1552-4604.1981.tb01749.x
232. Singh JA, Akhras KS, Shiozawa A. Comparative effectiveness of urate lowering with febuxostat versus allopurinol in gout: analyses from large U.S. managed care cohort. Arthritis Res Ther . (2015) 17 :120. doi: 10.1186/s13075-015-0624-3
233. Bridgeman MB, Chavez B. Febuxostat for the treatment of gout. Expert Opin Pharmacother . (2015) 16 :395–8. doi: 10.1517/14656566.2015.985588
234. Chohan S. Safety and efficacy of febuxostat treatment in subjects with gout and severe allopurinol adverse reactions. J Rheumatol . (2011) 38 :1957–9. doi: 10.3899/jrheum.110092
235. Miyata H, Takada T, Toyoda Y, Matsuo H, Ichida K, Suzuki H. Identification of febuxostat as a new strong ABCG2 inhibitor: potential applications and risks in clinical situations. Front Pharmacol . (2016) 7 :518. doi: 10.3389/fphar.2016.00518
236. Schumacher HR Jr, Becker MA, Wortmann RL, Macdonald PA, Hunt B, Streit J, et al. Effects of febuxostat versus allopurinol and placebo in reducing serum urate in subjects with hyperuricemia and gout: a 28-week, phase III, randomized, double-blind, parallel-group trial. Arthritis Rheum . (2008) 59 :1540–8. doi: 10.1002/art.24209
237. Paschou E, Gavriilaki E, Kalaitzoglou A, Papaioannou G, Tsompanakou A, Sabanis N. “Febuxostat hypersensitivity: another cause of DRESS syndrome in chronic kidney disease?”. Eur Ann Allergy Clin Immunol . (2016) 48 :251–5.
Google Scholar
238. White WB, Saag KG, Becker MA, Borer JS, Gorelick PB, Whelton A, et al. Cardiovascular Safety of Febuxostat or Allopurinol in Patients with Gout. N Engl J Med . (2018) 378 :1200–10. doi: 10.1056/NEJMoa1710895
239. Beard SM, von Scheele BG, Nuki G, Pearson IV. Cost-effectiveness of febuxostat in chronic gout. Eur J Health Econ . (2014) 15 :453–63. doi: 10.1007/s10198-014-0617-1
240. Gandhi PK, Gentry WM, Ma Q, Bottorff MB. Cost-effectiveness analysis of allopurinol versus febuxostat in chronic gout patients: a U.S. payer perspective. J Manag Care Spec Pharm . (2015) 21 :165–75. doi: 10.18553/jmcp.2015.21.2.165
241. Hosoya T, Ogawa Y, Hashimoto H, Ohashi T, Sakamoto R. Comparison of topiroxostat and allopurinol in Japanese hyperuricemic patients with or without gout: aphase 3, multicentre, randomized, double-blind, double-dummy, active-controlled, parallel-group study. J Clin Pharm Ther . (2016) 41 :290–7. doi: 10.1111/jcpt.12391
242. Horino T, Hatakeyama Y, Ichii O, Matsumoto T, Shimamura Y, Inoue K, et al. Effects of topiroxostat in hyperuricemic patients with chronic kidney disease. Clin Exp Nephrol . (2017) 22 :337–45. doi: 10.1007/s10157-017-1452-3
243. Sato T, Ashizawa N, Matsumoto K, Iwanaga T, Nakamura H, Inoue T, et al. Discovery of 3-(3-cyano-4-pyridyl)-5-(4-pyridyl)-1,2,4-triazole, FYX-051 - a xanthine oxidoreductase inhibitor for the treatment of hyperuricemia Bioorg Med Chem Lett . (2009) 19 :6225–9. doi: 10.1016/j.bmcl.2009.08.091
244. Matsumoto K, Okamoto K, Ashizawa N, Nishino T. FYX-051: a novel and potent hybrid-type inhibitor of xanthine oxidoreductase. J Pharmacol Exp Ther . (2011) 336 :95–103. doi: 10.1124/jpet.110.174540
245. Pittman JR., Bross MH. Diagnosis and management of gout. Am Fam Physician (1999) 59 :1799–806.
246. Perez-Ruiz F, Hernandez-Baldizon S, Herrero-Beites AM, Gonzalez-Gay MA. Risk factors associated with renal lithiasis during uricosuric treatment of hyperuricemia in patients with gout. Arthritis Care Res . (2010) 62 :1299–305. doi: 10.1002/acr.20221
247. Hamada T, Ichida K, Hosoyamada M, Mizuta E, Yanagihara K, Sonoyama K, et al. Uricosuric action of losartan via the inhibition of urate transporter 1 (URAT 1) in hypertensive patients. Am J Hypertens . (2008) 21 :1157–62. doi: 10.1038/ajh.2008.245
248. Lee M-HH, Graham GG, Williams KM, Day RO. A benefit-risk assessment of benzbromarone in the treatment of gout: was its withdrawal from the market in the best interest of patients? Drug Saf . (2008) 31 :643–65. doi: 10.2165/00002018-200831080-00002
249. Robbins N, Koch SE, Tranter M, Rubinstein J. The history and future of probenecid. Cardiovasc Toxicol . (2012) 12 :1–9. doi: 10.1007/s12012-011-9145-8
250. Pui K, Gow PJ, Dalbeth N. Efficacy and tolerability of probenecid as urate-lowering therapy in gout; clinical experience in high-prevalence population. J Rheumatol . (2013) 40 :872–6. doi: 10.3899/jrheum.121301
251. Reinders MK, van Roon EN, Jansen TLTA, Delsing J, Griep EN, Hoekstra M, et al. Efficacy and tolerability of urate-lowering drugs in gout: a randomised controlled trial of benzbromarone versus probenecid after failure of allopurinol. Ann Rheum Dis . (2009b) 68 :51–6. doi: 10.1136/ard.2007.083071
252. Reinders MK, Haagsma C, Jansen TLTA, van Roon EN, Delsing J, van de Laar MAFJ, et al. A randomised controlled trial on the efficacy and tolerability with dose escalation of allopurinol 300-600 mg/day versus benzbromarone 100-200 mg/day in patients with gout. Ann Rheum Dis . (2009a) 68 :892–7. doi: 10.1136/ard.2008.091462
253. Hisatome I, Tanaka Y, Kotake H, Kosaka H, Hirata N, Fujimoto Y, et al. Renal hypouricemia due to enhanced tubular secretion of urate associated with urolithiasis: successful treatment of urolithiasis by alkalization of urine K+, Na(+)-citrate. Nephron (1993) 65 :578–82. doi: 10.1159/000187567
254. Shen Z, Yeh LT, Wallach K, Zhu N, Kerr B, Gillen M. In Vitro and In Vivo interaction studies between lesinurad, a selective urate reabsorption inhibitor, and major liver or kidney transporters. Clin Drug Investig . (2016) 36 :443–52. doi: 10.1007/s40261-016-0386-y
255. Gillen M, Yang C, Wilson D, Valdez S, Lee C, Kerr B, et al. Evaluation of pharmacokinetic interactions between lesinurad, a new selective urate reabsorption inhibitor, and CYP enzyme substrates sildenafil, amlodipine, tolbutamide, and repaglinide. Clin Pharmacol Drug Dev . (2017) 6 :363–76. doi: 10.1002/cpdd.324
256. Shen Z, Tieu K, Wilson D, Bucci G, Gillen M, Lee C, et al. Evaluation of pharmacokinetic interactions between lesinurad, a new selective urate reabsorption inhibitor, and commonly used drugs for gout treatment. Clin Pharmacol Drug Dev . (2017) 6 :377–87. doi: 10.1002/cpdd.323
257. Fleischmann R, Kerr B, Yeh L-T, Suster M, Shen Z, Polvent E, et al. Pharmacodynamic, pharmacokinetic and tolerability evaluation of concomitant administration of lesinurad and febuxostat in gout patients with hyperuricaemia. Rheumatology (2014) 53 :2167–74. doi: 10.1093/rheumatology/ket487
258. Perez-Ruiz F, Sundy JS, Miner JN, Cravets M, Storgard C, Group RS. Lesinurad in combination with allopurinol: results of a phase 2, randomised, double-blind study in patients with gout with an inadequate response to allopurinol. Ann Rheum Dis . (2016) 75 :1074–80. doi: 10.1136/annrheumdis-2015-207919
259. Bardin T, Keenan RT, Khanna PP, Kopicko J, Fung M, Bhakta N, et al. Lesinurad in combination with allopurinol: a randomised, double-blind, placebo-controlled study in patients with gout with inadequate response to standard of care (the multinational CLEAR 2 study). Ann Rheum Dis . (2017) 76 :811–20. doi: 10.1136/annrheumdis-2016-209213
260. Dalbeth N, Jones G, Terkeltaub R, Khanna D, Kopicko J, Bhakta N, et al. Lesinurad, a selective uric acid reabsorption inhibitor, in combination with febuxostat in patients with tophaceous gout: findings of a phase III clinical trial. Arthritis Rheumatol . (2017) 69 :1903–1913. doi: 10.1002/art.40159
261. Saag KG, Fitz-Patrick D, Kopicko J, Fung M, Bhakta N, Adler S, et al. Lesinurad combined with allopurinol: a randomized, double-blind, placebo-controlled study in gout patients with an inadequate response to standard-of-care allopurinol (a US-Based Study). Arthritis Rheumatol . (2017) 69 :203–12. doi: 10.1002/art.39840
262. Tausche AK, Alten R, Dalbeth N, Kopicko J, Fung M, Adler S, et al. Lesinurad monotherapy in gout patients intolerant to a xanthine oxidase inhibitor: a 6 month phase 3 clinical trial and extension study. Rheumatology (2017) 56 :2170–8. doi: 10.1093/rheumatology/kex350
263. Hoy SM. Lesinurad: first global approval. Drugs (2016) 76 :509–16. doi: 10.1007/s40265-016-0550-y
264. Deeks E.D. Lesinurad: a review in hyperuricaemia of gout. Drugs Aging (2017) 34 :401–10. doi: 10.1007/s40266-017-0461-y
265. Robinson PC, Dalbeth N. Lesinurad for the treatment of hyperuricaemia in people with gout. Expert Opin Pharmacother . (2017) 18 :1875–81. doi: 10.1080/14656566.2017.1401609
266. Sherman MR, Saifer MGP, Perez-Ruiz F. PEG-uricase in the management of treatment-resistant gout and hyperuricemia. Adv. Drug Delivery Rev . (2008) 60 :59–68. doi: 10.1016/j.addr.2007.06.011
267. Sundy JS, Baraf HSB, Yood RA, Edwards NL, Gutierrez-Urena SR, Treadwell EL, et al. Efficacy and tolerability of pegloticase for the treatment of chronic gout in patients refractory to conventional treatment. Two randomized controlled trials. J Am Med Assoc . (2011) 306 :711–70. doi: 10.1001/jama.2011.1169
268. Taniguchi T, Ashizawa N, Matsumoto K, Iwanaga T. Enhancement of pharmacological effects of uricosuric agents by concomitant treatment with pyrazinamide in rats. Naunyn Schmiedebergs Arch Pharmacol . (2017) 390 :253–60. doi: 10.1007/s00210-016-1324-5
269. Taniguchi T, Ashizawa N, Matsumoto K, Iwanaga T, Saitoh K. Uricosuric agents decrease the plasma urate level in rats by concomitant treatment with topiroxostat, a novel xanthine oxidoreductase inhibitor J Pharmacy Pharmacol . (2016) 68 :76–83. doi: 10.1111/jphp.12490
270. Barranco C. Crystal arthritis Arhalofenate safely prevents gout flare. Nat Rev Rheumatol . (2016) 12 :252. doi: 10.1038/nrrheum.2016.53
271. Choi Y-J, Vanina L, Annette L, Vicena V, Abarca N, Rantz T, et al. Arhalofenate Is a Novel Dual-Acting Agent with Uricosuric and Anti-Inflammatory Properties . In: 2012 ACR/ARHP Annual Meeting. Hayward, CA (2012).
272. Dalbeth N, Lauterio TJ, Wolfe HR. Mechanism of action of colchicine in the treatment of gout. Clin Ther . (2014) 36 :1465–79. doi: 10.1016/j.clinthera.2014.07.017
273. Shen Z, Gillen M, Miner JN, Bucci G, Wilson DM, Hall JW. Pharmacokinetics, pharmacodynamics, and tolerability of verinurad, a selective uric acid reabsorption inhibitor, in healthy adult male subjects. Drug Des Devel Ther . (2017) 11 :2077–86. doi: 10.2147/DDDT.S140658
274. Moolenburgh JD, Reinders MK, Jansen TLTA. Rasburicase treatment in severe tophaceous gout: a novel therapeutic option. Clin Rheumatol . (2006) 25 :749–52. doi: 10.1007/s10067-005-0043-y
275. Bluestone R, Campion D, Klinenberg JR. Halofenate. Its selection and trial as a primary uricosuric agent. Arthritis Rheum . (1975) 18 (Suppl. 6):859–62. doi: 10.1002/art.1780180732
276. Poiley J, Steinberg AS, Choi YJ, Davis CS, Martin RL, McWherter CA, et al. A randomized, double-blind, active- and placebo-controlled efficacy and safety study of arhalofenate for reducing flare in patients with gout. Arthritis Rheumatol . (2016) 68 :2027–34. doi: 10.1002/art.39684
277. Steinberg AS, Vince BD, Choi YJ, Martin RL, McWherter CA, Boudes PF. The pharmacodynamics, pharmacokinetics, and safety of arhalofenate in combination with febuxostat when treating hyperuricemia associated with gout. J Rheumatol . (2017) 44 :374–9. doi: 10.3899/jrheum.161062
278. Diaz-Torne C, Perez-Herrero N, Perez-Ruiz F. New medications in development for the treatment of hyperuricemia of gout. Curr Opin Rheumatol . (2015) 27 :164–9. doi: 10.1097/BOR.0000000000000146
279. Fleischmann R, Winkle P, Miner J, Yan X, Hicks L, Valdez S, et al. AB0880 Pharmacodynamic effects and safety of verinurad (RDEA3170) in combination with allopurinol versus allopurinol alone in adults with gout: a phase 2a, open-label study. Ann Rheum Dis . (2017) 76 (Suppl. 2):1364. doi: 10.1136/annrheumdis-2017-eular.5429
280. Shiramoto M, Liu S, Shen Z, Hall J. THU0435 Pharmacodynamics, pharmacokinetics, and safety of verinurad (RDEA3170) in combination with febuxostat versus febuxostat alone and verinurad alone in japanese adults with gout or asymptomatic hyperuricemia: a phase 2A, open-label study. Ann Rheum Dis . (2017) 76 (Suppl. 2):371–2. doi: 10.1136/annrheumdis-2017-eular.5391
281. Ahn SO, Ohtomo S, Kiyokawa J, Nakagawa T, Yamane M, Lee KJ, et al. Stronger uricosuric effects of the novel selective URAT1 inhibitor UR-1102 lowered plasma urate in tufted capuchin monkeys to a greater extent than benzbromarone. J Pharmacol Exp Ther . (2016) 357 :157–66. doi: 10.1124/jpet.115.231647
282. Yoon S, Shin D, Jang I-J, Yu K-S, Lee H. Pharmacokinetics, pharmacodynamics, and tolerability of LC350189, a novel xanthine oxidase inhibitor, in healthy subjects. Drug Des Dev Ther . (2015) 9 :5033–49. doi: 10.2147/DDDT.S86884
283. Mandal AK, Mercado A, Foster A, Zandi-Nejad K, Mount DB. Uricosuric targets of tranilast. Pharmacol Res Perspect . (2017) 5 :e00291. doi: 10.1002/prp2.291
284. Dua P, Gurrell R, Kirby S, Sudworth M, Loudon PT. Acute kidney injury observed during phase 1 clinical trials of a novel xanthine oxidase/URAT1 dual inhibitor PF-06743649. Clin Rheumatol . (2016) 35 :2045–51. doi: 10.1007/s10067-016-3273-2
285. Bantia S, Parker C, Upshaw R, Cunningham A, Kotian P, Kilpatrick JM, et al. Potent orally bioavailable purine nucleoside phosphorylase inhibitor BCX-4208 induces apoptosis in B- and T-lymphocytes–a novel treatment approach for autoimmune diseases, organ transplantation and hematologic malignancies. Int Immunopharmacol . (2010) 10 :784–90. doi: 10.1016/j.intimp.2010.04.009
286. Grunebaum E, Cohen A, Roifman CM. Recent advances in understanding and managing adenosine deaminase and purine nucleoside phosphorylase deficiencies. Curr Opin Allergy Clin Immunol . (2013) 13 :630–8. doi: 10.1097/ACI.0000000000000006
287. Nguyen AD, Baysari MT, Kannangara DR, Tariq A, Lau AY, Westbrook JI, et al. Mobile applications to enhance self-management of gout. Int J Med Inform . (2016) 94 :67–74. doi: 10.1016/j.ijmedinf.2016.06.021
Keywords: URAT1, xanthine oxidase, crystal deposition, uric acid, kidney disease, hypertension, diabetes
Citation: Benn CL, Dua P, Gurrell R, Loudon P, Pike A, Storer RI and Vangjeli C (2018) Physiology of Hyperuricemia and Urate-Lowering Treatments. Front. Med . 5:160. doi: 10.3389/fmed.2018.00160
Received: 12 March 2018; Accepted: 08 May 2018; Published: 31 May 2018.
Reviewed by:
Copyright © 2018 Benn, Dua, Gurrell, Loudon, Pike, Storer and Vangjeli. This is an open-access article distributed under the terms of the Creative Commons Attribution License (CC BY) . The use, distribution or reproduction in other forums is permitted, provided the original author(s) and the copyright owner are credited and that the original publication in this journal is cited, in accordance with accepted academic practice. No use, distribution or reproduction is permitted which does not comply with these terms.
*Correspondence: Caroline L. Benn, [email protected] ; [email protected]
† Present Address: Caroline L. Benn, CNS biology, Astex Therapeutics, Cambridge, United Kingdom Ciara Vangjeli, Genomics plc, Oxford, United Kingdom
The Results of the URRAH (Uric Acid Right for Heart Health) Project: A Focus on Hyperuricemia in Relation to Cardiovascular and Kidney Disease and its Role in Metabolic Dysregulation
- Review article
- Open access
- Published: 04 October 2023
- Volume 30 , pages 411–425, ( 2023 )
Cite this article
You have full access to this open access article
- Alessandro Maloberti 1 , 2 na1 ,
- Alessandro Mengozzi 3 , 4 na1 ,
- Elisa Russo 5 ,
- Arrigo Francesco Giuseppe Cicero ORCID: orcid.org/0000-0002-4367-3884 6 , 7 ,
- Fabio Angeli 8 , 9 ,
- Enrico Agabiti Rosei 10 ,
- Carlo Maria Barbagallo 11 ,
- Bruno Bernardino 12 ,
- Michele Bombelli 2 , 13 ,
- Federica Cappelli 3 ,
- Edoardo Casiglia 14 ,
- Rosario Cianci 15 ,
- Michele Ciccarelli 16 ,
- Massimo Cirillo 17 ,
- Pietro Cirillo 18 ,
- Giovambattista Desideri 12 ,
- Lanfranco D’Elia 19 ,
- Raffaella Dell’Oro 2 , 20 ,
- Rita Facchetti 2 ,
- Claudio Ferri 12 ,
- Ferruccio Galletti 19 ,
- Cristina Giannattasio 1 , 2 ,
- Loreto Gesualdo 18 ,
- Guido Iaccarino 21 ,
- Luciano Lippa 22 ,
- Francesca Mallamaci 23 ,
- Stefano Masi 3 ,
- Maria Masulli 16 ,
- Alberto Mazza 24 ,
- Maria Lorenza Muiesan 10 ,
- Pietro Nazzaro 25 ,
- Gianfranco Parati 2 , 26 ,
- Paolo Palatini 14 ,
- Paolo Pauletto 27 ,
- Roberto Pontremoli 5 ,
- Nicola Riccardo Pugliese 3 ,
- Fosca Quarti-Trevano 2 , 20 ,
- Marcello Rattazzi 14 ,
- Gianpaolo Reboldi 28 ,
- Giulia Rivasi 29 ,
- Massimo Salvetti 10 ,
- Valerie Tikhonoff 14 ,
- Giuliano Tocci 30 , 31 ,
- Andrea Ungar 29 ,
- Paolo Verdecchia 32 ,
- Francesca Viazzi 5 ,
- Massimo Volpe 30 , 33 ,
- Agostino Virdis 3 ,
- Guido Grassi 2 , 20 ,
- Claudio Borghi 6 , 7 &
Working Group on Uric Acid and Cardiovascular Risk of the Italian Society of Hypertension (SIIA)
2277 Accesses
12 Citations
7 Altmetric
Explore all metrics
The relationship between Serum Uric Acid (UA) and Cardiovascular (CV) diseases has already been extensively evaluated, and it was found to be an independent predictor of all-cause and cardiovascular mortality but also acute coronary syndrome, stroke and heart failure. Similarly, also many papers have been published on the association between UA and kidney function, while less is known on the role of UA in metabolic derangement and, particularly, in metabolic syndrome. Despite the substantial number of publications on the topic, there are still some elements of doubt: (1) the better cut-off to be used to refine CV risk (also called CV cut-off); (2) the needing for a correction of UA values for kidney function; and (3) the better definition of its role in metabolic syndrome: is UA simply a marker, a bystander or a key pathological element of metabolic dysregulation?. The Uric acid Right for heArt Health (URRAH) project was designed by the Working Group on uric acid and CV risk of the Italian Society of Hypertension to answer the first question. After the first papers that individuates specific cut-off for different CV disease, subsequent articles have been published responding to the other relevant questions. This review will summarise most of the results obtained so far from the URRAH research project.
Similar content being viewed by others
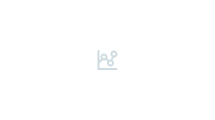
Exploration into Uric and Cardiovascular Disease: Uric Acid Right for heArt Health (URRAH) Project, A Study Protocol for a Retrospective Observational Study
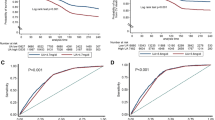
Hyperuricemia and Risk of Cardiovascular Outcomes: The Experience of the URRAH (Uric Acid Right for Heart Health) Project
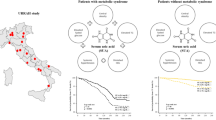
The importance of including uric acid in the definition of metabolic syndrome when assessing the mortality risk
Avoid common mistakes on your manuscript.
1 Introduction
A dramatically growing body of evidence suggests that serum uric acid (SUA) plays a relevant role in cardiovascular (CV) and metabolic disease incidence and clinical severity, acting either as an independent risk factor or synergistically with other known CV risk factors. Despite the established evidence, the threshold level of SUA able to significantly contribute to cardiovascular risk remains undefined [ 1 ]. Moreover, it is not yet clear if SUA is the best marker of purine metabolism and if purine dysmetabolism is per se a cardiometabolic risk factor or if SUA is an indirect marker of oxidative stress that is per se a cardiometabolic risk factor [ 2 ].
Anyway, the association of SUA and CV disease risk has also been largely investigated in Mendelian randomization analyses. It has been suggested that every 1-SD increase in genetically predicted SUA was associated with an increased risk of coronary heart disease (odds ratio 1.19 [95% CI 1.10–1.30]; P = 4 × 10 −5 ), peripheral artery disease (1.12 [95%CI 1.03–1.21]; P = 9 × 10 −3 ), and stroke (1.11 [95% CI 1.05–1.18]; P = 2 × 10 −4 ), where elevated blood pressure was estimated to mediate approximately one-third of the effect of urate on CV disease risk [ 3 ]. In a very large genome-wide study (N. 457,690 individuals), the Mendelian randomization analysis showed that elevated genetically determined serum urate levels were associated with increased risks of coronary heart disease in men but not in women [ 4 ].
Furthermore, recently, Moshkovits et al. have evaluated the impact of SUA on the risk of developing a composite of death, acute coronary syndrome, or stroke in 19,769 asymptomatic self-referred adults aged 40–79 years free of cardiovascular disease and diabetes (mean age 50 ± 8 years, 31% women) annually screened in a preventive healthcare setting. During a median follow-up of 6 years, 1658 (8%) subjects reached the study endpoint. Continuous net reclassification improvement analysis showed a 13% improvement in the accuracy of classification when high SUA was added to either pooled cohort equations (PCE) model or Systematic COronary Risk Evaluation 2 (SCORE2) model (P < 0.001 for both). In particular, Subgroup analyses showed a significant 16–20% improvement in the model performance among normal-weight and low-risk subjects (P < 0.001 for PCE; P = 0.026 and P < 0.001 for SCORE2, respectively) [ 5 ].
In this context, the Uric acid Right for heArt Health (URRAH) project has been designed to define, as primary objective, the SUA level above which the independent risk of cardiovascular disease increases in a significant manner in a large and well-characterized Mediterranean population sample [ 6 ]. However, after answering this first question, we find that such a database will be able to provide important information on many other still unanswered questions about SUA, such as modifying factors of the relationship with CV events and a better explanation of its role in kidney disease and metabolic derangements. This review describes the main results of the URRAH research project.
2 The URRAH Project
The protocol of this study has been described extensively in previous publications [ 7 ]. Briefly, this is a multicentre retrospective, observational cohort study which has involved the collection of data on (mainly hypertensive outpatients and subjects from general population with a median follow-up period of 10 years (up to 31 July 2017).
Data from participant’s centre were collected and has been included into a general database. The various cohorts included comes from Italian Centres of Hypertension, distributed in almost all the Italian regions and recognised by the Italian Society of Hypertension.
Inclusion criteria were the availability of at least one or serum UA levels determination and of complete information’s about demographics, CV risk factor (known history of arterial hypertension, diabetes mellitus, smoking habit, overweight/obesity defined through body mass index and waist circumference), previous CV events, CV drug therapies, blood pressure values, biochemical data (total and fractioned cholesterol, triglycerides and renal function estimated). From some centre also data on cardiac (Left Ventricular Hypertrophy – LVH) and renal (urinary albumin excretion) hypertension-mediated organ damage were reported.
At the end of the follow-up, the following hard endpoints (based on the International Classification of Diseases, Tenth Revision - ICD-10) has been evaluated: all-cause mortality, CV mortality, fatal and non-fatal acute myocardial infarction, coronary revascularization, fatal and non-fatal stroke and heart failure.
Depending on the aim and on the variable needed for the specific analysis total number of the subjects used could vary between the different sub-studies but, in general, the whole population is composed by 23,475 subjects that presents a mean age of 57 ± 15 years and of which 51% were males. The main characteristics of study population cohorts and the main results are summarized in Table 1 .
2.1 Hyperuricemia and Cardiovascular Diseases
In the URRAH study, whether SUA could be related to CV diseases has been extensively investigated with a particular interest in founding specific cut-offs. The first published work identified the cut-off most suitable for predicting total and CV mortality in the entire study population [ 7 ]. During a median follow-up time of 134 months (interquartile range 74–164), 1571 CV deaths (over 3279 all-cause death, 47.9%) were identified, and SUA significantly correlates in univariate analysis (Hazard Ratio – HR: 1.28; 95% CI 1.24–1.33, p < 0.001). In multivariable analysis with all the classic CV risk factors inserted as covariates, the associations were consistently significant; even better, the strength of the association increased (HR 2.08; 95% CI 1.46–2.97, p < 0.001).
As expected in the hypothesis of the project, a lower cut-off was founded when compared to the classic one (6 mg/dL for females and 7 mg/dL for males). From ROC curve analysis, the optimal cut point for CV mortality was 5.6 mg/dL. Furthermore, the addition of SUA values increases the predictivity of the Heart Score (Harrell’s C 0.780 vs. 0.754, p < 0.001) and correctly reclassified 40.06% of subjects without events over the Heart Score at the cost of a false negative association of 12.28%, providing a significant Net Reclassification Improvement of 0.27 (p < 0.001).
The second paper published [ 8 ] regards the possible cut-off values for fatal Myocardial Infarction (MI) prediction. 445 subjects experienced the endpoint of the present analysis with a significant association with SUA (HR: 1.381; 95% CI 1.096–1.758, p = 0.006). At ROC analysis, the best cut-off for fatal MI was 5.70 mg/dL. When gender-specific analyses were performed, the significant association was confirmed in women (HR: 0.154; 95% CI 1.105–2.075, p < 0.001) but not in men (HR 1.294, 95% CI 0.924–1.813, p = 0.1). The role of gender in the relationship between SUA and CV disease has been extensively discussed in a previous review of the URRAH study [ 9 ].
A further paper on the URRAH project focused on Heart Failure (HF) [ 10 ]. SUA was found to be a significant predictor of incident HF (HR: 1.29; 95% CI 1.23–1.359, p < 0.001) and of fatal HF (HR: 1.268; 95% CI 1.121–1.35, p < 0.001), as well. In this case, the most discriminant cut-offs for SUA were 5.34 mg/dL and 4.89 mg/dL, respectively. Our results are in accordance with other population studies and meta-analyses regarding the ability of SUA to predict HF development [ 11 , 12 ]. However, published paper results are more heterogeneous when SUA is evaluated in patients with HF. In these patients, SUA could act as a detrimental factor on left ventricular function and metabolism, but the opposite could also be true, i.e. the worst peripheral tissue vascularisation could increase SUA levels. In fact, SUA could result from increased purine degradation determined by hypoxia and tissue catabolism, which also determines an increased lactate release that reduces renal UA excretion [ 13 ]. Furthermore, HF is frequently associated with kidney impairment (and again a lower SUA clearance), and an increase in xanthine oxidase activity has been found in acute decompensated HF [ 14 ].
A problem interpreting the relationship between SUA and HF is the issue related to diuretic use. Diuretic treatments (especially thiazides) are able to determine an increased urate renal reabsorption that leads to hyperuricemia development. Diuretics are quite common in hypertensive patients and represent the principal therapeutic option to reduce congestion in HF patients. These secondary effects of diuretics were considered a benign problem, but data from the URRAH study argues against this hypothesis. In a specific analysis of the URRAH database, we found that 17.2% of individuals take diuretics, of whom 58% had SUA higher than the median value (4.8 mg/dL) [ 15 ]. Subjects with hyperuricemia using diuretic seems to present a higher prevalence of death for any cause (21.9 vs. 19.0%, p < 0.001) and first CV events (11.3 vs. 8.1%, p < 0.001) when compared with subjects with hyperuricemia without diuretic use while no differences were seen regarding CV deaths (5.1 vs. 4.1%, p = 0.013). However, at multivariable analysis (covariates: age, sex, systolic blood pressure, body mass index, glycemia, total and HDL cholesterol, smoking, CV therapies and estimated glomerular filtration rate), the above-mentioned findings were not confirmed for all three evaluated outcomes.
Since the central hypothesis linking UA to CV events is the role of xanthine oxidase as a trigger for oxidative stress, diuretic-induced reduction of UA renal excretion was thought to be not related to an increase in CV events. Although this study presents some limitations, it was the first to confirm that diuretic-related hyperuricemia was associated with a significant increase in CV diseases and mortality. The two main limitations were that we could not discriminate between patients with reduced uric acid excretion and those with uric acid overproduction (in fact, the presence of hyperuricemia during diuretic use does not automatically exclude the coexistence of increased production) but also that all the types of diuretic drugs were included in the present analysis. However, the effect on SUA level is more commonly observed with thiazides. Unfortunately, data on diuretic type were available only for a fraction of patients too small for a sub-group analysis.
To complete the data on the relationship between SUA and CV events, also an analysis on stroke was done [ 16 ]. SUA was associated, at multivariable regression analysis adjusted for confounders (age, sex, arterial hypertension, diabetes, chronic kidney disease, smoking habit, ethanol intake, body mass index, low-density lipoprotein cholesterol and use of diuretics) with an HR of 1.249 (95% CI 1.041–1.497, p = 0.016). A prognostic cut-off value of 4.79 mg/dL was identified as the best threshold.
Furthermore, an age-stratified survival analysis was performed in the older adults (≥ 65 years; n = 8000) of the URRAH study participants. While no independent association was found between patients older than 75 years and mortality (all-cause and CV), patients in the 65–74 year age group showed optimal discrimination using a dedicated cut-off of 4.8 mg/dL, which is valid for both All-Cause Mortality (ACM) and CV mortality (CVM): HR 1.45, (95% CI 1.23–1.68) and 1.46 (95% CI 1.20–1.79). Peculiarly, in participants older than 75, the relationship between SUA and mortality was described by J-shaped curves [ 17 ].
Two URRAH papers focused on factors that could modulate the SUA relationship with CV mortality. The first focused on heart rate and the possibility that SUA action on CV events is by sympathetic activity [ 18 ]. Heart rate is a well-known CV risk factor that directly damages the heart and the arterial wall, but it can also represent a marker of a sympathetic nervous system overdrive. At multivariable analysis, each unit increase in SUA determined an increase in the HR for CV mortality of 9.4%, while the increase was 5.3% for each heart rate unit increment. In categorical analysis, we have seen that hyperuricemia (SUA > 5.5 mg/dL) exerts a higher increase in HR for CV mortality in subjects with elevated heart rate. The HR for the relationship between SUA and CV mortality in subjects with a heart rate under the median value (71.3 bpm) was 1.38 (95% CI 1.20–1.59), while this increase to an HR of 2.09 (95% CI 1.75–2.51) in those over the median value. So, one could hypothesize that the sympathetic nervous system overactivity facilitates uric acid action on CV events.
Finally, the most recently published URRAH paper focused on the relationship of SUA with LVH and the possibility that this target organ damage modifies its relation with CV mortality [ 19 ]. In multiple regression analysis, SUA was significantly associated with Left Ventricular Mass Index in men and women (beta = 0.095 and 0.069, respectively, p < 0.001 for both analyses). Both SUA and LVH were, as expected, associated with CV death in multivariable models (hyperuricemia HR 1.751; 95% CI 1.394–2199, p = 0.001; LVH HR 2.050; 95% CI 1.576–2.668, p = 0.001). The combined presence of hyperuricemia (SUA > 5.1 mg/dL for females and > 5.6 mg/dL for males [ 7 ]) and LVH (LVMI > 95 g/m 2 for females and > 115 g/m 2 for males) significantly increase the HR for CV mortality when compared to those factors taken individually to a total HR of 3785 (95% CI 1.789–8.008) for women and 5.273 (95% CI 3.044–9.135) for men.
In conclusion, our data suggest that other factors could modulate the contribution of SUA to CV mortality. This is undoubtedly modulated by the presence of tachycardia and LVH, both of which increase the ability of SUA to detect CV disease.
Figure 1 resumes the results of the URRAH study in terms of the different cut-offs established for CV events while Fig. 2 summarize data on factors able to modifying that relationship.
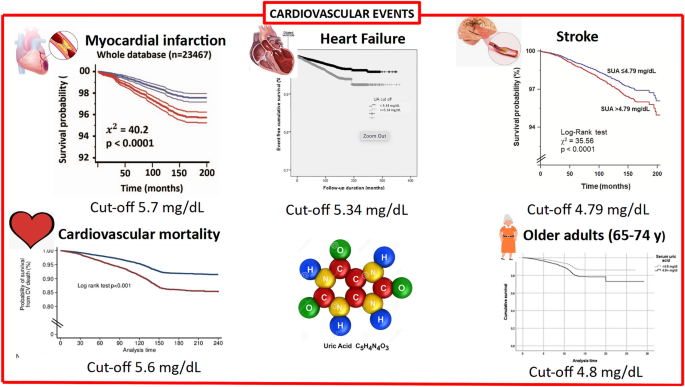
Findings from the URRAH study regarding the relationship between serum uric acid and cardiovascular mortality and events. Figure from references [ 7 , 8 , 10 , 16 ]
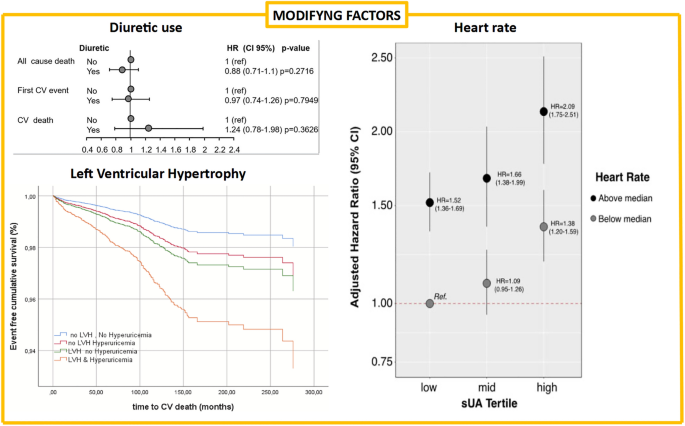
Findings from the URRAH study regarding factors able to modify the relationship between uric acid and cardiovascular events. Figure from references [ 15 , 17 , 18 ]
2.2 Hyperuricemia and Kidney Disease in Predicting Mortality
Glomerular filtration rate (GFR) is unquestionably one of the main determinants of SUA levels, thus hyperuricemia is a typical finding in chronic kidney disease (CKD) patients. Consistent evidence indicates that the relationship between hyperuricemia and CKD is bidirectional. As well a reduction in GFR can precede and lead to the development of hyperuricemia, increased SUA levels per se can adversely impact renal function [ 20 , 21 ]. However, how GFR and SUA levels are related and the appropriate threshold of SUA for defining asymptomatic hyperuricemia in the contest of CKD remain unclear. The URRAH database, with its large baseline cohort and relatively long-term follow-up [ 22 ], provided an ideal opportunity to investigate this interaction between SUA levels and CKD components (eGFR and albuminuria) and their impact in determining mortality.
A recent cross-sectional analysis [ 23 ] including 26,971 individuals from the URRAH database provided new insights into these complex relationships. The study showed the more significant the severity of the CKD stage, the higher the occurrence of hyperuricemia. Prevalence of hyperuricemia defined based on previously validated URRAH cut-offs specific for CVM and ACM was 32 and 57%, respectively, and increased significantly from 20 to 33% in subjects with eGFR > 90 ml/min to 60, and 80% in CKD 3b patients. Multiple logistic regression analyses indicated that the main covariates associated with hyperuricemia defined with CVM threshold were CKD stage, male gender, history of hypertension, and triglycerides (TG) levels.
Moreover, this was the largest population study in which the relationship between SUA levels and the presence of micro and macro-albuminuria have been investigated. Hyperuricemia was more likely present in patients with albuminuria (50.5%, 54.9%, and 57.1% in patients with normoalbuminuria, microalbuminuria and macroalbuminuria, respectively, p = 0.0062). Those with albuminuria showed higher SUA levels (5.18 ± 1.40 vs. 5.45 ± 1.56, p < 0.0001), more frequent use of allopurinol and a history of gout compared to those without albuminuria. Unexpectedly, in patients with GFR below 45 ml/min, the prevalence of hyperuricemia was lower in the presence of macroalbuminuria. While this finding is unforeseen, it may be related to a higher prevalence of individuals with diabetes among these patients with more severe kidney impairment. This data could be explained by an increase in glycosuria and a greater loss of uric acid in the urine reported in patients with decompensated diabetes, resulting in decreased SUA levels [ 24 ].
Despite the known great impact of CKD components (both reduced GFR and the presence of albuminuria) on CV and mortality risk [ 25 , 26 ], the interplay between SUA, GFR and albuminuria in causing mortality and the independent role of each one of these conditions on CV and mortality risk is a matter of research. The URRAH Study Group [ 27 ] tried to answer these questions with a longitudinal study. The cohort was composed of 21,963 patients who were followed for 9.8 years. A ROC curve analysis yielded plausible GFR stage-specific cut-off values of SUA for CVM (cut-off points of SUA = 4.1, 5.8 and 6.9 mg/dL in subjects with GFR > 90, 60–90 and < 60 ml/min/m 2 , respectively), and for ACM (cut-off points of SUA = 5.1, 4.8 and 6.8 mg/dL in subjects with GFR > 90, 60–90 and < 60 ml/min/1.73 m 2 , respectively). Results of interaction effect regression analysis indicated that SUA and GFR interplay in determining mortality. More interestingly, it was described for the first time as the independent predictive power of SUA tends to decrease along with the severity of renal impairment. This study concluded that high SUA levels are a risk factor for CV and all-cause mortality independently and additively to reduced GFR and the presence of albuminuria in patients at cardiovascular risk (Fig. 3 ).
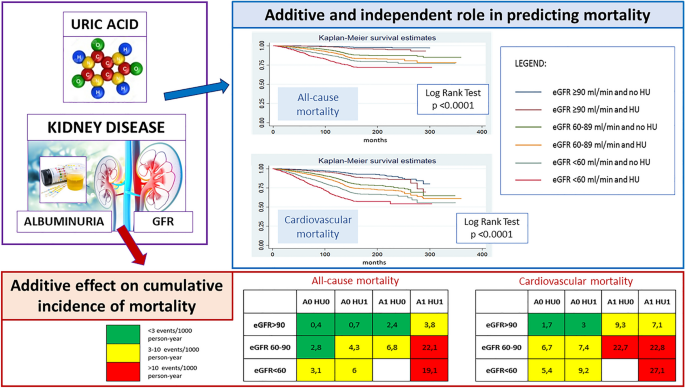
Hyperuricemia is a risk factor for cardiovascular and all-cause mortality independently and additively to reduced GFR and the presence of albuminuria. A Albuminuria, eGFR estimated Glomerular Filtration Rate, HU HyperUricemia
Altogether, these data suggest that in the context of greater global risk, as is the case when GFR is even slightly reduced, SUA becomes a significant correlate of unfavourable outcome only at serum concentration greater than what is observed in subjects with normal renal function. From a pathophysiological point of view, these data sustain the hypothesis that while hyperuricemia may result from reduced kidney clearance, uric acid perpetuates renal injury, leading to a progressive vicious cycle of CKD progression and after that to an increased CV and mortality risk. Several pathogenetic mechanisms explaining uric acid-mediated kidney and vascular damage have been hypothesized, including inflammation with cytokine release and oxidative stress [ 28 ], promotion of endothelial, vascular [ 29 ], and interstitial damage, upregulation of the renin–angiotensin aldosterone system, and changes in glomerular hemodynamics leading to glomerulosclerosis and fibrosis [ 30 ].
The findings of specific SUA levels predicting mortality in different CKD strata could also explain the relatively conflicting data previously reported in the literature on the relationship between hyperuricemia and unfavourable outcomes in the presence of CKD at different stages [ 31 ]. In fact, renal function may have acted as a confounder in the relationship between hyperuricemia and CV risk when data were analyzed in aggregate without adjusting for GFR values.
Because SUA is so strongly dependent on renal function, the URRAH Study Group determined the prognostic cut-off values for the ratio between SUA mg/dl and serum creatinine (sCr mg/dl ), assessing sCr as an adequate indicator of the kidney function (the addiction of GFR to the Cox models did not change the results significantly) [ 32 ]. This study identified a SUA/sCr cut-off predictive of CV risk for the first time. The SUA/sCr cut-off of 5.35, by indexing for renal function, proved to be a functional tool for identifying those patients with the highest risk of developing CV events during follow-up, with no significant differences between men and women. Therefore, this a-dimensional, purely numerical variable was proposed as a novel element that can be treated in the epidemiological field as a new variable for screening individuals at increased risk.
In summary, there is consistent evidence from URRAH data that SUA levels and CKD stages are closely related. For this reason, there is a need for a specific cut-off defining asymptomatic hyperuricemia in the presence of CKD. In particular, the proposal derived from the URRAH Project is to use a cut-off of SUA > 7.0 mg/dl for patients with CKD 3 or a cut-off of SUA adjusted for creatinine of 5.35. The second major point emerging from these studies is the independent and additive role of each condition (hyperuricemia, GFR reduction and albuminuria) in increasing mortality risk. This suggests that despite CKD being a major determinant of the presence and degree of hyperuricemia, these two conditions may have, at least in part, different pathogenetic mechanisms by which they both contribute to the excess of CV morbidity and mortality. Nevertheless, further translational research is needed.
2.3 Serum Uric Acid in the Context of Metabolic Dysregulation
The definition of a high-risk CV phenotype cannot ignore the individual metabolic profile. Impaired metabolic homeostasis defines ageing and cardiometabolic diseases such as dyslipidemia, obesity, type 2 diabetes (T2D) and arterial hypertension and drives CV risk. Moreover, it is involved in a deleterious interplay with other actors of detrimental CV evolution [ 33 ]. Uric acid is not exempt from this harmful connection, as it has a double-edged relationship with metabolic disorders. Elevated SUA is a common finding in metabolic diseases [ 34 ], and pathophysiological and clinical evidence supports a potential interaction between SUA, glucose and lipid metabolism [ 35 , 36 ]. Hyperglycaemia and altered levels of circulating lipoproteins have common molecular pathways of damage that are also shared with SUA. One of these is the activation of the NLRP3-inflammasome [ 33 ], which leads to the persistent low-grade systemic inflammatory response that is a hallmark of cardiometabolic disease [ 37 ]. The pro-oxidant activity that SUA acquires at higher concentrations can further disrupt the homeostatic balance, altering HDL metabolism and function [ 38 ]. Given the dramatic importance that metabolic diseases are gaining in the global health landscape [ 39 ], the URRAH study investigated, in metabolic subgroups among URRAH study participants, the relationship between uric acid and cardiometabolic diseases from a clinical perspective [ 40 , 41 , 42 , 43 ] (Fig. 4 ).
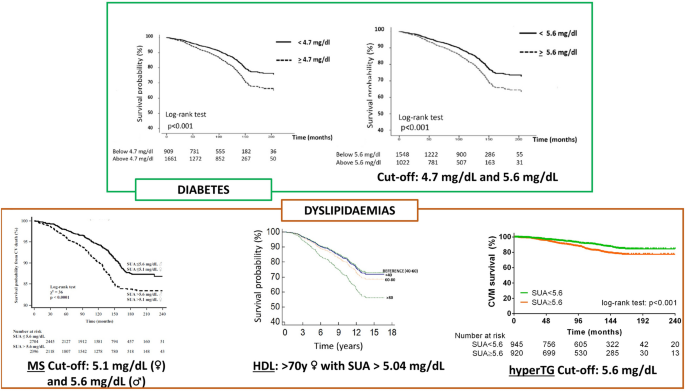
Findings from the URRAH study regarding the relationship of uric acid with diabetes and dyslipidemia. Figure from references [ 40 , 41 , 42 , 43 ]. MetS Metabolic syndrome, HDL High Density Lipoprotein, hyperTG hypertriglyceridemia
In another study in URRAH patients with diabetes (n = 2,570), the established thresholds of SUA identified for ACM (SUA ≥ 4.7 mg/dL) and CV mortality (SUA ≥ 5.6 mg/dL) [ 7 ] confirmed their predictive power [ 40 ]: HR 1.23, (95% CI 1.04–1.47) and 1.31 (95% CI, 1.03–1.66), respectively. Similar results were seen in a subpopulation (n = 8124) with different degrees of cardiometabolic damage (patients with obesity, arterial hypertension, T2D, either alone or combined) but without established cardiovascular disease (subjects with previous CV events, history of heart failure, systolic/diastolic blood pressure > 240/149 mmHg, fasting blood glucose > 350 mg/dl, or serum creatinine > 4 mg/dL were excluded). A total of 8,124 patients were included in the final analysis : HR 1.25, (95% CI 1.12–1.40) for ACM and 1.31 (95% CI 1.11–1.74) for CV mortality [ 42 ]. The association with CV mortality was also examined in a sub-cohort of patients selected based on the data availability for the metabolic syndrome (MS) parameters (n = 9589; n = 5100 met MS criteria), confirming that SUA ≥ 5.6 mg/dL was strongly associated with CV death: HR 1.79 (95% CI 1.15–2.79). In this context, it was also verified how including sex-specific SUA cut-offs in the MS definition improved CV mortality reclassification in patients with and without MS (net reclassification improvement of 7.1%) [ 43 ].
The URRAH study group also investigated the relationship between the different parameters of the metabolic profile and the clinical relevance of their interplay. SUA was found to be inversely correlated with HDL (r = −0.206) [ 41 ], with plasma glucose in patients with diabetes (r = −0.09) [ 40 ], and positively correlated with TG in patients without established CV disease (r = 0.332) [ 42 ] In female patients with high HDL (> 80 mg/dL), a specific SUA cut-off (4.96 mg/dL) was able to identify a higher mortality risk (HR 1.61; 95% CI 1.08–2.39). Notably, the inclusion of the BMI in the model reduced the strength of the association (HR 1.51; 95% CI, 1.00–2.27) [ 41 ]. Concerning TG and using the acknowledged SUA cut-offs [ 7 ], the interaction with SUA was significant regarding ACM and CV mortality, and no gender-specific effect was observed [ 42 ]. Although with the limitation of reduced sample size due to further stratification into distinct disease subgroups, an exploratory analysis supports the predictive power of SUA since the initial cardiometabolic derangement [ 42 ].
In conclusion, although limited by the observational nature of the URRAH study, all these results confirm the relevant association between SUA and impaired metabolic states. More importantly, they suggest that SUA retains its predictive power across the cardiometabolic spectrum and highlight a relevant adverse interplay with part of the lipid profile. Finally, the J-shaped curves found in patients over 75 years of age call for specific follow-up studies [ 39 ]. Given the longer life expectancy of the population and the dramatic increase in the prevalence of metabolic diseases [ 38 ], SUA, which is inexpensive and widely available in plasma, seems particularly relevant in the context of early risk stratification and possible therapeutic intervention.
3 Conclusions
Despite the availability of always more refined algorithms to predict incident CV diseases [ 44 ], a relatively large number of events remain unexpected. This could be due to different reasons, among them the lack of knowledge of some risk factors and the lack of attention to clearly emerging risk factors. Among them, SUA seems to be of particular interest. Increasing epidemiological evidence supports the need for further investigation of the determinants of cardiometabolic and renal risk associated with SUA and other purine metabolism biomarkers. In fact, from one side, suboptimal SUA levels are associated with several risk factors, organ damage and pathological conditions per se associated with an increased CV and metabolic risk, while on the other side, SUA reduction has not been yet clearly associated with a reduction of CV risk [ 45 ].
Thus, when interpreting epidemiological data on SUA as a risk factor, we have to consider that widely prevalent (and often underestimated) conditions like wrong lifestyle [ 46 ], overweight/obesity and insulin resistance [ 47 ] impair SUA levels at the same time increasing cardiometabolic risk. Conversely, some commonly used drugs reduced SUA levels and CV risk, such as losartan, fenofibrate and Sodium-glucose cotransporter-2 (SGLT2) inhibitors [ 48 ].
A recent network meta-analysis including 23 double-blind, placebo-controlled, randomized clinical trials carried out with different SUA lowering drugs concluded that allopurinol and febuxostat had significantly lower composite renal events (deterioration of renal function, end-stage renal disease, and initiation of renal replacement therapy) than placebo (Relative Risk - RR 0.39, 95% CI 0.23–0.66, and RR 0.68, 95% CI 0.46–0.99, respectively), but no apparent effect on major CV events [ 49 ]. A larger meta-analysis including 30 trials (N = 18,585) concluded that xanthine oxidase inhibitors produced a 6.0% reduction in relative risk for major adverse cardiovascular events, mainly because of the benefit attributed to allopurinol (RR: 0.61, 95% CI 0.46–0.80, I 2 = 21.0%), while febuxostat would have a more neutral effect (RR: 1.09, 95% CI 0.998–1.19, I 2 = 0.0%) [ 50 ]. These data should be interpreted cautiously since women and racial and ethnical minorities are underrepresented in controlled clinical trials testing SUA-lowering drugs [ 51 ], and it is well known that CV risk, SUA levels and the impact of SUA on CV risk are different in men and women, and in white versus other ethnicities. Besides, at least one part of the cardiovascular event preventive effects of the SGLT2 inhibitors have been attributed to their ability to improve SUA levels significantly [ 52 ].
In this context, data coming from large cohorts of unselected subjects and patients such as the URRAH one helps to more clearly understand the role of SUA as independent CV risk factors and the subcohorts of subjects where suboptimal SUA levels are associated with higher CV risk.
Data availability statement
Original data subsets related to single URRAH published analyses are available upon motivated inquire and approval by the URRAH steering committee.
Kuwabara M, Kodama T, Ae R, Kanbay M, Andres-Hernando A, Borghi C, Hisatome I, Lanaspa MA. Update in uric acid, hypertension, and cardiovascular diseases. Hypertens Res. 2023. https://doi.org/10.1038/s41440-023-01273-3 . ( Epub ahead of print ).
Article PubMed Google Scholar
Cicero AFG, Fogacci F, Di Micoli V, Angeloni C, Giovannini M, Borghi C. Purine metabolism dysfunctions: experimental methods of detection and diagnostic potential. Int J Mol Sci. 2023;24(8): 7027.
Article CAS PubMed PubMed Central Google Scholar
Gill D, Cameron AC, Burgess S, Li X, Doherty DJ, Karhunen V, Abdul-Rahim AH, Taylor-Rowan M, Zuber V, Tsao PS, Klarin D, VA Million Veteran Program, Evangelou E, Elliott P, Damrauer SM, Quinn TJ, Dehghan A, Theodoratou E, Dawson J, Tzoulaki I. Urate, blood pressure, and cardiovascular disease: evidence from mendelian randomization and meta-analysis of clinical trials. Hypertension. 2021;77(2):383–92.
Article CAS PubMed Google Scholar
Yang F, Lu Y, Chen S, Wang K, Hu T, Cui H. Sex-specific effect of serum urate levels on coronary heart disease and myocardial infarction prevention: a mendelian randomization study. Nutr Metab Cardiovasc Dis. 2022;32(5):1266–74.
Moshkovits Y, Tiosano S, Kaplan A, Kalstein M, Bayshtok G, Kivity S, Segev S, Grossman E, Segev A, Maor E, Fardman A. Serum uric acid significantly improves the accuracy of cardiovascular risk score models. Eur J Prev Cardiol. 2023;30(7):524–32.
Desideri G, Virdis A, Casiglia E, Borghi C, Working Group on Uric Acid and Cardiovascular Risk of the Italian Society of Hypertension. Exploration into uric and cardiovascular disease: uric acid right for heArt Health (URRAH) Project, a study protocol for a retrospective observational study. High Blood Press Cardiovasc Prev. 2018;25(2):197–202.
Virdis A, Masi S, Casiglia E, Tikhonoff V, Cicero AFG, Ungar A, et al. Identification of the uric acid thresholds predicting an increased total and cardiovascular mortality over 20 years. Hypertension. 2020;75(2):302–8.
Casiglia E, Tikhonoff V, Virdis A, Masi S, Barbagallo CM, Bombelli M, Bruno B, Cicero AFG, Cirillo M, Cirillo P, Desideri G, D’Elia L, Ferri C, Galletti F, Gesualdo L, Giannattasio C, Iaccarino G, Lippa L, Mallamaci F, Maloberti A, Mazza A, Muiesan ML, Nazzaro P, Palatini P, Parati G, Pontremoli R, Quarti-Trevano F, Rattazzi M, Rivasi G, Salvetti M, Tocci G, Ungar A, Verdecchia P, Viazzi F, Volpe M, Grassi G, Borghi C, Working Group on Uric Acid and Cardiovascular Risk of the Italian Society of Hypertension (SIIA). Serum uric acid and fatal myocardial infarction: detection of prognostic cut-off values: the URRAH (Uric Acid Right for Heart Health) study. J Hypertens. 2020;38(3):412–9.
Maloberti A, Giannattasio C, Bombelli M, Desideri G, Cicero AFG, Muiesan ML, Rosei EA, Salvetti M, Ungar A, Rivasi G, Pontremoli R, Viazzi F, Facchetti R, Ferri C, Bernardino B, Galletti F, D’Elia L, Palatini P, Casiglia E, Tikhonoff V, Barbagallo CM, Verdecchia P, Masi S, Mallamaci F, Cirillo M, Rattazzi M, Pauletto P, Cirillo P, Gesualdo L, Mazza A, Volpe M, Tocci G, Iaccarino G, Nazzaro P, Lippa L, Parati G, Dell’Oro R, Quarti-Trevano F, Grassi G, Virdis A, Borghi C, Working Group on Uric Acid and Cardiovascular Risk of the Italian Society of Hypertension (SIIA). Hyperuricemia and risk of cardiovascular outcomes: the experience of the URRAH (Uric Acid Right for Heart Health) project. High Blood Press Cardiovasc Prev. 2020;27(2):121–8.
Muiesan ML, Salvetti M, Virdis A, Masi S, Casiglia E, Tikhonoff V, Barbagallo CM, Bombelli M, Cicero AFG, Cirillo M, Cirillo P, Desideri G, D’Eliak L, Ferri C, Galletti F, Gesualdo L, Giannattasio C, Iaccarino G, Mallamaci F, Maloberti A, Mazza A, Nazzaro P, Palatini P, Parati G, Pontremoli R, Rattazzi M, Rivasi G, Tocci G, Ungar A, Verdecchia P, Viazzi F, Volpe M, Grassi G, Borghi C. From the working group on uric acid, cardiovascular risk of the Italian society of hypertension. Serum uric acid, predicts heart failure in a large Italian cohort: search for a cut-off value the URic acid right for heArt health study. J Hypertens. 2021;39(1):62–9.
Huang H, Huang B, Li Y, Huang Y, Li J, Yao H, Jing X, Chen J, Wang J. Uric acid and risk of heart failure: a systematic review and meta-analysis. Eur J Heart Fail. 2014;16(1):15–24.
Ekundayo OJ, Dell’Italia LJ, Sanders PW, Arnett D, Aban I, Love TE, Filippatos G, Anker SD, Lloyd-Jones DM, Bakris G, Mujib M, Ahmed A. Association between hyperuricemia and incident heart failure among older adults: a propensity-matched study. Int J Cardiol. 2010;142(3):279–87. https://doi.org/10.1016/j.ijcard.2009.01.010 .
Doehner W, Jankowska EA, Springer J, Lainscak M, Anker SD. Uric acid and xanthine oxidase in heart failure - emerging data and therapeutic implications. Int J Cardiol. 2016;213:15–9.
Okazaki H, Shirakabe A, Matsushita M, Shi-bata Y, Sawatani T, Uchiyama S, et al. Plasma xanthine oxidoreductase activity in patients with decompensated acute heart failure re-quiring intensive care. ESC Heart Fail. 2019;6(2):336–43.
Article PubMed PubMed Central Google Scholar
Maloberti A, Bombelli M, Facchetti R, Barbagallo CM, Bernardino B, Rosei EA, Casiglia E, Cicero AFG, Cirillo M, Cirillo P, Desideri G, D’elia L, Dell’Oro R, Ferri C, Galletti F, Giannattasio C, Loreto G, Iaccarino G, Lippa L, Mallamaci F, Masi S, Mazza A, Muiesan ML, Nazzaro P, Parati G, Palatini P, Pauletto P, Pontremoli R, Quarti-Trevano F, Rattazzi M, Rivasi G, Salvetti M, Tikhonoff V, Tocci G, Ungar A, Verdecchia P, Viazzi F, Volpe M, Virdis A, Grassi G, Borghi C, Working Group on Uric Acid. Cardiovascular Risk of the Italian society of hypertension (SIIA). Relationships between diuretic-related hyperuricemia and cardiovascular events: data from the URic acid right for heArt health study. J Hypertens. 2021;39(2):333–40.
Tikhonoff V, Casiglia E, Spinella P, Barbagallo CM, Bombelli M, Cicero AFG, Cirillo M, Cirillo P, Desideri G, D’elia L, Ferri C, Galletti F, Gesualdo L, Giannattasio C, Iaccarino G, Mallamaci F, Maloberti A, Masi S, Mazza A, Muiesan ML, Nazzaro P, Palatini P, Parati G, Pontremoli R, Quarti-Trevano F, Rattazzi M, Rivasi G, Salvetti M, Tocci G, Ungar A, Verdecchia P, Viazzi F, Virdis A, Volpe M, Grassi G, Borghi C, Working Group on Uric Acid and Cardiovascular Risk of the Italian Society of Hypertension (SIIA). Identification of a plausible serum uric acid cut-off value as prognostic marker of stroke: the uric acid right for heart health (URRAH) study. J Hum Hypertens. 2022;36(11):976–82.
Ungar A, Rivasi G, Di Bari M, Virdis A, Casiglia E, Masi S, et al. The association of uric acid with mortality modifies at old age: data from the uric acid right for heart health (URRAH) study. J Hypertens. 2022;40(4):704–11.
Palatini P, Parati G, Virdis A, Reboldi G, Masi S, Mengozzi A, Casiglia E, Tikhonoff V, Cicero AFG, Ungar A, Rivasi G, Salvetti M, Barbagallo CM, Bombelli M, Dell’Oro R, Bruno B, Lippa L, D’Elia L, Verdecchia P, Angeli F, Mallamaci F, Cirillo M, Rattazzi M, Cirillo P, Gesualdo L, Mazza A, Giannattasio C, Maloberti A, Volpe M, Tocci G, Georgiopoulos G, Iaccarino G, Nazzaro P, Galletti F, Ferri C, Desideri G, Viazzi F, Pontremoli R, Muiesan ML, Grassi G, Borghi C. High heart rate amplifies the risk of cardiovascular mortality associated with elevated uric acid. Eur J Prev Cardiol. 2021;14:zwab023. https://doi.org/10.1093/eurjpc/zwab023 . ( Epub ahead of print ).
Article Google Scholar
Muiesan ML, Agabiti Rosei C, Paini A, Casiglia E, Cirillo M, Grassi G, Iaccarino G, Mallamaci F, Maloberti A, Mazza A, Mengozzi A, Palatini P, Parati G, Reboldi G, Rivasi G, Russo E, Salvetti M, Tikhonoff V, Tocci G, Borghi C, Working Group on Uric Acid and Cardiovascular Risk of the Italian Society of Hypertension (SIIA). Serum uric acid and left ventricular mass index independently predict cardiovascular mortality: The uric acid right for heart health (URRAH) project. Eur J Intern Med. 2023:S0953-6205(23)00123-1. https://doi.org/10.1016/j.ejim.2023.04.010 . ( Epub ahead of print ).
Rincon-Choles H, Jolly SE, Arrigain S, Konig V, Schold JD, Nakhoul G, et al. Impact of uric acid levels on kidney disease progression. Am J Nephrol. 2017;46(4):315–22.
De Cosmo S, Viazzi F, Pacilli A, Giorda C, Ceriello A, Gentile S, et al. Serum uric acid and risk of CKD in type 2 diabetes. Clin J Am Soc Nephrol. 2015;10(11):1921–9.
Del Pinto R, Viazzi F, Pontremoli R, Ferri C, Carubbi F, Russo E. The URRAH study. Panminerva Med. 2021;63(4):416–23.
PubMed Google Scholar
Russo E, Viazzi F, Pontremoli R, Barbagallo CM, Bombelli M, Casiglia E, et al. Association of uric acid with kidney function and albuminuria: the uric acid right for heArt health (URRAH) project. J Nephrol. 2022;35(1):211–21.
Yan D, Tu Y, Jiang F, Wang J, Zhang R, Sun X, et al. Uric acid is independently associated with diabetic kidney disease: a cross-sectional study in a Chinese population. PLoS One. 2015;10(6): e0129797.
Matsushita K, Coresh J, Sang Y, Chalmers J, Fox C, Guallar E, et al. Estimated glomerular filtration rate and albuminuria for prediction of cardiovascular outcomes: a collaborative meta-analysis of individual participant data. Lancet Diabetes Endocrinol. 2015;3(7):514–25.
Luo Q, Xia X, Li B, Lin Z, Yu X, Huang F. Serum uric acid and cardiovascular mortality in chronic kidney disease: a meta-analysis. BMC Nephrol. 2019;20(1):18.
Russo E, Viazzi F, Pontremoli R, Barbagallo CM, Bombelli M, Casiglia E, et al. Serum uric acid and kidney disease measures independently predict cardiovascular and total mortality: the uric acid right for heart health (URRAH) project. Front Cardiovasc Med. 2021;8: 713652.
Milanesi S, Verzola D, Cappadona F, Bonino B, Murugavel A, Pontremoli R, et al. Uric acid and angiotensin II additively promote inflammation and oxidative stress in human proximal tubule cells by activation of toll-like receptor 4. J Cell Physiol. 2019;234(7):10868–76.
Russo E, Bertolotto M, Zanetti V, Picciotto D, Esposito P, Carbone F, et al. Role of uric acid in vascular remodeling: cytoskeleton changes and migration in VSMCs. Int J Mol Sci. 2023;24(3):2960.
Russo E, Verzola D, Cappadona F, Leoncini G, Garibotto G, Pontremoli R, et al. The role of uric acid in renal damage - a history of inflammatory pathways and vascular remodeling. Vessel Plus. 2021;5:15.
CAS Google Scholar
Russo E, Verzola D, Leoncini G, Cappadona F, Esposito P, Pontremoli R, et al. Treating hyperuricemia: the last word hasn’t been said yet. J Clin Med. 2021;10(4):819.
Casiglia E, Tikhonoff V, Virdis A, Grassi G, Angeli F, Barbagallo CM, et al. Serum uric acid/serum creatinine ratio as a predictor of cardiovascular events. Detection of prognostic cardiovascular cut-off values. J Hypertens. 2023;41(1):180–6.
Schunk SJ, Kleber ME, März W, Pang S, Zewinger S, Triem S, et al. Genetically determined NLRP3 inflammasome activation associates with systemic inflammation and cardiovascular mortality. Eur Heart J. 2021;42(18):1742–56.
Lurbe E, Torro MI, Alvarez-Pitti J, Redon J, Borghi C, Redon P. Uric acid is linked to cardiometabolic risk factors in overweight and obese youths. J Hypertens. 2018;36(9):1840–6.
Li B, Chen L, Hu X, Tan T, Yang J, Bao W, et al. Association of serum uric acid with all-cause and cardiovascular mortality in diabetes. Diabetes Care. 2023;46(2):425–33.
Latz E, Duewell P. NLRP3 inflammasome activation in inflammaging. Semin Immunol. 2018;40:61–73.
Franceschi C, Garagnani P, Parini P, Giuliani C, Santoro A. Inflammaging: a new immune-metabolic viewpoint for age-related diseases. Nat Rev Endocrinol. 2018;14(10):576–90.
Barter PJ, Rye KA. HDL cholesterol concentration or HDL function: which matters? Eur Heart J. 2017;38(32):2487–9.
Chew NWS, Ng CH, Tan DJH, Kong G, Lin C, Chin YH, et al. The global burden of metabolic disease: data from 2000 to 2019. Cell Metabol. 2023;35(3):414-428.e413.
Article CAS Google Scholar
Masulli M, D’Elia L, Angeli F, Barbagallo CM, Bilancio G, Bombelli M, et al. Serum uric acid levels threshold for mortality in diabetic individuals: the URic acid right for heArt health (URRAH) project. Nutr Metab Cardiovasc Dis. 2022;32(5):1245–52.
Palatini P, Virdis A, Masi S, Mengozzi A, Casiglia E, Tikhonoff V, et al. Hyperuricemia increases the risk of cardiovascular mortality associated with very high HdL-cholesterol level. Nutr Metab Cardiovasc Dis. 2022;33:323.
Mengozzi A, Pugliese NR, Desideri G, Masi S, Angeli F, Barbagallo CM, et al. Serum uric acid predicts all-cause and cardiovascular mortality independently of hypertriglyceridemia in cardiometabolic patients without established CV disease: a sub-analysis of the URic acid right for heArt health (URRAH) study. Metabolites. 2023;13:2.
Pugliese NR, Mengozzi A, Virdis A, Casiglia E, Tikhonoff V, Cicero AFG, et al. The importance of including uric acid in the definition of metabolic syndrome when assessing the mortality risk. Clin Res Cardiol. 2021;110(7):1073–82.
Graham IM, Di Angelantonio E, Huculeci R, European Society of Cardiology’s Cardiovascular Risk Collaboration (CRC). New way to SCORE risk: updates on the ESC scoring system and incorporation into ESC cardiovascular prevention guidelines. Curr Cardiol Rep. 2022;24(11):1679–84.
Li X, Meng X, Timofeeva M, Tzoulaki I, Tsilidis KK, Ioannidis JP, Campbell H, Theodoratou E. Serum uric acid levels and multiple health outcomes: umbrella review of evidence from observational studies, randomized controlled trials, and mendelian randomization studies. BMJ. 2017;357:j2376.
Yokose C, McCormick N, Choi HK. The role of diet in hyperuricemia and gout. Curr Opin Rheumatol. 2021;33(2):135–44.
Drozdz D, Alvarez-Pitti J, Wójcik M, Borghi C, Gabbianelli R, Mazur A, Herceg-Čavrak V, Lopez-Valcarcel BG, Brzeziński M, Lurbe E, Wühl E. Obesity and cardiometabolic risk factors: from childhood to adulthood. Nutrients. 2021;13(11): 4176.
Cicero AFG, Fogacci F, Kuwabara M, Borghi C. Therapeutic strategies for the treatment of chronic hyperuricemia: an evidence-based update. Medicina. 2021;57(1): 58.
Sapankaew T, Thadanipon K, Ruenroengbun N, Chaiyakittisopon K, Ingsathit A, Numthavaj P, Chaiyakunapruk N, McKay G, Attia J, Thakkinstian A. Efficacy and safety of urate-lowering agents in asymptomatic hyperuricemia: systematic review and network meta-analysis of randomized controlled trials. BMC Nephrol. 2022;23(1):223.
Ying H, Yuan H, Tang X, Guo W, Jiang R, Jiang C. Impact of serum uric acid lowering and contemporary uric acid-lowering therapies on cardiovascular outcomes: a systematic review and meta-analysis. Front Cardiovasc Med. 2021;8: 641062.
Fogacci F, Borghi C, Di Micoli A, Degli Esposti D, Cicero AFG. Inequalities in enrollment of women and racial minorities in trials testing uric acid lowering drugs. Nutr Metab Cardiovasc Dis. 2021;31(12):3305–13.
Yip ASY, Leong S, Teo YH, Teo YN, Syn NLX, See RM, Wee CF, Chong EY, Lee CH, Chan MY, Yeo TC, Wong RCC, Chai P, Sia CH. Effect of sodium-glucose cotransporter-2 (SGLT2) inhibitors on serum urate levels in patients with and without diabetes: a systematic review and meta-regression of 43 randomized controlled trials. Ther Adv Chronic Dis. 2022;13:20406223221083508.
Download references
Author information
Alessandro Maloberti and Alessandro Mengozzi share the first authorship.
Authors and Affiliations
Cardiology IV, “A.De Gasperis” Department, Ospedale Niguarda Ca’ Granda, Milan, Italy
Alessandro Maloberti & Cristina Giannattasio
School of Medicine and Surgery, Milano-Bicocca University, Milan, Italy
Alessandro Maloberti, Michele Bombelli, Raffaella Dell’Oro, Rita Facchetti, Cristina Giannattasio, Gianfranco Parati, Fosca Quarti-Trevano & Guido Grassi
Department of Clinical and Experimental Medicine, University of Pisa, Pisa, Italy
Alessandro Mengozzi, Federica Cappelli, Stefano Masi, Nicola Riccardo Pugliese & Agostino Virdis
Department of Cardiology, Center for Translational and Experimental Cardiology (CTEC), University Hospital Zurich, University of Zurich, Schlieren, Switzerland
Alessandro Mengozzi
Department of Internal Medicine, University of Genoa and IRCCS Ospedale Policlinico SanMartino, Genoa, Italy
Elisa Russo, Roberto Pontremoli & Francesca Viazzi
Hypertension and Cardiovascular Risk Research Group, Department of Medical and Surgical Science, Alma Mater Studiorum University of Bologna, Bologna, Italy
Arrigo Francesco Giuseppe Cicero & Claudio Borghi
Cardiovascular Medicine Unit, IRCCS AOU S. Orsola di Bologna, Pad. 25 - 1st Floor, Via Massarenti, 9, 40138, Bologna, Italy
Department of Medicine and Surgery, University of Insubria, Varese, Italy
Fabio Angeli
Department of Medicine and Cardiopulmonary Rehabilitation, Maugeri Care and Research Institutes, IRCCS Tradate, Varese, Italy
Department of Clinical and Experimental Sciences, University of Brescia, Brescia, Italy
Enrico Agabiti Rosei, Maria Lorenza Muiesan & Massimo Salvetti
Biomedical Department of Internal Medicine and Specialistics, University of Palermo, Palermo, Italy
Carlo Maria Barbagallo
Department of Life, Health and Environmental Sciences, University of L’Aquila, L’Aquila, Italy
Bruno Bernardino, Giovambattista Desideri & Claudio Ferri
Department of Internal Medicine, Pio XI Hospital of Desio, ASST Brianza, Desio, Italy
Michele Bombelli
Department of Medicine, University of Padua, Padua, Italy
Edoardo Casiglia, Paolo Palatini, Marcello Rattazzi & Valerie Tikhonoff
Department of Translational and Precision Medicine, University of Rome La Sapienza, Rome, Italy
Rosario Cianci
Department of Medicine, Surgery and Dentistry, University of Salerno, Salerno, Italy
Michele Ciccarelli & Maria Masulli
Department of Public Health, ‘‘Federico II’’ University of Naples, Naples, Italy
Massimo Cirillo
Nephrology, Dialysis and Transplantation Unit, Department of Emergency and Organ Transplantation, ‘‘Aldo Moro’’ University of Bari, Bari, Italy
Pietro Cirillo & Loreto Gesualdo
Department of Clinical Medicine and Surgery, ‘‘Federico II’’ University of Naples, Naples, Italy
Lanfranco D’Elia & Ferruccio Galletti
Clinica Medica, San Gerardo Hospital, Monza, Italy
Raffaella Dell’Oro, Fosca Quarti-Trevano & Guido Grassi
Department of Advanced Biomedical Sciences, ‘‘Federico II’’ University of Naples, Naples, Italy
Guido Iaccarino
Italian Society of General Medicine (SIMG), Avezzano, L’Aquila, Italy
Luciano Lippa
Reggio Cal Unit, Clinical Epidemiology of Renal Diseases and Hypertension, CNR-IFC, Reggio Calabria, Italy
Francesca Mallamaci
Department of Internal Medicine, Santa Maria della Misericordia General Hospital, AULSS 5 Polesana, Rovigo, Italy
Alberto Mazza
Department of Medical Basic Sciences, Neurosciences and Sense Organs, University of Bari Medical School, Bari, Italy
Pietro Nazzaro
Department of Cardiovascular, Neural and Metabolic Sciences, San Luca Hospital, IRCCS, Istituto Auxologico Italiano, Milan, Italy
Gianfranco Parati
Medicina Interna I, Ca’ Foncello University Hospital, Treviso, Italy
Paolo Pauletto
Department of Medical and Surgical Science, University of Perugia, 06100, Perugia, Italy
Gianpaolo Reboldi
Department of Geriatric and Intensive Care Medicine, Careggi Hospital and University of Florence, Florence, Italy
Giulia Rivasi & Andrea Ungar
Hypertension Unit, Division of Cardiology, Sant’Andrea Hospital, Rome, Italy
Giuliano Tocci & Massimo Volpe
Department of Clinical and Molecular Medicine, University of Rome Sapienza, Rome, Italy
Giuliano Tocci
Hospital S. Maria della Misericordia, Perugia, Italy
Paolo Verdecchia
IRCCS San Raffaele, Rome, Italy
Massimo Volpe
You can also search for this author in PubMed Google Scholar
Corresponding author
Correspondence to Arrigo Francesco Giuseppe Cicero .
Ethics declarations
Open access funding provided by Alma Mater Studiorum - Università di Bologna within the CRUI-CARE Agreement.
Conflict of Interest
The Authors declares that there is no conflict of interest.
Rights and permissions
Open Access This article is licensed under a Creative Commons Attribution-NonCommercial 4.0 International License, which permits any non-commercial use, sharing, adaptation, distribution and reproduction in any medium or format, as long as you give appropriate credit to the original author(s) and the source, provide a link to the Creative Commons licence, and indicate if changes were made. The images or other third party material in this article are included in the article's Creative Commons licence, unless indicated otherwise in a credit line to the material. If material is not included in the article's Creative Commons licence and your intended use is not permitted by statutory regulation or exceeds the permitted use, you will need to obtain permission directly from the copyright holder. To view a copy of this licence, visit http://creativecommons.org/licenses/by-nc/4.0/ .
Reprints and permissions
About this article
Maloberti, A., Mengozzi, A., Russo, E. et al. The Results of the URRAH (Uric Acid Right for Heart Health) Project: A Focus on Hyperuricemia in Relation to Cardiovascular and Kidney Disease and its Role in Metabolic Dysregulation. High Blood Press Cardiovasc Prev 30 , 411–425 (2023). https://doi.org/10.1007/s40292-023-00602-4
Download citation
Received : 21 July 2023
Accepted : 20 September 2023
Published : 04 October 2023
Issue Date : September 2023
DOI : https://doi.org/10.1007/s40292-023-00602-4
Share this article
Anyone you share the following link with will be able to read this content:
Sorry, a shareable link is not currently available for this article.
Provided by the Springer Nature SharedIt content-sharing initiative
- Cardiovascular diseases
- Kidney function
- Metabolic syndrome
- Metabolic derangement
- Find a journal
- Publish with us
- Track your research
- - Google Chrome
Intended for healthcare professionals
- Access provided by Google Indexer
- My email alerts
- BMA member login
- Username * Password * Forgot your log in details? Need to activate BMA Member Log In Log in via OpenAthens Log in via your institution

Search form
- Advanced search
- Search responses
- Search blogs
- Serum uric acid levels...
Serum uric acid levels and multiple health outcomes: umbrella review of evidence from observational studies, randomised controlled trials, and Mendelian randomisation studies
- Related content
- Peer review
This article has a correction. Please see:
- Serum uric acid levels and multiple health outcomes: umbrella review of evidence from observational studies, randomised controlled trials, and Mendelian randomisation studies - August 08, 2017
- Xue Li , PhD student 1 ,
- Xiangrui Meng , PhD student 1 ,
- Maria Timofeeva , statistical geneticist 2 ,
- Ioanna Tzoulaki , senior lecturer 3 ,
- Konstantinos K Tsilidis , assistant professor 3 4 ,
- John PA Ioannidis , professor 5 6 7 ,
- Harry Campbell , professor 1 ,
- Evropi Theodoratou , chancellor’s fellow 1 2
- 1 Centre for Global Health Research, Usher Institute of Population Health Sciences and Informatics, University of Edinburgh, Edinburgh EH8 9AG, UK
- 2 Colon Cancer Genetics Group, Medical Research Council Human Genetics Unit, Medical Research Council Institute of Genetics & Molecular Medicine, University of Edinburgh, Edinburgh, UK
- 3 Department of Epidemiology and Biostatistics, School of Public Health, Imperial College London, London, UK
- 4 Department of Hygiene and Epidemiology, University of Ioannina School of Medicine, Ioannina, Greece
- 5 Stanford Prevention Research Center, Stanford School of Medicine, Stanford, CA, USA
- 6 Department of Health Research and Policy, Stanford School of Medicine, Stanford, CA, USA
- 7 Department of Statistics, Stanford University, Stanford, CA, USA
- Correspondence to: E Theodoratou E.Theodoratou{at}ed.ac.uk
- Accepted 10 May 2017
Objective To map the diverse health outcomes associated with serum uric acid (SUA) levels.
Design Umbrella review.
Data sources Medline, Embase, Cochrane Database of Systematic Reviews, and screening of citations and references.
Eligibility criteria Systematic reviews and meta-analyses of observational studies that examined associations between SUA level and health outcomes, meta-analyses of randomised controlled trials that investigated health outcomes related to SUA lowering treatment, and Mendelian randomisation studies that explored the causal associations of SUA level with health outcomes.
Results 57 articles reporting 15 systematic reviews and144 meta-analyses of observational studies (76 unique outcomes), 8 articles reporting 31 meta-analyses of randomised controlled trials (20 unique outcomes), and 36 articles reporting 107 Mendelian randomisation studies (56 unique outcomes) met the eligibility criteria. Across all three study types, 136 unique health outcomes were reported. 16 unique outcomes in meta-analyses of observational studies had P<10 -6 , 8 unique outcomes in meta-analyses of randomised controlled trials had P<0.001, and 4 unique outcomes in Mendelian randomisation studies had P<0.01. Large between study heterogeneity was common (80% and 45% in meta-analyses of observational studies and of randomised controlled trials, respectively). 42 (55%) meta-analyses of observational studies and 7 (35%) meta-analyses of randomised controlled trials showed evidence of small study effects or excess significance bias. No associations from meta-analyses of observational studies were classified as convincing; five associations were classified as highly suggestive (increased risk of heart failure, hypertension, impaired fasting glucose or diabetes, chronic kidney disease, coronary heart disease mortality with high SUA levels). Only one outcome from randomised controlled trials (decreased risk of nephrolithiasis recurrence with SUA lowering treatment) had P<0.001, a 95% prediction interval excluding the null, and no large heterogeneity or bias. Only one outcome from Mendelian randomisation studies (increased risk of gout with high SUA levels) presented convincing evidence. Hypertension and chronic kidney disease showed concordant evidence in meta-analyses of observational studies, and in some (but not all) meta-analyses of randomised controlled trials with respective intermediate or surrogate outcomes, but they were not statistically significant in Mendelian randomisation studies.
Conclusion Despite a few hundred systematic reviews, meta-analyses, and Mendelian randomisation studies exploring 136 unique health outcomes, convincing evidence of a clear role of SUA level only exists for gout and nephrolithiasis.
Introduction
Uric acid was thought to be a biologically inert waste product from purine metabolism, until in the early 1800s it was discovered that an increased serum uric acid (SUA) level was the cause of gout. 1 Subsequently, associations of uric acid concentration with cardiovascular and renal disorders were also observed. 2 These associations were explored in several prospective studies but yielded conflicting results, and therefore the causal role of uric acid in these diseases was widely questioned. 3 4 5 6 It was argued that these associations are either confounded by other risk factors, such as obesity and hypertension, or are representative of reverse causality. 4 7 These inconclusive findings led to a shift of interest away from uric acid, and asymptomatic hyperuricemia was not considered as an indication for SUA lowering treatment in patients with cardiovascular and renal diseases. 8 9
New findings have fuelled enthusiasm to address this longstanding controversy. 10 Recent epidemiological studies have explored associations of uric acid with a wide range of conditions (cardiovascular diseases, metabolic syndrome, diabetes, and cancer) and some intermediate phenotypes or biomarkers. 11 In an attempt to understand the possible underlying mechanisms, laboratory studies have been carried out and found that uric acid is potentially involved in multiple biological processes, including oxidative stress, systemic inflammation, and intrahepatic fructose metabolism, all mechanisms that could be linked to the development of cardiovascular disease and metabolic syndrome. 12 13 14 Alternatively, uric acid level may only present a marker of high oxidative stress associated with increased xanthine oxidase activity, instead of being an active agent in the pathogenic processes. 15 Finally, taking into account the antioxidant properties of uric acid (acting as a free radical scavenger), its potential mechanistic roles in these disorders may be complex. 16
In view of the potential importance of uric acid, assessing the credibility of the observed evidence may have implications both for clinical practice and public health. It is recognised that different types of studies have specific strengths and weaknesses that can be complementary (see box 1). An umbrella review, which collects and evaluates evidence from multiple resources systematically, might therefore help clarify the composite literature. We carried out an umbrella review of meta-analyses of observational studies, meta-analyses of randomised controlled trials, and Mendelian randomisation studies on associations between SUA level and multiple health outcomes. In particular, we summarised the range of related health outcomes, presented the magnitude, direction, and significance of the reported associations and effects, assessed the potential biases, and identified which associations and effects have the most convincing evidence.
Box 1: Strengths and limitations of study types
Although none of the following study types are infallible, all are able to provide useful information about causal inference and can complement each other to achieve increasing certainty about causality
Observational studies
Aim to examine the association between an exposure and an outcome and to test whether the association is caused by chance, bias, or confounding
Typically are affected by residual confounding, undetected bias, or reverse causality, which may generate associations that are not reliable indicators of causality
Randomised controlled trials
An approach to obtain evidence of a causal effect of a treatment or intervention on a disease process
Eliminates many of the biases and confounding factors that are present in observational studies
Limitations include non-adherence to the assigned intervention, limited external validity, short term intervention effects, and non-retention, which can all render the results invalid or questionable
High costs and ethical concerns can also limit the application of the trials in scientific research
Mendelian randomisation studies
Provide a cost effective analogy to a randomised controlled trial by using genetic variants as proxies to test the causality of an association between exposure and outcome
Is not influenced by the confounding inherent in observational studies and not seriously affected by reverse causality, but does rely on several assumptions (the genetic instruments should be associated with the exposure of interest, they should not be associated with known confounders, and they should affect the outcome solely through the exposure) that can be hard to identify and control
May lack power when the proportion of trait variance explained by the genetic instruments is small
Literature search and selection criteria
We systematically searched Medline, Embase, and the Cochrane Database of Systematic Reviews from inception to 17 July 2016 using a comprehensive search strategy (see table S1 in the web appendix) to identify systematic reviews and meta-analyses of observational studies, meta-analyses of randomised controlled trials, and Mendelian randomisation studies. All identified publications went through a three step parallel review of title, abstract, and full text (performed by XL and XM) based on predefined inclusion and exclusion criteria.
We included systematic reviews and meta-analyses of observational studies that examined associations between serum uric acid (SUA) levels (or hyperuricemia) and health outcomes; meta-analyses of randomised controlled trials that investigated health outcomes related to SUA lowering treatment (intervention with one or a combination of two or more SUA lowering drugs versus placebo or no treatment), including xanthine oxidase inhibitors (allopurinol, febuxostat, or oxypurinol), uricosuric agents (probenecid, benzbromarone, thiazides, or citrates), and uricase analogues (pegloticase or rasburicase); and Mendelian randomisation studies that explored SUA (or hyperuricemia) associations in relation to health outcomes by using genetic instruments influencing SUA levels. The identified health outcomes included a wide range of diseases, intermediate phenotypes, and biomarkers. We excluded studies investigating associations between gout and health outcomes and meta-analyses of randomised controlled trials that used non-drug interventions, such as dietary or lifestyle interventions. We further excluded animal and laboratory studies, meta-analyses on the prevalence of gout and hyperuricemia, and meta-analyses of randomised controlled trials that focused on drug variables, safety, and effects of reducing SUA levels without investigating other health effects.
Data extraction
One investigator (XL) extracted data, which were checked by a second investigator (XM). For each eligible study, we extracted the PubMed identification number, lead author’s name, journal name, publication year, study population, number of studies included, and outcomes investigated. For meta-analyses investigating more than one health outcome, we recorded each outcome separately. For meta-analyses of observational studies and of randomised controlled trials, we extracted the reported summary risk estimates (risk ratio, odds ratio, hazard ratio, or mean difference) with the 95% confidence intervals and the corresponding number of case and control participants. Furthermore, for each unique outcome we extracted data from the individual component studies that were included in the meta-analyses for further analysis. This second level extraction included data on study design, number of cases, total number of participants, relative risk estimates, and 95% confidence intervals for each component study. When more than one meta-analysis existed for the same outcome in the same population, we extracted individual component data from the most recent and largest meta-analysis. In a few exceptions where the most recent was not also the largest meta-analysis, we explored the reason for this discrepancy. If the most recent included prospective studies and the largest one had fewer prospective studies plus some retrospective data, we kept the one with the largest amount of prospective data; otherwise we kept the largest meta-analysis. For Mendelian randomisation studies, we extracted data on study population, sample size, genetic instruments, the variance of SUA level explained by the genetic instruments (R 2 ) and Mendelian randomisation effect estimates (odds ratio, hazard ratio, mean difference, or regression coefficient β), standard deviation of SUA levels, and standard deviation of continuous outcomes.
Data analysis
For systematic reviews we performed descriptive analyses and presented the authors’ conclusions. For each unique meta-analysis of observational studies and of randomised controlled trials, we estimated several metrics, including the summary effect and 95% confidence intervals using a random effect model (DerSimonian Laird method) 17 ; the heterogeneity among studies (Q statistic and I 2 metric with 95% confidence intervals); the 95% prediction interval to predict the range of effect size that would be expected in a new original study, after accounting for both the heterogeneity among individual studies and the uncertainty of the summary effect estimated in the random effect model (the calculation of 95% prediction interval is based on the predicted distribution derived from a function of the degree of heterogeneity, number of studies included, and within study standard errors) 18 19 ; the presence of small study effects by using the Egger’s regression asymmetry test to investigate if small studies tend to give larger estimates of effect size than large studies (significance threshold P<0.10) 20 ; and the excess significance test to assess if the observed number (O) of studies with significant results was greater than the expected number (E) using the χ 2 test: A=[(O−E) 2 /E+(O−E) 2 /(n−E)] (significance threshold P<0.10). 21 22 For the excess significance test, we calculated the expected number (E) of studies with significant findings by using the sum of statistical power estimated for each component study. The statistical power of each component study was calculated with an algorithm that uses a non-central t distribution, by assuming the true effect size to be the same as that of the largest component study (with smallest variance) in the meta-analysis. 23 If the type of metric in a meta-analysis was mean difference, we firstly calculated Cohen’s d by weighing the pooled standard deviation based on the sample size of individual studies. We then transformed Cohen’s d, Hedges g, and other standardised mean difference metrics to odds ratios. 24 We compared the results reported in overlapping meta-analyses to evaluate their concordance in terms of the direction and statistical significance of the observed associations. All statistical analyses were conducted in Stata (StataCorp) version 14.0.
Owing to the extensive differences in genetic instruments used in the Mendelian randomisation studies we did not conduct quantitative syntheses. Instead, we performed and present here a descriptive analysis of the individual studies. When more than one Mendelian randomisation study was conducted for the same outcome, we compared the concordance of the findings for the direction and statistical significance of the reported association and retained the study with the largest number of cases and participants for further analysis and comparison. If all of the information required for calculation was provided (ie, sample size, number of cases, R 2 , estimates of association, standard deviation of continuous outcomes, and standard deviation of SUA levels), we performed a power calculation for the largest Mendelian randomisation studies by using the non-centrality parameter based approach. 25 For Mendelian randomisation studies with missing R 2 values, we performed a crude power estimation by using the R 2 values from other Mendelian randomisation studies that used the same genetic variants as instruments.
Credibility assessment
As previously proposed, 26 we classified evidence from meta-analyses of observational studies with nominally statistically significant summary results (P<0.05) into four categories (class I, II, III, and IV). Convincing (class I) evidence was assigned to associations with a statistical significance of P<10 −6 , included more than 1000 cases (or more than 20 000 participants for continuous outcomes), had the largest component study reporting a significant result (P<0.05), had a 95% prediction interval that excluded the null, did not have large heterogeneity (I 2 <50%), and showed no evidence of small study effects (P>0.10) and of excess significance bias (P>0.10). Highly suggestive (class II) evidence was assigned to associations that reported a significance of P<0.001, included more than 1000 cases (or more than 20 000 participants for continuous outcomes), and had the largest component study reporting a statistically significant result (P<0.05). Suggestive (class III) evidence was assigned to associations that reported a significance of P<0.01 with more than 1000 cases (or more than 20 000 participants for continuous outcomes). Weak (class IV) evidence was assigned to the remaining significant associations with P<0.05. For each association in the convincing or highly suggestive categories we reassessed the evidence after excluding the retrospective and case-control studies in an attempt to address reverse causality. Finally, for each association in the convincing category we reassessed the evidence after we examined each meta-analysis in depth by assessing the eligibility of the included studies as well as verifying the data used in the meta-analysis.
Evidence from meta-analyses of randomised controlled trials was assessed in terms of the significance of the summary effect (P<0.01, 0.01≤ P<0.05, P≥0.05), 95% prediction interval (excluding the null or not), and presence of large heterogeneity (I 2 >50%), small study effects (P>0.10), and excess significance (P>0.10). We also noted the conclusions from any evidence classification (GRADE 27 or equivalent system) applied by the original meta-analyses. Finally, we assessed the evidence from individual Mendelian randomisation studies for statistical significance of the effect estimate (P<0.01) and of the statistical power (>80%). 28
For overlapping outcomes that were investigated in meta-analyses of observational studies and/or meta-analyses of randomised controlled trials and/or individual Mendelian randomisation studies, we examined if the direction and statistical significance of the associations were reported concordantly across the different study types. We noted the overlapping outcomes that were graded as class I or II in meta-analyses of observational studies and had a 95% prediction interval excluding the null in meta-analyses of randomised controlled trials. For these outcomes we also presented the evidence from Mendelian randomisation studies if available.
Patient involvement
No patients were involved in setting the research question or the outcome measures, nor were they involved in developing plans for design or implementation of the study. No patients were asked to advise on interpretation or writing up of results. There are no plans to disseminate the results of the research to study participants or the relevant patient community.

Literature review
Overall, the parallel reviews identified 4608 publications across three databases. After applying the inclusion or exclusion criteria, 101 publications were selected for inclusion (fig 1 ⇓ ). Specifically, 15 systematic reviews and 144 meta-analyses of observational studies were reported in 57 articles 29 30 31 32 33 34 35 36 37 38 39 40 41 42 43 44 45 46 47 48 49 50 51 52 53 54 55 56 57 58 59 60 61 62 63 64 65 66 67 68 69 70 71 72 73 74 75 76 77 78 79 80 81 82 83 84 85 ; 31 meta-analyses of randomised controlled trials were reported in 8 articles 86 87 88 89 90 91 92 93 ; and 107 Mendelian randomisation studies were reported in 36 articles (see tables S2 to S5, respectively, in web appendix). 94 95 96 97 98 99 100 101 102 103 104 105 106 107 108 109 110 111 112 113 114 115 116 117 118 119 120 121 122 123 124 125 126 127 128 129 Across all three study types, 136 unique outcomes were reported.
Fig 1 Study flowchart
- Download figure
- Open in new tab
- Download powerpoint
Meta-analyses of observational studies
Overall, 144 meta-analyses of observational studies were identified (see table S3 in web appendix). The median number of studies included in meta-analyses was 5 (range 2-31), the median number of participants was 7932 (129-1 017 810), and the median number of cases was 1176 (49-34 370). More than one meta-analysis was conducted for 16 outcomes (see table S3 in web appendix). The direction and statistical significance of the reported associations in overlapping meta-analyses were concordant for 14 (88%) outcomes: atrial fibrillation incidence (n=3), 39 52 82 coronary heart disease (n=4), 41 72 76 83 hypertension incidence (n=3), 44 74 85 stroke incidence (n=2), 48 75 diabetes (n=3), 49 50 79 chronic kidney disease (n=3), 54 55 77 mild cognitive impairment (n=2), 58 80 Parkinson’s disease (n=3), 58 59 81 multiple sclerosis (n=2), 60 78 coronary heart disease mortality (n=3), 41 72 76 cardiovascular disease mortality (n=2), 65 84 stroke mortality (n=2), 48 75 all cause mortality in patients with heart failure (n=2), 43 67 and all cause mortality in the general population (n=2). 65 84 Discordance in the statistical significance was present for two outcomes: diabetic neuropathy (n=2) 51 53 and Alzheimer’s disease (n=4). 57 58 73 80
After removing the overlapping meta-analyses (which were conducted in the same population for the same outcome), 76 unique meta-analyses were retained. The meta-analyses reported a wide range of outcomes (table 1 ⇓ ): cardiovascular outcomes (n=13), diabetes related outcomes (n=9), kidney disorders (n=7), neurocognitive disorders (n=11), cancer outcomes (n=6), all cause or cause specific mortality (n=22), and other outcomes (n=8). Overall, 58 (76%) of the 76 non-overlapping meta-analyses reported nominally significant summary results (P<0.05). Figures 1 and 2 in the web appendix show the summary effects of the unique meta-analyses of observational studies. Of these, 12 (92%) meta-analyses in cardiovascular outcomes, 8 (89%) in diabetes related outcomes, all 7 (100%) in kidney disorders, 1 (9%) in neurocognitive disorders, 1 (17%) in cancer outcomes, 15 (68%) in all cause and cause specific mortality, and 6 (75%) in other outcomes reported summary estimates with P<0.05 and suggested that high levels of SUA were associated with an increased risk of disease. In addition, 7 (64%) meta-analyses in neurocognitive disorders and 1 (12%) in other outcomes (composite of adverse outcomes (death or major adverse cardiovascular event) in patients with acute ischaemic stroke) reported summary estimates with P<0.05 and suggested inverse associations with SUA level.
Health outcomes and evidence class reported in meta-analyses (MA) of observational studies
- View inline
We then applied our evidence classification criteria. Sixteen (21%) meta-analyses had P<10 −6 , 10 (13%) had a 95% prediction interval that excluded the null, 27 (36%) had more than 1000 cases (or more than 20 000 participants for continuous outcomes), 15 (20%) had no large heterogeneity (I 2 <50%), and 34 (45%) had neither small study effects nor excess significant bias. Based on these metrics, only one of 76 (1%) outcomes presented convincing evidence (class I: stroke mortality in general population), 7 (9%) outcomes presented highly suggestive evidence (class II: heart failure incidence, hypertension incidence, impaired fasting glucose or diabetes, chronic kidney disease incidence, coronary heart disease mortality, all cause mortality in patients with heart failure, and non-alcoholic fatty liver disease), and 9 (12%) outcomes presented suggestive evidence (class III: atrial fibrillation, coronary heart disease incidence, cardiovascular disease, prehypertension, medium term major adverse cardiac event, type 2 diabetes, cardiovascular disease mortality, chronic kidney disease mortality, death, or cardiac events). The remaining 41 (54%) statistically significant outcomes presented weak evidence (class IV).
We performed a thorough examination and reassessed the meta-analyses of stroke mortality 48 (class I) and found that data from the largest study were incorrect (the events represented stroke incidence cases rather than stroke deaths and the included study had not published data on stroke mortality). 130 Furthermore, the data from two individual studies reported comparisons of SUA categories that differed from other studies (the highest sextile versus the second or third sextile rather than the lowest), 131 132 and a fourth study had been using only data on ischaemic stroke deaths but missing the data on haemorrhagic stroke deaths. 133 When we excluded the stroke incidence study, used the proper comparison for the other two studies, and added the missing data in the fourth study, the association with stroke mortality was not statistically significant (table 2 ⇓ ). For the highly suggestive outcomes (class II), when we limited the data to prospective cohort studies, all associations retained their ranking, except for all cause mortality in patients with heart failure and non-alcoholic fatty liver disease, which were downgraded to class III (table X in the web appendix).
Reassessing the credibility of associations with class I and II evidence reported in meta-analyses (MA) of observational studies
Meta-analyses of randomised controlled trials
We identified 31 meta-analyses of randomised controlled trials on SUA lowering treatment from eight publications (see table S4 in web appendix). The median number of studies included in the meta-analyses was 5 (range 2-10) and the median number of participants was 216 (41-738). More than one meta-analysis was found for five outcomes (see table S4 in web appendix). The direction and statistical significance of the effects in overlapping meta-analyses were in concordance only for one (20%) outcome: serum creatinine level (n=2). 88 89 Discordance in either the direction and/or the statistical significance was found for the remaining four outcomes: glomerular filtration rate (n=2), 88 89 end stage kidney disease (n=2), 88 89 systolic blood pressure (n=2), 89 93 and diastolic blood pressure (n=2). 89 93
Twenty unique meta-analyses (table 3 ⇓ ) were identified for the outcomes in relation to kidney disorders (n=10), endothelial function (n=2), all cause and cause specific mortality (n=4), and other outcomes (n=4). Figure 3 in the web appendix shows the summary effects of the unique meta-analyses of randomised controlled trials. Overall, 12 (60%) reported a nominally significant summary result at P<0.05 (8 had P<0.001). Only three (15%) meta-analyses had a 95% prediction interval that excluded the null (two nephrolithiasis outcomes (with thiazide and citrate treatment) and one renal function outcome), 11 (55%) meta-analyses showed no large heterogeneity (I 2 <50%), and 13 (65%) meta-analyses showed neither small study effects nor excess significant bias.
Health outcomes reported in meta-analyses of randomised controlled trials
Only one outcome (recurrence of nephrolithiasis with citrates treatment) reported a P<0.001, had a 95% prediction interval excluding the null, and had no evidence of large heterogeneity or bias. In the original meta-analyses, the strength of evidence was graded collectively for three nephrolithiasis outcomes (thiazide, citrate, or allopurinol treatment) by using an approach conceptually similar to the GRADE ranking system, 134 and evidence for these three nephrolithiasis outcomes was graded as moderate.
A total of 107 Mendelian randomisation analyses were identified from 36 publications (see table S5 in web appendix). The median number of participants was 7158 (range 343-206 822) and median number of cases was 2225 (19-65 877). The proportion of variance in SUA level (R 2 ) explained by genetic instruments was 2-6%. More than one Mendelian randomisation study was identified for 14 outcomes (see table S5 in web appendix). Discordance in either the direction and/or the statistical significance of association among overlapping Mendelian randomisation existed for all the identified outcomes: body mass index (n=7), 95 96 101 102 110 115 121 bone mineral density in femoral neck (n=2), 97 98 coronary heart disease (n=5), 96 100 106 118 126 diastolic blood pressure (n=7), 96 101 106 110 119 121 124 systolic blood pressure (n=7), 96 101 106 110 119 121 124 metabolic syndrome (n=2), 107 120 glucose level (n=3), 96 106 121 triglyceride level (n=3), 96 121 123 diabetes (n=6), 96 99 105 100 122 127 serum creatinine level (n=2), 110 129 estimated glomerular filtration rate (n=5), 106 110 121 128 129 Parkinson’s disease (n=5), 111 112 116 117 125 memory performance (n=2), 114 and gout (n=3). 99 100 106
The 56 unique outcomes (table 4 ⇓ ) investigated in individual Mendelian randomisation studies belonged to the following categories: anthropometric variables (n=9), cardiovascular outcomes (n=15), kidney disorders (n=6), metabolic disorders (n=5), neurocognitive disorders (n=5), metabolites (n=11), all cause and cause specific mortality (n=3), and other outcomes (n=2). Only nine (16%) outcomes (diabetic macrovascular disease, arterial stiffness (internal diameter of carotid artery), adverse renal events, Parkinson’s disease, lifetime anxiety disorders, memory performance, cardiovascular disease mortality, sudden cardiac death, and gout) presented significant associations of P<0.05. Three Mendelian randomisation studies (on memory performance, Parkinson’s disease, and gout) reported discordant results in the direction and/or statistical significance in other Mendelian randomisation studies. Of note, only four outcomes (diabetic macrovascular disease, arterial stiffness (internal diameter of carotid artery), renal events, and gout) reported a P<0.01, and only that for gout was based on convincing evidence (P=3.55E-40, n=71 501, power >99%).
Health outcomes reported in Mendelian randomisation studies
Comparison of findings from meta-analyses
Table 5 ⇓ summarises the outcomes reported in meta-analyses of observational studies with highly suggestive evidence or meta-analyses of randomised controlled trials with 95% prediction intervals excluding the null. Among these outcomes, hypertension and chronic kidney disease showed concordant evidence between meta-analyses of observational studies and the selected (largest) meta-analyses of randomised controlled trials on their corresponding intermediate traits or surrogate outcomes (eg, systolic blood pressure, diastolic blood pressure, serum creatinine level, estimated glomerular filtration rate, and end stage renal disease) but had discordant evidence from Mendelian randomisation studies. Moreover, even for these outcomes there were additional meta-analyses of randomised controlled trials that had found discordant effects in terms of direction and/or statistical significance for all these intermediate traits or surrogate outcomes, with the exception of serum creatinine level. Heart failure, impaired fasting glucose or diabetes, and coronary heart disease mortality showed no evidence from meta-analyses of randomised controlled trials, and Mendelian randomisation studies reported discordant evidence on the corresponding outcomes, the intermediate traits, or the surrogate outcomes. Recurrence of nephrolithiasis was only reported in meta-analysis of randomised controlled trials, and no evidence was found from meta-analyses of observational studies or Mendelian randomisation studies.
Summary of evidence grading and comparison of multiple evidence
In this study, we provide a comprehensive overview of reported associations between serum uric acid (SUA) levels and a wide range of health outcomes by incorporating evidence from systematic reviews and meta-analyses of observational studies, meta-analyses of randomised controlled trials, and Mendelian randomisation studies. We also further evaluated the reported evidence by following criteria that we have previously applied to appraise the epidemiological credibility in several research specialties. 26 135 136 Our study comprised 76 unique meta-analyses of observational studies, 20 unique meta-analyses of randomised controlled trials, and 56 unique individual Mendelian randomisation studies, which overall covered 136 unique health outcomes.
Main findings and possible explanations
Most health outcomes that were reported to be associated with SUA level were identified from meta-analyses of observational studies, but after the application of our criteria none of them were classified as convincing (class I). Highly suggestive evidence (class II) existed for five health outcomes, including heart failure, hypertension, impaired fasting glucose or diabetes, chronic kidney disease, and coronary heart disease mortality in the general population. Notably, a large proportion (80%) of the examined meta-analyses displayed substantial heterogeneity (I 2 >50%), indicating that these associations should be interpreted with caution. Possible sources of the observed heterogeneity include the mixture of prospective, retrospective, or case-control studies and the mixture of different comparison groups, since some meta-analyses synthesised individual studies with diverse contrasted categories of SUA levels (eg, various choices of tertiles, quartiles, quintiles, or sextiles of SUA levels). Likewise, although the outcomes with class I or II evidence fulfilled the criteria of credibility assessment for meta-analyses of observational studies, it would be inadvisable to conclude causation on this basis alone, owing to the inherent limitations of unmeasured confounding, undetected bias, or reverse causality in observational studies. In relation to reverse causality for example, some of the associations that were initially classified as class II (eg, all cause mortality in patients with heart failure and non-alcoholic fatty liver disease), were no longer highly suggestive (and were downgraded to class III) when focusing on prospective observational data and excluding the retrospective studies.
Current evidence from meta-analyses of randomised controlled trials was limited to the beneficial effects of SUA lowering treatment on some intermediate traits or biomarkers related to cardiovascular and renal disorders (eg, blood pressure, endothelial functions, and renal function). However, when multiple meta-analyses of randomised controlled trials existed for traits or markers, often the results were not concordant in direction of effect and/or statistical significance. Although 12 health outcomes had P<0.05, only recurrence of nephrolithiasis with citrate treatment achieved P<0.001, with 95% prediction interval excluding the null. Two additional health outcomes (recurrence of nephrolithiasis using thiazides and end stage renal disease in patients with coronary heart disease using allopurinol) also had a 95% prediction interval excluding the null. Large heterogeneity and evidence of bias were common even in meta-analyses of randomised controlled trials (in 45% of meta-analyses and 35% of randomised controlled trials). When incorporating evidence from meta-analyses of randomised controlled trials with that from meta-analyses of observational studies, there was a notable gap, as health outcomes that were investigated in meta-analyses of observational studies and classified as class I or II have generally not been evaluated in meta-analyses of randomised controlled trials. In a few cases, data from randomised controlled trials on surrogate outcomes (eg, systolic blood pressure, diastolic blood pressure, and renal function tests) that corresponded to disease outcomes in observational studies (hypertension, chronic kidney disease) were available, but conclusions from extrapolation of surrogate outcomes, which were evaluated in short term trials, to long term clinical outcomes should be treated with caution.
As an alternative to randomised controlled trials, the Mendelian randomisation design has been developed for exploring the causal effect of biomarkers on health outcomes. Fifty six Mendelian randomisation studies were identified that explored the causal role of SUA in cardiovascular, metabolic, neurocognitive, and renal disorders or related traits and biomarkers. In contrast with the meta-analyses of observational studies where most of the results (76%) were significant at P<0.05, most (84%) health outcomes investigated in Mendelian randomisation studies were not statistically significant. The generally negative results across so many health outcomes suggest that the large effects have probably not been missed, but most of the included Mendelian randomisation studies could have been underpowered to detect modest effects. When retaining the largest Mendelian randomisation study for each health outcome, significant results with P<0.05 were only reported for nine health outcomes, and only four of these health outcomes (diabetic macrovascular disease, arterial stiffness (internal diameter of carotid artery), renal events, and gout) had P<0.01, whereas only the gout outcome was based on evidence from a Mendelian randomisation study with adequate power. Of the other five health outcomes with P<0.05, Parkinson’s disease and memory performance had at least one other Mendelian randomisation study that was not significant or had an association in the opposite direction.
Several instrumental variable assumptions need to be fulfilled for the results of a Mendelian randomisation analysis to be valid. The first assumption states that the genetic instrument should be strongly associated with the intermediate phenotype. SUA level has an evident heritable component with an overall heritability of 40-60%, 137 but the strength of genetic instruments used in Mendelian randomisation studies was small or moderate, accounting for only 2-6% of SUA variance. Currently, the proportion of SUA variance explained by all common genetic variants identified by a genome wide association study remains relatively small (7%). 138 This limits the power of genetic instruments to detect causal associations with SUA level. The second and third assumptions (the instrument is associated with the outcome through the studied exposure only and the genotype is independent of other factors that affect the outcome) are more difficult to evaluate given the largely unknown complexity and interconnectedness of biological pathways underlying the genetic variants related to SUA level. The included Mendelian randomisation studies tried to validate these assumptions either by excluding single nucleotide polymorphisms related to other known confounding factors, by excluding single nucleotide polymorphisms that had potential pleiotropic effects, or by applying new Mendelian randomisation methods to account for pleiotropic effects (eg, Egger Mendelian randomisation analysis or network Mendelian randomisation).
Clinical implications and future research
Current recommendations on the drug treatment of hyperuricemia are related to gout or nephrolithiasis. 8 Since a wide range of health outcomes has been identified to be associated with SUA level, a renewed interest in whether individuals with asymptomatic hyperuricemia should be treated with SUA lowering drugs for the prevention or treatment of associated cardiovascular and metabolic diseases. In this study we raised large uncertainty about the potential therapeutic benefits of an expansion of SUA lowering treatment. Although we identified some highly suggestive associations from observational studies, there was a lack of concordance with clinically relevant endpoints from randomised controlled trials or surrogate endpoints from Mendelian randomisation studies, and therefore evidence is insufficient to support any SUA lowering drug intervention for these outcomes. Furthermore, the adverse effects of SUA lowering drugs should be taken into consideration (eg, an estimated 0.1% of patients treated with allopurinol, the first line SUA lowering drug, develop allopurinol hypersensitivity syndrome, which can be life threatening). 9
Our study does not support one of the recommendations in the recently updated European League Against Rheumatism gout treatment guidelines, which suggest that SUA level <3.0 mg/dL is not recommended for gout management in the long term. 139 This recommendation is based on several observational studies in which low SUA levels were associated with increased risk of multiple neurological diseases, including Alzheimer’s disease and Parkinson’s disease. 140 141 142 However, in our umbrella review a number of meta-analyses reported nominally statistically significant associations of low SUA levels with increased risk of multiple neurological diseases, but several other meta-analyses (9 out of 28) did not support these findings. Moreover, our credibility assessment showed that the nominally significant associations were consistent with class IV evidence, and a causal effect has not consistently been established for any neurological disease in Mendelian randomisation studies. Therefore, there is no adequate evidence against lowering SUA levels in patients with gout because of an increased risk of neurological diseases.
For future research, efforts to address the limitations and caveats in current evidence will be beneficial. In particular, as the current clinical trials of SUA lowering treatment largely focus on the effect of allopurinol on some intermediate traits or biomarkers, the effect of SUA reduction on clinically relevant endpoints of the convincing and highly suggestive associations might be worth further investigation. In addition, efforts to evaluate whether other SUA lowering agents have the same effect as xanthine oxidase inhibitors will help to determine if these effects are truly due to the SUA reduction itself rather than the mechanisms of xanthine oxidase inhibition. Finally, noting the largely discordant evidence in Mendelian randomisation studies, better designed such studies with collaboration of large international consortiums might assist in deciding whether the lack of replication of highly suggestive findings of observational studies is owing to low power to detect moderate or small effects, or owing to truly negative effects.
Strengths and weaknesses of this review
The strengths of umbrella reviews have been described in detail. 26 135 136 Here we summarised and presented the evidence of the associations between SUA level and a wide spectrum of health related outcomes systematically and thoroughly by incorporating information from meta-analyses of observational studies, meta-analyses of randomised controlled trials, and Mendelian randomisation studies. We then calculated a number of additional metrics and applied well defined criteria to assess the credibility of the observed associations.
In relation to study weaknesses, umbrella reviews focus on existing meta-analyses and therefore outcomes that were not assessed in a meta-analysis are not included in the review. For example, we found no formal meta-analysis of observational studies on SUA level and urolithiasis or gout, even though these associations are well established. Although there are some differences in SUA levels between men and women, there is not sufficient evidence at a meta-analysis level and therefore we did not attempt to perform subgroup analyses by sex. To avoid subjectivity, we did not include reviews without explicit systematic literature searches, but this could limit the breadth of the results to some extent, if some non-systematic reviews cover questions that have not been addressed by systematic reviews. 143 144 Furthermore, we did not appraise the quality of the individual studies, since this should be the responsibility of the authors of the original meta-analysis and it was beyond the scope of the current umbrella review.
We adopted credibility assessment criteria, which were based on established tools for observational evidence, and their individual limitations have been summarised previously. 26 135 136 None of the components of these criteria provides firm proof of lack of reliability, but they cumulatively map the possibility that the results are susceptible to bias and uncertainty. Given the wide variety of study designs and populations considered in several of the meta-analyses, one might claim that large heterogeneity in particular may not necessarily be worrisome. However, considering it is difficult to differentiate the real heterogeneity from the heterogeneity that reflects some forms of bias or uncertainty, we applied I 2 <50% as one of the criteria for class I evidence (convincing) for meta-analyses of observational studies, so as to assign the top evidence grade only to associations that are most robust and without hints of bias. In most cases I 2 >50% indicates the presence of component studies with opposite effects or of component studies with and without statistically significant associations. However, nine meta-analyses of observational studies classified as class II, III, or IV had an I 2 >50%, with all component studies reporting a statistically significant association of the same direction. Only one of these nine meta-analyses (heart failure incidence) would be upgraded from class II to class I if we did not consider the heterogeneity criterion, since the other eight also failed additional class I criteria. No meta-analyses of randomised controlled trials had an I 2 >50% with all component studies reporting a statistically significant association with the same direction.
Finally, another limitation of the umbrella review approach is the use of existing meta-analyses taking their results at face value. Meta-analyses are known to have common flaws 145 and their results may also depend on choices made about what estimates to select from each primary study and how to represent them in the meta-analysis (eg, in what contrast of exposure levels). This may be a common problem when the factor of interest is continuous, as in the case of SUA level, and where different comparisons of levels of the risk factor may be selected to express risk. 146 We therefore decided to investigate any meta-analyses with seemingly convincing evidence in more detail. In this process, the only meta-analysis that seemed to achieve convincing evidence (class I: stroke mortality) was found to actually have major flaws. Recalculation of the results showed that the evidence was downgraded to not statistically significant. It is possible that similar thorough evaluations might have downgraded the credibility of some additional meta-analyses. In addition, we noted that many primary studies are represented in the calculations of meta-analyses by using only a small subset of the data of extreme groups (eg, as the risk ratio for an event in extreme quintiles of SUA levels). In these cases, the number of events pertinent to these extreme groups may be much fewer than the total number of events used in calculating the amount of evidence criteria. Therefore, some meta-analyses that seemingly include studies with more than 1000 cases may actually capture fewer than 1000 cases in the main calculations and thus their grading appraisal should have been weaker. These flaws and deficiencies are difficult to decipher without a thorough reconstruction of all observational meta-analyses, and they may explain why observational evidence for SUA associations generally did not show good concordance with evidence from randomised controlled trials and Mendelian randomisation studies in our umbrella evaluation.
Meta-analyses of observational data for SUA level and other risk factors need to be strengthened. For continuous putative risk factors such as SUA concentration, a consensus on the categorisation of levels of interest would be useful. This might be achieved by careful meta-analyses of individual level data in inclusive consortiums. This approach would allow a more accurate and reliable exploration of both linear and non-linear associations (eg, the possibility of U-shaped associations with increased risk at both very high and very low levels). Currently available data from meta-analyses do not allow for consistent handling and assessment of such non-linear relations. Conversely, data dredging using different categorisations of SUA levels for comparison is likely to fuel a literature with spurious associations. 147
This comprehensive umbrella review will help investigators to judge the relative priority of health outcomes related to SUA level for future research and clinical management of disease. In summary, despite a few hundred systematic reviews, meta-analyses, and Mendelian randomisation studies exploring 136 unique health outcomes, convincing evidence of a clear role of SUA level only exists for gout and nephrolithiasis. Concordant evidence between observational studies and randomised controlled trials existed for hypertension and chronic kidney disease, but a potential causal role of SUA level for these outcomes has not been verified by current Mendelian randomisation studies and even for these two outcomes not all meta-analyses of randomised controlled trials are concordant among themselves and with observational evidence. Therefore, the available evidence does not support any change in the existing clinical recommendations in relation to hyperuricemia.
What is already known on this topic
Observational studies suggest that high serum uric acid (SUA) levels are associated with multiple health outcomes, including cardiovascular and metabolic diseases (increased risk) or neurological diseases (decreased risk), yet it remains to be determined whether these observed associations are causal
Clinical trials of SUA lowering have shown that xanthine oxidase inhibition decreases blood pressure and improves renal function
There is still debate as to whether SUA level is simply a marker of xanthine oxidase activity or a causal factor involved in systemic inflammation
What this study adds
Of the 136 health outcomes related to SUA level that were examined in meta-analyses of observational studies, meta-analyses of randomised controlled trials, and Mendelian randomisation studies, convincing evidence of a clear association exists only for gout and nephrolithiasis
The available evidence does not support any change in the existing clinical recommendations in relation to hyperuricemia
Contributors: ET and HC conceived the study and JPAI contributed to the design. XL and XM performed the systematic review and data extraction. XL performed the statistical analysis. XL and ET wrote the manuscript. HC, JPAI, KKT, MT, and IT critically reviewed the manuscript and contributed important intellectual content. All authors have read and approved the final manuscript as submitted. ET is the guarantor.
Funding: XL and XM are supported by the China Scholarship Council. ET is supported by a CRUK Career Development Fellowship.
Competing interests: All authors have completed the ICMJE uniform disclosure form at www.icmje.org/coi_disclosure.pdf (available on request from the corresponding author) and declare: no support from any organisation for the submitted work; no financial relationships with any organisations that might have an interest in the submitted work in the previous three years; no other relationships or activities that could appear to have influenced the submitted work.
Ethical approval: Not required.
Data sharing: No additional data available.
Transparency: The manuscript’s guarantor (ET) affirms that the manuscript is an honest, accurate, and transparent account of the study being reported; that no important aspects of the study have been omitted; and that any discrepancies from the study as planned (and, if relevant, registered) have been explained.
This is an Open Access article distributed in accordance with the Creative Commons Attribution Non Commercial (CC BY-NC 4.0) license, which permits others to distribute, remix, adapt, build upon this work non-commercially, and license their derivative works on different terms, provided the original work is properly cited and the use is non-commercial. See: http://creativecommons.org/licenses/by-nc/4.0/ .
- ↵ Garrod AB. Observations on certain pathological conditions of the blood and urine, in gout, rheumatism, and Bright’s disease. Med Chir Trans 1848 ; 31 : 83 - 97 . doi:10.1177/095952874803100109 pmid:20895908 . OpenUrl
- ↵ Davis NS Jr. The cardio-vascular and renal relations and manifestations of gout. JAMA 1897 ; XXIX : 261 - 2 doi:10.1001/jama.1897.02440320005001a . OpenUrl
- ↵ Freedman DS, Williamson DF, Gunter EW, Byers T. Relation of serum uric acid to mortality and ischemic heart disease. The NHANES I Epidemiologic Follow-up Study. Am J Epidemiol 1995 ; 141 : 637 - 44 . doi:10.1093/oxfordjournals.aje.a117479 pmid:7702038 . OpenUrl
- ↵ Culleton BF, Larson MG, Kannel WB, Levy D. Serum uric acid and risk for cardiovascular disease and death: the Framingham Heart Study. Ann Intern Med 1999 ; 131 : 7 - 13 . doi:10.7326/0003-4819-131-1-199907060-00003 pmid:10391820 . OpenUrl
- ↵ Wannamethee SG, Shaper AG, Whincup PH. Serum urate and the risk of major coronary heart disease events. Heart 1997 ; 78 : 147 - 53 . doi:10.1136/hrt.78.2.147 pmid:9326988 . OpenUrl
- ↵ Vaccarino V, Krumholz HM. Risk factors for cardiovascular disease: one down, many more to evaluate. Ann Intern Med 1999 ; 131 : 62 - 3 . doi:10.7326/0003-4819-131-1-199907060-00012 pmid:10391817 . OpenUrl
- ↵ Feig DI, Kang D-H, Johnson RJ. Uric acid and cardiovascular risk. N Engl J Med 2008 ; 359 : 1811 - 21 . doi:10.1056/NEJMra0800885 pmid:18946066 . OpenUrl
- ↵ Khanna D, Fitzgerald JD, Khanna PP, et al. American College of Rheumatology. 2012 American College of Rheumatology guidelines for management of gout. Part 1: systematic nonpharmacologic and pharmacologic therapeutic approaches to hyperuricemia. Arthritis Care Res (Hoboken) 2012 ; 64 : 1431 - 46 . doi:10.1002/acr.21772 pmid:23024028 . OpenUrl
- ↵ Stamp L, Dalbeth N. Urate-lowering therapy for asymptomatic hyperuricaemia: A need for caution. Semin Arthritis Rheum 2016 . pmid:27591828 .
- ↵ Nakagawa T, Kang DH, Feig D, et al. Unearthing uric acid: an ancient factor with recently found significance in renal and cardiovascular disease. Kidney Int 2006 ; 69 : 1722 - 5 . doi:10.1038/sj.ki.5000391 pmid:16598194 . OpenUrl
- ↵ Soltani Z, Rasheed K, Kapusta DR, Reisin E. Potential role of uric acid in metabolic syndrome, hypertension, kidney injury, and cardiovascular diseases: is it time for reappraisal? Curr Hypertens Rep 2013 ; 15 : 175 - 81 . doi:10.1007/s11906-013-0344-5 pmid:23588856 . OpenUrl
- ↵ George J, Struthers AD. Role of urate, xanthine oxidase and the effects of allopurinol in vascular oxidative stress. Vasc Health Risk Manag 2009 ; 5 : 265 - 72 . doi:10.2147/VHRM.S4265 pmid:19436671 . OpenUrl
- ↵ Meotti FC, Jameson GN, Turner R, et al. Urate as a physiological substrate for myeloperoxidase: implications for hyperuricemia and inflammation. J Biol Chem 2011 ; 286 : 12901 - 11 . doi:10.1074/jbc.M110.172460 pmid:21266577 . OpenUrl
- ↵ Nakagawa T, Hu H, Zharikov S, et al. A causal role for uric acid in fructose-induced metabolic syndrome. Am J Physiol Renal Physiol 2006 ; 290 : F625 - 31 . doi:10.1152/ajprenal.00140.2005 pmid:16234313 . OpenUrl
- ↵ Pasalic D, Marinkovic N, Feher-Turkovic L. Uric acid as one of the important factors in multifactorial disorders--facts and controversies. Biochem Med (Zagreb) 2012 ; 22 : 63 - 75 . doi:10.11613/BM.2012.007 pmid:22384520 . OpenUrl
- ↵ Alvarez-Lario B, Macarrón-Vicente J. Is there anything good in uric acid? QJM 2011 ; 104 : 1015 - 24 . doi:10.1093/qjmed/hcr159 pmid:21908382 . OpenUrl
- ↵ DerSimonian R, Laird N. Meta-analysis in clinical trials. Control Clin Trials 1986 ; 7 : 177 - 88 . doi:10.1016/0197-2456(86)90046-2 pmid:3802833 . OpenUrl
- ↵ Higgins JP, Thompson SG, Spiegelhalter DJ. A re-evaluation of random-effects meta-analysis. J R Stat Soc Ser A Stat Soc 2009 ; 172 : 137 - 59 . doi:10.1111/j.1467-985X.2008.00552.x pmid:19381330 . OpenUrl
- ↵ Higgins JP. Commentary: Heterogeneity in meta-analysis should be expected and appropriately quantified. Int J Epidemiol 2008 ; 37 : 1158 - 60 . doi:10.1093/ije/dyn204 pmid:18832388 . OpenUrl
- ↵ Egger M, Davey Smith G, Schneider M, Minder C. Bias in meta-analysis detected by a simple, graphical test. BMJ 1997 ; 315 : 629 - 34 . doi:10.1136/bmj.315.7109.629 pmid:9310563 . OpenUrl
- ↵ Ioannidis JP. Excess significance bias in the literature on brain volume abnormalities. Arch Gen Psychiatry 2011 ; 68 : 773 - 80 . doi:10.1001/archgenpsychiatry.2011.28 pmid:21464342 . OpenUrl
- ↵ Ioannidis JP, Trikalinos TA. An exploratory test for an excess of significant findings. Clin Trials 2007 ; 4 : 245 - 53 . doi:10.1177/1740774507079441 pmid:17715249 . OpenUrl
- ↵ Ioannidis JPA. Clarifications on the application and interpretation of the test for excess significance and its extensions. J Math Psychol 2013 ; 57 : 184 - 7 doi:10.1016/j.jmp.2013.03.002 . OpenUrl
- ↵ Chinn S. A simple method for converting an odds ratio to effect size for use in meta-analysis. Stat Med 2000 ; 19 : 3127 - 31 . doi:10.1002/1097-0258(20001130)19:22<3127::AID-SIM784>3.0.CO;2-M pmid:11113947 . OpenUrl
- ↵ Brion MJ, Shakhbazov K, Visscher PM. Calculating statistical power in Mendelian randomization studies. Int J Epidemiol 2013 ; 42 : 1497 - 501 . doi:10.1093/ije/dyt179 pmid:24159078 . OpenUrl
- ↵ Bellou V, Belbasis L, Tzoulaki I, Evangelou E, Ioannidis JP. Environmental risk factors and Parkinson’s disease: An umbrella review of meta-analyses. Parkinsonism Relat Disord 2016 ; 23 : 1 - 9 . doi:10.1016/j.parkreldis.2015.12.008 pmid:26739246 . OpenUrl
- ↵ Kavanagh BP. The GRADE system for rating clinical guidelines. PLoS Med 2009 ; 6 : e1000094 . doi:10.1371/journal.pmed.1000094 pmid:19753107 . OpenUrl
- ↵ Pierce BL, Ahsan H, Vanderweele TJ. Power and instrument strength requirements for Mendelian randomization studies using multiple genetic variants. Int J Epidemiol 2011 ; 40 : 740 - 52 . doi:10.1093/ije/dyq151 pmid:20813862 . OpenUrl
- ↵ Baker JF, Krishnan E, Chen L, Schumacher HR. Serum uric acid and cardiovascular disease: recent developments, and where do they leave us? Am J Med 2005 ; 118 : 816 - 26 . doi:10.1016/j.amjmed.2005.03.043 pmid:16084170 . OpenUrl
- ↵ Strazzullo P, Puig JG. Uric acid and oxidative stress: relative impact on cardiovascular risk? Nutr Metab Cardiovasc Dis 2007 ; 17 : 409 - 14 . doi:10.1016/j.numecd.2007.02.011 pmid:17643880 . OpenUrl
- ↵ Barron E, Lara J, White M, Mathers JC. Blood-borne biomarkers of mortality risk: systematic review of cohort studies. PLoS One 2015 ; 10 : e0127550 . doi:10.1371/journal.pone.0127550 pmid:26039142 . OpenUrl
- ↵ Dimitroula HV, Hatzitolios AI, Karvounis HI. The role of uric acid in stroke: the issue remains unresolved. Neurologist 2008 ; 14 : 238 - 42 . doi:10.1097/NRL.0b013e31815c666b pmid:18617849 . OpenUrl
- ↵ Hwu CM, Lin KH. Uric acid and the development of hypertension. Med Sci Monit 2010 ; 16 : RA224 - 30 . pmid:20885365 . OpenUrl
- ↵ Avram Z, Krishnan E. Hyperuricaemia--where nephrology meets rheumatology. Rheumatology (Oxford) 2008 ; 47 : 960 - 4 . doi:10.1093/rheumatology/ken070 pmid:18443007 . OpenUrl
- ↵ Feig DI. Uric acid: a novel mediator and marker of risk in chronic kidney disease? Curr Opin Nephrol Hypertens 2009 ; 18 : 526 - 30 . doi:10.1097/MNH.0b013e328330d9d0 pmid:19654543 . OpenUrl
- ↵ Alonso A, Sovell KA. Gout, hyperuricemia, and Parkinson’s disease: a protective effect? Curr Rheumatol Rep 2010 ; 12 : 149 - 55 . doi:10.1007/s11926-010-0083-4 pmid:20425025 . OpenUrl
- ↵ Chang YT, Chang WN, Tsai NW, et al. The roles of biomarkers of oxidative stress and antioxidant in Alzheimer’s disease: a systematic review. Biomed Res Int 2014 ; 2014 : 182303 . doi:10.1155/2014/182303 pmid:24949424 . OpenUrl
- ↵ Cnossen JS, de Ruyter-Hanhijärvi H, van der Post JA, Mol BW, Khan KS, ter Riet G. Accuracy of serum uric acid determination in predicting pre-eclampsia: a systematic review. Acta Obstet Gynecol Scand 2006 ; 85 : 519 - 25 . doi:10.1080/00016340500342037 pmid:16755708 . OpenUrl
- ↵ Zhang CH, Huang DS, Shen D, et al. Association Between Serum Uric Acid Levels and Atrial Fibrillation Risk. Cell Physiol Biochem 2016 ; 38 : 1589 - 95 . doi:10.1159/000443099 pmid:27082929 . OpenUrl
- ↵ Zhao J, Liu T, Korantzopoulos P, et al. Association between serum uric acid and atrial fibrillation recurrence following catheter ablation: A meta-analysis. Int J Cardiol 2016 ; 204 : 103 - 5 . doi:10.1016/j.ijcard.2015.11.167 pmid:26655551 . OpenUrl
- ↵ Li M, Hu X, Fan Y, et al. Hyperuricemia and the risk for coronary heart disease morbidity and mortality a systematic review and dose-response meta-analysis. Sci Rep 2016 ; 6 : 19520 . doi:10.1038/srep19520 pmid:26814153 . OpenUrl
- ↵ Qin T, Zhou X, Wang J, et al. Hyperuricemia and the Prognosis of Hypertensive Patients: A Systematic Review and Meta-Analysis. J Clin Hypertens (Greenwich) 2016 ; 18 : 1268 - 78 . doi:10.1111/jch.12855 pmid:27247021 . OpenUrl
- ↵ Huang H, Huang B, Li Y, et al. Uric acid and risk of heart failure: a systematic review and meta-analysis. Eur J Heart Fail 2014 ; 16 : 15 - 24 . doi:10.1093/eurjhf/hft132 pmid:23933579 . OpenUrl
- ↵ Wang J, Qin T, Chen J, et al. Hyperuricemia and risk of incident hypertension: a systematic review and meta-analysis of observational studies. PLoS One 2014 ; 9 : e114259 . doi:10.1371/journal.pone.0114259 pmid:25437867 . OpenUrl
- ↵ Jiang M, Gong D, Fan Y. Serum uric acid levels and risk of prehypertension: a meta-analysis. Clin Chem Lab Med 2016 . pmid:27343474 .
- ↵ Song X, Wang Y, Hou X, et al. Association between hyperuricemia and clinical adverse outcomes after percutaneous coronary intervention: A meta-analysis. Int J Cardiol 2015 ; 201 : 658 - 62 . doi:10.1016/j.ijcard.2015.07.074 pmid:26363629 . OpenUrl
- ↵ Trkulja V, Car S. On-admission serum uric acid predicts outcomes after acute myocardial infarction: systematic review and meta-analysis of prognostic studies. Croat Med J 2012 ; 53 : 162 - 72 . doi:10.3325/cmj.2012.53.162 pmid:22522995 . OpenUrl
- ↵ Li M, Hou W, Zhang X, Hu L, Tang Z. Hyperuricemia and risk of stroke: a systematic review and meta-analysis of prospective studies. Atherosclerosis 2014 ; 232 : 265 - 70 . doi:10.1016/j.atherosclerosis.2013.11.051 pmid:24468137 . OpenUrl
- ↵ Kodama S, Saito K, Yachi Y, et al. Association between serum uric acid and development of type 2 diabetes. Diabetes Care 2009 ; 32 : 1737 - 42 . doi:10.2337/dc09-0288 pmid:19549729 . OpenUrl
- ↵ Jia Z, Zhang X, Kang S, Wu Y. Serum uric acid levels and incidence of impaired fasting glucose and type 2 diabetes mellitus: a meta-analysis of cohort studies. Diabetes Res Clin Pract 2013 ; 101 : 88 - 96 . doi:10.1016/j.diabres.2013.03.026 pmid:23608549 . OpenUrl
- ↵ Xu Y, Zhu J, Gao L, et al. Hyperuricemia as an independent predictor of vascular complications and mortality in type 2 diabetes patients: a meta-analysis. PLoS One 2013 ; 8 : e78206 . doi:10.1371/journal.pone.0078206 pmid:24205159 . OpenUrl
- ↵ Xu X, Du N, Wang R, Wang Y, Cai S. Hyperuricemia is independently associated with increased risk of atrial fibrillation: A meta-analysis of cohort studies. Int J Cardiol 2015 ; 184 : 699 - 702 . doi:10.1016/j.ijcard.2015.02.038 pmid:25777070 . OpenUrl
- ↵ Yu S, Chen Y, Hou X, et al. Serum Uric Acid Levels and Diabetic Peripheral Neuropathy in Type 2 Diabetes: a Systematic Review and Meta-analysis. Mol Neurobiol 2016 ; 53 : 1045 - 51 . doi:10.1007/s12035-014-9075-0 pmid:25579387 . OpenUrl
- ↵ Zhu P, Liu Y, Han L, Xu G, Ran JM. Serum uric acid is associated with incident chronic kidney disease in middle-aged populations: a meta-analysis of 15 cohort studies. PLoS One 2014 ; 9 : e100801 . doi:10.1371/journal.pone.0100801 pmid:24959886 . OpenUrl
- ↵ Li L, Yang C, Zhao Y, Zeng X, Liu F, Fu P. Is hyperuricemia an independent risk factor for new-onset chronic kidney disease?: A systematic review and meta-analysis based on observational cohort studies. BMC Nephrol 2014 ; 15 : 122 . doi:10.1186/1471-2369-15-122 pmid:25064611 . OpenUrl
- ↵ Huang Y, Li YL, Huang H, Wang L, Yuan WM, Li J. Effects of hyperuricemia on renal function of renal transplant recipients: a systematic review and meta-analysis of cohort studies. PLoS One 2012 ; 7 : e39457 . doi:10.1371/journal.pone.0039457 pmid:22745759 . OpenUrl
- ↵ Du N, Xu D, Hou X, et al. Inverse Association Between Serum Uric Acid Levels and Alzheimer’s Disease Risk. Mol Neurobiol 2016 ; 53 : 2594 - 9 . doi:10.1007/s12035-015-9271-6 pmid:26084440 . OpenUrl
- ↵ Khan A. T JQ, Dawson J. Serum uric acid level and cognitive function; A systematic review and meta-analysis. Cerebrovasc Dis 2013 ; 35 : 821 . OpenUrl
- ↵ Shen C, Guo Y, Luo W, Lin C, Ding M. Serum urate and the risk of Parkinson’s disease: results from a meta-analysis. Can J Neurol Sci 2013 ; 40 : 73 - 9 . doi:10.1017/S0317167100012981 pmid:23250131 . OpenUrl
- ↵ Wang L, Hu W, Wang J, Qian W, Xiao H. Low serum uric acid levels in patients with multiple sclerosis and neuromyelitis optica: An updated meta-analysis. Mult Scler Relat Disord 2016 ; 9 : 17 - 22 . doi:10.1016/j.msard.2016.05.008 pmid:27645338 . OpenUrl
- ↵ Abraham A, Drory VE. Influence of serum uric acid levels on prognosis and survival in amyotrophic lateral sclerosis: a meta-analysis. J Neurol 2014 ; 261 : 1133 - 8 . doi:10.1007/s00415-014-7331-x pmid:24699859 . OpenUrl
- ↵ Flatow J, Buckley P, Miller BJ. Meta-analysis of oxidative stress in schizophrenia. Biol Psychiatry 2013 ; 74 : 400 - 9 . doi:10.1016/j.biopsych.2013.03.018 pmid:23683390 . OpenUrl
- ↵ Bartoli F, Crocamo C, Mazza MG, Clerici M, Carrà G. Uric acid levels in subjects with bipolar disorder: A comparative meta-analysis. J Psychiatr Res 2016 ; 81 : 133 - 9 . doi:10.1016/j.jpsychires.2016.07.007 pmid:27442964 . OpenUrl
- ↵ Yan S, Zhang P, Xu W, et al. Serum Uric Acid Increases Risk of Cancer Incidence and Mortality: A Systematic Review and Meta-Analysis. Mediators Inflamm 2015 ; 2015 : 764250 . doi:10.1155/2015/764250 pmid:26504361 . OpenUrl
- ↵ Zhao G. Baseline serum uric acid level as a predictor of cardiovascular disease related mortality and all-cause mortality: A meta-analysis of prospective studies. Cardiology (Switzerland) 2014 ; 129 : 47 . OpenUrl
- ↵ Xia X, Luo Q, Li B, Lin Z, Yu X, Huang F. Serum uric acid and mortality in chronic kidney disease: A systematic review and meta-analysis. Metabolism 2016 ; 65 : 1326 - 41 . doi:10.1016/j.metabol.2016.05.009 pmid:27506740 . OpenUrl
- ↵ Tamariz L, Harzand A, Verma S, Jones J, Hare J. Uric acid as a predictor of mortality in congestive heart failure: A meta-analysis. J Am Coll Cardiol 2009 ; 53 : A169 . OpenUrl
- ↵ Yan L, Liu Z, Zhang C. Uric acid as a predictor of in-hospital mortality in acute myocardial infarction: a meta-analysis. Cell Biochem Biophys 2014 ; 70 : 1597 - 601 . doi:10.1007/s12013-014-0101-7 pmid:25015064 . OpenUrl
- ↵ Wang Z, Lin Y, Liu Y, et al. Serum Uric Acid Levels and Outcomes After Acute Ischemic Stroke. Mol Neurobiol 2016 ; 53 : 1753 - 9 . doi:10.1007/s12035-015-9134-1 pmid:25744569 . OpenUrl
- ↵ Li X, Miao X, Wang H, et al. Association of Serum Uric Acid Levels in Psoriasis: A Systematic Review and Meta-Analysis. Medicine (Baltimore) 2016 ; 95 : e3676 . doi:10.1097/MD.0000000000003676 pmid:27175702 . OpenUrl
- ↵ Zhou Y, Wei F, Fan Y. High serum uric acid and risk of nonalcoholic fatty liver disease: A systematic review and meta-analysis. Clin Biochem 2016 ; 49 : 636 - 42 . doi:10.1016/j.clinbiochem.2015.12.010 pmid:26738417 . OpenUrl
- ↵ Braga F, Pasqualetti S, Ferraro S, Panteghini M. Hyperuricemia (HUA) as an independent risk factor for coronary heart disease (CHD) in the general population: A systematic review and meta-analysis. . Clin Chem Lab Med 2015 ; 53 : S1199 . OpenUrl
- ↵ Chen X, Guo X, Huang R, Chen Y, Zheng Z, Shang H. Serum uric acid levels in patients with Alzheimer’s disease: a meta-analysis. PLoS One 2014 ; 9 : e94084 . doi:10.1371/journal.pone.0094084 pmid:24714617 . OpenUrl
- ↵ Grayson PC, Kim SY, LaValley M, Choi HK. Hyperuricemia and incident hypertension: a systematic review and meta-analysis. Arthritis Care Res (Hoboken) 2011 ; 63 : 102 - 10 . doi:10.1002/acr.20344 pmid:20824805 . OpenUrl
- ↵ Kim SY, Guevara JP, Kim KM, Choi HK, Heitjan DF, Albert DA. Hyperuricemia and risk of stroke: a systematic review and meta-analysis. Arthritis Rheum 2009 ; 61 : 885 - 92 . doi:10.1002/art.24612 pmid:19565556 . OpenUrl
- ↵ Kim SY, Guevara JP, Kim KM, Choi HK, Heitjan DF, Albert DA. Hyperuricemia and coronary heart disease: a systematic review and meta-analysis. Arthritis Care Res (Hoboken) 2010 ; 62 : 170 - 80 . pmid:20191515 . OpenUrl
- ↵ Li YL, Wang L, Li J, Huang Y, Yuan WM. [The correlation between uric acid and the incidence and prognosis of kidney diseases: a systematic review and meta-analysis of cohort studies]. Zhonghua Nei Ke Za Zhi 2011 ; 50 : 555 - 61 . pmid:22041264 . OpenUrl
- ↵ Liu B, Shen Y, Xiao K, Tang Y, Cen L, Wei J. Serum uric acid levels in patients with multiple sclerosis: a meta-analysis. Neurol Res 2012 ; 34 : 163 - 71 . pmid:22333889 . OpenUrl
- ↵ Lv Q, Meng XF, He FF, et al. High serum uric acid and increased risk of type 2 diabetes: a systemic review and meta-analysis of prospective cohort studies. PLoS One 2013 ; 8 : e56864 . doi:10.1371/journal.pone.0056864 pmid:23437258 . OpenUrl
- ↵ Schrag M, Mueller C, Zabel M, et al. Oxidative stress in blood in Alzheimer’s disease and mild cognitive impairment: a meta-analysis. Neurobiol Dis 2013 ; 59 : 100 - 10 . doi:10.1016/j.nbd.2013.07.005 pmid:23867235 . OpenUrl
- ↵ Shen L, Ji HF. Low uric acid levels in patients with Parkinson’s disease: evidence from meta-analysis. BMJ Open 2013 ; 3 : e003620 . doi:10.1136/bmjopen-2013-003620 pmid:24247326 . OpenUrl
- ↵ Tamariz L, Hernandez F, Bush A, Palacio A, Hare JM. Association between serum uric acid and atrial fibrillation: a systematic review and meta-analysis. Heart Rhythm 2014 ; 11 : 1102 - 8 . doi:10.1016/j.hrthm.2014.04.003 pmid:24709288 . OpenUrl
- ↵ Wheeler JG, Juzwishin KDM, Eiriksdottir G, Gudnason V, Danesh J. Serum uric acid and coronary heart disease in 9,458 incident cases and 155 084 controls: Prospective study and meta-analysis. PLoS Med 2005 ; 2 (3): 0236 - 43 . OpenUrl
- ↵ Yang Y, Fan Y, Li J, et al. Serum uric acid as a predictor for cardiovascular and all-cause mortality in women versus men. Int J Cardiol 2015 ; 185 : 125 - 8 . doi:10.1016/j.ijcard.2015.03.121 pmid:25791110 . OpenUrl
- ↵ Zhang W, Sun K, Yang Y, Zhang H, Hu FB, Hui R. Plasma uric acid and hypertension in a Chinese community: prospective study and metaanalysis. Clin Chem 2009 ; 55 : 2026 - 34 . doi:10.1373/clinchem.2009.124891 pmid:19729471 . OpenUrl
- ↵ Fink HA, Wilt TJ, Eidman KE, et al. Medical management to prevent recurrent nephrolithiasis in adults: a systematic review for an American College of Physicians Clinical Guideline. Ann Intern Med 2013 ; 158 : 535 - 43 . doi:10.7326/0003-4819-158-7-201304020-00005 pmid:23546565 . OpenUrl
- ↵ Wang H, Wei Y, Kong X, Xu D. Effects of urate-lowering therapy in hyperuricemia on slowing the progression of renal function: a meta-analysis. J Ren Nutr 2013 ; 23 : 389 - 96 . doi:10.1053/j.jrn.2012.08.005 pmid:23131573 . OpenUrl
- ↵ Zhang YF, He F, Ding HH, et al. Effect of uric-acid-lowering therapy on progression of chronic kidney disease: a meta-analysis. J Huazhong Univ Sci Technolog Med Sci 2014 ; 34 : 476 - 81 . doi:10.1007/s11596-014-1302-4 pmid:25135714 . OpenUrl
- ↵ Bose B, Badve SV, Hiremath SS, et al. Effects of uric acid-lowering therapy on renal outcomes: a systematic review and meta-analysis. Nephrol Dial Transplant 2014 ; 29 : 406 - 13 . doi:10.1093/ndt/gft378 pmid:24042021 . OpenUrl
- ↵ Higgins P, Dawson J, Lees KR, McArthur K, Quinn TJ, Walters MR. Xanthine oxidase inhibition for the treatment of cerebrovascular and cardiovascular disease: A systematic review and meta-analysis. Cerebrovasc Dis 2010 ; 29 : 321 . pmid:20130397 . OpenUrl
- ↵ Kanbay M, Siriopol D, Nistor I, et al. Effects of allopurinol on endothelial dysfunction: a meta-analysis. Am J Nephrol 2014 ; 39 : 348 - 56 . doi:10.1159/000360609 pmid:24751886 . OpenUrl
- ↵ Chaudhari T, McGuire W. Allopurinol for preventing mortality and morbidity in newborn infants with hypoxic-ischaemic encephalopathy. Cochrane Database Syst Rev 2012 ;( 7 ): CD006817 . doi:10.1002/14651858.CD006817.pub3
- ↵ Agarwal V, Hans N, Messerli FH. Effect of allopurinol on blood pressure: a systematic review and meta-analysis. J Clin Hypertens (Greenwich) 2013 ; 15 : 435 - 42 . doi:10.1111/j.1751-7176.2012.00701.x pmid:23730993 . OpenUrl
- ↵ Korostishevsky M, Steves CJ, Malkin I, Spector T, Williams FM, Livshits G. Genomics and metabolomics of muscular mass in a community-based sample of UK females. Eur J Hum Genet 2016 ; 24 : 277 - 83 . doi:10.1038/ejhg.2015.85 pmid:25898920 . OpenUrl
- ↵ Lyngdoh T, Vuistiner P, Marques-Vidal P, et al. Serum uric acid and adiposity: deciphering causality using a bidirectional Mendelian randomization approach. PLoS One 2012 ; 7 : e39321 . doi:10.1371/journal.pone.0039321 pmid:22723994 . OpenUrl
- ↵ White J, Sofat R, Hemani G, et al. International Consortium for Blood Pressure (ICBP) UCLEB (University College London-London School of Hygiene & Tropical Medicine-Edinburgh-Bristol Consortium. Plasma urate concentration and risk of coronary heart disease: a Mendelian randomisation analysis. Lancet Diabetes Endocrinol 2016 ; 4 : 327 - 36 . doi:10.1016/S2213-8587(15)00386-1 pmid:26781229 . OpenUrl
- ↵ Dalbeth N, Topless R, Flynn T, Cadzow M, Bolland MJ, Merriman TR. Mendelian randomization analysis to examine for a causal effect of urate on bone mineral density. J Bone Miner Res 2015 ; 30 : 985 - 91 . doi:10.1002/jbmr.2434 pmid:25502344 . OpenUrl
- ↵ Xiong A, Yao Q, He J, Fu W, Yu J, Zhang Z. No causal effect of serum urate on bone-related outcomes among a population of postmenopausal women and elderly men of Chinese Han ethnicity--a Mendelian randomization study. Osteoporos Int 2016 ; 27 : 1031 - 9 . doi:10.1007/s00198-015-3341-5 pmid:26588908 . OpenUrl
- ↵ Kleber ME, Delgado G, Grammer TB, et al. Uric Acid and Cardiovascular Events: A Mendelian Randomization Study. J Am Soc Nephrol 2015 ; 26 : 2831 - 8 . doi:10.1681/ASN.2014070660 pmid:25788527 . OpenUrl
- ↵ Keenan T, Zhao W, Rasheed A, et al. Causal Assessment of Serum Urate Levels in Cardiometabolic Diseases Through a Mendelian Randomization Study. J Am Coll Cardiol 2016 ; 67 : 407 - 16 . doi:10.1016/j.jacc.2015.10.086 pmid:26821629 . OpenUrl
- ↵ Palmer TM, Nordestgaard BG, Benn M, et al. Association of plasma uric acid with ischaemic heart disease and blood pressure: mendelian randomisation analysis of two large cohorts. BMJ 2013 ; 347 : f4262 . doi:10.1136/bmj.f4262 pmid:23869090 . OpenUrl
- ↵ Oikonen M, Wendelin-Saarenhovi M, Lyytikäinen LP, et al. Associations between serum uric acid and markers of subclinical atherosclerosis in young adults. The cardiovascular risk in Young Finns study. Atherosclerosis 2012 ; 223 : 497 - 503 . doi:10.1016/j.atherosclerosis.2012.05.036 pmid:22749515 . OpenUrl
- ↵ Yan D, Wang J, Jiang F, et al. A causal relationship between uric acid and diabetic macrovascular disease in Chinese type 2 diabetes patients: A Mendelian randomization analysis. Int J Cardiol 2016 ; 214 : 194 - 9 . doi:10.1016/j.ijcard.2016.03.206 pmid:27064641 . OpenUrl
- ↵ Mallamaci F, Testa A, Leonardis D, et al. A genetic marker of uric acid level, carotid atherosclerosis, and arterial stiffness: a family-based study. Am J Kidney Dis 2015 ; 65 : 294 - 302 . doi:10.1053/j.ajkd.2014.07.021 pmid:25301104 . OpenUrl
- ↵ Sluijs I, Holmes MV, van der Schouw YT, et al. InterAct Consortium. A Mendelian Randomization Study of Circulating Uric Acid and Type 2 Diabetes. Diabetes 2015 ; 64 : 3028 - 36 . doi:10.2337/db14-0742 pmid:25918230 . OpenUrl
- ↵ Yang Q, Köttgen A, Dehghan A, et al. Multiple genetic loci influence serum urate levels and their relationship with gout and cardiovascular disease risk factors. Circ Cardiovasc Genet 2010 ; 3 : 523 - 30 . doi:10.1161/CIRCGENETICS.109.934455 pmid:20884846 . OpenUrl
- ↵ Dai X, Yuan J, Yao P, et al. Association between serum uric acid and the metabolic syndrome among a middle- and old-age Chinese population. Eur J Epidemiol 2013 ; 28 : 669 - 76 . doi:10.1007/s10654-013-9829-4 pmid:23864233 . OpenUrl
- ↵ Greenberg KI, McAdams-DeMarco MA, Köttgen A, Appel LJ, Coresh J, Grams ME. Plasma Urate and Risk of a Hospital Stay with AKI: The Atherosclerosis Risk in Communities Study. Clin J Am Soc Nephrol 2015 ; 10 : 776 - 83 . doi:10.2215/CJN.05870614 pmid:25717072 . OpenUrl
- ↵ Testa A, Mallamaci F, Spoto B, et al. Association of a polymorphism in a gene encoding a urate transporter with CKD progression. Clin J Am Soc Nephrol 2014 ; 9 : 1059 - 65 . doi:10.2215/CJN.11041013 pmid:24742479 . OpenUrl
- ↵ Hughes K, Flynn T, de Zoysa J, Dalbeth N, Merriman TR. Mendelian randomization analysis associates increased serum urate, due to genetic variation in uric acid transporters, with improved renal function. Kidney Int 2014 ; 85 : 344 - 51 . doi:10.1038/ki.2013.353 pmid:24048376 . OpenUrl
- ↵ Gao J, Xu H, Huang X, Chen H. Short communication: genetic variations of SLC2A9 in relation to Parkinson’s disease. Transl Neurodegener 2013 ; 2 : 5 . doi:10.1186/2047-9158-2-5 pmid:23422251 . OpenUrl
- ↵ Facheris MF, Hicks AA, Minelli C, et al. Variation in the uric acid transporter gene SLC2A9 and its association with AAO of Parkinson’s disease. J Mol Neurosci 2011 ; 43 : 246 - 50 . doi:10.1007/s12031-010-9409-y pmid:20589538 . OpenUrl
- ↵ Lyngdoh T, Bochud M, Glaus J, et al. Associations of serum uric acid and SLC2A9 variant with depressive and anxiety disorders: a population-based study. PLoS One 2013 ; 8 : e76336 . doi:10.1371/journal.pone.0076336 pmid:24204615 . OpenUrl
- ↵ Houlihan LM, Wyatt ND, Harris SE, et al. Variation in the uric acid transporter gene (SLC2A9) and memory performance. Hum Mol Genet 2010 ; 19 : 2321 - 30 . doi:10.1093/hmg/ddq097 pmid:20197412 . OpenUrl
- ↵ Burgess S, Daniel RM, Butterworth AS, Thompson SG. EPIC-InterAct Consortium. Network Mendelian randomization: using genetic variants as instrumental variables to investigate mediation in causal pathways. Int J Epidemiol 2015 ; 44 : 484 - 95 . doi:10.1093/ije/dyu176 pmid:25150977 . OpenUrl
- ↵ González-Aramburu I, Sánchez-Juan P, Jesús S, et al. Genetic variability related to serum uric acid concentration and risk of Parkinson’s disease. Mov Disord 2013 ; 28 : 1737 - 40 . doi:10.1002/mds.25507 pmid:23712608 . OpenUrl
- ↵ González-Aramburu I, Sánchez-Juan P, Sierra M, et al. Serum uric acid and risk of dementia in Parkinson’s disease. Parkinsonism Relat Disord 2014 ; 20 : 637 - 9 . doi:10.1016/j.parkreldis.2014.02.023 pmid:24637121 . OpenUrl
- ↵ Han X, Gui L, Liu B, et al. Associations of the uric acid related genetic variants in SLC2A9 and ABCG2 loci with coronary heart disease risk. BMC Genet 2015 ; 16 : 4 . doi:10.1186/s12863-015-0162-7 pmid:25634581 . OpenUrl
- ↵ Mallamaci F, Testa A, Leonardis D, et al. A polymorphism in the major gene regulating serum uric acid associates with clinic SBP and the white-coat effect in a family-based study. J Hypertens 2014 ; 32 : 1621 - 8, discussion 1628 . doi:10.1097/HJH.0000000000000224 pmid:24805955 . OpenUrl
- ↵ McKeigue PM, Campbell H, Wild S, et al. Bayesian methods for instrumental variable analysis with genetic instruments (‘Mendelian randomization’): example with urate transporter SLC2A9 as an instrumental variable for effect of urate levels on metabolic syndrome. Int J Epidemiol 2010 ; 39 : 907 - 18 . doi:10.1093/ije/dyp397 pmid:20348110 . OpenUrl
- ↵ Parsa A, Brown E, Weir MR, et al. Genotype-based changes in serum uric acid affect blood pressure. Kidney Int 2012 ; 81 : 502 - 7 . doi:10.1038/ki.2011.414 pmid:22189840 . OpenUrl
- ↵ Pfister R, Barnes D, Luben R, et al. No evidence for a causal link between uric acid and type 2 diabetes: a Mendelian randomisation approach. Diabetologia 2011 ; 54 : 2561 - 9 . doi:10.1007/s00125-011-2235-0 pmid:21717115 . OpenUrl
- ↵ Rasheed H, Hughes K, Flynn TJ, Merriman TR. Mendelian randomization provides no evidence for a causal role of serum urate in increasing serum triglyceride levels. Circ Cardiovasc Genet 2014 ; 7 : 830 - 7 . doi:10.1161/CIRCGENETICS.114.000556 pmid:25249548 . OpenUrl
- ↵ Sedaghat S, Pazoki R, Uitterlinden AG, et al. Association of uric acid genetic risk score with blood pressure: the Rotterdam study. Hypertension 2014 ; 64 : 1061 - 6 . doi:10.1161/HYPERTENSIONAHA.114.03757 pmid:25185132 . OpenUrl
- ↵ Simon KC, Eberly S, Gao X, et al. Parkinson Study Group. Mendelian randomization of serum urate and parkinson disease progression. Ann Neurol 2014 ; 76 : 862 - 8 . doi:10.1002/ana.24281 pmid:25257975 . OpenUrl
- ↵ Stark K, Reinhard W, Grassl M, et al. Common polymorphisms influencing serum uric acid levels contribute to susceptibility to gout, but not to coronary artery disease. PLoS One 2009 ; 4 : e7729 . doi:10.1371/journal.pone.0007729 pmid:19890391 . OpenUrl
- ↵ Sun X, Zhang R, Jiang F, et al. Common variants related to serum uric acid concentrations are associated with glucose metabolism and insulin secretion in a Chinese population. PLoS One 2015 ; 10 : e0116714 . doi:10.1371/journal.pone.0116714 pmid:25617895 . OpenUrl
- ↵ Tabara Y, Kohara K, Kawamoto R, et al. Association of four genetic loci with uric acid levels and reduced renal function: the J-SHIPP Suita study. Am J Nephrol 2010 ; 32 : 279 - 86 . doi:10.1159/000318943 pmid:20714133 . OpenUrl
- ↵ Voruganti VS, Franceschini N, Haack K, et al. Replication of the effect of SLC2A9 genetic variation on serum uric acid levels in American Indians. Eur J Hum Genet 2014 ; 22 : 938 - 43 . doi:10.1038/ejhg.2013.264 pmid:24301058 . OpenUrl
- ↵ Holme I, Aastveit AH, Hammar N, Jungner I, Walldius G. Uric acid and risk of myocardial infarction, stroke and congestive heart failure in 417,734 men and women in the Apolipoprotein MOrtality RISk study (AMORIS). J Intern Med 2009 ; 266 : 558 - 70 . doi:10.1111/j.1365-2796.2009.02133.x pmid:19563390 . OpenUrl
- ↵ Mazza A, Pessina AC, Pavei A, Scarpa R, Tikhonoff V, Casiglia E. Predictors of stroke mortality in elderly people from the general population. The CArdiovascular STudy in the ELderly. Eur J Epidemiol 2001 ; 17 : 1097 - 104 . doi:10.1023/A:1021216713504 pmid:12530768 . OpenUrl
- ↵ Kuo CF, See LC, Yu KH, Chou IJ, Chiou MJ, Luo SF. Significance of serum uric acid levels on the risk of all-cause and cardiovascular mortality. Rheumatology (Oxford) 2013 ; 52 : 127 - 34 . doi:10.1093/rheumatology/kes223 pmid:22923756 . OpenUrl
- ↵ Chen JH, Chuang SY, Chen HJ, Yeh WT, Pan WH. Serum uric acid level as an independent risk factor for all-cause, cardiovascular, and ischemic stroke mortality: a Chinese cohort study. Arthritis Rheum 2009 ; 61 : 225 - 32 . doi:10.1002/art.24164 pmid:19177541 . OpenUrl
- ↵ Owens DK, Lohr KN, Atkins D, et al. AHRQ series paper 5: grading the strength of a body of evidence when comparing medical interventions--agency for healthcare research and quality and the effective health-care program. J Clin Epidemiol 2010 ; 63 : 513 - 23 . doi:10.1016/j.jclinepi.2009.03.009 pmid:19595577 . OpenUrl
- ↵ Theodoratou E, Tzoulaki I, Zgaga L, Ioannidis JP. Vitamin D and multiple health outcomes: umbrella review of systematic reviews and meta-analyses of observational studies and randomised trials. BMJ 2014 ; 348 : g2035 . doi:10.1136/bmj.g2035 pmid:24690624 . OpenUrl
- ↵ Belbasis L, Savvidou MD, Kanu C, Evangelou E, Tzoulaki I. Birth weight in relation to health and disease in later life: an umbrella review of systematic reviews and meta-analyses. BMC Med 2016 ; 14 : 147 . doi:10.1186/s12916-016-0692-5 pmid:27677312 . OpenUrl
- ↵ Krishnan E, Lessov-Schlaggar CN, Krasnow RE, Swan GE. Nature versus nurture in gout: a twin study. Am J Med 2012 ; 125 : 499 - 504 . doi:10.1016/j.amjmed.2011.11.010 pmid:22365026 . OpenUrl
- ↵ Köttgen A, Albrecht E, Teumer A, et al. LifeLines Cohort Study CARDIoGRAM Consortium DIAGRAM Consortium ICBP Consortium MAGIC Consortium. Genome-wide association analyses identify 18 new loci associated with serum urate concentrations. Nat Genet 2013 ; 45 : 145 - 54 . doi:10.1038/ng.2500 pmid:23263486 . OpenUrl
- ↵ Richette P, Doherty M, Pascual E, et al. 2016 updated EULAR evidence-based recommendations for the management of gout. Ann Rheum Dis 2017 ; 76 : 29 - 42 . doi:10.1136/annrheumdis-2016-209707 pmid:27457514 . OpenUrl
- ↵ Chen H, Mosley TH, Alonso A, Huang X. Plasma urate and Parkinson’s disease in the Atherosclerosis Risk in Communities (ARIC) study. Am J Epidemiol 2009 ; 169 : 1064 - 9 . doi:10.1093/aje/kwp033 pmid:19299404 . OpenUrl
- ↵ Weisskopf MG, O’Reilly E, Chen H, Schwarzschild MA, Ascherio A. Plasma urate and risk of Parkinson’s disease. Am J Epidemiol 2007 ; 166 : 561 - 7 . doi:10.1093/aje/kwm127 pmid:17584757 . OpenUrl
- ↵ Kim TS, Pae CU, Yoon SJ, et al. Decreased plasma antioxidants in patients with Alzheimer’s disease. Int J Geriatr Psychiatry 2006 ; 21 : 344 - 8 . doi:10.1002/gps.1469 pmid:16534775 . OpenUrl
- ↵ Feig DI, Madero M, Jalal DI, Sanchez-Lozada LG, Johnson RJ. Uric acid and the origins of hypertension. J Pediatr 2013 ; 162 : 896 - 902 . doi:10.1016/j.jpeds.2012.12.078 pmid:23403249 . OpenUrl
- ↵ Tamariz L, Hare JM. Xanthine oxidase inhibitors in heart failure: where do we go from here? Circulation 2015 ; 131 : 1741 - 4 . doi:10.1161/CIRCULATIONAHA.115.016379 pmid:25986446 . OpenUrl
- ↵ Ioannidis JP. The Mass Production of Redundant, Misleading, and Conflicted Systematic Reviews and Meta-analyses. Milbank Q 2016 ; 94 : 485 - 514 . doi:10.1111/1468-0009.12210 pmid:27620683 . OpenUrl
- ↵ Kavvoura FK, Liberopoulos G, Ioannidis JP. Selection in reported epidemiological risks: an empirical assessment. PLoS Med 2007 ; 4 : e79 . doi:10.1371/journal.pmed.0040079 pmid:17341129 . OpenUrl
- ↵ Ioannidis JP. Exposure-wide epidemiology: revisiting Bradford Hill. Stat Med 2016 ; 35 : 1749 - 62 . doi:10.1002/sim.6825 pmid:26646432 . OpenUrl
Progress of uric acid in cardiovascular disease
Affiliation.
- 1 Department of Cardiology, Affiliated Hospital of Guilin Medical University, Guilin, China.
- PMID: 38633361
- PMCID: PMC11019825
- DOI: 10.1097/XCE.0000000000000300
Due to the global prevalence of hyperuricemia (HUA), there is growing interest in research on uric acid (UA). HUA is a common condition that has various adverse consequences, including gout and kidney disease. However, recent studies have also implicated UA in the development of cardiovascular diseases (CVD) such as atrial fibrillation (AF) and coronary heart disease (CHD). Experimental and clinical research has extensively demonstrated the detrimental effects of elevated serum UA levels on cardiovascular health. Furthermore, serum UA levels have been identified as predictors of CVD outcomes following percutaneous coronary intervention (PCI) and catheter ablation. Additionally, the use of UA-lowering therapy holds important implications for the management of CVD. This review aims to consolidate the current evidence on the relationship between serum UA and CVD.
Keywords: atrial fibrillation; cardiovascular disease; coronary heart disease; hyperuricemia; uric acid.
Copyright © 2024 The Author(s). Published by Wolters Kluwer Health, Inc.
Publication types
- Open access
- Published: 22 April 2024
The influence of maternal prepregnancy weight and gestational weight gain on the umbilical cord blood metabolome: a case–control study
- Xianxian Yuan ORCID: orcid.org/0000-0001-8762-8471 1 ,
- Yuru Ma 1 ,
- Jia Wang 2 ,
- Yan Zhao 1 ,
- Wei Zheng 1 ,
- Ruihua Yang 1 ,
- Lirui Zhang 1 ,
- Xin Yan 1 &
- Guanghui Li ORCID: orcid.org/0000-0003-2290-1515 1
BMC Pregnancy and Childbirth volume 24 , Article number: 297 ( 2024 ) Cite this article
40 Accesses
Metrics details
Maternal overweight/obesity and excessive gestational weight gain (GWG) are frequently reported to be risk factors for obesity and other metabolic disorders in offspring. Cord blood metabolites provide information on fetal nutritional and metabolic health and could provide an early window of detection of potential health issues among newborns. The aim of the study was to explore the impact of maternal prepregnancy overweight/obesity and excessive GWG on cord blood metabolic profiles.
A case control study including 33 pairs of mothers with prepregnancy overweight/obesity and their neonates, 30 pairs of mothers with excessive GWG and their neonates, and 32 control mother-neonate pairs. Untargeted metabolomic profiling of umbilical cord blood samples were performed using UHPLC‒MS/MS.
Forty-six metabolites exhibited a significant increase and 60 metabolites exhibited a significant reduction in umbilical cord blood from overweight and obese mothers compared with mothers with normal body weight. Steroid hormone biosynthesis and neuroactive ligand‒receptor interactions were the two top-ranking pathways enriched with these metabolites ( P = 0.01 and 0.03, respectively). Compared with mothers with normal GWG, in mothers with excessive GWG, the levels of 63 metabolites were increased and those of 46 metabolites were decreased in umbilical cord blood. Biosynthesis of unsaturated fatty acids was the most altered pathway enriched with these metabolites ( P < 0.01).
Conclusions
Prepregnancy overweight and obesity affected the fetal steroid hormone biosynthesis pathway, while excessive GWG affected fetal fatty acid metabolism. This emphasizes the importance of preconception weight loss and maintaining an appropriate GWG, which are beneficial for the long-term metabolic health of offspring.
Peer Review reports
The obesity epidemic is an important public health problem in developed and developing countries [ 1 ] and is associated with the emergence of chronic noncommunicable diseases, including type 2 diabetes mellitus (T2DM), hypertension, cardiovascular disease, nonalcoholic fatty liver disease (NAFLD), and cancer [ 2 , 3 , 4 ]. Maternal obesity is the most common metabolic disturbance in pregnancy, and the prevalence of obesity among women of childbearing age is 7.1% ~ 31.9% in some countries [ 5 ]. In China, the prevalence of overweight and obesity has also increased rapidly in the past four decades. Based on Chinese criteria, the latest national prevalence estimates for 2015–2019 were 34.3% for overweight and 16.4% for obesity in adults (≥ 18 years of age) [ 6 ].
Increasing evidence implicates overnutrition in utero as a major determinant of the health of offspring during childhood and adulthood, which is compatible with the developmental origins of health and disease (DOHaD) framework [ 7 ]. Maternal obesity and excessive gestational weight gain (GWG) are important risk factors for several adverse maternal outcomes, including gestational diabetes and hypertensive disorders, fetal death, and preterm birth [ 8 , 9 , 10 ]. More importantly, they have negative implications for offspring, both perinatally and later in life. Evidence from cohort studies focusing on offspring development confirms the relationship between maternal obesity/excessive GWG and offspring obesity programming [ 11 , 12 , 13 ]. Currently, there is no unified mechanism to explain the adverse outcomes associated with maternal obesity and excessive GWG, which may be the independent and interactive effects of the obese maternal phenotype itself and the diet associated with this phenotype. In addition to genetic and environmental factors, metabolic programming may also lead to the intergenerational transmission of obesity through epigenetic mechanisms.
Metabolomics, which reflects the metabolic phenotype of human subjects and animals, is the profiling of metabolites in biofluids, cells and tissues using high-throughput platforms, such as mass spectrometry. It has unique potential in identifying biomarkers for predicting occurrence, severity, and progression of diseases, as well as exploring underlying mechanistic abnormalities [ 14 , 15 ]. Umbilical cord metabolites can provide information about fetal nutritional and metabolic health, and may provide an early window for detection of potential health issues in newborns [ 16 ]. Previous studies have reported differences in umbilical cord metabolite profiles associated with maternal obesity [ 17 , 18 ]. However, the results were inconsistent due to differences in sample sizes, ethnicity and region, and mass spectrometry. In addition, most studies have not considered the difference in the effects of prepregnancy body mass index (BMI) and GWG on cord blood metabolites.
To investigate the relationship between early metabolic programming and the increased incidence of metabolic diseases in offspring, we studied the associations between elevated prepregnancy BMI/excessive GWG and umbilical cord metabolic profiles. Another purpose of this study was to explore whether there were differences in the effects of prepregnancy overweight/obesity and excessive GWG on cord blood metabolites.
Study population
This was a hospital-based, case control study that included singleton pregnant women who received prenatal care and delivered vaginally at Beijing Obstetrics and Gynecology Hospital, Capital Medical University, from January 2022 to March 2022. We selected 33 pregnant women with a prepregnancy BMI ≥ 24.0 kg/m 2 regardless of their gestational weight gain as the overweight/obese group, 30 pregnant women with a prepregnancy BMI of 18.5–23.9 kg/m 2 and a GWG > 14.0 kg as the excessive GWG group, and 32 pregnant women with a BMI of 18.5–23.9 kg/m 2 and a GWG of 8.0–14.0 kg as the control group. The ages of the three groups were matched (± 1.0 years), and the prepregnancy BMIs of the excessive GWG and control groups were matched (± 1.0 kg/m 2 ).
The inclusion criteria were women with singleton pregnancies, those aged between 20 and 45 years, those with full-term delivery (gestational age ≥ 37 weeks), those with a prepregnancy BMI ≥ 18.5 kg/m 2 , those without prepregnancy diabetes mellitus (DM) or hypertension, and those without gestational diabetes mellitus (GDM). The exclusion criteria were women with multiple pregnancies, those less than 20 years or more than 45 years old, those with a prepregnancy BMI < 18.5 kg/m 2 , those with prepregnancy DM, hypertension or GDM, and those without cord blood samples.
We classified pregnant women into BMI categories based on Chinese guidelines [ 19 ]: normal weight (prepregnancy BMI 18.5–23.9 kg/m 2 ), overweight (prepregnancy BMI 24.0–27.9 kg/m 2 ), and obese (prepregnancy BMI ≥ 28.0 kg/m 2 ). GWG guideline concordance was defined by the 2021 Chinese Nutrition Society recommendations according to prepregnancy BMI. The upper limits of GWG for normal weight, overweight, and obesity were 14.0 kg, 11.0 kg, and 9.0 kg, respectively.
Ethical approval and written informed consent were obtained from all participants. The study has been performed according to the Declaration of Helsinki, and the procedures have been approved by the ethics committees of Beijing Obstetrics and Gynecology Hospital, Capital Medical University (2021-KY-037).
Sample and data collection
Maternal and neonatal clinical data were collected from the electronic medical records system of Beijing Obstetrics and Gynecology Hospital. Maternal clinical characteristics included age, height, prepregnancy and predelivery weight, education level, smoking and drinking status during pregnancy, parity, conception method, comorbidities and complications of pregnancy, family history of DM and hypertension, gestational age, mode of delivery, and biochemical results during pregnancy. Prepregnancy BMI was calculated as prepregnancy weight in kilograms divided by the square of height in meters. GWG was determined by subtracting the prepregnancy weight in kilograms from the predelivery weight in kilograms. GDM was defined using the IAPDSG’s diagnostic criteria at 24 to 28 +6 weeks gestation and the fasting glucose and 1- and 2-h glucose concentrations at the time of the oral glucose tolerance test (OGTT). Neonatal clinical characteristics included sex, birth weight and length. Macrosomia was defined as a birth weight of 4,000 g or more [ 20 ]. Low birth weight (LBW) was defined as a birth weight less than 2,500 g [ 21 ].
Umbilical cord blood samples were obtained by trained midwives after clamping the cord at delivery. Whole blood samples were collected in EDTA tubes, refrigerated for < 24 h, and centrifuged at 2,000 r.p.m. at 4 ℃ for 10 min. Plasma aliquots were stored at -80 ℃ until shipment on dry ice to Novogene, Inc. (Beijing, China) for untargeted metabolomic analysis.
Untargeted metabolomic analyses
Ultrahigh-performance liquid chromatography tandem mass spectrometry (UHPLC‒MS/MS) analyses were performed using a Vanquish UHPLC system (Thermo Fisher, Germany) coupled with an Orbitrap Q Exactive™ HF mass spectrometer (Thermo Fisher, Germany) at Novogene Co., Ltd. (Beijing, China). Detailed descriptions of the sample preparation, mass spectrometry and automated metabolite identification procedures are described in the Supplementary materials .
Statistical analysis
Clinical data statistical analysis.
Quantitative data are shown as the mean ± standard deviation (SD) or median (interquartile range), and categorical data are presented as percentages. The Mann‒Whitney U test, chi-square test, and general linear repeated-measures model were used to assess the differences between the control and study groups when appropriate. A P value < 0.05 was considered statistically significant. All analyses were performed using Statistical Package of Social Sciences version 25.0 (SPSS 25.0) for Windows (SPSS Inc).
Umbilical cord metabolome statistical analysis
These metabolites were annotated using the Human Metabolome Database (HMDB) ( https://hmdb.ca/metabolites ), LIPIDMaps database ( http://www.lipidmaps.org/ ), and Kyoto Encylopaedia of Genes and Genomes (KEGG) database ( https://www.genome.jp/kegg/pathway.html ). Principal component analysis (PCA) and partial least-squares discriminant analysis (PLS-DA) were performed at metaX. We applied univariate analysis ( T test) to calculate the statistical significance ( P value). Metabolites with a variable importance for the projection (VIP) > 1, a P value < 0.05 and a fold change (FC) ≥ 2 or FC ≤ 0.5 were considered to be differential metabolites. A false discovery rate (FDR) control was implemented to correct for multiple comparisons. The q -value in the FDR control was defined as the FDR analog of the P -value. In this study, the q -value was set at 0.2. For clustering heatmaps, the data were normalized using z scores of the intensity areas of differential metabolites and were plotted by the Pheatmap package in R language.
The correlations among differential metabolites were analyzed by cor () in R language (method = Pearson). Statistically significant correlations among differential metabolites were calculated by cor.mtest () in R language. A P value < 0.05 was considered statistically significant, and correlation plots were plotted by the corrplot package in R language. The functions of these metabolites and metabolic pathways were studied using the KEGG database. The metabolic pathway enrichment analysis of differential metabolites was performed when the ratio was satisfied by x/n > y/N, and the metabolic pathway was considered significantly enriched when P < 0.05.
Demographic characteristics of study participants
The demographic and clinical characteristics of the three population groups enrolled in the study are summarized in Table 1 . Mothers had no significant difference regarding their ages or gestational ages. Compared to the mothers in the excessive GWG and control groups, those in the prepregnancy overweight/obesity group had a significantly higher prepregnancy BMI (25.6 (24.5, 27.2) kg/m 2 ). However, there was no significant difference in prepregnancy BMI between mothers in the excessive GWG group (20.3 ± 1.2 kg/m 2 ) and mothers in the control group (20.6 ± 1.5 kg/m 2 ). Mothers in the excessive GWG group had the highest GWG (17.0 (15.5, 19.1) kg) among the three groups. The mean GWG of the mothers in the prepregnancy overweight/obesity group was 12.9 ± 3.8 kg, which was similar to that of the control group (11.8 ± 1.5 kg). It was noteworthy that among the 33 prepregnancy overweight/obese pregnant women, 20 of them had appropriate GWG, 1 had insufficient GWG, and 12 had excessive GWG. The proportion of mothers who underwent invitro fertilization and embryo transfer (IVF-ET) in the prepregnancy overweight/obesity group (15.2%) was significantly higher than that in the excessive GWG and control groups. There were no statistically significant differences in the proportions of pregnancy outcomes among the three groups, including preeclampsia, premature rupture of membranes, postpartum hemorrhage, macrosomia, and LBW. The babies in the three groups showed no significant difference regarding their birth weights or lengths.
The biochemical parameters of the mothers during pregnancy are shown in Table 2 . The levels of triglyceride (TG) and uric acid (UA) of mothers in the prepregnancy overweight/obesity group were significantly higher than those of the mothers in the excessive GWG and control groups in the first trimester. However, there was no significant difference in the blood glucose and lipid levels in the second and third trimesters of pregnancy among the three groups.
PCA and PLS-DA analysis of cord blood metabolites
Functional and taxonomic annotations of the identified metabolites included the HMDB classification annotations, LIPID MAPS classification annotations, and KEGG pathway annotations. Those cord blood metabolites included lipids and lipid-like molecules, organic acids and their derivatives, and organoheterocyclic compounds, which were mainly involved in metabolism. To better understand the structure of the cord blood metabolome in cases versus controls, we used unsupervised PCA to identify metabolites contributing the most to observed differences in the dataset. PCA did not clearly separate the three groups. We next used PLS-DA to identify metabolites that were predictive of case versus control status. PLS-DA clearly distinguished the cases from the controls (Fig. 1 ), the prepregnancy overweight/obesity group vs. the control group (R2Y = 0.82, Q2Y = 0.37; R2Y = 0.77, Q2Y = 0.13, respectively) (Fig. 1 A), and the excessive GWG group vs. the control group (R2Y = 0.76, Q2Y = 0.16; R2Y = 0.81, Q2Y = 0.41) (Fig. 1 B).
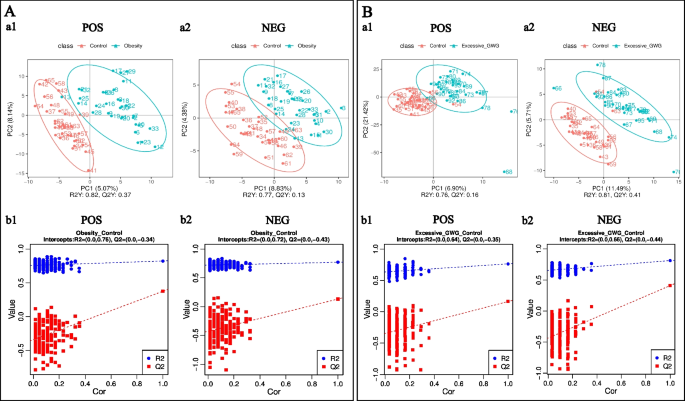
PLS-DA of identified cord blood metabolites. A the prepregnancy overweight/obesity group vs. the control group; B the excessive GWG group vs. the control group. (a) PLS-DA score. The horizontal coordinates are the score of the sample on the first principal component; the longitudinal coordinates are the score of the sample on the second principal component; R2Y represents the interpretation rate of the model, and Q2Y is used to evaluate the predictive ability of the PLS-DA model, and when R2Y is greater than Q2Y, it means that the model is well established. (b) PLS-DA valid. Horizontal coordinates represent the correlation between randomly grouped Y and the original group Y, and vertical coordinates represent the scores of R2 and Q2. (1) POS, positive metabolites; (2) NEG, negative metabolites
Maternal prepregnancy overweight/obesity
Screening differential metabolites according to a PLS-DA VIP > 1.0, a FC > 1.2 or < 0.833 and a P value < 0.05, a total of 106 cord blood metabolites (77 positive metabolites and 29 negative metabolites) differed between the prepregnancy overweight/obesity group and the control group. Compared with those in the control group, the levels of 46 metabolites (19 positive metabolites and 27 negative metabolites) were increased in the prepregnancy overweight/obesity group, among which octopamine was the metabolite with the largest increase, followed by (2S)-4-Oxo-2-phenyl-3,4-dihydro-2H-chromen-7-yl beta-D-glucopyranoside, N-tetradecanamide, stearamide, and methanandamide (Fig. 2 A). Compared with the control group, in the prepregnancy overweight/obesity group, there were 60 metabolites (58 positive metabolites and 2 negative metabolites) with reduced concentrations, among which senecionine was the metabolite with the largest decrease, followed by 3-(methylsulfonyl)-2H-chromen-2-one, methyl EudesMate, cuminaldehyde, and 2-(tert-butyl)-1,3-thiazolane-4-carboxylic acid (Fig. 2 A).
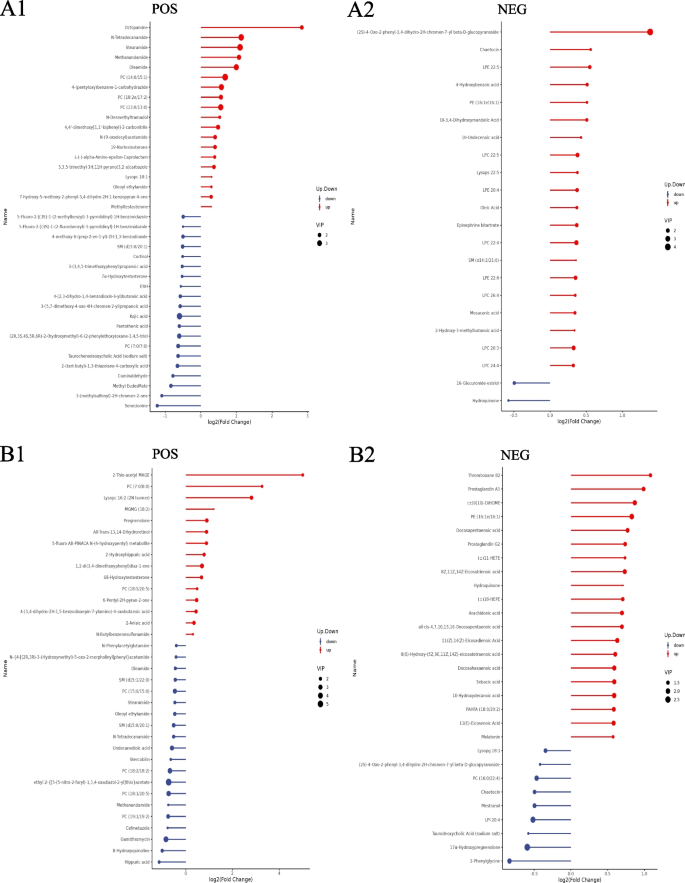
Stem plots of differential cord blood metabolites. A the prepregnancy overweight/obesity group vs. the control group; B the excessive GWG group vs. the control group. (1) positive metabolites; (2) negative metabolites. Notes: The color of the dot in the stem plots represents the upward and lower adjustment, the blue represents downward, and the red represents upward. The length of the rod represents the size of log2 (FC), and the size of the dot represents the size of the VIP value
A hierarchical analysis of the two groups of differential metabolites obtained was carried out, and the difference in metabolic expression patterns between the two groups and within the same comparison was obtained, which is shown in Fig. 3 . KEGG pathway analysis of differential cord blood metabolites associated with the prepregnancy overweight/obesity group versus the control group is shown in Table 3 and Fig. 4 A. The metabolite enrichment analysis revealed that steroid hormone biosynthesis ( P value = 0.01) and neuroactive ligand‒receptor interactions ( P value = 0.03) were the two pathways that were most altered between the prepregnancy overweight/obesity group and the control group. 19 metabolites were distributed in the pathway of steroid hormone biosynthesis, and 4 metabolites were distributed in the pathway of neuroactive ligand‒receptor interactions. In the steroid hormone biosynthesis pathway, the levels of corticosterone, 11-deoxycortisol, cortisol, testosterone, and 7α-hydroxytestosterone were decreased in the prepregnancy overweight/obesity group relative to those in the control group. In the neuroactive ligand‒receptor interaction pathway, the level of cortisol was decreased and the levels of trace amines were increased in the prepregnancy overweight/obesity group relative to the control group.
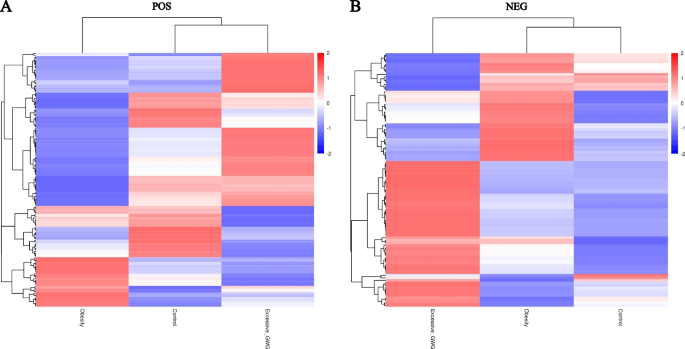
Clustering heat maps of differential cord blood metabolites of the three groups. A positive metabolites; B negative metabolites. Notes: Longitudinal clustering of samples and trans-verse clustering of metabolites. The shorter the clustering branches, the higher the similarity. Through horizontal comparison, we can see the relationship between groups of metabolite content clustering
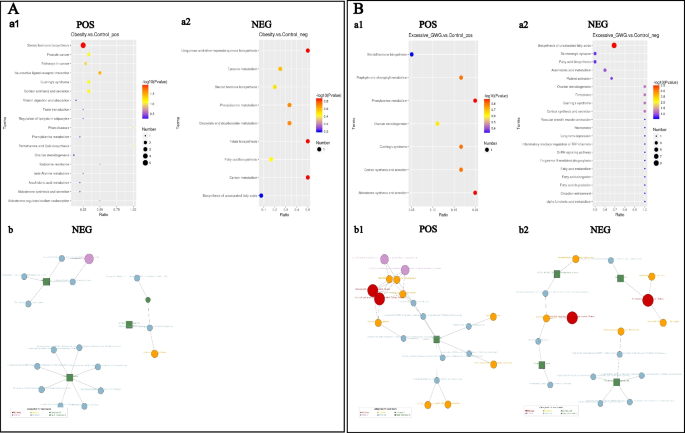
KEGG enrichment scatterplots (a) and net (b) of differential cord blood metabolites. A the prepregnancy overweight/obesity group vs. the control group; B the excessive GWG group vs. the control group. (1) positive metabolites; (2) negative metabolites. Notes: (a) The horizontal co-ordinates in the figure are x/y (the number of differential metabolites in the corresponding metabolic pathway/the total number of total metabolites identified in this pathway). The value represents the enrichment degree of differential metabolites in the pathway. The color of the point rep-resents the P -value of the hypergeometric test, and the size of the point represents the number of differential metabolites in the corresponding pathway. (b) The red dot represents a metabolic pathway, the yellow dot represents a substance-related regulatory enzyme information, the green dot represents the background substance of a metabolic pathway, the purple dot represents the molecular module information of a class of substances, the blue dot represents a substance chemical reaction, and the green square represents the differential substance obtained by this comparison
Maternal excessive GWG
A total of 109 cord blood metabolites (52 positive metabolites and 57 negative metabolites) differed between the excessive GWG group and the control group. Compared with the control group, in the excessive GWG group, there were 63 metabolites (15 positive metabolites and 48 negative metabolites) with increased concentrations, among which 2-thio-acetyl MAGE was the metabolite with the largest increase, followed by PC (7:0/8:0), lysopc 16:2 (2 N isomer), MGMG (18:2), and thromboxane B2 (Fig. 2 B). Compared with the levels in the control group, the levels of 46 metabolites (37 positive metabolites and 9 negative metabolites) in the excessive GWG group were reduced, among which hippuric acid had the largest decrease, followed by 8-hydroxyquinoline, gamithromycin, 2-phenylglycine, and cefmetazole (Fig. 2 B).
A hierarchical analysis of differential metabolites obtained in the two groups was carried out, and the difference in metabolic expression patterns between the two groups and within the same comparison was obtained, which is shown in Fig. 3 . KEGG pathway analysis of the cord blood metabolites associated with the excessive GWG group versus the control group is shown in Table 4 and Fig. 4 B. The metabolite enrichment analysis revealed that biosynthesis of unsaturated fatty acids was the most altered pathway between the excessive GWG and control groups ( P value < 0.01). There were 13 metabolites distributed in the enriched pathway. The levels of docosapentaenoic acid (DPA), docosahexaenoic acid (DHA), arachidonic acid, adrenic acid, palmitic acid, stearic acid, behenic acid, lignoceric acid, and erucic acid were increased in the excessive GWG group relative to those in the control group.
Our present study found that both maternal prepregnancy overweight/obesity and excessive GWG could affect umbilical cord blood metabolites, and they had different effects on these metabolites. Regardless of their gestational weight gain, the umbilical cord blood of prepregnancy overweight and obese mothers had 46 metabolites increased and 60 metabolites decreased compared with the umbilical cord blood of mothers with normal body weight and appropriate GWG. Steroid hormone biosynthesis and neuroactive ligand‒receptor interactions were the two top-ranking pathways enriched with these metabolites. Compared with mothers with normal prepregnancy BMI and appropriate GWG, in mothers with normal prepregnancy BMI but excessive GWG, the levels of 63 metabolites were increased and those of 46 metabolites were decreased in umbilical cord blood. Biosynthesis of unsaturated fatty acids was the most altered pathway enriched with these metabolites.
There were many differential metabolites in the cord blood between the prepregnancy overweight/obesity group and the control group and between the excessive GWG group and the control group. However, the roles of most of these differential metabolites are unknown. The levels of stearamide and methanandamide were increased in the prepregnancy overweight/obesity group. Stearamide, also known as octadecanamide or kemamide S, belongs to the class of organic compounds known as carboximidic acids. Stearamide, which is increased in the serum of patients with hepatic cirrhosis and sepsis, may be associated with the systemic inflammatory state [ 22 , 23 ]. Methanandamide is a stable analog of anandamide that participates in energy balance mainly by activating cannabinoid receptors. Methanandamide dose-dependently inhibits and excites tension-sensitive gastric vagal afferents (GVAs), which play a role in appetite regulation [ 24 ]. In mice fed a high-fat diet, only an inhibitory effect of methanandamide was observed, and GVA responses to tension were dampened [ 24 , 25 ]. These changes may contribute to the development and/or maintenance of obesity. Moreover, methanandamide can produce dose-related hypothermia and attenuate cocaine-induced hyperthermia by a cannabinoid 1-dopamine D2 receptor mechanism [ 26 ].
Metabolomic pathway analysis of the cord blood metabolite features in the prepregnancy overweight and obesity group identified two filtered significant pathways: steroid hormone biosynthesis and neuroactive ligand‒receptor interaction pathways. In the steroid hormone biosynthesis pathway, the levels of several glucocorticoids (including corticosterone, 11-deoxycortisol, cortisol, testosterone, and 7α-hydroxytestosterone) were decreased in the prepregnancy overweight/obesity group. In addition to the physiological role of glucocorticoids in the healthy neuroendocrine development and maturation of fetuses and babies, glucocorticoids are essential to human health by regulating different physiological events in mature organs and tissues, such as glucose metabolism, lipid biosynthesis and distribution, food intake, thermogenesis, and mood and learning patterns [ 27 ]. Glucocorticoids have been considered as a link between adverse early-life conditions and the development of metabolic disorders in later life [ 28 , 29 , 30 ]. However, there is still much controversy regarding the role of maternal obesity in the fetal–steroid hormone biosynthesis pathway. Studies of maternal obesity animal models showed that corticosterone and cortisol levels were increased in the offspring of obese mothers [ 31 , 32 ]. A study reported by Satu M Kumpulainen et al. showed that young adults born to mothers with higher early pregnancy BMIs show lower average levels of diurnal cortisol, especially in the morning [ 33 ]. Laura I. Stirrat et al. found that increased maternal BMI was associated with lower maternal cortisol, corticosterone, and 11-dehydrocorticosterone levels. However, there were no associations between maternal BMI and glucocorticoid levels in the cord blood [ 34 ]. The differences in the study protocols of these previous studies may explain the mixed findings, such as cortisol measured from peripheral blood, cord blood or saliva; variation in measurement time points; the number of samples. Although the effect of maternal obesity on fetal steroid hormone levels is controversial, dysregulation of glucocorticoids may be a plausible mechanism by which maternal obesity can increase the risk of metabolic disorders and mental health disorders in offspring.
The effect of excessive GWG on umbilical cord blood metabolites is different from that of maternal overweight and obesity. Compared with the control group, in the excessive GWG group, the level of thromboxane B2 was increased and the level of hippuric acid was decreased. Thromboxane B2, which is important in the platelet release reaction, is a stable, physiologically active compound formed in vivo from prostaglandin endoperoxides. Hippuric acid is an acyl glycine formed from the conjugation of benzoic acid with glycine. Several studies have confirmed that both thromboxane B2 and hippuric acid levels are associated with diet. Dietary fatty acids affect platelet thromboxane production [ 35 , 36 , 37 ]. In our study, several fatty acids (e.g., palmitic acid, stearic acid, behenic acid, and lignoceric acid) in the excessive GWG group were also increased, which may have led to the increase in thromboxane B2 levels. Hippuric acid can be detected after the consumption of whole grains and anthocyanin-rich bilberries [ 38 , 39 ]. A healthy diet intervention increased the signals for hippuric acid to incorporate polyunsaturated fatty acids [ 38 ], and the low level of hippuric acid was associated with lower fruit-vegetable intakes [ 39 ]. Maternal overnutrition and unhealthy dietary patterns are the main reasons for excessive GWG [ 40 , 41 ]. Therefore, we speculated that the differences in thromboxane B2 and hippuric acid between the excessive GWG and control groups were associated with maternal diet during pregnancy. The effect of these differential metabolites on the long-term metabolic health of offspring after birth needs further study.
Metabolomic pathway analysis of the cord blood metabolite features in the excessive GWG group identified that biosynthesis of unsaturated fatty acids was the filtered significant pathway. The levels of several fatty acids in this pathway were increased in the excessive GWG group, including long-chain saturated fatty acids (e.g., palmitic acid (C 16:0), stearic acid (C 18:0), behenic acid (C 22:0), and lignoceric acid (C 23:0)), monounsaturated fatty acids (erucic acid), and polyunsaturated fatty acids (e.g., DPA, DHA, arachidonic acid, and adrenic acid). Because perinatal fatty acid status can be influenced by maternal dietary modifications or supplementation [ 42 ], we speculated that maternal diet during pregnancy caused the difference in umbilical cord blood fatty acids between the excessive GWG and control groups. A large body of evidence from mechanistic studies supports the potential of fatty acids to influence later obesity. However, the possible mechanisms and observed relationships are complex and related to the types and patterns of fatty acids [ 43 , 44 ]. Maternal dietary fatty acids have been found to induce hypothalamic inflammation, cause epigenetic changes, and alter the mechanisms of energy control in offspring [ 43 ]. Evidence from cell culture and rodent studies showed that polyunsaturated fatty acids might serve several complex roles in fetuses, including the stimulation and/or inhibition regulation of adipocyte differentiation [ 44 ]. The questions of whether lower n-6 long-chain polyunsaturated fatty acid levels or higher n-3 long-chain polyunsaturated fatty acid levels are of more relevance and whether the long-term effects differ with different offspring ages remain [ 44 ]. Although there is a biologically plausible case for the relevance of perinatal fatty acid status in later obesity risk, available data in humans suggest that the influence of achievable modification of perinatal n-3/n-6 status is not sufficient to influence offspring obesity risk in the general population [ 45 ]. Further studies seem justified to clarify the reasons.
The advantage of our present study is that we simultaneously analyzed the effects of prepregnancy overweight/obesity and excessive GWG on cord blood metabolites and explored their differences. In addition, to exclude the effect of hyperglycemia on cord blood metabolites, both women with prepregnancy diabetes mellitus and gestational diabetes mellitus were excluded from our study. The limitation of our study is that it was a single-center study with a small sample, especially in the prepregnancy overweight/obesity group. In the future, we can expand the sample size and conduct a subgroup analysis of the prepregnancy overweight/obesity group and analyze the differences in the effects of different degrees of obesity on cord blood metabolites. The prepregnancy overweight/obesity group can be further divided into an appropriate GWG group and an excessive GWG group, and the differences in the effects of these two groups on umbilical cord blood metabolites can be analyzed. Moreover, the dietary pattern of the pregnant woman could affect the production of cord blood metabolites. We did not investigate the dietary patterns of the mothers in this study, which is another limitation of this study. In future studies, we should investigate maternal dietary patterns as a very important confounding variable.
In conclusion, our present study confirmed that both prepregnancy overweight/obesity and excessive GWG could affect umbilical cord blood metabolites, and they had different effects on these metabolites. Prepregnancy overweight and obesity affected the fetal steroid hormone biosynthesis pathway, while normal prepregnancy body weight but excessive GWG affected fetal fatty acid metabolism. This emphasizes the importance of preconception weight loss and maintaining an appropriate GWG, which are beneficial for the long-term metabolic health of offspring.
Availability of data and materials
Data sets generated during the current study are not publicly available but will be available from the corresponding author at a reasonable request. Responses to the request for the raw data will be judged by a committee including XXY and GHL.
Abbreviations
Excessive gestational weight gain
Ultrahigh-performance liquid chromatography tandem mass spectrometry
Type 2 diabetes mellitus
Nonalcoholic fatty liver disease
The developmental origins of health and disease
Body mass index
Diabetes mellitus
Gestational diabetes mellitus
Oral glucose tolerance test
Low birth weight
Standard deviation
The Human Metabolome Database
Kyoto Encylopaedia of Genes and Genomes
Principal component analysis
Partial least-squares discriminant analysis
Importance for the projection
Fold change
Invitro fertilization and embryo transfer
Triglyceride
Docosapentaenoic acid
Docosahexaenoic acid
Gastric vagal afferents
Collaborators GBDO, Afshin A, Forouzanfar MH, Reitsma MB, Sur P, Estep K, Lee A, Marczak L, Mokdad AH, Moradi-Lakeh M, et al. Health effects of overweight and obesity in 195 countries over 25 years. N Engl J Med. 2017;377(1):13–27.
Article Google Scholar
Bjerregaard LG, Jensen BW, Angquist L, Osler M, Sorensen TIA, Baker JL. Change in overweight from childhood to early adulthood and risk of type 2 diabetes. N Engl J Med. 2018;378(14):1302–12.
Article PubMed Google Scholar
Sharma V, Coleman S, Nixon J, Sharples L, Hamilton-Shield J, Rutter H, Bryant M. A systematic review and meta-analysis estimating the population prevalence of comorbidities in children and adolescents aged 5 to 18 years. Obes Rev. 2019;20(10):1341–9.
Article PubMed PubMed Central Google Scholar
Llewellyn A, Simmonds M, Owen CG, Woolacott N. Childhood obesity as a predictor of morbidity in adulthood: a systematic review and meta-analysis. Obes Rev. 2016;17(1):56–67.
Article CAS PubMed Google Scholar
Poston L, Caleyachetty R, Cnattingius S, Corvalan C, Uauy R, Herring S, Gillman MW. Preconceptional and maternal obesity: epidemiology and health consequences. Lancet Diabetes Endocrinol. 2016;4(12):1025–36.
Pan XF, Wang L, Pan A. Epidemiology and determinants of obesity in China. Lancet Diabetes Endocrinol. 2021;9(6):373–92.
Barker DJ. The developmental origins of adult disease. J Am Coll Nutr. 2004;23(6 Suppl):588S-595S.
LifeCycle Project-Maternal O, Childhood Outcomes Study G, Voerman E, Santos S, Inskip H, Amiano P, Barros H, Charles MA, Chatzi L, Chrousos GP, et al. Association of gestational weight gain with adverse maternal and infant outcomes. JAMA. 2019;321(17):1702–15.
Aune D, Saugstad OD, Henriksen T, Tonstad S. Maternal body mass index and the risk of fetal death, stillbirth, and infant death: a systematic review and meta-analysis. JAMA. 2014;311(15):1536–46.
Ukah UV, Bayrampour H, Sabr Y, Razaz N, Chan WS, Lim KI, Lisonkova S. Association between gestational weight gain and severe adverse birth outcomes in Washington State, US: a population-based retrospective cohort study, 2004–2013. PLoS Med. 2019;16(12):e1003009.
Starling AP, Brinton JT, Glueck DH, Shapiro AL, Harrod CS, Lynch AM, Siega-Riz AM, Dabelea D. Associations of maternal BMI and gestational weight gain with neonatal adiposity in the Healthy Start study. Am J Clin Nutr. 2015;101(2):302–9.
Voerman E, Santos S, Patro Golab B, Amiano P, Ballester F, Barros H, Bergstrom A, Charles MA, Chatzi L, Chevrier C, et al. Maternal body mass index, gestational weight gain, and the risk of overweight and obesity across childhood: an individual participant data meta-analysis. PLoS Med. 2019;16(2):e1002744.
Heslehurst N, Vieira R, Akhter Z, Bailey H, Slack E, Ngongalah L, Pemu A, Rankin J. The association between maternal body mass index and child obesity: a systematic review and meta-analysis. PLoS Med. 2019;16(6):e1002817.
Newgard CB. Metabolomics and metabolic diseases: where do we stand? Cell Metab. 2017;25(1):43–56.
Johnson CH, Ivanisevic J, Siuzdak G. Metabolomics: beyond biomarkers and towards mechanisms. Nat Rev Mol Cell Biol. 2016;17(7):451–9.
Article CAS PubMed PubMed Central Google Scholar
Hivert MF, Perng W, Watkins SM, Newgard CS, Kenny LC, Kristal BS, Patti ME, Isganaitis E, DeMeo DL, Oken E, et al. Metabolomics in the developmental origins of obesity and its cardiometabolic consequences. J Dev Orig Health Dis. 2015;6(2):65–78.
Schlueter RJ, Al-Akwaa FM, Benny PA, Gurary A, Xie G, Jia W, Chun SJ, Chern I, Garmire LX. Prepregnant obesity of mothers in a multiethnic cohort is associated with cord blood metabolomic changes in offspring. J Proteome Res. 2020;19(4):1361–74.
Shokry E, Marchioro L, Uhl O, Bermudez MG, Garcia-Santos JA, Segura MT, Campoy C, Koletzko B. Impact of maternal BMI and gestational diabetes mellitus on maternal and cord blood metabolome: results from the PREOBE cohort study. Acta Diabetol. 2019;56(4):421–30.
Chen C, Lu FC, Department of Disease Control Ministry of Health PRC. The guidelines for prevention and control of overweight and obesity in Chinese adults. Biomed Environ Sci. 2004;17(Suppl):1–36.
PubMed Google Scholar
The American College of Obstetricians and Gynecologists. Macrosomia: ACOG practice bulletin, number 216. Obstet Gynecol. 2020;135(1):e18–e35.
Goldenberg RL, Culhane JF. Low birth weight in the United States. Am J Clin Nutr. 2007;85(2):584S-590S.
Lian JS, Liu W, Hao SR, Guo YZ, Huang HJ, Chen DY, Xie Q, Pan XP, Xu W, Yuan WX, et al. A serum metabonomic study on the difference between alcohol- and HBV-induced liver cirrhosis by ultraperformance liquid chromatography coupled to mass spectrometry plus quadrupole time-of-flight mass spectrometry. Chin Med J (Engl). 2011;124(9):1367–73.
CAS PubMed Google Scholar
Ding W, Xu S, Zhou B, Zhou R, Liu P, Hui X, Long Y, Su L. Dynamic plasma lipidomic analysis revealed cholesterol ester and amides associated with sepsis development in critically Ill patients after cardiovascular surgery with cardiopulmonary bypass. J Pers Med. 2022;12(11):1838.
Christie S, O’Rielly R, Li H, Nunez-Salces M, Wittert GA, Page AJ. Modulatory effect of methanandamide on gastric vagal afferent satiety signals depends on nutritional status. J Physiol. 2020;598(11):2169–82.
Christie S, O’Rielly R, Li H, Wittert GA, Page AJ. High fat diet induced obesity alters endocannabinoid and ghrelin mediated regulation of components of the endocannabinoid system in nodose ganglia. Peptides. 2020;131:170371.
Rasmussen BA, Kim E, Unterwald EM, Rawls SM. Methanandamide attenuates cocaine-induced hyperthermia in rats by a cannabinoid CB1-dopamine D2 receptor mechanism. Brain Res. 2009;1260:7–14.
Facchi JC, Lima TAL, Oliveira LR, Costermani HO, Miranda GDS, de Oliveira JC. Perinatal programming of metabolic diseases: the role of glucocorticoids. Metabolism. 2020;104:154047.
Reynolds RM, Walker BR, Syddall HE, Andrew R, Wood PJ, Whorwood CB, Phillips DI. Altered control of cortisol secretion in adult men with low birth weight and cardiovascular risk factors. J Clin Endocrinol Metab. 2001;86(1):245–50.
Valtat B, Dupuis C, Zenaty D, Singh-Estivalet A, Tronche F, Breant B, Blondeau B. Genetic evidence of the programming of beta cell mass and function by glucocorticoids in mice. Diabetologia. 2011;54(2):350–9.
Jia Y, Li R, Cong R, Yang X, Sun Q, Parvizi N, Zhao R. Maternal low-protein diet affects epigenetic regulation of hepatic mitochondrial DNA transcription in a sex-specific manner in newborn piglets associated with GR binding to its promoter. PLoS ONE. 2013;8(5):e63855.
Rodriguez JS, Rodriguez-Gonzalez GL, Reyes-Castro LA, Ibanez C, Ramirez A, Chavira R, Larrea F, Nathanielsz PW, Zambrano E. Maternal obesity in the rat programs male offspring exploratory, learning and motivation behavior: prevention by dietary intervention pre-gestation or in gestation. Int J Dev Neurosci. 2012;30(2):75–81.
Tuersunjiang N, Odhiambo JF, Long NM, Shasa DR, Nathanielsz PW, Ford SP. Diet reduction to requirements in obese/overfed ewes from early gestation prevents glucose/insulin dysregulation and returns fetal adiposity and organ development to control levels. Am J Physiol Endocrinol Metab. 2013;305(7):E868-878.
Kumpulainen SM, Heinonen K, Kaseva N, Andersson S, Lano A, Reynolds RM, Wolke D, Kajantie E, Eriksson JG, Raikkonen K. Maternal early pregnancy body mass index and diurnal salivary cortisol in young adult offspring. Psychoneuroendocrinology. 2019;104:89–99.
Stirrat LI, Just G, Homer NZM, Andrew R, Norman JE, Reynolds RM. Glucocorticoids are lower at delivery in maternal, but not cord blood of obese pregnancies. Sci Rep. 2017;7(1):10263.
Prisco D, Filippini M, Francalanci I, Paniccia R, Gensini GF, Serneri GG. Effect of n-3 fatty acid ethyl ester supplementation on fatty acid composition of the single platelet phospholipids and on platelet functions. Metabolism. 1995;44(5):562–9.
Kaapa P, Uhari M, Nikkari T, Viinikka L, Ylikorkala O. Dietary fatty acids and platelet thromboxane production in puerperal women and their offspring. Am J Obstet Gynecol. 1986;155(1):146–9.
Teng KT, Chang CY, Kanthimathi MS, Tan AT, Nesaretnam K. Effects of amount and type of dietary fats on postprandial lipemia and thrombogenic markers in individuals with metabolic syndrome. Atherosclerosis. 2015;242(1):281–7.
Hanhineva K, Lankinen MA, Pedret A, Schwab U, Kolehmainen M, Paananen J, de Mello V, Sola R, Lehtonen M, Poutanen K, et al. Nontargeted metabolite profiling discriminates diet-specific biomarkers for consumption of whole grains, fatty fish, and bilberries in a randomized controlled trial. J Nutr. 2015;145(1):7–17.
Brunelli L, Davin A, Sestito G, Mimmi MC, De Simone G, Balducci C, Pansarasa O, Forloni G, Cereda C, Pastorelli R, et al. Plasmatic hippuric acid as a hallmark of frailty in an Italian cohort: the mediation effect of fruit-vegetable intake. J Gerontol A Biol Sci Med Sci. 2021;76(12):2081–9.
Ferreira LB, Lobo CV, Miranda A, Carvalho BDC, Santos LCD. Dietary patterns during pregnancy and gestational weight gain: a systematic review. Rev Bras Ginecol Obstet. 2022;44(5):540–7.
Tielemans MJ, Garcia AH, Peralta Santos A, Bramer WM, Luksa N, Luvizotto MJ, Moreira E, Topi G, de Jonge EA, Visser TL, et al. Macronutrient composition and gestational weight gain: a systematic review. Am J Clin Nutr. 2016;103(1):83–99.
Lewis RM, Wadsack C, Desoye G. Placental fatty acid transfer. Curr Opin Clin Nutr Metab Care. 2018;21(2):78–82.
Cesar HC, Pisani LP. Fatty-acid-mediated hypothalamic inflammation and epigenetic programming. J Nutr Biochem. 2017;42:1–6.
Demmelmair H, Koletzko B. Perinatal polyunsaturated fatty acid status and obesity risk. Nutrients. 2021;13(11):3882.
Hauner H, Brunner S. Early fatty acid exposure and later obesity risk. Curr Opin Clin Nutr Metab Care. 2015;18(2):113–7.
Download references
Acknowledgements
The authors thank the study participants for their involvement and research assistants for their help conducting the study.
This research was funded by the Beijing Natural Science Foundation, grant number 7214231.
Author information
Authors and affiliations.
Division of Endocrinology and Metabolism, Department of Obstetrics, Beijing Obstetrics and Gynecology Hospital, Capital Medical University, Beijing Maternal and Child Health Care Hospital, No. 251, Yaojiayuan Road, Chaoyang District, Beijing, 100026, China
Xianxian Yuan, Yuru Ma, Yan Zhao, Wei Zheng, Ruihua Yang, Lirui Zhang, Xin Yan & Guanghui Li
Department of Obstetrics and Gynecology, The Second Hospital of Jilin University, Changchun, 130041, Jilin, China
You can also search for this author in PubMed Google Scholar
Contributions
XXY designed the study. XXY, WZ, LRZ and XY analyzed the data. YRM, JW, YZ and RHY took part in data collection and management. XXY wrote the manuscript. XXY and GHL reviewed the manuscript and contributed to manuscript revision. All authors contributed to the article and approved the submitted version. All authors reviewed the manuscript.
Corresponding author
Correspondence to Guanghui Li .
Ethics declarations
Ethics approval and consent to participate.
This study has been performed in accordance with the Declaration of Helsinki and has been approved by the ethics committee of Beijing Obstetrics and Gynecology Hospital, Capital Medical University (2021-KY-037). Informed consent was obtained from all subjects involved in the study to publish this paper. All methods were carried out in accordance with relevant guidelines and regulations in the declaration.
Consent for publication
Not applicable.
Competing interests
The authors declare no competing interests.
Additional information
Publisher’s note.
Springer Nature remains neutral with regard to jurisdictional claims in published maps and institutional affiliations.
Supplementary Information
Supplementary material 1., rights and permissions.
Open Access This article is licensed under a Creative Commons Attribution 4.0 International License, which permits use, sharing, adaptation, distribution and reproduction in any medium or format, as long as you give appropriate credit to the original author(s) and the source, provide a link to the Creative Commons licence, and indicate if changes were made. The images or other third party material in this article are included in the article's Creative Commons licence, unless indicated otherwise in a credit line to the material. If material is not included in the article's Creative Commons licence and your intended use is not permitted by statutory regulation or exceeds the permitted use, you will need to obtain permission directly from the copyright holder. To view a copy of this licence, visit http://creativecommons.org/licenses/by/4.0/ . The Creative Commons Public Domain Dedication waiver ( http://creativecommons.org/publicdomain/zero/1.0/ ) applies to the data made available in this article, unless otherwise stated in a credit line to the data.
Reprints and permissions
About this article
Cite this article.
Yuan, X., Ma, Y., Wang, J. et al. The influence of maternal prepregnancy weight and gestational weight gain on the umbilical cord blood metabolome: a case–control study. BMC Pregnancy Childbirth 24 , 297 (2024). https://doi.org/10.1186/s12884-024-06507-x
Download citation
Received : 30 September 2023
Accepted : 11 April 2024
Published : 22 April 2024
DOI : https://doi.org/10.1186/s12884-024-06507-x
Share this article
Anyone you share the following link with will be able to read this content:
Sorry, a shareable link is not currently available for this article.
Provided by the Springer Nature SharedIt content-sharing initiative
- Maternal obesity
- Gestational weight gain
- Offspring health
- Metabolites
- Umbilical cord blood

An official website of the United States government
The .gov means it’s official. Federal government websites often end in .gov or .mil. Before sharing sensitive information, make sure you’re on a federal government site.
The site is secure. The https:// ensures that you are connecting to the official website and that any information you provide is encrypted and transmitted securely.
- Publications
- Account settings
Preview improvements coming to the PMC website in October 2024. Learn More or Try it out now .
- Advanced Search
- Journal List
- Int J Endocrinol
- v.2019; 2019

Effects of Uric Acid on Diabetes Mellitus and Its Chronic Complications
1 Department of Endocrinology, Zhongnan Hospital of Wuhan University, Wuhan, Hubei 430071, China
2 Department of Endocrinology, Affiliated Haikou Hospital of Xiangya Medical College, Central South University, Haikou, Hainan 570208, China
Yancheng Xu
With the deepening of the researches on uric acid, especially in the study of metabolic diseases, uric acid has been found to be closely related to obesity, metabolic syndrome, nonalcoholic fatty liver disease, diabetes, and other metabolic diseases. Uric acid causes a series of pathophysiological changes through inflammation, oxidative stress, vascular endothelial injury, and so on and thus subsequently promotes the occurrence and development of diseases. This review confirmed the positive correlation between uric acid and diabetes mellitus and its chronic complications through the pathogenesis and clinical studies aspects.
1. Introduction
In recent years, human intake of foods such as those with the umami flavor (rich in purines), high added sugar (sucrose), and high fructose corn syrup have increased dramatically [ 1 ]. Fructose is the main component of added sugar. Unlike other sugars, fructose can cause mitochondrial oxidative stress [ 2 , 3 ] and inhibits AMPK [ 4 ], and the subsequent intracellular ATP depletion [ 5 ] and nucleotide turnover lead to a significant increase in serum uric acid [ 6 ]. In addition to causing gout, many studies have shown that hyperuricemia is also closely related to cardiovascular diseases, metabolic syndrome, insulin resistance, and diabetes [ 7 , 8 ]. However, its function is a matter of debate [ 9 ]. Here, we reviewed the effects of hyperuricemia on diabetes and its complications and concluded that high levels of uric acid is closely related to diabetes and its chronic complications.
1.1. Uric Acid Formation
In the human body, uric acid is the ultimate product of purine metabolism ( Figure 1 [ 10 ]). It is generated in the liver. Purine nucleotides decompose to hypoxanthine and guanine, some of which can be recycled and phosphorylated into hypoxanthine nucleotides, while the remaining part is metabolized by xanthine dehydrogenase/oxidase (XDH/XO) enzymatic reaction to the terminal product uric acid. XDH/XO is mainly expressed in the parenchymal cells of the liver and small intestine. XDH has low reactivity and can be converted to XO. Uric acid production primarily depends on the amount of substrate and the activity of XO [ 11 ]. In the end, XDH/XO promotes the final steps in purine metabolism which convert hypoxanthine to xanthine and xanthine to UA [ 11 ]. The kidney also plays an important role in the regulation of blood uric acid levels. The circulating uric acid is easily filtered from the glomeruli into the renal tubule. About 90% of filtered UA is reabsorbed by the middle of the proximal convoluted tubule mainly by urate transporter 1 (URAT1) and glucose transporter 9 (GLUT9) [ 12 ], and the remaining excreted 10% is responsible for 60–70% of total body uric acid excretion [ 13 , 14 ]. A small amount of uric acid secreted in the intestine is responsible for 30–40% [ 14 ]. The production and excretion rate of uric acid is relatively constant in healthy people. Changes in the uric acid content in body fluids can reflect the state of metabolism, immunity, and other functions of the human body. If the body produces too much uric acid or the excretion mechanism is degraded, the body will retain excessive uric acid. Hyperuricemia was defined as the circulating uric acid levels of more than 5.7 mg/dl for women and 7.0 mg/dl for men [ 15 ]. When the blood uric acid concentration exceeds the norm, the human body fluid becomes acidic, which affects the normal function of the human cells, subsequently leading to metabolic disease in the long term [ 16 – 18 ].

Process of purine metabolism in humans.
2. Pathological Mechanism of Uric Acid on Diabetes and Its Chronic Complications
2.1. uric acid and diabetes.
At present, many studies have shown that the relevant pathological mechanisms include some aspects as follows ( Figure 2 ):
- Inflammation . Increased uric acid levels in the blood promoted the expression of interleukin-1 β (IL-1 β ), interleukin-6 (IL-6), tumor necrosis factor- α (TNF- α ) [ 19 ], and CRP production [ 20 ]. In animal studies, the activation of inflammation induced by UA decreases insulin sensitivity in mice [ 21 ], and infusion of UA into mice can increase TNF- α levels and activate the classical inflammatory pathway [ 22 ]. In human studies, serum UA was positively associated with TNF- α , interleukin-6 and C-reactive protein in healthy people [ 23 ].
- Oxidative Stress . Excessive uric acid will lead to an increase in reactive oxygen species (ROS) production, which leads to inflammation and dysfunction in the vessel [ 24 ]. UA is a powerful antioxidant that can remove superoxide and hydroxyl radicals in plasma, and UA has prooxidant effects in vascular tissue by increasing ROS production, such as H 2 O 2 [ 24 ]. UA-mediated oxidative stress-induced lipid peroxidation, DNA damage, and activation of inflammatory factors finally lead to cellular damage [ 24 ]. Oxidative stress also can affect the expression of insulin gene, causing a decrease in insulin secretion [ 25 ].
- Endothelial Dysfunction . Endothelial dysfunction is characterized by deficiencies in the synthesis and/or bioavailability of endothelium-derived NO [ 26 ]. In addition, UA reduces endothelial NO bioavailability in humans [ 27 ]. Uric acid inhibits proliferation and migration of endothelial cells and NO secretion [ 20 ]. UA can react with NO to form 6-aminouracil, UA-dependent ROS reacts with NO to form peroxynitrite, and UA can hold back L-arginine uptake and stimulate L-arginine degradation [ 6 ]. As a result of the effects of hyperglycemia and neurohormonal activation, UA levels are independently associated with endothelial dysfunction in animals and humans, thereby promoting hypertension [ 28 ].
- Inhibiting Insulin Pathway . UA directly inhibits the trigger of insulin signaling pathway by an ectonucleotide pyrophosphatase/phosphodiesterase 1 (ENPP1) recruitment at the receptor level [ 29 ].

Metabolism of uric acid leading to diabetes mellitus and its chronic complication.
All factors interference with glucose homeostasis and insulin sensitivity promotes the development of diabetes [ 30 – 32 ].
2.2. Uric Acid and Diabetic Chronic Complications
The aforementioned changes to diabetes are also directly related to the metabolic disorder: desulfation of glycosaminoglycans (GAGs) and formation of advanced glycation end products (AGE) and receptors (RAGE) [ 33 ]. It is widely believed that polyol bypass, protein kinase C, hexosamine activation, advanced glycosylation products (AGEs), increased hyperglycemia-induced mitochondria production of reactive oxygen species (ROS), inflammation, and endothelial dysfunction are the common pathogenic characteristics of chronic complications of diabetes mellitus [ 10 , 33 – 39 ], which mainly include macroangiopathy, microangiopathy, and neuropathy. Two other mechanisms are associated with chronic complications as follows ( Figure 2 ):
- Activation of RAAS . Uric acid can lead to the activation of the renin-angiotensin-aldosterone System (RAAS), through increasing the production of juxtaglomerular renin [ 40 ]. UA-induced ROS stimulated the increase of plasma angiotensin II which induced aldosterone release, leading to activation of RAAS [ 24 , 41 ]. RAAS activation induced afferent renal arteriolopathy and tubulointerstitial fibrosis in rodent models [ 42 ]. In diabetes, RAAS activation causes a range of pathological changes including vascular dysfunction, high intraglomerular pressure, inflammation, and so on, leading to cardiovascular and renal complications [ 43 ].
- Thrombus . Uric acid seems to trigger platelet adhesion and aggregation, thus favoring vascular thrombosis [ 44 ].
3. Epidemiology Studies
3.1. uric acid and diabetes.
The relationship between uric acid and diabetes has gradually become a hot topic of research, but controversy still exists. On the one hand, some study reported uric acid was not associated with diabetes. For example, Sluijs et al. [ 45 ] used a genetic score of 24 uric acid-related sites for Mendelian randomization studies, in the European prospective survey data—Cancer and Nutrition (EPIC) study, which was an interactive case-cohort study of vast number of subjects from eight European countries. In EPIC, after a mean of 10 years of follow-up, the results suggested that hyperuricemia was not salient associated with a higher risk of diabetes after adjusting for interference factors when their participant number was increased from 10,576 to 41,508. Similarly, a large prospective cohort study was performed by Li [ 46 ] who followed up 4412 nondiabetic patients for 4.7 years to study urate changes in glucose metabolism. They found the uric acid concentration was not related to an increased risk of type 2 diabetes mellitus (T2DM).
On the opposite hand, more clinical trials demonstrated uric acid was significantly associated with diabetes. For example, Bombelli et al. [ 47 , 48 ] randomly selected 3,200 northern Italian residents between the ages of 25 and 74 and found that increased uric acid resulted in an increased risk of impaired fasting glucose (IFG), and people with higher median UA levels may also develop metabolic syndrome and diabetes. In women, serum uric acid (SUA) levels in the normal range were associated with an increased risk of new-onset diabetes compared with women with low-normal values [ 49 ]. Older adults with high levels of uric acid (6.0 mg/dl for men and 5.5 mg/dl for women) were more susceptible to metabolic syndrome and T2DM, especially in the 75–84 years age group [ 50 ]. Serum UA was an important predictor of risk of metabolic syndrome, diabetes, and hypertension in adult males [ 51 ]. However, the relationship between blood UA and decreased insulin sensitivity in patients with type 1 diabetes mellitus is weaker than in healthy subjects [ 52 ].
Through reading a large number of literature and studies, we believe that uric acid is closely related to diabetes. Poor lipid metabolism in individuals with higher UA levels may lead to increased fasting and postprandial insulin levels, high-sensitivity C-reactive protein, hepatic insulin resistance index, and decreased glomerular filtration rate and skeletal muscle insulin sensitivity; high levels of SUA may impair liver insulin sensitivity and insulin clearance [ 53 ]. Perticone F [ 54 ] was documented when hypertensive NGT ≥ 155 mmHg, and UA is closely related to 1-h postload glucose during an oral glucose tolerance. We [ 55 ] analyzed the clinical characteristics and islet function index of 403 newly diagnosed patients with T2DM (mean age, 50.21 ± 13.34 years old; 62.5% male) and analyzed the SUA levels according to gender. Multivariate linear regression analysis showed that SUA had an independent effect on insulin secretion in female patients; the islet β -cell function of male was also affected by SUA, age, body mass index (BMI), and blood lipids; SUA correlated positively with insulin secretion and the insulin resistance index in male patients.
In terms of gestational diabetes, Leng [ 56 ] found that the SUA level is positively related with the risk of T2DM and prediabetes in the Tianjin region of China gestational diabetes mellitus (GDM) prevention planning data. In the group with GDM and impaired glucose tolerance (IGT), the mean SUA level was significantly increased in early pregnancy, and a UA level of 3.95 mg/dl could predict GDM with 60% specificity and 100% sensitivity [ 57 ].
3.2. Uric Acid and Diabetic Chronic Complications
3.2.1. uric acid and diabetic macrovascular disease.
Diabetic macroangiopathy refers to atherosclerosis of blood vessels such as the aorta, coronary artery, basilar artery, renal artery, and peripheral arteries, especially in the heart and cerebrovascular diseases, which is caused by dysfunction of endothelial cells, advanced glycation end product (AGEs/RAGEs) system, the hexosamine pathway, inflammation, oxidant stress, protein kinase (PKC), and polyol [ 34 – 37 ]. Some clinical studies have shown a positive correlation between uric acid and diabetic macroangiopathy. Yan et al. [ 58 ] used Mendelian randomized analysis to determine whether there is a causal relationship between UA and diabetic macrovascular disease and found that the prevalence of diabetic macrovascular disease was significantly higher in the hyperuricemia group than in the healthy population, suggesting that UA and diabetic macrovascular disease are related. Indeed, the link between female-weighted genetic risk score (GRS) and diabetic macrovascular disease was greater than expected. Hyperuricemia was also observed to be associated with an increased incidence of atrial fibrillation in hospitalized patients with T2DM [ 59 ]. Hyperuricemia can increase the risk of sudden atrial fibrillation by approximately four-fold [ 60 ] and is associated with cardiovascular mortality [ 61 ]. Cardiovascular and cerebrovascular diseases are mainly caused by ischemia and hypoxia resulting from coronary atherosclerosis. Du et al. [ 62 ] performed a meta-analysis of patients with T2DM to determine whether SUA levels were associated with cerebral infarction and calculated the ratio of means (RoM) for SUA and the average cerebral infarction or average diabetes control ratio of individual studies and then compared it with the calculated 95% confidence intervals. The results showed that higher SUA levels might lead to cerebral infarction in patients with T2DM. Wang et al. [ 63 ] used the “Comprehensive Diabetes Prevention and Control Study (CRPCD)” data to explore the relationship between SUA and ischemic stroke in patients with T2DM in China. A total of 19,442 participants were enrolled in a cross-sectional study. The SUA level was significantly higher in patients over 60 years of age than in people under 60 years of age. Serum UA levels were independently and positively correlated with ischemic stroke in patients under 60 years of age, and it was characterized by U-type association in patients over 60 years of age. We speculated that the incidence of other established stroke risk factors such as hypertension, dyslipidemia, and chronic kidney disease increased with age would made it difficult to establish UA as an independent role in stroke.
Diabetic hyperglycemia causes metabolic abnormalities, which can affect systemic organs. Diabetic foot is caused by peripheral vascular disease, peripheral (motor, sensory, and autonomic) neuropathy, and excessive mechanical stress (repetitive external or minor trauma) in diabetic patients, leading to the destruction and deformity of the soft tissue and bone joint system of the foot [ 64 ]. The pathogenesis is partly the same as diabetic vascular and neuropathy complications [ 65 ]. Uric acid can be used as an independent risk factor to assess the development of diabetic foot [ 66 ].
3.2.2. Uric Acid and Diabetic Microangiopathy
Diabetic microangiopathy is a specific complication of diabetes. The typical changes comprise microcirculatory disorders and microvascular basement membrane thickening, which mainly lead to diabetic nephropathy (DN) [ 51 ] and diabetic retinopathy (DR) [ 67 – 70 ].
(1) Uric Acid and Diabetic Nephropathy . Diabetic nephropathy is a long-standing microvascular complication of diabetes and is the leading cause of end-stage renal disease in developed countries [ 10 , 71 ]. As an inflammatory factor, UA increases oxidative stress and promotes the activation of the renin-angiotensin-aldosterone system (RAAS) [ 21 , 41 ]. Therefore, UA levels are associated with the occurrence and development of DN and are independent risk factors for early kidney disease [ 72 , 73 ], which help to predict microalbuminuria progression [ 74 ]. Serum UA and microalbuminuria levels were significantly positively correlated with renal disease in patients with T2DM [ 75 ]. Patients with higher SUA levels have poorer renal function, independent of glycated hemoglobin (HbA1c) or the duration of diabetes [ 76 ]. In T2DM, there is an independent and significant positive association between higher blood UA and an increased risk of a reduced glomerular filtration rate (eGFR) [ 77 ]. Blood UA levels greater than 5.5 mg/dl can predict chronic kidney disease of stage 3 and above in T2DM [ 78 ]. The level of SUA that protects against progression of type 2 diabetic nephropathy (diabetic kidney disease (DKD)) is lower than the current normal value. The optimal cut-off value is 377.5 µmol/l (6.3 mg/dl) for men and 309.0 μ mol/l (5.2 mg/dl) for women [ 79 ]. In Chinese patients with T2DM, UA-related alleles such as SLC2A9 rs11722228 (solute carrier family 2 member 9), SLC2A9 rs3775948, and ABCG2 rs2231142 (ATP binding cassette subfamily G member 2) may affect susceptibility to DKD [ 80 ]. Contrast-enhanced ultrasound (CEUS) was used to show renal microvascular hyperperfusion, with a decreased glomerular filtration rate and reduced UA excretion in patients with DKD [ 81 ]. Xanthine oxidase (XO) is a very important enzyme that is responsible for the conversion of sulfhydryl groups to UA. Elevation of UA by 1 μ mol/l enhanced the probability of albuminuria by 1.5%, and a rise in XO activity of 1 U/l also increased the probability of albuminuria by 1.5%. In diabetes, both XO and uric acid are independently associated with albuminuria [ 82 ].
In patients with type 1 diabetes without complications, higher UA levels are associated with lower GFR, which is due to UA-mediated increased resistance in afferent renal arteriole promoting the renal microcirculation ischemia [ 83 , 84 ].
In type 1 diabetes, kidney damage is more common in men whose SUA and creatinine concentrations and the albumin excretion rate are higher than those in female patients. Indeed, hyperglycemia adversely affects the activity of estrogen receptors (ER) and this may be gender-specific. The progression of renal disease in men with T1D is associated with a decline in free estradiol levels [ 85 ], and 17 β -estradiol shows antioxidant, antiapoptotic, and anti-inflammatory properties [ 86 ]. The SUA level in boys but not girls with T1D was positively correlated with subclinical inflammation marker levels (CRP, IL-6, TNF- α ), renal function indicators (albumin excretion rate, cystatin-C level), and blood pressure; it was negatively correlated with anti-inflammatory IL-10 [ 87 ].
(2) Uric Acid and Diabetic Retinopathy . Diabetic retinopathy (DR) is a specific fundus lesion that is the main cause of blindness in patients with diabetes [ 88 ]. Based on the changes of haemodynamics or vascular geometry, vascular injury is considered to be the prime motivator for the initiation and progression of DR, including pericytosis, platelet aggregation, thickening of basement membrane, and neuroglial damage [ 89 ]. The blood retinal barrier, as precondition to vision acuity, is vulnerable to injury during the progression of DR. This is a consequence of the interplay of AGE, hexosamine, polyol, inflammation, NO decline, oxidative stress, PKC, and RAS [ 38 ]. Uric acid is closely related to these pathological changes. Clinically, DR is classified into nonproliferative diabetic retinopathy (NPDR) (also known as simple type or background type) and proliferative diabetic retinopathy (PDR), according to whether or not retinal neovascularization occurs [ 90 ]. In Chinese patients with T2DM, reduction in urinary uric acid excretion (UUAE) is an independent risk factor for DR [ 91 ]. Elevated SUA levels are significantly associated with albuminuria and DR severity [ 92 ], but not with the retinal nerve fibre layer or macular thickness [ 93 ]. A study reported that increased SUA levels were associated with an increased severity of DR in Taiwan [ 94 ]. Kuwata [ 95 ] analyzed data from 1839 patients with T2DM in Japan by gender stratification and found that higher SUA levels were associated with an increased risk of DR in men, but not in women. The results showed sex hormones play an important role in the metabolism of uric acid, which deserved to discuss the specific mechanism further.
3.2.3. Uric Acid and Diabetic Peripheral Neuropathy
Diabetic neuropathy is one of the most common chronic complication of diabetes [ 96 ], characterized by damage to nerve glial cells, axons, and endothelial cells, and the morbidity from 30% to 50% in T2DM [ 97 ]. Diabetic peripheral neuropathy (DPN) is the main clinical manifestation of sensory and autonomic nerve symptoms, distal symmetry polyneuropathy, and motor neuropathy are the most common types of DPN [ 98 ]. The pathophysiology changes conclude polyol pathway, PKC activity, increased AGEs, oxidative stress (ROS), inflammation (IL-1 β , IL-6, TNF α , and COX-2), microvascular alterations (endothelial dysfunction), nerve degeneration and regrowth (MMPs, Schwann cells and ECM), and the changes of the blood-nerve barrier [ 39 , 99 , 100 ]. Lin et al. [ 101 ] observed significant differences in the ratio of motor and sensory nerve amplitude and conduction velocity (CV) parameters between groups with different blood UA levels (both P < 0.05). Blood UA levels were negatively correlated with the ratio of motor and sensory nerve amplitude and CV. Blood UA at 9 mg/dl and total cholesterol of 5.2 mmol/l were significantly associated with DPN in patients who had suffered from T2DM for more than 10 years. Yu et al. [ 102 ] performed a meta-analysis of 1388 patients with T2DM with peripheral neuropathy and in 4746 patients without peripheral neuropathy and showed that SUA levels were significantly elevated in patients with diabetes complicated with peripheral neuropathy and that increased hyperuricemia was related with increased risk of peripheral neuropathy.
4. Conclusion
Complex genetic and environmental factors contribute to causing diabetes, and chronic complications of diabetes may occur throughout the body. The pathogenesis of T2DM is complex, involving various interacting factors. Its increased incidence rate is a great concern worldwide. Hyperuricemia is closely related to the development of diabetes and its chronic complications. Many animal and human experiments have confirmed that UA mainly affects diabetes and its complications through inflammation, oxidative stress, endothelial function damage, and other effects. We call for further researches to explore the molecular mechanism, especially in the direct effect of uric acid on insulin secretion.
Acknowledgments
This study was supported by the National Natural Science Foundation of China (no. 81370872). The authors are indebted to the Department of Endocrinology, Zhongnan Hospital of Wuhan University, for providing suggestion during the preparation of the manuscript.
Conflicts of Interest
The authors declare that there are no conflicts of interest related with the publication of this paper.
Authors' Contributions
Qing Xiong and Jie Liu contributed equally to this work.
Thank you for visiting nature.com. You are using a browser version with limited support for CSS. To obtain the best experience, we recommend you use a more up to date browser (or turn off compatibility mode in Internet Explorer). In the meantime, to ensure continued support, we are displaying the site without styles and JavaScript.
- View all journals
- My Account Login
- Explore content
- About the journal
- Publish with us
- Sign up for alerts
- Open access
- Published: 24 March 2021
Detrimental effects of long-term elevated serum uric acid on cognitive function in rats
- Tian Tian 1 na1 ,
- Xi-run Liu 2 na1 ,
- Ting-ting Li 1 ,
- Zhi-chao Nie 1 ,
- Shuang-jing Li 1 ,
- Yan Tang 1 ,
- Cong-wei Gu 3 ,
- Wang-dong Xu 1 &
- Hong Jia 1 , 4
Scientific Reports volume 11 , Article number: 6732 ( 2021 ) Cite this article
5762 Accesses
14 Citations
1 Altmetric
Metrics details
- Cognitive neuroscience
- Learning and memory
Uric acid is a powerful antioxidant. However, its elevated levels in association with cardiovascular diseases predispose individuals to cognitive impairment. Uric acid’s effects on cognition may be related to its concentration and exposure period. We aimed to explore the effects of long-term elevated serum uric acid on cognitive function and hippocampus. Rats were randomly divided into four groups: NC, M1, M2 and M3 groups. Hyperuricemia was established in rats at week 6 and maintained until week 48 in groups M1, M2 and M3. The rats’ spatial learning and memory abilities were assessed by the Morris Water Maze test at weeks 0, 6, 16, 32, and 48. After week 48, we observed pathological changes in right hippocampal CA1 and CA3 regions, and measured levels of oxidative stress, inflammatory cytokines, and β-amyloid peptide of left hippocampus. Starting from week 6, the serum uric acid level of M3 group > M2 group, the serum uric acid level of M2 group > M1 group, and the serum uric acid level of M1 group > NC group. The rats in M3 and M2 groups had longer escape latencies, longer mean distances to the platform, more extensive pathological damage, stronger inflammation response, higher oxidative stress and β-amyloid peptide levels than those in NC group. No significant differences were observed between M1 and NC groups. In addition, we also found that oxidative stress significantly correlated with tumour necrosis factor-α and β-amyloid peptide. Long-term elevated serum uric acid was significantly associated with cognitive impairment risk. Oxidative stress, tumour necrosis factor-α and β-amyloid peptide may mediate the pathogenesis of the cognitive impairment induced by uric acid. The detrimental effect of elevated serum uric acid on cognitive function was probably expressed when the serum uric acid concentration reached a certain level.
Similar content being viewed by others
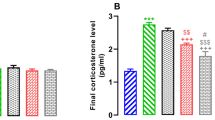
Vitamin D3 administration prevents memory deficit and alteration of biochemical parameters induced by unpredictable chronic mild stress in rats
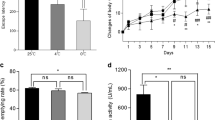
Drinking water temperature affects cognitive function and progression of Alzheimer’s disease in a mouse model
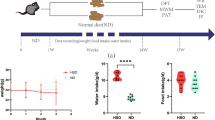
Dietary salt promotes cognition impairment through GLP-1R/mTOR/p70S6K signaling pathway
Introduction.
Uric acid is an end-product of purine metabolism generated during the enzymatic degradation of xanthine and excreted from the kidneys and bowels. Elevated serum uric acid (SUA) is associated with several cardiovascular diseases and often contributes to cognitive impairment morbidity 1 . Cognitive impairment is a chronic neurodegenerative condition characterized by poor learning and memory 2 . It is estimated that about 22% of the elderly > 70 years of age have some degree of cognitive impairment in the United States 3 . With the aging of our society, cognitive impairment is becoming a serious public health problem. More studies are required to identify the risk factors of cognitive impairment.
Recently, several studies investigated the association between uric acid and cognitive impairment with inconsistent results. In theory, uric acid is an endogenous antioxidant, accounting for about two-thirds of the plasma antioxidant power in humans, which is thought to exert a neuroprotective effect 4 . Findings from several studies support this hypothesis. Several cross-sectional or case–control studies have suggested that an increased SUA level is related to a decreased risk of cognitive dysfunction 5 , 6 , 7 . In a prospective study, a higher baseline SUA level was associated with subsequently enhanced cognitive performances, even in the special cognitive domain 8 , 9 . Similar results have also been demonstrated in the early stage of cognitive dysfunction. A large cross-sectional study on 2102 Chinese elderly individuals has shown a linear decrease of mild cognitive impairment prevalence with the increase of SUA levels 10 .
However, the antioxidant capacity of the uric acid remains questionable in several studies. The elevated SUA level has been linked to several chronic disease states, including obesity, metabolic syndrome, diabetes, hypertension, non-alcoholic fatty liver disease and cardiovascular events 11 , 12 , 13 , 14 . SUA decreased in gout patients treated with pegloticase (a recombinant uricase), but the plasma markers of oxidative stress remained unchanged 15 . These conflicting results indicate that higher SUA levels are related to poor cognitive performances. The Rotterdam Scan Study found that elevated SUA was related to white matter atrophy and worse cognition 16 . In a prospective cohort study, higher baseline SUA was associated with faster cognitive decline over time in a visual memory/visual construction ability test 17 .
Overall, the association between uric acid and cognitive impairment is complex. Findings from previous studies were inconsistent. Recently, in Huang et al. study, generalized additive models revealed a U-shaped carve relationship between SUA and cognitive function. Still, the further analysis found no significant positive association between increased mild cognitive impairment risk and elevated SUA among subjects whose SUA was above the cut-off point (388.63 µmol/L) 18 . The uric acid impact on cognitive function may go through a chronic development process. It may be impossible to observe the uric acid’s detrimental effect on cognition while in low concentration or during short-term exposure. In this study, we aimed to explore the effects of long-term elevated SUA on the cognitive function and hippocampus.
Ethics statement
The Ethics Committee for Animal Research of the Southwest Medical University approved the whole experiment. Animals were handled in strict accordance with good animal practice. During the experiment, all animal-related procedures were performed following relevant guidelines and regulations of the Ethics Committee for Animal Research of the Southwest Medical University. All procedures involving live animals were also performed observing the Animal Research: Reporting of In Vivo Experiments (ARRIVE) guidelines.
Thirty-two male Sprague-Dawley rats (213 ± 14.21 g weight, 8 months old) were provided by the Laboratory Animal centre of the Southwest Medical University. The rats were housed two per cage in clean cages under controlled temperature (23 ± 2 °C), relative humidity (55 ± 5%), and light–dark cycle (light, 08:00–20:00 h; dark, 20:00–08:00 h). Access to food and water was unrestricted. The animals were pacified before and after each experiment.
Hyperuricemia rats
To establish hyperuricemia models, rats were fed yeast and/or potassium oxonate diets 19 , 20 . Yeast contains abundant protein and a nucleotide, which can increase uric acid production. Potassium oxonate, a uricase inhibitor, can inhibit uric acid excretion. The combined application of yeast and potassium oxazinate improve the increasing of SUA levels. Rats were randomly divided into four groups of eight rats: NC group, M1 group, M2 group and M3 group. NC group was the control group with a normal diet. M1 group was the control group with yeast diet, and M1 group rats were fed a yeast diet containing 1% yeast per day. M2 group was the control group with a potassium oxonate diet, and M2 group rats were fed a potassium oxonate diet containing 0.025% potassium oxonate per day. M3 group was the positive group with yeast and potassium oxonate diet, and M3 group rats were fed a diet containing 1% of yeast and 0.025% potassium oxonate per day. We persistently provided yeast and potassium oxonate diets to rats. The rats were anesthetized by intraperitoneal injection of sodium pentobarbital 30 mg/kg, and blood (1 ml) was sampled from rat heart in vivo at weeks 0, 6, 16, 32 and 48. The blood was centrifuged for collect serum, the SUA level were measured by automatic biochemical analyzer (Beckman, USA).
Morris water maze test
The rats’ spatial learning and memory abilities were evaluated by the Morris water maze (MWM) test, as described previously 21 , before each blood draw. In the orientation-navigation test, each rat was allowed to swim and search for a platform within 120 s in 5 consecutive days. The escape latency, swimming speed and mean distance to the platform were recorded. If the rat failed to find the platform, the escape latency was recorded as 120 s. In the spatial probe test, the platform was removed on the 6th day. Each rat was allowed to swim within 120 s, and the frequency with which the rat passed the original platform quadrant was recorded. All trials were conducted between 10:00 a.m. and 13:00. The behavioural differences of the rats in each MWM test among the groups were evaluated. At week 48, the escape latencies and mean distances to the platform among groups showed a significant difference, and the rats stopped receiving food. The 48th-week indexes served as final data.
Histopathology
After week 48, the rats were anesthetized with 40 mg/kg pentobarbital sodium and then humanely killed to collect the hippocampus. The right hippocampus was fixed in 4% paraformaldehyde solution and cut into sections. The sections stained with haematoxylin–eosin (HE) were used to observe the CA1 and CA3 regions’ morphology and structure. The sections stained with terminal deoxynucleotidyl transferase-mediated nick end labelling (TUNEL) were used to monitor the CA1 and CA3 regions’ apoptotic cells. Apoptotic cells are pyramidal cells with prominent yellowish-brown granules or plaques in the nuclei. Three non-overlapping fields of CA1 and CA3 regions in each section were randomly selected to monitor the apoptotic cells and calculate the apoptosis indexes (apoptosis index = the number of apoptotic cells/the number of total cells × 100%) under a light microscope (400×), respectively.
An enzyme-linked immunosorbent assay (ELISA)
Left hippocampus tissue and iced physiological saline were mixed in a volume ratio of 1:9 and then added to a tissue homogenizer for homogenization. The homogenate was centrifuged at 4000 rpm for 15 min to obtain a supernatant used for the ELISA test.
The superoxide dismutase (SOD) levels were measured using an ELISA kit (Jiancheng, Nanjing, China) at an absorbance of 450 nm, and the detection limit was 0.5 U/ml. The malondialdehyde (MDA) levels were measured using an ELISA kit (Jiancheng, Nanjing, China) at an absorbance of 532 nm. The glutathione peroxidase (GSH-Px) levels were measured using an ELISA kit (Jiancheng, Nanjing, China) at an absorbance of 412 nm. The β-amyloid peptide (Aβ) levels were measured using an ELISA kit (Duqiao, Shanghai, China) at an absorbance of 450 nm, and the detection limit was 1.0 ng/ml. The interleukin-1β (IL-1β) levels were measured using an ELISA kit (Duqiao, Shanghai, China) at an absorbance of 450 nm, and the detection limit was 0.1 pg/ml. The tumour necrosis factor-α (TNF-α) levels were measured using an ELISA kit (Duqiao, Shanghai, China) at an absorbance of 450 nm, and the detection limit was 1.0 pg/ml. All experimental operations complied with the manufacturer’s protocol. All samples were measured in duplicates, and plates were read automatically using a full-wavelength microplate reader (Molecular Devices, USA). Concentration was calculated based on a standard linear curve.
Statistical analysis
SPSS 24.0 was used for all statistical analyses. Data with normal distribution were presented as mean value ± standard deviation and analysed with Analysis of variance. Variables without normal distribution were presented as median (25th percentile, 75th percentile) and analysed with the Kruskal–Wallis H test. Repeated measures analysis of variance was used to evaluate the difference of repeated measurement data among the groups. The associations between SUA, oxidative stress, inflammation cytokines and Aβ were analysed using Pearson’s correlation analysis. P-value < 0.05 was established as statistically significant. GraphPad 6.0 was used to draw graphs.
A rat with an SUA value > 110 µmol/L was considered a hyperuricemia rat 22 . The SUA levels in M3 and M2 groups were 148.24 ± 21.99 µmol/L and 142.56 ± 21.21 µmol/L, respectively, at week 6, and the SUA level in the M1 group was 136.93 ± 19.22 µmol/L at week 16, indicating that we successfully induced hyperuricemia rat models in the M3, M2 and M1 groups.
From week 6, the SUA levels in M3, M2 and M1 groups were persistently increasing. At week 6, the SUA levels of rats in the M3 group were higher than those in the M1 group ( P < 0.001) and NC group ( P < 0.001), the SUA levels of rats in the M2 group were higher than those in the M1 group ( P < 0.001) and NC group ( P < 0.001), the SUA levels of rats in the M1 group were higher than those in the NC group ( P < 0.001). At week 16, the SUA levels of rats in the M3 group were higher than those in the M1 group ( P = 0.004) and NC group ( P < 0.001), the SUA levels of rats in the M2 group were higher than those in the M1 group ( P = 0.025) and NC group ( P < 0.001), the rats’ SUA levels of the M1 group were higher than those in the NC group ( P < 0.001). At week 32, the rats SUA levels in the M3 group were higher than those in the M2 group ( P = 0.003), M1 group ( P < 0.001), and NC group ( P < 0.001), and the SUA levels of rats in the M2 group ( P < 0.001) and M1 group ( P < 0.001) were higher than those in the NC group. At week 48, the SUA concentrations of rats in the M3 group were higher than those in the M1 group ( P < 0.001) and NC group ( P < 0.001), and the SUA levels of rats in the M2 group were higher than those in the M1 group ( P = 0.013) and NC group ( P < 0.001), the SUA concentrations of rats in the M1 group were higher than those in NC group ( P < 0.001). In general, starting from week 6, the SUA level of the M3 group > M2 group, the SUA level of the M2 group > M1 group, and the SUA level of the M1 group > NC group (Fig. 1 a).
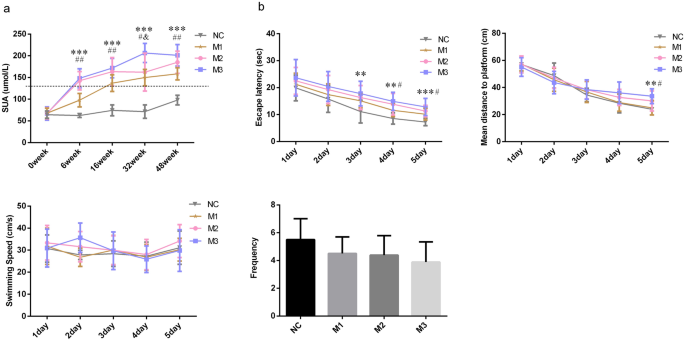
SUA levels and MWM indicators of rats. Data presented as mean ± standard deviation, n = 8 for each group. *P < 0.05 compared to the NC group, # P < 0.05 compared to the M1 group, and & P < 0.05 compared to the M2 group.
Morris water maze
The escape latency and mean distance to the platform can reflect the learning ability of the animal. At week 48, the reduction in escape latency and mean distance to the platform were observed in all rats in 5 days. But the performance comparison among the groups was difficult. The rats in the 4th group had comparable swimming speed (F = 0.708, P = 0.555). The escape latencies (F = 3.093, P = 0.043) and mean distances to the platform (F = 3.196, P = 0.039) in the four groups were different. The escape latencies in the M3 ( P = 0.007) and M2 ( P = 0.035) groups were more extended than that in the NC group. The rats in the M3 ( P = 0.023) and M2 ( P = 0.041) groups had worse performance in the mean distance to the platform than those in the NC group. The mean distance in the M1 ( P = 0.035) group was also shorter than that of the M3 group. The frequency of the rat passing the original platform quadrant indicated the degree of memory consolidation which had taken place after learning. The rats had comparable frequencies among groups (F = 1.896, P = 0.153), but the frequencies in M3, M2 and M1 groups were all lower than the NC group. In general, the rats in M3 and M2 groups had longer escape latencies and mean distances to the platform than those in the NC and M1 groups, indicating that the rats’ spatial learning ability in the M3 and M2 groups were worse than those in the NC and M1 groups (Fig. 1 b).
Histopathology analysis
The cell layer and number of CA1 and CA3 regions decreased considerably in the M3 group. Decreased cell layer and number of CA1 regions were also observed in the M2 group. The CA3 region cell gaps considerably increased, and the partial cells became smaller with concentrated cytoplasm and nuclei stained deeply in the M2 group. The cell gaps of the CA1 region slightly increased, and the CA1 region cell layer and number of partial cells also slightly decreased in the M1 group (Fig. 2 a).
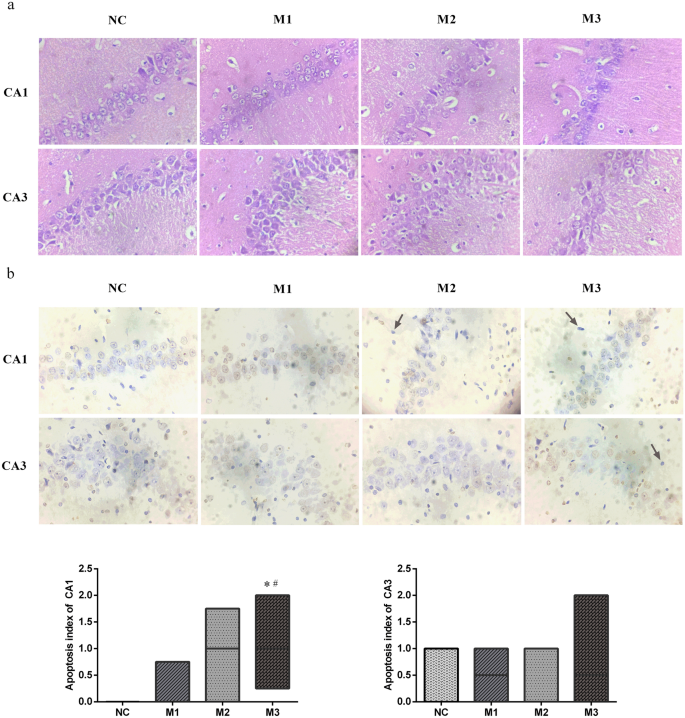
The pathological changes of hippocampal CA1 and CA3 regions of rats. ( a ) Haematoxylin–eosin staining, × 400. ( b ) terminal deoxynucleotidyl transferase nick end labelling, × 400. Apoptotic bodies were marked with arrows. Data performed as median (25th percentile, 75th percentile), n = 8 for each group. *P < 0.05 compared with the NC group, # P < 0.05 compared with the M1 group.
An apoptotic cell is defined as a pyramidal cell with prominent yellowish-brown granules or plaques in the nucleus. The apoptotic bodies were marked with arrows. In the CA1 regions, the apoptosis index of the M3 group was higher than those of M1 ( P = 0.043) and NC ( P = 0.009) groups. We could not find the statistical difference in the apoptosis index in the CA3 regions (H = 1.732, P = 0.630). Hence, we concluded that the CA1 region of the M3 group had more extensive pathological damage than those of the NC and M1 groups (Fig. 2 b).
Oxidative stress
SOD, GSH-Px and MDA are common indicators of oxidative stress. In this study, the different SOD (F = 9.045, P < 0.001) and MDA (F = 4.197, P = 0.014) levels of the hippocampus were observed among groups. The SOD levels in the M3 group were higher than those in the M1 ( P < 0.001) and NC group ( P < 0.001). SOD concentrations in the M2 group were higher than those in the M1 ( P = 0.003) and NC group ( P = 0.003). The MDA levels of the M3 group were greater than those of the M1 ( P = 0.007) and NC group ( P = 0.003). We did not find significant differences in GSH-Px levels among the groups (F = 0.254, P = 0.858). In general, rats in the M3 and M2 groups had higher of oxidative stress levels than those in the NC and M1 groups (Fig. 3 ).
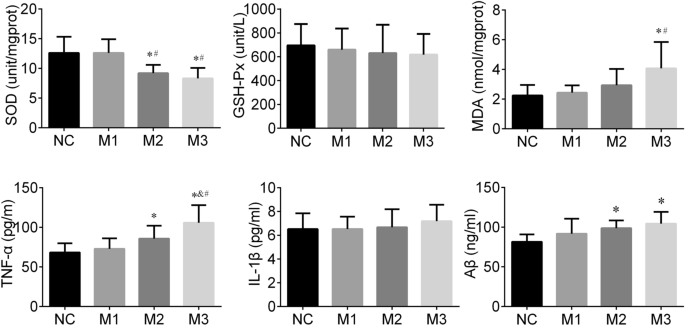
The hippocampal oxidative stress, inflammation cytokines and Aβ of rats. Data is calculated as mean ± standard deviation, n = 8 for each group.
Inflammation cytokines
IL-1β and TNF-α are known as classic inflammatory cytokines. In this study, the hippocampal TNF-α level in the M3 group was higher than those in M2 ( P = 0.021), M1 ( P < 0.001), and NC ( P < 0.001) groups. The TNF-α level of the hippocampus in the M2 ( P = 0.042) group was also greater than that in the NC group. We did not find a significant difference in IL-1β levels among groups (F = 0.454, P = 0.716). In general, the hippocampal tissues of the M3 and M2 groups had a stronger inflammation response than that of the NC and M1 group (Fig. 3 ).
Aβ has been reported to induce Alzheimer’s-like learning and memory impairments. In this study, the hippocampal Aβ level among groups showed differences (F = 4.141, P = 0.015), the Aβ levels in the M3 ( P = 0.002) and M2 ( P = 0.018) groups were higher than that of the NC group (Fig. 3 ).
Associations between SUA at week 48, oxidative stress, inflammation cytokines and Aβ
Pearson’s correlation analysis showed that there was a significantly negative correlation between SUA and SOD (r = − 0.552, P = 0.002), a significantly positive correlation between SUA and TNF-α (r = 0.542, P = 0.001), a significantly positive correlation between SUA and Aβ (r = 0.550, P = 0.001) (Fig. 4 a). Moreover, we also found that TNF-α was associated significantly with SOD (r = − 0.464, P = 0.007) and MDA (r = 0.368, P = 0.038), and Aβ was significantly correlated with SOD (r = -0.376, P = 0.034) (Fig. 4 b). We found that the higher the SUA level, the stronger was the response of oxidative stress and inflammation, and the higher the Aβ level. The stronger response to oxidative stress, the stronger the inflammatory response and the higher the Aβ level.
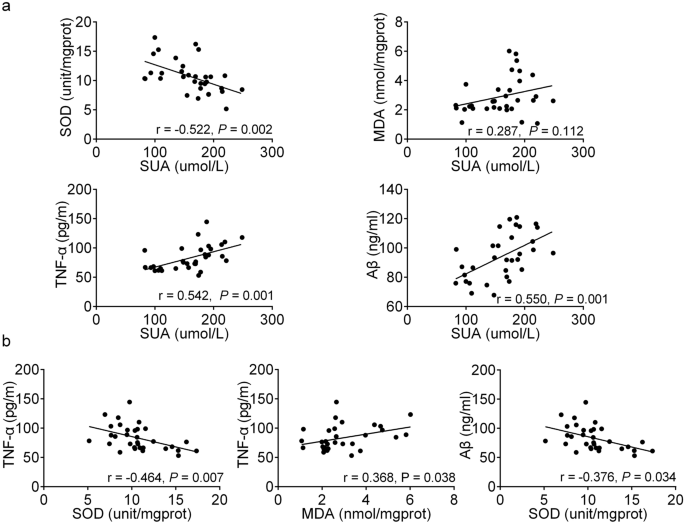
Associations between SUA at week 48, oxidative stress, inflammation cytokines and Aβ. n (subjects’ count) = 32.
The association between cognitive impairment, a chronic neurodegenerative disorder, and uric acid has been extensively studied, but the results are conflicting. Our data provided clear evidence for the detrimental impact of long-term elevated SUA on cognitive function. Long-term elevated SUA may induce oxidative stress and increase the expression of TNF-α and Aβ in the rat hippocampus.
MWM test is one of the most used methods for evaluating the rat’s spatial learning and memory abilities. The hippocampal CA1 and CA3 regions are the critical brain regions for learning and memory, and damage in this area may contribute to cognitive impairment 23 . In our study, the rats in M3 and M2 groups had longer escape latencies and mean distances to the platform than those of the NC group, indicating poor learning ability under long-term elevated SUA. The histopathology results also showed that the CA1 region of the M3 group had more damage than that of the NC group. These findings suggested the detrimental effect of long-term elevated SUA on cognition to a certain extent and were consistent with several previous studies. Vannorsdall et al. reported that high SUA concentration was related to cognitive impairment in healthy community-dwelling older women 24 . In a prospective cohort study, higher baseline SUA was associated with faster cognitive decline over time 17 . Also, elevated SUA was correlated with greater matter atrophy and cerebral ischemia, which might mediate the association between SUA and cognitive function 16 , 25 .
Cerebral tissue is susceptible to oxidative damage due to its high oxygen requirement for metabolism, high level of polyunsaturated fatty acids, and low concentration of antioxidant resource 4 , 26 . The exact mechanisms of how uric acid affects cognition remain unknown; however, oxidative stress is one of the critical factors contributing to the occurrence and development of neurodegenerative disorders 27 . The uric acid concentration in the cerebrospinal fluid depends mainly on the SUA concentration and an impaired blood–brain barrier 28 . Uric acid in serum could pass through the blood–brain barrier and be deposited at a significant level in the hippocampus 19 . Cerebral tissue also could produce uric acid under certain conditions of brain ischemia 29 . Uric acid stimulates the production of hydrogen peroxide 30 . The enzymatic degradation of xanthine also produces superoxide anions during the generation process of uric acid 24 . The imbalance between reactive oxygen species production and elimination could induce oxidative stress, which will eventually cause cell damage and apoptosis 31 . SOD and MAD are the commonly sensitive indexes of oxidative stress in a clinical setting 32 , 33 . In this study, we observed lower SOD levels and higher MDA levels of hippocampal regions in the M3 and M2 groups than those of the NC group. SUA level was significantly negatively correlated with SOD level, indicating the increased oxidative stress induced by uric acid. Our results were consistent with a previous study’s findings, which detected the hippocampal SOD activity in hyperuricemia rats and found uric acid decreased SOD activity by 19% 19 . Shimamura et al. demonstrated that oxidative stress correlated negatively with cognitive function and positively with postoperative ischemic lesions in carotid artery stenosis stenting 34 .
Hippocampal inflammation is considered to be a risk factor for neurodegenerative disorders 35 . It could trigger reactive gliosis involving the microglia and astroglia 19 . Reactive microglia and astroglia can release inflammation cytokines and chemokines, thereby enhancing the inflammatory response of the brain 36 . Long-term hippocampal modifications induced by inflammation have a crucial impact on brain excitability, associated with neurological dysfunction 37 . The inflammation responses also cause neuronal death and blockade of neurogenesis, thus leading to cognitive impairment 38 , 39 . In this study, we observed higher hippocampal TNF-α levels in M3 and M2 groups under sustained elevated SUA than those in the NC group, indicating the occurrence of hippocampal inflammation. This finding was consistent with previous studies. Shao et al. study showed that a high uric acid diet increased hippocampal inflammation and reactive gliosis in rodents 19 . Uric acid may induce hippocampal inflammation by activating TLR4/NF-κB signalling, enhancing the inflammatory cytokine gene expression and the upregulation of TNF-α and IL-1β levels 19 .
We also found that TNF-α level was significantly correlated with the hippocampal oxidative stress response in rats. Many research results suggest that the oxidative stress induced by uric acid is like a second messenger for mediators of inflammation 40 . Elevated uric acid can induce reactive oxygen species in vascular endothelial cells, and massive accumulation of reactive oxygen species can upregulate the expression of TNF-α and IL-6 41 . A recent study regarding the treatment of severe gouty arthritis with allopurinol and febuxostat found that MDA and nicotinamide adenine dinucleotide phosphate oxidase levels decreased when uric acid was reduced, suggesting oxidative stress may be involved in the inflammation induced by uric acid 42 .
Aβ consists of 39–42 amino acid residues and is generated from a sequent cleavage of amyloid precursor protein by β-secretase and γ-secretase 43 . Aβ is the major component of an amyloid plaque, one of the hallmarks of Alzheimer’s disease. Our study found Aβ level was negatively correlated with the SOD level and positively correlated with the SUA level. Zlokovic first proposed the idea that age-related vascular injury preceded neurodegeneration and cognitive impairment 44 . Uric acid affects the structure and function of the brain vascular system through oxidative stress and increases the risk of cerebral ischemia 45 . Cerebral ischemia causes endothelial cells to stimulate AβPP/β-secretase expression, leading to an increased Aβ production 46 . Meanwhile, impaired endothelial cells induce the abnormal transmembrane Aβ transport proteins expression, causing the abnormal Aβ transport proteins to cross the blood–brain barrier, resulting in over-deposition of hematogenous Aβ in the brain 47 . The soluble Aβ oligomers are the major cause of synaptic dysfunction and ultimately neurodegeneration 48 . Aβ plaques can also activate astrocytes and microglia. Activated astrocytes and microglia migrate and surround the plaque, release inflammation-associated proteins in the brain, thus causing neuroinflammation 49 , 50 . In addition, Aβ promotes oxidative stress and apoptosis, which leads to cognitive impairment. Elevated SUA levels may amplify these effects of Aβ 51 .
In this study, no significant differences in escape latencies, mean distances to the platform, levels of Aβ, oxidative stress and inflammation cytokines were observed between the M1 and NC groups, although the M1 group always had a higher SUA level. The pathological injuries in the hippocampal CA1 and CA3 regions of M1 group were also slight compared to the NC group. These results may indicate that the detrimental effect of elevated SUA on cognitive function was probably expressed when the SUA concentration reaches a certain level.
Our results in terms of histopathology, oxidative stress, TNF-α and Aβ in rat hippocampus provided relatively direct evidence for the detrimental effect of elevated SUA on cognitive function. However, several limitations should be noted. First, as we did not observe the histopathology, oxidative stress, inflammation cytokines and Aβ in the intermediate process due to the small sample size restriction, we could not identify the SUA concentration at which pathological changes of the hippocampus occurred. Second, we did not observe a statistical difference in frequencies among the groups, although rats with higher SUA had lower frequencies. However, the damage of the hippocampal CA1 and CA3 regions were evident in the M3 and M2 groups. A significant difference in frequencies might be registered if the sample size would be increased. Third, this study did not involve research of the signalling pathways between oxidative stress and TNF-α and Aβ in the hippocampus. Further research is needed to confirm our findings.
Long-term elevated serum uric acid was significantly associated with cognitive impairment risk. Oxidative stress, tumour necrosis factor-α and β-amyloid peptide may mediate the pathogenesis of the cognitive impairment induced by uric acid. The detrimental effect of elevated serum uric acid on cognitive function was probably expressed when the serum uric acid concentration reached a certain level.
Data availability
The data used to support the findings of this study are available from the corresponding authors upon request.
Abbreviations
Serum uric acid
Superoxide dismutase
Glutathione peroxidase
Malondialdehyde
Interleukin-1β
Tumor necrosis factor-α
β-Amyloid peptide
Borghi, C. et al. Serum uric acid and the risk of cardiovascular and renal disease. J. Hypertens. 33 , 1729–1741 (2015) ( discussion 1741 ).
Article CAS PubMed Google Scholar
Chang, K. W. et al. Modulation of the MAPKs pathways affects Aβ-induced cognitive deficits in Alzheimer’s disease via activation of α7nAChR. Neurobiol. Learn Mem. 168 , 107154 (2020).
Brookmeyer, R. et al. National estimates of the prevalence of Alzheimer’s disease in the United States. Alzheimers Dement. 7 , 61–73 (2011).
Article PubMed PubMed Central Google Scholar
Du, N. et al. Inverse association between serum uric acid levels and Alzheimer’s disease risk. Mol. Neurobiol. 53 , 2594–2599 (2016).
Tuven, B., Soysal, P., Unutmaz, G., Kaya, D. & Isik, A. T. Uric acid may be protective against cognitive impairment in older adults, but only in those without cardiovascular risk factors. Exp. Gerontol. 89 , 15–19 (2017).
Xiu, S. et al. Serum uric acid and impaired cognitive function in community-dwelling elderly in Beijing. Neurosci. Lett. 637 , 182–187 (2017).
Wu, Y. et al. Association of serum uric acid level with muscle strength and cognitive function among Chinese aged 50–74 years. Geriatr. Gerontol. Int. 13 , 672–677 (2013).
Article PubMed Google Scholar
Wang, T., Wu, Y., Sun, Y., Zhai, L. & Zhang, D. A prospective study on the association between uric acid and cognitive function among middle-aged and older Chinese. J. Alzheimers Dis. 58 , 79–86 (2017).
Molshatzki, N., Weinstein, G., Streifler, J. Y., Goldbourt, U. & Tanne, D. Serum uric acid and subsequent cognitive performance in patients with pre-existing cardiovascular disease. PLoS ONE 10 , e0120862 (2015).
Article PubMed PubMed Central CAS Google Scholar
Liu, M., Wang, J., Zeng, J. & He, Y. Relationship between serum uric acid level and mild cognitive impairment in Chinese community elderly. BMC Neurol. 17 , 146 (2017).
Bonakdaran, S. & Kharaqani, B. Association of serum uric acid and metabolic syndrome in type 2 diabetes. Curr. Diabetes Rev. 10 , 113–117 (2014).
Chen, Y. Y. et al. The association of uric acid with the risk of metabolic syndrome, arterial hypertension or diabetes in young subjects—An observational study. Clin. Chim. Acta 478 , 68–73 (2018).
Zheng, X. et al. Serum uric acid and non-alcoholic fatty liver disease in non-obesity Chinese adults. Lipids Health Dis. 16 , 202 (2017).
Borghi, C. et al. Serum uric acid levels are associated with cardiovascular risk score: A post hoc analysis of the EURIKA study. Int. J. Cardiol. 253 , 167–173 (2018).
Hershfield, M. S. et al. Treating gout with pegloticase, a PEGylated urate oxidase, provides insight into the importance of uric acid as an antioxidant in vivo. Proc. Natl. Acad. Sci. USA 107 , 14351–14356 (2010).
Article ADS CAS PubMed PubMed Central Google Scholar
Verhaaren, B. F. et al. The relation of uric acid to brain atrophy and cognition: The Rotterdam Scan Study. Neuroepidemiology 41 , 29–34 (2013).
Beydoun, M. A. et al. Serum uric acid and its association with longitudinal cognitive change among urban adults. J. Alzheimers Dis. 52 , 1415–1430 (2016).
Huang, R. et al. U-shaped association between serum uric acid levels and cognitive functions in patients with type 2 diabetes: A cross-sectional study. J. Alzheimers Dis. 69 , 135–144 (2019).
Shao, X. et al. Uric acid induces cognitive dysfunction through hippocampal inflammation in rodents and humans. J. Neurosci. 36 , 10990–11005 (2016).
Mazzali, M. et al. Elevated uric acid increases blood pressure in the rat by a novel crystal-independent mechanism. Hypertension 38 , 1101–1106 (2001).
Vorhees, C. V. & Williams, M. T. Morris water maze: Procedures for assessing spatial and related forms of learning and memory. Nat. Protoc. 1 , 848–858 (2006).
Li, Y. et al. Anti-hyperuricemic peptides derived from bonito hydrolysates based on in vivo hyperuricemic model and in vitro xanthine oxidase inhibitory activity. Peptides 107 , 45–53 (2018).
Qian, X. et al. Baicalein improves cognitive deficits and hippocampus impairments in temporal lobe epilepsy rats. Brain Res. 1714 , 111–118 (2019).
Vannorsdall, T. D., Kueider, A. M., Carlson, M. C. & Schretlen, D. J. Higher baseline serum uric acid is associated with poorer cognition but not rates of cognitive decline in women. Exp. Gerontol. 60 , 136–139 (2014).
Article CAS PubMed PubMed Central Google Scholar
Vannorsdall, T. D., Jinnah, H. A., Gordon, B., Kraut, M. & Schretlen, D. J. Cerebral ischemia mediates the effect of serum uric acid on cognitive function. Stroke 39 , 3418–3420 (2008).
Martinez-Finley, E. J., Avila, D. S., Chakraborty, S. & Aschner, M. Insights from Caenorhabditis elegans on the role of metals in neurodegenerative diseases. Metallomics 3 , 271–279 (2011).
Feng, Y. et al. The protective effect of astaxanthin on cognitive function via inhibition of oxidative stress and inflammation in the brains of chronic T2DM rats. Front. Pharmacol. 9 , 748 (2018).
Bowman, G. L., Shannon, J., Frei, B., Kaye, J. A. & Quinn, J. F. Uric acid as a CNS antioxidant. J. Alzheimers Dis. 19 , 1331–1336 (2010).
Heo, S. H. & Lee, S. H. High levels of serum uric acid are associated with silent brain infarction. J. Neurol. Sci. 297 , 6–10 (2010).
Ko, J. et al. Uric acid induced the phenotype transition of vascular endothelial cells via induction of oxidative stress and glycocalyx shedding. FASEB J. 33 , 13334–13345 (2019).
Song, C. & Zhao, X. Uric acid promotes oxidative stress and enhances vascular endothelial cell apoptosis in rats with middle cerebral artery occlusion. Biosci. Rep . 38 , BSR20170939 (2018).
Huang, C. et al. Lead-induced oxidative stress and antioxidant response provide insight into the tolerance of Phanerochaete chrysosporium to lead exposure. Chemosphere 187 , 70–77 (2017).
Article ADS CAS PubMed Google Scholar
Gol, M., Ghorbanian, D., Soltanpour, N., Faraji, J. & Pourghasem, M. Protective effect of raisin (currant) against spatial memory impairment and oxidative stress in Alzheimer disease model. Nutr. Neurosci. 22 , 110–118 (2019).
Shimamura, N. et al. Intra-arterial oxidative stress correlates negatively with cognitive function and positively with postoperative ischemic lesions in carotid artery stenosis stenting. J. Neurointerv. Surg. 10 , 440–445 (2018).
Jeon, B. T. et al. Resveratrol attenuates obesity-associated peripheral and central inflammation and improves memory deficit in mice fed a high-fat diet. Diabetes 61 , 1444–1454 (2012).
Yang, S. H. Cellular and molecular mediators of neuroinflammation in Alzheimer disease. Int. Neurourol. J. 23 , S54-62 (2019).
Boitard, C. et al. Impairment of hippocampal-dependent memory induced by juvenile high-fat diet intake is associated with enhanced hippocampal inflammation in rats. Brain Behav. Immun. 40 , 9–17 (2014).
Heneka, M. T. et al. Neuroinflammation in Alzheimer’s disease. Lancet Neurol. 14 , 388–405 (2015).
Ransohoff, R. M. How neuroinflammation contributes to neurodegeneration. Science 353 , 777–783 (2016).
Kaneko, C. et al. Fructose suppresses uric acid excretion to the intestinal lumen as a result of the induction of oxidative stress by NADPH oxidase activation. Biochim. Biophys. Acta Gen. Subj. 1861 , 559–566 (2017).
Zhou, Y., Zhao, M., Pu, Z., Xu, G. & Li, X. Relationship between oxidative stress and inflammation in hyperuricemia: Analysis based on asymptomatic young patients with primary hyperuricemia. Medicine (Baltimore) 97 , e13108 (2018).
Article CAS Google Scholar
Xie, H. et al. EGCG attenuates uric acid-induced inflammatory and oxidative stress responses by medicating the NOTCH pathway. Oxid. Med. Cell Longev. 2015 , 214836 (2015).
Choi, S. M. et al. Effects of flavonoid compounds on β-amyloid-peptide-induced neuronal death in cultured mouse cortical neurons. Chonnam. Med. J. 50 , 45–51 (2014).
Zhang, S. et al. Transplantation of in vitro cultured endothelial progenitor cells repairs the blood-brain barrier and improves cognitive function of APP/PS1 transgenic AD mice. J. Neurol. Sci. 387 , 6–15 (2018).
van de Haar, H. J. et al. Neurovascular unit impairment in early Alzheimer’s disease measured with magnetic resonance imaging. Neurobiol. Aging 45 , 190–196 (2016).
Cai, Z., Liu, Z., Xiao, M., Wang, C. & Tian, F. Chronic cerebral hypoperfusion promotes amyloid-beta pathogenesis via activating β/γ-secretases. Neurochem. Res. 42 , 3446–3455 (2017).
Provias, J. & Jeynes, B. The role of the blood–brain barrier in the pathogenesis of senile plaques in Alzheimer’s disease. Int. J. Alzheimers Dis. 2014 , 191863 (2014).
CAS PubMed PubMed Central Google Scholar
Tu, S., Okamoto, S., Lipton, S. A. & Xu, H. Oligomeric Aβ-induced synaptic dysfunction in Alzheimer’s disease. Mol. Neurodegener. 9 , 48 (2014).
Iversen, L. L., Mortishire-Smith, R. J., Pollack, S. J. & Shearman, M. S. The toxicity in vitro of beta-amyloid protein. Biochem. J. 311 (Pt 1), 1–16 (1995).
Meraz-Ríos, M. A., Toral-Rios, D., Franco-Bocanegra, D., Villeda-Hernández, J. & Campos-Peña, V. Inflammatory process in Alzheimer’s Disease. Front Integr Neurosci 7 , 59 (2013).
Desideri, G. et al. Uric acid amplifies Aβ amyloid effects involved in the cognitive dysfunction/dementia: evidences from an experimental model in vitro. J. Cell. Physiol. 232 , 1069–1078 (2017).
Download references
Acknowledgements
This work received a Grant from the Department of Science and Technology of the Sichuan Province [Grant number 2015JY0063]. Also, the authors wish to thank Ms Jia for her excellent editorial assistance in manuscript preparation.
Author information
These authors contributed equally: Tian Tian and Xi-run Liu.
Authors and Affiliations
School of Public Health, Southwest Medical University, Luzhou, 646000, China
Tian Tian, Ting-ting Li, Zhi-chao Nie, Shuang-jing Li, Yan Tang, Wang-dong Xu & Hong Jia
Clinical Drug Trial Institution, The Affiliated Hospital of Southwest Medical University, Luzhou, 646000, China
Laboratory Animal Centre, Southwest Medical University, Luzhou, 646000, China
Cong-wei Gu
Collaborative Innovation Centre for Prevention of Cardiovascular Research of Sichuan Province, Key Laboratory for Medical Electrophysiology of Ministry of Education, Luzhou, 646000, China
You can also search for this author in PubMed Google Scholar
Contributions
All authors have read and approved the final version of the manuscript. H.J., Y.T., C.G., W.X. were involved with the experimental design. X.L., T.T., T.L., Z.N., S.L. performed the animal experiment. T.T. wrote the manuscript.
Corresponding author
Correspondence to Hong Jia .
Ethics declarations
Competing interests.
The authors declare no competing interests.
Additional information
Publisher's note.
Springer Nature remains neutral with regard to jurisdictional claims in published maps and institutional affiliations.
Rights and permissions
Open Access This article is licensed under a Creative Commons Attribution 4.0 International License, which permits use, sharing, adaptation, distribution and reproduction in any medium or format, as long as you give appropriate credit to the original author(s) and the source, provide a link to the Creative Commons licence, and indicate if changes were made. The images or other third party material in this article are included in the article's Creative Commons licence, unless indicated otherwise in a credit line to the material. If material is not included in the article's Creative Commons licence and your intended use is not permitted by statutory regulation or exceeds the permitted use, you will need to obtain permission directly from the copyright holder. To view a copy of this licence, visit http://creativecommons.org/licenses/by/4.0/ .
Reprints and permissions
About this article
Cite this article.
Tian, T., Liu, Xr., Li, Tt. et al. Detrimental effects of long-term elevated serum uric acid on cognitive function in rats. Sci Rep 11 , 6732 (2021). https://doi.org/10.1038/s41598-021-86279-y
Download citation
Received : 04 December 2020
Accepted : 11 March 2021
Published : 24 March 2021
DOI : https://doi.org/10.1038/s41598-021-86279-y
Share this article
Anyone you share the following link with will be able to read this content:
Sorry, a shareable link is not currently available for this article.
Provided by the Springer Nature SharedIt content-sharing initiative
This article is cited by
Alcohol spiked with zolpidem and midazolam potentiates inflammation, oxidative stress and organ damage in a mouse model.
- Biwott Kipchumba
- Francis Gitonga
- Nyariki James Nyabuga
Forensic Toxicology (2024)
Higher serum uric acid is associated with poorer cognitive performance in healthy middle-aged people: a cross-sectional study
- Yousef Khaled
- Aya A. Abdelhamid
- Laiche Djouhri
Internal and Emergency Medicine (2023)
Liver-derived metabolites as signaling molecules in fatty liver disease
- Jin Rong Ow
- Philipp Kaldis
Cellular and Molecular Life Sciences (2023)
By submitting a comment you agree to abide by our Terms and Community Guidelines . If you find something abusive or that does not comply with our terms or guidelines please flag it as inappropriate.
Quick links
- Explore articles by subject
- Guide to authors
- Editorial policies
Sign up for the Nature Briefing newsletter — what matters in science, free to your inbox daily.


IMAGES
VIDEO
COMMENTS
Case study—Elevated uric acid and hypertension [1:10:55] "Patient H". Basics: 46, Male. Body type: Normal BMI. Hx: Prior to onboarding, patient was diagnosed with essential HTN, blood pressure ranging from 140/90 to 160/90 mmHg. He was treated with lisinopril (ACEi) bringing him into the 130/90 range (still higher than ideal for 46 year ...
Experimental studies show that uric acid is a potent activator of the renin-angiotensin-aldosterone system (RAAS). ... The case for uric acid-lowering treatment in patients with hyperuricaemia ...
However, the study failed to show any benefits from raising uric acid, suggesting that PD may lower uric acid or that uric acid is a biomarker of other processes relevant to PD . To assess the causality between uric acid and PD, a Mendelian randomization approach was used to systematically evaluate the inherent risk of lower urate levels and ...
In any case, the results ... 1.92) as the results from 8 cohort studies and serum uric acid levels elevated in patients with AF as the results from 29 studies .
A comparison of the top vs. bottom quartile of uric acid levels in our case-control study gave an OR of pre-eclampsia of 1.49 (1.16, 1.90), which is very similar to the results observed for uric ...
Introduction. Hyperuricemia is a common issue in daily clinical practice, estimated to occur in approximately 8.9% to 24.4% of the general population [1, 2].Although its definitions may differ slightly depending on laboratory norms and test methods, hyperuricemia is usually defined as serum uric acid (UA) concentration higher than 7 mg/dl [].Age- and sex-specific norms for serum UA are ...
Introduction. The role of serum uric acid (SUA) in the prediction of ischemic stroke has been a debated issue for the past few decades. A growing body of research suggests that there is a positive association between SUA and ischemic stroke [1,2].However, elevated SUA level is also found to be associated with a number of common risk factors of ischemic stroke like hypertension [], obesity ...
The authors speculate however that it is the activity of these uric acid transporters, rather than the serum urate levels, that are having the protective effect on renal function and that using fractional excretion of uric acid as the exposure in a Mendelian randomization study, instead of serum urate, would help to determine if this is the case.
The Uric acid Right for heArt Health (URRAH) project has been designed to define, as primary objective, the level of uricemia above which the independent risk of cardiovascular disease may increase in a significantly manner in a general Italian population. ... Indeed, the available epidemiological case studies are not homogeneous, and some ...
Design Nested case-control study. Setting UK general practice database, 2000-7. ... Diuretics increase the net reabsorption of uric acid in the proximal tubule of the nephron and thereby reduce urinary excretion and increase the risk of hyperuricaemia7 8 and gout.2 The increase in serum uric acid concentration and the risk of gout caused by ...
Purpose of Review This review aims to summarize recent evidence regarding the complex relationship between uric acid (UA), gout, and brain diseases. Recent Findings Observational studies have suggested that patients with hyperuricemia or gout might have a decreased risk of neurodegenerative diseases. Conversely, they may be at increased risk of cerebrovascular disease. Mendelian randomization ...
The relationship between Serum Uric Acid (UA) and Cardiovascular (CV) diseases has already been extensively evaluated, and it was found to be an independent predictor of all-cause and cardiovascular mortality but also acute coronary syndrome, stroke and heart failure. Similarly, also many papers have been published on the association between UA and kidney function, while less is known on the ...
Methods This was a case-control study based on 91 cases with COVID-19 and 273 age- and sex-matched healthy control subjects. ... Several experimental and clinical studies support a role for uric ...
Introduction. Uric acid was thought to be a biologically inert waste product from purine metabolism, until in the early 1800s it was discovered that an increased serum uric acid (SUA) level was the cause of gout.1 Subsequently, associations of uric acid concentration with cardiovascular and renal disorders were also observed.2 These associations were explored in several prospective studies but ...
Uric acid has antioxidant effects. Some studies have shown that low uric acid levels could be a risk factor for cardiometabolic diseases. In the Syst-Eur trial [], the CASTEL study [], and the ...
To quantify the association between maternal uric acid levels and pre-eclampsia risk in a large collection of primigravid women. A case-control study (1365 cases of pre-eclampsia and 1886 normotensive controls) was conducted. Pre-eclampsia was defined as blood pressure ≥ 140/90 mmHg and proteinuria …
Resistance (HOMA-IR) is widespread. Insulin resistance can lead to hyperuricemia by reducing the kidney ability to excrete urate, thus increasing sodium reabsorption. The aim of this study was to investigate the possible relationship between serum uric acid levels and insulin resistance. This was a retrospective case-control study. A total of 2530 applications in 2-year time were included in ...
Cohort study: Uric acid levels were significantly higher in patients with higher averaged, dopamine transporter binding. ... Du et al. analyzed 24 studies, including 21 case-control studies and 3 cohort studies, and found that patients with Alzheimer's disease had lower uric acid levels than healthy individuals ...
Due to the global prevalence of hyperuricemia (HUA), there is growing interest in research on uric acid (UA). HUA is a common condition that has various adverse consequences, including gout and kidney disease. However, recent studies have also implicated UA in the development of cardiovascular diseases (CVD) such as atrial fibrillation (AF) and ...
Some previous studies reported the sex-specific threshold values of uric acid for all-cause mortality: uric acid levels ≥8.5 mg/dL in a male population in Japan (n = 49,413) 17 and Korea (n ...
However, the study failed to show any benefits from raising uric acid, suggesting that PD may lower uric acid or that uric acid is a biomarker of other processes relevant to PD . To assess the causality between uric acid and PD, a Mendelian randomization approach was used to systematically evaluate the inherent risk of lower urate levels and ...
Association of Serum Uric Acid with Non-Valvular Atrial Fibrillation: A Retrospective Study in China. Hua-Jing Yuan 1 Shandong University of ... This was a cross-sectional retrospective design study. Patient case data were collected from the electronic medical record database of the Affiliated Hospital of Shandong University of Traditional ...
In the initial 7-day and 19-day proof-of-concept studies in mice, ALLN-346 oral therapy resulted in the normalization of urinary uric acid excretion and reduced hyperuricaemia by 44% when the drug ...
Hippuric acid is an acyl glycine formed from the conjugation of benzoic acid with glycine. Several studies have confirmed that both thromboxane B2 and hippuric acid levels are associated with diet. Dietary fatty acids affect platelet thromboxane production [35,36,37]. In our study, several fatty acids (e.g., palmitic acid, stearic acid, behenic ...
On the one hand, some study reported uric acid was not associated with diabetes. For example, Sluijs et al. used a genetic score of 24 uric acid-related sites for Mendelian randomization studies, in the European prospective survey data—Cancer and Nutrition (EPIC) study, which was an interactive case-cohort study of vast number of subjects ...
Uric acid's effects on cognition may be related to its concentration and exposure period. ... Several cross-sectional or case-control studies have suggested that an increased SUA level is ...