- Type 2 Diabetes
- Heart Disease
- Digestive Health
- Multiple Sclerosis
- COVID-19 Vaccines
- Occupational Therapy
- Healthy Aging
- Health Insurance
- Public Health
- Patient Rights
- Caregivers & Loved Ones
- End of Life Concerns
- Health News
- Thyroid Test Analyzer
- Doctor Discussion Guides
- Hemoglobin A1c Test Analyzer
- Lipid Test Analyzer
- Complete Blood Count (CBC) Analyzer
- What to Buy
- Editorial Process
- Meet Our Medical Expert Board

What Are Signs of Liver Disease?
- Risk Factors
- Symptoms by Stage
Many people with liver disease are entirely unaware of their condition, in part because the signs of liver problems are often subtle or nonexistent. In 2023, a review of the National Health and Nutrition Examination Survey (NHANES) found that 93% of people with fatty liver disease had no knowledge of their condition and that 8.8% were found to have advanced liver disease.
In most cases, the symptoms of liver disease are only apparent when there is significant and often irreversible liver damage. This is why it is important to know the early signs of liver disease and hepatitis (liver inflammation). This is especially true if you have risk factors for liver disease .
This article takes a closer look at the signs of liver disease, including the symptoms you are likely to experience at different stages of the disease. It also explains how liver disease is diagnosed and when you might consider screening in the absence of symptoms.
Illustration by Mira Norian for Verywell Health
Could You Have Liver Disease Symptoms?
Liver disease, also known as hepatic disease, is a general term that refers to any condition affecting the liver. There are acute forms of liver disease that develop abruptly and generally resolve with treatment and chronic forms that persist, causing progressive damage to the liver.
Chronic liver disease can progress asymptomatically (without symptoms) over years and only become apparent when irreversible damage is done.
Symptoms like fatigue, dark urine, and jaundice (yellowing of the skin and eyes) can develop with the onset of cirrhosis (extensive liver scarring) and get progressively worse as liver disease advances to end-stage liver disease and liver failure.
The three most common forms of chronic liver disease in the United States are:
- Viral hepatitis : Hepatitis B and hepatitis C are viral infections that can progress from acute to chronic infection in some people.
- Alcoholic liver disease (ALD) : This is a type of fatty liver disease, also known as alcoholic hepatosteatosis , caused by excessive alcohol use.
- Metabolic dysfunction-associated steatotic liver disease (MASLD) : This is a type of liver disease, formerly known as nonalcoholic fatty liver disease (NAFLD), caused by metabolic conditions like diabetes and obesity.
What makes these diseases so concerning is that only a handful of people are aware of their condition. According to the American Liver Foundation, no less than 100 million people in the United States currently have some form of liver disease, of whom only 1.8% are diagnosed.
Because the symptoms are often subtle or easily missed or attributed to other causes, it is important to understand not only the signs of liver disease but also the factors that place you at an increased risk.
In the United States, this includes:
- Drinking alcohol heavily
- Having obesity
- Having metabolic syndrome (a group of conditions including high blood pressure (hypertension) , high blood sugar, abnormal levels of cholesterol and fat in the blood , and excess abdominal fat that can lead to heart disease, diabetes, and stroke)
- Having type 2 diabetes
- Having high blood pressure
- Sharing needles and syringes (a major route of infection for hepatitis B and C)
Where Is My Liver Located?
The liver is the largest internal organ in the human body, roughly the size of a football. It is situated on the right side of the upper abdomen just below the ribcage and diaphragm (the fibrous muscle separating the abdominal and chest cavities).
The liver itself has no pain receptors. Any pain or discomfort felt is due to inflammation or injury of the membrane surrounding the liver, called the liver capsule.
How Liver Disease Symptoms Progress in Stages
Chronic liver diseases tend to progress silently over many years before noticeable symptoms develop.
With hepatitis B and C, it can take 10 to 20 years for an acute infection to progress to cirrhosis. ALD generally takes at least 20 years of heavy drinking before signs of alcoholic hepatitis appear, while MASLD can take anywhere from three to 20 years before a severe form of the disease called metabolic dysfunction-associated steatohepatitis (MASH) develops.
Although the timelines can vary, the stages of the disease and the sequence of symptoms remain largely the same. With chronic liver disease, the stages can be broadly categorized as fibrosis, cirrhosis, and liver failure.
While the underlying causes of chronic liver diseases differ, the one condition they all share is chronic inflammation . When the liver is involved, the inflammation is referred to as hepatitis .
Although inflammation is the body's way of controlling disease, it can cause significant damage if it persists unchecked. With hepatitis, the persistent, low-level inflammation can progressively damage liver cells and cause the formation of scar tissues known as fibrosis .
Under normal circumstances, the liver repairs itself by making new liver cells and attaching them to the web of connective tissues where the old cells died. However, with chronic inflammation, scar tissues can develop faster than new liver cells are produced.
Fibrosis itself causes no symptoms, and it can be difficult to diagnose as imaging tests often fail to detect any changes until the scarring is extensive. The only indication at this stage may be abnormalities in blood tests used to evaluate liver function.
As scar tissues increasingly supplant healthy tissues, the liver will start to lose its ability to perform key functions, including the production of bile for digestion, the storage of glucose for energy, the synthesis of coagulants for blood clotting, and the filtering of waste from the bloodstream.
When fibrosis is severe enough to cause the loss of liver function, you are said to have cirrhosis . This is the stage when symptoms start to develop.
During the early stage of cirrhosis when the liver is still functional—referred to as compensated cirrhosis —you may experience nonspecific signs of hepatitis (signs that could be caused by a number of conditions), including:
- General tiredness or fatigue
- Muscle pain ( myalgia )
- Joint pain ( arthralgia )
- Itchy skin ( pruritus )
- Low-grade fever
- Loss of appetite
- Dull pain in the upper right part of the abdomen
When the liver is no longer functional—a condition referred to as decompensated cirrhosis —more overt symptoms will develop, including:
- Clay-colored stool
- Nausea and vomiting
- Yellowing of the skin and eyes ( jaundice )
- A swollen liver ( hepatomegaly )
- Swelling in the legs, feet, or ankles ( edema )
Does Liver Disease Always End With Cirrhosis?
Not everyone with chronic liver disease will progress to advanced disease. In fact, only 20% to 30% of people with viral hepatitis progress to cirrhosis. Similarly, only 20% to 25% of people with ALD and 20% to 30% of people with MASLD experience severe progression leading to cirrhosis and liver failure.
Liver Failure
In rare cases, decompensated cirrhosis is partially reversible. In most instances, however, decompensation is the gateway to liver failure—the end stage of liver disease. This is the point at which the loss of liver function has become life-threatening.
With liver failure, severe complications will develop. While efforts can be made to sustain life, a liver transplant is ultimately the only means of survival.
In addition to symptoms of severe hepatitis, liver failure can also manifest with:
- Rapid heart rate ( tachycardia )
- Shortness of breath ( dyspnea )
- Vomiting of blood ( hematemesis )
- Decreased or no urine output ( oliguria )
- Bloody or tarry stools ( melena )
- Finger clubbing ( acropachy )
- Spider veins ( telangiectasia )
- Easy bruising ( ecchymosis )
- Fluid overload and swelling of the abdomen ( ascites )
- Confusion and seizures (caused by hepatic encephalopathy )
- Acute kidney failure (caused by hepatorenal syndrome )
- Hypovolemic shock (caused by severe variceal bleeding )
Does Cirrhosis Cause Cancer?
Cirrhosis can also increase the risk of hepatocellular carcinoma (HCC) , the most common form of liver cancer. Studies suggest that 80% to 90% of people with HCC have cirrhosis and that roughly 12% of people with cirrhosis will eventually develop HCC, usually within the span of 10 years.
Signs of Liver Disease in Someone Else
Signs of liver disease are as difficult to spot in someone else as in yourself. But this doesn't mean you shouldn't look, particularly if someone you love is older and has multiple health concerns.
The simple fact is that the risk of cirrhosis increases with age and that most diagnoses are made when the disease is advanced and damage to the liver is severe.
A 2019 study in JAMA Network Open suggests that the current median age of diagnosis for cirrhosis in the United States is 58 years. This is the stage of life—between ages 55 and 64—in which nearly half of all cirrhosis deaths occur.
Because older adults often juggle multiple chronic conditions, they may ignore or miss the early signs of liver disease when dealing with more overt illnesses like diabetes, heart disease, or chronic obstructive pulmonary disease (COPD).
This is why it is important to intervene if a loved one has signs of liver disease—or simply has risk factors for the disease with no overt signs or symptoms.
In the past, the American Association for the Study of Liver Diseases (AASLD) advised against routine screening for fatty liver disease due to uncertainties surrounding diagnostic tests and their long-term benefits. But in 2022, the group reversed its decision and now endorses screening of people suspected of having fatty liver disease.
Similarly, the American Gastroenterology Association (AGA) and the American Diabetes Association (ADA) now recommend the routine screening of people at risk of liver disease, including those with:
- Type 2 diabetes
- Excessive alcohol use
- Two or more signs of metabolic syndrome (such as abdominal obesity, high blood pressure, impaired fasting glucose , high triglycerides , and low levels of high-density lipoprotein (HDL) cholesterol , considered "good cholesterol")
The criteria outlined by the AASLD, AGA, and ADA cover a large proportion of the older adult population in the U.S., making it all the more reasonable to recommend screening if you have any suspicions about chronic liver disease in yourself or a loved one.
Steps to Getting Liver Disease Symptoms Treated
Often, the first sign of liver disease is seen on a liver function test (LFT) . This is a routine panel of blood tests that measure the levels of enzymes produced by the liver when it is under stress. Based on which enzymes are high or low, your healthcare provider may have clues as to which type of liver disease you have.
For example, with chronic viral hepatitis, alanine aminotransferase (ALT) levels remain elevated while aspartate aminotransferase (AST) levels are often normal. With alcoholic liver disease, the opposite may be true, while MASLD usually causes high levels of both AST and ALT.
Based on these early clues, your healthcare provider may order one of several imaging tests to check for abnormalities in your liver, including nodules, lesions, or tumors. Options include:
- Abdominal ultrasound : This noninvasive imaging tool bounces sound waves off your liver to create a detailed image of its shape, size, and structure.
- Computed tomography (CT) scan : This imaging technology composites multiple X-ray images to create three-dimensional (3D) "slices" of your liver.
- Magnetic resonance imaging (MRI) : This imaging technique produces highly detailed images without radiation and is especially good at detecting blockages in liver ducts and blood vessels.
- Transient elastography : This newer imaging tool, also known as a FibroScan, is highly sensitive to liver fibrosis and can measure the stiffness of your liver using a form of ultrasound.
In addition, your healthcare provider may order a blood test called a hepatitis virus panel that can detect current or past infection with hepatitis A, B, or C.
Another routine blood test called a complete blood count (CBC) may be especially useful in people with ALD, who commonly experience thrombocytopenia (low platelet count) and anemia (low red blood cell count).
If needed, a liver biopsy may be performed to obtain a small sample of liver tissue (usually with a hollow-core needle inserted through a small incision in your belly). A liver biopsy can definitively diagnose cirrhosis and how advanced the condition is.
If liver disease is diagnosed, medications may be prescribed along with lifestyle and dietary changes to slow disease progression, preserve liver function, and manage symptoms. The treatment may involve avoiding alcohol, eating a healthy diet, losing weight, and exercising regularly. Antiviral drugs can help manage chronic hepatitis B infection and even cure most cases of hepatitis C .
The signs of liver disease are often subtle or non-existent in the early stages and may continue to be so for many years until damage to your liver is severe. Symptoms like fatigue, dark urine, and jaundice can develop with the onset of cirrhosis and get progressively worse as it advances to end-stage liver disease and liver failure.
It is important to know the early signs of liver disease to avoid disease progression (and possibly reverse liver damage). Even in the absence of symptoms, older adults who drink excessively, have type 2 diabetes, or have signs of metabolic disorder may benefit from screening, given the high rate of undiagnosed liver disease in the United States.
Kim DS, Ye C, Ismail M, Pyrsopoulos NT. P0950 - Prevalence of fibrosis among US adults unaware of their nonalcoholic fatty liver disease: a 2017-2020 United States National Health and Nutrition Examination Surveys (NHANES) assessment . ACG 2023 Annual Scientific Meeting Abstracts . Vancouver, Canada: American College of Gastroenterology; October 22, 2023.
Wazir H, Abid M, Essani B, et al. Diagnosis and treatment of liver disease: current trends and future directions . Cureus. 2023;15(12):e49920. doi:10.7759/cureus.49920
American Liver Foundation. How many people have liver disease?
Canivet CM, Boursier J. Screening for liver fibrosis in the general population: where do we stand in 2022? Diagnostics (Basel). 2023;13(1):91. doi:10.3390/diagnostics13010091
Canadian Society of Intestinal Research. The liver - an amazing organ .
Klinge M, Coppler T, Liebschultz JM, et al. The assessment and management of pain in cirrhosis . Curr Hepatol Rep. 2018;17(1):42–51. doi:10.1007/s11901-018-0389-7
Lin J, Wu JF, Zhang HW, Cao GW. Virus-related liver cirrhosis: molecular basis and therapeutic options . World J Gastroenterol. 2014;20(21):6457–6469. doi:10.3748/wjg.v20.i21.6457
Hosseini N, Shor J, Szabo G. Alcoholic hepatitis: a review . Alcohol Alcohol. 2019;54(4):408–416. doi:10.1093/alcalc/agz036
Heyens LJM, Busschots D, Koek GH, Robaeys G, Francque S. Liver fibrosis in non-alcoholic fatty liver disease: from liver biopsy to non-invasive biomarkers in diagnosis and treatment. Front Med (Lausanne). 2021;8:615978. doi:10.3389/fmed.2021.615978
Berumen J, Baglieri J, Kisseleva T, Mekeel K. Liver fibrosis: pathophysiology and clinical implications . WIREs Mech Dis. 2021;13(1):e1499. doi:10.1002/wsbm.1499
L Kiss A. Inflammation in focus: the beginning and the end . Pathol Oncol Res. 2022 Jan 4;27:1610136. doi:10.3389/pore.2021.1610136
MedlinePlus. Hepatitis .
World Health Organization. Global hepatitis programme: treatment & care .
Mellinger JL. Epidemiology of alcohol use and alcoholic liver disease . Clin Liver Dis (Hoboken). 2019;13(5):136–139. doi:10.1002/cld.806
Fernando DH, Forbes JM, Angus PW, Herath CB. Development and progression of non-alcoholic fatty liver disease: the role of advanced glycation end products . Int J Mol Sci. 2019;20(20):5037. doi:10.3390/ijms20205037
Jagdish RK, Roy A, Kumar K, et al. Pathophysiology and management of liver cirrhosis: from portal hypertension to acute-on-chronic liver failure . Front Med . 2023;10:2023. doi:10.3389/fmed.2023.1060073
Mansour D, McPherson S. Management of decompensated cirrhosis . Clin Med (Lond). 2018;18(Suppl 2):s60–s65. doi:10.7861/clinmedicine.18-2s-s60
Bengtsson B, Widman L, Wahlin S, Stal P, Bjorkstrom NK, Hagstrom H. The risk of hepatocellular carcinoma in cirrhosis differs by etiology, age and sex: a Swedish nationwide population‐based cohort study . United European Gastroenterol J. 2022;10(5):465–476. doi:10.1002/ueg2.12238
Orman ES, Roberts A, Ghabril M, et al. Trends in characteristics, mortality, and other outcomes of patients with newly diagnosed cirrhosis . JAMA Netw Open. 2019;2(6):e196412. doi:10.1001/jamanetworkopen.2019.6412
Chen CM, Yoon YHL. Cirrhosis mortality trends . In: Surveillance Report #118 Liver Cirrhosis Mortality in the United States: National, State, and Regional Trends, 2000-2019 . Sterling, VA: National Institute on Alcohol Abuse and Alcoholism; 2022.
National Institute of Diabetes and Digestive and Kidney Diseases. Chronic hepatitis .
Torruellas C, French SW, Medici V. Diagnosis of alcoholic liver disease . World J Gastroenterol. 2014;20(33):11684–11699. doi:10.3748/wjg.v20.i33.11684
National Institute of Diabetes and Digestive and Kidney Diseases. Diagnosis of NAFLD & NASH .
MedlinePlus. Hepatitis virus panel .
National Institute of Diabetes and Digestive and Kidney Diseases. Diagnosis of cirrhosis .
National Institute of Diabetes and Digestive and Kidney Diseases. Treatment for cirrhosis .
By James Myhre & Dennis Sifris, MD Dr. Sifris is an HIV specialist and Medical Director of LifeSense Disease Management. Myhre is a journalist and HIV educator.
New focus for chronic liver disease care
D eveloping improved care models for decompensated liver disease is one of the major clinical challenges in gastroenterology and hepatology, and in a recent landmark study, published in Hepatology , researches from Flinders Medical Center and Flinders University provide evidence that improved models of care can benefit patients with decompensated cirrhosis.
Identifying improved care is of primary importance as the number of chronic liver failure cases at South Australia's public hospitals has increased more than three-fold in the past decade, while obesity-related liver disease is expected to become a modern epidemic by 2050. Nationally, more than 6 million Australians suffer from chronic liver disease, resulting in more than 7,000 deaths a year.
The study represents more than five years of work conducting a randomized controlled trial (RCT) led by Professor Alan Wigg from Flinders University's College of Medicine and Public Health and Flinders Medical Center. The RCT involved six Australian tertiary care hospitals across three states and represents one of the first investigator-initiated multicenter RCTs in Australian hepatology.
The primary aim of this randomized control trial was to assess the efficacy of a chronic disease management (CDM) model to reduce liver-related emergency admissions to hospitals. Secondary aims were to assess effects on quality-of-care and patient reported outcomes.
"Chronic disease management approaches appear to be a logical, evidence-based strategy and have been successfully applied in many non-liver settings," says Professor Wigg.
"We believe that CDM models for decompressed cirrhosis have great potential towards an improved standard of care in hepatology. However, we also need to accept that CDM models in decompensated cirrhosis may not be as effective as CDM in other chronic diseases due to the more complex and severe nature of this liver disorder."
The study's primary outcome showed that the liver-related emergency admissions rate was not significantly improved despite robust intervention, nor was any improvement in patient survival recorded.
However, the study did show several significant and clinically important benefits including reduced emergency admissions due to hepatic encephalopathy, one of the most frequent, preventable and expensive causes for admission in patients with decompensated cirrhosis. Improvements in quality of care and quality of life were also demonstrated in the CDM group.
The detailed work undertaken by the study also highlighted several significant shortcomings in the care of decompensated cirrhosis. While alcohol-related cirrhosis was the major cause of decompensated cirrhosis in about 70% of participants in the study, access for them to obtain high-quality alcohol addiction, mental health and supportive care services remained limited.
A lack of trained liver disease management nurses was also identified, with the few available trained liver nurses being employed part-time (three days per week) and required to manage up to 22 patients.
More information: Alan J Wigg et al, A randomized multicenter trial of a chronic disease management intervention for decompensated cirrhosis. The Australian Liver Failure (ALFIE) trial, Hepatology (2024). DOI: 10.1097/HEP.0000000000000862
Provided by Flinders University
- Open access
- Published: 08 May 2022
Mesenchymal stromal cells (MSCs) and their exosome in acute liver failure (ALF): a comprehensive review
- Samin Shokravi 1 ,
- Vitaliy Borisov 2 ,
- Burhan Abdullah Zaman 3 ,
- Firoozeh Niazvand 4 ,
- Raheleh Hazrati 5 ,
- Meysam Mohammadi Khah 6 ,
- Lakshmi Thangavelu 7 ,
- Sima Marzban 1 ,
- Armin Sohrabi 8 , 9 &
- Amir Zamani 10
Stem Cell Research & Therapy volume 13 , Article number: 192 ( 2022 ) Cite this article
4022 Accesses
22 Citations
2 Altmetric
Metrics details
Recently, mesenchymal stromal cells (MSCs) and their derivative exosome have become a promising approach in the context of liver diseases therapy, in particular, acute liver failure (ALF). In addition to their differentiation into hepatocytes in vivo, which is partially involved in liver regeneration, MSCs support liver regeneration as a result of their appreciated competencies, such as antiapoptotic, immunomodulatory, antifibrotic, and also antioxidant attributes. Further, MSCs-secreted molecules inspire hepatocyte proliferation in vivo, facilitating damaged tissue recovery in ALF. Given these properties, various MSCs-based approaches have evolved and resulted in encouraging outcomes in ALF animal models and also displayed safety and also modest efficacy in human studies, providing a new avenue for ALF therapy. Irrespective of MSCs-derived exosome, MSCs-based strategies in ALF include administration of native MSCs, genetically modified MSCs, pretreated MSCs, MSCs delivery using biomaterials, and also MSCs in combination with and other therapeutic molecules or modalities. Herein, we will deliver an overview regarding the therapeutic effects of the MSCs and their exosomes in ALF. As well, we will discuss recent progress in preclinical and clinical studies and current challenges in MSCs-based therapies in ALF, with a special focus on in vivo reports.
Introduction
Acute liver failure (ALF) is characterized by the occurrence of coagulopathy (international normalized ratio [INR] > 1.5) and any level of encephalopathy emerging 24 weeks following the occurrence of the first symptoms in patients who have no history of previous liver disease [ 1 ]. The timing and the level of clinical presentation can be classified into three types: hyperacute, acute, and subacute [ 2 ]. Hyperacute and acute types involve fulminant hepatic failure, while the subacute type is also named subfulminant [ 3 ]. Interestingly, the mortality rate among the patients whose hepatic encephalopathy appears 8 weeks after the onset of symptoms (fulminant hepatic failure) is lower than the patients with a more gradually evolving course [ 4 ]. Multiorgan failure (MOF) has proved to be the main cause of death (> 50%) from ALF, while intracranial hypertension (ICH) and infection are the other main causes of mortality in this patient population [ 5 ].
During last two decades, a diversity of stem cells, such as pluripotent stem cells (PSCs), mesenchymal stromal cells (MSCs), hepatic progenitor cells (HPCs), and hematopoietic stem cells (HSCs), has been used to treat liver diseases [ 6 , 7 , 8 ]. However, MSCs are the most common type used in research, since they pose no ethical challenges and can be obtained easily [ 9 , 10 ]. Results show that MSCs have the capability of differentiating more than once; moreover, they can self-renew. They can differentiate into an array of cell lineages, including hepatocyte-like cells (HLCs) [ 11 ]. MSCs are also characterized by other properties, such as anti-inflammatory, antiapoptosis, antifibrotic, antioxidant, blood vessel formation, improvement of tissue repair, and growth factor secretion [ 12 , 13 ]. Despite many preclinical and clinical investigations on MSCs used in treating ALF, it is still unknown what mechanism contributes to the therapeutic effect of MSCs. Besides, MSCs-exosomes have caught the attention of many researchers as a new cell-free method regarding the regeneration of the liver [ 14 , 15 ]. They have dissipated the worries concerning the direct application of MSC (e.g., immunogenicity and tumor formation [ 16 ]. Such exosomes encompass high frequencies of cytoplasmic and membrane proteins, including enzymes, transcription factors, lipids, ECM proteins. They also include nucleic acids, such as mitochondrial DNA (mtDNA), single-stranded DNA (ssDNA), double-stranded DNA (dsDNA), messenger RNA (mRNA), and microRNA (miRNA) [ 17 ]. The size of exosomes varies from 30 to 150 nm, and they can be transferred to other cells to do their functions. As a highly controlled process, the generation of exosome from the other organisms similar to themselves is comprised of three main steps: (1) formation of endocytic vesicles by the folding of the exterior area of the plasma membrane, (2) formation of multivesicular bodies (MVBs) by inward budding of the endosomal membrane, and (3) incorporation of established MVBs with the plasma membrane and secretion of the vesicular contents, called exosomes [ 14 , 18 ]. Exosomes elicit antioxidant effects and motivate target cells to trigger downstream signals. Moreover, they convey genetic material to target cells, leading to the suppression of inflammation and apoptosis. [ 19 , 20 ].
This review aims to give an overview of the present knowledge to elucidate mechanisms used by MSCs to underlie liver restoration in ALF. Another aim is to present a discussion of new developments in preclinical and clinical investigations on MSCs therapy in liver-associated diseases, with a particular focus on ALF.
Pathophysiology of ALF
Acetaminophen (APAP) has proved to be the main cause of ALF [ 21 ]. The following people are highly likely to experience ALF stimulated by APAP: alcoholic people who use APAP; people who suffer from malnutrition; or people who take medications that are believed to induce CYP450 enzymes (e.g., phenytoin, carbamazepine, or rifampin). Results of a study on 308 patients with severe liver disorder in the USA revealed APAP as the main cause of ALF in 40% of patients [ 22 ]. The other causes detected were as follows in the increasing order of prevalence:
Malignancy (1%)
Budd-Chiari Syndrome (2%)
Pregnancy (2%)
Wilson disease (3%)
Hepatitis A virus infection (4%)
Autoimmune hepatitis (4%)
Ischemic hepatitis (6%),
Hepatitis B virus infection (6%)
Idiosyncratic drug-induced liver injury (13%)
The causes of 17 percent of cases were not known [ 4 ].
Based on results, it is possible to categorize the ALF pathophysiology into two groups: pathophysiologies of liver problems involving a specific cause and pathophysiology concerning the appearance of secondary multiorgan failure (MOF) [ 23 ]. With regard to the pathophysiology of liver disorders, the results show that APAP toxicity is the main cause [ 24 ]. Secondary MOF often derives from the primary extensive pro-inflammatory effect, which leads to a pervasive inflammatory effect syndrome. Then, a deregulated anti-inflammatory response ensues [ 25 , 26 ].
It is not clear what mechanism causes the ongoing death of tissue when there is no ongoing injury. Oxidative stress results in the formation of reactive oxygen species (ROS). This, in turn, activates c-Jun N-terminal kinase (JNK) through a series of events [ 27 ]. Such activation may support disruption of normal mitochondrial function , which inspires more hepatocyte necrosis and damage associated molecular patterns (DAMPs) [ 28 , 29 ]. DAMPs bring about the activation of hepatic macrophages, resulting in the formation of the inflammasome [ 30 , 31 ]. Concisely, as complexes characterized by multiple proteins, inflammasomes receive the intracellular danger signals through NOD-like receptors (NLRs) [ 32 ]. The inflammasome effectively regulates the inflammatory response by eliciting a response to low-threshold signals. Toll-like receptors (TLRs) induction by DAMPs leads to the inflammasome activation, supporting the subsequent activation of caspase-1 and IL-1β secretion [ 33 , 34 ]. Researchers have identified the characteristics of the NLR family pyrin domain containing 3 (NLRP3) inflammasome belonging to the inflammasome family. NLRP3 inflammasome has three potential activation pathways: (1) ATP signal which occurs outside a cell, leading to potassium efflux and pannexin recruitment; (2) incorporation of crystalized cholesterol, uric acid or amyloid with lysosomal dysfunction after the ingestion and elimination of these particles; and (3) activation by reactive oxygen species (ROS) [ 33 , 35 , 36 ]. Investigations have examined the activation of inflammasome in APAP-induced ALF by a special focus on the contribution of the inflammasome to acute liver disorder [ 37 ]. It appears that DAMPs are released from necrotic hepatocytes and sinusoidal endothelial cells, leading to the activation of the inflammasome in the manner mentioned above.
The rationality of MSCs therapy in ALF
MSCs migration to damage tissue by interaction with several receptors and molecules, and thereby inducing liver recovery by various mechanisms has been proved (Fig. 1 ). Although the mechanisms of MSCs transplantation are still not entirely understood, a growing body of proof has indicated that their immunomodulation, differentiation, and antifibrotic capabilities play central roles in liver repair. Among them, anti-inflammatory potential of MSCs play most critical role. Although there is no clear evidence indicating the MSCs in vivo differentiation into hepatoid cells post-transplantation, MSCs can be differentiated into hepatocyte-like cells (HLCs) in vitro and then be infused. Of course, this process is time-consuming process with insufficient established HLCs, thereby hindering its therapeutic utility in clinic. However, there is some evidence indicating that replacing fetal bovine serum (FBS) with polyvinyl alcohol (PVA) might lead to improved differentiation ability [ 38 ]. In vivo, as only a small number of intravenously injected cells reach the liver, MSCs-mediated favored effects mainly depend on the secreted molecules rather than their direct effects or differentiation into hepatocytes post-transplantation [ 39 ].
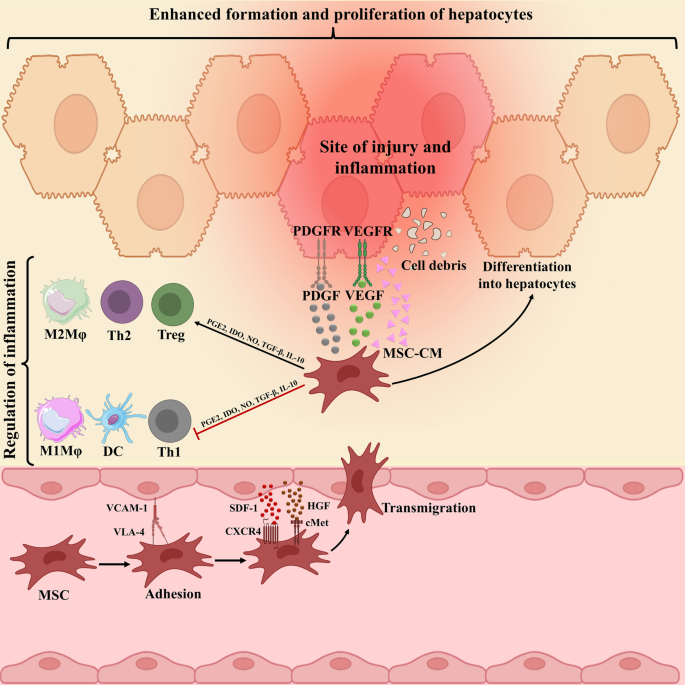
Underlying mechanism complicated in mesenchymal stromal cells (MSCs) migration to damaged liver tissue. The connections between CXCR4 and SDF-1ɑ, c-Met and HGF, and finally VLA-4 and VCAM-1 underlie cell to cell interaction between endothelial cells (ECs) and MSCs, which, in turn, facilitate MSCs migration to damaged liver tissue. Then MSCs secrete anti-inflammatory molecules, such as PGE2, IDO, TGF-β, IL-10, and NO to down-regulate inflammation. These molecules prompt the change of inflammatory to proliferating phase largely defined by the secretion of PDGF and VEGF, sustaining hepatocyte formation and proliferation
MSC anti-inflammatory properties
The hepatocyte loss is the first symptom and mechanism contributing to acute liver damage. It is still unclear what causes ongoing necrosis when there is no injury. ROS are produced in response to oxidative stress. This, in turn, activates c-Jun N-terminal kinase (JNK) through a series of events, resulting in mitochondrial dysfunctions. These events lead to a higher level of hepatocyte necrosis, as well as the expansion of DAMPs [ 40 ]. DAMPs stimulate the activation of hepatic macrophages and the formation of the inflammasome [ 41 ]. In the next stage, the release of pro-inflammatory cytokines eases the recruitment of a larger number of immune cells to the inflammation area, and so advances hepatocyte cell necrosis.
The majority of past investigations have indicated that MSCs play a therapeutic role in the treatment of liver dysfunction by releasing trophic factors and the factors modulating the immune system [ 42 ]. Although the role of MSCs in modulating the immune system is unclear, they might control the immune cells through the secretion of soluble factors and the contacts between cells. The regulation of adaptive and innate immune responses by MSCs is exerted by inhibiting T cells and dendritic cells (DCs), which leads to a reduction in the activation and growth of B cells [ 43 , 44 ]. This, in turn, enhances the formation of regulatory T (Treg) cells and prevents the growth and toxicity of natural killer (NK) cells induced by the chemotherapeutic molecules [ 45 ]. Also, transforming growth factor-beta (TGF-β) and interleukin 10 (IL-10) as crucial factors in the regulation of a large number of inflammatory cells [ 46 , 47 ]. Studies revealed a significant increase in the amounts of TGF-β and IL-10 in serum following the injection of UC-MSCs, but a significant decrease in the amounts of IL-6, tumor necrosis factor-alpha (TNF-α), and cytotoxic T lymphocytes (CTLs) was seen in peripheral blood [ 48 ]. This led to the restoration of liver function, as well as a reduction in the development of disease and the level of mortality. Furthermore, transient T cell apoptosis can be induced by BM-MSCs through the Fas ligand (FasL)-dependent pathway [ 49 ]. Then, macrophages are stimulated by apoptotic T cells to form high amounts of TGF-β, resulting in the up-regulation of Treg cells to trigger immune tolerance. MSCs can prevent cytotoxic CTLs and NK cells through the contact between cells and paracrine factors, including indoleamine 2,3-dioxygenase (IDO), TGF-β, and prostaglandin E2 (PGE2) [ 50 , 51 ]. Of course, TGF-β acts as a two-edged sword. It can weaken the immune system and thereby suppress liver inflammation [ 49 ]; on the other hand, it can increase liver fibrosis [ 52 ]. MSCs, in fact, can act as an immunomodulatory agent in reducing the inflammation of the body through up-regulating anti-inflammatory Treg cells and decreasing T helper 1 (Th1) and Th17 cells in ALF [ 53 ]. Moreover, the inflammation after MSC transplantation can be indirectly stimulated by up-regulating M2-type macrophages, leading to the secretion of a variety of anti-inflammatory factors, such as chemokine ligand 1 (CCL-1) and IL-10, up-regulation Th2, and Treg cells [ 54 ]. Also, MSCs play an important role in the reduction of B-cell growth through contact between cells and the secretion of soluble factors [ 55 ]. Finally, MSC transplantation can play an effective role in mitigating liver damage in ALF by decreasing the number and activity of neutrophils in both peripheral blood and the liver.
MSCs differentiate into HLCs
MSCs are characterized by their ability to proliferate and differentiate in vitro. For the first time, Friedenstein et al. procured MSCs in 1968 from the bone marrow (BM) [ 56 , 57 ]. After that, MSCs obtained from multiple sources, making them an excellent supply of multipotent cells for treatment of liver dysfunctions. A variety of methods are used to differentiate MSCs into HLCs [ 58 , 59 ]. Studies show that multiple signals contribute to the regulation of the cells’ behavior in a cooperative manner. Such signals are usually triggered by extracellular matrix (ECM), growth factors, and even juxtacrine signals [ 60 ]. Each one of the organs, as well as the developmental stage, is characterized by a specific regulated timing and distribution pattern of signals. As a result, achieving better results in the case of in vitro cultures requires establishing a type of environment that resembles the local environment. Based on the research previously done, it is possible to differentiate MSCs obtained from various sources into hepatocytes in the case of both mice and humans through the implementation of a variety of protocols and methods in vitro [ 61 , 62 ].
Hepatic differentiation protocol is known to be the most frequently used method for hepatic differentiation, which benefits from Iscove's Modified Dulbecco's Medium (IMDM), as well as cytokine cocktail. Epidermal growth factor (EGF) or fibroblast growth factors (FGF) trigger the MSCs to differentiate into endodermal cells during the early induction stage. EGF prompts the MSCs to proliferate by interfaces with EGF receptor (EGFR) [ 63 ]. Besides, FGF is a member of a bigger family that is comprised of seven polypeptides with similar characteristics [ 64 ]. This family plays an essential role in the primary stage of endodermal patterning [ 65 ]. In particular, FGF-4 and basic FGF (bFGF) are commonly used. Like EGF, FGF influences the growth rate of MSCs [ 66 ].
Generally, the differentiation of cells is stimulated by adding FGF, HGF, nicotinamide (NTA), and also insulin-transferrin-selenium (ITS) into cultures [ 67 ]. As a mesenchymal origin pleiotropic cytokine, hepatocyte growth factor (HGF) contributes to adjustment of growth, differentiation, and chemotactic migration of MSCs [ 68 ]. MSCs’ exposure to HGF for a short time causes the activation of c-Met receptors along with its downstream agents such as extracellular signal-regulated protein kinase (ERK)1/2, p38, mitogen-activated protein kinases (MAPKs), and phosphoinositide 3-kinase (PI3K) /Akt [ 69 , 70 ]. MSCs’ exposure to HGF for a long time will make changes in cytoskeletal arrangement; moreover, it results in the migration of cells and a notable decrease in proliferation. In addition, ITS and NTA promote the growth and survival of primary hepatocytes [ 71 ].
Despite the fact that MSCs can differentiate in culture through induction, the organ-specific microenvironment is the best technique, enabling MSCs differentiation into a certain cell type. The ability to express hepatocyte-specific genes is one of the specific characteristics of hepatic-differentiated cells, which can be affected by microenvironmental features [ 72 ]. Reports display that in the case of humans, the differentiation of the MSCs obtained from umbilical cord (UC) into HLCs occurs more quickly in the fibrotic liver microenvironment [ 73 ].
Other studies also show that the differentiation of MSCs into functional hepatocytes does not occur directly; rather, these cells initially differentiate into biliary epithelial cells (BEC)-like cells, followed by differentiation into HLCs [ 74 ]. However, according to the results of other investigations, MSCs transdifferentiation infrequently occurs after MSC infusion in animal models [ 75 ]. MSCs obtained from menstrual blood, for instance, turned out to prevent hepatic satellite cells (HSCs) activation and resultant liver fibrosis, leading to the improvement of liver function. Yet, very few transplanted MSCs differentiated into functional HLCs in vivo [ 76 ]. These results demonstrate that the therapeutic impact of MSCs is mediated mainly by indirect paracrine signaling.
MSCs antifibrotic properties
Thanks to their antifibrotic and immunosuppressive properties, MSCs play an important role in the treatment of fibrosis [ 77 ]. Also, fibrosis in not a common pathological signs of ALF; long-term liver damage mainly results in fibrosis. MSC transplantation could attenuate liver fibrosis by down-regulation of TGF-β1, Smad2, collagen type I, and smooth muscle alpha-actin (αSMA), reducing liver fibrosis regions in vivo [ 78 ]. Besides, BM-MSCs decreased hepatic collagen distribution by impairing the TGF-β/Smad signaling pathway in a cirrhosis rat models [ 79 ]. MSCs also ameliorated hepatic microvascular dysfunction and portal hypertension, which contribute to complications defining clinical decompensating [ 80 ]. Further, the expression of matrix metalloproteinase (MMP)-2, -9, -13, and -14 can be up-regulated by MSCs [ 81 ], which, in turn, attenuates liver fibrosis through degrading extracellular matrix (ECM) [ 82 ]. MSCs reinforce this effect by the down-regulation of the tissue inhibitors of matrix metalloproteinases (TIMPs). Importantly, there is an association between the balanced levels of MMPs and TIMP and fibrosis resolution [ 83 ]. Moreover, MSCs have both direct and indirect roles in inhibiting the activation and growth of hepatic satellite cells (HSCs) and thereby could inhibit collagen synthesis [ 84 ]. The direct interactional relationship between MSCs and HSCs helps to inhibit HSC proliferation by stimulating G0/G1 cell-cycle arrest. This is done by inhibiting the phosphorylation of ERK1/2 [ 85 ]. On the other hand, MSCs contain substantial levels of milk fat globule-EGF factor 8 (MFGE8). The MFGE8 reduces expression levels of TGF-β1 receptor on HSCs, thus strikingly fences primary human HSCs activation [ 86 ]. In co-culture conditions, MSCs also mainly impair α-smooth muscle actin (α-SMA) expression of HSCs, which is mediated, in part, by the activation of the Notch pathway [ 87 ]. The indirect secretion of some pivotal factors (IL-10, HGF, TGF-β, and TNF-α) by MSCs averts the growth of HSCs and reduces the formation of collagen. In contrast, HGF and NGF enhance the apoptosis of HSCs [ 88 , 89 ].
MSCs antioxidant properties
One of the events deriving from ROS is oxidative stress, which is known as a common driver in creating damage to the liver. Some of these damages include the liver failure, liver fibrosis, liver cirrhosis, viral hepatitis, and also hepatocellular carcinoma (HCC) [ 90 , 91 ]. The results of some investigations have revealed that MSCs play a strong mediatory antioxidant role in different animal models [ 92 , 93 ]. Oxidative liver injury is mostly caused by thioacetamide (TAA) or carbon tetrachloride (CCl4) as the most commonly used toxins. These types of toxins give rise to hepatocyte dysfunction through the peroxidation of lipid and proteins alkylation, nucleic acids, and lipids [ 94 , 95 ], resulting in the inflammatory response, hepatocellular injury, and liver fibrosis. Cell signaling and homeostasis require a low level of physiologic ROS formed by the mitochondrial respiration. MSCs have proved to have the capability of mitigating oxidative stress simulated by CCl4 and TAA in vivo [ 96 , 97 ]. Through enhancing the superoxide dismutase (SOD) expression and antioxidant response elements (AREs) stimulation, MSCs boost antioxidant and cytoprotective performance, leading to a reduction in hepatocyte apoptosis [ 98 , 99 ]. Due to their antioxidant role along with their role in modulating the immune systems, MSCs can be very useful in developing therapies for liver injuries.
The importance of MSCs-exosome as cell-free approach in ALF
Exosome is a main subtype of extracellular vesicles (EVs) with a diameter in the range of 40–150 nm. Exosome are mainly produced by a diversity of human cells, such as stem/stromal cells, immune cells, and tumor cells [ 100 ]. They include several biological components, more importantly, miRNAs, proteins, lipids and mRNAs, as cargo [ 101 , 102 ]. The production and secretion procedure of exosome consists of three chief steps: (1) creation of endocytic vesicles through invagination of the plasma membrane, (2) creation of multivesicular bodies (MVBs) upon endosomal membranes’ inward budding, and (3) incorporation of created MVBs with the plasma membrane and releases of the vesicular contents termed exosomes [ 14 , 18 ]. Then, the contents of exosomes are transferred to the recipient cells, and thereby modify physiological cells [ 15 ]. As a result of their great capabilities to elicit hepatoprotective effect, exosomes are recently been considered as a rational therapeutic option for liver failure, thereby circumventing comprehensions concerning stromal cells’ direct transplantation [ 103 , 104 ]. They are smaller and less complex compared with parent cells, and thereby easier to produce and store. Also, they exhibit no risk of tumor formation. Importantly, exosomes are less immunogenic than their parent cells due to their lower membrane-bound proteins. Recently, UC-MSCs-derived glutathione peroxidase1 (GPX1) enriched exosome showed the capacity to compromise oxidative stress as well as apoptosis in the hepatocyte, stimulating hepatoprotective effect in ALF rodent models [ 105 ]. Also, MSCs-derived exosome potently reduced inflammatory response in ALF animal models by impairment of IL-6-mediated signaling axis [ 106 ] and also down-regulation of NLRP3 pathway [ 107 ]. However, further studies are strongly needed to entirely elucidate how MSCs-derived exosomes exert their hepatoprotective influences in vivo.
MSCs in ALF (animal studies)
Native mscs.
MSCs-based treatments have shown huge potential for regenerating the liver and repairing its injury, which resulted from several liver disorders (Tables 1 , 2 ). In vivo, MSCs can migrate to damaged tissues and constrain the production of pro-inflammatory molecules (e.g., IL-1, IL-6, and TNF-ɑ) and ultimately potentiate liver cells growth. As described, the chief mechanism behind the MSCs-mediated positive effects is their immunoregulatory potential rather than direct differentiation into haptoid cells. These cells efficiently hinder the activation of both innate and adaptive immune system cells, such as neutrophils, macrophages, NK cells, DCs, monocytes, and also lymphocytes. Studies in liver failure animal models revealed that MSCs could transdifferentiate into albumin-expressing HLCs, and also may support normal hepatocytes proliferation in vivo upon fusion with them [ 108 ]. Findings have outlined the important roles of SDF-1/CXCR4 axis to ease MSCs migration to damaged tissue, sustaining liver rescue in ALF [ 108 ]. As well, injection of MSCs-derived hepatocyte into mice with liver failure ameliorated liver function, as evidenced by analysis of serum profile as well as biochemical factors rates [ 109 ]. Notably, the serum levels of TGF-β1 and IL-10 in transplanted animals were more prominent than in control animals [ 109 ]. Other studies displayed that pyroptosis, a unique shape of programmed cell death induced by penetrating inflammatory reaction, was suppressed by MSCs therapy in ALF preclinical model [ 110 ]. Accordingly, MSCs administration caused liver repair in C57BL/6 mice by up-regulation of IL-10 and concomitantly suppression of NLRP3 [ 110 ]. Given that NLRP3 inflammasome elicits liver failure through induction of procaspase-1 and pro-IL-1 β accompanied with the adjustment of downstream CD40-CD40L signaling, its inhibition as elicited by MSCs can enable liver recovery in ALF [ 111 ]. Besides, the study of the soluble factor produced by MSCs and their potent desired impacts in a rat model of ALF revealed that IL-10, which mainly is secreted by MSCs, has a central role in ALF recovery post-transplantation [ 112 ]. It was found that phosphorylated STAT3 diminished upon IL-10 injection and conversely STAT3 suppression abrogated IL-10-induced effects in vivo, reflecting the eminent role of STAT3 signaling in exerting IL-10-induced anti-inflammatory influences [ 112 ]. In addition to the IL-10, MSC-produced PGE2 could constrain apoptosis and simultaneously augment hepatocyte proliferation, thereby decreasing ALF [ 113 ]. In fact, PGE2 stimulated YAP activation and then activated YAP suppressed phosphatase and tensin homolog (PTEN) and consequently up-regulated mammalian target of rapamycin (mTOR), a foremost controller of cell growth. This axis in turn protected versus ALF through increasing hepatocyte proliferation [ 113 ]. Furthermore, there is clear evidence signifying that MSCs could modify phenotype and function of macrophages, adjust DCs either differentiation or maturation, and impede the T cell activities by the production of tumor necrosis factor-alpha-stimulated gene 6 (TSG-6) in ALF models [ 114 ]. TSG-6 mainly averts the inflammatory response as a result of suppressing P38 and JNK signaling axes, providing a suitable milieu for ALF rescue upon MSCs transplantation [ 115 ]. MSCs also can induce their favored influences by heme oxygenase (HO) 1, a rate-limiting enzyme in heme metabolism, which is noted as an effective antioxidative and cytoprotective molecule. Recently, it was proven that MSCs administration gave rise to HO-1 up-regulation, whereas suppressing HO-1 impaired MSCs-induced desired effects and also hepatocyte autophagy [ 116 ]. These favored effects upon MSCs therapy were most probably caused by PI3K/Akt signaling pathway-induced HO-1 up-regulation [ 116 ]. Also, Zhang et al. found that systemic administration of BM-MSCs into the ALF rat model attenuated ALF by up-regulation of the HO-1 expression and subsequent attenuation in neutrophil infiltration and activation [ 117 ]. This event finally reduced hepatocyte apoptosis and also improve their proliferation, culminating liver recovery. Similarly, the pivotal role of neutrophils in ALF pathogenesis has been clarified by other reports [ 118 ]. In the D-galactosamine-induced ALF animal model, the great number of neutrophils aggregated in the liver tissue along with promoted myeloperoxidase (MPO) activity and enhanced alanine aminotransferase (ALT) and aspartate aminotransferase (AST) serum levels are mainly detected [ 118 ]. Nonetheless, injection of BM-MSCs brought about functional recovery, which was documented by reduced ALT and AST levels and also improved survival rate in the treatment group compared with the control group (50% vs 12.5%). Notably, the intervention led to a robust decrease in the population of neutrophils in the liver, MPO function, and also the expression of pro-inflammatory factors, including TNF-α, IL-1β, interferon gamma (IFNγ) and CXC chemokine ligands 1/2 (CXCL1/2) [ 118 ]. In addition, in a monkey model of ALF, systemic administration of the MSCs derived from another source, unbiblical cord (UC), reduced hepatic aggregation and maturation of circulating monocytes and their IL-6 releases, resulted in prolonged survival [ 119 ]. UC-MSCs also could induce a reduction in ALF by provoking the endogenous liver regeneration in association with suppression of liver cell apoptosis by up-regulating HGF/c-Met signaling axis [ 120 ] or down-regulation of Notch and STAT1/STAT3 signaling [ 121 ]. The positive influences of MSC therapy on hepatocyte proliferation also may arise from activation of AKT/ glycogen synthase kinase 3 beta (GSK-3β)/β-catenin pathway and enhancement in glucose metabolism leading to improved survival rate in ALF animal model [ 122 ]. Interestingly, intraportal injection of MSCs showed superiority over hepatic intra-arterial, intravenous, and intrahepatic injection in terms of liver recovery rate in swine with ALF. Notably, the liver recovery might be attributable to down-regulation of caspase-3, up-regulation of apoptosis inhibitor survivin as well as activation of AKT and ERK axes [ 123 ]. On the other hand, another study revealed that systemic infusion of MSCs was more effective than the intraperitoneal (IP) injection to support liver recovery because of the more significant increase in expression levels of growth factor vascular endothelium growth factor (VEGF) [ 124 ]. Also, compared with BM-MSC, adipose tissue (AT)-derived MSCs displayed higher therapeutic capacities, as defined by estimation of ALT and AST levels post-transplantation in ALF murine model [ 125 ].
MSCs delivery using biomaterials
Present cell transplantation approaches are hindered via poor post-delivery survival, liver ECM and vasculature deterioration, and also difficulties in fusion into the host tissue [ 126 ]. As a result, scientists are persuaded to deliver MSCs within biomaterial structure to sustain the transplants’ viability and also potentiate MSCs long-standing activation in vivo [ 126 ].
Recent reports noted that BM-MSCs are valued options to co-culture with hepatocytes in poly (lactic acid-glycolic acid) (PLGA) scaffolds, enhancing the hepatocellular activities [ 127 ]. Administration of BM-MSCs and hepatocyte seeded PLGA scaffolds led to the considerably advanced cellular proliferation and conversely supported a striking reduction in ALT, AST, and total bilirubin in ALF preclinical models post-transplantation, ultimately leading to the prolonged survival [ 127 ]. Another study demonstrated that MSCs seeded PLGA scaffolds were survived for 3 weeks, and displayed more evident activities than MSCs injected by intravenous route, which was verified by lower mortality in vivo [ 128 ]. However, there was no significant alteration in hepatic inflammation and necrosis zones between the two applied interventions [ 128 ]. Also, poly L-lactic acid (PLLA) nanofiber scaffold could improve the hepatic differentiation of BM-MSCs [ 129 ]. In vitro, analysis exhibited that expression levels of liver-specific markers, more importantly, albumin and α-fetoprotein, were greater in differentiated cells on the nanofibers compared with differentiated cells in plates. These results deliver the proof of the theory that engineered PLLA scaffold could be an efficient alternative to augment MSCs differentiation into functional HLCs [ 129 ]. Besides, BM- and AT-MSC seeded regenerated silk fibroin (RSF) matrices potently differentiated into HLCs in vitro and also stimulated angiogenesis and restored liver functions in the ALF mice model in vivo [ 130 ].
Combination therapy with MSCs
A diversity of studies recently has focused on combination therapy with MSCs and other molecules or modalities to diminish ALF. Meanwhile, co-administration of MSCs with Icaritin, a well-known ingredient isolated from traditional Chinese medicine, resulted in promising outcomes in vivo [ 131 ]. Indeed, MSCs co-cultured with Icaritin improved survival, reduced serum levels of AST and ALT, and elicited histological variations in liver tissue more potently than MSCs alone. Importantly, the up-regulation of HGF/c-Met by Icaritin was found to be involved in MSCs-triggered antiapoptotic influences on hepatocytes, reflecting the potential of herbal extracts to promote MSC-mediated therapeutic impacts [ 131 ]. The addition of the granulocyte colony-stimulating factor (G-CSF) to UCB-MSCs also improved survival and reduced ROS and pro-inflammatory cytokines expressions in ALF murine model [ 132 ]. Also, intervention engendered a significant reduction in cell apoptosis in liver tissues more evidently than UCB-MSCs alone [ 132 ]. These findings were similar to previous reports displaying that G-CSF therapy alone could significantly attenuate short-term mortality in patients suffering from liver failure mainly by reducing inflammation concomitant with activating PI3K/Akt axis in hepatocytes [ 133 , 134 ]. In another study, thanks to the crucial role of IL-1 in the progress of ALF, Sang et al. evaluated possible synergetic effects of combined use of MSCs with 2 mg/kg interleukin-1 receptor antagonist (IL-1Ra) in vivo [ 135 ]. They found that treatment significantly attenuated liver cell apoptosis, improved their proliferation, and eventually enhanced animal survival. It is supposed that the observed effects were dependent on enhancement in AKT and also a reduction in nuclear factor (NF)-κB expression, potentiating liver cell proliferation [ 135 ]. Similarly, co-administration of MSCs plus IL-1Ra chitosan nanoparticles (NPs) was more effective than MSC transplantation alone for treating ALF [ 136 ]. IL-1Ra-loaded NPs administration by ear veins exhibited synergistic impacts with portal vein injection of MSC in a mini swine model of ALF by the hindrance of liver inflammation [ 136 ].
Pretreated MSCs
Current studies have verified that pretreatment with chemical agents, hypoxia, and also cytokine or chemokine in vitro can improve the therapeutic merits of MSCs upon transplantation in vivo [ 137 , 138 ]. Compared to native MSCs, pretreated MSCs largely demonstrate developed hepatogenic differentiation, homing capability, and survival and paracrine impacts.
In 2019, Nie et al. suggested that IL-1β pretreatment could circumvent the MSC's poor migration toward the injured region in ALF murine model [ 139 ]. Correspondingly, IL-1β-MSCs showed superiority over native MSCs respecting the survival time and liver function in vivo. Remarkably, IL-1β-MSCs suppressed liver cell apoptosis and necrosis and also provoked their proliferation. Preferred effects were most probably enticed by improved CXCR4 expression resulting from IL-1β pretreatment and thereby increased migration toward CXCL12 (SDF-1 α) in damage tissue [ 139 ]. Interestingly, pretreatment with injured liver tissue might improve MSCs homing and also their hepatogenic differentiation [ 140 ]. In vivo, transplantation of pretreated MSCs led to an enhancement in albumin, cytokeratin 8, 18, and antiapoptotic protein Bcl-xl levels, whereas supported a reduction in pro-apoptotic protein Bax and caspase-3 levels [ 140 ]. Likewise, short-term, but not long-term, sodium butyrate (NaB) treatment augmented hepatogenic differentiation of BM-MSCs and consequently alleviated liver injury in vivo, according to Li et al. reports [ 141 ].
Genetically modified MSCs
Genetically modified MSCs mainly are used to enhance their colonization rate post-transplantation, leading to ameliorated liver recovery in ALF. Meanwhile, genetically modified MSC to overexpress the CXCR4 gene demonstrated more appropriate migration capability toward SDF-1α and also induce better hepatoprotective impacts in vitro [ 142 ]. In ALF murine model, CXCR4-MSCs efficiently migrated to damaged tissue, and in turn, brought about prolonged survival and restored liver activity more prominent than native MSCs transplantation [ 142 ]. Besides, UCB-MSCs modified to overexpress vascular endothelial growth factor 165 (VEGF165), a strong pro-angiogenic factor, potentiated the UCB-MSCs multipotency and also resulted in better homing and colonization in liver tissues post-transplantation [ 143 ]. While both native UCB-MSCs and VEGF 165 -encoding UCB-MSC restored liver activity in the ALF rat model, modified stromal cells exhibited more desired therapeutic influences on ALF [ 143 ]. Given that IL-35 plays a pivotal role in Treg-induced immunoregulation, Wang et al. evaluated the therapeutic merits of IL-35 overexpressing MSCs in ALF [ 144 ]. They showed that modified stromal cells migrated to the damaged tissues and considerably reduced the necrosis zones of damaged livers. Moreover, IL-35-MSCs averted hepatocyte apoptosis through down-regulation of the FASL expression by immune cells. They also attenuated IFN-γ levels secreted by immune cells potently via targeting JAK1-STAT1/STAT4 signal pathway [ 144 ]. As described in previous sections, IL-1Ra elicits strong anti-inflammatory and antiapoptotic impacts on immune response in liver failure. Accordingly, Zheng and coworkers showed that transplantation of IL-1Ra-encoding amniotic fluid (AF)-MSCs by the portal vein in the ALF rat model led to reduced mortality as well as ameliorated liver activity [ 145 ].
MSCs-exosome in ALF
Exosomes are small membrane-bound EVs that are produced and then released by numerous types of cells, such as stem/stromal cells, immune cells, or tumor cells. Exosomes are comprised of a myriad of biological components, including proteins, lipids, mRNAs as well as miRNAs as cargo, which can be conveyed to the recipient cells [ 103 ]. Such cargo can adjust physiological cell functions and thereby adapt tissue microenvironment, and also inspire hepatocyte proliferation, reflecting their competencies to be described as a rational therapeutic option in liver diseases, such as ALF (Table 3 ). Reduced levels of miR-20a-5p accompanied with the enhanced level of CXCL8, most eminent neutrophil chemoattractants, are mainly observed in hepatocytes during ALF. But, BM-MSCs-exosome could improve miR-20a-5p expression and conversely attenuate CXCL8 levels in hepatocytes [ 146 ]. Also, systemic injection of UC-MSC-exosome (16 mg/kg) induced liver restoration in the ALF mice model [ 105 ]. It was found that glutathione peroxidase1 (GPX1) enriched exosome-mitigated oxidative stress and apoptosis in the hepatocyte, while the elimination of GPX1 led to the abrogated UC-MSCs-exosome-elicited hepatoprotective impacts in mice [ 105 ]. In addition, UC-MSC-exosomes potently modified the membranous expression of CD154 (or CD40 ligand) in intrahepatic CD4 + T cells, largely contributing to the inflammatory response in the liver [ 147 ]. The suppressive effect on CD154 expression and resultant inflammation was due to the existence of chaperonin containing TCP1 subunit 2 (CCT2) in these exosomes, which targets Ca2 + influx and down-regulates CD154 generation in CD4 + T cells [ 147 ]. In another study, Shao et al. screened the miRNAs in the MSCs-exosomes complicated in inhibition of IL-6-mediated signaling axis in ALF mice model. They showed that miR-455-3p was released by exosomes and efficiently instigated PI3K signaling, and in turn, sustained hepatocyte proliferation [ 106 ]. Also, IL-6 pretreated MSCs or exosomes exhibited higher levels of miR-455-3p compared with native MSCs or their derivative exosome. In fact, miR-455-3p-enriched exosomes suppressed macrophages activation, reduced local liver injury, and also diminish the expression of pro-inflammatory cytokines in vivo [ 106 ]. The miR-455-3p also could constrain activation of HSCs and liver fibrosis upon down-regulation of the heat shock protein (HSP) 47/TGF-β/Smad4 signaling pathway [ 148 ]. Importantly, C-reactive protein (CRP) enriched placenta-derived mesenchymal stromal cells (PD-MSCs)- exosome could up-regulate Wnt signaling pathway as well as angiogenesis in animal hepatocytes by interacting with endothelial cells [ 149 ]. Another study also revealed that rat BM-MSCs-exosome-rich fractionated secretome could bring about a hepatoprotective impact in ALF models mainly caused by diminished oxidative stress [ 150 ]. Similarly, transplantation of exosomes derived from menstrual blood-mesenchymal stromal cells (Men-SCs) that contained a diversity of cytokines, such as intercellular adhesion molecule-1 (ICAM-1 or CD54), angiopoietin-2, Axl, angiogenin, insulin-like growth factor-binding protein 6 (IGFBP-6), osteoprotegerin, IL-6, and IL-8, improved liver function in the ALF animal model [ 151 ]. Treatment resulted in improved survival rates as well as reserved hepatocyte apoptosis. Notably, attenuated numbers of neutrophils and also diminished levels of caspase-3 were evidenced post-transplantation, assuming that Men-SC-exosome can be a substitute treatment to support liver failure [ 151 ]. Pretreatment of UC-MSCs-exosome with TNF-α also enhanced exosome-induced hepatoprotective influence in the ALF mice model [ 107 ]. Pretreated exosomes led to the attenuated serum ALT, AST, and pro-inflammatory cytokines levels and concomitantly down-regulated stimulation of NLRP3 inflammasome. Molecular analysis revealed that miRNA-299-3p up-regulated in TNF-α-primed MSCs-exosome played an eminent role in the amelioration of liver damage in ALF by blocking the NLRP3 pathway [ 107 ]. Apart from its role in liver failure recovery, a miR-299-3p activity as a potent tumor suppressor has been documented in hepatocellular carcinoma by alleviating tumor size and venous infiltration [ 152 ].
MSCs-conditioned medium (CM) could also modify morphological characteristics of hepatocytes in the ALF model. Meanwhile, secretome achieved by cultivating MSCs with low oxygen content (10%) provoked more prominent hepatoprotective influence, and significantly reduced ALT and AST and also pro-inflammatory cytokines serum levels following injection in vivo [ 153 ]. In another study, Li and coworkers evaluated the therapeutic merits of CM from MSCs co-cultured with hepatocytes in the ALF rat model [ 154 ]. The apoptotic cells frequency was lower in CM derived from co-cultured cells than MSCs-CM or hepatocyte-CM. Also, CM derived from co-cultured cells strikingly alleviated liver injury and facilitated liver recovery, indicating the advantages of this strategy for liver failure therapy [ 154 ]. Also, silica magnetic graphene oxide (SMGO) could enhance the hypo protective influences of MSC-CM in ALF in vivo [ 155 ]. Meanwhile, administration of 300 μg/kg SMGO boosted MSC-CM competencies to avert necrosis, apoptosis, and inflammation of hepatocytes. Besides, SMGO therapy up-regulated the expression of VEGF and matrix metalloproteinase-9 (MMP-9) in vitro [ 155 ]. Another report also demonstrated that administration of CM from embryonic stem cell (ESC)-derived MSCs potentiated the proliferation of primary hepatocyte and improved IL-10 secretion from immune cells in vivo [ 156 ]. It appeared that such events might arouse because of the existence of VEGF in ESC-MSC-CM, which affect hepatocytes proliferation and migration, generating new avenues to cure ALF [ 156 ]. Likewise, MSC-CM sustained hepatocytes proliferation, reduced their apoptosis, compromised macrophages infiltration, elevated Th2 and Treg cells population, decreased levels of Th17 cells population, and eventually enabled HSCs death in ALF preclinical model [ 157 ]. The MSC-CM injection caused glycogen synthesis and storage recovery and also ameliorated ALF with no effect on Th1 cells [ 157 ]. Also, CM achieved from either amniotic fluid (AF)-MSCs or hepatic progenitor-like (HPL) cells derived from AF-MSCs thanks to the presence of IL-10, IL-1Ra, IL-13, and IL-27 stimulated liver recovery in the mice model with ALF [ 158 ].
MSCs in liver-associated conditions (clinical trials)
Several clinical trials have been accomplished or are ongoing to address the safety, feasibility and efficacy of MSCs therapy in liver-associated conditions, most frequently in liver failure and liver cirrhosis (Table 4 , Fig. 2 ). BM-MSCs and UC-MSCs are most commonly used types of cells. Of course, there is still no definite standard for which source of MSCs should be applied for clinical use. It seems that UC-MSCs are preferred for liver failure treatment as a result of higher differentiation capability. Also, the immunogenicity of UC-MSCs is lower than that of BM-MSCs [ 159 , 160 ]. Hence, autologous BM-MSCs and allogeneic UC-MSCs are highly preferred. On the other hand, poor proliferation, anti-inflammatory and self-renewal ability impedes AT-MSCs application in clinic [ 161 ]. Although intravenous injection is most used route, intraportal administration is evidently the optimal route for MSC therapy in liver-associated conditions due to the faster engraftment and the prohibited off-target accumulation. However, we must assess patients’ conditions and the potential risk of applying a particular route before choosing the administration route.
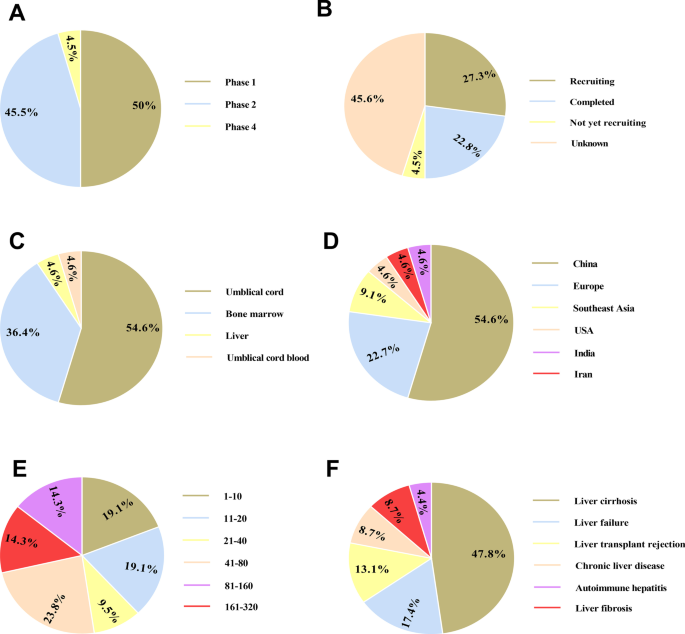
Clinical trials based on mesenchymal stromal cells (MSCs) therapy in liver-associated conditions registered in ClinicalTrials.gov (November 2021). The schematic demonstrates clinical depending on the study phase ( A ), study status ( B ), MSCs source ( C ), study location ( D ), participant number ( E ), and condition ( F )
Liver failure
A study of the safety and preliminary efficacy of UC-MSC transplantation (3 times at 4-week intervals) was carried out by Ming and colleagues [ 162 ]. They showed that the intervention had no unwanted effects, while attenuated total bilirubin and ALT levels, prolonged survival rate, and finally ameliorated liver functions, as evidenced by improved serum albumin, and prothrombin activity [ 162 ]. As well, intrasplenic and intrahepatic administration of autologous BM-MSCs derived hepatocyte inspired short-term amelioration in patient’s ascites, lower limb edema, and serum albumin [ 163 ]. Of course, defining the life span of the transplanted cells, and also determining the presence of long-term side effects is urgently required [ 163 ]. Moreover, another trial, which was accomplished from 2010 to 2013, indicated that systemic administration of allogeneic BM-MSCs could exert therapeutic benefits in patients suffering from HBV-related LF [ 164 ]. Meanwhile, stromal cell therapy augmented serum total bilirubin and ultimately promoted the 24-week survival rate by stimulating liver rescue concomitant with lessening the occurrences of stern infections compared with the control group (16.1% versus 33.3%) [ 164 ]. Likewise, other trials also exhibited that autologous BM-MSC transplantation was safe for chronic HBV-induced LF patients, as shown by the incidence of no serious intervention-related events and carcinoma during 192 weeks follow-up [ 165 ]. Also, the short-term outcome was promising; however, long-term efficacy was not clearly amended [ 165 ].
Liver cirrhosis
Cirrhosis is a late-stage liver disease in which healthy liver tissue is substituted with scar tissue and the liver is perpetually damaged. Liver transplantation is a standard therapeutic plan aiming to treat liver cirrhosis patients [ 166 ]. Meanwhile, MSCs have recently been noted as a possible therapeutic option to partially ameliorate liver function in this condition as a result of their appreciated antifibrotic and immunoregulatory attributes [ 55 ].
A phase 1 trial on 4 patients with decompensated liver cirrhosis verified the safety and feasibility of MSCs therapy [ 167 ]. Moreover, the life quality of all patients was ameliorated post-transplantation concerning the mean physical and mental component scales [ 167 ]. In primary biliary cirrhosis patients, UC-MSC injection by peripheral vein (3 times at 4-week intervals) exhibited no serious untoward effects [ 168 ]. Also, intervention caused a robust reduction in serum alkaline phosphatase (ALP) and γ-glutamyltransferase (GGT) levels compared to the control group during 4 years follow-up. Notwithstanding, no alteration was observed in levels of ALT, AST, total bilirubin, albumin, INR, or the prothrombin time activity. Thereby, comprehensive randomized controlled cohort trials are justified to authorize the clinical merits of UC-MSC transplantation [ 168 ]. In addition, injection of autologous BM-MSCs led to a partial amelioration of liver function in 25 Egyptian patients suffering from HCV-triggered liver cirrhosis, as evinced by improved prothrombin activity and serum albumin levels along with reduced bilirubin level [ 169 ]. In a similar condition, Amin et al. found that intrasplenic administration of autologous BM-MSCs potentiated liver function with attenuation in total bilirubin, AST, ALT, prothrombin time (PT), and also INR levels [ 170 ]. Autologous BM-MSCs therapy also inspired an improvement in liver function among HBV-related liver cirrhosis patients following transplantation [ 171 ]. This trial was conducted in 56 patients with HBV-induced liver cirrhosis, and results showed an enhancement in Treg/Th17 ratio post-transplantation during 24-week follow-up [ 171 ]. Consistently, mRNA levels of forkhead box protein P3 (FOXP3), an eminent Treg-associated transcription factor, strikingly were diminished, whereas retinoic acid-related orphan receptor gamma t (RORγt) expression levels which are tightly in association with Th17 cells were reduced. Further, the intervention resulted in an enhancement in TGF-β levels, while IL-17, TNF-α, and IL-6 were significantly decreased following transplantation [ 171 ]. In contrast to several cited trials implying that the autologous MSC therapy can be a safe and effective alternative for patients with liver cirrhosis [ 172 , 173 ], Mohamadnejad et al. noted that MSC infusion by peripheral vein had no advantageous result in cirrhotic patients [ 174 ]. Overall, large-scale studies are required to achieve reliable results concerning MSCs therapy in liver cirrhosis.
Potential risks of MSC transplantation
The treatment of liver dysfunctions through MSCs has been the central aim of several clinical and preclinical investigations. In this context, a few issues need to be dealt with cautiously (e.g., the possible emergence of carcinogenesis and the transmission of the virus). Different growth factors can be secreted by MSCs, and this may stimulate the growth of tumor cells and angiogenesis [ 175 , 176 ]. The past experimental investigations showed that the number of passages is a defining factor in rendering a tissue malignant or cancerous. Studies show that chromosome abnormalities may occur after more than three passages in the MSCs of mice [ 177 , 178 ]. Moreover, MSCs are likely to experience telomeric deletions after a multitude of passages. Despite the lack of any clinical reports on the malignant transformation of human MSCs, the follow-up period was not long enough for the formation of a tumor for most of them [ 179 ]. As a result, there need to be more studies on chromosomal integrity before MSCs transplantation to make sure that the procedure is completely safe.
Contrary to autotransplantation, allotransplantation can pose the danger of the spread of the virus to the patients [ 180 ]. Even though the spread of parvovirus B19 into BM cells was observed in vitro, there is no confirmed case of parvovirus B19-positive MSC-related viremia in humans. Yet, we do not know the spread of the herpes simplex virus (HSV) and cytomegalovirus (CMV) via MSCs in vivo. Owing to these facts, recipients, and donors of MSC are recommended to be screened for parvovirus B19, HSV, and CMV, as immunosuppressed patients are likely to catch infectious [ 181 ].
Enhancing the quantity of MSCs-secreted molecules
Now, restricted secretion of soluble mediators, such as exosome, from parental MSCs fences their wide-ranging application in clinics. Following some passages, MSCs mainly demonstrates abrogated competence to produce and then release soluble factor. Recent studies have indicated that tangential flow filtration (TFF) system-based tactics support the secretion of greater levels of vesicles from origin stromal cells than vesicle isolation by ultracentrifuge [ 182 ]. Further, ultrasonication of MSC-derived extracellular vesicles could improve their yields up to 20-fold [ 183 ]. Other proofs are indicating that three dimensional (3D) culture may facilities the incessant production of MSC-derived exosome [ 184 , 185 ]. Cultivation of MSCs in 3D cultures together with conventional either differential ultracentrifugation or TFF also could engender a higher quantity of MSCs-derived secretome [ 186 ]. Also, MSCs culture on particular biomaterials, such as alginate hydrogel [ 187 ] and avitene ultrafoam collagen ease generation of exosome with higher quantity and also potency [ 188 ]. As well, pretreatment of MSCs with hypoxia or various molecules, in particular cytokines or chemokines (e.g., IFN-γ, TNFα, IL-1β, IL-6, and TGF-β), gives largely rise to the secretion of vesicles with greater regenerative competencies [ 189 , 190 , 191 ].
Conclusion and future direction
Some investigations in preclinical models of liver diseases, such as ALF, have verified the MSC's unique competence to establish hepatocyte in vivo. Nonetheless, it seems that the therapeutic merits of MSCs largely rely on their aptitudes to secrete a myriad of factors, more importantly, cytokines, growth factors, and miRNAs, facilitating liver recovery. During last two decades, various clinical trials have been conducted to evaluate the capability of MSCs therapy in liver-associated conditions, such as ALF (Table 4 , Fig. 2 ); however, achieved outcomes are quite inconsistent. Given that autologous MSCs derived from elder patients or patients with obesity experienced abrogated proliferation and differentiation capability, using allogeneic cells in some cased is urgently required. In this circumstance, screening recipients and donors of MSC for parvovirus B19, HSV, and CMV are of paramount importance. Taken together, the providing of a universal MSC quality standard evaluation system is required.
To determine the mechanism contributed to MSCs therapy, it is urgently required to determine the protein, DNA and RNA secreted by MSCs. The proteomics and transcriptomics can play a pivotal role in evaluating the underlying mechanism. Notably, improving the frequency of cells homing to the damaged liver is the central point to potentiate the therapeutic impacts of MSCs. In fact, investigation of the homing attributes of MSCs is of paramount importance to augment the effective therapeutic quantity of such cells. In published clinical results, MSCs have been administrated into patients by several available routes, more frequently intravenous routes followed by intrahepatic injection (e.g., by the portal vein and hepatic artery). Also, intrasplenic injection has been applied in a few studies. Based on findings, a remarkable number of cells are trapped in the lungs upon systemic injection and thereby did not move to the liver afterward. Hence, finding better administration route is recommended to achieve significant outcome in vivo. Meanwhile, a study indicated that intraportal injection was more effective than hepatic intra-arterial injection and also intravenous injection to restore liver injury in vivo [ 123 ]. As well, it has been shown that portal vein injection has superiority over intrasplenic injection [ 192 ]. On the other hand, other reports exhibited that injection by the hepatic artery was not beneficial for the transdifferentiation of MSCs.
Among the recent clinical trials concerning the MSCs therapy for liver diseases (e.g., liver failure) treatment, the total number of MSCs employed was from 10 6 to 10 9 , irrespective of which method was applied to deliver the dose. The large range of doses applied is difficult to explicate as there are few reports including comparisons of several doses in the same clinical trial. Nonetheless, it seems that as few as 1 × 10 7 cells can be helpful based on recent published results.
In sum, although clinical trials have evidenced the safety and modest efficacy of short-term application of MSCs, further trials are warranted before MSCs application in clinical to treat ALF and other liver-associated conditions for optimizing administration routes as well as dosses.
Availability of data and materials
Not applicable.
Abbreviations
Mesenchymal stromal cells
Acute live failure
Hepatocyte growth factor
Tumor necrosis factor α
Interferon gamma
Vascular endothelial growth factor
Bone marrow
Umbilical cord
Adipose tissue
Transforming growth factor β
Aspartate aminotransferase
Alanine aminotransferase
Interleukin
Hadem J, Tacke F, Bruns T, Langgartner J, Strnad P, Denk GU, Fikatas P, Manns MP, Hofmann WP, Gerken G. Etiologies and outcomes of acute liver failure in Germany. Clin Gastroenterol Hepatol. 2012;10(6):664-9.e2.
Article PubMed Google Scholar
Lee WM, editor. Acute liver failure. Seminars in respiratory and critical care medicine. New York: Thieme Medical Publishers; 2012.
Google Scholar
Blackmore L, Bernal W. Acute liver failure. Clin Med. 2015;15(5):468.
Article Google Scholar
Patton H, Misel M, Gish RG. Acute liver failure in adults: an evidence-based management protocol for clinicians. Gastroenterol Hepatol. 2012;8(3):161–212.
Lee WM. Acute liver failure in the United States. Semin Liver Dis. 2003;23(3):217–26.
Article CAS PubMed Google Scholar
Tao Y-C, Wang M-L, Chen E-Q, Tang H. Stem cells transplantation in the treatment of patients with liver failure. Curr Stem Cell Res Ther. 2018;13(3):193–201.
Schacher FC, Martins Pezzi da Silva A, Silla LMDR, Álvares-da-Silva MR. Bone marrow mesenchymal stem cells in acute-on-chronic liver failure grades 2 and 3: a phase I-II randomized clinical trial. Can J Gastroenterol Hepatol. 2021;2021:1–9.
Choi J, Kang S, Kim B, So S, Han J, Kim G-N, Lee M-Y, Roh S, Lee J-Y, Oh SJ. Efficient hepatic differentiation and regeneration potential under xeno-free conditions using mass-producible amnion-derived mesenchymal stem cells. Stem Cell Res Ther. 2021;12(1):1–20.
Article CAS Google Scholar
Wang J-l, Ding H-r, Pan C-y, Shi X-l, Ren H-z. Mesenchymal stem cells ameliorate lipid metabolism through reducing mitochondrial damage of hepatocytes in the treatment of post-hepatectomy liver failure. Cell Death Dis. 2021;12(1):1–15.
Marofi F, Vahedi G, Hasanzadeh A, Salarinasab S, Arzhanga P, Khademi B, Farshdousti HM. Mesenchymal stem cells as the game-changing tools in the treatment of various organs disorders: Mirage or reality? J Cell Physiol. 2019;234(2):1268–88.
Saito Y, Ikemoto T, Morine Y, Shimada M. Current status of hepatocyte-like cell therapy from stem cells. Surg Today. 2021;51(3):340–9.
Markov A, Thangavelu L, Aravindhan S, Zekiy AO, Jarahian M, Chartrand MS, Pathak Y, Marofi F, Shamlou S, Hassanzadeh A. Mesenchymal stem/stromal cells as a valuable source for the treatment of immune-mediated disorders. Stem Cell Res Ther. 2021;12(1):1–30.
Tavakoli S, Ghaderi Jafarbeigloo HR, Shariati A, Jahangiryan A, Jadidi F, Jadidi Kouhbanani MA, Hassanzadeh A, Zamani M, Javidi K, Naimi A. Mesenchymal stromal cells; a new horizon in regenerative medicine. J Cell Physiol. 2020;235(12):9185–210.
Moghadasi S, Elveny M, Rahman HS, Suksatan W, Jalil AT, Abdelbasset WK, Yumashev AV, Shariatzadeh S, Motavalli R, Behzad F, et al. A paradigm shift in cell-free approach: the emerging role of MSCs-derived exosomes in regenerative medicine. J Transl Med. 2021;19(1):302.
Article CAS PubMed PubMed Central Google Scholar
Hassanzadeh A, Rahman HS, Markov A, Endjun JJ, Zekiy AO, Chartrand MS, Beheshtkhoo N, Kouhbanani MAJ, Marofi F, Nikoo M. Mesenchymal stem/stromal cell-derived exosomes in regenerative medicine and cancer; overview of development, challenges, and opportunities. Stem Cell Res Ther. 2021;12(1):1–22.
Golchin A, Hosseinzadeh S, Ardeshirylajimi A. The exosomes released from different cell types and their effects in wound healing. J Cell Biochem. 2018;119(7):5043–52.
Marote A, Teixeira FG, Mendes-Pinheiro B, Salgado AJ. MSCs-derived exosomes: cell-secreted nanovesicles with regenerative potential. Front Pharmacol. 2016;7:231.
Article PubMed PubMed Central Google Scholar
Jadli AS, Ballasy N, Edalat P, Patel VB. Inside (sight) of tiny communicator: exosome biogenesis, secretion, and uptake. Mol Cell Biochem. 2020;467(1):77–94.
Nakamura Y, Kita S, Tanaka Y, Fukuda S, Obata Y, Okita T, Nishida H, Takahashi Y, Kawachi Y, Tsugawa-Shimizu Y. Adiponectin stimulates exosome release to enhance mesenchymal stem-cell-driven therapy of heart failure in mice. Mol Ther. 2020;28(10):2203–19.
Psaraki A, Ntari L, Karakostas C, Korrou-Karava D, Roubelakis MG. Extracellular vesicles derived from mesenchymal stem/stromal cells: the regenerative impact in liver diseases. Hepatology. 2021;14:261–8.
Simmons OL, Meinzer C, Rule J, Lee WM. Liver transplantation for acetaminophen-induced acute liver failure: role of psychiatric comorbidity in listing decisions and outcomes. Dig Dis Sci. 2020;65(6):1861–8.
Ostapowicz G, Fontana RJ, Schiødt FV, Larson A, Davern TJ, Han SH, McCashland TM, Shakil AO, Hay JE, Hynan L, et al. Results of a prospective study of acute liver failure at 17 tertiary care centers in the United States. Ann Intern Med. 2002;137(12):947–54.
Dong V, Nanchal R, Karvellas CJ. Pathophysiology of acute liver failure. Nutr Clin Pract. 2020;35(1):24–9.
Riordan SM, Williams R. Acute liver failure: targeted artificial and hepatocyte-based support of liver regeneration and reversal of multiorgan failure. J Hepatol. 2000;32:63–76.
Chapel A, Bertho JM, Bensidhoum M, Fouillard L, Young RG, Frick J, Demarquay C, Cuvelier F, Mathieu E, Trompier F. Mesenchymal stem cells home to injured tissues when co-infused with hematopoietic cells to treat a radiation-induced multi-organ failure syndrome. J Gene Med. 2003;5(12):1028–38.
Bone RC. Sepsis, the sepsis syndrome, multi-organ failure: a plea for comparable definitions. Philadelphia: American College of Physicians; 1991.
Das J, Ghosh J, Manna P, Sil PC. Acetaminophen induced acute liver failure via oxidative stress and JNK activation: protective role of taurine by the suppression of cytochrome P450 2E1. Free Radic Res. 2010;44(3):340–55.
Jiang W-P, Deng J-S, Huang S-S, Wu S-H, Chen C-C, Liao J-C, Chen H-Y, Lin H-Y, Huang G-J. Sanghuangporus sanghuang mycelium prevents paracetamol-induced hepatotoxicity through regulating the MAPK/NF-κB, Keap1/Nrf2/HO-1, TLR4/PI3K/Akt, and CaMKKβ/LKB1/AMPK pathways and suppressing oxidative stress and inflammation. Antioxidants. 2021;10(6):897.
Shi S, Wang L, van der Laan LJ, Pan Q, Verstegen MM. Mitochondrial dysfunction and oxidative stress in liver transplantation and underlying diseases: new insights and therapeutics. Transplantation. 2021;105(11):2362–73.
Wu X, Dong L, Lin X, Li J. Relevance of the NLRP3 inflammasome in the pathogenesis of chronic liver disease. Front Immunol. 2017;8:1728.
Article PubMed PubMed Central CAS Google Scholar
Del Campo JA, Gallego P, Grande L. Role of inflammatory response in liver diseases: therapeutic strategies. World J Hepatol. 2018;10(1):1.
He K, Zhu X, Liu Y, Miao C, Wang T, Li P, Zhao L, Chen Y, Gong J, Cai C. Inhibition of NLRP3 inflammasome by thioredoxin-interacting protein in mouse Kupffer cells as a regulatory mechanism for non-alcoholic fatty liver disease development. Oncotarget. 2017;8(23):37657.
Zhan C, Lin G, Huang Y, Wang Z, Zeng F, Wu S. A dopamine-precursor-based nanoprodrug for in-situ drug release and treatment of acute liver failure by inhibiting NLRP3 inflammasome and facilitating liver regeneration. Biomaterials. 2021;268:120573.
Woolbright BL, Jaeschke H. Role of the inflammasome in acetaminophen-induced liver injury and acute liver failure. J Hepatol. 2017;66(4):836–48.
Zhao Q, Wu C-S, Fang Y, Qian Y, Wang H, Fan Y-C, Wang K. Glucocorticoid regulates NLRP3 in acute-on-chronic hepatitis B liver failure. Int J Med Sci. 2019;16(3):461.
Tao Y, Wang N, Qiu T, Sun X. The role of autophagy and NLRP3 inflammasome in liver fibrosis. BioMed Res Int. 2020;2020:1–8.
CAS Google Scholar
Li L, Shan S, Kang K, Zhang C, Kou R, Song F. The cross-talk of NLRP3 inflammasome activation and necroptotic hepatocyte death in acetaminophen-induced mice acute liver injury. Hum Exp Toxicol. 2021;40(4):673–84.
Choi J, Kang S, Kim B, So S, Han J, Kim G-N, Lee M-Y, Roh S, Lee J-Y, Oh SJ, et al. Efficient hepatic differentiation and regeneration potential under xeno-free conditions using mass-producible amnion-derived mesenchymal stem cells. Stem Cell Res Ther. 2021;12(1):569.
Zhang S, Yang Y, Fan L, Zhang F, Li L. The clinical application of mesenchymal stem cells in liver disease: the current situation and potential future. Ann Transl Med. 2020;8(8):565.
Du XX, Shi Y, Yang Y, Yu Y, Lou HG, Lv FF, Chen Z, Yang Q. DAMP molecular IL-33 augments monocytic inflammatory storm in hepatitis B-precipitated acute-on-chronic liver failure. Liver Int. 2018;38(2):229–38.
Li M, Ling T, Teng F, Hu C, Su Z, Zhang C, Li X, Zhao T, Mu X, Li Y. CD5L deficiency attenuate acetaminophen-induced liver damage in mice via regulation of JNK and ERK signaling pathway. Cell Death Discov. 2021;7(1):1–11.
Weiss ARR, Dahlke MH. Immunomodulation by mesenchymal stem cells (MSCs): mechanisms of action of living, apoptotic, and dead MSCs. Front Immunol. 2019;10:1191.
Song N, Scholtemeijer M, Shah K. Mesenchymal stem cell immunomodulation: mechanisms and therapeutic potential. Trends Pharmacol Sci. 2020;41:653–64.
Carreras-Planella L, Monguió-Tortajada M, Borràs FE, Franquesa M. Immunomodulatory effect of MSC on B cells is independent of secreted extracellular vesicles. Front Immunol. 2019;10:1288.
Sun S-J, Lai W-H, Jiang Y, Zhen Z, Wei R, Lian Q, Liao S-Y, Tse H-F. Immunomodulation by systemic administration of human-induced pluripotent stem cell-derived mesenchymal stromal cells to enhance the therapeutic efficacy of cell-based therapy for treatment of myocardial infarction. Theranostics. 2021;11(4):1641.
Bahroudi M, Bakhshi B, Soudi S, Najar-Peerayeh S. Immunomodulatory effects of mesenchymal stem cell-conditioned media on lipopolysaccharide of Vibrio cholerae as a vaccine candidate. Stem Cell Res Ther. 2021;12(1):1–14.
Putra A, Ridwan FB, Putridewi AI, Kustiyah AR, Wirastuti K, Sadyah NAC, Rosdiana I, Munir D. The role of TNF-α induced MSCs on suppressive inflammation by increasing TGF-β and IL-10. Open Access Maced J Med Sci. 2018;6(10):1779.
Wang L, Deng Z, Sun Y, Zhao Y, Li Y, Yang M, Yuan R, Liu Y, Qian Z, Zhou F. The study on the regulation of Th cells by MSCs through the JAK-STAT signaling pathway to protect naturally aged sepsis model rats. Front Immunol. 2021;13:820685. https://doi.org/10.3389/fimmu.2022.820685 .
Akiyama K, Chen C, Wang D, Xu X, Qu C, Yamaza T, Cai T, Chen W, Sun L, Shi S. Mesenchymal-stem-cell-induced immunoregulation involves FAS-ligand-/FAS-mediated T cell apoptosis. Cell Stem Cell. 2012;10(5):544–55.
Miloradovic D, Miloradovic D, Markovic BS, Acovic A, Harrell CR, Djonov V, Arsenijevic N, Volarevic V. The effects of mesenchymal stem cells on antimelanoma immunity depend on the timing of their administration. Stem Cells Int. 2020;2020:1–13.
Volarevic V, Nurkovic J, Arsenijevic N, Stojkovic M. Concise review: therapeutic potential of mesenchymal stem cells for the treatment of acute liver failure and cirrhosis. Stem Cells. 2014;32(11):2818–23.
Bataller R, Brenner DA. Liver fibrosis. J Clin Investig. 2005;115(2):209–18.
Hu C, Wu Z, Li L. Mesenchymal stromal cells promote liver regeneration through regulation of immune cells. Int J Biol Sci. 2020;16(5):893.
Huang B, Cheng X, Wang H, Huang W, Wang D, Zhang K, Zhang H, Xue Z, Da Y, Zhang N. Mesenchymal stem cells and their secreted molecules predominantly ameliorate fulminant hepatic failure and chronic liver fibrosis in mice respectively. J Transl Med. 2016;14(1):1–12.
Tsuchiya A, Takeuchi S, Watanabe T, Yoshida T, Nojiri S, Ogawa M, Terai S. Mesenchymal stem cell therapies for liver cirrhosis: MSCs as “conducting cells” for improvement of liver fibrosis and regeneration. Inflamm Regen. 2019;39(1):1–6.
Friedenstein A, Piatetzky-Shapiro I, Petrakova K. Osteogenesis in transplants of bone marrow cells. Development. 1966;16(3):381–90.
Friedenstein AJ, Deriglasova UF, Kulagina NN, Panasuk AF, Rudakowa SF, Luriá EA, Ruadkow IA. Precursors for fibroblasts in different populations of hematopoietic cells as detected by the in vitro colony assay method. Exp Hematol. 1974;2(2):83–92.
CAS PubMed Google Scholar
Ullah I, Seo K, Wi H, Kim Y, Lee S, Ock SA. Induction of the differentiation of porcine bone marrow mesenchymal stem cells into premature hepatocyte-like cells in an indirect coculture system with primary hepatocytes. Anim Cells Syst. 2020;24(5):289–98.
Ji R, Zhang N, You N, Li Q, Liu W, Jiang N, Liu J, Zhang H, Wang D, Tao K. The differentiation of MSCs into functional hepatocyte-like cells in a liver biomatrix scaffold and their transplantation into liver-fibrotic mice. Biomaterials. 2012;33(35):8995–9008.
Afshari A, Shamdani S, Uzan G, Naserian S, Azarpira N. Different approaches for transformation of mesenchymal stem cells into hepatocyte-like cells. Stem Cell Res Ther. 2020;11(1):1–14.
Afshari A, Shamdani S, Uzan G, Naserian S, Azarpira N. Different approaches for transformation of mesenchymal stem cells into hepatocyte-like cells. Stem Cell Res Ther. 2020;11(1):54.
Taléns-Visconti R, Bonora A, Jover R, Mirabet V, Carbonell F, Castell JV, Gómez-Lechón MJ. Hepatogenic differentiation of human mesenchymal stem cells from adipose tissue in comparison with bone marrow mesenchymal stem cells. World J Gastroenterol: WJG. 2006;12(36):5834.
Ye JS, Su XS, Stoltz JF, de Isla N, Zhang L. Signalling pathways involved in the process of mesenchymal stem cells differentiating into hepatocytes. Cell Prolif. 2015;48(2):157–65.
Popovici C, Roubin R, Coulier F, Birnbaum D. An evolutionary history of the FGF superfamily. BioEssays. 2005;27(8):849–57.
Battula VL, Bareiss PM, Treml S, Conrad S, Albert I, Hojak S, Abele H, Schewe B, Just L, Skutella T. Human placenta and bone marrow derived MSC cultured in serum-free, b-FGF-containing medium express cell surface frizzled-9 and SSEA-4 and give rise to multilineage differentiation. Differentiation. 2007;75(4):279–91.
Eom YW, Oh J-E, Lee JI, Baik SK, Rhee K-J, Shin HC, Kim YM, Ahn CM, Kong JH, Kim HS. The role of growth factors in maintenance of stemness in bone marrow-derived mesenchymal stem cells. Biochem Biophys Res Commun. 2014;445(1):16–22.
Wang Y, Yu X, Chen E, Li L. Liver-derived human mesenchymal stem cells: a novel therapeutic source for liver diseases. Stem Cell Res Ther. 2016;7(1):1–8.
Neuss S, Becher E, Wöltje M, Tietze L, Jahnen-Dechent W. Functional expression of HGF and HGF receptor/c-met in adult human mesenchymal stem cells suggests a role in cell mobilization, tissue repair, and wound healing. Stem Cells. 2004;22(3):405–14.
Park B-W, Jung S-H, Das S, Lee SM, Park J-H, Kim H, Hwang J-W, Lee S, Kim H-J, Kim H-Y. In vivo priming of human mesenchymal stem cells with hepatocyte growth factor–engineered mesenchymal stem cells promotes therapeutic potential for cardiac repair. Sci Adv. 2020;6(13):eaay6994.
Forte G, Minieri M, Cossa P, Antenucci D, Sala M, Gnocchi V, Fiaccavento R, Carotenuto F, De Vito P, Baldini PM. Hepatocyte growth factor effects on mesenchymal stem cells: proliferation, migration, and differentiation. Stem Cells. 2006;24(1):23–33.
Chivu M, Dima SO, Stancu CI, Dobrea C, Uscatescu V, Necula LG, Bleotu C, Tanase C, Albulescu R, Ardeleanu C. In vitro hepatic differentiation of human bone marrow mesenchymal stem cells under differential exposure to liver-specific factors. Transl Res. 2009;154(3):122–32.
Dai LJ, Li HY, Guan LX, Ritchie G, Zhou JX. The therapeutic potential of bone marrow-derived mesenchymal stem cells on hepatic cirrhosis. Stem Cell Res. 2009;2(1):16–25.
Yan Y, Xu W, Qian H, Si Y, Zhu W, Cao H, Zhou H, Mao F. Mesenchymal stem cells from human umbilical cords ameliorate mouse hepatic injury in vivo. Liver Int. 2009;29(3):356–65.
Zhang GZ, Sun HC, Zheng LB, Guo JB, Zhang XL. In vivo hepatic differentiation potential of human umbilical cord-derived mesenchymal stem cells: Therapeutic effect on liver fibrosis/cirrhosis. World J Gastroenterol. 2017;23(46):8152–68.
Tsiapalis D, O’Driscoll L. Mesenchymal stem cell derived extracellular vesicles for tissue engineering and regenerative medicine applications. Cells. 2020;9(4):991.
Article CAS PubMed Central Google Scholar
Chen L, Zhang C, Chen L, Wang X, Xiang B, Wu X, Guo Y, Mou X, Yuan L, Chen B, et al. Human menstrual blood-derived stem cells ameliorate liver fibrosis in mice by targeting hepatic stellate cells via paracrine mediators. Stem Cells Transl Med. 2017;6(1):272–84.
Choi JS, Jeong IS, Han JH, Cheon SH, Kim S-W. IL-10-secreting human MSCs generated by TALEN gene editing ameliorate liver fibrosis through enhanced anti-fibrotic activity. Biomater Sci. 2019;7(3):1078–87.
Xuan J, Feng W, An Z, Yang J, Xu H, Li J, Zhao Z, Wen W. Anti-tgf [beta]-1 receptor inhibitor mediates the efficacy of the human umbilical cord mesenchymal stem cells against liver fibrosis through tgf [beta]-1/smad pathway. Mol Cell Biochem. 2017;429(1–2):113.
Jang YO, Cho M-Y, Yun C-O, Baik SK, Park K-S, Cha S-K, Chang SJ, Kim MY, Lim YL, Kwon SO. Effect of function-enhanced mesenchymal stem cells infected with decorin-expressing adenovirus on hepatic fibrosis. Stem Cells Transl Med. 2016;5(9):1247–56.
Pietrosi G, Fernández-Iglesias A, Pampalone M, Ortega-Ribera M, Lozano JJ, García-Calderó H, Abad-Jordà L, Conaldi PG, Parolini O, Vizzini G. Human amniotic stem cells improve hepatic microvascular dysfunction and portal hypertension in cirrhotic rats. Liver Int. 2020;40(10):2500–14.
Kim M-D, Kim S-S, Cha H-Y, Jang S-H, Chang D-Y, Kim W, Suh-Kim H, Lee J-H. Therapeutic effect of hepatocyte growth factor-secreting mesenchymal stem cells in a rat model of liver fibrosis. Exp Mol Med. 2014;46(8):e110.
Rockel JS, Rabani R, Viswanathan S, editors. Anti-fibrotic mechanisms of exogenously-expanded mesenchymal stromal cells for fibrotic diseases. Semin Cell Dev Biol. 2020;101:87–103.
Motawi TM, Atta HM, Sadik NA, Azzam M. The therapeutic effects of bone marrow-derived mesenchymal stem cells and simvastatin in a rat model of liver fibrosis. Cell Biochem Biophys. 2014;68(1):111–25.
Raafat N, Aal SMA, Abdo FK, El Ghonaimy NM. Mesenchymal stem cells: in vivo therapeutic application ameliorates carbon tetrachloride induced liver fibrosis in rats. Int J Biochem Cell Biol. 2015;68:109–18.
Wang J, Bian C, Liao L, Zhu Y, Li J, Zeng L, Zhao RC. Inhibition of hepatic stellate cells proliferation by mesenchymal stem cells and the possible mechanisms. Hepatol Res. 2009;39(12):1219–28.
An SY, Jang YJ, Lim H-J, Han J, Lee J, Lee G, Park JY, Park S-Y, Kim JH, Do B-R. Milk fat globule-EGF factor 8, secreted by mesenchymal stem cells, protects against liver fibrosis in mice. Gastroenterology. 2017;152(5):1174–86.
Chen S, Lin N, Pan W, Hu K, Xu R. Activation of Notch1 signaling by marrow-derived mesenchymal stem cells through cell–cell contact inhibits proliferation of hepatic stellate cells. Life Sci. 2011;89(25–26):975–81.
Lee C, Kim M, Han J, Yoon M, Jung Y. Mesenchymal stem cells influence activation of hepatic stellate cells, and constitute a promising therapy for liver fibrosis. Biomedicines. 2021;9(11):1598.
Duman DG, Zibandeh N, Ugurlu MU, Celikel C, Akkoc T, Banzragch M, Genc D, Ozdogan O, Akkoc T. Mesenchymal stem cells suppress hepatic fibrosis accompanied by expanded intrahepatic natural killer cells in rat fibrosis model. Mol Biol Rep. 2019;46(3):2997–3008.
De Maria N, Colantonl A, Fagiuoli S, Liu G-J, Rogers BK, Farinati F, Van Thiel DH, Floyd RA. Association between reactive oxygen species and disease activity in chronic hepatitis C. Free Radic Biol Med. 1996;21(3):291–5.
Takemoto K, Hatano E, Iwaisako K, Takeiri M, Noma N, Ohmae S, Toriguchi K, Tanabe K, Tanaka H, Seo S. Necrostatin-1 protects against reactive oxygen species (ROS)-induced hepatotoxicity in acetaminophen-induced acute liver failure. FEBS Open Bio. 2014;4:777–87.
Choe G, Kim S-W, Park J, Park J, Kim S, Kim YS, Ahn Y, Jung D-W, Williams DR, Lee JY. Anti-oxidant activity reinforced reduced graphene oxide/alginate microgels: mesenchymal stem cell encapsulation and regeneration of infarcted hearts. Biomaterials. 2019;225:119513.
Dey R, Kemp K, Gray E, Rice C, Scolding N, Wilkins A. Human mesenchymal stem cells increase anti-oxidant defences in cells derived from patients with Friedreich’s ataxia. Cerebellum. 2012;11(4):861–71.
Murakami T, Nagamura Y, Hirano K. The recovering effect of betaine on carbon tetrachloride-induced liver injury. J Nutr Sci Vitaminol. 1998;44(2):249–55.
Mitsuhashi M, Morimura K, Wanibuchi H, Kiyota A, Wada S, Nakatani T, Fukushima S. Examination of the rat model of liver injury via thioacetamide (TAA) or carbon tetrachloride (CCl4). J Toxicol Pathol. 2004;17(3):219–22.
Eom YW, Shim KY, Baik SK. Mesenchymal stem cell therapy for liver fibrosis. Korean J Intern Med. 2015;30(5):580.
Raafat N, Abdel Aal SM, Abdo FK, El Ghonaimy NM. Mesenchymal stem cells: in vivo therapeutic application ameliorates carbon tetrachloride induced liver fibrosis in rats. Int J Biochem Cell Biol. 2015;68:109–18.
Cho KA, Woo SY, Seoh JY, Han HS, Ryu KH. Mesenchymal stem cells restore CCl4-induced liver injury by an antioxidative process. Cell Biol Int. 2012;36(12):1267–74.
Quintanilha LF, Takami T, Hirose Y, Fujisawa K, Murata Y, Yamamoto N, Goldenberg RC, Terai S, Sakaida I. Canine mesenchymal stem cells show antioxidant properties against thioacetamide-induced liver injury in vitro and in vivo. Hepatol Res. 2014;44(10):E206–17.
Phinney DG, Pittenger MF. Concise review: msc-derived exosomes for cell-free therapy. Stem Cells. 2017;35(4):851–8.
Razeghian E, Margiana R, Chupradit S, Bokov DO, Abdelbasset WK, Marofi F, Shariatzadeh S, Tosan F, Jarahian M. Mesenchymal stem/stromal cells as a vehicle for cytokine delivery: an emerging approach for tumor immunotherapy. Front Med. 2021;8:721174.
Rahbaran M, Baghini SS, Mardasi M, Razeghian E. MSCs modifies the proliferation of leukemia MOLT-4 cells and induces their apoptosis through up-regulating Bax, caspase-3, and-8, and down-regulating Bcl-2 expression. Ann Cancer Res Ther. 2021;29(1):79–84.
Zhang S, Hou Y, Yang J, Xie D, Jiang L, Hu H, Hu J, Luo C, Zhang Q. Application of mesenchymal stem cell exosomes and their drug-loading systems in acute liver failure. J Cell Mol Med. 2020;24(13):7082–93.
Jiang L, Zhang S, Hu H, Yang J, Wang X, Ma Y, Jiang J, Wang J, Zhong L, Chen M. Exosomes derived from human umbilical cord mesenchymal stem cells alleviate acute liver failure by reducing the activity of the NLRP3 inflammasome in macrophages. Biochem Biophys Res Commun. 2019;508(3):735–41.
Yan Y, Jiang W, Tan Y, Zou S, Zhang H, Mao F, Gong A, Qian H, Xu W. hucMSC exosome-derived GPX1 is required for the recovery of hepatic oxidant injury. Mol Ther. 2017;25(2):465–79.
Shao M, Xu Q, Wu Z, Chen Y, Shu Y, Cao X, Chen M, Zhang B, Zhou Y, Yao R. Exosomes derived from human umbilical cord mesenchymal stem cells ameliorate IL-6-induced acute liver injury through miR-455-3p. Stem Cell Res Ther. 2020;11(1):1–13.
Zhang S, Jiang L, Hu H, Wang H, Wang X, Jiang J, Ma Y, Yang J, Hou Y, Xie D, et al. Pretreatment of exosomes derived from hUCMSCs with TNF-α ameliorates acute liver failure by inhibiting the activation of NLRP3 in macrophage. Life Sci. 2020;246:117401.
Hao NB, Li CZ, Lü MH, Tang B, Wang SM, Wu YY, Liang GP, Yang SM. SDF-1/CXCR4 axis promotes MSCs to repair liver injury partially through trans-differentiation and fusion with hepatocytes. Stem Cells Int. 2015;2015:960387.
Zhang S, Zhu Z, Wang Y, Liu S, Zhao C, Guan W, Zhao Y. Therapeutic potential of Bama miniature pig adipose stem cells induced hepatocytes in a mouse model with acute liver failure. Cytotechnology. 2018;70(4):1131–41.
Wang J, Ren H, Yuan X, Ma H, Shi X, Ding Y. Interleukin-10 secreted by mesenchymal stem cells attenuates acute liver failure through inhibiting pyroptosis. Hepatol Res. 2018;48(3):E194-e202.
Ma HC, Wang X, Wu MN, Zhao X, Yuan XW, Shi XL. Interleukin-10 contributes to therapeutic effect of mesenchymal stem cells for acute liver failure via signal transducer and activator of transcription 3 signaling pathway. Chin Med J (Engl). 2016;129(8):967–75.
Liu Y, Ren H, Wang J, Yang F, Li J, Zhou Y, Yuan X, Zhu W, Shi X. Prostaglandin E(2) secreted by mesenchymal stem cells protects against acute liver failure via enhancing hepatocyte proliferation. FASEB J. 2019;33(2):2514–25.
Zhou JH, Lu X, Yan CL, Sheng XY, Cao HC. Mesenchymal stromal cell-dependent immunoregulation in chemically-induced acute liver failure. World J Stem Cells. 2021;13(3):208–20.
Liu L, Song H, Duan H, Chai J, Yang J, Li X, Yu Y, Zhang X, Hu X, Xiao M, et al. TSG-6 secreted by human umbilical cord-MSCs attenuates severe burn-induced excessive inflammation via inhibiting activations of P38 and JNK signaling. Sci Rep. 2016;6(1):30121.
Wang Y, Wang JL, Ma HC, Tang ZT, Ding HR, Shi XL. Mesenchymal stem cells increase heme oxygenase 1-activated autophagy in treatment of acute liver failure. Biochem Biophys Res Commun. 2019;508(3):682–9.
Zhang ZH, Zhu W, Ren HZ, Zhao X, Wang S, Ma HC, Shi XL. Mesenchymal stem cells increase expression of heme oxygenase-1 leading to anti-inflammatory activity in treatment of acute liver failure. Stem Cell Res Ther. 2017;8(1):70.
Zhao X, Shi XL, Zhang ZH, Ma HC, Yuan XW, Ding YT. Role of neutrophils in treatment of rats with D-galactosamine-induced acute liver failure with bone marrow mesenchymal stem cells. Zhonghua Gan Zang Bing Za Zhi. 2016;24(8):601–7.
Guo G, Zhuang X, Xu Q, Wu Z, Zhu Y, Zhou Y, Li Y, Lu Y, Zhang B, Talbot P, et al. Peripheral infusion of human umbilical cord mesenchymal stem cells rescues acute liver failure lethality in monkeys. Stem Cell Res Ther. 2019;10(1):84.
Zhang Y, Li Y, Li W, Cai J, Yue M, Jiang L, Xu R, Zhang L, Li J, Zhu C. Therapeutic effect of human umbilical cord mesenchymal stem cells at various passages on acute liver failure in rats. Stem Cells Int. 2018;2018:7159465.
He Y, Guo X, Lan T, Xia J, Wang J, Li B, Peng C, Chen Y, Hu X, Meng Z. Human umbilical cord-derived mesenchymal stem cells improve the function of liver in rats with acute-on-chronic liver failure via downregulating Notch and Stat1/Stat3 signaling. Stem Cell Res Ther. 2021;12(1):396.
Ding HR, Wang JL, Tang ZT, Wang Y, Zhou G, Liu Y, Ren HZ, Shi XL. Mesenchymal stem cells improve glycometabolism and liver regeneration in the treatment of post-hepatectomy liver failure. Front Physiol. 2019;10:412.
Sang JF, Shi XL, Han B, Huang T, Huang X, Ren HZ, Ding YT. Intraportal mesenchymal stem cell transplantation prevents acute liver failure through promoting cell proliferation and inhibiting apoptosis. Hepatobiliary Pancreat Dis Int. 2016;15(6):602–11.
Putra A, Rosdiana I, Darlan DM, Alif I, Hayuningtyas F, Wijaya I, Aryanti R, Makarim FR, Antari AD. Intravenous administration is the best route of mesenchymal stem cells migration in improving liver function enzyme of acute liver failure. Folia Med (Plovdiv). 2020;62(1):52–8.
Zare H, Jamshidi S, Dehghan MM, Saheli M, Piryaei A. Bone marrow or adipose tissue mesenchymal stem cells: comparison of the therapeutic potentials in mice model of acute liver failure. J Cell Biochem. 2018;119(7):5834–42.
Ali M, Payne SL. Biomaterial-based cell delivery strategies to promote liver regeneration. Biomater Res. 2021;25(1):5.
Liu M, Yang J, Hu W, Zhang S, Wang Y. Superior performance of co-cultured mesenchymal stem cells and hepatocytes in poly(lactic acid-glycolic acid) scaffolds for the treatment of acute liver failure. Biomed Mater. 2016;11(1):015008.
Article PubMed CAS Google Scholar
Kang HT, Jun DW, Jang K, Hoh JK, Lee JS, Saeed WK, Chae YJ, Lee JH. Effect of stem cell treatment on acute liver failure model using scaffold. Dig Dis Sci. 2019;64(3):781–91.
Ghaedi M, Soleimani M, Shabani I, Duan Y, Lotfi AS. Hepatic differentiation from human mesenchymal stem cells on a novel nanofiber scaffold. Cell Mol Biol Lett. 2012;17(1):89–106.
Xu L, Wang S, Sui X, Wang Y, Su Y, Huang L, Zhang Y, Chen Z, Chen Q, Du H, et al. Mesenchymal stem cell-seeded regenerated silk fibroin complex matrices for liver regeneration in an animal model of acute liver failure. ACS Appl Mater Interfaces. 2017;9(17):14716–23.
Wang L, Li S, Wang HY, Zeng J, Zhang ZZ, Lv DY, Kuang WH. In a rat model of acute liver failure, Icaritin improved the therapeutic effect of mesenchymal stem cells by activation of the hepatocyte growth factor/c-met pathway. Evid Based Complement Alternat Med. 2019;2019:4253846.
Chen H, Tang S, Liao J, Liu M, Lin Y. Therapeutic effect of human umbilical cord blood mesenchymal stem cells combined with G-CSF on rats with acute liver failure. Biochem Biophys Res Commun. 2019;517(4):670–6.
Chavez-Tapia NC, Mendiola-Pastrana I, Ornelas-Arroyo VJ, Noreña-Herrera C, Vidaña-Perez D, Delgado-Sanchez G, Uribe M, Barrientos-Gutierrez T. Granulocyte-colony stimulating factor for acute-on-chronic liver failure: systematic review and meta-analysis. Ann Hepatol. 2015;14(5):631–41.
Nam HH, Jun DW, Jang K, Saeed WK, Lee JS, Kang HT, Chae YJ. Granulocyte colony stimulating factor treatment in non-alcoholic fatty liver disease: beyond marrow cell mobilization. Oncotarget. 2017;8(58):97965–76.
Sang JF, Shi XL, Han B, Huang X, Huang T, Ren HZ, Ding YT. Combined mesenchymal stem cell transplantation and interleukin-1 receptor antagonism after partial hepatectomy. World J Gastroenterol. 2016;22(16):4120–35.
Xiao JQ, Shi XL, Tan JJ, Zhang L, Xu Q, Ding YT. A novel treatment regimen for acute liver failure based on a combination of mesenchymal stem cells transplantation and IL-lRa-loaded chitosan nanoparticles. Zhonghua Gan Zang Bing Za Zhi. 2013;21(4):308–14.
Nasiri E, Alizadeh A, Roushandeh AM, Gazor R, Hashemi-Firouzi N, Golipoor Z. Melatonin-pretreated adipose-derived mesenchymal stem cells efficeintly improved learning, memory, and cognition in an animal model of Alzheimer’s disease. Metab Brain Dis. 2019;34(4):1131–43.
Huang P, Wang L, Li Q, Tian X, Xu J, Xu J, Xiong Y, Chen G, Qian H, Jin C, et al. Atorvastatin enhances the therapeutic efficacy of mesenchymal stem cells-derived exosomes in acute myocardial infarction via up-regulating long non-coding RNA H19. Cardiovasc Res. 2020;116(2):353–67.
Nie H, An F, Mei J, Yang C, Zhan Q, Zhang Q. IL-1β Pretreatment improves the efficacy of mesenchymal stem cells on acute liver failure by enhancing CXCR4 expression. Stem Cells Int. 2020;2020:1498315.
Mohsin S, Shams S, Ali Nasir G, Khan M, Javaid Awan S, Khan SN, Riazuddin S. Enhanced hepatic differentiation of mesenchymal stem cells after pretreatment with injured liver tissue. Differentiation. 2011;81(1):42–8.
Li QY, Chen J, Luo YH, Zhang W, Xiao EH. Sodium Butyrate Pre-Treatment Enhance Differentiation of Bone Marrow Mesenchymal Stem Cells (BM-MSCs) into Hepatocytes and Improve Liver Injury. Curr Mol Med. 2021;1.
Ma HC, Shi XL, Ren HZ, Yuan XW, Ding YT. Targeted migration of mesenchymal stem cells modified with CXCR4 to acute failing liver improves liver regeneration. World J Gastroenterol. 2014;20(40):14884–94.
Chen H, Tang S, Liao J, Liu M, Lin Y. VEGF(165) gene-modified human umbilical cord blood mesenchymal stem cells protect against acute liver failure in rats. J Gene Med. 2021;23(10):e3369.
Wang W, Guo H, Li H, Yan Y, Wu C, Wang X, He X, Zhao N. Interleukin-35 gene-modified mesenchymal stem cells protect concanavalin A-induced fulminant hepatitis by decreasing the interferon gamma level. Hum Gene Ther. 2018;29(2):234–41.
Zheng YB, Zhang XH, Huang ZL, Lin CS, Lai J, Gu YR, Lin BL, Xie DY, Xie SB, Peng L, et al. Amniotic-fluid-derived mesenchymal stem cells overexpressing interleukin-1 receptor antagonist improve fulminant hepatic failure. PLoS ONE. 2012;7(7):e41392.
Zhang J, Gao J, Lin D, Xiong J, Wang J, Chen J, Lin B, Gao Z. Potential networks regulated by MSCs in acute-on-chronic liver failure: exosomal miRNAs and intracellular target genes. Front Genet. 2021;12:650536.
Zheng J, Lu T, Zhou C, Cai J, Zhang X, Liang J, Sui X, Chen X, Chen L, Sun Y, et al. Extracellular vesicles derived from human umbilical cord mesenchymal stem cells protect liver ischemia/reperfusion injury by reducing CD154 expression on CD4+ T cells via CCT2. Adv Sci (Weinh). 2020;7(18):1903746.
Wei S, Wang Q, Zhou H, Qiu J, Li C, Shi C, Zhou S, Liu R, Lu L. miR-455-3p alleviates hepatic stellate cell activation and liver fibrosis by suppressing HSF1 expression. Mol Ther Nucleic Acids. 2019;16:758–69.
Jun JH, Kim JY, Choi JH, Lim JY, Kim K, Kim GJ. Exosomes from placenta-derived mesenchymal stem cells are involved in liver regeneration in hepatic failure induced by bile duct ligation. Stem Cells Int. 2020;2020:5485738.
Damania A, Jaiman D, Teotia AK, Kumar A. Mesenchymal stromal cell-derived exosome-rich fractionated secretome confers a hepatoprotective effect in liver injury. Stem Cell Res Ther. 2018;9(1):31.
Chen L, Xiang B, Wang X, Xiang C. Exosomes derived from human menstrual blood-derived stem cells alleviate fulminant hepatic failure. Stem Cell Res Ther. 2017;8(1):9.
Dang S, Zhou J, Wang Z, Wang K, Dai S, He S. MiR-299-3p functions as a tumor suppressor via targeting Sirtuin 5 in hepatocellular carcinoma. Biomed Pharmacother. 2018;106:966–75.
Temnov A, Rogov K, Zhalimov V, Igor P, Pekov S, Bader A, Sklifas A, Giri S. The effect of a mesenchymal stem cell conditioned medium fraction on morphological characteristics of hepatocytes in acetaminophen-induced acute liver failure: a preliminary study. Hepat Med. 2019;11:89–96.
PubMed PubMed Central Google Scholar
Chen L, Zhang J, Yang L, Zhang G, Wang Y, Zhang S. The effects of conditioned medium derived from mesenchymal stem cells cocultured with hepatocytes on damaged hepatocytes and acute liver failure in rats. Stem Cells Int. 2018;2018:9156560.
Foroutan T, Kabiri F, Motamedi E. Silica magnetic graphene oxide improves the effects of stem cell-conditioned medium on acute liver failure. ACS Omega. 2021;6(33):21194–206.
Lotfinia M, Kadivar M, Piryaei A, Pournasr B, Sardari S, Sodeifi N, Sayahpour FA, Baharvand H. Effect of secreted molecules of human embryonic stem cell-derived mesenchymal stem cells on acute hepatic failure model. Stem Cells Dev. 2016;25(24):1898–908.
Huang B, Cheng X, Wang H, Huang W, la Ga HuZ, Wang D, Zhang K, Zhang H, Xue Z, Da Y, et al. Mesenchymal stem cells and their secreted molecules predominantly ameliorate fulminant hepatic failure and chronic liver fibrosis in mice respectively. J Transl Med. 2016;14:45.
Zagoura DS, Roubelakis MG, Bitsika V, Trohatou O, Pappa KI, Kapelouzou A, Antsaklis A, Anagnou NP. Therapeutic potential of a distinct population of human amniotic fluid mesenchymal stem cells and their secreted molecules in mice with acute hepatic failure. Gut. 2012;61(6):894–906.
Cho PS, Messina DJ, Hirsh EL, Chi N, Goldman SN, Lo DP, Harris IR, Popma SH, Sachs DH, Huang CA. Immunogenicity of umbilical cord tissue–derived cells. Blood. 2008;111(1):430–8.
Deuse T, Stubbendorff M, Tang-Quan K, Phillips N, Kay MA, Eiermann T, Phan TT, Volk H-D, Reichenspurner H, Robbins RC. Immunogenicity and immunomodulatory properties of umbilical cord lining mesenchymal stem cells. Cell Transplant. 2011;20(5):655–67.
Strioga M, Viswanathan S, Darinskas A, Slaby O, Michalek J. Same or not the same? Comparison of adipose tissue-derived versus bone marrow-derived mesenchymal stem and stromal cells. Stem Cells Dev. 2012;21(14):2724–52.
Shi M, Zhang Z, Xu R, Lin H, Fu J, Zou Z, Zhang A, Shi J, Chen L, Lv S, et al. Human mesenchymal stem cell transfusion is safe and improves liver function in acute-on-chronic liver failure patients. Stem Cells Transl Med. 2012;1(10):725–31.
Amer ME, El-Sayed SZ, El-Kheir WA, Gabr H, Gomaa AA, El-Noomani N, Hegazy M. Clinical and laboratory evaluation of patients with end-stage liver cell failure injected with bone marrow-derived hepatocyte-like cells. Eur J Gastroenterol Hepatol. 2011;23(10):936–41.
Lin BL, Chen JF, Qiu WH, Wang KW, Xie DY, Chen XY, Liu QL, Peng L, Li JG, Mei YY, et al. Allogeneic bone marrow-derived mesenchymal stromal cells for hepatitis B virus-related acute-on-chronic liver failure: a randomized controlled trial. Hepatology. 2017;66(1):209–19.
Peng L, Xie DY, Lin BL, Liu J, Zhu HP, Xie C, Zheng YB, Gao ZL. Autologous bone marrow mesenchymal stem cell transplantation in liver failure patients caused by hepatitis B: short-term and long-term outcomes. Hepatology. 2011;54(3):820–8.
Tsochatzis EA, Bosch J, Burroughs AK. Liver cirrhosis. Lancet. 2014;383(9930):1749–61.
Mohamadnejad M, Alimoghaddam K, Mohyeddin-Bonab M, Bagheri M, Bashtar M, Ghanaati H, Baharvand H, Ghavamzadeh A, Malekzadeh R. Phase 1 trial of autologous bone marrow mesenchymal stem cell transplantation in patients with decompensated liver cirrhosis. Arch Iran Med. 2007;10(4):459–66.
Wang L, Li J, Liu H, Li Y, Fu J, Sun Y, Xu R, Lin H, Wang S, Lv S, et al. Pilot study of umbilical cord-derived mesenchymal stem cell transfusion in patients with primary biliary cirrhosis. J Gastroenterol Hepatol. 2013;28(Suppl 1):85–92.
El-Ansary M, Abdel-Aziz I, Mogawer S, Abdel-Hamid S, Hammam O, Teaema S, Wahdan M. Phase II trial: undifferentiated versus differentiated autologous mesenchymal stem cells transplantation in Egyptian patients with HCV induced liver cirrhosis. Stem Cell Rev Rep. 2012;8(3):972–81.
Amin MA, Sabry D, Rashed LA, Aref WM, el-Ghobary MA, Farhan MS, Fouad HA, Youssef YA. Short-term evaluation of autologous transplantation of bone marrow-derived mesenchymal stem cells in patients with cirrhosis: Egyptian study. Clin Transplant. 2013;27(4):607–12.
Xu L, Gong Y, Wang B, Shi K, Hou Y, Wang L, Lin Z, Han Y, Lu L, Chen D, et al. Randomized trial of autologous bone marrow mesenchymal stem cells transplantation for hepatitis B virus cirrhosis: regulation of Treg/Th17 cells. J Gastroenterol Hepatol. 2014;29(8):1620–8.
Kharaziha P, Hellström PM, Noorinayer B, Farzaneh F, Aghajani K, Jafari F, Telkabadi M, Atashi A, Honardoost M, Zali MR, et al. Improvement of liver function in liver cirrhosis patients after autologous mesenchymal stem cell injection: a phase I-II clinical trial. Eur J Gastroenterol Hepatol. 2009;21(10):1199–205.
Liang J, Zhang H, Zhao C, Wang D, Ma X, Zhao S, Wang S, Niu L, Sun L. Effects of allogeneic mesenchymal stem cell transplantation in the treatment of liver cirrhosis caused by autoimmune diseases. Int J Rheum Dis. 2017;20(9):1219–26.
Mohamadnejad M, Alimoghaddam K, Bagheri M, Ashrafi M, Abdollahzadeh L, Akhlaghpoor S, Bashtar M, Ghavamzadeh A, Malekzadeh R. Randomized placebo-controlled trial of mesenchymal stem cell transplantation in decompensated cirrhosis. Liver Int. 2013;33(10):1490–6.
Ball SG, Shuttleworth CA, Kielty CM. Mesenchymal stem cells and neovascularization: role of platelet-derived growth factor receptors. J Cell Mol Med. 2007;11(5):1012–30.
Kim DH, Yoo KH, Choi KS, Choi J, Choi S-Y, Yang S-E, Yang Y-S, Im HJ, Kim KH, Jung HL. Gene expression profile of cytokine and growth factor during differentiation of bone marrow-derived mesenchymal stem cell. Cytokine. 2005;31(2):119–26.
Zhou YF, Bosch-Marce M, Okuyama H, Krishnamachary B, Kimura H, Zhang L, Huso DL, Semenza GL. Spontaneous transformation of cultured mouse bone marrow-derived stromal cells. Cancer Res. 2006;66(22):10849–54.
Røsland GV, Svendsen A, Torsvik A, Sobala E, McCormack E, Immervoll H, Mysliwietz J, Tonn JC, Goldbrunner R, Lønning PE, et al. Long-term cultures of bone marrow-derived human mesenchymal stem cells frequently undergo spontaneous malignant transformation. Cancer Res. 2009;69(13):5331–9.
Dahl J-A, Duggal S, Coulston N, Millar D, Melki J, Shahdadfar A, Brinchmann JE, Collas P. Genetic and epigenetic instability of human bone marrow mesenchymal stem cells expanded in autologous serum or fetal bovine serum. Int J Dev Biol. 2002;52(8):1033–42.
Zhang Z, Lin H, Shi M, Xu R, Fu J, Lv J, Chen L, Lv S, Li Y, Yu S, et al. Human umbilical cord mesenchymal stem cells improve liver function and ascites in decompensated liver cirrhosis patients. J Gastroenterol Hepatol. 2012;27(Suppl 2):112–20.
Kang SH, Kim MY, Eom YW, Baik SK. Mesenchymal stem cells for the treatment of liver disease: present and perspectives. Gut Liver. 2020;14(3):306–15.
Kim JY, Rhim W-K, Yoo Y-I, Kim D-S, Ko K-W, Heo Y, Park CG, Han DK. Defined MSC exosome with high yield and purity to improve regenerative activity. J Tissue Eng. 2021;12:20417314211008624.
Wang L, Abhange KK, Wen Y, Chen Y, Xue F, Wang G, Tong J, Zhu C, He X, Wan Y. Preparation of engineered extracellular vesicles derived from human umbilical cord mesenchymal stem cells with ultrasonication for skin rejuvenation. ACS Omega. 2019;4(27):22638–45.
Cao J, Wang B, Tang T, Lv L, Ding Z, Li Z, Hu R, Wei Q, Shen A, Fu Y. Three-dimensional culture of MSCs produces exosomes with improved yield and enhanced therapeutic efficacy for cisplatin-induced acute kidney injury. Stem Cell Res Ther. 2020;11(1):1–13.
Yan L, Wu X. Exosomes produced from 3D cultures of umbilical cord mesenchymal stem cells in a hollow-fiber bioreactor show improved osteochondral regeneration activity. Cell Biol Toxicol. 2020;36(2):165–78.
Haraszti RA, Miller R, Stoppato M, Sere YY, Coles A, Didiot M-C, Wollacott R, Sapp E, Dubuke ML, Li X. Exosomes produced from 3D cultures of MSCs by tangential flow filtration show higher yield and improved activity. Mol Ther. 2018;26(12):2838–47.
Qazi TH, Mooney DJ, Duda GN, Geissler S. Biomaterials that promote cell-cell interactions enhance the paracrine function of MSCs. Biomaterials. 2017;140:103–14.
Phan J, Kumar P, Hao D, Gao K, Farmer D, Wang A. Engineering mesenchymal stem cells to improve their exosome efficacy and yield for cell-free therapy. J Extracell Vesicles. 2018;7(1):1522236.
Lee B-C, Kang K-S. Functional enhancement strategies for immunomodulation of mesenchymal stem cells and their therapeutic application. Stem Cell Res Ther. 2020;11(1):1–10.
Cunningham CJ, Redondo-Castro E, Allan SM. The therapeutic potential of the mesenchymal stem cell secretome in ischaemic stroke. J Cereb Blood Flow Metab. 2018;38(8):1276–92.
Nakao Y, Fukuda T, Zhang Q, Sanui T, Shinjo T, Kou X, Chen C, Liu D, Watanabe Y, Hayashi C. Exosomes from TNF-α-treated human gingiva-derived MSCs enhance M2 macrophage polarization and inhibit periodontal bone loss. Acta Biomater. 2021;122:306–24.
Amer M-EM, El-Sayed SZ, Abou El-Kheir W, Gabr H, Gomaa AA, El-Noomani N, Hegazy M. Clinical and laboratory evaluation of patients with end-stage liver cell failure injected with bone marrow-derived hepatocyte-like cells. Eur J Gastroenterol Hepatol. 2011;23(10):936–41.
Saleh M, Taher M, Sohrabpour AA, Vaezi AA, Nasiri Toosi M, Kavianpour M, Ghazvinian Z, Abdolahi S, Verdi J. Perspective of placenta derived mesenchymal stem cells in acute liver failure. Cell Biosci. 2020;10:71.
Tautenhahn HM, Brückner S, Baumann S, Winkler S, Otto W, von Bergen M, Bartels M, Christ B. Attenuation of postoperative acute liver failure by mesenchymal stem cell treatment due to metabolic implications. Ann Surg. 2016;263(3):546–56.
Gilsanz C, Aller MA, Fuentes-Julian S, Prieto I, Blázquez-Martinez A, Argudo S, Fernández-Delgado J, Beleña J, Arias J, De Miguel MP. Adipose-derived mesenchymal stem cells slow disease progression of acute-on-chronic liver failure. Biomed Pharmacother. 2017;91:776–87.
Lin NC, Wu HH, Ho JH, Liu CS, Lee OK. Mesenchymal stem cells prolong survival and prevent lethal complications in a porcine model of fulminant liver failure. Xenotransplantation. 2019;26(6):e12542.
Wang J-L, Ding H-R, Pan C-Y, Shi X-L, Ren H-Z. Mesenchymal stem cells ameliorate lipid metabolism through reducing mitochondrial damage of hepatocytes in the treatment of post-hepatectomy liver failure. Cell Death Dis. 2021;12(1):111.
Amiri F, Molaei S, Bahadori M, Nasiri F, Deyhim MR, Jalili MA, Nourani MR, Habibi RM. Autophagy-modulated human bone marrow-derived mesenchymal stem cells accelerate liver restoration in mouse models of acute liver failure. Iran Biomed J. 2016;20(3):135–44.
Hao N-B, Li C-Z, Lü M-H, Tang B, Wang S-M, Wu Y-Y, Liang G-P, Yang S-M. SDF-1/CXCR4 axis promotes MSCs to repair liver injury partially through trans-differentiation and fusion with hepatocytes. Stem Cells Int. 2015;2015:960387.
Ramanathan R, Rupert S, Selvaraj S, Satyanesan J, Vennila R, Rajagopal S. Role of human Wharton’s Jelly derived mesenchymal stem cells (WJ-MSCs) for rescue of d-galactosamine induced acute liver injury in mice. J Clin Exp Hepatol. 2017;7(3):205–14.
Huang YJ, Chen P, Lee CY, Yang SY, Lin MT, Lee HS, Wu YM. Protection against acetaminophen-induced acute liver failure by omentum adipose tissue derived stem cells through the mediation of Nrf2 and cytochrome P450 expression. J Biomed Sci. 2016;23:5.
Zhang S, Chen L, Liu T, Zhang B, Xiang D, Wang Z, Wang Y. Human umbilical cord matrix stem cells efficiently rescue acute liver failure through paracrine effects rather than hepatic differentiation. Tissue Eng Part A. 2012;18(13–14):1352–64.
Liao H, Du S, Jiang T, Zheng M, Xiang Z, Yang J. UMSCs attenuate LPS/D-GalN-induced acute liver failure in mice by down-regulating the MyD88/NF-κB pathway. J Clin Transl Hepatol. 2021;9(5):690–701.
Milosavljevic N, Gazdic M, Simovic Markovic B, Arsenijevic A, Nurkovic J, Dolicanin Z, Djonov V, Lukic ML, Volarevic V. Mesenchymal stem cells attenuate acute liver injury by altering ratio between interleukin 17 producing and regulatory natural killer T cells. Liver Transpl. 2017;23(8):1040–50.
Fathy M, Okabe M, Saad Eldien HM, Yoshida T. AT-MSCs antifibrotic activity is improved by eugenol through modulation of TGF-β/Smad signaling pathway in rats. Molecules. 2020;25(2):348.
Zhao X, Shi X, Zhang Z, Ma H, Yuan X, Ding Y. Combined treatment with MSC transplantation and neutrophil depletion ameliorates D-GalN/LPS-induced acute liver failure in rats. Clin Res Hepatol Gastroenterol. 2016;40(6):730–8.
Kong D, Xu H, Chen M, Yu Y, Qian Y, Qin T, Tong Y, Xia Q, Hang H. Co-encapsulation of HNF4α overexpressing UMSCs and human primary hepatocytes ameliorates mouse acute liver failure. Stem Cell Res Ther. 2020;11(1):449.
Feng J, Yao W, Zhang Y, Xiang AP, Yuan D, Hei Z. Intravenous anesthetics enhance the ability of human bone marrow-derived mesenchymal stem cells to alleviate hepatic ischemia-reperfusion injury in a receptor-dependent manner. Cell Physiol Biochem. 2018;47(2):556–66.
Despeyroux A, Duret C, Gondeau C, Perez-Gracia E, Chuttoo L, de Boussac H, Briolotti P, Bony C, Noël D, Jorgensen C, et al. Mesenchymal stem cells seeded on a human amniotic membrane improve liver regeneration and mouse survival after extended hepatectomy. J Tissue Eng Regen Med. 2018;12(4):1062–73.
Parekkadan B, van Poll D, Suganuma K, Carter EA, Berthiaume F, Tilles AW, Yarmush ML. Mesenchymal stem cell-derived molecules reverse fulminant hepatic failure. PLoS ONE. 2007;2(9):e941.
Kantarcıoğlu M, Demirci H, Avcu F, Karslıoğlu Y, Babayiğit MA, Karaman B, Öztürk K, Gürel H, Akdoğan Kayhan M, Kaçar S, et al. Efficacy of autologous mesenchymal stem cell transplantation in patients with liver cirrhosis. Turk J Gastroenterol. 2015;26(3):244–50.
Jang YO, Kim YJ, Baik SK, Kim MY, Eom YW, Cho MY, Park HJ, Park SY, Kim BR, Kim JW, et al. Histological improvement following administration of autologous bone marrow-derived mesenchymal stem cells for alcoholic cirrhosis: a pilot study. Liver Int. 2014;34(1):33–41.
Salama H, Zekri AR, Medhat E, Al Alim SA, Ahmed OS, Bahnassy AA, Lotfy MM, Ahmed R, Musa S. Peripheral vein infusion of autologous mesenchymal stem cells in Egyptian HCV-positive patients with end-stage liver disease. Stem Cell Res Ther. 2014;5(3):70.
Fang X, Liu L, Dong J, Zhang J, Song H, Song Y, Huang Y, Cui X, Lin J, Chen C, et al. A study about immunomodulatory effect and efficacy and prognosis of human umbilical cord mesenchymal stem cells in patients with chronic hepatitis B-induced decompensated liver cirrhosis. J Gastroenterol Hepatol. 2018;33(4):774–80.
Zhang YC, Liu W, Fu BS, Wang GY, Li HB, Yi HM, Jiang N, Wang G, Zhang J, Yi SH, et al. Therapeutic potentials of umbilical cord-derived mesenchymal stromal cells for ischemic-type biliary lesions following liver transplantation. Cytotherapy. 2017;19(2):194–9.
Suk KT, Yoon JH, Kim MY, Kim CW, Kim JK, Park H, Hwang SG, Kim DJ, Lee BS, Lee SH, et al. Transplantation with autologous bone marrow-derived mesenchymal stem cells for alcoholic cirrhosis: phase 2 trial. Hepatology. 2016;64(6):2185–97.
Shi M, Liu Z, Wang Y, Xu R, Sun Y, Zhang M, Yu X, Wang H, Meng L, Su H, et al. A pilot study of mesenchymal stem cell therapy for acute liver allograft rejection. Stem Cells Transl Med. 2017;6(12):2053–61.
Detry O, Vandermeulen M, Delbouille MH, Somja J, Bletard N, Briquet A, Lechanteur C, Giet O, Baudoux E, Hannon M, et al. Infusion of mesenchymal stromal cells after deceased liver transplantation: a phase I-II, open-label, clinical study. J Hepatol. 2017;67(1):47–55.
Download references
Acknowledgements
No Funders.
Author information
Authors and affiliations.
Department of Research and Academic Affairs, Larkin Community Hospital, Miami, FL, USA
Samin Shokravi & Sima Marzban
I.M. Sechenov First Moscow State Medical University (Sechenov University), Moscow, Russian Federation
Vitaliy Borisov
Basic Sciences Department, College of Pharmacy, University of Duhok, Duhok, Kurdistan Region, Iraq
Burhan Abdullah Zaman
School of Medicine, Abadan University of Medical Sciences, Abadan, Iran
Firoozeh Niazvand
Department of Medicinal Chemistry, Pharmacy Faculty, Tabriz University of Medical Sciences, Tabriz, Iran
Raheleh Hazrati
Department of Oral and Maxillofacial Surgery, School of Dentistry, Shahid Beheshti University of Medical Sciences, Tehran, Iran
Meysam Mohammadi Khah
Department of Pharmacology, Saveetha Dental College, Saveetha Institute of Medical and Technical Science, Saveetha University, Chennai, India
Lakshmi Thangavelu
Student Research Committee, Tabriz University of Medical Sciences, Tabriz, Iran
Armin Sohrabi
Immunology Research Center, Tabriz University of Medical Sciences, Tabriz, Iran
Stem Cell Research Center, Tabriz University of Medical Sciences, Tabriz, Iran
Amir Zamani
You can also search for this author in PubMed Google Scholar
Contributions
All authors contributed to the conception and the main idea of the work. SS, BAZ, VB, FN, RH, MMK, LT, AS, and SM drafted the main text, figures, and tables. AZ and SM supervised the work and provided the comments and additional scientific information. SS and BAZ and AS also reviewed and revised the text. All authors read and approved the final manuscript.
Corresponding authors
Correspondence to Sima Marzban or Amir Zamani .
Ethics declarations
Ethics approval and consent to participate, consent for publication, competing interests.
The authors declare that they have no competing interests.
Additional information
Publisher's note.
Springer Nature remains neutral with regard to jurisdictional claims in published maps and institutional affiliations.

Rights and permissions
Open Access This article is licensed under a Creative Commons Attribution 4.0 International License, which permits use, sharing, adaptation, distribution and reproduction in any medium or format, as long as you give appropriate credit to the original author(s) and the source, provide a link to the Creative Commons licence, and indicate if changes were made. The images or other third party material in this article are included in the article's Creative Commons licence, unless indicated otherwise in a credit line to the material. If material is not included in the article's Creative Commons licence and your intended use is not permitted by statutory regulation or exceeds the permitted use, you will need to obtain permission directly from the copyright holder. To view a copy of this licence, visit http://creativecommons.org/licenses/by/4.0/ . The Creative Commons Public Domain Dedication waiver ( http://creativecommons.org/publicdomain/zero/1.0/ ) applies to the data made available in this article, unless otherwise stated in a credit line to the data.
Reprints and permissions
About this article
Cite this article.
Shokravi, S., Borisov, V., Zaman, B.A. et al. Mesenchymal stromal cells (MSCs) and their exosome in acute liver failure (ALF): a comprehensive review. Stem Cell Res Ther 13 , 192 (2022). https://doi.org/10.1186/s13287-022-02825-z
Download citation
Received : 19 November 2021
Accepted : 28 February 2022
Published : 08 May 2022
DOI : https://doi.org/10.1186/s13287-022-02825-z
Share this article
Anyone you share the following link with will be able to read this content:
Sorry, a shareable link is not currently available for this article.
Provided by the Springer Nature SharedIt content-sharing initiative
- Mesenchymal stromal cell (MSCs)
- Acute liver failure (ALF)
- Immunomodulation
Stem Cell Research & Therapy
ISSN: 1757-6512
- Submission enquiries: Access here and click Contact Us
- General enquiries: [email protected]

Never Before Exposed: Borassus Flabellifer’s Shadow on Liver Health
- Harshil Gadhiya Department of Pharmacology, School of Pharmacy, RK University, Rajkot-360020, Gujarat, India
- Riddhi Shingala Department of Pharmacology, School of Pharmacy, RK University, Rajkot-360020, Gujarat, India
- Dhirangi Gajipara Department of Pharmacology, School of Pharmacy, RK University, Rajkot-360020, Gujarat, India
- Yash Radhanpura Department of Pharmacology, School of Pharmacy, RK University, Rajkot-360020, Gujarat, India
- Pravin Tirgar Department of Pharmacology, School of Pharmacy, RK University, Rajkot-360020, Gujarat, India
The last stage of many hepatic diseases, including hepatitis, is cirrhosis. The liver's vital function in detoxifying xenobiotics—such as medications and toxic composites—is the subject of much research. Liver cirrhosis is the cause of alterations in the liver's architecture that mostly affect protein binding, blood circulation, and the enzymes that break down medicinal substances. The main reason why therapeutic metabolising enzymes disappear is because to liver towel loss. However, not all enzyme activity is decreased in tandem; some are only changed in one specific case. This case report focuses on the clinical features, diagnostic evaluation, and therapeutic concerns of a patient having liver cirrhosis. Further research and advancements in our comprehension of the pathogenesis and treatment of cirrhosis are necessary in order to enhance outcomes and minimise the burden of this illness on those who are impacted.
- Elbekai RH, Korashy HM, El-Kadi AO. The effect of liver cirrhosis on the regulation and expression of drug metabolizing enzymes. Current drug metabolism. 2004 Apr 1;5(2):157-67.
- Tsochatzis EA, Bosch J, Burroughs AK. Liver cirrhosis. The Lancet. 2014 May 17;383(9930):1749-61.
- Ginès P, Krag A, Abraldes JG, Solà E, Fabrellas N, Kamath PS. Liver cirrhosis. The Lancet. 2021 Oct 9;398(10308):1359-76.
- Ardizzone G, Arrigo A, Schellino MM, Stratta C, Valzan S, Skurzak S, Andruetto P, Panio A, Ballaris MA, Lavezzo B, Salizzoni M. Neurological complications of liver cirrhosis and orthotopic liver transplant. InTransplantation proceedings 2006 Apr 1 (Vol. 38, No. 3, pp. 789-792). Elsevier.
- Jerry A. A comprehensive review on the medicinal properties of Borassus flabellifer. Journal of Academia and Industrial Research. 2018 Dec;7(7):93-7.
- Krishnaveni TR, Arunachalam R, Chandrakumar M, Parthasarathi G, Nisha R. Potential review on palmyra (Borassus flabellifer L.). Advances in Research. 2020 Aug 4;21(9):29-40.
- Poordad FF. Presentation and complications associated with cirrhosis of the liver. Current medical research and opinion. 2015 May 4;31(5):925-37.
- Prieto O JE, Sánchez P S, Prieto O RG, Rojas D EL, González L, Mendivelso F. Clinical Characteristics and Decompensation in Patients with Liver Cirrhosis Treated at Two Hepatology Centers in Bogota DC from 2010 To 2014. Revista colombiana de Gastroenterología. 2016 Jan;31(1):1-8.
- Ge PS, Runyon BA. Treatment of patients with cirrhosis. New England Journal of Medicine. 2016 Aug 25;375(8):767-77.
How to Cite
- Endnote/Zotero/Mendeley (RIS)
Make a Submission
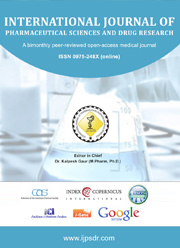
Citation Indices
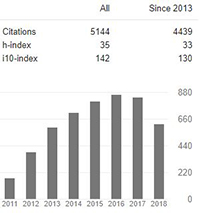
reject-rate
Quick Links
- Aims & Scope
- Editorial Policies
- Reviewer Guidelines
- Publication Ethics & Malpractice Statement
- Palgiarism Policy
- Retraction Policy
- Open Access Policy
- Processing Charges and Waiver Policy
Developed By


- News Releases
Study advances understanding of liver immune response in cirrhosis
Restoring LSECtin activity could be a therapeutic strategy for cirrhosis. The findings contribute to a better understanding of the immune mechanisms involved in cirrhosis and provide insights into possible treatment avenues for this condition
Universidad Miguel Hernandez de Elche
Researchers from UMH (Spain) leading the study.
Credit: UMH
The Department of Clinical Medicine at the Miguel Hernández University of Elche (UMH) leads research on the immune system's role in advanced chronic liver disease. Through various experiments on mouse cirrhosis models and human tissues, they have examined how the protein LSECtin interacts with the liver's immune response. The results show that decreasing LSECtin levels increases a specific type of immune cells in the liver that can negatively contribute to the disease progression. This suggests that restoring these levels could potentially restore altered liver functions in cirrhosis. The UMH's Hepatic and Intestinal Immunobiology Group, associated with IDIBE, and researchers from other Spanish institutions have published these findings in the scientific journal Liver International.
Some immune system cells specialized in recognizing pathogenic microorganisms such as bacteria, viruses, or fungi have so-called 'innate receptors.' These receptors are the first line of defense against infections, triggering rapid and general immune responses. Unlike adaptive receptors, which require a 'learning' process of the immune system, as with some vaccines, innate receptors do not change their specificity throughout an individual's life. The study led by the UMH focuses on type C lectins, a type of innate receptor relevant to cirrhosis.
Dr. Rubén Francés, director of the UMH's Hepatic and Intestinal Immunobiology Group and study leader, explains that liver cirrhosis is the final stage of various chronic liver diseases. During cirrhosis, there is an imbalance in the immune system, during which different innate receptors play a fundamental role in controlling inflammation. Hence, the interest in studying lectins.
Type C lectins help bind and process antigens from pathogens, promote the adhesion of leukocytes to enter liver tissue, and modulate T lymphocyte responses. One of these lectins, called LSECtin or (CLEC4G), is mainly present in the cells lining the liver's blood vessels. "LSECtin plays a key role in regulating the liver's immune system," explains the UMH researcher, "as its activity as an innate receptor in the endothelium modulates the response of these cells to microbial stimuli, they are usually exposed to and modulates the function of inflammatory lymphocytes through specific ligands."
The activation of innate receptors triggers a series of responses to eliminate pathogens and stimulate the immune system. "Therefore, they are essential for the early recognition of threats to the body," explains Francés.
UMH Immunology researcher Esther Caparrós Cayuela, co-leader of the study, explains how this research was carried out on mouse liver blood vessel cells: "Mediators of the immune system modulate the expression of LSECtin. When there is inflammation, the expression of this lectin decreases, while anti-inflammatory mediators activate it." Likewise, the team found a lower expression of LSECtin in both chronic liver damage models in mice and in cirrhotic patients.
The loss of this protein during pathology may contribute to the characteristic inflammation damage of cirrhosis. "In fact," says the UMH Department of Clinical Medicine researcher, "we found a close correlation between the loss of LSECtin and the recruitment of pro-inflammatory lymphocytes (called Th17) in cirrhotic livers."
Additionally, the team of researchers restored the activity of the LSECtin protein in cirrhotic animal cells by applying treatment with anti-inflammatory immune mediators. This achievement, explains Caparrós, suggests that the recovery of LSECtin could be a potential therapeutic strategy.
The UMH's Hepatic and Intestinal Immunobiology Group is part of the Biomedical Research Network in Hepatic and Digestive Diseases (CIBERehd) of the Carlos III Health Institute. In addition to Caparrós and Francés, researchers from the UMH Department of Clinical Medicine Sebastian Martínez López, Enrique Ángel Gomis, Paula Boix, and Isabel Gómez-Hurtado; UMH Pharmacology researcher Pedro Zapater; researchers from the August Pi i Sunyer Biomedical Research Institute (IDIBAPS) Anabel Fernández Iglesias and Jordi Gracia Sancho; University Complutense researcher Francisco Javier Cubero; and researcher from the Institute of Neuroscience, a joint center of the UMH and the Spanish National Research Council (CSIC), Javier Morante, have participated in the study.
This study has received funding from the Carlos III Health Institute, the Ministry of Science and Innovation, the HORIZON EUROPE Framework Programme, the Department of Innovation, Universities, Science, and Digital Society of the Valencian Community, the Community of Madrid, and the Agency for Management of University and Research Grants of the Government of Catalonia.
10.1111/liv.15836
Method of Research
Experimental study
Subject of Research
Human tissue samples
Article Title
Cirrhosis-downregulated LSECtin can be retrieved by cytokines, shifts the TLR-induced LSECs secretome and correlates with the hepatic Th response
Article Publication Date
31-Jan-2024
Disclaimer: AAAS and EurekAlert! are not responsible for the accuracy of news releases posted to EurekAlert! by contributing institutions or for the use of any information through the EurekAlert system.
Original Source
- Open access
- Published: 12 April 2024
Role of radiomics in staging liver fibrosis: a meta-analysis
- Xiao-min Wang 1 &
- Xiao-jing Zhang 2
BMC Medical Imaging volume 24 , Article number: 87 ( 2024 ) Cite this article
Metrics details
Fibrosis has important pathoetiological and prognostic roles in chronic liver disease. This study evaluates the role of radiomics in staging liver fibrosis.
After literature search in electronic databases (Embase, Ovid, Science Direct, Springer, and Web of Science), studies were selected by following precise eligibility criteria. The quality of included studies was assessed, and meta-analyses were performed to achieve pooled estimates of area under receiver-operator curve (AUROC), accuracy, sensitivity, and specificity of radiomics in staging liver fibrosis compared to histopathology.
Fifteen studies (3718 patients; age 47 years [95% confidence interval (CI): 42, 53]; 69% [95% CI: 65, 73] males) were included. AUROC values of radiomics for detecting significant fibrosis (F2-4), advanced fibrosis (F3-4), and cirrhosis (F4) were 0.91 [95%CI: 0.89, 0.94], 0.92 [95%CI: 0.90, 0.95], and 0.94 [95%CI: 0.93, 0.96] in training cohorts and 0.89 [95%CI: 0.83, 0.91], 0.89 [95%CI: 0.83, 0.94], and 0.93 [95%CI: 0.91, 0.95] in validation cohorts, respectively. For diagnosing significant fibrosis, advanced fibrosis, and cirrhosis the sensitivity of radiomics was 84.0% [95%CI: 76.1, 91.9], 86.9% [95%CI: 76.8, 97.0], and 92.7% [95%CI: 89.7, 95.7] in training cohorts, and 75.6% [95%CI: 67.7, 83.5], 80.0% [95%CI: 70.7, 89.3], and 92.0% [95%CI: 87.8, 96.1] in validation cohorts, respectively. Respective specificity was 88.6% [95% CI: 83.0, 94.2], 88.4% [95% CI: 81.9, 94.8], and 91.1% [95% CI: 86.8, 95.5] in training cohorts, and 86.8% [95% CI: 83.3, 90.3], 94.0% [95% CI: 89.5, 98.4], and 88.3% [95% CI: 84.4, 92.2] in validation cohorts. Limitations included use of several methods for feature selection and classification, less availability of studies evaluating a particular radiological modality, lack of a direct comparison between radiology and radiomics, and lack of external validation.
Although radiomics offers good diagnostic accuracy in detecting liver fibrosis, its role in clinical practice is not as clear at present due to comparability and validation constraints.
Peer Review reports
Introduction
Chronic liver disease is an important health concern due to high prevalence of metabolic dysfunction associated fatty liver disease (MAFLD), hepatitis B/C, and alcoholic liver disease. Whereas mortality due to liver disease has declined in some countries like the USA and China, some countries such as India and Mongolia still have higher mortality rates. Increasing trends in the prevalence are also noted in the United Kingdom and Russia [ 1 ]. In the USA, 1.8% of the population has a liver disease diagnosis [ 2 ]. In China, although the mortality rates have decreased, the prevalence of liver disease is increasing [ 3 ]. Globally, the incidence of non-alcoholic steatohepatitis-caused cirrhosis is increasing by 1.35% each year [ 4 ].
Liver fibrosis is a modifiable factor that is associated with worse health outcomes, transplants, and mortality [ 5 ]. Liver fibrosis may develop due to chronic viral infection, long-term alcohol use, or steatohepatitis. It is estimated that liver fibrosis affects 7.7% of individuals in the general population of the United States of America and obese individuals are at much higher risk [ 6 ]. The latest stage of fibrosis, cirrhosis, is the eleventh leading cause of mortality [ 1 ]. An increasing trend in mortality due to cirrhosis has been observed globally from 1990 to 2017 [ 7 ]. Several methods are available to measure liver fibrosis. The liver biopsy is considered diagnostic “gold standard” for staging liver fibrosis. The biopsy is a highly valuable tool but can be associated with complications, sampling errors, and between-observer variations in judgments. Fibrosis can be patchy so that not all parts of the liver contain fibrosis evenly, therefore, a biopsy may fail to capture some samples [ 8 ]. Among other methods, serum biomarkers may also help in detecting fibrosis. Radiological methods including magnetic resonance imaging (MRI) magnetic resonance elastography (MRE), computed tomography (CT), ultrasonography, and elastography also provide non-invasive means of measuring fibrosis [ 9 ].
Radiomics is a post-radiology process of high-throughput extraction of features from radiological images for conversion into mineable data involving complex processes of artificial intelligence such as machine learning, deep learning, and convolutional neural networks to maximize predictability. It is developed on the premise that pathophysiological tissues and organs contain much information that can be quantified and differentiated from normal tissues and organs. Extraction of a large number of features to form a database and then mining the data for analyses aids decision support leading to improved diagnostic accuracy and prognostic capability [ 10 , 11 , 12 ]. Radiomics takes texture as a spatial arrangement of predefined voxels through which complicated features of the image can be read and mathematical calculations of these arrangement characteristics differentiate normal from abnormal. The heterogeneity in the selected features reflects the heterogeneity of histopathological changes [ 13 ]. Radiomics features can be morphological, histogram, textural, and high-order features. Morphological features include the shape, size, and volume of the region of interest. Histogram is the plotting of pixel values against pixel frequency and can be used to describe many features such as magnitude, dispersion, asymmetry, peakedness, flatness, randomness, uniformity, etc. Textural features provide spatial relationships between neighboring pixels. High-order features are those acquired after applying filters to images [ 12 , 14 ].
Several reviews have described the role of radiomics in the diagnosis and staging of various types of cancers [ 15 , 16 ]. Among other clinical applications, radiomics has been found to be a valuable aid in cardiomyopathy [ 17 , 18 ], musculoskeletal diseases [ 19 , 20 ], neurological and psychiatric disorders [ 21 , 22 , 23 , 24 ], and liver diseases [ 25 ]. Several studies have reported the diagnostic accuracy indices of radiomics in diagnosing and staging liver fibrosis. However, there is no synthesis of these outcomes which are sometimes variable and even inconsistent. The present study aimed to evaluate the role of radiomics in diagnosing and staging liver fibrosis by conducting a systematic review of relevant studies and performing meta-analyses of statistical indices.
The present study was conducted by following PRISMA guidelines.
Inclusion and exclusion criteria
A study was included in the meta-analysis if a) it prospectively or retrospectively recruited patients with chronic liver disease who had histologically confirmed fibrosis in the liver; b) performed radiomic analyses based on any radiological modality to diagnose and/or differentiate fibrosis; and c) reported diagnostic accuracy indices of radiomics in diagnosing and differentiating liver fibrosis stages by in comparison with histopathology. Studies were excluded based on the following criteria: a study a) reported diagnostic performance of radiomics for liver fibrosis without adequate statistical data; b) reported the outcomes of pediatric patients; and c) reported the diagnostic accuracy of a combined clinical-radiological radiomics model.
Literature search
Electronic scientific databases (Embase, Ovid, Science Direct, Springer, and Web of Science) were searched for the identification of relevant studies using area-specific keywords. The primary search strategy was “Radiomics AND liver fibrosis OR cirrhosis AND diagnostic accuracy”. Secondary keywords were used in several other combinations with this primary string. The detailed literature search strategy is given in Appendix S1. After the identification of studies, reference lists of related articles were also screened for additional studies. The literature search encompassed peer-reviewed research articles published in English from the date of database inception till May 2023.
Data analysis
Data on the design and conduct of studies, patient demographics, clinical characteristics, fibrosis stage, radiomics design and analyses, and diagnostic accuracy outcome data were extracted from the research articles of respective studies and organized in data sheets. The quality of the included studies was assessed with the Quality Assessment of Diagnostic Accuracy Studies (QUADAS-2) scale. This scale assesses the quality of studies under the domains of risk of bias and applicability concerns by evaluating patient selection, index test, reference standard, and flow and timing. Diagnostic accuracy endpoints (Accuracy, area under receiver operator curve (AUROC), sensitivity, and specificity of various radiomics models compared to histologically proven fibrosis were extracted from the research articles of respective studies and pooled under random effects model using the point estimates and their 95% confidence intervals of these indices. Subgroup analyses were performed with respect to fibrosis stage (significant fibrosis; stages F2-4, advanced fibrosis; stages F3-4, and cirrhosis; stage F4) and with respect to the study cohort (training, test/validation). Statistical analyses were performed with Stata software (Stata Corporation, College Station, Texas, USA).
Fifteen studies [ 13 , 26 , 27 , 28 , 29 , 30 , 31 , 32 , 33 , 34 , 35 , 36 , 37 , 38 , 39 ] were included that were published between 2018 and 2023 (Fig. 1 ). In these studies, radiomic analyses were performed involving 3718 patients with chronic liver diseases including hepatitis and MAFLD. The age of these patients was 47.3 years [95% confidence interval (CI): 42.0, 52.5]. The proportion of males was 69% [95% CI: 65, 73]. Histologically (biopsy/surgical) confirmed fibrosis stage was F0 in 13% [95% CI: 7,20], F1 in 17% [95% CI: 13, 23], F2 in 21% [95% CI: 17,26], F3 in 16% [95% CI: 13,19], and F4 in 27% [95% CI: 23, 33] of the patients. Radiological modalities used in these studies were: MRI (5), CT (3), ultrasonography (2), positron emission tomography (1), shear-wave elastography (2), and MRE (2).
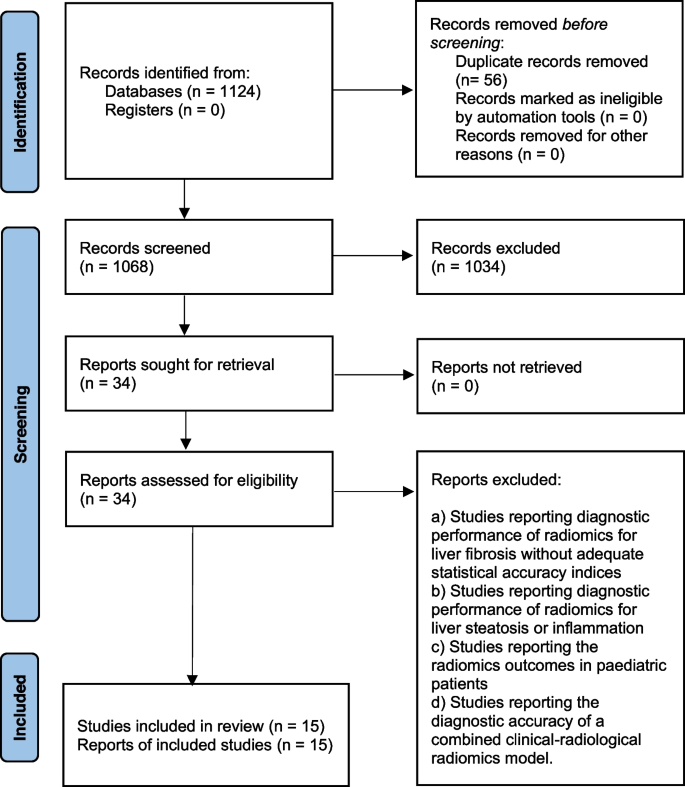
A flowchart of study screening and selection process
Important characteristics of the included studies are given in Table S 1 . The quality of the included studies was moderate in general according to the QUADAS-2 scale (Table S 2 ). The risk of bias was limited to the retrospective design of studies which could have introduced patient selection bias. Moreover, the interval between the index test and reference test was up to six months which might have impacted adequate flow and timing. Included studies also varied with regards to validation ranging from no validation [ 26 ], Five/Ten-fold cross-validation [ 27 , 30 , 32 , 36 , 39 ], and leave-one-out cross-validation [ 13 ] to internal validation [ 28 , 30 , 31 , 33 , 34 , 37 , 38 ], and internal and external validation [ 29 ]. There was no significant publication bias according to Egger’s test (Bias coefficient 0.114 [-11.80, 12.03]; p =0.984) or Begg’s test (Adjusted Kendall’s score -29±18; p =0.125) (Figure S 1 a and b).
The pooled AUROC value of radiomics for the diagnosis of any liver fibrosis was 0.878 [95% CI: 0.850, 0.906]. The AUROC values for the detection of significant fibrosis, advanced fibrosis, and cirrhosis were 0.914 [95% CI: 0.889, 0.938], 0.924 [95% CI: 0.901, 0.946], and 0.944 [95% CI: 0.929, 0.963] respectively in training cohorts, and 0.886 [95% CI: 0.826, 0.905], 0.887 [95% CI: 0.834, 0.939], and 0.930 [95% CI: 0.905, 0.954] respectively in test/validation cohorts (Figs. 2 and 3 ).
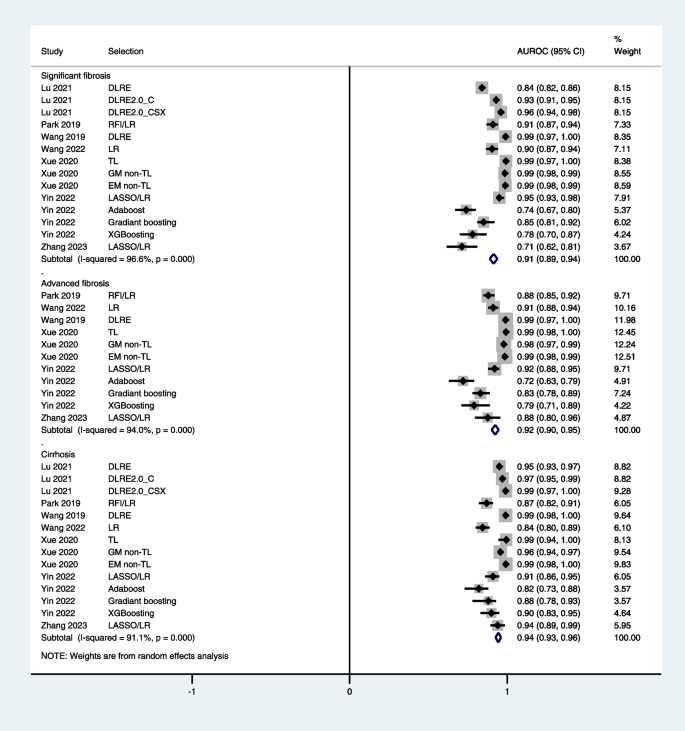
Forest graphs showing the outcomes of meta-analysis of AUROC values of radiomics in diagnosing fibrosis stages in training cohorts
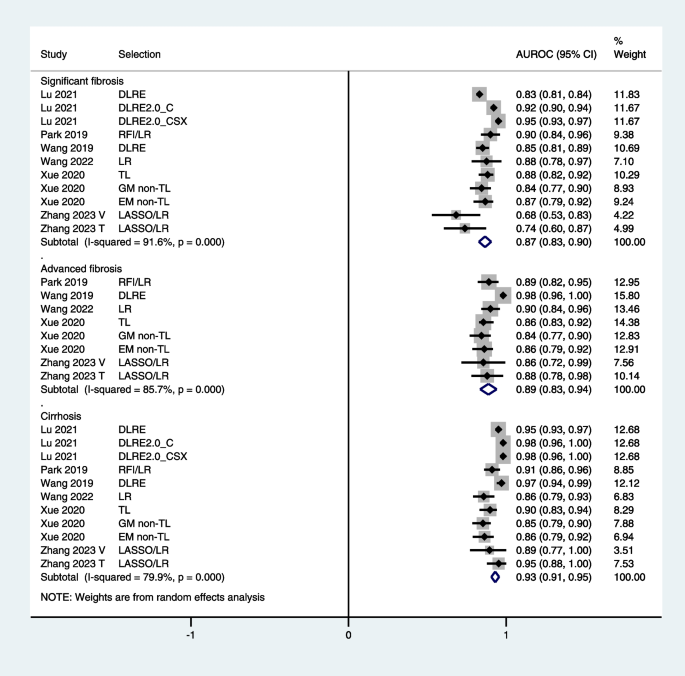
Forest graphs showing the outcomes of meta-analysis of AUROC values of radiomics in diagnosing fibrosis stages in test/validation cohorts
The pooled accuracy of radiomics in diagnosing any liver fibrosis was 83.5% [95% CI: 81.7, 85.4]. The accuracy values of radiomics in the detection of significant fibrosis, advanced fibrosis, and cirrhosis were 80.6% [95% CI: 76.2, 85.1], 83.5% [95% CI: 81.3, 85.8], and 81.6% [95% CI: 78.7, 84.5] respectively in training cohorts, and 77.0% [95% CI: 68.6, 85.5], 84.3% [95% CI: 79.8, 88.8], and 81.3% [95% CI: 77.0, 85.7] respectively in test/validation cohorts (Figures S 2 a and b).
In training cohorts, the sensitivity of radiomics in diagnosing significant fibrosis, advanced fibrosis, and cirrhosis was 84.0% [95% CI: 76.1, 91.9], 86.9% [95% CI: 76.8, 97.0], and 92.7% [95% CI: 89.7, 95.7] respectively (Fig. 4 ). In test/validation cohorts, the sensitivity of radiomics in diagnosing significant fibrosis, advanced fibrosis, and cirrhosis was 75.6% [95% CI: 67.7, 83.5], 80.0% [95% CI: 70.8, 89.3], and 92.0% [95% CI: 87.8, 96.1] respectively (Fig. 5 ).
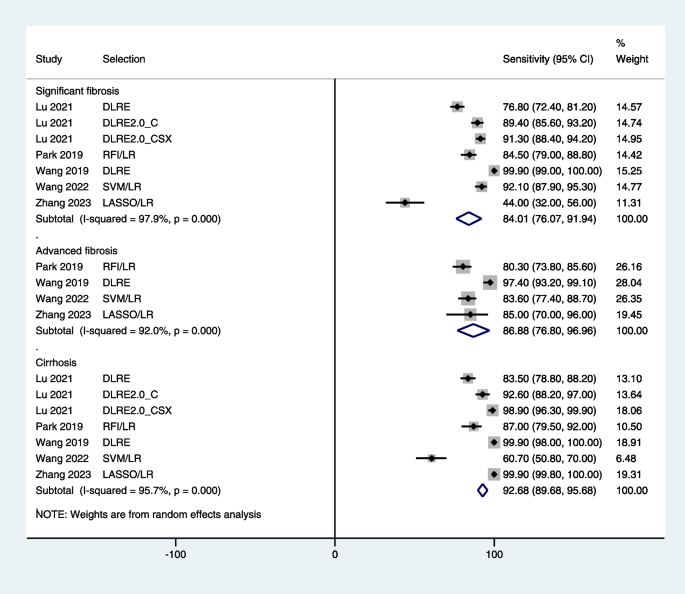
A forest graph showing the outcomes of meta-analysis of the sensitivity of radiomics in diagnosing fibrosis stages in training cohort
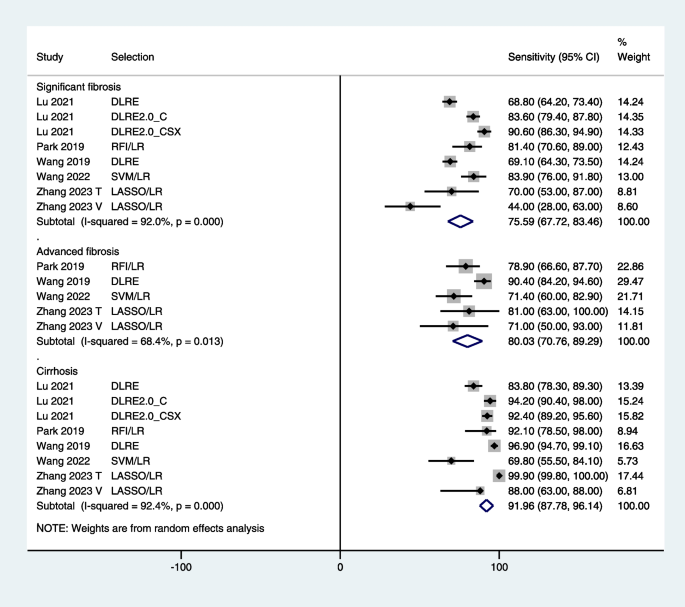
A forest graph showing the outcomes of meta-analysis of the sensitivity of radiomics in diagnosing fibrosis stages in test/validation cohorts
In training cohorts, the specificity of radiomics in diagnosing significant fibrosis, advanced fibrosis, and cirrhosis was 88.6% [95% CI: 83.0, 94.2], 88.4% [95% CI: 81.9, 94.8], and 91.1% [95% CI: 86.8, 95.5], whereas in test/validation cohorts, the specificity was 86.8% [95% CI: 83.3, 90.3], 94.0% [95% CI: 89.5, 98.4], and 88.3% [95% CI: 84.4, 92.2] respectively (Figure S 3 a and b).
Among the included studies, Hu et al. [ 27 ] who found thin-sliced CT images to yield better performance of radiomics than thick-sliced CT images reported AUROC values of 0.90 [95% CI: 0.84, 0.96] for F1 vs F2-4, 0.85 [95% CI: 0.78, 0.92] for F1-2 vs F3-4, and 0.94 [95% CI: 0.89, 0.97] for F1-3 vs F4 stages. Lan et al. [ 13 ] who studied MRE radiomics found AUROC values of 0.89 [95% CI: 0.84, 0.94] for F0 vs F1-4, 0.93 [95% CI: 0.89, 0.98] for F0-1 vs F2-4, 0.92 [95% CI: 0.88, 0.97] for F0-2 vs F3-4, and 0.95 [95% CI: 0.90, 0.997] for F0-3 vs F4 stages in their echo-planar images. They observed AUROC values of 0.89 [95% CI: 0.83, 0.94], 0.87 [95% CI: 0.81, 0.93], 0.89 [95% CI: 0.84, 0.95], and 0.94 [95% CI: 0.89, 0.997] for F0 vs F1-4, F0-1 vs F2-4, F0-2 vs F3-4 and F0-3 vs F4 stages respectively in their gradient recalled echo images.
This meta-analysis found that radiomics exhibits high accuracy in diagnosing and staging liver fibrosis. The AUROC values for the detection of significant fibrosis, advanced fibrosis, and cirrhosis were approximately 0.91, 0.92, and 0.94 in training cohorts and 0.89, 0.89, and 0.93 in validation cohorts, respectively. However, despite the good efficiency of radiomics in diagnosis and staging liver fibrosis observed herein, several factors make these findings inconclusive and dependent on future studies to refine this evidence. For example, several types of radiological modalities were used for radiomics in individual studies and a direct comparison of diagnostic performance between radiomics and radiology was mostly lacking. Moreover, various models were utilized for radiomics, and these studies lacked external validation.
Some studies that could not be included in this meta-analysis have also reported variable diagnostic performance of radiomics in staging liver fibrosis. Cui et al. [ 40 ] utilized multiphase CT-based radiomics to stage liver fibrosis and found the sensitivity of diagnosing significant fibrosis, advanced fibrosis, and cirrhosis to be 30-41%, 59-71%, and 84-87%, and the specificities being 84-90%, 71-79%, and 50-58% respectively. Duan et al. [ 41 ] observed better performance of ultrasound-based radiomics in diagnosing cirrhosis (AUROC 0.86) than advanced fibrosis (AUROC 0.77). Zhou et al. [ 42 ], also reported higher efficieny of ultrasound-based radiomics in diagnosing cirrhosis (AUROC 0.83-0.86) than significant fibrosis (AUROC 0.69-0.71) or advanced fibrosis (AUROC 0.67-0.72).
Many studies have shown that the diagnostic accuracy of radiomics is better than serological biomarkers [ 30 , 42 , 43 , 44 ]. Sim et al. [ 32 ] found better diagnostic performance of MRE radiomics (AUROC 0.97 [95% CI: 0.93, 1]) than clinical features (AUROC 0.91 [95% CI: 0.81, 0.97]) in diagnosing significant fibrosis. Wang et al. [ 34 ] also reported that the AUROC values for significant fibrosis, advanced fibrosis, and cirrhosis were higher with CT-based radiomics (AUROC 0.88, 0.90, and 0.86) than with either aspartate transaminase-to-platelet ratio (AUROC 0.69, 0.67, and 0.65) or Fibrosis-4 index (AUROC 0.71, 0.71, and 0.7) respectively. Xue et al. [ 35 ] also found better diagnostic performance of multimodal ultrasound radiomics (AUROC 0.9-0.93) than either aspartate transaminase-to-platelet ratio (AUROC 0.72-0.78) or Fibrosis-4 index (AUROC 0.69-0.75) in staging liver fibrosis. Zhao et al. [ 38 ] found better diagnostic efficiency of MRI radiomics (accuracy 0.8) than clinical markers (accuracy 0.68) in differentiating non-significant fibrosis from clinically significant fibrosis in the test cohort. Some authors have suggested that a model combining radiomics and clinical biomarkers may further improve the diagnostic accuracy of fibrosis [ 38 , 45 ].
Despite good diagnostic accuracy values observed for radiomics in liver fibrosis assessment in the present study, inconsistencies were observed in the outcomes of individual studies. Whereas Wang et al. [ 34 ] and Lan et al. [ 13 ] found radiomics better than radiological elastography in diagnosing advanced fibrosis and cirrhosis, Sim et al. [ 32 ] did not find a statistically significant difference. Lu et al. [ 29 ] and Sim et al. [ 32 ] found that radiomics distinguished well between significant fibrosis (F2-4) and non-significant fibrosis (F0-1). However, Zhang et al. [ 37 ] reported that radiomics was unable to distinguish between non-significant fibrosis and significant fibrosis. Zhao et al. [ 38 ] reported that a combined use of radiomics and clinical biomarkers performed better than radiomics alone, whereas Sim et al. [ 32 ] did not find a significant difference in performance between radiomics and combined use of radiomics, radiology, and clinical biomarkers.
We have observed that some diagnostic accuracy indices were slightly higher in training cohorts than in validation cohorts. Wang et al. [ 33 ] who found that the accuracy of deep learning radiomics of elastography for the diagnosis of significant fibrosis was lower in the validation cohort in comparison with the training cohort suggested that this could be because of the lower heterogeneity in F0 and F1 groups and can be overcome possibly by adapting multiple strategies for fibrosis classification. Lu et al. [ 29 ] found that AUROC values increased for their deep learning radiomics of elastography when datasets had a higher prevalence of patients with F0 and F1 stages. They suggested that the inclusion of higher proportions of patients with F0 and F1 stages can yield better accuracy because about 80% of patients with chronic hepatitis B have F0 or F1 stage in the general population. In the present study, the pooled percentages of patients with F0 and F1 stages were 13% and 17% respectively.
Fibrosis develops by the excessive deposition of extracellular matrix in the liver as a response to wound healing after which satellite cells activate, higher levels of alpha smooth muscle actins are produced, and collagen I/II are synthesized. Such processes increase the stiffness of the liver progressively and may lead to cirrhosis. Fibrosis is usually associated with the accumulation of collagen fibers, not well-defined portal vein walls, and irregular hepatic vein margins. Gray-scale ultrasound images capture such information to reflect the scattering of fine structures. Moreover, coarse echotexture and a mild increase in echogenicity of hepatic parenchyma are usually observed in cirrhosis [ 35 ].
So far, studies addressing radiomics lack robust validation in larger and clinically diverse settings which present reproducibility challenges. In the present review, we found that all except one study involved internal validation, and therefore, the synthesis of these outcomes remains inconclusive and dependent on future studies with larger sample sizes and better designs with special focus on external validation. Radiomics models without external validation are at increased risk of being specialized in specific radiographs that hamper generalizability due to overfitting [ 46 ].
Overfitting and multi-collinearity may affect radiomics models. During training, high-dimensional features may overfit and thus may yield optimistic outcomes. Moreover, traditional statistical models may not work adequately to deal with multicollinearity among textural features. To avoid this, it is suggested that the removal of unreliable or irrelevant features and the reduction of dimensions of predictors may yield better outcomes [ 30 , 31 ]. The sensitivity of AdaBoost to noisy data or outliers makes it more suitable for cases facing overfitting problems. A frequently used classifier, the Support Vector Machine, uses preselected nonlinear mapping to map input parameters in a high-dimensional feature space to optimize feature classification. Random forest unifies several weak predictor classifiers to make an accurate and stable predictor [ 28 ].
The AUROC is a performance metric to quantify the power of a model in discriminating cases from non-cases. An AUROC value can lie between 0 and 1. It combines the sensitivity and specificity of a marker/modality for the diagnosis of a precisely defined stage of fibrosis. Sensitivity is usually evaluated in patients with advanced fibrosis and specificity in non-advanced fibrosis [ 47 ]. However, the AUROC values can be biased if the fibrosis distribution in the study population differs from that of the whole population to which it is being applied [ 48 ]. Although biopsy is considered a gold standard for the diagnosis of fibrosis in the liver, it has a high rate of false positives and false negatives in comparison with the whole liver due to sampling error. An AUROC value of 0.82 for distinguishing between F2 and F1 when the entire liver was used as the reference index will inform approximately 20% error rate of the biopsy (false positive and false negative rates) compared with the entire liver. Thus, discordance in the staging of liver fibrosis between a modality such as radiomics and biopsy can be due to an error of the modality as well as due to an error of the biopsy [ 47 ].
Currently, the evidence regarding the role of radiomics in diagnosis of liver fibrosis and staging is constrained with several caveats. Quality of medical images acquired through different modalities may vary depending on several factors such as scanners, protocols, and personnel that can affect the reproducibility of radiomics output. A lack of standardization of image acquisition, preprocessing steps, extraction of features, and analyses also makes it difficult to compare radiomics outcomes of various studies performed under different settings. The etiology of fibrosis, progression, and the presence of comorbidities may also affect the accuracy of radiomics outcomes. Moreover, biological interpretation of radiomic features is lacking due to which it is difficult to associate radiomic features with histopathological characteristics.
Several limitations of the present study need to be considered while interpreting the outcomes of this review. An important limitation of the present study was the presence of high statistical heterogeneity in the meta-analyses. Although sources of heterogeneity could not be traced statistically, it is reasonable to assume that clinical and methodological heterogeneity might have played an influencing role. Authors utilized different methods for feature selection and classification, worked with a variety of software, and analyzed a highly variable number of features. Radiomics analyses were based on several radiological modalities and fewer studies were available to evaluate a particular modality in a pooled design. Most studies were retrospective in design due to which several types of biases could have been introduced. Inclusion and exclusion criteria differed substantially across the included studies that recruited several conditions of chronic liver disease including hepatitis B/C, autoimmune hepatitis, liver failure, early-stage cirrhosis, nonalcoholic fatty liver disease, and primary sclerosing cholangitis. Some studies could not be included because of the lack of variance data for diagnostic accuracy indices.
In this meta-analysis of 15 studies, the use of radiomics in staging liver fibrosis has been found to be associated with good diagnostic accuracy. However, the present-day outcome data are inconclusive regarding the use of radiomics in clinical practice owing to heterogeneity in methodology and outcomes of reviewed studies in which the radiomic evaluations were based on several radiological modalities subjected to a variety of analytical models yielding varying outcomes and lacking external validation. Non-invasiveness and the involvement of machine learning make radiomics an attractive option for decision support. The outcomes reported so far are promising and need to be validated in multicenter studies having larger datasets and better comparability and validation aspects in designs.
Availability of data and materials
The datasets used or analyzed during the current study are available from the corresponding author on reasonable request.
Cheemerla S, Balakrishnan M. Global epidemiology of chronic liver disease. Clin Liver Dis (Hoboken). 2021;17(5):365–70.
Article PubMed PubMed Central Google Scholar
Centers for disease control and prevention. national center for health statistics. fast stats homepage. Chronic liver disease. Available at: https://www.cdc.gov/nchs/fastats/liver-disease.htm . Last accessed on March 16, 2024.
Li M, Wang ZQ, Zhang L, Zheng H, Liu DW, Zhou MG. Burden of cirrhosis and other chronic liver diseases caused by specific etiologies in China, 1990–2016: Findings from the Global Burden of Disease Study 2016. Biomed Environ Sci. 2020;33(1):1–10.
PubMed Google Scholar
Zhai M, Liu Z, Long J, Zhou Q, Yang L, Zhou Q, et al. The incidence trends of liver cirrhosis caused by nonalcoholic steatohepatitis via the GBD study 2017. Sci Rep. 2021;11:5195. https://doi.org/10.1038/s41598-021-84577-z .
Article CAS PubMed PubMed Central Google Scholar
Angulo P, Kleiner DE, Dam-Larsen S, Adams LA, Bjornsson ES, Charatcharoenwitthaya P, et al. liver fibrosis, but no other histologic features, is associated with long-term outcomes of patients with nonalcoholic fatty liver disease. Gastroenterology. 2015;149(2):389–97.
Article PubMed Google Scholar
Choi YS, Beltran TA, Calder SA, Padilla CR, Berry-Caban CS, Salyer KR. Prevalence of hepatic steatosis and fibrosis in the United States. Metab Syndr Relat Disord. 2022;20(3):141–7.
GBD 2017 Cirrhosis Collaborators. The global, regional, and national burden of cirrhosis by cause in 195 countries and territories, 1990–2017: a systematic analysis for the Global Burden of Disease Study 2017. Lancet Gastroenterol Hepatol. 2020;5(3):245–66.
Article Google Scholar
Chu LC, Park S, Kawamoto S, Yuille AL, Hruban RH, Fishman EK. Current status of radiomics and deep learning in liver imaging. J Comput Assist Tomogr. 2021;45:343–51.
Manning DS, Afdhal NH. Diagnosis and quantitation of fibrosis. Gastroenterology. 2008;134(6):1670–81.
Article CAS PubMed Google Scholar
Gillies RJ, Kinahan PE, Hricak H. Radiomics: images are more than pictures, they are data. Radiology. 2016;278:563–77.
Lambin P, Leijenaar RTH, Deist TM, Peerlings J, de Jong EEC, van Timmeren J, et al. Radiomics: the bridge between medical imaging and personalized medicine. Nat Rev Clin Oncol. 2017;14(12):749–62. https://doi.org/10.1038/nrclinonc.2017.141 .
Scapicchio C, Gabelloni M, Barucci A, Cioni D, Saba L, Neri E. A deep look into radiomics. Radiol Med. 2021;126(10):1296–311.
Lan GY, Guo Y, Zhang XY, Cai XL, Shi Y. Value of radiomic analysis of data from magnetic resonance elastography for diagnosing fibrosis stages in patients with hepatitis B/C. Chin J Acad Radiol. 2019;1:74–84.
Park HJ, Park B, Lee SS. Radiomics and deep learning: hepatic applications. Korean J Radiol. 2020;21:387–401.
Liu Z, Wang S, Dong D, Wei J, Fang C, Zhou X, et al. The applications of radiomics in precision diagnosis and treatment of oncology: Opportunities and challenges. Theranostics. 2019;9(5):1303–22.
Zhang YP, Zhang XY, Cheng YT, Li B, Teng XZ, Zhang J, et al. Artificial intelligence-driven radiomics study in cancer: the role of feature engineering and modeling. Mil Med Res. 2023;10(1):22. https://doi.org/10.1186/s40779-023-00458-8 .
Neisius U, El-Rewaidy H, Nakamori S, Rodriguez J, Manning WJ, Nezafat R. Radiomic analysis of myocardial native T1 imaging discriminates between hypertensive heart disease and hypertrophic cardiomyopathy. JACC Cardiovasc Imaging. 2019;12(10):1946–54.
Neisius U, El-Rewaidy H, Kucukseymen S, Tsao CW, Mancio J, Nakamori S, et al. Texture signatures of native myocardial T1 as novel imaging markers for identification of hypertrophic cardiomyopathy patients without scar. J Magn Reson Imaging. 2020;52(3):906–19.
Cuadra MB, Favre J, Omoumi P. Quantification in musculoskeletal imaging using computational analysis and machine learning: Segmentation and radiomics. Semin Musculoskelet Radiol. 2020;24(1):50–64. https://doi.org/10.1055/s-0039-3400268 .
Chea P, Mandell JC. Current applications and future directions of deep learning in musculoskeletal radiology. Skeletal Radiol. 2020;49(2):183–97.
Park YW, Choi D, Lee J, Ahn SS, Lee SK, Lee SH, et al. Differentiating patients with schizophrenia from healthy controls by hippocampal subfields using radiomics. Schizophr Res. 2020;223:337–44.
Park YW, Choi YS, Kim SE, Choi D, Han K, Kim H, et al. Radiomics features of hippocampal regions in magnetic resonance imaging can differentiate medial temporal lobe epilepsy patients from healthy controls. Sci Rep. 2020;10:19567.
Bang M, Park YW, Eom J, Ahn SS, Kim J, Lee SK, et al. An interpretable radiomics model for the diagnosis of panic disorder with or without agoraphobia using magnetic resonance imaging. J Affect Disord. 2022;305:47–54.
Zhao K, Ding Y, Han Y, Fan Y, Alexander-Bloch AF, Han T, et al. Independent and reproducible hippocampal radiomic biomarkers for multisite Alzheimer’s disease: diagnosis, longitudinal progress and biological basis. Sci Bull. 2020;65:1103–13.
Article CAS Google Scholar
Liu F, Ning Z, Liu Y, Liu D, Tian J, Luo H, et al. Development and validation of a radiomics signature for clinically significant portal hypertension in cirrhosis (CHESS1701): a prospective multicenter study. EBioMedicine. 2018;36:151–8.
Chen ZW, Tang K, Zhao YF, Chen YZ, Tang LJ, Li G, et al. Radiomics based on fluoro-deoxyglucose positron emission tomography predicts liver fibrosis in biopsy-proven MAFLD: a pilot study. Int J Med Sci. 2021;18:3624–30.
Hu P, Chen L, Zhong Y, Lin Y, Yu X, Hu X, et al. Effects of slice thickness on CT radiomics features and models for staging liver fibrosis caused by chronic liver disease. Japanese J Radiol. 2022;40:1061–8.
Li W, Huang Y, Zhuang BW, Liu GJ, Hu HT, Li X, et al. Multiparametric ultrasomics of significant liver fibrosis: A machine learning-based analysis. Eur Radiol. 2019;29:1496–506.
Lu X, Zhou H, Wang K, Jin J, Meng F, Mu X, et al. Comparing radiomics models with different inputs for accurate diagnosis of significant fibrosis in chronic liver disease. Eur Radiol. 2021;31:8743–54.
Park HJ, Lee SS, Park B, Yun J, Sung YS, Shim WH, et al. Radiomics analysis of Gadoxetic Acid–enhanced MRI for staging liver fibrosis. Radiology. 2019;290:380–7.
Qiu QT, Zhang J, Duan JH, Wu SZ, Ding JL, Yin Y. Development and validation of radiomics model built by incorporating machine learning for identifying liver fibrosis and early-stage cirrhosis. Chin Med J. 2020;133(22):2653–9.
Sim KC, Kim MJ, Cho Y, Kim HJ, Park BJ, Sung DJ, et al. Diagnostic feasibility of magnetic resonance elastography radiomics analysis for the assessment of hepatic fibrosis in patients with nonalcoholic fatty liver disease. J Comput Assist Tomogr. 2022;46:505–13.
Wang K, Lu X, Zhou H, Gao Y, Zheng J, Tong M, et al. Deep learning Radiomics of shear wave elastography significantly improved diagnostic performance for assessing liver fibrosis in chronic hepatitis B: a prospective multicentre study. Gut. 2019;68:729–41.
Wang J, Tang S, Mao Y, Wu J, Xu S, Yue Q, et al. Radiomics analysis of contrast-enhanced CT for staging liver fibrosis: an update for image biomarker. Hepatol Int. 2022;16:627–39.
Xue LY, Jiang ZY, Fu TT, Wang QM, Zhu YL, Dai M, et al. Transfer learning radiomics based on multimodal ultrasound imaging for staging liver fibrosis. Eur Radiol. 2020;30:2973–83.
Yin Y, Yakar D, Dierckx RAJO, Mouridsen KB, Kwee TC, de Haas RJ. Combining hepatic and splenic CT radiomic features improves radiomic analysis performance for liver fibrosis staging. Diagnostics. 2022;12:550. https://doi.org/10.3390/diagnostics12020550 .
Zhang D, Cao Y, Sun Y, Xia Zhao X, Peng C, Zhao J, et al. Radiomics nomograms based on R2* mapping and clinical biomarkers for staging of liver fibrosis in patients with chronic hepatitis B: a single-center retrospective study. Eur Radiol. 2023;33:1653–67.
Zhao R, Zhao H, Ge YQ, Zhou FF, Wang LS, Yu HZ, et al. Usefulness of noncontrast MRI-based radiomics combined clinic biomarkers in stratification of liver fibrosis. Canadian J Gastroenterol Hepatol. 2022;2022:2249447. Article ID 2249447.
Zheng R, Shi C, Wang C, Shi N, Qiu T, Chen W, et al. Imaging-based staging of hepatic fibrosis in patients with hepatitis B: A dynamic radiomics model based on Gd-EOB-DTPA-enhanced MRI. Biomolecules. 2021;11:307.
Cui E, Long W, Wu J, Li Q, Ma C, Lei Y, et al. Predicting the stages of liver fibrosis with multiphase CT radiomics based on volumetric features. Abdom Radiol. 2021;46:3866–76.
Duan YY, Qin J, Qiu WQ, Li SY, Li C, Liu AS, et al. Performance of a generative adversarial network using ultrasound images to stage liver fibrosis and predict cirrhosis based on a deep-learning radiomics nomogram. Clin Radiol. 2022;77(10):e723–31.
Zhou Z, Zhang Z, Gao A, Tai DI, Wu S, Tsui PH. Liver fibrosis assessment using radiomics of ultrasound homodyned-K imaging based on the artificial neural network estimator. Ultrasonic Imaging. 2022;44(5–6):229–41.
Yasaka K, Akai H, Kunimatsu A, Abe O, Kiryu S. Liver fibrosis: deep convolutional neural network for staging by using gadoxetic acid–enhanced hepatobiliary phase MR images. Radiology. 2018;287:146–55.
Choi KJ, Jang JK, Lee SS, Sung YS, Shim WH, Kim HS, et al. Development and validation of a deep learning system for staging liver fibrosis by using contrast agent–enhanced CT images in the liver. Radiology. 2018;289:688–97.
Zheng W, Guo W, Xiong M, Chen X, Gao L, Song Y, et al. Clinic-radiological features and radiomics signatures based on Gd-BOPTA-enhanced MRI for predicting advanced liver fibrosis. European Radiology. 2023;33:633–44.
Yamada A, Kamagata K, Hirata K, Ito R, Nakaura T, Ueda D, et al. Clinical applications of artificial intelligence in liver imaging. Radiol Med. 2023;128(6):655–67.
Poynard T, Halfon P, Castera L, Charlotte F, Le Bail B, Munteanu M, et al. Variability of the area under the receiver operating characteristic curves in the diagnostic evaluation of liver fibrosis markers: impact of biopsy length and fragmentation. Aliment Pharmacol Ther. 2007;25(6):733–9.
Guha IN, Myers RP, Patel K, Talwalkar JA. Biomarkers of liver fibrosis: What lies beneath the receiver operating characteristic curve? Hepatology. 2011;54:1454–62.
Download references
Acknowledgements
Not applicable.
Author information
Authors and affiliations.
School of Medical Imaging, Tianjin Medical University, No.1, Guangdong Road, Hexi District, Tianjin, 300203, China
Xiao-min Wang
Department of Radiology, The First Medical Center, Chinese PLA General Hospital, Beijing, 100853, China
Xiao-jing Zhang
You can also search for this author in PubMed Google Scholar
Contributions
XMW: Literature search, data analysis, manuscript writing; manuscript review; XJZ: Literature search; manuscript editing.
Corresponding author
Correspondence to Xiao-min Wang .
Ethics declarations
Ethics approval and consent to participate, consent for publication, competing interests.
The authors declare no competing interests.
Additional information
Publisher’s note.
Springer Nature remains neutral with regard to jurisdictional claims in published maps and institutional affiliations.
Supplementary Information
Supplementary material 1., rights and permissions.
Open Access This article is licensed under a Creative Commons Attribution 4.0 International License, which permits use, sharing, adaptation, distribution and reproduction in any medium or format, as long as you give appropriate credit to the original author(s) and the source, provide a link to the Creative Commons licence, and indicate if changes were made. The images or other third party material in this article are included in the article's Creative Commons licence, unless indicated otherwise in a credit line to the material. If material is not included in the article's Creative Commons licence and your intended use is not permitted by statutory regulation or exceeds the permitted use, you will need to obtain permission directly from the copyright holder. To view a copy of this licence, visit http://creativecommons.org/licenses/by/4.0/ . The Creative Commons Public Domain Dedication waiver ( http://creativecommons.org/publicdomain/zero/1.0/ ) applies to the data made available in this article, unless otherwise stated in a credit line to the data.
Reprints and permissions
About this article
Cite this article.
Wang, Xm., Zhang, Xj. Role of radiomics in staging liver fibrosis: a meta-analysis. BMC Med Imaging 24 , 87 (2024). https://doi.org/10.1186/s12880-024-01272-x
Download citation
Received : 13 June 2023
Accepted : 10 April 2024
Published : 12 April 2024
DOI : https://doi.org/10.1186/s12880-024-01272-x
Share this article
Anyone you share the following link with will be able to read this content:
Sorry, a shareable link is not currently available for this article.
Provided by the Springer Nature SharedIt content-sharing initiative
BMC Medical Imaging
ISSN: 1471-2342
- General enquiries: [email protected]
SYSTEMATIC REVIEW article
Diagnostic accuracy of circulating mirnas to discriminate hepatocellular carcinoma from liver cirrhosis: a systematic review and meta-analysis provisionally accepted.
- 1 Wollo University, Ethiopia
- 2 Jigjiga University, Ethiopia
- 3 Dessie Hospital, Ethiopia
- 4 Debre Tabor University, Ethiopia
The final, formatted version of the article will be published soon.
Introduction: Hepatocellular carcinoma (HCC) and liver cirrhosis (LC) stand as the primary causes of global mortality. Given their profound impact, the development of highly sensitive and specific circulating diagnostic markers becomes imperative to effectively identify and differentiate between cirrhosis and HCC. Accurate diagnosis is paramount in guiding appropriate therapeutic interventions. Hence, this study endeavors to evaluate the potential of microRNAs (miRNAs) in discerning between HCC and LC.Method: This study followed the Preferred Reporting Items for Systematic Review and Meta-Analysis (PRISMA) guidelines, with the protocol officially registered on PROSPERO under the reference number CRD42023417494. A thorough search across multiple databases like PubMed, Embase, Scopus, Wiley Online Library, and Science Direct was conducted to identify relevant studies published from January 1, 2018, to August 10, 2023. The included studies underwent methodological quality assessment using the Quality Assessment of Diagnostic Accuracy Studies 2 (QADAS-2) tool. The synthesis of pooled sensitivity, specificity, and other relevant diagnostic parameters employed a random-effects model and was conducted using Stata 14.0. Heterogeneity was assessed using I 2 and Cochrane Q, with subsequent subgroup analysis and meta-regression performed to identify potential sources of observed heterogeneity. A sensitivity analysis was performed to assess the resilience of the findings. Furthermore, Deeks' funnel plot was employed to evaluate publication bias.In this meta-analysis, we included fifteen publications, encompassing 787 HCC patients and 784 LC patients. The combined sensitivity, specificity, positive likelihood ratio (PLR), negative likelihood ratio (NLR), diagnostic odds ratio (DOR), and area under the curve (AUC) values of miRNAs in differentiating HCC from LC were 0.84 (95% CI: 0.78-0.88), 0.79 (95% CI: 0.73-0.84), 3.9 (95% CI: 3.0-5.2), 0.21 (95% CI: 0.14-0.29), 19.44 (95% CI: 11-34), and 0.88 (95% CI: 0.85-0.91), respectively. The results of the subgroup analysis revealed that upregulated miRNA levels and miRNA assessments specifically for individuals of European descent exhibited superior diagnostic performance.The results of this study suggested that circulating miRNAs, especially those that are upregulated, have the potential to function as robust and promising biomarkers in the differentiation of HCC from LC.
Keywords: miRNAs, non-coding RNAs, diagnostic biomarkers, Hepatocellular Carcinoma, HCC, liver cancer, Liver Cirrhosis, Meta-analysis
Received: 21 Dec 2023; Accepted: 12 Apr 2024.
Copyright: © 2024 Alemayehu, Belete, Walle, Getu, Mulatie, Teshome, Anley, Weldehanna, Gedefie and Ebrahim. This is an open-access article distributed under the terms of the Creative Commons Attribution License (CC BY) . The use, distribution or reproduction in other forums is permitted, provided the original author(s) or licensor are credited and that the original publication in this journal is cited, in accordance with accepted academic practice. No use, distribution or reproduction is permitted which does not comply with these terms.
* Correspondence: Mx. Ermiyas Alemayehu, Wollo University, Dessie, Ethiopia
People also looked at
- Study Guides
- Homework Questions
CASE-STUDY-ON-COVID19

- Journal Introduction
- Editorial Board
- Style of Editorial Department
- Advertising Policies
- Instructions for Authors
- Editorial Policies
- Open Access Policy
- Publishing Ethics
- Publication Process Flow
- Peer Review Process
- Conflict of interest statement
- Statement of Human and Animal Rights
- Statement of Informed Consent and ethical approval
- Data Sharing Policy
- Statement of Copyright information
- Open Science Identifier (OSID) Recording
- Paper Template
- Handling method for withdrawal
- Online First
- Current Issue
- F5000 Articles
- Guide to foreign journals
- Industry information
- Video Classroom
- Guideline interpretation
- Share facebook twitter google linkedin
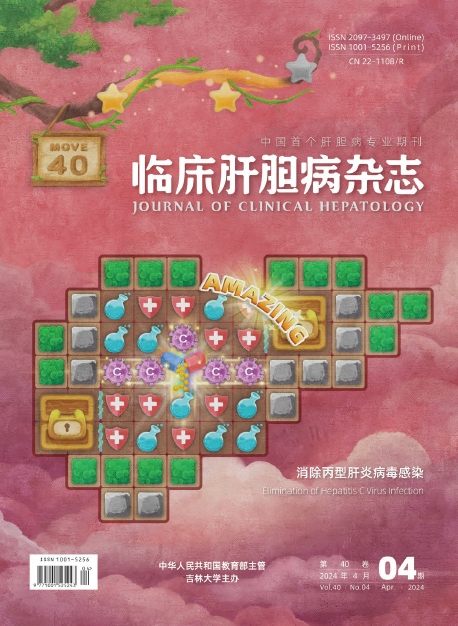
Changing trend of the disease burden of liver cirrhosis in China from 1990 to 2019
Doi: 10.12449/jch240414.
- Shanshan LIU 1a, 2 ,
- Xiaohui YU 1a ,
- Jianwei CAI 1b , , ,
Gastroenterology Department,The 940 Hospital of Joint Logistic Support Force of PLA Lanzhou 730050,China
Hepatobiliary Surgery Department,The 940 Hospital of Joint Logistic Support Force of PLA,Lanzhou 730050,China
The First Clinical Medical College of Gansu University of Chinese Medicine,Lanzhou 730000,China
Joint Support Force 940 Hospital In-hospital Project (2022yxky016) ;
Gansu Provincial Clinical Medical Research Center for Non infectious Liver Diseases (21JR7RA017)
- Accepted Date: 2023-10-16
- Published Date: 2024-04-11
- Liver cirrhosis ,
- Cost of Illness ,
- Forecasting ,
Proportional views
通讯作者: 陈斌, [email protected].
沈阳化工大学材料科学与工程学院 沈阳 110142
Figures( 6 ) / Tables( 4 )
Article Metrics
- PDF Downloads( 3 )
- Abstract views( 16 )
- HTML views( 9 )

The first journal specializing in hepatobiliary and pancreatic diseases in China
Supervisor:Ministry of Education of the People's Republic of China
Sponsor:Jilin University
Academic Support: Chinese Society of Hepatology,Chinese Medical Association
Address: 461 Xinjiang Road, Changchun
Submit:0431-88782044
Peer review:0431-88783542
Email: [email protected]
About Journal
Submission guideline, journal online, hepatobiliary college.
- All Guidelines
- Domestic guidelines
- Foreign guidelines
- Guideline translation

Export File
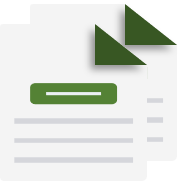
- Figure 1. Changes in the incidence, prevalence, and mortality of liver cirrhosis in China, the world, and different SDI regions from 1990 to 2019
- Figure 2. Changes in the proportion composition of YLL and YLD in Chinese population with liver cirrhosis from 1990 to 2019
- Figure 3. Age changes in the burden of liver cirrhosis in the Chinese population from 1990 to 2019
- Figure 4. Age-period-cohort model effect diagram of incidence of liver cirrhosis in China from 1990 to 2019
- Figure 5. Age-period-cohort model effect diagram of mortality of liver cirrhosis in China from 1990 to 2019
- Figure 6. Proportion of risk factors for liver cirrhosis disease burden in China from 1990 to 2019
Hepatic steatosis predicts metachronous liver metastasis in colorectal cancer patients: a nested case-control study and systematic review
Affiliations.
- 1 Department of Colorectal Surgery and Oncology (Key Laboratory of Cancer Prevention and Intervention, China National Ministry of Education, Key Laboratory of Molecular Biology in Medical Sciences, Zhejiang Province, China), The Second Affiliated Hospital, Zhejiang University School of Medicine 300th Yuanju Street, Hangzhou 310000, Zhejiang, China.
- 2 Center for Medical Research and Innovation in Digestive System Tumors 88th Jiefang Street, Hangzhou 310000, Zhejiang, China.
- 3 Zhejiang Provincial Clinical Research Center for CANCER 88th Jiefang Street, Hangzhou 310000, Zhejiang, China.
- 4 Cancer Center of Zhejiang University 88th Jiefang Street, Hangzhou 310000, Zhejiang, China.
- 5 Department of Radiation Oncology, Key Laboratory of Cancer Prevention and Intervention, Ministry of Education, The Second Affiliated Hospital, Zhejiang University School of Medicine 88th Jiefang Street, Hangzhou 310000, Zhejiang, China.
- PMID: 38590410
- PMCID: PMC10998736
- DOI: 10.62347/JHMS4303
Nearly twenty-five percent of colorectal cancer (CRC) patients develop metachronous colorectal liver metastasis (CRLM) after curative surgery. Hepatosteatosis is the most prevalent liver condition worldwide, but its impact on the incidence of metachronous CRLM is understudied. In the present study, we aimed to investigate the predictive value of hepatic steatosis on the development of metachronous CRLM. First, a nested case-control study was conducted, enrolling stage I to III CRC patients in the National Colorectal Cancer Cohort (NCRCC) database. Metachronous CRLM patients and recurrence-free patients were matched via propensity-score matching. Fatty liver was identified based on treatment-naïve CT scans and the degree of hepatic fibrosis was scored. Multivariable analysis was conducted to investigate the association between fatty liver and metachronous CRLM. In our database, a total of 414 patients were included. Metachronous CRLM patients had considerably higher rates of hepatic steatosis (30.9% versus 15.9%, P<0.001) and highly fibrotic liver (11.6% versus 2.9%, P=0.001) compared to recurrence-free patients. Multivariable analysis showed that fatty liver (odds ratios [OR]=1.99, 95% confidence interval [CI] 1.19-3.30, P=0.008) and fibrotic liver (OR=4.27, 95% CI 1.54-11.81, P=0.005) were associated with high risk of metachronous CRLM. Further, a systematic literature review was performed to assess available evidence on the association between hepatosteatosis and development of metachronous CRLM. In the systematic review, 1815 patients were pooled from eligible studies, and hepatic steatosis remained a significant risk factor for metachronous CRLM (OR=1.90, 95% CI 1.35-2.66, P<0.001, I 2 =25.3%). In conclusion, our data suggest that patients with a steatotic liver and a high fibrosis score at CRC diagnosis have elevated risk of developing metachronous CRLM.
Keywords: Hepatic steatosis; L/S ratio; colorectal cancer; metachronous liver metastasis; tumor stage.
AJCR Copyright © 2024.

IMAGES
VIDEO
COMMENTS
Cirrhosis can also increase the risk of hepatocellular carcinoma (HCC), the most common form of liver cancer. Studies suggest that 80% to 90% of people with HCC have cirrhosis and that roughly 12% of people with cirrhosis will eventually develop HCC, usually within the span of 10 years.
Dr. Rubén Francés, director of the UMH's Hepatic and Intestinal Immunobiology Group and study leader, explains that liver cirrhosis is the final stage of various chronic liver diseases. During ...
While alcohol-related cirrhosis was the major cause of decompensated cirrhosis in about 70% of participants in the study, access for them to obtain high-quality alcohol addiction, mental health ...
We identified 217 patients who underwent laparoscopic treatment of hepatocellular carcinoma with liver cirrhosis in the University of Hong Kong, Queen Mary Hospital, between 2000 and 2015. 112 patients had undergone percutaneous RFA, and 105 patients who had undergone LLR with similar were selected for comparison.
In a case report of two patients with HE, ... In addition, in our patient cohort HE was not a major clinical problem in patients with liver cirrhosis who received TIPS. To study the effects of TIPS application on the luminal microbiome, stool samples were collected before, shortly, and long after the TIPS application. ...
Acute liver failure (ALF) is characterized by the occurrence of coagulopathy (international normalized ratio [INR] > 1.5) and any level of encephalopathy emerging 24 weeks following the occurrence of the first symptoms in patients who have no history of previous liver disease [].The timing and the level of clinical presentation can be classified into three types: hyperacute, acute, and ...
The last stage of many hepatic diseases, including hepatitis, is cirrhosis. The liver's vital function in detoxifying xenobiotics—such as medications and toxic composites—is the subject of much research. Liver cirrhosis is the cause of alterations in the liver's architecture that mostly affect protein binding, blood circulation, and the enzymes that break down medicinal substances.
News Release 10-Apr-2024. Study advances understanding of liver immune response in cirrhosis. Restoring LSECtin activity could be a therapeutic strategy for cirrhosis. The findings contribute to a ...
Fibrosis has important pathoetiological and prognostic roles in chronic liver disease. This study evaluates the role of radiomics in staging liver fibrosis. After literature search in electronic databases (Embase, Ovid, Science Direct, Springer, and Web of Science), studies were selected by following precise eligibility criteria. The quality of included studies was assessed, and meta-analyses ...
When is a Liver Biopsy Still Needed to Make the Diagnosis . If there is more than one liver disease ; If diagnosis is in doubt ; Why Patients with NAFLD have a 10% Higher Mortality Rate . Cardiovascular disease ; Cirrhosis/hepatoma is not the main cause of early morbidity/mortality ; Standard Ways of Treating NAFLD . Exercise and weight loss
The presence of liver cirrhosis and the use of antibiotic therapy, but not the application of TIPS, were associated with a significant shift of the intestinal bacterial communities, showing a high impact on the microbiota of patients with liver cirrhosis. ... prospective study J Dig Dis. 2024 Apr 10. doi: 10.1111/1751-2980.13262.
Immunobiology Group and study leader, explains that liver cirrhosis is 2/4. the final stage of various chronic liver diseases. During cirrhosis, there is
Introduction: Hepatocellular carcinoma (HCC) and liver cirrhosis (LC) stand as the primary causes of global mortality. Given their profound impact, the development of highly sensitive and specific circulating diagnostic markers becomes imperative to effectively identify and differentiate between cirrhosis and HCC. Accurate diagnosis is paramount in guiding appropriate therapeutic interventions ...
In this case study, we report a COVID-19 patient with cirrhosis. On February 11, 2020, a 73-year-old woman came to the Fever Clinic of the First Hospital of Changsha, China. Ten minutes later, she was taken to the examination room and evaluated by a clinic doctor. The chief complaint of the patient was a fever—her body temperature peaked at ...
d to define outcomes associated with stage 1B in a nationwide US cohort of hospitalized cirrhosis patients with AKI. Hospitalized cirrhosis patients with AKI in the Cerner-Health-Facts database from January 2009 to September 2017 (n = 6250) were assessed for AKI stage 1 (≥1.5-2-fold increase in sCr from baseline) and were followed for 90 days for outcomes. The primary outcome was 90-day ...
Objective To investigate the prevalence of liver cirrhosis and the changing trend of the disease burden of liver cirrhosis in the Chinese population from 1990 to 2019, and to provide a data reference for formulating the prevention and treatment strategies for liver cirrhosis in China. Methods The Global Burden of Disease Study 2019 was used to collect the data on the incidence rate ...
To our knowledge, this is the first published case of pulmonary TM infection in an HIV-negative patient with liver cirrhosis. HIV-Negative Case of Talaromyces marneffei Pulmonary Infection with Liver Cirrhosis in China: A Case Report and Literature Review Infect Drug Resist. 2024 Apr 4:17:1333-1343. doi: 10.2147/IDR.S451880. ...
Hepatosteatosis is the most prevalent liver condition worldwide, but its impact on the incidence of metachronous CRLM is understudied. In the present study, … Hepatic steatosis predicts metachronous liver metastasis in colorectal cancer patients: a nested case-control study and systematic review Am J Cancer Res. 2024 Mar 15 ...
Liver/Kidney Disease Case Study. Press F11. Select menu option View > Enter Fullscreen. for full-screen mode. Edit • Print • Download • Embed • Share. JeopardyLabs. Which oral symptom is commonly associated with the medication Johnny is taking?, What Medication is NOT a part of Johnny's prescription medications? , How often does ...
301 Moved Permanently. openresty