Thank you for visiting nature.com. You are using a browser version with limited support for CSS. To obtain the best experience, we recommend you use a more up to date browser (or turn off compatibility mode in Internet Explorer). In the meantime, to ensure continued support, we are displaying the site without styles and JavaScript.
- View all journals
- Explore content
- About the journal
- Publish with us
- Sign up for alerts
- Perspective
- Published: 21 September 2018

Recent advances in the study of aggression
- Meghan E. Flanigan ORCID: orcid.org/0000-0002-3185-7459 1 &
- Scott J. Russo ORCID: orcid.org/0000-0002-6470-1805 1
Neuropsychopharmacology volume 44 , pages 241–244 ( 2019 ) Cite this article
7851 Accesses
34 Citations
15 Altmetric
Metrics details
- Social neuroscience
You have full access to this article via your institution.
Introduction
Aggression is an evolutionarily conserved behavior that controls social hierarchies and protects valuable resources like mates, food, and territory. In most cases, aggression is a normal and necessary component of social behavior. In humans, however, some forms of aggression are considered pathological behaviors that threaten lives, increase the likelihood of future psychiatric disease in victims and witnesses, and incur tremendous economic burdens on society [ 1 ]. Furthermore, while abnormal aggressive behavior is a symptom shared across a wide range of psychiatric and neurological diseases, there are few, if any, approved treatments aimed specifically at curbing it [ 2 ]. Despite the massive costs of violence on society, the pervasiveness of aggression among psychiatric patients, and our current lack of treatments, aggression historically has been understudied compared with other emotional behaviors in psychiatric patients [ 3 ]. While a recent surge in preclinical studies interrogating the neural circuitry underpinning aggression has provided important new findings regarding the brain regions, cell types, and neural ensembles governing specific components of this complex behavior, significant gaps in our understanding remain. In order to successfully develop novel treatments aimed at reducing aggression in a variety of patient populations, it is crucial that we address these gaps systematically, and with the consideration that aggressive behavior is influenced by an interconnected circuitry that integrates processes related to motivation, arousal, impulse control, memory, metabolism, sensory perception, and hormonal signaling, among others.
Recent advances in behavioral models of aggression
In order to investigate the neural circuit mechanisms underlying pathological aggressive behavior in humans, it is critical that researchers utilize animal models that fully capture the essential features of excessive human violence (see Table 1 ). Clinically, aggressive behavior is commonly classified as either proactive or reactive, and each of these classifications is generally associated with specific behavioral characteristics relevant to psychiatric disorders [ 4 ]. However, until recently, animal models of aggression have largely not distinguished between domains of behavior relevant to specific clinical subtypes of aggression. This may be an important reason for why our understanding of the neural circuitry of aggressive behavior remains tenuous.
Proactive aggression is commonly defined as aggression that is purposeful, goal-driven, and characterized by low emotional reactivity (hypoarousal). Under certain conditions, proactive aggression bears resemblance to drug addiction: aggression is sought compulsively despite adverse consequences (jail) and relapse to aggression following abstinence is common (recidivism) [ 5 ]. Consistent with this notion, researchers have recently developed animal models of aggression that are inspired by classical models of drug reward like self-administration and conditioned place preference (CPP) [ 6 , 7 , 8 , 9 ]. These models have been used to identify a handful of classical reward circuits that may be important for pathological aggression, and we argue for their widespread use in future investigations.
Reactive aggression is commonly defined as aggression that is impulsive, hostile, and characterized by high emotional reactivity (hyperarousal). Current animal models that are potentially relevant to reactive aggression include instigation/frustration models, alcohol exposure models, and anabolic steroid exposure models, as these models result in hyperarousal-associated aggression. However, it is important to note that in many humans and animal models, aggression cannot simply be defined as either proactive or reactive. Thus, it is important that animal models of aggression strive to recapitulate specific behavioral domains of proactive versus reactive aggression most relevant to those found in psychiatric patient populations.
Stepping outside of the VMH
A large portion of what we currently know about the circuitry governing aggression relates to the ventromedial hypothalamus (VMH), which was first identified as a site for driving the initiation of intermale attacks in the 1960s [ 10 ] (for review, see [ 11 ]). This focus has been driven by the idea that gaining a more detailed understanding of the control points for the expression of rodent species-typical aggressive behavior will promote the development of effective treatment strategies for humans displaying abnormal aggression [ 12 ]. Despite the appeal of this approach, there is scarce evidence that the VMH is involved in abnormal human aggression [ 4 ]. Rather, clinical neuroimaging studies have identified nuclei that are likely upstream of attack initiation nodes like the VMH as dysregulated in patients displaying aggression [ 13 ]. We argue that the wide range of aggressive behavior observed in human psychiatric patients is likely the result of aberrant activity within multiple neural circuits across the brain, each of which carry different streams of information that converge on attack initiation nuclei to control aggression. The development of effective treatments for aggression will require a deeper understanding of the functional connectivity and behavioral roles of each of these circuits in animal models that reflect the heterogeneity of aggressive behavior observed in human patients (see Table 1 ).
Influences of motivation on aggression
A growing number of studies in humans and animals illustrate that the propensity to carry out violent acts is influenced by the degree to which an individual finds aggression rewarding [ 14 ]. This suggests that neural circuits controlling the positive valence of aggressive social interactions are dysregulated in some patients displaying abnormal aggression, though it is unclear precisely how primary reward centers interact with aggression initiation circuitry. In support of this, neuroimaging studies in human psychiatric patients, particularly those with personality disorders, describe structural and functional abnormalities in key reward-related regions like the striatum that are correlated with aggression [ 15 , 16 ]. Consistent with human studies, recent investigations utilizing animal models of aggression CPP have identified functional roles for both the nucleus accumbens (NAc) [ 17 ] and the lateral habenula (LHb) [ 9 ] in the reinforcing effects of aggression. Interestingly, the VMH itself has also been reported to control aggression-seeking behavior in an operant model, indicating that this nucleus is not simply functioning as an on/off switch for attack, but may integrate information from primary reward centers to reinforce aggressive behavior [ 6 ]. A very recent study also found that the ventral premammillary nucleus (PMv) plays an important role in aggression and the establishment of social hierarchy via divergent projections to the supramammillary nucleus (SuM) and the VMH [ 18 ]. Although PMv inputs to the SuM promote aggression CPP without impacting aggression, PMv inputs to the VMH promote aggression without impacting aggression CPP. These results suggest that circuits controlling these two aspects of aggressive behavior (valence versus initiation) may be dissociable. Much work is required to determine how non-hypothalamic regions like the LHb and the NAc interface with the VMH or other aggression initiation circuits to promote aggression and its rewarding effects. Multisynaptic tracing and functional mapping studies will be important for determining this. In addition, we know that levels of aggression increase with repeated experience, a phenomenon akin to drug-induced sensitization, which is prominently regulated by reward circuits including the NAc and LHb. Thus, a key future question is how aggression experience shapes reward-related neural and behavioral responses to guide future aggressive interactions. Does aggression intensity mirror changes in the reward valence and is this associated with reward circuit plasticity? Understanding these important questions may be highly relevant for the treatment of extremely aggressive individuals exhibiting high rates of recidivism.
Influences of emotional reactivity and impulse control on aggression
Although some psychiatric patients exhibiting abnormal aggression do so because they find violence rewarding (termed proactive aggression, see previous section), others may do so because of inappropriate emotional reactivity to perceived social threats and poor impulse control (termed reactive aggression) [ 13 ]. Perhaps unsurprisingly, a large body of evidence suggests that the circuits controlling these two types of aggressive behavior differ significantly [ 4 ]. Clinical neuroimaging studies broadly suggest that reactive aggression involves simultaneous hypofunction of the medial prefrontal cortex (mPFC) and hyperfunction of the amygdala [ 13 ]. Activation of the amygdala and extended amygdala promotes aggression in a variety of animal models [ 19 ]. However, preclinical studies investigating the role of the mPFC in aggression appear conflicting. For example, there is evidence that optogenetic stimulation of the mPFC both reduces [ 20 ] and increases [ 21 , 22 , 23 ] aggression and dominance. These discrepant findings may be explained by differences in the animal models of aggression used. It is possible that mPFC hypofunction underlies reactive forms of aggression, whereas PFC hyperfunction underlies proactive forms of it. In addition, it may be that specific outputs from the mPFC play opposing roles in aggression such that broad manipulation of this nucleus provides inconsistent results. This idea is somewhat supported by a recent study that found differential roles for mPFC outputs to the mediobasal hypothalamus and lateral hypothalamus, which drive species-typical versus species atypical (escalated) aggression, respectively [ 24 ]. Future work should aim to functionally dissect the specific roles of mPFC cell types and their outputs in models of proactive versus reactive aggression. Furthermore, the downstream circuit mechanisms by which the mPFC and amygdala influence the initiation of aggression should be fully explored.
Influences of social context on aggression
Recent work suggests that circuits conveying information about social context influence the activity of attack nuclei. For example, the capacity for optogenetic stimulation of VMH neurons to initiate aggression is affected by whether residents and intruders are single or group housed prior to testing [ 25 ]. Although stimulation of VMH neurons is sufficient to drive aggression independently of pheromone sensing capabilities, gonadal hormone status, and physical cues indicating the presence of a conspecific in single-housed males, this manipulation is insufficient to initiate attack in group-housed males [ 25 , 26 ]. This suggests that VMH neurons may in fact be regulated by circuits conveying social context, such as those relevant to olfaction and ultrasonic vocalization. As VMH neurons have the capacity to distinguish male versus female conspecifics [ 27 ], it is possible that the VMH also encodes other social information about male conspecifics within a group, including their status in the social hierarchy, to control whether aggression is initiated. Mechanistic insights into this phenomenon may enable us to reduce violence in patients through the therapeutic normalization of circuits signaling whether aggression is appropriate in given social contexts.
Other factors influencing aggression
In many psychiatric patients, violent behaviors intensify during certain times of day, indicating that circadian circuits controlling arousal may be important modulators of VMH neurons driving aggression [ 28 ]. Though this effect has been well documented in clinical populations, only one recent study has investigated the relevant circuit mechanisms in an animal model of aggression [ 29 ]. Researchers found that there is indeed a daily rhythm of aggressive behavior in mice that resembles clinical patterns of aggression in psychiatric patients [ 29 ]. This rhythm appears to be controlled by a multisynaptic circuit from the suprachiasmatic nucleus to the VMH via the subventricular zone (SVZ). Inhibitory inputs to VMH neurons from the SVZ were found to be more active during the day than at night, thereby resulting in increased aggressive behavior in the early night. The findings of this study have potentially important implications for future studies on aggression, which will require the consideration of behavioral testing time in the design of experiments and interpretation of results. These results also suggest that psychiatric patients who display daily oscillations in aggressive behavior, such as those with Alzheimer’s disease or psychosis, may respond well to treatments targeting this circuit. It is very likely that other circuits conveying information about an individual’s internal state (hunger, thirst, etc.) are also playing roles in aggressive behavior, though these have yet to be explored.
Aggression in females
A central question that remains is whether the same circuits controlling aggression in males are involved in female aggression. Comparisons of the mechanisms of male and female aggression in animal models have classically proven difficult because of fundamental differences in the contexts in which males and females attack. However, a handful of recent studies have begun to investigate this. One recent study found that optogenetic activation of aromatase-positive neurons in the amygdala promotes both intermale aggression and maternal aggression [ 30 ]. Interestingly, optogenetic stimulation of VMH neurons promotes aggression in intact, but not ovariectomized females [ 25 , 31 , 32 ], suggesting that unlike in males, female gonadal hormone signaling is downstream of VMH. Moreover, although VMH neural ensembles encoding sexual versus aggressive behavior appear to largely overlap in males, these populations display little overlap in females [ 31 ]. These differences in VMH circuitry, as well as potential differences in the contributions of various inputs to VMH neurons of males and females, should be explored. It also remains unknown whether recent findings describing the capacity for male rodents to be highly reinforced by aggression extends to females. Considering the propensity for women, particularly those with neuropsychiatric disorders, to carry out premeditated violent acts [ 33 ], it is crucial that the field extends its efforts to interrogate underlying circuit mechanisms of reward, if any, in female animal models of aggression.
Conclusions
In order to develop more effective treatments for neuropsychiatric illnesses marked by heightened aggression, we must consider the fact that aggression symptoms in clinical populations take various forms, which likely involve different mechanisms and underlying circuitry. For example, while treatments for patients with personality disorders may require reducing the valence of aggression to prevent future violent acts, treatments for aggressive patients with Alzheimer’s disease may require a normalization of circadian dysfunction. Patients who display reactive aggression, such as those with Intermittent Explosive disorder, may require treatments that improve function in circuits controlling behavioral inhibition and top–down impulse control. Moreover, there are clear sex differences in aggression that we need to better understand before we can adequately address aggression circuitry in men versus women. Ultimately, our ability to develop personalized treatments for the broad array of aggressive disorders will require the systematic utilization of a variety of animal models, informed by studies of human illness, which recapitulate specific etiological factors driving aggressive behavior.
World Health Organization. World report on violence and health. Geneva, Switzerland; 2002.
Barlow K, Grenyer B, Ilkiw-Lavalle O. Prevalence and precipitants of aggression in psychiatric inpatient units. Aust NZ J Psychiatry. 2000;34:967–74.
Article CAS Google Scholar
Anderson DJ. Optogenetics, sex, and violence in the brain: implications for psychiatry. Biol Psychiatry. 2012;71:1081–9.
Article Google Scholar
Haller J. The neurobiology of abnormal manifestations of aggression--a review of hypothalamic mechanisms in cats, rodents, and humans. Brain Res Bull. 2013;93:97–109.
Golden SA, Shaham Y. Aggression addiction and relapse: a new frontier in psychiatry. Neuropsychopharmacology. 2018;43:224–5.
Falkner AL, Grosenick L, Davidson TJ, Deisseroth K, Lin D. Hypothalamic control of male aggression-seeking behavior. Nat Neurosci. 2016;19:596–604.
Golden SA, Heins C, Venniro M, Caprioli D, Zhang M, Epstein DH, et al. Compulsive addiction-like aggressive behavior in mice. Biol Psychiatry. 2017;82:239–48.
Golden SA, Aleyasin H, Heins R, Flanigan M, Heshmati M, Takahashi A, et al. Persistent conditioned place preference to aggression experience in adult male sexually-experienced CD-1 mice. Genes Brain Behav. 2017;16:44–55.
Golden SA, Heshmati M, Flanigan M, Christoffel DJ, Guise K, Pfau ML, et al. Basal forebrain projections to the lateral habenula modulate aggression reward. Nature. 2016;534:688–92.
Putkonen P. Attack elicited by forebrain and hypothalamic stimulation in the chicken. Experientia.1966;2:405–7.
Hashikawa Y, Hashikawa K, Falkner A, Lin D. Ventromedial hypothalamus and the generation of aggression. Front Syst Neurosci. 2017;11:94.
Siegel A, Bhatt S, Bhatt R, Zalcman SS. The neurobiological bases for development of pharmacological treatments of aggressive disorders. Curr Neuropharmacol. 2007;5:135–47.
Fanning JR, Keedy S, Berman ME, Lee R, Coccaro EF. Neural correlates of aggressive behavior in real time: a review of fMRI studies of laboratory reactive aggression. Curr Behav Neurosci Rep. 2017;4:138–50.
Aleyasin H FM, Golden SA,Takahashi A, Menard C, Pfau ML, Multer J, et al. Cell-type-specific role of ΔFosB in nucleus accumbens in modulating inter-male aggression. J Neurosci. 2018;38:5913–24.
Hoptman MJ, Volavka J, Czobor P, Gerig G, Chakos M, Blocher J, et al. Aggression and quantitative MRI measures of caudate in patients with chronic schizophrenia or schizoaffective disorder. J Neuropsychiatry Clin Neurosci. 2006;18:509–15.
Decety J, Michalska KJ, Akitsuki Y, Lahey BB. Atypical empathic responses in adolescents with aggressive conduct disorder: a functional MRI investigation. Biol Psychol. 2009;80:203–11.
Aleyasin H, Flanigan ME, Russo SJ. Neurocircuitry of aggression and aggression seeking behavior: nose poking into brain circuitry controlling aggression. Curr Opin Neurobiol. 2018;49:184–91.
Stagkourakis S, Spigolon G, Williams P, Protzmann J, Fisone G, Broberger C. A neural network for intermale aggression to establish social hierarchy. Nat Neurosci. 2018;21:834–42.
Zelikowsky M, Hui M, Karigo T, Choe A, Yang B, Blanco MR, et al. The neuropeptide Tac2 controls a distributed brain state induced by chronic social isolation stress. Cell. 2018;173:1265–79.
Takahashi A, Nagayasu K, Nishitani N, Kaneko S, Koide T. Control of intermale aggression by medial prefrontal cortex activation in the mouse. PLoS ONE. 2014;9:e94657.
Biro L, Sipos E, Bruzsik N, Farkas I, Zelena D, Balazsfi D, Toth M, Haller J. Task division within the prefrontal cortex: distinct neuron populations selectively control different aspects of aggressive behavior via the hypothalamus. J Neurosci. 2018;38:4065–75.
Wang F, Zhu J, Zhu H, Zhang Q, Lin Z, Hu H. Bidirectional control of social hierarchy by synaptic efficacy in medial prefrontal cortex. Science. 2011;334:693–7.
Zhou T, Zhu H, Fan Z, Wang F, Chen Y, Liang H, et al. History of winning remodels thalamo-PFC circuit to reinforce social dominance. Science. 2017;357:162–8.
Biro L, Sipos E, Bruzsik B, Farkas I, Zelena D, Balazsfi D, et al. Task division within the prefrontal cortex: distinct neuron populations selectively control different aspects of aggressive behavior via the hypothalamus. J Neurosci. 2018;38:4065–75.
Yang T, Yang CF, Chizari MD, Maheswaranathan N, Burke KJ Jr, Borius M, et al. Social control of hypothalamus-mediated male aggression. Neuron. 2017;95:955–70.
Lin D, Boyle MP, Dollar P, Lee H, Lein ES, Perona P, et al. Functional identification of an aggression locus in the mouse hypothalamus. Nature. 2011;470:221–6.
Remedios R, Kennedy A, Zelikowsky M, Grewe BF, Schnitzer MJ, Anderson DJ. Social behaviour shapes hypothalamic neural ensemble representations of conspecific sex. Nature. 2017;500:388–92.
Canevelli MVM, Trebbanstoni A, Sarli G, D’Antonio F, Tariciotti L, de Lena C, et al. Sundowning in dementia: clinical relevance, pathophysiological determinants, and therapeutic approaches. Front Med. 2016;3:73.
Todd WD, Fenselau H, Wang JL, Zhang R, Machado NL, Venner A, et al. A hypothalamic circuit for the circadian control of aggression. Nat Neurosci. 2018;21:717–24.
Unger EK, Burke KJ Jr., Yang CF, Bender KJ, Fuller PM, Shah NM. Medial amygdalar aromatase neurons regulate aggression in both sexes. Cell Rep. 2015;10:453–62.
Hashikawa K, Hashikawa Y, Tremblay R, Zhang J, Feng JE, Sabol A, et al. Esr1(+) cells in the ventromedial hypothalamus control female aggression. Nat Neurosci. 2017;20:1580–90.
Yang CF, Chiang MC, Grat DC, Prabhakaran M, Alvarado M, Juntti SA, et al. Sexually dimorphic neurons in the ventromedial hypothalamus govern mating in both sexes and aggression in males. Cell. 2013;153:896–909.
Putkonen H, Collander J, Honkasalo M-L, Lönnqvist J. Personality disorders and psychoses form two distinct subgroups of homicide among female offenders. J Forensic Psychiatry. 2001;12:300–12.
Haller J, van de Schraaf J, Kruk MR. Deviant forms of aggression in glucocorticoid hyporeactive rats: a model for ‘pathological’ aggression? J Neuroendocrinol. 2001;13:102–7.
Miczek KA, Weerts EM, Tornatzky W, DeBold JF, Vatne TM. Alcohol and “bursts” of aggressive behavior: ethological analysis of individual differences in rats. Psychopharmacol (Berl). 1992;107:551–63.
Gobrogge KL, Liu Y, Young LJ, Wang Z. Anterior hypothalamic vasopressin regulates pair-bonding and drug-induced aggression in a monogamous rodent. Proc Natl Acad Sci USA. 2009;106:19144–9.
Ricci LA, Grimes JM, Knyshevski I, Melloni RH. Repeated cocaine exposure during adolescence alters glutamic acid decarboxylase-65 (GAD65) immunoreactivity in hamster brain: correlation with offensive aggression. Brain Res. 2005;1035:131–8.
Toth M, Halasz J, Mikics E, Barsy B, Haller J. Early social deprivation induces disturbed social communication and violent aggression in adulthood. Behav Neurosci. 2008;122:849–54.
Ambar G, Chiavegatto S. Anabolic-androgenic steroid treatment induces behavioral disinhibition and downregulation of serotonin receptor messenger RNA in the prefrontal cortex and amygdala of male mice. Genes Brain Behav. 2009;8:161–73.
de Almeida RM, Miczek KA. Aggression escalated by social instigation or by discontinuation of reinforcement (“frustration”) in mice: inhibition by anpirtoline: a 5-HT1B receptor agonist. Neuropsychopharmacology. 2002;27:171–81.
Download references
Acknowledgements
The authors are supported by NIH grants 1R01MH114882-01 (SJR) and F31 MH111108-01A1 (MEF).
Author information
Authors and affiliations.
Department of Neuroscience, Center for Affective Neuroscience and Friedman Brain Institute, Icahn School of Medicine at Mount Sinai, One Gustav L. Levy Place, New York, NY, 10029, USA
Meghan E. Flanigan & Scott J. Russo
You can also search for this author in PubMed Google Scholar
Corresponding authors
Correspondence to Meghan E. Flanigan or Scott J. Russo .
Ethics declarations
Competing interests.
The authors declare no competing interests.
Additional information
Publisher's note: Springer Nature remains neutral with regard to jurisdictional claims in published maps and institutional affiliations.
Rights and permissions
Reprints and permissions
About this article
Cite this article.
Flanigan, M.E., Russo, S.J. Recent advances in the study of aggression. Neuropsychopharmacol 44 , 241–244 (2019). https://doi.org/10.1038/s41386-018-0226-2
Download citation
Received : 11 July 2018
Revised : 13 September 2018
Accepted : 16 September 2018
Published : 21 September 2018
Issue Date : January 2019
DOI : https://doi.org/10.1038/s41386-018-0226-2
Share this article
Anyone you share the following link with will be able to read this content:
Sorry, a shareable link is not currently available for this article.
Provided by the Springer Nature SharedIt content-sharing initiative
This article is cited by
Hypothalamic subunit volumes and relations to violence and psychopathy in male offenders with or without a psychotic disorder.
- Christina Bell
- Jaroslav Rokicki
- Unn K. Haukvik
European Archives of Psychiatry and Clinical Neuroscience (2024)
Neuroanatomical correlates of aggressiveness: a case–control voxel- and surface-based morphometric study
- Stephanie Seidenbecher
- Maria Schöne
- Thomas Frodl
Brain Structure and Function (2023)
Sex differences in appetitive and reactive aggression
- Antonio V. Aubry
- C. Joseph Burnett
- Scott J. Russo
Neuropsychopharmacology (2022)
Quick links
- Explore articles by subject
- Guide to authors
- Editorial policies

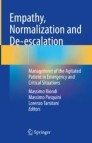
Empathy, Normalization and De-escalation pp 25–29 Cite as

Neurobiology of Aggression and Violence
- Francesco Saverio Bersani 4 ,
- Simone Mimun 4 &
- Roberto Delle Chiaie 4
- First Online: 29 January 2021
966 Accesses
Research has led to the conceptualization of various qualitatively different forms of human aggression, such as the so-called impulsive/defensive/affective aggression and non-impulsive/premeditated/offensive/predatory aggression. A growing amount of data is providing insight into the biological underpinnings of aggressiveness: at the molecular level, several mediators have been linked to such dimension, including serotonin, dopamine, norepinephrine, acetylcholine, glutamate, gamma-aminobutyric acid, vasopressin, oxytocin, opiates, cytokines, testosterone, and cortisol, while at the brain circuitry level, aggressive behaviour is thought to result from impaired complex relationships between cortical and sub-cortical structures. In the present chapter, an overview of the evidence related to clinical, genetic, molecular, and physiological characteristic associated with aggression is provided.
This is a preview of subscription content, log in via an institution .
Buying options
- Available as PDF
- Read on any device
- Instant download
- Own it forever
- Available as EPUB and PDF
- Compact, lightweight edition
- Dispatched in 3 to 5 business days
- Free shipping worldwide - see info
- Durable hardcover edition
Tax calculation will be finalised at checkout
Purchases are for personal use only
Vitiello B, Stoff DM. Subtypes of aggression and their relevance to child psychiatry. J Am Acad Child Adolesc Psychiatry. 1997;36(3):307–15.
Article CAS Google Scholar
Haller J. Normal and abnormal aggressions: definitions and operational approaches. In: Neurobiological bases of abnormal aggression and violent behaviour. Wien: Springer; 2014. p. 1–31.
Chapter Google Scholar
Siever LJ. Neurobiology of aggression and violence. Am J Psychiatry. 2008;165(4):429–42.
Article Google Scholar
Veroude K, et al. Genetics of aggressive behavior: an overview. Am J Med Genet B Neuropsychiatr Genet. 2016;171B(1):3–43.
Meloy JR. Empirical basis and forensic application of affective and predatory violence. Aust N Z J Psychiatry. 2006;40(6–7):539–47.
Bersani FS, Pasquini M. The “outer dimensions”: impulsivity, anger/aggressiveness, activation. In: Biondi M, Pasquini M, Picardi A, editors. Dimensional psychopathology. Cham: Springer International Publishing; 2018. p. 211–32.
Carpiniello B, Mencacci C, Vita A. Violence as a social, clinical, and forensic problem. In: Carpiniello B, Vita A, Mencacci C, editors. Violence and mental disorders. Cham: Springer Nature; 2020. p. 3–24.
Petit JR. Management of the acutely violent patient. Psychiatr Clin North Am. 2005;28(3):701–11, 710.
Brunner HG, et al. Abnormal behavior associated with a point mutation in the structural gene for monoamine oxidase A. Science. 1993;262(5133):578–80.
Manchia M, et al. Neurobiology of violence. In: Carpiniello B, Vita A, Mencacci C, editors. Violence and mental disorders. Cham: Springer Nature; 2020. p. 25–47.
Seo D, Patrick CJ, Kennealy PJ. Role of serotonin and dopamine system interactions in the neurobiology of impulsive aggression and its comorbidity with other clinical disorders. Aggress Violent Behav. 2008;13(5):383–95.
Wong JS, Gravel J. Do sex offenders have higher levels of testosterone? Results from a meta-analysis. Sex Abuse. 2018;30(2):147–68.
Archer J. The influence of testosterone on human aggression. Br J Psychol. 1991;82(Pt 1):1–28.
Haller J. Hormonal determinants. In: Neurobiological bases of abnormal aggression and violent behaviour. Wien: Springer; 2014. p. 33–68.
Coccaro EF. Association of C-reactive protein elevation with trait aggression and hostility in personality disordered subjects: a pilot study. J Psychiatr Res. 2006;40(5):460–5.
Coccaro EF, Lee R, Coussons-Read M. Elevated plasma inflammatory markers in individuals with intermittent explosive disorder and correlation with aggression in humans. JAMA Psychiat. 2014;71(2):158–65.
Montalvo-Ortiz JL, et al. Genome-wide DNA methylation changes associated with intermittent explosive disorder: a gene-based functional enrichment analysis. Int J Neuropsychopharmacol. 2018;21(1):12–20.
Halaris A, Leonard BE. Inflammation in psychiatry. Basel: Karger International; 2013.
Book Google Scholar
Haller J. Neural circuits subserving aggression: general models. In: Neurobiological bases of abnormal aggression and violent behaviour. Wien: Springer; 2014. p. 69–78.
Haller J. Focal points of aggression control. In: Neurobiological bases of abnormal aggression and violent behaviour. Wien: Springer; 2014. p. 79–144.
Damasio H, et al. The return of Phineas Gage: clues about the brain from the skull of a famous patient. Science. 1994;264(5162):1102–5.
Tomlinson MF, Brown M, Hoaken PNS. Recreational drug use and human aggressive behavior: a comprehensive review since 2003. Aggress Violent Behav. 2016;27:9–29.
Schifano F, et al. Substance-use disorders and violence. In: Carpiniello B, Vita A, Mencacci C, editors. Violence and mental disorders. Cham: Springer Nature; 2020. p. 95–114.
Veenema AH, Neumann ID. Neurobiological mechanisms of aggression and stress coping: a comparative study in mouse and rat selection lines. Brain Behav Evol. 2007;70:274–85.
Google Scholar
Download references
Author information
Authors and affiliations.
Department of Human Neurosciences, Sapienza University of Rome, Rome, Italy
Francesco Saverio Bersani, Simone Mimun & Roberto Delle Chiaie
You can also search for this author in PubMed Google Scholar
Corresponding author
Correspondence to Francesco Saverio Bersani .
Editor information
Editors and affiliations.
Massimo Biondi
Department of Human Neuroscience, Sapienza University of Rome, Rome, Italy
Massimo Pasquini
Lorenzo Tarsitani
Rights and permissions
Reprints and permissions
Copyright information
© 2021 Springer Nature Switzerland AG
About this chapter
Cite this chapter.
Bersani, F.S., Mimun, S., Delle Chiaie, R. (2021). Neurobiology of Aggression and Violence. In: Biondi, M., Pasquini, M., Tarsitani, L. (eds) Empathy, Normalization and De-escalation. Springer, Cham. https://doi.org/10.1007/978-3-030-65106-0_2
Download citation
DOI : https://doi.org/10.1007/978-3-030-65106-0_2
Published : 29 January 2021
Publisher Name : Springer, Cham
Print ISBN : 978-3-030-65105-3
Online ISBN : 978-3-030-65106-0
eBook Packages : Medicine Medicine (R0)
Share this chapter
Anyone you share the following link with will be able to read this content:
Sorry, a shareable link is not currently available for this article.
Provided by the Springer Nature SharedIt content-sharing initiative
- Publish with us
Policies and ethics
- Find a journal
- Track your research
JavaScript disabled
You have to enable JavaScript in your browser's settings in order to use the eReader.
Or try downloading the content offline
Did you know?
Reader environment loaded
Loading publication (313.3 KB)
Large documents might take a while
Neurobiology and Neural Circuits of Aggression
Affiliations.
- 1 Department of Neurobiology and Department of Neurosurgery of Second Affiliated Hospital, Key Laboratory for Biomedical Engineering of Education Ministry, Zhejiang University School of Medicine, Hangzhou, Zhejiang, China.
- 2 Department of Neurobiology and Department of Neurosurgery of Second Affiliated Hospital, Key Laboratory for Biomedical Engineering of Education Ministry, Zhejiang University School of Medicine, Hangzhou, Zhejiang, China. [email protected].
- 3 NHC and CAMS Key Laboratory of Medical Neurobiology, MOE Frontier Science Center for Brain Research and Brain Machine Integration, School of Brain Science and Brain Medicine, Zhejiang University, Hangzhou, Zhejiang, China. [email protected].
- PMID: 32852736
- DOI: 10.1007/978-981-15-7086-5_2
Aggression takes several forms and can be offensive or defensive. Aggression between animals of the same species or society aims to inflict harm upon another for the purpose of protecting a resource such as food, reproductive partners, territory, or status. This chapter explores the neurobiology of aggression. We summarize the behavior of aggression, rodent models of aggression, and the correlates of aggressive behavior in the context of neuroendocrinology, neurotransmitter systems, and neurocircuitry. Translational implications of rodent studies are briefly discussed, applying basic research to brain imaging data and therapeutic approaches to conditions where aggression is problematic.
Keywords: Aggression; Hypothalamus; Neural circuits.
Publication types
- Aggression*
- Brain / physiology*
- Models, Animal
- Neurobiology*
- Neurotransmitter Agents

An official website of the United States government
The .gov means it’s official. Federal government websites often end in .gov or .mil. Before sharing sensitive information, make sure you’re on a federal government site.
The site is secure. The https:// ensures that you are connecting to the official website and that any information you provide is encrypted and transmitted securely.
- Publications
- Account settings
Preview improvements coming to the PMC website in October 2024. Learn More or Try it out now .
- Advanced Search
- Journal List
- J Child Adolesc Psychopharmacol

The Neurobiology of Impulsive Aggression
This selective review provides a model of the neurobiology of impulsive aggression from a cognitive neuroscience perspective. It is argued that prototypical cases of impulsive aggression, those associated with anger, involve the recruitment of the acute threat response system structures; that is, the amygdala, hypothalamus, and periaqueductal gray. It is argued that whether the recruitment of these structures results in impulsive aggression or not reflects the functional roles of ventromedial frontal cortex and dorsomedial frontal and anterior insula cortex in response selection. It is also argued that impulsive aggression may occur because of impaired decision making. The aggression may not be accompanied by anger, but it will reflect disrupted evaluation of the rewards/benefits of the action.
Introduction
A ggression can be defined as behavior directed toward harming or injuring another living being who is motivated to avoid such treatment. It is a natural and adaptive part of the mammalian social behavioral repertoire. However, it can become maladaptive if it is exaggerated, persistent, or expressed out of context (Connor et al. 2006 ; Nelson and Trainor 2007 ). Aggressive and antisocial behaviors are the leading cause of all child and adolescent referrals to mental health clinicians (Berkowitz 1993 ). Each antisocial individual has been calculated to cost society up to 10 times more than their healthy counterparts in aggregate healthcare and social service expenditures (Nelson and Trainor 2007 ). Aggression, therefore, is a serious social concern and is an economic burden on society.
Impulsive, also known as reactive, aggression is contrasted with planned or instrumental aggression (Berkowitz 1993 ; Dodge et al. 1997 ). Instrumental aggression is goal directed (e.g., mugging for the purpose of stealing someone's wallet), whereas impulsive (reactive) aggression is initiated as a response to a provocation, without any identifiable goal (Blair 2010 ).
The ability to classify individual aggressive acts as impulsive or instrumental has been questioned however (Bushman and Anderson 2001 ). An example of this would be attempting to classify an incident involving someone shooting a person 5 days after discovering that that person had been having an affair with the shooter's spouse. There is a clear reactive component (anger and frustration); however, the action is planned and, as a gun is used, definitively instrumental. However a distinction can be made between the neural systems that mediate impulsive/reactive aggression to an intense threat and those involved in choosing among instrumental acts, including instrumental aggression. These neural systems will be considered. In addition, it will be argued that the systems involved in response choice also influence whether impulsive aggression is expressed.
It will be argued that: 1) There is a neural circuit that mediates the acute threat response (amygdala, hypothalamus, periaqueductal gray [PAG]) which, when activated to a sufficient degree, initiates impulsive aggression; 2) as a function of its role in representing action values and response choice, the ventromedial frontal cortex (vmPFC) partially determines whether acute threat systems activation results in impulsive aggression; and 3) vmPFC is implicated in reinforcement-based decision making. If vmPFC functioning is compromised, reinforcement-based decision making will be disrupted, leading to “impulsive” behavior including “impulsive” aggression. A fourth argument that will be made is that the dorsomedial frontal and anterior insula cortices are also involved in reinforcement-based decision making and also influence, together with vmPFC, whether impulsive aggression is expressed (see Fig. 1 ).

Systems implicated in impulsive aggression. The circuit running from the amygdala to the hypothalamus and from there to the periaqueductal gray is thought to mediate reactive aggression. The probability that activation of this circuit is expressed as reactive aggression is partly determined by systems implicated in reinforcement-based decision making including the ventromedial (vmPFC) and dorsomedial frontal (dmFC) and anterior insula cortices (AIC). The vmPFC is particularly important for representing the value of actions and objects. The dmFC is thought to use this value information to affect response choice (Hare, Camerer, Rangel, 2009 ), partly implemented through the AIC.
The Acute Threat Response
Animals demonstrate a gradated and instinctual response to threat. Distant threats induce freezing, then, as they draw closer, flight, and, finally, impulsive aggression when they are very close and escape is impossible (Blanchard et al. 1977). As such, impulsive aggression can involve unplanned, enraged attacks on the object perceived to be the threat source. Animal studies have indicated that impulsive aggression is mediated via a circuit that runs from the medial amygdala, largely via the stria terminalis to the medial hypothalamus and from there to the dorsal half of the PAG (Panksepp 1998 ; Gregg and Siegel 2001 ; Nelson and Trainor 2007 ; Lin et al. 2011 ). It has been argued that this circuit mediates impulsive aggression in humans also, not only to threat but also to frustration and social provocation ( Blair 2004 ). Three strands of data support this argument.
First, functional magnetic resonance imaging (fMRI) work with humans has shown that the increasing proximity of a threat is associated with increased activity within the amygdala, hypothalamus and PAG (Mobbs et al. 2007 , 2009 , 2010 ; Coker-Appiah et al. 2013 ). In addition, recent work has demonstrated that these regions also respond to frustrating stimuli. In this study, participants were blocked from obtaining a reward with levels of experienced frustration being parametrically varied by manipulating the participants' motivation to obtain the reward prior to blocking (Yu et al. 2014 ).
Second, there has been work with laboratory-based “models” of impulsive aggression: The Taylor Aggression Paradigm (TAP) (Taylor 1967 ), the Point Subtraction Aggression Paradigm (PSAP) (Cherek et al. 1997 ), and computationally similar social exchange paradigms (Strobel et al. 2011 ; White et al. 2013a , 2014a ). In these tasks, participants have the opportunity to retaliate to other individuals' actions (e.g., previous punishments [TAP], point removals [PSAP], or unfair allocations [social exchange]). In all cases, the participants' aggressive responses (retaliatory responses) are a function of provocation level (Cherek et al. 1997 ; White et al. 2014a ).
Before continuing, it is worth noting that whereas the TAP and PSAP (and retaliatory versions of social exchange) paradigms are considered to index impulsive aggression (Taylor 1967 ; Cherek et al. 1997 ), they do not simply index impulsive aggression. Unfair provocations initiate anger, a definitional feature of impulsive aggression (Berkowitz 1993 ; Cherek et al. 1997 ; Sanfey et al. 2003 ). However, they do not only elicit an instinctual response to threat or intruders. Rather, the participant plans a response, choosing how much to retaliate to the other individual (Cherek et al. 1997 ; White et al. 2014a ). As such, retaliatory behavior should involve activity within acute threat response systems and regions involved in response choice/decision making (discussed subsequently).
In line with the idea that impulsive retaliation will be associated with increased acute threat response system activity, retaliation on the TAP and in social exchange paradigms elicits activity within the amygdala, hypothalamus, and PAG. For example, high relative to low punishments to the competitor on the PSAP have been associated with increased activity within the amygdala and hypothalamus (extending proximal to the PAG) (Veit et al. 2010 ). Similarly, decisions to reject the proposer's unfair offers on social exchange paradigms are associated with increased activity within the PAG (Sanfey et al. 2003 ; Tabibnia et al. 2008 ; Corradi-Dell'Acqua et al. 2013 ). Moreover, the level of punishment delivered to an unfair partner is directly related to the level of PAG activity (Strobel et al. 2011 ; White et al. 2013a , 2014b ).
The third strand of data supporting the argument that the acute threat response systems (amygdala, hypothalamus, and PAG) mediate impulsive aggression comes from work with patient populations at increased risk for impulsive aggression. Given the literature briefly reviewed previously, it can be predicted that such patients will show heightened responsiveness in regions implicated in impulsive aggression to emotional provocation (Blair 2001 ) ; that is, the amygdala, hypothalamus, and PAG (Panksepp 1998 ; Gregg and Siegel 2001 ; Nelson and Trainor 2007 ; Lin et al. 2011 ). In line with this suggestion, patients with posttraumatic stress disorder (PTSD) (Shin et al. 2006 ), intermittent explosive disorder (Coccaro et al. 2007 ), severe mood dysregulation (Thomas et al. 2013 ), and borderline personality disorder (Hazlett et al. 2012 ), as well as impulsively aggressive spouse abusers (Lee et al. 2008 ), all with an increased risk for reactive aggression, all show increased amygdala responsiveness to threatening stimuli relative to comparison individuals. Moreover, a recent study reported a positive association between propensity for impulsive aggression and amygdala responses to fearful expressions in a large sample of individuals ( n =310) (Choe et al. 2015 ). However, none of these studies reported either increased responsiveness of the hypothalamus or the PAG. Although this lack likely reflects methodology, neither region is typically investigated in current fMRI work.
Determining the Behavioral Consequences of Acute Threat System Activation: The Role of the vmPFC
The acute threat circuitry is assumed to be regulated via frontal cortical regions, particularly the vmPFC. The dominant view is that the vmPFC inhibits (“puts the brakes on”) the aggressive responses mediated by the amygdala, hypothalamus, and PAG ( Nelson and Trainor 2007 ; Schiller and Delgado 2010 ; Diekhof et al. 2011 ; Etkin et al. 2011 ). Consistent with this view, some animal studies show that lesions of the vmPFC increase aggression (Izquierdo et al. 2005 ), and human patients with vmPFC lesions are at increased risk for impulsive aggression (Grafman et al. 1996 ). In addition, there has been a report that lesions of the vmPFC show increased amygdala responses to threatening stimuli relative to comparison individuals (Motzkin et al. 2015 ), although other studies report that patients with vmPFC lesions show typical transient reactions to emotional stimuli (Gillihan et al. 2011 ). Moreover, at first pass, the data from the studies of approaching threat also support the “brakes” view. Increasing activity within the PAG as the threat approached was associated with decreasing activity within the vmPFC (Mobbs et al. 2007 , 2009 , 2010 ). Moreover, increasing activity within the PAG during social exchange tasks, as punishment level delivered to an unfair partner increased, was also associated with decreasing activity within the vmPFC (White et al. 2013, 2014), although not always (Strobel et al. 2011 ).
But other data do not support a “brakes” function for the vmPFC. For example, the fMRI literature indicates that the vmPFC is not involved in emotional regulation (Buhle et al. 2014 ). Moreover, vmPFC lesions “protect” the individual from the development of PTSD/depression (Koenigs and Grafman 2009 ). Critically, animal studies demonstrate that vmPFC lesions suppress amygdala activity during decision- making paradigms (Schoenbaum et al. 2006 ) and decrease fear reaction to novel threat stimuli in macaques (Izquierdo et al. 2005 ). Moreover, although studies with patients at increased risk for emotional lability and impulsive aggression are often assumed to demonstrate disruption in the regulatory role of the PFC, the reality is that the data are inconsistent both with respect to whether an effect is shown and, if it is shown, with respect to what region of frontal cortex is implicated (Herpertz et al. 2001 ; Lee et al. 2008 , 2009 ; New et al. 2009 ).
Considerable work demonstrates that the vmPFC, through interactions with the amygdala/caudate, represents object or action value (Schoenbaum et al. 2011 ; O'Doherty et al. 2015 ). Therefore, rather than consider the vmPFC to be simply putting the brakes on the amygdala, it might be better to consider that it provides information on potential rewards and costs of future actions, so that optimal response choice can occur. The optimal choice might be freezing or fighting. According to this view, for example, vmPFC dysfunction reduces, not increases, amygdala responsiveness during decision making because the integrated functioning of these structures is allowing response choice (cf. Schoenbaum and Roesch 2005 ). There is an inverse relationship between PAG and vmPFC activity as a function of retaliatory punishment in the social exchange paradigms, because retaliation is associated with money lost to the participant and the vmPFC is representing this lost reward (White et al. 2013, 2014). Lesions of the vmPFC/orbital frontal cortex (OFC) increase impulsive aggression not because the aggressive response is disinhibited, but rather because the costs and benefits of engaging in impulsive aggression are not properly represented. This view places an instrumental slant on many instances of impulsive aggression; that is, although impulsive aggression may be an automatic response to an extreme threat, it may also be a selected response (as fear reactions to novel threat stimuli and responses on the TAP and PSAP are). In this regard, it is notable that the aggression shown by primates following OFC lesions correlates highly with the aggression shown to the primate by other primates (Bachevalier et al. 2011 ). In other words, the increased aggression may be just one reflection of poorer behavioral choices in the primate following the OFC lesion.
The Role of the vmPFC in Reinforcement-Based Decision Making
Instrumental aggression is, by definition, goal-directed antisocial behavior conducted to gain a favorable outcome (e.g., another individual's money) (Berkowitz 1993 ). As such, instrumental aggression is mediated by the neural architecture that processes instrumental actions generally (Blair et al. 2014 ). An important consideration is that whether or not an instrumental action is initiated depends upon reinforcement-based decision making.
An adequate review of the extensive literature on reinforcement-based decision making is beyond the scope of the current article, particularly given its focus on impulsive aggression (see instead Schoenbaum et al. 2011 ; O'Doherty 2012 ; Rangel and Clithero 2012 ). Core structures involved include the amygdala, vmPFC, dorsomedial frontal cortex (dmFC), anterior insula cortex (AIC) and striatum (Schoenbaum et al. 2011 ; O'Doherty 2012 ; Rangel and Clithero 2012 ;). It is argued that patients with psychopathic traits are at increased risk for instrumental aggression because of a failure to process other individuals' distress (Blair 2013 ). The individual with psychopathic traits is more likely to choose actions that harm others (including instrumental aggression) because the action's costs (in harm to others) are represented weakly (Blair 2013 ). Supporting this hypothesis, amygdala responsiveness to other individuals' fear expressions is inversely associated with instrumental aggression (Lozier et al. 2014 ).
Behavior, however, is often classified as impulsive when it is instrumental but initiated without an adequate processing of the costs/benefits of the action (the individual “impulsively” grabs the small, immediate reward rather than waiting for a period of time for the much greater reward [Mischel et al. 1989 ] or, as a forensic example, mugs an individual despite knowledge of that person's lack of financial resources). A notable task of propensity for this form of impulsiveness is the temporal discounting (TD) task (Mitchell 1999 ). In this task, participants are asked to choose between an immediate reward and a delayed reward of greater value. The smaller the amount of the immediate reward that the participants will accept in preference to a larger future reward reflects their level of impulsivity (Mitchell 1999 ).
The appropriate representation of future reward magnitude relies on the responsiveness of the striatum (nucleus accumbens) and vmPFC (for a review, see Peters and Buchel 2011 ). Lesions of the vmPFC increase impulsiveness on this task (Sellitto et al. 2010 ) and individuals showing greater impulsivity on the task show weaker striatal responsiveness to future rewards (e.g., Ballard and Knutson 2009 ). Consistent with previous findings of reduced representation of reward information within striatum and vmPFC in youth with conduct disorder (CD) (Finger et al. 2008 ; Crowley et al. 2010 ; Finger et al. 2011 ; White et al. 2013b ), youth with CD show increased impulsiveness on the TD task (White et al. 2014b ).
In short, failure to adequately represent rewards will result in impulsive behavior (i.e., poorly motivated behavioral choices) including, potentially, an increased risk for “impulsive” aggression.
The Role of Other Regions of the Cortex: Dorsomedial and Anterior Insula Cortices
It should be noted tha, studies have shown that frustration and social provocation evoke responses within the dmFC and AIC, as well as in the vmPFC (King-Casas et al. 2008 ; Rilling et al. 2008 ; Sanfey et al. 2008; Corradi-Dell'acqua et al. 2013 ; White et al. 2014a ; Yu et al. 2014 ). It is not typically suggested that these regions are involved in the regulation of the amygdala/PAG. Instead, these regions are implicated in the representation of outcomes and response choice, particularly the avoidance of suboptimal outcomes (Alexander and Brown 2011 ). The suggestion is that the dmPFC responds to unexpected outcomes (cf. Alexander and Brown 2011 ) and the AIC/inferior frontal gyrus orchestrates potentially necessary changes in behavioral response (cf. Blair and Cipolotti 2000 ; Budhani et al. 2007 ). The functional integrity of these structures can be indexed through “behavioral inhibition” tasks (e.g., the Stop and Go/No-Go tasks). Impaired performance on these tasks is associated with an increased risk for impulsive aggression (Young et al. 2009 ).
Conclusions
The goal of this review was to provide a brief overview of the neurobiology of impulsive aggression. In summary, the suggestion is that many cases of impulsive aggression, particularly those associated with anger, involve the recruitment of the acute threat response system (amygdala, hypothalamus, and PAG). It is suggested the impulsive aggressive response, mediated by the acute threat system, is modulated by the vmPFC. The argument is not that the vmPFC puts the “brakes” on the acute threat response but rather that it allows the representation of expected rewards and punishments associated with the action. This information is then utilized by other regions, perhaps particularly the dmFC in conjunction with the AIC, which will either initiate impulsive aggression or prevent it, depending upon reinforcement expectancies. The vmPFC, together with the dmFC/AIC, are involved in response choice generally. If they are compromised, behavior generally is more likely to be impulsive. There may be an increase in (impulsive) aggression as a result of this increased impulsivity.
Clinical Significance
An understanding of the neurobiology of aggression provides an underlying framework for clinical decision making with respect to aggressive patients. This literature stresses that decisions for the patient presenting with elevated instrumental aggression should be different from those presenting with impulsive aggression. If the patient is presenting with instrumental aggression, particularly if this is accompanied by high limited prosocial emotions, current interventions are likely to be less successful. If the patient is presenting with predominantly impulsive aggression, then the current literature particularly stresses interventions that might reduce acute threat response related activity and/or improve the role of the vmPFC in modulating behavior via reward expectation representation. Moreover, the literature therefore stresses that these functional processes should be considered treatment targets. It will be important to determine whether current and future interventions influence the functioning of these mechanisms.
Disclosures
No competing financial interests exist.
- Alexander WH, Brown JW: Medial prefrontal cortex as an action-outcome predictor . Nat Neurosci 14 :1338–1344, 2011 [ PMC free article ] [ PubMed ] [ Google Scholar ]
- Bachevalier J, Machado CJ, Kazama A: Behavioral outcomes of late-onset or early-onset orbital frontal cortex (areas 11/13) lesions in rhesus monkeys . Ann N Y Acad Sci 1239 :71–86, 2011 [ PMC free article ] [ PubMed ] [ Google Scholar ]
- Ballard K, Knutson B: Dissociable neural representations of future reward magnitude and delay during temporal discounting . Neuroimage 45 :143–150, 2009 [ PMC free article ] [ PubMed ] [ Google Scholar ]
- Berkowitz L: Aggression: Its causes, consequences, and control . Philadelphia:Temple University Press; 1993 [ Google Scholar ]
- Blair RJ: The neurobiology of psychopathic traits in youths . Nat Rev Neurosci 14 :786–799, 2013 [ PMC free article ] [ PubMed ] [ Google Scholar ]
- Blair RJR: Neuroimaging of psychopathy and antisocial behavior: A targeted review . Curr Psychiatry Rep 12 :76–82, 2010 [ PMC free article ] [ PubMed ] [ Google Scholar ]
- Blair RJR: The roles of orbital frontal cortex in the modulation of antisocial behavior . Brain Cogn 55 :198–208, 2004 [ PubMed ] [ Google Scholar ]
- Blair RJR. Neurocognitive models of aggression, the antisocial personality disorders, and psychopathy . J Neurol Neurosurg Psychiatry 71 :727–731, 2001 [ PMC free article ] [ PubMed ] [ Google Scholar ]
- Blair RJ, Cipolotti L: Impaired social response reversal. A case of 'acquired sociopathy' . Brain 123 ( Pt 6 ):1122–1141, 2000 [ PubMed ] [ Google Scholar ]
- Blair RJ, White SF, Meffert H, Hwang S: Disruptive behavior disorders: Taking an RDoC(ish) approach . Curr Top Behav Neurosci 16 :319–336, 2014 [ PubMed ] [ Google Scholar ]
- Budhani S, Marsh AA, Pine DS, Blair RJR: Neural correlates of response reversal: Considering acquisition . Neuroimage 34 :1754–1765, 2007 [ PubMed ] [ Google Scholar ]
- Buhle JT, Silvers JA, Wager TD, Lopez R, Onyemekwu C, Kober H, Weber J, Ochsner KN: Cognitive reappraisal of emotion: a meta-analysis of human neuroimaging studies . Cereb Cortex 24 :2981–2990, 2014 [ PMC free article ] [ PubMed ] [ Google Scholar ]
- Bushman BJ, Anderson CA: Is it time to pull the plug on the hostile versus instrumental aggression dichotomy? Psychol Rev 108 :273–279, 2001 [ PubMed ] [ Google Scholar ]
- Cherek DR, Moeller FG, Schnapp W, Dougherty DM: Studies of violent and nonviolent male parolees: I. Laboratory and psychometric measurements of aggression . Biol Psychiatry 41 :514–522, 1997 [ PubMed ] [ Google Scholar ]
- Choe DE, Shaw DS, Forbes EE: Maladaptive social information processing in childhood predicts young men's atypical amygdala reactivity to threat . J Child Psychol Psychiatry 56 :549–557, 2015 [ PMC free article ] [ PubMed ] [ Google Scholar ]
- Coccaro EF, McCloskey MS, Fitzgerald DA, Phan KL: Amygdala and orbitofrontal reactivity to social threat in individuals with impulsive aggression . Biol Psychiatry 62 :168–178, 2007 [ PubMed ] [ Google Scholar ]
- Coker–Appiah DS, White SF, Clanton R, Yang J, Martin A, Blair RJ: Looming animate and inanimate threats: The response of the amygdala and periaqueductal gray . Soc Neurosci 8 :621–630, 2013 [ PMC free article ] [ PubMed ] [ Google Scholar ]
- Connor DF, Carlson GA, Chang KD, Daniolos PT, Ferziger R, Findling RL, Hutchinson JG, Malone RP, Halperin JM, Plattner B, Post RM, Reynolds DL, Rogers KM, Saxena K, Steiner H: Juvenile maladaptive aggression: A review of prevention, treatment, and service configuration and a proposed research agenda . J Clin Psychiatry 67 :808–820, 2006 [ PubMed ] [ Google Scholar ]
- Corradi–Dell'Acqua C, Civai C, Rumiati RI, Fink GR: Disentangling self- and fairness-related neural mechanisms involved in the ultimatum game: An fMRI study . Soc Cogn Affect Neurosci 8 :424–431, 2013 [ PMC free article ] [ PubMed ] [ Google Scholar ]
- Crowley TJ, Dalwani MS, Mikulich–Gilbertson SK, Du YP, Lejuez CW, Raymond KM, Banich MT: Risky decisions and their consequences: Neural processing by boys with antisocial substance disorder . PLoS One 5 :e12835, 2010 [ PMC free article ] [ PubMed ] [ Google Scholar ]
- Diekhof EK, Geier K, Falkai P, Gruber O: Fear is only as deep as the mind allows. A coordinate-based meta-analysis of neuroimaging studies on the regulation of negative affect . Neuroimage 58 :275–285, 2011 [ PubMed ] [ Google Scholar ]
- Dodge KA, Lochman JE, Harnish JD, Bates JE, Pettit GS: Reactive and proactive aggression in school children and psychiatrically impaired chronically assaultive youth . J Abnorm Psychol 106 :37–51, 1997 [ PubMed ] [ Google Scholar ]
- Etkin A, Egner T, Kalisch R: Emotional processing in anterior cingulate and medial prefrontal cortex . Trends Cogn Sci 15 :85–93, 2011 [ PMC free article ] [ PubMed ] [ Google Scholar ]
- Finger EC, Marsh AA, Blair KS, Reid ME, Sims C, Ng P, Pine DS, Blair RJR: Disrupted reinforcement signaling in the orbital frontal cortex and caudate in youths with conduct disorder or oppositional defiant disorder and a high level of psychopathic traits . Am J Psychiatry 168 :834–841, 2011 [ PMC free article ] [ PubMed ] [ Google Scholar ]
- Finger EC, Marsh AA, Mitchell DG, Reid ME, Sims C, Budhani S, Kosson DS, Chen G, Towbin KE, Leibenluft E, Pine DS, Blair JR: Abnormal ventromedial prefrontal cortex function in children with psychopathic traits during reversal learning . Arch Gen Psychiatry 65 :586–594, 2008 [ PMC free article ] [ PubMed ] [ Google Scholar ]
- Gillihan SJ, Xia C, Padon AA, Heberlein AS, Farah MJ, Fellows LK: Contrasting roles for lateral and ventromedial prefrontal cortex in transient and dispositional affective experience . Soc Cogn Affect Neurosci 6 :128–137, 2011 [ PMC free article ] [ PubMed ] [ Google Scholar ]
- Grafman J, Schwab K, Warden D, Pridgen BS, Brown HR: Frontal lobe injuries, violence, and aggression: A report of the Vietnam head injury study . Neurology 46 :1231–1238, 1996 [ PubMed ] [ Google Scholar ]
- Gregg TR, Siegel A: Brain structures and neurotransmitters regulating aggression in cats: implications for human aggression . Prog Neuropsychopharmacol Biol Psychiatry 25 :91–140, 2001 [ PubMed ] [ Google Scholar ]
- Hare TA, Camerer CF, Rangel A: Self-control in decision-making involves the modulation of the vmPFC valuation system . Science 324 :646–648, 2009 [ PubMed ] [ Google Scholar ]
- Hazlett EA, Zhang J, New AS, Zelmanova Y, Goldstein KE, Haznedar MM, Meyerson D, Goodman M, Siever LJ, Chu KW: Potentiated amygdala response to repeated emotional pictures in borderline personality disorder . Biol Psychiatry 72 :448–456, 2012 [ PMC free article ] [ PubMed ] [ Google Scholar ]
- Herpertz SC, Dietrich TM, Wenning B, Krings T, Erberich SG, Willmes K, Thron A, Sass H: Evidence of abnormal amygdala functioning in borderline personality disorder: A functional MRI study . Biol Psychiatry 50 :292–298, 2001 [ PubMed ] [ Google Scholar ]
- Izquierdo A, Suda RK, Murray EA: Comparison of the effects of bilateral orbital prefrontal cortex lesions and amygdala lesions on emotional responses in rhesus monkeys . J Neurosci 25 :8534–8542, 2005 [ PMC free article ] [ PubMed ] [ Google Scholar ]
- King–Casas B, Sharp C, Lomax–Bream L, Lohrenz T, Fonagy P, Montague PR: The rupture and repair of cooperation in borderline personality disorder . Science 321 :806–810, 2008 [ PMC free article ] [ PubMed ] [ Google Scholar ]
- Koenigs M, Grafman J: Posttraumatic stress disorder: the role of medial prefrontal cortex and amygdala . Neuroscientist 15 :540–548, 2009 [ PMC free article ] [ PubMed ] [ Google Scholar ]
- Lee TMC, Chan S–C, Raine A: Hyperresponsivity to threat stimuli in domestic violence offenders: A functional magnetic resonance imaging study . J Clin Psychiatry 70 :36–45, 2009 [ PubMed ] [ Google Scholar ]
- Lee TMC, Chan S–C, Raine A: Strong limbic and weak frontal activation to aggressive stimuli in spouse abusers . Mol Psychiatry 13 :655–656, 2008 [ PubMed ] [ Google Scholar ]
- Lin D, Boyle MP, Dollar P, Lee H, Lein ES, Perona P, Anderson DJ: Functional identification of an aggression locus in the mouse hypothalamus . Nature 470 :221–226, 2011 [ PMC free article ] [ PubMed ] [ Google Scholar ]
- Lozier LM, Cardinale EM, VanMeter JW, Marsh AA: Mediation of the relationship between callous-unemotional traits and proactive aggression by amygdala response to fear among children with conduct problems . JAMA Psychiatry 71 :627–636, 2014 [ PMC free article ] [ PubMed ] [ Google Scholar ]
- Mischel W, Shoda Y, Rodriguez ML: Delay of gratification in children . Science 244 :933–938, 1989 [ PubMed ] [ Google Scholar ]
- Mitchell SH: Measures of impulsivity in cigarette smokers and non-smokers . Psychopharmacology (Berl) 146 :455–464, 1999 [ PubMed ] [ Google Scholar ]
- Mobbs D, Marchant JL, Hassabis D, Seymour B, Tan G, Gray M, Petrovic P, Dolan RJ, Frith CD: From threat to fear: The neural organization of defensive fear systems in humans . J Neurosci 29 :12236–12243, 2009 [ PMC free article ] [ PubMed ] [ Google Scholar ]
- Mobbs D, Petrovic P, Marchant JL, Hassabis D, Weiskopf N, Seymour B, Dolan RJ, Frith CD: When fear is near: Threat imminence elicits prefrontal-periacqueductal gray shifts in humans . Science 317 :1079–1083, 2007 [ PMC free article ] [ PubMed ] [ Google Scholar ]
- Mobbs D, Yu R, Rowe JB, Eich H, FeldmanHall O, Dalgleish T: Neural activity associated with monitoring the oscillating threat value of a tarantula . Proc Natl Acad Sci U S A 107 :20,582–20, 586, 2010 [ PMC free article ] [ PubMed ] [ Google Scholar ]
- Motzkin JC, Philippi CL, Wolf RC, Baskaya MK, Koenigs M: Ventromedial prefrontal cortex is critical for the regulation of amygdala activity in humans . Biol Psychiatry 77 :276–284, 2015 [ PMC free article ] [ PubMed ] [ Google Scholar ]
- Nelson RJ, Trainor BC: Neural mechanisms of aggression . Nat Rev Neurosci 8 :536–546, 2007 [ PubMed ] [ Google Scholar ]
- New AS, Hazlett EA, Newmark RE, Zhang J, Triebwasser J, Meyerson D, Lazarus S, Trisdorfer R, Goldstein KE, Goodman M, Koenigsberg HW, Flory JD, Siever LJ, Buchsbaum MS: Laboratory induced aggression: A positron emission tomograpy study of aggressive individuals with borderline personality disorder . Biol Psychiatry 66 :1107–1114, 2009 [ PMC free article ] [ PubMed ] [ Google Scholar ]
- O'Doherty JP: Beyond simple reinforcement learning: the computational neurobiology of reward-learning and valuation . Eur J Neurosci 35 :987–990, 2012 [ PubMed ] [ Google Scholar ]
- O'Doherty JP, Lee SW, McNamee D: The structure of reinforcement-learning mechanisms in the human brain . Curr Opin Behav Sci 1 :94–100, 2015 [ Google Scholar ]
- Panksepp J: Affective Neuroscience: The Foundations of Human and Animal Emotions . New York: Oxford University Press; 1998 [ Google Scholar ]
- Peters J, Buchel C: The neural mechanisms of inter-temporal decision-making: understanding variability . Trends Cogn Sci 15 :227–239, 2011 [ PubMed ] [ Google Scholar ]
- Rangel A, Clithero JA: Value normalization in decision making: theory and evidence . Curr Opin Neurobiol 22 :970–981, 2012 [ PMC free article ] [ PubMed ] [ Google Scholar ]
- Rilling JK, Goldsmith DR, Glenn AL, Jairam MR, Elfenbein HA, Dagenais JE, Murdock CD, Pagnoni G: The neural correlates of the affective response to unreciprocated cooperation . Neuropsychologia 46 :1256–1266, 2008 [ PubMed ] [ Google Scholar ]
- Sanfey AG, Rilling JK, Aronson JA, Nystrom LE, Cohen JD: The neural basis of economic decision-making in the Ultimatum Game . Science 300 :1755–1758, 2003 [ PubMed ] [ Google Scholar ]
- Schiller D, Delgado MR: Overlapping neural systems mediating extinction, reversal and regulation of fear . Trends Cogn Sci 14 :268–276, 2010 [ PMC free article ] [ PubMed ] [ Google Scholar ]
- Schoenbaum G, Roesch M: Orbitofrontal cortex, associative learning, and expectancies . Neuron 47 :633–636, 2005 [ PMC free article ] [ PubMed ] [ Google Scholar ]
- Schoenbaum G, Roesch MR, Stalnaker TA: Orbitofrontal cortex, decision-making and drug addiction . Trends Neurosci 29 :116–124, 2006 [ PMC free article ] [ PubMed ] [ Google Scholar ]
- Schoenbaum G, Takahashi Y, Liu TL, McDannald MA: Does the orbitofrontal cortex signal value? Ann N Y Acad Sci 1239 :87–99, 2011 [ PMC free article ] [ PubMed ] [ Google Scholar ]
- Sellitto M, Ciaramelli E, di Pellegrino G: Myopic discounting of future rewards after medial orbitofrontal damage in humans . J Neurosci 30 :16429–16436, 2010 [ PMC free article ] [ PubMed ] [ Google Scholar ]
- Shin LM, Rauch SL, Pitman RK: Amygdala, medial prefrontal cortex, and hippocampal function in PTSD . Ann N Y Acad Sci 1071 :67–79, 2006 [ PubMed ] [ Google Scholar ]
- Strobel A, Zimmermann J, Schmitz A, Reuter M, Lis S, Windmann S, Kirsch P: Beyond revenge: Neural and genetic bases of altruistic punishment . Neuroimage 54 :671–680, 2011 [ PubMed ] [ Google Scholar ]
- Tabibnia G, Satpute AB, Lieberman MD: The sunny side of fairness: Preference for fairness activates reward circuitry (and disregarding unfairness activates self-control circuitry) . Psychol Sci 19 :339–347, 2008 [ PubMed ] [ Google Scholar ]
- Taylor SP: Aggressive behavior and physiological arousal as a function of provocation and the tendency to inhibit aggression . J Pers 35 :297–310, 1967 [ PubMed ] [ Google Scholar ]
- Thomas LA, Kim P, Bones BL, Hinton KE, Milch HS, Reynolds RC, Adleman NE, Marsh AA, Blair RJ, Pine DS, Leibenluft E: Elevated amygdala responses to emotional faces in youths with chronic irritability or bipolar disorder . Neuroimage (Amst) 2 :637–645, 2013 [ PMC free article ] [ PubMed ] [ Google Scholar ]
- Veit R, Lotze M, Sewing S, Missenhardt H, Gaber T, Birbaumer N: Aberrant social and cerebral responding in a competitive reaction time paradigm in criminal psychopaths . Neuroimage 49 :3365–3372, 2010 [ PubMed ] [ Google Scholar ]
- White SF, Brislin SJ, Meffert H, Sinclair S, Blair RJR: Callous-unemotional traits modulate the neural response associated with punishing another individual during social exchange: A preliminary investigation . J Pers Disord 27 :99–112, 2013a [ PMC free article ] [ PubMed ] [ Google Scholar ]
- White SF, Brislin SJ, Sinclair S, Blair JR: Punishing unfairness: Rewarding or the organization of a reactively aggressive response? Hum Brain Mapp 35 :2137–2147, 2014a [ PMC free article ] [ PubMed ] [ Google Scholar ]
- White SF, Clanton R, Brislin SJ, Meffert H, Hwang S, Sinclair S, Blair RJ: Reward: Empirical contribution. Temporal discounting and conduct disorder in adolescents . J Pers Disord 28 :5–18, 2014b [ PMC free article ] [ PubMed ] [ Google Scholar ]
- White SF, Pope K, Sinclair S, Fowler KA, Brislin SJ, Williams WC, Pine DS, Blair RJ: Disrupted expected value and prediction error signaling in youths with disruptive behavior disorders during a passive avoidance task . Am J Psychiatry 170 :315–323, 2013b [ PMC free article ] [ PubMed ] [ Google Scholar ]
- Young SE, Friedman NP, Miyake A, Willcutt EG, Corley RP, Haberstick BC, Hewitt JK: Behavioral disinhibition: Liability for externalizing spectrum disorders and its genetic and environmental relation to response inhibition across adolescence . J Abnorm Psychol 118 :117–130, 2009 [ PMC free article ] [ PubMed ] [ Google Scholar ]
- Yu R, Mobbs D, Seymour B, Rowe JB, Calder AJ: The neural signature of escalating frustration in humans . Cortex 54 :165–178, 2014 [ PubMed ] [ Google Scholar ]

IMAGES
VIDEO
COMMENTS
Acts of violence account for an estimated 1.43 million deaths worldwide annually. While violence can occur in many contexts, individual acts of aggression account for the majority of instances. In some individuals, repetitive acts of aggression are grounded in an underlying neurobiological susceptibility that is just beginning to be understood.
This field of research is promoted by the development of high-resolution brain imaging techniques and high-throughput genomics analyses, both of which considerably developed after the 1990s. ... In this comprehensive review, we discuss the neurobiology of aggression in humans based on the results of neuroanatomy, neuroimaging, neurotransmitters ...
However, despite significant progress in research, the mechanisms through which these factors lead to aggressive behaviors are poorly understood. This review provides the current state of knowledge regarding the neurobiology of aggression and highlights recent evidence discussing its relationship with alcohol and trauma.
Aggression is an evolutionarily conserved behavior that controls social hierarchies and protects valuable resources like mates, food, and territory. In most cases, aggression is a normal and ...
Aggression and violence represent a significant public health concern and a clinical challenge for the mental healthcare provider. A great deal has been revealed regarding the neurobiology of violence and aggression, and an integration of this body of knowledge will ultimately serve to advance clinical diagnostics and therapeutic interventions.
Acts of violence account for an estimated 1.43 million deaths worldwide annually. While violence can occur in many contexts, individual acts of aggression account for the majority of instances. In some individuals, repetitive acts of aggression are grounded in an underlying neurobiological susceptibility that is just beginning to be understood. The failure of "top-down" control systems in ...
1. Aggression-history studies investigate the neural signatures of aggression-proneness. Aggression is evaluated by psychological and psychiatric inventories and criminal records. In their turn, brain mechanisms are investigated with a delay, by the methodologies that are briefly presented below.
The research on the relationship between testosterone and aggression in humans has mainly been stimulated by studies suggesting that (1) in several animal species, testosterone facilitates aggression; (2) testosterone is higher in men than in women, with men having higher levels of aggression than women; and (3) individuals with more aggressive ...
Thus, advances in the un-derstanding of the neurobiology of violence can contrib-ute meaningfully to a rational assessment and treatment of individuals with pathological aggression and a predis-position to violence. Received Nov. 16, 2007; revision received Jan. 11, 2008; accepted Jan. 14, 2008 (doi: 10.1176/appi.ajp.2008.07111774).
Recent advances in behavioral models of aggression. In order to investigate the neural circuit mechanisms underlying pathological aggressive behavior in humans, it is critical that researchers utilize animal models that fully capture the essential features of excessive human violence (see Table 1).Clinically, aggressive behavior is commonly classified as either proactive or reactive, and each ...
The Neurobiological Basis of Human Aggression: A Review on Genetic and Epigenetic Mechanisms. Am J Med Genet Part B 171B:650-675. in children and adolescents can have long-term negative effects on the individual development, especially with regard to interaction with peers and other social relationships.
Aggression and violence represent a significant public health concern and a clinical challenge for the mental healthcare provider. A great deal has been revealed regarding the neurobiology of violence and aggression, and an integration of this body of knowledge will ultimately serve to advance clinical diagnostics and therapeutic interventions ...
Social experience generates persistent aggressive states. •. Changes in experience-dependent sex hormones remodel local and long-range circuits in the brain's "social behavior network.". •. Circuit-level remodeling may change the relationship between neural activity and an aggression "threshold.".
The neurobiology of aggression. Aggression is an unlearned, not a learned, behaviour. If one needs to be convinced of this fact, he or she should observe the interaction of normal 2-year-olds for several minutes. It is likely that one child will in some way provoke the other, whether with the grabbing of a toy or an unintentional push, and then ...
Aggression can be conceptualized as any behavior, physical or verbal, that involves attacking another person or animal with the intent of causing harm, pain or injury. Because of its high prevalence worldwide, aggression has remained a central clinical and public safety issue. Aggression can be caused by several risk factors, including biological and psychological, such as genetics and mental ...
While violence can occur in many contexts, individual acts of aggression account for the majority of instances. In some individuals, repetitive acts of aggression are grounded in an underlying neurobiological susceptibility that is just beginning to be understood. The failure of "top-down" control systems in the prefrontal cortex to modulate ...
In this chapter, we review research that explores the roles of serotonin, norepinephrine, dopamine, glutamate, and γ-aminobutyric acid (GABA) in aggressive behavior. We highlight how maladaptive aggression is determined by neural activity in specific pathways and receptors in the serotonin system, which in turn are modulated by catecholamines ...
Research over the past few decades has also proposed a link between alcohol consumption and aggressive behaviors. Alcohol consumption can escalate aggressive behavior in humans, often leading to domestic violence or serious crimes. Converging lines of evidence have also shown that trauma and posttraumatic stress disorder (PTSD) could have a ...
Aggression comes in many forms: verbal, physical, or sexual; with or without a weapon; and impulsive or premeditated. The absence of a widely accepted definition for aggression and adequately validated scales to gauge the severity of aggressive acts and personality traits has been a problem for research in the neurobiology of aggression.
Over the past few decades, neurobiologists have identified neural circuits that promote and modulate aggression; however, far less is known regarding the motivational processes that drive aggression. Recent research suggests that aggression can activate reward centers in the brain to promote positive valence.
This chapter explores the neurobiology of aggression. We summarize the behavior of aggression, rodent models of aggression, and the correlates of aggressive behavior in the context of neuroendocrinology, neurotransmitter systems, and neurocircuitry. Translational implications of rodent studies are briefly discussed, applying basic research to ...
We provide key information on future research work and best ways to manage anger and reduce aggression. Importantly, future research should investigate how anger related behaviours is acquired and how stress impacts the development of anger. Keywords: Neural ... International Review of Neurobiology. 2010; 91:289-320. doi: 10.1016/S0074-7742 ...
Abstract. This selective review provides a model of the neurobiology of impulsive aggression from a cognitive neuroscience perspective. It is argued that prototypical cases of impulsive aggression, those associated with anger, involve the recruitment of the acute threat response system structures; that is, the amygdala, hypothalamus, and ...