Digital teaching and learning equipped with PD
What we learned from analyzing tech integration models.
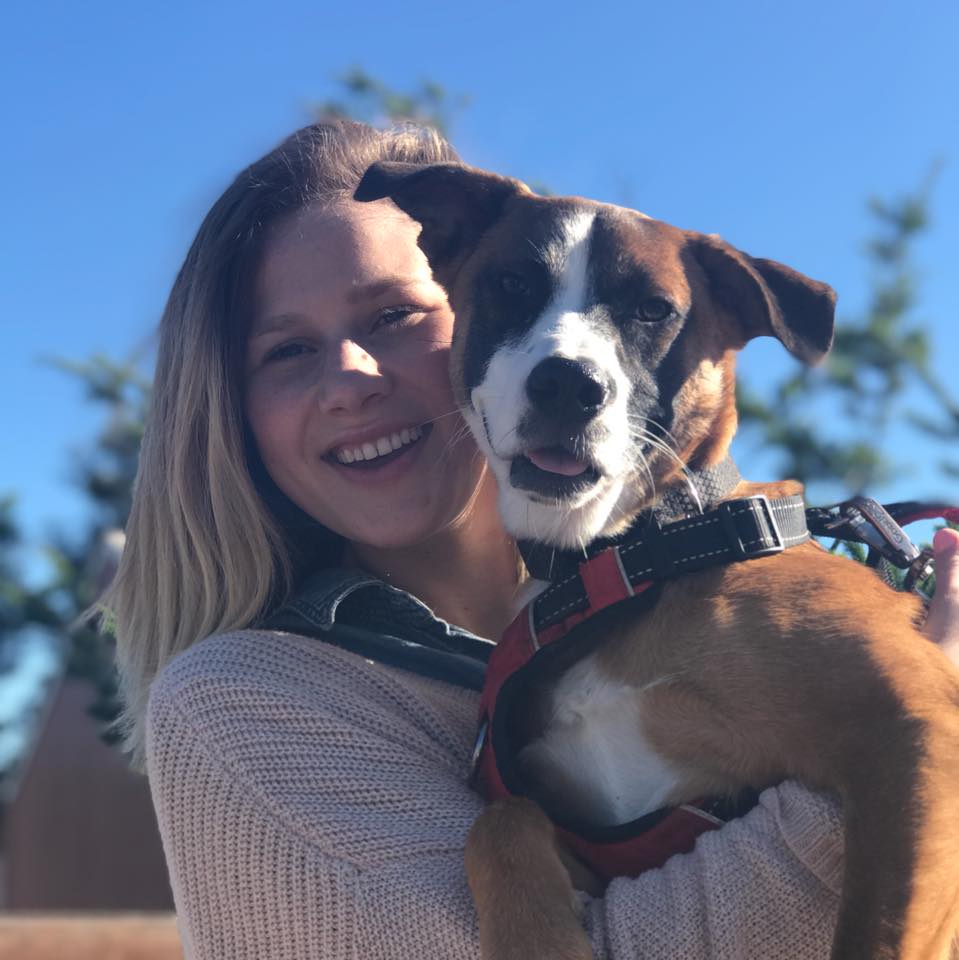
- Share on Twitter
- Share on LinkedIn
- Share on Facebook
- Send to Pinterest
- Share via Facebook
**This article is an excerpt from our whitepaper, The Tech Coach's Guide to Impactful Technology Integration in Schools .
What does tech integration that fosters deep learning look like? With abundant tech integration models, we decided to first look there to begin to conceptually understand what disruptive tech integration looks like. The different models offer insightful perspectives for approaching tech integration and creating meaningful learning experiences with digital tools. We’ve identified a few worthwhile models to include here and summarized their characteristics, benefits, goals, and disruptive qualities.
SAMR is a four-part framework developed by Dr. Ruben Puentedura that categorizes the level of integration on a four-part scale shortened to the acronym SAMR. The letters stand for Substitution, Augmentation, Modification, and Redefinition.
- Substitution : Technology directly substitutes a non-technical function, like viewing a document online rather than in print.
- Augmentation : Technology functionally improves a task while serving the same purpose, like having students perform research on a subject rather than reading pre-selected articles printed in advance.
- Modification : Technology redesigns the learning task altogether, like allowing students to post questions online and having their classmates address them.
- Redefinition : Technology creates entirely new learning experiences, like connecting with experts on a subject virtually and interviewing them to learn more on a topic.
Ultimately, the goal is to get to those deeper levels of tech integration in the classroom – modification then redefinition – rather than just substitution and augmentation. Though, substitution and augmentation should not be discarded and are effective in some cases.
To conceptualize the depth of integration and learning, SAMR can be aligned with Bloom’s Taxonomy as shown in the image below.
The lower levels of Bloom’s – remembering, understanding, and applying – fit with substitution and augmentation. As tech integration deepens, so do the ways we engage students with content, which aligns well with analyzing, evaluating, and creating.
What are the benefits of this model? SAMR focuses on the depth of technology in the classroom and the functional role it is playing in the context of student learning.
What is the goal of this model? Learning immersed: educators use technology to transform the very nature of learning.
What does this model teach us about disruption? Embodied in the approach is the disruptive capacity of technology to create authentic and student-led learning opportunities that utilize the adaptive, personalized, and interactive features specific to technology.

Technology Integration Matrix
Developed by the Florida Center for Instructional Technology (FCIT) in 2005, the Technology Integration Matrix , which is now in its third edition, is a 25-cell grid mapping the deployment of technology in the classroom alongside five characteristics for deep and meaningful learning.
The five phases of integration are entry, adoption, adaptation, infusion, and transformation.
- Entry : Technology is initially introduced in the teaching process.
- Adoption : Students are introduced to the functionality and process of different tools.
- Adaptation : Students independently use tools in self-directed ways.
- Infusion : Students identify the tools needed to satisfy the learning task at hand.
- Transformation : Technology opens up entirely new possibilities for learning.
The five characteristics of meaningful learning are active, collaborative, constructive, authentic, and goal directed.
- Active : Learning experiences are founded in the use of technology as opposed to the passive consumption of content through it.
- Collaborative : Learning centers on student collaboration and work between students.
- Constructive : Learning builds upon prior knowledge and is scaffolded in conjunction with students’ developing skills.
- Authentic : Learning is rooted in real-world contexts and extends beyond the bounds of the classroom.
- Goal-Directed : Learning goals are set by students, and they actively monitor their progress toward them.
Each phase of integration has a corresponding cell aligned to each characteristic, so teachers can see opportunities to make learning meaningful as they integrate technology.
What are the benefits of this model? The Technology Integration Matrix serves as a useful reference for teachers looking to integrate technology and set realistic goals at each phase. With specific examples and ideas for each cell in the matrix, teachers can be strategic as they plan the deployment of technology and build tech-driven learning environments in their classrooms.
What is the goal of this model? Learning bolstered: optimize tech integration for deep learning.
What does this model teach us about disruption? With alignment between the phases of integration and essential characteristics of effective learning, the matrix accentuates elements of active, collaborative, scaffolded, authentic, and student-directed learning through tech integration.
Instruction and Technology Integration Model
The Instruction and Technology Integration Model outlines key instructional modes in a blended learning environment. This model was created intentionally to answer ‘the how’ for tech integration. With a focus on closing the achievement gap and fostering equity in our education system, the model hones student engagement through effective tech integration in optimal learning environments.
The key modes are teacher-driven, group-driven, and student-driven, followed by High Impact Centers.
- Teacher-Driven : Teacher introduces new concepts and demonstrates to the class.
- Group-Driven : Teacher facilitates learning with technology and offers feedback to students.
- Group-Driven (Part 2) : Teacher facilitates learning with technology, and students work in differentiated groups.
- Student-Driven : Students work independently, and teacher provides feedback as needed.
- High Impact Centers : Students work in groups and independently in a project-based environment; the structure of the class depends on students’ abilities.
At each phase, the model offers the primary role, key actions, format, responsibilities, tech integration options, and research to support the approach.
What are the benefits of this model? The Instruction and Technology Integration Model offers concrete actions teachers can take to enhance learning through tech integration. With implementation examples, teachers are equipped to leverage this approach for their classrooms.
What is the goal of this model? Learning facilitated: guide students to understanding through constructive support that closes achievement gaps
What does this model teach us about disruption? The disruptive effect of blended learning with elements of collaboration, differentiation, and hands-on practice immerses students in accessible and impactful learning.
Three Tiers of Technology Integration Model
Originally released by Washington State’s Office of Public Instruction, the Three Tiers of Technology Integration illustrate the depth of technology in classrooms. The tiers cover the different functions of technology in learning environments and each progressively enhance the quality and depth of technology’s role: Teacher Focus on Productivity, Instructional Presentation and Student Productivity, and Powerful Student-Centered 21st Century Learning Environment.
- Teacher Focus on Productivity : Technology helps teachers enhance their productivity and supports with classroom management and administrative functions.
- Instructional Presentation and Student Productivity : Technology facilitates learning by delivering instructional content and providing tools to perform work.
- Powerful Student-Centered 21st Century Learning Environment : Technology engages students in collaborative and student-led learning experiences.
What are the benefits of this model? The tiers illustrate the different performative tasks technology supports in classrooms and the value it provides in each capacity.
What is the goal of this model? Learning modernized: leverage technology as a strategic tool for teaching and learning in the 21st century
What does this model teach us about disruption? By advancing how students learn through tech, the approach illustrates the disruptive capacity of technology in collaborative and student-led learning.
What makes tech integration impactful?

TPACK Model
TPACK is a three-part Venn diagram illustrating the diverse intersections of technological knowledge, pedagogical knowledge, and content knowledge:
Individual Sets:
- Technological Knowledge (TK) : How technology is selected and used, as well as the quality of content delivered through the tool
- Pedagogical Knowledge (PK) : How learning is planned and facilitated
- Content Knowledge (CK) : What teachers know about a particular subject or topic
Dual Intersections:
- Pedagogical-Content Knowledge (PCK) : The intersection of the pedagogical and content knowledge sets represents how instruction is facilitated in content areas. This used to be the standard approach to instructional design.
- Technological-Content Knowledge (TCK) : The intersection of the technological and content knowledge sets represents how technology is integrated in content areas.
- Technological-Pedagogical Knowledge (TPK) : The intersection of the technological and pedagogical knowledge sets is how technology is selected and managed throughout the learning experience.
Finally, the intersection of all three sets of knowledge bases is TPACK, which refers to the teachers’ selection and integration of digital tools to enhance the learning experience and provide opportunities for deep and lasting learning in content areas.
What are the benefits of this model? TPACK builds upon more traditional approaches to instruction, making tech integration an enhancement to existing instructional design processes. By blending technology with both content and pedagogy, TPACK helps teachers develop holistic and intentional strategies for the application of digital tools.
What is the goal of this model? Learning redesigned: develop tech-driven learning experiences optimized to fit pedagogical and content needs
What does this model teach us about disruption? Depending on the depth of tech integration, the model could involve any number of disruptive qualities to hone existing pedagogical approaches like active, authentic, and collaborative learning.
Definitive Qualities of Disruption
The above models share the common goal of deepening tech integration in the classroom and focus on how teachers can meaningfully build tech-driven learning experiences. Keep in mind that the models aren’t mutually exclusive; they can be followed in tandem, modified and combined, or just serve as #techinspo and references to spark ideas in your coffee-fueled classroom planning sessions.
What I find to be most important about the tech integration models we researched is they don’t talk about the types of tools educators need to be using; rather, they focus on the qualities a digital tool or program brings to the learning experience. In a lot of ways, it levels the playing field between Google Earth and VR goggles or 3D printers and – well – normal printers. Tech integration doesn’t mean having to have cutting-edge technology (but it doesn’t discount this either); it means finding opportunities to optimize, enhance, and transform learning.
We are seeing these opportunities, inspired by digital capabilities, take hold in education. Besides the aforementioned blended learning, there are others like project-based learning and maker education. And online instructional content is also popularizing computer-based learning. These and other approaches are all coming about in response to instructional shifts founded in technology and the need to design learning that builds conceptual knowledge and skills.
Tech integration’s goal must be to enhance and transform the learning process so that it is effective for today’s students and their futures. In whatever model, disruption is the North Star.
Related Articles
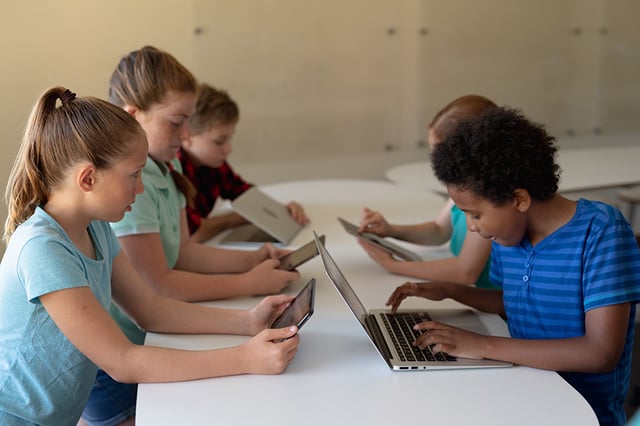
The Digital Use Divide in Education, the Digital Equity Series
The digital use divide concerns the nature of technology integration in student learning and whether it is used in passive or active ways.
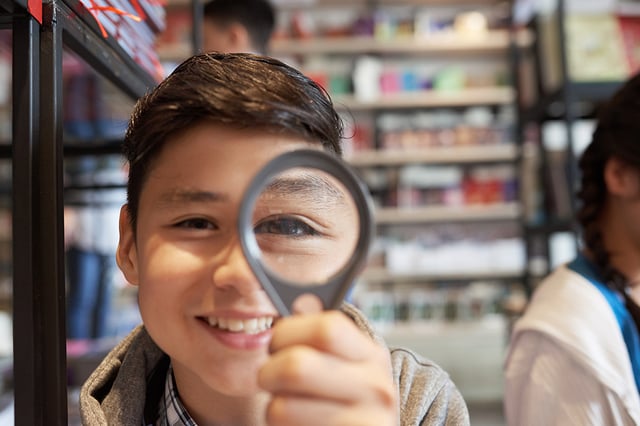
On the Quest for Relevance with Technology Integration
With technology integration, teachers must feel empowered to try, to learn from failure, and to invent new ways to teach with digital tools in the classroom.
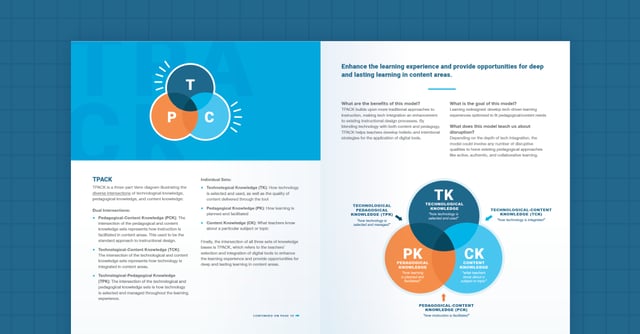
The Tech Coach's Guide to Impactful Technology Integration in Schools
This whitepaper defines effective tech integration for student learning and gives readers strategies to enhance their use of technology in the classroom.
Presented by
© Copyright 2023 equip by Learning.com
Cookie Settings
End of page. Back to Top
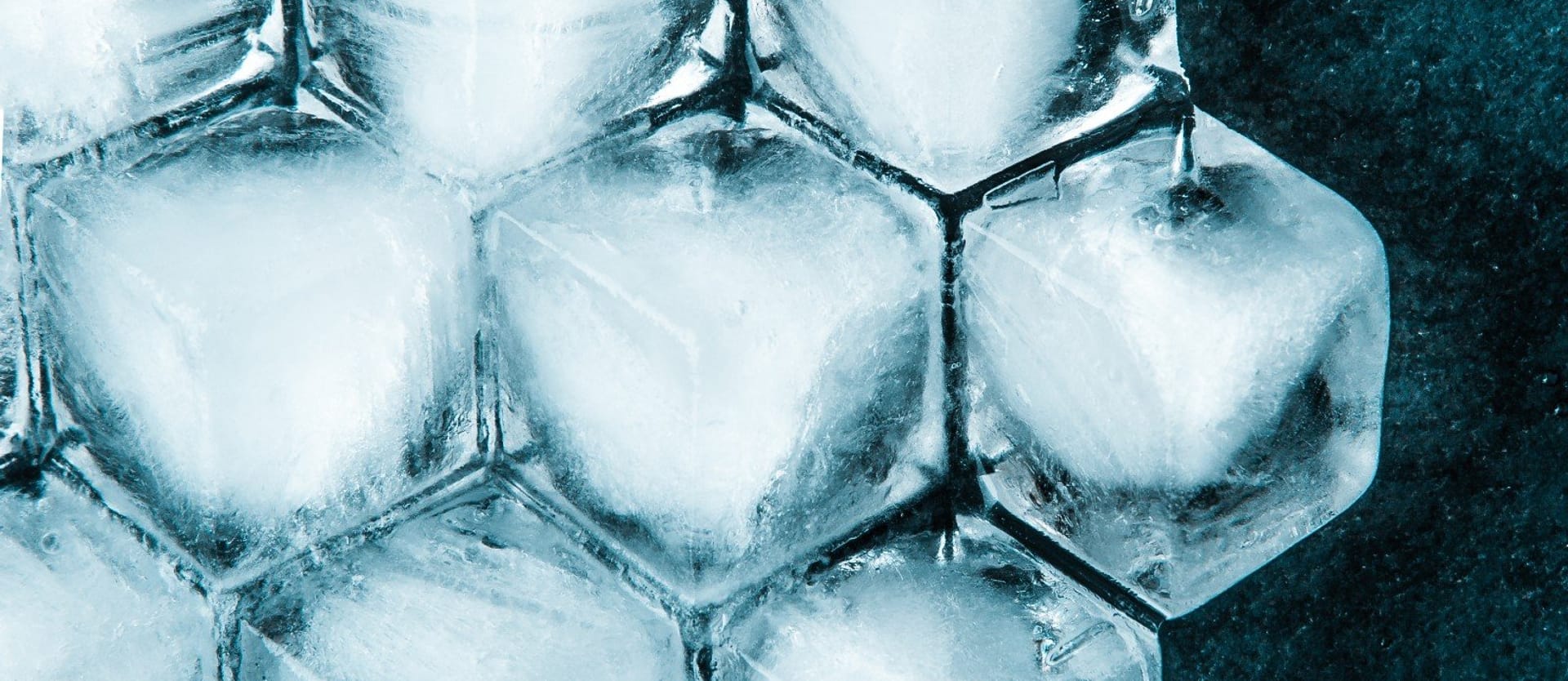
System Integration: Types, Approaches, and Implementation Steps
- 10 min read
- Business , Engineering
- 10 Mar, 2021
- No comments Share
What is system integration and when do you need it?
Legacy system integration, enterprise application integration (eai), third-party system integration, business-to-business integration, ways to connect systems, how to approach system integration, point-to-point model.
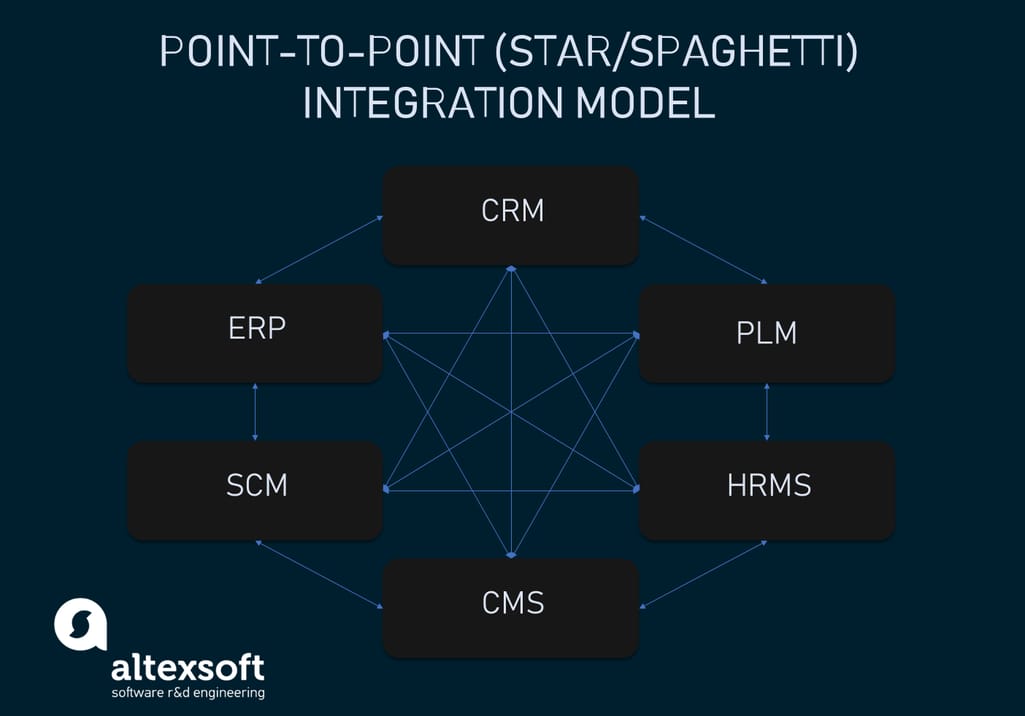
The point-to-point (star/spaghetti) integration architecture.
Hub-and-spoke model
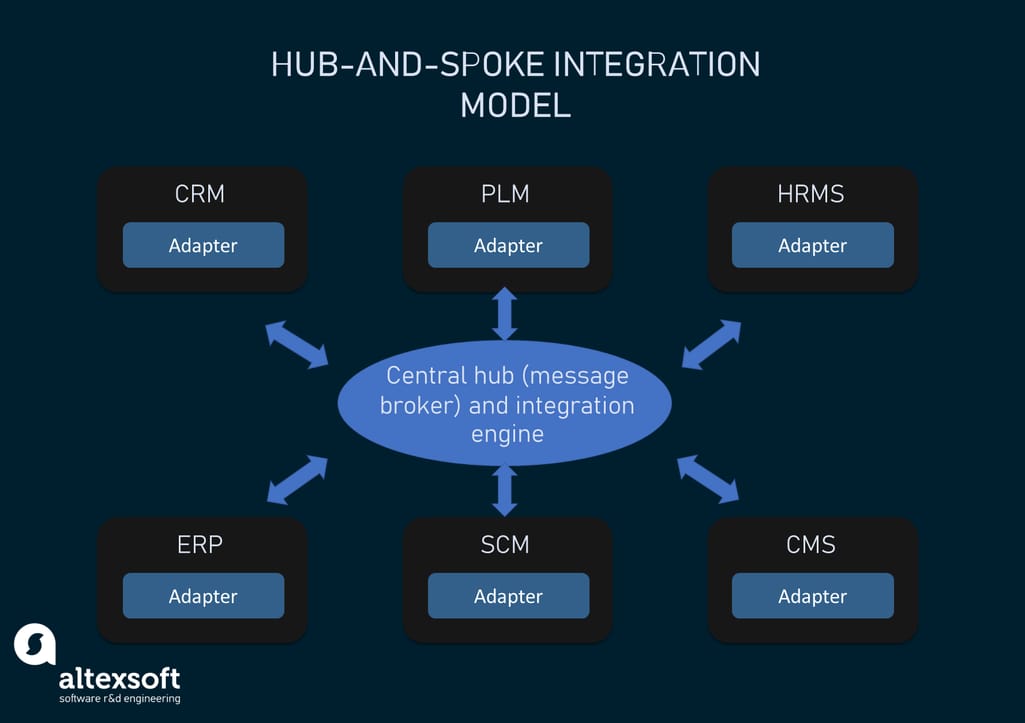
The hub-and-spoke integration architecture.
Enterprise Service Bus (ESB) model
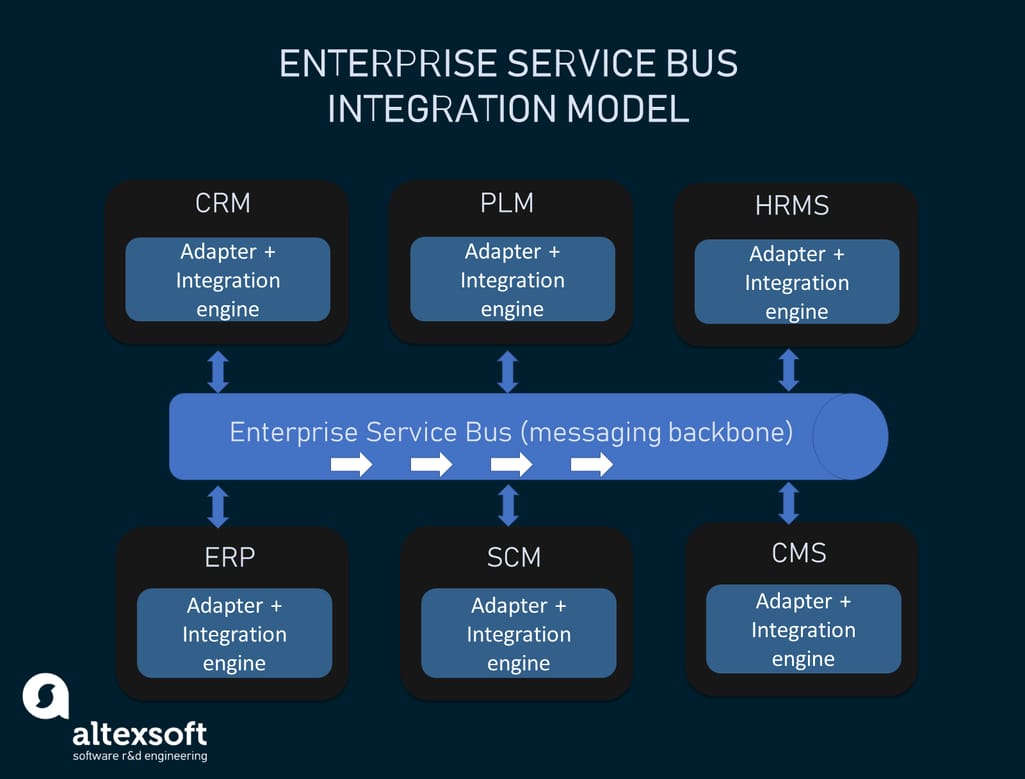
The enterprise service bus integration architecture.
Deployment options for integrated systems
Integration platform as a service (ipaas).
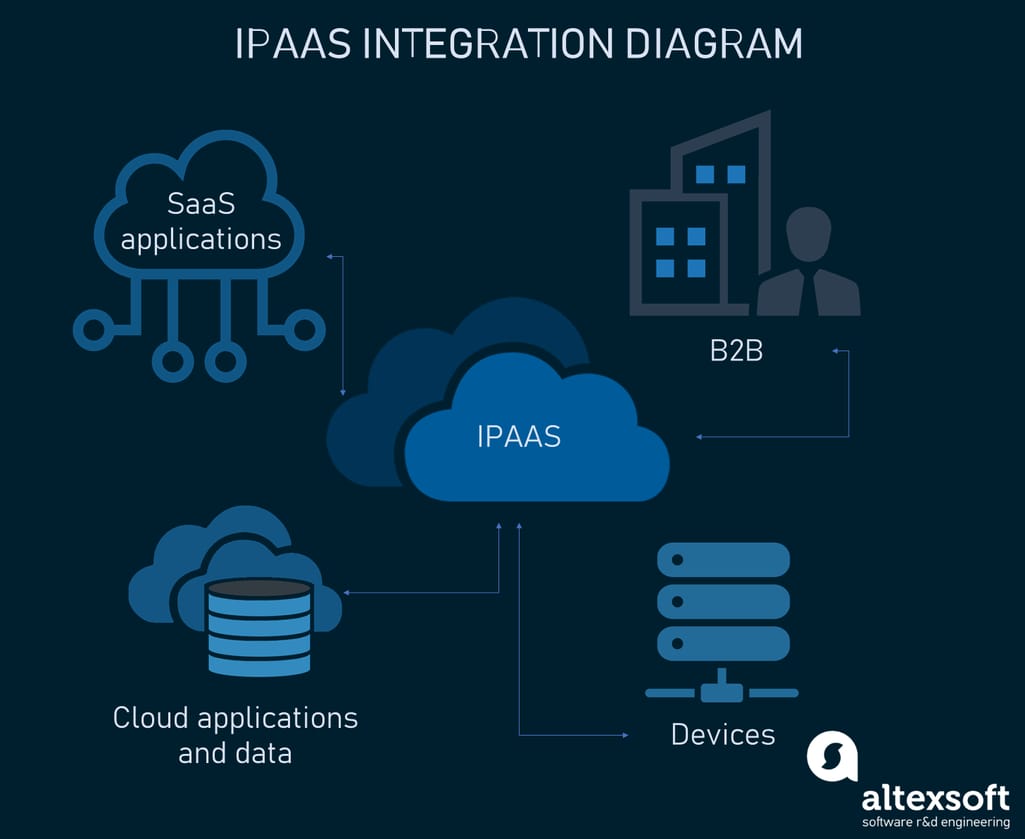
Simplified illustration of possible iPaaS connections.
Hybrid integration platform (HIP)
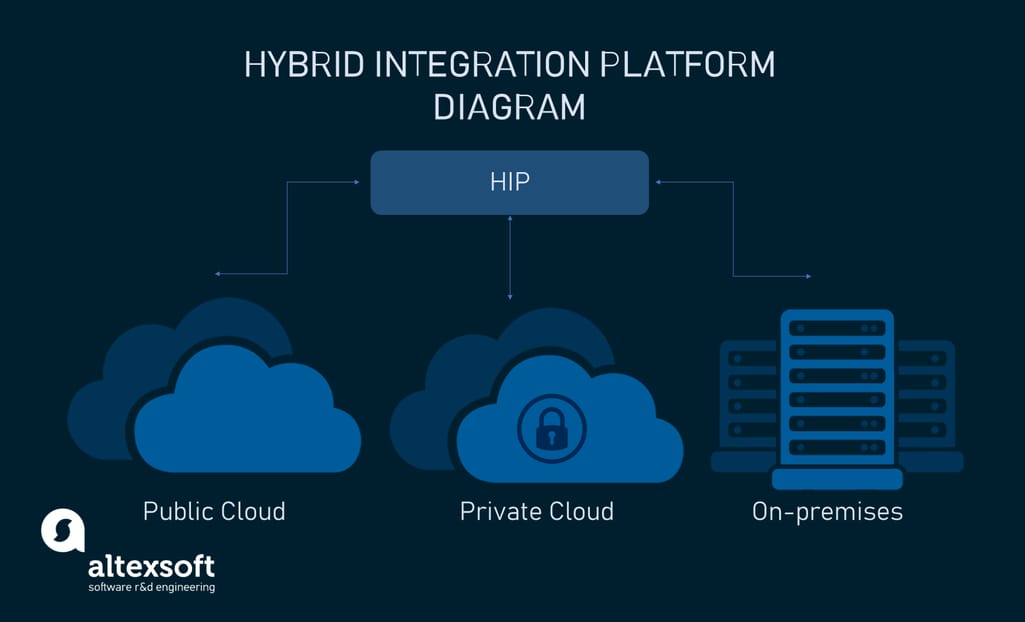
Hybrid integration platform diagram.
Key steps of system integration
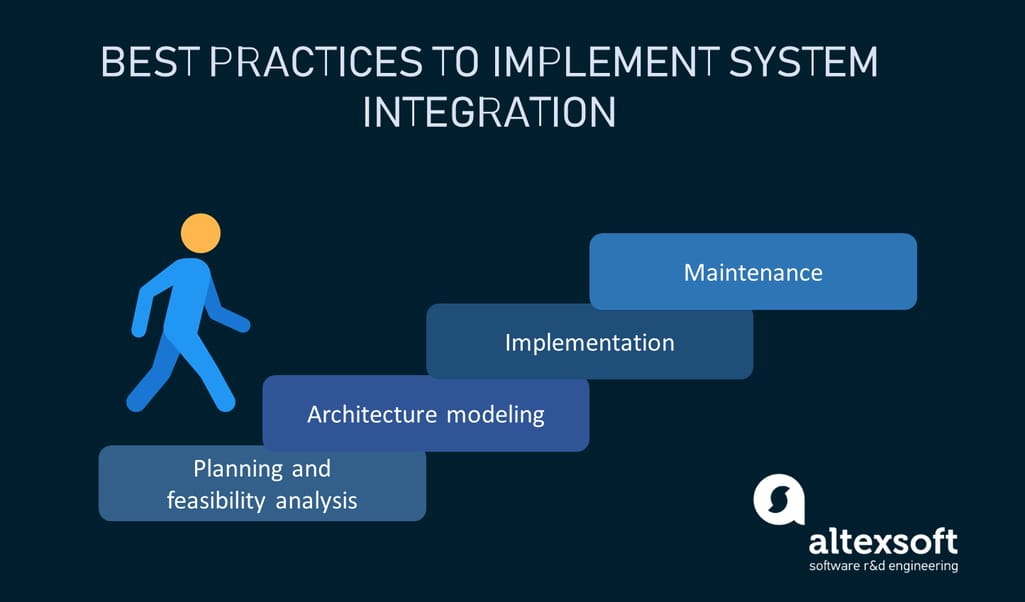
Steps to take to implement system integration.
Planning and feasibility analysis
Architecture modeling, implementation, maintenance, what is the role of system integrators.
Thank you for visiting nature.com. You are using a browser version with limited support for CSS. To obtain the best experience, we recommend you use a more up to date browser (or turn off compatibility mode in Internet Explorer). In the meantime, to ensure continued support, we are displaying the site without styles and JavaScript.
- View all journals
- My Account Login
- Explore content
- About the journal
- Publish with us
- Sign up for alerts
- Open access
- Published: 08 June 2021
An infrastructure with user-centered presentation data model for integrated management of materials data and services
- Shilong Liu 1 , 2 , 3 ,
- Yanjing Su ORCID: orcid.org/0000-0003-2773-4015 1 , 4 ,
- Haiqing Yin 1 , 5 ,
- Dawei Zhang ORCID: orcid.org/0000-0002-6546-6181 6 ,
- Jie He 1 , 2 , 3 ,
- Haiyou Huang ORCID: orcid.org/0000-0002-2801-2535 4 , 7 ,
- Xue Jiang 1 , 5 , 8 ,
- Xuan Wang 1 , 2 , 3 ,
- Haiyan Gong ORCID: orcid.org/0000-0003-1419-3301 1 , 2 , 3 ,
- Zhuang Li 1 , 2 , 3 ,
- Hao Xiu 1 , 2 , 3 ,
- Jiawang Wan 1 , 2 , 3 &
- Xiaotong Zhang ORCID: orcid.org/0000-0001-7600-7231 1 , 2 , 3
npj Computational Materials volume 7 , Article number: 88 ( 2021 ) Cite this article
3499 Accesses
16 Citations
1 Altmetric
Metrics details
- Computational methods
- Techniques and instrumentation
With scientific research in materials science becoming more data intensive and collaborative after the announcement of the Materials Genome Initiative, the need for modern data infrastructures that facilitate the sharing of materials data and analysis tools is compelling in the materials community. In this paper, we describe the challenges of developing such infrastructure and introduce an emerging architecture with high usability. We call this architecture the Materials Genome Engineering Databases (MGED). MGED provides cloud-hosted services with features to simplify the process of collecting datasets from diverse data providers, unify data representation forms with user-centered presentation data model, and accelerate data discovery with advanced search capabilities. MGED also provides a standard service management framework to enable finding and sharing of tools for analyzing and processing data. We describe MGED’s design, current status, and how MGED supports integrated management of shared data and services.
Similar content being viewed by others
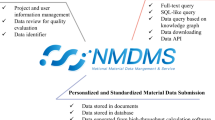
A repository for the publication and sharing of heterogeneous materials data
Haiyan Gong, Jie He, … Jiawang Wan
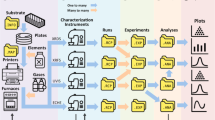
Tracking materials science data lineage to manage millions of materials experiments and analyses
Edwin Soedarmadji, Helge S. Stein, … John M. Gregoire
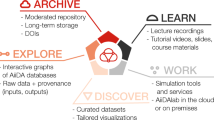
Materials Cloud, a platform for open computational science
Leopold Talirz, Snehal Kumbhar, … Nicola Marzari
Introduction
Increasingly, the materials community is acknowledging that the availability of vast data resources carries the potential to answer questions previously out of reach. Yet the lack of data infrastructure for preserving and sharing data has been a problem for decades. The need for such infrastructure was identified as early as the 1980s 1 , 2 . Since then, a large effort has been made in the materials community to establish materials databases and data repositories 3 , 4 , 5 , 6 . Some studies focus on specific subfield within materials science and develop corresponding databases. The Inorganic Crystal Structure Database is a comprehensive collection of crystal structure data of inorganic compounds containing more than 180,000 entries and covering the literature from 1913 7 , where all crystal structure information is uniformly represented in the well-established Crystallographic Information File 8 . The Materials Project of first-principles computation provides open web-based access to computed information on known and predicted materials as well as powerful analysis tools to inspire and design novel materials 9 , 10 , 11 . The Royal Society of Chemistry’s ChemSpider is a free chemical structure database providing fast text and structure search access to over 67 million structures from hundreds of data sources 12 . National Environmental Corrosion Platform of China focuses on corrosion data and includes five major databases and 13 topical corrosion databases, which contains over 18 million data of 600 materials 13 . National Materials Scientific Data Sharing Network of China provides access to massive materials data resources that are collected from more than 30 research institutes across China 14 . Such infrastructures effectively solve problems for the target fields but are not general enough to meet the needs of the broad materials community. Moreover, the isolated management of materials data leads to information silos and impedes the process of data discovery and reuse.
As materials discovery becomes more data intensive and collaborative, reliance on shared digital data in scientific research is becoming more commonplace 15 , 16 , 17 . Several reports on Integrated Computational Materials Engineering have continued to highlight such need 18 , 19 , 20 . In 2011, the United States announced the Materials Genome Initiative to encourage communities to develop infrastructure to halve the time and cost from materials discovery to application 21 . In addition to US efforts, there are other international projects, such as the Metallurgy Europe program 22 in Europe, the “Materials research by Information Integration” Initiative (MI 2 I) 23 in Japan and the Materials Genome Engineering (MGE) program in China that have been launched to develop such infrastructure.
China’s MGE program originated at the S14 Xiangshan Science Forum on System Engineering in Materials Science in December 2011. The Chinese materials community reached the consensus on collaborative development of shared platforms integrated with theoretical computing, databases and materials testing at the forum. Following this forum was a series of conferences held across the nation from 2012 to 2014 for detailed strategic planning 24 , 25 . In 2016, the MGE program was launched by the Chinese government to change the concept of materials research to the new model of theoretical prediction and experimental verification from the traditional model of experience-guided experiment. It encourages researchers to integrate technologies for high-throughput computing (HTC), high-throughput experimenting (HTE), and specialized databases, and to develop a centralized, intelligent data mining infrastructure to speed up materials discovery and innovation 26 .
The launch of the MGE program presents new challenges for modern data infrastructure. One is how to store and manage the ever-increasing amount of materials data with complex data types and structures. Better management of data benefits easier discovery and retrieval of datasets, better reproducibility, and reuse of the study results 27 , 28 , 29 , 30 , 31 , 32 . Another is how to integrate data analysis and data mining techniques to unlock the great potential of such materials data. As materials research is evolving to the fourth paradigm of scientific research as Materials 4.0 33 , 34 , great improvements in materials discovery have been achieved in applying machine learning techniques and big data methods to materials data 35 , 36 , 37 , 38 , 39 , 40 . Better integration between data and tools makes it convenient to discover the value of data.
To address these challenges, some studies take the approach to stand up very general materials data repositories that store as much data as possible without imposing strict restrictions on the structure or format. The Materials Commons provides open access to a broad range of materials data of experimental and simulation information, and allows collaboration through scientific workflows 41 . The Materials Data Facility provides data infrastructure resources and scalable shared data services to facilitate data publication and discovery 42 . The Materials Data Repository of National Institute of Standards and Technology provides a concrete mechanism for the interchange and reuse of research data on materials systems, which accepts data in any format 43 . These infrastructures provide a convenient means to preserve a wide variety of data, but do not enable straightforward searching and retrieving for data contents, or integrating with analysis tools due to the extreme heterogeneity in the stored data.
Some recent studies have recognized the importance of data standards. The Materials Data Curation System uses data and metadata models expressed as Extensible Markup Language (XML) Schema composed by researchers to dynamically generate data entry forms 44 . The Citrination platform has developed a hierarchical data structure called the Physical Information File that can accommodate complex materials data, ensuring that they are human searchable and machine readable for data mining 45 . These infrastructures provide a standardized data format to reduce the heterogeneity in the stored data, but enables only technical experts to manipulate these data formats due to the introduction of complex data types and structures.
After considering these previous efforts, we believe that the development of modern data infrastructure for MGE will hinge on two main technical requirements corresponding to integrated management of shared data and services:
The infrastructure needs to provide a user-centered presentation data model 46 for materials researchers to collect and normalize heterogeneous materials data from various data providers easily and efficiently.
The infrastructure needs to provide a service management framework of capabilities to integrate with various services and tools for analyzing and processing data, and cooperate with databases seamlessly, which enables service discovery and data reuse.
With these requirements in mind, we have developed the Materials Genome Engineering Databases (MGED), which is an emerging architecture with high usability. Serving as the materials data and service platform for MGE, MGED are differentiated from these previous efforts by our emerging architecture with high usability and user-centered dynamic container model (DCM). The architecture of MGED consists of four main systems: data collecting system (DCS), data exchange system (DES), hybrid data storage system (HDSS), and data service system (DSS). DCS collects and normalizes original datasets into standard container format constructed by DCM. DCM is a presentation data model that reflects the characteristics of materials data and allows effective user interaction with the database. DCM provides data schemas to represent the organization of experimental and computational data with associated metadata and data containers for the content of these data. Schemas are made from combinations of standard data types that encode data values and structures. These data types are well designed to have only ten kinds after consideration of suitableness for materials data and convenience for user interaction. Together with automation tools provided by DCS, MGED provide a low-overhead and convenient means to deposit heterogeneous materials data from various data providers. DES manages data mapping rules used for data parsing and reconstruction, and format transformation rules used for data exchange, storage, and service. HDSS is responsible for the management of storage technologies used in MGED and stores the data into corresponding databases according to its structural characteristics. DSS provides a fundamental services framework for search and discovery of data and a service integration framework for the management of various third-party analysis tools. DSS allows data and tools to be joined into a seamless integrated workflow to make data reuse and analysis more effectively. With the integrated management of shared data and tools, MGED provides researchers with an open and collaborative environment for quickly and conveniently preserving and analyzing data.
Architecture
MGED adopts a browser-server architecture using Python-based Django framework that allows users to easily access materials data and services on the platform through a browser. MGED is currently online and accessible at www.mgedata.cn and mged.nmdms.ustb.edu.cn .
Figure 1 provides a high-level view of MGED’s architecture. MGED has four main systems: DCS, DES, HDSS, and DSS. DCS collects and normalizes original datasets from multiple data providers into standard container format. DES manages data mapping rules used for data parse and reconstruction, and handles data transformation among formats used for exchange, storage, and service. HDSS combines several storage technologies and stores each category of data occurred on the platform separately in the corresponding database. DSS manages a variety of data services including fundamental services like data search and third-party enhanced services like data mining.
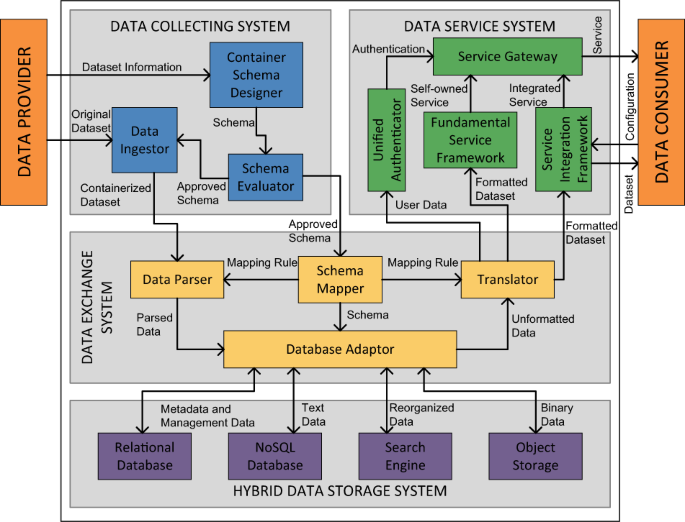
The architecture of MGED consists of four main systems: DCS, DES, HDSS, and DSS. DCS is responsible for collecting original datasets from multiple data providers and normalizing them into standard container format. DES manages data mapping rules and performs data transformation among different formats. HDSS stores each category of data into the corresponding database. DSS provides various data services through the fundamental services framework and the service integration framework.
Figure 1 also indicates two main materials data flows: the data colleting flow from data providers and data using flow from data consumers.
Data providers makes data available to themself or to others. Data providers contains end users, researchers, tools, and data platforms that provides diversified data. In the data colleting flow, a data provider customizes schemas to represent the exchange structure of original datasets through the container schema designer. Schemas then are evaluated by the schema evaluator. When approved they will be stored into databases in HDSS through the schema mapper and the database adaptor. Approved schemas will be used by the data ingestor to normalize and transform original datasets from the data provider to containerized datasets, which is the standard data format used in MGED. After normalization, containerized datasets will be parsed as components like metadata, textual materials data and binary files, and these components will be stored separately to appropriate databases by the database adaptor.
Data consumers receive the value output of MGED. Data consumers contains end users, researchers, tools, and data platforms that analyze collected data. A data consumer interacts with MGED through the services provided by the service gateway to get access to the information of interest. The data consumer initiate query commands through the search service in the fundamental service framework to look up datasets. The database adaptor will retrieve the search result from different databases and send it to the translator. The translator performs transformation to present the result in a format that the service expects. The service integration framework receives the formatted result and transmits it to the data consumer for subsequent analysis. The analysis result can be stored to some data provider for later sharing. These two data flows constitute a virtuous circle of data sharing and service sharing.
The data collecting system
In this section we provide an overview of the DCS. We analyzed the factors that need to be considered in the materials data collecting process and proposed the DCM that meets these requirements. Current implementations of DCS are based on DCM and contains the following components: container schema designer, data ingestor, and schema evaluator.
DCM is a user-centered presentation data model that reflects the user’s model of the data and allows effective user interaction with the database. Its name comes from the concept of containers in real life. A container in real life generally refers to a device for storing, packaging, and transporting a product. It usually has a fixed internal structure for fixing different types of products, such as a toolbox with corresponding shapes to fix hammers, scissors, and the like. In contrast, abstract containers in DCM are designed to have internal structure constructed dynamically from different types of basic structure. Therefore, DCM provides a way to store, wrap, and exchange data and enables users to customize schemas suitable for the structure of the data. DCM supports customization of attributes and structures. Users can arbitrarily choose attribute names without any restrictions in principle, but practically names in schemas that are publicly available on MGED should follow naming conventions of materials community. Attribute values can be restricted by data types. Structures can be customized by different combinations of data types.
As DCM plays a central role in MGED, its usability largely determines the usability of the platform. To this end, we developed the container schema designer to assist users in visually modifying existing schemas or creating entirely new schemas with built-in types, as illustrated in Fig. 2 . We take the data of shape memory alloys as an example and shows how the attributes and structure of the data are described through the graphical user interface (GUI) of the container schema designer.
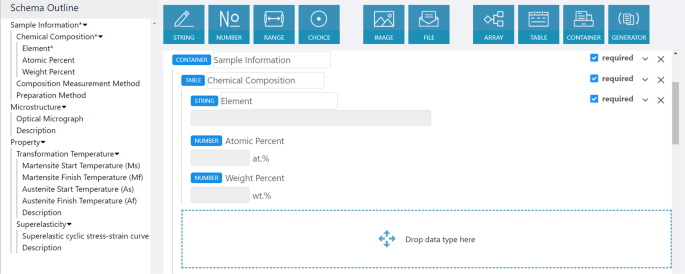
The designer allows users drag icons of data types and drop them to the dotted box to construct their schemas. The schema structure of the example data of shape memory alloys is shown in the Schema Outline.
Container schemas are created by users with great flexibility. Various schemas can be created to describe the same field of materials, which will reduce the quality of data normalization and increase the difficulty for users to discover and use data. Therefore, we have developed the schema evaluator to evaluate the quality of schemas. New schemas in a certain field will be evaluated by experts in that field from the materials expert database. With deep understanding of both materials and schemas, evaluation experts can correct the inappropriate materials terminology and structure in the schema. Approved schemas will be published on the platform.
DCS also contains convenient tools provided by the data ingestor that allow for collecting datasets from data providers and normalizing them into containerized datasets automatically to reduce users’ workload.
The data exchange system
In this section we outline the implementation architecture of DES. DES handles two exchange processes: the data persistence process that converts datasets from the container format to the database format, and the data retrieval process that converts datasets from the database format to the service format. DES consists of the following components: data parser, schema mapper, translator, and database adaptor.
In the data persistence process, the datasets are uploaded with information structured in a certain container format, such as XML, JavaScript Object Notation (JSON), or Excel. The container format is a data exchange format that facilitates the data collecting from data providers to MGED and data sharing from MGED to services. The uploaded containerized datasets then will be converted to the corresponding database format in HDSS to achieve high efficiency for search and retrieval. The schema mapper generates mapping rules from container format to database format. The data parser handles containerized datasets from the data ingestor and breaks down them into parts suitable for different databases. The database adaptor then connects to all databases in HSS and stores each part into proper database.
In the data retrieval process, the database adaptor performs data retrieval operation from databases by generating database query statements satisfying users’ search conditions. The retrieved container schema will be sent to the schema mapper for mapping rule generation. The retrieved unformatted data are transferred to the translator. The translator performs reconstruction to present the dataset in a format that the service expects.
The hybrid data storage system
HDSS is responsible for the management of storage technologies used in MGED. Data storage technologies are diverse and optimized for storing different categories of data. Figure 3 shows the categories of data stored in MGED from the perspective of storage. Management data include user account information, access privileges, and other miscellanies required for proper functionality. Scientific data include metadata like authors and digital object identifier (DOI) of the dataset, text data that structure information in text, and binary data that structure information in files. Therefore, HDSS has adopted multiple storage technologies to manage these data.
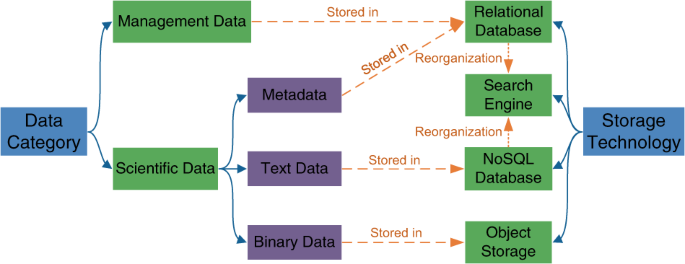
The data stored in MGED are categorized as management data or scientific data. The scientific data are categorized as metadata, text data, or binary data. Each type of data is stored in the corresponding storage technologies.
Figure 3 also shows the storage technologies used in HDSS and the corresponding category of data stored in them. A relational database is used to store metadata and management data that fit into the relational model. A NoSQL database is used to store heterogeneous text data that have no fixed schema. All binary data uploaded to MGED are persisted to an object storage. In addition, metadata and text data on the platform are reorganized and indexed in a search engine to enable complex queries. The current implementation of HDSS has adopted the well-known database systems as its backends. Specifically, PostgreSQL, MongoDB, MongoDB’s GridFS, and Elasticsearch are used as the corresponding backends of the relational database, the NoSQL database, the object storage, and the search engine. We are also improving HDSS to support more database systems.
The data service system
In this section we outline the implementation architecture of DSS. DSS consists of the following components: service gateway, unified authenticator, fundamental service framework, and service integration framework.
The service gateway provides a unified portal for data consumers to access services. It verifies requests from data consumers and distributes them to corresponding services.
The unified authenticator handles user authentication and authorization privilege verification. MGED and third-party tools have user management functions of their own. Because these systems are independent of each other, users need to repeatedly log in to use each of them. The unified authenticator provides an open authorization service that allows secure API authorization in a simple and standard method from third-party services, making it easy for users to use services with the account of MGED.
The fundamental service framework provides services that promotes data discovery and sharing. It mainly includes search and export service, digital identification service (DIS), and classification and statistics service.
The search service provides three kinds of search functions to enable users to make complex queries. The basic search function allows users to quickly locate the required datasets through metadata information like data titles, abstracts, owners, and keywords. The advanced search function based on container schemas allows users to impose constraints on data attributes of interest and accurately access the required datasets; the full-text search function allows users to use multiple keywords to obtain datasets that contain these keywords whether in metadata or attributes. Each piece of data in the search result will be represented in visual interface generated from the corresponding schema. As shown in Fig. 4 , the detailed information of the shape memory alloys is represented in the generated interface and the representation structure is the same as the structure described in the schema. Besides, the datasets in search results can be exported to JSON, XML, and EXCEL format for further research. The result datasets can also be exported with filters to select out only concerned attributes. We also provide data export APIs for integrated services.
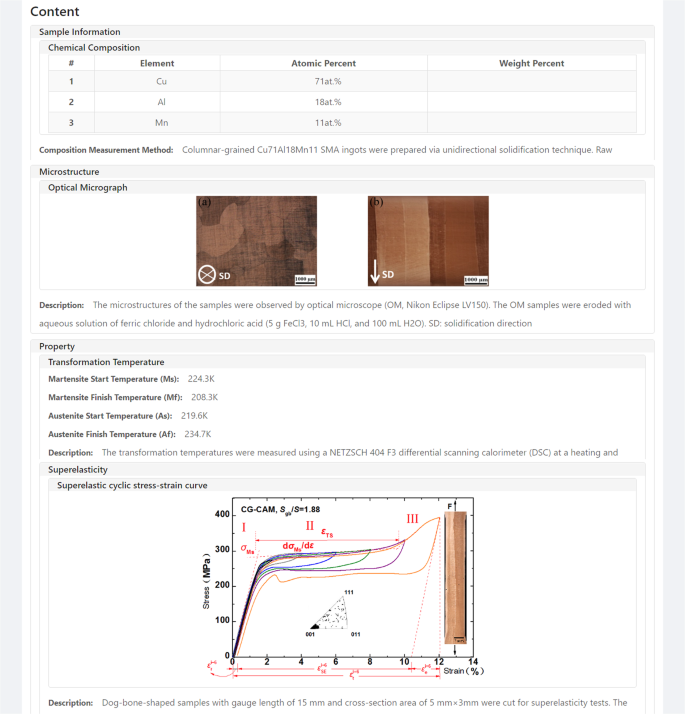
The data representation interface is generated dynamically according to the schema of the example data of shape memory alloys.
Datasets are uniquely identified by the DIS with a DOI that contains information about owners and location of the underlying dataset. Association of a digital identifier facilitates discovery and citation of the dataset.
In addition, we also provide a classification and statistics service to help users quickly understand the status of materials data on the platform, as shown in Fig. 5 . We divide materials science into different levels of fields and organize them into a category tree. Each piece of materials data on the platform is divided into a field in the tree. Statistics information of each field are shown in various visualization methods. Statistics information includes the total amount of data in MGED and separate amount of data in each field with their respective trends in data volume. Other information like the number of visits and downloads of each piece of data, popular fields, and rankings provides users detailed view to estimate hot data or fields. As of March 2021, there have been 7.3 million pieces of materials data in total collected through two website portals of MGED, including 20 major fields in the category tree. The top five fields with the most data are fields of special alloy, material thermodynamics/kinetics, catalytic materials, the first principle calculation, biomedical materials. We have also developed a simple data evaluation function that allows users to score each piece of data on the platform, which helps others judge data quality.
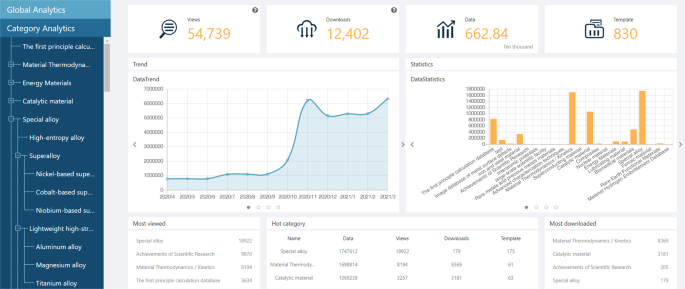
The fields of materials science are divided into a hierarchy in the category tree shown in the left box. The statistics information of each field is shown in various visualization methods in different white boxes on the right.
The service integration framework is responsible for integrating third-party computing and analysis tools for further research. Third-party online services can directly be integrated to MGED with an access portal in the service gateway and a dedicated API to transfer data. The offline service will be provided an introduction portal for users to download and use.
At present, the framework under development has integrated several services developed by cooperative teams in our project, such as MatCloud for HTC 47 , OCPMDM for data mining 48 , and the Interatomic Potentials Database for atomistic simulations. There have been some studies using data and services provided by MGED 49 , 50 , 51 . When the framework is fully developed and the integration process standard has been established, MGED will be open to all researchers in materials community and collaborates with them in development and integration of useful tools that improve data utilization, which promotes service sharing process and accelerates materials discovery.
To summarize, we have developed a modern materials data infrastructure, MGED, for scalable and robust integrated management of shared data and services for the materials science community. We have concluded from previous work that the development of modern infrastructure for MGE will hinge on two main technical requirements corresponding to integrated management of shared data and services: a user-centered presentation data model for easy and efficient data collecting and normalizing, and a service management framework capable of integrating various tools for analyzing and processing data. To address these requirements, we have developed our emerging architecture of MGED with high usability. In particular, we proposed a user-centered presentation data model, DCM, for materials researchers to get heterogeneous materials data into and out of MGED conveniently. DCM provides schemas to represent data and containers for the content of these data. Schemas consist of standard data types that describe data values and structures, which are designed not only to handle the heterogeneity of the data, but also to provide the convenience of user interaction. We also developed DSS to manage fundamental services for data search and discovery, and enhanced services provided by third-party for data analysis. DSS allows the stored data and integrated tools to be joined into a workflow to make data reuse and analysis more effectively. With the integrated management of shared data and tools, MGED provides researchers with an open and collaborative environment for quickly and conveniently preserving and analyzing data.
Characteristics and classification of materials data
Materials data providers are diverse and fragmented. Datasets they provide are typically heterogeneous and stored in different custom formats. We have developed DCS to communicate with data providers and collect datasets. In the design of DCS, decisions were made around technologies for the improvement of system usability. Data characteristics suitableness and operation convenience were the two mainly considered factors for usability.
Datasets collected from data providers need to be normalized into a common schema to enable accurate search and analysis. Meanwhile, the common schema should be suitable for materials data characteristics to reduce users’ cognitive burden and learning cost. We have analyzed a large amount of materials data and summarized their characteristics, as shown in Fig. 6 . Materials data are usually composed of a set of attributes with relationships in the abstract. Attributes are identified by their names. Values of attributes can be described in several different forms. These forms are called primitive data representations, such as a paragraph of text, a number, an interval, a list of numbers, or even files. The relationship between attributes is described by composite data representations, such as groups, hierarchy, or tables. Combination of attributes described by different data representations ultimately form a tree-like data structure. Then we developed the DCM to accommodate these characteristics.
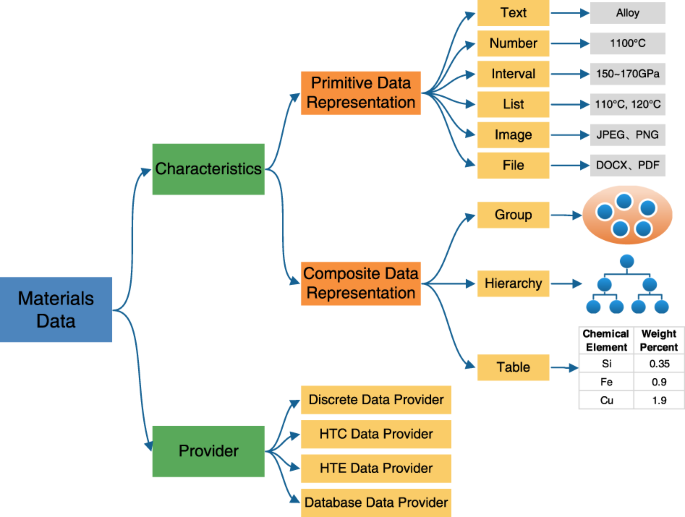
From the point of view of the characteristics of materials data, they can be characterized by primitive data representation forms and composite data representation forms. From another point of view of providers of materials data, they can be classified into four types based on the regularity of datasets stored in them.
At the same time, the data collecting process is time-consuming and laborious when researchers have to manually transfer original datasets to data infrastructure, which will reduce their motivation for sharing data. Therefore, DCS should contains convenient tools that allow for operation automation to reduce users’ physical burden. We have classified data providers into four categories based on the regularity of datasets stored in them: discrete data providers, HTC data providers, HTE data providers, and database data providers. A discrete data provider is a materials researcher who organizes materials data with self-defined formats. HTC data providers refer to various materials computing software. HTE data providers refer to experimental equipment such as scientific apparatus. A database data provider is a database that has already stored large volumes of materials data. We provide dedicated data collecting tools for each category with appropriate granularity of operation.
The dynamic container model
DCM contains two main components: container schemas and container instances. A container schema represents the abstract description of attributes and structures of a materials dataset. The colon symbol \(:\) is used to represent the relation between the attribute and the type. The type declaration expression \(x:T\) indicates that the type of the attribute \(x\) is \(T\) . A container schema \({\cal{S}}\) is a set of type declaration expressions and defined by \({\cal{S}} = \{ x_i:T_i^{i \in 1..n}\} = \{ x_1:T_1,x_2:T_2, \ldots ,x_n:T_n\}\) , where \(x_i\) is the attribute name and \(T_i\) the type name.
A container instance represents the abstract description of a piece of data in the dataset, which is constrained by the schema of the dataset and specifies the value of each attribute of the data. We represent the relation between the attribute and the value by the equal sign =. The assignment expression \(x = v\) indicates that the attribute \(x\) has a value of \(v\) at a certain moment. A container instance \({\cal{C}}\) is a set of assignment expressions and defined by \({\cal{C}} = \{ x_i = v_i^{i \in 1..n}\} = \{ x_1 = v_1,x_2 = v_2, \ldots ,x_n = v_n\}\) .
A schema together with several instances constrained by it constructs a containerized dataset, which is a normalized description of a materials dataset. A containerized dataset is defined as a pair \(\left( {{\cal{S}},{\cal{D}}} \right)\) where \({\cal{D}} = \{ C_i^{i \in 1..n}\} = \{ C_1,C_2, \ldots ,C_n\}\) .
The quantity and complexity of data types largely determine the functionality and usability of DCM. With analysis of materials data characteristics above, we define ten kinds of build-in types, including four primitive types, namely String, Number, Image, and File, and six composite types, that is Range, Choice, Array, Table, Container, and Generator.
Primitive types are basic components without internal structures. The type String represents a textual description. The type Number represents a numeric value. The type Image and File represent information in image formats and file formats separately. Considering the popularity of pictures in materials data and the requirement for subsequent image processing, we separate Image from File intentionally as an independent data type for high usability.
Composite types are constructed by combinations of built-in types. The type Range is composed of Number and represents an interval value of two numbers; the type Choice is composed of String and represents the text options that an attribute can take; the type Array is composed of an arbitrary built-in type T and indicates that an attribute should take an ordered list of values of T ; the type Generator, Container, and Table consist of a collection of fields which are labeled build-in types. They differ in the form of values that an attribute can take. An attribute of Generator can only take one value of some field in the collection. An attribute of Container can take one set of values of all fields in the collection. An attribute of Table can take any number of sets of values of all fields in the collection. Table 1 shows some examples of the type declaration form of each build-in type and the corresponding attribute assignment form.
The data collecting tools
The data ingestor is responsible for collecting datasets from data providers and normalizing them into containerized datasets. It contains several dedicated data collecting tools to assist with data collecting process.
For discrete data providers, we provide GUIs and application programming interfaces (APIs) with high usability. The interfaces for data import are generated dynamically from user-designed schemas. The data ingestor also allows for automated curation of multiple datasets via user scripts through the representational state transfer API.
For HTC data providers and HTE data providers, we are developing tools for data extraction and transformation to help users automatically submit data. Datasets generated by calculation software are often in some standard formats. Therefore, extraction rules for them can be obtained easily according to the structure of formats. Datasets from HTC data providers are relatively diverse in formats. Therefore, only necessary metadata information can be extracted, and the original datasets will be submitted together with extracted metadata for future analysis.
For database data providers, we are developing a migration tool to automatically exchange data. Datasets in databases are generally stored in normal forms. Schemas for datasets and mapping rules can be extracted and built by user scripts. With schemas and mapping rules, the migration tool can automatically recognize original datasets, collect, and convert them into containerized datasets.
The digital identification service
In response to the materials community trend toward open data, MGED allows researchers to group data into datasets and publish through the DIS. When a dataset is published, it is given additional descriptive metadata and a digital identifier. The descriptive metadata includes information about titles, authors, and belonged projects to indicate data contribution and promote participation. The identifier is generated automatically by DIS and resolvable to the location of the underlying data and metadata. Association of a digital identifier enables convenient sharing and citation for research data.
In practice, information contained in a dataset may be incomplete or inaccurate, which will reduce confidence and trust in shared datasets. DIS provides internal reviews by experts from our materials expert database to ensure that the dataset being published passes specific quality control checks. DIS currently supports DOI and we are developing a multiple identification framework that integrate other identifiers and provide APIs to meet the diverse needs of users.
Data availability
The data that support the findings of this study are available from the corresponding author upon reasonable request.
Code availability
The codes that support the findings of this study are available from the corresponding author upon reasonable request.
Westbrook, J. H. & Rumble, J. R., Jr. Computerized Materials Data Systems (National Bureau of Standards, 1983).
Cahn, R. W. The Coming of Materials Science (Pergamon, 2001).
Kalidindi, S. R. Data science and cyberinfrastructure: critical enablers for accelerated development of hierarchical materials. Int. Mater. Rev. 60 , 150–168 (2015).
Article CAS Google Scholar
Kalidindi, S. R. & De Graef, M. Materials data science: current status and future outlook. Annu. Rev. Mater. Res. 45 , 171–193 (2015).
Hill, J., Mannodi-Kanakkithodi, A., Ramprasad, R. & Meredig, B. Materials data infrastructure and materials informatics. in Computational Materials System Design (eds. Shin, D. & Saal, J.) 193–225 (Springer, 2018).
Warren, J. A. & Ward, C. H. Evolution of a materials data infrastructure. JOM 70 , 1652–1658 (2018).
Article Google Scholar
Belsky, A., Hellenbrandt, M., Karen, V. L. & Luksch, P. New developments in the inorganic crystal structure database (ICSD): accessibility in support of materials research and design. Acta Crystallogr. Sect. B 58 , 364–369 (2002).
Hall, S. R., Allen, F. H. & Brown, I. D. The crystallographic information file (CIF): a new standard archive file for crystallography. Acta Crystallogr. Sect. A 47 , 655–685 (1991).
Jain, A. et al. Commentary: the materials project: a materials genome approach to accelerating materials innovation. APL Mater. 1 , 011002 (2013).
Ong, S. P. et al. Python materials genomics (pymatgen): a robust, open-source python library for materials analysis. Comput. Mater. Sci. 68 , 314–319 (2013).
Ong, S. P. et al. The materials application programming interface (API): a simple, flexible and efficient API for materials data based on REpresentational State Transfer (REST) principles. Comput. Mater. Sci. 97 , 209–215 (2015).
Pence, H. E. & Williams, A. ChemSpider: an online chemical information resource. J. Chem. Educ. 87 , 1123–1124 (2010).
Li, X. Practice analysis about the sharing service of national materials environmental corrosion platform. China Sci. Technol. Resour. Rev. 50 , 101–107 (2018).
Google Scholar
Yin, H., Jaing, X., Zhang, R., Liu, G. & Qu, X. National materials scientific data sharing network and its application to innovative developmentof materials industries. China Sci. Technol. Resour. Rev. 48 , 58–65 (2016).
Ward, C. H., Warren, J. A. & Hanisch, R. J. Making materials science and engineering data more valuable research products. Integr. Mater. Manuf. Innov. 3 , 292–308 (2014).
Li, X. et al. Share corrosion data. Nature 527 , 441–442 (2015).
Jain, A., Persson, K. A. & Ceder, G. Research update: the materials genome initiative: data sharing and the impact of collaborative ab initio databases. APL Mater. 4 , 053102 (2016).
National Research Council. Materials Research to Meet 21st-Century Defense Needs (National Academies Press, 2003).
National Research Council. Accelerating Technology Transition: Bridging the Valley of Death for Materials and Processes in Defense Systems (National Academies Press, 2004).
National Research Council. Integrated Computational Materials Engineering: A Transformational Discipline for Improved Competitiveness and National Security (National Academies Press, 2008).
Holdren, J. P. Materials Genome Initiative for Global Competitiveness (National Science and Technology Council, 2011).
Jarvis, D. et al. Metallurgy Europe—A Renaissance Programme for 2012-2022 (European Science Foundation, 2012).
Japan Science and Technology Agency. “Materials research by Information Integration” Initiative. http://www.nims.go.jp/MII-I/en/ (2015).
Wang, H., Xiang, Y., Xiang, X. & Chen, L. Materials genome enables research and development revolution. Sci. Technol. Rev. 33 , 13–19 (2015).
Yin, H., Qu, X. & Xie, J. Analysis of the implementation and development of the Material Genome Initiative in Beijing. Adv. Mater. Ind. 1 , 27–29 (2014).
O’Meara, S. Materials science is helping to transform China into a high-tech economy. Nature 567 , S1–S5 (2019).
de Pablo, J. J., Jones, B., Kovacs, C. L., Ozolins, V. & Ramirez, A. P. The Materials Genome Initiative, the interplay of experiment, theory and computation. Curr. Opin. Solid State Mater. Sci. 18 , 99–117 (2014).
Olson, G. B. & Kuehmann, C. J. Materials genomics: from CALPHAD to flight. Scr. Mater. 70 , 25–30 (2014).
Sumpter, B. G., Vasudevan, R. K., Potok, T. & Kalinin, S. V. A bridge for accelerating materials by design. npj Comput. Mater. 1 , 15008 (2015).
Austin, T. Towards a digital infrastructure for engineering materials data. Mater. Disco. 3 , 1–12 (2016).
Pfeif, E. A. & Kroenlein, K. Perspective: data infrastructure for high throughput materials discovery. APL Mater. 4 , 053203 (2016).
The Minerals Metals & Materials Society (TMS). Building a Materials Data Infrastructure: Opening New Pathways to Discovery and Innovation in Science and Engineering (TMS, 2017).
Hey, T., Tansley, S. & Tolle, K. The Fourth Paradigm: Data-Intensive Scientific Discovery (Microsoft Research, 2009).
Jose, R. & Ramakrishna, S. Materials 4.0: materials big data enabled materials discovery. Appl. Mater. Today 10 , 127–132 (2018).
Raccuglia, P. et al. Machine-learning-assisted materials discovery using failed experiments. Nature 533 , 73–76 (2016).
Mueller, T., Kusne, A. G. & Ramprasad, R. Machine learning in materials science: recent progress and emerging applications. Rev. Comput. Chem. 29 , 186–273 (2016).
CAS Google Scholar
Ward, L., Agrawal, A., Choudhary, A. & Wolverton, C. A general-purpose machine learning framework for predicting properties of inorganic materials. npj Comput. Mater. 2 , 16028 (2016).
Liu, Y. et al. Materials discovery and design using machine learning. J. Mater. 3 , 159–177 (2017).
Ramprasad, R., Batra, R., Pilania, G., Mannodi-Kanakkithodi, A. & Kim, C. Machine learning in materials informatics: recent applications and prospects. npj Comput. Mater. 3 , 54 (2017).
Schmidt, J., Marques, M. R. G., Botti, S. & Marques, M. A. L. Recent advances and applications of machine learning in solid-state materials science. npj Comput. Mater. 5 , 83 (2019).
Puchala, B. et al. The materials commons: a collaboration platform and information repository for the global materials community. JOM 68 , 2035–2044 (2016).
Blaiszik, B. et al. The materials data facility: data services to advance materials science research. JOM 68 , 2045–2052 (2016).
Material Measurement Laboratory. NIST Materials Data Repository. https://materialsdata.nist.gov/ (2017).
Dima, A. et al. Informatics Infrastructure for the Materials Genome Initiative. JOM 68 , 2053–2064 (2016).
O’Mara, J., Meredig, B. & Michel, K. Materials data infrastructure: a case study of the citrination platform to examine data import, storage, and access. JOM 68 , 2031–2034 (2016).
Jagadish, H. V. et al. Making database systems usable. in Proceedings of the 2007 ACM SIGMOD International Conference on Management of Data. 13–24 (Association for Computing Machinery, 2007).
Yang, X. et al. MatCloud: a high-throughput computational infrastructure for integrated management of materials simulation, data and resources. Comput. Mater. Sci. 146 , 319–333 (2018).
Zhang, Q., Chang, D., Zhai, X. & Lu, W. OCPMDM: online computation platform for materials data mining. Chemom. Intell. Lab. Syst. 177 , 26–34 (2018).
Zhao, X. P., Huang, H. Y., Wen, C., Su, Y. J. & Qian, P. Accelerating the development of multi-component Cu-Al-based shape memory alloys with high elastocaloric property by machine learning. Comput. Mater. Sci. 176 , 109521 (2020).
Gao, X., Wang, L. & Yao, L. Porosity prediction of ceramic matrix composites based on random forest. IOP Conf. Ser. Mater. Sci. Eng. 768 , 052115 (2020).
Ma, B. et al. A fast algorithm for material image sequential stitching. Comput. Mater. Sci. 158 , 1–13 (2019).
Download references
Acknowledgements
The authors thank the Ministry of Science and Technology, the Ministry of Industry and Information Technology, and other relevant departments for their efforts in launching MGE program and their support for our projects and MGED. MGED is a collaboration among the University of Science and Technology Beijing (USTB), the Shanghai University (SHU), Tsinghua University (THU), Sichuan University (SCU), Southwest Jiaotong University (SWJTU), Beijing University of Technology (BJUT), Beijing Institute of Technology (BIT), the Central South University (CSU), Northwestern Polytechnical University (NPU), Shanghai Jiao Tong University (SJTU), the Computer Network Information Center (CNIC) of the Chinese Academy of Sciences (CAS), Ningbo Institute of Materials Technology and Engineering (NIMTE) of CAS, The Academy of Mathematics and Systems Science (AMSS) of CAS, the Central Iron and Steel Research Institute (CISRI), the Institute of Metal Research (IMR) of CAS, the Institute of Chemistry of CAS (ICCAS), the Ningbo Institute of Information Technology Application of CAS, the Institute of High Energy Physics (IHEP) of CAS, Beijing Computing Center, the National Supercomputer Center in Tianjin, Beijing Institute of Aeronautical Materials (BIAM) of the Aero Engine Corporation of China (AECC), and Tianjin Nanda General Data Technology Co., Ltd. This research was supported in part by the National Key Research and Development Program of China under Grant Nos. 2016YFB0700500 and 2018YFB0704300, the Fundamental Research Funds for the University of Science and Technology Beijing under Grant FRF-BD-19-012A, and the National Natural Science Foundation of China under Grant No. 61971031. We would like to thank the users of MGED for their support and feedback in improving the platform.
Author information
Authors and affiliations.
Beijing Advanced Innovation Center for Materials Genome Engineering, University of Science and Technology Beijing, Beijing, China
Shilong Liu, Yanjing Su, Haiqing Yin, Jie He, Xue Jiang, Xuan Wang, Haiyan Gong, Zhuang Li, Hao Xiu, Jiawang Wan & Xiaotong Zhang
School of Computer and Communication Engineering, University of Science and Technology Beijing, Beijing, China
Shilong Liu, Jie He, Xuan Wang, Haiyan Gong, Zhuang Li, Hao Xiu, Jiawang Wan & Xiaotong Zhang
Beijing Key Laboratory of Knowledge Engineering for Materials Science, Beijing, China
Corrosion and Protection Center, University of Science and Technology Beijing, Beijing, China
Yanjing Su & Haiyou Huang
Collaborative Innovation Center of Steel Technology, University of Science and Technology Beijing, Beijing, China
Haiqing Yin & Xue Jiang
National Materials Corrosion and Protection Data Center, Institute for Advanced Materials and Technology, University of Science and Technology Beijing, Beijing, China
- Dawei Zhang
Institute for Advanced Materials and Technology, University of Science and Technology Beijing, Beijing, China
Haiyou Huang
Beijing Key Laboratory of Materials Genome Engineering, University of Science and Technology Beijing, Beijing, China
You can also search for this author in PubMed Google Scholar
Contributions
S.L., Y.S., H.Y., J.H., X.J., X.W., and X.Z. participated in the design and discussion of the infrastructure architecture. S.L., X.W., H.G., Z.L., H.X., and J.W. developed and implemented the architecture. S.L formulated the problem and participated in the development of the data model with J.H. and X.Z. H.H. prepared the data used for the demonstration. S.L. prepared the initial draft of the paper. All authors contributed to the discussions and revisions of the paper.
Corresponding author
Correspondence to Xiaotong Zhang .
Ethics declarations
Competing interests.
A patent application (201710975996.1) on the storage method of early version of MGED has been submitted by University of Science and Technology Beijing with X.Z., S.L., Y.S., H.Y., X.J., and X.W. as inventors.
Additional information
Publisher’s note Springer Nature remains neutral with regard to jurisdictional claims in published maps and institutional affiliations.
Rights and permissions
Open Access This article is licensed under a Creative Commons Attribution 4.0 International License, which permits use, sharing, adaptation, distribution and reproduction in any medium or format, as long as you give appropriate credit to the original author(s) and the source, provide a link to the Creative Commons license, and indicate if changes were made. The images or other third party material in this article are included in the article’s Creative Commons license, unless indicated otherwise in a credit line to the material. If material is not included in the article’s Creative Commons license and your intended use is not permitted by statutory regulation or exceeds the permitted use, you will need to obtain permission directly from the copyright holder. To view a copy of this license, visit http://creativecommons.org/licenses/by/4.0/ .
Reprints and permissions
About this article
Cite this article.
Liu, S., Su, Y., Yin, H. et al. An infrastructure with user-centered presentation data model for integrated management of materials data and services. npj Comput Mater 7 , 88 (2021). https://doi.org/10.1038/s41524-021-00557-x
Download citation
Received : 09 September 2020
Accepted : 19 May 2021
Published : 08 June 2021
DOI : https://doi.org/10.1038/s41524-021-00557-x
Share this article
Anyone you share the following link with will be able to read this content:
Sorry, a shareable link is not currently available for this article.
Provided by the Springer Nature SharedIt content-sharing initiative
This article is cited by
Advances in machine learning- and artificial intelligence-assisted material design of steels.
- Guangfei Pan
- Feiyang Wang
- Xinping Mao
International Journal of Minerals, Metallurgy and Materials (2023)
Reviewing machine learning of corrosion prediction in a data-oriented perspective
- Leonardo Bertolucci Coelho
- Herman Terryn
npj Materials Degradation (2022)
- Haiyan Gong
- Jiawang Wan
Scientific Data (2022)
A review of the recent progress in battery informatics
npj Computational Materials (2022)
Quick links
- Explore articles by subject
- Guide to authors
- Editorial policies
Sign up for the Nature Briefing newsletter — what matters in science, free to your inbox daily.

- Integrate.io
Glossary of Terms
What is application integration.
Application integration is the process of enabling communication between two or more independent applications to reduce data redundancy and avoid information silos.
Why is Application Integration Necessary?
Very often, enterprises, such as banks and hospitals, have on-premise applications such as a customer relationship management software (CRM) or an enterprise resource planning software (ERP). However, to improve performance or to add new capabilities, they might deploy newer technologies, such as a cloud or a web application. These newer additions need to interoperate with older systems for smooth data flow. Application integration is the process of enabling this interoperability between the old and the new to achieve data consistency across an organization.
How is Application Integration Useful?
Imagine you run a food delivery business. If you have an integrated application architecture, data from your orders is transformed for your ERP system for processing, goes to your logistics department for delivery information, is transferred to your finance department for invoicing, and is also transformed for your CRM for customer information. All of this happens without any manual intervention.
In other words, application integration helps organizations improve overall business efficiency while reducing IT costs.
How is Application Integration Achieved?
Broadly speaking, application integration has four different levels, which vary in their complexity and functionality. These are:
- Presentation level integration : At the presentation level, different applications are presented as a single application with a common interface. It is achieved through middleware technology. Presentation level integration is, perhaps, the earliest kind of application integration. It is also known as screen scraping.
- Business process integration : This level of integration connects various business processes to common servers, which can be on-premise or cloud . Business process integration, largely, deals with how different applications will interact with each other. It is helpful in automating business processes, reducing human error, and improving overall efficiency.
- Data integration : One of the key processes for efficient application integration is data integration . It ensures that applications can talk to each other by way of exchanging data. Since different applications might output different data formats, data transformation is a key part of this integration level. Simply speaking, data is converted into a format that is understood by all applications. Data transformation is achieved either by writing custom code for all applications in an architecture, or deploying data connectors, which act as an intermediary between applications. Data connectors transform data output from different applications into a common language that is understood by all. They are a more scalable and efficient solution for data integration.
- Communications-level integration : This is the ultimate level in application integration. It is used to achieve business process and data integration. While data integration ensures that applications can talk to each other, communications-level integration defines how communication happens. It employs APIs (application program interface) to achieve the task. Different methods are available for communications-level integration viz. point-to-point approach, hub-and-spoke model, and an enterprise service bus (ESB).
In addition to different levels of integration, application integration also employs two different kinds of messaging for communication between applications and data sources. These are synchronous communication and asynchronous communication.
A guide to the nomenclature of data integration technology.
What is middleware.
Middleware software connects easily to other software, allowing assorted applications to communicate with each other. Discover how it benefits application developers.
What is Cloud Integration?
Cloud integration is the process of connecting separate data repositories and/or applications into one central platform. These repositories and applications could reside on different cloud platforms or a combination of...
[email protected] +1-888-884-6405
©2024 Integrate.io
- Solutions Home
- Release Notes
- Support & Resources
- Documentation
- Documentation API
- Service Status
- Privacy Policy
- Terms of Service
- Consent Preferences
- White Papers
Get the Integrate.io Newsletter
Choose your free trial, etl & reverse etl, formerly xplenty.
Low-code ETL with 220+ data transformations to prepare your data for insights and reporting.
Formerly FlyData
Replicate data to your warehouses giving you real-time access to all of your critical data.
API Generation
Formerly dreamfactory.
Generate a REST API on any data source in seconds to power data products.
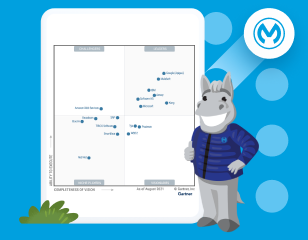
Gartner names MuleSoft a Leader
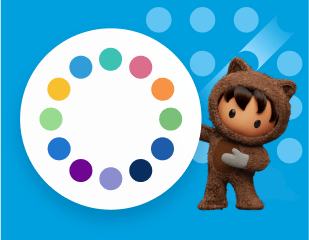
Unleash the power of Salesforce Customer 360 through integration
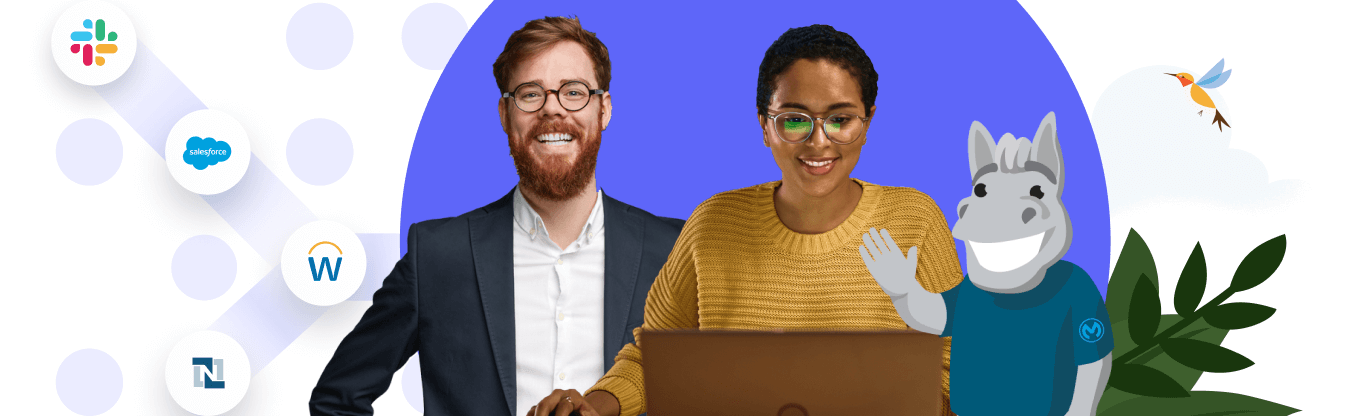
Anypoint Platform Fundamentals
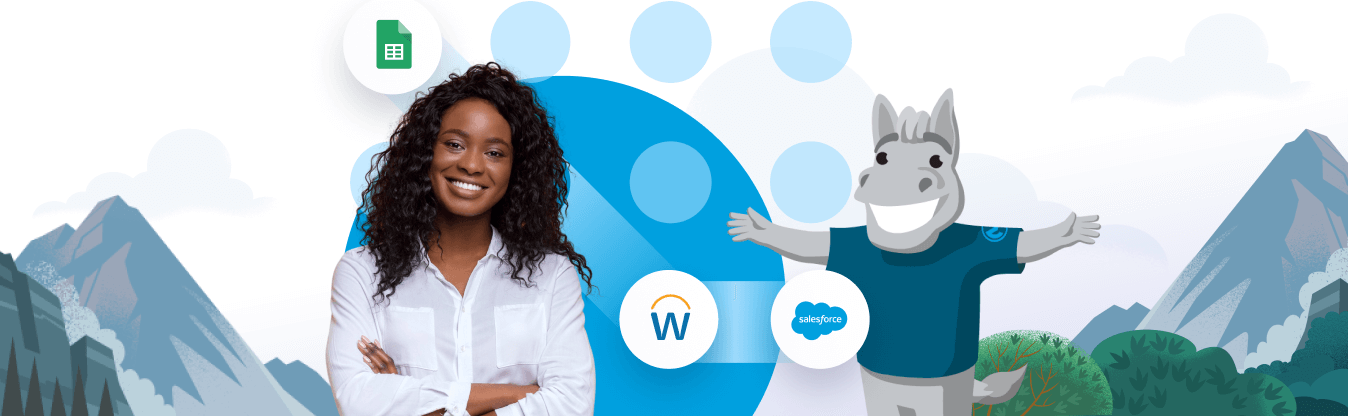
MuleSoft at World Tour
Deutschsprachig.
Diese Inhalte gibt es auch auf Deutsch. Um die Sprache zu ändern, klicken Sie auf das Symbol.
Deutsche Version
© Copyright 2024 Salesforce, Inc. All rights reserved .
- SOA resources
- Applications integration
Applications Integration
Synchronous vs. asynchronous communication in applications integration, what is applications integration.
Applications integration (or enterprise application integration) is the sharing of processes and data among different applications in an enterprise. For both small and large organizations alike, it has become a mission-critical priority to connect disparate applications and leverage application collaboration across the enterprise in order to improve overall business efficiency, enhance scalability, and reduce IT costs.
Before architecting an applications integration solution, however, it is crucial to understand the different levels of integration and in particular, how messages are exchanged in an applications integration environment. This article provides an overview of the different levels of integration - the presentation level, the business process level, the data level, and the communications level - and then examines communications-level integration in greater detail with a discussion of synchronous and asynchronous communication.
The Different Levels of Applications Integration
There are four different levels of applications integration. At the presentation level, integration is achieved by presenting several different applications as a single application with a common user interface (UI). Also known as “screen scraping,” this older approach to integration involves using middleware technology to collect the information that a user enters into a web page or some other user interface. Presentation-level integration was previously used to integrate applications that could not otherwise be connected, but applications integration technology has since evolved and become more sophisticated, making this approach less prevalent.
With business process integration, the logical processes required by an enterprise to conduct its business are mapped onto its IT assets, which often reside in different parts of the enterprise and increasingly, the cloud . By identifying individual actions in a workflow and approaching their IT assets as a meta-system (i.e. a system of systems), enterprises can use applications integration to define how individual applications will interact in order to automate crucial business processes, resulting in the faster delivery of goods and services to customers, reduced chances for human error, and lower operational costs.
Aside from business process integration, data integration is also required for successful applications integration. If an application can’t exchange and understand data from another application, inconsistencies can arise and business processes become less efficient. Data integration is achieved by either writing code that enables each application to understand data from other applications in the enterprise or by making use of an intermediate data format that can be interpreted by both sender and receiver applications. The latter approach is preferable over the former since it scales better as enterprise systems grow in size and complexity. In both cases, access, interpretation, and data transformation are important capabilities for successfully integrating data.
Underlying business process and data integration are communications-level integration. This refers to how different applications within an enterprise talk to each other, either through file transfer, request/reply methods, or messaging. In many cases, applications weren’t designed to communicate with each other, requiring technologies for enabling such communication. These include Application Programming Interfaces (APIs), which specify how applications can be called, and connectors that act as intermediaries between applications. At the communications level, it is also important to consider the architecture of interactions between applications, which can be integrated according to a point-to-point model, hub-and-spoke approach, or with an Enterprise Service Bus (ESB).
Getting the Message: Synchronous vs. Asynchronous Communication
Without effective communication, business processes and data cannot be properly integrated. Depending on an enterprise’s particular needs, communication can be either synchronous, asynchronous, or some combination of the two.
In synchronous communication, a sender application sends a request to a receiver application and must wait for a reply before it can continue with its processing. This pattern is typically used in scenarios where data requests need to be coordinated in a sequential manner.
In asynchronous communication, a sender application sends a message to a receiver application and continues its processing before receiving a response. In other words, the sender application does not depend on the receiver application to complete its processing. If multiple applications are being integrated in such a fashion, the sender application can complete its processing even if other subprocesses have not finished processing.
When designing applications integration solutions, asynchronous communication offers a number of advantages over synchronous communication, especially when it comes to services in SOA . In synchronous communication patterns, timeouts are more common when an application has to wait for responses from several other applications. This means that the availability of services increases since individual processes are not blocked out as frequently due to waiting on other subprocesses to complete. Subprocesses can, in fact, be executed in any order. Moreover, asynchronous communication allows for the loose coupling of applications, eliminating the need for connection management. This results in an applications integration solution that is more flexible, agile, and scalable - essential attributes for today’s enterprise systems.
Want to learn more? Explore Anypoint Platform , a unified, highly productive, hybrid platform for application integration.

You have been redirected
You have been redirected to this page because Servicetrace has been acquired by MuleSoft. Click here to learn more.
After 17 years of reporting on the API economy, ProgrammableWeb has made the decision to shut down operations. Click here to learn more.
Cette page est disponible en Français
Voir en Français
Verfügbar in Deutsch
Diese Seite gibt es auch auf Deutsch
Deutsche Seite
Stay on English page
Esta página está disponible en español
Ver en español
Essa página está disponível em Português
Veja-a em Português
このページは日本語でもご利用いただけます
- Design and discover event-driven APIs with the new AsyncAPI
- Synchronous and Asynchronous Throttling
- Asynchronous Messaging Patterns
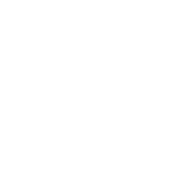
Introduction to the Technology Integration Matrix
Dr. James Welsh FCIT Director University of South Florida
This free technology integration model is used in many schools around the world. The TIM keeps the focus on effective, research-based pedagogy rather than solely on technology.
James gives a short history of the development of the Technology Integration Matrix and then explains the relationship of the five characteristics of meaningful learning environments (active, collaborative, constructive, authentic, and goal-directed) to the five levels of technology integration (entry, adoption, adaptation, infusion, and transformation).
James then discusses the underlying concepts of the TIM including teacher ownership of learning vs student ownership of learning, procedural understandings vs conceptual understandings, conventional use of technology tools vs their complex use, and the instructional focus on tools vs a focus on content.
Return to index of video presentations.
On Model Integration and Integration Modelling
Introduction to the Subject Area Integration Modelling
Cite this chapter
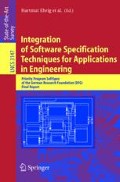
- Martin Große-Rhode 23
Part of the book series: Lecture Notes in Computer Science ((LNCS,volume 3147))
715 Accesses
1 Citations
Integration is a necessary activity in each software development process. Within a model-based approach also the models that are used must be integrated. Model integration has two dimensions: On the one hand, the model components that result from the decomposition of the system under consideration have to be put together. On the other hand, different views onto the system are distinguished and modelled separately in order to decrease the complexity of the development process; the according models also must be brought together again.
Integration models are meta-level definitions of model integration methods. Ideally they can be instantiated with arbitrary kinds of models and modelling languages, which then yields an integration method for these models. In this introduction integration modelling is put into perspective; integration models are presented in the following contributions.
This is a preview of subscription content, log in via an institution to check access.
Access this chapter
- Available as PDF
- Read on any device
- Instant download
- Own it forever
- Compact, lightweight edition
- Dispatched in 3 to 5 business days
- Free shipping worldwide - see info
Tax calculation will be finalised at checkout
Purchases are for personal use only
Institutional subscriptions
Unable to display preview. Download preview PDF.
Boiten, E.A., Bowman, H., Derrick, J., Linington, P.F.: Steen M.W.A. Viewpoint consistency in ODP. Computer Networks 34(3), 503–537 (2000)
Article Google Scholar
Bowman, H., Boiten, E.A., Derrick, J., Steen, M.W.A.: Strategies for consistency checking based on unification. Science of Computer Programming 33, 261–298 (1999)
Article MATH MathSciNet Google Scholar
Büssow, R., Dörr, H., Geisler, R., Grieskamp, W., Klar, M.: μ SZ – Ein Ansatz zur systematischen Verbindung von Z und Statecharts. Technical Report 96-32, Technische Universität Berlin (February 1996)
Google Scholar
Bohn, J., Damm, W., Klose, J., Moik, A., Wittke, H.: Modeling and validating train system applications using statemate and live sequence charts. In: Proc. Int. Conf. Integrated Design and Process Technology, IDPT 2002 (2002)
Büssow, R., Geisler, R., Klar, M.: Specifying safety-critical embedded systems with statecharts and Z: a case study. In: Astesiano, E. (ed.) ETAPS 1998 and FASE 1998. LNCS, vol. 1382, pp. 71–87. Springer, Heidelberg (1998)
Chapter Google Scholar
Burmester, S., Giese, H., Niere, J., Tichy, M., Wadsack, J.P., Wagner, L., Wendehals, R., Zündorf, A.: Tool integration at the meta-model level within the FUJABA tool suite. In: Proc. Workshop on Tool-Integration in System Development (TIS) at ESEC/FSE 2003, pp. 51–56 (2003)
Bishop, R.H.: Modern Control Systems Analysis and Design Using MATLAB and Simulink. Addison-Wesley, Reading (1997)
Brinksma, E. (ed.). Information processing systems – Open Systems Interconnection – LOTOS – A formal description technique based on the temporal ordering of observational behaviour. ISO 8807 (1989), International Standard
Bowman, H., Steen, M.W.A., Boiten, E.A., Derrick, J.: A formal framework for viewpoint consistency. Computing Laboratory Technical Report 22-99, University of Kent at Canterbury (December 1999), http://www.cs.ukc.ac.uk/people/staff/jd1/pubs.html
Damm, W., Harel, D.: Lscs: Breathing life into message sequence charts. Formal Methods in System Design 19(1), 45–80 (2001)
Article MATH Google Scholar
Enders, B.E., Goedicke, M., Heverhagen, T., Tracht, R., Tröpfner, P.: Towards an integration of different specification methods by using the viewpoint framework. In: Tanik, M.M., Ertas, A. (eds.) Proc. 5th World Conference on Integrated Design and Process Technology (IDPT 2000), Society for Design and Process Science (2000)
Egyed, A.: Heterogeneous View Integration and its Automation. PhD thesis, University of Southern California, Los Angeles (April 1999)
ISO/IEC 15437, Information Technology - Enhancements to LOTOS (2001)
Egyed, A., Medvidovic, N.: A formal approach to heterogeneous software modeling. In: Maibaum, T. (ed.) FASE 2000. LNCS, vol. 1783, pp. 178–192. Springer, Heidelberg (2000)
Fischer, C.: CSP–OZ: A combination of Object–Z and CSP. In: Bowman, H., Derrick, J. (eds.) Formal Methods for Open Object–Based Distributed Systems (FMOODS 1997), vol. 2, pp. 423–438. Chapman & Hall, Boca Raton (1997)
Fay, A., Jansen, L., Schnieder, E.: Towards a knowledge-based decision support system for high speed train traffic control. In: Proceedings of the World Congress on Railway Research (WCRR 1996). Association of American Railroads (1996)
Finkelstein, A., Kramer, J., Nuseibeh, B., Goedicke, M., Finkelstein, L.: Viewpoints: A framework for integrating multiple perspectives in system development. International Journal of Software Engineering and Knowledge Engineering 2(1), 31–58 (1992)
Goguen, J., Burstall, R.M.: Institutions: Abstract model theory for specification and programming. Journal of the ACM 39(1), 95–146 (1992)
Goedicke, M., Enders, B., Meyer, T., Taentzer, G.: Viewpoint–oriented software development: Tool support for integrating multiple perspectives by distributed graph transformations. In: Schwartzbach, M.I., Graf, S. (eds.) TACAS 2000. LNCS, vol. 1785, pp. 43–47. Springer, Heidelberg (2000)
Geisler, R., Klar, M., Mann, S.: Precise semantics of integrated modeling languages by formal metamodeling. In: Tanik, M.M., Ertas, A. (eds.) Proc. 5th World Conference on Integrated Design and Process Technology. Society for Design and Process Science (2000)
Giese, H., Kardos, M., Nickel, U.A.: Towards design verification and validation at multiple levels of abstraction. In: Proc. IFIP World Computer Congress, Stream 7 on Distributed and Parallel Embedded Systems (DIPES 2002), Montreal, Canada (August 2002)
Große–Rhode, M.: Semantic Integration of Heterogeneous Software Specifications. EATCS Monographs in Theoretical Computer Science. Springer, Heidelberg (2004)
Gruber, T.R.: A translation approach to portable ontologies. Knowledge Acquisition 5(2), 199–220 (1993)
Gurevich, Y.: Evolving algebras, a tutorial introduction. In: Bulletin of the EATCS 43, pp. 264–284. Springer, Heidelberg (1991)
Gurevich, Y.: Evolving algebra 1993. In: Börger, E. (ed.) Specification and Validation Methods, Oxford University Press, Oxford (1994)
Hendler, J., Berners-Lee, T., Miller, E.: Integrating applications on the semantic web. Journal of the Institute of Electrical Engineers of Japan 122(10), 676–680 (2002)
Jansen, L., Schnieder, E.: Traffic control systems case study: Problem description and a note on domain-based software specification. In: Ehrig, H., Große-Rhode, M., Orejas, F. (eds.) Proc. International Workshop on Integration of Specification Techniques with Applications in Engineering (INT 2000), pp. 41–47 (2000)
Klose, J., Lettrari, M.: Scenario-based monitoring and testing of realtime uml models. In: Gogolla, M., Kobryn, C. (eds.) UML 2001. LNCS, vol. 2185, p. 317. Springer, Heidelberg (2001)
The MathsWorks, http://www.mathworks.com/
Nickel, U.A., Kardos, M.: ASMs as integration platform towards integration of distributed production control systems at multiple levels of abstraction. In: Proc. 10th International Workshop on Abstract State Machines (2003)
ISO/IEC International Standard 10746, ITU–T recommendation X.901– X.904: Reference model of open distributed processing – Parts 1–4
Orejas, F., Pino, E.: On the integration of heterogeneous modular specifications. In: Ehrig, H., Damm, W., Desel, J., Große-Rhode, M., Reif, W., Schnieder, E., Westkämper, E. (eds.) INT 2004. LNCS, vol. 3147, pp. 582–601. Springer, Heidelberg (2004)
World Wide Web Consortium. Resource Description Format, http://www.w3.org/RDF/
Reisig, W.: Petri Nets and Algebraic Specifications. In: Rozenberg/Jensen (eds.) High Level Petri Nets (1991)
Reisig, W., Vautherin, J.: An algebraic approach to high level Petri nets. In: Proceedings of the 8th European Workshop on Petri Nets, Zaragoza (1987)
Schröter, G., Braatz, B., Klein, M.: Semantical integration of objectoriented viewpoint specification techniques. In: Ehrig, H., Damm, W., Desel, J., Große-Rhode, M., Reif, W., Schnieder, E., Westkämper, E. (eds.) INT 2004. LNCS, vol. 3147, pp. 602–626. Springer, Heidelberg (2004)
International Electrotechnical Commission. International Standard 61131, Programmable Controllers. Part 3: Programming Languages. IEC (1993)
Schnieder, E., Jansen, L.: Begriffsmodelle der Automatisierungstechnik – Basis effizienten Engineerings. In: Schnieder, E. (ed.) Engineering komplexer Automatisierungssysteme (EKA 2001), pp. 1–27 (2001)
World Wide Web Consortium. Semantic Web, http://www.w3.org/2001/sw/
Download references
Author information
Authors and affiliations.
Fraunhofer ISST Berlin, Germany
Martin Große-Rhode
You can also search for this author in PubMed Google Scholar
Editor information
Editors and affiliations.
Technische Universität Berlin, Germany
Hartmut Ehrig
OFFIS e.V., Escherweg 2, 26121, Oldenburg, Germany
Werner Damm
Department of Applied Computer Science, Catholic University of Eichstätt-Ingolstadt,
Lehrstuhl für Softwaretechnik und Programmiersprachen Institut für Informatik, Universität Augsburg, 86135, Augsburg, Germany
Wolfgang Reif
Institute for Traffic Safety and Automation Engineering, Technical University of Braunschweig, Braunschweig, Germany
Eckehard Schnieder
Institute of Industrial Manufacturing and Management (IFF), University of Stuttgart, Nobelstrasse 12, D-70569, Stuttgart, Germany
Engelbert Westkämper
Rights and permissions
Reprints and permissions
Copyright information
© 2004 Springer-Verlag Berlin Heidelberg
About this chapter
Große-Rhode, M. (2004). On Model Integration and Integration Modelling. In: Ehrig, H., et al. Integration of Software Specification Techniques for Applications in Engineering. Lecture Notes in Computer Science, vol 3147. Springer, Berlin, Heidelberg. https://doi.org/10.1007/978-3-540-27863-4_30
Download citation
DOI : https://doi.org/10.1007/978-3-540-27863-4_30
Publisher Name : Springer, Berlin, Heidelberg
Print ISBN : 978-3-540-23135-6
Online ISBN : 978-3-540-27863-4
eBook Packages : Springer Book Archive
Share this chapter
Anyone you share the following link with will be able to read this content:
Sorry, a shareable link is not currently available for this article.
Provided by the Springer Nature SharedIt content-sharing initiative
- Publish with us
Policies and ethics
- Find a journal
- Track your research
Home Blog Design Multimedia Presentation: Insights & Techniques to Maximize Engagement
Multimedia Presentation: Insights & Techniques to Maximize Engagement
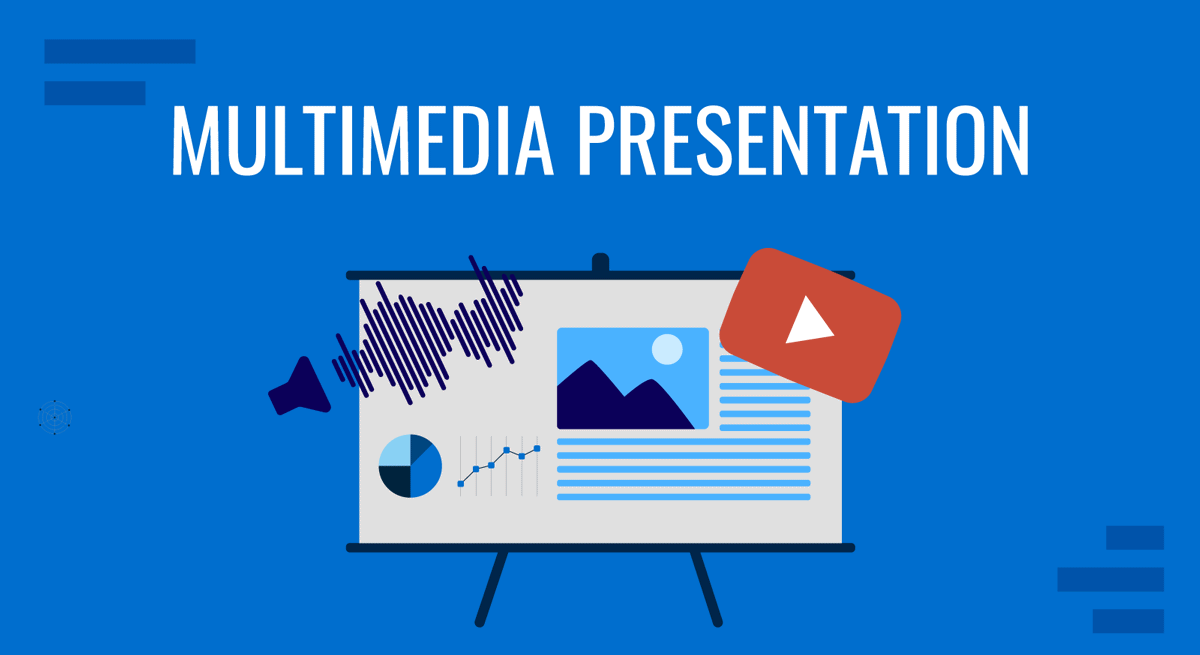
Effective public speaking is a crucial skill often overlooked. Multimedia presentations, such as digital stories, offer an opportunity to cultivate this skill. When appropriately executed, they blend visuals, text, and compelling speech in any setting. This article explores multimedia presentation, focusing on its definition, components, and the advantages it brings to the table.
Table of Contents
What is a Multimedia Presentation?
Types of mediums used in a multimedia presentation, advantages of multimedia presentation, how to create a multimedia presentation.
- How to Deliver a Multimedia Presentation?
Recommended Templates for Multimedia Presentations
Final words.
Multimedia originates from the combination of “multi” and “media,” where “multi” denotes “many” [1]. It represents a medium facilitating the seamless transfer of information between locations. Multimedia encompasses the computer-assisted integration of various elements like text, drawings, still and moving images (videos), graphics, audio, animation, and other forms of media [1]. It allows the expression, storage, communication, and digital information processing.
A multimedia presentation goes beyond traditional slides to convey information. It is a dynamic approach that enhances engagement and boosts information retention among the audience. With the use of technology, it requires thoughtful design, pacing, and interactive components. Multimedia presentations deliver information through various channels, offering a multi-sensorial experience. As a consequence, they accommodate different learning preferences.
To create a truly immersive experience, multimedia presentations leverage different mediums. Video clips offer dynamic visual content, infographics provide concise information, audio snippets add a layer of narration, and animations bring concepts to life. The synergy of these elements elevates the overall impact of the presentation.
Multimedia presentations employ diverse mediums to construct a comprehensive and immersive experience.
Video Clips
Video content consists of photographic images displayed in apparent motion at speeds ranging from 24 to 30 frames per second, and even higher values depending on the topic. The term “video” denotes a moving image accompanied by sound, commonly seen in television broadcasts [2]. Text can be incorporated into videos through captions or embedded in images, as seen in slide presentations. It provides a captivating element to the presentation.
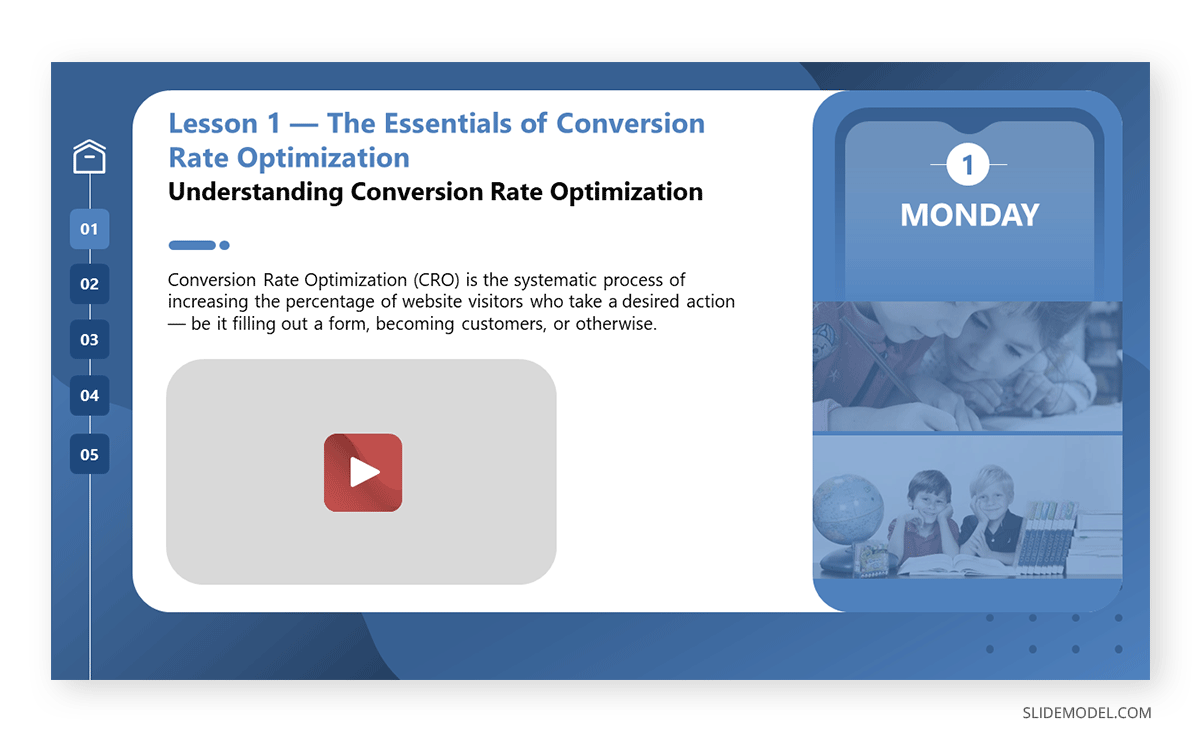
By incorporating motion and imagery, video clips enhance audience understanding and retention, as they can be introduced as a refreshing break during the course of the presentation. It offers the presenter a moment to regroup, especially in lengthy presentations. Videos can emotionally engage audiences through narratives, but their inclusion alone doesn’t guarantee benefits. For effective integration, videos have to follow the narrative of the speech the presenter is sharing. This implies that no random video can be added to a multimedia presentation, and presenters should also take extra care in unrequired effects that don’t contribute to the overall impact of the speech (i.e., abusing VFX or unprofessional transitions).
Now we know why and how videos help a presentation, those who are unfamiliar can learn how to embed a video on a Google Slide .
Infographics
Infographics contribute to the attractiveness of multimedia applications. In numerous situations, the audience prefers visuals over extensive text. It aids in the clarification of concepts and provides background information. In contrast to regular graphics, infographics are commonly acknowledged as a better medium to communicate data extracted from charts and graphs [3]. Their visually appealing format ensures clarity without overwhelming the viewer, making data more digestible.
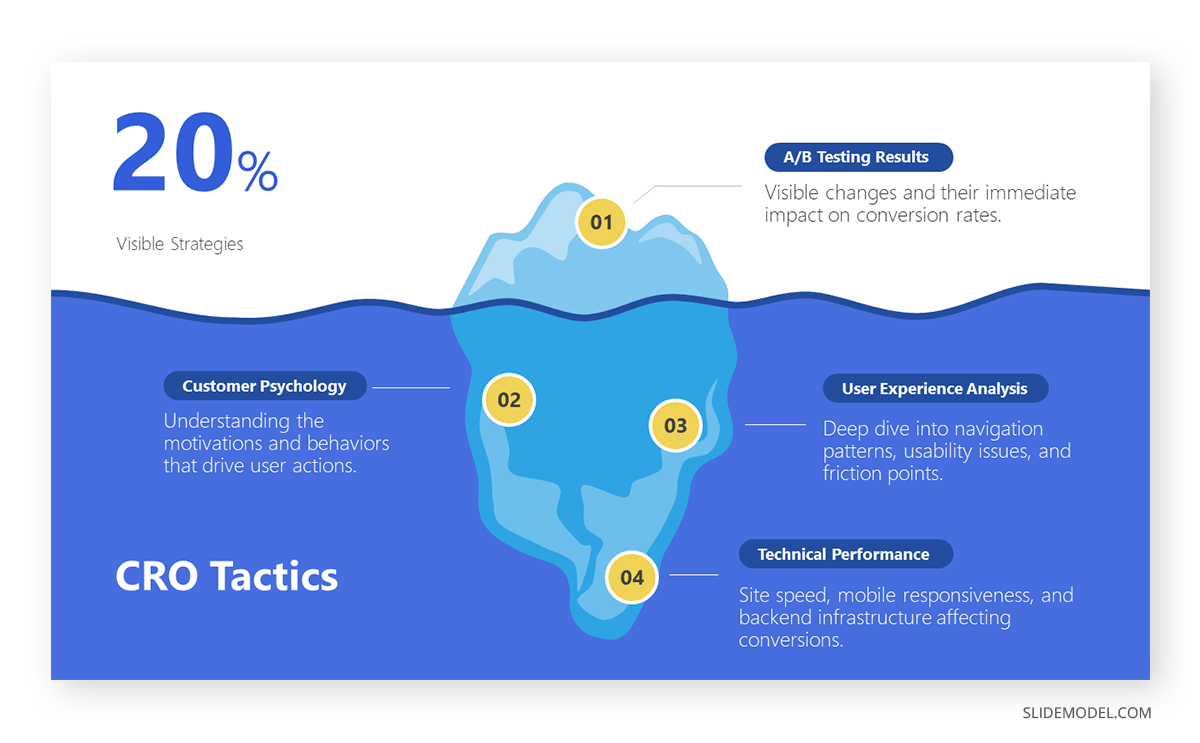
Text [4] is used in multimedia presentations because it is a familiar media channel. It includes characters of different sizes and styles, making words, phrases, and paragraphs. The text requires less effort compared to a video. But this doesn’t mean presenters shouldn’t care about formatting.
Different fonts and sizes in text matter. Using the wrong font shows a lack of professionalism. Multimedia uses text to share information or enhance other media. Therefore, fonts and sizes can be varied for a cohesive aesthetic.
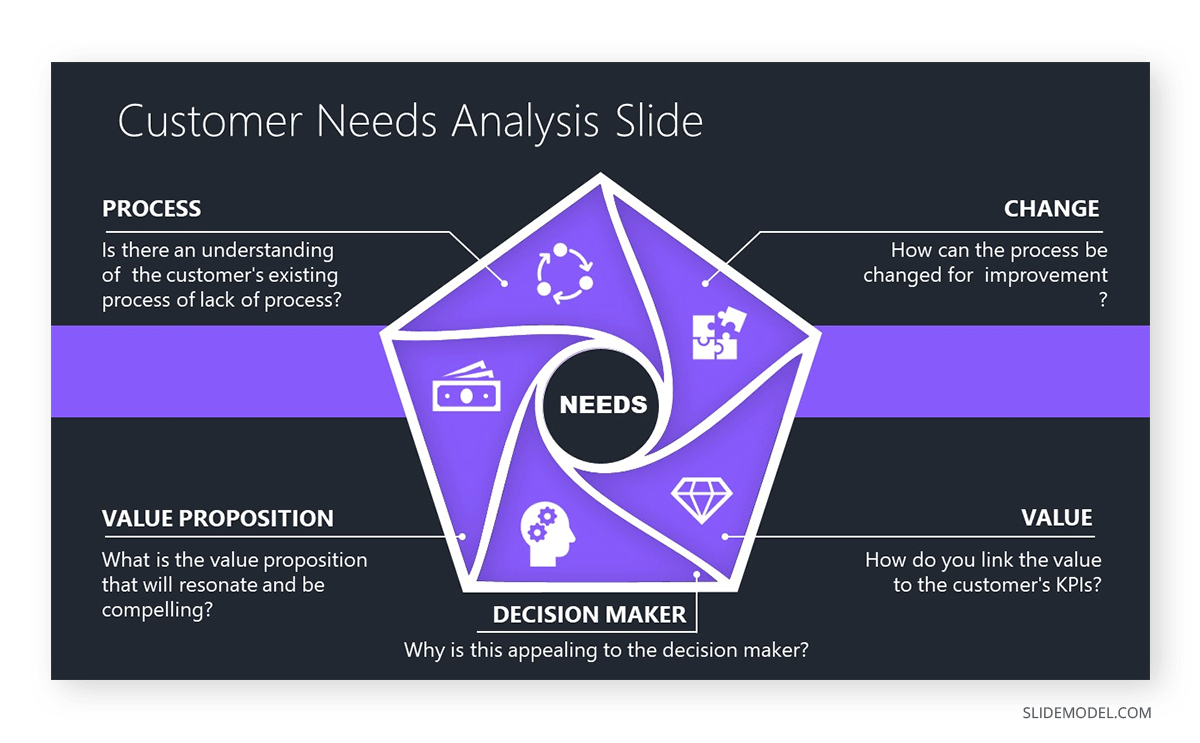
Graphic Design effects, like text on portrait, are mighty in presentations. This technique, featuring phrases alongside visuals of well-known public figures, enhances interest and reinforces key messages. Choosing the right colors and contrast in text design has a psychological appeal. It increases the audience’s interest.
Text design should also consider those with visual impairments. Hence, the text to be used has to follow specific guidelines. Multimedia follows this by presenting text in various formats. This helps everyone understand the message quickly. They make information accessible instantly.
Audio Snippets
Adding audio into a media presentation gives users information that may be challenging to convey through other mediums. Certain types of information, like a motivational speech or enlightening music, are best conveyed through audio. It is a common practice in the motivational presentation niche to use audio as a resource to accompany exercises, like meditational practices, group activities, and storytelling techniques in which the audience has to place themselves in the shoes of the “character” in the story, and the list goes on.
Research indicates that presenting information through multiple senses improves retention [6]. Importantly, audio can make communication accessible for users who present visual impairments.
Animation is a series of static images rapidly flipped through, creating the illusion of movement [5]. Animation involves making a still image appear to be in motion. It enhances the visual appeal of a presentation. Animation is used for illustrating concepts related to movement, such as playing a guitar or hitting a golf ball. These actions are challenging to depict accurately with static images or text alone.
Likewise, animated sequences can guide employees to respond correctly to different threat scenarios. For instance, showing threat scenarios through animation can enhance employees’ ability to recognize and respond to potential risks. Through movement and visual storytelling, animated templates contribute to a deeper understanding of intricate ideas.
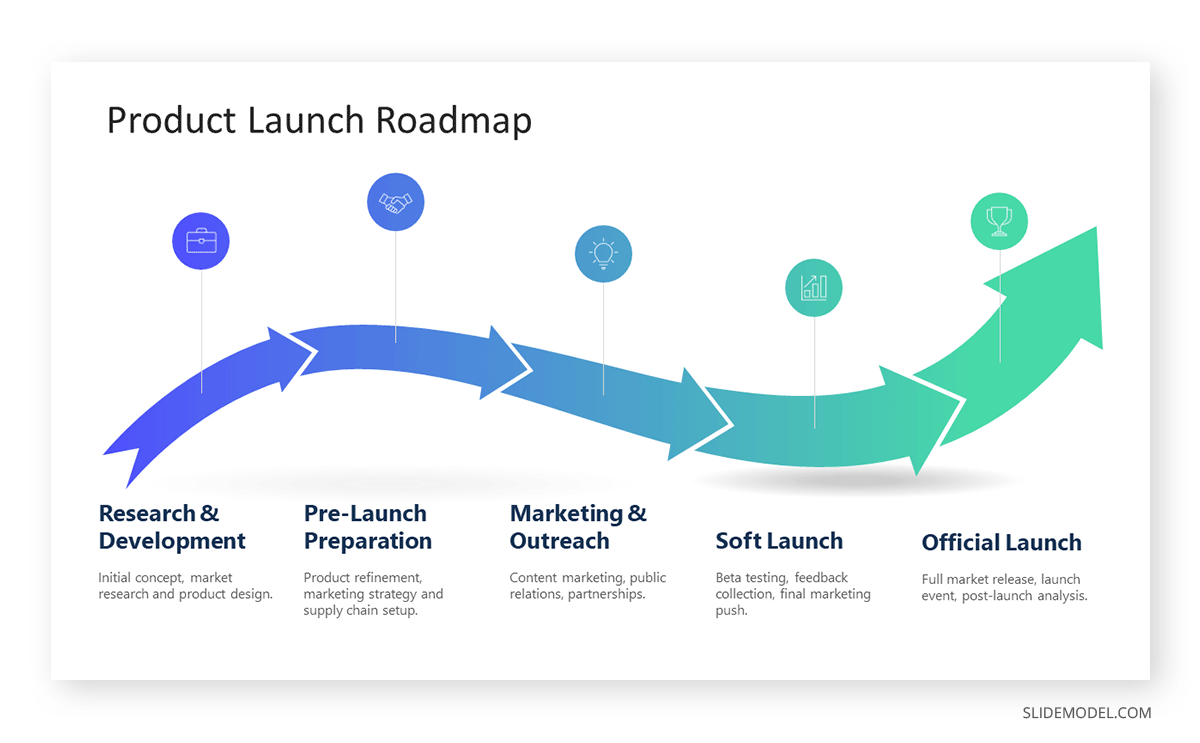
Multimedia presentations emerge as a powerful tool for effective communication, offering engagement, retention, and adaptability in professional and educational settings.
Enhanced Engagement
Multimedia presentations excel in captivating audiences through a customized approach to diverse learning preferences. Multimedia presentations stimulate interest and maintain active engagement by integrating visuals and audio.
Improved Information Retention
Research conducted by Saini and Baba underscores the efficacy of multimedia presentations in enhancing information retention [7]. The combination of visual and auditory stimuli helps understanding quickly. It improves the audience’s ability to grasp and remember key concepts.
Professional Appeal
In professional settings, adopting multimedia presentations contributes to elevated sophistication. This method showcases a commitment to deliver information dynamically and accessibly. Thus, presentation on multimedia positively reflects on both the presenter and the organization.
Flexibility in Communication
Multimedia presentations offer flexibility in conveying complex concepts . Incorporating various mediums allows presenters to adapt their communication style to suit the nature of the information. It makes it more accessible to a broader audience.
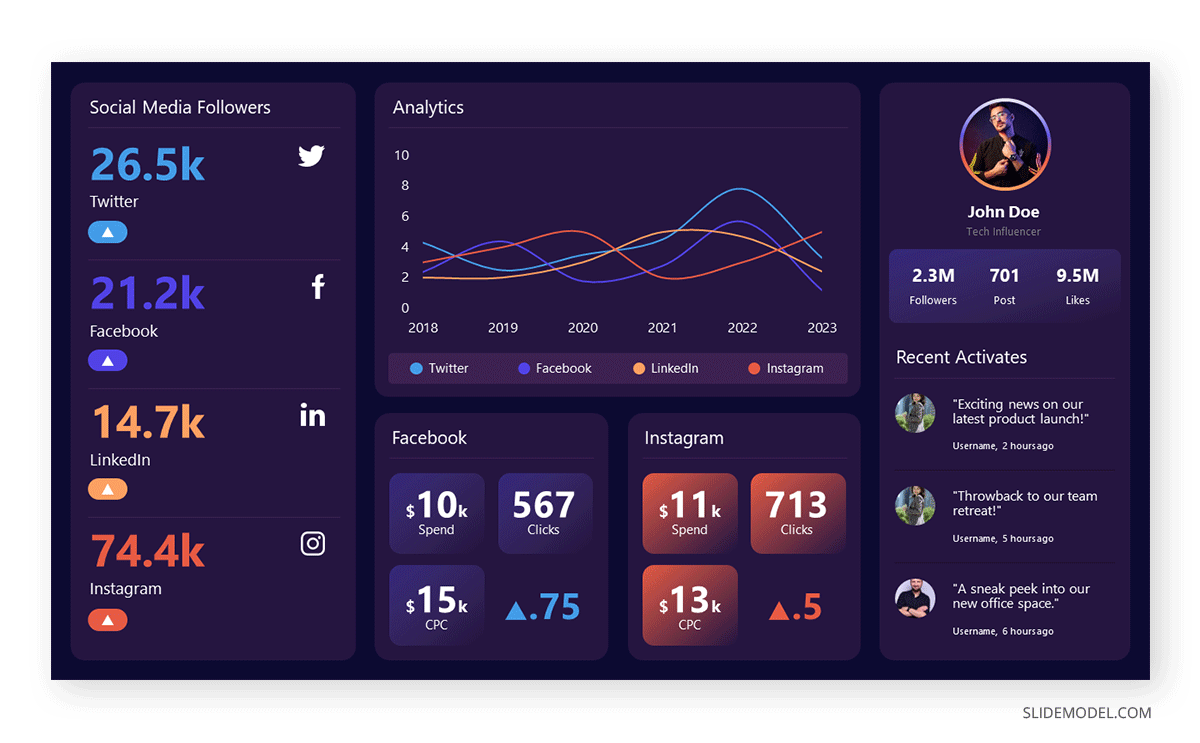
Increased Accessibility
The inclusion of diverse mediums enhances accessibility for a wider audience. Visual elements cater to visual learners, while auditory components appeal to those who learn best through listening. This inclusive approach ensures that the message resonates with different audiences.
Enhanced Persuasion
The dynamic nature of multimedia presentations facilitates a persuasive delivery . Engaging visuals, coupled with compelling narration, influence opinions. It effectively conveys the presenter’s message, making it more memorable and impactful.
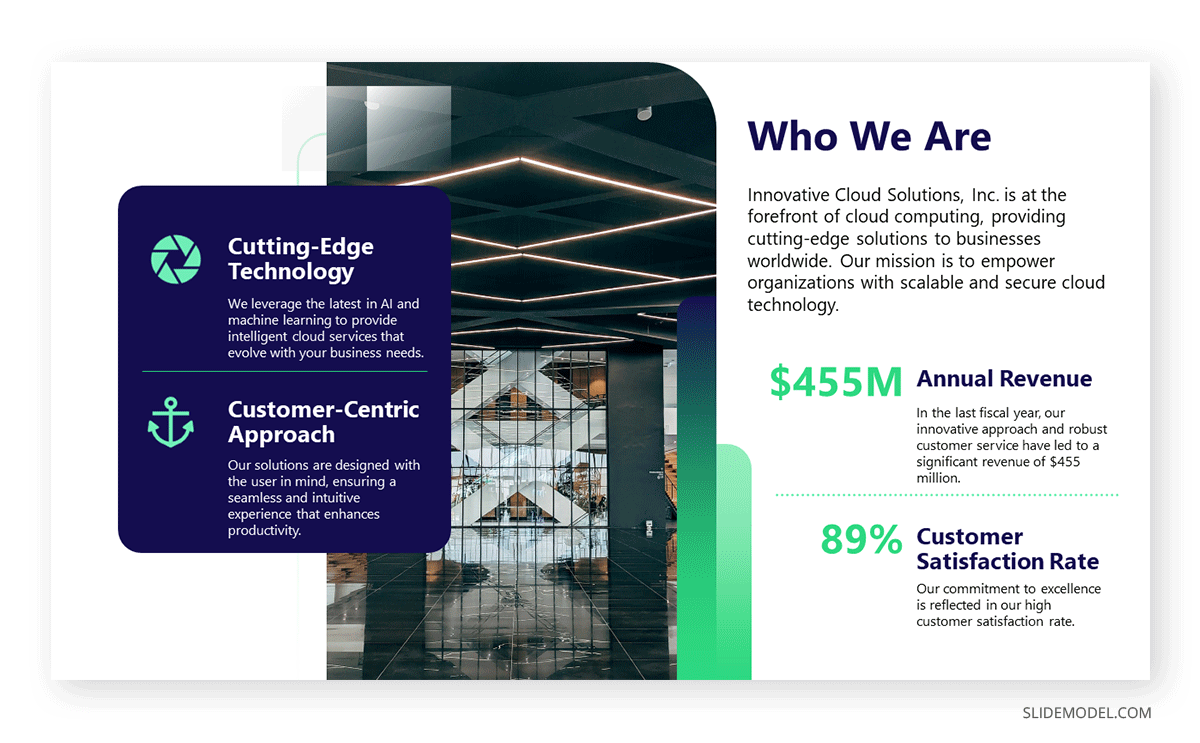
Real-time Demonstration
Multimedia presentations enable real-time demonstration of concepts and ideas. Whether showcasing product features or illustrating complex processes, integrating videos, animations, and live demonstrations leave a lasting impression.
Adaptability to Technological Advances
By using multimedia presentations, presenters showcase adaptability to technological advances [8]. Multimedia presentation aligns with contemporary communication trends. It positions the presenter as forward-thinking and responsive to evolving methods of information delivery.
Crafting an effective multimedia presentation involves thoughtful planning and execution. A well-structured and engaging multimedia presentation is created by following several steps
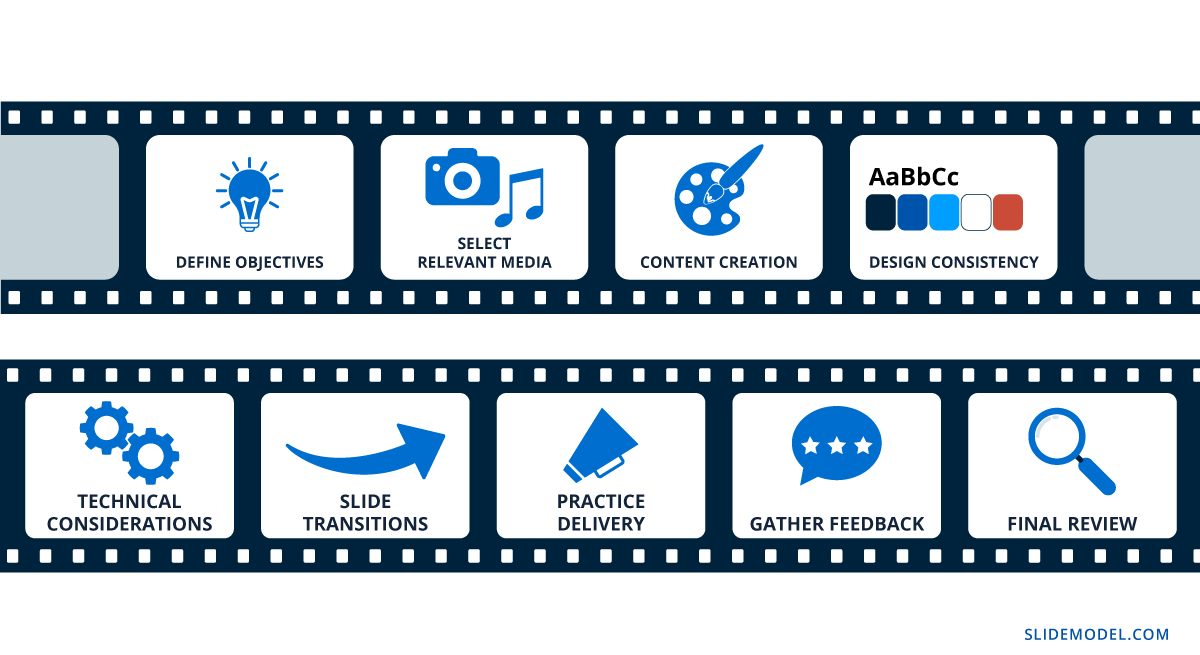
Define Presentation Objectives
The goals and objectives of the presentation must be clearly outlined [9]. A presenter should identify the key message that is conveyed to the audience. This initial step guides the selection of multimedia elements based on the nature of the content. For instance, data presentations involve the use of infographics. On the other hand, motivational presentations may require photos, illustrations, and videos. Likewise, Business presentations may include interactive graphs and infographics.
Audience Research
Understand the target audience to customize content. Choose multimedia elements that resonate with them [9]. Consider the audience’s demographics, preferences, and expectations to create a presentation that captures their interest.
Select Media Channels
Choose appropriate multimedia channels, such as GIFs, videos, infographics, and animated charts, per the defined objectives. Different media channels convey information in distinct ways. Selecting the right ones enhances the overall impact of the presentation. Otherwise, the presenter can learn about design ideas in detail from SlideModel.
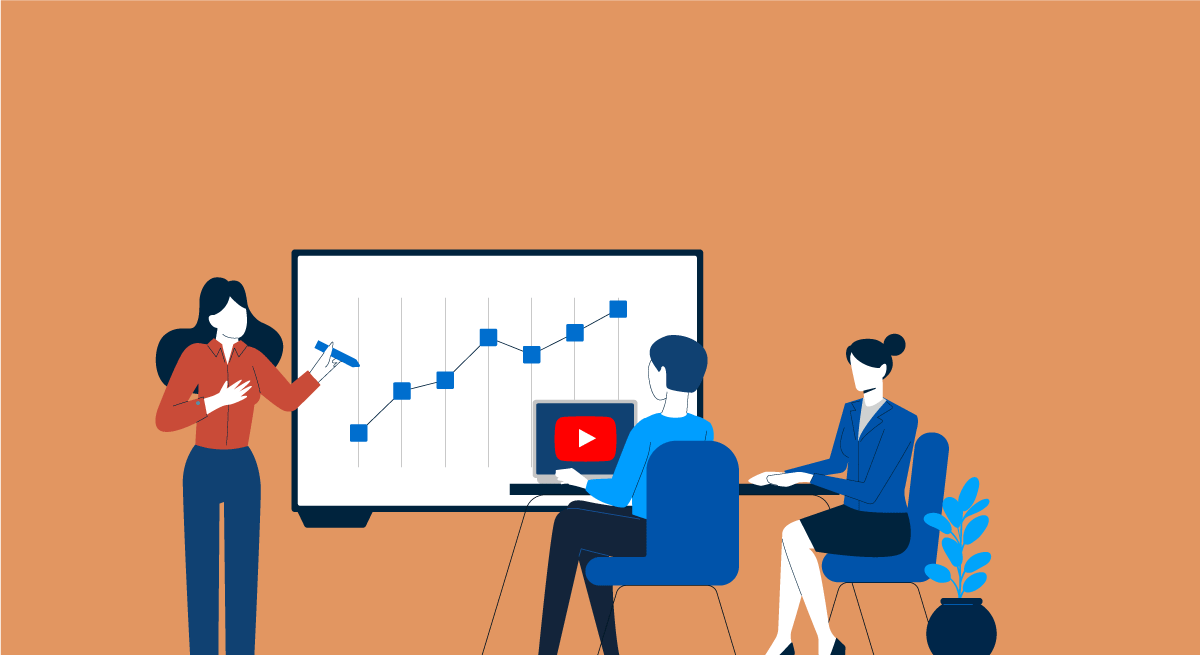
Write the Presentation Story
Develop a structured storyboard outlining the sequence of content, visuals, and multimedia elements. The story should have a logical flow. It captures the audience’s attention and maintains their interest throughout the presentation.
Select a SlideModel Template
Use a SlideModel template to maintain consistency and professionalism in design, including fonts, colors, and layout. Templates provide a cohesive visual identity. These templates reinforce the message and facilitate a clear understanding of the content. There are plenty of templates available that can assist in creating a multimedia presentation.
Create Narrations or Captions
Develop concise and focused content that supports the key message. This contributes to the overall narrative. Narrations provide spoken explanations, while captions offer written context. Narrations or captions should complement the visuals. They provide context and enhance the audience’s understanding.
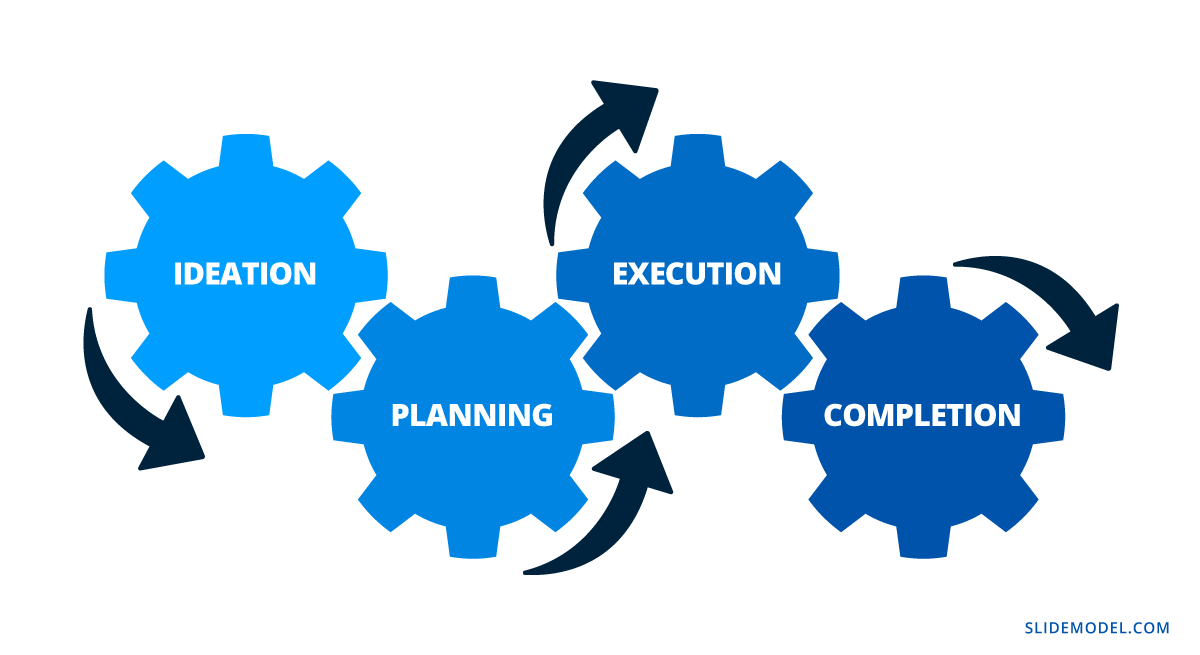
Create Deliverables
Deliverables include the finalized presentation file. It ensures compatibility with the chosen presentation software. Generate deliverables for the multimedia presentation. Optimize files for seamless integration and playback. Pay attention to file sizes and formats.
Transitions and Timings
Incorporate smooth slide transitions for a seamless flow between content. Avoid excessive transitions that may distract from the message. Set appropriate timings to maintain a well-paced presentation. Keep the audience engaged.
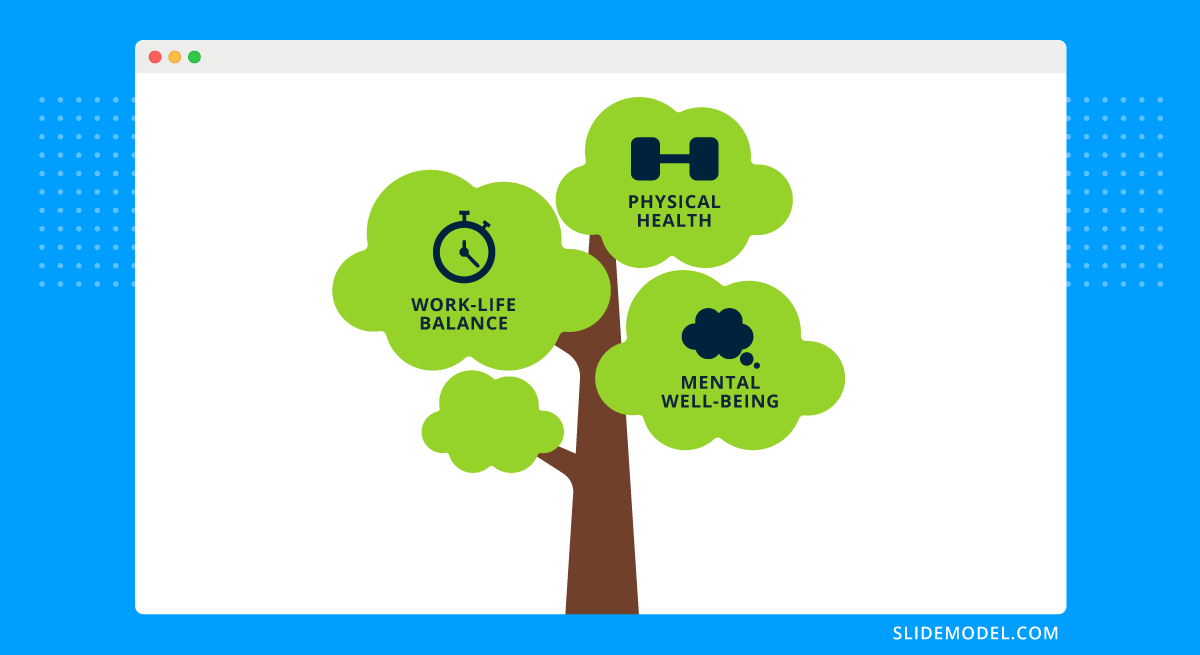
Speaker Notes
Prepare speaker notes to guide the presenter during the delivery. Attention must be paid to timing and the use of multimedia elements. Speaker notes serve as a roadmap. It ensures a confident and well-timed presentation.
How to Deliver a Multimedia Presentation
Delivering a multimedia presentation with impact involves a strategic approach.
Testing Presentation on Arrival
Prioritize testing upon arrival to prevent any technical inconveniences. Verify the compatibility of multimedia elements and the presentation software with the venue’s system. A smooth technical setup is essential for a flawless multimedia presentation.
Timing of Multimedia Elements
Study the timing of multimedia elements. Especially when introducing videos. Allocate time for a Q&A session post-presentation. This strategic timing ensures audience commitment, thus providing a platform for clarifications and discussions.
Avoid Inconsistent Perceptual Mediums
Leverage various perceptual channels, like hearing and vision, to enhance comprehension. Clarify infographics through spoken explanations. Steer clear of concurrent speech and sound sources to prevent interference and distractions. Seamless integration of multiple media sources is paramount for an effective presentation [10].
Professional Tone
Uphold a professional and authoritative tone throughout the presentation. Stay away from overly casual language to underscore the gravity of your message. Opt for a direct approach, aligning with the formality expected in a multimedia presentation.
Body Language
Pay attention to your body language, a critical aspect of multimedia presentations. Show confident and open postures. It reinforces the verbal communication. Effective body language contributes to the audience’s understanding.
Strategic Pauses for Multimedia Absorption
Introduce strategic pauses at key junctures to allow the audience to absorb multimedia information. Recognize that multimedia elements often require additional processing time. Strategic breaks enhance understanding. This prevents information overload and ensures a smooth flow throughout the presentation.
Visual Commitment through Multimedia
Pay close attention to the effective use of multimedia elements. Use body language to complement multimedia content. It will enhance the audience’s connection with the presentation. Effective visual engagement is crucial for a successful multimedia delivery.
1. Pitch Deck for Multimedia Presentation PowerPoint Template
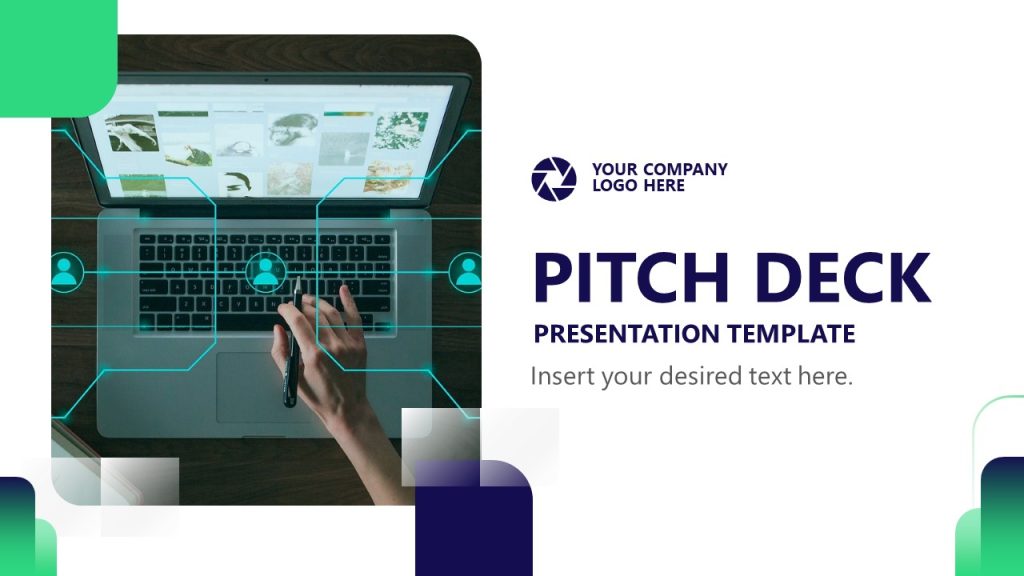
If you are looking for multimedia presentation examples, in this article, we used one slide of this investor pitch deck to showcase why graphics matter in first impressions. Customize this presentation template to meet the requirements of your company; it just takes a couple of clicks!
Use This Template
2. Multimedia Annual Report PowerPoint & Google Slides Template
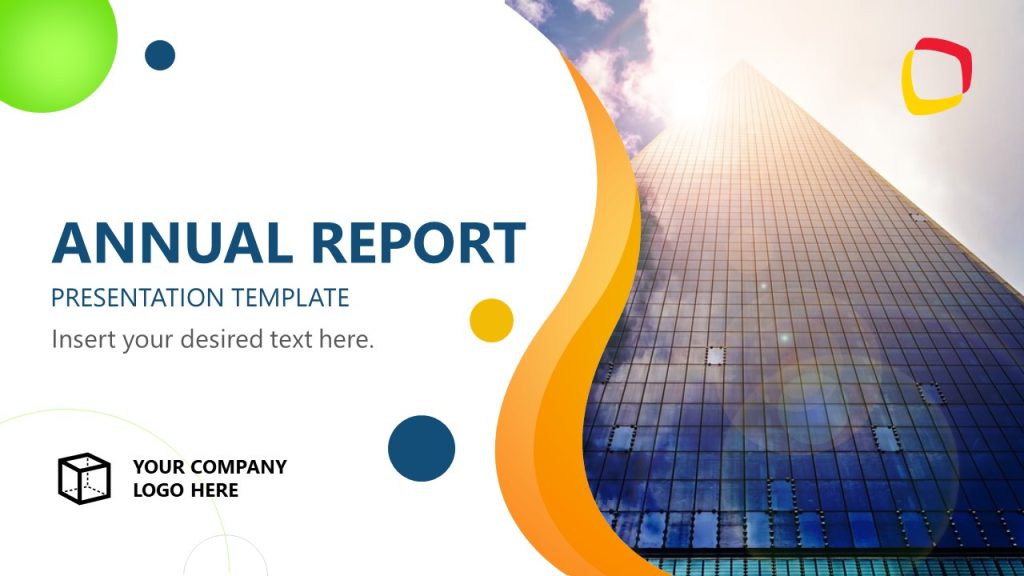
Rather than delivering a plain MS Excel sheet, create your report presentation by using this presentation on multimedia format template. Entirely customizable, you can adapt the presentation theme to any color scheme you choose. Download it today and make your annual report presentation the highlight of this year!
3. CV Template for Interview Meetings with Multimedia Effects
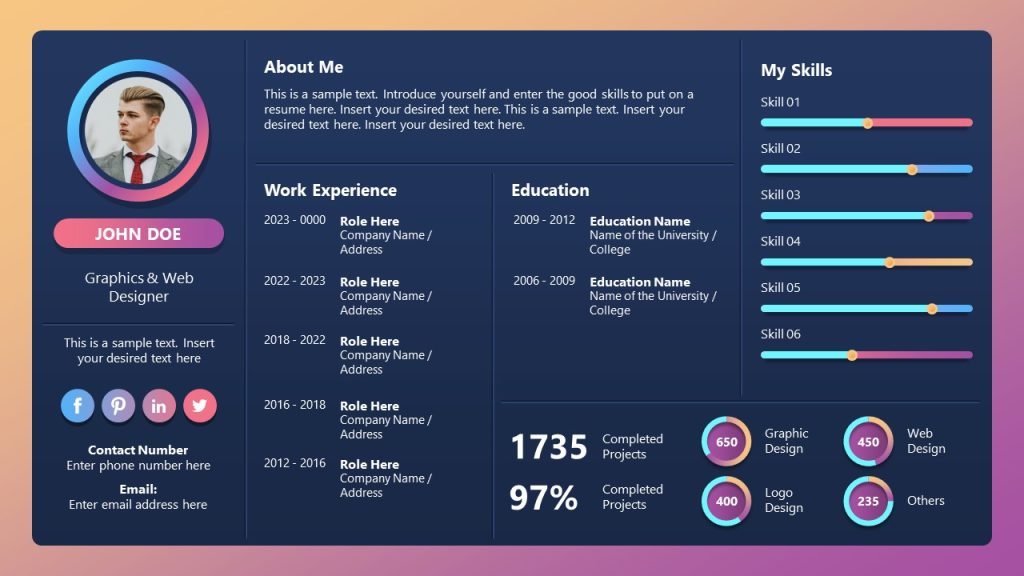
Long gone are the days in which resumes were a blank piece of paper with text and a bad-quality photo. Impress recruiters with a well-designed curriculum vitae by harnessing the advantages of multimedia presentations.
4. Collection of Infographic PPT Templates for Multimedia Presentations
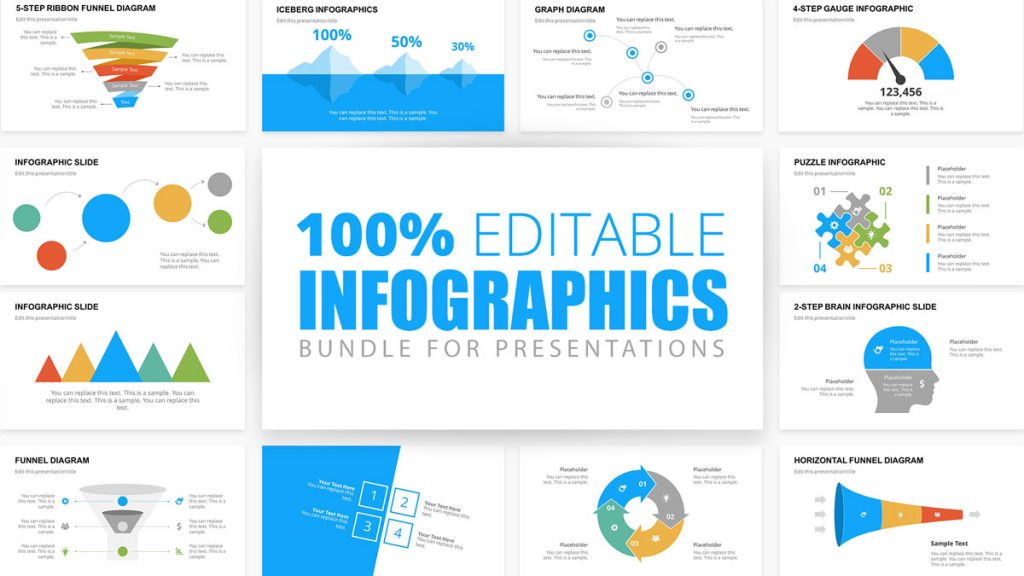
For those who don’t know where to start with infographics, this slide deck has it all. Bubbles, demographics, iceberg metaphor, funnels, and more. Access now and customize the graphics in this high-quality infographics template for PowerPoint – fully compatible with Google Slides and Keynote.
Multimedia presentations are at the forefront of the transformation of public speaking. Using various mediums, presenters create engaging experiences for their audience. Integrating multimedia elements in business or academia raises presentations to new heights.
[1] Li, Z.N., Drew, M.S. and Liu, J., 2004. Fundamentals of multimedia (pp. 253-265). Upper Saddle River (NJ): Pearson Prentice Hall. https://www.goodreads.com/book/show/57105399-fundamentals-of-multimedia
[2] Bowen, C., 2017. Grammar of the Edit . Routledge. https://www.goodreads.com/en/book/show/546377
[3] Siricharoen, W.V., 2013, May. Infographics: the new communication tools in digital age. In The international conference on e-technologies and business on the web (ebw2013) (Vol. 169174). https://www.researchgate.net/profile/Waralak-Siricharoen/publication/256504130_Infographics_the_new_communication_tools_in_digital_age/links/0c9605232e6f666b1f000000/Infographics-the-new-communication-tools-in-digital-age.pdf
[4] Malhotra, R. and Verma, N., 2020. An impact of using multimedia presentations on engineering education. Procedia Computer Science , 172 , pp.71-76.
[5] Pavithra, A., Aathilingam, M. and Prakash, S.M., 2018. Multimedia and its applications. International journal for research & development in technology , 10 (5), pp.271-276.
[6] Henmon, V.A.C., 1912. The relation between mode of presentation and retention. Psychological Review , 19 (2), p.79. https://ia600708.us.archive.org/view_archive.php?archive=/28/items/crossref-pre-1923-scholarly-works/10.1037%252Fh0072137.zip&file=10.1037%252Fh0072813.pdf
[7] Saini, G. and Baba, M.M., 2023. Psychological expedient of multimedia in blended learning and metamemory satisfaction. The Learning Organization . https://www.emerald.com/insight/content/doi/10.1108/TLO-11-2022-0130/full/html
[8] Lauer, C., 2009. Contending with terms: “Multimodal” and “multimedia” in the academic and public spheres. Computers and composition , 26 (4), pp.225-239. https://citeseerx.ist.psu.edu/document?repid=rep1&type=pdf&doi=1dd08158225c41e82243a7efe74e61c140293775
[9] Hosseini, Z. and Kamal, A., 2013. How to design effective multimedia presentations. In 7th International Symposium on Advances in Science and Technology (7th SASTech 2013) Pp (pp. 7-8).
[10] Sutcliffe, A.G., 1999. A design method for effective information delivery in multimedia presentations. New review of hypermedia and multimedia, 5(1), pp.29-58. https://doi.org/10.1080/13614569908914707
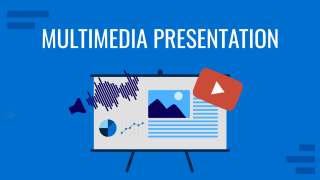
Like this article? Please share
Design, Presentation Approaches Filed under Design
Related Articles
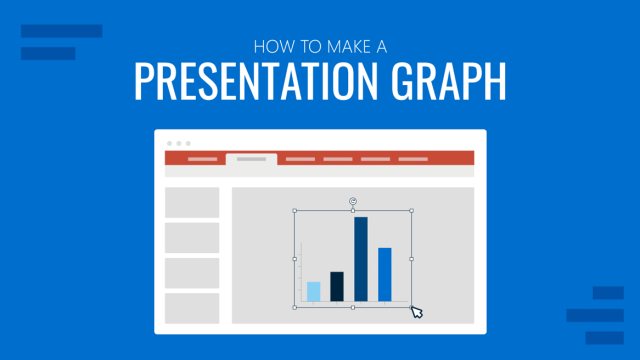
Filed under Design • March 27th, 2024
How to Make a Presentation Graph
Detailed step-by-step instructions to master the art of how to make a presentation graph in PowerPoint and Google Slides. Check it out!
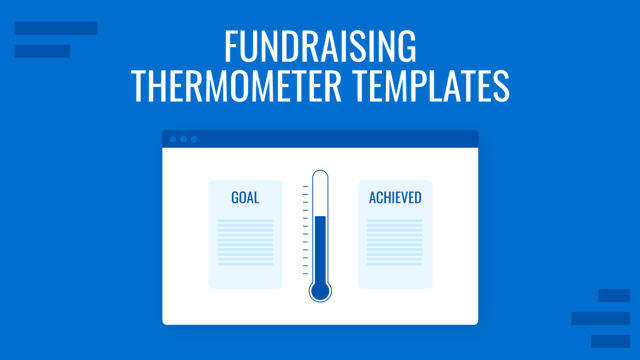
Filed under Presentation Ideas • February 29th, 2024
How to Make a Fundraising Presentation (with Thermometer Templates & Slides)
Meet a new framework to design fundraising presentations by harnessing the power of fundraising thermometer templates. Detailed guide with examples.
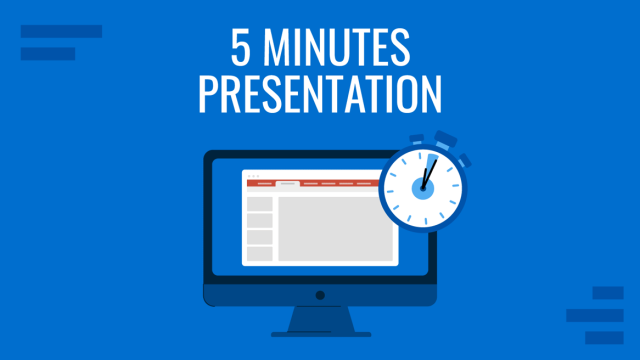
Filed under Presentation Ideas • February 15th, 2024
How to Create a 5 Minutes Presentation
Master the art of short-format speeches like the 5 minutes presentation with this article. Insights on content structure, audience engagement and more.
Leave a Reply
This browser is no longer supported.
Upgrade to Microsoft Edge to take advantage of the latest features, security updates, and technical support.
Data integration patterns for Microsoft industry clouds
- 6 contributors
Applies to:
Microsoft Cloud for Sustainability
Microsoft cloud for financial services, microsoft cloud for healthcare.
- Microsoft Cloud for Retail
Industry Data Models serve as the foundation for their respective Microsoft Industry Clouds. Depending on the data estate maturity level, integrating the solutions with other systems may be necessary.
Selecting the appropriate integration pattern is a crucial to ensure a successful implementation between Microsoft Industry Clouds solutions and external systems. This article presents the integration patterns, tools, and technologies relevant to integration, and the factors to consider when making decisions.
The need for integration
Third-party systems may have separate processes and even different business logic. If the third-party system uses the same underlying Industry Cloud common data model (CMD); the need for data transfer, synchronization, and programming to transform the data is eliminated.
We use data integration patterns in the following scenarios:
- Primary or transactional data that isn't the central part of a single, continuous management process. Data is synchronized between a process in one system to Microsoft industry cloud.
- Data is shared or exchanged between systems when needed for calculations.
- Data is shared or exchanged between systems, so actions in one system are reflected in the other.
- Aggregate data from a system with a detailed level of data is exchanged to a system with a higher-level representation of data.
How to select the right integration pattern
There are many technical options for integration development and each have its own pros and cons. To identify the right pattern for integration extension, you can consider the below factors and weigh each of them across the options:
Based on these factors, you can identify the integration pattern and choose the right tool or technology for implementation.
Integration patterns
In this section, we explore the following integration patterns that can be used while integrating with Dataverse.
Real-time/synchronous integration
Near real-time/asynchronous integration, batch integration, presentation layer integration.
In each pattern, a unique structure is presented, and it can be actualized by using one or more patterns. The subsequent sections provide insight into how these patterns are materialized with specific technologies, along with considerations and the appropriate scenarios in which to apply them.
Real-time integration is indispensable in scenarios where the source system requires instant or minimal latency responses to the data it sends. This requirement becomes crucial when the business use case mandates both the source and destination systems to consistently remain synchronized, ensuring uninterrupted data coherence between the two entities. Synchronous integration becomes essential when the destination system necessitates an immediate response to seamlessly proceed with an ongoing process, enabling timely execution of subsequent actions.
This form of integration is often synonymous with synchronous integration. The following diagram illustrates the prevalent pattern of synchronous integration, where Application A initiates a request to an Application B, and promptly receives a response, ensuring timely and responsive data exchange.
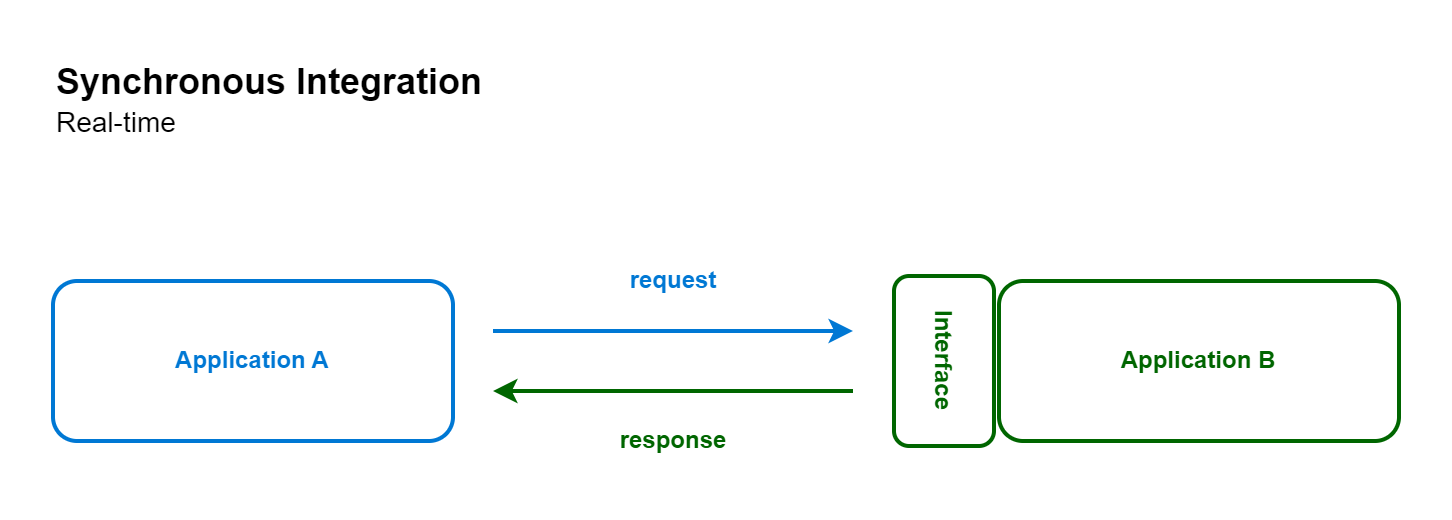
Some of the mentioned technology choices can be expanded to incorporate an intermediary system, serving as a relay to facilitate the transaction process. This relay option effectively separates the source and the target applications by managing the communication of requests and responses on their behalf.
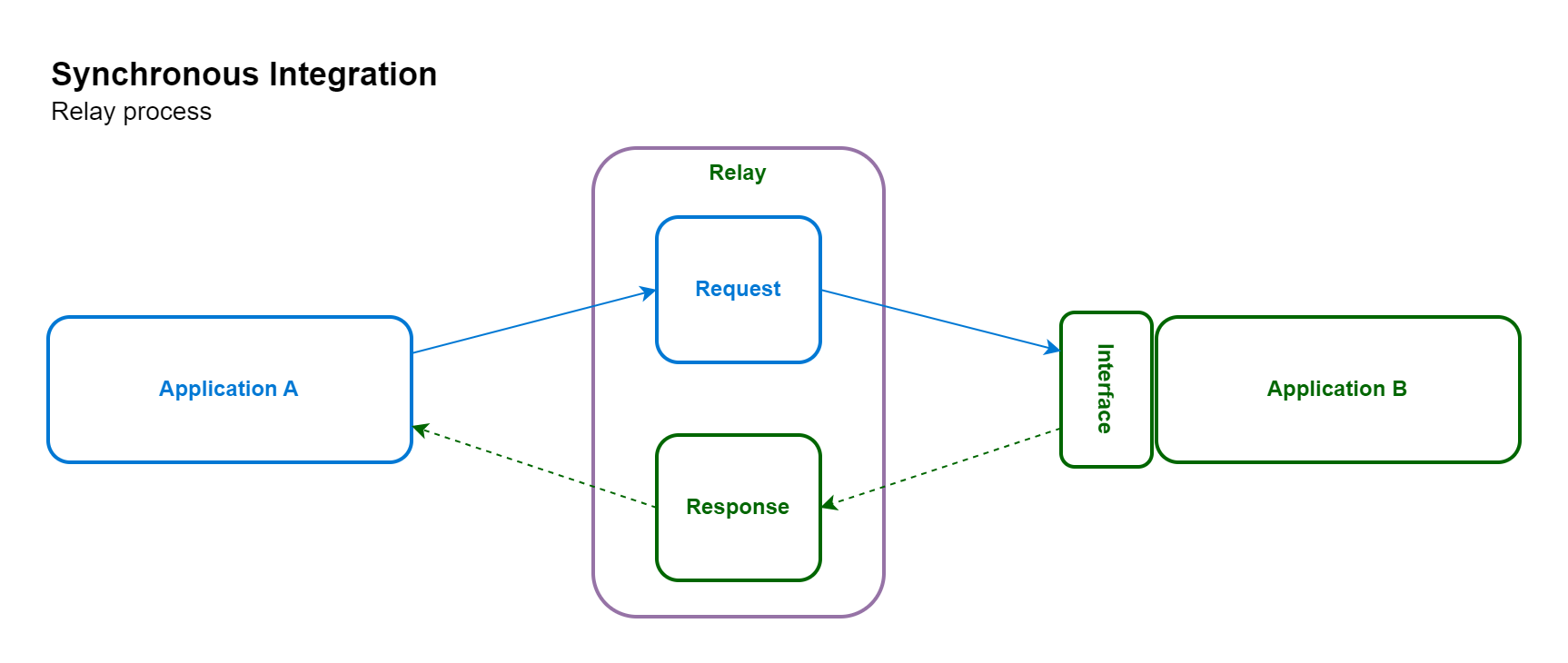
You can implement these synchronous data integration patterns with different technologies that are available in our industry cloud solutions. The following table provides best practice patterns on when to use them.
Asynchronous integration is recommended in scenarios where there's no immediate requirement for real-time responses in a business process or action. Typically, it's employed when there's a substantial volume of message communication between applications and systems. Asynchronous integration patterns ensure that communication between systems doesn't block or slow down processes, allowing each system to work independently and asynchronously. Some of the most common ways to implement asynchronous integrations are with message queues, publish-subscribe, and batch integrations. You can use these integrations separately or combined, depending on the requirements. They're often referred to collectively as event-driven architecture (EDA).
In the following message queue pattern, the sender adopts an event-driven framework, and the consumer creates a binding directly to an event. When the message is sent, the receiver is notified directly and receives the data contained in the event message.
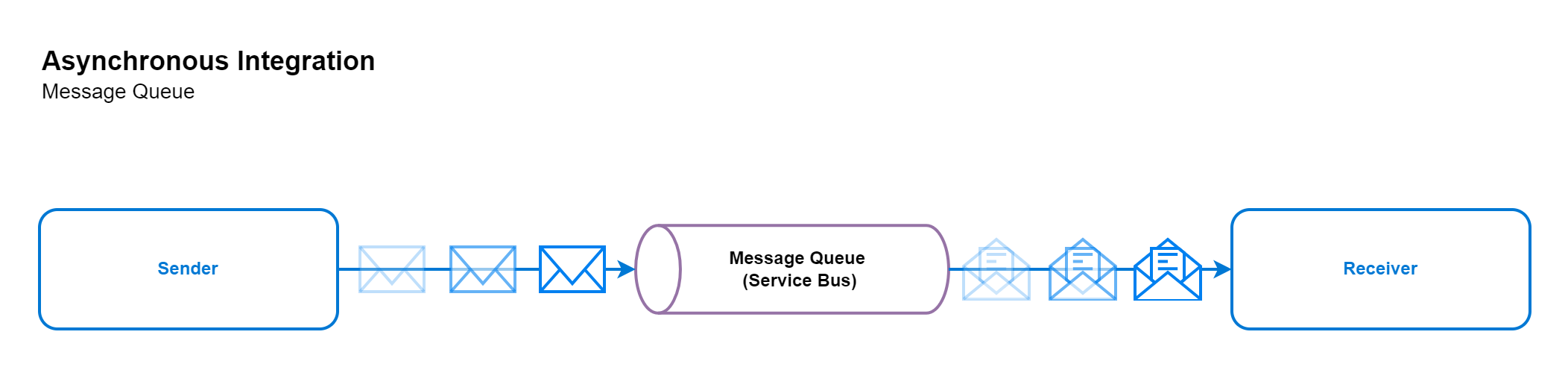
In the following publish-subscribe pattern, the publisher generates a message in a standardized published format and transmits it to a dedicated publish/subscribe channel, which can have one or more subscribers. Each subscriber is subscribed to a specific channel or topic, allowing it to receive and process the published message (event) as required. The publish-subscribe pattern is chosen for one-to-many communication scenarios, because multiple subscribers can independently receive and process the messages (events).
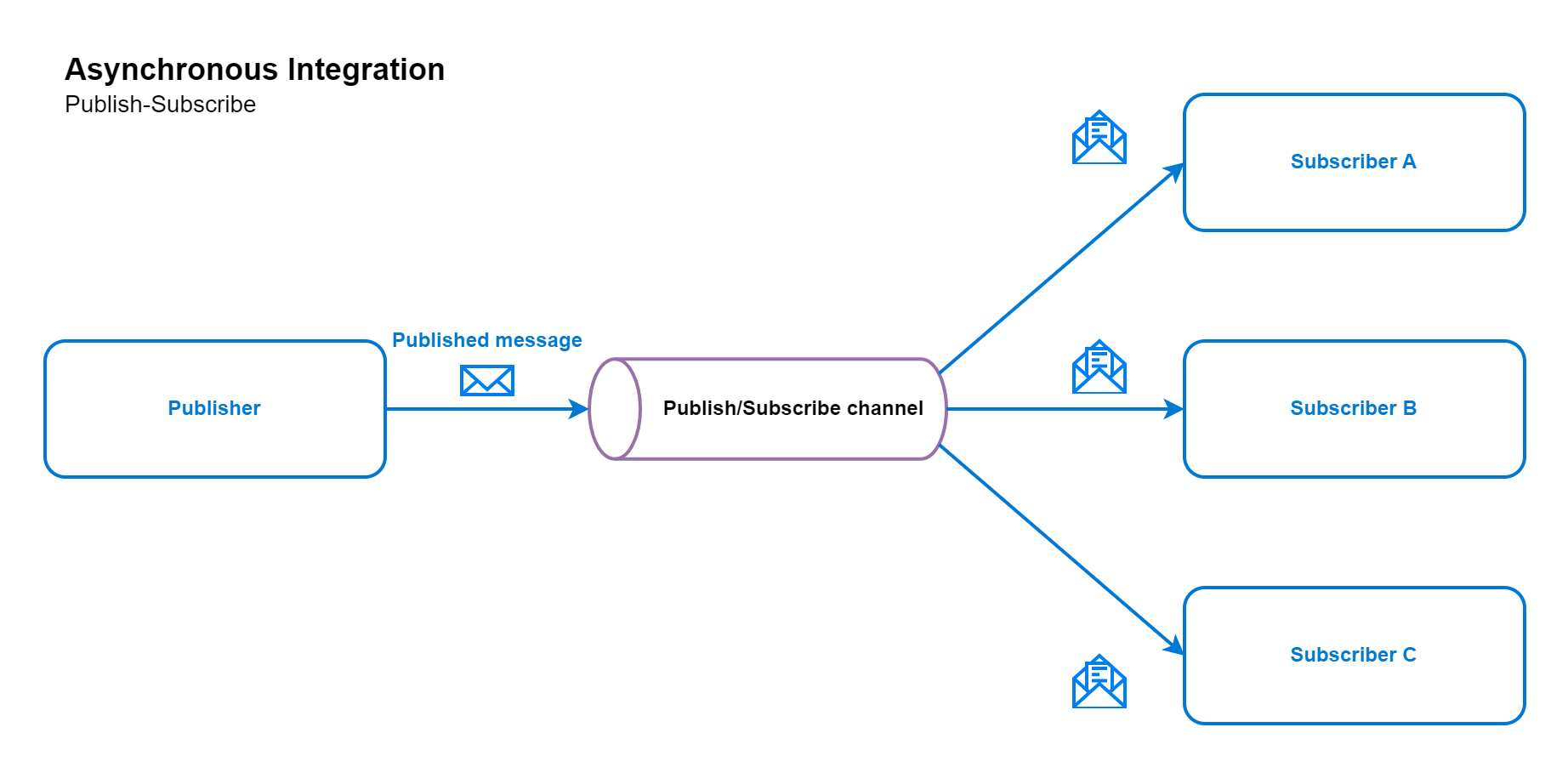
These asynchronous data integration patterns can be implemented with different options, the following table provides you with the available options and best practices on when to use them.
Batching is the practice of gathering and transporting a set of messages or records in a batch to limit chatter and overhead. Batch processing collects over a period of time data and then processes it in batches. This approach is useful when dealing with large volumes of data or when the processing requires significant resources. This pattern also scopes for replicating the master data to replica storage for analytics purposes.
Presentation or user interface integration is at the top level of system, this is what the user sees and interacts with. In certain use cases integration needs to happen at this level by combining the information from different systems or data sources and showing it in a single user interface. Model-driven applications is a component of this, it contributes to the comprehensive user experience by enabling data-driven interactions and facilitating seamless navigation within the integrated environment. Presentation integration is needed when there is a desire to maintain the existing business logic or application structure while enabling easy data aggregation, user interface customization, or enhancement of the user experience. Conversely, it does carry inherent constraints, including complexities in integration and maintenance, significant interdependence among integrated systems, potential performance implications, and considerations regarding data consistency.
- Enabling data aggregation
- User interface customization
- Enhanced user experience
Conversely, it has inherent constraints, including:
- Complexities in integration and maintenance
- Significant interdependence among integrated systems
- Potential performance implications
- Considerations regarding data consistency
Summary of integration patterns
In the world of software integration, there are various patterns and mechanisms available to exchange data between different systems. Each pattern has its own advantages and disadvantages, and choosing the right one can greatly affect the performance and efficiency of the integrated systems.
The following table summarizes these integration patterns: real-time or synchronous integration, asynchronous integration, batching integration, and presentation layer integration. You can explore the mechanisms, triggers, pros, cons, and use cases for each pattern, to help you make an informed decision when selecting an integration approach for your system.
- Cloud for Sustainability API (Preview)
- Generalized emission calculation API
- API reference overview for Environmental Credit Service (Preview)
- Operational Data Estate
- Overview of Dataverse healthcare APIs
- Azure Health Data Services documentation
- Data integration toolkit
- Manage FHIR data using Data integration toolkit
- Configure virtual health data tables
Coming soon: Throughout 2024 we will be phasing out GitHub Issues as the feedback mechanism for content and replacing it with a new feedback system. For more information see: https://aka.ms/ContentUserFeedback .
Submit and view feedback for
Additional resources

IMAGES
VIDEO
COMMENTS
The Instruction and Technology Integration Model offers concrete actions teachers can take to enhance learning through tech integration. With implementation examples, teachers are equipped to leverage this approach for their classrooms. ... Instructional Presentation and Student Productivity, and Powerful Student-Centered 21st Century Learning ...
The Presentation Integration pattern is sometimes disparagingly called screen scraping because the middleware collects (or scrapes ... An end-user security model typically enforces a fine-grained security scheme that includes the specific data records or fields that a user is permitted to see. This makes exposing the functions through ...
Presentation integration connects applications to give end users a unified view of the data. Among the three approaches to integration, this is the least complicated. ... If the data model changes, the integration code has to change accordingly. Connecting to the data tier is more suitable if separate logic, independent of the ones in the ...
The connected model of the integrated curriculum is the view through an opera glass, providing a close-up of the details, subtleties, and inter-connections within each sub-ject area. . . . The nested model views the curriculum through three-dimensional glasses, targeting multiple dimen-sions of a lesson. . . . The se-quenced model views the cur-
The Technology Integration Matrix (TIM) provides a framework for describing and targeting the use of technology to enhance learning. Videos from the Matrix are also available arranged by subject area or by grade level. This section includes background information on the development of the TIM.
This chapter focuses on a new design technique for the analysis and design of data integration processes. This technique uses a graphical process modeling view of data integration similar to the graphical view an entity-relationship diagram provides for data models. The Business Case for a New Design Process. There is a hypothesis to the issue ...
Point-to-point model. Point-to-point integration (P2P) is the architectural pattern in which every system is directly connected to all other systems and apps it needs to work in tandem and share information with. This model can be realized via APIs, webhooks, or custom code.
Enterprise application integration (EAI) is the process of connecting an organization's business applications, services, databases and other systems into an integrating framework that facilitates communications and interoperability. An EAI platform enables the seamless exchange of data, while automating business processes and workflows.
DCM is a user-centered presentation data model that reflects the user's model of the data and allows effective user interaction with the database. Its name comes from the concept of containers ...
3. The model The presentation model specifies the structure and graphical components of displays, how the components are connected to application data, the visual appearance of each component, and how the components are laid out. 3.1 Basic constructs The model consists of a part-whole hierarchy of graphical objects that is defined by
Presentation level integration: At the presentation level, different applications are presented as a single application with a common interface. It is achieved through middleware technology. ... Different methods are available for communications-level integration viz. point-to-point approach, hub-and-spoke model, and an enterprise service bus ...
These models include; • Presentation integration • Data integration and • Function Integration 15. Presentation integration model • allows the integration of new software through the existing presentations of the legacy software. • This is typically used to create a new user interface but may be used to integrate with other applications.
Logical data integration model definition—Produces a detailed representation of the data integration requirements at the data set (entity/table)level, which details the trans-formation rules and target logical data sets (entity/tables). These models are still consid-ered to be technology-independent.
Applications integration (or enterprise application integration) is the sharing of processes and data among different applications in an enterprise. For both small and large organizations alike, it has become a mission-critical priority to connect disparate applications and leverage application collaboration across the enterprise in order to ...
Conceptual Data Integration Models A conceptual data integration model is an implementation-free representation of the data integration requirements for the proposed system that will serve as a basis for "scoping" how they are to be satisfied and for project planning purposes in terms of source systems analysis, tasks and duration, and resources.
notion of presentation component, a loosely-coupled, coarse-grained module or application which includes a presentation layer (i.e. UI and logic to manage user interactions), that offers a ...
In this paper we propose a framework for the integration of stand-alone modules or applications, where integration occurs at the presentation layer. Hence, the final goal is to reduce the effort ...
This free technology integration model is used in many schools around the world. The TIM keeps the focus on effective, research-based pedagogy rather than solely on technology. James gives a short history of the development of the Technology Integration Matrix and then explains the relationship of the five characteristics of meaningful learning ...
Physical data integration model definition—Produces a detailed representation of the data integration specifications at the component level. They should be represented in terms of the component-based approach and be able to represent how the data will optimally flow through the data integration environment in the selected development technology.
Abstract. Integration is a necessary activity in each software development process. Within a model-based approach also the models that are used must be integrated. Model integration has two dimensions: On the one hand, the model components that result from the decomposition of the system under consideration have to be put together.
Multimedia Presentation: Insights & Techniques to Maximize Engagement. Design • December 28th, 2023. Effective public speaking is a crucial skill often overlooked. Multimedia presentations, such as digital stories, offer an opportunity to cultivate this skill. When appropriately executed, they blend visuals, text, and compelling speech in any ...
Model-driven applications is a component of this, it contributes to the comprehensive user experience by enabling data-driven interactions and facilitating seamless navigation within the integrated environment. ... Presentation integration is needed when there is a desire to maintain the existing business logic or application structure while ...
In my previous post, I briefly outlined a popular framework for integration of technology in the classroom, known as the SAMR model. It… · 4 min read · Jun 5, 2019