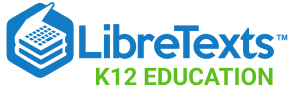
- school Campus Bookshelves
- menu_book Bookshelves
- perm_media Learning Objects
- login Login
- how_to_reg Request Instructor Account
- hub Instructor Commons
- Download Page (PDF)
- Download Full Book (PDF)
- Periodic Table
- Physics Constants
- Scientific Calculator
- Reference & Cite
- Tools expand_more
- Readability
selected template will load here
This action is not available.
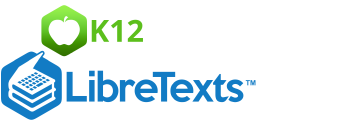

1.3: Developing Hypotheses
- Last updated
- Save as PDF
- Page ID 5311
- What is a hypothesis?
An educated guess? Is that what you learned a hypothesis is? Lots of people have learned that, but it’s not exactly right. So what is a hypothesis? There are two hypotheses listed below. They address a question about carbon dioxide in the atmosphere. Check out what those hypotheses are. Then we'll see what to do with them next.
Asking a New Question
First, we need to find a question that we want to answer. Let's start with the fact that atmospheric CO 2 has been increasing since 1958. This leads us to ask the following: why is atmospheric CO 2 increasing?
Possible Answers to the Question
A hypothesis is a reasonable explanation for a small range of phenomena. A hypothesis is limited in scope; it attempts to explain a single event or a fact. A hypothesis must be testable and falsifiable . In other words, we must be able to test it, and we must be able to disprove it.
Back to answering the question. Let's say we do some background research to learn the possible sources of carbon dioxide in the atmosphere. We discover that there are at least two (there are actually many more):
- CO 2 is released into the atmosphere by volcanoes when they erupt.
- CO 2 is released when fossil fuels are burned.
From these two facts, we can create two hypotheses; we will have multiple working hypotheses . We can test each of these hypotheses. We can prove either or both of these hypotheses false. In this case, it's even possible that both are true.
Hypothesis 1
Atmospheric CO 2 has increased over the past five decades because the amount of CO 2 gas released by volcanoes has increased.
Hypothesis 2
The increase in atmospheric CO 2 is due to the increase in the amount of fossil fuels that are being burned.
Usually, testing a hypothesis requires making observations or performing experiments. In this case, we will look into existing scientific literature to see if either of these hypotheses can be disproved, or if one or both can be supported by the data.
- A hypothesis is a reasonable explanation for a small range of phenomena.
- A scientific hypothesis must be both testable and falsifiable.
- If two or more hypotheses are being tested, they are called multiple working hypotheses.
- How is a hypothesis "a reasonable explanation”? Why is that a better definition than “an educated guess”?
- What if a hypothesis is shown to be wrong: Is the question the scientists are trying to answer a bad question?
- What are multiple working hypotheses? What are the two hypotheses proposed to answer the question in this concept?
Explore More
Use the resource below to answer the questions that follow.
- Why is it important to have a specific hypothesis?
- How can you test a scientific hypothesis?
- Write an example of a hypothesis, and explain how you would test it.
What is Earth Science?
The nature of science, lesson objectives.
- Identify the goal of science.
- Explain the importance of asking questions.
- Describe how scientists study the natural world.
- Explain how and why scientists collect data.
- Describe the three major types of scientific models.
- Explain how a scientific theory differs from a hypothesis.
- Describe appropriate safety precautions inside and outside the science laboratory.
- conceptual model
- dependent variable
- independent variable
- mathematical model
- physical model
- scientific method
Introduction
Science is a path to gaining knowledge about the natural world. The study of science also includes the body of knowledge that has been collected through scientific inquiry.
To conduct a scientific investigation, scientists ask testable questions. To answer those questions, they make systematic observations and carefully collect relevant evidence. Then they use logical reasoning and some imagination to develop hypotheses and explanations. Finally, scientists design and conduct experiments based on their hypotheses.
Goal of Science
Scientists seek to understand the natural world. Scientists begin with a question and then try to answer the question with evidence and logic. A scientific question must be testable. It does not rely on faith or opinion. Our understanding of natural Earth processes help us to understand why earthquakes occur where they do and to understand the consequences of adding excess greenhouse gases to our atmosphere.
Scientific research may be done to build knowledge or to solve problems. Scientific discoveries may lead to technological advances. Pure research often aids in the development of applied research. Sometimes the results of pure research may be applied long after the pure research was completed. Sometimes something unexpected is discovered while scientists are conducting their research.
Some ideas are not testable. For example, supernatural phenomena, such as stories of ghosts, werewolves, or vampires, cannot be tested. Look at this website to see why astrology is not scientific .
Scientists describe what they see, whether in nature or in a laboratory. Science is the realm of facts and observations. However, science does not make moral judgments, such as “It is bad that the volcano erupted” and opinions are not relevant to scientific inquiry. Scientists might enjoy studying tornadoes, but their opinion that tornadoes are exciting is not important to learning about them. Scientists increase our technological knowledge, but science does not determine how or if we use that knowledge. Scientists learned to build an atomic bomb, but scientists didn’t decide whether or when to use it. Scientists have accumulated data on warming temperatures. Their models have shown the likely causes of this warming. But although scientists are largely in agreement on the causes of global warming, they can’t force politicians or individuals to pass laws or change behaviors.
For science to work, scientists must make some assumptions. The rules of nature, whether simple or complex, are the same everywhere in the universe. Natural events, structures, and landforms have natural causes. Evidence from the natural world can be used to learn about those causes. The objects and events in nature can be understood through careful, systematic study. Scientific ideas can change if we gather new data or learn more. An idea, even one that is accepted today, may need to be changed slightly or be entirely replaced if new evidence is found that contradicts it. Scientific knowledge can withstand the test of time. Accepted ideas in science become more reliable as they survive more tests.
Scientific Method
You have probably learned that the scientific method is the way scientists approach their work. The scientific method is a series of steps that help to investigate a question. Scientists use data and evidence gathered from observations, experience, or experiments to answer their questions.
But scientific inquiry rarely proceeds in the same sequence of steps outlined by the scientific method. For example, the order of the steps might change because more questions arise from the data that is collected. Still, to come to verifiable conclusions, logical, repeatable steps of the scientific method must be followed, as seen in the Figure below .
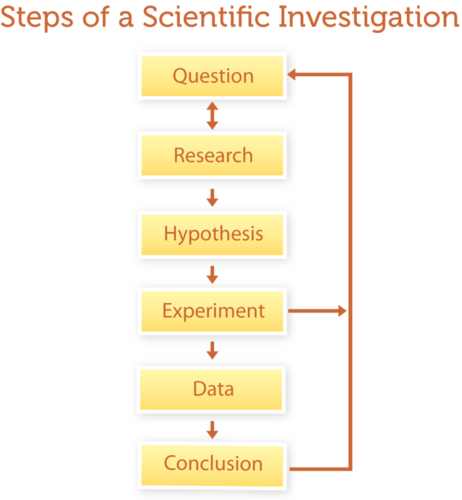
- Science Notes Posts
- Contact Science Notes
- Todd Helmenstine Biography
- Anne Helmenstine Biography
- Free Printable Periodic Tables (PDF and PNG)
- Periodic Table Wallpapers
- Interactive Periodic Table
- Periodic Table Posters
- How to Grow Crystals
- Chemistry Projects
- Fire and Flames Projects
- Holiday Science
- Chemistry Problems With Answers
- Physics Problems
- Unit Conversion Example Problems
- Chemistry Worksheets
- Biology Worksheets
- Periodic Table Worksheets
- Physical Science Worksheets
- Science Lab Worksheets
- My Amazon Books
Hypothesis Examples

A hypothesis is a prediction of the outcome of a test. It forms the basis for designing an experiment in the scientific method . A good hypothesis is testable, meaning it makes a prediction you can check with observation or experimentation. Here are different hypothesis examples.
Null Hypothesis Examples
The null hypothesis (H 0 ) is also known as the zero-difference or no-difference hypothesis. It predicts that changing one variable ( independent variable ) will have no effect on the variable being measured ( dependent variable ). Here are null hypothesis examples:
- Plant growth is unaffected by temperature.
- If you increase temperature, then solubility of salt will increase.
- Incidence of skin cancer is unrelated to ultraviolet light exposure.
- All brands of light bulb last equally long.
- Cats have no preference for the color of cat food.
- All daisies have the same number of petals.
Sometimes the null hypothesis shows there is a suspected correlation between two variables. For example, if you think plant growth is affected by temperature, you state the null hypothesis: “Plant growth is not affected by temperature.” Why do you do this, rather than say “If you change temperature, plant growth will be affected”? The answer is because it’s easier applying a statistical test that shows, with a high level of confidence, a null hypothesis is correct or incorrect.
Research Hypothesis Examples
A research hypothesis (H 1 ) is a type of hypothesis used to design an experiment. This type of hypothesis is often written as an if-then statement because it’s easy identifying the independent and dependent variables and seeing how one affects the other. If-then statements explore cause and effect. In other cases, the hypothesis shows a correlation between two variables. Here are some research hypothesis examples:
- If you leave the lights on, then it takes longer for people to fall asleep.
- If you refrigerate apples, they last longer before going bad.
- If you keep the curtains closed, then you need less electricity to heat or cool the house (the electric bill is lower).
- If you leave a bucket of water uncovered, then it evaporates more quickly.
- Goldfish lose their color if they are not exposed to light.
- Workers who take vacations are more productive than those who never take time off.
Is It Okay to Disprove a Hypothesis?
Yes! You may even choose to write your hypothesis in such a way that it can be disproved because it’s easier to prove a statement is wrong than to prove it is right. In other cases, if your prediction is incorrect, that doesn’t mean the science is bad. Revising a hypothesis is common. It demonstrates you learned something you did not know before you conducted the experiment.
Test yourself with a Scientific Method Quiz .
- Mellenbergh, G.J. (2008). Chapter 8: Research designs: Testing of research hypotheses. In H.J. Adèr & G.J. Mellenbergh (eds.), Advising on Research Methods: A Consultant’s Companion . Huizen, The Netherlands: Johannes van Kessel Publishing.
- Popper, Karl R. (1959). The Logic of Scientific Discovery . Hutchinson & Co. ISBN 3-1614-8410-X.
- Schick, Theodore; Vaughn, Lewis (2002). How to think about weird things: critical thinking for a New Age . Boston: McGraw-Hill Higher Education. ISBN 0-7674-2048-9.
- Tobi, Hilde; Kampen, Jarl K. (2018). “Research design: the methodology for interdisciplinary research framework”. Quality & Quantity . 52 (3): 1209–1225. doi: 10.1007/s11135-017-0513-8
Related Posts
Hypothesis Definition (Science)
- Chemical Laws
- Periodic Table
- Projects & Experiments
- Scientific Method
- Biochemistry
- Physical Chemistry
- Medical Chemistry
- Chemistry In Everyday Life
- Famous Chemists
- Activities for Kids
- Abbreviations & Acronyms
- Weather & Climate
- Ph.D., Biomedical Sciences, University of Tennessee at Knoxville
- B.A., Physics and Mathematics, Hastings College
A hypothesis is an explanation that is proposed for a phenomenon. Formulating a hypothesis is a step of the scientific method .
Alternate Spellings: plural: hypotheses
Examples: Upon observing that a lake appears blue under a blue sky, you might propose the hypothesis that the lake is blue because it is reflecting the sky. One alternate hypothesis would be that the lake is blue because water is blue.
Hypothesis Versus Theory
Although in common usage the terms hypothesis and theory are used interchangeably, the two words mean something different from each other in science. Like a hypothesis, a theory is testable and may be used to make predictions. However, a theory has been tested using the scientific method many times. Testing a hypothesis may, over time, lead to the formulation of a theory.
- Null Hypothesis Definition and Examples
- Hypothesis, Model, Theory, and Law
- Theory Definition in Science
- What Is a Testable Hypothesis?
- Scientific Hypothesis, Model, Theory, and Law
- Scientific Method Flow Chart
- What Is a Hypothesis? (Science)
- Why Ice is Blue
- How To Design a Science Fair Experiment
- Definition of a Hypothesis
- Six Steps of the Scientific Method
- What Are the Elements of a Good Hypothesis?
- Scientific Method Lesson Plan
- What 'Fail to Reject' Means in a Hypothesis Test
- Hypothesis Test for the Difference of Two Population Proportions
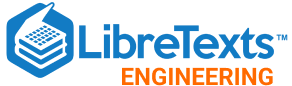
- school Campus Bookshelves
- menu_book Bookshelves
- perm_media Learning Objects
- login Login
- how_to_reg Request Instructor Account
- hub Instructor Commons
- Download Page (PDF)
- Download Full Book (PDF)
- Periodic Table
- Physics Constants
- Scientific Calculator
- Reference & Cite
- Tools expand_more
- Readability
selected template will load here
This action is not available.
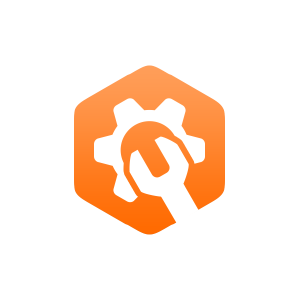
1.4: Environment and environmental science
- Last updated
- Save as PDF
- Page ID 12221
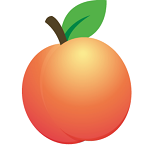
- Caralyn Zehnder, Kalina Manoylov, Samuel Mutiti, Christine Mutiti, Allison VandeVoort, & Donna Bennett
- Georgia College and State University via GALILEO Open Learning Materials
Viewed from space, Earth (Figure \(\PageIndex{1}\) ) offers no clues about the diversity of lifeforms that reside there. The first forms of life on Earth are thought to have been microorganisms that existed for billions of years in the ocean before plants and animals appeared. The mammals, birds, and plants so familiar to us are all relatively recent, originating 130 to 200 million years ago. Humans have inhabited this planet for only the last 2.5 million years, and only in the last 200,000 years have humans started looking like we do today. There are around 7.35 billion people today ( https://www.census.gov/popclock/ ).
.png?revision=1&size=bestfit&width=580&height=303)
The word environment describes living and nonliving surroundings relevant to organisms. It incorporates physical, chemical and biological factors and processes that determine the growth and survival of organisms, populations, and communities. All these components fit within the ecosystem concept as a way to organize all of the factors and processes that make up the environment. The ecosystem includes organisms and their environment within a specific area. Review the previous section for in-depth information regarding the Earth’s ecosystems. Today, human activities influence all of the Earth’s ecosystems.
Environmental science studies all aspects of the environment in an interdisciplinary way. This means that it requires the knowledge of various other subjects including biology, chemistry, physics, statistics, microbiology, biochemistry, geology, economics, law, sociology, etc. It is a relatively new field of study that has evolved from integrated use of many disciplines. Environmental engineering is one of the fastest growing and most complex disciplines of engineering. Environmental engineers solve problems and design systems using knowledge of environmental concepts and ecology, thereby providing solutions to various environmental problems. Environmentalism , in contrast, is a social movement through which citizens are involved in activism to further the protection of environmental landmarks and natural resources. This is not a field of science, but incorporates some aspects of environmental knowledge to advance conservation and sustainability efforts.
The Process of Science
Environmental science is a science, but what exactly is science? Science (from the Latin scientia, meaning “knowledge”) can be defined as all of the fields of study that attempt to comprehend the nature of the universe and all its parts. The scientific method is a method of research with defined steps that include experiments and careful observation. One of the most important aspects of this method is the testing of hypotheses by means of repeatable experiments. A hypothesis is a suggested explanation for an event, which can be tested. A theory is a tested and confirmed explanation for observations or phenomena that is supported by many repeated experiences and observations.
The scientific method
The scientific process typically starts with an observation (often a problem to be solved) that leads to a question. The scientific method consists of a series of well-defined steps. If a hypothesis is not supported by experimental data, a new hypothesis can be proposed. Let’s think about a simple problem that starts with an observation and apply the scientific method to solve the problem. One Monday morning, a student arrives in class and quickly discovers that the classroom is too warm. That is an observation that also describes a problem: the classroom is too warm. The student then asks a question: “Why is the classroom so warm?”
Proposing a Hypothesis
Recall that a hypothesis is a suggested explanation that can be tested. To solve a problem, several hypotheses may be proposed. For example, one hypothesis might be, “The classroom is warm because no one turned on the air conditioning.” But there could be other responses to the question, and therefore other hypotheses may be proposed. A second hypothesis might be, “The classroom is warm because there is a power failure, and so the air conditioning doesn’t work.” Once a hypothesis has been selected, the student can make a prediction. A prediction is similar to a hypothesis but it typically has the format “If . . . then . . . .” For example, the prediction for the first hypothesis might be, “If the student turns on the air conditioning, then the classroom will no longer be too warm.”
Testing a Hypothesis
A valid hypothesis must be testable. It should also be falsifiable, meaning that it can be disproven by experimental results. Importantly, science does not claim to “prove” anything because scientific understandings are always subject to modification with further information. This step — openness to disproving ideas — is what distinguishes sciences from non-sciences. The presence of the supernatural, for instance, is neither testable nor falsifiable.
To test a hypothesis, a researcher will conduct one or more experiments designed to eliminate, or disprove, the hypotheses. Each experiment will have one or more variables and one or more controls. A variable is any part of the experiment that can vary or change during the experiment. The independent variable is the variable that is manipulated throughout the course of the experiment. The dependent variable , or response variable is the variable by which we measure change in response to the independent variable. Ideally, all changes that we measure in the dependent variable are because of the manipulations we made to the independent variable. In most experiments, we will maintain one group that has had no experimental change made to it. This is the control group . It contains every feature of the experimental group except it is not given any manipulation. Therefore, if the results of the experimental group differ from the control group, the difference must be due to the hypothesized manipulation, rather than some outside factor. Look for the variables and controls in the examples that follow.
To test the hypothesis “The classroom is warm because no one turned on the air conditioning,” the student would find out if the air conditioning is on. If the air conditioning is turned on but does not work, there should be another reason, and this hypothesis should be rejected. To test the second hypothesis, the student could check if the lights in the classroom are functional. If so, there is no power failure and this hypothesis should be rejected. Each hypothesis should be tested by carrying out appropriate experiments. Be aware that rejecting one hypothesis does not determine whether or not the other hypotheses can be accepted; it simply eliminates one hypothesis that is not valid (Figure \(\PageIndex{2}\)). Using the scientific method, the hypotheses that are inconsistent with experimental data are rejected.
The scientific method may seem too rigid and structured. It is important to keep in mind that, although scientists often follow this sequence, there is flexibility. Sometimes an experiment leads to conclusions that favor a change in approach; often, an experiment brings entirely new scientific questions to the puzzle. Many times, science does not operate in a linear fashion; instead, scientists continually draw inferences and make generalizations, finding patterns as their research proceeds. Scientific reasoning is more complex than the scientific method alone suggests. Notice, too, that the scientific method can be applied to solving problems that aren’t necessarily scientific in nature.
.png?revision=1&size=bestfit&width=375&height=610)
If you're seeing this message, it means we're having trouble loading external resources on our website.
If you're behind a web filter, please make sure that the domains *.kastatic.org and *.kasandbox.org are unblocked.
To log in and use all the features of Khan Academy, please enable JavaScript in your browser.
Biology library
Course: biology library > unit 1, the scientific method.
- Controlled experiments
- The scientific method and experimental design
Introduction
- Make an observation.
- Ask a question.
- Form a hypothesis , or testable explanation.
- Make a prediction based on the hypothesis.
- Test the prediction.
- Iterate: use the results to make new hypotheses or predictions.
Scientific method example: Failure to toast
1. make an observation..
- Observation: the toaster won't toast.
2. Ask a question.
- Question: Why won't my toaster toast?
3. Propose a hypothesis.
- Hypothesis: Maybe the outlet is broken.
4. Make predictions.
- Prediction: If I plug the toaster into a different outlet, then it will toast the bread.
5. Test the predictions.
- Test of prediction: Plug the toaster into a different outlet and try again.
- If the toaster does toast, then the hypothesis is supported—likely correct.
- If the toaster doesn't toast, then the hypothesis is not supported—likely wrong.
Logical possibility
Practical possibility, building a body of evidence, 6. iterate..
- Iteration time!
- If the hypothesis was supported, we might do additional tests to confirm it, or revise it to be more specific. For instance, we might investigate why the outlet is broken.
- If the hypothesis was not supported, we would come up with a new hypothesis. For instance, the next hypothesis might be that there's a broken wire in the toaster.
Want to join the conversation?
- Upvote Button navigates to signup page
- Downvote Button navigates to signup page
- Flag Button navigates to signup page

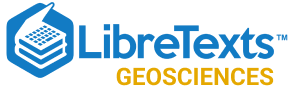
- school Campus Bookshelves
- menu_book Bookshelves
- perm_media Learning Objects
- login Login
- how_to_reg Request Instructor Account
- hub Instructor Commons
- Download Page (PDF)
- Download Full Book (PDF)
- Periodic Table
- Physics Constants
- Scientific Calculator
- Reference & Cite
- Tools expand_more
- Readability
selected template will load here
This action is not available.
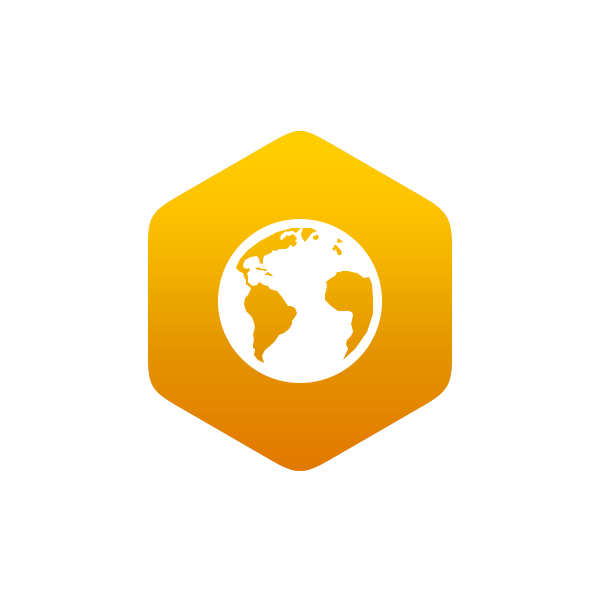
2.2: Earth Science and Its Branches
- Last updated
- Save as PDF
- Page ID 12673
Lesson Objectives
- Define and describe Earth Science as a general field with many branches.
- Identify the field of geology as a branch of Earth Science that deals with the solid part of the Earth.
- Describe the field of oceanography as a branch of Earth Science that has several subdivisions that deal with the various aspects of the ocean.
- Define the field of meteorology as a branch of Earth Science that deals with the atmosphere.
- Understand that astronomy is an extension of Earth Science that examines other parts of the solar system and universe.
- List some of the other branches of Earth Science, and how they relate to the study of the Earth.
Overview of Earth Science
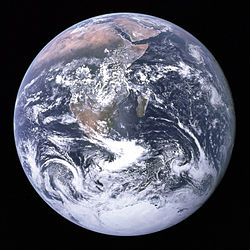
Figure 1.9 : Earth as seen from Apollo 17.
Earth is the mighty planet upon which we all live. Only recently have humans begun to understand the complexity of this planet. In fact, it was only a few hundred years ago that we discovered that Earth was just a tiny part of an enormous galaxy, which in turn is a small part of an even greater universe. Earth Science deals with any and all aspects of the Earth. Our Earth has molten lava, icy mountain peaks, steep canyons and towering waterfalls. Earth scientists study the atmosphere high above us as well as the planet’s core far beneath us. Earth scientists study parts of the Earth as big as continents and as small as the tiniest atom. In all its wonder, Earth scientists seek to understand the beautiful sphere on which we thrive (Figure 1.9).
Because the Earth is so large and science is so complex, Earth scientists specialize in studying just a small aspect of our Earth. Since all of the branches are connected together, specialists work together to answer complicated questions. Let’s look at some important branches of Earth Science.
Geology is the study of the solid matter that makes up Earth. Anything that is solid, like rocks, minerals, mountains, and canyons is part of geology. Geologists study the way that these objects formed, their composition, how they interact with one another, how they erode, and how humans can use them. Geology has so many branches that most geologists become specialists in one area. For example, a mineralogist studies the composition and structure of minerals such as halite (rock salt), quartz, calcite, and magnetite (Figure 1.10).
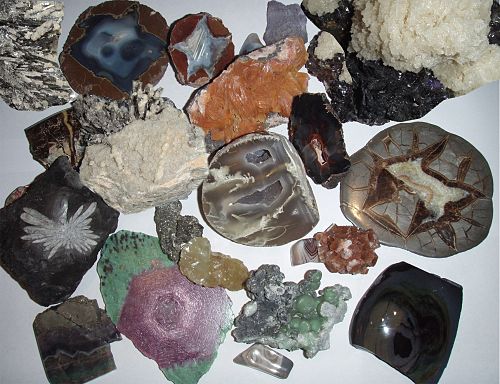
Figure 1.10 : Mineralogists focus on all kinds of minerals.
Figure 1.11 : Seismographs are used to measure earthquakes and pinpoint their origins.
A volcanologist braves the high temperatures and molten lava of volcanoes. Seismologists study earthquakes and the forces of the Earth that create them. Seismologists monitor earthquakes worldwide to help protect people and property from harm (Figure 1.11). Scientists interested in fossils are paleontologists, while scientists who compare other planets’ geologies to that of the Earth are called planetary geologists. There are geologists who only study the Moon. Some geologists look for petroleum, others are specialists on soil. Geochronologists study how old rocks are and determine how different rock layers formed. There are so many specialties in geology that there is probably an expert in almost anything you can think of related to the Earth (Figure 1.12).
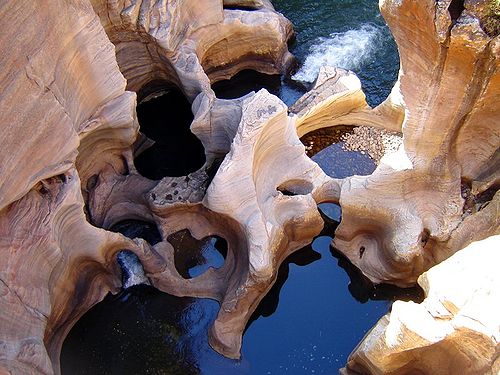
Figure 1.12 : Geology is the study of the solid Earth and its processes.
Oceanography
Oceanography is the study of everything in the ocean environment. More than 70% of the Earth’s surface is covered with water. Most of that water is found in the oceans. Recent technology has allowed us to go to the deepest parts of the ocean, yet much of the ocean remains truly unexplored. Some people call the ocean the last frontier. But it is a frontier already deeply influence by human activity. As the human population gets ever bigger, we are affecting the ocean in many ways. Populations of fish and other marine species have plummeted because of overfishing; contaminants are polluting the waters, and global warming caused by greenhouse gases is melting the thick ice caps. As ocean waters warm, the water expands and, along with the melting ice caps, causes sea levels to rise.
Climatologists help us understand the climate and how it will change in the future in response to global warming. Oceanographers study the vast seas and help us to understand all that happens in the water world. As with geology, there are many branches of oceanography. Physical oceanography is the study of the processes in the ocean itself, like waves and ocean currents (Figure 1.13). Marine geology uses geology to study ocean earthquakes, mountains, and trenches. Chemical oceanography studies the natural elements in ocean water and pollutants.
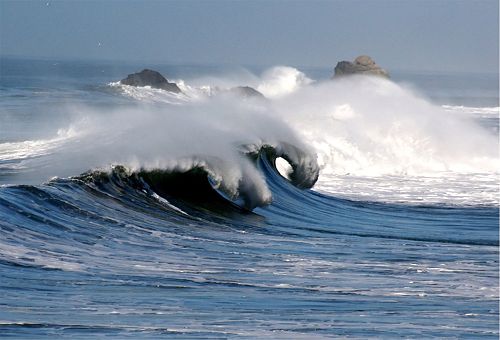
Figure 1.13 : Physical oceanography studies things like currents and waves.
Climatology and Meteorology
Meteorologists don’t study meteors — they study the atmosphere! Perhaps this branch of Earth Science is strangely named but it is very important to living creatures like humans. Meteorology includes the study of weather patterns, clouds, hurricanes, and tornadoes. Using modern technology like radars and satellites, meteorologists work to predict or forecast the weather. Because of more accurate forecasting techniques, meteorologists can help us to prepare for major storms, as well as help us know when we should go on picnics.
Climatologists and other atmospheric scientists study the whole atmosphere, which is a thin layer of gas that surrounds the Earth. Most of it is within about 10 – 11 kilometers of the Earth’s surface. Earth’s atmosphere is denser than Mars’s thin atmosphere, where the average temperature is -63° C, and not as thick as the dense atmosphere on Venus, where carbon dioxide in the atmosphere makes it hot and sulfuric acid rains in the upper atmosphere. The atmosphere on Earth is just dense enough to even out differences in temperature from the equator to the poles, and contains enough oxygen for animals to breathe.
Over the last several decades, climatologists studying the gases in our atmosphere have found that humans are putting higher levels of carbon dioxide into the air by burning fossil fuels (Figure 1.14). Normally, the atmosphere contains small amounts of carbon dioxide, however, with increases in the burning of fossil fuels more than normal amounts are present. These higher concentrations of carbon dioxide can lead to higher surface temperatures. Much of climate change science is based on the increases of greenhouse gases, like carbon dioxide, in the atmosphere and the effect those higher concentrations have on global temperatures. Climatologists can help us better understand the climate and how it may change in the future in response to different amounts of greenhouse gases and other factors (Figure 1.15).
Figure 1.14 : Man-made carbon dioxide released into the atmosphere has been linked to rises in atmospheric temperatures.
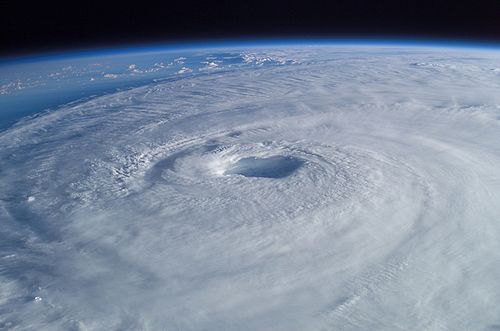
Figure 1.15: When hurricanes are accurately forecast by meteorologists, many lives can be saved.
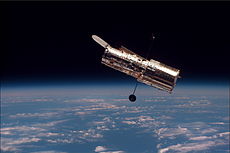
Figure 1.16 : The Hubble Space Telescope
Astronomers have proven that our Earth and solar system are not the only set of planets in the universe. As of June 2015, over a thousand planets outside our solar system had been discovered. Although no one can be sure how many there are, astronomers estimate that there are billions of other planets. In addition, the universe contains black holes, other galaxies, asteroids, comets, and nebula. As big as Earth seems to us, the entire universe is vastly greater. Our Earth is an infinitesimally small part of our universe.
Astronomers use resources on the Earth to study physical things beyond the Earth. They use a variety of instruments like optical telescopes and radio telescopes to see things far beyond what the human eye can see. Spacecraft travel great distances in space to send us information on faraway places, while telescopes in orbit observe astronomical bodies from the darkness of space (Figure 1.16).
Astronomers ask a wide variety of questions. Astronomers could study how an object or energy outside of Earth could affect us. An impact from an asteroid could have terrible effects for life on Earth. Strong bursts of energy from the sun, called solar flares, can knock out a power grid or disturb radio, television or cell phone communications. But astronomers ask bigger questions too. How was the universe created? Are there other planets on which we might live? Are there resources that we could use? Is there other life out there? Astronomy also relies on Earth Science, when scientists compare what we know about life on Earth to the chances of finding life beyond this planet.
Other Branches of Earth Science
Geology, oceanography, and meteorology represent a large part of Earth science, while astronomy represents science beyond Earth. However, there are still many smaller branches of science that deal with the Earth or interact greatly with Earth sciences. Most branches of science are connected with other branches of science in some way or another. A biologist who studies monkeys in rainforests must be concerned with the water cycle that brings the rain to the rainforests. She must understand the organic chemistry of the food the monkeys eat, as well as the behavior between the monkeys. She might examine the soil in which the trees of the rainforest grow. She must even understand the economy of the rainforest to understand reasons for its destruction. This is just one example of how all branches of science are connected.
Below are examples of a few branches of science that are directly related to Earth science. Environmental scientists study the ways that humans interact with the Earth and the effects of that interaction. We hope to find better ways of sustaining the environment. Biogeography is a branch of science that investigates changes in populations of organisms in relation to place over time. These scientists attempt to explain the causes of species’ movement in history. Ecologists focus on ecosystems, the complex relationship of all life forms and the environment in a given place (Figure 1.17). They try to predict the chain reactions that could occur when one part of the ecosystem is disrupted.
Figure 1.17 : In a marine ecosystem, coral, fish, and other sea life depend on each other for survival.
As opposed to an oceanographer, a limnologist studies inland waters like rivers and lakes. A hydrogeologist focuses on underground water found between soil and rock particles, while glaciologists study glaciers and ice.
None of these scientific endeavors would be possible without geographers who explore the features of the surface and work with cartographers, who make maps. Stratigraphy is another area of Earth science which examines layers of rock beneath the surface (Figure 1.18). This helps us to understand the geological history of the Earth. There is a branch of science for every interest and each is related to the others.
Figure 1.18 : Folded strata are layers in the rock that have bent over time. Stratigraphy attempts to explain these layers and the geologic history of the area.
Review Questions
- What are three major branches of Earth science?
- What branch of science deals with stars & galaxies beyond the Earth?
- List important functions of Earth scientists.
- What do you think is the focus of a meteorologist?
- An ecologist notices that an important coral reef is dying off. She believes that it has to do with some pollution from a local electric plant. What type of scientist might help her analyze the water for contamination?
- Design an experiment that you could conduct in any branch of Earth science. Identify the independent variable and dependent variable. What safety precautions would you have to take?
- Therefore, we must utilize the processes of System Science, in order to fully understand the Earth Systems and its variations as a whole.
Points to Consider
- Why is Earth science so important?
- Which branch of Earth science would you most like to explore?
- What is the biggest problem that we face today? Which Earth scientists may help us to solve the problem?
- What other branches of science or society are related to and necessary for Earth science?
- Located at : http://en.wikibooks.org/wiki/High_School_Earth_Science/Earth_Science_and_Its_Branches . License : CC BY-SA: Attribution-ShareAlike

Gaia theory: is it science yet?
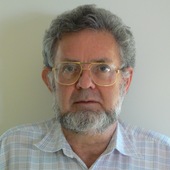
Honorary Senior Associate, Faculty of Science, The University of Melbourne
Disclosure statement
Ian Enting does not work for, consult, own shares in or receive funding from any company or organisation that would benefit from this article, and has disclosed no relevant affiliations beyond their academic appointment.
University of Melbourne provides funding as a founding partner of The Conversation AU.
View all partners
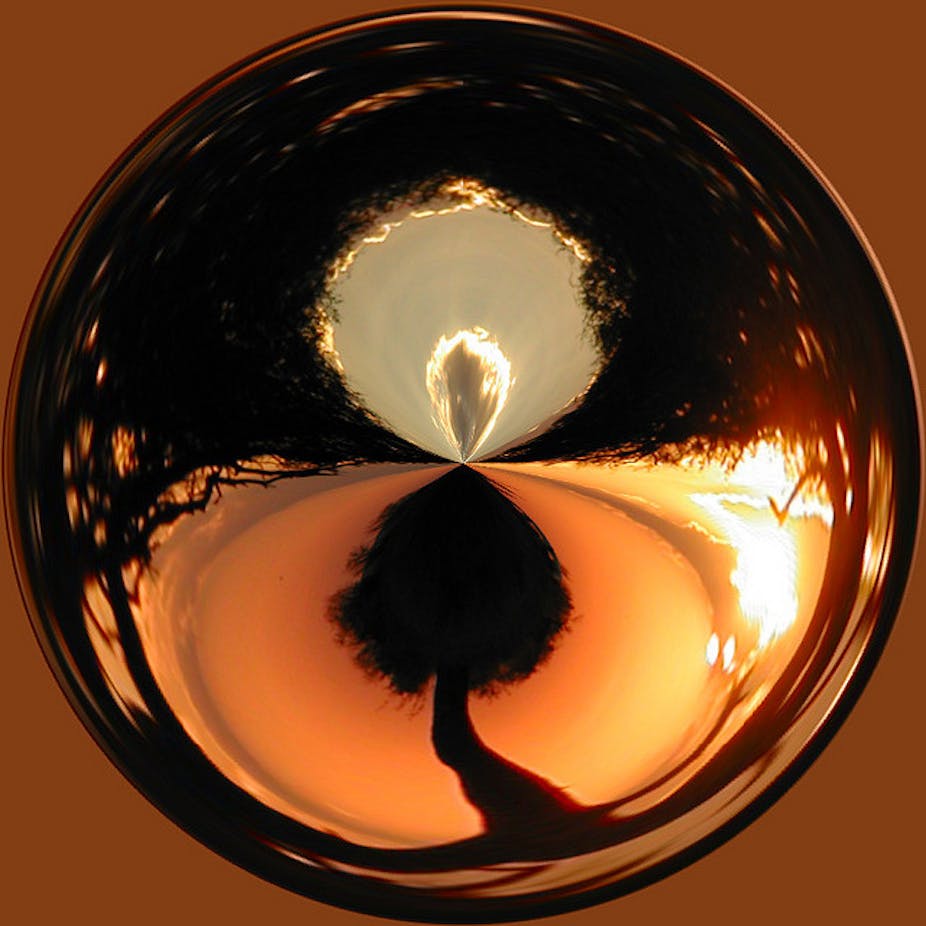
James Lovelock’s “ Gaia hypothesis ” has challenged conventional thinking about the nature of the earth as an integrated system. Gaia proposes that the earth acts like a living organism — that life is part of a self-regulating system, manipulating the physical and chemical environment to maintain the planet as a suitable home for life itself. Lovelock has developed this idea in a series of books, from “Gaia: A new look at life on earth” (1979) through to “Revenge of Gaia” (2006) and “The Vanishing Face of Gaia” (2009). He argues that as changes in the physical earth system occur, living systems respond so as to mitigate such changes.
How can a planet be alive?
In claiming that Gaia is “lifelike”, Lovelock notes the difficulty of defining life. He points out that a biological emphasis on (potential for) reproduction would, for example, exclude postmenopausal women. On the other hand, a physical emphasis on entropy reduction would include refrigerators. This leads Lovelock to emphasise physiological self-regulation as the defining characteristic of life-like systems - networks of interacting processes serve to regulate each other to preserve the functioning of the organism
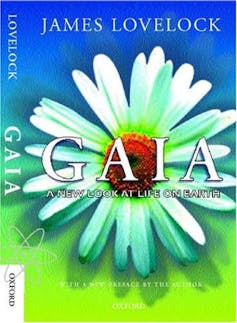
In discussing the concept of Gaia, Lovelock now distinguishes:
Gaia hypothesis : the original version — the Earth’s organisms regulate the physical and chemical components of the earth system so as to maintain the planet as an optimal habitat for life.
Gaia theory : the revision in response to critics — the combined physical, chemical and biological components of the earth system regulate the planet so as to maintain it as a habitat for life.
Various analyses have tried to distinguish between “weak” and “strong” Gaia, with weak Gaia differing little from conventional earth system science.
But isn’t Gaia for hippies?
The name Gaia has been widely used as a metaphor, as well co-opted for a large amount of pseudo-scientific baggage. This does not invalidate any underlying science any more than the majority of physics is invalidated by similar appropriation of terms such as “relativity”, “crystals”, “force fields” etc.
After stripping away such baggage, one has to confront the question: is what Lovelock is saying science and mysticism? While Lovelock has used the term “geophysiology” to avoid some of the mystical associations, he notes that all that has been achieved is that the term geophysiology now carries the same suspicion as the name Gaia.
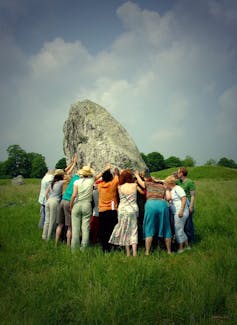
The confrontation between Gaian theory and “conventional” science is largely focused on a few key words: “Gaia is like a living organism … whose goal is to maintain the planet in state fit for life”.
A powerful argument against the Gaia hypothesis is the assertion (such as that made by Richard Dawkins in The Extended Phenotype ) that Gaia cannot arise from Darwinian evolution of life — the planet as a whole is not a unit of selection.
Dawkins can be answered by an anthropic argument (wherein observations of the physical universe must be compatible with the existence of the conscious life that observes it):
The emergence of Gaian self-regulation through the course of evolution is allegedly extremely improbable.
Nevertheless, the long-term survival of life on a planet without Gaian self-regulation may well be even more improbable.
Therefore, intelligent observers are most likely to find themselves on a planet with Gaian self-regulation.
Personally, I find this sort of argument unsatisfying. However, similar arguments seem to be needed to “explain” the physical universe — it is a very precise combination of physical constants that allows the existence of atoms heavier than hydrogen and helium. Anyway, if Gaian self-regulation has arisen by chance, one would still want to know how it works.
For me, one of the most intriguing possibilities is some form of “innate Gaia” — rather than being highly improbable, some degree of Gaian self-regulation is inevitable.
Writing in Nature Tim Lenton has proposed that if:
- the physical system is stable, and
- the biological system has self-increasing growth, and
- there is a physical optimum for growth
then the steady state will be whichever side of the optimum leads to negative feedbacks, thus enhancing the stability of the physical system. The “optimal for life” in the original Gaia hypothesis is replaced by “mutually enhanced stability of the physical and biological systems”.
A theory with gaps is still a theory
While Thomas Henry Huxley famously talked of “the slaying of a beautiful hypothesis by an ugly fact” such discrepancies can also mean that the “ugly facts” are being misinterpreted.
For example, the gap in Wegener’s account of continental drift was that the continents aren’t ploughing through the crust — they are being carried by the crust. The gap in Darwin’s argument 150 years ago was the implicit assumption of blending of characteristics, so that new traits would be diluted. Mendel’s experiments showed that this is not so. Working out the details has been the work of subsequent generations of population geneticists.
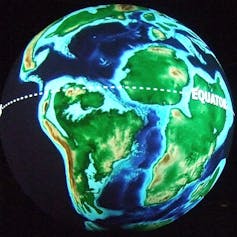
Returning to Dawkins’ argument as quoted above, the hidden assumption that may represent a weak point is the assumption of a single level of selection.
In Revenge of Gaia , Lovelock quotes William Hamilton: “Just as the observations of Copernicus needed a Newton to explain them, we need another Newton to explain how Darwinian evolution leads to a habitable planet.” This echoes Alfred Wegener: “The Newton of [continental] drift has not yet appeared. His absence need cause no anxiety.”
To summarise: gaps and discrepancies in a theory imply a case for serious further study, not necessarily a reason to panic and immediately abandon any consideration of the idea.
What does Gaia mean for humankind?
In his recent books, Lovelock argues that humanity is like an army with over-extended supply lines — there is no option but to retreat (allowing Gaia to recover). Depending on humanity’s choices the retreat could be comparable to the British from Dunkirk or Napoleon from Moscow. We can take control of population ourselves, or see it plummet as Gaia kills us off.
My interpretation of what Lovelock is proposing as the potential relation between Gaia and humanity is the 20th century concept of “Mutually Assured Destruction” rather than “revenge”.
These concerns seem to be based on Lovelock’s expectation of a third climate state. The last 500,000 years show an alternation between quasi-stable warm and cold states, flipping on a 100,000 year cycle.
Lovelock (using simple modelling described in Vanishing Face of Gaia) proposes that higher CO₂ will lead to a third, hotter, quasi-stable state. The proposed causal chain is: warming from more CO₂ → more stable oceans, less circulation → less nutrients at surface, so less algal production → less pumping of CO₂ into deep oceans → more CO₂ remains in the atmosphere, locking in the warming.
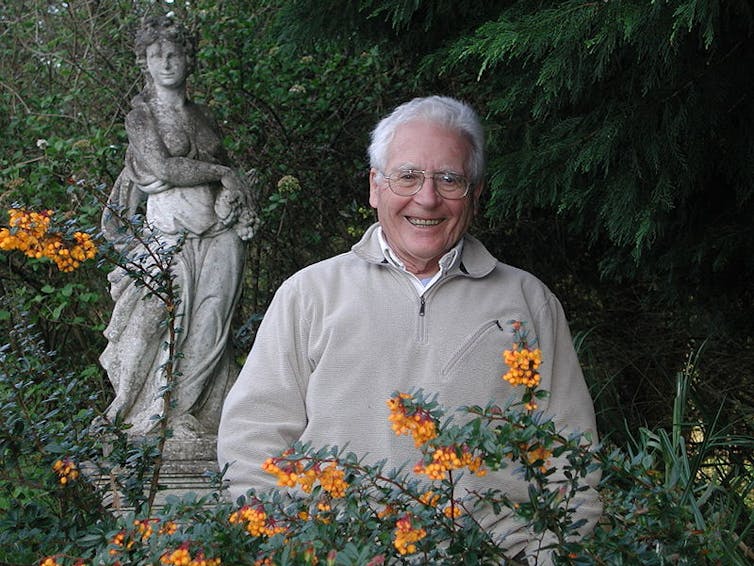
But is there real evidence for a “third climate state”? Apart from the general principle that once self-regulation of a system fails, the failure can be very abrupt, are the arguments really Gaian?
So does it work?
An “ideal” summary would answer the question: “Is Lovelock right? Does the Gaia concept describe how the earth works?” I hope you won’t be too disappointed if I fail to commit to an answer. Indeed the whole process of preparing my talk and then editing it for The Conversation would have been less fun if I had been working from a preconceived view.
At times Lovelock seems to equate “Gaia” with “earth system science” by asking “would you have bought The Vanishing Face of Earth System Science?” A more substantive question is to ask: “is the (strong) Gaia concept established science?”, to which the answer is “not yet, and maybe it never will be”.
We come back to the statement that for Gaia “we need another Newton…”. Would a complete theory be a matter of filling in the gaps, as 150 years of accumulating evidence has “filled in the details” in Darwinian evolution? Or would the survival of Gaian theory mean morphing into something different, in the way that continental drift morphed into plate tectonics?
My best guess is that if “strong” Gaian theory survives (with or without the name Gaia) it will be through some such similar transformation. The “innate Gaia” implied by negative feedbacks being an “automatic” consequence of an interaction between expanding life and a dissipative physical system may well be part of such a re-synthesis.
Assessing Lovelock’s role as a “key thinker” raises the question of whether, regardless of its validity, the Gaia hypothesis has had a positive influence on the development of earth system science. (Lovelock’s other contributions to science through instrumentation have been invaluable). If, as I do not, one equates Gaia to current earth system science then the question largely disappears — the implication is that the rest of science has caught up with Lovelock.
My view is that even though “strong Gaia” and probably “innate Gaia” currently lie beyond the boundaries of established science, Lovelock’s role in pushing the boundaries of thinking about the earth system has spurred the thinking of many in the emerging earth system science community. This is a valuable legacy, regardless of the ultimate fate of his ideas.
This article is based on a lecture delivered in April 2009 as part of The University of Melbourne series of public lectures on Key Thinkers.
- Gaia theory

Program Manager, Teaching & Learning Initiatives

Lecturer/Senior Lecturer, Earth System Science (School of Science)

Sydney Horizon Educators (Identified)

Deputy Social Media Producer

What Do We Mean by “Theory” in Science?
A theory is a carefully thought-out explanation for observations of the natural world that has been constructed using the scientific method, and which brings together many facts and hypotheses.
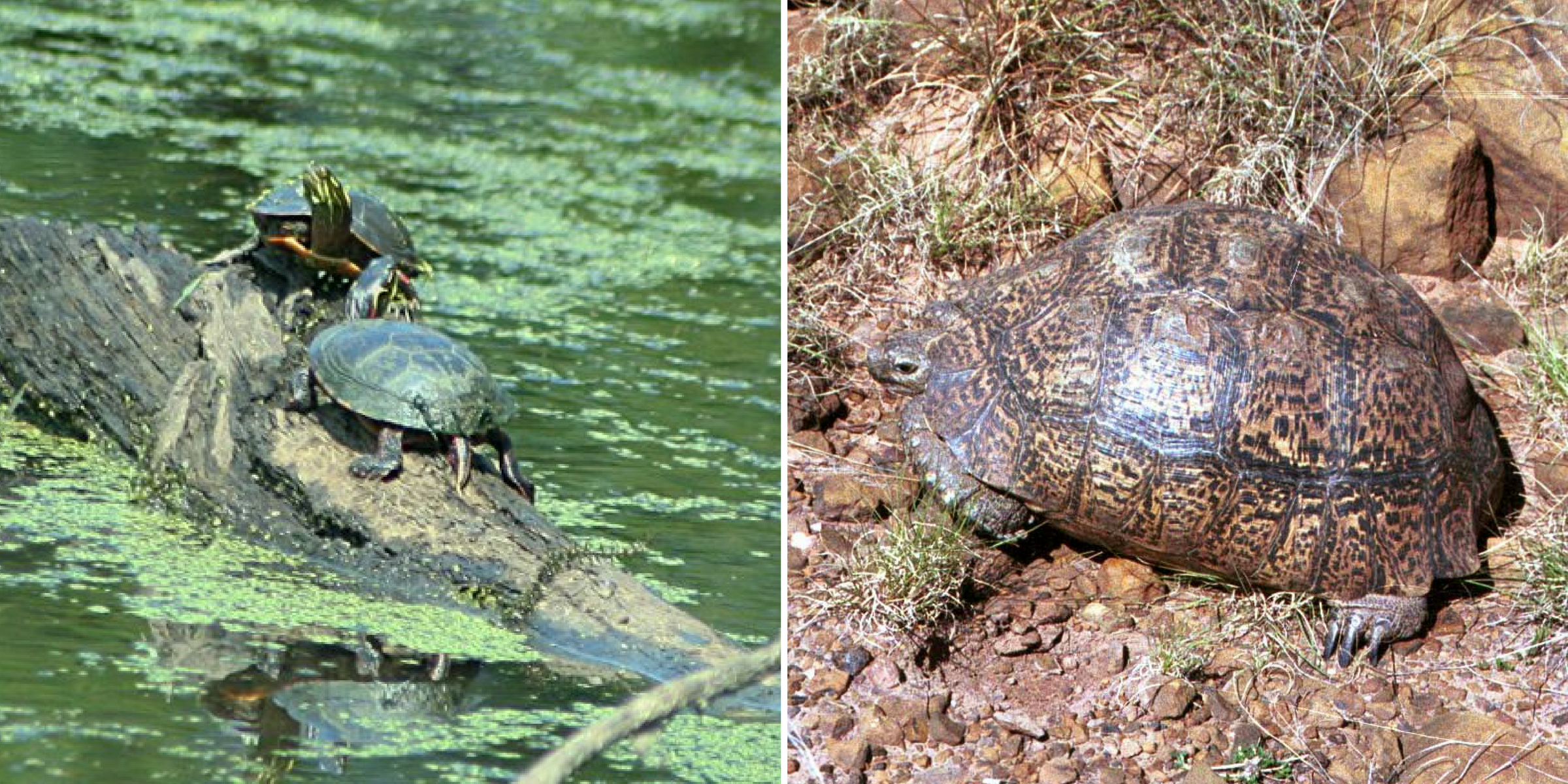
In a previous blog post, I talked about the definition of “fact” in a scientific context , and discussed how facts differ from hypotheses and theories. The latter two terms also are well worth looking at in more detail because they are used differently by scientists and the general public, which can cause confusion when scientists talk about their work.
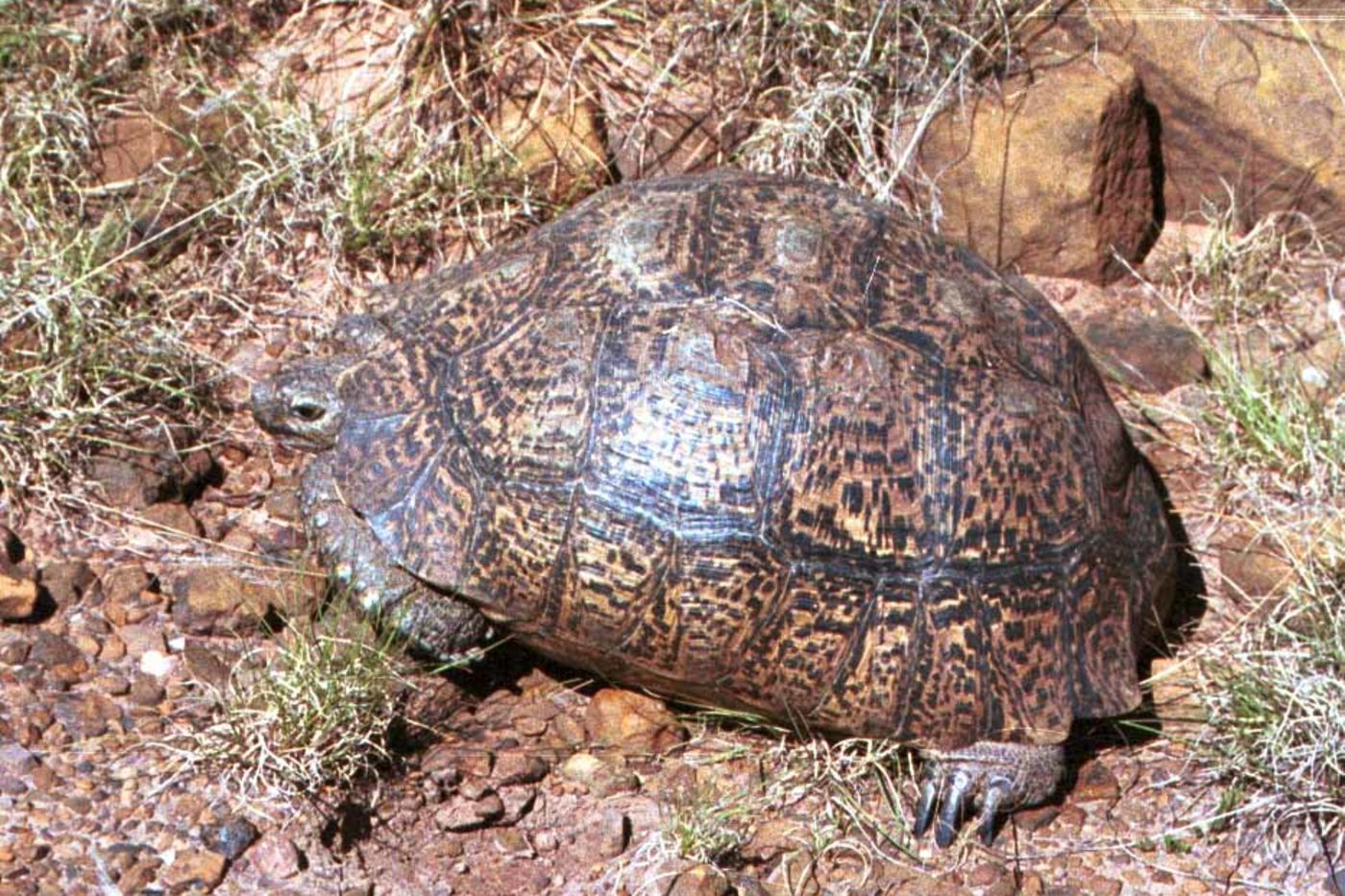
With these definitions in mind, a simplified version of the scientific process would be as follows. A scientist makes an observation of a natural phenomenon. She then devises a hypothesis about the explanation of the phenomenon, and she designs an experiment and/or collects additional data to test the hypothesis. If the test falsifies the hypothesis (i.e., shows that it is incorrect), she will have to develop a new hypothesis and test that. If the hypothesis is corroborated (i.e., not falsified) by the test, the scientist will retain it. If it survives additional scrutiny, she may eventually try to incorporate it into a larger theory that helps to explain her observed phenomenon and relate it to other phenomena.
That's all fairly abstract, so let's look at a concrete example involving some recent research I undertook with a group of collaborators. The theory of evolution states that the process of natural selection should work to optimize the function of an organism's parts if the changes increase the chances of the organism successfully producing offspring and the changes are heritable (i.e., can be passed down from generation to generation).
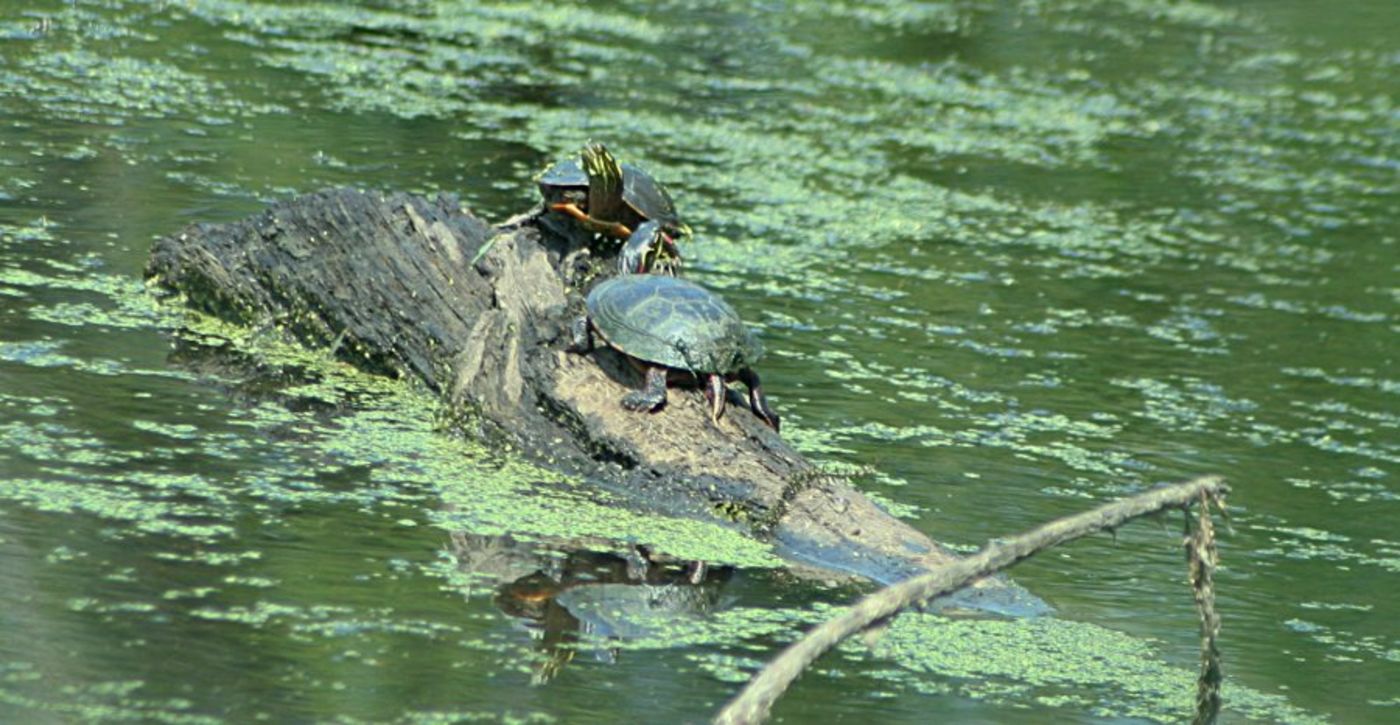
But what happens when there are multiple selective pressures at work? We might hypothesize that turtles that spend most of their time in water face a trade-off between having a strong shell and one that is streamlined (making them more efficient swimmers), whereas streamlining would be less important to turtles on land, allowing them to evolve stronger shells even if they aren’t very streamlined.
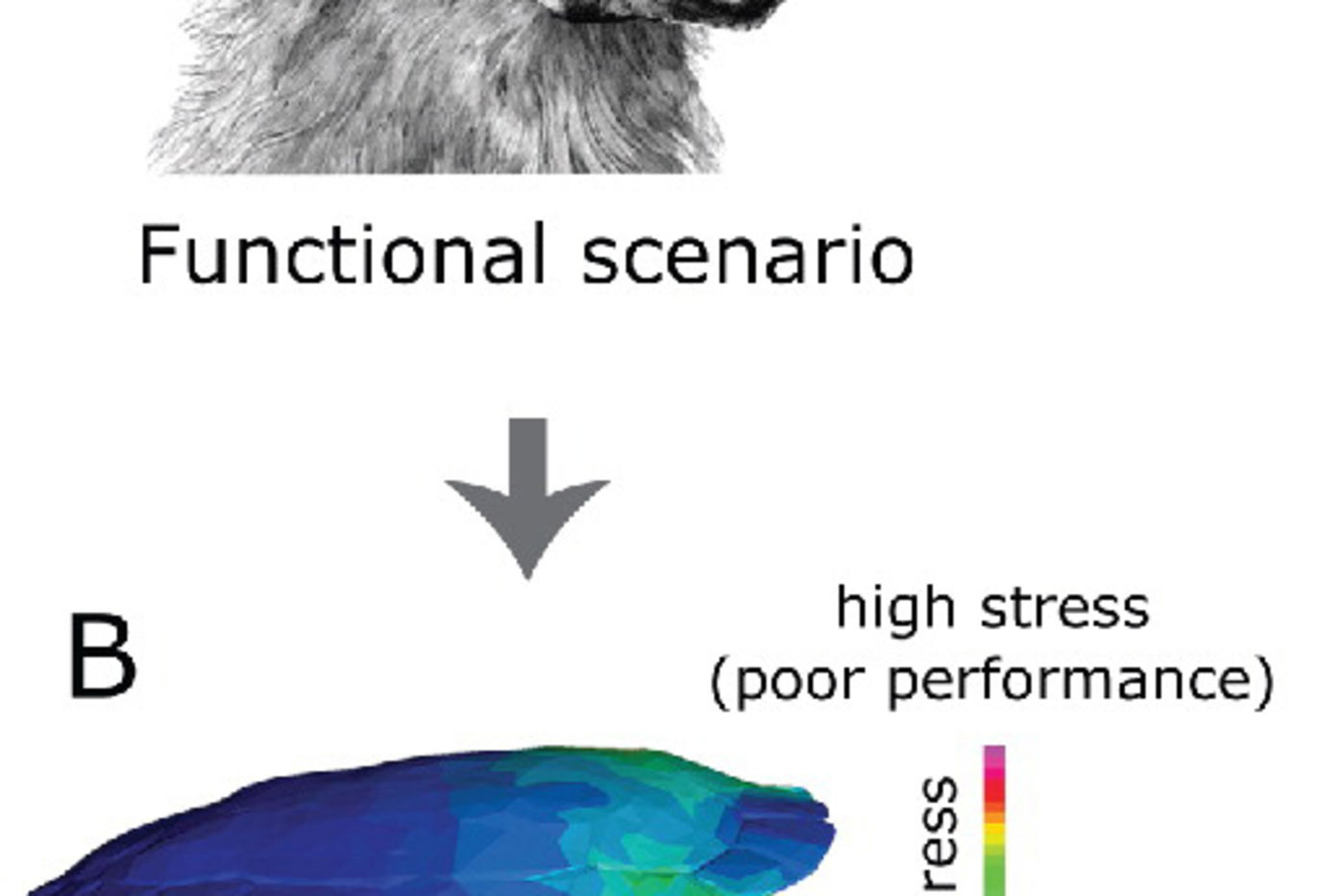
Our results corroborated our hypothesis that aquatic turtles are forced to make more of a trade-off between strength and streamlining than turtles that live on land. In general, the shell shapes of our aquatic turtles were more streamlined but weaker than those of our land turtles, and our mathematical model of natural selection indicated that selection for streamlining was acting more strongly on the aquatic species.
As with any idea in science, our results are open to further testing. For example, other researchers might develop a better model of natural selection that shows that our model was overly simplistic. Or they might collect data from more turtle species that shows that our results were based on a false pattern stemming from sampling too few species (we considered 47 species in our dataset, about 14% of living turtle species). For now, though, our results can be added as a piece of evidence that is consistent with the predictions of the large explanatory theory of evolution.
If you would like to learn more about this research, the scientific paper describing the work can be found in the Journal of Vertebrate Paleontology . You can see some of the turtle specimens that we used in this research in The Field Museum's exhibition Specimens: Unlocking the Secrets of Life , open through January 7, 2018.
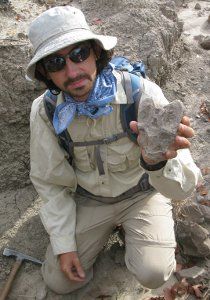
I am a paleobiologist interested in three main topics: 1) understanding the broad implications of the paleobiology and paleoecology of extinct terrestrial vertebrates, particularly in relation to large scale problems such as the evolution of herbivory and the nature of the end-Permian mass extinction; 2) using quantitative methods to document and interpret morphological evolution in fossil and extant vertebrates; and 3) tropic network-based approaches to paleoecology. To address these problems, I integrate data from a variety of biological and geological disciplines including biostratigraphy, anatomy, phylogenetic systematics and comparative methods, functional morphology, geometric morphometrics, and paleoecology.
A list of my publications can be found here.
More information on some of my research projects and other topics can be found on the fossil non-mammalian synapsid page.
Most of my research in vertebrate paleobiology focuses on anomodont therapsids, an extinct clade of non-mammalian synapsids ("mammal-like reptiles") that was one of the most diverse and successful groups of Permian and Triassic herbivores. Much of my dissertation research concentrated on reconstructing a detailed morphology-based phylogeny for Permian members of the clade, as well as using this as a framework for studying anomodont biogeography, the evolution of the group's distinctive feeding system, and anomodont-based biostratigraphic schemes. My more recent research on the group includes: species-level taxonomy of taxa such as Dicynodon , Dicynodontoides , Diictodon , Oudenodon , and Tropidostoma ; development of a higher-level taxonomy for anomodonts; testing whether anomodonts show morphological changes consistent with the hypothesis that end-Permian terrestrial vertebrate extinctions were caused by a rapid decline in atmospheric oxygen levels; descriptions of new or poorly-known anomodonts from Antarctica, Tanzania, and South Africa; and examination of the implications of high growth rates in anomodonts. Fieldwork is an important part of my paleontological research, and recent field areas include the Parnaíba Basin of Brazil , the Karoo Basin of South Africa, the Ruhuhu Basin of Tanzania , and the Luangwa Basin of Zambia. My collaborators and I have made important discoveries in the course of these field projects, including the first remains of dinocephalian synapsids from Tanzania and a dinosaur relative that implies that the two main lineages of archosaurs (one including crocodiles and their relatives and the other including birds and dinosaurs) were diversifying in the early Middle Triassic, only a few million years after the end-Permian extinction. Finally, the experience I have gained while studying Permian and Triassic terrestrial vertebrates forms the foundation for work I am now involved in using models of food webs to investigate how different kinds of biotic and abiotic perturbations could have caused extinctions in ancient communities.
Geometric morphometrics is the basis of most of my quantitative research on evolutionary morphology, and I have been using this technique to address several biological and paleontological questions. For example, I conducted a simulation-based study of how tectonic deformation influences our ability to extract biologically-relevant shape information from fossil specimens, and the effectiveness of different retrodeformation techniques. I also used the method to address taxonomic questions in biostratigraphically-important anomodont taxa, and I served as a co-advisor for a Ph.D. student at the University of Bristol who used geometric morphometrics and finite element analysis to examine the functional significance of skull shape variation in fossil and extant crocodiles. Focusing on more biological questions, I am currently working on a large geometric morphometric study of plastron shape in extant emydine turtles. To date, I have compiled a data set of over 1600 specimens belonging to nine species, and I am using these data to address causes of variation at both the intra- and interspecific level. Some of the main goals of the work are to examine whether plastron morphology reflects a phylogeographic signal identified using molecular data in Emys marmorata , whether the "miniaturized" turtles Glyptemys muhlenbergii and Clemmys guttata have ontogenies that differ from those of their larger relatives, and how habitat preference, phylogeny, and shell kinesis affect shell morphology.
A collaborative project that began during my time as a postdoctoral researcher at the California Academy of Sciences involves using using models of trophic networks to examine how disturbances can spread through communities and cause extinctions. Our model is based on ecological principles, and some of the main data that we are using are a series of Permian and Triassic communities from the Karoo Basin of South Africa. Our research has already shown that the latest Permian Karoo community was susceptible to collapse brought on by primary producer disruption, and that the earliest Triassic Karoo community was very unstable. Presently we are investigating the mechanics that underlie this instability, and we're planning to investigate how the perturbation resistance of communities as changed over time. We've also experimented with ways to use the model to estimate the magnitude and type of disruptions needed to cause observed extinction levels during the end-Permian extinction event in the Karoo. Then there's the research project I've been working on almost my whole life .
Morphology and the stratigraphic occurrences of fossil organisms provide distinct, but complementary information about evolutionary history. Therefore, it is important to consider both sources of information when reconstructing the phylogenetic relationships of organisms with a fossil record, and I am interested how these data sources can be used together in this process. In my empirical work on anomodont phylogeny, I have consistently examined the fit of my morphology-based phylogenetic hypotheses to the fossil record because simulation studies suggest that phylogenies which fit the record well are more likely to be correct. More theoretically, I developed a character-based approach to measuring the fit of phylogenies to the fossil record. I also have shown that measurements of the fit of phylogenetic hypotheses to the fossil record can provide insight into when the direct inclusion of stratigraphic data in the tree reconstruction process results in more accurate hypotheses. Most recently, I co-advised two masters students at the University of Bristol who are examined how our ability to accurately reconstruct a clade's phylogeny changes over the course of the clade's history.
Related content, Check this out
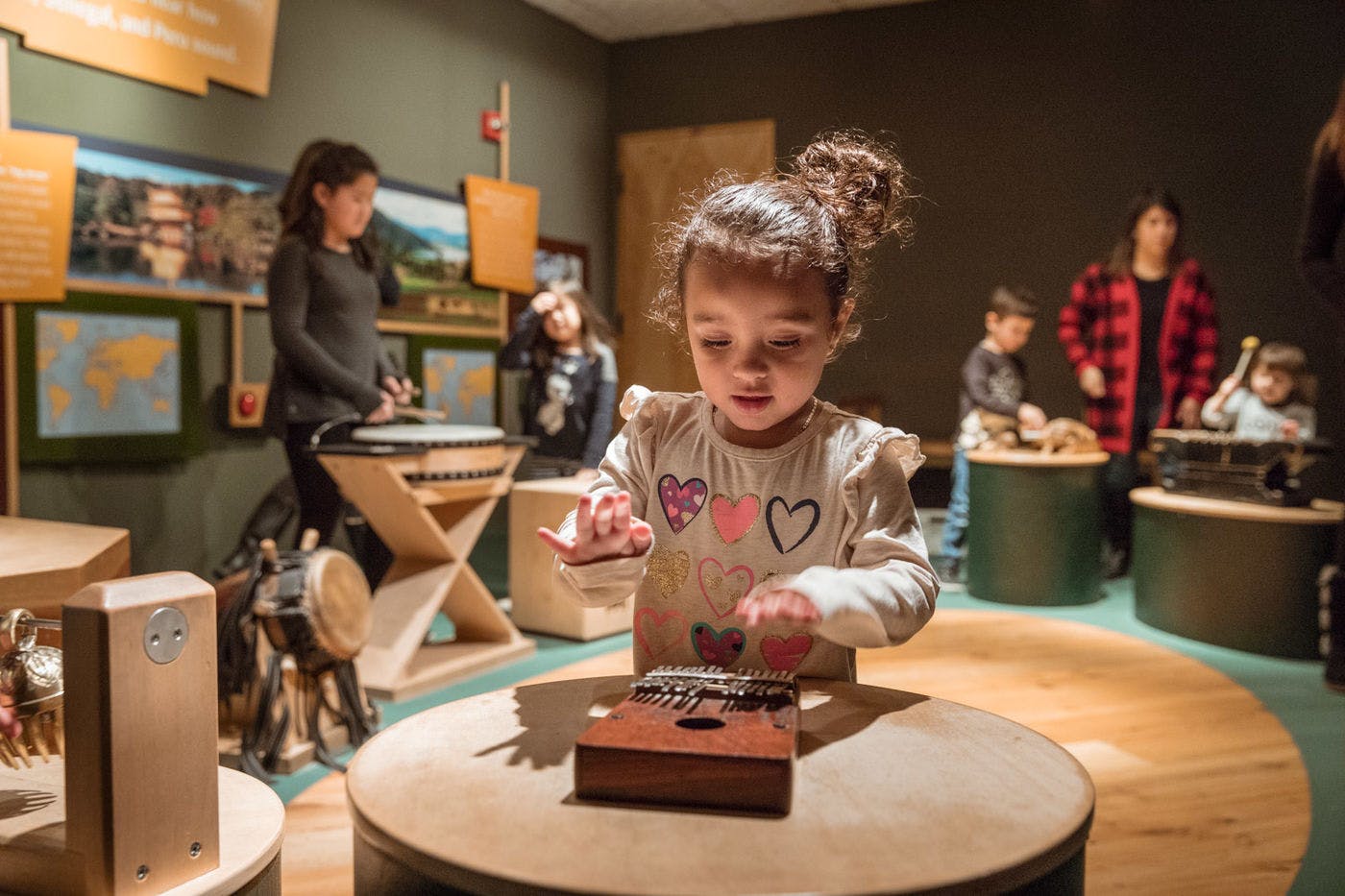
Thank you for visiting nature.com. You are using a browser version with limited support for CSS. To obtain the best experience, we recommend you use a more up to date browser (or turn off compatibility mode in Internet Explorer). In the meantime, to ensure continued support, we are displaying the site without styles and JavaScript.
- View all journals
- Explore content
- About the journal
- Publish with us
- Sign up for alerts
- Published: 19 April 2024
Asteroid Kamo‘oalewa’s journey from the lunar Giordano Bruno crater to Earth 1:1 resonance
- Yifei Jiao ORCID: orcid.org/0000-0003-1097-0521 1 ,
- Bin Cheng ORCID: orcid.org/0000-0002-8025-9113 1 ,
- Yukun Huang ORCID: orcid.org/0000-0003-1215-4130 1 , 2 ,
- Erik Asphaug ORCID: orcid.org/0000-0003-1002-2038 3 ,
- Brett Gladman 2 ,
- Renu Malhotra ORCID: orcid.org/0000-0002-1226-3305 3 ,
- Patrick Michel ORCID: orcid.org/0000-0002-0884-1993 4 ,
- Yang Yu ORCID: orcid.org/0000-0001-9329-7015 5 &
- Hexi Baoyin ORCID: orcid.org/0000-0002-6389-8677 1 , 6
Nature Astronomy ( 2024 ) Cite this article
538 Accesses
400 Altmetric
Metrics details
- Asteroids, comets and Kuiper belt
- Rings and moons
Among the nearly 30,000 known near-Earth asteroids (NEAs), only tens possess Earth co-orbital characteristics with semi-major axes ~1 au. In particular, 469219 Kamo‘oalewa (2016 HO3), an upcoming target of China’s Tianwen-2 asteroid sampling mission, exhibits a meta-stable 1:1 mean-motion resonance with Earth. Intriguingly, recent ground-based observations show that Kamo‘oalewa has spectroscopic characteristics similar to space-weathered lunar silicates, hinting at a lunar origin instead of an asteroidal one like the vast majority of NEAs. Here we use numerical simulations to demonstrate that Kamo‘oalewa’s physical and orbital properties are compatible with a fragment from a crater larger than 10–20 km formed on the Moon in the last few million years. The impact could have ejected sufficiently large fragments into heliocentric orbits, some of which could be transferred to Earth 1:1 resonance and persist today. This leads us to suggest the young lunar crater Giordano Bruno (22 km diameter, 1–10 Myr age) as the most likely source, linking a specific asteroid in space to its source crater on the Moon. The hypothesis will be tested by the Tianwen-2 mission when it returns a sample of Kamo‘oalewa. And the upcoming NEO Surveyor mission may help us to identify such a lunar-derived NEA population.
This is a preview of subscription content, access via your institution
Access options
Access Nature and 54 other Nature Portfolio journals
Get Nature+, our best-value online-access subscription
24,99 € / 30 days
cancel any time
Subscribe to this journal
Receive 12 digital issues and online access to articles
111,21 € per year
only 9,27 € per issue
Buy this article
- Purchase on Springer Link
- Instant access to full article PDF
Prices may be subject to local taxes which are calculated during checkout
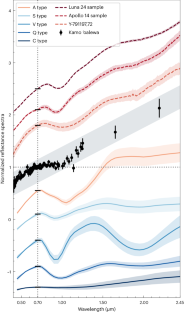
Similar content being viewed by others
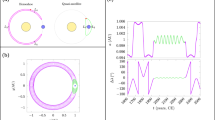
Lunar ejecta origin of near-Earth asteroid Kamo’oalewa is compatible with rare orbital pathways
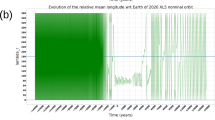
Orbital stability analysis and photometric characterization of the second Earth Trojan asteroid 2020 XL5
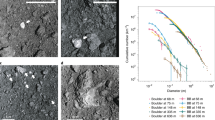
Collisional history of Ryugu’s parent body from bright surface boulders
Data availability.
The raw simulation data are available from the corresponding authors upon reasonable request. Source data are provided with this paper.
Code availability
The spectral classification code classy is available via GitHub at https://github.com/maxmahlke/classy (ref. 65 ). The N -body code REBOUND is an open-source package available via GitHub at https://github.com/hannorein/rebound (ref. 66 ). The impact code SPHSOL is available from the corresponding authors on reasonable request.
De la Fuente Marcos, C. & De la Fuente Marcos, R. Asteroid (469219) 2016 HO3, the smallest and closest Earth quasi-satellite. Mon. Not. R. Astron. Soc. 462 , 3441–3456 (2016).
Article ADS Google Scholar
Qi, Y. & Qiao, D. Co-orbital transition of 2016 HO3. Astrodynamics 7 , 3–14 (2023).
Sharkey, B. N. et al. Lunar-like silicate material forms the Earth quasi-satellite (469219) 2016 HO3 Kamo‘oalewa. Commun. Earth Environ. 2 , 231 (2021).
Gong, Y. et al. Cosmology from the Chinese space station optical survey (CSS-OS). Astrophys. J. 883 , 203 (2019).
Zhang, T., Xu, K. & Ding, X. China’s ambitions and challenges for asteroid–comet exploration. Nat. Astron 5 , 730–731 (2021).
Pieters, C. et al. Distinctive space weathering on Vesta from regolith mixing processes. Nature 491 , 79–82 (2012).
Milliken, R. The RELAB Spectral Library Bundle. NASA Planetary Data System https://doi.org/10.17189/1519032 (2020).
Nishiizumi, K. et al. Exposure histories of lunar meteorites: ALHA81005, MAC88104, MAC88105, and Y791197. Geochim. Cosmochim. Acta 55 , 3149–3155 (1991).
Binzel, R. et al. Compositional distributions and evolutionary processes for the near-Earth object population: results from the MIT–Hawaii Near-Earth Object Spectroscopic Survey (MITHNEOS). Icarus 324 , 41–76 (2019).
DeMeo, F. E., Binzel, R. P., Slivan, S. M. & Bus, S. J. An extension of the Bus asteroid taxonomy into the near-infrared. Icarus 202 , 160–180 (2009).
Migliorini, F. et al. Vesta fragments from v6 and 3:1 resonances: implications for V-type near-Earth asteroids and howardite, eucrite and diogenite meteorites. Meteorit. Planet. Sci. 32 , 903–916 (1997).
DeMeo, F. E. et al. Olivine-dominated A-type asteroids in the main belt: distribution, abundance and relation to families. Icarus 322 , 13–30 (2019).
Melosh, H. J. Impact Cratering: a Geologic Process (Oxford Univ. Press, 1989).
Bart, G. D. & Melosh, H. Distributions of boulders ejected from lunar craters. Icarus 209 , 337–357 (2010).
Horányi, M. et al. A permanent, asymmetric dust cloud around the Moon. Nature 522 , 324–326 (2015).
Singer, K. N., Jolliff, B. L. & McKinnon, W. B. Lunar secondary craters and estimated ejecta block sizes reveal a scale-dependent fragmentation trend. J. Geophys. Res. Planets 125 , e2019JE006313 (2020).
Hawke, B. R. et al. The origin of lunar crater rays. Icarus 170 , 1–16 (2004).
Gladman, B. J., Burns, J. A., Duncan, M. J. & Levison, H. F. The dynamical evolution of lunar impact ejecta. Icarus 118 , 302–321 (1995).
Castro-Cisneros, J. D., Malhotra, R. & Rosengren, A. J. Lunar ejecta origin of near-Earth asteroid Kamo‘oalewa is compatible with rare orbital pathways. Commun. Earth Environ. 4 , 372 (2023).
Yeomans, D. K. & Chamberlin, A. B. Comparing the Earth impact flux from comets and near-Earth asteroids. Acta Astronaut. 90 , 3–5 (2013).
Artemieva, N. & Shuvalov, V. Numerical simulation of high-velocity impact ejecta following falls of comets and asteroids onto the Moon. Sol. Syst. Res. 42 , 329–334 (2008).
Benz, W. & Asphaug, E. Simulations of brittle solids using smooth particle hydrodynamics. Comput. Phys. Commun. 87 , 253–265 (1995).
Grady, D. E. & Kipp, M. E. Continuum modelling of explosive fracture in oil shale. Int. J. Rock Mech. Min. Sci. 17 , 147–157 (1980).
Article Google Scholar
Melosh, H., Ryan, E. & Asphaug, E. Dynamic fragmentation in impacts: hydrocode simulation of laboratory impacts. J. Geophys. Res. Planets 97 , 14735–14759 (1992).
Schmidt, R. M. & Housen, K. R. Some recent advances in the scaling of impact and explosion cratering. Int. J. Impact Eng. 5 , 543–560 (1987).
Melosh, H. Impact ejection, spallation, and the origin of meteorites. Icarus 59 , 234–260 (1984).
Vokrouhlicky`, D. & Čapek, D. YORP-induced long-term evolution of the spin state of small asteroids and meteoroids: Rubincam’s approximation. Icarus 159 , 449–467 (2002).
Robbins, S. J. A new global database of lunar impact craters >1–2 km: 1. Crater locations and sizes, comparisons with published databases, and global analysis. J. Geophys. Res. Planets 124 , 871–892 (2019).
Barlow, N. Status report on crater databases for Mercury, the Moon, Mars, and Ganymede. In Proc. Third Planetary Data Workshop and The Planetary Geologic Mappers Annual Meeting , Abstract 7027 (Lunar and Planetary Institute, 2017).
Neukum, G., Ivanov, B. & Hartmann, W. Cratering records in the inner solar system in relation to the lunar reference system. Space Sci. Rev. 96 , 55–86 (2001).
Bottke, W., Nolan, M. C., Greenberg, R. & Kolvoord, R. A. in Hazards Due to Comets and Asteroids (ed. Gehrels T.) 337–357 (Univ. of Arizona Press, 1994).
Gladman, B., Michel, P. & Froeschlé, C. The near-Earth object population. Icarus 146 , 176–189 (2000).
Huang, Y. & Gladman, B. Four-billion year stability of the Earth–Mars belt. Mon. Not. R. Astron. Soc. 500 , 1151–1157 (2021).
Mazrouei, S., Ghent, R. R., Bottke, W. F., Parker, A. H. & Gernon, T. M. Earth and Moon impact flux increased at the end of the Paleozoic. Science 363 , 253–257 (2019).
Morota, T. et al. Formation age of the lunar crater Giordano Bruno. Meteorit. Planet. Sci. 44 , 1115–1120 (2009).
Drozd, R., Hohenberg, C., Morgan, C., Podosek, F. & Wroge, M. Cosmic-ray exposure history at Taurus–Littrow. In Proc. Lunar Science Conference , Abstract 1087 (Lunar and Planetary Institute, 1977).
Bhattacharya, S. & Saran, S. Enhanced hydration at Giordano Bruno crater on the far side of the Moon and its implications. In Proc. 48th Annual Lunar and Planetary Science Conference , Abstract 1780 (Lunar and Planetary Institute, 2017).
Basilevsky, A. & Head, J. Age of Giordano Bruno crater as deduced from the morphology of its secondaries at the Luna 24 landing site. Planet. Space Sci. 73 , 302–309 (2012).
König, B., Neukum, G. & Fechtig, H. Recent lunar cratering: absolute ages of Kepler, Aristarchus, Tycho. In Proc. Lunar Science Conference , Abstract 1190 (Lunar and Planetary Institute, 1977).
Harris, A. W., Boslough, M., Chapman, C. R., Drube, L. & Michel, P. in Asteroids IV (eds Bottke, W. F., DeMeo F. E. & Michel, P.) 835–854 (Univ. of Arizona Press, 2015).
Mainzer, A. et al. Near-Earth object Surveyor mission: data products and survey plan. In AAS/Division for Planetary Sciences Meeting Abstracts , Vol. 53 (American Astronomical Society, 2021).
Fritz, J. Impact ejection of lunar meteorites and the age of Giordano Bruno. Icarus 221 , 1183–1186 (2012).
Morais, M. & Morbidelli, A. The population of near-Earth asteroids in co-orbital motion with the Earth. Icarus 160 , 1–9 (2002).
Granvik, M. et al. Debiased orbit and absolute-magnitude distributions for near-Earth objects. Icarus 312 , 181–207 (2018).
DeMeo, F. E. & Carry, B. Solar System evolution from compositional mapping of the asteroid belt. Nature 505 , 629–634 (2014).
Delbo, M. et al. Alignment of fractures on Bennu’s boulders indicative of rapid asteroid surface evolution. Nat. Geosci. 15 , 453–457 (2022).
Zhang, Y. & Michel, P. Shapes, structures, and evolution of small bodies. Astrodynamics 5 , 293–329 (2021).
Cambioni, S. et al. Fine-regolith production on asteroids controlled by rock porosity. Nature 598 , 49–52 (2021).
Rozitis, B., MacLennan, E. & Emery, J. P. Cohesive forces prevent the rotational breakup of rubble-pile asteroid (29075) 1950 DA. Nature 512 , 174–176 (2014).
Lauretta, D. et al. The unexpected surface of asteroid (101955) Bennu. Nature 568 , 55–60 (2019).
Mahlke, M., Carry, B. & Mattei, P.-A. Asteroid taxonomy from cluster analysis of spectrometry and albedo. Astron. Astrophys. 665 , A26 (2022).
Jiao, Y., Yan, X., Cheng, B. & Baoyin, H. SPH–DEM modelling of hypervelocity impacts on rubble-pile asteroids. Mon. Not. R. Astron. Soc. 527 , 10348–10357 (2024).
Bui, H. H., Fukagawa, R., Sako, K. & Ohno, S. Lagrangian meshfree particles method (SPH) for large deformation and failure flows of geomaterial using elastic–plastic soil constitutive model. Int. J. Numer. Anal. Methods Geomech. 32 , 1537–1570 (2008).
Tillotson, J. H. Metallic Equations of State for Hypervelocity Impact, Technical Report (General Dynamics, 1962).
Collins, G. S., Melosh, H. J. & Ivanov, B. A. Modeling damage and deformation in impact simulations. Meteorit. Planet. Sci. 39 , 217–231 (2004).
Jutzi, M. SPH calculations of asteroid disruptions: the role of pressure dependent failure models. Planet. Space Sci. 107 , 3–9 (2015).
Rein, H. & Liu, S.-F. REBOUND: an open-source multi-purpose N -body code for collisional dynamics. Astron. Astrophys. 537 , A128 (2012).
Rein, H. et al. Hybrid symplectic integrators for planetary dynamics. Mon. Not. R. Astron. Soc. 485 , 5490–5497 (2019).
Rein, H. & Tamayo, D. WHFAST: a fast and unbiased implementation of a symplectic Wisdom–Holman integrator for long-term gravitational simulations. Mon. Not. R. Astron. Soc. 452 , 376–388 (2015).
Rein, H. & Spiegel, D. S. IAS15: a fast, adaptive, high-order integrator for gravitational dynamics, accurate to machine precision over a billion orbits. Mon. Not. R. Astron. Soc. 446 , 1424–1437 (2015).
Park, R. S., Folkner, W. M., Williams, J. G. & Boggs, D. H. The JPL planetary and lunar ephemerides DE440 and DE441. Astron. J. 161 , 105 (2021).
Delbo, M., Mueller, M., Emery, J., Rozitis, B. & Capria, M. in Asteroids IV (eds Bottke, W. F., DeMeo F. E. & Michel, P.) 107–128 (Univ. of Arizona Press, 2015).
Fenucci, M. & Novaković, B. The role of the Yarkovsky effect in the long-term dynamics of asteroid (469219) Kamo’oalewa. Astron. J. 162 , 227 (2021).
Burns, J. A., Lamy, P. L. & Soter, S. Radiation forces on small particles in the solar system. Icarus 40 , 1–48 (1979).
The classy code. GitHub https://github.com/maxmahlke/classy (2022).
The REBOUND code. GitHub https://github.com/hannorein/rebound (2024).
Connors, M., Wiegert, P. & Veillet, C. Earth’s Trojan asteroid. Nature 475 , 481–483 (2011).
Santana-Ros, T. et al. Orbital stability analysis and photometric characterization of the second Earth trojan asteroid 2020 XL 5 . Nat. Commun. 13 , 447 (2022).
Robinson, M. S. et al. Lunar Reconnaissance Orbiter Camera (LROC) instrument overview. Space Sci. Rev. 150 , 81–124 (2010).
Download references
Acknowledgements
B.C. is supported by the National Natural Science Foundation of China (no. 12202227) and the Postdoctoral Innovative Talent Support Program of China (no. BX20220164). This work is also supported by the National Natural Science Foundation of China under grant 62227901. We thank W. F. Bottke and others for valuable discussions on this work at the Asteroids, Comets, Meteors Conference 2023. We thank M. Connors, T. Santana-Ros and F. Ferrari for providing helpful comments to improve and clarify the manuscript. We acknowledge the use of imagery from Lunar QuickMap ( https://quickmap.lroc.asu.edu ), a collaboration between NASA, Arizona State University and Applied Coherent Technology Corp.
Author information
Authors and affiliations.
Tsinghua University, Beijing, China
Yifei Jiao, Bin Cheng, Yukun Huang & Hexi Baoyin
Department of Physics and Astronomy, University of British Columbia, Vancouver, British Columbia, Canada
Yukun Huang & Brett Gladman
Lunar and Planetary Laboratory, University of Arizona, Tucson, AZ, USA
Erik Asphaug & Renu Malhotra
Laboratoire Lagrange, Observatoire de la Côte d’Azur, Université Côte d’Azur, CNRS, Nice, France
Patrick Michel
Beihang University, Beijing, China
Inner Mongolia University of Technology, Inner Mongolia, China
Hexi Baoyin
You can also search for this author in PubMed Google Scholar
Contributions
Y.J. performed the SPH and N -body numerical simulations and analysed the numerical results. B.C. and H.B. initiated the project, designed the simulations and led the research. Y.H., B.G. and R.M. contributed to the discussion of the dynamical evolution of lunar ejecta and the spectral comparison. E.A., P.M. and Y.Y. contributed to the discussion of the lunar impact ejection process. All authors contributed to interpretation of the results and preparation of the paper.
Corresponding authors
Correspondence to Bin Cheng or Hexi Baoyin .
Ethics declarations
Competing interests.
The authors declare no competing interests.
Peer review
Peer review information.
Nature Astronomy thanks Martin Connors, Fabio Ferrari and Toni Santana-Ros for their contribution to the peer review of this work.
Additional information
Publisher’s note Springer Nature remains neutral with regard to jurisdictional claims in published maps and institutional affiliations.
Extended data
Extended data fig. 1 comparison of kamo‘oalewa’s spectral slope with bus-demeo asteroid taxonomies and lunar materials..
Due to errors in the infrared reflectance, Kamo‘oalewa’s slope possibly ranges from 76 to 101%/ μ m 3 . Typical asteroid slopes are plotted with the mean values and the standard deviations, based on 371 asteroid samples 10 . The slopes of lunar meteorite and samples, whose spectra have been shown in Fig. 1 , are measured using the online Bus-DeMeo taxonomy tool 10 .
Source data
Extended data fig. 2 spectral comparison using the mixture of common factor analysers (mcfa) model..
The latent scores are computed as a lower-dimensional representation of the reflectance spectrum, using the classy package 51 . The MCFA results suggest that Kamo‘oalewa is spectrally similar to lunar materials, but incompatible with any typical asteroid spectrum in public repositories.
Extended Data Fig. 3 Simulated rotation distribution of escaping SPH particles with L \({}_{\max }\) larger than 36 m, for a Kepler-sized crater forming event.
Here we use the SPH particle vorticity to approximate the rotation state of the sub-resolution fragments. The upper panel presents the cumulative fraction of these high-velocity and low-shocked particles versus the rotation period. Note that about 65% of these particles, when ejected, are spinning faster than Kamo‘oalewa which has a period of 28.3 min 3 . The lower panel is a boxplot of the period distribution, suggesting a median of about 6 minutes and an interquartile range (IQR, the box range from the first quartile Q1 to the third quartile Q3) from a few to several tens of minutes. The whiskers are bounded at Q1-1.5*IQR and Q3+1.5*IQR, with the flier points removed. There are 466 and 94 SPH particles used for k=10 30 m −3 and k=10 33 m −3 , respectively.
Extended Data Fig. 4 The location and topography of lunar crater Giordano Bruno.
Left is a map of the lunar farside using the Lunar QuickMap ( https://bit.ly/45Ftwjh ). Right is the topographic map of GB crater from the Lunar Reconnaissance Orbiter Camera (LROC) data 69 .
Extended Data Fig. 5 Initial condition of N-body simulations.
We start each set of simulation at a random lunar phase ϕ , which indicates the relative position of the Sun-Earth-Moon system, and launched 300 particles along a θ = 45 ∘ cone at random azimuths ζ and with a given velocity magnitude distribution v 0 (following a power law distribution ranging from 2.38 to 6.0 km/s, and with the power of -4.0 according to Supplementary Fig. 7 ).
Extended Data Fig. 6 GB ejecta delivered to Earth, normalized with the background lunar meteorite flux.
It has been estimated that the GB ejecta is comparable to the total lunar ejecta produced by other craters over 10 Myr 42 , thus the background lunar ejecta per Myr is about one-tenth of the GB total ejecta. Assuming the same delivery efficiency (Earth collision fraction over time) as the GB ejecta, we can integrate the product of the ejecta volume and the delivery efficiency of all previous craters, to estimate the background flux delivered to Earth per Myr. The result shows a ten-fold spike of GB meteorites than the background flux in the first Myr after GB formation (several million years ago). However, this spike is currently unobservable due to the short terrestrial preservation period of lunar meteorites, which only lasts a few hundred thousand years 8 . Presently, GB ejecta contributes to roughly 10% of the background flux of lunar meteorites, implying that our current collection of lunar meteorites likely contains only a handful of GB ejecta, for example, the possible GB meteorites Yamato-82192/82193/86032 42 .
Supplementary information
Supplementary information.
Supplementary Text, Figs. 1–8 and Tables 1–5.
Supplementary Data
Source Data for Supplementary Figs. 1–8.
Source Data Fig. 1
Statistical source data.
Source Data Fig. 2
Source data fig. 3, source data fig. 4, source data extended data fig. 1, source data extended data fig. 2, source data extended data fig. 3, source data extended data fig. 6, rights and permissions.
Springer Nature or its licensor (e.g. a society or other partner) holds exclusive rights to this article under a publishing agreement with the author(s) or other rightsholder(s); author self-archiving of the accepted manuscript version of this article is solely governed by the terms of such publishing agreement and applicable law.
Reprints and permissions
About this article
Cite this article.
Jiao, Y., Cheng, B., Huang, Y. et al. Asteroid Kamo‘oalewa’s journey from the lunar Giordano Bruno crater to Earth 1:1 resonance. Nat Astron (2024). https://doi.org/10.1038/s41550-024-02258-z
Download citation
Received : 24 July 2023
Accepted : 27 March 2024
Published : 19 April 2024
DOI : https://doi.org/10.1038/s41550-024-02258-z
Share this article
Anyone you share the following link with will be able to read this content:
Sorry, a shareable link is not currently available for this article.
Provided by the Springer Nature SharedIt content-sharing initiative
Quick links
- Explore articles by subject
- Guide to authors
- Editorial policies
Sign up for the Nature Briefing newsletter — what matters in science, free to your inbox daily.

- International edition
- Australia edition
- Europe edition
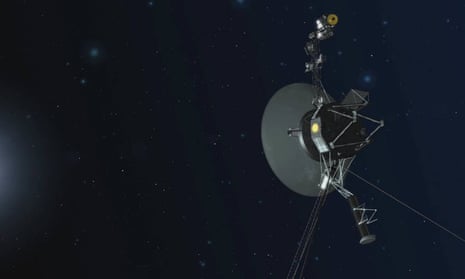
Voyager 1 transmitting data again after Nasa remotely fixes 46-year-old probe
Engineers spent months working to repair link with Earth’s most distant spacecraft, says space agency
Earth’s most distant spacecraft, Voyager 1, has started communicating properly again with Nasa after engineers worked for months to remotely fix the 46-year-old probe.
Nasa’s Jet Propulsion Laboratory (JPL), which makes and operates the agency’s robotic spacecraft, said in December that the probe – more than 15bn miles (24bn kilometres) away – was sending gibberish code back to Earth.
In an update released on Monday , JPL announced the mission team had managed “after some inventive sleuthing” to receive usable data about the health and status of Voyager 1’s engineering systems. “The next step is to enable the spacecraft to begin returning science data again,” JPL said. Despite the fault, Voyager 1 had operated normally throughout, it added.
Launched in 1977, Voyager 1 was designed with the primary goal of conducting close-up studies of Jupiter and Saturn in a five-year mission. However, its journey continued and the spacecraft is now approaching a half-century in operation.
Voyager 1 crossed into interstellar space in August 2012, making it the first human-made object to venture out of the solar system. It is currently travelling at 37,800mph (60,821km/h).
Hi, it's me. - V1 https://t.co/jgGFBfxIOe — NASA Voyager (@NASAVoyager) April 22, 2024
The recent problem was related to one of the spacecraft’s three onboard computers, which are responsible for packaging the science and engineering data before it is sent to Earth. Unable to repair a broken chip, the JPL team decided to move the corrupted code elsewhere, a tricky job considering the old technology.
The computers on Voyager 1 and its sister probe, Voyager 2, have less than 70 kilobytes of memory in total – the equivalent of a low-resolution computer image. They use old-fashioned digital tape to record data.
The fix was transmitted from Earth on 18 April but it took two days to assess if it had been successful as a radio signal takes about 22 and a half hours to reach Voyager 1 and another 22 and a half hours for a response to come back to Earth. “When the mission flight team heard back from the spacecraft on 20 April, they saw that the modification worked,” JPL said.
Alongside its announcement, JPL posted a photo of members of the Voyager flight team cheering and clapping in a conference room after receiving usable data again, with laptops, notebooks and doughnuts on the table in front of them.
The Retired Canadian astronaut Chris Hadfield, who flew two space shuttle missions and acted as commander of the International Space Station, compared the JPL mission to long-distance maintenance on a vintage car.
“Imagine a computer chip fails in your 1977 vehicle. Now imagine it’s in interstellar space, 15bn miles away,” Hadfield wrote on X . “Nasa’s Voyager probe just got fixed by this team of brilliant software mechanics.
Voyager 1 and 2 have made numerous scientific discoveries , including taking detailed recordings of Saturn and revealing that Jupiter also has rings, as well as active volcanism on one of its moons, Io. The probes later discovered 23 new moons around the outer planets.
As their trajectory takes them so far from the sun, the Voyager probes are unable to use solar panels, instead converting the heat produced from the natural radioactive decay of plutonium into electricity to power the spacecraft’s systems.
Nasa hopes to continue to collect data from the two Voyager spacecraft for several more years but engineers expect the probes will be too far out of range to communicate in about a decade, depending on how much power they can generate. Voyager 2 is slightly behind its twin and is moving slightly slower.
In roughly 40,000 years, the probes will pass relatively close, in astronomical terms, to two stars. Voyager 1 will come within 1.7 light years of a star in the constellation Ursa Minor, while Voyager 2 will come within a similar distance of a star called Ross 248 in the constellation of Andromeda.
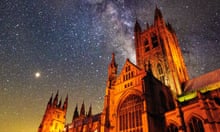
Cosmic cleaners: the scientists scouring English cathedral roofs for space dust
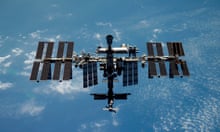
Russia acknowledges continuing air leak from its segment of space station
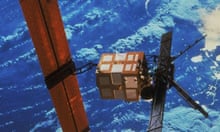
Uncontrolled European satellite falls to Earth after 30 years in orbit
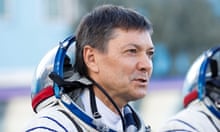
Cosmonaut Oleg Kononenko sets world record for most time spent in space
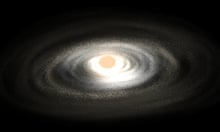
‘Old smokers’: astronomers discover giant ancient stars in Milky Way
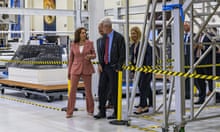
Nasa postpones plans to send humans to moon
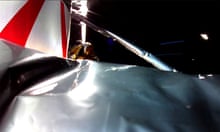
What happened to the Peregrine lander and what does it mean for moon missions?
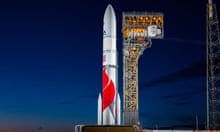
Peregrine 1 has ‘no chance’ of landing on moon due to fuel leak
Most viewed.
'Uncharted territory': El Niño to flip to La Niña in what could be the hottest year on record
A quick flip from El Niño to La Niña is coming soon, but what does that mean for the U.S.?
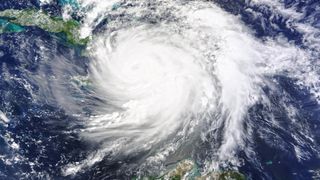
El Niño is likely to give way soon, ushering in a quick switch to its opposite atmospheric and ocean pattern, La Niña.
For the U.S., this climatological flip-flop will likely mean a greater risk of major hurricanes in the Atlantic as well as areas of drier-than-usual weather in the southern portions of the country. Globally, La Niña usually leads to declining temperatures, but the lag in when the effects take place means that 2024 will likely still be a top-five year for temperature in climate history, said Tom Di Liberto , a climate scientist at the National Oceanic and Atmospheric Administration (NOAA).
"All signs suggest that 2024 is going to be another warm year," Di Liberto told Live Science.
El Niño and La Niña describe opposing patterns in the trade winds that encircle the equator, blowing west from South America toward Asia. In a neutral year, when neither pattern is in play, these trade winds push warm water westward, which drives cool ocean water up from the depths to replace it.
When El Niño is in play, the trade winds weaken, so the eastern Pacific, along the west coast of North and South America, stays warmer. The effect, according to NOAA, is that the jet stream moves southward, drying Canada and the northern U.S. but bringing moisture to the southern portions of the U.S.
Related: Why don't hurricanes form at the equator?
In a La Niña year, the trade winds strengthen, pushing warm water toward Asia and increasing the upwelling of cold water off the Pacific coast of the Americas. The jet stream moves northward, drying the Southwest and Southeast and bringing wetter weather to the Pacific Northwest and the Great Lakes.
Sign up for the Live Science daily newsletter now
Get the world’s most fascinating discoveries delivered straight to your inbox.
The El Niño pattern has officially been active since June 2023 , but NOAA's Climate Prediction Center now reports that the pattern is weakening, with an 85% chance of a switch to neutral conditions before June. La Niña is then expected to roar back, with a 60% chance of La Niña conditions between June and August, the National Centers for Environmental Prediction reports.
"When it comes to El Niños of this strength, moderate to strong, it"s not uncommon to see these events end rapidly and then shift into La Niña rapidly," Di Liberto said.
Ocean measurements currently show warm surface temperatures in the Pacific, Di Liberto said, but below-average cold water beneath. Once that cold water hits the surface, the switch will happen quickly, he said.
The flip from El Niño to La Niña raises the risk of a strong upcoming hurricane season, said Alex DesRosiers , a doctoral candidate in atmospheric science at Colorado State University. During El Niño, rising heat from the eastern Pacific flows into the upper atmosphere, leading to stronger winds at high altitudes. This creates vertical wind shear — a difference in wind speed and direction at the surface versus higher in the atmosphere. And vertical wind shear, DesRosiers told Live Science, "can really act to tear apart hurricanes as they try to form."
During La Niña, the upper atmosphere winds calm, reducing wind shear. This allows the convection of warm, moist air from the ocean surface to form big storms.
"As we move into La Niña, the atmosphere becomes more supportive of allowing storms to bubble up and intensify," DesRosiers said.
— The surface of the ocean is now so hot it's broken every record since satellite measurements began
— Atlantic's hurricane alley is so hot from El Niño it could send 2024's storm season into overdrive
— We may need a new 'Category 6' hurricane level for winds over 192 mph, study suggests
As a result of the expected La Niña and current extremely warm Atlantic Ocean surface temperatures, CSU's Tropical Weather & Climate Research team is currently predicting a very active Atlantic hurricane season , with a forecast of 23 named storms (versus the average of 14.4) and five hurricanes of Category 3 or higher (versus the average of 3.2). This year may look similar to 2010 and 2020, both of which were busy storm seasons, although it's not guaranteed that strong storms will impact land, DesRosiers said.
All of these climatic patterns are taking place against a backdrop of rising ocean and surface temperatures. So, while La Niña usually brings cooler-than-average temperatures to the northern U.S., this region could still experience a scorching summer due to the background effects of climate change , Di Liberto said.
Similarly, although 2023 was an El Niño year, which should suppress hurricanes, it saw an above-average hurricane season, DesRosiers said. This busy storm season might be due, in part, to 2023 being the warmest year on record.
"With an Atlantic that is this warm," he said, "we're kind of in uncharted territory."
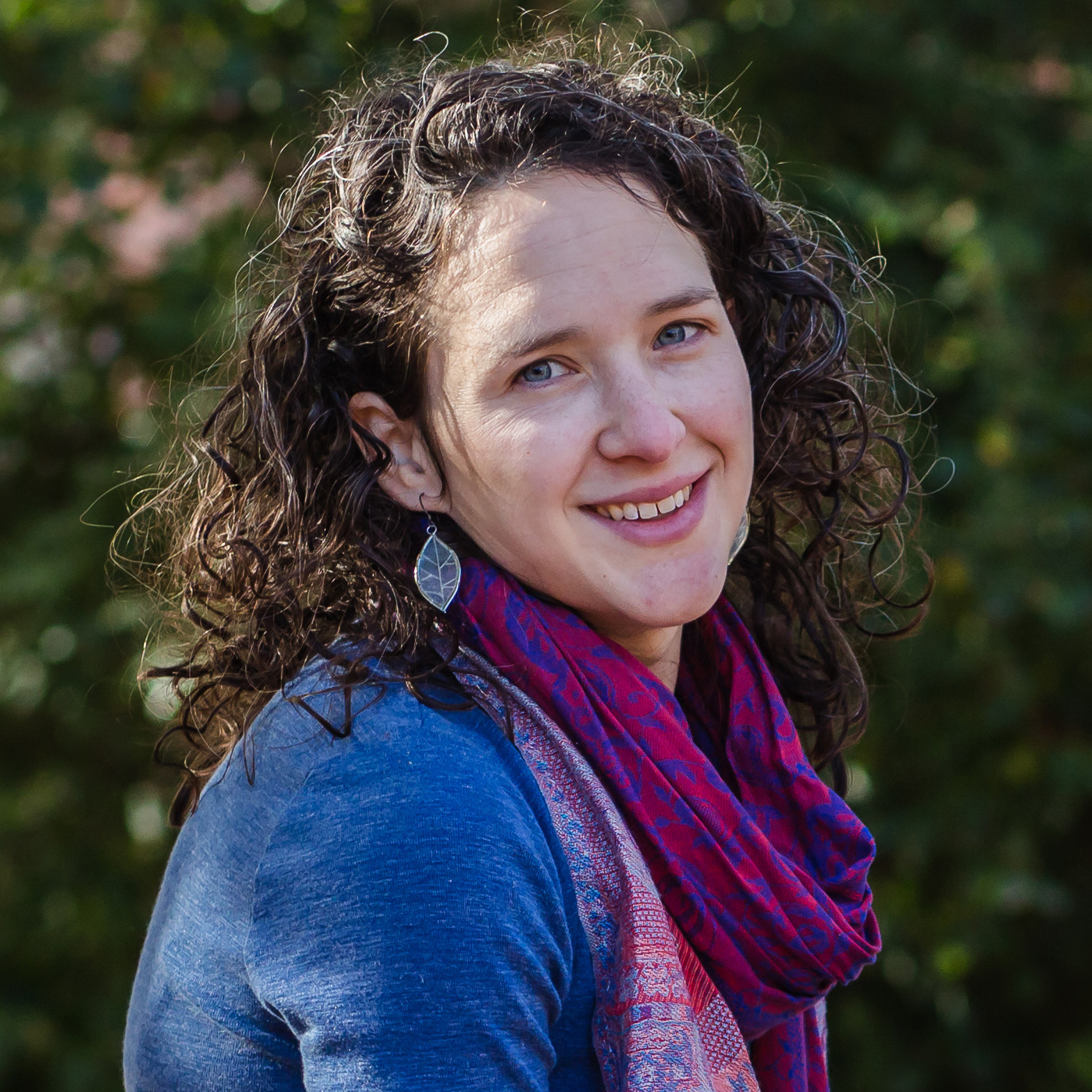
Stephanie Pappas is a contributing writer for Live Science, covering topics ranging from geoscience to archaeology to the human brain and behavior. She was previously a senior writer for Live Science but is now a freelancer based in Denver, Colorado, and regularly contributes to Scientific American and The Monitor, the monthly magazine of the American Psychological Association. Stephanie received a bachelor's degree in psychology from the University of South Carolina and a graduate certificate in science communication from the University of California, Santa Cruz.
Eerie, orange skies loom over Athens as dust storm engulfs southern Greece
Massive heat wave and a supercell thunderstorm caused deadly, baseball-size hailstones to rain down on Spain
Traces of hallucinogenic plants and chile peppers found at Maya ball court suggest rituals took place there
Most Popular
- 2 Giant, 82-foot lizard fish discovered on UK beach could be largest marine reptile ever found
- 3 Global 'time signals' subtly shifted as the total solar eclipse reshaped Earth's upper atmosphere, new data shows
- 4 'I nearly fell out of my chair': 1,800-year-old mini portrait of Alexander the Great found in a field in Denmark
- 5 NASA reveals 'glass-smooth lake of cooling lava' on surface of Jupiter's moon Io
- 2 Scientists find one of the oldest stars in the universe in a galaxy right next to ours
- 3 DNA analysis spanning 9 generations of people reveals marriage practices of mysterious warrior culture
- 4 5 catastrophic megathrust earthquakes led to the demise of the pre-Aztec city of Teotihuacan, new study suggests
- 5 Plato's burial place finally revealed after AI deciphers ancient scroll carbonized in Mount Vesuvius eruption

IMAGES
VIDEO
COMMENTS
scientific hypothesis, an idea that proposes a tentative explanation about a phenomenon or a narrow set of phenomena observed in the natural world.The two primary features of a scientific hypothesis are falsifiability and testability, which are reflected in an "If…then" statement summarizing the idea and in the ability to be supported or refuted through observation and experimentation.
Bibliography. A scientific hypothesis is a tentative, testable explanation for a phenomenon in the natural world. It's the initial building block in the scientific method. Many describe it as an ...
Write an example of a hypothesis, and explain how you would test it. This page titled 1.3: Developing Hypotheses is shared under a CK-12 license and was authored, remixed, and/or curated by CK-12 Foundation via source content that was edited to the style and standards of the LibreTexts platform; a detailed edit history is available upon request.
The hypothesis of Andreas Cellarius, showing the planetary motions in eccentric and epicyclical orbits.. A hypothesis (pl.: hypotheses) is a proposed explanation for a phenomenon.For a hypothesis to be a scientific hypothesis, the scientific method requires that one can test it. Scientists generally base scientific hypotheses on previous observations that cannot satisfactorily be explained ...
A hypothesis (plural hypotheses) is a proposed explanation for an observation. The definition depends on the subject. In science, a hypothesis is part of the scientific method. It is a prediction or explanation that is tested by an experiment. Observations and experiments may disprove a scientific hypothesis, but can never entirely prove one.
The Gaia hypothesis posits that the Earth is a self-regulating complex system involving the biosphere, the atmosphere, the hydrospheres and the pedosphere, tightly coupled as an evolving system. The hypothesis contends that this system as a whole, called Gaia, seeks a physical and chemical environment optimal for contemporary life.
The Earth formed roughly 4.5. . billion years ago, and life probably began between 3.5. . and 3.9. . billion years ago. The Oparin-Haldane hypothesis suggests that life arose gradually from inorganic molecules, with "building blocks" like amino acids forming first and then combining to make complex polymers.
Scientific Method. You have probably learned that the scientific method is the way scientists approach their work. The scientific method is a series of steps that help to investigate a question. Scientists use data and evidence gathered from observations, experience, or experiments to answer their questions.
Gaia hypothesis, model of the Earth in which its living and nonliving parts are viewed as a complex interacting system that can be thought of as a single organism. Developed c. 1972 largely by British chemist James E. Lovelock and U.S. biologist Lynn Margulis, the Gaia hypothesis is named for the Greek Earth goddess. It postulates that all living things have a regulatory effect on the Earth ...
A hypothesis proposes a relationship between the independent and dependent variable. A hypothesis is a prediction of the outcome of a test. It forms the basis for designing an experiment in the scientific method.A good hypothesis is testable, meaning it makes a prediction you can check with observation or experimentation.
Hypothesis Versus Theory . Although in common usage the terms hypothesis and theory are used interchangeably, the two words mean something different from each other in science. Like a hypothesis, a theory is testable and may be used to make predictions. However, a theory has been tested using the scientific method many times.
The Process of Science. The scientific method. Proposing a Hypothesis; Testing a Hypothesis; Viewed from space, Earth (Figure \(\PageIndex{1}\)) offers no clues about the diversity of lifeforms that reside there.The first forms of life on Earth are thought to have been microorganisms that existed for billions of years in the ocean before plants and animals appeared.
Theory vs. Hypothesis: Basics of the Scientific Method. Written by MasterClass. Last updated: Jun 7, 2021 • 2 min read. Though you may hear the terms "theory" and "hypothesis" used interchangeably, these two scientific terms have drastically different meanings in the world of science.
The scientific method. At the core of biology and other sciences lies a problem-solving approach called the scientific method. The scientific method has five basic steps, plus one feedback step: Make an observation. Ask a question. Form a hypothesis, or testable explanation. Make a prediction based on the hypothesis.
Earth Science deals with any and all aspects of the Earth. Our Earth has molten lava, icy mountain peaks, steep canyons and towering waterfalls. Earth scientists study the atmosphere high above us as well as the planet's core far beneath us. Earth scientists study parts of the Earth as big as continents and as small as the tiniest atom.
This does not mean theories are not meaningful. For a hypothesis to become a theory, scientists must conduct rigorous testing, typically across multiple disciplines by separate groups of scientists.
A hypothesis is the best answer to a question based on what is known. Scientists take that best answer and do experiments to see if it still makes sense or if a better answer can be made. When a scientist has a question they want to answer, they research what is already known about the topic. Then, they come up with their best answer to the ...
Gaia hypothesis: the original version — the Earth's organisms regulate the physical and chemical components of the earth system so as to maintain the planet as an optimal habitat for life.
Earth sciences, the fields of study concerned with the solid Earth, its waters, and the air that envelops it. Included are the geologic, hydrologic, and atmospheric sciences. The broad aim of the Earth sciences is to understand the present features and past evolution of Earth and to use this knowledge, where appropriate, for the benefit of humankind. . Thus, the basic concerns of the Earth ...
A hypothesis is an idea about how something works that can be tested using experiments. A prediction says what will happen in an experiment if the hypothesis is correct. Presenter 1: We are going ...
A hypothesis is a tentative, testable answer to a scientific question. Once a scientist has a scientific question she is interested in, the scientist reads up to find out what is already known on the topic. Then she uses that information to form a tentative answer to her scientific question. Sometimes people refer to the tentative answer as "an ...
A theory is a carefully thought-out explanation for observations of the natural world that has been constructed using the scientific method, and which brings together many facts and hypotheses. In a previous blog post, I talked about the definition of "fact" in a scientific context, and discussed how facts differ from hypotheses and ...
The expanding Earth or growing Earth hypothesis was a hypothesis arguing that the position and relative movement of continents is due at least partially to the volume of Earth increasing. Conversely, geophysical global cooling was the hypothesis that various features could be explained by Earth contracting.
The hypothesis will be tested by the Tianwen-2 mission when it returns a sample of Kamo'oalewa. ... exhibits a meta-stable 1:1 mean-motion resonance with Earth. Intriguingly, recent ground-based ...
Antarctic sea ice has been disappearing over the last several summers. Now, climate scientists are wondering whether it will ever come back.
Earth Day is a global event which aims to highlight the importance of protecting the environment. It takes place every year on 22 April. It was set up in 1970 by Gaylord Nelson, a US senator and ...
Earth's most distant spacecraft, Voyager 1, has started communicating properly again with Nasa after engineers worked for months to remotely fix the 46-year-old probe.. Nasa's Jet Propulsion ...
For the U.S., this climatological flip-flop will likely mean a greater risk of major hurricanes in the Atlantic as well as areas of drier-than-usual weather in the southern portions of the country.