- Our Mission

3 Simple Strategies to Improve Students’ Problem-Solving Skills
These strategies are designed to make sure students have a good understanding of problems before attempting to solve them.
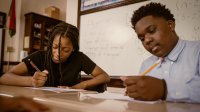
Research provides a striking revelation about problem solvers. The best problem solvers approach problems much differently than novices. For instance, one meta-study showed that when experts evaluate graphs , they tend to spend less time on tasks and answer choices and more time on evaluating the axes’ labels and the relationships of variables within the graphs. In other words, they spend more time up front making sense of the data before moving to addressing the task.
While slower in solving problems, experts use this additional up-front time to more efficiently and effectively solve the problem. In one study, researchers found that experts were much better at “information extraction” or pulling the information they needed to solve the problem later in the problem than novices. This was due to the fact that they started a problem-solving process by evaluating specific assumptions within problems, asking predictive questions, and then comparing and contrasting their predictions with results. For example, expert problem solvers look at the problem context and ask a number of questions:
- What do we know about the context of the problem?
- What assumptions are underlying the problem? What’s the story here?
- What qualitative and quantitative information is pertinent?
- What might the problem context be telling us? What questions arise from the information we are reading or reviewing?
- What are important trends and patterns?
As such, expert problem solvers don’t jump to the presented problem or rush to solutions. They invest the time necessary to make sense of the problem.
Now, think about your own students: Do they immediately jump to the question, or do they take time to understand the problem context? Do they identify the relevant variables, look for patterns, and then focus on the specific tasks?
If your students are struggling to develop the habit of sense-making in a problem- solving context, this is a perfect time to incorporate a few short and sharp strategies to support them.
3 Ways to Improve Student Problem-Solving
1. Slow reveal graphs: The brilliant strategy crafted by K–8 math specialist Jenna Laib and her colleagues provides teachers with an opportunity to gradually display complex graphical information and build students’ questioning, sense-making, and evaluating predictions.
For instance, in one third-grade class, students are given a bar graph without any labels or identifying information except for bars emerging from a horizontal line on the bottom of the slide. Over time, students learn about the categories on the x -axis (types of animals) and the quantities specified on the y -axis (number of baby teeth).
The graphs and the topics range in complexity from studying the standard deviation of temperatures in Antarctica to the use of scatterplots to compare working hours across OECD (Organization for Economic Cooperation and Development) countries. The website offers a number of graphs on Google Slides and suggests questions that teachers may ask students. Furthermore, this site allows teachers to search by type of graph (e.g., scatterplot) or topic (e.g., social justice).
2. Three reads: The three-reads strategy tasks students with evaluating a word problem in three different ways . First, students encounter a problem without having access to the question—for instance, “There are 20 kangaroos on the grassland. Three hop away.” Students are expected to discuss the context of the problem without emphasizing the quantities. For instance, a student may say, “We know that there are a total amount of kangaroos, and the total shrinks because some kangaroos hop away.”
Next, students discuss the important quantities and what questions may be generated. Finally, students receive and address the actual problem. Here they can both evaluate how close their predicted questions were from the actual questions and solve the actual problem.
To get started, consider using the numberless word problems on educator Brian Bushart’s site . For those teaching high school, consider using your own textbook word problems for this activity. Simply create three slides to present to students that include context (e.g., on the first slide state, “A salesman sold twice as much pears in the afternoon as in the morning”). The second slide would include quantities (e.g., “He sold 360 kilograms of pears”), and the third slide would include the actual question (e.g., “How many kilograms did he sell in the morning and how many in the afternoon?”). One additional suggestion for teams to consider is to have students solve the questions they generated before revealing the actual question.
3. Three-Act Tasks: Originally created by Dan Meyer, three-act tasks follow the three acts of a story . The first act is typically called the “setup,” followed by the “confrontation” and then the “resolution.”
This storyline process can be used in mathematics in which students encounter a contextual problem (e.g., a pool is being filled with soda). Here students work to identify the important aspects of the problem. During the second act, students build knowledge and skill to solve the problem (e.g., they learn how to calculate the volume of particular spaces). Finally, students solve the problem and evaluate their answers (e.g., how close were their calculations to the actual specifications of the pool and the amount of liquid that filled it).
Often, teachers add a fourth act (i.e., “the sequel”), in which students encounter a similar problem but in a different context (e.g., they have to estimate the volume of a lava lamp). There are also a number of elementary examples that have been developed by math teachers including GFletchy , which offers pre-kindergarten to middle school activities including counting squares , peas in a pod , and shark bait .
Students need to learn how to slow down and think through a problem context. The aforementioned strategies are quick ways teachers can begin to support students in developing the habits needed to effectively and efficiently tackle complex problem-solving.
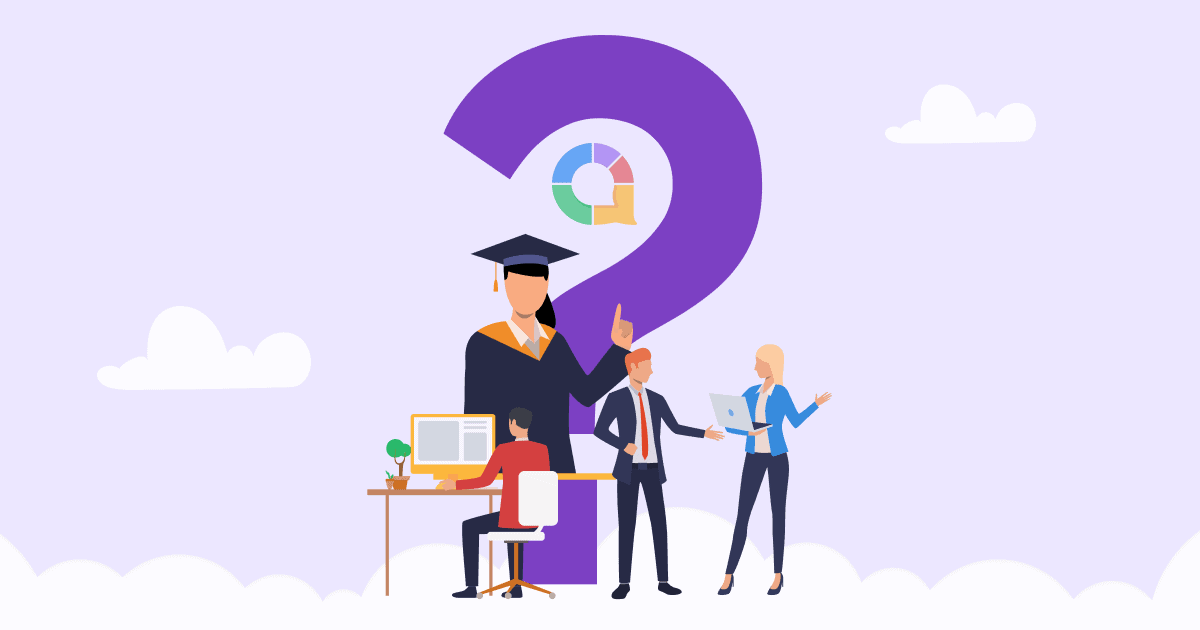
A Questionnaire Sample For Students | 45+ Questions With Tips
Jane Ng • 21 Mar 2024 • 8 min read
Questionnaires are an excellent method for gathering data and better understanding students’ opinions on school-related issues. It is especially useful for teachers, administrators, or researchers who want to gather valuable insights to improve their work. Or for students who need to share their feedback on their school experience.
However, coming up with the right questions can be a challenge. That’s why in today’s post, we provide questionnaire sample for students that you can use as a starting point for your own surveys.
Whether you are searching for output on a specific topic, or a general of how students are feeling, our sample questionnaire with 45+ Questions can help.
Table of Contents
- Grab Free Survey Tool Here!
- What Is A Questionnaire Sample For Students
Types Of Questionnaire Samples For Students
- 45+ Examples Of A Questionnaire Sample For Students
Tips For Conduct An Questionnaire Sample For Students
- Key Takeaways
Frequently Asked Questions
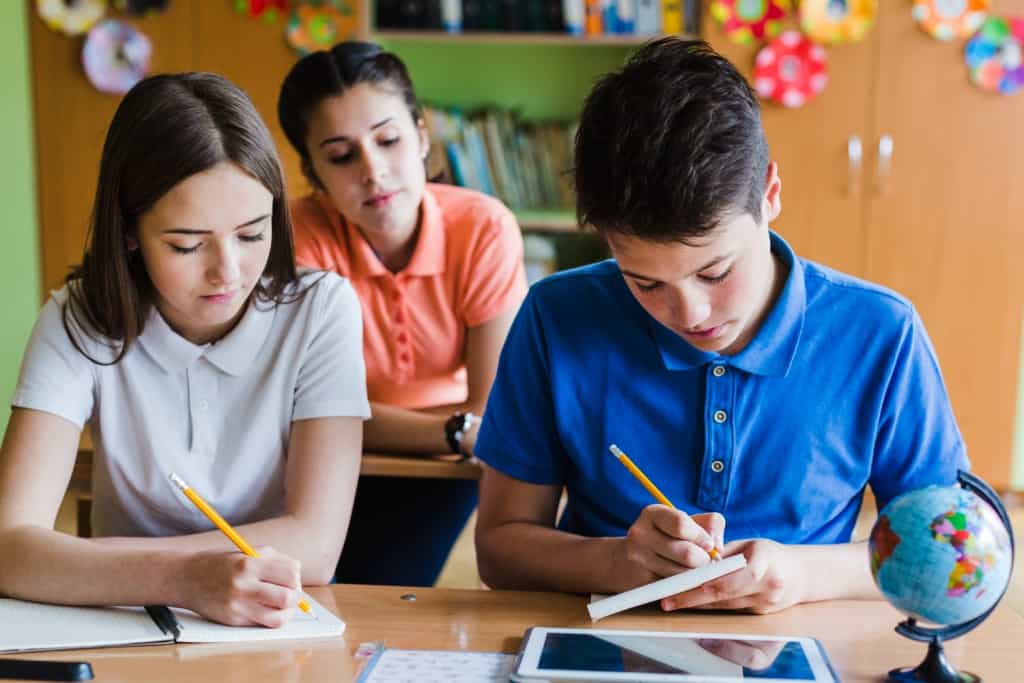
Grab the Free Survey Tool Now!
Questionnaires unlock a treasure trove of student voices! Top free survey tools let teachers, administrators, and researchers gather valuable feedback to improve the school experience. Students can also use questionnaires to share their perspectives, making everyone a part of positive change via creating classroom polling simple, just in a few steps!.
Unlock the full potential – try AhaSlides, for free now!
- AhaSlides Rating Scale | Free Survey Scale Creator
- AhaSlides Online Poll Maker | Top Survey Tool in 2024
- How to design questionnaires , 7 Key Strategies
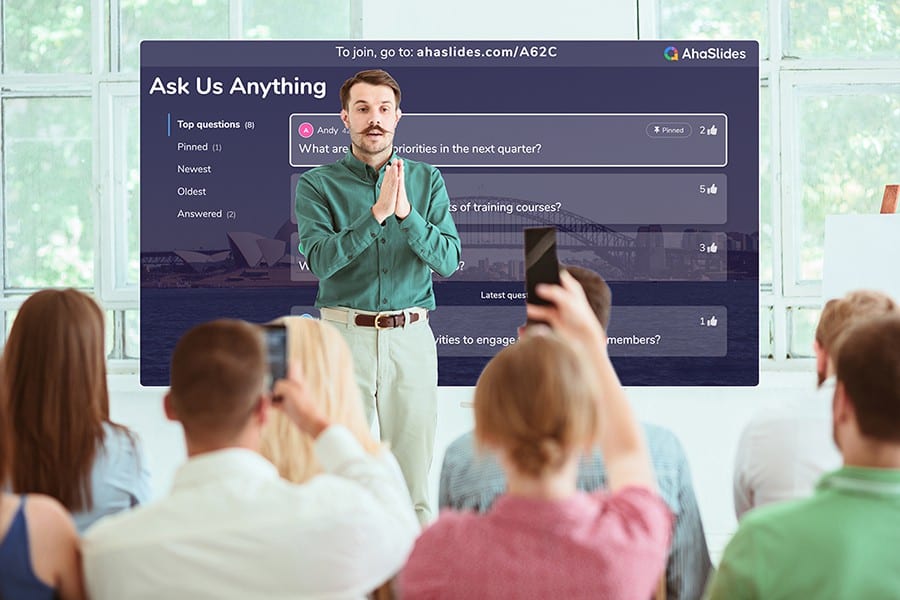
Know Your Class Better!
Use quiz and games on AhaSlides to create fun and interactive survey, to gather public opinions at work, in class or during small gathering
What Is A Questionnaire Sample For Students?
A Questionnaire Sample For Students is a pre-designed set of questions to collect insights and feedback from students.
Administrators, teachers, and researchers can create a questionnaire to get a deeper understanding of different aspects of student’s academic life.
It includes topics with questions, including academic performance questionnaires, teacher evaluations, school environments, mental health, and other important areas of students.
These questions are easy to answer and can be given in paper form or through online surveys. The results can be used to make decisions to improve the overall learning experience for students.
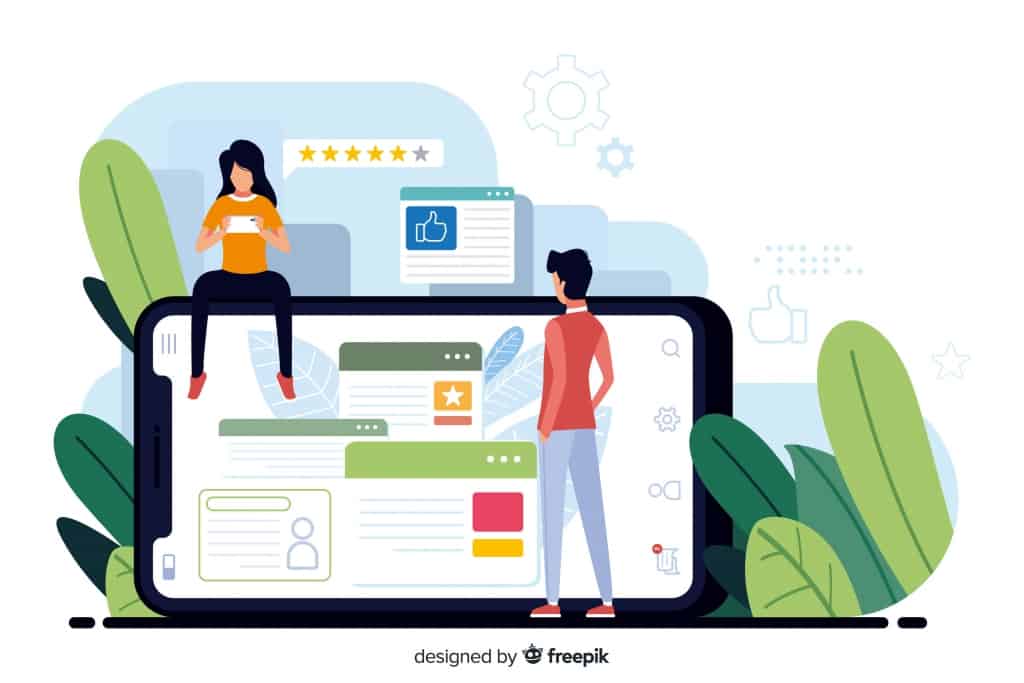
Depending on the purpose of the survey, there are several types of questionnaire samples for students. Here are the most common types:
- Academic Performance Questionnaire: A questionnaire sample aims to collect data on student’s academic performance, including grades, study habits, and learning preferences, or it could be research questionnaires samples.
- Teacher Evaluation Questionnaire : It aims to gather students’ feedback about their teachers’ performance, teaching styles, and effectiveness.
- School Environment Questionnaire: This includes questions to gather feedback about the school’s culture, student-teacher relationships, communication, and engagement.
- Mental Health and Bullying Questionnaire: This aims to gather information about students’ mental health and emotional well-being with a topic such as depression and anxiety, stress, suicide risk, bullying behaviors, help-seeking behaviors, etc.
- Career Aspirations Questionnaire: It aims to gather information about students’ career goals and aspirations, including their interests, skills, and plans.
- Getting to know your students questionnaire as the way to know your students better, both in class and during extracurricular activities.
🎊 Tips: Use Live Q&A to gather more feedbacks and opinions to improve brainstorming sessions !
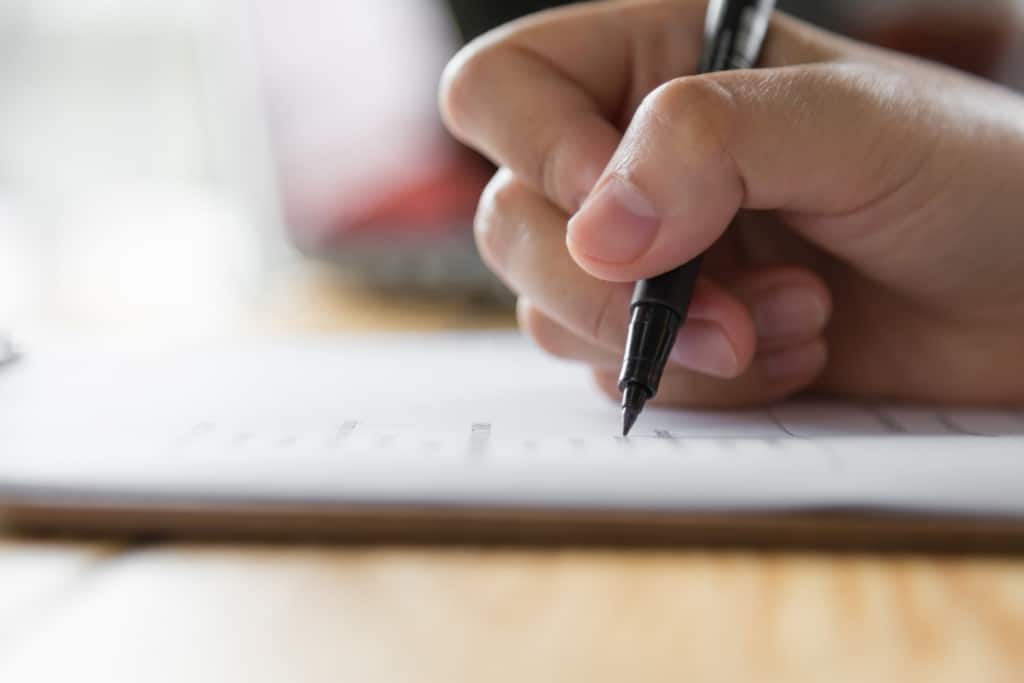
Examples Of A Questionnaire Sample For Students
Academic performance – a questionnaire sample for students.
Here are some examples in an academic performance questionnaire sample:
1/ How many hours do you typically study per week?
- Less than 5 hours
- 5-10 hours
- 10-15 hours
- 15-20 hours
2/ How often do you complete your homework on time?
- Always
- Sometimes
- Rarely
2/ How do you rate your study habits and time management skills?
- Excellent
- Good
3/ Can you focus in your class?
4/ What motivates you to learn more?
- Curiosity – I simply love to learn new things.
- Love of learning – I enjoy the process of learning and find it rewarding in and of itself.
- Love of a subject – I am passionate about a particular subject and want to learn more about it.
- Personal growth – I believe learning is essential for personal growth and development.
5/ How often do you seek help from your teacher when you are struggling with a subject?
- Almost always
6/ What resources do you use to support your learning, such as textbooks, online resources, or study groups?
7/ What aspects of the class do you like the most?
8/ What aspects of the class do you dislike the most?
9/ Do you have supportive classmates?
10/ What learning tips would you give to students in next year’s class?
Teacher Evaluation – A Questionnaire Sample For Students
Here are some potential questions you could use in a Teacher Evaluation Questionnaire:
1/ How well did the teacher communicate with students?
2/ How knowledgeable was the teacher in the subject matter?
- Very knowledgeable
- Moderately knowledgeable
- Somewhat knowledgeable
- Not knowledgeable
3/ How well did the teacher engage students in the learning process?
- Very engaging
- Moderately engaging
- Somewhat engaging
- Not engaging
4/ How easy to contact when the teacher is outside of class?
- Very approachable
- Moderately approachable
- Somewhat approachable
- Not approachable
5/ How effectively did the teacher use classroom technology (e.g. smartboard, online resources)?
6/ Does your teacher find you struggling with their subject?
7/ How well does your teacher respond to questions from students?
8/ What are the areas in which your teacher excelled?
9/ Are there any areas the teacher should improve?
10/ Overall, how would you rate the teacher?
School Environment – A Questionnaire Sample For Students
Here are some examples of questions in a School Environment Questionnaire:
1/ How safe do you feel in your school?
- Moderately safe
- Somewhat safe
2/ Is your school clean and well-maintained?
3/ How clean and well-maintained is your school?
- Very clean and well-maintained
- Moderately clean and well-maintained
- Somewhat clean and well-maintained
- Not clean and well-maintained
4/ Does your school prepare you for college or a career?
5/ Do school personnel have the necessary training and resources to keep students safe? What additional training or resources could be effective?
6/ How well does your school support students with special needs?
- Moderately well
- Somewhat well
7/ How inclusive is your school environment for students from diverse backgrounds?
8/ From 1 – 10, how would you rate your school environment?
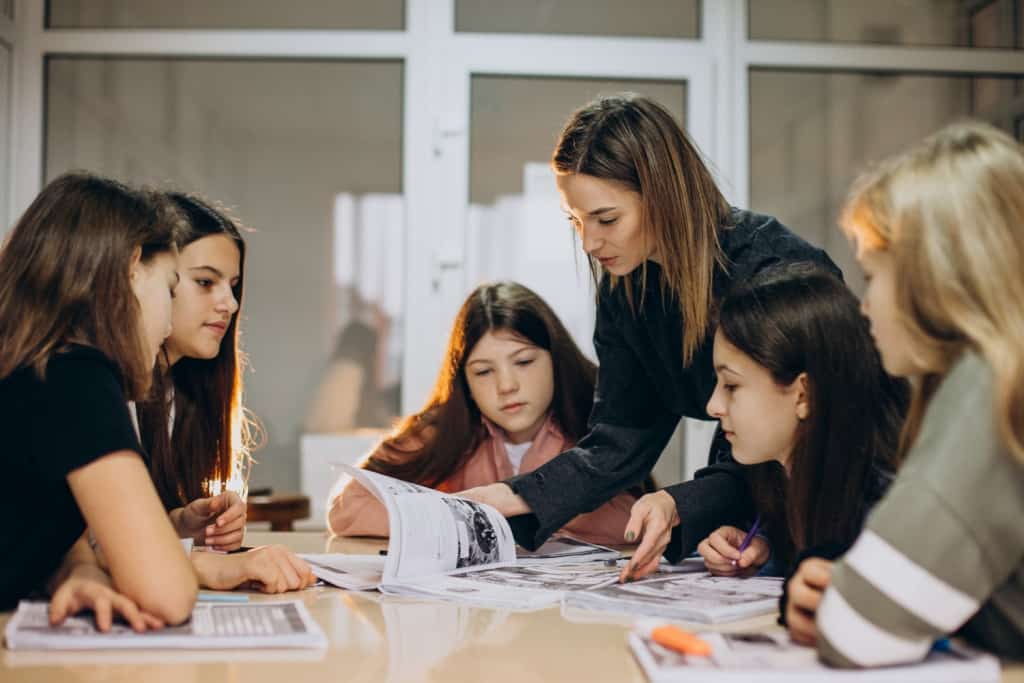
Mental Health and Bullying – A Questionnaire Sample For Students
These questions below can help teachers and school administrators understand how common mental illnesses and bullying are among students, as well as what types of support are required to tackle these issues.
1/ How frequently do you feel depressed or hopeless?
2/ How often do you feel anxious or stressed?
3/ Have you ever been subjected to school bullying?
4/ How frequently have you been the victim of bullying?
- A few times
- Several times
5/ Can you tell us about your bullying experience?
6/ What type(s) of bullying have you experienced?
- Verbal bullying (e.g. name-calling, teasing)
- Social bullying (e.g. exclusion, spreading rumors)
- Physical bullying (e.g. hitting, pushing)
- Cyberbullying (e.g. online harassment)
- All behaviors above
7/ If you have talked to someone, who did you talk to?
- Parent/Guardian
8/ How effectively do you think your school handles bullying?
9/ Have you ever tried to seek help for your mental health?
10/ Where did you go for help if you needed it?
- School counselor
- Outside therapist/counselor
- Doctor/Healthcare provider
- Parent/Guardian
11/ How well does your school, in your opinion, manage mental health issues?
12/ Is there anything else you would like to share about mental health or bullying in your school?
Career Aspirations Questionnaire – A Questionnaire Sample For Students
By gathering information about career aspirations, educators and counselors can provide tailored guidance and resources to help students navigate their desired careers.
1/ What are your career aspirations?
2/ How confident do you feel about achieving your career goals?
- Very confident
- Quite confident
- Somewhat confident
- Not confident at all
3/ Have you talked to anyone about your career aspirations?
4/ Have you participated in any career-related activities at school? What were they?
5/ How helpful have these activities been in shaping your career aspirations?
- Quite helpful
- Somewhat helpful
- Not helpful
6/ What obstacles do you think may stand in the way of achieving your career aspirations?
- Lack of finance
- Lack of access to educational resources
- Discrimination or bias
- Family responsibilities
- Other (please specify)
7/ What resources or support do you think would help pursue your career aspirations?
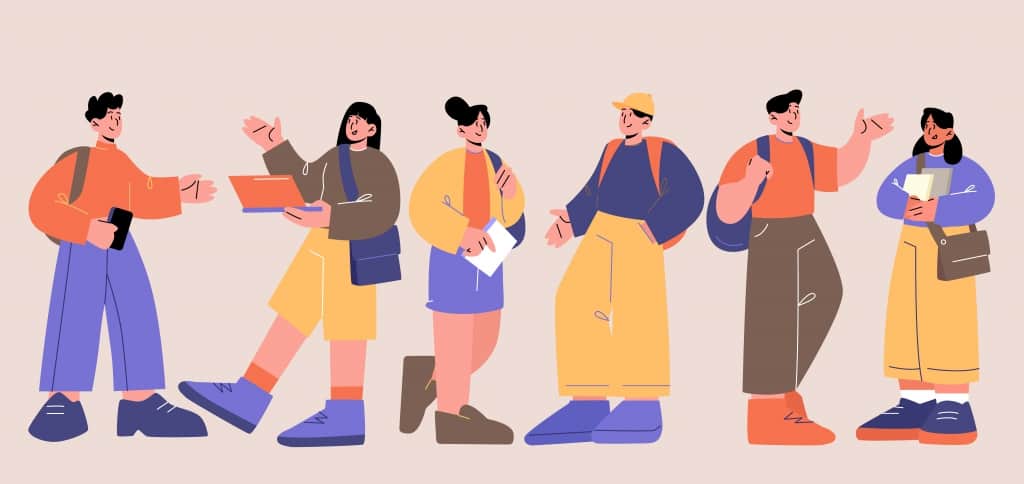
By following these tips, you can conduct a successful questionnaire sample for students that provides valuable insights:
- Clearly define the purpose and objectives of the questionnaire: Before you start, ensure that you’re aware of the information you want to collect and how you plan to use it.
- Use simple and clear language: Use language that is easy for students to understand and avoid using technical terms that could confuse them.
- Keep the questionnaire brief: To keep students’ attention, keep the questionnaire short and focus on the most important questions.
- Use a mix of question types: To gain a more thorough knowledge of student opinions, use different question forms, such as multiple-choice and open-ended questions .
- Offer incentives: Offering incentives, such as a small gift, can encourage student participation and provide honest feedback.
- Use a digital platform: Using a digital platform like AhaSlides will save you tons of time and effort, but still be able to ensure the effectiveness of your survey. With support from AhaSlides Live Question and Answer Feature and real-time quizzes and online poll maker , students can easily read, answer and interact with the questions live, so teachers would know how to improve for the upcoming surveys! AhaSlides also helps you to distribute, collect, and create reports and analyze data based on your previous live sessions!
Key Takeaways
Educators can gain insight into student perspectives on various topics, from academic performance to mental health and bullying by using a questionnaire sample for students.
In addition, with the right tools and strategies in place, you can make the most of this powerful method to create positive change in the lives of your students.
What is sample questionnaire format?
A questionnaire is series of questions, which is used to gather information from people and community.
Criterias of Effectiveness Questionnaire Sample?
A good questionnaire survey should be interesting, interactive, reliable, valid, succinct and super clear.
How many types of questionnaire?
Structured Questionnaire, Unstructured Questionnaire, Open-ended Questionnaire and Close-ended Questionnaire (Check out Closed-ended questions examples from AhaSlides)…
Where can I find the best research questionnaires samples?
It’s simple, you should visit a survey platform, like SurveyMonkey to explore a wide range of free questionnaire templates across various areas, including customer satisfaction, event feedback and employee engagement… to be inspired. Or, you also should re-visit the university library or professional associations to grab more academic knowledge to make sure that your research paper is on the right track!
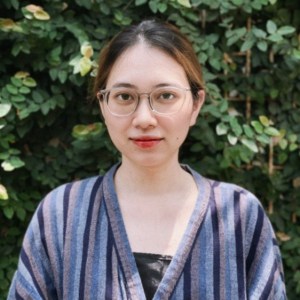
A writer who wants to create practical and valuable content for the audience
More from AhaSlides
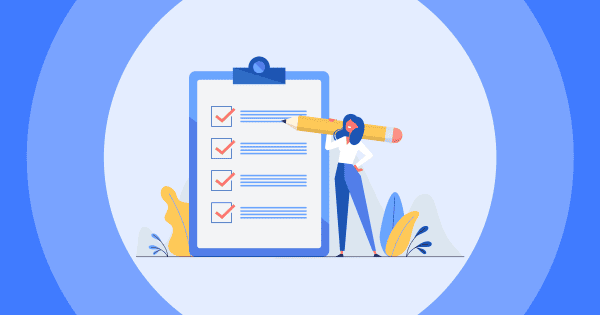
- Brain Development
- Childhood & Adolescence
- Diet & Lifestyle
- Emotions, Stress & Anxiety
- Learning & Memory
- Thinking & Awareness
- Alzheimer's & Dementia
- Childhood Disorders
- Immune System Disorders
- Mental Health
- Neurodegenerative Disorders
- Infectious Disease
- Neurological Disorders A-Z
- Body Systems
- Cells & Circuits
- Genes & Molecules
- The Arts & the Brain
- Law, Economics & Ethics
- Neuroscience in the News
- Supporting Research
- Tech & the Brain
- Animals in Research
- BRAIN Initiative
- Meet the Researcher
- Neuro-technologies
- Tools & Techniques
- Core Concepts
- For Educators
- Ask an Expert
- The Brain Facts Book

Test Your Problem-Solving Skills
Personalize your emails.
Personalize your monthly updates from BrainFacts.org by choosing the topics that you care about most!
Find a Neuroscientist
Engage local scientists to educate your community about the brain.
Image of the Week
Check out the Image of the Week Archive.
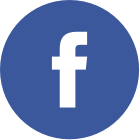
SUPPORTING PARTNERS
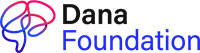
- Privacy Policy
- Accessibility Policy
- Terms and Conditions
- Manage Cookies
Some pages on this website provide links that require Adobe Reader to view.
Your browser is not supported
Sorry but it looks as if your browser is out of date. To get the best experience using our site we recommend that you upgrade or switch browsers.
Find a solution
- Skip to main content
- Skip to navigation
Take our Science Teaching Survey (15 minutes) and shape the future of education! Your insights matter.
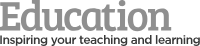
- Back to parent navigation item
- Collections
- Sustainability in chemistry
- Simple rules
- Teacher well-being hub
- Women in chemistry
- Global science
- Escape room activities
- Decolonising chemistry teaching
- Teaching science skills
- Post-lockdown teaching support
- Get the print issue
- RSC Education
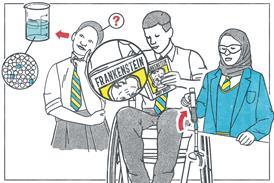
- More from navigation items
Take our Science Teaching Survey (15 minutes) and shape the future of education!
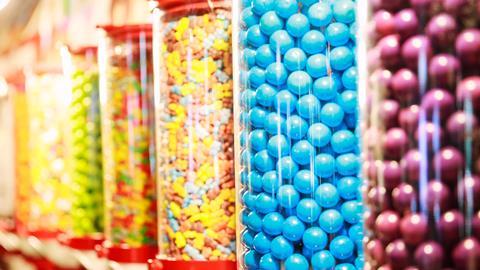
Source: © Sung Yoon Jo/Shutterstock
Practice makes perfect, but mix it up
By Fraser Scott 2022-03-24T08:30:00+00:00
- No comments
Does question bank design affect student performance?
We know it is important to provide students with repeated opportunities to practise solving questions. In a new study, researchers examined how the presentation and format of practice questions influences students’ problem-solving performance. The study revealed that mixed problem sets are better than questions arranged by topic.
There are two types of question practice teachers can give their students. The first, blocked practice, involves solving multiple problems of the same type, or about the same concept, before moving on to another. Practice worksheets and end-of-chapter questions in textbooks are often blocked practice type questions. They hone students’ algorithmic problem-solving skills, but at the expense of their conceptual understanding of the topic. The second, interleaved practice, shuffles between different types of questions in one session. It is more difficult, because students must identify the type of question being asked, or the concept it relates to, in addition to answering. Shuffled questions are thought to help with long-term learning and are similar to the questioning format in students’ exams.
The researchers investigated the effects of these two kinds of practice. They recruited 79 university students from general chemistry classes. They gave one group assignments with mixed questions and a control group assignments with questions organised into topics or chapters. They compared the groups’ performances through one pre-test and post-test after each of three problem-solving sessions.
Teaching tips
- Source or compile more mixed practice question banks, and avoid solely using topic-specific question sets from textbooks.
- To increase the difficulty and benefits of mixed problem question sets, do not include details identifying the relevant topics or chapters.
- Blocked practice still has a place. Certain topics, particularly those to do with numerical problem-solving, may require extensive blocked practice before students can engage with the benefits of interleaved practice.
Probing the problems
Rather than looking at overall scores, the researchers used a more detailed analysis. They broke each problem into the sub-problems, or steps, required to answer the problem. They then categorised students’ answers to the steps as successful, neutral or unsuccessful. Subcategories provided more insight into the students’ work. For example, the neutral category contained the subcategories ‘not required’, ‘did not know to do’ and ‘did something else’.
The study revealed that students in the interleaved-practice group increased their problem-solving success more than those in the blocked-practice group. Significantly, the achievement gap between the experimental and control groups widened as the study progressed. Following interleaved practice, students’ neutral codes decreased by about 70%, unsuccessful codes decreased by about 40%, and the successful codes increased by about 52%. Even if students were not able to complete the entire problem, they still improved at individual steps.
Importantly, even though A-, B- and C-grade students showed different levels of improvement, they all benefited from interleaved practice. Perhaps unexpectedly, B- and C-grade students improved the most. This might relate to their poorer conceptual understanding of topics or assessment literacy beforehand, which interleaved practice helps to develop.
Fraser Scott
O. Gulacar et al, Chem. Educ. Res. Pract., 2022, DOI: 10.1039/D1RP00334H
O. Gulacar et al, Chem. Educ. Res. Pract., 2022, 23 , 422–435 ( DOI: 10.1039/D1RP00334H )
- Action and context-based research
- Developing teaching practice
- Education research
- Problem solving
Related articles
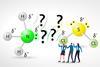
Understanding how students untangle intermolecular forces
2024-03-14T05:10:00Z By Fraser Scott
Discover how learners use electronegativity to predict the location of dipole−dipole interactions
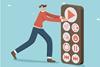
Why I use video to teach chemistry concepts
2024-02-27T08:17:00Z By Helen Rogerson
Helen Rogerson shares why and when videos are useful in the chemistry classroom
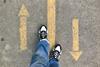
3 ways to boost knowledge transfer and retention
2024-02-20T05:00:00Z By David Read
Apply these evidence-informed cognitive processes to ensure your learners get ahead
No comments yet
Only registered users can comment on this article., more from education research.
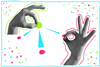
How visuospatial thinking boosts chemistry understanding
2024-04-18T06:07:00Z By David Read
Encourage your students to use their hands to help them get to grips with complex chemistry concepts
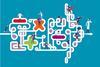
Boost maths skills to improve chemistry learning
2024-01-18T08:00:00Z By Fraser Scott
Use these evidence-based tips to help your learners get ahead with chemical calculations
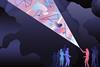
Support student sensemaking through directed dialogue
2023-12-19T09:27:00Z By David Read
Discover how to encourage effective classroom conversation to boost student understanding
- Contributors
- Print issue
- Email alerts
Site powered by Webvision Cloud
Problem-Solving Style Questionnaire
Table of Contents
Here in this post, we are sharing the “Problem-Solving Style Questionnaire”. You can read psychometric and Author information. We have thousands of Scales and questionnaires in our collection ( See Scales and Questionnaires ). You can demand us any scale and questionnaires related to psychology through our community , and we will provide you with a short time. Keep visiting Psychology Roots .
About Problem-Solving Style Questionnaire
Author details.
Thomas Cassidy and Christopher Long Parker
(I still confuse between above two. Some Resources mention 1st one and some on second one. Hope as researcher you can help to verify this)
Translation Availability
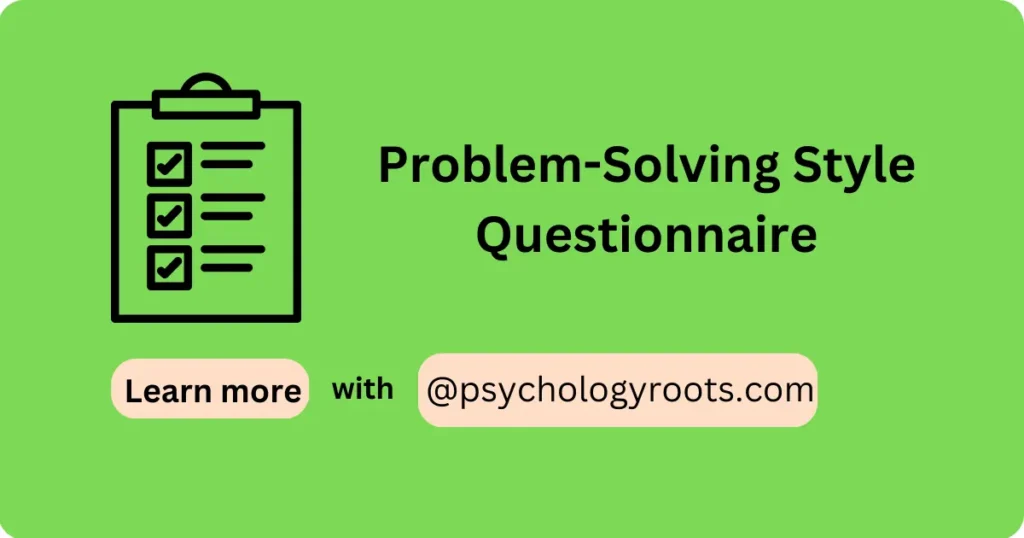
Background/Description
The Problem-Solving Style Questionnaire (PSSQ) is a self -report questionnaire that measures four dimensions of problem-solving style: sensing, intuitive, feeling, and thinking. It was developed by Thomas Cassidy and Christopher Long in 1996, based on Carl Jung ’s theory of psychological types.
The PSSQ is a widely used instrument for assessing problem-solving style in a variety of settings, including educational institutions, workplaces, and counseling offices. It has been shown to be a reliable and valid measure of problem-solving style, and it has been used in numerous research studies to investigate the relationship between problem-solving style and other variables, such as academic achievement , job performance, and mental health.
The PSSQ is a relatively short instrument, consisting of 20 items with five items for each dimension. Respondents rate each item on a five-point Likert scale, from 1 (strongly disagree) to 5 (strongly agree). The scores for each dimension are then summed to create a total score for that dimension.
The following is a brief interpretation of the four PSSQ dimensions:
- Sensing: People with a high sensing problem-solving style prefer to learn through concrete experiences and focus on practical details. They may also be good at hands-on tasks and troubleshooting problems.
- Intuitive: People with a high intuitive problem-solving style prefer to learn through abstract concepts and theories and focus on the big picture. They may also be good at thinking creatively and coming up with new ideas.
- Feeling: People with a high feeling problem-solving style prefer to make decisions based on personal values and emotions. They may also be good at empathizing with others and considering their needs .
- Thinking: People with a high thinking problem-solving style prefer to make decisions based on logic and objective reasoning. They may also be good at analyzing information and identifying patterns and trends.
It is important to note that everyone has a unique combination of problem-solving styles. There is no one “best” problem-solving style. The best way to solve a problem is to use the style that is most effective for the specific situation.
The PSSQ can be used in a variety of ways. It can be used to help individuals understand their own problem-solving style and to develop strategies for improving their problem-solving skills. It can also be used to identify individuals who may need additional support in problem-solving.
For example, a student who has a high intuitive problem-solving style may benefit from learning how to break down complex problems into smaller steps. A student who has a high sensing problem-solving style may benefit from learning how to see the big picture and generate ideas.
The PSSQ can also be used in the workplace to help employees understand their own problem-solving style and to develop teams with a variety of problem-solving styles. This can lead to more effective problem-solving and better decision-making.
Administration, Scoring and Interpretation
The Problem-Solving Style Questionnaire (PSSQ) can be administered in a variety of settings, including educational institutions, workplaces, and counseling offices. It is a relatively short instrument, so it can be administered individually or in groups.
To administer the PSSQ, simply provide respondents with a copy of the questionnaire and instruct them to read each item carefully and rate it on a five-point Likert scale, from 1 (strongly disagree) to 5 (strongly agree). Once respondents have completed the questionnaire, collect the questionnaires and score them.
To score the PSSQ, simply sum the responses to the five items for each dimension. The total score for each dimension ranges from 5 to 25. Higher scores indicate a stronger preference for that problem-solving style.
Here are some additional tips for administering the PSSQ:
- Provide respondents with a quiet and comfortable place to complete the questionnaire.
- Allow respondents enough time to complete the questionnaire without feeling rushed.
- Be sure to answer any questions that respondents may have about the questionnaire.
- Once respondents have completed the questionnaire, thank them for their time.
Reliability and Validity
The Problem-Solving Style Questionnaire (PSSQ) has been shown to be a reliable and valid measure of problem-solving style.
Reliability refers to the consistency of a measure. A reliable measure is one that produces similar results when administered to the same people at different times.
Validity refers to the accuracy of a measure. A valid measure is one that measures what it is intended to measure.
The PSSQ has been shown to be reliable in a number of studies. For example, Cassidy and Long (1996) reported that the PSSQ had a Cronbach’s alpha of .77, which is considered to be a good level of reliability .
The PSSQ has also been shown to be valid in a number of studies. For example, Cassidy and Long (1996) found that the PSSQ scores were correlated with other measures of problem-solving style, such as the Myers-Briggs Type Indicator (MBTI).
In addition, the PSSQ has been used in a number of research studies to investigate the relationship between problem-solving style and other variables, such as academic achievement , job performance, and mental health. The results of these studies suggest that the PSSQ is a valid measure of problem-solving style.
Available Versions
Ghodrati, M., Bavandian, L., Moghaddam, M. M., & Attaran, A. (2014). On the relationship between problem-solving trait and the performance on C-test. Theory and practice in language studies , 4 (5), 1093-1100.
Khan, M. J., Younas, T., & Ashraf, S. (2016). Problem Solving Styles as Predictor of Life Satisfaction Among University Students. Pakistan Journal of Psychological Research , 31 (1).
Important Link
- Video Guide
- How to Get Permission
- Sample Email for Permission
- Community Discussions
Scale File:
Frequently asked questions.
What is the Problem-Solving Style Questionnaire (PSSQ)? The PSSQ is a self -report questionnaire that measures four dimensions of problem-solving style: sensing, intuitive, feeling, and thinking.
What are the four dimensions of problem-solving style measured by the PSSQ? The four dimensions of problem-solving style measured by the PSSQ are: Sensing, Intuitive, Feeling, and Thinking.
How is the Problem-Solving Style Questionnaire scored? The PSSQ is scored by summing the responses to the five items for each dimension. The total score for each dimension ranges from 5 to 25. Higher scores indicate a stronger preference for that problem-solving style.
What are the benefits of using the Problem-Solving Style Questionnaire? The PSSQ can be used to help individuals and teams understand their problem-solving styles and develop strategies for improving their problem-solving skills. It can also be used to identify individuals who may need additional support in problem-solving.
Is the PSSQ a reliable and valid measure of problem-solving style? Yes, the PSSQ has been shown to be a reliable and valid measure of problem-solving style. It has been used in a number of research studies to investigate the relationship between problem-solving style and other variables, such as academic achievement , job performance, and mental health.
Please note that Psychology Roots does not have the right to grant permission for the use of any psychological scales or assessments listed on its website. To use any scale or assessment, you must obtain permission directly from the author or translator of the tool. Psychology Roots provides information about various tools and their administration procedures, but it is your responsibility to obtain proper permissions before using any scale or assessment. If you need further information about an author’s contact details, please submit a query to the Psychology Roots team.
Help Us Improve This Article
Have you discovered an inaccuracy? We put out great effort to give accurate and scientifically trustworthy information to our readers. Please notify us if you discover any typographical or grammatical errors. Make a comment. We acknowledge and appreciate your efforts.
Share With Us
If you have any scale or any material related to psychology kindly share it with us at [email protected] . We help others on behalf of you.
Psychology Roots
Problem solving styles scale, psychologist jobs in punjab special education department, related posts, ashworth scale, attachment style classification questionnaire for latency..., gender stereotype scale, arthritis impact measurement scale, argentinean scale on perceptions of relationships..., aggression questionnaire, leave a comment cancel reply.
You must be logged in to post a comment.
Adblock Detected
- Open access
- Published: 03 January 2020
Students’ problem-solving strategies in qualitative physics questions in a simulation-based formative assessment
- Mihwa Park ORCID: orcid.org/0000-0002-9549-9515 1
Disciplinary and Interdisciplinary Science Education Research volume 2 , Article number: 1 ( 2020 ) Cite this article
9497 Accesses
1 Citations
3 Altmetric
Metrics details
Previous studies on quantitative physics problem solving have been concerned with students’ using equations simply as a numerical computational tool. The current study started from a research question: “How do students solve conceptual physics questions in simulation-based formative assessments?” In the study, three first-year college students’ interview data were analyzed to characterize their problem-solving strategies in qualitative physics questions. Prior to the interview, the participating students completed four formative assessment tasks in physics integrating computer simulations and questions. The formative assessment questions were either constructed-response or two-tiered questions related to the simulations. When interviewing students, they were given two or three questions from each task and asked to think aloud about the questions. The findings showed that students still used equations to answer the qualitative questions, but the ways of using equations differed between students. The study found that when students were able to connect variables to a physical process and to interpret relationships among variables in an equation, equations were used as explanatory or conceptual understanding tools, not just as computational tools.
Introduction
Since the new U.S. science standards, Next Generation Science Standards (NGSS), were released (NGSS Lead States, 2013 ), science assessments have been moving towards revealing students’ reasoning and their ability to apply core scientific ideas in solving problems (National Research Council, 2014 ; Pellegrino, 2013 ). Underwood, Posey, Herrington, Carmel, and Cooper ( 2018 ) suggested types of questions aligned with three-dimensional learning in A Framework for K-12 Science Education (National Research Council, 2012 ). These questions include constructed-response (CR) questions and two-tiered questions. Underwood et al. ( 2018 ) also argued that questions should address core and cross-cutting ideas and ask students to consider how scientific phenomena occur so that they can construct explanations and engage in argumentation. The underlying assumption of this approach could be that qualitative explanation questions (i.e., questions that ask students to explain qualitatively) reveal students’ reasoning and understanding of core scientific concepts better than do traditional multiple-choice and simple-calculation questions. Numerous studies in physics education have examined students’ problem-solving strategies, including studies that have identified differences in the problem-solving strategies employed by experts and novices. Experts tend to start by using general scientific principles to analyze problems conceptually, while novices tend to start by selecting equations and plugging in numbers (Larkin, McDermott, Simon, & Simon, 1980 ; Maloney, 1994 ; Simon & Simon, 1978 ). Thus, giving students opportunities to reason qualitatively about problems could help them to think like experts (van Heuvelen, 1991 ).
Another way to enhance students’ conceptual understanding of scientific ideas could be using computer simulations, because computer simulations help students visualize scientific phenomena that cannot be easily and accurately observed in real life. Many empirical studies support integrating computer simulations into assessments in order to promote students’ engagement in exploring scientific phenomena (de Jong & van Joolingen, 1998 ) and their conceptual understanding (Rutten, van Joolingen, & van der Veen, 2012 ; Trundle & Bell, 2010 ). For example, Quellmalz, Timms, Silberglitt, and Buckley ( 2012 ) developed a simulation-based science assessment, and found that the assessment was effective to reveal students’ knowledge and to find evidence of students’ reasoning. In the current study, computer simulations and conceptual qualitative questions were incorporated as integral parts of formative assessment to reveal students’ problem-solving strategies in answering qualitative physics questions. Therefore, the current study investigated students’ problem-solving strategies in physics, which offered them opportunities to elicit their reasoning by qualitatively explaining what would happen and why it would happen about a given physical situation.
Students’ strategies to solving physics problems
Early research on physics problem solving identified differences between experts and novices in their problem-solving strategies. For example, experts’ knowledge is organized into structures; thus, they demonstrate the effective use of sophisticated strategies to solve problems (Gick, 1986 ). Conversely, novices tend to describe physics problems at best in terms of equations, and spontaneously use superficial analogies (Gick, 1986 ). Experts also effectively use the problem decomposition strategy: breaking down a problem into subproblems, then solving each subproblem and combining them to form the final solution (Dhillon, 1998 ). They also apply relevant principle and laws to solve problems (Chi, Feltovich, & Glaser, 1981 ; Dhillon, 1998 ). By contrast, novices start with selecting equations and cue into surface features (Chi et al., 1981 ). A common finding from studies on differences between experts and novices in problem solving (e.g., Chi et al., 1981 ; Dhillon, 1998 ; Gick, 1986 ; Larkin et al., 1980 ) is that experts demonstrate their expertise in conceptual analysis of the problems using scientific principles and laws, then translate the problem into relevant mathematical equations, while novices jump to mathematical manipulations without the prior process of conceptual analysis (Larkin et al., 1980 ).
Huffman ( 1997 ) incorporated the results of studies on the differences in problem solving between experts and novices to formulate explicit problem-solving procedures for students. The procedures include five steps: (a) performing a qualitative analysis of the problem situation; (b) translating the conceptual analysis into a simplified physics description; (c) translating the physics description into specific mathematical equations to plan the solution; (d) combining the equations according to the plan; and (e) evaluating the solution to ensure it is reasonable and complete (Huffman, 1997 ). In essence, the procedure is designed to ensure students will conceptually reason about the problem first, using relevant scientific principles and laws, before jumping to selecting mathematical equations.
It is possible that students’ problem-solving strategies are influenced by problem representations (verbal, mathematical, graphical, etc.). Kohl and Finkelstein ( 2006 ) investigated how problem representations and student performance were related, and found that student strategies to solve physics problems often varied with different representations. They also found that not only problem representations but a number of other things, including prior knowledge and experience in solving problems from their previous classes, also influenced students’ performance, especially in the case of low-performing students. When asking students not to calculate a science question but to explain it conceptually, a study found that they still used equations or numerical values to solve the problems, indicating that they translated a conceptual qualitative question into a quantitative one (De Cock, 2012 ). Although students may succeed in calculating values in physics problems, it doesn’t always mean that they have good conceptual understanding of the questions (McDermott, 1991 ).
While earlier studies have been concerned with students’ using equations without conceptual understanding when solving problems, mathematical modeling plays a critical role in the epistemology in physics (Redish, 2017 ). Redish emphasized the importance of connecting physical meaning to mathematical representation when solving problems, because in physics, mathematical equations are linked to physical systems, and an equation contains packed conceptual knowledge. Thus, in physics, equations are not only computational tools but also symbolic representations of logical reasoning (Redish, 2005 , 2017 ). As such, students are expected to incorporate mathematical equations into their intuition of the physical world to conceptualize the physical system (Redish & Smith, 2008 ). In a study of students’ quantitative problem solving, Kuo, Hull, Gupta, and Elby ( 2012 ) pointed out the importance of connecting mathematical symbols to conceptual reasoning. Their study was conducted based on an assumption that equations should be blended with conceptual meaning in physics, which turned the attention of researchers on problem solving from how students select equations to how they use the equations. Kuo et al. ( 2012 ) concluded that blending of mathematical operations with conceptual reasoning constitutes good problem solving; thus, this blended process should be a part of problem-solving expertise in physics.
Using computer simulations as an assessment tool
Given that visualization plays a central role in the conceptualization process of physics (Kozhevnikov, Motes, & Hegarty, 2007 ), previous studies have used computer simulations to visualize scientific phenomena, especially those that cannot be accurately observed in real life, and reported their positive effect on students’ learning outcomes (Ardac & Akaygun, 2004 ; Dori & Hameiri, 2003 ). Using computer simulation to facilitate student learning in science was found to be especially effective on student performance, motivation (Rutten et al., 2012 ), and conceptual change (Smetana & Bell, 2012 ).
Computer simulation can be used not only as an instructional tool but also as an assessment tool. For example, Park, Liu, and Waight ( 2017 ) developed computer simulations for U.S. high school chemistry classes to help students conceptualize scientific phenomena, and then integrated the simulations into formative assessments with questions related to the simulations. Quellmalz et al. ( 2012 ) and Srisawasdi and Panjaburee ( 2015 ) also embedded computer simulations into formative assessments for use in science classrooms, and demonstrated positive effects on students’ performance compared to students who experienced only traditional assessments (e.g., paper-and-pencil tests). While many empirical studies have been done to investigate problem-solving strategies of students, there is a lack in studies on students’ strategies to solve physics problems when computer simulations were used as a visual representation and conceptual explanation questions were asked to reveal the students’ reasoning. This study addresses the gap in the body of literature by investigating students’ strategies in solving conceptual explanation questions in a simulation-based formative assessment.
Research procedure and participants
In the study, computer simulations and formative assessment questions were integrated into a web-based formative assessment system for online administration, which allowed students to use it at their convenience (Park, 2019 ). The formative assessment questions were either CR or two-tiered questions related to the simulations. A two-tiered question consists of a simple multiple-choice (MC) question and a justification question for which students write a justification for their answer to the MC question. This format of question was suggested to diagnose possible misconceptions held by students (Treagust, 1985 ) and to provide information about students’ reasoning behind their selected responses (Gurel, Eryılmaz, & McDermott, 2015 ). Computer simulations were selected from the Physics Education Technology (PhET) project ( https://phet.colorado.edu/ ) and embedded into the formative assessment system. The assessments targeted students’ conceptual understanding in physics, thus they were not asked to calculate any values or to demonstrate their mathematical competence (Park, 2019 ). Specifically, the questions presented a scientific situation and asked students to predict what would happen; then the assessment system asked students to run a simulation, posing questions asking for explanation of the phenomena and comparison between their prior ideas and the observed phenomena. Figure 1 presents example questions and simulation for the energy conservation task. After answering the questions, students ran the simulation and responded to questions asking how the skater’s highest speed changed and why they think it happened using evidence found in the simulation.
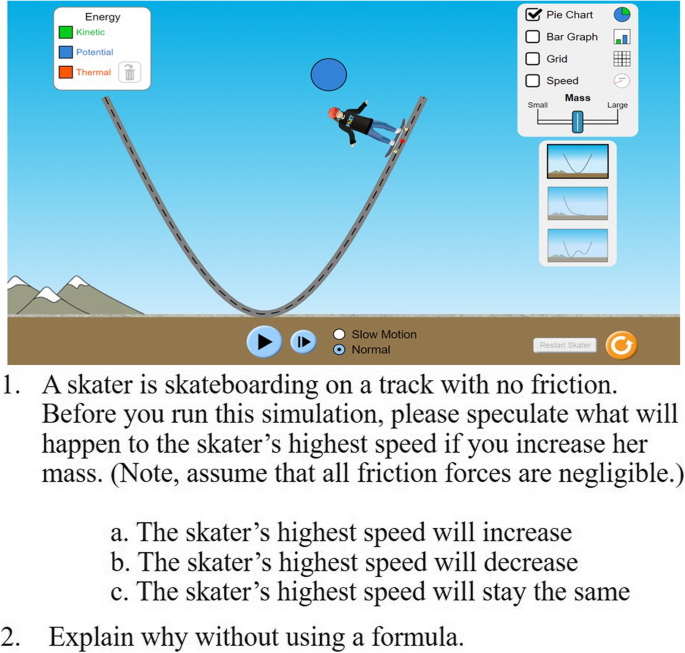
Energy conservation task example questions
Initially, first-year college students were recruited from a calculus-based, introductory level physics course at a large, public university in the United States; no particular demographic was targeted during recruitment. The physics course was offered to students majoring in subjects related to science or engineering and covered mechanics, including kinematics and conservation of energy, so simulations were selected to align with the course content. After selecting simulations from the PhET project, related formative assessment questions were created. As previously mentioned, the questions first asked students to predict what would happen in a given situation. In this case, verbal (expressed in writing) and pictorial representations (including images, diagrams, or graphs) describing the situation were shown on the screen (Fig. 1 ). Next, after the students answered the questions, the simulations were enabled for the students to run, and they were asked to explain the results. In total, four formative assessment tasks were developed and implemented online, and each task contained from 14 to 17 questions. Topics for the four tasks were (1) motion in two dimensions, (2) the laws of motion, (3) motion in one dimension and friction, and (4) conservation of energy. Descriptions of the four tasks are presented below.
Task 1: Students explore what factors will affect an object’s projectile motion when firing a cannon.
Task 2: Students create an applied force such as pulling against or pushing an object and observe how it makes the object move.
Task 3: Students explore the forces at work when a person tries to push a filing cabinet on a frictionless or frictional surface.
Task 4: Students explore a skater’s motion on different shapes of tracks and explore the relationship between the kinetic energy and thermal energy of the skater.
After the participating students completed the online implementations of the four tasks, an interview invitation email was sent to the students who had completed all four tasks, did not skip any questions, and did not answer a question with an off-task response, but included responses that needed further clarification. Initially, we invited six students to clarify and elaborate on their responses so we could better understand what they were thinking. When scoring students’ written responses, some responses needed further clarification. For example, students mentioned that in projectile motion, “mass is not relative to time”; “the greater angle will create a larger x component of velocity in a projectile motion”; or “an object’s speed is broken up evenly resulting in more air time”. In case of the energy conservation task, the responses needing more clarification were “the speed did not change because speed does not depend on mass” or “because a skater’s total energy increases with increase in mass, her speed does not change”. Those responses were not clear to the author. Thus, the author decided to invite them to clarify their responses. During the interviews, the students’ verbal responses inspired the author to explore differences in their problem-solving strategies to answer conceptual physics questions. Three students especially, Alex, Christopher, and Blake (all pseudonyms), demonstrated noticeable differences in their problem-solving strategies; therefore, they are the focus of the analysis in the current study.
Interview context and protocols
Semi-structured interviews were conducted to investigate students’ reasoning when responding to conceptual physics questions. To this end, the students were given two or three questions from each task and asked to think aloud about the questions. After they verbally answered each question, they were given their original written responses to see if their answers had changed, and if so, to explain why. When students used mathematical equations or graphs in their explanations, they were asked to explain why they used those particular strategies and how the strategies helped them to answer the questions. Some example interview questions were; “Please read the question. Will you tell me your answer for the question?”, “How did you answer this question?”, “Could you clarify what this means?”, and “What did you mean by (specific terms that students used)?” Students were interviewed individually by two interviewers. The interviews, which took place in an interview room located at their university, each lasted an hour.
While the interviews were going on, the author wrote memos about the students’ strategies to answer the given questions and their misconceptions about science. Interviews were audio recorded and transcribed verbatim. The transcripts were initially analyzed to prepare and organize data into emergent themes. In this process, the memos were also used. As a result, three initial themes were developed: 1) students’ strategies to answer problems, 2) effects of the assessment on students’ learning, and 3) students’ misconceptions about science. In the study, the first theme—strategies to answer problems—was made a focus in the next level of analysis, as the students demonstrated noticeable differences in using equations to answer conceptual physics problems. After choosing the theme as a main focus, the author analyzed it by open coding the relevant parts of the transcripts of the individual student interviews (interviews about Tasks 1–3) to formulate possible characterizations of students’ problem-solving strategies, especially when they were using equations. The author constantly compared the characterizations to integrate and refine them (Strauss & Corbin, 1998 ). After that, the rest of each individual student interview transcript (interviews about Task 4) was analyzed, using the same categories to confirm the findings. Students’ drawings (i.e., graphs) used to explain their reasoning were also considered as a data source (Creswell, 2016 ). Once characterizations in students’ use of equations in qualitative physics question were identified and compared across cases, the analysis results were given to a physics education researcher to seek an external check (Creswell & Miller, 2000 ).
Previous studies on expert and novice problem-solving strategies were reflected in the design of the formative assessment questions. Specifically, it was hypothesized that conceptual explanation questions would help students think about the questions more conceptually, so that they would start to solve them using scientific concepts and laws. Therefore, short written questions in the tasks asked the students to explain or to justify their answers without using a formula. Nonetheless, when we were interviewing students, we found that they preferred to use equations and mathematical concepts when explaining physical situations. Although the three participating students commonly used equations or mathematical concepts in their explanations, how they used the equations or mathematical concepts differed. Detailed findings are presented below in three subsections representing patterns in problem-solving strategies. Formative assessment Tasks 1, 2, and 3 were designed to address the topic of Motion and Force, while Task 4 covered the topic of Energy Conservation. We analyzed interview data by these two topics. Note that two terms—formula and equation—were not differentiated in the analysis of data; instead, they were considered synonyms.
Alex’s case – using equations as a conceptual understanding tool
Motion and force.
When interviewing Alex, we asked him what would happen if a person pushed a box, then let it go (Task 2). He said, “If it is frictionless, the box will move forever with a constant velocity, and if friction exists, the speed will decrease and eventually the box will stop.” This answer was very similar to his original written response. Next, we asked what would happen to the box’s motion after another box was placed on top of it. Alex said, “I don’t know how to explain this without a formula.” Because the original questions had asked students not to use formulas, he assumed that he was not allowed to use one in this explanation, and obviously he was struggling to explain without it. We told him to use formulas whenever he wanted, and he quickly jumped into using one.
Alex: Resultant force equals mass times acceleration, so if you have a bigger mass. Uh, if the resultant force was 50N, that’s the force you applied, and then you had 10N in friction, for example, then the resultant force is 40. You had, if you had 20kg, the acceleration would be 2. If you had 50kg, the acceleration would be 4 over 5, which is 0.8, which is less than 2. So, the more mass you have the smaller the acceleration is going to be, as a result of the resultant force equals ma equation.
In this statement, Alex explained what would happen in the given situation with algebraic solutions, using F = ma equation, and concluded that mass would affect the object’s acceleration, as he demonstrated. He further described how the eq. ( F = ma ) helped him to explain the given physical situation.
Alex: If you use the formula, then it makes it much easier, because in real life, you never see something moving without friction, so it just clouds your judgment a bit.
In this statement, Alex described the role of equation for him as a conceptual understanding tool, especially in an ideal situation that is not observable in real life. This was something the author had not initially expected from the students during their interviews. When we asked Alex the next question in Task 3, his answer further supported the finding that equations helped him understand physical situations. Specifically, we asked, in a situation when a person was pushing a cabinet on either a frictionless or a frictional surface, what would happen to the cabinet’s motion and why.
Alex: The normal force is, the gravitational force cancels out the y , so the only thing acting on the—in the x -direction, which is the direction being pushed is the applied force, so as small of a force you apply to it, it’s still going to move it because there’s nothing opposing it…if there was friction, I agree that it won’t move. Because the friction, the friction is the coefficient of friction times the normal force, so, since it’s a really big object, it’s going to have a significant amount of friction acting on it.
In his verbal explanation, Alex used a mathematical concept and an equation to explain the given phenomenon, using the vector concept for two components of force and a mathematical equation for frictional force. Obviously, he found equations useful to make sense of physical situations and to explain his understanding to others. Notably, he started his answer by referring to the formula for kinetic friction force and used the formula as a tool to explain why the cabinet wouldn’t move on a frictional surface. His explanation again demonstrated that equations and mathematical concepts were useful to understanding and interpreting scientific phenomena, and not only as a simple computational tool, at least for Alex.
Conservation of energy
Task 4 was designed to investigate students’ conceptions of mechanical energy and its conservation. We asked Alex, when a skater is skateboarding on a track with no friction, what would happen to the skater’s highest speed as the skater’s mass increases? He again asked us if he could use equations. We confirmed that he was allowed to use equations anytime he wanted. Then he immediately started writing equations on the board (see Fig. 2 ). While he was writing, he explained each variable involved in the equations:
Alex: So, her initial, so, um, at the start, her initial energy is mgh + ½ mv 0 2 and then her final [writing on board] mgh + ½ mv f 2 , but the smaller thing to do is that they [mass] all cancel out, so the mass is really, it doesn’t play a role in the height or the velocity. And then, if you wanted to see how the conversion of energy works, if you were initially starting at the maximum height, whatever that is, you could do ½ mv 2 . At the start, her velocity is 0, at the top, so this cancels out, if we’re analyzing it at the bottom, which is her max speed, then this [ h ] is 0, and then you just do gh = v 2 . To find her velocity. Just looking at this, there’s no mass in this, so it doesn’t matter [the skater’s speed]. When you actually work it out, all the masses cancel out, so it doesn’t matter what the mass is, in reality, when you actually calculate it.
This response was different than his original written response to the same question: “If the skater has a larger mass, she will in turn have a larger gravitational potential energy since GPE [gravitational potential energy] has a direct relationship to mass. As a result and according to the principles of conservation of energy, the KE [kinetic energy] will be greater and thus the velocity will be greater.” In this original written response, Alex included a typical misconception that heavier objects fall faster (e.g., Gunstone, Champagne, & Klopfer, 1981 ; Lazonder & Ehrenhard, 2014 ); “If the skater has a larger mass…thus the velocity will be greater” (in his written response). This was the only case of a misconception found in Alex’s written responses. Notably, when he was using equations, he deduced that “it doesn’t matter what the mass is, in reality, when you actually calculate it” from his step-by-step problem-solving procedure using algebraic solutions. Although he solved the problem using equations through algebraic computation, he explained how the object’s velocity and height would change as the object moved: “At the start, her velocity is 0, at the top, so this cancels out, if we’re analyzing it at the bottom, which is her max speed, then this [ h ] is 0, and then you just do gh = v 2 .” Then he connected conceptual meaning to the equation: “Just looking at this, there’s no mass in this, so it doesn’t matter [the skater’s speed].” This confirmed that for Alex, equations were the first tool to make sense of physical situation. In other words, when he applied an equation to a physical situation, he considered variables related to specific situations, then connected conceptual meaning to the variables, which indicated that for him, equations played a role in analyzing and understanding physical situation.
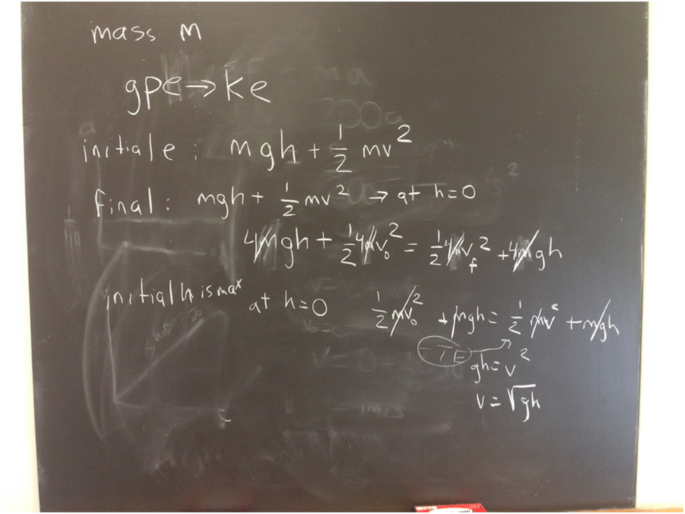
Alex’s explanation
Christopher’s case – using equations as an explanatory tool
We asked Christopher a question—which tank shell would go farther when the initial angles for two tank shells were different (Task 1). In his original written response, he mentioned that “tank A (initial angle: 45 degree)’s speed is broken up more evenly and this results in more air time which leads to more distance covered in the x axis as well.” This answer was similar to Christopher’s thinking-aloud response, so we asked him to elaborate on what he meant by “speed is broken up more evenly.” Below is his response.
Christopher: Because the velocity is a vector quantity, the speed is still the same, but the velocity, the x and y axis are going to be more evenly split [for Tank A, with a 45-degree initial angle], whereas for Tank B [10-degree initial angle] it would have been almost all in the x axis and close to none in the y , so it wouldn’t get that much air time because the force of gravity still stays the same.
As seen in his response, Christopher deduced his answer from a mathematical concept (vector in this case) explaining why the 45-degree shell would have a greater horizontal range than the 10-degree shell one. His problem-solving strategy in the next questions (questions from Tasks 2 and 3) further confirmed that he used mathematical concepts and equations to explain physical situations. For example, when asked to compare two situations from Task 2—a person pushes a box and lets it go, and after placing another box on top of that, a person pushes both boxes and lets them go—Christopher immediately used F = ma and explained the situation.
Christopher: The velocity and the speed will be decreased because, when applying force, force is mass times acceleration. So, if it would be the same exact force with a higher mass, then the acceleration would have to go down significantly in order to keep the same number [force]. So, because of this, it wouldn’t speed up as much, so it would have a lower velocity after the force was applied [compared to the previous situation]. While you are pushing, the acceleration is constant. And if they let it go, there is no acceleration. Then speed will stay the same.
In his statement, he referred to F = ma , and explained why the box’s acceleration would be smaller when its mass increased using algebraic solutions, which is similar to Alex’s case. The difference is that Christopher’s explanation contained an interpretation of the relationship among velocity, acceleration, and applied force: “So, because of this, it wouldn’t speed up as much, so it would have a lower velocity after the force was applied.” This implies that Christopher did not just use the equation as a computational tool, but linked meanings to variables (force, velocity, mass, and acceleration) and interpreted a relationship among them. When we asked him a question from Task 3—when a person is pushing a cabinet, how will the cabinet’s velocity change after passing over the frictionless surface and traveling onto the surface with friction?—his answer reconfirmed that he considered the relationship among variables and gave conceptual meaning not only to the variables but also to the relationship, and used a mathematical concept as an important tool to interpret a physical situation.
Christopher: So, the velocity is 100% dependent on the acceleration, which depends on the force, and then in this scenario, it is the force at first, it has a much higher total net force in the x direction, whereas later on it decreases [on a frictional surface], but there’s still a positive net force in the x direction, so it will continue. The reason why it continues to speed up is because the acceleration is still positive. ‘Cause mass can’t really be negative so that [acceleration] is the only variable [to determine the change of velocity]. So, that’s why velocity continues to increase, it’s just not as much as before.
In his statement, Christopher did not interpret an individual variable separately; rather, he first considered the relationship between force, velocity, and acceleration using the concept of vector and scalar quantity (e.g., mass is not a vector quantity), and explained how each variable was influenced by the other variables’ changes. From the statements above, it is clear that Christopher reasoned through a physical process by interpreting relationships among variables and attaching conceptual meaning to the relationship and the variables.
When we asked Christopher about change in the skater’s highest speed when the skater’s mass increased, his original written and oral responses contained the common answer that the skater’s highest speed would stay the same because gravity acts on all objects equally: “the downward acceleration will be the same.” We further asked him about how total mechanical energy changes. His response is below.
Christopher: Her [the skater’s] mechanical energy would increase because the velocity would stay the same for kinetic, but the mass would go up, so it would make the answer higher. And it’s probably easier to think of it with GPE, can I use the formula to it?
Then he drew a formula on board (Fig. 3 ), and explained why the total mechanical energy would change.
Christopher: This is mg. Since these two [ gh, ½ ] stay the same for both cases, they can be canceled out. So then, these are the only variables in ME (mechanical energy), so if this [ m ] increases, then the whole system[’s energy] will increase, but it won’t change this [ v ] in the specific scenario. If you were to use the equations, once you were to set them equal to each other and solve for the final answer for each, they would still be the same, even though the mass is higher. But because it’s multiplied, you can cancel it [ m ] on both sides for that specific scenario, so it mainly just depends on the constant ½ and then the variable of height and the final velocity which would be the same for this case.
In his response, Christopher first explained the physical situation using the concept of energy and considered the situation as a system: “Her [the skater’s] mechanical energy would increase,” and “so if this [ m ] increases, then the whole system[‘s energy] will increase.” In order to prove why mass doesn’t affect the skater’s speed, he used an equation as an explanatory tool—“And it’s probably easier to think of it with GPE, can I use the formula to it?”—and showed that mass doesn’t affect the skater’s speed: “You can cancel it [ m ] on both sides for that specific scenario.” A noticeable difference from Alex’s approach is that Christopher used equations to prove his claim and to explain it in an easier way, while Alex used equations to make sense of the situation. In other words, equations were in play mainly as explanatory tools for Christopher, whereas they acted as conceptual understanding tools for Alex. Similarly to his previous responses to questions in Motion and Force, Christopher again demonstrated that he considered how all variables were related each other in the system, and attached meaning to the relationship and variables. Interestingly, he often used the phrase “specific scenario,” so we asked what it meant. Below is his response.
Christopher: The equations don’t really help because even though I see it and it’s in my head, but it’s not really useful if I don’t know the scenario. If it’s some problems, I know, are purposefully shaped to muddle it up, and make it purposefully confusing, but usually, when you run the scenario, in a program or in your head, it kind of takes out that confusing stuff.
The above response illustrated that Christopher conceptually interpreted the physical situation first, then translated equations into the physical situation. This strategy shared a commonality with Alex’s in that both students used equations in their explanations and connected how variables in the equations changed as the specific physical situation changed. At the same time, there was a difference between the two students. Christopher’s strategy started with an analysis of the situation, creating a physical scenario and then translating equations into the physical situation, while Alex mentioned relevant equations first, then connected them to the physical situation.
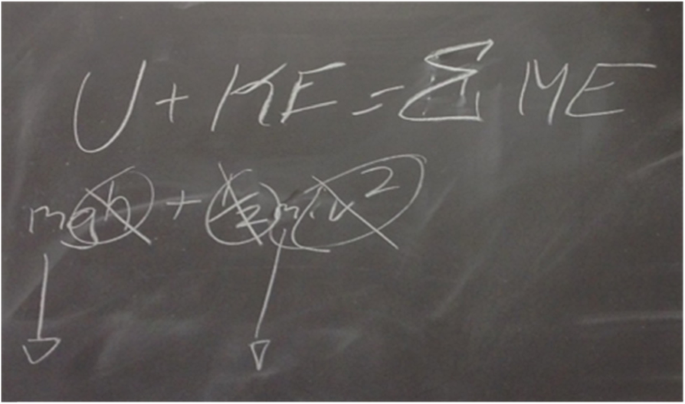
Christopher’s explanation. Note: ME = mechanical energy
Blake’s case – using equations as a computational tool
When we asked Blake which one would go farther when shot from a cannon, a tank shell or a baseball (when air resistance was negligible; Task 1), her original written response and her thinking-aloud response were similar: the mass of an object is not relative to its motion. When we asked her to explain why, she said:
Blake: Because I don’t see kg on the units at all [in the simulation]. kg is the unit for mass, kilograms, so, it’s not written as kg/m/s or something. You could easily compare it with units and mass is not part of the unit.
Her response was interesting in that she used the unit of velocity rather than acceleration. Also, she did not show her conceptual understanding of physical variables and their relationship as Christopher had done. We further asked her what factors should be changed to maximize the horizontal range of the projectile object, in order to elicit her reasoning about a projectile motion. Below is her response.
Blake: You need to throw it faster. Um, because, if you look at gun for example. It’s a really high velocity. So, you just see it going like straight because it’s just high velocity. And, um, if, if I’m throwing this phone, maximum distance it could go is like here [tosses phone, not very far]. Angle? I think…like the maximum distance for x axis and y axis is 45 degrees, but I think it should be a little lower. Around 45 but plus or minus 5 degrees, so like 40 degrees.
Interviewer: Why would you say that?
Blake: It doesn’t get that much time for vertical velocity, but the horizontal velocity will be faster.
In her response, Blake used real-life examples—shooting a gun and throwing a phone—as analogies to reason how to increase the horizontal range of a projectile object. However, when she threw the phone, she tossed it, which started it with a different initial angle from that of a bullet shot from a gun : “You just see it going like straight because it’s just high velocity.” Although she considered two directions of velocity when determining the optimal initial angle, she did not provide a scientifically reasonable explanation for why the initial angle should be lower than 45 degrees. It might be that Blake had learned that 45 degrees is the angle used to maximize range, but that she thought velocity would be more critical than the angle to determine the range, especially that the x -component of velocity would more important than the y -component because an object will fly faster horizontally than vertically when the x- component is greater. Thus, she lowered the initial angle a little bit. In the above statements, Blake did not demonstrate that she could consider the relationship between variables and link conceptual meanings to them (e.g., “Because I don’t see kg on the units at all” and “It doesn’t get that much time for vertical velocity, but the horizontal velocity will be faster”).
For the next question, we asked what would happen to the box’s motion after another box was placed on top of it. She said, “It would still be constant and stay at constant velocity in that motion.” We asked the question again, to clarify if she understood it.
Blake: Yeah. The velocity would be the same. After you let it go. So it will be at constant speed. And the force is proportional to the...wait, well acceleration is proportional to force and mass.
In her response, Blake attempted to apply Newton’s second law ( F = ma ), as the other two students had; however, she didn’t realize that acceleration is inversely proportional to mass, and therefore the velocity would be changed by the different acceleration. As a result, her response involved a misconception that mass doesn’t affect the speed of an object. In other words, she demonstrated her lack of understanding of the relationships between the variables (acceleration, velocity, mass, and force) involved in the situation. Her response to the questions confirmed that she explained scientific phenomena using variables in equations but failed to recognize the relationships among them. Instead she focused on individual variables, e.g., how acceleration will change as force changes, but did not explain how that would change velocity. She also did not explain how two components of velocity affect an object’s motion. Interestingly, she also used the unit of variable to justify her answer without applying conceptual meanings to it. For Blake, equations and units seemed to play important roles in explaining physical situations, but her connection of equations to physical situations was, at best, based on interpretations of individual variables.
When we asked Blake about change in the skater’s highest speed when the skater’s mass increased, her original written response was that her highest speed would increase because the mass of the skater would require more energy. When we interviewed her, her answer was different from her original response.
Blake: I think it should stay the same. I was thinking of the formula.
When we asked her to explain in more detail, she wrote an equation on the board (Fig. 4 ) and explained what it meant.
Blake: The highest point, because there won’t be any kinetic energy. And it’ll be mgh . Also ½ mv 2 and it [ m ] cancels out. It was exactly the same. The speed was the same. But—wasn’t there a bar graph [in the simulation]? Well, the total energy was bigger [in the simulation]. The total energy. But the total energy was same—no bigger.
Similarly to Alex, Blake used an equation to explain that the skater’s speed wouldn’t change because v doesn’t contain m after canceling out. However, she did not describe why kinetic energy is zero at the highest point and why potential energy is zero at the bottom. It might be that she just did not mention this, but it was obvious that she did not understand how the object’s mass affected the system: “But the total energy was same—no bigger.” We further asked her how the total mechanical energy of the skater would change when the skater’s mass increased. This time, she said, “Well, the total energy was bigger. ‘Cause energy depends on mass and either height or speed of a person.” As seen in the response, she thought of variables in equations of gravitational potential energy ( mgh ) and kinetic energy ( \( \frac{1}{2} \) mv 2 ). When asked why she previously had said the total mechanical energy would be the same, she answered, “because energy is always conserved.” This illustrated her misconception that the amount of energy should always be the same regardless of mass; however, when she considered variables in equations of PE and KE, she answered the question accurately. Throughout the interview, we found that Blake’s strategy to solve questions was consistent across different tasks; she used formulas and units as her first approach. However, a difference between Blake and the other two students is that although she used equations and variables, she did not explain how the variables influenced each other; and how they would change as a specific situation changed. In other words, she did not translate equations into physical situations nor link conceptual meanings to the variables and the relationships between them. The findings showed that for Blake, equations were more likely used as a simple computational tool.

Blake’s explanation
Disconnection of students’ problem-solving strategies from physics lecture
The three students all mentioned that they liked simulation-based questions. Alex said that the questions themselves made him think a lot, and running simulations also made him think more deeply: “Beforehand it [the task] just seems really simple, so you don’t put much thought into it. That’s easy. Just write it down, but then, once you run it, it makes you think about it more. So that’s cool too.”
As seen in his responses to the questions in the tasks, Alex used equations as conceptual understanding tools consistently across tasks. When we asked if he had learned this approach from his physics course, he said that his physics class heavily focused on solving problems but mostly by just reading off equations and plugging in numbers.
Alex: Physics is not about reading equations and stuff off a slide. It’s about working things by hand, and my professor, he has all the solutions to the problems in the book. He had them on a clear sheet of paper, and a Sharpie and then, so if he has problem 20, he puts problem 20 on the projector, and then he put that clean sheet there, and then he points to, oh here I did “v = a + blah blah,” so that’s really not effective at all in my opinion.
Christopher mentioned that the formative assessment would be very helpful for a lot of students, because it showed physical scenario. His physics class was more formula-based, with activities such as showing a formula and plugging in numbers to demonstrate how to solve a physics problem, which Christopher felt was disconnected from how he learned science. As he demonstrated, he learned best when he created a physical scenario, then translated it into equations. Alex also mentioned that physics is “about working things by hand,” which implies that he emphasized linking problem-solving procedures to physical situations. In Blake’s case, she mentioned that “It will help students to learn the concept better, but I think students will hate it [the formative assessment] because students will be like, ‘I don’t have time for this. It’s just that I am like too busy for this.’” In sum, the three students had a common opinion that the simulation-based formative assessment had helped them understand the given physical situation better, but the reasons why they liked it differed, as did their problem-solving strategies.
Discussion and conclusion
Previous studies on problem solving were concerned with students’ using equations simply as numerical computational tools by plugging in numbers. While experts tend to start with a conceptual analysis of problems using scientific principles and laws, novices start by selecting and manipulating equations without conceptual analysis (Larkin et al., 1980 ). The difference in solving problems might be more obvious in quantitative questions, in which a mathematically framed physics question may prompt students to use equations without conceptual understanding (Kohl & Finkelstein, 2006 ). The current study started from the research question “How do students solve conceptual physics questions in simulation-based formative assessments?” The findings showed that the students still used equations to answer the questions. However, their utilizations of equations were different. For example, Alex’s and Christopher’s strategies involved using equations to explain or interpret the given physical situation. To do so, they connected variables to physical situations and provided meanings to the variables and the relationships among the variables. Blake, however, used equations and units as tools to find answers for the questions without a clear connection of the variables and equations to the given physical situations. Christopher’s strategy was especially noticeable in that he used equations as effective explanatory tools for a physical situation. He started an analysis of the physical situation, then translated equations into the situation by creating a physical scenario in a system, such as how variables change as the situation changes, and how the variables are related to each other within the physical system. Alex’s explanations illustrated that he utilized equations to understand a physical situation. The difference between him and Christopher is that Alex used equations as major tools to analyze and understand the situation, while Christopher used them to effectively and easily explain the situation. Noticeably, Alex used algebraic computation processes using an equation to understand a given physical process.
Kuo et al. ( 2012 ) argued that linking conceptual reasoning to mathematical formalism indicates a more expert level of understanding and demonstrates robust solutions integrating conceptual and symbolic reasoning. They found that students used equations not just as computational tools but as tools to find conceptual shortcuts to solve physics problems. Although Kuo et al.’s study focused on quantitative problem solving, the current study revealed a similar finding where questions were created qualitatively without asking any calculations. Another difference from Kuo et al.’s study is that they provided an equation to students first, then asked them to explain the equation and apply the equation to a physical situation, whereas the current study provided a physical situation without any equations. As a conclusion, the current study supports that equations can be important in conceptualizing a physical situation by connecting conceptual meanings to equations. Therefore, mathematical equations can be used alternatively in problem solving (Kuo et al., 2012 ). Redish and Smith ( 2008 ) also illuminated the power of equations in solving physics problems and making sense of physical systems when students are able to link physical scenarios to mathematical equations. Thus, the connection of physical meaning to equations should be emphasized in teaching and learning physics in order to help students to conceptualize physical system (Redish & Smith, 2008 ).
Previous studies of quantitative physics problem solving have focused on using equations first when solving a physics question without a conceptual analysis of the problem situation, which indicated equations were in play as a simple computational tool. Although, the current study found a similar case, in which a student used equations as a simple computational tool, we also found that students used equations as a conceptual understanding or an effective explanatory tool. Indeed, using equations helped Alex realize his misconception and explain the situation accurately. While previous studies have emphasized performing a conceptual analysis first using scientific principles when solving a problem, this study argues the positive roles of using equations when it includes a connection between the equations and the physical situation. Therefore, this study contributes to the literature on physics problem solving in that equations can be used for students as tools for a conceptual understanding and as an explanatory tool. In this study, Christopher’s strategy was closer to the strategy used by experts, since he visualized a given situation to analyze by creating a physical scenario, then connected the relevant equations to the situation to explain the physical scenario. On the other hand, Alex used equations first to answer questions by connecting variables to the physical process through an algebraic solution process. Especially for Alex, equations facilitated his physical understanding of the problem and ability to explain the physical process. Although Alex and Blake used equations primarily as tools to answer questions, Blake did not demonstrate her interpretations of variables or the relationships among them in equations; nor did she connect variables to a physical situation. This indicated that her utilization of equations was closer to simple computational tools.
In conclusion, mathematical equations in physics were important when students were conceptually explaining a physical situation. It was revealed that using equations helped them explain a physical situation with more scientifically normative ideas. However, the ways they used equations differed between students. An equation could be an explanatory tool, a conceptual understanding tool, or a computational tool. The essence of the findings was that when students were able to connect variables to a physical process and to interpret relationships among variables in an equation, equations were in play as tools in understanding and explaining a physical situation. On the other hand, without interpretations of variables and connections to a physical situation, equations only served as simple computational tools. The study also found that students’ strategies to answer questions, especially conceptual ones, did not change with different topics in physics.
Implications and study limitations
As some students pointed out, their physics lectures demonstrated how to solve quantitative questions using equations as computational tools. As Christopher’s problem solving strategy was similar to the strategy used by experts, we suggest that his strategy be reflected in teaching physics. To be more specific, physics educators may provide an opportunity for students to visualize the physics phenomena. They could use models or computer simulations to help this procedure. Second, they should emphasize how equations are used to explain the phenomena as a “conceptual shortcut” (Kuo et al., 2012 , p. 39) by connecting equations and variables to the physical situation. In other words, as Alex and Christopher demonstrated, if physics instructions emphasize connections between physical meanings and mathematical expressions, it help students understand physical phenomenon. As we consider physics instructors as experts, perhaps in some cases their expert level of using equations was not reflected in their teaching. A future study topic would be to investigate the reason for the gap between physics experts’ strategies in solving physics problems and their teaching practices when demonstrating how to solve physics problems.
Although the findings of this study suggest an alternative way of using equations as an explanatory or a conceptual analysis tool for a physical situation, the findings might not be generalizable because the study context was limited to an introductory level physics course. Also, it is possible that topics for the tasks (kinematics and mechanical energy conservation) involving several equations might have influenced students’ strategies in answering questions. However, Redish ( 2017 ) emphasizes that a goal of physics is to create mathematical modeling (equations) that can predict and explain physical phenomena. Consequently, mathematical equations are included in physics topics and taught extensively in physics instruction especially in high school and college. We argue that students’ understanding of mathematical modeling in physics should not be considered as a following step after conceptual understanding of scientific principles. Instead, we support the claim that blending of physical meaning with mathematical operations should be emphasized in teaching physics (Kuo et al., 2012 ; Redish, 2005 , 2017 ). We also suggest that future studies should investigate how students’ strategies to answer questions are different in other topics, such as thermodynamics or electricity and magnetism.
Availability of data and materials
Interview data are not available for public.
Abbreviations
Constructed-Response
Gravitational Potential Energy
Kinetic Energy
Next Generation Science Standards
Ardac, D., & Akaygun, S. (2004). Effectiveness of multimedia-based instruction that emphasizes molecular representations on students’ understanding of chemical change. Journal of Research in Science Teaching , 41 (4), 317–337.
Article Google Scholar
Chi, M. T. H., Feltovich, P. J., & Glaser, R. (1981). Categorization and representation of physics problems by experts and novices. Cognitive Science , 5 , 121–152.
Creswell, J. W. (2016). 30 essential skills for the qualitative researcher . Thousand Oaks: Sage.
Google Scholar
Creswell, J. W., & Miller, D. L. (2000). Determining validity in qualitative inquiry. Theory Into Practice , 39 , 124–130.
De Cock, M. (2012). Representation use and strategy choice in physics problem solving. Physical Review Special Topics - Physics Education Research , 8 (2), 020117.
de Jong, T., & van Joolingen, W. R. (1998). Scientific discovery learning with computer simulations of conceptual domains. Review of Educational Research , 68 (2), 179–201.
Dhillon, A. S. (1998). Individual differences within problem-solving strategies used in physics. Science Education , 82 (3), 379–405.
Dori, Y. J., & Hameiri, M. (2003). Multidimensional analysis system for quantitative chemistry problems: symbol, macro, micro, and process aspects. Journal of Research in Science Teaching , 40 (3), 278–302.
Gick, M. L. (1986). Problem-solving strategies. Educational Psychologist , 21 (1–2), 99–120. https://doi.org/10.1080/00461520.1986.9653026 .
Gunstone, R. F., Champagne, A. B., & Klopfer, L. E. (1981). Instruction for understanding: a case study: learning research and development center . Pittsburgh: University of Pittsburgh.
Gurel, D. K., Eryılmaz, A., & McDermott, L. C. (2015). A review and comparison of diagnostic instruments to identify students’ misconceptions in science. Eurasia Journal of Mathematics, Science & Technology Education , 11 (5), 989–1008.
Huffman, D. (1997). Effect of explicit problem solving instruction on high school students’ problem-solving performance and conceptual understanding of physics. Journal of Research in Science Teaching , 34 (6), 551–570.
Kohl, P. B., & Finkelstein, N. D. (2006). Effects of representation on students solving physics problems. Physical Review Special Topics - Physics Education Research , 2 (1), 010106.
Kozhevnikov, M., Motes, M. A., & Hegarty, M. (2007). Spatial visualization in physics problem solving. Cognitive Science , 31 (4), 549–579.
Kuo, E., Hull, M. M., Gupta, A., & Elby, A. (2012). How students blend conceptual and formal mathematical reasoning in solving physics problems. Science Education , 97 (1), 32–57.
Larkin, J. H., McDermott, J., Simon, D. P., & Simon, H. A. (1980). Expert and novice performance in solving physics problems. Science , 208 (4450), 1335–1342.
Lazonder, A. W., & Ehrenhard, S. (2014). Relative effectiveness of physical and virtual manipulatives for conceptual change in science: how falling objects fall. Journal of Computer Assisted Learning , 30 (2), 110–120.
Maloney, D. P. (1994). Research on problem solving: physics. In D. L. Gabel (Ed.), Handbook of research in science teaching and learning , (pp. 327–354). New York: Macmillan.
McDermott, L. C. (1991). Millikan lecture 1990: What we teach and what is learned—closing the gap. American Journal of Physics , 59 , 301–315.
National Research Council (2012). A framework for K-12 science education: practices, crosscutting concepts, and core ideas . Washington, DC: National Academies Press.
National Research Council (2014). Developing assessments for the next generation science standards. Committee on developing assessments of science proficiency in K-12. In W. J. W. Pellegrino, M. R. Wilson, J. A. Koenig, & A. S. Beatty (Eds.), Board on testing and assessment and board on science education. Division of behavioral and social sciences and education . Washington, DC: The National Academies Press.
NGSS Lead States. (2013). Next Generation Science Standards: For states, by states. Washington, DC: The National Academies Press.
Park, M. (2019). Effects of simulation-based formative assessments on students’ conceptions in physics. Eurasia Journal of Mathematics, Science and Technology Education , 15 (7), em1722.
Park, M., Liu, X., & Waight, N. (2017). Development of the connected chemistry as formative assessment pedagogy for high school chemistry teaching. Journal of Chemical Education , 94 (3), 273–281.
Pellegrino, J. W. (2013). Proficiency in science: Assessment challenges and opportunities. Science , 340 (6130), 320–323.
Quellmalz, E. S., Timms, M. J., Silberglitt, M. D., & Buckley, B. C. (2012). Science assessments for all: integrating science simulations into balanced state science assessment systems. Journal of Research in Science Teaching , 49 (3), 363–393.
Redish, E. F. (2005). Problem solving and the use of math in physics courses . Delhi: Proceedings of the Conference, World View on Physics Education in 2005: focusing on change.
Redish, E. F. (2017). Analysing the competency of mathematical modelling in physics. In T. Greczyło, & E. Dębowska (Eds.), Key competences in physics teaching and learning , (pp. 25–40). Chum: Springer International Publishing.
Chapter Google Scholar
Redish, E. F., & Smith, K. A. (2008). Looking beyond content: skill development for engineers. Journal of Engineering Education , 97 (3), 295–307.
Rutten, N., van Joolingen, W. R., & van der Veen, J. T. (2012). The learning effects of computer simulations in science education. Computers & Education , 58 (1), 136–153.
Simon, D. P., & Simon, H. A. (1978). Individual differences in solving physics problems. In R. S. Sigler (Ed.), Children’s thinking: what develops? (pp. 325–348). Hillsdale: Erlbaum.
Smetana, L., & Bell, R. L. (2012). Computer simulations to support science instruction and learning: a critical review of the literature. International Journal of Science Education , 34 (9), 1337–1370.
Srisawasdi, N., & Panjaburee, P. (2015). Exploring effectiveness of simulation-based inquiry learning in science with integration of formative assessment. Journal of Computers in Education , 2 (3), 323–352.
Strauss, A. L., & Corbin, J. (1998). Basics of qualitative research: grounded theory procedures and techniques . Newbury Park: Sage.
Treagust, D. F. (1985). Diagnostic tests to evaluate students’ misconceptions in science . French Lick Springs: Paper presented at the annual meeting of the National Association for research in science teaching (NARST).
Trundle, K. C., & Bell, R. L. (2010). The use of a computer simulation to promote conceptual change: a quasi-experimental study. Computers & Education , 54 (4), 1078–1088.
Underwood, S. M., Posey, L. A., Herrington, D. G., Carmel, J. H., & Cooper, M. M. (2018). Adapting assessment tasks to support three-dimensional learning. Journal of Chemical Education , 95 , 207–217.
van Heuvelen, A. (1991). Learning to think like a physicist: a review of research based instructional strategies. American Journal of Physics , 59 , 891–897.
Download references
Acknowledgements
Author information, authors and affiliations.
Department of Curriculum and Instruction, College of Education, Texas Tech University, Box 41071, Lubbock, TX, 79409-1071, USA
You can also search for this author in PubMed Google Scholar

Contributions
First and Corresponding author. Dr. MP (100% contribution). Dr. Park designed the study, collected and analyzed data, and wrote the manuscript entirely. The author read and approved the final manuscript.
Corresponding author
Correspondence to Mihwa Park .
Ethics declarations
Competing interests.
The author declares that he/she has no competing interests
Additional information
Publisher’s note.
Springer Nature remains neutral with regard to jurisdictional claims in published maps and institutional affiliations.
Rights and permissions
Open Access This article is distributed under the terms of the Creative Commons Attribution 4.0 International License ( http://creativecommons.org/licenses/by/4.0/ ), which permits unrestricted use, distribution, and reproduction in any medium, provided you give appropriate credit to the original author(s) and the source, provide a link to the Creative Commons license, and indicate if changes were made.
Reprints and permissions
About this article
Cite this article.
Park, M. Students’ problem-solving strategies in qualitative physics questions in a simulation-based formative assessment. Discip Interdscip Sci Educ Res 2 , 1 (2020). https://doi.org/10.1186/s43031-019-0019-4
Download citation
Received : 14 June 2019
Accepted : 21 November 2019
Published : 03 January 2020
DOI : https://doi.org/10.1186/s43031-019-0019-4
Share this article
Anyone you share the following link with will be able to read this content:
Sorry, a shareable link is not currently available for this article.
Provided by the Springer Nature SharedIt content-sharing initiative
- Formative assessment
- Physics education
- Problem solving

71+ Free Social Problem-Solving Scenarios
Do you have kiddos who struggle with their social problem-solving skills? Teach your students the simple process of how to solve a problem along with having them review how well their solution worked or didn’t work.
Why Teach Problem Solving Skills?
Learning to problem solve is an essential skill that is used not only throughout childhood but also into adulthood. Social problem solving is the ability to change or adapt to undesirable situations that arise throughout our day.
On a daily basis, a child will encounter social problems that they will need to solve.
Anything from:
- arguing with another student
- to hurting a friend’s feelings
- to having a difficult conversation
- working with others

Start with Small Problems
Many of the “problems” children encounter are often small problems which the child may be over-reacting to, such as wanting a different coloring crayon or wanting to be first in line, however, these small problems are still very real to the child.
Practicing problem-solving with these small problems can be a great learning opportunity. Children can practice problem-solving with a small problem which can help them learn how to handle bigger problems in the future.
Problem Solving Importance
Social problem-solving skills are critical to a child’s social interactions, personal and professional relationships. A child’s ability to handle change, cope with stress, and handle challenges improves with a child’s ability to successfully solve social problems.
The ultimate goal is that the child will be able to solve social problems all on their own, but until they can independently solve a problem they will need to learn how to communicate and self-advocate to positively solve their problems.
Steps to Problem Solving
Children can be taught how to problem solve through a guided process of breaking down the problem and using simple steps to solve the problem.
Learning specific steps to problem-solving can allow children to remember how to solve a problem when they become overwhelmed or stressed.
Although learning to solve a problem independently can take some time and practice it is well worth the investment to have a child who can eventually solve most social situations in a positive manner on their own.
What we learnt about solving problems is don't freak out, if one thing doesn't work , try something else out. And work together as a team. #melthammathsweek #MELTHAMPUPILVOICE @problemsolveit pic.twitter.com/iVm1Im4Aue — yr6melthamce (@yr6melthamce) February 4, 2019
Problem Solving Form
Teach your students the 4 steps to becoming a social problem-solver.
- Identify the problem. For instance, start by having your student identify the social problem.
- Create three solutions. Also, have your student come up with three different solutions that they could use to solve the problem that they identified.
- Identify the consequences. Then, identify the consequence for each individual solution.
- Pick the best solution. Lastly, have your student identify which of their three solutions is the best choice Then have your student put into words why they think that solution is the best solution.
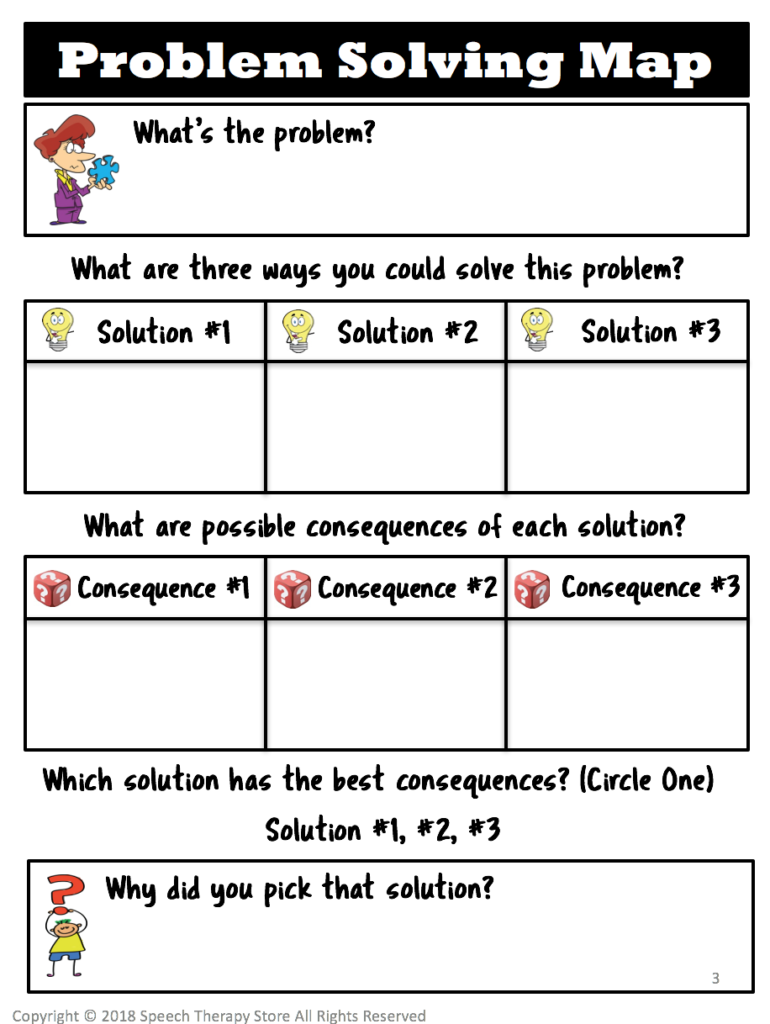
Problem Solving Review Form
After your students go through the social problem-solver have them use the social problem-solving review form.
- What happened. For instance, after your student tried their solution have them explain what happened next.
- Review the results. Also, have your student identify whether or not their solution got them the results they wanted.
- Use this solution again. Furthermore, have your student identify whether or not they would use this solution again in the future to solve the same or similar problem.
- What would you do differently? Finally, have your student explain what they would do differently if they didn’t get the results they wanted or if they wouldn’t use that solution again in the future.
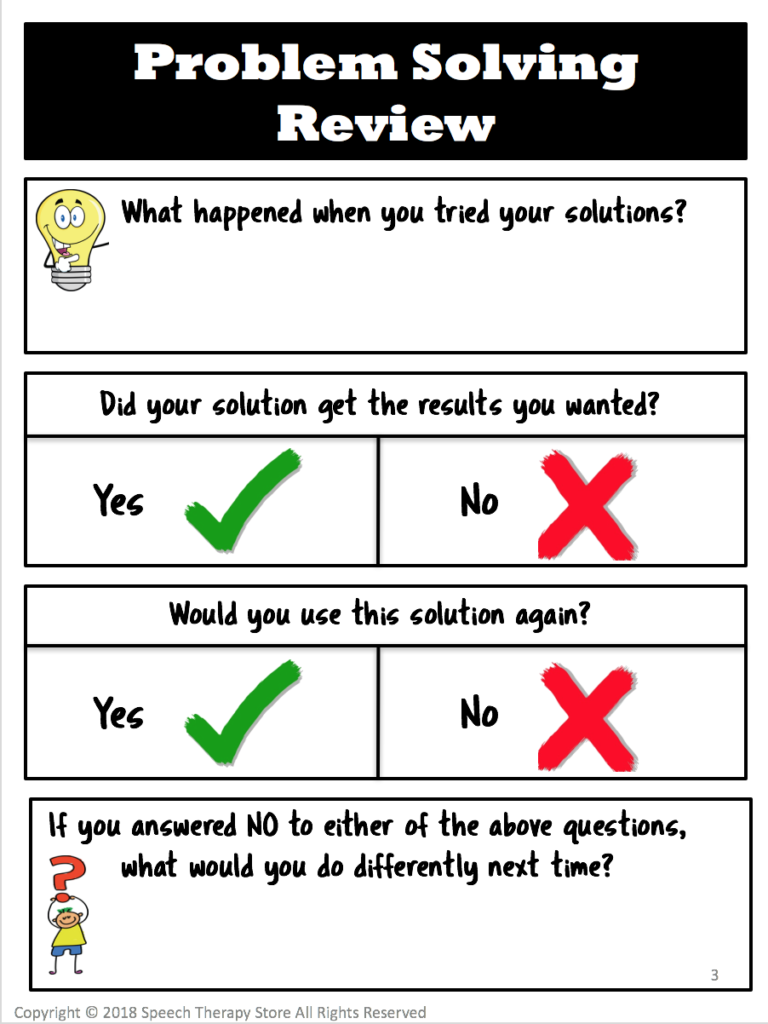
71+ Social Problem Scenarios + 6 Blank Scenarios
Use the 71 social problem-solving scenarios to have your students get great experience practicing how to solve a social problem.
Also, included are 6 blank scenarios. Then laminate them so you can use them over and over again. Therefore, create social problems that the student experiences and needs help solving.

Wordless Video teaching Problem Solving
Watch this super cute wordless animation with your students and have them discuss the problem they see and how to best solve the problem.
Use this as a fun practice example to get your students started towards learning how to problem-solve.
Demonstrate Through Modeling
Model and discuss empathy.
First and foremost, children need to understand how another person might be feeling in a given situation in order to become a good social problem solver. The student needs to learn how to “stand in someone else’s shoes” for a little bit.
One way you can work on this skill is during the reading time you can focus on how a particular character in the story might be feeling.
Ask questions, such as:
- “How do they feel right now?”
- “How would you feel in that same situation?”
- “Why do you think they feel that way?”
Model Problem-Solving Skills as the Teacher
When you are faced with a problem you can solve the problem by thinking aloud for the students to hear how you solve a problem.
You can state the problem, then come up with possible solutions, then identify the possible consequences to each solution, then pick and explain why a solution is the best option.
For example, you could say, “I was hoping to take the class outside for a stress walk around the track before the reading test, but the problem is that it is raining outside. I could still take you outside, but then you will get wet, or we could walk the halls, but then we’d have to be really quiet because there are other classes learning, or we could just skip the walk and take the reading test, but then you might not do as well on the test. I think based on all of those solutions the best solution will be to walk the hallway, but you guys will have to promise to be quiet so that we don’t disrupt other classes.
Modeling the problem-solving process can be very helpful for the students to watch, observe, and later implement themselves.
Teach Communication
Have students communicate how they are feeling.
Teaching your students to share their emotions in a respectful way can improve their ability to problem-solve.
Have students use an “I” sentence frame, such as, “I feel _____ (insert feeling word) when _____ (identify what made you feel that way).”
For example, “I felt sad when Jackson broke my favorite pencil” or “I was mad when I wasn’t picked to be first in line.”
This way students can communicate how they are feeling using honest and open communication. Teaching students to appropriately communicate their emotions can help solve some social problems from the beginning.
Encourage Independency
Encourage your student to problem solve.
If your student is struggling to problem solve independently encourage them to do so using open-ended questions.
- “How could you fix this problem?”
- “What would be a fair solution?”
- “What would happen if you used that solution?”
Let the Student try to Problem Solve Independently
Give your students the space to try and solve their own problems using the guided strategies. Try not to come running to their rescue for every little problem.
Some problems are small and a great opportunity for the student to learn and practice. If an adult does all of the problem solving for a student then what are they really learning?
Give your students the time and space they need to practice solving small problems on their own. Of course, if it is a bigger or more serious problem then have an adult help guide the problem-solving process.
Tell an Adult
Remind your students that there are still some problems that are too big for them to solve on their own and that it is okay to get help from an adult to solve big problems.
For example, if the student doesn’t feel safe, someone is being hurt physically or emotionally, or if they tried to solve a problem independently but it didn’t work and they need help. Let them know that it’s okay to tell an adult.
Teach How to Disagree and How to Make Up
Discuss how to disagree respectfully.
Remind your student that they won’t always agree with their teacher, friends, classmate, or parents and that’s okay. Even the people we like might have different opinions, interests, and likes than we do.
However, even if we disagree with someone we should still treat them with respect. Treating someone with respect means to not call them names, ignore them, yell or hit them. It means that you do try to create solutions that both parties can agree with and to apologize when we hurt others’ feelings.
Role-Play How to Make Up
Practice in everyday life how to make up after a social problem .
Students are really having to stretch their brains today. It's @NSPCC #NumberDay and @problemsolveit are challenging Y9 and 10 to solve the escape room boxes. It's not as easy as it looks! The promise of a few sweet treats for the winners seems to be helping though! pic.twitter.com/AxRRJnJIv2 — CongletonHS (@CongletonHS) February 2, 2018
Be sure to get your free social problem solver today below! I hope you and your students love this freebie.
Have your students use task card scenarios to help them identify how they and others might feel in different social scenarios. Be sure to discuss the problem, identify possible solutions, identify the consequences of those possible solutions, and then based on those consequences pick the best solution.
Make social problem-solving a game by telling the students that they are social detectives and that it is their job to use what they know about social rules to help them identify the possible and best solutions.
Start practicing today with 71+ free social problem social task cards! Do your students need more practice?
Be sure to check out my other freebie for 31 wordless animated videos to teach problem-solving and so much more.
Make Problem Solving Easier with this Freebie!
Download yours today to get started.
Get More Problem Solving Time Saving Materials
Next, be sure to check out the following time-saving materials to continue to teach your students how to solve their social problems in addition to this freebie.
Weekly Social Pragmatics Homework
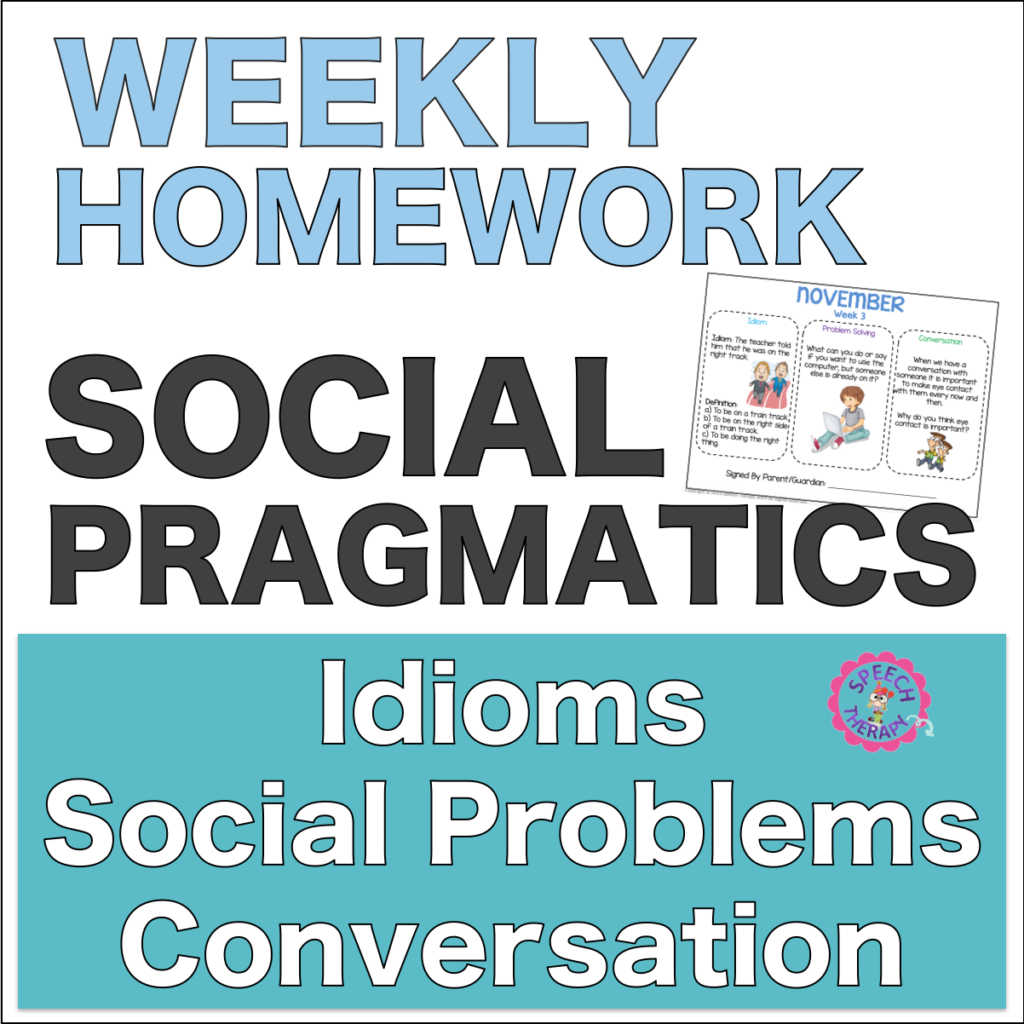
- Weekly problem-solving. Send home a weekly homework page that includes a problem-solving scenario plus an idiom and a conversational practice scenario.

Restorative Justice Problem Solving Flip Book

- Restorative justice graphic visual. Use this graphic visual to help your student restore a social relationship after a social problem.

Self-Advocating Role-Play Scenarios

- Self-advocating in high school. Teach your high schoolers the process to self-advocate for what they need.
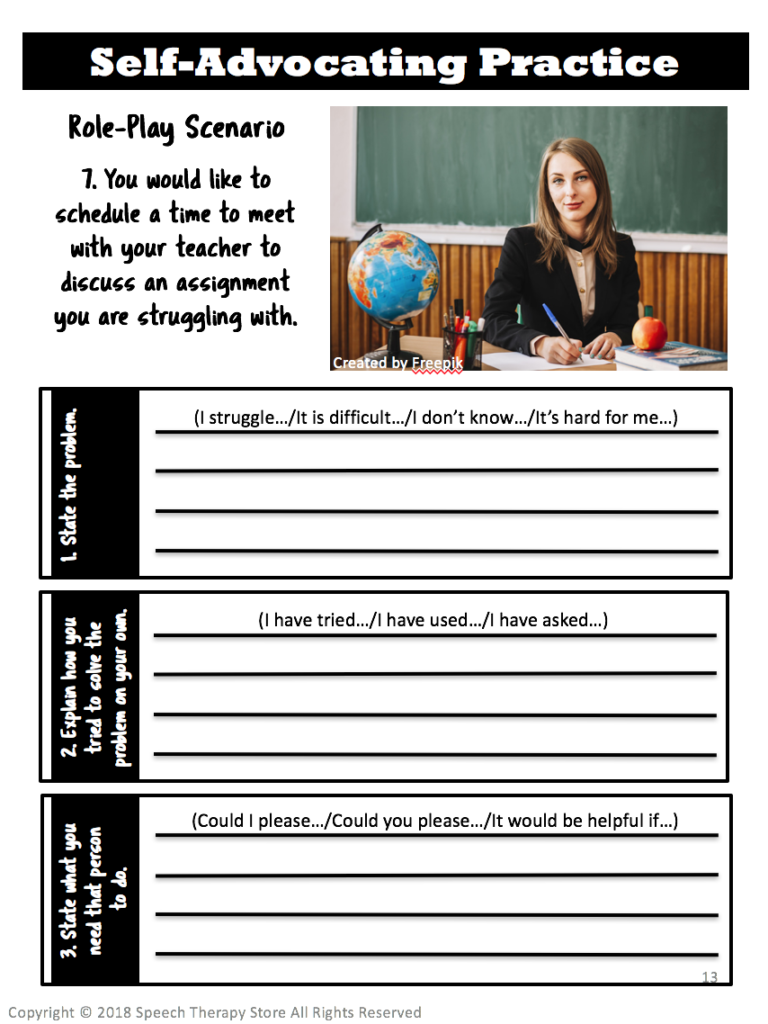
5th-12th Grade Life Skills Problem Solving

- Life skills problem-solving. In addition, this life skills differentiated bundle includes a problem-solving lesson plan.

I recommend you read Problem Solving Wheel: Help Kids Solve Their Own Problems , 61+ Free Fillable SLP Planner Pages 2020-2021 , 430+ Free Multisyllabic Words List Activity Bundle , or 432+ Free IEP Goal Bank to Save You Time posts because they include freebies as well and who doesn’t want more freebies!
Got questions? Leave a comment. Let’s chat!
Monday 30th of January 2023
Hello! I have entered my name and email twice (yesterday & today) to receive to 71+ Free Social Problem-Solving Senarios, but I have not received anything yet. Not even an email back to mine in order to subcribe. Thanks for your help! Tracy
Melissa Berg
Tuesday 31st of January 2023
Hi Tracy, Thanks so much for reaching out! Sorry about that. We went ahead and sent you an email with the PDF attached. Wishing you all my best, Melissa
Problem Solving Skills
Tuesday 30th of August 2022
I truly love your site. Excellent colors, theme and writing. Thanks for sharing.
Laura Ricca
Monday 11th of April 2022
Tuesday 12th of April 2022
Hi Laura, I'm glad you found this resource helpful. Melissa
Modified Mental Health and Suicide Prevention - Speech Therapy Store
Monday 11th of May 2020
[…] 71+ FREE SOCIAL PROBLEM-SOLVING SCENARIOS […]
Problem Solving Wheel: Help Kids Solve Their Own Problems - Speech Therapy Store
Monday 4th of May 2020
[…] 71+ Free Social Problem Solving Task Cards Scenarios […]
- Join Mind Tools
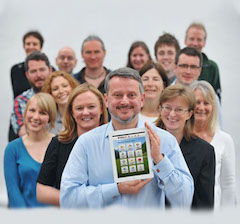
How Good Is Your Problem Solving?

© iStockphoto Entienou
Use a systematic approach.
Good problem solving skills are fundamentally important if you're going to be successful in your career.
But problems are something that we don't particularly like.
They're time-consuming.
They muscle their way into already packed schedules.
They force us to think about an uncertain future.
And they never seem to go away!
That's why, when faced with problems, most of us try to eliminate them as quickly as possible. But have you ever chosen the easiest or most obvious solution – and then realized that you have entirely missed a much better solution? Or have you found yourself fixing just the symptoms of a problem, only for the situation to get much worse?
To be an effective problem-solver, you need to be systematic and logical in your approach. This quiz helps you assess your current approach to problem solving. By improving this, you'll make better overall decisions. And as you increase your confidence with solving problems, you'll be less likely to rush to the first solution – which may not necessarily be the best one.
Once you've completed the quiz, we'll direct you to tools and resources that can help you make the most of your problem-solving skills.
How Good Are You at Solving Problems?
Instructions.
For each statement, click the button in the column that best describes you. Please answer questions as you actually are (rather than how you think you should be), and don't worry if some questions seem to score in the 'wrong direction'. When you are finished, please click the 'Calculate My Total' button at the bottom of the test.
Your last quiz results are shown.
You last completed this quiz on , at .
Score Interpretation
Answering these questions should have helped you recognize the key steps associated with effective problem solving.
This quiz is based on Dr Min Basadur's Simplexity Thinking problem-solving model. This eight-step process follows the circular pattern shown below, within which current problems are solved and new problems are identified on an ongoing basis. This assessment has not been validated and is intended for illustrative purposes only.
Figure 1 – The Simplexity Thinking Process
Reproduced with permission from Dr Min Basadur from "The Power of Innovation: How to Make Innovation a Part of Life & How to Put Creative Solutions to Work" Copyright ©1995
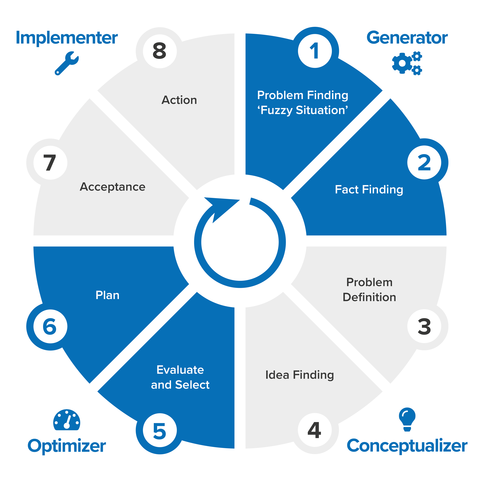
Below, we outline the tools and strategies you can use for each stage of the problem-solving process. Enjoy exploring these stages!
Step 1: Find the Problem
(Questions 7, 12)
Some problems are very obvious, however others are not so easily identified. As part of an effective problem-solving process, you need to look actively for problems – even when things seem to be running fine. Proactive problem solving helps you avoid emergencies and allows you to be calm and in control when issues arise.
These techniques can help you do this:
- PEST Analysis helps you pick up changes to your environment that you should be paying attention to. Make sure too that you're watching changes in customer needs and market dynamics, and that you're monitoring trends that are relevant to your industry.
- Risk Analysis helps you identify significant business risks.
- Failure Modes and Effects Analysis helps you identify possible points of failure in your business process, so that you can fix these before problems arise.
- After Action Reviews help you scan recent performance to identify things that can be done better in the future.
- Where you have several problems to solve, our articles on Prioritization and Pareto Analysis help you think about which ones you should focus on first.
Step 2: Find the Facts
(Questions 10, 14)
After identifying a potential problem, you need information. What factors contribute to the problem? Who is involved with it? What solutions have been tried before? What do others think about the problem?
If you move forward to find a solution too quickly, you risk relying on imperfect information that's based on assumptions and limited perspectives, so make sure that you research the problem thoroughly.
Step 3: Define the Problem
(Questions 3, 9)
Now that you understand the problem, define it clearly and completely. Writing a clear problem definition forces you to establish specific boundaries for the problem. This keeps the scope from growing too large, and it helps you stay focused on the main issues.
A great tool to use at this stage is CATWOE . With this process, you analyze potential problems by looking at them from six perspectives, those of its Customers; Actors (people within the organization); the Transformation, or business process; the World-view, or top-down view of what's going on; the Owner; and the wider organizational Environment. By looking at a situation from these perspectives, you can open your mind and come to a much sharper and more comprehensive definition of the problem.
Cause and Effect Analysis is another good tool to use here, as it helps you think about the many different factors that can contribute to a problem. This helps you separate the symptoms of a problem from its fundamental causes.
Step 4: Find Ideas
(Questions 4, 13)
With a clear problem definition, start generating ideas for a solution. The key here is to be flexible in the way you approach a problem. You want to be able to see it from as many perspectives as possible. Looking for patterns or common elements in different parts of the problem can sometimes help. You can also use metaphors and analogies to help analyze the problem, discover similarities to other issues, and think of solutions based on those similarities.
Traditional brainstorming and reverse brainstorming are very useful here. By taking the time to generate a range of creative solutions to the problem, you'll significantly increase the likelihood that you'll find the best possible solution, not just a semi-adequate one. Where appropriate, involve people with different viewpoints to expand the volume of ideas generated.
Don't evaluate your ideas until step 5. If you do, this will limit your creativity at too early a stage.
Step 5: Select and Evaluate
(Questions 6, 15)
After finding ideas, you'll have many options that must be evaluated. It's tempting at this stage to charge in and start discarding ideas immediately. However, if you do this without first determining the criteria for a good solution, you risk rejecting an alternative that has real potential.
Decide what elements are needed for a realistic and practical solution, and think about the criteria you'll use to choose between potential solutions.
Paired Comparison Analysis , Decision Matrix Analysis and Risk Analysis are useful techniques here, as are many of the specialist resources available within our Decision-Making section . Enjoy exploring these!
Step 6: Plan
(Questions 1, 16)
You might think that choosing a solution is the end of a problem-solving process. In fact, it's simply the start of the next phase in problem solving: implementation. This involves lots of planning and preparation. If you haven't already developed a full Risk Analysis in the evaluation phase, do so now. It's important to know what to be prepared for as you begin to roll out your proposed solution.
The type of planning that you need to do depends on the size of the implementation project that you need to set up. For small projects, all you'll often need are Action Plans that outline who will do what, when, and how. Larger projects need more sophisticated approaches – you'll find out more about these in the Mind Tools Project Management section. And for projects that affect many other people, you'll need to think about Change Management as well.
Here, it can be useful to conduct an Impact Analysis to help you identify potential resistance as well as alert you to problems you may not have anticipated. Force Field Analysis will also help you uncover the various pressures for and against your proposed solution. Once you've done the detailed planning, it can also be useful at this stage to make a final Go/No-Go Decision , making sure that it's actually worth going ahead with the selected option.
Step 7: Sell the Idea
(Questions 5, 8)
As part of the planning process, you must convince other stakeholders that your solution is the best one. You'll likely meet with resistance, so before you try to “sell” your idea, make sure you've considered all the consequences.
As you begin communicating your plan, listen to what people say, and make changes as necessary. The better the overall solution meets everyone's needs, the greater its positive impact will be! For more tips on selling your idea, read our article on Creating a Value Proposition and use our Sell Your Idea Bite-Sized Training session.
Step 8: Act
(Questions 2, 11)
Finally, once you've convinced your key stakeholders that your proposed solution is worth running with, you can move on to the implementation stage. This is the exciting and rewarding part of problem solving, which makes the whole process seem worthwhile.
This action stage is an end, but it's also a beginning: once you've completed your implementation, it's time to move into the next cycle of problem solving by returning to the scanning stage. By doing this, you'll continue improving your organization as you move into the future.
Problem solving is an exceptionally important workplace skill.
Being a competent and confident problem solver will create many opportunities for you. By using a well-developed model like Simplexity Thinking for solving problems, you can approach the process systematically, and be comfortable that the decisions you make are solid.
Given the unpredictable nature of problems, it's very reassuring to know that, by following a structured plan, you've done everything you can to resolve the problem to the best of your ability.
This site teaches you the skills you need for a happy and successful career; and this is just one of many tools and resources that you'll find here at Mind Tools. Subscribe to our free newsletter , or join the Mind Tools Club and really supercharge your career!
Rate this resource
The Mind Tools Club gives you exclusive tips and tools to boost your career - plus a friendly community and support from our career coaches!
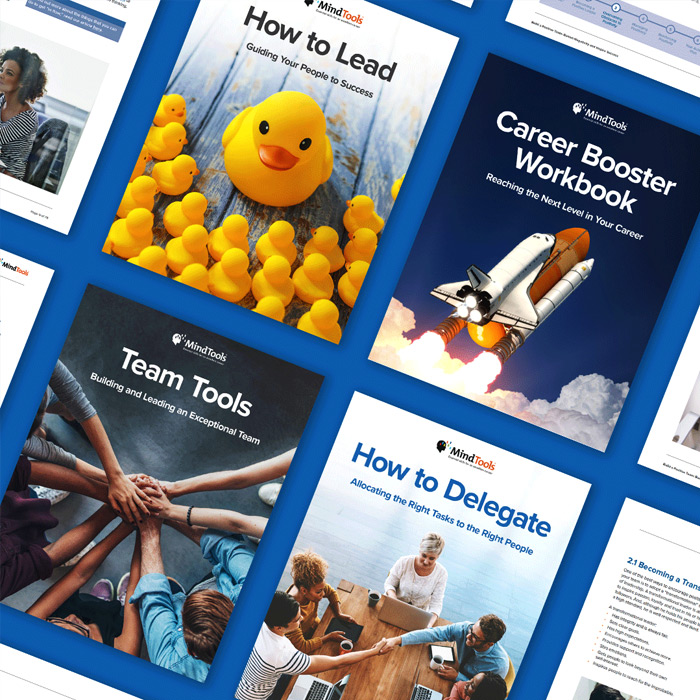
Comments (220)
- Over a month ago Sonia_H wrote Hi PANGGA, This is great news! Thanks for sharing your experience. We hope these 8 steps outlined will help you in multiple ways. ~Sonia Mind Tools Coach
- Over a month ago PANGGA wrote Thank you for this mind tool. I got to know my skills in solving problem. It will serve as my guide on facing and solving problem that I might encounter.
- Over a month ago Sarah_H wrote Wow, thanks for your very detailed feedback HardipG. The Mind Tools team will take a look at your feedback and suggestions for improvement. Best wishes, Sarah Mind Tools Coach
Please wait...
- WV App Login
- Site Search
- Report Templates
- Speech Helpers
- SLP Resources
- Top 10 Tips
- Getting an Eval
- Certified SLP
- How to Say the R Sound
- 0-18 Months
- 18-36 Months
- 18-30 Months
- 30-36 Months
- 10-11 Years
- Articulation
- Cleft Palate
- Phonological
- Dysphagia Causes
- Dysphagia Treatment
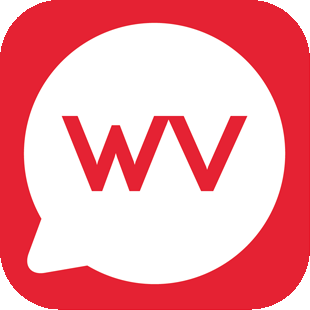
30 Problem Solving Scenarios for Speech Therapy Practice
As promised here are the words for your unlimited use .
If you know others who can use our lists ...
... please share this page using our site share buttons.
Explore Our Goal Reaching, Client Centered Products
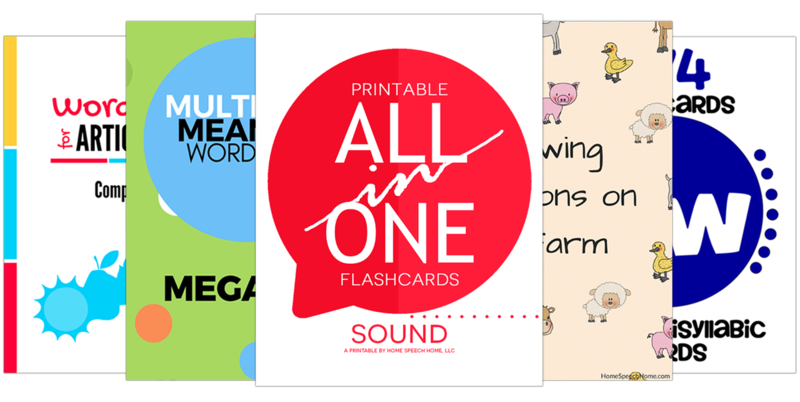
SEE ALSO: Houston We Have a Problem! Activities for Problem Solving
Problem solving scenarios.
- Your friends came over to your house for a movie night. One of your friends brought another friend so there are more people than you planned for. You want to pass out the drinks but you only have five cans of soda and you need 6 for everyone to have one. What could you do?
- After basketball practice you go back to the locker room with your team to shower and change. When you are done dressing, you can't find your shoes. What could you do?
- You have been waiting all day for lunch to come because you are starving. Finally class gets over and you get to go to lunch. Except when you go to get to your lunch, it's not there. You probably left it at home. What could you do?
- There is a guy in your class who is always mean to you. He always bumps you when he walks by and he calls you names. He knocks stuff out of your hands and makes you feel stupid. You don't think you can take it anymore. What could you do?
- You really want to invite this new girl/guy to come to your birthday party, but you have never talked to them before. You are worried they will say no. What could you do?
- You rode the bus to school today and on the way in people are pointing and laughing at you. You go in the bathroom and see that you have pink gum all over the back of your pants. What could you do?
- You wake up and see that your alarm never went off. So you are starting your morning 15 minutes later than you planned. It is a really important day at school and you cannot be late. What could you do?
- You are giving a group presentation in front of class and it's your turn to talk. All of the sudden you sneeze. You cover it with your hand, but now your hand is full of stuff you sneezed out. What could you do?
- You are eating dinner at a fancy restaurant with your parents and their friends. You have a really messy dinner and accidentally flip a noodle into the lady's lap. They are busy talking and don't notice it. What could you do?
- You are taking a test and there is no talking allowed. You are writing your answers on the paper and your pencil breaks. What could you do?
- You are taking a test and the guy behind you asks you for help. He wants to know what you put for question number two. What could you do?
- You are at a birthday party and you have waited in line for a long time for your turn to hit the pinata. It is finally going to be your turn and it looks like the next hit will break the pinata. But you suddenly have to go to the bathroom. What could you do?
- You are hanging outside with your friend and she decides to pick your neighbor's flowers. She gives you the pretty handful of flowers and right then your neighbor opens the door. She asks you why you picked her flowers. What could you do?
- You borrowed your sister's skates one day without asking and they broke while you were using them. What could you do?
- You are eating at a friend's house and the mom piles your plate full of food. It looks really good and you want to eat it all but you can't because you just ate a snack. What could you do so you don't hurt her feelings?
SEE ALSO: The Best Free App for Speech Therapy

- Your teacher was working at her desk. You wanted to ask her a question, but she didn't see your hand raised. What should you do?
- You started to do your work, but you weren't sure if you were doing it right. What should you do?
- You were playing tether-ball and were the champion so far. In the next game, you slightly touched the rope. Only one student saw you touch the rope. What will you do?
- The teacher is giving directions, but your friend sitting next to you keeps talking. You can't hear the directions. What should you do?
- You didn't do your homework. Your teacher was upset with you. What should you do?
- You finished eating and felt a burp coming. What are you going to do?
- You were waiting to swing. When it was your turn, another boy jumped in front of you and took the swing. What would you do?
- You waited a long time, but your mom didn't come to pick you up after school. What should you do?
- A bully threatened to beat you up after school. What should you do?
- A boy on the playground keeps pushing you and making you mad. What would you do?
- You were sitting in class doing your work and you hear the fire alarm. What should you do?
- An adult you didn't know came on to the playground and asked if you would help look for his lost dog. What would you do?
- You forgot your lunch at home. What would you do?
- The person sitting behind you keeps tapping your chair with his foot. What should you do?
- You finished your work early. What should you do?
This list of functional words was professionally selected to be the most useful for a child or adult who has difficulty with problem solving scenarios.
We encourage you to use this list when practicing at home.
Home practice will make progress toward meeting individual language goals much faster.
Speech-Language Pathologists (SLPs) are only able to see students/clients 30-60 mins (or less) per week. This is not enough time or practice for someone to handle Problem solving scenarios.
Every day that your loved one goes without practice it becomes more difficult to help them.
SEE ALSO: The Best Books for Speech Therapy Practice
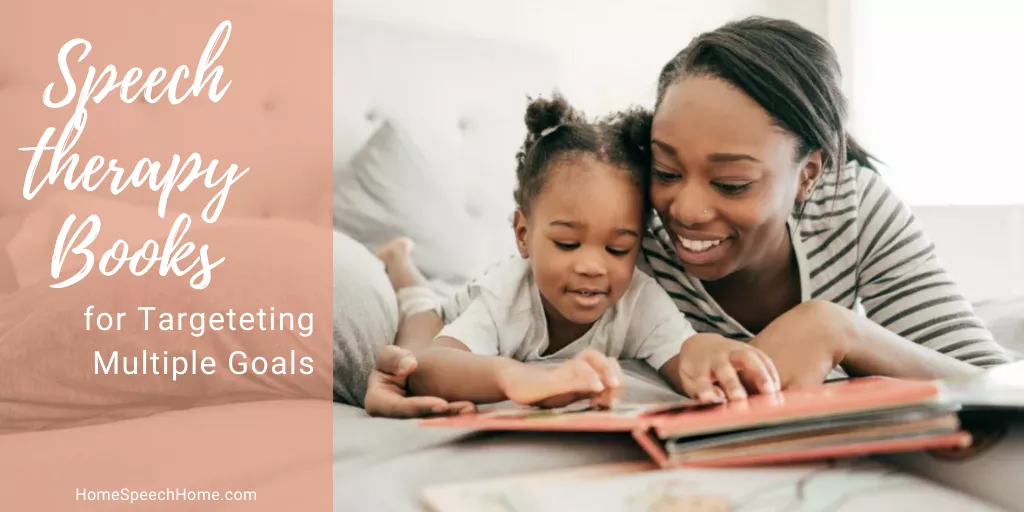
We know life is busy , but if you're reading this you're probably someone who cares about helping their loved one as much as you can.
Practice 5-10 minutes whenever you can, but try to do it on a consistent basis (daily).
Please, please, please use this list to practice.
It will be a great benefit to you and your loved one's progress.
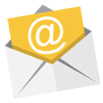
Freebies, Activities, and Specials, Oh My! Sign up for Terrific Therapy Activity Emails
See Past Email Examples
Your information is 100% private & never shared .
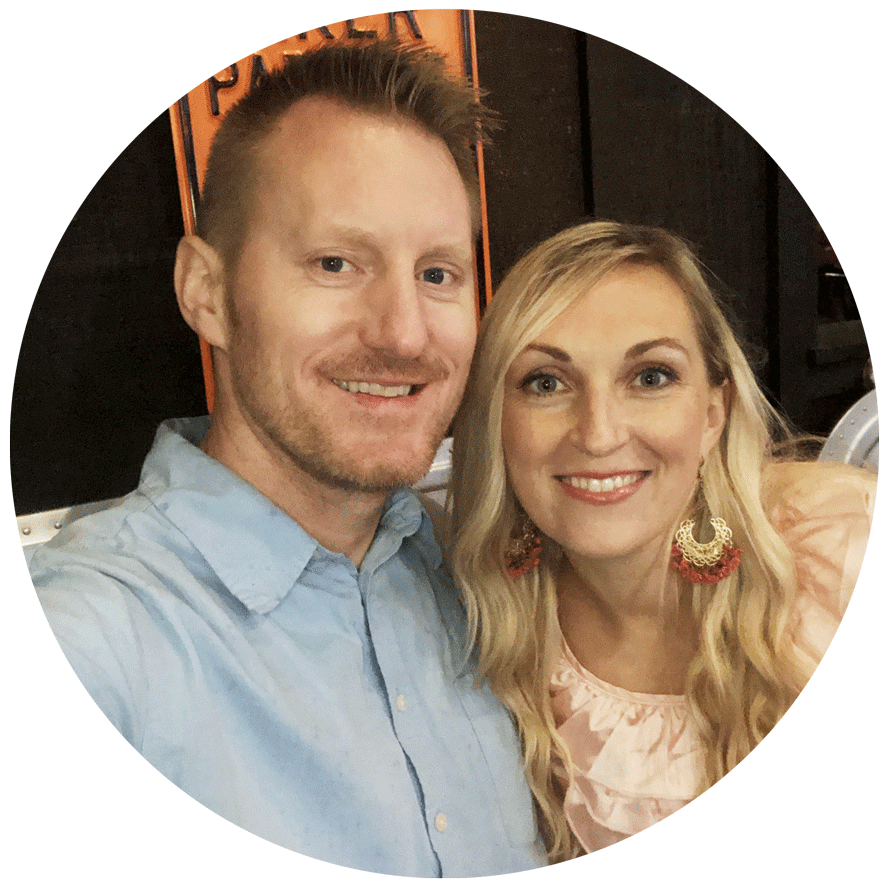
Hi! We're Luke and Hollie.
We are both MS CCC-SLPs and fell in love while studying for our degrees. Since then we have done everything together - graduated, worked, and started a family. We spend most of our time with our family and the rest making this site for you.
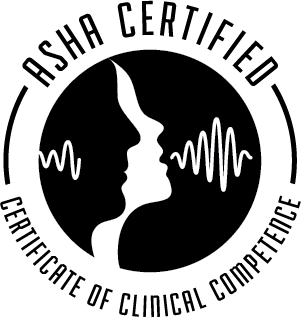
Top Free Resources
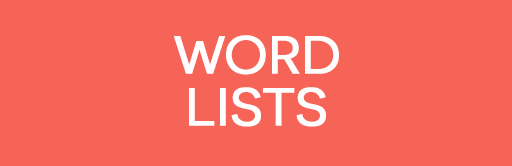
Word Vault Essential
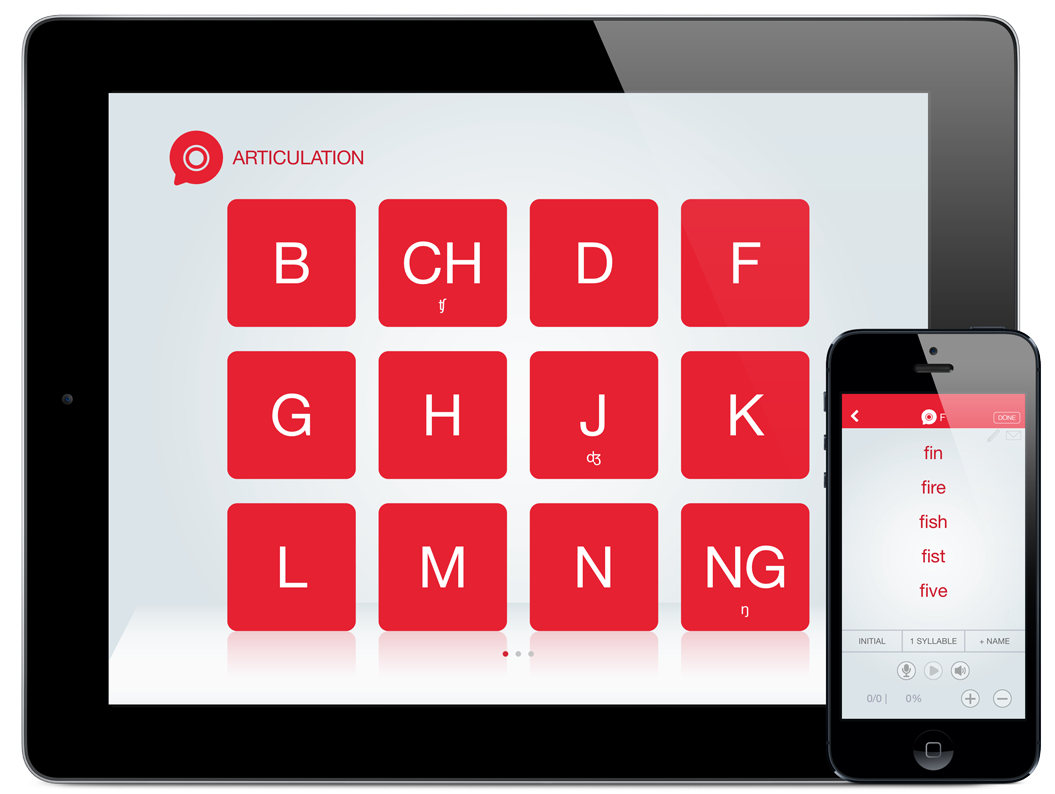
# 1 Chronological Age Calculator
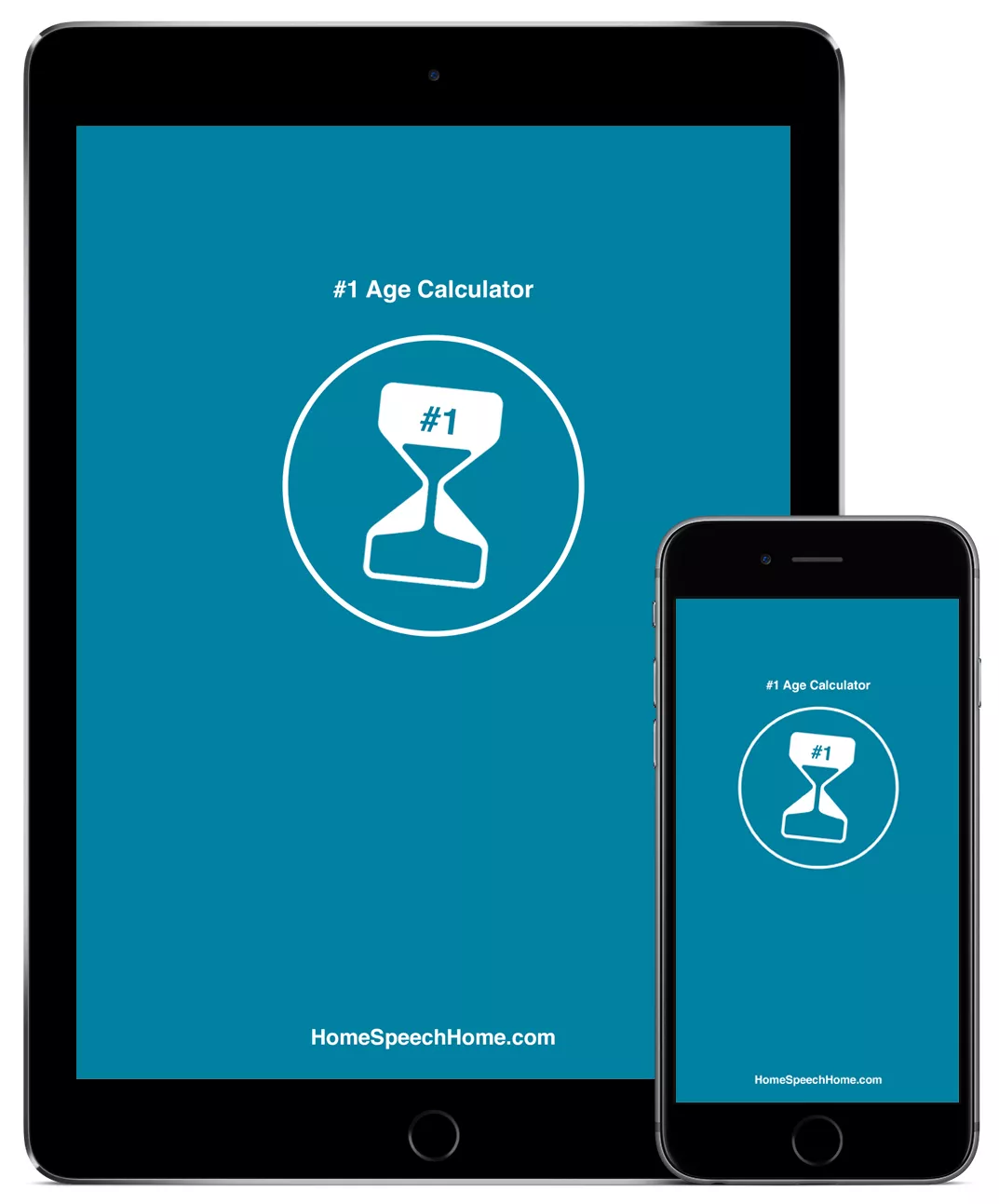
Popular Materials
All in one printable flashcards.
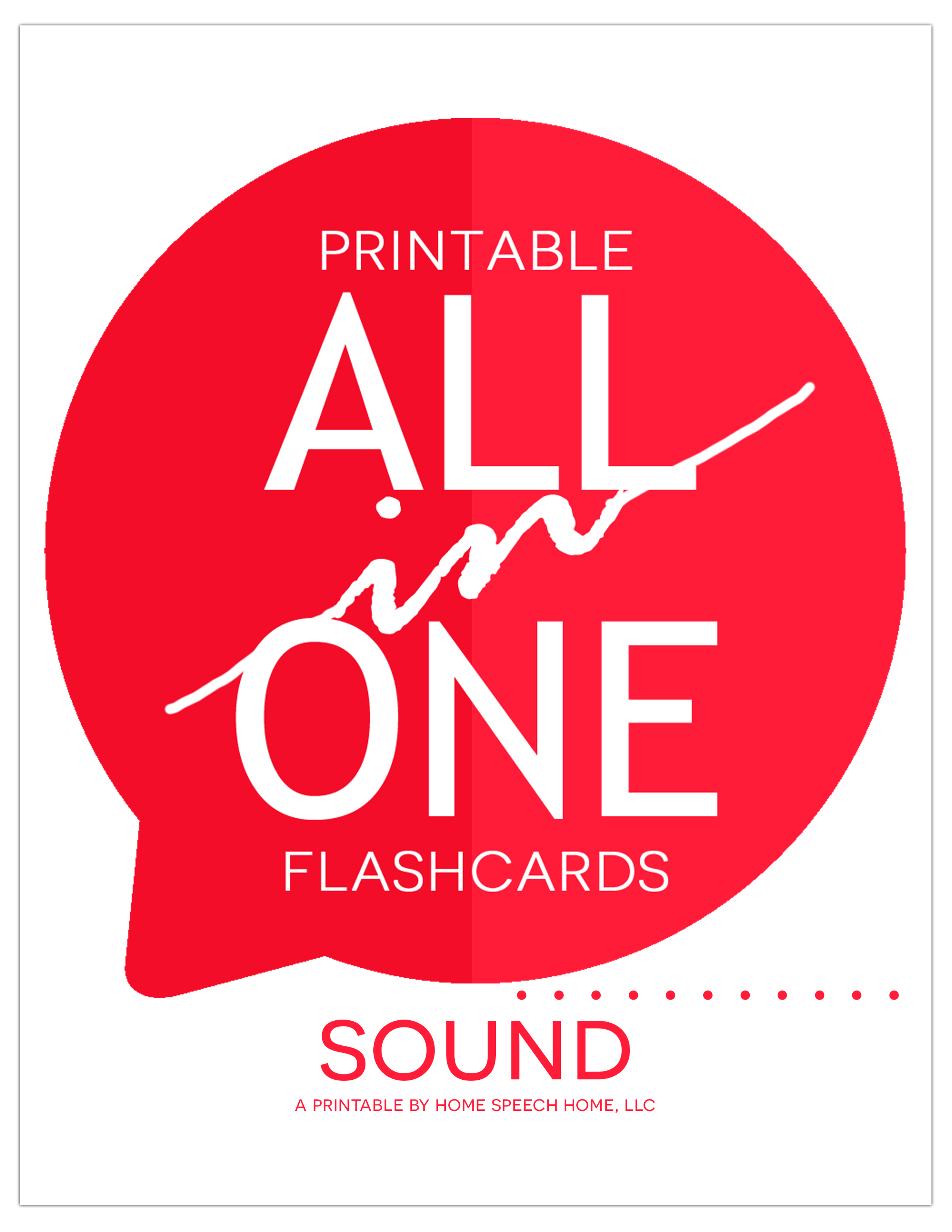
Multiple Meaning Word Mega Pack
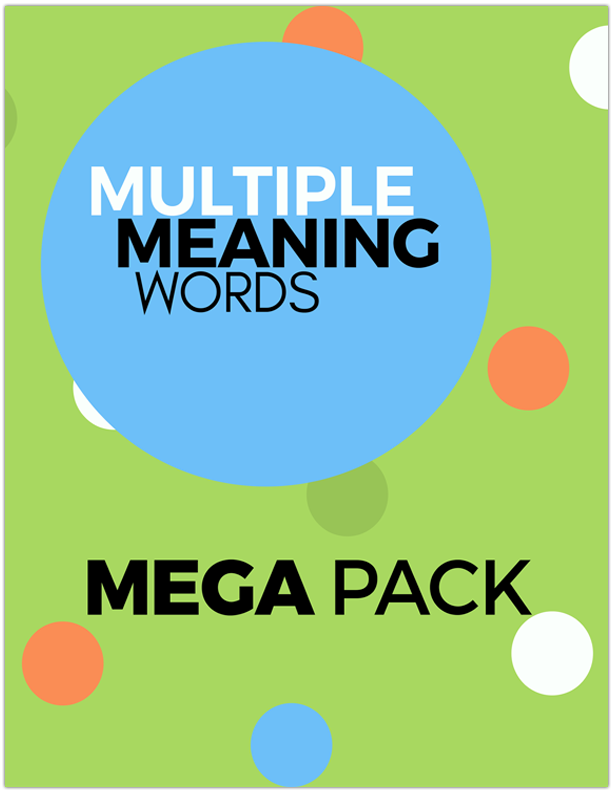
Complete Articulation Word Search
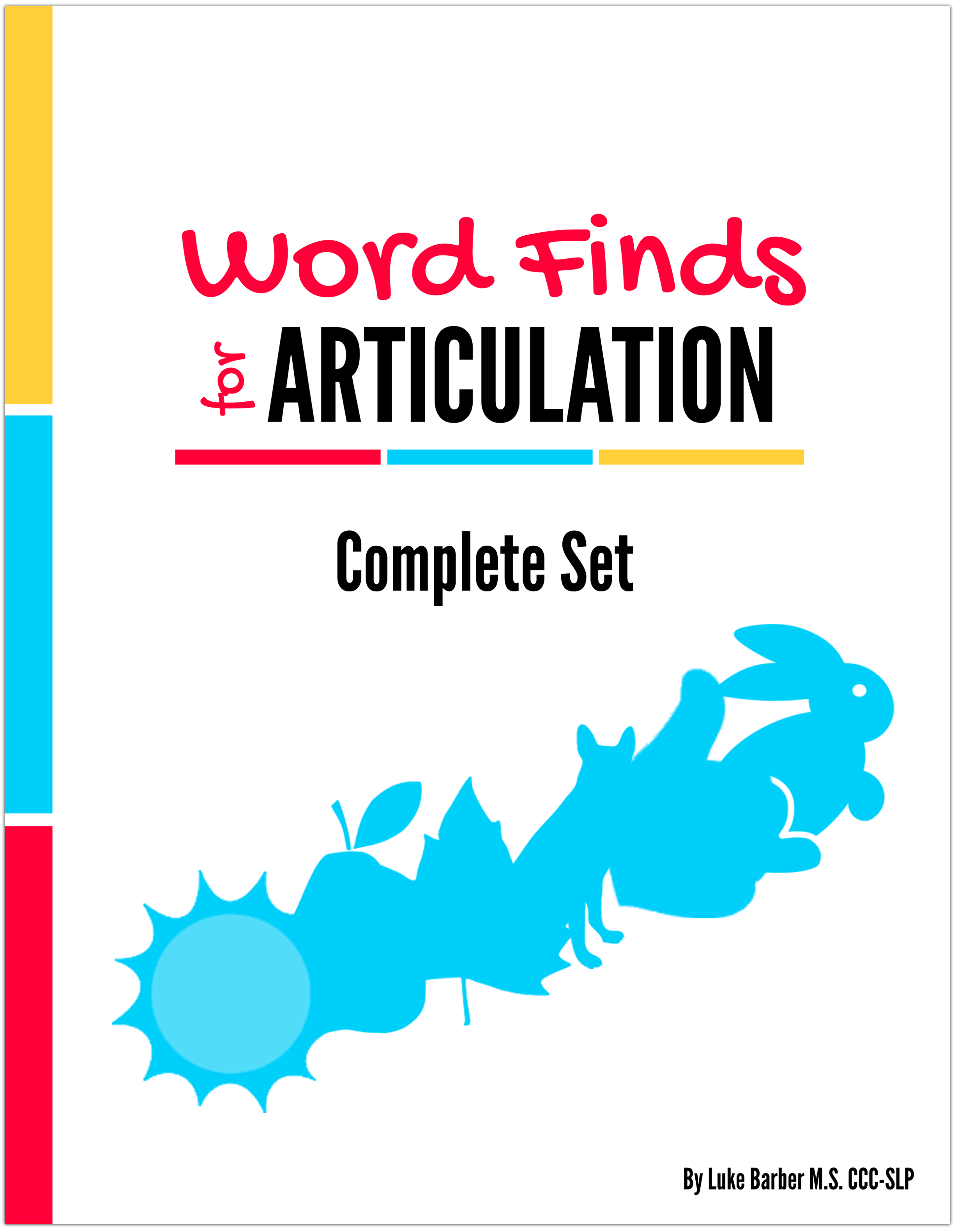
New! 111 Articulation Stories
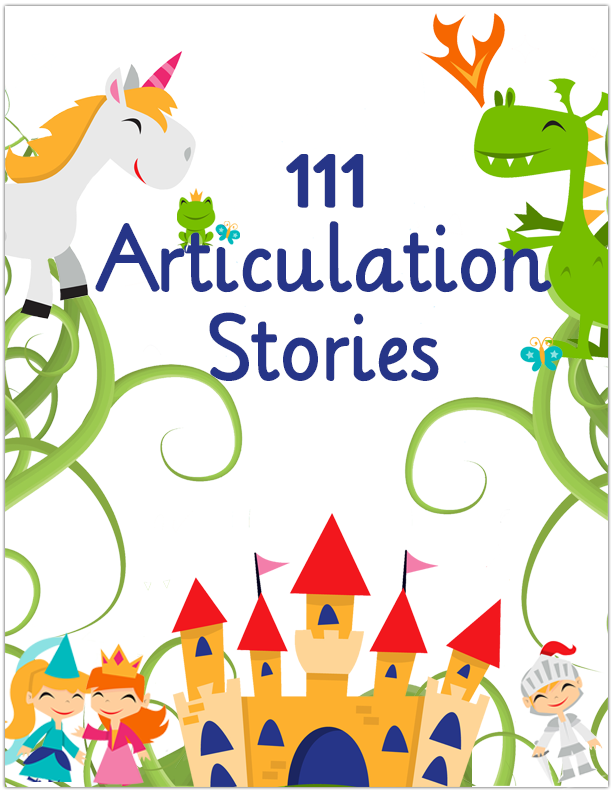
Teaching the Sound Books
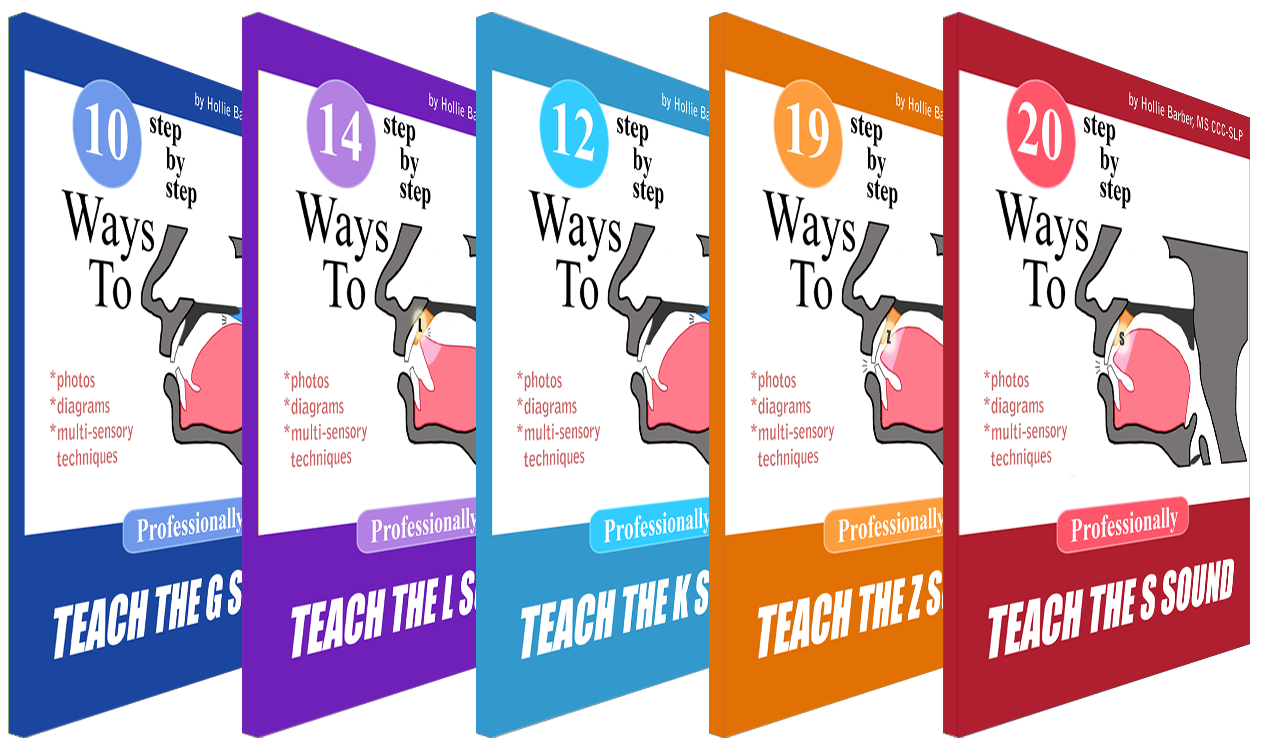
Multi-Syllabic Words Flashcards
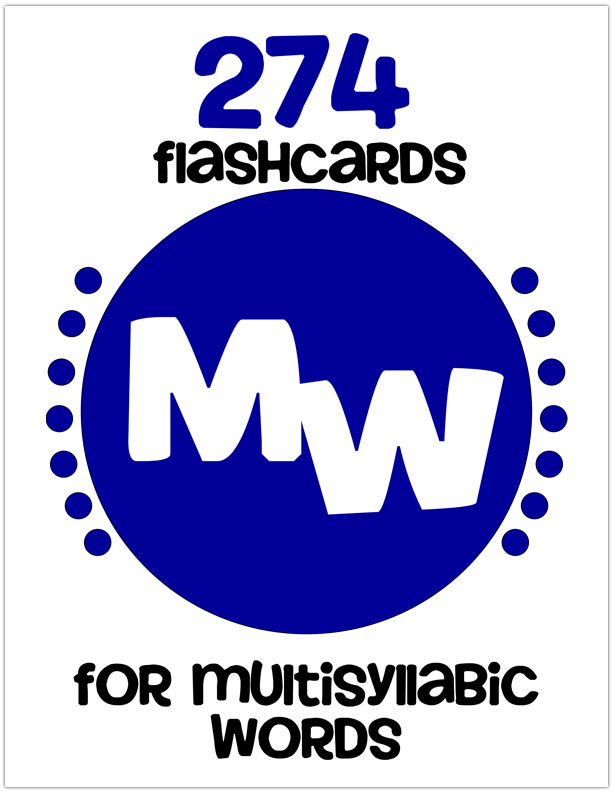
Apps to Save You Time & Help Your Clients
Articulation therapy + pirate adventures = awesomeness.

This App Will Get Your Kids Talking
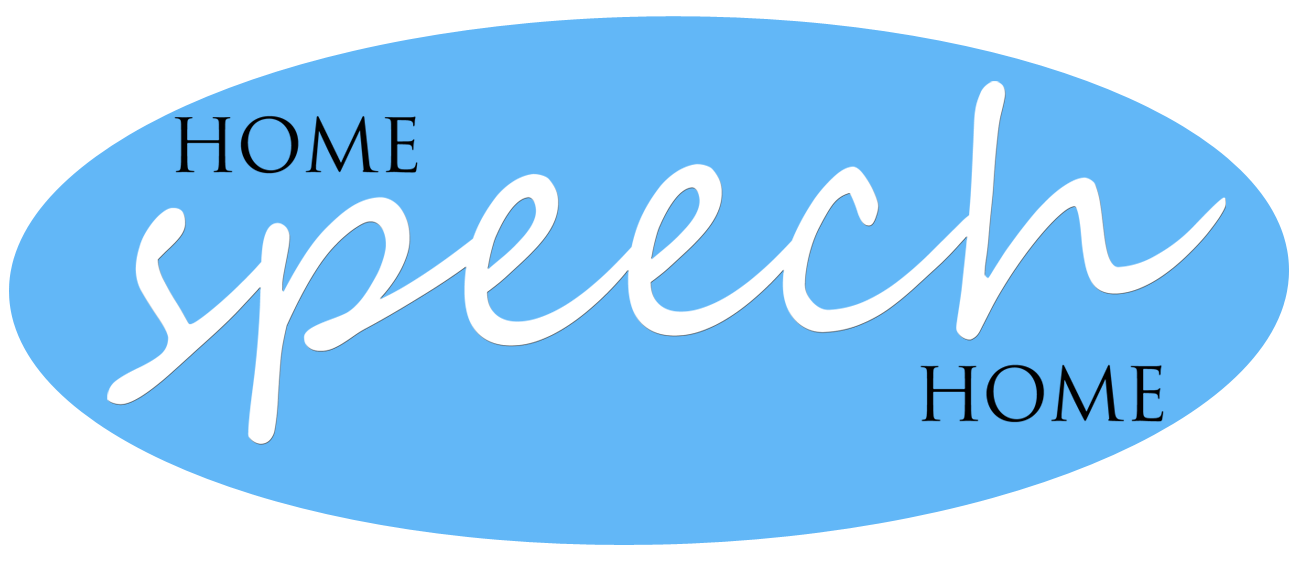
Image Credits
Copyright © 2010 –
HomeSpeechHome.com | All Rights Reserved
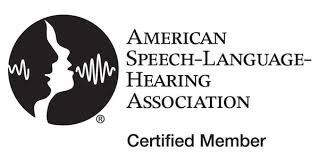
This website contains affiliate links, meaning if you buy something from them we may make some money (at no cost to you). By using our affiliate links, you are helping to support our site which is a U.S.-based, family-run small business :)
- The Vice Chancellor and Dean
- Facts and Figures
- Our Departments
- Zachry Engineering Education Complex
- Advising and Support
- Degree Programs
- Engineering Academies
- Online Degrees by Department
- Online Courses
- Engineering Global Programs
- Admissions and Aid
- Undergraduate Admissions
- Graduate Admissions
- Transfer Students
- Entry to a Major
- Explore Engineering Career Paths
- Visit With Us
- Student Life
- Find Your Community
- Get Creative
- Interact with Industry
- Solve Problems
- SuSu and Mark A. Fischer '72 Engineering Design Center
- Meloy Engineering Innovation and Entrepreneurship Program
- Undergraduate Research
- Autonomy and Robotics
- Education and Training Research
- Energy Systems and Services Research
- Health Care Research
- Infrastructure Research
- Materials and Manufacturing Research
- National Security and Safety Research
- Space Engineering
- Partner With Us
- PK-12 and Educators
- Researchers
- Reach Our Divisions
Problems Worth Solving Empowers Student Entrepreneurship
April 24, 2024 By Abriana Ciabattari
- Campus Community
- Current Students
- Entrepreneurship
The Meloy Engineering Innovation and Entrepreneurship Program hosted the second annual Problems Worth Solving, a College of Engineering pitch competition that invites Aggies of all majors to explore critical problems that could potentially serve as the basis for new startups or investments.
The Problems Worth Solving Competition does not ask students to find a solution to their problem; rather, students focus on pitching the significance of the problem itself. In this unique event format, participants navigate a critical element of entrepreneurship: the user experience.
The contest requires participants to submit an application of short answers and a 60-second video pitching their problem. The top five finalists are invited to present five-minute problem pitches live in front of judges.
The leading participants in this year's competition placed significant emphasis on customer discovery interviews and embraced a human-centered design approach by engaging in meaningful dialogue with the communities most impacted by the problems they chose to address.

Public health student Diana Salha won first place in the competition and a $2,500 award. Her pitch centered around themes from over 200 personal interviews with patients who were admitted to the hospital for routine surgery and contracted catheter-associated urinary tract infections (CAUTI), leaving them with the life-altering after-effects of sepsis. CAUTIs are the most common hospital-acquired infection in the U.S., yet as urinalysis tests are not often conducted until symptoms arise, it is incredibly hard to catch the infection before early sepsis.
“I think it’s really important to know how to tell a story in a concise and eloquent manner, and that can be really difficult sometimes,” explained Salha. “The team at Problems Worth Solving really helped a lot in getting me to the point where I could tell an effective story. The mentorship they brought throughout the process of this competition has given me skills that I will be able to use throughout my career.”
In addition to the first-place title, Salha earned the distinction of being a guest sponsor for a 2024-25 Aggies Invent centered around identifying solutions to her winning problem. Salha will have the opportunity to present the problem statements, offer guidance throughout the event and serve as one of the judges.

Pre-med computer engineering student Nathan Fernandes was awarded second place and $2,000 for presenting the problem of denied medical insurance claims. Insurance companies deny billions of claims annually in the U.S., and denied claims often remain unresolved, forcing doctors to increase the cost of services to the patient. Reimbursements commonly face delays and private practices lack the resources to employ specialized billing staff, exacerbating the situation.
“I almost didn’t apply to Problems Worth Solving because I was afraid I couldn’t do it, or that my ideas weren’t good enough,” Fernandes reflected. “With great mentors, I was able to see that my ideas are truly valuable. Overcoming imposter syndrome and gaining this confidence in myself is key to any endeavor I take part in, and I’m really grateful for this opportunity to do so.”

Alberto Rodriguez, a civil engineering master’s student with ten years of experience as an active-duty naval officer, was awarded third place and a $1,500 prize for his pitch surrounding the threat of unmanned aerial vehicles (UAVs) on international trade. UAVs are difficult to detect and expensive to combat, and they contribute to rising disruptions in the supply chain, which impacts the world economy and puts troops at risk.
“What we are seeing today is an unprecedented use of UAV technology aimed at harming global trade,” asserted Rodriguez. “Preserving trade is vital to preserving the American way of life. This problem is near and dear to me and is certainly a problem worth solving.”
Winning fourth place, doctoral chemical engineering student Aakansha Shaji presented a widespread problem found in her research: the complications associated with the Helicobacter pylori (H. pylori) bacterium. A chronic H. pylori infection can develop into gastritis, ulcers and stomach cancer, and most antibiotics are resistant to the bacteria.
“There is a long and rich history of investigators trying to understand how this bacterium causes extreme complications,” said Shaji. “Despite their greatest efforts, fighting it seems like an elusive dream.”
Fifth-place winner, biomedical engineering student Suchitaa Sawhney, shed light on the threats of antimicrobial resistance, a prevailing problem in the medical industry. This phenomenon occurs as germs and bacteria evolve to resist treatments, a cycle exacerbated by the persistent use of antibiotics.
Three honorable mentions — Reid Smith, Digvijaysinh Barad and Sam Rice — were awarded $250 each.
Problems Worth Solving is a unique event that offers students a platform to pitch problems that they’re passionate about, and often, ones that form the very foundation of their collegiate research endeavors.
“I wrote my college application essays about this problem,” Sawhney explained. “Now, as someone entering the healthcare field next year, this issue hits very close to home. It’s been my mission for the past four or five years — it’s imperative that we find a solution.”
- Facebook Facebook
- Twitter Twitter
- LinkedIn LinkedIn
- Email Email
- Print Print
- Share full article
For more audio journalism and storytelling, download New York Times Audio , a new iOS app available for news subscribers.

- April 26, 2024 • 21:50 Harvey Weinstein Conviction Thrown Out
- April 25, 2024 • 40:33 The Crackdown on Student Protesters
- April 24, 2024 • 32:18 Is $60 Billion Enough to Save Ukraine?
- April 23, 2024 • 30:30 A Salacious Conspiracy or Just 34 Pieces of Paper?
- April 22, 2024 • 24:30 The Evolving Danger of the New Bird Flu
- April 19, 2024 • 30:42 The Supreme Court Takes Up Homelessness
- April 18, 2024 • 30:07 The Opening Days of Trump’s First Criminal Trial
- April 17, 2024 • 24:52 Are ‘Forever Chemicals’ a Forever Problem?
- April 16, 2024 • 29:29 A.I.’s Original Sin
- April 15, 2024 • 24:07 Iran’s Unprecedented Attack on Israel
- April 14, 2024 • 46:17 The Sunday Read: ‘What I Saw Working at The National Enquirer During Donald Trump’s Rise’
- April 12, 2024 • 34:23 How One Family Lost $900,000 in a Timeshare Scam
Harvey Weinstein Conviction Thrown Out
New york’s highest appeals court has overturned the movie producer’s 2020 conviction for sex crimes, which was a landmark in the #metoo movement..
Hosted by Katrin Bennhold
Featuring Jodi Kantor
Produced by Nina Feldman , Rikki Novetsky and Carlos Prieto
Edited by M.J. Davis Lin and Liz O. Baylen
Original music by Dan Powell and Elisheba Ittoop
Engineered by Chris Wood
Listen and follow The Daily Apple Podcasts | Spotify | Amazon Music
When the Hollywood producer Harvey Weinstein was convicted of sex crimes four years ago, it was celebrated as a watershed moment for the #MeToo movement. Yesterday, New York’s highest court of appeals overturned that conviction.
Jodi Kantor, one of the reporters who broke the story of the abuse allegations against Mr. Weinstein in 2017, explains what this ruling means for him and for #MeToo.
On today’s episode

Jodi Kantor , an investigative reporter for The New York Times.

Background reading
The verdict against Harvey Weinstein was overturned by the New York Court of Appeals.
Here’s why the conviction was fragile from the start .
There are a lot of ways to listen to The Daily. Here’s how.
We aim to make transcripts available the next workday after an episode’s publication. You can find them at the top of the page.
The Daily is made by Rachel Quester, Lynsea Garrison, Clare Toeniskoetter, Paige Cowett, Michael Simon Johnson, Brad Fisher, Chris Wood, Jessica Cheung, Stella Tan, Alexandra Leigh Young, Lisa Chow, Eric Krupke, Marc Georges, Luke Vander Ploeg, M.J. Davis Lin, Dan Powell, Sydney Harper, Mike Benoist, Liz O. Baylen, Asthaa Chaturvedi, Rachelle Bonja, Diana Nguyen, Marion Lozano, Corey Schreppel, Rob Szypko, Elisheba Ittoop, Mooj Zadie, Patricia Willens, Rowan Niemisto, Jody Becker, Rikki Novetsky, John Ketchum, Nina Feldman, Will Reid, Carlos Prieto, Ben Calhoun, Susan Lee, Lexie Diao, Mary Wilson, Alex Stern, Dan Farrell, Sophia Lanman, Shannon Lin, Diane Wong, Devon Taylor, Alyssa Moxley, Summer Thomad, Olivia Natt, Daniel Ramirez and Brendan Klinkenberg.
Our theme music is by Jim Brunberg and Ben Landsverk of Wonderly. Special thanks to Sam Dolnick, Paula Szuchman, Lisa Tobin, Larissa Anderson, Julia Simon, Sofia Milan, Mahima Chablani, Elizabeth Davis-Moorer, Jeffrey Miranda, Renan Borelli, Maddy Masiello, Isabella Anderson and Nina Lassam.
Katrin Bennhold is the Berlin bureau chief. A former Nieman fellow at Harvard University, she previously reported from London and Paris, covering a range of topics from the rise of populism to gender. More about Katrin Bennhold
Jodi Kantor is a Pulitzer Prize-winning investigative reporter and co-author of “She Said,” which recounts how she and Megan Twohey broke the story of sexual abuse allegations against Harvey Weinstein, helping to ignite the #MeToo movement. Instagram • More about Jodi Kantor
Advertisement

IMAGES
VIDEO
COMMENTS
Problem-Solving Prior to Instruction" (EDU-2019-3907). Questionnaires to assess students' incoming cognitive and motivational characteristics (administered before the problem-solving phase) Effort Regulation (5-point Likert scale from "strongly disagree" to "strongly agree") 1.
3. Three-Act Tasks: Originally created by Dan Meyer, three-act tasks follow the three acts of a story. The first act is typically called the "setup," followed by the "confrontation" and then the "resolution.". This storyline process can be used in mathematics in which students encounter a contextual problem (e.g., a pool is being ...
This allows students to be lifelong learners and more flexible and adaptable in the future. -Dr. Steven Rougas, Director of the Doctoring Program, Alpert Medical School. Problem solving is a "goal-oriented" process that includes creating and manipulating problems as mental models (Jonassen, 2000). Brown faculty from a variety of disciplines ...
Here are the most common types: Academic Performance Questionnaire: A questionnaire sample aims to collect data on student's academic performance, including grades, study habits, and learning preferences, or it could be research questionnaires samples. Teacher Evaluation Questionnaire: It aims to gather students' feedback about their ...
The article presents the construction process and psychometric properties of the Critical Thinking Questionnaire (CThQ). ... approaches to provide students with more choices, problem-solving, and ...
Test Your Problem-Solving Skills. Personalize Your Emails Personalize your monthly updates from BrainFacts.org by choosing the topics that you care about most! Sign Up Find a Neuroscientist Engage local scientists to educate your community about the brain. ...
in this process, problem posing, is revealed and added by Gonzales (1994) who claims that it is one of the most significant and critical ways to acquire a deeper comprehension of the PS process. In this step, the student amends the problem just solved to obtain a similar one. 1.2. Mathematical Problem-solving
After solving the problem, the students filled out a self-monitoring questionnaire that requested them to reflect retrospectively on the metacognitive strategies they employed for the problem. In ...
In a new study, researchers examined how the presentation and format of practice questions influences students' problem-solving performance. The study revealed that mixed problem sets are better than questions arranged by topic. There are two types of question practice teachers can give their students. The first, blocked practice, involves ...
assessments strategies in enhancing students' problem solving skills, and we therefore remained with 20 studies that describe the assessment strategies in enhancing students' mathematical problem-solving skills. The remaining studies were published from 1997 until 2020. Besides the above-prescribed criteria, we only
Self-assessment, in the education framework, is a methodology that motivates students to play an active role in reviewing their performance. It is defined as "the evaluation or judgment of 'the worth' of one's performance and the identification of one's strengths and weaknesses with a view to improving one's learning outcomes". The goal of this research is to study the ...
The Problem-Solving Style Questionnaire (PSSQ) is a self -report questionnaire that measures four dimensions of problem-solving style: sensing, intuitive, feeling, and thinking. It was developed by Thomas Cassidy and Christopher Long in 1996, based on Carl Jung 's theory of psychological types.
Problem solving skills, student perception of skills, confidence, transfer. Introduction In his Partnership for 21st Century Learning Framework, Ken Kay (2010) invokes certain key skills today's students should develop: critical thinking and problem solving, creativity and innovation, and collaboration and communication. Problem solving skills
A number of research projects in educational assessment reveal that students struggle when it comes to accomplishing problem-solving tasks in Mathematics. Such a struggle is primarily due to the ...
Previous studies on quantitative physics problem solving have been concerned with students' using equations simply as a numerical computational tool. The current study started from a research question: "How do students solve conceptual physics questions in simulation-based formative assessments?" In the study, three first-year college students' interview data were analyzed to ...
Student Student's solution to the problem The student's approach to solving the problem Leyla 9X6=54 (1stsolution) 9+6=15 (2ndsolution) 7+6=13 15+13=28 to the solution. She was sure of the correctness of her She defined what was required as the sum of the ages of Can and Ece six years later.
71+ Social Problem Scenarios + 6 Blank Scenarios. Use the 71 social problem-solving scenarios to have your students get great experience practicing how to solve a social problem. Also, included are 6 blank scenarios. Then laminate them so you can use them over and over again. Therefore, create social problems that the student experiences and ...
Step 1: Find the Problem. (Questions 7, 12) Your score is 0 out of 0. Some problems are very obvious, however others are not so easily identified. As part of an effective problem-solving process, you need to look actively for problems - even when things seem to be running fine.
Home practice will make progress toward meeting individual language goals much faster. Speech-Language Pathologists (SLPs) are only able to see students/clients 30-60 mins (or less) per week. This is not enough time or practice for someone to handle Problem solving scenarios. Every day that your loved one goes without practice it becomes more ...
The game contains problem-solving questions, grouped into three levels of difficulty, and was assessed for its gender-related impact on students' interest and problem-solving skills.
A thinking skills questionnaire QUESTIONS Thinking skills and problem-solving skills are critically essential as we face an uncertain and opportunity-filled future. As lower-skilled and repetitive jobs disappear (e.g. cleaners, factory workers, laundry workers, etc) and working from home becomes more popular, new types of jobs are being created
Dereli-İman, E. (2013). The social problem-solving questionnaire: Evaluation of psychometric properties among Turkish primary school students. Egitim ... adaptation of the social problem-solving scale into Turkish for students in the first stage of primary school, and it analyzes the relationship between social problem solving and aggression.
It is entirely reasonable to feel unsure of how to support students with dyslexia in solving mathematics word problems. Mathematics difficulties often co-occur with reading disabilities (Willcutt et al., 2013), and the nature of word problems can make them a particularly difficult challenge for students with dyslexia.Word problems require orchestrating multiple skills and knowledge sources in ...
The Problems Worth Solving Competition does not ask students to find a solution to their problem; rather, students focus on pitching the significance of the problem itself. In this unique event format, participants navigate a critical element of entrepreneurship: the user experience.
Solving complex problems is crucial for navigating the 21st century's development. The primary measure of academic accomplishment required in both educational and occupational settings and daily life is this competency. However, especially when learning mathematics, students still have relatively limited skills in addressing complex problems. Including students' experiences and knowledge from ...
Students who feel strongly about the Palestinian cause feel like the point is disruption, that something so big, and immediate, and urgent is happening that they need to get in the faces of their ...
Darmawan & Ramlah's research (2021) with the article title analysis of students' mathematical problem-solving abilities in solving TIMSS questions based on Polya stages. Based on the research above, it can be concluded that research related to students' problem-solving abilities is often the object of research.
Harvey Weinstein Conviction Thrown Out New York's highest appeals court has overturned the movie producer's 2020 conviction for sex crimes, which was a landmark in the #MeToo movement.