Prenatal stress: Effects on fetal and child brain development
Affiliations.
- 1 Institute of Psychiatry, Psychology and Neuroscience, King's College London, London, United Kingdom; Perinatal Imaging and Health, Centre for the Developing Brain, School of Biomedical Engineering and Imaging Sciences, King's College London, London, United Kingdom.
- 2 Institute of Psychiatry, Psychology and Neuroscience, King's College London, London, United Kingdom; National Female Hormone Clinic, Maudsley Hospital, SLaM NHS Foundation Trust, London, United Kingdom.
- 3 Visiting Professor of Perinatal Psychobiology, Imperial College London, London, United Kingdom. Electronic address: [email protected].
- PMID: 32204831
- DOI: 10.1016/bs.irn.2019.11.002
The impact of stress on brain health begins in the womb. Both animal and human studies have found that prenatal maternal stress affects the brain and behavior of the offspring. Stressful life events, exposure to a natural disaster, and symptoms of maternal anxiety and depression increase the risk for the child having a range of emotional, behavioral and/or cognitive problems in later life. These include depression, anxiety, Attention Deficit Hyperactivity Disorder (ADHD), and/or conduct disorders. There is an increased risk for other outcomes also, including preterm delivery and reduced telomere length, possibly indicative of an accelerated life history. The causal role of prenatal maternal stress on the etiology of the neurodevelopmental disorders is supported by large population cohorts, which have controlled for a wide range of potential confounders, including postnatal maternal mood. More recently, research has begun to explore the biological correlates and mediators of these findings. These studies suggest that the hypothalamic pituitary adrenal (HPA) axis plays a role in mediating the effects of maternal stress on the fetal brain. Further, in vivo brain imaging research reports that maternal stress is associated with changes in limbic and frontotemporal networks, and the functional and microstructural connections linking them. The structural changes include cortical thinning and an enlarged amygdala. While these studies have been conducted on smaller sample sizes and could not control for many confounders, the observed brain changes do plausibly underlie many of the emotional, behavioral and cognitive changes found to be associated with prenatal stress.
Keywords: Brain; Cortisol; Fetus; MRI; Prenatal; Psychopathology; Stress.
© 2020 Elsevier Inc. All rights reserved.

Publication types
- Amygdala* / diagnostic imaging
- Amygdala* / growth & development
- Amygdala* / pathology
- Cerebral Cortex* / diagnostic imaging
- Cerebral Cortex* / growth & development
- Cerebral Cortex* / pathology
- Hydrocortisone / metabolism*
- Hypothalamo-Hypophyseal System* / metabolism
- Hypothalamo-Hypophyseal System* / physiopathology
- Mental Disorders* / etiology
- Mental Disorders* / metabolism
- Mental Disorders* / pathology
- Mental Disorders* / physiopathology
- Neurodevelopmental Disorders* / etiology
- Neurodevelopmental Disorders* / metabolism
- Neurodevelopmental Disorders* / pathology
- Neurodevelopmental Disorders* / physiopathology
- Neuroimaging*
- Prenatal Exposure Delayed Effects* / metabolism
- Prenatal Exposure Delayed Effects* / pathology
- Prenatal Exposure Delayed Effects* / physiopathology
- Stress, Psychological* / complications
- Stress, Psychological* / metabolism
- Stress, Psychological* / pathology
- Stress, Psychological* / physiopathology
- Hydrocortisone
- Subject List
- Take a Tour
- For Authors
- Subscriber Services
- Publications
- African American Studies
- African Studies
- American Literature
- Anthropology
- Architecture Planning and Preservation
- Art History
- Atlantic History
- Biblical Studies
- British and Irish Literature
- Childhood Studies
- Chinese Studies
- Cinema and Media Studies
- Communication
- Criminology
- Environmental Science
- Evolutionary Biology
- International Law
- International Relations
- Islamic Studies
- Jewish Studies
- Latin American Studies
- Latino Studies
- Linguistics
- Literary and Critical Theory
- Medieval Studies
- Military History
- Political Science
- Public Health
- Renaissance and Reformation
- Social Work
- Urban Studies
- Victorian Literature
- Browse All Subjects
How to Subscribe
- Free Trials
In This Article Expand or collapse the "in this article" section Prenatal Development
Introduction, general overviews.
- Fetal Brain Anatomy
- Fetal Sensation
- Fetal Temperament
- Fetal Learning and Memory
- Fetal Programming
- Exposure to Nicotine
- Exposure to Alcohol
- Exposure to Antipsychotic and Psychotropic Drugs
- Exposure to Illegal Drugs
- Polydrug Exposure
- Exposure to Maternal Depression and Anxiety
- Exposure to Maternal Stress and Distress
- Exposure to Maternal Weight Gain and Obesity
- Influence of Prenatal Attachment
- Moderators of Prenatal Development
Related Articles Expand or collapse the "related articles" section about
About related articles close popup.
Lorem Ipsum Sit Dolor Amet
Vestibulum ante ipsum primis in faucibus orci luctus et ultrices posuere cubilia Curae; Aliquam ligula odio, euismod ut aliquam et, vestibulum nec risus. Nulla viverra, arcu et iaculis consequat, justo diam ornare tellus, semper ultrices tellus nunc eu tellus.
- Developmental Psychology (Cognitive)
- Developmental Psychology (Social)
- Emerging Adulthood
- Life-Span Development
- Twin Studies
Other Subject Areas
Forthcoming articles expand or collapse the "forthcoming articles" section.
- Data Visualization
- Remote Work
- Workforce Training Evaluation
- Find more forthcoming articles...
- Export Citations
- Share This Facebook LinkedIn Twitter
Prenatal Development by Victoria Molfese , Amanda Prokasky , Kathleen Moritz Rudasill , Ibrahim Hakki Acar , Xiaoqing Tu , Kate Sirota , Brian Keiser LAST REVIEWED: 27 November 2013 LAST MODIFIED: 27 November 2013 DOI: 10.1093/obo/9780199828340-0135
For decades, researchers have investigated how events in the prenatal period impact women and their infants. These studies, particularly by researchers in the medical, neuroscience, and behavioral science fields, led to discoveries of important information regarding the prenatal events that were strongly associated with mortality (or death) and morbidity (or incidences of injury, pathology and abnormalities/anomalies, and neurobehavioral sequelae) in the neonatal and infancy periods. Among the many common findings from early research studies, two are particularly noteworthy. First, maternal and fetal risk conditions arising in the prenatal period do not do so in isolation. Sameroff and Chandler characterized this as a “continuum of reproductive casualty,” in which several risks become linked together and affect events during pregnancy, outcomes at birth, and in infant and child development in subsequent years (Sameroff, Arnold. J., and Michael J. Chandler. 1975. Reproductive risk and the continuum of caretaker casualty. In Review of Child Development Research . Vol. 4. Edited by Francis D. Horowitz, 187–244. Chicago: University of Chicago Press). Second, the impacts of these risk events on outcomes were found to vary, and not all pregnancies nor all neonates and infants were impacted to the same extent, if at all. Indeed, Sameroff and Chandler addressed the variability or uncertainty of impacts of prenatal events by adding a “continuum of caretaking casualty” to their model to include the important roles of family, society, and the environment. This resulting “transactional model of development” brought attention to the importance of genetic, biological, and environmental interactions before and after birth on the outcomes observed in neonates, infants, and children. Across time, research interests in prenatal and perinatal risks and their impacts on neonates, infants, and children have expanded to the extent that many variables heavily researched in the 1970s, 1980s, and 1990s are no longer hot topics. Instead, advances in research and meta-analysis designs, statistical and data modeling, new technologies, and multidisciplinary collaborations are enabling investigations that were either not attempted in the past or only to a limited extent.
The authors would like to thank Jayden Nord for his invaluable input and assistance on this article.
The framework for the publications included in this article is built from the writings of several authors whose works have important implications for understanding prenatal development and infant outcomes. These writings in particular have addressed the relation between gene-environment interactions, sometimes now framed as fetal programming, or the resetting of genetic programming during the fetal period due to environmental variables. There is evidence that fetal programming can change outcomes in the neonatal period and across the life span. While discussions of gene-environment interactions are not new, what is new are the efforts of researchers in different fields to understand whether and how genetic influences set trajectories for outcomes that may be moderated by environmental variables. Classic publications on genetic and environmental interactions include Waddington 1957 , describing an “epigenetic landscape” depicting how the environment can influence the path of development set in motion by genes. Waddington’s theoretical and research contributions are well described by in the biography Slack 2002 and in McLearn 1970 , in a chapter on genetics and behavioral development. From these publications we have a better understanding of the application of fetal programming—an application to frame reconsiderations of preterm infant care, as described in Schug, et al. 2012 ; and a broader understanding of the continuum of prenatal development and infant outcomes, as presented in Hopkins and Johnson 2005 . These publications, plus Sameroff and Chandler 1975 , frame this article’s focus on research investigations of fetal development, evidence of fetal programming, and the influence of prenatal events on infant outcomes, such as prenatal exposures to maternal drug use, mental health problems, obstetric events such as weight grain, and mother-infant attachment. Finally, there is a section on multivariate designs enabling investigations of the effects of moderating or mediating variables in studies of prenatal development and infant outcomes. Together, these publications reflect current pathways in research on prenatal development.
Hopkins, Brian, and Scott P. Johnson, eds. 2005. Prenatal Development of Postnatal Functions . Westport, CT: Praeger.
The seven chapters in this book focus on the continuity between prenatal events and postnatal outcomes. Chapters on the sensory and vestibular systems, brain structure and function, and the impacts of maternal anxiety and stress link theories and findings related to fetal programming, showing that early life events are detectable across the life span.
McLearn, Gearld. 1970. Genetic influences on behavior and development. In Carmichael’s manual of child psychology . 3d ed. Edited by Paul H. Mussen, 39–76. New York: Wiley.
This chapter addresses behavior genetics and includes a description of Waddington’s epigenetic landscape, complete with a graphic representation and examples from animal and human research. Although research citations are dated, there is a clear presentation on the basics of genetics, and behavior genetics intended to inform researchers focusing on human development.
Sameroff, Arnold J., and Michael J. Chandler. 1975. Reproductive risk and the continuum of caretaker casualty. In Review of Child Development Research . Vol. 4. Edited by Francis D. Horowitz, M. Hetherington, S. Scarr-Salapatek, and G. Siegel, 187–244. Chicago: Univ. of Chicago Press.
This is a classic chapter focused on variables in the prenatal and perinatal periods that create risks for pregnancy outcomes and the development of neonates and infants. Research is reviewed on several reproductive risks and stressors as well as examples of research illustrating how caretaking casualty negatively impacts infant outcomes.
Schug, Thaddeus T., Adrian Erlebacher, Sarah Leibowitz, et al. 2012. Fetal programming and environmental exposures: Implications for prenatal care and preterm birth. Annals of the New York Academy of Sciences 1276:37–46.
DOI: 10.1111/nyas.12003
The chapters herein present findings from multiple fields relevant to fetal programming through investigations of gene-gene and gene-environment interactions (or epigenetics) during the prenatal period. The conference goal is to use research findings to improve understanding of preterm birth and improve obstetric care. Available online for purchase or by subscription.
Slack, Jonathan M. W. 2002. Conrad Hal Waddington: The last Renaissance biologist? Nature Reviews Genetics 3:889–895.
DOI: 10.1038/nrg933
This is a biography of the life and works of Conrad Waddington and with relevance to current research on the human genome. Details of Waddington’s work preceding and succeeding the publication of The Strategy of the Genes that introduces the “epigenetic landscape” helps put Waddington’s work into perspective. Available online for purchase or by subscription.
Waddington, Conrad H. 1957. The Strategy of the Genes . New York: Macmillian.
This classic work has complex but detailed descriptions of the epigenetic landscape. The pathways (“chreodes” or “creodes”) and the paths (“canalization”) of cell change are described along with mechanisms by which perturbations can occur. How genetic variation and environmental influences reach levels necessary for pathway changes are described.
back to top
Users without a subscription are not able to see the full content on this page. Please subscribe or login .
Oxford Bibliographies Online is available by subscription and perpetual access to institutions. For more information or to contact an Oxford Sales Representative click here .
- About Psychology »
- Meet the Editorial Board »
- Abnormal Psychology
- Academic Assessment
- Acculturation and Health
- Action Regulation Theory
- Action Research
- Addictive Behavior
- Adolescence
- Adoption, Social, Psychological, and Evolutionary Perspect...
- Advanced Theory of Mind
- Affective Forecasting
- Affirmative Action
- Ageism at Work
- Allport, Gordon
- Alzheimer’s Disease
- Ambulatory Assessment in Behavioral Science
- Analysis of Covariance (ANCOVA)
- Animal Behavior
- Animal Learning
- Anxiety Disorders
- Art and Aesthetics, Psychology of
- Artificial Intelligence, Machine Learning, and Psychology
- Assessment and Clinical Applications of Individual Differe...
- Attachment in Social and Emotional Development across the ...
- Attention-Deficit/Hyperactivity Disorder (ADHD) in Adults
- Attention-Deficit/Hyperactivity Disorder (ADHD) in Childre...
- Attitudinal Ambivalence
- Attraction in Close Relationships
- Attribution Theory
- Authoritarian Personality
- Bayesian Statistical Methods in Psychology
- Behavior Therapy, Rational Emotive
- Behavioral Economics
- Behavioral Genetics
- Belief Perseverance
- Bereavement and Grief
- Biological Psychology
- Birth Order
- Body Image in Men and Women
- Bystander Effect
- Categorical Data Analysis in Psychology
- Childhood and Adolescence, Peer Victimization and Bullying...
- Clark, Mamie Phipps
- Clinical Neuropsychology
- Clinical Psychology
- Cognitive Consistency Theories
- Cognitive Dissonance Theory
- Cognitive Neuroscience
- Communication, Nonverbal Cues and
- Comparative Psychology
- Competence to Stand Trial: Restoration Services
- Competency to Stand Trial
- Computational Psychology
- Conflict Management in the Workplace
- Conformity, Compliance, and Obedience
- Consciousness
- Coping Processes
- Correspondence Analysis in Psychology
- Counseling Psychology
- Creativity at Work
- Critical Thinking
- Cross-Cultural Psychology
- Cultural Psychology
- Daily Life, Research Methods for Studying
- Data Science Methods for Psychology
- Data Sharing in Psychology
- Death and Dying
- Deceiving and Detecting Deceit
- Defensive Processes
- Depressive Disorders
- Development, Prenatal
- Diagnostic and Statistical Manual of Mental Disorders (DSM...
- Discrimination
- Dissociative Disorders
- Drugs and Behavior
- Eating Disorders
- Ecological Psychology
- Educational Settings, Assessment of Thinking in
- Effect Size
- Embodiment and Embodied Cognition
- Emotional Intelligence
- Empathy and Altruism
- Employee Stress and Well-Being
- Environmental Neuroscience and Environmental Psychology
- Ethics in Psychological Practice
- Event Perception
- Evolutionary Psychology
- Expansive Posture
- Experimental Existential Psychology
- Exploratory Data Analysis
- Eyewitness Testimony
- Eysenck, Hans
- Factor Analysis
- Festinger, Leon
- Five-Factor Model of Personality
- Flynn Effect, The
- Forensic Psychology
- Forgiveness
- Friendships, Children's
- Fundamental Attribution Error/Correspondence Bias
- Gambler's Fallacy
- Game Theory and Psychology
- Geropsychology, Clinical
- Global Mental Health
- Habit Formation and Behavior Change
- Health Psychology
- Health Psychology Research and Practice, Measurement in
- Heider, Fritz
- Heuristics and Biases
- History of Psychology
- Human Factors
- Humanistic Psychology
- Implicit Association Test (IAT)
- Industrial and Organizational Psychology
- Inferential Statistics in Psychology
- Insanity Defense, The
- Intelligence
- Intelligence, Crystallized and Fluid
- Intercultural Psychology
- Intergroup Conflict
- International Classification of Diseases and Related Healt...
- International Psychology
- Interviewing in Forensic Settings
- Intimate Partner Violence, Psychological Perspectives on
- Introversion–Extraversion
- Item Response Theory
- Law, Psychology and
- Lazarus, Richard
- Learned Helplessness
- Learning Theory
- Learning versus Performance
- LGBTQ+ Romantic Relationships
- Lie Detection in a Forensic Context
- Locus of Control
- Loneliness and Health
- Mathematical Psychology
- Meaning in Life
- Mechanisms and Processes of Peer Contagion
- Media Violence, Psychological Perspectives on
- Mediation Analysis
- Memories, Autobiographical
- Memories, Flashbulb
- Memories, Repressed and Recovered
- Memory, False
- Memory, Human
- Memory, Implicit versus Explicit
- Memory in Educational Settings
- Memory, Semantic
- Meta-Analysis
- Metacognition
- Metaphor, Psychological Perspectives on
- Microaggressions
- Military Psychology
- Mindfulness
- Mindfulness and Education
- Minnesota Multiphasic Personality Inventory (MMPI)
- Money, Psychology of
- Moral Conviction
- Moral Development
- Moral Psychology
- Moral Reasoning
- Nature versus Nurture Debate in Psychology
- Neuroscience of Associative Learning
- Nonergodicity in Psychology and Neuroscience
- Nonparametric Statistical Analysis in Psychology
- Observational (Non-Randomized) Studies
- Obsessive-Complusive Disorder (OCD)
- Occupational Health Psychology
- Olfaction, Human
- Operant Conditioning
- Optimism and Pessimism
- Organizational Justice
- Parenting Stress
- Parenting Styles
- Parents' Beliefs about Children
- Path Models
- Peace Psychology
- Perception, Person
- Performance Appraisal
- Personality and Health
- Personality Disorders
- Personality Psychology
- Person-Centered and Experiential Psychotherapies: From Car...
- Phenomenological Psychology
- Placebo Effects in Psychology
- Play Behavior
- Positive Psychological Capital (PsyCap)
- Positive Psychology
- Posttraumatic Stress Disorder (PTSD)
- Prejudice and Stereotyping
- Pretrial Publicity
- Prisoner's Dilemma
- Problem Solving and Decision Making
- Procrastination
- Prosocial Behavior
- Prosocial Spending and Well-Being
- Protocol Analysis
- Psycholinguistics
- Psychological Literacy
- Psychological Perspectives on Food and Eating
- Psychology, Political
- Psychoneuroimmunology
- Psychophysics, Visual
- Psychotherapy
- Psychotic Disorders
- Publication Bias in Psychology
- Reasoning, Counterfactual
- Rehabilitation Psychology
- Relationships
- Reliability–Contemporary Psychometric Conceptions
- Religion, Psychology and
- Replication Initiatives in Psychology
- Research Methods
- Risk Taking
- Role of the Expert Witness in Forensic Psychology, The
- Sample Size Planning for Statistical Power and Accurate Es...
- Schizophrenic Disorders
- School Psychology
- School Psychology, Counseling Services in
- Self, Gender and
- Self, Psychology of the
- Self-Construal
- Self-Control
- Self-Deception
- Self-Determination Theory
- Self-Efficacy
- Self-Esteem
- Self-Monitoring
- Self-Regulation in Educational Settings
- Self-Report Tests, Measures, and Inventories in Clinical P...
- Sensation Seeking
- Sex and Gender
- Sexual Minority Parenting
- Sexual Orientation
- Signal Detection Theory and its Applications
- Simpson's Paradox in Psychology
- Single People
- Single-Case Experimental Designs
- Skinner, B.F.
- Sleep and Dreaming
- Small Groups
- Social Class and Social Status
- Social Cognition
- Social Neuroscience
- Social Support
- Social Touch and Massage Therapy Research
- Somatoform Disorders
- Spatial Attention
- Sports Psychology
- Stanford Prison Experiment (SPE): Icon and Controversy
- Stereotype Threat
- Stereotypes
- Stress and Coping, Psychology of
- Student Success in College
- Subjective Wellbeing Homeostasis
- Taste, Psychological Perspectives on
- Teaching of Psychology
- Terror Management Theory
- Testing and Assessment
- The Concept of Validity in Psychological Assessment
- The Neuroscience of Emotion Regulation
- The Reasoned Action Approach and the Theories of Reasoned ...
- The Weapon Focus Effect in Eyewitness Memory
- Theory of Mind
- Therapy, Cognitive-Behavioral
- Thinking Skills in Educational Settings
- Time Perception
- Trait Perspective
- Trauma Psychology
- Type A Behavior Pattern (Coronary Prone Personality)
- Unconscious Processes
- Video Games and Violent Content
- Virtues and Character Strengths
- Women and Science, Technology, Engineering, and Math (STEM...
- Women, Psychology of
- Work Well-Being
- Wundt, Wilhelm
- Privacy Policy
- Cookie Policy
- Legal Notice
- Accessibility
Powered by:
- [66.249.64.20|45.133.227.243]
- 45.133.227.243
Thank you for visiting nature.com. You are using a browser version with limited support for CSS. To obtain the best experience, we recommend you use a more up to date browser (or turn off compatibility mode in Internet Explorer). In the meantime, to ensure continued support, we are displaying the site without styles and JavaScript.
- View all journals
- My Account Login
- Explore content
- About the journal
- Publish with us
- Sign up for alerts
- Open access
- Published: 29 June 2021
Interactive relations between maternal prenatal stress, fetal brain connectivity, and gestational age at delivery
- Moriah E. Thomason ORCID: orcid.org/0000-0001-9745-1147 1 , 2 , 3 ,
- Jasmine L. Hect 4 ,
- Rebecca Waller 5 &
- Paul Curtin 6
Neuropsychopharmacology volume 46 , pages 1839–1847 ( 2021 ) Cite this article
6362 Accesses
30 Citations
37 Altmetric
Metrics details
- Neural patterning
- Risk factors
Studies reporting significant associations between maternal prenatal stress and child outcomes are frequently confounded by correlates of prenatal stress that influence the postnatal rearing environment. The major objective of this study is to identify whether maternal prenatal stress is associated with variation in human brain functional connectivity prior to birth. We utilized fetal fMRI in 118 fetuses [48 female; mean age 32.9 weeks ( SD = 3.87)] to evaluate this association and further addressed whether fetal neural differences were related to maternal health behaviors, social support, or birth outcomes. Community detection was used to empirically define networks and enrichment was used to isolate differential within- or between-network connectivity effects. Significance for χ 2 enrichment was determined by randomly permuting the subject pairing of fetal brain connectivity and maternal stress values 10,000 times. Mixtures modelling was used to test whether fetal neural differences were related to maternal health behaviors, social support, or birth outcomes. Increased maternal prenatal negative affect/stress was associated with alterations in fetal frontoparietal, striatal, and temporoparietal connectivity (β = 0.82, p < 0.001). Follow-up analysis demonstrated that these associations were stronger in women with better health behaviors, more positive interpersonal support, and lower overall stress (β = 0.16, p = 0.02). Additionally, magnitude of stress-related differences in neural connectivity was marginally correlated with younger gestational age at delivery (β = −0.18, p = 0.05). This is the first evidence that negative affect/stress during pregnancy is reflected in functional network differences in the human brain in utero, and also provides information about how positive interpersonal and health behaviors could mitigate prenatal brain programming.
Similar content being viewed by others
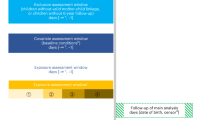
Maternal diabetes and risk of attention-deficit/hyperactivity disorder in offspring in a multinational cohort of 3.6 million mother–child pairs
Adrienne Y. L. Chan, Le Gao, … Ian C. K. Wong
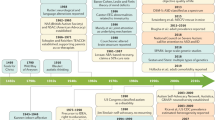
Autism spectrum disorder
Catherine Lord, Traolach S. Brugha, … Jeremy Veenstra-VanderWeele
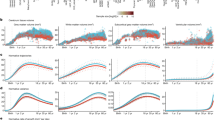
Brain charts for the human lifespan
R. A. I. Bethlehem, J. Seidlitz, … A. F. Alexander-Bloch
Introduction
Children born to mothers who endure heightened psychological or physiological stress during pregnancy may experience negative consequences as a result of these early programming events. Injurious effects of prenatal stress can cut across domains, increasing risk for neuropsychiatric and neurodevelopmental disorders [ 1 , 2 , 3 , 4 ], altering hormonal [ 5 , 6 , 7 ] and physiological [ 8 , 9 , 10 ] bodily responses, and increasing susceptibility to a range of disease processes [ 11 , 12 , 13 , 14 ]. Studies of fetal behavior and physiology suggest that maternal prenatal stress may influence fetal brain development even before birth [ 15 , 16 , 17 ]. Furthermore, negative consequences of early stress exposure appear to be lasting, affecting even the prevalence of adult neurodegenerative disorders [ 18 ] thus shaping long-term outcomes and potentially contributing to the transfer of risk to subsequent generations [ 19 , 20 ].
A long-standing challenge for this crucial area of research is that children born to mothers exposed to high levels of prenatal stress experience more birth complications [ 21 ], and are more frequently reared in high-stress environments [ 22 ], confounding our ability to conclude from postnatal brain measurements that the fetal brain is altered before birth. Animal studies show that prenatal stress results in shorter and less complex dendrites, hypomyelination, and altered synaptogenesis [ 23 , 24 , 25 , 26 ]. Postnatal studies in neonates and children corroborate these observations showing that stress alters newborn functional neural connectivity [ 27 , 28 , 29 ] and child structural brain development [ 30 ]. Importantly, differences in child brain structure have been shown to mediate, in part, the association between prenatal stress and affective problems in childhood [ 31 ]. While such findings suggest that prenatal stress alters the fetal brain, examining these outcomes postnatally represents a major limitation. Prospective evidence that the fetal brain is altered is needed to provide stronger evidence that maternal stress during pregnancy impacts the human brain in utero.
The present study addresses this gap by leveraging emergent functional magnetic resonance imaging (fMRI) techniques to evaluate, for the first time, whether and how variation in maternal stress relates to human fetal brain system organization. Resting-state functional connectivity (RSFC) fMRI has recently been adapted to study fetal brain functional network development [ 32 , 33 ]. This technique relies on recording spontaneous functional signals across the whole brain, and then evaluating covariation and interaction between signals over time. Brain regions that demonstrate coordinated activity are considered “functionally connected”, which has been shown to have an anatomical basis [ 34 , 35 , 36 ]. This technique has borne rapid insight into the global coordination of fetal brain activity and the wide scale architecture of brain networks. There is now evidence that RSFC develops initially in utero [ 37 , 38 , 39 , 40 , 41 ], is altered in fetuses with atypical neuroanatomy [ 42 ], differs between the sexes [ 43 ], relates to infant outcomes [ 44 ], and is prospectively diminished in fetuses born preterm [ 45 ].
The primary objective of this study is to establish whether maternal prenatal stress relates to changes in the child’s brain before birth; the second is to determine whether psychosocial support and health behaviors affect this association; and the third is to test whether this neurobiological embedding of stress relates to how early a child will be born. There is limited data on normative processes of fetal brain functional network development, as such, our hypotheses are focused on regions central to programming large-scale fetal functional network architecture and commonly implicated in psychiatric and neurodevelopmental diseases [ 39 , 46 , 47 ]. Specifically, we hypothesize that maternal prenatal negative affect/stress (NAS) will be associated with altered connectivity of high-order association cortices, specifically prefrontal and parietal regions, and with connectivity of the insular and temporal regions. In addition, because the effects of stress vary widely from person to person, we further hypothesized that higher levels of social support and adaptive health behaviors would moderate the impact of maternal NAS on child prenatal brain development. Finally, motivated by prior work that has linked prenatal stress to premature delivery [ 48 , 49 , 50 ], we hypothesized that greater magnitude of association between maternal NAS and fetal brain connectivity effects would relate to younger age at delivery.
Materials and methods
Participants.
The fetal neuroimaging sample consisted of 118 cases (48 female), with mother mean age 25.1 years ( SD = 4.5). Exclusions for participation included presence of suspected fetal central nervous system abnormality as determined by 20-week ultrasound and/or contraindication for MRI (e.g., pacemaker, ferromagnetic material in mother’s body, claustrophobia). The mean age of fetuses at the time of MRI was 32.9 weeks GA ( SD = 3.87; range 26–39 weeks), and mean age at birth was 39.4 weeks GA ( SD = 1.1). One hundred five cases in this sample have been studied in prior functional connectivity studies [ 39 , 45 , 51 ] and 118 of these cases were studied in a recent investigation of prenatal sex differences [ 43 ]. Ultrasound examination administered by a referring physician was performed within 1 week of MRI examination to determine fetal GA. All women provided written informed consent before undergoing MRI examination. Participation was approved by Human Investigation Committee of Wayne State University. Study participants were followed longitudinally to assure that they developed no complications during pregnancy. Sociodemographic characteristics and birth outcomes in mother–child dyads ( N = 118) are provided in SI Appendix, Table S1 and a summary table for all case exclusions is provided in SI Appendix, Table S2 .
Definition of maternal prenatal stress
Conceptualization and measurement of stress has changed over time [ 52 ]. Prior studies of the effects of intrauterine stress have used biological measures such as salivary cortisol and self-report questionnaires of anxiety, depression, and stress [ 30 , 31 , 53 ]. Here, we empirically derived a single factor representing prenatal Negative Affect and Stress (NAS) and in supplementary analyses tested the association of this factor and all subscales with salivary cortisol in a subset of mothers for which cortisol measurements were available. The primary advantage of this approach is reducing the number of statistical tests performed across inherently co-linear measures, while maintaining individual measure loadings [ 54 ]. Maternal stress during pregnancy was assessed using summary scores from five scales that assessed internalizing problems and stress: the Center for Epidemiological Studies Depression Scale CES-D; [ 55 ] the State Trait Anxiety Inventory (Trait) STAI-T [ 56 ]; the Penn State Worry Questionnaire PSWQ [ 57 ]; the Perceived Stress Scale PSST [ 58 ]; and the Satisfaction with Life Scale SWLS [ 59 ]. Descriptive statistics for prenatal affect and stress scales are provided in SI Appendix, Table S3 . To account for variance in stress exposure and negative affect, as well as minimize the number of models run, we used factor analysis in in Mplus vs. 7.2 [ 60 ] to derive a maternal NAS index. Specifically, in a random half of the sample, we subjected the five scales to exploratory factor analysis (EFA) requesting models with one and two factors. EFA established that these scales loaded best onto a single factor (CFI = .98, TLI = .96, RMSEA = .08, SRMR = .03; all factor loadings, p < .001). We next subjected the scales to confirmatory factor analysis (CFA) in the other random half of the sample to validate the model fit. Again, the five scales showed high loadings and good fit to a one-factor model ( n = 99, CFI = .98, TLI = .97, RMSEA = .06, SRMR = .03; factor loadings, p < .001). We thus reran the CFA in the full sample to obtain individual NAS scores within the full sample. The model showed good fit to the data (χ 2 = 10.23, p = 0.07, df = 5; CFI = 0.98; TLI = 0.96; RMSEA = 0.07; SRMR = 0.03) with each scale loading significantly on the NAS factor ( p < .001). Individual factor loadings are provided in Fig. 1 .
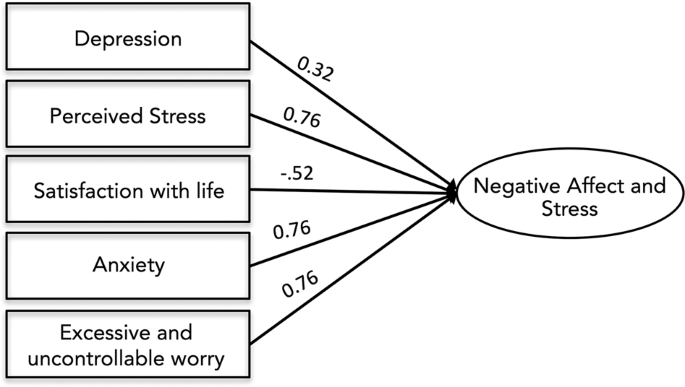
All factor loading scores were significant at <0.001. Model showed excellent fit to the data: χ 2 = 10.23, p = 0.07, df = 5; CFI = 0.98; TLI = 0.96; RMSEA = 0.07; SRMR = 0.03. Measures included the Center for Epidemiological Studies Depression Scale (CES-D), the State Trait Anxiety Inventory (STAI), the Penn State Worry Questionnaire (PSWQ), the Perceived Stress Scale (PSST), and the Satisfaction with Life Scale (SWLS).
Functional data preprocessing
Time frames corresponding to periods of minimal head motion in the fetus were identified using FSL image viewer [ 61 ]. Brainsuite [ 62 ] was used to manually generate 3D masks for single reference images drawn from time periods, or segments, of fetal movement quiescence. Masks were binarized and applied only to frames corresponding to their select segment, and only those data were retained for further analyses. Each segment was manually reoriented, realigned to the mean BOLD volume, resampled to 2 mm isotropic voxels, and normalized to a 32-week fetal brain template [ 63 ] using affine transformation in Statistical Parametric Mapping (SPM8) [ 64 ] software implemented in MATLAB. Motion parameters for each low-motion segment were checked to ensure only segments that consisted of at least 20 sec (10 frames) of low motion were retained in subsequent processing steps. The level of censoring applied, 1 mm mean XYZ and 1.5° mean PYR, has been reported previously [ 39 , 45 , 51 ]. Application of these quality assurance steps resulted in elimination of mean = 56% frames per participant. The resulting sample was 118 cases with an average of 159 frames (SD = 42). Average translational and rotational motion across subjects ranged from 0.01 to 0.52 mm and 0.6° to 1.1°, respectively. In a final step, to correct for variation in normalization across segments within-participant, normalized images were concatenated into one run, realigned to the mean BOLD volume, and smoothed with a 4 mm FWHM Gaussian kernel.
Functional brain segmentation
A spatially constrained group level clustering approach [ 65 ] was used to parcellate the area of a 32-week GA fetal template brain [ 63 ] into 197 spatially contiguous, similarly sized ROIs (SI Appendix, Fig. S1 ). This method utilizes 4D fetal data normalized to 32-week fetal template space and produces functionally homogenous clusters by assessing voxel timeseries similarity in a given dataset, using Pearson correlations, then iteratively merging voxels that showed maximal within-cluster similarity and minimal between-cluster similarity. Next, it identifies the most representative clusters of voxels using a normalized cut algorithm [ 66 ] and performs group level clustering. This approach yields ROIs that span the full extent of cortical, subcortical and cerebellar regions and is representative of observed functional connectivity patterns in this fetal sample, after application of motion correction, normalization, and concatenation steps. This is an established data-driven approach to parcellation [ 39 , 43 , 67 , 68 ], useful in the human fetal brain where priors are limited and developmental change is exceedingly rapid. ROIs were then classified by hemisphere, by lobe, and by coordinates corresponding to center of mass.
Derivation of fetal brain networks
CONN functional connectivity toolbox (v14n) [ 69 ] was used to generate Pearson correlations matrices between these 197 ROIs for each subject. Processing included linear detrending, nuisance regression using aCompCor of five principal components extracted from a 32-week fetal atlas white matter and CSF mask, six head motion parameters, and band-pass filtering at 0.008–0.09 Hz. To create a subnetwork model of the fetal brain, the complete set of unique n = 19,306 ROI-pair functional connectivity (FC) (Fisher-z) values for data from all participants were averaged, producing a 197 × 197 connectivity matrix (Fig. 2 ). The set of averaged correlations across subjects was thresholded and binarized at multiple Fisher-z values, corresponding to edge density sparseness thresholds ranging from 1 to 10% of all possible surviving connections at steps of 0.1%, to generate 91 total adjacency matrices. Connections between ROI pairs separated by <10 mm were removed to minimize the effects of blurring in the spatially normalized fMRI data. The Infomap community detection algorithm [ 70 ] was used to assign ROIs to neural subnetworks based on maximization of within-module random walks applied to adjacency matrices at each threshold. Solutions for each threshold were combined using an automated consensus procedure to provide a single model of the community structure by maximizing the normalized mutual information of groups of neighboring solutions and then maximizing modularity [ 71 ]. This network solution enabled network-pair-level analyses for the full group (Fig. 2 ) and reduced the overall number of possible comparisons by more than 150-fold.
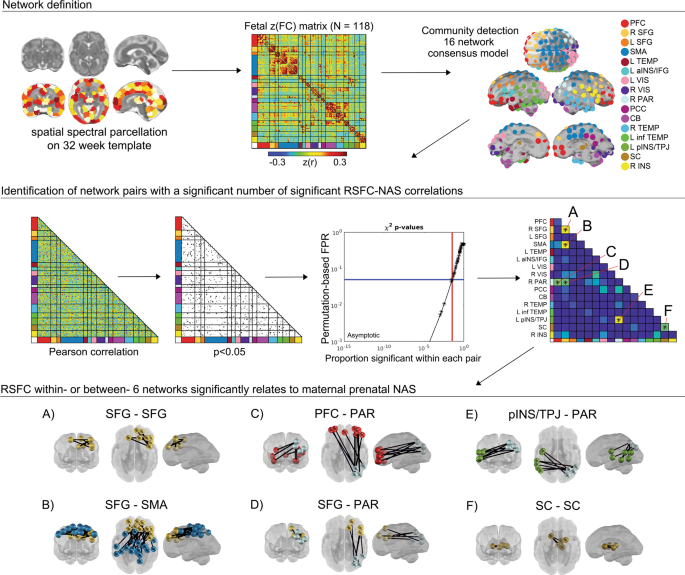
Community detection analysis generated a 16 functional network consensus model from a matrix of 197 functionally defined regions. Enrichment was performed to assess the degree to which NAS correlated edges (Pearson p < 0.05) in a network pair were overrepresented, tested using a permutation-based estimated false positive rate (FPR). The result was six network pairs with differences in connectivity related to maternal prenatal NAS ( A – F ). Within and between-network pairs enriched with significant ROI–ROI resting-state functional connectivity (RSFC) are displayed in the bottom panel, displayed on a 32-week human fetal brain cortical surface. PFC prefrontal cortex, SFG superior frontal gyrus, SMA somatomotor area, aINS anterior insula, IFG inferior frontal gyrus, VIS Visual, PAR parietal, PCC posterior cingulate cortex, CB cerebellum, inf TEMP inferior temporal, pINS posterior insula, TPJ temporoparietal junction, SC subcortical gray matter.
Examination of NAS-related differences in fetal functional connectivity
Exploratory and confirmatory factor analyses were used to generate a single multi-measure factor assessing maternal prenatal negative affect/stress (NAS). Analyses and factor loadings are provided in the Supplemental Material . The resulting NAS score was adjusted for gestational age at scan by computing residual values using the regression NAS–GA + error and used for all subsequent analyses. Enrichment analyses were performed to identify within- or between- network pairs with a significant clustering of strong RSFC-NAS correlations. This enrichment approach has recently been applied to functional neuroimaging studies of fetuses and infants [ 43 , 68 , 71 , 72 ], and is an adaptation of methods used in large-scale genome association studies [ 73 , 74 , 75 ]. The approach applies a p-threshold of 0.05 to all ROI-pair correlations in the group Fisher-z-transformed FC matrix then uses a Chi-square test to assess the level clustering of strong FC-NAS correlations for within- and between-network ROI pairs. Significance for χ 2 enrichment is determined by randomly permuting the subject pairing of FC and NAS values 10,000 times [ 71 , 73 ]. Only networks that were significantly enriched for FC-NAS (χ 2 , df = 1, p < 0.05) were treated as significant findings.
Mixtures-modeling of brain-stress relationships
To explore whether the associations between maternal NAS scores and fetal brain connectivity were buffered by the effects of social support or adaptive health behaviors, we first used exploratory factor analyses to reduce dimensionality across ten measures of social environment and health behavior. Analysis of scree plots with actual and resampled data and Bartlett’s test for sphericity both confirmed that these variables were best summarized as three factors, one linked to health (“Health Engagement”; HE), one to family conflict/cohesion (“Family Systems”; FS) and a third that was a mixture of sleep, medical adherence, substance use, and ECR-R interpersonal avoidance (“Health and Adult Relationship”; HAR) (SI Appendix, Fig. S2 ). Next, to further reduce dimensionality, we applied a mixtures-based modeling strategy, weighted quantile sum (WQS) regression [ 76 , 77 ], to construct a single empirically-estimated connectivity index (i.e., WQS stress-connectivity index), summarizing the overall magnitude of RSFC effects in a given fetal brain. In a third step, initial univariate models were constructed to evaluate relationships between the WQS stress-connectivity index and maternal NAS scores without additional health and social support factors. Finally, the models were rerun adding the health and social support factors to examine both effect modification and to test for interaction effects. In all models, a robust cross-validation strategy was applied, such that the data were divided into training (40% of data) and validation (60%) sets, with model weights estimated in the training set before application to the validation set, both to avoid over-fitting and ensure the generalizability of results. Additional information about WQS model estimation is provided in Supplemental Material .
Evaluation of prenatal NAS, fetal brain FC, and gestational age at delivery
We evaluated relationships between maternal prenatal NAS, fetal brain, and age at delivery. Standard linear models were used to evaluate these relationships, using either maternal stress, individual between-network connectivity values, or the stress-connectivity WQS mixture, derived as above-described, as predictors.
Fetal brain targets of maternal NAS
Community detection analysis generated a 16 functional network consensus model (Fig. 2 ) that became the basis for isolating significant relationships between maternal prenatal NAS and between and within network RSFC. Results of enrichment analysis demonstrated that effects of maternal prenatal NAS were evident in six fetal network pairings. Specifically, maternal prenatal NAS factor scores were related to variation in FC values between the (i) superior frontal and sensorimotor networks (SFG–SMA), (ii) a left posterior insula/temporoparietal junction and a right superior parietal network (pINS/TPJ–PAR), (iii) superior frontal and parietal networks (SFG–PAR), and (iv) prefrontal and parietal networks (PFC–PAR). In addition, maternal prenatal NAS scores were related to FC within the (v) subcortical striatal network (SC–SC), and the (vi) superior frontal gyrus network (SFG–SFG). Significant between and within network effects were confirmed with χ 2 tests for independence. A summary of connections comprising significant enrichment results is provided in Table 1 and between-network positive and negative significant connections are visually represented in Fig. 3 .
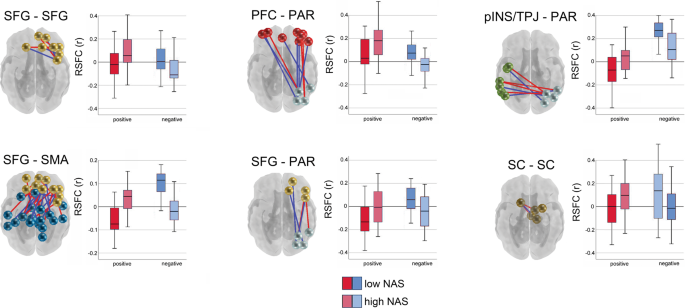
Connections that are stronger in fetuses of mothers with higher NASF are plotted in red, and the reverse is plotted in blue. Boxplots denote global mean centered r-values for the top and bottom NASF quartiles, averaged across positive or negative network edges within each network pair. SFG superior frontal gyrus, SMA somatomotor network, SC subcortical gray matter, pINS/TPJ posterior insula/ temporoparietal junction, PAR parietal, PFC prefrontal cortex.
Moderation of effects by social support and health behaviors
We further evaluated relationships between fetal connectivity and maternal stress in a mixtures-based strategy. A weighted quantile sum (WQS) model replicated findings from enrichment and χ 2 tests, showing that NAS and connectivity across the 6 networks was significant as a mixture (β = 0.82, p < 0.001) and highlighting that the strongest associations were in connectivity of SFG–SMA, pINS/TPJ-PC, and SFG–PAR network pairs (SI Appendix, Fig. S3 ). Next, we tested for moderation by examining potential interactions in WQS models that included health and social support factors. We found no significant associations between NAS and factors corresponding to diet, exercise (factor HE ), and family conflict/cohesion (factor FS ), but found that a factor comprising sleep, medical adherence, substance avoidance, and low interpersonal avoidance (factor HAR ), significantly moderated (β = 0.16, p = 0.02) the relationship between the maternal prenatal NAS and the WQS stress-connectivity index. The effect was such that participants with lower HAR scores had weaker associations with the stress-connectivity index, but higher stress overall. In the context of this interaction, connectivity between PFC–PAR and SFG–SMA networks were most significantly related to HAR ( p < 0.000; SI Appendix, Table S4 ).
Maternal NAS-related brain FC relates to gestational age at delivery
Maternal NAS scores were associated with younger gestational age at delivery (β = −0.36, p = 0.003), in agreement with prior literature [ 48 , 50 ]. Here, we extended our analysis of stress-connectivity indices to consider potential associations between stress correlates in the fetal brain and fetal gestational age at birth. Our findings indicated a marginally significant negative association (β = −0.18, p = 0.05) between the omnibus WQS stress-connectivity index and gestational age at delivery. That is, a single measure reflecting the effect of stress on neural connectivity in each fetus was associated with how early that fetus was born (see Fig. 4 ). Given the marginal significance of this relationship, we pursued follow-up analyses with traditional linear models to investigate the association between NAS and length of gestation in each network pair. Applying Holm–Bonferroni correction to six tests of connectivity to gestational age at birth, we observed significant associations between gestational age at delivery and connectivity between SFG–PAR (β = −2.46, p = 0.008), pINS/TPJ–PAR (β = −2.68, p = 0.007), and SFG–SMA (β = −3.52, p = 0.016) network pairs (Table 2 ).
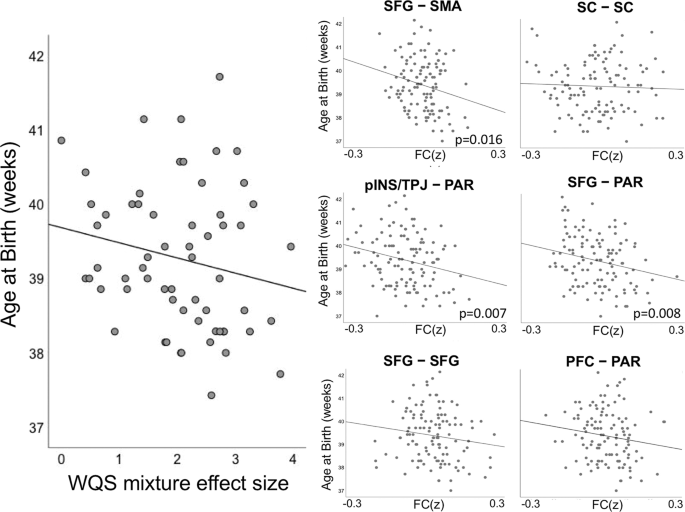
The left panel depicts the negative association (β = −0.18, p = 0.05) between the WQS stress-connectivity index and gestational age at delivery. Thus, stress-related changes in fetal connectivity are significantly associated with shorter gestational periods. The right panels depict average connectivity across network edges significantly associated with maternal stress for each subject for each of the six significant network pairs (FC(z)). Significant associations between strength of stress-connectivity effect and gestational age at delivery was observed in the SFG–PAR (β = −2.46, p = 0.008), pINS/TPJ–PAR (β = −2.68, p = 0.007), and SFG–SMA (β = −3.52, p = 0.016) network pairs.
Evaluation of potential confounds
Tests of associations between maternal demographics, data quality control (QC) parameters, and maternal NAS residuals used in the enrichment analysis were largely not significant, suggesting that several factors with potential to confound observed stress-brain mixtures do not appear to have influenced analyses performed in this dataset. Maternal NAS residual scores were not related to maternal age (r = 0.018, p = .85), gross income (r = −0.061, p = 0.55), average translational motion (r = −0.109, p = 0.24), or frame count (r = 0.123, p = 0.18). However, maternal NAS residual scores were significantly related to average rotational motion (r = −0.185, p = 0.045). Consequently, we performed additional analyses testing the relationship between motion and significant between-network connectivity effects across subjects. Connectivity values were not related to motion for the majority of network pairs, but a correlation was observed between rotational motion and SFG–SMA connectivity (r = −0.24, p = 0.009). This correlation does not surpass Bonferroni correction for multiple tests, but is nonetheless highlighted and reported in Appendix, Table S5 . As an additional step, we confirmed that motion measures and the overall WQS connectivity index were not significantly related, WQS and XYZ motion, r = 0.008, p = .951, and WQS and PYR motion, r = −0.165, p = .203. Additional contextual variables of interest in our analyses, specifically social support and health behaviors, did have the expected relationship with maternal NAS: NAS scores were negatively correlated with prenatal ECR-R avoidance (r = −0.46, p < 0.001), diet (r = −0.23, p = 0.016), medical adherence (r = −0.29, p = 0.001), and sleep (r = −0.34, p < 0.001), and positively associated with substance abuse (r = 0.41, p < 0.001). Maternal NAS was not related to other social support and health behaviors subscales: ECR-R anxiety, FES-R cohesion, FES-R expressiveness, FES-R conflict, exercise.
From a developmental origins of health and disease (DOHaD) perspective, this research provides a framework to evaluate the very early embedding of biological risk in social and behavioral contexts [ 78 , 79 ]. Research in neonates and young children has suggested that prenatal stress hormones act on various signaling receptors that interact with genetic/epigenetic factors and additional environmental input to influence brain development prior to birth [ 80 ]. Animal models of early life stress have bolstered this view, providing evidence that mouse pups stressed in the first weeks of life (equivalent to human third trimester) show shorter and less complex dendrites, hypomyelination, and altered synaptogenesis [ 23 , 24 , 25 , 26 ]. However, whether or not neurodevelopmental trajectories begin to be altered in the womb has remained a long-standing question in the field of human prenatal stress programming. This study provides evidence that maternal prenatal stress and negative affect are associated with alterations in human brain networks before birth. We report differences in fetal system-level dynamics (i.e., functional connectivity) thus extending models of biological embedding of stress by propelling us back to the most delicate time in human brain maturation, where change is more rapid than at any other time in the life course.
Alterations in fetal functional connectivity were apparent between superior frontal and motor regions and in cross-hemispheric connectivity of posterior insula and temporoparietal brain regions (pINS/TPJ–PAR). These observations are of interest given that connectivity between these regions increases with advancing fetal age [ 40 , 81 ], and abnormal connectivity in these regions has been reported among fetuses and neonates born preterm [ 45 , 82 , 83 ]. Such studies confirm the importance of connectional processes taking place between these regions during the fetal period and lead to suggestion that changes to these processes may have long-ranging effects. Interestingly, temporo-insular regions have also been implicated in the generation of spontaneous functional activity during late stages of fetal development [ 46 , 47 ], leading to suggestions that these spontaneous functional bursts of activity may be foundational to establishing the organizational properties of the human functional connectome architecture [ 46 ].
We also observed significant differences in RSFC between anterior and posterior frontal and parietal regions (SFG–PAR; PFC–PAR) and connectivity within the superior frontal gyrus (SFG–SFG) in fetuses exposed to greater stress in utero. Research in neonates and infants confirms that these networks are evident early in human life [ 84 ], and research in adults suggests a potential transdiagnostic role of disrupted frontoparietal network connectivity in human affective, psychiatric, and neurological disease [ 85 ]. Further, alterations in frontoparietal networks have been shown to be experientially dependent, as evidenced in longitudinal studies of acute psychosocial stress exposure [ 86 ] and cross-sectional investigations of RSFC among individuals with childhood trauma exposure [ 87 ]. We also observed right laterality in our stress-related frontoparietal RSFC differences. While few, if any, studies of emotional psychopathology have specifically addressed laterality of frontoparietal RSFC disruptions, the important role of the right hemisphere in processing emotions is widely studied [ 88 ]. Further, animal models of induced depression have reported laterality in differential gene expression in frontoparietal regions, specifically, right lateralized effects in animals that show reduced resilience to stress exposure [ 89 ]. Convergence of our human fetal results with these known priors suggests that further examination of both early childhood onset of affect-related frontoparietal disturbance, including examinations of laterality, are warranted.
In addition, we observed prenatal maternal stress-related differences in RSFC within a fetal subnetwork encompassing areas of the striatum. The effect of stress on the striatum has been shown to relate to neuronal microstructure [ 90 ], gene and neurochemical expression [ 91 ], and connectivity [ 92 , 93 , 94 ]. Studies both in humans and in animals confirm that restructuring of the striatum following stress has behavioral relevance, most strongly impacting domains of social behavior, decision-making, affective valuation, and risk for internalizing illness [ 90 , 91 , 92 , 93 ]. It is possible that prenatal variation in striatal subnetwork functional connectivity predisposes individuals to long-term processing differences across these and other related behavioral domains. Our study provides evidence to support this claim, and suggests that combined changes in the striatum, insulo-temporal and frontoparietal brain systems may underlie long-term stress-related behavioral effects. It is noteworthy that across network pairs the observed relationships between maternal NAS and fetal functional connectivity were comprised of a mix of both positive and negative associations, without clear directionality. That is, both augmented and diminished network connectivity was observed. This finding is not unexpected when studying subnetworks comprised of numerous nodes that are separated in space and/or expansive. This approach has the advantage of enabling examination of whole-brain connectivity, which is particularly favorable in the fetal brain where priors are presently rather limited. However, a natural next step will be to address directionality within specific circuits and at finer resolution.
Individual responses to stress vary widely. A question we sought to address after confirming the existence of prenatal alterations in human brain RSFC was whether specific protective variables explained variation in maternal NAS-related brain differences. The unique sample recruited for this study was predominately low-income, unpartnered, minority women drawn from a community with elevated levels of stress and violence. This is an important population to work with both because this is a population with considerable need, and because meta-analysis indicates associations between maternal prenatal stress and child outcomes are strongest in high-risk groups [ 95 ]. It is known that there are specific traits, such as internal locus of control, social skills, exercise, maternal mindfulness, optimism, and ego development that predict more resilient outcomes [ 96 , 97 , 98 , 99 ]. For example, Christianson and colleagues showed that exercise mitigates the expression of stressor-induced anxiety; [ 100 ] Young and colleagues showed that responses to stress are attenuated by strong male bonds in wild macaques; [ 101 ] and Wellman and colleagues showed individual differences in the effects of stress on REM in Wistar rats and suggest that sleep may be an important biomarker of stress resilience and vulnerability [ 102 ]. We found that a latent factor comprising social support and health, specifically, sleep, medical adherence, avoiding alcohol and cigarettes, and romantic closeness, was a significant modifier of the association between maternal stress and fetal functional connectivity (SI Appendix, Fig. S4 ), such that stress had reduced impact in fetuses of less healthy mothers. Although seemingly counterintuitive, these findings are consistent with evidence that attenuated stress, physiological, and immune responses occur under conditions of chronic stress [ 103 , 104 ]. That is, when prenatal conditions are more challenging, it may adaptive for the fetus to be less sensitive to potential modifiers, such as stress. In contrast, under less challenging prenatal conditions, stress may have potential to have greater effect. From a public health standpoint, the main effects of NAS on the fetal brain continue to point to specific interventional targets for reducing the effects of prenatal stress programming, including sleep and relationship support, ease of access to medical care, and resources targeting reduced substance use. One caveat of the current study is narrow focus on high-risk women; it will be important for future research to evaluate replication of these observations in samples with varied sociodemographic composition.
Finally, we discovered that a greater overall magnitude in the association between maternal NAS and fetal functional brain alteration was associated with a shorter gestational course. Prior studies have reported a negative association between maternal stress and fetal age at delivery, but to our knowledge this is the first study to report that the magnitude of response to stress in the fetal brain may additionally explain variability in birth outcomes. Importantly, these data alone do not suggest that changes in the fetal brain mediate early delivery. Instead, the relative magnitude of the association between maternal prenatal stress on fetal neurobiology is likely reflective of unobserved factors that impact delivery timing, including broader stress-related alterations in perinatal biology and physiology. Here, these associations were observed in the 3 weeks prior to delivery. It will be important for future work to test whether interactions between stress, timing of delivery and fetal brain FC extends to fetuses subsequently born preterm.
In research that considers offspring correlates of maternal stress, negative outcomes are frequently emphasized. However, in the original Barker hypothesis [ 105 ] in utero adaptations are oriented around promoting fetal/offspring survival. Within that context, fetal brain differences reported here may reflect adaptations of the fetus to best meet the challenges of the harsh environment contributing to maternal stress. The present study does not include assessment of child outcomes, which limits ability to address the relevance of fetal brain adaptations to future child well-being. Furthermore, maternal stress is not easily decoupled from confounding contextual and health factors. As an example, we did not gather information about illicit and prescription drug use and are thus unable to evaluate potential contribution of those factors to observed effects. An additional consideration in studies that examine offspring correlates of maternal prenatal stress is that mother and fetus share genetic liability that is important in the patterning of neural circuitry. Overall, in studies such as this, attribution about causality and directionality are not warranted, and much remains to be done to understand how the specific intrauterine signaling factors promote or hinder optimal welfare of the future child.
Overall, our data suggest that widespread differences in the fetal brain are related to maternal self-reported stress and negative affect during pregnancy. The findings advance prior human studies that have demonstrated associations between prospective measures of psychological and biological indices of stress and brain structure and function [ 27 , 28 , 29 , 30 , 31 , 106 ]. Empirical evidence presented here, along with data presented in those influential prior studies, support the notion that excess stress in utero has the potential to affect neural development with implications for future health across the lifespan.
Davis EP, Sandman CA. Prenatal psychobiological predictors of anxiety risk in preadolescent children. Psychoneuroendocrinology. 2012;37:1224–33.
Article PubMed PubMed Central Google Scholar
Walder DJ, Laplante DP, Sousa-Pires A, Veru F, Brunet A, King S. Prenatal maternal stress predicts autism traits in 6½ year-old children: Project Ice Storm. Psychiatry Res. 2014;219:353–60.
Article PubMed Google Scholar
Lahti M, Savolainen K, Tuovinen S, Pesonen AK, Lahti J, Heinonen K, et al. Maternal depressive symptoms during and after pregnancy and psychiatric problems in children. J Am Acad Child Adolesc Psychiatry. 2017;56:30–39. e37
Van den Bergh BRH, Marcoen A. High antenatal maternal anxiety is related to ADHD symptoms, externalizing problems, and anxiety in 8- and 9-year-olds. Child Dev. 2004;75:1085–97.
Karlen J, Frostell A, Theodorsson E, Faresjo T, Ludvigsson J. Maternal influence on child HPA axis: a prospective study of cortisol levels in hair. Pediatrics. 2013;7:7–e1340.
Google Scholar
Davis EP, Glynn LM, Waffarn F, Sandman CA. Prenatal maternal stress programs infant stress regulation. J Child Psychol Psychiatry. 2011;52:119–29.
Smith JT, Waddell BJ. Increased fetal glucocorticoid exposure delays puberty onset in postnatal life. Endocrinology. 2000;141:2422–8.
Article CAS PubMed Google Scholar
Monk C, Fifer WP, Myers MM, Bagiella E, Duong JK, Chen IS, et al. Fetal heart rate reactivity differs by women’s psychiatric status during psychological stress, but not paced breathing. Dev Psychobiol. 2011;53:221–33.
de Weerth C, Buitelaar JK, Beijers R. Infant cortisol and behavioral habituation to weekly maternal separations: Links with maternal prenatal cortisol and psychosocial stress. Psychoneuroendocrinology. 2013;38:2863–74.
Article PubMed CAS Google Scholar
Braeken MA, Kemp AH, Outhred T, Otte RA, Monsieur GJ, Jones A, et al. Pregnant mothers with resolved anxiety disorders and their offspring have reduced heart rate variability: implications for the health of children. PLoS ONE. 2013;8:e83186.
Article PubMed PubMed Central CAS Google Scholar
Entringer S, Buss C, Wadhwa PD. Prenatal stress and developmental programming of human health and disease risk: concepts and integration of empirical findings. Curr Opin Endocrinol Diabetes Obes. 2010;17:507–16.
Beijers R, Jansen J, Riksen-Walraven M, de Weerth C. Maternal prenatal anxiety and stress predict infant illnesses and health complaints. Pediatrics. 2010;126:e401–409.
O'connor TG, Winter MA, Hunn J, Carnahan J, Pressman EK, Glover V, et al. Prenatal maternal anxiety predicts reduced adaptive immunity in infants. Brain Behav Immun. 2013;32:21–8.
Article CAS PubMed PubMed Central Google Scholar
Cookson H, Granell R, Joinson C, Ben-Shlomo Y, Henderson AJ. Mothers’ anxiety during pregnancy is associated with asthma in their children. J Allergy Clin Immunol. 2009;123:847–53. e811
Monk C, Sloan RP, Myers MM, Ellman L, Werner E, Jeon J, et al. Fetal heart rate reactivity differs by women’s psychiatric status: an early marker for developmental risk? J Am Acad Child Adolesc Psychiatry. 2004;43:283–90.
DiPietro JA, Costigan KA, Gurewitsch ED. Fetal response to induced maternal stress. Early Hum Dev. 2003;74:125–38.
Van den Bergh BRH. The influence of maternal emotions during pregnancy on fetal and neonatal behavior. J Perinat Prenat Psychol Health. 1990;5:119–30.
Faa G, Marcialis MA, Ravarino A, Piras M, Pintus MC, Fanos V. Fetal programming of the human brain: is there a link with insurgence of neurodegenerative disorders in adulthood? Curr Med Chem. 2014;21:3854–76.
Cents RA, Tiemeier H, Luijk MP, Jaddoe VW, Hofman A, Verhulst FC, et al. Grandparental anxiety and depression predict young children’s internalizing and externalizing problems: the generation R study. J Affect Disord. 2011;128:95–105.
Wehmer F, Porter RH, Scales B. Pre-mating and pregnancy stress in rats affects behaviour of grandpups. Nature. 1970;227:622.
Ding X-X, Wu YL, Xu SJ, Zhu RP, Jia XM, Zhang SF, et al. Maternal anxiety during pregnancy and adverse birth outcomes: A systematic review and meta-analysis of prospective cohort studies. J Affect Disord. 2014;159:103–10.
Heron J, O’Connor TG, Evans J, Golding J, Glover V. The course of anxiety and depression through pregnancy and the postpartum in a community sample. J Affect Disord. 2004;80:65–73.
Bock J, Murmu MS, Biala Y, Weinstock M, Braun K. Prenatal stress and neonatal handling induce sex-specific changes in dendritic complexity and dendritic spine density in hippocampal subregions of prepubertal rats. Neuroscience. 2011;193:34–43.
Afadlal S, Polaboon N, Surakul P, Govitrapong P, Jutapakdeegul N. Prenatal stress alters presynaptic marker proteins in the hippocampus of rat pups. Neurosci Lett. 2010;470:24–7.
Bennett GA, Palliser HK, Shaw JC, Walker D, Hirst JJ. Prenatal stress alters hippocampal neuroglia and increases anxiety in childhood. Dev Neurosci. 2015;37:533–45.
Suzuki A, Iinuma M, Hayashi S, Sato Y, Azuma K, Kubo KY. Maternal chewing during prenatal stress ameliorates stress-induced hypomyelination, synaptic alterations, and learning impairment in mouse offspring. Brain Res. 2016;1651:36–43.
Scheinost D, Kwon SH, Lacadie C, Sze G, Sinha R, Constable RT, et al. Prenatal stress alters amygdala functional connectivity in preterm neonates. NeuroImage Clin. 2016;12:381–8.
Posner J, Cha J, Roy AK, Peterson BS, Bansal R, Gustafsson HC, et al. Alterations in amygdala-prefrontal circuits in infants exposed to prenatal maternal depression. Transl Psychiatry. 2016;6:e935.
Qiu A, Anh TT, Li Y, Chen H, Rifkin-Graboi A, Broekman BF, et al. Prenatal maternal depression alters amygdala functional connectivity in 6-month-old infants. Transl Psychiatry. 2015;5:e508.
Buss C, Davis EP, Muftuler LT, Head K, Sandman CA. High pregnancy anxiety during mid-gestation is associated with decreased gray matter density in 6-9-year-old children. Psychoneuroendocrinology. 2010;35:141–53.
Buss C, Davis EP, Shahbaba B, Pruessner JC, Head K, Sandman CA. Maternal cortisol over the course of pregnancy and subsequent child amygdala and hippocampus volumes and affective problems. Proc Natl Acad Sci USA. 2012;109:E1312–1319.
Anderson AL, Thomason ME. Functional plasticity before the cradle: a review of neural functional imaging in the human fetus. Neurosci Biobehav Rev 2013;37:2220–32.
van den Heuvel MI, Thomason ME. Functional connectivity of the human brain in utero. Trends Cogn Sci. 2016;20:931–9.
Johnston JM, Vaishnavi SN, Smyth MD, Zhang D, He BJ, Zempel JM, et al. Loss of resting interhemispheric functional connectivity after complete section of the corpus callosum. J Neurosci. 2008;28:6453–8.
Hagmann P, Cammoun L, Gigandet X, Meuli R, Honey CJ, Wedeen VJ, et al. Mapping the structural core of human cerebral cortex. PLoS Biol. 2008;6:e159.
van den Heuvel MP, Sporns O. An anatomical substrate for integration among functional networks in human cortex. J Neurosci. 2013;33:14489–14500.
Jakab A, Schwartz E, Kasprian G, Gruber GM, Prayer D, Schöpf V, et al. Fetal functional imaging portrays heterogeneous development of emerging human brain networks. Front Hum Neurosci. 2014;8:852.
Schopf V, Kasprian G, Brugger PC, Prayer D. Watching the fetal brain at ‘rest’. Int J Dev Neurosci. 2012;30:11–7.
Thomason, M, Brown, JA, Dassanayake, MT, Shastri, R, Marusak, HA, Hernandez-Andrade, E, et al. Intrinsic functional brain architecture derived from graph theoretical analysis in the human fetus. PLoS ONE. 2014:9;e94423. https://doi.org/10.1371/journal.pone.0094423 .
Thomason, M, Dassanayake, MT, Shen, S, Katkuri, Y, Alexis, M, Anderson, AL et al. Cross-hemispheric functional connectivity in the human fetal brain. Sci Transl Med. 2013;5:173ra24. https://doi.org/10.1126/scitranslmed.3004978 .
Thomason ME, Grove LE, Lozon TA Jr, Vila AM, Ye Y, Nye MJ, et al. Age-related increases in long-range connectivity in fetal functional neural connectivity networks in utero. Dev Cogn Neurosci. 2015;11:96–104.
Jakab A, Kasprian G, Schwartz E, Gruber GM, Mitter C, Prayer D, et al. Disrupted developmental organization of the structural connectome in fetuses with corpus callosum agenesis. Neuroimage. 2015;111:277–88.
Wheelock MD, Hect JL, Hernandez-Andrade E, Hassan SS, Romero R, Eggebrecht AT, et al. Sex differences in functional connectivity during fetal brain development. Dev Cogn Neurosci. 2019;36:100632.
Thomason ME, Hect J, Waller R, Manning JH, Stacks AM, Beeghly M, et al. Prenatal neural origins of infant motor development: associations between fetal brain and infant motor development. Dev Psychopathol. 2018;30:763–72.
Thomason ME, Scheinost D, Manning JH, Grove LE, Hect J, Marshall N, et al. Weak functional connectivity in the human fetal brain prior to preterm birth. Sci Rep. 2017;7:39286.
Thomason ME. Structured spontaneity: building circuits in the human prenatal brain. Trends Neurosci. 2018;41:1–3.
Arichi T, Whitehead K, Barone G, Pressler R, Padormo F, David Edwards A, et al. Localization of spontaneous bursting neuronal activity in the preterm human brain with simultaneous EEG-fMRI. eLife. 2017;6:e27814.
Grigoriadis S, VonderPorten EH, Mamisashvili L, Tomlinson G, Dennis CL, Koren G, et al. The impact of maternal depression during pregnancy on perinatal outcomes: a systematic review and meta-analysis. J Clin Psychiatry. 2013;74:e321–341.
Khalesi ZB, Bokaie M. The association between pregnancy-specific anxiety and preterm birth: a cohort study. Afr Health Sci. 2018;18:569–75.
Pesonen AK, Lahti M, Kuusinen T, Tuovinen S, Villa P, Hämäläinen E, et al. Maternal prenatal positive affect, depressive and anxiety symptoms and birth outcomes: the PREDO study. PLoS ONE. 2016;11:e0150058.
van den Heuvel MI, Turk E, Manning JH, Hect J, Hernandez-Andrade E, et al. Hubs in the human fetal brain network. Dev Cogn Neurosci. 2018;30:108–15.
Slavich GM. Life stress and health: a review of conceptual issues and recent findings. Teach Psychol. 2016;43:346–55.
Wu Y, Lu YC, Jacobs M, Pradhan S, Kapse K, Zhao L, et al. Association of prenatal maternal psychological distress with fetal brain growth, metabolism, and cortical maturation. JAMA Netw Open. 2020;3:e1919940.
van den Heuvel MI, Hect JL, Smarr BL, Qawasmeh T, Kriegsfeld LJ, Barcelona J, et al. The influence of maternal stress during pregnancy on Toddlers’ sleep problems: the role of cortico-cerebellar connectivity in utero . Sci Rep. 2021;11:2228.
Radloff LS. The CES-D Scale: a self-report depression scale for research in the general population. Appl Psychol Meas. 1977;1:385–401.
Article Google Scholar
Spielberger, CD. State-trait anxiety inventory: a comprehensive bibliography. Palo Alto: Consulting Psychologists Press; 1984.
Meyer TJ, Miller ML, Metzger RL, Borkovec TD. Development and validation of the Penn State Worry Questionnaire. Behav Res Ther. 1990;28:487–95.
Cohen S, Kamarck T, Mermelstein R. A global measure of perceived stress. J Health Soc Behav. 1983;24:385–96.
Diener E, Emmons RA, Larsen RJ, Griffin S. The satisfaction with life scale. J Personal Assess. 1985;49:71–5.
Article CAS Google Scholar
Muthén, LK & Muthén, BO. Mplus User’s Guide. Los Angeles: Muthén & Muthén; 2014.
FSL. FMRIB Software Library. http://www.fmrib.ox.ac.uk/fsl/ . 2000.
Shattuck DW, Leahy RM. BrainSuite: an automated cortical surface identification tool. Med Image Anal. 2002;6:129–42.
Serag A, Aljabar P, Ball G, Counsell SJ, Boardman JP, Rutherford MA, et al. Construction of a consistent high-definition spatio-temporal atlas of the developing brain using adaptive kernel regression. Neuroimage. 2012;59:2255–65.
Friston K. Statistical Parametric Mapping 8 from the Wellcome Trust Centre for Neuroimaging. http://www.fil.ion.ucl.ac.uk/spm/ . 2009.
Craddock RC, James GA, Holtzheimer PE 3rd, Hu XP, Mayberg HS. A whole brain fMRI atlas generated via spatially constrained spectral clustering. Hum Brain Mapp. 2012;33:1914–28.
van den Heuvel M, Mandl R, Hulshoff Pol H. Normalized cut group clustering of resting-state FMRI data. PLoS ONE. 2008;3:e2001.
Norr, ME, Hect, JL, Lenniger, CJ, Van den Heuvel, M & Thomason, ME. An examination of maternal prenatal BMI and human fetal brain development. J Child Psychol Psychiatry. 2021;62:458–69. https://doi.org/10.1111/jcpp.13301 .
Thomason ME, Hect JL, Rauh VA, Trentacosta C, Wheelock MD, Eggebrecht AT, et al. Prenatal lead exposure impacts cross-hemispheric and long-range connectivity in the human fetal brain. Neuroimage. 2019;191:186–92.
Whitfield-Gabrieli S, Nieto-Castanon A. Conn: a functional connectivity toolbox for correlated and anticorrelated brain networks. Brain Connect. 2012;2:125–41.
Rosvall M, Bergstrom C. Maps of random walks on complex networks reaveal community structure. PNAS. 2008;105:1118–23.
Eggebrecht AT, Elison JT, Feczko E, Todorov A, Wolff JJ, Kandala S, et al. Joint attention and brain functional connectivity in infants and Toddlers. Cereb Cortex. 2017;27:1709–20.
Wheelock MD, Austin NC, Bora S, Eggebrecht AT, Melzer TR, Woodward LJ, et al. Altered functional network connectivity relates to motor development in children born very preterm. Neuroimage. 2018;183:574–83.
Backes C, Rühle F, Stoll M, Haas J, Frese K, Franke A, et al. Systematic permutation testing in GWAS pathway analyses: identification of genetic networks in dilated cardiomyopathy and ulcerative colitis. BMC Genomics. 2014;15:622.
Rivals I, Personnaz L, Taing L, Potier MC. Enrichment or depletion of a GO category within a class of genes: which test? Bioinformatics. 2007;23:401–7.
Khatri P, Sirota M, Butte AJ. Ten years of pathway analysis: current approaches and outstanding challenges. PLoS Comput Biol. 2012;8:e1002375.
Carrico C, Gennings C, Wheeler D, Factor-Litvak P. Characterization of weighted quantile sum regression for highly correlated data in a risk analysis setting. J Agric Biol Environ Stat. 2015;20:100–20. https://doi.org/10.1007/s13253-014-0180-3 .
Curtin P, Kellogg J, Cech N, Gennings, C. A random subset implementation of weighted quantile sum (WQS RS) regression for analysis of high-dimensional mixtures. Commun Stat Simul Comput. 2019:1119–34. https://doi.org/10.1080/03610918.2019.1577971 .
Barnes MD, Heaton TL, Goates MC, Packer JM. Intersystem implications of the developmental origins of health and disease: advancing health promotion in the 21st century. Healthcare. 2016;4:45. https://doi.org/10.3390/healthcare4030045 .
Gluckman PD, Hanson MA, Buklijas T. A conceptual framework for the developmental origins of health and disease. J Dev Orig Health Dis. 2010;1:6–18.
Monk C, Feng T, Lee S, Krupska I, Champagne FA, Tycko B. Distress during pregnancy: epigenetic regulation of placenta glucocorticoid-related genes and fetal neurobehavior. Am J Psychiatry. 2016;173:705–13.
Takahashi E, Folkerth RD, Galaburda AM, Grant PE. Emerging cerebral connectivity in the human fetal brain: an MR tractography study. Cereb Cortex. 2012;22:455–64.
Batalle D, Muñoz-Moreno E, Tornador C, Bargallo N, Deco G, Eixarch E, et al. Altered resting-state whole-brain functional networks of neonates with intrauterine growth restriction. Cortex. 2016;77:119–31.
Young JM, Vandewouw MM, Morgan BR, Smith ML, Sled JG, Taylor MJ. Altered white matter development in children born very preterm. Brain Struct Funct. 2018;223:2129–41.
Gao W, Alcauter S, Smith JK, Gilmore JH, Lin W. Development of human brain cortical network architecture during infancy. Brain Struct Funct. 2015;220:1173–86.
Baker JT, Dillon DG, Patrick LM, Roffman JL, Brady RO Jr, Pizzagalli DA, et al. Functional connectomics of affective and psychotic pathology. Proc Natl Acad Sci USA. 2019;116:9050–9. https://doi.org/10.1073/pnas.1820780116 .
Liston C, McEwen BS, Casey BJ. Psychosocial stress reversibly disrupts prefrontal processing and attentional control. Proc Natl Acad Sci USA. 2009;106:912–7.
Yu M, Linn KA, Shinohara RT, Oathes DJ, Cook PA, Duprat R, et al. Childhood trauma history is linked to abnormal brain connectivity in major depression. Proc Natl Acad Sci USA. 2019;116:8582–90.
Gainotti G. The role of the right hemisphere in emotional and behavioral disorders of patients with frontotemporal lobar degeneration: an updated review. Front Aging Neurosci. 2019;11:55.
Farhang S, Barar J, Fakhari A, Mesgariabbasi M, Khani S, Omidi Y, et al. Asymmetrical expression of BDNF and NTRK3 genes in frontoparietal cortex of stress-resilient rats in an animal model of depression. Synapse. 2014;68:387–93.
Taylor SB, Anglin JM, Paode PR, Riggert AG, Olive MF, Conrad CD. Chronic stress may facilitate the recruitment of habit- and addiction-related neurocircuitries through neuronal restructuring of the striatum. Neuroscience. 2014;280:231–42.
Valuskova P, Farar V, Janisova K, Ondicova K, Mravec B, Kvetnansky R, et al. Brain region-specific effects of immobilization stress on cholinesterases in mice. Stress. 2017;20:36–43.
Fareri DS, Tottenham N. Effects of early life stress on amygdala and striatal development. Dev Cogn Neurosci. 2016;19:233–47.
Vogel S, Klumpers F, Schröder TN, Oplaat KT, Krugers HJ, Oitzl MS, et al. Stress induces a shift towards striatum-dependent stimulus-response learning via the mineralocorticoid receptor. Neuropsychopharmacology. 2017;42:1262–71.
Thomason ME, Marusak HA, Tocco MA, Vila AM, McGarragle O, Rosenberg DR, et al. Altered amygdala connectivity in urban youth exposed to trauma. Soc Cogn Affect Neurosci. 2015;10:1460–8. https://doi.org/10.1093/scan/nsv030 .
Bussières EL, Tarabulsy GM, Pearson J, Tessier R, Forest JC, Giguère Y. Maternal prenatal stress and infant birth weight and gestational age: a meta-analysis of prospective studies. Dev Rev. 2015;36:179–99.
Luthar SS. Vulnerability and resilience: a study of high-risk adolescents. Child Dev. 1991;62:600–16.
van den Heuvel MI, Johannes MA, Henrichs J, Van den Bergh BRH. Maternal mindfulness during pregnancy and infant socio-emotional development and temperament: the mediating role of maternal anxiety. Early Hum Dev. 2015;91:103–8.
Braeken MAKA, Jones A, Otte RA, Nyklíček I, Van den Bergh BRH. Potential benefits of mindfulness during pregnancy on maternal autonomic nervous system function and infant development. Psychophysiology. 2017;54:279–88.
Lobel M, DeVincent C, Kaminer A, Meyer B. The impact of prenatal maternal stress and optimistic disposition on birth outcomes in medically high-risk women. Health Psychol. 2000;19:551–3.
Christianson JP, Greenwood BN. Stress-protective neural circuits: not all roads lead through the prefrontal cortex. Stress. 2014;17:1–12.
Young C, Majolo B, Heistermann M, Schulke O, Ostner J. Responses to social and environmental stress are attenuated by strong male bonds in wild macaques. Proc Natl Acad Sci USA. 2014;111:18195–18200.
Wellman LL, Fitzpatrick ME, Hallum OY, Sutton AM, Williams BL, Sanford LD. Individual differences in animal stress models: considering resilience, vulnerability, and the amygdala in mediating the effects of stress and conditioned fear on sleep. Sleep. 2016;39:1293–303.
Barnum CJ, Pace TW, Hu F, Neigh GN, Tansey MG. Psychological stress in adolescent and adult mice increases neuroinflammation and attenuates the response to LPS challenge. J Neuroinflammation. 2012;9:9.
Bustos SG, Giachero M, Maldonado H, Molina VA. Previous stress attenuates the susceptibility to Midazolam’s disruptive effect on fear memory reconsolidation: influence of pre-reactivation D-cycloserine administration. Neuropsychopharmacology. 2010;35:1097–108.
Barker DJ. The fetal and infant origins of adult disease. BMJ. 1990;301:1111.
Lautarescu A, Pecheva D, Nosarti C, Nihouarn J, Zhang H, Victor S, et al. Maternal prenatal stress is associated with altered uncinate fasciculus microstructure in premature neonates. Biol Psychiatry. 2019;87:559–69.
Download references
Acknowledgements
We thank Adam Eggebrecht, Muriah Wheelock, David Shattuck, and Prantik Kundu for contributions and advice in developing methodology for analyzing fetal fMRI data. We thank Pavan Jella and Sophia Neuenfeldt for their assistance in data acquisition and thank Toni Lewis and Claudia Espinoza-Heredia for assistance with data management and sharing. Importantly, we thank participant families who generously shared their time and expressed interest in helping future babies to achieve their best possible health outcomes.
This project was supported by awards from the National Institutes of Health, MH110793, DA050287, MH122447, and ES032294.
Author information
Authors and affiliations.
Department of Child and Adolescent Psychiatry, New York University Medical Center, New York, NY, USA
Moriah E. Thomason
Department of Population Health, New York University Medical Center, New York, NY, USA
Neuroscience Institute, NYU Langone Health, New York, NY, USA
Medical Scientist Training Program, University of Pittsburgh & Carnegie Mellon University, Pittsburgh, PA, USA
Jasmine L. Hect
Department of Psychology, University of Pennsylvania, Philadelphia, PA, USA
Rebecca Waller
Department of Environmental Medicine and Public Health, Icahn School of Medicine at Mount Sinai, New York, NY, USA
Paul Curtin
You can also search for this author in PubMed Google Scholar
Contributions
MT and PC conceived and designed the experiments. MT and JH performed the experiments. MT, JH, RW, and PC analyzed the data. MT contributed materials/analysis tools. MT, RW, and PC wrote the paper.
Corresponding author
Correspondence to Moriah E. Thomason .
Ethics declarations
Competing interests.
The authors declare no competing interests.
Additional information
Publisher’s note Springer Nature remains neutral with regard to jurisdictional claims in published maps and institutional affiliations.
Supplementary information
Supplemental material, rights and permissions.
Open Access This article is licensed under a Creative Commons Attribution 4.0 International License, which permits use, sharing, adaptation, distribution and reproduction in any medium or format, as long as you give appropriate credit to the original author(s) and the source, provide a link to the Creative Commons license, and indicate if changes were made. The images or other third party material in this article are included in the article’s Creative Commons license, unless indicated otherwise in a credit line to the material. If material is not included in the article’s Creative Commons license and your intended use is not permitted by statutory regulation or exceeds the permitted use, you will need to obtain permission directly from the copyright holder. To view a copy of this license, visit http://creativecommons.org/licenses/by/4.0/ .
Reprints and permissions
About this article
Cite this article.
Thomason, M.E., Hect, J.L., Waller, R. et al. Interactive relations between maternal prenatal stress, fetal brain connectivity, and gestational age at delivery. Neuropsychopharmacol. 46 , 1839–1847 (2021). https://doi.org/10.1038/s41386-021-01066-7
Download citation
Received : 18 January 2021
Revised : 03 June 2021
Accepted : 08 June 2021
Published : 29 June 2021
Issue Date : September 2021
DOI : https://doi.org/10.1038/s41386-021-01066-7
Share this article
Anyone you share the following link with will be able to read this content:
Sorry, a shareable link is not currently available for this article.
Provided by the Springer Nature SharedIt content-sharing initiative
This article is cited by
Brain structural and functional outcomes in the offspring of women experiencing psychological distress during pregnancy.
- Josepheen De Asis-Cruz
- Catherine Limperopoulos
Molecular Psychiatry (2024)
The neural exposome influences the preterm fetal-to-neonatal connectome
- Mark S. Scher
Pediatric Research (2024)

Functional changes in neural mechanisms underlying post-traumatic stress disorder in World Trade Center responders
- Azzurra Invernizzi
- Elza Rechtman
- Megan K. Horton
Translational Psychiatry (2023)
Deletion of ARGLU1 causes global defects in alternative splicing in vivo and mouse cortical malformations primarily via apoptosis
- Fenyong Yao
- Shisheng Huang
Cell Death & Disease (2023)
Maternal anxiety during pregnancy is associated with weaker prefrontal functional connectivity in adult offspring
- Marion I. van den Heuvel
- Bea R.H. Van den Bergh
Brain Imaging and Behavior (2023)
Quick links
- Explore articles by subject
- Guide to authors
- Editorial policies

Click through the PLOS taxonomy to find articles in your field.
For more information about PLOS Subject Areas, click here .
Loading metrics
Open Access
Peer-reviewed
Research Article
The Effect of Prenatal and Childhood Development on Hearing, Vision and Cognition in Adulthood
* E-mail: [email protected]
Affiliation School of Psychological Sciences, University of Manchester, Manchester, United Kingdom
Affiliation Population Health Sciences and Ophthalmology and Visual Sciences, School of Medicine and Public Health, University of Wisconsin, Madison, Wisconsin, United States of America
Affiliations Cincinnati Children’s Hospital Medical Center and Department of Otolaryngology, University of Cincinnati College of Medicine, Cincinnati, Ohio, United States of America, NIHR Nottingham Hearing Biomedical Research Unit, Nottingham, United Kingdom, MRC Institute of Hearing Research, Nottingham, United Kingdom
Affiliations NIHR Nottingham Hearing Biomedical Research Unit, Nottingham, United Kingdom, Otology and Hearing Group, Division of Clinical Neuroscience, School of Medicine, University of Nottingham, Nottingham, United Kingdom
Affiliations NIHR Nottingham Hearing Biomedical Research Unit, Nottingham, United Kingdom, MRC Institute of Hearing Research, Nottingham, United Kingdom, Otology and Hearing Group, Division of Clinical Neuroscience, School of Medicine, University of Nottingham, Nottingham, United Kingdom
Affiliations School of Psychological Sciences, University of Manchester, Manchester, United Kingdom, Central Manchester Universities Hospitals NHS Foundation Trust, Manchester, United Kingdom
- Piers Dawes,
- Karen J. Cruickshanks,
- David R. Moore,
- Heather Fortnum,
- Mark Edmondson-Jones,
- Abby McCormack,
- Kevin J. Munro
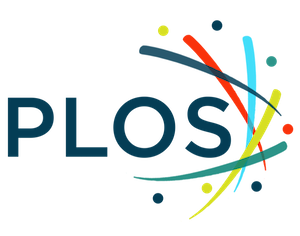
- Published: August 24, 2015
- https://doi.org/10.1371/journal.pone.0136590
- Reader Comments
It is unclear what the contribution of prenatal versus childhood development is for adult cognitive and sensory function and age-related decline in function. We examined hearing, vision and cognitive function in adulthood according to self-reported birth weight (an index of prenatal development) and adult height (an index of early childhood development). Subsets (N = 37,505 to 433,390) of the UK Biobank resource were analysed according to visual and hearing acuity, reaction time and fluid IQ. Sensory and cognitive performance was reassessed after ~4 years (N = 2,438 to 17,659). In statistical modelling including age, sex, socioeconomic status, educational level, smoking, maternal smoking and comorbid disease, adult height was positively associated with sensory and cognitive function (partial correlations; pr 0.05 to 0.12, p < 0.001). Within the normal range of birth weight (10 th to 90 th percentile), there was a positive association between birth weight and sensory and cognitive function ( pr 0.06 to 0.14, p < 0.001). Neither adult height nor birth weight was associated with change in sensory or cognitive function. These results suggest that adverse prenatal and childhood experiences are a risk for poorer sensory and cognitive function and earlier development of sensory and cognitive impairment in adulthood. This finding could have significant implications for preventing sensory and cognitive impairment in older age.
Citation: Dawes P, Cruickshanks KJ, Moore DR, Fortnum H, Edmondson-Jones M, McCormack A, et al. (2015) The Effect of Prenatal and Childhood Development on Hearing, Vision and Cognition in Adulthood. PLoS ONE 10(8): e0136590. https://doi.org/10.1371/journal.pone.0136590
Editor: Martin Walter, Leibniz Institute for Neurobiology, GERMANY
Received: April 28, 2015; Accepted: August 5, 2015; Published: August 24, 2015
Copyright: © 2015 Dawes et al. This is an open access article distributed under the terms of the Creative Commons Attribution License , which permits unrestricted use, distribution, and reproduction in any medium, provided the original author and source are credited
Data Availability: Data are available from the UK Biobank for researchers who meet the criteria for access to confidential data. For details of how to access to UK Biobank data, see http://www.ukbiobank.ac.uk/scientists-3/ or email [email protected] .
Funding: The authors received no specific funding for this work.
Competing interests: The authors have declared that no competing interests exist.
Introduction
Prenatal and early childhood development have a critical effect on long-term health in adulthood [ 1 ]. Early development may affect adult susceptibility to a range of non-communicable disease including cardiovascular disease [ 2 ] and diabetes [ 3 ]. The aim of this study was to examine the associations between prenatal and early childhood development with cognitive, vision and hearing function in middle age. Commonly used indexes of prenatal development include birth weight and other measures of body size at birth. Measures of body size at birth represent an indirect, summative measure of influences on the developing foetus [ 4 ]. In contrast to measures of body size at birth (an index of prenatal exposure), measures of adult leg length and height are sensitive to environmental factors and nutrition in early childhood that impact on growth [ 5 ].
Birth weight and post-natal growth during early childhood and adolescence are positively associated with cognitive ability in childhood [ 6 – 17 ]. There is less research on whether early life development affects cognitive performance in adulthood and old age, and the findings in the existing literature are mixed. Some studies of middle aged and older adults did not detect reliable associations between early life development and cognitive function in adulthood and older age [ 18 – 20 ]. One possible explanation may be that early life development exerts a stronger influence on cognition in childhood than later in life. Richards and colleagues [ 16 ] assessed cognitive function in 3,900 participants at ages 8, 11, 15, 26 and 43 years. Birth weight was positively associated with cognitive ability at 8, 11, 15 and 26 years, though there was no association at 43 years. Other studies do support associations between both prenatal [ 21 , 22 ] and childhood [ 22 , 23 ] development and adult cognitive function. In summary, there is inconsistent evidence that pre-natal growth may affect cognitive ability in adulthood. Previous research findings are also inconsistent in relation to early life development and age-related change in cognitive function. Martyn et al [ 19 ] and Shenkin et al [ 20 ] reported no association between any anthropometric parameter at birth and estimated cognitive decline. Gale and colleagues [ 24 ] found associations between cognitive decline and adult head circumference, but not head circumference at birth.
To date, most research attention has focused on the long-term cognitive outcomes of low birth weight babies and birth weight within the normal range. However, there is also evidence that unusually large babies may have poorer cognitive outcomes. Large babies are defined as being outside the normal range of distribution according to weight, head circumference or length (i.e. greater than the 90 th percentile or 97 th percentile, depending on the cut-point used [ 25 ]). Maternal diabetes and obesity are associated with higher likelihood of a large baby [ 26 ]. Cesur and Rashad [ 27 ] analysed two longitudinal data sets, examining patterns of academic performance in junior school for a combined sample of 19,280 children. Children who had either low or high birth weight had poorer academic performance compared to children within the normal range of birth weight. Given increasing rates of diabetes and obesity globally [ 28 , 29 ] and parallel evidence of increasing numbers of babies who are large for gestational age [ 30 ], adverse developmental outcomes associated with overweight babies is likely to assume increased importance.
Few studies have investigated associations between early life development and hearing and visual sensory function. Birth weight was reported to be associated with likelihood of having state registration for hearing impairment [ 31 ], hearing and visual acuity [ 32 ] and self-reported hearing problems [ 33 ]. Sayer et al [ 34 ] reported that reduced growth in childhood (but not birth weight) was associated with lens opacity (but not visual acuity) and poorer hearing levels when assessed around age 67 years. Barrenas [ 35 ] reported that adult height was associated with hearing, and concluded that adverse early life experience may convey increased susceptibility to hearing loss in adulthood. However, not all studies support associations between prenatal and/or childhood development and adult sensory function; Ecob and colleagues [ 36 ] reported no association between birth weight or BMI during childhood and hearing assessed at age 45 years.
It may be that the association between indices of early life development and adult cognition and sensory function is due to confounding; factors including socio-economic status or birth trauma may account for the association, despite attempts to control statistically for potential confounds. However, there is evidence from experimental studies with animal models that supports observational findings in humans for the influence of early life exposures on development of cognitive and sensory systems [ 37 ]. It is plausible that there is a direct causal relationship between early development and neurosensory function in adulthood, although the mechanism is unclear. It may be that under-nutrition impacts on development of the brain and sensory organs [ 38 ], or there may be alterations in gene expression that affect cognitive and sensory function [ 39 – 41 ]. Alternatively, glucocorticoid hormones or growth factors may be modulated by early experience and impact on neurosensory development [ 35 , 42 – 44 ]. It is also possible that the effect of early development on cognitive and sensory function is via increased susceptibility to diabetes and cardiovascular disease; adverse early development is associated with increased risk of cardiovascular disease and diabetes [ 2 , 3 ], and cognitive decline and poor hearing and visual function are independently associated with cardiovascular disease and diabetes [ 45 – 48 ].
It is unclear what the relative contribution of prenatal versus childhood development is for adult cognitive and sensory function and decline in function with age. In the present study, we examined hearing, vision and cognitive function in middle age according to birth weight (an index of prenatal development) and adult height (an index of early childhood development) in a very large sample of middle aged people, thereby increasing power for detecting small effects later in life. We also examined change in hearing, vision and cognitive function longitudinally over ~4 years as a function of birth weight and adult height. We hypothesized that i) cognitive and sensory function would be associated with birth weight, with poorer performance for low and high birth weight and better performance for larger babies within the normal range; ii) taller adults would have better cognitive and sensory function than shorter adults; iii) low and high birth weights and short adult height would be associated with a greater decline in sensory and cognitive function.
The UK Biobank sample
This study utilised data from the UK Biobank resource [ 49 ]. UK Biobank is an open access resource that is open to bona fide scientists undertaking health-related research that is in the public good. Approved scientists from the UK and overseas and from academia, government, charity and commercial companies can use the Resource (for details of access, see http://www.ukbiobank.ac.uk/scientists-3/ ). Ethical approval for UK Biobank was obtained from the National Health Service North West Multi-centre Research Ethics Committee. During 2006–2010, 503,325 participants were recruited, with a response rate of 5.47%. As data collection proceeded, additional measures were added to the test protocol. Thus, different numbers of participants completed each measure ( Table 1 ). For the analysis of visual acuity, participants were excluded if they required glasses for distance viewing, but had completed visual acuity testing without them. Participants attended a UK Biobank assessment centre and provided informed consent. Participants completed a 90 minute assessment that included i) a computerised questionnaire on lifestyle, environment and medical history, ii) physical measures including hearing and vision testing. A description of the procedure and additional data collected may be found elsewhere ( http://www.ukbiobank.ac.uk/ ). Data on sex, ethnicity and area of residence were collected for each participant. Areas of residence were translated to Townsend deprivation scores to provide a proxy measure of socioeconomic status. Townsend scores are widely used in health studies, and are applicable across the countries of the UK [ 50 ]. Townsend scores for small geographical areas (Electoral wards in England, Wales and Northern Ireland, postal sectors in Scotland) are based on four variables including employment, car ownership, home ownership and household occupancy. Each variable is translated to a z-score relative to the national level. Z-scores are then summed to give a single deprivation score for each area. Lower Townsend scores represent areas associated with more affluent socioeconomic status.
- PPT PowerPoint slide
- PNG larger image
- TIFF original image
https://doi.org/10.1371/journal.pone.0136590.t001
During 2012 and 2013, 17,819 participants were recruited for repeat assessment, with a response rate of 21%. All baseline and physical measures were repeated, including hearing, vision and cognition. The average interval between baseline testing and repeat assessment was 4 years, and ranged between 2 and 7 years. (For further details of the repeat assessment, see http://biobank.ctsu.ox.ac.uk/~bbdatan/Repeat_assessment_doc_v1.0.pdf ).
Birth weight and adult height
Self-reported birth weight was recorded for those participants who reported that they knew their own birth weight. Self-reported birth weight is a valid measure of birth weight [ 51 , 52 ]. Participants’ standing height was measured in centimeters using a Seca 202 telescopic measuring rod ( http://www.seca.com/ ).
Hearing was assessed with the Digit Triplet Test (DTT[ 53 ]), a speech-in-noise test that is used internationally for large-scale hearing screening. A detailed description of the DTT is provided elsewhere ( http://biobank.ctsu.ox.ac.uk/crystal/label.cgi?id=100049 ). Briefly, fifteen sets of three monosyllabic digits were presented over circumaural headphones (Sennheiser HD-25). Participants first set the volume of the stimuli to a comfortable level. Each ear was tested separately with the order of testing randomised across participants. Digits were presented in a background of noise shaped to match the spectrum of the speech stimuli. Noise levels varied adaptively, contingent on recognition of the three digits, to estimate the signal-to-noise ratio (SNR) for a criterion performance of 50% correct. The speech recognition threshold was calculated from the mean SNR for the last eight triplets. Lower (more negative) scores represent better performance. The recognition threshold for the better ear was used as the index of hearing.
Visual acuity testing was based on reading high contrast letters with the participant seated at a distance of 4 metres. Visual acuity measures were completed on both eyes. Participants wore glasses or contact lenses that were normally worn for distance viewing. Visual acuity scores were determined as the logMAR size at which 3 out of the 5 letters presented were read correctly. A detailed description of visual acuity testing is provided elsewhere ( http://biobank.ctsu.ox.ac.uk/crystal/refer.cgi?id=100250 ). Visual acuity for the better eye was used as the index of vision.
Cognitive tests
Cognitive tests were completed via a computerised touchscreen interface. A detailed description of cognitive testing is reported elsewhere ( http://biobank.ctsu.ox.ac.uk/crystal/label.cgi?id=100026 ). Processing speed testing was based on the card game ‘Snap’. Participants were shown two pairs of cards out of a set of 12 pairs. If both cards displayed a matching symbol, participants pressed a response button as quickly as possible using their dominant hand. Reaction time was taken as the average time to correctly respond to a matching pair. Fluid intelligence (IQ) (the capacity for logical thought and problem solving, independent of acquired knowledge) was estimated via multiple choice responses to questions such as "Bud is to Flower as Child is to?" Participants had 2 minutes to complete as many questions as possible out of 13. Correct responses scored 1 point, while questions that were answered incorrectly or that were not completed within the time limit scored zero.
Cardiovascular disease, hypertension, cholesterol and diabetes
Cardiovascular disease was identified on the basis of participant report of any cardiovascular problems, including heart attack, stroke, heart failure, angina, transient ischemic attack, intermittent claudication, arterial embolism or deep venous thrombosis. Hypertension was identified if the participant reported that they had high blood pressure, currently took medication for high blood pressure, or had a measured systolic blood pressure greater than 140 mm Hg or diastolic pressure greater than 90 mm Hg. High cholesterol was identified if the participant reported that they had high cholesterol, or that they were currently taking medication for high cholesterol. Diabetes was identified if the participant reported that they had Type 1 or Type 2 diabetes, or that they took insulin for diabetes.
Education level, smoking and maternal smoking
Education level was categorised according to the highest self-reported level of study (University or college degree or other professional qualification; A/AS level, O level, GCSE or vocational qualification; no qualification post primary school). Maternal smoking was recorded in response to the question "Did your mother smoke regularly around the time when you were born?" Smoking status was based on self-report of previous or current tobacco smoking (current/previous/never).
Data analysis
Participants were fractionally ranked by birth weight and adult height separately for males and females. Fractional ranks were then pooled across sexes to provide percentile ranks by birth weight and by height within the sample independent of sex. Plots of reaction time, IQ, hearing and vision acuity by percentile rank for birth weight and adult height were generated. To index change in performance over time, performance at the baseline assessment was subtracted from performance on repeat assessment. Change in performance was then divided by the time between baseline and repeat assessment to provide a figure for the average annual change in performance for the hearing, vision and cognitive tests.
To analyse the association between adult height and hearing, vision and cognition, a linear regression model was applied with cognitive or sensory performance as the dependent variable, adult height as the independent variable, with age, sex, Townsend deprivation index quintile, educational level, smoking, diabetes, cardiovascular disease, hypertension, high cholesterol and maternal smoking as possible confounds. Similar analyses were carried out to analyse the association between adult height and change in hearing, vision and cognition.
To analyse the association between birth weight and hearing, vision and cognition, linear regression models were applied for those participants within the normal range (10 th to 90 th percentile) of birth weight with age, sex, Townsend deprivation index quintile, educational level, smoking, diabetes, cardiovascular disease, hypertension, high cholesterol and maternal smoking as possible confounds. Similar analyses were carried out to analyse the association between birth weight and change in hearing, vision and cognition. To compare the smallest and largest extremes of the distribution by birth weight, the top and bottom 3% were compared to the 3% of the sample in the middle of the distribution (i.e. +/- 1.5% of the sample either side of the 50 percentile). An ANOVA model was applied, hearing, vision and cognition as the dependent variable and group (bottom, middle or top 3% of the distribution) as the independent variable in the model, with the covariates age, sex, Townsend deprivation index quintile, educational level, smoking, diabetes, cardiovascular disease, hypertension, high cholesterol and maternal smoking. All analyses were performed with SPSS version 20.0 ( http://www-01.ibm.com/software/analytics/spss/ ).
Table 1 shows the number and demographic characteristics of the subsets of participants who completed the hearing, vision and cognitive tests and for whom birth weight or adult height data were available. Table 2 describes the average birth weight and height for males and females in each birth weight and height percentile.
https://doi.org/10.1371/journal.pone.0136590.t002
Cognitive and sensory function in adulthood
Adult height..
Fig 1 (left hand side, ‘1a. Adult height’) shows hearing, vision and cognitive performance by percentiles of adult height. Regression models including height, sex, age, Townsend quintile, smoking status, cardiovascular disease, diabetes, hypertension, high cholesterol and maternal smoking and hearing, vision, reaction time and IQ were all statistically significant (Hearing: r 2 = 0.06, F(11,120783) = 756, p < 0.001; Vision: r 2 = 0.08, F(11,54922) = 402, p < 0.001; Reaction time: r 2 = 0.10, F(11,358036) = 3642, p < 0.001; IQ: r 2 = 0.16, F(11,120857) = 2073, p < 0.001). Adult height was a significant contributor to each regression model ( Table 3 , left hand side ‘3a. Function in adulthood’). Partial correlations ( pr ) for height controlling for sex, age, Townsend quintile, smoking status, cardiovascular disease, diabetes, hypertension, high cholesterol and maternal smoking were: hearing 0.06; vision 0.06, reaction time 0.05; IQ 0.12.
https://doi.org/10.1371/journal.pone.0136590.g001
https://doi.org/10.1371/journal.pone.0136590.t003
Birth weight.
Fig 1 (right hand side, ‘1b. Birth weight’) shows hearing, vision and cognitive performance by birth weight percentile. Within the normal range of birth weight (10 th to 90 th percentile), regression models including birth weight, sex, age, Townsend quintile, smoking status, cardiovascular disease, diabetes, hypertension, hypercholesterolemia and maternal smoking and hearing, vision, reaction time and IQ were all statistically significant (Hearing: r 2 = 0.06, F(11,55158) = 781, p < 0.001; Vision: r 2 = 0.07, F(11,25737) = 187, p < 0.001; Reaction time: r 2 = 0.11, F(11,163368) = 1808, p < 0.001; IQ: r 2 = 0.14, F(11,55586) = 837, p < 0.001). Birth weight made a statistically significant contribution to each model ( Table 3 , left hand side ‘3a. Function in adulthood’). Partial correlations ( pr ) for birth weight controlling for sex, age, Townsend quintile, smoking status, cardiovascular disease, diabetes, hypertension, cholesterol and maternal smoking were: hearing 0.04; vision 0.01, reaction time 0.01; IQ 0.12.
The top and bottom 3% by birth weight were compared with the middle 3% (centred on the 50 th percentile). For an ANOVA including birth weight category (low, middle, high) and potential confounds there were significant differences in hearing, vision, reaction time and IQ across birth weight category ( Table 4 ). Bonferroni post-hoc comparisons revealed that for hearing, vision, reaction time and IQ, the middle category had significantly better performance than both the low and high categories (both p < 0.001). The high category had significantly better performance than the low category for hearing and IQ ( p = 0.01; p < 0.001). There was no difference between the low and high categories in reaction time or vision ( p = 0.93; p = 0.43).
https://doi.org/10.1371/journal.pone.0136590.t004
Change in sensory and cognitive function
Fig 2 (left hand side, ‘2a. Adult height’) shows the annual change in hearing, vision, reaction time and IQ by percentile of adult height. For all figures, positive scores correspond to improvement in performance while negative scores correspond to worse performance over time. For vision and reaction time, there was a uniform pattern of worse performance over time. For hearing, the lowest height group showed a tendency for improved performance. For IQ, there was a tendency for improved performance across almost all percentile groups.
https://doi.org/10.1371/journal.pone.0136590.g002
Regression models ( Table 3 , right hand side ‘3b. 4-year change in function’) including height, sex, age, Townsend quintile, smoking status, cardiovascular disease, diabetes, hypertension, high cholesterol and maternal smoking and change in hearing, vision, reaction time and IQ were statistically significant for change in hearing, reaction time and IQ (Hearing: r 2 = 0.01, F(11,3768) = 3.43, p < 0.001; Reaction time: r 2 = 0.004, F(11,11372) = 4.20, p < 0.001; Fluid IQ: r 2 = 0.03, F(11,3875) = 9.5, p < 0.001). The regression model for change in visual acuity was not statistically significant ( r 2 = 0.01, F(11,2079) = 0.99, p = 0.45). Height was not a significant contributor to any model. For hearing, older age and lower educational attainment was associated with improved function. Older age and high cholesterol was associated with slower reaction time at reassessment. More deprived Townsend quintile and lower educational attainment was associated with improved IQ over time.
Fig 2 (right hand side, ‘2b. Birth weight’) shows the annual change in hearing, vision, reaction time and IQ by percentile of birth weight. Patterns of change were similar to those for adult height. There was a tendency for worse performance over time for hearing, vision and reaction time across the range of birth weight. Small improvements were evident for fluid IQ. Within the normal range of birth weight (10 th to 90 th percentile), regression models including birth weight, sex, age, Townsend quintile, smoking status, cardiovascular disease, diabetes, hypertension, cholesterol and maternal smoking were statistically significant for change in hearing, reaction time and IQ (Hearing: r 2 = 0.013, F(11,1767) = 2.17, p = 0.01; Reaction time: r 2 = 0.076, F(11,5004) = 2.62, p = 0.002; IQ: r 2 = 0.032, F(11,1825) = 5.42, p < 0.001). The model for change in vision was not statistically significant ( r 2 = 0.01, F(1,1027) = 0.89, p = 0.55). Birth weight was not a significant contributor to any model ( Table 3 , right hand side ‘3b. 4-year change in function’). The top and bottom 3% by birth weight were compared with the middle 3% (centred on the 50 th percentile). In ANOVA including birth weight category (low, middle, high) and potential confounders, there were no significant differences in birth weight category for change in performance ( Table 5 ).
https://doi.org/10.1371/journal.pone.0136590.t005
In statistical models that included age, sex, socioeconomic status, educational level, diabetes, cardiovascular disease, hypertension, high cholesterol, smoking status and maternal smoking, adult height was linearly related to hearing, vision and cognitive function in middle age, with taller adults having better function. Environmental factors and nutrition in early childhood (indexed by adult height) are thus related to sensory and cognitive function in middle age. Birth weight showed a different pattern of association. Within the normal range (10 th to 90 th percentile), larger birth weight was associated with better hearing, vision and cognitive function. Participants with both the smallest and the largest birth weights had significantly poorer function than those within the normal range. Adult cognitive and sensory function are therefore related to prenatal experience (indexed by birth weight). It is striking that effects of early development persist into middle age, after decades of life experiences that impact on cognitive and sensory functioning. Early life factors may also interact with environmental exposures during the lifespan to increase susceptibility to cognitive and sensory impairment [ 54 ]. Common influence by early life factors may explain the observation that cognitive and sensory performance are associated, independently of age [ 55 ].
On reassessment after ~4 years, improvements in the IQ and the hearing measure were apparent. Performance on the vision and reaction time tasks showed a more expected pattern of decline in performance. Practice effects are well-known in studies of cognitive aging and may affect some tests more than others [ 56 ], and it is problematic to dis-entangle practice effects from age-related changes in performance. Neither adult height nor birth weight were consistently associated with change in sensory or cognitive function over time. Prenatal and early childhood development were associated with the level of sensory and cognitive functioning in adulthood, but not to the rate of change in function with age, as indexed by the measures used in the present study. Note that adverse early life experience may still be a risk for earlier development of sensory and cognitive impairment. Although the rate of change with age may be similar, those who begin from a lower level of performance will reach the threshold of impairment sooner (cf the concept of ‘cognitive reserve’ and brain ageing [ 57 ]). This finding may have significant implications for preventing sensory and cognitive impairment in older age; modelling suggests that even relatively modest delays in cognitive decline would substantially reduce the number of cases of dementia in the population [ 58 ]. The same may be true for hearing and vision impairment. Consistent with previous research, indices of early life development were associated with a small amount of variance in cognitive and sensory performance. For example, across the range of adult height, the effect on hearing corresponded to a 1 dB difference in signal to noise ratio for speech recognition (the mean difference in signal to noise ratio for speech recognition between the ages of 40 and 69 years is 1.2 dB [ 59 ]), a 0.08 logMAR difference in visual acuity, a difference of 67 ms in reaction time and 1.7 points on a 13-point scale of fluid IQ. Even if modest in size, the effects of prenatal and childhood development are universal and may thus be significant determinants of cognitive and sensory aging at a population level [ 37 ]. Shifts in the mean level of function within a population have dramatic effects on the numbers of people that fall within the range of clinical impairment. For example, a decrease in the mean IQ of the population by 5 points on a standard distribution would double the number of people with an IQ <70 [ 37 ]. The effect of early development on cognitive aging has been recognised as a research priority [ 60 , 61 ]. Effects of early development in relation to vision and hearing impairment in adulthood and old age may warrant similar attention.
The present study has some limitations. The study was observational with cross-sectional and longitudinal measurement, and it is not possible to establish a causal link between early development factors and adult cognitive and sensory function. We attempted to control statistically for potential confounds such as socioeconomic status, educational level, age, comorbid disease, smoking and maternal smoking. However, it is possible that unmeasured confounds or insufficient control of measured confounds could impact results. For example, no information was available on gestational age or parental body size. Ideally, body size measures at birth should be corrected for parental size and gestational age in order to distinguish between growth appropriate for gestational age and growth restriction [ 62 ], though the necessary data are often unavailable. Even with correction for gestational age and parental size, body size at birth does not allow insight into the aetiology of low birth weight, for example whether due to acute injury resulting in impaired growth or slow growth from conception [ 63 ]. The UK Biobank sample had a low response rate (5.47%) and includes a higher proportion of white, female and less deprived people than in the general UK population. However, due to the very large and inclusive nature of the sample, associations between risk factors and health outcomes can be made with confidence [ 49 ]. Different numbers of participants completed the different tests and participated in the reassessment. In particular, reassessment included lower numbers of participants than the baseline assessment, so the analysis of change in cognitive and sensory function is statistically lower-powered than the cross-sectional analysis. However, sample sizes for reassessment remained sufficiently large (2,438 to 17,659, depending on the measures) and compare favourably with previously published studies with much smaller samples.
Sensory and cognitive impairments in older age are a substantial and increasing source of poor quality of life and economic costs globally [ 64 ]. As with other diseases that are linked to early development, cognitive and sensory function appears to be influenced by the full range of development; not just those within the extremely deprived range, but also those within the normal range. In models of infectious disease, individuals remain healthy until they contract the disease. In contrast, non-communicable diseases like diabetes, cardiovascular disease, cognitive impairment and sensory impairment develop gradually over the life course. Early development may programme a metabolic trajectory for the lifespan which interacts with accumulated challenges to health (such as obesity, smoking or hypertension [ 35 ]) and age-related declines in physiological plasticity [ 65 ]. One implication of this model is that the effectiveness of interventions in adulthood are relatively limited, while intervention in a developmentally more plastic period is likely to have a much larger impact in altering the metabolic trajectory and preventing disease [ 65 ]. In order to reduce the burden of cognitive decline and sensory impairment, future research attention may centre on identifying avenues for intervention to optimise foetal growth and childhood development [ 62 ]. Public health policy should also take into account the importance of focusing on interventions during early life, with potentially greater effect than attempting to mitigate cognitive decline and sensory impairment in adulthood and older age.
Acknowledgments
The views expressed are those of the author(s) and not necessarily those of the NHS, the NIHR or the Department of Health. This research was facilitated by Manchester Biomedical Research Centre. This research was conducted using the UK Biobank Resource.
Author Contributions
Conceived and designed the experiments: PD KJC KJM DRM. Analyzed the data: PD MEJ AM DRM HF KJM. Contributed reagents/materials/analysis tools: PD MEJ. Wrote the paper: PD KJC DRM HF MEJ AM KJM.
- View Article
- Google Scholar
- PubMed/NCBI
- 4. Kline J, Stein Z, Susser M (1989) Conception to birth: epidemiology of prenatal development. New York: Oxford University Press.
- 27. Cesur R, Rashad I (2008) High birth weight and cognitive outcomes. Cambridge, MA: National Bureau of Economic Research. 1–30 p.
- 58. Vickland V, Morris T, Draper B, Low L, Brodaty H (2012) Modelling the impact of interventions to delay the onset of dementia in Australia. A report for Alzheimer’s Australia. Alzheimer's Australia.
- 60. European Parliament (2011) Futurage. A roadmap for European ageing research.
Academia.edu no longer supports Internet Explorer.
To browse Academia.edu and the wider internet faster and more securely, please take a few seconds to upgrade your browser .
- We're Hiring!
- Help Center
Prenatal Development
- Most Cited Papers
- Most Downloaded Papers
- Newest Papers
- Save to Library
- Last »
- Prenatal and Perinatal Psychology Follow Following
- Brain and Cognitive Development Follow Following
- Early Childhood Development Follow Following
- Child and adolescent mental health Follow Following
- Non Invasive Prenatal Diagnosis Follow Following
- Prenatal screening Follow Following
- Prenatal Care Follow Following
- Digit Ratio Follow Following
- Prenatal Care, Epidemiology Follow Following
- Prenatal Stress Follow Following
Enter the email address you signed up with and we'll email you a reset link.
- Academia.edu Publishing
- We're Hiring!
- Help Center
- Find new research papers in:
- Health Sciences
- Earth Sciences
- Cognitive Science
- Mathematics
- Computer Science
- Academia ©2024

Child Development and Learning Studies Lab • Designed by M. Sami Gürdal •
Özyeğin University
Prenatal Development
Prenatal development period includes the growth and development process of the zygote, which is formed by the union of sperm and egg in the womb (Shiel Jr., 2018). First 4 months of the baby in the womb is a vital period regarding the beginning of the formation of organs. During this period, new features such as the onset of the heartbeat and then the emergence of the head, ears, and eyes, and along with this, their tiny arms and legs gradually begin to become evident. The most important developing system at this period is the nervous system. In this system, firstly the head grows, the cartilage skeletal system emerges and then the muscles are formed, and shaped. At the end of the eight weeks, the embryo begins to look like a human-being. The reason for this is that all facial features and organs develop in this period. Also, the existing features after the baby (fetus) reaches the fourth month, the arms, legs, and brain features continue to develop in more detail (O’Rahilly & Müller, 2008). The fact that most of the congenital developmental disorders emerge in these first 4 months shows how important this stage is (O’Rahilly & Müller, 2008). In the prenatal period, babies begin to hear some sounds in the womb, such as the mother’s voice and heartbeat. These sounds are very important for the maturation of the baby’s sensory development in the future (Webb et all., 2015).
Prenatal development has an important role in our lives due as it is a factor that affects our health in childhood and even adulthood. In this period, pregnant mothers should pay attention to their health in order to contribute to the development of the baby in terms of cognitive, visual, and auditory functions since the baby in the womb begins to be directly affected by the mother’s experiences (Dawes et al., 2015). If the mother does not eat healthy during pregnancy, the baby is likely to be born prematurely with low weight and height because of this situation and at the same time, malnutrition, along with poor environmental conditions, causes development of cognitive dysfunctions in infants (Dawes et al., 2015). In a study by Richards et al. (2001), on cognitive dysfunctions, the cognitive functions of the participants from childhood to adulthood were investigated. As a result of this research, it was found that birth weight and cognitive ability were significantly correlated. Briefly, we can say that birth weight and the growth environment after birth are important factors for the healthy development of a child’s cognitive abilities. Finally, prenatal development has a significant impact on the auditory and visual sensory functions of the baby in the womb, and birth weight was found to be positively associated with hearing and visual functions (Olsen et al., 2001).
The prenatal period is also important for the emotional development of babies. The emotional state of pregnant mothers directly affects their babies (O’Connor et al., 2003). It has been revealed that the mother’s prenatal stress causes the baby’s emotional disorders, and this effect may be long-lasting (Desocio, 2018). However, not all stress is harmful. Some stress and anxiety during pregnancy can be considered as “normal” (Desocio, 2018). In fact, some types of stress and anxiety, called positive stress, increase temporary stress hormones, but with healthy coping strategies, this returns to normal levels. This has facilitated adaptation during the developmental transition of pregnancy (Shonkoff et al., 2009). On the other hand, it has been observed that pregnant women who have supportive relationships around them can tolerate moderate and severe stresses more easily (Shonkoff et al., 2009). However, in the absence of supportive relationships, toxic stress may be experienced depending on the intensity, frequency, and duration of stress (cited in Shonkoff et al., 2009). The health and development of both babies and mothers who are exposed to this stress during pregnancy are adversely affected (Dunkel-Shetter & Tanner, 2012). For example, it has been found that women who are exposed to severe psychosocial stress factors such as losing someone important to them in the first months of pregnancy, being exposed to extreme trauma, poverty, discrimination, unemployment and/or living in harsh conditions, are found to be at greater risk of giving birth prematurely and having a low birth weight of the baby (Dunkel-Shetter & Tanner, 2012). In addition, maternal stress experienced in the first months of pregnancy has a significant effect on the brain development of the child (Sandman et al., 2012). For example, in a study (Sandman et al., 2012), it was observed that when the children of mothers who experienced psychosocial stress in the first months of pregnancy become 5 and 8 years old, there were structural differences in their brain development compared to other children. These structural differences in their brain regions are related to the reduction of factors involved in numerous cognitive functions such as attention, problem-solving, memory, learning, and language development (Sandman et al., 2012). These studies have shown that the first trimester of pregnancy is a sensitive and critical period in terms of changes in mental development, therefore stresses experienced during this period increase the tendency to develop a serious mental disorder in later life (DeSocio, 2018).
The health of the baby and mother depends on the use and behavior of prenatal health services, such as the support they receive before and during birth and maternity care. Because this care can be used for early detection of birth complications or high-risk pregnancies. Therefore, it can initiate prevention or intervention. It has been shown in the study that with the prenatal care, the risks of low birth weight and infant mortality were greatly reduced. (Henze, 2004).
At the same time, common symptoms experienced during this period are anxiety, fatigue, sleepiness, indecision, and frequent mood changes ranging from depressive reactions to excitement. Our priority will be the activities that the mother will do to keep her psychological health stable during pregnancy. These can be the activities like yoga, exercise, meditation, and walking. Overall, these methods can be a good way to relieve feelings of stress and frustration. Another method is to find a support group where the mother can share her interests with different mothers, which can also help her get through this period in a healthier way. Sharing common interests and common concerns in these groups will not make the mother feel lonely, and knowing other mothers who have these similar problems will keep her mood healthier. When these methods are insufficient, if the mother feels anxious or depressed, a doctor or psychological support should be sought. The sooner the treatment or therapy process is started, the better the mother will feel. Getting the support of a therapist can be an appropriate way to deal with stress and depression and to reduce tension during pregnancy. All these approaches will positively affect the psychological health of the mother and, naturally, the development of the baby in many ways.
Written by Beril Berksu, Zeynep Şişman, Elif Cilmeli, Çağla Sarıga, Zeynep Yalçıntaş, and İbrahim Hakkı Acar
Click for PDF file and references
Leave a Reply Cancel reply
Your email address will not be published. Required fields are marked *
Post Comment
Related Posts
Supporting children during the covid-19 pandemic, mindful parenting and child development, temperement in early childhood, maternal well-being and child development.
Begin typing your search term above and press enter to search. Press ESC to cancel.

An official website of the United States government
The .gov means it’s official. Federal government websites often end in .gov or .mil. Before sharing sensitive information, make sure you’re on a federal government site.
The site is secure. The https:// ensures that you are connecting to the official website and that any information you provide is encrypted and transmitted securely.
- Publications
- Account settings
Preview improvements coming to the PMC website in October 2024. Learn More or Try it out now .
- Advanced Search
- Journal List
- J Pediatr Genet
- v.4(2); 2015 Jun

Prenatal Exposures and Short and Long Term Developmental Outcomes
Identifying human teratogens: an update.
1 Department of Medical Genetics, University of British Columbia, Vancouver, BC, Canada
Christina D. Chambers
2 Department of Pediatrics and Family Medicine and Public Health, University of California, San Diego, La Jolla, California, United States
A human teratogen is an agent that alters the growth or structure of the developing embryo or fetus, thereby causing birth defects. The first human teratogen identified in 1941 by an ophthalmologist, Norman Gregg, was maternal rubella infection in pregnancy, which produced a triad of defects (cataracts, heart malformations, and deafness) in the infants. 1 Following the identification of thalidomide, an antinauseant, as a major human teratogen causing severe birth defects in 1961, 2 research in teratology began to expand and there was increased awareness of the possible teratogenic impact of maternal exposures during pregnancy.
Several factors that determine the teratogenicity of an exposure have been set forth as the principles of teratology by Wilson, 3 guiding researchers in the study and understanding of teratogenic agents. These include, but are not limited to, the following: Abnormal development produced by a teratogenic exposure is manifested as death, malformation(s), growth retardation, or a functional disorder. These include neurologic impairments, such as mental retardation, and long-term effects on cognition and behavior that may appear later in childhood. A second principle of teratology states that susceptibility to teratogenesis varies with the developmental stage at the time of exposure, and a third claims that manifestations of abnormal development depend on dose and duration of a teratogenic exposure. These indicate that not all exposures deemed as teratogenic are actually teratogenic all the time; the timing and dose of a particular exposure during pregnancy often determine the kind and extent of its teratogenic potential. The embryonic period, during which organogenesis takes place, occurs between implantation at around 14 days to around 60 days postconception. This is usually the most sensitive period to teratogenesis when exposure to a teratogenic agent has the greatest likelihood of producing a malformation. For example, administration of many established major teratogenic drugs, such as isotretinoin, valproic acid, warfarin, or high-dose methotrexate, in specific gestational windows in the first trimester is associated with a high risk of having a baby with a congenital malformation, but the risk significantly decreases in the second or third trimesters of pregnancy. In some cases, several periods of susceptibility may exist for a single organ, such as in the case of craniosynostosis, an abnormality that occurs as a result of premature fusing of cranial sutures. Also, for some teratogens, a level of exposure exists below which probably no demonstrated harmful embryonic effect occurs, such as in the case of methotrexate, a folic acid antagonist. 4
Another teratology principle states that susceptibility to a teratogenic exposure also depends on the fetal and maternal genotype. Genetic predisposition to the effects of several teratogenic exposures has been intensively studied. For example, embryonic exposure to cigarette smoke has been established as a risk factor for orofacial clefts 5 and gastroschisis, 6 but even higher risks were found for cleft palate among the offspring of maternal smokers in the presence of a rare transforming growth factor α ( TGFA ) genotype 7 or a variation in the nitric oxide synthase ( NOS3 ) gene in the infant. 8 The genetic basis underlying susceptibility to fetal alcohol syndrome and abnormal glucose metabolism-induced birth defects have also been linked to certain maternal and fetal gene variants. 9 Specifically with regard to drug metabolism, genetic variation in maternal metabolism of certain antiepileptic medications may predispose her infant to the development of birth defects. 9 10
Experimental animal teratology studies performed on rodents are critical in determining the risk or safety in pregnancy of a particular agent, and are usually used by industry and regulatory agencies as a first step before a specific agent is approved for marketing, such as in the case of medications. However, it is sometimes difficult to extrapolate results from available animal studies to humans in part due to the relevance of the dose utilized and in part due to species differences in response to a medication. Experimental teratology studies or randomized controlled trials in human pregnancy are rarely permitted because of ethical reasons. Therefore, to determine whether an exposure is teratogenic in humans, observational studies are usually the method that is used to evaluate risk or safety. If these are performed at all, often the results are not available until many years after an agent has been marketed for use. 11 These include descriptive studies such as case reports, which are published reports of individual cases of birth defects in an infant exposed prenatally to the agent of interest, raising hypotheses about potential causality, or clinical case series, which track several affected infants with known common exposure histories, and determine whether a characteristic malformation pattern exists. Many major human teratogens, such as thalidomide, isotretinoin, rubella, and fetal alcohol syndrome, have been identified using clinical series.
Analytical observational studies, on the other hand, include cohort studies, which measure the frequencies of outcomes in exposed and unexposed (control) pregnancies. They can be prospective in nature, whereby exposures are recorded during pregnancy without knowledge of the outcomes. Data for prospective cohort studies can be obtained either for an entire population in a comprehensive and ongoing fashion (population-based) or through more focused studies relying on volunteers, such as through the efforts of one or more teratogen information services (exposure cohort). Retrospective record-linkage cohort studies are usually collected for administrative purposes (e.g., as prescription records or hospital discharge summaries) at the time a medical service is provided and then subsequently electronically linked to birth outcomes on a case-by-case basis. The third type of analytical epidemiological studies is case-control studies, which measure the frequency of exposure in the pregnancies of mothers of babies with (cases) or without (controls) birth defects. Exposure information in case-control studies is usually collected retrospectively through interviews or questionnaires given to mothers. These investigations are very useful for studying rare outcomes such as specific birth defects but may be limited by recall bias. Reproducibility of associations of a certain teratogenic exposure with an adverse outcome, using different types of observational studies, has been crucial in providing evidence in support of causality for many teratogens, particularly those with more moderate magnitude of effects.
Our goal for this special issue in the Journal of Pediatric Genetics is to present readers with emerging new data on prenatal exposures affecting short- and long-term developmental outcomes that may help guide clinicians and patients in considering the risks and benefits of various treatments. We begin with an update on the teratogenicity of maternal mycophenolate mofetil, an immunosuppressant that is frequently used in patients who have received solid organ transplants. In their paper, Coscia et al provide recent evidence from the literature, namely case reports and case series, of a recurrent pattern of malformations associated with maternal mycophenolate mofetil treatment during the first trimester of pregnancy. The authors strongly advise that pregnancy should be avoided while on this medication, and that providers and their patients consider modification of treatment prior to conception or when an unplanned pregnancy is discovered.
The next article in this issue by Yazdy et al reviews recent data regarding the effect on the fetus of maternal use in pregnancy of the most common prescription pain medications, opioid analgesics. The authors report on first-trimester opioid use and an increased risk of birth defects, including neural tube defects and congenital heart defects. The authors also review adverse fetal effects associated with maternal treatment anytime in pregnancy, such as poor fetal growth, preterm birth, and neonatal abstinence syndrome. It is therefore important that health care providers discuss these recent findings with their patients and explain the potential risks and benefits of taking these medications during pregnancy.
Teratogenic exposures are not necessarily always medications, infections, chemicals, or physical agents that a woman may encounter in her pregnancy. The teratogenic exposure may also be a preexisting chronic or physical condition, such as excess body weight. Iessa and Berard's update on prepregnancy maternal obesity in pregnancy summarizes interesting data on potential associations with birth defects, and other adverse outcomes, such as macrosomia or fetal death. This review also presents evidence on maternal obesity and the possible association with poor neurodevelopmental outcomes.
The next two articles of the special issue specifically focus on the effect of psychotropic and antiepileptic drugs (AEDs) on long-term cognitive and behavioral phenotypes in the offspring. The most frequently used class of antidepressant medications, selective serotonin reuptake inhibitors (SSRIs), has been a hot topic of interest over the past 10 year with regard to their use during pregnancy. Research results in the field have been widely conflicting, though most studies agree that a small teratogenic effect of these medications cannot be ruled out. Many AEDs, on the other hand, are established teratogens that adversely affect embryonic development when administered early in pregnancy. The papers in this special issue by Boukhris and Berard, and Gerard and Meador that cover these two drug classes review recent investigations into the effect of SSRIs and AEDs on neurodevelopment, with specific focus on autism spectrum disorders. The authors also touch on possible mechanisms of cognitive teratogenesis with regard to genetic susceptibility to SSRIs and AEDs. In the final paper in this special issue, Alwan and Hamamy present a review of maternal iron deficiency during pregnancy, suggesting that it may increase the risk for several adverse outcomes, such as fetal growth restriction, obesity, and high blood pressure in the offspring later in life, as well as neurobehavioral abnormalities.
Prenatal And Infant Development Research Paper

Sample Prenatal And Infant Development Research Paper. Browse other research paper examples and check the list of research paper topics for more inspiration. If you need a research paper written according to all the academic standards, you can always turn to our experienced writers for help. This is how your paper can get an A! Feel free to contact our research paper writing service for professional assistance. We offer high-quality assignments for reasonable rates.
Infancy extends from birth through 2 years of age. It has long been regarded as a special period of life in which numerous important transformations of structure take place, and the essential processes of information exchange with the world are laid down. Over this period, the infant is transformed from a condition of utter dependence on the caregiver for survival to a condition of relative independence or life-preserving agency—a transformation accompanied by marked physical, behavioral, and cognitive changes. While no characterization of this transition is entirely satisfactory, several elements set the infant apart from children of other ages. Despite disclaimers that what happens very early in life can be reversed or improved upon, most developmental psychologists celebrate the very considerable and enduring influence of early experience. This research paper paints a broad picture of infant development, its prenatal antecedents, and its relation to the behavior of older children and adults.
Academic Writing, Editing, Proofreading, And Problem Solving Services
Get 10% off with 24start discount code, 1. prenatal development, 1.1 physical and cognitive development.
The ‘prenatal period’ extends from conception to birth. During the ‘germinal stage’ (fertilization–2 weeks) and ‘embryonic stage’ (2–8 weeks), the fertilized egg rapidly divides and attaches to the uterine wall, the major organs and systems of the body differentiate, and interconnections form between the developing components of the central and peripheral nervous systems. During the fetal stage (8 weeks–birth), the organism grows rapidly and the physical features that identify it as human become increasingly apparent.
During the prenatal period, the mechanisms that will facilitate the organism’s adaptation postnatally become functionally mature. Infants born prematurely, for example, who readily learn responses that increase the amount of quiet sleep, reveal this anticipatory maturation. The characteristics of the mother’s voice are also learned during the prenatal period. After birth, hungry infants orient and root in the direction of their mother’s voice, thereby increasing their efficiency of breast-feeding irrespective of whether the mother is holding them on the left or right. Newborns will also learn to activate a tape-recording of their mother’s voice—but not of another woman’s voice—by sucking non-nutritively on a modified nipple. Moreover, ‘prenatal learning’ is highly specific: newborns will suck harder to listen to their mother reading a Dr. Seuss passage that she had read aloud daily during the last 6 weeks of gestation than to hear her reading a novel Dr. Seuss passage (DeCasper and Spence 1986). This specificity continues postnatally throughout the first half year. Similar learning prenatally familiarizes organisms with the linguistic environment into which they eventually will be born.
1.2 The Jacksonian Principle
The development and dissolution of prenatal motor function is described by the ‘Jacksonian principle,’ which states that the last reflexes to develop are the first to disappear when the organism undergoes demise, whereas the first to develop are the last to disappear (Jackson 1884, cited in Taylor 1958). The contralateral (avoiding) reflex, which moves a stimulated site away from a tactile stimulus, is supplanted weeks later by an ipsilateral reflex (Hooker 1952). This sequence is reversed by fetal anoxia. The reversion occurs because reflexes that develop first are well practiced and require less oxygen to be initiated than reflexes that are more recent. Similarly, the order in which sensory functions develop (tactile, vestibular, thermal, chemical, auditory, and visual) is subsequently reversed during injury, disease, or aging.
2. Mileposts Of Infant Development
2.1 zero–6 months.
Birth marks the organism’s transition from a parasitic, waterborne existence in a thermally constant environment with constant access to nutrition to an in-dependent, land-dwelling existence in a desiccating and cold environment with discontinuous access to food. The occasion of birth is unremarkable, important primarily for triggering the onset of the ‘regulatory mechanisms’ (respiration, blood flow, digestion) that are critical for this transition. These regulatory arrangements and their interactions are the major constraints on the newborn’s behavior and the environmental conditions it can tolerate (Adolph 1968). The evolutionary requirement of all newborn mammals is to grow, which necessitates maximizing energy intake and minimizing energy expenditure. Not surprisingly, therefore, newborns primarily eat and sleep. Early postnatal learning also reflects this necessity: Newborns readily learn where and when food is accessible. Also, because full-term newborns do not physiologically thermo regulate before 4–9 weeks of age, they will not learn energetically costly responses that compete with behavioral thermoregulation.
Over the first 3 months of life, the birth weight of the infant doubles. During this period, brain development is very rapid and influenced appreciably by the kinds of experience to which the infant has been subjected. While most information about dendrite proliferation, the directionality of neuronal connections, cell death, and the effects of deprivation on neural and behavioral development comes from mammals other than humans, the universality of the effects described by neuroanatomists and neuropsychologists is impressive.
In the period following birth, much of the infant’s behavior is reflexive, that is, specific stimuli call forth specific reactions (e.g., touches to the mouth elicit rooting and mouthing, abrasions of the skin elicit retraction of the injured body part). This early behavior is principally under the control of the subcortical portions of the brain, and the elicited behaviors are typically swift and obligatory.
Between 2 and 4 months of age, cortical maturation—especially dendritic proliferation—is increasingly evident, and neonatal reflexes disappear or weaken. Also during this period, activity undergoes a major transition from elicited to voluntary. The developmental sequence in which reflexes emerged during the prenatal period is recapitulated during this transitional period, and the sequence in which the postnatal reflexes appear is similarly reversed when the organism is challenged (Humphrey 1969). The postnatal repetition of fetal activity sequences is again due to the lower oxygen requirement of more primitive, hence more practiced, reflexes. Practicing neonatal reflexes reduces their oxygen requirement and speeds the transition.
The transitional process has been implicated in the sudden infant death syndrome (SIDS). McGraw (1943) characterized the period when reflexes are neither reliably elicited nor under voluntary control as a period of ‘disorganized behavior.’ The transitional period of the respiratory occlusion reflex corresponds to the time when SIDS is most likely to occur (95 percent of all crib deaths occur between 2–5 months). By 6 months, the occlusion reflex has come under cortically mediated voluntary control, and the infant is once again able to defend successfully against respiratory occlusion (Lipsitt 1979).
Also, over the first 3 months of age the behavioral repertoire of the infant sharply expands. Infants can acquire both classically conditioned and operantly conditioned responses at birth and can remember what they have learned for a surprisingly long time (Lipsitt 1969). An eyeblink reflex that is classically conditioned at 10 days of age, for example, is remembered for at least 10 days. Newborns can habituate to a repeatedly presented stimulus and discriminate it from others that they have not previously encountered. Imitation, a particularly efficient form of learning, can be demonstrated within hours of birth. Not only can newborns imitate an adult’s facial gestures immediately after they were modeled, but by 6 weeks of age, infants can imitate facial gestures after a 24-hour delay. Piaget (1962) described early facial imitation as stimulus-bound and reflexive and hypothesized that it disappeared along with many other neonatal reflexes. However, it was later shown not to disappear (e.g., Meltzoff and Moore 1994). Infants’ early imitation of facial expressions foreshadows their later dyadic interactions with adults and siblings.
By 3 months of age, infants can acquire arbitrary categories, learn the serial order of an arbitrary list, and pick up incidental information about events in their visual surround—including information about correlated features (‘what goes with what’). Although some aspects of visual processing (e.g., accommodation and convergence, and feature search) are adult-like by 3–4 months, others (e.g., visual acuity and conjunction search) do not achieve adult levels for 1 year. Infants can detect depth by 2–3 months but do not fear depth until they begin to crawl. They can also recognize faces of live adults after 24 hours by 6 weeks of age and can recognize them from photos by 5 months.
Observers often remark that infants blossom into ‘social creatures’ between 2 and 4 months of age, cooing and smiling widely at individuals who interact with them, reaching to other persons, and intensely watching the behavior of others. By their fourth month, infants follow conversations between adults with their eyes, differentiate social from nonsocial objects (which they previously did not), and imitate vocalizations. Not surprisingly, social stimuli are highly effective in establishing and maintaining behavior at this time. The features that define a stimulus as social, however, change with age. Although newborns differentiate between facial expressions and prefer to look at faces than at other visual stimuli, this preference is not based on a social dimension but on contrast and movement—critical features of the human face which influence infants’ scanning patterns over the first 8 weeks. By 4 weeks of age, however, infants display greater pupillary dilation to social than nonsocial stimuli; by 6 weeks, they display differential cardiac responses to mothers and strangers; and by 12 weeks, they display differential pupillary responses to them and smile more frequently at the mother than at a stranger.
2.2 Six To 12 Months
During the second half-year, a normal infant becomes a walker, a speaker, and a social being who responds emotionally to parents and differentially to familiar persons and strangers. The simultaneous appearance of strong attachment to caregivers and wariness of unfamiliar individuals at 9–10 months of age is one of the most consistent benchmarks of this period (Ainsworth 1979). At the same time, infants begin to look at the target of a pointing finger rather than at the finger per se and begin to exhibit social referencing—a strategy by which infants assess the emotional valence of a new situation or person by checking how the caregiver has responded and responding in kind.
For a half-century, another developmental benchmark was attainment at 10 months of object permanence—the concept that an unseen object or person continues to exist. In the classic object search task, infants younger than 10 months consistently failed to retrieve an object they had just watched an adult hide. New paradigms using looking instead of reaching measures, however, have now revealed that even 3month-olds can locate where an object was hidden.
The types of learning of which infants are capable and their understanding of the physical world rapidly expands over the last half-year. Infants can individuate objects, solve problems requiring causal reasoning, form learning sets (‘learning how to learn’), comprehend some spoken speech, repeat a couple of syllables and perhaps produce a word, plan and execute a detour, and track objects through occlusion—a finding that has fueled heated debate over whether infants can count. Before infants begin to crawl (7–9 months), they have already learned what happens in what places. Once infants can locomote, they begin to form a cognitive map or representation of the spatial relationships between these places, which facilitates navigation from one place to another.
2.3 Twelve To 24 Months
Over this period, infants’ linguistic, motoric, and cognitive skills continue to improve and broaden. Between 12 and 18 months of age, for example, infants can use landmarks to locate objects instead of using their own body as a reference point, and between 18 and 24 months, they can use geometric cues in the environment to reorient. Although 18 months was set as the minimum age for deferred imitation of actions on an object (Piaget 1962), the use of tasks particularly suited to younger infants has lowered this age to 6 months. Still, infants’ ability to imitate televised demonstrations lags well behind their ability to imitate live demonstrations. The same live demonstration that 6-month-olds can imitate after 24 hours cannot be imitated from television before 18 months, although 15-month-olds can imitate some televised actions after 24 hours if the viewing conditions are more naturalistic. Generally speaking, the pervasiveness of reports that infants’ cognitive and motoric performance is superior in natural settings calls into question most if not all minimum age limits that have been imposed by laboratory tests.
Within the last five years, research on the ontogeny of memory has revealed that retention increases linearly over the infancy period, and reminders protract retention even longer. At all ages, a single reactivation or reinstatement reminder, for example, doubles the life of a memory—a remarkable result given that memories of older infants are initially so long. The finding that a few reinstatements can maintain a memory acquired at 6 months through 2 years of age reveals that early experiences can be enduring and raises new questions about infantile amnesia (adults’ inability to recollect experiences from early child-hood). The two memory systems (declarative, non- declarative) found in older children and normal adults are also functional by 3 months (Rovee-Collier et al. 2001).
3. Risk Factors And Individual Differences
In recent years, advances in the successful delivery and survival of very small and premature babies, often born in jeopardy, have fueled new debate over the importance of early experience. Because the lower threshold for survival is very new, it is inevitable that time must pass before the numerous longitudinal studies, now under way, will yield critical information about the relation between specific prenatal deficits and neonatal hazards and particular developmental outcomes. Such investigations are unlikely to rule out the possibility that some affected infants will be indistinguishable from their age-matched peers in developmental competencies. It is a question of proportions and resiliency.
Although infants born 38 weeks after conception are, on average, less mature than those born at 40 weeks are, the level of physiological maturity can vary among infants born at 40 weeks by as much as 3–4 weeks. Sex differences are also present at birth, with girls being 1–2 weeks ahead of boys in neurological maturation. In normal infants, some of this variability disappears by the end of the first year, but many individual differences persist, the full range and significance of which are not yet fully appreciated.
Early attempts to predict later intellectual performance from infant behavior focused on sensory and motor abilities and was unsuccessful. Modest-to-good correlations have now been found between measures of information processing beginning at 5–6 months (e.g., habituation rate, novelty preference, 1-week retention ratios) and scores on various intelligence tests at 3–5 years. The Fagan Test of Infant Intelligence (Fagan and Detterman 1992), which correlates later intelligence with infant novelty preference scores, is sensitive to early exposure to environmental toxins, substances of abuse, and nutritional deficits and supplements, as well as to maternal HIV infection, intraventricular hemorrhage, bronchopulmonary dysplasia, genetic anomalies, and various neurological abnormalities.
4. Past Trends And Future Trajectory
The origins of research on infant development can be traced to baby diaries published by Darwin and Preyer in the late nineteenth century. Over the first half of the twentieth century, detailed diaries of their own children’s behavior stimulated Morgan, Stern, Guillaume, Valentine, and Piaget to propose theoretical accounts of early cognitive development (Wallace et al. 1994). At the same time, Pavlov’s seminal studies spawned longitudinal studies of classical conditioning with infants in Russia and other Eastern-block countries. These early studies provided the first systematic data on infant learning and documented the sequential onset of sensory function from the age at which stimuli from different modalities became effective conditioned stimuli. During this period, Western researchers produced only scattered reports of infant conditioning and retention, emotional development, activity, and color vision.
In 1958, federal funding of a long-term prospective study of infant development ushered in the era of modern infancy research. Using sophisticated apparatus and innovative methods, researchers at 12 sites across the US began to systematically document the infant’s basic sensory and learning abilities. These initial inquiries sparked subsequent generations of research on all aspects of perceptual, cognitive, and behavioral development across the entire span of infancy. By the end of the twentieth century, a large amount of developmental data was amassed on infant attention, perception, cognition, motor skills, and the sequellae of many early risk conditions.
The twenty-first century will witness intensive research that elaborates this knowledge and challenges other long-held beliefs about infant development. Also in this century, advances in cognitive and behavioral neuroscience should facilitate attempts to relate developmental changes in infant cognition to specific changes in the developing brain. This effort will require the development of new, noninvasive techniques and the adaptation of current ones (e.g., functional magnetic resonance imaging, positron emission topography) for use with very young infants. Finally, methodological advances should allow new insights into prenatal development and its contribution to postnatal development.
Bibliography:
- Adolph E F 1968 Origins of Physiological Regulations. Academic Press, New York
- Ainsworth M D S 1979 Attachment as related to mother-infant interaction. In: Rosenblatt J S, Hinde R A, Beer C, Busnel M (eds.) Advances in the Study of Behavior. Academic Press, New York, Vol. 9
- DeCasper A J, Spence M J 1986 Prenatal maternal speech influences newborns’ perception of speech sounds. Infant Behavior and Development 9: 133–50
- Fagan J F III, Detterman D K 1992 The Fagan Test of Infant Intelligence: A technical summary. Journal of Applied Developmental Psychology 13: 173–93
- Hooker D 1952 The Prenatal Origin of Behavior. University of Kansas Press, Lawrence, KS
- Humphrey T 1969 Postnatal repetition of human prenatal activity sequences with some suggestions of their neuroanatomical basis. In: Robinson R J (ed.) Brain and Early Behaviour: Development in the fetus and infant. Academic Press, London
- Lipsitt L P 1969 Learning capacities of the human infant. In: Robinson R J (ed.) Brain and Early Behaviour: Development in the fetus and infant. Academic Press, London
- Lipsitt L P 1979 Critical conditions in infancy: A psychological perspective. American Psychologist 34: 973–80
- McGraw M B 1943 The Neuromuscular Maturation of the Human Infant. Columbia University Press, New York
- Meltzoff A N, Moore M K 1994 Imitation, memory, and the representation of persons. Infant Behavior and Development 17: 83–99
- Piaget J 1962 Play, Dreams, and Imitation in Children. Routledge and Kegan Paul, London
- Rovee-Collier C, Barr R (in press) Infant cognition. In: Wixted J (ed.) Stevens’ Handbook of Experimental Psychology. Wiley New York, Vol. 4
- Rovee-Collier C K, Hayne H, Colombo M 2001 The Development of Implicit and Explicit Memory. Benjamins Publishing, Amsterdam
- Smotherman W P, Robinson S R 1988 Behavior of the Fetus. Telford Press, Caldwell, NJ
- Taylor J E (ed.) 1958 Selected Writings of John Hughlings Jackson. Hodder and Stoughton, London, Vol. 2
- Wallace D B, Franklin M B, Keegan R T 1994 The observing eye: A century of baby diaries. Human Development 37: 1–29
ORDER HIGH QUALITY CUSTOM PAPER

EDITORIAL article
This article is part of the research topic.
Embryo Development and Selection: Advances in Genetics
Editorial: Embryo Development and Selection: Advances in Genetics Provisionally Accepted
- 1 Guilin Medical University, China
- 2 Hainan Medical University, China
The final, formatted version of the article will be published soon.
Assisted reproductive technologies (ART) are being utilized with increasing frequency across the globe to support individuals who face challenges in conceiving naturally. ART procedures facilitate pregnancy by extracting eggs from a woman's ovaries, fusing them with sperm in a laboratory setting, and subsequently reintroducing them into the woman's body. The efficacy of ART is subject to a multitude of factors, encompassing genetic considerations, historical medical and reproductive data, the specific medications employed during ART treatment, and any complications that may arise during pregnancy. The focus of this research topic is the selection of embryos during the ART process, and an exploration of the factors that influence embryo development and the overall success rate of ART. 1. Abu et al. evaluated the impact of supplementing a single-dose GnRH agonist to the standard progestogen regimen for luteal phase support in IVF treatments. Compared to using progestogens alone, they found that adding a GnRH agonist improved the overall IVF outcomes.2. Andreescu has conducted a review on the dysregulation of immune responses between the mother and fetus, a factor that heightens the risk of embryo rejection and reproductive failure.This paper provides an exhaustive overview of the current literature on the influence of Calcineurin inhibitors and anti-TNF treatment in enhancing the live birth rate post embryo transfer. It concludes that the suppression of immunological rejection and the promotion of immunological tolerance are vital in safeguarding embryos and averting immunological assaults. Therefore, it is important to exercise caution while selecting use of any immunosuppressive therapy in pregnancy. indicates that the risks associated with angular pregnancy following ART may not be as severe as previously thought. With regular, close monitoring, the majority of these cases can be managed expectantly, often resulting in live births. 5. Zhu and colleagues conducted a study to identify the risk factors that influence the occurrence of heterotopic pregnancies following IVF-ET. They also examined the outcomes of pregnancies after surgical intervention for heterotopic pregnancies. Their research indicates that a history of ectopic pregnancy, multiple abortions, tubal infertility, and multiple-embryo transfer may significantly increase the risk of a subsequent heterotopic pregnancy after IVF-ET.The study also found that for patients with heterotopic pregnancies who undergo surgery, factors such as a shorter operation duration, a smaller ectopic mass, and a location in the ampulla of the fallopian tube are associated with a more favorable reproductive prognosis. IVF success rates are influenced by a multitude of factors, including genetic considerations, historical medical and reproductive data, the types of medications used during ART treatment, and complications that may arise during pregnancy. The research papers discussed here offer significant insights into improving the success rates of IVF. They underscore the efficacy of a combined treatment approach using N-Acetylcysteine and Gonadotropins, which has been shown to enhance ovarian response to superovulation drugs and improve the quality of blastocysts in older women. The papers also identify surgical time, the size of ectopic masses, and their location in the ampulla of the fallopian tube as key factors in determining the reproductive prognosis in patients with ectopic pregnancies. Additionally, the use of GnRH agonists in the luteal phase has been found to increase IVF success rates. The papers also discuss the evaluation of cornual pregnancy following ART. The aim of these studies is to reduce the risks associated with assisted reproduction and increase pregnancy rates, thereby providing invaluable information for clinical IVF centers. The referenced studies investigate the influence of several factors on the success rate of IVF. These factors encompass the patient's medical history, the treatment protocol, the medications administered during treatment, and complications encountered during pregnancy.The insights and research outcomes presented are of immense value for improving the success rates of assisted reproductive techniques.
Keywords: assisted reproductive technologies, Pregnancy rates, Embryo development, Embryo selection, Influence factor
Received: 13 Apr 2024; Accepted: 22 Apr 2024.
Copyright: © 2024 Liu and Ma. This is an open-access article distributed under the terms of the Creative Commons Attribution License (CC BY) . The use, distribution or reproduction in other forums is permitted, provided the original author(s) or licensor are credited and that the original publication in this journal is cited, in accordance with accepted academic practice. No use, distribution or reproduction is permitted which does not comply with these terms.
* Correspondence: Mx. Yanlin Ma, Hainan Medical University, Haikou, 571199, Hainan Province, China
People also looked at

IMAGES
VIDEO
COMMENTS
Introduction. Research in the Developmental Origins of Health and Disease has highlighted effects of maternal stress during pregnancy on long-term health outcomes among offspring [1-6].This reflects in part the effects of maternal stress hormones such as cortisol, which is important in promoting fetal neurodevelopment but which, at high levels, might have adverse effects that vary by infant ...
In Review of Child Development Research. Vol. 4. Edited by Francis D. Horowitz, 187-244. ... Across time, research interests in prenatal and perinatal risks and their impacts on neonates ...
Introduction Past research has shown relationships between stress during pregnancy, and related psychosocial health measures such as anxiety and depressive symptoms, with infant, child, and adult outcomes. However, most research is from high-income countries. We conducted a scoping review to identify research studies on prenatal stress and outcomes of the pregnancy or offspring in low- and ...
The impact of stress on brain health begins in the womb. Both animal and human studies have found that prenatal maternal stress affects the brain and behavior of the offspring. Stressful life events, exposure to a natural disaster, and symptoms of maternal anxiety and depression increase the risk for the child having a range of emotional ...
Naive knowledge of prenatal development. The literature on children's naïve ideas about prenatal development is scarce. Most studies focus on either conception (e.g., Bernstein and Cowan, 1975), inheritance (Springer and Keil, 1989), or birth models (e.g., Nagy, 1953; Kreitler and Kreitler, 1966).Discussing the creation of babies, Kreitler and Kreitler argued that 4- and 5-year-olds' views ...
Edited by Francis D. Horowitz, M. Hetherington, S. Scarr-Salapatek, and G. Siegel, 187-244. Chicago: Univ. of Chicago Press. This is a classic chapter focused on variables in the prenatal and perinatal periods that create risks for pregnancy outcomes and the development of neonates and infants. Research is reviewed on several reproductive ...
We utilized fetal fMRI in 118 fetuses [48 female; mean age 32.9 weeks (SD = 3.87)] to evaluate this association and further addressed whether fetal neural differences were related to maternal ...
Histological research materials cover in more detail the embryonic and pre-fetal periods of development, while data on the fetal period are presented mainly by macro-preparations and schemes [82,83,84,85,86]. Published data on human brain development at different fetal stages are not systematized; they relate to individual aspects of ...
Recent morphological data on human brain development are quite fragmentary. However, they are highly requested for a number of medical practices, educational programs, and fundamental research in the fields of embryology, cytology and histology, neurology, physiology, path anatomy, neonatology, and others. This paper provides the initial information on the new online Human Prenatal Brain ...
DOI: 10.1080/87565640902964508 This empirical study investigates the impacts of maternal depression and anxiety during and after pregnancy and infants' neuromotor development at 3 months. High prenatal maternal anxiety and depression impacted infant outcomes. Available online for purchase or by subscription.
anatomy, neonatology, and others. This paper provides the initial information on the new online. Human Prenatal Brain Development Atlas (HBDA). The Atlas will start with forebrain annotated ...
Introduction. Prenatal and early childhood development have a critical effect on long-term health in adulthood [].Early development may affect adult susceptibility to a range of non-communicable disease including cardiovascular disease [] and diabetes [].The aim of this study was to examine the associations between prenatal and early childhood development with cognitive, vision and hearing ...
Abstract. This paper summarizes the current status of research on prenatal environmental influences. The effects of maternal smoking, diet, alcohol and drug use on fetal development are examined ...
Prenatal development includes a vast amount of changes that begin with conception, as a sperm cell from the father merges with the ovum (egg) from the mother.This produces a zygote, which starts with only 1 cell, and 23 chromosomes from each of the parents.There are three periods of development that occur from zygote to fetus. These are the germinal, embryonic, and fetal periods, as follows:
Fetal development research in context: Seventy-five years of influence of the Fels Longitudinal Study ... it is the purpose of the present paper to deal with the measurement of fetal activity, and of its use as a criterion of individual differences in behavior". (Richards et al., 1938, p 69)
pathologies. Populations most vulnerable to prenatal stress are African-Americans and individuals of low socioeconomic status. Methods. The Pregnancy Risk Assessment Monitoring System (PRAMS) research project, a collaboration between the Centers for Disease Control and Prevention and individual state health departments, was utilized for this study.
Based on former research in naïve biology and taking into consideration RR theory ( Karmiloff-Smith, 1990 ), we predict children to have the following mental models of prenatal development. I) A no change model, predicting that a fetus has the same shape and equivalent bodily functions as a newborn.
A growing body of research also indicates that prenatal influences contribute to the development of psychiatric disorders such as anxiety, depression and externalizing behavior problems (Davis, Glynn, Waffarn, & Sandman, 2011).The lasting impact on the offspring's brain and behavior is still...
PDF | On Dec 1, 2016, Muhammad Tahir and others published Factors Affecting Prenatal Development : An Analysis from the Sunnah and Contemporary Psychology | Find, read and cite all the research ...
Prenatal Development. Prenatal development period includes the growth and development process of the zygote, which is formed by the union of sperm and egg in the womb (Shiel Jr., 2018). First 4 months of the baby in the womb is a vital period regarding the beginning of the formation of organs. During this period, new features such as the onset ...
A human teratogen is an agent that alters the growth or structure of the developing embryo or fetus, thereby causing birth defects. The first human teratogen identified in 1941 by an ophthalmologist, Norman Gregg, was maternal rubella infection in pregnancy, which produced a triad of defects (cataracts, heart malformations, and deafness) in the infants. 1 Following the identification of ...
1.1 Physical And Cognitive Development. The 'prenatal period' extends from conception to birth. During the 'germinal stage' (fertilization-2 weeks) and 'embryonic stage' (2-8 weeks), the fertilized egg rapidly divides and attaches to the uterine wall, the major organs and systems of the body differentiate, and interconnections ...
By the end of the second month, your baby is about 1 inch long and. weighs about 1/30 of an ounce. At about 6 weeks, your baby's heart beat can usually be detected. After the 8th week, your baby ...
The focus of this research topic is the selection of embryos during the ART process, and an exploration of the factors that influence embryo development and the overall success rate of ART.1. Abu et al. evaluated the impact of supplementing a single-dose GnRH agonist to the standard progestogen regimen for luteal phase support in IVF treatments.