MIT Technology Review
- Newsletters

How we transform to a fully decarbonized world
A world powered by electricity from abundant, renewable resources is now within reach.
- Deb Chachra archive page
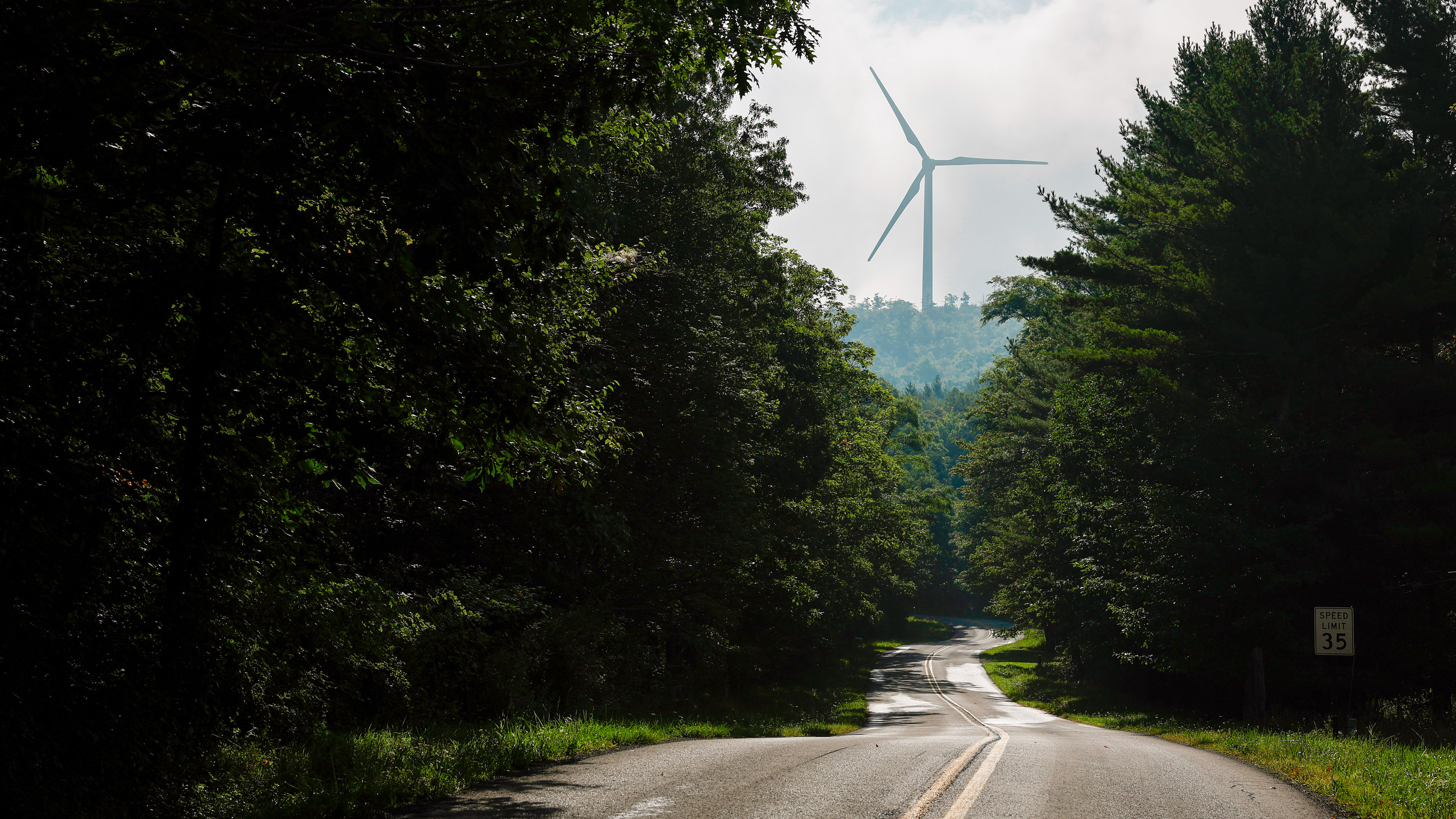
In 1856, Napoleon III commissioned a baby rattle for his newborn son, to be made from one of the most precious metals known at the time: light, silvery, and corrosion-resistant aluminum. Despite its abundance—it’s the third most common element in Earth’s crust—the metal wasn’t isolated until 1824, and the complexity and cost of the process made the rattle a gift fit for a prince. It wasn’t until 1886 that two young researchers, on opposite sides of the Atlantic, developed the method that is still used for refining aluminum commercially. The Hall-Héroult process is extraordinarily energy intensive: the chemically modified ore is dissolved into a high-temperature bath of molten minerals, and an electrical current is passed through it to separate the metallic aluminum. It’s also intrinsically energy intensive: part of the reason the metal was isolated only relatively recently is because aluminum atoms bind so tightly to oxygen. No amount of clever engineering will change that physical reality. The astronomical growth in worldwide aluminum production over the last century was made possible by the build-out of the energy infrastructure necessary to power commercial refineries, and to do so in a way that was economically viable. In the US, that was facilitated by the massive hydroelectricity projects built by the federal government as part of Franklin D. Roosevelt’s New Deal, closely followed by World War II and the immense mobilization of resources it entailed: aluminum was the material of choice for the thousands and thousands of aircraft that rolled off wartime assembly lines as fast as others were shot down. Within a century, the metal went from precious and rare to ubiquitous and literally disposable.
Just as much as technological breakthroughs, it’s that availability of energy that has shaped our material world. The exponential rise in fossil-fuel usage over the past century and a half has powered novel, energy-intensive modes of extracting, processing, and consuming matter, at unprecedented scale. But now, the cumulative environmental, health, and social impacts—in economics terms, the negative externalities—of this approach have become unignorable. We can see them nearly everywhere we look, from the health effects of living near highways or oil refineries to the ever-growing issue of plastic, textile, and electronic waste.
We’re accustomed to thinking about the energy transition as a way of solving the environmental problem of climate change. We need energy to meet human needs—for protection from the elements (whether as warmth or cooling), fuel for cooking, artificial light, social needs like mobility and communication, and more. Decarbonizing our energy systems means meeting these needs without burning fossil fuels and releasing greenhouse gases into the atmosphere. Largely as a result of public investment in clean-energy research and development, a world powered by electricity from abundant, renewable, nonpolluting sources is now within reach.
Just as much as technological breakthroughs, it’s the availability of energy that has shaped our material world
What is much less appreciated is that this shift also has the potential to power a transformation in our relationship with matter and materials, enabling us to address the environmental problem of pollution and waste. That won’t happen by accident, any more than the growth of these industries in the 20th century was an accident. In order to reach this future, we need to understand, research, invest in, and build it. Every joule of electricity that comes from fossil fuels means paying for what’s burned to produce it. In fact, because of the inefficiency of thermal generation, it means paying for many more joules of heat.
Energy generation from renewable sources has capital and operating costs, of course, but minimal, incremental ones. That’s because the input energy arrives as wind or sunlight, not as boxcars of coal. In the big picture, this means that in a fully decarbonized world, all energy will be closer to hydroelectricity in its economics: while it may never quite be “too cheap to meter,” it may indeed be too cheap to reliably generate a profit on an open energy market. This is a problem for investor-owned energy infrastructure, but it’s potentially transformative for community-owned systems (including public utilities, nonprofit electricity cooperatives, or local microgrids), where cheaper and more abundant energy can power a just transition and a new economy.
Twentieth-century investments in energy infrastructure, like the New Deal’s Rural Electrification Act of 1936 and its counterparts worldwide, formed the basis for the global industrial economy. If we can achieve a similar scale of commitment to renewable energy—prioritizing abundance and access over profit—it will lead to another jump in what’s possible in the material world, where what was previously unthinkably expensive becomes quotidian reality. For example, just like refining aluminum, desalinating seawater is intrinsically energy intensive. But in a world with cheap, clean electricity, residents of coastal cities could get a reliable supply of drinking water from oceanside water treatment plants instead of contested freshwater sources.
Desalination is not the only energy-intensive process that would become viable. Aluminum, glass, and steel are among the most recycled materials in part because so much energy is needed to make them from their raw precursors that recovery is economically worthwhile. In contrast, plastics—in their near infinite variety—don’t lend themselves to mechanical recycling except in a handful of cases. Effectively recycling plastics means breaking them down into their chemical building blocks, ready to be put together into new forms. And since most plastics will burn to produce heat, going in the opposite direction—reassembling those carbon atoms into new plastics—requires a significant input of energy. It’s always been easier, cheaper, and more profitable to just dump the waste into landfills and make new plastics out of freshly extracted oil and gas. But if the energy came from inexpensive renewables, the whole economic equation of making plastics could change. Carbon dioxide could be pulled from the air and transformed into useful polymers using energy from the sun, with the waste plastic decomposed into raw materials so the process could begin again.
If this sounds familiar, it’s because it’s how plants work. But, just like Hall and Héroult’s breakthrough for aluminum, new processes would require both energy and technological innovation. Decades of research have gone into creating new kinds of plastics from fossil fuels, and only a proportionally tiny amount into what happens to those plastics at the end of their lives. But now numerous companies, including Twelve, are building on new research to do just this kind of transformation, using renewably sourced energy to turn water and atmospheric carbon dioxide back into hydrocarbons, in the form of fuel and materials.
Prioritizing abundance and access over profit will lead to another jump in what’s possible.
Finally, it’s not just about plastic. If we succeed in building a world of even cheaper and more abundant energy but we again use it to supercharge extraction, consumption, and disposal, then we might “solve” the pressing crisis around energy while worsening the multiple environmental crises posed by pollution. Instead, we can think about community-led investments in energy infrastructure as spinning up a new industrial system in which clean, inexpensive renewable energy makes it possible to recover a broad range of materials. That would cut out the enormous costs of primary extraction and disposal, including environmental depredation and geopolitical conflict.
Building momentum as fast as we can will limit the materials bill for the huge changes that decarbonization will entail, like replacing combustion-powered vehicles with their electric equivalents. This is already happening with companies like Ascend Elements , currently building a facility in Hopkinsville, Kentucky, to produce materials for new batteries from recycled lithium batteries. It’s financed by more than half a billion dollars of recent private investment that builds on $480 million in Department of Energy grants, and the work is based on fundamental research that was supported by the National Science Foundation. As more and more clean, renewable energy comes online, we need to continue with policies that support research and development on the new technologies required to recover all kinds of materials—together with regulations that account for the true costs of extraction and disposal. This will facilitate not just an energy transition but also a matter transition, ensuring that the industrial sector aligns with the health of our planet.
Climate change and energy
The problem with plug-in hybrids their drivers..
Plug-in hybrids are often sold as a transition to EVs, but new data from Europe shows we’re still underestimating the emissions they produce.
- Casey Crownhart archive page
Harvard has halted its long-planned atmospheric geoengineering experiment
The decision follows years of controversy and the departure of one of the program’s key researchers.
- James Temple archive page
Decarbonizing production of energy is a quick win
Clean technologies, including carbon management platforms, enable the global energy industry to play a crucial role in the transition to net zero.
- ADNOC archive page
The hard lessons of Harvard’s failed geoengineering experiment
Some observers argue the end of SCoPEx should mark the end of such proposals. Others say any future experiments should proceed in markedly different ways.
Stay connected
Get the latest updates from mit technology review.
Discover special offers, top stories, upcoming events, and more.
Thank you for submitting your email!
It looks like something went wrong.
We’re having trouble saving your preferences. Try refreshing this page and updating them one more time. If you continue to get this message, reach out to us at [email protected] with a list of newsletters you’d like to receive.
Towards Sustainable Energy: A Systematic Review of Renewable Energy Sources, Technologies, and Public Opinions
Ieee account.
- Change Username/Password
- Update Address
Purchase Details
- Payment Options
- Order History
- View Purchased Documents
Profile Information
- Communications Preferences
- Profession and Education
- Technical Interests
- US & Canada: +1 800 678 4333
- Worldwide: +1 732 981 0060
- Contact & Support
- About IEEE Xplore
- Accessibility
- Terms of Use
- Nondiscrimination Policy
- Privacy & Opting Out of Cookies
A not-for-profit organization, IEEE is the world's largest technical professional organization dedicated to advancing technology for the benefit of humanity. © Copyright 2024 IEEE - All rights reserved. Use of this web site signifies your agreement to the terms and conditions.
Notification: View the latest site access restrictions, updates, and resources related to the coronavirus (COVID-19) »
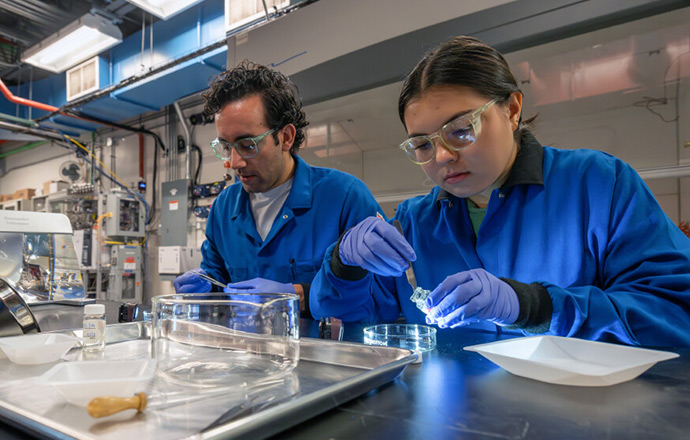
The (In)Visible Plastic Pollution Problem
NREL researchers are on a mission to develop renewable-energy-powered technologies to detect, quantify, and collect plastic from U.S. waterways.
The National Renewable Energy Laboratory (NREL) is transforming energy through research, development, commercialization, and deployment of renewable energy and energy efficiency technologies.
Partner with us to accelerate the transition of renewable energy and energy efficiency technologies to the marketplace.
Data and Tools
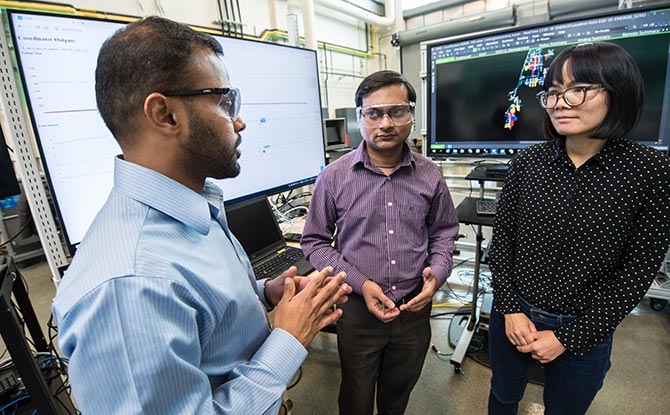
Find NREL-developed data sets, maps, models, and tools used for the analysis of energy and energy efficiency technologies.
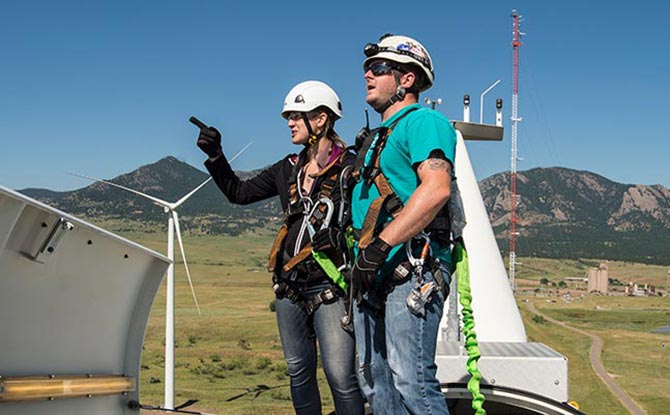
Explore our current job postings, which include research positions and other career opportunities in a variety of fields.
Energy Basics
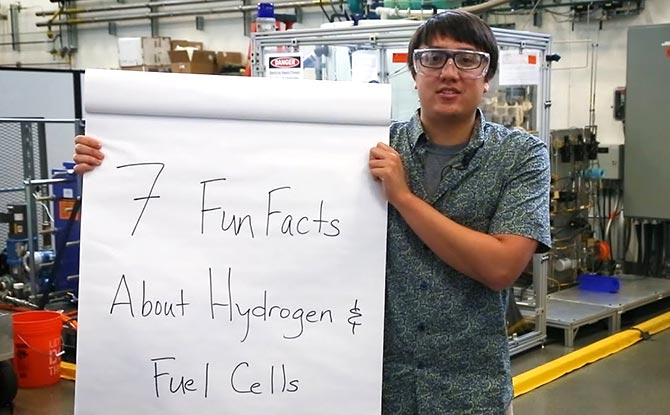
Learn about the basics of our research areas—bioenergy, geothermal, hydrogen, solar, transportation, wind, and water.
2024 JISEA Annual Meeting Convenes Diverse Clean Energy Voices To Address Emerging Challenges
April 26, 2024
NREL Keeps Getting Greener
April 25, 2024
News Release: NREL Proof of Concept Shows Path to Easier Recycling of Solar Modules
NREL Researchers Outline Path Forward for Tandem Solar Cells
Popular Publications
- Federal Aviation Administration Vertiport Electrical Infrastructure Study
- Fall 2023 Solar Industry Update
- Long-Duration Energy Storage: Resiliency for Military Installations
- LA100 Equity Strategies. Executive Summary
- 2023 State of Innovation: Emerging Climate Technologies Primed for Private Investment
Research Areas
Advanced Manufacturing
Chemistry and Nanoscience
Computational Science
Energy Analysis
Energy Security and Resilience
Energy Storage
Grid Modernization
Hydrogen and Fuel Cells
Integrated Energy Solutions
International Activities
Materials Science
State, Local, and Tribal Governments
Transportation and Mobility
Text version
Suggestions or feedback?
MIT News | Massachusetts Institute of Technology
- Machine learning
- Social justice
- Black holes
- Classes and programs
Departments
- Aeronautics and Astronautics
- Brain and Cognitive Sciences
- Architecture
- Political Science
- Mechanical Engineering
Centers, Labs, & Programs
- Abdul Latif Jameel Poverty Action Lab (J-PAL)
- Picower Institute for Learning and Memory
- Lincoln Laboratory
- School of Architecture + Planning
- School of Engineering
- School of Humanities, Arts, and Social Sciences
- Sloan School of Management
- School of Science
- MIT Schwarzman College of Computing
Study reveals a reaction at the heart of many renewable energy technologies
Press contact :, media download.
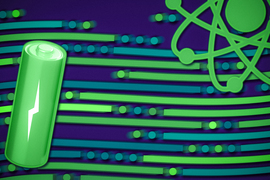
*Terms of Use:
Images for download on the MIT News office website are made available to non-commercial entities, press and the general public under a Creative Commons Attribution Non-Commercial No Derivatives license . You may not alter the images provided, other than to crop them to size. A credit line must be used when reproducing images; if one is not provided below, credit the images to "MIT."
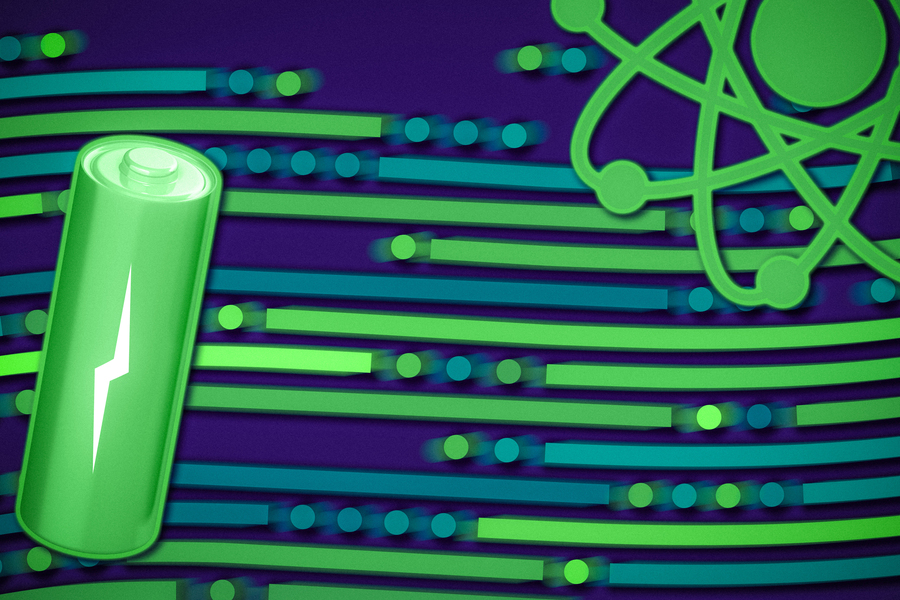
Previous image Next image
A key chemical reaction — in which the movement of protons between the surface of an electrode and an electrolyte drives an electric current — is a critical step in many energy technologies, including fuel cells and the electrolyzers used to produce hydrogen gas.
For the first time, MIT chemists have mapped out in detail how these proton-coupled electron transfers happen at an electrode surface. Their results could help researchers design more efficient fuel cells, batteries, or other energy technologies.
“Our advance in this paper was studying and understanding the nature of how these electrons and protons couple at a surface site, which is relevant for catalytic reactions that are important in the context of energy conversion devices or catalytic reactions,” says Yogesh Surendranath, a professor of chemistry and chemical engineering at MIT and the senior author of the study.
Among their findings, the researchers were able to trace exactly how changes in the pH of the electrolyte solution surrounding an electrode affect the rate of proton motion and electron flow within the electrode.
MIT graduate student Noah Lewis is the lead author of the paper , which appears today in Nature Chemistry . Ryan Bisbey, a former MIT postdoc; Karl Westendorff, an MIT graduate student; and Alexander Soudackov, a research scientist at Yale University, are also authors of the paper.
Passing protons
Proton-coupled electron transfer occurs when a molecule, often water or an acid, transfers a proton to another molecule or to an electrode surface, which stimulates the proton acceptor to also take up an electron. This kind of reaction has been harnessed for many energy applications.
“These proton-coupled electron transfer reactions are ubiquitous. They are often key steps in catalytic mechanisms, and are particularly important for energy conversion processes such as hydrogen generation or fuel cell catalysis,” Surendranath says.
In a hydrogen-generating electrolyzer, this approach is used to remove protons from water and add electrons to the protons to form hydrogen gas. In a fuel cell, electricity is generated when protons and electrons are removed from hydrogen gas and added to oxygen to form water.
Proton-coupled electron transfer is common in many other types of chemical reactions, for example, carbon dioxide reduction (the conversion of carbon dioxide into chemical fuels by adding electrons and protons). Scientists have learned a great deal about how these reactions occur when the proton acceptors are molecules, because they can precisely control the structure of each molecule and observe how electrons and protons pass between them. However, when proton-coupled electron transfer occurs at the surface of an electrode, the process is much more difficult to study because electrode surfaces are usually very heterogenous, with many different sites that a proton could potentially bind to.
To overcome that obstacle, the MIT team developed a way to design electrode surfaces that gives them much more precise control over the composition of the electrode surface. Their electrodes consist of sheets of graphene with organic, ring-containing compounds attached to the surface. At the end of each of these organic molecules is a negatively charged oxygen ion that can accept protons from the surrounding solution, which causes an electron to flow from the circuit into the graphitic surface.
“We can create an electrode that doesn’t consist of a wide diversity of sites but is a uniform array of a single type of very well-defined sites that can each bind a proton with the same affinity,” Surendranath says. “Since we have these very well-defined sites, what this allowed us to do was really unravel the kinetics of these processes.”
Using this system, the researchers were able to measure the flow of electrical current to the electrodes, which allowed them to calculate the rate of proton transfer to the oxygen ion at the surface at equilibrium — the state when the rates of proton donation to the surface and proton transfer back to solution from the surface are equal. They found that the pH of the surrounding solution has a significant effect on this rate: The highest rates occurred at the extreme ends of the pH scale — pH 0, the most acidic, and pH 14, the most basic.
To explain these results, researchers developed a model based on two possible reactions that can occur at the electrode. In the first, hydronium ions (H 3 O + ), which are in high concentration in strongly acidic solutions, deliver protons to the surface oxygen ions, generating water. In the second, water delivers protons to the surface oxygen ions, generating hydroxide ions (OH - ), which are in high concentration in strongly basic solutions.
However, the rate at pH 0 is about four times faster than the rate at pH 14, in part because hydronium gives up protons at a faster rate than water.
A reaction to reconsider
The researchers also discovered, to their surprise, that the two reactions have equal rates not at neutral pH 7, where hydronium and hydroxide concentrations are equal, but at pH 10, where the concentration of hydroxide ions is 1 million times that of hydronium. The model suggests this is because the forward reaction involving proton donation from hydronium or water contributes more to the overall rate than the backward reaction involving proton removal by water or hydroxide.
Existing models of how these reactions occur at electrode surfaces assume that the forward and backward reactions contribute equally to the overall rate, so the new findings suggest that those models may need to be reconsidered, the researchers say.
“That’s the default assumption, that the forward and reverse reactions contribute equally to the reaction rate,” Surendranath says. “Our finding is really eye-opening because it means that the assumption that people are using to analyze everything from fuel cell catalysis to hydrogen evolution may be something we need to revisit.”
The researchers are now using their experimental setup to study how adding different types of ions to the electrolyte solution surrounding the electrode may speed up or slow down the rate of proton-coupled electron flow.
“With our system, we know that our sites are constant and not affecting each other, so we can read out what the change in the solution is doing to the reaction at the surface,” Lewis says.
The research was funded by the U.S. Department of Energy Office of Basic Energy Sciences.
Share this news article on:
Related links.
- Yogesh Surendranath
- Department of Chemistry
Related Topics
- Energy storage
- Sustainability
- Renewable energy
Related Articles
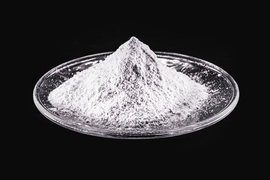
A more sustainable way to generate phosphorus
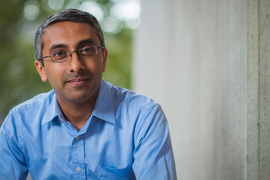
Yogesh Surendranath wants to decarbonize our energy systems
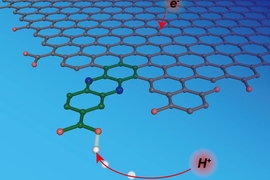
Thermodynamic insights could lead to better catalysts
Previous item Next item
More MIT News
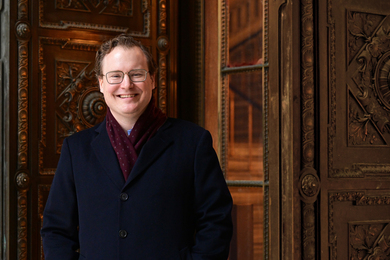
Exploring the history of data-driven arguments in public life
Read full story →
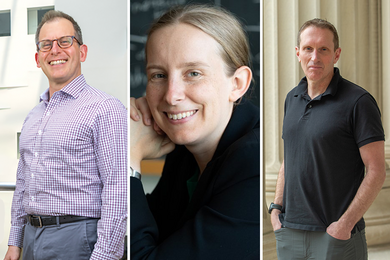
Three from MIT awarded 2024 Guggenheim Fellowships
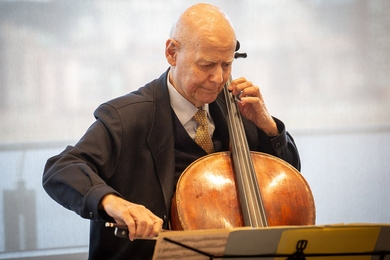
A musical life: Carlos Prieto ’59 in conversation and concert
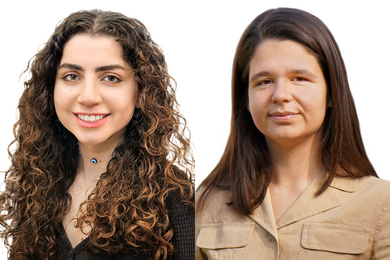
Two from MIT awarded 2024 Paul and Daisy Soros Fellowships for New Americans
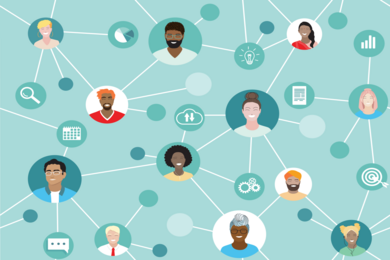
MIT Emerging Talent opens pathways for underserved global learners
The MIT Edgerton Center’s third annual showcase dazzles onlookers
- More news on MIT News homepage →
Massachusetts Institute of Technology 77 Massachusetts Avenue, Cambridge, MA, USA
- Map (opens in new window)
- Events (opens in new window)
- People (opens in new window)
- Careers (opens in new window)
- Accessibility
- Social Media Hub
- MIT on Facebook
- MIT on YouTube
- MIT on Instagram
Create an account
Create a free IEA account to download our reports or subcribe to a paid service.
R&D and technology innovation
- Introduction
- Key findings
- Overview and 2020 update
- Upstream oil and gas investment
- Midstream and downstream oil and gas investment
- Biofuels investment
- Coal supply investment
- Overview of power investment
- Final investment decisions
- Implications of power investment
- Trends in renewable power costs and investments
- Networks and battery storage
- Overview of energy efficiency investment trends
- Trends in end-use markets
- Cross-sector trends in energy finance
- Sectoral trends in energy finance
- Role of institutional investors in energy investment
- Sustainable finance and energy investment
- Investment in energy R&D
Trends in investment for technology innovation
- Methodology
Cite report
IEA (2020), World Energy Investment 2020 , IEA, Paris https://www.iea.org/reports/world-energy-investment-2020, Licence: CC BY 4.0
Share this report
- Share on Twitter Twitter
- Share on Facebook Facebook
- Share on LinkedIn LinkedIn
- Share on Email Email
- Share on Print Print
Report options
R&d and technology innovation, investment in energy r&d, government energy r&d spending grew by 3% in 2019, with robust expansion in europe and the united states alongside steady spending in china as the 13th five year plan nears its end, spending on energy r&d by national governments and the european union, 2014-2019, at 80%, more public energy r&d went to low-carbon technologies in 2019 than the prior year, but the near-term outlook depends on the inclusion of clean energy r&d in recovery measures.
Government energy R&D spending in 2019 grew by 3% to USD 30 billion in 2019, and was mostly directed to low-carbon energy technologies. While the growth rate in 2019 was below that of the previous two years, it remained above the annual average since 2014. It also reflects the multi‑annual nature of many government R&D budgets, which follow cyclical trends within budget periods. For example, a main reason for the weaker growth in 2019 was the stabilisation of China’s public energy R&D spending, yet this is closely tied to the Five-Year Plan (FYP) framework in China. Certain research programmes, especially those related to coal technologies, have passed their peak spending under the 13th FYP and are now analysing results and planning for the next funding period, from 2021 to 2025. There are indications that low-carbon technologies, including hydrogen, could receive a boost in the next period.
Growth was robust in Europe and the United States; spending on public energy R&D rose by 7% in both economies, above the recent annual trend. Looking ahead, increasing energy R&D has been a central feature of policy discussions in the European Union as part of the European Green Deal and the expanded R&D programme Horizon Europe. In the United States, Congress will decide the level of funding for 2021 by October and this will be influenced by considerations relating to economic heath and economic stimulus. Several proposals to increase energy R&D are currently circulating in Washington, including a bipartisan American Energy Innovation Act with provisions to establish new R&D programmes. At 0.06%, Japan has one of the highest ratios of public energy R&D spending to GDP, alongside China, and spending there was constant with respect to GDP in 2019.
In 2019, around 80% of all public energy R&D spending was on low-carbon technologies – energy efficiency, CCUS, renewables, nuclear, hydrogen, energy storage and cross-cutting issues such as smart grids. With 6% growth, spending on low-carbon technologies rose faster than total public energy R&D spending, reaching USD 25 billion in 2019. In China, the low-carbon component of energy R&D grew by 10% in 2019, with big increases in R&D for energy efficiency and hydrogen in particular, driving up the global total. However, to some extent this year-on-year growth in 2019 reflects a slower start to 13th FYP spending compared with fossil fuel technologies, not an unfolding trend. Major governments are increasing energy research investments, as they pledged to do in 2015 under the Mission Innovation initiative.
While it is too early to determine the impact of the Covid-19 pandemic on public energy R&D, the risks are clear. R&D practitioners may find it difficult to execute funded projects in 2020, and public budgets will be under pressure. Experience from the 2008 financial crisis indicates that budgets are likely to be fixed in 2020, most likely seeing reductions in 2022‑23. In wealthier countries, cuts may be modest or nonexistent as governments pursue countercyclical R&D policy through stimulus measures. In 2009‑11, the American Recovery and Reinvestment Act raised annual energy R&D spending by 75% compared with 2006‑08, before it fell back in 2012‑14. If global public R&D were raised by 75% in coming years, it would add USD 18 billion of funding. In emerging markets, on the other hand, R&D budgets and value chains are less resilient and the public sector is more dominant. This poses a risk to the development of appropriate clean energy technologies for countries expected to grow their energy sectors most in coming decades.
Corporate energy R&D spending grew 3% in 2019, with little growth in the leading sectors of oil and gas and automotive, both of which could restrain R&D as revenue drops in 2020-2021
Growth rates for revenue and r&d for selected sectors and the european union, 2007-2012, global reported corporate energy r&d spending in selected sectors, 2010-2019, the push for electro-mobility and cleaner cars has boosted overall corporate energy r&d since 2010, while renewables grew the fastest, at 74%, and oil and gas grew the least, at 9%.
Companies active in energy technology sectors over the last decade have increased their total annual energy R&D spending by around 40% since 2010, based on our analysis of the latest available data from annual reports. The total energy R&D spending of this sample reached around USD 90 billion in 2019, 3% higher than in 2018. The multi‑year trend traces two periods averaging growth of over 5% (2010‑13 and 2015‑18), preceded by the global financial crisis and divided by the economic impact of the oil price collapse of 2014, which caused a 10% drop in the R&D spending of oil and gas companies over two years. In each case, the periods of higher growth could therefore have been responses to disruptions. While it is hard to draw conclusions from a single year, the apparent slowdown in 2019 could have reflected a return to the longer-term trend. That issue will not be answered in 2020, however, as the impacts of the Covid-19 pandemic will almost certainly lead to a reduction in corporate energy R&D in 2020‑21.
Across the period, companies active in renewable energy technologies represent a particularly bright and resilient story. These companies spent 74% more on R&D between 2010 and 2019, adding over USD 2.5 billion to efforts to improve their technologies, complementing over USD 4 billion spent on renewables R&D by governments.
Automakers – who typically have much higher R&D budgets than energy companies in absolute terms and as a share of revenue – continue to increase their spending as government policies and competitive pressures drive increased focus on energy efficiency and electric vehicles. However, the data suggest they may be facing a balancing act between a weaker outlook for car sales revenue and the need to invest in new vehicles and manufacturing supply chains.
Despite securing a higher share of sales from more profitable cars such as SUVs, the lower margins on EVs – an area of expected strong future growth – means that investments in new production lines are expected to stretch balance sheets. Energy-related automotive R&D is estimated to have stabilised between 2018 and 2019 after several years of growth, and this sector is a key factor in the overall stagnation. This reflects wider cuts to R&D in the automotive supply chain in 2019 that offset growth from the major carmakers. VW Group, for example, increased overall R&D to USD 16 billion, or 7% of revenue. Anticipated cuts to R&D spending in 2020‑21 could be a setback to fuel economy improvements that are needed to accompany the shift to larger vehicles (see Energy End Use and Efficiency section) and electrification.
The financial crisis of 2008 and the oil price collapse of 2014 provide some insight into the likely response of companies to the impacts of the Covid-19 pandemic. In 2009‑10 the total R&D spending of major sectors held up well relative to revenues, with the exception of the automotive sector. However, the electricity supply and renewables sectors were the only ones not to experience slower growth or cuts to R&D budgets in this period. After 2014, oil and gas company R&D took four years to return to growth and remains below the 2013 level.
While R&D spending is likely to suffer, it can be expected to be much less affected than capital expenditures, as companies seek to retain R&D staff and capabilities, and complete ongoing projects. As in 2009, the outcome will be policy-dependent, with tax incentives and R&D-specific loans being proposed for inclusion in stimulus packages. Still, for the large-scale demonstration of technologies, such as CCUS, cuts to investment could be more damaging than those to R&D.
Venture capital investment remained robust in 2019, with more diversification of sectors and countries for energy technology start-ups. Storage and hydrogen saw the most growth.
Global early-stage venture capital investment in energy technology companies, 2010-2019, while policies in major economies supported vc investment in 2019, private-led scale-up of innovative energy technologies could face major headwinds over the next two years.
Total equity investment in energy technology start-ups, including growth equity, by all investor types, stood at USD 16.5 billion in 2019. Of this, early-stage venture capital (VC) (seed, series A and series B), which supports innovative firms through their highest risk stages, is estimated to have been USD 4 billion. These sums are lower than those spent on energy R&D by governments and companies, but this private risk capital plays an important role by enabling market creation and scale-up of the most market-ready technologies. The total reported deal value in 2019 was 7% below that of 2018, but the last two years are well above the decadal average.
Other indicators also show the market is maturing. Compared with recent years, 2019 early-stage VC was spread across more technology areas. While low-carbon transport (mostly EVs and charging) was 35% of this, its share was much lower than in recent years. Time will tell whether this represents consolidation at the end of a period of exuberance marking the start of EV deployment. Other sectors grew significantly, notably energy storage, hydrogen and fuel cells, but also bioenergy and solar. Combined, they largely offset the USD 1.5 billion decline in transport. Higher levels of growth equity, despite lower follow-on deals, provide another indication that investors see storage and hydrogen as having high growth potential. Notable start-ups completing funding rounds included energy storage company Energy Vault (USD 110 million), biomethane producer Bioenergy DevCo (USD 106 million), Jiangsu Guofu Hydrogen (USD 60 million) and battery pack maker Romeo Power (USD 88 million). Among the deals for hydrogen technologies, most were for firms with novel hydrogen production devices, such as pressurised or photocatalytic electrolysers.
Start-ups receiving funding also diversified geographically. At 19%, Europe had its highest share of reported deal value in four years, while China, at 22%, had its lowest share since 2016. The US share declined to 41% in 2019 compared with 45% in 2018. By contrast, India increased its share to 12% in 2019, from an average of 3% over the previous decade. Notable Indian energy start-ups completing funding rounds included Ola Electric Mobility (USD 250 million), Hero Future Energies (USD 150 million) and Ecozen Solutions (USD 6 million).
The average disclosed deal value for energy tech start-ups was 10% lower in 2019 than 2018, but at USD 12 million, it remains higher than at any point over the previous decade. Deal size is particularly high in China, with start-ups there sometimes raising hundreds of millions of dollars in a single funding round, such as Hozon Automobile (USD 450 million) and Enovate Motors (USD 300 million). However, European and US early-stage VC deal sizes are also at all-time highs. This indicates investor confidence in new energy technologies to play a disruptive and profitable role in the energy sector in the next decade.
While diversification of sectors and geography, plus rising deal value, were good-news stories for 2019, the near-term outlook is very different. Early-stage energy VC deals were still on a par with 2018‑19 levels in Q1 2020, but lower activity was evident in China and global declines are expected in Q2-4 due to financial risks, working restrictions and policy uncertainty. When including later-stage deals such as growth equity, which tend to be larger, Q1 2020 activity was 50% lower than in recent years. Some countries have already included support for start-ups in stimulus announcements, including France and the United Kingdom.
Corporate investment in energy start-ups is still led by digital firms, but with a larger role for car companies. Sources of VC funding are slowly diversifying geographically.
Share of domestic investors in countries' vc activity, 2010-2019, corporate venture capital and growth equity by sector of investor, 2010-2019, the globalisation of energy technology vc indicates a maturing sector with corporate and financial investors alike seeking opportunities in funding the best start-ups around the world.
Corporate investments in energy technology start-ups, including corporate venture capital, reached a new high in 2019, at around USD 5 billion. The strong annual increase was driven by a small number of very large growth-equity rounds, notably in low-carbon transport. Large corporations continue to see a strategic case for direct investment in innovative, nimble technology players. Companies inside and outside the energy sector are using corporate VC as part of a flexible and open energy innovation strategy.
Traditional energy actors (i.e. fossil fuels, utilities, IPPs, energy equipment and services) account for a decreasing share of investments; about 23% in 2016‑19 compared with 49% over 2012 to 2015. The notable spending by electrical equipment manufacturers in electricity storage and EVs, which drove the trend in 2018, was absent. Oil and gas companies accounted for roughly 50% of investments by traditional energy actors but at USD 290 million, their spending was less than in 2017 and 2018. Deals involved start-ups in bioenergy (e.g. Shell investing in Punjab Renewable Energy Systems), CCUS (Chevron in Carbon Engineering), energy storage (Eni in Form Energy), hydrogen (Total in Sunfire) and solar (Equinor in Yellow Door Energy and Oxford PV).
Conversely, the share of non‑traditional actors in corporate investments for energy start-ups rose again. The strong investment presence of the ICT and electronics sectors since the mid-2010s was maintained with nearly USD 2 billion in 2019, mostly in low-carbon transport, energy storage and efficiency, including for data centres.
Of the nearly USD 5.9 billion of VC and other equity invested in low-carbon transport start-ups in 2019 in total, USD 3 billion was from corporate investors, of which USD 1 billion came from the transport sector and USD 1.8 billion from the ICT sector. Electric pickup truck producer Rivian raised over USD 3 billion in 2019 from investors including Ford Motor Company and Amazon. Electric vehicle manufacturer Weltmeister Motor secured USD 450 million in a growth-equity deal led by ICT company Baidu.
A country’s VC landscape is defined by its investors as well as its start-ups. For example, four-fifths of the investment in US-based start-ups comes from US investors. This share has declined slightly in recent years, but not as steeply as the share of investment received by Europe-based start-ups from Europe-based investors. That share is now closer to 70%, similar to that of China, which has dipped from over 90% since the middle of the last decade.
While most governments seek to keep the domestic gains from innovation, there are also benefits to attracting overseas finance to local start-ups. World-class start-ups attract investment from all over the world and many VC funds scour the globe for talent. Corporate VC, particularly from multinationals, tends to have a global scope because it seeks opportunities with the best business fit, and often with local market knowledge. The rising share of inward VC in China, Europe and the United States may indicate a more globalised and efficient environment for energy technology entrepreneur finance. Investment in start-ups from Australia, India and Israel comes largely from overseas, reflecting the ability of their entrepreneurs to compete for capital given their relatively smaller domestic VC sectors.
A record capacity of electrolysers to produce hydrogen was added in 2019, supported by vehicles in Europe and industry in China, with a far bigger wave of projects on its way
Capacity of electrolysers for hydrogen production by commissioning year by regions, 2010-2020, capacity of electrolysers for hydrogen production by commissioning year and intended use of hydrogen, 2010-2020, but with demand concentrated in hard-hit sectors, a planned 2020 surge faces new challenges.
In recent years, capacity additions of water electrolysers to produce hydrogen have expanded rapidly, from 2 MW e in 2010 to 25 MW e in 2019. This activity reflects surging interest in hydrogen as an alternative to fossil fuels in a diverse range of uses, from powering vehicles to storing electricity, refining oil, heating homes, and producing synthetic fuels such as methane or ammonia.
Electrolysers use electricity to split water into oxygen and hydrogen, which generates no CO 2 emissions at the point of use. The powering of electrolysers by low-carbon electricity or applying CCUS to hydrogen production from fossil fuels can support low-carbon hydrogen at the point of production. No new CCUS-equipped hydrogen production – each plant equivalent to 100 MW e to 600 MW e of electrolysers – has been added since 2016 but several are planned, mostly in Europe.
Investment has surged in the past two years. Electrolysers installed in 2019 represent capital expenditure of around USD 40 million, while those in construction may be worth over ten times more. Electrolysers have grown in scale, from below 0.5 MW e on average in 2010 to 6 MW e, and quoted costs for newer designs have halved. A 10 MW e facility began operation in Japan in March, and a 20 MW e plant is in construction in Canada, both for vehicles, and potentially industrial uses too. Several hundred MW e of announced projects seek financial close this year or next; over 600 MW e could be commissioned through 2021, nearly three times the total additions since 2010. Two electrolyser factories, 1 GW e and 360 MW e, are in construction in the United Kingdom and Norway, with others under development in China.
However, many of these investments could face delays related to Covid-19 restrictions, revised capital expenditure plans and weaker hydrogen demand. The automotive, oil and gas, and steel industries, among others, may all review the economics of electrolysis hydrogen applications in light of weaker revenues and lower coal and gas prices.
To date, much demand for electrolysis hydrogen – some two to five times more expensive than that from fossil fuels today – has been supported by one-off government initiatives. Project developers expect more predictable demand ahead, arising from industrial emissions reduction commitments (e.g. by oil refiners), and policy incentives that promote clean hydrogen. These factors depend on the evolution of the current downturn, as well as government efforts to include in stimulus measures policies that support demand and supply of hydrogen. In this light, the vehicles sector has been the most dynamic route for hydrogen uptake, based often on hydrogen from natural gas or coal without CCUS. At the end of 2019, 23 350 fuel-cell electric vehicles (FCEVs) were on the road, nearly half registered in 2019 alone, while hydrogen refuelling stations increased by 20% to 460. Most activity has come in Japan and the United States. While blending hydrogen into the gas grid, to deliver low-carbon heat for buildings, is also mentioned in policy debates (see Energy End Use and Efficiency section), just 0.6 MW e was added for this dedicated purpose in 2019 and 2020.
China represents a major new factor in the development of hydrogen. From minimal levels in 2018, China is aiming for around 150 MW e in operation by 2021, mostly for mobility, with increased signs of public support. In April 2020, Hebei province approved USD 1.2 billion of projects for hydrogen equipment manufacturing, filling stations, fuel cells and hydrogen production, including electrolysis (Xu and Singh, 2020). Sales of FCEVs climbed from a few units in 2017 to almost 4 400 in 2019; China now leads hydrogen bus and truck deployment.
Will industrial policy help spur venture capital for hydrogen and speed commercialisation?
The landscape of hydrogen technologies and companies is in flux. Industrial uses and the gas grid are becoming more important target markets, China is playing a larger role in technology development, and there is not yet a dominant technology design.
Several countries seek to become technology leaders in hydrogen and are developing policies and financial measures to support strategic investment. This is reflected in the diversity of countries from which the hydrogen start-ups attracting most early-stage VC come.
In addition to a push in China, the Netherlands published a Climate Act in 2019 with specific hydrogen targets and, in May 2020, the Australian government announced up to USD 200 million for a new hydrogen fund (CEFC, 2020). A number of countries, including Portugal, have indicated possible support for hydrogen as part of economic recovery measures, while Germany’s national strategy was delayed to accommodate the new economic context.
In 2019, there were more early-stage VC deals for hydrogen start-ups than in any previous year, with over USD 110 million invested in 25 deals. The largest deals were for hydrogen production systems, especially novel technologies, including pressurised electrolysers, saltwater splitting, anion electrolyte membranes and photocatalytic reactors. Few of these firms are proposing the current market-leading technology of alkaline electrolysers, or the more expensive, more flexible challenger polymer electrolyte membrane electrolysers, which are popular for new demonstration projects. This shows that competition between electrolyser technologies is not yet settled.
Among growth equity deals, which tend to involve less technology innovation risk, mobility was a key target market in 2019.
Largest early-stage VC deals in hydrogen start-ups in 2019
Largest growth equity deals in hydrogen firms in 2019, while ccus investment was modest in 2019, new announcements emerged from the united states, where 15 projects seek to enter construction by 2024 to qualify for the 45q tax credit, total capacities of 15 announced us co2 capture projects targeting final investment decision by the end of 2023, 80% of these projects plan to sell the captured co2 to the oil industry for storage via enhanced oil recovery, but the current market turmoil could threaten their timelines.
Capital spending on CCUS projects remained modest in 2019, at under USD 1 billion, mostly on projects that have been under development for several years. The first two CCUS trains of the Gorgon LNG project in Australia began operation, following delays since construction began in 2009. It is now the world’s largest dedicated geological CO 2 storage facility (i.e. the CO 2 is not used for enhanced oil recovery [EOR]). It will be able to store 4 Mt of CO 2 per year when the third train starts, which is due in 2020, and if the LNG plant runs at full capacity. Four other CCUS projects are in construction, two in Canada and two in China. While no FIDs have been taken since 2017, in 2020 three oil and gas firms stated a willingness to invest USD 700 million to develop CO 2 storage in Norway by 2024, pending public funding (Equinor, 2020).
Investment signals for CCUS in the United States improved following the extension and reform of the 45Q tax credit in 2017. Companies have since been waiting for regulatory clarification before committing to building, and some of this – on construction criteria – was published in early 2020. Fifteen projects are now reportedly targeting 45Q support, pushing the global number of large-scale projects in development over 30, the highest since 2014. Depending on the size and sector, each project could invest USD 100 million to USD 1 000 million if successful.
Section 45Q of the US tax code provides up to USD 50 (inflation adjusted) per tonne of CO 2 sent to geological storage, or up to USD 35 per tonne used for EOR, for up to 12 years, if the effectiveness of storage is monitored and construction begins by 1 January 2024. Like other production-based incentives, 45Q incentivises investors to identify cost-effective projects developed by any promoter, though the credit is best monetised by bigger players with larger tax burdens.
Alongside 45Q, California’s Low Carbon Fuel Standard (LCFS) has emerged as a key complementary policy for projects linked to biofuels production, DAC or EOR. Globally, 45Q and LCFS are trailblazers for DAC support. The 2019 CCUS protocol clarifies how projects can benefit from LCFS credits – which traded at around USD 180 per tonne of CO 2 in 2019 – and requires monitoring of stored CO 2 for 100 years.
Among projects targeting 45Q support, some of the closest to investment decision and operation are those that aim to capture high-purity CO 2 that is currently vented from bioethanol production or natural gas processing. These are among the lowest-cost options for capturing CO 2 and the projects plan to pair this with EOR, which can be the CO 2 storage option that is quickest to bring into operation. It often requires only a connecting pipeline to an existing EOR operation.
However, the current oil price downturn and sharp cuts to upstream investment programmes suggest EOR operators may be reluctant to sign new contracts (see Fuel Supply section). Project sponsors such as ExxonMobil and Occidental Petroleum have cut capital budgets by over 30% in 2020. While some discussion of an extension of the 45Q deadline (beyond 2024) and increasing the value of credits emerged before the crisis, no decisions have been made to adjust the policy.
The largest US announcements are associated with coal and natural gas-fired power plants, with CO 2 capture capacities of 1 Mt to 6 Mt per year. Several of these projects are looking to sell the CO 2 for EOR. Given the likely challenges to securing CO 2 off-take contracts over the coming two and a half years, dedicated geological CO 2 storage options may become more attractive than before.
Subscription successful
Thank you for subscribing. You can unsubscribe at any time by clicking the link at the bottom of any IEA newsletter.
Renewable and Energy Efficiency Technologies Overview
- Strategic Energy Analysis Center
Research output : NREL › Presentation
Publication series
Nrel publication number.
- NREL/PR-6A20-81120
- nuclear-renewable hybrid energy systems
- renewable energy
This output contributes to the following UN Sustainable Development Goals (SDGs)
Access to Document
- https://www.nrel.gov/docs/fy22osti/81120.pdf
Fingerprint
- Renewables Engineering 100%
- Energy Conservation Engineering 100%
- Energy Efficiency Engineering 100%
- Renewable Energy Engineering 100%
- Electricity Grid Engineering 66%
- Generation Technology Engineering 33%
- Energy Technology Engineering 33%
- Renewable Conversion Engineering 33%
T1 - Renewable and Energy Efficiency Technologies Overview
AU - Ruth, Mark
N2 - Renewable energy and energy efficiency technologies are growing rapidly and, with increased penetration, are increasingly impacting how the electricity grid operates. This presentation outlines a number of the technologies and describes the research, development, and deployment the National Renewable Energy Laboratory is partaking on them. It also provides data on the growth of renewable electricity generation technologies over the last decade and how their increasing generation impacts grid operations. It is intended for an audience familiar with energy technologies but who are not experts in either renewable energy or the electricity grid such as attendees at the virtual course nuclear-renewable integrated systems.
AB - Renewable energy and energy efficiency technologies are growing rapidly and, with increased penetration, are increasingly impacting how the electricity grid operates. This presentation outlines a number of the technologies and describes the research, development, and deployment the National Renewable Energy Laboratory is partaking on them. It also provides data on the growth of renewable electricity generation technologies over the last decade and how their increasing generation impacts grid operations. It is intended for an audience familiar with energy technologies but who are not experts in either renewable energy or the electricity grid such as attendees at the virtual course nuclear-renewable integrated systems.
KW - nuclear-renewable hybrid energy systems
KW - renewable energy
M3 - Presentation
T3 - Presented at the Joint ICTP-IAEA Course on Nuclear-Renewable Integrated Energy Systems: Phenomenology, Research and Development, 4-8 October 2021
- ENVIRONMENT
Renewable energy, explained
Solar, wind, hydroelectric, biomass, and geothermal power can provide energy without the planet-warming effects of fossil fuels.
In any discussion about climate change , renewable energy usually tops the list of changes the world can implement to stave off the worst effects of rising temperatures. That's because renewable energy sources such as solar and wind don't emit carbon dioxide and other greenhouse gases that contribute to global warming .
Clean energy has far more to recommend it than just being "green." The growing sector creates jobs , makes electric grids more resilient, expands energy access in developing countries, and helps lower energy bills. All of those factors have contributed to a renewable energy renaissance in recent years, with wind and solar setting new records for electricity generation .
For the past 150 years or so, humans have relied heavily on coal, oil, and other fossil fuels to power everything from light bulbs to cars to factories. Fossil fuels are embedded in nearly everything we do, and as a result, the greenhouse gases released from the burning of those fuels have reached historically high levels .
As greenhouse gases trap heat in the atmosphere that would otherwise escape into space, average temperatures on the surface are rising . Global warming is one symptom of climate change, the term scientists now prefer to describe the complex shifts affecting our planet’s weather and climate systems. Climate change encompasses not only rising average temperatures but also extreme weather events, shifting wildlife populations and habitats, rising seas , and a range of other impacts .
Of course, renewables—like any source of energy—have their own trade-offs and associated debates. One of them centers on the definition of renewable energy. Strictly speaking, renewable energy is just what you might think: perpetually available, or as the U.S. Energy Information Administration puts it, " virtually inexhaustible ." But "renewable" doesn't necessarily mean sustainable, as opponents of corn-based ethanol or large hydropower dams often argue. It also doesn't encompass other low- or zero-emissions resources that have their own advocates, including energy efficiency and nuclear power.
Types of renewable energy sources
Hydropower: For centuries, people have harnessed the energy of river currents, using dams to control water flow. Hydropower is the world's biggest source of renewable energy by far, with China, Brazil, Canada, the U.S., and Russia the leading hydropower producers . While hydropower is theoretically a clean energy source replenished by rain and snow, it also has several drawbacks.
FREE BONUS ISSUE
Large dams can disrupt river ecosystems and surrounding communities , harming wildlife and displacing residents. Hydropower generation is vulnerable to silt buildup, which can compromise capacity and harm equipment. Drought can also cause problems. In the western U.S., carbon dioxide emissions over a 15-year period were 100 megatons higher than they normally would have been, according to a 2018 study , as utilities turned to coal and gas to replace hydropower lost to drought. Even hydropower at full capacity bears its own emissions problems, as decaying organic material in reservoirs releases methane.
Dams aren't the only way to use water for power: Tidal and wave energy projects around the world aim to capture the ocean's natural rhythms. Marine energy projects currently generate an estimated 500 megawatts of power —less than one percent of all renewables—but the potential is far greater. Programs like Scotland’s Saltire Prize have encouraged innovation in this area.
Wind: Harnessing the wind as a source of energy started more than 7,000 years ago . Now, electricity-generating wind turbines are proliferating around the globe, and China, the U.S., and Germany are the leading wind energy producers. From 2001 to 2017 , cumulative wind capacity around the world increased to more than 539,000 megawatts from 23,900 mw—more than 22 fold.
Some people may object to how wind turbines look on the horizon and to how they sound, but wind energy, whose prices are declining , is proving too valuable a resource to deny. While most wind power comes from onshore turbines, offshore projects are appearing too, with the most in the U.K. and Germany. The first U.S. offshore wind farm opened in 2016 in Rhode Island, and other offshore projects are gaining momentum . Another problem with wind turbines is that they’re a danger for birds and bats, killing hundreds of thousands annually , not as many as from glass collisions and other threats like habitat loss and invasive species, but enough that engineers are working on solutions to make them safer for flying wildlife.
You May Also Like
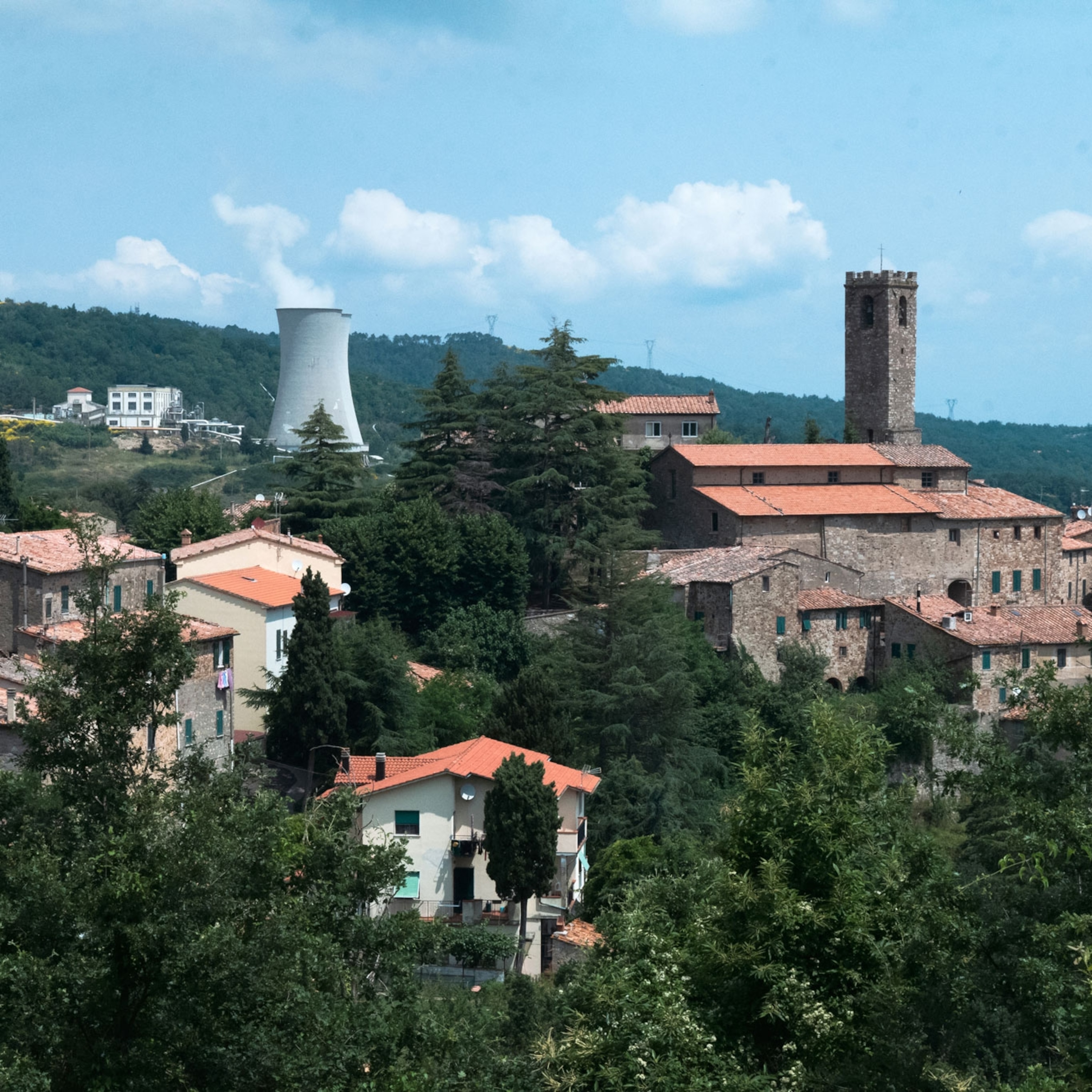
Can energy harnessed from Earth’s interior help power the world?
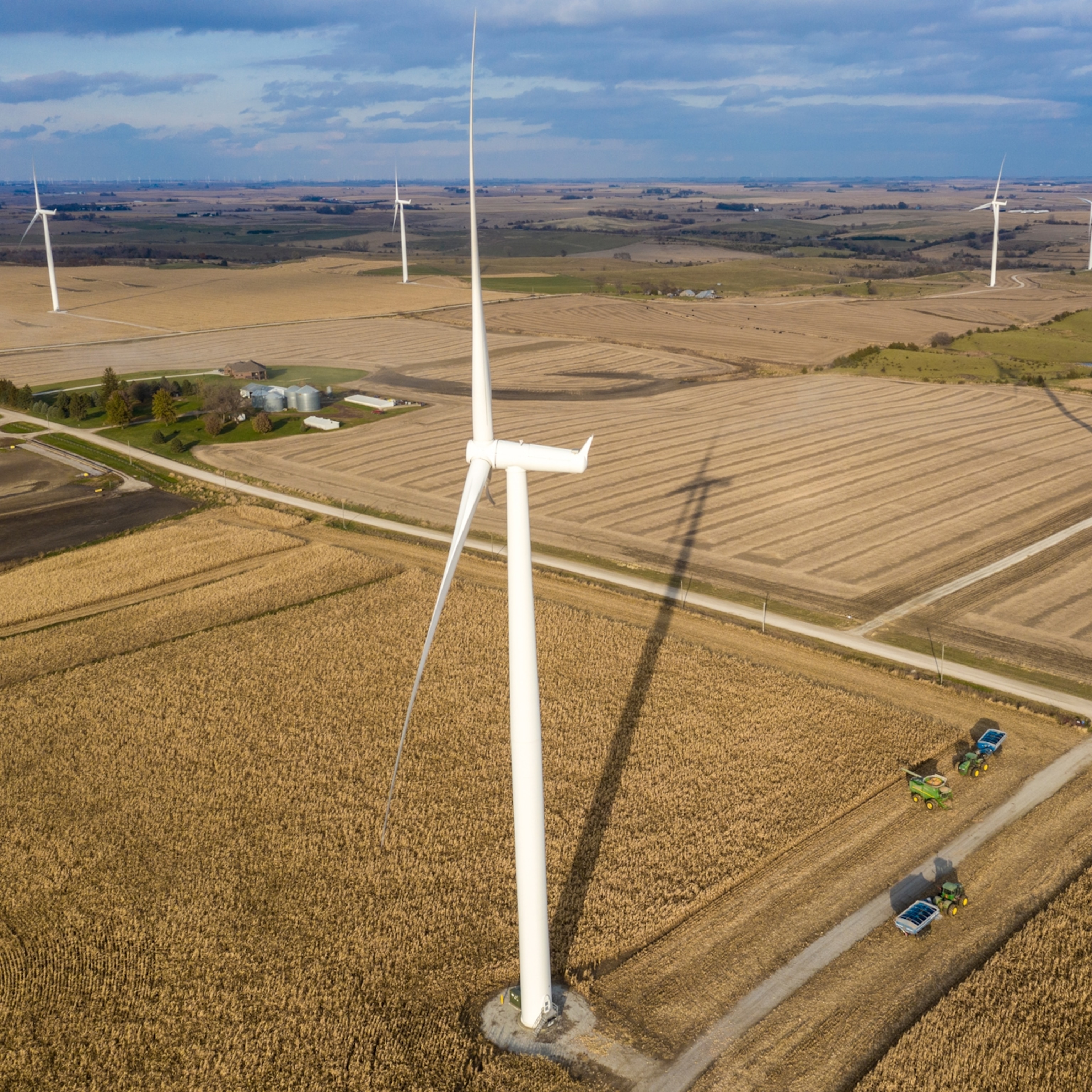
How the historic climate bill will dramatically reduce U.S. emissions
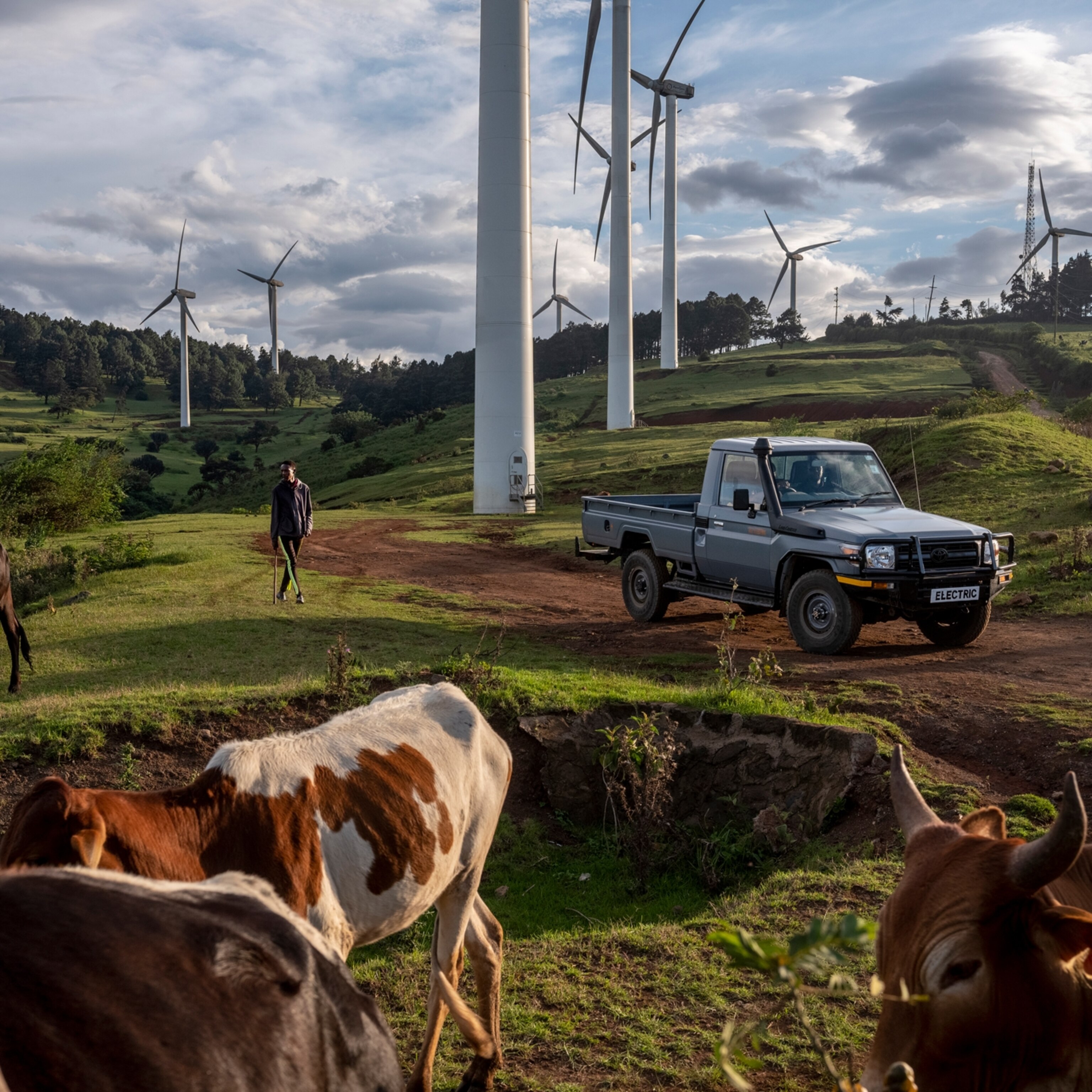
5 environmental victories from 2021 that offer hope
Solar: From home rooftops to utility-scale farms, solar power is reshaping energy markets around the world. In the decade from 2007 and 2017 the world's total installed energy capacity from photovoltaic panels increased a whopping 4,300 percent .
In addition to solar panels, which convert the sun's light to electricity, concentrating solar power (CSP) plants use mirrors to concentrate the sun's heat, deriving thermal energy instead. China, Japan, and the U.S. are leading the solar transformation, but solar still has a long way to go, accounting for around two percent of the total electricity generated in the U.S. in 2017. Solar thermal energy is also being used worldwide for hot water, heating, and cooling.
Biomass: Biomass energy includes biofuels such as ethanol and biodiesel , wood and wood waste, biogas from landfills, and municipal solid waste. Like solar power, biomass is a flexible energy source, able to fuel vehicles, heat buildings, and produce electricity. But biomass can raise thorny issues.
Critics of corn-based ethanol , for example, say it competes with the food market for corn and supports the same harmful agricultural practices that have led to toxic algae blooms and other environmental hazards. Similarly, debates have erupted over whether it's a good idea to ship wood pellets from U.S. forests over to Europe so that it can be burned for electricity. Meanwhile, scientists and companies are working on ways to more efficiently convert corn stover , wastewater sludge , and other biomass sources into energy, aiming to extract value from material that would otherwise go to waste.
Geothermal: Used for thousands of years in some countries for cooking and heating, geothermal energy is derived from the Earth’s internal heat . On a large scale, underground reservoirs of steam and hot water can be tapped through wells that can go a mile deep or more to generate electricity. On a smaller scale, some buildings have geothermal heat pumps that use temperature differences several feet below ground for heating and cooling. Unlike solar and wind energy, geothermal energy is always available, but it has side effects that need to be managed, such as the rotten egg smell that can accompany released hydrogen sulfide.

Ways to boost renewable energy
Cities, states, and federal governments around the world are instituting policies aimed at increasing renewable energy. At least 29 U.S. states have set renewable portfolio standards —policies that mandate a certain percentage of energy from renewable sources, More than 100 cities worldwide now boast at least 70 percent renewable energy, and still others are making commitments to reach 100 percent . Other policies that could encourage renewable energy growth include carbon pricing, fuel economy standards, and building efficiency standards. Corporations are making a difference too, purchasing record amounts of renewable power in 2018.
Wonder whether your state could ever be powered by 100 percent renewables? No matter where you live, scientist Mark Jacobson believes it's possible. That vision is laid out here , and while his analysis is not without critics , it punctuates a reality with which the world must now reckon. Even without climate change, fossil fuels are a finite resource, and if we want our lease on the planet to be renewed, our energy will have to be renewable.
Related Topics
- SUSTAINABILITY
- RENEWABLE ENERGY
- GEOTHERMAL ENERGY
- SOLAR POWER
- HYDROELECTRIC POWER
- CLIMATE CHANGE
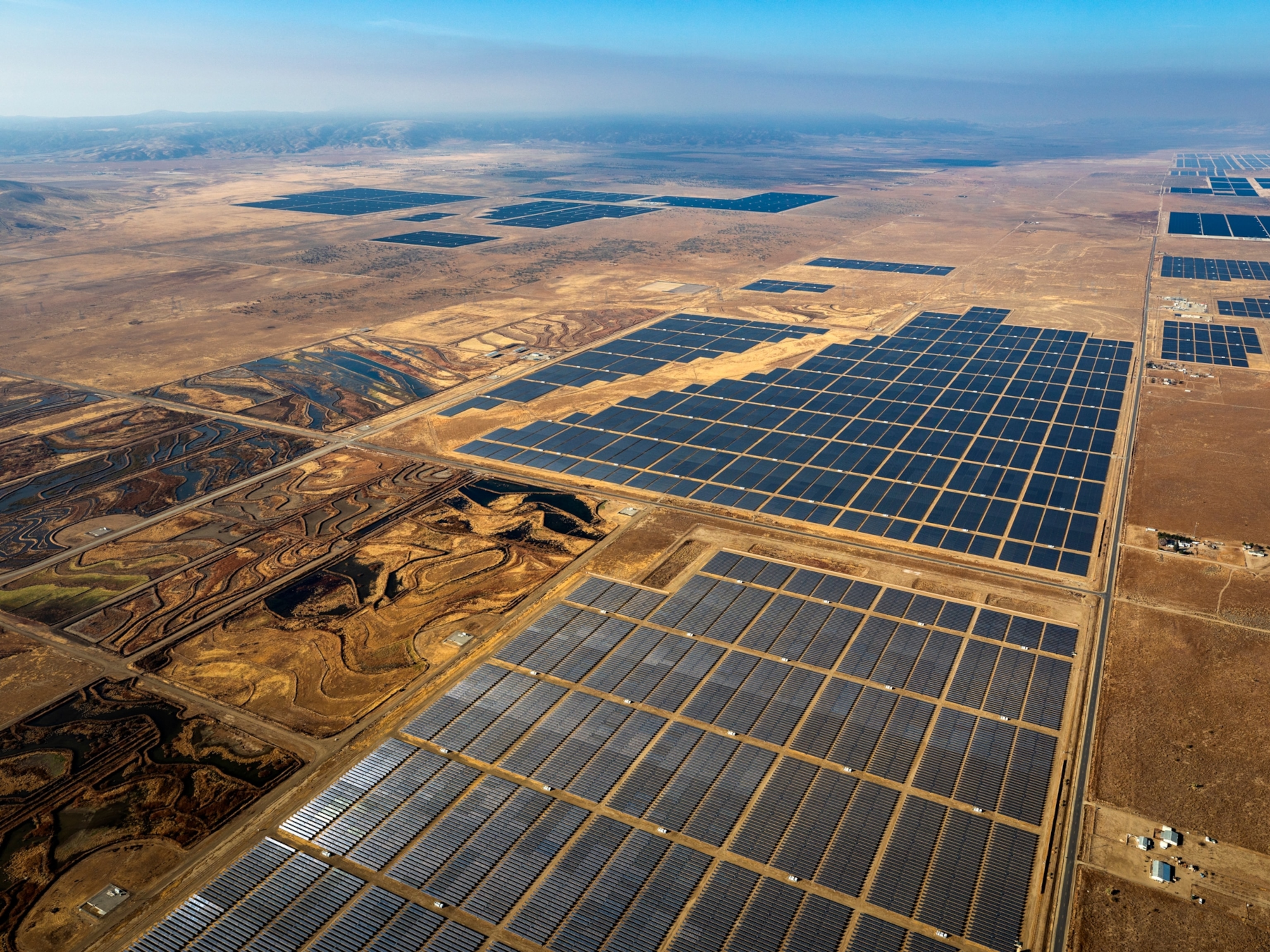
Activists fear a new threat to biodiversity—renewable energy
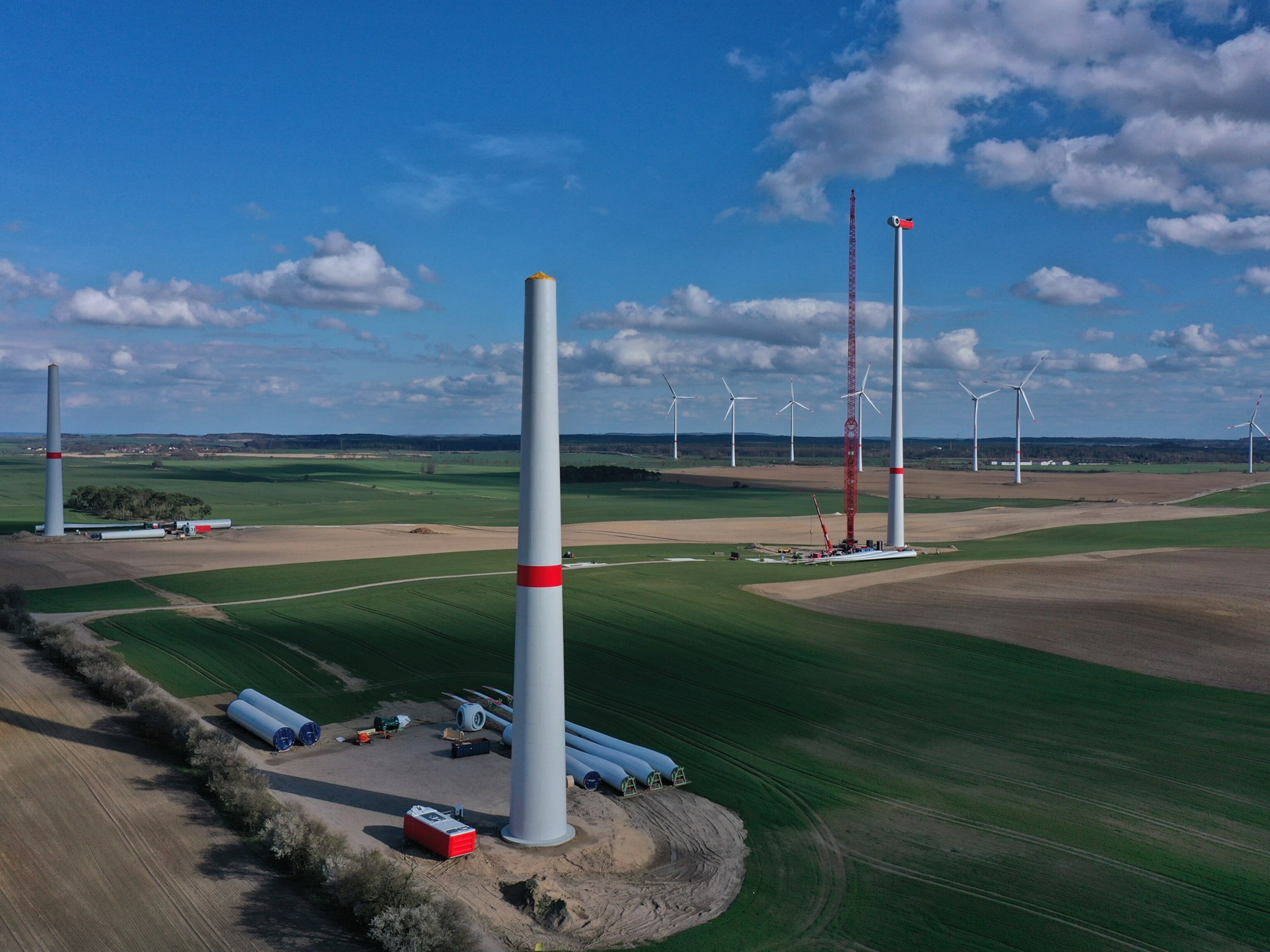
How the Ukraine war is accelerating Germany's renewable energy transition
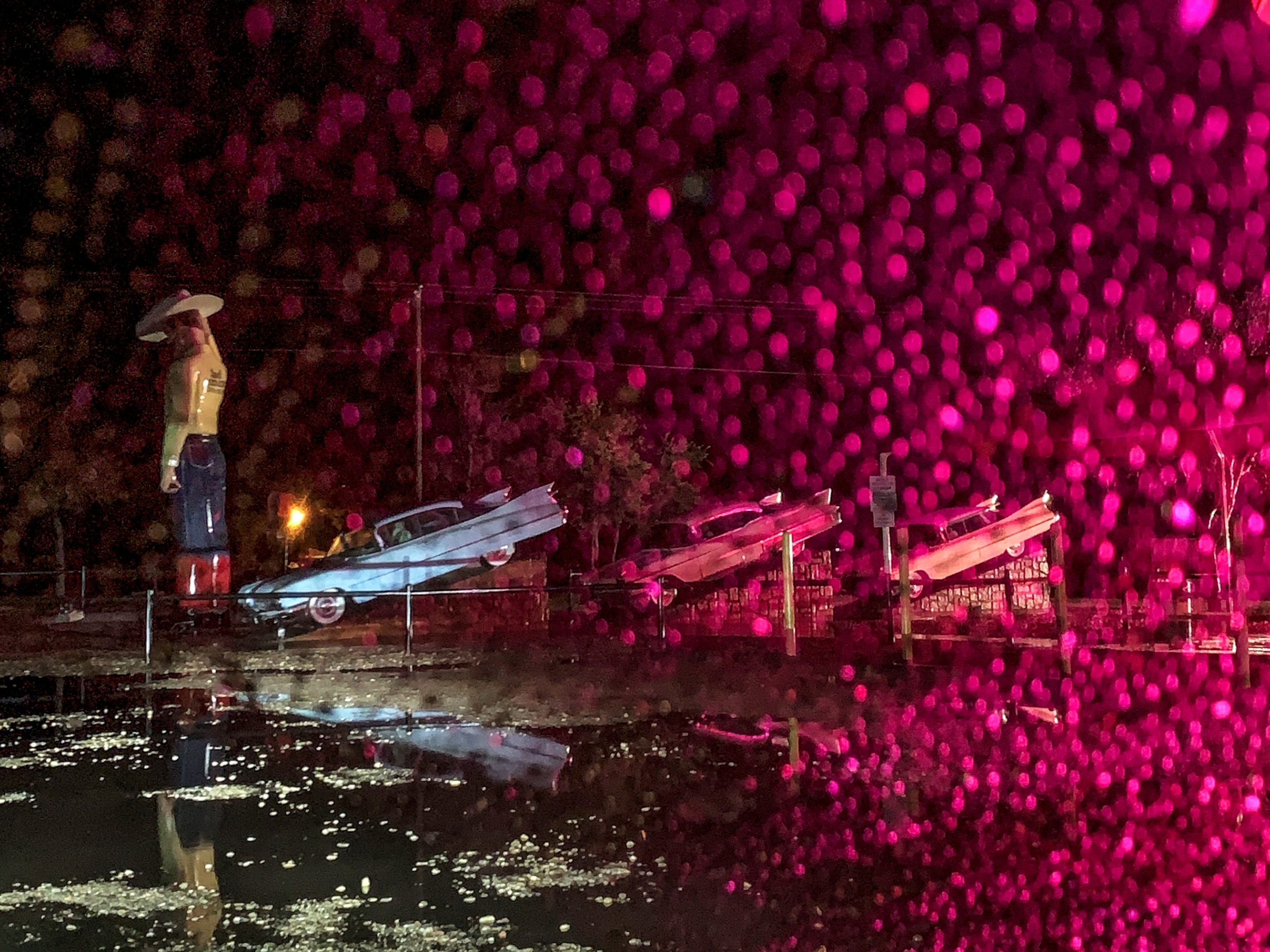
We took the Great American Road Trip—in electric cars
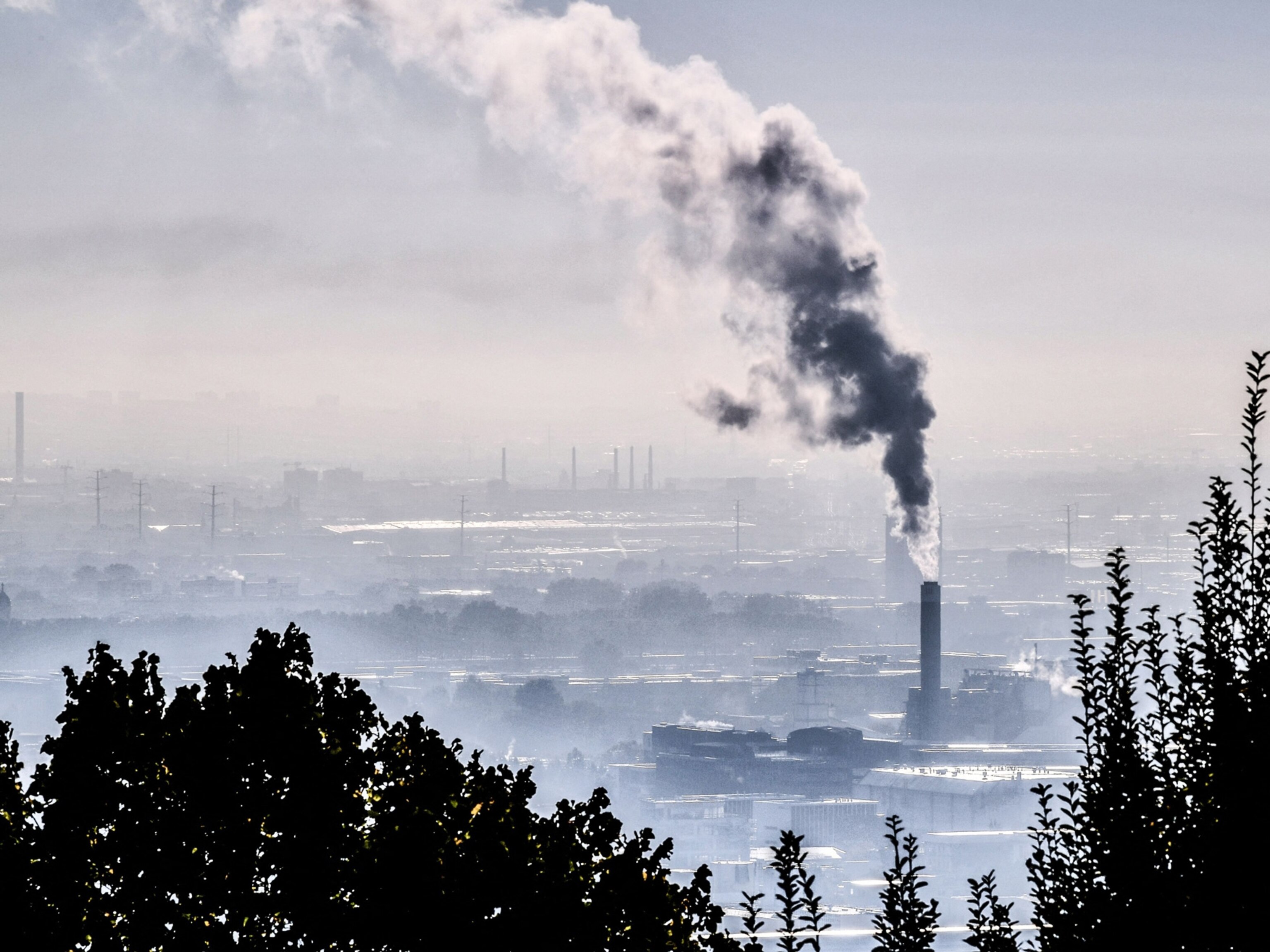
What’s at stake at COP26—the crucial global climate summit
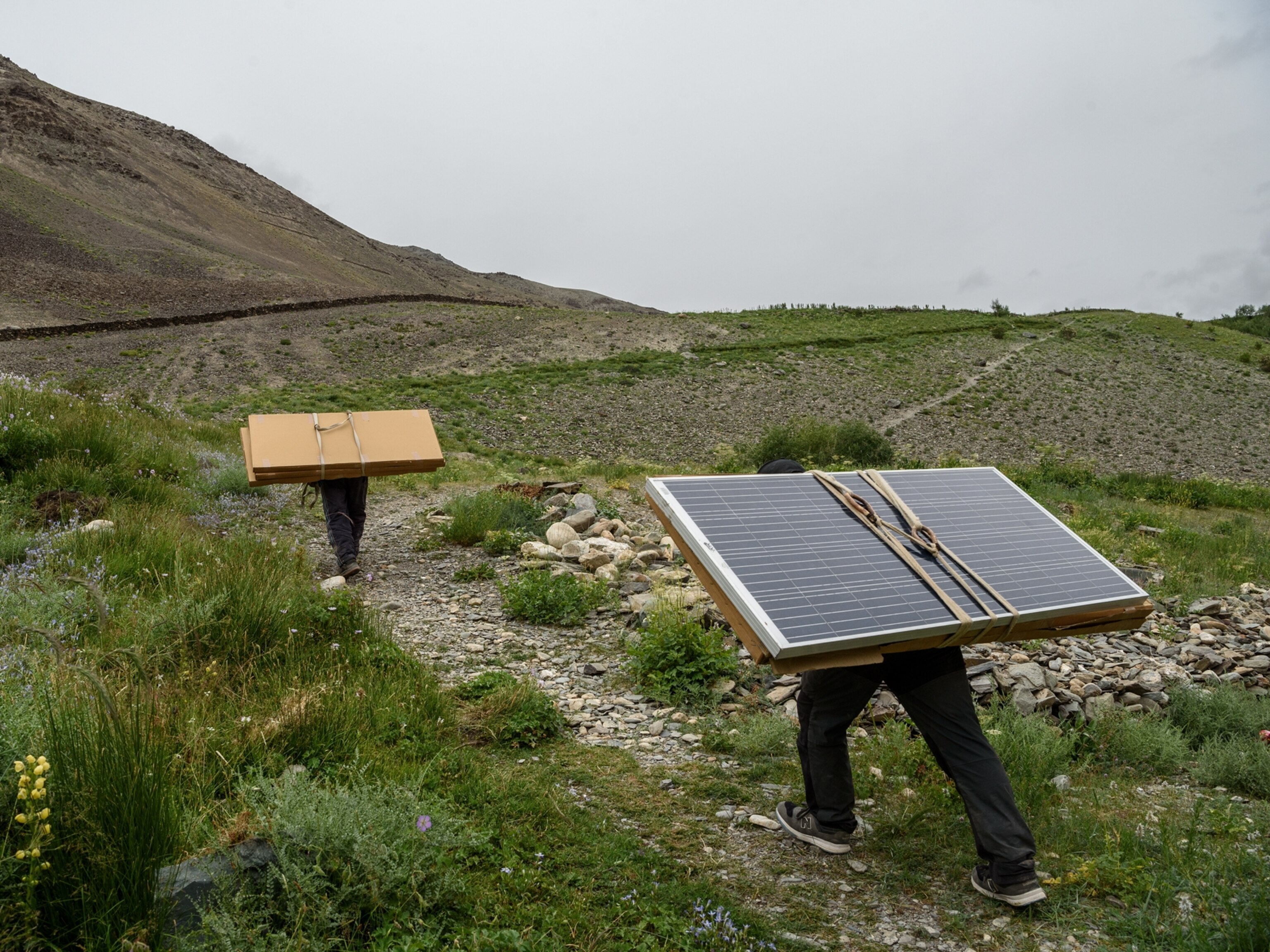
India bets its energy future on solar—in ways both small and big
- Environment
- Perpetual Planet
History & Culture
- History & Culture
- History Magazine
- Mind, Body, Wonder
- Paid Content
- Terms of Use
- Privacy Policy
- Your US State Privacy Rights
- Children's Online Privacy Policy
- Interest-Based Ads
- About Nielsen Measurement
- Do Not Sell or Share My Personal Information
- Nat Geo Home
- Attend a Live Event
- Book a Trip
- Inspire Your Kids
- Shop Nat Geo
- Visit the D.C. Museum
- Learn About Our Impact
- Support Our Mission
- Advertise With Us
- Customer Service
- Renew Subscription
- Manage Your Subscription
- Work at Nat Geo
- Sign Up for Our Newsletters
- Contribute to Protect the Planet
Copyright © 1996-2015 National Geographic Society Copyright © 2015-2024 National Geographic Partners, LLC. All rights reserved
Renewable-energy development in a net-zero world
The rapid maturation of wind and solar power has been nothing short of astonishing. Not long ago, the development of new solar and wind farms was typically driven by small regional players, and the cost was significantly higher than that of a coal plant. Today, the cost of renewables has plummeted, and many solar and wind projects are undertaken by large multinational companies, which often also announce staggering development targets.
About the authors
This article is a collaborative effort by Florian Heineke, Nadine Janecke, Holger Klärner, Florian Kühn , Humayun Tai , and Raffael Winter , representing views from McKinsey’s Electric Power & Natural Gas Practice.
Over the past decade, the growth of renewable energy has consistently and dramatically outperformed nearly all expectations (Exhibit 1). Upward corrections of estimates have become something of a ritual.
But this growth story is just getting started. As countries aim to reach ambitious decarbonization targets, renewable energy—led by wind and solar—is poised to become the backbone of the world’s power supply. Along with capacity additions from major energy providers, new types of players are entering the market (Exhibit 2). Today’s fast followers include major oil and gas companies, which aim to shift their business models to profit from the increased demand for renewables and the electrification of vehicles, and private-equity players and institutional investors that make renewable energy a central component of their investment strategy. Leaders in the shipping industry are investing in renewables to enable the production of hydrogen and ammonia as zero-emission fuel sources; steel manufacturers are eyeing green hydrogen to decarbonize their steel production, with renewables providing the green electricity for the process. Car manufacturing companies are also striking renewable-energy deals to help power their operations and manufacturing, as well as making investments in wind and solar projects.
McKinsey estimates that by 2026, global renewable-electricity capacity will rise more than 80 percent from 2020 levels (to more than 5,022 gigawatts). 1 Global Energy Perspective 2022 , McKinsey, April 2022. Of this growth, two-thirds will come from wind and solar, an increase of 150 percent (3,404 gigawatts). By 2035, renewables will generate 60 percent of the world’s electricity. 2 Global Energy Perspective 2022 , McKinsey, April 2022. But even these projections might be too low. Three years ago, we looked at advances made by renewable energy and asked, “How much faster can they grow?” 3 “ Rethinking the renewable strategy for an age of global competition ,” McKinsey, October 11, 2019. The answer is: faster than you think they can.
Three core capabilities for wind and solar developers
This race to build additional solar and wind capacity increases the pressure on developers to execute efficiently and heightens competition for finite resources. Still, the three winning capabilities we identified three years ago as important for building or expanding a renewables business are even more critical now. They form the bedrock required to tackle upcoming challenges:
- Value-chain excellence. As competition intensifies and government support for renewables subsides, strong capabilities across the entire value chain are the required cost of admission. For instance, gaining access to scarce amounts of attractive land will require differentiation in project origination and development. As margins squeeze and operators’ exposure to risk increases, ambitious companies will want to explore new, profitable offtake markets for their electricity, such as data centers or hydrogen electrolyzers for industrial production.
- Economies of scale and skill. Driven by the rapid scaling of the renewables industry, many players have built efficient operating models. However, finding employees with the necessary skills and capabilities, particularly in high-demand areas such as project development and engineering, is becoming a bottleneck for growth ambitions.
- Agile operating model. Agility and speed will be key in finding innovative ways to integrate partners and in establishing robust, high-performing supply chains. They will also enable businesses to shift resources quickly to the biggest value pools and respond to changes in the landscape, such as shifting regulations or price volatility.
Four challenges that will define the new era of renewable energy
Leveraging these capabilities as a strong foundation, successful renewables developers must navigate an increasingly complex and competitive landscape. Specifically, they will have to focus on and address four emerging challenges:
- A scarcity of top-quality land. Developers are in a constant scramble to identify new sites with increasing speed. Our analysis in Germany, a country aiming to nearly double its share of electricity coming from renewables by 2030, offers a glimpse into the constraints. Of the 51 percent of the country’s land that is potentially suitable for onshore wind farms, regulatory, environmental, and technical constraints eliminate all but 9 percent. 4 McKinsey land use optimization model. Meeting capacity targets will mean adding wind turbines to 4 to 6 percent of the country, giving developers very little room for error.
- A blue-collar and white-collar labor shortage. Across economies, the “Great Attrition” is making it difficult for companies to find and keep employees. Since April 2021, 20 million to 25 million US workers have quit their jobs, and 40 percent of employees globally say they are at least somewhat likely to leave their current position in the next three to six months. 5 Aaron De Smet, Bonnie Dowling, Bryan Hancock, and Bill Schaninger, “ The Great Attrition is making hiring harder. Are you searching the right talent pools? ,” McKinsey Quarterly , July 13, 2022; Table 4. Quits levels and rates by industry and region, seasonally adjusted, US Bureau of Labor Statistics, updated October 4, 2022. This environment presents a particularly acute challenge for industries such as renewable energy, where specific technical expertise and experience are crucial elements of success. For instance, our analysis suggests that between now and 2030, the global renewables industry will need an additional 1.1 million blue-collar workers to develop and construct wind and solar plants, and another 1.7 million to operate and maintain them. 6 Renewable energy benefits: Leveraging local capacity for onshore wind , International Renewable Energy Agency (IRENA), 2017; Renewable energy benefits: Leveraging local capacity for offshore wind , IRENA, 2018; Renewable energy benefits: Leveraging local capacity for solar PV , IRENA, 2017. This includes construction laborers, electricians, truck and semitrailer drivers, and operating engineers.
- Supply chain pressures. The soaring cost of steel, manufacturing disruptions caused by extended lockdowns in China, and transportation backlogs at ports are already making it difficult for wind and solar developers to complete projects in their pipeline on time and on budget. Some of these pressures will abate as others move to the forefront. For instance, many of the raw materials needed to manufacture solar panels and wind turbines are projected to be in short supply. This includes nickel, copper, and rare earth metals such as neodymium and praseodymium, which are indispensable for the creation of magnets used in wind turbine generators.
- Pressure on profits and volatility of returns in the short term. The increasing number of players moving into the renewable-development space, combined with reduced levels of government support and higher costs of materials, technology, and financing, is putting pressure on returns. At the same time, an all-time-high price volatility creates uncertainty and market risk.
Renewables developers will need to act decisively to prepare for these upcoming challenges. In a series of future articles, we provide detailed insights on each of these pressures and share potential ways players can take action.
Florian Heineke is a consultant in McKinsey’s Frankfurt office; Nadine Janecke is an associate partner in the Hamburg office; Holger Klärner is a partner in the Berlin office; Florian Kühn is a partner in the Oslo office; Humayun Tai is a senior partner in the New York office; and Raffael Winter is a partner in the Düsseldorf office.
The authors wish to thank Nadia Christakou, Florent Erbar, David Frankel, Emil Hosius, Anna Kemp, Nadine Palmowski, Andreas Schlosser, Sophia Spitzer, Christian Staudt, and Jakub Zivansky for their contributions to this article.
Explore a career with us
Related articles.
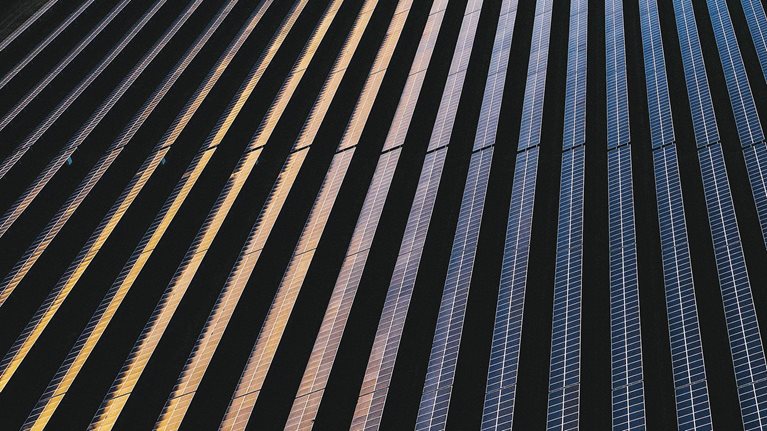
Building resilient supply chains for the European energy transition
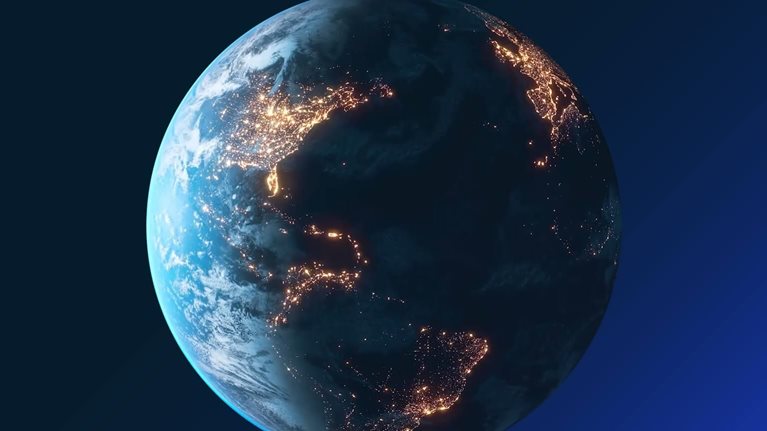
Global Energy Perspective 2022

The power sector’s net-zero transition: Scaling up renewables and infrastructure
5 smart renewable energy innovations
Fast and effective renewable energy innovation is critical to meeting climate goals. Image: REUTERS/Nathan Frandino
.chakra .wef-1c7l3mo{-webkit-transition:all 0.15s ease-out;transition:all 0.15s ease-out;cursor:pointer;-webkit-text-decoration:none;text-decoration:none;outline:none;color:inherit;}.chakra .wef-1c7l3mo:hover,.chakra .wef-1c7l3mo[data-hover]{-webkit-text-decoration:underline;text-decoration:underline;}.chakra .wef-1c7l3mo:focus,.chakra .wef-1c7l3mo[data-focus]{box-shadow:0 0 0 3px rgba(168,203,251,0.5);} Douglas Broom
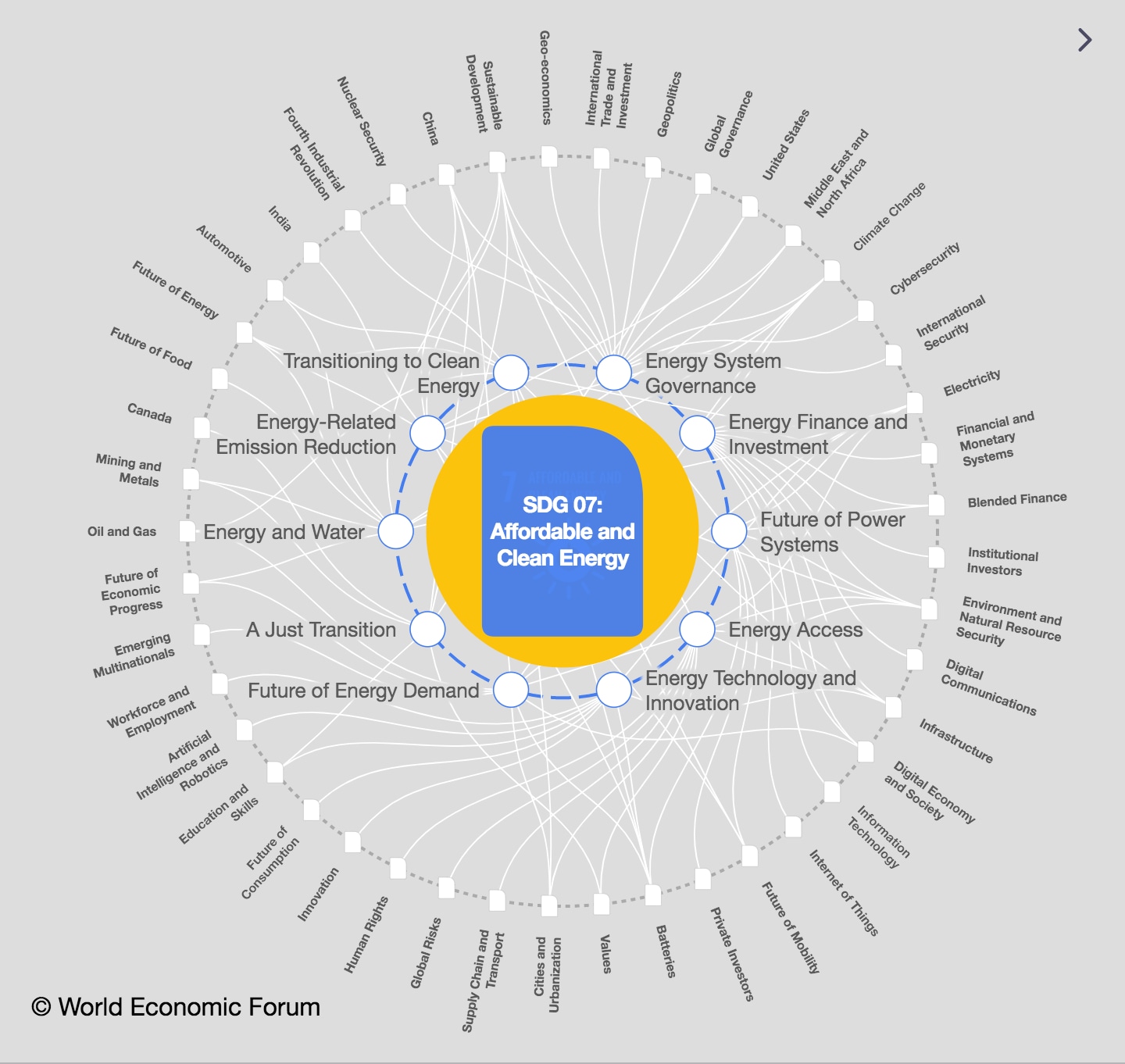
.chakra .wef-9dduvl{margin-top:16px;margin-bottom:16px;line-height:1.388;font-size:1.25rem;}@media screen and (min-width:56.5rem){.chakra .wef-9dduvl{font-size:1.125rem;}} Explore and monitor how .chakra .wef-15eoq1r{margin-top:16px;margin-bottom:16px;line-height:1.388;font-size:1.25rem;color:#F7DB5E;}@media screen and (min-width:56.5rem){.chakra .wef-15eoq1r{font-size:1.125rem;}} SDG 07: Affordable and Clean Energy is affecting economies, industries and global issues
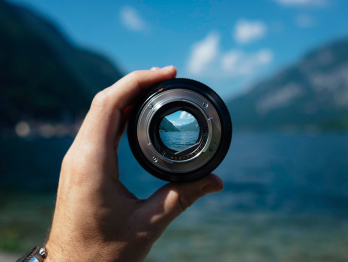
.chakra .wef-1nk5u5d{margin-top:16px;margin-bottom:16px;line-height:1.388;color:#2846F8;font-size:1.25rem;}@media screen and (min-width:56.5rem){.chakra .wef-1nk5u5d{font-size:1.125rem;}} Get involved with our crowdsourced digital platform to deliver impact at scale
Stay up to date:, tech and innovation.
Listen to the article
This article was first published on 16 May 2023 and was updated on 21 September 2023.
- Progress on the global energy transition has seen only "marginal growth" in the past three years, according to a World Economic Forum report.
- Fast and effective renewable energy innovation is critical to meeting climate goals.
- Here are five solutions that could help countries meet emissions targets.
The need for renewable energy innovation has never been greater.
In its 2023 report, Fostering Effective Energy Transition , the World Economic Forum says that 95% of countries have improved their total Energy Transition Index score over the past decade , but there has been only "marginal growth" in the past three years.
The Global Risks Report 2023 ranked failure to mitigate climate change as one of the most severe threats in the next two years, while climate- and nature- related risks lead the rankings by severity over the long term.
The World Economic Forum’s Centre for Nature and Climate is a multistakeholder platform that seeks to safeguard our global commons and drive systems transformation. It is accelerating action on climate change towards a net-zero, nature-positive future.
Learn more about our impact:
- Scaling up green technologies: Through a partnership with the US Special Presidential Envoy for Climate, John Kerry, and over 65 global businesses, the First Movers Coalition has committed $12 billion in purchase commitments for green technologies to decarbonize the cement and concrete industry.
- 1 trillion trees: Over 90 global companies have committed to conserve, restore and grow more than 8 billion trees in 65 countries through the 1t.org initiative – which aims to achieve 1 trillion trees by 2030.
- Sustainable food production: Our Food Action Alliance is engaging 40 partners who are working on 29 flagship initiatives to provide healthy, nutritious, and safe foods in ways that safeguard our planet. In Vietnam, it supported the upskilling of 2.2 million farmers and aims to provide 20 million farmers with the skills to learn and adapt to new agricultural standards.
- Eliminating plastic pollution: Our Global Plastic Action Partnership is bringing together governments, businesses and civil society to shape a more sustainable world through the eradication of plastic pollution. In Ghana, more than 2,000 waste pickers are making an impact cleaning up beaches, drains and other sites.
- Protecting the ocean: Our 2030 Water Resources Group has facilitated almost $1 billion to finance water-related programmes , growing into a network of more than 1,000 partners and operating in 14 countries/states.
- Circular economy: Our SCALE 360 initiative is reducing the environmental impacts of value chains within the fashion, food, plastics and electronics industries, positively impacting over 100,000 people in 60 circular economy interventions globally.
Want to know more about our centre’s impact or get involved? Contact us .
Greenhouse gas emissions need to be almost halved by 2030 if warming is to be limited to 1.5°C, warns the Intergovernmental Panel on Climate Change in its Sixth Assessment Report.
So, it’s encouraging that innovators continue to pioneer fresh approaches that are making the goal of switching the world to renewable energy more achievable. Here are five such energy innovations.
Solar and wind power working together
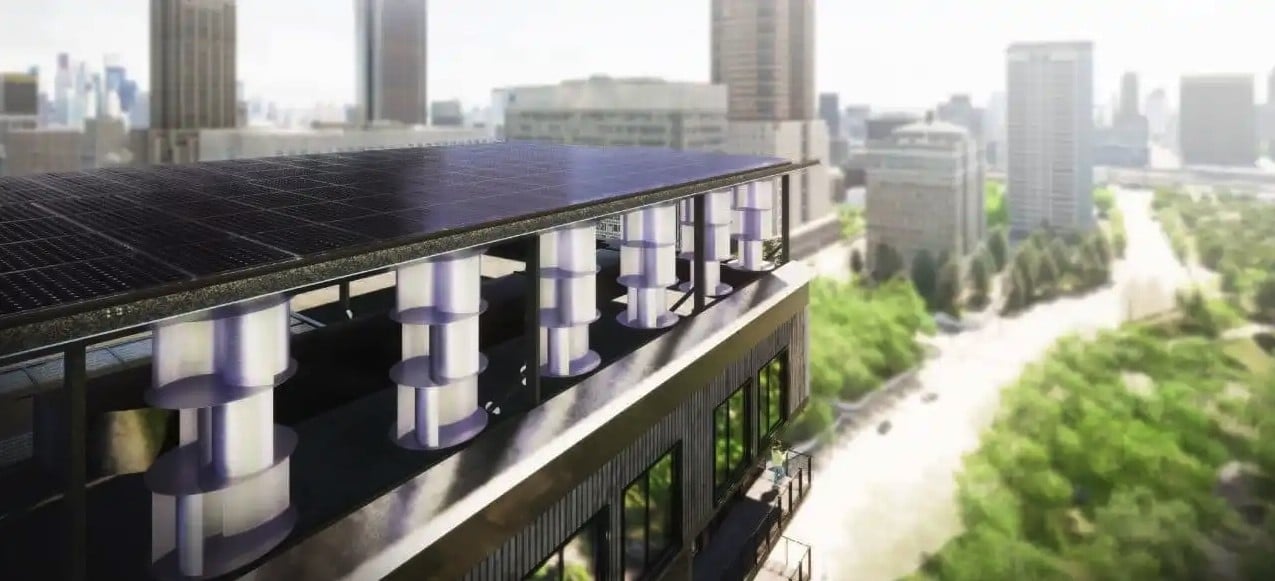
It’s tempting to think that renewable energy installations need to be either solar or wind powered. But French start-up Unéole has come up with a small-scale, easy to install solution that uses sun and wind power in a single unit .
Designed to be used on the flat roofs of offices and apartment buildings, the platform uses multiple wind turbines under a photovoltaic roof to create a silent solution that produces 40% more energy than a pure solar system and can generate power round the clock.
These turbines never turn
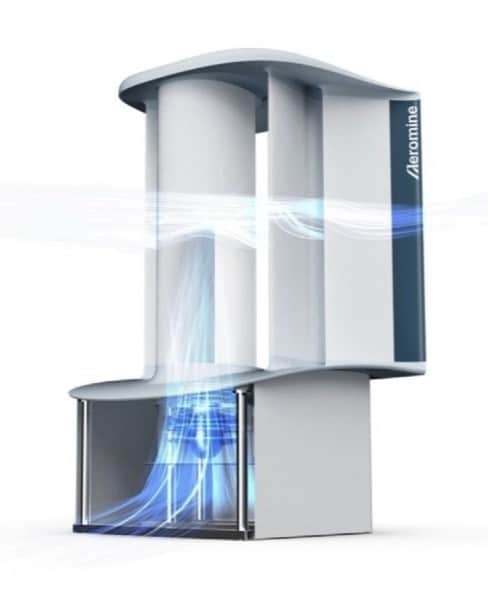
Wind power doesn’t have to mean huge turbines. A US start-up has invented a system that uses three-metre tall wind generators with no external moving parts . Sitting on the edge of roofs, Aeromine uses the natural airflow up the front of the building to generate power.
The system’s aerodynamic fins guide fast-rising air past an internal turbine, which the company claims produces 50% more power than other sustainable options. Combined with rooftop solar and battery storage, it can meet 100% of a building’s needs, the company says.
Have you read?
Solar to dominate us energy mix in 2023. here's what you need to know about the global energy transition this week, can europe’s rush for renewables solve its energy crisis, renewables will be world’s top electricity source within three years, iea data reveals, solar canals.
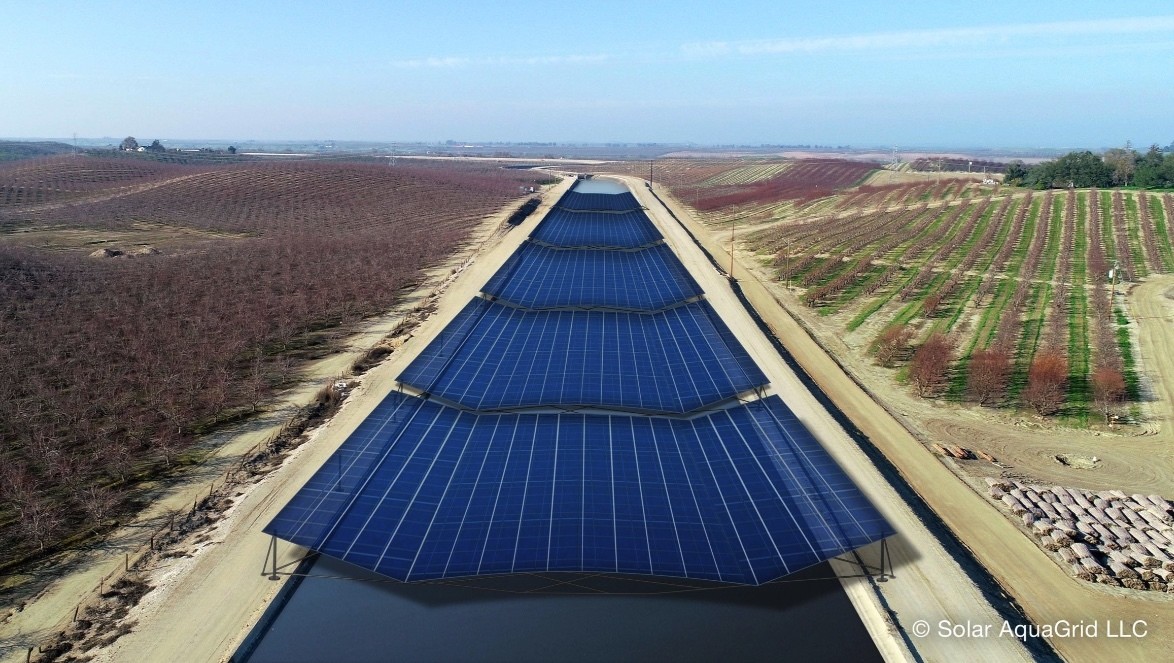
California is prone to droughts . The first 22 years of this century were the state’s driest period since the year 800 , prompting fears of a megadrought. The problem has been made more acute because the state’s water distribution system uses open canals.
Start-up SolarAquaGrid is trialling a scheme to roof over the canals with solar panels generating power and cutting evaporation. If all 6,400 km of the state’s canals were fitted, it’s forecast to save 283 billion litres of water a year and generate power for 9.4 million homes.
Solar power windows
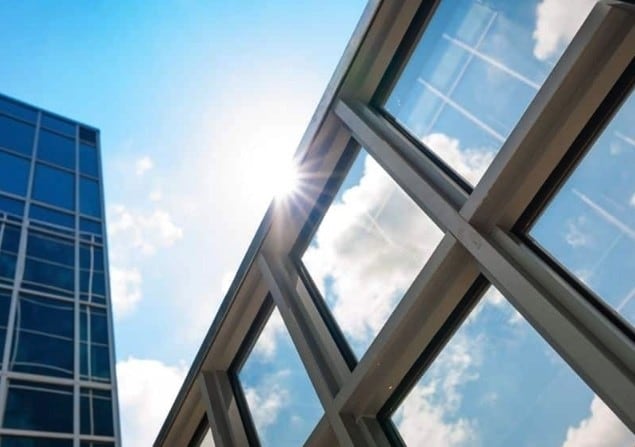
The windows in the image above are also solar panels . This transparent renewable energy source has been developed by California-based Ubiquitous Technology which says it could revolutionize solar power.
The glass is treated to allow visible light, what we see, to pass through it while absorbing and converting invisible ultraviolet and infrared light into electricity. The company says the solar windows can generate up to 30% of a building's power needs.
Making water from air
With water scarcity likely to be an issue for two-thirds of the world's population by 2025, finding alternative sources is vital. US start-up Source is providing one option. It has created off-grid "hydropanels" that can turn air into water .
Fans inside the panels pull water vapour out of the air, which in turn is turned into liquid water that can be mineralized ready for use as drinking water.
One hydropanel could eliminate the need for 54,000 single-use plastic water bottles over its 15-year lifespan, the company says.
So far, Source has installed panels in 50 countries and has projects under way to provide water in hard-to-reach areas.
Don't miss any update on this topic
Create a free account and access your personalized content collection with our latest publications and analyses.
License and Republishing
World Economic Forum articles may be republished in accordance with the Creative Commons Attribution-NonCommercial-NoDerivatives 4.0 International Public License, and in accordance with our Terms of Use.
The views expressed in this article are those of the author alone and not the World Economic Forum.
Related topics:
The agenda .chakra .wef-n7bacu{margin-top:16px;margin-bottom:16px;line-height:1.388;font-weight:400;} weekly.
A weekly update of the most important issues driving the global agenda
.chakra .wef-1dtnjt5{display:-webkit-box;display:-webkit-flex;display:-ms-flexbox;display:flex;-webkit-align-items:center;-webkit-box-align:center;-ms-flex-align:center;align-items:center;-webkit-flex-wrap:wrap;-ms-flex-wrap:wrap;flex-wrap:wrap;} More on Forum Institutional .chakra .wef-nr1rr4{display:-webkit-inline-box;display:-webkit-inline-flex;display:-ms-inline-flexbox;display:inline-flex;white-space:normal;vertical-align:middle;text-transform:uppercase;font-size:0.75rem;border-radius:0.25rem;font-weight:700;-webkit-align-items:center;-webkit-box-align:center;-ms-flex-align:center;align-items:center;line-height:1.2;-webkit-letter-spacing:1.25px;-moz-letter-spacing:1.25px;-ms-letter-spacing:1.25px;letter-spacing:1.25px;background:none;padding:0px;color:#B3B3B3;-webkit-box-decoration-break:clone;box-decoration-break:clone;-webkit-box-decoration-break:clone;}@media screen and (min-width:37.5rem){.chakra .wef-nr1rr4{font-size:0.875rem;}}@media screen and (min-width:56.5rem){.chakra .wef-nr1rr4{font-size:1rem;}} See all

Day 2 #SpecialMeeting24: Key insights and what to know
Gayle Markovitz
April 28, 2024
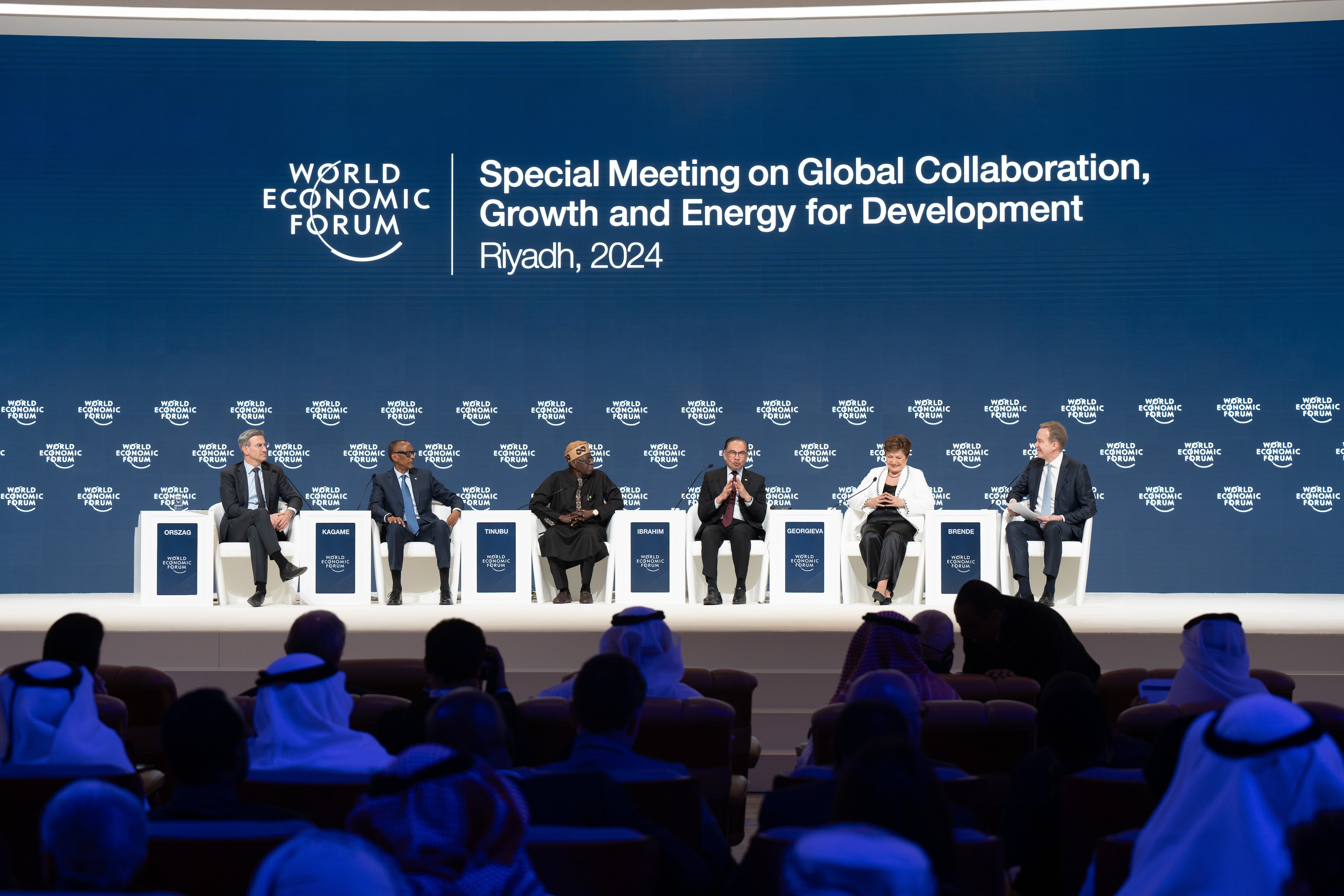
Day 1 #SpecialMeeting24: Key insights and what just happened
April 27, 2024
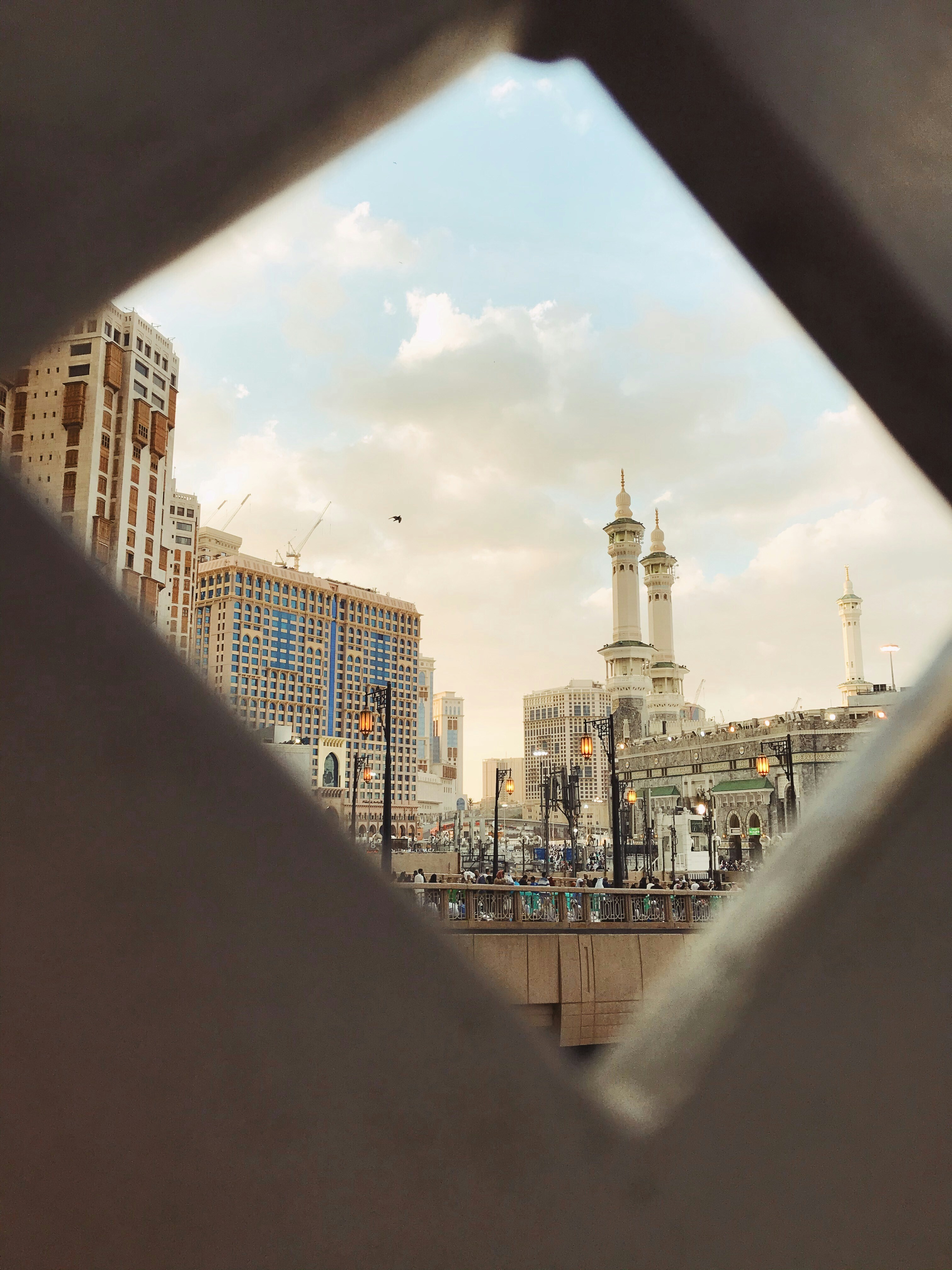
#SpecialMeeting24: What to know about the programme and who's coming
Mirek Dušek and Maroun Kairouz
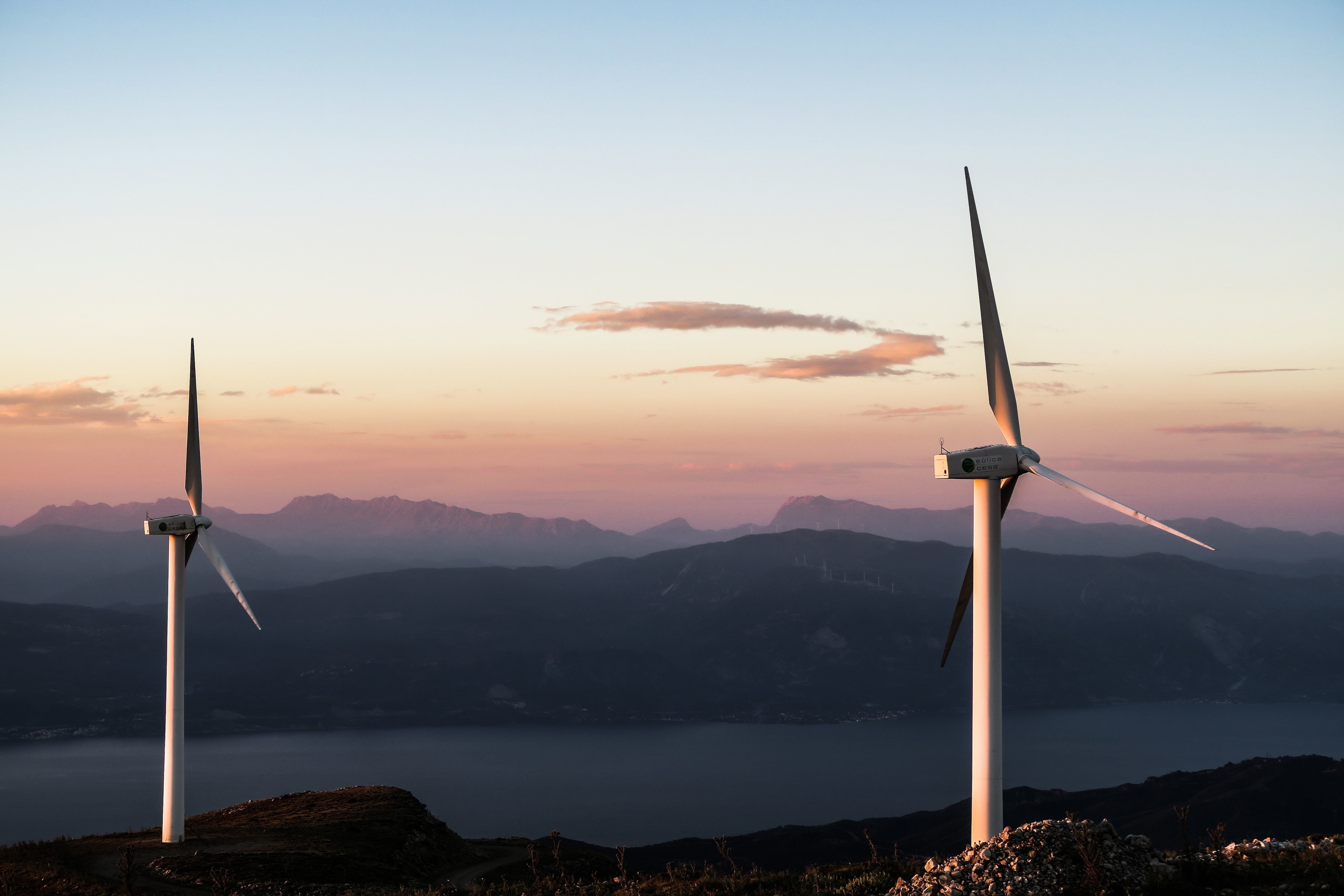
Climate finance: What are debt-for-nature swaps and how can they help countries?
Kate Whiting
April 26, 2024
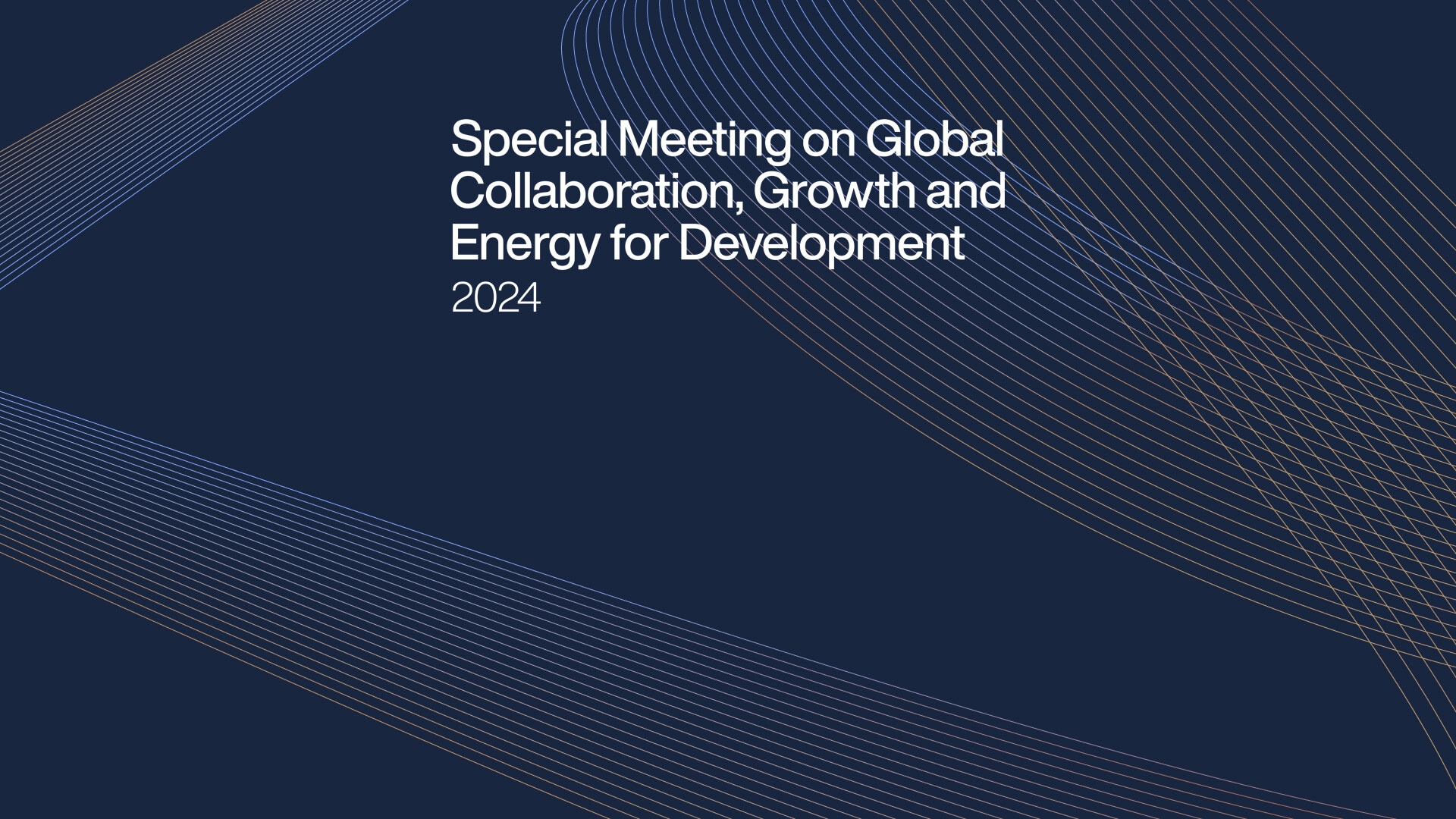
What to expect at the Special Meeting on Global Collaboration, Growth and Energy for Development
Spencer Feingold and Gayle Markovitz
April 19, 2024
From 'Quit-Tok' to proximity bias, here are 11 buzzwords from the world of hybrid work
April 17, 2024
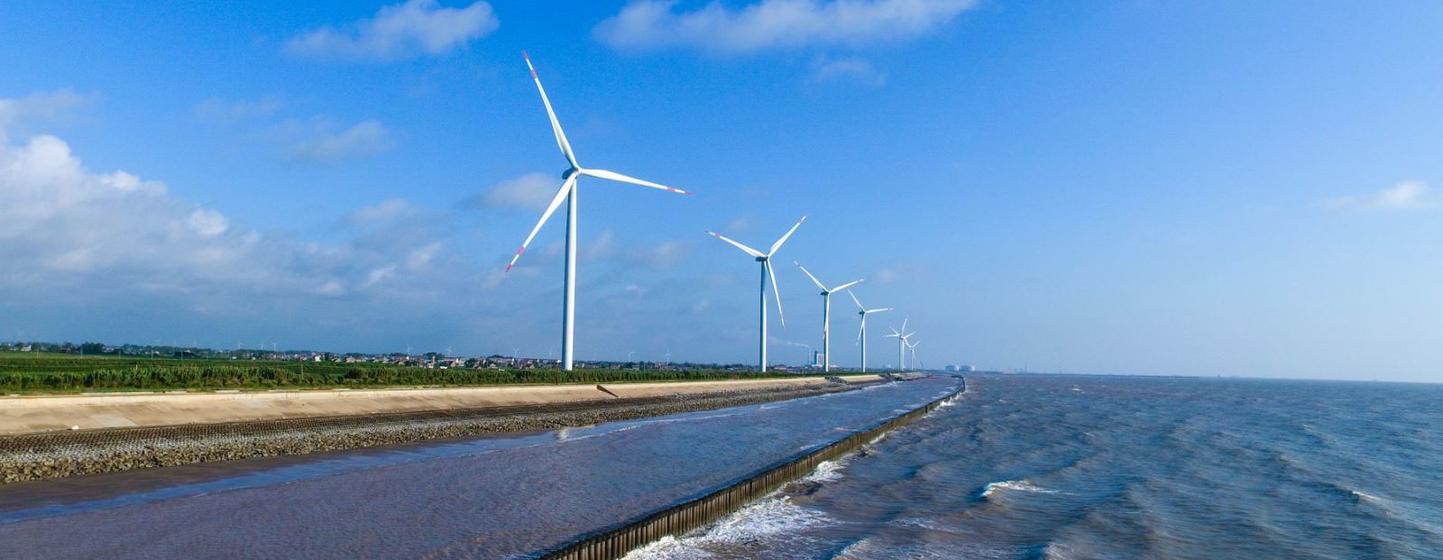
Renewable energy transforming the landscape
Facebook Twitter Print Email
Renewable energy, if supported by governments, can “truly change the landscape” in terms of achieving equitable access to affordable and clean energy, but only if they can move from “commitment to action”, according to the Director-General of the International Renewable Energy Agency (IRENA).
Renewable energy is generally defined as any energy source that is continuously replenished. It includes solar and wind power as well as bioenergy (organic matter burned as fuel) and hydroelectric power.
IRENA* chief Francesco La Camera spoke to UN News ahead of a special meeting on Friday on transitioning to sustainable sources of energy which is taking place at the UN Headquarters in New York as part of the first ever Sustainability Week .
Ensuring access to affordable reliable, sustainable and modern energy for all people, wherever they are in the world, is the aim of Sustainable Development Goal 7 (SDG 7).
UN News: What challenges have you faced when trying to persuade governments, international organisations and other stakeholders to embrace renewable energy?
Francesco La Camera: There are no difficulties in persuading governments to adopt renewable energy, but from the commitments to the action, there is always something lagging.
What is important in relation to the countries, with our members, is to support them in finding the right way to translate commitment into action. I think this is the challenge we have to face: how we can move to tripling renewable installation capacity by 2030? Now what is at stake is how we can really achieve this goal.
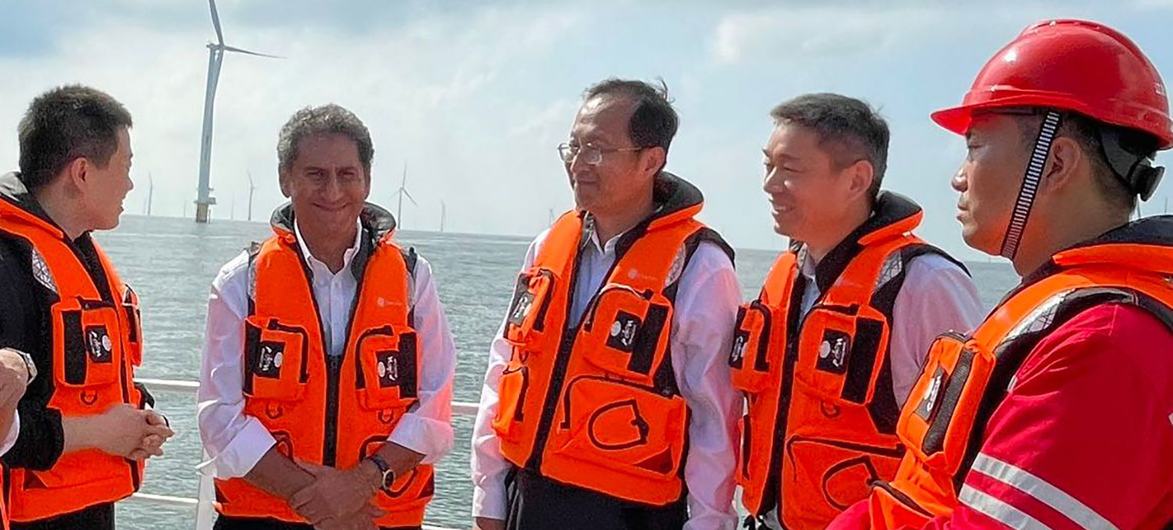
UN News: How can these challenges be overcome to ensure that countries commit and take action?
Francesco La Camera: All the countries have made commitments. We have to rewrite the way international cooperation works. In this respect, all different entities involved must make an effort.
For example, at IRENA, we have been working with President William Ruto of Kenya to forge a partnership to accelerate the renewable energy deployment in Africa. This initiative – Accelerated Partnership for Renewables in Africa’ (APRA) – was launched during the first Africa Climate Summit in Nairobi last year, and a joint statement was signed by leaders of APRA at COP28 to drive the renewable energy transition as a strategic solution to energy access, security and green growth in Africa.
We now have seven African countries, including Kenya, as well as developed countries such as Denmark, Germany, the US, and we also have the [United Arab Emirates] UAE involved. This is an example of how we are trying to rewrite the landscape of international cooperation. We are building the plan and supporting these countries in creating their own plans for fostering renewables. Together we transform to a new international cooperation mechanism to turn their plans into reality.
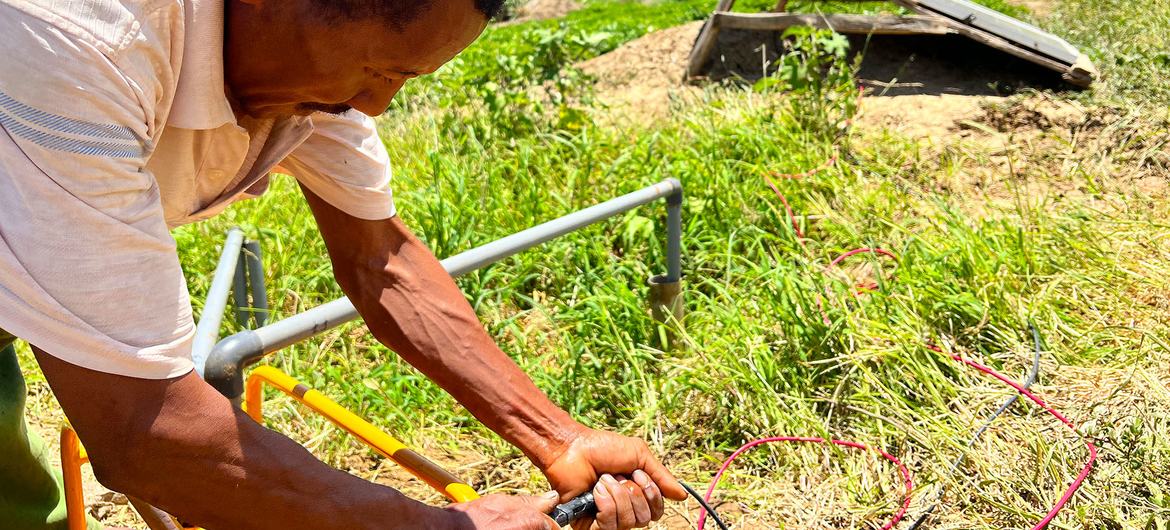
UN News: Are there notable differences in approaches, commitments and reactions between developing and developed countries when it comes to the energy transition?
Francesco La Camera: The developed world has to change the system. But, the developing countries can leap forward and transition directly to a new energy system as there is a lack of real energy systems. The main difference lies in the status of the energy system in these different parts of world, which is reflected largely in the existing inequality.
The other aspect is that the developed countries may have the tools, instruments and financial resources to drive the changes.
The developing world needs support in many aspects. Countries require financial and technological support to exchange experiences and technology. These are barriers that need to be overcome today to speed up the transition, especially in Africa.
In this respect, Africa is probably the most important powerhouse in the world for renewable energy and green hydrogen [a clean and renewable energy carrier]. But, Africa lacks the infrastructure to make this potential beneficial to its people, which would also benefit the world. Infrastructure such as ports, pipelines and civil infrastructure are decisive and crucial.
UN News: Could you give us an example of a site visit where you witnessed the critical role of renewable energy in achieving SDG 7 by 2030?
Francesco La Camera: One example that impressed me was Mauritius, where our support for solar panel installation in private houses, private buildings and public buildings has been truly transforming the landscape, giving a big push for achieving SDG 7.
UN News: Do you think the examples you mentioned can be replicated elsewhere in the world?
Francesco La Camera: To speed up the transition, we need to overcome some structural barriers that exist today. Infrastructure is the first barrier to overcome. Without efficient electricity and without providing storage interconnectivity, flexibility and balancing the grids, we cannot progress. Modernising and building infrastructure where it is absent is the top priority.
There are also the problems linking to the existing legal framework. The market is still designed in a way that does not favour the deployment of renewables. There are still a lot of subsidies for fossil fuel projects which I think should be tackled immediately.
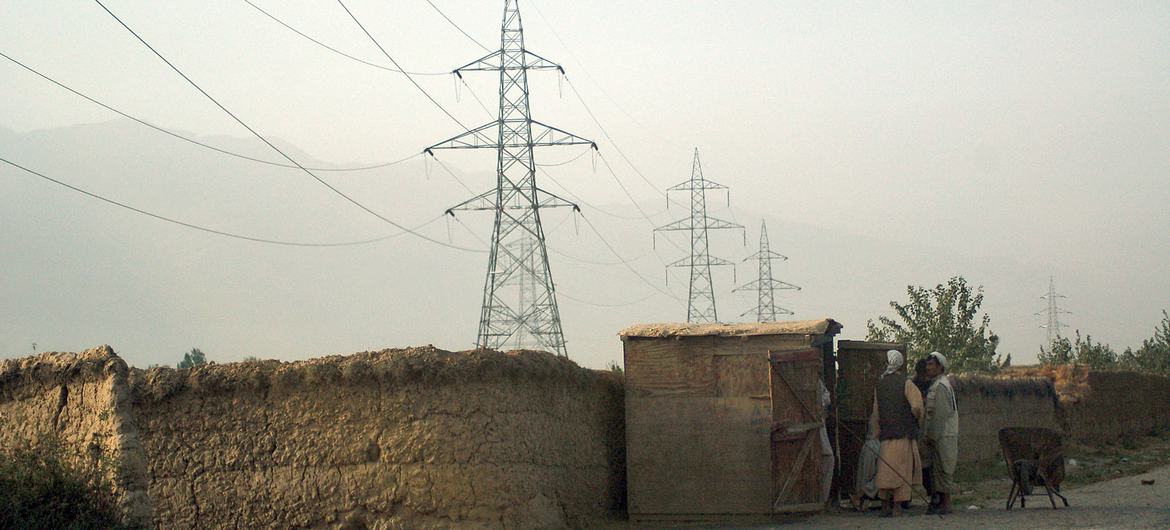
Additionally, power purchase agreements are designed in a way that discourages renewable energy development. Market pricing mechanisms often do not support renewables because renewables need long-term contracts for stability and security in the electricity provided and the cost to be paid.
Finally, we need skilled professionals and a skillful workforce to be deployed on the ground.
We have to overcome these three barriers if we truly want the energy system to accelerate the transition from fossil fuels, as called for at COP28 in Dubai a few months ago.
UN News: How can normal citizens contribute to the renewable energy transition?
Francesco La Camera: We are striving to be more efficient in all our choices, but what is more important is the legal environment where everyone feels compelled to take action. We cannot only call for the moral imperatives. Society also makes an easier and simpler environment for people to make the right choices in terms of efficiency and energy conservation.
This interview has been edited for length and clarity.
* IRENA is an intergovernmental agency aiming to support countries in their transition to a sustainable energy future. Earlier this year on 26 January, the UN observed the first International Day of Clean Energy, which coincides IRENA’s founding anniversary.
College of Engineering
New approach could make reusing captured carbon far cheaper, less energy-intensive.
A team led by Marta Hatzell designed a new electrochemical reactor to seamlessly integrate into direct air capture systems and turn CO 2 into useful raw materials.
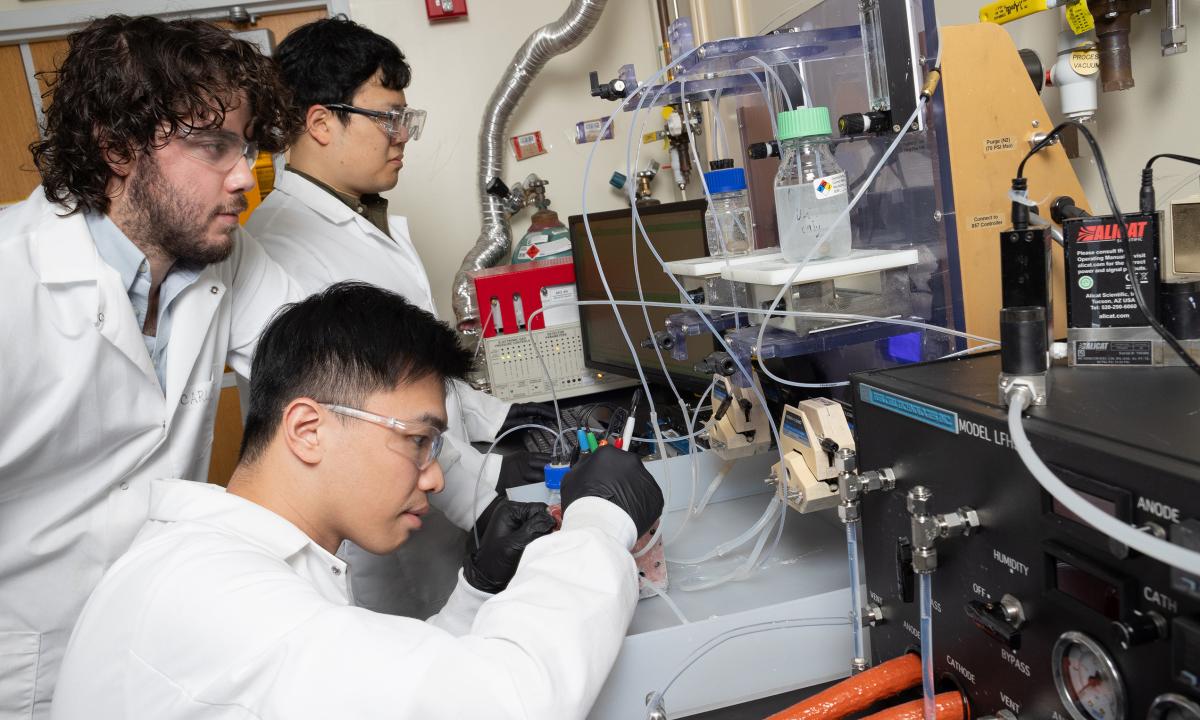
A new electrochemical reactor design developed with Marta Hatzell by postdoctoral scholar Hakhyeon Song (middle) and Ph.D. students Carlos Fernández and Po-Wei Huang (seated) converts carbon dioxide removed from the air into useful raw material. Their approach is cheaper and simpler while requiring less energy, making it a promising tool to improve the economics of direct air capture systems. (Photo: Candler Hobbs)
Engineers at Georgia Tech have designed a process that converts carbon dioxide removed from the air into useful raw material that could be used for new plastics, chemicals, or fuels.
Their approach dramatically reduces the cost and energy required for these direct air capture (DAC) systems, helping improve the economics of a process the researchers said will be critical to addressing climate change.
The key is a new kind of catalyst and electrochemical reactor design that can be easily integrated into existing DAC systems to produce useful carbon monoxide (CO) gas. It’s one of the most efficient such design ever described in scientific literature, according to lead researcher Marta Hatzell and her team. They published details April 16 in Energy and Environmental Science , a top journal for energy-related research.
“All of my team’s research projects focus on decarbonization, which I care about because of climate change, but this one in particular has the opportunity to make an impact and move toward commercialization more quickly,” said Hatzell, associate professor in the George W. Woodruff School of Mechanical Engineering and the School of Chemical and Biomolecular Engineering . “That’s why publishing our work is important, to help get this technology out into the real world.”
Typically, the DAC process involves pulling carbon dioxide out of the air using some kind of chemical or material that wants to grab the CO 2 molecules. To release that captured carbon — to store it underground, for example, or process it for productive reuse — requires significant energy and complicated, expensive systems. Along the way, those systems usually lose some of the CO 2 , often only using half of the carbon they’ve removed from the air or less.
Hatzell’s team is focused on improving an approach that uses a liquid alkaline solution called KOH to capture the carbon in a DAC system. The KOH turns the gas CO 2 into bicarbonates, which eventually have to be separated again.
The Georgia Tech design avoids that expensive, energy-intensive step altogether.
Working with Jihun Oh’s lab at the Korea Advanced Institute of Science and Technology, researchers created a new nickel-based catalyst and paired it with a bipolar membrane electrode assembly. Their setup uses electricity to extract CO 2 from the bicarbonates right next to the catalyst, which then converts it to carbon monoxide gas.
That’s the secret sauce of the system designed by Hatzell, postdoctoral scholar Hakhyeon Song, and Ph.D. students Carlos Fernández and Po-Wei Huang: It combines two steps into one.
“We're capturing the CO 2 into carbonates, which is a spontaneous process and doesn’t take much energy. And we’re getting rid of the desorption process and all of that energy expenditure,” Fernández said. “We save about 90% of the energy in the capture process, and about 50% of the capital cost.”
Their setup also is extremely efficient at using all of the CO 2 that moves through the reactor, according to Song. This is vastly better than systems that keep the carbon dioxide as a gas throughout the separation process.
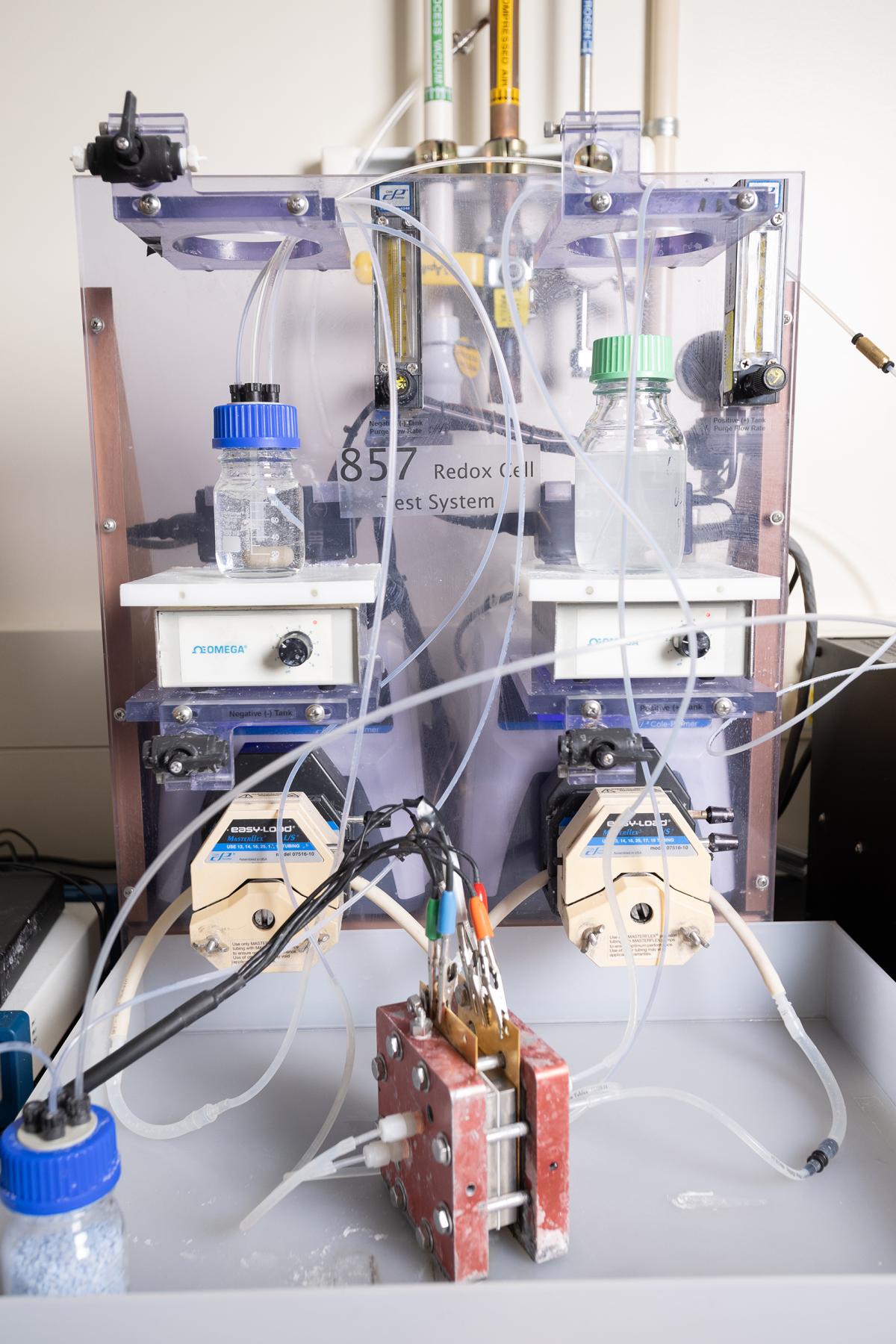
The experimental setup researchers in Marta Hatzell’s lab used to test their new electrochemical reactor for carbon capture. (Photo: Candler Hobbs)
“We’re twice as efficient. Our CO 2 utilization efficiency is almost 70%, but the gas-phase system is 35%,” Song said. “The maximum CO 2 utilization in gas-based systems is theoretically 50%. But in our case, our maximum efficiency is 100%.”
In another important advance, the team’s catalyst works well in an acidic environment, which has been a limitation of existing systems using bipolar membranes. When the reactor layer with the catalyst turns acidic, another chemical process called a hydrogen evolution reaction occurs that competes with the reaction that reduces CO 2 to CO. The new nickel-based catalyst suppresses this interference.
Producing carbon monoxide from the CO 2 scrubbed from the air is a complicated, intensive process. But if done economically, the resulting raw material could be linked to existing chemical processes and turned into new useful products.
Making those connections is next on the team’s plate. CO can become the basis for plastics, important industrial chemicals like ethylene, and maybe even jet fuel one day.
“That's why we chose CO,” Fernández said. “Other products are harder to make, and CO is a good base for any carbon chemical. You can go from CO to almost anything through thermochemical processes.”
About the Research
Citation: Song, H., Fernandez, C. A., Choi, H., Huang, P. W., Oh, J., & Hatzell, M. C. (2024). Integrated carbon capture and CO production from bicarbonates through bipolar membrane electrolysis. Energy & Environmental Science . https://doi.org/10.1039/D4EE00048J
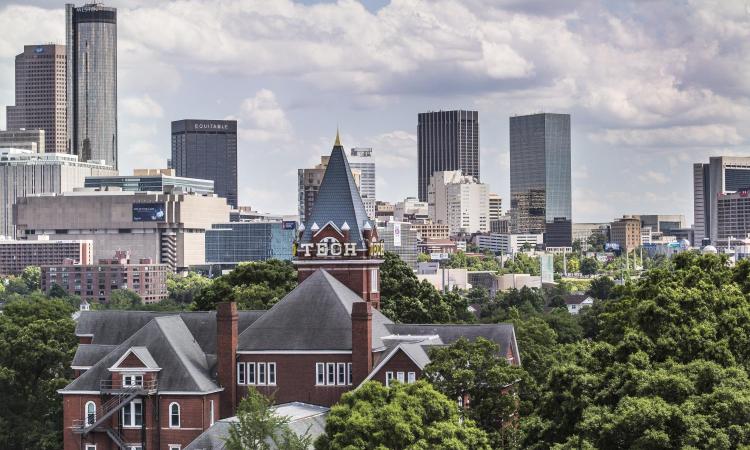
Preeminence in Research
Related content.
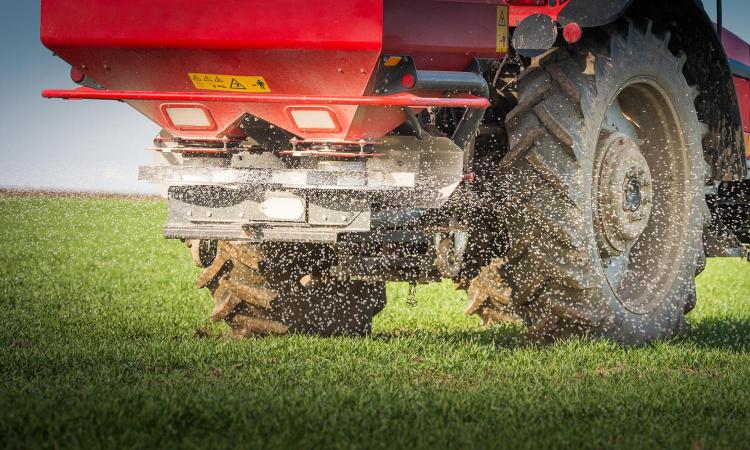
Photochemistry and a New Catalyst Could Make Fertilizer More Sustainable
New insight into the role of carbon in a low-temperature, light-based reaction may help create ammonia for fertilizer while a new catalyst offers a path to recycling the runoff.
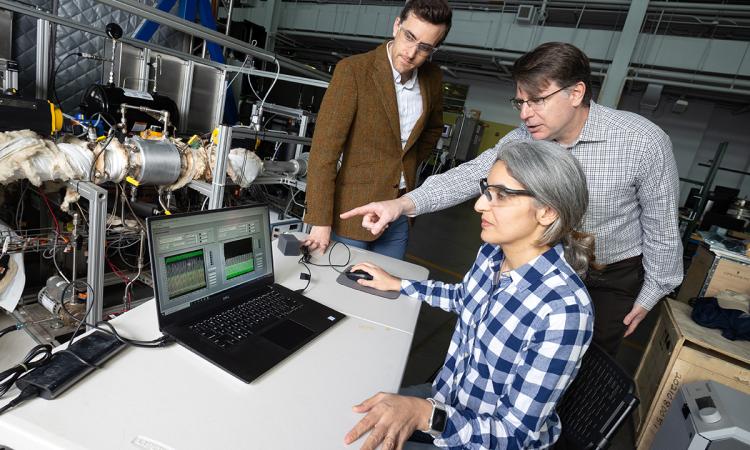
Cutting Emissions Isn’t Enough. We Need to Scrub Carbon Directly from the Air
New Direct Air Capture Center will leverage Georgia Tech’s leadership in a burgeoning field.
Higher interest rates pose risk to renewable sector, hurting energy transition, say analysts
- Medium Text
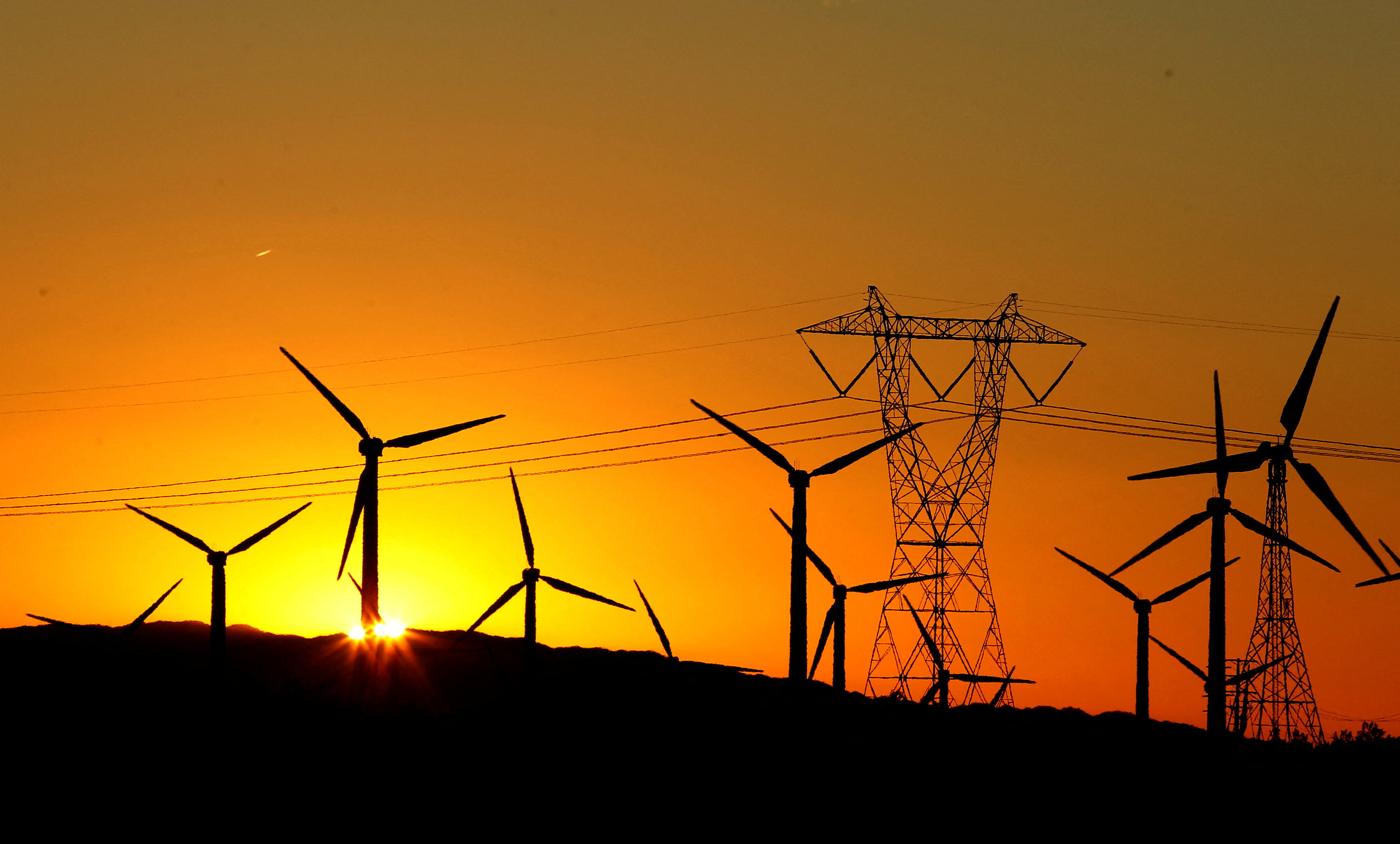
Sign up here.
Reporting by Seher Dareen and Mrinalika Roy in Bengaluru; Editing by Tasim Zahid
Our Standards: The Thomson Reuters Trust Principles. New Tab , opens new tab
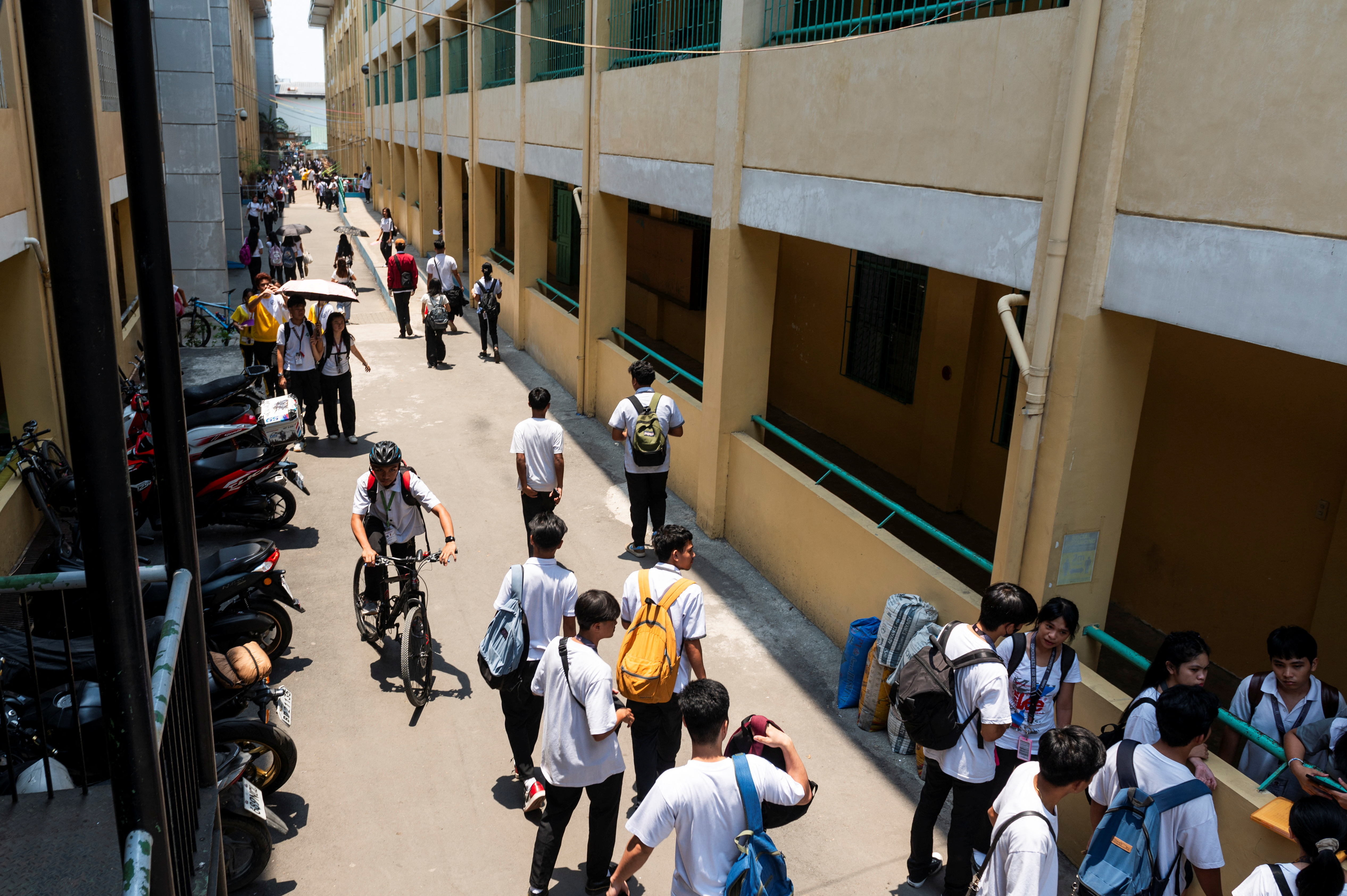
Sustainability Chevron
Philippine students suffer in wilting heat, thwarting education efforts.
Sweltering heat in the Philippines can curb farm production, disrupt water and power and weigh on businesses, but it also takes a toll on students, hampering the Southeast Asian nation's efforts to catch up to its neighbours in education.
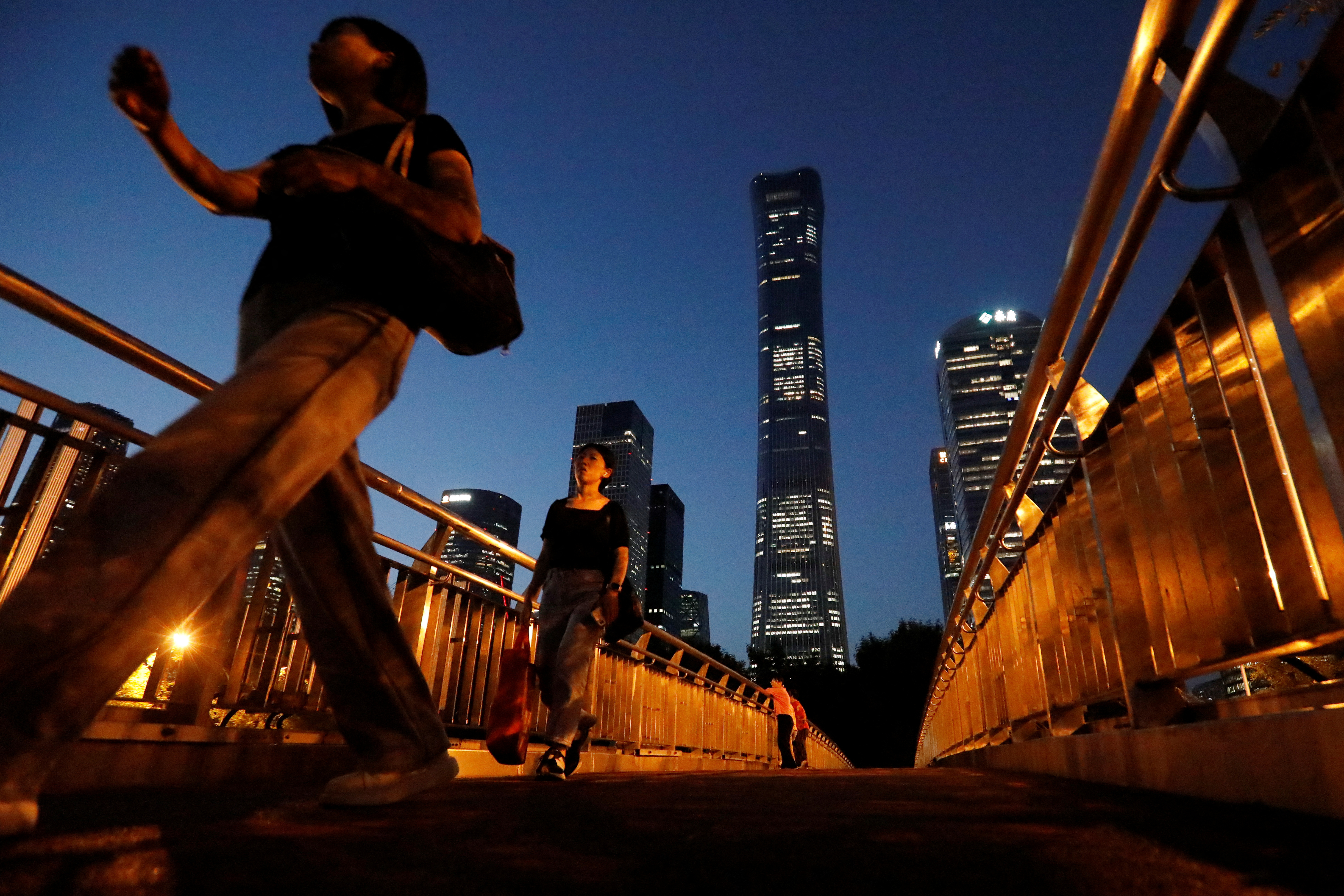
Thank you for visiting nature.com. You are using a browser version with limited support for CSS. To obtain the best experience, we recommend you use a more up to date browser (or turn off compatibility mode in Internet Explorer). In the meantime, to ensure continued support, we are displaying the site without styles and JavaScript.
- View all journals
- Explore content
- About the journal
- Publish with us
- Sign up for alerts
- Review Article
- Published: 27 May 2019
Public perceptions of and responses to new energy technologies
- Hilary S. Boudet ORCID: orcid.org/0000-0002-8096-9107 1
Nature Energy volume 4 , pages 446–455 ( 2019 ) Cite this article
8853 Accesses
254 Citations
45 Altmetric
Metrics details
- Energy and society
- Environmental social sciences
Energy’s central place in economic, political and social systems—and the broad impacts that energy choices have on the natural world and public health—mean that new technologies often spur public reactions. Understanding these public responses and their drivers is important, as public support can influence new technology adoption and deployment. Here I review the literature on public perceptions of and responses to a wide range of new energy technologies. Unlike previous reviews that tend to focus on particular technologies or types of technologies, this Review covers both large-scale energy infrastructure projects, such as utility-scale wind and solar, fossil fuel extraction and marine renewables, as well as small-scale, ‘consumer-facing’ technologies such as electric vehicles, rooftop solar and smart meters. This approach reveals broad trends that may facilitate communication between policymakers, technologists and the public, and support the transition to a more sustainable energy system.
This is a preview of subscription content, access via your institution
Access options
Access Nature and 54 other Nature Portfolio journals
Get Nature+, our best-value online-access subscription
24,99 € / 30 days
cancel any time
Subscribe to this journal
Receive 12 digital issues and online access to articles
111,21 € per year
only 9,27 € per issue
Buy this article
- Purchase on Springer Link
- Instant access to full article PDF
Prices may be subject to local taxes which are calculated during checkout
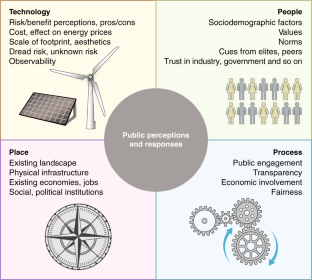
Similar content being viewed by others
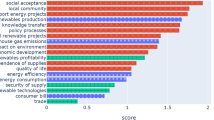
Social innovation supports inclusive and accelerated energy transitions with appropriate governance
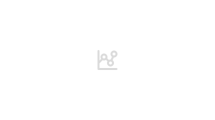
An agenda for future Social Sciences and Humanities research on energy efficiency: 100 priority research questions
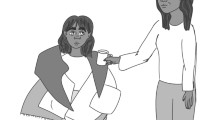
The importance of social relations in shaping energy demand
IPCC Global Warming of 1.5 °C (eds Masson-Delmotte, V. et al.) (World Meteorological Organization, 2018).
Ansolabehere, S. & Konisky, D. M. Cheap and Clean: How Americans Think about Energy in the Age of Global Warming (MIT Press, 2014).
Rand, J. & Hoen, B. Thirty years of North American wind energy acceptance research: What have we learned? Energy Res. Soc. Sci. 29 , 135–148 (2017).
Google Scholar
Hess, D. J. & Coley, J. S. Wireless smart meters and public acceptance: the environment, limited choices, and precautionary politics. Public Underst. Sci. 23 , 688–702 (2014).
Slovic, P., Fischhoff, B. & Lichtenstein, S. Why study risk perception? Risk Anal. 2 , 83–93 (2006).
Bauer, M. W. Editorial. Public Underst. Sci. 18 , 378–382 (2009).
Clarke, C. E. et al. Public opinion on energy development: the interplay of issue framing, top-of-mind associations, and political ideology. Energy Policy 81 , 131–140 (2015).
Yin, R. K. Case Study Research and Applications: Design and Methods (SAGE, 2017).
Ellis, G., Barry, J. & Robinson, C. Many ways to say ‘no’, different ways to say ‘yes’: applying Q-Methodology to understand public acceptance of wind farm proposals. J. Environ. Plann. Manag. 50 , 517–551 (2007).
Hui, I., Cain, B. E. & Dabiri, J. O. Public receptiveness of vertical axis wind turbines. Energy Policy 112 , 258–271 (2018).
Rothensee, M. in The Internet of Things Vol. 4952 (eds Floerkemeier, C. et al.) 123–139 (Springer, 2008).
Thomas, M., Partridge, T., Harthorn, B. H. & Pidgeon, N. Deliberating the perceived risks, benefits, and societal implications of shale gas and oil extraction by hydraulic fracturing in the US and UK. Nat. Energy 2 , 17054 (2017).
Balta-Ozkan, N., Davidson, R., Bicket, M. & Whitmarsh, L. The development of smart homes market in the UK. Energy 60 , 361–372 (2013).
Klick, H. & Smith, E. R. A. N. Public understanding of and support for wind power in the United States. Renew . Energy 35 , 1585–1591 (2010).
Thomas, M. et al. Public perceptions of hydraulic fracturing for shale gas and oil in the United States and Canada. Wiley Interdiscip. Rev. Clim. Change 8 , e450 (2017).
Wiersma, B. & Devine-Wright, P. Public engagement with offshore renewable energy: a critical review. Wiley Interdiscip. Rev. Clim. Change 5 , 493–507 (2014).
Spence, A., Demski, C., Butler, C., Parkhill, K. & Pidgeon, N. Public perceptions of demand-side management and a smarter energy future. Nat. Clim. Change 5 , 550–554 (2015).
Carpini, M. X. D. & Keeter, S. What Americans Know about Politics and Why It Matters (Yale Univ. Press, 1996).
Boudet, H. et al. “Fracking” controversy and communication: using national survey data to understand public perceptions of hydraulic fracturing. Energy Policy 65 , 57–67 (2014).
Boudet, H. S., Zanocco, C. M., Howe, P. D. & Clarke, C. E. The effect of geographic proximity to unconventional oil and gas development on public support for hydraulic fracturing. Risk Anal. 38 , 1871–1890 (2018).
Energy and Climate Change Public Attitudes Tracker: Wave 25 Summary Report (Department for Business, Energy and Industrial Strategy, 2018); https://www.gov.uk/government/statistics/energy-and-climate-change-public-attitudes-tracker-wave-25
Raimi, K. T. & Carrico, A. R. Understanding and beliefs about smart energy technology. Energy Res. Soc. Sci. 12 , 68–74 (2016).
Krause, R. M., Carley, S. R., Lane, B. W. & Graham, J. D. Perception and reality: public knowledge of plug-in electric vehicles in 21 U.S. cities. Energy Policy 63 , 433–440 (2013).
Hargreaves, T., Nye, M. & Burgess, J. Making energy visible: a qualitative field study of how householders interact with feedback from smart energy monitors. Energy Policy 38 , 6111–6119 (2010).
Smale, R., van Vliet, B. & Spaargaren, G. When social practices meet smart grids: flexibility, grid management, and domestic consumption in The Netherlands. Energy Res. Soc. Sci. 34 , 132–140 (2017).
Devine-Wright, P. Beyond NIMBYism: towards an integrated framework for understanding public perceptions of wind energy. Wind Energy 8 , 125–139 (2005).
Espeland, W. N. The Struggle for Water: Politics, Rationality, and Identity in the American Southwest (Univ. Chicago Press, 1998).
Whitmarsh, L. et al. UK public perceptions of shale gas hydraulic fracturing: the role of audience, message and contextual factors on risk perceptions and policy support. Appl. Energy 160 , 419–430 (2015).
Ipsos MORI Quantitative Research into Public Awareness, Attitudes, and Experience of Smart Meters: Wave 4 (Department of Energy and Climate Change, 2014); https://assets.publishing.service.gov.uk/government/uploads/system/uploads/attachment_data/file/277045/key_findings_summary_quantitative_sm_public_attitudes_research_wave_4.pdf
Carlisle, J. E., Kane, S. L., Solan, D. & Joe, J. C. Support for solar energy: examining sense of place and utility-scale development in California. Energy Res. Soc. Sci. 3 , 124–130 (2014).
Besley, J. C. & McComas, K. A. Something old and something new: comparing views about nanotechnology and nuclear energy. J. Risk Res. 18 , 215–231 (2014).
Ho, S. S. et al. Science literacy or value predisposition? A meta-analysis of factors predicting public perceptions of benefits, risks, and acceptance of nuclear energy. Environ. Commun. 13 , 457–471 (2018).
Stedman, R. C., Evensen, D., O’Hara, S. & Humphrey, M. Comparing the relationship between knowledge and support for hydraulic fracturing between residents of the United States and the United Kingdom. Energy Res. Soc. Sci. 20 , 142–148 (2016).
Stoutenborough, J. W. & Vedlitz, A. The role of scientific knowledge in the public’s perceptions of energy technology risks. Energy Policy 96 , 206–216 (2016).
Jacquet, J. B. Landowner attitudes toward natural gas and wind farm development in northern Pennsylvania. Energy Policy 50 , 677–688 (2012).
Krause, R. M., Carley, S. R., Warren, D. C., Rupp, J. A. & Graham, J. D. “Not in (or under) my backyard”: geographic proximity and public acceptance of carbon capture and storage facilities. Risk Anal. 34 , 529–540 (2014).
Vasi, I. B., Walker, E. T., Johnson, J. S. & Tan, H. F. “No fracking way!” Documentary film, discursive opportunity, and local opposition against hydraulic fracturing in the United States, 2010 to 2013. Am. Sociol. Rev. 80 , 934–959 (2015).
Druckman, J. N. & Bolsen, T. Framing, motivated reasoning, and opinions about emergent technologies. J. Commun. 61 , 659–688 (2011).
Scheufele, D. A. & Lewenstein, B. V. The public and nanotechnology: how citizens make sense of emerging technologies. J. Nanopart. Res. 7 , 659–667 (2005).
van der Linden, S. A conceptual critique of the cultural cognition thesis. Sci. Commun. 38 , 128–138 (2016).
Oltra, C. et al. Public responses to CO 2 storage sites: lessons from five European cases. Energy Environ. 23 , 227–248 (2012).
Slovic, P. Perception of risk. Science 236 , 280–285 (1987).
Stoutenborough, J. W., Vedlitz, A. & Liu, X. The influence of specific risk perceptions on public policy support: an examination of energy policy. Ann. Am. Acad. Pol. Soc. Sci. 658 , 102–120 (2015).
Rogers, E. M. Diffusion of Innovations 5th edn (Free Press, 2003).
Rezvani, Z., Jansson, J. & Bodin, J. Advances in consumer electric vehicle adoption research: a review and research agenda. Transp. Res. D. 34 , 122–136 (2015).
Schelly, C. Residential solar electricity adoption: What motivates, and what matters? A case study of early adopters. Energy Res. Soc. Sci. 2 , 183–191 (2014).
Wolske, K. S., Stern, P. C. & Dietz, T. Explaining interest in adopting residential solar photovoltaic systems in the United States: toward an integration of behavioral theories. Energy Res. Soc. Sci. 25 , 134–151 (2017).
Haggett, C. Over the sea and far away? A consideration of the planning, politics and public perception of offshore wind farms. J. Environ. Policy Plann. 10 , 289–306 (2008).
Selma, L., Seigo, O., Dohle, S. & Siegrist, M. Public perception of carbon capture and storage (CCS): a review. Renew. Sustain. Energy Rev. 38 , 848–863 (2014).
Jenkins, K., McCauley, D., Heffron, R., Stephan, H. & Rehner, R. Energy justice: a conceptual review. Energy Res. Soc. Sci. 11 , 174–182 (2016).
Cotton, M. Shale gas — community relations: NIMBY or not? Integrating social factors into shale gas community engagements. Nat. Gas. Electr. 29 , 8–12 (2013).
Sierzchula, W., Bakker, S., Maat, K. & van Wee, B. The influence of financial incentives and other socio-economic factors on electric vehicle adoption. Energy Policy 68 , 183–194 (2014).
Geels, F. W., Berkhout, F. & van Vuuren, D. P. Bridging analytical approaches for low-carbon transitions. Nat. Clim. Change 6 , 576–583 (2016).
Howe, P. D. & Mathieu, J. L. Age and perceived benefits are associated with willingness to participate in an electric load control program. Preprint at https://doi.org/10.31235/osf.io/rpg46 (2018).
Sigrin, B., Pless, J. & Drury, E. Diffusion into new markets: evolving customer segments in the solar photovoltaics market. Environ. Res. Lett. 10 , 084001 (2015).
Finucane, M. L., Slovic, P., Mertz, C. K., Flynn, J. & Satterfield, T. A. Gender, race, and perceived risk: the ‘white male’ effect. Health Risk Soc. 2 , 159–172 (2000).
McCright, A. M. & Dunlap, R. E. Bringing ideology in: the conservative white male effect on worry about environmental problems in the USA. J. Risk Res. 16 , 211–226 (2013).
Jacques, P. J., Dunlap, R. E. & Freeman, M. The organisation of denial: conservative think tanks and environmental scepticism. Environ. Polit. 17 , 349–385 (2008).
Farrell, J. Corporate funding and ideological polarization about climate change. Proc. Natl Acad. Sci. USA 113 , 92–97 (2016).
Davis, C. & Fisk, J. M. Energy abundance or environmental worries? Analyzing public support for fracking in the United States. Rev. Policy Res. 31 , 1–16 (2014).
Clarke, C. E. et al. How geographic distance and political ideology interact to influence public perception of unconventional oil/natural gas development. Energy Policy 97 , 301–309 (2016).
Brown, E., Hartman, K., Borick, C. P., Rabe, B. G. & Ivacko, T. M. The National Surveys on Energy and Environment Public Opinion on Fracking: Perspectives from Michigan and Pennsylvania (May 2013) (Center for Local, State, and Urban Policy, 2013).
O’Connor, C. D. & Fredericks, K. Citizen perceptions of fracking: the risks and opportunities of natural gas development in Canada. Energy Res. Soc. Sci. 42 , 61–69 (2018).
Gravelle, T. B. & Lachapelle, E. Politics, proximity and the pipeline: mapping public attitudes toward Keystone XL. Energy Policy 83 , 99–108 (2015).
Baiocchi-Wagner, E. A. & Talley, A. E. The role of family communication in individual health attitudes and behaviors concerning diet and physical activity. Health Commun. 28 , 193–205 (2013).
Howell, E. L. et al. How do U.S. state residents form opinions about ‘fracking’ in social contexts? A multilevel analysis. Energy Policy 106 , 345–355 (2017).
Graziano, M. & Gillingham, K. Spatial patterns of solar photovoltaic system adoption: the influence of neighbors and the built environment. J. Econ. Geogr. 15 , 815–839 (2015).
Meckling, J. & Nahm, J. The politics of technology bans: industrial policy competition and green goals for the auto industry. Energy Policy 126 , 470–479 (2019).
Bell, S. E. & York, R. Community economic identity: the coal industry and ideology construction in West Virginia. Rural Sociol. 75 , 111–143 (2010).
Vasi, I. B. Winds of Change: The Environmental Movement and the Global Development of the Wind Energy Industry . (Oxford Univ. Press, 2011).
Boudet, H. S. From NIMBY to NIABY: regional mobilization against liquefied natural gas in the United States. Environ. Polit. 20 , 786–806 (2011).
Evensen, D. & Stedman, R. Beliefs about impacts matter little for attitudes on shale gas development. Energy Policy 109 , 10–21 (2017).
Batel, S. & Devine-Wright, P. Towards a better understanding of people’s responses to renewable energy technologies: insights from social representations theory. Public Underst. Sci. 24 , 311–325 (2015).
Moscovici, S. in Social Cognition (ed. Forgas, J.) 181–209 (Academic Press, 1981).
Bugden, D., Evensen, D. & Stedman, R. A drill by any other name: social representations, framing, and legacies of natural resource extraction in the fracking industry. Energy Res. Soc. Sci. 29 , 62–71 (2017).
McAdam, D. & Boudet, H. Putting Social Movements in their Place: Explaining Opposition to Energy Projects in the United States, 2000–2005 (Cambridge Univ. Press, 2012).
Boudet, H., Bugden, D., Zanocco, C. & Maibach, E. The effect of industry activities on public support for ‘fracking’. Environ. Polit. 25 , 593–612 (2016).
Giordono, L. S., Boudet, H. S., Karmazina, A., Taylor, C. L. & Steel, B. S. Opposition “overblown”? Community response to wind energy siting in the western United States. Energy Res. Soc. Sci. 43 , 119–131 (2018).
Cotton, M. & Devine-Wright, P. Putting pylons into place: a UK case study of public perspectives on the impacts of high voltage overhead transmission lines. J. Environ. Plann. Manag. 56 , 1225–1245 (2013).
Yu, J., Wang, Z., Majumdar, A. & Rajagopal, R. DeepSolar: A machine learning framework to efficiently construct a solar deployment database in the United States. Joule 2 , 2605–2617 (2018).
Sovacool, B. K., Kivimaa, P., Hielscher, S. & Jenkins, K. Vulnerability and resistance in the United Kingdom’s smart meter transition. Energy Policy 109 , 767–781 (2017).
Devine-Wright, P. Place attachment and public acceptance of renewable energy: a tidal energy case study. J. Environ. Psychol. 31 , 336–343 (2011).
Devine-Wright, P. Rethinking NIMBYism: the role of place attachment and place identity in explaining place-protective action. J. Community Appl. Soc. Psychol. 19 , 426–441 (2009).
Wright, R. A. & Boudet, H. S. To act or not to act: context, capability, and community response to environmental risk. Am. J. Sociol. 118 , 728–777 (2012).
Unruh, G. C. Understanding carbon lock-in. Energy Policy 28 , 817–830 (2000).
Stedman, R. C. Toward a social psychology of place: predicting behavior from place-based cognitions, attitude, and identity. Environ. Behav. 34 , 561–581 (2002).
Jacquet, J. B. & Stedman, R. C. The risk of social-psychological disruption as an impact of energy development and environmental change. J. Environ. Plann. Manag. 57 , 1285–1304 (2014).
Vorkinn, M. & Riese, H. Environmental concern in a local context: the significance of place attachment. Environ. Behav. 33 , 249–263 (2001).
Devine-Wright, P. & Howes, Y. Disruption to place attachment and the protection of restorative environments: a wind energy case study. J. Environ. Psychol. 30 , 271–280 (2010).
McLachlan, C. ‘You don’t do a chemistry experiment in your best china’: symbolic interpretations of place and technology in a wave energy case. Energy Policy 37 , 5342–5350 (2009).
Willow, A. J., Zak, R., Vilaplana, D. & Sheeley, D. The contested landscape of unconventional energy development: a report from Ohio’s shale gas country. J. Environ. Stud. Sci. 4 , 56–64 (2014).
Wolsink, M. Social acceptance revisited: gaps, questionable trends, and an auspicious perspective. Energy Res. Soc. Sci. 46 , 287–295 (2018).
Wüstenhagen, R., Wolsink, M. & Bürer, M. J. Social acceptance of renewable energy innovation: an introduction to the concept. Energy Policy 35 , 2683–2691 (2007).
Bomberg, E. Shale we drill? Discourse dynamics in UK fracking debates. J. Environ. Policy Plann. 19 , 72–88 (2017).
Whitton, J., Brasier, K., Charnley-Parry, I. & Cotton, M. Shale gas governance in the United Kingdom and the United States: opportunities for public participation and the implications for social justice. Energy Res. Soc. Sci. 26 , 11–22 (2017).
Strupeit, L. & Palm, A. Overcoming barriers to renewable energy diffusion: business models for customer-sited solar photovoltaics in Japan, Germany and the United States. J. Clean. Prod. 123 , 124–136 (2016).
Beierle, T. C. & Cayford, J. Democracy in Practice: Public Participation in Environmental Decisions (Routledge, 2002).
Agterbosch, S., Meertens, R. M. & Vermeulen, W. J. The relative importance of social and institutional conditions in the planning of wind power projects. Renew. Sustain. Energy Rev. 13 , 393–405 (2009).
Firestone, J. et al. Reconsidering barriers to wind power projects: community engagement, developer transparency and place. J. Environ. Policy Plann. 20 , 370–386 (2017).
Garvin, D. A. & Roberto, M. A. What you don’t know about making decisions. Harv. Bus. Rev. 79 , 108–119 (2001).
Bell, D., Gray, T. & Haggett, C. The ‘social gap’ in wind farm siting decisions: explanations and policy responses. Environ. Polit. 14 , 460–477 (2005).
Jegen, M. & Philion, X. D. Power and smart meters: a political perspective on the social acceptance of energy projects. Can. Public Adm. 60 , 68–88 (2017).
Boudet, H. S. An “insiteful” comparison: contentious politics in liquefied natural gas facility siting in the U.S. MIT Proj. 11 , 47–76 (2016).
McAdam, D. et al. “Site fights”: explaining opposition to pipeline projects in the developing world. Sociol. Forum 25 , 410–427 (2010).
Eaton, E. & Kinchy, A. Quiet voices in the fracking debate: ambivalence, nonmobilization, and individual action in two extractive communities (Saskatchewan and Pennsylvania). Energy Res. Soc. Sci. 20 , 22–30 (2016).
Boudet, H. S. & Ortolano, L. A tale of two sitings: contentious politics in liquefied natural gas facility siting in California. J. Plann. Educ. Res. 30 , 5–21 (2010).
Arnstein, S. R. A ladder of citizen participation. J. Am. Inst. Plann. 35 , 216–224 (1969).
Gilley, B. Authoritarian environmentalism and China’s response to climate change. Environ. Polit. 21 , 287–307 (2012).
Yang, C.-J. Launching strategy for electric vehicles: lessons from China and Taiwan. Technol. Forecast. Soc. Change 77 , 831–834 (2010).
Aczel, M. R. & Makuch, K. E. The lay of the land: the public, participation and policy in China’s fracking frenzy. Extr. Ind. Soc. 5 , 508–514 (2018).
Sher, C. & Wu, C. Fracking in China: community impacts and public support of shale gas development. J. Contemp. China 27 , 626–641 (2018).
Yu, C.-H., Huang, S.-K., Qin, P. & Chen, X. Local residents’ risk perceptions in response to shale gas exploitation: evidence from China. Energy Policy 113 , 123–134 (2018).
Jaspal, R., Nerlich, B. & Lemańcyzk, S. Fracking in the Polish press: geopolitics and national identity. Energy Policy 74 , 253–261 (2014).
Lis, A. Co-production of shale gas publics in Poland and the negotiation of state citizens relations. Extr. Ind. Soc. 5 , 673–681 (2018).
Haggerty, J. H., Kroepsch, A. C., Walsh, K. B., Smith, K. K. & Bowen, D. W. Geographies of impact and the impacts of geography: unconventional oil and gas in the American West. Extr. Ind. Soc. 5 , 619–633 (2018).
Stoffle, R. W., Stone, J. V. & Heeringa, S. G. Mapping risk perception shadows: defining the locally affected population for a low-level radioactive waste facility in Michigan. Environ. Prof. 15 , 316–333 (1993).
Pasqualetti, M. J. Wind energy landscapes: society and technology in the California Desert. Soc. Nat. Resour. 14 , 689–699 (2001).
Visschers, V. H. M. & Siegrist, M. Find the differences and the similarities: relating perceived benefits, perceived costs and protected values to acceptance of five energy technologies. J. Environ. Psychol. 40 , 117–130 (2014).
Lesbirel, S. H. NIMBY Politics in Japan: Energy Siting and the Management of Environmental Conflict (Cornell Univ. Press, 1998).
Fraune, C. & Knodt, M. Sustainable energy transformations in an age of populism, post-truth politics, and local resistance. Energy Res. Soc. Sci. 43 , 1–7 (2018).
Ajzen, I. The theory of planned behavior. Organ. Behav. Hum. Decis. Process. 50 , 179–211 (1991).
Stern, P. C. Toward a coherent theory of environmentally significant behavior. J. Soc. Issues 56 , 407–424 (2000).
Shove, E., Pantzar, M. & Watson, M. The Dynamics of Social Practice: Everyday Life and How it Changes (SAGE, 2012).
Shove, E. & Walker, G. What is energy for? Social practice and energy demand. Theory Cult. Soc. 31 , 41–58 (2014).
Strengers, Y. Peak electricity demand and social practice theories: reframing the role of change agents in the energy sector. Energy Policy 44 , 226–234 (2012).
Shove, E. Beyond the ABC: climate change policy and theories of social change. Environ. Plann. A 42 , 1273–1285 (2010).
Strengers, Y. in Smart Energy Technologies in Everyday Life: Smart Utopia? (ed. Strengers, Y.) 34–52 (Palgrave Macmillan, 2013).
Devine-Wright, P. et al. A conceptual framework for understanding the social acceptance of energy infrastructure: insights from energy storage. Energy Policy 107 , 27–31 (2017).
Download references
Acknowledgements
I thank J. Flora and D. Schaffer for their thoughtful insights.
Author information
Authors and affiliations.
School of Public Policy, Oregon State University, Corvallis, OR, USA
Hilary S. Boudet
You can also search for this author in PubMed Google Scholar
Corresponding author
Correspondence to Hilary S. Boudet .
Ethics declarations
Competing interests.
The author declares no competing interests.
Additional information
Publisher’s note: Springer Nature remains neutral with regard to jurisdictional claims in published maps and institutional affiliations.
Rights and permissions
Reprints and permissions
About this article
Cite this article.
Boudet, H.S. Public perceptions of and responses to new energy technologies. Nat Energy 4 , 446–455 (2019). https://doi.org/10.1038/s41560-019-0399-x
Download citation
Received : 05 June 2018
Accepted : 24 April 2019
Published : 27 May 2019
Issue Date : June 2019
DOI : https://doi.org/10.1038/s41560-019-0399-x
Share this article
Anyone you share the following link with will be able to read this content:
Sorry, a shareable link is not currently available for this article.
Provided by the Springer Nature SharedIt content-sharing initiative
This article is cited by
Socio-environmental factors and solar housing system adoption: moderating effect of attitude.
- Ahsen Maqsoom
- Muhammad Hammad
- Tahira Nazir
Innovative Infrastructure Solutions (2024)
Machine learning reduces soft costs for residential solar photovoltaics
- Changgui Dong
- Gregory Nemet
- Eric O’Shaughnessy
Scientific Reports (2023)
Perception spillover from fracking onto public perceptions of novel energy technologies
- Steve Westlake
- Conor H. D. John
Nature Energy (2023)
A framework to centre justice in energy transition innovations
- Patricia Romero-Lankao
- Nicole Rosner
- Scott Schnur
Energy-space concept for the transition to a low-carbon energy society
- Indre Siksnelyte-Butkiene
- Dalia Streimikiene
- Tomas Balezentis
Environment, Development and Sustainability (2023)
Quick links
- Explore articles by subject
- Guide to authors
- Editorial policies
Sign up for the Nature Briefing: Anthropocene newsletter — what matters in anthropocene research, free to your inbox weekly.


IMAGES
VIDEO
COMMENTS
As more and more clean, renewable energy comes online, we need to continue with policies that support research and development on the new technologies required to recover all kinds of materials ...
Renewable Energy Technologies "Renewable energy technologies" is an umbrella term that stands for energy production using a renewable energy source like solar, wind, water (hydro and tidal), biomass (biofuels and wastes), and geothermal heat. ... Energy research and development and demonstration projects should be encouraged to improve ...
Impact of global heterogeneity of renewable energy supply on heavy industrial production and green value chains. A new study of low-carbon value chains of basic materials (steel and chemicals ...
This study reveals that: 1. Global research in the new energy field is in a period of accelerated growth, with solar energy, energy storage and hydrogen energy receiving extensive attention from ...
The use of renewable energy resources, such as solar, wind, and biomass will not diminish their availability. Sunlight being a constant source of energy is used to meet the ever-increasing energy need. This review discusses the world's energy needs, renewable energy technologies for domestic use, and highlights public opinions on renewable energy. A systematic review of the literature was ...
Renewable energy's share of total global energy consumption was just 19.1% in 2020, according to the latest UN tracking report, but one-third of that came from burning resources such as wood.
Shijing Sun, a research scientist in Buonassisi's group, used a combination of machine learning and high-throughput experiments to quickly assess and test perovskite solar cells. ... there is a push to develop more robust energy storage systems for renewable technologies. Storing energy for later use when resources aren't supplying a ...
The National Renewable Energy Laboratory ... our research has largely confirmed the key conclusions from RE Futures and, in some ways, identified that it might have been a conservative snapshot of the future. ... the cost of clean energy technologies has declined faster than anyone expected or was estimated in RE Futures, as shown here with ...
Our research explores energy systems and technologies—and the science behind them—for a future powered by affordable, abundant, and clean energy. This research supports our vision of transforming today's energy challenges into tomorrow's solutions. Learn more about NREL's vision and its three focus areas: integrated energy pathways ...
NREL researchers are on a mission to develop renewable-energy-powered technologies to detect, quantify, and collect plastic from U.S. waterways. The National Renewable Energy Laboratory (NREL) is transforming energy through research, development, commercialization, and deployment of renewable energy and energy efficiency technologies.
The U.S. Department of Energy's 17 national laboratories conduct research and help bring renewable energy technologies to market. Renewable Energy at Home Homeowners and renters can use clean energy at home by buying green power, installing renewable energy systems to generate electricity, or using renewable resources for water and space ...
Study reveals a reaction at the heart of many renewable energy technologies Study reveals a reaction at the heart of many renewable energy technologies ... Ryan Bisbey, a former MIT postdoc; Karl Westendorff, an MIT graduate student; and Alexander Soudackov, a research scientist at Yale University, are also authors of the paper. Passing protons.
The pace of deployment of some clean energy technologies - such as solar PV and electric vehicles - shows what can be achieved with sufficient ambition and policy action, but faster change is urgently needed across most components of the energy system to achieve net zero emissions by 2050, according to the IEA's latest evaluation of global progress.
Major governments are increasing energy research investments, as they pledged to do in 2015 under the Mission Innovation initiative. ... Across the period, companies active in renewable energy technologies represent a particularly bright and resilient story. These companies spent 74% more on R&D between 2010 and 2019, adding over USD 2.5 ...
Renewable energy and energy efficiency technologies are growing rapidly and, with increased penetration, are increasingly impacting how the electricity grid operates. This presentation outlines a number of the technologies and describes the research, development, and deployment the National Renewable Energy Laboratory is partaking on them.
In contrast, renewable energy sources accounted for nearly 20 percent of global energy consumption at the beginning of the 21st century, largely from traditional uses of biomass such as wood for heating and cooking.By 2015 about 16 percent of the world's total electricity came from large hydroelectric power plants, whereas other types of renewable energy (such as solar, wind, and geothermal ...
Risk Analysis is an international journal publishing new developments, empirical research and commentaries on a wide range of topics in the field of risk analysis. Abstract The allocation of budgets for renewable energy (RE) technology is significantly influenced by geopolitical risks (GPRs), reflecting the intricate interplay among global ...
Nature Reviews Materials (2024) Transitioning from fossil fuels to renewable energy sources is a critical global challenge; it demands advances — at the materials, devices and systems levels ...
Hydropower is the world's biggest source of renewable energy by far, with China, Brazil, Canada, the U.S., and Russia the leading hydropower producers. While hydropower is theoretically a clean ...
2. McKinsey estimates that by 2026, global renewable-electricity capacity will rise more than 80 percent from 2020 levels (to more than 5,022 gigawatts). 1 Of this growth, two-thirds will come from wind and solar, an increase of 150 percent (3,404 gigawatts).
This paper investigates the evolving landscape of blockchain technology in renewable energy. The study, based on a Scopus database search on 21 February 2024, reveals a growing trend in scholarly output, predominantly in engineering, energy, and computer science. The diverse range of source types and global contributions, led by China, reflects the interdisciplinary nature of this field.
Scaling up green technologies: Through a partnership with the US Special Presidential Envoy for Climate, John Kerry, and over 65 global businesses, the First Movers Coalition has committed $12 billion in purchase commitments for green technologies to decarbonize the cement and concrete industry. 1 trillion trees: Over 90 global companies have committed to conserve, restore and grow more than 8 ...
Countries require financial and technological support to exchange experiences and technology. These are barriers that need to be overcome today to speed up the transition, especially in Africa. In this respect, Africa is probably the most important powerhouse in the world for renewable energy and green hydrogen [a clean and renewable energy ...
By assessing scientific publication in renewable energy, including solar, wind, biomass and geothermal energy, as well as new energy system technologies, such as advanced nuclear energy, hydrogen ...
A new electrochemical reactor design developed with Marta Hatzell by postdoctoral scholar Hakhyeon Song (middle) and Ph.D. students Carlos Fernández and Po-Wei Huang (seated) converts carbon dioxide removed from the air into useful raw material. Their approach is cheaper and simpler while requiring less energy, making it a promising tool to improve the economics of direct air capture systems.
Authored by Brian McIntosh - Research Director, Power and Renewables at Wood Mackenzie Electricity demand is set to surge over the coming decades as addressing climate change becomes a key focus ...
The U.S. Department of Energy (DOE) Bioenergy Technologies Office (BETO) hosted the 2023 Project Peer Review in April 2023 in Denver, Colorado. During the event, new research and development (R&D) projects from 11 technology areas within BETO's portfolio were presented to external subject-matter experts from industry, academia, and federal agencies. Experts reviewed the research and provided ...
Therefore, the promoting environmental-friendly business activities by inculcating various initiatives, for instance, Renewable Energy Initiatives (REI) such as the production, consumption, and distribution of renewable sources of energy, use of electric vehicles, production and distribution of RE products for instance solar panels, solar roofs ...
Over 80% of planned new energy installations in the U.S. in 2023 were from sources like battery, solar, and wind, according to data from the U.S. Energy Information Administration.
I have highlighted four categories of factors researchers have identified as shaping public perceptions of and responses to new energy technologies—technology, people, place and process (Fig. 1 ...