A Methodological Approach for the Study of Domes
- Open access
- Published: 02 October 2020
- Volume 22 , pages 983–1013, ( 2020 )

Cite this article
You have full access to this open access article
- Carlo Bianchini ORCID: orcid.org/0000-0002-1195-1755 1
2651 Accesses
7 Citations
2 Altmetric
Explore all metrics
Domes still represents a prickly subject, large dimensions coupled with a limited accessibility making their study difficult and time-consuming. Although throughout history this problem has been tackled in different ways, relevant methodological and operational issues have until now limited the success of these efforts. Digital technologies have radically changed this scenario. 3D active and passive capturing systems currently allow for a dense and accurate surveying while modelling software provides powerful tools to build virtual counterparts of surfaces and to investigate their geometric properties. The aim of this paper is to present three different projects developed by a research group in the Department of History, Representation and Restoration of Architecture—Sapienza University of Rome. Based on their results, the group has set up and tested a protocol that can guide users from acquisition through modelling and, finally, to the reading of the geometric properties of domes.
Similar content being viewed by others
Baroque Topologies: Novel Approaches to Analysis and Representation of the Baroque Interior in the Era of Big Data
The hermitage of sant’alberto in butrio: methodologies of integrated survey between tradition and digital innovation.
3D Modelling of Heritage Objects: Representation, Engagement and Performativity of the Virtual Realm
Avoid common mistakes on your manuscript.
Introduction
The study of domes still represents a thorny subject. In fact, large dimensions coupled with a limited accessibility make the study of their shapes and geometry a traditionally difficult task (Rondelet 1802 –17; Leroy 1845 ). Although throughout history this problem has been tackled in different ways (Letaroully 1840 –55; Emerson and Van Nice 1943 ; Dorffner et al. 2000 ; Hidaka and Satō 2004 ), relevant methodological and operational issues have until now limited the success of these efforts.
Digital technologies have radically changed this scenario. On one hand, 3D active capturing systems (Light Detection and Ranging, LIDAR) and passive ones (Structure from Motion, SfM) currently allow for a dense and accurate surveying of surfaces (Bianchini and Russo 2018 ); on the other, modelling software provides powerful tools to build virtual counterparts (3D models) of these surfaces as well as to investigate their geometric properties (Migliari 2008 ).
The aim of this paper is to present three different projects developed in the last decade by a research group belonging to the Department of History, Representation and Restoration of Architecture (DSDRA)—Sapienza University of Rome. Based on the results of these activities, our group has set up and tested a protocol that can guide users from the acquisition phase through the modelling and, eventually, to the reading of the geometric properties of domes. However, this chain of activities must not be regarded as a mere application of different technologies but instead as the operational display of a rigorous scientific methodology (Bianchini 2014 ) that guides the construction of a ‘knowledge system’ suitable for collecting, interpreting and storing information. The components of this system are of various types: first quantitative (essentially resulting from surveying operations), but also qualitative. While the former can be channelled through a rigorous scientific approach, the latter mainly draw on the investigator’s subjective sensitivity and interpretative skill. Nonetheless, this last is often a key ingredient for achieving levels of understanding that are denied to simple measurement (Ribichini 2015 ; Chiavoni 2018 ; Inglese et al. 2019 ).
This duality between measuring and interpretation in the study of built artefacts has also had a strong impact on the hypothesis discussed and the result presented in this paper. Even if very briefly, we cannot afford to bypass some of the epistemological implications of this approach, namely to reference the qualitative component (interpretation) to the concept of irrefutability in the sense proposed by Karl Popper ( 1963 ). On the other hand, we must reference the quantitative one (measuring) to the bimillennial disciplinary context of survey, certainly one of the most powerful tools developed by scholars (archaeologist, architects, historians, etc.) that ‘distinguish those who know from those who remain on the surface, even so-called “cultured”, of knowledge’. Footnote 1 It actually implies the idea of measurement, that is to say, the possibility of turning into quantity some qualities of the studied phenomenon (in this case an artefact, a building, a site, etc.) by using the ratio between the measured quantity and an appropriate unit of measure. Thanks to this method, we can build the model of the complex phenomenon we are investigating as a simplified version of the original.
The Epistemological Framework
Quite apart from the theoretical framework, the application of this approach to built artefacts, and especially to domes, strictly depends on the available measurement technologies. For centuries, the limited possibilities offered by instruments have restricted the number of measurable points. Therefore, any survey campaign required a preliminary accurate design in order to select and then acquire (measure) the really significant points.
In recent decades, instead, all 3D capturing technologies (LIDAR, SfM) have somehow inverted this order so that selection nowadays follows acquisition. Therefore, the term ‘survey’ refers not only to the measuring campaign, but also to a very structured process that leads to the construction of 2D or 3D models starting from a real object (Docci et al. 2011 ; Bianchini 2012 ; Gaiani 2012 ). This workflow can be broken down into several different tasks of which, certainly, the acquisition phase Footnote 2 represents the first step. All those that follow—namely selection, interpretation and representation of the acquired data—actually lead to 2D and 3D models that somehow concur in adding to our knowledge of the phenomenon, i.e., the built artefacts we are investigating (Fig. 1 ).
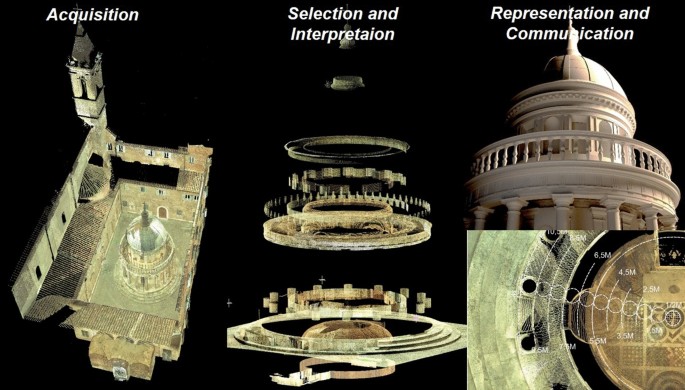
The phases of the survey workflow
Referring to the traditional survey approach, the tasks of acquisition, selection, interpretation and representation of data are genetically tangled together. Massive acquisition technologies have instead separated all steps: capturing has become the only onsite activity, while selection, interpretation, and representation of data are now carried out later and generally offsite (Bianchini 2014 ). This last remark is in our opinion very valuable, because it allows for a revisiting of the entire matter in the light of the scientific method, also putting the survey process to the test of Karl Popper’s “principle of falsifiability”, still the benchmark for evaluating scientific theories. Footnote 3 The capturing phase seems to be highly compatible with the validation workflow consolidated within the scientific community. Instead, selection, interpretation and representation cannot satisfy these requirements and must thus always be considered subjective and irrefutable in the terms set out by Popper.
However, it is within the selection and interpretation phases that the model is produced. It is mainly a geometric model, i.e., a simplified reading of the objective multi-dimensional complexity referring to its geometric essence made of points, lines and surfaces. The real object and its virtual substitute are thus bound together by a biunivocal correspondence that can be used to simulate different operations as if they were made directly on the object itself.
From a strictly epistemological point of view, such a model can be considered the result of a selection carried out by a subject aiming at extracting some of the potentially infinite information embedded into an object. The selected data, though, are not the result of a random or automatic processing. On the contrary, they are the result of an interpretation, a subjective reading that strictly complies with rules established by the subject himself/herself. In other words, starting from the selected set of data, we always tend to demonstrate a hypothesis we have formulated beforehand even if subconsciously.
However, at this stage the model produced is, so to speak, for personal use only, existing mainly in the mind of the subject and often incoherently on scattered supports (such as sketches on napkins). Hence, representation is the method to transform this personal model so to make it understandable to others. This phase actually translates the “raw” model into a shareable one, applying a codification that relies on descriptive geometry and in particular on the science of representation (Docci and Migliari 1992 ).
This whole process has been traditionally rooted in the following principles:
human beings have an ability, innate or acquired, to conceive the qualities of physical space;
of the n qualities of a physical space, the geometric qualities optimize the operations of interaction between subject and space;
space can be effectively manipulated and modified thanks to the correspondence between the real object and its geometric abstraction (geometric model);
the geometric model, through the process of representation becomes a 2D graphic model or a 3D virtual model.
While for the 2D graphic models we can refer to the millennia-old tradition of drawing (Docci 1997 ), the 3D virtual models are only the 25-year-old products of the so-called “digital revolution” and of its wide “bundle” of hardware and software tools that nowadays allow for an actual interaction between our real world and its virtual version.
Digital modelling systems represent a real novelty in comparison with the traditional graphic workflow: while for the 2D graphic models the process establishes a biunivocal correspondence between the object and its graphic representation by means of projection and section operations, 3D modelling software creates instead an entire 3D virtual space.
Therefore, more than producing a mere representation of the object, any 3D modelling software provides the possibility to interact with a digital environment that, initially empty, is step by step populated by elements that together build a virtual replica of the object.
This process establishes a direct correspondence between the physical and the virtual space: to each material point P r Footnote 4 identified by its coordinates x r ,y r ,z r in the real space corresponds to a virtual point P v univocally identified by the Cartesian triplet x v ,y v ,z v (Fig. 2 ).
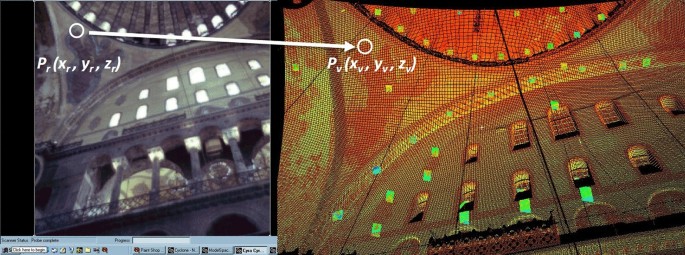
Correspondence between physical and virtual space
Consequently, the digital environment created by 3D modelling software provides an actual spatial framework for all the subsequent constructions.
Therefore, the relevance of 2D/3D models exceeds the mere production of their final and perhaps more evident outputs (drawings, images). Their vocation is instead exquisitely heuristic, providing ‘the way of recognizing, from an exact description, the forms of bodies, and of deducing from them all the truths which result both from their form and from their respective positions” Footnote 5 and the possibility “… to realize in all directions, at small scale on paper, or in large scale in an area or on a plaster, the measurements and shapes of a part of a building”. Footnote 6
Methodology
The theoretical background discussed so far actually represents the backbone of the methodology we have applied to some of the most relevant domed structures in the world.
As mentioned in the very first lines of this paper, domes are difficult to investigate. Throughout history, many methods have been applied to measure their surfaces and to assess their geometry and shape. Despite these efforts, some “intrinsic” constraints have always limited the success of these tasks: on one hand, the surveying campaigns used to produce small and inaccurate datasets; on the other, the tools for the investigation of surfaces were rich from a theoretical standpoint but very poor from an operational one. The combination of the two has generally prevented the achievement of reliable results. Besides, domes are quite difficult even to reach: their large dimension and troublesome position in the fabric is very often coupled with a scant accessibility of their upper levels that deeply affects the implementation of direct surveying procedures. Topographic measurements are also limited by the same logistic problems but, accepting the adoption of a very time-comsuming workflow, they have represented a significant jump forward in the study of domes, as witnessed by the work on Hagia Sophia in Istanbul (Emerson and Van Nice 1943 ; Mainstone 1988 ).
Stereophotogrammetry (Fondelli 1992 ) seemed for a while able to solve many of these issues. This technique allows for the construction of a “virtual model” of an object using a coordinated pair of photographic shots. Complex hardware (now obsolete) operated by skilled users could lead to the measuring and drawing in a CAD 3D space of single points, lines and polylines, simply by exploring the virtual model visually. However, the clear improvements introduced by stereophotogrammetry in terms of operational capabilities and accuracy were differently applicable to domes shapes. Due to the dependence on the coverage and position of photographic acquisitions, the accuracy of measurement was generally high in correspondence of the keystone, but dramatically decreasing in moving towards the level of the springing (Bianchini and Paolini 2003 ).
Instead, LIDAR and SfM systems in the multiple configurations that we can observe nowadays (stable, portable, flying, diving) produce dense and accurate datasets in the form of point clouds. Yet, this “capturing power” would have been actually irrelevant without the parallel development of 3D modelling systems that grants us the possibility not only to do better what we used to do, but above all to do what was simply impossible before.
The samples presented in sections that follow (the domes of the Hagia Sophia in Istanbul, San Carlo ai Catinari in Rome, and St. Peter’s Basilica in the Vatican City) intend to present both a demonstrator of the workflow discussed so far and some interesting results in the geometric study of surfaces.
Hagia Sophia in Istanbul
‘Glory to God, who deemed me worthy to finish this construction. O Solomon, thus have I prevailed over you.’ With these words Emperor Justinian, together with the Patriarch inaugurated Hagia Sophia church on 27 December 537 ad , only 5 years after the laying of the first stone.
Hagia Sophia is undoubtedly the product of the Emperor’s will: inspired by Justinian’s new-found faith, this unprecedented construction is also more prosaically driven by the political need to explicitly embody in this building his imperial power. That is why the Emperor invested enormous resources and personal energy in this endeavour. He chose carefully his team of designers (the Greek mathematician and architect Anthemius of Tralles assisted by Isidorus the Elder of Miletus) but he personally supervised the work imposing strict working conditions. Ten thousand workers, divided into two competing, “militarized” teams, accomplished the construction task in an unprecedented time. This speed, however, undermined the stability of the building from the very beginning and determined a first major collapse of its dome some 20 years after the dedication of the church. A new covering, less hollow than Antemius’, was then rebuilt by Isidorus the Younger of Miletus.
The overall design of Hagia Sophia is based on a huge square with sides of approximately 62 m. Another smaller, concentric square (side of about 31 m, or 100 Byzantine feet) is contoured at its corners by four large piers that support the central dome. The transition from square to the springing circumference of the dome is solved by inserting four spherical pendentives, another great invention of Antemius, that will become an absolute paradigm of Western architecture. Transformed in a mosque by Mehmed the Conqueror after Constantinople fall in 1453, at the beginning of the twentieth century Ataturk changed its function into a museum but, very recently, it has been re-converted into a mosque.
The huge dome that soars above its central space, certainly its most remarkable element, has also proven over time to be its Achilles’ heel. The irregular surface we can currently observe visiting the monument is thus the result of the layered and often incoherent reconstructions that followed the three major collapses that occurred over time.
We approached the study of this structure basing on a general point cloud of about 100 million points Footnote 7 (Fig. 3 ), spanning from a minimum density of 4 × 4 cm to a maximum of 2 × 2 mm. The processing of this dataset allowed for the construction of a number of 2D and 3D models aimed at assessing the dimensions and alignments of the structures bearing the dome.
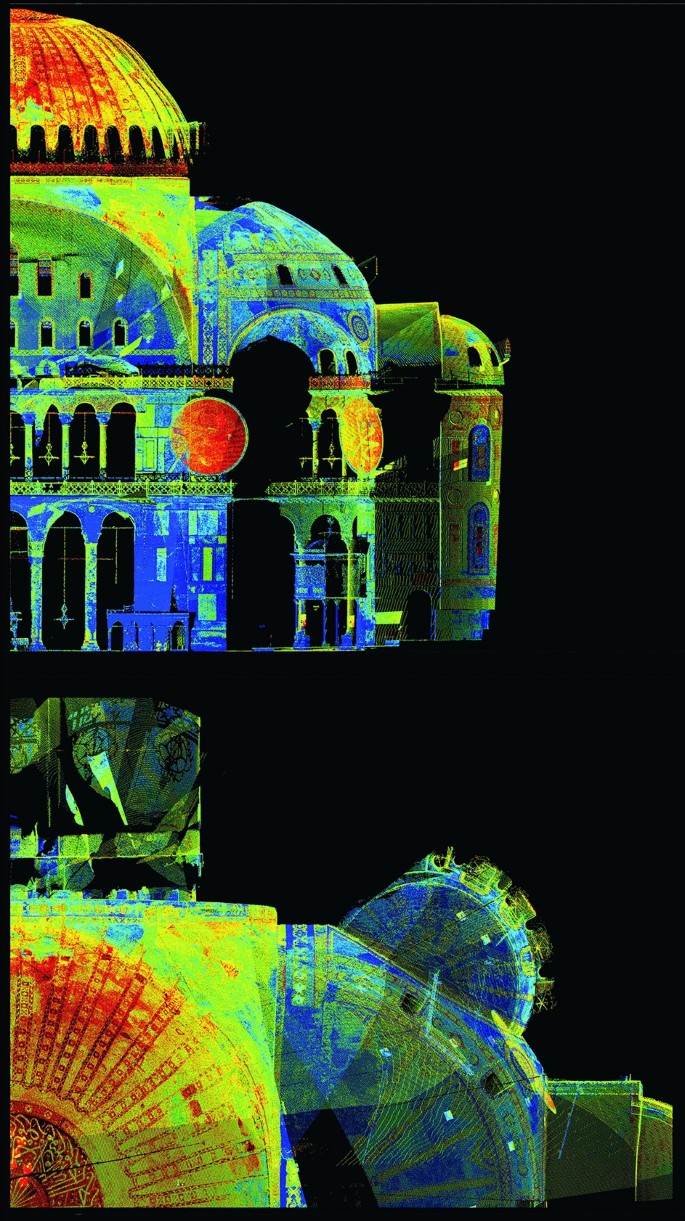
Hagia Sophia’s general point cloud
We focused, in particular, on the four main piers, on the two large arches located east and west along the main axis of the nave and, finally, on the pair of arches and subarches facing respectively north and south.
The first phase investigated the shape of the arches bearing the dome along the east and west axis. We started by sectioning the point cloud along ten vertical planes perpendicular to the axis of each arch (Fig. 4 ). The set of 3D points intersected along each plane was then interpolated in order to trace the best fitting circumference to assume as the most probable profile used in the construction.
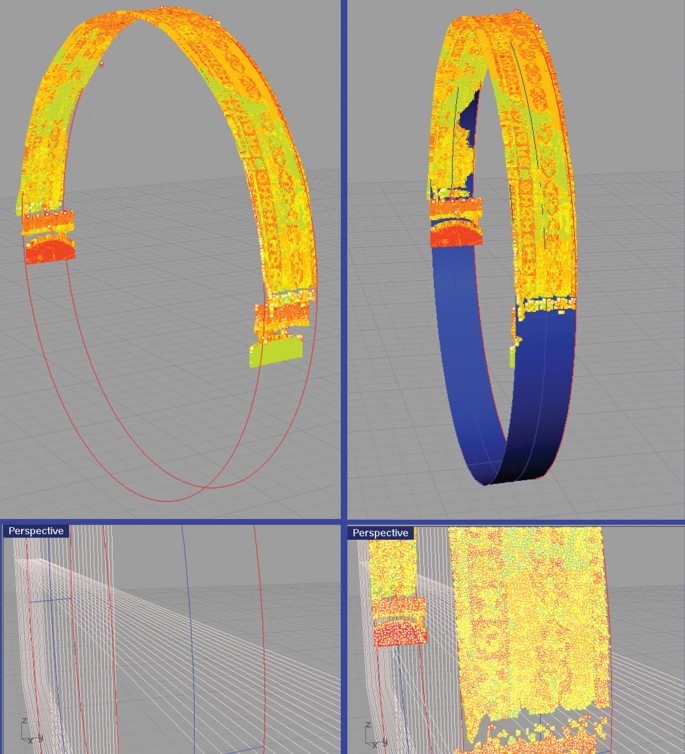
Study of the geometry of the arches bearing the dome
This operation revealed an unexpected geometry: the intrados of neither east nor west arches correspond to a cylindrical surface, but are instead portions of a truncated circular cone. The outer and inner semicircles delimiting each arch have been built using different radii: the largest, facing the dome, measure 16.81 m while the other is 16.50 m long (Fig. 5 ).
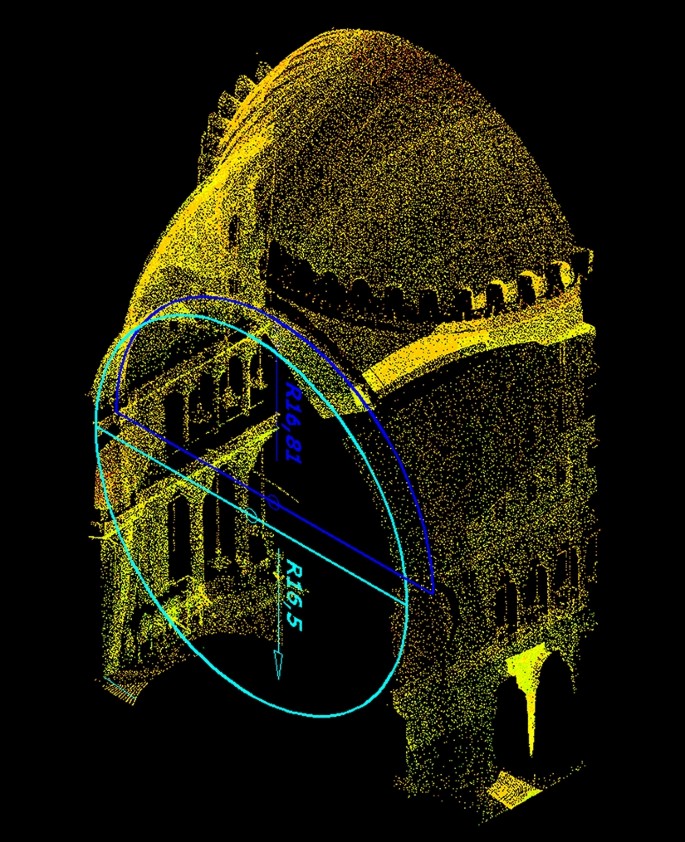
The shape of the intrados of the arches
The reason for this difference is not clear; it is too great and regular to be unintentional but still too small to be noted without measuring. Nevertheless, it could be explained either in terms of “smoothing” the too sharp transition between the intrados of the arches and the dome, or as an optical correction conceived by the designer.
Other interesting findings refer to the north and south walls, both clearly deformed over time by the thrust of the dome. The north wall is bending considerably outwards: 66 cm in the portion between the ground floor and the upper cornice of the matroneum, reaching a total incline of approximately 1 m just below the springing level of the dome.
The dome is actually the element that has been subjected to the greatest transformations. Originally designed by Anthemius of Tralles as a very hollow portion of a sphere, it collapsed for a combination of reasons in 558 ad . Rebuilt by Isidore of Miletus, again based upon a sphere but with a much higher profile, the dome suffered two other partial collapses in 989 and 1346 caused by major earthquakes. The structure we see today is then the result of the layering of these reconstructions visibly carried out in an approximate way by a work force not skilled enough to handle properly the problem.
Our study started by sectioning the point cloud of the dome using 60 horizontal planes at 25 cm intervals (Fig. 6 ). We then selected 45 of them as representative of the most evident deformations. Likewise, we constructed a number of radial sections passing through the vertical axis of the sphere and the middle of the ribs that compose the intrados of the dome (Fig. 7 ).
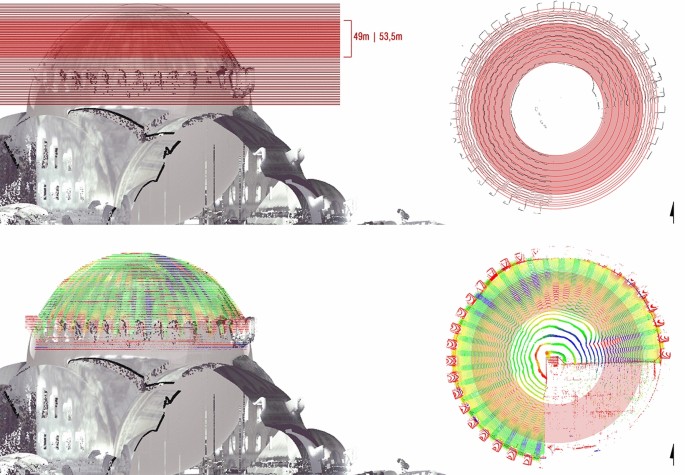
The 2D study of Hagia Sophia dome: horizontal sectioning
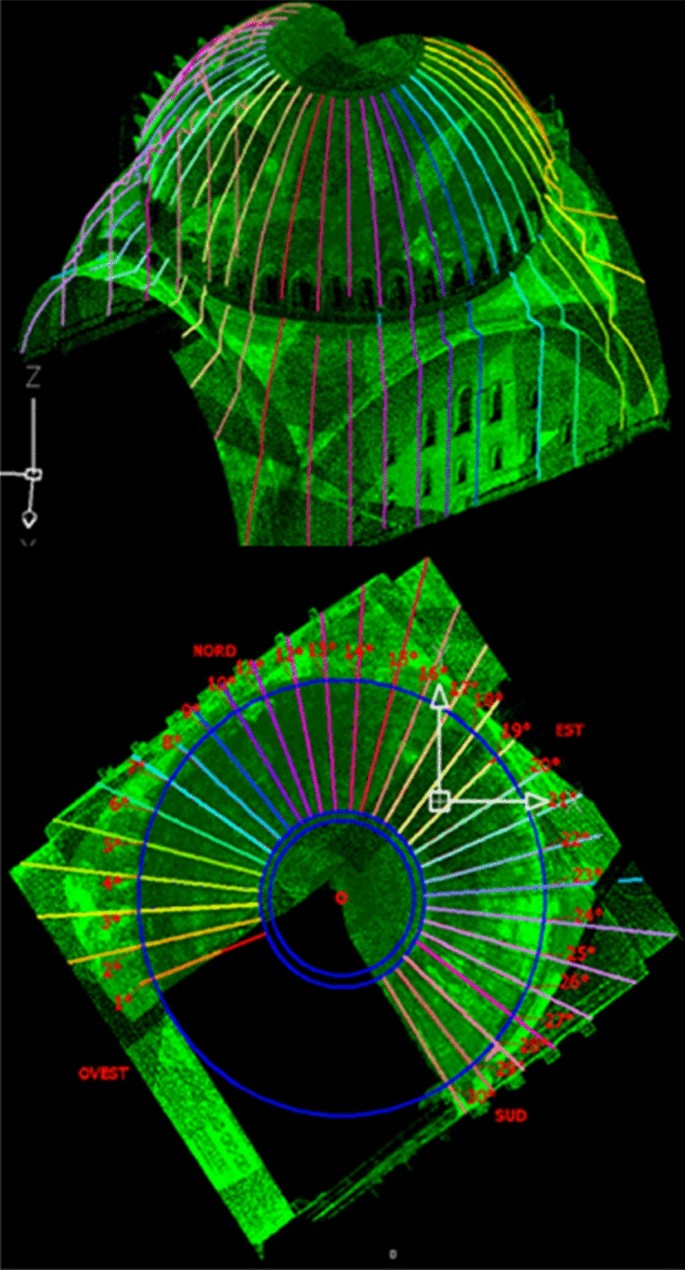
The 2D study of Hagia Sophia dome: vertical sectioning
This set of horizontal and vertical curves showed the location and degree of unevenness of the surface: from the unmistakable re-stitching along the north-east diagonal, to the deformations in the interval between 53.5 and 49 m above ground level. Finally, other remarkable differences were found at the level of the springing of the dome along the east and southeast directions.
Ancient Byzantine building techniques undoubtedly played a key role in both the construction of this masterpiece of Western architecture but also in creating its main weak points. The hollow shape of the first two domes (and their consequent huge thrust outwards) not only affected the dome’s stability, but also undermined the solidity of the four main piers bearing the covering. The building phase itself, too quick to ensure the correct curing of the masonry, decreased on one hand the bearing capacity of the piers (and walls too) while on the other it caused important settling already evident during the construction.
In spite of the fact that the piers were made of solid stone blocks up to the level of the matroneum, still their deformation is indeed quite evident: the thrust of the east and west transversal arches made the piers lean outwards, widening them at the level of the springing by approximately 60 cm (Fig. 8 ).
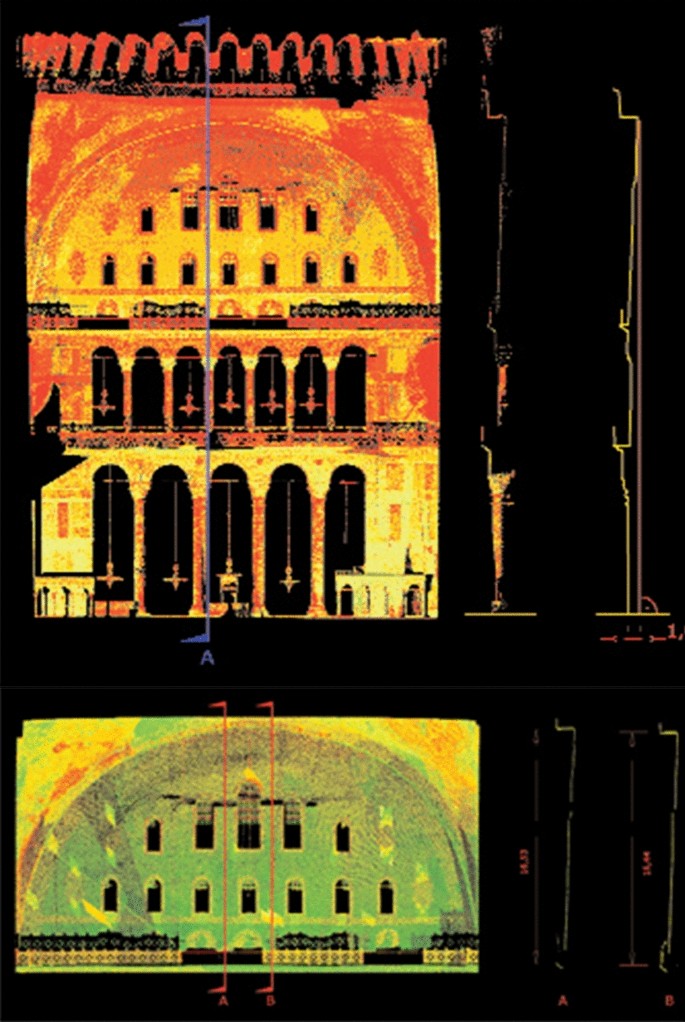
The 2D study of Hagia Sophia’s great walls
All these conclusions belong to the “to do better what we used to do” class; in other words we have taken advantage of traditional 2D graphic models, as many other scholars have done for a long time (Emerson and Van Nice 1943 ; Sanpaolesi 1978 ; Mainstone 1988 ; Dorffner et al. 2000 ; Hidaka and Sato 2004 ). It is time now to shift to the “to do what simply was impossible before” class, namely to show the results of our 3D investigation (Bianchini and Paolini 2003 ; Docci 2003 ; Hoffmann and Theocharis 2016 ).
The first question we addressed was the reconstruction of the original shape of the dome, obviously not Anthemius’ sphere (lost forever) but instead that of Isodore. Working directly on the 3D point cloud, we decided to search for the spherical surface best fitting our point cloud. However, taking into account the actual “umbrella” shape of the intrados, we choose to build two different spheres: one interpolating the points lying on the inner ribs, the other considering those belonging to the outer sails (Fig. 9 ).
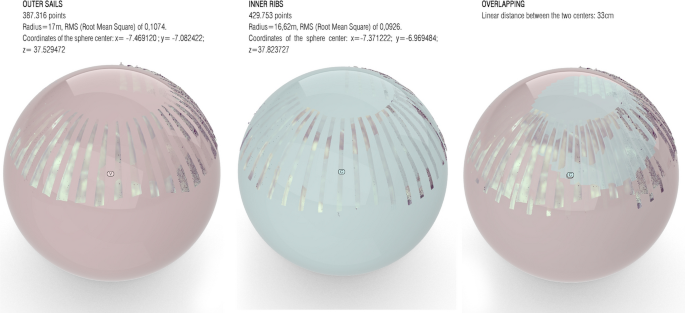
The search for the best-fitting spheres of sails and ribs
In the first case we selected a set of almost 430,000 points that led to a sphere with a radius of 16.62 m, while in the second the points chosen were about 380,000 and the radius of the generated sphere was of 17.00 m. Assuming the springing plane of the dome at 41.18 m from ground level, the distance between this plane and the spheres’s centers were respectively of 1.85 m and 2.15 m. Finally, the centres were displaced 0.33 m one from the other and approximately aligned with the top of the arches bearing the dome (Figs. 10 , 11 ).
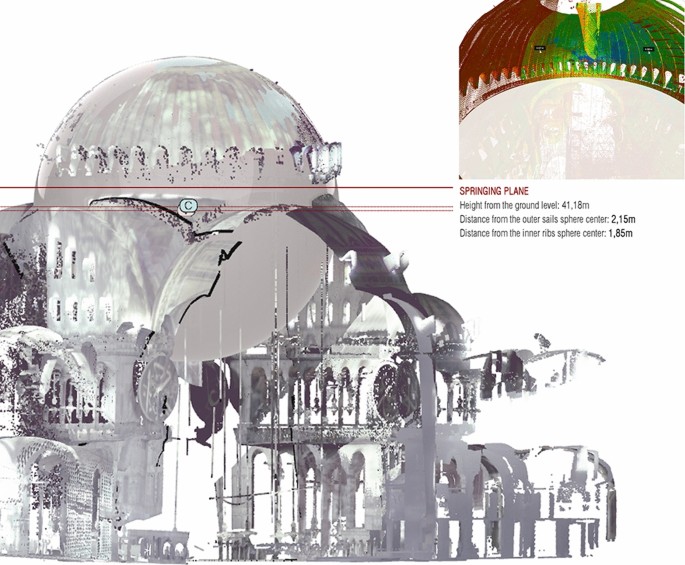
Hagia Sophia ideal sphere: comparison between the ribs and sails interpolated surfaces
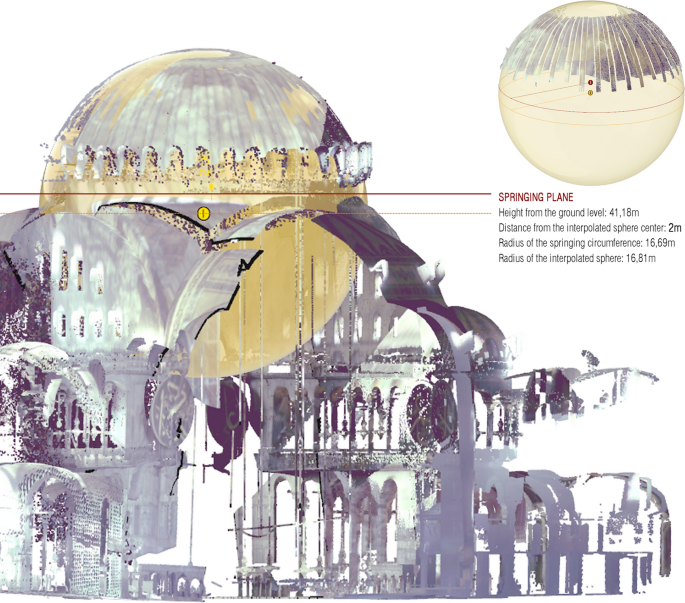
Hagia Sophia ideal sphere: centre, radius, springing circumference
In this framework, we could assume as the “ideal” sphere the average one derived from the combination of the previous two. This surface shows the following features: radius = 16.81 (54 Byzantine feet ± 4 cm); distance of the centre from the springing plane = 2.00 m. It is interesting to note that the radius of this sphere actually coincides with that of the great arches we discussed above. However, without overestimating the value of this coincidence (too many “subjective” choices to become a real proof of anything), it nonetheless suggests again a very refined (and overall simple) proportional control set up by the designers at the very beginning of this huge project. Furthermore, it also evokes more technical matters directly connected with the construction process in terms of the reutilization for the upper dome of the provisional structures (such as centrings) used for the lower arches. The circumference at the springing of this spherical dome measures 104.81 m and has a diameter of 33.38 m (106 Byzantine feet).
We then adopted this interpolated shape as a benchmark for making a comparison between the “ideal” geometry of Hagia Sophia’s dome and its actual configuration. We could thus verify that the circumference at the springing has deformed into an irregular oval with the east–west diameter measuring 32.46 m while the north–south is 31.65 m long (Fig. 12 ). Many remarkable offsets between the ideal line and the surveyed profile concentrate along the north–south direction perpendicular to the main axis of the building. This fact is probably due to the different degrees of rigidity of the bearing structures. The semi-domes coupled to the east–west arches seem to have balanced the thrust of the dome more efficiently than the simple arches enclosing the north and south walls.
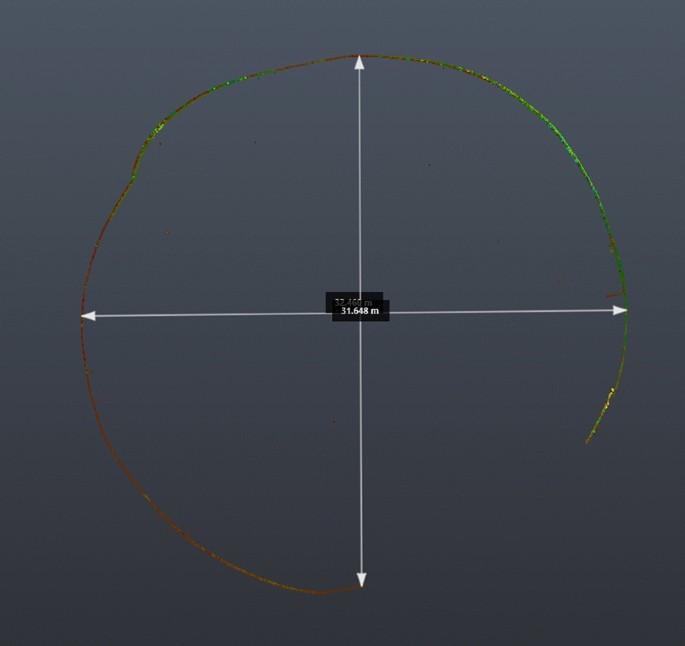
Evidence of the deformation leading to an irregular oval shape
As a result, many ribs on the intrados of the dome are presently crooked (in some points by up to 80–100 cm) with deformations that concentrate in two directions, spanning some 45° on both sides of the main east–west axis. This configuration matches quite well with the documented reconstruction of approximately one quarter of the dome after its collapse in 1346 (Fig. 13 ).
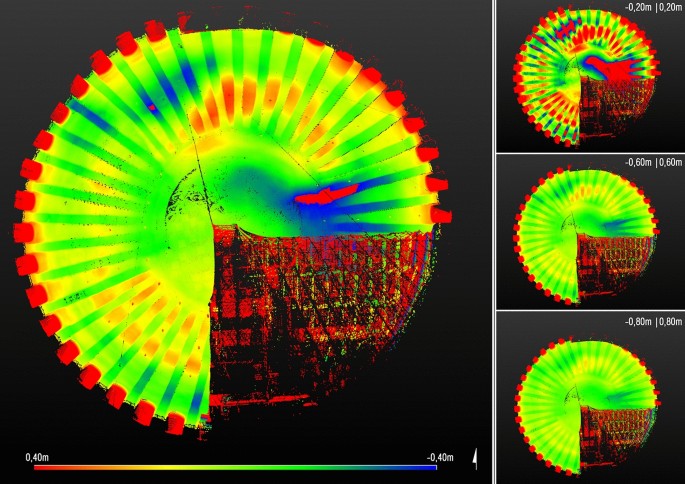
Deformation of the intrados surface
The final step of this 3D analysis was the reconstruction of a model that could represent the actual geometry of the intrados. On the basis of all sections made and, obviously, on the 3D point cloud we thus built a NURBS surface in which all the deformations discussed above are visible, as well as the current general layout of the intrados. This type of model could help not only further investigations but also suggest to non-specialists the “transformations” suffered by the structure over the centuries (Fig. 14 ).
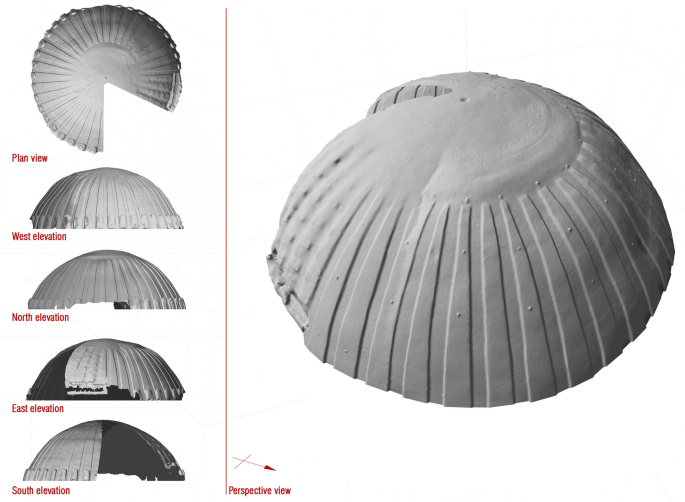
The superficial 3D model of the dome of Hagia Sophia
Church of St. Carlo ai Catinari
The Church of San Carlo ai Catinari (Rendina 2000 ), dedicated to St. Carlo Borromeo, was built during the first decades of the seventeenth century to a design by Rosato Rosati. Owing to some structural problems, Pope Pius IX ordered the restoration of the entire building and especially of its dome (the third largest in Rome after St. Peter’s Basilica and Sant’Andrea della Valle), which had heavily suffered from weather damages as well as from the French artillery’s bombing of 1849 during the battle against the Repubblica Romana for the control of Rome (Fig. 15 ).
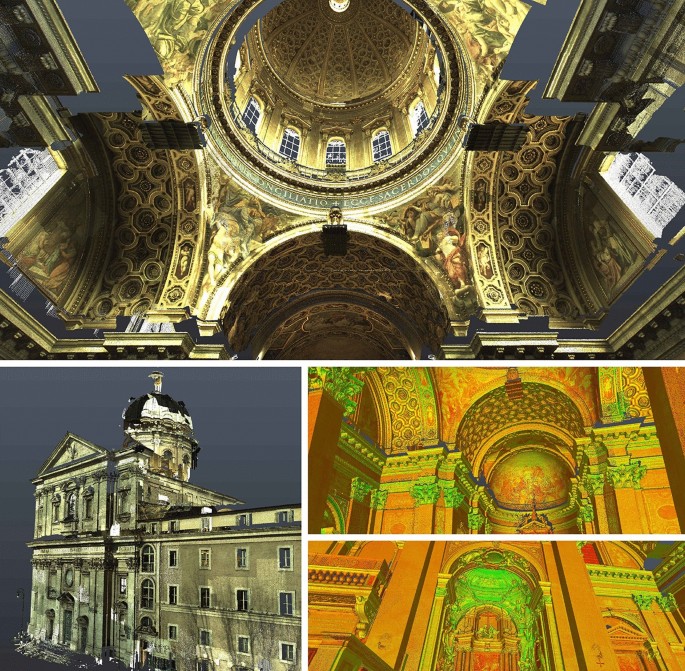
Texturized point cloud of San Carlo ai Catinari
The geometry of this dome and of its bearing structures (drum and piers) was first investigated by our team in 2006 and then again in 2018 Footnote 8 in order to identify the possible damages caused by the earthquakes that had struck the centre of Italy in that period. In contrast to Hagia Sophia, San Carlo’s dome and drum did not show any evident deformation or irregular geometry, and appeared instead both regular and homogenous. We could say, then, that our task was to assess the level of this regularity: in other words, we aimed at identifying and measuring all the superficial deformations and misalignments revealed by our 3D capturing systems.
Our reference dataset was again the general point cloud, while our ideal shape for benchmarking was a surface of revolution generated by the rotation of a 2D curve around a central vertical axis (for the dome) and a cylinder (for the drum). Therefore, we actually organized our workflow so as to define position and geometry of both these elements according to the protocol tested in Hagia Sophia. Thus, the dome and the drum were sectioned with a set of horizontal planes at intervals of 60 cm (Fig. 16 ).
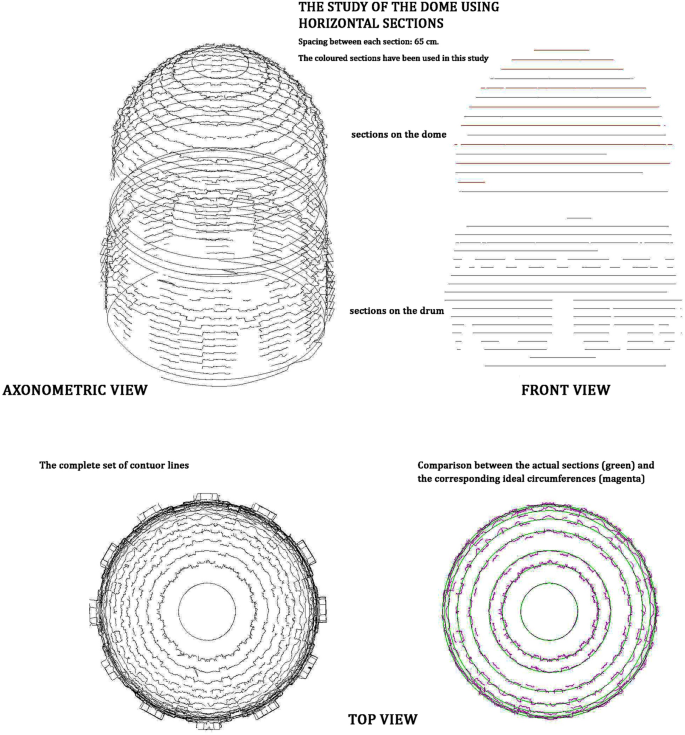
2D study of the dome and drum of San Carlo: horizontal sectioning
This slicing operation produced a corresponding number of horizontal profiles representing the progression of surfaces’ layout from the base of the drum to the top of the dome. As the decorated intrados is enriched by progressively narrowing deep coffers, we assumed their bottoms as reference profiles for the following constructions of circles. The benchmark geometry of the system (a surface of revolution for the dome, cylinder for the drum) obviously implies that all curves generated would approximate a circle.
Our comparison between the ideal lines and those cutting the bottom of the coffers confirmed this hypothesis, with differences less than ± 1 cm and pretty close to the instrumental level of accuracy (± 0.6 cm). However, we had to assess the vertical alignment of all these circles. Therefore, we first considered the horizontal circumferences at the lantern and springing level of the dome. Having projected the centre of the former onto the latter, we measured the offset between these points, obtaining a value of 2 cm, well under our most optimistic expectations both for our geometric model and the static condition of San Carlo’s dome.
We thus assumed the straight line between the centres of the upper and lower circles as the vertical axis of the dome and the drum and baseline for a family of vertical planes radially slicing the two (Fig. 17 ). This set of planes eventually generated several vertical profiles of the dome and the drum. They were not exactly the same, obviously, but their differences were not that significant and led us to conclude that the geometry of the structure was still quite conserved in comparison with the original surface of revolution. In particular, the model of the dome showed that it approximates quite closely a spherical surface, except for the portion right under the lantern, which denotes a slight increase in the inclination of the curve, a correction that may have been intended to mitigate the thrust of the structure on the lower drum. The centre of the sphere lies a few centimetres beneath the cornice located at the level of the springing of the dome.
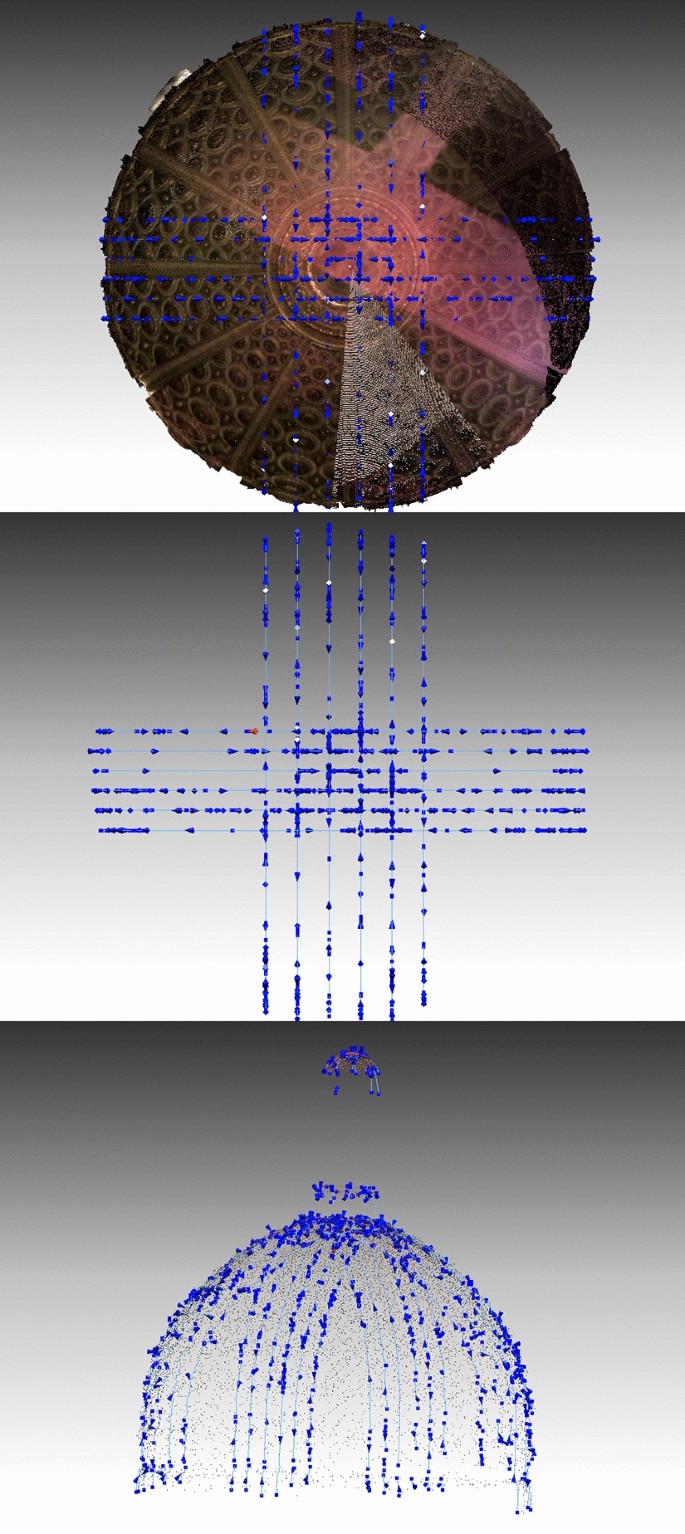
2D study of the dome and drum of San Carlo: the vertical sections
The geometric model resulting from the processing described in the previous section proved very useful for studying the “stability in relation to the shape” of the dome and drum of San Carlo. The reliable reconstruction of some key geometric elements (profiles, axis, position of the centres of curvature, etc.) allowed for an assessment of symmetries, rotations and displacements which, passed on to structural experts, effectively contributed to understanding the current condition of the church.
St. Peter’s Basilica in the Vatican City
It would be impossible in a few lines to provide even a synthetic description of the long and winding process leading to the greatest dome of Christianity (Di Stefano 1980 ; Spagnesi 2003 ). However, it is worth mentioning that the “final” project by Michelangelo simply erased the previous idea of Antonio da Sangallo the Younger, which we can only fully appreciate thanks to the huge wooden model he realized while chief architect of the Fabbrica di San Pietro (1520–1546).
Michelangelo’s dome (Fig. 18 ) is a double-domed structure that takes advantage of the herring-bone brick laying pattern (already used by Brunelleschi for Santa Maria del Fiore) for both its structural features and the consequent reduction of provisional structures such as centrings. After Michelangelo’s death in 1564, Giacomo Della Porta and Domenico Fontana continued the construction, reinterpreting in some way the original design. They modified the curvature of the dome and positioned into the masonry the first great iron chains to compensate for the thrust of the shell (Fontana 1694 ). In the middle of the eighteenth century, a similar intervention was carried out by Poleni in order to respond to the visible cracks crossing the dome and the drum (Rocchi 2009 ; Carusi 2010 ).
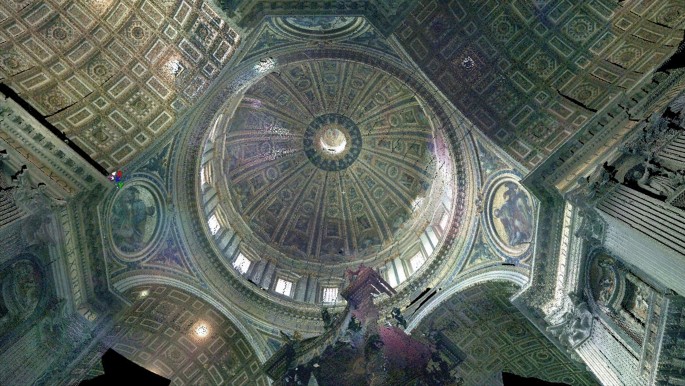
St. Peter’s in the Vatican City: texturized point cloud
Many “self-defining” surveys have been published since the construction of the dome. However, in our opinion only the work of Letaroully seems to be consistent enough to be considered an actual survey. With all the limits of a not very refined procedure, overlaying our profiles with the drawings of his Édifices de Rome moderne (Letaroully 1840 –55), we have found a very good correspondence.
We could say that both Hagia Sophia and San Carlo represented preliminary tests for the challenging task described in the next lines: the study of St. Peter’s dome. Despite the different dimensions and nature of the double-dome structure, the approach used for St. Peter was not much different from that used in the first two cases: the 3D point cloud Footnote 9 was sectioned horizontally and vertically, obtaining in the first case “parallels” and in the second “meridians”. As in the case of St. Carlo, our aim was to fix the geometry of the system, identify possible deformations, and assess its verticality. When our work was concluded, we were to have passed our model and results to the structural engineers of the group for further investigations. We focused our surveying campaign on the area of the basilica covered by the dome: the four main piers, the drum and finally the inner and outer domes. Several acquisitions were performed at different levels (ground floor, first and second cornices, lantern) and, by a lucky coincidence, Footnote 10 we succeeded in capturing a portion of the inner surfaces of the double dome (Fig. 19 ).
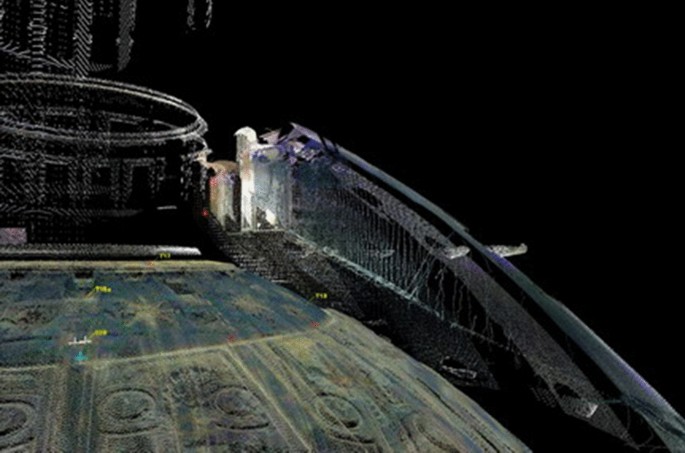
Portion of the inner surfaces of the double dome
The first element investigated was the drum starting with two horizontal sections (Fig. 20 ): the first almost at its top and the second at its base (respectively at 70 m and 58.35 m above the floor of the Basilica).
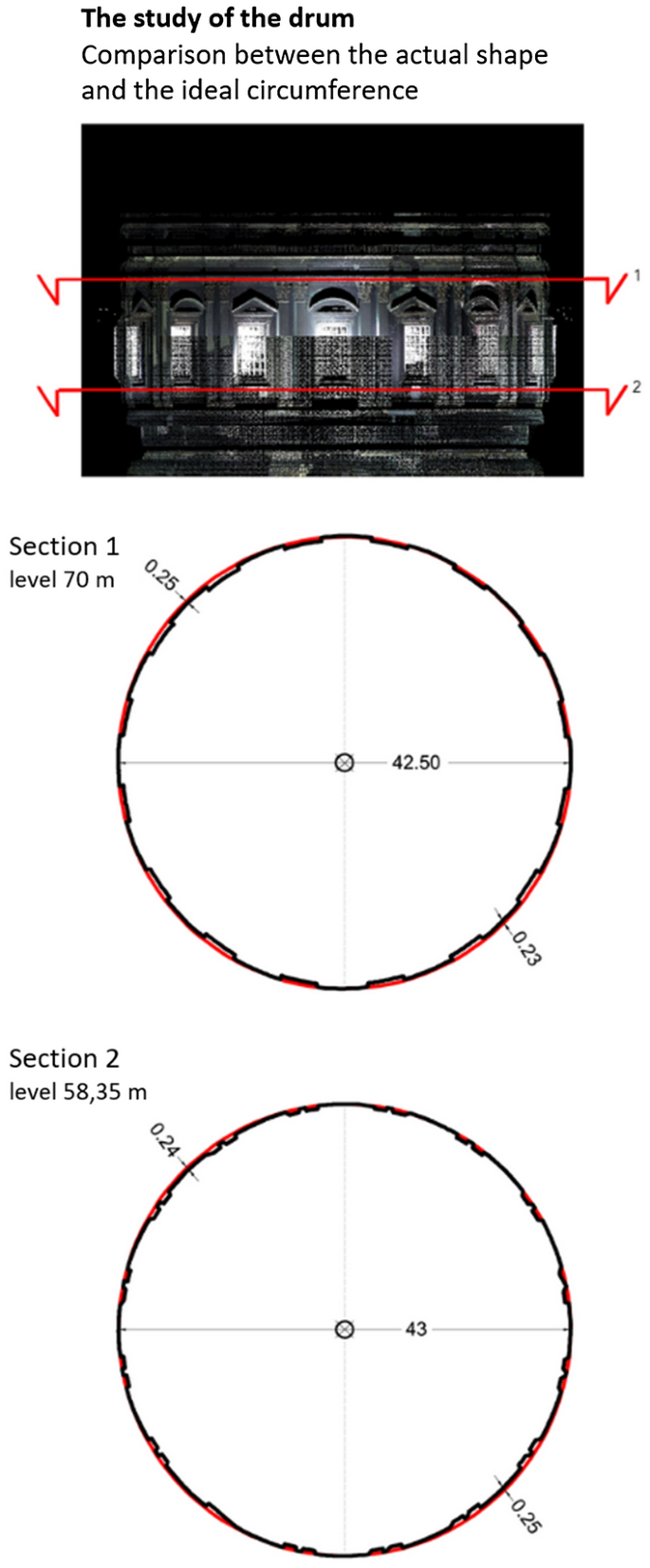
2D study of the drum of St. Peter: horizontal sectioning
From these curves we could determine the diameter of the cylindrical surface in correspondence of the external walls in which open the great windows of the drum. Despite some minor offsets and two major ones, varying between 23 and 25 cm along the diagonal aligned with the so-called Pier of Saint Veronica (south-west corner beyond the transept), still these irregularities did not appear relevant enough to contradict the hypothesis that these profiles closely approximated circles.
In this framework, the lower diameter (or, if you like, the width of the drum at the level of its springing) measures 43.00 m, decreasing to 42.50 m at the opposite end. There is thus a slight outwards tapering of the drum at its bottom, probably due to a well-known settlement of its foundations.
The study of the dome surface proceeded according to the workflow already presented in the previous sections: seven horizontal sections were generated in this case (between 77.50 m and 98.00 m above the ground floor level) and compared with the corresponding “ideal” circumferences. However, for better understanding, we chose on this occasion to consider two circles for each level: an inner one (shown in red in the figures) constructed through the ribs and one more external (shown in blue) passing instead for the bottom of the coffers (Fig. 21 ).
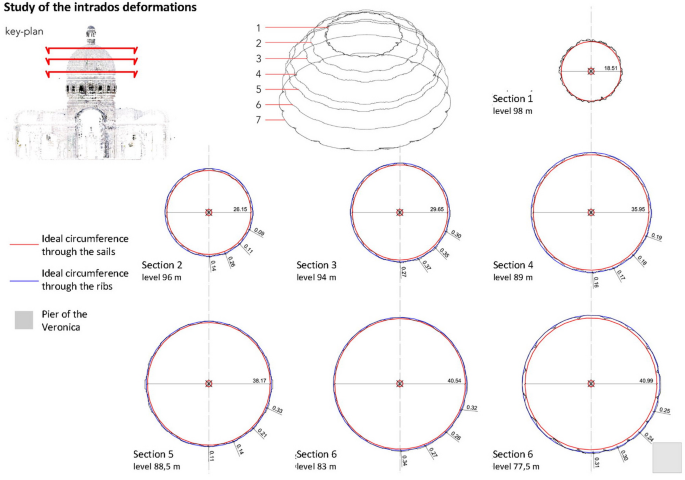
2D study of the dome of St. Peter: horizontal sectioning
As for the drum, also in this case we generally registered offsets spanning from a few centimetres to a maximum of 20 cm, always on the outer section. The only exception is the curve drawn at 94 m, where we measured again the highest offset value (37 cm) right above the Pier of Saint Veronica. However, considering the long and complex chaining interventions on the dome and the rich decorations applied to the intrados, in our opinion these values do not affect the overall geometry of the curves. The step after this focused on the horizontality of the main structures, namely cornices (first and second) and the base of the lantern: in this case the differences we found were consistently under 5 cm.
All the previous constructions led to the tracing of the vertical axis of the surface of revolution that represents our ideal model of the dome (as in San Carlo). The sectioning of the point cloud with a family of radial vertical planes generated a set of meridian profiles, one for each rib and one for each wedged portion. Furthermore, for the part “in between” the domes that we had the chance to capture, these curves were complemented by other two profiles lying respectively on the extrados of the inner dome and on the intrados of the outer one (Fig. 22 ).
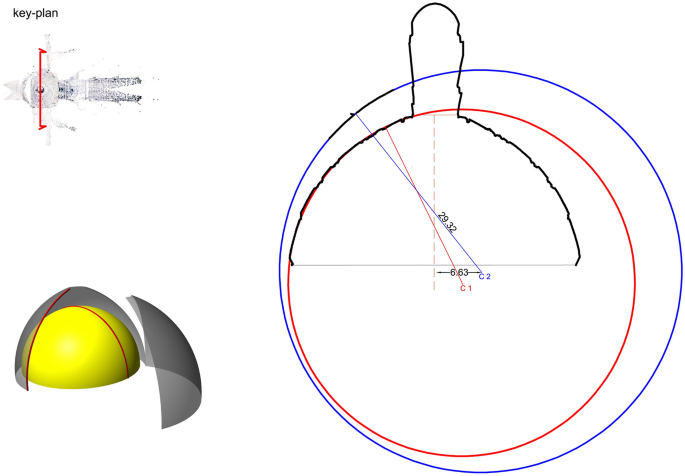
The circles considered in order to understand the difference between the actual and the ideal circumferences
The study of all these lines revealed that they closely approximate circular arcs, thus suggesting a precise 3D positioning of their centres.
Further analysis dealt with the profile of the entire dome. We chose then two pairs of curves that, cut on the wedged surface, lay on the same vertical plane and were aligned with two opposite piers. These compose two perpendicular sections of the entire dome and reveal that the diameters of these diagonal profiles of the inner dome differ only by some 15 cm, always in correspondence with the Pier of St. Veronica (Fig. 23 ). It is interesting to note that the centres of the profiles lay below the springing line and were offset by 5.35 m with respect to the vertical axis of the dome (Fig. 24 ). Similar analysis have regarded the extrados of the inner dome and the intrados of the outer one (Fig. 25 ).
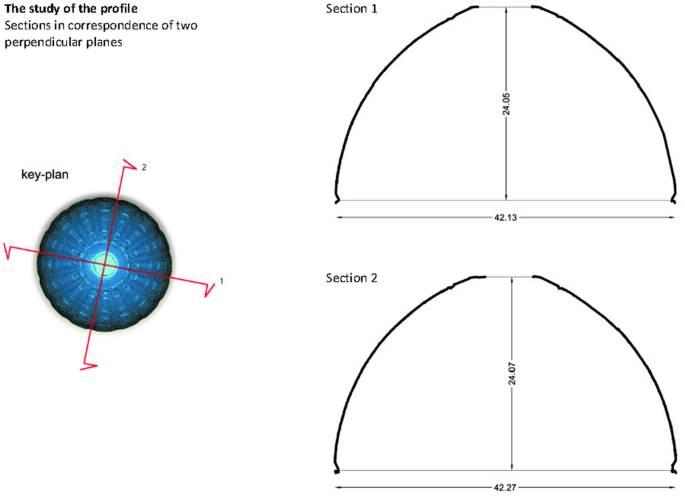
2D study of the dome of St. Peter: sections through the ribs
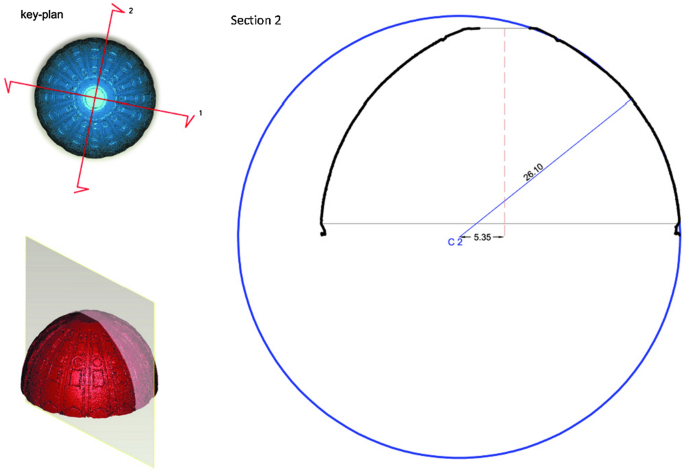
2D study of the dome of St. Peter: radial profiles
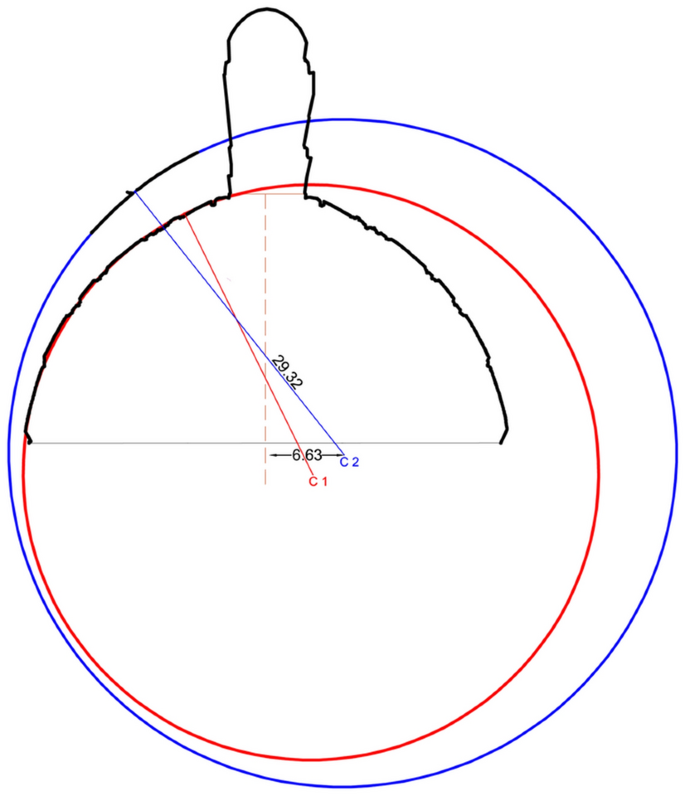
2D study of the dome of St. Peter: offset of the centres of the extrados of the inner dome and the intrados of the outer one
The 3D modelling phase completed our work also in this case. Taking into account the overall regularity of the geometry, we started by modelling one section of the dome between two ribs. In contrast to Hagia Sophia, in this case we had to distinguish the architectural elements (ribs, cornices, arches, etc.) from the decorative ones (statues, stuccos, etc.). For the former we used a geometric modelling, namely a constructive approach in which all elements were modelled directly using NURBS generative primitives and tools (Fig. 26 ). For the latter, instead, due to their lack of any geometric footprint, we chose instead to “simply” interpolate the point cloud into a mesh surface (Fig. 27 ).
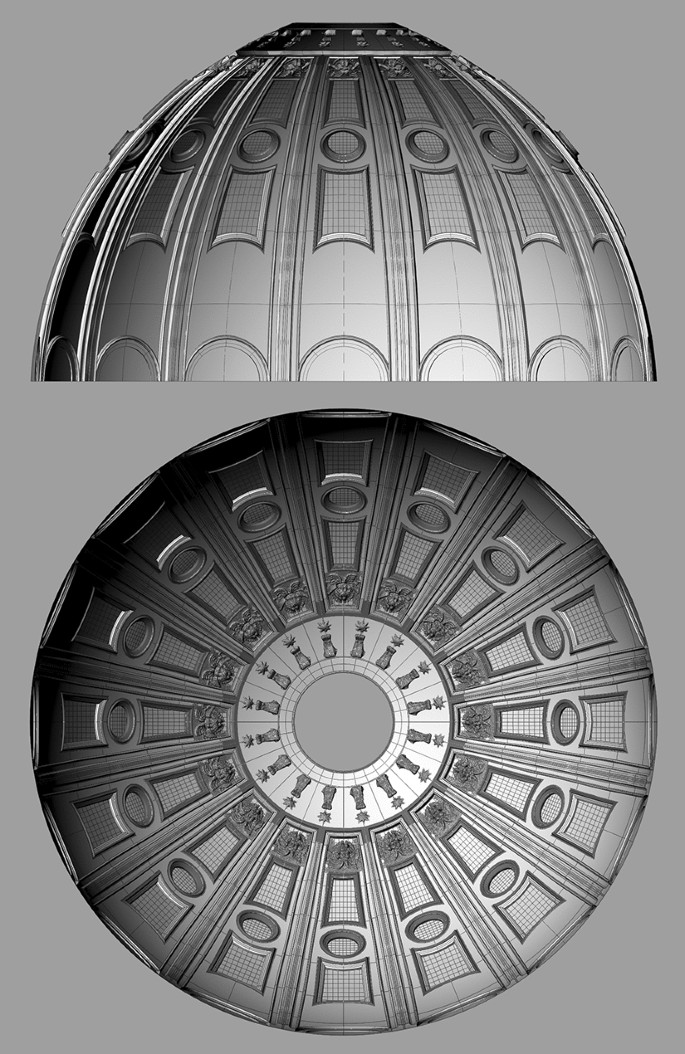
3D study of the dome of St. Peter: reconstruction of the intrados
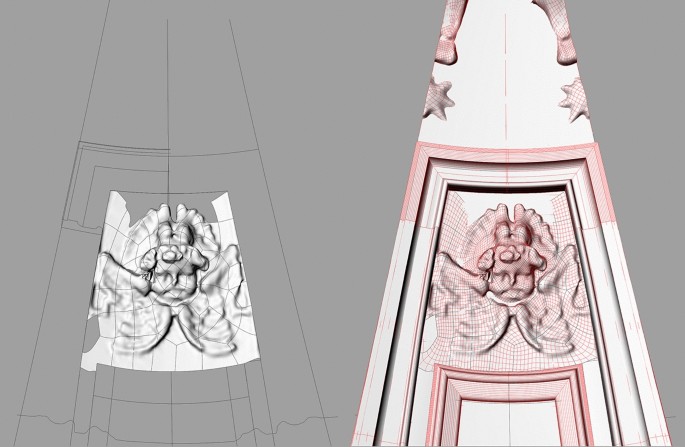
3D study of the dome of St. Peter: model of the decorative elements
Conclusions
Domes are thorny subjects indeed but the technologies we can use nowadays certainly have radically changed the approach to this problem. Nevertheless, the 3D capturing systems (accurate, fast and massive) provide data that have very little intelligence inside and represent only the first step of that complex workflow which transforms data into information thanks to a process of selection and interpretation. This is a high-end ability presently reserved to human beings, but which could, in perspective, be extended and taught to some automatic systems too. However, many of the secrets domes have so far concealed can be revealed thanks to these systems, also effectively contributing to their conservation. The more deeply this task is rooted in the scientific method, the better the results will be. It is hoped that the work presented in this paper is able to outline a replicable operative protocol in the study of domes that could guide scholars and even professionals through this difficult activity. In this framework, the three cases illustrated would represent the necessary tests for our protocol or, in a more optimistic vision, some “good practice” in the study of domes. However, we must not give the impression that our study is the first to investigate the metric and geometric features of the three domes presented in this paper. On the contrary, we must compare our conclusions with a vast body of literature with different level of concordance.
With regard to Hagia Sophia, there is an actual correspondence between the 2D geometry presented by Emerson and Van Nice ( 1943 ), Mainstone ( 1988 ), Hikada and Satō ( 2004 ) and ours. Instead, very different is the 3D side of the study, where instead the results are novel in comparison with the earlier works, and also represent an evolution of our previous study (Bianchini and Paolini 2003 ). San Carlo ai Catinari, for reasons we have not been able to clarify, has been much less studied and our survey appears to be the first systematic one carried out at least in the last 100 years. Instead, for the dome of St. Peter’s we perhaps have too much literature but, again, very little data to refer to: the majority of published works are based on partial measuring and local surveys without any systematic reference. The only exception seems to be the work of Letaroully, which, in our opinion, might be the result of a systematic campaign. In this case too, then, our work could be considered somehow unprecedented for the technology used and for the analyses carried out. In any case it has been the first to geometrically connect the inner and outer domes.
Sanpaolesi ( 1973 : 62): … distingue i conoscitori da coloro che restano alla superficie, anche cosiddetta colta, della conoscenza .
In the literature we also find the terms ‘surveying’, ‘measuring’, and ‘capturing’ for this phase.
With this principle, Popper sought to resolve the impasse that arose between Russell’s fruitless attempts to construct “complete” logical deductive systems, and the cataclysm that swept through epistemological thinking following Kurt Gödel’s proof of the Incompleteness Theorem. Popper, well aware of the inherent inadequacy of the tools that human beings have for cognizing reality and that, in the final analysis, it is substantially impossible to provide “positive” proof that any given statement is true, shifts the centre of gravity of knowledge from proving that something is true to showing that it is false. For Popper, a theory is scientific only if it is possible to devise experiments that demonstrate its inadequacy, i.e., that refute it as false. This approach has been revealed as highly profitable in terms of advancing of knowledge: if a theory withstands an attempt of falsification, it will be stronger, more general and thus closer to the truth; conversely, if the attempt succeeds, an aspect will be revealed that the theory was unable to explain, and a new and stimulating line of research will thus be opened up.
With the term material point we refer to the small objectual area that is synthetized in the model by a single geometric point. For instance, if we consider a 3D laser scanner, the dimensions of this area span from some millimeters to few tenths of a millimeter depending on the instrumental resolution.
Monge ( 1811 : 1): … la manière de reconnaître, d’après une description exacte, les formes des corps, et d'en déduire toutes les vérités qui résultent et de leur forme et de leurs positions respectives …
Rondelet ( 1802 –1817: Tome II, 75): … se rendre compte dans tous les sens, en petit sur le papier, ou en grand sur une aire ou un enduit, des mesures et des formes d’une partie d’édifice .
It is worth underlining that the point cloud analyzed in this study is the product of a “scientific” joint venture between our group from DSDRA and the team from the Institut für Kunstgeschichte der Universität Bern led by Volker Hoffmann and Nikolaos Theocharis ( 2016 ). As a quarter of the central area of Hagia Sophia is almost permanently occupied by a huge scaffolding used for the restoration of the dome, which prevents a complete capturing of its intrados, we decided to share our “individual” clouds in order to improve the quality and quantity of available data. Although we can read only very few points in a relevant portion of the dome (only those captured by the beams filtering through the scaffolding), this unconventional exchange of data is, in our opinion, a valuable good practice in the study of complex structures.
This work is based on two different 3D point clouds. The first, acquired in 2006 with a Leica HDS 3000, counts about 70 million points. The second one, captured in 2018 with a FARO 405, is made of more than 500 million points. Both have been coupled with a topographic campaign to ensure the highest control on the quality of the final model. Furthermore, in 2018 a digital photogrammetric surveying took place together with the scanning, adding some 100 million points to the final model, which was also texturized with high resolution images. The average uncertainty of the final numeric model was under 1 cm.
The point cloud of about 100 million points was acquired with a Leica HDS 3000 and coupled with a topographic campaign to ensure the highest control on the quality of the final model. Only the 3D cloud captured from the lantern window was not accompanied by a topographic reference, due to time constraints. The average uncertainty of the final numeric model was under 1 cm.
We were able to take advantage of an intervention of maintenance (substitution of a broken pane of glass) to scan the intrados of the dome from the inside of the gap between the inner and outer domes, thus allowing the common registration of both set of points.
Bianchini, Carlo. 2012. Rilievo e metodo scientifico | Survey and scientific method. In: Elogio della teoria. Identità delle discipline del disegno e del rilievo | In praise of theory. The fundamentals of the disciplines of representation and survey (Proceedings, 34° Convegno internazione dei docent della rappresentazione), 391–400. Rome: Gangemi Editore.
Bianchini, Carlo. 2014. Survey, modeling, interpretation as multidisciplinary components of a Knowledge System. SCIRES-IT-SCIentific RESearch and Information Technology 4(1): 15–24.
Bianchini, Carlo and Priscilla Paolini. 2003. Rilievo per il restauro e la messa in sicurezza di Hagia Sophia a Istanbul: prime sperimentazioni. Disegnare idee immagini | Drawing ideas images 26: 20–31.
Bianchini, Carlo and Michele Russo. 2018. Massive 3D acquisition of CH. In: 3rd Digital Heritage International Congress (DigitalHERITAGE) held jointly with 2018 24th International Conference on Virtual Systems & Multimedia (VSMM 2018), 1–8. https://doi.org/10.1109/DigitalHeritage.2018.8810069.
Carusi, Marta. 2010. La struttura portante della cupola di Della Porta: 1588–2010. Annali di architettura: Rivista del Centro Internazionale di Studi di Architettura Andrea Palladio di Vicenza 22: 125–150.
Chiavoni, Emanuela. 2018. Rome and Its Domes: Drawing, Art and Architecture . Periodica Polytechnica Architecture 49(1): 72–76.
Di Stefano, Roberto. 1980. La cupola di San Pietro: storia della costruzione e dei restauri . Naples: Edizioni scientifiche italiane.
Docci, Mario (ed). 1997. Il Disegno di progetto dalle origini al XVIII secolo . Rome: Gangemi.
Docci, Mario. 2003. Hagia Sophia. Analisi del rilevamento interno | The survey of the interior of Hagia Sophia. Disegnare idee immagini | Drawing, Ideas images 26: 32–45.
Docci, Mario, Carlo Bianchini, Alfonso Ippolito. 2011. Papers for a theory of Architectural survey | Contributi per una teoria del rilevamento architettonico. Disegnare Idee Immagini | Drawing ideas images 42: 34–41.
Docci, Mario and Riccardo Migliari. 1992. Scienza della rappresentazione . Rome: La Nuova Italia Scientifica.
Dorffner Lionel, Karl Kraus, Josef Tschannerl, Orhan Altan, Sitki Külür, Gönül Toz. 2000. Hagia Sophia—Photogrammetric record of a world cultural heritage. International Archives of Photogrammetry and Remote Sensing 33(B5/1; PART 5): 172–175.
Emerson, William and Robert L. Van Nice. 1943. Hagia Sophia, Istanbul: Preliminary Report of a Recent Examination of the Structure. American Journal of Archaeology 47(4): 403–436.
Fondelli, Mario. 1992. Trattato di fotogrammetria urbana e architettonica . Bari: Laterza.
Fontana, Carlo. 1694. Il Tempio Vaticano . Rome: Giovanni Francesco Buagni.
Gaiani, Marco 2012. Per una revisione critica della teoria del rilievo dopo l’avvento dei mezzi digitali. | Towards a critical rethinking of the theory of surveying following the advent of digital media. In: Elogio della teoria. Identità delle discipline del disegno e del rilievo | In praise of theory. The fundamentals of the disciplines of representation and survey (Proceedings, 34° Convegno internazione dei docent della rappresentazione), 375–382. Rome: Gangemi Editore, 2012.
Hidaka, Ken’ichirō and Tatsuki Satō (eds.). 2004. Architectural-Structural Survey of Hagia Sophia . Tokyo: Chūō-Kōron Bijutsu Shuppan.
Hoffmann, Volker and Nikolaos Theocharis. 2016. Hagia Sophia 3D . Edition Topoi. https://doi.org/10.17171/2-6.
Inglese, Carlo, Mario Docci, Alfonso Ippolito. 2019. Archaeological Heritage: Representation between Material and Immaterial. In: Analysis, Conservation, and Restoration of Tangible and Intangible Cultural Heritage , eds. Carlo Inglese, Mario Docci, Alfonso Ippolito, 1–22. IGI Global.
Leroy, Charles-François-Antoine. 1845. Traité de Stéréotomie . Paris: Gauthier-Villars.
Letaroully, P. M. 1840–55. Édifices de Rome moderne: ou recueil des palais, maisons, églises, couvents, et autres monuments publics et particuliers les plus remarquables de la ville de Rome , 3 vols. Liége: D. Avanzo.
Mainstone Rowland J. 1988. Hagia Sophia: Architecture, Structure and Liturgy of Justinian’s Great Church . London: Thames and Hudson.
Migliari, Riccardo. 2008. Descriptive Geometry in the Era of Computers. In: Abitare Virtuale significa rappresentare , ed. Maurizio Unali, 14–31. Rome: Kappa.
Monge, Gaspard. 1811. Géométrie Descriptive . Paris: Klostermann.
Popper, Karl. 1963. Conjectures and Refutations: The Growth of Scientific Knowledge . London Routledge.
Rendina Claudio. 2000. Le Chiese di Roma . Rome: Newton & Compton Editori.
Ribichini Luca. 2015. Sant’Ivo alla Sapienza: Faith and Reason | Sant’Ivo alla Sapienza tra Fede e Ragione. Disegnare idee immagini | Drawing ideas image s 50: 12–23.
Rocchi, Paolo (ed). 2009. Nuove Ricerche sulla Gran Cupola del Tempio Vaticano . Rome: PreProgetti Editore.
Rondelet, Jean-Baptiste. 1802–1817. Traité théorique et pratique de l’art de bâtir . Paris: Chez l’auteur.
Sanpaolesi, Piero. 1973. Discorso sulla metodologia del restauro dei monumenti . Florence: Editrice Edam.
Sanpaolesi, Piero. 1978. La chiesa di S. Sofia a Costantinopoli . Rome: Officina Edizioni.
Spagnesi, Gianfranco. 2003. Roma: la Basilica di San Pietro, il borgo e la città . Milan: Jaca Book.
Download references
Acknowledgements
The results presented in this paper can be considered as the most recent product of an almost 20-year-long activity carried out by a research team from the DSDRA—Sapienza University of Rome of which the author is part. In this framework, it is worth mentioning the composition of the different crews and the institutions and people providing their support and help. For the Hagia Sophia project: The DSDRA team: Prof. Mario Docci, Prof. Alfonso Ippolito, Prof. Priscilla Paolini, Arch. Marika Griffo. UNIMED and in particular it’s former General Secretary, the late-lamented Prof. Franco Rizzi, and his deputy Roberto Albergoni. The Boğaziçi University of Istanbul (the late-lamented Prof. Gunhan Danisman) and, finally, the Direction of the Hagia Sophia Museum. Prof. Hoffman and Prof. Theocaris from Institut für Kunstgeschichte der Universität Bern for their availability in sharing their data with our team. For the San Carlo ai Catinari project: The DSDRA team: Prof. Mario Docci, Prof. Alfonso Ippolito, Prof. Carlo Inglese, Geom. Marco Di Giovanni, Arch. Martina Attenni, Arch. Marika Griffo. Prof. Paolo Rocchi and his team. For the St. Peter in the Vatican City project: The DSDRA team: Prof. Mario Docci, Prof. Alfonso Ippolito, Prof. Priscilla Paolini, Arch. Kostantin Brandenburg, Geom Marco Di Giovanni. Prof. Paolo Rocchi and his team. The Veneranda Fabbrica di San Pietro and in particular Mons. Angelo Comastri, Arch. Luca Virgilio, Dr. Pietro Zander. All images in this paper are by the author.
Open access funding provided by Università degli Studi di Roma La Sapienza within the CRUI-CARE Agreement.
Author information
Authors and affiliations.
Department of History, Representation and Restoration of Architecture, Sapienza Università di Roma, Piazza Borghese, 9, Rome, Italy
Carlo Bianchini
You can also search for this author in PubMed Google Scholar
Corresponding author
Correspondence to Carlo Bianchini .
Additional information
Publisher's note.
Springer Nature remains neutral with regard to jurisdictional claims in published maps and institutional affiliations.
Rights and permissions
This article is published under an open access license. Please check the 'Copyright Information' section either on this page or in the PDF for details of this license and what re-use is permitted. If your intended use exceeds what is permitted by the license or if you are unable to locate the licence and re-use information, please contact the Rights and Permissions team .
About this article
Bianchini, C. A Methodological Approach for the Study of Domes. Nexus Netw J 22 , 983–1013 (2020). https://doi.org/10.1007/s00004-020-00526-9
Download citation
Accepted : 16 September 2020
Published : 02 October 2020
Issue Date : December 2020
DOI : https://doi.org/10.1007/s00004-020-00526-9
Share this article
Anyone you share the following link with will be able to read this content:
Sorry, a shareable link is not currently available for this article.
Provided by the Springer Nature SharedIt content-sharing initiative
- Hagia Sophia
- San Carlo ai Catinari
- St. Peter’s
- Find a journal
- Publish with us
- Track your research
- Reference Manager
- Simple TEXT file
People also looked at
Perspective article, a case study on dome structure of composite structural insulation panels with ansys simulations.

- College of Civil Engineering and Architecture, Henan University, Kaifeng, China
Thin-shell dome structures are conventionally built with concrete. However, the building process is time-consuming and of high labor cost. Composite Structural Insulation Panels (CSIPs) have recently been regarded to be suitable for large-span civil structures, in terms of its light weight, easy processing, and mass production. Hence, this paper explores the feasibility of CSIPs domes applied in large-span dome structures. Compared with the performance of thin-shell concrete domes, the stiffness, strength, and stability of CSIPs domes are studied by means of refined finite element analysis. Results demonstrate CSIPs dome possesses good dynamic performance, bears larger nonlinear loads, and even copes with stress loads under earthquakes. Moreover, the stiffness should be handled in the onsite applications to fulfil the safety requirements.
Introduction
Dome structure is one of the most important structural forms in the history of architectural development, which not only meets the demand for large-span architecture, but also enhances the aesthetics of architecture ( Mu et al., 2017 ). And dome structures are often used in public buildings such as stadiums, conference halls, convention centers, botanical gardens, and industrial buildings. Initially dome structures were built by stone, wood, concrete and other materials. Until 1811, the dome structure with steel was developed ( Cui et al., 2001 ). From the 1920s onwards, thin-shell concrete domes were favored by most architects for their reasonable and effective form of force ( Popov, 1991 ). However, thin-shell concrete domes were very time-consuming and slow in construction, and gradually were replaced by new dome structures such as ordinary steel, high-strength steel, aluminum alloy, and cable dome structures. Thus, it can be found that the innovation of building materials has promoted the development of dome structures.
In addition, due to the wide application of dome structure in practical engineering, scholars have conducted extensive research on the performance of dome structures. In terms of the relationship between dome structures and architectural function, Fan (2001) proposed that dome structures could not only meet the need of architectural function for large space, but also be an important factor of art expression. In terms of the rationality of dome structure, Qian et al. (1996) investigated the mechanics and working mechanism of domes and proposed that the most reasonable dome structure should be the thin-shell structure. Yang (2010) mentioned that span was an important factor affecting thin-shell domes. In the analysis of dome structures with different materials, Noh (2005) stated that although the thin-shell concrete structure was a structure with excellent load-bearing performance, the high sensitivity of the shell to initial defects would lead to buckling damage. Zou (2019) stated that the cast-in-place concrete dome structure formwork and its support system were relatively complex, difficult to construct and costly. Dong and Yuan (2008) concluded that it was necessary to improve cable dome construction system in terms of new systems and materials. In terms of research methods, Lan et al. (2017) proved ANSYS software simulations could be used to analyse the performance of thin-shell dome. In summary, the thin-shell dome structure can meet the demand of buildings for large-span space and architectural aesthetics, but the structural rationality, economic factors and construction of different material domes will restrict the development and application of dome structure.
Currently, the Composite Structural Insulation Panels (CSIPs) using E-glass/polypropylene (PP) laminate as the panel and expanded polystyrene (EPS) foam as the core material, as shown in Figure 1A , are receiving more and more attentions. Firstly, due to the high strength-to-weight ratio, excellent impact resistance and high durability, CSIPs panels can take up the bending and axial stresses and prevent buckling or twisting. Secondly, with the application of core materials and its high R-value, CSIPs have better thermal resistance and resistance to shear stress. Thirdly, the fabrication process of CSIPs, as shown in Figure 1B , is more efficient and time-saving, and a large number of high-quality CSIPs can be produced in a short period of time. Finally, the CSIPs panels can be spliced by polyvinyl chloride (PVC) connectors to ensure overall performance. Based on the factors affecting the dome structure, CSIPs are the ideal alternative materials for thin-shell concrete dome structures. However, only a few scholars, including Mousa and Uddin (2010) , Uddin and Du (2014) , Du et al. (2019) , and Yang et al. (2017) , have conducted research on the mechanical properties and improvement of CSIPs, and no scholars conducted research on the application of CSIPs in dome structures. Therefore, on the basis of existing research, CSIPs domes are studied by comparing with thin-shell concrete domes through numerical simulations in this paper.
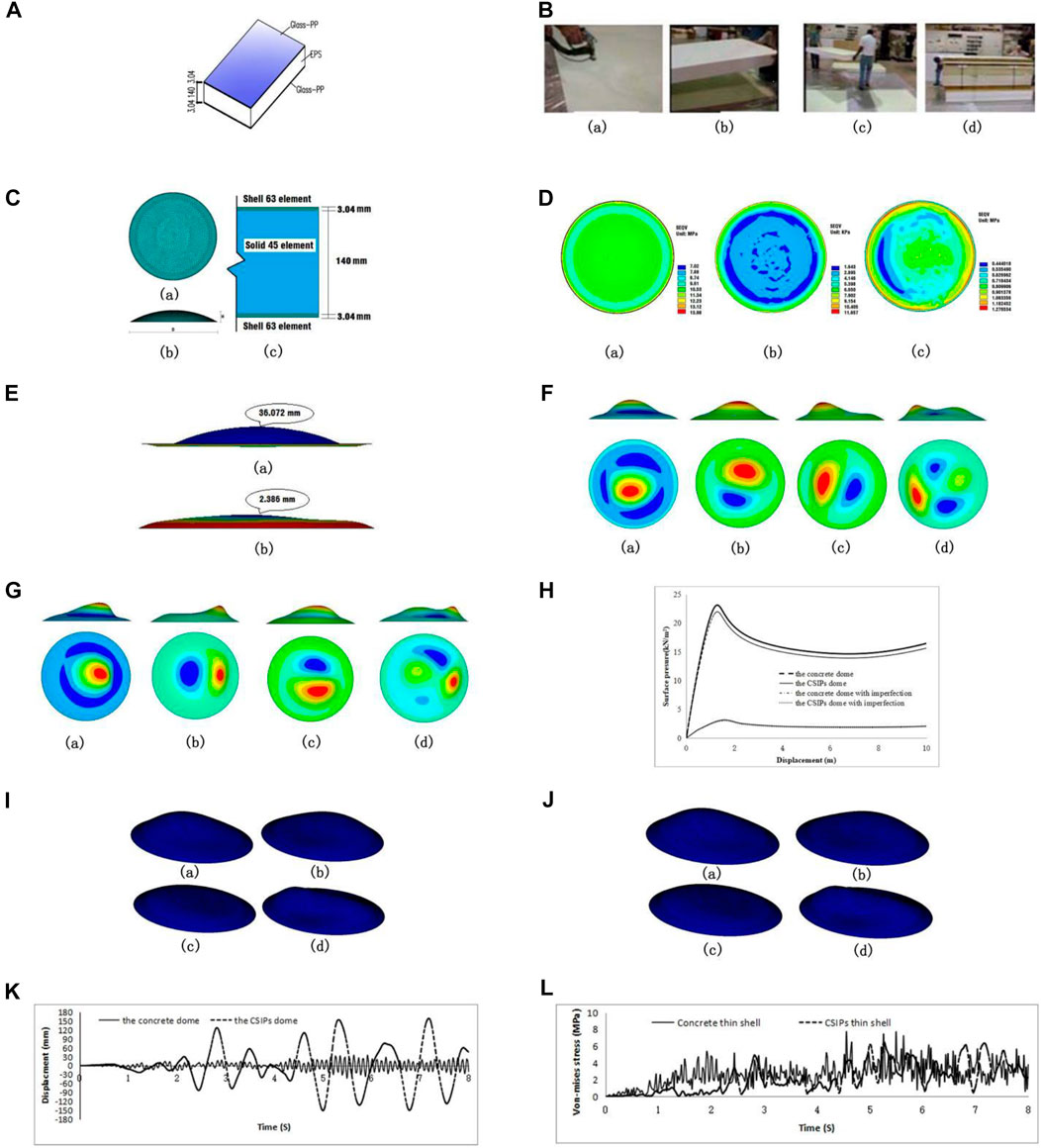
FIGURE 1 . (A) Schematic diagram of CSIPs (unit: mm); (B) Manufacturing of CSIPs: (a) spraying hot-melt adhesive bonding agent; (b) placing the foam core on the lower panel sheet; (3) making a piece of completed CSIP; (d) storing the fabricated CSIPs panels; (C) Structural parameters of the finite element model of CSIPs dome: (a) plan; (b) elevation; (c) cross-section; (D) Von-Mises stress: (a) GFRP of CSIPs dome; (b) EPS of CSIPs dome (c) thin-shell concrete dome; (E) Vertical displacements: (a) vertical displacement of CSIPs dome; (b) vertical displacement of thin-shell concrete dome; (F) Top 4 buckling mode shapes of CSIPs dome: (a) first order; (b) second order; (c) third order; (d) fourth order; (G) Top 4 buckling mode shapes of thin-shell concrete dome: (a) first order; (b) second order; (c) third order; (d) fourth order; (H) Vertical displacement versus load curve at the center point; (I) Mode shapes and frequencies of CSIPs dome: (a) first order, f = 1. 1,033; (b) second order, f = 1.1064; (c) third order, f = 1.1307. (d) fourth order, f = 1.5323; (J) Mode shape and frequencies of thin-shell concrete dome: (a) first order, f = 12.065; (b) second order, f = 12,141; (c) third order, f = 12.680; (d) fourth order, f = 16.503; (K) Time-displacement curve of center point under EL-Centro seismic wave; (L) Time-stress curve of element with Maximum Von-mises stress under EL-Centro seismic wave.
Research methodology
Refinement of finite element analysis.
The thin-shell dome structure, including CSIPs domes and thin-shell concrete domes, is an elastic system, which reduces the destructive effect of external load on the structure by deformation. However, the thin-shell dome structure will be feasible for buildings only when the structural stability is guaranteed. The structure stability usually includes the geometric stability of the structure, the displacement of the structure and the elastic stability of the structure ( Qian and Yang, 2003 ). The main analysis method of current dome structure is to divide the whole structure into many very small unit elements when the dome structure can be assumed to be a homogeneous continuum structure. Then the strength, stiffness and stability of dome structure can be studied from static analysis, eigenvalue buckling analysis and modal analysis with finite element analysis software ( Shen and Chen, 1999 ). The above-mentioned studies are carried out under ideal conditions, but in actual engineering dome structure often has large deflections and imperfect initial defects, so the performance of the dome structure should be studied by geometric nonlinear analysis to maintain structural stability ( Lin and Shen, 1996 ). In addition, as dome structure is a space structure with obvious performance of deformation, studies show that both horizontal and vertical seismic forces would have a large effect on domes ( Cao and Zhang, 1998 ). Therefore, the dynamic stability of domes under multidimensional seismic forces should be analyzed. Furthermore, the Time-history analysis under earthquakes has the advantage of analyzing the sequence of plastic hinges, and the weakness of the structure, so it is usually used to analyze the dynamic stability of dome structures ( Deng and Zhu, 2002 ).
In this paper, ANSYS 14.0 software is used to analyze performance of CSIPs dome and thin-shell concrete dome. The research program is divided into three processes: selection of the basic dome research model, establishment of the finite element model, and comparative analysis of the strength, stiffness, and stability of the two research domes.
1) The selection of the basic dome research model. The model selection should be representative, which means the calculation should be reduced as much as possible under the condition of ensuring accuracy and the simulation should be able to fully reflect the distribution of the strain state of the dome structures.
2) Establish the finite element model. ANSYS14.0 was used to establish the basic dome research models with uniform loading. The calculation is chosen to stop after the dome reaches the ultimate load in order to save the calculation time. Finally, all the data obtained from the analysis are extracted for analysis.
3) Compare and analyze the performance of the domes with different material parameters. Analyze the performance of CSIPs domes and thin-shell concrete domes in terms of strength, stiffness and stability by comparing the analysis results in five aspects, including static analysis, eigenvalue buckling analysis, geometrical non-linear analysis, modal analysis, and Time-history analysis under earthquakes.
Finite element analysis research model
The research models shown in Figure 1C are geometrically identical CSIPs dome and thin-shell concrete dome. 3.04 mm thickness faceplates were selected for the E-glass/polypropylene laminate and the properties of the laminate and expanded polystyrene (EPS) foam cores are shown in Table 1 (A and B), respectively. Table 1 (C) lists the material properties of thin-shell concrete. The structural parameters of both domes are the same: the span is 60 m, the thickness is 146.08 mm, the vector-to-span ratio is 0.134.
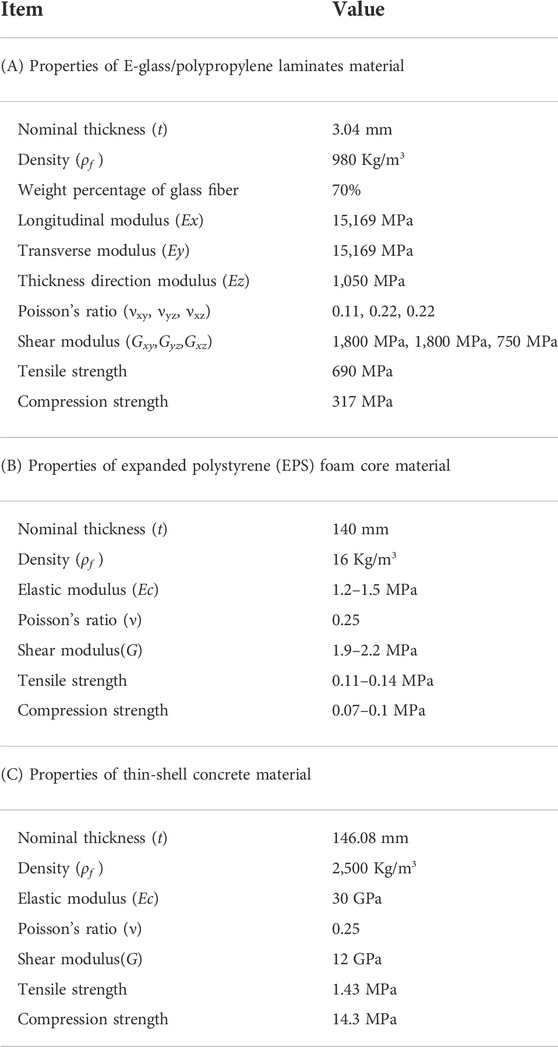
TABLE 1 . Properties of analysis models.
It is assumed that both domes are fixed on supports and there is no relative slip between the faceplates and core materials in CSIPs dome model. The appropriate amount of shell elements could help to improve the efficiency and to ensure the analysis accuracy in ANSYS ( Lan et al., 2017 ). In CSIPs dome model, 26,820 elements are analyzed, compared to 16,260 elements of the thin-shell concrete model we used. In this analysis, the faceplates of the CSIPs dome were simulated using Shell63 elements of the ANSYS while the standard Solid45 elements were used for the EPS foam core and concrete.
Results and discussion
Comparative study of static analysis.
Static analysis refers to the static load response of the structure, which responds to the stiffness and load-bearing capacity of the structure. Static analysis is fundamental in structural research. In this paper, in addition to considering the self-weight of the structure, a uniform load of 1 KN/m 2 was used for the external forces of the dome. Figure 1D demonstrates that the Von-Mises stress on the adjacent area of the faceplates in CSIPs dome has a maximum value of 13.98 MPa, while the maximum Von-Mises stress on the expanded polystyrene (EPS) foam core is only 11.66 KPa. The Von-Mises stress on the thin-shell concrete dome has a maximum value of 1.28 MPa. This indicates that the faces plates have high compression strength which can bear majority of the external load.
Moreover, the maximum vertical displacement of the CSIPs dome is 36.072 mm, which is much larger than that of thin-shell concrete dome of 2.386 mm ( Figure 1E ). This indicates that the E-glass/polypropylene laminate and the expanded polystyrene (EPS) foam core, which have a smaller modulus of elasticity, caused the structural stiffness of CSIPs dome to be lower than that of thin-shell concrete dome. Nevertheless, the maximum displacement of the CSIPs dome is about 1/1,663 of the span, which still meets the range allowed by the code. Further analysis shows that, if only gravity is considered, the displacements of CSIPs dome and thin-shell concrete dome are 1.928 mm and 1.889 mm which are close to each other. It indicates that the vertical stiffness of both domes is close when only self-weight is considered.
From the results of the static analysis, the CSIPs dome has the same performance of bearing the external load with thin-shell concrete dome. However, the stiffness of CSIPs dome is much less than that of thin-shell concrete dome. Therefore, for large-span dome structures using CSIPs, it is particularly important to consider the stiffness and to make the structural displacement within the scope of normative permission.
Comparative study of eigenvalue buckling analysis
The eigenvalue buckling analysis can be used to predict the theoretical strength (bifurcation point) of an ideal linear elastic structure. Figures 1F,G , show the first four buckling modes. They present the same buckling modes performance of CSIPs dome and thin-shell concrete dome. The first order buckling mode shapes of both domes are depressed downward in the central region of the dome. The shapes of the second and third order buckling modes are both half-shell up-convex and half-shell down-concave states.
The results of the eigenvalue buckling analysis show that the CSIPs dome has a similar buckling mode shape to the thin-shell concrete dome. It further shows that CSIPs material is suitable for replacing thin-shell concrete material to construct large-span domes and will not lead to significant changes in the stability of domes.
Comparative study of geometrical non-linear analysis
Practical situations such as large deflections and initial defect imperfections can be integrated by non-linear analysis. In ANSYS 14.0, the geometry updated after eigenvalue buckling analysis is usually used to consider imperfections. In terms of geometric defects, the most dangerous geometrically defective structures are configured with first order buckling mode shapes; and 1/1,000 of the span is the maximum deformation value that can be accepted for geometric defects.
Figure 1H presents the results of the geometric non-linear analysis of CSIPs dome and thin-shell concrete dome. From the results, it can be seen that CSIPs dome is subjected to fewer external loads than that of thin-shell concrete dome. The non-linear limit load on CSIPs dome is a uniform surface pressure with a pressure value of 3.17 KN/m 2 , while the non-linear limit load on thin-shell concrete dome has a value of 23.1 KN/m 2 . This is caused by the weak expanded polystyrene (EPS) foam core, which does not provide sufficient shear resistance. Further, it can be found that the initial geometric defect reduced the limit load of CSIPs dome from 3.17 KN/m 2 to 3.04 KN/m 2 with a percentage reduction of 4.1%, respectively, and reduced the limit load of thin-shell concrete dome from 23.1 KN/m 2 to 21.9 KN/m 2 with a percentage reduction of 5.2%. It indicates that the initial geometric defects have a relatively small effect on both domes to withstand external loads.
Comparative study of modal analysis
The modal analysis could determine the vibration characteristics of a structure. Figures 1I,J show the top 4 modal shapes and frequencies of CSIPs dome and thin-shell concrete dome, respectively.
Figures 1I,J show that the fourth order mode shapes of the CSIPs dome and thin-shell concrete dome are similar. The first order and second order modal shapes are both characterized by one half of the shell is convex upwards, and the other half is concave downwards. The third order and fourth order modal shapes are symmetric vertical vibrations with a two-wave shape. This indicates that the dynamic performance of CSIPs dome is stable and reliable. In addition, the frequencies of CSIPs dome are much smaller than those of thin-shell concrete dome. The fundamental frequencies of both domes are 1.1033 and 12.065, respectively, which are mainly caused by the low material elastic modulus and relatively low stiffness of the CSIPs domes.
Comparative study of time-history analysis under earthquakes
In this study, Firstly, the damping values and seismic load directions are brought into the system, and secondly, use the following equations to calculate the α and β coefficients of Rayleigh damping.
In which ζ represents the constant damping ratio, ζ of CSIPs and thin-shell concrete respectively equal 0.02 and 0.05 ( Zhang et al., 2021 ), α represents the mass matrix multiplier, β represents the stiffness matrix multiplier, and ω represents the structural frequency got from the modalities analysis. Thirdly, vertical acceleration seismic waves with a peak value of 0.2 g were applied to each structural model and linear elastic material models were considered simultaneously.
Figure 1K shows that the maximum dynamic displacement of CSIPs dome is 151.0 mm and the thin-shell concrete dome is 42.3 mm. The deviations between the two values are large, with the former being about 3.6 times larger than the latter. This is due to the lower modulus of elasticity of the CSIPs material. Further, it can be found that the maximum Von-mises stress of the CSIPs dome is smaller than that of the thin-shell concrete dome. The values are 6.45 MPa and 7.72 MPa, respectively ( Figure 1L ). The former is a little lower than the latter. Finally, the above values indicate that CSIPs domes have better dynamic performance and can better cope with the stress loads under earthquakes.
This paper explores the feasibility of CSIPs domes applied in large-span dome structures. Compared with the performance of thin-shell concrete domes, the stiffness, strength, and stability of CSIPs domes are studied by means of refined finite element analysis. The following conclusion were obtained.
1) Through static analysis, it can be seen that, under the uniform load, the Von-Mises maximum stress value of CSIPs dome is slightly larger than that of thin-shell concrete dome. However, the displacement of CSIPs dome is larger than that of thin-shell concrete dome. This is caused mainly because the CSIPs dome has an advantage of lighter weight. Therefore, for large-span dome structures using CSIPs, it is particularly important to consider the stiffness and to make the structural displacement within the scope of normative permission.
2) The results of eigenvalue buckling analysis show that the buckling mode shapes of CSIPs dome and thin-shell concrete dome are the same, and the modal analysis results show that the first fourth order mode shapes of CSIPs dome and thin-shell concrete dome are also similar. It indicates that CSIPs material can replace the traditional thin-shell concrete material to build the dome and the stability and dynamic of the dome structures will not be significantly changed.
3) The nonlinear analysis of the geometry shows that the initial geometric defects have a relatively small effect on the surface pressure of CSIPs dome and thin-shell concrete dome. The surface pressure of CSIPs dome is much less than that of thin-shell concrete dome. This is due to the fact that the CSIPs core is weak and does not provide sufficient shear resistance. Hence, we can conclude that the overall performance of CSIPs dome is better than that of thin-shell concrete dome.
4) The results of the Time-history analysis under earthquake show that the displacement of CSIPs dome under seismic forces is 3.6 times more than that of thin-shell concrete dome. But the maximum Von-mises stress of CSIPs dome is less than that of thin-shell concrete dome. The reason of the results is that the weight of CSIPs dome is less than that of thin-shell concrete dome. Therefore it is safe to conclude that the CSIPs dome has better dynamic stability under seismic forces.
Furthermore, in this paper, only the selected single model is analyzed in details in the aspect of finite element analysis. Due to the limits of the research level and simulation calculation conditions, the following problems remain to be studied:
1) This study is based on only a single dome model for preliminary analysis between different materials, different spans, rise-span ratio, shell thickness and boundary conditions were not compared.
2) The research is a preliminary analysis under the premise of static stability. Various defects generated during the construction process often have a greater impact on the stability of domes. The influence of various defects in the actual construction on domes needs further analysis.
Data availability statement
The raw data supporting the conclusion of this article will be made available by the authors, without undue reservation.
Author contributions
CL conceived the idea and wrote the manuscript. LZ reviewed the manuscript. CL and LZ were involved the manuscript discussion and correction.
This research was supported by the National Science Foundation in China (NSFC) as a part of the research project (U1704141) and the project was supported by the Foundation of Zhejiang Provincial Key Laboratory of Space Structures (202106).
Conflict of interest
The authors declare that the research was conducted in the absence of any commercial or financial relationships that could be construed as a potential conflict of interest.
Publisher’s note
All claims expressed in this article are solely those of the authors and do not necessarily represent those of their affiliated organizations, or those of the publisher, the editors and the reviewers. Any product that may be evaluated in this article, or claim that may be made by its manufacturer, is not guaranteed or endorsed by the publisher.
Cao, Z., and Zhang, Y. G. (1998). Seismic response analysis of single-layer spherical mesh shell. Build. Struct. 8, 40–43. doi:10.19701/j.jzjg.1998.08.011
CrossRef Full Text | Google Scholar
Cui, X. Q., Guo, Y. L., and Zhu, Z. Y. (2001). Dome structural system. Spat. Struct. 7 (1), 3–10. doi:10.13849/j.issn.1006-6578.2001.01.001
Deng, J., and Zhu, Y. P. (2002). A review of research methods on seismic performance of mesh and shell structures. Constr. Budg. 1, 37–38. doi:10.13993/j.cnki.jzyys.2002.01.012
Dong, S. L., and Yuan, X. F. (2008). Advances in research on cable domes. J. Zhejiang Univ. Eng. Sci. 42 (1), 1–7. doi:10.3785/j.issn.1008-973X.2008.01.001
Du, W. F., Liu, Q., Zhou, Z. Y., and Uddin, N. (2019). Experimental investigation of innovative composite folded thin cylindrical concrete shell structures. Thin-Walled Struct. 137 (1), 224–230. doi:10.1016/j.tws.2019.01.014
Fan, Z. H. (2001). Artistic form of expression of structure in architecture. J. Beijing Inst. Civ. Eng. Archit. 17 (1), 59–64. doi:10.3969/j.issn.1004-6011.2001.01.011
Lan, Y. Q., Xue, S. D., Li, X. Y., and Geng, L. (2017). Analysis on static behavior of levy rigid bracing domes. Spatital Struct. 23 (2), 22–29. doi:10.13849/j.issn.1006-6578.2017.02.022
Lin, Y. J., and Shen, S. Z. (1996). Stability of K6 single layer reticulated shells. J. Harbin Univ. C. E. Archit. 30 (2), 39–44.
Google Scholar
Mousa, M., and Uddin, N. (2010). Debonding of composites structural insulated sandwich panels. J. Reinf. Plast. Compos. 29, 3380–3391. doi:10.1177/0731684410380990
Mu, Z. G., Yang, Y. Q., Ge, P., and Zhang, X. Y. (2017). “Analysis on the economic impact factors of the long-span spatial structure type selection,” in Proceedings of the 26th National Conference on Structural Engineering , Changsha, China , February 2, 2017 , 75–82.
Noh, H. C. (2005). Ultimate strength of large scale reinforced concrete thin shell structures. Thin-Walled Struct. 43 (9), 1418–1443. doi:10.1016/j.tws.2005.04.004
Popov, E. P. (1991). Progress in analysis and design of RC shells. Eng. Struct. 13 (2), 128–143. doi:10.1016/0141-0296(91)90047-g
Qian, R. J., Shen, Z. Y., Xia, S. H., and Hong, X. Q. (1996). Structure concepts and mechanisms of tensegrity system. Spat. Struct. 2 (3), 2–7. doi:10.13849/j.issn.1006-6578.1996.03.001
Qian, R. J., and Yang, L. P. (2003). Analysis, design and construction of tensioned structures . Nanjing: Southeast University Press , 160.
Shen, S. Z., and Chen, X. (1999). Stability of the mesh shell structure . Peking: Science Press , 88–92.
Uddin, N., and Du, W. F. (2014). New thin shells made of composite structural insulated panels. J. Reinf. Plastics Compos. 33 (21), 1954–1965. doi:10.1177/0731684414549492
Yang, L. L. (2010). Structural response changes of thin-shell domes of different spans under seismic effects. Mass Technology 11, 19.
Yang, X., Du, W. F., Liu, C. Y., and Sun, Y. (2017). Mechanical properties and experimental researches of new CSIPs sandwich panels. J. Henan Univ. Sci. 47 (3), 358–365. doi:10.15991/j.cnki.411100.2017.03.014
Zhang, F., Du, W. F., and Zhang, H. (2021). A novel bionic-based substructure division method for topology optimization. Structures 29 (4), 937–946. doi:10.1016/j.istruc.2020.11.061
Zou, S. W. (2019). Discussion on construction technology of dome structure. Constr. Budg. 11, 85–88. doi:10.13993/j.cnki.jzyys.2019.11.023
Keywords: composite structural insulation panels, large-span domes, numerical simulation, simulation analysis, application
Citation: Liang C and Zhu L (2022) A case study on dome structure of composite structural insulation panels with ANSYS simulations. Front. Mater. 9:1072600. doi: 10.3389/fmats.2022.1072600
Received: 17 October 2022; Accepted: 18 November 2022; Published: 30 November 2022.
Reviewed by:
Copyright © 2022 Liang and Zhu. This is an open-access article distributed under the terms of the Creative Commons Attribution License (CC BY). The use, distribution or reproduction in other forums is permitted, provided the original author(s) and the copyright owner(s) are credited and that the original publication in this journal is cited, in accordance with accepted academic practice. No use, distribution or reproduction is permitted which does not comply with these terms.
*Correspondence: Liming Zhu, [email protected]
- Current Students
Journal Highlights Math Research by Professor and Student
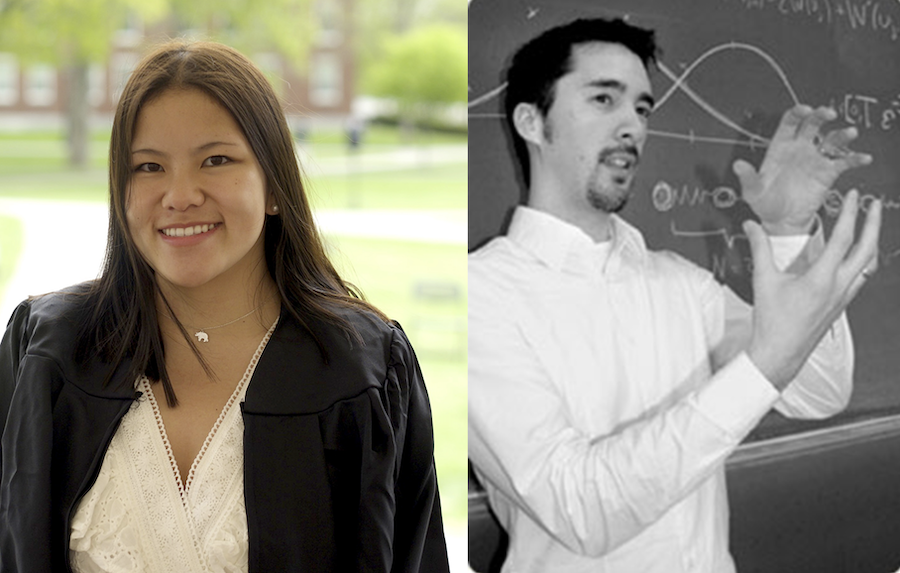
The paper , "Modulation instability and wavenumber bandgap breathers in a time layered phononic lattice," is the result of a yearslong collaboration of Chong, Wallace, and two researchers at CalTech.
Their project focused on how pressure waves can be manipulated by materials. It began in 2021 with a theoretical component—which Wallace worked on for her honors theses, " Instability in a Time-Modulated Lattice .” She is now wrapping up a master's degree in applied mathematics at Columbia University.
Wallace said she reached out to Chong in 2021 to inquire whether she could assist him with a research project. She had excelled in his ordinary differential equations and partial differential equations classes, Chong said, and he was just launching a new research program. So he invited her to work as his research assistant in the summer, a partnership that extended through her senior year.
The work Wallace completed in the first stage of the research program was followed by numerical and experimental simulations, conducted by Chong and his Caltech teammates, which was then backed up with rigorous mathematical proofs in a follow-up paper. “It is a nice spectrum that goes from Evy's analytical computations to computer simulations, the laboratory, and to mathematical proof,” Chong said.
New Insights
Their April 11 paper is garnering attention because it opens new ground in the field of physical energy. Their project specifically focused on understanding how pressure waves are affected by media that varies over time. (Pressure waves, of which the most commonly known is a sound wave, are set off when a mass or object is compressed or decompressed by an external force, such as an explosion.)
Traditionally, researchers have experimented with pressure waves using media that vary in space while they remain constant in time.
For those who are not familiar with his field, Chong came up with a helpful analogy to explain his research, comparing the time-varying media he and Wallace studied to a floor, and the waves passing over the media to a person walking across that floor. “A traditional floor might have a pattern of alternating spots of rough and smooth areas,” he began. “As you’re walking across the floor, how your feet touch the ground will affect how you walk along it.”
In contrast, a medium that changes over time resembles a wacky floor in an amusement park fun house. Rather than having both smooth and rough patches, it is entirely smooth one moment before shifting to a rough surface, switching back and forth between these two patterns. “How you walk through that medium will be different than walking across a traditional floor,” Chong said.
Wallace's original calculations showed exactly where this system would be unstable, which is desirable. When a system is stable, “it means the amplitude of the wave doesn't grow,” Chong said, whereas an unstable system results in a wave that amplifies quite a bit.
“What Evy did was to tell us exactly what material parameters would be needed for that to happen, an exact formula for when and under what circumstances you get interesting instability,” he said. “Having a formula that exact is quite rare. Usually you have to approximate it or do some numerical simulations.”
By the way, the materials they studied aren't linoleum or wood panels or anything most of us would recognize as typical flooring materials. “Our experiment consists of magnets,” Chong said. They looked at variables such as the distance and strength of magnets, as well as the effect of dampening from air friction. “Each of those properties has a parameter that you can tune, so having a formula saying this combination of parameters leads to amplification is very helpful.”
What is of particular interest to researchers working in this area is how to maintain the force of a wave or signal, because over time, all waves degrade. Each electrical circuit, for example, needs an amplifier to work, Chong said.
“So when talking about the applications of our work, our system would act as an amplifier,” he added. “Based on what Evy did, you would know exactly how to make the material so that the wave would amplify.”
Possible applications
Though Chong and his team are working on a basic research level, in the long term, understanding the systems they are studying could lead to innovations in “acoustic logic,” Chong explained, which is like the logic underlying electrical circuits, but which don't use electricity but rather pressure waves. They could even help create a situation where “acoustic circuits” could operate with the use of ambient vibrations only, and thus avoid detection from sensors.
“We’re still doing work that is more fundamental,” Chong said. “But understanding how these systems behave could contribute to that the much-longer project of having a goal like that.”
Wallace's undergraduate work with Chong led to a research collaboration with a Columbia faculty member. With him, Wallace expanded Chong's one-dimensional study to two dimensions.
Starting this type of high-level research at Bowdoin, Wallace said, “synthesized everything I had learned at Bowdoin and learned about math in general.” She continued, “Doing research allowed me to take what I learned out of the classroom and apply it to something I could see in the the real world, and see how it would be very important and relevant.”
The journal, which is open access, and Bowdoin College provided support to cover the publication fees via the Fletcher Family Research Grant. Wallace's research was supported by a National Science Foundation grant and the Bowdoin Kaufman Family Fellowship .
Related Stories
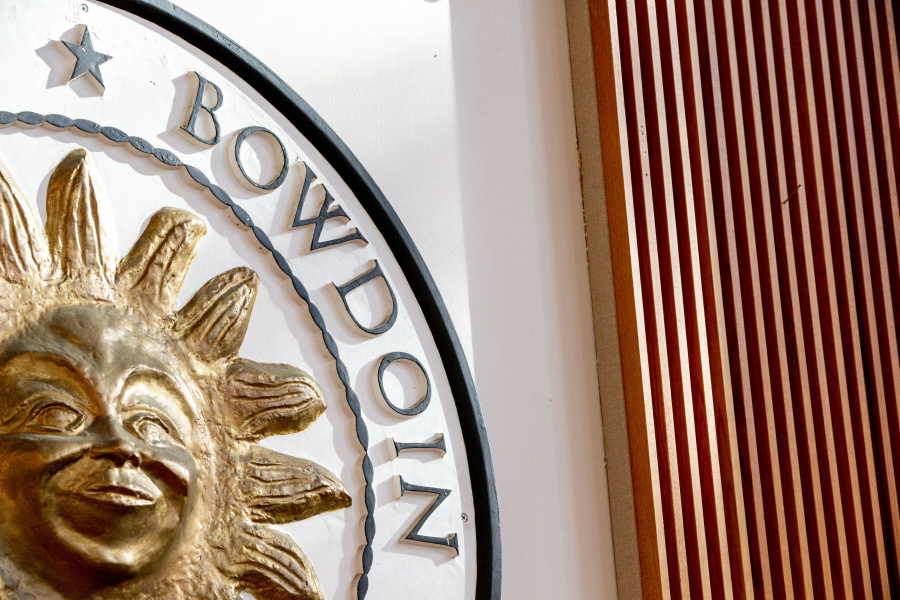
- Make a Gift
- Request Info
- Offices & Services A-Z
- Accessibility

Biostatistics Graduate Program
Julia thome is first author of public health reports paper.
Posted by duthip1 on Wednesday, May 29, 2024 in News .
Congratulations to PhD candidate Julia Thome on the publication of Reporting of Child Maltreatment During the COVID-19 Pandemic in a Southern State in the United States in Public Health Reports last week, online ahead of print. The paper was co-authored by associate professor Rameela Raman and colleagues at the Vanderbilt Center of Excellence for Children in State Custody, which is within the Vanderbilt Department of Psychiatry & Behavioral Sciences. Thome, Raman, and the other members of this team studied how COVID-19 stay-at-home orders may have affected trends in child maltreatment allegations across different socioeconomic groups.
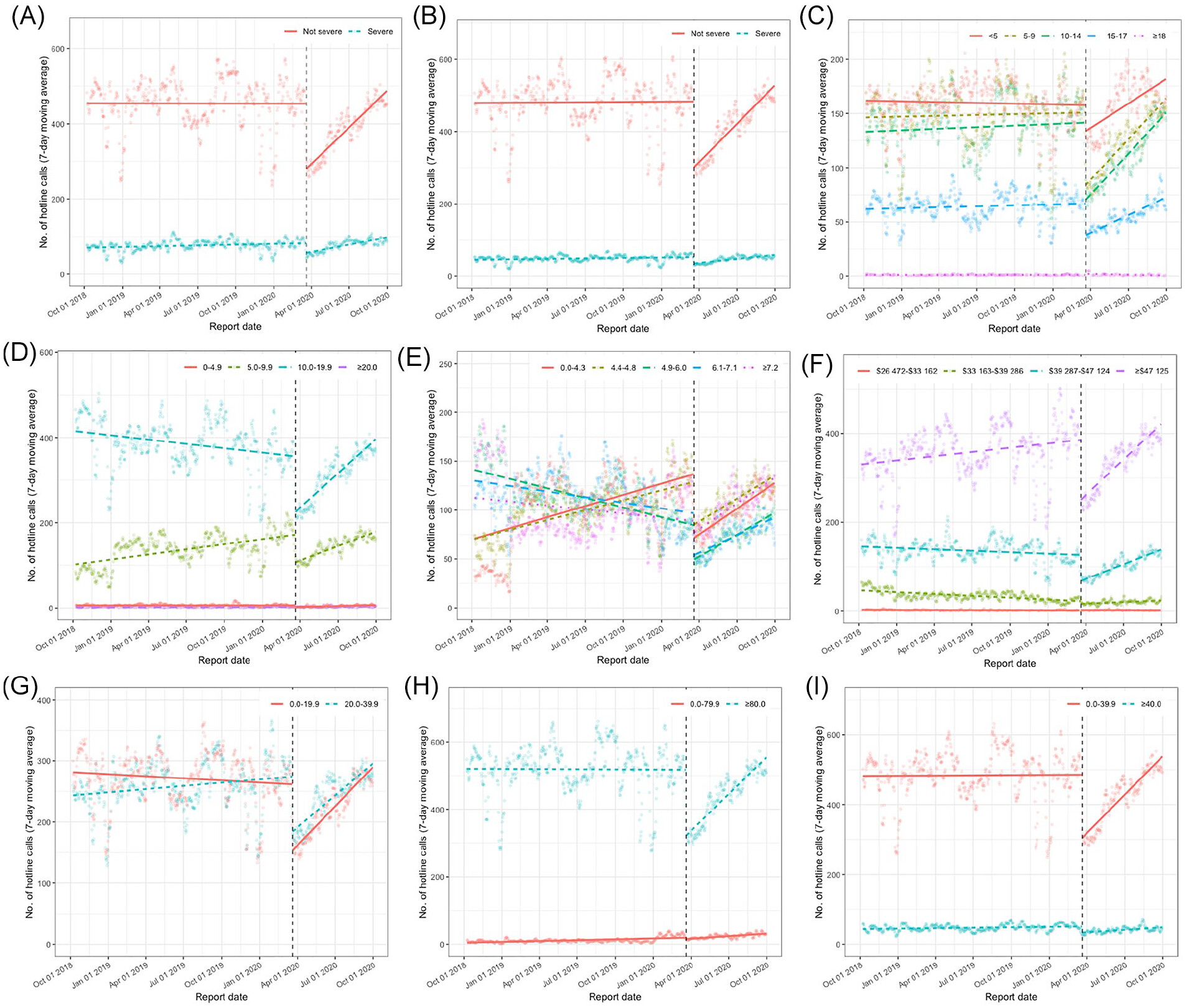
Tags: child abuse , child maltreatment , child neglect , COVID-19 , hotline calls , publications
Leave a Response
You must be logged in to post a comment
Academia.edu no longer supports Internet Explorer.
To browse Academia.edu and the wider internet faster and more securely, please take a few seconds to upgrade your browser .
- We're Hiring!
- Help Center
- Most Cited Papers
- Most Downloaded Papers
- Newest Papers
- Save to Library
- Indian Climate and Vegetation Follow Following
- Landsape Follow Following
- Barrier Free Design Follow Following
- Interiors Follow Following
- Arches Follow Following
- Gemoetric Patterns Follow Following
- Accessibility and universal design (Architecture) Follow Following
- Environmental Philosophy Follow Following
- Mosque Architecture Follow Following
- Islamic Calligraphy Follow Following
Enter the email address you signed up with and we'll email you a reset link.
- Academia.edu Publishing
- We're Hiring!
- Help Center
- Find new research papers in:
- Health Sciences
- Earth Sciences
- Cognitive Science
- Mathematics
- Computer Science
- Academia ©2024
Purdue Hospitality and Tourism Management graduate student wins best paper award for innovative technology research
Written By: Rebecca Hoffa, [email protected]
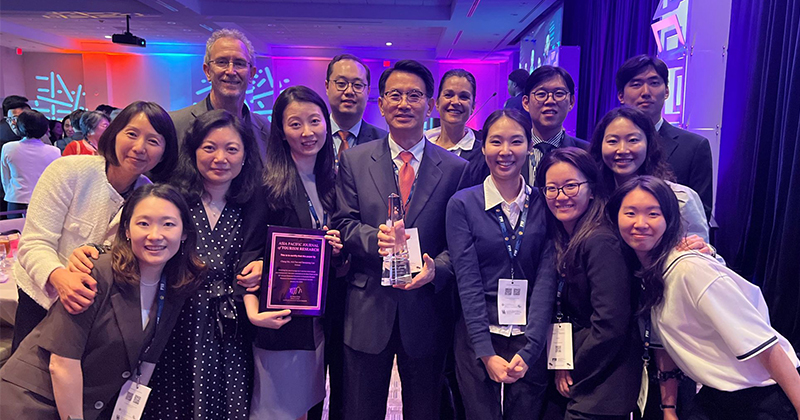
Evita Ma poses with the fellow Purdue HTM attendees at the 29th Annual Graduate Education and Graduate Student Research Conference in Hospitality and Tourism in January. (Photo provided)
As Starship robots deliver food to hungry Boilermakers across Purdue University’s West Lafayette campus, their presence is often welcomed like that of a neighborhood pet — students are often seen helping them when they get stuck or smiling and moving out of their way when they meet them on the sidewalk. When Alei (Aileen) Fan , associate professor in the White Lodging-J.W. Marriott, Jr. School of Hospitality and Tourism Management (HTM) and an expert in service innovation and experience design, helped one along its way one day, she was met with a low-tone, male voice saying, “Thank you.” This contradicted the “cute” vision Fan had in her mind of the robots being like her dog at home.
When Fan relayed the experience to her PhD student Chang (Evita) Ma , the College of Health and Human Sciences graduate student was inspired to investigate deeper how the combination of appearance and voice impacted the consumer’s overall experience in the service encounter.
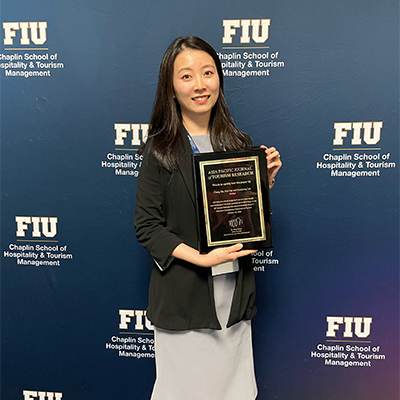
Evita Ma poses with her best paper award at the 29th Annual Graduate Education and Graduate Student Research Conference in Hospitality and Tourism. (Photo provided)
These efforts culminated in winning a best paper award at the 29th Annual Graduate Education and Graduate Student Research Conference in Hospitality and Tourism in January.
“I’m really honored,” Ma said. “As far as I know, for the past three years or so, no one from our school has gotten the award, so it was a pleasure to have that. It’s very competitive — over the 130-some presentations, I was selected as one of the four winners.”
The study looked at how people’s reactions differed when comparing matched, or congruent, voice and appearance and mismatched, or incongruent, voice and appearance. The researchers found that depending on the robot’s function, people tended to be either more or less accepting of when a robot’s voice does not match its appearance.
In a utilitarian scenario where the consumer really only desires the robot to perform a job function and doesn’t care as much about having an emotional connection, people often prefer the congruent voice and appearance because they feel the incongruent one is not well-designed or not capable of performing their desired outcome. When consumers are in a hedonic scenario where they don’t care as much about the functions the robot is performing but simply wish to have a connection or engage with the robot, the congruency doesn’t matter as much, and some of the surprising elements, such as the mismatched voice and appearance, may attract people to engage with it.
“We as human beings actually view things holistically,” Ma said. “We don’t separate the different parts.”
Considering how these distinct factors work together to shape consumer perceptions could ultimately shape the characteristics of future service robots to improve service encounters across the industry.
“It’s a timely topic,” Fan said. “Whether you like it or not, technology takes up a lot of our lives. This research provided practical guidelines for industries and companies on how to design different robots to better serve our customer.”
This study resulted as a product of Ma’s study “Decoding the Shared Pathways of Consumer Technology Experience in Hospitality and Tourism: A Meta-Analysis,” which examined the literature currently available to investigate the different types of technology and how humans interact with them. Ma ultimately noticed a gap in papers that combined elements — many simply looked at appearance or voice independently.
“Very few of the papers actually combine all the different subtle elements together; they just focus on one single perspective like appearance or voice,” Ma said. “So, I began looking at: What is the combination between those? Our initial idea was we wanted to know how the combination of design elements of service robots impacts the customer’s reactions and feelings of the service in different scenarios.”
Prior to coming to Purdue, Ma spent four years gaining industry experience at the Hospitality Financial and Technology Professionals nonprofit in Hong Kong, where she made many connections on the technology side of the industry and solidified her interest in coming to a very technology-focused university to earn her PhD.
“Purdue has such a strong background in terms of technology and engineering, so that was also one of the reasons I wanted to keep focusing on that for my research area,” Ma said. “After taking a class with Dr. Fan, I decided to join her team.”
Beyond research, Ma has fully immersed herself in the teaching and engagement areas of graduate student life as well. Ma teaches two 200-level marketing courses to undergraduate HTM students, where she’s maximized opportunities for the students to engage in experiential education. She’s also working on curriculum development with HTM faculty and has become involved in several graduate student organizations, including the Purdue Graduate Student Government, where she is a senator.
After graduating from the program, Ma plans to pursue a faculty position that allows her to combine her passion for research with her love for teaching and service.
“She’s really the star student,” Fan said. “When we evaluate a PhD student, there are three aspects: research, teaching and service. Evita is excellent in all of these.”
A new future of work: The race to deploy AI and raise skills in Europe and beyond
At a glance.
Amid tightening labor markets and a slowdown in productivity growth, Europe and the United States face shifts in labor demand, spurred by AI and automation. Our updated modeling of the future of work finds that demand for workers in STEM-related, healthcare, and other high-skill professions would rise, while demand for occupations such as office workers, production workers, and customer service representatives would decline. By 2030, in a midpoint adoption scenario, up to 30 percent of current hours worked could be automated, accelerated by generative AI (gen AI). Efforts to achieve net-zero emissions, an aging workforce, and growth in e-commerce, as well as infrastructure and technology spending and overall economic growth, could also shift employment demand.
By 2030, Europe could require up to 12 million occupational transitions, double the prepandemic pace. In the United States, required transitions could reach almost 12 million, in line with the prepandemic norm. Both regions navigated even higher levels of labor market shifts at the height of the COVID-19 period, suggesting that they can handle this scale of future job transitions. The pace of occupational change is broadly similar among countries in Europe, although the specific mix reflects their economic variations.
Businesses will need a major skills upgrade. Demand for technological and social and emotional skills could rise as demand for physical and manual and higher cognitive skills stabilizes. Surveyed executives in Europe and the United States expressed a need not only for advanced IT and data analytics but also for critical thinking, creativity, and teaching and training—skills they report as currently being in short supply. Companies plan to focus on retraining workers, more than hiring or subcontracting, to meet skill needs.
Workers with lower wages face challenges of redeployment as demand reweights toward occupations with higher wages in both Europe and the United States. Occupations with lower wages are likely to see reductions in demand, and workers will need to acquire new skills to transition to better-paying work. If that doesn’t happen, there is a risk of a more polarized labor market, with more higher-wage jobs than workers and too many workers for existing lower-wage jobs.
Choices made today could revive productivity growth while creating better societal outcomes. Embracing the path of accelerated technology adoption with proactive worker redeployment could help Europe achieve an annual productivity growth rate of up to 3 percent through 2030. However, slow adoption would limit that to 0.3 percent, closer to today’s level of productivity growth in Western Europe. Slow worker redeployment would leave millions unable to participate productively in the future of work.

Demand will change for a range of occupations through 2030, including growth in STEM- and healthcare-related occupations, among others
This report focuses on labor markets in nine major economies in the European Union along with the United Kingdom, in comparison with the United States. Technology, including most recently the rise of gen AI, along with other factors, will spur changes in the pattern of labor demand through 2030. Our study, which uses an updated version of the McKinsey Global Institute future of work model, seeks to quantify the occupational transitions that will be required and the changing nature of demand for different types of jobs and skills.
Our methodology
We used methodology consistent with other McKinsey Global Institute reports on the future of work to model trends of job changes at the level of occupations, activities, and skills. For this report, we focused our analysis on the 2022–30 period.
Our model estimates net changes in employment demand by sector and occupation; we also estimate occupational transitions, or the net number of workers that need to change in each type of occupation, based on which occupations face declining demand by 2030 relative to current employment in 2022. We included ten countries in Europe: nine EU members—the Czech Republic, Denmark, France, Germany, Italy, Netherlands, Poland, Spain, and Sweden—and the United Kingdom. For the United States, we build on estimates published in our 2023 report Generative AI and the future of work in America.
We included multiple drivers in our modeling: automation potential, net-zero transition, e-commerce growth, remote work adoption, increases in income, aging populations, technology investments, and infrastructure investments.
Two scenarios are used to bookend the work-automation model: “late” and “early.” For Europe, we modeled a “faster” scenario and a “slower” one. For the faster scenario, we use the midpoint—the arithmetical average between our late and early scenarios. For the slower scenario, we use a “mid late” trajectory, an arithmetical average between a late adoption scenario and the midpoint scenario. For the United States, we use the midpoint scenario, based on our earlier research.
We also estimate the productivity effects of automation, using GDP per full-time-equivalent (FTE) employee as the measure of productivity. We assumed that workers displaced by automation rejoin the workforce at 2022 productivity levels, net of automation, and in line with the expected 2030 occupational mix.
Amid tightening labor markets and a slowdown in productivity growth, Europe and the United States face shifts in labor demand, spurred not only by AI and automation but also by other trends, including efforts to achieve net-zero emissions, an aging population, infrastructure spending, technology investments, and growth in e-commerce, among others (see sidebar, “Our methodology”).
Our analysis finds that demand for occupations such as health professionals and other STEM-related professionals would grow by 17 to 30 percent between 2022 and 2030, (Exhibit 1).
By contrast, demand for workers in food services, production work, customer services, sales, and office support—all of which declined over the 2012–22 period—would continue to decline until 2030. These jobs involve a high share of repetitive tasks, data collection, and elementary data processing—all activities that automated systems can handle efficiently.
Up to 30 percent of hours worked could be automated by 2030, boosted by gen AI, leading to millions of required occupational transitions
By 2030, our analysis finds that about 27 percent of current hours worked in Europe and 30 percent of hours worked in the United States could be automated, accelerated by gen AI. Our model suggests that roughly 20 percent of hours worked could still be automated even without gen AI, implying a significant acceleration.
These trends will play out in labor markets in the form of workers needing to change occupations. By 2030, under the faster adoption scenario we modeled, Europe could require up to 12.0 million occupational transitions, affecting 6.5 percent of current employment. That is double the prepandemic pace (Exhibit 2). Under a slower scenario we modeled for Europe, the number of occupational transitions needed would amount to 8.5 million, affecting 4.6 percent of current employment. In the United States, required transitions could reach almost 12.0 million, affecting 7.5 percent of current employment. Unlike Europe, this magnitude of transitions is broadly in line with the prepandemic norm.
Both regions navigated even higher levels of labor market shifts at the height of the COVID-19 period. While these were abrupt and painful to many, given the forced nature of the shifts, the experience suggests that both regions have the ability to handle this scale of future job transitions.

Businesses will need a major skills upgrade
The occupational transitions noted above herald substantial shifts in workforce skills in a future in which automation and AI are integrated into the workplace (Exhibit 3). Workers use multiple skills to perform a given task, but for the purposes of our quantification, we identified the predominant skill used.
Demand for technological skills could see substantial growth in Europe and in the United States (increases of 25 percent and 29 percent, respectively, in hours worked by 2030 compared to 2022) under our midpoint scenario of automation adoption (which is the faster scenario for Europe).
Demand for social and emotional skills could rise by 11 percent in Europe and by 14 percent in the United States. Underlying this increase is higher demand for roles requiring interpersonal empathy and leadership skills. These skills are crucial in healthcare and managerial roles in an evolving economy that demands greater adaptability and flexibility.
Conversely, demand for work in which basic cognitive skills predominate is expected to decline by 14 percent. Basic cognitive skills are required primarily in office support or customer service roles, which are highly susceptible to being automated by AI. Among work characterized by these basic cognitive skills experiencing significant drops in demand are basic data processing and literacy, numeracy, and communication.
Demand for work in which higher cognitive skills predominate could also decline slightly, according to our analysis. While creativity is expected to remain highly sought after, with a potential increase of 12 percent by 2030, work activities characterized by other advanced cognitive skills such as advanced literacy and writing, along with quantitative and statistical skills, could decline by 19 percent.
Demand for physical and manual skills, on the other hand, could remain roughly level with the present. These skills remain the largest share of workforce skills, representing about 30 percent of total hours worked in 2022. Growth in demand for these skills between 2022 and 2030 could come from the build-out of infrastructure and higher investment in low-emissions sectors, while declines would be in line with continued automation in production work.
Business executives report skills shortages today and expect them to worsen
A survey we conducted of C-suite executives in five countries shows that companies are already grappling with skills challenges, including a skills mismatch, particularly in technological, higher cognitive, and social and emotional skills: about one-third of the more than 1,100 respondents report a shortfall in these critical areas. At the same time, a notable number of executives say they have enough employees with basic cognitive skills and, to a lesser extent, physical and manual skills.
Within technological skills, companies in our survey reported that their most significant shortages are in advanced IT skills and programming, advanced data analysis, and mathematical skills. Among higher cognitive skills, significant shortfalls are seen in critical thinking and problem structuring and in complex information processing. About 40 percent of the executives surveyed pointed to a shortage of workers with these skills, which are needed for working alongside new technologies (Exhibit 4).

Companies see retraining as key to acquiring needed skills and adapting to the new work landscape
Surveyed executives expect significant changes to their workforce skill levels and worry about not finding the right skills by 2030. More than one in four survey respondents said that failing to capture the needed skills could directly harm financial performance and indirectly impede their efforts to leverage the value from AI.
To acquire the skills they need, companies have three main options: retraining, hiring, and contracting workers. Our survey suggests that executives are looking at all three options, with retraining the most widely reported tactic planned to address the skills mismatch: on average, out of companies that mentioned retraining as one of their tactics to address skills mismatch, executives said they would retrain 32 percent of their workforce. The scale of retraining needs varies in degree. For example, respondents in the automotive industry expect 36 percent of their workforce to be retrained, compared with 28 percent in the financial services industry. Out of those who have mentioned hiring or contracting as their tactics to address the skills mismatch, executives surveyed said they would hire an average of 23 percent of their workforce and contract an average of 18 percent.
Occupational transitions will affect high-, medium-, and low-wage workers differently
All ten European countries we examined for this report may see increasing demand for top-earning occupations. By contrast, workers in the two lowest-wage-bracket occupations could be three to five times more likely to have to change occupations compared to the top wage earners, our analysis finds. The disparity is much higher in the United States, where workers in the two lowest-wage-bracket occupations are up to 14 times more likely to face occupational shifts than the highest earners. In Europe, the middle-wage population could be twice as affected by occupational transitions as the same population in United States, representing 7.3 percent of the working population who might face occupational transitions.
Enhancing human capital at the same time as deploying the technology rapidly could boost annual productivity growth
About quantumblack, ai by mckinsey.
QuantumBlack, McKinsey’s AI arm, helps companies transform using the power of technology, technical expertise, and industry experts. With thousands of practitioners at QuantumBlack (data engineers, data scientists, product managers, designers, and software engineers) and McKinsey (industry and domain experts), we are working to solve the world’s most important AI challenges. QuantumBlack Labs is our center of technology development and client innovation, which has been driving cutting-edge advancements and developments in AI through locations across the globe.
Organizations and policy makers have choices to make; the way they approach AI and automation, along with human capital augmentation, will affect economic and societal outcomes.
We have attempted to quantify at a high level the potential effects of different stances to AI deployment on productivity in Europe. Our analysis considers two dimensions. The first is the adoption rate of AI and automation technologies. We consider the faster scenario and the late scenario for technology adoption. Faster adoption would unlock greater productivity growth potential but also, potentially, more short-term labor disruption than the late scenario.
The second dimension we consider is the level of automated worker time that is redeployed into the economy. This represents the ability to redeploy the time gained by automation and productivity gains (for example, new tasks and job creation). This could vary depending on the success of worker training programs and strategies to match demand and supply in labor markets.
We based our analysis on two potential scenarios: either all displaced workers would be able to fully rejoin the economy at a similar productivity level as in 2022 or only some 80 percent of the automated workers’ time will be redeployed into the economy.
Exhibit 5 illustrates the various outcomes in terms of annual productivity growth rate. The top-right quadrant illustrates the highest economy-wide productivity, with an annual productivity growth rate of up to 3.1 percent. It requires fast adoption of technologies as well as full redeployment of displaced workers. The top-left quadrant also demonstrates technology adoption on a fast trajectory and shows a relatively high productivity growth rate (up to 2.5 percent). However, about 6.0 percent of total hours worked (equivalent to 10.2 million people not working) would not be redeployed in the economy. Finally, the two bottom quadrants depict the failure to adopt AI and automation, leading to limited productivity gains and translating into limited labor market disruptions.

Four priorities for companies
The adoption of automation technologies will be decisive in protecting businesses’ competitive advantage in an automation and AI era. To ensure successful deployment at a company level, business leaders can embrace four priorities.
Understand the potential. Leaders need to understand the potential of these technologies, notably including how AI and gen AI can augment and automate work. This includes estimating both the total capacity that these technologies could free up and their impact on role composition and skills requirements. Understanding this allows business leaders to frame their end-to-end strategy and adoption goals with regard to these technologies.
Plan a strategic workforce shift. Once they understand the potential of automation technologies, leaders need to plan the company’s shift toward readiness for the automation and AI era. This requires sizing the workforce and skill needs, based on strategically identified use cases, to assess the potential future talent gap. From this analysis will flow details about the extent of recruitment of new talent, upskilling, or reskilling of the current workforce that is needed, as well as where to redeploy freed capacity to more value-added tasks.
Prioritize people development. To ensure that the right talent is on hand to sustain the company strategy during all transformation phases, leaders could consider strengthening their capabilities to identify, attract, and recruit future AI and gen AI leaders in a tight market. They will also likely need to accelerate the building of AI and gen AI capabilities in the workforce. Nontechnical talent will also need training to adapt to the changing skills environment. Finally, leaders could deploy an HR strategy and operating model to fit the post–gen AI workforce.
Pursue the executive-education journey on automation technologies. Leaders also need to undertake their own education journey on automation technologies to maximize their contributions to their companies during the coming transformation. This includes empowering senior managers to explore automation technologies implications and subsequently role model to others, as well as bringing all company leaders together to create a dedicated road map to drive business and employee value.
AI and the toolbox of advanced new technologies are evolving at a breathtaking pace. For companies and policy makers, these technologies are highly compelling because they promise a range of benefits, including higher productivity, which could lift growth and prosperity. Yet, as this report has sought to illustrate, making full use of the advantages on offer will also require paying attention to the critical element of human capital. In the best-case scenario, workers’ skills will develop and adapt to new technological challenges. Achieving this goal in our new technological age will be highly challenging—but the benefits will be great.
Eric Hazan is a McKinsey senior partner based in Paris; Anu Madgavkar and Michael Chui are McKinsey Global Institute partners based in New Jersey and San Francisco, respectively; Sven Smit is chair of the McKinsey Global Institute and a McKinsey senior partner based in Amsterdam; Dana Maor is a McKinsey senior partner based in Tel Aviv; Gurneet Singh Dandona is an associate partner and a senior expert based in New York; and Roland Huyghues-Despointes is a consultant based in Paris.
Explore a career with us
Related articles.

Generative AI and the future of work in America

The economic potential of generative AI: The next productivity frontier
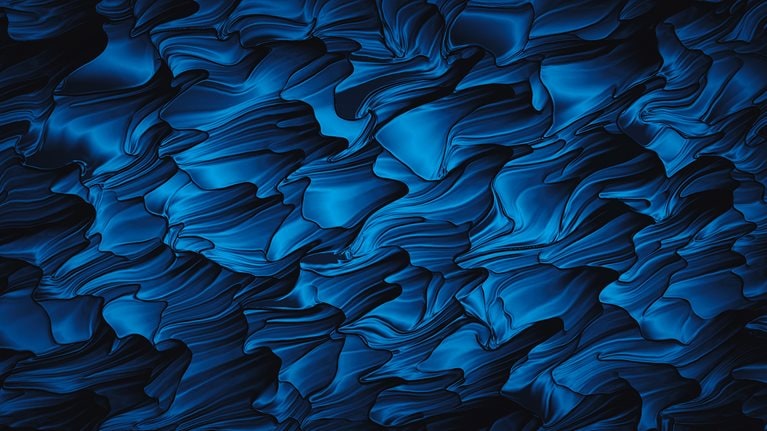
What every CEO should know about generative AI
Suggestions or feedback?
MIT News | Massachusetts Institute of Technology
- Machine learning
- Social justice
- Black holes
- Classes and programs
Departments
- Aeronautics and Astronautics
- Brain and Cognitive Sciences
- Architecture
- Political Science
- Mechanical Engineering
Centers, Labs, & Programs
- Abdul Latif Jameel Poverty Action Lab (J-PAL)
- Picower Institute for Learning and Memory
- Lincoln Laboratory
- School of Architecture + Planning
- School of Engineering
- School of Humanities, Arts, and Social Sciences
- Sloan School of Management
- School of Science
- MIT Schwarzman College of Computing
Scientists identify mechanism behind drug resistance in malaria parasite
Press contact :.
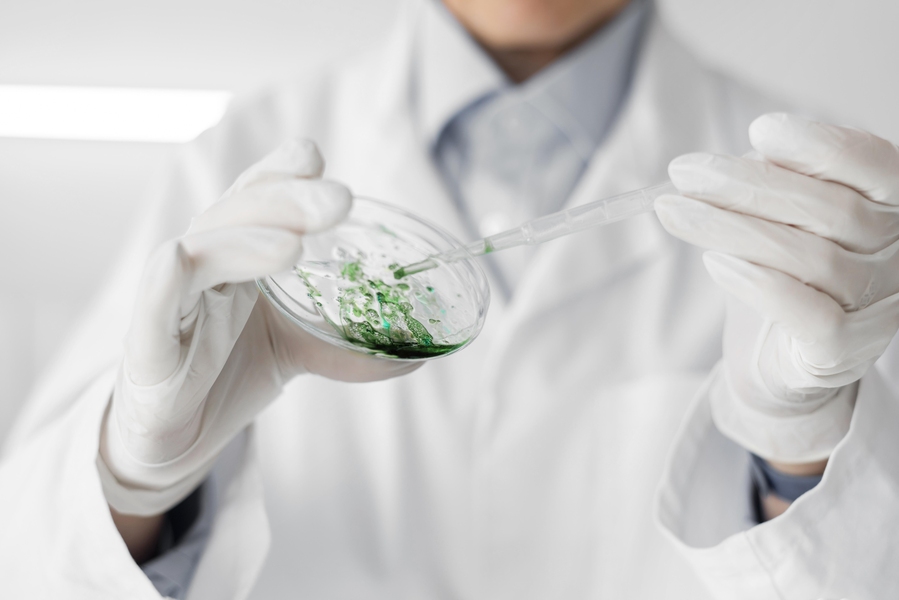
Previous image Next image
Share this news article on:
Related links.
- Peter Dedon
- Antimicrobial Resistance Interdisciplinary Research Group
- Singapore-MIT Alliance for Research and Technology (SMART)
- Department of Biological Engineering
Related Topics
- Drug resistance
- Antibiotics
- Biological engineering
- Drug development
- International initiatives
- Collaboration
Related Articles
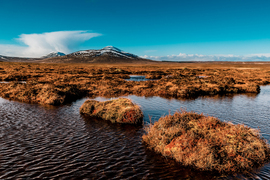
Satellite-based method measures carbon in peat bogs
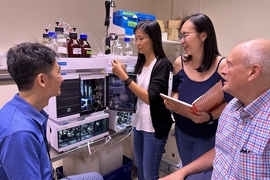
Newly discovered bacterial communication system aids antimicrobial resistance
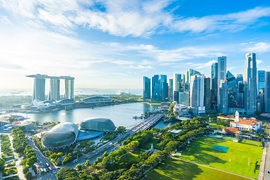
SMART launches research group to advance AI, automation, and the future of work
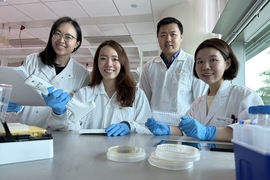
A novel combination therapy counters antibiotic-resistant Mycobacterium abscessus infections
Previous item Next item
More MIT News
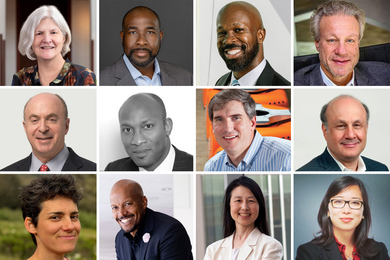
MIT Corporation elects 10 term members, two life members
Read full story →
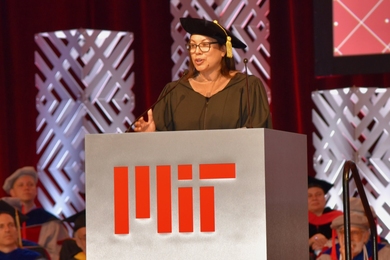
Diane Hoskins ’79: How going off-track can lead new SA+P graduates to become integrators of ideas
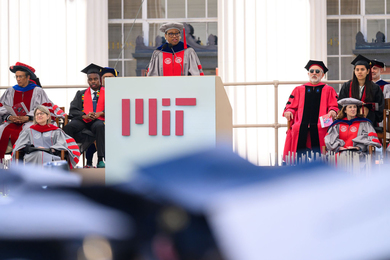
Chancellor Melissa Nobles’ address to MIT’s undergraduate Class of 2024
Noubar Afeyan PhD ’87 gives new MIT graduates a special assignment
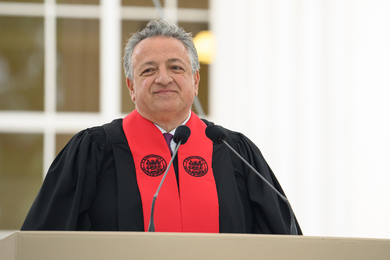
Commencement address by Noubar Afeyan PhD ’87
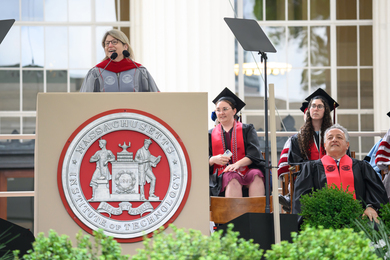
President Sally Kornbluth’s charge to the Class of 2024
- More news on MIT News homepage →
Massachusetts Institute of Technology 77 Massachusetts Avenue, Cambridge, MA, USA
- Map (opens in new window)
- Events (opens in new window)
- People (opens in new window)
- Careers (opens in new window)
- Accessibility
- Social Media Hub
- MIT on Facebook
- MIT on YouTube
- MIT on Instagram

IMAGES
VIDEO
COMMENTS
The study of domes still represents a thorny subject. In fact, large dimensions coupled with a limited accessibility make the study of their shapes and geometry a traditionally difficult task (Rondelet 1802-17; Leroy 1845).Although throughout history this problem has been tackled in different ways (Letaroully 1840-55; Emerson and Van Nice 1943; Dorffner et al. 2000; Hidaka and Satō 2004 ...
Study of Dome structures with specific Focus on Monolithic. and Geodesic Domes for Housing. Riya Anna Abraham, G. Kesava Chandran. 1 3rd Year B.Tech, Civil Engineering, Manipal Institute of ...
A dome-covered house is an example of designing sustainable buildings by learning from the optimized biological forms from the nature. The paper presents a 3-D thermal and air flow (3D-TAF) model ...
The address: 23 Ismail Alkbany St, From Tay ran St, Naser-City, Cairo, Egypt. Telephone Number: 002 02 27270353 Mobile: 002 0122488 5337 Email: Maged_alyyossef@ yahoo.com. POWER OF DOMES IN ...
This paper aims to determine which type of reticulated dome is superior in terms of material efficiency by comparing the minimized weight of different dome types, taking into account stress and buckling constraints. The study includes hemispherical Schwedler, Kiewitt, and geodesic domes with a diameter of 16m, and a gravity load of 2kNm−2.
International Journal of Research Publication and Reviews, Vol 4, no 9, pp 3485-3488 September 2023 3486 Mihailescu and Sundaram's study in 2009 delves into the significance of quality control during the construction of domes. The research not only addresses construction processes but also highlights considerations for waterproofing and insulation.
Journal of Geophysical Research: Solid Earth is a premier AGU geophysics journal, publishing research articles from across the Earth sciences that significantly advance the field. Abstract The surface deformation field measured at volcanic domes provides insights into the effects of magmatic processes, gravity- and gas-driven processes, and the ...
The experimental research described in this paper has contributed to the understanding of the system's structural behaviour, and has allowed further validation of the feasibility of the construction technology and its application to doubly-curved vaults. This validation has been achieved by building and load-testing two composite sail domes.
Our paper concludes that both geodesic and monolithic domes are sustainable structures for housing and points out that further research and investigation needs to be undertaken on the same. Keywords—Geodesic domes, Geodesic spheres, Monolithic domes, Flat Roofs, Airforming, Shell, Geotangent, Surface Area.
In this paper, ANSYS 14.0 software is used to analyze performance of CSIPs dome and thin-shell concrete dome. The research program is divided into three processes: selection of the basic dome research model, establishment of the finite element model, and comparative analysis of the strength, stiffness, and stability of the two research domes.
Dome is a hollow semi-spherical self-supporting structural element that is held in compression by gravity acting on the structure. In contrast to the use of domes as roof structures, this paper presents the analysis of structural domes for housing construction. Their light-weightiness, efficiency, and cost-effectiveness is much more advantageous owing to curved surfaces and enhanced use of ...
International Research Journal of Engineering and Technology (IRJET) e-ISSN: 2395-0056 Volume: 09 Issue: 07 | July 2022 www.irjet.net p-ISSN: 2395-0072 Review on Comparative Study on Behaviour of Various Dome Structures for Different Parameters Mr. Aniket G. Sawant 1, Prof. Pandurang S. Patil 2 1PG Student, Dept. of Civil Engineering, Rajarambapu Institute of Technology, Maharashtra, India ...
In this research paper, the analysis of steel dome is examined using computer software E-tabs. This paper conducts a deep study of major features of structural analysis of E-tab software. E-tab software has the analysis of its own features option, output option, limitation, and advantages. Dome is one of the powerful architectural representations.
a lot in the design and construction of geodesic domes. V. K. Dogra (2) has described a step-by step procedure for the construction of geodesic domes with A4 size waste papers. Still the geometry of the geodesic dome is a riddle to many of the engineers and architects. In this paper the geometry of the geodesic domes, based on two types of ...
Monolithic dome is a rounded building structure typically made of concrete and rigid steel rods. It consists of an outer airtight form, polyurethane foam insulation and reinforced concrete. The ...
Research Paper On Domes. Decent Essays. 746 Words. 3 Pages. Open Document. In the beginning a lot of people thought they were going to die. Hi I'm Maddie Baker and I am a survivor of a horrible event. In 2020 all the national newsgroups were afraid their systems were picking up evidence of a 75 mile wide meteorite coming towards Earth.
In 2022, IOPP retracted nearly 500 papers from conference proceedings after the PPS flagged tortured phrases in the papers. When Eggleton and her team investigated, they found reams of other problems—fake identity, citation cartels in which researchers insert irrelevant references to one another, and even entirely fabricated research.
We are proposing a brand new architecture and a fabrication technology that can support the scalability requirements of a hardware system for a quantum computer," says Linsen Li, an electrical engineering and computer science (EECS) graduate student and lead author of a paper on this architecture.
The paper, "Modulation instability and wavenumber bandgap breathers in a time layered phononic lattice," is the result of a yearslong collaboration of Chong, Wallace, and two researchers at CalTech.. Their project focused on how pressure waves can be manipulated by materials. It began in 2021 with a theoretical component—which Wallace worked on for her honors theses, "Instability in a Time ...
Julia Thome is first author of Public Health Reports paper. Posted by duthip1 on Wednesday, May 29, 2024 in News.. Congratulations to PhD candidate Julia Thome on the publication of Reporting of Child Maltreatment During the COVID-19 Pandemic in a Southern State in the United States in Public Health Reports last week, online ahead of print. The paper was co-authored by associate professor ...
View Domes Research Papers on Academia.edu for free.
These efforts culminated in winning a best paper award at the 29th Annual Graduate Education and Graduate Student Research Conference in Hospitality and Tourism in January. "I'm really honored," Ma said. "As far as I know, for the past three years or so, no one from our school has gotten the award, so it was a pleasure to have that.
Specifically the "geodesic dome" structure is the most attractive structure which provides more space. Geodesic is a Latin word where "geo" means earth and "desic" means dividing. It ...
Our analysis finds that demand for occupations such as health professionals and other STEM-related professionals would grow by 17 to 30 percent between 2022 and 2030, (Exhibit 1). Exhibit 1. [email protected]. By contrast, demand for workers in food services, production work, customer services, sales, and office ...
This publication is the first peer-reviewed quantum computing paper from the Cleveland Clinic-IBM Discovery Accelerator partnership. For decades, researchers have leveraged computational approaches to predict protein structures. A protein folds itself into a structure that determines how it functions and binds to other molecules in the body.
In a paper titled "tRNA modification reprogramming contributes to artemisinin resistance in Plasmodium falciparum", published in the journal Nature Microbiology, researchers from SMART's Antimicrobial Resistance (AMR) interdisciplinary research group documented their discovery: A change in a single tRNA, a small RNA molecule that is involved in translating genetic information from RNA to ...