- Research article
- Open access
- Published: 23 December 2021

Synthesis, antibacterial and antioxidant activities of Thiazole-based Schiff base derivatives: a combined experimental and computational study
- Fitsum Lemilemu 1 ,
- Mamaru Bitew 1 ,
- Taye B. Demissie 2 ,
- Rajalakshmanan Eswaramoorthy 3 &
- Milkyas Endale 1
BMC Chemistry volume 15 , Article number: 67 ( 2021 ) Cite this article
7683 Accesses
33 Citations
4 Altmetric
Metrics details
Thiazole-based Schiff base compounds display significant pharmacological potential with an ability to modulate the activity of many enzymes involved in metabolism. They also demonstrated to have antibacterial, antifungal, anti-inflammatory, antioxidant, and antiproliferative activities. In this work, conventional and green approaches using ZnO nanoparticles as catalyst were used to synthesize thiazole-based Schiff base compounds.
Among the synthesized compounds, 11 showed good activities towards Gram-negative E. coli (14.40 ± 0.04), and Gram-positive S. aureus (15.00 ± 0.01 mm), respectively, at 200 μg/mL compared to amoxicillin (18.00 ± 0.01 mm and 17.00 ± 0.04). Compounds 7 and 9 displayed better DPPH radical scavenging potency with IC 50 values of 3.6 and 3.65 μg/mL, respectively, compared to ascorbic acid (3.91 μg/mL). The binding affinity of the synthesized compounds against DNA gyrase B is within − 7.5 to − 6.0 kcal/mol, compared to amoxicillin (− 6.1 kcal/mol). The highest binding affinity was achieved for compounds 9 and 11 (− 6.9, and − 7.5 kcal/mol, respectively). Compounds 7 and 9 displayed the binding affinity values of − 5.3 to − 5.2 kcal/mol, respectively, against human peroxiredoxin 5. These values are higher than that of ascorbic acid (− 4.9 kcal/mol), in good agreement with the experimental findings. In silico cytotoxicity predictions showed that the synthesized compounds Lethal Dose (LD 50 ) value are class three (50 ≤ LD 50 ≤ 300), indicating that the compounds could be categorized under toxic class. Density functional theory calculations showed that the synthesized compounds have small band gap energies ranging from 1.795 to 2.242 eV, demonstrating that the compounds have good reactivities.
Conclusions
The synthesized compounds showed moderate to high antibacterial and antioxidant activities. The in vitro antibacterial activity and molecular docking analysis showed that compound 11 is a promising antibacterial therapeutics agent against E. coli, whereas compounds 7 and 9 were found to be promising antioxidant agents. Moreover, the green synthesis approach using ZnO nanoparticles as catalyst was found to be a very efficient method to synthesize biologically active compounds compared to the conventional method.
Peer Review reports
Introduction
The design and synthesis of organic compounds for medicinal applications is an important area of medicinal chemistry. When the organic compounds are complexed with transition metals, there has been observed an increase in the potency of therapeutic compounds [ 1 , 2 , 3 , 4 , 5 ]. Medicinal inorganic chemistry is also becoming the most attractive and growing research area with great scope to explore the potential of Schiff base compounds and their metal complexes for therapeutic applications [ 3 , 6 ]. In this aspect, many Schiff base compounds have been synthesized since 1864 [ 7 ], with wide areas of biological applications [ 7 , 8 , 9 , 10 , 11 ]. They are important building blocks for new materials with promising electronic, mechanical or biological properties [ 12 ]. They have attracted a great deal of interest in recent times for the design of new biologically significant compounds. More than 60% of all the known organic compounds are heterocyclic compounds [ 13 , 14 ]. However, the range of easily accessible and suitable functionalized heterocyclic building blocks for the synthesis of structurally diverse libraries of therapeutics is limited. Compounds containing azomethine group (–C=N–), Schiff bases (SBs), are usually synthesized by condensation of primary amines and active carbonyl groups [ 15 ]. Such compounds display promising pharmacological properties as anti-bacterial [ 16 ], antifungal [ 17 ], anti-cancer [ 18 , 19 ], antioxidant [ 19 , 20 ], antimalarial [ 21 ] anti-inflammatory [ 22 ], antiviral [ 23 ] and anti-proliferative properties [ 24 ]. They have been utilized as synthons to prepare biologically and industrially active compounds via ring closure, cycloaddition, and replacement reactions [ 15 , 25 ]. The pharmacophore potential of SBs is because of their ability to form complex compounds with metal ions in the active center of many enzymes involved in metabolism [ 16 ]. Moreover, the nitrogen atom of imine (–C=N–) is suggested to form a hydrogen bond with the active centers of cell constituents and thereby interfering the normal cell processes [ 2 , 9 ]. Thiazole moiety is one of the important pharmacophores in drug discovery and development with a wide range of therapeutic targets including antibacterial [ 26 ], anti-diabetic [ 18 , 19 ], anti-cancer [ 27 ], anti-inflammatory [ 22 ] and antiviral [ 23 , 28 ]. Although the exact biochemical mechanism of the antioxidant and antibacterial activities of thiazole-based Schiff base compounds are not well explored, the likely mechanism for their antioxidant activities have been suggested to be due to their ability to donate hydrogen to free radicals, ability to bind to reversible oxygen redox system from biochemical reactions, and their potential to deactivate many cellular enzymes. More importantly, their ability to inhibit aminoacyl-tRNA synthesis pathways is suggested as the possible mechanism to act as antibacterial agents [ 10 , 11 ].
In the present work, we report five thiazole-based Schiff bases designed and synthesized using conventional and green synthesis methods. The absorption, distribution, metabolism, excretion, and toxicity (ADMET) profile predictions, DFT calculations, molecular docking study against DNA gyrase B and human peroxiredoxin 5, antibacterial and antioxidant activities of the five thiazole-based Schiff base derivatives are also presented.
Experimental
Five thiazole-based Schiff bases were designed by altering the substituents on the phenyl ring of acetophenone and benzaldehyde (Scheme 1 ). Benzaldehyde (99% AR), 4-nitrobenzaldehyde (99% AR), 4-hydroxybenzaldehyde (98.8), acetophenone (AR), 4-nitroacetophenone (98%), 2-hydroxy acetophenone (95%), thiourea (99% AR), iodine beads, ethanol (absolute 99.8%), glacial acetic acid (99.5%), methanol (98.8% AR), ethyl acetate (99.5% AR), diethyl ether, sodium thiosulphate (99.5%), sodium carbonate anhydrous (99.5%), DPPH (Sigma-Aldrich) and others were purchased from Loba Chemie PVT Ltd, Addis Ababa (Neway PLC), Ethiopia. The synthesized compounds were purified by recrystallization and silica gel column chromatography (using silica gel 60–120 mesh size). Thin Layer Chromatography (TLC) profile was monitored using thin layer chromatography plate (Merck Silica gel 60 F254) and visualized using UV–Vis lamp at 254 and 365 nm. Melting points were determined using open capillary tubes (Thiele tube) filled in oil, where the melting points were measured in a range of values. NMR ( 1 H and 13 C) spectra were recorded using Bruker Avance 400 MHz spectrometer using deuterated chloroform (CDCl 3 ) and dimethyl sulfoxide (DMSO- d 6 ) solvents. Absorption spectra were recorded using double beam UV–Visible spectrophotometer (model 2201, India). FTIR spectra were recorded in KBr from 4000 to 400 cm −1 using Fourier-Transform Infrared Spectrometer (FTIR, Shimadzu Corporation, Japan).
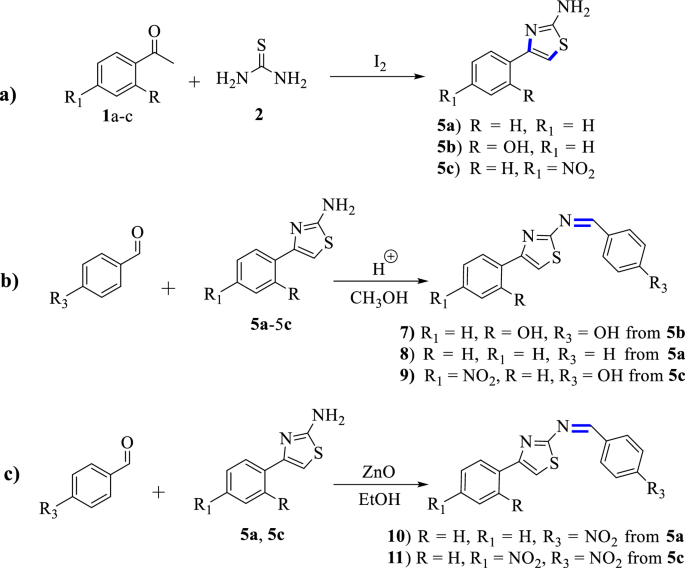
General schemes for the synthesis of Schiff base-based thiazole and its derivatives: a synthetic steps for the intermediate products, b conventional synthetic steps, and c green synthetic steps. Note that 7 , 8 and 9 were synthesized from 5b , 5a and 5c , respectively, whereas 10 and 11 were synthesized from 5a and 5c, respectively
Synthesis procedures for compounds 5a–c
Thiourea (40 mmol, 2 molar equiv.) was mixed with iodine beads (20 mmol, 1 molar equiv.) in a mortar and the mixture was homogenized with a pestle [ 29 ]. The solids were scratched off into a 250 mL round-bottom flask with a magnetic stirring bar and mixed with the corresponding acetophenone, 2-hydroxy acetophenone and 4-nitro acetophenone (20 mmol, 1 molar equiv.) (Scheme 1 , Path a ) [ 30 , 31 ]. The mixture was refluxed and stirred for 3 h at 100 °C in an oil bath under air-cooled condenser. Aqueous solution of sodium thiosulfate pentahydrate (5%) was added (with concomitant mechanical disruption of the solid matrix) in sufficient amount to reduce the remaining iodine (until brown colour disappeared) [ 29 , 31 ]. The suspension was filtered off and carefully washed with ~20 mL of diethyl ether (caution: product partially soluble) to remove the residues of unreacted acetophenone and its derivatives [ 29 , 31 ]. The crude product was dissolved in hot water, filtered, and the hot filtrate was adjusted to pH = 9 using aqueous Na 2 CO 3 anhydrous [ 31 ]. The products were collected by suction filtration. The physical properties are presented in Additional file 1 : Table S1.
Synthesis of thiazole-based Schiff bases (7–9)
A solution of phenyl thiazole (25 mmol) and benzaldehyde and its derivatives (25 mmol) were mixed in methanol (40 mL) (Scheme 1 , Path b ) [ 32 ]. The reaction mixture was refluxed for 5 h at 65 °C. The mixture was left to stand at room temperature overnight and concentrated using rotary evaporator. The residue was washed with n -hexane and filtered. Then it was hydrolyzed in water and extracted with EtOAc. Powders of the compounds ( 7 – 9 ) were obtained after drying with Na 2 SO 4 [ 33 ]. The physical properties of the synthesized compounds are presented in Additional file 1 : Table S1.
Green synthesis of Schiff bases (10 and 11)
A solution of acetophenonethiazole (0.4 g, 2.3 mmol) and p -nitrobenzaldehyde (0.34 g, 2.3 mmol), and p -nitroacetophenone thiazole (0.3 g, 13.5 mmol) and p -nitrobenzaldehyde (0.205 g, 13.5 mmol) (Scheme 1 , Path c ) [ 34 ] were mixed in absolute ethanol (30 mL) and refluxed for 4 h in the presence of 15% ZnO nanoparticles load at 78 °C for compounds 10 and 11 , respectively. The mixture was poured into 150 mL of crushed ice, while stirring with glass road and powders obtained were washed with cold ethanol and diethyl ether to remove unreacted amine, aldehyde and its derivatives [ 33 ] (Scheme 1 ) . The physical properties of the synthesized compounds are presented in Additional file 1 : Table S1.
Antibacterial activity
In vitro antibacterial activities of the test compounds against multi drug resistant bacterial strains were studied by disk diffusion assay [ 35 ]. The media was prepared by dissolving 38 g of Mueller Hinton agar (MHA) medium in 1000 mL of distilled water and autoclaved at 121 °C for 15 min. The autoclaved medium was poured into sterile plates (20–25 mL/plate) and allowed to solidify under sterile condition at room temperature.
The bacterial cultures were inoculated into the nutrient broth (inoculation medium) and incubated overnight at 37 °C [ 36 , 37 ]. Inoculated medium containing a 24 h grown culture was added aseptically to the nutrient medium and mixed systematically to get an even distribution. The solution was poured into ~20 mL of sterile MHA in sterile culture plates and allowed to attain room temperature [ 36 , 37 ]. Sterile agar-disc diffusion previously soaked in a known concentration (100 μg/mL and 200 μg/mL per disc) of the synthesized compound and standard drugs were prepared in DMSO using nutrient agar tubes and carefully placed at the center of the labeled seeded plate [ 36 , 37 ]. Mueller–Hinton sterile agar plates were seeded with indicator bacterial strains (1.3 × 10 8 cfu/mL) and allowed to stay at 37 °C for 3 h. Sterile filter paper disks with a diameter of 6 mm were placed over these plates. Finally, the plates were incubated at 37 °C for 24 h [ 35 , 36 , 37 ].
The mean inhibition zones were measured with a ruler and compared with the positive control (a disk containing amoxicillin) of the same concentration in millimeter. DMSO was used as a negative control during the whole test. The mean inhibition zone (MIZ) was expressed as mean value ± standard deviation.
Bacterial culture
The bacterial species, isolated from stored stool specimens and identified according to biochemical tests, were obtained from Adama Public Health Research & Referral Laboratory Center, Ethiopia. It is important to note that no patient was involved during specimen collection. The species were then cultured overnight using Eosin Methylene Blue (EMB) agar [ 37 ]. Gram-negative strains ( Escherichia coli (ATCC25922) and Pseudomonas aeruginosa (ATCC27853)) and Gram-positive strains ( Staphylococcus aureus (ATCC25923) and Streptococcus pyogenes (ATCC19615)) were used to test the activities of the synthesized compounds. Different concentrations were prepared from the synthesized compounds by dissolving 5 mg of each compound in 5 mL DMSO to make 1 mg/mL of standard solution. The experiment was performed in triplicates. The standard solution was serially diluted to furnish 100 μg/mL and 200 μg/mL samples for each synthesized compound. The concentrations of each sample were incorporated into sterile blank paper discs and dried at 37 °C [ 38 ].
Antioxidant activity
In vitro antioxidant activities of the synthesized compounds were assessed according to a reported method [ 39 ]. In brief, 0.01 mg/mL (0.001% (w/v)) solution of DPPH in methanol and DMSO was prepared, 1 mL of this solution was poured into 4 mL of the synthesized samples in methanol to furnish four different concentrations (1.25, 2.5, 5, 10 μg/mL) [ 40 ]. The control was made by adding 1 mL of DPPH solution to 4 mL of methanol or 4 mL of DMSO. Absorbance was measured against blank at λ max of 517 nm using UV–Visible Spectrophotometer (SM-1600 Spectrophotometer, India) [ 41 ]. Ascorbic acid was used as a positive control. The activity was expressed in IC 50 in which the concentration of the compounds is required to give a 50% decrease in absorbance compared to that of the control solution. The percentage inhibition of the synthesized compounds against DPPH was calculated using [ 42 ]:
where A control is the absorbance of the control, A sample is the absorbance of the test compounds.
Pharmacokinetic studies
Physicochemical properties, drug likeness and pharmacokinetic properties (ADME) of the designed thiazole-based Schiff base compounds were determined using the SwissADME Web server [ 43 ]. The percent absorption (%Abs) of the synthesized compounds was calculated using the formula %Abs = 109–0.345 TPSA [ 44 ]. The toxicity profile of the synthesized compounds was predicted using ProTox-II Web tool [ 45 ].
Density functional theory study
Geometry optimizations and frequency calculations of the synthesized compounds ( 7–11 ) were performed using the Gaussian 16 program package [ 46 ] and the results were visualized using GaussView 06 software. Density functional theory (DFT) and time dependent density functional theory (TD-DFT) calculations were performed using the B3LYP hybrid functional [ 47 , 48 , 49 ] together with 6–311++G(d,p) basis set [ 49 ]. Grimme’s dispersion correction [ 50 ] was employed to treat non-bonding interactions during the calculations. Such a combination of functional and basis sets has been used in previous studies [ 51 , 52 ]. Solvent effects were corrected using the polarizable continuum model in its integral equation formalism (IEFPCM) [ 53 ] together with either methanol or DMSO solvents to mimic the experimental conditions. The transition states were calculated using the quadratic synchronous transit (QST3) method, which requires not only an initial guess for the transition-state geometry, but also the optimized structures of the reactants and products. The optimized transition state structures were confirmed by the presence of an appropriate single imaginary vibrational frequency. The changes in Gibbs free energies were all calculated at 298.15 K and 1 atm.
The optimized geometries were confirmed to be real minima without any imaginary vibrational frequency by performing vibrational frequency calculations. The frontier molecular orbitals (highest occupied molecular orbital, HOMO, and lowest unoccupied molecular orbital, LUMO), energy gap (Δ E = E LUMO − E HOMO ), electronegativity ( χ = − ½ ( E HOMO + E LUMO )), electronic chemical potential ( μ = ½ ( E HOMO + E LUMO ) = − χ ), global chemical hardness ( η = ½ ( E LUMO − E HOMO )), global softness (σ = 1/2η), global electrophilicity index ( ω = μ 2 /2η ), and nucleophilicity index ( Nu = 1/ ω ) , dipole moment, natural atomic charges (NAC) and molecular electrostatic potential (MEP) of the synthesized compounds were also calculated and analyzed at the same level of theory [ 54 ]. The polarizability unites were converted from au into angstrom (au/1.88973), and then to Bohr 3 (angstrom * 0.529177) using standard conversion factors.
- Molecular docking
Molecular docking studies of the synthesized compounds ( 7 – 11 ) against target proteins (human peroxiredoxin 5 PDB ID: 1HD2 and E. coli DNA gyrase B PDB ID: 6F86) were performed using AutoDock Vina 4.2 (MGL tools 1.5.7) following standard protocol [ 55 , 56 ]. The three- and two-dimensional construction of the compounds, energy minimization of the synthesized compounds, docking simulations, crystal structures of the receptor molecules ( E. coli DNA gyrase B, PDB ID: 6F86 [ 57 ]; and human peroxiredoxin 5, PDB ID: 1HD2 [ 58 ], protein preparation, docking algorithm and ligand representations were conducted following the same procedure and protocols reported in our previous work [ 59 , 60 ].
Results and discussion
Synthesis and reaction mechanisms.
Five novel compounds were synthesized and characterized using spectroscopic methods with yields from 68.3 to 83.3%. Shorter reaction time was achieved for ZnO nanoparticle catalyzed approach compared to the conventional glacial acetic acid catalyzed reactions under reflux conditions. Physical properties and NMR spectral data of the synthesized compounds are presented in supporting information (Additional file 1 : Figs. S1–S10). The change in Gibbs free energies of the reaction mechanisms for the synthesis of compounds 3 and 7 using the conventional method was analyzed from DFT calculations. The results are presented in Fig. 1 .
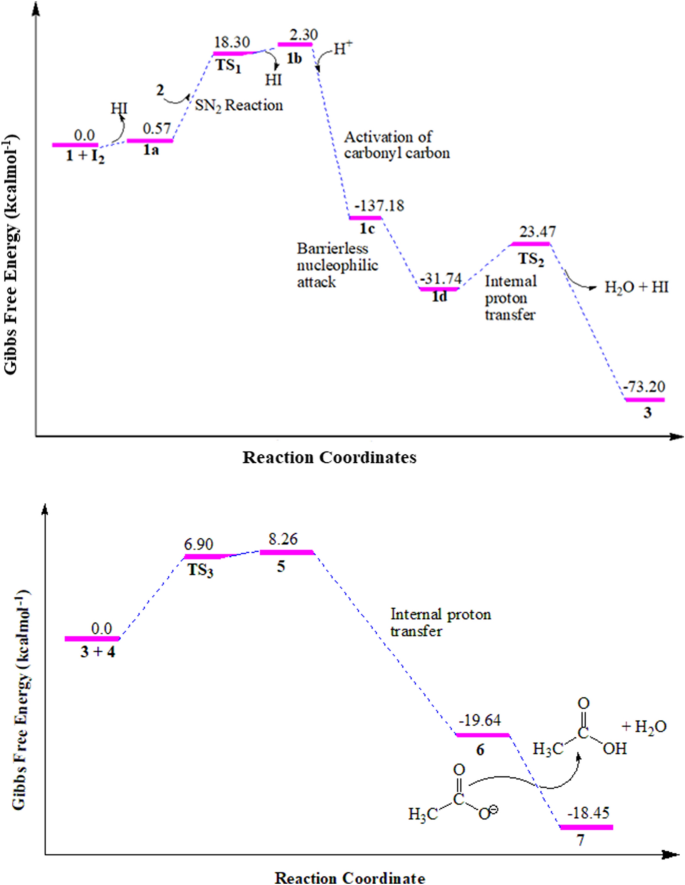
Free energy profile for the conventional synthesis of compounds 3 (top) and 7 (bottom). Note that the change in energies were calculated with respect to the reaction paths.
Free energy profile of the intermediates and the transitions states involved in the reaction mechanisms are presented in Fig. 1 . The S N 2 reaction of 1a with 2 in a neat condition resulted in the formation of the first transition state (TS 1 ) with 18.3 kcal/mol energy barrier. The removal of HI from TS 1 gives unstable intermediate 1b (2.3 kcal/mol). The HI is further used to activate the carbonyl carbon, which yields a very stable intermediate 1c (− 137.18 kcal/mol). Cyclization of compound 1c through a barrierless nucleophilic attack yields the formation of 1d. Internal proton transfer from the imine to hydroxyl group followed by the abstraction of a proton by I − takes the reaction to the formation of the second transition state (TS 2 ) with an energy barrier of 23.47 kcal/mol . The concomitant removal of water and hydrogen molecules from TS 2 yields the formation of compound 3 with a change in Gibbs free energy of − 73.20 kcal/mol (Fig. 1 ), assuring the formation of a stable reaction intermediate.
The nucleophilic addition of compound 3 and 4 in the presence of acetic acid gives the formation of the third transition state (TS 3 ) with activation energy barrier of 6.9 kcal/mol which gives the formation of compound 5 (1.36 kcal/mol). The internal proton transfer from the amine of imine was suggested to form compound 6 (− 19.64 kcal/mol) which yields the target compound 7 (− 18.45 kcal/mol) via deprotonation and dehydration (Fig. 1 ). Overall, the formation of compound 7 follows a radical reaction to generate α-halo carbonyl compounds, nucleophilic substitution, and nucleophilic addition reaction mechanisms. The summarized plausible reaction mechanism, based on the change in Gibbs free energies of the reaction steps, for the synthesis of 3 and 7 is presented in Fig. 2 .
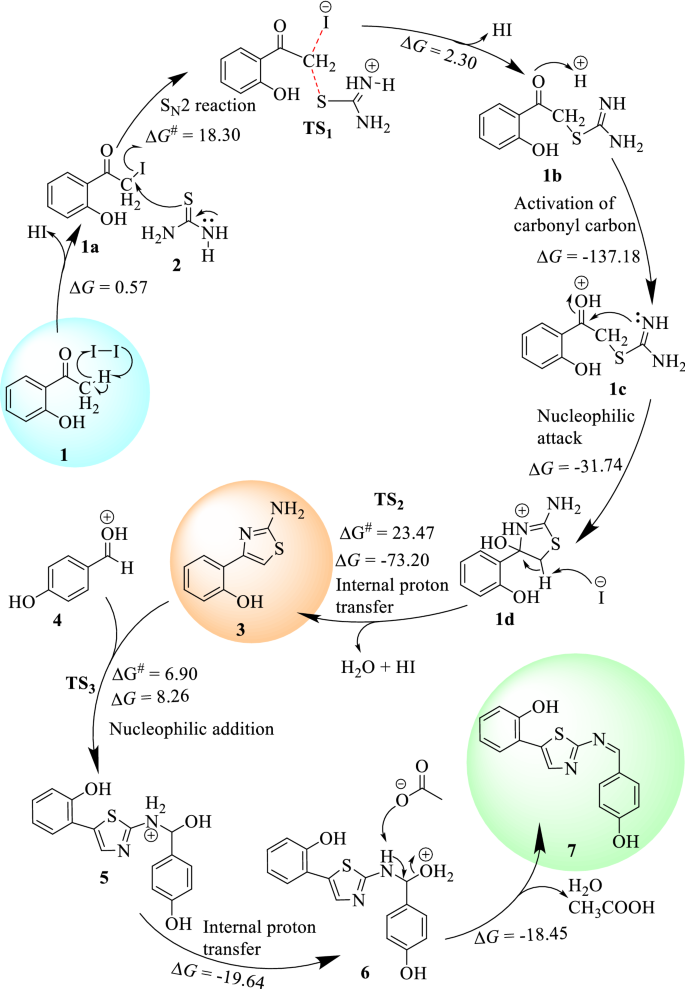
The plausible reaction mechanism of compounds 3 and 7 calculated using B3LYP-GD3/6-311+ +G(d,p)/Methanol. At the start of the reaction, it proceeds via an S N 2 reaction mechanism followed by the formation of first transition state (TS 1 ). The second (TS 2 ) and third (TS 3 ) transition states follow nucleophilic reactions
The experimental FTIR spectra of compounds 7 – 10 are presented in Fig. 3 , whereas that of 11 is presented in Additional file 1 : Fig. S10. To further support the synthesis of the compounds, we compared the experimental and DFT calculated IR vibrational spectra of the five compounds. The results are in good agreement with each other (Table 1 ). The absence of amine peaks and the appearance of a peak (experimental/theoretical) at 1616/1618 cm −1 , 1632/1661 cm −1 , 1632/1582 cm −1 , and 1608/1657 cm −1 indicates the characteristic peaks for C=N stretching of the imine groups of compounds 7–10 (Fig. 3 ; Table 1 ).
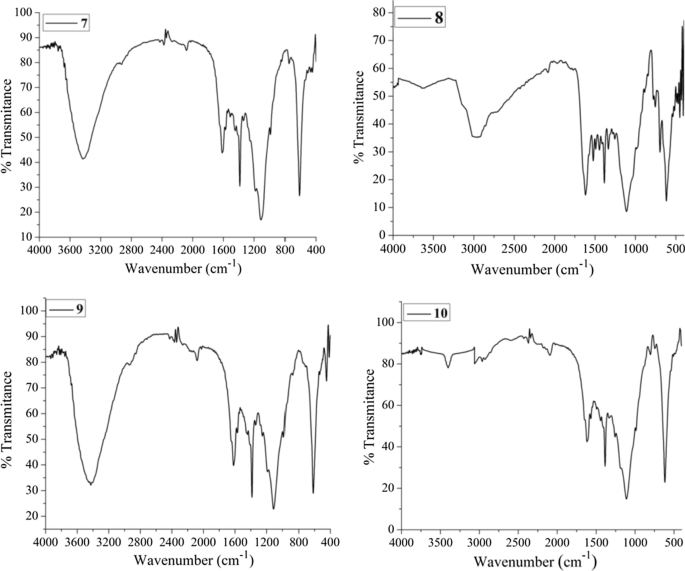
FTIR spectra of the synthesized compound 7 – 10
UV–Vis absorption spectra of the synthesized compounds were recorded from 200 to 800 nm at room temperature using methanol (for 8 and 9 ), and DMSO (for 7 , 10 and 11 ) (Fig. 4 ). The computational/experimental maximum absorption bands (Fig. 4 ) for the synthesized compounds appeared at 221/260 nm, 203/214 nm, 215/260 nm, 210/269 nm, and 218/276 nm for compounds 7–11 , respectively. These λ max absorption bands are attributed to the transition of electrons from π to π* orbitals as the HOMO–LUMO systems are mainly localized over the π-system of the imine part of the molecule (vide infra), in agreement with previous reports [ 61 ] (Additional file 1 : Fig. S11). Even though satisfactory results are obtained from the DFT calculations, there are differences between the experimental and calculated maximum absorption wavelengths for some of the compounds. This can be attributed to missing treatment of molecular packing forces in the computational method.
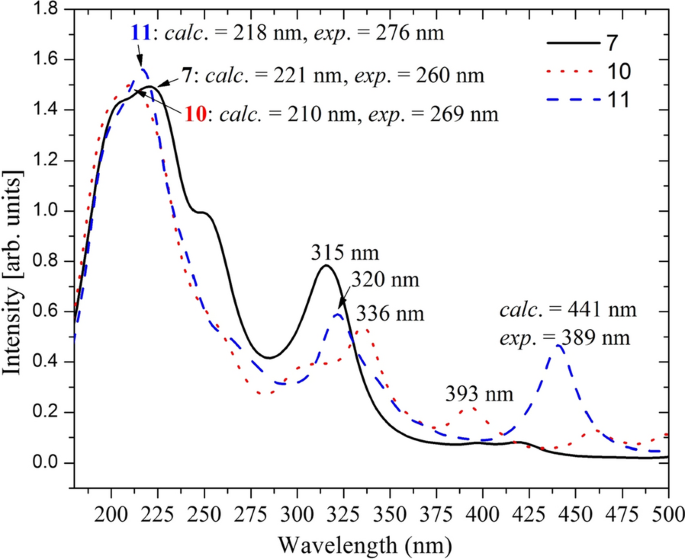
Comparison of the experimental absorption wavelengths with the corresponding B3LYP-GD3/6-311++G(d,p)/PCM/DMSO calculated results. The calculated spectra were red-shifted by 30 nm for better comparison with the experimental results
Thiazole containing compounds were proved to have potent antimicrobial activity against multidrug-resistant strains of S. aureus [ 62 ]. In this work, in vitro antibacterial activities of the synthesized compounds were done against four clinical bacterial isolates (Fig. 5 ). The synthesized compounds were less active against Gram-negative rather than Gram-positive bacteria. Compounds 10 and 11 displayed good activities against E. coli with MIZ of 10.50 ± 0.02 and 14.40 ± 0.04 mm diameter, respectively, compared with amoxicillin (18.00 ± 0.01 mm) at 200 μg/mL (Table 2 ). Compounds 8 and 11 showed good activities against S. aureus , while compounds 7 , 9 and 10 showed good activities against S. pyogenes (Table 2 ).
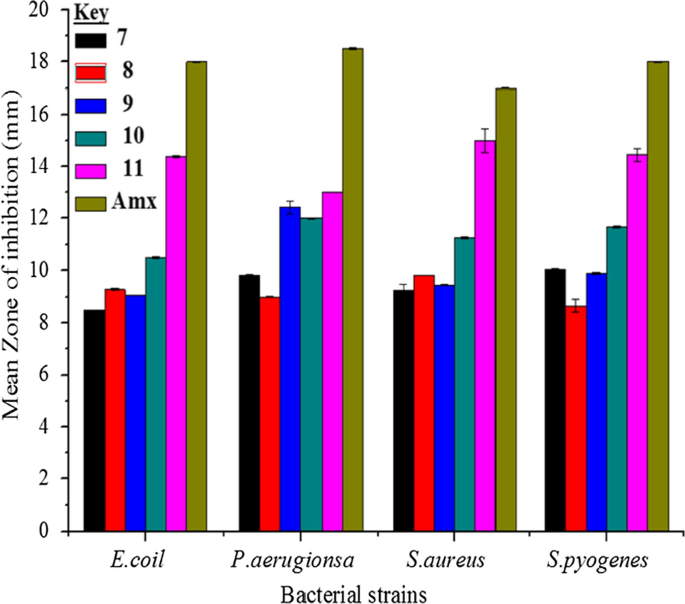
Mean inhibition zone of the synthesized compounds in mm (mean ± SD) at 200 µg/mL. The inset stands for the type of compounds
Compound 11 showed strong antibacterial activity against Gram-negative ( P. aeruginosa ) and Gram-positive ( S. aureus ) with MIZ of 13.00 ± 0.02 and 15.00 ± 0.01 mm compared with amoxicillin (18.50 ± 0.45 and 17.00 ± 0.04 mm), respectively, at 200 μg/mL (Table 2 ; Fig. 5 ). This suggests that compound 11 is potentially a promising therapeutic antibacterial agent. The good activity exhibited by compound 11 compared to others ( 7 – 10 ) is due to the appearance of electron withdrawing groups (–NO 2 ) in the structure of the compound that would create localized electron deficient sites which can facilitate a feasible condition for interaction with biomolecules (proteins, amino acids, nucleic acids, and enzymes) [ 56 , 57 , 58 ]. The high antibacterial activity of compound 11 can also be attributed to its high dipole moment (vide infra) [ 63 ].
Oxidative stress is a cause of cancer as well as neurological and cardiovascular diseases which are the leading causes of death [ 41 ]. Compounds 7–11 were evaluated for their antioxidant activities using DPPH assay. Compounds 7 (80.89%, IC 50 value 3.6) and 9 (70%, IC 50 value 3.65 μg/mL) (Table 3 ; Additional file 1 : Figs. S12, S13) showed promising antioxidant potential at concentration of 10 μg/mL compared to ascorbic acid (91.2%, IC 50 value 3.98 μg/mL). The high antioxidant activity of compound 7 could be due to electron donating –OH substituents [ 64 , 65 ]. The quantum mechanical descriptors of the compounds support these experimental results (vide infra).
In silico pharmacokinetics (ADME) and drug likeness studies analysis
To shed more light on the in vitro analysis of the antibacterial and antioxidant activities of the synthesized compounds, in silico pharmacokinetics and drug-likeness studies were conducted. The results are presented in Additional file 1 : Tables S2, S3. The molecular weight of the molecules ≤500), the number of hydrogen bond acceptors (≤10), number of hydrogen bond donors (≤5) and the lipophilicity (iLogP) values ≤5) were used to follow Lipinski’s rule of five for drug like molecular nature of a molecule [ 66 ]. The SwissADME predicted results showed that the synthesized compounds ( 7 – 11 ) satisfy Lipinski’s rule of five [ 43 ] with zero violations for their drug like molecular nature (Table 4 ).
The SwissADME predicted LogP values are in the range from 2.10 to 3.07 assuring optimal lipophilicity of the synthesized compounds. In addition, TPSA of the synthesized molecules were used to predict percent absorption descriptors. The predicted TPSA value (in Å 2 ) are in the range from 39.99 to 145.13 inferring very good intestinal absorption of compounds 7 – 10, and relatively poor intestinal absorption for compound 11 . It has been suggested that molecules with a TPSA of 140 Å 2 and above would be poorly absorbed (< 10% fractional absorption), while compounds with a TPSA 60 Å 2 would be well absorbed (> 90%) [ 67 ]. High degree of absorption was predicted for compounds 7 – 10 . The predicted human skin permeability coefficients (logKp) values of the synthesized compounds for their human stratum corneum penetration were found to be in the range of − 4.37 to − 5.59 cm/s, inferring low skin permeability [ 43 ]. A relatively less skin permeant was predicted for compound 7 .
Pharmacokinetically, high GI absorption for 7 , 8 and 9 , BBB permeate for 8 were predicted. None of the synthesized compounds were predicted as Pg-p substrate (Additional file 1 : Table S2). SwissADME predicted results also suggested that compound 9 is a better active therapeutic agent compared to other synthesized compounds. Besides, the interaction of therapeutic molecules with cytochromes P450 (CYP) isoforms as substrate of these enzymes has been proposed to screen a therapeutically active molecule. It is reported that the inhibition of CYP isoenzymes is certainly one major cause of pharmacokinetics-related drug-drug interactions [ 68 , 69 ], thereby leading to toxic/adverse effects due to the lower clearance and accumulation of the drug or its metabolites [ 70 ]. In this work, except to CYP1A2 isoform, most of the synthesized compounds were substrates of (CYP) isoforms. The results further suggest that the synthesized compounds have good drug-like molecular nature.
Absence of toxicity data is one of the life threatening factors for choosing a compound as a therapeutic candidate [ 71 ]. Herein, the organ toxicity (hepatotoxicity) and toxicological endpoints (carcinogenicity, immunotoxicity, mutagenicity and cytotoxicity) of the five compounds were predicted. The ProTox-II predicted organ toxicity results showed that all the synthesized compounds show hepatotoxicity and mutagenicity toxicological endpoint. Besides, all the compounds show no toxicity towards immunotoxicity toxicological endpoint. Carcinogenicity toxicological endpoints were predicted for compounds 7 , 9 , 10 and 11 (Additional file 1 : Table S3). The results showed that LD 50 values of the synthesized compounds are class three (50 ≤ LD 50 ≤ 300), indicating that they are toxic [ 45 ].
Quantum mechanical descriptors
It is known that high eigenvalue of HOMO and small band gap energy between HOMO and LUMO of a compound are associated with its high antioxidant activity [ 72 ]. Having this in mind, we analyzed the quantum mechanical descriptors of all the compounds. The results are presented in Table 5 . The HOMO and LUMO frontier molecular orbitals were used to analyze the relative reactivity and describe the wave function distribution of the molecules. The energy gaps (in eV ) were calculated to be 2.242, 1.795, 2.095, 1.805 and 2.181 for compounds 7–11 , respectively (Table 5 ). The results indicated that the synthesized compounds have good reactivity. The results further revealed that compound 8 has the minimum HOMO–LUMO energy gap signifying that it has high chemical reactivity and sizable intramolecular charge transfer.
Compounds 9–11 showed large electronegativity values 5.194 eV, 5.188 eV and 5.3425 eV, respectively. The structural differences brought the observed electronegativity differences between compounds 10 (single –NO 2 substituent) and 11 (double –NO 2 substituent) (Table 5 ). The presence of electron donating hydroxyl substituent in compound 7 reduced its electronegativity (4.759 eV) relative to its structurally analogous compound 8 (5.074 eV). On the other hand, relatively large dipole moment values were calculated for compound 9 (14.629 Debye) and compound 11 (10.164 Debye) inferring potentially good antimicrobial activity of the synthesized compounds (Additional file 1 : Fig. S14).
The DFT calculated HOMO–LUMO band gap energy (Fig. 6 ) showed the role of the substituents in the reactivity of the synthesized compounds. The presence of two electron donating hydroxyl groups in compound 7 ( E g = 2.242 eV) decreases its relative reactivity to its structurally analogous compound 8 (1.795 eV). The effect of substituent difference for compound 8 (no NO 2 ), 9 (one NO 2 ) and 11 (two NO 2 ) was also observed in their band gap energies (1.795 eV, 2.095 eV and 2.181 eV, respectively).
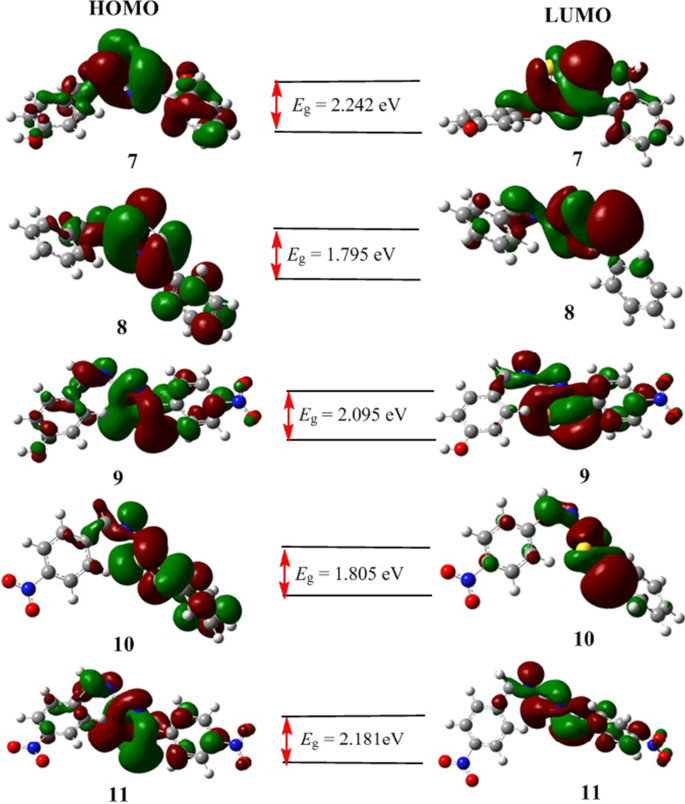
HOMO–LUMO distributions of the synthesized compounds ( 7 – 11 ). E g represents for the band gap in electron volts (eV)
The wave function distribution for HOMO and LUMO orbitals of compounds 8 and 11 is from uniform (in 8 ) distribution to the central part of the molecule (in 11 ) as the number of –NO 2 substituent increases. The localization of electron density is concentrated to the thiazole part of the molecules, decreasing the uniform localization as the number of NO 2 increases from compound 8 to compound 11 inferring the potential interaction part of the compounds is the thiazole part.
Molecular electrostatic potential
The molecular electrostatic potential (MEP) surfaces of the synthesized compounds ( 7–11 ) were plotted from the optimized structures of the compounds at the same level of theory. The MEP maps of the synthesized compounds ( 7 and 10 ) are presented in Fig. 7 and Additional file 1 : Figs. S15–S17. The most negative electrostatic potential region of compound 7 is mainly localized over the thiazole, over the phenyl part of the thiazole for 8 and 10 , whereas on the thiazole and nitro parts of the phenyl thiazole unit of 9 , and the nitro part of phenyl thiazole of compound 11 . These MEP results suggest that the specified regions are the possible binding sites. The dipole moments of the synthesized compounds are found to project to the regions where electrostatic potential region is localized (Additional file 1 : Fig. S17).
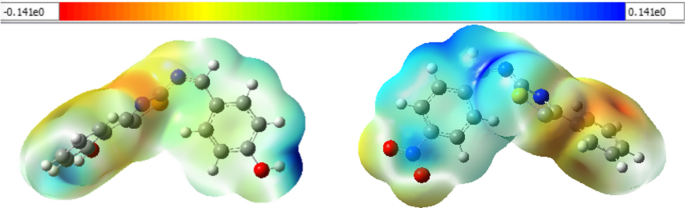
Molecular electrostatic potential map of compound 7 (left) and 10 (right). Negative regions are represented by red, orange, and yellow colors with a decrease in electrostatic potential (yellow → red), whereas positive regions are illustrated by green and blue colors
Natural atomic charge analysis
The natural atomic charges (NAC) were calculated using B3LYP-GD3/6–311++G(d,p) level of calculation for compounds 7 and 10 (Additional file 1 : Fig. S14). The synthesized compounds have nitrogen and sulfur heteroatoms. The oxygen atom of the hydroxy substituents in 7 bear the highest negative charge for the phenyl and benzyl oxygen atoms (− 0.677 and − 0.673, respectively). The thiazole nitrogen of 7 and 10 have the highest negative charges (− 0.53 and − 0.548) relative to the imine nitrogen (− 0.493 and − 0.413), respectively. The sulfur atom of the thiazole unit bears positive natural atomic charge of 0.331 and 0.379, respectively, for compounds 7 and 10 . These charge analyses suggest the presence of charge transfer from sulfur to methine of the thiazole part of the compounds.
Molecular docking score analysis
Most antibacterial agents act by targeting key components of bacterial metabolism: cell-walls, DNA-directed RNA polymerase, protein synthesis and modification, enzymes and DNA gyrase B [ 63 , 73 ]. To further support the in vitro antibacterial and antioxidant activities of the compounds, molecular docking studies of the five synthesized novel compounds with the binding sites of E. coli DNA gyrase B (PDB ID: 6F86) [ 57 ] were performed in order to predict the protein–ligand interactions. The results are presented in Figs. 8 and 9 and Additional file 1 : Figs. S18–S21. The results showed that the compounds have high binding affinity (− 7.5 to − 6 kcal/mol). The higher binding affinity of compounds 9 and 11 (− 6.9 and − 7.5 kcal/mol, respectively) compared to the standard drug amoxicillin (− 6.1 kcal/mol) suggests that the compounds are promising antibacterial agents against E. coli (Table 6 ) [ 71 ]. The higher binding affinity obtained for compounds 9 and 11 is because the compounds have similar residual interactions profile with the amino acid residues Ile-78 (Table 6 ). Moreover, compounds 7 and 8 have similar residual amino acid (Ala-4, Asp-73, Glu-50, Ile-78 and Thr-165) interactions and comparable binding affinities (Additional file 1 : Figs. S18, S19). The hydrogen bond interactions of compound 11 with Asn-46, Val-120, Ser-121 and residual hydrophobic interaction with Glu-50, Ile-78 (Fig. 8 ; Additional file 1 : Figs. S18–S21) also indicated that compound 11 has lower binding affinities, mainly due to the strong electron withdrawing ability of the nitro group of the compound [ 74 ]. The findings obtained from the molecular docking analysis (large negative binding affinity) further support the experimental and DFT results (large dipole moment). The overall analysis showed that all the compounds, except 8 , are active against E. coli (Table 2 ). Comparison with the positive control also suggests that the synthesized compounds are found to be promising antibacterial agents compared to amoxicillin.
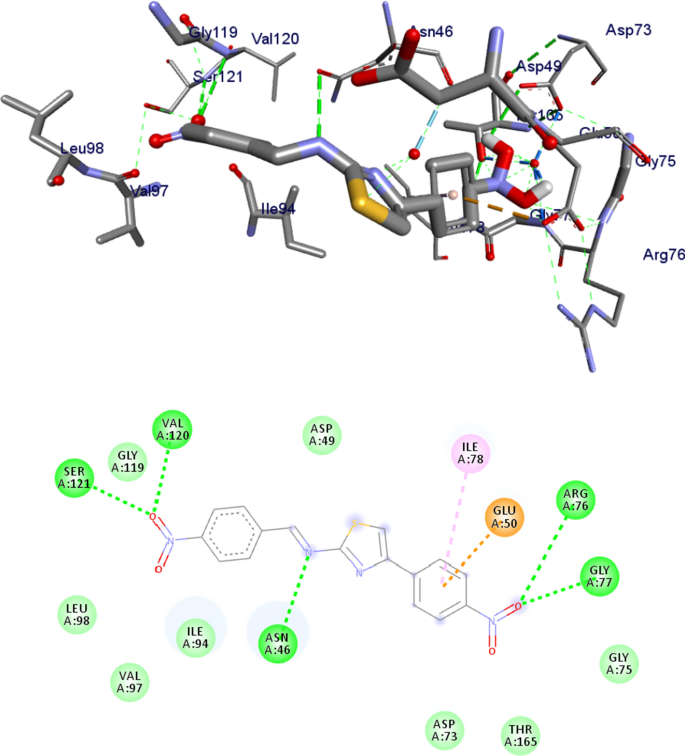
Possible binding interaction of compound 11 against E. coli DNA gyrase B (PDB ID: 6F86)
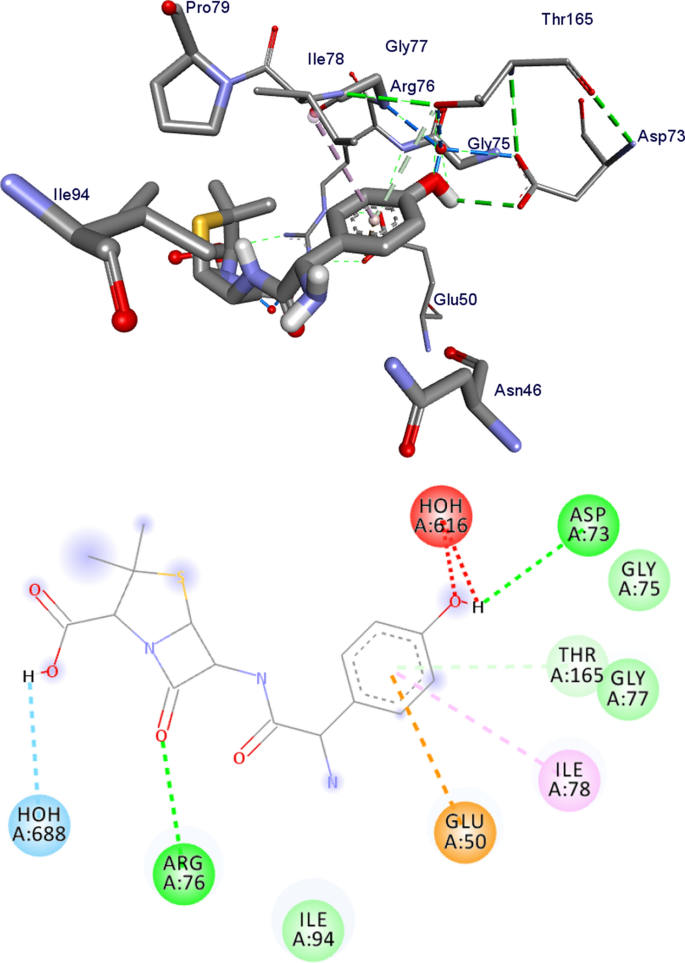
Possible binding interaction of amoxicillin against E. coli DNA gyrase B (PDB ID: 6F86)
Molecular docking against human peroxiredoxin 5
The molecular docking analysis of the synthesized compounds was also carried out to investigate their binding and interaction patterns with human peroxiredoxin 5 (PDB ID: 1HD2) [ 58 ]. The in silico study of compounds 7 – 11 showed minimum binding affinities within the binding pocket of human peroxiredoxin 5, ranging from − 5.0 to − 5.3 kcal/mol (Additional file 1 : Table S4, Figs. S22–S27). Compound 7 (− 5.3 kcal/mol) displayed the higher binding affinity values compared to ascorbic acid (− 4.9 kcal/mol) (Additional file 1 : Figs. S22–S27). As discussed above, the higher antioxidant activity of compound 7 is because of the high energy of HOMO. This is in good agreement with the experimental findings (vide supra) and suggests that compound 7 has potentially good antioxidant activity.
In the present study, we reported conventional and green synthesis of five thiazole-based Schiff base derivatives together with their ADMET profile predictions, DFT calculated results, molecular docking study against E. coli DNA gyrase B and human peroxiredoxin 5, in vitro antibacterial and antioxidant activities. The structures of the synthesized compounds were determined using combined spectroscopic techniques (UV-Vis, FTIR, 1 H NMR and 13 C NMR). The results were further supported by DFT calculations. Among the synthesized compounds, compound 11 displayed a promising biological activity. Compounds 7 and 9 showed better DPPH radical scavenging potency. The molecular docking results indicated that compounds 9 and 11 exhibited good interaction with DNA gyrase B. The experimental and computational studies on the antibacterial and antioxidant activities are in good agreement. Compound 7 displayed enhanced minimum binding affinity against human peroxiredoxin 5 compared to ascorbic acid. In silico pharmacokinetics studies showed that all synthesized compounds are biologically significant obeying Lipinski rule of 5. The in silico cytotoxicity predictions revealed that the LD 50 values of the synthesized compounds are class three (50 ≤ LD 50 ≤ 300). The experimental and computational results of the present study also suggest that compound 11 is a promising antibacterial agent against E. coli , whereas compound 7 was found to possess promising antioxidant potentials. However, further study is recommended to synthesize a series of related thiazole-based Schiff base derivatives for functional group inclusions, structure activity relationship, and in vivo toxicity studies to develop lead antioxidant and antibacterial agents.
Availability of data and materials
The datasets supporting the findings of this article are all presented in the main manuscript. Additional data which further support the findings are presented in the Additional files.
Prajapat P. Role of organic, medicinal & pharmaceutical chemistry in drug design: introduction. J Nanomed Res. 2018;7(2):69–70.
Google Scholar
Orvig C, Abrams MJ. Medicinal inorganic chemistry: introduction. Chem Rev. 1999;99(9):2201–4.
Article CAS PubMed Google Scholar
Jones MR, Duncan D, Storr T. Introduction to Ligand Design in Medicinal Inorganic Chemistry: John Wiley & Sons Ltd.: Chichester, West Sussex, PO19 8SQ, United Kingdom. 2014
Sumrra SH, Habiba U, Zafar W, Imran M, Chohan ZH. A review on the efficacy and medicinal applications of metal-based triazole derivatives. J Coord Chem. 2020;73(20–22):2838–77.
Article CAS Google Scholar
Zafar W, Sumrra SH, Chohan ZH. A review: pharmacological aspects of metal based 1,2,4-triazole derived Schiff bases. Eur J Med Chem. 2021;222:113602.
Varol M. The importance of metal-based drugs in medicinal inorganic chemistry to improve life quality of patients. J Appl Pharm. 2016;8(1):1–2.
Article Google Scholar
Hussain Z, Yousif E, Ahmed A, Altaie A. Synthesis and characterization of Schiff’s bases of sulfamethoxazole. Org Med Chem Lett. 2014;4(1):1–4.
Article PubMed PubMed Central CAS Google Scholar
More M, Joshi P, Mishra Y, Khanna P. Metal complexes driven from Schiff bases and semicarbazones for biomedical and allied applications: a review. Mater Today Chem. 2019;14:100195.
Article CAS PubMed PubMed Central Google Scholar
Vashi K, Naik H. Synthesis of novel Schiff base and azetidinone derivatives and their antibacterial activity. E-J Chem (Online). 2004;1(5):272–5.
Al-Amiery AA, Al-Majedy YK, Ibrahim HH, Al-Tamimi AA. Antioxidant, antimicrobial, and theoretical studies of the thiosemicarbazone derivative Schiff base 2-(2-imino-1-methylimidazolidin-4-ylidene) hydrazinecarbothioamide (IMHC). Org Med Chem Lett. 2012;2(1):1–7.
Jain AK, Singla R, Shrivastava B. Thiazole: a remarkable antimicrobial and antioxidant agents. Pharmacologyonline. 2011;2:1072–84.
Sharma PK, Amin A, Kumar M. A review: medicinally important nitrogen sulphur containing heterocycles. Open Med Chem J. 2020;14(1):49–64.
Farouk Elsadek M, Mohamed Ahmed B, Fawzi Farahat M. An overview on synthetic 2-aminothiazole-based compounds associated with four biological activities. Molecules. 2021;26(5):1449.
Heravi MM, Zadsirjan V. Prescribed drugs containing nitrogen heterocycles: an overview. RSC Adv. 2020;10(72):44247–311.
Sadigova S, Magerramov A, Allakhverdiev M. Synthesis of Schiff bases and oxazolidines from 2-amino-4-phenylthiazole. Russ J Org Chem. 2008;44(12):1821–3.
Login CC, Bâldea I, Tiperciuc B, Benedec D, Vodnar DC, Decea N, Suciu Ş. A novel Thiazolyl Schiff base: antibacterial and antifungal effects and in vitro oxidative stress modulation on human endothelial cells. Oxid Med Cell Longev. 2019;2019:1–11.
Bharti S, Nath G, Tilak R, Singh S. Synthesis, anti-bacterial and anti-fungal activities of some novel Schiff bases containing 2, 4-disubstituted thiazole ring. Eur J Med Chem. 2010;45(2):651–60.
Makawana JA, Sangani CB, Lin L, Zhu H-L. Schiff’s base derivatives bearing nitroimidazole and quinoline nuclei: new class of anticancer agents and potential EGFR tyrosine kinase inhibitors. Bioorg Med Chem Lett. 2014;24(7):1734–6.
de Santana TI, de Oliveira BM, de Moraes Gomes PAT, da Cruz ACN, da Silva TG, Leite ACL. Synthesis, anticancer activity and mechanism of action of new thiazole derivatives. Eur J Med Chem. 2018;144:874–86.
Article PubMed CAS Google Scholar
Taha M, Ismail NH, Jamil W, Yousuf S, Jaafar FM, Ali MI, Kashif SM, Hussain E. Synthesis, evaluation of antioxidant activity and crystal structure of 2, 4-dimethylbenzoylhydrazones. Molecules. 2013;18(9):10912–29.
Harpstrite SE, Collins SD, Oksman A, Goldberg DE, Sharma V. Synthesis, characterization, and antimalarial activity of novel schiff-base-phenol and naphthalene-amine ligands. Med Chem. 2008;4(4):392–5.
Geronikaki A, Hadjipavlou-Litina D, Amourgianou M. Novel thiazolyl, thiazolinyl and benzothiazolyl Schiff bases as possible lipoxygenase’s inhibitors and anti-inflammatory agents. Il Farmaco. 2003;58(7):489–95.
Décor A, Grand-Maître C, Hucke O, O’Meara J, Kuhn C, Constantineau-Forget L, Brochu C, Malenfant E, Bertrand-Laperle M, Bordeleau J. Design, synthesis and biological evaluation of novel aminothiazoles as antiviral compounds acting against human rhinovirus. Bioorg Med Chem Lett. 2013;23(13):3841–7.
Nassar MY, Aly HM, Moustafa ME, Abdelrahman EA. Synthesis, characterization and biological activity of new 3-substitued-4-amino-5-hydrazino-1, 2, 4-triazole schiff bases and their Cu (II) complexes: a new approach to CuO nanoparticles for photocatalytic degradation of methylene blue dye. J Inorg Organomet Polym Mater. 2017;27(5):1220–33.
Kajal A, Bala S, Kamboj S, Sharma N, Saini V. Schiff bases: a versatile pharmacophore. J Catal. 2013;2013:1–14.
Chen Y-Y, Gopala L, Bheemanaboina RRY, Liu H-B, Cheng Y, Geng R-X, Zhou C-H. Novel naphthalimide aminothiazoles as potential multitargeting antimicrobial agents. ACS Med Chem Lett. 2017;8(12):1331–5.
Zhang Z-H, Wu H-M, Deng S-N, Cai X-Y, Yao Y, Mwenda MC, Wang J-Y, Cai D, Chen Y. Design, synthesis, and anticancer activities of novel 2-amino-4-phenylthiazole scaffold containing amide moieties. J Chem. 2018;2018.
Ejaz S, Nadeem H, Paracha RZ, Sarwar S, Ejaz S. Designing, synthesis and characterization of 2-aminothiazole-4-carboxylate Schiff bases; antimicrobial evaluation against multidrug resistant strains and molecular docking. BMC Chem. 2019;13(1):1–13.
Zitko J, Jand’ourek O, Paterová P, Navrátilová L, Kuneš J, Vinšová J, Doležal M. Design, synthesis and antimycobacterial activity of hybrid molecules combining pyrazinamide with a 4-phenylthiazol-2-amine scaffold. MedChemComm. 2018;9(4):685–96.
Aldelfy Z, Alshamkhani Z, Al-Assadi M. 2-Hydroxybenzylidene-4-(4-SubstitutedPhenyl)-2-amino thiazole and their Pt (II) complexes: synthesis, characterization and biological study. Egypt J Chem. 2019;62(10):1851–67.
Koppireddi S, Komsani JR, Avula S, Pombala S, Vasamsetti S, Kotamraju S, Yadla R. Novel 2-(2, 4-dioxo-1, 3-thiazolidin-5-yl) acetamides as antioxidant and/or anti-inflammatory compounds. Eur J Med Chem. 2013;66:305–13.
Lipkin A, Smirnov V. Synthesis of azomethines from 2-amino-4-phenylthiazole. Chem Heterocycl. 1968;4(3):423–423.
Sakarya H, Görgün K, Öğretir C. Synthesis and characterization of novel substituted N -benzothiazole-2-yl-acetamides. Arab J Chem. 2016;9:S1314–9.
Amorim CR, Pavani TF, Lopes AF, Duque MD, Mengarda AC, Silva MP, de Moraes J, Rando DG. Schiff bases of 4-phenyl-2-aminothiazoles as hits to new antischistosomals: synthesis, in vitro, in vivo and in silico studies. Eur J Pharm Sci. 2020;150:105371.
Ommenya F, Nyawade E, Andala D, Kinyua J. Synthesis, characterization and antibacterial activity of Schiff base, 4-Chloro-2-{(E)-[(4-fluorophenyl) imino] methyl} phenol metal (II) complexes. J Chem. 2020;2020:1–8.
Burdass D, Grainger J, Hurst J (2016) Basic practical microbiology—a manual. Charles Darwin House, 12 Roger Street, London WC1N 2JU, UK: Microbiology Society.
Tamene D, Endale M. Antibacterial activity of coumarins and carbazole alkaloid from roots of clausena anisata. Adv Pharmacol Sci. 2019;2019:1–8.
Piotto S, Sessa L, Diana R, Torrens G, Juan C, Caruso U, Iannelli P. Synthesis and antimicrobial studies of new antibacterial azo-compounds active against staphylococcus aureus and listeria monocytogenes. Molecules. 2017;22(8):1372.
Article PubMed Central CAS Google Scholar
Paduch R, Woźniak A. The effect of Lamium album extract on cultivated human corneal epithelial cells (10.014 prsv-t). J Ophthalmic Vis Res. 2015;10(3):229.
Article PubMed PubMed Central Google Scholar
Ćavar S, Kovač F, Maksimović M. Synthesis and antioxidant activity of selected 4-methylcoumarins. Food Chem. 2009;117(1):135–42.
Mukhia R, Basistha B, Chhetri DR. Variation in antioxidant activity of a rattan species, Plectocomia himalayana Griff. by DPPH assay based on two different methods of methanol extraction. Res J Pharmacogn Phytochem. 2018;10(2):175–8.
Sahu RK, Kar M, Routray R. DPPH free radical scavenging activity of some leafy vegetables used by tribals of Odisha, India. J Med Plants. 2013;1(4):21–7.
Daina A, Michielin O, Zoete V. SwissADME: a free web tool to evaluate pharmacokinetics, drug-likeness and medicinal chemistry friendliness of small molecules. Sci Rep. 2017;7(1):1–13.
Remko M. Theoretical study of molecular structure, pKa, lipophilicity, solubility, absorption, and polar surface area of some hypoglycemic agents. J Mol Struct. 2009;897(1–3):73–82.
Banerjee P, Eckert AO, Schrey AK, Preissner R. ProTox-II: a webserver for the prediction of toxicity of chemicals. Nucleic Acids Res Spec Publ. 2018;46(W1):W257–63.
Frisch MJ, Trucks GW, Schlegel HB, Scuseria GE, Robb MA, Cheeseman JR, Scalmani G, Barone V, Petersson GA, Nakatsuji H, Li X, Caricato M, Marenich AV, Bloino J, Janesko BG, Gomperts R, Mennucci B, Hratchian HP, Ortiz JV, Izmaylov AF, Sonnenberg JL, Williams, Ding F, Lipparini F, Egidi F, Goings J, Peng B, Petrone A, Henderson T, Ranasinghe D, Zakrzewski VG, Gao J, Rega N, Zheng G, Liang W, Hada M, Ehara M, Toyota K, Fukuda R, Hasegawa J, Ishida M, Nakajima T, Honda Y, Kitao O, Nakai H, Vreven T, Throssell K, Montgomery Jr. JA, Peralta JE, Ogliaro F, Bearpark MJ, Heyd JJ, Brothers EN, Kudin KN, Staroverov VN, Keith TA, Kobayashi R, Normand J, Raghavachari K, Rendell AP, Burant JC, Iyengar SS, Tomasi J, Cossi M, Millam JM, Klene M, Adamo C, Cammi R, Ochterski JW, Martin RL, Morokuma K, Farkas O, Foresman JB, Fox DJ: Gaussian 16 Rev. C.01. In . Wallingford CT: Gaussian Inc.; 2016.
Lee C, Yang W, Parr RG. Development of the Colle-Salvetti correlation-energy formula into a functional of the electron density. Phys Rev B. 1988;37(2):785.
Stephens PJ, Devlin FJ, Chabalowski CF, Frisch MJ. Ab initio calculation of vibrational absorption and circular dichroism spectra using density functional force fields. J Phys Chem. 1994;98(45):11623–7.
Beck AD. Density-functional thermochemistry. III. The role of exact exchange. J Chem Phys. 1993;98(7):5648–5646.
Grimme S, Furche F, Ahlrichs R. An improved method for density functional calculations of the frequency-dependent optical rotation. Chem Phys Lett. 2002;361(3–4):321–8.
Demissie TB, Hansen JH. Mechanism and site selectivity in visible-light photocatalyzed C–H functionalization: insights from DFT calculations. J Org Chem. 2016;81(16):7110–20.
Demissie TB, Sundar MS, Thangavel K, Andrushchenko V, Bedekar AV, Bouř P. Origins of optical activity in an oxo-helicene: experimental and computational studies. ACS Omega. 2021;6(3):2420–8.
Tomasi J, Mennucci B, Cammi R. Quantum mechanical continuum solvation models. Chem Rev. 2005;105(8):2999–3094.
Ismael M, Abdel-Mawgoud A-MM, Rabia MK, Abdou A. Design and synthesis of three Fe (III) mixed-ligand complexes: exploration of their biological and phenoxazinone synthase-like activities. Inorg Chim Acta. 2020;505:119443.
Seeliger D, de Groot BL. Ligand docking and binding site analysis with PyMOL and Autodock/Vina. J Comput Aided Mol Des. 2010;24(5):417–22.
Trott O, Olson A. Software news and updates Gabedit—a graphical user interface for computational chemistry softwares. J Comput Chem. 2010;31:455–61.
CAS PubMed PubMed Central Google Scholar
Narramore S, Stevenson CE, Maxwell A, Lawson DM, Fishwick CW. New insights into the binding mode of pyridine-3-carboxamide inhibitors of E. coli DNA gyrase. Bioorg Med Chem. 2019;27(16):3546–50.
Declercq J-P, Evrard C, Clippe A, Vander Stricht D, Bernard A, Knoops B. Crystal structure of human peroxiredoxin 5, a novel type of mammalian peroxiredoxin at 1.5 Å resolution. J Mol Biol. 2001;311(4):751–9.
Eswaramoorthy R, Hailekiros H, Kedir F, Endale M. In silico molecular docking, DFT analysis and ADMET studies of Carbazole Alkaloid and Coumarins from Roots of Clausena anisata: a potent inhibitor for quorum sensing. Adv Appl Bioinform Chem. 2021;14:13.
PubMed PubMed Central Google Scholar
Galma W, Endale M, Getaneh E, Eswaramoorthy R, Assefa T, Melaku Y. Antibacterial and antioxidant activities of extracts and isolated compounds from the roots extract of Cucumis prophetarum and in silico study on DNA gyrase and human peroxiredoxin 5. BMC Chem. 2021;15(1):1–17.
Ermiş E. Synthesis, spectroscopic characterization and DFT calculations of novel Schiff base containing thiophene ring. J Mol Struct. 2018;1156:91–104.
Mohammad H, Mayhoub AS, Cushman M, Seleem MN. Anti-biofilm activity and synergism of novel thiazole compounds with glycopeptide antibiotics against multidrug-resistant staphylococci. J Antibiot Res. 2015;68(4):259–66.
Salihović M, Pazalja M, Halilović SŠ, Veljović E, Mahmutović-Dizdarević I, Roca S, Novaković I, Trifunović S. Synthesis, characterization, antimicrobial activity and DFT study of some novel Schiff bases. J Mol Struct. 2021;1241:130670.
Kumar N, Gusain A, Kumar J, Singh R, Hota PK. Anti-oxidation properties of 2-substituted furan derivatives: a mechanistic study. J Lumin. 2021;230:117725.
Saqib M, Iqbal S, Mahmood A, Akram R. Theoretical investigation for exploring the antioxidant potential of chlorogenic acid: a density functional theory study. Int J Food Prop. 2016;19(4):745–51.
Lipinski CA, Lombardo F, Dominy BW, Feeney PJ. Experimental and computational approaches to estimate solubility and permeability in drug discovery and development settings. Adv Drug Deliv Rev. 1997;23(1–3):3–25.
Clark DE. Rapid calculation of polar molecular surface area and its application to the prediction of transport phenomena. 1. Prediction of intestinal absorption. J Pharm Sci. 1999;88(8):807–14.
Hollenberg PF. Characteristics and common properties of inhibitors, inducers, and activators of CYP enzymes. Drug Metab Rev. 2002;34(1–2):17–35.
Huang S-M, Strong JM, Zhang L, Reynolds KS, Nallani S, Temple R, Abraham S, Al Habet S, Baweja RK, Burckart GJ. Drug interactions/review: new era in drug interaction evaluation: US Food and Drug Administration update on CYP enzymes, transporters, and the guidance process. J Clin Pharmacol. 2008;48(6):662–71.
Kirchmair J, Göller AH, Lang D, Kunze J, Testa B, Wilson ID, Glen RC, Schneider G. Predicting drug metabolism: experiment and/or computation? Nat Rev Drug Discov. 2015;14(6):387–404.
Sadeghi M, Moradi M, Madanchi H, Johari B. In silico study of garlic ( Allium sativum L.)-derived compounds molecular interactions with α-glucosidase. In Silico Pharmacol. 2021;9(1):1–8.
Jean Nono H, Bikélé Mama D, Ghogomu JN, Younang E. A DFT study of structural and bonding properties of complexes obtained from first-row transition metal chelation by 3-alkyl-4-phenylacetylamino-4, 5-dihydro-1H-1, 2, 4-triazol-5-one and its derivatives. Bioinorg Chem Appl. 2017;2017:1–15.
Nepali K, Lee H-Y, Liou J-P. Nitro-group-containing drugs. J Med Chem. 2018;62(6):2851–93.
Mital A. Synthetic nitroimidazoles: biological activities and mutagenicity relationships. Sci Pharm. 2009;77(3):497–520.
Download references
Acknowledgements
The authors would like to acknowledge the Department of Applied Chemistry, School of Applied Natural Science, Adama Science and Technology University, Adama, Ethiopia, for offering MSc scholarship to FL. The Adama Public Health Research & Referral Laboratory Center, Ethiopia is greatly acknowledged for their generous support. TBD acknowledges the Department of Chemistry, University of Botswana for the research facilities. Computational resources were supplied by the project “e-Infrastruktura CZ” (e-INFRA CZ ID:90140) supported by the Ministry of Education, Youth and Sports of the Czech Republic.
No funding was received for this research work from outside source.
Author information
Authors and affiliations.
Department of Applied Chemistry, Adama Science and Technology University, P.O. Box 1888, Adama, Ethiopia
Fitsum Lemilemu, Mamaru Bitew & Milkyas Endale
Department of Chemistry, University of Botswana, Notwane Rd, P/bag UB 00704, Gaborone, Botswana
Taye B. Demissie
Department of Biomaterials, Saveetha Dental College and Hospital, Saveetha University, Chennai, 600 077, India
Rajalakshmanan Eswaramoorthy
You can also search for this author in PubMed Google Scholar
Contributions
FL designed, conducted the experimental work, analyzed the experimental data and drafted the manuscript. ME designed, supervised the entire experimental work, participated in data analysis, interpretation, and manuscript critical review. TBD and MB carried out the DFT calculations and participated in data analysis, interpretations, and manuscript critical review. RE and MB took part in the in silico calculations, the molecular docking calculations, and analysis. All authors read and approved the final manuscript.
Corresponding authors
Correspondence to Fitsum Lemilemu or Milkyas Endale .
Ethics declarations
Ethics approval and consent to participate.
Not applicable.
Consent for publication
Competing interests.
The authors declare no conflicts of interest.
Additional information
Publisher's note.
Springer Nature remains neutral with regard to jurisdictional claims in published maps and institutional affiliations.
Supplementary Information
Additional file 1:.
Spectral data of the synthesized compounds ( 1 H NMR, 13 C NMR, FTIR and UV–Vis spectra), comparison of experimental and calculated absorption spectra, Autodock Vina conformations of compounds 7 — 11 against E. coli DNA gyrase B, NAC, MEP and human peroxiredoxin 5 binding domains, DFT optimized geometries (xyz files), and QM descriptors are included within the additional files.
Rights and permissions
Open Access This article is licensed under a Creative Commons Attribution 4.0 International License, which permits use, sharing, adaptation, distribution and reproduction in any medium or format, as long as you give appropriate credit to the original author(s) and the source, provide a link to the Creative Commons licence, and indicate if changes were made. The images or other third party material in this article are included in the article's Creative Commons licence, unless indicated otherwise in a credit line to the material. If material is not included in the article's Creative Commons licence and your intended use is not permitted by statutory regulation or exceeds the permitted use, you will need to obtain permission directly from the copyright holder. To view a copy of this licence, visit http://creativecommons.org/licenses/by/4.0/ . The Creative Commons Public Domain Dedication waiver ( http://creativecommons.org/publicdomain/zero/1.0/ ) applies to the data made available in this article, unless otherwise stated in a credit line to the data.
Reprints and permissions
About this article
Cite this article.
Lemilemu, F., Bitew, M., Demissie, T.B. et al. Synthesis, antibacterial and antioxidant activities of Thiazole-based Schiff base derivatives: a combined experimental and computational study. BMC Chemistry 15 , 67 (2021). https://doi.org/10.1186/s13065-021-00791-w
Download citation
Received : 09 August 2021
Accepted : 04 December 2021
Published : 23 December 2021
DOI : https://doi.org/10.1186/s13065-021-00791-w
Share this article
Anyone you share the following link with will be able to read this content:
Sorry, a shareable link is not currently available for this article.
Provided by the Springer Nature SharedIt content-sharing initiative
- Schiff base
- Antibacterial
- Antioxidant
- Drug likeness
- DFT analysis

BMC Chemistry
ISSN: 2661-801X
- Submission enquiries: [email protected]
- General enquiries: [email protected]

An official website of the United States government
The .gov means it’s official. Federal government websites often end in .gov or .mil. Before sharing sensitive information, make sure you’re on a federal government site.
The site is secure. The https:// ensures that you are connecting to the official website and that any information you provide is encrypted and transmitted securely.
- Publications
- Account settings
Preview improvements coming to the PMC website in October 2024. Learn More or Try it out now .
- Advanced Search
- Journal List
- Antibiotics (Basel)

A Review on the Antimicrobial Activity of Schiff Bases: Data Collection and Recent Studies
Jessica ceramella.
1 Department of Pharmacy, Health and Nutritional Sciences, University of Calabria, 87036 Arcavacata di Rende, Italy; [email protected] (J.C.); [email protected] (D.I.); [email protected] (F.C.); [email protected] (R.L.); [email protected] (M.S.S.)
Domenico Iacopetta
Alessia catalano.
2 Department of Pharmacy-Drug Sciences, University of Bari “Aldo Moro”, 70126 Bari, Italy
Francesca Cirillo
Rosamaria lappano, maria stefania sinicropi, associated data.
Not applicable.
Schiff bases (SBs) have extensive applications in different fields such as analytical, inorganic and organic chemistry. They are used as dyes, catalysts, polymer stabilizers, luminescence chemosensors, catalyzers in the fixation of CO 2 biolubricant additives and have been suggested for solar energy applications as well. Further, a wide range of pharmacological and biological applications, such as antimalarial, antiproliferative, analgesic, anti-inflammatory, antiviral, antipyretic, antibacterial and antifungal uses, emphasize the need for SB synthesis. Several SBs conjugated with chitosan have been studied in order to enhance the antibacterial activity of chitosan. Moreover, the use of the nanoparticles of SBs may improve their antimicrobial effects. Herein, we provide an analytical overview of the antibacterial and antifungal properties of SBs and chitosan-based SBs as well as SBs-functionalized nanoparticles. The most relevant and recent literature was reviewed for this purpose.
1. Introduction
Schiff bases (SBs) are organic compounds characterized by an imine or azomethine group (>C=N–) that are widely used as pigments and dyes, catalysts, polymer stabilizers [ 1 ], luminescence chemosensors [ 2 ] and intermediates in organic synthesis [ 3 ]. SBs can also be used as corrosion inhibitors for different metal–electrolyte systems, since they adsorb and form a corrosion-mitigating surface film through their electron-rich centers, including the imine moiety. In fact, this moiety can offer strong bonding with metallic ions because of its π-acceptor properties [ 4 , 5 , 6 ]. Moreover, several studies addressed the tribological activities of SBs and their role as biolubricant additives [ 7 , 8 , 9 , 10 ]. Additionally, the use of SBs as catalysts in fixation of CO 2 to mitigate its accumulation in the atmosphere has been widely described [ 11 ]. SBs have also been studied in the carbohydrate research field in relation to Amadori products [ 12 ] and in material chemistry for applications in photoactive solar energy [ 13 , 14 ] and vitrimers [ 15 ]. Moreover, chemical reactions involving SBs may be found in sensors used to analyze illicit drugs and determine analytes in seized samples [ 16 ]. The exceptional popularity of SBs in organic chemistry can be attributed to their simple synthesis techniques, which use cheap materials. In fact, different synthetic routes continue to be described in the literature [ 17 , 18 , 19 ]. In biological chemistry, SBs have demonstrated a broad range of biological activities [ 20 ], including antimalarial, antiproliferative [ 21 ], analgesic, anti-inflammatory [ 22 ], antiviral, antipyretic, antifungal [ 23 ] and antibacterial [ 24 ] properties ( Figure 1 ). The imine or azomethine group (>C=N–) seems to be critical for their biological activities.

Main properties and uses of Schiff bases.
Recently, evidence on anticancer effects elicited by SBs has been provided [ 25 , 26 ]. It is worth noting that, in most cases, higher activity has been reported for SBs–metals complexes, rather than SBs alone [ 27 , 28 ]. Indeed, the presence of unpaired electrons on the nitrogen atom of the azomethine moiety gives strong chelating ability to SBs. SBs are now considered interesting ligands for coordination chemistry, thanks to their chelating ability along with, the convenience of separation and flexibility on the C=N group [ 29 ]. In recent years, the phenomenon of antibiotic resistance in hospitals, communities and environment has increasingly grown. It is known that the overuse of antibiotics has led to an increase in bacterial resistance [ 30 ] and, in turn, to decreasing efficiency of the rare available antibiotics [ 31 , 32 ]. It is noteworthy that, also in this case, the complexation of SBs with metals generally leads to enhanced antibacterial and antifungal effects in comparison with free SBs [ 33 , 34 , 35 ]. Recently, a novel and green cellulose-based antibacterial complex of an SB with copper was fabricated. Its antibacterial activity against Escherichia coli and Staphylococcus aureus increased by 472% and 823%, respectively, in comparison to the SB ligand [ 36 ]. However, SBs complexes are not totally devoid of disadvantages. For instance, when using SBs as homogeneous catalysts there can be reduction in the activation level in the process, demanding the recovery and recycling of catalyst and metal contaminations in products [ 37 ]. Moreover, the keto–enolic tautomeric isomerism that may occur at the double bond C=N, in the presence of ortho -hydroxy groups, may lead to different activities for the two tautomers [ 38 ]. Considerable interest has also been recently devoted to chitosan-based Schiff bases (CBSs) for their application in various fields such as biology, catalysis, sensors and water treatment [ 39 ]. Chitosan is a polysaccharide of natural origin, obtained from marine crustaceans, mollusks, insects and fungi. Of note, chitosan has shown antitumor, antiulcer, immunostimulatory, antidiabetic, antioxidant and antibacterial activities [ 40 ]. Recently, chitosan and its derivatives have also received attention as adsorbents for heavy metal ions and their removal from wastewater [ 41 , 42 , 43 ]. Nanoparticles represent an excellent platform for a broad range of biological and biomedical applications. Schiff base-functionalized nanoparticles have often been used for the determination of heavy metal ions [ 44 ]. Recently, the synthesis of core–shell (Fe 3 O 4 @SiO 2 ) magnetic nanoparticles functionalized using an SB ligand for uranium extraction has also been reported [ 45 ]. Currently, nanoparticles may represent a promising approach for fighting antimicrobial resistance [ 46 ]. In this scenario, and given the need to identify new therapeutics in the antimicrobial field, this narrative review aims at highlighting the recent studies describing the molecular mechanisms involved in the antibacterial and antifungal effects elicited by SBs and CBSs as well as SB- and CBS-based nanoparticles.
2. Schiff Bases as Antimicrobial Agents
The antibacterial and antifungal activity of some SBs has been reported. The minimal inhibitory concentration (MIC) and/or inhibition zone diameter (IZD) are given.
2.1. Schiff Bases with Antibacterial Activity
A recent work by Hassan et al. (2019) [ 47 ] on SBs derived from 5-aminopyrazoles, namely 5-(benzylideneamino)-3-(4-methoxyphenylamino)- N -phenyl-1 H -pyrazole-4-carboxamides, showed their antimicrobial activity against multidrug-resistant bacteria (MDRB). In particular, compounds 1 – 3 ( Table 1 ) were more active than ciprofloxacin against Gram-positive Staphylococcus epidermidis (MIC = 7.81 µg/mL versus 15.62 µg/mL of ciprofloxacin), while compound 4 had the same activity of the reference against Enterococcus faecalis (MIC = 7.81 µg/mL), which is a Gram-positive organism responsible for serious infections in humans [ 48 ]. Interestingly, compounds 2 and 3 were also active against Gram-negative Acinetobacter baumanni , showing the same MIC values of ciprofloxacin (MIC = 15.62 µg/mL). Moreover, in silico ADMET studies were carried out. Enzymatic assays and molecular docking revealed that compound 3 exerted a strong inhibitory activity against S. aureus DNA gyrase and dihydrofolate reductase kinases.
Schiff bases with antibacterial activity.
Recently, Gümüş et al. (2020) [ 49 ] described two series of SB derivatives with anthracene- and pyrene-based units, which were synthesized and evaluated for their antibacterial activity against Gram-negative Bacillus cereus, E. coli and Pseudomonas aeruginosa . These experiments were performed by using the disk diffusion method taking tetracycline (30 μg) and streptomycin (10 μg) discs as positive controls. DNA binding activities were also tested by agarose gel electrophoresis, in which DNA molecules behave in accordance with their mass, charge and shape. As a result of binding the compounds to calf thymus DNA (CT-DNA), free DNA moves faster on the gel as it is smaller in respect to bound DNA. Of note, compounds 5 and 6 ( E )-2-((anthracen-1-ylimino)methyl)quinolin-8-ol and ( E )-2-((pyren-1-ylimino)methyl)pyridin-3-ol, respectively, which were found to be stably bound to CT-DNA, showed antibacterial activity against Bacillus cereus and E. coli .
Erturk et al. (2020) [ 50 ] synthesized two SBs, namely 4-(((8-hydroxyquinolin-2-yl)methylene)amino)-1,5-dimethyl-2-phenyl-1,2-dihydro-3 H -pyrazol-3-one and 4-(((10-chloroanthracen-9-yl)methylene)amino)-1,5-dimethyl-2-phenyl-1,2-dihydro-3 H -pyrazol-3-one ( 7 and 8 , respectively), that were studied for their antimicrobial and antioxidant properties. Both compounds proved high antibacterial activity against Gram-positive bacteria Micrococcus luteus and S. aureus (MIC = 25 μg/mL and 12.5 μg/mL, respectively, versus MIC = 100 μg/mL and 12.5 μg/mL of ampicillin). In addition, compound 8 exhibited high antifungal potential against fungi Aspergillus niger (MIC = 12.5 μg/mL versus MIC = 12.5 μg/mL of nystatin). They also showed antiproliferative activity against the MCF-7 human breast cancer cell line (IC 50 < 0.1 mM and IC 50 = 0.14 mM for 7 and 8 , respectively).
In the study of Mishra et al. (2020) [ 51 ] the synthesis and antibacterial evaluation of two SB ligands comprising benzothiazole derivatives, namely ( N , N′ , E , N , N′E )- N , N ′-(1,3-phenylenebis(methanylylidene))bis(5-nitrobenzo[d]thiazoL2-amine ( 9 ) and ( N , N′ , E , N , N′E )- N , N ′-(1,3-phenylene-bis(methanylylidene))bis(5-methylthiazo-L2-amine ( 10 ) and their lanthanide (III) complexes were described. Ligands showed antibacterial activity against S. aureus causing skin infection and food poisoning and pimple-causing Propionic bacteria acnes . Of note, the complexes were more active than SBs alone.
Yusuf et al. (2020) [ 52 ] reported the synthesis and antimicrobial evaluation of three SBs, ( E )-1-(2-nitrophenyl)- N -( o -tolyl)methanimine ( 11 ), ( E )-2-isopropyl- N -(2-nitrobenzylidene)aniline ( 12 ) and ( E )-2-((2-nitrobenzylidene)amino)phenol ( 13 ). Compounds 11 – 13 were tested against E. coli , Salmonella typhimurium and P. aeruginosa as shown in Table 1 . Then, compounds 11 – 13 were docked into the active site pocket as observed experimentally of crystalized structures from E. coli , S. typhimurium , P. aeruginosa and S. aureus. Compound 12 showed the highest inhibitory activity against the Gram-negative bacteria S. thyphymurium and P. aeruginosa (MIC = 15.625 µg/mL and 7.81 µg/mL, respectively) compared to the reference drug (chloramphenicol: MIC = 31.25 µg/mL and 62.50 µg/mL, respectively).
Interesting findings on P. aeruginosa were also reported by Chemchem et al. (2020) [ 53 ] for twelve isatin SBs obtained by green synthesis. The compounds were studied against standard and clinical bacterial strains by the agar-well diffusion method. Compound 14 and 15 revealed the lowest MIC (625 μg/mL) against standard S. aureus 25923 and Klebsiella pneumoniae 700,603, respectively, while the reference compound colistin was inactive. When tested against P. aeruginosa clinical bacterial strains, compounds 16 and 14 were the most active, showing an MIC value of 78 μg/mL with a large IZD (24 mm and 15 mm, respectively), compared to the antibiotic fosfomycin (IZD = 16 mm). QSAR studies showed that increased hydrophobic character associated with decreased dipole moment led to higher antibacterial activity against the standard K. pneumoniae strain.
Warad et al. (2020) [ 54 ] reported the synthesis of three SBs and their antibacterial evaluation by means of the disk diffusion test. The compounds showed low activity with respect to gentamicin. The most interesting compound was 2-(piperazin-1-yl)- N -(thiophen-2-ylmethylene)-ethanamine ( 17 ). The most sensitive isolates to this compound were Gram-positive S. aureus and methicillin-resistant S. aureus (MRSA), as shown in Table 1 , compared to gentamicin (IZD = 25 and 24 mm, respectively) and P. aeruginosa (gentamicin, IZD = 16 mm).
Bayeh at al. (2020) [ 55 ] described the synthesis of three SBs and their antimicrobial activities compared to ciprofloxacin and chloramphenicol, as references. Among the SBs, 18 showed higher activity (IZD = 32 mm) than the references against Gram-positive S. epidermidis (IZD = 24 and 26.7 mm for ciprofloxacin and chloramphenicol, respectively) and against Gram-negative P. aeruginosa (IZD = 21.3 and 27.3 mm for ciprofloxacin and chloramphenicol, respectively), whereas compound 19 was more active against S. aureus (IZD = 32.5 mm) in respect to both ciprofloxacin and chloramphenicol (IZD = 24 and 26.3 mm, respectively).
Anwar et al. (2020) [ 56 ] described the synthesis of SBs of amikacin and their screening for in vitro antibacterial activity by the well diffusion method against Bacillus megaterium, Bacillus subtilis, Stenotrophomonas maltophilia, S. aureus, M. luteus, Serratia marcescens and E. coli. The authors concluded that derivatives of amikacin with aromatic rings were more active antibacterials than those with an aliphatic side chain. The most interesting compounds were 20 and 21 , which showed higher activity against S. aureus (IZD = 37 and 321 mm, respectively) than amikacin (IZD = 29 mm) and E. coli (IZD = 37 and 38 mm versus amikacin IZD = 25 mm).
Salihović et al. (2021) [ 57 ] described the synthesis of two SBs deriving from l -cysteine, namely 2-((2-chlorobenzylidene)amino)-3-mercaptopropanoic acid ( 22 ) and 3-mercapto-2-((2-methoxybenzylidene)amino)propanoic acid ( 23 ), and an antimicrobial activity evaluation against five Gram-positive ( S. aureus, B. subtilis, Clostridium sporogenes, M. luteus and Microccocus flavus ), five Gram-negative ( E. coli, P. aeruginosa, Proteus hauseri, K. pneumoniae, Salmonella enterica and subsp. enterica serovar Enteritidis) standard bacterial strains and some yeasts ( A. brasiliensis, S. cerevisiae and C. albicans ). Compound 22 showed higher antimicrobial activity against all tested bacteria (MIC = 1.284 mM), while compound 23 showed lower activity (MIC = 2.612 mM) compared to amikacin (MIC between 0.08 and 0.111 mM). The higher antifungal activity was found against A. brasiliensis for both compounds (MIC = 1.284 mM and 1.306 mM, respectively) versus amphotericin B (MIC = 0.044 mM).
Srinivasan et al. (2021) [ 58 ] described the synthesis and antibacterial evaluation of two pyrene-based SBs, 4-[(5-pyren-1-yl-thiophen-2-ylmethylene)-amino]-phenol ( 24 ) and 4-[(4-pyren-1-yl-benzylidene)-amino]-phenol ( 25 ), against two strains of P. aeruginosa, 9027 and 27853. The authors determined that 24 was more active as an antibacterial when compared to 25 ; however, MIC or IZD values were not given. The treatment with compound 24 showed a minimum effect on the cell viability at a concentration range from 100 to 500 μg/mL. It was only found to be cytotoxic, i.e., almost 70% cell growth inhibition was evident, at a high concentration of 24 , i.e., 1000 μg/mL.
Sumrra et al. (2021) [ 59 ] reported a study on two mono-SBs, 4-[(5-amino-1 H -1,2,4-triazol-3-yl)imino]methylbenzene-1,3-diol ( 26 ) and 2-[(5-amino-1 H -1,2,4-triazol-3-yl)imino]methyl}-6-methoxyphenol ( 27 ), and one bis-SBs, 2,2′-{1 H -1,2,4-triazole-3,5-diylbis [nitrilomethylylidene]}bis(6-methoxyphenol) ( 28 ), along with their metal complexes. An antibacterial activity evaluation was carried out for five bacterial strains ( Halomonas halophila, Chromohalobacter israelensis, E. coli, Chromohalobacter salexigens and Halomonas salina ). They exhibited antibacterial activity, and 26 was found to be more active against C. salexigens and H. salina and 27 was found to be more active against E. coli and C. salexigens , while 28 showed higher activity against E. coli and H. salina. Streptomycin, which was used as a reference, showed IZD values between 20 and 23 mm.
Ragi et al. (2021) [ 60 ] recently studied two SBs, 2,2′-(5,5-dimethylcyclohexane-1,3-diylidene)bis(azan-1-yl-1-ylidene)diphenol ( 29 ) and N , N ′-(5,5-dimethylcyclohexane-1,3-diylidene)dianiline ( 30 ), against S. aureus and its target proteins after pre-filtering the drug-like properties of the compounds using the Lipinski rule of five. The compounds showed activity similar to that of ampicillin against S. aureus (IZD = 26 and 25 mm, respectively, versus 30 mm of ampicillin, all measured at 500 μg disc −1 concentration). The mechanisms by which these molecules can inhibit the growth of S. aureus were established by molecular docking studies on six different target proteins of S. aureus : sortase-A, clumping factor A (ClfA), dihydrofolate reductase (DHFR), DNA gyrase, undecaprenyl diphosphate synthase (UPPS) and dehydrosqualene synthase (CrtM) (PDB ID: 1T2P, 1N67, 2W9S, 3U2D, 4H8E and 2ZCO, respectively). Both SBs showed good binding affinity for the target protein DHFR, although in different sites. Thus, the authors suggested that the appreciable growth-inhibitory power exerted by compounds DmChDp and DmChDa against S. aureus could be related to the deactivation of dihydrofolate reductase [ 58 ].
Singhal et al. (2021) [ 61 ] studied 15 novel bis indole-based SBs as antibacterials. Comparative analyses against S. aureus and E. coli showed a higher inhibition of bis-SBs than the reference drug ciprofloxacin and their mono counterparts, the mono-SBs. The hexyl linker-based bis-SBs 31 – 33 depicted higher antibacterial action at 50 μg/mL, and the inhibitory effect increased with the increasing number of carbon atoms on the linker chain. Specifically, bis-SB 33 was the most active, showing MIC values of 37 and 34 mm against E. coli and S. aureus , respectively, compared to ciprofloxacin (IZD = 14 mm and 17 mm, respectively). Molecular docking studies on this compound were carried out with CT-DNA (PDBID:1BNA) and SARS-CoV-2 Mpro (3CL protease, PDBID:6LU7) via ultraviolet-visible and fluorescence spectroscopy techniques. Compound 33 proved its efficacy as a potential DNA binder and antiviral agent.
A recent study by Aragón-Muriel et al. (2021) [ 62 ] investigated the antibacterial potential of four benzimidazole-based Schiff bases and their metal complexes against two Gram-positive strains ( S. aureus 25923, Listeria monocytogenes 19115) and two Gram-negative strains ( E. coli 25922, P. aeruginosa 27583). As generally observed, the activity of the complexes was higher than that of SBs alone. Moreover, isomerism in SBs seemed to play a crucial role, as is often the case for compounds with biological activity [ 63 ]. The major activity found in the study by Aragón-Muriel et al. (2021) [ 62 ] was shown by 34 and 35 (MIC = 250 ng/mL against S. aureus versus 0.5 ng/mL of ciprofloxacin). Despite the low activity of SBs, this study provided novel insights into the mechanisms through which the compound exerts antibacterial action, suggesting that interactions with the bacterial membrane or interactions with DNA could be involved. The types of interactions that occurred between nucleic acid and new compounds was studied by UV/V spectrophotometry. Differences can be found in the tautomers deriving from the keto–enol equilibrium ( Figure 2 ). The interaction of DNA with these systems would result in the stabilization of one of the tautomers when a DNA–compound complex is formed. The interaction with DNA seemed to favor the keto form of 34 , while the union of 35 with DNA strands stabilized the enol form of 35 .

Keto–enol equilibrium of 34 and 35 SBs.
2.2. Schiff Bases with Antifungal Activity
All the data described in this paragraph are summarized in Table 2 . Some studies did not report MICs or IZDs but did provide inhibitory indexes (IIs).
Schiff bases with antifungal activity.
Magalhães et al. (2020) [ 64 ] described the synthesis and the in vitro antifungal evaluation of 23 cinnamyl SBs. Six of them showed antifungal activities against strains of Candida , Aspergillus , Fonsecaea and, specifically, Cryptococcus species. Compounds 36 and 37 showed MIC values more than two-fold lower than that of the reference fluconazole against all the Cryptococcus neoformans strains (MIC = 1.33 µg/mL and 1.4 µg/mL, respectively, versus fluconazole, 5.2 µg/mL) and Cryptococcus gattii strains (MIC = 5.3 µg/mL and 2.8 µg/mL, respectively, versus fluconazole, 9.2 µg/mL), while cinnamyl SB 38 was as potent as fluconazole against all strains from both Cryptococcus species. No significant cytotoxic effects were observed for SBs against human lung (MRC-5, human fetal lung fibroblast), kidney (HEK 293, human embryonic kidney) or red blood cells, all presenting selective indexes higher than 10.
Chen et al. (2020) [ 65 ] described a structural modification of inulin by SBs in order to improve its biological activity. The antifungal studies against three kinds of plant pathogenic fungi ( Botrytis cinerea, Fusarium oxysporum f. sp. cucumerium Owen and Phomopsis asparagi ), according to Guo’s method [ 66 ], showed that synthetic inulin derivatives demonstrated a broad antifungal spectrum. At 1.6 mg/mL, the IIs of 39 were 93%, 83% and 82% against B. cinerea, F. oxysporum f. sp. cucumerium Owen and P. asparagi , respectively. The lipophilic characteristics of 39 due to the presence of the benzene ring and the acetyl groups allow this compound to easily penetrate into the cell, leading to apoptosis. Moreover, the new inulin derivatives modified with the Schiff bases showed an excellent metal binding property, suppressing the growth of microbes by their interaction with cellular components.
A recent study by Hamad et al. [ 67 ] described the synthesis of SBs of sulphonamides and their antifungal activity against multidrug-resistant Candida auris , an emerging fungal pathogen with a high mortality rate, which is difficult to identify and carries considerable risks of healthcare outbreaks [ 68 ]. In 2020, diverse cases of candidemia outbreaks related to COVID-19 in intensive care units in hospitals and severe fungal coinfections have been reported worldwide [ 69 ]. The SBs of sulphonamides were first tested against a multispecies panel of Candida strains ( C. auris TDG1912 , Candida albicans NCPF3281 and NCPF3179, Candida glabrata NCPF8018, Candida krusei NCPF3876, Candida tropicalis NCPF8760 and Candida parapsilosis NCPF3209). Compound 40 showed interesting MIC values against almost all the strains in the range of 4–32 µg/mL (versus 0.5–>128 µg/mL of fluconazole). Thus, it was tested against a larger panel of multidrug-resistant C. auris strains (TDG2506, TDG2512, TDG1102, TDG2211, NCPF8984, NCPF8977 and NCPF8971), and its antifungal activity was confirmed with MICs of 4–16 µg/mL, which are very low considering that in four cases (TDG2506, TDG1102, TDG2211 and NCPF8984) the MIC value for fluconazole was ≥128 µg/mL. Compound 41 showed the same results of 40 only on the extended panel [ 64 ].
Bhagwatrao Biradar et al. (2021) [ 70 ] reported the synthesis of SBs derived from dehydroacetic acid (DHA) and evaluated their antimicrobial activity. The most interesting compounds were 42, showing an IZD = 19 mm against A. niger (griseofulvin IZD = 22 mm) and 43 against C. albicans (IZD = 21 mm versus griseofulvin, IZD = 25 mm).
3. Chitosan-Based Schiff Bases (CBSs)
Chitosan ( N -deacetylated product of chitin) is a natural biopolymer composed of β- d -glucosamine and N -acetyl-β- d -glucosamine units with a 1,4-linkage. Chitosan is an attractive material because of its properties such as immunological activity, biocompatibility, low toxicity and biodegradability. Chitosan is insoluble in water and most organic solvents, which seriously limits its application. CBSs result from the chemical modification of chitosan via imine functionalization (RR′C=N-R″; R = alkyl/aryl, R′ = H/alkyl/aryl and R″ = chitosan ring). They are usually synthesized by the Schiff condensation reaction between chitosan’s amino groups and carbonyl compounds with the removal of water molecules. The antibacterial applications of chitosan derivatives are numerous [ 71 , 72 ]. Recently, two chitosan Schiff bases were used for the removal of Cr(VI) from wastewater with high efficiency [ 73 , 74 ]. Antimicrobial studies of CBSs are reported in Table 3 .
Structure and antibacterial activity of chitosan-based Schiff bases.
Barbosa et al. (2017) [ 75 ] described several biopolymeric Schiff bases of chitosan and different salicylaldehydes and their complexes with palladium(II) and platinum(II). They were tested for antimicrobial activity in vitro against two common bacterial and fungal plant pathogens, Pseudomonas syringae pv. tomato and Fusarium graminearum , respectively, and for their antiproliferative activity against human MCF-7 breast cancer cells. Compared to the unmodified chitosan, CBSs and their complexes had higher antibacterial effects against P. syringae but were highly toxic against MCF-7 cells. In particular, the MIC value of 44 was 25 µg/mL (versus >50 µg/mL of purified chitosan). The antifungal effects against F. graminearum were less pronounced compared to the nonmodified chitosan ( 44 , MIC = 50 µg/mL versus 30 µg/mL of purified chitosan), suggesting diverse modes of action for the two fungal species.
Wei et al. (2019) [ 76 ] synthesized eight CBSs and quaternary ammonium salts in order to improve the antioxidant and antifungal activity of chitosan. The antifungal activity against F. oxysporum f. sp. cucumerium , B. cinerea and F. oxysporum f. sp. niveum was evaluated using a mycelium growth rate test. CBSs exhibited enhanced antifungal activity when compared to chitosan, especially at 1.0 mg/mL. Chitosan II was 16.9% at 1.0 mg/mL. The most interesting were 6-[4-(2,3-dihydroxyl-benzimide) pyridine] acetyl-2- N , N , N -trimethyl-chitosan chloride ( 45 ) and 6-[4-(2,3,4-trihydroxyl-benzimide) pyridine] acetyl-2- N , N , N -trimethyl-chitosan chloride ( 46 ), which showed IIs >90.0% at 1.0 mg/mL against F. oxysporum f. sp. cucumerium and B. cinerea . The chitosan derivatives also showed stronger antioxidant activity than chitosan. It was suggested that the higher density of the positive charge contributes to the antifungal activity. The positive charge could interact with the anionic substances, such as glucan, mannan, proteins and lipids to form a lipophilic layer around the cell, which could prevent nutrient exchange in the cells. The authors posited that the higher antifungal activity of 45 and 46 might be due to the presence of phenolic groups.
Hassan et al. (2018) [ 77 ] described the ability of two antimicrobial chitosan SBs, 47 and 48 , to boost the antimicrobial activity of native chitosan against Gram-positive bacteria ( S. aureus and B. cereus ), Gram-negative bacteria ( E. coli , P. aeruginosa and Salmonella sp.) and C. albicans fungal species. The antimicrobial activity of SB 47 was significantly higher than that of SB 48 and chitosan (IZD = 11.5, 12.6, 13, 13.9, 13.6 and 11.4 mm (against E. coli , P. aeruginosa , Salmonella spp. and S. aureus , C. albicans ) using erythromycin (IZD = 11 and 12.6 mm against E. coli and Salmonella spp.) and nystatine (IZD = 15.2 mm against C. albicans ) as reference drugs. The highest concentration of compound 47 could inhibit the growth of Gram-positive bacteria up to 99%, whereas compound 48 recorded the maximum inhibition rate against Gram-positive bacteria, by approximately 82%. The higher action of compound 47 on Gram-positive bacteria than Gram-negative bacteria, which appeared at 250 μg/mL, was attributed to the difference of the cell wall structures. The cytotoxicity of the developed materials was estimated by MTT assay in fibroblast cells. The cellular toxicity of SBs I and II at 200 mg were 5.2% and 6.3%, respectively, thus substantiating their safety.
Ali et al. (2018) [ 78 ] studied a chitosan derivative, a methyl acrylate chitosan-bearing p -nitrobenzaldehyde ( 49 ) Schiff base, and examined its antibacterial activity against MDR Gram-positive bacteria MDR S. aureus (MDR-SA) and MDR Gram-negative bacteria MDR P. aeruginosa (MDR-PA), MDR K. pneumoniae (MDR-KP) and MDR E. coli (MDR-EC) by using the agar-well diffusion method. The chitosan derivative 49 showed significantly higher antibacterial activity against MDR-SA and MDR-PA compared to other bacteria. MDR S. aureus 4 (MDR-SA-04) and MDR P. aeruginosa 9 (MDR-PA-09) strains were found to be more susceptible Gram-positive and Gram-negative bacterial strains to 49 , respectively. The highest mean IZDs ranged from 15.0 ± 0.23 to 28.5 ± 0.03 mm and from 10.5 ± 0.03 to 22.1 ± 0.05 mm, respectively. On the other hand, 49 revealed the highest antibacterial activity, showing an MIC value of 6.25 μg/mL for both bacterial strains. Moreover, it exhibited antibiofilm activity against MDR-PA-09, as assessed by using a microtiter plate. The compound also showed antioxidant and anti-inflammatory activities, as determined by the 2,2-diphenyl-2-picrylhydrazyl (DPPH) method and by albumin denaturation, membrane stabilization of red blood cells assay and proteinase inhibition methods.
Recently, Wei et al. (2021) [ 79 ] reported another work regarding the bis-Schiff bases of chitosan derivatives tested for their antifungal action against three plant pathogenic fungi ( B. cinerea , Fusarium oxysporum f. sp. cucumerinum and Fusarium oxysporum f. sp. Niveum ). The results show that the bis-Schiff bases of chitosan showed higher antifungal activity than chitosan, especially at 1.0 mg/mL. Specifically, derivatives bearing halogeno-benzenes ( 50 – 52 ) showed IIs higher than 95% at 1.0 mg/mL against B. cinerea (versus chitosan 23.8%), and this high activity was attributed to the halogen with stronger electron-withdrawing properties.
4. Nanoformulations and Nanomedicines
Nanotechnology has recently emerged as a very interesting field of research with countless biomedical science applications. It is described as the design, characterization and application of structures, devices and systems by monitoring shape and size at a nanometer scale (1 nm to 100 nm) [ 80 ]. Silver, gold and copper nanoparticles have been widely described for their anticancer properties [ 81 ], and silver and gold nanoparticles have also shown antibacterial and antiviral activities [ 82 , 83 ]. In addition, experimental evidence regarding nanoparticles with Schiff bases for the diagnosis and treatment of tumors have been also reported. Some prodrug nanomedicines deriving from Schiff bases with doxorubicin and polyethylene glycol (PEG), one of the most widely used hydrophilic polymer approved by Food and Drug Administration (FDA) with negligible toxicity, have been described for their antitumor activity [ 84 ]. Moreover, the functionalization of chitosan nanoparticles with the anticancer 5-fluorouracil to obtain chitosan nanocarriers through Schiff base formation has been studied [ 85 ].
4.1. Nanoparticles of Schiff Bases
Several studies on the ability of nanoparticles to enhance antibacterial activities have also been reported. Several nanocojugates, i.e., Schiff base complexes with zinc conjugated to silver nanoparticles, showed excellent antimicrobial activity against S. aureus [ 86 ]. A recent work by Minhaz et al. (2020) [ 87 ] compared the activity of an SB ( 53 ) and silver nanoparticles of this compound ( 53-AgNPs ), studied as nanoprobes. The latter exhibited significant antibacterial and anticancer activities and was also used as sensitive protocol for the detection and quantification of heavy metal Hg(II) in tap water. Regarding antibacterial activity, the IZDs values for 53 were 8.0, 9.0, 7.0 and 7.0 mm against S. aureus , B. subtilis , P. aeruginosa and E. coli , respectively, and 53-AgNPs achieved IZD values of 18.0, 18.0, 10.0 and 10.0 mm, respectively (values for vancomycin were 18.0, 25.0, 12.0 and 13.0 mm, respectively). Cytotoxicity results show that 53-AgNPs at a concentration of 100 mg/mL induced DU-145 cell toxicity and caused 94% cell death after treatment up to 72 h. Moreover, in normal cells (L-929) death rate was lower (up to 1.5%) as compared to cancer cells, and this was indicative of a selective action of this compound.
4.2. Nanoparticles of Schiff Bases with Chitosan
Biomaterials based on chitosan modified with nanoparticles have shown promising results as antibacterials [ 88 , 89 , 90 ]. In fact, chitosan-based nanoparticles have several advantages including efficiency, cost-effectiveness, biocompatibility, biodegradability, non-toxicity and non-immunogenicity [ 91 ].
Abdel-Monem et al. (2020) [ 92 ] described the synthesis of nanocomposites of salicylaldehyde and anisaldehyde carboxymethyl chitosan–Schiff bases ( 54 and 55 , respectively, Table 4 ) also loaded with silver nanoparticles ( 54-AgNO 3 and 55-AgNO 3 ) and studied their antibacterial activities against Gram-positive bacteria ( B. subtilis , S. aureus and Streptococcus faecalis ) and Gram-negative bacteria ( E. coli , Neisseria gonorrhoeae and P. aeruginosa ). CMCs derivatives presented remarkable antimicrobial activity, which was increased using silver (AgNO 3 5%). Ampicillin was used as a reference (IZD = 20, 18, 18, 22, 20 and 17 mm against B. subtilis , S. aureus , S. faecalis , E. coli , N. gonorrhoeae and P. aeruginosa , respectively). SB 54 was more active than SB 55 . Moreover, 54-AgNO 3 was more active than 55-AgNO 3 . Interestingly, compound 54-AgNO 3 was even more active against P. aeruginosa than the reference (ampicillin; IZD = 17 mm). Cytotoxic activity was evaluated against liver HePG2 and breast MCF7 cancer cells. CMC-SBs showed lower cytotoxicity than carboxymethyl chitosan against both tumor cell lines. The cytotoxicity was even lower when considering CMC-SBs silver nanoparticles.
Structure and antibacterial activity of nanoparticles of SBs and CBSs.
Recently, a CSB ( 56 ) and its CSB-NiFe nanocomposite ( 56-NiFe ) were tested as antimicrobials against two Gram-positive ( S. aureus and B. cereus ) and two Gram-negative ( E. coli and P. aeruginosa ) bacteria by the agar-well diffusion method [ 93 ]. The results obtained show that the antibacterial activities of the compounds 56 and 56-NiFe were stronger than that of pure chitosan. The authors postulated that the compounds show weak antibacterial activity against P. aeruginosa , medium activity against B. cereus and good activity against S. aureus and E. coli . However, the MIC and/or IZD values are not given.
SBs are inexpensive compounds and easy to synthesize. They display structural and electronic features that enable their application in a large number of research fields, such as analytical, inorganic and organic chemistry. Moreover, their antibacterial, antifungal, anticancer, urease inhibitor and antioxidant effects have been widely reported. Additionally, SBs have shown antiglycation activities, anti-inflammatory, antitumor, antiviral, antipyretic and anti-HIV-1 abilities. Nowadays, the need for more effective antibacterial and antifungal therapies is pressing due to the high mortality rates that are associated with bacterial and fungal diseases as well as the growing number of multidrug-resistant strains. This review summarizes the antimicrobial effects of SBs as described in the recent literature and highlights the importance of CSBs. Chitosan is a product of the deacetylation of chitin, which is widely found in nature. It has proved antibacterial activity, good biodegradation, outstanding biocompatibility, is non-toxic and has excellent chemical and physical properties. As a result, chitosan and its derivatives have been widely tested in the antimicrobial field, showing antibacterial activity against Gram-positive and Gram-negative bacteria as well as antifungal potential. Interestingly, chitosan nanoparticles exhibit antibacterial activity. The most recent studies on CBSs and their nanoparticles as antibacterials have been herein summarized. It is worth mentioning that antibacterial and antitumor activities are generally higher when organic compounds, including SBs, are complexed with metals. Hence, the antimicrobial action of SBs reviewed herein may be taken into account to favor the development of novel metal complexes with improved antimicrobial capacities. Finally, the antibacterial activity of SBs, along with their known anticorrosive potential, might also represent great potential for their future application in several types of surgeries.
Acknowledgments
We would like to thank the special award, namely “Department of Excellence 2018–2022” (Italian Law 232/2016) at the Department of Pharmacy, Health and Nutritional Sciences of the University of Calabria (Italy) and the “Sistema Integrato di Laboratori per L’Ambiente—(SILA) PONa3_00341”.
Abbreviations
Author contributions.
Conceptualization, R.L. and M.S.S.; writing—original draft preparation, J.C.; writing—review and editing D.I. and A.C.; data curation, F.C.; supervision, M.S.S. All authors have read and agreed to the published version of the manuscript.
This research received no external funding.
Institutional Review Board Statement
Informed consent statement, data availability statement, conflicts of interest.
The authors declare no conflict of interest.
Publisher’s Note: MDPI stays neutral with regard to jurisdictional claims in published maps and institutional affiliations.
Academia.edu no longer supports Internet Explorer.
To browse Academia.edu and the wider internet faster and more securely, please take a few seconds to upgrade your browser .
Enter the email address you signed up with and we'll email you a reset link.
- We're Hiring!
- Help Center
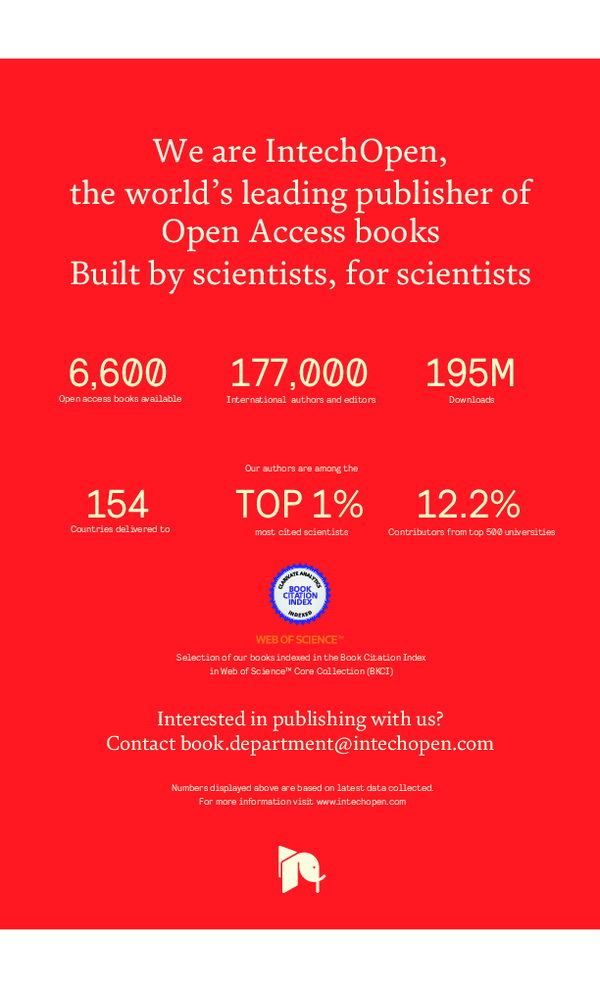
Schiff Bases and Their Metal Complexes: Synthesis, Structural Characteristics and Applications

Schiff Base in Organic, Inorganic and Physical Chemistry [Working Title]
The development of Schiff base was a major step forward in the area of coordination chemistry. Schiff bases, a class of organic compounds, carry the imine or azomethine (>C=N–) functional group. Schiff bases played an influencing role in the development of coordination chemistry and were a key point in the development of inorganic, bioinorganic chemistry and optical materials. Schiff bases, widely used in inorganic, organic, and analytical chemistry, account for a significant portion of the more commonly employed classes of organic molecules. The ability of Schiff base ligands to form stable metal complexes with a wide range of transition and other metal ions makes them extremely useful. Condensation of a primary amine with an aldehyde or ketone yields a Schiff bases. In this chapter, we focused on introducing Schiff bases, classified them and their metal complexes, and discussed several synthesis methods, including conventional and green approaches. This chapter also elaborated ...
Related Papers
KISHOR DANAO
Schiff bases are the condensation products of primary amines and carbonyl compounds, which are becoming more and more significant. Schiff bases are imine or azomethine (–C=N–) functional group containing compounds that are produced through a nucleophile addition process. Excellent chelators called Schiff bases have a place in both qualitative and quantitative analysis of metals in aqueous media. Schiff bases were discovered to be auxiliary scaffolds and adaptable pharmacophore for the creation and production of numerous bioactive leads compounds, and this special quality made them accessible for a wide range of biological applications. Schiff bases exhibit significant biological properties including analgesic, anti-inflammatory, antibacterial, anticonvulsant, anti-tubercular, anticancer, antioxidant, anthelmintic antiglycation, and antidepressant activities. In situ cross-linked hydrogel systems are created using the Schiff bases, which are frequently utilized in coordination, organ...
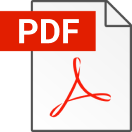
Stability of Coordination Compounds [Working Title]
Biointerface Research in Applied Chemistry
Ibrahim Khan
Schiff bases are chemical compounds formed from the condensation reaction of aldehydes or ketones with amines. These compounds are majorly used in industries and also have significant biological activities, including antioxidant, antibacterial, antifungal, antiviral, antitumor, and anti-inflammatory. The majority of these compounds show excellent catalytic activities. Schiff bases are considered to be the most versatile ligands as they form complexes with the metal atoms. They are called privileged ligands because these compounds can be synthesized simply by condensation. The complexes of copper, zinc, and cadmium are the essential metallic complexes of these compounds. Schiff bases and its metallic complexes are instrumental in scavenging the free radicals and thus protect living bodies from the adverse effects of these radicals. Due to the recent exhaustive study on Schiff bases, it becomes an independent filed of research in chemistry. These compounds are widely investigated; how...
Ionic Liquids - Recent Progress in Synthesis, Characterization and Application [Working Title]
Biswajit Sinha
IntechOpen eBooks
Adebimpe Adesina
Ekhlass Uddin
Schiff base ligands and their metal complexes representing one of the most versatile and long standing topics of immense interest in coordination chemistry. Schiff bases and their metal complexes is fascinating, because it reveals a great richness of structural, physico-chemical and catalytic properties. Investigation of such compounds is essential to precise and understand structure-property relationships in order to optimize and improve their use in a wide range of fields, including catalysis, supramolecular chemistry, magnetism, electrochemistry, nanoscience, energy materials, optical and redox properties of metal complexes with those of the organic materialsand biological applications. This review presents the contemporary research development of the field, with emphasis on the fundamental concepts, catalytic, medicinal, fluorescent, biological and few miscellaneous applications of various functional metal Schiff base complexes. This review that contains 190 references should act as a source of information to researchers interested to work in this domain and stimulate further investigation in this fascinating area of Schiff base coordination compounds.
Ahmed Mohammed Abu-dief Mohammed
Schiff bases and their complexes are versatile compounds synthesized from the condensation of an amino compound with carbonyl compounds and widely used for industrial purposes and also exhibit a broad range of biological activities including antifungal, anti-bacterial, antimalarial, antiproliferative, anti-inflammatory, antiviral, and antipyretic properties. Many Schiff base complexes show excellent catalytic activity in various reactions and in the presence of moisture. Over the past few years, there have been many reports on their applications in homogeneous and heterogeneous catalysis. The high thermal and moisture stabilities of many Schiff base complexes were useful attributes for their application as catalysts in reactions involving at high temperatures. The activity is usually increased by complexation therefore to understand the properties of both ligands and metal can lead to the synthesis of highly active compounds. The influence of certain metals on the biological activity of these compounds and their intrinsic chemical interest as multidentate ligands has prompted a considerable increase in the study of their coordination behaviour. Development of a new chemotherapeutic Schiff bases and their metal complexes is now attracting the attention of medicinal chemists. This review compiles examples of the most promising applied Schiff bases and their complexes in different areas.
Nevin Turan
The work include the synthesis, spectroscopic characterization and as well as catalytic activities of Fe(II), Zn(II), Ru(II), and Pd(II) complexes with a novel Schiff base ligand derived from methyl 2amino-5,5,7,7-tetramethyl-4,5,6,7-tetrahydrobenzo[b]thiophene-3-carboxylate and salicylaldehyde. Spectroscopic techniques including IR, UV–Vis, NMR, and mass analysis as well as elemental analysis and magnetic susceptibility measurement were used to identify the compounds. Based on elemental and spectral studies octahedral geometry was proposed to Fe(II), Zn(II) and Ru(II) complexes and square planar geometry was proposed to Pd(II) complex. To improve more efficient and stable catalysts, we wanted to study if there is any influence the catalytic activity of ruthenium complex for the transfer hydrogenation of ketones and we wanted to examine the catalytic activities of palladium complex in Suzuki C-C cross coupling reactions under various conditions.
Chemical and Process Engineering Research
Shilashi Badasa Oljira
The Schiff base ligand was synthesized from 2- hyhdroxybenzohydrazide and benzaldehyde. The imines, very well known and popular as Schiff Bases. Recent developments on their “metallo-imines” variants have been described. The applications of Schiff bases in organic synthesis is recognizable Schiff bases are aldehyde or ketone like compounds in which the carbonyl group is replaced by an imine or azomethine group. They are widely used for industrial purposes and also exhibit a broad range of biological activities. The IR spectral data reveal that the ligand behaves as bidentate with ON donor atom sequence towards central metal ion. Keywords : Bidentate Schiff base, Antibacterial activity, IR spectral data
Journal of Metallic Material Research
Nisha Saxena
Bacterial infections are a major cause for impulsive deaths in human beings.Bacterial infections of the respiratory, gastrointestinal and central nervoussystem account for the majority of cases of sudden casualties. Readily availabledrugs are getting ineffective by each passing day as the mutation is veryfast in these pathogenic microbes resulting in drug resistance. The growingresistance of bacteria necessitates the development of new and effectivecompounds of desired characteristics that could bar the rapid development ofbacterial cell inside of the host body. Along with cellular resistance for clinicalantibiotics, co-bacterial infections during microbial attacks (viz. virus, fungus,protozoans etc.) also demand for some novel antibacterial drugs having highefficacy and minimal side effects on human body. These antibiotics shouldalso be compatible with remedies ongoing for core microbial infections. So,in demand of search for effective antibacterial moieties, the scope of transitio...
RELATED PAPERS
Journal of Molecular Structure
Eduardo França
Deu Muhendislik Fakultesi Fen ve Muhendislik
Kenan BULDURUN
Schiff Base Ligands and its Metal Complexes for Bio-medical Applications [Working Title]
Eduardo Brambila
BioMed Research International
Ayodele Odularu
Earthline Journal of Chemical Sciences
mohammed adamu
Vikas Borge
Mahbub Alam
Bioinorganic Chemistry and Applications
Spectrochimica Acta Part A: Molecular and Biomolecular Spectroscopy
Vadde Ravinder
Current medicinal chemistry
Luciano Saso
narjala jyothi
Journal of Basic and Applied Research in Biomedicine , felix N sunday
Journal of Coordination Chemistry
Sayeda Samina Ahmed
International Research Journal of Pure and Applied Chemistry
Salihu Sani
Journal of Molecular Catalysis A: Chemical
Paula Oliveira
International Journal of Pharmacy and Pharmaceutical Sciences
International Journal of Pharmacy and Pharmaceutical Sciences (IJPPS)
Ashish Asatkar
Baghdad Science Journal
Isam Al-Karkhi
Journal of Chemistry: Education Research and Practice
mohsen mostafa
NIGERIAN ANNALS OF PURE AND APPLIED SCIENCES
Agbese Samuel , Gideon Adamu Shallangwa
Journal of Drug Delivery and Therapeutics
College Of Basic Education Research Journal
Dr.maher alta'yy
Egyptian Journal of Chemistry
Mohamad M Shakdofa
Manohar Lokhande
Trends in Sciences
Mohd Abdul Fatah Abdul Manan
Zenodo (CERN European Organization for Nuclear Research)
Gajanan Dongare
Ali Al-shaheri
IP International Journal of Comprehensive and Advanced Pharmacology
Senthil Kumar Raju
Carbohydrate Polymers
Rosa Carballo
Luca Rigamonti
Science Journal of Chemistry
Motahar Hossain
Coordination Chemistry Reviews
Salvador Celedón
prateek tyagi
Asian Journal of Chemistry
felix N sunday
European Journal of Inorganic Chemistry
Fabio Marchetti , Carlo Nervi , C. Di Nicola
Organic Chemistry International
Dr . G. VASUKI Gnanasambandam
Inorganica Chimica Acta
Subhas Debnath
Polycyclic Aromatic Compounds
Basavarajaiah S M
RELATED TOPICS
- We're Hiring!
- Help Center
- Find new research papers in:
- Health Sciences
- Earth Sciences
- Cognitive Science
- Mathematics
- Computer Science
- Academia ©2024
The state of AI in early 2024: Gen AI adoption spikes and starts to generate value
If 2023 was the year the world discovered generative AI (gen AI) , 2024 is the year organizations truly began using—and deriving business value from—this new technology. In the latest McKinsey Global Survey on AI, 65 percent of respondents report that their organizations are regularly using gen AI, nearly double the percentage from our previous survey just ten months ago. Respondents’ expectations for gen AI’s impact remain as high as they were last year , with three-quarters predicting that gen AI will lead to significant or disruptive change in their industries in the years ahead.
About the authors
This article is a collaborative effort by Alex Singla , Alexander Sukharevsky , Lareina Yee , and Michael Chui , with Bryce Hall , representing views from QuantumBlack, AI by McKinsey, and McKinsey Digital.
Organizations are already seeing material benefits from gen AI use, reporting both cost decreases and revenue jumps in the business units deploying the technology. The survey also provides insights into the kinds of risks presented by gen AI—most notably, inaccuracy—as well as the emerging practices of top performers to mitigate those challenges and capture value.
AI adoption surges
Interest in generative AI has also brightened the spotlight on a broader set of AI capabilities. For the past six years, AI adoption by respondents’ organizations has hovered at about 50 percent. This year, the survey finds that adoption has jumped to 72 percent (Exhibit 1). And the interest is truly global in scope. Our 2023 survey found that AI adoption did not reach 66 percent in any region; however, this year more than two-thirds of respondents in nearly every region say their organizations are using AI. 1 Organizations based in Central and South America are the exception, with 58 percent of respondents working for organizations based in Central and South America reporting AI adoption. Looking by industry, the biggest increase in adoption can be found in professional services. 2 Includes respondents working for organizations focused on human resources, legal services, management consulting, market research, R&D, tax preparation, and training.
Also, responses suggest that companies are now using AI in more parts of the business. Half of respondents say their organizations have adopted AI in two or more business functions, up from less than a third of respondents in 2023 (Exhibit 2).
Gen AI adoption is most common in the functions where it can create the most value
Most respondents now report that their organizations—and they as individuals—are using gen AI. Sixty-five percent of respondents say their organizations are regularly using gen AI in at least one business function, up from one-third last year. The average organization using gen AI is doing so in two functions, most often in marketing and sales and in product and service development—two functions in which previous research determined that gen AI adoption could generate the most value 3 “ The economic potential of generative AI: The next productivity frontier ,” McKinsey, June 14, 2023. —as well as in IT (Exhibit 3). The biggest increase from 2023 is found in marketing and sales, where reported adoption has more than doubled. Yet across functions, only two use cases, both within marketing and sales, are reported by 15 percent or more of respondents.
Gen AI also is weaving its way into respondents’ personal lives. Compared with 2023, respondents are much more likely to be using gen AI at work and even more likely to be using gen AI both at work and in their personal lives (Exhibit 4). The survey finds upticks in gen AI use across all regions, with the largest increases in Asia–Pacific and Greater China. Respondents at the highest seniority levels, meanwhile, show larger jumps in the use of gen Al tools for work and outside of work compared with their midlevel-management peers. Looking at specific industries, respondents working in energy and materials and in professional services report the largest increase in gen AI use.
Investments in gen AI and analytical AI are beginning to create value
The latest survey also shows how different industries are budgeting for gen AI. Responses suggest that, in many industries, organizations are about equally as likely to be investing more than 5 percent of their digital budgets in gen AI as they are in nongenerative, analytical-AI solutions (Exhibit 5). Yet in most industries, larger shares of respondents report that their organizations spend more than 20 percent on analytical AI than on gen AI. Looking ahead, most respondents—67 percent—expect their organizations to invest more in AI over the next three years.
Where are those investments paying off? For the first time, our latest survey explored the value created by gen AI use by business function. The function in which the largest share of respondents report seeing cost decreases is human resources. Respondents most commonly report meaningful revenue increases (of more than 5 percent) in supply chain and inventory management (Exhibit 6). For analytical AI, respondents most often report seeing cost benefits in service operations—in line with what we found last year —as well as meaningful revenue increases from AI use in marketing and sales.
Inaccuracy: The most recognized and experienced risk of gen AI use
As businesses begin to see the benefits of gen AI, they’re also recognizing the diverse risks associated with the technology. These can range from data management risks such as data privacy, bias, or intellectual property (IP) infringement to model management risks, which tend to focus on inaccurate output or lack of explainability. A third big risk category is security and incorrect use.
Respondents to the latest survey are more likely than they were last year to say their organizations consider inaccuracy and IP infringement to be relevant to their use of gen AI, and about half continue to view cybersecurity as a risk (Exhibit 7).
Conversely, respondents are less likely than they were last year to say their organizations consider workforce and labor displacement to be relevant risks and are not increasing efforts to mitigate them.
In fact, inaccuracy— which can affect use cases across the gen AI value chain , ranging from customer journeys and summarization to coding and creative content—is the only risk that respondents are significantly more likely than last year to say their organizations are actively working to mitigate.
Some organizations have already experienced negative consequences from the use of gen AI, with 44 percent of respondents saying their organizations have experienced at least one consequence (Exhibit 8). Respondents most often report inaccuracy as a risk that has affected their organizations, followed by cybersecurity and explainability.
Our previous research has found that there are several elements of governance that can help in scaling gen AI use responsibly, yet few respondents report having these risk-related practices in place. 4 “ Implementing generative AI with speed and safety ,” McKinsey Quarterly , March 13, 2024. For example, just 18 percent say their organizations have an enterprise-wide council or board with the authority to make decisions involving responsible AI governance, and only one-third say gen AI risk awareness and risk mitigation controls are required skill sets for technical talent.
Bringing gen AI capabilities to bear
The latest survey also sought to understand how, and how quickly, organizations are deploying these new gen AI tools. We have found three archetypes for implementing gen AI solutions : takers use off-the-shelf, publicly available solutions; shapers customize those tools with proprietary data and systems; and makers develop their own foundation models from scratch. 5 “ Technology’s generational moment with generative AI: A CIO and CTO guide ,” McKinsey, July 11, 2023. Across most industries, the survey results suggest that organizations are finding off-the-shelf offerings applicable to their business needs—though many are pursuing opportunities to customize models or even develop their own (Exhibit 9). About half of reported gen AI uses within respondents’ business functions are utilizing off-the-shelf, publicly available models or tools, with little or no customization. Respondents in energy and materials, technology, and media and telecommunications are more likely to report significant customization or tuning of publicly available models or developing their own proprietary models to address specific business needs.
Respondents most often report that their organizations required one to four months from the start of a project to put gen AI into production, though the time it takes varies by business function (Exhibit 10). It also depends upon the approach for acquiring those capabilities. Not surprisingly, reported uses of highly customized or proprietary models are 1.5 times more likely than off-the-shelf, publicly available models to take five months or more to implement.
Gen AI high performers are excelling despite facing challenges
Gen AI is a new technology, and organizations are still early in the journey of pursuing its opportunities and scaling it across functions. So it’s little surprise that only a small subset of respondents (46 out of 876) report that a meaningful share of their organizations’ EBIT can be attributed to their deployment of gen AI. Still, these gen AI leaders are worth examining closely. These, after all, are the early movers, who already attribute more than 10 percent of their organizations’ EBIT to their use of gen AI. Forty-two percent of these high performers say more than 20 percent of their EBIT is attributable to their use of nongenerative, analytical AI, and they span industries and regions—though most are at organizations with less than $1 billion in annual revenue. The AI-related practices at these organizations can offer guidance to those looking to create value from gen AI adoption at their own organizations.
To start, gen AI high performers are using gen AI in more business functions—an average of three functions, while others average two. They, like other organizations, are most likely to use gen AI in marketing and sales and product or service development, but they’re much more likely than others to use gen AI solutions in risk, legal, and compliance; in strategy and corporate finance; and in supply chain and inventory management. They’re more than three times as likely as others to be using gen AI in activities ranging from processing of accounting documents and risk assessment to R&D testing and pricing and promotions. While, overall, about half of reported gen AI applications within business functions are utilizing publicly available models or tools, gen AI high performers are less likely to use those off-the-shelf options than to either implement significantly customized versions of those tools or to develop their own proprietary foundation models.
What else are these high performers doing differently? For one thing, they are paying more attention to gen-AI-related risks. Perhaps because they are further along on their journeys, they are more likely than others to say their organizations have experienced every negative consequence from gen AI we asked about, from cybersecurity and personal privacy to explainability and IP infringement. Given that, they are more likely than others to report that their organizations consider those risks, as well as regulatory compliance, environmental impacts, and political stability, to be relevant to their gen AI use, and they say they take steps to mitigate more risks than others do.
Gen AI high performers are also much more likely to say their organizations follow a set of risk-related best practices (Exhibit 11). For example, they are nearly twice as likely as others to involve the legal function and embed risk reviews early on in the development of gen AI solutions—that is, to “ shift left .” They’re also much more likely than others to employ a wide range of other best practices, from strategy-related practices to those related to scaling.
In addition to experiencing the risks of gen AI adoption, high performers have encountered other challenges that can serve as warnings to others (Exhibit 12). Seventy percent say they have experienced difficulties with data, including defining processes for data governance, developing the ability to quickly integrate data into AI models, and an insufficient amount of training data, highlighting the essential role that data play in capturing value. High performers are also more likely than others to report experiencing challenges with their operating models, such as implementing agile ways of working and effective sprint performance management.
About the research
The online survey was in the field from February 22 to March 5, 2024, and garnered responses from 1,363 participants representing the full range of regions, industries, company sizes, functional specialties, and tenures. Of those respondents, 981 said their organizations had adopted AI in at least one business function, and 878 said their organizations were regularly using gen AI in at least one function. To adjust for differences in response rates, the data are weighted by the contribution of each respondent’s nation to global GDP.
Alex Singla and Alexander Sukharevsky are global coleaders of QuantumBlack, AI by McKinsey, and senior partners in McKinsey’s Chicago and London offices, respectively; Lareina Yee is a senior partner in the Bay Area office, where Michael Chui , a McKinsey Global Institute partner, is a partner; and Bryce Hall is an associate partner in the Washington, DC, office.
They wish to thank Kaitlin Noe, Larry Kanter, Mallika Jhamb, and Shinjini Srivastava for their contributions to this work.
This article was edited by Heather Hanselman, a senior editor in McKinsey’s Atlanta office.
Explore a career with us
Related articles.

Moving past gen AI’s honeymoon phase: Seven hard truths for CIOs to get from pilot to scale
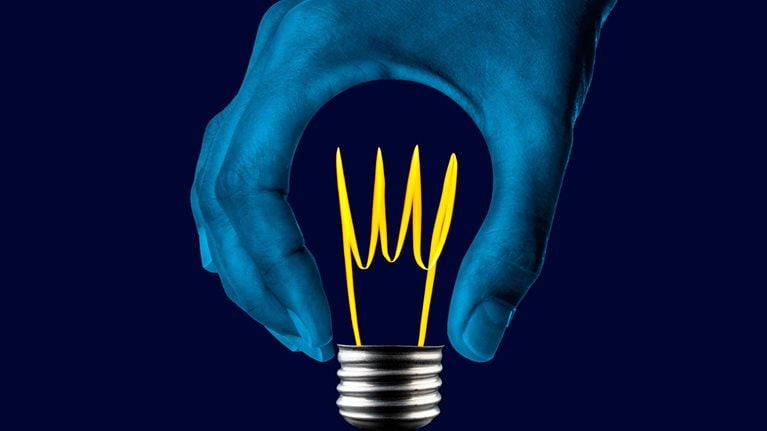
A generative AI reset: Rewiring to turn potential into value in 2024

Implementing generative AI with speed and safety

IMAGES
VIDEO
COMMENTS
An experimental research study was conducted to synthesize schiff base compounds using m-Nitrobenzaldehyde and p-Chloroaniline. The nitro group in the schiff base was then reduced into an amino group.
Abstract. Three new series of biologically active amino substituted Schiff bases with general formula, R1N=CHR2. Here R1 = 2-amino-benzthiazole, 4-amino-salicylic acid and 4-aminophenol. R2 =4 ...
Abstract. Schiff bases are a vast group of compounds characterized by the presence of a double bond linking carbon and nitrogen atoms, the versatility of which is generated in the many ways to combine a variety of alkyl or aryl substituents. Compounds of this type are both found in nature and synthesized in the laboratory.
PDF | Schiff base metal complexes are derived by the condensation reaction of amine and aldehydic groups. ... several research papers have been published with novel green methodologies utilizing ...
The second portion of the paper discussed Schiff's research on how aldehydes and ketones react with primary amines. Schiff noticed that this reaction produced an entirely novel category of compounds that he called "Schiff bases". ... Methods of synthesis for Schiff Base Ligand and Metal Complex. a) ... View PDF View article Google Scholar ...
SB modified NPs are applied as biosensor and adsorbent for the removal of heavy metals from aqueous solution [33], [34].Research conducted by J. Zhao et al., has showed that modification of magnetic iron oxide NPs with SB has exhibited dominating removal of heavy metals behavior over its mother NPs [35].Furthermore, Schiff base modified NPs are used in electrocatalysis, catalytic dye ...
Pharmaceuticals 2022, 15, 454 3 of 15 Scheme 1. Synthesis of Schiff base L and its LnIII complexes (La-Lc). 2.2. Spectral Investigations of the Ligand (L) Using 1H-NMR and 13C-NMR The 1H-NMR spectrum data of Schiff base L in acetonitrile solvent revealed seven signals, the most significant of which was at 8.68 ppm, which corresponds to the azomethine
Background Thiazole-based Schiff base compounds display significant pharmacological potential with an ability to modulate the activity of many enzymes involved in metabolism. They also demonstrated to have antibacterial, antifungal, anti-inflammatory, antioxidant, and antiproliferative activities. In this work, conventional and green approaches using ZnO nanoparticles as catalyst were used to ...
The wide range of biological studies of the Schiff bases are now attracting the attention of researchers which can lead to the identification of promising lead compounds. This review consists of the recent developments and various methodologies to synthesize Schiff base as well as their biological activities covering the last 20 years. Keywords
A Schiff base behaves as a Flexi-dentate ligand and commonly co-ordinates through the O atom of the de -pronated phenolic group and the N atom of azomethine group.[3] In Schiff base azomethane nitrogen and other donor atoms like oxygen play a vital role in co-ordination chemistry. Hence an attempt is made to study the interaction of reduced ...
Chemical and Process Engineering Research www.iiste.org ISSN 2224-7467 (Paper) ISSN 2225-0913 (Online) Vol.35, 2015 84 A Review: An Overview on Synthesis of Some Schiff bases and there Metal Complexes with Anti-Microbial Activity ... Azomethine linkage of Schiff base play an important role in medical field with so many ... (Paper) ISSN 2225 ...
Introduction. In the year 1864, Hugo Schiff was the fir st to synthesize Schiff' s base under. azeotropic distillation by using aldeh yde or ket one and primary amine. They can be. considered a ...
Schiff bases (SBs) have extensive applications in different fields such as analytical, inorganic and organic chemistry. They are used as dyes, catalysts, polymer stabilizers, luminescence chemosensors, catalyzers in the fixation of CO 2 biolubricant additives and have been suggested for solar energy applications as well. Further, a wide range of pharmacological and biological applications ...
Coumarin-based Schiff base and its earth metal complexes [75] have been used to treat pests (Tribolium castaneu) and worms (Meloidogyne incognita). 4.5 Analytical applications Schiff bases have been used as analytical probes or reagents by researchers. These are used to analyze primary amines, carbonyl compounds, and functional groups.
Hugo Schiff initially characterized Schiff base around 160 years ago [1], since then, it has been known by his name, "Schiff".Although discovered in coordination chemistry almost 100 years, Schiff bases represent a key role as ligands which are among the most commonly utilized organic substances [2].They are used as dyes and pigments, catalysts, organic synthesis intermediates, and polymer ...
This work highlights the relevance of metal complexation strategy to stabilize the ligands and improve their bioactivity in Schiff base complexes, which have been screen for their antibacterial activity against Gram negative and positive bacteria and antifungal activity showing promising antibacterial and biological activity. Expand. 14. PDF.
The development of Schiff base was a major step forward in the area of coordination chemistry. Schiff bases, a class of organic compounds, carry the imine or azomethine (>C=N-) functional group ...
Schiff bases are a vast group of compounds characterized by the presence of a double bond linking carbon and nitrogen atoms, the versatility of which is generated in the many ways to combine a variety of alkyl or aryl substituents. Compounds of this type are both found in nature and synthesized in the laboratory. For years, Schiff bases have been greatly inspiring to many chemists and biochemists.
2.3 Synthesis of Schiff base ligand (HL) The Schiff base ligand (HL) was prepared by refluxing the ambroxol drug (6.61 mmol, 2.5 g), dissolved in ethanol, with 2-quinoline carbaldehyde (6.61 mmol, 1.04 g). The resulting Schiff base was stirred under reflux for about 2h. During that, a reddish brown solid compound
corresponding Ru(III)-Schi base complexes. is indicates that hydrogenation of the C=N bond of Schi base ligands led to a modi cation of the coordination environment of the central Ru(III) cations. Nair et al. [ ]reported that Co(II), Ni(II), Cu(II), and Zn(II) complexes of the Schi base derived from indole--carboxaldehyde and m -
To determine the potential of Schiff bases to form complexes with transition metal ions, azo-methine is a crucial component. 1, 2 Schiff bases are essential in the biological field due to the ...
We report the synthesis and crystal structures of three new copper(II) Schiff-base complexes. The complexes have been characterized by elemental analysis and Fourier transform infrared (FT-IR) and UV-visible spectroscopies. The X-ray diffraction (XRD) analysis reveals that complexes 1 and 3 crystallize in a monoclinic space group C2/c and 2 in a triclinic space group P1̅, each adopting a ...
If 2023 was the year the world discovered generative AI (gen AI), 2024 is the year organizations truly began using—and deriving business value from—this new technology. In the latest McKinsey Global Survey on AI, 65 percent of respondents report that their organizations are regularly using gen AI, nearly double the percentage from our ...
PDF | On Jan 1, 2014, K. Brodowska and others published Schiff bases - Interesting range of applications in various fields of science | Find, read and cite all the research you need on ResearchGate