We have a new app!
Take the Access library with you wherever you go—easy access to books, videos, images, podcasts, personalized features, and more.
Download the Access App here: iOS and Android . Learn more here!
- Remote Access
- Save figures into PowerPoint
- Download tables as PDFs


Chapter 6. Genetic Disorders
- Download Chapter PDF
Disclaimer: These citations have been automatically generated based on the information we have and it may not be 100% accurate. Please consult the latest official manual style if you have any questions regarding the format accuracy.
Download citation file:
- Search Book
Jump to a Section
- Autosomal Dominant Disorders
- Autosomal Recessive Disorders
- Lysosomal Storage Disorders
- Glycogen Storage Disorders
- Genetic Conditions with X-Linked Inheritance Pattern
- Genetic Conditions with Mixed Inheritance Patterns
- Additional Genetic Abnormalities
- Sex Chromosome Abnormalities
- Diseases of Genomic Imprinting
- Sexual Differentiation Disorders
- Full Chapter
- Supplementary Content
There are three major types of mutations: (1) genome mutations, which involve loss or gain of an entire chromosome; (2) chromosomal mutations, which involve alterations in one or more chromosomes that are usually identifiable by karyotyping; and (3) gene mutations, which are partial or complete deletion of the gene or alteration of the base. Genome mutations usually result in death of the fetus, or death during infancy or early childhood.
Many diseases have a genetic component, albeit without a specific identifiable gene mutation. Such conditions are said to have a multifactorial inheritance pattern. Examples of such diseases include coronary artery disease, hypertension, gout, and diabetes mellitus.
When discussing genetic diseases, some definitions are important to remember: (1) hereditary or familial, a condition derived from parents (i.e., a condition that is transmitted in the germ line); and (2) congenital, a condition that is present at birth. Not all hereditary conditions are congenital, and not all congenital conditions are hereditary. Some hereditary conditions are manifested at the time of birth or shortly thereafter, and many manifest later in life.
The overall effects of the mutation of a single gene include (1) an enzyme defect; (2) defects in membrane receptors and/or transport system; (3) alterations in structure, function, or quantity of nonenzymatic protein; or (4) mutations resulting in unusual reactions to drugs. An enzyme defect can cause accumulation of substrate, a metabolic block resulting in a decreased amount of needed end product, or failure to inactivate a tissue-damaging substrate.
Overview: In general, autosomal dominant disorders have reduced penetrance and variable expressivity. They usually do not encode enzymes because a loss of up to 50% of an enzyme's activity can be compensated for by activity of the enzyme encoded by the normal allele ( Table 6-1 ).
LDL, low-density lipoprotein; APC, adenomatous polyposis coli.
Familial Hypercholesterolemia
Mutation: Low-density lipoprotein receptor gene ( LDL ); there are more than 100 known mutations.
Mechanism: The LDL receptor recognizes apolipoprotein B100 or apolipoprotein E; therefore, a mutation of the receptor results in impaired uptake of cholesterol into cells.
Manifestations of familial hypercholesterolemia
- Elevated cholesterol level: Heterozygotes have half the normal amount of LDL receptors and two to three times the normal level of cholesterol; homozygotes have five or more times the normal level of cholesterol.
- Tendon sheath xanthomas, corneal arcus, and xanthelasma.
- Early atherosclerosis and its consequences; homozygotes usually die of cardiovascular disease before the age of 30 years.
Familial Polyposis ...
Sign in or create a free Access profile below to access even more exclusive content.
With an Access profile, you can save and manage favorites from your personal dashboard, complete case quizzes, review Q&A, and take these feature on the go with our Access app.
Pop-up div Successfully Displayed
This div only appears when the trigger link is hovered over. Otherwise it is hidden from view.
Please Wait
- Patient Care & Health Information
- Diseases & Conditions
- Down syndrome
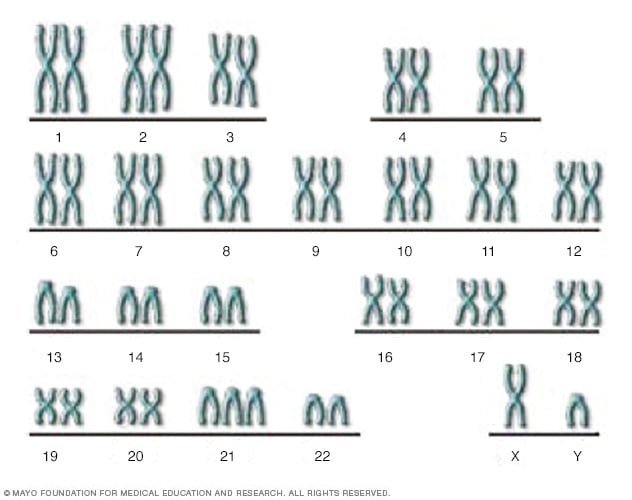
- The genetic basis of Down syndrome
There are 23 pairs of chromosomes, for a total of 46. Half the chromosomes come from the egg (the mother) and half come from the sperm (the father). This XY chromosome pair includes the X chromosome from the egg and the Y chromosome from the sperm. In Down syndrome, there is an additional copy of chromosome 21, resulting in three copies instead of the normal two copies.
Down syndrome is a genetic disorder caused when abnormal cell division results in an extra full or partial copy of chromosome 21. This extra genetic material causes the developmental changes and physical features of Down syndrome.
Down syndrome varies in severity among individuals, causing lifelong intellectual disability and developmental delays. It's the most common genetic chromosomal disorder and cause of learning disabilities in children. It also commonly causes other medical abnormalities, including heart and gastrointestinal disorders.
Better understanding of Down syndrome and early interventions can greatly increase the quality of life for children and adults with this disorder and help them live fulfilling lives.
Products & Services
- A Book: Mayo Clinic Family Health Book, 5th Edition
- Newsletter: Mayo Clinic Health Letter — Digital Edition
Each person with Down syndrome is an individual — intellectual and developmental problems may be mild, moderate or severe. Some people are healthy while others have significant health problems such as serious heart defects.
Children and adults with Down syndrome have distinct facial features. Though not all people with Down syndrome have the same features, some of the more common features include:
- Flattened face
- Protruding tongue
- Upward slanting eye lids (palpebral fissures)
- Unusually shaped or small ears
- Poor muscle tone
- Broad, short hands with a single crease in the palm
- Relatively short fingers and small hands and feet
- Excessive flexibility
- Tiny white spots on the colored part (iris) of the eye called Brushfield's spots
- Short height
Infants with Down syndrome may be average size, but typically they grow slowly and remain shorter than other children the same age.
Intellectual disabilities
Most children with Down syndrome have mild to moderate cognitive impairment. Language is delayed, and both short and long-term memory is affected.
When to see a doctor
Children with Down syndrome usually are diagnosed before or at birth. However, if you have any questions regarding your pregnancy or your child's growth and development, talk with your doctor.
There is a problem with information submitted for this request. Review/update the information highlighted below and resubmit the form.
From Mayo Clinic to your inbox
Sign up for free and stay up to date on research advancements, health tips, current health topics, and expertise on managing health. Click here for an email preview.
Error Email field is required
Error Include a valid email address
To provide you with the most relevant and helpful information, and understand which information is beneficial, we may combine your email and website usage information with other information we have about you. If you are a Mayo Clinic patient, this could include protected health information. If we combine this information with your protected health information, we will treat all of that information as protected health information and will only use or disclose that information as set forth in our notice of privacy practices. You may opt-out of email communications at any time by clicking on the unsubscribe link in the e-mail.
Thank you for subscribing!
You'll soon start receiving the latest Mayo Clinic health information you requested in your inbox.
Sorry something went wrong with your subscription
Please, try again in a couple of minutes
Human cells normally contain 23 pairs of chromosomes. One chromosome in each pair comes from your father, the other from your mother.
Down syndrome results when abnormal cell division involving chromosome 21 occurs. These cell division abnormalities result in an extra partial or full chromosome 21. This extra genetic material is responsible for the characteristic features and developmental problems of Down syndrome. Any one of three genetic variations can cause Down syndrome:
- Trisomy 21. About 95 percent of the time, Down syndrome is caused by trisomy 21 — the person has three copies of chromosome 21, instead of the usual two copies, in all cells. This is caused by abnormal cell division during the development of the sperm cell or the egg cell.
- Mosaic Down syndrome. In this rare form of Down syndrome, a person has only some cells with an extra copy of chromosome 21. This mosaic of normal and abnormal cells is caused by abnormal cell division after fertilization.
- Translocation Down syndrome. Down syndrome can also occur when a portion of chromosome 21 becomes attached (translocated) onto another chromosome, before or at conception. These children have the usual two copies of chromosome 21, but they also have additional genetic material from chromosome 21 attached to another chromosome.
There are no known behavioral or environmental factors that cause Down syndrome.
Is it inherited?
Most of the time, Down syndrome isn't inherited. It's caused by a mistake in cell division during early development of the fetus.
Translocation Down syndrome can be passed from parent to child. However, only about 3 to 4 percent of children with Down syndrome have translocation and only some of them inherited it from one of their parents.
When balanced translocations are inherited, the mother or father has some rearranged genetic material from chromosome 21 on another chromosome, but no extra genetic material. This means he or she has no signs or symptoms of Down syndrome, but can pass an unbalanced translocation on to children, causing Down syndrome in the children.
Risk factors
Some parents have a greater risk of having a baby with Down syndrome. Risk factors include:
- Advancing maternal age. A woman's chances of giving birth to a child with Down syndrome increase with age because older eggs have a greater risk of improper chromosome division. A woman's risk of conceiving a child with Down syndrome increases after 35 years of age. However, most children with Down syndrome are born to women under age 35 because younger women have far more babies.
- Being carriers of the genetic translocation for Down syndrome. Both men and women can pass the genetic translocation for Down syndrome on to their children.
- Having had one child with Down syndrome. Parents who have one child with Down syndrome and parents who have a translocation themselves are at an increased risk of having another child with Down syndrome. A genetic counselor can help parents assess the risk of having a second child with Down syndrome.
Complications
People with Down syndrome can have a variety of complications, some of which become more prominent as they get older. These complications can include:
- Heart defects. About half the children with Down syndrome are born with some type of congenital heart defect. These heart problems can be life-threatening and may require surgery in early infancy.
- Gastrointestinal (GI) defects. GI abnormalities occur in some children with Down syndrome and may include abnormalities of the intestines, esophagus, trachea and anus. The risk of developing digestive problems, such as GI blockage, heartburn (gastroesophageal reflux) or celiac disease, may be increased.
- Immune disorders. Because of abnormalities in their immune systems, people with Down syndrome are at increased risk of developing autoimmune disorders, some forms of cancer, and infectious diseases, such as pneumonia.
- Sleep apnea. Because of soft tissue and skeletal changes that lead to the obstruction of their airways, children and adults with Down syndrome are at greater risk of obstructive sleep apnea.
- Obesity. People with Down syndrome have a greater tendency to be obese compared with the general population.
- Spinal problems. Some people with Down syndrome may have a misalignment of the top two vertebrae in the neck (atlantoaxial instability). This condition puts them at risk of serious injury to the spinal cord from overextension of the neck.
- Leukemia. Young children with Down syndrome have an increased risk of leukemia.
- Dementia. People with Down syndrome have a greatly increased risk of dementia — signs and symptoms may begin around age 50. Having Down syndrome also increases the risk of developing Alzheimer's disease.
- Other problems. Down syndrome may also be associated with other health conditions, including endocrine problems, dental problems, seizures, ear infections, and hearing and vision problems.
For people with Down syndrome, getting routine medical care and treating issues when needed can help with maintaining a healthy lifestyle.
Life expectancy
Life spans have increased dramatically for people with Down syndrome. Today, someone with Down syndrome can expect to live more than 60 years, depending on the severity of health problems.
There's no way to prevent Down syndrome. If you're at high risk of having a child with Down syndrome or you already have one child with Down syndrome, you may want to consult a genetic counselor before becoming pregnant.
A genetic counselor can help you understand your chances of having a child with Down syndrome. He or she can also explain the prenatal tests that are available and help explain the pros and cons of testing.
- What is Down syndrome? National Down Syndrome Society. http://www.ndss.org/down-syndrome/what-is-down-syndrome/. Accessed Dec. 16, 2016.
- Down syndrome fact sheet. National Down Syndrome Society. http://www.ndss.org/Down-Syndrome/Down-Syndrome-Facts/. Accessed Dec. 16, 2016.
- Messerlian GM, et al. Down syndrome: Overview of prenatal screening. http://www.uptodate.com/home. Accessed Dec. 16, 2016.
- National Library of Medicine. Down syndrome. Genetics Home Reference. https://ghr.nlm.nih.gov/condition/down-syndrome. Accessed Dec. 16, 2016.
- Facts about Down syndrome. Centers for Disease Control and Prevention. https://www.cdc.gov/ncbddd/birthdefects/downsyndrome.html. Accessed Dec. 16, 2016.
- Down syndrome. Eunice Kennedy Shriver National Institute of Child Health and Human Development. https://www.nichd.nih.gov/health/topics/down/conditioninfo/Pages/default.aspx. Accessed Dec. 16, 2016.
- Frequently asked questions. Prenatal genetic diagnostic tests. FAQ164. Pregnancy. American College of Obstetricians and Gynecologists. https://www.acog.org/-/media/For-Patients/faq164.pdf?dmc=1&ts=20161216T1208042192. Accessed Dec. 16, 2016.
- Ostermaier KK. Down syndrome: Management. http://www.uptodate.com/home. Accessed Dec. 22, 2016.
- Ostermaier KK. Down syndrome: Clinical features and diagnosis. http://www.uptodate.com/home. Accessed Jan. 10, 2017.
- Gabbe SG, et al., eds. Genetic screening and prenatal genetic diagnosis. In: Obstetrics: Normal and Problem Pregnancies. 7th ed. Philadelphia, Pa.: Saunders Elsevier; 2017.
- Rink BD, et al. Screening for fetal aneuploidy. Seminars in Perinatology. 2016;40:35.
- Bunt CW, et al. The role of the family physician in the care of children with Down syndrome. American Family Physician. 2014;90:851.
- Butler Tobah YS (expert opinion). Mayo Clinic, Rochester, Minn. Jan. 26, 2017.
Associated Procedures
- Amniocentesis
- Genetic testing
- Symptoms & causes
- Diagnosis & treatment
- Doctors & departments
Mayo Clinic does not endorse companies or products. Advertising revenue supports our not-for-profit mission.
- Opportunities
Mayo Clinic Press
Check out these best-sellers and special offers on books and newsletters from Mayo Clinic Press .
- Mayo Clinic on Incontinence - Mayo Clinic Press Mayo Clinic on Incontinence
- The Essential Diabetes Book - Mayo Clinic Press The Essential Diabetes Book
- Mayo Clinic on Hearing and Balance - Mayo Clinic Press Mayo Clinic on Hearing and Balance
- FREE Mayo Clinic Diet Assessment - Mayo Clinic Press FREE Mayo Clinic Diet Assessment
- Mayo Clinic Health Letter - FREE book - Mayo Clinic Press Mayo Clinic Health Letter - FREE book
Your gift holds great power – donate today!
Make your tax-deductible gift and be a part of the cutting-edge research and care that's changing medicine.

A .gov website belongs to an official government organization in the United States.
A lock ( ) or https:// means you've safely connected to the .gov website. Share sensitive information only on official, secure websites.
- Genetics Basics
- Family Health History
- About Cascade Testing
Genetic Testing
What to know.
Genetic testing looks for changes in your DNA that can inform your medical care. Talk to your healthcare provider about whether genetic testing is right for you.
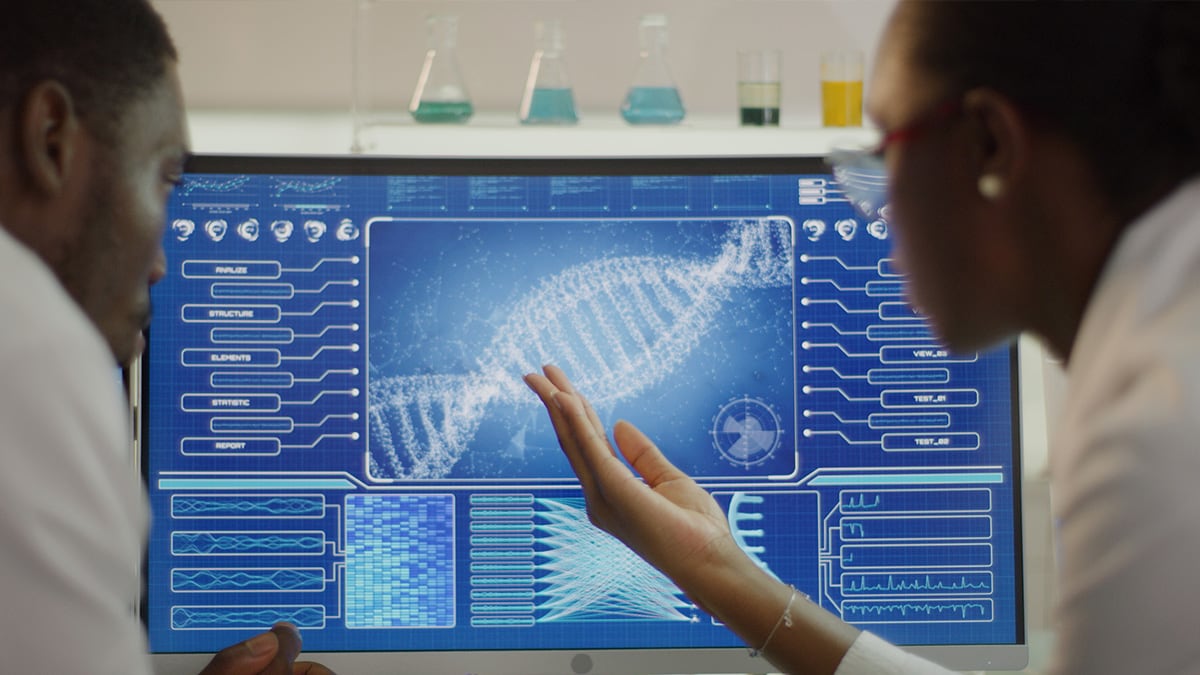
Genetic testing looks for genetic changes, sometimes called mutations or variants, in your DNA. Some genetic changes can cause disease or other health conditions. You inherit your DNA from your parents, so any genetic changes they have can be passed down to you.
Genetic testing can give you information to help guide the decisions you make about the medical care you or your family member receives. For example, genetic testing can provide a diagnosis for a genetic condition such as fragile X syndrome or information about your risk of developing cancer. There are many different kinds of genetic tests. Genetic tests use a blood or spit sample, and results are usually ready in a few weeks.
Because we share DNA with our family members, if testing shows that you have a genetic change, your family members may have the same genetic change. Genetic counseling before genetic testing can help make sure you are the right person in your family to get a genetic test and can help make sure you're getting the right test. Genetic counseling after genetic testing can help make sure you understand your results.
Reasons for genetic testing
There are several reasons to consider getting a genetic test, including the following:
- To learn whether you have a genetic condition that runs in your family, before you have symptoms
- To learn whether you could have a child with a genetic condition
- To diagnose a genetic condition if you or your child has symptoms
- To understand and guide your cancer prevention or treatment plan
After learning more about genetic testing, you can decide if it's right for you.
Types of genetic tests
There are many different kinds of genetic tests. There is no single genetic test that can detect all genetic conditions. Your healthcare provider can help you decide what genetic test is right for you based on your medical and family history and the condition for which you're being tested.
Single gene testing
Single gene tests look for genetic changes in only one gene. Your healthcare provider might recommend single gene testing if you or your child have symptoms of a specific condition or syndrome that is usually or always caused by changes in the same gene. Examples include Duchenne muscular dystrophy and sickle cell disease . If there is a known genetic change (mutation) in your family, your healthcare provider might recommend single gene testing to check for that change.
Genetic testing panels
Genetic testing panels look for genetic changes in many genes in the same test. Your healthcare provider might recommend a genetic testing panel if you or your child have symptoms of a disease or condition that can be caused by genetic changes in many different genes. Examples include primary immunodeficiency , cerebral palsy , and epilepsy . Your healthcare provider might also recommend a genetic testing panel to check for genetic changes that make you more likely to develop a disease such as breast cancer or colorectal (colon) cancer , especially if the disease runs in your family but a specific genetic change that might be causing the disease in family members has not yet been identified.
Large-scale genomic testing
There are two different kinds of large-scale genomic tests, which look for genetic changes throughout a person's DNA:
- Exome sequencing looks at all the genes in the DNA (whole exome) or just the genes that are related to medical conditions (clinical exome).
- Whole genome sequencing is the largest genetic test and looks at all of a person's DNA, not just the genes.
Your healthcare provider might recommend exome sequencing or whole genome sequencing if you or your child has a complex medical condition, or if other testing has not found a genetic cause for a disease or condition. For example, your healthcare provider might recommend whole genome sequencing if you have a rare disorder that appears to have a genetic cause, but none has been found using other tests. 1 2 If your child has autism spectrum disorder, your healthcare provider might recommend whole exome sequencing if other testing does not find a cause. 3
Large-scale genetic tests can have findings unrelated to why the test was ordered in the first place. These are called secondary findings, and they can include genetic changes related to adult-onset conditions in children. For example, a child may have exome or genome sequencing to provide a genetic diagnosis for autism spectrum disorder, and a secondary finding is that they have a genetic change that makes them more likely to develop breast cancer as an adult.
Clinical versus direct-to-consumer genetic tests
Clinical genetic tests are different from direct-to-consumer (DTC) genetic tests. Your healthcare provider orders clinical genetic tests for a specific medical reason—you cannot order them on your own. In contrast, you can buy DTC tests online or in a store, for example, to learn more about your ancestry. DTC test results can be used to make decisions about lifestyle choices or identify issues to discuss with your healthcare provider. However, DTC tests cannot determine for certain whether or not you will get a disease. Nor should these tests be used alone to make decisions about your treatment or medical care or in place of clinical genetic testing.
Other tests
Some tests check for changes other than gene changes.
Chromosomes
DNA is packaged into structures called chromosomes. Some tests look for changes in chromosomes rather than in genes. For example, your healthcare provider might recommend a type of chromosome testing called a chromosomal microarray if your child has autism spectrum disorder.
Gene expression
Genes are expressed, or turned on, at different levels in different types of cells. Gene expression tests compare these levels between normal cells and diseased cells, because knowing about the difference can provide important information for treating the disease. For example, these tests can guide chemotherapy treatment for breast cancer.
Understanding test results
Genetic counseling is important to help you understand what the results of your test mean for you. What results mean for you can depend on your family health history, age, and other factors.
- Find a genetic counselor in your area through the National Society of Genetic Counselors.
- Find a medical geneticist (genetic healthcare provider) in your area through the American College of Medical Genetics and Genomics.
- A guide for the diagnosis of rare and undiagnosed disease: beyond the exome . Genome Medicine. 2022 Feb 28; 14(23). Marwaha S, Knowles JW, Ashley EA.
- Genome sequencing as a diagnostic test . CMAJ. 2021 Oct 25; 193(42): E1626–E1629. Costain G, Cohn RD, Scherer SW, Marshall CR.
- Identification, Evaluation, and Management of Children With Autism Spectrum Disorder . Pediatrics. 2020 Jan; 145 (1). Hyman SL, Levy SE, Myers SM.
Genomics and Your Health
Learn more about genomics and its importance for your health
Thank you for visiting nature.com. You are using a browser version with limited support for CSS. To obtain the best experience, we recommend you use a more up to date browser (or turn off compatibility mode in Internet Explorer). In the meantime, to ensure continued support, we are displaying the site without styles and JavaScript.
- View all journals
- Explore content
- About the journal
- Publish with us
- Sign up for alerts
- RESEARCH BRIEFINGS
- 14 May 2024
Mega study charts how genetic variants affect metabolism
This is a summary of: Karjalainen, M. K. et al . Genome-wide characterization of circulating metabolic biomarkers. Nature 628 , 130–138 (2024) .
Access options
Access Nature and 54 other Nature Portfolio journals
Get Nature+, our best-value online-access subscription
24,99 € / 30 days
cancel any time
Subscribe to this journal
Receive 51 print issues and online access
185,98 € per year
only 3,65 € per issue
Rent or buy this article
Prices vary by article type
Prices may be subject to local taxes which are calculated during checkout
doi: https://doi.org/10.1038/d41586-024-00458-1
Figure 1 is published under a CC BY 4.0 licence.
Kettunen, J. et al. Nature Commun. 7 , 11122 (2016).
Article PubMed Google Scholar
Richardson, T. G. et al. PLoS Biol. 20 , e3001547 (2022).
Holmes, M. V., Ala-Korpela, M. & Smith, G. D. Nature Rev. Cardiol. 14 , 577–590 (2017).
Soininen, P. et al. Analyst 134 , 1781–1785 (2009).
Download references
Reprints and permissions
Related Articles
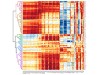
Software tools identify forgotten genes
Technology Feature 24 MAY 24
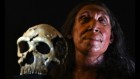
Neanderthal–human baby-making was recent — and brief
News 24 MAY 24
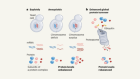
Cells cope with altered chromosome numbers by enhancing protein breakdown
News & Views 22 MAY 24
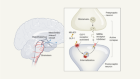
Dual-action obesity drug rewires brain circuits for appetite
News & Views 15 MAY 24
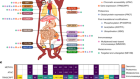
Temporal dynamics of the multi-omic response to endurance exercise training
Article 01 MAY 24
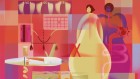
Obesity drugs aren’t always forever. What happens when you quit?
News Feature 16 APR 24
Professor, Division Director, Translational and Clinical Pharmacology
Cincinnati Children’s seeks a director of the Division of Translational and Clinical Pharmacology.
Cincinnati, Ohio
Cincinnati Children's Hospital & Medical Center
Data Analyst for Gene Regulation as an Academic Functional Specialist
The Rheinische Friedrich-Wilhelms-Universität Bonn is an international research university with a broad spectrum of subjects. With 200 years of his...
53113, Bonn (DE)
Rheinische Friedrich-Wilhelms-Universität
Recruitment of Global Talent at the Institute of Zoology, Chinese Academy of Sciences (IOZ, CAS)
The Institute of Zoology (IOZ), Chinese Academy of Sciences (CAS), is seeking global talents around the world.
Beijing, China
Institute of Zoology, Chinese Academy of Sciences (IOZ, CAS)
Full Professorship (W3) in “Organic Environmental Geochemistry (f/m/d)
The Institute of Earth Sciences within the Faculty of Chemistry and Earth Sciences at Heidelberg University invites applications for a FULL PROFE...
Heidelberg, Brandenburg (DE)
Universität Heidelberg
Postdoctoral scholarship in Structural biology of neurodegeneration
A 2-year fellowship in multidisciplinary project combining molecular, structural and cell biology approaches to understand neurodegenerative disease
Umeå, Sweden
Umeå University
Sign up for the Nature Briefing newsletter — what matters in science, free to your inbox daily.
Quick links
- Explore articles by subject
- Guide to authors
- Editorial policies
Click through the PLOS taxonomy to find articles in your field.
For more information about PLOS Subject Areas, click here .
Loading metrics
Open Access
Peer-reviewed
Research Article
Genetic effects and causal association analyses of 14 common conditions/diseases in multimorbidity patterns
Roles Data curation, Formal analysis, Writing – original draft
Affiliations Collaborative Innovation Center for Bone and Immunology between Sihong Hospital and Soochow University, Center for Genetic Epidemiology and Genomics, School of Public Health, Suzhou Medical College of Soochow University, Suzhou, Jiangsu P. R. China, Department of Orthopedics, Sihong Hospital, Suzhou, Jiangsu, P. R. China
Roles Conceptualization, Data curation, Formal analysis
Roles Conceptualization, Formal analysis
Affiliation Jiangsu Key Laboratory of Preventive and Translational Medicine for Geriatric Diseases, Soochow University, Suzhou, Jiangsu, P. R. China
Roles Writing – original draft, Writing – review & editing
* E-mail: [email protected] (SFL); [email protected] (PH)

Roles Writing – review & editing
Affiliations Collaborative Innovation Center for Bone and Immunology between Sihong Hospital and Soochow University, Center for Genetic Epidemiology and Genomics, School of Public Health, Suzhou Medical College of Soochow University, Suzhou, Jiangsu P. R. China, Department of Orthopedics, Sihong Hospital, Suzhou, Jiangsu, P. R. China, Changzhou Geriatric Hospital Affiliated to Soochow University, Changzhou, China
- Ting Fu,
- Yi-Qun Yang,
- Chang-Hua Tang,
- Pei He,
- Shu-Feng Lei
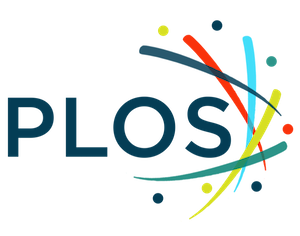
- Published: May 16, 2024
- https://doi.org/10.1371/journal.pone.0300740
- Reader Comments
Multimorbidity has become an important health challenge in the aging population. Accumulated evidence has shown that multimorbidity has complex association patterns, but the further mechanisms underlying the association patterns are largely unknown.
Summary statistics of 14 conditions/diseases were available from the genome-wide association study (GWAS). Linkage disequilibrium score regression analysis (LDSC) was applied to estimate the genetic correlations. Pleiotropic SNPs between two genetically correlated traits were detected using pleiotropic analysis under the composite null hypothesis (PLACO). PLACO-identified SNPs were mapped to genes by Functional Mapping and Annotation of Genome-Wide Association Studies (FUMA), and gene set enrichment analysis and tissue differential expression were performed for the pleiotropic genes. Two-sample Mendelian randomization analyses assessed the bidirectional causality between conditions/diseases.
LDSC analyses revealed the genetic correlations for 20 pairs based on different two-disease combinations of 14 conditions/diseases, and genetic correlations for 10 pairs were significant after Bonferroni adjustment ( P <0.05/91 = 5.49E-04). Significant pleiotropic SNPs were detected for 11 pairs of correlated conditions/diseases. The corresponding pleiotropic genes were differentially expressed in the brain, nerves, heart, and blood vessels and enriched in gluconeogenesis and drug metabolism, biotransformation, and neurons. Comprehensive causal analyses showed strong causality between hypertension, stroke, and high cholesterol, which drive the development of multiple diseases.
Conclusions
This study highlighted the complex mechanisms underlying the association patterns that include the shared genetic components and causal effects among the 14 conditions/diseases. These findings have important implications for guiding the early diagnosis, management, and treatment of comorbidities.
Citation: Fu T, Yang Y-Q, Tang C-H, He P, Lei S-F (2024) Genetic effects and causal association analyses of 14 common conditions/diseases in multimorbidity patterns. PLoS ONE 19(5): e0300740. https://doi.org/10.1371/journal.pone.0300740
Editor: Chunyu Liu, State University of New York Upstate Medical University, UNITED STATES
Received: October 24, 2023; Accepted: March 4, 2024; Published: May 16, 2024
Copyright: © 2024 Fu et al. This is an open access article distributed under the terms of the Creative Commons Attribution License , which permits unrestricted use, distribution, and reproduction in any medium, provided the original author and source are credited.
Data Availability: All relevant data are within the manuscript and its Supporting Information files.
Funding: The author(s) received no specific funding for this work.
Competing interests: The authors have declared that no competing interests exist.
Introduction
Multimorbidity is a coexistence state of two or more medical conditions/diseases [ 1 , 2 ], which commonly occurs in older adults. The estimated prevalence of multimorbidity was 67.8% in older adults aged 65 years and older by 2035 in the UK [ 3 ]. These chronic diseases mainly include long-term mental conditions/diseases, physical noncommunicable diseases of long duration, and long-term chronic diseases of the musculoskeletal system. Comorbidity substantially increases the medical and socioeconomic burden. Especially during the COVID-19 pandemic, individuals with multimorbidity have been at higher risk of infection and adverse outcomes [ 4 ].
The pathogenesis underlying the occurrence of multimorbidity is probably related to aging, heredity, and other factors (e.g., socioeconomic deprivation) [ 5 ]. In the comorbidity patterns, the correlations among the diseases are complex, and some diseases may be the driving factors of other diseases. For example, some chronic diseases contribute to the progression of other conditions/diseases, with slight clinical symptoms and undetectable symptoms, especially for older persons [ 6 ]. Diabetes and hypertension are the most common chronic diseases in Spanish people aged over 65 years with dementia [ 7 ]. In the observational study, Bendayan et al. ranked 14 common diseases to older adults in descending order of prevalence, with hypertension, heart disease, depression, and asthma [ 8 ]. A study that included 0.5 billion Chinese adults has identified four multimorbidity patterns, including cardiometabolic multimorbidity (diabetes, stroke and hypertension), cancer, psychiatric problems (neurasthenia) and arthritis [ 9 ]. A review study suggests that depression, hypertension, diabetes, arthritis and asthma can easily co-exist with other diseases [ 10 ]. However, in traditional cohort studies, the analysis of the association between diseases is easily affected by many confounding factors that are difficult to control or unknown, leading to unreliable results. For example, Poblador-Plou et al. studied multimorbidity patterns in people over 65 years of age in Spain [ 7 ]. They found that diabetes and hypertension were the most common chronic diseases in people with dementia. However, when they identified multimorbidity patterns, the patterns associated with dementia did not include diabetes or hypertension, but include Parkinson’s disease, osteoporosis, thyroid disease, anxiety, and neurosis. Therefore, in multimorbidity patterns, the complex correlation patterns and the underlying mechanisms for the correlations are still largely unknown.
Therefore, this study selected 14 conditions/diseases that were the main diseases experienced by middle and older age individuals and the major sources of long-standing illnesses for people aged 65 years and older in other national surveys (details can be found in https://www.elsa-project.ac.uk/data-and-documentation ). We performed systemic analyses (e.g., linkage disequilibrium score regression (LDSC) [ 11 ], the pleiotropic analysis method under the composite original hypothesis (PLACO) [ 12 ], and mapping and functional annotation of pleiotropic SNPs were conducted by FUMA) [ 13 ] to detect the genetic correlations and the underlying pleiotropic genes and the driving conditions/diseases by inferring the causal relations (Mendelian randomization analysis) [ 14 ] among 14 conditions/diseases. Understanding the genetic components shared by them and the causal association patterns is essential for elucidating phenotypic relationships and disease pathology, which could help to provide basic data for the prevention, diagnosis, and treatment of these conditions/diseases.
Methods and materials
Gwas summary statistics for 14 conditions/diseases.
Following the selection of the conditions/diseases in the English Longitudinal Study of Aging (ELSA) [ 8 ]. The study also selected the fourteen conditions/diseases, including hypertension, type 2 diabetes, cancer, chronic obstructive pulmonary disease, heart problems, stroke, psychiatric problems (mental health problems ever diagnosed by a professional: Anxiety, nerves or generalized anxiety disorder), arthritis, asthma, high cholesterol, cataracts, Parkinson’s disease, hip fracture, and depression. There are no ethical issues involved in this study and "ethical approval and consent to participate" is not applicable. The statistics of 14 conditions/diseases were downloaded from the GWAS ( Table 1 ) .
- PPT PowerPoint slide
- PNG larger image
- TIFF original image
https://doi.org/10.1371/journal.pone.0300740.t001
Genetic correlation analysis for each pair of condition/disease–LDSC.
LDSC can estimate heritability and confounding bias between SNPs [ 15 ], and its principle and algorithm are well described [ 16 ]. We assessed the genetic correlation between two phenotypes by using LDSC ( https://github.com/bulik/ldsc ). LD scores could be calculated from the European samples in the 1000 Genomes Project as a reference panel [ 17 ]. Quality controls were implemented on the SNPs before LDSC analysis to filter out unqualified SNPs (e.g., nonbiallelic SNPs, stranded-ambiguous SNPs, duplicate SNPs, SNPs without rs numbers, SNPs with minor allele frequencies<0.01, SNPs located in the major histocompatibility complex (MHC) region with a complex LD structure (HLA region markers) and SNPs that were not in or whose alleles did not match Phase 3 of the 1000 Genomes Project) [ 18 ].
Pleiotropic analysis under the composite hypothesis.
The functional annotation of pleiotropic SNPs
Afterward, we functionally mapped and annotated the PLACO-screened pleiotropic SNPs using the online tool Functional Mapping and Annotation (FUMA, https://fuma.ctglab.nl/ .) [ 13 ]. Independent significant SNPs were identified according to the default thresholds of P <5E-08 and r 2 <0.6 in FUMA. These SNPs are further represented by lead SNPs, which are a subset of the independent significant SNPs if the SNP had r 2 < 0.1. Then, we used positional mapping of deleterious coding SNPs and expression quantitative trait loci (eQTLs) to map independent significant SNPs to genes. SNPs were mapped to certain genes if the combined annotation-dependent depletion (CADD) score was >12.37 [ 13 , 20 ]; otherwise, they were filtered. For eQTL mapping, all independent significant SNPs in LD were mapped to eQTLs in gene tissue expression (GTEx) v8 tissue types.
Finally, gene set enrichment analysis (GSEA) was undertaken to test the possible biological mechanisms of pleiotropic genes. Hypergeometric tests are conducted to assess the possible biological functions of genes of interest. A series of pathway enrichment analyses, including the Molecular Signatures Database (MSigDB) [ 21 ] and KEGG [ 22 ], were used. The expression (transcripts per million) of prioritized genes in different tissues was estimated from GTEx v8 following winsorization at 50 and log2 transformation [ 23 ]. The mRNA expression data were first normalized, and then, for each gene, Student’s two-sided t test was performed per tissue against all other tissues. The fold change (FC) of the gene expression level was utilized to represent the differential expression between different tissues. The adjusted p value < 0.05 ( P bon<0.05) and absolute log fold change 0.58 were defined as a DEG set in a given tissue.
Two-sample Mendelian randomization for inferring causal relationships among different conditions/diseases
Mendelian randomization (MR) uses genetic variants as instrumental variables to determine whether an observational association between a risk factor and an outcome is consistent with a causal effect [ 24 ]. Based on GWAS summary statistics, Mendelian randomization (MR) analyses were performed to detect potential causality between the 14 diseases. For instrumental variable (IV) selection, independent significant (IVs) ( P <5E-08) and LD r 2 <0.01 within 10Mb based on the 1000Genomes Project phase 3 Europeans were kept [ 25 ]. Besides, we incorporated the F score to prevent weak instrument IVs (SNPs with F<10 were excluded) [ 26 ]. The inverse variance weighted method was applied to estimate the causal association between various diseases (IVW P <0.05), with a supplementary analysis of the weighted median, weight mode, and MR‒Egger. To address multiple hypothesis testing, we estimated the false discovery rate (FDR) adjusted p values, in the main IVW MR analyses, using the sequential p value approach proposed by Benjamini & Hochberg [ 27 ]. For the trait pairs with heterogeneity, we used IVW analysis with different models. Cochran Q statistics confirm the robustness of the MR estimates [ 28 ]. All analyses were performed by using the R package “TwoSampleMR” (version 0.5.6).
Linkage disequilibrium score regression analysis (LDSC)
LDSC estimated the genetic correlations among the 14 phenotypes ( S1 Table in S2 File ). Twenty pairs of conditions/diseases showed significant genetic correlations ( P <0.05). The negative and positive coefficients ranged from -0.20~-0.08 and 0.16~0.75, respectively ( Table 2 ). Among the 20 studied pairs, genetic correlations for 10 pairs were significant after Bonferroni adjustment ( P <0.05/91 = 5.49E-04) ( Fig 1A ). We found that hypertension was significant genetic correlations with 5 diseases/conditions. It was positively genetically correlated with other 4 diseases/conditions, including T2D (rg = 0.23, P = 1.3E-08), stroke (rg = 0.49, P = 1.2E-21), high cholesterol (rg = 0.62, P = 3.2E-39) and depression (rg = 0.37, P = 1.3E-07). Besides, hypertension had a negative correlation with asthma (rg = -0.15, P = 2.0E-04). High cholesterol was positively genetically correlated with T2D (rg = 0.21, P = 4.7E-06), stroke (rg = 0.26, P = 2.05E-08) and depression (rg = 0.23, P = 1.0E-04). Depression was positively genetically correlated with psychiatric problems (mainly referring to anxiety and nerves) (rg = 0.75, P = 1.5E-16) and negatively correlated with asthma (rg = -0.20, P = 1.0E-04), respectively ( Table 2 and Fig 1A ).
(A) LDSC results. Square color indicates r(g), blue indicates a positive correlation, and red indicates a negative correlation. Square size indicates Bonferroni-adjusted p value, * indicates P < 5.49E-04. Psychiatric problems: Mental health problems ever diagnosed by a professional: Anxiety, nerves or generalized anxiety disorder. (B) Differential expression levels of identified pleiotropic genes between 11 pairs of phenotypes in GTEx v8 54 tissue types. The -log10 (P value) in the graph refers to the probability of hypergeometric testing. Red bars denote tissues that remained significantly prominent after Bonferroni correction (P<0.05). (C) The shared enrichment pathways and/or GO terms. In the Sankey bubble diagram, the first column on the left side indicates each phenotypic pairwise combination. The second column represents the gene set enrichment analysis of the genes associated with the combination, in which different phenotypic combinations identified the same enrichment signal. The bubble diagram on the right side shows the genes enriched in the corresponding gene set. Positional gene sets are sets of genes that are categorized based on their different locations on the chromosome. Psychiatric problems: Mental health problems ever diagnosed by a professional: Anxiety, nerves or generalized anxiety disorder. (D) Network plot obtained from two-sample Mendelian randomization analysis for the 14 diseases/conditions (IVW P <0.05). The starting point of the connecting line represents exposure, and the end point of the arrow indicates the outcome. COPD: Chronic obstructive pulmonary disease. Psychiatric problems: Mental health problems ever diagnosed by a professional: Anxiety, nerves or generalized anxiety disorder.
https://doi.org/10.1371/journal.pone.0300740.g001
https://doi.org/10.1371/journal.pone.0300740.t002
Functional annotation for pleiotropic SNPs and corresponding genes
Furthermore, PLACO was used to investigate pleiotropic SNPs for the significant pairs in the LDSC analysis (i.e., 20 condition pairs in Table 2 ). The results showed that 11/20 pairs of correlated traits had pleiotropic SNPs ( S2 Table in S2 File ). We further applied FUMA for functional annotation of the relevant SNPs. For the 11 pairs, a total of 8,927 lead SNPs were selected ( S3 Table in S2 File and S1 Fig in S1 File ). For the pleiotropic genes of 11 pairs, we performed tissue differential expression analysis in GTEx v8 54 tissue types and showed that the pleiotropic genes were mainly enriched in the brain, nerves, kidney, heart, and blood vessels, particularly for these up-regulated pleiotropic genes ( Fig 1B ), indicating that these genes probably play important biological functions. We also performed enrichment analysis for each significant pair ( S4 Table in S2 File ) and identified a total of 21 common sets of genes involved in at least two pairs. Seven significant KEGG pathways were shared pathways, such as drug metabolism cytochrome p450 and porphyrin and chlorophyll metabolism ( Fig 1C ).
For a more detailed explanation of the pleiotropic SNPs and corresponding genes, we further divided these associated traits into five subgroups.
Hypertension-associated subgroup.
The hypertension-associated group consisted of hypertension-psychiatric problems (anxiety and nerves), hypertension-arthritis, hypertension-asthma, and hypertension-high cholesterol. The pleiotropic SNPs identified by PLACO analysis were mapped to 1,030 pleiotropic genes ( S5 Table in S2 File ). The gene ontology (GO) gene sets showed that remarkably strong enrichment signals in the glucuronation and metabolism for the biological process (BP) terms. (i.e., GO_FLAVONOID_GLUCURONIDATION, P adj = 1.36E-04; GO_URONIC_ACID_METABOLIC_PROCESS, P adj = 5.58E-03;); For the KEGG, which them mainly were abundant in glucuronate interconversions and metabolism, (i.e., KEGG_PENTOSE_AND_GLUCURONIDATE_INTERCONVERSION, P adj = 5.99E-08; KEGG_STARCH_AND_SUCROSE_METABOLISM, P adj = 1.25E-04.) ( S6 Table in S2 File and S2 Fig in S1 File ). We found that the identified pleiotropic genes were significantly enriched in the brain, kidney, heart, and nerve tissue ( S3A Fig in S1 File ).
T2D-associated subgroup. A total of 1,582 unique pleiotropic genes were identified in the T2D-related cluster, which included T2D-cancer, T2D-asthma, and T2D-high cholesterol ( S7 Table in S2 File ). We found that the 1,582 genes showed strong enrichment signals in gene sets related to oncogenic transcription factors and the biocarta pathway ( S8 Table in S2 File ). Pleiotropic genes were mainly concentrated in brain tissue, down-regulated in the kidney and spleen, and up-regulated in vascular tissue and muscle ( S3B Fig in S1 File ).
Psychiatric problems-related group.
The psychiatric problems-associated group mainly refers to phenotypes associated with anxiety and nerves, including asthma and high cholesterol. A total of 1,455 unique pleiotropic genes were identified by positional mapping and eQTL mapping ( S9 Table in S2 File ). For the biological process (BP) terms of GO, which them mainly were associated with adhesion pathways (i.e., GO_BIOLOGICAL_ADHESION, P adj = 1.36E-04; GO_CELL_CELL_ADHESION, P adj = 4.22E-06). For the molecular function (MF) terms of GO, these gene sets were primarily involved in synapses and neurotransmission pathways (i.e., GO_SYNAPSE, P adj = 2.32E-03; GO_NEURON_PART, P adj = 1.72E-02 ( S10 Table in S2 File and S4 Fig in S1 File ), which accumulate in brain and nerve tissues. (S3C Fig in S1 File ) .
High cholesterol-depression subgroup.
A total of 303 unique genes were identified by positional mapping and eQTL mapping between high cholesterol and depression ( S11 Table in S2 File ). These genes were enriched in diseases or mutants of signal transduction and associated with apoptosis ( S12 Table in S2 File and S5 Fig in S1 File ). However, they did not show significant differences in 30 tissues (S3D Fig in S1 File ) .
Parkinson’s disease-hip fracture subgroup.
There were 985 unique putative pleiotropic genes detected between Parkinson’s disease and hip fracture via two mapping methods ( S13 Table in S2 File ). The Gene Ontology (GO) gene sets showed that 985 unique genes had significant enrichment signals in neurons and cells (e.g., GO_REGULATION_OF_CELL_PROJECTION_ORGANIZATION, P adj = 1.15E-04; GO_CELL_PROJECTION_ORGANIZATION, P adj = 2.13E-04) (S14 Table in S2 File and S6 Fig in S1 File ) . Interestingly, differential expression of these unique genes was significantly enriched in the prostate (S3E Fig in S1 File ).
Causal associations among 14 conditions/diseases inferred by Mendelian randomization analysis
Finally, our study systematically analyzed the bidirectional causal relationships among 14 conditions/diseases. Of all 182 examined associations, 82 pairs had instrumental variables with F-values larger than 10 ( S15 Table in S2 File ). Among these 82 pairs, we found 13 pairs with significant causal effects (IVW P <0.05). Notably, IVW analysis demonstrated that the three phenotypes (hypertension, stroke, and high cholesterol) not only displayed significant bidirectional causal associations among them but also showed unidirectional causal associations from these three phenotypes to others, which indicated that they increased the risk of other diseases (Figs 1D and 2 ) . After adjustments of P -values, eight pairs of traits still had significantly causal relationships: hypertension→stroke ( P adj = 2.73E-22), hypertension→high cholesterol ( P adj = 1.11E-19), T2D→high cholesterol ( P adj = 1.67E-03), stroke→hypertension ( P adj = 1.03E-02), high cholesterol→hypertension ( P adj = 6.01E-10), high cholesterol→cancer ( P adj = 4.20E-02), high cholesterol→stroke ( P adj = 4.56E-04), asthma→COPD ( P adj = 2.75E-05). However, there was no significant causal relationship between T2D and hypertension ( P adj = 5.26E-02) and when stroke was the exposure and high cholesterol was the outcome, the P adj = 9.68E-02, suggesting that the causality between high cholesterol and stroke may be unidirectional ( S16 Table in S2 File ). In addition, MR‒Egger regression analyses showed no potential pleiotropy (all P intercept > 0.05), and Cochran’s Q test indicated that heterogeneity was present in more than half (61.54%) of 13 pairs. For disease pairs with heterogeneity ( P heter<0.05), the P-value of IVW with random effects was selected; for those without heterogeneity, the P-value of IVW with fixed effects was selected ( P heter>0.05) ( S17 Table in S2 File ). Scatter plots of the MR results are provided in the supplementary materials ( S7 Fig in S1 File ).
It contained the ratio of exposure to outcome (OR) with a 95% confidence interval (95% Cl). Blue squares indicate ORs, and the area of the square indicates the size of the OR value; the larger the OR value is, the larger the area of the square.
https://doi.org/10.1371/journal.pone.0300740.g002
Since the phenotypic correlations were probably caused by shared genetic effects and/or significant causal effects, we summarized the underlying mechanisms for the 24 studied pairs ( Table 3 ). The phenotypic correlations for six pairs (hypertension & T2D, hypertension & stroke, hypertension & high cholesterol, T2D & high cholesterol, stroke & high cholesterol, and stroke & Parkinson’s disease) were probably caused by both shared genetic effects and significant causal effects, but for other pairs, their correlations were probably caused by either shared genetic effects or significant causal effects. Hypertension, stroke, and high cholesterol have been frequently observed to be associated with other conditions/diseases.
https://doi.org/10.1371/journal.pone.0300740.t003
This study performed systemic analyses to detect the shared genetic and causal effects of 14 common conditions/diseases in multimorbidity patterns. LDSC analysis showed that the 20 studied pairs were genetically correlated, indicating that genetic effects were shared by the 14 conditions/diseases. The pleiotropic SNPs identified by PLACO and the corresponding mapping genes overlapped among these studied phenotypic pairs and were enriched in some pathways and/or GO terms with important biological functions. Compared to others, hypertension, stroke, and high cholesterol were observed to be causally/genetically associated with other phenotypes at a high frequency, and they most likely serve as the driving factors for other conditions/diseases.
Previous GWASs have shown that numerous genomic loci are significantly associated with a large diversity of traits [ 29 ] Extensive pleiotropic effects were identified as a possible explanation for comorbidity. This finding pointed to potential common genetic mechanisms between diseases. Most importantly, we found that hypertension, stroke, and high cholesterol were associated with more than one phenotype, emphasizing their prominence in the comorbidity pattern [ 30 ]. Among ~380 chronic disease combinations identified in older adults, hypertension was present in almost every combination of prevalent diseases [ 31 ].
Functional annotation analysis showed that putative pleiotropic genes of 11 pairs of traits were involved in biological processes such as biotransformation (e.g., glucuronate pathway, drug metabolism). The glucuronate pathway is indispensable for the physiological function of the body and has the functions of detoxification and excretion, and the metabolism of many drugs depends on it [ 32 ]. Several chronic inflammatory diseases (such as metabolic syndrome, T2D, and cardiovascular disease) could affect glucose metabolism [ 33 ]. We identified 985 pleiotropic genes in Parkinson’s disease and hip fracture that were involved in the development of neurons. Previous studies have shown that neuronal damage in Parkinson’s disease leads to symptoms such as tremors and muscle stiffness, which contribute to an increased number of falls and are also associated with fracture risk [ 34 ]. The above findings suggest that neuronal regulation plays an important role in the pathophysiology of both Parkinson’s disease and hip fracture.
The pleiotropic genes were mainly enriched in the brain, nerves, kidney, heart, and blood vessels. Previous evidence supports that vascular dysfunction is a common feature of several chronic diseases [ 35 ]. For example, hypertension, T2D, and kidney diseases were proven to be associated with cerebrovascular damage [ 36 ]. Chronic inflammatory diseases could induce higher chemokine production and activate intrarenal macrophages and dendritic cells in rats [ 37 ]. This suggested that the kidneys may be involved. In addition, we found that some disease pairs shared common biological functions. Seven KEGG pathways were associated with different trait pairs. The pathways involved in drug metabolism by cytochrome p450 and the metabolism of xenobiotics by cytochrome p450 were related to the balance between the oxidative stress system and antioxidant capacity and might play a vital role in hypertension-related conditions/diseases [ 38 ]. In addition, another study found that both hypertension and high cholesterol in animal models lead to increased systemic and vascular oxidative stress, resulting in reduced vasodilatation [ 39 ]. The oxidative system is closely associated with hypertension and T2D [ 40 ]. Based on the above evidence, we speculated that the dysregulation of the oxidative stress system may represent the major characteristics of the pathological process of hypertension-related diseases. The metabolism of porphyrin and chlorophyll and the metabolism of ascorbic acid and erythrosine in two trait pairs (hypertension—psychiatric problems and psychiatric problems—high cholesterol) may be newly discovered pathways because they have not been previously reported (psychiatric problems mainly refer to anxiety and nerves).
The LDSC analysis showed that hypertension was genetically related to T2D. The Mendelian randomization method demonstrated that T2D had a unidirectional causal effect on hypertension. Previous MR analyses have shown similar results. Zhu et al. used community-based disease GWAS data (p = 0.44 for SBP→T2D and p = 0.20 for DBP→T2D) [ 41 ], which showed no causal relations from hypertension to T2D, which was in line with our results with no adjustment. However, after correction, there was no causal effect of T2D on hypertension, thus, caution should be exercised when considering the causality of the two phenotypes. A cross-sectional study showed that clinically significant depressive symptoms were associated with elevated blood pressure, but they could not determine any causal relationship between hypertension and depression [ 42 ]. Our MR analysis showed that there was no causal association between them. In comparison, LDSC showed a strong genetic correlation between them (r(g) = 0.37, P = 1.30E-07). Evidence suggests that endothelial dysfunction plays an important role in the pathobiology of depression [ 43 ]. The vascular endothelium regulates vascular homeostasis and is a key factor in vascular health [ 44 ]. Therefore, we speculate that hypertension could lead to decreased vascular function and endothelial damage, which increases the risk of developing depression.
Hypertension and high cholesterol are common clinical comorbidities that have been previously described [ 45 ]. Hernández et al. analyzed the chronic combination pattern of 6,101 older adults aged 50 years and older and found that hypertension and high cholesterol were the most common coexisting conditions/diseases [ 46 ]. The 267 pleiotropic genes shared between them were mainly enriched in the synthesis and degradation of fibrinogen complexes, which have been reported to play an important role in high cholesterol patients with hypertension [ 47 ]. There also existed bidirectional causality between hypertension and stroke. Both the LDSC and MR results suggest that there are shared genetic determinants between the two phenotypes. A meta-analysis revealed that for every 10 mmHg increase in systolic blood pressure, the risk of stroke increased by 22%, and mortality increased by 56% [ 48 ]. This may be attributed to the possibility that hypertension accelerates the atherosclerotic process, thus increasing the probability of stroke. We confirmed the association from a genetic perspective.
We noticed that no pleiotropic SNPs/genes were found between hypertension and stroke or between stroke and high cholesterol. The possible reason is that we conducted SNP filtering under stricter thresholds (P<5E-08), which helped increase the efficacy of the test but also led to the removal of real pleiotropic SNPs. Moreover, the pleiotropy of complex traits/diseases is an essential basis for genetic correlations between diseases [ 49 ]. Hypertension, T2D, and high cholesterol seem to occupy an influential position in comorbidity patterns, which are presumably the dominant diseases to study the patterns of other conditions/diseases. Due to the uncertainty that all confounding factors have been identified, observational trials studying associations between diseases are susceptible to interference by many external factors, such as medications [ 50 ]. For example, certain diseases are bad outcomes for the treatment of specific diseases (e.g., clozapine and olanzapine, used for treating psychiatric disorders, can lead to insulin sensitivity and lipid metabolism changes, which increase the risk of diabetes and cardiovascular disease) [ 50 ]. However, this co-occurrence is not a genetic association of the diseases themselves but rather an iatrogenic condition resulting from psychiatric medications. Therefore, even if a strong statistical association between exposure and outcome is observed, causal conclusions should be drawn with caution. The present study assessed the relationship between diseases by using an associated genetic variation, which is usually irrespective of environmental or lifestyle factors and could strengthen causal inference. An in-depth analysis of correlations between the disease/conditions from the perspective of genetic variation, identification of pleiotropic genes and biological pathways, and causal effects was conducted to provide genetic insights for observational studies.
However, there are two limitations to our study. First, the summary data in our study only represent European populations; thus, caution should be taken when generalizing the findings to other ethnic groups (e.g., East Asians). Second, although we used multiple MR approaches to mitigate confounding due to pleiotropy, residual bias cannot be entirely ruled out, as it is an established limitation of the MR methodology [ 51 ].
The high phenotypic correlations for the 14 conditions/diseases in the multimorbidity patterns were caused by shared genetic effects and/or significant causal effects. Compared to others, hypertension, stroke, and high cholesterol were most frequently observed to be causally/genetically associated with other phenotypes, and they most likely serve as the driving factors for other conditions/diseases. These findings may provide basic data for the prevention, diagnosis, and treatment of these conditions/diseases.
Supporting information
S1 checklist. human participants research checklist..
https://doi.org/10.1371/journal.pone.0300740.s001
https://doi.org/10.1371/journal.pone.0300740.s002
https://doi.org/10.1371/journal.pone.0300740.s003
Acknowledgments
We thank all the studies for making the summary association statistics data publicly available.
- View Article
- PubMed/NCBI
- Google Scholar
Got any suggestions?
We want to hear from you! Send us a message and help improve Slidesgo
Top searches
Trending searches
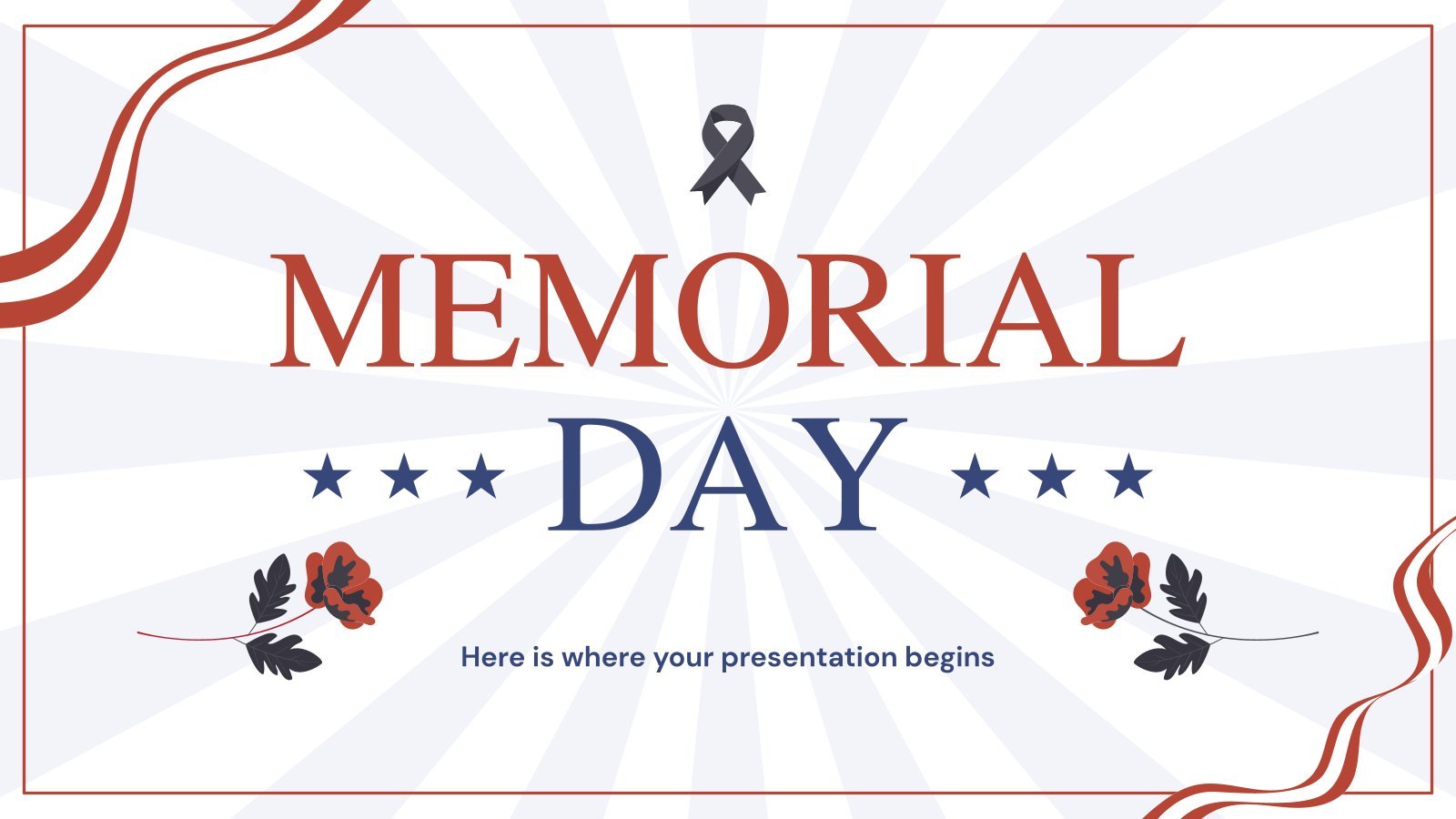
memorial day
12 templates
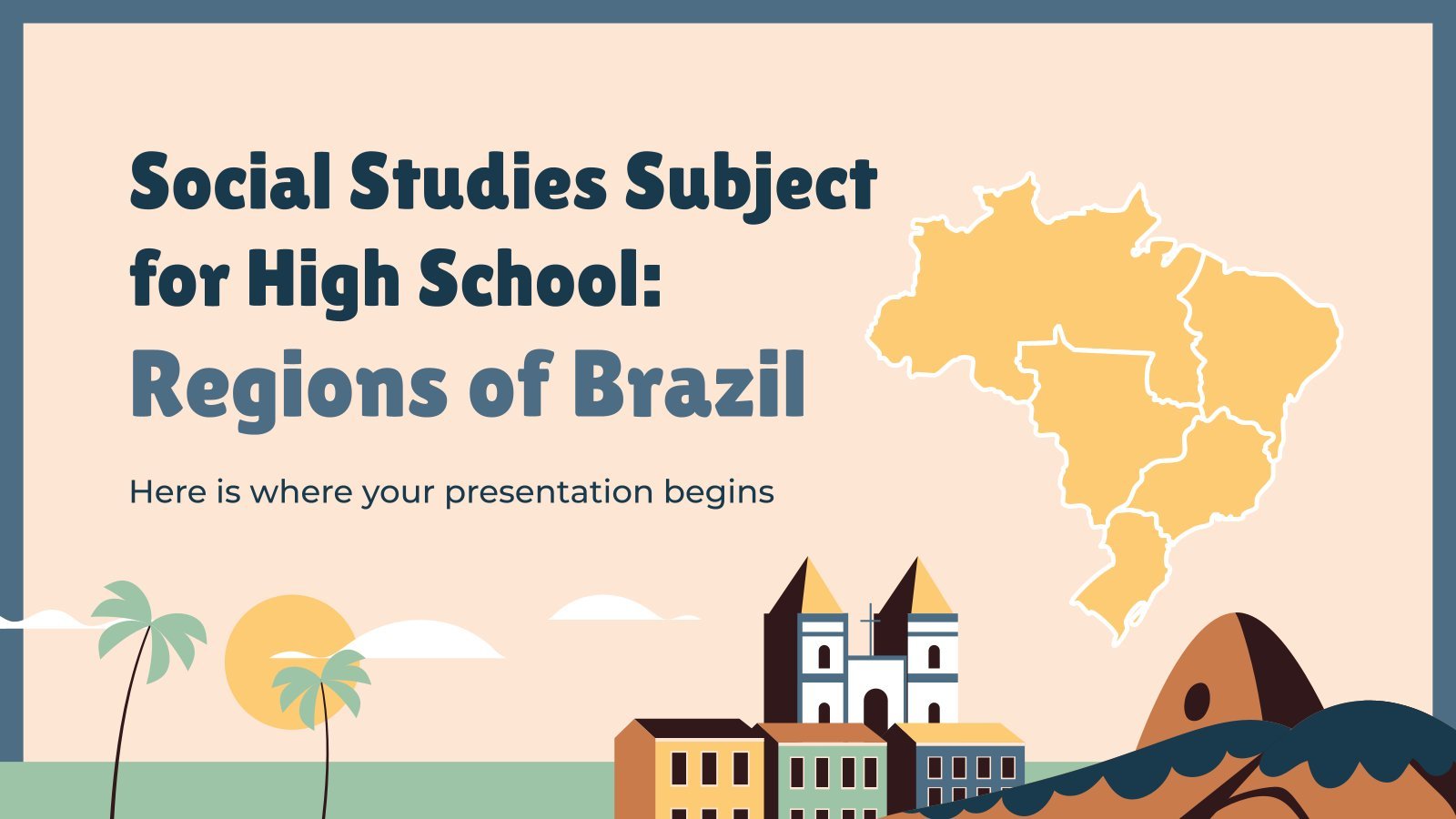
66 templates
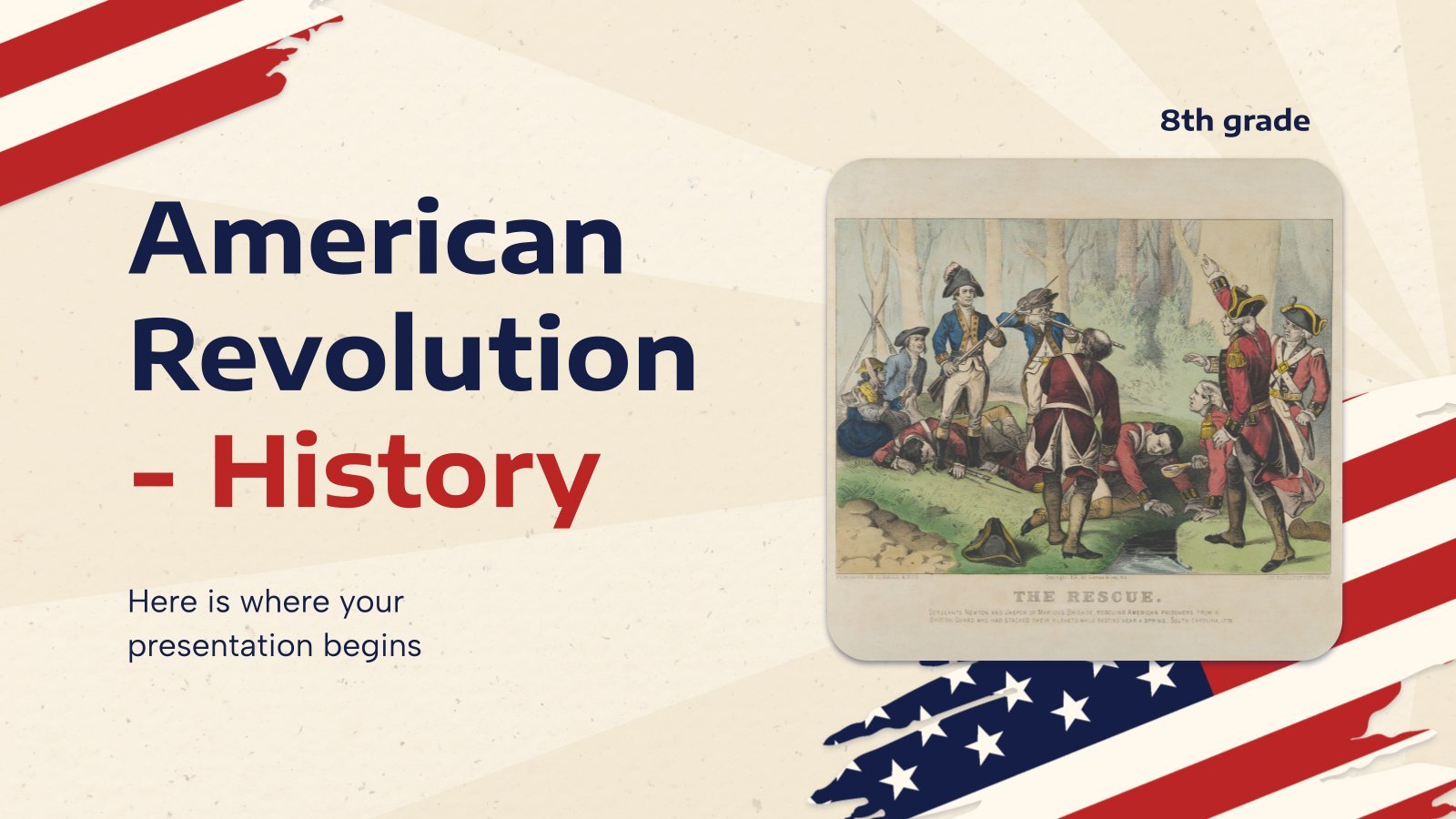
american history
75 templates
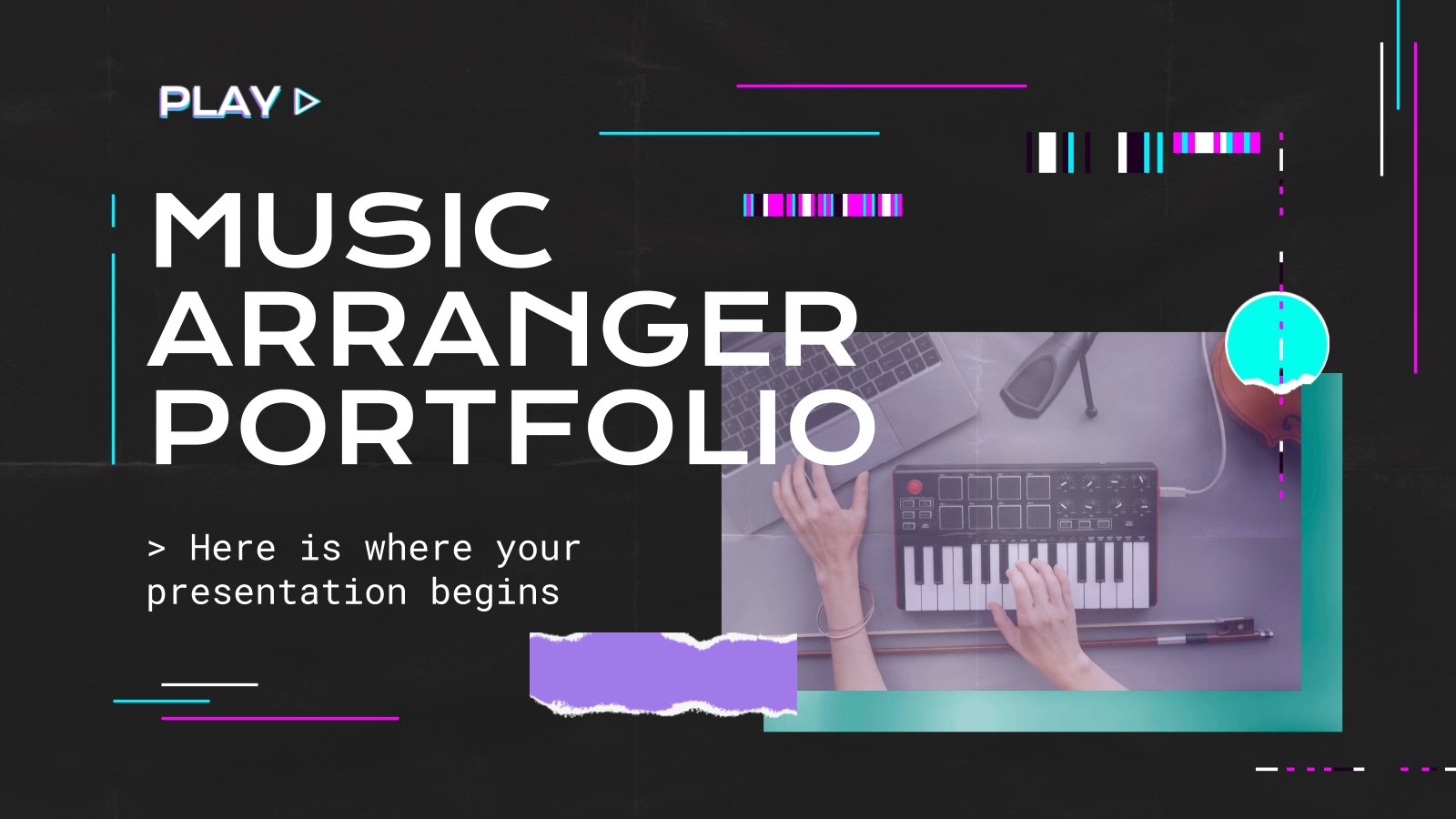
music video
21 templates
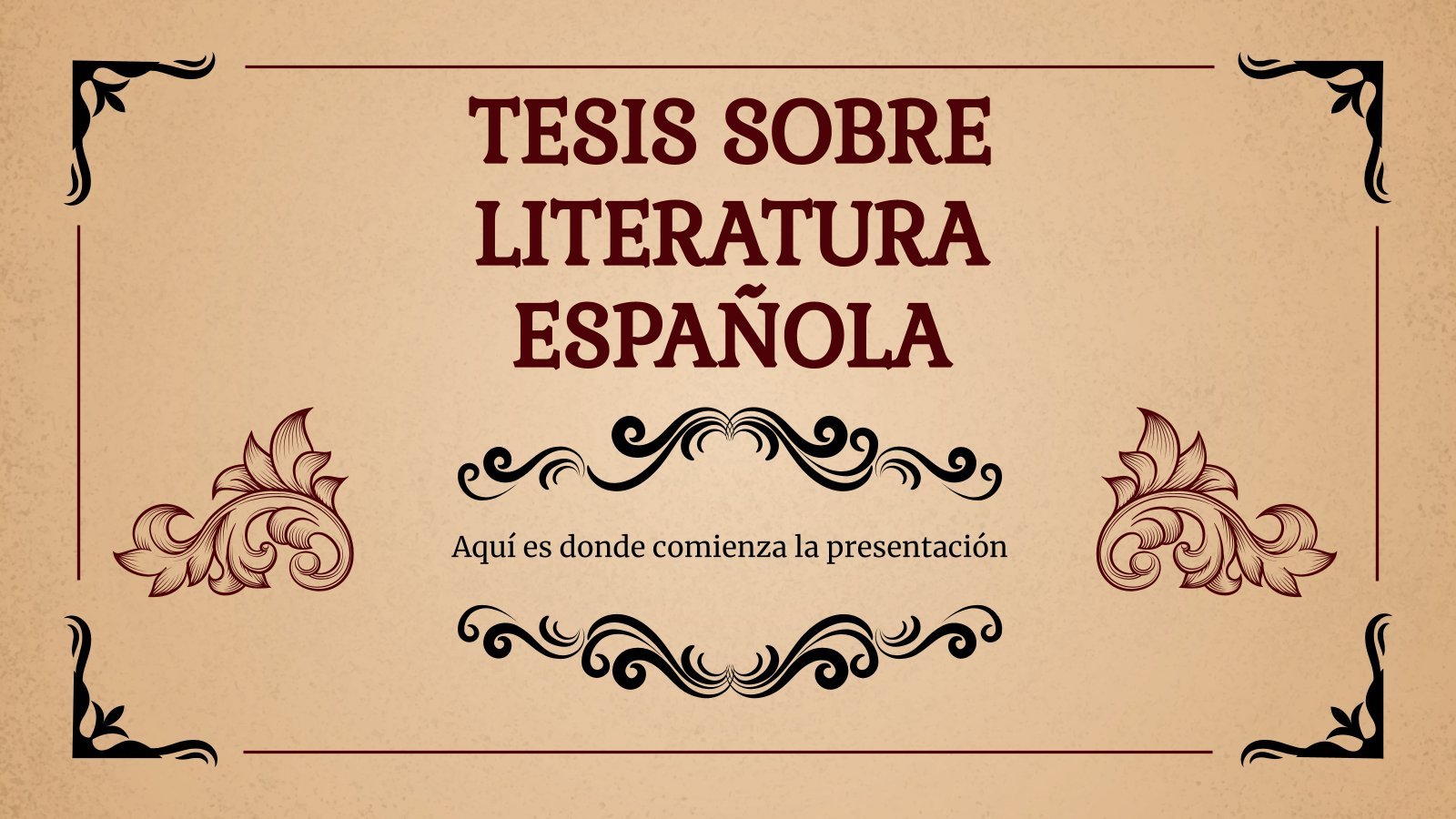
150 templates
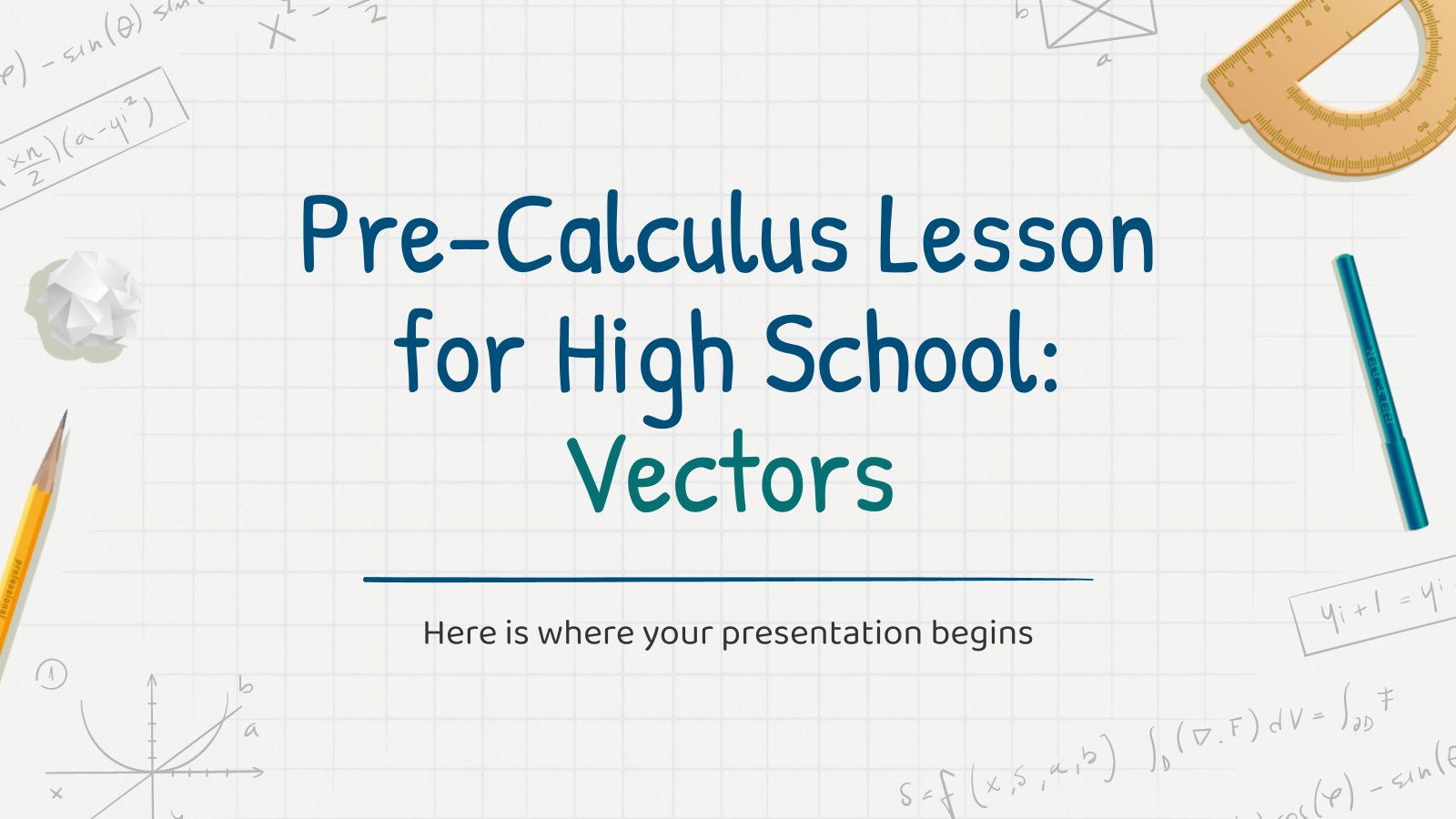

Disease Presentation templates
Use these free professional presentation templates to talk about a disease, its risks, symptoms, detection, and treatment options. work in google slides or powerpoint and impress the medical community..
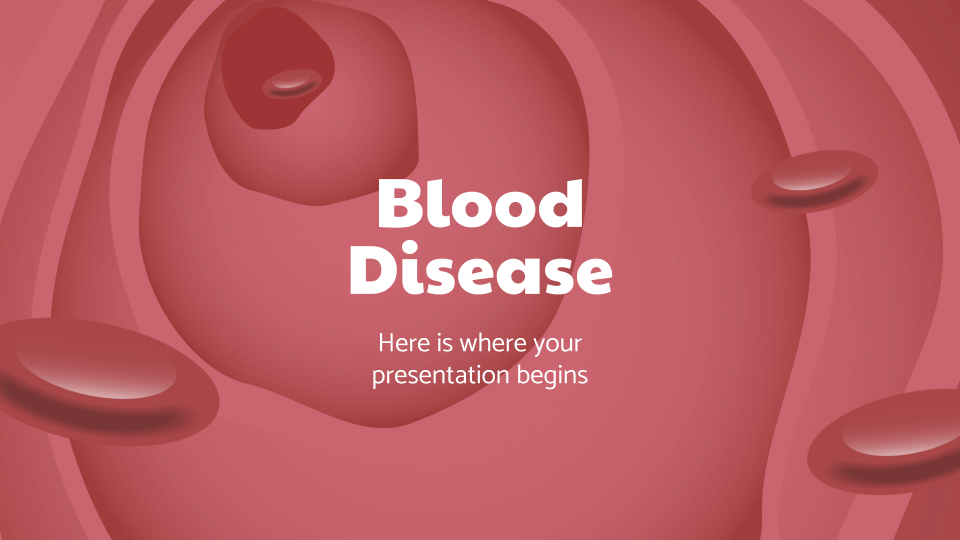
It seems that you like this template!
Premium template.
Unlock this template and gain unlimited access
Blood Disease
Diseases and illnesses are true woes of life. Educate your audience about blood disease with Slidesgo’s latest medical presentation. It has everything you need to explain its diagnosis process, pathology, treatment, and more.
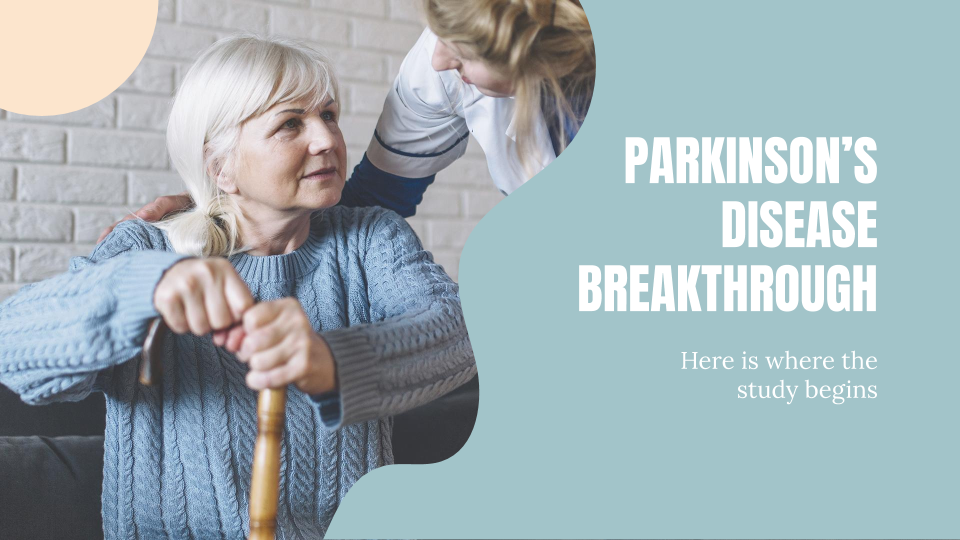
Parkinson's Disease Breakthrough
Currently, one of the most notorious degenerative disorders is Parkinson’s disease, for which there is no cure yet. If you’re a member of the medical community and need to present the latest breakthrough on this, we can help you with this template.
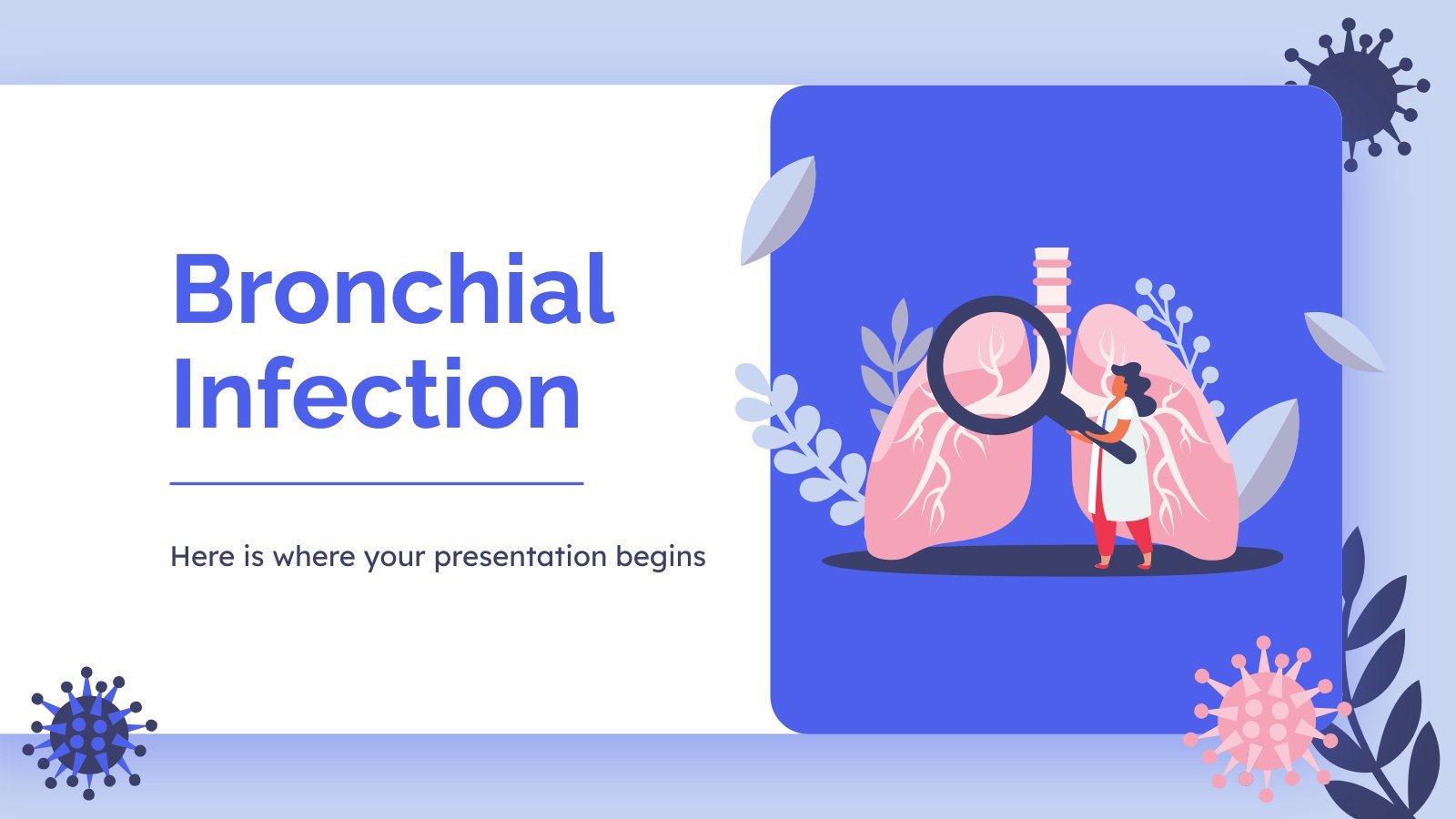
Bronchial Infection
Download the Bronchial Infection presentation for PowerPoint or Google Slides. Taking care of yourself and of those around you is key! By learning about various illnesses and how they are spread, people can get a better understanding of them and make informed decisions about eating, exercise, and seeking medical attention....
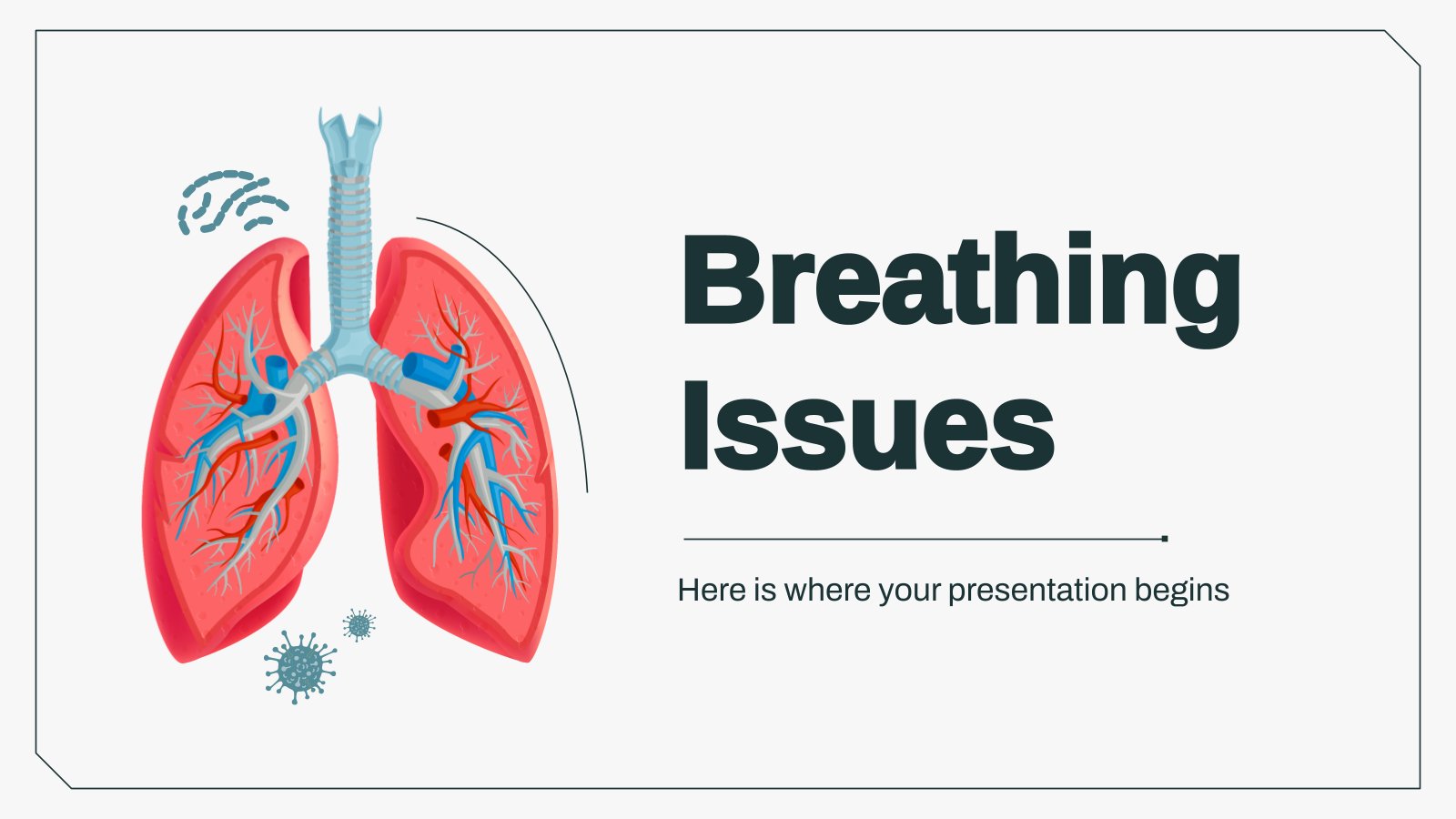
Breathing Issues
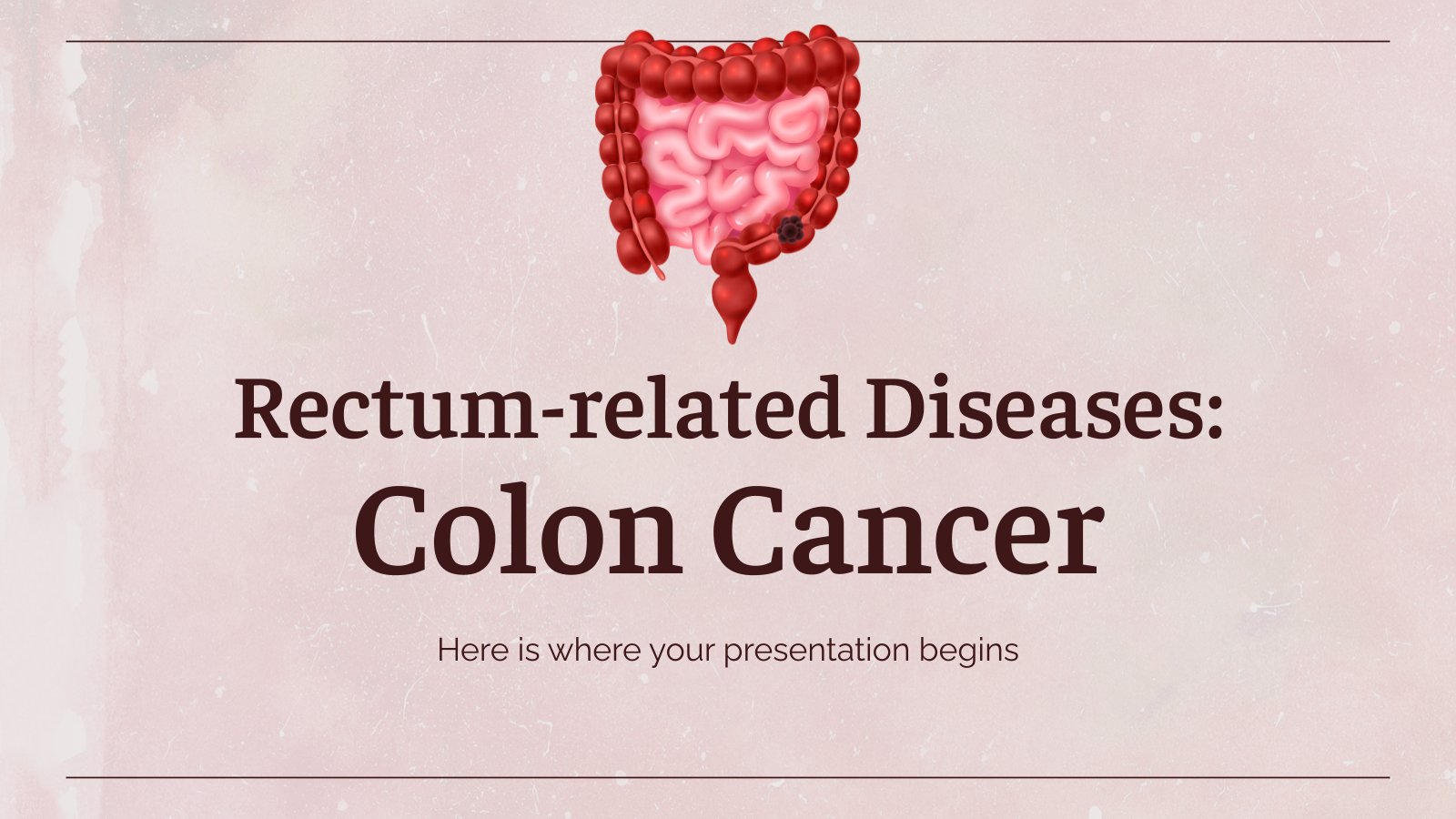
Rectum-related Diseases: Colon Cancer
Download the "Rectum-related Diseases: Colon Cancer" presentation for PowerPoint or Google Slides. Taking care of yourself and of those around you is key! By learning about various illnesses and how they are spread, people can get a better understanding of them and make informed decisions about eating, exercise, and seeking...
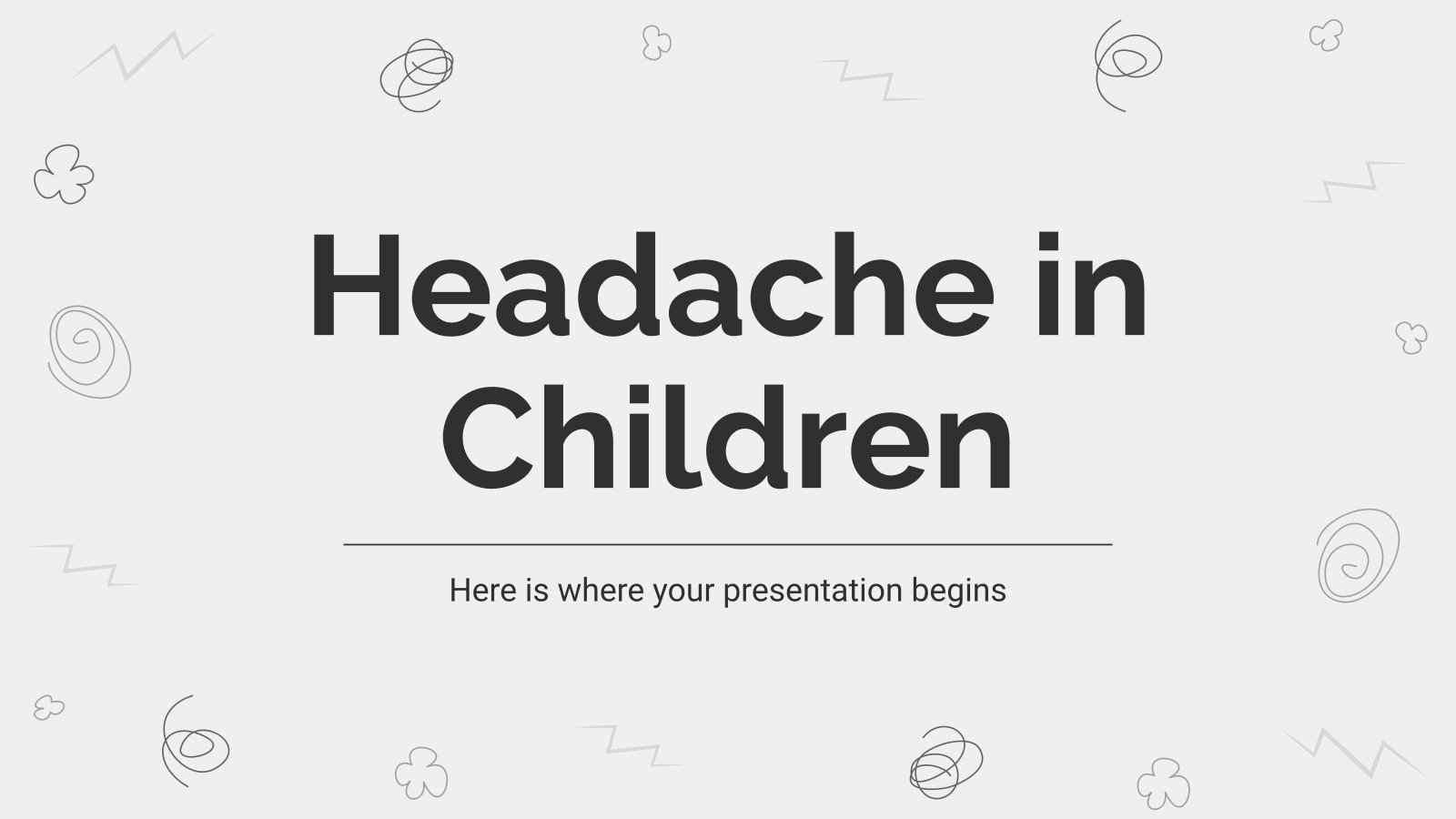
Headache in Children
Download the "Headache in Children" presentation for PowerPoint or Google Slides. Taking care of yourself and of those around you is key! By learning about various illnesses and how they are spread, people can get a better understanding of them and make informed decisions about eating, exercise, and seeking medical...
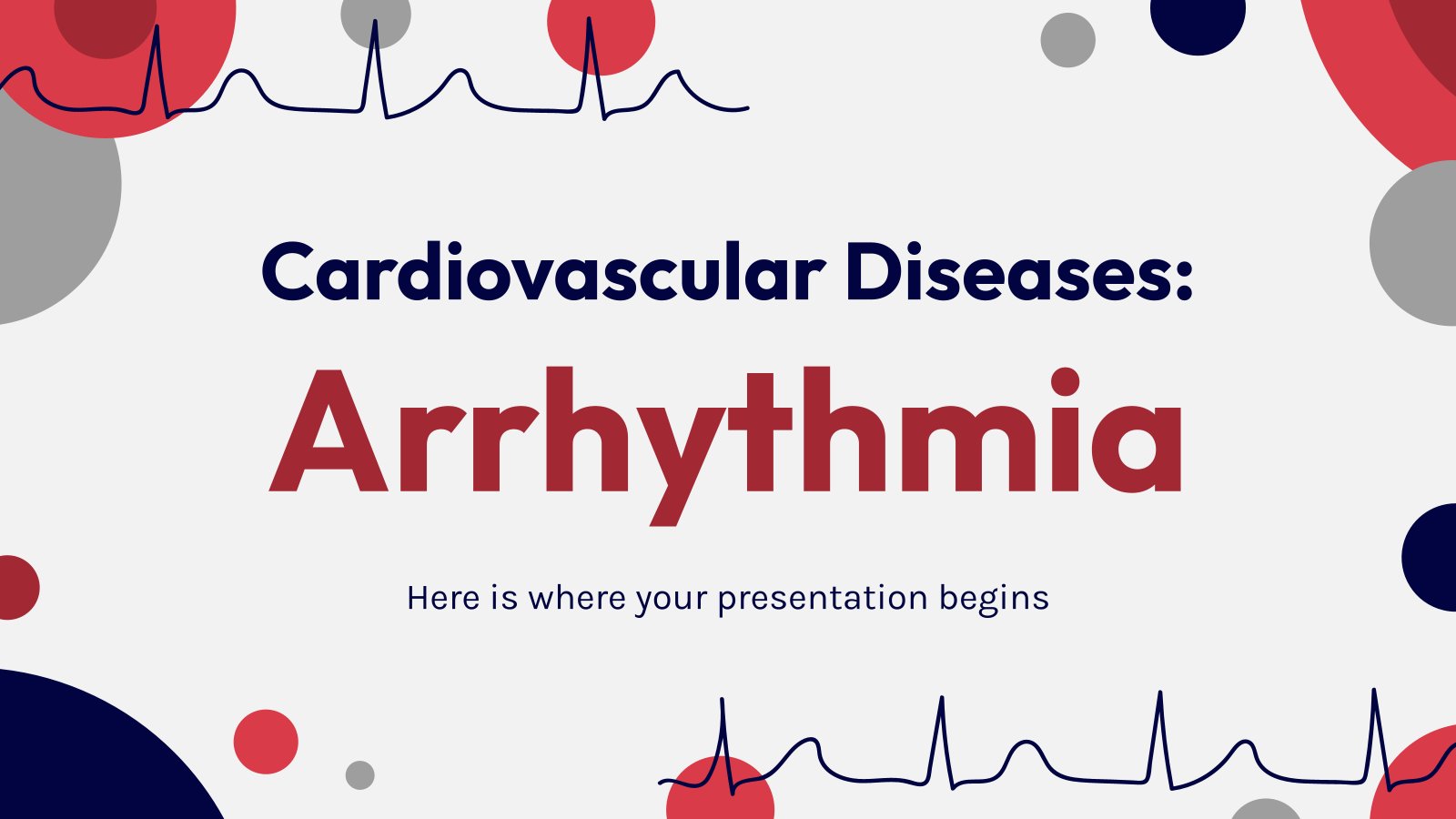
Cardiovascular Diseases: Arrhythmia
Boom, boom. You hear it? It’s you heart sending blood to every inch of your body, it has always done it and it will for the rest of your life. But sometimes, boom might be a bit too fast, or too slow. Arrythmia happens when the pounding rhythm of your...
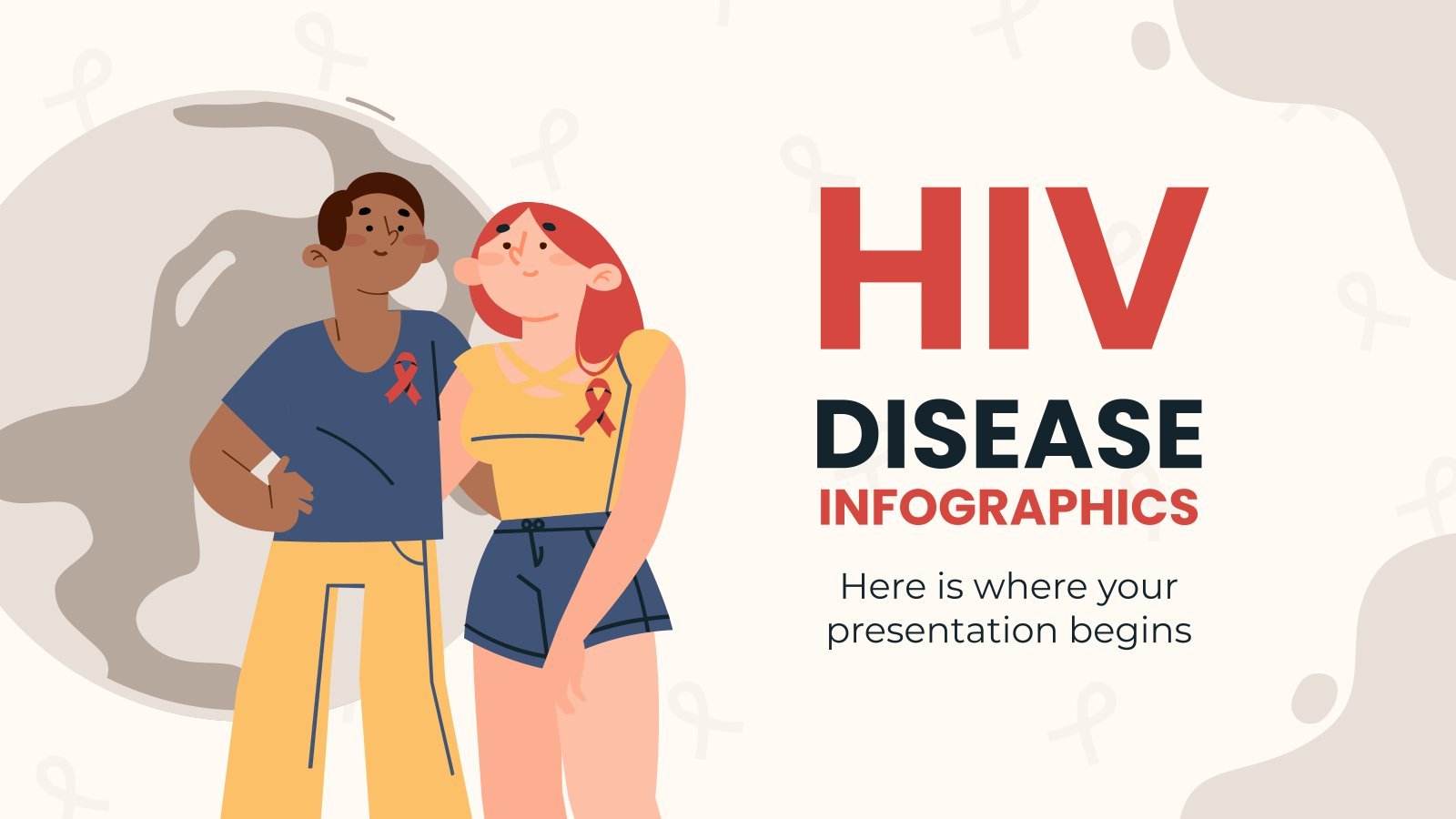
HIV Disease Infographics
Download the HIV Disease Infographics template for PowerPoint or Google Slides and discover the power of infographics. An infographic resource gives you the ability to showcase your content in a more visual way, which will make it easier for your audience to understand your topic. Slidesgo infographics like this set...
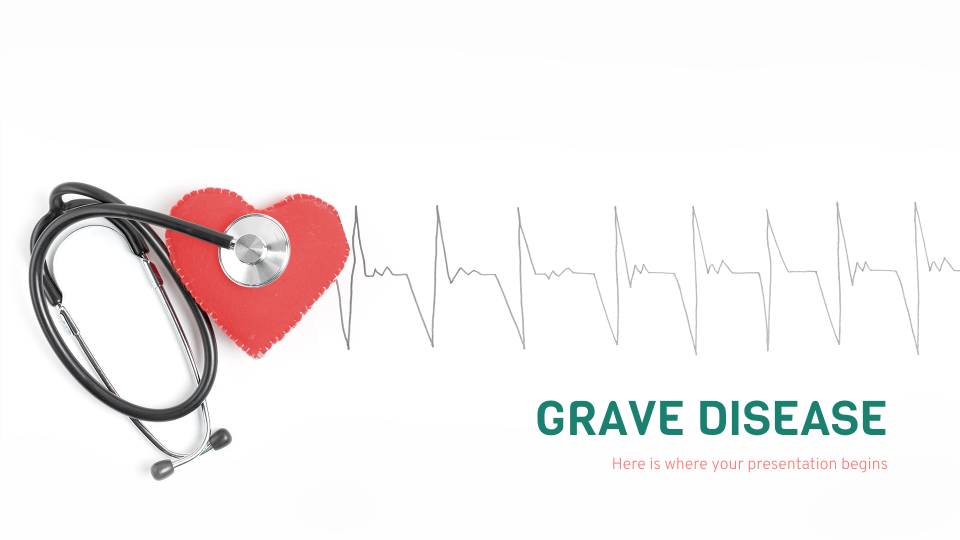
Grave Disease
Contribute to the medical community and share your findings and information on a grave disease with the use of this new template. Its slide design, simple and clean, makes it visually appealing and interesting, so you can talk about the pathology, the diagnosis, the treatment and everything needed for an...
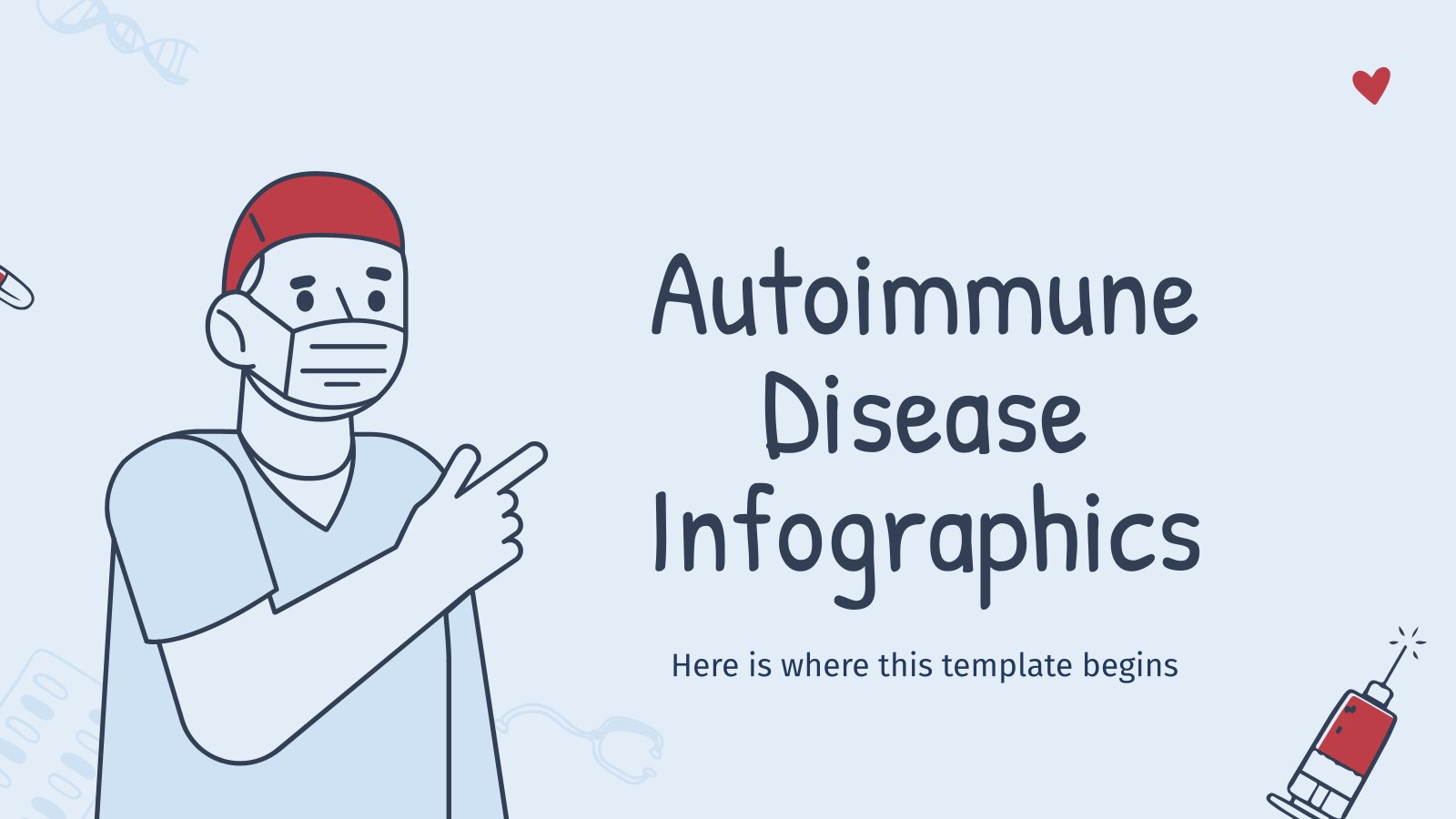
Autoimmune Disease Infographics
Infographics help people understand complex concepts easier. That’s why they are so commonly used in medicine! Booklets, posters, images… even in textbooks and presentations! If you want to speak about research on autoimmune diseases, this set of infographics will help you visualize ideas better. In addition, this pack comes together...
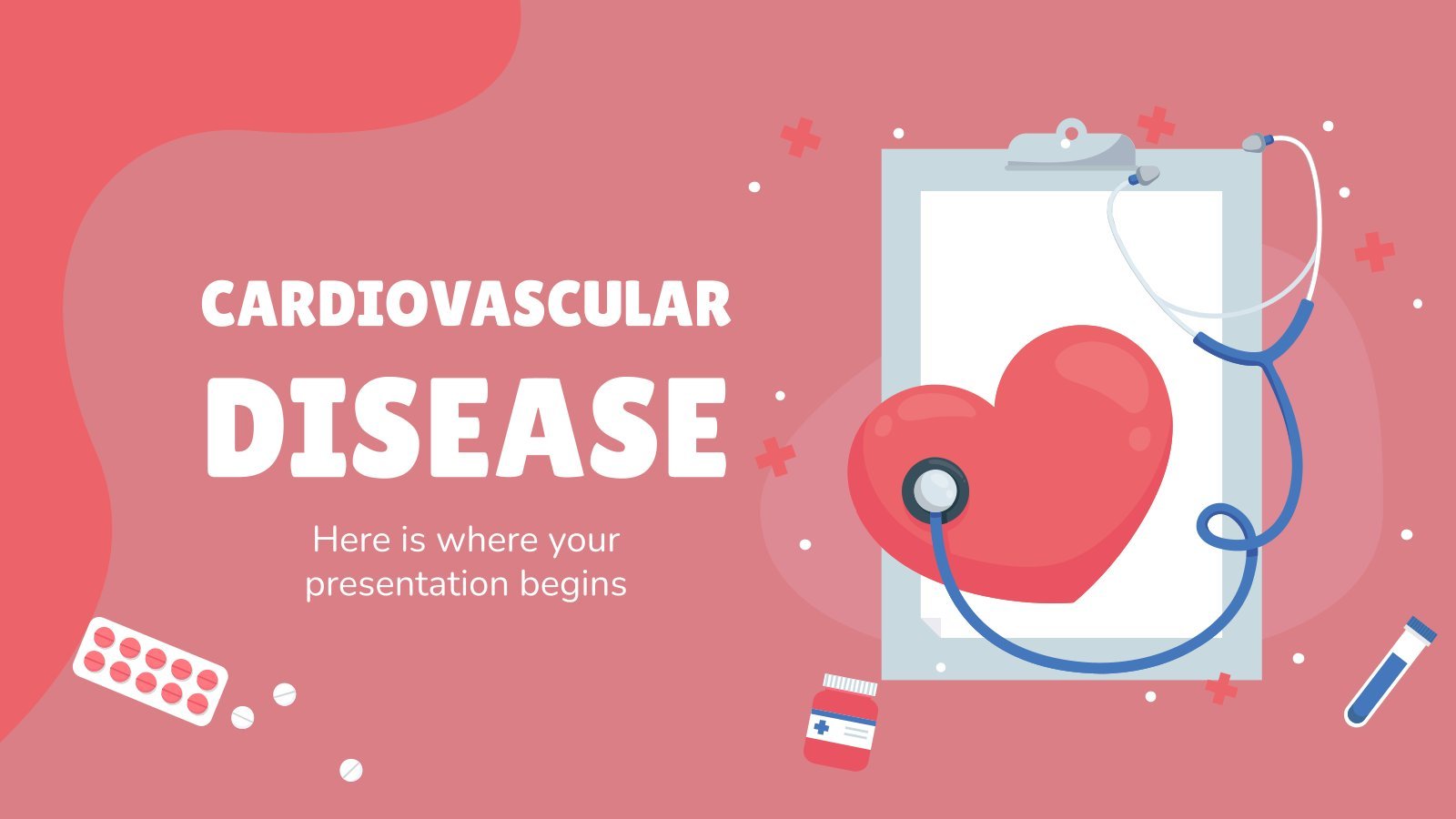
Cardiovascular Disease
It’s time to talk about cardiovascular diseases. Provide some data about them using this creative template full of illustrations of hearts, body diagrams, tables. We have also added useful sections to share data about the diagnosis, recommendations, pathology, treatment and processes.
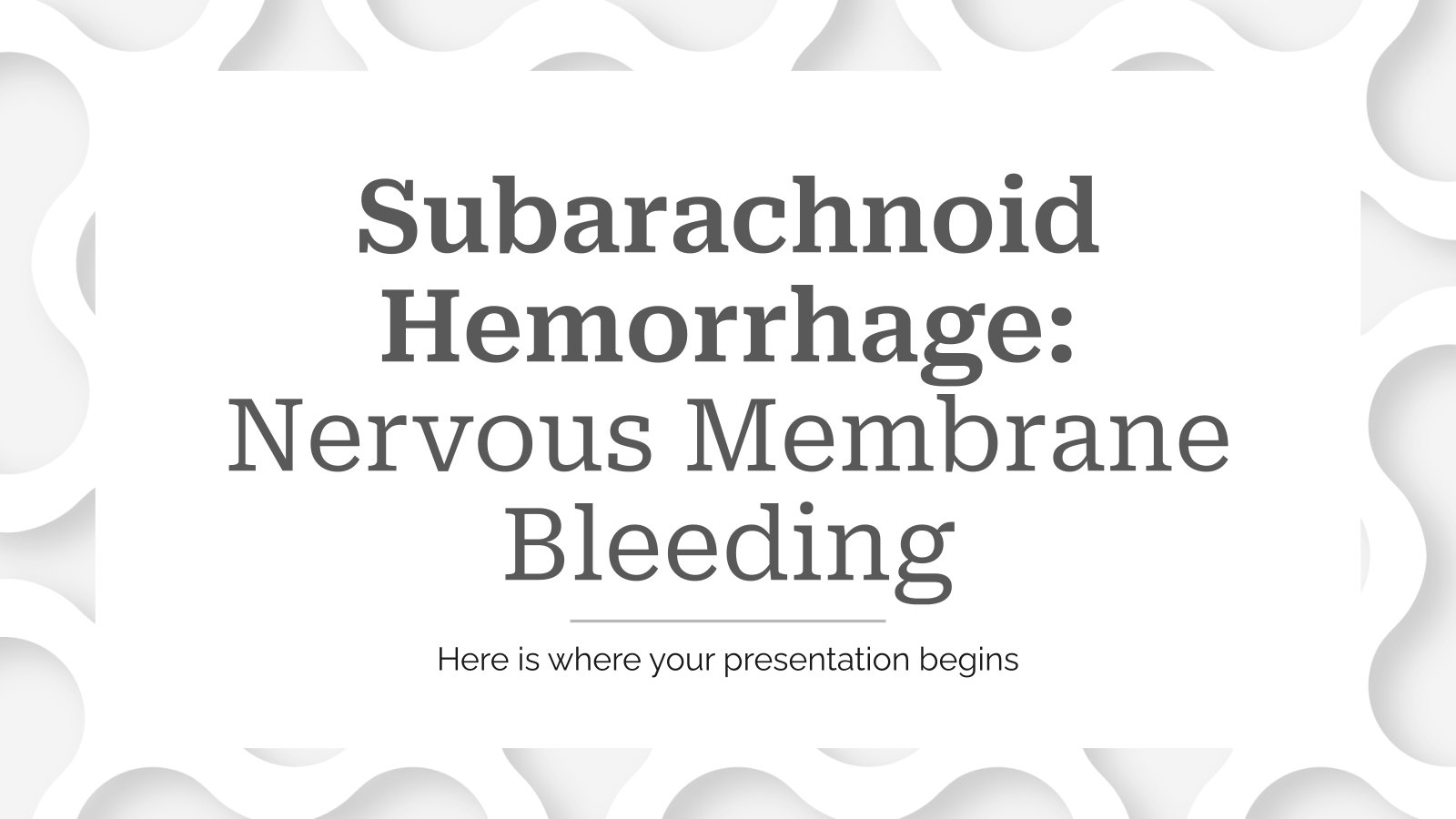
Subarachnoid Hemorrhage: Nervous Membrane Bleeding
Download the Subarachnoid Hemorrhage: Nervous Membrane Bleeding presentation for PowerPoint or Google Slides. Taking care of yourself and of those around you is key! By learning about various illnesses and how they are spread, people can get a better understanding of them and make informed decisions about eating, exercise, and...
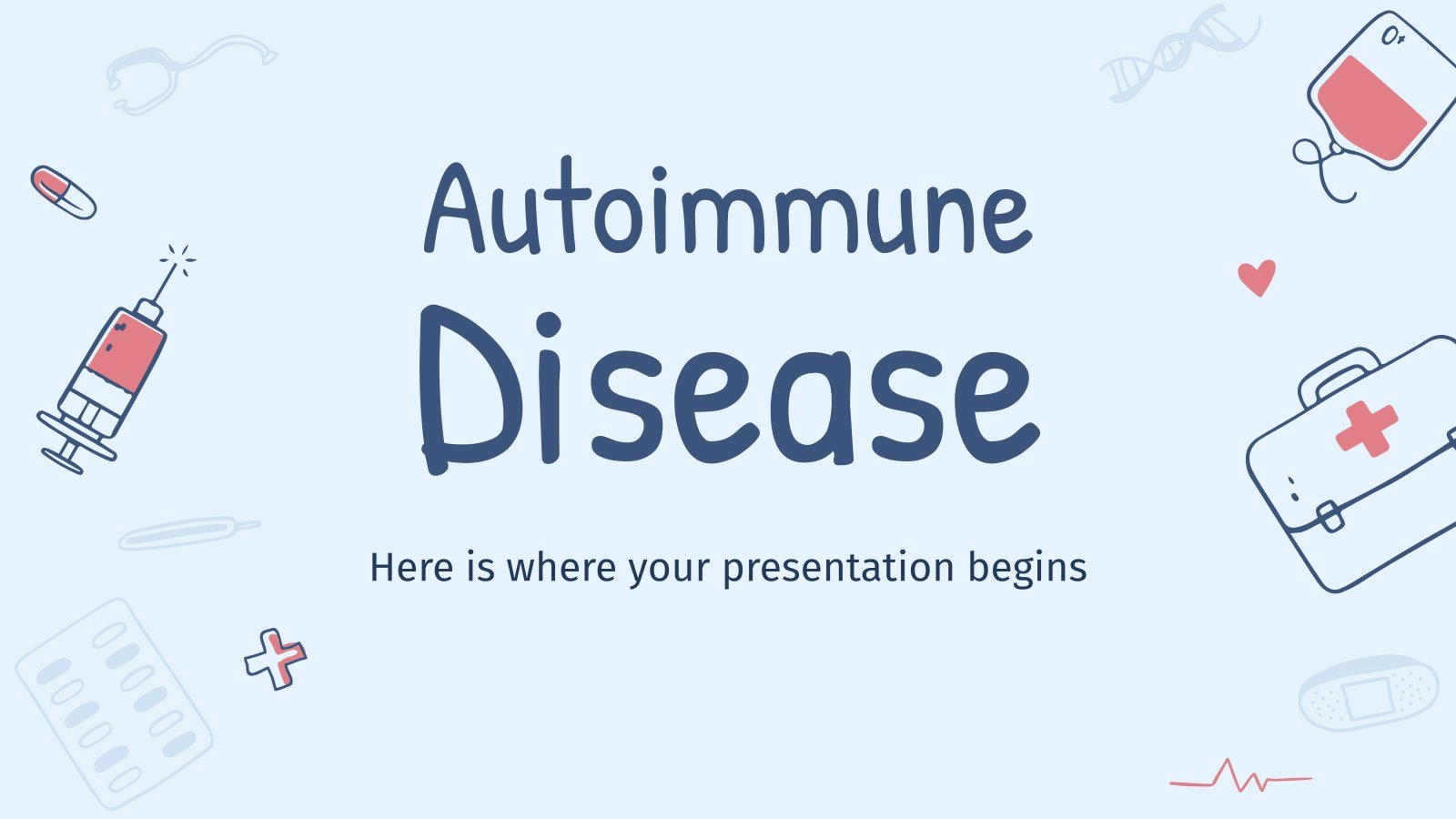
Autoimmune Disease
We have created this new template for presentations about an autoimmune disease. Its friendly design and overall look facilitates the reading and the understanding of the symptoms, treatment, recommendations and other essential data. Use the specific slides for that information and modify the maps, tables and graphs to provide more...
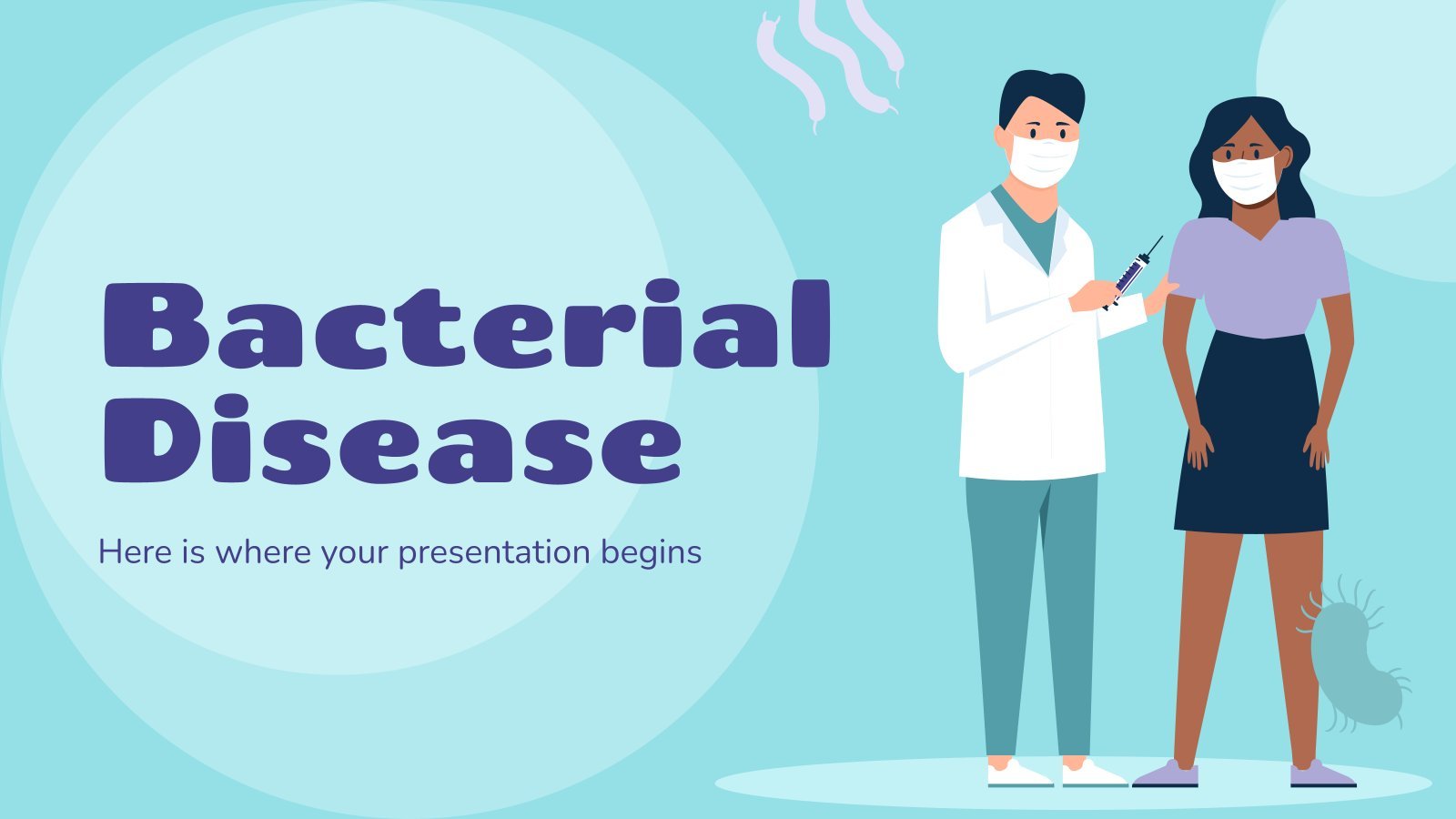
Bacterial Disease
There are many diseases caused by pathogenic bacteria, so this presentation can be useful if you want to focus on one and provide as much information about it as possible. As a template, it offers many examples of layouts in which you can describe things such as symptoms, recommendations, treatment,...
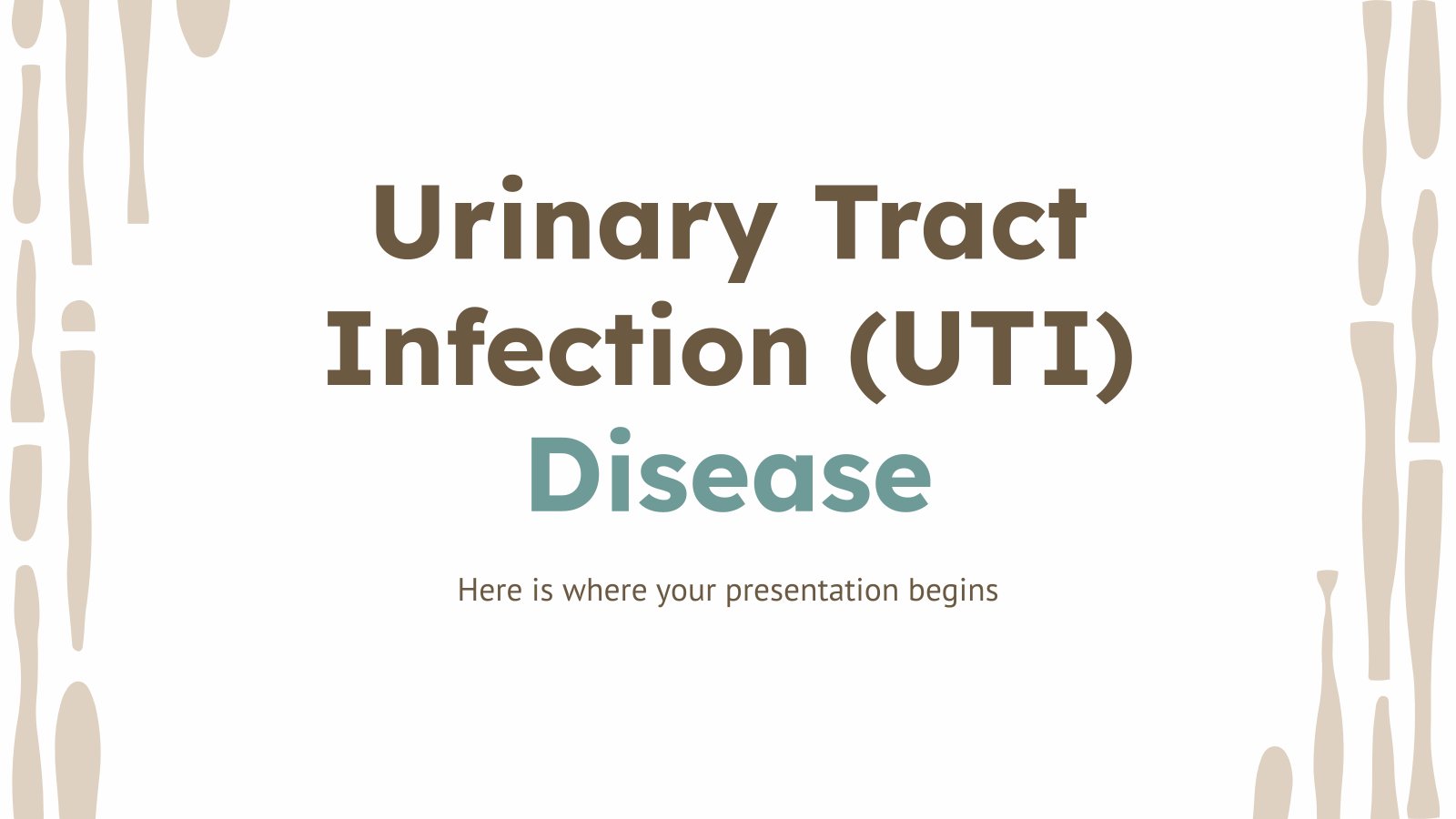
Urinary Tract Infection (UTI) Disease
Download the "Urinary Tract Infection (UTI) Disease" presentation for PowerPoint or Google Slides. Taking care of yourself and of those around you is key! By learning about various illnesses and how they are spread, people can get a better understanding of them and make informed decisions about eating, exercise, and...
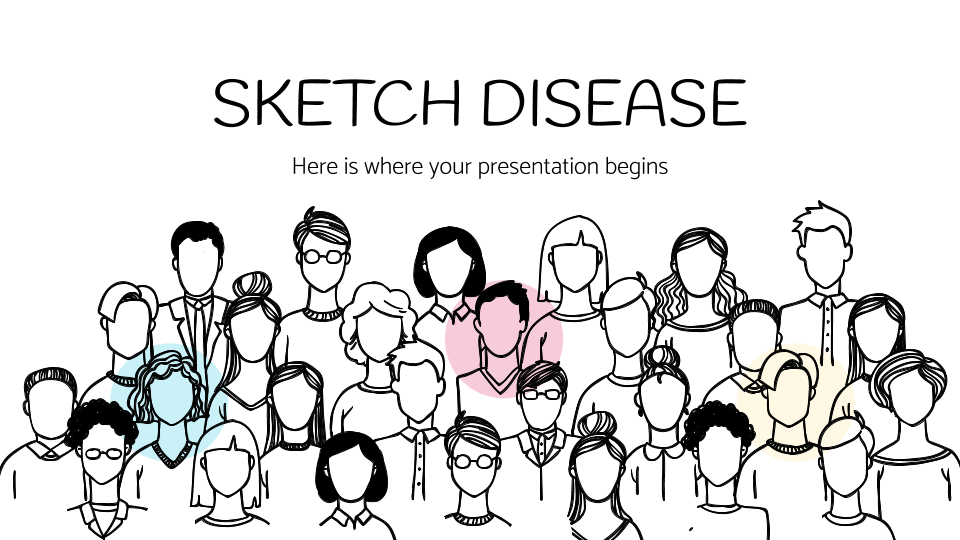
Sketch Disease
Prevention is better than cure. It’s important to get educated about medical conditions as early as possible. Perk up the normally somber theme of illnesses and diseases with Slidesgo’s latest disease presentation template.

Coronary Heart Disease
Coronary heart disease (CHD) is a very common complication that blocks the arteries of the heart. It is caused by the accumulation of fats in your bloodstream and can cause chest pains, shortness of breath, feeling weak or sick… You might also have heard about atherosclerosis. It’s a synonym for...
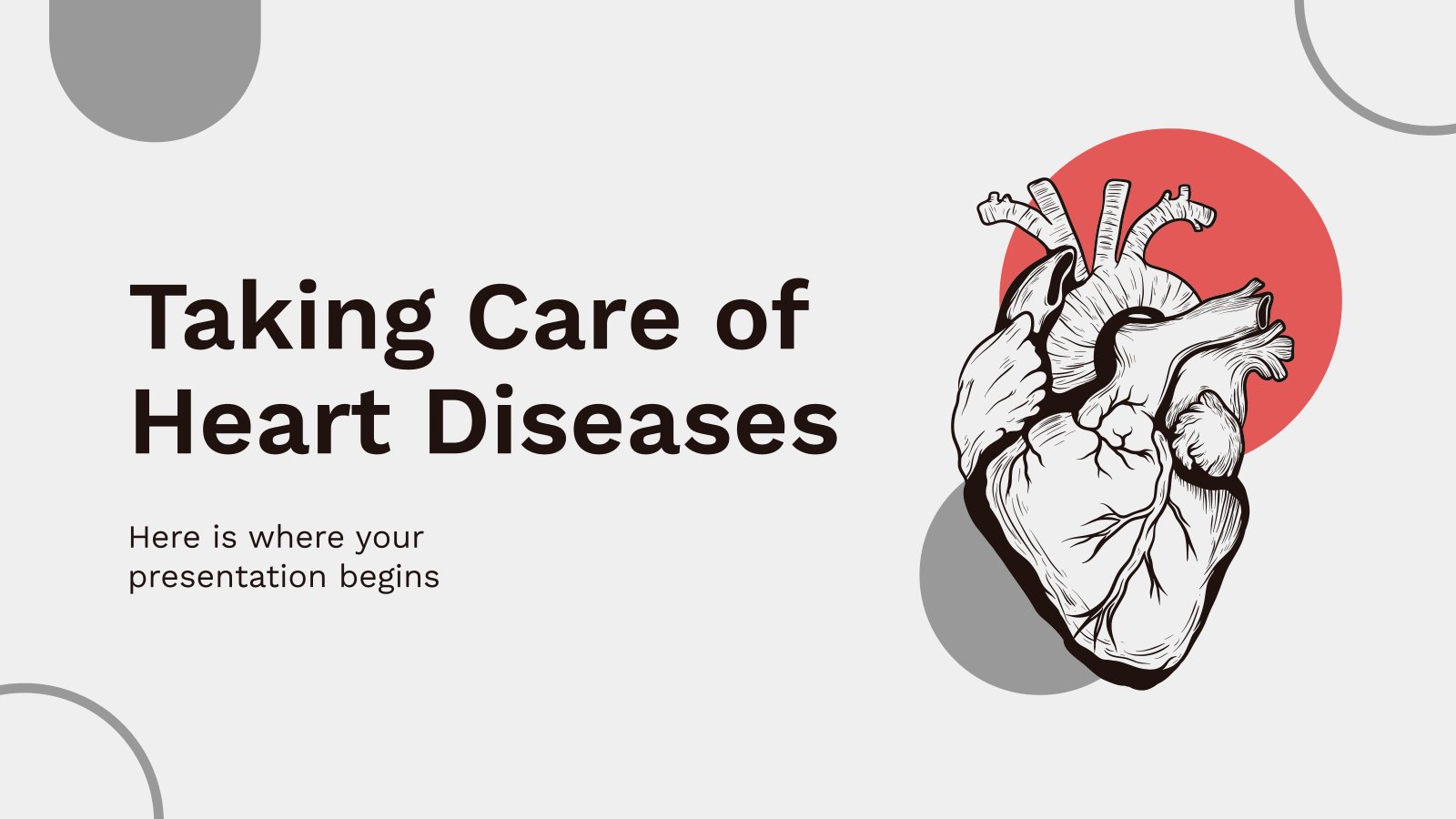
Taking Care of Heart Diseases
Download the "Taking Care of Heart Diseases" presentation for PowerPoint or Google Slides. Taking care of yourself and of those around you is key! By learning about various illnesses and how they are spread, people can get a better understanding of them and make informed decisions about eating, exercise, and...
- Page 1 of 46
Great presentations, faster
Slidesgo for Google Slides :
The easy way to wow
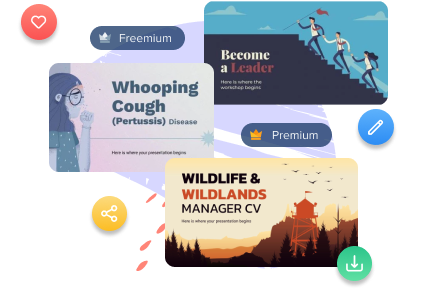
Register for free and start editing online
Map Your Show
What PAs Should Know About the Genetics of Autism
Originally Aired - Monday, May 20 • 8:00 AM - 9:00 AM
Your account does not have access to this session.
0">Resources
Create or Log in to My Conference Planner to see Videos and Resources.
{{chatBodyContent}}
This Session Has Not Started Yet
Be sure to come back after the session starts to have access to session resources.
Location: George R. Brown Convention Center, Level 3, 371
Event Information
This presentation is intended for practicing PAs who want to learn more about the overlap between genetics and autism spectrum disorder. The goal is to help PAs “think genetically” when evaluating and seeing patients with autism spectrum disorder. The intricacies of a genetics evaluation will be reviewed to help PAs better understand, appreciate, and utilize a genetics consult. The history, definition, diagnosis, epidemiology, and etiology of autism spectrum disorder will be discussed. Selected genetic disorders associated with autism spectrum disorder will also be reviewed. Briefly, options for genetic testing will be discussed as well as genetic counseling and consenting for genetic testing.
At the conclusion of this session, participants should be able to: • Discuss the history, definition, diagnosis, epidemiology, and etiology of autism spectrum disorder • Recognize patients with autism spectrum disorder who may benefit from a genetics evaluation • Examine the genetic etiology of selected genetic disorders associated with autism spectrum disorder
CME Number: H24214
Create or Log in to My Conference Planner to add notes .

Accreditation
- AAPA Category 1 CME – 1

An official website of the United States government
The .gov means it’s official. Federal government websites often end in .gov or .mil. Before sharing sensitive information, make sure you’re on a federal government site.
The site is secure. The https:// ensures that you are connecting to the official website and that any information you provide is encrypted and transmitted securely.
- Publications
- Account settings
Preview improvements coming to the PMC website in October 2024. Learn More or Try it out now .
- Advanced Search
- Journal List
- Int J Mol Sci
- PMC10889342

Unraveling the Genetic Landscape of Neurological Disorders: Insights into Pathogenesis, Techniques for Variant Identification, and Therapeutic Approaches
Zeba firdaus.
1 Department of Internal Medicine, Mayo Clinic, Rochester, MN 55905, USA; [email protected]
2 Department of Biochemistry and Molecular Biology, Mayo Clinic, Rochester, MN 55905, USA
Xiaogang Li
Genetic abnormalities play a crucial role in the development of neurodegenerative disorders (NDDs). Genetic exploration has indeed contributed to unraveling the molecular complexities responsible for the etiology and progression of various NDDs. The intricate nature of rare and common variants in NDDs contributes to a limited understanding of the genetic risk factors associated with them. Advancements in next-generation sequencing have made whole-genome sequencing and whole-exome sequencing possible, allowing the identification of rare variants with substantial effects, and improving the understanding of both Mendelian and complex neurological conditions. The resurgence of gene therapy holds the promise of targeting the etiology of diseases and ensuring a sustained correction. This approach is particularly enticing for neurodegenerative diseases, where traditional pharmacological methods have fallen short. In the context of our exploration of the genetic epidemiology of the three most prevalent NDDs—amyotrophic lateral sclerosis, Alzheimer’s disease, and Parkinson’s disease, our primary goal is to underscore the progress made in the development of next-generation sequencing. This progress aims to enhance our understanding of the disease mechanisms and explore gene-based therapies for NDDs. Throughout this review, we focus on genetic variations, methodologies for their identification, the associated pathophysiology, and the promising potential of gene therapy. Ultimately, our objective is to provide a comprehensive and forward-looking perspective on the emerging research arena of NDDs.
1. Introduction
With an increase in the aging population, neurodegenerative disorders (NDDs) are among the major health issues in the modern world. It is estimated that they will become the second leading cause of death globally by 2050, surpassing cancer [ 1 ]. Neurodegenerative disorders that are chronic and progressive exhibit a distinct pattern of neuron loss in motor, sensory, or cognitive systems [ 2 ]. The common symptoms of NDDs include impairment of the motor system, sensory network, cognitive function, memory, and abstract thinking that could appear during the disease progression [ 3 ]. Alzheimer’s disease (AD), Parkinson’s disease (PD), and amyotrophic lateral sclerosis (ALS) take the forefront among neurodegenerative disorders, resulting in the unfortunate demise of thousands of Americans. Moreover, the patient count is anticipated to rise steadily in the upcoming decades [ 2 , 4 ].
NDDs primarily emerge during late adulthood and are frequently associated with the accumulation of protein aggregates. For instance, AD is predominantly characterized by the aggregation of amyloid β and tau proteins, while PD and ALS are characterized by the accumulation of α-synuclein [ 5 ] and TDP43 [ 6 ], respectively ( Figure 1 ). While the initial diagnosis of an NDD is typically based on clinical presentation, definitive confirming requires post-mortem pathological analysis to identify the specific protein aggregates. Moreover, the presentation of NDDs varies widely, and it is increasingly recognized that the diagnosis exists on a spectrum, with a greater prevalence of mixed pathology and overlapping clinical features than previously acknowledged [ 5 , 7 ]. Understanding the underlying causes and pathological processes of neurological diseases stands as one of the most critical challenges in the fields of medical and biological sciences due to their relatively high prevalence, largely unknown mechanisms, and significant impact on affected individuals, families, and society.

Abnormal protein deposition in affected brain regions during AD, PD, and ALS. In AD, cerebral cortex and hippocampus is predominantly affected and identified by amyloid plaque deposition, and a-synuclein deposition in basal ganglia is a hallmark of PD. In ALS, the brain stem and spinal cord are affected with the accumulation of TDP-43 aggregates.
NDDs are influenced by a wide range of genetic factors, from straightforward direct predisposition in diseases like Huntington’s disease and spinocerebellar atrophy to more complex roles in diseases like AD and PD [ 8 , 9 ]. A number of causative genes for familial forms of NDDs have been identified; these genes are inherited as Mendelian traits [ 10 ], and their discovery has advanced knowledge of the molecular mechanisms underlying the distinctive neuronal degeneration that distinguishes each disorder. Only a small percentage of ALS, AD, and PD cases (5–10%) are familial, while the vast majority (>90%) are sporadic [ 11 ], most likely due to complex interactions between genetic and environmental factors in addition to the slow, sustained neuronal dysfunction caused by aging.
This review is primarily dedicated to dissecting the complex web of genetic changes in neurological disorders such as ALS, AD, and PD. Our investigation extends to the methodologies employed for pinpointing these alterations. Through this review, we aspire to paint a broad and forward-looking canvas, capturing the dynamic landscape of research in neurodegenerative diseases.
2. Genetic Techniques in Addressing Neurodegenerative Disorders
In the realm of molecular genetics and genetic epidemiology, disease-related genes are typically classified into two principal groups: causative genes and susceptibility genes [ 12 ]. Through the identification of the causal or susceptibility genes for each neurological disorder, along with the utilization of transgenic techniques, our grasp of the fundamental molecular mechanism has improved, creating a pathway for the exploration of potential therapeutic targets. In this section, we will discuss different cutting-edge high throughput technologies to identify the genetic variation in neurodegenerative disorders ( Table 1 ).
Comparative analysis of genetic techniques, their advantages, and limitations.
Many neurological disorders are known to have substantial genetic factors contributing to their development [ 13 ]. Recent progress in the fields of genome technologies, encompassing genome-wide association studies (GWASs) and next-generation sequencing (NGS) technology, has substantially enhanced our insights into the genetic factors contributing to these diseases [ 14 ]. In the case of rare Mendelian disorders, NGS exhibits the capacity to unveil novel genes harboring mutations that intricately underpin the phenotypic expressions [ 14 ]. GWAS, based on the common disease–common variant hypothesis, aims to elucidate how common genetic variability is associated with the development of prevalent diseases [ 15 ]. Most common neurological diseases like AD, PD, and ALS can be studied using both approaches. GWAS works well for sporadic cases (without a family history), while NGS-based studies are best for cases with a strong family connection, indicating a likely genetic inheritance [ 16 ].
Genome-wide association studies (GWASs) have successfully revealed numerous susceptibility genes for common diseases such as diabetes as well as NDD, the odds ratios associated with these risk alleles are generally low and account for only a small proportion of estimated heritability [ 17 ]. The theoretical framework for a GWAS is the ‘common disease—common variant hypothesis’, in which common diseases are attributable in part to allelic variants present in more than 1–5% of the population [ 18 ]. It is assumed that risk alleles with a large effect size may be rare in frequency and hard to detect with GWASs employing common single nucleotide polymorphisms (SNPs). The current experience with GWASs strongly suggests that rarer variants that are hard to detect with GWASs may account for the ‘missing’ heritability [ 19 ]. This suggests the presence of rare variants (found in less than 5% of the population) between the extremes of the frequency spectrum. Such rare variants may have large effect sizes as genetic risk factors for diseases. Thus, we need a paradigm shift from the ‘common disease—common variants hypothesis’ to a ‘common disease—multiple rare variants hypotheses’ to identify disease-relevant alleles with large effect sizes [ 20 , 21 ]. These rare variants, although not causative, typically have a significant impact, and GWASs using common SNPs struggle to capture them due to their large effect size [ 22 ].
The advancement in next generation sequencing technologies has notably enhanced the efficiency and affordability of both whole exome sequencing (WES) and whole genome sequencing (WGS) in the recent past [ 23 ]. In contrast to the extended timeframe and high expenses associated with first-generation sequencing, specifically Sanger sequencing which required several years and millions of dollars to sequence an entire diploid human genome, an NGS platform can achieve this sequencing within a few weeks, at a modest cost [ 24 ]. Furthermore, NGS technology has facilitated the discovery of rare variants with substantial effects, revealing missense or nonsense single-base substitutions, as well as small insertions or deletions. These findings bear significant implications for risk prediction, diagnosis, and the treatment of neurological diseases [ 14 ].
Despite the vast array of mutations identified through next-generation sequencing (NGS), its short-read lengths (150–300 bp) and limited representation in GC-rich/poor areas poses challenges in resolving expansions beyond several kilobases [ 25 ]. In comparison to NGS, two prevalent long-read sequencers referred as LRS, including single-molecule real-time (SMRT) sequencing developed by Pacific Biosciences (PacBio), Menlo Park, CA, USA [ 26 ] and nanopore sequencing by Oxford Nanopore Technologies (ONTs), Oxford Science Park, Oxford, UK [ 27 ], present an alternative method for sequencing single DNA molecules in real time ( Figure 2 ). The reads generated by these platforms are exceptionally long, extending over several tens of kilobases and capturing entire repetitive regions [ 28 ]. Additionally, there is a potential to diminish the guanine-cytosine (GC) bias to a lesser degree and attain a more even genome coverage without the necessity for PCR amplification, unlike NGS [ 28 , 29 ]. In the realm of targeted sequencing, a noteworthy advancement is the integration of clustered regularly interspaced short palindromic repeats/CRISPR-associated 9 (CRISPR/Cas9)-mediated amplification-free enrichment with long-read sequencing (LRS), presenting an essential tool for targeted sequencing [ 30 ]. The distinctive attributes of LRS render it highly appropriate for unraveling neurodegenerative diseases, particularly in instances where NGS outcomes are uninformative.

A simplified flowchart depicting the streamlined pathways involved in next generation genome sequencing.
In a more recent development within the human domain, the telomere-to-telomere consortium utilized long-read whole-genome sequencing (WGS) to successfully sequence the very first “complete human genome” [ 31 , 32 ]. The initiative commenced with the aim of constructing a human reference genome devoid of any gaps; thus, researchers utilized both PacBio and ONT technologies to meticulously sequence every facet of the genome, including the historically elusive telomeres and centromeres [ 31 , 32 ]. Long-read whole-genome sequencing (WGS) stands out for its capability of navigating through intricate genomic regions, facilitating the identification of structural variations like insertions, deletions, inversions, translocations, expansions, and copy number variations [ 33 , 34 ]. Short-read sequencing, with its typically shorter read lengths, struggles to capture these structural variations adequately [ 34 ]. The exploration of structural variation through long-read WGS may shed light on some of the unexplained heritability in ALS [ 35 ]. Until now, only one study has delved into long-read whole-genome sequencing (WGS) within the realm of ALS, specifically targeting C9orf72 repeat expansions. Utilizing the ONT MinION (Oxford Science Park, Oxford, UK), no reads covering the C9orf72 expansion were identified, while PacBio SMRT sequencing yielded an 8× coverage of the expansion [ 35 ]. Notably, there have been no reported large-scale association studies in ALS integrating long-read WGS.
3. Genetic Mutations and Corresponding Cellular Alterations in Neurodegenerative Disorders
In this section, we will delve into the cellular mechanisms of pathogenicity linked to genetic variations, focusing specifically on neurodegenerative disorders such as ALS, AD, and PD. Additionally, other neurodegenerative diseases are also touched on briefly in this section to provide a comprehensive overview of this expansive field.
3.1. Amyotrophic Lateral Sclerosis (ALS)
ALS is a fatal neurodegenerative disease that predominantly affects motor neurons in the brain, brainstem, and spinal cord [ 6 ]. The term “amyotrophy” refers to the loss of muscles and “lateral sclerosis” refers to the loss of axons and muscle in the lateral spinal cord columns. ALS causes gradual voluntary muscle weakness that spreads to neighboring body parts, usually resulting in death from respiratory failure within 2–4 years of diagnosis. In addition to motor neuron loss, the neuropathological features include intracellular cytoplasmic inclusions of eosinophilic Bunina bodies and ubiquitinated TDP-43 [ 6 ]. Furthermore, there is significant variation in disease symptoms, with frontotemporal dementia (FTD) occurring in about 15% of patients, and cognitive and behavioral changes occurring in up to 60% of patients [ 6 ].
3.1.1. Genetic and Pathological Overlap between ALS and FTD
ALS has a close association with frontotemporal dementia (FTD), sharing a common molecular etiology with this condition [ 36 ]. Roughly 15% of individuals diagnosed with FTD develop motor neuron disease (MND), while conversely, up to 50% of those with MND exhibit clear signs of cognitive defects [ 37 ]. Numerous studies have revealed clinical, pathological, and genetic similarities between these conditions. Consequently, they are now viewed as two expressions of a single disease continuum, known as either the ALS-FTD spectrum or the FTD-ALS spectrum. The broader term, frontotemporal lobar degeneration, is linked with motor neuron disorders under the name FTLD-MND [ 36 ]. ALS is a degenerative MND marked by progressive muscle atrophy, paralysis, and ultimately, death typically occurring within 3–5 years of symptom onset due to respiratory failure. On the other hand, FTD manifests with alterations in social behavior and/or language skills at disease onset, stemming from neurodegeneration in the frontal and temporal lobes, culminating in death within 3–12 years of symptom onset [ 38 ].
Despite the heterogeneous clinical phenotypes observed in FTD and ALS, implying potentially divergent underlying biological mechanisms, it is widely acknowledged that these diseases share significant clinical, genetic, and neuropathological similarities [ 39 ]. The prevalent mutations implicated in disease causation are found in chromosome 9 open reading frame 72 ( C9ORF72 ) and progranulin ( GRN ), both leading to TDP-43 neuropathology, as well as in microtubule-associated protein tau ( MAPT ), which leads to tau neuropathology [ 40 ]. The leading factors responsible for ALS, FTD, or their co-occurrence (ALS-FTD), in a familial context, are commonly the pathogenic hexanucleotide repeat expansions in C9ORF72 [ 41 ]. Approximately 25–40% of familial cases of ALS and FTD exhibit this mutation, while 5–7% of sporadic cases also test positive for pathogenic expansions in C9ORF72 [ 41 ]. In addition to C9ORF72 , mutations in several other genes, such as TARDBP , SQSTM1 , VCP , FUS , TBK1 , CHCHD10 , and UBQLN2 , have been identified in association with both ALS and FTD [ 34 , 37 ]. For patients with ALS and FTD who possess these particular mutations, excluding FUS, the prevalent pathological feature is the presence of ubiquitinated protein deposits primarily consisting of TDP-43 [ 36 ]. The reason why mutations in the same genes lead to distinct clinical syndromes despite similar neuropathology remains unclear and could be attributed to variations in mutation localization affecting downstream processes, as well as potential influences from modifying genetic and/or environmental factors. However, the presence of shared genetic factors in FTD and ALS suggests the existence of common molecular mechanisms driving disease pathology, to some degree. Ongoing efforts in developing novel disease-modifying treatments for both ALS and FTD patients are targeting specific molecular subtypes.
3.1.2. Epidemiology
The risk of ALS increases with aging and is highest between the ages of 60 and 79 [ 42 ]. It is unclear whether the incidence of ALS has changed in the last few decades, but it is expected to rise as the population ages. According to a meta-analysis, the standardized global incidence of ALS is only 168 per 100,000 person-years of follow-up, but this varies by region [ 42 ]. In populations with a predominance of European ancestry, like those in Europe and North America, the incidence ranges from 1.71 per 100,000 to 1.89 per 100,000 and may even be higher in population-based studies [ 43 ]. Asian populations have lower incidences, varying from 0.73 per 100,000 in south Asia to 0·94 per 100,000 in west Asia, whereas Oceania universally has the highest incidence (2.25 per 100,000) [ 43 ]. Additionally, incidence differs by sex, with a standardization male-to-female ratio of 1.35 that is influenced by the age of onset. Genetics also play a role; heritability is higher in mother–daughter pairs, whereas the most common known ALS risk gene, C9orf72 , lowers the onset age in men versus women [ 43 ]. Thus, ALS is caused by complex interactions between age, gender, and genetics, which have implications for preclinical and clinical research.
Even though there are several known genetic risks for ALS, approximately 85% of cases do not have a single genetic cause [ 44 ]; thus, the pathophysiology of the disease remains unknown, delaying the development of effective therapies. Till now, there are only two effective drugs available: riluzole and edaravone [ 45 ]. Recently, two additional drugs were approved by the FDA that include Toferson (the first gene therapy for ALS) [ 46 ] and Relyvrio [ 47 ]. Non-pharmacological multidisciplinary care, for example, the use of early non-invasive ventilation and feeding tube insertion, can improve patient outcomes to a certain extent before significant weight loss [ 6 ]. Due to the scarcity of treatments, researchers have focused their efforts on the complex genetics of ALS and the associated pathomechanism [ 48 ].
3.1.3. Genetic Causes and Risk Factor
ALS is typically classified as familial or sporadic. This straightforward division, however, ignores the disease’s complex genetic architecture, which is characterized by gene penetrance, heritability, and inheritance (monogenic, oligogenic, and polygenic). Only 10–15% of individuals have Mendelian ALS, though it has incomplete penetrance in most families [ 49 ]. In the remaining 85%, large GWASs may be able to find rare variants, i.e., mutations found in a single family that may affect disease risk and phenotypic presentation [ 50 ]. Ancestral European (i.e., European, American, Canadian, and Australian) and Asian populations are the main sources of the current knowledge of validated genes for ALS [ 51 ]. Although at least 40 genes have been linked to the disease, 4 genes, namely C9orf72 , SOD1 , TARDBP (coding for TDP-43), and FUS , account for roughly 48% of familial cases and 5% of sporadic cases in European populations, and significantly contribute to the pathophysiology of disease [ 52 ].
- Superoxide dismutase ( SOD1 )
In 1993, the identification of the SOD1 gene as the causative factor for the familial form of ALS was a major advancement in this field [ 53 ]. SOD1 (Cu-Zn SOD) is a 32 kda homodimeric protein, comprising 153 amino acids and containing one copper and one Zn binding site, present abundantly in the nucleus, cytosol and mitochondria [ 54 ]. Currently, more than 200 mutations are reported in this gene [ 55 ]. SOD1 plays antioxidant role in the cellular system by lowering the concentration of reactive oxygen species (ROS), and also converting ROS into oxygen and hydrogen peroxide (H 2 O 2 ) [ 56 ].
Patients with a SOD1 -mutation have clinical traits like early onset, longer disease duration, and motor symptoms that typically start in the lower limbs, with rare occurrences of cognitive disturbances [ 57 ]. The discovery of SOD1 in ALS has significantly added to the understanding of the disease, in particular with the development of transgenic models. Mutated SOD1 triggers ROS production and causes oxidative stress and abnormal iron metabolism, but the detailed molecular mechanism is still to be elucidated [ 58 ]. The mutated SOD1 contains an exposed N-terminal short domain, the derlin-1-binding region (DBR), that initiates endoplasmic reticulum stress. In ALS, SOD1 mutation induces a conformational change that may lead to motor neuron toxicity [ 59 ].
Excitotoxicity (glutamate-mediated neurotoxicity) is a possible pathogenic mechanism for ALS, which explains why the glutamate release inhibitor riluzole shows modest therapeutic efficiency in this disorder [ 60 ]. Even though this is debatable in SOD1 ALS patients, excitotoxicity may result from a selective loss of the glutamate transporter-1 (GLT1), excessive glutamate efflux, or toxicity of glial cells, the consequences of which are disturbances in neuronal calcium homeostasis [ 58 , 61 ]. Mitochondrial dysfunction has also been observed in SOD1 -mutated ALS animal models; an increase in the production of oxidative stressors such as nitric oxide, superoxide, and peroxynitrite, as well as a decrease in the ability of mitochondria to synthesize adenosine triphosphate (ATP) are mitochondrial-based mechanisms causing motor dysfunction [ 62 ]. Depolarization is a crucial phenomenon in axonal transports. Motor neurons are particularly sensitive to energy reduction because it disturbs ionic Na + /K + pumps and quickly results in a slow depolarization with hyperexcitability due to the progressive loss of Na + and K + reversal potentials [ 63 ]. The depolarization is further worsened by persistent sodium channels (pNa + ) and raises intracellular calcium that triggers apoptotic pathways [ 63 ]. Interestingly, Riluzole is a pNa + blocker and this property may also play a role in its neuroprotective effects [ 64 ].
Recently, some authors have proposed the ‘prion-like propagation’ of mutant SOD1 misfolding and motor neuron degeneration along corticospinal pathways [ 65 ]. The concept driving this hypothesis is that a mutant or wild-type misfolded protein spreads along anatomical pathways and transmits its abnormal misfolding properties to native proteins, causing toxic aggregation. These properties have been reported in vitro for SOD1 , TARDBP , FUS , and C9ORF72 , but in vivo only for SOD1 so far [ 66 ].
Moreover, the genome-wide data showed that the disease progression and severity are correlated with SOD1 point mutations, like the p.A4V mutation is associated with severe disease symptoms and p.H46R shows slow progression [ 67 , 68 ]. In homozygous cases, a fast disease progression is reported in the p.L126S mutation [ 69 ], which is relatively slow in heterozygous cases [ 70 ]. Recently, another SOD1 (p.L144S) variation in an Iranian family was reported as severe in a homozygous condition [ 71 ]. The relatively common mutation p.N86S shows low penetrance and phenotypic diversity even within families [ 72 ].
- Chromosome 9 open reading frame 72 ( C9ORF72 )
The C9ORF72 gene is present at the 9p21 locus of chromosome 9. In 2011, a massive GGGGCC (G4C2) hexanucleotide repeat expansion mutation (HREM) within intron 1 of C9ORF72 was recognized as a pathogenic mutation in ALS [ 73 ]. Nearly 30 G4C2 HREMs have been reported in healthy individuals, whereas more than 70 repeats are speculated in ALS patients [ 73 ]. Presymptomatic carriers of the C9ORF72 expansion mutation have earlier brain atrophy (especially focal atrophy of the left supramarginal gyrus) and cognitive alterations than healthy controls of similar ages [ 74 ]. A few theories have been put forth to explain how C9ORF72 contributes to the emergence of C9ORF72 -related disorders. The first theory says that a decrease in the C9ORF72 protein level inhibits endosomal trafficking and perturbs endocytosis, which results in impaired autophagy; however, C9ORF72 knockout mice do not exhibit motor neurodegeneration [ 75 ]. Another theory postulates that the massive G4C2 HREMs could be neurotoxic by forming length-dependent RNA foci that would enclose RNA binding proteins and shut down the RNA processing system [ 76 ]. According to a third hypothesis, the C9/ALS pathogenicity is mediated by dipeptide repeats (DPRs) which are derived from repeat-associated non-AUG translation of G4C2 (or G2C4) RNA in five different DPRs called ‘polyGA’, ‘polyGP’, ‘polyGR’, ‘polyPA’, and ‘polyPR’ [ 77 ]. The aggregated DPRs have been reported in the motor neuron of an ALS patient, but their role in disease progression is not clear [ 77 ]. C9ORF72 expansion mutations result in susceptibility to Ca 2+ permeable-amino-3-hydroxy-5-methyl-4-isoxazolepropionic acid (AMPA) receptor-mediated excitotoxicity and cause motor neuron degeneration [ 78 ]. C9ORF72 repeat expansion results in mutant protein and haploinsufficiency from the wild-type allele. Therefore, in C9ORF72 ALS patients, haploinsufficiency for C9ORF72 activity results in neurodegeneration, which is caused by at least two mechanisms: accumulation of glutamate receptors (which results in excitotoxicity) and impaired clearance of neurotoxic DRPs derived from the repeat expansion. Additionally, C9ORF72 expansion RNA transcripts aggregate into toxic RNA foci, blocking RNA-binding proteins and altering RNA metabolism. The abnormal translation of C9ORF72 transcript expansions produces toxic dipeptide repeats, e.g., poly proline-arginine repeats (poly-PRs) and poly glycine-arginine repeats (poly-GRs) [ 79 ].
In 2008, TARDBP (encoding TDP-43) was recognized as a causative gene of ALS [ 80 ]. TDP-43 aggregates in the cytoplasm of motor neurons were reported in approximately 90% of sporadic and familial (with C9ORF27 mutation) cases, and hence considered as a key pathological feature in ALS. The cytoplasmic aggregates of TDP-43 are characterized by abnormal phosphorylation, truncation, and mislocalization. The accumulation of abnormal TDP-43 or loss of function in physiological TDP-43 causes neurodegeneration in the ALS disease. TDP-43 propagates in a prion-like fashion along the motor pathway [ 81 ]. Moreover, even if TDP-43 is common in both ALS and FTD, in ALS, the inclusions are circumferential (curved) in contrast to FTD where the inclusions are rounded.
Familial ALS with TARDBP mutations is more common in the limbs and has a wider age range of onset. Among these mutations, the p.G376D mutation has a notably fast disease progression, taking less than 1.5 years from onset to death [ 82 ]. The p. G298S is another mutation considered as short-lived [ 57 ], whereas the p.A315T mutation has a longer disease course of 8–10 years [ 57 ].
TARDBP -coded TDP-43 is a ribonucleoprotein involved in exon splicing, gene transcription, mRNA stability, mRNA biosynthesis, and the formation of nuclear bodies [ 83 ]. The C-terminal region of TDP-43, which is rich in Gln/Asn-sequence like prions, is capable of binding directly to several ribonucleoproteins mediating protein–protein interactions and splicing repression [ 84 ]. TDP-43 loses the ability to mediate splicing repression when its C-terminal region is lacking [ 84 ]. TDP-43 accumulation results in RNA instability that triggers apoptosis by disrupting the pathways for energy production and protein synthesis [ 85 ]. TDP-43 cytoplasmic inclusions are nearly the most common feature, reported in about 97% cases of ALS [ 48 ]. It is a nuclear DNA/RNA binding protein that mislocalized to the cytoplasm and underwent significant post-translational modification or truncation in patients with ALS. TDP-43 mislocalization impairs the RNA splicing of stathmin-2, a protein required for microtubule stability, lowering stathmin-2 concentration, and impairing axonal growth and motor neuron function [ 86 ].
FUS was identified as a causative gene for ALS in 2009 [ 87 ]. In the early onset of ALS, the frequency of FUS mutation is high because of de novo mutation [ 88 ]. The FUS mutations typically manifest in patients in their 30s or 40s, with upper extremity or cervical onset and a fast-progressing disease course of 2 years [ 89 ]. In contrast, p.Q519E and p.S513P are reported mutations showing older age of onset and slow disease progression [ 55 ].
TDP-43 neuronal inclusions are widely accepted pathological hallmarks of both ALS and FTD; however, it has been demonstrated that 10% of FTD patients also have inclusions in their neuronal and glial cells that are immunoreactive for FUS but lack TDP-43 inclusions [ 90 ]. The cytoplasmic FUS inclusions comprise RNAs and proteins (from suspended translation units) and promote cell survival under stressed environments by redistributing translational resources. FUS are also involved in synaptic plasticity and dendritic integrity of neurons [ 91 ]. In the presence of mutated FUS , the function of stress granules (FUS inclusions) is compromised and results in motor neuron dysfunction [ 92 ].
3.1.4. Additional Risk Loci from Genome-Wide Association Studies
In recent years, new genes for ALS have been identified, including TBK1 , NEK1 , CCNF , C21ORF2 (also known as CFAP410 ), ANXA11 , TIA1 , KIF5A , GLT8D1 , LGALSL , and DNAJC7 , which have highlighted significant recurrent pathways and opened new research directions [ 48 ]. Importantly, the genes linked to ALS have a different degree of pathogenicity and susceptibility risk. Highly penetrant mutations (e.g., in TARDBP , SOD1 , and FUS ) usually cause disease, whereas some variants associated with ALS (e.g., ANG , ATXN2 , and DCTN ) do not necessarily cause the disease, rather increase the risk of its development [ 48 ]. Moreover, even causative mutations are not completely penetrant, and interactions with the environment alter the risk of disease development. A recently published GWAS, including 29,612 patients with ALS and 122,656 controls, identified 15 risk loci ( Table 2 ) [ 93 ]. Among the newly discovered genes in ALS, TIA1 participates in RNA metabolism; TBK1 , CCNF , and NEK1 are involved in proteostasis or autophagy; and ANXA11 , C21orf2 , and KIF5A are involved in cytoskeletal or trafficking defects [ 94 ]. The mechanism of neurodegeneration mediated by DNAJC7 , GLT8D1 , and LGALSL is not clear. It is speculated that DNAJC7 , a heat shock protein co-chaperone, may be involved in proteostasis or autophagy, and GLT8D1 , a glycosyltransferase, may interfere in ganglioside biosynthesis and O-linked β-N-acetylglucosamine modification [ 48 ]. The cellular role of galectin-related protein (encoded by LGALSL ) is unknown; however, galectins are galactoside-binding proteins.
Risk loci identified in cross-ancestry genome-wide association study (GWAS), the associated function and disease mechanism in amyotrophic lateral sclerosis.
Thus, the identification of novel genes for ALS may open unexplored research fields and pathological mechanisms [ 48 ]. Despite significant progress in ALS research, the pathophysiology is still only partially understood. However, as we obtain more insights into the genetic architecture of ALS, the molecular mechanisms by which various mutations converge on recurrently dysregulated nervous system pathways are being discovered. Impaired RNA metabolism, trafficking defects, autophagy, mitochondrial dysfunction, and compromised DNA repair are the most common pathological pathways reported in ALS patients [ 79 ]. Among the most prevalent ALS genes, mutant C9ORF72 , TARDBP , and FUS all impair RNA metabolism; C9ORF72 repeat expansions, TARDBP , and SOD1 cause defects in protein homoeostasis and autophagy.
3.2. Alzheimer’s Disease (AD)
Alois Alzheimer, on 3 November 1906, in the 37th Meeting of the South-West German Psychiatrists, reported an unusual case study involving a “peculiar severe disease process of the cerebral cortex” and this study was later published in 1907 [ 109 ]. Alzheimer described a 51-year-old woman with paranoia, progressive sleep and memory disturbance, aggression, and confusion in his seminal paper. Less than 5 years after she passed away, a neuropathologic examination of her brain revealed the plaques and tangles that are now considered as the main pathological feature of the disease [ 110 ]. With shared underlying neuropathologic changes, AD is now understood to be a heterogeneous and polygenic group of both hereditary and sporadic neurodegenerative disorders. Even though there are many different variants of AD, most cases still have a clinical presentation like that of the original case, albeit with a later age of onset. A clinical diagnosis is frequently made based on initial memory dysfunction that later stretches to affect multiple cognitive domains. A neuropathologic diagnosis of AD requires the presence of both amyloid beta (Aβ) plaques outside neuronal cell, and neurofibrillary tangles (NFTs) inside the neurons, which can now be easily identified using immunohistochemical stains such as Aβ and tau, respectively [ 111 ]. Alzheimer’s initial discoveries laid the groundwork for the current clinical and neuropathologic diagnostic criteria for AD; however, over time, our understanding of the underlying genetics has led to changes in the conceptualization and characterization of this disease.
The Aβ plaques and NFTs had previously been reported in the brains of elderly subjects both with and without dementia, and some researchers considered them a part of the aging process [ 112 ]. What set Alzheimer’s case apart was that it occurred in a relatively young individual; therefore, AD was defined as a separate disease from what was observed in older people. In the second half of the 20th century, however, AD became redefined as a clinically heterogeneous disease, united by a common underlying set of pathologic changes in the brain that could affect more commonly older but also younger adults [ 113 ].
Based on an age cutoff of 65 years, AD is commonly classified as either early onset (EOAD) or late onset (LOAD) [ 114 ]. LOAD cases have some genetic risk factors but often occur sporadically and are by far the most common. On the contrary, EOAD is responsible for only 5–10% of all AD cases [ 115 ]. In general, two types of inheritance patterns have been noticed in EOAD: mendelian (mEOAD) patterns and nonmendelian (nmEOAD) patterns. mEOAD forms are fully penetrant, with an autosomal dominant inheritance pattern and frequent mutations in APP , PSEN1 , and PSEN2 . nmEOAD, on the other hand, is sporadic or has irregular inheritance patterns (i.e., inheritance patterns that are not obviously autosomal dominant, or with highly variable age at onset, including LOAD). The genetic etiology of nmEOAD is unknown, but it is widely assumed to be polygenic and multifactorial in nature [ 114 ].
3.2.1. Epidemiology
AD is the most prevalent type of dementia, accounting for 50–75% of all cases [ 114 ]. According to current estimates, 5.4 million Americans have AD; by the middle of the next century, that number is expected to quadruple, largely due to population aging [ 116 ]. It is estimated that by 2050, a new case of AD will be diagnosed every 33 s, resulting in nearly a million new cases each year [ 116 ]. After age 65, the incidence of AD rises exponentially with age and doubles every five years. Currently, the disease affects one in nine people over the age of 65 and one in three people over the age of 85 [ 116 ]. While deaths from heart disease, prostate cancer, and stroke have decreased over the past ten years, the number of deaths from AD have increased, making it the sixth leading cause of mortality in the United States. By the year 2050, it is anticipated that the total cost of health-care, long-term care, and hospice services for those with dementia will have increased from the current USD 290 billion to more than USD 1.1 trillion [ 116 ].
While the field still has challenges in defining the etiology, diagnosis, and treatment of AD, significant progress has been made in our comprehension of the role of genetics in LOAD as well as in the sporadic and familial EOAD forms. It is a consensus that a precision medicine strategy will be necessary to treat this complicated and multifactorial disease, which requires a highly sophisticated understanding of its genetic frameworks.
3.2.2. Genetic Causes and Risk Factors
In 1991, Goate et al. discovered a missense mutation on exon 17 of the APP gene that changed amino acid 717 from valine to isoleucine (p. Val717Ile) [ 117 ]. The mutation is known as the London mutation because it was first identified in an English family. Following this initial finding, Chartier-Harlin et al. and Murrell et al. discovered different mutations (V717G and V717F, respectively) in the same amino acid in additional families with AD [ 118 , 119 ]. Despite being in ethnically Romanian kindred, the mutation discovered by Murrell et al. became known as the Indiana mutation. In 1993, Mullan et al. identified two large Swedish families with EOAD who had a double mutation (K670N/M671L) in the N terminus of APP called the Swedish mutation [ 120 ]. Since then, numerous additional APP mutations have been found, directing research towards the APP processing pathways [ 121 ].
The known APP mutations were unable to account for the presence of EOAD in several families. The first non- APP mutations in the presenilin 1 ( PSEN1 ) gene were discovered in multiple ethnic families in 1995 [ 122 ]. These included PSEN1 C410Y (Ashkenazi Jewish), H163R (American and French Canadian), M146L (Italian), L286V (German), and A246E (Anglo-Saxon–Celt). Shortly after this finding, Campion et al. found six novel PSEN1 mutations in eight additional families, all of which were in highly conserved regions of the gene [ 123 ]. Since then, numerous additional PSEN1 mutations have been identified [ 124 ]. A third case of the PSEN1 variant, Y389H, has been identified with EOAD in a Korean patient [ 125 ]. The identification of presenilin 2 ( PSEN2 ), a gene homologous to PSEN1 on chromosome 1, was the next major genetic discovery in AD. Rogaev et al. [ 126 ] discovered two different mutations that were associated with familial AD namely M239V in Italian kindred and N141I in a Volga German pedigree, while Levy-Lahad et al. [ 127 ] also discovered the N141I mutation in Volga German kindred. With the advancement in sequencing technology, Finckh et al. sequenced individuals with EOAD and discovered additional mutations in PSEN1 (F105L) and PSEN2 (T122P and M239I), respectively [ 128 ]. Furthermore, known mutations in these three genes, APP , PSEN1 , and PSEN2 , account for only about 1% of autosomal dominantly inherited AD [ 129 ], but their identification has resulted in a significant advancement in understanding of the pathophysiology of AD. According to estimates, between 55–75% of LOAD cases are heritable. In contrast to EOAD, no causal genes for LOAD have been found; instead, several risk genes have been identified [ 114 ].
The gene APOE on chromosome 19 encodes apolipoprotein E, and unlike all other mammals, humans have three prevalent alleles (ε2, ε3, and ε4). Pericak-Vance et al., using an affected-member-pedigree linkage analysis, discovered that the patients’ genomic markers were linked to chromosome 19 (HAS 19) rather than to the previously identified chromosome 21 locus [ 130 ]. Simultaneously, Namba et al. found that apoE was localized to NFTs and Aβ deposits in the brain of AD patients [ 131 ]. Strittmatter et al. used an in vitro assay in 1993 to demonstrate that apoE binds to Aβ with high avidity and that APOE-4 was more prevalent in LOAD patients than in unrelated, age-matched controls [ 132 ]. Following this, Corder et al. evaluated 42 LOAD families and discovered a gene dosage effect, resulting in approximately a 3-fold higher risk in APOE ε4/ε4 genotype (homozygous) compared to those with a single APOE ε4 allele [ 133 ]. Even though the frequencies of APOE genotypes vary across ethnic groups, the ε4 allele has been repeatedly linked to an increased risk of AD. Interestingly, in a large Colombian EOAD cohort, a carrier of the PSEN1 (E280A) mutation also had two copies of the rare APOE3 Christchurch mutation (R136S), and she did not experience cognitive deficits until her 70s, nearly three decades after the usual age of onset [ 134 ]. This discovery has sparked interest in the potential protective benefits of this mutation.
Following the discovery of the gene dosage-dependent risk of APOE ε4 with LOAD, researchers investigated other genes in the endocytic and cellular recycling pathways. Scherzer et al. discovered six genes that exhibited differential expression in AD patients using a DNA microarray screen [ 135 ]. One of those genes was SORL1 , which encodes a neuronal apoE receptor. Six SNPs were found by Rogaeva et al. to be significantly associated with AD in two different regions of the SORL1 gene using previously identified SNPs in these pathways [ 136 ]. Intronic variants in SORL1 have been linked to familial and sporadic AD, according to Lee et al. [ 137 ], and the TGen data set and an urban multiethnic community have confirmed these findings [ 135 ]. These intronic variants are thought to be in regulatory sequences and may affect SORL1′s physiological role in APP processing.
Multiple NDDs have been linked to NFTs which are encoded by microtubule-associated protein tau ( MAPT ). Several missense mutations, insertions, deletions, and splice-site mutations were found in the MAPT gene, which was initially studied in relation to FTD [ 138 ]. Roks et al. performed an association study using the A169 polymorphism in exon 9 and the (CA) n-repeat polymorphism in intron 9 and discovered no mutations causally associated with EOAD [ 139 ]. Baker et al. sequenced the MAPT gene to look for an association between MAPT and progressive supranuclear palsy (PSP) [ 140 ]. They discovered a series of SNPs that were completely out of sync with one another, revealing two extended haplotypes (H1 and H2) that covered the entire MAPT gene. Further research showed that Caucasian patients with tauopathies have an overrepresentation of the H1 haplotype [ 141 ]. However, subsequent research on the MAPT haplotype and AD has produced mixed results, with some studies finding a link between the H1 haplotype and AD and others failing to support these findings [ 142 ]. Since the H2 haplotype is almost exclusively found in Caucasian populations, Sun and Jia evaluated only the H1 haplotype in a Chinese Han population and discovered an SNP within the promoter of MAPT [ 143 ]. Comparing patients with sporadic AD from the Chinese Han ethnic group to healthy controls revealed that the 347C allele was overrepresented and when tested in cell lines, the SNP (347C/C versus 347 G/G) significantly increased transcriptional activity, upregulating gene expression [ 143 ].
Triggering receptor expressed on myeloid cells 2 ( TREM2 ) is a receptor of the innate immune system expressed on microglia, macrophages, dendritic cells, and encoded by TREM2 [ 144 ]. TREM2 mutations were discovered in the early 2000s to cause polycystic lipomembranous osteodysplasia with sclerosing leukoencephalopathy (PLOSL), also known as Nasu–Hakola disease [ 145 ]. Presenile dementia with neurological abnormalities is among the prominent symptoms of PLOSL. However, TREM2 was not thought to be a potential candidate gene for dementia until TREM2 mutations were discovered in a Lebanese family with early-onset dementia but no PLOSL [ 146 ]. Following the discovery of TREM2 ’s potential role in early onset dementia, Guerreiro et al. studied whole exome/genome sequencing of 281 AD patients and 504 healthy controls. They observed a disproportionate number of variants in exon 2 of TREM2 in AD patients compared to healthy controls, and these variants increased the AD risk in a heterozygous state [ 147 ]. So far, 46 genetic variants in TREM2 have been investigated in relation to LOAD. Within each population, these variants cause a roughly 2–4-fold increase in the risk of developing LOAD [ 148 ]. Additionally, TREM2 variants have been linked to ALS, PD, and FTD indicating that altered TREM2 function may indirectly increase the risk of neurodegeneration, possibly through dysfunctional microglia [ 149 ].
ABCA7 is a membrane-bound protein associated with transporting lipids across the cell membrane through the utilization of energy derived from ATP. It plays pivotal roles in three key cellular processes: regulating cholesterol metabolism, managing phospholipids, and facilitating phagocytosis [ 150 , 151 ]. ABCA7 facilitates the formation of lipid and exchangeable apolipoproteins, including apolipoprotein E (apoE), into high-density lipoprotein (HDL) particles, which are then discharged into the extracellular environment. This process, known as cell lipid efflux, ultimately results in the removal of lipids from the cells [ 152 ]. ABCA7 is involved in AD pathogenesis, contributing to the clearance of Aβ and the transport of the amyloid-β protein precursor (AβPP) [ 151 ]. Disruptions in the ABCA7 gene due to mutations can impair one or more functions of the protein, potentially leading to the development of the neuropathology associated with AD. However, mutations in ABCA7 do not have uniform effects, and the precise alterations in ABCA7 function resulting from these mutations associated with AD risk remain elusive. Understanding these variations is particularly crucial due to the significant impact of ABCA7-related AD risk among African American/Black adults [ 153 ]. In mouse models of AD, the absence of ABCA7 leads to elevated levels of Aβ in the brain, either by enhancing Aβ production or impairing its clearance [ 153 ]. Both mouse Abca7 knockouts and human carriers of the ABCA7 AD-risk allele, who do not have AD, generally demonstrate minor behavioral and cognitive alterations [ 154 ].
3.2.3. Additional Risk Loci from Genome-Wide Association Studies (GWASs)
GWASs have been a more recent method for determining genetic susceptibility to AD. A total of 695 genes have been found to affect the risk of LOAD through 1395 GWASs and 320 meta-analyses [ 155 ]. According to the GWASs, the top 10 genes that are most strongly linked to the risk of LOAD are APOE , BIN1 , CLU , ABCA7 , CR1 , PICALM , MS4A6A , CD33 , MS4AE , and CD2AP [ 155 ]. Several of these studies combined multiple GWAS data sets with the idea that a common disease might present with a common variant, necessitating a large number of samples. A more recent, two-stage GWAS involving 111,326 clinically diagnosed/“proxy” AD cases and 677,663 controls was recently published. They discovered 75 risk loci ( Table 3 ), 42 of which were novel at the time of the study [ 156 ].
Risk loci identified in cross-ancestry genome-wide association study (GWAS), the associated function and disease mechanism in Alzheimer’s disease.
To validate CLU , CR1 , and PICALM as risk loci for LOAD, Jun et al. examined the SNPs within each gene in various populations (Caucasian, African American, Arab, and Caribbean Hispanic), as well as in a combined data set [ 224 ]. They stated that the CLU , CR1 , and PICALM loci in this population are susceptibility loci for AD. Additionally, the authors examined these SNPs collectively with APOE genotypes and discovered that the association with PICALM was only seen in patients who had the APOE 4 allele, whereas the CLU was only present in patients lacking the APOE 4 allele [ 224 ]. Kunkle et al. confirmed many previously identified genome-wide significant loci in 94,437 LOAD patients ( CR1 , BIN1 , INPP5D , HLA-DRB1 , TREM2 , CD2AP , NYAP1 , EPHA1 , PTK2B , CLU , SPI1 , MS4A2 , PICALM , SORL1 , FERMT2, SLC24A4 , ABCA7 , APOE , and CASS4 ) [ 225 ]. These genes may have a pleiotropic connection with traits associated with AD. The authors further compared the genetic makeup of LOAD to 792 other human illnesses, traits, and behaviors to further explore this theory [ 225 ]. They confirmed the findings of Marioni et al. that the genetic architecture of LOAD is more strongly associated with a maternal family history of AD than with a paternal family history of AD [ 226 ]. Kunkle et al. performed a pathway analysis for common and rare variants found in LOAD patients to better understand how all these genetic risk loci may contribute to AD. The majority of genes belonged to the gene ontology pathway for immune response activation; the assembly of protein–lipid complexes was the most significant common variant pathway, while tau protein binding was the most significant rare variant pathway [ 225 ].
Overall, NFTs and amyloid plaque deposition are considered as the major pathway in AD progression. It is noteworthy that newly discovered genetic risk factors are frequently first assessed in relation to established pathways; therefore, much research is needed to understand the underlying mechanisms in AD progression which may also avail novel therapeutic options.
3.3. Parkinson’s Disease (PD)
PD is a complicated and progressive neurodegenerative disorder first described by James Parkinson in his 1817 publication, “Essay on the Shaking Palsy” [ 227 ]. In that essay, Dr. Parkinson expressed hope by writing, “there appears to be sufficient reason for hoping that some remedial process may ere long be discovered, by which, at least, the progress of the disease may be stopped” [ 227 ]. Until now, no definitive neuroprotective therapy for PD has been developed after more than 200 years.
However, significant advances in our understanding of the molecular basis of neurodegeneration in PD have been made in recent years, bringing us closer to developing effective disease-modifying treatments. Pathologically, PD is characterized by the loss of dopaminergic neurons and deposition of α-synuclein aggregates (Lewy bodies) in the substantia nigra pars compacta (SN) located in the midbrain [ 228 ]. Additional brain areas and nondopaminergic neurons are also included in PD pathology. However, recent research has indicated that the loss of dopaminergic terminals in the basal ganglia, rather than neurons in the SN, is critical for the onset of motor symptoms [ 229 ]. Moreover, symptomatically, the disease is characterized by resting tremors, cogwheel rigidity, bradykinesia, autonomic dysfunction, and cognitive decline. Additionally, anosmia, constipation, depression, and rapid eye movement (REM) sleep behavior disorder could be noticed long before the motor dysfunction in PD patients [ 230 ].
3.3.1. Epidemiology
According to health-care utilization estimates, the annual incidence of PD ranges from 5/100,000 to more than 35/100,000 new cases [ 231 ]. Age is a significant risk factor, and the prevalence of PD increases with age. The prevalence of PD is anticipated to rise sharply as the world’s population ages, doubling in the following two decades [ 232 ]. In a meta-analysis of four populations in North America, it was found that prevalence increased from less than 1% of men and women aged 45–54 to 4% of men and 2% of women aged 85 or more [ 229 ]. Along with this increase, the societal and economic burden of PD will also rise unless more effective treatment options, or prevention methods, are discovered.
Most PD cases are likely to have a multifactorial etiology caused by the interaction of environmental and genetic factors. PD risk may be increased by head trauma and toxic chemical exposure, while it may be decreased by certain lifestyle choices. Although 5–10% cases of PD can be linked directly to identifiable mutations in particular genes, most PD patients lack these mutations [ 233 ]. The most prevalent PD-linked genetic mutations also have incomplete penetrance, suggesting the involvement of additional genetic or environmental factors. Simon et al. (2020) compared the prevalence rate in monozygotic and dizygotic twins and found that the heritability of PD is only 30%, implying that most of the PD risk is related to environmental and behavioral factors [ 233 ].
3.3.2. Genetic Causes and Risk Factors
PD genetics provides an important window into the disease’s mechanism by highlighting specific molecular pathways and their cellular effects. The intricate genetics of PD are outlined in this section, with an emphasis on the genes that have been most extensively studied.
SNCA (PARK1/4) is a five-exon gene present on chromosome 4 (4q22.1) and is one of three paralogs that comprise the synuclein family. Synucleins are presynaptic proteins and include α-synucleins, β-synucleins, and γ-synucleins encoded by SNCA , SNCB , and SNCG, respectively [ 234 ]. SNCA -encoded α-synuclein is a 14-kDa protein and expressed ubiquitously in the brain. It has been shown to promote the long-term maintenance of the presynaptic compartment, aid in the formation of SNARE complexes, and control the release of dopaminergic vesicles [ 234 ]. β-Synuclein shares 78% structural homology with α-synuclein and is co-expressed with α-synuclein throughout the brain, including the presynaptic region. Meanwhile, γ-synuclein exhibits 67% homology and is found in the peripheral nervous system, specific central neuronal subtypes, and in breast, colon, and pancreatic cancers [ 234 ]. Even though only α-synuclein is thought to be involved in the pathophysiology of PD, patients with DLB (dementia with Lewy bodies) have been found to have lower levels of the SNCB transcript, and in vitro studies indicate that β-synuclein may antagonize α-synuclein-induced aggregation and toxicity [ 235 ]. Point mutations in SNCA were discovered to be the first cause of autosomal dominant PD [ 236 ], leading to the discovery that α-synuclein is the most abundant protein component of Lewy bodies (LBs) [ 237 ]. Other reported pathogenic SNCA variants, in addition to p.A53T, are p.A30P, p.E46K, and p.G51D. These missense mutations are all found in the membrane-binding region of α-synuclein [ 238 ].
Additionally, there is intrafamilial heterogeneity, which affects how people with SNCA mutations present clinically. Generally, all patients have an early onset of the disease (as young as 19 years old), with the majority of cases developing symptoms when patients are 40 to 60 years old [ 239 ]. All patients present with the traditional motor symptoms of PD. However, patients with p.A53T, p.E46K, or p.G51D mutations also have early cognitive impairment. Pathologically, these patients exhibit widespread LB accumulation, SN degeneration, cortical thinning (p.A53T), and involvement of the motor cortex, among other features (p.G51D) [ 239 ].
A disease-causing triplication of the wild-type SNCA locus was described shortly after the discovery of these point mutations in a family with autosomal dominant parkinsonian motor signs, early onset of symptoms (mean age 36 years), and prominent cognitive defects [ 240 ]. Complete loss of the SN and severe cortical and hippocampal atrophy were present in the four patients from this family who underwent neuropathological examination [ 240 ]. Later, more than 19 families have been reported with autosomal dominant disease caused by duplications in wild-type SNCA [ 241 ]. The identification of these families provides several important insights into the pathophysiology of PD. It first suggests that increases in wild-type α-synuclein levels are enough to trigger PD pathogenesis. Second, the disease symptoms are linked to gene dosage, with SNCA triplication patients experiencing symptoms sooner than duplication patients [ 242 ]. Thirdly, this implies that PD risk may be raised by less penetrant SNCA variants that result in small changes in α-synuclein levels. This latter hypothesis is supported by a study published in 2016, which discovered a variant in the SNCA enhancer linked to an elevated risk of PD, that reduces the binding of transcriptional repressors and increases SNCA expression [ 243 ].
Autosomal dominant PD is also caused by variations in LRRK2 ( PARK8 ) [ 244 ]. The age of disease onset differs between LRRK2 pathogenic variants and families [ 242 ]. Leucine-rich repeat kinase 2 (LRRK2) is a 253-kDa protein, encoded by the LRRK2 gene and expressed in the brain, lungs, kidneys, and immune system [ 239 ]. It has both GTPase and kinase activity, with the GTPase activity being carried out by a Ras of complex proteins (ROC)/C-terminal of Ras (COR) and the kinase activity being carried out by the kinase domain [ 245 ]. The remaining domains of this protein participate in interacting with other proteins. Up to 1% of all cases of sporadic PD are caused by mutations in LRRK2 , the second most frequent genetic risk factor. LRRK2 variants account for 29% of familial PD in Ashkenazi Jews [ 246 ] and 70% of PD in North Africans [ 247 ]. All the highly penetrant LRRK2 variants are thought to increase the kinase activity directly or indirectly. Later, a clinical report documented an increased LRRK2 kinase activity in sporadic PD patient brains and provided additional evidence to support this hypothesis [ 248 ]. This, coupled with the absence of any PD-like phenotypes in Lrrk2 −/− mice, has led to the hypothesis that elevated kinase activity is what causes LRRK2 to have a pathogenic effect [ 249 ]. LRRK2 -PD patients have a clinical phenotype like sporadic PD, but the pathology varies [ 250 ]. Given the complexity of how LRRK2 mutations affect the biology of the protein, it is perhaps not surprising that different mutations cause different pathological phenotypes or that they cause incomplete penetrance.
VPS35 (PARK17) encodes vacuolar protein sorting 35 (VPS35), a heteropentameric protein complex that is responsible for retrograde protein sorting from the endosome to the cell membrane and trans-Golgi network, and vice versa. The c.1858G>A (p.D620N) variant, which is found primarily in Caucasian populations, accounts for an estimated 0.1–1% of patients with familial PD and causes autosomal dominant PD with high but incomplete penetrance [ 251 ]. Patients with VPS35 p.D620N present clinical symptoms similar to those seen in idiopathic PD, with an average age of onset ranging from 48.3 to 53 years old, depending on the family [ 252 ]. It remains to be seen whether this variant has a dominantly negative effect, a toxic gain-of-function, or another mechanism. Patients with VPS35 variants may or may not have α-synuclein pathology. This is because the only data available are based on a single autopsy with only a few pathological investigations [ 251 ].
GBA is the gene that encodes β-glucocerebrosidase (GCase), a lysosomal enzyme that degrades glucosylceramide (GluCer) into glucose and ceramide. Homozygous GBA mutations cause Gaucher’s disease, a recessive lysosomal storage disorder (GD) [ 253 ]. This disease primarily affects the liver, spleen, and bones, but it can also affect the central nervous system. GD patients are classified based on brain involvement, with type 1 patients having no neurological symptoms and types 2 and 3 experiencing neurological symptoms [ 253 ].
However, depending on the variant and population studied, heterozygous GBA mutation carriers have a 5–8-fold increased risk of PD [ 253 ]. The risk of PD or DLB is increased by GBA variants in heterozygosity that result in GD in homozygosity [ 254 ]. GBA variants are the most prevalent genetic risk factor for PD. Two GBA variants, namely E365K and T408M, raise the risk of but do not result in GD [ 255 , 256 ]. Clinically, people with PD having mild GBA mutations exhibit motor symptoms that are like those of idiopathic PD and respond to levodopa quickly. The clinical symptoms, however, are related to the relevant variant, with some patients showing early disease onset like sleep disorders, dementia, and a higher frequency of psychiatric symptoms such as depression and anxiety [ 257 ]. The brains of these patients show typical neuropathological features of PD, such as nigral degeneration and LB deposition throughout the brain [ 257 ].
3.3.3. Additional Risk Loci from Genome-Wide Association Studies
In 2009, the first GWAS loci for PD were identified using information from about 5000 patients and 9000 healthy controls [ 258 ]. To date, the largest GWAS of PD was carried out including the study of 7.8 M SNPs in 37.7 K cases, 18.6 K UK Biobank (Cheshire, UK) proxy-cases (having a first degree relative with PD), and 1.4 M healthy controls. Consequently, 90 distinct independent risk signals ( Table 4 ) were identified in 78 genomic regions across the entire genome, including 38 novel independent risk signals in 37 loci [ 259 ].
Risk loci identified in cross-ancestry genome-wide association study (GWAS), the associated function and disease mechanism in Parkinson’s disease.
Numerous GWAS loci have been found to be close to the so-called monogenic PD gene ( SNCA , LRRK2 , GBA , and VPS35 ); these regions are referred to as pleomorphic risk loci [ 326 ]. PD can be caused by rare coding variants at these genes, but more common variants—often non-coding and with a smaller effect size—can also raise the risk of developing the condition. Additionally, some loci are near genes that contribute to the development of additional conditions, including MAPT , GRN , NEK1 linked to FTD and ALS, and NOD1 linked to Crohn’s disease and Blau syndrome [ 326 ]. According to estimates, the heritable portion of PD caused by common genetic variability is approximately 22% [ 327 ], and the GWAS loci discovered to date only account for a small portion of this. So, there are still a ton of undiscovered risk variants.
Overall, understanding the genetic factors influencing PD risk, onset, and progression is critical for developing treatments that can slow or stop disease progression. Many genes and GWAS loci that contribute to the development of PD have been identified so far. The search for genetic risk factors must continue, and a concerted, cooperative effort must be made to comprehend the implications of these findings on the molecular and biological levels.
Based upon the discussion so far, it is clear that genetic factors play a significant role in increasing the risk of neurodegenerative diseases and influencing the expression of disease characteristics; however, only about 10% cases of NDDs are considered to have familial form of disease, and among these cases only a fraction can be attributed to known, rare, highly penetrant genetic variants. Similarly, while genome-wide association studies (GWASs) have made notable progress in identifying common GWAS-SNPs in cohort with neurodegenerative disease, despite the advancement, these variants account for only a small portion of heritable risk [ 328 , 329 ]. Even after combining the effect of both Mendelian large-effect rare mutations and common disease-associated SNPs, a considerable portion of heritability across NDDs remains unexplained [ 330 , 331 ], suggesting its multifactorial nature and the involvement of environmental factors.
As a result, identifying particular risk factors, relevant biomarkers, prospective new therapeutic targets and agents, and even definitive diagnoses remain difficult. Pathological brain features during neurodegeneration demonstrate a significant overlap across distinct forms of neurodegenerative disorders. There is currently no diagnostic test that can clearly establish the existence, absence, or categorization of a neurodegenerative disorder. The underlying mechanism is another unsolved subject in the majority of neurodegenerative disorders. Most can be identified by intracellular protein deposits; however, it is unclear whether this is a key mechanism or a result of another disrupted cell function. There are numerous proposed pathways of neurodegeneration, including primary impacts of protein homeostasis, disrupted protein degradation, mitochondrial dysfunction, and so on ( Figure 3 ). It is critical to better understand the disease pathophysiology to enhance early diagnosis and the development of disease-modifying medicines.

This pictorial illustration aims to provide a detailed overview of protein aggregation, the intricate molecular mechanism involved, and the resultant damage to neurons in common neurodegenerative conditions.
3.3.4. Other Neurodegenerative Disorders
Huntington’s disease (HD) manifests as a rare monogenic neurodegenerative condition marked by motor, cognitive, and psychiatric deficits. These symptoms typically arise in patients aged 30–50 years and death occurs approximately 10–15 years after the onset of clinical symptoms [ 332 ]. Huntington’s disease (HD) results from the expansion of a CAG repeat region in exon 1 of the huntingtin (HTT) gene, situated on chromosome 4, surpassing a pathogenic threshold of at least 37 CAGs. Inheritance of 40 or more CAGs within this region is linked with 100% disease penetrance [ 332 ]. While Huntington’s disease (HD) is primarily attributed to inheriting at least one mutant HTT allele, there is growing recognition of additional genetic factors that influence the age-of-onset and severity of HD. These factors are currently under investigation as potential targets for therapeutic interventions [ 333 ]. The expansion of CAG repeats results in the production of mutant HTT (mHTT) proteins containing elongated N-terminal polyQ tracts. These expanded tracts directly induce misfolding of the mHTT protein [ 334 ]. Misfolded mHTT proteins aggregate into amyloid structure, forming intranuclear inclusion bodies, which are a distinctive diagnostic hallmark observed in the brain of individuals with HD [ 335 ]. The misfolding and aggregation of HTT disturb various cellular processes, supported by significant evidence suggesting that oligomers and/or insoluble fibrils of mHTT play a direct role in causing neurodegeneration in HD [ 334 ]. HTT knockout is embryonic lethal, while conditional knockout induces progressive neurodegenerative phenotypes in adult mice. This highlights the indispensable role of HTT expression in the normal development of the CNS [ 336 ].
Prion diseases represent a distinctive and uncommon set of neurodegenerative disorders found naturally in both humans and various animal species. Creutzfeldt-Jakob disease (CJD) in humans, scrapie in sheep and goats, bovine spongiform encephalopathy (BSE) in cattle, and chronic wasting disease (CWD) in cervids are just a few examples of well-known forms of prion disease [ 337 ]. Both human and animal prion diseases share a common pathology, featuring spongiform degeneration in the grey matter regions of the brain, reactive proliferation of glial populations, neuronal loss, and the accumulation of a misfolded and disease-associated form of the prion protein known as PrP Sc in the CNS [ 338 ]. Human prion diseases, like other neurodegenerative disorders, primarily occur as sporadic or genetic conditions. Notably, sporadic CJD is the most prevalent form, constituting roughly 85% of all human prion diseases [ 337 ]. The revelation that prion diseases are linked to the conversion of the normal host-encoded cellular prion protein (PrPC) into a misfolded form (PrPSc) through post-translational modification, regardless of nucleic acid involvement, is recognized as the protein-only hypothesis [ 338 ]. A polymorphism at codon 129 (c129) within the prion protein gene (PRNP) significantly impacts both susceptibility to and the clinical characteristics of human prion diseases [ 338 ].
Multiple sclerosis (MS) is a chronic inflammatory and neurodegenerative disease of the CNS, mediated by the immune system [ 339 ]. MS stands as a primary contributor to disability among young adults and is estimated to impact approximately 2.8 million individuals globally [ 340 ]. GWASs have uncovered over 230 risk alleles associated with MS, the majority of which are genes involved in regulating the immune system [ 341 ]. The initial event triggering symptomatic pathology involves the infiltration of peripheral immune cells previously sensitized to elements of the myelin sheath. A key characteristic of MS is the formation of focal inflammatory and demyelinating lesions, primarily occurring in white matter regions of the brain, optic nerve, and spinal cord, although lesions in intracortical and deep gray matter are also observed [ 339 ]. T and B lymphocytes, along with macrophages, predominantly migrate from the periphery into the CNS parenchyma, resulting in the emergence of perivascular demyelination, subependymal/pial demyelination, and neuroaxonal degeneration. Inflammatory demyelination and compromised healing ultimately lead to axonal transection, resulting in permanent neurodegeneration and clinical disability [ 339 ]. MS is characterized by three primary disease courses: relapsing–remitting (RRMS), primary progressive (PPMS), and secondary progressive (SPMS). In each clinical course, patients may encounter fluctuating disease activity, manifested by the emergence of new or worsening neurological dysfunction [ 342 ].
4. Gene Therapy for Neurodegenerative Diseases
In concept, gene therapy is a simple and clear-cut process. It entails treating a disease through the introduction of a transgene that either replaces or repairs a malfunctioning gene [ 343 ]. In practical application, the process is significantly more intricate, requiring optimization of various factors like selecting the appropriate vector, optimizing the delivery method, and carefully choosing the transgene are pivotal in practice. The dynamics between the host’s immune system and the vector or transgene can introduce further complexities to the overall therapeutic approach. In neurodegenerative diseases, the intricacies of the target tissue add an additional layer of complexity.
In the domain of gene therapy vectors, there are two primary classifications: viral and non-viral [ 344 ]. Viral vectors utilize the inherent infective properties of viruses, undergoing genetic modifications to eliminate replicative genes, making them suitable for clinical applications [ 344 ]. In addressing neurodegenerative diseases, the prominent viral vectors include adeno-associated viruses (AAVs) and lentiviruses [ 345 ]. Both AAVs and lentiviral vectors can effectively infect dividing and non-dividing cells. However, a key distinction lies in the fact that lentiviruses integrate into the host genome, whereas AAVs do not [ 345 ]. Integration provides enduring and stable expression but simultaneously introduces the potential risk of integrational mutagenesis. Even though AAVs do not integrate, they can still facilitate persistent gene expression in nondividing cells [ 345 ]. Non-viral vectors typically involve either naked plasmid DNA or complexes with cationic lipids or polymers. These vectors exert a localized impact and demand a higher therapeutic dosage compared to viral vectors [ 346 ]. Generally, non-viral delivery results in only temporary gene expression, often inadequate for effectively addressing chronic neurodegenerative conditions [ 346 ].
Choosing the delivery route is crucial, especially for the central nervous system (CNS). Remote delivery achieved through intravenous injection, as a non-invasive approach, is advantageous. However, the blood–brain barrier (BBB) stands as a substantial barricade impeding the entry of most vectors into the CNS [ 347 ]. Therefore, the significant discovery that AAV9 can penetrate the blood–brain barrier (BBB) is notable [ 348 ]. Remote delivery poses certain drawbacks, including an elevated risk of off-target effects and the necessity for a larger dose to attain a therapeutic level in the target tissue. Conversely, direct delivery to the CNS mitigates off-target effects and lowers the necessary dosage of the gene therapy vector [ 347 ]. In the central nervous system (CNS), this can be achieved through intraparenchymal injection (directly into the brain or spinal cord) or by injecting into the cerebrospinal fluid (CSF), either through intracerebroventricular (ICV) or intrathecal routes [ 347 ].
The resurgence of gene therapy brings with it the enticing promise of not only targeting the fundamental causes of disease but also providing enduring corrections [ 349 ]. Severe combined immune deficiency (SCID) was the first clinical success with gene therapy. SCID is the most severe human inborn errors of immunity, with absent T and B lymphocyte function, making the infant susceptible to life-threatening infections with high mortality in the absence of treatment [ 350 ]. Additionally, Casgevy is the first FDA-approved gene therapy to utilize a type of novel genome-editing technology (CRISPR-Cas9) for the treatment of sickle cell disease (SCD) in patients 12 years and older [ 351 ]. In neurodegenerative diseases, spinal muscular atrophy (SMA) was the first to be cured with the exogenous introduction of SMN1 gene to children under two years of age [ 352 ].
Embracing various forms of genome manipulation, this approach is particularly attractive in the domain of neurodegenerative diseases, where traditional pharmacological interventions have faced consistent challenges [ 345 ]. In contrast to organs where achieving therapeutic concentrations through repeated doses is more feasible, most agents administered peripherally encounter difficulties in efficiently crossing the blood–brain barrier when targeting the brain. Hence, the notion of a singular, enduring intervention, often termed “one and done”, becomes particularly attractive when addressing diseases impacting the central nervous system (CNS) [ 353 ]. In the following discussion, we will briefly explore relevant technologies for therapeutics targeting neurodegenerative diseases.
5. Gene Expression
5.1. the exogenous introduction of genes into the cns.
The most direct approach in gene therapy involves the exogenous introduction of genes into the CNS. These introduced genes have the potential to rectify the loss of gene function caused by pathological mutations, such as the instance of the SMN1 gene in spinal muscular atrophy [ 352 ].
5.2. DNA Editing
The clinical adoption of DNA-editing tools is underway, with their ability to modify gene expression or address pathogenic mutations [ 354 ]. In broad terms, two crucial elements come into play: DNA-binding domains designed to identify specific genomic sequences and nucleases that create double-stranded breaks (DSBs). DSB repair is facilitated by nonhomologous end-joining (NHEJ), an endogenous mechanism prone to errors, resulting in the insertion and deletion of sequences (INDELs) within the reading frame [ 354 ]. Typically, this leads to frameshift mutations and premature termination codons (PTCs), ultimately causing the targeted gene to be knocked out. In an alternative scenario, when an external template is available, intrinsic homology-dependent repair (HDR) mechanisms come into play to introduce desired sequences or point mutations into the host genome [ 354 ]. Three fundamental DNA-editing nucleases include zinc-finger nucleases (ZFNs), transcription activator-like effector nucleases (TALENs), and CRISPR-associated nucleases [ 355 ]. The structure of ZFNs involves two essential segments: a DNA-binding domain composed of three–six zinc fingers, each with the capability to recognize three DNA base-pairs in the host genome, and a DNA-cleaving domain originating from the endonuclease Fok1 [ 355 ]. Given that Fok1 functions as a dimer, the design involves two ZFNs binding to opposite strands of the intended genomic DNA. This configuration enables the Fok1 domains to dimerize and initiate DNA cleavage [ 355 ]. In the case of the transcription activator-like effector nucleases (TALENs), they incorporate a sequence of transcription activator-like effectors (TALEs) along with the Fok1 DNA-cleavage domain [ 356 ]. The TALE polypeptide is made up of 33 or 34 amino acids, and within this structure, residues 12 and 13 are responsible for recognizing a specific DNA base [ 356 ].
In the synthetic CRISPR system, a custom-designed guide RNA (gRNA) is employed to direct a Cas9 nuclease to specific locations on the host DNA ( Figure 4 ). Another prerequisite involves the presence of protospacer adjacent motif (PAM) sites, which are scattered throughout the genome [ 357 ]. For the frequently used Cas9, streptococcus pyogenes Cas9 (SpCas9), the corresponding guide RNA (gRNA) is comprised of a CRISPR RNA (crRNA) recognizing the genomic target sequence and a trans-activating CRISPR RNA (tracrRNA) that facilitates the recruitment of Cas9 [ 357 ]. Following the introduction of DSBs by Cas9 and subsequent repair through NHEJ, INDELs commonly lead to a shift in the reading frame, introducing a PTC [ 358 ]. Typically, mRNAs containing these mutated PTCs undergo degradation via nonsense-mediated decay (NMD), an intrinsic surveillance mechanism, resulting in the depletion of the corresponding protein. Theoretically, CRISPR-guided HDR offers the potential to introduce specific point mutations or insertions into the native genome using donor templates (the flow-chart is shown in Figure 5 ). However, the frequency of HDR-mediated repair is generally lower than that of NHEJ in most cells, restricting the scope of recombination [ 353 ]. Additionally, HDR naturally transpires during mitotic recombination, limiting its application primarily to postmitotic neurons. Despite these challenges, ongoing strategies are being developed to enhance HDR efficiency in the brain [ 359 ].

This illustration provides an overview of CRISPR-Cas9-mediated gene editing. The Cas9 enzyme is triggered by attaching to guide RNA (gRNA) and then binding to the corresponding genomic sequence just before the 3-nucleotide PAM sequence. After binding, Cas9 induces a double-strand break, and the DNA is repaired using either the NHEJ or HDR pathway, leading to an edited gene sequence.

This visual illustration delineates the critical steps involved in the CRISPR-Cas9 gene editing process including the design of guide RNA, formation of the CRISPR-Cas9 complex, target DNA recognition, and the introduction of modifications.
5.3. CRISPR-Mediated Base Editing and Prime Editing
The prevalent genetic disorders in human pathologies, encompassing inherited neurodegenerative diseases, are attributed to single-base pair point mutations [ 360 ]. DNA base editors are hybrid proteins formed by the fusion of a dCas9 or nCas9 with a deaminase protein, enabling the deamination and modification of cytidine or adenine base pairs ( Figure 6 ). Cytosine base editors facilitate the transformation of C:G to T:A, while adenine base editors alter A:T to G:C [ 361 , 362 ]. The effective use of base editing encounters limitations due to the necessity for appropriate PAM sequences and the occurrence of undesired nucleotide substitutions in the vicinity of the target site, arising from the broad activity range of deaminases. Recent Cas9 variations have been created exhibiting increased PAM compatibility and cytidine deaminase variants with more restricted activity windows [ 183 ]. Overcoming the obstacles associated with the large size of the base editors for AAV packaging in in vivo delivery, a recent study employed dual AAVs. These dual AAVs transported split base editors, which were subsequently reassembled in situ through trans-splicing inteins [ 363 ].

The flow chart details the sequential steps in the CRISPR-mediated base editing process, encompassing the design of guide RNA, the formation of the CRISPR base editing complex, targeted base pair modification, and the final edited genetic sequence.
Off-target effects, particularly undesired RNA editing, have been documented in the context of base editing [ 364 ]. Nevertheless, more recent versions of base editors have been reported to demonstrate reduced off-target activity [ 365 ]. The concept of prime editing ( Figure 7 ) broadens the scope of base editing, offering expanded genomic targeting, more possibilities for genetic alterations, and enhanced precision. The prime-editing guide RNA (pegRNA) is designed to encompass both the typical DNA-targeting sequence and the desired editing sequence, allowing for the substitution of targeted genomic DNA nucleotides [ 366 ]. The associated nCas9 is coupled with a reverse transcriptase, initiating the reverse transcription of pegRNA ( Figure 8 ). This process transfers the encoded genetic information from the pegRNA, encompassing insertions, deletions, and base conversions, to the targeted genome [ 366 ].

This illustrative guide delineates the critical stages in the CRISPR-mediated prime editing process including the design of prime editing guide RNA (pegRNA), formation of the CRISPR prime editing complex (reverse transcriptase and Cas9 nickase), precise editing of the target sequence and repair, and the ultimate generation of the edited genetic information.

CRISPR-mediated transcriptional regulation by adding nuclease-deactivated cas9 (dcas9) to inhibit ( A ) or activate ( B ) transcription of targeted gene. CRISPR-mediated base editing involves a dCas9 or nickase Cas9 (nCas9) to bind with adenosine or cytosine ( C ) deaminase that changes AT to GC or CG to TA. The prime editing ( D ) involves nCas9 fused with RT that transcribes a part of pegRNA at target site.
6. Genetic Therapy for AD
One of the initial clinical trials in gene therapy for Alzheimer’s disease involved introducing the gene encoding nerve growth factor (NGF) to the cholinergic nucleus basalis of Meynert. This was accomplished through direct, bilateral injections of AAV2 into the brain [ 367 ]. NGF, as an intrinsic neurotrophic factor, governs the growth and viability of cholinergic neurons by functionally activating the TRKA receptor [ 368 ]. Studies conducted in preclinical animal models, including non-human primates (NHPs), demonstrated the favorable impact of administering external NGF, thereby establishing a rationale for human trials [ 369 ]. Despite the safety and sustained expression of AAV2–NGF observed in Phase 1/2 clinical trials for mild-to-moderate AD, there was no obvious improvement in cognitive function [ 370 ]. Furthermore, analysis of autopsy brains from this study revealed that the administered AAV2–NGF did not reach the intended cholinergic neurons in the nucleus basalis of Meynert [ 371 ]. Consequently, the effectiveness of this therapeutic approach remains uncertain.
6.1. Targeting MAPT
Utilizing antisense oligonucleotides (ASOs) against the MAPT gene, which encodes tau, researchers have effectively reduced MAPT mRNA and tau protein levels in animal models. These ASOs demonstrate the ability to mitigate tau phosphorylation and aggregation, prevent neuronal death, and extend survival in transgenic mice expressing a human mutant (P301S) tau. Moreover, they contribute to a reduction in CNS tau levels in NHPs [ 372 ]. This strategy is presently being tested in a clinical trial and reported more than 50% reduction in tau synthesis in the CNS of mild AD patients ( {"type":"clinical-trial","attrs":{"text":"NCT03186989","term_id":"NCT03186989"}} NCT03186989 ) [ 372 ]. In an alternative approach, ASO-mediated exon skipping can effectively lower MAPT mRNA and tau protein levels in both cellular and in vivo settings [ 373 ]. Emerging evidence indicates that tau is involved in regulating presynaptic function [ 374 ] and the acute knockdown of tau in adult mice led to learning and memory deficits [ 375 ].
6.2. Targeting APOE
Human susceptibility to Alzheimer’s disease is linked to three apolipoprotein E (APOE) polymorphic alleles—E2, E3, and E4. APOE4 is recognized as the primary risk factor for sporadic Alzheimer’s disease, while APOE2 is associated with a protective effect [ 376 ]. Research with animal models implies an association between APOE4 and the progression of both amyloid beta and tau pathology [ 377 ]. A gene therapy approach involves increasing the levels of protective APOE2 in the brain. Indeed, expressing APOE2 through viral vectors attenuates Aβ pathology in a mouse model that relies on amyloid [ 378 ]. A Phase 1 trial involving the delivery of AAV–APOE2, administered into the cerebrospinal fluid through intracisternal injections, is anticipated to commence shortly ( {"type":"clinical-trial","attrs":{"text":"NCT03634007","term_id":"NCT03634007"}} NCT03634007 ). Human APOE4 and APOE3 exhibit a distinction in only one amino acid residue at position 112 [ 379 ]. While current technology faces limitations in producing widespread single-nucleotide changes in the brain, research has demonstrated that converting APOE4 to APOE3 through gene editing in induced pluripotent stem cells (iPSCs) or cerebral organoids can alleviate AD-associated phenotypes [ 380 ]. This approach holds promise as a potential option for gene therapy in the future.
6.3. Targeting APP
The APP gene plays a pivotal role in both sporadic and familial AD, making the silencing or modulation of APP an attractive prospect for gene therapy. Employing CRISPR–Cas9 technology, a recent investigation selectively deactivated mutant familial APP Swedish alleles in both cellular and in vivo settings, without impacting the corresponding wild-type alleles [ 381 ]. In an alternate study, antisense oligonucleotides (ASOs) were employed to omit the penultimate exon (exon 17) of APP [ 382 ]. The γ-secretase cleavage site and a substantial portion of the transmembrane domain of APP are encoded by exon 17. Deleting this exon results in the removal of the membrane-anchoring segment of APP, leading to a decrease in Aβ secretion in both cellular and in vivo settings [ 382 ]. Nonetheless, the elimination of the membrane-anchoring segment of APP is anticipated to result in the loss of its physiological functions. In a recent investigation, a CRISPR–Cas9 DNA-excision approach based on NHEJ was employed to introduce PTCs in the last exon (exon 18) of APP. This resulted in the truncation of the last approximately 36 amino acids and the removal of a pentapeptide YENPTY endocytic motif that initiates the amyloidogenic pathway [ 383 ]. Regulatory mechanisms in transcription render the last exon impervious to NMD. Consequently, the presence of PTCs in this region does not lead to mRNA degradation; instead, it causes protein truncations. This method carries the additional benefit of preserving the integrity of the amino terminus and transmembrane domains of APP, which are thought to contribute to axonal and synaptic physiology. As a result, there is no observable impact on physiological functions [ 383 ].
7. Gene Therapy for PD
7.1. modulating neuronal signaling.
Enhancing dopaminergic signaling in Parkinson’s disease (PD) has been achieved through gene therapies utilizing adeno-associated viruses (AAVs). The regulation of the dopamine synthesis pathway involves three key enzymes—GTP cyclohydrolase 1 (GCH1), tyrosine hydroxylase (TH), and aromatic amino acid DOPA decarboxylase (AADC). Clinical trials involving AAV2– AADC have shown safe, sustained expression over a period of up to 4 years, and a modest amelioration of symptoms [ 384 ]. A more precise administration of AAV2– AADC in non-human primates (NHPs) through real-time MRI guidance demonstrated safety and good tolerability, as evidenced by the trial results ( {"type":"clinical-trial","attrs":{"text":"NCT03065192","term_id":"NCT03065192"}} NCT03065192 ) [ 385 ]. Furthermore, a gene therapy utilizing lentivirus, known as ProSavin, was designed to deliver all three rate-limiting enzymes (TH, AADC, and GCH1). The therapy demonstrated positive tolerability during Phase 1/2 trials, and subsequent examinations indicated moderate improvements in motor function [ 386 ]. The decline in dopaminergic tonus within the striatum in PD results in an overactive glutamatergic subthalamic nucleus (STN). Preclinical studies demonstrated that enhancing GABA activation via the subthalamic overexpression of glutamate acid decarboxylase (GAD), an enzyme involved in GABA synthesis, effectively alleviated PD-like symptoms [ 387 ]. In Phase 1/2 clinical trials, the administration of AAV2– GAD into the STN demonstrated both good tolerability and improvement in symptoms associated with PD that persisted for a year [ 388 ].
7.2. Targeting Disease Genes—SNCA, GBA, and LRRK2
In rodent models of PD, the use of short hairpin RNA (shRNA) or antisense oligonucleotides (ASOs) to knockdown α-synuclein has proven effective in preventing neurodegeneration [ 389 , 390 ]. Additionally, CRISPR-mediated gene silencing of α-synuclein boosted the cell viability in dopaminergic neurons derived from human-induced pluripotent stem cells (iPSCs) sourced from a patient with PD [ 391 ]. However, unresolved concerns have been noted in some in vivo studies regarding physiological phenotypes following the knockdown of α-synuclein [ 392 ].
Administering AAV– GBA1 directly into the brain resulted in a reduction in α-synuclein levels and pathology in rodent models [ 393 ]. When GBA1 was diffusely delivered to the brain through intravenous injections of AAV-PHP.B– GBA1 in an A53T α-synuclein mouse model, it not only alleviated α-synuclein pathology but also contributed to substantial behavioral recovery and an extension of lifespan [ 394 ]. A recent Phase 1/2 clinical trial ( {"type":"clinical-trial","attrs":{"text":"NCT04127578","term_id":"NCT04127578"}} NCT04127578 ) is underway to treat individuals with PD. The approach involves the intracisternal injection of AAV9– GBA1 directly into the cerebrospinal fluid.
The expression of LRRK2 in the lungs, kidneys, and spleen implies that a general inhibition of LRRK2 may give rise to pathological modifications in these specific tissues [ 395 ]. Hence, in this context, gene therapy proves beneficial by selectively inhibiting LRRK2 in the brain. The successful attenuation of mRNA and protein levels through the intracerebral injection of LRRK2 ASOs was achieved without noticeable phenotypic alterations in the kidneys or lungs [ 396 ]. Intrathecal injections of BIIB094, an ASO targeting LRRK2 developed by Ionis Pharmaceuticals, is currently in a Phase 1 clinical trial for individuals with PD ( {"type":"clinical-trial","attrs":{"text":"NCT03976349","term_id":"NCT03976349"}} NCT03976349 ).
8. Gene Therapy for ALS
Targeting sod1.
Administering ASO– SOD1 into the cerebrospinal fluid (CSF) effectively diminished SOD1 levels and significantly prolonged survival in a transgenic rat model expressing mutated human SOD1 [ 397 ]. Furthermore, the intrathecal administration of ASO– SOD1 has been well-tolerated among patients with ALS exhibiting SOD1 mutations [ 398 ]. A follow-up Phase 1/2 study demonstrated that the administration of ASO– SOD1 resulted in a reduction in SOD1 protein levels in CSF [ 399 ] and a Phase 3 trial is currently underway (Tofersen). Additionally, RNA interference (RNAi) and CRISPR technology have been employed to downregulate mutant SOD1; a one-time intrathecal or intravenous injection of AAV- SOD1 -sgRNA results in reduction in SOD1 levels, enhanced motor function, and significantly extended the lifespan of mice having mutant SOD1 [ 400 ]. Recently, a proof-of-concept investigation demonstrated the feasibility of intrathecally delivering AAVs expressing microRNAs against SOD1 in two patients with SOD1 -related ALS. Postmortem analysis in one patient revealed the effective suppression of SOD1 in the spinal cord, affirming the potential success of this approach [ 401 ]. The intracerebroventricular injection of ASO– C9orf72 resulted in a reduction in C9orf72 mRNA foci and cognitive improvement in a transgenic mouse model with 450 G4C2 repeats in the C9orf72 gene [ 402 ]. An ongoing Phase 1 clinical trial is investigating the intrathecal administration of ASO– C9orf72 in patients diagnosed with C9orf72 -linked ALS.
9. Conclusions and Future Perspectives
The discussion so far has concluded that the neurodegenerative diseases discussed in this review, including AD, PD, and ALS, share several epidemiologic and genetic features. First, they all have an etiologic dichotomy, with more common late-onset forms and less common familial forms. It is possible (and likely) that a significant proportion of cases that were previously thought to be sporadic and nonfamilial will ultimately be found to result from disease-causing mutations or genetic risk factors (like APOE-ε4 in AD). Second, in some instances, identical mutations and polymorphisms have been linked to and associated with a variety of clinically and neuropathologically distinct disease entities. GWASs have played a significant role in this effort because they have enabled the discovery of novel genetic associations that are not based on prior knowledge. Unfortunately, GWAS findings, so far, have explained only a small proportion of the heritability of complex diseases, making genetic risk prediction tests for these diseases currently unfeasible. Overcoming the constraints of GWAS, NGS platforms like WGS and WES have revealed a plethora of rare variants that are pivotal in understanding complex neurological diseases and Mendelian neurological conditions.
In the past, tackling the pervasive pathology of neurodegenerative diseases through gene therapy was deemed challenging. However, breakthroughs in vector technologies now enable the broad delivery of genes into the CNS. Coupled with modern genome-manipulation tools, gene-based therapies hold the potential to revolutionize the clinical management of both inherited and sporadic neurodegenerative diseases—conditions marked by their devastating impact and a current lack of effective disease-modifying treatments. Nevertheless, numerous challenges persist, and the pivotal transition into a new era requires systematic advancements in gene-delivery vector development, comprehensive safety assessments of contemporary gene manipulation tools, and transparent collaboration among various stakeholders.
Acknowledgments
X.L. acknowledges support from a grant from the PKD Foundation.
Funding Statement
This research was funded by the National Institutes of Health (R01 DK129241 and DK126662).
Author Contributions
Conceptualization, Z.F. and X.L.; writing the manuscript, Z.F.; provision of suggestions and supervision, X.L. All authors have read and agreed to the published version of the manuscript.
Conflicts of Interest
The authors declare no conflicts of interest.
Disclaimer/Publisher’s Note: The statements, opinions and data contained in all publications are solely those of the individual author(s) and contributor(s) and not of MDPI and/or the editor(s). MDPI and/or the editor(s) disclaim responsibility for any injury to people or property resulting from any ideas, methods, instructions or products referred to in the content.

IMAGES
VIDEO
COMMENTS
Genetic disorders occur when a mutation affects your genes or chromosomes. Some disorders cause symptoms at birth, while others develop over time. Genetic testing can help you learn more about the likelihood of experiencing a genetic disorder. If you or a loved one have a genetic disorder, it's important to seek care from an experienced ...
What to know. Genetic disorders are health problems that happen because of some type of abnormality in a person's genetic material. There are several types of genetic disorders. Some disorders are caused by a genetic change (mutation) in a single gene; some are caused by an abnormality in one of the chromosomes; and some are complex, involving ...
Definition. Mutations in DNA can result in genetic disorders. Genetic disorders are conditions that occur as a result of changes to or mutations in DNA within the body's cells. Most cells in the ...
human genetic disease, any of the diseases and disorders that are caused by mutations in one or more genes.. With the increasing ability to control infectious and nutritional diseases in developed countries, there has come the realization that genetic diseases are a major cause of disability, death, and human tragedy. Rare, indeed, is the family that is entirely free of any known genetic disorder.
A genetic disorder is a disease caused in whole or in part by a change in the DNA sequence away from the normal sequence. Genetic disorders can be caused by a mutation in one gene (monogenic disorder), by mutations in multiple genes (multifactorial inheritance disorder), by a combination of gene mutations and environmental factors, or by damage to chromosomes (changes in the number or ...
When most people consider the genetic basis of disease, they might think about the rare, single gene disorders, such as cystic fibrosis (CF), phenylketonuria or haemophilia, or perhaps even cancers with a clear heritable component (for example, inherited predisposition to breast cancer). ... In conclusion, the presentation and severity of ...
1. Principle of Segregation: Two members of a gene pair segregate from each other in the formation of gametes; half the gametes carry one allele, and the other half carry the other allele What it means: each gene has two copies (alleles) and a parent will give only one copy to a child. The other parent will give another copy, and thus the child ...
Genetics Presentation templates ... Genetic Diseases Download the "Genetic Diseases" presentation for PowerPoint or Google Slides. Taking care of yourself and of those around you is key! By learning about various illnesses and how they are spread, people can get a better understanding of them and make informed decisions about eating, exercise ...
Abstract. Genetic diseases cause numerous complex and intractable pathologies. DNA sequences encoding each human's complexity and many disease risks are contained in the mitochondrial genome, nuclear genome, and microbial metagenome. Diagnosis of these diseases has unified around applications of next-generation DNA sequencing.
Diagram featuring examples of a disease located on each chromosome. A genetic disorder is a health problem caused by one or more abnormalities in the genome.It can be caused by a mutation in a single gene (monogenic) or multiple genes (polygenic) or by a chromosomal abnormality.Although polygenic disorders are the most common, the term is mostly used when discussing disorders with a single ...
All diseases have a genetic component. However, the extent to which genes contribute to disease varies and much remains to be learned. Advances in understanding the genetic mechanisms behind these disease enables the development of early diagnostic tests, new treatments, or interventions to prevent disease onset or minimize disease severity. This chapter provides information about the ...
When discussing genetic diseases, some definitions are important to remember: (1) hereditary or familial, a condition derived from parents (i.e., a condition that is transmitted in the germ line); and (2) congenital, a condition that is present at birth. Not all hereditary conditions are congenital, and not all congenital conditions are hereditary.
A primary goal of human genetics is to identify DNA sequence variants that influence biomedical traits, particularly those related to the onset and progression of human disease. Over the past 25 ...
Down syndrome is a genetic disorder caused when abnormal cell division results in an extra full or partial copy of chromosome 21. This extra genetic material causes the developmental changes and physical features of Down syndrome. Down syndrome varies in severity among individuals, causing lifelong intellectual disability and developmental ...
Table 1, Table 2 are not exhaustive for all presentations of known genetic disorders. For example, no patients with complaints of visual changes were reported in our sample. Marfan syndrome and Stickler syndrome, both disorders of connective tissue, may be associated with retinal detachment. Leber optic atrophy may present with sudden or ...
Jun 28, 2014 • Download as PPT, PDF •. 273 likes • 158,568 views. AI-enhanced description. Chhabi Acharya. This document discusses genetic disorders, which are illnesses caused by abnormalities in a person's genome. It begins by introducing genetic disorders and explaining that most are rare. It then discusses the history of genetics ...
What it is. Genetic testing looks for genetic changes, sometimes called mutations or variants, in your DNA. Some genetic changes can cause disease or other health conditions. You inherit your DNA from your parents, so any genetic changes they have can be passed down to you. Keep Reading: Genetics Basics. Genetic testing can give you information ...
This ppt describes about patterns of inheritance, classification of genetic disorders, genetic testing, and few disorders like down syndrome. Healthcare. 1 of 74. Genetic Disorders.ppt - Download as a PDF or view online for free.
Download the "Genetic Diseases" presentation for PowerPoint or Google Slides. Taking care of yourself and of those around you is key! By learning about various illnesses and how they are spread, people can get a better understanding of them and make informed decisions about eating, exercise, and seeking medical attention. This Google Slides ...
The clinical presentations of genetic disorders vary widely, from an isolated (major) congenital anomaly (CA) or multiple malformations (MCA) to more subtle clinical signs or symptoms. The diagnostic pathway is often long and requires extensive evaluations that may be invasive and costly . Diagnosis of most genetic disorders in neonatal and ...
Toda y, the number of genetic diseases is arou nd 10000 conditions, a ecting to 6%-8% of all populations. life expectancy, or improve qualities of life. These therapies are diverse, using drugs ...
The study provided detailed metabolic profiles of genetic variants associated with lipoproteins and lipids, enhancing our understanding of lipid loci at a granular level. We also observed genetic ...
Introduction. Multimorbidity is a coexistence state of two or more medical conditions/diseases [1, 2], which commonly occurs in older adults.The estimated prevalence of multimorbidity was 67.8% in older adults aged 65 years and older by 2035 in the UK [].These chronic diseases mainly include long-term mental conditions/diseases, physical noncommunicable diseases of long duration, and long-term ...
May 23, 2024. Schizophrenia is a complex disease with variable presentations. The diverse nature of this mental health disorder has made understanding the mechanisms that cause the disease, and subsequently developing effective treatments, especially challenging. In a new study published May 23 in Science, a team led by McLean Hospital ...
The start of 2022 is an inflection point in the development of diagnostics and treatments for rare genetic diseases in prenatal, pediatric, and adult individuals-the theme of this special issue. ... Phenotype expansion and its antecedent—very incomplete knowledge of the breadth of clinical presentations of childhood genetic diseases—are ...
Perk up the normally somber theme of illnesses and diseases with Slidesgo's latest disease presentation template. Medical. 16:9 / Like . Download . Download and open in Google Slides . Download as a PowerPoint file ...
Genetic testing performed by next-generation sequencing using a kidney disease gene panel identified a novel non-canonical splice site variant in the PHEX gene. ... Ars, E.; Torra, R. Rare diseases, rare presentations: Recognizing atypical inherited kidney disease phenotypes in the age of genomics. Clin. Kidney J. 2017, 10, 586-593. [Google ...
Selected genetic disorders associated with autism spectrum disorder will also be reviewed. Briefly, options for genetic testing will be discussed as well as genetic counseling and consenting for genetic testing. This presentation is intended for practicing PAs who want to learn more about the overlap between genetics and autism spectrum ...
NDDs are influenced by a wide range of genetic factors, from straightforward direct predisposition in diseases like Huntington's disease and spinocerebellar atrophy to more complex roles in diseases like AD and PD [8,9].A number of causative genes for familial forms of NDDs have been identified; these genes are inherited as Mendelian traits [], and their discovery has advanced knowledge of ...