

Problems and Problem Solving in Chemistry Education: Analysing Data, Looking for Patterns and Making Deductions
Problem solving is central to the teaching and learning of chemistry at secondary, tertiary and post-tertiary levels of education, opening to students and professional chemists alike a whole new world for analysing data, looking for patterns and making deductions. As an important higher-order thinking skill, problem solving also constitutes a major research field in science education. Relevant education research is an ongoing process, with recent developments occurring not only in the area of quantitative/computational problems, but also in qualitative problem solving.
The following situations are considered, some general, others with a focus on specific areas of chemistry: quantitative problems, qualitative reasoning, metacognition and resource activation, deconstructing the problem-solving process, an overview of the working memory hypothesis, reasoning with the electron-pushing formalism, scaffolding organic synthesis skills, spectroscopy for structural characterization in organic chemistry, enzyme kinetics, problem solving in the academic chemistry laboratory, chemistry problem-solving in context, team-based/active learning, technology for molecular representations, IR spectra simulation, and computational quantum chemistry tools. The book concludes with methodological and epistemological issues in problem solving research and other perspectives in problem solving in chemistry.
With a foreword by George Bodner.
- Cite Icon Cite
Problems and Problem Solving in Chemistry Education: Analysing Data, Looking for Patterns and Making Deductions, The Royal Society of Chemistry, 2021.
Download citation file:
- Ris (Zotero)
- Reference Manager
Digital access
Print format, table of contents.
- Front Matter
- Acknowledgments
- Author Biographies
- Chapter 1: Introduction − The Many Types and Kinds of Chemistry Problems p1-14 By Georgios Tsaparlis Georgios Tsaparlis University of Ioannina, Department of Chemistry Ioannina Greece [email protected] Search for other works by this author on: This Site PubMed Google Scholar Abstract Open the PDF Link PDF for Chapter 1: Introduction − The Many Types and Kinds of Chemistry Problems in another window
- Chapter 2: Qualitative Reasoning in Problem-solving in Chemistry p15-37 By Vicente Talanquer Vicente Talanquer Department of Chemistry and Biochemistry, University of Arizona Tucson AZ 85721 USA [email protected] Search for other works by this author on: This Site PubMed Google Scholar Abstract Open the PDF Link PDF for Chapter 2: Qualitative Reasoning in Problem-solving in Chemistry in another window
- Chapter 3: Scaffolding Metacognition and Resource Activation During Problem Solving: A Continuum Perspective p38-67 By Nicole Graulich ; Nicole Graulich Justus-Liebig-Universität Gießen Germany Search for other works by this author on: This Site PubMed Google Scholar Axel Langner ; Axel Langner Justus-Liebig-Universität Gießen Germany Search for other works by this author on: This Site PubMed Google Scholar Kimberly Vo ; Kimberly Vo Monash University Australia [email protected] Search for other works by this author on: This Site PubMed Google Scholar Elizabeth Yuriev Elizabeth Yuriev Monash University Australia [email protected] Search for other works by this author on: This Site PubMed Google Scholar Abstract Open the PDF Link PDF for Chapter 3: Scaffolding Metacognition and Resource Activation During Problem Solving: A Continuum Perspective in another window
- Chapter 4: Deconstructing the Problem-solving Process: Beneath Assigned Points and Beyond Traditional Assessment p68-92 By Ozcan Gulacar ; Ozcan Gulacar University of California, Davis, Department of Chemistry One Shields Avenue Davis CA 95616 USA [email protected] Search for other works by this author on: This Site PubMed Google Scholar Charlie Cox ; Charlie Cox Duke University, Department of Chemistry Box 90346, 128 Science Drive Durham NC 27708-0346 USA Search for other works by this author on: This Site PubMed Google Scholar Herb Fynewever Herb Fynewever Calvin University, Department of Chemistry 3201 Burton SE Grand Rapids MI 49546 USA Search for other works by this author on: This Site PubMed Google Scholar Abstract Open the PDF Link PDF for Chapter 4: Deconstructing the Problem-solving Process: Beneath Assigned Points and Beyond Traditional Assessment in another window
- Chapter 5: It Depends on the Problem and on the Solver: An Overview of the Working Memory Overload Hypothesis, Its Applicability and Its Limitations p93-126 By Georgios Tsaparlis Georgios Tsaparlis University of Ioannina, Department of Chemistry Ioannina Greece [email protected] Search for other works by this author on: This Site PubMed Google Scholar Abstract Open the PDF Link PDF for Chapter 5: It Depends on the Problem and on the Solver: An Overview of the Working Memory Overload Hypothesis, Its Applicability and Its Limitations in another window
- Chapter 6: Mechanistic Reasoning Using the Electron-pushing Formalism p127-144 By Gautam Bhattacharyya Gautam Bhattacharyya Missouri State University, Department of Chemistry 901 South National Avenue Springfield MO 65897 USA [email protected] Search for other works by this author on: This Site PubMed Google Scholar Abstract Open the PDF Link PDF for Chapter 6: Mechanistic Reasoning Using the Electron-pushing Formalism in another window
- Chapter 7: Scaffolding Synthesis Skills in Organic Chemistry p145-165 By Alison B. Flynn Alison B. Flynn Department of Chemistry and Biomolecular Sciences, University of Ottawa 10 Marie Curie Ottawa Ontario K1N 6N5 Canada [email protected] Search for other works by this author on: This Site PubMed Google Scholar Abstract Open the PDF Link PDF for Chapter 7: Scaffolding Synthesis Skills in Organic Chemistry in another window
- Chapter 8: Problem Solving Using NMR and IR Spectroscopy for Structural Characterization in Organic Chemistry p166-198 By Megan C. Connor ; Megan C. Connor Department of Chemistry, University of Michigan Ann Arbor Michigan USA [email protected] Search for other works by this author on: This Site PubMed Google Scholar Ginger V. Shultz Ginger V. Shultz Department of Chemistry, University of Michigan Ann Arbor Michigan USA [email protected] Search for other works by this author on: This Site PubMed Google Scholar Abstract Open the PDF Link PDF for Chapter 8: Problem Solving Using NMR and IR Spectroscopy for Structural Characterization in Organic Chemistry in another window
- Chapter 9: Assessing System Ontology in Biochemistry: Analysis of Students’ Problem Solving in Enzyme Kinetics p199-216 By Jon-Marc G. Rodriguez ; Jon-Marc G. Rodriguez University of Iowa, Department of Chemistry E355 Chemistry Building Iowa City Iowa 52242-1294 USA [email protected] Search for other works by this author on: This Site PubMed Google Scholar Sven J. Philips ; Sven J. Philips Purdue University, Department of Chemistry 560 Oval Drive West Lafayette IN 47907 USA Search for other works by this author on: This Site PubMed Google Scholar Nicholas P. Hux ; Nicholas P. Hux Purdue University, Department of Chemistry 560 Oval Drive West Lafayette IN 47907 USA Search for other works by this author on: This Site PubMed Google Scholar Marcy H. Towns Marcy H. Towns Purdue University, Department of Chemistry 560 Oval Drive West Lafayette IN 47907 USA Search for other works by this author on: This Site PubMed Google Scholar Abstract Open the PDF Link PDF for Chapter 9: Assessing System Ontology in Biochemistry: Analysis of Students’ Problem Solving in Enzyme Kinetics in another window
- Chapter 10: Problem Solving in the Chemistry Teaching Laboratory: Is This Something That Happens? p217-252 By Ian Hawkins ; Ian Hawkins Welch College Gallatin TN 37066 USA Search for other works by this author on: This Site PubMed Google Scholar Vichuda K. Hunter ; Vichuda K. Hunter Middle Tennessee State University, Department of Chemistry PO Box 68 Murfreesboro TN 37132 USA [email protected] Search for other works by this author on: This Site PubMed Google Scholar Michael J. Sanger ; Michael J. Sanger Middle Tennessee State University, Department of Chemistry PO Box 68 Murfreesboro TN 37132 USA [email protected] Search for other works by this author on: This Site PubMed Google Scholar Amy J. Phelps Amy J. Phelps Middle Tennessee State University, Department of Chemistry PO Box 68 Murfreesboro TN 37132 USA [email protected] Search for other works by this author on: This Site PubMed Google Scholar Abstract Open the PDF Link PDF for Chapter 10: Problem Solving in the Chemistry Teaching Laboratory: Is This Something That Happens? in another window
- Chapter 11: Problems and Problem Solving in the Light of Context-based Chemistry p253-278 By Karolina Broman Karolina Broman Umeå University Sweden [email protected] Search for other works by this author on: This Site PubMed Google Scholar Abstract Open the PDF Link PDF for Chapter 11: Problems and Problem Solving in the Light of Context-based Chemistry in another window
- Chapter 12: Using Team Based Learning to Promote Problem Solving Through Active Learning p279-319 By Natalie J. Capel ; Natalie J. Capel Keele University UK [email protected] Search for other works by this author on: This Site PubMed Google Scholar Laura M. Hancock ; Laura M. Hancock Keele University UK [email protected] Search for other works by this author on: This Site PubMed Google Scholar Chloe Howe ; Chloe Howe Keele University UK [email protected] Search for other works by this author on: This Site PubMed Google Scholar Graeme R. Jones ; Graeme R. Jones Keele University UK [email protected] Search for other works by this author on: This Site PubMed Google Scholar Tess R. Phillips ; Tess R. Phillips Keele University UK [email protected] Search for other works by this author on: This Site PubMed Google Scholar Daniela Plana Daniela Plana Keele University UK [email protected] Search for other works by this author on: This Site PubMed Google Scholar Abstract Open the PDF Link PDF for Chapter 12: Using Team Based Learning to Promote Problem Solving Through Active Learning in another window
- Chapter 13: Technology, Molecular Representations, and Student Understanding in Chemistry p321-339 By Jack D. Polifka ; Jack D. Polifka Department of Chemistry, Human Computer Interaction Program, Iowa State University Ames IA 50011 USA [email protected] Search for other works by this author on: This Site PubMed Google Scholar John Y. Baluyut ; John Y. Baluyut Math and Science Division, University of Providence Great Falls MT, 59405 USA Search for other works by this author on: This Site PubMed Google Scholar Thomas A. Holme Thomas A. Holme Department of Chemistry, Human Computer Interaction Program, Iowa State University Ames IA 50011 USA [email protected] Search for other works by this author on: This Site PubMed Google Scholar Abstract Open the PDF Link PDF for Chapter 13: Technology, Molecular Representations, and Student Understanding in Chemistry in another window
- Chapter 14: An Educational Software for Supporting Students’ Learning of IR Spectral Interpretation p340-360 By Maria Limniou ; Maria Limniou School of Psychology, University of Liverpool UK [email protected] Search for other works by this author on: This Site PubMed Google Scholar Nikos Papadopoulos ; Nikos Papadopoulos Department of Chemistry, Aristotle University of Thessaloniki Greece Search for other works by this author on: This Site PubMed Google Scholar Dimitris Gavril ; Dimitris Gavril Department of Chemistry, Aristotle University of Thessaloniki Greece Search for other works by this author on: This Site PubMed Google Scholar Aikaterini Touni ; Aikaterini Touni Department of Chemistry, Aristotle University of Thessaloniki Greece Search for other works by this author on: This Site PubMed Google Scholar Markella Chatziapostolidou Markella Chatziapostolidou Department of Chemistry, Aristotle University of Thessaloniki Greece Search for other works by this author on: This Site PubMed Google Scholar Abstract Open the PDF Link PDF for Chapter 14: An Educational Software for Supporting Students’ Learning of IR Spectral Interpretation in another window
- Chapter 15: Exploring Chemistry Problems with Computational Quantum Chemistry Tools in the Undergraduate Chemistry Curriculum p361-384 By Michael P. Sigalas Michael P. Sigalas Aristotle University of Thessaloniki, Laboratory of Quantum and Computational Chemistry, Department of Chemistry Thessaloniki 54124 Greece [email protected] Search for other works by this author on: This Site PubMed Google Scholar Abstract Open the PDF Link PDF for Chapter 15: Exploring Chemistry Problems with Computational Quantum Chemistry Tools in the Undergraduate Chemistry Curriculum in another window
- Chapter 16: Methodological and Epistemological Issues in Science Education Problem-solving Research: Linear and Nonlinear Paradigms p385-413 By Dimitrios Stamovlasis ; Dimitrios Stamovlasis Aristotle University of Thessaloniki Thessaloniki Greece [email protected] Search for other works by this author on: This Site PubMed Google Scholar Julie Vaiopoulou Julie Vaiopoulou Democritus University of Thrace Alexandroupolis Greece [email protected] University of Nicosia Nicosia Cyprus Search for other works by this author on: This Site PubMed Google Scholar Abstract Open the PDF Link PDF for Chapter 16: Methodological and Epistemological Issues in Science Education Problem-solving Research: Linear and Nonlinear Paradigms in another window
- Chapter 17: Issues, Problems and Solutions: Summing It All Up p414-444 By Georgios Tsaparlis Georgios Tsaparlis University of Ioannina, Department of Chemistry Ioannina Greece [email protected] Search for other works by this author on: This Site PubMed Google Scholar Abstract Open the PDF Link PDF for Chapter 17: Issues, Problems and Solutions: Summing It All Up in another window
- Chapter 18: Postscript – Two Issues for Provocative Thought: (a) The Potential Synergy Between HOTS and LOTS (b) When Problem Solving Might Descend to Chaos Dynamics p445-456 By Georgios Tsaparlis Georgios Tsaparlis University of Ioannina, Department of Chemistry Ioannina Greece [email protected] Search for other works by this author on: This Site PubMed Google Scholar Abstract Open the PDF Link PDF for Chapter 18: Postscript – Two Issues for Provocative Thought: (a) The Potential Synergy Between HOTS and LOTS (b) When Problem Solving Might Descend to Chaos Dynamics in another window
- Subject Index p457-467 Open the PDF Link PDF for Subject Index in another window
Advertisement
- Campaigning and outreach
- News and events
- Awards and funding
- Privacy policy
- Journals and databases
- Locations and contacts
- Membership and professional community
- Teaching and learning
- Help and legal
- Cookie policy
- Terms and conditions
- Get Adobe Acrobat Reader
- Registered charity number: 207890
- © Royal Society of Chemistry 2023
This Feature Is Available To Subscribers Only
Sign In or Create an Account
What is the Scientific Method: How does it work and why is it important?
The scientific method is a systematic process involving steps like defining questions, forming hypotheses, conducting experiments, and analyzing data. It minimizes biases and enables replicable research, leading to groundbreaking discoveries like Einstein's theory of relativity, penicillin, and the structure of DNA. This ongoing approach promotes reason, evidence, and the pursuit of truth in science.
Updated on November 18, 2023

Beginning in elementary school, we are exposed to the scientific method and taught how to put it into practice. As a tool for learning, it prepares children to think logically and use reasoning when seeking answers to questions.
Rather than jumping to conclusions, the scientific method gives us a recipe for exploring the world through observation and trial and error. We use it regularly, sometimes knowingly in academics or research, and sometimes subconsciously in our daily lives.
In this article we will refresh our memories on the particulars of the scientific method, discussing where it comes from, which elements comprise it, and how it is put into practice. Then, we will consider the importance of the scientific method, who uses it and under what circumstances.
What is the scientific method?
The scientific method is a dynamic process that involves objectively investigating questions through observation and experimentation . Applicable to all scientific disciplines, this systematic approach to answering questions is more accurately described as a flexible set of principles than as a fixed series of steps.
The following representations of the scientific method illustrate how it can be both condensed into broad categories and also expanded to reveal more and more details of the process. These graphics capture the adaptability that makes this concept universally valuable as it is relevant and accessible not only across age groups and educational levels but also within various contexts.

Steps in the scientific method
While the scientific method is versatile in form and function, it encompasses a collection of principles that create a logical progression to the process of problem solving:
- Define a question : Constructing a clear and precise problem statement that identifies the main question or goal of the investigation is the first step. The wording must lend itself to experimentation by posing a question that is both testable and measurable.
- Gather information and resources : Researching the topic in question to find out what is already known and what types of related questions others are asking is the next step in this process. This background information is vital to gaining a full understanding of the subject and in determining the best design for experiments.
- Form a hypothesis : Composing a concise statement that identifies specific variables and potential results, which can then be tested, is a crucial step that must be completed before any experimentation. An imperfection in the composition of a hypothesis can result in weaknesses to the entire design of an experiment.
- Perform the experiments : Testing the hypothesis by performing replicable experiments and collecting resultant data is another fundamental step of the scientific method. By controlling some elements of an experiment while purposely manipulating others, cause and effect relationships are established.
- Analyze the data : Interpreting the experimental process and results by recognizing trends in the data is a necessary step for comprehending its meaning and supporting the conclusions. Drawing inferences through this systematic process lends substantive evidence for either supporting or rejecting the hypothesis.
- Report the results : Sharing the outcomes of an experiment, through an essay, presentation, graphic, or journal article, is often regarded as a final step in this process. Detailing the project's design, methods, and results not only promotes transparency and replicability but also adds to the body of knowledge for future research.
- Retest the hypothesis : Repeating experiments to see if a hypothesis holds up in all cases is a step that is manifested through varying scenarios. Sometimes a researcher immediately checks their own work or replicates it at a future time, or another researcher will repeat the experiments to further test the hypothesis.

Where did the scientific method come from?
Oftentimes, ancient peoples attempted to answer questions about the unknown by:
- Making simple observations
- Discussing the possibilities with others deemed worthy of a debate
- Drawing conclusions based on dominant opinions and preexisting beliefs
For example, take Greek and Roman mythology. Myths were used to explain everything from the seasons and stars to the sun and death itself.
However, as societies began to grow through advancements in agriculture and language, ancient civilizations like Egypt and Babylonia shifted to a more rational analysis for understanding the natural world. They increasingly employed empirical methods of observation and experimentation that would one day evolve into the scientific method .
In the 4th century, Aristotle, considered the Father of Science by many, suggested these elements , which closely resemble the contemporary scientific method, as part of his approach for conducting science:
- Study what others have written about the subject.
- Look for the general consensus about the subject.
- Perform a systematic study of everything even partially related to the topic.

By continuing to emphasize systematic observation and controlled experiments, scholars such as Al-Kindi and Ibn al-Haytham helped expand this concept throughout the Islamic Golden Age .
In his 1620 treatise, Novum Organum , Sir Francis Bacon codified the scientific method, arguing not only that hypotheses must be tested through experiments but also that the results must be replicated to establish a truth. Coming at the height of the Scientific Revolution, this text made the scientific method accessible to European thinkers like Galileo and Isaac Newton who then put the method into practice.
As science modernized in the 19th century, the scientific method became more formalized, leading to significant breakthroughs in fields such as evolution and germ theory. Today, it continues to evolve, underpinning scientific progress in diverse areas like quantum mechanics, genetics, and artificial intelligence.
Why is the scientific method important?
The history of the scientific method illustrates how the concept developed out of a need to find objective answers to scientific questions by overcoming biases based on fear, religion, power, and cultural norms. This still holds true today.
By implementing this standardized approach to conducting experiments, the impacts of researchers’ personal opinions and preconceived notions are minimized. The organized manner of the scientific method prevents these and other mistakes while promoting the replicability and transparency necessary for solid scientific research.
The importance of the scientific method is best observed through its successes, for example:
- “ Albert Einstein stands out among modern physicists as the scientist who not only formulated a theory of revolutionary significance but also had the genius to reflect in a conscious and technical way on the scientific method he was using.” Devising a hypothesis based on the prevailing understanding of Newtonian physics eventually led Einstein to devise the theory of general relativity .
- Howard Florey “Perhaps the most useful lesson which has come out of the work on penicillin has been the demonstration that success in this field depends on the development and coordinated use of technical methods.” After discovering a mold that prevented the growth of Staphylococcus bacteria, Dr. Alexander Flemimg designed experiments to identify and reproduce it in the lab, thus leading to the development of penicillin .
- James D. Watson “Every time you understand something, religion becomes less likely. Only with the discovery of the double helix and the ensuing genetic revolution have we had grounds for thinking that the powers held traditionally to be the exclusive property of the gods might one day be ours. . . .” By using wire models to conceive a structure for DNA, Watson and Crick crafted a hypothesis for testing combinations of amino acids, X-ray diffraction images, and the current research in atomic physics, resulting in the discovery of DNA’s double helix structure .
Final thoughts
As the cases exemplify, the scientific method is never truly completed, but rather started and restarted. It gave these researchers a structured process that was easily replicated, modified, and built upon.
While the scientific method may “end” in one context, it never literally ends. When a hypothesis, design, methods, and experiments are revisited, the scientific method simply picks up where it left off. Each time a researcher builds upon previous knowledge, the scientific method is restored with the pieces of past efforts.
By guiding researchers towards objective results based on transparency and reproducibility, the scientific method acts as a defense against bias, superstition, and preconceived notions. As we embrace the scientific method's enduring principles, we ensure that our quest for knowledge remains firmly rooted in reason, evidence, and the pursuit of truth.

The AJE Team
See our "Privacy Policy"
Problem-Solving in Chemistry
Cite this chapter.
- George M. Bodner 19 &
- J. Dudley Herron 20
Part of the book series: Science & Technology Education Library ((CTISE,volume 17))
1933 Accesses
15 Citations
2 Altmetric
This is a preview of subscription content, log in via an institution to check access.
Access this chapter
- Available as PDF
- Read on any device
- Instant download
- Own it forever
- Available as EPUB and PDF
- Compact, lightweight edition
- Dispatched in 3 to 5 business days
- Free shipping worldwide - see info
- Durable hardcover edition
Tax calculation will be finalised at checkout
Purchases are for personal use only
Institutional subscriptions
Unable to display preview. Download preview PDF.
Ashmore, A.D, Frazer, M.J. & Casey, R.J. (1979). Problem-solving and problem-solving networks in chemistry. Journal of Chemical Education , 56, 377–379.
Article CAS Google Scholar
Asieba, F.O. & Egbugara, O.U. (1993). Evaluation of pupils’ chemical problem-solving skills using a problem-solving model. Journal of Chemical Education , 70, 38–39.
Article Google Scholar
Bodner, G.M. & McMillen, T.L.B. (1986). Cognitive restructuring as an early Stage in problem-solving. Journal of Research in Science Teaching , 23, 727–737
Bodner, G.M. (1987). The role of algorithms in teaching problem-solving. Journal of Chemical Education , 64, 513–514
Bodner, G.M. (1991). Toward a unified theory of problem-solving: A view from chemistry.’ In Toward a unified theory of problem-solving: Views from the content domain. M.U. Smith, (Ed.). Hillsdale, NJ: Lawrence Erlbaum Associates.
Google Scholar
Bodner, G.M. & Pardue, H.L. (1995). Chemistry: An experimental science , (2 nd ed.) New York: Wiley.
Bodner, G.M. & Domin, D.S. (2000). Mental models: The role of representations in problem-solving in chemistry. University Chemistry Education , 4, 24–30.
Bowen, C.W. (1990). Representational systems used by graduate students while problem-solving in organic synthesis. Journal of Research in Science Teaching , 27, 351–370.
Bowen, C.W. & Bodner, G.M. (1991). Problem-solving processes used by students in organic synthesis. International Journal of Science Education , 13, 58–143
Bunce, D.M. & Heikkinen, H. (1986). The effects of an explicit problem-solving approach on mathematical chemistry achievement. Journal of Research in Science Teaching , 23, 11–20.
Bunce, D.M., Gabel, D.L. & Samuael, J.V. (1991). Enhancing chemistry problem-solving achievement using problem categorization. Journal of Research in Science Teaching , 28, 505–521.
Camacho, M., & Good R. (1989). Problem-solving and chemical equilibrium: successful versus unsuccessful performance. Journal of Research in Science Teaching , 26, 251–272.
Carter, C.S., LaRussa, M.A., Bodner, G.M. (1987). A study of two measures of spatial ability as predictors of success in different levels of general chemistry. Journal of Research in Science Teaching , 24, 645–657
Chi, M.T.H., Feltovich, P.J., & Glaser, R. (1981). Categorization and representation of physics problems by experts and novices. Cognitive Science , 5, 121–152.
de Astudillo, L.R., & Niaz, M. (1996). Reasoning strategies used by students to solve stoichiometry problems and its relationship to alternative conceptions prior knowledge and cognitive variables. Journal of Science Education Technology , 5, 131–140.
Dewey, J. (1910). How to think . Boston: Heath.
Book Google Scholar
Dods, R.F. (1996). A problem-based learning design for teaching biochemistry. Journal of Chemical Education , 73, 225–228.
Dods, R.F. (1997). An action research study of the effectiveness of problem based learning in promoting the acquisition and retention of knowledge. Journal of Education of the Gifted , 20:423–437.
Domin, D.S., & Bodner G. (submitted). M. Differences in the number and types of representations constructed by successful and unsuccessful problem solvers. Journal of Research in Science Teaching .
Ehrlich, E., Flexner, S.B., Carruth, G., & Hawkins, J.M. (1980). Oxford American Dictionary . Oxford: Oxford University Press.
Fasching, J.L., & Erickson, B.L. (1985). Group discussions in the chemistry classroom and the problem-solving skills of students. Journal of Chemical Education , 62, 842–848.
Frank, D.V., Baker C.A., & Herron, J.D. (1987). Should students always use algorithms to solve problems? Journal of Chemical Education , 64, 514–515
Friedel, A.W., Gabel, D.L., & Samuel, J. (1990). Using analogs for chemistry problem-solving: Does it increase understanding? School Science and Mathematics , 90, 674–682.
Gabel, D.L., & Sherwood R.D. (1983). Facilitating problem-solving in high school chemistry. Journal of Research in Science Teaching , 20, 163–177
Gabel, D.L., Sherwood, R.D., & Enochs L. (1984). Problem-solving skills of high school chemistry students. Journal of Research in Science Teaching , 21, 221–233
Gabel, D.L., & Samuel, K.V. (1986). High school students’ ability to solve molarity problems and their analog counterparts. Journal of Research in Science Teaching, 23 , 165–176
Gardner, D. & Bodner, G.M. (2001). Students’ conceptions of physical chemistry:. Studies of graduate and undergraduate students . Paper presented at the meeting of the National Association for Research in Science Teaching, St. Louis, MO.
Hanson, D. & Wolfskill, T. (2000). Process workshops-a new model for instruction. Journal of Chemical Education , 77, 120–130
Hayes, J. (1980). The complete problem solver . Philadelphia: The Franklin Institute.
Hesse, J.J. & Anderson C.W. (1992). Students’ conceptions of chemical change. Journal of Research in Science Teaching , 29, 277–299
Heyworth, R.M. (1999). Procedural and conceptual knowledge of expert and novice students for the solving of a basic problem in chemistry. International Journal of Science Education , 21, 195–211
Herron, J.D. (1996). The chemistry classroom: Formulas for successful teaching , Washington, DC: American Chemical Society.
Herron, J.D., & Greenbowe, T.J. (1986). What can we do about Sue: a case study of competence. Journal of Chemical Education , 63, 526–531.
Holtzlaw, H.F., Robinson, W.R., & Nebergall, W.H. (1984). General chemistry (7th ed.) Lexington: D.C. Heath.
Huffman, D. (1997). Effect of explicit problem-solving instruction on high school students’ PS performance and conceptual understanding of physics. Journal of Research in Science Teaching , 34, 551–570.
Johnstone, A.H., & El-Banna H. (1986). Capacities, demands and processes — a predictive model for science education. Education in Chemistry , 23, 80–84.
CAS Google Scholar
Kumar. D.D. (1993). Assessment of expert-novice chemistry problem-solving using hypercard: Early findings. Journal of Science Education Technology , 2, 481–485.
Lave, J. & Wenger, E. (1991). Situated learning: Legitimate peripheral. participation . Cambridge: Cambridge University Press.
Larkin, J., McDermott, J., Simon, D.P. & Simon, H. A. (1980). Models of competence in solving physics problems. Science , 208, 1335–1342
Larkin, J.H. & Rainard, B. (1984). A research methodology for studying how people think. Journal of Research in Science Teaching , 21, 235–254.
Lee, K.L., & Fensham, P. (1996). General strategy for solving high school electrochemistry problems. International Journal of Science Education , 18, 543–555.
Lee, K.L., Goh, N.K., Chia, L.S., & Chin, C. (1996). Cognitive variables in problem-solving in chemistry: A revisited study. Science Education , 80, 691–710.
Lesh, R., Hoover, M., Hole, B., Kelly, A. & Post, T. (2000). Principles for developing thought-revealing activities for students and teachers. In A. Kelly & R. Lesh (Eds.), Handbook of research design in mathematics and science education (pp. 591–645). Mahwah: Lawrence Erlbaum.
Lythcott, J. (1990). Problem-solving and requisite knowledge of chemistry. Journal of Chemical Education, 67 , 248–252.
Mason, D.S., Shell, D.F. & Crawley, F.E. (1997). Differences in problem-solving by nonscience majors in introductory chemistry on paired algorithmic-conceptual problems. Journal of Research in Science Teaching , 34, 905–924.
Nakhleh, M.B. (1993). Are our students conceptual thinkers or algorithmic problem solvers? Journal of Chemical Education , 70, 52–55.
Nakhleh, M.B., & Mitchell R.C. (1993). Concept learning versus problem-solving: there is a difference. Journal of Chemical Education , 70, 190–192.
Niaz, M. (1988). Manipulation of M Demand of chemistry problems and its effect on student performance: A neo-Piagetian study. Journal of Research in Science Teaching , 25, 643–657.
Niaz, M. (1989). The relationship between m-demand, algorithms, and problem-solving: A neo-Piagetian analysis. Journal of Chemical Education , 66, 422–424.
Niaz, M., Robinson W.R. (1992). Manipulation of logical structure of chemistry problems and its effect on student performance. Journal of Research in Science Teaching , 29:211–226
Niaz, M. (1995). Progressive transitions from algorithmic to conceptual understanding in student ability to solve chemistry problems: A Lakatosian interpretation. Science Education , 79, 19–36.
Niaz, M. (1995). Cognitive conflict as a teaching strategy in solving chemistry problems: A dialectic-constructivist perspective. Journal of Research in Science Teaching , 32, 959–970.
Niaz, M. (1996). How students circumvent problem-solving strategies that require greater cognitive complexity. Journal of College Science Teaching , 15, 361–63.
Noh, T., & Sharmann, L. C. (1997). Instructional influence of a molecular-level pictorial presentation of matter on students’ conceptions and problem-solving ability. Journal of Research in Science Teaching , 34, 199–217.
Nurrenbern, S.C., & Pickering, M. (1987). Conceptual learning versus problem-solving: is there a difference? Journal of Chemical Education , 64, 508–510.
Pendley, B.D., Bretz, R.L., & Novak, J.D. (1994). Concept maps as a tool to assess learning in chemistry. Journal of Chemical Education , 71, 9–15.
Pestel, B. (1993). Teaching problem-solving without modeling through ‘thinking aloud pair problem-solving’. Science Education , 77, 83–94.
Phelps, A. J. (1996). Teaching to enhance problem-solving: It’s more than the numbers. Journal of Chemical Education , 73, 301–304.
Pickering, M. (1990). Further studies on concept learning versus problem-solving. Journal of Chemical Education , 67, 254–255.
Polya, G. (1946). How to solve it: a New Aspect of mathematical method . Princeton, NJ: Princeton University Press.
Pribyl, J.R., & Bodner, G.M. (1987). Spatial ability and its role in organic chemistry: a study of four organic courses. Journal of Research in Science Teaching , 24, 229–240.
Robinson, W.R., & Niaz, M. (1991). Performance based on instruction by lecture or by interaction and its relationship to cognitive variables. International Journal of Science Education , 13, 203–215.
Ross, M. & Fulton, R. (1994). Active learning strategies in the analytical chemistry. classroom. Journal of Chemical Education , 71, 141–143.
Sawrey, B.A. (1990). Concept learning versus problem-solving: revisited. Journal of Chemical Education , 67, 253–254.
Shibley, I.A. Jr., & Zimmaro, D.M. (2002). The influence of collaborative learning on student attitude and performance in an introductory chemistry lab. Journal of Chemical Education , 79, 745–748.
Smith, C.A., Powell, S.C. & Wood, E.J. (1995). Problem based learning and problem-solving skills. Biochemical Education , 23, 149–152.
Smith, K.J. & Metz, P.A. (1996). Evaluating student understanding of solution chemistry through microscopic representations. Journal of Chemical Education , 73, 233–235.
Smith, M.U. & Good, R. (1984). Problem-solving and classical genetics: successful versus unsuccessful performance. Journal of Research in Science Teaching , 21, 895–912.
Smith, M.U. (1988). Toward a unified theory of problem-solving: a view from biology. Paper presented at the annual meeting of the American Educational Research Association in New Orleans.
Staver, J.R. & Lumpe, A.T. (1995). Two investigations of students’ understanding of the mole concept and its use in problem-solving. Journal of Research in Science Teaching , 32, 177–193.
Staver, J. & Jacks, T. (1988). The influence of cognitive reasoning level cognitive restructuring ability disembedding ability working memory capacity and prior knowledge on students performance on balancing equations by inspection. Journal of Research in Science Teaching , 25, 63–775.
Thomas, P.L., & Schwenz, R.W. (1998). College physical chemistry students’ conceptions of equilibrium and fundamental thermodynamics. Journal of Research in Science Teaching , 35, 1151–1160.
Tingle, J.B., & Good, R. Effects of cooperative grouping on stoichiometric problem-solving in high school chemistry. Journal of Research in Science Teaching , 27, 671–683.
Towns, M., & Grant, E. (1998). ‘I believe I will go out of this class actually knowing something:’ Cooperative learning activities in physical chemistry. Journal of Research in Science Teaching , 34, 819–835.
Treagust, D.F. (1988). The development and use of diagnostic instruments to evaluate students’ misconceptions in science. International Journal of Science Education , 10, 159–169.
Tsaparlis, G., Kousathana, M., & Niaz, M. (1998). Molecular-equilibrium problems: manipulation of logical structure and of m demand and their effect on student performance. Science Education , 82, 437–454.
Voska, K.W., & Heikkinen, H.W. (2000). Identification and analysis of student conceptions used to solve chemical equilibrium problems. Journal of Research in Science Teaching , 37, 160–176.
Wheatley, G. H. (1984). Problem-solving in school mathematics. MEPS Technical Report 84.01, School Mathematics and Science Center, Purdue University, West Lafayette, IN.
Whimby, A (1984). The key to higher order thinking is precise processing. Educational Leadership , 42, 66–70.
Woods, D.R. (1989a). Developing students’ problem-solving skills. Journal of College Science Teaching , 19, 108–110.
Woods, D.R. (1989b). Developing students’ problem-solving skills. Journal of College Science Teaching , 19, 176–179.
Yarroch, W.L. (1985). Students’ understanding of chemical equation balancing. Journal of Research in Science Teaching , 22, 449–459.
Download references
Author information
Authors and affiliations.
Purdue University, USA
George M. Bodner
Morehead State University, USA
J. Dudley Herron
You can also search for this author in PubMed Google Scholar
Editor information
Editors and affiliations.
The University of Reading, UK
John K. Gilbert
Utrecht University, The Netherlands
Onno De Jong
Federal University of Minas Gerias, Brazil
Rosária Justi
Curtin University of Technology, Australia
David F. Treagust
Leiden University, The Netherlands
Jan H. Van Driel
Rights and permissions
Reprints and permissions
Copyright information
© 2002 Kluwer Academic Publishers
About this chapter
Bodner, G.M., Herron, J.D. (2002). Problem-Solving in Chemistry. In: Gilbert, J.K., De Jong, O., Justi, R., Treagust, D.F., Van Driel, J.H. (eds) Chemical Education: Towards Research-based Practice. Science & Technology Education Library, vol 17. Springer, Dordrecht. https://doi.org/10.1007/0-306-47977-X_11
Download citation
DOI : https://doi.org/10.1007/0-306-47977-X_11
Publisher Name : Springer, Dordrecht
Print ISBN : 978-1-4020-1112-2
Online ISBN : 978-0-306-47977-9
eBook Packages : Springer Book Archive
Share this chapter
Anyone you share the following link with will be able to read this content:
Sorry, a shareable link is not currently available for this article.
Provided by the Springer Nature SharedIt content-sharing initiative
- Publish with us
Policies and ethics
- Find a journal
- Track your research

Want to create or adapt books like this? Learn more about how Pressbooks supports open publishing practices.
3 Chemical Problem Solving Strategies
Video Transcript
Unit Analysis and Problem Solving
Original quantity x conversion factor = equivalent quantity for example converting between length units given that 1 meter = 39.37 inches conversion factors or the same relationship, just invert as necessary to give you the units you need, calculations: using unit analysis.
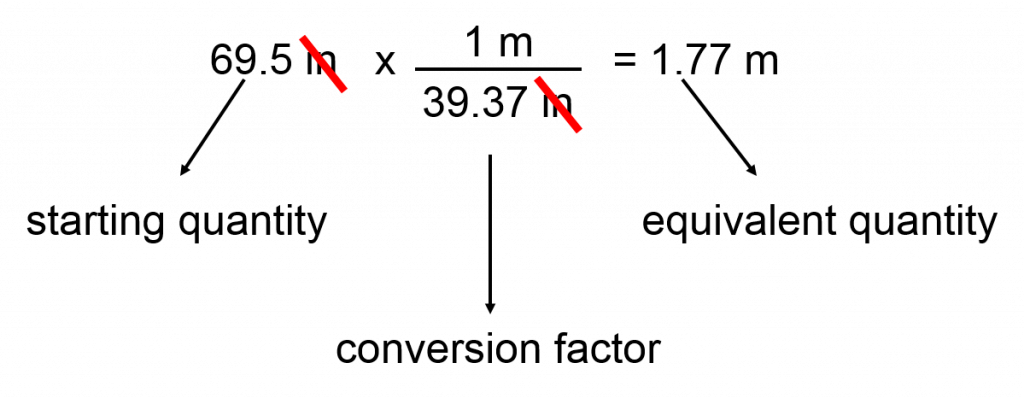
The more you use the “long method” of converting units, the fewer errors you will make!
Problem Solving Examples
How many moles of oxygen atoms are there in a 10 ml volume of water, convert volume of water to moles of oxygen.

= There are 0.55 moles of oxygen atoms. Always Check Units!
Problems set, below are two documents. one is practice problems, the second is the same problems with solutions. they can be downloaded and changed to suit your needs..
Be Prepared! Everything you should know for 1st year Chemistry Copyright © by Andrew Vreugdenhil and Kelly Wright is licensed under a Creative Commons Attribution 4.0 International License , except where otherwise noted.

Share This Book
Your browser is not supported
Sorry but it looks as if your browser is out of date. To get the best experience using our site we recommend that you upgrade or switch browsers.
Find a solution
- Skip to main content
- Skip to navigation
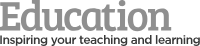
- Back to parent navigation item
- Collections
- Sustainability in chemistry
- Simple rules
- Teacher well-being hub
- Women in chemistry
- Global science
- Escape room activities
- Decolonising chemistry teaching
- Teaching science skills
- Post-lockdown teaching support
- Get the print issue
- RSC Education
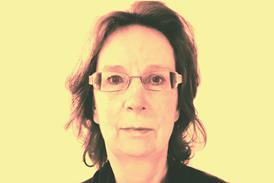
- More from navigation items
Getting the most out of the UK Chemistry Olympiad
- 2 Your complete guide to the UK Chemistry Olympiad
- 3 What you and your students will gain from the Olympiad
- 4 ‘It’s a really enjoyable experience and good preparation for A-level’
- 5 Join the problem-solving set
- 6 ‘Having a go at something really difficult builds confidence’
- 7 Questions, questions, questions …
Your complete guide to the UK Chemistry Olympiad

- No comments
Find out how your learners can take part in this valuable experience that goes beyond purely academic pursuits
The UK Chemistry Olympiad gives aspiring young chemists a chance to explore chemistry beyond the post-16 curriculum. Each year, the four top-scoring participants from the UK competition go on to represent the UK at the International Chemistry Olympiad – in 2025, this will take place in the United Arab Emirates.
Registration
Your school can register on the our website from September to early January. The recommend minimum age for participants is 16. You must be signed up to Teach Chemistry to register.
Your school can register on the Royal Society of Chemistry Education website ( rsc.li/3Vg21Lv ) from September to early January. The recommend minimum age for participants is 16. Make sure you’ve renewed your Teach Chemistry membership to register.
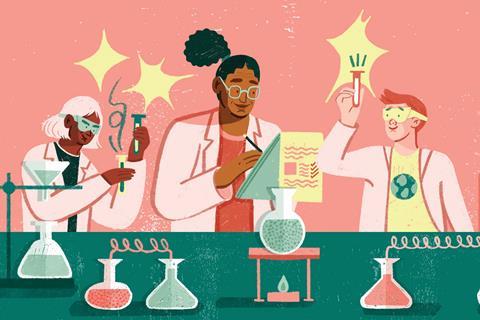
Source: © Olivia Waller/Folio Art
Once you’ve registered your school, it’s time to start preparing learners for the written exam and to ready yourself for the marking
This is a written exam that learners take at their own schools in late January. In 2024, 14,915 learners participated in this round. Be prepared, because you grade your own students’ papers and submit the marks to the RSC. Approximately two-thirds of participants receive a bronze, silver or gold certificate for their efforts.
Even a seemingly low score can result in an award … 10 marks out of 82 can earn a bronze certificate
The working group behind the UK Chemistry Olympiad selects approximately 30 top-scoring students from Round one to compete in Round two. They strive to achieve a mix of gender, year groups and types of school among the Round two participants.
How to prepare pupils for Round one
Bolstering problem-solving skills is the most important thing you can do to prepare your learners for the UK Chemistry Olympiad, and taking them over past questions from the UK Chemistry Olympiad is a vital part of this. You’ll find previous questions and mark schemes on the RSC Education website. Explore these during extracurricular STEM clubs – find out how other teachers do it or fold them into your curriculum as extension tasks.
Bolstering problem-solving skills is the most important thing you can do to prepare your learners for the UK Chemistry Olympiad, and taking them over past questions from the UK Chemistry Olympiad is a vital part of this. You’ll find previous questions and mark schemes on the RSC Education website ( rsc.li/3WNFDu5 ). Explore these during extracurricular STEM clubs – find out how other teachers do it on page 21 or fold them into your curriculum as extension tasks.
You will also need to expose your learners to some areas of chemistry that regularly come up on the UK Chemistry Olympiad papers but are not covered – or are not covered in sufficient depth – on curriculums. Use the RSC’s explainers to help introduce your students to these topics.
You will also need to expose your learners to some areas of chemistry that regularly come up on the UK Chemistry Olympiad papers but are not covered – or are not covered in sufficient depth – on curriculums. Use the RSC’s explainers to help introduce your students to these topics ( rsc.li/4bSOor8 ).
Confidence is key. You may need to help your learners gain sufficient confidence to take on questions that appear impossible at first glance. Explain questions in Round one are designed to be hard, and that even a seemingly low score can result in an award. In 2024, for instance, students needed 10 marks out of 82 to earn a bronze certificate. Use the introductory question resource to help build confidence; practice papers for the Cambridge Chemistry Challenge are also helpful for this purpose.
Confidence is key. You may need to help your learners gain sufficient confidence to take on questions that appear impossible at first glance. Explain questions in Round one are designed to be hard, and that even a seemingly low score can result in an award. In 2024, for instance, students needed 10 marks out of 82 to earn a bronze certificate. Use the introductory question resource ( rsc.li/4bLNXi3 ) to help build confidence; practice papers for the Cambridge Chemistry Challenge are also helpful for this purpose ( bit.ly/3UI20OQ ).
Access the free RSC UK Chemistry Olympiad resources , which you can encourage your learners to explore on their own. Visit our UK Olympiad webpages for more details.
Visit edu.rsc.org/enrichment/uk-chemistry-olympiad to access the free RSC UK Chemistry Olympiad resources, which you can encourage your learners to explore on their own. You can also encourage your learners to explore all these resources on their own – visit our UK Olympiad webpages for more details.
This takes place over a long weekend during the Easter school holidays at a UK university chemistry department. It involves theoretical and practical instruction on topics identified as important for that year’s international competition.
The weekend starts with a group dinner, and the next day participants receive lab training and complete a practical test. On the third day, students attend lectures on topics they won’t have encountered at school and then sit a theory exam.
On the final day, the working group announce the four students selected to represent the UK at the International Chemistry Olympiad (IChO) .
How to prepare pupils for Round two
Before Round two, participants receive a textbook – with a list of chapters to read – and samples of past questions. Help your students by going through this information with them.
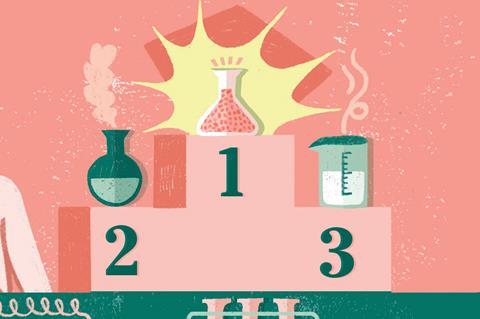
Being selected for the International Chemistry Olympiad is an honour, and it’s also when training starts in earnest
International Chemistry Olympiad
The International Chemistry Olympiad (IChO) takes place in July each year and involves around 90 countries. To prepare the UK Olympiad team, the UK Olympiad working group hosts online study sessions and two in-person training events. Over a weekend in May and a week in late June/early July, participants practise practical skills, test their problem-solving abilities and receive additional theoretical instruction. Participants also take a mock exam at the end of the training week.
Olympiad successes
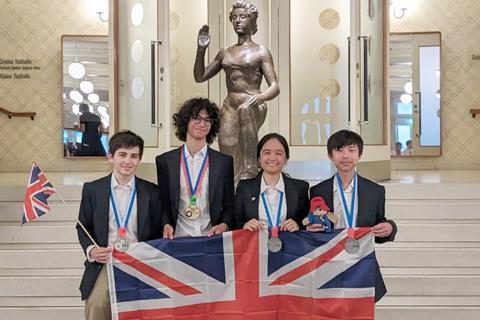
Since the UK team started participating in the International Chemistry Olympiad in 1984, it has accumulated 17 gold medals, 70 silver medals and 66 bronze medals. In 2023, Kiran Desai-Kinvig, Kiran Diamond, Perth Saritsiri and Patrick Fung (left to right) brought home gold and silver medals from the IChO in Switzerland.
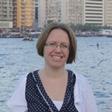
More from Nina Notman
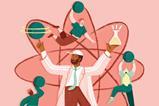
Why you and your students should get involved in the Olympiad
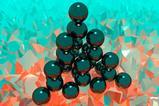
New method grows larger diamonds
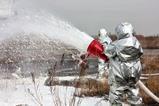
PFAS levels in surface and groundwater exposed
- Competitions
- Prizes and awards
- Problem solving
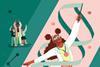
What you and your students will gain from the Olympiad
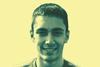
‘It’s a really enjoyable experience and good preparation for A-level’
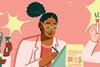
Join the problem-solving set
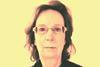
‘Having a go at something really difficult builds confidence’
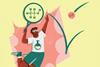
Questions, questions, questions …
Related articles.
2024-06-05T07:00:00Z
It’s the competition with something for every learner and teacher. Discover the benefits of participation here
Simply the best – classic questions from UK Chemistry Olympiads
2024-06-05T07:00:00Z By Lynne Maxwell
From cheese to pop cans, favourite questions from the competition
How a teacher uses the UK Chemistry Olympiad to boost their learners’ outcomes
No comments yet
Only registered users can comment on this article., more from feature.
2024-06-05T07:00:00Z By Nina Notman
Challenge your learners and ignite a passion for chemistry
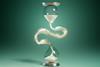
Longer classes: what’s the verdict?
2024-05-20T08:00:00Z By Angeli Mehta
Discover why some schools have switched to fewer, longer lessons in a school day – and how it’s going
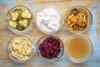
Why fermented foods are good for your gut – and your teaching
2024-04-15T05:30:00Z By Emma Davies
From kimchi to kefir, tuck into the complex chemistry of fermentation and its health potential
- Contributors
- Print issue
- Email alerts
Site powered by Webvision Cloud

COMMENTS
The scientific method, as developed by Bacon and others, involves several steps: Ask a question - identify the problem to be considered. Make observations - gather data that pertains to the question. Propose an explanation (a hypothesis) for the observations. Make new observations to test the hypothesis further.
Problem solving is a complex set of activities, processes, and behaviors for which various models have been used at various times. Specifically, "problem solving is a process by which the learner discovers a combination of previously learned rules that they can apply to achieve a solution to a new situation (that is, the problem)". 2 Zoller identifies problem solving, along with critical ...
In doing so, they are using the scientific method. 1.2: Scientific Approach for Solving Problems is shared under a not declared license and was authored, remixed, and/or curated by LibreTexts. Chemists expand their knowledge by making observations, carrying out experiments, and testing hypotheses to develop laws to summarize their results and ...
2.E: Measurement and Problem Solving (Exercises) Exercises for Chapter 2 of Tro's Introductory Chemistry textmap. Chemistry, like all sciences, is quantitative. It concerns quantities, things that have amounts and units. Dealing with quantities and relating them to one another is very important in chemistry. In ….
on problem solving for several reasons. First, problem solving is what chemists do, regardless of whether they work in the area of synthesis, spectroscopy, theory, analysis, or the characterization of compounds. Second, it was clear that individuals who were successful in chemistry courses either developed good problem solving skills — more or
Explain the role of modeling in chemistry. 1.1.3. Evaluate the statement, "Scientific models reveal what is true about the world." 1.1.4. ... Explain why orderly problem solving is important in chemistry. 3.3.4. Summarize the process of problem solving. 3.3.5.
The following situations are considered, some general, others with a focus on specific areas of chemistry: quantitative problems, qualitative reasoning, metacognition and resource activation, deconstructing the problem-solving process, an overview of the working memory hypothesis, reasoning with the electron-pushing formalism, scaffolding ...
Description. Chapters. This book is the revised edition of Understanding Basic Chemistry Through Problem Solving published in 2015. It is in a series of Understanding Chemistry books, which deals with Basic Chemistry using the problem solving approach. Written for students taking either the university of Cambridge O-level examinations or the ...
The literature on problem-solving in chemistry is complicated by the absence of agreement on the meaning such basic terms as 'problem' and 'problem ... subgoals, (4) selecting what they recognised as important information from the translation statements obtained in the first three processes, ( 5) retrieving rules or facts from memory, (6 ...
Problem solving is a process of exploration. Like. historical explorers, students embarking on a problem need to equip themselves with as much knowledge as possible about the territory to be explored. Most university students are reasonably good at acquiring knowledge, if by "knowledge" one means "a compendium of facts".
Dimensional analysis in chemistry is an orderly problem solving process that establishes relationships between different units of measurement. The existence of this relationship allows a ...
The book is written in such a way as to guide the reader through the understanding and applications of essential chemical concepts using the problem-solving approach. The authors have also retained the popular discourse feature from their previous two books — Understanding Advanced Physical Inorganic Chemistry and Understanding Advanced ...
Problem solving in any area is a very complex process. It involves an understanding of the language in which the problem is stated, the interpretation of what is given in the problem and what is sought, an understanding of the science concepts involved in the solution, and the ability to perform mathematical operations if these are involved in ...
Written for students taking either the University of Cambridge O-level examinations or the GCSE examinations, this guidebook covers essential topics and concepts under stipulated chemistry syllabi. The topics are explored through an explanatory and inquiry-based approach. They are interrelated and easy to understand, with succinct explanations ...
2.6: Problem-Solving Strategies is shared under a not declared license and was authored, remixed, and/or curated by LibreTexts. The conversion factor works because of the relationship, not because it is has a value of one. Once we have established that a relationship exists, it is no longer necessary to memorize a ….
Article. Research Process. The scientific method is a systematic process involving steps like defining questions, forming hypotheses, conducting experiments, and analyzing data. It minimizes biases and enables replicable research, leading to groundbreaking discoveries like Einstein's theory of relativity, penicillin, and the structure of DNA.
Mental models: The role of representations in problem-solving in chemistry. University Chemistry Education, 4, 24-30. Google Scholar. Bowen, C.W. (1990). Representational systems used by graduate students while problem-solving in organic synthesis. Journal of Research in Science Teaching, 27, 351-370.
Unit analysis helps avoid errors in a multi-step calculation and provides the units for the final answer. 1) Write the units with every number you include in a series of calculations. 2) String your calculations together as a series of multiplications or divisions before doing any math. 3) Cancel your units to see the calculation evolve.
Bolstering problem-solving skills is the most important thing you can do to prepare your learners for the UK Chemistry Olympiad, and taking them over past questions from the UK Chemistry Olympiad is a vital part of this. You'll find previous questions and mark schemes on the RSC Education website (rsc.li/3WNFDu5). Explore these during ...
These pages present some stoichiometry problems and strategies for solving them. The pages recommend a problem solving strategy then show you how to work through each step of the problem. As you work through the problems, you will notice that: The current step is displayed in bold. Information used in the current step is highlighted in red.
Explain why orderly problem solving is important in chemistry. List each of the steps in chemical problem solving. Demonstrate how to use formulas in problem solving. Describe how to check the reasonableness of an answer. Use the problem solving methods discussed in this section to obtain a correct answer to a mathematical problem. Chapter 4 ...
Solution. Since density = mass volume, we need to divide the mass in grams by the volume in milliliters. In general: the number of units of B = the number of units of A × unit conversion factor. The necessary conversion factors are given in Table 1.7.1: 1 lb = 453.59 g; 1 L = 1.0567 qt; 1 L = 1,000 mL.
Chemistry (updated 5/10/21) Explain how a scientist's worldview affects how he interprets evidence. Justify the statement, "I am able to serve God as a chemist." Describe how a chemist solves problems. Explain the phrase "Think Safety" in the context of the chemistry laboratory. Define chemistry. Explain the role of modeling in chemistry.
Chemists measure the properties of matter and express these measurements as quantities. A quantity is an amount of something and consists of a number and a unit. The number indicates how many (or how much), and the unit describes the scale of measurement. For example, when a distance is reported as "5 miles," the quantity has been expressed ...