Updates on COVID-19 for Grad Students and Postdocs
Graduate program in stem cell biology & regenerative medicine, stanford is a world leader in stem cell research and regenerative medicine. central discoveries in stem cell biology – tissue stem cells and their use for regenerative therapies, transdifferentiation into mature cell-types, isolation of cancerous stem cells, and stem cell signaling pathways – were made by stanford faculty and students. our mission is to train the next generation of stem cell scientists..
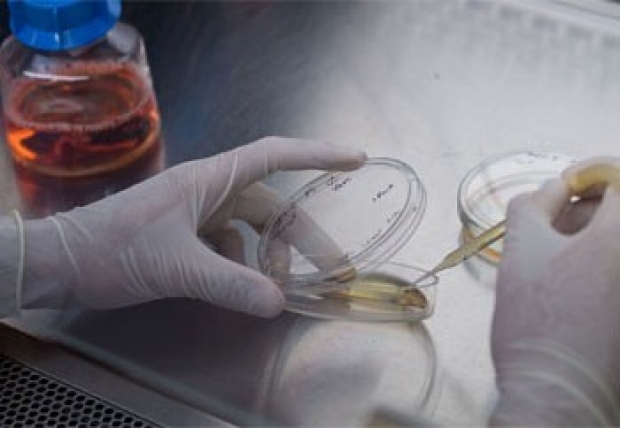

About the SCBRM Graduate Program
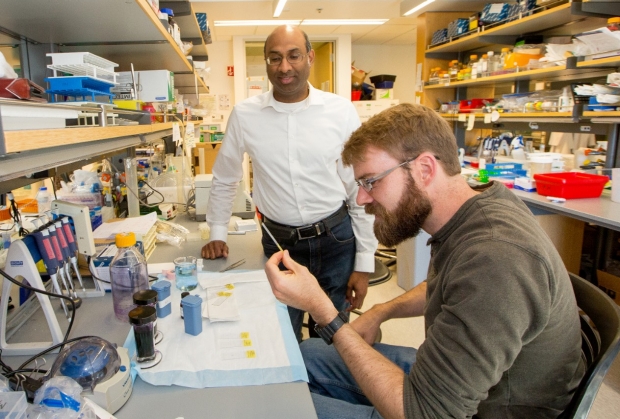
Unique Opportunities for Medical Students

Stem Cell PhD Program
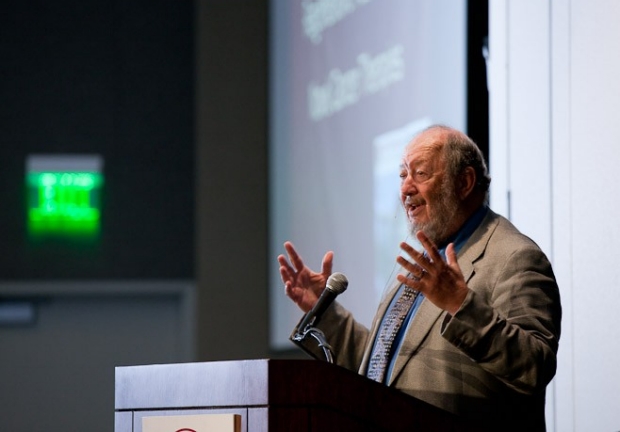
Faculty and Their Research Interests
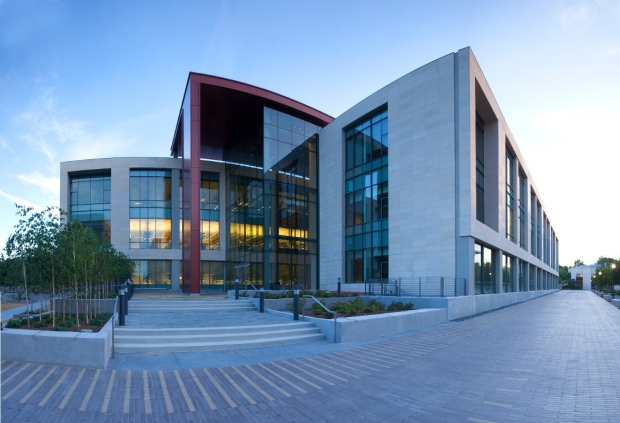
Application Process
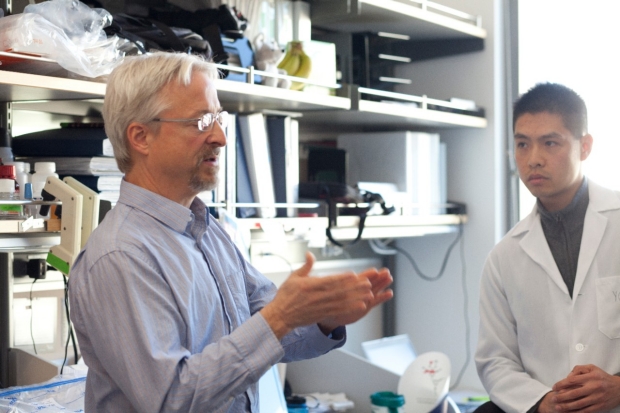
Curriculum and Handbooks
Learn about the many ways to support the institute for Stem Cell Biology and Regenerative Medicine
- Duke University »
- Pratt School of Engineering »
- Degree Program
- Enrollment and Graduation Rates
- Career Outcomes
- Campus Tours
- How to Apply
- Double Majors
- BME Design Fellows
- For Current Students
- 4+1: BSE+Master's Degree
- Degree Programs
- Concentrations
- Certificates
- PhD Program
- Meet Our Students
- Certificates & Training Programs
- Major Research Programs
- Centers & Initiatives
- Research News
- Faculty Profiles
- Awards & Recognition
- About the Partnership
- Proposal Process
- Oversight Committee
- Vision & Mission
- Facts & Stats
- Serving Society
- Our History
- Community Resources
- DEIC Across Duke BME
- Report a Concern
- Email Newsletter
- Media Coverage
- Driving Directions

Tissue Engineering and Regenerative Medicine
Research in tissue engineering and regenerative medicine seeks to replace or regenerate diseased or damaged tissues, organs, and cells – a challenging endeavor, but one that has tremendous potential for the practice of medicine.
Technologies under investigation range from biomaterial/cell constructs for repairing various tissues and organs, to stem cell therapies, to immune therapies. Our work in this area is highly multidisciplinary, combining materials science, cell biology, clinical science, immunology, stem cell biology, genome science, and others.
Accordingly, researchers in this area within Duke BME are broadly interactive with departments throughout the university including Duke University Medical Center clinical departments, the Duke University School of Medicine departments of Cell Biology and Immunology, the Duke Department of Chemistry, and others. This community is also supported by centers and programs such as Regeneration Next and the Center for Biomolecular and Tissue Engineering (CBTE) .
Primary Faculty

Nenad Bursac
Professor of Biomedical Engineering
Research Interests: Embryonic and adult stem cell therapies for heart and muscle disease; cardiac and skeletal muscle tissue engineering; cardiac electrophysiology and arrhythmias; genetic modifications of stem and somatic cells; micropatterning of proteins and hydrogels.

Pranam D. Chatterjee
Assistant Professor of Biomedical Engineering
Research Interests: Integration of computational and experimental methodologies to design novel proteins for applications in genome editing, targeted protein modulation, and reproductive bioengineering

Joel Collier
Theodore Kennedy Professor of Biomedical Engineering
Research Interests: The design of biomaterials for a range of biomedical applications, with a focus on understanding and controlling adaptive immune responses. Most materials investigated are created from molecular assemblies- proteins, peptides or bioconjugates that self-organize into useful…

Sharon Gerecht
Paul M. Gross Distinguished Professor of Biomedical Engineering
Research Interests: stem cells, biomaterials, hypoxia, blood vessels, physics of cancer, regenerative medicine

Charles Gersbach
John W. Strohbehn Distinguished Professor of Biomedical Engineering
Research Interests: Gene therapy, genomics and epigenomics, biomolecular and cellular engineering, regenerative medicine, and synthetic biology.
John Wirthlin Hickey

Samira Musah
Assistant Professor in the Department of Biomedical Engineering
Research Interests: Induced pluripotent stem cells (iPS cells), disease mechanisms, regenerative medicine, molecular and cellular basis of human kidney development and disease, organ engineering, patient-specific disease models, biomarkers, therapeutic discovery, tissue and organ transplantation,…

Tatiana Segura
Research Interests: The design of biomaterials to promote endogenous repair and reducing inflammation through the design of the geometry of the material, and delivering genes, proteins and drugs.

George A. Truskey
R. Eugene and Susie E. Goodson Distinguished Professor of Biomedical Engineering
Research Interests: Cardiovascular tissue engineering, mechanisms of atherogenesis, cell adhesion, and cell biomechanics.

Shyni Varghese
Professor of Biomedical Engineering, Mechanical Engineering & Materials Science and Orthopaedics
Research Interests: Musculoskeletal tissue repair, disease biophysics and organ-on-a-chip technology
Secondary Faculty

Geoffrey Steven Ginsburg
Adjunct Professor in the Department of Medicine

Cynthia Ann Toth
Joseph A.C. Wadsworth Distinguished Professor of Ophthalmology

Stefan Zauscher
Professor in the Thomas Lord Department of Mechanical Engineering and Materials Science
Research Interests: Nano-mechanical and nano-tribological characterization (elasticity, friction, adhesion) of materials including organic thin films; self-assembled monolayers, polymeric gels, and cellulosics; Fabrication of polymeric nanostructures by scanning probe lithography; Colloidal probe…
Adjunct Faculty
Jennifer L West
Adjunct Professor of Biomedical Engineering
Research Interests: Biomaterials, nanotechnology and tissue engineering that involves the synthesis, development, and application of novel biofunctional materials, and the use of biomaterials and engineering approaches to study biological problems.
Faculty Emeritus

William M. Reichert
Professor Emeritus of Biomedical Engineering
Research Interests: Biosensors, protein mediated cell adhesion, and wound healing.
- Student/Faculty Portal
- Learning Hub (Brightspace)
- Continuous Professional Development
Regenerative Sciences
Regenerative sciences track.
faculty spanning multidisciplinary departments
education in discovery, clinical translation, and application of regenerative solutions
Guaranteed 5-year internal fellowship
includes full tuition, stipend and benefits
Seeking to spur development of innovative medical breakthroughs, Mayo Clinic Graduate School of Biomedical Sciences, in partnership with the Center for Regenerative Biotherapeutics , started one of the nation's first doctoral research training programs in regenerative sciences.
Regenerative medicine is transforming clinical practice with the development of new therapies, treatments and surgeries for patients with chronic conditions, debilitating injuries and degenerative diseases. Advances in developmental and cell biology, immunology, and other fields unlock new opportunities for innovative breakthroughs for the next generation of regenerative diagnostic and therapeutic medical solutions.
The Regenerative Sciences (REGS) Ph.D. track at Mayo Clinic is a transdisciplinary Ph.D. Program designed to prepare the next generation of scientists to accelerate the discovery, translation, and application of cutting-edge regenerative diagnostics and therapeutics. The REGS Ph.D. track builds on the existing Mayo Clinic Regenerative Sciences Training Program (RSTP) to now offer in-depth curriculum and advanced training opportunities.
The Regenerative Sciences Track places a significant emphasis on laboratory-based research training. Laboratory research is complemented with both core and track-specific courses, as well as advanced courses on current topics in regenerative science and medicine.
The regenerative sciences curriculum encompasses the full spectrum of regenerative science topics, including molecular and cell biology, stem cell biology, developmental biology, tissue engineering, biomaterials and nanomedicine, genome editing and gene therapies, regulatory and translational science, product development, biomanufacturing, entrepreneurship and more.
Students in Regenerative Sciences join a close-knit community of learners, are provided unique hands-on- experiences and collaborate with some of the brightest minds in the field.
See the full Regenerative Sciences Track curriculum (PDF)
Graduates of the Regenerative Sciences Ph.D. track will be integral to forming the multidisciplinary workforce needed to drive the future of health care at Mayo Clinic and across the world.
Learn more: What is Regenerative Medicine - Mayo Clinic Radio
Focus areas
- Molecular and epigenetic mechanisms of stem and progenitor cell proliferation and differentiation, as well as tissue degeneration and regeneration
- Immune responses to viral insult and tissue healing
- Gene editing for cell therapy applications and to alter disease progression
- Extracellular vesicles in disease progression and for tissue regeneration
- Tissue engineering and bioengineering of novel therapies, including 3-D printing, electrospinning, and advanced biomanufacturing
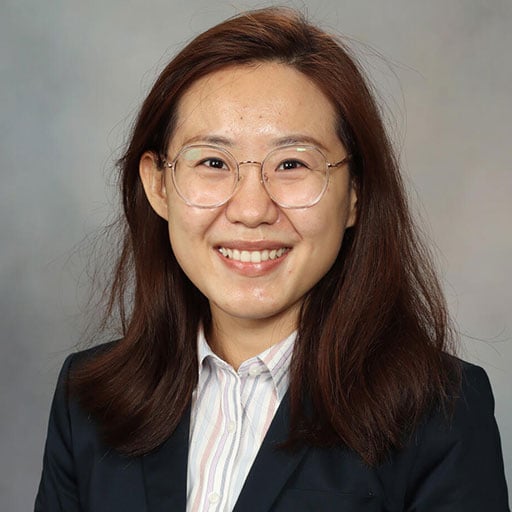
Mayo Clinic is an incredible place for doctoral training in regenerative science. The interdisciplinary strategy here allows research and courses to be tailored according to each student’s interests and ability. Moreover, Mayo Clinic provides a wealth resource to develop collaborations within the institution, which will offer students more ways to communicate and promote students to achieve their personal goals.
Shan Gao Ph.D. student, Regenerative Sciences Track
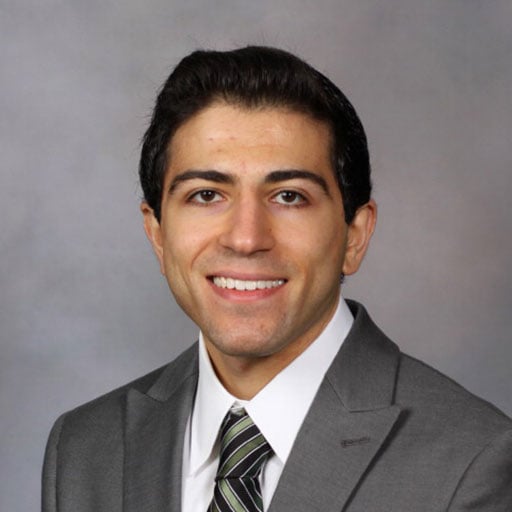
Mayo Clinic provides unparalleled access to world-renowned clinicians and researchers all focused on clinically relevant research. Mayo Clinic’s Center for Regenerative Medicine permeates throughout the institution. Thus, the REGS program gives students the necessary experience and knowledge to drive future research in restoring form and function in any field of medicine.
Armin Garmany M.D.-Ph.D. student, Regenerative Sciences Track

The study of Regenerative Sciences (REGS) at Mayo Clinic is unparalleled. Students are funded to study cutting-edge biomedical science in their domain of interest with plentiful opportunities to translate benchside discoveries to the patient bedside and beyond. I chose Mayo Clinic's REGS program to join its community of researchers, practitioners, and entrepreneurs who everyday advance the science and practice of regenerative medicine and bring new regenerative solutions to the world.
Samuel Buchl Ph.D. student, Regenerative Sciences Track
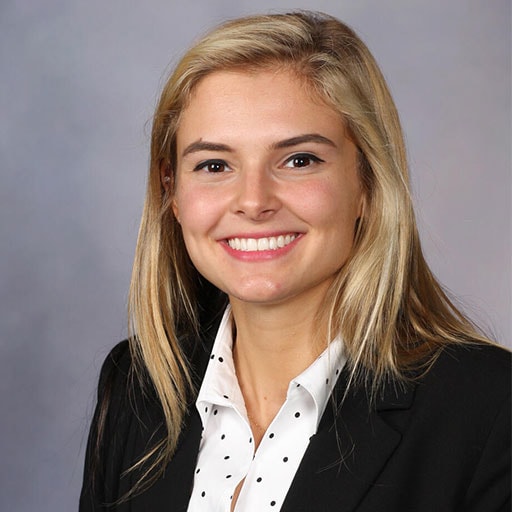
The Regenerative Sciences Ph.D. track at Mayo Clinic thoroughly equips students to be leaders in biomedical research through an unmatched curriculum of multidisciplinary science and world-class research training. REGS is a collaborative and supportive program in a promising field of medicine that provides the foundational skills to pipeline research to patient care.
Delaney Liskey Ph.D. student, Regenerative Sciences Track
Thesis topics
Current students thesis topics.
- "Targeted Regenerative Therapies for Heart Failure Susceptibility," Armin Garmany (Mentor: Andre Terzic, M.D., Ph.D.)
- "Novel Look Into the Crude Stromal Vascular Fraction (SVF) from Human Adipose-Derived Tissue and Its Role in Regulating the Self-Renewing Capacity of Brain Tumor-Initiating Cells," Rawan Alkharboosh (Mentor: Alfredo Quinones-Hinojosa, M.D.)
- "Tissue Quality in Existing and Emerging Treatments for Osteoarthritis," Katherine Arnold (Mentor: Jennifer Westendorf, Ph.D.)
- "Harnessing the Mesenchymal Stem Cell Secretome to Target Alpha-Synuclein-Associated Dysfunction in Parkinson's Disease," Jeremy Burgess (Mentor: Pamela McLean, Ph.D.)
- "Retinal Neuroprotection Properties of an ATP-Sensitive Potassium Channel Opener," Catherine Knier (Mentor: Michael Fautsch, Ph.D.)
- "Towards a Subcutaneous Combination Biodevice for the Treatment of Type 1 Diabetes," Ethan Law (Mentor: Quinn Peterson, Ph.D.)
- "Modulation of CART Cell Activation to Enhance Antitumor Response via CRISPR-mediated Gene Editing and Combined Immunotherapy," Claudia Manriquez Roman (Mentor: Saad Kenderian, M.B., Ch.B.)
- "Systems Biology for Engineering Regenerative Immunotherapies in Precision Neuro-oncology," Dileep Monie (Mentors: Hu Li, Ph.D. and Richard Vile, Ph.D.
- "APOE2 Effects on Central and Peripheral Vasculature," Francis Shue (Mentor: Guojun Bu, Ph.D.)
- "Engineering of Antiviral Extracellular Vesicles," Amanda Terlap (Mentor: Atta Behfar, M.D., Ph.D.)
- "Glycome of Breast Cancer-Derived Extracellular Vesicles in Metastasis," Sierra Walker (Mentor: Joy Wolfram, Ph.D.)
- "Bidirectional Interactions Between Stem Cell Populations of the Subventricular Zone and Glioblastoma," Emily Norton (Mentor: Hugo Guerrero Cazares, M.D., Ph.D.)
- "Measles Virus Vector for Gene Editing and Reprogramming of Human Fibroblasts," Ramya Rallabandi (Mentor: Patricia Devaux, Ph.D.)
- "Precise Genetic Engineering of Human Primary Cells for Cell Therapy-Based Applications," (Mentor: Stephen Ekker, Ph.D.)
Recent graduates thesis topics
- "Epigenetic Control of the Architectural and Trophic Functions of Mesenchymal Stem Cells in Musculoskeletal Tissue Regeneration Therapies," (Mentor: Andre van Wijnen, Ph.D.)
- "Metabolic Regulation of Muscle Stem Cells," (Mentor: Jason Doles, Ph.D.)
- "Purified Exosome Product Enhances Neovascularization in Peripheral Arterial Disease," (Mentors: Atta Behfar, M.D., Ph.D. and Andre Terzic, M.D., Ph.D.)
- "Antigen Presentation by CNS-Resident Microglia and Macrophages is Required for Antigen-Specific CD8 T Cell Responses in the Brain Following Viral Challenge," (Mentor: Aaron Johnson, Ph.D.)
Meet the director
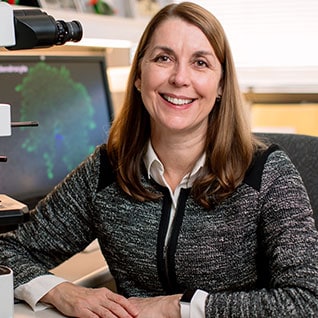
Training opportunities extend from fundamental science principles through laboratory skills and hands-on experiences. Students will also have the opportunity to develop an understanding of national and international regulatory agencies, and business requirements and procedures needed to implement the discovery, translation, application pipeline for new regenerative technologies.
We are excited to provide a program of training that will serve as an incubator to develop the next generation of leaders in regenerative science and medicine.
Isobel Scarisbrick, Ph.D. Regenerative Sciences Track Director Professor of Physical Medicine & Rehabilitation Phone: 507-284-0124 Email: [email protected] See research interests
Browse a list of Regenerative Sciences Track faculty members
Frontier research and innovative teaching at the interface of biology and medicine
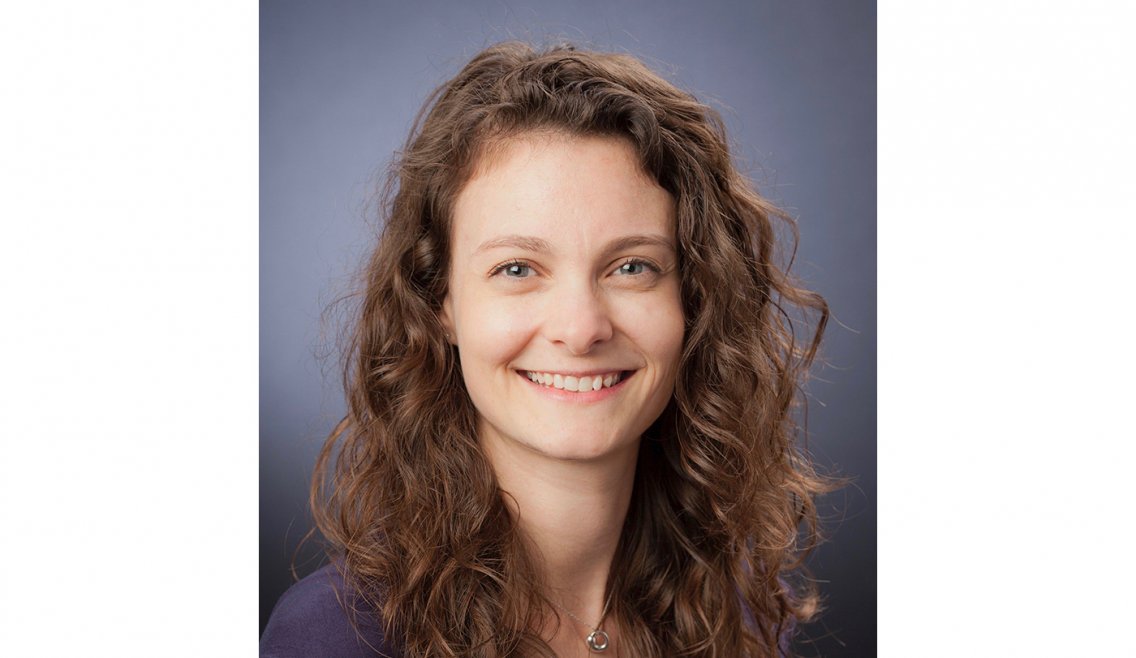
HSCRB Faculty Recognized for their Excellence in Undergraduate Teaching
Two hscrb faculty members were awarded honors for their work instructing undergraduates..
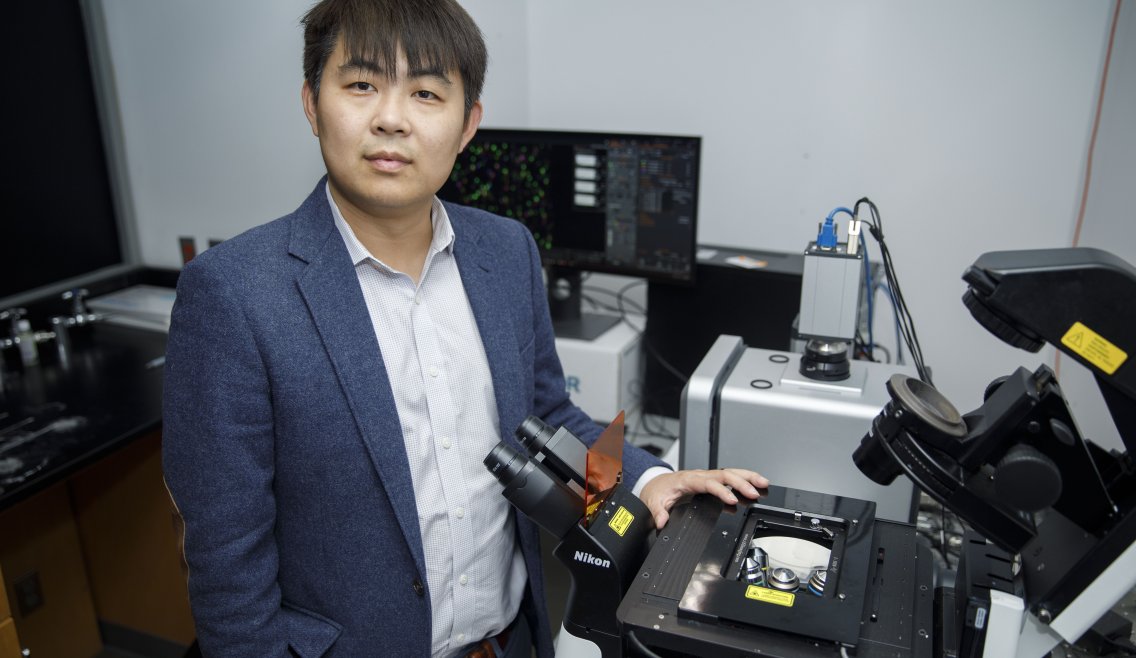
Fei Chen Wins 2024 Star-Friedman Challenge for Promising Scientific Research
The challenge provides seed funding for novel research in the physical or social sciences, with an emphasis on new directions that might not otherwise be supported through traditional funding channels., hscrb research, combating disease and tissue degeneration and improving human health., related links.
- Our Research
- Faculty and Labs
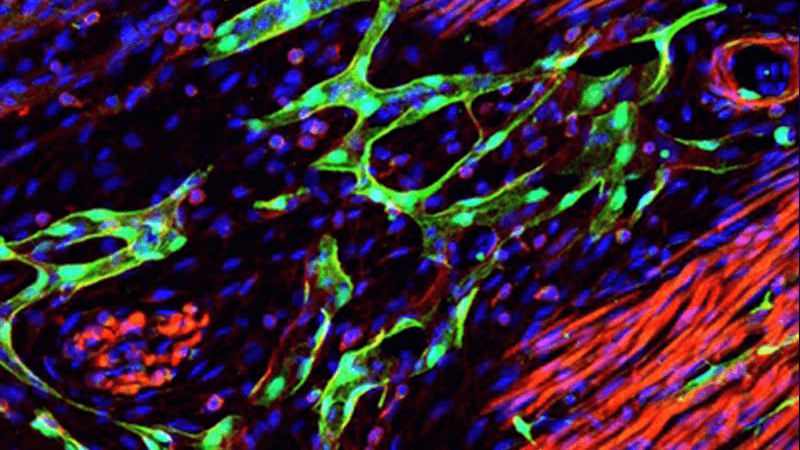
HSCRB Education
The best place to learn about stem cell science, at the graduate and undergraduate level..
- Undergraduate Students
- Graduate Students
HSCRB Introduces New Postdoc Professional Development Series
- Architecture
- Engineering
- Fashion & Textiles
- Social Science
Building for a future that has yet to be defined.
- Sidney Kimmel Medical College
- College of Architecture & The Built Environment
- Kanbar College of Design, Engineering & Commerce
- College of Health Professions
- College of Humanities & Sciences
College of Life Sciences
- College of Nursing
- College of Pharmacy
- College of Population Health
- College of Rehabilitation Sciences
- Institute of Emerging Health Professions
- Continuing & Professional Studies
- Jefferson Institute for Bioprocessing
Prepare yourself for what’s yet to come.
- Request Information
- Schedule a Visit
- Take a Virtual Tour
- Apply for Admission
- Financing Your Education
Contact Admissions
Start your Jefferson journey today.
- Financial Aid Office
- Student Accounts Office
Expert Center City and East Falls staffs are here to help.
Each of our locations offers a unique learning environment.
- Research Research Research Overview Applied Research Basic Research Clinical Research Labs & Service Centers Research Resources
- News News The Nexus News Archive
Cell Biology & Regenerative Medicine
Doctor of Philosophy
4 - 5 Years
Call to Actions
Contact information.
- Co-Director, Cell Biology & Regenerative Medicine PhD Program
- Associate Professor
Program Information
1020 Locust Street M-46 JAH Philadelphia , Pennsylvania 19107
- 215-951-2800
Connect with Us
The PhD Graduate Program in Cell Biology & Regenerative Medicine (CBRM) provides students with the background, training, and experience necessary to launch a career as an independent scientific investigator that significantly contributes to the advancement of human health. Faculty in our research program use the tools of molecular and cellular biology, tissue engineering, genetic animal models, advanced imaging techniques, bioinformatics, and computational modeling to uncover novel therapeutic strategies to address musculoskeletal injuries, tissue repair and regeneration, cardiovascular diseases, fibrosis, vision disorders, mitochondrial diseases, neurodegenerative conditions, and more.
Program Overview
The Cell Biology & Regenerative Medicine (CBRM) program is seeking students with a strong interest and background in science and engineering, particularly cell biology, developmental biology, molecular biology, biomedical engineering, bioengineering, biochemical engineering, quantitative biology, and related fields. Students are offered foundational coursework in their first year followed by a combination of electives tailored to their research interests and career aspirations. Students will develop competencies in tissue engineering, imaging for cell biology, bioinformatics, extracellular matrix biology and cell signaling. Formal training in grant proposal and scientific writing as well as weekly research discussion groups complete the student research and academic experience.
The CBRM program brings together faculty members across disciplines to offer students opportunities to craft their thesis research in a vibrant scientific environment with state-of the-art facilities. Students' research and education is fully supported through NIH training grants, endowed fellowships, investigator-initiated research grants, or individual graduate fellowships. The students also receive financial support to present their research findings at regional, national, and international scientific conferences. Graduates of the program have gone on to successful research careers in both academia and industry.
Here are the major research themes within the program:
- Musculoskeletal Biology
- Liver Disease, Surgery and Transplantation
- Cardiovascular Disease
- Mitochondrial Metabolism & Pathology
- Neurodegenerative & Vision Disorders
- Fibrosis & Matrix Biology
- Computational & Systems Biology

Student Group
A low student-to-faculty ratio is maintained to ensure an optimal learning environment for our students. The student body is diverse. The Graduate Student Association (GSA) coordinates many cultural and recreational activities, as well organizing community projects.
Additional Program Offerings

KEY APPLICATION AREA
Tissue Engineering & Regenerative Medicine
Research in tissue engineering and regenerative medicine encompasses all aspects of the research and development continuum from mechanistic studies to translational approaches. Collaborative efforts with colleagues at Rice and the Texas Medical Center address unmet clinical needs for a plethora of tissues ranging from bone to cartilage to heart valve to inner ear.
Specific areas of interest include structure and function relationships in living tissues, synthesis and fabrication of biomimetic materials and extracellular matrix constructs, combinations of biomaterials with cell populations for modulating cell function and guiding tissue growth, stem cell programming, drug and gene delivery systems for tissue induction and regeneration, 3D printing and bioprinting, and bioreactor designs for cell culture and disease modeling.
Rice BIOE researchers working in this key application area:
Caleb bashor, phd, faculty profile | laboratory website, jane grande-allen, phd, isaac hilton, phd, kevin mchugh, phd, antonios mikos, phd, jordan miller, phd, robert raphael, phd, omid veiseh, phd.

- Schools & departments

Regenerative Medicine PhD
Awards: PhD
Study modes: Full-time, Part-time
Funding opportunities
Programme website: Regenerative Medicine
Upcoming Introduction to Postgraduate Study and Research events
Join us online on the 19th June or 26th June to learn more about studying and researching at Edinburgh.
Choose your event and register
Research profile
Research excellence.
The Centre for Regenerative Medicine (CRM) is a world leading research centre based at the University of Edinburgh’s Institute for Regeneration and Repair.
Our scientists and clinicians study stem cells, disease and tissue repair to advance human health. By better understanding how stem cells are controlled and how diseases develop in a lab environment, we hope to find new ways to treat patients.
Our research is aimed at developing new treatments for major diseases including cancer, heart disease, diabetes, degenerative diseases such as multiple sclerosis and Parkinson's disease, and liver failure.
The Centre houses 25 research groups and has a staff of more than 270 scientists, graduate students, support and ancillary staff.
Research themes
Our work is currently organised into five themes. To promote collaboration within the Centre, we adopt a flexible approach to these themes, with each Principal Investigator (PI) having one or more secondary affiliations.
Two themes focus on fundamental research:
- pluripotency and iPS
- lineage and cell specification
The other three themes aim to translate fundamental research discoveries into clinical programmes relevant to brain, blood and liver diseases and to tissue repair.
The Centre has strong collaborative links to other centres within the University, such as the Euan MacDonald Centre for MND Research, the MS Centre and the Roslin Institute.
We also invest in technological development in all areas.
Training and support
Training within the Centre is provided through a structured series of seminars and literature reviews, in addition to the laboratory and scientific research skills training provided to you by your supervisors.
Many of our PhD students are involved in collaborative projects that provide cross-disciplinary experience and/or promote translation into the biotechnology or clinical fields.
How will I learn?
Our programme includes short courses taught by basic and clinical stem cell scientists, providing a state-of-the-art theoretical background in a variety of areas relating to regenerative medicine including:
- developmental biology
- pluripotent and tissue stem cell biology
- degeneration and regeneration of adult tissues
- genetic engineering
- bioinformatics
We provide specialist lectures and short practical modules covering key technologies, including:
- DNA analysis and genetic engineering
- flow cytometry
In Year 1, you will participate in a weekly Centre for Regenerative Medicine ( CRM ) Postgraduate Discussion Group led by CRM group leaders. These discussion groups aim to widen your knowledge of stem cell and regenerative medicine research and to enhance your ability to critically review the literature in this field.
In addition to the taught components and research project, you will participate in a number of activities, including:
- regular lab meetings of your research group
- an internal seminar series
- seminars by visiting national and international speakers
- Journal Club
- poster presentations
- Three Minute Thesis presentation session.
Generic and transferable skills training is provided through the University's Institute for Academic Development (IAD).
- Institute of Academic Development
Since 2011, the Centre has been housed in a new, specially designed building that provides high quality research facilities, including:
- state of the art centralised cell culture facility for isolation and culture of primary and established cell lines including embryonic and induced pluripotent stem cells
- clinical-grade GMP cell culture facility
- specific pathogen free animal facility
- transgenic service covering derivation and provision of mouse embryonic stem cells, blastocyst injection, morula aggregation and production of defined genetic alterations
- ultrasound micro-injection equipment
- flow cytometry service consisting of a suite of cell sorters and analysers operated by facility staff that can be operated by users following comprehensive training
- a recently established single cell genomic analysis service using a 10x Genomics Chromium Controller
- quantitative real-time polymerase chain reaction equipment
- Fluidigm Biomark and CellPrep for single cell transcriptomics
Imaging facilities
We also have imaging facilities, including:
- standard compound microscopy
- widefield, confocal, and lightsheet microscopes
- high-content and timelapse imaging
The facility has dedicated imaging managers and offers two high-end workstations for bio-image processing and analysis.
Take a virtual tour of our facilities at the Centre for Regenerative Medicine:
- Virtual tour
Entry requirements
These entry requirements are for the 2024/25 academic year and requirements for future academic years may differ. Entry requirements for the 2025/26 academic year will be published on 1 Oct 2024.
A UK 2:1 honours degree or its international equivalent.
International qualifications
Check whether your international qualifications meet our general entry requirements:
- Entry requirements by country
- English language requirements
Regardless of your nationality or country of residence, you must demonstrate a level of English language competency at a level that will enable you to succeed in your studies.
English language tests
We accept the following English language qualifications at the grades specified:
- IELTS Academic: total 6.5 with at least 6.0 in each component. We do not accept IELTS One Skill Retake to meet our English language requirements.
- TOEFL-iBT (including Home Edition): total 92 with at least 20 in each component. We do not accept TOEFL MyBest Score to meet our English language requirements.
- C1 Advanced ( CAE ) / C2 Proficiency ( CPE ): total 176 with at least 169 in each component.
- Trinity ISE : ISE II with distinctions in all four components.
- PTE Academic: total 62 with at least 59 in each component.
Your English language qualification must be no more than three and a half years old from the start date of the programme you are applying to study, unless you are using IELTS , TOEFL, Trinity ISE or PTE , in which case it must be no more than two years old.
Degrees taught and assessed in English
We also accept an undergraduate or postgraduate degree that has been taught and assessed in English in a majority English speaking country, as defined by UK Visas and Immigration:
- UKVI list of majority English speaking countries
We also accept a degree that has been taught and assessed in English from a university on our list of approved universities in non-majority English speaking countries (non-MESC).
- Approved universities in non-MESC
If you are not a national of a majority English speaking country, then your degree must be no more than five years old* at the beginning of your programme of study. (*Revised 05 March 2024 to extend degree validity to five years.)
Find out more about our language requirements:
Fees and costs
Additional programme costs.
Most laboratories require a bench fee of up to £5,000 per year. This cost can be covered in Research Council studentships.
Living costs
You will be responsible for covering living costs for the duration of your studies.
Tuition fees
Scholarships and funding, featured funding.
- College of Medicine & Veterinary Medicine funding opportunities
UK government postgraduate loans
If you live in the UK, you may be able to apply for a postgraduate loan from one of the UK’s governments.
The type and amount of financial support you are eligible for will depend on your programme, the duration of your studies, and your residency status.
Programmes studied on a part-time intermittent basis are not eligible.
- UK government and other external funding
Other funding opportunities
Search for scholarships and funding opportunities:
- Search for funding
Further information
- Postgraduate Administrator, Kelly Douglas
- Phone: +44 (0)131 651 9500
- Contact: [email protected]
- Centre for Regenerative Medicine
- Institute for Regeneration and Repair
- The University of Edinburgh
- Little France
- Programme: Regenerative Medicine
- School: Edinburgh Medical School: Clinical Sciences
- College: Medicine & Veterinary Medicine
Select your programme and preferred start date to begin your application.
PhD Regenerative Medicine - 3 Years (Full-time)
Phd regenerative medicine - 6 years (part-time), application deadlines.
We encourage you to apply at least one month prior to entry so that we have enough time to process your application. If you are also applying for funding or will require a visa then we strongly recommend you apply as early as possible.
- How to apply
You must submit two references with your application.
Before making your application, you must make contact with a potential supervisor to discuss your research proposal. Further information on making a research degree application can be found on the College website:
- How to apply for a research degree
Find out more about the general application process for postgraduate programmes:
- Sponsored Article
Brush Up: Tissue Engineering and Regenerative Medicine
A new frontier in repairing organ damage.
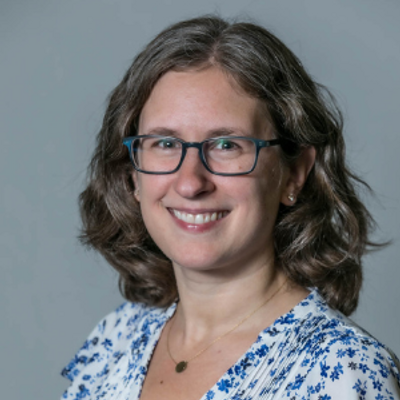
Jen has a PhD in human genetics from the University of California, Los Angeles where she is currently a project scientist. She enjoys teaching and communicating complex scientific concepts to a wide audience.
View full profile.
Learn about our editorial policies.
What Is Regenerative Medicine? Regenerative medicine replaces tissue or organs that are damaged by trauma, disease, or congenital disorders. This is different from more traditional therapies that treat the symptoms of tissue damage. There are three main concentrations within the field of regenerative medicine: tissue engineering, cellular therapy, and artificial organs. The use of tissue engineering in regenerative medicine, known as TERM, is an active area of research that involves creating functional tissue through the combination of cells, scaffolds, and growth factors to restore normal biological function. 1 Clinicians treat millions of patients with tissue engineered regenerative devices. So far, the most successful tissue regeneration therapies occur in soft tissues such as skin, cartilage, and corneal tissues.
Using Tissue Engineering to Regenerate Damaged Tissue
How does tissue engineering work?
During healthy tissue development, cells build and surround themselves with an extracellular matrix. This matrix, or scaffold, contains structural proteins and acts as a reservoir for signaling molecules that cells use to communicate and organize themselves into functional complexes or tissues.
The overall goal of tissue engineering in the context of regenerative medicine is to establish a 3D cell or biomaterial complex that functions similarly to the in vivo tissue extracellular matrix. In general, tissue engineering involves the design and implantation of a scaffold that is biologically compatible with the area to be regenerated. New cells are then either attracted to or grown directly onto the scaffold. 2 The FDA has approved engineered artificial cartilage and skin therapies, and researchers are developing many other therapies for different tissues and disorders (see table below).
Scaffolds in tissue engineering Scientists seed scaffolds with their desired cell type during or following implantation. Alternatively, they may add growth factors to the scaffold and wait until the structure is populated by the surrounding tissue.
Choosing a scaffold type and source for tissue engineering is imperative for regenerating functional tissue. Pore size and overall architecture are important variables to consider when designing a scaffold. Pores play a crucial role in tissue regeneration because they allow for the exchange of nutrition and oxygen with surrounding tissue as well as expulsion of waste products and vascularization. The overall architecture of the tissue is important for exposing surfaces for cell attachment as well as mechanical cell stimuli.
Scaffolds can be natural or synthetic. Natural scaffolds are derived from donor tissues where the cells are chemically removed, leaving only the extracellular matrix. Natural scaffolds can either come from a patient or a healthy donor, and they have the advantage of retaining the unique structural and functional architecture of complex tissues. Researchers can also create natural scaffolds in vitro, such as those made from collagen and Matrigel, which are comprised of basement membrane proteins. 3,4
Scientists can develop synthetic scaffolds from various polymers, including polyglycolic acid (PGA), polylactic acid (PLA), and poly(lactide-co-glycolide) acid (PLGA). Scaffolds made with these polymers are flexible and porous, making them ideal structures for cellular integration. Synthetic scaffolds are also biodegradable, with different polymers degrading at different times, allowing damaged tissue to regenerate without the use of permanent prosthetic implants. Synthetic scaffolds also have consistent structures between replicates as they are generated in a laboratory; however, they can cause inflammation in the recipient more readily than natural scaffolds. 5
3D printing in tissue engineering With recent progress in 3D printing methods, researchers create complex synthetic scaffold structures with more consistent architecture and pore sizes. Hydrogel materials, such as alginate hydrogel and gelatin, are typically used in 3D printing due to their effective crosslinking and biocompatible properties. 6
Stem cells in tissue engineering and regenerative therapy Mesenchymal, embryonic, and induced pluripotent stem cells effectively promote damaged tissue regeneration. However, in many tissues, transplanted stem cells have poor survival and differentiation capabilities. The development of stem cell technology in combination with tissue engineering techniques, such as scaffolds and the addition of growth factors, has allowed researchers to improve the viability and proliferation of stem cells in regenerative medicine. 7
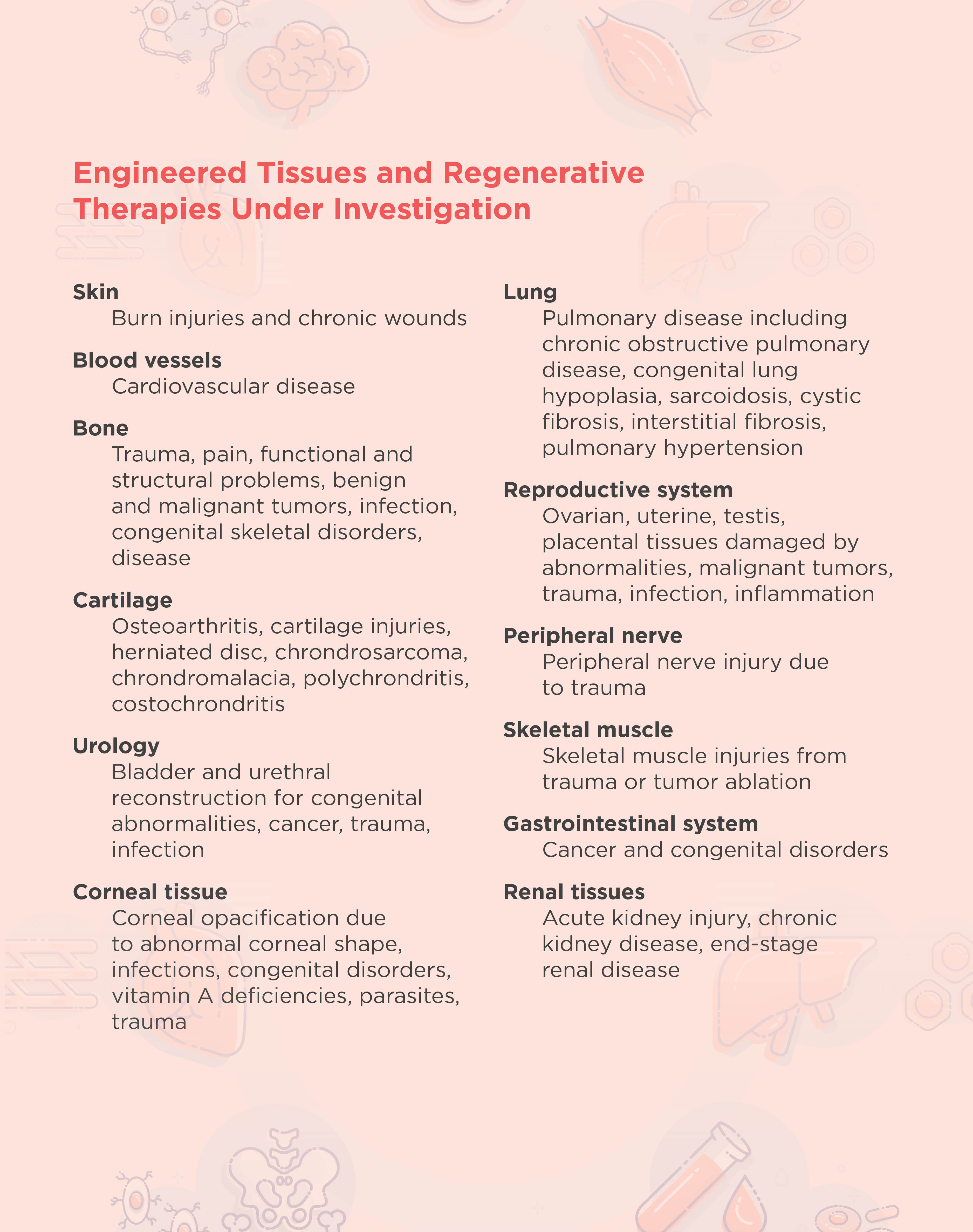
2. F. Han et al., “Tissue engineering and regenerative medicine: Achievements, future, and sustainability in Asia,” Front Bioeng Biotechnol , 8:83, 2020.
3. S. Sundaram et al., “Tissue engineering and regenerative medicine” in Rossi's Principles of Transfusion Medicine . Fifth edition. T.L. Simon, ed., New York, N.Y.: John Wiley & Sons Inc., 2016, pp. 488-504.
4. C. Motta et al., “Tissue engineering and regenerative medicine” in Rossi's Principles of Transfusion Medicine . Sixth edition. T.L. Simon, ed., New York, N.Y.: John Wiley & Sons Inc., 2022, pp. 648-660.
5. Y. Li et al., “The effect of mechanical loads on the degradation of aliphatic biodegradable polyesters,” Regen Biomater , 4:179-190, 2017.
6. Z. Yazdanpanah et al., “3D bioprinted scaffolds for bone tissue engineering: State-of-the-art and emerging technologies,” Front Bioeng Biotechnol , 10:824156, 2022.
7. S.G. Kwon et al., “Recent advances in stem cell therapeutics and tissue engineering strategies,” Biomater Res , 22:36, 2018.
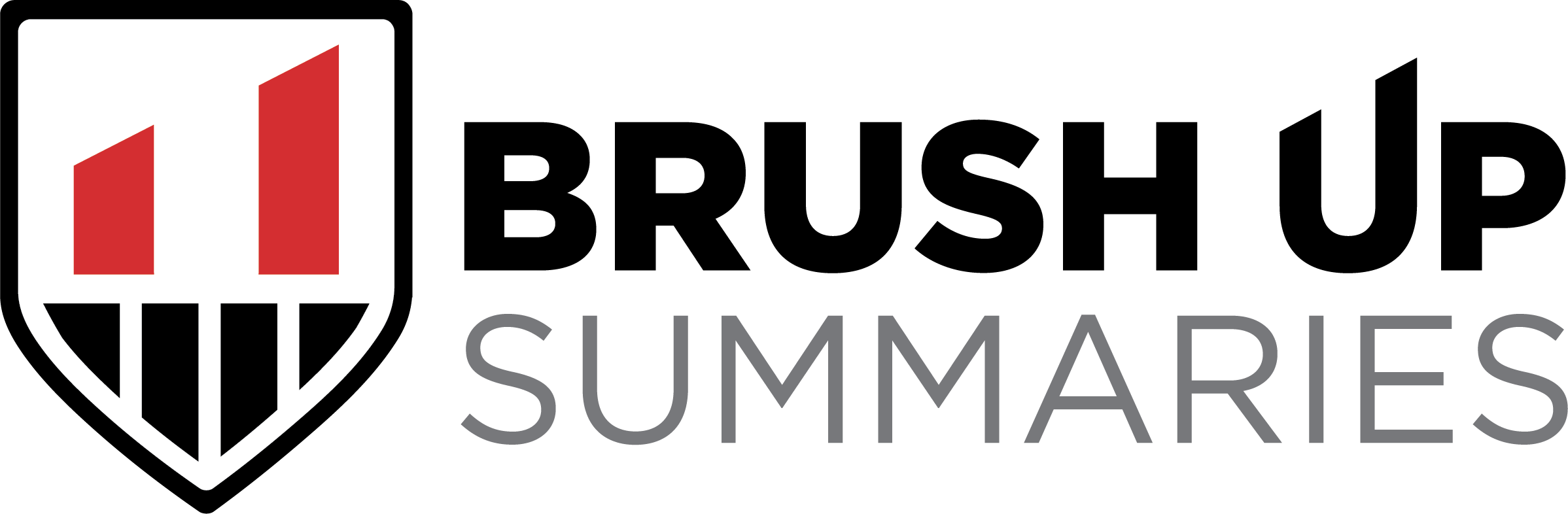
- Graduate School
- Medical, health and life sciences
- Medical biotechnology
Regenerative medicine (including stem cells and tissue engineering)
Parent category, research centres, institutes & clusters in regenerative medicine (including stem cells and tissue engineering).
- BC Regenerative Medicine - BCRegMed
Graduate Degree Programs in Regenerative medicine (including stem cells and tissue engineering)
Master of engineering in biomedical engineering (meng).
Biomedical Engineers apply their knowledge in engineering, biology, and medicine to healthcare and medical device industries. Biomedical Engineering is a distinct field that encompasses engineering disciplines, biology, life sciences, medicine, clinical applications, and the improvement of human...
UBC Researchers conducting research in Regenerative medicine (including stem cells and tissue engineering)
Mcnagny, kelly marshall, school of biomedical engineering, department of medical genetics, faculty of medicine.
Faculty (G+PS eligible/member)
Cellular immunology; Regenerative medicine (including stem cells and tissue engineering); Stem Cells; Immunology; Inflammation; Mouse models of human disease; Tissue degeneration/regeneration; Cancer; innate immune response; kidney function; Biologics and therapeutics
Rana, MD Mohosin
Faculty of medicine.
Postdoctoral Fellow
Regenerative medicine (including stem cells and tissue engineering); Material Sciences; Polymer chemistry; Tissue Engineering; Organ Engineering; Nanotechnology; Regenerative medicine; microfluidics; Bioengineering
Student & Alumni Stories in Regenerative medicine (including stem cells and tissue engineering)

Tanya Bennet
Doctor of Philosophy in Biomedical Engineering (PhD)
Airway-On-A-Chip: Development and In Vitro Validation of a Microfluidic Cell Culture Model For Chronic Obstructive Pulmonary Disease

Omar Bashth
Uncovering the Role of Human Stem Cell Competition in Pluripotency and Lineage Commitment
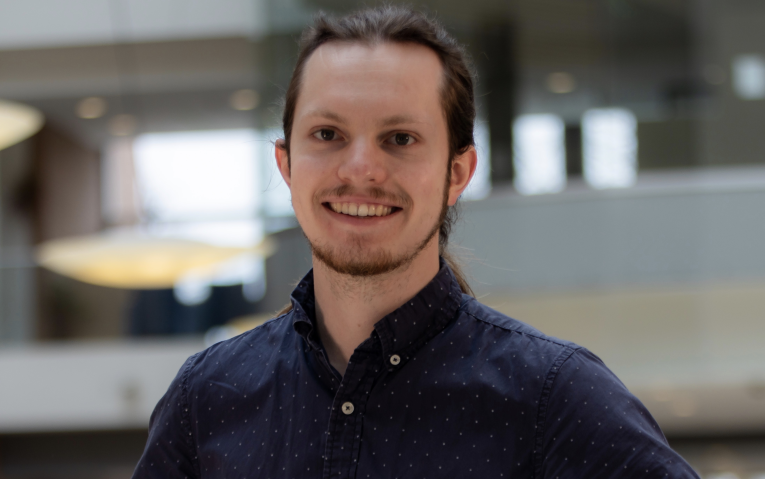
Alexander Garner
Doctor of Philosophy in Cell and Developmental Biology (PhD)
Investigating how pancreatic islet architecture impacts function
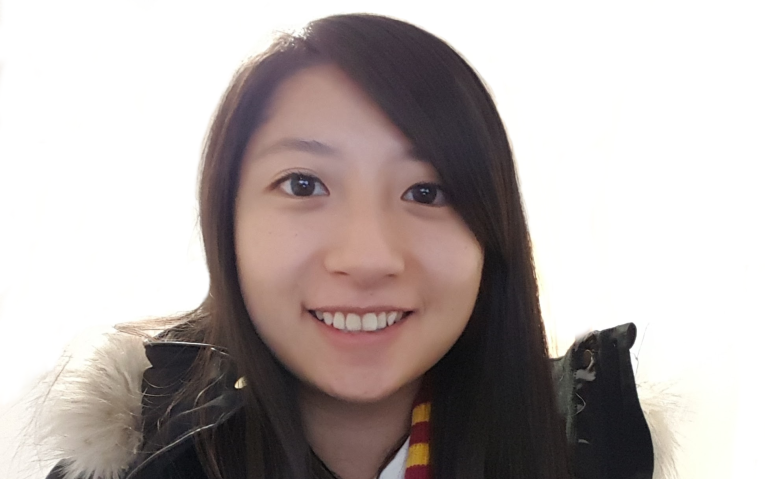
Doctor of Philosophy in Experimental Medicine (PhD)
Mutations in the giant protein titin and risk of heart rhythm disorders
French Name
French description.
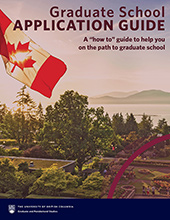
Read tips on applying, reference letters, statement of interest, reaching out to prospective supervisors, interviews and more in our Application Guide!
- Why Grad School at UBC?
- Graduate Degree Programs
- Application & Admission
- Info Sessions
- Research Supervisors
- Research Projects
- Indigenous Students
- International Students
- Tuition, Fees & Cost of Living
- Newly Admitted
- Student Status & Classification
- Student Responsibilities
- Supervision & Advising
- Managing your Program
- Health, Wellbeing and Safety
- Professional Development
- Dissertation & Thesis Preparation
- Final Doctoral Exam
- Final Dissertation & Thesis Submission
- Life in Vancouver
- Vancouver Campus
- Graduate Student Spaces
- Graduate Life Centre
- Life as a Grad Student
- Graduate Student Ambassadors
- Meet our Students
- Award Opportunities
- Award Guidelines
- Minimum Funding Policy for PhD Students
- Killam Awards & Fellowships
- Policies & Procedures
- Information for Supervisors
- Dean's Message
- Leadership Team
- Strategic Plan & Priorities
- Vision & Mission
- Equity, Diversity & Inclusion
- Initiatives, Plans & Reports
- Graduate Education Analysis & Research
- Media Enquiries
- Newsletters
- Giving to Graduate Studies
Strategic Priorities
- Strategic Plan 2019-2024
- Improving Student Funding
- Promoting Excellence in Graduate Programs
- Enhancing Graduate Supervision
- Advancing Indigenous Inclusion
- Supporting Student Development and Success
- Reimagining Graduate Education
- Enriching the Student Experience
Initiatives
- Public Scholars Initiative
- 3 Minute Thesis (3MT)
- PhD Career Outcomes
Tissue Engineering and Regenerative Medicine
- Indexed and included in PubMed/MEDLINE with all articles from 2019 searchable.
- Promotes sharing and dissemination of knowledge among members and provides insights into new research trends.
- Aims to stimulate interdisciplinary research integrating information from biology, medicine, pharmacology, and more.
- Covers a wide array of issues in polymer chemistry, natural science, engineering, molecular biology, genomics, cytology, medical science, etc.
- Listed in the Science Citation Index-Expanded (SCI-E) and published bimonthly.
- Chong-Su Cho
Societies and partnerships
- Korean Tissue Engineering and Regenerative Medicine (opens in a new tab)
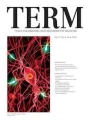
Latest issue
Volume 21, Issue 4
Latest articles
Lgr5 modulates differentiated phenotypes of chondrocytes through pi3k/akt signaling pathway.
- Tianyu Zhang
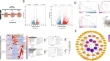
CA1 Modulates the Osteogenic Differentiation of Dental Follicle Stem Cells by Activating the BMP Signaling Pathway In Vitro
- Jin-ze Zhao
- Ying-Ying Ge
- Wen-lin Xiao
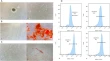
Hyaluronic Acid Based Adipose Tissue-Derived Extracellular Matrix Scaffold in Wound Healing: Histological and Immunohistochemical Study
- Dong Yeon Kim
- Eunjeong Ko
- Young Joon Jun
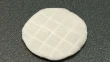
Development of Cell Culture Platforms for Study of Trabecular Meshwork Cells and Glaucoma Development
- Kook In Youn
- Kwang Hoon Song
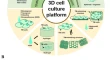
Reducing Nogo-B Improves Hepatic Fibrosis by Inhibiting BACe1-Mediated Autophagy
- YingJie Zhuang
- ZhengYi Liu
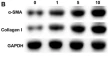
Journal updates
Special announcement.
Now indexed in PubMed/MEDLINE
We are pleased to announce that Tissue Engineering and Regenerative Medicine has been accepted for indexing and inclusion in PubMed/MEDLINE. All articles published from 2019 will be indexed and searchable in PubMed.
Please visit the PubMed website ( http://www.ncbi.nlm.nih.gov/pubmed/ ) for more information.
Journal information
- Chemical Abstracts Service (CAS)
- EI Compendex
- Google Scholar
- Japanese Science and Technology Agency (JST)
- Korea Citation Index (KCI)
- OCLC WorldCat Discovery Service
- PubMedCentral
- Science Citation Index Expanded (SCIE)
- TD Net Discovery Service
- UGC-CARE List (India)
Rights and permissions
Editorial policies
© Korean Tissue Engineering and Regenerative Medicine Society
- Find a journal
- Publish with us
- Track your research
- Undergraduate Courses
- Postgraduate Taught Courses
- Professional, Part-time and Evening Courses
- PhDs and Research Masters
- Online Courses
- Micro-credentials
- How to Apply
- Fees & Funding
- Modes of Study
- Scholarships
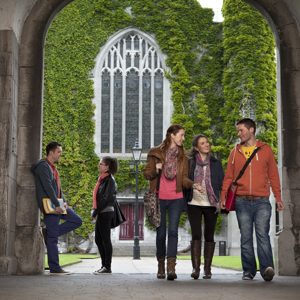
Choosing a course is one of the most important decisions you'll ever make! View our courses and see what our students and lecturers have to say about the courses you are interested in at the links below.
View Courses
- Accommodation Advisory Service
- Campus Activities
- Student Support
- Study Abroad
- International Office
- Mature Students
- Students with Disabilities
- Student Ambassador Programme
- For Parents and Guardians
- Access Student Information
- Life in Galway
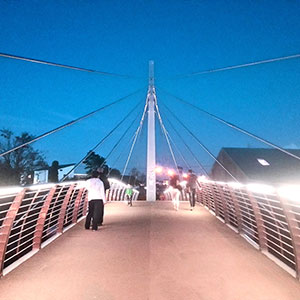
University Life
Each year more than 4,000 choose University of Galway as their University of choice. Find out what life at University of Galway is all about here.
Read about life at University of Galway
- News & Events
- Strategy 2020-2025
- Cois Coiribe (Publication)
- University Leadership
- Sustainability - National SDG Champion
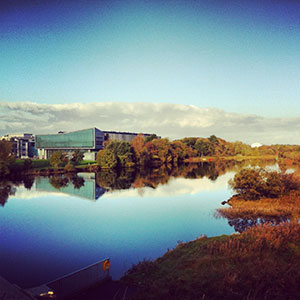
About University of Galway
Since 1845, University of Galway has been sharing the highest quality teaching and research with Ireland and the world. Find out what makes our University so special – from our distinguished history to the latest news and campus developments.
About University of Galway
- Adult Learning and Professional Development
- College of Arts, Social Sciences, & Celtic Studies
- College of Business, Public Policy and Law
- College of Medicine, Nursing & Health Sciences
- College of Science and Engineering
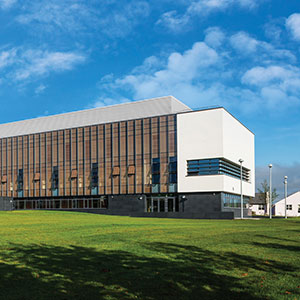
Colleges & Schools
University of Galway has earned international recognition as a research-led university with a commitment to top quality teaching across a range of key areas of expertise.
Colleges and Schools
Research Areas
- Research Office
- Innovation Office
- Researcher Development Centre
- Research Community Portal
- Research centres, institutes, and units
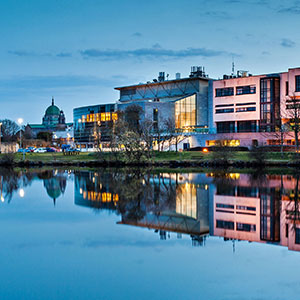
Research & Innovation
University of Galway’s vibrant research community take on some of the most pressing challenges of our times.
- Career Development Centre (for Employers)
- Business Innovation Centre
- Conference & Event Centre
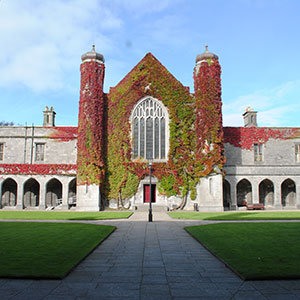
Guiding Breakthrough Research at University of Galway
We explore and facilitate commercial opportunities for the research community at University of Galway, as well as facilitating industry partnership.
- Latest News
- Alumni Services
- Cois Coiribe
- Alumni Awards
- Follow our Social Channels
- Update Your Details
- Upcoming Alumni Events
- Previous Alumni Events
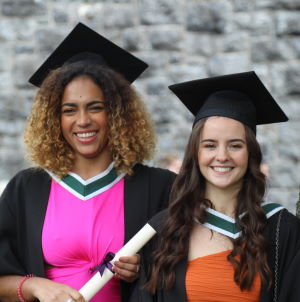
Alumni & Friends
There are 128,000 University of Galway alumni worldwide. Stay connected to your alumni community! Join our social networks and update your details online.
- About Engagement
- Learning with Community
- Community Partnerships
- Research with Communities
- University of Sanctuary
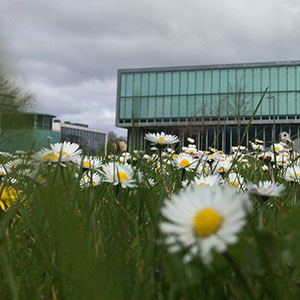

Community Engagement
At University of Galway, we believe that the best learning takes place when you apply what you learn in a real world context. That's why many of our courses include work placements or community projects.
Real Learning
Gateway Pages
- Prospective Students
- Current Students
- Ollscoil na Gaillimhe
- A High Contrast
- Registration
- Office 365 (Email)
- Student Registry Helpdesk
- Fees & Grants
- Exam Timetables
- Academic Skills Hub
- Student Services
- Student Volunteering
- Students' Union
- Financial System (Agresso)
- Academic Records
- Human Resources
- Academic Terms Dates
- Information Solutions & Services (IT Services)
- Buildings & Estates
- Service Desk
- Colleges & Schools
Biomedical Engineering and Regenerative Medicine (Structured PhD)
- Find a Supervisor/PhD Project
- Choosing a Research Programme
- Structured Programmes
- Non-Structured Programmes
- Research Scholarships
Course Overview
Course outline, course fees.
This Structured PhD programme spans the Biomedical Engineering and Regenerative Medicine domains and aims to become a leading international graduate education programme in the field, in which Irelandis already taking a strong international role. The overall objective is to develop PhD graduates who will produce ground-breaking scientific results benefiting human health and contributing to economic growth, and who will, in turn, lead research and development in these fields in industry and academia. BME&RM combines a PhD research project with a unique experiential learning and didactic programme, resulting in an unparalleled learning experience for the student in terms of its combination of world-class research and focused clinical and industrial interaction.
The BME&RM programme will be delivered by a core partnership of institutions: National University of Ireland Galway, University of Limerick and University College Cork, linked with a wider consortium of partner institutions nationally and internationally: Galway-Mayo Institute of Technology, Institute of Technology Sligo, University of Ulster, Queen’s University Belfast, Georgia Institute of Technology, University of Pittsburgh, Duke University, Irish Medical Devices Association (IMDA), Rice University, Mayo Clinic, RWTH Aachen University and Georgia Tech Ireland.
Programmes Available
(Structured Phd) Biomedical Engineering and Regenerative Medicine—4 years (full-time) Applications are made online via the NUI Galway Postgraduate Applications System .
Learning Outcomes
Entry requirements.
More Information can be obtained from the Mechanical and Biomedical Engineering website:
http://www.nuigalway.ie/mechbio/bmerm/
Who’s Suited to This Course
Current research projects.
Current Project
Current funded research opportunity
Work placement, related student organisations, career opportunities, find a supervisor / phd project.
If you are still looking for a potential supervisor or PhD project or would like to identify the key research interests of our academic staff and researchers, you can use our online portal to help in that search
Curriculum Information
Glossary of terms, year 1 (60 credits), year 2 (60 credits), year 3 (60 credits), year 4 (60 credits), year 5 (60 credits), year 6 (60 credits), year 7 (0 credits), year 8 (0 credits), year 9 (0 credits), year 10 (0 credits), year 11 (0 credits), year 12 (0 credits), year 13 (0 credits), year 14 (0 credits), year 15 (0 credits), current projects.
Areas of Interest:
- Functional Biomaterials
- Mechanics of Biological Cells, Tissues and Systems
- Bioelectronics and Rehabilitation Engineering
- Regenerative Orthobiologics and Neurotherapeutics
- Regenerative Cardiovascular Therapeutics
Researcher Profiles
Professor Peter McHugh http://ncbes.eurhost.net/biomechanics-1.aspx Professor Gearoid O’Laighin http://ncbes.eurhost.net/bioelectronics.aspx Professor Timothy O’Brien www.remedi.ie Professor Frank Barry www.remedi.ie Professor Abhay Pandit www.nfb.ie
Fees: Non EU
Extra information.
EU Part time: Year 1 €4,250 p.a. (€4,390 including levy) 2024/25
All students, irrespective of funding, must pay the student levy of €140.
Professor Peter McHugh T: +353 91 493 152 F: +353 91 563 991 E: [email protected] W: Biomedical Engineering People

What Our Students Say
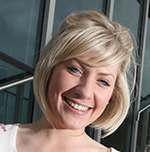
Ríona Ní Ghriallais | Biomedical Engineering PhD
For my research, I investigate medical devices for the femoral artery, the major artery of the leg. I became interested in research after completing my final year project in the fourth year of my undergraduate Biomedical Engineering degree. Studying Biomedical Engineering at NUI Galway introduced me to the fascinating world of medical devices. The degree provided me with the fundamental skills set required to begin a career as an engineer along with the opportunity to study a broad range of subjects. From this I found those that interested me the most, which led me towards choosing the topic of my research work.
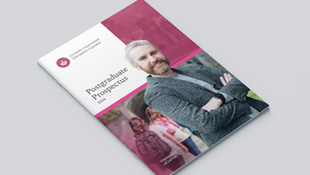
Postgraduate Prospectus 2024 PDF (3.3MB)
Manage Cookies
Some features need cookies to work properly. Cookies also let us (a) remember your preferences, (b) collect anonymous usage statistics, and (c) see how well our online ads are working.
No personal data is stored on these cookies but, under EU law, we still need to ask you this every 6 months. To learn more about our use of cookies, view our Privacy Policy .
Founded in 1845, we've been inspiring students for over 175 years. University of Galway has earned international recognition as a research-led university with a commitment to top quality teaching.
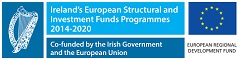
University of Galway, University Road, Galway, Ireland H91 TK33 T. +353 91 524411
Get Directions Send Us an Email
Twitter Instagram Facebook YouTube LinkedIn RSS
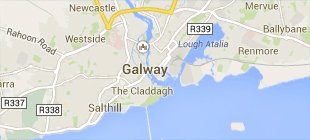
© 2023 University of Galway. All Rights Reserved. Server AWS University of Galway is a registered charity. RCN 20002107
- Privacy & Cookies
- Contact & Enquiries
- Accessibility

An official website of the United States government
Here’s how you know
Official websites use .gov A .gov website belongs to an official government organization in the United States.
Secure .gov websites use HTTPS A lock ( Lock A locked padlock ) or https:// means you’ve safely connected to the .gov website. Share sensitive information only on official, secure websites.

- Grants & Funding
- Grant Programs
Tissue Engineering & Regenerative Medicine Research Program
Staff contacts.
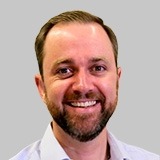
Regenerative Medicine
18 Dec 2023 Turning the Tap Back On
07 Jan 2021 Stem Cell Treatment Corrects Skull Shape and Restores Brain Function in Mouse Model of Childhood Disorder
28 Jan 2021 Surgical Adhesive Inspired by Slug Slime
30 Sep 2019 How Does a Mouse’s Tooth Grow?
19 Nov 2018 The Quest to Understand Dental Stem Cells
15 Oct 2018 Human Skeletal Stem Cell Identified
Grantee News
10 Apr 2024 UM Research Discovers New Way to Generate Human Cartilage
26 Apr 2023 Do Spiny Mice Hold the Key to Regenerative Healing? UK Study Explores
17 Apr 2023 Healing the Unhealable: New Approach Helps Bones Mend Themselves
22 Mar 2023 Researchers Find Key to Healing Muscle Injuries in Elderly
09 Jan 2022 A Crowning Achievement in Understanding Head Development
18 Nov 2021 A Stunning 3D Map of Blood Vessels and Cells in a Mouse Skull Could Help Scientists Make New Bones
07 Sep 2021 Nerve Repair, With Help From Stem Cells
06 Oct 2021 Massage Doesn’t Just Make Muscles Feel Better, it Makes Them Heal Faster and Stronger
26 Apr 2021 Skin and Bones Repaired by Bioprinting during Surgery
14 May 2018 First Description of mEAK-7 Gene Could Suggest Path toward Therapies for Cancer, Other Diseases
Funding Opportunities & Notices
Previously funded grants.
NIH RePORTER - Research Portfolio Online Reporting Tool (RePORT)

An official website of the United States government
The .gov means it’s official. Federal government websites often end in .gov or .mil. Before sharing sensitive information, make sure you’re on a federal government site.
The site is secure. The https:// ensures that you are connecting to the official website and that any information you provide is encrypted and transmitted securely.
- Publications
- Account settings
Preview improvements coming to the PMC website in October 2024. Learn More or Try it out now .
- Advanced Search
- Journal List
- Tissue Eng Part B Rev

Tissue Engineering and Regenerative Medicine: Recent Innovations and the Transition to Translation
Matthew b. fisher.
1 McKay Orthopaedic Research Laboratory, Department of Orthopaedic Surgery, Perelman School of Medicine, University of Pennsylvania, Philadelphia, Pennsylvania.
3 Philadelphia Veterans Administration Medical Center, Philadelphia, Pennsylvania.
Robert L. Mauck
2 Department of Bioengineering, School of Engineering and Applied Science, University of Pennsylvania, Philadelphia, Pennsylvania.
The field of tissue engineering and regenerative medicine (TERM) has exploded in the last decade. In this Year (or so) in Review, we highlight some of the high impact advances within the field over the past several years. Using the past as our guide and starting with an objective premise, we attempt so to identify recent “hot topics” and transformative publications within the field. Through this process, several key themes emerged: (1) tissue engineering: grafts and materials, (2) regenerative medicine: scaffolds and factors that control endogenous tissue formation, (3) clinical trials, and (4) novel cell sources: induced pluripotent stem cells. Within these focus areas, we summarize the highly impactful articles that emerged from our objective analysis and review additional recent publications to augment and expand upon these key themes. Finally, we discuss where the TERM field may be headed and how to monitor such a broad-based and ever-expanding community.
Scope and Aim of This Review
The objective of this “Year in Review” article is to identify and recount significant recent events in the broad discipline of tissue engineering and regenerative medicine (TERM). This builds from the foundation established by Dr. Michael Lysaght, whose data-driven publications first defined our field and the early challenges and opportunities in it, identified for us when the “end of the beginning” had occurred, and detailed the ups and the downs in our translational and commercial aspirations. 1 – 5 In constructing this review, some of the questions that we asked were “What constitutes the TERM field?” and “How would one write a review that both captures the events of today and presages the events of tomorrow?” Moreover, since reviews abound, we wished to write a review that could add to the literature instead of duplicating it. Indeed, we recognized from the outset that we are by definition limited by our own small world-view and specialization (both of the authors being orthopedically inclined tissue engineers), and that this might limit our ability to capture (and subsequently detail) the incredibly diversity of our field.
To answer these questions and to capture the breadth of the TERM field, we started with an objective premise. Specifically, we developed a framework to identify the occurrence of a “transformative event” (i.e., publication) in the TERM domain. As will be detailed below, the sheer number of publications in this area is overwhelming, and it is oftentimes very difficult to separate the “wheat from the chaff,” particularly in publications that are outside one's own small area of expertise. While there are of course certain key signals that may indicate that an event of significance has occurred—for example, publication in a high impact factor journal and/or dissemination in the lay press—quite often, the most impactful publications in our field arise from the primordial TERM soup without much fanfare, but are quickly taken up as a new path forward by the field. In order to establish objective metrics by which to identify such important contributions, we first gathered numerical data on the history of TERM publications, and from this, identified specific criteria that could provide an early reading on the “impact trajectory” for any work, even very soon after its original publication. We then applied these metrics to the literature of the last few years, and used the outcomes of this analysis to define and populate the substance of this review article. Our methods of analysis are detailed in the following section, and the content we identified is then parsed into specific categories and reviewed with respect to ongoing work.
Methods of Review
The first challenge in conducting this review was the sheer number of recent publications in the TERM field. The origins of the terms “tissue engineering” and “regenerative medicine” have been previously discussed in this journal, 1 with the former coming into common parlance in the mid-to-late 1980s and the latter gaining momentum around the turn of the 21st century. Since their definition, the TERM field has grown substantially. A simple search for “tissue engineering” or “regenerative medicine” within a commonly used database (Scopus ® ; Elsevier) reveals over 40,000 hits to date, with more than 28,000 original articles and 6000 review articles. The number of TERM articles continues to rise ( Fig. 1 ) with nearly 4000 original articles published in 2010, compared to a mere 360 a decade earlier. This can be partially attributed to the increasing use of the same common terminology, particularly for the more recent “regenerative medicine.” Still, there is no doubt that our field is expanding and capturing a larger portion of the work done across the biomedical sciences. More importantly, it makes a brute force review of the field impossible.

The rise of tissue engineering and regenerative medicine (TERM). (Results obtained via Scopus ® search using key words “tissue engineering” OR “regenerative medicine”). Color images available online at www.liebertpub.com/teb
This brings us to our second challenge, namely, how to objectively decide which recent articles to include in this review. The most objective measure of an article's impact (in our un-objective opinion) is the number of times it has been cited. However, each impactful article must be read by the field, digested, and inspire new experiments (and possibly obtain funding for said experiments), which then need to be completed and published. This process creates a “citation lag time” of 1–3 years. Indicators which allow a more immediate sense of an article's impact include a journal's impact factor, “buzz” within the field, online views or downloads, and press releases or newspaper/periodical stories, but lack somewhat in objectivity.
Our challenge then was to develop a framework in which to organize recent advances in the TERM field given both the abundance of literature and the need for objective metrics to evaluate impact. To do so, we first examined impactful articles from previous years to determine if there were early and common trends among these articles. We limited our initial TERM search to original articles (excluding reviews) and organized these by the number of times cited. Examining the top 20 most cited articles in the TERM field ( Table 1 ), 6 – 25 a few trends become clear. First, a high number of citations early (within 2 years) generally indicated that the article would be impactful later as well (5 years). A typical example is the article by Zuk et al. 7 published in Tissue Engineering in 2001, which first described the isolation and characterization of adipose-derived progenitor cells. Second, in the year of publication, there is substantial variability due to articles being published early or later in the year ( Fig. 2 ). By 1–2 years postpublication, this variability sharply decreases. Thus, even though the number of citations varies widely early on, the numbers tend to converge for impactful articles. This means however, that there may be impactful articles published in 2012, which we cannot isolate at this time. As such, we have expanded our review to include years 2010 and 2011, since we feel we can better identify key publications in this more expansive time frame using citations as an objective measure.

Number of citations each year postpublication (as a percentage of total TERM articles in that year) for the top 20 TERM publications. Data presented as box and whiskers plot featuring median, interquartile range, and minimum/maximum (Langer and Vacanti 6 excluded as an outlier).
Top Cited Tissue Engineering and Regenerative Medicine Articles
Search conducted on 12/3/2012 using Scopus ® . Excludes Reviews as defined in Scopus ® . Citation numbers are given as number of citations within a given year postpublication. Percentages represent number of citations divided by the number of TERM publications in that year. Article by Takahashi and Yamanaka 85 did not appear within search parameters, but is provided given its seminal nature.
n/a, data unavailable; TERM, tissue engineering and regenerative medicine.
We then applied this analysis to TERM articles published in 2010–2012. Examining the top 50 publications in terms of citations (See Fig. 3 for a scatter plot in each year and domain), one can appreciate the distribution in times cited. Using the Grubbs' test for outliers (circled on these plots), one can then clearly identify publications that have separated from the pack and represent a study that has had broad impact on the TERM field in a short period of time. Within this set of studies, we identified several key themes in the TERM literature. These are: Tissue Engineering: Grafts and Materials, Regenerative Medicine: Scaffolds and Factors that Control Endogenous Tissue Formation, Clinical Trials, and Novel Cell Sources: Induced Pluripotent Stem Cells. These categories will form the basis for the next sections. In each area, one or more highly impactful articles will be highlighted, and a discussion of recent publications will be included to augment and expand upon these key themes that are clearly emerging in the TERM field. Finally, we will conclude with a discussion on where the TERM field may be headed and how to monitor such an ever-expanding community.

Top 50 TE and RM publications for 2010–2012 with the outliers circled. These publications formed the basis of this review and defined discussion categories.
Recent Advances in TERM
Tissue engineering: grafts and materials.
When first conceived, many engineers considered that tissue engineering would involve the de novo engineering of new tissues for implantations, using starting materials and methods drawn from chemical engineering, biomaterials science, and mechanical engineering principles. However, some of the most high profile recent studies on tissue engineering have focused on translating relatively simple approaches into preclinical and clinical studies. For example, in 2009 the field was stimulated by the first report of an engineered airway based on a cadaveric decellularized implant in a human patient, 26 one of the first instances of a tissue engineering approach saving a life. Since then, replacements based on this idea have increased in complexity, including engineered lungs, livers, and vascular grafts. A common theme in many of these approaches is the use of decellularized extracellular matrix (ECM) to serve as a scaffold for cell seeding. The rationale is that a decellularized ECM maintains a niche, which can serve to maintain cell phenotype and encourage production of tissue specific matrix and functional properties. This type of approach is not radical, as decellularized tissues have been used as allografts in surgical replacement procedures for decades, and pioneering work by Badylak and colleagues 27 , 28 has defined the regenerative potential of these ECM-based implants.
Two recent notable examples in this area that emerged from our objective analysis were studies by Petersen et al. 29 and Ott et al. 30 Both of these studies reported data on the use of decellularized lung tissue as a scaffold for viable lung replacement ( Fig. 4A ). In general, cell seeding of decellularized ECM from donor animals and culture allowing both media and air exchange maintained cell viability and allowed the production of lung-specific matrix ( Fig. 4B ) and other molecules, including surfactant protein precursors. When used for short-term replacement (several hours) of the left lung in adult rats, blood perfusion and ventilation were achieved with the tissue-engineered graft. Petersen et al. 29 reported lower oxygen levels compared to normal; however, complete hemoglobin saturation was achieved. Ott et al. 30 further noted the presence of pulmonary secretions, suggesting functionality. Yet, the transplanted lungs required higher pressure to be fully inflated and had fluid buildup, suggestive of pulmonary edema. While additional preclinical studies are necessary to show the longer-term viability and efficacy of such approaches, these results are unquestionably promising with regard to ECM-based scaffolds for lung replacement.

Use of decellularized tissue matrix for TE applications. Whole lungs can be readily decellularized through perfusion techniques [right upper lobe (RUL), right middle lobe (RML)] (A) . When implanted in vivo , such tissues are perfused by red blood cells and maintain partial function in the short-term (B) . Recellularized liver matrix also maintains similar structure and cellular viability and phenotype (C) . Scale bar=100 μm. Decellularized tissue-engineered vascular grafts maintain patency when implanted and allow repopulation with native cells [graft (g), carotid artery (ca), adventitia (a)] (D) . White arrowhead points out alpha-smooth muscle actin-positive cell. Scale bars=100 μm. (Adapted from Petersen et al. , 29 Ott et al. , 30 Uygun et al. , 31 and Dahl et al. , 33 [with permission from AAAS and MacMillan Publishers, Ltd]).
These same principles have also been applied to liver regeneration, for example in a recent publication by Uygun et al. , 31 which also emerged from our objective screen. In this study, rat liver tissue was decellularized and perfused with primary rat hepatocytes. Cell viability was maintained over 5 days in culture, and albumin and urea production increased with time, with albumin production reaching 20% of normal. Vascularized grafts were then implanted and perfused in vivo , following a nephrectomy in recipient rats. After 8 h, cells maintained viability at preoperative levels (∼80% viability) and remained histologically similar to the native tissue ( Fig. 4C ). Here too, additional preclinical work is required to fully investigate the potential of such ECM-based cell-seeded grafts, but this early work is quite promising.
While vascular autografts are quite common, some have considered devitalized allografts (from cadaveric tissue) as an alternative source, and have shown good mechanics and biocompatibility through this decellularization process. 32 As an alternative to removing cells from native tissue, Dahl et al. 33 recently took an interesting tissue engineering approach to create vascular grafts. Here, the authors isolated human smooth muscle cells and cultured them on tubular polyglycolic acid scaffolds ( Fig. 4D ). Over a period of several months, the grafts possessed suture retention strength, burst pressure, and compliance similar to native tissues. These “engineered” grafts were then decellularized and stored for up to 1 year without an appreciable loss in mechanical function. In a model of arteriovenous bypass in baboons, the grafts remained functional for up to 6 months, with little fibrosis, calcification, or thickening, and 88% of the grafts remained patent and mechanically stable. In a canine model of carotid or coronary bypass, these grafts preseeded with endothelial cells remained 83% patent at 1 month with only one occlusion. Although little immunogenicity was noted and all grafts remodeled to possess a biochemical composition more similar to native tissue, longer-term studies and direct comparison to the clinical gold standard will be needed to fully validate this approach. This work is quite interesting, however, as it replaces traditional cadaveric grafts with those generated through “traditional” TE approaches (cells coupled to biomaterials and grown in vitro ), which have several potential advantages. It will be interesting to see whether this novel concept is adopted across the spectrum of organ/tissue replacement in TERM.
Stemming from the publications noted above that met our objective criteria for inclusion in this review, considerable work has been carried out to expand this exciting new TERM focus area. For instance, recent studies have examined how other cell types, such as mesenchymal stem cells, can colonize and integrate within decellularized lung tissues. 34 In cardiac applications, methods have been developed to enhance decellularization while preserving vascular beds in thick tissue slices. 35 Similarly, bone allograft processing techniques have recently been optimized to support mesenchymal cell attachment and mineral deposition. 36 Investigators have also begun to explore how such tissues can be re-enervated, for example in skeletal muscle preparations, guiding both vascular health as well as neural connectivity to the host. 37 These and other publications support the growing interest and increasing complexity in organ/native ECM-based tissue replacement approaches.
In addition to these ECM-derived approaches, there of course remains active development of novel biomaterial scaffolds. Progress in the past year has included electrospun materials that foster cellular colonization 38 , 39 and can direct depth dependent and anatomic reconstitution of cell and matrix organization. 40 , 41 Other studies have built off of the acellular concept, imbuing acellular materials with molecules that attract progenitor cells to the wound interface. 42 Still other studies have developed materials that can optimally deliver and sequester cells within a repair environment, based on nano-scale surface topography. 43 In terms of vascularization, progress has been made in both engineered artificial vascular networks 44 as well as via the development of advanced materials that allow endothelial cells to remodel and build their own vascular network. 45 Finally, while quite a lot of work focuses on decellularized matrices and cell-seeded biomaterials, significant progress continues in the formation of almost completely cell based tissue constructs, even for load bearing structures. 46 This activity, premised upon both “traditional” and emerging themes, points to continued innovation and expansion of TERM applications for the repair and replacement of ever more complex systems.
Regenerative medicine: scaffolds and factors that control endogenous tissue formation
Over the past decade, it is clear that regenerative medicine approaches have increased in popularity for a variety of reasons. Cellular therapies remain a large focus, and the efficacy of several cell types have been evaluated in vivo . In addition, considerable efforts in regenerative biology have focused on the developmental origins of stem and progenitor cells, and on elucidating how they persist (or don't) in adult organisms. Another major focus has been on the development of biomaterials to either release bioactive factors to aid in the healing response and/or provide a scaffold that can promote appropriate tissue formation. This generally acellular approach seeks to foster repair by optimizing the response of endogenous progenitor cell pools, rather than cell delivery itself.
For example, one interesting recent study that emerged from our objective analysis highlighted the multiple roles that a scaffold may play in promoting the regenerative response in the case of bone healing in a rat model. This study, by Kolambkar et al. , 47 employed a combined scaffold using a tubular mesh made from electrospun poly(ɛ-caprolactone) nanofibers that wrapped around the bone defect and helped to localize a peptide-modified alginate hydrogel injected to fill the defect and deliver recombinant bone morphogenetic protein-2 (rhBMP-2) ( Fig. 5 ). With delivery of rhBMP-2, bony bridging occurred consistently (as assessed by micro-computed tomography), but did not occur with the scaffolds alone, showing the importance of growth factor delivery. In addition, macroscopic perforations in the nanofiber mesh seemed to accelerate repair, resulting in a twofold increase in the torsional stiffness of the healing bone. Revascularization of the defects was not increased, and the authors suggest that the perforations allowed endogenous progenitor cells to infiltrate and positively influence repair. Thus, when designing scaffolds for regenerative medicine applications, there are many factors to consider which may ultimately impact their success, even for relatively simple tissues such as ligaments and tendons. 48

Bioscaffolds for bone regeneration. Nanofibers with macroscopic perforations and alginate releasing bone morphogenetic protein-2 (BMP-2) were placed within a critically sized bone defect (A) . Micro-computed tomography images showing increased bone formation with scaffold treatment (B) . (Adapted from Kolambkar et al. 47 [with permission from Elsevier]).
For methods such as that noted above to be successful, the endogenous progenitor pool must be both local to the defect site and of sufficient number/capacity as to mount a repair response. In a recent study by Jaskelioff et al. 49 (which also emerged from our objective analysis), the authors investigated how stem cell depletion is related to genome damage. Specifically, they tested the hypothesis that declining organ function may be eliminated or even reversed by engineering progenitor cells to reactivate endogenous telomerase activity. Their findings showed a marked reversal of degenerative neuronal and sensory phenotypes in adult mice, supporting the notion that maintenance of progenitor cell pools, or rescuing progenitor cell activity, can lead to wide scale tissue and organ rejuvenation.
Along a similar line, investigators have queried normal regenerative processes in amphibians and mammals to define the role of endogenous stem cell populations in regeneration. For instance, our objective screen of the literature picked up a recent publication by Porrello et al. , 50 who studied cardiac regeneration in mice. These investigators found, quite strikingly, that while cardiac regeneration can occur early (1 day after birth), this capacity is lost by 7 days after birth. This early healing was characterized as “regenerative,” with a lack of fibrosis and normal heart function achieved after 2 months. Conversely, just a week later, the response to the same injury was characterized by a repair response, with scar tissue formation, much like one would see in mature animals. This disjunction between fetal and adult regenerative response may offer new insight into regenerative therapies across a range of diseases and injuries. More generally, the study of injury mechanisms, and how they interface and activate endogenous progenitor cell populations, is an active and growing area of inquiry, 51 , 52 with a particular focus on elucidating how progenitor cells interact with cells of the immune system. 53 – 55
In addition to this age dependent change in regenerative capacity, considerable work has focused on identifying precisely which pool of stem cells actively participates in endogenous repair/regeneration processes. Work by Rinkevich et al. 56 showed via lineage tracing that those cells that reconstitute the mouse digit are lineage restricted. That is, the stem cell pool that forms cartilage and bone for instance is different than the cell population that forms other adjacent tissues (muscle and skin). Understanding how, when, and where these different progenitor cell populations are operative will inform ongoing TERM studies aimed at recruiting and manipulating these endogenous regenerative cell sources. Studying atypical resolution of injury and/or repair in other mammalian species may shed additional light on regenerative processes. For example, the recent report on “skin shedding” and regeneration in an African spiny mouse, 57 while certainly atypical, may provide new directions for TERM investigators. While these models may sometimes be far afield from the actual application of regenerative strategies (arising as they often do from developmental biology studies), they build a basic science framework from which novel technologies may grow. Moreover, they define the theoretical and basic science limits on what will be possible with endogenous tissue engineering/regenerative medicine.
This area of regenerative medicine is continually expanding. For instance, in a high profile article from 2010, Lee et al. showed that whole joint cartilage repair could be achieved in a rabbit model in which a polymeric joint prosthesis was implanted, with endogenous cells recruited to via inclusion of transforming growth factor beta (TGF-β). 58 Along these same lines, Shah et al. reported the use of nanofibers made from peptide amphiphile molecules which have high binding affinity for TGF-β and showed the potential for cartilage regeneration in rabbits. 59 Still others have turned to novel methods, such as control through magnetic fields, to permit superior release of factors in vivo . 60 In some cases, rather than delivering a specific growth factor, investigators have utilized the broader paracrine activities of injected cells, particularly stem cells, to advance regeneration. Indeed, this paracrine effect is thought by many to be one of the dominant mechanisms by which injected stem cells offer a therapeutic benefit. 61 – 64 It is important to point out that these factors, whether they derive from the scaffold or are released by implanted cells, can influence the regenerative environment in nonintuitive ways. For instance, a recent report detailed how growth factors themselves can bind to pro-inflammatory cytokines to prevent arthritis in mice, 65 allowing for the possibility of simultaneous anabolic and anti-catabolic functionality.
In the biomaterials domain of regenerative medicine, much attention has been paid to the advancement of material design through the promotion of endogenous stem cell differentiation toward a specific phenotype of interest by modulating physical attributes of the scaffold (including stiffness, topography, and porosity). 66 – 70 Others have suggested that stem cells with a specific propensity towards a defined lineage may have different physical properties 71 and have used these differences to select for a specific cell phenotype from a heterogeneous population prior to implantation. 72 , 73 Thus advanced materials, which both select refined populations and direct their differentiation, may provide a mechanism to achieve improved in vivo regeneration.
Clinical trials
TERM approaches are also making their way into clinical practice, with a number of clinical studies published over the past few years. For instance, a tissue engineering approach was used to restore function in a small cohort of boys with large urethral defects. 74 Biopsies of bladder tissue were obtained, and smooth muscle and epithelial cells were isolated and cultured. The cells were then seeded onto tubular poly (glycolic acid)-poly (lactic-co-glycolic acid) scaffolds ( Fig. 6A ). Following culture for 1 week to ensure cell viability and matrix production, grafts were used for urethral reconstruction. The authors report that all five patients maintained functional flow rates at 36–72 months postimplantation and that biopsies confirmed tissue organization similar to the native tissue. This approach shows promise for traumatic or congenital injuries to the urethra where simple repair or on-lay grafts are insufficient.

Recent clinical applications of TERM approaches. A tissue-engineered urethra can maintain function in patients in the long-term (A) . Injection of cardiosphere-derived stem cells can reduce scar size in patients postinfarction (B) . (Adapted from Raya-Rivera et al. 74 and Makkar et al. 75 [with permission from Elsevier]). CDC, cardiosphere-derived cells.
In addition to tissue replacement, much interest has focused on the perfusion of cells into the heart for the treatment of myocardial infarction, and several clinical studies have recently reported initial results. One phase I prospective, randomized, controlled trial (RCT) aimed to treat infarction with progenitor cells (called cardiosphere-derived autologous stem cells). 75 Autologous endomyocardial cells were obtained and cultured using a specific culture method, including culture in nonadherent plates to form three-dimensional (3D) cardiospheres in order to obtain a population positive for CD105 and negative for CD45 ( Fig. 6B ). Although some adverse events were reported with implantation of these cells, by 6 months, a significant reduction in scar mass and increase in viable heart mass and wall thickness was found. However, no differences in functional measurements, such as ejection fraction could be determined at this early time point. Nevertheless, the reduction in scar tissue and increase of viable tissue with this treatment supported movement on to a phase 2 study. Other recent studies injecting bone marrow-derived progenitor cells also lend support to the injection of stem cells to enhance function following myocardial infarction and failure of coronary stents. 76
In other applications, autologous stem cell therapy has been used to reverse corneal destruction due to burns. In one study, 77 autologous limbal stem cells were cultured on fibrin to treat corneal damage in over 100 patients. At 10 years follow-up, more than 75% of patients had a restored corneal epithelium layer. Clinical success was correlated to the percentage of functional stem cells (holoclone-forming) observed in culture. Specifically, if cultures contained more than 3% holoclone-forming cells, clinical success was found in 78% of patients. On the other hand, if less than 3% holoclone-forming cells were found, success was seen in only 11% of patients. This study shows the potential for limbal stem cells for corneal repair; however, additional randomized controlled clinical trials are needed.
Another quite popular focus of clinical trials in recent years has revolved around the use of platelet-rich plasma (PRP) to treat orthopedic sports injuries, with both considerable interest and criticism abounding in the literature. Several randomized control trials are underway, and early results have been recently published. Peerbooms et al. first reported the beneficial effects of PRP over corticosteroid injections for the treatment of lateral epicondylitis in a double-blind RCT. 78 At 1 year, marked improvements were seen in both patient reported outcomes as well as functional scores, and more recent data suggests these results persist up to 2 years. 79
Others have reported on the limited impact of PRP or platelet-rich fibrin matrix (PRFM) for other types of injuries, such as rotator cuff repairs or Achilles tendinopathy. 80 – 82 In addition to these studies, another recent study showed that blood elements (including platelets) can induce a pro-inflammatory response. 83 Thus, while such platelet-based treatments may offer some promise, much research, both clinical and basic science, is still needed to evaluate the impact of PRP and PRFM. Complications include variable patient demographics, injury types and previous treatment, and potential differences between the many PRP and PRFM products currently on the market. Together, these clinical and preclinical studies may soon resolve these issues, and may provide a valuable adjuvant to repair in certain clinical scenarios.
In the coming years, it is likely that there will be a dramatic increase in the number of published clinical studies that utilize TERM approaches. Indeed, the number of registered clinical trials in the U.S. featuring either “tissue engineering” and/or “regenerative medicine” has increased from 38 in 2007 to 83 in 2011. These translational studies will inform the field as to the true efficacy of TERM approaches in clinical practice and will help to refine work across the research space, from in vitro development to preclinical models.
Novel cell sources: induced pluripotent stem cells
While the work above quite often involves the delivery of adult stem cells, significant interest remains in the development and application of newly emergent progenitor cell sources. For example, research into the use of embryonic stem cells (ESCs) for TERM applications continues unabated. A recent study by Marolt et al. showed, for example, that culture of human ESCs on decellularized bone matrix in a perfusion bioreactor 84 resulted in formation of a stable bone-like tissue. Quite interestingly, when these tissue constructs were implanted subcutaneously in immunodeficient mice, teratomas formed with implantation of undifferentiated human ESCs but not with implantation of ESC-derived bone. This work illustrates the continued development of ESC-based therapies in TERM, as well as the potential limitations in such work when differentiation is not adequately controlled.
The past several years have also borne witness to a truly impactful (if not the most impactful) development in TERM. That is, the discovery and characterization of induced pluripotent stem cells (iPSCs), first defined by Takahashi and Yamanaka in 2006, 85 describing how differentiated adult cells could be reprogrammed back to an embryonic state through the presence of only four transcription factors (Oct-4, Sox-2, c-Myc, and Klf4). The original article detailing this technology has already been cited over 4400 times to date! The impact of this work cannot be overstated, as was evidenced by the awarding of the Nobel Prize in Medicine to Dr. Yamanaka this past year, only 6 years after publication of his seminal article. Research surrounding the use of iPSCs has seen a rapid and exponential growth, and interested readers are directed to a number of fine reviews on this topic. 86 – 89 Instead, we will highlight some of the most highly impactful articles found through our objective analysis to provide a general sense of the immense progress in this area.
While the original iPSC “cocktail” was quite simple, recent work has focused on further refining and simplifying this process. Instead of ectopic expression of several transcription factors, an alternative approach is to express specific microRNAs (miRNAs). In a recent study, expression of the miR302/367 cluster via viral transfection was able to reprogram both mouse embryonic fibroblasts and human foreskin and dermal fibroblasts with a several fold increase in efficiency relative to the typical transcription factors. 90 Another major issue limiting translation of iPSC technology has been the need for viral infection to introduce the necessary transcription factors for reprogramming, which raises regulatory concerns due to genomic integration or mutagenesis and is relatively inefficient. Alternatively, synthetic mRNAs can be used directly to reprogram differentiated cells into iPSCs. 91 This approach was much more efficient (two orders of magnitude higher than viral transfection). Due to the short activity span of such treatments, however, multiple injections would be needed clinically. The authors also note that RNA-derived iPSCs had global transcriptional patterns that were more similar to human ESCs, suggesting that this reprogramming process may yield cells of higher quality. Direct transfection of miRNAs without a viral carrier can also allow reprogramming of mouse and human somatic cells into iPSCs. 92 Another way to create iPSCs is to create heterokaryons (fused cells) from mouse ESCs and human fibroblasts, which allows reprogramming with 70% efficiency within 1 day and allows study of the molecular mechanisms of reprogramming. 93
Others have tested the hypothesis that differentiated cells can be reprogrammed in situ , abrogating the need for in vitro manipulation. For example, Qian et al. injected a retrovirus expressing the Yamanaka transcription factors to reprogram adult resident nonmyocytes in the murine heart into iPSCs. 94 Analysis of single cells revealed ventricular cardiomyocyte-like action potentials, beating upon electrical stimulation, and evidence of electrical coupling. In an injury model (coronary ligation), retroviral treatment modestly attenuated cardiac dysfunction for up to 3 months. Although iPSC induction did not fully restore function following injury, this study shows that in vivo reprogramming could have therapeutic potential.
Along these lines, there has been considerable interest in direct reprogramming of one differentiated cell into another. For example, it has recently been shown that retroviral expression of a set of transcription factors can reprogram cardiac fibroblasts into myocyte-like cells both in vitro and in vivo in a mouse model. 95 Following induction of myocardial infarction, injected retroviruses led to a substantial improvement in functional outcomes, such as ejection fraction, for up to 12 weeks relative to controls. More recently, cardiac fibroblasts have been reprogrammed into myocytes via expression of specific miRNAs. 96 Huang et al. directly induced mouse tail-tip fibroblasts into hepatocyte-like (iHep) cells. 97 When transplanted ectopically in vivo in a model of liver failure, these iHep cells significantly improved survivability (∼40%) at 8 weeks relative to fibroblasts (0%); however, survivability was still less than true hepatocytes (∼90%). Similarly, expression of a combination of three genes has been shown to convert mouse embryonic and postnatal fibroblasts into neurons in vitro. 98 These studies, and many others like them, indicate the very rapid developments and innovations that are occurring in this sphere of regenerative medicine.
As iPSCs become more widely available and adopted by the TERM community, the transition to translation of this cell type has already started. However, some caution must be taken in this process, as there remain several issues that could limit their eventual clinical potential. For instance, while some studies have focused in the in vivo applications of these cells in situations of organ repair, issues have arisen related to the immunogenicity of autologous cell-derived iPSCs that are reprogrammed in vitro and reimplanted. In an inbred mouse model, “autologous” ESCs failed to illicit an immune response, while “autologous” embryonic fibroblasts reprogrammed into iPSCs were mostly rejected by the host and featured T cell infiltration. 99 The authors of this study attributed these findings to abnormal gene expression in a portion of the iPSCs. While this is certainly a potential reason, additional work is required to fully understand this behavior.
An additional persistent concern with iPSCs is the variability in their differentiation pathways, most likely arising from incomplete or aberrant reprogramming of the parent population. 100 In response, Boulting et al. have recently suggested the use of a set of validated cell lines to expedite research findings and to better standardize results. 101 Other investigators have developed novel processing methods to isolate “pure” populations of iPSC for particular applications. For instance, work by Diekman et al. 102 showed that passage through a chondrogenic “filter,” that is, selecting subpopulations that originally differentiate towards the chondrogenic lineage most robustly, enabled the isolation of sub-populations of iPSCs that had improved cartilage formation when placed back into 3D culture conditions ( Fig. 7 ). These differentiation-based selection criteria for iPSC will undoubtedly become more complex and refined in future work. For example, such sorting could be coupled with concepts demonstrated in the work of Gilbert et al. , 103 who showed that the matrix elasticity on which progenitor cells are plated can influence the retention or loss of stemness. Similarly, soluble factors, sometimes derived from high throughput screening of chemical libraries, could be used to further refine these cell populations, as well as enforce their differentiation trajectories in vitro and in vivo. 104 , 105 These and other microenvironmental inputs will likely be used to tune iPSC differentiation specificity, efficacy, and fidelity over the coming years to forward specific TERM applications and translation of this cell type on to preclinical and clinical applications.

Induced pluripotent stem cells (iPSCs) can produce cartilage-like matrix. Collagen immunohistochemistry for matrix made by passage 2 iPSCs sorted via expression of type II collagen (GFP+). (Adapted from Diekman et al. 102 [with permission from the National Academy of Sciences]).
Concluding Remarks and Discussion
Writing a Year in Review that captures the present state and future directions of any field of inquiry is no easy task, especially when that field is as diverse and ever-changing as TERM. In fact, since its inception, the TERM field has been characterized in some sense by its lack of definition and its incredible breadth. Indeed, it is precisely this breadth and diversity that drew so many of us into the field in the first place. Many seemingly discordant lines of research have now become intertwined in TERM and constitute the fabric of our field, with these concepts arising from the blurring of boundaries between traditional disciplines. While this point is sometimes easy to forget, much of what we now consider commonplace in TERM was only a short time ago separated by barriers of dogma and discipline. As these lines continue to blur, and multi-disciplinary research becomes more the rule than the exception, our field is experiencing tremendous growth. The unlimited potential of this work has captured the attention of the lay public (as evidenced by the recent 3 day, front-page New York Times profile on the TERM field 106 ). Moreover, in almost every aspect of the field as evidenced in this review, we are beginning to witness the critical stage of transition to translation.
While quite exciting for all in involved, rapid growth of any field is not without its drawbacks. Specifically, the pace of growth is now so fast that it impossible for most of us to keep up with the field as a whole, or even a small subset of it. For example, a TERM search specific to “cartilage” returns more than 450 articles published in 2011 alone, meaning that one would need to read more than one article per day just to stay abreast of this small portion of the TERM terrain. It is difficult (amongst all this noise) to recognize and separate the truly important contributions from those that are less so. In addition to the sheer numbers of physical publications in the TERM field, the number of outlets through which research findings are being disseminated is also expanding. The scientific community is increasingly exploiting new mechanisms to achieve faster publication (i.e., all electronic journals). In the absence of other, faster objective metrics, the increased use of editorials, reviews, letters to the editor, etc. might provide a way to increase credibility and interest quickly in emerging themes in TERM, as well as mitigate the “citation lag time” commonly found, even for important articles.
In this review, we attempted to both engage the diversity and to encapsulate the ever-expanding TERM field by “letting the numbers speak for themselves,” using the impact trajectories of the last several years to provide a framework for discussion. In using these objective criteria to guide us, we found considerable innovation in a number of traditional TERM fields, but also new ideas that are beginning to take hold in emerging focal areas. For instance, in the realm of tissue replacement, we are now seeing not just scaffolds of ever-increasing complexity derived from standard engineering methods, but also complex scaffolds predicated on natural designs (and native tissues themselves, once decellularized). In the broader field of regenerative medicine, we are seeing developmental biology begin to address not just the formation of tissues, but the specific role that endogenous stem cells play in both generative and regenerative processes. Integrating these basic science findings with novel materials that specifically recruit endogenous populations may provide a next wave in smart biomaterials for tissue repair. Likewise, new cell sources, most prominently iPSCs, have come to the fore, making autologous cell-based therapies for any tissue a real possibility. Finally, our objective screen showed that ours is truly a translational field, and that TERM advances are being reduced to clinical practice at an ever-increasing rate. Furthermore, as we are by and large quantitative scientists, both the quantitative nature of these outcome measures and levels of evidence in support of these applications are advancing as well. Together, these advances are now beginning to change the lives of small subsets of the population, and in the future, these novel approaches will be able to address a host of diseases and instances of tissue degeneration that were heretofore untreatable. Here's hoping that the next Year in Review will continue to find such exciting activity, and further illustrate the growing impact of TERM technologies on improving human health.
Acknowledgments
We gratefully acknowledge support from the National Institutes of Health (R01EB02425, R01EB008722, R01AR056624), the Veterans' Administration (I01RX000174, I01RX000700), the Musculoskeletal Transplant Foundation (Junior Investigator Grant), the Orthopedic Research and Education Foundation, the Penn Center for Musculoskeletal Disorders (P30AR050950), and the Penn Institute for Regenerative Medicine.
Disclosure Statement
No competing financial interests exist.
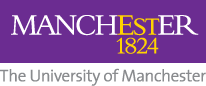
Alternatively, use our A–Z index
Attend an open day
Download our course brochure
Discover more about Medicine at Manchester
MSc Tissue Engineering for Regenerative Medicine / Overview
Year of entry: 2024
- View full page
We require an honours degree (minimum Upper Second) or overseas equivalent in:
- biological sciences
- medical sciences
- biomedical materials
- veterinary medicine
- pharmacology
Full entry requirements
Please apply via our online application form. See the application and selection section for details of the supporting documents we require.
We recommend that you apply as early as possible. We reserve the right to close applications if the course is full.
Course options
Course overview.
- Learn how to research strategies to repair, replace and ultimately regenerate various tissues and organs to solve major clinical problems.
- Gain a comprehensive insight into topical issues such as stem cells, design and characterisation of biomaterials and nanomaterials (including graphene), biofabrication (including 3D bioprinting), cell and gene therapies, commercialisation and clinical translation of regenerative therapies.
- Prepare for PhD study, specialist clinical training or a career in related industries, including pharmaceutical, biotechnology and regenerative medicine sectors.
- Study at a university ranked 8th in the UK and among the top 30 in the world for Medicine (QS Rankings 2023).
For entry in the academic year beginning September 2024, the tuition fees are as follows:
- MSc (full-time) UK students (per annum): £14,500 International, including EU, students (per annum): £34,500
Further information for EU students can be found on our dedicated EU page.
The fees quoted above will be fully inclusive for the course tuition, administration and computational costs during your studies.
All fees for entry will be subject to yearly review and incremental rises per annum are also likely over the duration of courses lasting more than a year for UK students (fees are typically fixed for international students for the course duration at the year of entry).
For general fees information please visit postgraduate fees. Always contact the department if you are unsure which fee applies to your qualification award and method of attendance.
Policy on additional costs
All students should normally be able to complete their programme of study without incurring additional study costs over and above the tuition fee for that programme. Any unavoidable additional compulsory costs totalling more than 1% of the annual home undergraduate fee per annum, regardless of whether the programme in question is undergraduate or postgraduate taught, will be made clear to you at the point of application. Further information can be found in the University's Policy on additional costs incurred by students on undergraduate and postgraduate taught programmes (PDF document, 91KB).
Scholarships/sponsorships
Contact details, courses in related subject areas.
Use the links below to view lists of courses in related subject areas.
- Anatomical Science
- Biological Sciences
- Biomedical Sciences
- Biochemistry
- Molecular Biology
- Nanoscience
Regulated by the Office for Students
The University of Manchester is regulated by the Office for Students (OfS). The OfS aims to help students succeed in Higher Education by ensuring they receive excellent information and guidance, get high quality education that prepares them for the future and by protecting their interests. More information can be found at the OfS website .
You can find regulations and policies relating to student life at The University of Manchester, including our Degree Regulations and Complaints Procedure, on our regulations website .
Tissue Engineering and Regenerative Medicine (MSc)
- Duration: 1 year
- Mode: Full time
Find out more about studying here as a postgraduate at our next Open Day .
Why study this course
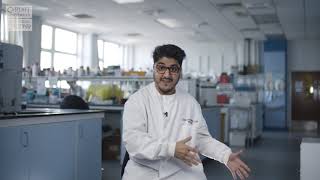
The Programme provides in-depth training in this branch of biomedical science, including stem cell biology, biomaterials and tissue/organ engineering.
First of its kind in the UK
Our course was the first of its kind in the UK , building on Cardiff University’s research excellence and critical mass of tissue engineering researchers.
Our interdisciplinary network
Teaching is delivered by academics across the University.
Visit clinical settings
Opportunities to visit clinical settings and local industrial partners to speak with clinicians with keen interests in tissue engineering and repair.
Research project
The MSc combines both taught modules and a 5-month research project which can be chosen by you. Previous student projects have been in research areas such as stem cell biology, bone, skin, oral tissues and drug delivery.
1+3 year programme
Students with scholarships/funding support have the option to apply for the MSc as a 1+3 year programme of study , continuing into a PhD programme within the School upon obtaining a Master’s degree qualification
With an ageing population and chronic diseases on the rise, there is a need for more effective medical treatments and therefore a need for trained tissue engineering researchers to deliver these technologies.
Tissue engineering and regenerative medicine is an ever-emerging interdisciplinary field of biomedical research; which combines life sciences, biology and engineering, to progress the repair, replacement and enhancement of diseased and damaged tissues.
It’s a field that has the potential to deliver treatments for diseases, injuries and disorders where currently people have no options.
The Programme provides in-depth training in this branch of biomedical science, including stem cell biology, biomaterials and tissue/organ engineering. The MSc offers a balanced combination of theory and practice; and can serve either as preparation for a PhD or as a self-contained master’s qualification.
The programme is both lecture and laboratory based ; benefiting from taught modules that cover areas such as Cellular & Molecular Biology and Stem Cells and Regenerative Medicine and culminating in a hands-on 5-month research project . It also includes several opportunities to visit relevant clinical settings and local industrial partners.
Students are taught by academics from across the School of Biosciences, Dentistry, Medicine, Optometry and Pharmacy. You will therefore study within a multidisciplinary community with internationally excellent academic, research and clinical expertise not available at many other institutions.
Based at The University Hospital of Wales (the 3 rd largest university hospital in the UK), you will develop technical laboratory skills and will be presented with a number of opportunities to extend your communication and presentation skills, both written and orally.
You will acquire a number of specific skills, such as the ability to collect, analyse and interpret a range of complex quantitative and qualitative data. You will also develop a number of valuable laboratory research-based skills, through the completion of a 5-month research project of your choice*, which will be presented as a poster.
Graduates from this Programme will have a broad spectrum of knowledge and a variety of skills, making them highly attractive both to potential employers and research establishments in this fast-developing sector.
*subject to supervisor status.
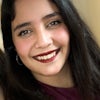
After finishing my Bachelor's degree in Biochemistry in Spain, I was looking for a more specific and challenging field. I got interested in the development of tissues by 3D bioprinting and so I chose to focus on this. The facilities were incredibly good, and I found all the staff really helpful and always open to questions and new ideas. In my project, I focused on the development of a new fibrin-based bioink that will hopefully help in the creation of a human skin model. The design of the composition of this bioink was challenging as there was not any previous work done with it in the model of bioprinter used in the lab; this is what made the project even more interesting and exciting for me. I would totally recommend this course to any person with a high interest in research and willing to focus their future career in a developing field as it will offer many opportunities.
Where you'll study
School of Dentistry
We are the only dental school in Wales, providing unique and important leadership in dental research, teaching and patient care.
- About us Chevron right
- Research at the School of Dentistry Chevron right
- Academic staff Chevron right
- Telephone +44 (0)29 2074 2544/8797
- Marker Heath Park, Cardiff, CF14 4XY
Admissions criteria
In order to be considered for an offer for this programme you will need to meet all of the entry requirements. Your application will not be progressed if the information and evidence listed is not provided.
With your online application you will need to provide:
- A copy of your degree certificate and transcripts which show you have achieved a 2:2 honours degree in a relevant subject area such as Bioengineering, Biology, Dentistry, Life Sciences, Medical Engineering, or Medicine, or an equivalent international degree. If your degree certificate or result is pending, please upload any interim transcripts or provisional certificates.
- A copy of your IELTS certificate with an overall score of 6.5 with 5.5 in all subskills, or evidence of an accepted equivalent. Please include the date of your expected test if this qualification is pending. If you have alternative acceptable evidence, such as an undergraduate degree studied in the UK, please supply this in place of an IELTS.
- Two signed and dated academic or professional/employer references which demonstrate your suitability for the programme.
- A personal statement which outlines your reasons for applying to the programme and how your academic experience, skills or long-term goals make you the ideal candidate for the programme.
- An up-to-date CV which includes your full academic and work history.
Application Deadline
The application deadline is 30 June 2024. If you submit an application after this date, we will only consider it if places are still available.
Selection process
We will review your application and if you meet the entry requirements, we will invite you to an interview. Following interview, offers will be made to the highest scoring candidates.
Find out more about English language requirements .
Criminal convictions
You are not required to complete a DBS (Disclosure Barring Service) check or provide a Certificate of Good Conduct to study this course.
If you are currently subject to any licence condition or monitoring restriction that could affect your ability to successfully complete your studies, you will be required to disclose your criminal record. Conditions include, but are not limited to:
- access to computers or devices that can store images
- use of internet and communication tools/devices
- freedom of movement
- contact with people related to Cardiff University.
Course structure
MSc Tissue Engineering and Regenerative Medicine commences in September each year with Stage 1 , a 6-month, taught component.
Stage 1 is taught almost entirely at a small group teaching level, supported by laboratory sessions, interactive workshops and tutorials, in addition to visits to relevant hospital clinics and local companies involved in producing tissue engineering and repair therapies. Modules are assessed by various written assignments, presentations and formal examinations.
On completing Stage 1, students undertake a 5-month, laboratory-based research project between April-September ( Stage 2 ). Projects are chosen by students from topics supplied by academic supervisors across the University. Previous student projects have been in research areas such as embryonic or mesenchymal stem cell biology; cartilage, bone, skin or oral tissue repair; fibrosis; and biomaterials and drug delivery. Stage 2 culminates in the submission of an MSc Dissertation, based on MSc Project findings
The modules shown are an example of the typical curriculum and will be reviewed prior to the 2024/25 academic year. The final modules will be published by September 2024.
The MSc in Tissue Engineering and Regenerative Medicine is a one year, full-time Programme. The MSc Programme commences in September each year with Stage 1 , a 6-month, taught component involving four compulsory Modules.
On completing Stage 1, students undertake a 5-month, laboratory-based research project, between April-September ( Stage 2 ). Stage 2 culminates in the submission of an MSc Dissertation, based on MSc Project findings.
The University is committed to providing a wide range of module options where possible, but please be aware that whilst every effort is made to offer choice this may be limited in certain circumstances. This is due to the fact that some modules have limited numbers of places available, which are allocated on a first-come, first-served basis, while others have minimum student numbers required before they will run, to ensure that an appropriate quality of education can be delivered; some modules require students to have already taken particular subjects, and others are core or required on the programme you are taking. Modules may also be limited due to timetable clashes, and although the University works to minimise disruption to choice, we advise you to seek advice from the relevant School on the module choices available.
Learning and assessment
How will i be taught.
Teaching is delivered via lectures, laboratory sessions, interactive workshops and tutorials, in addition to visits to relevant hospital clinics, such as orthopaedics, nephrology and dermatology, and local companies involved in producing tissue engineering and repair therapies.
This Programme is based within the School of Dentistry and taught by academic staff from across Cardiff University and by external speakers.
All taught modules within the Programme are compulsory and students are expected to attend all lectures, laboratory sessions and other timetabled sessions. Students will receive supervision to help them complete the dissertation, but are also expected to engage in considerable independent study. Dissertation topics are normally chosen by the students from a list of options proposed by academic staff in areas relevant to the MSc in Tissue Engineering.
How will I be assessed?
The four taught Modules within the Programme are assessed through in-course assessments, including:
- Extended essays
- Oral presentations
- Poster presentations
- Statistical assignments
- Critical appraisals
Dissertation (no more than 20,000 words).
How will I be supported?
Blackboard, on which students will find course materials and links to related materials. Students will be supervised when undertaking their dissertation. Supervision will include scheduled regular meetings to discuss progress, provide advice and guidance; and provide written feedback on draft dissertation contents.
Feedback Students will receive written feedback on all assessments, in addition to oral feedback on assessed oral/poster presentations
What skills will I practise and develop?
The Learning Outcomes for this Programme describe what you will be able to do as a result of your study at Cardiff University. They will help you to understand what is expected of you.
The Learning Outcomes for this Programme can be found below:
Knowledge & Understanding:
On successful completion of the Programme you will be able to demonstrate:
- Systematic understanding of the multi-disciplinary nature of tissue engineering and regenerative medicine and the need for integration of knowledge from different fields.
- An understanding of the cellular/tissue alterations which occur during various disease states and ageing in humans.
- A comprehensive knowledge of the core principles of tissue engineering and regenerative medicine.
- An understanding of the physical and biochemical techniques available for the assessment and development of tissue engineered and regenerative medicine products for clinical use.
Intellectual Skills:
On successful completion of the Programme you will be able to:
- Critically evaluate knowledge at the forefront of a range of disciplines, such as stem cell biology, biomaterials and tissue/organ engineering.
- Utilise knowledge and scientific evidence-based methods in the assessment and solution of challenges in the field of tissue engineering and regenerative medicine.
Professional Practical Skills:
- Collect, evaluate, synthesise and interpret qualitative and quantitative data in a variety of ways; and identify means to gather new data where required.
Transferable/Key Skills:
- Analytical skills
- Communication and presentation skills
The MSc tissue engineering course is a fantastic course teaching the ethical, social, clinical and bimolecular aspects of modern tissue engineering and translational research with a final six month dissertation project in a world class laboratory. The course attracts students from all over the world and provides many opportunities to engage in a supportive and friendly environment. For me, this course was vital for my progression to becoming an independent researcher. I found that the lectures were really engaging, allowing me to develop my knowledge of tissue engineering. I was then able to apply this to my laboratory project. For me the project was the best part of this course, as it allowed me to develop key laboratory skills and undertake novel research on wound healing in a world class facility.
Tuition fees for 2024 entry
Your tuition fees and how you pay them will depend on your fee status. Your fee status could be home, island or overseas.
Learn how we decide your fee status
Fees for home status
Students from the eu, eea and switzerland.
If you are an EU, EEA or Swiss national, your tuition fees for 2024/25 be in line with the overseas fees for international students, unless you qualify for home fee status. UKCISA have provided information about Brexit and tuition fees .
Fees for island status
Learn more about the postgraduate fees for students from the Channel Islands or the Isle of Man .
Fees for overseas status
More information about tuition fees and deposits , including for part-time and continuing students.
Financial support
Financial support may be available to individuals who meet certain criteria. For more information visit our funding section. Please note that these sources of financial support are limited and therefore not everyone who meets the criteria are guaranteed to receive the support.
Additional costs
There are no additional costs that need to be considered.
Will I need any specific equipment to study this course/programme?
No specific equipment is required to study on this programme.
Living costs
We’re based in one of the UK’s most affordable cities. Find out more about living costs in Cardiff .
Master's Scholarships
An award open to UK students intending to study one of our taught master’s degrees.
Postgraduate loans
If you are starting your master’s degree in September 2024 or later, you may be able to apply for a postgraduate loan to support your study at Cardiff University.
Alumni Discount
The alumni discount is available for Cardiff University graduates who are planning to start an eligible master's in 2024/25.
Careers and placements
After successfully completing this MSc, you should have a broad spectrum of knowledge and a variety of skills, making you highly attractive both to potential employers and research establishments.
Since its introduction in 2006, 95% of our MSc graduates have progressed onto career paths highly relevant to tissue engineering and regenerative medicine. These include PhDs within Cardiff and at other UK, EU and USA Universities, Graduate-Entry Medicine, Specialist Registrar Training, Teaching, and positions in Industry and Clinical Laboratory settings.
You will have the opportunity to attend clinical attachments, in areas such as orthopaedics, nephrology and dermatology. Furthermore, you will also have the opportunity to visit local companies involved in producing tissue engineering and repair therapies for clinical use. These include Cell Therapy Ltd., Reneuron plc, Biomonde Ltd., and MBI Wales Ltd.
Open Day visits
Make an enquiry, international, discover more.
Search for your courses
Related searches: Dentistry
HESA Data: Copyright Higher Education Statistics Agency Limited 2021. The Higher Education Statistics Agency Limited cannot accept responsibility for any inferences or conclusions derived by third parties from its data. Data is from the latest Graduate Outcomes Survey 2019/20, published by HESA in June 2022.
Module information
Postgraduate
Be part of a thriving postgraduate community in a university known internationally for outstanding research and teaching.
Postgraduate prospectus 2024

Download a copy of our prospectus, school and subject brochures, and other guides.
Order or download
Get in touch if you have a question about studying with us.
- Português Br
- Journalist Pass
3D bioprinting: transforming medical images into human tissue
Susan Buckles
Share this:
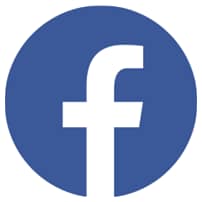
Mayo Clinic researchers are using technology to produce tissue models of different body parts to study damaged or diseased tissues and organs. They envision a day when a 3D bioprinter could mold living cells into a therapy or cure for complex disorders.
"3D bioprinters are just like the 3D printers that print small plastic toys or parts. Instead of using hard plastics or metals to construct a part or prototype, 3D bioprinters use biocompatible materials containing living cells to print three dimensional structures of tissue that could be used to improve human health," says Kevin Dicker, Ph.D., a bioprinting expert and a process development staff scientist for the Center for Regenerative Biotherapeutics in Arizona. "Bioprinters are tools to accelerate research in the field of tissue engineering."
3D bioprinting holds intrigue among researchers for its potential to study disease progression and screen new treatments for conditions such as end-stage organ failure, cartilage defects and atopic dermatitis, a skin disease also known as eczema. Dr. Dicker and the process development team are working to establish standard operating procedures for biomanufacturing tissue for testing in early-stage clinical trials. This pioneering work at Mayo Clinic is aimed at integrating tissue engineering into clinically relevant therapies that could be studied in clinical trials.
Dr. Dicker utilizes a 3D bioprinter to study tissue engineering.
Mayo Clinic's Center for Regenerative Biotherapeutics supports research to advance tissue engineering and cell and gene therapies to clinical care for patients with rare and complex diseases.
Bioprinting with living cells
The 3D bioprinter utilizes a digital blueprint of a design from medical imaging, such as MRI or CT scans, and then gets to work. This powerful, high-tech tool precisely places bioinks composed of living cells, hydrogels, biomaterials and growth factors in a layer-by-layer fashion. The final 3D tissue model it produces can mimic the structure, mechanics and physiology of human organs, muscle or cartilage.
"You can formulate a bioink composed of gelatin-like materials which can be printed into a desired shape. In addition, we can encapsulate living cells into that gelatin-like bioink," says Dr. Dicker. "This type of tissue engineering is an emerging technology in regenerative medicine that has the potential to transform laboratory research and clinical practice by bioengineering replacements for damaged or diseased tissue."
Bioprinting organs
The complex tissue structures that come from the 3D bioprinter have allowed researchers to study ways to bioprint human organs. Mayo Clinic has developed the capability of bioprinting skin to mimic inflammatory skin disease. This bioprinted skin model is being studied in the lab of Saranya Wyles, M.D., Ph.D. , to test treatments and understand disease progression for conditions such as atopic dermatitis (eczema).
"We have created the first-in-kind human skin model with functional epidermis and dermis, recapitulating human skin. This 3D model gives us a humanlike model for studying inflammatory skin conditions. It accurately recreates patient-specific disease and provides the ability to test new therapies," says Dr. Wyles.
Besides its use for disease models, this emerging technology is being explored for manufacturing human tissue and organs.
"The ultimate goal is to someday be able to print organs and tissue on demand. However, we aren’t quite there yet," says Dr. Dicker. "We hope to advance this technology as a solution to the global shortage of donor organs. If we are able to bioprint functional kidneys, for example, that would be a huge relief on the healthcare system."
Bioprinting implants
In Arizona, the research team of David Lott, M.D. , is developing 3D bioprinted implants for the larynx or trachea. The implants could be used to replace damaged or diseased portions of the organ while maintaining the healthy tissue. "The 3D bioprinter has the ability to construct complex tissue which has both stiff cartilage and soft tissue regions. This enables us to print vocal folds, soft tissue responsible for vocalization, and the surrounding cartilage within the larynx," says Dr. Dicker. "We hope to develop a 3D bioprinted implant for patients who need a part of their larynx removed because of disease or trauma."
Challenges to bioprinting
While 3D Bioprinting holds a high potential, Mayo Clinic and other research institutions still have challenges to overcome. In order for a bioprinted organ to function, it must have a connection to blood, oxygen and nutrients. Researchers have struggled to grow a network of capillaries and blood vessels in the bioprinted structures at scale that would deliver those vital elements. Another challenge is how to integrate the bioprinted tissues with the human body while preventing rejection of the implant.
3D bioprinting has the potential to transform healthcare, but more years of research are needed to fully understand and apply this technology.
Related stories:
Electrospinning biotherapies of tomorrow
Bringing regenerative technology of the future to patients today
- Becoming a doctor: Mayo Clinic Alix School of Medicine students graduate in Arizona Mayo Clinic Minute: Ticks and the diseases they carry
Related Articles
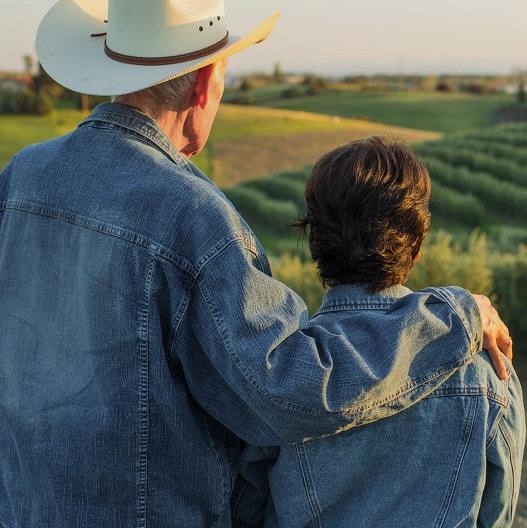

Institute for Stem Cell & Regenerative Medicine
Meet the 2024 tietze award winners.
ISCRM Associate Director Nate Sniadecki, PhD , Professor of Mechanical Engineering and Hichem Tasfaout, PhD , Acting Assistant Professor, Neurology have received prestigious awards from the John H. Tietze Foundation Trust that will help fuel promising research underway in their labs.
Engineering 3D Tendons

Much of the research on tendon healing has relied on studies with rodents, but there is a biological difference between rodent cells and human cells. With funding from the 2024 John H. Tietze Stem Cell Scientist Award, Dr. Nate Sniadecki and his lab will develop an in vitro, mechanobiological model of human tendons. These engineered tendon models have the potential to be used to test new therapies, model genetic diseases, or build replacement tissue materials.
The award will enable the researchers to, we will examine the maturation of stem cell-derived tenocytes in a mechanobiological 3D environment and recapitulate the bone-tendon interface (enthesis) using a suspended open microfluidics-based tissue engineering approach. The lab will explore whether tendon cells cultured in 3D mature faster than they do in 2D environments and examine how closely these engineered tendons resemble human tissues.
Importantly, development of a sophisticated ETC platform will enable future studies in the Sniadecki lab on tendon injury mechanisms and surgical engraftment studies for tissue replacement therapy.
Testing a New Way to Treat Muscle Disorders

The Jaconette L. Tietze Young Scientist Award will enable Dr. Hichem Tasfaout to study how AAV vectors could best be used to treat Ryanodine Receptor 1-related myopathies, a family of disorder that present muscle weakness, motor delay resulting in difficulties walking and climbing stairs, scoliosis, muscle pain (myalgia), muscle cramps, and fatigue.
RYR1 disorders are caused by genetic mutations in the RYR1 gene, which abolish the expression of RYR1. Functional copies of the faulty RYR1 gene can, in theory, be delivered to the affected muscles using AAV vectors, if the healthy gene could be packaged into the relatively small vector.
Funding from the Tietze Award will allow Dr. Tasfaout to investigate a new gene delivery method that take advantage of a system, developed in the Chamberlain Lab , known as SIMPLI-GT. This technology enables the expression of large proteins by directing several protein fragments to affected muscle cells and then fusing them into one functional protein using small molecules named split intein. This novel gene therapy approach aims to express the missing RYR1 protein and, if successful, would benefit all patients with RYR1-myopathies.
About the Tietze Research Awards
John H. Tietze Stem Cell Scientist Award
The John H. Tietze Stem Cell Scientist Award is a one-year award of $50,000 to support the research of any UW FACULTY member of ISCRM who is pursuing novel preliminary experiments, where the grant might provide sufficient stimulus to enable the research to advance to the point of being competitive for external funding. The research should involve or be relevant to some aspect of stem or progenitor cell biology or therapies.
Jaconette L. Tietze Young Scientist Award
The Jaconette L. Tietze Young Scientist Award is also for one year of support of $25,000. Preference will be given a junior faculty (including Acting Instructor level) located at the UW who has not yet received major external funding (such as an R01). The research should involve or be relevant to some aspect of stem or progenitor cell biology or therapies.
- Accessibility Options:
- Skip to Content
- Skip to Search
- Skip to footer
- Office of Disability Services
- Request Assistance
- 305-284-2374
- High Contrast
- School of Architecture
- College of Arts and Sciences
- Miami Herbert Business School
- School of Communication
- School of Education and Human Development
- College of Engineering
- School of Law
- Rosenstiel School of Marine, Atmospheric, and Earth Science
- Miller School of Medicine
- Frost School of Music
- School of Nursing and Health Studies
- The Graduate School
- Division of Continuing and International Education
- People Search
- Class Search
- IT Help and Support
- Privacy Statement
- Student Life
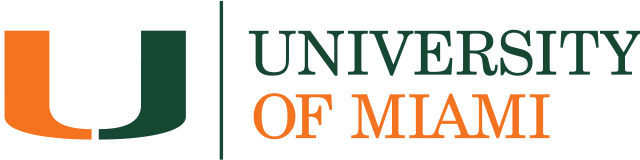
- Search Site
- Main College
- College News
Biomedical Engineering
- Chemical, Environmental and Materials Engineering
- Civil and Architectural Engineering
- Electrical and Computing Engineering
- Industrial Engineering
- Mechanical and Aerospace Engineering
- Letter from the Chair
- Department Clusters
- Undergraduate
- Imaging, Optics, & Lasers
- Biomechanics, Microfluidics, Biomaterials and Tissue
- Neural Engineering, Signals, & Instrumentation
- News and Events
- Advisory Board
- PhD Students
- MD/PhD Program
- Medical Physics
- PhD Program
- Listening Exercise
- Communications
Master's in Biomedical Engineering
The MS program in Biomedical Engineering (BME) welcomes students from diverse backgrounds, including
- students with undergraduate degrees in biomedical engineering and other engineering disciplines who seek advanced professional training or specialization in a particular area of biomedical engineering;
- students with an undergraduate degree in Physics, Mathematics, Computer Science, Chemistry, Biology or other fields of natural or health science who seek to diversify their career opportunities by acquiring an engineering degree
- students who are preparing for admission to advanced health-related or other professional programs such as medical school.
- professional engineers with undergraduate degrees in other disciplines who plan to enter the field of biomedical engineering.
Students enrolled in the MS program acquire the skills required to solve complex problems at the interface of engineering and medicine or biology. Graduates are prepared for successful careers in the biomedical industry, academia, or government (FDA, US Patent Office), or for further study in doctoral or health-related programs.
The interdisciplinary nature of the department and our strong ties with the University of Miami Miller School of Medicine provides students with many opportunity to collaborate with clinicians and researchers at several world-renowned research and clinical centers, including the Bascom Palmer Eye Institute, The Miami Project to Cure Paralysis, the Diabetes Research Institute, the University of Miami Ear Institute, the Biomedical Nanotechnology Institute (BioNIUM), the Sylvester Comprehensive Cancer Center, and the Miami Veterans Administration Research Service.
There are three broad fields of graduate study and research:
- Imaging and medical physics
- Neural engineering
- Regenerative engineering
Industry Advisory Board
The MS program is informed by an industry advisory board with significant experience in medicine, business, government and technology. To view the advisory board, click here .
Students enrolled in the MS in Biomedical Engineering must successfully complete 27 credits and a three-credit design or research project (BME706).
Required Courses – 12 Credits
At least two of the following three courses
Electives – 18 credits
Medical imaging, neural engineering, regenerative engineering.
Graduate Bulletin
To learn more about the MS in Biomedical Engineering at the University of Miami - College of Engineering, please fill out the form below and one of our team members will get back to you as soon as possible.
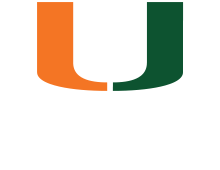
- 1251 Memorial Drive McArthur Engineering Building Coral Gables , FL 33146
- 305-284-2445 305-284-2445
- Academic Calendar
- Alumni & Friends
- Medical Center
- Hurricane Sports
- Parking & Transportation
- social-facebook
- social-twitter
- social-youtube
- social-instagram
Copyright: 2024 University of Miami. All Rights Reserved. Emergency Information Privacy Statement & Legal Notices
Individuals with disabilities who experience any technology-based barriers accessing the University’s websites or services can visit the Office of Workplace Equity and Inclusion .
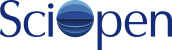
Logging off
Therapeutic nucleic acids in regenerative medicine and tissue repair.

1 Center for Nanomedicine and Department of Anesthesiology, Brigham and Women’s Hospital, Harvard Medical School, Boston, Massachusetts 02115, United States

In the vanguard of biomedical innovation, regenerative medicine emerges as a transformative paradigm, concentrating its efforts on rectifying tissue deficits and functional aberrations in patients through the strategic augmentation of endogenous cellular processes. Central to this approach is nucleic acid therapy, which offers a novel pathway for tissue regeneration by modulating key signaling pathways. As one of the most effective ways to regulate cell function, nucleic acids are crucial for various tissue regeneration; however, their in vivo therapeutic applications face substantial challenges, including nuclease degradation, cell membrane impermeability, and targeted intracellular transport. Biomaterial-based gene delivery systems offer a solution for stable and localized drug delivery by enabling the controlled overexpression of therapeutic nucleic acids, producing functional regulatory agents. This review presents examples of nucleic acid applications in regenerative medicine, highlighting the synergy between nucleic acids and biomaterial technologies. It underlines the importance of nucleic acid delivery techniques, the choice of therapeutic nucleic acids, and biomaterials for advancing tissue repair. The latest developments in designing nucleic acid biomaterial-based delivery vehicles are explored, the mechanism of nucleic acids in tissue regeneration is elucidated, and their limitations are discussed while considering future directions for the clinical translation of nucleic acid-based therapeutics.

FullText for HTML: https://doi.org/.....
Publication history
© Tsinghua University Press 2024
Rights and permissions
Reprints and Permission requests may be sought directly from editorial office. Email: [email protected]

京ICP备 10035462号-42

Tissue Engineering & Biomaterials Laboratory
Fischell department of bioengineering, yearly archives: 2023, robert choe successfully defends dissertation and earns phd.

These past few months, Robert Choe has been working hard to prepare for his dissertation defense. A defense is the last step any graduate student needs to complete to PhD. This is where they present the work they have done over the past five years and show how they completed their thesis in front of a committee of professors. The PhD candidate must “defend” their work to the committee to prove they made a significant difference in their field.
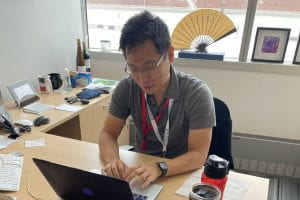
On Wednesday October 4th, Robert successfully presented his dissertation defense and was awarded his PhD! Robert’s work focused on the development of an implantable osteochondral scaffold for cartilage engineering. Robert leveraged the unique capabilities of 3D printing to build computational models of a broad range of osteochondral scaffolds, identifying the critical parameters that support a strong and resilient bone – cartilage interface. Robert then examined the proper cell delivery strategy for the bone phase of the 3D printed scaffolds, defining a strategy for osteoblast – osteoclast coculture for bone tissue development and maintenance. Robert’s work has already been communicated in 5 journal articles and numerous conference presentations. After BIOE, Robert will return to his DMD roots to take an associate prosthodontist position at ClearChoice Dental Implant Centers. Congratulations again to Robert on both his outstanding work and incredible efforts!
John P. Fisher Elected to BMES Board of Directors
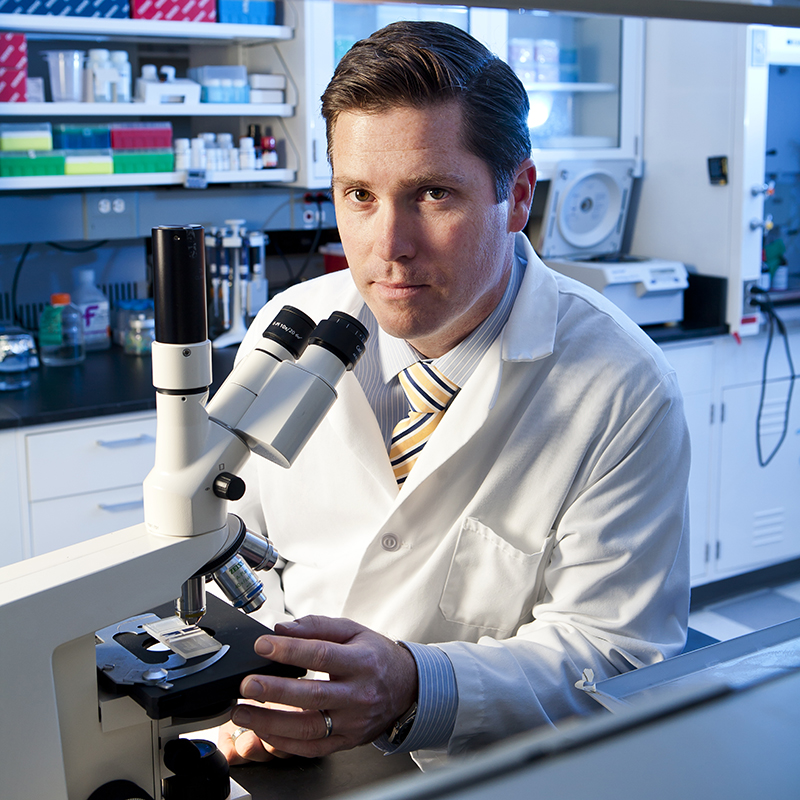
Congratulations to Dr. Fisher as The Biomedical Engineering Society (BMES) announced his election to its Board of Directors beginning this fall. Fisher is a MPower Professor, Distinguished-Scholar Teacher, Fischell Family Distinguished Professor, and Department Chair in the Fischell Department of Bioengineering (BIOE) at the University of Maryland (UMD).
Fisher has been an active member of BMES for more than 20 years, having joined the society in 2003 and been named a Fellow of BMES in 2016. He has served in various roles in the society, including abstract reviewer, session chair, and track chair in numerous Annual BMES Meetings.
“I am thrilled to continue supporting BMES as a member of the BMES Board of Directors and to give back to the society that has provided us with so many opportunities over the years.” John P. Fisher
Established in 1968, BMES is the world’s leading professional society for students, faculty, researchers, and industry professionals in biomedical engineering. With over 6,800 members, the society is committed to fostering an inclusive community to advance human health through education, discovery, and translation.
BMES hosts an Annual Meeting that brings together over five thousand biomedical engineers each year and puts on more than 2,500 scientific presentations, including talks on cancer treatment, heart disease, women’s health, global health disparities, and more.
In 2018, Fisher was the Co-Chair of the BMES Annual Meeting in Atlanta, Georgia, spearheading the large celebration of theBMES 50th Anniversary.
In addition to his work with BMES, Fisher has served in numerous member and leadership positions within partner societies, including the American Institute for Medical and Biological Engineering (AIMBE); the International Academy of Medical and Biological Engineering (IAMBE); the International Society for Biofabrication (ISBF); the Society for Biomaterials (SFB); and the Tissue Engineering and Regenerative Medicine International Society (TERMIS).
In many of his society positions, as well as in his role at UMD, Fisher has worked closely with historically underserved and underrepresented students, encouraging their participation in and passion for STEM careers.
“Recent events in our country and across the world have presented a number of challenges to our society and within our scientific communities—challenges around health, equity, and economics,” says Fisher.
In his new role on the BMES Board of Directors, he proposes to address these challenges with “continued, enhanced, and robust vigilance to support the work of the students, trainees, and young investigators of BMES. From reduced financial burdens to mentorship and expanded opportunities to share their work with the broader scientific community, our commitment to the next generation of biomaterial scientists and engineers is particularly needed at this critical juncture.”
Fisher currently serves as Past-President of the Americas Chapter of TERMIS and was the Co-Chair of the 2014 TERMIS-Americas Annual Meeting. In his TERMIS leadership roles, Fisher led efforts to broaden the TERMIS-Americas Chapter from North America to the entire Americas, established the Diversity, Equity, and Inclusion (DEI) Committee within the chapter, and participated in a delegation to South Africa to launch Africa’s first tissue engineering society.
In addition, Dr. Fisher currently serves as the Co-Editor-in-Chief of the journal Tissue Engineering while serving on the editorial boards of key biomedical engineering journals such as the Journal of Biomedical Materials Research Part A ; Bioprinting ; Biofabrication ; and the Journal of Tissue Engineering & Regenerative Medicine .
Story provided by the Fischell Department of Bioengineering.
University of Otago Collaboration Visit

Shannon McLoughlin, a PhD Candidate in Professor John Fisher’s Tissue Engineering and Biomaterials Lab (TEBL), traveled to University of Otago in New Zealand to work with Associate Professor Jaydee Cabral on 3D printing nipple areolar complex (NAC) scaffolds for mastectomy patients. Dr. Cabral recently received a grant from the Royal Society of New Zealand Catalyst Fund for this collaborative work with Dr. Fisher’s lab. The proposal focuses on the use of 3D printing to generate vascularized NAC constructs and culturing them in 3D printed bioreactor systems. Shannon worked with an undergraduate honor’s student Emma Jackson on bioink synthesis, endothelial cell culture, and 3D bioprinting on the GeSIM Bioscaffolder. In addition to working in Dr. Cabral’s lab, she attended Queenstown Research Week Molecular Biology Meeting to present the lab’s work on “Biofabrication Strategies for the Generation of Bone Tissue Interfaces.” Outside of the lab, they explored Queenstown and Dunedin, learned about the rich history and culture of New Zealand, and even saw some penguins on the Peninsula!

Story written by Shannon McLoughlin
2023 Fischell Fellowship in Biomedical Engineering
On Friday, August 25, more than 80 Fischell Department of Bioengineering graduate students, faculty, and staff celebrated at the University of Maryland Golf Course for the sixth annual EPIC Retreat where the 2023 Fischell Fellows were announced.
The Fischell Fellowship is a remarkable opportunity for graduate students pursuing research and product design within the biomedical industry. But what does it mean to be a Fischell Fellow? The Fellowship is given to individuals for recognition of their work and achievements and provides them with more than just the ability to fund their Ph.D. projects. This fellowship encourages recipients to submit invention disclosures for their research in order to push their scientific findings toward commercialization, enabling their discoveries to help people across the world.
This year, the Department announced the two recipients of the 2023 Fischell Fellowship in Biomedical Engineering as David Boegner and Shannon McLoughlin. Shannon is a fifth year PhD student conducting her research here in the Tissue Engineering and Biomaterials Laboratory. Her research involves enhancing extrusion bioprinting resolution to generate thin membranous tissue structures, such as the periosteum.
“I’m incredibly honored to be selected for this award and am extremely grateful to the Department for supporting me throughout my final year,”
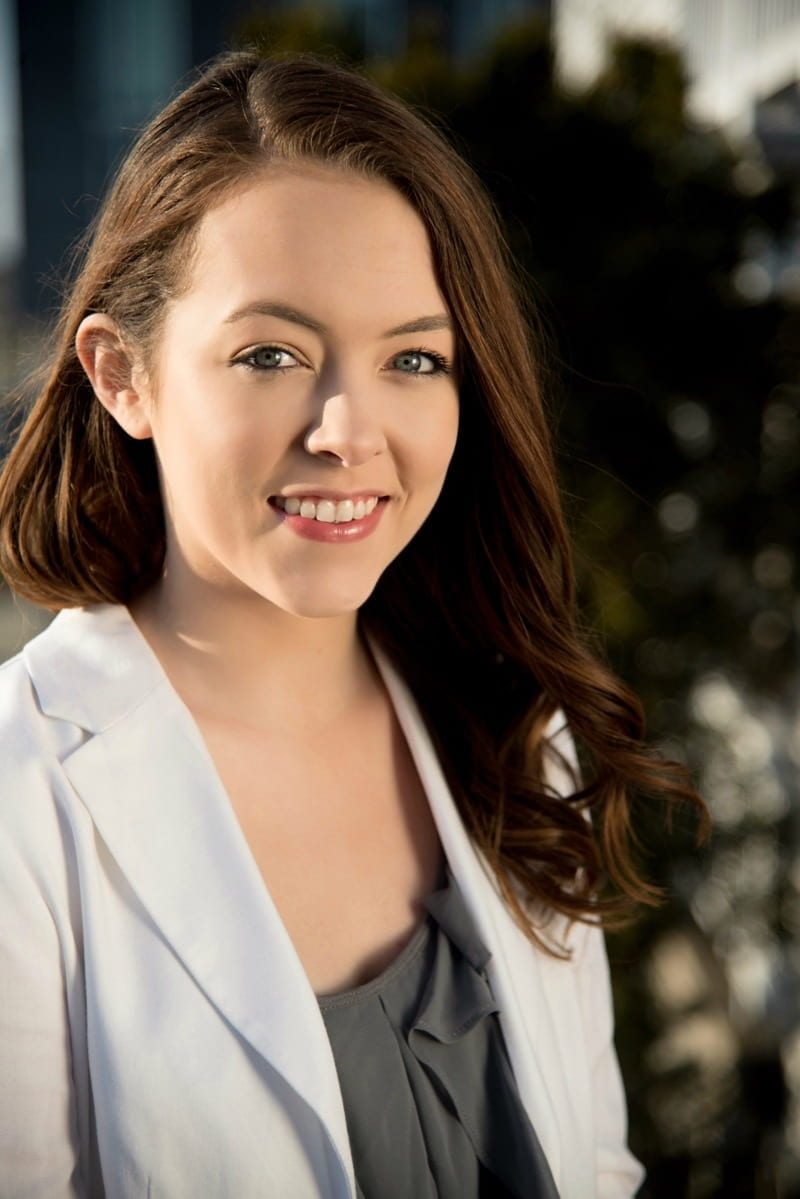
“This fellowship has given me the opportunity to focus on the translational capacity of my research so that the promise of the technologies I’ve developed in the lab can become a clinical reality.” Shannon McLoughlin
BIOE Student Researchers Receive 2023 SFB Awards

The Fischell Department of Bioengineering (BIOE) left a mark at this year’s Society For Biomaterials (SFB) 2023 Annual Meeting and Exposition. On April 19, 2023, senior Blake Kuzemchak and recent alum Erfan Jabari were awarded the SFB 2023 Outstanding Undergraduate Student Research Award and the C. William Hall Scholarship, respectively. Kuzemchak and Jabari have both worked in BIOE Chair Dr. John Fisher’s Tissue Engineering & Biomaterials Lab (TEBL).
On receiving his award, Kuzemchak said that it felt great to be recognized for his efforts. However, the most valuable part of this experience, he said, was learning to write and publish a lead-author manuscript. It gives him a head start for what is coming in graduate school.
Kuzemchak is interested in studying regenerative medicine and bionics for amputees. Specifically, he wants to develop neural interfaces that take advantage of the regenerative properties of peripheral nerves to make a far more robust nerve recording device than what the field offers now. Amputees could control more advanced bionics with a more natural feel with this new recording scheme. In fact, these neural implants could, in general, allow the average person to interface their nervous system directly with machines rather than through physical touch.
Kuzemchak enjoyed working in Fisher’s lab and was given a lot of independence after training—a rare opportunity for undergraduate researchers.
“I have an excellent graduate student mentor, Robert Choe , who helped me understand the key principles of tissue engineering and the workflow of research to shape my raw passion into productive research,” he said. “Robert pushed me to be independent and creative in my work, which I used to publish my manuscript. It has been, and continues to be, a rewarding experience working in the TEBL.”
This project started as proposed by Fisher and turned into one of his projects. His research was on developing a material that mimics the electrical properties of skin that can be 3D printed on demand into complex structures. This can be used to calibrate Bioimpedance Spectroscopy (BIS) devices that take advantage of the electrical properties of tissue to identify skin cancer. Typical natural materials cannot be readily 3D printed to recapitulate complex tissue and tumor geometries, which can often skew results for BIS devices. However, the semi-synthetic material he developed and characterized is 3D printable and maintains similar electrical properties to the skin.
Jabari is a recent BIOE graduate and has been a member of TEBL since 2019. He has assisted with osteochondral bioprinting studies and completed an honors thesis in bone regeneration. These experiences helped him better grasp the exciting role bioengineering plays in solving medicine’s most complex challenges and reinforced his interest in regenerative medicine.
Jabari noted that to be nominated by Fisher and to receive this scholarship award were exciting accomplishments. It was encouraging for his previous undergraduate thesis and the planned National Institute of Dental and Craniofacial Research (NIDCR)/TEBL post-bac research endeavors to be celebrated by SFB.
“During my junior and senior years, I completed an honors thesis that explored the role of hematopoietic osteoclasts in bone formation as a tissue engineering strategy for defect repair,” he explained. “The human mesenchymal stromal cell/osteoclast coculture system developed in this work elucidates the anabolic coupling capacity of osteoclasts and provides a platform for future investigations of osteoclast-directed bone formation.”
Jabari plans to utilize the skills he gained from this experience to develop transplantable bone marrow models and investigate hematopoietic stem cell mechanobiology as an NIDCR/TEBL post-bac.
Story from the Fischell Department of Bioengineering.
- Search News and Events
- News overview
- Media relations
World-class collaboration receives €37.5 million for regenerative medicine using smart materials
TU/e professor Carlijn Bouten is contributing to the DRIVE-RM consortium with her department and her knowledge of regenerating the body's own cardiovascular materials.
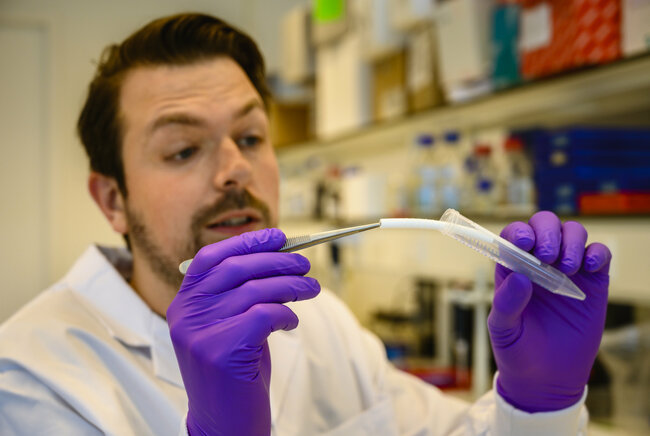
The DRIVE-RM consortium, led by Professor of Experimental Nephrology Marianne Verhaar from UMC Utrecht, has been awarded €37.5 million under the prestigious NWO SUMMIT program. The SUMMIT grant recognizes world-class collaborations, while further strengthening these partnerships. The DRIVE-RM collaboration involves UMC Utrecht, Utrecht University, Eindhoven University of Technology, Maastricht University, and the Hubrecht Institute, focusing on smart materials that assist the body in healing.
Regenerative medicine involves repairing or replacing damaged tissues and organs by leveraging the body's own healing processes. DRIVE-RM specifically focuses on material-driven regeneration, using intelligent, lifelike, or even living materials that can prompt the human body to generate new tissue.
This approach could radically change the treatment of chronic diseases such as kidney failure, heart failure, and worn joints in the future.
Recognition as world leaders
The NWO SUMMIT program targets top-tier collaborations and facilitates world-class breakthroughs. The project leader of DRIVE-RM is Marianne Verhaar, nephrologist and professor of Experimental Nephrology at UMC Utrecht.
Verhaar states, “Over the past 20 years, we have established strong collaborative efforts in regenerative medicine in the Netherlands with several large, innovative, and successful projects like the MDR Gravitation consortium. The SUMMIT grant is a wonderful recognition of this work and underscores the importance of regenerative medicine as a leading field in the Netherlands.”
Five know more than one
The involved institutes have been collaborating for some time, resulting in valuable innovations. One example is a synthetic, biodegradable blood vessel that aids the body in regenerating blood vessels. “With the consortium, we developed a blood vessel made of a degradable material that is gradually replaced by the body's own tissue,” says Verhaar.
“It can function immediately as a blood vessel and does not lose functionality as the body takes over. This innovation beautifully combines biology and technology. Now, we will see if it also works well in patients.”
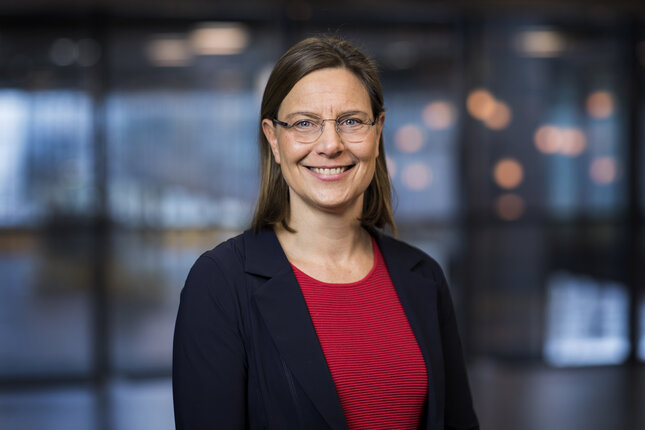
Bringing together techniques and disciplines
DRIVE-RM is led by five outstanding scientists, all experienced in leading innovative research projects. In addition to Verhaar, who focuses on kidney and vascular regeneration, the team contains Carlijn Bouten (TU/e, cardiovascular regeneration and tissue technology), Jos Malda (Utrecht University and UMC Utrecht, biofabrication and bone/cartilage regeneration), Jeroen Bakkers (Hubrecht Institute and UMC Utrecht, regenerative biology and heart regeneration), and Clemens van Blitterswijk (Maastricht University, tissue technology and biomaterials).
The DRIVE-RM project takes a multidisciplinary approach, integrating for example Utrecht innovations such as organoids and 4D bioprinting with the technical and material expertise of TU/e and Maastricht University.
This leads to new insights in directing repair processes from the level of individual cells to whole organs. These combinations are essential for customizing treatments tailored to individual patients and their specific conditions.
A new approach is needed
The number of people with chronic diseases is increasing significantly, mainly due to aging populations. This leads to human suffering and high healthcare costs. “Regenerative medicine offers a promising opportunity to effectively treat chronic conditions by encouraging the body to heal itself,” continues Verhaar.
“Our scientists aim to fully understand the repair processes of tissues and organs in the patient and then direct this with implantable smart materials.” The DRIVE-RM consortium focuses on developing new treatments for heart failure, kidney failure, and disorders of bones, cartilage, and joints.
“Besides advancing scientific research and technological innovation, DRIVE-RM also provides a platform for collaboration with clinicians to make the translation to clinical practice,” says Verhaar, “and with ethicists to address ethical issues surrounding regenerative therapies.”
Together with the involved Health Technology Assessment experts, this provides a framework that ensures these advanced interventions remain affordable and accessible.
Enabling future generations of scientists
The DRIVE-RM consortium places great emphasis on maintaining a healthy, inclusive, and diverse research culture and training the next generation—a key goal of the SUMMIT program. Therefore, the team explicitly includes emerging talents who will continue to strengthen Dutch research in regenerative medicine.
Verhaar concludes, “Ultimately, DRIVE-RM is more than just a research initiative; it is a vision for the future of healthcare. A future in which chronic diseases can truly be cured. By collaborating with leading academic institutions, DRIVE-RM aims to make these visionary treatments a reality.”
The first round of SUMMIT grants
The DRIVE-RM project is one of five collaborations supported with a SUMMIT grant. Read NWO's announcement for an overview of the five applications.
Cell-matrix interactions in cardiovascular tissue regeneration
The research group investigates the interplay between living cells and the mechanobiological cues originating from the extracellular matrix under conditions of tissue growth, adaptation, regeneration and degeneration. For this, the group uses ‘living’ model systems (cell, cell-matrix, engineered tissue, native tissue) to quantify and understand these aspects at different levels of complexity.
“We investigate how living cells shape and adapt their own environment in order to rationally design regenerative implants for the heart,” says professor Carlijn Bouten.
The obtained fundamental knowledge is translated into engineering approaches to restore or regenerate living tissues, in particular for the human heart. A prominent example is the development of a synthetic, biodegradable heart valve prosthesis that seduces the body to create a new, living heart valve at the site of implantation. Along this line, novel developments concentrate on the in situ tissue engineering of coronary arteries and vascular access grafts.
- More on this Biomedical Engineering research group
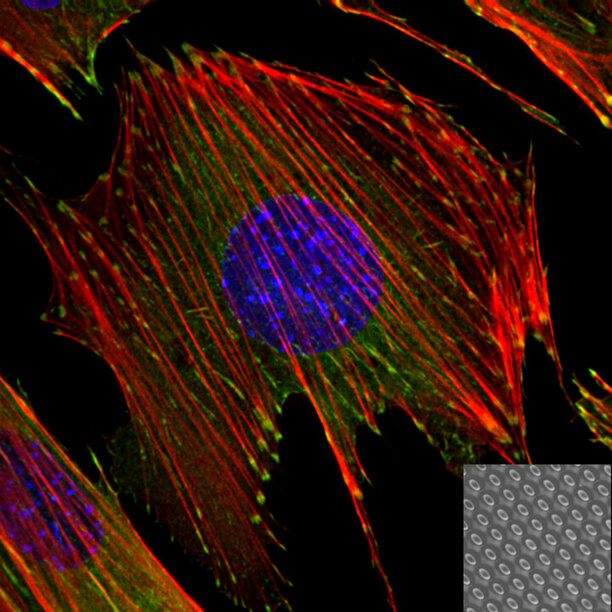
More on Health
- All the news on Health
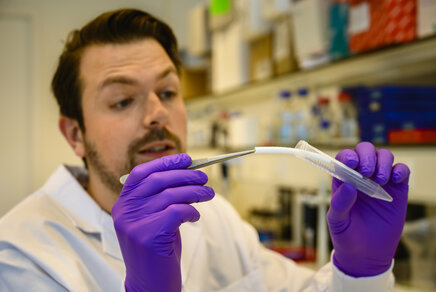
Latest news
- News Overview
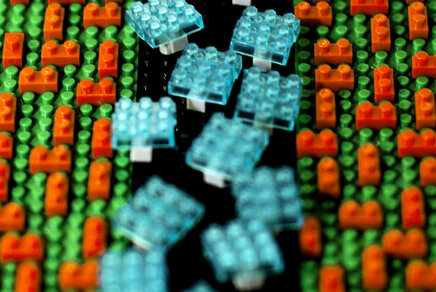
Keep following us
Newsletter research.
Sign up for our bimonthly newsletter that brings you the latest in groundbreaking research from TU/e.
TU/e podcasts
The Dutch podcast Sound of Science discusses the latest scientific discoveries and the role of technology in society.
Be part of our community and stay up to date on everything that happens at TU/e by following us on LinkedIn.
Be the first to know the latest TU/e news via our X channel.
Instagram - Research
Follow our latest research news via our research channel on Instagram.
On our YouTube channel you find the latest videos and animations about research, education and working at TU/e.
City of Hope Awarded $5.4-Million Grant to Create New Stem Cell Laboratory
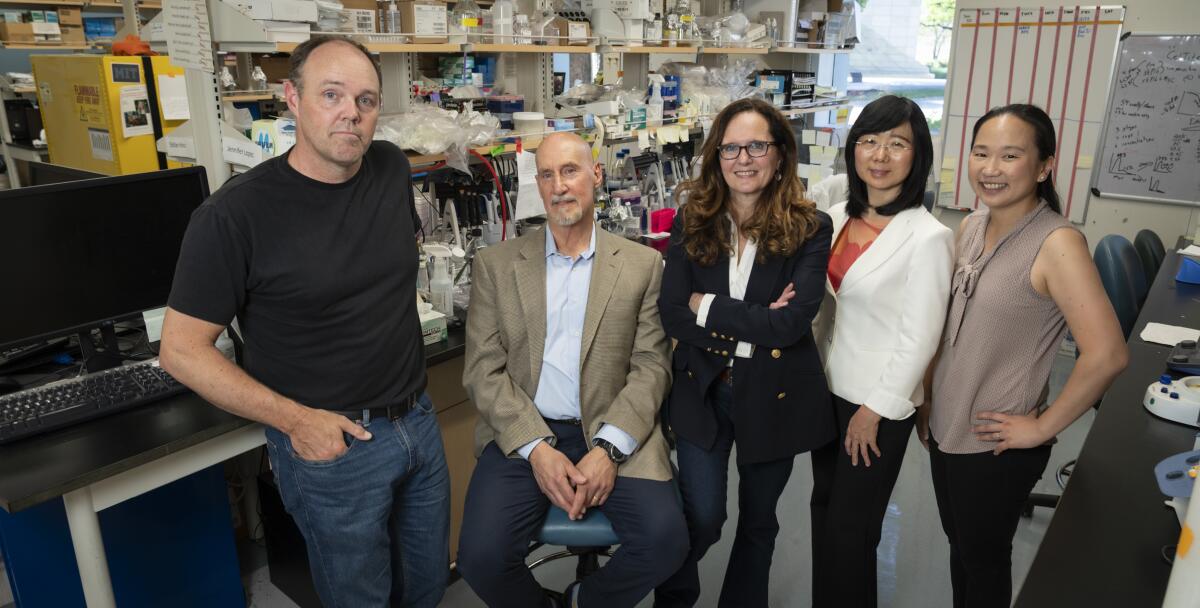
- Show more sharing options
- Copy Link URL Copied!
City of Hope, one of the largest cancer research and treatment organizations in the United States, has been awarded $5.4 million from the California Institute for Regenerative Medicine (CIRM) to build and fund a stem cell research laboratory on its Duarte campus that will further expand its scientific capabilities.
The mission of the unique Stem Cell-Based Disease Modeling Laboratory is two-fold. First, it will advance stem cell-based disease modeling to spur innovation in regenerative medicine. The laboratory leverages City of Hope’s infrastructure and expertise using human cell-derived organoids – a key focus in current efforts to create disease models relevant to understanding biological mechanisms that often lead to new therapies. In particular, the laboratory will supply healthy and cancerous stem cell-based models in brain, heart and breast tissue to the region’s scientists for research.
Second, it will stimulate stem cell research in medically underserved communities by increasing scientists’ access to specialized laboratory equipment services and training and by educating students about regenerative medicine.
“Our new laboratory will expand access to state-of-the-art disease models to researchers at City of Hope and our neighboring institutions, enabling them to pursue impactful scientific questions and to accelerate innovation in stem cell therapies,” said program director Nadia Carlesso, Ph.D., City of Hope’s chair of stem cell biology and regenerative medicine. “This will accelerate research in regenerative medicine, benefiting patients and researchers throughout California. We also aim to plant the seed for future careers in regenerative medicine by educating students about the field.”
Carlesso’s team will focus on Southern California regions, such as the Inland Empire, which is home to some of the fastest-growing, low-income communities that face greater health issues and have access to fewer physicians.
“It is important to emphasize that innovative biomedical research flourishes when people with different perspectives, experience and skills are empowered to explore new ideas and to work collaboratively and inclusively,” Carlesso said. “Thus, recruiting, training, retaining and nurturing a workforce representing all dimensions of diversity is critical for the development and implementation of leading-edge therapies that can reach underserved populations.”
To this end, a 22-person educational team will educate community physicians, researchers and students on how to use stem cell disease models with the goal of cultivating California’s future workforce in regenerative medicine. These programs will partner with higher education institutes in the Inland Empire and with K-12 school districts in Duarte, Monrovia, Charter Oak and Azusa as well as provide hands-on training to undergraduate/graduate students, postdoctoral fellows, researchers and others.
“We are eager to introduce more people to the exciting world of stem cell research and shape their vision for future jobs in science,” said co-program director John Termini, Ph.D., professor of cancer biology and molecular medicine at City of Hope. “Our workshops will explore how to apply the field’s techniques and examine diverse approaches to solving medical problems.”
“Long-term activities supported by the laboratory will accelerate research in regenerative medicine, benefiting patients and researchers throughout California,” said Carlesso, who is also associate director of basic research at the Gehr Family Center for Leukemia Research at Beckman Research Institute of City of Hope.
Led by Carlesso and Termini, the laboratory team includes Michael Barish, Ph.D., professor of stem cell biology and regenerative medicine; Mark LaBarge, Ph.D., professor of population sciences; June-Wha Rhee, M.D., assistant professor of cardiology; and Yanhong Shi, Ph.D., chair of neurodegenerative diseases and Herbert Horvitz Professor in Neuroscience.
The grant to Carlesso’s team adds to the more than $212 million that the Beckman Research Institute at City of Hope has received from CIRM to date, indicating the state-funded agency’s support of City of Hope’s long-standing leadership in stem cell-related therapies.
As a biomedical institution, City of Hope holds deep expertise in developmental and stem cell biology, resulting in strong clinical programs in bone marrow transplantation, cancer immunotherapy and gene therapy research to correct genetic defects as well as cell replacement and tissue regeneration strategies to potentially treat diabetes.
The Stem Cell-Based Disease Modeling Laboratory will operate in a renovated space that will assemble state-of-the-art instruments and technologies and centralize the generation and establishment of organoid models. It joins a wealth of City of Hope core resources, including Biostatistics & Mathematical Oncology and Gene Editing and Viral Vector cores, to both advance science and train others in subspecialized scientific areas.

IMAGES
VIDEO
COMMENTS
Stanford is a world leader in stem cell research and regenerative medicine. Central discoveries in stem cell biology - tissue stem cells and their use for regenerative therapies, transdifferentiation into mature cell-types, isolation of cancerous stem cells, and stem cell signaling pathways - were made by Stanford faculty and students.
Research in tissue engineering and regenerative medicine seeks to replace or regenerate diseased or damaged tissues, organs, and cells - a challenging endeavor, but one that has tremendous potential for the practice of medicine. Technologies under investigation range from biomaterial/cell constructs for repairing various tissues and organs ...
Seeking to spur development of innovative medical breakthroughs, Mayo Clinic Graduate School of Biomedical Sciences, in partnership with the Center for Regenerative Biotherapeutics, started one of the nation's first doctoral research training programs in regenerative sciences.. Regenerative medicine is transforming clinical practice with the development of new therapies, treatments and ...
Combating disease and tissue degeneration and improving human health. This is the ultimate goal of HSCRB's research, whether it is focused on the most basic level of cellular development or screening chemical compounds for potential drugs. Our mission is to push the frontiers of stem cell and regenerative biology, illuminating the workings of ...
The PhD Graduate Program in Cell Biology & Regenerative Medicine (CBRM) provides students with the background, training, and experience necessary to launch a career as an independent scientific investigator that significantly contributes to the advancement of human health. Faculty in our research program use the tools of molecular and cellular biology, tissue engineering, genetic animal models ...
Ying Zheng, PhD (Bioengineering) Dr. Zheng's research focuses on understanding and engineering the fundamental structure and functions in living tissue and organ systems from nanometer, micrometer to centimeter scale. These are the faculty members that are specialized in tissue engineering at the Institute for Stem Cell & Regenerative Medicine.
Tissue Engineering &. Regenerative Medicine. Research in tissue engineering and regenerative medicine encompasses all aspects of the research and development continuum from mechanistic studies to translational approaches. Collaborative efforts with colleagues at Rice and the Texas Medical Center address unmet clinical needs for a plethora of ...
Regenerative Medicine is a multidisciplinary field developing next-generation therapies that aim to augment, repair, replace or regenerate tissues and organs. This field can be broadly defined by three overlapping technology domains: cell therapy, gene therapy, and tissue engineering.
The PhD track in Tissue Engineering and Regenerative Medicine (TERM) prepares the students for a career in cutting edge research and development of cell- and biomaterial-based medical products. The MPE track introduces students to the use of cells, materials, biochemical and biomechanical factors in the development of functional substitutes ...
The Centre for Regenerative Medicine (CRM) is a world leading research centre based at the University of Edinburgh's Institute for Regeneration and Repair. Our scientists and clinicians study stem cells, disease and tissue repair to advance human health. By better understanding how stem cells are controlled and how diseases develop in a lab ...
The use of tissue engineering in regenerative medicine, known as TERM, is an active area of research that involves creating functional tissue through the combination of cells, scaffolds, and growth factors to restore normal biological function. 1 Clinicians treat millions of patients with tissue engineered regenerative devices.
Graduate Degree Programs in Regenerative medicine (including stem cells and tissue engineering) Master of Engineering in Biomedical Engineering (MEng) Biomedical Engineers apply their knowledge in engineering, biology, and medicine to healthcare and medical device industries.
Tissue Engineering and Regenerative Medicine is a platform for the advancement and dissemination of research and technologies related to its field. Indexed and included in PubMed/MEDLINE with all articles from 2019 searchable. Promotes sharing and dissemination of knowledge among members and provides insights into new research trends.
Course Overview. This Structured PhD programme spans the Biomedical Engineering and Regenerative Medicine domains and aims to become a leading international graduate education programme in the field, in which Irelandis already taking a strong international role. The overall objective is to develop PhD graduates who will produce ground-breaking ...
The Tissue Engineering and Regenerative Medicine Program supports basic and translational research on employing bioengineering- and stem cell biology- based approaches for the reconstruction, repair, and regeneration of dental, oral, and craniofacial (DOC) tissues damaged because of disease or injury.
3D printing of scaffolds for tissue engineering and regenerative medicine. Brunel University London Mechanical and Automotive Engineering. This project aims to develop multifunctional novel bioactive tissue scaffolds using 3D printing, biomaterials science and controlled drug delivery systems to enhance tissue regeneration.
3D printing of scaffolds for tissue engineering and regenerative medicine. Brunel University London Mechanical and Automotive Engineering. This project aims to develop multifunctional novel bioactive tissue scaffolds using 3D printing, biomaterials science and controlled drug delivery systems to enhance tissue regeneration.
Methods of Review. The first challenge in conducting this review was the sheer number of recent publications in the TERM field. The origins of the terms "tissue engineering" and "regenerative medicine" have been previously discussed in this journal, 1 with the former coming into common parlance in the mid-to-late 1980s and the latter gaining momentum around the turn of the 21st century.
Search Funded PhD Projects, Programmes & Scholarships in Engineering, Biomedical Engineering, regenerative medicine. Search for PhD funding, scholarships & studentships in the UK, Europe and around the world. ... Hydrogel-based three-dimensional (3D) printing has garnered significant interest in various biomedical fields like tissue engineering ...
Our MSc Tissue Engineering for Regenerative Medicine looks at regenerating and ... commercialisation and clinical translation of regenerative therapies. Prepare for PhD study, specialist clinical training or a career in related industries, including pharmaceutical, biotechnology and regenerative medicine sectors. Study at a university ranked ...
The MSc in Tissue Engineering and Regenerative Medicine is a one year, full-time Programme. The MSc Programme commences in September each year with Stage 1, a 6-month, taught component involving four compulsory Modules. On completing Stage 1, students undertake a 5-month, laboratory-based research project, between April-September ( Stage 2 ).
In addition, we can encapsulate living cells into that gelatin-like bioink," says Dr. Dicker. "This type of tissue engineering is an emerging technology in regenerative medicine that has the potential to transform laboratory research and clinical practice by bioengineering replacements for damaged or diseased tissue." Bioprinting organs
With funding from the 2024 John H. Tietze Stem Cell Scientist Award, Dr. Nate Sniadecki and his lab will develop an in vitro, mechanobiological model of human tendons. These engineered tendon models have the potential to be used to test new therapies, model genetic diseases, or build replacement tissue materials.
Self-Assembling Peptide RADA16: A Promising Scaffold for Tissue Engineering and Regenerative Medicine. Xin Yao 1 Department of Orthopaedics, Lanzhou University Second Hospital, Lanzhou 730030, Gansu, ... nontoxic degradation products and ease of modification make it an ideal scaffold for tissue engineering. RADA16 is a good delivery vehicle for ...
There are three broad fields of graduate study and research: Imaging and medical physics; Neural engineering; Regenerative engineering; Industry Advisory Board. The MS program is informed by an industry advisory board with significant experience in medicine, business, government and technology. To view the advisory board, click here. Curriculum
In the vanguard of biomedical innovation, regenerative medicine emerges as a transformative paradigm, concentrating its efforts on rectifying tissue deficits and functional aberrations in patients through the strategic augmentation of endogenous cellular processes. Central to this approach is nucleic acid therapy, which offers a novel pathway for tissue regeneration by modulating key signaling ...
The PhD candidate must "defend" their work to the committee to prove they made a significant difference in their field. 01010101. ... (ISBF); the Society for Biomaterials (SFB); and the Tissue Engineering and Regenerative Medicine International Society (TERMIS). ...
Decellularization and characterization of camel pericardium as a new scaffold for tissue engineering and regenerative medicine. Muhammad Mehdi Jafari Sorkhdehi, Ali Doostmohammadi, ... Department of Tissue Engineering and Applied Cell Sciences, School of Medicine, Semnan University of Medical Sciences, Semnan, Iran.
Regenerative medicine involves repairing or replacing damaged tissues and organs by leveraging the body's own healing processes. DRIVE-RM specifically focuses on material-driven regeneration, using intelligent, lifelike, or even living materials that can prompt the human body to generate new tissue.
City of Hope, one of the largest cancer research and treatment organizations in the United States, has been awarded $5.4 million from the California Institute for Regenerative Medicine (CIRM) to ...