
An official website of the United States government
The .gov means it’s official. Federal government websites often end in .gov or .mil. Before sharing sensitive information, make sure you’re on a federal government site.
The site is secure. The https:// ensures that you are connecting to the official website and that any information you provide is encrypted and transmitted securely.
- Publications
- Account settings
Preview improvements coming to the PMC website in October 2024. Learn More or Try it out now .
- Advanced Search
- Journal List
- HHS Author Manuscripts


Challenges to Sustainable Safe Drinking Water: A Case Study of Water Quality and Use across Seasons in Rural Communities in Limpopo Province, South Africa
Joshua n. edokpayi.
1 Department of Hydrology and Water Resources, University of Venda, Thohoyandou 0950, South Africa; [email protected]
2 Department of Civil and Environmental Engineering, University of Virginia, Charlottesville, VA 22904, USA; ude.qud@drelhak (D.M.K.); moc.liamg@320hlc (C.L.H.); ude.ainigriv@sm4rfc (C.R.); ude.ainigriv@e9saj (J.A.S.)
Elizabeth T. Rogawski
3 Department of Public Health Sciences, University of Virginia, Charlottesville, VA 22908, USA; ude.ainigriv@m5rte
4 Division of Infectious Diseases & International Health, University of Virginia, Charlottesville, VA 22908, USA; ude.ainigriv.ccm.liamcsh@v8dr
David M. Kahler
5 Center for Environmental Research and Education, Duquesne University, Pittsburgh, PA 15282, USA
Courtney L. Hill
Catherine reynolds.
6 School of Civil and Environmental Engineering, Georgia Institute of Technology, Atlanta, GA 30332, USA
Emanuel Nyathi
7 Department of Animal Science, University of Venda, Thohoyandou 0950, South Africa; [email protected]
James A. Smith
John o. odiyo, amidou samie.
8 Department of Microbiology, University of Venda, Thohoyandou 0950, South Africa; [email protected] (A.S.); [email protected] (P.B.)
Pascal Bessong
Rebecca dillingham.
Author Contributions: Conceived and designed the experiments: J.N.E., E.T.R., D.M.K., C.L.H. Performed the experiments: J.N.E., E.T.R., D.M.K., C.L.H., C.R., E.N. Contributed reagents/materials/analysis tools: P.B., E.N., A.S., R.D., J.A.S., J.O.O. Analyzed the data: J.N.E., E.T.R., D.M.K., C.L.H. Wrote the paper: J.N.E., E.T.R., D.M.K., C.L.H. Participated in the editing of the manuscript: J.N.E., E.T.R., D.M.K., C.L.H., P.B., A.S., R.D., J.A.S., J.O.O., E.N., C.R.
Associated Data
Table S2: Membrane-filtration results for E. Coli and total coliforms of water sources,
Table S3: Anion concentrations (mg/L) of water sources,
Table S4: Major metal concentrations (mg/L) of water sources,
Table S5: Trace metal concentrations μg/L) of water sources.
Consumption of microbial-contaminated water can result in diarrheal illnesses and enteropathy with the heaviest impact upon children below the age of five. We aimed to provide a comprehensive analysis of water quality in a low-resource setting in Limpopo province, South Africa. Surveys were conducted in 405 households in rural communities of Limpopo province to determine their water-use practices, perceptions of water quality, and household water-treatment methods. Drinking water samples were tested from households for microbiological contamination. Water from potential natural sources were tested for physicochemical and microbiological quality in the dry and wet seasons. Most households had their primary water source piped into their yard or used an intermittent public tap. Approximately one third of caregivers perceived that they could get sick from drinking water. All natural water sources tested positive for fecal contamination at some point during each season. The treated municipal supply never tested positive for fecal contamination; however, the treated system does not reach all residents in the valley; furthermore, frequent shutdowns of the treatment systems and intermittent distribution make the treated water unreliable. The increased water quantity in the wet season correlates with increased treated water from municipal taps and a decrease in the average contaminant levels in household water. This research suggests that wet season increases in water quantity result in more treated water in the region and that is reflected in residents’ water-use practices.
1. Introduction
Clean and safe drinking water is vital for human health and can reduce the burden of common illnesses, such as diarrheal disease, especially in young children. Unfortunately, in 2010, it was estimated that 1.8 billion people globally drank water that was not safe [ 1 ]. This scenario is most common in developing countries, and the problem is exacerbated in rural areas [ 1 ]. Significant amounts of time are spent by adults and school children upon water abstraction from various sources [ 2 , 3 ]. It is estimated that, in developing countries, women (64%) and girls (8%) spend billions of hours a year collecting water [ 1 ]. The erratic supply of safe drinking and domestic water often affects good hygiene practices. In most developing countries of the world, inadequate supplies of drinking water can contribute to the underage death of children in the region [ 4 – 10 ].
Storage of collected water from rivers, springs, community stand-pipes, and boreholes is a common practice in communities that lack potable water supplies piped into their homes. Even when water is piped into the home, it is often not available on a continuous basis, and water storage is still necessary. Water is stored in various containers which include jerry cans, buckets, drums, basins and local pots [ 11 – 13 ]. It has been reported that when collection of water from sources of high quality is possible, contamination during transport, handling and storage and poor hygienic practices often results and can cause poor health outcomes [ 11 , 13 – 15 ].
South Africa is a semi-arid country that has limited water resources, and the provision of adequate water-supply systems remains a great challenge. In some of the major cities, access to clean and safe drinking water is comparable to what is found in other developed cities, but this is not the case in some cities, towns and most villages where there is constant erratic supply of potable water, and in some cases, there is no water supply system [ 16 ]. Although access to clean and safe drinking water is stipulated as a constitutional right for all South Africans in the country’s constitution [ 17 , 18 ], sustainable access to a potable water supply by millions of South Africans is lacking.
Residents of communities with inadequate water supply are left with no alternative other than to find local sources of drinking water for themselves. Rural areas are the most affected, and residents resort to the collection of water from wells, ponds, springs, lakes, rivers and rainwater harvesting to meet their domestic water needs [ 19 – 24 ]. Water from such sources is often consumed without any form of treatment [ 12 , 19 , 21 ]. However, these alternative sources of drinking water are often vulnerable to point and non-point sources of pollution and are contaminated frequently by fecal matter [ 5 , 19 , 25 ]. A report by the South African Council for Scientific and Industrial Research clearly showed that almost 2.11 million people in South Africa lack access to any safe water infrastructure. The consumption of water from such unimproved sources without treatment constitutes a major public health risk [ 26 ].
Consumption of contaminated drinking water is a cause of diarrheal disease, a leading cause of child mortality in developing countries with about 700,000 deaths of children under the age of 5 reported in 2011 [ 10 , 27 ]. In South Africa, diarrhea is one of the leading causes of death among young children, and this problem is worst in children infected with HIV (Human Immunodeficiency Virus).
The health risks associated with the consumption of unsafe drinking water are not only related to infectious diseases but also to other environmental components such as fluoride, arsenic, lead, cadmium, nitrates and mercury. Excessive consumption of these substances from contaminated drinking water can lead to cancer, dental and skeletal fluorosis, acute nausea, memory lapses, renal failure, anemia, stunted growth, fetal abnormalities and skin rashes [ 16 , 28 ]. Groundwater contamination with high arsenic concentrations have been reported in Bangladesh, and high fluoride concentrations have been reported in the drinking water from various provinces in South Africa [ 28 – 34 ].
Temporary seasonal variations have been reported to influence the levels of contaminants in various water sources differently. The key environmental drivers across the wet and dry seasons include: volume of water, flow, frequency of rainfall events, storm run-off, evaporation and point sources of pollution [ 35 , 36 ]. An increase in storm-water run-off within a river catchment may increase the level of contaminants due to land-use activities. Increased water volume could lead to a decrease in the concentration of contaminants due to the dilution effect. A low incidence of rainfall and high evaporation can cause a contaminant to concentrate in water. Very few water-quality parameters such as turbidity are expected to be higher in the wet season. Other parameters can vary depending on the key environmental drivers. There is paucity of data on the effect of change across seasons on water-use practices among household in rural areas of developing countries.
The geographic area for this study is located 35 km north of Thohoyandou, in Limpopo Province, South Africa. The area is primarily agricultural, such that water contamination by nitrates is a potential concern. In addition, mining operations in the area may contaminate water sources with heavy metals.
The significance of this study lies in the broad characterization of water-quality parameters that could affect human health, which is not restricted to microbiological analysis. In a rural community, the primary concern of drinking water is the microbiological quality of the water and chemical constituents are often considered not as problematic. This study was designed to evaluate a broad spectrum of water-quality constituents of natural water sources and household drinking water used by residents of rural communities in Limpopo Province. We also aimed to determine how water sources and collection practices change between dry and wet seasons within a one-year sampling period.
2. Materials and Methods
2.1. study design.
A baseline census of 10 villages in the Thulamela Municipality of Limpopo Province was completed to identify all households in which there was at least one healthy child under 3 years of age in the household, the child’s caregiver was at least 16 years of age, and the household did not have a permanent, engineered water-treatment system. 415 households that met these eligibility criteria were enrolled for the purposes of a water-treatment intervention trial. The baseline assessment of water-quality and use practices is reported here. Caregivers of the child under 3 years of age were given a questionnaire concerning demographics, socioeconomic status, water-use practices, sanitation and hygiene practices, and perceptions of water quality and health. In addition, a sample of drinking water was taken from a random selection of 25% of the total enrolled households in the dry (June–August 2016) and wet seasons (January–February 2017). The participant population was sorted by community, as a surrogate for water supply, and one-third from each community was randomly selected by a random number generated within Microsoft Excel (Seattle, WA, USA), which was sampled. The protocol used was approved by the Research Ethics Committee at the University of Venda (SMNS/15/MBY/27/0502) and the Institutional Review Board for Health Sciences Research at the University of Virginia (IRB-HSR #18662). Written informed consent was obtained from all participants and consent documentation was made available in English and Tshivenda. The majority of the baseline surveys were conducted in the dry season (approximately April to October). Six-months later, follow-on surveys were conducted at the height of the wet season (approximately November to March; however, the height of the season in 2016–17 was January to March).
2.2. Regional Description of the Study Area
The communities are located in a valley in the Vhembe District of Limpopo Province, South Africa ( Figure 1 ). The valley surrounds the Mutale River in the Soutpansberg Mountains and is located around 22°47′34′′ S and 30°27′01′′ E, in a tropical environment that exhibits a unimodal dry/wet seasonality ( Figure 2 ). In recent years, the area has received annual precipitation between 400 mm and 1100 mm; more importantly, the timing of the precipitation is highly variable ( Figure 2 ). Specifically, in 2010, the annual precipitation was about 750 mm; however, the majority of the precipitation came in March while, traditionally, the wet season begins earlier, in September or October. The year 2011 had the highest precipitation in the six-year period and had the majority of the rainfall in November. The years 2012 and 2015 began with a typical precipitation pattern; however, the rainfall did not continue as it did in 2013 and 2014. Annual temperature of the area also varies, with the highest temperature always recorded in the wet season ( Figure 3 ). There has been much variability of temperature in past years; however, this is beyond the scope of this study. The abbreviations used in Figure 1 and other figures, including the supplementary data and the type of the various water sources used in this study, are shown in Table 1 .

Map of the study area. The communities are all located within the Mutale River watershed. The rivers are indicated in blue, villages outlined in purple, environmental samples in blue squares, tributaries in green circles (which have intermittent flow), watershed boundary in orange. This heavily agricultural area has cultivated areas along both sides of thee Mutale River for the vast majority of the region; the area is shown with green outlines. There are two identified brick-processing areas shown in brown rectangles. Unfortunately, some sites are so close that the markers overlap (as with CR and IR). The location of the community supplies (CA, CB, and CC) are not shown to protect the privacy of those villages. See supplemental information for Google Earth files.

Precipitation trends in the study area. ( a ) Annual precipitation by hydrologic year. Data quality are presented on a scale of zero to unity where the quantity shown represents the proportion of missing or unreliable data in a year; ( b ) Cumulative precipitation for the last five complete years; ( c ) Average monthly precipitation calculated for years with greater than 90% reliable data (bottom right). All data are presented by the standard Southern hemisphere hydrologic year from July to June numbered with the ending year. Data are from the Nwanedzi Natural Reserve at the Luphephe Dam (17 km from the study area) and fire available through the Republic of South Africa, Department of Water and Sanitation, Hydrologic Services ( http://www.dwa.gov.za/Hydrology/ ).

The mean monthly temperature in the region recorded at Punda Milia. ( a ) Mean monthly temperature based on the means from 1962–1984; ( b ) Mean monthly temperature record. Data are available from the National Oceanic and Aviation Administration (U.S.), National Climatic Data Center, Climate Data Online service ( https://www.ncdc.noaa.gov/cdo-web/ ).
Abbreviations, water sources and type.
Agriculture occupies tine greatest land cover in the valley. Mogt households are engaged in some level of farming. Crops cultivated include maize and vegetables, and tree fruits include mangos and citrus fruits. Livestock is prevalent in the area with chickens, goats, and cattle. Smaller animals typically remain closer to households and larger animals graze throughout the region without boundaries. There are several brick-making facilities in the valley that include excavation, brick-forming and drying.
2.3. Water Sources
Drinking water in the study communities is available from a number of municipal and natural sources. The primary source of drinking water for seven of the villages is treated, municipal water. Two of the villages have community-level boreholes, storage tanks, and distribution tanks. An additional village has a borehole as well; however, residents report that, since its installation, the system has never supplied water.
The water for the treatment facility is drawn from behind a weir in the Mutale River and pumped to a retention basin. The water then undergoes standard treatment that includes pH adjustment, flocculation, settling, filtration, and chlorine disinfection. Water is then pumped to two elevated tanks that supply several adjacent regions, including the study area. Specifically, Branch 1 supplies Tshandama, Pile, Mutodani, Tshapasha and Tshibvumo; Branch 2 supplies an intermediary tank that in turn serves Matshavhawe, Muledane and Thongwe. Households can pay for a metered yard connection for the water used; these yard connections can be connected to household plumbing at the household’s discretion. The treated municipal water service is intermittent. Service in Tshandama and Pile was observed to be constant during the wet season and for only about two to three days per week during the dry season. Service in the remaining communities is two to four days per week during the wet season and about two days per week during the dry season. Furthermore, for the past two years, major repairs in the dry season caused the treated municipal water to cease completely. Households typically stored water for the periods when the treated municipal water was off; however, when the municipal water was unavailable for longer periods or not on the anticipated schedule, households obtained water from natural sources. The community-level boreholes provided water almost constantly but were subject to failure and delays in repairs.
Aside from the municipal sources, many residents of three villages have access to a community installed and operated distribution system that delivers water from the adjacent ephemeral rivers throughout the community (CA, CB, and CC). These systems are constructed with 50 mm to 70 mm (5 to 7 × 10 −2 m) high-density polyethylene pipes. Even these community-level schemes provide water on a schedule and sometimes require repair. Another common source of water for the community is springs. These shallow groundwater sources are common in the valley; however, there are communities that do not have a nearby spring. Some springs have had a pipe placed at the outlet to keep the spring open and facilitate filling containers. Researchers did not observe any constructions around the springs to properly isolate them from further contamination, and they are, therefore, not improved water sources. Pit latrines are common in every household throughout the region. Source (TS) is located near these communities while other springs (OS, LS) are located in agricultural areas. Boreholes provide deep groundwater supplies but require a pump. Such systems provide water as long as there is power for the pump and the well is deep enough to withstand seasonal variations. The two clinics in the study area surveyed each relied on a borehole for their water supply. Some residents also collected water directly from the river. The Mutale River is a perennial river; however, the ephemeral rivers, the Tshiombedi, Madade, Pfaleni, and Tshala Rivers, do not flow in the dry season all the way to the floor of the valley. The Tshala River has a diversion to a lined irrigation canal that always carries water, but there is very little flow that remains in the natural channel.
2.4. Water Sampling
The team of community health workers (CHW) that had previously conducted the MAL-ED (Malnutrition and Enteric Diseases) study in the same region [ 37 ] were recruited to assist with the data collection for this study; specifically, the regional description and water sources. These CHWs have an intimate knowledge of the communities as they are residents and have conducted health research in the area. The CHWs provided information on the location and condition of the various water sources in the study communities.
Water sources were tested during two intensive study periods: one in the dry season (June–August, 2016) and the other in the wet season (January–February, 2017). Water sources for investigation were selected based on identification from resident community health workers. Single samples were taken from all 28 identified drinking water sources in the 10 villages and three days of repeated samples were taken from six sources, which represented a range of sources (e.g., surface, borehole, shallow ground, pond, and municipal treated) in the dry season. Single samples of 17 of the original sources and three days of repeated samples were taken from five sources in the wet season, six months later. Some sources were not resampled because the routes to the sources were flooded, and these sources were likely infrequently used during the wet season due to blocked pathways. The wet and dry season measurements gave two different scenarios for water-use behaviors and allowed the researchers to measure representative water-quality parameters.
2.5. Measurement of Physicochemical Parameters
Physicochemical parameters of source water samples were measured in the field by a YSI Professional Plus meter (YSI Inc., Yellow Springs, OH, USA) for pH, dissolved oxygen and conductivity. The probes and meter was calibrated according to the manufacturer’s instructions. Turbidity was measured in the field with an Orbeco-Hellige portable turbidimeter (Orbeco Hellige, Sarasota, FL, USA) (U.S. Environmental Protection Agency method 180.1) [ 38 ]. The turbidimeter was calibrated according to the manufacturer’s instructions. Measured levels were compared to the South African water-quality standards in the regulations [ 39 ], pursuant to the Water Services Act of 1997.
2.6. Microbiological Water-Quality Analysis
Escherichia coli ( E. coli ) and total coliform bacteria were measured in both source and household water samples by membrane filtration according to U.S. Environmental Protection Agency method 10,029 [ 40 ]. Sample cups of the manifold were immersed in a hot-water bath at 100 °C for 15 min. Reverse osmosis water was flushed through the apparatus to cool the sample cups. Paper filter disks of 47 mm (4.7 × 10 −2 m) diameter and 0.45 μm (4.5 × 10 −7 m) pore size (EMD Millipore, Billerica, MA, USA) were removed from their sterile, individual packages and transferred to the surface of the manifold with forceps with an aseptic technique. Blank tests were run with reverse osmosis dilution water. Two dilutions were tested: full-strength (100 mL sample) and 10 −2 (1 mL sample with 99 mL of sterile dilution water) were passed through the filters; this provides a range of zero to 30,000 CFU/100 mL (colony forming units) for both E. coli and total coliforms. The filter paper was placed in a sterile petri dish with absorbent pad with 2 mL (2 × 10 −6 m 3 ) of selective growth media solution (m-ColiBlue24, EMD Millipore, Billerica, MA, USA). The samples were incubated at 35 °C (308.15 K) for 23–25 h. Colonies were counted on the full-strength sample. If colonies exceeded 300 (the maximum valid count), the dilution count was used. In all tests, the dilution value was expected to be within 10 −2 of the full-strength value and the sample was discarded otherwise.
The distribution of the household bacteria levels was evaluated by the (chi square) χ 2 goodness-of-fit test for various subsets of the data. Subsets of the data were then compared by an unpaired Student’s t-test for statistical significance; specifically, wet versus dry season levels as well as any other subsets that could demonstrate differences within the data.
2.7. Major Metals Analysis
A Thermo ICap 6200 Inductively Coupled Plasma Atomic Emission Spectrometer (ICP-AES, Chemetix Pty Ltd., Johannesburg, South Africa) was used to analyze the major metals in the various samples. The National Institute of Standards and Technology traceable standards (NIST, Gaithersburg, MD, USA) purchased from Inorganic Ventures (INORGANIC VENTURES 300 Technology Drive Christiansburg, Christiansburg, VA, USA) were used to calibrate the instrument for the quantification of selected metals. A NIST-traceable quality control standard from De Bruyn Spectroscopic Solutions, Bryanston, South Africa, were analyzed to verify the accuracy of the calibration before sample analysis, as well as throughout the analysis to monitor drift.
2.8. Trace Metals Analysis
Trace elements were analyzed in source water samples using an Agilent 7900 Quadrupole inductively coupled plasma mass spectrometer (ICP-MS) (Chemetix Pty Ltd., Johannesburg, South Africa). Samples were introduced via a 0.4 mL/min (7 × 10 −9 m 3 s −1 ) micro-mist nebulizer into a Peltier-cooled spray chamber at a temperature of 2 °C (275.15 K), with a carrier gas flow of 1.05 L/min (1.75 × 10 −5 m 3 s −1 ). The elements V, Cr, Mn, Fe, Co, Ni, Cu, Zn, As, Se were analyzed under He-collision mode to remove polyatomic interferences. NIST-traceable standards was used to calibrate the instrument. A NIST-traceable quality control standard of a separate supplier to the main calibration standards was analyzed to verify the accuracy of the calibration before sample analysis.
2.9. Anion Analysis
The anions were analyzed in source-water samples as stated in Edokpayi et al. [ 41 ]. Briefly, an Ion Chromatograph (Metrohm, Johannesburg, South Africa) was used to analyze the concentrations of fluoride, bromide, nitrates, chloride and sulfate. Calibration standards in the range of 1–20 mg/L were prepared from 100 mg/L stock solution containing all the test elements. Prior to analysis, the samples were filtered with a 0.45 μm (4.5 × 10 −7 m) syringe filter. Eluent for the sample run was prepared from sodium bicarbonate and sodium carbonate. A 50 mmol/L sulphuric acid with a flow rate of 0.5 mL/min (8 × 10 −9 m 3 s −1 ) was used as suppressant.
3.1. Socio-Demographic Characteristics of Enrolled Households
We included 405 enrolled households who completed the baseline questionnaire. The majority of caregivers were the mothers (n = 342, 84.4%, median age = 27 years) or grandmothers (n = 51, 12.6%, median age = 50 years) of a young child in the household. Almost all the caregivers had completed at least secondary school education (n = 371, 91.6%). Median monthly income for the entire household was USD$106 (interquartile range (IQR): 71–156). Access to improved sanitation was high. 373 (n = 92.1%) households used an improved pit latrine, and only 19 (n = 4.7%) reported open defecation. However, few households (n = 35,8.6%) reported having a designated place to wash hands near their toilet, and only 29% (n = 119) reported always using soap when washing hands.
Most households had their primary water source ( Table 2 ) piped into their or their neighbor’s yard (dry: n = 226, 62.3%; wet: n = 241, 67.5%) or used a public tap (dry: n = 69, 19.0%; wet: n = 74, 20.7%). A minority (dry: n = 40, 11.0%; wet: n = 19, 5.3%) collected their water directly from rivers, lined canals, or springs. Water was collected by adult women in most households, and it was reported to take a median of 10 min (IQR, both seasons: 5–30) to go to their water source, collect water, and come back in one trip. Three quarters (n = 270, 74.4%) reported that their water source was not continually available in the dry season and two-thirds (n = 234, 65.5%) in the wet season. Almost half (48.9%) reported interruptions in availability that lasted at least 7 days in the dry season and 32.8% in the wet season. Households stored water during interruptions and/or collected water from alternative sources (dry: n = 133, 36.6%; wet: n = 115, 32.2%), which were surface water or shallow groundwater sources (e.g., rivers, lined canals, or springs).
Primary drinking-water sources reported among 363 and 357 households in the study area in the dry and wet seasons, respectively.
Household water was most frequently stored in jerry cans or plastic buckets (n = 363, 89.7%), while 25 households stored water in large drums or plastic tanks (6.2%). Most households reported that their drinking water containers were covered (n = 329, 81.2%), but most used a cup with a handle (n = 281, 69.4%) or their hands (n = 93, 23.0%) for water collection ( Table 3 ). Only 13.3% (n = 54) households reported treating their water, mainly by boiling (n = 22), chlorine (n = 15), or letting the water stand and settle (n = 11).
Mode of water collection from storage containers.
Approximately one-third of caregivers (n = 114, 28.2%) perceived that one can get sick from drinking water (n = 114, 28.2%), and cited diarrhea, schistosomiasis, cholera, fever, vomiting, ear infections, malnutrition, rash, flu and malaria as specific illnesses associated with water. Despite these perceptions, the majority were satisfied with their current water source (n = 297, 73.3%). Those who were unsatisfied cited reasons of insufficient quantity (n = 75), shared water supply (n = 65), uncleanliness (n = 73), cloudiness (n = 47), and bad odor or taste (n = 38).
3.2. Physicochemical and Microbiological Characteristics of the Water Sources
pH and conductivity values ranged between 5.5–7.3 and 24–405 μS/cm in the wet season and 5.8–8.7 and 8–402 μS/cm in the dry season ( Table S1 ). Both pH and conductivity levels were within the recommended limits of the World Health Organization (WHO) for drinking water. The microbiological results and turbidity of the sources tested are presented in Figures Figures4 4 and and5, 5 , and Table S2 , respectively. Microbiological data show contamination with E. coli , a fecal coliform that is potentially pathogenic, and other coliform bacteria.

Membrane filtration results for ( a ) E. coli and ( b ) other coliforms. Data are presented for wet and dry seasons. The four ephemeral rivers (*) have no dry season data because they had no flow; all other sources have the results reported, some of which are zero or near-zero. South African National Standard (SANS 241:1-2015) set the limit of 0 CFU/100 mL for E. coli and 10 CFU/100 mL for total coliforms (CFU/10 −4 m 3 ). Ephemeral rivers that do not flow all the way into the valley are indicated (*) in the dry season.

Turbidity of the water sources in the study area. Two to three measurements were taken during an intensive study period from 13 January 2017 to 4 February 2017 in the wet season and three to four measurements from 5 June 2016 to 15 July 2016 in the dry season. The median measurement of the values is reported here. Ephemeral rivers that do not flow all the way into the valley are indicated (*) in the dry season.
Municipal treated water never showed any detectable colony-forming units (CFU) in a 100 mL sample for E. coli , which is within the Soufh African regulation [ 39 ]. In the wet season, other coliform bacteriaweae detected in the treated wtter (a median valueof 10 CFU/100 mL wac recorded).
Household sample of stored water ( Figure 6 ) show that bacterial contamination levels ranged from no detectable colonies lo the maximum detection level of our protocol of 30,000 CFU/100 mL. There is a trend that total colitorm levels ere lower (during the wet season than the dry season. In the wet season, some communities within the sturdy area had access to constant municipal treated water as monitored by researcher verification of public tap-watcr availebJlity. Othet communities had intermittent access to municipal treated water. Of these honseholds, those that had constant access to treated water at or near their household did have less total coliform in their stored water than those with intermittent services ( Figure 7 ). This neglects the communities that are outside of the municipal treated-water servic e area.

Box-and-whisker plot of total coliform measurements of stored, untreated water in study households in the wet (n = 95) and dry (n = 103) seasons. The box-and-whisker plot indicates the mean (diamond), first, second, and third quartiles (box), and minimum and maximum (whiskers).

Box-and-whisker plot of total coliform measurements of stored water in the wet season in study households in communities that had verified continuous access to municipal treated water versus verified intermittent access.
The total coliform from households in communities with verified continuous treated water had a log-normal distribution (verified by 99%, α = 1 significance level, χ 2 goodness-of-fit test) and were statistically significantly lower (α =1 significance level) than those from households in communities with verified intermittent treated water. Unfortunately, due to the low number of samples from intermittent households, a χ 2 goodness-of-fit test was not meaningful.
3.3. Anion Concentrations
Major anions investigated in the various water sources fell within the recommended guideline values from the WHO [ 42 ]. Fluoride concentrations ranged from below the detection limit (bdl) to 0.82 mg/L in the dry season and to 1.48 mg/L ( Table S3 ) in the wet season. Fluoride levels fell below the threshold limit for fluoride in drinking water from the WHO (1.5 mg/L). Nitrates were also observed within the limit of drinking water, between bdl–17.48 mg/L and bdl–9.72 mg/L in the dry and wet seasons, respectively. Chloride, sulfate and phosphate levels were also present in moderate levels in the various water sources; however, a relatively high concentration of chloride of 462.9 mg/L was determined in the Mutale River in the wet season.
3.4. Trace and Major Elements Composition
Major metals in the various water sources in both seasons complied with the recommended limits of SANS and WHO in drinking water [ 39 , 42 ]. Sodium concentrations in the range of 3.14–41.03 mg/L and 3.02–15.34 mg/L were measured in the wet and the dry seasons, respectively ( Table S4 ). Low values of potassium were measured. Calcium levels ranged between 0.66–33.91 mg/L and 0.53–27.39 mg/L, in the wet and dry seasons, respectively. Low levels of magnesium were also found. Most of the water sources can be classified as soft water owing to the low levels of calcium and magnesium. Aluminium (Al) concentration ranged between 39.18–438 μg/L ( Figure 8 ). Two of the water sources which are community-based water supply systems recorded high levels of Al which exceeded the aesthetic permissible levels of drinking water; others fell within this limit. Similarly, the levels of iron (Fe) varied between 37.30–1354 mg/L and 35.21–1262 mg/L in the wet and the dry seasons, respectively ( Figure 9 ). Some of the sources showed high Fe concentration which exceeded the aesthetic permissible limit of WHO in drinking water [ 42 ]. Two community-based water systems had higher levels of Fe in the wet season as well as the major river in the region (Mutale River) for which high Fe levels were observed in both seasons. One of the clinic boreholes also recorded high levels of Fe above the permissible aesthetic value of (300 mg/L) in both seasons. Temporary seasonal variation was significant only in the levels of Fe and Al. In the wet season, their levels were generally higher than in the dry season. Some other trace metals of concern like Pb, Hg, As, Cd, Cr, Ni, Cu, Mn, Sr were all present at low levels that were below their recommended limits in drinking water for both seasons ( Table S5 ).

Aluminum, measured by an inductively coupled plasma mass spectrometer (ICP-MS), concentration for natural sources in the study area in the wet and dry seasons. The SANS 241 standard is shown (an operational standard is intended for treated water). Sources marked with * are intermittent sources and had no dry-season sample. Other sources have measured concentrations; although they may be too low to plot.

Iron, measured by an ICP-MS, concentration for natural sources in the study area in the wet and dry seasons. The SANS 241 standard is shown. Sources marked with * are intermittent sources and had no dry-season sample. Other sources have measured concentrations; although they may be too low to plot.
4. Discussion
This study provides a comprehensive description of water quality and drinking-water use across seasons in a low-resource community in rural South Africa, including a variety of water sources, ranging from the municipal tap to natural sources and a combination of both when the municipal tap was intermittently available.
Water sources in the study area, aside from the municipal tap, were highly contaminated with E. coli in both the wet and dry seasons; that is, E. coli was above the South African standard (acute health) of 0 CFU/100 mL. It is particularly important to note that E. coli was detected in the boreholes used for water at the local clinics, implying inadequate access to potable water for potentially immunocompromised patients. While the municipal treated water met the E. coli detection limit, the municipal tap did not always fall within the standards of turbidity (≤1 NTU operational and ≤5 NTU aesthetic) and total coliform (≤10 CFU/100 mL) [ 39 ]. These are not direct health risks; however, both measurements can be used to judge the efficacy of the treatment process and suggest that treatment may not have removed other pathogens that were not directly tested, such as protozoan parasites.
While the microbiological contamination of the drinking-water sources was not acceptable, the chemical constituents fell within the South African guidelines [ 39 ]. Calcium, sodium, magnesium and potassium were present in low levels and their concentrations complied with regulatory standards of SANS [ 39 ] and WHO [ 42 ]. Some metals (cadmium, mercury, arsenic and lead) known to be carcinogenic, mutagenic and teratogenic, causing various acute and chronic diseases to humans even at trace levels in drinking water, were investigated and found to be present in very low concentrations that could be of no health risk to the consumers of the various water resources in the region. However, some other metals, such as Al and Fe, were higher in some of the water sources; yet these were still well below the health guidelines for the respective constituent (recommended health levels from SANS and WHO are given as Al < 0.9 mg/L, Fe < 2 mg/L). At these levels, they do not present a health risk but could impart color and significant taste to the water thereby affecting its aesthetic value. Water sources from the community water-supply systems and one of the clinic boreholes recorded higher levels of Al and Fe. The other metals evaluated (copper, zinc, nickel, chromium, Se and Mn) were present in low levels that complied with their recommended limits in drinking water [ 39 , 42 ].
Fluoridation of drinking water is a common practice for oral health in many countries [ 43 ]. The required level of fluoride to reduce incidences of dental caries is in the range of 0.6–0.8 mg/L; however, levels above 1.5 mg/L are associated with dental and skeletal fluorosis [ 43 – 45 ]. The likelihood of fluorosis as a result of high concentration of fluoride is low in these communities, but there could be a high incidence of dental caries since fluoride levels below 0.6 mg/L were measured and some of the water sources did not have fluoride concentrations detectable by the instrument. The National Children’s Health Survey conducted in South Africa showed that 60.3% of children in the age group of 6 years have dental caries. Approximately a third (31.3%) of children aged 4–5 years in Limpopo province have reported cases of dental caries [ 44 , 45 ].
Chloride levels in the water sources do not cause any significant risk to the users except imparting taste to the water for some of the sources that recorded chloride levels above 300 mg/L. Although the study area is characterized by farming activities, the nitrate concentrations measured do not present any health risks. Therefore, the occurrence of methemoglobinemia or blue-baby syndrome as a result of high nitrate levels is unlikely. Other anions were present in moderate levels that would also not constitute any health risks. The levels of all the anions determined in the various sources were lower than the recommended guidelines of WHO [ 42 ].
The microbiological analysis of environmental water sources revealed several trends. Without exception in these samples, bacterial levels in the wet season were higher than in the dry season. This may be caused by greater runoff or infiltration, which carries bacteria from contaminated sources to these water bodies. The upward trend in bacteria in the municipal treated water is not explained by an increase in runoff, but may be due to higher turbidity of the intake for the municipal treated water in the wet season. The treatment facility workers reported to the researchers that they were unable to monitor the quality of the treated water due to instrument failure during the wet season surveillance period.
Water stored in the household showed that the mean total coliform in the wet season was lower than that in the dry season. This trend is opposite to what was observed in the source, or environmental samples. This difference may be explained by the greater availability of treated water in the wet season versus the dry season for approximately 40% of the sampled households ( Figure 7 ). In addition, it is possible that families try to save water during the dry season and do not reject residual water, while the rainy season allows easier washing of the container and for it to be filled with fresh water more regularly.
In the wet season, two communities had consistently treated water available from household connections (usually a tap somewhere in a fence-in yard) or public taps. While the municipal treated water was of lower quality in the wet season than the dry season, the quality was significantly better than most environmental sources.
Another potential explanation is that residents stored their water within their households for a shorter time, which is supported by the use data that showed interruptions in supply were more common and for longer duration in the dry season. The quality of the water stored in households with continuous supply versus intermittent supply also suggests that water availability may play a role in household water quality. This is consistent with research that demonstrates that intermittent water supply introduces contamination into the distribution system in comparison with continuous supply [ 46 ]. Intermittent supply of water may also result in greater quantity and duration of storage at household level, which could increase the likelihood of contamination.
While it has been shown that the quality of water used for drinking in these villages does not meet South African standards, this problem is confounded by evidence from surveys indicating that residents believe they have high-quality water and, therefore, do not use any form of treatment. In the rare case that they do, it is by letting the water stand and settle or by boiling. In addition, even if treated water is collected, there is a risk of recontamination during storage and again when using a cup held by a hand to retrieve water from storage devices, which was common in surveyed homes. In addition, there was little to no detectable residual chlorine in the municipal tap water to prevent recontamination. A previous study performed in an adjacent community showed higher household treatment levels; however, this may have been due to intervention studies in that community (the community in question was excluded from this study because of previous interventions) [ 47 ]. The study also concurred that boiling was the most common method employed.
Given that most of the water from the various sources in this community is contaminated and not treated, there is a high risk of enteric disease in the community. Lack of access to adequate water and sanitation cause exposure to pathogens through water, excreta, toxins, and water-collection and storage pathways, resulting in immense health impacts on communities [ 48 ]. A large burden of death and disability due to lack of access to clean water and sanitation is specifically associated with diarrheal diseases, intestinal helminths, schistosomiasis and trachoma [ 49 ]. While it was found in this study that the study area has a high prevalence of improved sanitation, the likelihood of poor water quality due to intermittent supply and lack of treatment poses a risk of the adverse health effects described. In a previous longitudinal cohort study of children in these villages, most children were exclusively breastfed for only a month or less, and 50% of children had at least one enteropathogen detected in a non-diarrheal stool by three months of age [ 50 ]. Furthermore, the burden of diarrhea was 0.66 episodes per child-year in the first 2 years of life, and stunting prevalence (length-for-age z-score less than −2) in the cohort increased from 12.4% at birth to 35.7% at 24 months [ 50 ]. It is likely that contaminated water contributed to the observed pathogen burden and stunting prevalence in these communities. In summary, microbiological contamination of the drinking water is high in the study area, and risk from other chemical constituents is low. Therefore, engineered solutions should focus more on improving the microbiological quality of the drinking water.
The intermittent supply in municipal tap water, inadequate water quality from alternative sources, and the risk of recontamination during storage suggest a need for a low-cost, point-of-use water-treatment solution to be used at the household level in these communities. Access to clean drinking water will contribute to improving the health of young children who are at highest risk of the morbidity and mortality associated with waterborne diseases. Such an intervention may go beyond the prevention of diarrhea by impacting long-term outcomes such as environmental enteropathy, poor growth and cognitive impairment, which have been associated with long-term exposure to enteropathogens [ 51 ]. This is supported by a recent finding that access to improved water and sanitation was associated with improvements on a receptive vocabulary test at 1, 5 and 8 years of age among Peruvian, Ethiopian, Vietnamese and Indian children [ 52 ]. The implementation of point-of-use water treatment devices would ensure that water is safe to drink before consumption in the homes of these villages, improving child health and development.
5. Conclusions
This study was comprehensive in the assessment of all aspects of water quality and corresponding water-use practices in rural areas of Limpopo Province. The results obtained indicate that microbiological water quality is more likely to have adverse effect on the consumers of natural water without adequate treatment, as E. coli was determined in all the natural water sources. Local needs assessments are critical to understanding local variability in water quality and developing appropriate interventions. Interventions to ensure clean and safe drinking water in rural areas of Limpopo province should, first and foremost, consider microbiological contamination as a priority. Risk-assessment studies of the impact of water quality on human health is, therefore, recommended.
Supplementary Material
Tables s1 through s5.
Table S1: Physical characteristics of water sources. Two to three measurements were taken during an intensive study period from 13 January 2017 to 4 February 2017 in the wet season, and three to four measurements from 5 June 2016 to 15 July 2016 in the dry season. The median measurement of the values is reported here. Sites with missing samples, such as ephemeral rivers that do not flow all the way into the valley in the dry season, are indicated (*). Sites with missing data due to instrument failure are indicated (#). Values that were below the detection limit are indicated (bdl). South African regulation (SANS 241:1-2015) and the World Health Organization Recommended Guidelines for Drinking Water Quality (Fourth Edition) are listed; parameters not listed are indicated (nl),
Acknowledgments:
This project was funded by the Fogarty International Center (FIC) of the National Institutes of Health (NIH) (Award Number D43 TW009359), National Science Foundation (NSF) (Award Number CBET-1438619), the Center for Global Health at the University of Virginia (CGH), and the University of Virginia’s Jefferson Public Fellows (JPC) program. The content is solely the responsibility of the authors and does not represent the official views of the funders. The authors also acknowledge the tireless work of the community field workers who undertook interventions and collected all of the survey data. The authors also acknowledge A. Gaylord, N. Khuliso, S. Mammburu, K. McCain and E. Stinger, who performed much of the water-quality analysis and T. Singh, who supported the laboratory analysis for inorganic materials.
Supplementary Materials: The following are available online at www.mdpi.com/s1 ,
Conflicts of Interest: The authors declare no conflict of interest.
- Show search
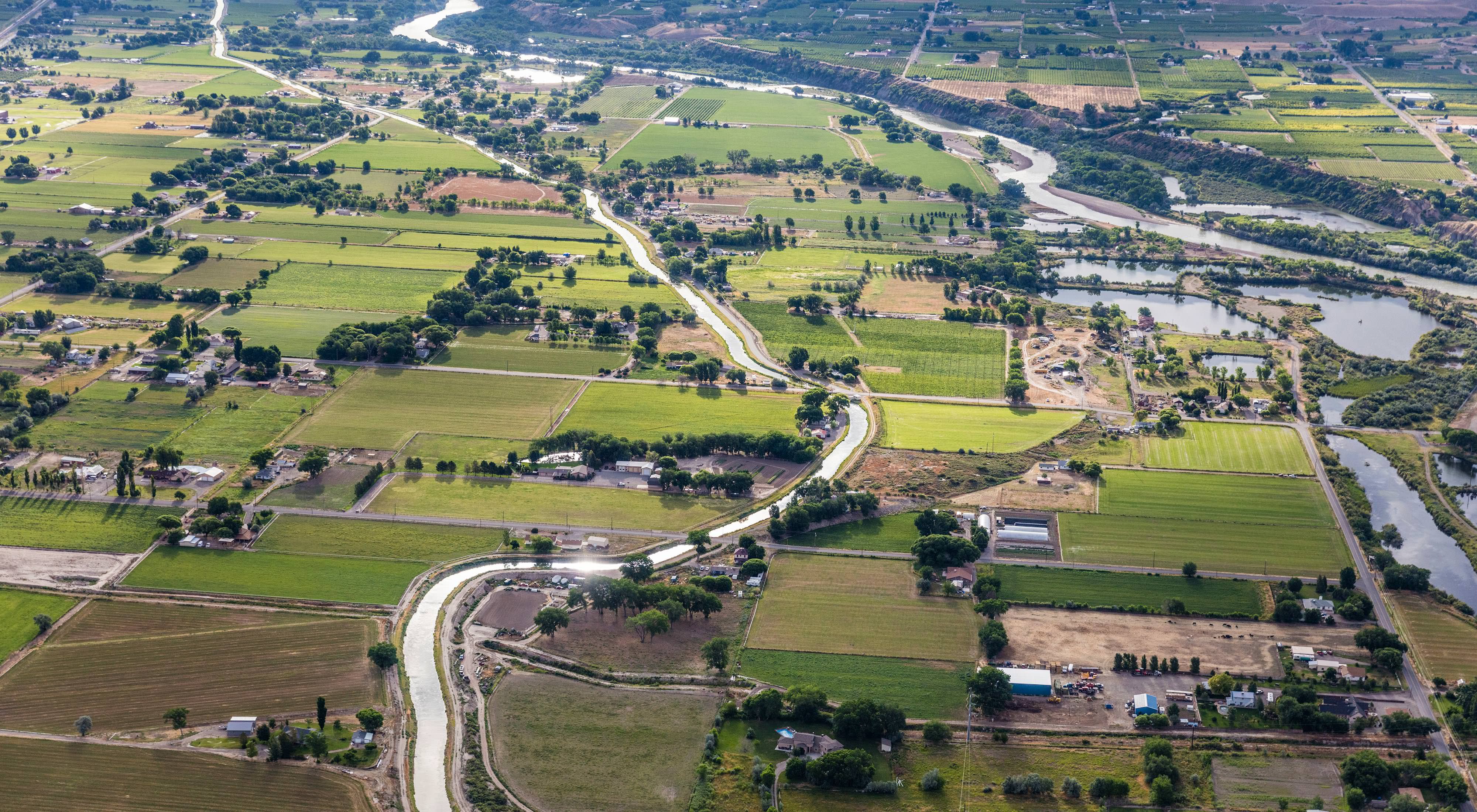
Food & Water Stories
Solutions to Address Water Scarcity in the U.S.
More than half the nation has regularly experienced droughts since 2000.
February 13, 2020 | Last updated March 31, 2022
- Agriculture
- Groundwater
Freshwater is essential for all life on Earth. Yet, only 2.5 percent of Earth’s vast water resources are fresh, and less than 1 percent of that is easily accessible for people and nature. This limited resource must be managed wisely, particularly in the face of climate change, growing populations, and increasing demands from multiple sectors. To meet this challenge, The Nature Conservancy works to reduce demands for water among the biggest users, improve the flexibility of water governance and management, and advance financial incentives and tools that enable users—like irrigation districts and cities—to transfer water, which will provide water security for the environment as well.
The benefits of healthy, sustainable water resources:
- Supply clean drinking water.
- Grow our food and are used to raise livestock.
- Produce the goods we use.
- Drive economic and social benefits.
- Sustain healthy rivers, lakes, springs and associated habitats that support diverse wildlife.
- Provide for recreation and tourism. And can be harnessed to produce clean hydroelectric power.
Freshwater Challenges in the U.S.
More than half the continental U.S. has regularly experienced drought conditions over the past two decades.
Because of climate change, experts predict precipitation will likely decline 20 to 25 percent by 2100 in much of the West.
Since 2000, the Colorado River Basin— which supplies water to 40 million people—has experienced historic drought conditions.
Water scarcity isn’t limited to Western states. In 2014, 40 of 50 state water managers expected shortages in some portion of their states over the next 10 years.
Our Freshwater Conservation Strategies
Our approach to protecting freshwater involves a combination of water funds, promoting incentives, collaborating with water users, and advancing sound water policies and funding.
We invest in water markets and water transactions with cities, irrigation districts and other water users to demonstrate flexible water use and transfers that include water for environmental needs.
We promote and incentivize innovative approaches that reduce demands and improve infrastructure to move and store water more efficiently.
We collaborate with farmers, ranchers and corporations to reduce water use at all levels in the agricultural supply chain.
We advance local, state and federal policies that enable water managers to effectively meet the needs of people and nature. We also work to secure state and federal funding that supports sustainable water practices and agreements.
We demonstrate our work through more than 70 projects across the nation. Currently, approximately 90 percent of these are located in the western U.S., including TNC’s Colorado River Program . The remaining projects are located east of the Mississippi River in regions that are beginning to experience water scarcity more frequently and intensely.
Finding Solutions to Water Scarcity in Agriculture
Agriculture accounts for 70 percent of the planet’s freshwater withdrawals annually. This presents a tremendous opportunity to work with farmers and agriculture supply chain companies to ensure the sustainability of the food we eat and the water upon which it relies. In the U.S., irrigation accounts for more than 80 percent of total water consumptive use and up to 94 percent of consumption in regions most prone to water scarcity. Below are two case studies that demonstrate how TNC is working with farmers to help reduce water use.
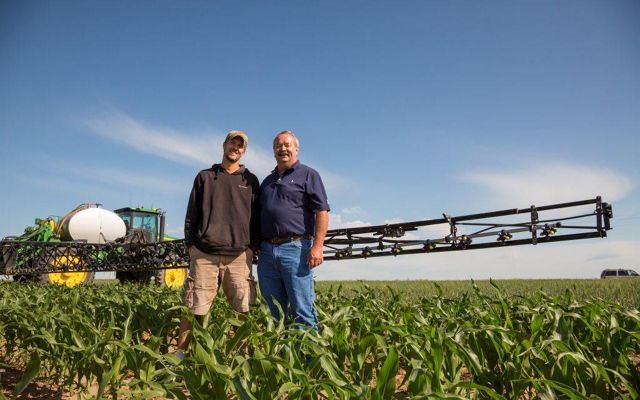
Case Study 1: Saving Water, Securing the Nation's Food
Agricultural irrigation accounts for 90 percent of the consumptive water use in Nebraska. There are 7 million acres of irrigated land in the Platte River Valley alone. Here, The Nature Conservancy recognized a chance to conserve water at a large scale and reached out to landowners interested in new water-saving irrigation technologies. These efforts evolved into a collaboration with Coca-Cola, John Deere, McDonalds and the World Wildlife Fund to launch the Western Nebraska Irrigation Project in 2014.
TNC connected 11 farmers who collectively manage 8,000 acres with technology providers to install soil moisture probes, pivot telemetry and weather stations to help reduce water use. The farmers were then provided support that enabled them to micromanage irrigation and reduce the amount of water pumped from the underlying aquifer by 20 percent. The project site included quality historic water-use records, which enabled researchers to measure change. Over a three-year period, the farmers saved over a billion gallons of water—enough to fill more than 110,000 semi tanker trucks.
Less groundwater pumping not only helps secure the resiliency of the underlying aquifer, it also saves the farmers time and money. One participating farmer reported “the probes paid for themselves easily each year,” while another noted the technology saved time because he didn’t have to drive to his field to check conditions.
The project’s success spurred interest in Central Nebraska, where TNC teamed with Nestlé Purina and Cargill to launch a second irrigation project in 2018. Here, researchers estimated that 20 participating farmers saved at least 120 million gallons of water in the first year. In March 2020, the project reached its enrollment target of 50 farmers, with many reporting that the technology and training has helped them save time and money because of less pumping.
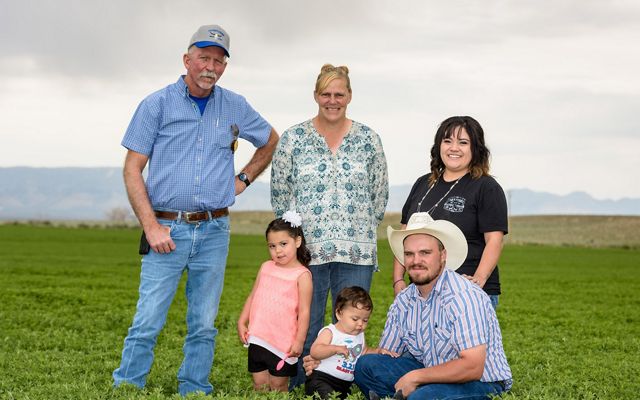
Case Study 2: Price River, Utah—Water Wise Solutions for People and Nature
TNC is working with a wide range of partners in the Price River watershed to enhance water use in ways that benefit agricultural operations while improving flows. Since 2016, TNC has worked with farmers to test water-saving methods that could inform drought contingency plans in the Colorado River’s Upper Basin. Working with farmers and other partners, TNC is exploring temporary, voluntary and compensated measures—like water banking—to help reduce the risk of water shortages for all.
Quote : Kevin Cotner
My family has farmed here for three generations. Working with TNC gives me a chance to help improve my agricultural operations and the wildlife habitat here that I care so much about.
Kevin Cotner
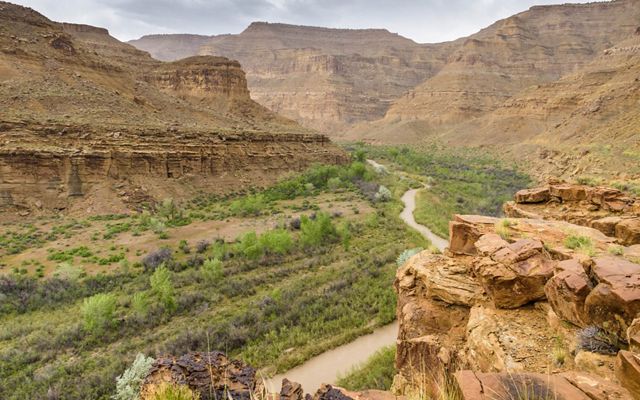
Agriculture uses about 80 percent of the water in the Colorado River Basin, with large flows moving from rivers to fields through irrigation ditches, canals and other structures. The infrastructure that moves irrigation water is often old, leaky and inefficient. To address this challenge, TNC works with water users to improve the delivery and timing of irrigation water through updated canal lining and piping. On the Price River, TNC has negotiated an innovative water-management agreement with a canal company to enhance flows and agriculture. The agreement upgrades the company’s infrastructure and benefits six rare fish species in the lower Price. Water that isn’t delivered to shareholders is stored in an adjacent reservoir for strategic releases in late summer when levels in the river typically drop. While these measures increase water for the environment, they can also benefit farm productivity and help enhance the security of water for agriculture.
Take Action
You can advance TNC’s efforts to secure clean fresh water around the world. Make a difference today!
More Water Stories
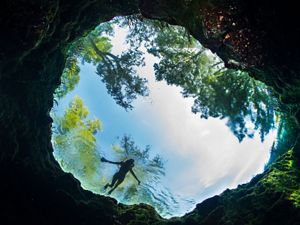
Groundwater: Our Most Valuable Hidden Resource
Though it's out of sight, groundwater is critical for biodiversity, growing food and other needs for a healthy planet. See what The Nature Conservancy is doing to safeguard this hidden resource.
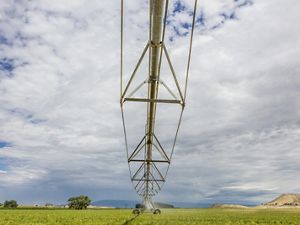
Demand Management in the Colorado River Basin
State Drought Contingency Plans in the Colorado River Basin are using demand management to reduce water use at critical times and compensate users for saving water.
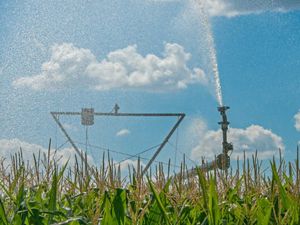
Western Nebraska Irrigation Project
A three-year pilot project with Nebraska farmers has yielded big water savings on the Platte River.
Suggestions or feedback?
MIT News | Massachusetts Institute of Technology
- Machine learning
- Social justice
- Black holes
- Classes and programs

Departments
- Aeronautics and Astronautics
- Brain and Cognitive Sciences
- Architecture
- Political Science
- Mechanical Engineering
Centers, Labs, & Programs
- Abdul Latif Jameel Poverty Action Lab (J-PAL)
- Picower Institute for Learning and Memory
- Lincoln Laboratory
- School of Architecture + Planning
- School of Engineering
- School of Humanities, Arts, and Social Sciences
- Sloan School of Management
- School of Science
- MIT Schwarzman College of Computing
Case study suggests new approach to urban water supply
Press contact :, media download.
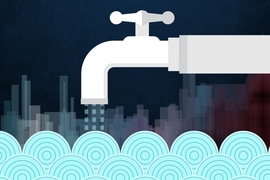
*Terms of Use:
Images for download on the MIT News office website are made available to non-commercial entities, press and the general public under a Creative Commons Attribution Non-Commercial No Derivatives license . You may not alter the images provided, other than to crop them to size. A credit line must be used when reproducing images; if one is not provided below, credit the images to "MIT."
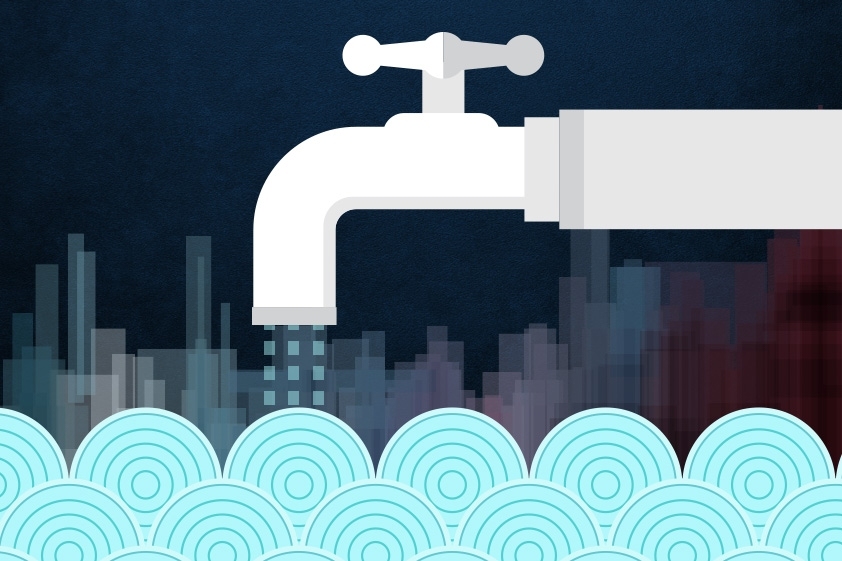
Previous image Next image
If you live in the developed world, safe water is usually just a faucet-turn away. And yet, global warming, drought conditions, and population growth in coming decades could change that, ushering in an era of uncertain access to water.
Now an MIT-based research team has evaluated those potential problems and, based on a case study in Australia, suggested an alternate approach to water planning. In a new paper, the researchers find there is often a strong case for building relatively modest, incremental additions to water infrastructure in advanced countries, rather than expensive larger-scale projects that may be needed only rarely.
More specifically, the study looks at the city of Melbourne, where a 12-year drought from 1997 to 2009 led to construction of a $5 billion facility, the Victorian Desalination Plant. It was approved in 2007 and opened in 2012 — at a time when the drought had already receded. As a result, the plant has barely been used, and its inactivity, combined with its hefty price tag, has generated considerable controversy.
As an alternative, the study suggests, smaller, modular desalination plants could have met Melbourne’s needs at a lower price.
“If you build too much infrastructure, you’re building hundreds of millions or billions of dollars in assets you might not need,” says Sarah Fletcher, a PhD candidate in MIT’s Institute for Data, Systems, and Society (IDSS), who is the lead author of the new paper.
To be sure, Fletcher adds, “You don’t want to be in a situation where you have less water supply than you have demand.” As such, the study does not argue that a single solution applies to all cases, but presents a new method for pinpointing the best plan — and notes that in many cases, “moderate investment increases, together with flexible infrastructure design, can mitigate water-shortage risk significantly.”
The new paper, “Water Supply Infrastructure Planning: Decision-Making Framework to Classify Multiple Uncertainties and Evaluate Flexible Design,” was recently published online in the Journal of Water Resources Planning and Management , and will appear in the October 2017 print volume.
The co-authors are Fletcher, who is also affiliated with MIT’s Joint Program on the Science and Policy of Global Change; Marco Miotti, a PhD student in IDSS; Jaichander Swaminathan, a PhD student in MIT’s Department of Mechanical Engineering; Magdalena Klemun, a PhD student in IDSS; Kenneth Strzepek, a research scientist at the MIT Joint Program on the Science and Policy of Global Change and an emeritus professor of engineering at the University of Colorado; and Afreen Siddiqi, a research scientist in IDSS.
Siddiqi visited Melbourne during its historic drought and learned from local experts about the city’s challenging water-supply problem. The genesis of the current study comes from Siddiqi’s investigation into the Melbourne case and assessment that the complex problem of urban water security lies at the intersection of engineering design and strategic planning.
The MIT team’s new framework for water-supply analysis incorporates several uncertainties that policymakers must confront in these cases, and runs large numbers of simulations of water availability over a 30-year period. It then presents planners with a decision tree about which infrastructure options are best calibrated to their needs.
The significant uncertainties include climate change and its effects on rainfall, as well as the impact of water shortages and population growth.
In studying the Melbourne case, the researchers looked at six infrastructure alternatives, including multiple types of desalination plants and a possible new pipeline to more-distant sources, and combinations of these things.
“The main methodological contribution for the paper is this framework to look at different uncertainties of different types and put that all together in one piece of analysis,” Fletcher says.
The results highlight a vexing problem in water-access planning: Shortages can be acute, but they may last for relatively short periods of time.
For instance, the team ran 100,000 simulations of 30-year conditions in Melbourne and found that in 80 percent of all years, there would be no water shortages at all. And yet, for the years where drought conditions did hold, large water shortages were more common than minor water shortages.
As a result, when costs were factored into the analysis, simply building no new infrastructure was the best option around 50 percent of the time. However, doing nothing was also the “worst-performing alternative” around 30 percent of the time.
That’s why the option of building smaller desalination plants can make sense. The Melboune plant that was built can produce 150 million cubic meters of water per year. But in the MIT team’s simulations, building a desalination plant half that size usually works well: It was the best-performing option in 20 percent of the simulations, and in the top three of 90 percent of the simulations. It was never, in all 100,000 simulations, the worst or second-worst-performing option.
Moreover, Fletcher points out, building smaller at first gives planners the ability to bring a new plant online more quickly and then scale up if needed.
“You only build a certain number of modules in the beginning, and you can add a certain number later,” Fletcher says. “That’s different than building a small plant and then another small plant. You’re being proactive and planning to adapt in the future.”
So thinking small, in this scenario, make considerable sense. But as the researchers acknowledge, the exact results of their study would likely vary from region to region, depending on all the climate and population factors that affect water supply.
Even so, they think their new study framework can at least help planners make the case that building on a smaller scale may position cities and countries best in the long run. Or, as Siddiqi puts it, “building on a smaller scale, but planning big” may be the optimal approach.
“We’re used to building large-scale desalination plants, and there’s less of history of building more modular plants,” Fletcher says. “It’s challenging because these are large investments with long lifetimes. But if you think of a modular plant as an insurance policy against drought, maybe you want to have it around.”
Share this news article on:
Related links.
- Paper: “Water Supply Infrastructure Planning: Decision-Making Framework to Classify Multiple Uncertainties and Evaluate Flexible Design”
- Institute for Data, Systems, and Society
- Joint Program on the Science and Policy of Global Change
Related Topics
- Climate change
- Infrastructure
- Desalination
- Sustainability
Related Articles
![case study on water supply “For China, it looks like industrial growth [has the greatest impact on water shortages] as people get wealthier,” Charles Fant says. “In India, population growth has a huge effect. It varies by region.”](https://news.mit.edu/sites/default/files/styles/news_article__archive/public/images/201603/MIT-WaterChina.jpg?itok=in3EX01z)
Water problems in Asia’s future?
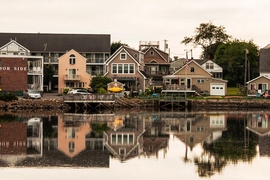
Game for climate adaptation
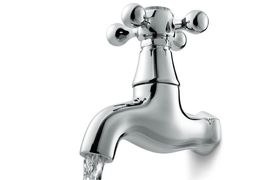
The high value of water
Previous item Next item
More MIT News
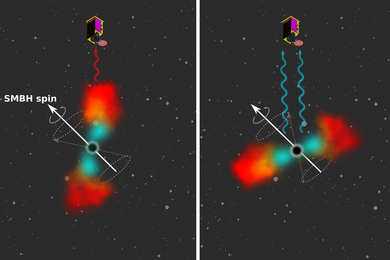
Using wobbling stellar material, astronomers measure the spin of a supermassive black hole for the first time
Read full story →
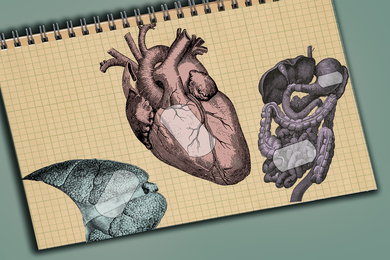
Adhesive coatings can prevent scarring around medical implants
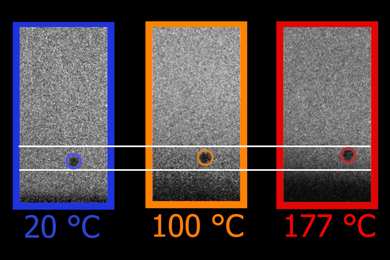
Study: Under extreme impacts, metals get stronger when heated
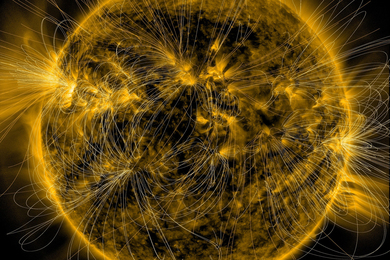
The origin of the sun’s magnetic field could lie close to its surface
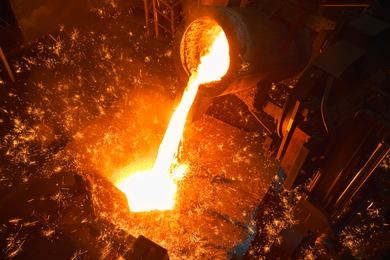
Making steel with electricity
H2 underground
- More news on MIT News homepage →
Massachusetts Institute of Technology 77 Massachusetts Avenue, Cambridge, MA, USA
- Map (opens in new window)
- Events (opens in new window)
- People (opens in new window)
- Careers (opens in new window)
- Accessibility
- Social Media Hub
- MIT on Facebook
- MIT on YouTube
- MIT on Instagram
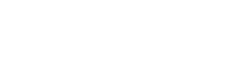
U.S. Climate Resilience Toolkit
- Steps to Resilience
- Case Studies
- Addressing Water Supply Risks from Flooding and Drought
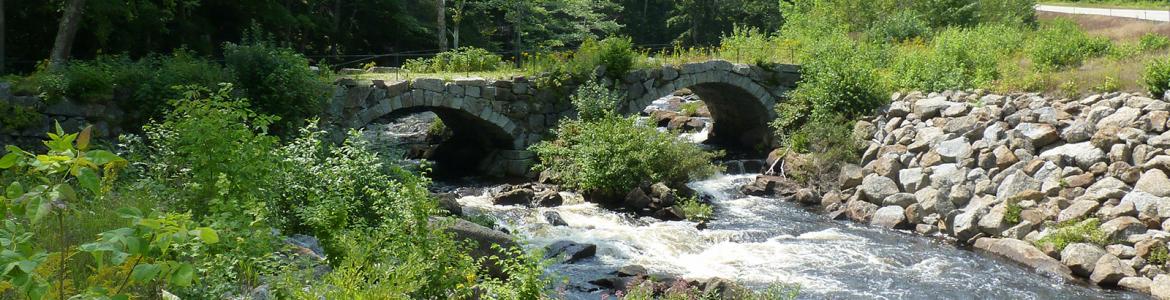
Extreme weather can threaten water supply
Flowing rivers reduced to dry channels. Dairy cows that produce less milk. And residents who normally rely on well water filling up plastic jugs from hoses at public libraries and fire stations.
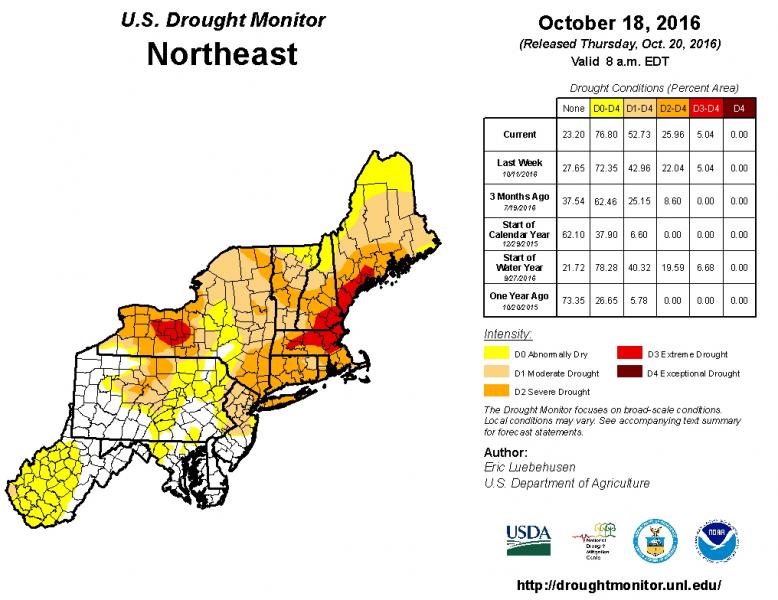
The map shows drought conditions—ranging from "abnormally dry" to "extreme drought"—in the Northeast during the fall of 2016. Click on the image for a larger view.
This was the scene in New Hampshire during the fall of 2016, when a severe drought struck areas of the Northeast. Though a formal attribution study of the drought—a study that can tell us how much of the credit or risk for an event should go to global warming and how much should go to natural weather patterns or random climate variability—has not yet been completed, some water-supply professionals are concerned that the drought may be connected to climate change.
The Third National Climate Assessment released in 2014 notes that drought and heavier storms will increase in the Northeast as the planet continues to warm. As evidence, the report cites that precipitation falling during “very heavy precipitation events” increased by more than 70 percent between 1958 and 2010 in the region. It also notes that increased temperatures—which cause greater evaporation and earlier spring and winter snow melt—will bring more droughts.
The summer of 2016, therefore, with its dismal rainfall and many 90°-plus days, seemed like a harbinger of what’s to come. The drought stressed both natural water resources and those of city water managers, raising concerns that the region’s water sector will become less resilient over time.
Water supply vulnerabilities
Main Street in downtown Keene, New Hampshire.
Keene, New Hampshire, has been a leader in climate change planning and mitigation. For nearly a decade, city officials in the picturesque hamlet have been planning for climate change and the extreme weather it brings, putting special emphasis on efforts to protect their water supply.
For instance, they’ve identified and prohibited further development in and around the city’s 200-year floodplain, and they’ve also adopted new design standards that require extra space for snow stacking during winter. In addition, they’ve conducted numerous vulnerability assessments, as well as updated capital improvement and hazard mitigation plans.
Still, one of the key vulnerabilities that city leaders needed to explore further was the future of their water assets. The city gets its water from two reservoirs, built in 1910 and 1931, that are located outside the city limits, and from two well-fields that supplement its surface water supply. Studies have shown that prolonged drought combined with more intense storms and large amounts of run-off can impact the overall volume, turbidity, and total organic carbon (TOC) concentration of municipalities’ water supplies.
Risk assessment tools for water managers
To better prepare for extreme weather events and their impact on the water supply, Keene water department officials Donna Hanscom, Aaron Costa, and Ben Crowder, along with a group of water department staff, spent six months in 2016—from February through July—working with officials from the U.S. Environmental Protection Agency (EPA). Through a series of calls, webinars, and one in-person event, agency officials and city water managers walked through a climate change risk assessment process.
A key component of the process was using the EPA’s Climate Resilience Evaluation and Awareness Tool (CREAT)—specialized software designed for water managers. Users can apply pre-packaged climate data within the tool, or they can add their own climate data for improved accuracy.
When selecting their data, Hanscom, Costa, and Crowder realized that one of CREAT’s weather stations is directly on a dam within the city limits. Even though this data was highly localized, they wanted to make their dataset even more specific by including additional statistics that measured precipitation from different perspectives. To do that, they included statistics from a 100-year and 500-year storm dataset, as well as monthly precipitation measurements, in addition to the pre-set annual precipitation dataset. At the end of the exercise, CREAT generated a comparison of the potential costs of climate change impacts versus costs of implementing adaptation measures.
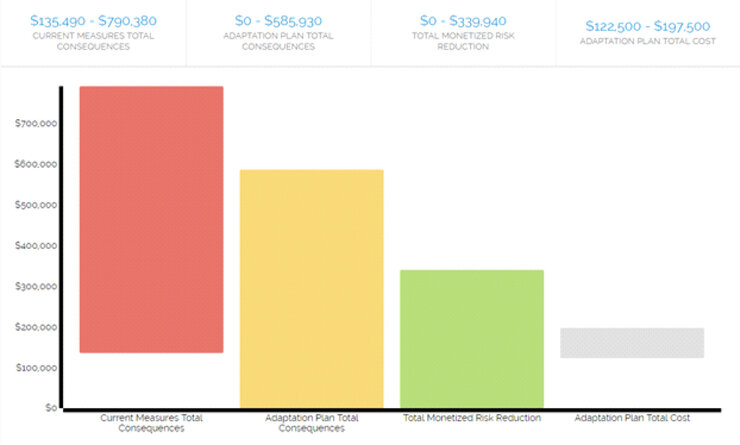
An overview of the CREAT assessment results for the Keene exercise.
In a one-and-a-half day exercise, Keene’s water utility managers and engineers were able to assess the utility’s vulnerability to extreme rainfall events—both a 100-year and a 500-year storm scenario—as well as prolonged periods of drought on their reservoir’s turbidity and total organic carbon levels. The results raised some concern in the water department, because they have already seen their TOC concentrations double during high rainfall events. Increased TOC levels require more chemical treatments to clean the water, which produces extra waste.
Planning for implementation
Keene’s water utility is planning to implement some of the adaptation strategies identified through the CREAT process in the coming years. For example, Keene is working on a watershed management plan to analyze the effectiveness of efficiency and conservation measures during drought. They are also analyzing overall water yield. Additionally, the team is looking at alternative treatments to address turbidity and TOC problems, and to reduce the amount of water lost in sewers due to frequent cleanings of the filters. Finally, Keene plans to focus on the extreme weather effects related to wastewater in a future assessment using CREAT.
Alyssa Hall, U.S. Environmental Protection Agency, and Nancy Averett, freelance writer.
Ellen Wagner. CC-BY-NC-ND 2.0, https://creativecommons.org/licenses/by-nc-nd/2.0/ , via Flickr

Water Supply Challenges in Rural Areas: A Case Study from Central Kazakhstan
Affiliations.
- 1 Department of Public Health, Karaganda Medical University, Gogol Street 40, Karaganda 100008, Kazakhstan. [email protected].
- 2 Division of Water Resources Engineering, Lund University, Box 118, SE-221 00 Lund, Sweden. [email protected].
- 3 Center for Middle Eastern Studies, Lund University, Box 221, SE-221 00 Lund, Sweden. [email protected].
- 4 Division of Water Resources Engineering, Lund University, Box 118, SE-221 00 Lund, Sweden. [email protected].
- 5 Department of Public Health, Karaganda Medical University, Gogol Street 40, Karaganda 100008, Kazakhstan. [email protected].
- 6 Department of Public Health, Karaganda Medical University, Gogol Street 40, Karaganda 100008, Kazakhstan. [email protected].
- PMID: 30813591
- PMCID: PMC6427320
- DOI: 10.3390/ijerph16050688
Rural water supplies have traditionally been overshadowed by urban ones. That must now change, as the Sustainable Development Goals calls for water for all. The objective of the paper is to assess the current access to and the perceived water quality in villages with various types of water supply. The survey was carried out during July⁻December 2017 in four villages in central Kazakhstan. Overall, 1369 randomly selected households were interviewed. The results revealed that even though villagers were provided with tap water, significant numbers used alternative sources. There were three reasons for this situation: residents' doubts regarding the tap water quality; use of other sources out of habit; and availability of cheaper or free sources. Another problem concerned the volume of water consumption, which dropped sharply with decreased quality or inconvenience of sources used by households. Moreover, people gave a poor estimate to the quality and reliability of water from wells, open sources and tankered water. The paper suggests that as well decentralization of water management as monitoring of both water supply and water use are essential measures. There must be a tailor-made approach to each village for achieving the Sustainable Development Goal of providing rural Kazakhstan with safe water.
Keywords: access to water; drinking water sources; perceived water quality; reliability of water supply systems; rural area; volume of water consumption.
- Drinking Water / standards
- Rural Population
- Surveys and Questionnaires
- Water Quality
- Water Supply / standards
- Water Supply / statistics & numerical data*
- Water Wells
- Drinking Water
Water and Disasters—A Case Study of India
- First Online: 21 May 2024
Cite this chapter
- Rahul Kumar 4 &
- Anil Kumar Dikshit 4
Part of the book series: Society of Earth Scientists Series ((SESS))
Natural disasters frequency has increased over the past decades. Water is the force behind every physical and chemical process on earth, and disasters are no exception. Water is the reason behind nearly 90% of the disasters. India has braved 360 natural disasters between the years 2000–2020, affecting almost 1120 million people. Floods and storms have been the most predominant disasters in India for 21 years, affecting more than 424 million people. These disasters lead to substantial loss of life and property and render the affected population homeless many times with little or no resources for survival. The impacts of natural disasters like floods, drought, tsunami and cyclones upon water sources was investigated through literature to understand the various scenarios presented by these disasters. The effects on water supply system were also examined. Studying these scenarios helps determine the mitigation measures of disasters and make technologies that can be useful in these situations.
This is a preview of subscription content, log in via an institution to check access.
Access this chapter
- Available as PDF
- Read on any device
- Instant download
- Own it forever
- Available as EPUB and PDF
- Durable hardcover edition
- Dispatched in 3 to 5 business days
- Free shipping worldwide - see info
Tax calculation will be finalised at checkout
Purchases are for personal use only
Institutional subscriptions
Adikari Y, Yoshitani J (2009) Global trends in water-related disasters: an insight for policymakers. United Nations World Water Assess. Program. Publ. pp 1–24
Google Scholar
Barik SK, Muduli PR, Mohanty B, Behera AT, Mallick S, Das A, Samal RN, Rastogi G, Pattnaik AK (2017) Spatio-temporal variability and the impact of Phailin on water quality of Chilika lagoon. Cont Shelf Res 136:39–56. https://doi.org/10.1016/j.csr.2017.01.019
Article Google Scholar
Chandrasekharan H, Sarangi A, Nagarajan M, Singh VP, Rao DUM, Stalin P, Natarajan K, Chandrasekaran B, Anbazhagan S (2008) Variability of soil-water quality due to Tsunami-2004 in the coastal belt of Nagapattinam district Tamilnadu. J Environ Manage 89:63–72. https://doi.org/10.1016/j.jenvman.2007.01.051
Article CAS Google Scholar
CRED (2020) EM-DAT The international disaster database. https://www.emdat.be/ . Last Accessed 31 Dec 2020
Dhawde R, Surve N, Macaden R, Wennberg AC, Seifert-Dähnn I, Ghadge A, Birdi T (2018) Physicochemical and bacteriological analysis of water quality in drought prone areas of Pune and Satara districts of Maharashtra, India. Environ—MDPI 5:1–20. https://doi.org/10.3390/environments5050061
Grayman WM (2011) Water-related disasters: a review and commentary. Front Earth Sci 5:371–377. https://doi.org/10.1007/s11707-011-0205-y
Kamalanandhini M, Kalaivizhi R, Golda Percy VP, Srividhya S, Dheepak S, Thiyaneshwaran KK (2019) Effect of flood event on water quality. Rasayan J Chem 12:849–854. https://doi.org/10.31788/RJC.2019.1225232
Kamalanandhini M, Annadurai R, Dheepak S, Deepak P, Metilda JE (2021) Assessment of hydrological drought condition and its impact on water quality-a case study in parts of Chengalpattu district, Tamil Nadu, India. Rasayan J Chem 14:51–57. https://doi.org/10.31788/RJC.2021.1416095
Mishra A, Alnahit A, Campbell B (2021) Impact of land uses, drought, flood, wildfire, and cascading events on water quality and microbial communities: a review and analysis. J Hydrol 596:125707. https://doi.org/10.1016/j.jhydrol.2020.125707
Mitra A, Halder P, Banerjee K (2011) Changes of selected hydrological parameters in Hooghly estuary in response to a severe tropical cyclone (Aila) Indian. J Mar Sci 40:32–36
CAS Google Scholar
PAHO (2002) Emergencies and disasters in drinking water supply and sewerage systems : guidelines for effective response. Washington DC, USA
Saha A, Salim SM, Sudheesan D, Suresh VR, Nag SK, Panikkar P, Das BK (2020) Impacts of a massive flood event on the physico-chemistry and water quality of river Pampa in Western Ghats of India. Int J Environ Anal Chem 00:1–19. https://doi.org/10.1080/03067319.2020.1843026
Shen G, Hwang SN (2019) Spatial–Temporal snapshots of global natural disaster impacts revealed from EM-DAT for 1900–2015. Geomatics Nat Hazards Risk 10:912–934. https://doi.org/10.1080/19475705.2018.1552630
Singh VS (2008) Impact of the earthquake and Tsunami of December 26, 2004, on the groundwater regime at Neill Island (south Andaman). J Environ Manage 89:58–62. https://doi.org/10.1016/j.jenvman.2007.01.049
Srinivas H, Nakagawa Y (2008) Environmental implications for disaster preparedness: lessons Learnt from the Indian Ocean Tsunami. J Environ Manage 89:4–13. https://doi.org/10.1016/j.jenvman.2007.01.054
Violette, S, Boulicot G, Gorelick SM (2009) Tsunami-induced groundwater salinization in southeastern India. Comptes Rendus—Geosci 341:339–346. https://doi.org/10.1016/j.crte.2008.11.013
Young S, Balluz L, Malilay J (2004) Natural and technologic hazardous material releases during and after natural disasters: a review. Sci Total Environ 322:3–20. https://doi.org/10.1016/S0048-9697(03)00446-7
Download references
Author information
Authors and affiliations.
Environmental Science and Engineering Department, Indian Institute of Technology Bombay, Mumbai, 400076, India
Rahul Kumar & Anil Kumar Dikshit
You can also search for this author in PubMed Google Scholar
Corresponding author
Correspondence to Rahul Kumar .
Editor information
Editors and affiliations.
Civil Engineering, National Institute of Technology, Kurukshetra, Kurukshetra, India
Krishna Kumar Singh
Civil Engineering, Indian Institute of Technology, Roorkee, Haridwar, Uttarakhand, India
Chandra Shekhar Prasad Ojha
Rights and permissions
Reprints and permissions
Copyright information
© 2024 The Author(s), under exclusive license to Springer Nature Switzerland AG
About this chapter
Kumar, R., Dikshit, A.K. (2024). Water and Disasters—A Case Study of India. In: Singh, K.K., Prasad Ojha, C.S. (eds) Sustainable Management of Land, Water and Pollution of Built-up Area. Society of Earth Scientists Series. Springer, Cham. https://doi.org/10.1007/978-3-031-56176-4_11
Download citation
DOI : https://doi.org/10.1007/978-3-031-56176-4_11
Published : 21 May 2024
Publisher Name : Springer, Cham
Print ISBN : 978-3-031-56175-7
Online ISBN : 978-3-031-56176-4
eBook Packages : Earth and Environmental Science Earth and Environmental Science (R0)
Share this chapter
Anyone you share the following link with will be able to read this content:
Sorry, a shareable link is not currently available for this article.
Provided by the Springer Nature SharedIt content-sharing initiative
- Publish with us
Policies and ethics
- Find a journal
- Track your research
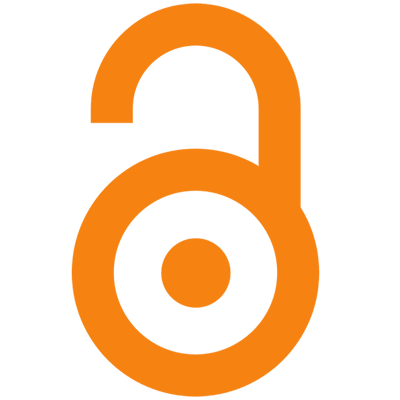
Toward a water-sensitive precinct with stormwater harvesting: a case study in South Africa

- Article contents
- Figures & tables
- Supplementary Data
- Open the PDF for in another window
- Guest Access
- Cite Icon Cite
- Permissions
- Search Site
Malesela Michael Mogano , John Okedi; Toward a water-sensitive precinct with stormwater harvesting: a case study in South Africa. Water Supply 2024; ws2024116. doi: https://doi.org/10.2166/ws.2024.116
Download citation file:
- Ris (Zotero)
- Reference Manager
The study assessed static management, i.e., without planned periodic maintenance of the storage (no sediment dredging) denoted as SC1, or with maintenance denoted as SC2. The second approach was dynamic management based on applying Real-Time Control (RTC) techniques to scenario SC1 and scenario SC2 denoted as RTC-1 and RTC-2, respectively. The dynamic management of the UCT dam with RTC-1 and RTC-2 approaches increase yield by 2.1 and 1.1 ml, respectively. Additionally, RTC-1 and RTC-2 approaches increase volumetric reliability by 5.3 and 2.5%, respectively, while maintaining the required level of service of a stormwater harvesting system. SC1 and SC2 results in water savings of up to 21.15 and 21.45 ZAR/kl, respectively, while RTC-1 and RTC-2 could save up to 19.35 and 19.45 ZAR/kl. Thus, static configurations results in water savings approximately 9% in comparison to RTC. In addition, static configurations harvested stormwater at a relatively lowest unit cost in comparison to RTC configurations. Notwithstanding this finding, the RTC system exhibits a great potential in reshaping the stormwater harvesting system to simultaneously deliver water conservation and stormwater management.
Assessed the extent to which stormwater harvesting could contribute to ‘net zero’, i.e., transitioning the University of Cape Town toward a ‘water-sensitive precinct’.
Impact of planned periodic maintenance in enhancing harvested stormwater volume.
Benefit of Real-Time Control (RTC) in enhancing harvested stormwater volume
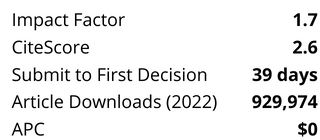
Affiliations
- ISSN 1606-9749 EISSN 1607-0798
- Open Access
- Collections
- Subscriptions
- Subscribe to Open
- Editorial Services
- Rights and Permissions
- Sign Up for Our Mailing List
- IWA Publishing
- Republic – Export Building, Units 1.04 & 1.05
- 1 Clove Crescent
- London, E14 2BA, UK
- Telephone: +44 208 054 8208
- Fax: +44 207 654 5555
- IWAPublishing.com
- IWA-network.org
- IWA-connect.org
- Cookie Policy
- Terms & Conditions
- Get Adobe Acrobat Reader
- ©Copyright 2024 IWA Publishing
This Feature Is Available To Subscribers Only
Sign In or Create an Account
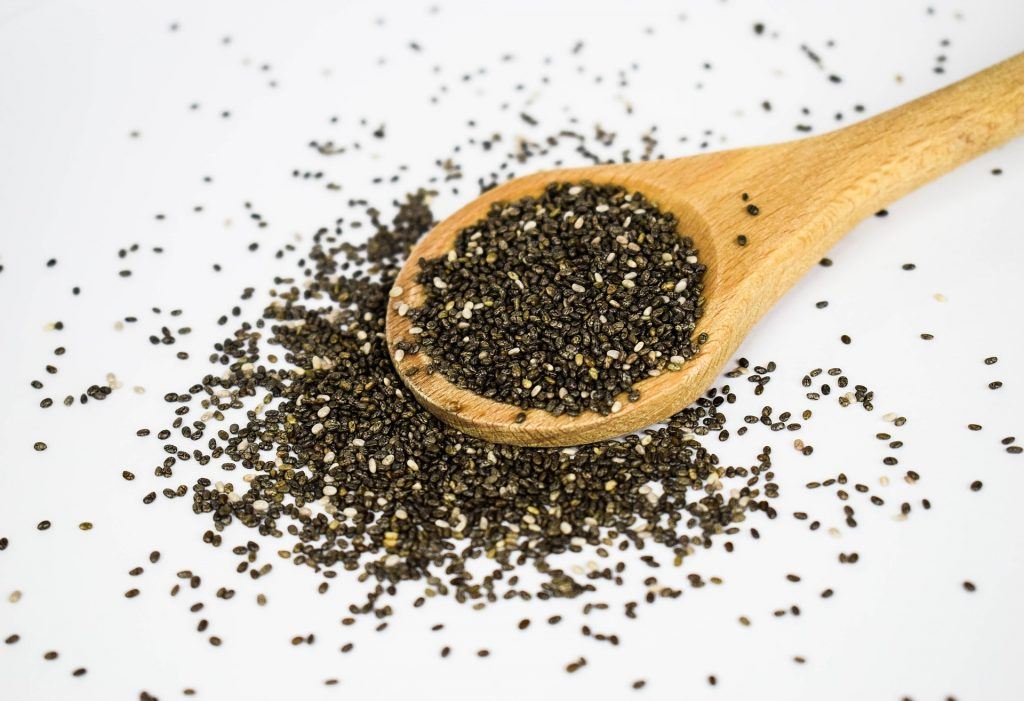
“Ch-ch-ch-chia” may be a familiar jingle if you grew up during the 1980s. Little did we know that those popular ceramic pets sprouting grass “hair” were a foretelling of the even greater success their seeds would have in edible form 25 years later. Chia seeds are often referred to as a “ superfood ” or functional food—unregulated terms more useful in the marketing sphere than by nutrition experts who understand that there is no magic bullet or replacement for a healthful dietary pattern that relies on a variety of nutritious foods.
Functional foods are touted to offer benefits beyond their nutritional value, such as lowering cholesterol or improving gut health. Not only listed as such, chia seeds are also used as a functional ingredient, added to less nutritious items like baked pastries and snacks, to improve their appeal to health-conscious consumers. Health claims about chia seeds include reducing appetite and weight, lowering triglycerides, and improving blood sugar levels in type 2 diabetes.
Chia seeds come from the plant Salvia hispanica L. , and were at one time a major food crop in Mexico and Guatemala. Cultivated as a food source as early as 3500 BC, it was offered to Aztec gods in religious ceremonies. According to industry reports, the chia seed market is projected to reach more than 2 billion USD in sales by 2022. [1]
- Polyunsaturated fat , as omega-3 fatty acids
Two tablespoons of chia seeds (1 ounce or 28 grams) contain about 140 calories, 4 grams of protein, 11 grams of fiber, 7 grams of unsaturated fat, 18% RDA for calcium, and trace minerals including zinc and copper. They are the richest plant source of omega-3 fatty acids. Chia seeds are a complete protein, containing all nine essential amino acids that cannot be made by the body. [2]
Chia Seeds and Health
Chia seeds contain several components that, when eaten as part of a balanced plant-rich diet, may prevent the development of various chronic diseases. Of particular interest by researchers is chia seeds’ high content of alpha-linolenic (ALA) fatty acids. Sixty percent of the oil in chia seeds is from these omega-3 fatty acids. [2] However, available research has been more favorable towards a diet containing omega-3-rich foods rather than on chia seeds alone.
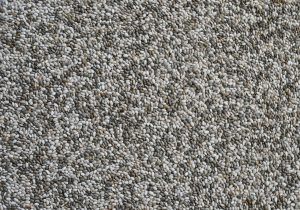
A large Chinese cohort of more than 63,000 individuals found that those with the highest intakes of omega-3 fatty acids from seafood and plant sources had a 17% reduced risk of cardiovascular mortality compared with those who had the lowest intakes. [3] Few cohort studies include ALA plant sources of omega-3, but these oils are fairly common in the Asian diet. The Nurses’ Health Study found a 40% reduced risk of sudden cardiac death in women who ate the highest amounts of ALA. [4] The Cardiovascular Health Study cohort with more than 5000 men and women, ages 65 years and older, found a 50% lower risk of fatal ischemic heart disease with higher intakes of ALA. [5] Food sources of ALA in these studies included whole grains, cooking oils, legumes, and soy.
Animal studies have shown that chia seeds can beneficially affect cholesterol levels, weight loss, and increased satiety. [6] However, literature reviews and controlled trials in humans have not shown a specific benefit of chia seeds on cardiovascular risk factors including body weight, blood pressure, lipid levels, blood sugar, and inflammation. [6-8] These findings affirm that chia seeds do not act alone to benefit human health but may contribute to disease prevention when incorporated as part of a varied plant-rich diet and other healthy lifestyle behaviors.
- People often wonder if chia seeds should be eaten ground instead of whole. The surface of chia seeds is delicate and easily breaks apart when exposed to moisture, so they are typically prepared with liquid foods (as seen with the recipe ideas below). In this way, they are absorbed and digested well in their whole form, unlike flax seeds. If eating the seeds dry, choosing ground chia seeds may help to improve absorption.
- Chia seeds last for 4-5 years without refrigeration. Store in a cool, dry spot.
- Chia Gel : Chia seeds absorb water quickly (up to 10 times their weight in liquid!). Place ¼ cup seeds in 1 cup liquid, stir well, and cover. Allow to sit for about 15-20 minutes until the texture changes to a soft gelatin. Store in refrigerator for up to one week. Add to smoothies and soups to boost nutrient value and create a thicker, more satisfying consistency.
- Chia Pudding : To make a dessert variation, mix ¼ cup of seeds with one cup of liquid such as milk (almond, soy, or dairy all work) or 100% fruit juice. Allow to sit for at least 15 minutes refrigerated. Add nuts, chopped fresh fruit, or cinnamon if desired.
- Chia Sprouts : Place chia seeds in a single layer (use only about a teaspoon to allow enough space to grow) in a terracotta saucer or unglazed clay dish. Spray the seeds with water several times and cover with plastic wrap or a clear glass dish. Put in a sunny spot. Spray morning and evening until green sprouts appear, about 3-7 days. Use these microgreens to garnish salads and sandwiches.
- Egg Replacer : This may be used to replace whole eggs in baking. For 1 whole egg, mix 1 tablespoon of whole chia seeds or 2 teaspoons ground chia seeds with 3 tablespoons water. Allow to sit for at least 5 minutes or until the mixture thickens to the consistency of a raw scrambled egg.
Chia seeds are a highly versatile ingredient. They have little if any distinctive flavor, so they don’t compete with other flavors in a dish. They also soften in the presence of liquid and become a less detectable texture. Commercially, they are added to cereals, crackers, beverages, breads, and other baked goods to boost their nutritional value. Basically, chia seeds can be added as long as there is moisture to hold the seed in place.
- Sprinkle a few teaspoons into breakfast cereal (hot or cold) salads, soups, or stews.
- Stir into salad dressings, sauces, marinades, or cake/muffin/bread batter.
- Use chia gel as a thickener added to smoothies, puddings, and soups (stir the gel into these foods after they are prepped or cooked).
More recipe ideas and serving suggestions featuring chia seeds:
- Quinoa Chia Edamame Veggie Burger
Did You Know?
- Chia seeds come in black and white varieties, but there is no difference in nutritional content.
- There are a few rare cautions when eating chia seeds. A case report presented at the American College of Gastroenterology Annual Scientific Meeting in 2014 made headlines describing a patient who ate dry chia seeds followed by a glass of water. The seeds expanded in the esophagus and caused a blockage. Because they quickly swell after absorbing liquid, it is advised to eat chia seeds that have already been soaked in liquid or are served with a moist food, such as oatmeal or yogurt. Do not eat dry chia seeds by themselves. People who have dysphagia, a condition that causes difficulty in swallowing (as was the case with this patient) or other digestive issues, should eat chia seeds with care.
- Mordor Intelligence. Global Chia Seeds Market – Analysis of Growth, Trends and Forecast (2017-2022) . Accessed 12/4/2017.
- Suri, S., Passi, J. S., Goyat, J. Chia Seed (Salvia Hispanica L.) – A New Age Functional Food. 4 th International Conference on Recent Innovations in Science Engineering and Management . March 20, 2016.
- Koh AS, Pan A, Wang R, Odegaard AO, Pereira MA, Yuan JM, Koh WP. The association between dietary omega-3 fatty acids and cardiovascular death: the Singapore Chinese Health Study. Eur J Prev Cardiol . 2015 Mar;22(3):364-72.
- Albert CM, Oh K, Whang W, Manson JE, Chae CU, Stampfer MJ, Willett WC, Hu FB. Dietary α-linolenic acid intake and risk of sudden cardiac death and coronary heart disease. Circulation . 2005 Nov 22;112(21):3232-8.
- Lemaitre RN, King IB, Mozaffarian D, Kuller LH, Tracy RP, Siscovick DS. n− 3 Polyunsaturated fatty acids, fatal ischemic heart disease, and nonfatal myocardial infarction in older adults: the Cardiovascular Health Study. Am J Clin Nutr . 2003 Feb 1;77(2):319-25.
- de Souza Ferreira C, de Sousa Fomes LD, Espirito Santo da Silva G, Rosa G. Effect of chia seed (Salvia hispanica L.) consumption on cardiovascular risk factors in humans: a systematic review. Nutricion hospitalaria . 2015;32(5).
- Nieman DC, Gillitt N, Jin F, Henson DA, Kennerly K, Shanely RA, Ore B, Su M, Schwartz S. Chia seed supplementation and disease risk factors in overweight women: a metabolomics investigation. J Altern Complement Med . 2012 Jul 1;18(7):700-8.
- Nieman DC, Cayea EJ, Austin MD, Henson DA, McAnulty SR, Jin F. Chia seed does not promote weight loss or alter disease risk factors in overweight adults. Nutr Res . 2009 Jun 1;29(6):414-8.
Terms of Use
The contents of this website are for educational purposes and are not intended to offer personal medical advice. You should seek the advice of your physician or other qualified health provider with any questions you may have regarding a medical condition. Never disregard professional medical advice or delay in seeking it because of something you have read on this website. The Nutrition Source does not recommend or endorse any products.

COMMENTS
Therefore, engineered solutions should focus more on improving the microbiological quality of the drinking water. The intermittent supply in municipal tap water, inadequate water quality from alternative sources, and the risk of recontamination during storage suggest a need for a low-cost, point-of-use water-treatment solution to be used at the ...
The benefits of healthy, sustainable water resources: Supply clean drinking water. Grow our food and are used to raise livestock. Produce the goods we use. Drive economic and social benefits. Sustain healthy rivers, lakes, springs and associated habitats that support diverse wildlife. ... Case Study 1: Saving Water, Securing the Nation's Food.
ABSTRACT. In Nelatur Village, which is the case study, the present system of water supply is intermittent supply and a tree. system or dead end system network is a dopted for water distribution ...
Description. The Eastern area of Tucuman province was faced to significant problems of "unsafe water" and inappropriate drinking water supply system. The shallow wells were contaminated by arsenic and other harmful pollutants. Several studies were conducted to solve the problem of insufficient drinking water supply mainly in small rural ...
1.3 The Water Supply Project 1.3.1 Economic Rationale and Role of Economic Analysis 12. The main rationale for Bank operations is the failure of markets to adequately provide what society wants. This is particularly true in the water supply sector. The provision of basic water supply services to poorer population groups
In 15 years, PPWSA managed to increase water production by around 440%, expand the distribution network by 557%, increase pressure in the system by 1260%, and reduce unaccounted-for water from 72% to 6.2%. Also, by 2008, PPWSA's customer base had grown by more than 660%.
But as the researchers acknowledge, the exact results of their study would likely vary from region to region, depending on all the climate and population factors that affect water supply. Even so, they think their new study framework can at least help planners make the case that building on a smaller scale may position cities and countries best ...
We take, as a case study, public water use and supply in Wales. The analysis shows how the proposed method, using both the quantity input-output model and the associated price dual, can be used to ...
Rural water supplies have traditionally been overshadowed by urban ones. That must now change, as the Sustainable Development Goals calls for water for all. The objective of the paper is to assess the current access to and the perceived water quality in villages with various types of water supply. The survey was carried out during July-December 2017 in four villages in central Kazakhstan ...
CASE STUDY Water company Aquanet S.A. is responsible for the water supply in the city of Pozna ń (550,000 inhabitants), in the Midwest of Poland. Like most water supply companies in Poland, Aquanet manually operated the water treatment and pumping facilities by operators. In 2011 Aquanet
Summary of Conservation Case Studies. water rates, a public education program, a high-efficiency plumbing program, landscaping programs, and large-use programs. drawdown so that the level of water demand should stay constant until 2005. Peak demand is down 14% from 1990.
Household water supply can cause different environmental impacts associated with the consumption of energy and materials, the generation of waste, and other inputs and outputs necessary to treat and distribute water. These impacts depend on the population's consumption patterns, due to the potential availability of different water sources. In this work, the environmental impacts of water ...
The city gets its water from two reservoirs, built in 1910 and 1931, that are located outside the city limits, and from two well-fields that supplement its surface water supply. Studies have shown that prolonged drought combined with more intense storms and large amounts of run-off can impact the overall volume, turbidity, and total organic ...
The presented case studies include the following eight water resources engineering problems: analysis of combined sewer overflow, flood control, agricultural water supply, water quality management, complex water resources management policy analysis, water dispute resolution, urban water management, reservoir management and rural water supply ...
Bay Water's official website and the U.S. EIA website. In this case study, the average water production rate of conventional water supply is 99 million gallons of water per day, which equals 374,755.8 m3/day; and the average water production rate of desalination water supply is 25 million gallons of water per day, which equals to 94,635.3 m3 ...
Abstract. Water constraint is a global problem that afflicts both developing and developed countries. More than a fourth, 2.1 out of 7.5 billion people worldwide still lack safely managed drinking ...
The water supply system is one of the important hydraulic infrastructures in providing water to consumers. In Nepal, most of the water supply systems are managed by the water supply users' committee (WSUC). In the present study, it is aimed to evaluate its institutional performance as the system reaches close to the end of design periods. Three water supply systems located in the three ...
Dual water systems are becoming an important urban water management strategy as more utilities adopt increasingly integrated approaches that enable matching source water quality to the intended use, more efficient use of resources, use of nontraditional local water sources, and more resilient systems.
The growing population has resulted in the need for new alternatives that guarantee water supply to the population. Among the alternatives, there is the individual system for capturing and utilizing rainwater. The objective of this article was to test a municipal policy that makes it mandatory to implement the system and to attest how much it can optimize the current public water supply system ...
The objective of the paper is to assess the current access to and the perceived water quality in villages with various types of water supply. The survey was carried out during July⁻December 2017 in four villages in central Kazakhstan. Overall, 1369 randomly selected households were interviewed. The results revealed that even though villagers ...
The objective of the case study is to show how Thai Quality Standard on assessing village water supply systems (QSVS) - based on WHO Drinking Water Quality Guidelines - were used to develop a self-assessment tool. The new tool allows the collection of structural and non-structural components of village water supply
The water supply system and sewerage system is affected due to damage to the infrastructure of the treatment plant, ... Dheepak S, Deepak P, Metilda JE (2021) Assessment of hydrological drought condition and its impact on water quality-a case study in parts of Chengalpattu district, Tamil Nadu, India. Rasayan J Chem 14:51-57. https://doi.org ...
An Evaluation Model for Measuring Customer Satisfaction Levels in a Water Supply Domain: Case Study—Water Supply in Hamedan. Water Policy 2011, 13, 490-505. [Google Scholar] Al-Ghuraiz, Y.; Enshassi, A. Customers' Satisfaction with Water Supply Service in the Gaza Strip. Build. Environ. 2005, 41, 1243-1250. [Google ...
The study assessed static management, i.e., without planned periodic maintenance of the storage (no sediment dredging) denoted as SC1, or with maintenance denoted as SC2. The second approach was dynamic management based on applying Real-Time Control (RTC) techniques to scenario SC1 and scenario SC2 denoted as RTC-1 and RTC-2, respectively.
In the present study, during a series of numerical simulations, the process of dimensional optimization of the solar still along with the optimization of the thermoelectric cooling system features connected to it was studied. This method, which is one of the new methods of water production, can be considered as one of the solutions for sustainable water supply to the dominant rural population ...
This study deals with the prediction of recurring failures in water supply networks, a complex and costly task, but essential for the effective maintenance of these vital infrastructures. Using historical failure data provided by Companhia de Água e Esgotos da Paraíba (CAGEPA), the research focuses on predicting the time until the next failure at specific points in the network.
Chia Gel: Chia seeds absorb water quickly (up to 10 times their weight in liquid!). Place ¼ cup seeds in 1 cup liquid, stir well, and cover. Place ¼ cup seeds in 1 cup liquid, stir well, and cover. Allow to sit for about 15-20 minutes until the texture changes to a soft gelatin.