Understanding Drug Use and Addiction DrugFacts
Many people don't understand why or how other people become addicted to drugs. They may mistakenly think that those who use drugs lack moral principles or willpower and that they could stop their drug use simply by choosing to. In reality, drug addiction is a complex disease, and quitting usually takes more than good intentions or a strong will. Drugs change the brain in ways that make quitting hard, even for those who want to. Fortunately, researchers know more than ever about how drugs affect the brain and have found treatments that can help people recover from drug addiction and lead productive lives.

What Is drug addiction?
Addiction is a chronic disease characterized by drug seeking and use that is compulsive, or difficult to control, despite harmful consequences. The initial decision to take drugs is voluntary for most people, but repeated drug use can lead to brain changes that challenge an addicted person’s self-control and interfere with their ability to resist intense urges to take drugs. These brain changes can be persistent, which is why drug addiction is considered a "relapsing" disease—people in recovery from drug use disorders are at increased risk for returning to drug use even after years of not taking the drug.
It's common for a person to relapse, but relapse doesn't mean that treatment doesn’t work. As with other chronic health conditions, treatment should be ongoing and should be adjusted based on how the patient responds. Treatment plans need to be reviewed often and modified to fit the patient’s changing needs.
Video: Why are Drugs So Hard to Quit?
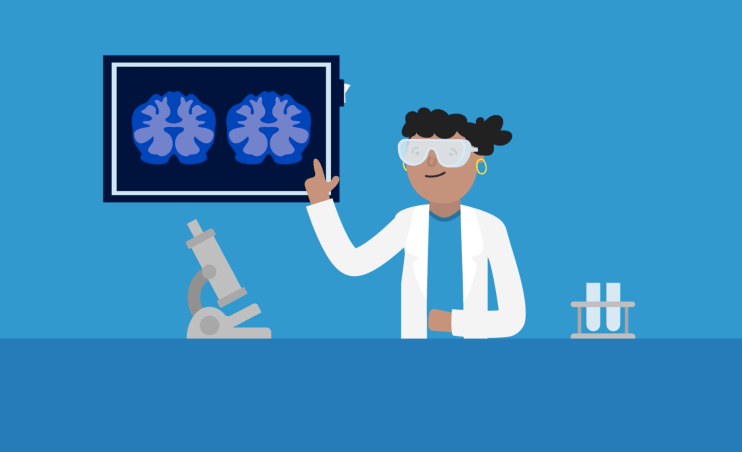
What happens to the brain when a person takes drugs?
Most drugs affect the brain's "reward circuit," causing euphoria as well as flooding it with the chemical messenger dopamine. A properly functioning reward system motivates a person to repeat behaviors needed to thrive, such as eating and spending time with loved ones. Surges of dopamine in the reward circuit cause the reinforcement of pleasurable but unhealthy behaviors like taking drugs, leading people to repeat the behavior again and again.
As a person continues to use drugs, the brain adapts by reducing the ability of cells in the reward circuit to respond to it. This reduces the high that the person feels compared to the high they felt when first taking the drug—an effect known as tolerance. They might take more of the drug to try and achieve the same high. These brain adaptations often lead to the person becoming less and less able to derive pleasure from other things they once enjoyed, like food, sex, or social activities.
Long-term use also causes changes in other brain chemical systems and circuits as well, affecting functions that include:
- decision-making
Despite being aware of these harmful outcomes, many people who use drugs continue to take them, which is the nature of addiction.
Why do some people become addicted to drugs while others don't?
No one factor can predict if a person will become addicted to drugs. A combination of factors influences risk for addiction. The more risk factors a person has, the greater the chance that taking drugs can lead to addiction. For example:
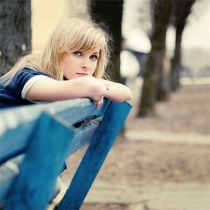
- Biology . The genes that people are born with account for about half of a person's risk for addiction. Gender, ethnicity, and the presence of other mental disorders may also influence risk for drug use and addiction.
- Environment . A person’s environment includes many different influences, from family and friends to economic status and general quality of life. Factors such as peer pressure, physical and sexual abuse, early exposure to drugs, stress, and parental guidance can greatly affect a person’s likelihood of drug use and addiction.
- Development . Genetic and environmental factors interact with critical developmental stages in a person’s life to affect addiction risk. Although taking drugs at any age can lead to addiction, the earlier that drug use begins, the more likely it will progress to addiction. This is particularly problematic for teens. Because areas in their brains that control decision-making, judgment, and self-control are still developing, teens may be especially prone to risky behaviors, including trying drugs.
Can drug addiction be cured or prevented?
As with most other chronic diseases, such as diabetes, asthma, or heart disease, treatment for drug addiction generally isn’t a cure. However, addiction is treatable and can be successfully managed. People who are recovering from an addiction will be at risk for relapse for years and possibly for their whole lives. Research shows that combining addiction treatment medicines with behavioral therapy ensures the best chance of success for most patients. Treatment approaches tailored to each patient’s drug use patterns and any co-occurring medical, mental, and social problems can lead to continued recovery.
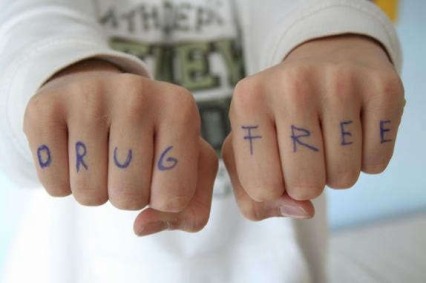
More good news is that drug use and addiction are preventable. Results from NIDA-funded research have shown that prevention programs involving families, schools, communities, and the media are effective for preventing or reducing drug use and addiction. Although personal events and cultural factors affect drug use trends, when young people view drug use as harmful, they tend to decrease their drug taking. Therefore, education and outreach are key in helping people understand the possible risks of drug use. Teachers, parents, and health care providers have crucial roles in educating young people and preventing drug use and addiction.
Points to Remember
- Drug addiction is a chronic disease characterized by drug seeking and use that is compulsive, or difficult to control, despite harmful consequences.
- Brain changes that occur over time with drug use challenge an addicted person’s self-control and interfere with their ability to resist intense urges to take drugs. This is why drug addiction is also a relapsing disease.
- Relapse is the return to drug use after an attempt to stop. Relapse indicates the need for more or different treatment.
- Most drugs affect the brain's reward circuit by flooding it with the chemical messenger dopamine. Surges of dopamine in the reward circuit cause the reinforcement of pleasurable but unhealthy activities, leading people to repeat the behavior again and again.
- Over time, the brain adjusts to the excess dopamine, which reduces the high that the person feels compared to the high they felt when first taking the drug—an effect known as tolerance. They might take more of the drug, trying to achieve the same dopamine high.
- No single factor can predict whether a person will become addicted to drugs. A combination of genetic, environmental, and developmental factors influences risk for addiction. The more risk factors a person has, the greater the chance that taking drugs can lead to addiction.
- Drug addiction is treatable and can be successfully managed.
- More good news is that drug use and addiction are preventable. Teachers, parents, and health care providers have crucial roles in educating young people and preventing drug use and addiction.
For information about understanding drug use and addiction, visit:
- www.nida.nih.gov/publications/drugs-brains-behavior-science-addiction/drug-abuse-addiction
For more information about the costs of drug abuse to the United States, visit:
- www.nida.nih.gov/related-topics/trends-statistics#costs
For more information about prevention, visit:
- www.nida.nih.gov/related-topics/prevention
For more information about treatment, visit:
- www.nida.nih.gov/related-topics/treatment
To find a publicly funded treatment center in your state, call 1-800-662-HELP or visit:
- https://findtreatment.samhsa.gov/
This publication is available for your use and may be reproduced in its entirety without permission from NIDA. Citation of the source is appreciated, using the following language: Source: National Institute on Drug Abuse; National Institutes of Health; U.S. Department of Health and Human Services.
- U.S. Department of Health & Human Services

- Virtual Tour
- Staff Directory
- En Español
You are here
The nih almanac, national institute on drug abuse (nida).
- Important Events
- Legislative Chronology
The National Institute on Drug Abuse (NIDA) is the lead federal agency supporting scientific research on drug use and addiction. NIDA’s mission is to advance science on drug use and addiction and to apply that knowledge to improve individual and public health through:
- Strategically supporting and conducting basic, clinical, and epidemiological research on drug use, its consequences, and the underlying neurobiological, behavioral, and social mechanisms involved.
- Ensuring the effective translation, implementation, and dissemination of scientific research findings to improve the prevention and treatment of SUDs, reduce the harms associated with drug use, guide policies, enhance public awareness of addiction as a chronic but treatable medical illness, and reduce stigma.
In line with this mission, NIDA works to ensure that the following research priorities are addressed across institute programs and initiatives:
- understanding drugs, the brain, and behavior
- developing and testing novel prevention, treatment, harm reduction, and recovery support strategies
- accelerating research on the intersection of substance use, HIV, and related comorbidities
- improving implementation of evidence-based strategies in real-world settings
- translating research into innovative health applications
NIDA also works to ensure that cross-cutting priorities are reflected across institute programs and initiatives, including:
- training the next generation of scientists
- identifying and developing approaches to reduce stigma
- understanding sex, sexual orientation, and gender differences
- identifying and developing approaches to reduce health disparities
- understanding interactions between substance use, HIV, and other comorbidities
- leveraging data science and analytics to understand real-world complexity
- developing personalized interventions informed by people with lived experience
Important Events in NIDA History
1974 — Congress establishes NIDA as the federal focal point for biomedical research on the nature and extent of substance use and substance use disorders.
1975 — NIDA supports the first nationally representative survey of adolescent and young adult substance use and attitudes. The ongoing Monitoring the Future (MTF) Survey tracks trends in past-year, past-month, and lifetime substance use among 12 th graders.
1979 — The NIDA Intramural Research Program (IRP) (formerly the Addiction Research Center) moves to Baltimore’s Johns Hopkins Bayview Medical Center (then, the Francis Scott Key Medical Center) from Lexington, KY.
NIDA-supported scientists identify the brain chemical dynorphin . Its discovery, along with other parts of the natural opioid system in the brain, revolutionizes the addiction field.
1980 — NIDA-supported researchers release data showing caregivers and teachers who receive training in supporting their children’s social and emotional needs (the Raising Healthy Children intervention) raise young people with better behavioral and health outcomes, benefits that are passed to their offspring.
1984 — Nicotine replacement therapy is developed by NIDA-supported researchers
1991 — The Monitoring the Future Survey expands to include 8th and 10th graders.
NIDA researchers clone the dopamine transporter , cocaine's primary site of action in the brain.
1992 — NIDA-supported research isolates anandamide , a chemical in the brain that interacts with the active ingredient in cannabis, delta-9-tetrahydrocannabinol (THC). In addition, the researchers discover that anandamide also plays a role in other brain activities, such as pain relief, sedation, memory, and cognition.
NIDA researchers demonstrate the effectiveness of sublingual buprenorphine for the treatment of opioid use disorder .
NIDA joins the National Institutes of Health (NIH).
1993 — The NIDA Medications Development Program obtains approval from the U.S. Food and Drug Administration (FDA) for levomethadyl acetate (LAAM), the first medication approved in a decade for the treatment of opioid use disorder.
1998 — As part of a team including the Pasteur Institute, Karolinska Institute and Glaxo Wellcome Geneva, a NIDA-supported researcher identifies a brain receptor activated by nicotine use. The finding identifies a potential target for smoking cessation treatment.
Researchers in NIDA's Intramural Research Program discover that the addiction pathway for cocaine is more complicated than previously understood. Researchers tested the theory that dopamine and serotonin were key to the euphoria associated with cocaine use, discovering that other factors may be involved in its rewarding effects.
NIDA scientists identify a chemical known as [D-Ala2,D-leu5]enkephalin (DADLE) that may help reduce long-term nerve damage from methamphetamine use. This has important implications for treating people with substance use disorder and the side effects of long-term substance use.
NIDA research leads to the FDA approval of buproprion for smoking cessation.
1999 — NIDA-supported researchers report the first clinical cases of cannabis withdrawal . Two scientific studies suggest that irritability, stomach pain, and withdrawal from social interactions may be symptoms of quitting cannabis after ongoing use. The findings suggest that effective treatments to alleviate these symptoms are needed. They also suggest that continued use of cannabis may be an effort to alleviate unpleasant withdrawal symptoms.
NIDA awards grants to the first five regional sites under its new Clinical Trials Network (CTN) . The network continues to conduct clinical research into medications for substance use disorders and to bring updated science to communities and healthcare offices.
Using brain scans and tracking cerebral phosphate metabolites, NIDA-supported researchers show that longer term methadone treatment is associated with brain recovery .
Research supported by NIDA identifies a way for scientists to estimate how likely an opioid is to cause tolerance and have the potential to cause addiction. The scale, called relative activity versus endocytosis or RAVE measure , calculates how effective different opioids are regulating the signaling of the opioid receptors they activate in the brain. It assesses the opioid’s ability to trigger cells to remove opioid receptors from the cell membrane, a process called internalization.
NIDA-supported research finds that HIV can survive in used syringes for four weeks or more after use. Specifically, the model estimates that blood containing HIV could survive in the razor-thin space inside the needle of the syringe.
NIDA-supported research identifies brain processes that increase the rewarding effects of cocaine the more a person uses it, a change thought to play an important role in the development of cocaine craving and addiction.
Researchers analyze the health care costs of substance use benefits and find substance use treatment is cost-effective . In this analysis, researchers found that changing even stringent limits on annual substance use benefits had only a small absolute effect on overall insurance costs under managed care, even though a large percentage of substance use patients were affected.
2000 — Researchers demonstrate that contingency management for cocaine use disorder can have long-lasting effects and serve as a valuable role in treating addictions. Patients in this study who received vouchers for having cocaine negative urine tests were more likely to have sustained cocaine abstinence during outpatient treatment than a comparative group that received incentives regardless of urine test results.
In a study partially funded by NIDA, researchers merge technologies from the imaging and genetic fields to discover that the number of brain dopamine D2 receptors an individual has may correlate directly with the amount of euphoria experienced while taking methylphenidate, a mild stimulant.
2001 — NIDA-supported animal research shows a single exposure to cocaine induces changes in brain cells that are very similar to long-term potentiation, a process that plays an important role in associating experiences with feelings and motivations.
2002 — NIDA-supported research leads to the FDA approval of buprenorphine and buprenorphine/naloxone , the first medications for opioid use disorder that can be prescribed in an office setting.
2006 — NIDA-supported research leads to the FDA approval of varenicline for tobacco use disorder
NIDA-supported research demonstrates that methadone is an effective means of reducing heroin use and criminal behavior among opioid-dependent individuals awaiting entry into a comprehensive methadone treatment program.
2008 — NIDA's Monitoring the Future Survey reveals an increased misuse of prescription medications and over-the-counter cough syrups among high school seniors, accounting for 8 of the top 13 drug categories reported by this group.
2009 — NIDA launches a comprehensive Physicians Outreach Initiative, NIDAMED , which gives medical professionals tools and resources to screen their patients for tobacco, alcohol, illicit, and nonmedical prescription drug use, including an interactive online drug use screening tool.
2010 — NIDA launches its first annual National Drug Facts Week (NDFW), a health observance that inspires dialogue about the science of drug use and addiction among youth. It provides an opportunity for scientists, students, educators, healthcare providers, and community partners to help advance addiction science and address youth drug and alcohol use in communities and nationwide.
2011 — The Population Assessment of Tobacco and Health (PATH) Study launches. A joint effort of NIDA and the U.S. Food and Drug Administration, PATH is a national longitudinal study of tobacco use and how it affects the health of people in the United States
2012 — A NIDA-supported seminal study demonstrates that scaling up HIV treatment in people who inject drugs results in reductions in community-level viral load and HIV transmission.
2013 — NIDA, The National Institute on Alcohol Abuse and Alcoholism (NIAAA), and The National Cancer Institute (NCI) form the Collaborative Research on Addiction at NIH (CRAN) . The partnership integrates resources and expertise to advance the science and treatment of substance abuse and addiction.
2014 — NIDA announces the development of an innovative National Drug Early Warning System (NDEWS) to monitor emerging nationwide new and emerging drug trends to help public health experts respond quickly.
2015 — NIDA-supported research leads to FDA approval of the first naloxone nasal spray .
NIDA launches the Adolescent Brain Cognitive Development (ABCD) Study . This study follows more than 11,800 children from ages 9-10 through adolescence into young adulthood. By integrating neuroimaging with genetics, neuropsychological, behavioral, and other health assessments, the study aims to shed light on how substance use and other experiences during adolescence affect brain development and later health outcomes such as drug use and addiction.
2016 — NIDA-supported research leads to FDA approval of a buprenorphine implant to improve treatment retention and reduce burdens associated with frequent dosing.
2017 — NIDA-supported research leads to the development of ReSET-O, the first FDA-approved smartphone app to deliver behavioral treatment for opioid use disorder.
NIDA-supported research develops Flowlytics , a cloud-based data analysis software that allows a health care facility to track its inventory of controlled substances, such as opioids, helping to prevent drug theft (also known as drug diversion). The patented software can detect potential drug diversion incidents earlier than previous methods.
2018 — The Helping to End Addiction Long-Term Initiative® or NIH HEAL Initiative® is launched to speed scientific solutions to stem the national opioid public health crisis. NIDA is leading HEAL-supported research aimed at preventing and treating opioid misuse and addiction.
NIDA-supported research leads to the FDA approval of lofexidine , the first non-opioid treatment for opioid withdrawal.
Researchers develop a biosensor to detect opioid receptor activation. Using this method, researchers are able to show that opioid receptors function in different cell locations depending on the type of opioid.
2019 — Researchers identify a brain receptor with anti-opioid activity, a discovery that opens the door to new way to improve opioid safety.
Through investment in its Small Business Innovation Research (SBIR) and Small Business Technology Transfer (STTR) programs, NIDA supports development of FDA-regulated therapeutic and diagnostic devices, mobile health and general wellness products, research tools, and health IT solutions. These include:
- Prapela ™, a hospital bassinet pad that delivers gentle, random vibrations to treat newborns who were exposed to opioids before birth. The bassinet pad helps improve newborns’ breathing and heart rate.
- OpenBeds , a HIPAA-compliant, cloud-based platform that facilitates referrals and coordination among medical and mental health providers, social services, and substance use programs.
- RelieVRx , the first VR-based therapeutic to receive FDA authorization for chronic lower back pain and the first to obtain a unique HCPS Level II code from the Centers for Medicare and Medicaid Services (CMS).
- SecondChance , which detects changes in a person’s breathing that may indicate a drug overdose or monitor other clinical indications.
- Boulder , a digital platform that delivers comprehensive treatment for OUD.
2020 — A study partially funded by NIDA finds why some people with HIV are able to maintain suppressed viral loads for years without ART. The study is chosen as a runner-up in Science magazine’s 2020 Breakthrough of the Year.
With funding from the HEAL Initiative, NIDA's Clinical Trials Network expands , adding five new nodes across the country.
Monitoring the Future Study and Population Assessment of Tobacco and Health (PATH) study data show that flavored e-cigarette products particularly appeal to youth. The data informs a 2020 U.S. Food and Drug Administration (FDA) policy prioritizing enforcement against certain unauthorized flavored cartridge-based products.
Biobot Analytics , another SBIR grantee, pioneers the commercial application of wastewater technology to monitor the presence of drugs in communities.
The National Drug Early Warning System (NDEWS), a network of 18 sentinel sites that monitors patterns of drug use across the nation, incorporates real-time surveillance and harnesses its network to collect data on substance use-related consequences of COVID-19.
2021 — With partial funding from the Helping to End Addiction Long-term ® Initiative, or NIH HEAL Initiative ® , NIDA launches the HEALthy Brain and Child Development (HBCD) Study to better understand very early brain development and the effects of environmental factors such as maternal substance use during pregnancy.
A study finds that a combination of oral bupropion and injectable naltrexone reduces methamphetamine use and cravings people with methamphetamine use disorder.
SBIR-funded Spark Biomedical develops a wearable treatment technology intended for the relief of opioid withdrawal symptoms.
A meta-analysis of studies shows that medication treatment for opioid use disorder (MOUD) is associated with greater adherence to antiretroviral therapy (ART) and HIV viral suppression.
2022 — NIDA collaborates with the Centers for Disease Control and Prevention and other government agencies for a study that shows the expansion of telehealth services during the COVID-19 pandemic was associated with people staying in treatment longer and reducing their risk of medically treated overdose.
A study finds that people with opioid use disorder who were incarcerated and received a medication approved to treat opioid use disorder, known as buprenorphine, were less likely to face rearrest and reconviction after release than those who did not receive the medication.
NIDA research contributes to a new U.S. Preventive Services Task Force recommendation on pre-exposure prophylaxis (PrEP) for the prevention of HIV for those at increased risk, including people who inject drugs.
2023 — A study under the NIH Heal Initiative shows that initiating buprenorphine treatment in the ER is safe, effective and does not trigger withdrawal in people with opioid use disorder. Clinician concern over this type of withdrawal can be a barrier to using this treatment.
Researchers pinpoint areas in the genome associated with general addiction risk, as well as the risk of specific substance use disorders – namely, alcohol, nicotine, cannabis, and opioid use disorders.
NIDA-supported research leads to FDA approval of nalmefene nasal spray , which can be used to treat fentanyl overdose.
NIDA Legislative Chronology
1966 — P.L. 89-793, the Narcotic Addict Rehabilitation Act, provided for increased Federal efforts in the rehabilitation and treatment of addiction to narcotics (limited to opiates).
1970 — P.L. 91-513, the Comprehensive Drug Abuse Prevention and Control Act, replaced the USPHS Act's definition of "narcotic addict" with a definition of "drug dependent person" to authorize treatment for both narcotic addiction and other drug problems.
1972 — P.L. 92-255, the Drug Abuse Office and Treatment Act, created a Special Action Office for Drug Abuse Prevention (SAODAP) in the Executive Office of the President, and authorized the establishment of NIDA within the Department to become operational in 1974. In cooperation with other Federal agencies, especially the National Institute of Mental Health's (NIMH) Division of Narcotic Addiction and Drug Abuse (DNADA), SAODAP established a national network of multi-modality drug use treatment programs.
1974 — P.L. 93-282, the Comprehensive Alcohol Abuse and Alcoholism Prevention, Treatment, and Rehabilitation Act Amendments, created the Alcohol, Drug Abuse, and Mental Health Administration (ADAMHA), which was charged with supervising and coordinating the functions of NIMH, NIDA, and NIAAA. Programs and responsibilities of DNADA and SAODAP were moved to NIDA. Section 204 of this law, enacted and effective on May 14, 1974, gave NIDA a permanent statutory basis, and established NIDA as a freestanding Institute.
1979 — P.L. 96-181, the Drug Abuse Prevention, Rehabilitation, and Treatment Act, mandated that at least 7% in FY 1980 and 10% in FY 1981 of NIDA's Community Programs budget be spent on prevention.
1981 — P.L. 97-35, the Omnibus Budget Reconciliation Act, repealed NIDA's formula grants and Community Programs project grants and contracts authorities, and established the Alcohol, Drug Abuse, and Mental Health Services (ADMS) Block Grant program, giving more control of treatment and prevention services to the states.
1986 — P.L. 99-570, the Anti-Drug Abuse Act of 1986, increased the Block Grant and created a substance use treatment enhancement. The Act also provided increased funds for all NIDA research, particularly AIDS research.
Executive Order 12564 mandated a drug-free Federal workplace program. NIDA became the lead agency, creating its Office of Workplace Initiatives.
1987 — P.L. 100-71, Supplemental Appropriations Act of 1987, required HHS (NIDA) to publish guidelines in the Federal Register for Federal drug testing.
1988 — P.L. 100-690, the Anti-Drug Abuse Act of 1988, established the Office of National Drug Control Policy (ONDCP) in the Executive Office of the President and authorized funds for Federal, state, and local law enforcement, school-based drug prevention efforts, and drug use treatment with special emphasis on people injecting drugs and at higher risk for acquiring HIV.
1989 and 1990 — P.L. 101-166 and P.L. 101-517, the Departments of Labor, HHS, and Education Appropriations Acts for FY 1990 and 1991, contained identical prohibitions precluding the use of funds provided under these enactments to carry out any program of distributing sterile needles.
1992 — P.L. 102-321, the ADAMHA Reorganization Act, transferred NIDA to NIH; earmarked 15% of the Institute's research appropriation for health services research; established a Medication Development Program within NIDA; provided authority to designate Drug Abuse Research Centers for interdisciplinary research on drug use and related biomedical, behavioral, and social issues; and created an Office on AIDS at NIDA.
P.L. 102-394, the Departments of Labor, HHS, and Education FY 1993 Appropriations Act, provided that up to $2 million of NIDA research funds be available to carry out section 706 of P.L. 102-321, which required the HHS Secretary, acting through NIDA, to request a National Academy of Sciences study of U.S. programs that provide both sterile hypodermic needles and bleach.
1993 — P.L. 103-112, the Department of Labor, HHS and Education FY 1994 Appropriations Act, prohibited the use of funds under the Act for any further implementation of section 706 of P.L. 102-321 (see above) and any program for distributing sterile needles.
1994 and 1996 — P.L. 103-333, the Departments of Labor, HHS, and Education Appropriations Act for FY 1995; P.L. 104-134, the Omnibus Consolidated Rescissions and Appropriations Act for FY 1996; and P.L. 104-208, the Omnibus Consolidated Appropriations Act for FY 1997 — each prohibited use of any funds provided in the enactments to carry out any program of distributing sterile needles.
1997 — P.L. 105-78, the Departments of Labor, HHS, and Education Appropriation Act for FY 1998, continued prior restrictions on needle-exchange programs through March 31, 1998, permitting funding thereafter of those programs meeting certain statutory requirements including criteria of the HHS Secretary.
1998 — P.L. 105-277, the Omnibus Consolidated and Emergency Supplemental Appropriations Act-1999, restored the general prohibition on funds for needle exchange programs; statutorily reestablished ONDCP in the Executive Office of the President with significantly expanded authority over drug control agencies; and required ONDCP to conduct a 4-year (FYs 1999-2002) national anti-drug media campaign aimed at youth.
1999 — P.L. 106-113, the Consolidated Appropriations Act-2000, continued the ban on funding of sterile needle and syringe exchange programs; prohibited use of appropriated funds for promotion of legalization of any Schedule I controlled substance; and postponed termination of NIDA's triennial report until 5/15/2000.
2000 — P.L. 106-554, the Consolidated Appropriations Act-2001, authorized the Director of NIH to negotiate a long-term lease for research facilities at Baltimore's Bayview Campus, and continued prior prohibitions on funding of sterile needle/syringe exchange programs and on promotion of legalization of Schedule I controlled substances.
P.L. 106-310, the Children's Health Act of 2000, repealed the Narcotic Addict Rehabilitation Act of 1966 [P.L. 89-793]; waived certain requirements of the Controlled Substances Act to permit qualified physicians to engage in office-based treatment of opioid addiction; and authorized expansion of NIDA research on methamphetamine and increased emphasis on ecstasy research.
2001 — P.L. 107-116, the Departments of Labor, HHS, and Education FY 2002 Appropriations Act, continued prior prohibitions on funding of sterile needle and syringe exchange programs and on legalization of Schedule I controlled substances.
2002 — Title II of P.L. 107-273, the Drug Abuse Education, Prevention, and Treatment Act of 2002, authorized NIDA expansion of interdisciplinary research and clinical trials with treatment centers of the National Drug Abuse Treatment Clinical Trials Network; and required a NIDA study on development of medications for amphetamine/methamphetamine addiction.
2003 — Division G of P.L. 108-7, the Departments of Labor, HHS, and Education FY 2003 Appropriations Act, continued prior prohibitions on funding of sterile needle and syringe exchange programs and on legalization of Schedule I controlled substances.
2004 — P.L. 108-358, the Anabolic Steroids Control Act of 2004, significantly expanded the list of anabolic steroids classified as controlled substances; required a review of Federal sentencing guidelines; and authorized $15 million, for each of the next fiscal years through 2009, for educational programs in schools to highlight the dangers of steroids, with preference given to programs deemed effective by NIDA.
2005 — P.L. 109-56 amended the Controlled Substances Act to lift the patient limitations imposed on medical practitioners in group practices regarding the prescribing of drug addiction treatments. Section 2013 of P.L. 109-59, the Safe, Accountable, Flexible, Efficient Transportation Equity Act, directed the Secretary of Transportation to advise and coordinate with other Federal agencies to address driving under the influence of controlled substances and, in cooperation with NIH (NIDA), to submit a report to Congress on drug-impaired driving.
2006 — P.L. 109-469, the U.S. Office of National Drug Control Policy (ONDCP) Reauthorization Act of 2006, in section 1102, amended the Controlled Substances Act to further relax the patient limitations on provision of drug addiction treatments, allowing medical practitioners to notify the HHS Secretary of need and intent to treat up to 100 patients. Section 1120 required the ONDCP Director to consult with NIH (NIDA) and the National Academy of Sciences in making policy relating to syringe exchange programs.
2006 — P.L. 109-482 (H.R. 6164) , the National Institutes of Health Reform Act of 2006, reaffirmed certain organizational authorities of the NIH Director including establishing, abolishing, and reorganizing national research institutes. It established the Scientific Management Review Board (SMRB) to advise the NIH Director on the use of these organizational authorities. In 2009, the SMRB began discussions about how to optimize research into substance use, and addiction at the NIH. In 2010, the SMRB voted in favor of recommending to the NIH Director the establishment of a new institute for substance use, and addiction-related research and the dissolution of NIAAA and NIDA.
2008 — P.L. 110-199, Second Chance Act of 2007, reauthorized and rewrote provisions of the 1968 Omnibus Crime Control and Safe Streets Act to expand reentry services for offenders. Required the Attorney General (1) to consult with NIDA (and SAMHSA) regarding performance outcome measures and data collection related to substance use and mental health services [sec.101 (k)]; and (2) in consultation with NIDA to conduct a study on the use and effectiveness of funding aftercare services for offenders completing substance use programs while incarcerated [sec. 102 (c)]. Permitted the U.S. Attorney General in consultation with NIDA to make research grants to evaluate the effectiveness of depot naltrexone for treatment of heroin addiction [sec. 244 (a)].
2009 — P.L. 111-117, the Consolidated Appropriations Act, changed federal law regarding potential funding for syringe exchange programs. The Act states: "None of the funds contained in this Act may be used to distribute any needle or syringe for the purpose of preventing the spread of blood borne pathogens in any location that has been determined by the local public health or local law enforcement authorities to be inappropriate for such distribution."; Thus, syringe exchange for this purpose is allowed unless public health or law enforcement authorities choose, at the local level, to prevent it. This change could result in additional research proposals, and thus funding, for syringe exchange-related research projects.
2010 — P.L. 111-148, The Patient Protection and Affordable Care Act, was signed into Law on March 23, 2010. This landmark legislation includes many of the provisions originally included in The Paul Wellstone and Pete Domenici Mental Health Parity and Addiction Equity Act, passed as part of the Emergency Economic Stabilization Act in October of 2008. Implementation of this legislation continues to develop and is designed to help ensure that all Americans have access to mental health and addiction treatment. The parity language prohibits health insurers from placing discriminatory restrictions on such treatment and bars health plans from charging higher copayments, coinsurance, deductibles, and maximum out-of-pocket limits and imposing lower day and visit limits on mental health and addiction care.
2011 — P.L. 112—74, the Consolidated Appropriations Act, rescinded the change made by P.L. 111-117 regarding potential funding for syringe exchange programs. The law now reads, "Notwithstanding any other provision of this Act, no funds appropriated in this Act shall be used to carry out any program of distributing sterile needles or syringes for the hypodermic injection of any illegal drug." It is so far unclear how these changes might affect research in this area.
2015 — The Consolidated Appropriations Act, 2016 changed the rules again for federal funding related to syringe exchange programs. While current law continues the prohibition on the use of federal funds for the actual purchase of syringes or sterile needles, it does allow existing programs in hard-hit communities to access federal funds for other program elements, including substance use counseling and referral to treatment, that support communities in their drive to end the cycle of addiction.
2016 — Comprehensive Addiction and Recovery Act (CARA), enacted on July 22, 2016, aims to address the national epidemic of opioid overdose by creating and expanding federal grant programs to include, but not limited to: expanding access to opioid overdose reversal drugs, by supporting the purchase and distribution of such medications and training for first responders; increasing awareness and educating the public regarding the misuse of prescription opioids; reauthorizing the National All Schedules Prescription Electronic Reporting (NASPER) Act, which provides grants to states to support and improve interoperability of PDMPs; and establishing an HHS-led task force to consolidate federal best practices for pain management.
21 St Century Cures Act was signed into law on December 13, 2016. The legislation provides NIH with critical tools and resources to advance biomedical research across the scientific spectrum, from foundational basic research studies to advanced clinical trials of promising new therapies. Importantly, the Cures Act provides NIH with improved flexibility and resources needed to accomplish its mission to improve the health of Americans. Most notably, the Cures Act implements measures to: alleviate administrative burdens that can prolong the start of clinical trials; improve privacy protections for research volunteers; encourage inclusion of diverse populations represented in clinical research; and open up new NIH funding opportunities for young investigator.
2018 — The “Substance Use-Disorder Prevention that Promotes Opioid Recovery and Treatment for Patients and Communities Act of 2018 (the SUPPORT Act),” became law on October 24, 2018. The legislation included provisions to strengthen the behavioral health workforce through increasing addiction medicine education; standardizing the delivery of addiction medicine; expanding access to high-quality, evidence-based care; and increasing coverage of addiction medicine services in a way that facilitates the delivery of coordinated and comprehensive treatment.
2022 — The Mainstreaming Addiction Treatment (MAT) Act and The Medication Access and Training Expansion (MATE) Act of the Consolidated Appropriations Act, 2023, were signed into law on December 29, 2022. The MAT Act allows any clinician registered to prescribe Schedule III substances the ability to treat opioid use disorder patients with the effective medication buprenorphine, without requiring the additional reporting steps—previously known as the X-waiver—that had been a barrier to provision of this care. The MATE Act requires physicians, including residents and fellows, and other health care professionals who prescribe controlled substances, to complete a one-time-only eight hours of training on the treatment and management of patients with opioid or other substance use disorders.
Biographical Sketch of NIDA Director, Nora D. Volkow, M.D.

Nora D. Volkow, M.D., is Director of the National Institute on Drug Abuse (NIDA) at the National Institutes of Health. NIDA is the world’s largest funder of scientific research on the health aspects of drug use and addiction.
Dr. Volkow's work has been instrumental in demonstrating that drug addiction is a brain disorder. As a research psychiatrist, Dr. Volkow pioneered the use of brain imaging to investigate how substance use affects brain functions. In particular, her studies have documented how changes in the dopamine system affect the functions of brain regions involved with reward and self-control in addiction. She has also made important contributions to the neurobiology of obesity, ADHD, and aging.
Dr. Volkow was born in Mexico and earned her medical degree from the National University of Mexico in Mexico City, where she received the Robins Award for best medical student of her generation. Her psychiatric residency was at New York University, where she earned a Laughlin Fellowship from The American College of Psychiatrists as one of 10 outstanding psychiatric residents in the United States.
Much of her professional career was spent at the Department of Energy’s Brookhaven National Laboratory in Upton, New York, where she held several leadership positions including Director of Nuclear Medicine, Chairman of the Medical Department, and Associate Laboratory Director for Life Sciences. Dr. Volkow was also a professor in the Department of Psychiatry and Associate Dean of the Medical School at The State University of New York at Stony Brook.
Dr. Volkow has published almost a thousand peer-reviewed articles, written 113 book chapters, manuscripts and articles, co-edited "Neuroscience in the 21st Century" and edited four books on neuroscience and brain imaging for mental and substance use disorders.
She received a Nathan Davis Award for Outstanding Government Service, was a Samuel J. Heyman Service to America Medal (Sammies) finalist and is a member of the National Academy of Medicine and the Association of American Physicians. Dr. Volkow received the International Prize from the French Institute of Health and Medical Research for her pioneering work in brain imaging and addiction science; was awarded the Carnegie Prize in Mind and Brain Sciences from Carnegie Mellon University; and was inducted into the Children and Adults with Attention-Deficit/Hyperactivity Disorder (CHADD) Hall of Fame. She was named one of Time magazine's "Top 100 People Who Shape Our World"; one of "20 People to Watch" by Newsweek magazine; Washingtonian magazine’s "100 Most Powerful Women"; "Innovator of the Year" by U.S. News & World Report; and one of "34 Leaders Who Are Changing Health Care" by Fortune magazine.
NIDA Directors
NIDA’s organizational structure comprises the Office of the NIDA Director , the Office of Management , the Office of Science Policy and Communications , the Intramural Research Program , the Division of Extramural Research , and three extramural funding divisions: the Division of Neuroscience and Behavior ; the Division of Therapeutics and Medical Consequences ; and the Division of Epidemiology, Services and Prevention Research . NIDA is also home to the Center for Clinical Trials Network , which manages the National Drug Abuse Treatment Clinical Trials Network ; the Office of Translational Initiatives and Program Innovations , which provides leadership to speed the translation of research discoveries into health applications; and the HIV Research Program , which is responsible for developing, planning, and coordinating high-priority research at the intersection of HIV, substance use, and SUDs. Through a wide range of programs, workshops, and funding mechanisms, the Office of Research Training, Diversity, and Disparities supports researchers at multiple stages of their careers, aims to enhance the diversity of the addiction science workforce, and promotes research to address health inequities.
Through grants and contracts awarded to investigators at research institutions around the country and overseas, as well as through its Intramural Research Program, NIDA addresses the most fundamental and essential questions about substance use. This includes detecting and responding to emerging substance use trends, understanding how drugs work in the brain and body, identifying social determinants of substance use risk and SUDs, and developing and testing new approaches to prevention, treatment, and recovery.
Learn more about NIDA Research Programs and Activities .
This page last reviewed on March 6, 2024
Connect with Us
- More Social Media from NIH
Thank you for visiting nature.com. You are using a browser version with limited support for CSS. To obtain the best experience, we recommend you use a more up to date browser (or turn off compatibility mode in Internet Explorer). In the meantime, to ensure continued support, we are displaying the site without styles and JavaScript.
- View all journals
- My Account Login
- Explore content
- About the journal
- Publish with us
- Sign up for alerts
- Review Article
- Open access
- Published: 12 August 2021
Drug addiction: from bench to bedside
- Julian Cheron 1 &
- Alban de Kerchove d’Exaerde ORCID: orcid.org/0000-0002-0682-5877 1
Translational Psychiatry volume 11 , Article number: 424 ( 2021 ) Cite this article
15k Accesses
24 Citations
1 Altmetric
Metrics details
- Molecular neuroscience
Drug addiction is responsible for millions of deaths per year around the world. Still, its management as a chronic disease is shadowed by misconceptions from the general public. Indeed, drug consumers are often labelled as “weak”, “immoral” or “depraved”. Consequently, drug addiction is often perceived as an individual problem and not societal. In technical terms, drug addiction is defined as a chronic, relapsing disease resulting from sustained effects of drugs on the brain. Through a better characterisation of the cerebral circuits involved, and the long-term modifications of the brain induced by addictive drugs administrations, first, we might be able to change the way the general public see the patient who is suffering from drug addiction, and second, we might be able to find new treatments to normalise the altered brain homeostasis. In this review, we synthetise the contribution of fundamental research to the understanding drug addiction and its contribution to potential novel therapeutics. Mostly based on drug-induced modifications of synaptic plasticity and epigenetic mechanisms (and their behavioural correlates) and after demonstration of their reversibility, we tried to highlight promising therapeutics. We also underline the specific temporal dynamics and psychosocial aspects of this complex psychiatric disease adding parameters to be considered in clinical trials and paving the way to test new therapeutic venues.
Similar content being viewed by others
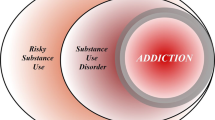
Addiction as a brain disease revised: why it still matters, and the need for consilience
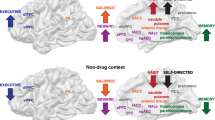
The neurobiology of drug addiction: cross-species insights into the dysfunction and recovery of the prefrontal cortex
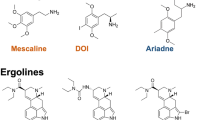
Mechanisms and molecular targets surrounding the potential therapeutic effects of psychedelics
Introduction.
Drug addiction including smoking, alcohol and illicit drug use is indirectly or directly responsible for 11.8 million deaths each year in the world [ 1 ]. According to the Global Burden of Disease study, this number is higher than deaths from cancer and accounts for a fifth of all deaths around the world [ 1 ].
Drug addiction is defined as a chronic, relapsing disease that results from the prolonged effects of drugs on the brain. Similarly to other neuropsychiatric diseases, drug addiction is intermingled with behavioural and social aspects that are equally important parts of the disease, complicating the overall therapeutic approach. Actually, it is only recently, in the beginning of the 21st century, that we started to see “the drug-addict” as someone suffering from a disease and whose brain has been altered fundamentally by drugs [ 2 ]. Therefore, the most effective treatment approaches include biological, behavioural and social-context components. Based on the latest scientific advances, treatment and management of drug addiction patients point towards a personalised strategy [ 3 ]. However, there are very few objective and effective strategies for treating drug addiction. Without the mandatory mechanistic basic knowledge on drug addiction, the development of new therapeutic strategies is postponed.
The neurobiological circuits and mechanisms that support compulsive seeking and consumption of drugs with addictive potential are partially known. They comprise a progressive shift in the involvement of ventral to dorsal and medial to lateral striatal circuitry [ 4 , 5 ], along with molecular and cellular adaptations to drugs of abuse exposure. They include neuronal and synaptic plasticity and modifications in gene expression, in part through epigenetic mechanisms [ 6 ]. Notably, drug-induced neuronal modifications can also occur in non-pathological processes, underlying the fact that drugs of abuse hijack normal adaptive changes in the brain [ 7 ]. Indeed, laboratory and clinical observations suggest that addiction is driven by the usurpation of neuronal processes that normally serve reward-related learning and memory. Most of the modifications that have been shown to be involved in a state of addiction (modified gene transcription, epigenetics, neuronal plasticity and neurotrophic mechanisms) are also associated with physiological forms of behavioural memory in murine model such as spatial memory, fear conditioning and operant conditioning [ 7 , 8 ].
We know that only a proportion of individuals (depending of the drug type) will develop drug addiction after several exposures [ 9 ]. This individual vulnerability is probably linked to both genetic and environmental factors [ 10 ]. Drug addiction is highly polygenic, as hundreds of genetic variations combined result in variable vulnerability [ 11 , 12 ]. Several types of environmental factors have been characterised and interact with an individual genetic background [ 12 , 13 ]. Psychosocial stress is one of the factors, but the most important one, is by far, the exposure to drugs of abuse. Usually, drug abuse starts with a ‘gateway’ drug (mostly socially driven) catapulting the individual vulnerability to other drugs of abuse [ 14 ].
During the last three decades, combine effort has been dedicated to identify brain regions and molecular pathways involved in the development of addiction to drugs of abuse. Here, we will focus on experimental approaches that helped to provide a clearer picture on the physiopathology of drug addiction guiding therapeutic opportunities.
Converging actions on brain reward pathway elicit its remodelling
The circuit at the centre of the disease is the mesolimbic pathway, also referred as the reward pathway (Fig. 1 ). The mesolimbic pathway includes dopaminergic neurons in the ventral tegmental area (VTA) of the midbrain and their targets in the limbic forebrain, especially the nucleus accumbens (NAc), a major component of the ventral striatum. The GABA medium-sized spiny neurons (MSNs, ~95% of striatal neurons), which are targets of glutamatergic and dopaminergic inputs, form two pathways [ 15 ]. The dopamine D1 receptor–positive (D1R) striatonigral MSNs project to the medial globus pallidus and substantia nigra pars reticulata (direct pathway) and coexpress dopamine D1 receptors and substance P, whereas D2R striatopallidal MSNs project to the lateral globus pallidus (indirect pathway) and coexpress dopamine D2 receptor, adenosine A2A receptor and enkephalin [ 16 , 17 ]. Through different initial mechanisms, drugs of abuse increase the release of dopamine in the NAc from the VTA [ 18 , 19 , 20 ]. This VTA-NAc pathway could be seen as primum movens for the acute rewarding effects of all drugs of abuse. Regardless that drugs of abuse have distinct protein targets and mechanisms of action, in the end, the main addiction-related modifications are common to nearly all drugs of abuse and converge on the VTA and NAc with common acute functional effects [ 21 ]. It is schematically conjectured, that when stimulated by dopamine, cells in the NAc produce feelings of reward and satisfaction [ 22 ]. The physiological function of this response is to facilitate the motivation for basic biological goal-directed behaviours as survival, social interaction and reproduction. By artificially causing a build-up of dopamine in the NAc, drugs of abuse generate an artificial reward effect [ 22 ]. As all drugs of abuse increase dopaminergic transmission to the NAc after acute administration, they also produce shared modifications in the mesolimbic system after chronic exposure. They include (i) hypofunction of the dopamine pathway that is seen as a major contributor to the negative emotional symptoms associated to drug withdrawal, leading to drug craving and relapse, and (ii) drug-induced adaptations in glutamatergic afferents to the NAc [ 23 , 24 ]. Clearly, these modifications in the mesolimbic system after the exposure to drugs of abuse is oversimplified. The hypofunction of dopaminergic system hypothesis is self-fulfilling in that research work has principally focused on dopamine to the exclusion of other neurotransmitters. Actually, some drug of abuse reinforcement appears to be independent of the mesocorticolimbic dopamine system (e.g. opioids [ 25 ], nicotine [ 26 ]), but support self-administration by imitating the effect of dopamine in the nucleus accumbens [ 21 , 27 , 28 ].
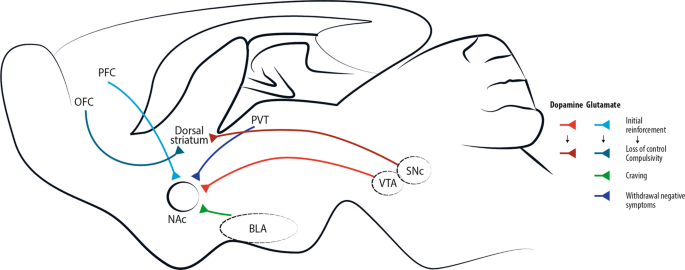
Addictive drugs of different types have a common effect of increasing levels of dopamine released by neurons projecting from the ventral tegmental area (VTA). This effect is central for initial drug reinforcement. Notably, drug taking with initial reinforcement involves a potentiation of the projection from prefrontal cortex (PFC) to nucleus accumbens (NAc), while other glutamatergic projections are mostly involved in craving, like basolateral amygdala (BLA)-NAc projection, or in withdrawal/negative symptoms, like paraventricular thalamus (PVT)-NAc projection. With increasing administration of drugs of abuse and progressive shift toward compulsive abuse, the dorsal (dorso-lateral) striatum seems more and more implicated, with dopaminergic cells involved shifting progressively from the VTA to the substantia nigra pars compacta (SNc) [ 4 ]. Recently, data acquired through optogenetic dopamine neuron self-stimulation suggested prominent synaptic strengthening of the orbitofrontal cortex (OFC) to dorsal (dorso-medial) striatum projection in compulsive mice [ 31 ].
Drug addiction is conceptually defined as a three-stages cycle: (1) consumption/binge/intoxication, (2) withdrawal with its negative affect and (3) craving stage (Fig. 2 ) [ 27 ]. Animal models and human imaging studies have exposed the different brain areas involved in each of these stages. Briefly, the VTA-NAc (for reinforcement) and dorsal striatum (for stimulus-response habits) are important for the consumption/binge/intoxication stage, the extended amygdala with the hypothalamus and the brainstem in the withdrawal stage and cortical areas, the dorsal striatum, the hippocampus and the basolateral amygdala in the craving stage (Fig. 2 ).
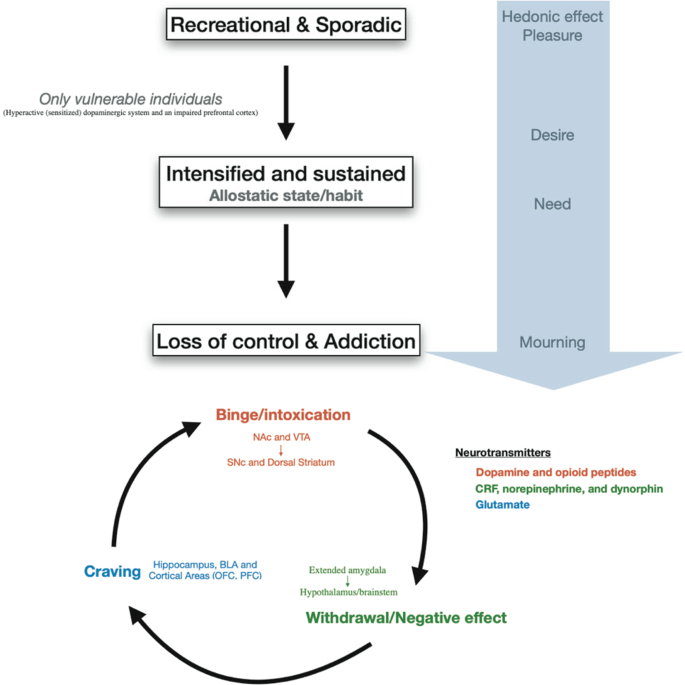
Progression to addiction is defined as a transition between three consecutive phases [ 252 ]: (1) Recreational, sporadic drug taking, in which drug of abuse administration is occasional and one activity among many other distractions of the individual. (2) Intensified and sustained drug use, in which drug administration strengthens and becomes the principal recreational activity of the individual; at this phase drug taking becomes a habit. (3) Loss of control of drug use and addiction, in which drug seeking and taking are now the principal activity of the patient. The first phase can occur to every person as drugs of abuse hijack the same brain circuit as natural rewards. The second phase occurs only in vulnerable users. The phase of addiction seems to be due to a second vulnerable trait with loss of control and compulsivity. Three stages of addiction are described [ 27 ]: (1) Binge/intoxication stage: reinforcing effects of drugs may initially use mainly dopamine and opioid peptides in the nucleus accumbens (NAc) and involves the ventral tegmental area (VTA). Subsequently, cue–response habits develop and includes the substantia nigra pars compacta (SNc) and the dorsal striatum. (2) Withdrawal/negative affect stage: the negative emotional state of withdrawal may involve the extended amygdala with corticotropin-releasing factor (CRF), norepinephrine and dynorphin as key neurotransmitters. Main projections of the extended amygdala consist of the hypothalamus and brainstem. (3) Craving stage: this stage includes conditioned reinforcement in the basolateral amygdala (BLA) and contextual processes in the hippocampus. This is controlled by cortical areas (prefrontal cortex (PFC) and orbitofrontal cortex (OFC)). A key neurotransmitter involved in the craving stage is glutamate.
The progression of drug addiction begins with the first exposure, mostly when the drug is taken voluntarily for its recreational and hedonic effect, and progressively consolidates during repeated but still controlled drug use. While administration intensifies along with loss of control over drug intake, drug use becomes habitual and compulsive in vulnerable individuals [ 4 , 29 , 30 ] (Fig. 2 ). This progression from voluntary drug intake to habitual and compulsive use represents a progression from ventromedial to more dorsolateral regions of the striatum and from prefrontal cortex (PFC) to orbitofrontal (OFC) and more global cortical region [ 4 , 31 ] (Fig. 1 ).
Synaptic plasticity
Brain plasticity is a fascinating capacity allowing appropriate modification of the neural activity in response to new experiences and environmental stimuli [ 32 ]. Modifying the synaptic strength between neurons is widely assumed to be the mechanism by which memory is encoded and stored in the brain [ 7 ]. Hence, it is appealing to hypothesise that drugs of abuse cause long-term alterations on behaviour by changing synaptic plasticity in key brain circuits [ 4 , 7 , 32 ].
Drugs of abuse such as cocaine induce specific synaptic plasticity in the mesolimbic circuitry. One single injection of an addictive drug can already modify the excitatory synaptic strengths in the VTA. Indeed, it has been extensively shown that the AMPA/NMDA ratio is increased in VTA dopamine neurons after one dose of cocaine and that some glutamate AMPA receptor 2 (GluA2)-containing AMPA receptors (AMPARs) are exchanged for GluA2-lacking ones [ 33 , 34 ]. At the same time, NMDA receptor (NMDAR) function decreases. All these elements cause an impairment in eliciting long-term potentiation (LTP). Different types of synaptic plasticity in VTA dopamine neurons induced by rewarding and aversive experiences are comprehensively reviewed by Pignatelli and Bonci [ 35 ]. Midbrain dopamine neurons are central in the mesolimbic circuitry for both natural rewards and drugs of abuse [ 18 , 36 ]. The VTA is known to be a central hub integrating numerous inhibitory inputs as GABAergic synapses represents 50–80% of all synapses onto VTA dopamine neurons [ 37 , 38 , 39 ]. GABAergic inhibition of dopamine neurons is mediated by both fast ionotropic GABA A receptors and slow metabotropic GABA B receptors [ 40 ].
In 2017, Edwards et al. [ 41 ] showed that the principal monosynaptic projection to VTA dopamine neurons arising from the NAc [ 42 ] inhibits the firing of dopamine neurons via activation of GABA B receptors, whereas local VTA inhibitory interneurons inhibits dopamine neurons through GABA A receptors. Today, it is well established that pharmacological activation of GABA B receptors (e.g. by baclofen) reduces cue-associated cocaine craving as well as reduce cocaine use in humans [ 43 , 44 , 45 ] and it reduces rewarding and reinforcing effects of cocaine on animal models [ 46 , 47 , 48 , 49 ]. Edwards et al. report [ 41 ] indicates that the therapeutic effects of baclofen might pass through VTA dopamine neurons’ GABA B receptors. Intrathecal Baclofen is an effective and safe long-term treatment used worldwide to treat severe spasticity [ 50 , 51 ]. Oral baclofen is less effective and has significant rates of side effects, like sedation, somnolence, vertigo and headache especially when prescribed off-label for drug addiction (because higher doses are commonly used) [ 51 , 52 ] . . Indeed, contrasting results on the effect of baclofen in reducing alcohol craving [ 53 , 54 , 55 ] and cocaine dependence [ 43 , 56 ] were probably due to different severity of alcohol dependence of the enroled patients. This is way higher dose are tested and often prescribed off-label for drug addiction [ 55 ]. Thus, self-poisoning that could lead to severe toxicity and death represents one the major concern of baclofen use in drug addiction. Therefore, baclofen should be prescribed with caution and close monitoring [ 52 , 57 ].
Together with drug of abuse-induced LTP at excitatory synapses, plasticity of GABAergic inhibitory synapse in the VTA also have an impact on the firing rate of VTA neurons, at least following opioid [ 58 ] and cocaine administration [ 59 ]. Normally, NMDA activation, during excitatory LTP (induced by high-frequency stimulation), leads to the release of NO that will activate guanylate cyclase in adjacent GABAergic terminals, which in turn, leads to increase in GABA release. This presynaptic NMDA receptor-dependent GABAergic LTP heterosynaptic plasticity, is named LTP GABA . Nugent el al. [ 58 ] showed that opioids blocks LTP GABA through a disruption of the coupling between nitric oxide (NO) and guanylate cyclase. The incapability of GABAergic synapses to potentiate after morphine or cocaine administration may promote LTP of glutamatergic synapses [ 58 , 59 ]. The early loss of inhibitory control combined with potentiation of glutamatergic synapses on dopaminergic neurons might represent adaptations that increase vulnerability to addiction [ 58 , 59 ]. Furthermore, GABA A receptor modulators modify the addictive drugs effects [ 60 , 61 ], and targeting these receptors might be seen as an effective therapeutic strategy but precluded by many side effects among which dependence itself [ 62 , 63 , 64 ].
In addition to the discovery of LTP GABA , Nugent’s group showed that morphine is also able to modulate a form of postsynaptic LTD (LTD GABA ) at GABAergic synapses onto VTA dopamine neurons. Remarkably, after a single administration of morphine, LTD GABA was absent in slices from morphine-treated rats while unaffected in slices from saline-treated rats, indicating a bidirectional control of morphine on GABAergic synaptic plasticity in the VTA [ 65 ]. This absence of LTD GABA is suggested to result from an occlusion effect due to prior morphine-induced decrease in GABAergic synaptic strength through potentiation of glutamatergic transmission and mediated by endocannabinoid signalling [ 66 ]. It is also possible that morphine alters the ability of synapses to exhibit evoked LTP or LTD in the VTA. Previous experiences such as exposure to drugs of abuse, stress, visual or sensory deprivation can change the ability of synapses to undergo subsequent plasticity in response to LTP and LTD induction protocols. This concept of modification of plasticity capability is referred as metaplasticity [ 67 ].
In the NAc, chronic exposure to addictive drugs induces specific synaptic changes that are different from those of the VTA, including a decrease of the AMPA/NMDA ratio as some AMPARs are endocytosed. This leads to a depressed synapse (sometimes referred as long-term depression (LTD) like state), where NMDAR-dependent LTD is reduced or, in some experiments, abolished [ 68 , 69 ]. Highlighting the importance of temporal aspects, studies of withdrawal period after chronic administration of cocaine, showed that synaptic AMPAR levels increase during the first week of withdrawal and persist elevated for weeks [ 70 , 71 , 72 ]. It is established that cocaine challenge transiently decreases AMPAR surface expression, while AMPARs recover back to upregulated levels within a week, with a continuous increase during what is known to be the incubation of craving stage [ 73 ].
The abstinence period after withdrawal is of particular interest considering the classical progression of the disease, the chance of relapse and the opportunity for new therapeutic targets. A seemingly counterintuitive concept named ‘incubation of cocaine craving’ was introduced by Grimm et al. [ 74 ] who modelled cocaine-craving behaviour by using rats trained to press a lever to receive an injection of cocaine and were then forced in a withdrawal period where cocaine reward was no longer given. This concept of ‘incubation’ did not originate in drug addiction research but came from a four-stage model of the creative process proposed by Graham Wallas in 1926 [ 75 ]. Consistent with clinical observations in humans [ 76 , 77 , 78 ], they showed that relapse was progressively stronger over 2 months of cocaine withdrawal and suggest that a craving syndrome progresses or ‘incubates’ during the first 2 months of cocaine abstinence, and probably lasts for longer [ 74 ]. Subsequently, it was shown that this increase was due to the addition of new AMPARs lacking GluA2 and that these new receptors mediate the ‘incubation of cocaine craving’ [ 72 ]. Conrad et al. [ 72 ] showed that after extended withdrawal from cocaine, addition of synaptic AMPARs together with the increased conductance of GluA2-lacking AMPARs triggers higher sensitivity of NAc neurons to cocaine-related cues, leading to a strengthening of drug craving syndrome and relapse. In line with these results, it was suggested that as soon as abstinence is reached, the risk of relapse might be reduced if GluA2-lacking AMPARs were inactivated or removed from NAc synapses. It was thus proposed that GluA2-lacking AMPARs could be a new target for drug development for the treatment of cocaine addiction. While these calcium permeable AMPARs are also critical for the pathogenesis of numerous other neurological disorders (including epilepsy [ 79 ], fragile X syndrome [ 80 ], amyotrophic lateral sclerosis [ 81 ], Parkinson’s [ 82 ] and Alzheimer’s [ 83 ] diseases), developing drugs that specifically target them and not calcium-impermeable AMPARs, which are critical for normal CNS function, is challenging [ 84 ] (Fig. 3 ).
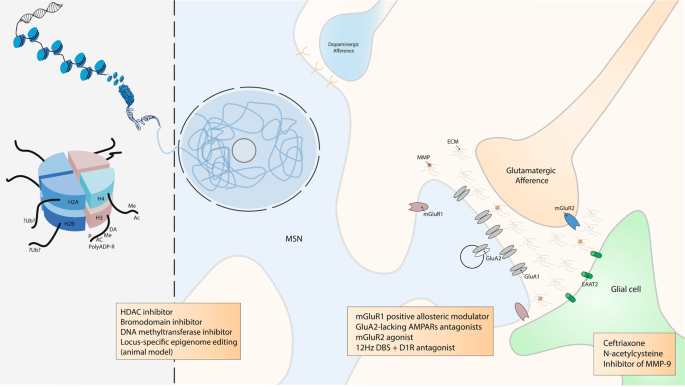
DNA is packaged inside nuclei with the help of histones. These are positively charged proteins that strongly adhere to negatively charged DNA and form complexes called nucleosomes. Each nucleosome is composed of DNA wound around histone octomers (H2A, H2B, H3 and H4). Nucleosomes fold up to form chromatin fibre, which forms loops compressed and folded to produce fibres, which are coiled into the chromatid of a chromosome. Only by loosening compacted chromatin, the DNA of a specific gene can be made accessible to transcription. Some of these drug-induced modifications at the chromatin level are extremely stable and sustain the drug of abuse-induced long-term behaviours. Among them, histone post-translational modifications (PTMs) are known to be causally involved in drug-induced behaviours [ 194 ]. PTMs include acetylation (Ac), methylation (Me), phosphorylation (P), ADP ribosylation (PolyADP-R) and dopaminylation (DA), among a growing list of newly discovered modifications [ 162 , 172 ]. For example, while ubiquitylation (Ub) of H2A is known to be a key interactor of H3 methylation [ 253 ], its supposed role in drug addiction is still unknown. At this epigenetic level, some drugs were demonstrated to have an influence on drug-induces behaviours such as histone deacetylase (HDAC), bromodomain and DNA methyltransferase inhibitors. Locus-specific epigenome editing is now encouraging as a new field of investigation as it might help to the discovery of new specific and causal drug of abuse targets. Overview of the tetrapartite glutamatergic synapse composed of a medium spiny neuron (MSN), a glutamatergic projection, a glial cell and the extracellular matrix (ECM). Here, we focused on synaptic potentiation after drug of abuse administration with the addition at the post-synaptic membrane of glutamate AMPA receptor 2 (GluA2) lacking AMPA receptors (AMPARs). This mechanism might be reduced by metabotropic glutamate receptor 1 (mGluR1) positive allosteric modulator or more directly by GluA2-lacking AMPARs antagonists. In the same way, it was also shown that presynaptic mGluR2 agonists can potentially abolish drug seeking and impair craving incubation. Optogenetically-inspired 12 Hz deep brain stimulation (DBS) in the nucleus accumbens can also be a promising novel therapeutic for addiction. Finally, ceftriaxone, N-acetylcysteine, and inhibitor of matrix metalloproteases 9 (MMP-9), mainly through their action on glial cell and the ECM, are very interesting molecules that may be added in the addiction therapeutic arsenal.
Inspired by previous work performed in the VTA showing that metabotropic glutamate receptor 1 (mGluR1) LTD induces removal of GluA2-lacking AMPARs from synapses [ 33 , 34 ], Loweth et al. [ 85 ] demonstrated that synaptic GluA2-lacking AMPAR decrease could be accomplished by in vivo evoked mGluR1 LTD in the NAc. More importantly, their group showed that after prolonged cocaine or methamphetamine withdrawal, systemic injection of a mGluR1 positive allosteric modulator attenuated the expression of incubated craving by reducing GluA2-lacking AMPARs in the NAc [ 85 , 86 ]. These results suggest a strategy in which abstinent methamphetamine or cocaine users could use a systemically active compound to protect themselves against cue-induced relapse.
These latter studies were conducted without differentiating between D1 receptor D1R MSNs and D2R MSNs. In 2014, Pascoli et al. [ 87 ] demonstrated that this increase in the strength of excitatory afferents was exclusively related to D1R MSNs. Interestingly, the type of drug-evoked plasticity involved is also dependent on the input. It has been shown that even in the same D1R MSN a synapse connecting the PFC to the NAc increases its strength by inserting GluA2-lacking AMPARs whereas a synapse connecting the ventral hippocampus to the NAc increases the AMPA/NMDA ratio by inserting GluA2-containing AMPARs [ 87 ].
Besides operant self-administration, all these long-term synaptic modifications also underlie behavioural changes associated with drugs of abuse, such as locomotor sensitisation [ 88 , 89 ]. Locomotor sensitisation is a behavioural protocol used to model drug-induced behaviour [ 90 , 91 ]. In rodents, repeated cocaine injection induces gradually increased locomotor activity; after 5 days of consecutive injections, the locomotor response reaches a ceiling level. This state lasts for months after cocaine withdrawal [ 91 ]. As an experimental model, locomotor sensitisation is linked with increased tendency to self-administer psychostimulants [ 92 , 93 ] and with reinstatement of previously extinguished self-administration [ 94 , 95 ]. Whereas the existence of psychomotor sensitisation in humans is discussed [ 96 , 97 ], it is a key aspect of vulnerability to drug addiction and relapse, specifically drug craving or compulsive drug-seeking behaviour [ 91 , 98 , 99 ]. Still, locomotor sensitisation can be dissociated from the rewarding effect of a drug of abuse and conditioned place preference or self-administration are more appropriate experimental paradigms to test this aspect [ 100 , 101 , 102 ]. Even if drug-induced locomotor sensitisation is unclearly present in humans, as an animal model it offers a clear readout to understand the mechanisms by which drugs of abuse induce long-term brain modifications [ 91 ].
Furthermore, it has been elegantly demonstrated that optogenetic stimulation of the excitatory projections to the NAc is able to reverse cocaine and alcohol-evoked plasticity [ 87 , 88 , 89 ]. Briefly, applying a NMDAR or mGluR1-dependent LTD on cortico-accumbal glutamatergic synapses, before a drug of abuse administration, diminishes its effect. In another study, Luscher’s team took advantage of the knowledge, obtained from optogenetic in vivo experiment in rodents, to implement a novel deep brain stimulation (DBS) protocol that abolishes behavioural sensitization to cocaine (and thus that would be efficient during the relapse phase) [ 103 ]. Basically, the idea is to manipulate synaptic plasticity in the NAc to reverse pathological synaptic transmission and its associated behaviours following exposure to drugs of abuse. In this study, as a therapeutic use of optogenetic tools in humans is for now inapplicable [ 104 ], the authors reversed cocaine-evoked plasticity and thus drug-induced behaviours by using DBS instead of optogenetic. Indeed, DBS is routinely used in clinic and a new DBS protocol can easily be translationally implemented to the human therapeutics [ 105 , 106 ]. They refined the classical high-frequency DBS protocol (that has no sustained effect on cocaine sensitization, probably because it does not affect synaptic plasticity) by applying a low frequency stimulation (12 Hz to equal the one used in the optogenetic endocannabinoid- dependent LTD protocol) in the NAc together with the administration of a D1R antagonist necessary to unmask the mGluR-dependent LTD in D1R MSNs as demonstrated previously [ 107 ] (Fig. 3 , see section on clinical treatment for broader discussion on DBS).
Kalivas’ group showed in 2009 [ 108 ] that after extended withdrawal from chronic cocaine self-administration, cocaine-induced metaplasticity at the excitatory synapses in the NAc that impairs the ability of PFC stimulation to produce LTP or LTD in NAc MSNs. They also showed that N-acetylcysteine reverses cocaine-induced metaplasticity, allowing the induction of both LTP and LTD and that N-acetylcysteine decreases cocaine-relapse in a rodent model. We are currently awaiting the results of a randomised and control study that is testing newly detoxified (and therefore abstinent) hospitalised patients who received a 3–4 week course of treatment, in order determine if N-acetylcysteine can be a useful medication candidate to avoid relapse in patients with cocaine dependence (NCT03423667).
GABAergic D1R and D2R MSNs, equally compose and are mosaically intermingled throughout the striatum [ 109 ]. As explained above, D1R and D2R MSNs send axonal projections outside the striatum, forming the two main output pathways, respectively the direct and indirect pathways [ 16 , 17 ]. In a certainly oversimplified model, the activation of the D1R MSNs result in facilitation of locomotion, reward, and reinforcement while the activation of D2R MSNs result in opposing effects [ 110 , 111 , 112 , 113 ]. In addition to the long-range projections, these neurons form short-range synaptic connections with one another within the striatum, and because they consist of inhibitory collaterals, a mechanism known as lateral inhibition [ 114 , 115 , 116 , 117 ]. Interestingly, these connections are not symmetrical, with D2R MSNs forming more synaptic connections on D1R MSNs [ 115 , 117 ]. Through this previously understudied collateral transmission, Dobbs et al. [ 115 ] presented a novel mechanism by which cocaine exerts its stimulant effect: cocaine, by blocking DAT receptors enhance levels of dopamine and subsequently activating D2Rs, causes a suppression of lateral inhibition and thus disinhibition of D1R MSNs in the NAc which in turn promotes locomotion [ 115 ]. Furthermore, Alvarez’ group suggested that constitutive low D2R levels, through imbalanced lateral inhibition, might pre-sensitised D1R MSNs, facilitate behavioural plasticity to repeated cocaine and promotes an addiction vulnerable phenotype [ 116 ].
The characterisation of the role of glia and the extracellular matrix (ECM) in drug-induced synaptic plasticity is an exciting emerging field of drug addiction research as it comes with promising new therapeutic possibilitiess [ 118 , 119 , 120 ]. Mulholland et al. [ 118 ] summarised and emphasised the role of the ECM and of astroglial cells in the regulation of synaptic plasticity. Of great interest, restoring downregulated glutamate transporter 1 (EAAT2) with ceftriaxone reduces drug seeking in animal models [ 121 , 122 ]. Matrix metalloproteases (MMP) are important regulators of the ECM and contribute to synaptic plasticity [ 123 ]. Inhibiting their activity result in suppression of the reinstatement of cocaine conditioned place preference [ 124 ] and selectively inhibiting MMP-9 prevents cue- and cocaine-induced reinstatement of cocaine self-administration [ 119 ]; these results open additional therapeutic possibilities with the use of inhibitors of MMP-9 as an innovative targeted approach [ 119 , 124 , 125 ] (Fig. 3 ). Still, at our knowledge, there are no randomised controlled study currently investigating these ECM-related drugs.
Drugs of abuse-induced modifications in glutamatergic nuclei targeting the NAc, or the VTA and essential part of the reward circuit, are less studied than cortico-striatal synapses despite the fact that they play a crucial role in the development of drug addiction. Indeed, in the OFC and PFC, chronic alcohol exposure significantly increases LTP in pyramidal neurons [ 126 , 127 ]. Kazanetz et al. [ 128 ] showed that repeated cocaine injections impair endocannabinoid-LTD and mGluR2/3-LTD in the PFC. They postulated that this might mechanistically participate in the induction of a postsynaptic, observed LTP-like phenomenon with an enhanced AMPA/NMDA ratio. It was also demonstrated that neurons of the infralimbic cortex present a decrease in mGluR2 [ 129 ]. In addition, alcohol-dependent rats exhibit an escalation of ethanol seeking, which was abolished by restoring mGluR2 expression in the infralimbic cortex via viral-mediated gene transfer [ 129 ]. Notably, mGluR2 agonist was shown to impair the incubation of cocaine craving [ 130 ] and to attenuate reinstatement of cocaine-seeking [ 131 , 132 ](Fig. 3 ). Recently, Caprioli et al. [ 133 ] extensively reviewed preclinical studies on allosteric modulators of mGluRs on animal models of drug addiction and their potential translational implications. The results reviewed [ 133 ] indicate an remarkable effect of allosteric modulators of presynaptic mGluR2 and possibly mGluR7, supporting the idea that these compounds should be tested as potential medications for addiction treatments.
Besides the PFC, other brain regions appear to be key areas in drug addiction as the paraventricular thalamus (PVT) - a central hub for cortical, sensory and limbic information [ 134 , 135 , 136 , 137 , 138 , 139 , 140 ]. In 2016, Zhu et al. [ 141 ] showed that chronic morphine administration potentiates excitatory synapses between the PVT and D2R MSNs via insertion of GluA2-lacking AMPARs. Remarkably, in vivo optogenetic depotentiation at these synapses abolishes morphine withdrawal symptoms. In a recent paper, projections from the PVT to the NAc were shown to be critical for augmentation of heroin seeking in food-restricted rats [ 142 ] (Fig. 1 ). Actually, Otis et al. [ 143 ] demonstrated that the PVT is an integrative hub for reward seeking behaviour and that PVT-NAc neurons integrate different inputs from the PFC and the lateral hypothalamus to precisely guide reward seeking behaviour. In a recent review, De Groote et al. [ 140 ] focused on the new advances in the understanding of the roles of the PVT-NAc connections in motivated behaviours, highlighting their implications in drug addiction.
Drug addiction-related genes and transcriptomic regulation
Modifications in gene expression contribute to the long-lasting effect sustaining drug addiction; thanks to gene-expression arrays, RNA-sequencing and candidate gene approaches, the specific genes and their regulatory transcriptomic mechanisms involved in drug addiction development and maintenance are now better understood.

Drug addiction-related genes
For example, the use of conditional gene knockout in mice emphasises the importance of monoamine membrane transporters (dopamine transporter, and serotonin transporter) [ 144 , 145 ] and of mGluRs [ 146 , 147 ]. As new animal models of drug addiction, these approaches are also useful to better characterise fine-tuning of important pathways involved in addiction. For example, a scaffold protein known as Maged1 has been shown to be involved in cocaine reward and reinforcement [ 148 ]. We demonstrated that Maged1 inactivation impairs drug-evoked dopamine release and glutamatergic synaptic plasticity in the NAc. Inactivation of Maged1 in mice was able to abolish behavioural sensitization to cocaine as well as cocaine conditioned place preference and operant self-administration behaviours [ 148 ]. This sole genetic alteration, causally linked to a strong alteration of drug-induced behaviours, impairs (at least) two core neuronal mechanisms leading to addictive behaviours: (1) cocaine-evoked release of dopamine in the NAc and (2) NAc plasticity, with a reduced AMPA/NMDA ratio and a resistance to LTD. Actually, it seems that, after Maged1 inactivation, the excitatory synapses in the NAc shift to a depressed state. Our hypothesis is that, in line with the previously discussed in vivo optogenetic induced LTD, this impairment could be a key factor for the significant decrease in sensitization to psychostimulants [ 87 , 103 , 148 ]. Actually, it seems that placing neurons in a state of ‘presensitization’ is able to prevent drug-induced sensitization itself [ 148 , 149 ]. Our group is now trying to understand what are the cellular and molecular pathways directly altered by Maged1 inactivation and responsible for this strong anti-addictive drug phenotype. Remarkably, the promoter of Maged1 was found in a list of 213 promoters that co-precipitate with acetylated histones and with the activated form of cAMP response element binding protein (CREB) after chronic drug taking [ 150 ]. In line with this result, preliminary and unpublished results from our laboratory point out a specific epigenetic mechanism, in parallel with an alteration of synaptic plasticity in excitatory projection to the NAc, that would link Maged1 to its major effect on drug-induced behaviours. This selected gene approach is of great interest in refining our knowledge of pathways hijacked by addictive drugs. Using cell sorting of D1R MSNs and D2R MSNs as described previously [ 151 ], our group also identified the G-protein-regulated inducer of neurite outgrowth 3 (GPRIN3) in both MSN populations but strikingly more expressed in D2R MSNs [ 149 ]. The GPRIN family (GPRIN1, GPRIN2 and GPRIN3) are Gαi/o-regulated proteins suggested to intermediate the communication between GPCRs and the sequential intracellular target [ 152 ]. Indeed, GPRIN1 and GPRIN2 have been described as alternative (to adenylyl cyclase) mediators of GPCRs signalling but GPRIN3 had a much less defined role [ 152 , 153 ]. To understand the role of GPRIN3 in the pathophysiology of the D2R-indirect pathway, we induced a D2R-MSNs-specific knockdown (KD) of GPRIN3 using small hairpin RNA and lentiviruses [ 151 , 154 ]. We first observed a significant increase in distal branching, the points of convergence between glutamate and dopamine synapses in MSNs [ 155 ] and also key targets of cocaine, which itself promotes increase in distal branching in the NAc of mice [ 156 , 157 , 158 , 159 ]. Thus, we tested the cocaine acute effect and locomotor sensitization and observed a decrease in cocaine-induced hyperlocomotion after inactivation of GPRIN3 using a CRISP/Cas9 approach. The significant increase in distal branching in GPRIN3 D2R-MSNs KD corroborates our hypothesis that the lack of GPRIN3 induces a ‘presensitization process’, able to change the targets of cocaine and therefore altering its effects [ 149 ]. Finally, we provide the first evidence that GPRIN3 partners with D2R in the striatum and modulates cocaine-induced behaviours [ 149 ].
Transcriptomic and epigenetic regulations
Epigenetics is a broad field and has multiple definitions that comprise several biochemical mechanisms (including DNA methylation and histone modifications) sustaining modifications in gene expression throughout the lifecycle of an organism without mutations of the DNA itself [ 160 , 161 , 162 ]. Epigenetics can be considered as the process through which environment (and normal development) interacts with an individual’s genome to determine all phenotypic traits, in health and disease. Stable modifications in gene expression are also said to be ‘epigenetic’, because they are heritable in the short term (through mitosis) [ 160 ] and in some cases trans-generationally, thus, providing a potential mechanism for environmental influences to be passed from parents to offspring [ 163 , 164 , 165 ]. Handel and Romagopalan [ 163 ] mentioned that “epigenetics allows the peaceful co-existence of Darwinian and Lamarckian evolution”. Such trans-generational epigenetic inheritance of drug addiction vulnerability remains debatable [ 161 ], but has been increasingly studied for the last 20 years [ 166 , 167 ]. Some epigenetic changes are very stable, an thus mediate both drug addiction susceptibility and drug-induced brain alterations that underlie the development of drug addiction [ 161 ].
As the NAc is seen as the central hub of drug addiction, with the notion that chronic drug use induces long-lasting structural, electrophysiological and transcriptional changes in the NAc, researchers are mostly targeting epigenetic modifications in NAc cells. Still, considering initial reports of cocaine-induced epigenetic modifications [ 168 , 169 ], it might be relevant to study further epigenetic changes in other regions such as glutamatergic inputs to the NAc, and further in the VTA, as they are implicated in the physiopathology of drug addiction [ 170 , 171 ] as mentioned above.
To date, the three main epigenetic mechanisms consist of (1) DNA methylation, (2) action of the non-coding RNAs and (3) histone post-translational modifications (PTMs). As an illustrative example, we will focus here only on histone PTMs. PTMs of histone residues on their N-terminal tails, that protrude from the nucleosome core, control chromatin condensation and the switch between euchromatin and heterochromatin and thus DNA-accessibility and gene expressions. PTMs include acetylation, methylation, phosphorylation, ADP ribosylation, ubiquitylation and sumoylation, among a growing list of newly discovered modifications [ 162 , 172 ].
Among these PTMs, the most studied is the acetylation of H3 and H4, that is increased in the NAc after chronic exposure to drugs of abuse [ 150 , 173 , 174 ]. This increase in global acetylation levels is the result of drug-induced alterations in the balance of histone acetyltransferase and histone deacetylase (HDAC) function and is associated with gene activation. CREB-binding protein, a histone acetyltransferase critical to memory processes [ 175 ], is required for cocaine-induced increases in histone acetylation in the NAc [ 176 ].
Fifteen years ago, Tsankova et al. [ 177 ] showed that imipramine, a monoamine reuptake inhibitor used for decades to treat depression, was effective through histone remodelling in depression and highlight the therapeutic potential for chromatin regulation with histone methylation and deacetylation inhibitors in depression. Nevertheless, like with synaptic plasticity (see above), discovering a drug that would interfere with epigenetic mechanisms and thus decrease drugs of abuse effect faces temporal aspects issues [ 173 , 176 , 178 , 179 , 180 , 181 , 182 ]. Indeed, timing has a strong impact considering conflicting results obtained after experimental manipulations of histone acetylation. An acute administration of HDAC inhibitors systemically or directly into the NAc, promotes behavioural responses to the drugs. However, prolonged administration decreases cocaine behavioural effects. In 2013, adding a new layer of complexity, Kennedy et al. provided comprehension to this time-dependent regulation [ 183 ]. Remarkably, they showed that prolonged intraNAc administration (but not acute administration) of a HDAC inhibitor attenuated cocaine behavioural effects by inducing a form of repressive histone methylation. This study showed, for the first time, cross-talk among different types of histone modifications [ 183 ]. Besides cross-talk between different epigenetic modifications, multiple modifications work in parallel and there is often a decoupling between an observed modification at a specific locus and its final transcription [ 161 ]. Decoding these chromatin marks will be a future challenging field. Like with HDAC inhibitors, there are promising findings based on the use of DNA methyltransferases inhibitor [ 184 , 185 ] (Fig. 3 ). Though, the main issue with these new potential treatments for drug addiction is their lack of specificity. One of the key challenge for the pharmaceutical industry will be to generate small molecules with more specific targets [ 6 ].
While histone acetylation and methylation are increasingly studied, an important field of future investigation will be to understand the other drug-induced histone PTMs. It already seems that chronic cocaine alters levels of histone phosphorylation [ 174 , 186 , 187 ], and poly-ADP ribosylation [ 188 ]. Recently, an unexpected role for the intracellular dopamine in VTA has been revealed, showing that DA interacts with chromatin to initiate a new form of epigenetic regulation called dopaminylation [ 189 ] (see Table 1 for a summary of cocaine-related epigenetic modifications).
Further studies showed that histone PTMs that occur in the NAc after chronic drug administration are locus specific [ 150 , 190 , 191 ]. Even though, drugs of abuse alter global levels of multiple histone PTMs, such as increased histone acetylation or decreased methylation in the NAc, genome-wide studies have confirmed that a greater number of genomic sites show increased acetylation [ 150 ] or decreased methylation [ 190 , 191 ]. Conversely, hundreds of genes show opposite or no changes in these same PTMs after drug exposure. What defines whether, and in which direction, a specific gene is modified in the context of a global histone PTM is an intriguing and unsolved question [ 161 ]. These genome-wide studies (ChIp on chip or ChIpSeq) are nowadays fundamental to understand where PTMs and other epigenetic modifications are deposited. This will be fundamental to guide new therapeutics.
Actually, with new tools such as zinc finger proteins (ZFPs) DNA-binding domains and, more recently, RNA-guided CRISPR/dCas9 (drastically easier to design) [ 192 , 193 ], it is now possible to control epigenetic modifications at a single gene in a specific type of cell in a specific brain region [ 162 ]. Heller et al. demonstrated that gene-targeted epigenetic editing (targeted to the Fosb [ 194 ] and Cdk5 [ 195 ] locus with ZFP technology) can alter drug-related behaviours [ 194 , 195 ]. This represents crucial evidence that gene-specific changes to the epigenome are not simply correlated, but rather causal, in regulating transcriptional responses to drugs of abuse administrations. These new results of “causal epigenomics” are very encouraging as they open the way to precise translational therapeutic approaches for drug addiction and other CNS diseases.
Linking epigenetics and synaptic plasticity
Today, most studies investigate synaptic plasticity and epigenetic as two distinct fields and it is not clear how these research topics are connected to each other. Understanding how epigenetics is connected to synaptic plasticity is an emerging research issue [ 6 ].
Of course, bridging epigenetic mechanisms with synaptic plasticity is not limited to drug addiction field. For example, in 2011, Monsey et al. [ 196 ] elegantly demonstrated that DNA methylation and histone H3 acetylation regulate auditory fear conditioning and its related synaptic plasticity in the amygdala. In 2014, Massart et al. [ 197 ] suggested that sleep deprivation induces epigenetic modification (alteration in DNA methylation and hydroxymethylation) that triggers synaptic plasticity modifications by changing expression of plasticity related genes.
Regarding drug addiction, some epigenetic marks seem fundamental and upstream as illustrated by HDAC inhibitors effect on drug-induced synaptic and behavioural modifications [ 178 , 198 , 199 , 200 ]. Additionally, Maze et al. [ 201 ] demonstrated morphological plasticity induced by cocaine through the histone methyltransferase G9a. Again advocating for causal epigenetic, Authement et al. [ 66 ] demonstrated that HDAC inhibition locally in the VTA is sufficient to reverse epigenetic modifications and synaptic plasticity changes after morphine administration.
Two transcription factors implicated in addiction exemplify this bridging attempt: CREB and ∆FosB (a truncated form of the FosB gene) are both activated by several drugs of abuse [ 202 ]. CREB activation occurs in both subtypes of NAc MSNs (D1R and D2R), while ∆FosB activation is limited to D1R MSNs in response to all drugs of abuse except for opioids, which remarkably induce the protein in both MSNs [ 203 ]. Expression of active CREB in NAc MSNs increases their excitability [ 204 ] and underlies drug-induced long-term synaptic plasticity and associated changes in dendritic spine plasticity [ 205 ]. ∆FosB is also linked to synaptic plasticity but evokes contrasting effects on the two MSN subtypes, with increased AMPA receptor function induced in D1R MSNs and decreased AMPA receptor function induced in D2R MSNs [ 206 ]. Renthal et al. [ 150 ] unravelled CREB and ∆FosB target genes and observed that these genes are mainly involved in neuronal excitability and synaptic function. Moreover, as already briefly discussed above, CREB and ∆FosB action have also been related to multiple epigenetic regulations, including histone acetylation and methylation [ 150 ]. Besides, a novel mechanism for bridging the gap between epigenetic control of transcription and synapse plasticity might be seen in microRNAs [ 207 ]. The most studied miRNA in the context of synaptic plasticity is miR-132 and is known to be CREB-dependent [ 208 ]. In the striatum, miR-212 targets the epigenetic regulator methyl CpG binding protein 2 (MeCP2). MeCP2 acts as a transcriptional repressor through recruitment of histone deacetylases to methylated DNA segments [ 209 , 210 ].
Clinical treatments for drug addiction
Besides psychosocial interventions [ 211 ] such as cognitive behavioural therapy, the most widely used treatment for drug addiction involves agonist-like medication, a solution inadequately called replacement or substitution therapy [ 212 ]. This type of treatment has been successfully implemented in the daily practice for opioid use disorder (e.g.: methadone, buprenorphine) [ 213 ] and tobacco use disorder (e.g.: nicotine patch or gum, varenicline) [ 214 ]. Currently, this agonist-like treatment is also promising for psychostimulant use disorder [ 215 ]. Still, considering the addictive drug-like effect, the risk of abuse, misuse and diversion, replacement therapy should be prescribed with caution [ 215 , 216 ].
Recently, a randomised and control study on a cocaine vaccine failed to show an effectiveness but instead raised an important issue: immunised subjects may have increased their cocaine use to overcome the competitive anti-cocaine antibody inhibition [ 217 ]. Even though significant improvements have been developed for immunopharmacotherapies for psychostimulant addiction over the last decade, very few candidates have been evaluated so far in clinical trials [ 218 ]. These considerations are some of the reasons why other treatments for drug addiction should emerge with the help of neurobiological research [ 219 ].
Following successful subthalamic nucleus DBS for Parkinson’s disease [ 220 , 221 , 222 ], DBS was investigated for diverse psychiatric diseases including depression [ 223 ], obsessive-compulsive disorder [ 224 ] and Tourette syndrome [ 225 ]. Today, indications for DBS are enlarging, with several positive case reports and small cases series that studied NAc DBS for drug addiction. The first studies showing potential positive effects on drug addiction were reports on application of NAc DBS primarily intended for other medication-refractory neuropsychiatric disorder where a comorbid drug addiction was unexpectedly resolved [ 226 , 227 ]. For DBS treatment in drug addiction, it seems that clinical empirical results led to further bench investigations and refinement [ 88 , 103 , 228 , 229 , 230 , 231 ], or at least, clinical and animal studies evolved in parallel with poor connectivity between the two.
Afterwards, many case reports and small cases series studied NAc DBS being used primarily for drug addiction, all showing encouraging decreases in drug use [ 232 , 233 , 234 , 235 , 236 , 237 ]. However, these studies are limited by their descriptive nature, inconstant follow-up, multiple publication bias, small patient numbers and lack of blinded stimulation and standardised outcome measures. At this stage, additional preclinical and clinical research are needed to clarify the role of DBS in the treatment of drug addiction [ 237 ]. Currently, randomised and control clinical studies are conducted (NCT01245075).
In a recent review, Sanna et al. [ 238 ] highlighted how repetitive transcranial magnetic stimulation (rTMS) confirms the hypodopaminergic hypothesis of drug addiction. While enhancing dopaminergic function through direct or indirect pharmacological approaches does not significantly alleviate symptoms, in numerous studies, and has not yielded a single FDA-approved medication [ 239 ], rTMS might indirectly modulate the dopaminergic system. Many rTMS studies stimulate the dorsolateral PFC [ 240 , 241 ] that projects to the VTA and thus induces an increase in dopamine release in the synaptic cleft in the NAc [ 55 , 242 , 243 ]. Nevertheless, considering the heterogeneity of methods used in rTMS studies during the last 10 years [ 238 ], protocols and guidelines, were recently suggested by an international network of experts in neuromodulation and addiction to improve homogeneity of studies [ 244 ]. From this report, it is clear that multiple technical details for optimal stimulation need further investigations that might be achieved through preclinical studies. For example, low frequency (but not high-frequency) rTMS before methamphetamine exposure in rats blocked drug-induced conditioned place preference [ 245 ]. Being non-invasive, with insignificant side effects, rTMS could be seen as a great opportunity for drug addiction treatment. We are currently waiting for the results of a randomised and control study that aims at determine if, in heavy alcohol users, a single session of TMS can lower a patient’s craving and brain response to alcohol cues (NCT02939313).
Interesting views of clinical treatments for drug addiction are discussed in some other reviews [ 212 , 215 , 216 , 219 ]. The clinical impact of new treatments also depends on their translation into clinical practice which is mainly promoted by the pharmaceutical industry [ 219 ]. Indeed, even when an effective treatment is identified through basic research, it is commonly challenging to translate it to clinical practice, as illustrated by naltrexone as a treatment of alcoholism [ 219 ]. Another example of problematic translation to clinic is illustrated by modafinil, a treatment that has been reported to attenuate cocaine euphoria but for which larger clinical randomised and controlled studies showed controversial results [ 246 , 247 ].
Future directions
Drug addiction is a brain disease strongly influenced by environment and psychosocial aspects. The psychosocial conditions in which it has developed are extremely important. Exposure to conditioned cues can be a central issue in causing drug cravings and relapses, even after successful treatment, and thus they have to be minimised [ 2 , 74 , 77 ]. The pathophysiological aspects are particularly unsteady. For instance, as discussed in this review and in other ones [ 73 , 248 ], synaptic plasticity is dynamically altered after psychostimulant administration, so that a treatment could have opposite effects depending on timing aspects of the administration protocol. In addition, a prolonged treatment may involve compensatory mechanisms, giving unexpected results (e.g.: when HDAC inhibitors and psychostimulants are both administered acutely, they have synergistic effects through hyperacetylation and thus transcriptional activation of psychostimulant-regulated target genes. Conversely, when a drug of abuse is given in the context of chronic HDAC inhibitor, compensatory mechanisms may promote acetylated histone to the promoters of genes responsible for inducing histone methylation and thus chromatin condensation and gene repression, all of which, in turn, gave opposite effect [ 183 ]). Thus, the evolution through the different stages of the disease has to be taken into account [ 249 ] and treatment must follow them. These two aspects have to be incorporated in a holistic treatment strategy. Besides, studying combination of different cutting-edge approaches, with animal models of addiction, such as targeted rTMS or DBS with more systemic epigenetic modulation might show a better restoration of altered synaptic transmission and decrease the probability of relapse in drug addiction. Basically, drug addiction is a disease that seems to be difficult to treat preventively but it is more conceivable to help patients that would be in an abstinence stage not to experience relapse of their disease. As addiction is chronic and relapsing, a good treatment outcome is a significant reduction of drug administration and long periods of withdrawal, with only sporadic relapses [ 2 ].
It is clear that the main issues for optimal therapeutic management of this specific psychiatric disease belong to its dynamic complexity, diverse temporal evolution and undeniably psychosocial aspects. In this review, we focused mostly on the effects of drugs of abuse on synaptic plasticity and epigenetic modifications. Nowadays, these two subfields are mostly studied separately and the understanding of how these two main addictive drug-induced brain modifications interact might be fundamental for addiction research [ 6 ]. Indeed, argument for clinical trials for new treatments emerge from fundamental behavioural studies that should be implemented in a global approach to the addicted patient.
Conclusions
Here, we highlight, from a vast fundamental literature (mainly based on rodent models), promising therapeutics that would potentially treat drug addiction. Based on effect, on synaptic plasticity and epigenetic mechanisms, treatments such as GluA2-lacking AMPAR antagonists [ 72 , 84 ], mGluR1 positive allosteric modulator [ 85 ], NAc 12Hz-DBS [ 103 ] (in line with other promising neuromodulation therapeutics such as rTMS or transcranial direct current stimulation [ 250 ]), N-acetylcysteine [ 108 ], HDAC inhibitors [ 183 ] or even (in very early stages of investigation) CRISPR/dCas9 epigenetic editing [ 194 , 195 ] could be potential candidates for human randomised clinical trials (Fig. 3 ).
Finally, it is fundamental to consider the specific clinical aspects of the disease that would help to develop a personalised-treatment strategy. Indeed, after going from the bench to the bedside it will also be essential to assess the reversed route.
Roth GA, Abate D, Abate KH, Abay SM, Abbafati C, Abbasi N, et al. Global, regional, and national age-sex-specific mortality for 282 causes of death in 195 countries and territories, 1980–2017: a systematic analysis for the Global Burden of Disease Study 2017. Lancet. 2018;392:1736–88.
Article Google Scholar
Leshner AI. Addiction is a brain disease, and it matters. Science. 1997;278:45–47.
Article CAS PubMed Google Scholar
Nielsen DA, Nielsen EM, Dasari T, Spellicy CJ. Pharmacogenetics of addiction therapy. Methods Mol Biol. 2014;1175:589–624.
Article PubMed CAS Google Scholar
Everitt BJ & Robbins TW. Drug addiction: updating actions to habits to compulsions ten years on. Annu Rev Psychol. 2015;67:23–50.
Article PubMed Google Scholar
Belin D, Everitt BJ. Cocaine seeking habits depend upon dopamine-dependent serial connectivity linking the ventral with the dorsal striatum. Neuron. 2008;57:432–41.
Nestler EJ, Lüscher C. The molecular basis of drug addiction: linking epigenetic to synaptic and circuit mechanisms. Neuron. 2019;102:48–59.
Article CAS PubMed PubMed Central Google Scholar
Nestler EJ. Cellular basis of memory for addiction. Dialogues Clin Neurosci. 2013;15:431–43.
Article PubMed PubMed Central Google Scholar
Nestler EJ. Molecular basis of long-term plasticity underlying addiction. Nat Rev Neurosci. 2001;2:119–28.
Anthony JC, Warner LA, Kessler RC. Comparative epidemiology of dependence on tobacco, alcohol, controlled substances, and inhalants: basic findings from the National Comorbidity Survey. Exp Clin Psychopharmacol. 1994;2:244–68.
Egervari G, Ciccocioppo R, Jentsch JD, Hurd YL. Shaping vulnerability to addiction – the contribution of behavior, neural circuits and molecular mechanisms. Neurosci Biobehav Rev. 2018;85:117–25.
Li MD, Burmeister M. New insights into the genetics of addiction. Nat. Rev. Genet. 2009;10:225–31.
Kreek MJ, Nielsen DA, Butelman ER, LaForge KS. Genetic influences on impulsivity, risk taking, stress responsivity and vulnerability to drug abuse and addiction. Nat Neurosci. 2005;8:1450–7.
Shohat S, Amelan A, Shifman S. Convergence and divergence in the genetics of psychiatric disorders from pathways to developmental stages. Biol Psychiatry. 2021;89:32–40.
Kandel, DB. Stages and Pathways of Drug Involvement: Examining the Gateway Hypothesis (Cambridge University Press, 2002).
Gerfen CR, Engber TM, Mahan LC, Susel Z, Chase TN, Monsma FJ Jr, et al. D1 and D2 dopamine receptor-regulated gene expression of striatonigral and striatopallidal neurons. Science. 1990;250:1429–32.
Schiffmann SN, Fisone G, Moresco R, Cunha RA, Ferré S. Adenosine A2A receptors and basal ganglia physiology. Prog. Neurobiol. 2007;83:277–92.
Gerfen CR, Surmeier DJ. Modulation of striatal projection systems by dopamine. Annu Rev Neurosci. 2011;34:441–66.
Di Chiara G, Imperato A. Drugs abused by humans preferentially increase synaptic dopamine concentrations in the mesolimbic system of freely moving rats. Proc Natl Acad Sci USA. 1988;85:5274–8.
Tanda G, Pontieri FE, Chiara GD. Cannabinoid and heroin activation of mesolimbic dopamine transmission by a common µ1 opioid receptor mechanism. Science. 1997;276:2048–50.
Pontieri FE, Tanda G, Orzi F, Chiara GD. Effects of nicotine on the nucleus accumbens and similarity to those of addictive drugs. Nature. 1996;382:255–7.
Nestler EJ. Is there a common molecular pathway for addiction? Nat Neurosci. 2005;8:1445–9.
Nestler EJ. The neurobiology of cocaine addiction. Sci Pract Perspect. 2005;3:4–10.
Kalivas PW, Volkow N, Seamans J. Unmanageable motivation in addiction: a pathology in prefrontal-accumbens glutamate transmission. Neuron. 2005;45:647–50.
Volkow ND, Fowler JS, Wang G-J, Swanson JM. Dopamine in drug abuse and addiction: results from imaging studies and treatment implications. Mol Psychiatry. 2004;9:557–69.
Chen SA, O'Dell LE, Hoefer ME, Greenwell TN, Zorrilla EP, Koob GF. Unlimited access to heroin self-administration: independent motivational markers of opiate dependence. Neuropsychopharmacology. 2006;31:2692–707.
Laviolette SR, Alexson TO, van der Kooy D. Lesions of the tegmental pedunculopontine nucleus block the rewarding effects and reveal the aversive effects of nicotine in the ventral tegmental area. J. Neurosci. 2002;22:8653–60.
Koob GF, Volkow ND. Neurocircuitry of addiction. Neuropsychopharmacology. 2010;35:217–38.
Pierce RC, Kumaresan V. The mesolimbic dopamine system: The final common pathway for the reinforcing effect of drugs of abuse? Neurosci Biobehav Rev. 2006;30:215–38.
Belin-Rauscent A, Fouyssac M, Bonci A, Belin D. How preclinical models evolved to resemble the diagnostic criteria of drug addiction. Biol. Psychiatry. 2016;79:39–46.
Piazza PV, Deroche-Gamonet V. A multistep general theory of transition to addiction. Psychopharmacology. 2013;229:387–413.
Pascoli V, Hiver A, Van Zessen R, Loureiro M, Achargui R, Harada M, et al. Stochastic synaptic plasticity underlying compulsion in a model of addiction. Nature. 2018;564:366–71.
Kauer JA, Malenka RC. Synaptic plasticity and addiction. Nat Rev Neurosci. 2007;8:844–58.
Mameli M, Balland B, Luján R, Lüscher C. Rapid synthesis and synaptic insertion of GluR2 for mGluR-LTD in the ventral tegmental area. Science. 2007;317:530–3.
Bellone C, Lüscher C. Cocaine triggered AMPA receptor redistribution is reversed in vivo by mGluR-dependent long-term depression. Nat Neurosci. 2006;9:636–41.
Pignatelli M, Bonci A. Role of dopamine neurons in reward and aversion: a synaptic plasticity perspective. Neuron. 2015;86:1145–57.
Stuber GD, Klanker M, de Ridder B, Bowers MS, Joosten RN, Feenstra MG, et al. Reward-predictive cues enhance excitatory synaptic strength onto midbrain dopamine neurons. Science. 2008;321:1690–2.
Bolam JP, Smith Y. The GABA and substance P input to dopaminergic neurones in the substantia nigra of the rat. Brain Res. 1990;529:57–78.
Henny P, Brown MT, Northrop A, Faunes M, Ungless MA, Magill PJ, et al. Structural correlates of heterogeneous in vivo activity of midbrain dopaminergic neurons. Nat Neurosci. 2012;15:613–9.
Bayer VE, Pickel VM. GABA-labeled terminals form proportionally more synapses with dopaminergic neurons containing low densities of tyrosine hydroxylase-immunoreactivity in rat ventral tegmental area. Brain Res. 1991;559:44–55.
Johnson SW, North RA. Two types of neurone in the rat ventral tegmental area and their synaptic inputs. J Physiol. 1992;450:455–68.
Edwards NJ, Tejeda HA, Pignatelli M, Zhang S, McDevitt RA, Wu J, et al. Circuit specificity in the inhibitory architecture of the VTA regulates cocaine-induced behavior. Nat Neurosci. 2017;20:438–48.
Menegas W, Bergan JF, Ogawa SK, Isogai Y, Umadevi Venkataraju K, Osten P, et al. Dopamine neurons projecting to the posterior striatum form an anatomically distinct subclass. eLife. 2015;4:e10032.
Ling W, Shoptaw S, Majewska D. Baclofen as a cocaine anti-craving medication: a preliminary clinical study. Neuropsychopharmacology. 1998;18:403–4.
CAS PubMed Google Scholar
Young KA, Franklin TR, Roberts DC, Jagannathan K, Suh JJ, Wetherill RR, et al. Nipping cue reactivity in the bud: baclofen prevents limbic activation elicited by subliminal drug cues. J Neurosci. 2014;34:5038–43.
Article PubMed PubMed Central CAS Google Scholar
Vocci FJ, Elkashef A. Pharmacotherapy and other treatments for cocaine abuse and dependence. Curr Opin Psychiatry. 2005;18:265–70.
Roberts DC, Andrews MM, Vickers GJ. Baclofen attenuates the reinforcing effects of cocaine in rats. Neuropsychopharmacology. 1996;15:417–23.
Slattery DA, Markou A, Froestl W, Cryan JF. The GABA B receptor-positive modulator GS39783 and the GABA B receptor agonist baclofen attenuate the reward-facilitating effects of cocaine: intracranial self-stimulation studies in the rat. Neuropsychopharmacology. 2005;30:2065–72.
Hotsenpiller G, Wolf ME. Baclofen attenuates conditioned locomotion to cues associated with cocaine administration and stabilizes extracellular glutamate levels in rat nucleus accumbens. Neuroscience. 2003;118:123–34.
Brebner K, Phelan R, Roberts DCS. Intra-VTA baclofen attenuates cocaine self-administration on a progressive ratio schedule of reinforcement. Pharmacol Biochem Behav. 2000;66:857–62.
Penn RD, Savoy SM, Corcos D, Latash M, Gottlieb G, Parke B, et al. Intrathecal baclofen for severe spinal spasticity. N Engl J Med. 1989;320:1517–21.
Sammaraiee Y, Yardley M, Keenan L, Buchanan K, Stevenson V, Farrell R. Intrathecal baclofen for multiple sclerosis related spasticity: a twenty year experience. Mult Scler Relat Disord. 2019;27:95–100.
Reynoard J, Schmitt C, Torrents R, Simon N. Toxicological considerations in the prescription of baclofen for the treatment of substance use disorders. Expert Opin Drug Metab Toxicol. 2020;16:309–17.
Garbutt JC, Kampov-Polevoy AB, Gallop R, Kalka-Juhl L, Flannery BA. Efficacy and safety of baclofen for alcohol dependence: a randomized, double-blind, placebo-controlled trial. Alcohol Clin Exp Res. 2010;34:1849–57.
Yamini D, Lee SH, Avanesyan A, Walter M, Runyon B. Utilization of baclofen in maintenance of alcohol abstinence in patients with alcohol dependence and alcoholic hepatitis with or without cirrhosis. Alcohol Alcohol. 2014;49:453–6.
Addolorato G, Leggio L, Hopf FW, Diana M, Bonci A. Novel therapeutic strategies for alcohol and drug addiction: focus on GABA, ion channels and transcranial magnetic stimulation. Neuropsychopharmacology. 2012;37:163–77.
Kahn R, Biswas K, Childress AR, Shoptaw S, Fudala PJ, Gorgon L, et al. Multi-center trial of baclofen for abstinence initiation in severe cocaine-dependent individuals. Drug Alcohol Depend. 2009;103:59–64.
Dore GM, Lo K, Juckes L, Bezyan S, Latt N. Clinical experience with baclofen in the management of alcohol-dependent patients with psychiatric comorbidity: a selected case series. Alcohol Alcohol. 2011;46:714–20.
Nugent FS, Penick EC, Kauer JA. Opioids block long-term potentiation of inhibitory synapses. Nature. 2007;446:1086–90.
Liu Q, Pu L, Poo M. Repeated cocaine exposure in vivo facilitates LTP induction in midbrain dopamine neurons. Nature. 2005;437:1027–31.
Barrett AC, Negus SS, Mello NK, Caine SB. Effect of GABA agonists and GABA-A receptor modulators on cocaine- and food-maintained responding and cocaine discrimination in rats. J Pharmacol Exp Ther. 2005;315:858–71.
Brodie JD, Figueroa E, Dewey SL. Treating cocaine addiction: from preclinical to clinical trial experience with γ-vinyl GABA. Synapse. 2003;50:261–5.
Barker JS, Hines RM. Regulation of GABAA receptor subunit expression in substance use disorders. Int J Mol Sci. 2020;21:4445.
Article CAS PubMed Central Google Scholar
Tan KR, Rudolph U, Lüscher C. Hooked on benzodiazepines: GABAA receptor subtypes and addiction. Trends Neurosci. 2011;34:188–97.
Brands B, Blake J, Marsh DC, Sproule B, Jeyapalan R, Li S. The impact of benzodiazepine use on methadone maintenance treatment outcomes. J Addict Dis. 2008;27:37–48.
Dacher M, Nugent FS. Morphine-induced modulation of LTD at GABAergic synapses in the ventral tegmental area. Neuropharmacology. 2011;61:1166–71.
Authement ME, Langlois LD, Kassis H, Gouty S, Dacher M, Shepard RD, et al. Morphine-induced synaptic plasticity in the VTA is reversed by HDAC inhibition. J Neurophysiol. 2016;116:1093–103.
Abraham WC, Bear MF. Metaplasticity: the plasticity of synaptic plasticity. Trends Neurosci. 1996;19:126–30.
Martin M, Chen BT, Hopf FW, Bowers MS, Bonci A. Cocaine self-administration selectively abolishes LTD in the core of the nucleus accumbens. Nat. Neurosci. 2006;9:868–9.
Thomas MJ, Beurrier C, Bonci A, Malenka RC. Long-term depression in the nucleus accumbens: a neural correlate of behavioral sensitization to cocaine. Nat. Neurosci. 2001;4:1217–23.
Boudreau AC, Wolf ME. Behavioral sensitization to cocaine is associated with increased AMPA receptor surface expression in the nucleus accumbens. J. Neurosci. 2005;25:9144–51.
Ferrario CR, Li X, Wang X, Reimers JM, Uejima JL, Wolf ME. The role of glutamate receptor redistribution in locomotor sensitization to cocaine. Neuropsychopharmacology. 2010;35:818–33.
Conrad KL, Tseng KY, Uejima JL, Reimers JM, Heng LJ, Shaham Y, et al. Formation of accumbens GluR2-lacking AMPA receptors mediates incubation of cocaine craving. Nature. 2008;454:118–21. https://doi.org/10.1038/nature06995 .
Wolf ME, Tseng KY. Calcium-permeable AMPA receptors in the VTA and nucleus accumbens after cocaine exposure:when, how, and why? Front Mol Neurosci. 2012;5:72.
Grimm JW, Hope BT, Wise RA, Shaham Y. Neuroadaptation. Incubation of cocaine craving after withdrawal. Nature. 2001;412:141–2.
Sadler-Smith E. Wallas’ four-stage model of the creative process: more than meets the eye? Creat Res J. 2015;27:342–52.
Gawin FH, Kleber HD. Abstinence symptomatology and psychiatric diagnosis in cocaine abusers. Clinical observations. Arch Gen Psychiatry. 1986;43:107–13.
Childress AR, Mozley PD, McElgin W, Fitzgerald J, Reivich M, O'Brien CP. Limbic activation during cue-induced cocaine craving. Am J Psychiatry. 1999;156:11–18.
Parvaz MA, Moeller SJ, Goldstein RZ. Incubation of cue-induced craving in adults addicted to cocaine measured by electroencephalography. JAMA Psychiatry. 2016;73:1127–34.
Lippman-Bell JJ, Zhou C, Sun H, Feske JS, Jensen FE. Early-life seizures alter synaptic calcium-permeable AMPA receptor function and plasticity. Mol Cell Neurosci. 2016;76:11–20.
Achuta VS, Möykkynen T, Peteri UK, Turconi G, Rivera C, Keinänen K, et al. Functional changes of AMPA responses in human induced pluripotent stem cell-derived neural progenitors in fragile X syndrome. Sci Sÿignal. 2018;11:eaan8784.
Article CAS Google Scholar
Selvaraj BT, Livesey MR, Zhao C, Gregory JM, James OT, Cleary EM, et al. C9ORF72 repeat expansion causes vulnerability of motor neurons to Ca 2+ -permeable AMPA receptor-mediated excitotoxicity. Nat Commun. 2018;9:347.
Kobylecki C, Cenci MA, Crossman AR, Ravenscroft P. Calcium-permeable AMPA receptors are involved in the induction and expression of l-DOPA-induced dyskinesia in Parkinson’s disease. J Neurochem. 2010;114:499–511.
Whitehead G, Regan P, Whitcomb DJ, Cho K. Ca2+-permeable AMPA receptor: a new perspective on amyloid-beta mediated pathophysiology of Alzheimer’s disease. Neuropharmacology. 2017;112:221–7.
Twomey EC, Yelshanskaya MV, Vassilevski AA, Sobolevsky AI. Mechanisms of channel block in calcium-permeable AMPA receptors. Neuron. 2018;99:956–.e4.
Loweth JA, Scheyer AF, Milovanovic M, LaCrosse AL, Flores-Barrera E, Werner CT, et al. Synaptic depression via mGluR1 positive allosteric modulation suppresses cue-induced cocaine craving. Nat. Neurosci. 2014;17:73–80.
Scheyer AF, Loweth JA, Christian DT, Uejima J, Rabei R, Le T, et al. AMPA receptor plasticity in accumbens core contributes to incubation of methamphetamine craving. Biol. Psychiatry. 2016;80:661–70.
Pascoli V, Terrier J, Espallergues J, Valjent E, O'Connor EC, Lüscher C. Contrasting forms of cocaine-evoked plasticity control components of relapse. Nature. 2014;509:459–64.
Ma T, Cheng Y, Roltsch Hellard E, Wang X, Lu J, Gao X, et al. Bidirectional and long-lasting control of alcohol-seeking behavior by corticostriatal LTP and LTD. Nat. Neurosci. 2018;21:373–83.
Pascoli V, Turiault M, Lüscher C. Reversal of cocaine-evoked synaptic potentiation resets drug-induced adaptive behaviour. Nature. 2012;481:71–75.
Valjent E, Bertran-Gonzalez J, Aubier B, Greengard P, Hervé D, Girault JA. Mechanisms of locomotor sensitization to drugs of abuse in a two-injection protocol. Neuropsychopharmacology 2010;35:401–15.
Robinson TE, Berridge KC. The neural basis of drug craving: an incentive-sensitization theory of addiction. Brain Res Brain Res Rev. 1993;18:247–91.
Schenk S, Partridge B. Sensitization and tolerance in psychostimulant self-administration. Pharmacol Biochem Behav. 1997;57:543–50.
Vezina P, Lorrain DS, Arnold GM, Austin JD, Suto N. Sensitization of midbrain dopamine neuron reactivity promotes the pursuit of amphetamine. J Neurosci. 2002;22:4654–62.
De Vries TJ, Schoffelmeer AN, Binnekade R, Mulder AH, Vanderschuren LJ. Drug-induced reinstatement of heroin- and cocaine-seeking behaviour following long-term extinction is associated with expression of behavioural sensitization. Eur J Neurosci. 1998;10:3565–71.
Suto N, Tanabe LM, Austin JD, Creekmore E, Pham CT, Vezina P. Previous exposure to psychostimulants enhances the reinstatement of cocaine seeking by nucleus accumbens AMPA. Neuropsychopharmacology. 2004;29:2149–59.
Vorspan F, de Witt P, Zerdazi EH, Karsinti E, Ksouda K, Icick R, et al. Chronic exposure to cocaine is associated with persistent behavioral disturbances. A cross-sectional dimensional study in outpatients with multiple substance use disorders. Psychopharmacology. 2020;237:3399–407.
Vorspan F, Icick R, Mekdad N, Courtin C, Bloch V, Bellivier F, et al. Translational study of the whole transcriptome in rats and genetic polymorphisms in humans identifies LRP1B and VPS13A as key genes involved in tolerance to cocaine-induced motor disturbances. Transl Psychiatry. 2020;10:381.
Vanderschuren LJ, Kalivas PW. Alterations in dopaminergic and glutamatergic transmission in the induction and expression of behavioral sensitization: a critical review of preclinical studies. Psychopharmacology. 2000;151:99–120.
Vezina P, Leyton M. Conditioned cues and the expression of stimulant sensitization in animals and humans. Neuropharmacology. 2009;56:160–8.
Tzschentke TM. REVIEW ON CPP: measuring reward with the conditioned place preference (CPP) paradigm: update of the last decade. Addict Biol. 2007;12:227–462.
Bardo MT, Bevins RA. Conditioned place preference: what does it add to our preclinical understanding of drug reward? Psychopharmacology. 2000;153:31–43.
Itzhak Y, Martin JL. Cocaine-induced conditioned place preference in mice: induction, extinction and reinstatement by related psychostimulants. Neuropsychopharmacology. 2002;26:130–4.
Creed M, Pascoli VJ, Lüscher C. Refining deep brain stimulation to emulate optogenetic treatment of synaptic pathology. Science. 2015;347:659–64.
Shen Y, Campbell RE, Côté DC, Paquet M-E. Challenges for therapeutic applications of opsin-based optogenetic tools in humans. Front Neural Circuits. 2020;14:41.
Vorspan F, Mallet L, Corvol J-C, Pelissolo A, Lépine J-P. Treating addictions with deep brain stimulation is premature but well-controlled clinical trials should be performed. Addict Abingdon Engl. 2011;106:1535–6.
Mahoney JJ, Hanlon CA, Marshalek PJ, Rezai AR, Krinke L. Transcranial magnetic stimulation, deep brain stimulation, and other forms of neuromodulation for substance use disorders: review of modalities and implications for treatment. J Neurol Sci. 2020;418:117149.
Shen W, Flajolet M, Greengard P, Surmeier DJ. Dichotomous dopaminergic control of striatal synaptic plasticity. Science. 2008;321:848–51.
Moussawi K, Pacchioni A, Moran M, Olive MF, Gass JT, Lavin A, et al. N -Acetylcysteine reverses cocaine-induced metaplasticity. Nat Neurosci. 2009;12:182–9.
Gerfen CR. The neostriatal mosaic: multiple levels of compartmental organization. Trends Neurosci. 1992;15:133–9.
Vicente AM, Galvão-Ferreira P, Tecuapetla F, Costa RM. Direct and indirect dorsolateral striatum pathways reinforce different action strategies. Curr Biol. 2016;26:R267–269.
Freeze BS, Kravitz AV, Hammack N, Berke JD, Kreitzer AC. Control of basal ganglia output by direct and indirect pathway projection neurons. J Neurosci. 2013;33:18531–9.
Durieux PF, Schiffmann SN, de Kerchove d’Exaerde A. Differential regulation of motor control and response to dopaminergic drugs by D1R and D2R neurons in distinct dorsal striatum subregions. EMBO J. 2012;31:640–53.
Cox J, Witten IB. Striatal circuits for reward learning and decision-making. Nat Rev Neurosci. 2019;20:482–94.
Lalchandani RR, van der Goes M-S, Partridge JG, Vicini S. Dopamine D2 receptors regulate collateral inhibition between striatal medium spiny neurons. J Neurosci. 2013;33:14075–86.
Dobbs LK, Kaplan AR, Lemos JC, Matsui A, Rubinstein M, Alvarez VA. Dopamine regulation of lateral inhibition between striatal neurons gates the stimulant actions of cocaine. Neuron. 2016;90:1100–13.
Dobbs LK, Kaplan AR, Bock R, Phamluong K, Shin JH, Bocarsly ME, et al. D1 receptor hypersensitivity in mice with low striatal D2 receptors facilitates select cocaine behaviors. Neuropsychopharmacology. 2019;44:805–16.
Tecuapetla F, Koós T, Tepper JM, Kabbani N, Yeckel MF. Differential dopaminergic modulation of neostriatal synaptic connections of striatopallidal axon collaterals. J Neurosci. 2009;29:8977–90.
Mulholland PJ, Chandler LJ, Kalivas PW. Signals from the fourth dimension regulate drug relapse. Trends Neurosci. 2016;39:472–85.
Smith AC, Kupchik YM, Scofield MD, Gipson CD, Wiggins A, Thomas CA, et al. Synaptic plasticity mediating cocaine relapse requires matrix metalloproteinases. Nat Neurosci. 2014;17:1655–7.
Moussawi K, Zhou W, Shen H, Reichel CM, See RE, Carr DB, et al. Reversing cocaine-induced synaptic potentiation provides enduring protection from relapse. Proc Natl Acad Sci USA. 2011;108:385–90.
Abulseoud OA, Camsari UM, Ruby CL, Kasasbeh A, Choi S, Choi DS. Attenuation of ethanol withdrawal by ceftriaxone-induced upregulation of glutamate transporter EAAT2. Neuropsychopharmacology. 2014;39:1674–84.
Knackstedt LA, Melendez RI, Kalivas PW. Ceftriaxone restores glutamate homeostasis and prevents relapse to cocaine seeking. Biol Psychiatry. 2010;67:81–84.
Huntley GW. Synaptic circuit remodelling by matrix metalloproteinases in health and disease. Nat. Rev. Neurosci. 2012;13:743–57.
Brown TE, Forquer MR, Harding JW, Wright JW, Sorg BA. Increase in matrix metalloproteinase-9 levels in the rat medial prefrontal cortex after cocaine reinstatement of conditioned place preference. Synapse. 2008;62:886–9.
Mizoguchi H, Yamada K, Mouri A, Niwa M, Mizuno T, Noda Y, et al. Role of matrix metalloproteinase and tissue inhibitor of MMP in methamphetamine-induced behavioral sensitization and reward: implications for dopamine receptor down-regulation and dopamine release. J Neurochem. 2007;102:1548–60.
Kroener S, Mulholland PJ, New NN, Gass JT, Becker HC, Chandler LJ. Chronic alcohol exposure alters behavioral and synaptic plasticity of the rodent prefrontal cortex. PLoS ONE. 2012;7:e37541.
Nimitvilai S, Lopez MF, Mulholland PJ, Woodward JJ. Chronic intermittent ethanol exposure enhances the excitability and synaptic plasticity of lateral orbitofrontal cortex neurons and induces a tolerance to the acute inhibitory actions of ethanol. Neuropsychopharmacology. 2016;41:1112–27.
Kasanetz F, Lafourcade M, Deroche-Gamonet V, Revest JM, Berson N, Balado E, et al. Prefrontal synaptic markers of cocaine addiction-like behavior in rats. Mol Psychiatry. 2013;18:729–37.
Meinhardt MW, Hansson AC, Perreau-Lenz S, Bauder-Wenz C, Stählin O, Heilig M, et al. Rescue of infralimbic mGluR2 deficit restores control over drug-seeking behavior in alcohol dependence. J Neurosci. 2013;33:2794–806.
Lu L, Uejima JL, Gray SM, Bossert JM, Shaham Y. Systemic and central amygdala injections of the mGluR2/3 agonist LY379268 attenuate the expression of incubation of cocaine craving. Biol Psychiatry. 2007;61:591–8.
Baptista MAS, Martin-Fardon R, Weiss F. Preferential effects of the metabotropic glutamate 2/3 receptor agonist LY379268 on conditioned reinstatement versus primary reinforcement: comparison between cocaine and a potent conventional reinforcer. J Neurosci. 2004;24:4723–7.
Peters J, Kalivas PW. The group II metabotropic glutamate receptor agonist, LY379268, inhibits both cocaine- and food-seeking behavior in rats. Psychopharmacology. 2006;186:143–9.
Caprioli D, Justinova Z, Venniro M, Shaham Y. Effect of novel allosteric modulators of metabotropic glutamate receptors on drug self-administration and relapse: a review of preclinical studies and their clinical implications. Biol Psychiatry. 2018;84:180–92.
Clark AM et al. Dopamine D2 receptors in the paraventricular thalamus attenuate cocaine locomotor sensitization. eNeuro. 2017;4:ENEURO.0227-17.2017.
Dong X, Li S, Kirouac GJ. Collateralization of projections from the paraventricular nucleus of the thalamus to the nucleus accumbens, bed nucleus of the stria terminalis, and central nucleus of the amygdala. Brain Struct. Funct. 2017;222:3927–43.
Huang AS, Mitchell JA, Haber SN, Alia-Klein N & Goldstein RZ. The thalamus in drug addiction: from rodents to humans. Philos Trans R Soc Lond B Biol Sci. 2018;373:20170028.
Perez SM, Lodge DJ. Convergent inputs from the hippocampus and thalamus to the nucleus accumbens regulate dopamine neuron activity. J Neurosci. 2018;38:10607–18.
Kelley AE, Baldo BA, Pratt WE. A proposed hypothalamic–thalamic–striatal axis for the integration of energy balance, arousal, and food reward. J Comp Neurol. 2005;493:72–85.
Penzo MA & Gao C. The paraventricular nucleus of the thalamus: an integrative node underlying homeostatic behavior. Trends Neurosci. 2021. https://doi.org/10.1016/j.tins.2021.03.001 .
De Groote A & de Kerchove d'Exaerde A. Thalamo-Nucleus Accumbens projections in motivated behaviors and addiction. Front Syst Neurosci. 2021;15:711350.
Zhu Y, Wienecke CFR, Nachtrab G, Chen X. A thalamic input to the nucleus accumbens mediates opiate dependence. Nature. 2016;530:219–22.
Chisholm A, Rizzo D, Fortin É, Moman V, Quteishat N, Romano A, et al. Assessing the role of corticothalamic and thalamo-accumbens projections in the augmentation of heroin seeking in chronically food-restricted rats. J Neurosci. 2021;41:354–65.
Otis JM, Zhu M, Namboodiri V, Cook CA, Kosyk O, Matan AM, et al. Paraventricular thalamus projection neurons integrate cortical and hypothalamic signals for cue-reward processing. Neuron. 2019;103:423–.e4.
Giros B, Jaber M, Jones SR, Wightman RM, Caron MG. Hyperlocomotion and indifference to cocaine and amphetamine in mice lacking the dopamine transporter. Nature. 1996;379:606–12.
Sora I, Hall FS, Andrews AM, Itokawa M, Li XF, Wei HB, et al. Molecular mechanisms of cocaine reward: combined dopamine and serotonin transporter knockouts eliminate cocaine place preference. Proc Natl Acad Sci USA. 2001;98:5300–5.
Yang H-J, Zhang HY, Bi GH, He Y, Gao JT, Xi ZX. Deletion of type 2 metabotropic glutamate receptor decreases sensitivity to cocaine reward in rats. Cell Rep. 2017;20:319–32.
Chiamulera C, Epping-Jordan MP, Zocchi A, Marcon C, Cottiny C, Tacconi S, et al. Reinforcing and locomotor stimulant effects of cocaine are absent in mGluR5 null mutant mice. Nat Neurosci. 2001;4:873–4.
De Backer J-F, et al. Deletion of Maged1 in mice abolishes locomotor and reinforcing effects of cocaine. EMBO Rep. 2018;19:e45089.
Karadurmus D, Rial D, De Backer JF, Communi D, de Kerchove d'Exaerde A, Schiffmann SN. GPRIN3 controls neuronal excitability, morphology, and striatal-dependent behaviors in the indirect pathway of the striatum. J. Neurosci. 2019;39:7513–28.
Renthal W, Kumar A, Xiao G, Wilkinson M, Covington HE, Maze I, et al. Genome-wide analysis of chromatin regulation by cocaine reveals a role for sirtuins. Neuron. 2009;62:335–48.
Ena SL, De Backer J-F, Schiffmann SN, de Kerchove d’Exaerde A. FACS array profiling identifies Ecto-5’ nucleotidase as a striatopallidal neuron-specific gene involved in striatal-dependent learning. J Neurosci. 2013;33:8794–809.
Iida, N & Kozasa, T. 29 - Identification and biochemical analysis of GRIN1 and GRIN2. In: Siderovski DP, editor. Methods in Enzymology. Academic Press; 2004;390:475–83.
Mototani Y, Okamura T, Goto M, Shimizu Y, Yanobu-Takanashi R, Ito A, et al. Role of G protein-regulated inducer of neurite outgrowth 3 (GRIN3) in β-arrestin 2-Akt signaling and dopaminergic behaviors. Pflügers Arch. 2018;470:937–47.
Ventura A, Meissner A, Dillon CP, McManus M, Sharp PA, Van Parijs L, et al. Cre-lox-regulated conditional RNA interference from transgenes. Proc Natl Acad Sci USA. 2004;101:10380–5.
David Smith A, Paul Bolam J. The neural network of the basal ganglia as revealed by the study of synaptic connections of identified neurones. Trends Neurosci. 1990;13:259–65.
Robinson TE, Kolb B. Alterations in the morphology of dendrites and dendritic spines in the nucleus accumbens and prefrontal cortex following repeated treatment with amphetamine or cocaine. Eur J Neurosci. 1999;11:1598–604.
Spencer S, Garcia-Keller C, Roberts-Wolfe D, Heinsbroek JA, Mulvaney M, Sorrell A, et al. Cocaine use reverses striatal plasticity produced during cocaine seeking. Biol Psychiatry. 2017;81:616–24.
MacAskill AF, Cassel JM, Carter AG. Cocaine exposure reorganizes cell type– and input-specific connectivity in the nucleus accumbens. Nat Neurosci. 2014;17:1198–207.
Dos Santos M, Cahill EN, Bo GD, Vanhoutte P, Caboche J, Giros B, et al. Cocaine increases dopaminergic connectivity in the nucleus accumbens. Brain Struct Funct. 2018;223:913–23.
Jaenisch R, Bird A. Epigenetic regulation of gene expression: how the genome integrates intrinsic and environmental signals. Nat Genet. 2003;33:245–54.
Nestler EJ. Epigenetic mechanisms of drug addiction. Neuropharmacology. 2014;76:259–68.
Hamilton PJ, Nestler EJ. Epigenetics and addiction. Curr Opin Neurobiol. 2019;59:128–36.
Handel AE, Ramagopalan SV. Is Lamarckian evolution relevant to medicine? BMC Med Genet. 2010;11:73.
Jablonka E & Lamb MJ. Epigenetic Inheritance And Evolution: The Lamarckian Dimension. Oxford University Press; 1999.
Springer JT & Holley D. An Introduction To Zoology: Investigating The Animal World. Jones & Bartlett Learning; 2013.
Vassoler FM, White SL, Schmidt HD, Sadri-Vakili G, Pierce RC. Epigenetic inheritance of a cocaine-resistance phenotype. Nat. Neurosci. 2013;16:42–47.
Goldberg LR, Gould TJ. Multigenerational and transgenerational effects of paternal exposure to drugs of abuse on behavioral and neural function. Eur J Neurosci. 2019;50:2453–66.
Sadri-Vakili G, Kumaresan V, Schmidt HD, Famous KR, Chawla P, Vassoler FM, et al. Cocaine-induced chromatin remodeling increases brain-derived neurotrophic factor transcription in the rat medial prefrontal cortex, which alters the reinforcing efficacy of cocaine. J Neurosci. 2010;30:11735–44.
Schmidt HD, Sangrey GR, Darnell SB, Schassburger RL, Cha JH, Pierce RC, et al. Increased brain-derived neurotrophic factor (BDNF) expression in the ventral tegmental area during cocaine abstinence is associated with increased histone acetylation at BDNF exon I-containing promoters. J Neurochem. 2012;120:202–9.
Gavin DP, et al. Stable histone methylation changes at proteoglycan network genes following ethanol exposure. Front Genet. 2018;9:346.
Sadakierska-Chudy A, Frankowska M, Jastrzębska J, Wydra K, Miszkiel J, Sanak M, et al. Cocaine administration and its withdrawal enhance the expression of genes encoding histone-modifying enzymes and histone acetylation in the rat prefrontal cortex. Neurotox Res. 2017;32:1–10.
Allis CD, Jenuwein T. The molecular hallmarks of epigenetic control. Nat Rev Genet. 2016;17:487–500.
Wang L, Lv Z, Hu Z, Sheng J, Hui B, Sun J, et al. Chronic cocaine-induced H3 acetylation and transcriptional activation of CaMKIIα in the nucleus accumbens is critical for motivation for drug reinforcement. Neuropsychopharmacology. 2010;35:913–28.
Brami-Cherrier K. Parsing molecular and behavioral effects of cocaine in mitogen- and stress-activated protein kinase-1-deficient mice. J Neurosci. 2005;25:11444–54.
Barrett RM, Wood MA. Beyond transcription factors: the role of chromatin modifying enzymes in regulating transcription required for memory. Learn Mem. 2008;15:460–7.
Malvaez M, Mhillaj E, Matheos DP, Palmery M, Wood MA. CBP in the nucleus accumbens regulates cocaine-induced histone acetylation and is critical for cocaine-associated behaviors. J Neurosci. 2011;31:16941–8.
Tsankova NM, Berton O, Renthal W, Kumar A, Neve RL, Nestler EJ. Sustained hippocampal chromatin regulation in a mouse model of depression and antidepressant action. Nat Neurosci. 2006;9:519–25.
Kumar A, Choi KH, Renthal W, Tsankova NM, Theobald DE, Truong HT, et al. Chromatin remodeling is a key mechanism underlying cocaine-induced plasticity in striatum. Neuron. 2005;48:303–14.
Sun J, Wang L, Jiang B, Hui B, Lv Z, Ma L. The effects of sodium butyrate, an inhibitor of histone deacetylase, on the cocaine- and sucrose-maintained self-administration in rats. Neurosci Lett. 2008;441:72–76.
Romieu P, Host L, Gobaille S, Sandner G, Aunis D, Zwiller J. Histone deacetylase inhibitors decrease cocaine but not sucrose self-administration in rats. J. Neurosci. 2008;28:9342–8.
Levine AA, Guan Z, Barco A, Xu S, Kandel ER, Schwartz JH. CREB-binding protein controls response to cocaine by acetylating histones at the fosB promoter in the mouse striatum. Proc Natl Acad Sci USA. 2005;102:19186–91.
Kalda A, Heidmets L-T, Shen H-Y, Zharkovsky A, Chen J-F. Histone deacetylase inhibitors modulates the induction and expression of amphetamine-induced behavioral sensitization partially through an associated learning of the environment in mice. Behav. Brain Res. 2007;181:76–84.
Kennedy PJ, Feng J, Robison AJ, Maze I, Badimon A, Mouzon E, et al. Class I HDAC inhibition blocks cocaine-induced plasticity by targeted changes in histone methylation. Nat Neurosci. 2013;16:434–40.
LaPlant Q, Vialou V, Covington HE, Dumitriu D, Feng J, Warren BL, et al. Dnmt3a regulates emotional behavior and spine plasticity in the nucleus accumbens. Nat Neurosci. 2010;13:1137–43.
Massart R, Barnea R, Dikshtein Y, Suderman M, Meir O, Hallett M, et al. Role of DNA methylation in the nucleus accumbens in incubation of cocaine craving. J Neurosci. 2015;35:8042–58.
Bertran-Gonzalez J, Bosch C, Maroteaux M, Matamales M, Hervé D, Valjent E, et al. Opposing patterns of signaling activation in dopamine D1 and D2 receptor-expressing striatal neurons in response to cocaine and haloperidol. J Neurosci. 2008;28:5671–85.
Brami‐Cherrier K, Roze E, Girault J-A, Betuing S, Caboche J. Role of the ERK/MSK1 signalling pathway in chromatin remodelling and brain responses to drugs of abuse. J Neurochem. 2009;108:1323–35.
Scobie KN, Damez-Werno D, Sun H, Shao N, Gancarz A, Panganiban CH, et al. Essential role of poly(ADP-ribosyl)ation in cocaine action. Proc Natl Acad Sci USA. 2014;111:2005–10.
Lepack AE, Werner CT, Stewart AF, Fulton SL, Zhong P, Farrelly LA, et al. Dopaminylation of histone H3 in ventral tegmental area regulates cocaine seeking. Science. 2020;368:197–201.
Sun H, Maze I, Dietz DM, Scobie KN, Kennedy PJ, Damez-Werno D, et al. Morphine epigenomically regulates behavior through alterations in histone H3 lysine 9 dimethylation in the nucleus accumbens. J Neurosci. 2012;32:17454–64.
Maze I, Feng J, Wilkinson MB, Sun H, Shen L, Nestler EJ. Cocaine dynamically regulates heterochromatin and repetitive element unsilencing in nucleus accumbens. Proc Natl Acad Sci USA. 2011;108:3035–40.
Liu XS, Wu H, Ji X, Stelzer Y, Wu X, Czauderna S, et al. Editing DNA methylation in the mammalian genome. Cell. 2016;167:233–247.e17.
Liao H-K, Hatanaka F, Araoka T, Reddy P, Wu MZ, Sui Y, et al. In vivo target gene activation via CRISPR/Cas9-mediated trans-epigenetic modulation. Cell. 2017;171:1495–1507.e15.
Heller EA, Cates HM, Peña CJ, Sun H, Shao N, Feng J, et al. Locus-specific epigenetic remodeling controls addiction- and depression-related behaviors. Nat. Neurosci. 2014;17:1720–7.
Heller EA, Hamilton PJ, Burek DD, Lombroso SI, Peña CJ, Neve RL, et al. Targeted epigenetic remodeling of the Cdk5 gene in nucleus accumbens regulates cocaine- and stress-evoked behavior. J Neurosci. 2016;36:4690–7.
Monsey MS, Ota KT, Akingbade IF, Hong ES, Schafe GE. Epigenetic alterations are critical for fear memory consolidation and synaptic plasticity in the lateral amygdala. PLoS ONE. 2011;6:e19958.
Massart R, Freyburger M, Suderman M, Paquet J, El Helou J, Belanger-Nelson E, et al. The genome-wide landscape of DNA methylation and hydroxymethylation in response to sleep deprivation impacts on synaptic plasticity genes. Transl Psychiatry. 2014;4:e347–e347.
Kyzar EJ, Pandey SC. Molecular mechanisms of synaptic remodeling in alcoholism. Neurosci Lett. 2015;601:11–19.
Rogge GA, Wood MA. The role of histone acetylation in cocaine-induced neural plasticity and behavior. Neuropsychopharmacology. 2013;38:94–110.
Kyzar EJ, Floreani C, Teppen TL & Pandey SC. Adolescent alcohol exposure: burden of epigenetic reprogramming, synaptic remodeling, and adult psychopathology. Front. Neurosci. 2016;10:222.
Maze I, Covington HE, Dietz DM, LaPlant Q, Renthal W, Russo SJ, et al. Essential role of the histone methyltransferase G9a in cocaine-induced plasticity. Science. 2010;327:213–6.
Robison AJ, Nestler EJ. Transcriptional and epigenetic mechanisms of addiction. Nat Rev Neurosci. 2011;12:623–37.
Lobo MK, Zaman S, Damez-Werno DM, Koo JW, Bagot RC, DiNieri JA, et al. ΔFosB induction in striatal medium spiny neuron subtypes in response to chronic pharmacological, emotional, and optogenetic stimuli. J Neurosci. 2013;33:18381–95.
Huang YH, Lin Y, Brown TE, Han MH, Saal DB, Neve RL, et al. CREB modulates excitability of nucleus accumbens neurons. Nat Neurosci. 2006;9:475–7.
Murphy DD, Segal M. Morphological plasticity of dendritic spines in central neurons is mediated by activation of cAMP response element binding protein. Proc Natl Acad Sci USA. 1997;94:1482–7.
Grueter BA, Robison AJ, Neve RL, Nestler EJ, Malenka RC. ∆FosB differentially modulates nucleus accumbens direct and indirect pathway function. Proc Natl Acad Sci USA. 2013;110:1923–8.
Smith ACW, Kenny PJ. MicroRNAs regulate synaptic plasticity underlying drug addiction. Genes Brain Behav. 2018;17:e12424.
Nudelman AS, DiRocco DP, Lambert TJ, Garelick MG, Le J, Nathanson NM, et al. Neuronal activity rapidly induces transcription of the CREB-regulated microRNA-132, in vivo. Hippocampus. 2010;20:492–8.
CAS PubMed PubMed Central Google Scholar
Yasui DH, Peddada S, Bieda MC, Vallero RO, Hogart A, Nagarajan RP, et al. Integrated epigenomic analyses of neuronal MeCP2 reveal a role for long-range interaction with active genes. Proc Natl Acad Sci USA. 2007;104:19416–21.
Chahrour M, Jung SY, Shaw C, Zhou X, Wong ST, Qin J, et al. MeCP2, a key contributor to neurological disease, activates and represses transcription. Science. 2008;320:1224–9.
De Crescenzo F, Ciabattini M, D'Alò GL, De Giorgi R, Del Giovane C, Cassar C, et al. Comparative efficacy and acceptability of psychosocial interventions for individuals with cocaine and amphetamine addiction: a systematic review and network meta-analysis. PLOS Med. 2018;15:e1002715.
Phillips KA, Epstein DH, Preston KL. Psychostimulant addiction treatment. Neuropharmacology. 2014;87:150–60.
Mattick RP, Breen C, Kimber J & Davoli M. Methadone maintenance therapy versus no opioid replacement therapy for opioid dependence. Cochrane Database Syst. Rev. 2009;CD002209. https://doi.org/10.1002/14651858.CD002209.pub2 .
Hartmann‐Boyce J, Chepkin SC, Ye W, Bullen C & Lancaster T. Nicotine replacement therapy versus control for smoking cessation. Cochrane Database Syst Rev. 2018. https://doi.org/10.1002/14651858.CD000146.pub5 .
Tardelli VS, Bisaga A, Arcadepani FB, Gerra G, Levin FR, Fidalgo TM. Prescription psychostimulants for the treatment of stimulant use disorder: a systematic review and meta-analysis. Psychopharmacology. 2020;237:2233–55.
Darke S & Farrell M. Which medications are suitable for agonist drug maintenance? Addiction. 2016;111:767–74.
Kosten TR, Domingo CB, Shorter D, Orson F, Green C, Somoza E, et al. Vaccine for cocaine dependence: a randomized double-blind placebo-controlled efficacy trial. Drug Alcohol Depend. 2014;140:42–47.
Hossain MK, Hassanzadeganroudsari M, Nurgali K, Apostolopoulos V. Vaccine development against methamphetamine drug addiction. Expert Rev Vaccines. 2020;19:1105–14.
Dackis C, O’Brien C. Neurobiology of addiction: treatment and public policy ramifications. Nat Neurosci. 2005;8:1431–6.
Benabid AL, Pollak P, Gervason C, Hoffmann D, Gao DM, Hommel M, et al. Long-term suppression of tremor by chronic stimulation of the ventral intermediate thalamic nucleus. Lancet. 1991;337:403–6.
Bergman H, Wichmann T, DeLong MR. Reversal of experimental parkinsonism by lesions of the subthalamic nucleus. Science. 1990;249:1436–8.
Limousin P, Pollak P, Benazzouz A, Hoffmann D, Le Bas JF, Broussolle E, et al. Effect on parkinsonian signs and symptoms of bilateral subthalamic nucleus stimulation. Lancet. 1995;345:91–95.
Johansen-Berg H, Gutman DA, Behrens TE, Matthews PM, Rushworth MF, Katz E, et al. Anatomical connectivity of the subgenual cingulate region targeted with deep brain stimulation for treatment-resistant depression. Cereb Cortex. 2008;1991:1374–83.
Greenberg BD, Malone DA, Friehs GM, Rezai AR, Kubu CS, Malloy PF, et al. Three-year outcomes in deep brain stimulation for highly resistant obsessive-compulsive disorder. Neuropsychopharmacology. 2006;31:2384–93.
Porta M, Brambilla A, Cavanna AE, Servello D, Sassi M, Rickards H, et al. Thalamic deep brain stimulation for treatment-refractory Tourette syndrome: two-year outcome. Neurology. 2009;73:1375–80.
Kuhn J, Lenartz D, Huff W, Lee S, Koulousakis A, Klosterkoetter J, et al. Remission of alcohol dependency following deep brain stimulation of the nucleus accumbens: valuable therapeutic implications? J Neurol Neurosurg Psychiatry. 2007;78:1152–3.
Mantione M, van de Brink W, Schuurman PR, Denys D. Smoking cessation and weight loss after chronic deep brain stimulation of the nucleus accumbens: therapeutic and research implications: case report. Neurosurgery. 2010;66:E218.
Levy D, Shabat-Simon M, Shalev U, Barnea-Ygael N, Cooper A, Zangen A. Repeated electrical stimulation of reward-related brain regions affects cocaine but not “natural” reinforcement. J Neurosci. 2007;27:14179–89.
Liu H-Y, Jin J, Tang JS, Sun WX, Jia H, Yang XP, et al. Chronic deep brain stimulation in the rat nucleus accumbens and its effect on morphine reinforcement. Addict Biol. 2008;13:40–46.
Vassoler FM, Schmidt HD, Gerard ME, Famous KR, Ciraulo DA, Kornetsky C, et al. Deep brain stimulation of the nucleus accumbens shell attenuates cocaine priming-induced reinstatement of drug seeking in rats. J Neurosci. 2008;28:8735–9.
Batra V, Tran TL, Caputo J, Guerin GF, Goeders NE, Wilden J. Intermittent bilateral deep brain stimulation of the nucleus accumbens shell reduces intravenous methamphetamine intake and seeking in Wistar rats. J Neurosurg. 2017;126:1339–50.
Heinze H-J, et al. Counteracting incentive sensitization in severe alcohol dependence using deep brain stimulation of the nucleus accumbens: clinical and basic science aspects. Front Hum Neurosci. 2009;3:22.
Kuhn J, Gründler TO, Bauer R, Huff W, Fischer AG, Lenartz D, et al. Successful deep brain stimulation of the nucleus accumbens in severe alcohol dependence is associated with changed performance monitoring. Addict Biol. 2011;16:620–3.
Kuhn J, Möller M, Treppmann JF, Bartsch C, Lenartz D, Gruendler TO, et al. Deep brain stimulation of the nucleus accumbens and its usefulness in severe opioid addiction. Mol Psychiatry. 2014;19:145–6.
Valencia-Alfonso C-E, Luigjes J, Smolders R, Cohen MX, Levar N, Mazaheri A, et al. Effective deep brain stimulation in heroin addiction: a case report with complementary intracranial electroencephalogram. Biol Psychiatry. 2012;71:e35–37.
Voges J, Müller U, Bogerts B, Münte T, Heinze H-J. Deep brain stimulation surgery for alcohol addiction. World Neurosurg. 2013;80:S28.e21–31.
Wang TR, Moosa S, Dallapiazza RF, Elias WJ, Lynch WJ. Deep brain stimulation for the treatment of drug addiction. Neurosurg Focus. 2018;45:E11.
Sanna A, Fattore L, Badas P, Corona G, Diana M. The hypodopaminergic state ten years after: transcranial magnetic stimulation as a tool to test the dopamine hypothesis of drug addiction. Curr Opin Pharmacol. 2021;56:61–67.
Verrico CD, Haile CN, Newton TF, Kosten TR, Garza RDL. Pharmacotherapeutics for substance-use disorders: a focus on dopaminergic medications. Expert Opin Investig Drugs. 2013;22:1549–68.
Amiaz R, Levy D, Vainiger D, Grunhaus L, Zangen A. Repeated high-frequency transcranial magnetic stimulation over the dorsolateral prefrontal cortex reduces cigarette craving and consumption. Addiction. 2009;104:653–60.
Pettorruso M, Di Giuda D, Martinotti G, Cocciolillo F, De Risio L, Montemitro C, et al. Dopaminergic and clinical correlates of high-frequency repetitive transcranial magnetic stimulation in gambling addiction: a SPECT case study. Addict Behav. 2019;93:246–9.
Cho SS, Strafella AP. rTMS of the left dorsolateral prefrontal cortex modulates dopamine release in the ipsilateral anterior cingulate cortex and orbitofrontal cortex. PLoS ONE. 2009;4:e6725.
Strafella AP, Paus T, Fraraccio M, Dagher A. Striatal dopamine release induced by repetitive transcranial magnetic stimulation of the human motor cortex. Brain. 2003;126:2609–15.
Ekhtiari H, Tavakoli H, Addolorato G, Baeken C, Bonci A, Campanella S, et al. Transcranial electrical and magnetic stimulation (tES and TMS) for addiction medicine: a consensus paper on the present state of the science and the road ahead. Neurosci Biobehav Rev. 2019;104:118–40.
Wu X-Q, Zan GY, Ju YY, Chen TZ, Guo LB, Jiao DL, et al. Low-frequency repetitive transcranial magnetic stimulation inhibits the development of methamphetamine-induced conditioned place preference. Behav Brain Res. 2018;353:129–36.
Dackis CA, Kampman KM, Lynch KG, Plebani JG, Pettinati HM, Sparkman T, et al. A double-blind, placebo-controlled trial of modafinil for cocaine dependence. J Subst Abus Treat. 2012;43:303–12.
Shearer J, Darke S, Rodgers C, Slade T, van Beek I, Lewis J, et al. A double-blind, placebo-controlled trial of modafinil (200 mg/day) for methamphetamine dependence. Addiction. 2009;104:224–33.
Chiamulera C, Piva A, Abraham WC. Glutamate receptors and metaplasticity in addiction. Curr Opin Pharmacol. 2021;56:39–45.
Le Moal M, Koob GF. Drug addiction: pathways to the disease and pathophysiological perspectives. Eur Neuropsychopharmacol. 2007;17:377–93.
Luigjes J, Segrave R, de Joode N, Figee M, Denys D. Efficacy of invasive and non-invasive brain modulation interventions for addiction. Neuropsychol Rev. 2019;29:116–38.
Jordi E, Heiman M, Marion-Poll L, Guermonprez P, Cheng SK, Nairn AC, Greengard P, Girault J-A. Proceedings of the National Academy of Sciences . 2013;110:9511-16. https://doi.org/10.1073/pnas.1307116110 .
Wang H, Wang L, Erdjument-Bromage H, Vidal M, Tempst P, Jones RS, et al. Role of histone H2A ubiquitination in Polycomb silencing. Nature. 2004;431:873–8.
Download references
Acknowledgements
We thank Michele Zoli, Romain Icick and Daniel Rial for helpful comments and corrections on the manuscript. Julian Cheron is supported by a fellowship of the FRS-FNRS (Belgium). Alban de Kerchove d´Exaerde is a Research Director of the FRS-FNRS. FRS-FNRS (Belgium). Fondation Simone et Pierre Clerdent, Fondation ULB, supported this study.
Author information
Authors and affiliations.
Laboratory of Neurophysiology, ULB Neuroscience Institute, Université Libre de Bruxelles (ULB), Brussels, B-1070, Belgium
Julian Cheron & Alban de Kerchove d’Exaerde
You can also search for this author in PubMed Google Scholar
Contributions
JC and AKE wrote the paper and AKE supervised all the work.
Corresponding author
Correspondence to Alban de Kerchove d’Exaerde .
Ethics declarations
Competing interests.
The authors declare no competing interests.
Additional information
Publisher’s note Springer Nature remains neutral with regard to jurisdictional claims in published maps and institutional affiliations.
Rights and permissions
Open Access This article is licensed under a Creative Commons Attribution 4.0 International License, which permits use, sharing, adaptation, distribution and reproduction in any medium or format, as long as you give appropriate credit to the original author(s) and the source, provide a link to the Creative Commons license, and indicate if changes were made. The images or other third party material in this article are included in the article’s Creative Commons license, unless indicated otherwise in a credit line to the material. If material is not included in the article’s Creative Commons license and your intended use is not permitted by statutory regulation or exceeds the permitted use, you will need to obtain permission directly from the copyright holder. To view a copy of this license, visit http://creativecommons.org/licenses/by/4.0/ .
Reprints and permissions
About this article
Cite this article.
Cheron, J., Kerchove d’Exaerde, A.d. Drug addiction: from bench to bedside. Transl Psychiatry 11 , 424 (2021). https://doi.org/10.1038/s41398-021-01542-0
Download citation
Received : 05 April 2021
Revised : 14 July 2021
Accepted : 23 July 2021
Published : 12 August 2021
DOI : https://doi.org/10.1038/s41398-021-01542-0
Share this article
Anyone you share the following link with will be able to read this content:
Sorry, a shareable link is not currently available for this article.
Provided by the Springer Nature SharedIt content-sharing initiative
This article is cited by
Snp-based and haplotype-based genome-wide association on drug dependence in han chinese.
- Yangyang Liu
BMC Genomics (2024)
USP7/Maged1-mediated H2A monoubiquitination in the paraventricular thalamus: an epigenetic mechanism involved in cocaine use disorder
- Julian Cheron
- Leonardo Beccari
- Alban de Kerchove d’Exaerde
Nature Communications (2023)
Methylation and expression quantitative trait locus rs6296 in the HTR1B gene is associated with susceptibility to opioid use disorder
- Jianbo Zhang
Psychopharmacology (2022)
Quick links
- Explore articles by subject
- Guide to authors
- Editorial policies


An official website of the United States government
Here’s how you know
Official websites use .gov A .gov website belongs to an official government organization in the United States.
Secure .gov websites use HTTPS A lock ( Lock Locked padlock icon ) or https:// means you’ve safely connected to the .gov website. Share sensitive information only on official, secure websites.

In Crisis? Call or Text 988
Your browser is not supported
Switch to Chrome, Edge, Firefox or Safari
Page title Principles of Drug Addiction Treatment: A Research-Based Guide (Third Edition)

Other Resources Like This
Last Updated: 12/12/2023

COMMENTS
Learn more about clinical research and how to participate in a NIDA-funded or NIDA-conducted clinical trial. NIDA's mission is to advance science on the causes and consequences of drug use and addiction and to apply that knowledge to improve individual and public health.
Drugs, Brains, and Behavior: The Science of Addiction. Preface. How Science Has Revolutionized the Understanding of Drug Addiction. For much of the past century, scientists studying drugs and drug use labored in the shadows of powerful myths and misconceptions about the nature of addiction.
Neuropsychopharmacology 46 , 1715–1723 ( 2021) Cite this article. 87k Accesses. 100 Citations. 321 Altmetric. Metrics. Abstract. The view that substance addiction is a brain disease, although...
Drugs change the brain in ways that make quitting hard, even for those who want to. Fortunately, researchers know more than ever about how drugs affect the brain and have found treatments that can help people recover from drug addiction and lead productive lives. What Is drug addiction?
The National Institute on Drug Abuse (NIDA) is the lead federal agency supporting scientific research on drug use and addiction. NIDA’s mission is to advance science on drug use and addiction and to apply that knowledge to improve individual and public health through:
Drug addiction is defined as a chronic, relapsing disease that results from the prolonged effects of drugs on the brain. Similarly to other neuropsychiatric diseases, drug addiction is...
Scientific studies on addiction have led to the development of a number of medications for pharmacological interventions, along with other nonpharmacotherapies including behavioral, cognitive, and social interventions (see the comprehensive reviews in this collection).
Science. 19 Apr 2024. Drug addictions are a leading global cause of health and economic burden, with opioids responsible for 80% of drug use–related deaths ( 1 ). Persistent drug use is accompanied by profound motivational reprioritization ( 2 ), with decision-making skewed toward drug use at the expense of other activities ( 3 ), often with ...
Addiction is the key process that underlies substance use disorders, and research using animal models and humans has revealed important insights into the neural circuits and molecules that mediate addiction.
Principles of Drug Addiction Treatment: A Research-Based Guide (Third Edition) This update of the National Institute on Drug Abuse’s Principles of Drug Addiction Treatment is intended to address addiction to a wide variety of drugs, including nicotine, alcohol, and illicit and prescription drugs. Authoring Agency.