Loading metrics
Open Access

Bioinformatics Projects Supporting Life-Sciences Learning in High Schools
Affiliation Instituto Gulbenkian de Ciência, Oeiras, Portugal
Affiliation Escola Secundária Stuart de Carvalhais, Queluz, Portugal
* E-mail: [email protected]
- Isabel Marques,
- Paulo Almeida,
- Renato Alves,
- Maria João Dias,
- Ana Godinho,
- José B. Pereira-Leal
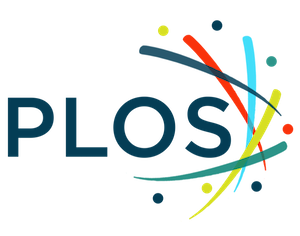
Published: January 23, 2014
- https://doi.org/10.1371/journal.pcbi.1003404
- Reader Comments
The interdisciplinary nature of bioinformatics makes it an ideal framework to develop activities enabling enquiry-based learning. We describe here the development and implementation of a pilot project to use bioinformatics-based research activities in high schools, called “Bioinformatics@school.” It includes web-based research projects that students can pursue alone or under teacher supervision and a teacher training program. The project is organized so as to enable discussion of key results between students and teachers. After successful trials in two high schools, as measured by questionnaires, interviews, and assessment of knowledge acquisition, the project is expanding by the action of the teachers involved, who are helping us develop more content and are recruiting more teachers and schools.
Citation: Marques I, Almeida P, Alves R, Dias MJ, Godinho A, Pereira-Leal JB (2014) Bioinformatics Projects Supporting Life-Sciences Learning in High Schools. PLoS Comput Biol 10(1): e1003404. https://doi.org/10.1371/journal.pcbi.1003404
Editor: Fran Lewitter, Whitehead Institute, United States of America
Copyright: © 2014 Marques et al. This is an open-access article distributed under the terms of the Creative Commons Attribution License , which permits unrestricted use, distribution, and reproduction in any medium, provided the original author and source are credited.
Funding: This work was funded by the Instituto Gulbenkian de Ciência. The funders had no role in the preparation of the manuscript.
Competing interests: The authors have declared that no competing interests exist.
Background and Motivation
Our lives are increasingly touched by science and technology, from the everyday activities of browsing the internet, taking a prescription drug, etc., to major societal discussions involving, for example, genetically modified foods, cloning, or stem cells. It is therefore imperative that we engage young people in science. We witnessed in the past shrinking numbers of students choosing science degrees for their university education [1] . This trend seems, however, to have been inverted both in Europe and in the United States [2] , [3] . A recent study points to the development of new and more attractive curricula and teaching methods as the driver for this increased interest [3] . In light of the growing evidence of a direct link between attitudes towards science and the way science is taught [1] , there is increasing recognition of the need to couple the traditional teacher-centred “deductive approach” to the learner-centred “inductive approach,” relying on observation, experimentation, and teacher guidance in constructing students' knowledge. This “bottom-up” approach, called enquiry-based learning (also known as problem-based learning or case-based learning) [4] recapitulates the scientific process (raising questions, collecting data, reasoning, reviewing evidence, drawing conclusions, and discussing results), thus promoting both ideas of science (scientific concepts) and ideas about science (process, practices, and critical thinking), i.e., about the Nature of Science (NOS).
Bioinformatics is a discipline at the intersection of biology, computer science, information science, mathematics, and to some extent also of chemistry and physics. It developed in response to the increasingly complex data types and relationships in biological research, addressing the need to manage and interpret biological information. This interdisciplinary nature makes bioinformatics an ideal framework to engage high school students, as it illustrates the interplay between different scientific areas, while touching on many aspects that are relevant to the younger generations—health, environment, etc. This has been recognized by many others who have implemented bioinformatics-training programs. Examples are a web-based, problem-oriented approach aimed at introducing students to bioinformatics [5] and the use of bioinformatics activities as a way to teach evolution [6] or notions of polymorphisms in the context of human genetic variation and disease [7] . Bioinformatics has also integrated with wet-lab activities in initiatives like the student-aimed “Cus-Mi-Bio” project [8] , which include gene finding activities, or in projects aimed at high school and college teachers, such as the ones at the Dolan DNA learning centre of Cold Spring Harbor Laboratory involving plant genome annotation [9] . More recently, activities that aim to introduce high school students to bioinformatics itself have also been reported [10] , and, as of 2012, an exercise using Basic Local Alignment Search Tool (BLAST) has been included on the Advanced Placement, high school biology, national test in the US ( http://apcentral.collegeboard.com/apc/members/courses/teachers_corner/218954.html ). Note, however, that these are likely isolated cases rather than the norm, as a survey revealed that in 2008 bioinformatics was still absent from the classroom in the US [11] , and likely elsewhere.
The “Bioinformatics@school” Program
We run a Bioinformatics Core at the Instituto Gulbenkian de Ciência, in Portugal, that has long been engaged in outreach activities. In 2007, we decided to implement a genomics/bioinformatics activity that would enable enquiry-based learning; link to the national curricula in biology in secondary education; introduce students to bioinformatics, genomics, and molecular biology, areas that underlie many of the key debates and products in our societies; foster active learning, making use of technologies that younger generations are increasingly comfortable with; and help teachers incorporate the latest advances in science into their teaching. We developed a prototype system that we describe the following components of here: its development, implementation, and the results of nearly five years of activity.
We developed and implemented a framework for the use of bioinformatics-based research projects in high schools to support the life-sciences curricula, which we named, in Portuguese, “Bioinformática na escola,” loosely translating to “Bioinformatics@school.” It consists of research projects that may be conducted independently by high school students of different ages, either under direct teacher supervision or as homework. Each work unit in a research project is designed to be carried out in 90 minutes, which is a standard class length in Portuguese high schools. We implemented it as a web portal ( Figure 1A, 1B )— www.bioinformatica-na-escola.org . Although primarily written in Portuguese, the site makes use of external, freely accessible bioinformatics tools and databases available in English. This is not a problem for Portuguese high school students that typically start learning English at the age of nine. Because of the dependency on external sites, we have ensured that students are given alternative access to any data on which progression to the following activity depends.
- PPT PowerPoint slide
- PNG larger image
- TIFF original image
( A ) Screenshots of the home page. ( B ) Screenshots of exercises pages.
https://doi.org/10.1371/journal.pcbi.1003404.g001
The whole program is structured as a set of projects with open-ended questions. A project may have a single activity or several, each having focused questions. Answering these focused questions enables students to discuss and/or solve the project's main question. Individual activities in the multi-activity projects were designed to also be used independently (discussed below). The concept lends itself both to classroom use, individually or in pairs, or as homework. We designed individual activities to explore specific concepts that are part of the school curriculum and the projects to be coherent with the curriculum of specific age groups, with the active collaboration of teachers in choosing the topics.
Projects are organized as follows. Once a project is selected, the student has access to a page that summarizes the problem to be solved and a link to the first activity. As the student enters one activity, s/he is presented with a sequential series of pages, each giving some background information on the specific problem the student has to follow and a brief description of the bioinformatics resources/tools to be used. At the end of each activity, the student is taken to a summary page (“now you know that…”) with an overview of the basic concepts that were addressed in the activity. All pages include links to additional information on key concepts, mostly on Wikipedia ( www.wikipedia.org ), including explanations about the resources and algorithms used in the analysis. Once the activity/final activity is complete, the student is taken to a summary page that reviews the key concepts of the project as a whole and a series of questions that act as primers for discussion amongst students and with the teacher(s) (see Figure 1A, 1B for screenshots). Table 1 summarizes the questions, concepts, and software and resources that are covered in each individual activity of “Vision,” the first multi-activity project that we have implemented in the Bioinformatics@school portal (further detailed in Text S1 ). Its implementation in schools is discussed below.
https://doi.org/10.1371/journal.pcbi.1003404.t001
Implementing “Bioinformatics@school”
Iterative development of project modules.
We started to develop “Bioinformatics@school” as a pilot project in 2007, in close collaboration with high school students and teachers. The first stage of the project consisted of identifying the topics within the high school curricula that would be amenable to bioinformatics treatment, as well as the ideal school year for the pilot to be developed. We chose 12th grade biology, in the last year of high school in the Portuguese educational system, as their curricula included multiple themes that were ideal to address using bioinformatics (such as genes, genomes, genetics, evolution, mutation, etc.), and these students would all have had several years of English language schooling (discussed above). The next phase of the project consisted in the enrollment of schools. Two secondary schools located in the Lisbon area were recruited, representing two different demographics. Escola Secundária Miguel Torga (ESMT), in Queluz, is a large suburban school that covers a variety of social strata, while Escola Secundária Quinta do Marquês (ESQM), in Oeiras, is located in a high income area with high levels of graduates and post-graduates. We engaged seven 12th grade Biology teachers, two from ESMQ and five from ESMT. One hundred and fifty students were involved in this initial pilot phase, representing multiple science-related career ambitions, ranging from engineering, health, biology, psychology, sports, etc.
We conceived the general framework described above and developed the first full project consisting of five activities, aimed at understanding how animals see different colours (“Vision” project, Table 1 ). We chose this question because we believed it to be sufficiently intriguing and relevant to engage the students (natural variants cause differential colour perception between species and between different people), but also for practical reasons—the biology of light detection via opsins is well understood, as is the 3D structure of opsins. The aim of the project is to motivate a discussion about evolution, molecular mechanisms, and disease, all inferred from bioinformatics analysis, while helping teachers and students engage with specific topics of the Life Sciences curriculum via the individual bioinformatics activities.
An innovative aspect of this project was the collaboration between scientists, teachers, and students on different aspects of the development, implementation, and testing—a three-way dialogue with continual updating in response to feedback of students and teachers. The development was iterative, first within our Bioinformatics Unit, and then in discussions with teachers. Once a first prototype was in place, one of us (IM) went to the schools to guide the students in the first activities of the project, with the help of the teacher. Student feedback was then used to improve the activities, in terms of rationale, language, and presentation.
Teacher training
Keeping up to date with the rapid developments in genomics and bioinformatics represents a challenge for high school teachers, particularly when many may have completed their training decades ago. In fact, in our experience, bioinformatics is a novel subject area to most Portuguese high school teachers. This led us to implement a parallel teacher-training program, again co-developed with the first set of teachers. Teachers were trained by bioinformatics experts, with the main goal of training to guide the students in the bioinformatics-based projects and to understand the basics of the bioinformatics methods and resources underlying each activity. We developed a teacher's manual that described the activities step by step and provided additional background information for the teacher to be comfortable with all the concepts in each activity. The teacher training consisted of having the teachers follow the same activities as the students, with the help of the teacher manual and under the supervision of a bioinformatician. We have expanded the teacher training to include seminars about applications of bioinformatics to human health, biotechnology, etc. A typical teacher training program lasts about 25 hours.
Extending the Program and Sustainability
After the successful pilot stage in 2007 the project has expanded to other geographical areas of Portugal. Thirty three new schools have joined the program, some via previously engaged teachers who took the program with them when they moved to a new school, others by new teachers who contacted us, after hearing about the project, and asked us to help them implement it in their schools. In total, schools of 11 municipalities in four Portuguese districts are currently following the program ( Figure 2A ). On their own initiative, some teachers have adapted the individual activities within the “Vision” project for use with younger students. They have also picked individual or subsets of activities and re-used them with different genes/systems, combining them in novel ways, to create new projects. They have also engaged with us to develop new projects (“Tasting Bitter”) and activities (“Tree of Life”). Furthermore, teachers are recruiting and training new teachers to use our activities. Interestingly, we observed that teachers tailored the activities to their own teaching style, some engaging the students almost at every mouse click, whereas others would only focus on explaining the basic ideas at the beginning and then discussing the outcomes at the end.
( A ) Map of schools participating, coloured by year of joining the project. ( B ) Summary of responses to confidential questionnaire. ( C ) Knowledge acquisition—each dot represents one class and the average score that students in that class achieved in the test before and after finishing the “Vision” project. ( D ) Confidence—each dot represents one class and the percent of answers that students in that class answered True or False, as opposed to answering “I don't know,” before and after finishing the “Vision” project.
https://doi.org/10.1371/journal.pcbi.1003404.g002
One aspect that worried us from early on was how to motivate teachers to engage with projects like ours when they are overwhelmed with teaching and administrative work. We realized that certification of the training is important for career progression within the Portuguese public educational system. We invested in having the project certified for teachers' continuous professional development by the national educational authorities (Conselho Científico-Pedagógico da Formação Contínua), thus making engagement with Bioinformatics@school even more appealing to the teachers. Recently we established a partnership with a teacher training centre (Centro de Formação Lezíria - Oeste) to enable other teachers in another Portuguese region to receive training in Bioinformatics activities and further promote the decentralization of “Bioinformatics@school.”
We have, thus, reason to believe that the use of the Bioinformatics@school platform is spreading on its own, with a dynamic beyond the ability of the small staff at the Bioinformatics Core that developed it.
Impact Assessment
We wished to evaluate how students and teachers perceive the program and to what extent it is an effective learning tool. These are independent questions that we addressed using different approaches. Conversations with students participating in the program suggested that they were motivated to participate in “hands-on” activities We implemented a simple confidential questionnaire to capture students' views beyond anecdotal opinions, that was given to 150 students (two schools, seven classes), during the implementation phase of the project. The results are shown in Figure 2B and reveal that the majority of the students found the approach used in this project more motivating than traditional teaching methods (58%), and enjoyed participating in it (60%). About 80% considered it had not been a waste of time and 80% would recommend the project to next year's colleagues. This type of questionnaire is useful in gauging attitudes towards the program, but it has caveats, namely that the students at this stage were very involved with the development of the Bioinformatics@school project and may be overly positive because of that. In addition, it gives no information about student learning. To address this, we devised a simple test on the concepts explored in the program, with “True/False/I Don't Know” answers ( Table S1 ). We asked four classrooms to take the test before and after the activities (this test was irrelevant for their grades). Plotting the percentage of correct answers per student before and after the activities ( Figure 2C ) revealed a dramatic increase in the proportion of correct answers, indicating that students actually gain knowledge. One surprising result was that the students appeared more confident after doing the activities: they increasingly answered the test questions as false or true, rarely using “I don't know” ( Figure 2D ). Since most of the concepts in our activities are part of the school curricula and were being covered in class by their teachers, we speculate that the decrease in “I don't know” answers may indicate that students are less afraid of venturing answers to scientific questions after doing the activities. Fear of science (“too complicated”) has been pointed out as a reason for the decreasing number of students pursuing scientific degrees [1] . This is an exciting finding that we will need to specifically evaluate further in the future. Regarding the teachers, we developed the whole program in close collaboration with them and obtained continuous feedback on the content and presentation. Although we have not as yet conducted a systematic evaluation of teachers' views about the program, the continuous contact with the currently more than seventy teachers involved suggests to us that this is a useful teaching/learning tool. In particular, teachers mention that these activities allow them to overcome the lack of laboratory-based practicals associated with some of the content in the curricula, like genetics and molecular biology. The fact that the program is spreading, with new teachers and schools recruited by word of mouth by the teachers themselves, underscores its interest and usefulness to teachers.
Discussion and Future Directions
In summary, we implemented a set of bioinformatics multi-activity research projects designed to enable enquiry-based learning in high schools. Assessment of this project has shown that students find it enjoyable and teachers believe it to be useful as a teaching aid. Objective assessment of knowledge acquisition revealed a clear positive effect both in knowledge and confidence of the students. Teachers have taken the initiative to adapt the activities to their own teaching settings and are also recruiting other teachers, which gives us further confidence in the usefulness of this project.
We have focused the projects on addressing specific biological questions, to serve the Life Sciences curriculum. This means that we don't explore the algorithmic or technological side of bioinformatics. For the future, we hope to engage teachers from mathematics, information technology, physics, and chemistry to develop projects that can serve the curricula of those particular subjects.
Recently, Form and Lewitter proposed a simple set of ten rules to guide the use of bioinformatics in high schools [12] . While these were not available at the time we were developing this project, it is interesting to note that we independently “discovered” several of these principles. We implemented individual activities with clear, simple goals (rule 1) that built on each other (rule 4), enabling students to “discover” concepts on their own (rules 5 and 8). Throughout this project we were always mindful that these activities need to serve the pre-existing curricula (Rule 3). In the future we would like to have multiple projects serving the same concepts that would allow students in each class to choose an individual project (rule 6: personalization) that they could then present and contrast to other projects pursued by their colleagues (rule 10: produce a product). We would like to develop a mapping of activities to concepts in the curricula, so that it becomes even easier for teachers to mix and match the individual activities to different contexts, thus using our project as a means to empower the teachers. Based on our experience in setting up this program, we would like to suggest two additional “simple rules” that we believe to be important when developing contents to be used in high schools:
- Engage teachers and students in the development of the activities, as a means of empowering them and ensuring that the end product meets all the cognitive and pedagogical requirements (e.g., engage the teachers in choosing the specific topics of the curricula that would benefit from bioinformatics-based projects as well as to advise on time or practical constraints on their use in the school setting; engage both teachers and students to identify weak/unappealing points in the contents and formats of the activities and to suggest better solutions, etc.).
- Evaluate the impact of the activities on engagement/enthusiasm for science and, in particular, on knowledge acquisition, as demonstrated effectiveness is the best way to get bioinformatics into the classroom. In our opinion, perpetuating useless activities just for the sake of their perceived modernity is more likely to harm the use of bioinformatics as a tool for high school science education than to advance it.
Our program was developed in Portuguese as it is targeted at Portuguese students. While this gives us potential access to a universe of more than 200 million Portuguese speakers worldwide, it is hard to use by speakers of other languages. We have started translating the whole set of activities into English, thus making Bioinformatics@school accessible to a much larger target audience. Equally, besides developing novel activities, we would like to adapt those from successful experiments elsewhere, and in due time will contact their authors directly. In this regard, the existence of a central repository of bioinformatics exercises to be used in high schools, with clear explanations according to pre-defined standards and mapping to specific concepts, would facilitate the adoption of bioinformatics in high schools. Developing standards and repositories should come naturally to the bioinformatics community!
Supporting Information
Questionnaire for impact assessment.
https://doi.org/10.1371/journal.pcbi.1003404.s001
Activities in the “Vision” project.
https://doi.org/10.1371/journal.pcbi.1003404.s002
Acknowledgments
We wish to thank all the high school teachers who have engaged with the Bioinformatics@school project, in particular Lurdes Louro (ESMT, Queluz), Filomena Delgado (ESQM, Oeiras), and Teresa Palma (Escola Secundária de Camões [ESdeC], Lisboa). We wish also to thank for their generosity and enthusiasm the initial batch of students from ESQM and ESMT who helped us develop ever better activities. Finally, we thank João Garcia and Gil Neto at the IGC, who provided invaluable IT support. We also wish to thank the Instituto Gulbenkian de Ciência for hosting this program.
- View Article
- Google Scholar
- 2. Kang K (2012) Graduate Enrollment in Science and Engineering Grew Substantially in the Past Decade but Slowed in 2010. National Center for Science and Engineering Studies. Available: http://www.nsf.gov/statistics/infbrief/nsf12317/ . Accessed 20 December 2013.
- 3. Kearney C (2010) Efforts to Increase Students' Interest in Pursuing Mathematics, Science and Technology Studies and Careers. Wastiau P, Gras-Velázquez A, Grečnerová B, Baptista R, editors. Brussels: European Schoolnet. Available: http://cms.eun.org/shared/data/pdf/spice_kearney_mst_report_nov2010.pdf . Accessed 20 December 2013.

An official website of the United States government
The .gov means it’s official. Federal government websites often end in .gov or .mil. Before sharing sensitive information, make sure you’re on a federal government site.
The site is secure. The https:// ensures that you are connecting to the official website and that any information you provide is encrypted and transmitted securely.
- Publications
- Account settings
Preview improvements coming to the PMC website in October 2024. Learn More or Try it out now .
- Advanced Search
- Journal List
- J Community Genet
- v.12(4); 2021 Oct

Supporting teachers to use genomics as a context in the classroom: an evaluation of learning resources for high school biology
Lauren mcknight.
1 Kinghorn Centre of Clinical Genomics, Garvan Institute of Medical Research, Darlinghurst, NSW Australia
2 School of Biotechnology and Biomolecular Sciences, University of New South Wales, Sydney, NSW Australia
Angela Pearce
Amanda willis.
3 St Vincent’s Clinical School, UNSW Medicine and Health, University of New South Wales, Sydney, NSW Australia
Mary-Anne Young
Bronwyn terrill, associated data.
Resources evaluated in this paper are available from www.garvan.org.au/kccg-teachers .
Not applicable.
As genomics becomes embedded into healthcare, public genomic health literacy is critical to support decision-making for personal and family health decisions and enable citizens to engage with related social issues. School science education has the potential to establish the foundations of genetic and genomic literacy. The concept of literacy extends beyond conceptual understanding of biological principles to familiarity with the applications and implications of genetics, critical thinking skills, and socioscientific reasoning. We developed and evaluated a suite of resources for teaching genetics and genomics in the Australian senior biology syllabus for students aged 16–18 years. The aim was to increase teachers’ knowledge and confidence to teach genetic and genomic content, and their capacity to develop robust genetic literacy in their students. Resources, including an inquiry-based task and five associated lesson plans, were developed and made freely available to teachers online. Evaluation was undertaken between December 2019 and March 2020 with a post-use survey emailed to teachers who had accessed the resources. The 56 teachers who responded rated the resources as high quality, engaging, and well-aligned with the syllabus. Teachers who used the resources self-reported increases in their knowledge and confidence in teaching. They also perceived positive outcomes in their students, reporting that the resources deepened their students understanding of genetic concepts, helped them to consider social and ethical issues, and developed their higher order thinking skills. Findings may inform future interactions with high schools to improve genetic literacy.
Supplementary Information
The online version contains supplementary material available at 10.1007/s12687-021-00550-3.
Introduction
Community awareness and understanding of genetics and its applications are important for the implementation of genomics into healthcare (Green and Guyer 2011 ; Ricciardi and Boccia 2017 ).
There have been significant international research efforts exploring the best ways to incorporate the emerging science of genomics into genetics education (Airey et al. 2019 ). In light of the varying definitions in the literature (Haury and Nehm 2012 ; Airey et al. 2019 ), we will consider genetics as an overarching term, genomics as the study of an organism’s complete set of genetic information, and genomic applications as the real-world use of genetic information generated through genomic technologies. Familiarity with genetics and genomics can facilitate personal informed consent in research and genomic data sharing (Middleton et al. 2020 ) as well as participation in civic discussions around applications of genomics in health and other settings (Hilton et al. 2011 ; Yacoubian 2018 ). However, studies consistently show low awareness and understanding of genetic concepts among the general population (Chapman et al. 2019 ; Haga et al. 2013 ; Ong et al. 2018 ).
Genetic literacy
Genetic literacy can be defined as “sufficient knowledge and appreciation of genomics principles to allow informed decision making for personal well-being and effective participation in social decisions on genetic issues” (Syurina et al. 2011 ). High school science has been identified as an opportunity to inform health and scientific literacy and specifically, genetic literacy (Boerwinkel and Waarlo 2011 ; Stern and Kampourakis 2017 ; Whitley et al. 2020 ). Boerwinkel et al. ( 2017 ) proposed three types of knowledge required for genetic literacy in non-scientists: key conceptual knowledge; sociocultural knowledge about contemporary applications and implications of genetics; and epistemic knowledge such as higher order thinking skills and critical literacy. These components align with earlier frameworks of socioscientific issues (SSI)-based science education (Sadler et al. 2007 ; Zeidler 2015 ), in which learning is centred around contemporary, complex, and controversial issues that require both scientific and moral reasoning.
Evidence-based educational approaches
Although conflicting definitions of scientific literacy and conflicting educational philosophies exist globally (Zeidler 2014 ; Zeidler et al. 2019 ), the Australian high school curriculum (for students 12–18 years of age) supports inclusion of SSI in science teaching along with student inquiry (Goodrum and Rennie 2007 ; National Curriculum Board 2008 ). The science syllabuses in Australia are underpinned by a set of general capabilities, each with a detailed continuum of learning (Australian Curriculum and Assessment & Reporting Authority 2021 ). One of these is “critical and creative thinking”, which includes the higher order thinking skills such as inquiry, problem solving, reflecting, and evaluating. Authentic student inquiry in real-world contexts has been well established as an effective method and practice of teaching for science and related issues (Goodrum and Rennie 2007 ; Nam and Chen 2017 ; Rönnebeck et al. 2016 ; Sadler et al. 2007 ), particularly in genetics (Brush et al. 2016 ; Wells 2017 ). Effective inquiry-based learning is supported by elements of student choice (Dorfman et al. 2017 ) and scaffolding, which refers to activities and supports that intentionally develop required skills (Belland et al. 2015 ; Brush and Saye 2002 ).
Neither SSI-based nor inquiry-based approaches to science education are new, although there remain barriers to widespread implementation (Aivelo and Uitto 2019 , Fitzgerald et al 2019 ). High school teachers have traditionally found genetics and genomics difficult to learn and teach (Kidman 2010 ; Steele and Aubusson 2004 ), and report a lack of support and resources (Goodrum and Rennie 2007 ). The need for teacher professional development (TPD) and teacher-focused resources has been emphasised to support teaching genetics (Larue et al. 2018 ; Stern and Kampourakis 2017 ). Links between school science teaching and scientific research institutes have been encouraged as means to engage students in science and to increase teacher confidence and capacity (Goodrum and Rennie 2007 ; Tytler et al. 2015 ).
Additional evidence-based educational approaches to support genetic literacy include developing students’ argumentation skills (Dawson and Venville 2013 ) and their ability to apply “system thinking” to reconcile the molecular and heredity conceptualisations of genetics (Duncan and Reiser 2007 ; Haskel-Ittah and Yarden 2018 ).
Context and resource development
In this paper, we describe the development and evaluation of a suite of contemporary genetics and genomics-focused resources to support high school science teachers. The aim was to increase teachers’ knowledge and confidence, and their capacity to develop robust genetic literacy and critical thinking skills in senior biology students, aged 16–18 years. It was essential to align the resources with local curricula and incorporate evidence-based approaches to genetics education. We aimed to support an inquiry-based approach, aligning with trends in teaching methods and practice as well as a newly introduced requirement of the state-wide biology syllabus for 15-hour depth studies. Depth studies are student-led projects that expand on an area of the curriculum and are tied to mandatory assessment of skills in scientific inquiry (NSW Education Standards Authority 2017 ; Eaton et al. 2019 ).
In 2018, a new senior biology syllabus was introduced into the Australian state of New South Wales (NSW). In May 2018, we distributed a needs assessment survey to local science teachers, which included questions on self-rated confidence to teach genetics content, perceived needs for content updates and resources, and preferences for style of teaching support. The survey was emailed to a convenience sample of eight known teachers and posted to existing local science teachers’ Facebook groups with thousands of members. Fifty-three science teachers responded, who taught in different locations (metropolitan, regional, rural) and school settings (public, religious, independent). However, these respondents may represent teachers who were particularly motivated or interested in genetics teaching. Teacher responses identified strong interest in resources designed specifically for senior biology including depth studies and lesson plans. Needs related to newer syllabus content, such as gene/environment interactions and population genetics, with more than half of the teachers indicating a need for resources and content updates in DNA sequencing and medical applications of genetic technologies.
Based on this identified set of needs and an audit of the resources teachers reported using, a complementary suite of resources was developed by a clinical genomics education team within an Australian medical research institute (MRI), in consultation with practising biology teachers. A program logic model was applied (based on Nisselle et al. 2019 ) to prioritise, develop, and evaluate the resources. Full details of the resource development are reported separately and the resources are available at http://www.garvan.org.au/kccg-teachers .
The lesson plans could be used as stand-alone curriculum-aligned activities, or in support of an extended student inquiry task (depth study) titled “Genetic Technologies, DNA & Disease” (see Table Table1). 1 ). The depth study allowed students to choose study topic areas within a guiding structure that encouraged consideration of SSI and critical thinking. The authentic context for the task was based on the ACCE model (analytic validity, clinical validity, clinical utility, and associated ethical, legal, and social implications) for evaluating genetic tests (Pitini et al. 2018 ). This approach was simplified for a student audience as an evaluation of risks and benefits.
Description of resources
The resources were made freely available via a website ( www.garvan.org.au/kccg-teachers ), with suggested answers for activities accessed via Dropbox™ on request by teachers. The resources were presented in workshops for 152 teachers at TPD conferences in 2018–2019, both off- and on-site at the MRI. They were also shared in a Facebook group for biology teachers within the state (NSW). Google analytics indicated that the resource pages received 8,598 visits in the period, and 278 teachers requested Dropbox access in this time.
Evaluation method
The collection of evaluation data was for quality improvement purposes and does not reflect human research for the purposes of human research ethics committee review (National Health and Medical Research Council 2014 ).
Evaluation design
A survey was developed to assess the intended outcomes of the resources as identified in the program logic framework. These included increased teacher knowledge, confidence, and skills, and positive outcomes for students. The survey (see Online Resource 1 ) contained six sections: participant characteristics; resource usage and modification; resource utility and quality (Likert scales); student learning outcomes (Likert scales); overall teacher outcomes (Likert scales); and open questions. The survey was piloted with four teachers who sense- and error-checked the content.
Data was collected using a post-only survey design in two parts: a short primary survey covering all resources for teachers who had interacted with the resources, and a more in-depth survey focussing on the depth study for teachers who had expressed written or verbal interest in providing feedback on this resource. Teachers who requested access to the resources during 2018–2019 were invited by email to complete an online survey in December 2019, regardless of whether they had used the activities. The primary survey (see Online Resource 1 ) was sent to 241 teachers, and 56 responses were received. Of the 37 teachers who had indicated interest in providing feedback on the depth study, nine teachers responded. These responses were not included in the quantitative analysis, but responses to open questions (see Online Resource 2 ) were used to supplement the evaluation.
Data analysis
Quantitative data from the primary survey was analysed by weighting responses from 1 (strongly disagree) to 5 (strongly agree) for the purpose of statistical comparison using IBM SPSS Statistics software. Descriptive statistics were used to provide a snapshot of the sample and describe the distribution of answers to survey items. Correlation analysis was conducted to assess relationships between variables. Group differences were assessed using chi square analysis (categorical data) or independent samples t test (weighted scores were treated as continuous variables for ease of analysis) and p was set at 0.05. Open answer questions from the primary survey were coded using content analysis by two researchers (AP, LM) and are described along with illustrative quotes. Identifiers for the quotes indicate whether the teacher used the depth study (DS) or only lesson plans (LP). Quotes from the nine teachers who responded to the detailed survey about the depth study (DS30-38) are included where they add further insight.
Evaluation results
Survey respondent characteristics.
Fifty-six teachers responded to the primary survey (response rate 23%). Table Table2 2 summarises their demographic data. All three school sectors in the state—public (45%), independent (38%), and Catholic (18%)—were represented in responses. The majority were from the capital city and metropolitan areas (66%) with 20% from regional and 9% from rural schools. These profiles aligned with averages for the state, other than an overrepresentation of independent schools, which employ 22% of teachers (Australian Bureau of Statistics 2019 ). Teachers taught an average of 19 senior biology students, and about half of the teachers had sole responsibility for planning assessment tasks, with the others having shared responsibility. Forty-five percent of respondents had attended a TPD workshop related to the resources.
Description of respondents’ characteristics ( N = 56)
Teacher engagement with resources
The majority of teachers (82%) had used at least one of the resources in their biology classes, and most had read the resources that were not used (Fig. 1 ). Teachers who used resources as part of the depth study (60%) used an average of 3.3 of the 5 lesson plans, compared to 1.9 for those who used only lesson plans. Each lesson plan was used by about half of the respondents, except for the ethics-focused activity “Genetic Testing Issue Scan” which was used by only 35%. Many teachers modified the resources for use in their classroom. Of teachers who used each resource, the proportion who modified ranged from 15% for Tiny Genome to 65% for the depth study. The Tiny Genome and Research Matrix lesson plans were specifically mentioned by multiple teachers in an open question about the “most successful aspect” of the resources.

Proportion of respondents who indicated interacting with each resource, either by using them in the classroom as written (black bars); using them in the classroom with modification (grey bars); or reading them (hatched bars)
Evaluation of resource quality
Overall, teachers rated the resources highly. Most teachers (94%) agreed or strongly agreed that the resources were high quality, aligned with the syllabus, and adaptable (see Online Resource 3 ). Eighty-five percent agreed that the resources were easy to access and use, and 78% agreed that the materials were appropriately pitched for their students. The quality of the resources was reflected in teachers’ comments about the “most successful aspect” of the resources including the currency and relevance of the resources, their professional presentation, and the way the resources were structured and aligned to the syllabus. For example:
“The way it was specifically written to meet syllabus requirements”. (DS12) “All the supporting activities to consolidate and scaffold for understanding”. (DS38)
The lower scores relating to ease of use and being appropriately pitched for students were supported by comments regarding the most challenging aspect, which frequently related to the difficulty of the tasks and the need to modify resources for lower ability students. For example:
“The high level of these resources was at times challenging”. (DS18)
Likewise, many responses to the question “what else would you need to make the resources most helpful” centred on simplification, either of length, difficulty, or the instructions associated with the resources. For example:
“Making tasks accessible to the full range of student ability and engagement”. (LP5)
Outcomes for students
Teachers reported positive outcomes for students after they used the resources (Fig. 2 ). Most teachers agreed or strongly agreed that the resources were relevant and engaging (97%), were easy for students to follow (87%), and deepened student’s understanding of genetic concepts (91%). There was also strong agreement that the resources supported the consideration of ethics and social issues (93%), developed higher order thinking skills (95%), and prepared students for future decision-making (87%). The lowest scoring variable related to preparing students for their final exams (66% agreement). There was a strong correlation between agreement that the resources helped students consider ethical and social issues and that they developed higher order thinking ( r = 0.79, p < 0.001). Teachers who agreed that the resources developed higher order thinking were also more likely to agree that the resources deepened students’ conceptual understanding ( r = 0.63, p < 0.001) and prepared them for future decision-making ( r = 0.64, p < 0.001).

Responses to questions regarding outcomes for students ( n = 47). For each statement, respondents selected either strongly agree (black bars), agree (grey bars), unsure (diagonally hatched bars), disagree (unfilled bars), or strongly disagree (horizontally hatched bars)
Echoing the quantitative findings, the development of higher order thinking skills was frequently mentioned by teachers in the “most successful aspect” responses. This included references to development of analytical thinking, problem solving, and information processing skills. For example:
“Good development of student analytical skills in Tiny Genome Task”. (LP5) “Stretching student’s thinking beyond ‘right’ and ‘wrong’ answers”. (LP14) “Disease matrix forced students to process information”. (DS2) “we had already been teaching them about mutations etc., but suddenly the light bulbs went on”. (DS34)
Teachers also commented that the resources were interesting, relevant, and engaging for students, while also deepening their understanding of genetic concepts. They commented on the “current”, “high tech” content, reflecting its development by a research institute. For example:
“Current up to date material which engaged students and made them think”. (DS20)
Respondents to the detailed survey also emphasised the relevance of the resources, including the importance of the connection with an MRI.
“Many students rated it as the highlight of Biology. It was relevant to their lives, interesting and really useful for their understanding of genetics”. (DS34) “I feel the link to a real genetics research organisation made it meaningful to the students”. (DS38)
Outcomes for teachers
Most teachers felt that they had the background knowledge to use the resources (93%), and they also agreed that using the resources (as well as attending any associated TPD) increased both their background knowledge (96%) and their confidence in teaching genetics (90%) (Fig. 3 ). There was a significant positive correlation between agreement with increased knowledge and increased confidence ( r = 0.84, p < 0.001). Respondents also indicated that they felt supported in teaching genetics (89%) and would likely use the resources again next year (91%). Only 44% agreed that the resources lightened their workload. Seventy percent of teachers indicated that the resources resulted in spending more time on social and ethical considerations. Mean weighted scores for this variable were higher among teachers who used the depth study ( M = 4.45) compared to those who used only lesson plans ( M = 3.57) [ t (51) = 2.01, p = 0.050].

Responses to questions regarding outcomes for teachers ( n = 54). For each statement, respondents selected either strongly agree (black bars), agree (grey bars), unsure (diagonally hatched bars), disagree (unfilled bars), or strongly disagree (horizontally hatched bars)
Teachers who agreed that the resources decreased their workload (44%) rated the resources more highly on multiple quality indicators compared to those who were unsure or disagreed with this statement. These data were supported by comments from teachers including:
“…saved me a lot of time as I did not need to research suitable genetic diseases myself. The marking rubric and outline for the task were excellent”. (DS8) “As students worked through scaffold at different pace, it allowed me to target support as needed”. (DS19)
The greatest differences in mean weighted scores related to the resources being appropriately pitched ( M = 4.48 vs M = 3.77, t (51) = 2.80, p = 0.002), deepening students’ understanding of genetics ( M = 4.55 vs M = 4.00, t (43) = 3.27, p = 0.002), and whether the teacher felt supported ( M = 4.57 vs M = 3.91, t (52) = 3.45, p = 0.001). There was no significant difference in the number of resources used or modified between teachers who agreed that their workload was reduced with those who did not. These data were supported by teachers commenting that they found the amount of information overwhelming. For example:
“Almost an overload of resources—picking and choosing which ones I would use and simplifying for the purposes of our task was a bit of a challenge initially”. (DS8) “all was nice but it took time for us teachers to get on top of all the info provided including knowledge on the genetic diseases”. (DS33)
There were no significant differences in mean weighted scores for any of the variables when comparing teachers who attended TPD workshops and those who did not. However, in open comments, multiple teachers suggested that additional TPD opportunities, school support, or time for TPD would be needed to make the resources most helpful. Indeed, comments relating to time restraints were common across both the challenges and needs open questions, both in relation to workload and also teaching time. For example:
“we just do not have that amount of time (even in the depth study …) to do all that work”. (DS15)
Teacher outcomes identified in the “most successful aspect” responses related to feeling supported, and having access to answers and reference materials. The nine teachers who responded to the detailed depth study-focussed survey identified ways in which the resources differed from their usual teaching approach. They described taking a more student-driven approach with opportunities for active learning, as well as placing a greater emphasis on ethics. For example:
“the group discussions were great. I usually do this stuff ad hoc. But the cards and other stimulus were great. To make the discussion happen and everyone had to be in it”. (DS30) “I wouldn't normally use role play in my classroom but I think it worked rather well so I'm planning to do it again”. (DS36)
A suite of resources was developed for teachers of senior biology that supported the teaching of human genetics and genomics and associated SSI within the redesigned senior biology syllabus in NSW, Australia. Resources included a student-led inquiry task or “depth study”, and modular, adaptable lesson plans. These were widely accessed, and implemented by many teachers beginning in 2019. The 56 teachers who responded to an evaluation survey reported that the resources were high quality, easy to use and adapt, and well-aligned with the syllabus. Teachers felt that using the resources supported their students to understand genetic concepts, consider social and ethical issues, and develop higher order thinking skills. Teachers also reported feeling supported in their teaching of genetics and increases in their confidence and background knowledge. In open comments, respondents emphasised the engaging, relevant, and authentic nature of the resources. Suggestions were also made to include options to simplify the resources, to make them more accessible to students of differing ability levels.
Teachers valued that the resources were both interesting and engaging but also well-aligned with the syllabus. The link with the MRI was directly mentioned in one comment as making the learning meaningful to students, and the currency and relevancy of the resources were also appreciated by multiple teachers. This dynamic has been framed as “bridging communities of practice” and has been shown to have benefits for students, teachers, and scientists (Tytler et al. 2015 , 2017 ). Medical research institutes (MRIs) and universities are well placed to support secondary teachers with current, relevant, and authentic contexts for teaching scientific concepts (Haury and Nehm 2012 ). In the case of these resources, educators employed by the MRI served in the role Tytler et al. ( 2017 ) call “brokers”, bridging the gap between the scientific developments in clinical genomics and the requirements of high school curriculum and assessment.
Teachers reported that the resources enhanced students’ understanding of genetic concepts as well as their higher order thinking skills. The depth study aligns closely with the SSI teaching and learning (SSI-TL) framework of Sadler et al. ( 2017 ), in which a contemporary issue is used as the stimulus for exploring science content as well as socioscientific reasoning. SSI-based approaches to science education specifically focus on critical thinking skills, but have also been demonstrated to support effective development of conceptual knowledge (Sadler et al. 2016 ). The depth study also aligns with frameworks of inquiry-based learning, particularly elements of critique and evaluation as emphasised by the National Research Council ( 2012 ). Some elements of inquiry are mandated by the syllabus requirements for depth studies (NSW Education Standards Authority 2017 ) including students’ choice in developing and evaluating their own inquiry questions and communicating the results of their investigation. There is recognised synergy between SSI- and inquiry-based approaches (Evans and Dolin 2018 ), and they have previously been combined to enhance student engagement with science for citizenship (Amos and Levinson 2019 ). Supporting student argumentation in SSI and open inquiry are two areas that have been identified as important for targeted professional development (Carson and Dawson 2016 ; Gulamhussein 2013 ). Teachers’ suggestions of additional professional development to support implementation of these resources are consistent with this identified need.
In addition to increases in background knowledge and confidence, teachers indicated shifts in their teaching approach as a result of using the resources, such as the inclusion of more active learning and class discussions. However, not all teachers agreed that they spent longer on social and ethical issues than they would have otherwise. It was not clear if this reflected a lack of focus on these aspects of the resources or a suggestion that responding teachers already valued and prioritised this aspect of teaching. Teacher confidence and preferences are known to vary widely in this area (Aivelo and Uitto 2019 ); thus, a potential future direction for research might be to examine the extent to which teachers were able to support and assess the development of socioscientific reasoning. Although uptake of the ethics-focussed lesson plan was lower than others, the depth study focused on balancing risks and benefits and supporting claims with evidence, which are key components of socioscientific reasoning (Karahan and Roehrig 2017 ), and is also found in ethical thinking frameworks (Buntting and Jones 2020 ).
Some findings from this evaluation may be transferrable to future interactions between scientific institutes or professions and teachers. Specifically, teachers confirmed that they value modular, curriculum-aligned resources and require adaptability to a range of student ability levels. Student engagement and interest is prioritised by teachers, and inclusion of some simplified ready-to-go versions of resources may increase their effectiveness and usage. Teacher workload is already high and bound by time restraints, and some teachers reported feeling overwhelmed by the volume of resources. Clarity should be a focus for both teacher- and student-facing resources. Although there were no significant differences found in this evaluation between teachers who attended teacher professional development and those who do not, multiple teachers expressed a need for time to familiarise themselves both the content and resources and TPD may help to fulfil this requirement.
Limitations of the evaluation methodology include the self-selecting group of participants and survey responders. Teachers who accessed the resources may represent those who would be most likely to value them, and those who used them most successfully may have been more likely to respond to the survey. The strengths and weaknesses of the resources identified by the evaluation are likely to represent genuine enablers and barriers to effectiveness. Teacher outcomes were self-reported, and student outcomes were evaluated only via their teachers’ perspectives, so social desirability bias cannot be ruled out. Future directions for evaluation of such resources could include direct measurement of student genetic literacy and socioscientific reasoning. If the required resources were available to support willing teachers, validated scales could be administered for each of these (Carver et al. 2017 ; Romine et al. 2017 ; Todd et al. 2017 ).
The resources developed relate to real-life applications of genetics and promoted active learning and authentic inquiry into the risks and benefits of genomic medicine. The resources aligned with the NSW biology syllabus as well as the three types of knowledge considered essential for genetic literacy (conceptual, sociocultural, and epistemic). Using a program logic approach, our evaluation established that the resources met the intended aims of producing high-quality and effective learning resources. The resources increased teacher confidence and knowledge and supported teachers to develop student genetic literacy, including higher order thinking skills, consideration of social and ethical issues, and deeper understanding of genetics concepts. Suggested improvements such as the inclusion of simplified options and additional teacher professional development may improve teacher familiarity with both the content and the resources. This would further enhance the resources’ usefulness and impact on the genetic literacy of Australian senior biology students.
Below is the link to the electronic supplementary material.
Acknowledgements
We would like to thank all of the teachers who contributed to the development and evaluation of the resources. In particular, we would like to thank John Duggan for his initial work on depth studies, and Jenni Hallett, Hannah Hindmarsh, and Aflal Sahabdeen for allowing the resources to be trialled in their classrooms.
Author contribution
Lauren McKnight and Bronwyn Terrill developed the resources. All authors contributed to the conception and design of the evaluation. Data collection and analysis were performed by Angela Pearce, Lauren McKnight, and Bronwyn Terrill. The first draft of the manuscript was written by Lauren McKnight and all authors reviewed the manuscript. All authors read and approved the final manuscript.
This evaluative study was supported by funding from the Kinghorn Foundation.
Data availability
Code availability, declarations.
Lauren McKnight is currently serving on the council of the Science Teachers Association of New South Wales, Australia (STANSW). B.T, A.P., A.W., and M-A.Y declare no competing interests. All educational materials and professional development were provided free of charge to teachers.
Publisher's Note
Springer Nature remains neutral with regard to jurisdictional claims in published maps and institutional affiliations.
- Airey J, Moore A, Davies R, Speakman D, Bennett J (2019) Viewed as a whole: syntheses of research evidence and teaching and learning support resources related to genomics education in schools. Wellcome Genome Campus, Hinxton. https://publicengagement.wellcomeconnectingscience.org/landscape-analysis-of-genomics-education-in-schools
- Aivelo T, Uitto A. Teachers’ choice of content and consideration of controversial and sensitive issues in teaching of secondary school genetics. Int J Sci Educ. 2019; 41 :2716–2735. doi: 10.1080/09500693.2019.1694195. [ CrossRef ] [ Google Scholar ]
- Amos R, Levinson R. Socio-scientific inquiry-based learning: an approach for engaging with the 2030 Sustainable Development Goals through school science. Int J Develop Educ Global Learn. 2019; 11 :29–49. doi: 10.18546/ijdegl.11.1.03. [ CrossRef ] [ Google Scholar ]
- Australian Bureau of Statistics (2019) Schools. https://www.abs.gov.au/statistics/people/education/schools/2019
- Australian Curriculum Assessment and Reporting Authority Critical and Creative Thinking learning continuum 2021 https://www.australiancurriculum.edu.au/media/1072/general-capabilities-creative-and-critical-thinking-learning-continuum.pdf . Accessed May 2021
- Belland BR, Gu J, Armbrust S, Cook B. Scaffolding argumentation about water quality: a mixed-method study in a rural middle school. Education Tech Research Dev. 2015; 63 :325–353. doi: 10.1007/s11423-015-9373-x. [ CrossRef ] [ Google Scholar ]
- Boerwinkel DJ, Waarlo AJ, editors. Genetics education for decision making. Freudenthal Institute for Science and Mathematics Education: Utrecht University, Utrecht, The Netherlands; 2011. [ Google Scholar ]
- Boerwinkel DJ, Yarden A, Waarlo AJ. Reaching a consensus on the definition of genetic literacy that is required from a twenty-first-century citizen. Sci Educ-Netherlands. 2017; 26 :1087–1114. doi: 10.1007/s11191-017-9934-y. [ CrossRef ] [ Google Scholar ]
- Brush T, Shin S, Shin S, Jung J, Gensic J, Glazewski K (2016) Design and implementation of a technology-supported socioscientific inquiry unit in high school biology. Int J Des Learn 7 10.14434/ijdl.v7i2.20124
- Brush TA, Saye JW (2002) A summary of research exploring hard and soft scaffolding for teachers and students using a multimedia supported learning environment. J Interact Online Learn 1:1–12 http://www.ncolr.org/jiol/issues/pdf/1.2.3.pdf
- Buntting C, Jones A (2020) Using Biotechnology to Develop Values Discourse in School Science. In Corrigan D, Buntting C, Fitzgerald A, Jones A (eds) Values in Science Education. Springer, Cham. 10.1007/978-3-030-42172-4_7
- Carson K, Dawson V (2016) A teacher professional development model for teaching socioscientific issues. Teaching science 62:28 https://asta.edu.au/generic/file-widget/download/id/1068
- Carver RB, Castera J, Gericke N, Evangelista NA, El-Hani CN (2017) young adults' belief in genetic determinism, and knowledge and attitudes towards modern genetics and genomics: the PUGGS questionnaire. PLoS One 12 10.1371/journal.pone.0169808 [ PMC free article ] [ PubMed ]
- Chapman R, Likhanov M, Selita F, Zakharov I, Smith-Woolley E, Kovas Y. New literacy challenge for the twenty-first century: genetic knowledge is poor even among well educated. J Community Genet. 2019; 10 :73–84. doi: 10.1007/s12687-018-0363-7. [ PMC free article ] [ PubMed ] [ CrossRef ] [ Google Scholar ]
- Dawson V, Carson K. Introducing argumentation about climate change socioscientific issues in a disadvantaged school. Res Sci Ed. 2018 doi: 10.1007/s11165-018-9715-x. [ CrossRef ] [ Google Scholar ]
- Dawson V, Venville G. Introducing high school biology students to argumentation about socioscientific issues. Can J Sci Math Technol Educ. 2013; 13 :356–372. doi: 10.1080/14926156.2013.845322. [ CrossRef ] [ Google Scholar ]
- Dorfman B-S, Issachar H, Zion M. Yesterday’s students in today’s world—open and guided inquiry through the eyes of graduated high school biology students. Res Sci Ed. 2017 doi: 10.1007/s11165-017-9683-6. [ CrossRef ] [ Google Scholar ]
- Duncan RG, Reiser BJ. Reasoning across ontologically distinct levels: students' understandings of molecular genetics. J Res Sci Teach. 2007; 44 :938–959. doi: 10.1002/tea.20186. [ CrossRef ] [ Google Scholar ]
- Eaton AC, Delaney S, Schultz M. Situating sustainable development within secondary chemistry education via systems thinking: a depth study approach. J Chem Educ. 2019 doi: 10.1021/acs.jchemed.9b00266. [ CrossRef ] [ Google Scholar ]
- Evans R, Dolin J (2018) Taking advantage of the synergy between scientific literacy goals, inquiry-based methods and self-efficacy to change science teaching. In: Tsivitanidou OE, Gray P, Rybska E, Louca L, Constantinou CP (eds) Professional development for inquiry-based science teaching and learning. Springer International Publishing, Cham, pp 105–120. 10.1007/978-3-319-91406-0_6
- Fitzgerald M, Danaia L, McKinnon DH. Barriers inhibiting inquiry-based science teaching and potential solutions: perceptions of positively inclined early adopters. Res Sci Ed. 2019; 49 :543–566. doi: 10.1007/s11165-017-9623-5. [ CrossRef ] [ Google Scholar ]
- Goodrum D, Rennie LJ (2007) The national action plan. Australian School Science Education National Action Plan, 2008–2012, vol 1, Australian Government Department of Education Science and Training, Canberra. https://apo.org.au/sites/default/files/resource-files/2007-09/apo-nid4048.pdf
- Green ED, Guyer MS. Charting a course for genomic medicine from base pairs to bedside. Nature. 2011; 470 :204–213. doi: 10.1038/nature09764. [ PubMed ] [ CrossRef ] [ Google Scholar ]
- Gulamhussein A (2013) Teaching the teachers: effective professional development in an era of high stakes accountability. Center for Public Education. https://www.academia.edu/28440314/Teaching_Effective_Professional_Development_in_an_Era_of_High_Stakes_Accountability_READ_THE_REPORT_Center_for_Public_Education
- Haga SB, Barry WT, Mills R, Ginsburg GS, Svetkey L, Sullivan J, Willard HF. Public knowledge of and attitudes toward genetics and genetic testing. Genet Test Mol Biomarkers. 2013; 17 :327–335. doi: 10.1089/gtmb.2012.0350. [ PMC free article ] [ PubMed ] [ CrossRef ] [ Google Scholar ]
- Haskel-Ittah M, Yarden A (2018) Students' conception of genetic phenomena and its effect on their ability to understand the underlying mechanism. CBE Life Sci Educ 17 10.1187/cbe.18-01-0014 [ PMC free article ] [ PubMed ]
- Haury DL, Nehm RH (2012) the global challenge of genomics education: a path to the future. in: genomics applications for the developing world. Springer New York, pp 311–333. 10.1007/978-1-4614-2182-5_19
- Hilton A, Nichols K, Kanasa H. Developing tomorrow’s decision-makers: opportunities for biotechnology education research. Aust Educ Res. 2011; 38 :449–465. doi: 10.1007/s13384-011-0039-3. [ CrossRef ] [ Google Scholar ]
- Karahan E, Roehrig G. secondary school students’ understanding of science and their socioscientific reasoning. Res Sci Ed. 2017; 47 :755–782. doi: 10.1007/s11165-016-9527-9. [ CrossRef ] [ Google Scholar ]
- Kidman G. What is an ‘interesting curriculum’ for biotechnology education? Students and teachers opposing views. Res Sci Ed. 2010; 40 :353–373. doi: 10.1007/s11165-009-9125-1. [ CrossRef ] [ Google Scholar ]
- Larue KM, McKernan MP, Bass KM, Wray CG (2018) Teaching the genome generation: bringing modern human genetics into the classroom through teacher professional development. J STEM Outreach 1 10.15695/jstem/v1i1.12 [ PMC free article ] [ PubMed ]
- Middleton A, et al. Global public perceptions of genomic data sharing: what shapes the willingness to donate DNA and health data? Am J Hum Genet. 2020; 107 :743–752. doi: 10.1016/j.ajhg.2020.08.023. [ PMC free article ] [ PubMed ] [ CrossRef ] [ Google Scholar ]
- Nam Y, Chen Y-C. Promoting argumentative practice in socio-scientific issues through a science inquiry activity. Eurasia J Math Sci Technol. 2017; 13 :3431–3461. doi: 10.12973/eurasia.2017.00737a. [ CrossRef ] [ Google Scholar ]
- National Curriculum Board (2008) National science curriculum: framing paper. Melbourne, Australia https://docs.acara.edu.au/resources/National_Science_Curriculum-Framing_paper.pdf
- National Health and Medical Research Council (2014) Ethical Considerations in Quality Assurance and Evaluation Activities. https://www.nhmrc.gov.au/about-us/resources/ethicalconsiderations-quality-assurance-and-evaluation-activities
- National Research Council . A framework for K-12 science education: practices, crosscutting concepts, and core ideas. Washington, DC: National Academy Press; 2012. [ Google Scholar ]
- Nisselle A et al. (2019) Ensuring best practice in genomic education and evaluation: a program logic approach. Front Genet 10 10.3389/fgene.2019.01057 [ PMC free article ] [ PubMed ]
- NSW Education Standards Authority (2017) Biology, Stage 6 Syllabus. NSW Syllabus for the Australian Curriculum, Sydney. https://educationstandards.nsw.edu.au/wps/portal/nesa/11-12/stage-6-learning-areas/stage-6-science/biology-2017
- Ong R, et al. Measuring the impact of genetic knowledge on intentions and attitudes of the community towards expanded preconception carrier screening. J Med Genet. 2018; 55 :744–752. doi: 10.1136/jmedgenet-2018-105362. [ PubMed ] [ CrossRef ] [ Google Scholar ]
- Pitini E, et al. How is genetic testing evaluated? A systematic review of the literature. Eur J Hum Genet. 2018; 26 :605–615. doi: 10.1038/s41431-018-0095-5. [ PMC free article ] [ PubMed ] [ CrossRef ] [ Google Scholar ]
- Ricciardi W, Boccia S. New challenges of public health: bringing the future of personalised healthcare into focus. Eur J Public Health. 2017; 27 :36–39. doi: 10.1093/eurpub/ckx164. [ PubMed ] [ CrossRef ] [ Google Scholar ]
- Romine WL, Sadler TD, Kinslow AT. Assessment of scientific literacy: development and validation of the Quantitative Assessment of Socio-Scientific Reasoning (QuASSR) J Res Sci Teach. 2017; 54 :274–295. doi: 10.1002/tea.21368. [ CrossRef ] [ Google Scholar ]
- Rönnebeck S, Bernholt S, Ropohl M. Searching for a common ground – a literature review of empirical research on scientific inquiry activities. Stud Sci Educ. 2016; 52 :161–197. doi: 10.1080/03057267.2016.1206351. [ CrossRef ] [ Google Scholar ]
- Sadler TD, Barab SA, Scott B. What do students gain by engaging in socioscientific inquiry? Res Sci Ed. 2007; 37 :371–391. doi: 10.1007/s11165-006-9030-9. [ CrossRef ] [ Google Scholar ]
- Sadler TD, Foulk JA, Friedrichsen PJ. Evolution of a model for socio-scientific issue teaching and learning. Int J Educ Math Sci Technol. 2017; 5 :75–87. doi: 10.18404/ijemst.55999. [ CrossRef ] [ Google Scholar ]
- Sadler TD, Romine WL, Topçu MS. Learning science content through socio-scientific issues-based instruction: a multi-level assessment study. Int J Sci Educ. 2016; 38 :1622–1635. doi: 10.1080/09500693.2016.1204481. [ CrossRef ] [ Google Scholar ]
- Steele F, Aubusson P. The challenge in teaching biotechnology. Res Sci Ed. 2004; 34 :365–387. doi: 10.1007/s11165-004-0842-1. [ CrossRef ] [ Google Scholar ]
- Stern F, Kampourakis K. Teaching for genetics literacy in the post-genomic era. Stud Sci Educ. 2017; 53 :193–225. doi: 10.1080/03057267.2017.1392731. [ CrossRef ] [ Google Scholar ]
- Syurina EV, Brankovic I, Probst-Hensch N, Brand A. Genome-based health literacy: a new challenge for public health genomics. Public Health Genomics. 2011; 14 :201–210. doi: 10.1159/000324238. [ PubMed ] [ CrossRef ] [ Google Scholar ]
- Todd A, Romine WL, Cook Whitt K. Development and validation of the learning progression–based assessment of modern genetics in a high school context. Sci Educ. 2017; 101 :32–65. doi: 10.1002/sce.21252. [ CrossRef ] [ Google Scholar ]
- Tytler R, Symington D, Cripps Clark J. Community-school collaborations in science: towards improved outcomes through better understanding of boundary issues. Int J Sci Math Educ. 2017; 15 :643–661. doi: 10.1007/s10763-015-9711-9. [ CrossRef ] [ Google Scholar ]
- Tytler R et al. (2015) Evaluating the model & outcomes of the Scientists and Mathematicians in Schools program 2015. Deakin University, Burwood, Victoria. https://www.csiro.au/en/Education/Programs/STEM-Professionals-in-Schools/How-the-program-works/Program-evaluation
- Verhoeff RP, Waarlo AJ, Boersma KT. Systems modelling and the development of coherent understanding of cell biology. Int J Sci Educ. 2008; 30 :543–568. doi: 10.1080/09500690701237780. [ CrossRef ] [ Google Scholar ]
- Wells P (2017) Adopting a student-inquiry stance for teaching genetics: a 10-year autoethnographic analysis. The Morning Watch: Educational and Social Analysis 45 https://journals.library.mun.ca/ojs/index.php/mwatch/article/view/1937
- Whitley KV, Tueller JA, Weber KS. Genomics education in the era of personal genomics: academic, professional, and public considerations. Int J Mol Sci. 2020; 21 :768. doi: 10.3390/ijms21030768. [ PMC free article ] [ PubMed ] [ CrossRef ] [ Google Scholar ]
- Yacoubian HA. Scientific literacy for democratic decision-making. Int J Sci Educ. 2018; 40 :308–327. doi: 10.1080/09500693.2017.1420266. [ CrossRef ] [ Google Scholar ]
- Zeidler DL. STEM education: a deficit framework for the twenty first century? A sociocultural socioscientific response. Cult Stud Sci Educ. 2014; 11 :11–26. doi: 10.1007/s11422-014-9578-z. [ CrossRef ] [ Google Scholar ]
- Zeidler DL (2015) Socioscientific Issues. In: Gunstone R (ed) Encyclopedia of Science Education. Springer Netherlands, pp 998–1003. 10.1007/978-94-007-2150-0_314
- Zeidler DL, Herman BC, Sadler TD (2019) New directions in socioscientific issues research. DISER 1. 10.1186/s43031-019-0008-7
Livingston High School Prepares Students for a Future in Biotech with Virtual Labs
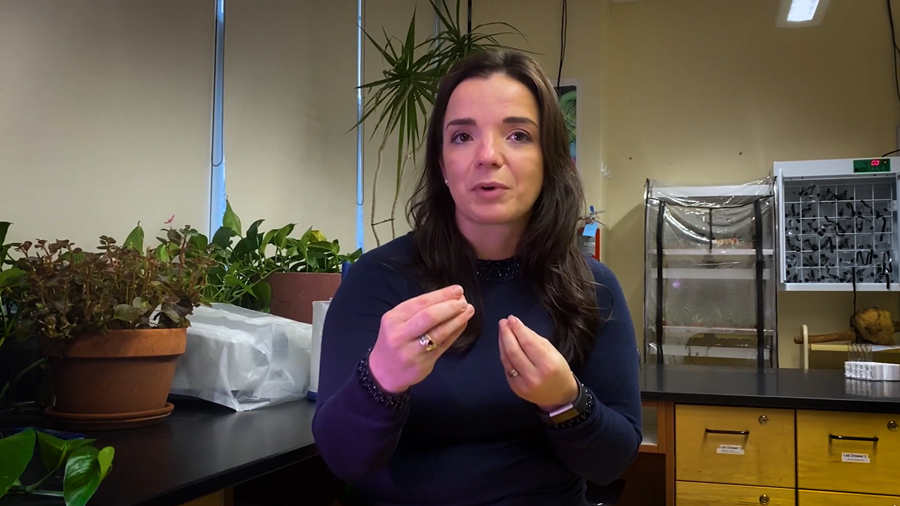
Watch Video
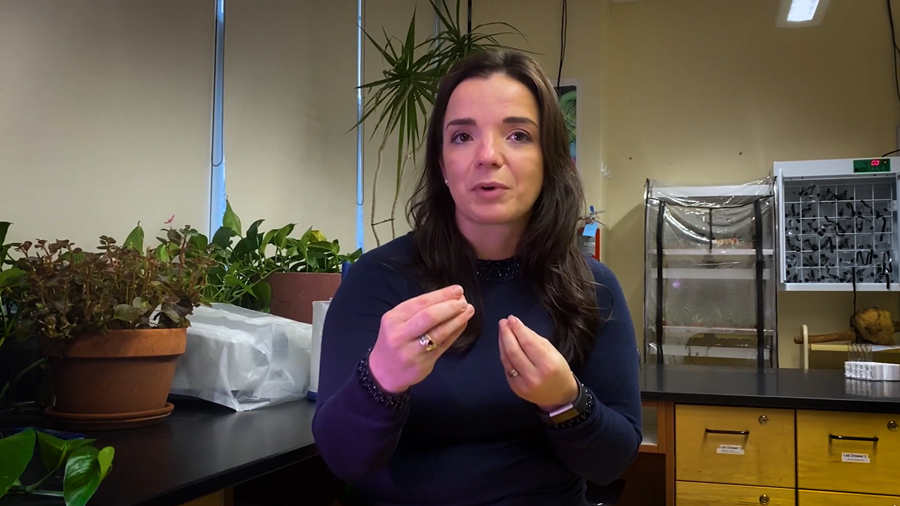
When Diane Sigales was asked to design a Biotech class for Livingston High School in New Jersey, she knew that she didn’t want to settle for less. Discover how Diane used virtual labs to give her students skills beyond the limits of what she thought was possible.
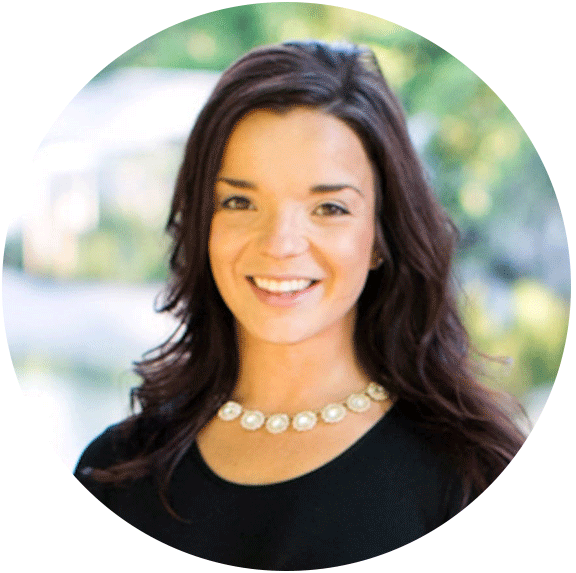
Giving students time in Labster to explore prior to the wet lab is invaluable. I can spend time with everyone because those skills were already practiced the night before with Labster.
Diane Sigales
Biotech Teacher
Diane wanted to give her students a real education in Biotech, but quickly found herself limited by the lab she could afford. Much of the breadth of skills and equipment needed to teach Biotech was beyond the scope of the typical time, budget, and resources available at the high school level.
Diane discovered Labster’s virtual labs and quickly found a library of engaging biotech simulations. She deployed Labster to introduce, practice, and review lab techniques that would have otherwise cost millions while inspiring students with interactive lessons that placed them in the shoes of scientists.
What I love most about Labster are the skills and types of labs that are available. I don’t have the funds for it, I don’t have the space for it — I could never give that to my students.
Diane was not only able to maximize time in wet labs due to practice simulations, but she also noticed a powerful impact on learning outcomes. She remarked that many students remembered what they had learned even a year later, often referencing scenarios they encountered in simulations. For Diane, the highlight was an email from a student who had gone on to get a job in their university lab as a freshman due to the skills they had learned in Labster.
Interview with Diane Sigales: Biotech Teacher
Diane Sigales teaches Biotech, an advanced course designed to set kids on an accelerated path towards a Biotech career, at Livingston High School.
What is your favorite thing about Labster?
What I love most about Labster is the skills and the types of Labs that are available–I don’t have the funds for it, I don’t have the space for it– I could never give that to my students.
How do you use virtual labs?
Most of the time I’m assigning Labster outside of class as either a homework they do before a lab or after a lab. Giving students time in Labster to explore prior to the wet lab is invaluable. I can spend time with everyone because those skills were already practiced the night before with Labster.
How does Labster help you target learning gaps?
After the students run a lab, Labster has a dashboard that has everything– you’re getting feedback on exactly what the students did right, and exactly what the students did wrong. It’s a great tool to know exactly which students need help with exactly which skills.
How does Labster help students understand the material better?
Students can take a virtual lab as many times as they need to, which allows them the comfort of knowing that the first time they work through a problem is not when it's graded. That really allows students to learn from their mistakes.
How important is it for students to have fun while they learn?
Students having fun while learning is essential to them truly mastering what they are trying to do. If you have a student that hates your class, they’re not going to come in excited. When students experience the gamification of Labster, they are engaged, they are excited, they are motivated to learn, which makes your job as a teacher that much easier.
What have you seen in terms of lasting impact on learning outcomes?
My student I have for my second year– they still remember those simulations. One of my students sent me an email after he graduated. He got a job as a freshman in a research lab at his college, because he did not have to be taught how to do the research.
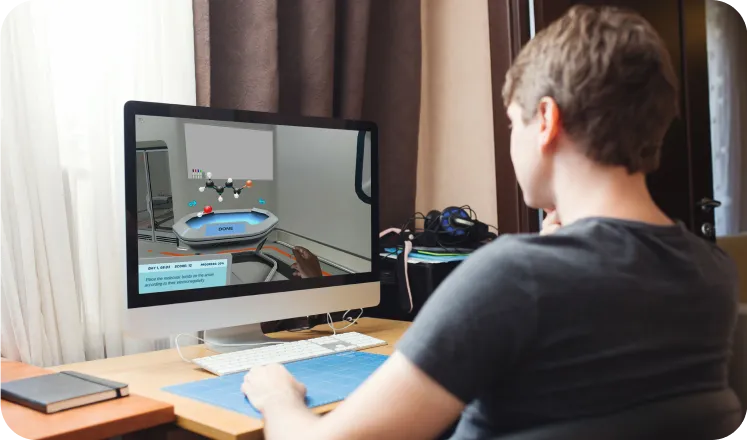
Explore more.
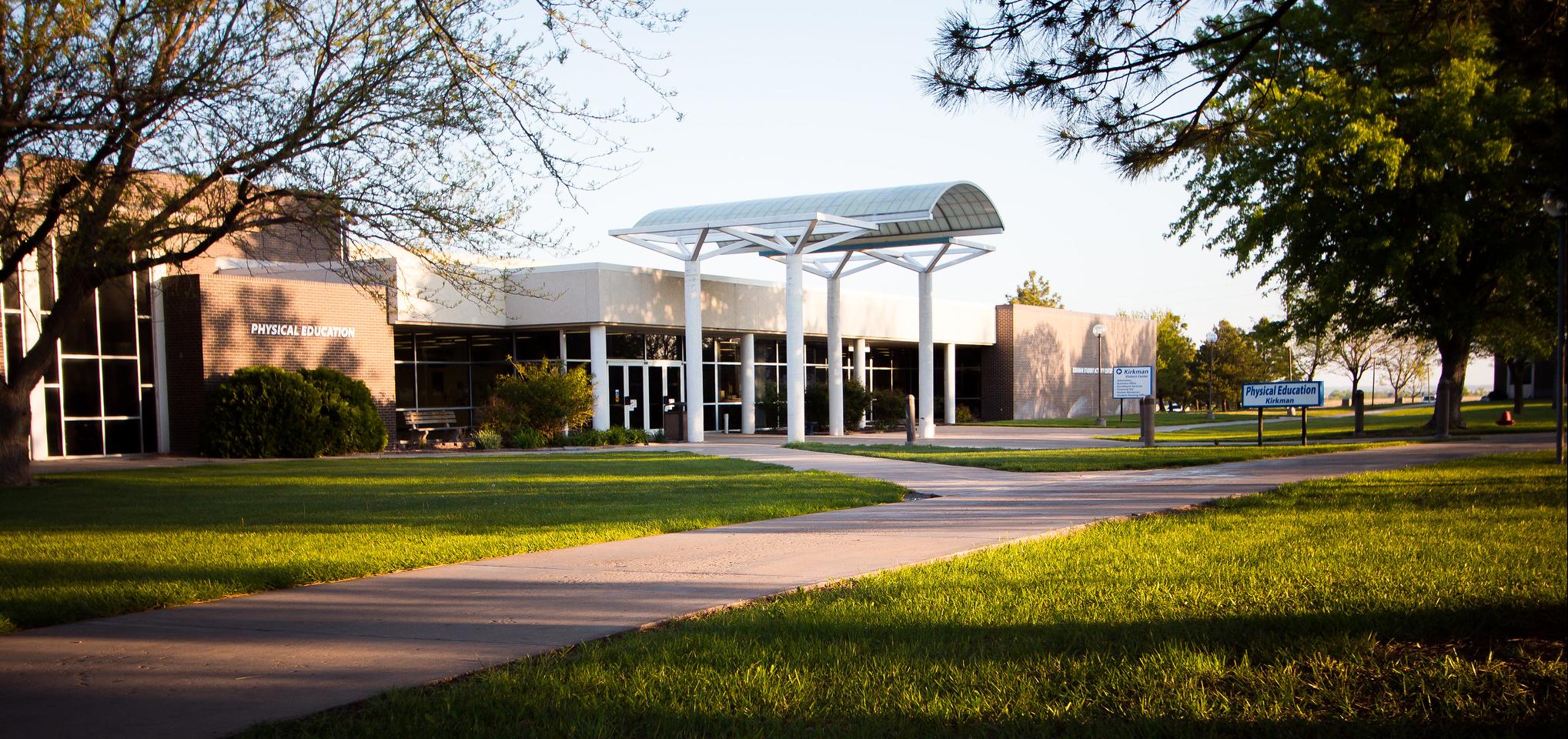
Barton Community College
Barton Community College Helps Students Step into the Shoes of a Scientist with Labster
.jpg)
The Centre for Distance Learning and Innovation (CDLI)
Labster Prepares High School Chemistry Students for Further STEM Studies & the Real World
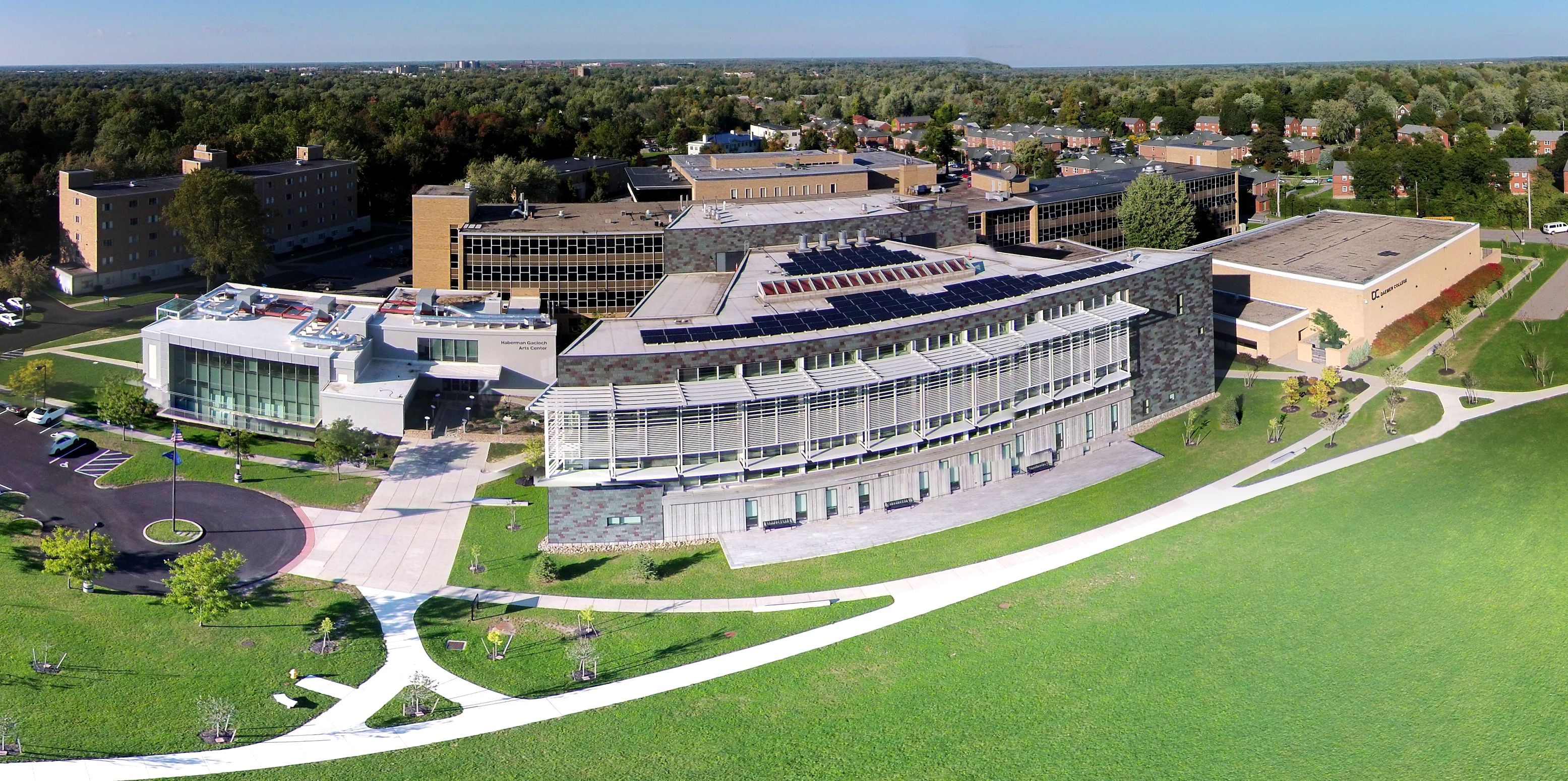
Daemen University
Daemen University Uses Labster to Inspire Curiosity-Driven Learning in Anxious Students
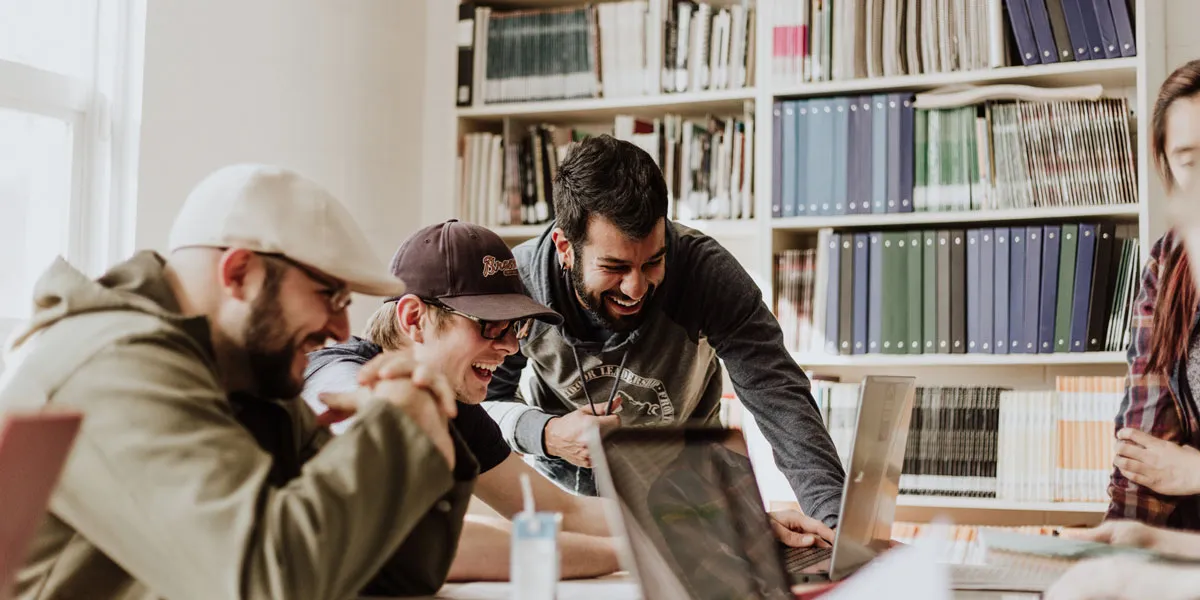
Discover The Most Immersive Digital Learning Platform.
Request a demo to discover how Labster helps high schools and universities enhance student success.


- Fisher Scientific
- Education Products
- Biotechnology Resources for Education
- The Importance of Biotechnology
The Case for Teaching Biotech in High Schools
Imagine providing your students with the foundation to create prosthetic limbs for combat veterans, design algae-powered cars, or solve crimes using forensics. Teaching biotechnology does just that while simultaneously instilling a highly desired skill set. Whether students continue their education with associate degrees, doctorates, or something in between, the rapidly expanding biotech market has many career options available at each education level.
Biotechnology education helps students apply the theories they learned in biology, chemistry, physics, and math to solve real-world problems. Additionally, biotechnology teaches highly sought-after soft skills like critical thinking, problem-solving, collaboration, and communication, which makes students more desirable candidates for jobs after graduation.
Even students who choose to pursue careers outside of traditional biotech roles like lab technician or microbiologist can benefit from early exposure to biotechnology courses. Many large biotech companies employ individuals with training in biotechnology to assist with financial analysis, marketing, and supply chain management. Helping students understand how real-world problems are solved at the benchtop, by the bedside, and in the office can open their eyes to many career opportunities they may not have previously considered.
Biotechnology provides a way for teachers to think outside the box and an opportunity to collaborate with local colleges, universities, and biotech companies. Partnerships with community colleges may offer students college credit. Additionally, partnering with a community college may help to support your program with shared supplies that reduce the costs of implementing new biotech programs.
Further reading:
- Biospace.com
References:
- Building it big in Texas: Community college program trains biotech workforce , National Science Foundation
- Best Biotechnology Degrees , College Choice
- Educational Institutions Highlight Biotech Through Partnerships and Programs , BioSpace
- How to Start a Program , AC2 Bio-Link Biotech Mentor Teacher Network
Quick Links
- Implementing a Biotechnology Program
- New to Biotechnology
- Contact Us for More Information
Biotech Products
- Classroom Equipment and Supplies
- Classroom DNA, RNA, and PCR Applications
- Classroom Gene Technology
- Classroom Immunology
- Classroom Teacher Resources
The views and opinions presented are courtesy of Ellyn Daugherty, educator and author of Biotechnology: Science for the New Millennium and do not necessarily mirror the views and opinions of Thermo Fisher Scientific. Thermo Fisher assumes no liability arising from use of information presented by Ellyn Daugherty and this site. All products shown are for education use only, not for diagnostic purposes.

Beyond Benign
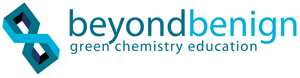
Green Chemistry Education
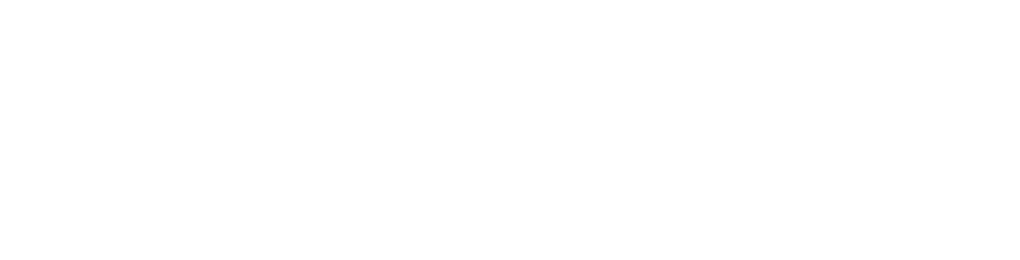

Lesson 01 – What’s up with Gena? Introduction to Biotech Case Study
June 22, 2017
Back to Topics
Elementary School Middle School High School Higher Ed
Students are challenged to formulate a hypothesis for what is wrong with a patient named Gena based on her family history and complaints of sickness.
Download Lesson
Whats_up_with_Gena.docx
For K-12 Teachers
For University Faculty
For College Students
What is Green Chemistry
Our Approach
Beyond Benign 18 Church Street P.O. Box 1016 Wilmington, MA 01887 info@beyondbenign.org
Sign Up for News
Subscribe to our email list

Search form
- Sign up for news
Cases in Industry Practice in Biotechnology

Authors : V. Celeste Carter and Kristen Hershbell Charles
These instructional materials were developed in collaboration with industry partners and utilize a case-method approach with hands-on laboratory components. The case studies focus on promoting student understanding of and facility with workplace skills needed to function efficiently, effectively, and safely in entry-level biotechnology careers.The case studies allow students to read or view a real-life situation that models a dilemma faced by a technician in industry. These cases provide a forum for problem-based learning in which the dilemmas faced by the technician may not have a "right" or "wrong" solution. The students discuss the case and struggle with the issues themselves and then apply their knowledge in a hands-on laboratory exercise.
The case studies and laboratory procedures are appropriate for high school, community college, and university levels. They are primarily targeted at educational institutions with programs or course offerings in biotechnology. The case studies and labs assume a basic knowledge of laboratory skills (e.g., preparing molar solutions, general safety measures, how to operate standard laboratory equipment such as micropipettors and centrifuges). In addition, basic knowledge of general and molecular biology is helpful, although the cases and labs are accompanied by appendices that provide necessary information about the fundamental concepts presented in each case.The students read and discuss the case and struggle with the embedded issues themselves in this real-world context--thereby sharpening the students' own knowledge of and facility with similar issues that they will likely face once in the workforce. Instructors facilitate these discussions using a "KWL" approach (the KWL approach engages students in responding to the following series of questions: What do we K now? What do we W ant to know? What have we L earned?). Most materials are available through the links below. Facilitators guides are provided with course-in-a-box access.
These cases were created with the help of the following industry partners: the Stanford Human Genome Center, Kaiser Permanente, CV Therapeutics, Lifescan, Stratagene, Inc., Inhale, Inc., and Bio101.
*Course-in-a-Box access : To view additional materials, log in to your InnovATEBIO account . If you are a biotech faculty member at a college or high school, join InnovATEBIO to get an account. Note - it may take a few days to verify your faculty position and set up the account.
Log in to view the following case studies:
I. It's as Easy as Pulling a Hair
II. Lighten Up
III. Enzyme Endurance
IV. Ultra Bad Hair Day
V. Under Pressure
VI. Exercise Your Options
Open Materials
The attachments can be downloaded without logging in.

Site design and copyright Digital World Biology®
DNA Profiling Activity

- DNA & RNA
- Biotechnology
- Patterns of Inheritance
- Bioinformatics
Resource Type
- Case Studies
Description
This multipart activity is designed to give students a firm understanding of genetic profiling using short tandem repeats (STRs), which is a process used by forensic labs around the world.
In Part 1 of this activity, students learn the basics of DNA profiling, including the structure and inheritance of STRs. In Part 2, students learn how DNA profiles are compiled with STRs that are typically used in forensic investigations. In Part 3, they work through a case study involving a robbery and build a DNA profile that can be compared to one constructed from a DNA sample left by a suspect at the scene of the crime. Throughout, analysis questions walk students through calculations on allele frequency and probability (using real data from national databases), providing opportunities for formative assessments of students’ understanding of DNA fingerprinting applications.
This activity can be used on its own or could be followed by downloading and unzipping the accompanying case studies in which students apply what they have learned to solve four cases: 1) twins switched at birth, 2) revisiting evidence from a crime for which the accused was sentenced to life in prison, 3) identifying a missing person, and 4) identifying victims of an earthquake.
The “Resource Google Folder” link directs to a Google Drive folder of resource documents in the Google Docs format. Not all downloadable documents for the resource may be available in this format. The Google Drive folder is set as “View Only”; to save a copy of a document in this folder to your Google Drive, open that document, then select File → “Make a copy.” These documents can be copied, modified, and distributed online following the Terms of Use listed in the “Details” section below, including crediting BioInteractive.
Student Learning Targets
- Interpret electrophoresis results by distinguishing DNA fragments by length and determining whether individuals are homozygous or heterozygous at different STR loci.
- Calculate allele frequencies and the probability of generating a match, at random, at one or more loci using allele frequency data.
Estimated Time
allele, DNA profile, electrophoresis, flanking sequence, genetic fingerprint, heterozygote, homozygote, locus, polymerase chain reaction (PCR), primer, repeat unit, short tandem repeat (STR)
Primary Literature
Hill, Carolyn R., David L. Duewer, Margaret C. Kline, Michael D. Coble, and John M. Butler. “U.S. population data for 29 autosomal STR loci.” Forensic Science International: Genetics 7, 3 (2013): e82–e83. http://dx.doi.org/10.1016/j.fsigen.2012.12.004 .
Jeffreys, Alec J. “Genetic Fingerprinting.” Nature Medicine 11,10 (2005): 1035–1039. https://doi.org/10.1038/nm1005-1035 .
Terms of Use
<p>Please see the <a href=" https://www.hhmi.org/terms-of-use" ; target="_blank">Terms of Use</a> for information on how this resource can be used.</p>
Version History
Curriculum connections, ngss (2013).
HS-LS3-1, HS-LS3-3; SEP2, SEP4, SEP5
AP Biology (2019)
IST-1.I, IST-1.K, IST-1.P, SYI-3.C, EVO-1.K; SP2, SP4, SP5
IB Biology (2016)
2.7, 3.5, 7.1, B.5
Common Core (2010)
ELA.RST.9–12.4, ELA.RST.9–12.7, ELA.WHST.9–12.1 Math.N-Q.1, Math.A-CED.1, Math.A-REI.3, Math.S-IC.1; MP1, MP3, MP4
Vision and Change (2009)
CC3, CC5; DP1, DP2, DP3, DP6
Explore Related Content
Other related resources.

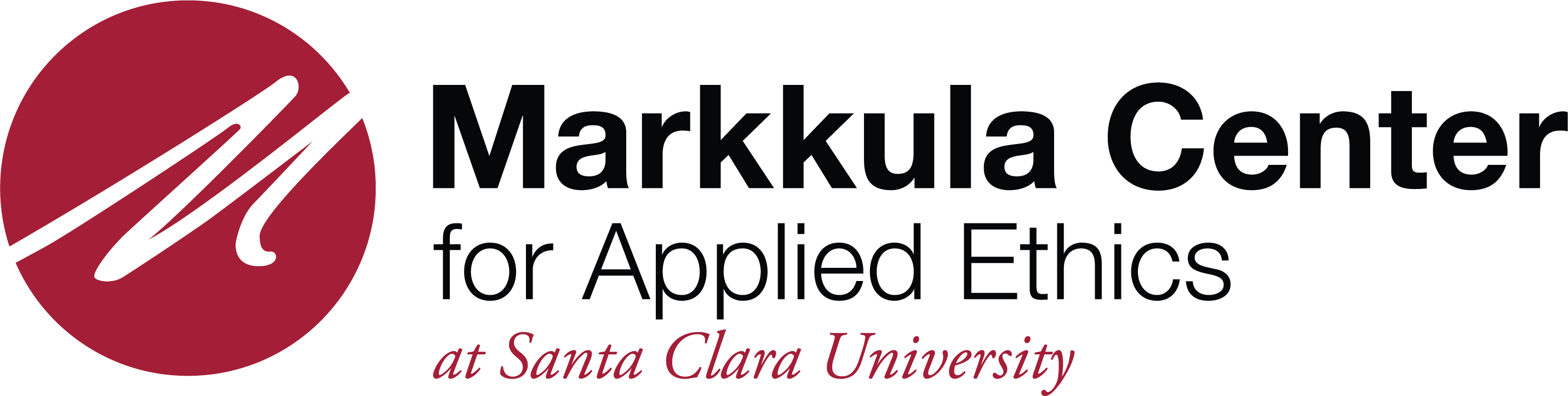
Case Studies: Thinking Ethically about Cutting Edge Biotechnology
- Markkula Center for Applied Ethics
- Focus Areas
- Bioethics Resources
- Bioethics Cases
These case studies represent interesting situations that a bioengineer, biologist or biochemist may encounter in their professional lives. By thinking about and discussing them, students can cultivate ethical patterns of thought prior to leaving academia, professionals can carefully consider the ethical implications of their work, and the public can consider what is at stake in living on the cutting edge.
DNA Strands
Madeline Eiken w as a Hackworth Fellow for the 2018-2019 school year. She graduated from Santa Clara University in 2019 where she majored in bioengineering and minored in chemistry. She was awarded the Ethics Center Markkula Prize in June 2019. She currently works at Allosource, a tissue bank in Colorado.
Ethics asks us to carefully consider what is right-making and wrong-making about what we do and the choices we make.
We live at a time of increasing technological power, a time when ethics compels all of us to meaningfully engage with science and technology to create a just world in which all can thrive. Our ethical considerations include a variety of stakeholders, so it is important that the general public, as well as scientists, engage in discussion about ethical issues. Ethics gives non-scientists a reason to care about complex scientific advances, and it gives scientists a reason to engage in the public square. Jennifer Doudna, one of the inventors of CRISPR genome editing technology, said that the social implications of this technology “yanked her out of the ivory tower” and spurred her to engage with the ethical and social implications of her work.
The three case studies here represent interesting situations that a bioengineer, biologist or biochemist may encounter in their professional lives. Students in these disciplines must be prepared to engage with ethical issues that arise during their education and in their future professional lives. By thinking about and discussing these case studies, students can cultivate ethical patterns of thought prior to leaving academia and professionals can carefully consider the ethical implications of their work. These case studies are accessible to non-scientists as well, enabling us all to think carefully about potential applications of current cutting-edge technology and practices.
As you consider the ethical issues displayed in these cases, you may want to use an ethics framework such as A Framework for Ethical Decision Making available from the Markkula Center for Applied Ethics.
My hope is that these case studies and the questions embedded in them generate rigorous thought and lively conversation, whether in the classroom, at the lab bench, or around the dinner table.
Up, Up, and Away: Clinical Trials Go International What are the ethical implications of conducting clinical trials in developing countries?
CRISPR: Next-Gen Mosquito Zapper? Is it ethical to eliminate native populations of disease-carrying pests through genetic manipulation?
The Case of the Missing Kidneys While organ donation is necessary to alleviate suffering and save lives, questions of autonomy, coercion, and the yuk factor deserve careful consideration as we seek to increase supply in the face of unrelenting demand.

Global Dimensions of Intellectual Property Rights in Science and Technology (1993)
Chapter: 13 biotechnology case study, 13 biotechnology case study.
GEORGE B. RATHMANN
I want to describe a bit of the history of the biotechnology field to give you a strong sense of the importance of this field, not just in itself but as a prelude to a new technology as it develops over the next century. I then relate that history to some questions that have been raised and finally relate my conclusions with respect to biotechnology to the objectives of the conference.
As rocky as the road for biotechnology was in the United States, what we see coming up on the world scene is much more difficult, much more serious. We desperately need a legal system to solve the problems, and it is our hope that there are ways of dealing with these issues.
The biotech era really dawned when Watson and Crick defined the structure of deoxyribonucleic acid (DNA). As with many world-shattering discoveries, this was simple and concise—a publication of one page outlining the structure of DNA ( Nature, April 25, 1953, p. 737). They also had the vision to say it would affect not only how we looked at deoxyribonucleic acid, but how we looked at life itself and our ability to understand living systems. There would be products, there would be opportunities, and there would be new insights that would be most important. All that was recognized in a one-page article.
As important and earth shaking as that was, from the standpoint of the commercialization of biotechnology, something nearly as important occurred on June 17, 1980, when the Supreme Court ruled that live organisms could be patented. It was well recognized as important at the time, but I think few
people realized how important it was for launching the commercialization of biotechnology.
In that patent, Dr. Ananda Chakrabarty, who was at G.E. at the time, claimed an organism that would digest oil. The invention was never commercialized, but it told the world that this field was going to be important and there were going to be commercial opportunities. An investment in trying to understand the biochemistry of life would pay off in the sense that the intellectual property could be protected. Within four months (October 14, 1980), the biotechnology company Genentech went public and jolted Wall Street with a rise in its stock price from $35 to $71 1/4. So it is clear that as of that date, biotechnology assumed increasing commercial importance.
At that time, in October 1980, I was looking at the opportunity to start a biotech company called Amgen and we were putting out a document that we hoped would raise $15 million. Partly because of Genentech's success, we were able to raise $19 million—with only a scientific advisory board, one employee, and promises for two future hires. So it certainly had a profound effect on whether Amgen would ever be. As a matter of fact, within a year, Amgen, Genetics Institute, Immunex, Genetics Systems, Chiron, and many others companies were formed. Within two years, more than 100 companies were formed as this era was launched.
Now, the Chakrabarty decision made it look simple: life forms were patentable. Genentech, Cetus and many others afterwards launched public offerings, recognizing the commercial potential that biotechnology would lead to new discoveries of valuable intellectual property, which could be protected by patents. In reality, it was not quite that simple and the launchings were not that consistent.
Venture capital funds vacillated quite a bit, although after 1980 there was a very substantial influx of venture capital ( Figure 13-1 ). There were periods when it went down, and periods when it went up. Although these look like gigantic numbers, remember it takes about a quarter of a billion dollars to bring a pharmaceutical product to market. It probably takes more than that to commercialize something important in agriculture, food, or other areas. So this flow of venture capital was actually inadequate to keep it going. Of course, the public made the difference, but it can be seen that this was not exactly a consistent, reliable source of funds, either.
If we smooth everything out, the market value of biotechnology stocks moved dramatically from 1980, when it was literally zero, to 1991, when it was more than $35 billion ( Figure 13-2 ). Those of us in the industry saw some very serious bumps in that curve. In 1987 some biotech companies lost 30-40 percent of the value of the company in a matter of a few days. When you finally smooth everything out, it looks a lot simpler and surer than it felt.
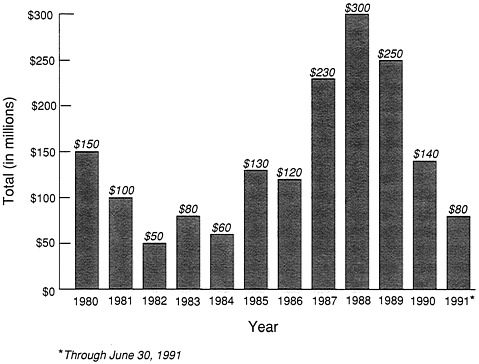
FIGURE 13-1 Venture Capital Disbursements in Biotechnology
Source: Venture Economics and Ernst and Young
Figure 13-3 shows the amount of capital raised through public stock offerings. In 1991, more money was raised in six months than for many years, and as a matter of fact, when the total figures came in for the year they exceeded $4 billion—equal to all the money that had been raised in the previous years since the launching of commercial biotechnology. Of course, the big news is $550 million in initial public offerings. Those are new companies whose survival may mean wonderful improvements to our lives around the world. At the same time they will be facing some of the rocky roads that the earlier companies faced. So we can see that it is not a steady, easy trip.
Product sales in the industry today have reached about $8 billion and are expected to reach $20 billion by the year 2000. That may be a very conservative figure. The drug industry worldwide by that time will be well over $200 billion, and biotechnology is contributing roughly half of the most important products today. By the time the year 2000 comes around, biotechnology-derived products could be even more important. Of course there should be many other parts of the biotech industry that are commercially interesting by that time.
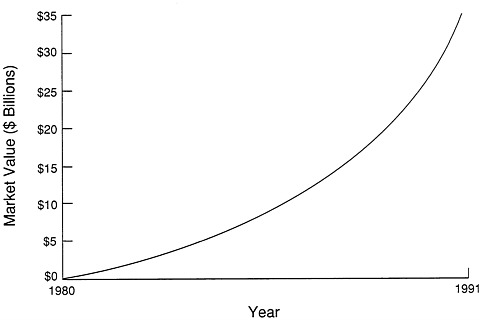
FIGURE 13-2 Market Value of Biotechnology Stocks

FIGURE 13-3 Amount of Capital Raised Through Initial Public Offerings and Other Public Offerings
Source: Paine Webber and Ernst and Young
So we are looking at something of great importance to the economy of the country and to international trade, which is discussed below.
I was asked by the National Research Council to address several questions. The first was, What adjustments in intellectual property rights have been made? Well, of course, the first is the allowance of claims to living organisms. The United States certainly led the way there. It was a very important opportunity that organisms that produced a pharmaceutical material could be claimed in patents. We had something tangible to claim even if the product being produced was already known or already had been defined.
One of the things that has been evolving over the last few years, and certainly in 1987 had a pretty dismal outlook, is referred to as In re Durden . This case implied that just because you have a novel starting material on which you carry out a process to produce another material, the process is not automatically patentable. That case was often interpreted much more severely to mean that unless the process is highly inventive, mere novelty because of novel starting materials does not make it patentable. So it was not possible in 1987 to get claims to the process that was going to produce, for example, in Amgen's case, erythropoietin by using a novel organism.
Because inventors could not claim the process, they had a very serious problem. They could not invoke any rights at all against companies who used their organism overseas, produced the product, and brought it in. They did not have a final product claim; they did not have a process claim; and there was no mechanism for protecting against the direct theft of the organism overseas—copying it, or following the teachings of the patent, and then just shipping the product to the United States.
However, an evolution has occurred since then. Certainly, a lot of process claims have now been granted. There is a bill authored by Congressman Boucher that would give guidance to the Patent Office to make sure it issues those claims. Without those claims, the organism patent is meaningless with respect to overseas competition. What if the overseas country does not issue the organism patent? The organism has only one purpose—to produce the protein, so the inventor is left with no protection against importation. Amazingly enough, the inventor is protected from infringement in the United States by U.S. companies but is unable to stop foreign infringement and U.S. importation. The trade implications are clear.
This has been a very serious problem that is now being addressed. Yet there are still concerns from people who wonder if it is really "fair" to keep foreign companies from bringing their products into the United States. They ask, "Isn't that protectionism?" This a very strange interpretation of fairness. I think these inventions are clearly being copied and misappropriated by foreign companies. Changes may or may not move smoothly, but these issues should be resolved in the next few years, and more and more compa-
nies are availing themselves of the process protection, though some opportunities have been abandoned after In re Durden objections.
There have been great differences in the interpretation of the scope of claims. My initial discussion is limited to the United States because global issues have really only come into play in the least five years. Even in the United States, the scope of claims has been quite a difficult issue with which to deal. The questions stated are, If the claims are too broad, doesn't it mean we are inhibiting the diffusion of technology? If the claims are too narrow, doesn't it mean that the inventor really is disadvantaged? I could say a lot about that, but in actual fact I will cite the record. A Boston court in the United States leaned toward a pretty narrow interpretation of the claims. In a Delaware court, a jury decided that the Genentech case should be very broadly interpreted and cover structures quite different from the ones that were defined in the patent simply because all the rest were straightforward once the patent teachings were available. So these are still issues, but I think we will move toward a pretty clear understanding over the next few years.
The effect on biotechnology advancement has not been smooth even in this country. Patent uncertainty has encouraged second entrants, who then plead that since they made such a significant investment, believing they were not going to be prevented from manufacturing the product, the terms of the claims of the patents should be relaxed. This has certainly been an expensive mistake in many cases.
Major delays in issuance of patents have prevented some innovators from pushing their products as rapidly as they could, because they feared that they might never have coverage and once they proved the success of the product, it could be duplicated relatively readily. I think many of us in the business got a lot of encouragement from the Orphan Drug Act, because that act suggested that we at least could get six years of protection if we were the first to have a product approved for an orphan indication. If we never received adequate patent protection, we still might be able to recoup our investments, which was very comforting. There has been a lot of controversy about the Orphan Drug Act and whether it should serve as a kind of substitute for the Patent Act. Nevertheless, it helped an embryonic biotechnology industry raise money and sustain its early critical momentum.
Finally, patents played a key role in attracting pharmaceutical companies' investments. These were very important for some companies in the early days. Even though the pharmaceutical companies were not the innovators, they certainly helped support many new biotechnology companies and they clearly needed the confidence of patent exclusivity.
As stated in congressional testimony by Dr. P. Roy Vagelos, Chairman of Merck & Co., "To sustain their ability to discover and develop products which form the basis of American competitiveness, U.S. pharmaceutical
companies count on renewed government support ... in strengthening international protection of intellectual property rights." We can illustrate that perhaps even more significantly in the biotech industry.
For example, in 1986 a pharmaceutical product would cost about $94 million and take somewhere between 10 and 20 years before entering the market. Some kind of protection is certainly required before that kind of investment is made. The figure today is $240 million. That number has been challenged by Congress and looked at many ways by the Office of Technology Assessment (OTA); the latest OTA study says that costs may often be that high, although sometimes they may be lower. However, it does not require a lot of arithmetic to figure this out. The pharmaceutical industry in this country alone spends about $10 billion on R&D per year, and about 30 new products—30 new molecular entities—are approved each year. That comes out to be more than $300 million invested for each success.
In fact, there are at most only four or five new therapeutic products approved each year that are important and if you divide by that, you arrive at astronomical figures for important new therapeutics. Also, all this investment is required years before you can enter the market and start to get a return. So this certainly fits the pattern of something that requires protection, and patents look like the way to do it.
In 1986 the average development time of a new pharmaceutical product was 10 years. The interesting thing is that biotechnology has compressed that time. Because of the rational design of these products, their remarkable efficiency and safety profile, and the understanding and cooperation of the U.S. Food and Drug Administration, the average development time is about four to seven years today for biotechnology products, which is a big help. However, it is still a long time and a large investment.
So let us review how biotechnology was commercialized. What happened is not particularly logical, not what anyone would have deduced sitting around a table trying to decide what was going to happen. When a biotech company decided it wanted to launch a product, it had to build a company to launch the product. All the different stages and structures had to be built—the vectors and expression systems, purifications, scale-up, manufacturing, clinical testing, regulatory submissions, and marketing. Surprisingly enough, almost all of these things were in place in major pharmaceutical companies, yet almost every single important invention was done by independent biotechnology companies. That is the fact; that is what we have to deal with. How were they able to do all this, why would they be the first to do it, and was it effective? Is it not terribly inefficient to have to create a company for each new product? That is what was done.
Small, start-up biotechnology companies were responsible for many miracle drugs. For example, Amgen developed erythropoietin, and we now know that 10 milligrams per year, one-fiftieth of an aspirin tablet, will
prevent 20 or more transfusions for people that are deficient in erythropoietin—and there are many more. Chiron produced the answer to hepatitis C, which is something that has plagued society and challenged scientists for more than 30 years—a well-defined disease about which nothing could be done. Cetus discovered ways of amplifying genes. Individual inventors, individual small companies, are pioneering and finding important new molecules and insights that are changing the way medicine is practiced today. This was done in a way that perhaps was hardly predictable—small, independent companies got started and did this all on their own—but this is exactly what happened. Sometimes it occurred with the support of large companies, but none of the key innovations and developments throughout the field were made by the large companies.
As I said, it was a fairly rocky road. I think that is important. The fragility of a new technology and the need for immediate action are more critical than making long-range plans to do wonderful things over long periods of time. These companies are fragile and their viability is always in question. Their survival is in jeopardy at all times. Take 1989 as an example. Headlines blared, "Clouds gather over the biotech field." Interestingly enough, firms were stumbling on regulation and patent problems. The patent situation looked very confused at that time. It was very difficult again to get financing, and the feeling was that many companies would go out of business and some did.
If we look at the number of financings, we see what has faced this emerging technology—and will probably apply to every new technology—big financing surges, dry spells, big surges. The dry spell in 1984 and 1985 seemed to last forever. We learned it can take eight quarters before you see another chance to raise money. When 1987 came along, the stock market wilted, and 1988, 1989, and 1990—one after another—were all very bad years. Of course, 1991 salvaged a lot of companies, but those were dangerous times for fragile, embryonic businesses.
So some protection is required. There is no question that patent protection fits the need in terms of the large investment required over a long period of time. The question is always asked, however, whether keeping the inventions secret would work. Well, it doesn't. Once the gene has been described, it is trivial to produce the product. Even if the gene is not described anywhere, once the structure is out, once the product is available even in clinical trials, the structure can be determined and often easily duplicated at a much lower cost. The cost is even lower because the copier only has to copy winners. He does not have to duplicate the losers. The copier avoids the major investments that the innovator had to make.
So international protection becomes the issue today. The problems in obtaining worldwide protection are difficult. There are many countries that do not honor the patent system. Surprisingly, countries that do not have
strong patent systems (e.g., China, India, Argentina, Brazil) are not troublesome to the biotech field, although the pharmaceutical industry has expressed concern. However, international trade competition with countries that purport to have a patent system is a very serious issue.
For example, Japan is a strong competitor. In Japan, patent flooding surrounds innovator's patents. The Japanese patent office grants narrow patents instead of broad ones. I think it is pretty obvious to those in this industry that small companies need broad patents. If you are going to try to compete in the marketplace with giants, you had better know that you have some reasonable protection against obvious duplication or partial duplication. The Japanese system has not produced many biotechnology innovations and has not produced biotechnology companies. Our problems with the Japanese system are narrow patents, sometimes taking 10 or more years to issue, and patent flooding, which surrounds the inventor's contribution and forces him to join up with a large, entrenched Japanese company to survive.
To summarize, developing countries have concerned some industries, but they have not been competitive in biotechnology. Europe has awarded strong patents that afford U.S. innovators reasonable protection. Japan has been a very serious issue. Today we see two companies in Japan enjoying the products of Amgen—two products approaching a billion dollars in sales, at prices two to four times that of the products in this country, guaranteeing high profits. It is very easy to see what is going to happen over the long term. Those companies are going to be able to invade other countries in the field of biotechnology and be very active participants in trade.
The question then is, Can the United States dictate or influence international patent practices? Well, somehow it has to. This sounds unfair to some, but it is equally unfair to have misappropriation of intellectual property.
We know the history of what happened: Japan behind, Japan even, Japan ahead. The outlook is very serious. If we think back about that 20year period around the 1960s when U.S. patents were not being upheld, that may have been why it was easy for the Japanese to move in and take over the territory.
Now, for future challenges: The federal government's patenting of the genome was a hypothetical question until a short time ago. Would this be serious? It has now become a very practical question. The U.S. Patent Office is currently examining the NIH's application for patents on certain gene sequences. In the meantime, the Industrial Biotechnology Association has held discussions with Reid Adler of the National Institutes of Health (NIH), biotech executives and administration officials who are examining this issue. What should the NIH do with respect to all of these gene patents? A good start is to provide a forum between industry, NIH and other inter-
ested parties to see if we can understand whether these patents should be applied for, whether they should be issued, and if issued, how they should be handled.
Finally, can patents be issued faster? The U.S. Patent and Trademark Office's numbers on the average time of application pendency are very strange and not helpful. The Patent Office has always figured out ways to say it is doing things in two years when, in fact, there has not been a useful biotech patent that has taken less than four years, and usually five. If we cannot get meaningful numbers, I don't think the problem can be solved. I think the Patent Office is misleading all of us.
In terms of the conference objectives, I would like to close with these thoughts concerning a few final issues: First, with respect to international perception of the importance of intellectual property rights, the world acknowledges that the United States was the pioneer in biotechnology, and that it was done by risk capital, as well as federal support of R&D, originally. The positive contribution to human welfare is acknowledged worldwide. That does not mean that all the countries in the world want to give strong patent protection for biotechnology, which is a very difficult issue.
Second, with respect to biotechnology patents, in the United States, the road has been rocky but reasonably satisfactory. Worldwide protection will ultimately be critical. It is sad that this did not occur long ago. Because of this lack, we are seeing companies in foreign countries appropriating U.S. technology to get started.
Finally, with respect to conflict resolution, the most precious resource of a budding new industry or budding new technology is time. The solutions have to be time sensitive. Grandiose solutions that involve 60 or 70 countries, and take years and years, will mean that a lot of the companies will fail before the solutions are in place. I think people should be aware of that.
I would remind you of one last thing. This is an industry of small companies. If you look at the profile of public biotechnology companies, only 13 percent have more than 300 employees, and none have more than 2,000 employees. If we look at all biotech companies (publicly and privately held), there are only 3 percent with more than 300 employees. We are dealing with a very, very broad-based, small-company business and my remarks apply as well to my firm, ICOS, which we started within the last year, as well as to the largest biotech companies, which are still relatively small. These are the companies seeking patent protection. Strong protection can hardly ''disadvantage small companies" as some critics suggest.
As technological developments multiply around the globe—even as the patenting of human genes comes under serious discussion—nations, companies, and researchers find themselves in conflict over intellectual property rights (IPRs). Now, an international group of experts presents the first multidisciplinary look at IPRs in an age of explosive growth in science and technology.
This thought-provoking volume offers an update on current international IPR negotiations and includes case studies on software, computer chips, optoelectronics, and biotechnology—areas characterized by high development cost and easy reproducibility. The volume covers these and other issues:
- Modern economic theory as a basis for approaching international IPRs.
- U.S. intellectual property practices versus those in Japan, India, the European Community, and the developing and newly industrializing countries.
- Trends in science and technology and how they affect IPRs.
- Pros and cons of a uniform international IPRs regime versus a system reflecting national differences.
READ FREE ONLINE
Welcome to OpenBook!
You're looking at OpenBook, NAP.edu's online reading room since 1999. Based on feedback from you, our users, we've made some improvements that make it easier than ever to read thousands of publications on our website.
Do you want to take a quick tour of the OpenBook's features?
Show this book's table of contents , where you can jump to any chapter by name.
...or use these buttons to go back to the previous chapter or skip to the next one.
Jump up to the previous page or down to the next one. Also, you can type in a page number and press Enter to go directly to that page in the book.
Switch between the Original Pages , where you can read the report as it appeared in print, and Text Pages for the web version, where you can highlight and search the text.
To search the entire text of this book, type in your search term here and press Enter .
Share a link to this book page on your preferred social network or via email.
View our suggested citation for this chapter.
Ready to take your reading offline? Click here to buy this book in print or download it as a free PDF, if available.
Get Email Updates
Do you enjoy reading reports from the Academies online for free ? Sign up for email notifications and we'll let you know about new publications in your areas of interest when they're released.

Translate this page
Hundreds of scientific articles. written for kids. approved by scientists. free., search or filter, search articles, reading level.
- Elementary school (2)
- Lower high school (40)
- Middle school (31)
- Upper high school (11)
Scientific Topic
- Biodiversity and Conservation (11)
- Biology (8)
- Chemistry (1)
- Energy and Climate (10)
- Food and Agriculture (3)
- Health and Medicine (22)
- Paleoscience (1)
- Physical Science (1)
- Pollution (2)
- Social Science (15)
- Technology (2)
- Water Resources (7)
- All NGSS standards 3-5-ETS1 (1) HS-ESS2 (4) HS-ESS3 (9) HS-ETS1 (3) HS-LS1 (16) HS-LS2 (10) HS-LS3 (6) HS-LS4 (6) HS-PS3 (1) HS-PS4 (1) MS-ESS1 (1) MS-ESS2 (3) MS-ESS3 (12) MS-ETS1 (1) MS-LS1 (14) MS-LS2 (10) MS-LS3 (4) MS-LS4 (2)
- All AP Environmental Science topics 1. The Living World: Ecosystems (9) 2. The Living World: Biodiversity (8) 3. Populations (18) 4. Earth Systems and Resources (2) 5. Land and Water Use (11) 6. Energy Resources and Consumption (3) 7. Atmospheric Pollution (1) 8. Aquatic and Terrestrial Pollution (7) 9. Global Change (16)
- All IB Biology topics 02. Molecular biology (5) 03. Genetics (5) 04. Ecology (5) 05. Evolution and biodiversity (2) 06. Human physiology (19) 08. Metabolism, cell respiration and photosynthesis (1) 10. Genetics and evolution (2) 11. Animal physiology (4) A. Neurobiology and behaviour (7) B. Biotechnology and bioinformatics (15) D. Human physiology (6)
- All Scientific Methods Agricultural yield data (6) Bacterial culture (1) Case study (47) Cell culture (5) Chromatography (2) Climate scenarios (25) Community jury (1) Controlled blinded study (2) Correlational study (1) CRISPR (1) CT (1) Data extrapolation (45) Data reconstruction (19) Data validation (36) DNA sequencing (37) ELISA (6) Experiment (137) Expert committee (1) Field study (41) Fluorescence imaging (11) Gene editing (11) GIS (3) GPS tracking (12) High resolution filming (1) Life cycle assessment (2) Mark-release-recapture (2) Mass spectrometry (11) Meta-analysis (4) Microscopy (22) Observation (47) Onsite study (1) PCR (Polymerase chain reaction) (16) Policy analysis (23) Proxy data (22) Radiocarbon dating (2) Remote sensing (1) Representative sampling (85) Risk analysis (9) Scientific modeling (118) Spectroscopy (1) Survey research (28) Systematic review (20) Tomography scans (2) Tree core sampling (4) X-ray scanner (2)
- All Scientific Figures Bar graph (7) Box and whisker plot (2) Data table (9) Histogram (4) Line graph (6) Map (15) Microscopy image (4) Pictograph (17) Pie chart (1) Scatter plot (2) Time series graph (11) Venn diagram (1)
- Research Location Africa (8) Asia (10) Australia (1) Europe (12) North America (19) Ocean (6) South America (2) Space (1)
- All Scientist Affiliations CDC (1) Cedars-Sinai Medical Center Los Angeles (1) Centre for Communicable Diseases Bangladesh (1) Colorado State University (1) Cornell University (1) Harvard University (2) Hebrew University of Jerusalem (1) Imperial College London (3) Indian Institute of Technology (1) INRS (1) Johannes Kepler University (1) Johns Hopkins University (1) Katholieke Universiteit Leuven (1) Lancaster University (2) LSHTM (1) McGill University (1) Monash University (1) NASA (1) National Clinical Research Center for Respiratory Diseases (1) Natural Resources Canada (1) Pennsylvania State University (1) Rutgers University (1) San Diego State University (1) Seattle Pacific University (1) Stanford University (3) Stockholm University (1) UC Berkeley (2) UC Davis (1) UC Los Angeles (1) University of Chicago (1) University of Copenhagen (1) University of Helsinki (1) University of Lausanne (1) University of Montreal (1) University of Northern Colorado (1) University of Oxford (2) University of Pittsburgh (1) University of Plymouth (1) University of South Carolina (1) University of South Florida (1) University of Southern Denmark (1) University of Texas (1) University of Tokyo (1) University of Toulouse (1) University of Tübingen (1) University of Washington (2) Wildlife Conservation Society (1) Yale University (1)
- All Additional Languages Afrikaans (0) Arabic (1) Bengali (1) Bulgarian (6) Burmese (0) Chinese (1) Danish (1) Dutch (1) French (3) German (1) Greek (0) Italian (0) Korean (0) Lao (1) Mongolian (0) Portuguese (1) Russian (0) Spanish (6) Swedish (1)
Articles by Publication Date
Where was the research.

How does fear speech spread on social media?
+ Audio version of article + Links to lessons on identifying hate speech and misinformation + Link to lessons on how kids can navigate social media + Article translated into Spanish

Why are women hunters important?
+ Audio version of article + Links to lessons about gender roles and equity + Blackboard video version of article

How can super-recognizers help police investigations?
+ Audio version of article + Links to lessons on facial recognition and technology from Khan Academy + Link to test if you are a super recognizer + Article translated into Spanish

Why is it so hard to bring back extinct species?
+ Audio version of article + Curated links to de-extinction activities + Link to DNA Interactive

How can black rats change the behavior of reef fish?
+ Audio version of article + Lesson idea video of activities about invasive species + Link to HHMI activity about trophic cascades

Can a robotic arm be controlled by the brain?
+ Lesson Idea video tutorial to build simple robots + Two reading levels available + Audio version of article

What was the impact of a delayed second COVID-19 vaccine?
+ Link to data analysis virus activity with HHMI + Curated links to lessons about viruses and infectious diseases + Audio version of article

How are aging and gut bacteria related?
+ Curated links to lessons on the human microbiome by Teach Genetics + Audio version of article + Article translated into Bulgarian

How can we protect the seabed from storms?
+ Audio version of article + Links to marine protected areas and sustainable fishing lesson plans + Introductory video on climate change and storms

How do chemicals affect animals (and their kids)?
+ Audio version of article + Links to epigenetics lesson plans + Introductory video on endocrine disrupting chemicals
Popular Articles

Why do measles survivors get sicker?

How can we know about dinosaurs’ social lives?

How does dark energy affect galaxies?
Article collections.

4 Scientific Articles about AI and ChatGPT

Socially Important Scientific Research in 2024 Election Year

Gross and Disgusting Science Articles Collection

High School Intro Biology Collection
Popular lesson ideas.

Ask-A-Scientist E3: Dr. María-Teresa Ramírez-Herrera, Earth scientist

Data Graphing Activity for 4th-7th Graders

SJK Academy: Research Recap Video Tutorials

SJK Recommends: The World’s Best STEM Resources. Free.
Learn more about us, funding support by:.

Disclaimer: Funders play no role in article selection and writing.
Featured by:

- Students/Parents
- Administrators
- PowerSchool Tips and Tricks
- Parents Association
- School Profile

- Forms & Documents
- Bell Schedules
- Club Schedule
- School Counselors
- Health Office
- Anti-Bullying (HIB)
- Senior Mentorship
- National Honor Society
- School News
- Working Papers
School Email List

- Summer Office Hours
- Summer Homework
- Summer Camp Registration
- How to Survive Finals: A Biotech Guide
- NJ School Performance Report 2017-18
- 2019-20 Governor's STEM Scholars
- Finding Resilience in the Face of Mental Health Challenges
- AP Testing Info May 2019
- RPI Medal Class of 2020
- Rising Junior Night 2019
- Social Media Manager Needed
- Join us on Facebook!
- Fall Parent Social
- Big thanks to all those who made our Spring Social a success!
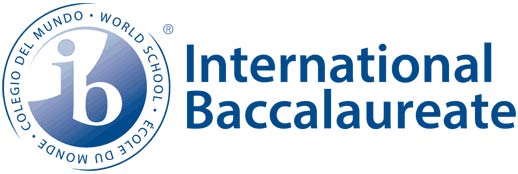
Mission Statement
Biotechnology High School, an International Baccalaureate World School, integrates life science, technology, and engineering into a rigorous curriculum that inspires students toward open-minded participation in the global community and prepares them for higher education and leadership in an increasingly demanding workplace through scholarly research, original investigations, and interactive partnerships.
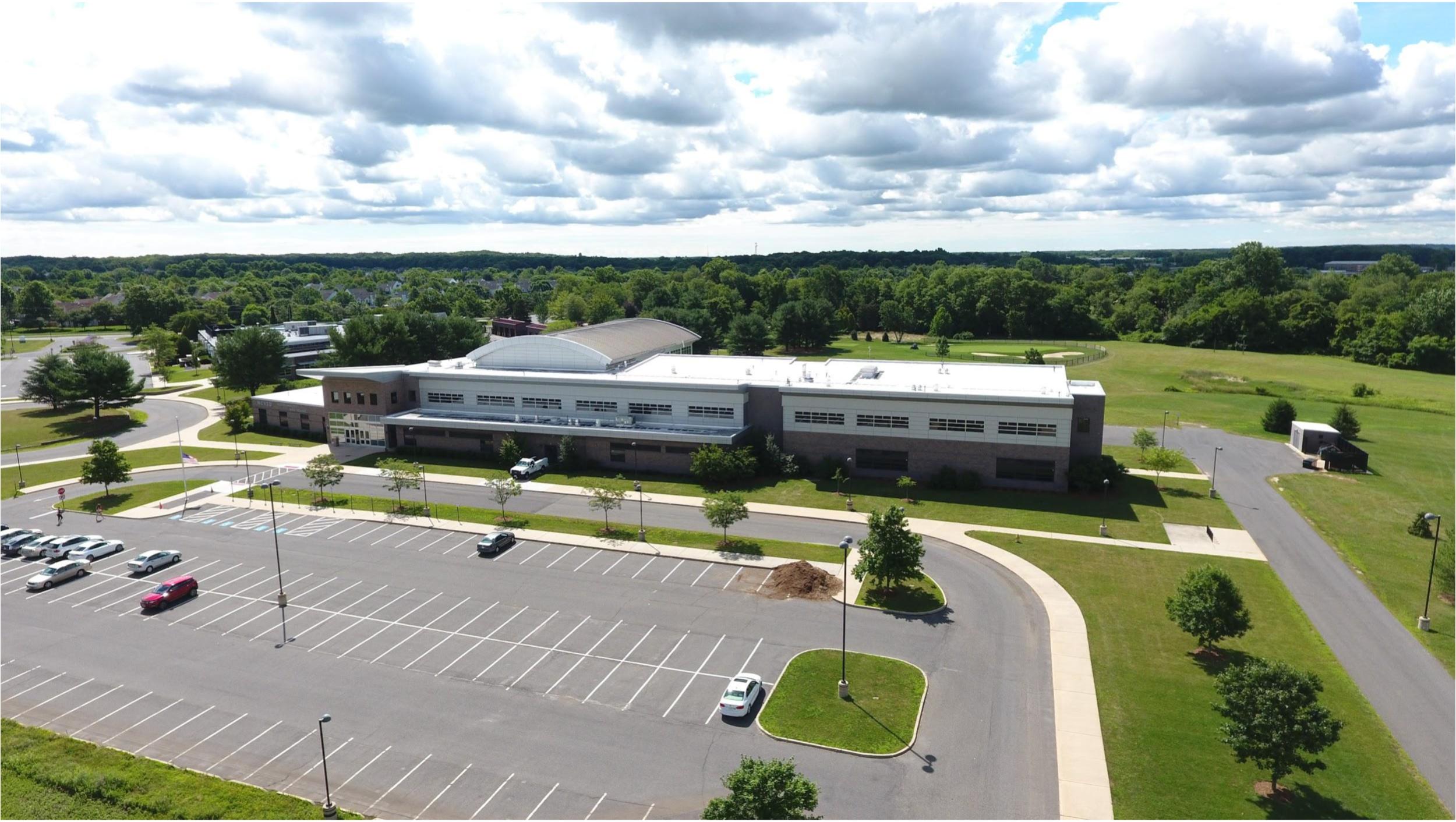
BTHS Information
- BTHS Student Handbook 2023- 2024
- Opening Letter & Forms 2023-2024
- MCVSD 2023-2024 Calendar
- 2023-2024 Bollinger Student Accident Insurance
- 2023-2024 Free & Reduced Lunch Forms
- 2023-2024 Free & Reduced Lunch Forms (Spanish)
- Nutri-Serve Food Service
- September Menu
- October Menu
- November Menu
- December Menu
- January Menu
- February Menu
- NJ School Performance Report
School Calendar
School Year 2023-2024
School Year 2024-2025
Tech Support Contact Chain
If your student is having a challenge with technology, please try the following to resolve the problem:
- Contact the classroom teacher
- Contact the student's school counselor
- Contact the BTHS Main Office - [email protected]
SCHOOL STORE
Biotechnology High School PSFA Online Store (company.site)
MCVDS Purchasing Manual
MCVSD Purchasing Manual
NJDOE’s State School Climate Coordinator ([email protected])
HIB Form (Spanish)
Non-Discrimination Clause: The Monmouth County Vocational School District does not discriminate on the basis of race, creed, color, national origin, ancestry, nationality, marital or domestic partnership or civil union status, sex, pregnancy, gender identity or expression, affectional or sexual orientation, reprisal or retaliation for prior civil rights activity, religion, age, disability, or socioeconomic status.

IMAGES
VIDEO
COMMENTS
The questionnaire developed was piloted in two high school classes (n = 43 students; Figure 2), which, as recommended by several authors (Treece and Treece, 1982; Connelly, 2008; Johanson and Brooks, 2010), represent slightly over 10% of the universe of students included in the main research study. This procedure allowed to ensure that the ...
The interdisciplinary nature of bioinformatics makes it an ideal framework to develop activities enabling enquiry-based learning. We describe here the development and implementation of a pilot project to use bioinformatics-based research activities in high schools, called "Bioinformatics@school." It includes web-based research projects that students can pursue alone or under teacher ...
Lesson plans from NYU Langone's High School Bioethics Project serve as guides for teachers who want to incorporate an in-depth analysis of bioethical issues and debates into their life sciences, social sciences, public policy, or theology courses. They are evidence-based tools that teachers can use to lead classes and discussions on topics in ...
High school science has been identified as an opportunity to inform health and scientific literacy and specifically, ... (Verhoeff et al. 2008), and example case studies: ... Using Biotechnology to Develop Values Discourse in School Science. In Corrigan D, Buntting C, Fitzgerald A, Jones A (eds) Values in Science Education. Springer, Cham. 10. ...
Saved By. 120 Members. This playlist can be used to teach several core topics in cell biology and genetics by connecting students with case studies and multimedia resources about cancer. The topics covered include the cell cycle, cancer, biotechnology, and genetic disease. By completing the resources in this playlist, students will be able to:
The aim of this study was to examine the development of understandings and attitudes about biotechnology processes as students progress through high school. In a cross-sectional case study, data ...
Case Studies. Livingston High School Prepares Students for a Future in Biotech with Virtual Labs. Livingston High School. Watch Video. When Diane Sigales was asked to design a Biotech class for Livingston High School in New Jersey, she knew that she didn't want to settle for less. Discover how Diane used virtual labs to give her students ...
The Case for Teaching Biotech in High Schools. Imagine providing your students with the foundation to create prosthetic limbs for combat veterans, design algae-powered cars, or solve crimes using forensics. Teaching biotechnology does just that while simultaneously instilling a highly desired skill set. Whether students continue their education ...
The Effect of Applying Biotechnology Skills Through Case-Study Learning on Increasing Biology Student's Knowledge, Attitudes and Interest Related to Biotechnology Fields Jody Bertram Biology Teacher J.W. Mitchell High School 2323 Little Road Trinity, Fl 34655 [email protected]
Teaching nonscience majors topics in biotechnology through case studies is the focus of this research. Our Biotechnology, Environment, and Related Issues module, developed within the Science for All framework, is aimed at elevating the level of students' scientific and technological literacy and their higher order thinking skills. The research goal was to investigate nonscience major students ...
Many examples of modern biotechnology depend on the ability to analyze, manipulate, and cut and paste pieces of DNA. Approaches for the sequencing and manipulation of DNA are sometimes referred to as DNA technology 4 .For example, for the cystic fibrosis gene therapy trial, researchers used DNA manipulation techniques to insert the chloride channel gene into a piece of carrier DNA (a ...
Download. Lesson 01 - What's up with Gena? Introduction to Biotech Case Study. Students are challenged to formulate a hypothesis for what is wrong with a patient named Gena based on her family history and complaints of sickness. Download Lesson. Whats_up_with_Gena.docx.
The case studies and laboratory procedures are appropriate for high school, community college, and university levels. ... They are primarily targeted at educational institutions with programs or course offerings in biotechnology. The case studies and labs assume a basic knowledge of laboratory skills (e.g., preparing molar solutions, general ...
High School Bioethics Project Learning Scenarios. Learning scenarios from NYU Langone's High School Bioethics Project are basic resources that teachers can use to supplement their curricula. Some are short case vignettes, while others include background material, discussion questions, multimedia selections, and suggestions for further reading.
In Part 1 of this activity, students learn the basics of DNA profiling, including the structure and inheritance of STRs. In Part 2, students learn how DNA profiles are compiled with STRs that are typically used in forensic investigations. In Part 3, they work through a case study involving a robbery and build a DNA profile that can be compared ...
Bioethics. Bioethics Resources. Bioethics Cases. Find case studies on topics in health care and biotechnology ethics, including end-of-life care, clinical ethics, pandemics, culturally competent care, vulnerable patient populations, and other topics in bioethics. (For permission to reprint cases, submit requests to [email protected] .)
CASE STUDY APPLICATIONS HIGH SCHOOL BIOETHICS CURRICULUM PROJECT KENNEDY INSTITUTE OF ETHICS, GEORGETOWN UNIVERSITY ... BIOTECHNOLOGY • Biotech I (TIERRA ... Sociology (Junior High) • Sociology (High School) Genie, The Wild Child: Research or Exploitation? Atomic Testing at Bikini Island .
These case studies represent interesting situations that a bioengineer, biologist or biochemist may encounter in their professional lives. By thinking about and discussing them, students can cultivate ethical patterns of thought prior to leaving academia, professionals can carefully consider the ethical implications of their work, and the public can consider what is at stake in living on the ...
program for high-school biotechnology majors, focusing on two case studies of lessons in which students were expected to formulate their research questions. Results: In-depth examination of students' questions, written during the two lessons, revealed that only in one of the lessons students' ability to ask research questions improved.
This thought-provoking volume offers an update on current international IPR negotiations and includes case studies on software, computer chips, optoelectronics, and biotechnology—areas characterized by high development cost and easy reproducibility. The volume covers these and other issues:
Elementary school (2) Lower high school (40) Middle school (31) Upper high school (11) Scientific Topic. Biodiversity and Conservation (11) Biology (8) Chemistry (1) Energy and Climate (10) Food and Agriculture (3) Health and Medicine (22) Paleoscience (1) Physical Science (1) Pollution (2) Social Science (15) Technology (2) Water Resources (7)
This study reports on the attitudes towards biotechnology of 905, 15-16 year-old students from 11 Western Australian schools. Students were asked to read 15 statements about biotechnology processes and to draw a line to separate what they considered 'acceptable' statements from those they considered 'unacceptable'.
Mission Statement. Biotechnology High School, an International Baccalaureate World School, integrates life science, technology, and engineering into a rigorous curriculum that inspires students toward open-minded participation in the global community and prepares them for higher education and leadership in an increasingly demanding workplace through scholarly research, original investigations ...
Voters to decide whether Fani Willis and judge in Georgia Trump election case keep their jobs ... California high school senior earns 122 college acceptances, $5.3M in scholarships in pursuit of ...
The high school science sequence at St. Anselm's includes Chemistry in grade 9, Physics in grade 10, AP Biology in grade 11, and a variety of electives, including AP Physics, in grade 12. Physics classes meet daily for 40 minutes, with a double lab period once per week (for a total of six meeting periods per section per week).