Recent Literature Review on Coumarin Hybrids as Potential Anticancer Agents
Affiliations.
- 1 Department of Pharmaceutical Chemistry, Discipline of Pharmaceutical Sciences, College of Health Sciences, University of KwaZulu- Natal (Westville), Durban-4000, South Africa.
- 2 School of Chemistry and Physics, University of KwaZulu Natal, P/Bag X54001, Westville, Durban 4000, South Africa.
- 3 Department of Chemistry, School of Science, GITAM Deemed to be University, Hyderabad, Telangana 502329, India.
- PMID: 35440315
- DOI: 10.2174/1871520622666220418143438
Cancer is considered one of the leading causes of death globally, especially patients with lung, pancreatic, or brain tumors are most likely to die of cancer, and patients with prostate and breast cancer are at a high risk of noncancer death. As a result, there is ongoing research regarding developing new, safe, and efficient anticancer agents. Coumarin-based naturally occurring compounds possess a broad spectrum of activity in medicinal chemistry, such as anticancer, anti-inflammatory, antimicrobial, antioxidant agents, etc. Many researchers have synthesized coumarinbased novel therapeutic agents via molecular hybridization technique, which offers an excellent opportunity to develop novel compounds with improved biological activities by incorporating two or more pharmacophores. This review aims to shed light on the recent developments of coumarin-based anticancer hybrid derivatives and their Structure-Activity Relationships (SAR). This review serves as a medium that medicinal chemists could utilize to design and synthesize coumarin derivatives with significant pharmacological value as future anticancer agents.
Keywords: Coumarin; SAR; anticancer agent; broad spectrum of activity; molecular hybridization; therapeutic agent.
Copyright© Bentham Science Publishers; For any queries, please email at [email protected].

Publication types
- Research Support, Non-U.S. Gov't
- Anti-Infective Agents* / pharmacology
- Antineoplastic Agents* / chemistry
- Antineoplastic Agents* / pharmacology
- Breast Neoplasms* / drug therapy
- Coumarins / chemistry
- Molecular Structure
- Structure-Activity Relationship
- Antineoplastic Agents
- Anti-Infective Agents
- Open supplemental data
- Reference Manager
- Simple TEXT file
People also looked at
Review article, coumarin-1,2,3-triazole hybrids as leading-edge anticancer agents.
- Laboratory of Chemistry, Department of Applied Sciences, Rajkiya Engineering College (Affiliated with Dr. A.P.J. Abdul Kalam Technical University Lucknow), Churk, India
Cancer is one of the most feared and dreaded diseases across the world. In clinical practice, a variety of anticancer agents of natural, semi-synthetic and synthetic origin exist, but they suffer from side effects and drug resistance, so they are insufficient to combat the disease. Coumarins are bicyclic benzene-pyrone-fused phytomolecules with a wide range of biological effects, including powerful anticancer activity on numerous cell lines. Additionally, they serve as an adaptable synthetic scaffold and research hub for medicinal chemists. On the other hand, triazoles are nitrogen-containing heterocycles having remarkable pharmacological effects including anticancer activities. Due to a better compatibility with the human metabolic system, the synthesis of nature inspired hybrid compounds as anticancer agents for a wide range of activity and fewer side effects is at the forefront of current research. In the last decade, huge research has been published on coumarin-1,2,3-triazole hybrids showing potent anticancer activities on various types of cancer. This review offers a recent, thorough literature compilation of contemporary research on the development of hybrid compounds based on coumarin-1,2,3-triazoles as potential anticancer leads throughout the previous 10 years.
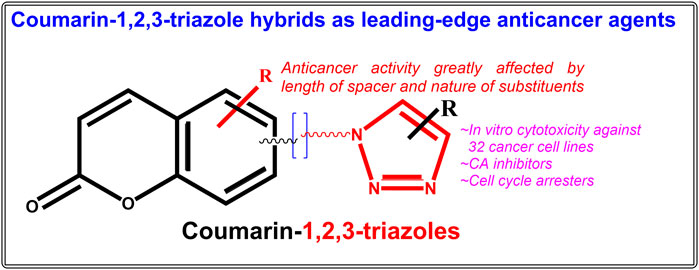
Introduction
Cancer is the rapid creation of abnormal cells in the body, growing beyond their usual boundaries ( Cooper and Hausman, 2007a ; 2007b ). The cancer cells can spread to other organs by invading the adjoining parts of the body, the process is called metastasis, which may be considered as the primary cause of death due to cancer ( Cooper and Hausman, 2007b ). Most of the cancer occurs in the form of malignant tumors with a few notable exceptions, such as leukemia ( Cooper and Hausman, 2007a ; 2007b ). The common type of cancer, known as “sarcoma”, which affects the epithelial cells lining internal organs or the skin, includes lung cancer, skin cancer, pancreatic cancer, ovarian cancer, etc. The other forms of cancer are lymphoma, melanoma, myeloma and mixed type cancers ( Cooper and Hausman, 2007a ; New Global Cancer Data: GLOBOCAN, 2018 ). Across the world, the lung, prostate, colorectal, stomach and liver cancer are the most common types of cancer in men, whereas, breast, colorectal, lung, cervical and thyroid cancer are the most common among women ( Bray et al., 2018 ; WHO, 2021 ). Out of total deaths across the globe in 2018, the one-sixth, accounting for an estimated 9.6 million were due to cancer ( Bray et al., 2018 ). In United States, the projected new cancer cases and cancer deaths are 1,918,030 and 609,360 respectively, for the year 2022; specifying 350 deaths per day from lung cancer, the leading cause of cancer death ( Siegel et al., 2022 ). In 2040, it is anticipated that there would be 29.5 million new instances of cancer per year and 16.4 million cancer-related deaths ( National Cancer Institute, 2021 ). The common methods of cancer treatment are chemotherapy, radiation therapy or surgery with chemotherapy ( Schirrmacher, 1985 ; Zugazagoitia et al., 2016 ). One or more ways of combinatorial treatment may be applied simultaneously based on the level of advancement in cancer. Some other therapies are hormone therapy, hyperthermia, immunotherapy, photodynamic therapy, stem cell transplant, surgery targeted therapy ( Schirrmacher, 1985 ; Zugazagoitia et al., 2016 ). However, still the chemotherapy is the essential mode of cancer treatment. Although the burden of cancer is increasing globally, it is having a nearly unaffordable impact on the medical infrastructure in low- and middle-income nations, which is why the death rate from cancer is so high ( Sung et al., 2021 ; Siegel et al., 2022 ).
Although there are many anticancer medications in use, the number of adverse effects and the emergence of resistance have rendered the current anticancer medications ineffective ( New Global Cancer Data: GLOBOCAN, 2018 ). New chemotherapeutic drugs are always required for the treatment of diverse cancer kinds. The drugs based on natural products have always been a great choice for a medicinal chemist for the treatment of a variety of diseases and illness because of their syncing with human metabolic system ( Dwivedi et al., 2014 ; Saxena et al., 2018 ; Upadhyay, 2019 ). More than half of the drugs approved by United States Food and Drug Administration (USFDA) are either natural product or their prototype in form of semi-synthetic or synthetic drug ( Upadhyay et al., 2014 , 2020 ; Newman and Cragg, 2020 ; Atanasov et al., 2021 ). Only 37% of the 974 small molecules that were developed as novel anticancer drugs between 1981 and 2006 were actually synthetic; the remainder were either naturally occurring, their derivatives, or modelled after natural products ( Cragg and Newman, 2009 ). Of the 175 small molecules used as anticancer medications between the years of the 1940s and 2010, 131 (74.8%) are non-synthetic and 85 (48.6%) are truly either natural compounds or directly derived from them ( Newman and Cragg, 2012 ). In this way, natural ingredients have served as medications for practically all prevalent illnesses and disabilities either directly or indirectly, and the process is still ongoing ( Cragg and Newman, 2009 ; Newman and Cragg, 2012 ). With improvements in anticancer drug development and discovery over the past few decades, the FDA has approved more than 100 drugs for cancer treatments ( Blagosklonny, 2004 ; Kinch, 2014 ). Currently available plant-based anticancer drugs fall into four categories: vinca alkaloids (vinblastine, vincristine, and vindesine), epipodophyllotoxins (etoposide and teniposide), taxanes (paclitaxel and docetaxel), and camptothecin derivatives (camptotecin and irinotecan) ( Desai et al., 2008 ; Zaid et al., 2017 ; Yedjou et al., 2019 ). Based on their modes of action, these drugs can be broadly divided into two basic categories: cytotoxic and targeted agents ( Masui et al., 2013 ; Winkler et al., 2014 ).
Both coumarin and 1,2,3-triazole moieties are reported to possess a diverse range of pharmacological activities including anticancer, anti-HIV, antimalarial, anti-tubercular, anti-microbial, anti-inflammatory, antioxidant, antiviral, and diabetic activities ( Stefanachi et al., 2018 ; Bozorov et al., 2019 ). In the past 10 years, many biologically active pharmacophores with coumarin-1,2,3-triazole moiety have been created by synthetic chemists in an effort to obtain multi-targeted single molecules ( Emami and Dadashpour, 2015 ; Upadhyay, 2021 ). The numerous advancements and uses of coumarin-1,2,3-triazole hybrid compounds as potential bioactive leads have already been outlined in a number of reviews ( Emami and Dadashpour, 2015 ; Fan et al., 2018 ; Al-Warhi et al., 2020 ; Song et al., 2020 ; Alam, 2022 ). Significant work has been done in recent years on the design, synthesis, and assessment of numerous hybrid compounds based on coumarin-1,2,3-triazoles as anticancer agents, the compilation of which may generate important findings for the development of novel anticancer drugs. An effort has been made to outline recent advancements in the development of hybrid compounds based on coumarin-1,2,3-triazoles as anticancer agents in this stand-alone review. The original research articles were searched in the Pubmed and Google Scholar databases under the keywords “Coumarin-1,2,3-triazole hybrid molecules as anticancer agents”, “Coumarin-1,2,3-triazole + anticancer’, Coumarin-1,2,3-triazole + antiproliferative” etc. The considered range of publication year was January 2012-July 2022, under which more than twenty-five original research articles on various coumarin-1,2,3-triazole hybrids with anticancer activity on a total of thirty-two cancer cell lines were finally taken in consideration. The current review will enable researchers to develop novel anticancer coumarin-1,2,3-triazole hybrids that are far more precise and have greater activity.
Coumarin and 1,2,3-triazole: The scaffolds with broad range biological activities
Coumarin (2H-1-Benzopyran-2-one) are phenolic compounds which is association of β -pyrone rings with benzene, widely distributed in plants bacteria and fungi ( Abyshev et al., 2006 ; Katsori and Hadjipavlou-Litina, 2014 ). Fundamentally, the benzopyrones have been split into two groups: benzo-α-pyrones, which are the primary class of coumarin, and benzo- γ -pyrones, which mostly contain flavonoids ( Lacy and O’Kennedy, 2004 ; Lacy, 2005 ). In plants, most of the benzopyrones are reported to exist in the form of glycosides making them highly polar ( Matos et al., 2015 ). Coumarins are a varied class of naturally occurring pharmacophores with a wide range of bioactivities viz. anti-inflammatory, antioxidant, antinociceptive, hepatoprotective, antithrombotic, antiviral, antimicrobial, antituberculosis, anticancer, antidepressant, antihyperlipidemic, anti-Alzheimer, anticholinesterase, and antiviral activities ( Upadhyay et al., 2012 ; Katsori and Hadjipavlou-Litina, 2014 ; Kraljević et al., 2016 ; Singh et al., 2019 ; Wang et al., 2020 ). There are numerous clinically effective compounds, both natural and synthetic, containing coumarin scaffolds ( Emami and Dadashpour, 2015 ; Upadhyay, 2019 ) ( Figure 1 ). Coumarins are reported to possess anticancer activity with minimal side effects ( Musa et al., 2008 ; Stefanachi et al., 2018 ; Rawat and Vijaya Bhaskar Reddy, 2022 ). They have the ability to regulate a wide variety of cellular pathways involved in cancer, including those involved in kinase inhibition, cell cycle arrest, angiogenesis, heat shock protein (HSP90) inhibition, telomerase inhibition, antimitotic activity, carbonic anhydrase inhibition, monocarboxylate transporters inhibition, aromatase inhibition, and sulfatase inhibition, each of which can be explored for specific anticancer activity ( Thakur et al., 2015 ; Goud et al., 2020 ; Wu et al., 2020 ).
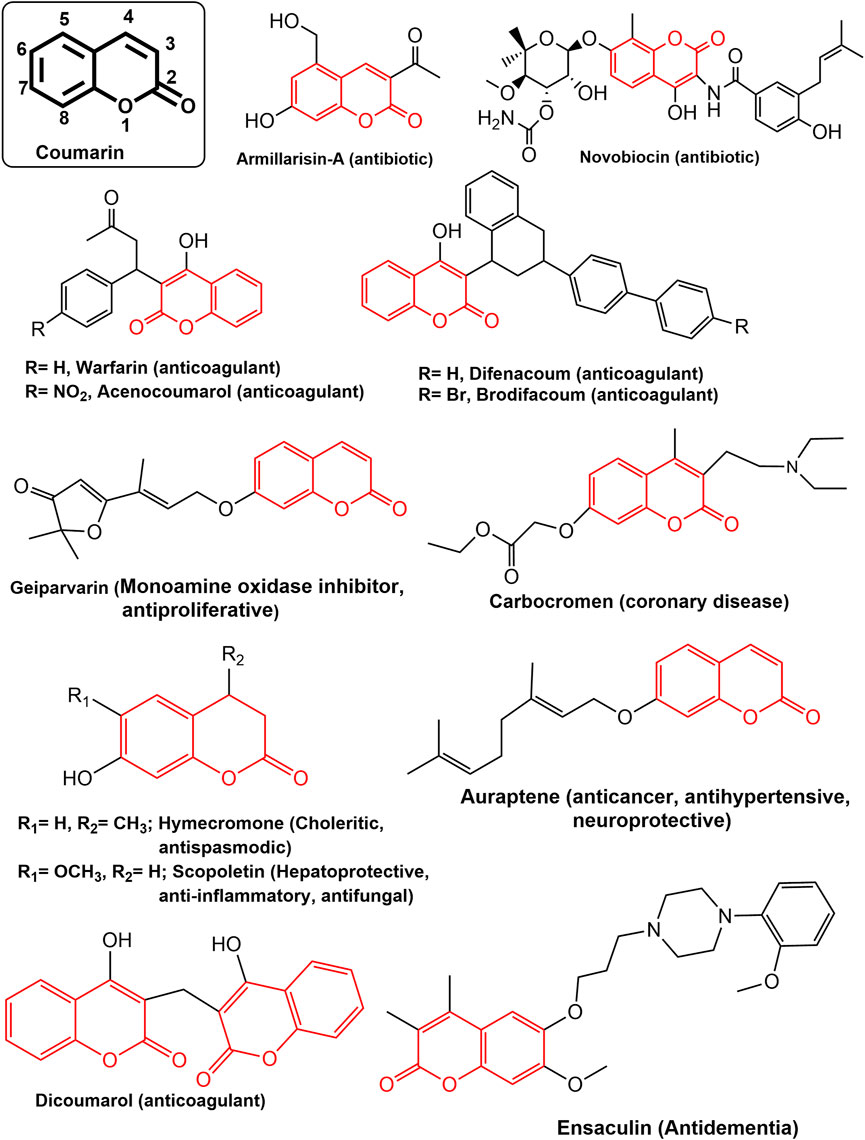
FIGURE 1 . Some notable drugs with coumarin moiety.
Nitrogen-based heterocyclic secondary metabolites are widely reported from plants, fungi, algae etc. Numerous heterocyclic compounds with nitrogen have been found to have a variety of pharmacological effects, including anti-cancer, anti-HIV, anti-malarial, anti-tubercular, anti-microbial, and antidiabetic properties ( Joule, 2016 ; Shang et al., 2018 ; Gopalakrishnan et al., 2021 ). Because nitrogen can interact in a variety of ways with biological targets, both naturally occurring and synthesized nitrogen-based heterocyclic molecules have drawn the interest of medicinal chemists ( Kerru et al., 2020 ; Upadhyay, 2021 ). The importance of nitrogen-based heterocycles can be judged not only in the form of approximately countless published research articles but also in the form of clinical molecules in the therapy of various diseases and debilities ( Kerru et al., 2020 ; Gopalakrishnan et al., 2021 ). A lot of representative nitrogen heterocycles viz. Ciprofloxacin, Tazobactum, Cefatrizine (antibiotic); Oxiconazole, Clotrimazole (Antifungal); Celecoxib (Anti-inflammatory), Rimonabant (Anti-obesity), Difenamizole (Anti-analgesic), Fezolamine (Antidepressant), Bedaquiline (Anti-TB), Pitavastatin (Cholesterol-lowering agent), Gefitinib (Growth factor receptor (EGFR) tyrosine kinase inhibitor), Ezetimibe (Cholesterol absorption inhibitor), Clavulanic acid (β-Lactamase inhibitor) are in regular clinical practice ( Kerru et al., 2020 ). Some of the noticeable nitrogen heterocycle-based molecules as anticancer drugs are Erlotinib, Lapatinib, Ibrutinib, Capecitabine, Folinic acid, Monastrol, Dacarbazine, and Carboxyamidotriazole ( Perabo et al., 2004 ; Romero et al., 2004 ; Dorababu, 2020 ; Kerru et al., 2020 ; Upadhyay, 2021 ). Amongst all the nitrogen-based molecules, the 1,2,3-triazole is a privileged moiety in biologically active leads putting its importance in the field of medicinal chemistry ( Upadhyay, 2021 ). Figure 2 shows a few examples of typical pharmaceuticals with a 1,2,3-triazole moiety. Due to the triazole unit’s strong dipole properties driven by the presence of three nitrogen atoms, a wide range of biological targets can be bound to it with a high affinity ( Liang et al., 2021 ). The 1,2,3-triazoles have been claimed to have a variety of pharmacological effects as well as anticancer effects through a variety of mechanisms of action ( Liang et al., 2021 ; Alam, 2022 ). Their anticancer property is due to inhibition of the enzymes that contribute to the development of this deadly disease, such as the carbonic anhydrases (CAs), thymidylate synthase (TS), aromatase, tryptophan-2,3-dioxygenase (TDO), vascular endothelial growth factor receptor (VEGFR), and epidermal growth factor receptor (EGFR) ( Perabo et al., 2004 ; Liang et al., 2021 ).
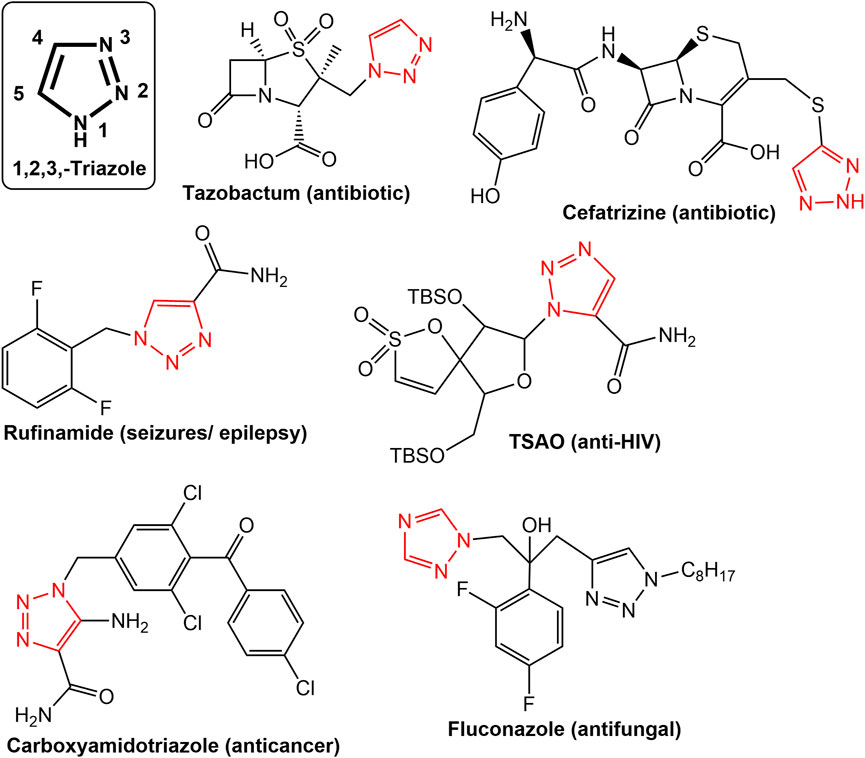
FIGURE 2 . Some notable drugs with triazole moiety.
Coumarin-1,2,3-triazole hybrid molecules as anticancer agents
Combining biologically relevant moieties with different modes of action to create hybrid therapeutic molecules is a brilliant strategy that may lead to the development of drugs with improved pharmacological properties for the treatment of complicated disorders, such as cancer ( Shaveta et al., 2016 ; Mohamed and Abuo-Rahma, 2020 ). Hybrid drugs can affect several targets involved in the growth of cancer cells at once, and as a result, they have received a lot of attention in recent years ( Xu et al., 2019a ; Bozorov et al., 2019 ; Liang et al., 2021 ; Alam, 2022 ). Recently there is a burst in the synthesis and assessment of coumarin-1,2,3-triazole based anticancer hybrid molecules. The major anticancer hybrid molecules covered in this review are simple coumarin-1,2,3-triazoles, chalcone-coumarin-1,2,3-triazoles, 1,2,3-triazole tethered C5-curcuminoid coumarin hybrids, 1,2,3-triazole tethered isatin-coumarin hybrids, morpholines linked coumarin-1,2,3-triazole hybrids, tetraethylene glycol-tethered isatin–1,2,3-triazole–coumarin hybrids, coumarin-1,2,3-triazole-podophyllotoxin hybrids, α, β -unsaturated carbonyl linked coumarin-triazole hybrids, and 1,2,3-triazole-coumarin-glycosyl hybrids.
Duan et al. Synthesised a series of coumarin-1,2,3-triazole-dithiocarbamate hybrids, which displayed good anticancer activity against human gastric carcinoma (MGC-803) and human breast cancer (MCF-7) cell lines, but all the derivatives were inactive against esophageal cancer (EC-109) cell line (IC 50 > 128 µM). The substitution of coumarin moiety with 1,2,3-triazole-dithiocarbamate residue at position 4 (derivative 1 ) led to potent anticancer activity against the MGC-803 (IC 50 4.96 µM), MCF-7 (IC 50 10.44 µM) and prostate cancer (PC-3) cell line (IC 50 36.84 µM), while the substitution with same residue at position 7 led to molecules with decreased anticancer activity (derivatives 2a-b ) in range of 67.05 µM–90.38 µM ( Duan et al., 2013 ). A series of chalcone-coumarin derivatives linked by the 1,2,3-triazole ring ( 3 – 9 ) were prepared and assessed for cytotoxic activity against human liver bile duct (HuCCA-1), human hepatocellular (HepG2), adenocarcinomic human alveolar basal epithelial (A549) and human malignant T-lymphoblastic (MOLT-3) cancer cell lines by Pingaew et al. Most of the synthesized hybrids, except for hybrid 5 , exhibited cytotoxicity against MOLT-3 cell line without affecting normal cells. SAR study systematically disclosed the effect of substitution pattern in both rings (A & B) of chalcone moiety. The hybrids with, 3/4 triazole at ring A and 2,3-di-OMe at ring B show potent HepG2 inhibitory effect as evident from IC 50 values 15.70, 8.18 and 4.26 µM for compounds 4 , 5 and 7 respectively. Meantime, hybrids with 3/4 triazole at ring A and tri-OMe substitution at ring B ( 8 , 9 ) were shown to lose the cytotoxic effect against HuCCA-1, HepG2, A549 except for 8 , which showed IC 50 6.13 µM against HuCCA-1 ( Pingaew et al., 2014 ). A series of 4-(1,2,3-triazol-1-yl)-coumarin derivatives were synthesized and evaluated for their anticancer activity against MCF-7, A549, and colorectal (SW480) human cancer cell lines. Most of the molecules exhibited significant antiproliferative activities. Compounds 10a-d exhibited IC 50 values of 9.45, >50, 36.83 and 1.72 respectively against MCF-7 cell line, from which SAR may be established that–CH 2 O–bridge at C-4 position of 1,2,3-triazole nucleus on phenyl moiety is the best linker for cytotoxic activity ( Zhang et al., 2014 ). Further, a close observation on 2/4 substituted phenoxy derivatives 11–23 (IC 50 values 34.14, 28.91, 24.82, 12.67, 2.04, 1.92, 11.41, 7.00, 3.13, 5.84, 8.56, 4.62, and 5.89 μM respectively against MCF-7 cell line) reveals that the anticancer action is enhanced by the presence of a hydrogen bond acceptor, such as a fluorine atom at the C-4 position of the phenoxy moiety and a methoxy group at the C-7 position of coumarin ( Zhang et al., 2014 ). Amongst all, the 4-(4-((4-fluorophenoxy) methyl)-1,2,3-triazol-1-yl)-7-methoxycoumarin ( 23) was found potentially active against all the tested cancer cell lines with IC 50 values of 5.89, 1.99 and 0.52 μM against MCF-7, SW480 and A549 respectively. In further studies, the compound 23 was found to possess ability to arrest the cell-cycle at G2/M phase ( Zhang et al., 2014 ). A series of 2-(4-R-triazolyl)-substituted 3-oxo-2,3-dihydrofurocoumarins was synthesized and evaluated against acute lymphoblastic leukaemia (CEM-13), membrane-type 4 (MT-4), myeloid leukaemia (U-937) human cancer cell lines using MTT assays. Most of the synthesized hybrids were active against the tested cell lines. It was observed that the selective cytotoxic activity to cancer cell lines interestingly increased in hybrid 24 bearing the 4-hydroxy-3-methoxybenzamidomethyl moiety on the triazole ring which showed 50% cytotoxic dose (CTD50) values 9.00, 10.00 and 8.00 µM respectively against CEM-13, MT-4, U-937 cancer cell lines. The hybrid 24 showed higher binding affinity (-9.2 kcal/mol) to active sites of the anticancer target PDE4B (Phosphodiesterase-4B), comparable to Rolipram (-8.3 kcal/mol), the standard drug ( Lipeeva et al., 2015 ). The structure of coumarin-1,2,3-triazole hybrids 1 – 24 have been depicted in Figure 3 .
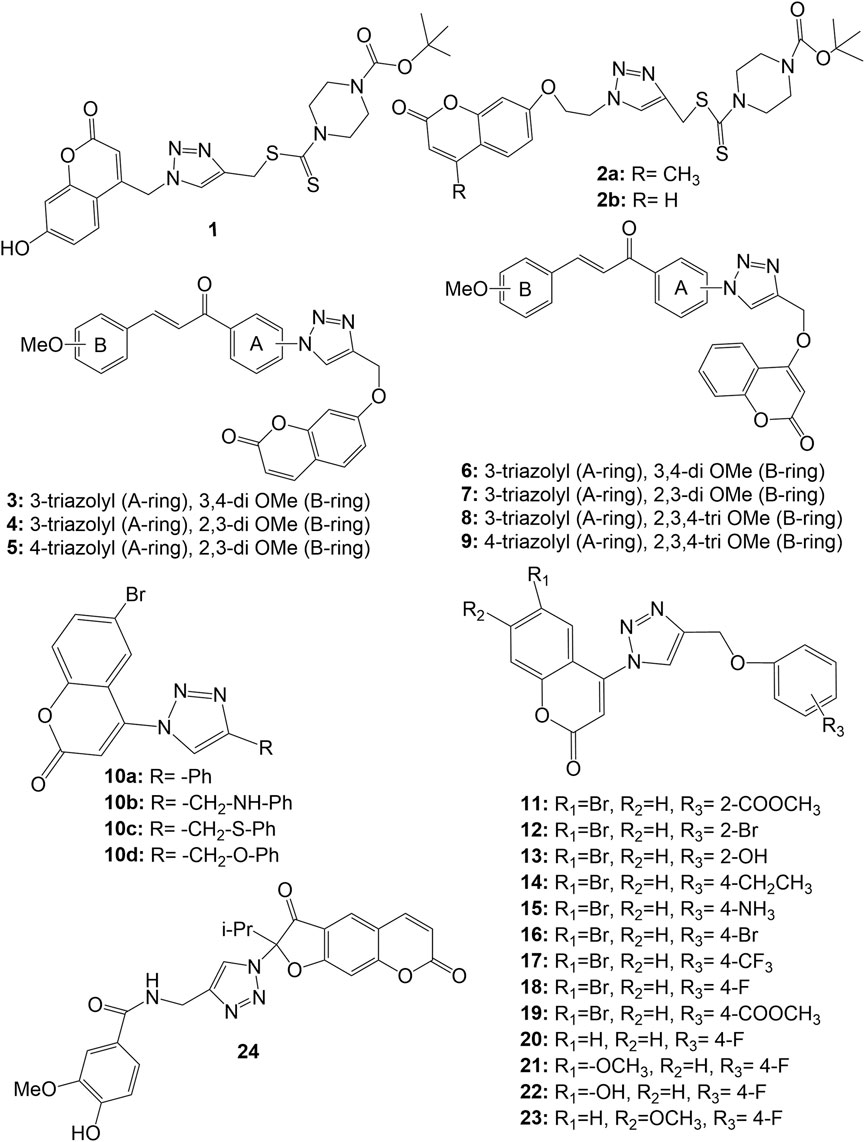
FIGURE 3 . Structure of coumarin-1,2,3-triazole-dithiocarbamate hybrids ( 1 , 2 ), 1,2,3-triazole linked chalcone-coumarin hybrids ( 3 – 9 ), 4-(1,2,3-triazol-1-yl)-coumarin hybrids ( 10 – 23 ), and 2-(4-R-triazolyl)-substituted 3-oxo-2,3-dihydrofurocoumarin hybrid ( 24 ).
A series of 1,2,3-triazole tethered coumarin 4-substituted alkyl, phenyl, alkylphenyl, dithiocarbamate, benzenesulfonamide, and benzofused heterocycle subunit were designed and synthesized by Kraljevic et al. for the evaluation of in vitro antiproliferative activity against human cancer cell lines A549, HepG2, CFPAC-1 (ductal pancreatic), HeLa (cervical cancer) and SW620 (colorectal cancer). Most of the compounds showed inhibitory effects against A549 and HeLa cell lines (IC 50 ≤ 30 μM). It was noticed that there is a strong correlation between lipophilicity and antiproliferative activities, suggesting that lipophilic 1,2,3-triazole-coumarin hybrids with the subunits phenylethyl ( 25 ), 3,5-difluorophenyl ( 26 ), 5-iodoindole ( 27 ) and benzimidazole ( 28 and 29 ) ( Figure 4 ) may very well have cytostatic potential ( Kraljević et al., 2016 ). The 7-methylcoumarin–1,2,3-triazole–2-methylbenzimidazole hybrid 28 showed maximum cytotoxicity against HepG2 cells with IC 50 value of 0.9 μM with high selectivity (SI = 50). The strong antiproliferative activity of 28 may be related to its suppression of 5-lipoxygenase (5-LO) activity and disruption of sphingolipid signalling by interfering with intracellular acid ceramidase (ASAH) activity ( Kraljević et al., 2016 ). Singh et al. synthesized 1,2,3-triazole tethered C5-curcuminoid coumarin bifunctional hybrids and evaluated them against THP-1 (acute monocytic leukemia), COLO-205 (colon), HCT-116 (colon) and PC-3 human cancer cell lines. The hybrid compounds 30a-b and 31 ( Figure 4 ) exhibited cytotoxicity against THP-1, COLO-205, and HCT-116 with IC 50 values ranging from 0.82 to 4.68, 2.34–6.78, and 4.48–9.95 μM respectively. The hybrid compounds, however, had no effect on the prostate cancer cell line (PC-3). It has been observed that the anticancer effect depends on the presence of a two-carbon spacer between the coumarin and triazole molecule ( Singh et al., 2016 ). In another strategy to design novel anticancer hybrid molecules, the authors synthesized a series of 1,2,3-triazole tethered isatin-coumarin hybrids by applying click chemistry approach and evaluated their cytotoxic potential. The compounds 32–44 ( Figure 4 ) displayed significant cytotoxicity against THP-1 (IC 50 values 0.73, 1.99, 5.47, 6.43, 7.01, 8.57, 5.03, 5.76, 6.99, 7.14, 5.12, 7.10, 7.56 µM respectively), COLO-205 (IC 50 values 3.45, 6.67, 8.87, 10.53, 12.01, 12.97, 5.71, 8.88, 10.66, 12.99, 7.67, 9.11, 13.14 respectively), and HCT-116 (IC 50 values 3.04, 5.41, 5.77, 8.09, 8.99, 9.54, 5.18, 7.26, 9.66, 11.46, 8.05, 10.44, 12.76 respectively) human cancer cell lines. The most potent compound 32 displayed IC 50 0.73, 3.45 and 3.04 µM against THP-1, COLO-205, and HCT-116 respectively, and also endowed with most prominent tubulin polymerization inhibition potential with an IC 50 value of 1.06 μM. Compound 33 having fluoro substitution on isatin, exhibited IC 50 1.99, 6.67, and 5.41 µM against THP-1, COLO-205, and HCT-116 respectively. SAR indicates that both increase in length of carbon-bridge connecting isatin moiety with triazole ring, and substitution on isatin retards the cytotoxic potential ( Singh et al., 2017 ). Goud et. al . synthesized a series of morpholine linked coumarin-1,2,3-triazole hybrids and evaluated against five human cancer cell lines, namely, bone (MG-63), lung (A549), breast (MDA-MB-231), colon (HCT-15) and liver (HepG2), using MTT assay. Among the synthesized, compounds 45–53 ( Figure 4 ) showed far better activity than the standard drug cisplatin against MG-63 and A549 cancer cell lines except 47 (IC 50 19.47 µM) against MG-63. The compounds 46 and 50 showed better cytotoxic activity than cisplatin against MDA-MB-231, HCT-15, and HepG2 cancer cell lines than cisplatin. Among all, compound 50 was found most potent, showing IC 50 values 0.80, 2.97, 4.05, 3.93, and 7.19 µM against MG-63, A549, HCT-15, MDA-MB-231, and HepG2 respectively. Meantime, cisplatin showed IC 50 17.42, 24.15, 19.45, 16.97, and 25.73 µM against MG-63, A549, HCT-15, MDA-MB-231, and HepG2 respectively. It was concluded that dimethylmorpholine derivatives with an electron-withdrawing group viz. 50 and 51 are more cytotoxic than simple morpholine derivatives like 45 , 46, and 48 . The compound 50 induced sub-G1 phase arrest, increased apoptosis, and promoted the production of reactive oxygen species justifying the potential cytotoxic activity ( Goud et al., 2019 ). In vitro anticancer activities of several isatin-1,2,3 triazole-tethered coumarin hybrids were investigated against HepG2, Hela, A549, DU145 (prostate cancer), SKOV3 (ovarian carcinoma), MCF-7, and drug-resistant MCF-7/DOX (doxorubicin-resistant MCF-7) human cancer cell lines. The hybrids 54–55 ( Figure 4 ) showed weak to moderate (IC 50 17.96 to > 50 μM) in vitro anticancer activities. SAR indicated that hydrogen-bond donor–NOH group at C-3, and electron-donating methyl group at C-5 positions of isatin motif could significantly enhance the anticancer activity as evident in case of hybrid 55 ( Diao et al., 2019 ).
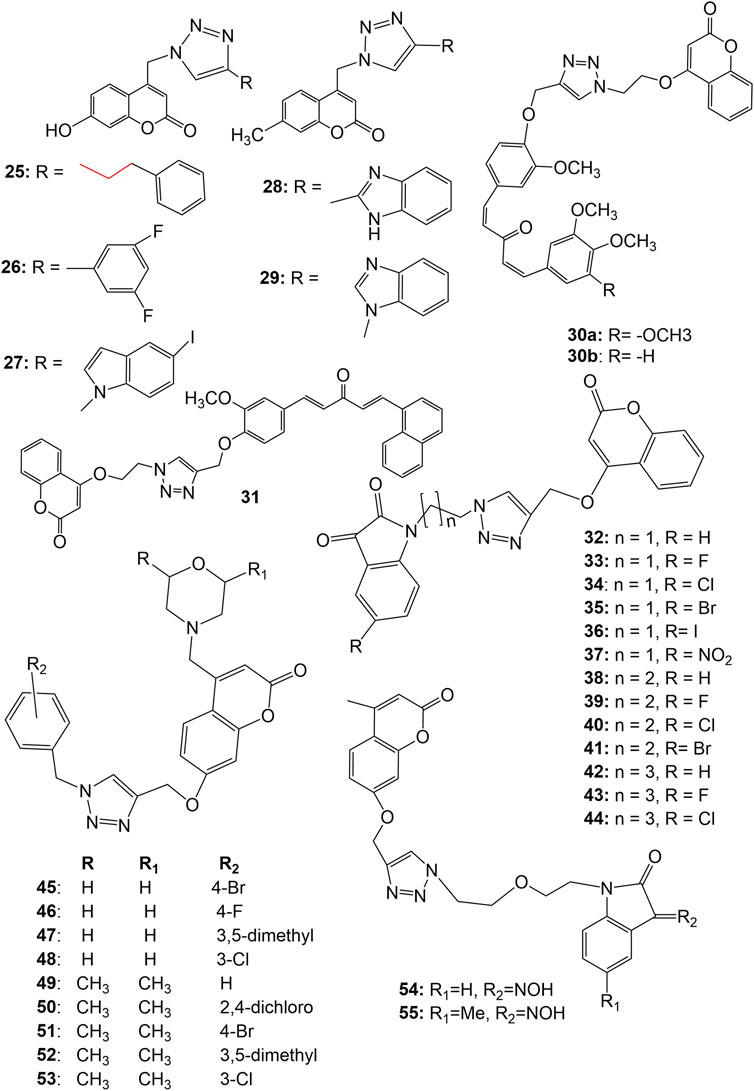
FIGURE 4 . Structure of 1,2,3-triazole tethered coumarin 4-substituted hybrids ( 25 – 29 ), 1,2,3-triazole tethered C5-curcuminoid coumarin hybrids ( 30 – 31 ), isatin-1,2,3 triazole-4-coumarin hybrids ( 32 – 44 ), morpholines linked coumarin-1,2,3-triazole hybrids ( 45 – 53 ), isatin-1,2,3 triazole-7-coumarin hybrids ( 54 – 55 ).
Xu et al. synthesized a series of tetraethylene glycol-tethered isatin–1,2,3-triazole–coumarin hybrids and assessed for in vitro cytotoxic activities against seven human cancer cell lines, namely HepG2, Hela, A549, DU145 (prostate), SKOV3 (ovarian adenocarcinoma), MCF-7 and MCF-7/DOX. Weak to moderate anticancer activity were displayed by the hybrid compounds. The hybrids 56–58 ( Figure 5 ) were found active against all the tested cell lines in a range of IC 50 values 20.09–49.24 µM. The SAR indicated that the electron withdrawing group at C-5 position of isatin motif enhances the anticancer activity ( Xu et al., 2019b ). Hao et al. synthesized a series of coumarin-1,2,3-triazole-podophyllotoxin hybrid molecules using the click reaction, and evaluated for their cytotoxic potential against A549, HepG2, HeLa and LoVo (colorectal) cancer cell lines using the MTT assay. Most of the hybrids exhibited equivalent or slightly weaker cytotoxic activities in all the 4 cell lines compared with that of etoposide (VP-16), the standard drug. The hybrids 59 exhibited IC 50 8.6, 9.3, 15.2, and 8.7; while 60 exhibited IC 50 13.3, 13.6, 19.8, and 7.3 μM against A549, HepG2, HeLa, and LoVo cells, respectively. Among all, hybrid 61 exhibited the most potent cytotoxicity, with IC 50 values of 17.5, 9.9, 9.7, and 4.9 μM against A549, HepG2, HeLa, and LoVo cells, respectively, which was far better than the standard VP-16 (IC 50 25.6, 10.5, 12.2, and 14.9 μM against A549, HepG2, HeLa, and LoVo cells, respectively). Additional research showed that compound 61 entered into the DNA of LoVo cells, selectively inhibited Topo IIβ than Topo IIα, arrested the cell cycle in the G1 phase, and disrupted microtubule organisation. It was observed that 1,2,3-triazole-4-methylamine is a more suitable linker than 1,2,3-triazole-4-methyloxy in this series conjugates ( Hao et al., 2019 ). Narsimha et al. synthesized various hybrid molecules using 3-fluoro-4-morpholinophenyl-1,2,3-triazolyl moiety. Among them the hybrid having 7-hydroxy-4-methylcoumarin at fourth position of 1,2,3-triazole ( 62 ), exhibited potent activity against both MCF-7 and HeLa cell lines with IC 50 values 3.12 and 2.77 μM, respectively. The cytotoxic activity of 62 was comparable to the standard drug doxorubicin (IC 50 values 2.63 μM in MCF-7 and 1.23 μM in HeLa cells). The cytotoxic activity of the hybrid having 4-hydroxycoumarin at fourth position of 1,2,3-triazole ( 63 ) also exhibited significant cytotoxicity against the two cancer cell lines MCF-7 and HeLa with IC 50 values 5.19 and 12.42 μM, respectively. Both the compounds were almost inactive (IC 50 values 58.11 and 66.38 μM respectively) against HEK-293 cancer cell lines ( Narsimha et al., 2020 ). Coumarin-tagged β -lactam triazole hybrids ( 64a-b ) showed moderate cytotoxic activity against MCF-7 cancer cell lines with IC 50 values of 53.55 and 58.62 μM, respectively. Both the derivatives showed high binding affinity (binding energies −11.3 and −10.9 kcal/mol respectively) with the target estrogen receptor-α (ER-α) ( Dhawan et al., 2020 ). A range of bifunctional molecular hybrids based on uracil and coumarin, roped with 1,2,3-triazole moiety were synthesized and assessed against six human cancer cell lines, namely Colo-205, MCF-7, A549, PA-1 (ovarian), PC-3 (prostate) and Hela cells by Sulforhodamine B assay. The synthesized molecules ( 65–68 ) were potentially active against MCF-7 cancer cell proliferation ( Sanduja et al., 2020 ). The compounds 65a - f , 66a - e , 67a - c and 68a - b showed 50% cell growth inhibitory concentration (GI 50 ) 1.55–6.88 µM, even better than the standard drug 5-Fluorouracil (GI 50 5.28 µM). The compounds 67d , 67e , 68c , 68d , and 68e ( Figure 5 ) also exhibited potential activity against MCF-7 cancer cell line with GI 50 values 7.75, 9.77, 7.29, 9.33, and 12.24 µM respectively. Amongst all, 65a (with a fluorine atom as R with two carbon chain length between triazole and coumarin moieties) was found to be a most potent hybrid (GI 50 = 1.55 µM). The hybrid 65a strongly inhibited the proliferation of MCF-7 cells by deferring the G2/M phase in cell cycle analysis studies. Further, in attempt to gain insight on interactions between 65a and the tubulin protein, docking studies were performed. The results indicated that 65a fit well at the interface of α2 and β1 subunits of tubulin (vinblastine binding site) and is stabilized by hydrogen bonding as well as van der Waal’s interactions suggesting it a potential lead for the discovery and development of novel treatments of breast cancer ( Sanduja et al., 2020 ). Noticeable that, hybrids with substituted uracil (electron withdrawing or electron donating) were more active than the hybrids with unsubstituted uracil. With the length of the chain increasing, the hybrid molecules’ activity reduced. Fluorine-substituted hybrids had the strong activity, and halogen substitution at C5 of uracil was found necessary for the activity ( Sanduja et al., 2020 ). A series of α, β -unsaturated carbonyl linked coumarin-1,2,3-triazole hybrids, 69–71 were synthesized by microwave irradiation conditions and were screened in vitro for their anticancer activity. The hybrid 70 was found most potent, exhibiting IC 50 10.538 and 9.845 µM respectively against PC-3 and DU-145 cell lines. The hybrids 69 and 71 were equally effective against PC-3 cell lines (IC 50 16.254 and 16.652 µM respectively) ( Vagish et al., 2021 ). Figure 5 shows the structure of the coumarin-1,2,3-triazole hybrids 56 – 71 , and Supplementary Tables S1, S2 of the supplementary material contains in vitro cytotoxicity potential of these hybrids against various cancer cell lines.
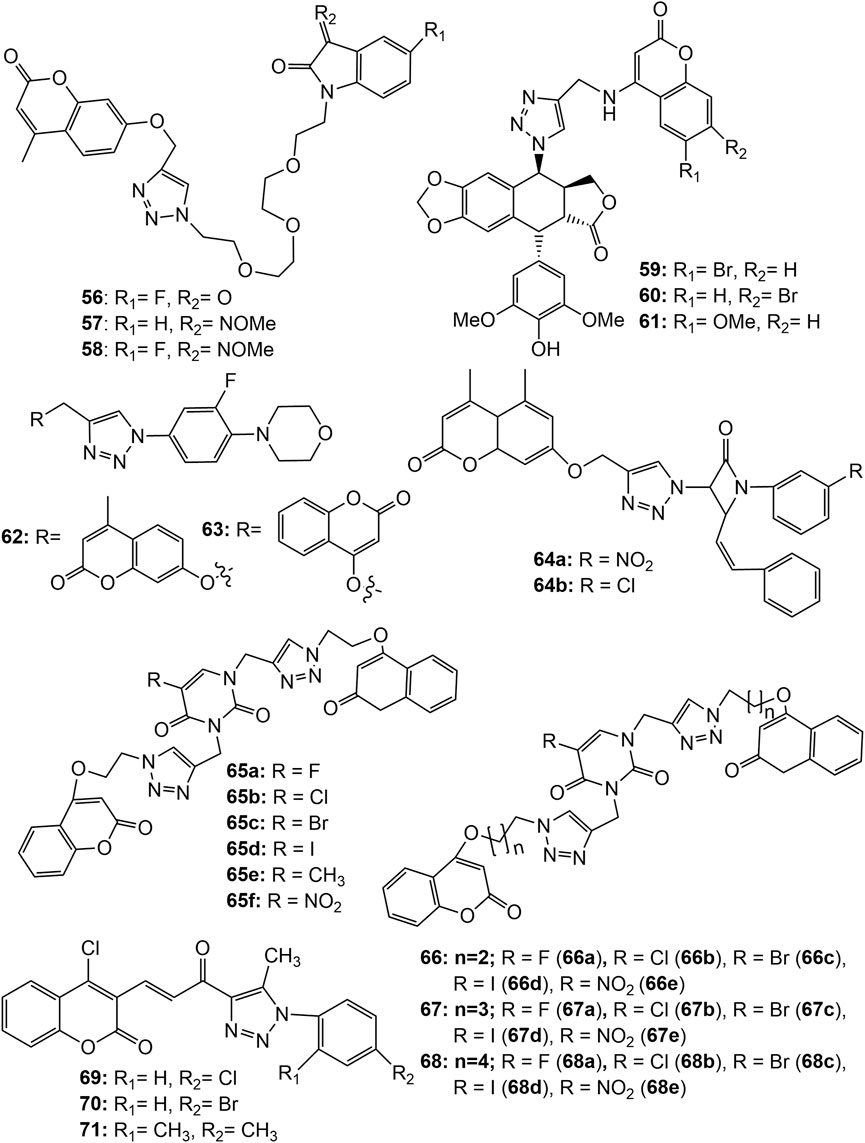
FIGURE 5 . Structure of tetraethylene glycol-tethered isatin–1,2,3-triazole–coumarin hybrids ( 56 – 58 ), coumarin-1,2,3-triazole-podophyllotoxin hybrids ( 59 – 61 ), 3-fluoro-4-morpholinophenyl-1,2,3-triazolyl coumarin hybrids ( 62 – 63 ), Coumarin-tagged β -lactam triazole hybrid ( 64 ), 1,2,3-triazole roped uracil-coumarin bifunctional hybrids ( 65 – 68 ), α, β -unsaturated carbonyl linked coumarin-1,2,3-triazole hybrids ( 69 – 71 ).
The Zinc-binding enzymes called human carbonic anhydrases (hCAs) catalyse the reversible hydration of carbon dioxide to bicarbonate ( Kumar et al., 2021 ). Various hCA isoforms have been isolated, and are reported to play crucial physiological roles. The over expressing of hCA isoforms, I, II, IX, and XII is found in maintaining neutral intracellular pH necessary for tumour cells to tolerate hypoxic conditions, hence, hCA inhibitors may be a good anticancer agent ( Pastorek et al., 2008 ; Singh et al., 2018 ; Supuran, 2018 ; Al-Warhi et al., 2020 ). Many hCA IX/XII inhibitors belonging to the coumarin and sulfocoumarin classes completed a successful Phase I clinical trial for the treatment of advanced, metastatic solid tumors ( Pastorek et al., 2008 ). In an attempt to search novel hCA inhibitors, a series of bis-coumarin derivatives linked with 1,2,3-triazole ring and alkyl chain ( 72a-d ) ( Figure 6 ) were synthesized. The derivatives showed selective inhibitory activity against hCA isoforms IX and XII. Among them 72c showed highest hCA IX inhibition (Ki = 144.6 nM) while 72b showed the highest hCA XII inhibition (Ki = 71.5 nM). The derivatives showed potent in vitro cytotoxicity (IC 50 0.383–13.552 µM) against renal adenocarcinoma (769P), HepG2 and MDA-MB-231 cell lines. The derivative 72a showed the strongest cytotoxic effect against HepG2 with an IC 50 value of 0.383 μM, which is almost 2-fold more than that of standard drug Doxorubicin ( Kurt et al., 2019 ). The results were fully supported by in silico binding energies with hCA isoform in docking studies ( Kurt et al., 2019 ). Another series of hybrids comprising coumarin-1,2,3-triazole-benzaldehyde and coumarin-1,2,3-triazole-sulphonamide were synthesized and evaluated for their hCA I, II, IX, and XII inhibition potential. All the coumarin-1,2,3-triazole-benzaldehyde hybrids ( 73–76 ) ( Figure 6 ) were not inhibiting hCA I, II and XII (Ki > 10,000 nM) except 73 which showed strong inhibition to XII (Ki = 60.9 nM). The hybrid 73 was also a potential inhibitor of IX (Ki = 127.5 nM) and showed strong cytotoxic effect against HT-29 (human colorectal adenocarcinoma) cancer cell line exhibiting IC 50 value of 18.89 µM. The hybrids 74 , 75 , and 76 showed IC 50 values 13.3, 9.03, and 7.47 µM respectively against HT-29 cancer cell lines ( Zengin Kurt et al., 2019 ). The coumarin-1,2,3-triazole-sulphonamide hybrids ( 77–80 ) ( Figure 6 ) were found to be potential inhibitors of hCA II, IX, and XII at nanomolar levels of Ki. Further, hybrids, 77 , 78 , 79 and 80 showed IC 50 values 14.29, 23.34, 17.7, and 17.01 µM respectively. Noticeable that except 80 , rest of the derivatives were toxic to healthy HEK293T embryonic kidney cell lines, hence hybrid 80 may be a promising anticancer drug candidate ( Zengin Kurt et al., 2019 ). Recently, a series of 6-coumarin-linked 4-anilinomethyl-1,2,3-triazole was synthesized and evaluated for their inhibitory potential against various hCA isoforms. Compounds 81a-e ( Figure 6 ) exhibited the best inhibitory profiles (Ki < 100 nM) against both against CA IX, and XII ( Thacker et al., 2021 ). New analogues of triazole-coumarin-glycosyl hybrids were synthesized and screened for their cytotoxic activities against Paca-2 (pancreatic), Mel-501 (female malignant), PC-3 (prostate) and A-375 (malignant human melanoma) cancer cell lines. The hybrids 82–84 ( Figure 6 ) possessed potent cytotoxic activity showing 94.7–99.6% inhibition of cell growth. The hybrid molecules 82 , 83 , and 84 exhibited IC 50 14.6, 33.4 and 16.9 µM respectively against Paca-2, and 16.7, 65.1 and 4.1 µM respectively against Mel-501 cancer cell lines. The in vitro cytotoxicity of 82 and 84 against Paca-2 were far better than the standard drug Doxorubicin (IC 50 19.4 µM) ( El-Sayed et al., 2022 ). However, the results were much more promising for coumarin-tetrazole derivative synthesized by the authors which displayed potent cytotoxic activity, broad superior inhibitory activity and high in silico binding affinity against anticancer enzymes EGFR (epidermal growth factor receptor), VEGFR-2 (Vascular endothelial growth factor receptor 2) and Cyclin dependent kinase 2 (CDK-2)/cyclin A2 Kinases ( El-Sayed et al., 2022 ).
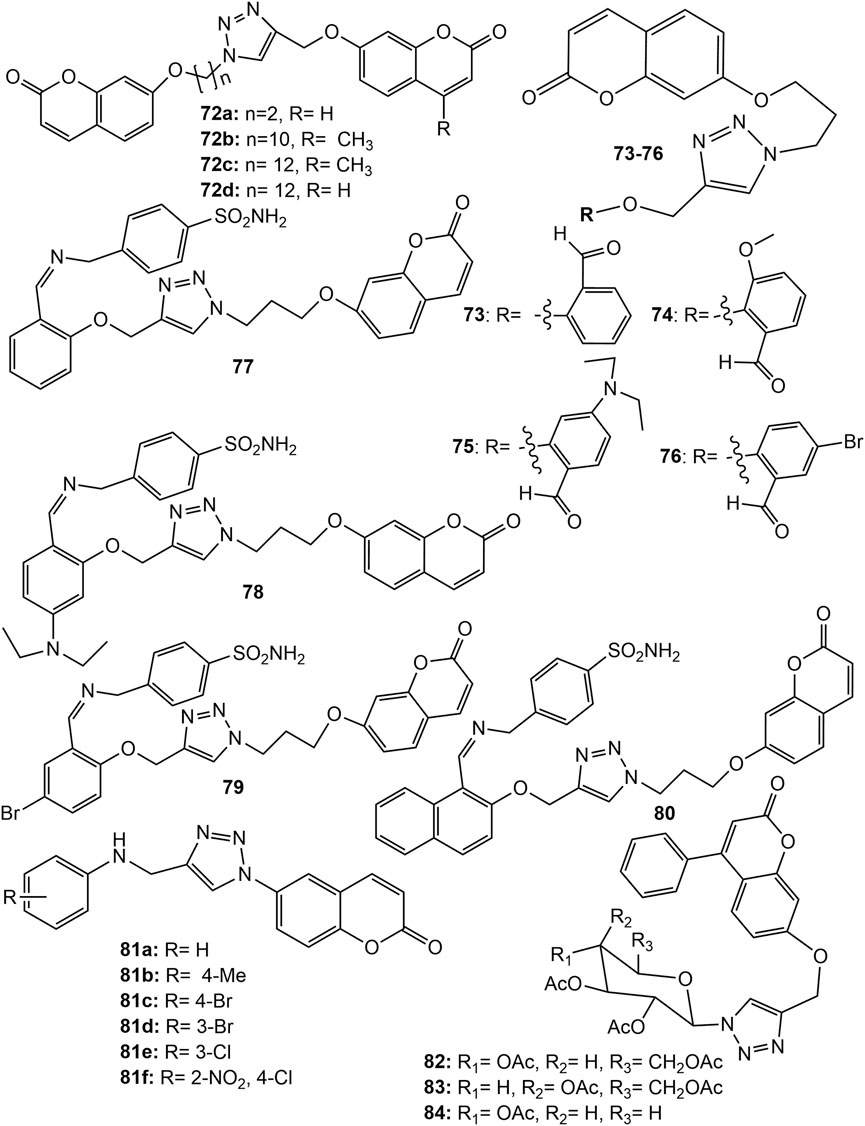
FIGURE 6 . Structure of 1,2,3-triazole linked bis-coumarin hybrid ( 72 ), coumarin-1,2,3-triazole-benzaldehyde hybrids ( 73 – 76 ), coumarin-1,2,3-triazole-sulphonamide hybrids ( 77 – 80 ), 6-coumarin-linked 4-anilinomethyl-1,2,3-triazole hybrid ( 81 ), 1,2,3-triazole-coumarin-glycosyl hybrids ( 82 – 84 ).
Overall SAR could be established for most potent coumarin-1,2,3-triazole anticancer hybrids. Numerous attempts have been made for modifying different positions of coumarin nucleus resulting in a large number of compounds having a diverse mechanism of actions. Most of the efforts have been made onF modifications at positions 4 and 7 of coumarin moiety. Attachment of 1,2,3-triazole moiety at positions 4 and 7 of coumarin seems crucial for anticancer activity. A further substitution of triazole moiety by aromatic/heteroaromatic benzo-fused rings with methylene linker enhances the anticancer activity. The SAR for some of the pharmacophores with potential cytotoxic activity have been depicted in Figure 7 .
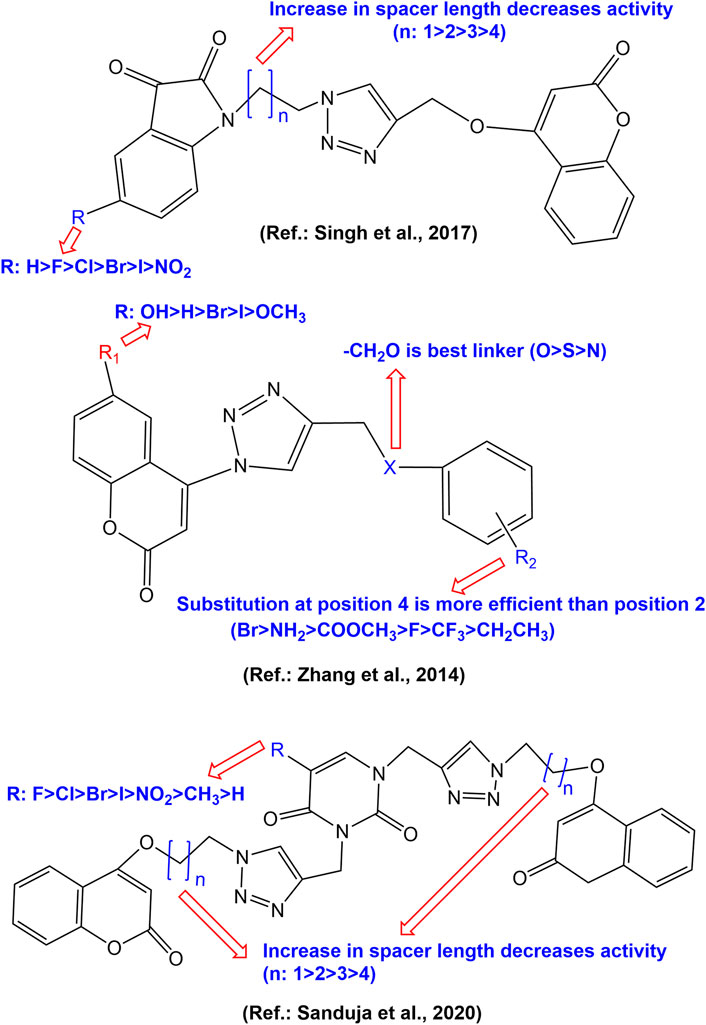
FIGURE 7 . Structure activity relationship of some notable coumarin-1,2,3-triazole scaffolds.
Millions of individuals worldwide are impacted by cancer each year, making it one of the major causes of mortality. Although both coumarin and 1,2,3-triazole moieties have proven anticancer properties, the single target drug therapies are insufficient for the treatment of cancer. The design of hybrid anticancer drugs by combining coumarin and 1,2,3-triazole moieties may produce a drug with a broader range of action acting simultaneously on multiple targets. Various coumarin-1,2,3-triazole hybrids discussed in this review have shown potential anticancer activities in terms of IC 50 comparable to standard clinical drugs against various cancer cell lines. The anticancer activity of potentially active hybrids has been further reported to be justified by selective inhibition of different enzymes such as EGFR, VEGFR-2 and CDK-2/cyclin A2 Kinases, Topo IIβ, and Topo IIα, involved in the progression of cancer, arrest of the cell cycle in G1 phase, and disturbing microtubule organization. Due to much better anticancer activity than clinically used reference drugs and interesting preliminary reports on mode of action, great opportunity can be seen for some of the hybrids viz. 46 , 50 , 61 , 65 – 68 reported in this review, hence further studies are recommended to explore them as a potential multi-targeted anticancer drug for clinical use. The present review represents an avenue for medicinal chemists to design and develop novel coumarin-1,2,3-triazole anticancer hybrids with better efficacy and fewer side effects.
Author contributions
KM and HU conceptualized, collected the information, designed and wrote the manuscript.
Acknowledgments
The authors thank Prof. Geetam Singh Tomar, Director, Rajkiya Engineering College Sonbhadra for the facilities and constructive support.
Conflict of interest
The authors declare that the research was conducted in the absence of any commercial or financial relationships that could be construed as a potential conflict of interest.
Publisher’s note
All claims expressed in this article are solely those of the authors and do not necessarily represent those of their affiliated organizations, or those of the publisher, the editors and the reviewers. Any product that may be evaluated in this article, or claim that may be made by its manufacturer, is not guaranteed or endorsed by the publisher.
Supplementary material
The Supplementary Material for this article can be found online at: https://www.frontiersin.org/articles/10.3389/fddsv.2022.1072448/full#supplementary-material
Abyshev, A. Z., Gindin, V. A., Semenov, E. v., Agaev, E. M., Abdulla-Zade, A. A., and Guseinov, A. B. (2006). Structure and biological properties of 2H-1-benzopyran-2-one (coumarin) derivatives. Pharm. Chem. J. 40, 607–610. doi:10.1007/s11094-006-0203-7
CrossRef Full Text | Google Scholar
Al-Warhi, T., Sabt, A., Elkaeed, E. B., and Eldehna, W. M. (2020). Recent advancements of coumarin-based anticancer agents: An up-to-date review. Bioorg. Chem. 103, 104163. doi:10.1016/j.bioorg.2020.104163
PubMed Abstract | CrossRef Full Text | Google Scholar
Alam, M. M. (2022). 1, 2, 3-triazole hybrids as anticancer agents: A review. Arch. Pharm. 355, e2100158. doi:10.1002/ardp.202100158
Atanasov, A. G., Zotchev, S. B., Dirsch, V. M., Orhan, I. E., Banach, M., Rollinger, J. M., et al. (2021). Natural products in drug discovery: Advances and opportunities. Nat. Rev. Drug Discov. 20, 200–216. doi:10.1038/s41573-020-00114-z
Blagosklonny, M. v. (2004). Analysis of FDA approved anticancer drugs reveals the future of cancer therapy. Cell Cycle 3, 1033–1040. doi:10.4161/cc.3.8.1023
Bozorov, K., Zhao, J., and Aisa, H. A. (2019). 1, 2, 3-Triazole-containing hybrids as leads in medicinal chemistry: A recent overview. Bioorg. Med. Chem. 27, 3511–3531. doi:10.1016/j.bmc.2019.07.005
Bray, F., Ferlay, J., Soerjomataram, I., Siegel, R. L., Torre, L. A., and Jemal, A. (2018). Global cancer statistics 2018: GLOBOCAN estimates of incidence and mortality worldwide for 36 cancers in 185 countries. Ca. Cancer J. Clin. 68, 394–424. doi:10.3322/caac.21492
Cooper, G. M., and Hausman, R. E. (2007a). The cell: A molecular approach . 2nd Edition.
Google Scholar
Cooper, G. M., and Hausman, R. E. (2007b). The development and causes of cancer. The cell: A molecular approach .
Cragg, G. M., and Newman, D. J. (2009). Nature: A vital source of leads for anticancer drug development. Phytochem. Rev. 8, 313–331. doi:10.1007/s11101-009-9123-y
Desai, A., Qazi, G., Ganju, R., El-Tamer, M., Singh, J., Saxena, A., et al. (2008). Medicinal plants and cancer chemoprevention. Curr. Drug Metab. 9, 581–591. doi:10.2174/138920008785821657
Dhawan, S., Awolade, P., Kisten, P., Cele, N., Pillay, A. S., Saha, S. T., et al. (2020). Synthesis, cytotoxicity and antimicrobial evaluation of new coumarin-tagged β-lactam triazole hybrid. Chem. Biodivers. 17, e1900462. doi:10.1002/cbdv.201900462
Diao, Q. P., Guo, H., and Wang, G. Q. (2019). Design, synthesis, and in vitro anticancer activities of diethylene glycol tethered isatin-1, 2, 3-triazole-coumarin hybrids. J. Heterocycl. Chem. 56, 1667–1671. doi:10.1002/jhet.3538
Dorababu, A. (2020). Report on recently (2017–20) designed quinoline-based human cancer cell growth inhibitors. ChemistrySelect 5, 13902–13915. doi:10.1002/slct.202003888
Duan, Y. C., Ma, Y. C., Zhang, E., Shi, X. J., Wang, M. M., Ye, X. W., et al. (2013). Design and synthesis of novel 1, 2, 3-triazole-dithiocarbamate hybrids as potential anticancer agents. Eur. J. Med. Chem. 62, 11–19. doi:10.1016/j.ejmech.2012.12.046
Dwivedi, G. R., Upadhyay, H. C., Yadav, D. K., Singh, V., Srivastava, S. K., Khan, F., et al. (2014). 4-Hydroxy-α-tetralone and its derivative as drug resistance reversal agents in multi drug resistant Escherichia coli . Chem. Biol. Drug Des. 83, 482–492. doi:10.1111/cbdd.12263
El-Sayed, W. A., Alminderej, F. M., Mounier, M. M., Nossier, E. S., Saleh, S. M., and Kassem, A. F. (2022). Novel 1, 2, 3-triazole-coumarin hybrid glycosides and their tetrazolyl analogues: Design, anticancer evaluation and molecular docking targeting EGFR, VEGFR-2 and CDK-2. Molecules 27, 2047. doi:10.3390/molecules27072047
Emami, S., and Dadashpour, S. (2015). Current developments of coumarin-based anti-cancer agents in medicinal chemistry. Eur. J. Med. Chem. 102, 611–630. doi:10.1016/j.ejmech.2015.08.033
Fan, Y. L., Ke, X., and Liu, M. (2018). Coumarin–triazole hybrids and their biological activities. J. Heterocycl. Chem. 55, 791–802. doi:10.1002/jhet.3112
Gopalakrishnan, A. K., Angamaly, S. A., and Velayudhan, M. P. (2021). An insight into the biological properties of imidazole-based schiff bases: A review. ChemistrySelect 6, 10918–10947. doi:10.1002/slct.202102619
Goud, N. S., Kumar, P., and Bharath, R. D. (2020). Recent developments of target based coumarin derivatives as potential anticancer agents. Mini Rev. Med. Chem. 20, 1754–1766. doi:10.2174/1389557520666200510000718
Goud, N. S., Pooladanda, V., Mahammad, G. S., Jakkula, P., Gatreddi, S., Qureshi, I. A., et al. (2019). Synthesis and biological evaluation of morpholines linked coumarin–triazole hybrids as anticancer agents. Chem. Biol. Drug Des. 94, 1919–1929. doi:10.1111/cbdd.13578
Hao, S. Y., Feng, S. L., Wang, X. R., Wang, Z., Chen, S. W., and Hui, L. (2019). Novel conjugates of podophyllotoxin and coumarin: Synthesis, cytotoxicities, cell cycle arrest, binding CT DNA and inhibition of Topo IIβ. Bioorg. Med. Chem. Lett. 29, 2129–2135. doi:10.1016/j.bmcl.2019.06.063
Joule, J. A. (2016). Natural products containing nitrogen heterocycles—some highlights 1990–2015. Adv. Heterocycl. Chem. 119, 81–106. doi:10.1016/BS.AIHCH.2015.10.005
Katsori, A. M., and Hadjipavlou-Litina, D. (2014). Coumarin derivatives: An updated patent review (2012-2014). Expert Opin. Ther. Pat. 24, 1323–1347. doi:10.1517/13543776.2014.972368
Kerru, N., Gummidi, L., Maddila, S., Gangu, K. K., and Jonnalagadda, S. B. (2020). A review on recent advances in nitrogen-containing molecules and their biological applications. Molecules 25, E1909. doi:10.3390/molecules25081909
Kinch, M. S., Patridge, E., Plummer, M., and Hoyer, D. (2014). An analysis of FDA-approved drugs for infectious disease: Antibacterial agents. Drug Discov. Today 19, 1283–1287. doi:10.1016/j.drudis.2014.07.005
Kraljević, T. G., Harej, A., Sedić, M., Pavelić, S. K., Stepanić, V., Drenjančević, D., et al. (2016). Synthesis, in vitro anticancer and antibacterial activities and in silico studies of new 4-substituted 1, 2, 3-triazole–coumarin hybrids. Eur. J. Med. Chem. 124, 794–808. doi:10.1016/j.ejmech.2016.08.062
Kumar, S., Rulhania, S., Jaswal, S., and Monga, V. (2021). Recent advances in the medicinal chemistry of carbonic anhydrase inhibitors. Eur. J. Med. Chem. 209, 112923. doi:10.1016/j.ejmech.2020.112923
Kurt, B. Z., Dag, A., Doğan, B., Durdagi, S., Angeli, A., Nocentini, A., et al. (2019). Synthesis, biological activity and multiscale molecular modeling studies of bis-coumarins as selective carbonic anhydrase IX and XII inhibitors with effective cytotoxicity against hepatocellular carcinoma. Bioorg. Chem. 87, 838–850. doi:10.1016/j.bioorg.2019.03.003
Lacy, A., and O'Kennedy, R. (2005). Studies on coumarins and coumarin-related compounds to determine their therapeutic role in the treatment of cancer. Curr. Pharm. Des. 10, 3797–3811. doi:10.2174/1381612043382693
Lacy, A., and O’Kennedy, R. (2004). Studies on coumarins and coumarin-related compounds to determine their therapeutic role in the treatment of cancer. Curr. Pharm. Des. 10, 3797–3811. doi:10.2174/1381612043382693
Liang, T., Sun, X., Li, W., Hou, G., and Gao, F. (2021). 1, 2, 3-triazole-containing compounds as anti–lung cancer agents: Current developments, mechanisms of action, and structure–activity relationship. Front. Pharmacol. 12, 661173. doi:10.3389/fphar.2021.661173
Lipeeva, A. v., Pokrovsky, M. A., Baev, D. S., Shakirov, M. M., Bagryanskaya, I. Y., Tolstikova, T. G., et al. (2015). Synthesis of 1 H -1, 2, 3-triazole linked aryl(arylamidomethyl) - dihydrofurocoumarin hybrids and analysis of their cytotoxicity. Eur. J. Med. Chem. 100, 119–128. doi:10.1016/j.ejmech.2015.05.016
Masui, K., Gini, B., Wykosky, J., Zanca, C., Mischel, P. S., Furnari, F. B., et al. (2013). A tale of two approaches: Complementary mechanisms of cytotoxic and targeted therapy resistance may inform next-generation cancer treatments. Carcinogenesis 34, 725–738. doi:10.1093/carcin/bgt086
Matos, M. J., Santana, L., Uriarte, E., Abreu, O. A., Molina, E., and Yordi, E. G. (2015). “Coumarins — an important class of phytochemicals,” in Phytochemicals - isolation, characterisation and role in human health . doi:10.5772/59982
Mohamed, M. F. A., and Abuo-Rahma, G. E. D. A. (2020). Molecular targets and anticancer activity of quinoline-chalcone hybrids: Literature review. RSC Adv. 10, 31139–31155. doi:10.1039/d0ra05594h
Musa, M., Cooperwood, J., and Khan, M. O. (2008). A review of coumarin derivatives in pharmacotherapy of breast cancer. Curr. Med. Chem. 15, 2664–2679. doi:10.2174/092986708786242877
Narsimha, S., Nukala, S. K., Savitha Jyostna, T., Ravinder, M., Srinivasa Rao, M., and Vasudeva Reddy, N. (2020). One-pot synthesis and biological evaluation of novel 4-[3-fluoro-4-(morpholin-4-yl)]phenyl-1H-1, 2, 3-triazole derivatives as potent antibacterial and anticancer agents. J. Heterocycl. Chem. 57, 1655–1665. doi:10.1002/jhet.3890
National Cancer Institute (2021). Cancer statistics - national cancer Institute . Availableat: https://www.cancer.gov/about-cancer/understanding/statistics. October 3 .
New Global Cancer Data: GLOBOCAN (2018). New global cancer Data: GLOBOCAN 2018. The global cancer observatory .
Newman, D. J., and Cragg, G. M. (2012). Natural products as sources of new drugs over the 30 years from 1981 to 2010. J. Nat. Prod. 75, 311–335. doi:10.1021/np200906s
Newman, D. J., and Cragg, G. M. (2020). Natural products as sources of new drugs over the nearly four decades from 01/1981 to 09/2019. J. Nat. Prod. 83, 770–803. doi:10.1021/acs.jnatprod.9b01285
Pastorek, J., Pastorekova, S., and Zatovicova, M. (2008). Cancer-associated carbonic anhydrases and their inhibition. Curr. Pharm. Des. 14, 685–698. doi:10.2174/138161208783877893
Perabo, F. G. E., Wirger, A., Kamp, S., Lindner, H., Schmidt, D. H., Müller, S. C., et al. (2004). Carboxyamido-triazole (CAI), a signal transduction inhibitor induces growth inhibition and apoptosis in bladder cancer cells by modulation of Bcl-2. Anticancer Res. 24, 2869–2877.
PubMed Abstract | Google Scholar
Pingaew, R., Saekee, A., Mandi, P., Nantasenamat, C., Prachayasittikul, S., Ruchirawat, S., et al. (2014). Synthesis, biological evaluation and molecular docking of novel chalcone-coumarin hybrids as anticancer and antimalarial agents. Eur. J. Med. Chem. 85, 65–76. doi:10.1016/j.ejmech.2014.07.087
Rawat, A., and Vijaya Bhaskar Reddy, A. (2022). Recent advances on anticancer activity of coumarin derivatives. Eur. J. Med. Chem. Rep. 5, 100038. doi:10.1016/j.ejmcr.2022.100038
Romero, D. H., Heredia, V. E. T., García-Barradas, O., López, M. E. M., Pavón, E. S., Narasimhan, B., et al. (2004). Recent advancement in imidazole as anti cancer agents : A review. Phosphorus Sulfur Silicon Relat. Elem. doi:10.1016/j.tet.2004.03.016
Sanduja, M., Gupta, J., Singh, H., Pagare, P. P., and Rana, A. (2020). Uracil-coumarin based hybrid molecules as potent anti-cancer and anti-bacterial agents. J. Saudi Chem. Soc. 24, 251–266. doi:10.1016/j.jscs.2019.12.001
Saxena, A., Upadhyay, H. C., Cheema, H. S., Srivastava, S. K., Darokar, M. P., and Bawankule, D. U. (2018). Antimalarial activity of phytol derivatives: In vitro and in vivo study. Med. Chem. Res. 27, 1345–1354. doi:10.1007/s00044-017-2132-2
Schirrmacher, V. (1985). Cancer metastasis: Experimental approaches, theoretical concepts, and impacts for treatment strategies. Adv. Cancer Res. 43, 1–73. doi:10.1016/S0065-230X(08)60942-2
Shang, X. F., Morris-Natschke, S. L., Yang, G. Z., Liu, Y. Q., Guo, X., Xu, X. S., et al. (2018). Biologically active quinoline and quinazoline alkaloids part II. Med. Res. Rev. 38, 1614–1660. doi:10.1002/med.21492
Shaveta, , Mishra, S., and Singh, P. (2016). Hybrid molecules: The privileged scaffolds for various pharmaceuticals. Eur. J. Med. Chem. 124, 500–536. doi:10.1016/j.ejmech.2016.08.039
Siegel, R. L., Miller, K. D., Fuchs, H. E., and Jemal, A. (2022). Cancer statistics, 2022. Ca. Cancer J. Clin. 72, 7–33. doi:10.3322/caac.21708
Singh, H., Kumar, M., Nepali, K., Gupta, M. K., Saxena, A. K., Sharma, S., et al. (2016). Triazole tethered C5-curcuminoid-coumarin based molecular hybrids as novel antitubulin agents: Design, synthesis, biological investigation and docking studies. Eur. J. Med. Chem. 116, 102–115. doi:10.1016/j.ejmech.2016.03.050
Singh, H., Singh, J. V., Bhagat, K., Gulati, H. K., Sanduja, M., Kumar, N., et al. (2019). Rational approaches, design strategies, structure activity relationship and mechanistic insights for therapeutic coumarin hybrids. Bioorg. Med. Chem. 27, 3477–3510. doi:10.1016/j.bmc.2019.06.033
Singh, H., Singh, J. v., Gupta, M. K., Saxena, A. K., Sharma, S., Nepali, K., et al. (2017). Triazole tethered isatin-coumarin based molecular hybrids as novel antitubulin agents: Design, synthesis, biological investigation and docking studies. Bioorg. Med. Chem. Lett. 27, 3974–3979. doi:10.1016/j.bmcl.2017.07.069
Singh, S., Lomelino, C. L., Mboge, M. Y., Frost, S. C., and McKenna, R. (2018). Cancer drug development of carbonic anhydrase inhibitors beyond the active site. Molecules 23, E1045. doi:10.3390/molecules23051045
Song, X. F., Fan, J., Liu, L., Liu, X. F., and Gao, F. (2020). Coumarin derivatives with anticancer activities: An update. Arch. Pharm. 353, e2000025. doi:10.1002/ardp.202000025
Stefanachi, A., Leonetti, F., Pisani, L., Catto, M., and Carotti, A. (2018). Coumarin: A natural, privileged and versatile scaffold for bioactive compounds. Molecules 23, E250. doi:10.3390/molecules23020250
Sung, H., Ferlay, J., Siegel, R. L., Laversanne, M., Soerjomataram, I., Jemal, A., et al. (2021). Global cancer statistics 2020: GLOBOCAN estimates of incidence and mortality worldwide for 36 cancers in 185 countries. Ca. Cancer J. Clin. 71, 209–249. doi:10.3322/caac.21660
Supuran, C. T. (2018). Carbonic anhydrase inhibitors as emerging agents for the treatment and imaging of hypoxic tumors. Expert Opin. Investig. Drugs 27, 963–970. doi:10.1080/13543784.2018.1548608
Thacker, P. S., Tiwari, P. L., Angeli, A., Srikanth, D., Swain, B., Arifuddin, M., et al. (2021). Synthesis and biological evaluation of coumarin-linked 4-anilinomethyl-1, 2, 3-triazoles as potent inhibitors of carbonic anhydrases ix and xiii involved in tumorigenesis. Metabolites 11, 225. doi:10.3390/metabo11040225
Thakur, A., Singla, R., and Jaitak, V. (2015). Coumarins as anticancer agents: A review on synthetic strategies, mechanism of action and SAR studies. Eur. J. Med. Chem. 101, 476–495. doi:10.1016/j.ejmech.2015.07.010
Upadhyay, H. C. (2021). Coumarin-1, 2, 3-triazole hybrid molecules: An emerging scaffold for combating drug resistance. Curr. Top. Med. Chem. 21, 737–752. doi:10.2174/1568026621666210303145759
Upadhyay, H. C., Dwivedi, G. R., Roy, S., Sharma, A., Darokar, M. P., and Srivastava, S. K. (2014). Phytol derivatives as drug resistance reversal agents. ChemMedChem 9, 1860–1868. doi:10.1002/cmdc.201402027
Upadhyay, H. C., Jaiswal, N., Tamrakar, A. K., Srivastava, A. K., Gupta, N., and Srivastava, S. K. (2012). Antihyperglycemic agents from Ammannia multiflora. Nat. Product. Commun. 7, 1934578X1200700–900. doi:10.1177/1934578x1200700724
Upadhyay, H. C. (2019). Medicinal chemistry of alternative therapeutics: Novelty and hopes with genus ammannia. Curr. Top. Med. Chem. 19, 784–794. doi:10.2174/1568026619666190412101047
Upadhyay, H. C., Singh, M., Prakash, O., Khan, F., Srivastava, S. K., and Bawankule, D. U. (2020). QSAR, ADME and docking guided semi-synthesis and in vitro evaluation of 4-hydroxy-α-tetralone analogs for anti-inflammatory activity. SN Appl. Sci. 2, 2069. doi:10.1007/s42452-020-03798-5
Vagish, C. B., Kumara, K., Vivek, H. K., Bharath, S., Lokanath, N. K., and Ajay Kumar, K. (2021). Coumarin-triazole hybrids: Design, microwave-assisted synthesis, crystal and molecular structure, theoretical and computational studies and screening for their anticancer potentials against PC-3 and DU-145. J. Mol. Struct. 1230, 129899. doi:10.1016/j.molstruc.2021.129899
Wang, G., Sun, S., Wu, B., and Liu, J. (2020). Coumarins as potential anti-drug resistant cancer agents: A mini review. Curr. Top. Med. Chem. 21, 1725–1736. doi:10.2174/1568026620999201113110041
WHO (2021). WHO | Cancer - Fact sheet N°297.
Winkler, G. C., Barle, E. L., Galati, G., and Kluwe, W. M. (2014). Functional differentiation of cytotoxic cancer drugs and targeted cancer therapeutics. Regul. Toxicol. Pharmacol. 70, 46–53. doi:10.1016/j.yrtph.2014.06.012
Wu, Y., Xu, J., Liu, Y., Zeng, Y., and Wu, G. (2020). A review on anti-tumor mechanisms of coumarins. Front. Oncol. 10, 592853. doi:10.3389/fonc.2020.592853
Xu, Z., Zhao, S. J., and Liu, Y. (2019a). 1, 2, 3-Triazole-containing hybrids as potential anticancer agents: Current developments, action mechanisms and structure-activity relationships. Eur. J. Med. Chem. 183, 111700. doi:10.1016/j.ejmech.2019.111700
Xu, Z., Zhao, S. J., Lv, Z. S., Gao, F., Wang, Y. L., Zhang, F., et al. (2019b). Design, synthesis, and evaluation of tetraethylene glycol-tethered isatin–1, 2, 3-triazole–coumarin hybrids as novel anticancer agents. J. Heterocycl. Chem. 56, 1127–1132. doi:10.1002/jhet.3475
Yedjou, C. G., Mbemi, A. T., Noubissi, F., Tchounwou, S. S., Tsabang, N., Payton, M., et al. (2019). Prostate cancer disparity, chemoprevention, and treatment by specific medicinal plants. Nutrients 11, E336. doi:10.3390/nu11020336
Zaid, H., Silbermann, M., Amash, A., Gincel, D., Abdel-Sattar, E., and Sarikahya, N. B. (2017). Medicinal plants and natural active compounds for cancer chemoprevention/chemotherapy. Evid. Based. Complement. Altern. Med. 2017, 7952417. doi:10.1155/2017/7952417
Zengin Kurt, B., Sonmez, F., Ozturk, D., Akdemir, A., Angeli, A., and Supuran, C. T. (2019). Synthesis of coumarin-sulfonamide derivatives and determination of their cytotoxicity, carbonic anhydrase inhibitory and molecular docking studies. Eur. J. Med. Chem. 183, 111702. doi:10.1016/j.ejmech.2019.111702
Zhang, W., Li, Z., Zhou, M., Wu, F., Hou, X., Luo, H., et al. (2014). Synthesis and biological evaluation of 4-(1, 2, 3-triazol-1-yl)coumarin derivatives as potential antitumor agents. Bioorg. Med. Chem. Lett. 24, 799–807. doi:10.1016/j.bmcl.2013.12.095
Zugazagoitia, J., Guedes, C., Ponce, S., Ferrer, I., Molina-Pinelo, S., and Paz-Ares, L. (2016). Current challenges in cancer treatment. Clin. Ther. 38, 1551–1566. doi:10.1016/j.clinthera.2016.03.026
Keywords: chemotherapy, drug resistance, hybrid molecules, coumarin, triazole, anticancer, anticytotoxic, apoptosis
Citation: Mishra KN and Upadhyay HC (2022) Coumarin-1,2,3-triazole hybrids as leading-edge anticancer agents. Front. Drug. Discov. 2:1072448. doi: 10.3389/fddsv.2022.1072448
Received: 17 October 2022; Accepted: 22 November 2022; Published: 06 December 2022.
Reviewed by:
Copyright © 2022 Mishra and Upadhyay. This is an open-access article distributed under the terms of the Creative Commons Attribution License (CC BY). The use, distribution or reproduction in other forums is permitted, provided the original author(s) and the copyright owner(s) are credited and that the original publication in this journal is cited, in accordance with accepted academic practice. No use, distribution or reproduction is permitted which does not comply with these terms.
*Correspondence: Harish C. Upadhyay, [email protected]
This article is part of the Research Topic
Discovery of EGFR Tyrosine Kinase Inhibitors for Cancer Treatment
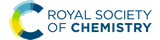
Latest developments in coumarin-based anticancer agents: mechanism of action and structure–activity relationship studies

First published on 20th October 2023
Many researchers around the world are working on the development of novel anticancer drugs with different mechanisms of action. In this case, coumarin is a highly promising pharmacophore for the development of novel anticancer drugs. Besides, the hybridization of this moiety with other anticancer pharmacophores has emerged as a potent breakthrough in the treatment of cancer to decrease its side effects and increase its efficiency. This review aims to provide a comprehensive overview of the recent development of coumarin derivatives and their application as novel anticancer drugs. Herein, we highlight and describe the largest number of research works reported in this field from 2015 to August 2023, along with their mechanisms of action and structure–activity relationship studies, making this review different from the other review articles published on this topic to date.
1. Introduction
Around 80% of the approved anticancer drugs are natural products. 3 Coumarin (2 H -1-benzopyran-2-one, Fig. 1 ) and its derivatives possess a wide variety of pharmacological properties, such as anti-inflammatory, 4,5 antibacterial, 6–9 antiviral, 10 antioxidant, 11–13 antirhombotic, 14 anti-Alzheimer, 15,16 and anticancer 17,18 activities. Accordingly, many researchers have thoroughly investigated the various mechanisms of action of different classes of coumarin-based anticancer agents, such as alkylating agents, angiogenesis inhibitors, kinase inhibitors, topoisomerase inhibitors, telomerase inhibitors, antimitotic activity, human carbonic anhydrase inhibitors, aromatase inhibitors, monocarboxylate transporter inhibitors, and hormonal antagonists.
In the search for novel anticancer drugs, natural products are always a major source. Many coumarin derivatives derived from natural sources show potential in vitro and in vivo anticancer activity. 19–22 Moreover, the concept of molecular hybridization, where two or more potential pharmacophores are combined into a single molecular framework, may provide fruitful results in the treatment with the coumarin moiety given that it may lead to the generation of new anticancer drugs with low toxicity, improved specificity, and enhanced effectivity. 23
In this review, we aim to provide an overview of the recent developments of coumarin-derived hybrids as potentially important anticancer drugs, focusing on their structure–activity relationship and mechanisms of action and highlighting articles published between 2015 and August 2023. In contrast to other review articles published on this topic, our article presents the greatest number of examples reported in this field during the past 8 years. Although the recently published reviews presented an interesting overview regarding the progress of coumarin-based anticancer agents in the past, 24,25 they only discussed a selected number of coumarin hybrids, not describing this topic as extensively as done herein. However, in this context, recently Cardona-Galeano and co-workers provided an interesting bibliometric analysis of coumarin hybrids. 26 In another review article, Al-Warhi et al. discussed a group of coumarin anticancer hybrids based on their mechanism of action. 27
2. Coumarin hybrids
2.1. coumarin-artemisinin hybrids.
The coumarin-artemisinin hybrid 1a ( Fig. 3 ) showed potential activities against four cancer cell lines, i.e. , HepG2 (IC 50 = 3.05 ± 1.60 μM), Hep3B (IC 50 = 3.76 ± 1.76 μM), A2780 (IC 50 = 5.82 ± 2.28 μM), and OVCAR-3 (IC 50 = 4.60 ± 1.81 μM). It was also reported that hybrid 1a is more potent than hybrids 1b and c , which has a linker between the piperazinyl and carbonyl groups. Fluorescence images revealed that the hybrids localized mainly in the mitochondria and their enhanced potency is due to their strong ability to accumulate in the mitochondria, which enhances the intracellular reactive oxygen species (ROS) level and triggers cell death. 31
Four series of twenty coumarin-artemisinin hybrids were developed using click chemistry, which showed efficient activity (IC 50 = 0.05–125.40 μM) when assessed (MTT assay) under normoxic or anoxic conditions against three cancer cell lines (HCT-116, MDA-MB-231, and HT-29). 32,33 It is worth noting that click chemistry has a wide application in medicinal chemistry. 34–37 The structure–activity relationship (SAR) studies showed that the 3-chloro and 4-methyl substituents in the coumarin moiety exhibited greater activity, whereas the 3-ethoxycarbonyl group in the coumarin ring exhibited reduced effectivity. It was also found that all these hybrids exhibited greater activity against the HT-29 cancer cell line under anoxic conditions. The first series of compounds ( 2a–e ) ( Fig. 4 ) exhibited IC 50 values in the range of 0.05–91.21 μM, while the hybrids in the second ( 3a–e ), third ( 4a–e ), and fourth ( 5a–e ) series showed IC 50 values in the range of 1.22–120.72 μM, 2.46–125.40 μM, and 0.43 > 100 μM, respectively. It was examined that the cytotoxic activities of most of these targeted compounds are 1–10-fold greater under anoxic conditions than that under normoxic conditions.
The potential activity of the hybrids when the linker is moved from the C-7 position to the C-4 position of the coumarin moiety was also examined and compared to doxorubicin (DOX) and DHA. 38 A total of thirty novel hybrids were designed and developed and their cytotoxicity against four cancer cell lines (HCT-116, MDA-MB- 231, HT-29, and A-549) was investigated. It was proven that the series of compounds 10 (IC 50 = 1.282 > 100 μM) and 11 (IC 50 = 0.039–93.53 μM) showed a better cytotoxicity effect than the others, indicating that the 4-oxygen group in the coumarin ring as a part of the linker can enhance the potency ( Fig. 5 ). The compounds in series 8 (8.57 > 100 μM) and 9 (9.33 > 100 μM) also showed significant activity against the HT-29 cancer cell line. In contrast, the cytotoxicity effect of compounds in series 6 and 7 was not very promising.
These hybrids inhibited the proliferation of HT-29 cells, arrested their G0/G1 phase, reduced the migration of tumor cells, and induced both apoptosis and ferroptosis in the HT-29 cancer cell line.
2.2. Coumarin-azole hybrids
Three coumarin–1,2,3-triazole hybrids ( 12a–c ) ( Fig. 7 ) were synthesized and examined for their potent activity against three cancer cell lines (PC3, MGC803, and HepG2). 42 Among them, 12c was found to be the most effective with IC 50 values of 0.34 ± 0.04 μM, 0.13 ± 0.01 μM, and 1.74 ± 0.54 μM against PC3, MGC803, and HepG2 cancer cell lines, respectively. This hybrid was found to inhibit MGC803 cell growth, induce G2/M phase arrest and apoptosis, and regulate the expression of apoptosis-related proteins.
Thirty-two novel coumarin–1,2,3-triazole hybrids ( Fig. 8 ) were designed and synthesized via the eco-friendly copper( I ) catalyzed Huisgen 1,3-dipolar cycloaddition under microwave irradiation and their potency was evaluated against five cancer cell lines (A549, HepG2, CFPAC-1, HeLa, and SW620). 43 Among them, the hybrids containing phenylethyl ( 13a ) and 3,5-difluorophenyl ( 13b ) showed the maximum potent activity against the A549 cell line (IC 50 = 24.78 μM and 21.06 μM, respectively). Also, 13c containing 5-iodoindole exhibited significant potency against the HepG2 cancer cell line with an IC 50 value of 8.57 μM. Compound 13d was highlighted as a lead with the highest cytotoxicity against the HepG2 cell line and an IC 50 value of 0.90 μM. This antiproliferative activity of 13d is due to the suppression of 5-lipoxygenase activity and perturbation of sphingolipid signaling by interfering with intracellular acid ceramidase activity. It induced cell death by early apoptosis.
A series of other coumarin–1,2,3-triazole hybrids was developed via a similar procedure to that previously mentioned and their antiproliferative activities against three cancer cell lines (HeLa, CaCo-2, and K562) and normal kidney MDCK1 cells were investigated. 44 Among them, compounds 14a–d ( Fig. 9 ) showed the most pronounced activity, although they were also found to be cytotoxic against normal MDCK1 cells. 14c possessed high activity against the K562 cell line with an IC 50 of value 17.9 ± 5.0 μM, whereas 14d showed significant activity against CaCo-2 cell lines with IC 50 value 9.7 ± 1.3 μM.
Fifteen amide-containing coumarin–1,2,3-triazole hybrids were synthesized and tested in vitro for their anticancer activity against the MDA-MB-231 cancer cell line under both normoxic and hypoxic conditions. 45 Among them, compound 15a ( Fig. 10 ) showed the maximum effectivity against the MDA-MB-231 cell line both under hypoxia (IC 50 = 0.03 μM) and normoxia (IC 50 = 1.34 μM), and it was proven to be more potent than doxorubicin (IC 50 = 0.60 μM under hypoxia and IC 50 = 1.07 μM under normoxia), cis -platin (IC 50 = 4.68 μM under hypoxia and IC 50 = 7.87 μM under normoxia), and hydroxycoumarin (IC 50 ≥ 100 μM under hypoxia and IC 50 ≥ 100 μM under normoxia). Compound 15b also showed moderate activity under hypoxia with an IC 50 value of 0.25 μM. Molecular docking analysis revealed that the anticancer activity of 15a is attributed to its potential to inhibit carbonic anhydrase IX.
A total of fifteen coumarin-tagged β-lactam 1,2,3-triazole hybrids was designed and their anticancer activities evaluated against three cancer cell lines (A549, MCF-7, and MDA-MB-231) together with the HEK-293 normal cell line. 46 Among them, 16a and 16b ( Fig. 11 ) showed prominent antiproliferative activity against the MCF-7 cell line with IC 50 = 53.55 and 58.62 μM, respectively. Molecular docking studies revealed that these two compounds target estrogen receptor-α.
Some other coumarin fused 1,2,3-triazole macrocycles were also developed and found to be active against cancer cells. 47–50 The most active hybrid 17 ( Fig. 12 ) with IC 50 = 49 μM only showed moderate activity against the MCF-7 cancer cell line.
Four coumarin–triazole hybrids were chosen and examined for their cytotoxic activity on several cancer cell lines and their in vitro toxicity was evaluated on the 3T3 (healthy fibroblasts) cell line. 51 They all showed significant cytotoxic activity against the MCF7 breast cancer cell line with an IC 50 value lower than that of cisplatin, while 18c ( Fig. 13 ) was the best among them with IC 50 = 2.66 μM.
A 1,2,3-triazole-tagged glycoside of 4-hydroxy coumarin base ( 19 ) ( Fig. 14 ) was synthesized utilizing click chemistry and its cytotoxicity was tested against liver cancer cell lines. 52 The IC 50 value was found to be 106.81 μg mL −1 .
1,2,4-Triazole has been evaluated as a novel anticancer, 53,54 antifungal, 55 and antibacterial 56 activities. This nucleus is stable to metabolic degradation and is target-specific with a broad spectrum of pharmacological activities. Furthermore, given that it is polar, it can enhance the solubility of the ligand and improve its activity. Thus, this system is a likely choice for the preparation of novel anticancer agents.
Eighteen coumarin–1,2,4-triazole hybrids were synthesized under microwave irradiation and conventional heating techniques. 57 The compounds were investigated for their anticancer activities against four cancer cell lines (BT20 human breast carcinoma, SK-Me1 128 melanoma, DU-145 prostate carcinoma, and A549 lung carcinoma) and HFC normal cell line together with the evaluation of the selectivity index (SI). Among the hybrids, 20a ( Fig. 15 ) showed the highest potency against the BT20 cell line with an IC 50 value of 6.4 μg mL −1 and SI = 5.2. 20b was proven to be the most effective against the DU-145 (IC 50 = 3.7 μg mL −1 and SI = 9.9) and SK-Me1 128 cell lines (IC 50 = 12.3 μg mL −1 and SI = 3.0). 20c was the most effective against the A549 cancer cell line (IC 50 = 7.5 μg mL −1 and SI = 4.2). The hybrids showed comparable activity to the reference cis -platin, but in general, they were not superior to the reference against the tested cancer cell lines.
Four coumarin–benzimidazole hybrids 22a–d ( Fig. 17 ) were screened for their anticancer activity via the evaluation of their GI 50 values against fourteen cancer cell lines, including AGS, KATO-III, SNU-1 (stomach cancer), SKOV3, OVCAR-8 (ovarian cancer), BXPC-3, PANC-1 (pancreatic cancer), T24 (bladder cancer), WiDr (colon cancer), HePG2 (liver cancer), SN12C (lung cancer), K562 (leukemia), MCF-7 (breast cancer) and HeLa. 59 They were all fairly potent against most of the cell lines excluding MCF-7. Compound 22a possessed maximum activity against thirteen of the fourteen cell lines (GI 50 below 0.41 μmol L −1 ). Further investigation showed that the hybrids have potent activity in inhibiting the PI3K-AKT-mTOR pathway and inducing cancer cell apoptosis.
A new series of coumarin–benzimidazole hybrids was designed and their anticancer activity was investigated in HeLa and HT29 cancer cell lines. 60 Among them, 23a and 23b ( Fig. 18 ) showed good potency against the HeLa cell line with GI 50 values of 36.2 and 35.3, respectively, whereas 23a and 23c possessed marked activity against the HT 29 cell line.
A series of imidazo[1,2- a ]pyrazine–coumarin hybrids was synthesized via the Suzuki–Miyaura coupling reaction and screened for their in vitro anticancer activity against sixty human cancer cell lines. 61 Among them, compounds 24a and 24b ( Fig. 19 ) showed a broad spectrum of activity against most of the cell lines and found to be more active than 5-fluorouracil.
A novel series of coumarin–imidazo[1,2- a ]pyridine derivatives was developed using silver( I )-catalyzed Groebke–Blackburn–Bienaymé multicomponent reaction and their antitumor activity was analyzed against three cancer cell lines (MCF-7, MDAMB-231, and Ishikawa). 62 Among them, compounds 25a and 25b ( Fig. 20 ) showed the maximum potency. Also, 25b having IC 50 = 14.12 ± 3.69 μM against MDA-MB-231 not only induced apoptosis in cells but also induced cell cycle arrest at the G0|G1 phase in the cell. It is worth noting that we recently published some interesting review articles regarding multicomponent reactions. 63,64
A novel series of coumarin–benzimidazole hybrids was obtained and their growth inhibitory effect ( in vitro ) was studied against six cancer cell lines (A549, H460, HT29, MKN-45, U87MG, and SMMC-7721) using foretinib as the standard reference. 65 The studies indicated that compounds 26a–d ( Fig. 21 ) were the most potent against the six cancer cell lines, while compound 26a was very toxic and 26b was harmful against the tested organism. Among the non-toxic compounds, compound 26d showed the highest potency against the A549 (IC 50 = 0.28 ± 0.04 μM) cancer cell line.
Another coumarin-tagged benzimidazole derivative, 2-(2-oxo-2 H -chromen-3-yl)- N -phenyl-1 H- benzo[ d ]imidazole-1-carbothioamide ( 27 ) ( Fig. 22 ), was synthesized and its activity was investigated in three cancer cell lines (MCF-7, NCI-H460, and SF-268). 66 This compound showed almost similar activity to the reference doxorubicin but also toxicity in normal cell lines.
Eight platinum( II ) complexes with a coumarin–benzimidazole hybrid were synthesized and their biological activity was evaluated against several cancer cell lines (HeLa, Hep-G2, and SK-OV-3/DDP). 67 Among them, compound 28 ( Fig. 23 ) showed excellent potency against the SK-OV-3/DDP cell line with IC 50 = 1.01 ± 0.27 μM and was better in comparison to the reference cis -platin. Further investigation indicated that this compound induced apoptosis in SK-OV-3/DDP cells via mitochondria dysfunction signaling pathways and was a telomerase inhibitor targeting c-myc promoter elements.
Two other platinum( II ) complexes, 29a and 29b ( Fig. 24 ), with a coumarin–benzimidazole moiety also possessed excellent anticancer activity against SK-OV-3/DDP cell lines with IC 50 values of 10.3 ± 0.3 and 0.5 ± 0.2 μM, respectively. 68 Cytotoxic mechanism studies indicated that these two complexes act similarly to the previous platinum-based complex by inhibiting cell cycle progression at the G2/M phase and changing the expression of cell cycle-related proteins.
Three ruthenium( II ) complexes with a coumarin–benzimidazole hybrid were designed and their antitumor efficiency was studied. 69 Among them, compound 30 ( Fig. 25 ) showed marked antitumor activity against the NCI-H460 cancer cell line (IC 50 = 0.30 ± 0.02 μM) with high selectivity. MTT assay studies revealed that this complex induced apoptosis via telomerase inhibition.
A new series of novel 3-benzylcoumarin imidazolium salts was prepared together with the evaluation of their anticancer properties against five cancer cell lines (HL-60, SMMC-7721, A-549, MCF-7, and SW-480). 70 Among them, compound 31a ( Fig. 26 ) showed the highest efficiency with IC 50 values in the range of 2.04–4.51 μM against five human tumor cell lines. Compound 31b was more selective to the SW-480 cell line with an IC 50 value 40.0-fold lower than DDP. SAR studies indicated that compound 31a can cause G0/G1 phase cell cycle arrest and apoptosis in the SMMC-7721 cell line.
A large series of coumarin-substituted benzimidazolium salts ( 32 ) ( Fig. 27 ) was developed and their cytotoxic properties studied against PC-3 and A2780 cancer cell lines. 71 All the salts showed moderate activity and were less active than docetaxel.
A novel series of sterically encumbered silver( I )-N-heterocyclic carbene complexes with coumarin–benzimidazole hybrids was designed and their cytotoxic activities studied against A549 and H1975 cancer cell lines. 72 Complexes 33a–d ( Fig. 28 ) showed promising activity against the above-mentioned cell lines, while complex 33e possessed a promising drug window with the IC 50 value of 13.7 ± 2.70 and 14.5 ± 1.20 μM against the H1975 and A549 cancer cell lines, respectively.
A new series of coumarin–pyrazole hybrids ( 34a–d ) ( Fig. 29 ) was synthesized and their activity screened against two cancer cell lines, i.e. , Hep-G2 and MCF-7. 73 However, they were not very pharmacologically important given that they were all were less effective than the reference cis -platin and possessed moderate activity against the above-mentioned two cell lines.
A series of twenty-two coumarin–pyrazole hybrids was designed and their antiproliferative activities studied in vitro against four cancer cell lines (HepG2, SMMC-7721, U87, and H1299). 74 Among them, compound 35 showed excellent anticancer activity against all the cell lines with IC 50 values of 2.96 ± 0.25, 2.08 ± 0.32, 3.85 ± 0.41, and 5.36 ± 0.60 μM against the HepG2, SMMC-7721, U87, and H1299 cancer cell lines, respectively. SAR studies revealed that hybrid 35 ( Fig. 30 ) displayed significant anti-metastasis effects by inhibiting cell migration and invasion in the highly metastatic SMMC-7721 cell line and dose-dependent reversed TGF-β1-induced epithelial-mesenchymal transition (EMT). Also, 35 showed low acute toxicity and possible tumor growth inhibitory properties against the SMMC-7721 cell line in vivo .
A new series of fifteen coumarin–pyrazole hybrids was synthesized via a one-pot multicomponent Vilsmeier–Haack reaction with good yield and their anticancer activities were screened against three cancer cell lines, i.e. , DU-145, MCF-7, and HeLa. 75 All the derivatives exhibited appreciable cytotoxic activity but were not better than the reference doxorubicin. Compounds 36a and 36b ( Fig. 31 ) showed good activity against the HeLa cell line with IC 50 values of 5.75 and 6.25 μM, respectively.
A similar series of coumarin–pyrazole hybrids was designed and their anticancer properties screened against five cancer cell lines (L1210, CEM, DU- 145, HeLa, and MCF-7). 76 Among them, compound 37a ( Fig. 32 ) possessed the maximum potency against the DU-145 cell line with an IC 50 value of 7 ± 1 μM, while compound 37b showed the maximum efficiency against the MCF-7 cell line with an IC 50 value of 8 ± 2 μM.
A novel group of coumarin-tagged 1,3,4-oxadizaole hybrids was prepared and their biological activities studied against the MDA-MB-231 and MCF-7 breast cancer cell lines. 77 Compound 38a ( Fig. 33 ) showed excellent cytotoxicity against the MCF-7 cell line with an IC 50 value of less than 5 μM, whereas compounds 38b and 38c possessed significant potency against the MDA-MB-231 cell line with an IC 50 value of 7.07 μM for both of them. Docking studies revealed that the stronger binding affinity of the designed derivatives is due to the presence of a sulfone unit attached to the substituted benzyl group in their pharmacophores. It is worth noting that oxadiazolyl sulfones are also an emerging tool in bioconjugation methodologies. 78,79
A library of twenty coumarin–1,3,4-oxadiazole conjugates was synthesized and analyzed for their inhibitory activity against the four physiologically relevant human carbonic anhydrase (hCA) isoforms CA I, CA II, CA IX, and CA XII. 80 Among them, compounds 39a and 39b ( Fig. 34 ) exhibited significant inhibition in lower micromolar potency against hCA XII ( K i of 0.16 μM) and hCA IX ( K i of 2.34 μM), respectively. Hence, these two compounds can serve as promising leads for designing novel anticancer agents by acting through hCA IX and XII inhibition. Besides their pharmaceutical properties, thioethers and thioesters are very useful building blocks in synthetic methodologies, providing unique pathways for building new molecules. 81
A novel series of coumarin–thiazole hybrids was designed and tested by employing human colon (DLD-1) and liver cancer cell lines (HepG2). 86 Among the nine compounds, 40a ( Fig. 35 ) was the most effective against DLD-1 with an EC 50 value of 5.79 μM, while compound 40b showed the maximum potency against the HepG2 cell line with an EC 50 value of 3.70 μM. The designed compounds act by blocking Hsp90 function and were determined to be valuable C-terminal Hsp90 inhibitors.
A similar series of coumarin–thiazole conjugates was developed and their cytotoxic activity examined against three human cancer cell lines, i.e. , MCF-7, HepG2 and SW480. 87 All the compounds showed moderate to low activity against the above-mentioned cell lines but none of them were more potent than the reference etoposide. Compound 41a ( Fig. 36 ) showed significant efficiency (IC 50 values of 7.5 ± 0.7, 16.9 ± 0.7, and 13.0 ± 0.6 μg mL −1 against MCF-7, HepG2, and SW480, respectively). Compound 41b possessed the maximum potency against the HepG2 cell line with IC 50 = 12.2 ± 2.3 μg mL −1 .
A novel series of thiazolopyrazolyl coumarin derivatives was designed and their cytotoxicity examined against four cancer cell lines (MCF-7, A549, PC3, and HepG2) together with a normal cell line, HFB4. 88 Among the synthesized hybrids, compounds 42a–e ( Fig. 37 ) showed significant activity towards the MCF-7 cell line (IC 50 = 5.41–10.75 μM) together with a low cytotoxic effect on the normal cell line. Several theoretical and experimental studies revealed the molecular mechanisms that control breast carcinoma metastasis. The mechanistic effectiveness in cell cycle progression, apoptotic induction, and gene regulation was analyzed for compound 42e due to its significant cytotoxicity against MCF-7 and potent VEGFR-2 inhibition. Flow cytometric analysis showed that compound 42e induced cell growth cessation at the G2/M phase and enhanced the percentage of cells in the pre-G1 phase, stimulating the apoptotic death of MCF-7 cells. Furthermore, real-time PCR assay showed that compound 42e upregulated p53 gene expression and elevated the Bax/Bcl-2 ratio. Moreover, the apoptotic induction of MCF-7 breast cancer cells was enhanced effectively through the activation of caspase-7 and 9 by compound 42e . Therefore, 42e can be considered a potent lead for the development of anti-breast cancer candidates.
A set of coumarin–thiazole hybrids 43a–d ( Fig. 38 ) showed significant potency against HeLa cells, which was observed to be more potent than the reference doxorubicin. 89 Compound 43d possessed maximum antiproliferative activity against the cancer cell lines with an IC 50 value of 0.0091 ± 0.0007 cM. The cell cycle investigation showed that compound 43b led to cell cycle cessation at the G0/G1 phase, indicating that the CDK2/E1 complex can be the plausible biological target. The RT-PCR gene expression assay showed that compound 43b increased the levels of the nuclear CDK2 regulators P21 and P27 by 2.30- and 5.7-fold, respectively. The ELISA technique showed also that compound 43b led to the remarkable activation of caspase-9 and -3, inducing cell apoptosis. The molecular docking study for 43a–d rationalized their superior CDK2 inhibitory activity through their hydrogen bonding and hydrophobic interactions with the key amino acids in the CDK2 binding site.
Twelve novel 1-thiazolyl-5-coumarin-3-yl-pyrazole derivatives were developed via a one-pot multicomponent reaction and their anticancer activity was investigated on two cancer cell lines (HepG2 and MCF7). 90 Compound 44a ( Fig. 39 ) showed significant cytotoxic activity against the HepG2 cell line with an IC 50 value of 3.74 ± 0.02 μM and 44b possessed significant activity against MCF-7 with an IC 50 value of 4.03 ± 0.02 μM. 44c was potent against both the HepG2 and MCF-7 cell lines with an IC 50 value of 3.06 ± 0.01 μM and 4.42 ± 0.02 μM, respectively.
Coumarin–benzothiazole hybrids were screened for their antitumor activity at a single dose (10 μm) against a panel of 60 cancer cell lines. 91 The most active compound 45 ( Fig. 40 ) was further screened at a five-dose level. It displayed half-maximal growth inhibition (GI50) values of 0.24 and 0.33 μm against the central nervous system (CNS) cancer (SNB-75) and ovarian cancer (OVCAR-4) cell lines, respectively.
Compounds 46a and 46b ( Fig. 41 ) were tested against the most common secondary ALK mutants such as L1196M, G1269A, and G1202R. 92 Compound 46a showed potent inhibitory activities against three ALK mutants, L1196M, G1269A, and G1202R, with IC 50 of 0.27 μM, 0.30 μM, and 0.59 μM, respectively. Compound 46b displayed an IC 50 value of 0.45 μM for L1196M and compatible enzymatic inhibitory activity against G1269A and G1202R with that against WT ALK.
A series of novel coumarin derivatives having 1,2,4-triazolo[3,4- b ][1,3,4]thiadiazole moieties was developed and analyzed in vitro for their anticancer activity against the HCT116 cell line. 93 Compound 47 ( Fig. 42 ) possessed significant anticancer activity with an IC 50 value of 2.656 μM. Molecular docking studies suggested its possible interaction with tyrosine kinases (CDK2).
A novel series of coumarin–thiadiazole hybrids was developed using nucleophilic substitution reaction and their anticancer activity was tested against HCT-116, MCF-7, and HepG2 but none of them were more potent than the reference cis -platin. 94 Compound 48a ( Fig. 43 ) showed maximum potency against the HCT-116 and MCF-7 cell lines with IC 50 values of 30.7 and 54.9 μg mL −1 , respectively, while 48b was the most potent against HepG2 with an IC 50 value of 24.9 μg mL −1 .
A series of 2-(3-substituted-4-methyl-2-oxo-2 H -chromen-7-yloxy)-2-methylpropanoic acid derivatives was developed by base-catalyzed dehydrohalogenative cyclization followed by Hantzsch synthesis and their in vitro cytotoxicity examined against the MCF-7, MDA-231, and HT29 cancer cell lines. 95 Thiazole derivative 49 ( Fig. 44 ) possessed the maximum potency against the MDA-231 and MCF-7 cell lines with IC 50 values of 4.84 ± 0.17 and 2.39 ± 0.03 μM, respectively.
A series of coumarin-3-yl-thiazol-3-yl-1,2,4-triazolin-3-ones was synthesized using a microwave-assisted multicomponent protocol and their anticancer activity investigated against four cancer cell lines, including A549, MDA-MBA-231, HeLa and K562. 96 Most of the derivatives showed better or comparative cytotoxic effects against all the cancer cell lines compared to the reference doxorubicin. Among them, compound 50a ( Fig. 45 ) was more potent against three of the four cancer cell lines with IC 50 = 0.16 μM against MDA-MBA-231, IC 50 = 0.17 μM against A549, IC 50 = 0.31 μM against K562, and IC 50 = 0.25 μM against the HeLa cell line. Compound 50b showed the maximum efficiency against the HeLa cell line with IC 50 = 0.21 μM.
The anticancer activity of a series of coumarin–thiazole derivatives was screened in vitro against two cancer cell lines (HeLa and COS-7) together with a normal cell line (W138). 97 Three compounds, 51a–c ( Fig. 46 ), showed better cytotoxic ability than doxorubicin. Also, 51c possessed the best potency against the HeLa cell line with IC 50 = 1.29 μM, while 51b and 51c showed significant cytotoxicity against COS-7 (IC 50 = 1.96 and 1.66 μM, respectively). In silico studies revealed that the compounds meet the optimal needs for good oral absorption with no expected toxicity hazards.
The coumarin–aminothiazole hybrids were examined for their cytotoxic activity against the HCT-116 and HT-29 cancer cell lines. 98 Among them, compounds 52a–e ( Fig. 47 ) were effective against both cell lines with IC 50 values in the range of 0.25 to 0.38 μM. However, compound 52d was the most potent with IC 50 = 0.25 ± 0.004 μM against HT-29 and IC 50 = 0.26 ± 0.016 μM against HCT-116. Further biological investigation of 52a using Western blotting, caspase activity, glucose uptake, ROS production, and NADPH/NADP levels showed the ability of this lead drug candidate to induce cancer cell death via energy restriction. Moreover, the assessment of the synergistic activity of 52a with cisplatin showed promising outcomes.
A series of nine novel acridine–thiazole bridged coumarin derivatives was prepared and evaluated for their in vitro antiproliferative activity on MDA-MB-231, A-549, and HT29 cell lines. 99 All the compounds showed a significant cytotoxicity effect but none of them were more potent than the reference cisplatin. Compound 53a ( Fig. 48 ) showed the maximum efficacy against the MDA-MB-231 cell line with IC 50 = 8.03 ± 0.81 μM. Compound 53b was significantly active against A-549 and HT-29 with IC 50 values 5.18 ± 1.04 μM and 23.09 ± 1.17 μM, respectively.
Several coumarin–thiazole derivatives were designed and synthesized and their cytotoxicity assessed on MCF-7 cancer cell lines using sorafenib as the reference drug. 100 Among them, 54a and 54b ( Fig. 49 ) demonstrated higher anticancer activities (IC 50 = 10.5 ± 0.71 and 11.2 ± 0.80 μM, respectively) than sorafenib (IC 50 = 5.10 ± 0.49 μM). These hybrids are thought to inhibit the vascular endothelial growth factor receptor (VEGFR-2) signaling system.
A series of coumarin–isoxazole derivatives was synthesized from imidoyl chlorides and various substituted 4-(prop-2-yn-1-yloxy)-2 H -chromen-2-one and their biological activity evaluated against the HepG2 cell line. 102 Among them, 56 ( Fig. 51 ) possessed the best activity (IC 50 = 12.85 μM L −1 ) against the HepG2 cell line. Its toxicity against Vero cells (IC 50 = 144.32 μM L −1 ) was lower than that of cis -platin (IC 50 = 28.63 μM L −1 ). It was observed that the presence of Cl or Br at the 6-position of the coumarin moiety enhances the bioactivity. The docking result showed that the compound can fruitfully interact with the protein.
A series of coumarin–thiazolidin-2,4-dione hybrids was tested for their anticancer activity against the MCF-7, HeLa, HT29, A549, and PC3 cancer cell lines, but none of them were more effective than the reference doxorubicin. 103 Among them, compound 57 ( Fig. 52 ) was the most potent against the MCF-7, HeLa, and A549 cell lines with IC 50 values in the range of 0.95 to 3.20 μM.
A novel series of coumarin–thiazolidinone derivatives was synthesized using the coupling methodology and their in vitro cytotoxicity screened against the MCF-7 cancer cell line. 104 Among them, compounds 58a and 58b ( Fig. 53 ) possessed noteworthy activity with IC 50 values of 15.65 ± 0.28 μg mL −1 and 12.15 ± 0.05 μg mL −1 , respectively. The structure–activity relationship studies indicated that the presence of an electron-releasing methoxy group increases the cytotoxic activity. Kinase inhibition and suitable binding are responsible for their significant biological property.
2.3. Coumarin–furoxan hybrids
A series of sixteen furoxan-based coumarin derivatives was synthesized and their antiproliferative activities investigated against several cancer cell lines including A549, HeLa, A2780, A2780/CDDP, and HUVEC. 105 They all showed moderate to excellent anticancer activity against the above-mentioned cell lines, but compound 59 ( Fig. 55 ) was observed to be the most potent with IC 50 values of 0.024, 0.053, 0.014, 0.062, and 0.034 μM, respectively. This compound was again investigated for its biological activity against some drug-resistant cell lines (MDA-MB-231, MDA-MB-231/Gem, SKOV3, and SKOV3/CDDP) and proved to be very effective against them also. Furthermore, 55 inhibited the growth of A2780 in vivo and displayed lower toxicity on non-tumorigenesis T29. Preliminary pharmacological studies revealed that 59 acts by inducing apoptosis, arresting the cell cycle at the G2/M phase in the A2780 cell line, and disrupting the phosphorylation of MEK1 and ERK1. Compound 59 was further studied to reveal its potential for apoptosis and autophagy induction in lung adenocarcinoma cells. 106 The cytotoxicity and apoptosis of A549 and H1299 cells induced by compound 59 were detected by MTT, microscopy, and western blot analysis. Significant growth inhibition and caspase-dependent apoptosis were observed in the compound 59 -treated A549 and H1299 cells. Then, it was confirmed that this compound induced autophagy by autophagosome formation, upregulated the expression of autophagy-related protein LC3-II, and autophagic flux. Importantly, abolishing autophagy using inhibitors and ATG5 siRNA enhanced the cytotoxicity of compound 59 , indicating the cytoprotective role of autophagy in lung adenocarcinoma. Further mechanistic investigations suggested that the Akt/mTOR and Erk signaling pathways contributed to autophagy induction by compound 59 .
Five phenylsulfonylfuroxan-merging 3-benzyl coumarins were designed and evaluated for their anticancer activities. 107 Among them, compound 60 ( Fig. 56 ) showed the most potent antiproliferation activities with IC 50 values ranging from 0.5 nM to 143 nM against nine drug-sensitive (HeLa, SKOV3, A549, OVCA429, OVCA433, A2780, MDA-MB-231, MCF-7 and KB) and four drug-resistant cancer cell lines (A2780/CDDP, MDA-MB-231/Gem, MCF-7/ADR, and KB-V). Preliminary pharmacological studies revealed that compound 60 acts by inducing early apoptosis and affecting the cell cycle. Furthermore, it gave 559- and 294-fold selectivity antiproliferation activity in the P-gp overexpressed drug-resistant cancer cell lines MCF-7/ADR and KB-V compared to the drug-sensitive MCF-7 and KB, implying that this compound may have an extra mechanism of anti-MDR-cancer with P-gp overexpression. Here, compound 60 contains fluorine. It is noteworthy to mention that fluorine-containing drugs are tremendously important and are successfully being used in the treatment of many diseases, e.g. , multiple myeloma, lymphoma, HIV, chronic heart failure, chronic myeloid leukemia, (ANCA)-associated vasculitis, migraines, von Hippel–Lindau disease, and non-small cell lung cancer. 108–115
A novel series of eleven furoxan–coumarin hybrids was developed and their antiproliferative activity studied on five human cancer cell lines including HeLa, SW620, HepG2, HCT116, and MCF7. 116 Among them, compounds 61a–c ( Fig. 57 ) showed potent anticancer activity and some evaluated to be more potent than the reference doxorubicin. Compound 61a was the most potent against the HepG2 cell line with IC 50 = 3.86 μM, while compound 61b showed the maximum activity against the SW620 and HCT116 cell lines with IC 50 values of 1.86 and 3.46 μM, respectively. Compound 61c was observed to be the most effective against the HeLa and MCF7 cell lines with IC 50 values of 0.88 and 0.61 μM, respectively.
A series of furoxan conjugates of N , N -dialkyl carboxy coumarins was developed as potential anticancer agents and tested for their in vitro antiproliferative activities on various cell lines (MDA-MB-231, 4T1, WiDr, MCF10A, and HDFa cell lines). 117 Among them, compound 62 ( Fig. 58 ) showed the highest potency with IC 50 values in the range of 0.02 to 38.6 μM. The in vitro mechanistic studies indicated that these compound generated substantial nitric oxide, inhibited colony formation, and caused apoptosis in cancer cells.
2.4. Coumarin–pyridine/pyrimidine hybrids
Similarly, pyrimidine ( Fig. 59 ), which is structurally related to pyridine, the building unit of DNA and RNA, has also been found to possess marked pharmacological effects.
The coumarin–pyridine hybrids 63a–c ( Fig. 60 ) showed weak to moderate activity against the A549 cancer cell line with IC 50 values in the range of 34.2 > 80 μM. 118 The compounds were found to potently inhibit in vitro microtubule formation via a substoichiometric mode such as CA-4.
A novel series of thirteen coumarin–pyridine derivatives was designed and their cytotoxic activities examined on four cancer cell lines including K562, HeLa, A549, and MCF7. 119 Among them, compounds 64a–e ( Fig. 61 ) showed the maximum potency against the MCF7, A549, HeLa, and K562 ( 64d and e ) cell lines with IC 50 values of 2.56 ± 0.17, 4.38 ± 0.09, 2.17 ± 0.45, 1.66 ± 0.09 and 1.66 ± 0.15 μM, respectively. Further investigation revealed that compounds 64a and 64c were much more potent PI3K inhibitors than S14161 and BENC-511 (reference). In addition, 64a was more selective to PI3Kα/β over PI3Kδ/γ, while 64c was a selective PI3Kα/β/δ inhibitor. 64c could also suppress the phosphorylation of Akt and induce K562 cell apoptosis.
Some other coumarin–pyridine hybrids such as 65a and b ( Fig. 62 ) (IC 50 : 69.1–377.8 mM against both A549 and MCF-7 cancer cell lines, MTT assay) were also active against the tested cancer cell lines, but most of them were much less potent than the references. 120–124
A novel series of fifteen coumarin–pyridine hybrids was synthesized via a one-pot four-component coupling reaction under a neat microwave method and their antiproliferative properties investigated against several cancer cell lines. 125 Among them, compounds 66a and 66b ( Fig. 63 ) exhibited promising anticancer activity at low concentrations (10 −5 M) against the NCI-60 cell line. These two potent anticancer molecules were screened for their CT-DNA cleavage and fluorescence quenching with BSA transport protein.
The similar synthesis of coumarin–pyridine conjugates was accomplished via conventional heating and microwave irradiation and the designed compounds were tested for their in vitro cytotoxicity. 126 The preliminary screening results showed that most of the compounds had moderate cytotoxic activity against the HCT-116 and MCF-7 cell lines, although compound 67 ( Fig. 64 ) exhibited potent activity against both cell lines with IC 50 values of 9.9 ± 0.82 and 14.1 ± 1.14 μM, respectively, which was comparable with the standard drug 5-fluorouracil.
A series of coumarin–pyridine hybrids was synthesized and their anticancer activity evaluated against the MCF-7, HCT-116, HepG-2, and A549 human cancer cell lines. 127 Among them, compounds 68a–c ( Fig. 65 ) showed the most potent growth inhibitory activities with IC 50 values in the range of 1.1 to 2.4 μM against the MCF-7 cell line. Flow cytometric analysis revealed that these compounds induced cell cycle arrest in the G2/M phase followed by apoptotic cell death. Furthermore, the activity of caspase-3 in MCF-7 cells was tested. The results indicated that compounds 68a–c increased the caspase-3 activity significantly compared to the control group.
Several thio/furo-fused pyridine moieties having a coumarin scaffold were synthesized using an FeCl 3 -catalyzed modified Pictet–Spengler reaction as the crucial final step and their biological activities were evaluated against three cancer cell lines including DU145, B16F10, and MCF-7. 128 Compound 69a ( Fig. 66 ) possessed significant anticancer activity against the DU145 and B16F10 cell lines with IC 50 values of 20.88 and 12.98 μM, respectively, whereas compound 69b was the most potent against the MCF-7 cell line (IC 50 = 8.00 μM).
A series of twelve coumarin–pyrimidine conjugates was synthesized under microwave irradiation and their cytotoxic activities evaluated against the A-549 and MDA-MB-231 cancer cell lines. 129 Some of them were observed to be more potent than the reference cis -platin. Compound 70a ( Fig. 67 ) showed the maximum potency against A549 with IC 50 = 2.15 ± 0.12 μM, while 70b was the most potent against the MCF-7 cell line with IC 50 = 2.23 ± 0.19 μM. The DNA cleavage study by the gel electrophoresis method revealed that compounds 70a and b inhibited the growth of the pathogenic organism by cleaving the genome given that no traces of DNA were found.
Coumarin–pyrimidine hybrid 71 ( Fig. 68 ) showed significant antiproliferative activity against the HePG2 and MCF-7 cancer cell lines with IC 50 values of 5.5 ± 0.19 and 6.9 ± 0.38 μg mL −1 , respectively. 130
A series of 2-phenylpyrimidine coumarin derivatives with potential telomerase-inhibiting activity was designed and synthesized and all the compounds were screened for their antiproliferative activity against the CNE2, KB, and Cal27 cell lines in vitro . 131 Among them, compound 72 ( Fig. 69 ) exhibited the best activity (IC 50 = 1.92 ± 0.13, 3.72 ± 0.54, and 1.97 ± 0.51 against the CNE2, KB, and Cal27 cell lines, respectively). Flow cytometry revealed that this compound can inhibit CNE2 proliferation. The molecular docking results indicated that compound 72 bonded with telomerase reverse transcriptase (TERT) through multiple interactions, including hydrogen bonding and hydrophobic interactions.
A series of C4–C4′ biscoumarin–pyrimidine hybrids was synthesized via S N 2 reaction of substituted 4-bromomethyl coumarin with thymine and screened for in vitro anticancer activity against C6 rat glioma cells. 132 Among the screened compounds, compound 73 ( Fig. 70 ) was recognized to be the best antiproliferative candidate, having an IC 50 value of 4.85 μM. All the compounds were found to be nontoxic toward healthy human embryonic kidney cells (HEK293). Furthermore, compound 73 displayed strong binding interactions with the drug carrier protein, human serum albumin, and exhibited good solution stability at biological pH conditions.
A fascinating family of low-molecular-weight coumarins (COUPYs) was developed, in which the carbonyl group of the lactone function of the classical coumarin scaffold was replaced by cyano(4-pyridine/pyrimidine)methylene moieties, and investigated as potential photodynamic therapy (PTT) anticancer tools. 133 Among them, three compounds, 74a–c ( Fig. 71 ), exhibited effective in vitro anticancer activities upon visible-light irradiation under both normoxia and hypoxia (phototherapeutic index of up to 71) and minimal toxicity toward normal cells. In addition, their cytotoxicity was also evaluated in non-tumorigenic ovarian tissue-derived cells (CHO) to determine their differential selectivity for cancer cell lines. Compound 74a showed excellent cytotoxicity against the HeLa and A2780 cell lines with IC 50 of values 0.19 ± 0.03, 0.09 ± 0.02 μM, respectively, with a selectivity factor of 15.6. Furthermore, compounds 74b and 74c were also effective against HeLa and A2780 with IC 50 values 1.1 ± 0.1 and 1.1 ± 0.3 μM, respectively, but their selectivity factor was low, and even lower than the reference cis -platin. Acting as excellent theranostic agents targeting mitochondria, the mechanism of action of these compounds was investigated in detail in HeLa cells. The generation of cytotoxic ROS and induction of apoptosis and/or autophagy were identified as the cell death modes triggered after irradiation with low doses of visible light.
Coumarin-tagged pyrimidine scaffold 75 ( Fig. 72 ) was found to selectively impede the proliferation of HER2-positive BC cells. 134 It induced DNA damage and apoptosis in HER-2-positive BC cells more effectively compared to HER-2 negative BC cells. In silico and theoretical calculations revealed that compound 75 could interact with c-Jun N-terminal kinase (JNK), and in vitro studies showed that it increased JNK phosphorylation through ROS generation.
A novel library of coumarin-furo[2,3- d ]pyrimidinone hybrid derivatives was synthesized and assessed for their antiproliferative activities against the HepG2 and HeLa cell lines in vitro . 135 Compound 76a ( Fig. 73 ) showed maximum potency against HepG2 with an IC 50 value of 4.87 μmol L −1 . The kinase activity assay revealed that compound 76a may be a multi-target inhibitor. Alternatively, compound 76b was the most potent against the HeLa cell line with an IC 50 value of 6.47 μmol L −1 . The structure–activity relationship study showed that a more bulky and electro-positive group at the C-2 position the of furo[2,3- d ]pyrimidinone ring enhanced the bioactivity.
2.5. Coumarin–indole/isatin hybrids
A series of indole-incorporated thiazolyl coumarins was developed and evaluated for their anticancer activities in vitro . 141 Among them, compound 77 (NSC: 768621/1) ( Fig. 75 ) showed excellent antiproliferative properties against the full panel of 60 human tumor cell lines. The five dose-level activity results revealed that compound 77 was active against all the cell lines. It showed potent activity against CCRFCEM (GI 50 : 0.33 μM), NCI-H522 (GI 50 : 1.03 μM), HCT116 (GI 50 : 1.60 μM), SF-539 (GI 50 : 1.58 μM), MALME-3 M (GI 50 : 1.59 μM), OVCAR-3 (GI 50 : 1.16 μM), UO-31 (GI 50 : 0.76 μM), PC-3 (GI 50 : 0.82 μM) and BT-549 (GI 50 : 1.13 μM).
6-(6-Fluoro-1 H -indol-2-yl)-7-hydroxy-4,8-dimethyl-2 H -chromen-2-one ( 78 ), a coumarin–indole conjugate ( Fig. 76 ), showed the highest level of antimitotic activity with mean GI 50 /TGI values of 3.28/13.24 mM and certain sensitivity profile towards the non-small cell lung cancer cell line HOP-92 (GI50/TGI/LC50 values 0.95/4.17/29.9 mM). 142
Another series of coumarin–indole derivatives was synthesized and their cytotoxic activities investigated in vitro against an MCF-7 cancer cell line together with a normal cell line. 143 Among the characterized compounds, 79 ( Fig. 77 ) showed the maximum potency against the MCF-7 cell line with IC 50 = 7.4 μM. Flow cytometric cell cycle analysis of 79 exhibited the apoptotic mode of cell death due to cell cycle arrest in the G2/M phase.
Further investigation revealed that hybrid 80 ( Fig. 78 ) with a bromine atom in position-7 of the coumarin ring displayed excellent dose-dependent cytotoxic activity with high selectivity for MCF-7 cells in the MTT assay. 144 Flow cytometry analysis of 80 showed cell cycle arrest in the S phase and the accumulation of cells in the subG1 phase. The apoptotic mode of cell death induced by 80 was further confirmed by annexin-V staining assay. The wound healing assay revealed a profound impairment in the migration of MCF-7 cells presumably due to the down-regulation of Bcl-2 and Bcl-xL proteins induced by 80 , as observed in the immunoblotting analysis. 80 was found to bind favorably to Bcl-2 and Bcl-xL in the docking simulation analysis, suggesting that it is a probable small molecule Bcl-2/Bcl-xL inhibitor and a potential lead for breast cancer chemotherapy with apoptotic and anti-metastatic properties.
A new series of indole–triazole–coumarin hybrids was developed via copper( I )-catalyzed [3 + 2] azide–alkyne cycloaddition and showed excellent binding affinity towards CDK2 kinase with cytotoxicity against the human breast cancer cell line MCF-7. 145 The IC 50 value (17.5 μM) and binding affinity (−11.2 kcal mol −1 ) obtained for 81 ( Fig. 79 ) against MCF-7 cells are promising and it can act as a lead to generate more potential anticancer moieties.
Another coumarin–indole hybrid, 3-(1-(5-(3-(1 H -indol-3-yl)propyl)-1,3,4-thiadiazol-2-ylimino)ethyl)-6-bromo-2 H -chromen-2-one (IPTBC) ( 82 ) ( Fig. 80 ), exhibited dose-dependent cytotoxicity in breast adenocarcinoma (MCF-7) cells. 146 This compound induced cell apoptosis through the active involvement of caspases.
The coumarin-β-carboline system 83 ( Fig. 81 ) showed antiproliferative activity against the HeLa cell line with a GI 50 value of 23.4 μg mL −1 . 147 In silico studies indicated the binding properties of 83 with the kinesin spindle protein (KSP) and tubulin protein. Gel electrophoresis studies revealed that compound 83 completely cleaved the CT-DNA.
A novel series of twelve ethylene glycol-tethered coumarin–isatin hybrids were designed and evaluated for their in vitro anticancer activities against HepG2, HeLa, A549, DU145, SKOV3, and MCF-7, and drug-resistant MCF-7/DOX (doxorubicin-resistant MCF7) by SRB assay. 148 Among them, compound 84a showed the maximum potency against HepG2, A549, DU145, MCF-7, and MCF-7/DOX with IC 50 values of 10.28, 10.92, 20.80, 11.29, and 14.45 μM, respectively. Compounds 84b and 84c possessed the highest efficiency against the HeLa and SKOV3 cell lines with IC 50 values of 11.54 and 18.63 μM, respectively ( Fig. 82 ).
Another series of twelve ethylene glycol-tethered coumarin–isatin hybrids was developed and examined for their in vitro cytotoxic activities against HepG2, HeLa, A549, DU145, SKOV3, and MCF-7 as well as drug-resistant MCF-7/DOX (doxorubicin-resistant MCF-7) human cancer cell lines. 149 Most of them had very little or no anticancer activities. Compound 85 ( Fig. 83 ) showed moderate activity against the SKOV3, and MCF-7 and MCF-7/DOX cell lines with IC 50 values of 23.76, 11.90, and 18.85 μM, respectively.
A series of twelve diethylene glycol-tethered isatin–1,2,3-triazole–coumarin hybrids was synthesized and investigated for their in vitro anticancer activities against HepG2, HeLa, A549, DU145, SKOV3, MCF-7, and MCF-7/DOX human cancer cell lines. 150 Among them, compound 86a ( Fig. 84 ) showed excellent potency against six of the seven cell lines (IC 50 values of 19.89, 21.32, 18.67, 31.50, 17.96, and 15.46 μM against HepG2, HeLa, A549, DU145, SKOV3, and MCF-7/DOX cell lines, respectively). Compound 86b was most effective against the MCF-7 cell line with IC 50 = 28.74 μM.
A similar study was carried out on tetraethylene glycol-tethered isatin–1,2,3-triazole–coumarin hybrids. 151 Among them, compound 87 ( Fig. 85 ) possessed the maximum anticancer activity (IC 50 values of 26.11, 25.49, 28.74, 33.42, 35.28, 29.25, and 20.09 against HepG2, HeLa, A549, DU145, SKOV3, MCF-7, and MCF-7/DOX, respectively).
A sulphonic acid-functionalized nitrogen sulfur co-doped graphite (SO 3 H-NSG)-based catalyst was prepared by coating the surface of carbon with a sulphonic acid-bearing ionic liquid, which was used for the synthesis of biologically active coumarin-substituted bis(indolyl)methanes that were finally evaluated for their toxicity and anticancer properties. 152 The cytotoxicities of compounds 88a–d ( Fig. 86 ) were investigated against the human breast carcinoma cell line (MCF-7), osteosarcoma cell line (HOS), and normal kidney epithelial cell line (NKE) by the Amber blue reduction assay. Among them, 88a–c showed significant cytotoxicity towards the breast cancer cell line with IC 50 of 19.75, 28.04, and 21.19 μM, respectively, whereas 88d was practically nontoxic. Similarly, 88a , b , and d showed significant cytotoxicity towards the osteosarcoma cell line with IC 50 of 20.76, 8.75, and 12.23 μM, respectively, whereas 88c was practically nontoxic. These compounds were also evaluated for their possible cytotoxicity against normal human cell lines (NKE). However, they did not significantly affect the growth of normal human kidney cells (IC 50 values of >50 μM).
A novel family of coumarin–indole derivatives was synthesized and evaluated as tubulin polymerization inhibitors targeting the colchicine binding site. 153 Among them, compound MY-413 ( 89 ) ( Fig. 87 ) displayed the most potent inhibitory activities against the gastric cancer cell line MGC-803 with an IC 50 value of 0.011 μM. Furthermore, the IC 50 values of compound 89 on fifteen cancer cell lines were lower than 100 nM and the IC 50 values of 9 cancer cell lines were less than 50 nM. Compound 89 effectively inhibited tubulin polymerization (IC 50 = 2.46 μM) by binding to the colchicine site. Screening for the inhibitory effects of the compound on 61 kinases revealed that compound MY-413 could inhibit MAPK 39 pathway-related kinases. Because of the inhibitory effects of compound MY-413 on tubulin polymerization and MAPK pathways, compound 89 induced cell apoptosis, arrested the cell cycle in the G2/M phase, induced cell proliferation inhibition and cell migration inhibition in the gastric cancer cell lines MGC-803 and HGC-27. In addition, compound MY-413 could significantly inhibit tumor growth in MGC-803 with tumor growth inhibition (TGI) rates of 70% (15 mg kg −1 ), 45% and 80% (30 mg kg −1 ) without obvious toxicity. Consistent with the in vitro results, compound MY-413 also inhibited the MAPK signaling pathway, and induced apoptosis and proliferation inhibition in vivo .
2.6. Coumarin–chalcone hybrids
A novel series of coumarin–chalcone hybrids was synthesized and evaluated for anti-proliferative activity against the estrogen receptor-positive MCF-7 and negative MDA-MB-435 breast cancer cell lines. 161 Compounds 90a–c ( Fig. 89 ) showed significant potency against the MCF-7 cell line with GI 50 values of <10, 18.9, and 32.5 μg mL −1 , respectively. Moreover, compound 90a was observed to be superior to N -methyl nitrosourea in vivo in terms of latency (5.5 weeks vs. 4.5 weeks) and reducing the tumor burden (3.1 vs. 4.45) and volume (3.3 mm 3 vs. 4.8 mm 3 ), suggesting that it can act as a lead for the generation of more potent anticancer candidates.
Coumarin–chalcone hybrids 91a–c ( Fig. 89 ) showed anticancer activity against the HepG2 and leukemia K562 cell lines. 162 Compound 91a was the most potent against HepG2 with IC 50 = 0.65 μM, while 91b showed the maximum efficiency against leukemia K562 with IC 50 = 0.93 μM. Furthermore, cell cycle analysis of 91a showed the activation of apoptotic signals as a result of cell cycle arrest in the G2/M phase ( Fig. 90 ).
Another coumarin–chalcone hybrid ( 92 ) ( Fig. 91 ), which is structurally similar to 90 , was synthesized and its anticancer activity was investigated against the T47D breast cancer cell line and cervix cancer cell line HeLa. 163 This compound has an IC 50 of 0.90 μM for the T47D breast cancer cell line and 2.32 μM for the HeLa cervix cancer cell line.
A novel series of coumarin–chalcone conjugates and their NO hybrids was designed and their antiproliferative properties investigated against the MCF-7 cancer cell line. 164 The coumarin–chalcone hybrid 93 ( Fig. 92 ) showed the maximum potency with an IC 50 value of 9.62 μg mL −1 . Among the NO hybrids, compound 94 was the most effective with an IC 50 value of 20.9 μg mL −1 .
S009-131 ( 95 ) ( Fig. 93 ), a coumarin–chalcone hybrid, possessed anti-proliferative and anti-tumor effects by triggering apoptosis. 165 S009-131 caused DNA damage by potential binding to the minor groove, which led to the phosphorylation and activation of ATM and DNA-PK, but not ATR. S009-131 induced the DNA-damage-response-triggered activation of p53 through phosphorylation at its key residues.
A series of novel coumarin–chalcone derivatives containing a urea moiety was developed and screened for their in vitro antiproliferative activities against cancer cell lines (H4IIE and HepG2). 166 In addition, the compounds were also tested on a normal cell line (CHO). Among them, compound 96a ( Fig. 94 ) exhibited the maximum potency against H4IIE compared to sorafenib with an IC 50 value of 1.62 ± 0.57 μM. Compound 96b also showed better inhibition against HepG2 than sorafenib with an IC 50 value of 2.326 ± 0.23 μM. Particularly, 96a induced H4IIE apoptosis and arrested cell cycle in the S phase.
A series of coumarin–chalcone hybrids was synthesized as selenoprotein thioredoxin reductase (TrxRs) inhibitors. 167 Most of them exhibited enhanced anticancer activity compared to xanthohumol (Xn). Among them, compound 97 ( Fig. 95 ) (IC 50 = 3.6 μM), a fluorescence agent, down-regulated the expression of TrxR and remarkably induced ROS accumulation to activate the mitochondrial apoptosis pathway. Furthermore, it inhibited cancer cell metastasis and abolished the colony formation ability of cancer cells.
A new series of thirteen coumarin-yl–chalcone derivatives was synthesized and in silico studies were performed to predict the anticancer activity of the compounds against Src, Alb tyrosine kinase, and homology model protein (PDB ID: 4csv ). 168 Derivatives 98a and b ( Fig. 96 ) showed moderate binding energies. The in vitro cytotoxic activity was analyzed for these two compounds against three human cell lines, including A549, Jurkat, and MCF-7. The results indicated that the hybrids displayed significant anticancer activity but are less cytotoxic than the standard imatinib.
A water-soluble chemo-sensor ( 99 ) ( Fig. 97 ) consisting of a chalcone–coumarin framework, which displayed excellent selectivity and sensitivity towards Al 3+ ions, showed significant anticancer activity against the MCF-7 cancer cell line with an IC 50 value of 15.38 μM. 169
2.7. Coumarin-imine hybrids
A similar series of nine coumarin-imine hybrids ( 101a–i ) ( Fig. 99 ) was synthesized and their antiproliferative profile evaluated against fibroblast cell lines and A549 cancer cell line. 171 The percentage of viable cells was determined at different concentrations in the range of 12.5 to 200 μg mL −1 . In terms of the WST-1 results, the concentrations of the compounds did not have a prominent effect on cell mortality in the cell line. For the fibroblast cells, the results were significant for only 101a and 101b . However, for the other samples ( 101c–i ), increasing the concentrations of the compounds caused an increase in cell death.
A series of coumarin–hydrazone hybrids was designed and evaluated for their anticancer activities against four cancer cell lines. 172 Among them, compound 102 ( Fig. 100 ) showed the most potency with IC 50 = 2.9 ± 0.4, 5.3 ± 1.1, 7.2 ± 0.9, and 9.1 ± 1.2 μM against the HL-60, KE-37, K-562, and MDA-MB-231 cell lines, respectively.
2.8. Coumarin-sulfonamide/sulfamate/sulfonate hybrids
Coumarin-sulfonamide hybrid 104 ( Fig. 102 ) was designed to obtain novel dual inhibitors of COX-2 and 5-LOX. 174 Compound 104 (IC 50 = 0.23 ± 0.16 μM for COX-2, 0.87 ± 0.07 μM for 5-LOX, and 4.48 ± 0.57 μM against A549) showed preliminary superiority compared with the positive controls celecoxib (IC 50 = 0.41 ± 0.28 μM for COX-2 and 7.68 ± 0.55 μM against A549) and zileuton (IC 50 = 1.35 ± 0.24 μM for 5-LOX). Further investigation confirmed that 104 could induce apoptosis and cell cycle arrest at the G2 phase in a dose-dependent manner in human non-small cell lung cancer A549 cells.
Different novel sets of coumarin-6-sulfonamide derivatives were synthesized and their growth inhibitory activity evaluated in vitro towards the proliferation of three cancer cell lines, i.e. , HepG2, MCF-7, and Caco-2. 175 Compounds 105a and 105b ( Fig. 103 ) emerged as the most active members against HepG2 cells (IC 50 = 3.48 ± 0.28 and 5.03 ± 0.39 mM, respectively). These compounds could induce apoptosis in HepG2 cells, as demonstrated by the upregulation of Bax and downregulation of Bcl-2, besides boosting the caspase-3 levels. Besides, compound 105a induced a significant increase in the percentage of cells at pre-G1 by 6.4-fold, with concurrent significant arrest in the G2-M phase by 5.4-fold compared to the control. Also, 105a displayed a significant increase in the percentage of annexin V-FITC-positive apoptotic cells from 1.75% to 13.76%.
Coumarin-sulfonamide hybrid 106 ( Fig. 104 ) showed excellent antiproliferative properties against the MCF-7 cancer cell line with IC 50 = 2.53 μM, together with the selective index (SI) of 59.26. 176
Coumarin-sulfonamide hybrid 107 ( Fig. 105 ), synthesized as a new chemotype of BRD4 inhibitors, possessed excellent cytotoxic ability against A549 (IC 50 = 4.63 μM), HepG2 (IC 50 = 4.75 μM), PANC-1 (IC 50 = 7.02 μM), and SGC-7901 (IC 50 = 6.39 μM) cell lines. 177 Moreover, compound 107 exhibited potent BRD4 binding affinity and cell proliferation inhibitory activity, and especially displayed a favorable PK profile with high oral bioavailability ( F = 49.38%) and metabolic stability ( T 1/2 = 4.2 h), meaningfully making it a promising lead compound for further drug development.
A novel series of coumarin-sulfamate hybrids was prepared as potential STS inhibitors. 178 The inhibitory effects of the synthesized compounds were tested on STS isolated from the human placenta and against estrogen receptor-(ER)-positive MCF-7 and T47D cells, as well as ER-negative MDA-MB-231 and SkBr3 cancer cell lines. Among the synthesized compounds, 108a and 108b ( Fig. 106 ) showed the highest inhibitory effect in enzymatic STS assays, both with IC 50 values of 0.18 μM. Compound 108b exhibited the highest potency against the MCF-7 and T47D cell lines (15.9 μM and 8.7 μM, respectively).
Potent bicyclic nonsteroidal sulfamate-based active-site-directed inhibitors of the enzyme steroid sulfatase (STS), an emerging target in the treatment of post-menopausal hormone-dependent diseases, including breast cancer, were designed. 179 The compounds were examined for STS inhibition in intact MCF-7 breast cancer cells and in placental microsomes. 3-Hexyl-4-methylcoumarin-7- O -sulfamate 109a and 3-benzyl-4-methylcoumarin-7- O -sulfamate 109b ( Fig. 107 ) were particularly effective inhibitors with IC 50 values of 0.68 and 1 nM in intact MCF-7 cells and 8 and 32 nM for placental microsomal STS, respectively. They were docked in the STS active site for comparison with estrone 3- O -sulfamate and irosustat, showing their sulfamate group close to the catalytic hydrated formylglycine residue and their pendant group lying between the hydrophobic side-chains of L103, F178, and F488.
A series of STAT3 inhibitors was developed and their anti-proliferative activity against four cancer cells investigated. 180 Among them, compound 110 ( Fig. 108 ) was the most potent with IC 50 = 1.43 ± 0.30, 1.89 ± 0.42, 2.88 ± 0.69, and 3.33 ± 0.23 μM against the MDA-MB-231, HCT-116, HepG2, and MCF-7 cancer cell lines, respectively. STAT3 phosphorylation was inhibited by compound 110 at both Tyr705 and Ser727 residues. Compound 110 inhibited STAT3 phosphorylation, whereas it did not influence the phosphorylation levels of STAT1, 26 JAK2, Src, and Erk1/2, indicating its good selectivity. Moreover, compound 110 down-regulated the expression of the STAT3-target genes Bcl-2 and cyclin D1, increased ROS production, and remarkably reduced the mitochondrial membrane potential to induce the mitochondrial apoptotic pathway. It also suppressed breast cancer 4T1-implanted tumor growth in vivo .
Fourteen new cycloalkane-fused tricyclic coumarin sulfonate derivatives were developed and evaluated for in vitro anticancer activity against the NCI-57 cancer cell line panel of nine different cancer types. 181 Among the compounds, 111a , 111b , and 111c ( Fig. 109 ) showed the highest activities. Compound 111b exerted the highest percentage of growth inhibition (91.91%) against the SNB-75 CNS cancer cell line at 10 μM concentration and was more active than carmustine against this cell line. Compound 111a also showed strong activity against HT29 colon, ACHN renal, and PC-3 prostate cancer cell lines. Furthermore, compound 111c was selective toward the HT29 colon cancer cell line.
A series of coumarin-sulfonamide hybrids was synthesized by the condensation reaction of appropriate N -heteroaryl-4-amino benzenesulfonamide with derivatives of 3-acetyl coumarin and their antiproliferative property was screened against the MDA-MB-231, MIA PaCa-2, and H357 cancer cell lines. 182 Among them, compound 112 ( Fig. 110 ) demonstrated significant activity against the MDA-MB-231 cell line (IC 50 = 7.78 ± 3.78 μM) and H357 cell line (IC 50 = 8.68 ± 1.10 μM) after 72 h.
2.9. Dihydroxycoumarins
A novel series of coumarin-based nonsteroidal-type fluorescence ligands for drug–target binding imaging was designed and developed. 183 Among the synthesized compounds, 113 ( Fig. 111 ) showed potent antiproliferative activity against the MCF-7 cancer cell line with IC 50 = 16.1 ± 0.7 μM and against MDA-MB-453 with IC 50 = 8.03 ± 0.6 μM. Furthermore, compound 113 could cross the cell membrane, localize, and image drug–target interaction in real time without cell washing.
A shikonin derivative, PMMB232 ( 114 ) ( Fig. 112 ), showed antiproliferation activity with an IC 50 value of 3.25 ± 0.35 μM. 184 Further, the treatment of HeLa cells with a variety of concentrations of 114 resulted in a dose-dependent event marked by apoptosis. To identify the detailed role and mechanism of PMMB232 in the progression of human cervical cancer, the expression of HIF-1α and E-cadherin in HeLa cells was detected. The results revealed that expression of HIF-1α was downregulated, while E-cadherin protein was upregulated. Meanwhile, glycolysis-related protein PDK1 decreased in the HeLa cells. Conversely, the expression of PDH-E1α was upregulated. The docking simulation results further indicated that PMMB232 can be well bound to HIF-1α.
Six 4-hydroxycoumarin derivatives were synthesized and their cytotoxic activities were investigated against four cancer cell lines (SMMC-7721, Bel-7402, MHCC97, and Hep3B). 185 Compound 115 ( Fig. 113 ) showed the maximum potency with IC 50 values = 6 ± 1.4, 8 ± 2.0, 7 ± 1.7, 9 ± 2.0 μM against SMMC-7721, Bel-7402, MHCC97 and Hep3B, respectively.
A series of nine coumarin derivatives was synthesized and their anticancer activity tested against four human breast cancer cells in vitro using the MTT assay. 186 Among them, compound 116 ( Fig. 114 ) showed the maximum potency with IC 50 = 25.3 ± 2.3, 15.2 ± 2.4, 25.7 ± 2.2, and 20.2 ± 3.0 μM against the MDA-MB-231, MDA-MB-468, Hst578, and HCC1937 cell lines, respectively.
A series of novel 4,7-dihydroxycoumarin-based acryloylcyanohydrazone derivatives was synthesized and evaluated for their antiproliferative activity against four different cancer cell lines (A549, HeLa, SKNSH, and MCF7). 187 Compound, 117 ( Fig. 115 ) was the most active with IC 50 values of 4.31 ± 0.04, 5.14 ± 0.16, 6.09 ± 0.32, and 3.42 ± 0.52 μM against A549, HeLa, SKNSH, and MCF7, respectively. Further results revealed that compound 117 induced cell cycle arrest at the G2/M phase and inhibited tubulin polymerization. The experimental data from the tubulin polymerization inhibition assay was validated by the molecular docking technique and the results exhibited strong hydrogen bonding interactions with amino acids (ASN-101, TYR-224, ASN-228, and LYS-254) of tubulin.
2.10. Anilinocoumarin hybrids
A series of novel 4-substituted coumarin derivatives was synthesized and their antiproliferative activity toward a panel of tumor cell lines was investigated. 188 Among them, compounds 118a–g ( Fig. 116 ) showed potent antiproliferative ability. 118f was the most potent (IC 50 values = 7–47 nM) and retained full activity in multidrug-resistant cancer cells. 118f caused G2/M phase arrest and interacted with the colchicine-binding site in tubulin, reducing the cell migration and disrupting capillary-like tube formation in HUVEC cells. Importantly, compound 118f significantly and dose-dependently reduced tumor growth in four xenograft models including paclitaxel-sensitive and resistant ovarian tumors (A2780s and A2780/T) and adriamycin-sensitive and resistant breast tumors (MCF-7 and MCF-7/ADR, respectively).
Eighteen selective ERα modulators (SERMs) were designed and their biological activity investigated against MCF-7 and Ishikawa cell lines. 189 The piperidyl-substituted compounds such as 119a and 119b ( Fig. 117 ) demonstrated strong ERα binding affinities and excellent anti-proliferative activities. Compound 119b displayed the most potent ERα binding affinity with an RBA value of 2.83%, while 119a exhibited the best anti-proliferative activity against MCF-7 cells with an IC 50 value of 4.52 ± 2.47 μM.
A novel series of 3-( N -substituted)aminocoumarins was developed rapidly and efficiently and their antiproliferative activity examined against human cancer cell lines. 190 Compound 120 ( Fig. 118 ) showed excellent anticancer activity against MT-4, MDA-MB-231, and MCF-7 cancer cell lines with GI 50 values of 12.6 ± 0.9, 11.8 ± 1.1 and 10.5 ± 1.2 μM, respectively.
Various 3-substituted 4-anilino-coumarin derivatives were designed and synthesized and their in vitro cytotoxicity screening performed against MCF-7, HepG2, HCT116, and Panc-1 cancer cell lines by the MTT assay. 191 Most of the synthesized compounds exhibited comparable anti-proliferative activity to the positive control 5-fluorouracil against the four tested cancer cell lines. Among the different substituents at the C-3 position the of coumarin scaffold, the 3-trifluoroacetyl group showed the most promising results. Especially, compounds 121a ( Fig. 119 ) (IC 50 = 16.57, 5.45, 4.42 and 5.16 μM) and 121b (IC 50 = 20.14, 6.71, 4.62 and 5.62 μM) showed excellent anti-proliferative activities on MCF-7, HepG2, HCT116 and Panc-1 cell lines, respectively. In addition, cell cycle analysis and apoptosis activation revealed that 121a induced G2/M phase arrest and apoptosis in MCF-7 cells in a dose-dependent manner. The low toxicity of compounds 121a and 121b was observed against human umbilical vein endothelial cells (HUVECs), suggesting their acceptable safety profiles in normal cells. Furthermore, the results of in silico ADME studies indicated that both 121a and 121b exhibited good pharmacokinetic properties.
A series of substituted aminomethyl benzocoumarin derivatives was synthesized and tested for their anticancer activity against the A549, MCF7, and A375 cancer cell lines. 192 Among them, the anilinocoumarin compound 122 ( Fig. 120 ) showed excellent growth inhibitory activity against the A549, MCF7, and A375 cancer cell lines with IC 50 values of 4.29 μM, 5.17 μM, and 9.02 μM, respectively. Compound 122 was also found to be quite promising at very low concentrations as an anticancer agent against the MCF7 and A549 cell lines.
Twenty-five coumarin-based derivatives were developed and investigated for their in vitro anticancer activity against the MCF-7 breast and PC-3 prostate cancer cell lines and further assessed for their in vitro VEGFR-2 kinase inhibitory activity. 193 Among them, compound 123 ( Fig. 121 ) (IC 50 = 1.24 μM) exhibited exceptional activities superior to the positive control staurosporine (IC 50 = 8.81 μM). Further study revealed that compound 123 was capable of inducing preG1 apoptosis, cell growth arrest at the G2/M phase, and activating caspase-9. A molecular docking study suggested that the most active anti-VEGFR-2 derivative 123 demonstrated the ability to interact with the key amino acids in the target VEGFR-2 kinase binding site.
A series of substituted coumarins was synthesized directly from coumarins and azides in the presence of Pr(OTf) 3 without any additives or ligands and the cytotoxic activity of the compounds was tested against the MGC-803, A549, and NCI-H460 cancer cell lines. 194 Compound 124 ( Fig. 122 ) showed maximum potency having IC 50 values of 10.19 ± 1.12, 8.75 ± 1.10, and 9.25 ± 1.28 μM against the MGC-803, A549, and NCI-H460 cell lines, respectively.
2.11. Coumarin-metal hybrids
A series of tailored novobiocin–ferrocene conjugates was prepared and investigated for in vitro anticancer activity against the MDA-MB-231 breast cancer line. 196 They all showed moderate antiproliferative character. Compounds 125a and 125b ( Fig. 124 ) showed maximum potency with IC 50 values of 11.7 and 11.8 μM, respectively.
The coumarin–ferrocene hybrid 126 ( Fig. 125 ) showed good anticancer activity when examined against the HCC38 cancer cell line with IC 50 = 1.06 μM. 197
Seven novel coumarin–ferrocene conjugates were synthesized and their biological activities thoroughly investigated against several human cancer cell lines. 198 Most of the hybrids showed moderate and good activity compared to the reference adriamycin. Compound 127a ( Fig. 126 ) showed good potency against the BIU-87 and MCF-7 cancer cell lines with the IC 50 values of 1.09 and 12.10 μM, respectively, while 127b was the most effective against SGC-7901 with IC 50 = 3.56 μM.
A series of novel organoplatinum( II ) complexes was designed and their cytotoxic effects on various cancer cell lines and drug-resistant cancer cell lines examined. 200 Among them, complex 129 showed marked potency against the HeLa and A549/DDP cell lines with IC 50 values of 0.15 ± 0.09 and 0.10 ± 0.05 μM, respectively ( Fig. 128 ).
Three coumarin-appended phosphorescent cyclometalated iridium( III ) complexes, 130a–c ( Fig. 129 ), were explored as mitochondria-targeted theranostic anticancer agents. 201 All three complexes could specifically target mitochondria and show better antiproliferative activities than cisplatin against various cancer cells including cisplatin-resistant cells. They could penetrate human cervical carcinoma (HeLa) cells quickly and efficiently, and carried out theranostic functions by simultaneously inducing and monitoring the morphological changes in the mitochondria. The mechanism studies showed that they exert their anticancer efficacy by initiating a cascade of events related to mitochondrial dysfunction. Genome-wide transcriptional and connectivity map analyses revealed that the cytotoxicity of complex 130c is associated with pathways involved in mitochondrial dysfunction and apoptosis.
Three families, namely isoselenocyanate, selenocarbamates, and selenoureas, were designed and tagged with the coumarin moiety, and their antiproliferative properties were analyzed. 202 Among them, selenourea 131 ( Fig. 130 ) showed significant cytotoxic activities with GI 50 values of 3.0 ± 0.5, 2.3 ± 0.7, 5.2 ± 0.4, 2.9 ± 1.1, and 3.8 ± 1.4 μM against the A549, HBL-100, HeLa, T-47D, WiDr cell lines, respectively. Moreover, dimer 132 also showed prominent cytotoxic activities with GI 50 values of 3.2 ± 0.7, 3.5 ± 1.4, 2.9 ± 0.1, 3.6 ± 0.4, and 4.5 ± 1.0 μM against the above-mentioned cancer cell lines, respectively.
Three new gold( I )-coumarin-based trackable therapeutic complexes ( 133a–c ) ( Fig. 131 ) were synthesized and their antiproliferative properties on several types of cancer cell lines including colon, breast, and prostate investigated. 203 They all displayed moderate anticancer activities against MDAMB-231, PC3, SW480, and HEK293T.
The zinc( II ) complex of 3-acetylcoumarin thiosemicarbazone ( 134 ) ( Fig. 132 ) showed significant cytotoxicity against human liver carcinoma (HepG-2) and lymphoblastoid multiple myeloma (IM-9) cell lines with the IC 50 value of 25 μg mL −1 . 204 The spectroscopic results suggested that the complex interacted with CT-DNA through the intercalative binding mode.
Two new copper( II ) ( 135 ) and nickel( II ) ( 136 ) ( Fig. 133 ) complexes with a new coumarin derivative were synthesized and their cytotoxic activities determined by the MTT assay. 205 The results showed that the designed drugs have significant cytotoxic activity against the HepG2, HL60, and PC3 cell lines. Cell apoptosis was detected by annexin V/PI flow cytometry and the results showed that the two complexes can induce apoptosis of the three human tumor cells.
A Co( II ) complex of coumarin [Co(CUAP)(H 2 O) 2 Cl] ( 137 ) ( Fig. 134 ) exhibited significant antiproliferative properties against the MCF-7 and K-562 cancer cell lines with IC 50 values of less than 10 μg mL −1 . 206
A Ru( III ) complex synthesized from a coumarin derivative ( 138 ) ( Fig. 135 ) was observed to be potent against the MCF-7 cancer cell line with an IC 50 value of less than 10 μg mL −1 . 207
Besides these findings, various metal-based coumarin complexes show significant cytotoxic activities against various cancer cell lines and this strategy is becoming increasingly important, which has a bright prospect. 208
2.12. Miscellaneous coumarin hybrids
A series of hydrazide–hydrazone and amide-substituted coumarin derivatives was synthesized and evaluated in vitro for their antitumor activity. 210 Among them, compound 140a ( Fig. 137 ) showed the maximum potency against the Panc-1 cell line with IC 50 = 0.129 ± 0.019 μM and selectivity ratio of >387.60. Compound 140b possessed significant effectivity against the HepG2 cell line with IC 50 = 4.892 ± 0.086 μM and selectivity ratio of >10.22. Compound 140c showed marked potency against CCRF cells with IC 50 = 3.108 ± 0.439 μM and selectivity ratio of >16.09. Besides, compound 140d was effective against all the cell lines with IC 50 values of 5.449 ± 1.380, 9.417 ± 0.548, and 7.448 ± 4.579 against Panc-1, HepG2, and CCRF cells with the corresponding selective ratio of >9.18, >5.31, and >6.71, respectively.
Tacrine-coumarin hybrids 141a and 141b ( Fig. 138 ) showed significant anti-metabolic activity against the 4T1 cell line, with IC 50 values of 5.7 μM and 7.0 μM, respectively, while compound 141a also showed promising activity against the MCF-7 cell line, with an IC 50 value of 6.0 μM. 211
A series of quinoline and thiazole-containing coumarin analogs was developed and their cytotoxic ability examined on mouse leukemic cells. 212 The result indicated that 142 ( Fig. 139 ) showed potent activity against EAC and DLA cells in the MTT assay (15.3 μM), trypan blue (15.6 μM), and LDH (14.2 μM) leak assay with 5-fluorouracil as the standard. The experimental data showed that compound 142 induced apoptotic cell death by activating apoptotic factors such as caspase-8 &-3, CAD, cleaved PARP, γ-H2AX, and by degrading genomic DNA of cancer cells, and thereby decreasing the ascitic tumor development in mice. The molecular docking study revealed that compound 142 has a very good interaction with caspase 3 protein by binding with the Arg 207 amino acid through a hydrogen bond.
An interesting fact can be nicely shown from the work by Zwergel et al. 213 The antiproliferative property of a particular coumarin-based hybrid can be significantly enhanced by slight modification in the coumarin moiety ( Fig. 140 , Table 1 ).
The styryl coumarin hybrids 3-SC1 ( 144a ), 7-SC2 ( 144b ), and 7-SC3 ( 144c ) ( Fig. 141 ) decreased the cell viability of SW480 in a time- and concentration-dependent manner (IC 50 -SW480/48 h = 6.92; 1.01 and 5.33 μM, respectively) with high selectivity indices after 48 h of treatment (>400; 67.8 and 7.2, respectively). 214 Among them, the most active molecule 7-SC2 induced a greater production of ROS in comparison with the control ( p < 0.05) together with a significant increase in the expression of p53 and caspase-3, and a significant reduction in the production of interleukin-6 of SW480 cells. When colon carcinogenesis was induced in Balb/c mice by intraperitoneal injection of azoxymethane, a significant reduction ( p < 0.05) in the number of preneoplastic lesions of the mice treated with styryl coumarin hybrid 7-SC2 was observed in the control group. Moreover, no side effects were associated with the administration of the compound. All these in vitro results and the effective reduction of preneoplastic lesions in vivo suggest that styryl coumarin 7-SC2 induces apoptosis in primary tumor cells and implies its potential ability at the early post-initiation phases of colon carcinogenesis.
A series of methylene thio-linked coumarin derivatives was prepared by the reaction of substituted 4-(bromomethyl)-2 H -chromen-2-one with various heterocyclic mercapto compounds via S N 2 reactions in the presence of K 2 CO 3 as a catalyst and their in vitro anticancer activity screened against the MCF-7 cancer cell line. 215 Compound 145 ( Fig. 142 ) with a methoxy-substituted benzimidazole ring was found to be the most effective with an IC 50 value of 0.18 μM.
Twenty porphyrin–coumarin compounds were synthesized via the condensation reaction of porphyrins with coumarin derivatives and their cytotoxic activity evaluated against A549 and HepG2 cells under light irradiation. 216 The structure–activity relationship studies indicated that the coumarin derivatives with shorter alkyl chains to porphyrins exhibited both photodynamic therapy (PDT) and chemotherapy. Moreover, the insertion of metal Zn in the porphyrins also increased the PDT effect of the compounds. Compound 146 ( Fig. 143 ) was found to be the most effective against the HepG2 cell line with an IC 50 value of 67.66 ± 0.61 μmol L −1 . Alternatively, the Zn-containing compound 147 exhibited the maximum potency against the A549 cell line with an IC 50 value of 52.37 ± 1.17 μmol L −1 (both under light irradiation).
Besides the above-mentioned coumarin hybrids, coumarin-hydroxamic acid, 217 coumarin–ergosterol peroxide, 218 coumarin–formonontin, 219 coumarin–costunolide, 220 coumarin–curcuminoid, 221 coumarin–furan, 222 coumarin–maltol, 223 coumarin–carbazole, 224 coumarin–quinazoline, 225 coumarin-steroidal, 226,227 coumarin-sugar, 228–232 coumarin-thiazin-2-thione, 233 coumarin–thiophosphate, 234 coumarin-triazolothiadiazines, 235 N -heterocyclocoumarin, 236 hydroxymercapto-methylcoumarin, 237 triazole-tethered coumarin–isatin, 238 and others 239–241 also showed certain anticancer activities.
In 2015, some triazole-modified coumarin-based compounds ( 148 ) were patented, which were proposed to be used in the treatment of various cancers such as breast and prostate cancer. 242 The general structure of the compounds is shown in Fig. 144 .
In 2016, various coumarin derivatives 149a–c with substituents at the 6-position with five or more than five carbon atoms were patented, which were used to treat pancreatic cancer. 243 The structures of some of the compounds are shown in Fig. 145 .
In 2017, the design of different coumarin–gossypol hybrids with antitumor activities was patented, 244 which had two different general structures ( 150a and 150b ), as shown in Fig. 146 .
In 2018, the method for the synthesis of a library of selenium-containing coumarin derivatives ( 151 ) was patented. 245 The general structure of the compounds is presented in Fig. 147 . These compounds were proposed to prevent primary cancer.
In 2019, a patent was filed for the use of terpenic coumarin derivatives as potential anticancer agents with high selectivity and low side effects. 246 The general structures of two types of these derivatives are shown in Fig. 148 .
In 2020, a patent was filed for several novel 4-phenyl-coumarin derivatives. 247 The compounds were prepared and used as specific mitochondrial RNA polymerase inhibitors for cancer treatment. The general structure of compound 153 is presented in Fig. 149 .
In 2022, the preparation of some coumarin derivatives ( 154 ) used for their anticancer and antioxidant properties was patented. 248 The general structure of the compounds is shown in Fig. 150 .
4. Conclusion
The above-mentioned studies also revealed that coumarin azole derivatives bind directly to DNA, which can lead to further improvement in oxidative stress. Coumarin-metal hybrids will also flourish to synthesize new drugs.
We hope, in the future, that the problems of side effects and toxicity regarding the coumarin scaffold will be overcome and coumarin-based anticancer drugs will be available in the market to fight against cancer and help cost-effectively eradicate cancer.
Conflicts of interest
- World Health Organization, Cancer , https://www.who.int/news-room/fact-sheets/detail/cancer Search PubMed .
- R. L. Siegel, K. D. Miller, H. E. Fuchs and A. Jemal, Ca-Cancer J. Clin. , 2022, 72 , 7–33 CrossRef .
- D. C. G. A. Pinto and A. M. S. Silva, Curr. Top. Med. Chem. , 2017, 17 , 3190–3198 CAS .
- M.-B. Nayeli, H.-R. Maribel, J.-F. Enrique, B.-P. Rafael, A.-F. Margarita, F.-M. Macrina, M.-D. Ivan and G.-C. Manasés, Nat. Prod. Res. , 2020, 34 , 3244–3248 CrossRef CAS PubMed .
- C. Mu, M. Wu and Z. Li, Chem. Biodiversity , 2019, 16 , 1800559 CrossRef .
- Y. Hu, Y. Shen, X. Wu, X. Tu and G.-X. Wang, Eur. J. Med. Chem. , 2018, 143 , 958–969 CrossRef CAS PubMed .
- K. Bhagat, J. Bhagat, M. K. Gupta, J. V. Singh, H. K. Gulati, A. Singh, K. Kaur, G. Kaur, S. Sharma, A. Rana, H. Singh, S. Sharma and P. M. Singh Bedi, ACS Omega , 2019, 4 , 8720–8730 CrossRef CAS .
- A. Singh, J. V. Singh, A. Rana, K. Bhagat, H. K. Gulati, R. Kumar, R. Salwan, K. Bhagat, G. Kaur, N. Singh, R. Kumar, H. Singh, S. Sharma and P. M. S. Bedi, ACS Omega , 2019, 4 , 11673–11684 CrossRef CAS PubMed .
- B. M. Chougala, S. Samundeeswari, M. Holiyachi, N. S. Naik, L. A. Shastri, S. Dodamani, S. Jalalpure, S. R. Dixit, S. D. Joshi and V. A. Sunagar, Eur. J. Med. Chem. , 2018, 143 , 1744–1756 CrossRef CAS PubMed .
- M. Z. Hassan, H. Osman, M. A. Ali and M. J. Ahsan, Eur. J. Med. Chem. , 2016, 123 , 236–255 CrossRef CAS .
- Y. Al-Majedy, A. Al-Amiery, A. A. Kadhum and A. BakarMohamad, Syst. Rev. Pharm. , 2016, 8 , 24–30 CrossRef .
- Y. Al-Majedy, D. Al-Duhaidahawi, K. Al-Azawi, A. Al-Amiery, A. Kadhum and A. Mohamad, Molecules , 2016, 21 , 135 CrossRef PubMed .
- R. Nagamallu, B. Srinivasan, M. B. Ningappa and A. K. Kariyappa, Bioorg. Med. Chem. Lett. , 2016, 26 , 690–694 CrossRef CAS PubMed .
- K. Kasperkiewicz, M. B. Ponczek and E. Budzisz, Pharmacol. Rep. , 2018, 70 , 1057–1064 CrossRef CAS PubMed .
- S.-S. Xie, J.-S. Lan, X. Wang, Z.-M. Wang, N. Jiang, F. Li, J.-J. Wu, J. Wang and L.-Y. Kong, Bioorg. Med. Chem. , 2016, 24 , 1528–1539 CrossRef CAS PubMed .
- N. Jiang, Q. Huang, J. Liu, N. Liang, Q. Li, Q. Li and S.-S. Xie, Eur. J. Med. Chem. , 2018, 146 , 287–298 CrossRef CAS PubMed .
- L. Zhang and Z. Xu, Eur. J. Med. Chem. , 2019, 181 , 111587 CrossRef CAS PubMed .
- X. Song, J. Fan, L. Liu, X. Liu and F. Gao, Arch. Pharm. , 2020, 353 , e2000025 CrossRef PubMed .
- L. Huang, Z.-L. Feng, Y.-T. Wang and L.-G. Lin, Chin. J. Nat. Med. , 2017, 15 , 881–888 CAS .
- J. C. J. M. D. S. Menezes and M. F. Diederich, Eur. J. Med. Chem. , 2019, 182 , 111637 CrossRef CAS PubMed .
- Q. Ma, J.-G. Jiang, X. Yuan, K. Qiu and W. Zhu, Food Chem. Toxicol. , 2019, 125 , 422–429 CrossRef CAS PubMed .
- T. Kubrak, M. Czop, P. Kołodziej, M. Ziaja-Sołtys, J. Bogucki, A. Makuch-Kocka, D. Aebisher, J. Kocki and A. Bogucka-Kocka, Molecules , 2019, 24 , 1824 CrossRef CAS .
- H. Singh, J. V. Singh, K. Bhagat, H. K. Gulati, M. Sanduja, N. Kumar, N. Kinarivala and S. Sharma, Bioorg. Med. Chem. , 2019, 27 , 3477–3510 CrossRef CAS PubMed .
- A. Rawat and A. Vijaya Bhaskar Reddy, Eur. J. Med. Chem. Rep. , 2022, 5 , 100038 CAS .
- R. Bhatia, A. Singh, B. Kumar and R. K. Rawal, in Key Heterocyclic Cores for Smart Anticancer Drug–Design Part II , Bentham Science Publishers, 2022, pp. 35–78 Search PubMed .
- W. C. Galeano, H. R. Malule and D. G. Ríos, J. Appl. Pharm. Sci. , 2023, 13 , 204–212 Search PubMed .
- T. Al-Warhi, A. Sabt, E. B. Elkaeed and W. M. Eldehna, Bioorg. Chem. , 2020, 103 , 104163 CrossRef CAS PubMed .
- Y. Tu, Angew. Chem., Int. Ed. , 2016, 55 , 10210–10226 CrossRef CAS PubMed .
- X. Liu, J. Cao, G. Huang, Q. Zhao and J. Shen, Curr. Top. Med. Chem. , 2019, 19 , 205–222 CrossRef CAS PubMed .
- B. Zhang, Arch. Pharm. , 2020, 353 (2) DOI: 10.1002/ardp.201900240 .
- X. Zhang, Q. Ba, Z. Gu, D. Guo, Y. Zhou, Y. Xu, H. Wang, D. Ye and H. Liu, Chem. – Eur. J. , 2015, 21 , 17415–17421 CrossRef CAS .
- Y. Tian, Z. Liang, H. Xu, Y. Mou and C. Guo, Molecules , 2016, 21 , 758 CrossRef .
- H. Yu, Z. Hou, Y. Tian, Y. Mou and C. Guo, Eur. J. Med. Chem. , 2018, 151 , 434–449 CrossRef CAS .
- D. Bauer, S. M. Sarrett, J. S. Lewis and B. M. Zeglis, Nat. Protoc. , 2023, 18 , 1659–1668 CrossRef CAS PubMed .
- A. Makarem, M. K. Sarvestani, K. D. Klika and K. Kopka, Synlett , 2019, 30 , 1795–1798 CrossRef CAS .
- A. Makarem, K. D. Klika, G. Litau, Y. Remde and K. Kopka, J. Org. Chem. , 2019, 84 , 7501–7508 CrossRef CAS PubMed .
- K. D. Klika, R. Alsalim, M. Eftekhari and A. Makarem, Dalton Trans. , 2022, 51 , 12436–12441 RSC .
- H. Yu, Z. Hou, X. Yang, Y. Mou and C. Guo, Molecules , 2019, 24 , 1672 CrossRef CAS PubMed .
- D. Rani, V. Garg and R. Dutt, Anti-Cancer Agents Med. Chem. , 2021, 21 , 1957–1976 CrossRef CAS PubMed .
- B. Zhang, Eur. J. Med. Chem. , 2019, 168 , 357–372 CrossRef CAS PubMed .
- F. Gao, T. Wang, J. Xiao and G. Huang, Eur. J. Med. Chem. , 2019, 173 , 274–281 CrossRef CAS PubMed .
- D.-J. Fu, P. Li, B.-W. Wu, X.-X. Cui, C.-B. Zhao and S.-Y. Zhang, Eur. J. Med. Chem. , 2019, 165 , 309–322 CrossRef CAS .
- T. G. Kraljević, A. Harej, M. Sedić, S. K. Pavelić, V. Stepanić, D. Drenjančević, J. Talapko and S. Raić-Malić, Eur. J. Med. Chem. , 2016, 124 , 794–808 CrossRef PubMed .
- A. Bistrović, N. Stipaničev, T. Opačak-Bernardi, M. Jukić, S. Martinez, L. Glavaš-Obrovac and S. Raić-Malić, New J. Chem. , 2017, 41 , 7531–7543 RSC .
- R. An, Z. Hou, J.-T. Li, H.-N. Yu, Y.-H. Mou and C. Guo, Molecules , 2018, 23 , 2281 CrossRef PubMed .
- S. Dhawan, P. Awolade, P. Kisten, N. Cele, A. Pillay, S. Saha, M. Kaur, S. B. Jonnalagadda and P. Singh, Chem. Biodiversity , 2020, 17 DOI: 10.1002/cbdv.201900462 .
- I. Ansary, H. Roy, A. Das, D. Mitra and A. K. Bandyopadhyay, ChemistrySelect , 2019, 4 , 3486–3494 CrossRef CAS .
- P. J. Raj and D. Bahulayan, Tetrahedron Lett. , 2017, 58 , 2122–2126 CrossRef CAS .
- S. Chekir, M. Debbabi, A. Regazzetti, D. Dargère, O. Laprévote, H. Ben Jannet and R. Gharbi, Bioorg. Chem. , 2018, 80 , 189–194 CrossRef CAS PubMed .
- S. Sinha, A. P. Kumaran, D. Mishra and P. Paira, Bioorg. Med. Chem. Lett. , 2016, 26 , 5557–5561 CrossRef CAS PubMed .
- L. V. Augsten, G. Göethel, B. Gauer, M. Feiffer Charão, G. von Poser, R. F. S. Canto, M. D. Arbo, V. L. Eifler-Lima and S. C. Garcia, RSC Med. Chem. , 2023, 14 , 869–879 RSC .
- R. W. Adam and E. H. Zimam, J. Popul. Ther. Clin. Pharmacol. , 2023, 30 (9), 345–356 Search PubMed .
- P. Kaur and A. Chawla, Int. Res. J. Pharm. , 2017, 8 , 10–29 CrossRef CAS .
- R. Kaur, A. Ranjan Dwivedi, B. Kumar and V. Kumar, Anti-Cancer Agents Med. Chem. , 2016, 16 , 465–489 CrossRef CAS PubMed .
- M. K. Kathiravan, A. B. Salake, A. S. Chothe, P. B. Dudhe, R. P. Watode, M. S. Mukta and S. Gadhwe, Bioorg. Med. Chem. , 2012, 20 , 5678–5698 CrossRef CAS .
- J. Zhang, S. Wang, Y. Ba and Z. Xu, Eur. J. Med. Chem. , 2019, 174 , 1–8 CrossRef CAS PubMed .
- B. Kahveci, F. Yılmaz, E. Menteşe and S. Ülker, Arch. Pharm. , 2017, 350 , 1600369 CrossRef PubMed .
- S. S. Alghamdi, R. S. Suliman, K. Almutairi, K. Kahtani and D. Aljatli, Drug Des., Dev. Ther. , 2021, 15 , 3289–3312 CrossRef PubMed .
- H. Liu, Y. Wang, A. Sharma, R. Mao, N. Jiang, B. Dun and J.-X. She, Anti-Cancer Drugs , 2015, 26 , 667–677 CrossRef CAS PubMed .
- M. Holiyachi, S. L. Shastri, B. M. Chougala, L. A. Shastri, S. D. Joshi, S. R. Dixit, H. Nagarajaiah and V. A. Sunagar, ChemistrySelect , 2016, 1 , 4638–4644 CrossRef CAS .
- R. Goel, V. Luxami and K. Paul, RSC Adv. , 2015, 5 , 37887–37895 RSC .
- K. V. Sashidhara, L. R. Singh, D. Choudhary, A. Arun, S. Gupta, S. Adhikary, G. R. Palnati, R. Konwar and R. Trivedi, RSC Adv. , 2016, 6 , 80037–80048 RSC .
- S. Imeni, A. Makarem and R. Javahershenas, Asian J. Org. Chem. , 2023, 12 DOI: 10.1002/ajoc.202300303 .
- R. Javahershenas and S. Nikzat, RSC Adv. , 2023, 13 , 16619–16629 RSC .
- R. M. Mohareb, A. E. M. Abdallah and A. A. Mohamed, Chem. Pharm. Bull. , 2018, 66 , 309–318 CrossRef CAS PubMed .
- R. M. Mohareb and M. S. Gamaan, Bull. Chem. Soc. Ethiop. , 2018, 32 , 541 CrossRef .
- T. Meng, Q.-P. Qin, Z.-R. Wang, L.-T. Peng, H.-H. Zou, Z.-Y. Gan, M.-X. Tan, K. Wang and F.-P. Liang, J. Inorg. Biochem. , 2018, 189 , 143–150 CrossRef CAS PubMed .
- Q.-P. Qin, S.-L. Wang, M.-X. Tan, Z.-F. Wang, X.-L. Huang, Q.-M. Wei, B.-B. Shi, B.-Q. Zou and H. Liang, Metallomics , 2018, 10 , 1160–1169 CrossRef CAS PubMed .
- Q.-P. Qin, Z.-F. Wang, X.-L. Huang, M.-X. Tan, B.-B. Shi and H. Liang, ACS Med. Chem. Lett. , 2019, 10 , 936–940 CrossRef CAS .
- X.-Q. Wang, X.-B. Chen, P.-T. Ye, Z.-X. Yang, M.-J. Bai, S.-Y. Duan, Y. Li and X.-D. Yang, Bioorg. Med. Chem. Lett. , 2020, 30 , 126896 CrossRef CAS PubMed .
- M. O. Karataş, S. Tekin, B. Alici and S. Sandal, J. Chem. Sci. , 2019, 131 , 69 CrossRef .
- G. Achar, C. R. Shahini, S. A. Patil, J. G. Małecki, S.-H. Pan, A. Lan, X.-R. Chen and S. Budagumpi, J. Inorg. Biochem. , 2018, 183 , 43–57 CrossRef CAS PubMed .
- K. R. A. Abdellatif, M. A. Abdelgawad, H. A. H. Elshemy, N. M. Kahk and D. M. El Amir, Lett. Drug Des. Discovery , 2017, 14 , 773–781 CAS .
- H. Dai, M. Huang, J. Qian, J. Liu, C. Meng, Y. Li, G. Ming, T. Zhang, S. Wang, Y. Shi, Y. Yao, S. Ge, Y. Zhang and Y. Ling, Eur. J. Med. Chem. , 2019, 166 , 470–479 CrossRef CAS PubMed .
- K. Vaarla, R. K. Kesharwani, K. Santosh, R. R. Vedula, S. Kotamraju and M. K. Toopurani, Bioorg. Med. Chem. Lett. , 2015, 25 , 5797–5803 CrossRef CAS PubMed .
- K. Vaarla, S. Karnewar, D. Panuganti, S. R. Peddi, R. R. Vedula, V. Manga and S. Kotamraju, ChemistrySelect , 2019, 4 , 4324–4330 CrossRef CAS .
- S. Dhawan, N. Kerru, P. Awolade, A. Singh-Pillay, S. T. Saha, M. Kaur, S. B. Jonnalagadda and P. Singh, Bioorg. Med. Chem. , 2018, 26 , 5612–5623 CrossRef CAS PubMed .
- P. Adumeau, M. Davydova and B. M. Zeglis, Bioconjugate Chem. , 2018, 29 , 1364–1372 CrossRef CAS PubMed .
- K. D. Klika, C. Da Pieve, K. Kopka, G. Smith and A. Makarem, Org. Biomol. Chem. , 2021, 19 , 1722–1726 RSC .
- S. G. Narella, M. G. Shaik, A. Mohammed, M. Alvala, A. Angeli and C. T. Supuran, Bioorg. Chem. , 2019, 87 , 765–772 CrossRef CAS .
- B. Akhlaghinia and A. Makarem, J. Sulfur Chem. , 2011, 32 , 575–581 CrossRef CAS .
- M. K. Kumawat, Curr. Drug Discovery Technol. , 2018, 15 , 196–200 CrossRef CAS PubMed .
- Y. Li, J. Geng, Y. Liu, S. Yu and G. Zhao, ChemMedChem , 2013, 8 , 27–41 CrossRef CAS .
- P. K. N. Sarangi, J. Sahoo, B. D. Swain, S. K. Paidesetty and G. P. Mohanta, Indian Drugs , 2016, 53 , 5–11 Search PubMed .
- A. Aliabadi, Anti-Cancer Agents Med. Chem. , 2016, 16 , 1301–1314 CrossRef CAS PubMed .
- İ. Koca, M. Gumuş, A. Ozgur, A. Disli and Y. Tutar, Anti-Cancer Agents Med. Chem. , 2015, 15 , 916–930 CrossRef PubMed .
- A. Ayati, T. Oghabi Bakhshaiesh, S. Moghimi, R. Esmaeili, K. Majidzadeh-A, M. Safavi, L. Firoozpour, S. Emami and A. Foroumadi, Eur. J. Med. Chem. , 2018, 155 , 483–491 CrossRef CAS .
- T. K. Mohamed, R. Z. Batran, S. A. Elseginy, M. M. Ali and A. E. Mahmoud, Bioorg. Chem. , 2019, 85 , 253–273 CrossRef CAS PubMed .
- S. S. Abd El-Karim, Y. M. Syam, A. M. El Kerdawy and T. M. Abdelghany, Bioorg. Chem. , 2019, 86 , 80–96 CrossRef CAS PubMed .
- R. Velpula, R. Deshineni, R. Gali and R. Bavantula, Res. Chem. Intermed. , 2016, 42 , 1729–1740 CrossRef CAS .
- M. T. Gabr, Heterocycl. Commun. , 2018, 24 , 243–247 CrossRef CAS .
- S. Mah, J. Jang, D. Song, Y. Shin, M. Latif, Y. Jung and S. Hong, Org. Biomol. Chem. , 2019, 17 , 186–194 RSC .
- L. H. Al-Wahaibi, H. M. Abu-Melha and D. A. Ibrahim, J. Chem. , 2018, 2018 , 1–8 CrossRef .
- K. M. Amin, A. M. Taha, R. F. George, N. M. Mohamed and F. F. Elsenduny, Arch. Pharm. , 2018, 351 , 1700199 CrossRef PubMed .
- K. Venkata Sairam, B. M. Gurupadayya, B. I. Vishwanathan, R. S. Chandan and D. K. Nagesha, RSC Adv. , 2016, 6 , 98816–98828 RSC .
- S. K. J. Shaikh, M. S. Sannaikar, M. N. Kumbar, P. K. Bayannavar, R. R. Kamble, S. R. Inamdar and S. D. Joshi, ChemistrySelect , 2018, 3 , 4448–4462 CrossRef CAS .
- M. T. Gabr, N. S. El-Gohary, E. R. El-Bendary, M. M. El-Kerdawy and N. Ni, EXCLI J. , 2017, 16 , 1114–1131 Search PubMed .
- F. Hersi, H. A. Omar, R. A. Al-Qawasmeh, Z. Ahmad, A. M. Jaber, D. M. Zaher and T. H. Al-Tel, Sci. Rep. , 2020, 10 , 2893 CrossRef CAS PubMed .
- S. G. Mane, K. S. Katagi, N. S. Kadam, M. C. Akki and S. D. Joshi, Polycyclic Aromat. Compd. , 2022, 42 , 371–390 CrossRef CAS .
- T. Z. Abolibda, M. Fathalla, B. Farag, M. E. A. Zaki and S. M. Gomha, Molecules , 2023, 28 , 689 CrossRef CAS PubMed .
- G. S. Lingaraju, K. S. Balaji, S. Jayarama, S. M. Anil, K. R. Kiran and M. P. Sadashiva, Bioorg. Med. Chem. Lett. , 2018, 28 , 3606–3612 CrossRef CAS PubMed .
- L.-M. Jia, S.-L. Huang, W.-G. Pan, Y.-H. Huang and P. Luo, J. Mol. Struct. , 2023, 1290 , 135892 CrossRef CAS .
- M. B. Palkar, S. S. Jalalpure, R. A. Rane, H. M. Patel, M. S. Shaikh, G. A. Hampannavar, W. S. Alwan, G. S. Bolakatti and R. Karpoormath, Anti-Cancer Agents Med. Chem. , 2015, 15 , 970–979 CrossRef CAS PubMed .
- R. R. Manda, R. V. Nadh, T. L. Viveka, G. Angajala and V. Aruna, J. Mol. Struct. , 2023, 1285 , 135453 CrossRef CAS .
- M.-M. Liu, X.-Y. Chen, Y.-Q. Huang, P. Feng, Y.-L. Guo, G. Yang and Y. Chen, J. Med. Chem. , 2014, 57 , 9343–9356 CrossRef CAS PubMed .
- Q. Wang, Y. Guo, S. Jiang, M. Dong, K. Kuerban, J. Li, M. Feng, Y. Chen and L. Ye, Phytomedicine , 2018, 39 , 160–167 CrossRef CAS PubMed .
- Y. Guo, Y. Wang, H. Li, K. Wang, Q. Wan, J. Li, Y. Zhou and Y. Chen, ACS Med. Chem. Lett. , 2018, 9 , 502–506 CrossRef CAS PubMed .
- J. He, Z. Li, G. Dhawan, W. Zhang, A. E. Sorochinsky, G. Butler, V. A. Soloshonok and J. Han, Chin. Chem. Lett. , 2023, 34 , 107578 CrossRef CAS .
- Y. Yu, A. Liu, G. Dhawan, H. Mei, W. Zhang, K. Izawa, V. A. Soloshonok and J. Han, Chin. Chem. Lett. , 2021, 32 , 3342–3354 CrossRef CAS .
- H. Mei, A. M. Remete, Y. Zou, H. Moriwaki, S. Fustero, L. Kiss, V. A. Soloshonok and J. Han, Chin. Chem. Lett. , 2020, 31 , 2401–2413 CrossRef CAS .
- Q. Wang, J. Han, A. Sorochinsky, A. Landa, G. Butler and V. A. Soloshonok, Pharmaceuticals , 2022, 15 , 999 CrossRef CAS PubMed .
- J. Han, L. Kiss, H. Mei, A. M. Remete, M. Ponikvar-Svet, D. M. Sedgwick, R. Roman, S. Fustero, H. Moriwaki and V. A. Soloshonok, Chem. Rev. , 2021, 121 , 4678–4742 CrossRef CAS PubMed .
- J. Han, A. M. Remete, L. S. Dobson, L. Kiss, K. Izawa, H. Moriwaki, V. A. Soloshonok and D. O'Hagan, J. Fluorine Chem. , 2020, 239 , 109639 CrossRef CAS .
- H. Mei, J. Han, S. White, D. J. Graham, K. Izawa, T. Sato, S. Fustero, N. A. Meanwell and V. A. Soloshonok, Chem. – Eur. J. , 2020, 26 , 11349–11390 CrossRef CAS PubMed .
- C. Da Pieve, A. Makarem, S. Turnock, J. Maczynska, G. Smith and G. Kramer-Marek, Molecules , 2020, 25 , 1562 CrossRef CAS PubMed .
- Z. Zhang, Z.-W. Bai, Y. Ling, L.-Q. He, P. Huang, H.-X. Gu and R.-F. Hu, Med. Chem. Res. , 2018, 27 , 1198–1205 CrossRef CAS .
- G. L. Nelson, C. T. Ronayne, L. N. Solano, S. K. Jonnalagadda, S. Jonnalagadda, T. J. Schumacher, Z. S. Gardner, H. Palle, C. Mani, J. Rumbley and V. R. Mereddy, Bioorg. Med. Chem. Lett. , 2021, 52 , 128411 CrossRef CAS PubMed .
- P. Mutai, G. Breuzard, A. Pagano, D. Allegro, V. Peyrot and K. Chibale, Bioorg. Med. Chem. , 2017, 25 , 1652–1665 CrossRef CAS PubMed .
- C.-C. Ma and Z.-P. Liu, Anti-Cancer Agents Med. Chem. , 2017, 17 , 395–403 CrossRef CAS PubMed .
- S. D. Durgapal, R. Soni, S. Umar, B. Suresh and S. S. Soman, Chem. Biol. Drug Des. , 2018, 92 , 1279–1287 CrossRef CAS PubMed .
- K. Ostrowska, D. Grzeszczuk, D. Maciejewska, I. Młynarczuk-Biały, A. Czajkowska, A. Sztokfisz, Ł. Dobrzycki and H. Kruszewska, Monatsh. Chem. , 2016, 147 , 1615–1627 CrossRef CAS .
- S. D. Naik, K. M. Hosamani and S. K. Vootla, Chem. Data Collect. , 2018, 15–16 , 207–222 CrossRef .
- A. A. Fadda, K. S. Mohamed, H. M. Refat and E. E. El-Bialy, Heterocycles , 2015, 91 , 134 CrossRef CAS .
- R. M. Mohareb and N. Y. Megally Abdo, Chem. Pharm. Bull. , 2015, 63 , 678–687 CrossRef CAS PubMed .
- B. M. Chougala, S. S, M. Holiyachi, N. S. Naik, L. A. Shastri, S. Dodamani, S. Jalalpure, S. R. Dixit, S. D. Joshi and V. A. Sunagar, ChemistrySelect , 2017, 2 , 5234–5242 CrossRef CAS .
- A. M. El-Naggar, M. M. Hemdan and S. R. Atta-Allah, J. Heterocycl. Chem. , 2017, 54 , 3519–3526 CrossRef CAS .
- E. A. Fayed, R. Sabour, M. F. Harras and A. B. M. Mehany, Med. Chem. Res. , 2019, 28 , 1284–1297 CrossRef CAS .
- T. U. Kumar, Y. Bobde, S. Pulya, K. Rangan, B. Ghosh and A. Bhattacharya, ChemistrySelect , 2019, 4 , 10726–10730 CrossRef CAS .
- K. M. Hosamani, D. S. Reddy and H. C. Devarajegowda, RSC Adv. , 2015, 5 , 11261–11271 RSC .
- S. A. Morsy, A. A. Farahat, M. N. A. Nasr and A. S. Tantawy, Saudi Pharm. J. , 2017, 25 , 873–883 CrossRef PubMed .
- N. Lv, M. Sun, C. Liu and J. Li, Bioorg. Med. Chem. Lett. , 2017, 27 , 4578–4581 CrossRef CAS PubMed .
- D. S. Reddy, M. Kongot, V. Singh, M. A. Siddiquee, R. Patel, N. K. Singhal, F. Avecilla and A. Kumar, Arch. Pharm. , 2021, 354 DOI: 10.1002/ardp.202000181 .
- E. Ortega-Forte, A. Rovira, A. Gandioso, J. Bonelli, M. Bosch, J. Ruiz and V. Marchán, J. Med. Chem. , 2021, 64 , 17209–17220 CrossRef CAS PubMed .
- N. Y. Kim, D. Vishwanath, Z. Xi, O. Nagaraja, A. Swamynayaka, K. Kumar Harish, S. Basappa, M. Madegowda, V. Pandey, G. Sethi, P. E. Lobie, K. S. Ahn and B. Basappa, Molecules , 2023, 28 , 3450 CrossRef CAS PubMed .
- Y. Jin, S. He, F. Wu, C. Luo, J. Ma and Y. Hu, Eur. J. Pharm. Sci. , 2023, 188 , 106520 CrossRef CAS PubMed .
- A. Kumari and R. K. Singh, Bioorg. Chem. , 2019, 89 , 103021 CrossRef CAS PubMed .
- A. Kumari and R. K. Singh, Bioorg. Chem. , 2020, 96 , 103578 CrossRef CAS PubMed .
- H. Guo, Eur. J. Med. Chem. , 2019, 164 , 678–688 CrossRef CAS PubMed .
- Z. Xu, S. Zhang, C. Gao, J. Fan, F. Zhao, Z.-S. Lv and L.-S. Feng, Chin. Chem. Lett. , 2017, 28 , 159–167 CrossRef CAS .
- S. A. Patil, R. Patil and D. D. Miller, Future Med. Chem. , 2012, 4 , 2085–2115 CrossRef CAS .
- R. Gali, J. Banothu, R. Gondru, R. Bavantula, Y. Velivela and P. A. Crooks, Bioorg. Med. Chem. Lett. , 2015, 25 , 106–112 CrossRef CAS PubMed .
- O. Galayev, Y. Garazd, M. Garazd and R. Lesyk, Eur. J. Med. Chem. , 2015, 105 , 171–181 CrossRef CAS .
- P. R. Kamath, D. Sunil, A. A. Ajees, K. S. R. Pai and S. Das, Bioorg. Chem. , 2015, 63 , 101–109 CrossRef CAS PubMed .
- P. R. Kamath, D. Sunil, A. A. Ajees, K. S. R. Pai and S. Biswas, Eur. J. Med. Chem. , 2016, 120 , 134–147 CrossRef CAS PubMed .
- R. Pathoor and D. Bahulayan, New J. Chem. , 2018, 42 , 6810–6816 RSC .
- P. R. Kamath, D. Sunil, M. M. Joseph, A. A. Abdul Salam and S. T.T., Eur. J. Med. Chem. , 2017, 136 , 442–451 CrossRef CAS PubMed .
- S. Samundeeswari, M. V. Kulkarni, S. D. Joshi, S. R. Dixit, S. Jayakumar and R. M. Ezhilarasi, ChemistrySelect , 2016, 1 , 5019–5024 CrossRef .
- Y. Fan, Z. Huang and M. Liu, J. Heterocycl. Chem. , 2018, 55 , 2722–2726 CrossRef CAS .
- Z. Xu, S.-J. Zhao, J.-L. Deng, Q. Wang, Z.-S. Lv and Y.-L. Fan, J. Heterocycl. Chem. , 2019, 56 , 400–405 CrossRef CAS .
- Q. Diao, H. Guo and G. Wang, J. Heterocycl. Chem. , 2019, 56 , 1667–1671 CrossRef CAS .
- Z. Xu, S. Zhao, Z. Lv, F. Gao, Y. Wang, F. Zhang, L. Bai, J. Deng, Q. Wang and Y. Fan, J. Heterocycl. Chem. , 2019, 56 , 1127–1132 CrossRef CAS .
- V. Singh, A. Dogra, J. Das, P. Manna and N. Gupta, FlatChem , 2021, 29 , 100279 CrossRef CAS .
- J. Song, Y.-F. Guan, W.-B. Liu, C.-H. Song, X.-Y. Tian, T. Zhu, X.-J. Fu, Y.-Q. Qi and S.-Y. Zhang, Eur. J. Med. Chem. , 2022, 238 , 14467 CrossRef PubMed .
- V. R. Yadav, S. Prasad, B. Sung and B. B. Aggarwal, Int. Immunopharmacol. , 2011, 11 , 295–309 CrossRef CAS PubMed .
- M. Pilatova, L. Varinska, P. Perjesi, M. Sarissky, L. Mirossay, P. Solar, A. Ostro and J. Mojzis, Toxicol. In Vitro , 2010, 24 , 1347–1355 CrossRef CAS PubMed .
- Y. Luo, R. Song, Y. Li, S. Zhang, Z.-J. Liu, J. Fu and H.-L. Zhu, Bioorg. Med. Chem. Lett. , 2012, 22 , 3039–3043 CrossRef CAS PubMed .
- A. Kamal, S. Prabhakar, M. Janaki Ramaiah, P. Venkat Reddy, C. Ratna Reddy, A. Mallareddy, N. Shankaraiah, T. Lakshmi Narayan Reddy, S. N. C. V. L. Pushpavalli and M. Pal-Bhadra, Eur. J. Med. Chem. , 2011, 46 , 3820–3831 CrossRef CAS PubMed .
- U. M. Kocyigit, Y. Budak, F. Eligüzel, P. Taslimi, D. Kılıç, İ. Gulçin and M. Ceylan, Arch. Pharm. , 2017, 350 , 1700198 CrossRef PubMed .
- U. M. Kocyigit, Y. Budak, M. B. Gürdere, F. Ertürk, B. Yencilek, P. Taslimi, İ. Gülçin and M. Ceylan, Arch. Physiol. Biochem. , 2018, 124 , 61–68 CrossRef CAS PubMed .
- S. Burmaoglu, A. O. Yilmaz, M. F. Polat, R. Kaya, İ. Gulcin and O. Algul, Bioorg. Chem. , 2019, 85 , 191–197 CrossRef CAS PubMed .
- S. N. Mokale, A. Begum, N. S. Sakle, V. R. Shelke and S. A. Bhavale, Biomed. Pharmacother. , 2017, 89 , 966–972 CrossRef CAS PubMed .
- H. A. H. Elshemy and M. A. Zaki, Bioorg. Med. Chem. , 2017, 25 , 1066–1075 CrossRef CAS PubMed .
- H. Suwito, H. Hardiyanti, K. Ul Haq, A. Kristanti and M. Khasanah, Mol. Ther. , 2018, 2018 , M1027 Search PubMed .
- H. A. El-Sherief, G. E.-D. A. Abuo-Rahma, M. E. Shoman, E. A. Beshr and R. M. Abdel-baky, Med. Chem. Res. , 2017, 26 , 3077–3090 CrossRef CAS .
- R. Ashraf, Hamidullah, M. Hasanain, P. Pandey, M. Maheshwari, L. R. Singh, M. Q. Siddiqui, R. Konwar, K. V. Sashidhara and J. Sarkar, Sci. Rep. , 2017, 7 , 45287 CrossRef CAS PubMed .
- B. Z. Kurt, N. Ozten Kandas, A. Dag, F. Sonmez and M. Kucukislamoglu, Arabian J. Chem. , 2020, 13 , 1120–1129 CrossRef CAS .
- Y. Wang, W. Zhang, J. Dong and J. Gao, Bioorg. Chem. , 2020, 95 , 103530 CrossRef CAS PubMed .
- K. Sathish Kumar, V. Kotra, P. K. Kola, CH. B. Praveena Devi, N. Anusha, B. Hari Babu, S. F. Adil, M. R. Shaik, M. Khan, A. Al-Warthan, O. Alduhaish and M. Mujahid Alam, Saudi J. Biol. Sci. , 2021, 28 , 386–394 CrossRef CAS PubMed .
- Y.-H. Chan, G.-Y. Yeap, Shanmugapriya and S. Sasidharan, Tetrahedron , 2022, 103 , 132568 CrossRef CAS .
- M. A. Motaleb and A. A. Selim, Bioorg. Chem. , 2019, 82 , 145–155 CrossRef CAS PubMed .
- I. Yahaya, M. Chemchem, B. Aydıner, N. Seferoğlu, F. Erva Tepe, L. Açık, N. Aytuna Çerçi, M. Türk and Z. Seferoğlu, J. Photochem. Photobiol., A , 2019, 368 , 296–306 CrossRef CAS .
- V. T. Angelova, N. G. Vassilev, B. Nikolova-Mladenova, J. Vitas, R. Malbaša, G. Momekov, M. Djukic and L. Saso, Med. Chem. Res. , 2016, 25 , 2082–2092 CrossRef CAS .
- X.-Y. Lu, Z.-C. Wang, S.-Z. Ren, F.-Q. Shen, R.-J. Man and H.-L. Zhu, Bioorg. Med. Chem. Lett. , 2016, 26 , 3491–3498 CrossRef CAS PubMed .
- F.-Q. Shen, Z.-C. Wang, S.-Y. Wu, S.-Z. Ren, R.-J. Man, B.-Z. Wang and H.-L. Zhu, Bioorg. Med. Chem. Lett. , 2017, 27 , 3653–3660 CrossRef CAS PubMed .
- A. Sabt, O. M. Abdelhafez, R. S. El-Haggar, H. M. F. Madkour, W. M. Eldehna, E. E.-D. A. M. El-Khrisy, M. A. Abdel-Rahman and L. A. Rashed, J. Enzyme Inhib. Med. Chem. , 2018, 33 , 1095–1107 CrossRef CAS PubMed .
- K. F. Debbabi, S. A. Al-Harbi, H. M. Al-Saidi, E. H. Aljuhani, S. M. Abd El-Gilil and M. S. Bashandy, J. Enzyme Inhib. Med. Chem. , 2016, 31 , 7–19 CrossRef CAS PubMed .
- Z. Zhang, L. Gu, B. Wang, W. Huang, Y. Zhang, Z. Ma, S. Zeng and Z. Shen, J. Enzyme Inhib. Med. Chem. , 2019, 34 , 808–817 CrossRef CAS PubMed .
- M. Daśko, M. Przybyłowska, J. Rachon, M. Masłyk, K. Kubiński, M. Misiak, A. Składanowski and S. Demkowicz, Eur. J. Med. Chem. , 2017, 128 , 79–87 CrossRef PubMed .
- D. Ganeshapillai, L. W. L. Woo, M. P. Thomas, A. Purohit and B. V. L. Potter, ACS Omega , 2018, 3 , 10748–10772 CrossRef CAS PubMed .
- G. Cai, W. Yu, D. Song, W. Zhang, J. Guo, J. Zhu, Y. Ren and L. Kong, Eur. J. Med. Chem. , 2019, 174 , 236–251 CrossRef CAS PubMed .
- M. I. El-Gamal, D. Baek and C.-H. Oh, Bull. Korean Chem. Soc. , 2016, 37 , 184–191 CrossRef CAS .
- M. Mahapatra, P. Mohapatra, S. K. Sahoo, A. K. Bishoyi, R. N. Padhy and S. K. Paidesetty, J. Mol. Struct. , 2023, 1283 , 135190 CrossRef CAS .
- L. Yang, Z. Hu, J. Luo, C. Tang, S. Zhang, W. Ning, C. Dong, J. Huang, X. Liu and H.-B. Zhou, Bioorg. Med. Chem. , 2017, 25 , 3531–3539 CrossRef CAS PubMed .
- H.-W. Han, C.-S. Zheng, S.-J. Chu, W.-X. Sun, L.-J. Han, R.-W. Yang, J.-L. Qi, G.-H. Lu, X.-M. Wang and Y.-H. Yang, Biomed. Pharmacother. , 2018, 97 , 656–666 CrossRef CAS PubMed .
- H. Han, C. Li, Z.-F. Zhang and G.-F. An, Main Group Chem. , 2019, 18 , 193–201 CAS .
- H. Han, Z.-F. Zhang, J.-F. Zhang and B. Zhang, Main Group Chem. , 2019, 18 , 71–79 CAS .
- P. Govindaiah, N. Dumala, P. Grover and M. Jaya Prakash, Bioorg. Med. Chem. Lett. , 2019, 29 , 1819–1824 CrossRef CAS PubMed .
- D. Cao, Y. Liu, W. Yan, C. Wang, P. Bai, T. Wang, M. Tang, X. Wang, Z. Yang, B. Ma, L. Ma, L. Lei, F. Wang, B. Xu, Y. Zhou, T. Yang and L. Chen, J. Med. Chem. , 2016, 59 , 5721–5739 CrossRef CAS PubMed .
- G. Luo, M. Chen, W. Lyu, R. Zhao, Q. Xu, Q. You and H. Xiang, Bioorg. Med. Chem. Lett. , 2017, 27 , 2668–2673 CrossRef CAS PubMed .
- A. V. Lipeeva, D. O. Zakharov, Y. V. Gatilov, M. A. Pokrovskii, A. G. Pokrovskii and E. E. Shults, ChemistrySelect , 2019, 4 , 10197–10201 CrossRef CAS .
- G. Luo, M. Muyaba, W. Lyu, Z. Tang, R. Zhao, Q. Xu, Q. You and H. Xiang, Bioorg. Med. Chem. Lett. , 2017, 27 , 867–874 CrossRef CAS PubMed .
- R. Soni, S. Umar, N. N. Shah, S. Balkrishnan and S. S. Soman, J. Heterocycl. Chem. , 2017, 54 , 2501–2510 CrossRef CAS .
- E. Y. Ahmed, N. A. Abdel Latif, M. F. El-Mansy, W. S. Elserwy and O. M. Abdelhafez, Bioorg. Med. Chem. , 2020, 28 , 115328 CrossRef CAS PubMed .
- J. Li, D. Hu, X. Liang, Y.-C. Wang, H.-S. Wang and Y. Pan, J. Org. Chem. , 2017, 82 , 9006–9011 CrossRef CAS PubMed .
- R. Wang, H. Chen, W. Yan, M. Zheng, T. Zhang and Y. Zhang, Eur. J. Med. Chem. , 2020, 190 , 112109 CrossRef CAS PubMed .
- M. Mbaba, J.-A. de la Mare, J. N. Sterrenberg, D. Kajewole, S. Maharaj, A. L. Edkins, M. Isaacs, H. C. Hoppe and S. D. Khanye, JBIC, J. Biol. Inorg. Chem. , 2019, 24 , 139–149 CrossRef CAS PubMed .
- M. Mbaba, A. N. Mabhula, N. Boel, A. L. Edkins, M. Isaacs, H. C. Hoppe and S. D. Khanye, J. Inorg. Biochem. , 2017, 172 , 88–93 CrossRef CAS PubMed .
- J.-N. Wei, Z.-D. Jia, Y.-Q. Zhou, P.-H. Chen, B. Li, N. Zhang, X.-Q. Hao, Y. Xu and B. Zhang, J. Organomet. Chem. , 2019, 902 , 120968 CrossRef CAS .
- H. Wang, L. Hu, W. Du, X. Tian, Q. Zhang, Z. Hu, L. Luo, H. Zhou, J. Wu and Y. Tian, ACS Biomater. Sci. Eng. , 2017, 3 , 836–842 CrossRef CAS PubMed .
- Q.-P. Qin, Z.-F. Wang, X.-L. Huang, M.-X. Tan, B.-Q. Zou and H. Liang, Eur. J. Med. Chem. , 2019, 184 , 111751 CrossRef CAS PubMed .
- R.-R. Ye, C.-P. Tan, L.-N. Ji and Z.-W. Mao, Dalton Trans. , 2016, 45 , 13042–13051 RSC .
- I. Lagunes, P. Begines, A. Silva, A. R. Galán, A. Puerta, M. X. Fernandes, I. Maya, J. G. Fernández-Bolaños, Ó. López and J. M. Padrón, Eur. J. Med. Chem. , 2019, 179 , 493–501 CrossRef CAS PubMed .
- A. Trommenschlager, F. Chotard, B. Bertrand, S. Amor, P. Richard, A. Bettaïeb, C. Paul, J.-L. Connat, P. Le Gendre and E. Bodio, ChemMedChem , 2018, 13 , 2408–2414 CrossRef CAS PubMed .
- K. N. A. Rahman, J. Haribabu, C. Balachandran, N. S. P. Bhuvanesh, R. Karvembu and A. Sreekanth, Polyhedron , 2017, 135 , 26–35 CrossRef CAS .
- T. Zhu, Y. Wang, W. Ding, J. Xu, R. Chen, J. Xie, W. Zhu, L. Jia and T. Ma, Chem. Biol. Drug Des. , 2015, 85 , 385–393 CrossRef CAS PubMed .
- N. Sunitha, C. I. S. Raj and B. S. Kumari, J. Mol. Struct. , 2023, 1285 , 135443 CrossRef CAS .
- M. Sumi, N. T. Nevaditha and B. Sindhu Kumari, Bioorg. Chem. , 2023, 136 , 106555 CrossRef CAS PubMed .
- S. Balcıoğlu, M. Olgun Karataş, B. Ateş, B. Alıcı and İ. Özdemir, Bioorg. Med. Chem. Lett. , 2020, 30 , 126805 CrossRef PubMed .
- L. H. Ramdani, O. Talhi, C. Decombat, M. Vermerie, A. Berry, A. Silva, K. Bachari, M.-P. Vasson, L. Delort and F. Caldefie-Chézet, Anticancer Res. , 2019, 39 , 6107–6114 CrossRef CAS PubMed .
- T. Nasr, S. Bondock, H. M. Rashed, W. Fayad, M. Youns and T. M. Sakr, Eur. J. Med. Chem. , 2018, 151 , 723–739 CrossRef CAS PubMed .
- Z. Solárová, M. Kello, S. Hamuľaková, L. Mirossay and P. Solár, Acta Chim. Slov. , 2018, 65 , 875–881 CrossRef .
- T. Prashanth, B. R. V. Avin, P. Thirusangu, V. L. Ranganatha, B. T. Prabhakar, J. N. N. Sharath Chandra and S. A. Khanum, Biomed. Pharmacother. , 2019, 112 , 108707 CrossRef CAS PubMed .
- C. Zwergel, B. Czepukojc, E. Evain-Bana, Z. Xu, G. Stazi, M. Mori, A. Patsilinakos, A. Mai, B. Botta, R. Ragno, D. Bagrel, G. Kirsch, P. Meiser, C. Jacob, M. Montenarh and S. Valente, Eur. J. Med. Chem. , 2017, 134 , 316–333 CrossRef CAS PubMed .
- A. Herrera-R, T. W. Naranjo, M. E. Maldonado, G. Moreno-Q, A. Yepes and W. Cardona-G, Med. Chem. Res. , 2020, 29 , 377–395 CrossRef CAS .
- P. R. Kadam, Y. D. Bodke, M. B, I. Pushpavathi, N. D. Satyanarayan and B. N. Nippu, J. Mol. Struct. , 2023, 1278 , 134918 CrossRef CAS .
- Z. Tian, L. Shi, Z. Liu, H. Li, L. Yang and Y. Liu, J. Mol. Struct. , 2023, 1288 , 135826 CrossRef CAS .
- F. Yang, N. Zhao, J. Song, K. Zhu, C. Jiang, P. Shan and H. Zhang, Molecules , 2019, 24 , 2569 CrossRef CAS PubMed .
- M. Bu, H. Li, H. Wang, J. Wang, Y. Lin and Y. Ma, Molecules , 2019, 24 , 3307 CrossRef PubMed .
- J.-N. Yao, X.-X. Zhang, Y.-Z. Zhang, J.-H. Li, D.-Y. Zhao, B. Gao, H.-N. Zhou, S.-L. Gao and L.-F. Zhang, Invest. New Drugs , 2019, 37 , 1300–1308 CrossRef CAS PubMed .
- Ch. Pavan Kumar, A. Devi, P. Ashok Yadav, R. Rao Vadaparthi, G. Shankaraiah, P. Sowjanya, N. Jain and K. Suresh Babu, J. Asian Nat. Prod. Res. , 2016, 18 , 1063–1078 CrossRef CAS PubMed .
- H. Singh, M. Kumar, K. Nepali, M. K. Gupta, A. K. Saxena, S. Sharma and P. M. S. Bedi, Eur. J. Med. Chem. , 2016, 116 , 102–115 CrossRef CAS PubMed .
- B. M. Chougala, S. L. Shastri, M. Holiyachi, L. A. Shastri, S. S. More and K. V. Ramesh, Med. Chem. Res. , 2015, 24 , 4128–4138 CrossRef CAS .
- S. Koparde, K. M. Hosamani, D. A. Barretto and S. D. Joshi, Chem. Data Collect. , 2018, 15–16 , 41–53 CrossRef .
- M. Patel, N. Pandey, J. Timaniya, P. Parikh, A. Chauhan, N. Jain and K. Patel, RSC Adv. , 2021, 11 , 27627–27644 RSC .
- S. Vodnala, A. K. D. Bhavani, R. Kamutam, V. G. M. Naidu, Promila and C. Prabhakar, Bioorg Med Chem Lett , 2016, 26 , 3973–3977 CrossRef CAS PubMed .
- M. Bu, T. Cao, H. Li, M. Guo, B. B. Yang, C. Zeng and L. Hu, ChemMedChem , 2017, 12 , 466–474 CrossRef CAS PubMed .
- B. Yu, P.-P. Qi, X.-J. Shi, R. Huang, H. Guo, Y.-C. Zheng, D.-Q. Yu and H.-M. Liu, Eur. J. Med. Chem. , 2016, 117 , 241–255 CrossRef CAS PubMed .
- H. Zhu, L. Yu, J. Liu, M. Wang, T. Zhang and F. Qiu, Chin. Herb. Med. , 2019, 11 , 113–115 CrossRef .
- Z.-M. Feng, Z.-Z. Liu, K. Xu, Y.-N. Yang, J.-S. Jiang and P.-C. Zhang, ChemInform , 2016, 47 DOI: 10.1002/chin.201633189 .
- K. B. Pal, M. Mahanti, X. Huang, S. Persson, A. P. Sundin, F. R. Zetterberg, S. Oredsson, H. Leffler and U. J. Nilsson, Org. Biomol. Chem. , 2018, 16 , 6295–6305 RSC .
- E. S. Moustafa, N. F. Swilam, O. B. Ghanem, A. N. Hashim, M. A. Nawwar, U. Lindequist and M. W. Linscheid, Pharmazie , 2018, 73 , 241–243 CAS .
- L.-L. Lou, R. Guo, Z.-Y. Cheng, P. Zhao, G.-D. Yao, X.-B. Wang, X.-X. Huang and S.-J. Song, Phytochem. Lett. , 2018, 24 , 15–20 CrossRef CAS .
- A. Sharma, S. Gudala, S. R. Ambati, S. penta, Y. Bomma, V. R. Janapala, A. Jha and A. Kumar, J. Chin. Chem. Soc. , 2018, 65 , 810–821 CrossRef CAS .
- W. Kozak, M. Daśko, M. Masłyk, B. Gielniewski, J. Rachon and S. Demkowicz, J. Asian Nat. Prod. Res. , 2015, 17 , 1091–1096 CrossRef CAS PubMed .
- A. Ibrar, S. Zaib, F. Jabeen, J. Iqbal and A. Saeed, Arch. Pharm. , 2016, 349 , 553–565 CrossRef CAS PubMed .
- K. Wada, M. Goto, K.-H. Lee and H. Yamashita, Chem. Pharm. Bull. , 2023, 71 , 52–57 CrossRef CAS PubMed .
- E. F. Bruna-Haupt, M. D. Perretti, H. A. Garro, R. Carrillo, F. Machín, I. Lorenzo-Castrillejo, L. Gutiérrez, E. G. Vega-Hissi, M. Mamberto, M. Menacho-Marquez, C. O. Fernández, C. García and C. R. Pungitore, ACS Omega , 2023, 8 , 26479–26496 CrossRef CAS PubMed .
- H. Singh, J. V. Singh, M. K. Gupta, A. K. Saxena, S. Sharma, K. Nepali and P. M. S. Bedi, Bioorg. Med. Chem. Lett. , 2017, 27 , 3974–3979 CrossRef CAS PubMed .
- A. S. Abd-El-Aziz, A. T. Alsaggaf, R. M. Okasha, H. E. A. Ahmed, R. Bissessur, A. A. Abdelghani and T. H. Afifi, ChemistrySelect , 2016, 1 , 5025–5033 CrossRef .
- M. Patel and K. Patel, Heterocycl. Commun. , 2019, 25 , 146–151 CAS .
- S. Prateeptongkum, W. Mahavorasirikul and N. Duangdee, ARKIVOC , 2018, 2018 , 73–85 Search PubMed .
- J. Zhao, H. Zhao and B. S. J. Blagg, WO2015/192099Al, 2015.
- D. Carrico-Moniz, US Pat. , US9388155BI, 2016 Search PubMed .
- C. Liang, D. Tian, M. Jia, X. Wang, X. Xie and D. Zhang, US Pat. , US9758503BI, 2017 Search PubMed .
- P. Arsenjans, J. Vasiljeva, I. Domracheva, I. Shestakova and I. Kalvins, PCT Int. Appl. WO2018/015788Al, 2018.
- F. Tosun and M. Miski, PCT Int. Appl. WO2019/209232A2, 2019.
- R. D. Lucrezia, T. Bergbrede, P. Nussbaumer, U. Koch, B. Klebl, A. Choidas, A. Unger, N. Larsson, M. Falkenberg-Gustafsson and C. M. Gustafsson, US Pat. , US2020/0031794Al, 2020 Search PubMed .
- A. R. Temmanaboyina, PCT Int. Appl. WO2022/238741Al, 2022.

An official website of the United States government
The .gov means it’s official. Federal government websites often end in .gov or .mil. Before sharing sensitive information, make sure you’re on a federal government site.
The site is secure. The https:// ensures that you are connecting to the official website and that any information you provide is encrypted and transmitted securely.
- Publications
- Account settings
Preview improvements coming to the PMC website in October 2024. Learn More or Try it out now .
- Advanced Search
- Journal List
- Pharmaceuticals (Basel)
- PMC10675815

Recent Progress in Synthesis, POM Analyses and SAR of Coumarin-Hybrids as Potential Anti-HIV Agents—A Mini Review
Mustapha suleiman.
1 Department of Chemistry, Faculty of Science, Universiti Teknologi Malaysia, Johor Bahru 81310, Johor, Malaysia; moc.liamg@700egsam
2 Department of Chemistry, Sokoto State University, Birnin Kebbi Road, Sokoto 852101, Nigeria
Faisal A. Almalki
3 Department of Pharmaceutical Chemistry, Faculty of Pharmacy, Umm Al-Qura University, Mecca 21955, Saudi Arabia; moc.liamg@2lasiafiklam (F.A.A.); [email protected] (T.B.H.)
Taibi Ben Hadda
4 Laboratory of Applied Chemistry & Environment, Faculty of Sciences, Mohammed Premier University, MB 524, Oujda 60000, Morocco
Sarkar M. A. Kawsar
5 Laboratory of Carbohydrate and Nucleoside Chemistry, Department of Chemistry, University of Chittagong, Chittagong 4331, Bangladesh; db.ca.uc@raswaka
Subhash Chander
6 Amity Institute of Phytochemistry & Phytomedicine, Amity University Uttar Pradesh, Noida 201313, India; moc.liamg@repininiashsahbus
Sankaranarayanan Murugesan
7 Medicinal Chemistry Research Laboratory, Birla Institute of Technology & Science Pilani (BITS Pilani), Pilani Campus, Pilani 333031, India; ni.ca.inalip-stib.inalip@nasegurum
Ajmal R. Bhat
8 Department of Chemistry, R.T.M. Nagpur University, Nagpur 440033, India; moc.liamg@lamjatahb

Andrey Bogoyavlenskiy
9 Research and Production Center for Microbiology and Virology, Almaty 050010, Kazakhstan
Joazaizulfazli Jamalis
Associated data.
The data and materials supporting the findings or analyses in their study are all readily accessible upon request.
The human immunodeficiency virus (HIV) is the primary cause of acquired immune deficiency syndrome (AIDS), one of the deadliest pandemic diseases. Various mechanisms and procedures have been pursued to synthesise several anti-HIV agents, but due to the severe side effects and multidrug resistance spawning from the treatment of HIV/AIDS using highly active retroviral therapy (HAART), it has become imperative to design and synthesise novel anti-HIV agents. Literature has shown that natural sources, particularly the plant kingdom, can release important metabolites that have several biological, mechanistic and structural representations similar to chemically synthesised compounds. Certainly, compounds from natural and ethnomedicinal sources have proven to be effective in the management of HIV/AIDS with low toxicity, fewer side effects and affordability. From plants, fungi and bacteria, coumarin can be obtained, which is a secondary metabolite and is well known for its actions in different stages of the HIV replication cycle: protease, integrase and reverse transcriptase (RT) inhibition, cell membrane fusion and viral host attachment. These, among other reasons, are why coumarin moieties will be the basis of a good building block for the development of potent anti-HIV agents. This review aims to outline the synthetic pathways, structure–activity relationship (SAR) and POM analyses of coumarin hybrids with anti-HIV activity, detailing articles published between 2000 and 2023.
1. Introduction
For over thirty years, human immunodeficiency virus type 1 (HIV-1) infection has been a major global health problem, affecting more than 37 million people worldwide [ 1 ]. Acquired immunodeficiency syndrome (AIDS), which is a result of the human immunodeficiency virus (HIV), provokes fatal infections and numerous related diseases. The human immunodeficiency virus is characterised by the destruction of the immune system, which leads to improper functioning of the human immune system, the latter being characterised by the ability to fight any incoming infection and resulting in the well-being of the body [ 2 ]. HIV is a lentivirus, otherwise known as a “slow” virus, which ultimately causes acquired immunodeficiency syndrome (HIV-1 and HIV-2). This is commonly known as acquired immunodeficiency syndrome (AIDS) [ 1 , 3 ]. According to the World Health Organisation (WHO), approximately 38 million people worldwide have been infected with HIV, and about 2 million new cases are diagnosed each year [ 4 ].
One of the well-known methods, Petra/Osiris/Molinspiration (POM) analysis, has been used frequently to create two-dimensional models to discover and suggest the kind of pharmacophore site that influences the biological activity with a change in the chemical. POM can predict molecule biological activities and depict the relationships between steric/electrostatic properties and biological activity as pharmacophore sites [ 5 ].
2. Bibliometric Analysis
There are only a few linked publications available in the WOS database about the keywords we used for the search. Keywords such as “coumarin”, “coumarin-hybrids”, “HIV”, and “SAR” were used to search for the topic search criteria on 3 September 2023, and 1302 research items were discovered from 2000 to 2023.
Recently, the development of various anti-HIV therapies has changed AIDS infection from a deadly disease to a more manageable yet chronic ailment. Such agents include nucleoside and nucleotide reverse transcriptase inhibitors, non-nucleoside reverse transcriptase inhibitors, protease inhibitors, fusion inhibitors, and co-receptor inhibitors. The treatment, however, of HIV infection has experienced many challenges, ranging from the severe side effects experienced to the mutation of the virus; these two have been lingering problems in the therapy of the disease, making the development of novel anti-HIV drugs necessary [ 6 ]. In response to the issue, scientists have developed novel anti-HIV agents that can effectively address the resistance and consequent toxicity problems reported by some anti-HIV agents currently used to treat HIV/AIDS. Due to this, “highly active antiretroviral therapy,” also known by the acronym (HAART), has become a new treatment [ 5 , 7 ], which has yielded a positive response from patients as reported by [ 8 , 9 ]. Antiretroviral therapy (ART) or combined antiretroviral therapy (cART) are other terms for this treatment regimen. The co-administration of different drugs that inhibit viral replication by distinct methods, so that the propagation of a virus resistant to a single agent is stopped by the action of the other agents in the combined regimen, is a major component of HAART. This therapy, HAART” is a type of medication that involves the simultaneous usage and administration of both non-nucleoside HIV-1 RT inhibitors and some protease inhibitors to increase the drug’s efficacy as reported by Olomola et al. [ 10 ]. Reports have shown that typical tenofovir–emtricitabine combination (nucleoside reverse transcriptase inhibitors) in combination with integrase strand transfer inhibitors or non-nucleoside reverse transcriptase inhibitors is used as a standard treatment for treatment-naive patients [ 11 , 12 , 13 ]. Another and most important goal of the HAART regimen is to reduce HIV transmission to others. In certain studies, the HAART regimen has been shown to lower the risk of sexual transmission to partners to practically zero [ 14 ]. Mother-to-child transmission during breastfeeding and pregnancy has also been drastically reduced [ 15 , 16 , 17 ]. Although highly active antiretroviral therapy (HAART) enables long-term control of virus replication in many individuals, it is not without unintended side effects, some of which are already emerging in older populations receiving long-term treatment [ 18 ].
Natural products have been used to treat viral illnesses for thousands of years and are increasingly important in medication research and development [ 19 ]. The exploration of medicinal plants and natural products that might yield affordable and effective therapeutic agents in the management of HIV/AIDS is a response to the declaration by the World Health Organisation (WHO) in 1989 [ 20 , 21 ]. Natural coumarins ( Figure 1 ) are classified into several types depending on their chemical diversity and complexity: simple coumarins ( 1 ), furanocoumarins ( 2 ), pyranocoumarins ( 3 ), biscoumarins ( 4 ), and additional coumarins such as phenylcoumarins ( 5 ) [ 22 , 23 , 24 ]. Coumarins 1 (2 H -chromen-2-one or 2 H -1-benzopyran-2-one), secondary metabolites that belong to the family of benzopyrones, are found in a variety of plant parts, such as roots, seeds, nuts, flowers, and fruit [ 25 , 26 ].

Structure of different coumarins.
It has been established that compounds possessing a coumarin moiety have exhibited a variety of intriguing and exciting activities due to their varying pharmacological properties, such as anti-bacterial activity [ 4 , 24 , 25 ], anti-cancer [ 4 , 25 , 26 ], anti-coagulant [ 4 , 27 ], anti-oxidant [ 28 ], anti-fungal [ 4 , 29 , 30 , 31 ], anti-tubercular [ 4 , 32 , 33 ] and most importantly for this research, anti-viral and anti-HIV activity [ 4 , 34 , 35 ].
Coumarin possesses the ability to perform several important interactions with diverse proteins, enzymes and receptors, ranging from hydrogen bonding, Van der Waals forces, chelation activities, hydrophobic interaction, and many more, which makes coumarin and its derivatives have a vast application as inhibitors of HIV protease: an integrase (IN) inhibitor, an inhibitor of viral DNA replication, a viral protein regulator (VPR) and also as an inhibitor of reverse transcriptase (RT). The ability to exert and manifest multiple anti-HIV activities as well as having the potential to overcome persistent resistance is a prominent property of coumarin and its derivatives [ 4 , 34 ]. Hybridization of molecules involves the combination of more than one pharmacophore to produce one hybrid compound that could inhibit HIV by single or multiple mechanisms and counterbalance the side effects that manifest because of the usage of a particular compound, or different hybrids that provide a novel anti-HIV activity with a much better result [ 4 , 36 ].
Many studies have shown that coumarin and the analogues of coumarin have exhibited a range of antiviral characteristics and more specifically act as HIV-1 inhibitors [ 2 , 4 , 5 , 34 , 35 ]. To the best of our knowledge, there has never been a comprehensive review that has detailed the coumarin hybrids with anti-HIV potential. This review aims to provide a comprehensive analysis of coumarin hybrids, focusing on their synthesis, biological activities, and structure–activity relationship (SAR). Specifically, it will explore their potential as anti-HIV agents, with a particular emphasis on articles published between 2000 and 2023.
3. Coumarin Hybrids
In 2004, Lan Xie and colleagues [ 36 ] conducted a study in which they synthesised hydroxymethyl (3′ R ,4′ R )-3′,4′-di-O-( S )-camphanoyl-(+)-cis-khellactone (DCK) derivatives. The aim was to enhance the oral bioavailability and water solubility of dicamphanoyl khelactone analogues [ 37 ] while evaluating their potential to inhibit HIV-1 replication in CD4 cells. These DCK derivatives belong to the category of pyranocoumarins, which are considered anti-HIV inhibitors and can be classified into four distinct structural classes.
The synthesis process for the analogues began with the preparation of methylated DCKs from methylated 7-hydroxycoumarin, as reported [ 38 , 39 ]. The methylated DCKs ( 6 , 7 and 8 ) were treated with N -bromosuccinimide to yield 3-bromomethyl ( 6a and 7a ) and 6-bromomethyl ( 8a ) substituted DCKs with a product yield range of 67–77%. Depending on the quantity of bromosuccinimide used, small amounts of 3- or 6-dibromoethyl compounds were also formed. By employing sodium acetate, the bromomethyl-DCK derivatives ( 6a , 7a and 8a ) were reacted with acetic anhydride to generate the corresponding acetoxymethyl-DCK derivatives ( 6b , 7b and 8b ) with 79–84% as the product yield. Subsequent acidic hydrolysis of these compounds produced the corresponding hydroxymethyl-DCK derivatives ( 6c , 7c and 8c ) and the yield was found to be above 85%. Further treatment of 6a and 8a with hexanemethylenetetramine, followed by hydrolysis, led to the formation of aminomethyl DCK ( 6d and 8d ). Additionally, the treatment of compound 6a with diethylamine resulted in the production of 3-dimethylaminomethyl DCK ( 6e ) with 71% yield of product [ 36 ], as illustrated in Scheme 1 .

Synthetic pathways for the synthesis of hydroxymethyl DCK analogues. Conditions and reagents: ( i ) N -bromosuccinimide in benzene, reflux; ( ii ) NaOAc, acetic anhydride, reflux; ( iii ) reflux, HCl (2N) in EtOH; ( iv ) diethylamine in toluene, reflux; ( v ) hexanemethylenetetramine in CHCl 3 , reflux; ( vi ) EtOH, HCl (2N), 100 °C.
The anti-HIV activity of all the newly synthesised analogues was tested using 4-methyl-DCK, DCK and AZT (zidovudine/azidothymidine) as the reference drugs. Based on the biological studies, the EC 50 values of compounds 7c and 6c (0.004 and 0.029 µM) were the most active compounds when compared to AZT and DCK standards. Compounds 6b , 7b and 6a showed EC 50 values better than those of AZT but similar to the values of DCK. The excellent potency exhibited by compound 7a with 0.00011 µM, as the EC 50 value is worth noting, was more potent than the 4-methyl-DCK standard. Moderate anti-viral activity was observed in the compounds 8a , 8c and 8d but even then, they exhibited better potency than AZT, although not as potent as DCK and the 4-methyl-DCK standards.
The structure–activity relationship (SAR) of the synthesised compounds indicates that bromomethyl, hydroxymethyl, or acetoxymethyl groups at the 3-position show better or similar activity than seen in compound 7a . A negative effect was seen in compounds that possess amino moieties, as seen in compounds 6d and 6e , which is an indication that the anti-HIV activity is not favoured by a diethylaminomethyl or aminomethyl group at the 3-position. The SAR can be generalised by saying favourability in anti-HIV activity is better in the 3-position of DCK but not the 6-position ( Figure 2 ).

SAR representation of hydroxymethyl DCK analogues.
Al-Soud et al. [ 39 ] synthesised a new hybrid of 1H-1,2,4-triazolylcoumarin due to the enormous biological activities exhibited by 1,2,4-triazole [ 40 ] and coumarin [ 41 ]. The synthetic pathway for the synthesis of triazolylcoumarin hybrids is shown in Scheme 2 , starting with 7-hydroxycoumarin 9 . 2-Chloroacetontrile was treated with 7-hydroxycoumarin 9 to prepare 2-(2 H -benzopyran-7-yloxy)acetonitrile 10 with 82% as the reaction yield, which was used as the precursor to synthesise 1,2,4-triazole in DMF containing K 2 CO 3 . On treatment with SbCl 5 at −60 °C, intermediate 12 was afforded from α,α′-dichloroazo compounds 11 . The colour of the mixture changes from orange to brown at around −30 °C, an indication that a cycloaddition reaction has occurred between nitrile 10 and cumulenes 12a – e to generate inseparable 1,2,4-triazolium hexachloroantimonates 13a – e . A spontaneous rearrangement occurred from compounds 13a – e to the intermediate 14a – e with an increase in temperature above −30 °C. The coumarin salts 15a – e were produced from 14a-e via 1,2-migration and the subsequent elimination of the CMe 2 Cl group. In the presence of NaHCO 3 and aqueous NH 3 , an in situ deprotonation of coumarin salts 15a – e afforded the desired compound 16a – e with a percentage yield of 72–85% [ 39 ].

Synthetic route for the synthesis of 1,2,4-triazolylcoumarin hybrid. Reagents and conditions: ( i ) ClCH 2 CN; ( ii ) CH 2 Cl 2 , SbCl 5 , −60 °C; ( iii ) −60 °C to 23 °C, CH 2 Cl 2 ; ( iv ) NH 3 , NaHCO 3 , MeCN, 2 h, 0 °C. 11a – 16a R 1 = R 2 = CH 3 ; b R 1 = R 2 = Et; c R 1 = R 2 = Et; d R 1 = CH 3 , R 2 = i- Pr, e R 1 = R 2 = (CH 2 ) 5 .
To further explore the potentiality of coumarin with 1,2,4 triazole, the scientists also synthesised another analogue following the same route as depicted in their previous work shown in Scheme 2 above. However, in this case, the research was extended on coumarin derivatives with 1,2,4-triazoles by adding a cyanomethyl group in position 7 of the backbone, so the resulting starting material was now (2-(5-methoxy-4-methyl-2 H -benzopyran-7-yloxy) acetonitrile) 16 . Scheme 3 illustrates the cycloaddition reaction that occurs between cumulenes 12a – e and compound 2-(5-methoxy-4-methyl-2 H -benzopyran-7-yloxy) acetonitrile. Compounds 17a and b were synthesised in 85 and 75% yield.

Synthetic pathway for the synthesis of novel hybrid 17. Reagents and conditions: ( i ) NH 3 , NaHCO 3 , MeCN, 2 h, 0 °C.
The anti-HIV activity of the newly synthesised compounds was carried out on compounds 10 , 16a – e and 17b using the HIV-2 ROD strain and the III B strain for HIV-1. The inhibition was monitored in MT-4 cells by virus-induced cytopathy (in vitro). A very weak inhibitory activity was noticed in all the tested compounds. Both HIV-2 and HIV-1 replication were not inhibited by the compounds. A promising activity was noticed in compound 17b with IC 50 value >0.17 µM, selectivity index of <1.0 and CC 50 of 0.17 µg/mol. The suggestion obtained from the SAR studies is that a higher anti-HIV activity was exteriorized by coumarin hybrids possessing carbon–coumarin linkage than the corresponding series of hybrids possessing oxygen linkage ( Figure 3 ).

SAR representation of 1H-1,2,4-triazolycoumarin hybrids.
Still on the hunt for a novel and potent anti-HIV drug, Trivedi et al. synthesised new coumarinyl chalcone analogues from two closely structurally related coumarins: 4-hydroxy-6-chloro-7-methylcoumarin and 4-hydroxy-8-isopropyl-5-methylcoumarin. The duo were their respective chalcones. The synthetic procedure is depicted in Scheme 4 . The starting materials used for this synthesis are malonic acid 19 and phenol 18 . In the presence of anhydrous ZnCl 2 , Lewis acid and phosphorus oxychloride (POCl 3 ), substituted coumarins 20 are prepared by the condensation of appropriately substituted malonic acid 19 and phenol 18 . The resulting substituted coumarin is acetylated using POCl 3 and glacial acetic acid as acetylating agents. The reaction proceeds to completion with the reaction with piperidine and CHCl 3 to produce the target compounds 23a – i and 24a – i with a percentage yield range of 69–79% [ 42 ].

A synthesis pathway for the synthesis of 23a – i and 24a – i . Reaction and condition; ( i ) POCl 3 , anhydrous ZnCl 2 , 70 °C; ( ii ) POCl 3 , glacial acetic acid; ( iii ) piperidine, CHCl 3 , 80 °C.
Using MT-4 cells, the antiviral activity screening of all the synthesised compounds was carried out against HIV-1 (IIIB) and HIV-2 (ROD) using zidovudine (AZT) as the reference drug for comparison purposes. Unfortunately, all the synthesised compounds exhibited no anti-HIV activity against all the viruses evaluated. Each activity showed a cut-off concentration of ≥5-fold lower than the cytotoxic concentration. The structure–activity relationship is presented in Figure 4 .

SAR presentation of new coumarinyl chalcone analogues 23a – i and 24a – i.
As a result of the mass interest garnered recently by thiourea as an interesting moiety for the design of novel and potent anti-HIV compounds, the hybridization of s-triazine derivatives, coumarin derivatives, and phenyl ethyl amine derivatives has been performed by Patel et al. to synthesise novel PETT (phenyl ethyl thiazolyl thiourea) analogues as possible novel HIV inhibitors. The synthesis of the novel PETT analogues ( Scheme 5 ) was initiated by the condensation of 2,4,6-trichloro-1,3,5-s-triazine 25 with 4-hydroxycoumarin 26 in the presence of acetone and 10% NaHCO 3 to yield compound 27 as an intermediate. The intermediate formed is then further condensed with 3,4-dimethoxy phenyl ethyl thiourea 28 to afford an important intermediate 29 , which further undergoes substitution with various substituted phenyl thiourea/ureas ( 30a – o/31a – o ) to produce the derivatives ( 32a – o ) and ( 33a – o ) of 3,4-dimethoxy phenyl ethyl-1,3,5-triazinyl thiourea with yield ranges of 53–79% and 50–75%, respectively [ 43 ].

A synthetic pathway for the synthesis of PETT analogues.
The MTT (4,5-dimethyl thiazol-2-yl)-2,5-diphenyl tetrazolium bromide) method for the screening of antiviral activity was used for the biological activity to determine the inhibition of replication of HIV. The anti-HIV screening of these derivatives shows no selectivity for HIV-1 and HIV-2. Compound 30h shows an EC 50 value of >124 µM/g mL which indicates poor activity. A moderate activity against both HIV-1 and HIV-2 was noticed in compound 31k with a selectivity index of 3 for both HIV-1 and HIV-2. Compounds 31c , 31h and 31n with S.I of 9,3 and 11, respectively, against HIV-1 were also observed. So generally, both analogues exert poor potency and selectivity.
The structure–activity relationship (SAR) analysis ( Figure 5 ) reveals that the poor activity of the synthesised analogue may be a result of the fact that the heterocyclic ring of the synthesised analogues contains a bulky triazine group. Overcrowding is also observed in the structure, which is a result of the ether linkage with the 4-hydroxycoumarin present, which disturbs the overall structure of the synthesised hybrids.

SAR representation of PETT analogues.
In the year 2008, Reddy et al. [ 44 ], on the basis of the anti-HIV activity exhibited by Saquinavir 34 as the first inhibitor of protease to inhibit both HIV-2 and HIV-1 proteases and also the anti-HIV potency of (+)-calanolide A 35 , which is a pyranocoumarin derivative, prompted them to synthesise a new substituted N 1 -[1-benzyl-3-(3- ter t-butylcarbamoyl-octahydroisoquinolin-2yl)-2-hydroxypropyl]-2-[(2-oxo-2 H -chromene-3-carbonyl)amino] succinimide as a novel anti-HIV agent.

The synthesis ( Scheme 6 ) began with the condensation of 2-(3-amino-2-hydroxy-4-phenyl-butyl) decahydroisoquinoline-3-carboxylic acid tert -butylamide 36 with N -benzyloxy carbonyl- L -asparagine 37 using triethylamine in dry hydrofuran for 24 h at room temperature and dicyclohexylcarbodiimide (DCC). The reaction was then deprotected with 5% Pd-C and hydrogen to prepare 2-amino- N 1 -[l-benzyl-3-(l- tert -butylcarbamoyloctahydroisoquinolin-2-yl)2-hydroxypropyl] succinamide 38 . Using the appropriate coumarin-3-carboxylic acid ( 39a – h ), compound 38 was then condensed in the presence of triethylamine for 24 h at room temperature in dry hydrofuran and dicyclohexylcarbodiimide (DCC) to afford the product N 1 -[1-benzyl-3-(3- ter t-butylcarbamoyl-octahydroisoquinolin-2yl)-2-hydroxy- propyl]-2-[(2-oxo-2 H -chromene-3-carbonyl)amino] succinimide 40.

Synthetic pathway for the synthesis of hybrid 40a – h. Reagents and conditions: ( i ) THF, DCC, TEA, 24 h, 20–25 °C, N 2 ; ( ii ) EtOAc, H 2 , 5% Pd/C, 20–25 °C, 48 h; ( iii ) THF, DCC, TEA, 20–25 °C, 24 h, N 2 .
MT-4 cells were used to monitor the inhibition of the induced cytopathic effect, and the activity was estimated by the MTT method. Using azidothymidine (AZT) as the reference drug, the synthesised compounds 40a – h were tested for anti-HIV activity against HIV-1 and HIV-2. Compound 40g exhibited greater activity against both HIV-1 and HIV-2 infections, but compound 40c showcased poor anti-HIV activity when tested against both HIV-1 and HIV-2. Moderate activity against both HIV-1 and HIV-2 was manifested by compounds 40d and 40f , while compounds 40e and 40h showed no activity against HIV-1 and HIV-2.
In summary, the structure–activity relationship (SAR) for compounds 40b , 40d , 40f , and 40g demonstrate that appropriate substitutions at positions 6 and 8 of the coumarin ring are necessary for the anti-HIV activity of the synthesized compounds.
Compound 40g shows that the chlorine atom at position 8 of the coumarin ring enhanced the anti-HIV activity of the hybrids ( Figure 6 ).

SAR representation of hybrids 40a – h.
In the year 2008, Al-Soud and co-workers [ 45 ] synthesised coumarin hybrids on the basis of the anti-HIV activity exhibited by coumarin derivatives and also the anti-HIV activity displayed by capravirine 41 with an EC 50 value of 0.0014 µg mL −1 , CC 50 value of 11 µg mL −1 and SI (selectivity index) value of 7857, which is a compound containing an imidazole moiety [ 45 , 46 , 47 ]. The standard drug used for comparison in this research is efavirenz which has an EC 50 of 0.003 and CC 50 of 40 µg mL −1 respectively.

The starting material for the synthesis was compound 42 . The two 4-bromomethylcoumarins 43 and 44 interacted with 42 in dimethyl formamide (DMF) to give the corresponding derivatives 45 and 46 with 43% and 46% yields, respectively. Following purification, Scheme 7 illustrates the synthesis of compounds 48 and 49 by treating 47 in DMF with 43 and 44 , with yields of 70 and 78%, respectively.

A synthetic pathway for the synthesis of 45 , 46 , 48 and 49 .
Al-Soud et al. [ 45 ] further synthesised another coumarin derivative that contains benzothiazole and benzoxazole moieties ( Scheme 8 ). The derivatives of benzoxazole 50 gave the compound 52 with 95% yield in the presence of NaH in DMF. 2-Chloroacetylchloride reacted with compound 51 to afford compound 53 (67% yield), which was treated with potassium phthalimide in the presence of K 2 CO 3 to produce compound 54 (90% yield). Treatment of the resulting mixture with hydrazine hydrate converted it to the corresponding amine 55 (82% yield). Coumarin sulfonyl chloride 56 was used to sulfonate compound 55 in the presence of triethylamine to give the sulphonamide derivative 57 as the final product with 71% yield.

Synthesis of compound 57. Reagents: ( i ) chloroacetyl chloride; ( ii ) K 2 CO 3 , potassium phthalimide; ( iii ) hydrazine hydrate.
Still on the work of Al-Soud, Scheme 9 depicts the synthesis of imidazole analogues 59 and 60 . In the presence of benzofuran, the imidazole derivatives 59 and 60 with a yield of 63 and 48%, respectively were synthesised by the treatment of 42 and 47 with 58 in the presence of NaH in DMF.

Synthesis of compounds 59 and 60 .
Using in vitro anti-HIV assay, compounds 45 , 46 , 48 , 49 , 59 and 60 were screened against both HIV-1 (strain III B ) and HIV-2 (strain ROD) in MT-4 (human T- lymphocyte) cells. The result shows no inhibition at CC 50 higher than EC 50 with the standard anti-HIV agents capravirine and efavirenz (EFV). However excellent activity when compared to other compounds was displayed by compounds 48 and 49 . HIV-1 inhibition was shown by compound 48 with an EC 50 value of 1.22 µg/mL and an EC 50 value of 0.51 µg/mL for HIV-2 inhibition. For compound 49 , HIV-1 inhibition was noted as possessing an EC 50 value of 1.45 µg/mL and HIV-2 inhibition with an EC 50 value of 1.78 µg/mL [ 45 ].
Based on the anti-HIV activity exhibited by s-triazine (probably as a result of the possession of the characteristics of Het–NH–Ph–U motif derivatives [ 48 ], 2-coumarin-4-yloxy-4,6-substituted-s-triazine derivatives were synthesised and their in vitro anti-HIV activities were tested, as reported by [ 49 ].
As shown in Scheme 10 , 2,4,6-trichloro-1,3,5-s-triazine 61 was condensed with 4-hydroxycoumarin 26 to afford an intermediate 62 (85% yield). In the presence of NaHCO 3 , the intermediate 62 reacted with 3,4-dimethoxyphenylethylamine 63 to form compound 64 (72% yield). To prepare the novel 3,4-dimethoxyphenylethyl-1,3,5-triazinyl amine derivatives 67a – m with a percentage yield range of 56–74% and 68a – m with a percentage yield of 55–75%, variously substituted phenyl urea/thiourea compounds 65a – m and 66a – m were reacted with intermediate 64 in the presence of NaHCO 3 in dioxane.

Synthetic pathway for hybrids 67a – m and 68a – m . Reagents and conditions: ( i ) 10% NaHCO 3 /acetone ( ii ) acetone/10% NaHCO 3 ( iii ) 10% NaHCO 3 /dioxane.
Another synthetic pathway ( Scheme 11 ) showed the replacement of two chlorine atoms on intermediate 62 , where intermediate 62 was reacted with glycine ethyl ester hydrochloride 69 to afford an important intermediate 70 (60% yield). On further treatment of 70 with various aryl ureas, new compounds 71a – m were formed with the yield range of 54–69%, and several aryl amines afforded 72a – m with a yield range of 56–67%.

Synthesis of compounds 71a – m and 72a – m . Reagents and conditions: ( i ) 10% NaHCO 3 /acetone ( ii ) acetone/10% NaHCO 3 ( iii ) 10% NaHCO 3 /dioxane.
To determine the ability of the synthesised compounds to inhibit the replication of HIV-1 (IIIB) and HIV-2, MT-4 cells were used for the screening utilising the in vitro screening method. For the purpose of comparison, nevirapine was used as a standard reference drug. To further test the efficacy of the synthesised compounds, the compounds that proved to be potent were also tested against HIV-RT double mutant strains (K103N and Y181C) using efavirenz as the standard reference drug. Compounds 68d and 68l were found to be active against HIV-1 with IC 50 values of 8.33 µg/mL and 10 µg/mL, respectively. For the modified analogues, compound 68d , with an IC 50 value of 1.07 µg/mL and SI of 4.3, was found to be the most potent of the synthesised compounds. A significant inhibition potential was noticed in compounds 66g and 66j with IC 50 values of 6.86 µg/mL and 7.16 µg/mL, respectively, and a common SI of 7.
The structure–activity relationship (SAR) indicates that compound 68d , with a methyl group at position 4 (4-CH 3 ), and compound 68c , which possesses an ethoxy group at position 4 (4-OEt) displayed selective inhibition of HIV-1. The SAR studies on the modified compounds ( 65 and 66a – m ) also deduced that compounds 66d and 66g , which have para-substituted chloro and methyl groups, exhibited a selective inhibition of HIV-1. The highest potency against HIV-1 was observed in compound 66d with a para-substituted methyl group (4-CH 3 ). As part of the research programme embarked on by Olomola et al. [ 10 ] for the design, synthesis and evaluation of coumarin derivatives, a group of coumarin hybrids was designed that contain both triazolothymidine and coumarin moieties as potential dual-action RT and HIV-1 PR inhibitors.
The synthesis started with the production of the corresponding adducts 75a – e ( Scheme 12 ), which were afforded by the DABCO (1,4-diazabicyclo[2.2.2]octane/triethylenediamine) catalysed Baylis–Hillman reaction of t-butyl acrylate 74 and the salicylaldehyde 73a – e . Compounds 76a – e 3-(chloromethyl)coumarins were formed from the cyclisation of 75a – e with HCl-AcOH. Propargylamine 77 further reacted with 76a – e in THF to give the corresponding 78a – e in a yield range of 52–80%. In the presence of copper (II) sulphate and ascorbic acid, the cycloaddition of 78a – e with azidothymidine 79 catalysed by Cu(I), resulted in the analogues 80a – e as the final hybrid with the yield range of 64–75% [ 50 ].

Synthesis of AZT-coumarin derivatives 80a – e . Reagents and conditions ( i ) CHCl 3 , DABCO, rt; ( ii ) AcOH, HCl, reflux; ( iii ) THF, propargylamine; ( iv ) Cu 2 SO 4 .5H 2 O, C 6 H 7 NaO 6 (sodium ascorbate), H 2 O-THF.
To screen the anti-HIV activity of the synthesised compounds, commercially available HIV-1 RT and HIV-1 PR kits were utilised. Promising anti-HIV activity against HIV-1 PR was apparent on the synthesised analogues with an IC 50 value range of 21–29 µM which is as much as two or three times higher than the IC 50 value of ritonavir with an IC 50 value of 9.85 µM. When compared with the IC 50 value of AZT 73, compounds 80a and 80b show comparable inhibitory activity.
The structure–activity relationship (SAR) reveals that the unsubstituted analogue ( 80a) and the analogue ( 80b ), which contain a bromine atom at the C-6 position of coumarin, are essential for the anti-HIV activity. However, a significant decrease in activity is observed in the compound that contains a chlorine atom at the C-6 position and an alkoxy group at the C-8 position of coumarin ( Figure 7 ).

SAR representation of hybrids 80a – e .
Interestingly, in their quest to design and synthesise a potent compound with HIV-1 protease inhibition potential, Olomola et al. [ 10 ] further synthesised a new series of coumarin derivatives in the form of N -benzylated amido group derivatives 85a – e . The synthesis of the modified analogues 85a – e is illustrated in Scheme 13 . The hybrids of 80a – e and 85a – e possess a common intermediate, 76a – e . To form the secondary amine 82a – e , the intermediate 76a – e was reacted with benzylamine 81 . The corresponding chloroacetamide 83a – e (78–98% yield) was afforded by the acylation of 82a – e with chloroacetyl chloride, and subsequently, in THF, the resulting compound was reacted with propargylamine to give the alkynylated product 84a – e (79–86% yield). Finally, with azidothymidine (AZT) 79 , compounds 84a – e underwent cycloaddition in the presence of copper (II) sulphate and ascorbic acid to afford the hybrid 85a – e with an isolated yield range of 70–80%.

Synthetic pathways for the synthesis of coumarin–AZT hybrids 85a – e . Reagents and condition: ( i ) CHCl 3 , DABCO, rt; ( ii ) AcOH, HCl, reflux.
Under a variety of conditions, the hybrids 85a – e were screened for HIV-1 RT and HIV-1 PR inhibition. Compounds 85a – e showed an inhibition potential of up to 99% of HIV-1 RT. The compounds also showed HIV-1 PR inhibition, but not like that of the corresponding 80a – e . They concluded that all the newly synthesised analogues 85a – e exhibited a better IC 50 value than azidothymidine 79 . The most active compounds against HIV-1 PR and HIV-1 RT were determined to be compounds 85a and 85b . From the anti-HIV result, the structure–activity relationship (SAR) revealed that the introduction of –OMe and –OEt (alkoxy groups) at the C-8 position of the coumarin will enhance the activity ( Figure 8 ).

SAR representation of hybrids 85a – e .
In 2018, new diazocoumarin derivatives were designed and synthesised by Livani and co-workers to study the anti-HIV activity (integrase inhibition) of the synthesised analogues [ 51 ]. The anti-HIV activity evidenced in the bis-azo compound and coumarin scaffold was the reason for the hybridization, and so the 5-(halo-substituted) benzylthio-1,3,4-oxadiazole moiety and coumarin core were fused together to form the newly designed compound. Using ethanol and 4-aminobenzoic acid 86 , ethyl aminobenzoate 87 (74% yield) was first prepared via Fischer esterification using sulphuric acid as a catalyst. In absolute ethanol and excess hydrazine hydrate under reflux, ethyl 4-aminobenzoate 87 was converted to 4-aminobenzohydrazide 88 with a yield of 82%. In the presence of alcoholic potash and carbon disulfide, the mercaptho-1,3,4-oxadiazole ring 89 was closed on the carbohydrazide intermediate. In the presence of 10% NaOH as a base and methanol as a solvent, an S -alkylation or benzylation reaction was performed with alkyl or substituted benzyl halides to afford 90a – h derivatives with a product yield range of 55–87%. In 10% sodium nitrite and 6M HCl, the diazonium salts of compound 91a – h were prepared. Through the coupling reaction of 4-hydroxycoumarin 92 with the diazonium salts, the final derivatives 93a – h were obtained in the range of 58–81% product yield ( Scheme 14 ).

Synthetic pathways for the synthesis of diazocoumarin derivatives. Reagents and condition: ( i ) H 2 SO 4 , ethanol, reflux, 24 h ( ii ) NH 2 -NH 2 .OH, ethanol (6 eq.), reflux, 96 h ( iii ) CS 2 , ethanol, KOH, reflux, 4h ( iv ) 10% aq. NaOH, methanol, substituted aryl/alkyl halides, r.t ( v ) 10% sodium nitrite, 6 M HCl, 0–5 °C ( vi ) 10% aq. Na 2 CO 3 , stir, 0–5 °C.
Via the single-cycle replication method, compounds 93a – h were assayed for anti-HIV activity, which was measured as the inhibition rate (%) of HIV-1 in P24 expression in the Hela cell culture. At 100 µM concentration, all compounds 93a – h showed anti-HIV activity in the range of 5–79%. Compound 93a , with an inhibition rate of 79%, and compound 87f , with cell viability of 52%, were superior to azidothymidine (AZT), with 58% viability. The results indicate that compound 87f with 4-chlorobenzyl possesses the best anti-HIV activity.
The structure–activity relationships (SAR) indicate that compound 87c tolerates propyl substitution reasonably well, which shows similar inhibition to that of compound 93e . Also, compound 93d shows that introducing an unsubstituted benzyl group leads to a drastic reduction in activity. So, it was concluded that compound 93f with a 4-chlorobenzyl group at C-5 shows the best anti-HIV activity ( Figure 9 ).

SAR representation of hybrids 93a – h .
Jesumoroti et al. [ 52 ] designed and synthesised a series of novel N ′-(3-hydroxybenzoyl)-2-oxo-2 H -chromene-3-carbohydrazide derivatives as potential HIV-1 integrase inhibitors by employing an approach called “scaffold-hopping”. The hybrids were produced by the combination of the coumarin moiety and hydrazide with the hope that 3-acyl-coumarin could mimic the diketo-acid moiety of raltegravir.
Adapting the Knoevenagel condensation reaction process, ethyl 2-oxo-2 H -chromene-3-carboxylate analogues 89a – e were produced by synthesising the acid precursors 91a – e from the condensation of salicylic aldehyde 88a – e with diethyl malonate. Compounds 90a-e were formed by the process of alkaline hydrolysis using NaOH. To afford the key intermediates, compounds 90a – e were reacted with oxalyl chloride to form 91a – e . Using H 2 SO 4 , esterification of substituted salicylic acid 92a – d in methanol produced 2-hydroxybenzoate derivatives 93a – d which on further hydrazinolysis afforded substituted 2-hydroxy benzohydrazides 94a – d . Finally, in the presence of saturated Na 2 CO 3 , stirring 94a – d with 91a – e overnight produced 95a – t with a variable yield range of 38–83% (attributed to the substitution pattern on the two rings ( Scheme 15 ).

Synthetic pathways for synthesis of coumarin-3-carbohydrazide derivatives. Reagents and conditions: ( i ) piperidine, diethyl malonate, ethanol, CH 3 COOH, 80 °C, 12 h ( ii ) ethanol, NaOH, reflux ( iii ) oxalyl chloride, reflux, 8 h. ( iv ) CH 3 OH, Conc. H 2 SO 4 , reflux, 24 h ( v ) 65% N 2 H 4 .H 2 O, 80–90 °C, overnight. ( vi ) Na 2 CO 3 , room temperature, overnight.
Chicoric acid was used as a standard in evaluating the HIV-1 IN inhibition of coumarin-3-carbohydrazide 98a – t in the nanomolar range. The result of the HIV-1 IN activity showed that both hybrids 98a and 98c have an inhibition ability (IC 50 = 14 nM) comparable to that of standard chicoric acid (IC 50 = 10 nM). Structure–activity relationship (SAR) analysis revealed that the highest HIV-1 IN inhibiting activity was observed in hybrids that contain bromo- or chloro-substituents on ring A of the coumarin moiety while also having only the OH group on the salicyl moiety (ring C). The introduction of the methoxy group into the system decreased the HIV-1 IN activity. So generally, SAR revealed that the introduction of the chloro-group at the C-5 position (R 2 ) of the phenyl group and the general introduction of the halogen atom in the C-6 position (R 1 ) of the coumarin moiety enhanced the activity ( Figure 10 ).

SAR representation of hybrids 98a – t .
To achieve multiple inhibitions of virally coded enzymatic functions, coumarin-based scaffolds were exploited to synthesise sixteen novel 4-hydroxy-2 H ,5 H -pyrano (3,2- c ) chromene-2,5-dione hybrids [ 53 ]. The theoretical binding affinity of all the synthesised hybrids was calculated via modelling studies on both RT-associated ribonuclease H (RNase H) and HIV-1 IN sites. The compounds were later subjected to a biological assay to determine RNase H inhibitors and dual HIV-1 IN inhibitor hybrids.
The reaction of malonic acid and phenol in the presence of phosphorus oxychloride and zinc chloride afforded the 4-hydroxycoumarin derivatives. The further treatment of these 4-hydroxycoumarin derivatives with zinc chloride, malonic acid and phosphorus oxychloride resulted in the production of 4-hydroxy-2-methylenepyrano [3,2- c ] chromene-2,5-dione ( 102 – 106 ). Compounds ( 102 – 106 ) were further acylated to give various acetyl-substituted compounds ( 107 – 110 ). Compounds ( 107 – 110 ) were further reacted with sodium metal and ethyl acetate to afford 4-hydroxy-3-(3-oxobutanoyl) pyrano [3,2- c ] chromene-2,5-dione ( 111 – 113 ). The acidic hydrolysis of compounds ( 111 – 113 ) afforded hybrid 114 . Using phenyl hydrazine and 3,4-diaminobenzophenone, compounds ( 111 – 113 ) underwent cyclization to form 3-(7-benzoyl-3 H -benzo [b] [ 1 , 4 ] diazepin-2-yl)-4-hydroxypyrano [3,2- c ] chromene-2,5-dione 115 and 116 and 4-hydroxy-3-(5-methyl-1-phenyl-1 H -pyrazol-3-yl) pyrano [3,2- c ] chromene-2,5-dione 117 , respectively, in good yield of 56–82% ( Scheme 16 ).

Synthetic pathways for the synthesis of hybrids 116 and 117 . Reagents and conditions: ( i ) POCl 3 , ZnCl 2 ; ( ii ) POCl 3 , ZnCl 2 ( iii ) gla CH 3 COOH, POCl 3 ( iv ) ethylacetate, Na; ( v ) Ethanol, HCl ( vi ) 3,4-DABP ( vii ) POCl 3 , 1,2-dichloromethane, Pd-C/H 2 ( viii ) Ph-NHNH 2 .
The molecular docking studies against the RNase H protein active site indicated that compounds 105 , 103 , 104 , 101 and 99 have the best binding affinity against the protein. Important hydrophobic interactions with binding pocket residues were also observed in the active site of the protein. The most interesting derivative was compound 105 for its ability to inhibit both RNase H and HIV-1 IN in the low micromolar range. To maintain excellent potency against PR while obtaining RT inhibition, coumarin moieties were fused into HIV-1 protease inhibitors to produce a more potent hybrid according to “portmanteau inhibitors” or designed multifunctional ligands (DMLs). Zhu and co-workers designed various coumarin hybrids with different linkers that exhibited weak inhibition of RT and excellent potency against PR [ 54 ].
The synthetic procedures began with the production of amine derivatives ( 122 – 125 ) which were afforded from commercially available (2 S , 3 S )1,2-epoxy-3-(boc-amino)-4-phenylbutane as shown in Scheme 17 a. Scheme 17 b depicts the synthetic pathways for synthesising coumarin–amide hybrids 123a – 125i , which proceed from the coupling of amines 123 – 125 with coumarin acids 122a – d under an EDCI/HOBt/DMAP-mediated coupling method. The synthesis of coumarin–carbamate hybrids 123j – 124k shown in Scheme 17 c shows the reaction of amines 123 , 124 with hydroxycoumarins 122j , 122k using bis(trichloromethyl) carbonate (BTC) as a condensing agent adapting to a one-pot reaction. The refluxing of chlorocoumarin 122 and amines 123 – 125 using DIEA as a catalyst afforded the target coumarin–amine hybrids 123 – 125l with a wide variation in the yield ranging from 22–98%, as shown in Scheme 17 d.

Molecular structure of compounds 126a – 128i . ( a ) A synthetic pathway for the synthesis of amine derivatives. Reagents and conditions: ( i ) CH 3 CN, i -BuNH 2 , 80 °C, 6 h; ( ii ) DIEA, aryl sulfonyl chloride, DMAP(Cat.), THF, 0 °C~r.t, 3–5 h; ( iii ) CH 2 Cl 2 -CF 3 COOH (1:1), 0 °C~r.t, 3 h; ( iv ) 50 psi, H 2 (gas), 10% Pd/C, CH 3 OH, r.t, 2 h. ( b ) Synthetic pathways for the synthesis of coumarin–amide hybrids 126a – 128i . Reagents and conditions: ( i ) HOBt, EDCI, anhydrous DMF, DMAP, Argon, 0 °C~r.t, 3 h. ( c ) Synthetic pathways for the synthesis of coumarin–carbide hybrids 126j – 128k . Reagents and condition: ( i ) Anhydrous DCM, DIEA, anhydrous THF, 0 °C~r.t, 1.5 h. ( d ) Synthetic pathways for the synthesis of coumarin–amine hybrids 123 – 125l . Reagents and conditions: ( i ) anhydrous EtOH, DIEA, reflux, 7 h.
RT activity and the HIV-1 PR assay were used to test all the synthesised hybrids. Coumarin–amide hybrids show PR inhibition with an IC 50 value range of (298.4 nM–0.40 nM) which indicates that the derivatives are active PR inhibitors except for hybrids 127h and 127i (557 nM and 563 nM, respectively). A four-fold activity with an IC 50 value of 0.40 nM was observed in hybrid 128a , indicating the best activity. A comparable potency as darunavir (1.72 ± 0.73 nm) was noticed in hybrid 126e with an IC 50 value of 1.62 nM, and a 54.46% inhibition ratio against WT HIV-1 was also noticed at a concentration of 100 nM. Generally, the synthesised hybrids show better inhibitory activity against PR than RT inhibition. Hybrid 128b with an IC 50 value of 75.25 µM against RT revealed its weakness when compared to the potency of efavirenz with IC 50 of 0.091 ± 0.008 µM.
4. Coumarin Hybrids with Weak Activity against HIV Infections
Through the synthesis of modified aminocoumarin as a leaving group, 7-amino-4-carbamoylmethylcoumarin (ACC) was synthesised via the solid-phase synthetic procedure for the inhibition of HIV-PR. However, relatively weak activity was observed in all the synthesised hybrids [ 55 ]. 6,6,10,10-Tetramethyl-6 H ,10 H -dipyranocoumarin (dipetalactone) also exhibited no anti-HIV activity (using HIV-1 strain IIIB of human immunodeficiency virus type 1). The molecular docking studies revealed weak to no significant amino acid interactions occurring within the binding pocket of the HIV proteins, which demonstrated its weak activity [ 56 ]. Drzewiecka et al. suggested that if only a methyl group is substituted in the dipyranocoumarin system, the compound will have no biological activity [ 56 ]. Novel chromeno–chromenones exhibit weak anti-HIV activity and were synthesised by the reaction of indole catalysed by L -pyroline and 4-hydroxycoumarin [ 57 ]. The synthetic pathway ( Scheme 18 ) depicts the reaction between the coumarin derivative and the indole. Some derivatives of coumarins have been synthesised and have exhibited weak to no activity against HIV [ 58 , 59 ].

Synthesis of novel chromeno–-chromenone hybrids. Reagents and conditions: ( i ) EtOH, L -proline, reflux, 12–14 h, 78–90%.
5. POM Analyses: Identification of Anti-HIV Pharmacophore Sites
The first point of the conclusion is that comparing the activities of molecules as cited above by calculating chemical parameters and using theoretical calculations has now become much easier. Developing technology and breakthroughs have improved both programmes and computers. The use of DFT theory and docking analysis is a robust tool that provides valuable information on the chemical, electronic, and physical properties of molecules, allowing us to explain their biological activities; however, only several of these techniques are effective in some situations. This is especially true when the prominent, active one is a metabolite, not the parent molecule. Hence, the theoretical study of prodrugs is a mistake, and this should be taken into consideration as well as stopped. As an alternative solution, we developed the POM (Petra/Osiris/Molinspiration) theory in collaboration with the NCI and TAACF of the USA [ 60 , 61 , 62 , 63 , 64 ].
The POM theory, invented by the group of Taibi Ben Hadda in collaboration with the American National Cancer Institute (NCI) and Tunisian–American Association for Cancer Research and Training Foundation (TAACF), led us to real success in the pharmacology and drug design fields [ 65 , 66 , 67 , 68 , 69 , 70 , 71 , 72 ]. Here we treat the coumarin moiety of the selected compounds to clarify the origin of their antiviral activity and to identify their pharmacophore sites according to the POM organigram ( Figure 11 ).
Current research has been encouraged by the potential pharmacological properties of coumarin compounds. As an extension of our study, it has also focused more on the identification of novel antiviral heterocyclic compounds for therapeutic purposes [ 73 ]. The objective of this research is to evaluate a series of hybrid coumarin congeners for their activities against HIV infections. Moreover, POM analyses were conducted to explain the experimental results of biological activity [ 65 , 69 , 70 , 71 , 72 , 74 , 75 ].

The Concept and Applications of POM Theory in the identification and optimisation of pharmacophore sites of various classes of drugs developed by Prof. T. Ben Hadda (the principal inventor of POM Theory) in collaboration with the NCI and TAACF of the USA [ 76 , 77 ].
The identification of the type of pharmacophore sites of these compounds was derived from the physical and chemical properties of the metabolites of the tested coumarin-hybrid by using the bioinformatics POM platform. Although the mechanism of the ring opening of coumarin was not well clarified, it appeared that the first step essentially seems to be the formation of the enolate from (A), resulting in the formation of the hemiacetal (B). The hemiacetal, being very unstable, opens immediately to a new formation (C) ( Figure 12 ).

Opening/closing ring of coumarin moiety [ 75 ].
The probable mechanism of the opening/closing ring reaction was described previously after 1980 [ 75 ]. Unfortunately, no chemist or pharmacologist, until now, has indicated the impact and importance of these processes on the bioactivity of coumarin. Therefore, it was for the first time that we attempted to do it, and hopefully it will be of benefit in the future. In contrast to all substituents (R 1 , R 3 , R 4 , R 5 and R 6 ), the sole and exceptional substituent is R 1 , which plays a crucial role in the arrangement of the antiviral pharmacophore site according to POM theory ( Figure 11 and Figure 13 ). Once again, POM theory works, gives more clarification, and helps in the drug design of new coumarin-hybrid candidates, allowing for more efficiency and selectivity.

Identification of antiviral pharmacophore sites of coumarin-hybrid compounds.
6. Conclusions and Future Viewpoint
Although it still has its own drawbacks and difficulties, the introduction of the HAART regimen has changed the dynamics of HIV disease, converting it from a nearly fatal illness into a chronic but seemingly stable condition. These setbacks among other reasons, made medicinal chemists and scientists plunge headfirst into the field of drug discovery due to the obvious necessity of developing a novel anti-HIV drug candidate having less prevalent complications than the HAART regimen. HAART has reduced the deadly impact of AIDS/HIV from its original status as a deadly disease to what is now considered a manageable infection and consequently scaled down the far-reaching destruction of the ailment throughout the world. Unfortunately, some patients suffer various side effects as well as the development of multi-drug resistance, thus inhibiting a smooth treatment for patients.
Natural sources, which are generally applicable to various infections, are currently one of the most common sources of medication. This led to the exploration of the coumarin moiety due to its versatility and druggability, as seen in the development of warfarin (anti-coagulants), methoxsalen (anti-dermatosis), novobiocin (antibiotics), etc. (+)-Calanolide A, a clinically-evaluated coumarin derivative, inhibits RT, IN, PR, Tat, and Vpr. The review considered in great detail the many reactions and pathways used in synthesis that resulted in the development of numerous coumarin hybrids with anti-HIV activity.
This compilation has shown that coumarin hybrids possess multiple anti-HIV mechanisms or can mitigate side effects, making their hybridization an effective strategy for developing novel agents with high potency against drug-resistant HIV strains and low toxicity compared to that experienced by patients under the current regime of HAART. Several potent hybrids have been designed and synthesised using coumarin synthesis methods. They can reduce toxicity and atone for the hybrid’s negative effects when combined with anti-HIV actions of coumarin hybrids. The structure–activity relationship of the synthesised hybrids will assist in understanding the correlation between their structural properties and anti-HIV activity, enabling the development of more effective anti-HIV hybrids.
Acknowledgments
The authors would like to acknowledge the Deanship of Scientific Research at Umm Al-Qura University, for supporting this work by Grant code: (23UQU4290462DSR1).
Abbreviations
Funding statement.
This work was supported by Grant No. BR10965178 of Ministry of Education and Science of the Republic of Kazakhstan (A. Bogoyavlenskiy, from Almaty, Kazakhstan). J. Jamalis wish to thank the Ministry of Higher Education (MOHE) Malaysia for funding this research under the Fundamental Research Grant Scheme (FRGS/1/2022/STG04/UTM/02/4).
Author Contributions
J.J., A.B., S.C., S.M.—supervision, investigation and critical review; F.A.A., T.B.H., S.M.A.K., A.R.B.—methodology, software’s and revision; M.S., original draft. All authors critically reviewed and approved the manuscript before submission. All authors have read and agreed to the published version of the manuscript.
Institutional Review Board Statement
Not applicable.
Informed Consent Statement
Data availability statement, conflicts of interest.
The authors declare no conflict of interest.
Disclaimer/Publisher’s Note: The statements, opinions and data contained in all publications are solely those of the individual author(s) and contributor(s) and not of MDPI and/or the editor(s). MDPI and/or the editor(s) disclaim responsibility for any injury to people or property resulting from any ideas, methods, instructions or products referred to in the content.

Anti-Cancer Agents in Medicinal Chemistry
ISSN (Print): 1871-5206 ISSN (Online): 1875-5992
Recent Literature Review on Coumarin Hybrids as Potential Anticancer Agents
- Department of Pharmaceutical Chemistry, Discipline of Pharmaceutical Sciences, College of Health Sciences, University of KwaZulu- Natal (Westville), Durban-4000, South Africa
- School of Chemistry and Physics, University of KwaZulu Natal, P/Bag X54001, Westville, Durban 4000, South Africa
- Department of Chemistry, School of Science, GITAM Deemed to be University, Hyderabad, Telangana 502329, India

Volume 23, Issue 2, 2023
Published on: 20 August, 2022
Page: [142 - 163] Pages: 22
DOI: 10.2174/1871520622666220418143438
Cancer is considered one of the leading causes of death globally, especially patients with lung, pancreatic, or brain tumors are most likely to die of cancer, and patients with prostate and breast cancer are at a high risk of noncancer death. As a result, there is ongoing research regarding developing new, safe, and efficient anticancer agents. Coumarin-based naturally occurring compounds possess a broad spectrum of activity in medicinal chemistry, such as anticancer, anti-inflammatory, antimicrobial, antioxidant agents, etc. Many researchers have synthesized coumarinbased novel therapeutic agents via molecular hybridization technique, which offers an excellent opportunity to develop novel compounds with improved biological activities by incorporating two or more pharmacophores. This review aims to shed light on the recent developments of coumarin-based anticancer hybrid derivatives and their Structure-Activity Relationships (SAR). This review serves as a medium that medicinal chemists could utilize to design and synthesize coumarin derivatives with significant pharmacological value as future anticancer agents.
Keywords: Coumarin , molecular hybridization , anticancer agent , therapeutic agent , broad spectrum of activity , SAR.
Graphical Abstract

Title: Recent Literature Review on Coumarin Hybrids as Potential Anticancer Agents
Volume: 23 Issue: 2
Author(s): Baji Baba Shaik, Naresh Kumar Katari*, Pule Seboletswe, Rambabu Gundla, Narva Deshwar Kushwaha, Vishal Kumar, Parvesh Singh, Rajshekhar Karpoormath and Muhammad D. Bala*
Abstract: Cancer is considered one of the leading causes of death globally, especially patients with lung, pancreatic, or brain tumors are most likely to die of cancer, and patients with prostate and breast cancer are at a high risk of noncancer death. As a result, there is ongoing research regarding developing new, safe, and efficient anticancer agents. Coumarin-based naturally occurring compounds possess a broad spectrum of activity in medicinal chemistry, such as anticancer, anti-inflammatory, antimicrobial, antioxidant agents, etc. Many researchers have synthesized coumarinbased novel therapeutic agents via molecular hybridization technique, which offers an excellent opportunity to develop novel compounds with improved biological activities by incorporating two or more pharmacophores. This review aims to shed light on the recent developments of coumarin-based anticancer hybrid derivatives and their Structure-Activity Relationships (SAR). This review serves as a medium that medicinal chemists could utilize to design and synthesize coumarin derivatives with significant pharmacological value as future anticancer agents.
Export Options
About this article.
Cite this article as:
Shaik Baba Baji, Katari Kumar Naresh*, Seboletswe Pule, Gundla Rambabu, Kushwaha Deshwar Narva, Kumar Vishal, Singh Parvesh, Karpoormath Rajshekhar and Bala D. Muhammad*, Recent Literature Review on Coumarin Hybrids as Potential Anticancer Agents, Anti-Cancer Agents in Medicinal Chemistry 2023; 23 (2) . https://dx.doi.org/10.2174/1871520622666220418143438
Call for Papers in Thematic Issues
Induction of cell death in cancer cells by modulating telomerase activity using small molecule drugs.
Telomeres are distinctive but short stretches present at the corners of chromosomes and aid in stabilizing chromosomal makeup. Resynthesis of telomeres supported by the activity of reverse transcriptase ribonucleoprotein complex telomerase. There is no any telomerase activity in human somatic cells, but the stem cells and germ cells undergone telomerase ... read more
Signaling and enzymatic modulators in cancer treatment
Cancer accounts for nearly 10 million deaths in 2022 and is considered the leading cause of worldwide mortality. Cancer outcome can be improved through an appropriate screening and early detection and through an efficient clinical treatment. Chemotherapy, radiotherapy and surgery are the most important approach for the treatment of several ... read more
Related Journals
Anti-Inflammatory & Anti-Allergy Agents in Medicinal Chemistry
Current Analytical Chemistry
Current Computer-Aided Drug Design
Current Bioactive Compounds

Current Cancer Drug Targets

Combinatorial Chemistry & High Throughput Screening
Current Cancer Therapy Reviews
Current Diabetes Reviews
Current Drug Safety

Current Drug Targets
Related Books

Drug Addiction Mechanisms in the Brain

Botanicals and Natural Bioactives: Prevention and Treatment of Diseases

Software and Programming Tools in Pharmaceutical Research

Objective Pharmaceutics: A Comprehensive Compilation of Questions and Answers for Pharmaceutics Exam Prep

Biosurfactants: A Boon to Healthcare, Agriculture & Environmental Sustainability

Medicinal Chemistry of Drugs Affecting Cardiovascular and Endocrine Systems

Frontiers in Clinical Drug Research - Anti-Cancer Agents

The Management of Metastatic Triple-Negative Breast Cancer: An Integrated and Expeditionary Approach

Advances in Dye Degradation

Medicinal Plants, Phytomedicines and Traditional Herbal Remedies for Drug Discovery and Development against COVID-19

- About Journal
- Editorial Board
- Journal Insight
- Current Issue
- Volumes /Issues
- Author Guidelines
- Graphical Abstracts
- Fabricating and Stating False Information
- Research Misconduct
- Post Publication Discussions and Corrections
- Publishing Ethics and Rectitude
- Increase Visibility of Your Article
- Archiving Policies
- Peer Review Workflow
- Order Your Article Before Print
- Promote Your Article
- Manuscript Transfer Facility
- Editorial Policies
- Allegations from Whistleblowers
- Forthcoming Thematic Issues
- Guest Editor Guidelines
- Editorial Management
- Ethical Guidelines for New Editors
- Reviewer Guidelines
- Abstract Ahead of Print 9
- Article(s) in Press 5
- Free Online Copy
- Most Cited Articles
- Most Accessed Articles
- Highlighted Article
- Most Popular Articles
- Editor's Choice
- Thematic Issues
- Open Access Articles
- Open Access Funding
- Library Recommendation
- Trial Requests
- Advertise With Us
- Meet the Executive Guest Editor(s)
- Brand Ambassador
- Author's Comment & Reviews
- New Journals 2023
- New Journals 2024
- Alert Subscription
Restricted Access Panel
Recent pharmacological insights about imidazole hybrids: a comprehensive review
- Review Article
- Published: 13 May 2024
Cite this article
- Samet Poyraz 1 ,
- Metin Yıldırım ORCID: orcid.org/0000-0003-1346-312X 2 &
- Mehmet Ersatir 3
18 Accesses
Explore all metrics
In this review, we evaluated the biological activities of hybrid molecules incorporating imidazoles—a cornerstone in medicinal chemistry known for their broad pharmacological spectrum, attributed to the chemical characteristics of their nitrogen atoms. In contrast to earlier reviews, which have concentrated their attention only on a single biological activity that is connected with these hybrid molecules, this review brings together the findings of a variety of investigations that cover a wide range of significant activities including antibacterial, antifungal, antituberculosis, antiviral, anticancer, antioxidant, antidiabetic, anti-inflammatory, and analgesic effects, along with the inhibition of cholinesterase, carbonic anhydrase, and monoamine oxidase (MAO) enzymes. Furthermore, we examined significant pharmacophores such as triazole, thiazole, indole, pyrazole, quinoline, sulfonamide, pyridine, chalcone, coumarin, pyrrole, and pyrrolidine, integrated into imidazole hybrids. Molecular docking studies and structure-activity relationship (SAR) discussions provide insight into the interactions of imidazole hybrids with key enzymes and receptors. This work aspires to contribute valuable insights into the development of novel imidazole hybrids, aiming to address critical health challenges of our era.
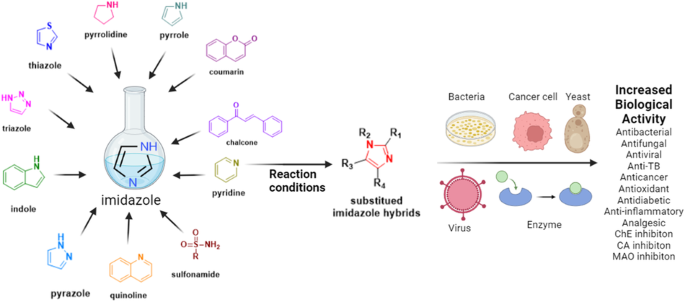
This is a preview of subscription content, log in via an institution to check access.
Access this article
Price includes VAT (Russian Federation)
Instant access to the full article PDF.
Rent this article via DeepDyve
Institutional subscriptions
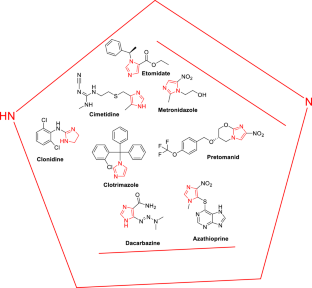
Similar content being viewed by others
Overview on Biological Activities of Imidazole Derivatives
Development of drugs based on imidazole and benzimidazole bioactive heterocycles: recent advances and future directions.
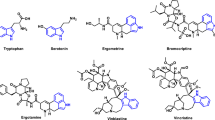
Recent progress in biologically active indole hybrids: a mini review
Newman DJ, Cragg GM. Natural products as sources of new drugs over the nearly four decades from 01/1981 to 09/2019. J Nat Prod. 2020;83:770–803. https://doi.org/10.1021/acs.jnatprod.9b01285 .
Article CAS PubMed Google Scholar
Farha MA, Brown ED. Drug repurposing for antimicrobial discovery. Nat Microbiol. 2019;4:565–77. https://doi.org/10.1038/s41564-019-0357-1 .
Salam MA, Al-Amin MY, Salam MT, Pawar JS, Akhter N, Rabaan AA, et al. Antimicrobial resistance: a growing serious threat for global public health. Healthcare. 2023;11:1946 https://doi.org/10.3390/healthcare11131946 .
Article PubMed PubMed Central Google Scholar
Tang KWK, Millar BC, Moore JE. Antimicrobial Resistance (AMR). Br J Biomed Sci. 2023;80. https://doi.org/10.3389/bjbs.2023.11387 .
Samreen, Ahmad I, Malak HA, Abulreesh HH. Environmental antimicrobial resistance and its drivers: a potential threat to public health. J Glob Antimicrob Resist. 2021;27:101–11. https://doi.org/10.1016/j.jgar.2021.08.001 .
WHO. Global tuberculosis report. 2023. https://iris.who.int/bitstream/handle/10665/373828/9789240083851-eng.pdf?sequence=1 .
WHO. Global cancer burden growing, amidst mounting need for services. 2024. https://www.who.int/news/item/01-02-2024-global-cancer-burden-growing--amidst-mounting-need-for-services .
Fotie J. Editorial for the Special Issue “Advances in the Development of Anticancer Drugs”. Int J Mol Sci. 2024;25:641. https://doi.org/10.3390/ijms25010641 .
Hantzsch A. Untersuchungen über Azole. Allgemeine Bemerkungen über Azole. Justus Liebigs Annalen der Chemie. 1888;249:1–6. https://doi.org/10.1002/jlac.18882490102 .
Article Google Scholar
Verma A, Joshi S, Singh D. Imidazole: having versatile biological activities. J Chem. 2013;2013:1–12. https://doi.org/10.1155/2013/329412 .
Article CAS Google Scholar
Banerjee S, Mukherjee S, Nath P, Mukherjee A, Mukherjee S, Ashok Kumar SK, et al. A critical review of benzimidazole: sky-high objectives towards the lead molecule to predict the future in medicinal chemistry. Results Chem. 2023;6:101013. https://doi.org/10.1016/j.rechem.2023.101013 .
Richaud A, Barba-Behrens N, Méndez F. Chemical reactivity of the imidazole: a semblance of pyridine and pyrrole? Organ Lett. 2011;13:972–5. https://doi.org/10.1021/ol103011h .
Cragg PJ. Supramolecular Chemistry: from biological inspiration to biomedical applications. 1st ed. Springer Dordrecht; 2010. https://doi.org/10.1007/978-90-481-2582-1 .
Peng X-M, Cai G-X, Zhou C-H. Recent developments in Azole compounds as antibacterial and antifungal agents. Curr Top Med Chem. 2013;13:1963–2010. https://doi.org/10.2174/15680266113139990125 .
Mendogralo EY, Nesterova LY, Nasibullina ER, Shcherbakov RO, Myasnikov DA, Tkachenko AG, et al. Synthesis, antimicrobial and antibiofilm activities, and molecular docking investigations of 2-(1H-Indol-3-yl)-1H-benzo[d]imidazole derivatives. Molecules. 2023;28:7095. https://doi.org/10.3390/molecules28207095 .
Article CAS PubMed PubMed Central Google Scholar
Sadeghian S, Bekhradi F, Mansouri F, Razmi R, Mansouri SG, Poustforoosh A, et al. Imidazole derivatives as novel and potent antifungal agents: Synthesis, biological evaluation, molecular docking study, molecular dynamic simulation and ADME prediction. J Mol Struct. 2024;1302:137447. https://doi.org/10.1016/j.molstruc.2023.137447 .
Straszak D, Siwek A, Głuch-Lutwin M, Mordyl B, Kołaczkowski M, Pietrzak A, et al. Modulation of the MOP receptor (μ Opioid Receptor) by Imidazo[1,2-a]imidazole-5,6-Diones: in search of the elucidation of the mechanism of action. Molecules. 2022;27:2930. https://doi.org/10.3390/molecules27092930 .
Serrao E, Xu Z-L, Debnath B, Christ F, Debyser Z, Long Y-Q, et al. Discovery of a novel 5-carbonyl-1H-imidazole-4-carboxamide class of inhibitors of the HIV-1 integrase–LEDGF/p75 interaction. Bioorgan Med Chem. 2013;21:5963–72. https://doi.org/10.1016/j.bmc.2013.07.047 .
Pattanayak P, Behera K, Behera PR. Synthetic clubbing of 2-Methyl-5-Nitroimidazole with 5(6)-Substituted-1H-Benzimidazole as potential antimicrobial and anthelmintic agents. Pharm Chem J. 2023;57:227–33. https://doi.org/10.1007/s11094-023-02872-7 .
El-Sofany WI, El-sayed WA, Abd-Rabou AA, El-Shahat M. Synthesis of new imidazole-triazole-glycoside hybrids as anti-breast cancer candidates. J Mol Struct. 2022;1270:133942 https://doi.org/10.1016/j.molstruc.2022.133942 .
Soumyashree DK, Keri RS, Reddy DS, MSK, Naik L, Kumar A et al. Design, synthesis, single-crystal X-ray and docking studies of imidazopyridine analogues as potent anti-TB agents. J Mol Struct. 2024;1295. https://doi.org/10.1016/j.molstruc.2023.136540 .
Nikitina PA, Basanova EI, Nikolaenkova EB, Os'kina IA, Serova OA, Bormotov NI, et al. Synthesis of esters and amides of 2-aryl-1-hydroxy-4-methyl-1H-imidazole-5-carboxylic acids and study of their antiviral activity against orthopoxviruses. Bioorgan Med Chem Lett. 2023;79:129080. https://doi.org/10.1016/j.bmcl.2022.129080 .
Marzouk AA, Bass AKA, Ahmed MS, Abdelhamid AA, Elshaier YAMM, Salman AMM, et al. Design, synthesis and anticonvulsant activity of new imidazolidindione and imidazole derivatives. Bioorgan Chem. 2020;101:104020. https://doi.org/10.1016/j.bioorg.2020.104020 .
Kumar R, Singh R, das Chagas Almeida A, da Trindade Granato J, de Oliveira Lemos AS, Kumar K, et al. Imidazo[1,2-a]pyrimidine as a new antileishmanial pharmacophore against leishmania amazonensis promastigotes and amastigotes. ACS Omega. 2023;8:40613–21. https://doi.org/10.1021/acsomega.3c05441 .
Rajesh R, Manikandan A, Sivakumar A, Ramasubbu C, Nagaraju N. Substituted methoxybenzyl-sulfonyl- 1H -benzo[d]imidazoles evaluated as effective H + /K + -ATPase inhibitors and anti-ulcer therapeutics. Eur J Med Chem. 2017;139:454–60. https://doi.org/10.1016/j.ejmech.2017.08.001 .
Ma B, Tan W, Zhang J, Mi Y, Miao Q, Guo Z. Preparation and characterization of chitosan derivatives bearing imidazole ring with antioxidant, antibacterial, and antifungal activities. Starch - Stärke. 2023;75. https://doi.org/10.1002/star.202200204 .
Awasthi A, Rahman MA, Bhagavan Raju M. Synthesis, in silico studies, and in vitro anti-inflammatory activity of novel imidazole derivatives targeting p38 MAP kinase. ACS Omega. 2023;8:17788–99. https://doi.org/10.1021/acsomega.3c00605 .
Hussain R, Rehman W, Khan S, Maalik A, Hefnawy M, Alanazi AS, et al. Imidazopyridine-based thiazole derivatives as potential antidiabetic agents: synthesis, in vitro bioactivity, and in silico molecular modeling approach. Pharmaceuticals. 2023;16:1288. https://doi.org/10.3390/ph16091288 .
Sultanova RM, Khusnutdinova NS, Borisova YG, Raskildina GZ, Meshcheryakova SA, Samorodov AV, et al. Synthesis and antiplatelet activity of 2-substituted imidazolines. Russian Chem Bull. 2023;72:1711–6. https://doi.org/10.1007/s11172-023-3951-6 .
Chaudhry F, Munir R, Ashraf M, Mehr-Un-Nisa N, Huma R, Malik N, et al. Exploring facile synthesis and cholinesterase inhibiting potential of heteroaryl substituted imidazole derivatives for the treatment of Alzheimer’s disease. Arabian J Chem. 2023;16:104384. https://doi.org/10.1016/j.arabjc.2022.104384 .
Karakaya A, Çevik UA, Erçetin T, Özkay Y, Kaplancıklı ZA. Synthesis of Imidazole-Thiazole Derivatives as Acetylcholinesterase and Butyrylcholinesterase Inhibitory Activities. Pharma Chem J. 2023;57:1439–43. https://doi.org/10.1007/s11094-023-03007-8 .
Chagas RS, Otsuka FAM, Pineda MAR, Salinas RK, Marana SR. Mechanism of imidazole inhibition of a GH1 β‐glucosidase. FEBS Open Biol. 2023;13:912–25. https://doi.org/10.1002/2211-5463.13595 .
Campos‐Fernández L, Ortiz‐Muñiz R, Cortés‐Barberena E, Mares‐Sámano S, Garduño‐Juárez R, Soriano‐Correa C. Imidazole and nitroimidazole derivatives as NADH‐fumarate reductase inhibitors: Density functional theory studies, homology modeling, and molecular docking. J Comput Chem. 2022;43:1573–95. https://doi.org/10.1002/jcc.26959 .
Dekate SM, Hatzade KM, Ghatole AM. Imidazole–Thiazole Hybrid: Synthesis, Brain Penetration, Cytochrome P450 Enzyme and Gastrointestinal Absorption Study. Iranian J Sci. 2023;47:1081–96. https://doi.org/10.1007/s40995-023-01496-6 .
Almatary AM, Husseiny WME, Selim KB, Eisa HMH. Nitroimidazole-sulfonamides as carbonic anhydrase IX and XII inhibitors targeting tumor hypoxia: design, synthesis, molecular docking and molecular dynamics simulation. J Mol Struct. 2022;1264:133260. https://doi.org/10.1016/j.molstruc.2022.133260 .
Noriega-Iribe E, Díaz-Rubio L, Estolano-Cobián A, Barajas-Carrillo VW, Padrón JM, Salazar-Aranda R, et al. In vitro and in silico screening of 2,4,5-Trisubstituted Imidazole derivatives as potential xanthine oxidase and acetylcholinesterase inhibitors, antioxidant, and antiproliferative agents. Appl Sci. 2020;10:2889. https://doi.org/10.3390/app10082889 .
Bashir B, Riaz N, Ejaz SA, Saleem M, Iqbal A, Mahmood HMK et al. Parsing p-tolyloxy-1,3,4-oxadiazolepropanamides as 15-lipoxygenase inhibitors prop up by in vitro and in silico profiling including structure determination. J Mol Struct. 2023;1275. https://doi.org/10.1016/j.molstruc.2022.134664 .
Shahin AI, Zaib S, Zaraei SO, Kedia RA, Anbar HS, Younas MT, et al. Design and synthesis of novel anti-urease imidazothiazole derivatives with promising antibacterial activity against Helicobacter pylori. Plos One. 2023;18:0286684. https://doi.org/10.1371/journal.pone.0286684 .
Osmaniye D, Levent S, Sağlık BN, Karaduman AB, Özkay Y, Kaplancıklı ZA. Novel imidazole derivatives as potential aromatase and monoamine oxidase-B inhibitors against breast cancer. N J Chem. 2022;46:7442–51. https://doi.org/10.1039/d2nj00424k .
Drugbank. Imidazole based drugs https://go.drugbank.com/categories/DBCAT002246 .
Keam SJ. Pretomanid: first approval. Drugs. 2019;79:1797–803. https://doi.org/10.1007/s40265-019-01207-9 .
Article PubMed Google Scholar
Sotgiu G, Pontali E, Centis R, D’Ambrosio L, Migliori GB. Delamanid (OPC-67683) for treatment of multi-drug-resistant tuberculosis. Expert Rev Anti-infective Ther. 2015;13:305–15. https://doi.org/10.1586/14787210.2015.1011127 .
Ali I, Lone MN, Aboul-Enein HY. Imidazoles as potential anticancer agents. Med Chem Commun. 2017;8:1742–73. https://doi.org/10.1039/c7md00067g .
Sharma P, LaRosa C, Antwi J, Govindarajan R, Werbovetz KA. Imidazoles as potential anticancer agents: an update on recent studies. Molecules. 2021;26:4213. https://doi.org/10.3390/molecules26144213 .
Zhang L, Peng X-M, Damu GLV, Geng R-X, Zhou C-H. Comprehensive review in current developments of imidazole-based medicinal chemistry. Med Res Rev. 2014;34:340–437. https://doi.org/10.1002/med.21290 .
Ivasiv V, Albertini C, Gonçalves AE, Rossi M, Bolognesi ML. Molecular hybridization as a tool for designing multitarget drug candidates for complex diseases. Curr Top Med Chem. 2019;19:1694–711. https://doi.org/10.2174/1568026619666190619115735 .
Borcea AM, Ionuț I, Crișan O, Oniga O. An overview of the synthesis and antimicrobial, antiprotozoal, and antitumor activity of thiazole and bisthiazole derivatives. Molecules. 2021;26:624. https://doi.org/10.3390/molecules26030624 .
Zhang Z, Shu B, Zhang Y, Deora GS, Li QS. 2,4,5-Trisubstituted thiazole: a privileged scaffold in drug design and activity improvement. Curr Top Med Chem. 2020;20:2535–77. https://doi.org/10.2174/1568026620999200917153856 .
Sharma S, Mittal N, Banik BK. Chemistry and therapeutic aspect of triazole: insight into the structure-activity relationship. Curr Pharm Des. 2023;29:2702–20. https://doi.org/10.2174/0113816128271288231023045049 .
Kaur B, Venugopal S, Verma A, Sahu SK, Wadhwa P, Kumar D, et al. Recent developments in the synthesis and anticancer activity of indole and its derivatives. Curr Org Synth. 2023;20:376–94. https://doi.org/10.2174/1570179419666220509215722 .
Li T, Xu H. Recent progress of bioactivities, mechanisms of action, total synthesis, structural modifications and structure-activity relationships of indole derivatives: a review. Mini Rev Med Chem. 2022;22:2702–25. https://doi.org/10.2174/1389557522666220330123538 .
Ahmad S, Alam O, Naim MJ, Shaquiquzzaman M, Alam MM, Iqbal M. Pyrrole: an insight into recent pharmacological advances with structure activity relationship. Eur J Med Chem. 2018;157:527–61. https://doi.org/10.1016/j.ejmech.2018.08.002 .
Ganesh BH, Raj AG, Aruchamy B, Nanjan P, Drago C, Ramani P. Pyrrole: a decisive scaffold for the development of therapeutic agents and structure-activity relationship. ChemMedChem. 2024;19:e202300447. https://doi.org/10.1002/cmdc.202300447 .
Poyraz S, Döndaş HA, Döndaş NY, Sansano JM. Recent insights about pyrrolidine core skeletons in pharmacology. Front Pharmacol. 2023;14:1239658. https://doi.org/10.3389/fphar.2023.1239658 .
De S, Kumar S K A, Shah SK, Kazi S, Sarkar N, Banerjee S, et al. Pyridine: the scaffolds with significant clinical diversity. RSC Adv. 2022;12:15385–406. https://doi.org/10.1039/d2ra01571d .
Elebiju OF, Ajani OO, Oduselu GO, Ogunnupebi TA, Adebiyi E. Recent advances in functionalized quinoline scaffolds and hybrids-Exceptional pharmacophore in therapeutic medicine. Front Chem. 2022;10:1074331. https://doi.org/10.3389/fchem.2022.1074331 .
Sharma S, Singh K, Singh S. Synthetic strategies for quinoline based derivatives as potential bioactive heterocycles. Curr Org Synth. 2023;20:606–29. https://doi.org/10.2174/1570179420666221004143910 .
Alam MA. Pyrazole: an emerging privileged scaffold in drug discovery. Future Med Chem. 2023;15:2011–23. https://doi.org/10.4155/fmc-2023-0207 .
Rajendran G, Bhanu D, Aruchamy B, Ramani P, Pandurangan N, Bobba KN, et al. Chalcone: a promising bioactive scaffold in medicinal chemistry. Pharmaceuticals. 2022;15:1250. https://doi.org/10.3390/ph15101250 .
Yildirim M, Poyraz S, Ersatir M. Recent advances on biologically active coumarin-based hybrid compounds. Med Chem Res. 2023;32:617–42. https://doi.org/10.1007/s00044-023-03025-x .
Alghamdi SS, Suliman RS, Almutairi K, Kahtani K, Aljatli D. Imidazole as a promising medicinal scaffold: current status and future direction. Drug Design Dev Ther. 2021;ume 15:3289–312. https://doi.org/10.2147/dddt.S307113 .
Dong H-R, Wu J-G, Huo G-Y. Design, synthesis and biological studies of some new imidazole-1,2,3-triazole hybrid derivatives. J Mol Struct. 2022;1256:132516. https://doi.org/10.1016/j.molstruc.2022.132516 .
Chauhan S, Verma V, Kumar D, Kumar A. Facile synthesis, antimicrobial activity and molecular docking of Novel 2,4,5‐Trisubstituted‐1H‐Imidazole–Triazole Hybrid Compounds. J Heterocyclic Chem. 2019;56:2571–9. https://doi.org/10.1002/jhet.3655 .
Subhashini NJP, Praveen Kumar E, Gurrapu N, Yerragunta V. Design and synthesis of imidazolo-1, 2,3-triazoles hybrid compounds by microwave-assisted method: evaluation as an antioxidant and antimicrobial agents and molecular docking studies. J Mol Struct. 2019;1180:618–28. https://doi.org/10.1016/j.molstruc.2018.11.029 .
Pradeep Kumar CB, Prathibha BS, Prasad KNN, Raghu MS, Prashanth MK, Jayanna BK, et al. Click synthesis of 1,2,3-triazole based imidazoles: antitubercular evaluation, molecular docking and HSA binding studies. Bioorgan Med Chem Lett. 2021;36:127810. https://doi.org/10.1016/j.bmcl.2021.127810 .
Samala R, Nukala SK, Manchal R, Nagavelli VR, Narsimha S. Synthesis and biological evaluation of coumarine-imidazo[1,2-c][1,2,3]triazoles: PEG-400 mediated one-pot reaction under ultrasonic irradiation. J Mol Struct. 2023;1290:135944. https://doi.org/10.1016/j.molstruc.2023.135944 .
Gariganti N, Loke SK, Pagadala E, Chinta P, Poola B, Chetti P, et al. Design, synthesis, anticancer activity of new amide derivatives derived from 1,2,3-triazole-benzofuran hybrids: An insights from molecular docking, molecular dynamics simulation and DFT studies. J Mol Struct. 2023;1273:134250. https://doi.org/10.1016/j.molstruc.2022.134250 .
Devi M, Kumar P, Singh R, Sindhu J, Kataria R. Design, synthesis, spectroscopic characterization, single crystal X-ray analysis, in vitro α-amylase inhibition assay, DPPH free radical evaluation and computational studies of naphtho[2,3-d]imidazole-4,9-dione appended 1,2,3-triazoles. Eur J Med Chem. 2023;250:115230. https://doi.org/10.1016/j.ejmech.2023.115230 .
Asgari MS, Mohammadi-Khanaposhtani M, Sharafi Z, Faramarzi MA, Rastegar H, Nasli Esfahani E, et al. Design and synthesis of 4,5-diphenyl-imidazol-1,2,3-triazole hybrids as new anti-diabetic agents: in vitro α-glucosidase inhibition, kinetic and docking studies. Mol Divers. 2020;25:877–88. https://doi.org/10.1007/s11030-020-10072-8 .
Al-blewi F, Shaikh SA, Naqvi A, Aljohani F, Aouad MR, Ihmaid S, et al. Design and Synthesis of Novel Imidazole Derivatives Possessing Triazole Pharmacophore with Potent Anticancer Activity, and In Silico ADMET with GSK-3β Molecular Docking Investigations. Int J Mol Sci. 2021;22:1162. https://doi.org/10.3390/ijms22031162 .
Chirra N, Shanigarapu V, Abburi NP, Sinegubova EO, Pedapati RK, Esaulkova YL, et al. Synthesis and antiviral activity of novel imidazo[2,1‐b]thiazoles coupled with morpholine and thiomorpholines. J Heterocycl Chemi. 2024;61:496–505. https://doi.org/10.1002/jhet.4778 .
Saini R, Dharavath N, Malladi SR. Design, synthesis, and antimicrobial activity of novel Isoxazolyl Imidazo[2,1‐b]Thiazole libraries. J Heterocycl Chem. 2022;59:1888–906. https://doi.org/10.1002/jhet.4524 .
Osmaniye D, Bozkurt NB, Kurban B, Yardımcı GB, Ozkay Y, Kaplancıklı ZA. Synthesis of Imidazole‐2,3‐dihydrothiazole Compounds as VEGFR‐2 inhibitors and their support with in silico studies. Chem Biodivers. 2023;20:202300944. https://doi.org/10.1002/cbdv.202300944 .
Dadou S, Altay A, Baydere C, Anouar EH, Türkmenoğlu B, Koudad Met al. Chalcone-based imidazo[2,1-b thiazole derivatives: synthesis, crystal structure, potent anticancer activity, and computational studies. Journal of Biomolecular Structure and Dynamics. 2023:1-16. https://doi.org/10.1080/07391102.2023.2280756 .
Singh R, Kumar R, Roy A, Behera PM, Atri AK, Kumar K, et al. Imidazo[2,1-b]thiazole based indoleamine-2,3-dioxygenase 1 (IDO1) inhibitor: structure based design, synthesis, bio-evaluation and docking studies. Bioorgan Med Chem Lett. 2023;96:129532. https://doi.org/10.1016/j.bmcl.2023.129532 .
Sabry MA, Ghaly MA, Maarouf AR, El-Subbagh HI. New thiazole-based derivatives as EGFR/HER2 and DHFR inhibitors: Synthesis, molecular modeling simulations and anticancer activity. Eur J Med Chem. 2022;241:114661. https://doi.org/10.1016/j.ejmech.2022.114661 .
Gadekar PK, Urunkar G, Roychowdhury A, Sharma R, Bose J, Khanna S, et al. Design, synthesis and biological evaluation of 2,3-dihydroimidazo[2,1-b]thiazoles as dual EGFR and IGF1R inhibitors. Bioorgan Chem. 2021;115:105151. https://doi.org/10.1016/j.bioorg.2021.105151 .
Jasiewicz B, Babijczuk K, Warżajtis B, Rychlewska U, Starzyk J, Cofta G, et al. Indole Derivatives Bearing Imidazole, Benzothiazole-2-Thione or Benzoxazole-2-Thione Moieties—synthesis, structure and evaluation of their cytoprotective, antioxidant, antibacterial and fungicidal activities. Molecules. 2023;28:708. https://doi.org/10.3390/molecules28020708 .
Gao Y, Huang D-C, Liu C, Song Z-L, Liu J-R, Guo S-K, et al. Streptochlorin analogues as potential antifungal agents: Design, synthesis, antifungal activity and molecular docking study. Bioorgan Med Chem. 2021;35:116073. https://doi.org/10.1016/j.bmc.2021.116073 .
Li Z-Z, Tangadanchu VKR, Battini N, Bheemanaboina RRY, Zang Z-L, Zhang S-L, et al. Indole-nitroimidazole conjugates as efficient manipulators to decrease the genes expression of methicillin-resistant Staphylococcus aureus. Eur J Med Chem. 2019;179:723–35. https://doi.org/10.1016/j.ejmech.2019.06.093 .
Wang Q, Arnst KE, Wang Y, Kumar G, Ma D, White SW, et al. Structure-guided design, synthesis, and biological evaluation of (2-(1H-Indol-3-yl)-1H-imidazol-4-yl)(3,4,5-trimethoxyphenyl) Methanone (ABI-231) analogues targeting the colchicine binding site in tubulin. J Med Chem. 2019;62:6734–50. https://doi.org/10.1021/acs.jmedchem.9b00706 .
Hogendorf AS, Hogendorf A, Popiołek-Barczyk K, Ciechanowska A, Mika J, Satała G, et al. Fluorinated indole-imidazole conjugates: Selective orally bioavailable 5-HT7 receptor low-basicity agonists, potential neuropathic painkillers. Eur J Med Chem. 2019;170:261–75. https://doi.org/10.1016/j.ejmech.2019.03.017 .
Alshabani LA, Kumar A, Willcocks SJ, Srithiran G, Bhakta S, Estrada DF, et al. Synthesis, biological evaluation and computational studies of pyrazole derivatives as Mycobacterium tuberculosis CYP121A1 inhibitors. RSC Med Chem. 2022;13:1350–60. https://doi.org/10.1039/d2md00155a .
El-Shahat M, El-Sofany WI, Soliman A-GA, Hasanin M. Newly synthesized imidazolotriazole, imidazolotriazine, and imidazole-pyrazole hybrid derivatives as promising antimicrobial agents. J Mol Struct. 2022;1250:131727. https://doi.org/10.1016/j.molstruc.2021.131727 .
Panchani NM, Kapadiya KM, Joshi HS. A green approach for the catalyst‐free synthesis of imidazole‐bearing pyrazole moiety using PEG‐400 as an efficient recyclable medium as potential anti‐tubercular and anti‐microbial agents. J Heterocycl Chem. 2022;59:2084–92. https://doi.org/10.1002/jhet.4543 .
Punia S, Verma V, Kumar D, Kumar A, Deswal L. Facile synthesis, antimicrobial evaluation and molecular docking studies of pyrazole-imidazole-triazole hybrids. J Mol Struct. 2021;1223:129216. https://doi.org/10.1016/j.molstruc.2020.129216 .
Zinad DS, Mahal A, Shareef OA. Antifungal activity and theoretical study of synthesized pyrazole-imidazole hybrids. IOP Conference Ser Mater Sci Eng. 2020;770:012053. https://doi.org/10.1088/1757-899x/770/1/012053 .
Kishk SM, McLean KJ, Sood S, Smith D, Evans JWD, Helal MA, et al. Design and synthesis of imidazole and triazole pyrazoles as mycobacterium tuberculosis CYP121A1 inhibitors. ChemistryOpen. 2019;8:995–1011. https://doi.org/10.1002/open.201900227 .
Prasad P, Kalola AG, Patel MP. Microwave assisted one-pot synthetic route to imidazo[1,2-a]pyrimidine derivatives of imidazo/triazole clubbed pyrazole and their pharmacological screening. N J Chem. 2018;42:12666–76. https://doi.org/10.1039/c8nj00670a .
Chaudhry F, Shahid W, al-Rashida M, Ashraf M, Ali Munawar M, Ain Khan M. Synthesis of imidazole-pyrazole conjugates bearing aryl spacer and exploring their enzyme inhibition potentials. Bioorgan Chem. 2021;108:104686. https://doi.org/10.1016/j.bioorg.2021.104686 .
Chaudhry F, Naureen S, Ashraf M, Al-Rashida M, Jahan B, Munawar MA, et al. Imidazole-pyrazole hybrids: synthesis, characterization and in-vitro bioevaluation against α-glucosidase enzyme with molecular docking studies. Bioorganic Chem. 2019;82:267–73. https://doi.org/10.1016/j.bioorg.2018.10.047 .
Zheng Y-G, Wang J-A, Meng L, Pei X, Zhang L, An L, et al. Design, synthesis, biological activity evaluation of 3-(4-phenyl-1H-imidazol-2-yl)-1H-pyrazole derivatives as potent JAK 2/3 and aurora A/B kinases multi-targeted inhibitors. Eur J Med Chem. 2021;209:112934. https://doi.org/10.1016/j.ejmech.2020.112934 .
Silva RC, De Freitas A, Vicente B, Midlej V, dos Santos MS. Exploring novel pyrazole-nitroimidazole hybrids: Synthesis and antiprotozoal activity against the human pathogen trichomonas vaginalis. Bioorgan Med Chem. 2024;102:117679. https://doi.org/10.1016/j.bmc.2024.117679 .
Roy D, Anas M, Manhas A, Saha S, Kumar N, Panda G. Synthesis, biological evaluation, Structure − Activity relationship studies of quinoline-imidazole derivatives as potent antimalarial agents. Bioorgan Chem. 2022;121:105671. https://doi.org/10.1016/j.bioorg.2022.105671 .
Diaconu D, Antoci V, Mangalagiu V, Amariucai-Mantu D, Mangalagiu II. Quinoline–imidazole/benzimidazole derivatives as dual-/multi-targeting hybrids inhibitors with anticancer and antimicrobial activity. Sci Rep. 2022;12:16988. https://doi.org/10.1038/s41598-022-21435-6 .
Somashekara B, Vijayakumar GR. Synthesis, antioxidant and antibacterial activities of quinoline incorporated 2,4,5-trisubstituted imidazole derivatives. Indian J Chem -Section B. 2021;60. https://doi.org/10.56042/ijcb.v60i12.38865 .
Shobhashana PG, Prasad P, Kalola AG, Patel MP. Synthesis of imidazole derivatives bearing quinoline nucleus catalysed by can and their antimicrobial, antitubercular and molecular docking studies. Res J Life Sci Bioinform Pharm Chem Sci. 2018;4. https://doi.org/10.26479/2018.0403.15 .
Kondaparla S, Manhas A, Dola VR, Srivastava K, Puri SK, Katti SB. Design, synthesis and antiplasmodial activity of novel imidazole derivatives based on 7-chloro-4-aminoquinoline. Bioorgan Chem. 2018;80:204–11. https://doi.org/10.1016/j.bioorg.2018.06.012 .
Kardile RA, Sarkate AP, Lokwani DK, Tiwari SV, Azad R, Thopate SR. Design, synthesis, and biological evaluation of novel quinoline derivatives as small molecule mutant EGFR inhibitors targeting resistance in NSCLC: In vitro screening and ADME predictions. Eur J Med Chem. 2023;245:114889. https://doi.org/10.1016/j.ejmech.2022.114889 .
Kundu B, Das SK, Paul Chowdhuri S, Pal S, Sarkar D, Ghosh A, et al. Discovery and mechanistic study of tailor-made quinoline derivatives as topoisomerase 1 poison with potent anticancer activity. J Med Chem. 2019;62:3428–46. https://doi.org/10.1021/acs.jmedchem.8b01938 .
Xing J, Zhang R, Jiang X, Hu T, Wang X, Qiao G, et al. Rational design of 5-((1H-imidazol-1-yl)methyl)quinolin-8-ol derivatives as novel bromodomain-containing protein 4 inhibitors. Eur J Med Chem. 2019;163:281–94. https://doi.org/10.1016/j.ejmech.2018.11.018 .
Subashini G, Vidhya K, Arasakumar T, Angayarkanni J, Murugesh E, Saravanan A, et al. Quinoline‐based imidazole derivative as heme Oxygenase‐1 Inhibitor: A Strategy for Cancer Treatment. ChemistrySelect. 2018;3:3680–6. https://doi.org/10.1002/slct.201800173 .
Xiao Z, Lei F, Chen X, Wang X, Cao L, Ye K et al. Design, synthesis, and antitumor evaluation of quinoline‐imidazole derivatives. Archiv der Pharmazie. 2018;351. https://doi.org/10.1002/ardp.201700407 .
Azmi A, Noori M, Khalili Ghomi M, Nazari Montazer M, Iraji A, Dastyafteh N, et al. Alpha-glucosidase inhibitory and hypoglycemic effects of imidazole-bearing thioquinoline derivatives with different substituents: In silico, in vitro, and in vivo evaluations. Bioorganic Chem. 2024;144:107106. https://doi.org/10.1016/j.bioorg.2024.107106 .
Balandis B, Kavaliauskas P, Grybaitė B, Petraitis V, Petraitienė R, Naing E, et al. Synthesis of Novel Benzenesulfonamide-Bearing Functionalized Imidazole Derivatives as Novel Candidates Targeting Multidrug-Resistant Mycobacterium abscessus Complex. Microorganisms. 2023;11:935. https://doi.org/10.3390/microorganisms11040935 .
Pradhan S, Prasad R, Sinha C, Sen P. Molecular modeling of potent novel sulfonamide derivatives as non-peptide small molecule anti-COVID 19 agents. J Biomol Struct Dyn. 2021;40:7129–42. https://doi.org/10.1080/07391102.2021.1897043 .
Hu Y, Pan G, Yang Z, Li T, Wang J, Ansari MF, et al. Novel Schiff base-bridged multi-component sulfonamide imidazole hybrids as potentially highly selective DNA-targeting membrane active repressors against methicillin-resistant Staphylococcus aureus. Bioorganic Chem. 2021;107:104575. https://doi.org/10.1016/j.bioorg.2020.104575 .
Żołnowska B, Sławiński J, Belka M, Bączek T, Chojnacki J, Kawiak A. Novel 2-alkythio-4-chloro-N-[imino(heteroaryl)methyl]benzenesulfonamide Derivatives: Synthesis, Molecular Structure, Anticancer Activity and Metabolic Stability. Int J Mol Sci. 2023;24:9768. https://doi.org/10.3390/ijms24119768 .
Alghamdi EM, Alamshany ZM, El Hamd MA, Taher ES, Farrag El‐Behairy M, Norcott PL et al. Anticancer activities of tetrasubstituted imidazole‐pyrimidine‐sulfonamide hybrids as inhibitors of EGFR Mutants. ChemMedChem. 2023;18. https://doi.org/10.1002/cmdc.202200641 .
Ali EMH, Abdel-Maksoud MS, Ammar UM, Mersal KI, Ho Yoo K, Jooryeong P, et al. Design, synthesis, and biological evaluation of novel imidazole derivatives possessing terminal sulphonamides as potential BRAFV600Einhibitors. Bioorganic Chem. 2021;106:104508. https://doi.org/10.1016/j.bioorg.2020.104508 .
Maji M, Acharya S, Bhattacharya I, Gupta A, Mukherjee A. Effect of an Imidazole-Containing Schiff Base of an Aromatic Sulfonamide on the Cytotoxic Efficacy of N,N-Coordinated Half-Sandwich Ruthenium(II) p-Cymene Complexes. Inorg Chem. 2021;60:4744–54. https://doi.org/10.1021/acs.inorgchem.0c03706 .
Al-Wahaibi LH, Youssif BGM, Taher ES, Abdelazeem AH, Abdelhamid AA, Marzouk AA. Design, synthesis, biological evaluation, and computational studies of Novel Tri-Aryl Imidazole-Benzene Sulfonamide Hybrids as Promising Selective Carbonic Anhydrase IX and XII Inhibitors. Molecules. 2021;26:4718. https://doi.org/10.3390/molecules26164718 .
Taher ES, Marzouk AA, Abd‐Allah WH, Giovannuzzi S, Ibrahim TS, Supuran CT et al. Tailored Tetrasubstituted Imidazole Carrying the Benzenesulfonamide Fragments as Selective Human Carbonic Anhydrase IX/XII Inhibitors. ChemMedChem. 2024. https://doi.org/10.1002/cmdc.202400004 .
Dende SK, Korupolu RB, Leleti KR. Design and Synthesis of Sulfonamide‐Attached 2‐(Isoxazol‐3‐yl)‐1H‐imidazoles as Anticancer Agents. ChemistrySelect. 2020;5:7919–22. https://doi.org/10.1002/slct.202001449 .
Altaib M, Doganc F, Kaşkatepe B, Göker H. Synthesis of some new 2-(substituted-phenyl)imidazo[4,5-c] and [4,5-b]pyridine derivatives and their antimicrobial activities. Mol Divers. 2023. https://doi.org/10.1007/s11030-023-10715-6 .
Xu WB, Meng YQ, Sun J, Yang YX, Li WX, Wang MY, et al. Synthesis and Antibacterial Activity Evaluation of Imidazole Derivatives Containing 6‐Methylpyridine Moiety. Chem Biodivers. 2023;20:202300105. https://doi.org/10.1002/cbdv.202300105 .
Antoci V, Cucu D, Zbancioc G, Moldoveanu C, Mangalagiu V, Amariucai-Mantu D, et al. Bis-(imidazole/benzimidazole)-pyridine derivatives: synthesis, structure and antimycobacterial activity. Future Med Chem. 2020;12:207–22. https://doi.org/10.4155/fmc-2019-0063 .
Rejinthala S, Endoori S, Thumma V, Mondal T New Imidazo[4,5‐c]pyridine‐piperidine Hybrids as Potential Anti‐cancer Agents and In‐Silico Studies. ChemistrySelect. 2024;9. https://doi.org/10.1002/slct.202303299 .
Aruchamy B, Drago C, Russo V, Pitari GM, Ramani P, Aneesh TP, et al. Imidazole-pyridine hybrids as potent anti-cancer agents. Eur J Pharm Sci. 2023;180:106323. https://doi.org/10.1016/j.ejps.2022.106323 .
Ali EMH, Mersal KI, Ammar UM, Zaraei S-O, Abdel-Maksoud MS, El-Gamal MI, et al. Structural optimization of 4-(imidazol-5-yl)pyridine derivatives affords broad-spectrum anticancer agents with selective B-RAFV600E/p38α kinase inhibitory activity: synthesis, in vitro assays and in silico study. Eur J Pharm Sci. 2022;171:106115. https://doi.org/10.1016/j.ejps.2022.106115 .
Umar AB, Uzairu A, Shallangwa GA, Uba S. Computational evaluation of potent 2-(1H-imidazol-2-yl) pyridine derivatives as potential V600E-BRAF inhibitors. Egyptian J Med Human Genet. 2020;21:67. https://doi.org/10.1186/s43042-020-00111-2 .
Wu YZ, Ying HZ, Xu L, Cheng G, Chen J, Hu YZ et al. Design, synthesis, and molecular docking study of 3H‐imidazole[4,5‐c]pyridine derivatives as CDK2 inhibitors. Archiv der Pharmazie. 2018;351. https://doi.org/10.1002/ardp.201700381 .
Ali EMH, Abdel-Maksoud MS, Hassan RM, Mersal KI, Ammar UM, Se-In C, et al. Design, synthesis and anti-inflammatory activity of imidazol-5-yl pyridine derivatives as p38α/MAPK14 inhibitor. Bioorgan Med Chem. 2021;31:115969. https://doi.org/10.1016/j.bmc.2020.115969 .
Osmaniye D, Kaya Cavusoglu B, Saglik B, Levent S, Acar Cevik U, Atli O, et al. Synthesis and anticandidal activity of new imidazole-chalcones. Molecules. 2018;23:831. https://doi.org/10.3390/molecules23040831 .
Rahimzadeh Oskuei S, Mirzaei S, Reza Jafari-Nik M, Hadizadeh F, Eisvand F, Mosaffa F, et al. Design, synthesis and biological evaluation of novel imidazole-chalcone derivatives as potential anticancer agents and tubulin polymerization inhibitors. Bioorgan Chem. 2021;112:104904. https://doi.org/10.1016/j.bioorg.2021.104904 .
Sasidharan R, Baek SC, Sreedharannair Leelabaiamma M, Kim H, Mathew B. Imidazole bearing chalcones as a new class of monoamine oxidase inhibitors. Biomed Pharmacother. 2018;106:8–13. https://doi.org/10.1016/j.biopha.2018.06.064 .
Karaj E, Dlamini S, Koranne R, Sindi SH, Perera L, Taylor WR, et al. Pharmacophore optimization of imidazole chalcones to modulate microtubule dynamics. Bioorgan Chem. 2022;122:105700. https://doi.org/10.1016/j.bioorg.2022.105700 .
Holiyachi M, Samundeeswari S, Chougala BM, Naik NS, Madar J, Shastri LA, et al. Design and synthesis of coumarin–imidazole hybrid and phenyl-imidazoloacrylates as potent antimicrobial and antiinflammatory agents. Monatshefte für Chemie - Chemical Monthly. 2018;149:595–609. https://doi.org/10.1007/s00706-017-2079-5 .
Hu Y, Shen Y, Wu X, Tu X, Wang G-X. Synthesis and biological evaluation of coumarin derivatives containing imidazole skeleton as potential antibacterial agents. Eur J Med Chem. 2018;143:958–69. https://doi.org/10.1016/j.ejmech.2017.11.100 .
Ashok D, Ramakrishna K, Nagaraju N, Reddy MR, Dharavath R, Sarasija M. Microwave-Assisted Synthesis of Substituted 2-(2H-Chromen-3-yl)-5-phenyl-1H-imidazole Based Coumarin Derivatives and Their Antimicrobial Activity. Russ J Gen Chem. 2021;91:711–6. https://doi.org/10.1134/s1070363221040216 .
Almansour AI, Arumugam N, Kumar RS, Kotresha D, Manohar TS, Venketesh S. Design, synthesis and cholinesterase inhibitory activity of novel spiropyrrolidine tethered imidazole heterocyclic hybrids. Bioorgan Med Chem Lett. 2020;30:126789. https://doi.org/10.1016/j.bmcl.2019.126789 .
Zhang M, Ding Y, Qin H-X, Xu Z-G, Lan H-T, Yang D-L, et al. One-pot synthesis of substituted pyrrole–imidazole derivatives with anticancer activity. Mol Divers. 2019;24:1177–84. https://doi.org/10.1007/s11030-019-09982-z .
Poyraz S, Döndaş HA, Sansano JM, Belveren S, Yamali C, Ülger M, et al. N-Benzoylthiourea-pyrrolidine carboxylic acid derivatives bearing an imidazole moiety: Synthesis, characterization, crystal structure, in vitro ChEs inhibition, and antituberculosis, antibacterial, antifungal studies. J Mol Struct. 2023;1273:134303. https://doi.org/10.1016/j.molstruc.2022.134303 .
Download references
Author information
Authors and affiliations.
Department of Analytical Chemistry, Mersin University, Faculty of Pharmacy, Mersin, Türkiye
Samet Poyraz
Department of Biochemistry, Harran University, Faculty of Pharmacy, Sanliurfa, Türkiye
Metin Yıldırım
Department of Chemistry, Cukurova University, Faculty of Art and Science, Adana, Türkiye
Mehmet Ersatir
You can also search for this author in PubMed Google Scholar
Corresponding author
Correspondence to Metin Yıldırım .
Ethics declarations
Conflict of interest.
The authors declare no competing interests.
Additional information
Publisher’s note Springer Nature remains neutral with regard to jurisdictional claims in published maps and institutional affiliations.
Rights and permissions
Springer Nature or its licensor (e.g. a society or other partner) holds exclusive rights to this article under a publishing agreement with the author(s) or other rightsholder(s); author self-archiving of the accepted manuscript version of this article is solely governed by the terms of such publishing agreement and applicable law.
Reprints and permissions
About this article
Poyraz, S., Yıldırım, M. & Ersatir, M. Recent pharmacological insights about imidazole hybrids: a comprehensive review. Med Chem Res (2024). https://doi.org/10.1007/s00044-024-03230-2
Download citation
Received : 13 February 2024
Accepted : 22 April 2024
Published : 13 May 2024
DOI : https://doi.org/10.1007/s00044-024-03230-2
Share this article
Anyone you share the following link with will be able to read this content:
Sorry, a shareable link is not currently available for this article.
Provided by the Springer Nature SharedIt content-sharing initiative
- Imidazole derivatives
- Hybrid molecules
- Pharmacological activity
- Molecular docking
- Structure-activity relationship (SAR)
- Find a journal
- Publish with us
- Track your research

IMAGES
VIDEO
COMMENTS
This review aims to shed light on the recent developments of coumarin-based anticancer hybrid derivatives and their Structure-Activity Relationships (SAR). This review serves as a medium that medicinal chemists could utilize to design and synthesize coumarin derivatives with significant pharmacological value as future anticancer agents.
Request PDF | Recent Literature Review on Coumarin Hybrids as Potential Anticancer Agents | Cancer is considered as one of the leading causes of death in the world, especially patients with lung ...
This review article covers the recent developments of coumarin derivatives that are biologically active against various cancer cell lines. ... In some literature, Coumarin based benzamide 39 as the best HDAC inhibitor ... Design synthesis and biological evaluation of coumarin-chalcone hybrids as potential anticancer agents. Bioorg. Chem., 95 ...
This review aims to shed light on the recent developments of coumarin-based anticancer hybrid derivatives and their Structure-Activity Relationships (SAR). This review serves as a medium that medicinal chemists could utilize to design and synthesize coumarin derivatives with significant pharmacological value as future anticancer agents.
This review offers a recent, thorough literature compilation of contemporary research on the development of hybrid compounds based on coumarin-1,2,3-triazoles as potential anticancer leads throughout the previous 10 years. ... S., and Dadashpour, S. (2015). Current developments of coumarin-based anti-cancer agents in medicinal chemistry. Eur ...
This review sheds light on the recent developments on coumarin based anticancer hybrid derivatives and their structure-activity relationships (SAR) and serves as a medium that medicinal chemists could utilise for the design and synthesis of coumarIn derivatives with significant pharmacological value as future anticancer agents. Cancer is considered as one of the leading causes of death in the ...
The purpose of this review article is to provide an emphasis on the recent developments of coumarin-containing hybrids as potential anticancer agents with insights into their structure-activity relationship (SAR) and action mechanisms, covering articles published between 2015 and 2019. 2. Coumarin-artemisinin hybrids.
Title:Recent Literature Review on Coumarin Hybrids as Potential Anticancer Agents Volume: 23 Issue: 2 Author(s): Baji Baba Shaik, Naresh Kumar Katari*, Pule Seboletswe, Rambabu Gundla, Narva Deshwar Kushwaha, Vishal Kumar, Parvesh Singh, Rajshekhar Karpoormath and Muhammad D. Bala*
In this review, we attempted to cover the biological and pharmacological properties of coumarin hybrid compounds synthesized in recent years (from 2015 to 2023). This study is expected to encourage scientists about the need for new potential bioactive coumarin hybrids to overcome challen-ging health disorders.
Coumarin hybrids have been designed and synthesized as an important new strategy in the field of medicinal chemistry. Coumarin core has been shown to form various compounds to combat several diseases including microbial, cancer, inflammatory, and neurodegenerative disorders. Recently, a number of coumarin-based drugs (e.g., coumadin, acenocoumarol, dicoumarol, phenprocoumon, and novobiocin ...
Coumarin hybrids have been successfully used as potential anticancer agents and many international patents have been filed in this regard. In 2015, some triazole-modified coumarin-based compounds ( 148 ) were patented, which were proposed to be used in the treatment of various cancers such as breast and prostate cancer. 242 The general ...
This review aims to shed light on the recent developments of coumarin-based anticancer hybrid derivatives and their Structure-Activity Relationships (SAR). This review serves as a medium that medicinal chemists could utilize to design and synthesize coumarin derivatives with significant pharmacological value as future anticancer agents. Keywords:
This review aims to summarize the recent advances made towards the development of coumarin-containing hybrids as potential anticancer agents, covering articles published between 2015 and 2019, and ...
Coumarin-1,2,3-triazole hybrids as leading-edge anticancer agents. This review offers a recent, thorough literature compilation of contemporary research on the development of hybrid compounds based on coumarin-1,2,3-triazoles as potential anticancer leads throughout the previous 10 years. Expand.
This review will focus on the recent development of coumarin hybrids as potential anticancer agents covering articles published from 2019 to 2020. Cancer can invade or spread to almost all parts of the body. The increasing morbidity and high mortality of cancer create a great demand for the development of novel anticancer drugs. Coumarin derivatives are ubiquitous in nature and can readily ...
These, among other reasons, are why coumarin moieties will be the basis of a good building block for the development of potent anti-HIV agents. This review aims to outline the synthetic pathways, structure-activity relationship (SAR) and POM analyses of coumarin hybrids with anti-HIV activity, detailing articles published between 2000 and 2023.
The progress in the field of cytotoxic compounds based on coumarin-pyrazoline hybrids is presented in this literature review. Most of these hybrids have been obtained via ring closure of the pyrazoline moiety through the reaction of coumarin-containing chalcone analogs with hydrazine and its derivatives. A small number of coumarin-pyrazoline hybrids have been also prepared through ...
Cancer is considered one of the leading causes of death globally, especially patients with lung, pancreatic, or brain tumors are most likely to die of cancer, and patients with prostate and breast cancer are at a high risk of noncancer death. As a result, there is ongoing research regarding developing new, safe, and efficient anticancer agents. Coumarin-based naturally occurring compounds ...
This review offers a recent, thorough literature compilation of contemporary research on the development of hybrid compounds based on coumarin-1,2,3-triazoles as potential anticancer leads throughout the previous 10 years. Cancer is one of the most feared and dreaded diseases across the world. In clinical practice, a variety of anticancer agents of natural, semi-synthetic and synthetic origin ...
Hence, this review is a comprehensive report on hybrid compounds containing carvacrol and its isomer, thymol, with potent anticancer and antibacterial agents reported between 2020 and 2024. Furthermore, their structural activity relationship (SAR) and recommended future strategies to further enhance their therapeutic effects will be discussed.
Coumarin-benzimidazole hybrids: A review of developments in medicinal chemistry. ... 1,3,4-Oxadiazole-containing hybrids as potential anticancer agents: Recent developments, mechanism of action and structure-activity relationships ... AI-powered research tool for scientific literature, based at the Allen Institute for AI. Learn More.
Based on the acquired data, compounds 7a-e were considered to be potentially effective anticancer agents. Devi et al. designed and synthesized naphtho[2,3-d]imidazole-4,9-dione 1,2,3-triazole hybrids 8 (Fig. 2) as α-amylase enzyme inhibitors and potential antioxidant agents. The α-amylase activity of the synthesized molecules was assessed by ...