Advertisement

Key questions for understanding drivers of biodiversity-ecosystem service relationships across spatial scales
- Perspective
- Open access
- Published: 14 February 2024
- Volume 39 , article number 36 , ( 2024 )
Cite this article
You have full access to this open access article
- Matthew G. E. Mitchell ORCID: orcid.org/0000-0002-3490-9314 1 ,
- Jiangxiao Qiu 2 ,
- Bradley J. Cardinale 3 ,
- Kai M. A. Chan ORCID: orcid.org/0000-0002-7804-3276 4 ,
- Felix Eigenbrod 5 ,
- María R. Felipe-Lucia 6 ,
- Aerin L. Jacob 7 , 8 ,
- Matthew S. Jones 9 &
- Laura J. Sonter 10
1945 Accesses
1 Altmetric
Explore all metrics
Biodiversity loss is predicted to have significant impacts on ecosystem services based on previous ecological work at small spatial and temporal scales. However, scaling up understanding of biodiversity-ecosystem service (BES) relationships to broader scales is difficult since ecosystem services emerge from complex interactions between ecosystems, people, and technology.
In order to inform and direct future BES research, identify and categorise the ecological and social-ecological drivers operating at different spatial scales that could strengthen or weaken BES relationships.
We developed a conceptual framework to understand the potential drivers across spatial scales that could affect BES relationships and then categorized these drivers to synthesize the current state of knowledge.
Our conceptual framework identifies ecological/supply-side and social-ecological/demand-side drivers, and cross-scale interactions that influence BES relationships at different scales. Different combinations of these drivers in different contexts will lead to a variety of strengths, shape, and directionality in BES relationships across spatial scales.
Conclusions
We put forward four predictions about the spatial scales that the effects of biodiversity, ecosystem service management, ecosystem co-production, and abiotic linkages or effects will be most evident on BES relationships and use these to propose future directions to best advance BES research across scales.
Similar content being viewed by others
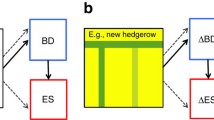
Disaggregating the evidence linking biodiversity and ecosystem services
Establishment of a comprehensive indicator system for the assessment of biodiversity and ecosystem services, how does habitat fragmentation affect the biodiversity and ecosystem functioning relationship.
Avoid common mistakes on your manuscript.
Introduction
Earth’s ecosystems and species are currently undergoing a mass extinction event due to human activities (IPBES 2019 ), with many ecosystem services crucial for human well-being also being degraded or lost, often due to the same drivers (e.g., land-use change, overexploitation, climate change; (IPBES 2019 )). While there is evidence that biodiversity loss can impact the provision of many ecosystem services (Balvanera et al. 2006 ; Cardinale et al. 2012 ; Bennett et al. 2015 ; IPBES 2019 ), it is not always the primary driver (Srivastava and Vellend 2005 ). This is because biodiversity-ecosystem service (BES) relationships depend on both ecological and socioecological processes that operate and interact across spatial and temporal scales (Scholes et al. 2013 ; Provost et al. 2023 ). Therefore, the scales at which biodiversity effects are the strongest or most evident for a specific ecosystem service may be different from the scales at which the effects of human activities on that same ecosystem service are strongest. Currently, it is unclear how the importance of these ecological and social drivers vary across scales and impact observed BES relationships. This is a key and urgent knowledge gap both fundamentally but also for conservation actions, especially since policies intended to simultaneously safeguard biodiversity and ecosystem services (e.g., UN Sustainable Development Goals; Kunming-Montreal Global Biodiversity Framework) are applied across multiple spatial scales and jurisdictional boundaries.
Foundational experimental and theoretical work on the mechanistic links between biodiversity and ecosystem functioning (BEF) has provided strong evidence that increasing diversity (usually species richness (Balvanera et al. 2014 ) but increasingly evenness, functional, or trait diversity (Díaz et al. 2007 ; Lavorel et al. 2013 ; Gross et al. 2017 ) – is required to provide increasing numbers or levels of ecosystem functions (Isbell et al. 2011 ; Tilman et al. 2014 ; O’Connor et al. 2017 ). Mechanistically, there are several biological processes (e.g., niche complementarity, selection effects/competition, facilitation, or mass ratio effects (Tilman et al. 2014 )) that lead to a greater efficiency in the capture of resources, biomass production, and decomposition and recycling of nutrients as diversity increases (Loreau 2010 ). In turn, these increases in functions can presumably result in greater ecosystem service supply and multifunctionality (Soliveres et al. 2016 ; Hautier et al. 2018 ; Provost et al. 2023 ). However, most of this research has been conducted using small-scale experiments spatially (e.g., 1-100 m 2 )(Gonzalez et al. 2020 ; Qiu and Cardinale 2020 ). Additionally, over 30 years of research in landscape ecology have shown the critical importance of spatial scaling and the influences of spatial scale on fluxes of organisms, materials and energy across the landscape (Wiens and Milne 1989 ), on feedbacks and interactions across systems (Peters et al. 2004 ) and on hierarchies of scale in understanding dynamics of landscapes (O’Neill et al. 1989 ). Consequently, it is clear that spatial scale is likely to affect BEF relationships, which has led to increasing attention both conceptually and empirically.
Over the past two decades, a growing number of BEF studies have begun to investigate whether and how biodiversity effects vary (i.e., amplify, weaken, or remain relatively constant) in ecosystems across a range of spatial scales, and the extent to which knowledge from small-scale experiments can be extrapolated and scaled up to inform conservation at landscape scales (Isbell et al. 2017 ; Gonzalez et al. 2020 ). For example, new empirical studies and syntheses at broader spatial scales (e.g., ecosystems and landscapes) have explored how biodiversity affects ecosystem functioning, with a particular focus on biomass production in larger and more natural systems (e.g., forests, grasslands, marine) (Grace et al. 2007 ; Mora et al. 2011 ; Duffy et al. 2017 ; Felipe-Lucia et al. 2020 ; Provost et al. 2023 ). These studies have revealed that after statistically controlling for confounding abiotic factors, biodiversity effects are common in nature and likely more pronounced in real landscapes than previously reported from controlled experiments (Duffy et al. 2017 ). Nevertheless, these studies thus far have mainly focused on how biodiversity affects biological control or how biomass impacts crop yield, carbon sequestration, and fodder yield (Cardinale et al. 2012 ) and generally fail to incorporate social-ecological dynamics, although this is starting to be addressed (e.g., Provost et al. 2023 ).
While the research described above has focused primarily on ecological processes, ecosystem service provision across scales can also be impacted by social drivers (e.g. demand/preferences), anthropogenic capitals (e.g., technologies, human and social capital), and more (e.g., management actions, investments). For example, food provision from croplands often depends on synthetic inputs and mechanisation at the farm scale that, in turn, can alter the relationships between food production and biodiversity at landscape scales (Provost et al. 2023 ). Similarly, recreation at the local scale often requires accessible infrastructure (trails, parking), or land use change that can in some cases lead to biodiversity loss at both local and regional scales (Miller et al. 2022 ; Virtanen et al. 2023 ). Thus, as the scale of observation varies, changes in the relative importance of ecosystem service supply and demand could produce more variable outcomes for observed BES relationships. One potential outcome is that the direct effects of biodiversity on ecosystem service provision might become less straightforward and more difficult to observe and quantify at broad spatial scales (Balvanera et al. 2014 ; Ricketts et al. 2016 ).
Consequently, different from BEF work, understanding BES relationships across scales is almost certainly complex because: (1) the different processes by which biodiversity affects different ecosystem services are likely service- (Balvanera et al. 2014 ) and scale-dependent (Gonzalez et al. 2020 ); and (2) other capitals (e.g., human and social capitals), in addition to natural capital, also play an important role in ecosystem service provision at different scales (Palomo et al. 2016 ; Barraclough et al. 2022 ) and can also influence the effects of biodiversity on specific services. Thus, across spatial scales it currently remains substantially challenging to predict which ecological, social, or socio-ecological processes are operating or are most important for determining BES relationships for different ecosystem services.
Here, in this Perspective paper, we develop a conceptual framework to help identify and categorise the different ecological and social-ecological drivers operating at different spatial scales, or across scales, that might strengthen or weaken BES relationships. Changes in spatial scales involve both extent and resolution; here we include both but emphasise changes in spatial extent. Based on our framework, we then synthesize the literature to understand what the current state of knowledge is around drivers of BES relationships across scales in order to identify knowledge gaps. Our paper does not intend to provide an exhaustive list of drivers or a full and complete literature review of the field, nor is our framework capable of capturing all possibilities, given the social-ecological complexities as previously elaborated. Instead, we use the framework to help formulate hypotheses and identify research questions that we believe will help inform future research needs to improve understanding of BES relationships across scales.
Conceptual framework of BES relationships across spatial scales
We present our heuristic conceptual framework in Fig. 1 . Following the ecosystem service cascade model (Potschin and Haines-Young 2011 ), species ( S1 , S2 , etc.) that makeup a community or ecosystem contribute to ecosystem functions ( F1 , F2 ) that produce an ecosystem service ( ES1 , Fig. 1 Box A). As spatial scale (e.g., the scale of observation) increases, observed environmental heterogeneity is assumed to increase, leading to more niche opportunities and increased species observed at broader scales (Fig. 1 Box B). Following an ecological model of service provision, these additional species contribute to greater ecosystem function ( F1 ) and additional functions ( F2 ), and thus increased ecosystem service provision becomes apparent (Fig. 1 Box B, Arrow 1 ). In our example, this broader scale is therefore more appropriate for evaluating this specific BES relationship since it encompasses the complete suite of species and functions that contribute to ecosystem service provision. As a result of these ecological dynamics, observed relationships between spatial scale and the strength or importance of biodiversity on ecosystem service provision will increase and saturate as spatial scale increases (Fig. 1 Box C), but will also vary in shape and strength across locations or contexts (different dashed lines) due to the level and scale of environmental heterogeneity in the system, the amount of functional redundancy between the species present, stochasticity in community assembly, as well as the strength and directions of species interactions, complementarity, or sampling effects (Fig. 1 Box B, Arrow 2 ).
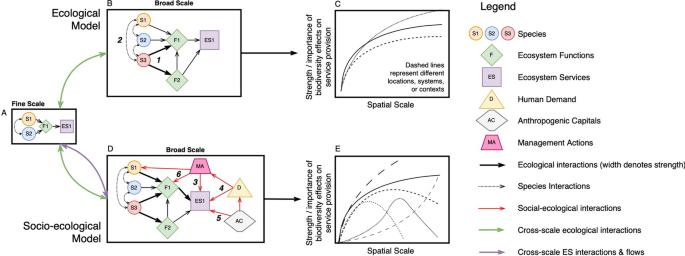
Potential pathways and relationships between biodiversity and ecosystem service provision with increasing spatial scale, considering either a simple ecological (top row) or more complex socio-ecological view (bottom row) of ecosystem service provision (see “ Conceptual Framework ” section for full details). For simplicity we consider only two functions and one service while in reality numerous functions contribute to and interact to provide multiple services. We have also excluded effects of abiotic variables on functions and services. We also focus on one potential driver (increasing extent of observation increases the number of niche opportunities and allows additional species to contribute to a function that leads to an ecosystem service) and do not include other potential drivers (e.g., changes in population sizes, reduced selection effects, changes in relative impacts of biodiversity versus abiotic drivers)
However, when a more socio-ecological model of ecosystem service provision is considered that incorporates human demand, management actions and anthropogenic capitals (Fig. 1 Box D), BES relationships across scales could vary even more substantially (Fig. 1 Box E). This arises because in addition to ecological effects across scales, changes in management actions ( MA ) to increase a focal service (Fig. 1 Box D, Arrow 3 ), changes in human demand ( D ) for specific ecosystem services (Fig. 1 Box D, Arrow 4 ), and changes in anthropogenic capitals ( AC – human, social, physical, and financial capitals; Fig. 1 Box D, Arrow 5 ) that in turn influence human demand for services, management actions, and ultimately ecosystem service provision, can change. In particular, management actions can themselves impact species (e.g., population sizes) or ecosystem functions (Fig. 1 Box D, Arrow 6 ), therefore influencing service provision. Given these more complex socio-ecological interactions across spatial scales, we predict that this will lead to more varied relationships between biodiversity and ecosystem services across spatial scales, including both stronger and weaker relationships, or ones where BES relationships approach zero, at broad spatial scales (Fig. 1 Box E).
In addition to ecological and socio-ecological interactions, cross-scale interactions (Fig. 1 ) are also likely to impact BES relationships. Cross-scale interactions are defined as synergistic and/or antagonistic interactions among multiple environmental drivers across multiple spatial scales (Darling and Côté 2008 ). Cross-scale interactions can be driven by: (1) effects of biophysical connections; (2) effects of dominant abiotic, land use, or management drivers; (3) combined effects of biophysical linkages and dominant drivers; and (4) artificial scale influences (Qiu et al. 2018 ). These cross-scale interactions can lead to positive or negative relationships between ecosystem services (Bennett et al. 2009 ), and likely a variety of context-dependent effects of management actions and biodiversity on ecosystem service provision across scales (Spake et al. 2019 ). For example, intensive agriculture practices are a management action that leads to increased local or field-level food provision but negatively affects water quality at broader watershed scales downstream (Rodríguez et al. 2006 ). Similarly, competing land uses (e.g., agriculture versus working forests versus conservation areas) provide different suites of services at different scales (Felipe-Lucia et al. 2014 ; Dade et al. 2019 ). Further, the effect of common drivers of services may be context-specific due to cross-scale interactions, both in terms of identity and strength. For example, in some parts of England increasing forest cover by planting trees can increase risk of invasive species colonisation as new forests increase connectivity while in other locations this is not the case (Spake et al. 2019 ).
From our conceptual framework what arises is the existence of a wide variety of different and distinct pathways and drivers that could affect BES relationships across scales, especially when the full suite of socio-ecological drivers and dynamics are considered (Fig. 1 Boxes D & E). In the next section of the paper, we attempt to provide an overview of what is currently known about these pathways at single scales and across scales.
Ecological or supply-side pathways
Current understanding of species-area relationships (SAR) predicts that the total number of species (species richness) encountered will increase as the extent or area of observation increases (Turner and Tjørve 2005 ). How might this impact BES relationships across spatial scales? In this section we identify several possible ecological or ecosystem service supply-side pathways that link biodiversity to service provision and which likely vary across spatial scales (Table 1 ).
- Species complementarity
Species differ in their use of limiting resources, thereby reducing interspecific relative to intraspecific competition (Tilman 1999 ). As the observed spatial scale increases, environmental heterogeneity and niche variety also tend to increase, leading to increasing total numbers of species, higher overall ecosystem functioning (Cardinale et al. 2004 ; Tscharntke et al. 2005 ; Reich et al. 2012 ; Thompson et al. 2018 ) and potentially increased service provision underpinned by these functions. Species complementarity (Fig. 1 Box B) is predicted to be especially important for productivity-underpinned services and has been observed at singles scales for forage, bioenergy, timber production (Gamfeldt et al. 2013 ; Isbell et al. 2018 ), and other services such as pest control (Dainese et al. 2017 ). While changes in the strength or importance of complementarity for services across scales have not been fully explored (Dimitrakopoulos and Schmid 2004 ; Qiu and Cardinale 2020 ), stronger effects at broader spatial scales (e.g. ecological community and regional scales) have been found for marine biomass (Bracken et al. 2017 ) and forest biomass production (Luo et al. 2019 ).
Sampling or species identity effects
Sampling or species identify effects refer to the fact that more diverse communities have a higher probability of including species with particularly effective functional traits that enable competitive dominance in the community (Cardinale et al. 2004 ), thus enhancing overall ecosystem functioning (Hector et al. 2002 ; Slade et al. 2019 ). Hence, as scale of observation increases and more species are encountered, there is an increased chance that the species disproportionately important for the ecosystem functions that underlie an ecosystem service will be present (Fig. 1 Box B Arrow 1). Empirical examples of the importance of specific species at single scales include pollination services (i.e., existence of particularly effective pollinators) (Albor et al. 2019 ), and recreational services (i.e., existence of iconic and/or rare species) (Dee et al. 2019 ; Lavorel et al. 2020 ). Across spatial scales, one example involves disease regulation (i.e., existence or loss of important host species (Ostfeld and LoGiudice 2003 ).
Contrastingly, some ecosystem services in specific contexts are related to individual species, often those that are most abundant or that are the most functionally important for a specific ecosystem service. For example, the abundance of common and dominant pollinator species, rather than pollinator richness overall, can be most important for pollination delivery (Winfree et al. 2015 ); monocultures of specific tree species can maximise individual services (Himes et al. 2020 ), although not multiple services at the same time (Gamfeldt et al. 2013 ); and for many cultural or regulating services individual or small groups of species are key (Bastian 2013 ). Thus, as the spatial scale of observation increases and environmental heterogeneity and biodiversity increase (Stein et al. 2014 ), while rare species may be encountered driving increased species richness, these rare species may not contribute significantly to ecosystem service provision (Winfree et al. 2015 ). Thus, BES relationships may not change significantly across spatial scales or may instead vary depending on the exact species-abundance variation that occurs across scales.
Species interactions
Species interactions (i.e., competition or facilitation) can alter the efficiency by which ecological communities utilise resources and/or the total magnitude of resource use (Bruno et al. 2003 ). As broader scales contain greater numbers of species, this could lead to changes in the relative strength or direction of species interactions with both positive and negative impacts for service provision (Fig. 1 Box B Arrow 2 ). For example, increasing the scale of observation could lead to increased probability of encountering positive species interactions that enhance ecosystem functions and associated services, leading to stronger positive BES relationships. Examples of positive species interactions occurring at greater spatial scales include oyster reefs and associated aquaculture production and wave attenuation services (Reeves et al. 2020 ), plant-plant facilitation for coastal wetlands and their services (Zhang and Shao 2013 ), and positive interactions between frugivorous birds for seed dispersal (García and Martínez 2012 ). Conversely, relative abundances of species and the strength of competitive interactions between species will also vary across spatial scales, which can also influence service provision and potentially weaken BES relationships. For example, if combinations of species with strong interspecific competition are more likely to be encountered at broader scales, this could reduce the per capita contribution of each species to ecosystem functioning and ultimately the provision of individual services (Slade et al. 2019 ). Further, while increased diversity at broader scales could increase overall service multifunctionality, it could also lead to decreased provision of individual services (Fanin et al. 2018 ) and thus weaken individual BES relationships.
Abiotic conditions
While biodiversity can contribute positively to the ecosystem functions that underlie ecosystem services, at the same time other environmental drivers also impact ecosystem services. For example, both abiotic drivers (e.g., rainfall and temperature) and biotic drivers (e.g., productivity) are known to impact soil organic carbon and climate regulation (Gaitán et al. 2019 ). A recent review of biodiversity impacts on ecosystem functioning (Plas 2019 ) found that abiotic drivers were more important than biodiversity effects for 66% of the cases examined, although this depended on the function or service in question and requires additional empirical evidence. Yet, in other cases biodiversity has been shown to be equal to or stronger than other abiotic variables (Paquette and Messier 2011 ; Hooper et al. 2012 ). In those cases where abiotic conditions vary more than levels of biodiversity at broader spatial scales and these abiotic conditions also strongly impact service provision, this could weaken BES relationships at larger spatial scales. At the same time, abiotic conditions and species diversity are often highly correlated (Stein et al. 2014 ), and therefore separating abiotic effects from other ecological effects is likely difficult. Therefore, strong BES relationships may not always indicate that species diversity is the strongest driver of service provision.
Cross-scale ecological interactions
Some of the ecological processes relevant to one ecosystem service that are positively influenced by species diversity can themselves have impacts on additional ecosystem functions at different spatial scales that underlie other services (Fig. 1 Cross-scale ecological interaction arrows). Thus, as processes such as species complementarity or sampling effects become stronger at broader spatial scales, cross-scale ecological feedbacks could strengthen BES relationships and lead to synergies (e.g., positive relationships) between different ecosystem services. For example, increased species diversity can increase primary production by vegetation (see Species Complementarity above) and subsequently soil organic carbon and climate regulation. In turn, higher soil organic carbon, by increasing soil fertility and soil water-holding capacity, can create a positive feedback loop with species richness and productivity, especially at broader regional or biome spatial scales (Chen et al. 2018 ). Another example relates to landscape structure and the presence of interspersed or connected patches of habitat more capable of supporting populations of mobile organisms and the ecosystem services they provide (Kremen et al. 2007 ; Riva and Fahrig 2023 ). Thus, at fine scales there may be negative relationships between food production and other services provided by natural habitats (pollination, pest control, recreation), but these may shift to positive relationships at landscape or regional scales as natural habitats provide connectivity and resilience to the ecological processes that underlie food production (Tscharntke et al. 2005 ; Dainese et al. 2019 ).
Socio-ecological or demand-side pathways
Ecosystem services emerge from complex interactions between people and nature, involving numerous pathways and inputs, both natural and anthropogenic, that can impact final service provision. This complexity and diversity in ecosystem service pathways, which occur across spatial scales, can result in varied BES relationships, and in many cases (but not all) decouple ecosystem service provision from biodiversity change at broader spatial scales. However, for many of the examples below there is, as of yet, little existing empirical work to support the dynamics we propose.
- Ecosystem service demand
Ecosystem service provision not only depends on the capacity of ecosystems to supply a service, but also on the level of demand for that service by people (Villamagna et al. 2013 ; Mitchell et al. 2015 ). At what scale demand is generated for different services can vary because the scale at which the relevant social-ecological interaction or stakeholder/organisation/institution operates and initiates management actions also vary (Hein et al. 2006 ; Geijzendorffer and Roche 2014 ; Lindborg et al. 2017 ). For example, demand for pollination is generated at the scale of agricultural fields or landscapes, for wild medicinal plants at the level of individuals or institutions, whereas demand for outdoor recreation often occurs at regional scales (Geijzendorffer and Roche 2014 ). Thus, incorporating demand into the quantification of ecosystem services across scales has the potential to significantly alter BES relationships (Fig. 1 . Box D Arrow 4 ). In particular, if the biodiversity or species most related to the provision of a particular ecosystem service increase across spatial scales whereas relative demand for that service decreases, this could lead to negative BES relationships at broader landscape spatial scales (Watson et al. 2019 ). For example, demand at the field or farm scale for agriculturally-relevant services like pollination or pest control might be high, leading to positive BES relationships (Schulp et al. 2014 ). However, as spatial scale increases, a shift from agricultural- to forest-dominated land cover could occur, leading to lower overall demand and provision for these two services at landscape scales despite potentially increased pollinator diversity at this scale.
- Ecosystem service co-production
Similar but distinct to changes in ecosystem service demand across scales, the nature of the co-production of services across scales can also shift and change (Fig. 1 Box D Arrow 5 ). Ecosystem services depend on a number of capitals, including natural, human, social, physical/manufactured, and economic capitals (Palomo et al. 2016 ). For example, agricultural production depends on soil fertility and primary production (natural capitals), as well as farming infrastructure and technology (manufactured capital), farming knowledge (human capital), and the institutions that support and govern agriculture (social capital). However, the contribution of these different capitals can shift across scales, for example moving from service provision being determined predominantly by natural capital and biodiversity, to being influenced to a greater degree by human or manufactured capitals (Kachler et al. 2023 ). For example, at local scales communities can depend on local fisheries as their food source, whose abundance is influenced by the diversity of fish (Stuart-Smith et al. 2013 ) and local knowledge of fishing techniques and stewardship, whereas at larger scales (e.g., metropolitan or continental scales), people may shift towards dependence on supplies from more remote aquaculture or fish farm infrastructures (Deutsch et al. 2007 ) as trade networks facilitate access to more distant ecosystems. Thus, shifts away from natural capital contributions across these zones could weaken the link between biodiversity and ecosystem service provision.
Cross-scale ecosystem service flows
Ecosystem services are not always produced in situ, but instead can be supplied in one location and then deliver benefits in another via complex ecosystem service flows (Fig. 1 Cross-scale ES interactions & flows) between areas of service supply and use (Costanza 2008 ; Mitchell et al. 2021 ). For example, regulation of freshwater amounts and quality often occur in upstream watersheds or ecosystems while the benefits are delivered to downstream beneficiaries (Brauman et al. 2007 ; Bagstad et al. 2014 ). Because of this, the biodiversity present in the location where a particular ecosystem service is being used or delivered to beneficiaries may not be directly influencing service provision due to such flows, in which case strong BES relationships may not be detected at fine scales. In other cases, as the spatial scale of the study increases (e.g., to the scale of watersheds or ‘servicesheds’), these BES relationships will become apparent. For example, relationships exist between prairie potholes, waterfowl populations, and the recreational services of bird watching and hunting at broad scales but not fine scales (Bagstad et al. 2019 ). Further, different spatial flows and ecosystem services dynamics can be evident across spatial scales (Andersson et al. 2015 ). For example, the cultural benefits of monarch butterflies in the USA and Canada are provided at a broad scale by overwintering habitat in Mexico, but also at finer scales between rural areas and urban residents (Semmens et al. 2018 ). Unfortunately, consistent methods to quantify these flows (Koellner et al. 2019 ; Chalkiadakis et al. 2022 ) as well as specific studies investigating the scale of ecosystem service flow and BES relationships are rare.
Four approaches to advance knowledge on BES relationships across spatial scales
From our review above, it is evident that a variety of ecological and socio-ecological drivers exist that could either strengthen or weaken BES relationships across scales. So how best to move forward and understand BES relationships? Here, we briefly introduce four propositions that could be used as a starting point to better understand the scale-dependent dynamics of BES relationships and use these to formulate hypotheses, design empirical investigations, and develop predictions about the spatial and conceptual scales at which different processes will be most important for BES relationships (Fig. 2 ).
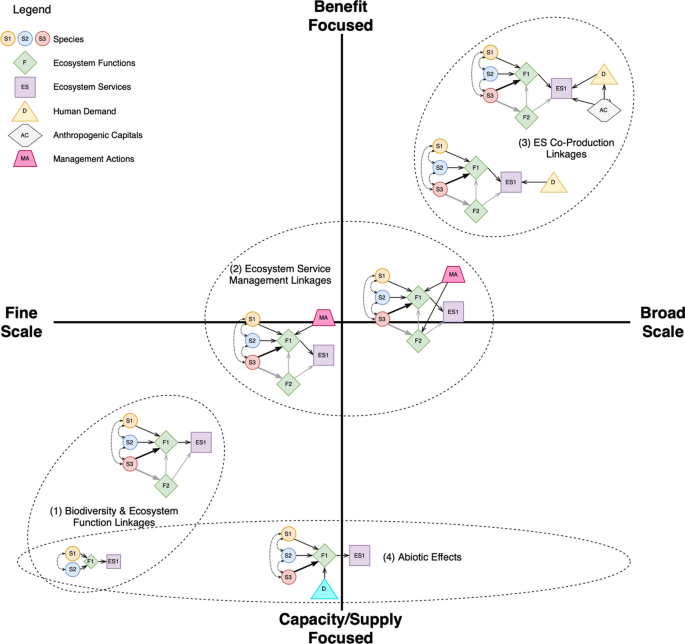
Predicted two-dimensional conceptual scales at which different ecosystem service dynamics will be most important for influencing BES relationships across scales. We expect that (1) ecological linkages involving species and ecosystem functions which generally strengthen BES relationships will be most important at fine spatial scales and when studies focus on service capacity or supply, (2) human management actions will be most important at intermediate spatial and conceptual scales, and (3) that ecosystem service co-production and shifts in human demand (D) for specific services will be most important at broad scales and when studies focus on measuring actual ecosystem service benefits. We also expect abiotic effects (AB) to be most important at a variety of spatial scales but mainly when the focus is on the capacity/supply of services
Proposition 1
Ecological BES linkages or drivers will have the greatest importance on ecosystem service supply at fine spatial scales. It is at these fine scales where abiotic conditions and social-ecological contexts are relatively uniform with less spatial heterogeneity across sample locations, allowing ecological processes to dominate and alter the supply of ecosystem services. Thus, we predict that ecological drivers including complementarity, sampling effects, and species interactions are more likely to be strongest at fine spatial scales (e.g., plots, fields, patches) and also when the nature of ES quantification is supply/capacity-focused (e.g., biomass, productivity, nutrient cycling). As spatial scale increases and other variables important to service provision such as human demand and human capitals vary, or when measures of service provision better incorporate actual or realised benefits, we expect overall weaker or more variable BES relationships.
Proposition 2
Human management actions will have the greatest influence on BES relationships at intermediate spatial scales (e.g., ecosystem, landscape) . This expectation arises from the fact that many natural resource and land use decisions, as well as the consequences of human management actions, occur primarily at ecosystem, watershed, or landscape scales where changes to land cover and landscape structure become evident. Thus, at these intermediate spatial scales, changes to landscape structure—by altering species dispersal and biodiversity levels, as well as socio-ecological interactions and ecosystem service flows (Mitchell et al. 2015 )—will alter ecosystem service provision and therefore the strength of BES relationships.
Proposition 3
Ecosystem service co-production and demand will have their greatest influence on BES relationships at broad (regional, national, global) scales and when quantification of ES is more benefit-focused . While differences in environmental values or technologies that drive ecosystem service demand do occur sometimes at the individual, farm/field, or property level, we expect that these processes are most likely to exert substantial influences on BES relationships at much broader scales. For example, when investigating BES relationships across biomes, regions, or countries where the environmental conditions, institutions, or cultural norms have resulted in fundamentally different social-ecological dynamics that contribute to ecosystem service provision. In this case, the spatial scales at which these effects become most important may vary depending on how human systems of governance, economics, or institutions are organized (i.e., size of nation-states varies significantly).
Proposition 4
Effects of abiotic drivers on BES relationships will be evident at varied scales depending on the scale of abiotic variation and species turnover, and when studies focus on service capacity/supply . Abiotic conditions change at a variety of scales, from the continental with gradients of temperature or precipitation to the local where soil conditions, topography, and land use can vary across short distances. Thus, effects of abiotic conditions on BES relationships will vary depending on the ecosystem service- and study system-specific context with respect to relevant species turnover with abiotic variables, the scales at which specific abiotic drivers operate, as well as how the scale of observation of the study compares to the scale of abiotic heterogeneity. In addition, since we expect that human demand and changes in anthropogenic capitals can help decouple service provision from abiotic variables, we expect that studies focused primarily on the supply-side of ecosystem services will observe abiotic effects on BES relationships.
Key future directions for understanding BES relationships across scales
In this section, we highlight several key future research directions that can advance BES research across scales. First, because the scale at which BES relationships will be strongest or most relevant likely changes depending on the ecosystem service(s) in question, considerable thought should be put into determining the most effective scales at which to measure services and biodiversity. This includes an understanding of the relevant scales at which a service is provided or contributes to human wellbeing, an awareness of the organizational hierarchies that are present across scales (e.g., communities, ecosystems, biomes or municipalities, states, and countries) that influence service provision, identification of emergent processes at broader scales that could influence BES relationships (e.g., connectivity, landscape heterogeneity, disturbance regimes), as well as the specific spatial scales at which species or functional diversity varies and contributes to service provision for a given context. Second, given the social-ecological nature of ecosystem services, it is important to go beyond measuring ecosystem functions or biomass to quantify actual ecosystem service benefits, such as the amount of calories produced/consumed, quantity of clean water supplied, or quantity and quality of time spent in recreational opportunities. In particular, incorporating or reconciling measurements of ecosystem service supply with demand that are directly pertinent to human well-being, especially for regulating services, will be a fruitful avenue of future research to better understand how service demand, anthropogenic capital, and human values alter BES relationships. Such understanding could also provide more leverage for translating knowledge into management actions. Third, efforts are needed to quantify, measure, and map biodiversity, ecosystem functions, and services across scales. While some research initiatives and monitoring programs have increasingly recognized the importance of multi-scale studies (ResNet, Biodiversity Exploratories), most studies tend to be focused on individual scales. There is a great potential to explicitly build consistent, multi-scale measurements into existing research infrastructures, especially with the increasing adoption of Earth Observations into ecosystem service and biodiversity assessments (Ramirez-Reyes et al. 2019 ). Such multi-scale research can also be achieved through multi-researcher large-scale projects or research networks (e.g., ResNet, GeoBON). Fourth, research is needed to build multi-scale BES models using current knowledge and modelling frameworks. While there are a plethora of models or model ensembles (e.g., ARIES, InVEST, Co$ting Nature, SWAT) for quantifying and assessing ecosystem services suitable for varied scales (e.g., local, regional watersheds, or continental assessments; (Vigerstol and Aukema 2011 ; Bagstad et al. 2013 )), there are much fewer models that can characterise and predict the influences of biodiversity on ES across scales. One exception is mathematical or theoretical models for population or community dynamics. Hence, there is an important gap to bridge that: (1) integrates current knowledge of different drivers and underlying BES relationships into dynamic ES models; (2) integrates current ecosystem service assessment models with biodiversity or community dynamics models; and (3) integrates ecological/biological models with social models, to incorporate the entire system of dynamics and feedbacks that determine service provision. Lastly, while we focused mainly on spatial scales, which is where most of the literature is currently grounded, we also expect that temporal scales could alter and moderate effects of biodiversity on ecosystem functions and therefore service provision (Reich et al. 2012 ; Isbell et al. 2017 ; Qiu and Cardinale 2020 ). In particular, increased species diversity and functional redundancy could influence ecosystem function and service provision stability, thus leading to more reliable ecosystem service provisioning (Biggs et al. 2020 ). However, there is very limited research thus far exploring the importance and processes through which biodiversity and ES relationships change over time. This limits our ability to understand influences of time lags or temporal scales in mediating BES relationships, as well as feedbacks between biodiversity, ES, management and other human actions.
A large number of potential drivers can contribute to BES relationships observed across spatial scales. While understanding the spatial dynamics of biodiversity and ecosystem services is central to identifying actions that can conserve both species and the benefits upon which people depend, few studies have explicitly investigated these BES relationships across spatial scales from a social-ecological lens. Identifying key variables to measure across spatial scales, integrating broad-scale biodiversity and ecosystem service data through the use of new Earth observation and modelling tools, and explicitly incorporating different spatial, institutional, and temporal scales into ecosystem service research are all required to fill this gap. Improving understanding of BES relationships across scales is critical as global conservation efforts accelerate and the need to identify actions at multiple scales that can safeguard biodiversity and maintain or increase ecosystem services grows.
Data availability
The datasets generated during and/or analysed during the current study are available from the corresponding author on reasonable request.
Albor C, García-Franco JG, Parra‐Tabla V et al (2019) Taxonomic and functional diversity of the co‐flowering community differentially affect Cakile edentula pollination at different spatial scales. J Ecol 107:2167–2181
Article Google Scholar
Andersson E, McPhearson T, Kremer P et al (2015) Scale and context dependence of ecosystem service providing units. Ecosyst Serv 12:157–164
Bagstad KJ, Semmens DJ, Waage S, Winthrop R (2013) A comparative assessment of decision-support tools for ecosystem services quantification and valuation. Ecosyst Serv 5:27–39
Bagstad K, Villa F, Batker D et al (2014) From theoretical to actual ecosystem services: mapping beneficiaries and spatial flows in ecosystem service assessments. Ecol Soc. https://doi.org/10.5751/es-06523-190264
Bagstad KJ, Semmens DJ, Diffendorfer JE et al (2019) Ecosystem service flows from a migratory species: spatial subsidies of the northern pintail. Ambio 48:61–73
Article ADS PubMed Google Scholar
Balvanera P, Pfisterer AB, Buchmann N et al (2006) Quantifying the evidence for biodiversity effects on ecosystem functioning and services. Ecol Lett 9:1146–1156
Article PubMed Google Scholar
Balvanera P, Siddique I, Dee L et al (2014) Linking Biodiversity and Ecosystem Services: current uncertainties and the necessary next steps. Bioscience 64:49–57
Barraclough AD, Cusens J, Måren IE (2022) Mapping stakeholder networks for the co-production of multiple ecosystem services: a novel mixed-methods approach. Ecosyst Serv 56:101461
Bastian O (2013) The role of biodiversity in supporting ecosystem services in Natura 2000 sites. Ecol Indic 24:12–22
Bennett EM, Peterson GD, Gordon LJ (2009) Understanding relationships among multiple ecosystem services. Ecol Lett 12:1394–1404
Bennett EM, Cramer W, Begossi A et al (2015) Linking biodiversity, ecosystem services, and human well-being: three challenges for designing research for sustainability. Curr Opin Env Sust 14:76–85
Biggs CR, Yeager LA, Bolser DG et al (2020) Does functional redundancy affect ecological stability and resilience? A review and meta-analysis. Ecosphere. https://doi.org/10.1002/ecs2.3184
Bracken MES, Douglass JG, Perini V, Trussell GC (2017) Spatial scale mediates the effects of biodiversity on marine primary producers. Ecology 98:1434–1443
Brauman KA, Daily GC, Duarte TK, Mooney HA (2007) The Nature and Value of Ecosystem Services: an overview highlighting Hydrologic services. Annu Rev Env Resour 32:67–98
Bruno JF, Stachowicz JJ, Bertness MD (2003) Inclusion of facilitation into ecological theory. Trends Ecol Evol 18:119–125
Bu W, Huang J, Xu H et al (2019) Plant functional traits are the mediators in regulating effects of abiotic site conditions on aboveground carbon stock-evidence from a 30 ha tropical forest plot. Front Plant Sci 9:1958
Article PubMed PubMed Central Google Scholar
Cardinale BJ, Ives AR, Inchausti P (2004) Effects of species diversity on the primary productivity of ecosystems: extending our spatial and temporal scales of inference. Oikos 104:437–450
Article ADS Google Scholar
Cardinale BJ, Duffy JE, Gonzalez A et al (2012) Biodiversity loss and its impact on humanity. Nature 486:59–67
Article ADS CAS PubMed Google Scholar
Chalkiadakis C, Drakou EG, Kraak M-J (2022) Ecosystem service flows: a systematic literature review of marine systems. Ecosyst Serv 54:101412
Chen S, Wang W, Xu W et al (2018) Plant diversity enhances productivity and soil carbon storage. Proc Natl Acad Sci 115:4027–4032
Article ADS CAS PubMed PubMed Central Google Scholar
Costanza R (2008) Ecosystem services: multiple classification systems are needed. Biol Conserv 141:350–352
Crouzat E, Mouchet M, Turkelboom F et al (2015) Assessing bundles of ecosystem services from regional to landscape scale: insights from the French Alps. J Appl Ecol 52:1145–1155
Dade MC, Mitchell MGE, McAlpine CA, Rhodes JR (2019) Assessing ecosystem service trade-offs and synergies: the need for a more mechanistic approach. Ambio 48:1116–1128
Dainese M, Schneider G, Krauss J, Steffan-Dewenter I (2017) Complementarity among natural enemies enhances pest suppression. Sci Rep-uk 7:8172
Dainese M, Martin EA, Aizen MA et al (2019) A global synthesis reveals biodiversity-mediated benefits for crop production. Sci Adv. https://doi.org/10.1126/sciadv.aax0121
Darling ES, Côté IM (2008) Quantifying the evidence for ecological synergies. Ecol Lett 11:1278–1286
Dee LE, Cowles J, Isbell F et al (2019) When do ecosystem services depend on rare species? Trends Ecol Evol 34:746–758
Deutsch L, Gräslund S, Folke C et al (2007) Feeding aquaculture growth through globalization: Exploitation of Marine ecosystems for fishmeal. Global Environ Change 17:238–249
Díaz S, Lavorel S, de Bello F et al (2007) Incorporating plant functional diversity effects in ecosystem service assessments. Proc Natl Acad Sci 104:20684–20689
Article ADS PubMed PubMed Central Google Scholar
Dimitrakopoulos PG, Schmid B (2004) Biodiversity effects increase linearly with biotope space. Ecol Lett 7:574–583
Doi H, Katano I, Negishi JN et al (2013) Effects of biodiversity, habitat structure, and water quality on recreational use of rivers. Ecosphere 4:1–11
Donadi S, Austin ÅN, Bergström U et al (2017) A cross-scale trophic cascade from large predatory fish to algae in coastal ecosystems. Proc Royal Soc B Biological Sci 284:20170045
Duffy JE, Godwin CM, Cardinale BJ (2017) Biodiversity effects in the wild are common and as strong as key drivers of productivity. Nature 549:261–264
Fanin N, Gundale MJ, Farrell M et al (2018) Consistent effects of biodiversity loss on multifunctionality across contrasting ecosystems. Nat Ecol Evol 2:269–278
Felipe-Lucia M, Comín F, Bennett E (2014) Interactions among ecosystem services across land uses in a floodplain agroecosystem. Ecol Soc. https://doi.org/10.5751/es-06249-190120
Felipe-Lucia MR, Soliveres S, Penone C et al (2020) Land-use intensity alters networks between biodiversity, ecosystem functions, and services. Proc Natl Acad Sci 117:28140–28149
Gaitán JJ, Maestre FT, Bran DE et al (2019) Biotic and Abiotic Drivers of Topsoil Organic Carbon Concentration in Drylands have similar effects at Regional and Global scales. Ecosystems 22:1445–1456
Gamfeldt L, Snäll T, Bagchi R et al (2013) Higher levels of multiple ecosystem services are found in forests with more tree species. Nat Commun 4:1340
García D, Martínez D (2012) Species richness matters for the quality of ecosystem services: a test using seed dispersal by frugivorous birds. Proc Royal Soc B Biological Sci 279:3106–3113
García-Nieto AP, García-Llorente M, Iniesta-Arandia I, Martín-López B (2013) Mapping forest ecosystem services: from providing units to beneficiaries. Ecosyst Serv 4:126–138
Geijzendorffer IR, Roche PK (2014) The relevant scales of ecosystem services demand. Ecosyst Serv 10:49–51
Gonzalez A, Germain RM, Srivastava DS et al (2020) Scaling-up biodiversity‐ecosystem functioning research. Ecol Lett 23:757–776
Grace JB, Anderson TM, Smith MD et al (2007) Does species diversity limit productivity in natural grassland communities? Ecol Lett 10:680–689
Gross N, Bagousse-Pinguet YL, Liancourt P et al (2017) Functional trait diversity maximizes ecosystem multifunctionality. Nat Ecol Evol 1:0132
Hao M, Zhang C, Zhao X, von Gadow K (2018) Functional and phylogenetic diversity determine woody productivity in a temperate forest. Ecol Evol 8:2395–2406
Hautier Y, Isbell F, Borer ET et al (2018) Local loss and spatial homogenization of plant diversity reduce ecosystem multifunctionality. Nat Ecol Evol 2:50–56
Hector A, Bazeley-White E, Loreau M et al (2002) Overyielding in grassland communities: testing the sampling effect hypothesis with replicated biodiversity experiments. Ecol Lett 5:502–511
Hein L, van Koppen K, de Groot RS, van Ierland EC (2006) Spatial scales, stakeholders and the valuation of ecosystem services. Ecol Econ 57:209–228
Hertzog LR, Boonyarittichaikij R, Dekeukeleire D et al (2019) Forest fragmentation modulates effects of tree species richness and composition on ecosystem multifunctionality. Ecology 100:e02653
Himes A, Puettmann K, Muraca B (2020) Trade-offs between ecosystem services along gradients of tree species diversity and values. Ecosyst Serv 44:101133
Hooper DU, Adair EC, Cardinale BJ et al (2012) A global synthesis reveals biodiversity loss as a major driver of ecosystem change. Nature 486:105–108
IPBES (2019) Global Assessment Report on Biodiversity and Ecosystem Services
Isbell F, Calcagno V, Hector A et al (2011) High plant diversity is needed to maintain ecosystem services. Nature 477:199–202
Isbell F, Gonzalez A, Loreau M et al (2017) Linking the influence and dependence of people on biodiversity across scales. Nature 546:65–72
Isbell F, Cowles J, Dee LE et al (2018) Quantifying effects of biodiversity on ecosystem functioning across times and places. Ecol Lett 21:763–778
Kachler J, Isaac R, Martín-López B et al (2023) Co‐production of nature’s contributions to people: what evidence is out there? People Nat 5:1119–1134
Koellner T, Bonn A, Arnhold S et al (2019) Guidance for assessing interregional ecosystem service flows. Ecol Indic 105:92–106
Kremen C, Williams NM, Aizen MA et al (2007) Pollination and other ecosystem services produced by mobile organisms: a conceptual framework for the effects of land-use change. Ecol Lett 10:299–314
Lavorel S, Storkey J, Bardgett RD et al (2013) A novel framework for linking functional diversity of plants with other trophic levels for the quantification of ecosystem services. J Veg Sci 24:942–948
Lavorel S, Rey P-L, Grigulis K et al (2020) Interactions between outdoor recreation and iconic terrestrial vertebrates in two French alpine national parks. Ecosyst Serv 45:101155
Lindborg R, Gordon LJ, Malinga R et al (2017) How spatial scale shapes the generation and management of multiple ecosystem services. Ecosphere. https://doi.org/10.1002/ecs2.1741
Loreau M (2010) Linking biodiversity and ecosystems: towards a unifying ecological theory. Philosophical Trans Royal Soc B Biol Sci 365:49–60
Luck GW, Harrington R, Harrison PA et al (2009) Quantifying the contribution of organisms to the provision of Ecosystem services. Bioscience 59:223–235
Luo Y, Cadotte MW, Burgess KS et al (2019) Greater than the sum of the parts: how the species composition in different forest strata influence ecosystem function. Ecol Lett 22:1449–1461
Metzger JP, Villarreal-Rosas J, Suárez-Castro AF et al (2021) Considering landscape-level processes in ecosystem service assessments. Sci Total Environ 796:149028
Miller AB, Blahna DJ, Morse WC et al (2022) From recreation ecology to a recreation ecosystem: a framework accounting for social-ecological systems. J Outdoor Recreat Tour 38:100455
Mitchell MGE, Suarez-Castro AF, Martinez-Harms M et al (2015) Reframing landscape fragmentation’s effects on ecosystem services. Trends Ecol Evol 30:190–198
Mitchell MGE, Schuster R, Jacob AL et al (2021) Identifying key ecosystem service providing areas to inform national-scale conservation planning. Environ Res Lett 16:014038
Article ADS CAS Google Scholar
Mora C, Aburto-Oropeza O, Bocos AA et al (2011) Global human footprint on the linkage between Biodiversity and Ecosystem Functioning in reef fishes. Plos Biol 9:e1000606
Article CAS PubMed PubMed Central Google Scholar
Mulder CPH, Uliassi DD, Doak DF (2001) Physical stress and diversity-productivity relationships: the role of positive interactions. Proc Natl Acad Sci 98:6704–6708
O’Connor MI, Gonzalez A, Byrnes JEK et al (2017) A general biodiversity–function relationship is mediated by trophic level. Oikos 126:18–31
O’Neill RV, Johnson AR, King AW (1989) A hierarchical framework for the analysis of scale. Landsc Ecol 3:193–205
Ostfeld RS, LoGiudice K (2003) COMMUNITY DISASSEMBLY, BIODIVERSITY LOSS, AND THE EROSION OF AN ECOSYSTEM SERVICE. Ecology 84:1421–1427
Palliwoda J, Fischer J, Felipe-Lucia MR et al (2021) Ecosystem service coproduction across the zones of biosphere reserves in Europe. Ecosyst People 17:491–506
Palomo I, Felipe-Lucia MR, Bennett EM et al (2016) Chapter six disentangling the pathways and effects of Ecosystem Service Co-production. Adv Ecol Res 54:245–283
Paquette A, Messier C (2011) The effect of biodiversity on tree productivity: from temperate to boreal forests. Glob Ecol Biogeogr 20:170–180
Peters DPC, Pielke RA, Bestelmeyer BT et al (2004) Cross-scale interactions, nonlinearities, and forecasting catastrophic events. Proc Natl Acad Sci United States Am 101:15130–15135
Potschin MB, Haines-Young RH (2011) Ecosystem services. Prog Phys Geog 35:575–594
Provost GL, Schenk NV, Penone C et al (2023) The supply of multiple ecosystem services requires biodiversity across spatial scales. Nat Ecol Evol 7:236–249
PubMed Google Scholar
Qiu J, Cardinale BJ (2020) Scaling up biodiversity–ecosystem function relationships across space and over time. Ecology 101:e03166
Qiu J, Carpenter SR, Booth EG et al (2018) Understanding relationships among ecosystem services across spatial scales and over time. Environ Res Lett 13:054020
Ramirez-Reyes C, Brauman KA, Chaplin-Kramer R et al (2019) Reimagining the potential of Earth observations for ecosystem service assessments. Sci Total Environ 665:1053–1063
Raudsepp-Hearne C, Peterson G (2016) Scale and ecosystem services: how do observation, management, and analysis shift with scale—lessons from Québec. Ecol Soc. https://doi.org/10.5751/es-08605-210316
Raymond CM, Bryan BA, MacDonald DH et al (2009) Mapping community values for natural capital and ecosystem services. Ecol Econ 68:1301–1315
Redlich S, Zhang J, Benjamin C et al (2022) Disentangling effects of climate and land use on biodiversity and ecosystem services—A multi-scale experimental design. Methods Ecol Evol 13:514–527
Reeves SE, Renzi JJ, Fobert EK et al (2020) Facilitating better outcomes: how positive species interactions can improve oyster reef restoration. Front Mar Sci 7:656
Reich PB, Tilman D, Isbell F et al (2012) Impacts of biodiversity loss escalate through time as redundancy fades. Science 336:589–592
Ricketts TH, Watson KB, Koh I et al (2016) Disaggregating the evidence linking biodiversity and ecosystem services. Nat Commun 7:13106
Riper CJV, Kyle GT (2014) Capturing multiple values of ecosystem services shaped by environmental worldviews: a spatial analysis. J Environ Manage 145:374–384
Riva F, Fahrig L (2023) Landscape-scale habitat fragmentation is positively related to biodiversity, despite patch‐scale ecosystem decay. Ecol Lett 26:268–277
Rodríguez JP, Beard TD Jr, Bennett EM et al (2006) Trade-offs across space, time, and ecosystem services. Ecol Soc. https://doi.org/10.5751/es-01667-110128
Scholes R, Reyers B, Biggs R et al (2013) Multi-scale and cross-scale assessments of social–ecological systems and their ecosystem services. Curr Opin Env Sust 5:16–25
Scholte SSK, Daams M, Farjon H et al (2018) Mapping recreation as an ecosystem service: considering scale, interregional differences and the influence of physical attributes. Landsc Urban Plan 175:149–160
Schulp CJE, Lautenbach S, Verburg PH (2014) Quantifying and mapping ecosystem services: demand and supply of pollination in the European Union. Ecol Indic 36:131–141
Semmens DJ, Diffendorfer JE, Bagstad KJ et al (2018) Quantifying ecosystem service flows at multiple scales across the range of a long-distance migratory species. Ecosyst Serv 31:255–264
Slade EM, Kirwan L, Bell T et al (2017) The importance of species identity and interactions for multifunctionality depends on how ecosystem functions are valued. Ecology 98:2626–2639
Slade EM, Bagchi R, Keller N, Philipson CD (2019) When do more species maximize more Ecosystem services? Trends Plant Sci 24:790–793
Article CAS PubMed Google Scholar
Soliveres S, van der Plas F, Manning P et al (2016) Biodiversity at multiple trophic levels is needed for ecosystem multifunctionality. Nature 536:456–459
Spake R, Bellamy C, Graham LJ et al (2019) An analytical framework for spatially targeted management of natural capital. Nat Sustain 2:90–97
Srivastava DS, Vellend M (2005) Biodiversity-ecosystem function research: is it relevant to Conservation? Annu Rev Ecol Evol Syst 36:267–294
Stein A, Gerstner K, Kreft H (2014) Environmental heterogeneity as a universal driver of species richness across taxa, biomes and spatial scales. Ecol Lett 17:866–880
Stuart-Smith RD, Bates AE, Lefcheck JS et al (2013) Integrating abundance and functional traits reveals new global hotspots of fish diversity. Nature 501:539–542
Thompson PL, Isbell F, Loreau M et al (2018) The strength of the biodiversity–ecosystem function relationship depends on spatial scale. Proc Royal Soc B 285:20180038
Tilman D (1999) The ecological consequences of changes in Biodiversity: a search for General principles. Ecology 80:1455–1474
Google Scholar
Tilman D, Isbell F, Cowles JM (2014) Biodiversity and Ecosystem Functioning. Annu Rev Ecol Evol Syst 45:1–23
Tribot A-S, Deter J, Mouquet N (2018) Integrating the aesthetic value of landscapes and biological diversity. Proc R Soc B 285:20180971
Tscharntke T, Klein AM, Kruess A et al (2005) Landscape perspectives on agricultural intensification and biodiversity–ecosystem service management. Ecol Lett 8:857–874
Turner WR, Tjørve E (2005) Scale-dependence in species‐area relationships. Ecography 28:721–730
van der Meulen ES, Braat LC, Brils JM (2016) Abiotic flows should be inherent part of ecosystem services classification. Ecosyst Serv 19:1–5
van der Plas F (2019) Biodiversity and ecosystem functioning in naturally assembled communities. Biol Rev 94:1220–1245
Vigerstol KL, Aukema JE (2011) A comparison of tools for modeling freshwater ecosystem services. J Environ Manage 92:2403–2409
Villamagna AM, Angermeier PL, Bennett EM (2013) Capacity, pressure, demand, and flow: a conceptual framework for analyzing ecosystem service provision and delivery. Ecol Complex 15:114–121
Virtanen EA, Kallio N, Nurmi M et al (2023) Recreational land use contributes to the loss of marine biodiversity. People Nat. https://doi.org/10.1002/pan3.10444
Watson KB, Galford GL, Sonter LJ et al (2019) Effects of human demand on conservation planning for biodiversity and ecosystem services. Conserv Biol 33:942–952
Wiens JA, Milne BT (1989) Scaling of ‘landscapes’ in landscape ecology, or, landscape ecology from a beetle’s perspective. Landsc Ecol 3:87–96
Wilby A, Thomas MB (2002) Natural enemy diversity and pest control: patterns of pest emergence with agricultural intensification. Ecol Lett 5:353–360
Winfree R, Fox JW, Williams NM et al (2015) Abundance of common species, not species richness, drives delivery of a real-world ecosystem service. Ecol Lett 18:626–635
Zhang L, Shao H (2013) Direct plant–plant facilitation in coastal wetlands: a review. Estuar Coast Shelf Sci 119:1–6
Download references
Acknowledgements
The ideas that formed the foundation for this paper were conceived of in part at an organized symposium entitled “Understanding the relationships between biodiversity and ecosystem service supply, demand, and provision” at the 2017 Ecological Society of America conference in Portland, Oregon, USA. We thank the conference organizers for facilitating this symposium.
This research was supported by an NSERC Postdoctoral Fellowship to MGEM.
Author information
Authors and affiliations.
Faculty of Land and Food Systems, University of British Columbia, 2357 Main Mall, Vancouver, BC, V6T 1Z4, Canada
Matthew G. E. Mitchell
School of Forest, Fisheries, and Geomatics Sciences, Fort Lauderdale Research and Education Center, University of Florida, Davie, FL, USA
Jiangxiao Qiu
Department of Ecosystem Science and Management, Pennsylvania State University, University Park, State College, PA, USA
Bradley J. Cardinale
Institute for Resources, Environment and Sustainability, University of British Columbia, Vancouver, BC, Canada
Kai M. A. Chan
School of Geography and Environmental Science, University of Southampton, Southampton, UK
Felix Eigenbrod
Departamento de Conservación de la Biodiversidad y Restauración Ecológica, CSIC - Instituto Pirenaico de Ecología (IPE, CSIC), Jaca (Huesca), Spain
María R. Felipe-Lucia
Nature Conservancy of Canada, Victoria, BC, Canada
Aerin L. Jacob
University of Northern British Columbia, Prince George, BC, Canada
Cascade Agroecology, Leavenworth, WA, USA
Matthew S. Jones
School of the Environment, The University of Queensland, St Lucia, QLD, Australia
Laura J. Sonter
You can also search for this author in PubMed Google Scholar
Contributions
MGEM and JQ led the study conception and design with contributions from all other authors and wrote the first draft of the manuscript. All authors commented on previous versions of the manuscript and read and approved the final manuscript.
Corresponding author
Correspondence to Matthew G. E. Mitchell .
Ethics declarations
Competing interests.
The authors have no relevant financial or non-financial interests to disclose.
Additional information
Publisher’s note.
Springer Nature remains neutral with regard to jurisdictional claims in published maps and institutional affiliations.
Rights and permissions
Open Access This article is licensed under a Creative Commons Attribution 4.0 International License, which permits use, sharing, adaptation, distribution and reproduction in any medium or format, as long as you give appropriate credit to the original author(s) and the source, provide a link to the Creative Commons licence, and indicate if changes were made. The images or other third party material in this article are included in the article's Creative Commons licence, unless indicated otherwise in a credit line to the material. If material is not included in the article's Creative Commons licence and your intended use is not permitted by statutory regulation or exceeds the permitted use, you will need to obtain permission directly from the copyright holder. To view a copy of this licence, visit http://creativecommons.org/licenses/by/4.0/ .
Reprints and permissions
About this article
Mitchell, M.G.E., Qiu, J., Cardinale, B.J. et al. Key questions for understanding drivers of biodiversity-ecosystem service relationships across spatial scales. Landsc Ecol 39 , 36 (2024). https://doi.org/10.1007/s10980-024-01842-y
Download citation
Received : 20 July 2023
Accepted : 10 January 2024
Published : 14 February 2024
DOI : https://doi.org/10.1007/s10980-024-01842-y
Share this article
Anyone you share the following link with will be able to read this content:
Sorry, a shareable link is not currently available for this article.
Provided by the Springer Nature SharedIt content-sharing initiative
- Spatial scale
- Sampling effects
- Cross-scale interactions
- Find a journal
- Publish with us
- Track your research

An official website of the United States government
The .gov means it’s official. Federal government websites often end in .gov or .mil. Before sharing sensitive information, make sure you’re on a federal government site.
The site is secure. The https:// ensures that you are connecting to the official website and that any information you provide is encrypted and transmitted securely.
- Publications
- Account settings
Preview improvements coming to the PMC website in October 2024. Learn More or Try it out now .
- Advanced Search
- Journal List
- Springer Nature - PMC COVID-19 Collection

Climate change and its impact on biodiversity and human welfare
K. r. shivanna.
Ashoka Trust for Research in Ecology and the Environment, Srirampura, Jakkur Post, Bengaluru, 560064 India
Climate change refers to the long-term changes in temperature and weather due to human activities. Increase in average global temperature and extreme and unpredictable weather are the most common manifestations of climate change. In recent years, it has acquired the importance of global emergency and affecting not only the wellbeing of humans but also the sustainability of other lifeforms. Enormous increase in the emission of greenhouse gases (CO 2 , methane and nitrous oxide) in recent decades largely due to burning of coal and fossil fuels, and deforestation are the main drivers of climate change. Marked increase in the frequency and intensity of natural disasters, rise in sea level, decrease in crop productivity and loss of biodiversity are the main consequences of climate change. Obvious mitigation measures include significant reduction in the emission of greenhouse gases and increase in the forest cover of the landmass. Conference of Parties (COP 21), held in Paris in 2015 adapted, as a legally binding treaty, to limit global warming to well below 2 °C, preferably to 1.5 °C by 2100, compared to pre-industrial levels. However, under the present emission scenario, the world is heading for a 3–4 °C warming by the end of the century. This was discussed further in COP 26 held in Glasgow in November 2021; many countries pledged to reach net zero carbon emission by 2050 and to end deforestation, essential requirements to keep 1.5 °C target. However, even with implementation of these pledges, the rise is expected to be around 2.4 °C. Additional measures are urgently needed to realize the goal of limiting temperature rise to 1.5 °C and to sustain biodiversity and human welfare.
Introduction
Climate change refers to long-term changes in local, global or regional temperature and weather due to human activities. For 1000s of years, the relationship between lifeforms and the weather have been in a delicate balance conducive for the existence of all lifeforms on this Planet. After the industrial revolution (1850) this balance is gradually changing and the change has become apparent from the middle of the twentieth century. Now it has become a major threat to the wellbeing of humans and the sustainability of biodiversity. An increase in average global temperature, and extreme and unpredictable weather are the most common manifestations of climate change. It has now acquired the importance of global emergency. According to the report of the latest Intergovernmental Panel for Climate Change (AR6 Climate Change 2021 ), human-induced climate change as is prevalent now is unprecedented at least in the last 2000 years and is intensifying in every region across the globe. In this review the drivers of climate change, its impact on human wellbeing and biodiversity, and mitigation measures being taken at global level are briefly discussed.
Drivers of climate change
Emission of green-house gases.
Steady increase in the emission of greenhouse gases (GHGs) due to human activities has been the primary driver for climate change. The principal greenhouse gases are carbon dioxide (76%), methane (16%), and to a limited extent nitrous oxide (2%). Until recent decades, the temperature of the atmosphere was maintained within a reasonable range as some of the sunlight that hits the earth was reflected back into the space while the rest becomes heat that keeps the earth and the atmosphere warm enough for the sustenance of life forms. Accumulation of greenhouse gases combine with water vapour to form a transparent layer in the atmosphere that traps infrared radiation (net heat energy) emitted from the Earth’s surface and reradiates it back to Earth’s surface, thus contributing to the increasing temperature (greenhouse effect). Methane is 25 times and nitrous oxide 300 times more potent than CO 2 in trapping heat. Until 2019, the US, UK, European Union, Canada, Australia, Japan and Russia were the major CO 2 producers and were responsible for 61% of world’s emissions. Now, China produces the maximum amount of CO 2 (27%) followed by USA (11%) and India (6.6%); on per capita basis, however, India stands ninth.
The emission of GHGs is largely due to the burning of fossil fuels (coal, oil and natural gas) for automobiles and industries which result in carbon emissions during their extraction as well as consumption. The amount of CO 2 in the atmosphere before the industrial revolution used to be around 280 ppm and now it has increased to 412 ppm (as of 2019). Increase in the atmospheric temperature also leads to an increase in the temperature of the ocean. The oceans play an important role in the global carbon cycle and remove about 25% of the carbon dioxide emitted by human activities. Further, some CO 2 dissolves in the ocean water releasing carbonic acid which increases the acidity of the sea water. Rising ocean temperatures and acidification not only reduce their capacity to act as carbon sinks but also affect ocean ecosystems and the populations that relay on them.
Increasing demand for meat and milk has led to a significant increase in the population of livestock and conversion of enormous amount of the land to pasture and farm land to raise livestock. Ruminant animals (largely cows, buffaloes and sheep) produce large amounts of methane when they digest food (through enteric fermentation by microbes), adding to the greenhouse gases in the atmosphere (Sejiyan et al. 2016 ). To produce 1 kg of meat it requires 7 kg of grain and between 5000 and 20,000 L of water whereas to produce 1 kg of wheat it requires between 500 and 4000 L of water (Pimentel and Pimentel 2003 ). Anaerobic fermentation of livestock manure also produces methane. According to Patrick Brown, our animal farming industry needs to be changed; using readily available plant ingredients, the nutritional value of any type of meat can be matched with about one twentieth of the cost (See Leeming 2021 ).
The main natural source of nitrous oxide released to the atmosphere (60%) comes from the activity of microbes on nitrogen-based organic material from uncultivated soil and waste water. The remaining nitrous oxide comes from human activities, particularly agriculture. Application of nitrogenous fertilizers to crop plants is a routine practice to increase the yield; many of the farmers tend to apply more than the required amount. However, it results in nitrous oxide emissions from the soil through nitrification and denitrification processes by microbes. Both synthetic and organic fertilizers increase the amount of nitrogen available in the soil to microbial action leading to the release of nitrous oxide. Organic fertilizers, however, release nitrogen more slowly than synthetic ones so that most of it gets absorbed by the plants as they become available. Synthetic fertilizers release nitrogen rapidly which cannot be used by plants right away, thus making the excess nitrogen available to microbes to convert to nitrous oxide. Presently CO 2 concentration in the atmosphere is higher than at any time in at least 2 million years, and methane and nitrous oxide are higher than at any time in the last 800,000 years (AR6 Climate Change 2021 ).
Permafrost (permanently frozen soil), widespread in Arctic regions of Siberia, Canada, Greenland, Alaska, and Tibetan plateau contains large quantities of organic carbon in the top soil leftover from dead plants that could not be decomposed or rot away due to the cold. Global warming-induced thawing of permafrost facilitates decomposition of this material by microbes thus releasing additional amount of carbon dioxide and methane to the atmosphere.
Deforestation
Limited deforestation in early part of human civilization was the result of subsistence farming; farmers used to cut down trees to grow crops for consumption of their families and local population. In preindustrial period also, there was a balance between the amount of CO 2 emitted through various processes and the amount absorbed by the plants. Forests are the main sinks of atmospheric CO 2 . After the industrial revolution, the trend began to change; increasing proportion of deforestation is being driven by the demands of urbanization, industrial activities and large-scale agriculture. A new satellite map has indicated that field crops have been extended to one million additional km 2 of land over the last two decades and about half of this newly extended land has replaced forests and other ecosystems (Potapov et al. 2021 ).
In recent decades the demands on forest to grow plantation crops such as oil palm, coffee, tea and rubber, and for cattle ranching and mining have increased enormously thus reducing the forest cover. According to the World Wildlife Fund (WWF), over 43 million hectares of forest was lost between 2004 and 2017 out of 377 million hectares monitored around the world (Pacheco et al. 2021 ). Amazon Rain Forest is the largest tropical rain forest of the world and covers over 5 million km 2 . It is undergoing extensive degradation and has reached its highest point in recent years. According to National Geographic, about 17% of Amazon rain forest has been destroyed over the past 50 years and is increasing in recent years; during the last 1 year it has lost over 10,000 km 2 . In most of the countries the forest cover is less than 33%, considered necessary. For example, India’s forest and tree cover is only about 24.56% of the geographical area (Indian State Forest Report 2019 ).
Impacts of climate change
Increase in atmospheric temperature has serious consequences on biodiversity and ecosystems, and human wellbeing. The most important evidences of climate change is the long term data available on the CO 2 levels, global temperature and weather patterns. The impacts of climate change in the coming decades are based on published models on the basis of the analysis of the available data. Comparison of the performance of climate models published between 1970 and 2007 in projecting global mean surface temperature and associated changes with actual observations have shown that the models were consistent in predicting global warming in the years after publication (Hausfather et al. 2019 ). This correlation between predicted models and actual data indicates that the models are indeed reliable in accurately predicting the global warming and its impacts on weather pattern in the coming decades and their consequences on biodiversity and human welfare.
Weather pattern and natural disasters
One of the obvious changes observed in recent years is the extreme and unpredictable weather, and an increase in the frequency and intensity of natural disasters. Brazil’s south central region saw one of the worst droughts in 2021with the result many major reservoirs reached < 20% capacity, seriously affecting farming and energy generation (Getirana et al. 2021 ). In earlier decades, it was possible to predict with reasonable certainty annual weather pattern including the beginning and ending of monsoon rains; farmers could plan sowing periods of their crops in synchrony with the prevailing weather. Now the weather pattern is changing almost every year and the farmers are suffering huge losses. Similarly the extent of annual rainfall and the locations associated with heavy and scanty rainfall are no more predictable with certainty. Many areas which were associated with scanty rainfall have started getting much heavier rains and the extent of rainfall is getting reduced in areas traditionally associated with heavy rainfall. Similarly the period and the extent of snowfall in temperate regions have also become highly variable.
Increase in the frequency and intensity of natural disasters such as floods and droughts, cyclones, hurricanes and typhoons, and wildfires have become very obvious. Top five countries affected by climate change in 2021 include Japan, Philippines, Germany, Madagascar and India. Apart from causing death of a large number of humans and other animals, economic losses suffered by both urban and rural populations have been enormous. Deadly floods and landslides during 2020 forced about 12 million people leave their homes in India, Nepal and Bangladesh. According to World Meteorological Organization’s comprehensive report published in August 2021 (WMO-No.1267), climate change related disasters have increased by a factor of five over the last 50 years; however, the number of deaths and economic losses were reduced to 2 million and US$ 3.64 trillion respectively, due to improved warning and disaster management. More than 91% of these deaths happened in developing countries. Largest human losses were brought about by droughts, storms, floods and extreme temperatures. The report highlights that the number of weather, climate and water-extremes will become more frequent and severe as a result of climate change.
Global warming enhances the drying of organic matter in forests, thus increasing the risks of wildfires. Wildfires have become very common in recent years, particularly is some countries such as Western United States, Southern Europe and Australia, and are becoming more frequent and widespread. They have become frequent in India also and a large number of them have been recorded in several states. According to European Space Agency, fire affected an estimated four million km 2 of Earth’s land each year. Wildfires also release large amounts of carbon dioxide, carbon monoxide, and fine particulate matter into the atmosphere causing air pollution and consequent health problems. In 2021, wildfires around the world, emitted 1.76 billion tonnes of carbon (European Union’s Copernicus Atmospheric Monitoring Service). In Australia, more than a billion native animals reported to have been killed during 2020 fires, and some species and ecosystems may never recover (OXFAM International 2021 ).
Sea level rise
Global warming is causing mean sea level to rise in two ways. On one hand, the melting of the glaciers, the polar ice cap and the Atlantic ice shelf are adding water to the ocean and on the other hand the volume of the ocean is expanding as the water warms. Incomplete combustion of fossil fuels, biofuels and biomass releases tiny particles of carbon (< 2.5 µm), referred to as black carbon. While suspended in the air (before they settle down on earth’s surface) black carbon particles absorb sun’s heat 1000s of times more effectively than CO 2 thus contributing to global warming. When black particles get deposited over snow, glaciers or ice caps, they enhance their melting further adding to the rise in sea level. Global mean sea level has risen faster since 1900 than over any preceding century in at least the last 3000 years. Between 2006 and 2016, the rate of sea-level rise was 2.5 times faster than it was for almost the whole of the twentieth century (OXFAM International 2021 ). Precise data gathered from satellite radar measurements reveal an accelerating rise of 7.5 cm from 1993 to 2017, an average of 31 mm per decade (WCRP Global Sea Level Budget Group 2018 ).
Snow accounts for almost all current precipitation in the Arctic region. However, it continues to warm four times faster than the rest of the world as the melting ice uncovers darker land or ocean beneath, which absorbs more sunlight causing more heating. The latest projections indicate more rapid warming and sea ice loss in the Arctic region by the end of the century than predicted in previous projections (McCrystall et al. 2021 ). It also indicates that the transition from snow to rain-dominated Arctic in the summer and autumn is likely to occur decades earlier than estimated. In fact this transition has already begun; rain fell at Greenland’s highest summit (3216 m) on 14 August 2021 for several hours for the first time on record and air temperature remained above freezing for about 9 h (National Snow and Ice Sheet Centre Today, August 18, 2021).
In the annual meeting of the American Geophysical Union (13 December 2021) researchers warned that rapid melting and deterioration of one of western Antarctica’s biggest glaciers, roughly the size of Florida, Thwaites (often called as Doomsday Glacier), could lead to ice shelf’s complete collapse in just a few years. It holds enough water to raise sea level over 65 cm. Thwaites glacier is holding the entire West Antarctic ice sheet and is being undermined from underneath by warm water linked to the climate change. Melting of Thwaites could eventually lead to the loss of the entire West Antarctic Ice Sheet, which locks up 3.3 m of global sea level rise. Such doomsday may be coming sooner than expected (see Voosen 2021 ). If this happens, its consequences on human tragedy and biodiversity loss are beyond imagination.
The Himalayan mountain range is considered to hold the world’s third largest amount of glacier ice after Arctic and Antarctic regions. It is considered as Asian water tower (Immerzeel et al. 2020 ); the meltwater from the Himalayan glaciers provide the source of fresh water to nearly 2 billion people living along the mountain valleys and lowlands around the Himalayas. These glaciers are melting at unprecedented rates. Recently King et al. ( 2021 ) studied 79 glaciers close to Mt. Everest by analysing mass-change measurements from satellite archives and reported that the rate of ice loss from glaciers consistently increased since the early 1960s. This loss is likely to increase in the coming years due to further warming. In another study, a tenfold acceleration in ice loss was observed across the Himalayas than the average rate in recent decades over the past centuries (Lee et al. 2021 ). Melting of glaciers also results in drying up of perennial rivers in summer leading to the water scarcity for billions of humans and animals, and food and energy production downstream. See level rise and melting of glaciers feeding the rivers could lead to migration of huge population, creating additional problems. Even when the increase in global temperature rise is limited to 1.5 °C (discussed later), it generates a global sea-level rise between 1.7 and 3.2 feet by 2100. If it increases to 2 °C, the result could be more catastrophic leading to the submergence of a large number of islands, and flooding and submergence of vast coastal areas, saltwater intrusion into surface waters and groundwater, and increased soil erosion. A number of islands of Maldives for example, would get submerged as 80% of its land area is located less than one meter above the sea level. The biodiversity in such islands and coastal areas becomes extinct. China, Vietnam, Fiji, Japan, Indonesia, India and Bangladesh are considered to be the most at risk. Sundarbans National Park (UNESCO world heritage Site), the world’s largest Mangrove Forest spread over 140,000 hectares across India and Bangladesh, is the habitat for Royal Bengal Tiger and several other animal species. The area has already lost 12% of its shoreline in the last four decades by rising see level; it is likely to be completely submerged. Jakarta in Indonesia is the fastest sinking city in the world; the city has already sunk 2.5 m in the last 10 years and by 2050, most of it would be submerged. In Europe also, about three quarters of all cities will be affected by rising sea levels, especially in the Netherlands, Spain, Belgium, Greece and Italy. The entire city of Venice may get submerged (Anonymous 2018 ). In USA, New York City and Miami would be particularly vulnerable.
Crop productivity and human health
Many studies have indicated that climate change is driving increasing losses in crop productivity (Zhu et al. 2021 ). The models on global yield loss for wheat, maize and rice indicate an increase in yield losses by 10 to 25% per degree Celsius warming (Deutsch et al. 2018 ). Bras et al. ( 2021 ) reported that heatwave and drought roughly tripled crop losses over the last 50 years, from − 2.2% (1964–1990) to − 7.3% (1991–2015). Overall, the loss in crop production from climate-driven abiotic stresses may exceed US$ 170 billion year –1 and represents a major threat to global food security (Razaaq et al. 2021 ). Analysis of annual field trials of common wheat in California from 1985 to 2019 (35 years), during which the global atmospheric CO 2 concentration increased by 19%, revealed that the yield declined by 13% (Bloom and Plant 2021 ). Apart from crop yield, climate change is reported to result in the decline of nutritional value of food grains (Jagermeyr et al. 2021 ). For example, rising atmospheric CO 2 concentration reduces the amounts of proteins, minerals and vitamins in rice (Zhu et al. 2018 ). This may be true in other cereal crops also. As rice supplies 25% of all global calories, this would greatly affect the food and nutritional security of predominantly rice growing countries. Climate change would also increase the prevalence of insect pests adding to the yield loss of crops. The prevailing floods and droughts also affect food production significantly. Global warming also affects crop productivity through its impact on pollinators. Insect pollinators contribute to crop production in 75% of the leading food crops (Rader et al. 2013 ). Climate change contributes significantly to the decline in density and diversity of pollinators (Shivanna 2020 ; Shivanna et al. 2020 ). Under high as well as low temperatures, bees spend less time in foraging (Heinrich 1979 ) adding additional constraints to pollination efficiency of crop species.
The IPCC Third Assessment Report (Climate change 2001: The scientific basis – IPCC) concluded that the poorest countries would be hardest hit with reductions in crop yields in most tropical and sub-tropical regions due to increased temperature, decreased water availability and new or changed insect pest incidence. Rising ocean temperatures and ocean acidification affect marine ecosystems. Loss of fish habitats is modifying the distribution and productivity of both marine and freshwater species thus affecting the sustainability of fisheries and populations dependent on them (Salvatteci 2022 ).
Air pollution is considered as the major environmental risk of climate change due to its impact on public health causing increasing morbidity and mortality (Manisalidis 2020 ). Particulate matter, carbon monoxide, nitrogen oxide, and sulphur dioxide are the major air pollutants. They cause respiratory problems such as asthma and bronchiolitis and lung cancer. Recent studies have indicated that exposure to air pollution is linked to methylation of immunoregulatory genes, altered immune cell profiles and increased blood pressure in children (Prunicki et al. 2021 ). In another study wildfire smoke has been reported to be more harmful to humans than automobiles emissions (Aquilera et al. 2021 ). Stubble burning (intentional incineration of stubbles by farmers after crop harvest) has been a common practice in some parts of South Asia particularly in India; it releases large amount of toxic gases such as carbon monoxide and methane and causes serious damage to the environment and health (Abdurrahman et al. 2020 ). It also affects soil fertility by destroying the nutrients and microbes of the soil. Attempts are being made to use alternative methods to prevent this practice.
A number of diseases such as zika fever, dengue and chikungunya are transmitted by Aedes mosquitoes and are now largely restricted to the monsoon season. Global warming facilitates their spread in time and space thus exposing new populations and regions for extended period to these diseases. Lyme disease caused by a bacterium is transmitted through the bite of the infected blacklegged ticks. It is one of the most common disease in the US. The cases of Lyme disease have tripled in the past two decades. Recent studies have suggested that variable winter conditions due to climate change could increase tick’s activity thus increasing the infections (see Pennisi 2022 ).
Biodiversity
Biodiversity and associated ecoservices are the basic requirements for human livelihood and for maintenance of ecological balance in Nature. Documentation of biodiversity, and its accelerating loss and urgent need for its conservation have become the main concern for humanity since several decades (Wilson and Peter 1988 ; Wilson 2016 ; Heywood 2017 ; IPBES 2019 ; Genes and Dirzo 2021 ; Shivanna and Sanjappa 2021 ). It is difficult to analyse the loss of biodiversity exclusively due to climate change as other human-induced environmental changes such as habitat loss and degradation, overexploitation of bioresources and introduction of alien species also interact with climate change and affect biodiversity and ecosystems. In recent decades there has been a massive loss of biodiversity leading to initiation of the sixth mass extinction crisis due to human-induced environmental changes. These details are not discussed here; they are dealt in detail in many other reviews (Leech and Crick 2007 ; Sodhi and Ehrlich 2010 ; Lenzen et al. 2012 ; Dirzo and Raven 2003 ; Raven 2020 ; Ceballos et al. 2015 ; Beckman et al. 2020 ; Shivanna 2020 ; Negrutiu et al. 2020 ; Soroye et al. 2020 ; Wagner 2020 , 2021 ; Anonymous 2021 ; Zattara and Aizen 2021 ).
Terrestrial species
There are several effects on biodiversity caused largely by climate change. Maxwell et al. ( 2019 ) reviewed 519 studies on ecological responses to extreme climate events (cyclones, droughts, floods, cold waves and heat waves) between 1941 and 2015 covering amphibians, birds, fish, invertebrates, mammals, reptiles and plants. Negative ecological responses have been reported for 57% of all documented groups including 31 cases of local extirpations and 25% of population decline.
Increase in temperature impacts two aspects of growth and development in plants and animals. One of them is a shift in distributional range of species and the other is the shift in phenological events. Plant and animal species have adapted to their native habitat over 1000s of years. As the temperature gets warmer in their native habitat, species tend to move to higher altitudes and towards the poles in search of suitable temperature and other environmental conditions. There are a number of reports on climate change-induced shifts in the distributional range of both plant and animal species (Grabherr et al. 1994 ; Cleland et al. 2007 ; Parmesan and Yohe 2003 ; Beckage et al. 2008 ; Pimm 2009 ; Miller-Rushing et al. 2010 ; Lovejoy and Hannah 2005 ; Lobell et al. 2011 ). Many species may not be able to keep pace with the changing weather conditions and thus lag behind leading to their eventual extinction. Long-term observations extending for over 100 years have shown that many species of bumblebees in North-America and Europe are not keeping up with the changing climate and are disappearing from the southern portions of their range (Kerr et al. 2015 ). Most of the flowering plants depend on animals for seed dispersal (Beckman et al. 2020 ). Defaunation induced by climate change and other environmental disturbances has reduced long-distance seed dispersal. Prediction of dispersal function for fleshly-fruited species has already reduced the capacity of plants to track climate change by 60%, thus severely affecting their range shifts (Fricke et al. 2022 ).
Climate change induced shifts in species would threaten their sustenance even in protected areas as they hold a large number of species with small distributional range (Velasquez-Tibata et al. 2013 ). Pautasso ( 2012 ) has highlighted the sensitivity of European birds to the impacts of climate change in their phenology (breeding time), migration patterns, species distribution and abundance. Metasequoia glyptostroboides is one of the critically endangered species with extremely small populations distributed in South-Central China. Zhao et al. ( 2020 ) analysed detailed meteorological and phenological data from 1960 to 2016 and confirmed that climate warming has altered the phenology and compressed the climatically suitable habitat of this species. Their studies revealed that the temperature during the last 57 years has increased significantly with the expansion of the length of growing season of this species. Climatically suitable area of the species has contracted at the rate of 370.8 km 2 per decade and the lower and upper elevation limits shrunk by 27 m over the last 57 years.
The other impact of climate change on plant and animal species has been in their phenological shift. Phenology is the timing of recurring seasonal events; it is a sort of Nature’s calendar for plants and animals. In flowering plants, various reproductive events such as the timing of flowering, fruiting, their intensity, and longevity are important phenological events, and in animals some of the phenological events include building of nests in birds, migration of animal species, timing of egg laying and development of the larva, pupa and adult in insects. Phenological events of both plants and animals are generally fixed in specific time of the year as they are based on environmental cues such as temperature, light, precipitation and snow melt. Phenological timings of species are the results of adaptations over 100 s of years to the prevailing environment. Wherever there is a mutualism between plants and animals, there is a synchrony between the two partners. For example in flowering plants, flowering is associated with the availability of pollinators and fruiting is associated with the availability of seed dispersers and optimal conditions for seed germination and seedling establishment. In animals also, phenological events are adapted to suit normal growth and reproduction. In temperate regions, melting of ice initiates leafing in plants; this is followed by the flowering in the spring. Similarly, warming of the climate before the spring induces hatching of the hibernating insects which feed on newly developed foliage. Insects emerge and ready to pollinate the flowers by the time the plants bloom.
The dates of celebration of the cherry blossom festival, an important cultural event in Japan that coincides with the peak of flowering period of this species and for which > 1000 years of historical records are available, has shown advances in the dates of the festival in recent decades (Primack et al. 2009 ). The records between 1971 and 2000 showed that the trees flowered an average of 7 days earlier than all the earlier years (Allen et al. 2013 ). These advances were correlated with increasing temperature over the years. Spring temperatures in the Red River valley, North Dakota, USA have extended the period of the growing season of plants significantly over the years. Flowering times, for which data are available from1910 to1961, have been shown to be sensitive to at least one variable related to temperature or precipitation for 75% of the 178 species investigated (Dunnell and Traverse 2011 ). The first flowering time has been significantly shifted earlier or later over the last 4 years of their study in 5–15% of the observed species relative to the previous century. Rhododendron arboretum , one of the central Himalayan tree species, flowers from early February to mid-March. Generalized additive model using real-time field observations (2009–2011) and herbarium records (1893–2003) indicated 88–97 days of early flowering in this species over the last 100 years (Gaira et al. 2014 ). This early flowering was correlated with an increase in the temperature.
One of the consequences of a shift in the distributional range of species and phenological timings is the possible uncoupling of synchronization between the time of flowering of plant species and availability of its pollinators (see Gerard et al. 2020 ). When a plant species migrates, its pollinator may not be able to migrate; similarly when a pollinator migrates, the plant species on which it depends for sustenance may not migrate. Memmott et al. ( 2007 ) explored potential disruption of pollination services due to climate change using a network of 1420 pollinators and 429 plant species by simulating consequences of phenological shifts that can be expected with doubling of atmospheric CO 2 . They reported phenological shifts which reduced available floral resources to 17–50% of all pollinator species. A long-term study since the mid-1970s in the Mediterranean Basin has indicated that unlike the synchrony present in the earlier decades between the flowering of plant species and their pollinators, insect phenoevents during the last decade showed a steeper advance than those of plants (Gordo and Sanz 2005 ). Similar asynchrony has been reported between the flowering of Lathyrus and one of its pollinators, Hoplitis fulgida (Forrest and Thomson 2011 ). Asynchrony between flowering and appearance of pollinator has also been reported in a few other cases (Kudo and Ida 2013 ; Kudo 2014 ). Such asynchrony could affect the sustenance of plant and/or pollinator species in the new environment.
Marine species
Amongst the marine species, corals are the most affected groups due to the rise in temperature and acidity of oceans. Corals live in a symbiotic relationship with algae which provide colour and photosynthates to the corals. Corals are extremely sensitive to heat and acidity; even an increase of 2–3°F of ocean water above normal results in expulsion of the symbiotic algae from their tissues leading to their bleaching (Hoegh-Guldberg et al. 2017 ). When this bleached condition continues for several weeks, corals die. Nearly one-third of the Great Barrier Reef, the world’s largest coral reef system that sustains huge Australian tourism industry, has died as a result of global warming (Hughes et al. 2018 ). According to the experts the reef will be unrecognizable in another 50 years if greenhouse gas emissions continue at the current rate.
According to UNESCO, coral reefs in all 29 reef-containing World Heritage sites would cease to exist as functioning ecosystems by the end of this century if greenhouse gas emissions continue to be emitted at the present rate (Elena et al. 2020 ). Recent assessment of the risk of ecosystem collapse to coral reefs of the Western Indian Ocean, covering about 5% of the global total, range from critically endangered to vulnerable (Obura et al. 2021 ). Coral reefs provide suitable habitat for thousands of other species, including sharks, turtles and whales. If corals die, the whole ecosystem will get disrupted.
Melting of ice in Arctic region due to global warming is threatening the survival of native animals such as polar bear, Arctic fox and Arctic wolf. Rising of sea level also leads to the extinction of a large number of endangered and endemic plant and animal species in submerged coastal areas and islands. Over 180,000 islands around the globe contain 20% of the world’s biodiversity. Bellard et al. ( 2013 ) assessed consequences of sea level rise of 1–6 m for 10 insular biodiversity hotspots and their endemic species at the risk of potential extinction. Their study revealed that 6 to19% of the 4447 islands would be entirely submerged depending on the rise of sea level; three of them, the Caribbean islands, the Philippines and Sundaland, displayed the most significant hotspots representing a potential threat for 300 endemic species. According to the Centre for Biological Diversity ( 2013 ) 233 federally protected threatened and endangered species in 23 coastal states are threatened if rising sea is unchecked. Recently more than 100 Aquatic Science Societies representing over 80,000 scientists from seven continents sounded climate alarm (Bonar 2021 ). They have highlighted the effects of climate change on marine and aquatic ecosystems and have called on the world leaders and public to undertake mitigation measures to protect and sustain aquatic systems and theirs services.
Mitigation measures
The principal mitigation measures against climate change are obvious; they include significant reduction in greenhouse gas emission, prevention of deforestation and increase in the forest cover. To reduce greenhouse gas emission, use of coal and fossil fuels needs to be reduced markedly. As climate change is a global challenge, local solutions confined to one or a few countries do not work; we need global efforts. Many attempts are being made to achieve these objectives at the global level since many decades. Mitigation measures are largely at the level of diplomatic negotiations involving states and international organizations, Governments and some nongovernmental organizations. The Intergovernmental Panel on Climate Change (IPCC) was established by the United Nations Environment Programme (UNEP) and the World Meteorological Organization (WMO) in 1988. Its mandate was to provide political leaders with periodic scientific assessments concerning climate change, its implications and risks, and also to put forward adaptation and mitigation strategies. In 1992 more than 1700 World scientists, including the majority of living Nobel laureates gave the first Warning to Humanity about climate change and associated problems. They expressed concern about potential damage to the Planet Earth by human-induced environmental changes such as climate change, continued human population growth, forest loss, biodiversity loss and ozone depletion. Conference of Parties (COP) of the UN Convention on Climate Change was established in 1992 under the United Nations Framework Convention on Climate Change (UNFCCC) to discuss global response to climate change. Its first meeting (COP 1) was held in Berlin in March 1995 and is being held every year since then. The Fifth Assessment Report of the IPCC, released in November 2014, projected an increase in the mean global temperature of 3.7 to 4.8 °C by 2100, relative to preindustrial levels (1850), in the absence of new policies to mitigate climate change; it highlighted that such an increase would have serious consequences. This prediction compelled the participating countries at the COP 21 held in Paris in December 2015 to negotiate effective ways and means of reducing carbon emissions. In this meeting the goal to limit global warming to well below 2 °C, preferably to 1.5 °C, compared to preindustrial levels was adapted by 196 participating countries as a legally binding treaty on climate change. It also mandated review of progress every 5 years and the development of a fund containing $100 billion by 2020, which would be replenished annually, to help developing countries to adopt non-greenhouse-gas-producing technologies.
In 2017, after 25 years after the first warning, 15,354 world scientists from 184 countries gave ‘second warning to humanity’ (Ripple et al. 2017 ). They emphasized that with the exception of stabilizing the stratospheric ozone layer, humanity has failed to make sufficient progress in solving these environmental challenges, and alarmingly, most of them are getting far worse. Analysis of Warren et al. ( 2018 ) on a global scale on the effects of climate change on the distribution of insects, vertebrates and plants indicated that even with 2 °C temperature increase, approximately 18% of insects, 16% of plants and 8% of vertebrates species are projected to loose > 50% geographic range; this falls to 6% for insects, 8% for plants and 4% for vertebrates when temperature increase is reduced to 1.5 °C.
UN Report on climate change (prepared by > 90 authors from 40 countries after examining 6000 scientific publications) released in October 2018 in South Korea also gave serious warning to the world. Some of the salient features of this report were:
- Overshooting 1.5 °C will be disastrous. It will have devastating effects on ecosystems, communities and economies. By 2040 there could be global food shortages, the inundation of coastal cities and a refugee crisis unlike the world has ever seen.
- Even 1.5 °C warming would rise sea levels by 26–77 cm by 2100; 2 °C would add another 10 cm which would affect another 10 million people living in coastal regions.
- Coral reefs are projected to decline 70–90% even at 1.5 °C. At 2 °C, 99% of the reefs would be ravaged.
- Storms, floods, droughts and forest fires would increase in intensity and frequency.
- The world has already warmed by about 1 °C since preindustrial times. We are currently heading for about 3–4 °C of warming by 2100.
- Unless rapid and deep reductions in CO 2 and other greenhouse gas emissions occur in the coming decades, achieving the goals of the 2015 Paris Agreement will be beyond reach.
- To keep 1.5 °C target, coal’s share of global electricity generation must be cut from the present 37% to no more than 2% by 2050. Renewable power must be greatly expanded. Net CO 2 emissions must come down by 45% (from 2010 levels) by 2030 and reach net zero (emissions of greenhouse gases no more than the amount removed from the atmosphere) around 2050.
This report awakened the world Governments about the seriousness of the climate change. The COP 26 meeting which was to be held in 2020 had to be postponed due to Covid-19 pandemic. The first part of the sixth report of IPCC was released in August 2021 (AR6 Climate Change 2021 ), just before the postponed COP 26 meeting was to be held; it highlighted that the threshold warming of 1.5 °C (the target of keeping the warming by the end of the century) would reach in the next 20 years itself and if the present trends continue, it would reach 2.7 °C by the end of the century.
Under this predicted climate emergency (see Ripple et al. 2020 ), COP 26 meeting was held in Glasgow, Scotland between October 31 and November 12, 2021. Nearly 200 countries participated in this meeting. The main aim of the COP 26 was finalization of the rules and procedures for implementation of the Paris agreement to keep the temperature increase to 1.5 °C. A number of countries including USA and European Union pledged to reach net zero carbon emission by 2050. China pledged to reach net zero emissions by 2060 and India by 2070. India also committed to reduce the use of fossil fuels by 40% by 2030. More than 100 countries committed to reduce worldwide methane emissions by 30% (of 2020 levels) by 2030 and to end deforestation by 2030. The average atmospheric concentration of methane reached a record 1900 ppb in September 2021; it was 1638 ppb in 1983 (US National Oceanic and Atmospheric Administration), highlighting the importance of acting on pledges made at the COP 26.
One of the limitations of COP meetings has been nonadherence of the commitment made by developed countries at Paris meeting to transfer US $100 billion annually to developing and poor countries to support climate mitigation and loss of damage, through 2025; only Germany, Norway and Sweden are paying their share. Several experts feel that the adoption of the Glasgow Climate Pact was weaker than expected. According to the assessment of Climate Action Tracker, a non-profit independent global analysis platform, emission reduction commitments by countries still lead to 2.4 °C warming by 2100. However, a positive outcome of the meeting was that it has kept alive the hopes of achieving the 1.5 °C goal by opening the options for further discussion in the coming COP meetings. Apart from implementation of mitigation pledges made by countries, it is also important to pay attention to climate adaptation since the negative effects of climate change will continue for decades or longer (AR6 Climate Change 2021 ). Investment in early warning is an important means of climate adaptation, which is lacking in many parts of Africa and Latin America.
Conclusions
Climate change has now become the fastest growing global threat to human welfare. The world has realized the responsibility of the present generation as it is considered to be the last generation capable of taking effective measures to reverse its impact. If it fails, human civilization is likely to be doomed beyond recovery. As emphasized by many organizations, the climate crisis is inherently unfair; poorer countries will suffer its consequences more than others. India is one amongst the nine countries identified to be seriously affected by climate change. According to a WHO analysis ( 2016 ) India could face more than 25% of all global climate-related deaths by 2050 due to decreasing food availability. China is expected to face the highest number of per capita food insecurity deaths. Bhutan, a small Himalayan kingdom with 60% forest cover, is the most net negative carbon emission country; its GHG emission is less than the amount removed from the atmosphere. Other countries should aim to emulate Bhutan as early as possible.
A number of other options have been suggested to trap atmospheric carbon dioxide (Climate change mitigation—Wikipedia). Carbon storage through sequestration of organic carbon by deep-rooted grasses has been one such approach (Fisher et al. 1994 ). Several studies from Africa have indicated that introduction of Brachiaria grasses in semi-arid tropics can help to increase not only carbon stock in the soil but also yield greater economic returns (Gichangi et al. 2017 ). Recently a new seed bank, ‘Future Seeds’ was dedicated at Palmira, Columbia to store world’s largest collection of beans, cassava, and tropical forage grasses for the use of breeders to create better performing and climate-resistant crops (Stokstad 2022 ). Brachiaria humidicola is one of the tropical forage grass stored in this seed bank for its potential benefit in carbon sequestration. Lavania and Lavania ( 2009 ) have suggested vetiver ( Vetiveria zizanioides ), a C 4 perennial grass, with massive fibrous root system that can grow up to 3 m into the soil in 1 year, as a potential species for this purpose. Vetiver is estimated to produce 20–30 tonnes of root dry matter per hectare annually and holds the potential of adding 1 kg atmospheric CO 2 annually to the soil carbon pool per m 2 surface area. Carbon dioxide capture and storage is another such potential approach. At present it is too expensive and this approach may have to wait until improvement of the technology, reduction in the cost and feasibility of transfer of the technology to developing countries (IPCC Special Report on carbon dioxide capture and storage 2005 ).
There has been some discussion on the role of climate change on speciation (Levin 2019 ; Gao et al. 2020 ). Some evolutionary biologists have observed that the rate of speciation has accelerated in the recent past due to climate change and would continue to increase in the coming decades (Thomas 2015 ; Levin 2019 ; Gao et al. 2020 ). They propose that auto- and allo-polyploidy are going to be the primary modes of speciation in the next 500 years (Levin 2019 , see also Gao 2019 , Villa et al. 2022 ). However, extinction of species imposed by climate change may excel positive impact on plant speciation via polyploidy (Gao et al. 2020 ). The question is will climate change induce higher level of polyploidy and other genetic changes in crop species also that would promote evolution of new genotypes to sustain productivity and quality of food grains? If so, it would ameliorate, to some extent, food and nutritional insecurity of humans especially in the developing world.
Effective implementation of the pledges made by different countries in COP 26 and actions to be taken in the coming COP meetings are going to be crucial and determine humanity’s success or failure in tackling climate change emergency. COP 26 climate pact to cut greenhouse gas emissions, end of deforestation and shift to sustainable transport is certainly more ambitious then earlier COPs. There are also many other positive signals for reducing fossil fuels. Scientists have started using more precise monitoring equipment to collect more reliable environmental data, and more options are being developed by researchers on renewable and alternate energy sources, and to capture carbon from industries or from the air (Chandler D, MIT News 24 Oct 2019, Swain F, BBC Future Planet, 12 March 2021). Scotland has become coal-free and Costa Rica has achieved 99% renewable energy. India has reduced the use of fossil fuel by 40% of it installed capacity, 8 years ahead of its commitment at the COP 26.
Further, people are becoming more conscious to reduce carbon emission by following climate-friendly technologies. Human sufferings associated with an increase in natural disasters throughout the world have focussed public attention on climate change as never before. They also realise the benefits of improved air quality by reducing consumption of coal and fossil fuels on health and ecosystems. The demand for electric vehicles is steadily growing. Reforestation is being carried out in a large scale in many countries. Recent studies across a range of tree plantations and native forests in 53 countries have revealed that carbon storage, soil erosion control, water conservation and biodiversity benefits are delivered better from native forests compared to monoculture tree plantations, although the latter yielded more wood (Hua et al. 2022 ). This has to be kept in mind in reforestation programmes. Hopefully the world will be able to realize the goal of limiting the temperature rise to 1.5 °C by the end of the century and humanity would learn to live in harmony with Nature.
Declarations
The author declares no conflict of interest.
- Abdurrahman MI, Chaki S, Saini G. Stubble burning: effects on health & environment, regulations and management practices. Environ. Adv. 2020 doi: 10.1016/j.envadv.2020.100011. [ CrossRef ] [ Google Scholar ]
- Allen JM, Terres MA, Katsuki T, et al. Modelling daily flowering probabilities: expected impact of climate change on Japanese cherry phenology. Global Change Biol. 2013; 20 :1251–1263. doi: 10.1111/gcb.12364. [ PubMed ] [ CrossRef ] [ Google Scholar ]
- Anonymous (2018) https://www.euronews.com/2018/02/02/rising-sea-levels-threat-a-shrinking-european-coastline-in-2100
- Anonymous (2021) Special Issue. Global decline in the Anthropocene. Proc Natl Acad Sci, USA 118: No 2
- Aquilera R, Curringham TW, Gershunov A, Benmarhnia T. Wildfire smoke impacts respiratory health more than fine particles from other sources: observational evidence from Southern California. Nat. Commun. 2021 doi: 10.1038/s41467-021-21708-0. [ PMC free article ] [ PubMed ] [ CrossRef ] [ Google Scholar ]
- AR6 Climate Change: The sixth assessment report on climate change. IPCC, Geneva (2021). https://www.ipcc.ch/report/ar6/wg1/
- Beckage B, Osborne B, Gavin DG, et al. A rapid upward shift of a forest ecotone during 40 years of warming in the Green Mountains of Vermont. Proc. Natl. Acad. Sci. USA. 2008; 105 :4197–4202. doi: 10.1073/pnas.0708921105. [ PMC free article ] [ PubMed ] [ CrossRef ] [ Google Scholar ]
- Beckman NG, Aslan CE, Rogers HS. The role of seed dispersal in plant populations: perspectives and advances in a changing world. AoB Plants. 2020 doi: 10.1093/aobpla/plaa010. [ PMC free article ] [ PubMed ] [ CrossRef ] [ Google Scholar ]
- Bellard C, Leclerc C, Courchamp F. Impact of sea level rise on the 10 insular biodiversity hotspots. Global Ecol. Biogeogr. 2013 doi: 10.1111/geb.12093. [ CrossRef ] [ Google Scholar ]
- Bloom AJ, Plant RC. Wheat grain yield decreased over the past 35 years, but protein content did not change. J. Exptl. Bot. 2021; 72 :6811–6821. doi: 10.1093/jxb/erab343. [ PubMed ] [ CrossRef ] [ Google Scholar ]
- Bonar SA. More than 111 aquatic-science societies sound climate alarm. Nature. 2021; 589 :352. doi: 10.1038/d41586-021-00107-x. [ PubMed ] [ CrossRef ] [ Google Scholar ]
- Bras TA, Seixas J, Nuno C, Jonas J. Severity of drought and heatwave crop losses tripled over the last five decades in Europe. Environ. Res. Lett. 2021 doi: 10.1088/1748-9326/abf004. [ CrossRef ] [ Google Scholar ]
- Ceballos G, Ehrlich PP, Barnosky AD, et al. Accelerated modern human-induced species losses: Entering the sixth mass extinction. Sci. Adv. 2015; 1 :e1400253. doi: 10.1126/sciadv.1400253. [ PMC free article ] [ PubMed ] [ CrossRef ] [ Google Scholar ]
- Centre for Biological Diversity: Deadly Waters: How Rising Seas Threaten 233 Endangered Species. (2013) https://www.biologicaldiversity.org/campaigns/sea-level_rise/pdfs/Sea_Level_Rise_Report_2013_web.pdf
- Cleland EE, Chuine I, Menzel A, et al. Shifting plant phenology in response to global change. Trends Ecol. Evol. 2007; 22 :357–365. doi: 10.1016/j.tree.2007.04.003. [ PubMed ] [ CrossRef ] [ Google Scholar ]
- Deutsch CA, Tewksbury JJ, Tigchelaar M, et al. Increase in crop losses to insect pests in a warming climate. Science. 2018; 361 :916–919. doi: 10.1126/science.aat3466. [ PubMed ] [ CrossRef ] [ Google Scholar ]
- Dirzo R, Raven PH. Global state of biodiversity and loss. Ann. Rev. Environ. Res. 2003; 28 :137–167. doi: 10.1146/annurev.energy.28.050302.105532. [ CrossRef ] [ Google Scholar ]
- Dunnell KL, Travers SE. Shifts in the flowering phenology of the northern Great Plains: patterns over 100 years. Am. J. Bot. 2011; 98 :935–945. doi: 10.3732/ajb.1000363. [ PubMed ] [ CrossRef ] [ Google Scholar ]
- Elena O, Matthew E-S, Matea O, et al. A conservation assessment of all natural World Heritage sites. Gland: IUCN; 2020. IUCN World Heritage Outlook 3. [ Google Scholar ]
- Fisher MJ, Rao IM, Ayarza MA, et al. Carbon storage by introduced deep-rooted grasses in the South American Savannas. Nature. 1994; 371 :236–238. doi: 10.1038/371236a0. [ CrossRef ] [ Google Scholar ]
- Forrest JRK, Thomson JD. An examination of synchrony between insect emergence and flowering in the Rocky Mountain meadows. Ecol. Monogr. 2011; 81 :469–491. doi: 10.1890/10-1885.1. [ CrossRef ] [ Google Scholar ]
- Fricke EC, Ordonez A, Rogers HS, et al. The effects of defaunation on plants’ capacity to track climate change. Science. 2022; 375 :210–214. doi: 10.1126/science.abk3510. [ PubMed ] [ CrossRef ] [ Google Scholar ]
- Gaira KS, Rawal R, Rawat B, Bhatt ID. Impact of climate change on the flowering of Rhododendron arboreum in central Himalaya, India. Curr. Sci. 2014; 106 :1735–1738. [ Google Scholar ]
- Gao JG. Dominant plant speciation types. A commentary on: plant speciation in the age of climate change. Ann. Bot. 2019; 124 :iv–vi. doi: 10.1093/aob/mcz174. [ PMC free article ] [ PubMed ] [ CrossRef ] [ Google Scholar ]
- Gao JG, Liu H, Wang N, et al. Plant extinction excels plant speciation in the Anthropocene. BMC Plant Biol. 2020; 20 :430. doi: 10.1186/s12870-020-02646-3. [ PMC free article ] [ PubMed ] [ CrossRef ] [ Google Scholar ]
- Genes L, Dirzo R. Restoration of plant–animal interactions in terrestrial ecosystems. Biol. Conserv. 2021 doi: 10.1016/j.biocon.2021.109393. [ CrossRef ] [ Google Scholar ]
- Gerard M, Vanderplanck M, Wood T, Michez D. Global warming and plant-pollinator mismatches. Emerg. Top. Life Sci. 2020; 4 :77–86. doi: 10.1042/ETLS20190139. [ PMC free article ] [ PubMed ] [ CrossRef ] [ Google Scholar ]
- Getirana A, Libonati R, Cataldi M. Brazil is in water crisis—it needs a drought plan. Nature. 2021; 600 :218–220. doi: 10.1038/d41586-021-03625-w. [ PubMed ] [ CrossRef ] [ Google Scholar ]
- Gichangi EM, Njarui DMG, Gatheru M. Plant shoots and roots biomass of Brachiaria grasses and their effect on soil carbon in the semi-arid tropics of Kenya. Trop. Subtrop. Agroecosyst. 2017; 20 :65–74. [ Google Scholar ]
- Gordo O, Sanz JJ. Phenology and climate change: a long-term study in a Mediterranean locality. Oecologia. 2005; 146 :484–495. doi: 10.1007/s00442-005-0240-z. [ PubMed ] [ CrossRef ] [ Google Scholar ]
- Grabherr G, Gottfried M, Pauli H. Climate effects on mountain plants. Nature. 1994; 369 :448. doi: 10.1038/369448a0. [ PubMed ] [ CrossRef ] [ Google Scholar ]
- Hausfather Z, Drake HF, Abbott T, Schmidt GA. Evaluating the performance of past climate model projections. Geophys. Res. Lett. 2019 doi: 10.1029/2019GL085378. [ CrossRef ] [ Google Scholar ]
- Heinrich B. Keeping a cool head: honeybee thermoregulation. Science. 1979; 205 :1269–1271. doi: 10.1126/science.205.4412.1269. [ PubMed ] [ CrossRef ] [ Google Scholar ]
- Heywood VH. Plant conservation in the Anthropocene: challenges and future prospects. Plant Divers. 2017; 39 :314–330. doi: 10.1016/j.pld.2017.10.004. [ PMC free article ] [ PubMed ] [ CrossRef ] [ Google Scholar ]
- Hoegh-Guldberg O, Poloczanska ES, Skirving W, Dove S. Coral Reef Ecosystems under climate change and ocean acidification. Front. Mar. Sci. 2017; 4 :158. doi: 10.3389/fmars.2017.00158. [ CrossRef ] [ Google Scholar ]
- Hua F, Bruijnzeel LA, Meli P, et al. The biodiversity and ecosystem service contributions and trade-offs of forest restoration approaches. Science. 2022 doi: 10.1126/science.abl4649. [ PubMed ] [ CrossRef ] [ Google Scholar ]
- Hughes TP, Kerry TJ, Baird AH, et al. Global warming transforms coral reef assemblages. Nature. 2018; 556 :492–496. doi: 10.1038/s41586-018-0041-2. [ PubMed ] [ CrossRef ] [ Google Scholar ]
- Immerzeel WW, Lutz AF, Andrade M, et al. Importance and vulnerability of the world’s water towers. Nature. 2020; 577 :364–369. doi: 10.1038/s41586-019-1822-y. [ PubMed ] [ CrossRef ] [ Google Scholar ]
- IPBES: The intergovernmental science-policy platform on biodiversity and ecosystem services. In: Sustainable development goals. IPBES, Bonn (2019)
- IPCC: IPCC special report on carbon dioxide capture and storage (2005). https://www.ipcc.ch›2018/03›srccs_wholereport-1
- Indian State Forest Report: Forest Survey of India (2019). https://www.drishtiias.com
- Jägermeyr J, Müller C, Ruane AC, et al. Climate impacts on global agriculture emerge earlier in new generation of climate and crop models. Nat. Food. 2021 doi: 10.1038/s43016-021-00400-y. [ CrossRef ] [ Google Scholar ]
- Kerr JT, Pinder A, Galpern P, et al. Climate impacts on bumblebees coverage across continents. Science. 2015; 349 :177–180. doi: 10.1126/science.aaa7031. [ PubMed ] [ CrossRef ] [ Google Scholar ]
- King O, Bhattacharya A, Ghuffar S, Tait A, et al. Six decades of glacier mass changes around Mt. Everest are revealed by historical and contemporary Images. One Earth. 2021 doi: 10.1016/j.oneear.2020.10.019. [ CrossRef ] [ Google Scholar ]
- Kudo G. Vulnerability of phenological synchrony between plants and pollinators in an alpine ecosystem. Ecol. Res. 2014; 29 :571–581. doi: 10.1007/s11284-013-1108-z. [ CrossRef ] [ Google Scholar ]
- Kudo G, Ida TY. Early onset of spring increases the mismatch between plants and pollinators. Ecology. 2013; 94 :2311–2320. doi: 10.1890/12-2003.1. [ PubMed ] [ CrossRef ] [ Google Scholar ]
- Lavania UC, Lavania S. Sequestration of atmospheric carbon into subsoil horizons through deep-rooted grasses-vetiver grass model. Curr. Sci. 2009; 97 :618–619. [ Google Scholar ]
- Lee E, Carrivick JL, Quincey DJ, et al. Accelerated mass loss of Himalayan glaciers since the little ice age. Sci. Rep. 2021; 11 :24284. doi: 10.1038/s41598-021-03805-8. [ PMC free article ] [ PubMed ] [ CrossRef ] [ Google Scholar ]
- Leech DI, Crick HQP. Influence of climate change on the abundance, distribution and phenology of woodland bird species in temperate regions. Ibis. 2007; 149 (Suppl. 2):128–145. doi: 10.1111/j.1474-919X.2007.00729.x. [ CrossRef ] [ Google Scholar ]
- Leeming J. Meet the food pioneer whose meat replacements are rocking the gravy boat. Nature. 2021; 590 :176. doi: 10.1038/d41586-021-00264-z. [ PubMed ] [ CrossRef ] [ Google Scholar ]
- Lenzen M, Moran D, Kanemoto K, et al. International trade drives biodiversity threats in developing nations. Nature. 2012; 486 :109–112. doi: 10.1038/nature11145. [ PubMed ] [ CrossRef ] [ Google Scholar ]
- Levin DA. Plant speciation in the age of climate change. Ann. Bot. 2019; 124 :769–775. doi: 10.1093/aob/mcz108. [ PMC free article ] [ PubMed ] [ CrossRef ] [ Google Scholar ]
- Lobell DB, Schlenker W, Costa-Roberts J. Climate trends and global crop production since 1980. Science. 2011; 333 :616–620. doi: 10.1126/science.1204531. [ PubMed ] [ CrossRef ] [ Google Scholar ]
- Lovejoy TE, Hannah L, editors. Biodiversity and climate change: transforming the biosphere. New Haven, London: Yale University Press; 2005. [ Google Scholar ]
- Manisalidis I, Stavropoulou E, Stavropoulos A, Bezirtzoglou E. Environmental and health impacts of air pollution: a review. Front. Public Health. 2020 doi: 10.3389/fpubh.2020.00014. [ PMC free article ] [ PubMed ] [ CrossRef ] [ Google Scholar ]
- Maxwell SL, Butt N, Maron M, et al. Conservation implications of ecological responses to extreme weather and climate events. Divers. Distrib. 2019; 25 :613–625. doi: 10.1111/ddi.12878. [ CrossRef ] [ Google Scholar ]
- McCrystall MR, Stroeve J, Serreze M, et al. New climate models reveal faster and larger increases in Arctic precipitation than previously projected. Nat. Commun. 2021 doi: 10.1038/s41467-021-27031-y. [ PMC free article ] [ PubMed ] [ CrossRef ] [ Google Scholar ]
- Memmott J, Craze PE, Waser NM, Price MV. Global warming and disruption of plant-pollinator interactions. Ecol. Lett. 2007; 10 :710–717. doi: 10.1111/j.1461-0248.2007.01061.x. [ PubMed ] [ CrossRef ] [ Google Scholar ]
- Miller-Rushing A, Hoye TH, Inouye D, Post E. The effects of phenological mismatches on demography. Philos. Trans. R. Soc. B. 2010; 365 :3177–3186. doi: 10.1098/rstb.2010.0148. [ PMC free article ] [ PubMed ] [ CrossRef ] [ Google Scholar ]
- Negrutiu I, Frohlich MW, Hamant O. Flowering plants in the Anthropocene: a political agenda. Trends Plant. Sci. 2020; 25 :349–368. doi: 10.1016/j.tplants.2019.12.008. [ PubMed ] [ CrossRef ] [ Google Scholar ]
- Obura D, Gudka M, Samoilys M, et al. Vulnerability to collapse of coral reef ecosystems in the Western Indian Ocean. Nat. Sustain. 2021; 5 :104–113. doi: 10.1038/s41893-021-00817-0. [ CrossRef ] [ Google Scholar ]
- OXFAM International: 5 natural disasters that beg for climate action. (2021) https://www.oxfam.org/en/5-natural-disasters-beg-climate-action
- Pacheco P, Mo K, Dudley N, et al. Deforestation fronts: drivers and responses in a changing world. Gland: WWF; 2021. [ Google Scholar ]
- Parmesan C, Yohe G. A globally coherent fingerprint of climate change impacts across natural systems. Nature. 2003; 421 :37–42. doi: 10.1038/nature01286. [ PubMed ] [ CrossRef ] [ Google Scholar ]
- Pautasso M. Observed impacts of climate change on terrestrial birds in Europe: an overview. Ital. J. Zool. 2012; 79 :296–314. doi: 10.1080/11250003.2011.627381. [ CrossRef ] [ Google Scholar ]
- Pennisi E. Lyme-carrying ticks live longer—and could spread farther—thanks to warmer winters. Science. 2022 doi: 10.1126/science.acz9985. [ CrossRef ] [ Google Scholar ]
- Pimentel D, Pimentel M. Sustainability of meat-based and plant-based diets and the environment. Am. J. Clin. Nutr. 2003; 78 :660S–663S. doi: 10.1093/ajcn/78.3.660S. [ PubMed ] [ CrossRef ] [ Google Scholar ]
- Pimm SL. Climate disruption and biodiversity. Curr. Biol. 2009; 19 :R595–R601. doi: 10.1016/j.cub.2009.05.055. [ PubMed ] [ CrossRef ] [ Google Scholar ]
- Potapov P, Turubanova S, Hansen MC, et al. Global maps of cropland extent and change show accelerated cropland expansion in the twenty-first century. Nat. Food. 2021 doi: 10.1038/s43016-021-00429-z. [ CrossRef ] [ Google Scholar ]
- Primack RB, Higuchi H, Miller-Rushing AJ. The impact of climate change on cherry trees and other species in Japan. Biol. Conserv. 2009; 142 :1943–1949. doi: 10.1016/j.biocon.2009.03.016. [ CrossRef ] [ Google Scholar ]
- Pruniki M, Cauwenberghs N, Lee J, et al. Air pollution exposure is linked with methylation of immunoregulatory genes, altered immune cell profiles, and increased blood pressure in children. Sci. Rep. 2021; 11 :4067. doi: 10.1038/s41598-021-83577-3. [ PMC free article ] [ PubMed ] [ CrossRef ] [ Google Scholar ]
- Rader R, Reilly J, Bartomeus I, Winfree R. Native bees buffer the negative impact of climate warming on honey bee pollination of watermelon crops. Global Change Biol. 2013; 19 :3103–3110. doi: 10.1111/gcb.12264. [ PubMed ] [ CrossRef ] [ Google Scholar ]
- Raven PH. Biological extinction and climate change. In: Al-Delaimy WK, Ramanathan V, SánchezSorondo M, editors. Health of people, health of planet and our responsibility. Cham: Springer; 2020. pp. 11–20. [ Google Scholar ]
- Razzaq A, Wani SH, Saleem F, et al. Rewilding crops for climate resilience: economic analysis and de novo domestication strategies. J Exptl Bot. 2021; 72 :6123–6139. doi: 10.1093/jxb/erab276. [ PubMed ] [ CrossRef ] [ Google Scholar ]
- Ripple WJ, Wolf C, Newsome TM, et al. World scientists’ warning to humanity: a second notice. Bioscience. 2017; 67 :1026–1028. doi: 10.1093/biosci/bix125. [ CrossRef ] [ Google Scholar ]
- Ripple WJ, Wolf C, Newsome TM, et al. World Scientists’ warning of a climate emergency. Bioscience. 2020; 70 :8–12. doi: 10.1093/biosci/biz152. [ CrossRef ] [ Google Scholar ]
- Salvatteci R, Schneider RR, Field EGD, et al. Smaller fish species in a warm and oxygen-poor Humboldt Current system. Science. 2022; 375 :101–104. doi: 10.1126/science.abj0270. [ PubMed ] [ CrossRef ] [ Google Scholar ]
- Sejiyan V, Bhatta R, Malik PK, et al. Livestock as sources of greenhouse gases and its significance to climate change. In: Moya BL, Pous J, et al., editors. Greenhouse gases. London: IntechOpen; 2016. [ Google Scholar ]
- Shivanna KR. The sixth mass extinction crisis and its impact on biodiversity and human welfare. Resonance. 2020; 25 :93–109. doi: 10.1007/s12045-019-0924-z. [ CrossRef ] [ Google Scholar ]
- Shivanna KR, Sanjappa M. Conservation of endemic and threatened flowering plants: challenges and priorities for India. J. Indian Bot. Soc. 2021; 101 :269–290. [ Google Scholar ]
- Shivanna KR, Tandon R, Koul M. ‘Global pollinator crisis’ and its impact on crop productivity and sustenance of biodiversity. In: Tandon R, Shivanna KR, Koul M, editors. Reproductive ecology of flowering plants: patterns and processes. Singapore: Springer; 2020. pp. 395–413. [ Google Scholar ]
- Sodhi NS, Ehrlich PR, editors. Conservation biology for all. Oxford: Oxford University Press; 2010. [ Google Scholar ]
- Soroye P, Newworld T, Kerr J. Climate change contributes to widespread declines among bumble bees across continents. Science. 2020; 367 :685–688. doi: 10.1126/science.aax8591. [ PubMed ] [ CrossRef ] [ Google Scholar ]
- Stokstad E. World’s largest bean and cassava collection gets a striking new home: “Future Seeds” gene bank will help plant breeders create new varieties of crops and carbon-storing grasses. ScienceInsider. 2022 doi: 10.1126/science.abq1510. [ CrossRef ] [ Google Scholar ]
- Thomas CD. Rapid acceleration of plant speciation during the Anthropocene. Trends Ecol. Evol. 2015; 30 :448–455. doi: 10.1016/j.tree.2015.05.009. [ PubMed ] [ CrossRef ] [ Google Scholar ]
- Velasquez-Tibata J, Salaman P, Catherine H, Graham CH. Effects of climate change on species distribution, community structure, and conservation of birds in protected areas in Colombia. Reg. Environ. Change. 2013; 13 :235–248. doi: 10.1007/s10113-012-032. [ CrossRef ] [ Google Scholar ]
- Villa S, Montagna M, Pierce S. Endemism in recently diverged angiosperms is associated with polyploidy. Plant Ecol. 2022 doi: 10.1007/s11258-022-01223-y9-y. [ CrossRef ] [ Google Scholar ]
- Voosen P. Key Antarctic ice shelf is within years of failure. Science. 2021; 374 :1420–1421. doi: 10.1126/science.acz9833. [ PubMed ] [ CrossRef ] [ Google Scholar ]
- Wagner DL. Insect decline in the Anthropocese. Ann. Rev. Entomol. 2020; 65 :457–480. doi: 10.1146/annurev-ento-011019-025151. [ PubMed ] [ CrossRef ] [ Google Scholar ]
- Wagner, D.L., Grames, E.M., Forister, M.L. et al.: Insect decline in the Anthropocene: Death by a thousand cuts. Proc. Natl. Acad. Sci. USA 118 , e2023989118 (2021). 10.1073/pnas.2023989118 [ PMC free article ] [ PubMed ]
- Warren R, Price J, Graham E, et al. The projected effect on insects, vertebrates and plants of limiting global warming to 1.5°C rather than 2°C. Science. 2018; 360 :791–795. doi: 10.1126/science.aar3646. [ PubMed ] [ CrossRef ] [ Google Scholar ]
- WCRP Global Sea Level Budget Group Global sea-level budget 1993–present. Earth Syst. Sci. Data. 2018; 10 :1551–1590. doi: 10.5194/essd-10-1551-2018. [ CrossRef ] [ Google Scholar ]
- WHO analysis . Monitoring health for the sustainable development goals. Geneva: WHO; 2016. World Health Statistics 2016. [ Google Scholar ]
- Wilson EO. Half-earth: our planet’s fight for life. New York: Liveright/Norton; 2016. [ Google Scholar ]
- Wilson EO, Peter FM, editors. Biodiversity. Washington DC: National Academy Press; 1988. [ PubMed ] [ Google Scholar ]
- Zattara EE, Aizen MA. Worldwide occurrence of records suggest a global decline in bee species richness. One Earth. 2021; 4 :114–123. doi: 10.1016/j.oneear.2020.12.005. [ CrossRef ] [ Google Scholar ]
- Zhao Z, Wang Y, Zang Z, et al. Climate warming has changed phenology and compressed the climatically suitable habitat of Metasequoia Glyptostroboides over the last half century. Global Ecol. Conserv. 2020; 23 :e01140. doi: 10.1016/j.gecco.2020.e01140. [ CrossRef ] [ Google Scholar ]
- Zhu C, Kobayashi K, Loladze I, et al. Carbon dioxide (CO 2 ) levels this century will alter the protein, micronutrients, and vitamin content of rice grains with potential health consequences for the poorest rice-dependent countries. Sci. Adv. 2018; 4 :eaaq012. doi: 10.1126/sciadv.aaq1012. [ PMC free article ] [ PubMed ] [ CrossRef ] [ Google Scholar ]
- Zhu T, Flavio C, De Lima F, De Smet I. The heat is on: how crop growth, development, and yield respond to high temperature. J. Exptl. Bot. 2021; 72 :7359–7373. doi: 10.1093/jxb/erab308. [ PubMed ] [ CrossRef ] [ Google Scholar ]
Click through the PLOS taxonomy to find articles in your field.
For more information about PLOS Subject Areas, click here .
Loading metrics
Open Access
Peer-reviewed
Research Article
Research on Biodiversity and Climate Change at a Distance: Collaboration Networks between Europe and Latin America and the Caribbean
* E-mail: [email protected]
Affiliations Institut de Recherche pour le Développement (IRD), UR 072, EGCE, UPR 9034, CNRS 91198 Gif-sur-Yvette Cedex, France and Université Paris-Sud 11, 91405, Orsay Cedex, France, Pontificia Universidad Católica del Ecuador, Facultad de Ciencias Exactas y Naturales, Av. 12 de Octubre y Roca, Quito, Ecuador
Affiliation Institut de Recherche pour le Développement (IRD), 44 bld de Dunkerque, 13572, Marseille, France
Affiliation Institut de Recherche pour le Développement (IRD), UMR PALOC, MNHN, Paris, 75005, France
Affiliations Microbial Ecology Centre, INRA (UMR 1418), CNRS (UMR 5557), Université de Lyon, Université Lyon1, 43 bd du 11 nov 1918, Villeurbanne, France, BiodivERsA, Fondation pour la Recherche sur la Biodiversité (FRB), 195 rue Saint Jacques, 75005, Paris, France
- Olivier Dangles,
- Jean Loirat,
- Claire Freour,
- Sandrine Serre,
- Jean Vacher,
- Xavier Le Roux
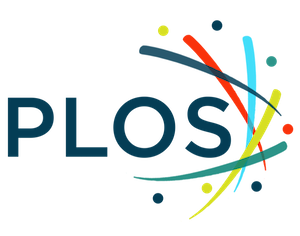
- Published: June 15, 2016
- https://doi.org/10.1371/journal.pone.0157441
- Reader Comments
Biodiversity loss and climate change are both globally significant issues that must be addressed through collaboration across countries and disciplines. With the December 2015 COP21 climate conference in Paris and the recent creation of the Intergovernmental Platform on Biodiversity and Ecosystem Services (IPBES), it has become critical to evaluate the capacity for global research networks to develop at the interface between biodiversity and climate change. In the context of the European Union (EU) strategy to stand as a world leader in tackling global challenges, the European Commission has promoted ties between the EU and Latin America and the Caribbean (LAC) in science, technology and innovation. However, it is not clear how these significant interactions impact scientific cooperation at the interface of biodiversity and climate change. We looked at research collaborations between two major regions—the European Research Area (ERA) and LAC—that addressed both biodiversity and climate change. We analysed the temporal evolution of these collaborations, whether they were led by ERA or LAC teams, and which research domains they covered. We surveyed publications listed on the Web of Science that were authored by researchers from both the ERA and LAC and that were published between 2003 and 2013. We also run similar analyses on other topics and other continents to provide baseline comparisons. Our results revealed a steady increase in scientific co-authorships between ERA and LAC countries as a result of the increasingly complex web of relationships that has been weaved among scientists from the two regions. The ERA-LAC co-authorship increase for biodiversity and climate change was higher than those reported for other topics and for collaboration with other continents. We also found strong differences in international collaboration patterns within the LAC: co-publications were fewest from researchers in low- and lower-middle-income countries and most prevalent from researchers in emerging countries like Mexico and Brazil. Overall, interdisciplinary publications represented 25.8% of all publications at the interface of biodiversity and climate change in the ERA-LAC network. Further scientific collaborations should be promoted 1) to prevent less developed countries from being isolated from the global cooperation network, 2) to ensure that scientists from these countries are trained to lead visible and recognized biodiversity and climate change research, and 3) to develop common study models that better integrate multiple scientific disciplines and better support decision-making.
Citation: Dangles O, Loirat J, Freour C, Serre S, Vacher J, Le Roux X (2016) Research on Biodiversity and Climate Change at a Distance: Collaboration Networks between Europe and Latin America and the Caribbean. PLoS ONE 11(6): e0157441. https://doi.org/10.1371/journal.pone.0157441
Editor: Robert Guralnick, University of Colorado, UNITED STATES
Received: December 2, 2015; Accepted: May 30, 2016; Published: June 15, 2016
Copyright: © 2016 Dangles et al. This is an open access article distributed under the terms of the Creative Commons Attribution License , which permits unrestricted use, distribution, and reproduction in any medium, provided the original author and source are credited.
Data Availability: All relevant data are within the paper and its Supporting Information files.
Funding: This research was funded by the ALCUE-Net project (FP7 funded project; Latin America, Caribbean and European Union Network on Research and Innovation; see www.alcuenet.eu ), in collaboration with the ERA-NET BiodivERsA (Horizon2020 funded project; see www.biodiversa.org ).
Competing interests: ALCUE-Net and BiodivERsA are initiatives gathering programmers and funders of research in many domains, including the biodiversity and climate change interface. ALCUE-Net is promoting collaboration between Europe and Latin America and the Caribbean for environmental sciences, while BiodivERsA is promoting pan-European research on biodiversity, including its international competitiveness and visibility. This requires mapping activities such as the bibliographic analysis made in the current study. This does not alter the authors' adherence to PLOS ONE policies on sharing data and materials.
Introduction
There is an increasing need to address global issues like biodiversity loss and climate change [ 1 ]. However, both issues are particularly complex, and addressing them requires skills and research inputs that span countries and disciplines [ 2 ]. Interdisciplinary teams have largely been promoted by funding programmes that direct research to development and politically desired goals [ 3 ]. Though collaborative research teams are common in studies of global, multi-faceted problems, international interdisciplinary research networks are not easy to build [ 4 ]. In particular, creating an effective international, interdisciplinary team is not as simple as bringing together a group of scientists from different disciplines and countries. It requires the development of common research languages, methods and models across both team members and countries [ 5 ].
Europe is the major donor for research and development cooperation in Latin America and the Caribbean (LAC), is the largest foreign direct investor in LAC, and is a key promoter of regional integration [ 6 ]. In the context of the EU strategy to stand as a world leader in tackling global challenges, the European Commission (EC) has indeed strongly promoted ties between the EU and LAC in science, technology and innovation. More than 750 LAC-based researchers have been funded through collaborative projects with European partners for a total €100 million through the 7th Framework Program (FP7) for research and innovation (2007–2013, www.eeas.europa.eu/lac ; see [ 7 ] for more details). As an example, CLARIS-LPB project (2008–2012 http://www.claris-eu.org/ ) brought 9 partners from LAC and 11 from EU together, to build possible scenarios on climate change on La Plata Basin and design adaptation strategies. The main areas of common interest promoted by the FP7 were renewable energies, climate services, bio-economy, marine research, ICT and health. Following the adoption by the EU-LAC Madrid Summit of the Joint Initiative for Research and Innovation (JIRI) in 2010, the EC has intensified its support for policy dialogue on science and technology. In particular, this led to the launch of the ALCUE-Net project (2013-2017- www.alcuenet.eu ) with the main objective to establish a bi-regional platform between actors involved in research and innovation, relevant stakeholders from the public and private sectors, and civil society so that a long-standing EU-LAC dialogue on science and technology could be implemented. The EC also supported the ERANet-LAC ( http://eranet-lac.eu ), which is a network of the EU and LAC Countries strengthening the bi-regional partnership in science, technology and innovation by planning and implementing concrete joint activities such as joint calls for research projects.
Beyond European programmes and initiatives, collaborative research between the ERA and LAC at the interface of biodiversity loss and climate change is also spurred by bilateral schemes that promote research between one European country and one LAC country. For instance, Brazil and Mexico were among the 14 countries for which the French National Research Agency (ANR) dedicated a bilateral scheme in 2014. For several years, the German Research Foundation (DFG) has operated a joint funding program with the Brazilian partner organisations FAPESP in the state of São Paulo and FAPEMIG in the state of Minas Gerais based on bilateral cooperation agreements.
However, it is not clear how these European, trans-continental and bilateral research programmes impact scientific cooperation at the interface of biodiversity and climate change. Political leaders in the European Union (EU) and in LAC have long recognised the importance of incorporating scientific knowledge into proposed solutions to the increasingly complex challenges that biodiversity loss and climate change pose to society at both local and global scales [ 8 , 9 ]. More knowledge-based solutions to these challenges are needed with the recent creation of the Intergovernmental Platform on Biodiversity and Ecosystem Services (IPBES), the debates associated with the December 2015 Climate Change Conference in Paris (COP21), and the need for the European Research Area (ERA) to explore nature-based solutions [ 10 ] and climate services [ 11 ]. ERA and LAC countries together host a great proportion of the world’s biodiversity [ 12 ] and are expected to experience significantly different and less predictable climates over time [ 13 ]. Therefore, cooperation at the interface of biodiversity and climate change should interest both, and demand holistic and regional approaches. But the outcome of the different research programmes developed for promoting ERA-LAC research collaboration remains to be evaluated, in particular for hot topics like the interactions between biodiversity and climate change.
Bibliographic analyses are useful for quantifying bi-regional cooperation in scientific research [ 14 , 15 ]. In particular, as scientific publications are the product of collaboration among researchers and institutions, they can provide an overview on the structure and dynamics of research networks. Therefore, the results of efforts to promote international research collaboration can be assessed by analysing networks of co-authors and characteristics of collaborations, such as temporal trends, and the geographic areas and research domains that they cover. Here, we analysed publications involving authors from the ERA and LAC published between 2003 and 2013 to study bi-regional research collaboration at the interface between biodiversity and climate change. We evaluated the relative importance of ERA-LAC collaboration compared to collaborations with other regions, the temporal evolution of the importance of ERA-LAC collaboration, the nationality of corresponding authors, and the type of research domain covered. We discuss the implications of our study to guide further development of ERA-LAC research collaborations in the future.

Materials and Methods
Bibliographic review.
Using the Web of Science version of the Thomson Reuters citation databases (WoS, webofknowledge.com), we searched the peer-reviewed literature (articles and reviews) published between 2003 and 2013 that focused on biodiversity, climate change and both together, and identified papers originating from ERA and LAC countries. The Web of Science platform draws from several online databases, three of which were particularly relevant for our search: two from the core collection (Science Citation Index, SCI: 7,100 journals; Social Science Citation Index, SSCI: 2,100 journals, etc.) and one from the Arts & Humanities Citation Index (AHCI: 1,700 journals). We found only four additional references by using other databases, including SciELO (mainly for South America), Scopus, Social Science Research Network, BioOne and MUSE.
We retrieved all publications with at least one author from a country within LAC (33 countries: Antigua and Barbuda, Argentina, Bahamas, Barbados, Belize, Bolivia, Brazil, Chile, Colombia, Costa Rica, Cuba, Dominica, Dominican Republic, Ecuador, El Salvador, Grenada, Guatemala, Guyana, Jamaica, Haiti, Honduras, Mexico, Nicaragua, Panama, Paraguay, Peru, Saint Kitts and Nevis, Saint Lucia, Saint Vincent and the Grenadines, Suriname, Trinidad and Tobago, Uruguay, Venezuela) and all publications with at least one author from a country within the ERA (28 countries from the EU plus 14 associate members: Austria, Belgium, Bulgaria, Croatia, Czech Republic, Cyprus, Denmark, Estonia, Finland, France, Germany, Greece, Hungary, Ireland, Italy, Latvia, Lithuania, Luxembourg, Malta, the Netherlands, Portugal, Poland, Romania, Slovakia, Slovenia, Spain, Sweden, United Kingdom, plus Albania, Bosnia, Faroe Islands, Israel, Iceland, Liechtenstein, Macedonia, Montenegro, Moldova, Norway, Serbia, Switzerland, Turkey). The publications with at least one author from a LAC country and at least one author from an ERA country were considered an ERA-LAC co-publication, as were publications authored by at least one scientist who was affiliated with organizations in both the ERA and LAC. To obtain baseline comparisons, we also performed a search for other continents, i.e. North America, Africa, Asia and Oceania.
As biodiversity is a broad, multi-faceted concept, we used the following keyword profile to identify biodiversity-related publications: TOPIC = (biodivers*, biological diversity, species richness, species diversity, taxonom*, phylogen*, animal diversity, mammal diversity, bird diversity, fish diversity, reptile diversity, amphibian diversity, frog diversity, insect diversity, plant diversity, weed diversity, microbial diversity, bacteria* diversity, fung* diversity, virus diversity, ecosystem diversity, habitat diversity, landscape diversity, biological conservation, species conservation, habitat conservation, genetic resource*, functional diversity, functional trait*, invasive species, biological invasion*, functional type*, functional group*). To search for publications on climate change, we used the following keywords: TOPIC = (climat* chang*, global warming, climat* variab*, climat* warming, extreme event*). We cross-referenced the two searches to identify publications at the interface of biodiversity and climate change and checked that all articles were related to these two specific topics. To obtain baseline comparisons, we also performed a search for other topics, i.e. all the publications not using any of the above-mentioned keywords.
All records were imported into an Excel dynamic database. Each record was tagged with its corresponding WoS section, SCI, SSCI or AHCI, a repeatable attribute as a relatively high level of record duplication exists between the three sections. The database was cleaned to avoid duplications and remove errors and inconsistencies (for example, in the country name, in the affiliations and address fields, see S1 Table ).
Data analysis
Co-publication network analysis..
We used the author addresses listed in the papers to determine the international networks of researchers. For each paper, we recorded information on all authors and identified the collaborating countries. Finally, we computed a triangular matrix to identify the strength of the links between each pair of countries based on the number of papers co-authored by these countries. Co-publication network mapping and analysis were performed using the open source Gephi software ([ 16 ], http://gephi.org ).
Mapping and co-authoring network analysis.
We performed two-dimensional spatial mapping of ERA-LAC co-authorship networks working at the interface of biodiversity and climate change using the Force-Atlas 2 algorithm in Gelphi. This algorithm creates a visual representation of nodes (countries) connected by edges (based on co-authorship) according to the following rules: 1) node size represents the number of publications, 2) all nodes are attracted to the center (the country with the highest number of publications), 3) all nodes repel each other (to prevent visual overlapping of the nodes), 4) all nodes that are connected by an edge attract each other, according to the weight of the edge (the number of publications with co-authorship between the two countries/regions). Two nodes are thus spatially closer if there are many collaborative publications. To examine the dynamics of the development of ERA-LAC co-authorship networks that were working at the interface of biodiversity and climate change over the last 11 years, we built maps for two different periods: 2003–2008 and 2008–2013.
We also created two-dimensional maps of the co-publication networks at the global level, looking at collaborations between the ERA, LAC, other European countries, North America, Africa, Asia, Oceania. As these networks were drawn on a world map background, only node size and edge weight were represented.
Authorship, themes, ecosystems, and interdisciplinarity.
To assess how involved different scientific communities were in ERA-LAC collaborations on issues related to biodiversity and climate change, we also computed 1) the proportion of ERA-LAC publications at the interface of biodiversity and climate change that correspond to five major scientific disciplines defined by the WoS (biological sciences, earth sciences, technology, human sciences, medicine), 2) several themes in each discipline (for example, within earth sciences we identified the following themes: geography, geology, oceanography, atmospheric sciences, and water resources). Several themes could be attributed to a single publication (this occurred for about 35% of all publications). For publications classified as ‘biological science’, which made up most of the records, we also identified whether the study was performed in terrestrial, marine or freshwater ecosystems, and whether the study had been performed within an LAC country or within the ERA. Better-resolution ecosystem units (e.g. corals, coastal zone, pelagic zone, mountains, dry forest, rainforest etc.) were not included in our analysis as 1) many articles spanned several ecosystem units (e.g., survey of disease vectors, global monitoring of birds), 2) several articles concerned systems that were related to several ecosystem units (e.g., glacial streams and riparian vegetation, forest and ponds of the Pantanal), 3) many studies were conducted in very singular ecosystems occurring only a few times in the database (e.g., cloud forest, paramos, altiplano deserts). Therefore, any analysis on ecosystem specificities would have been poorly relevant. In addition, we used a Venn diagram to visualize the interdisciplinarity of the ERA-LAC research on biodiversity and climate change, distinguishing three large disciplinary fields: biological, earth and human sciences.
Number of ERA-LAC publications on biodiversity and climate change, and temporal trends
The number of peer-reviewed publications that arose from collaborations between ERA and LAC countries on biodiversity, climate change and at the interface of biodiversity and climate change all increased exponentially between 2003 and 2013 ( Fig 1A ), with the highest exponential coefficients for the biodiversity/climate change interface (biodiversity/climate change = 0.28; climate change = 0.22; biodiversity = 0.15). As a control trend, the exponential coefficient for the publication number between ERA and LAC on all topics other than biodiversity and climate change was only of 0.087 during the same period. The total number of ERA-LAC co-publications at the interface of biodiversity and climate change between 2003 and 2013 was 500. The proportion of publications corresponding to this interface as compared to the sum of publications on ‘biodiversity’ plus ‘climate change’ increased 3-fold over the last decade from 2.2% to 6.9% (significant linear increase, R2 = 0.81, p < 0.01, Fig 1B ). In parallel, a 8-fold increase was observed for the proportion of publications on the biodiversity/climate change interface regarding the sum of publications on all other topics (dash line, R2 = 0.92, p < 0.01, Fig 1B ).
- PPT PowerPoint slide
- PNG larger image
- TIFF original image
(A) Temporal trend of the number of scientific publications (log scale) involving collaboration between Latin America/Caribbean (LAC) and the European Research Area (ERA) during the last decade: (White dots) climate change; (White squares) biodiversity; (Black dots) biodiversity and climate change; (Black triangles) all other topics. (B) Temporal evolution of the annual proportion of the total number of publications on biodiversity plus those on climate change (black dots, black line) and of the total number of publications on all other topics (white dots, dashed line) that were at the interface of biodiversity and climate change over the 10 year period studied.
https://doi.org/10.1371/journal.pone.0157441.g001
ERA-LAC collaboration and its importance at the global scale
At a global scale, ERA-LAC co-publications represented 7.9% of the total number of publications at the interface of biodiversity and climate change between 2003 and 2013, co-publications between the ERA and North America representing 22.9%. Between 2003 and 2013, Europeans authored 2.8 times more publications at the interface of biodiversity and climate change than did LAC scientists (3706 vs. 1312, respectively).
Europeans published studies at the interface of biodiversity and climate change mainly through intra-European networks (54.8% of all ERA publications on this topic), and to a lesser extent with North America (18.2%; Fig 2A ). ERA-LAC collaborations (500 publications) were comparable to those between the ERA and Africa (467 publications), Asia (543 publications) and Oceania (631 publications).
Data concern publications the interface of biodiversity and climate change as compared with other transcontinental collaborations on this topic for (A) the ERA and (B) LAC. The arrows in the maps represent the level of co-publication between two continents (node sizes are not comparable between the two panels). The pies on the right provide the values of the different links, including collaborations between countries within each region.
https://doi.org/10.1371/journal.pone.0157441.g002
LAC scientists published studies at the interface of biodiversity and climate change equally with the ERA, North America and other LAC countries (27.0 to 29.2%), and they rarely published with colleagues from Oceania, Asia and Africa ( Fig 2B ). The number of publications at the interface of biodiversity and climate change that arose from collaborations between ERA countries and North America, Oceania, Africa, Asia and other European countries all increased between 2003 and 2013 ( Fig 3A ), but the exponential coefficients were lower (0.21–0.24) than those calculated for the ERA-LAC collaboration (0.28). Moreover, for LAC countries, the collaboration with Oceania, Africa, Asia and European countries other than ERA, only slightly increased over the 2003–2013 period (exponential coefficient range = 0.09–0.19).
Temporal variation in the number of (Top) ERA and (Bottom) LAC publications on biodiversity X climate change that involved co-publications with either Africa, Oceania, Asia, Europe (non-ERA) or North America. The dashed line corresponds to the temporal trend observed for the ERA-LAC publications (see Fig 1A ).
https://doi.org/10.1371/journal.pone.0157441.g003
ERA-LAC collaboration network, and its temporal evolution
The proportion of ERA-LAC co-publications at the interface of biodiversity and climate change increased from around 3% in 2003 to 8% in 2013 of total ERA publications (significant linear increase: R 2 = 0.44, p < 0.05) and from around 25% to 40% of total LAC publications over the same period ( Fig 4 ) (R 2 = 0.41, p < 0.05). The increase in collaborative ERA-LAC papers at the interface of biodiversity and climate change (4.8 more papers in 2008–2013 than in 2003–2008) over time was associated with an expanded and more complex network of co-author links ( Fig 5 ). On average, ERA and LAC countries increased their number of papers between the two time periods by a factor of 5.1 and 4.4, respectively. Brazil played an increasingly important role in the network, producing an average of 8.5 more papers between 2008 and 2013 than it did between 2003 and 2008. Several ERA countries increased their influence between the two periods as well: Sweden (30-fold increase), Portugal (25) and Italy (8.7). While the core of the collaboration network included Brazil, Argentina, Mexico, UK, Spain, France, Germany, the Netherlands and Switzerland for both periods, by the second time period that core also included Chile, Venezuela, Ecuador, Colombia, Portugal, Italy and Denmark ( Fig 5 ).
Temporal variation in the proportion of (A) the ERA publications that involve co-publications with LAC, and (B) the LAC publications that involve co-publications with ERA. The topic considered is the interface of biodiversity and climate change.
https://doi.org/10.1371/journal.pone.0157441.g004
Cooperation network between countries from the ERA (in blue) and LAC (in red) on biodiversity and climate change together during (Top) 2003–2008 and (Bottom) 2008–2013. Disc size corresponds to the weighted degree (number of countries to which a country is linked, weighted by the number of publications).
https://doi.org/10.1371/journal.pone.0157441.g005
Overall, a country’s influence in cooperative networks with the other countries (betweenness centrality) increased with the number of publications produced, i.e. countries with fewer publications generally had lower betweenness centrality coefficients ( Fig 6 ). However, countries like, above all Italy and Mexico, but also Bolivia, the Netherlands and Spain had a higher betweenness centrality index than their publication number would suggest they should ( Fig 6 ), while others, like the UK, Germany, France and Brazil (and to a lesser extent Chile) had a lower betweenness centrality score than might be expected based on their publication numbers.
(A) countries from the ERA and (B) countries from the LAC. For each variable and each panel, values are normalized by the maximum value observed.
https://doi.org/10.1371/journal.pone.0157441.g006
Leading authorship
Overall, ERA researchers were corresponding authors for 50% of the ERA-LAC publications at the interface of biodiversity and climate change, while 30% were LAC researchers ( Fig 7 ). ERA authors based in the UK, Germany and Spain were the most common for ERA-led ERA-LAC publications. LAC authors based in Brazil and Mexico were most common for LAC-led ERA-LAC publications ( Fig 7 ).
https://doi.org/10.1371/journal.pone.0157441.g007
No ERA-LAC authors, institutions or projects contributed disproportionally to the bulk of publication (PIE = 0.91, 0.87 and 0.89, respectively). The most prolific authors, institutions and projects accounted to a maximum of 2.0, 3.8 and 3.1% of all publications, respectively).
Themes addressed, disciplines mobilized and interdisciplinarity level
Biological science was by far (63%) the most common theme among the ERA-LAC publications at the interface of biodiversity and climate change, followed by earth sciences (14%), technology sciences (13%) and human sciences (8%) ( Fig 8 ). The most common scientific domains within these themes were ecology (250 publications), biodiversity conservation (82 publications), science and technology (72 publications) and physical geography (44 publications). Publications in domains of high socio-economic relevance, such as agriculture, fisheries or tropical diseases, were rare.
The inset (top right) details the environments studied, indicating whether or not the study sites were on LAC territory. Note that a given discipline (e.g., geography) can be classified into two different domains (e.g., earth sciences and human sciences)
https://doi.org/10.1371/journal.pone.0157441.g008
Overall, 85% of all studies in biological sciences were conducted in sites/organisms within LAC territories, mainly in terrestrial and freshwater environments, and to a lesser extent, marine environments ( Fig 8 —inset). Interdisciplinary publications, at the interface of biodiversity and climate change, represented about one fourth (25.8%) of all publications in the ERA-LAC network ( Fig 9 ). Publications that spanned both biological and earth sciences were most common (105 publications) followed by those arising from both human and earth science (50 publications), biological and human sciences (46 publications) and biological, human, and earth sciences (36 publications).
Circle diameter is proportional (log-scale) to the total number of articles.
https://doi.org/10.1371/journal.pone.0157441.g009
Within the LAC region is an exceptionally rich diversity of species and ecosystems. There is already severe pressure on the capacity of these species and ecosystems to deliver essential services to society [ 19 , 20 , 21 ]. While sound management of biological resources may strengthen the economies of the region, mismanagement in some countries is accelerating environmental degradation, food and water insecurity, and health and social problems [ 22 ]. The additional challenges imposed by climate change require the scientific community to join forces in documenting the climate change-induced alterations to ecosystems, increasing understanding of the biological underpinnings of ecosystem services (in particular, those linked to climate regulation, and climate change effects on services) and ecosystem-based adaptation [ 23 ], and developing other approaches for maintaining and restoring resilient socio-ecological systems in the face of climate change [ 24 ]. Our analysis shows that most ERA-LAC studies focus on sites/organisms within LAC territories, which demonstrates that ERA-LAC collaboration mainly aims at better studying issues related to biodiversity and climate change in this biodiversity-rich and climate-sensitive region.
Growth in the number of papers at the interface of biodiversity and climate change has been exponential over the last decade, indicating that scientists in the ERA and LAC have recognized the importance of studying the links between biodiversity and climate change. The proportion of publications at the interface of biodiversity and climate change and of publications involving ERA-LAC partners has also significantly increased between 2008 and 2013 than it did between 2003 and 2008. This increase in ERA-LAC publication in biodiversity and climate change was higher than that for other topics and with other regions of the world, even North America. In addition, although EU development policy aims to support Latin American regional integration [ 25 ], our study reveal strong differences in international collaboration patterns within LAC: there were much fewer co-publications with low- and lower-middle-income countries and much more with emerging countries like Mexico and Brazil.
These two main characteristics of the ERA-LAC collaborations at the interface of biodiversity and climate change, i.e. growth in collaborations and marked differences in representation among countries, have already been reported in other studies on international scientific cooperation, both between ERA-LAC regions [ 15 ] and worldwide [ 24 ]. Various theories have been proposed to explain the rise of international scientific cooperation [ 24 ]. Among them, the development of ‘big science’, including global biodiversity projects (e.g., GLORIA, CTFS), historical relationships like former colonial ties (e.g. within the Spanish-speaking communities), and development of national research funding programmes (e.g., in Brazil or Ecuador) have likely contributed to the expansion of the ERA-LAC co-publication network working at the interface of biodiversity and climate change. Moreover, it is noteworthy that the EU program for research and innovation has been opened to international cooperation under various schemes (bilateral, multilateral), thus encouraging international research projects to emerge, as in the case of ERA-LAC collaboration. Bilateral science funding and development policy are therefore also at play to explain the increase in ERA-LAC collaboration and associated number of papers in the study period. These contribute to integrating research cooperation and policy into development cooperation processes, although it has to be differentiated from the official EU international cooperation and development programs (e.g., EuropeAid), which has less to do with scientific research policy.
The increase in scientific co-authorships between ERA-LAC countries is also likely an outcome of the increasingly complex web of relationships that has been weaved between scientists of the two regions [ 15 ]. While some countries like Mexico, Italy and the Netherlands relied heavily on international and regional collaboration to boost scientific production, others like Brazil and the UK appear to have another strategy by engaging in bilateral collaboration with selected countries or states [ 15 ]. Overall, countries like Brazil, France, the UK and Germany mostly develop bilateral cooperation with poor regional integration, and further efforts should be made to foster cooperation within LAC if regional integration is viewed as a key target. While no individual ERA country reached levels of co-authorship like that between the United States of America and LAC, the ERA as a whole equalled North America’s level of scientific partnerships with LAC. This pattern probably illustrates comparable economic units and funding flows between North America/ERA and LAC [ 7 ].
Many issues addressed by the scientific community studying biodiversity and climate change together (e.g., the study of reef system’s resilience to climate change or adaption to changing fishery resources) requires an interdisciplinary approach that includes life, earth and social sciences. Our study found that about one fourth of all studies on biodiversity and climate change within the ERA-LAC cooperation network were interdisciplinary. These levels may be underestimated, as our publication sample was not selected on terms that might have pulled in more human science papers. Moreover, within the large categories are also many disciplines and important interdisciplinarity which may be overlooked. While our study cannot conclude on the importance of interdisciplinarity in the ERA-LAC network, this issue is central to sound research on biodiversity and climate change and would merit further investigations. As evidenced in a recent ALCUE-Net meeting (see http://alcuenet.eu/meeting-events.php?event=TkRRNA ), a major barrier to the scientific cooperation between biodiversity and climate change communities seems to be the discrepancy in the data formats, resolution, uncertainties, and access of each community. To improve understanding and collaboration between both communities, their dialogue should be strengthened through capacity building activities and cooperation projects so that biodiversity community’s expectations could be better identified and climate models limitations better assessed. Moreover, although the internal disciplinary differentiation of science motivates linkages among research teams in specific research domains at the international level [ 26 ], the scientific community of both regions must put more effort into developing common study models and systems. This would help integrate a greater breadth of expertise, foster participatory science, and ultimately provide knowledge to decision makers consistently with the frameworks of the Intergovernmental Panel on Climate Change (IPCC) and IPBES.
Over the last twenty years, the ERA and LAC countries have committed themselves to consolidating their links through a strategic partnership. A major objective of these reinforced links is often to build countries’ capacity. However our analysis shows that while most ERA-LAC studies are performed on sites located in LAC territories only one third of the corresponding publications are led by LAC scientists. However, the relationship between capacity building and publication authorship is not straightforward and the poor leadership of LAC scientists may also illustrate the ERA origins of the funding and lack of research coordination in the LAC region. A second major objective is to promote academic excellence and productivity. Further parameters like journal impact factors and corresponding author metrics could be incorporated into bibliometric analyses to assess whether ERA-LAC collaboration has developed in a way that fulfils this goal. A third major objective of reinforced ERA-LAC links is to properly study the interplay of biodiversity and climate change issues over the entire LAC area. However, our results indicate that ERA-LAC collaboration is biased towards a few, emerging countries, with most studies focused on sites in Brazil and Mexico (which is a powerful actor of intra-LAC cooperation), and there is a need to better tailor cooperation with low- and mid-income countries by conducting more targeted collaborations for R&D studies on biodiversity and climate change. However, strengthening the connection between less developed countries and the global cooperation network will not be easy. For example, Bolivia appears in our analysis only as a peripheral link to the global network through regional hubs, but it has very limited academic resources in biodiversity and climate change science. The limitations of climate model downscaling (for example in the Andean region, [ 13 ]) and the fragmented information available on the conservation status of many South American species [ 27 ] illustrate the urgent need for ERA-LAC initiatives at the interface of climate change and biodiversity to integrate a truly regional approach. As it is unlikely that such an initiative would come from individual countries, the EU faces a key challenge in promoting regional scientific cooperation in LAC countries. It is a daunting task, but the recent calls launched by ERA-LAC networking projects such as the ERANet-LAC ( http://eranet-lac.eu , €6 millions in 2015–2016) are promising advances to address this issue.
Supporting Information
S1 table. complete database of publications used for the analysis..
https://doi.org/10.1371/journal.pone.0157441.s001
Acknowledgments
We are grateful to Sharilynn Wardrop, Eleanor O'Rourke, Carlos Ereño and two anonymous reviewers for useful comments on a previous version of this manuscript.
Author Contributions
Conceived and designed the experiments: OD XL. Performed the experiments: OD JL SS. Analyzed the data: JL SS. Contributed reagents/materials/analysis tools: JL SS. Wrote the paper: OD JV XL CF.
- View Article
- Google Scholar
- 2. Hannah L. Climate change biology. Academic Press; 2010.
- 3. Weingart P. A Short History of Knowledge Formations. In: Frodemann R, Thomson Klein J, Mitcham C, editors. The Oxford Handbook of Interdisciplinarity. Oxford: Oxford University Press; 2010. pp. 3–14.
- 4. Frodeman R, Klein JT, Mitcham C. The Oxford handbook of interdisciplinarity. Oxford University Press; 2010.
- 5. Valdez AC, Schaar AK, Ziefle M, Holzinger A, Jeschke S, Brecher C. Using mixed node publication network graphs for analyzing success in interdisciplinary teams. Active Media Technology: Springer Berlin Heidelberg. 2012. pp. 606–617.
- 7. CEPAL NU. The European Union and Latin America and the Caribbean in the new economic and social context. Edition ECLAC; 2015.
- 8. European Commission. Towards the EU-LAC Knowledge area—Scientific and technological cooperation between Latin America, the Caribbean and the European Union for sustainable development and social inclusion, Luxembourg, Publications Office of the European Union; 2010.
- 11. European Union. A European research and innovation roadmap for climate services, Luxembourg, Publications Office of the European Union; 2015.
- 12. Groombridge B, Jenkins M. World atlas of biodiversity: earth's living resources in the 21st century. University of California Press; 2002.
- 13. Pachauri RK, Allen MR, Barros VR, Broome J, Cramer W, Christ R et al. Climate Change 2014: Synthesis Report. Contribution of Working Groups I, II and III to the Fifth Assessment Report of the Intergovernmental Panel on Climate Change; 2014.
- 15. Russel JM, Ainsworth S. Mapping S&T Collaboration between Latin America and Europe: Bibliometric Analysis of Co-authorships (1984–2007) In Gaillard J, Arvanitis R, editors. Research Collaboration between Europe and Latin America: Mapping and Understanding partnership, Edition des Archives contemporaines; 2014. pp 49–77.
- PubMed/NCBI
- 21. Martínez R, Jørgensen PM, Tiessen H. Climate change and biodiversity in the tropical Andes. São José dos Campos: Inter-American Institute for Global Change Research. 2011.
- 23. Swanson T. Global action for biodiversity: an international framework for implementing the convention on biological diversity. Routledge; 2013.
- 25. European Commission. A stronger partnership between the European Union and Latin America. Luxembourg: Office for Official Publications of the European Communities; 2006.
- 27. UNEP. State of Biodiversity in Latin America and the Caribbean, UNEP report; 2010.
Thank you for visiting nature.com. You are using a browser version with limited support for CSS. To obtain the best experience, we recommend you use a more up to date browser (or turn off compatibility mode in Internet Explorer). In the meantime, to ensure continued support, we are displaying the site without styles and JavaScript.
- View all journals
- Explore content
- About the journal
- Publish with us
- Sign up for alerts
- Perspective
- Published: 13 May 2021
Biodiversity conservation as a promising frontier for behavioural science
- Kristian Steensen Nielsen ORCID: orcid.org/0000-0002-8395-4007 1 ,
- Theresa M. Marteau ORCID: orcid.org/0000-0003-3025-1129 2 ,
- Jan M. Bauer 3 ,
- Richard B. Bradbury ORCID: orcid.org/0000-0002-1245-2763 1 , 4 ,
- Steven Broad ORCID: orcid.org/0000-0002-1826-6400 5 ,
- Gayle Burgess 5 ,
- Mark Burgman 6 ,
- Hilary Byerly ORCID: orcid.org/0000-0002-7445-2099 7 ,
- Susan Clayton 8 ,
- Dulce Espelosin 9 ,
- Paul J. Ferraro ORCID: orcid.org/0000-0002-4777-5108 10 ,
- Brendan Fisher 11 , 12 ,
- Emma E. Garnett ORCID: orcid.org/0000-0002-1664-9029 1 , 13 ,
- Julia P. G. Jones 14 ,
- Mark Otieno 15 , 16 ,
- Stephen Polasky ORCID: orcid.org/0000-0003-4934-2434 17 , 18 ,
- Taylor H. Ricketts 11 , 12 ,
- Rosie Trevelyan 19 ,
- Sander van der Linden ORCID: orcid.org/0000-0002-0269-1744 20 ,
- Diogo Veríssimo 21 &
- Andrew Balmford 1
Nature Human Behaviour volume 5 , pages 550–556 ( 2021 ) Cite this article
23k Accesses
52 Citations
123 Altmetric
Metrics details
- Environmental studies
- Human behaviour
- Psychology and behaviour
- Sustainability
Human activities are degrading ecosystems worldwide, posing existential threats for biodiversity and humankind. Slowing and reversing this degradation will require profound and widespread changes to human behaviour. Behavioural scientists are therefore well placed to contribute intellectual leadership in this area. This Perspective aims to stimulate a marked increase in the amount and breadth of behavioural research addressing this challenge. First, we describe the importance of the biodiversity crisis for human and non-human prosperity and the central role of human behaviour in reversing this decline. Next, we discuss key gaps in our understanding of how to achieve behaviour change for biodiversity conservation and suggest how to identify key behaviour changes and actors capable of improving biodiversity outcomes. Finally, we outline the core components for building a robust evidence base and suggest priority research questions for behavioural scientists to explore in opening a new frontier of behavioural science for the benefit of nature and human wellbeing.
Similar content being viewed by others
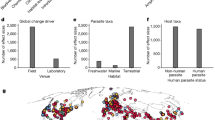
A meta-analysis on global change drivers and the risk of infectious disease
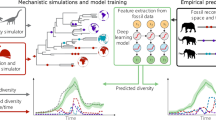
DeepDive: estimating global biodiversity patterns through time using deep learning
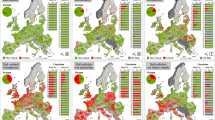
A unifying modelling of multiple land degradation pathways in Europe
A recent global synthesis estimates that 75% of Earth’s land surface has been fundamentally altered by human activities, 66% of the ocean has been negatively affected, and 85% of wetland areas have been lost 1 . The combined effects of land-use change and habitat fragmentation, overharvesting, invasive species, pollution and climate change have resulted in an average decline in monitored populations of vertebrates of nearly 70% since 1970 and extinction rates that are orders of magnitude higher than the average seen in the geological record 2 , 3 , 4 . The threats to species are so severe that there is growing scientific consensus that we are entering the sixth mass extinction—the fifth being the Cretaceous–Paleogene extinction event 66 million years ago that eliminated all non-avian dinosaurs 5 .
The rapid degradation of ecosystems and associated loss of species is of profound importance for at least three reasons. First, there are powerful moral arguments that people should not cause the avoidable extinction of perhaps one million or more species 6 . It is beyond the scope of this paper to describe such arguments, but philosophers have discussed the ethics of biodiversity conservation 7 , 8 , 9 and social scientists have identified public support for assigning moral value to nature 10 , 11 , 12 . Second, human prosperity depends on wild habitats and species for a host of essential benefits, from climate regulation, biogeochemical and flood regulation to food production and the maintenance of mental wellbeing 13 , 14 . Their deterioration thus presents an existential challenge 1 . Third, evidence suggests that pandemics resulting from greater disease transmission between humans and wild animals 15 , 16 will become more regular features of the future unless our interactions with wild species changes fundamentally 15 , 17 , 18 , 19 , 20 . The COVID-19 pandemic—with devastating effects on societies and economies worldwide—most probably emerged from interactions between people and wild animals in China and illustrates the unforeseen consequences that can arise from human encroachment into wild habitats and from poorly regulated exploitation of biodiversity 17 , 21 .
Humanity’s impacts on biodiversity are the result of our actions, from unsustainable wildlife harvesting to the rising demand for environmentally damaging foods 1 , 22 , 23 , 24 , 25 . Importantly, these actions are undertaken by actors in myriad roles—including consumers, producers and policymakers—who directly or indirectly impact ecosystems and wild species 26 . For example, the rapid clearance of the Amazon is driven by the actions of consumers across the globe who eat beef, regional policymakers who undervalue forest retention, and ultimately local ranchers who are incentivised to convert forest to pasture 27 , 28 . Similarly, the illegal trade in wildlife (for example, rhino horn, pangolin scales, tiger bones and elephant ivory) involves suppliers who hunt the animals, intermediaries (and perhaps corrupt enforcement agents) who facilitate trade and transport the products to market, and domestic and international consumers 24 , 29 , 30 , 31 . The impacts of people’s behaviour on biodiversity are of course not only manifest in less developed countries. For example, the continued illegal persecution of birds of prey in UK uplands is the result of choices by some gamekeepers to shoot and poison raptors to limit their predation of red grouse, by some hunters to pay exceptionally high prices for large daily ‘bags’ of grouse, and by policymakers to resist attempts at tighter regulation of the shooting industry 32 .
Because human activities are responsible for driving ecosystem decline, reversing current trends will require profound and persistent changes to human behaviour across actors and scales 33 . Despite its critical importance, the science of behaviour change has not been a principal focus of research in conservation science and is rarely applied in practical efforts to address major threats to biodiversity (for example, habitat loss and degradation, overharvesting of resources and species, and invasive species) 33 , 34 , 35 , 36 , 37 (A.B. et al., manuscript in preparation). Conservation scientists (defined broadly to include researchers across the natural and social sciences seeking to understand and mitigate these threats) have generally been slow to incorporate evidence from behavioural science into their theories and interventions 33 , 36 , 38 , 39 , 40 , 41 . Conversely, biodiversity conservation has also not been a strong focus of study for behavioural scientists (defined broadly to include those engaged in the scientific study of behaviour across diverse disciplines, including psychology, sociology, economics, anthropology and political science). One exception is research on common-pool resource management and commons dilemmas, which has a long history tracing back to the 1970s 42 , 43 , 44 , 45 . This research tradition has tackled issues closely linked to biodiversity conservation and foreshadows many contemporary and interdisciplinary analyses. More recently, social-marketing techniques have been used to tackle a variety of biodiversity problems and their potential is increasingly recognised 46 , 47 , 48 , 49 , 50 . For example, a recent study in the Philippines, Indonesia and Brazil used locally tailored social-marketing campaigns to shift social norms and increase sustainable fishing among communities of small-scale fisheries 50 . But while the number of successful applications of behavioural science to biodiversity conservation is increasing, they remain rare and often suffer from methodological limitations 51 . The conservation evidence base is consequently patchy and generally poorly informed by behavioural science 36 , 52 .
Meanwhile, in other contexts, behavioural science has made substantial gains in understanding how to encourage prosocial behaviour, including actions that ultimately affect biodiversity outcomes. A growing body of research related to climate change suggests the importance of social norms, risk communication, emotion and choice architecture in changing behaviour 53 , 54 , 55 , 56 , 57 . Behavioural science has been incorporated into some public efforts to encourage sustainable land management in the United States and the European Union 58 , 59 , 60 , 61 , 62 . Nevertheless, there are still few applications of behavioural science to explicitly address the most important proximate causes of biodiversity loss. Behavioural insights from research related to climate change, land management, consumer behaviour, voting, collective action and programme enrolment can inform the multi-scale approach needed to deliver effective biodiversity conservation, but this research has not been systematically linked to address biodiversity conservation problems. Moreover, the literature is heavily focused on households and is not well-developed for other important actors 57 , 63 . We therefore see unrealised potential for behavioural science to address the escalating biodiversity crisis.
Increasing scientific engagement
Behavioural scientists might be motivated to become engaged in biodiversity conservation research for at least three reasons. First, biodiversity conservation is essential for the long-term prosperity of people and nature. Its particular characteristics (see below) mean that it would be unhelpful simply to adopt behaviour-change interventions found effective in other domains: indeed, these do not necessarily generalize to biodiversity conservation 52 , 64 . Instead, the field offers a new arena for exploring important research questions and for testing novel interventions. Behavioural science research that focuses specifically on biodiversity conservation can contribute to the mitigation of a global and existential threat.
Second, engaging in biodiversity conservation research offers behavioural scientists a chance to investigate theories and interventions in new contexts and populations 65 , 66 , 67 . A key requirement for increasing the generalizability of behavioural science is to ramp up research activities outside North America, Australia and Europe 68 , 69 . Due to the importance of the tropics for biodiversity, the focus of many conservation interventions is in Africa, Latin America and Asia, providing opportunities to test theory and interventions in contexts which are less ‘WEIRD’ (western, educated, industrialized, rich and democratic). A related challenge is the need to shift behaviours of many different kinds of actors. Behaviour-change interventions in other sectors have been criticised for being too narrowly focused on end-users 70 , 71 : Conservation problems provide opportunities for targeting the behaviours of a far broader array of stakeholders. Moreover, conserving biodiversity often requires coordinated action across local, national and global actors, heterogeneous cultures and divergent financial interests, with the benefits of conservation commonly accruing to geographically and psychologically distant communities and indeed non-human species.
Finally, conservation scientists and practitioners are keen to collaborate more with behavioural scientists 72 , 73 . An increasing number of conservation scientists and practitioners recognise the need for stronger integration with behavioural science in order to design interventions that are grounded in greater understanding of the social, motivational and contextual drivers of people’s actions 33 , 39 , 74 , 75 . Naturally, as with all interdisciplinary collaborations, these collaborations will have their challenges 75 . However, recent examples show that effective collaborations can produce novel and mutually beneficial research that suggests practical routes to achieving behaviour change for biodiversity conservation 50 , 64 , 76 , 77 , 78 .
The remainder of this Perspective seeks to encourage greater engagement of behavioural scientists in conservation-targeted research and practice. We first highlight the diversity of actors involved in threats to biodiversity and the scope of behaviour changes required. In doing so, we propose routes to identifying key behaviour changes and prioritising among them on the basis of their potential for improving biodiversity outcomes. We suggest research questions for better understanding how to influence different actors’ behaviours and for improving conservation interventions, and close by making recommendations for how to expand the conservation evidence base systematically.
Identifying key actors and behaviour changes
Threats to biodiversity are rarely caused by a single action of a single actor. Rather, they typically result from multiple behaviours by multiple actors over large spatial and temporal scales 36 , 79 . It can thus be very challenging to identify those behaviour changes with the greatest promise of being achieved and of positively impacting biodiversity. Doing so requires specifying conservation targets (e.g., particular populations or ecosystems), and then systematically considering the proximate causes and underlying drivers of threats to them, the actors involved (for example, producers and consumers), and the harmful behaviours performed by those actors 26 , 39 , 45 , 80 .
The proximate threats to wild species and the places they live can be categorised into four main groups: habitat loss and degradation, overharvesting, invasive species, and climate change and pollution 81 , 82 , 83 . These threats also interact, with species or ecosystems commonly impacted by multiple threats, sometimes with amplifying effects. For example, the spread of some invasive plants is thought to be exacerbated by elevated nitrogen deposition and atmospheric CO 2 concentrations 84 , 85 . Proximate threats are driven by broader societal processes, including rising demand for food and consumer goods, weak local, national and international institutions that struggle to ensure the protection of public goods (including against corrupt actors), population growth and the growing disconnect of people from nature due to increasing urbanization and indoor recreation 86 . Many of the interventions conservationists deploy to tackle proximate threats, such as removing invasive species, restoring wetlands or propagating threatened species in captivity, are not primarily about changing people’s behaviour (although even in these examples those carrying out the management actions must be trained and incentivised, and behaviours must change if these threats are not to recur). However, given the pervasive importance of human activities in conservation problems, many interventions do involve attempts to alter behaviour. If behavioural science is to improve the effectiveness of these efforts, an important first step is to identify the main actors responsible for a given threat and the changes in their behaviour that might be required to alleviate it.
One tool for mapping the actors and behaviours impacting a conservation target is to build a threat chain (A.B. et al., manuscript in preparation). This is a simplified summary of knowledge of the reasons for the unfavourable status of a species or ecosystem, from changes in ecological dynamics to the socioeconomic mechanisms thought to be responsible, and their underlying drivers. Once this putative causal chain has been constructed, the main actors in the chain can be identified, along with changes in their behaviour that might potentially reduce the particular threat. Where conservation targets are impacted by multiple threats this process can be repeated, with the likely impact of different behaviour changes compared across threats in order to identify the most promising interventions for delivering those changes.
Using Amazon deforestation (as an example of habitat loss) for illustration 27 , 28 (Fig. 1 , red boxes), the extirpation of forest-dependent species and ecosystem processes resulting from conversion to pasture has been caused (inter alia) by a combination of rising global demand for beef, poor pasture and livestock management, the absence of incentives for forest retention and the practice of establishing de facto land tenure via forest clearance. Underlying drivers include weak governance at multiple levels and rising per capita demand for beef among a growing population in Brazil and beyond. Potential behaviour changes that might be targeted to reduce deforestation (blue boxes) include increased enforcement of forest protection legislation by government agencies, improved pasture and stock management by ranchers, a reduction in per capita demand for beef among domestic and international consumers, and an accelerated decline in human population growth in high-consumption countries.
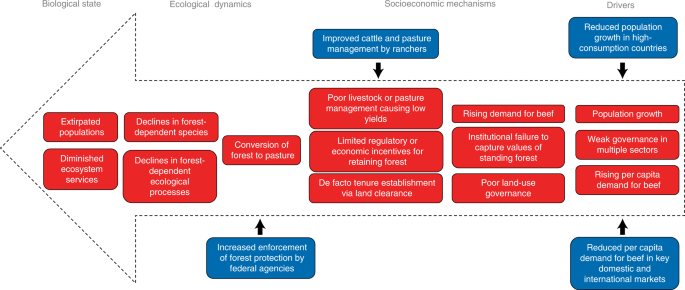
This example characterizes (in red boxes) the threat to the Amazon forest from conversion to cattle pasture. Potentially beneficial changes in the behaviours are in blue boxes. This threat chain addresses only one of several interacting threats impacting the conservation target. The threat chain model is adapted from Balmford et al. 26 .
As a heuristic, we conducted this threat-mapping exercise for 12 examples chosen to represent different threat processes and the diversity of ecological and socioeconomic contexts in which they arise (A.B. et al., manuscript in preparation). We identified nine main clusters of actors (rows in Fig. 2 ), classified by how their behaviour impacts conservation targets. Producers and extractors of natural resources, conservation managers and consumers are commonly identified as targets for behaviour-change interventions in conservation and other sectors. However, we also identified other actor groupings, including manufacturers and sellers, investors, policymakers, voters, communicators and lobbyists, all of whom may have considerable—usually indirect—influences on conservation outcomes, yet are commonly overlooked when it comes to behaviour-change interventions. Because our clusters of actors are operationally defined, they align well with the diversity of behaviour changes we identified (Fig. 2 , right column), including reducing consumers’ purchases of high-footprint items and directing investors’ investments towards less damaging production technologies. Our clusters can also be mapped onto more conventional organisational groups (such as citizens or businesses; Fig. 2 , ‘Actor—defined by group’ columns), but because such organizational groups impact conservation targets in heterogeneous ways, their correspondence with behaviour changes is much weaker than for our typology.
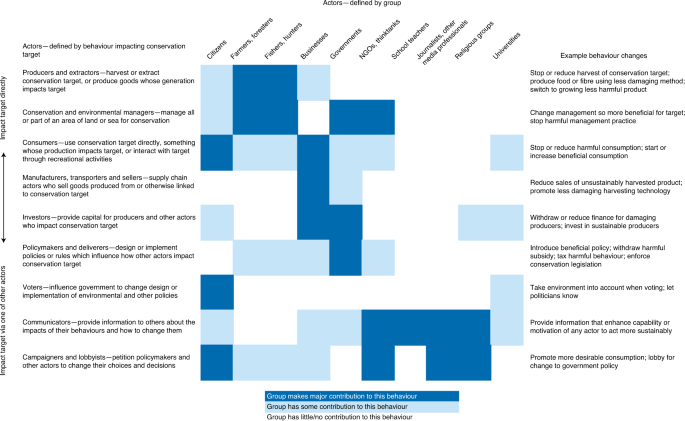
Actors classified according to their behavioural impacts on conservation targets (rows) and by their organizational affiliation. NGO, non-governmental organization.
Prioritising behaviour changes
After examining all major threats to a given conservation target and identifying promising behaviour changes involving specified actors, the next step is to prioritise behaviour changes and, in turn, the interventions potentially capable of achieving them. We suggest this should focus on two main characteristics that together determine the impact of behaviour-change interventions 57 , 87 . The first is the target behaviour’s potential, if changed, to improve the state of the conservation objective (by analogy with the climate change literature, its technical potential). In the Amazon example (Fig. 1 ), both enforcing forest protection laws and providing herd management support that is conditional on ranchers stopping clearance might be considered to have greater technical potential than slowing population growth in beef-consuming countries (which may have only limited effect if per capita demand continues to rise). Prioritising behaviours for research and intervention on the basis of their technical potential—considered an omission in behavioural science contributions to climate change mitigation 57 , 88 , 89 , 90 —ensures that resources and efforts are allocated toward the behaviours with the greatest potential to effectively mitigate biodiversity threats.
The second aspect to consider in prioritization is the behaviour’s plasticity, which refers to the degree to which a target behaviour can be changed by a specified intervention 57 . For example, to what extent can behaviour-change interventions increase the share of plant-based food in overseas or Brazilian diets, or improve the cattle and pasture management of Amazonian farmers? Due to the current paucity of conservation-focused behaviour-change interventions, good estimates of behavioural plasticity will often be lacking. Instead, it will often be necessary to use evidence from interventions targeting comparable behaviours relating to other actors, contexts or domains until more direct data become available 87 . Although considerations of technical potential and behavioural plasticity should guide the selection of behaviours to study and intervene against, we note that additional considerations may become pertinent when selecting interventions for implementation (for example, feasibility, stakeholder support and costs) 91 , 92 , 93 .
Given the range of actors involved in causing ecosystem change and the complexity of their behaviour, standalone behaviour-change interventions are unlikely to effectively mitigate a biodiversity threat (as illustrated in Fig. 1 ). Individual-level interventions—for example, targeting specific farmers, manufacturers, or investors—may well form an important part of the solution, but they will usually be insufficient on their own. For example, successfully incentivising ranchers in one Amazonian municipality to retain their remaining forests will be of little benefit to biodiversity if prevailing market failure or weak institutions continue to incentivise forest clearance elsewhere. Tackling more systemic drivers, such as environmentally damaging subsidy regimes, corporate interests, poor governance and persistent norms, also necessitates population-level interventions that can alter economic systems, institutional systems and physical infrastructure. Importantly, the intent here is not to undermine the legitimacy of individual-level interventions—quite the contrary. Systemic changes also cannot be achieved without individual-level behaviour changes and support 57 , 94 , 95 . Different levels of intervention must work in concert, which requires a holistic understanding of the determinants of human behaviour.
Building a robust evidence base
Generating evidence on behaviour-change interventions for biodiversity conservation demands a mix of methods, including experimental and observational studies using quantitative and qualitative techniques 96 , 97 , 98 . Critically, to build an evidence base, these studies must be based on mapping and synthesizing the existing literature 99 . They also need to be embedded in relevant conceptual or theoretical frameworks, coupled with a theory of change, and designed with the statistical power to answer the study questions. This might include, for example, taking a systems perspective 98 , as well as using a taxonomy or typology of interventions 100 , 101 .
Behavioural responses and the effectiveness of interventions are likely to vary between social and cultural contexts. Assessing the effect size of interventions in different settings will be key to building a robust evidence base that has global application. Improving the cross-cultural profile of behavioural science evidence is thus imperative, and particularly so for biodiversity conservation, where many problems are centred outside Europe and North America. Achieving this will, however, be challenging given that the research capacity in behavioural science remains low in high-income countries and even lower elsewhere. International partnerships will therefore be an important strand of building capacity across regions.
Emergent research questions
Given that behavioural science research into conservation-related problems is still in its infancy, many important questions remain unanswered. In this final section, we outline four higher-order questions that we believe could impact the effectiveness of interventions aimed at reducing people’s negative impacts on biodiversity, natural habitats and the services provided by ecosystems. While these questions can apply to prosocial behaviour more broadly, we believe that there is considerable merit in tackling them within the context of biodiversity conservation, in part through devising and testing novel interventions in the field. This will necessitate close collaboration between behavioural scientists and conservation scientists and practitioners.
The first research question deals with prioritization. As with climate change interventions, there is a clear need for a more systematic understanding of the technical potential of different behaviour changes: which ones, if delivered, would be most likely to reduce a threat and thereby enhance the status of the conservation target, taking into account other threats it faces 80 , 91 ? Given the focus of many recent environmental interventions on appealing, tractable but relatively low-impact behaviour changes (for example, eating more locally grown food or avoiding plastic drinking straws), such prioritization is badly needed 88 , 90 . One challenge in identifying priorities may be the complexity of conservation outcomes: estimating probable impacts of behaviour changes on highly interconnected ecosystems may be more difficult than impacts on greenhouse gas levels 80 , but we suggest that this is a surmountable problem. A further consideration here is how far a behaviour change addressing one conservation issue might reduce (or indeed increase) threats to other conservation targets 102 .
The remaining research questions are all aimed at improving our understanding of the plasticity of priority behaviours (that is, those with high technical potential to improve biodiversity outcomes 91 ). Our second suggested question is which interventions work best to alter priority behaviours, and how does this vary across contexts? One key aspect is exploring how the suitability of behaviour-change interventions varies with the level of deliberation and perceived importance of the decision being made. Consider contrasting interventions aimed at increasing how often consumers buy sustainably (rather than unsustainably) sourced fish. For someone making a weekly shopping trip such a choice may be performed with limited deliberation, which means that interventions targeting automatic decision-making processes may be effective 103 . However, for other actors, such as supply-chain managers making bulk purchases for supermarkets, different interventions—perhaps motivated by limiting reputational risk—will probably be required. At the level of decision makers designing national or international fisheries policy, other sorts of interventions 104 —potentially linked to cessation or realignment of taxpayer subsidies—might need to be considered.
This example also illustrates our third suggested research question: how does the effectiveness of behaviour-change interventions vary with the financial and psychological costs of the change for the target actor? Differences in motivation will be important here. In some instances, actors may benefit directly from pro-conservation behaviour (for example, because eating more sustainably sourced fish aligns with health values, or keeping their pet cat indoors reduces its risk of injury). But sometimes those choices may carry costs (for example, sustainable seafood may be more expensive or difficult to source). In the case of the supermarket chains, there may be financial and administrative costs to switching suppliers, at least over the short term. Policymakers will also face strong lobbying pressure to continue to support the policy status quo. Clearly, different interventions will be needed across such diverse contexts. Varied interventions may also be needed within actor groups. For example, supermarket chains may differ in their motivations, knowledge, demographics and other interests in ways that warrant different types of behaviour-change interventions.
Lastly, how can practitioners design interventions to ensure that behaviour changes persist over the long term? Although many intervention studies do not evaluate persistence over time, those that do commonly observe that effectiveness wanes 105 , 106 , 107 . In some contexts, it might be possible to design one-off interventions with long-lasting effects, but in others, delivering lasting change may necessitate recurring rounds of intervention or the repeated introduction of novel interventions. Better understanding the persistence of intervention effects will be key to sustaining beneficial behaviour change.
Many more questions will emerge as this field develops. Addressing them will require fresh partnerships and continued commitment to work across disciplines and in unfamiliar circumstances. Such partnerships may follow recommendations for interdisciplinary collaborations around biodiversity conservation 108 , 109 or be inspired by existing programmes and networks (some of which collaborate closely with practitioners), such as the Cambridge Conservation Initiative, Center for Behavioral and Experimental Agri-environmental Research, and Science for Nature and People Partnership. We submit that there are few other opportunities where behavioural scientists have such potential to tackle one of the great challenges of our age. We hope this Perspective can help inspire this critical work.
Summary for Policymakers of the Global Assessment Report on Biodiversity and Ecosystem Services of the Intergovernmental Science-Policy Platform on Biodiversity and Ecosystem Services http://rid.unrn.edu.ar/handle/20.500.12049/4223 (IPBES Secretariat, 2019).
Maxwell, S. L., Fuller, R. A., Brooks, T. M. & Watson, J. E. M. Biodiversity: The ravages of guns, nets and bulldozers. Nature 536 , 143–145 (2016).
Article CAS PubMed Google Scholar
Living Planet Report 2020—Bending the Curve of Biodiversity Loss (WWF, 2020).
Ceballos, G. et al. Accelerated modern human-induced species losses: entering the sixth mass extinction. Sci. Adv. 1 , e1400253 (2015).
Article PubMed PubMed Central Google Scholar
Ceballos, G., Ehrlich, P. R. & Dirzo, R. Biological annihilation via the ongoing sixth mass extinction signaled by vertebrate population losses and declines. Proc. Natl Acad. Sci. USA 114 , E6089–E6096 (2017).
Article CAS PubMed PubMed Central Google Scholar
Tilman, D. et al. Future threats to biodiversity and pathways to their prevention. Nature 546 , 73–81 (2017).
Partridge, E. Nature as a moral resource. Environ. Ethics 6 , 101–130 (1984).
Article Google Scholar
Ehrenfeld, D. in Biodiversity (ed. Wilson, E. O.) 212–216 (National Academy Press, 1988).
Taylor, P. W. The ethics of respect for nature. Environ. Ethics 3 , 197–218 (1981).
Clayton, S. in The Virtues of Sustainability (ed. Kawall, J.) Ch. 1 (Oxford Univ. Press, 2021).
Feinberg, M. & Willer, R. The moral roots of environmental attitudes. Psychol. Sci. 24 , 56–62 (2013).
Article PubMed Google Scholar
Kellert, S. R. The Value of Life: Biological Diversity and Human Society (Island Press, 1997).
Bratman, G. N. et al. Nature and mental health: an ecosystem service perspective. Sci. Adv. 5 , eaax0903 (2019).
Díaz, S. et al. Assessing nature’s contributions to people. Science 359 , 270–272 (2018).
Gibb, R. et al. Zoonotic host diversity increases in human-dominated ecosystems. Nature 584 , 398–402 (2020).
Balmford, A., Fisher, B., Mace, G. M., Wilcove, D. S. & Balmford, B. COVID-19: Analogues and lessons for tackling the extinction and climate crises. Curr. Biol. 30 , R969–R971 (2020).
Dobson, A. P. et al. Ecology and economics for pandemic prevention. Science 369 , 379–381 (2020).
Allen, T. et al. Global hotspots and correlates of emerging zoonotic diseases. Nat. Commun. 8 , 1124 (2017).
Morens, D. M., Daszak, P., Markel, H. & Taubenberger, J. K. Pandemic COVID-19 joins history’s pandemic legion. mBio 11 , e00812-20 (2020).
Wilkinson, D. A., Marshall, J. C., French, N. P., Hayman, D. T. S. & Wilkinson, D. A. Habitat fragmentation, biodiversity loss and the risk of novel infectious disease emergence. J. R. Soc. Interface 15 , 20180403 (2018).
Mallapaty, S. Where did COVID come from? WHO investigation begins but faces challenges. Nature 587 , 341–342 (2020).
Tilman, D. & Clark, M. Global diets link environmental sustainability and human health. Nature 515 , 518–522 (2014).
Poore, J. & Nemecek, T. Reducing food’s environmental impacts through producers and consumers. Science 992 , 987–992 (2018).
‘t Sas-Rolfes, M., Challender, D. W. S., Hinsley, A., Veríssimo, D. & Milner-Gulland, E. J. Illegal wildlife trade: scale, processes, and governance. Annu. Rev. Environ. Resour. 44 , 201–230 (2019).
Dietz, T. Drivers of human stress on the environment in the twenty-first century. Annu. Rev. Environ. Resour. 42 , 189–213 (2017).
Balmford, A. et al. Capturing the many dimensions of threat: comment on Salafsky et al. Conserv. Biol. 23 , 482–487 (2009).
Nepstad, D. C., Stickler, C. M. & Almeida, O. T. Globalization of the Amazon soy and beef industries: opportunities for conservation. Conserv. Biol. 20 , 1595–1603 (2006).
zu Ermgassen, E. K. H. J. et al. Results from on-the-ground efforts to promote sustainable cattle ranching in the Brazilian Amazon. Sustainability 10 , 1301 (2018).
MacFarlane, D. et al. Reducing demand for overexploited wildlife products: Lessons from systematic reviews from outside conservation science. Preprint at OSF https://osf.io/preprints/8935b/ (2020).
Thomas-Walters, L. et al. Motivations for the use and consumption of wildlife products. Conserv. Biol. 35 , 483–491 (2021).
Johnson, C. K. et al. Global shifts in mammalian population trends reveal key predictors of virus spillover risk. Proc. R. Soc. B 287 , 20192736 (2020).
Avery, M. Inglorious: Conflict in the Uplands (Bloomsbury, 2015).
Cowling, R. M. Let’s get serious about human behavior and conservation. Conserv. Lett. 7 , 147–148 (2014).
Marselle, M., Turbe, A., Shwartz, A., Bonn, A. & Colléony, A. Addressing behavior in pollinator conservation policies to combat the implementation gap. Conserv. Biol. 35 , 610–622 (2021).
Saunders, C. D. The emerging field of conservation psychology. Hum. Ecol. Rev. 10 , 137–149 (2003).
Google Scholar
Selinske, M. J. et al. Revisiting the promise of conservation psychology. Conserv. Biol. 32 , 1464–1468 (2018).
Schultz, P. W. Conservation means behavior. Conserv. Biol. 25 , 1080–1083 (2011).
Cinner, J. How behavioral science can help conservation. Science 362 , 889–891 (2018).
Reddy, S. M. W. et al. Advancing conservation by understanding and influencing human behavior. Conserv. Lett. 10 , 248–256 (2017).
Burgess, G. Powers of persuasion? Conservation communications, behavioural change and reducing demand for illegal wildlife products. TRAFFIC Bull. 28 , 65–73 (2016).
Kidd, L. R. et al. Messaging matters: A systematic review of the conservation messaging literature. Biol. Conserv. 236 , 92–99 (2019).
Ostrom, E. et al. The Drama of the Commons (National Academy Press, 2002).
Dietz, T., Ostrom, E. & Stern, P. C. The struggle to govern the commons. Science 302 , 1907–1912 (2003).
Ostrom, E. Governing the Commons: The evolution of Institutions for Collective Action (Cambridge Univ. Press, 1990).
Stern, P. C., Young, O. R. & Druckman, D. E. Global Environmental Change: Understanding the Human Dimensions (National Academy Press, 1992).
Veríssimo, D. The past, present, and future of using social marketing to conserve biodiversity. Soc. Mar. Q. 25 , 3–8 (2019).
Veríssimo, D. et al. Does it work for biodiversity? Experiences and challenges in the evaluation of social marketing campaigns. Soc. Mar. Q. 24 , 18–34 (2018).
Green, K. M., Crawford, B. A., Williamson, K. A. & DeWan, A. A. A meta-analysis of social marketing campaigns to improve global conservation outcomes. Soc. Mar. Q. 25 , 69–87 (2019).
DeWan, A., Green, K., Li, X. & Hayden, D. Using social marketing tools to increase fuel-efficient stove adoption for conservation of the golden snub-nosed monkey, Gansu Province, China. Conserv. Evid. 10 , 32–36 (2013).
McDonald, G. et al. Catalyzing sustainable fisheries management though behavior change interventions. Conserv. Biol. 35 , 1176–1189 (2020).
Veríssimo, D. & Wan, A. K. Y. Characterizing efforts to reduce consumer demand for wildlife products. Conserv. Biol. 33 , 623–633 (2019).
Byerly, H. et al. Nudging pro-environmental behavior: evidence and opportunities. Front. Ecol. Environ. 16 , 159–168 (2018).
van der Linden, S., Maibach, E. & Leiserowitz, A. Improving public engagement with climate change: five ‘best practice’ insights from psychological science. Perspect. Psychol. Sci. 10 , 758–763 (2015).
Chapman, D. A., Lickel, B. & Markowitz, E. M. Reassessing emotion in climate change communication. Nat. Clim. Chang. 7 , 850–852 (2017).
Nisa, C. F., Bélanger, J. J., Schumpe, B. M. & Faller, D. G. Meta-analysis of randomised controlled trials testing behavioural interventions to promote household action on climate change. Nat. Commun. 10 , 4545 (2019).
Valkengoed, A. M. Van & Steg, L. Meta-analyses of factors motivating climate change adaptation behaviour. Nat. Clim. Chang. 9 , 158–163 (2019).
Nielsen, K. S. et al. How psychology can help limit climate change. Am. Psychol. 76 , 130–144 (2021).
Dessart, F. J., Barreiro-Hurlé, J. & van Bavel, R. Behavioural factors affecting the adoption of sustainable farming practices: a policy-oriented review. Eur. Rev. Agric. Econ. 46 , 417–471 (2019).
Palm-Forster, L. H., Ferraro, P. J., Janusch, N., Vossler, C. A. & Messer, K. D. Behavioral and experimental agri-environmental research: methodological challenges, literature gaps, and recommendations. Environ. Resour. Econ. 73 , 719–742 (2019).
Wallen, K. E. & Kyle, G. T. The efficacy of message frames on recreational boaters’ aquatic invasive species mitigation behavioral intentions. Hum. Dimens. Wildl. 23 , 297–312 (2018).
Metcalf, A. L., Angle, J. W., Phelan, C. N., Muth, B. A. & Finley, J. C. More ‘bank’ for the buck: microtargeting and normative appeals to increase social marketing efficiency. Soc. Mar. Q. 25 , 26–39 (2019).
Green, K. M., DeWan, A., Arias, A. B. & Hayden, D. Driving adoption of payments for ecosystem services through social marketing, Veracruz, Mexico. Conserv. Evid. 10 , 48–52 (2013).
Stern, P. C. & Dietz, T. A broader social science research agenda on sustainability: Nongovernmental influences on climate footprints. Energy Res. Soc. Sci. 60 , 101401 (2020).
Niemiec, R. M., Sekar, S., Gonzalez, M. & Mertens, A. The influence of message framing on public beliefs and behaviors related to species reintroduction. Biol. Conserv. 248 , 108522 (2020).
Watts, D. J. Should social science be more solution-oriented?. Nat. Hum. Behav. 1 , 0015 (2017).
Berkman, E. T. & Wilson, S. M. So useful as a good theory? The practicality crisis in (social) psychological theory. Perspect. Psychol. Sci . https://doi.org/10.1177/1745691620969650 (2021).
Giner-Sorolla, R. From crisis of evidence to a ‘crisis’ of relevance? Incentive-based answers for social psychology’s perennial relevance worries. Eur. Rev. Soc. Psychol. 30 , 1–38 (2019).
Henrich, J., Heine, S. J. & Norenzayan, A. The weirdest people in the world? Behav. Brain Sci. 33 , 61–83 (2010).
Rad, M. S., Martingano, A. J. & Ginges, J. Toward a psychology of Homo sapiens : making psychological science more representative of the human population. Proc. Natl Acad. Sci. USA 115 , 11401–11405 (2018).
Ewert, B. Moving beyond the obsession with nudging individual behaviour: towards a broader understanding of behavioural public policy. Public Policy Adm. 35 , 337–360 (2020).
Bhargava, B. S. & Loewenstein, G. Behavioral economics and public policy 102: beyond nudging. Am. Econ. Rev. 105 , 396–401 (2015).
Wright, A. J. et al. Competitive outreach in the 21st century: why we need conservation marketing. Ocean Coast. Manag. 115 , 41–48 (2015).
Veríssimo, D. & McKinley, E. Introducing conservation marketing: why should the devil have all the best tunes? Oryx 50 , 14 (2016).
Nilsson, D., Fielding, K. & Dean, A. J. Achieving conservation impact by shifting focus from human attitudes to behaviors. Conserv. Biol. 34 , 93–102 (2020).
Bennett, N. J. et al. Mainstreaming the social sciences in conservation. Conserv. Biol. 31 , 56–66 (2017).
Nelson, K. M., Partelow, S. & Schlüter, A. Nudging tourists to donate for conservation: experimental evidence on soliciting voluntary contributions for coastal management. J. Environ. Manag. 237 , 30–43 (2019).
Niemiec, R. M., Willer, R., Ardoin, N. M. & Brewer, F. K. Motivating landowners to recruit neighbors for private land conservation. Conserv. Biol. 33 , 930–941 (2019).
Byerly, H., D’Amato, A. W., Hagenbuch, S. & Fisher, B. Social influence and forest habitat conservation: experimental evidence from Vermont’s maple producers. Conserv. Sci. Pract. 1 , e98 (2019).
Amel, E., Manning, C., Scott, B. & Koger, S. Beyond the roots of human inaction: fostering collective effort toward ecosystem conservation. Science 279 , 275–279 (2017).
Selinske, M. J. et al. Identifying and prioritizing human behaviors that benefit biodiversity. Conserv. Sci. Pr. 2 , e249 (2020).
Diamond, J. M. in Extinctions (ed. Nitecki, M. H.) 191–246 (Univ. Chicago Press, 1984).
Millennium Ecosystem Assessment. Ecosystems and Human Well-being: Synthesis (Island Press, 2005).
Wilson, E. O. The Future of Life (Vintage, 2002).
Dukes, J. S. & Mooney, H. A. Does global change increase the success of biological invaders? Trends Ecol. Evol. 14 , 135–139 (1999).
Smith, S. D. et al. Elevated CO 2 increases productivity and invasive species success in an arid ecosystem. Nature 408 , 79–82 (2000).
Díaz, S. et al. Pervasive human-driven decline of life on Earth points to the need for transformative change. Science 366 , eaax3100 (2019).
Dietz, T., Gardner, G. T., Gilligan, J., Stern, P. C. & Vandenbergh, M. P. Household actions can provide a behavioral wedge to rapidly reduce U.S. carbon emissions. Proc. Natl Acad. Sci. USA 106 , 18452–18456 (2009).
Stern, P. C. Toward a coherent theory of environmentally significant behavior. J. Soc. Issues 56 , 407–424 (2000).
Stern, P. C. Contributions of psychology to limiting climate change. Am. Psychol. 66 , 303–314 (2011).
Nielsen, K. S., Cologna, V., Lange, F., Brick, C. & Stern, P. C. The case for impact-focused environmental psychology. J. Environ. Psychol . https://doi.org/10.1016/j.jenvp.2021.101559 (2021).
Nielsen, K. S. et al. Improving climate change mitigation analysis: a framework for examining feasibility. One Earth 3 , 325–336 (2020).
Vandenbergh, M. P. & Gilligan, J. M. Beyond Politics (Cambridge Univ. Press, 2017).
Selinske, M. J. et al. We have a steak in it: eliciting interventions to reduce beef consumption and its impact on biodiversity. Conserv. Lett. 13 , e12721 (2020).
Seto, K. C. et al. Carbon lock-in: types, causes, and policy implications. Annu. Rev. Environ. Resour. 41 , 425–452 (2016).
Marteau, T. M. Towards environmentally sustainable human behaviour: targeting non-conscious and conscious processes for effective and acceptable policies. Philos. Trans. R. Soc. A 375 , 20160371 (2017).
Ogilvie, D. et al. Using natural experimental studies to guide public health action: turning the evidence-based medicine paradigm on its head. J. Epidemiol. Community Health 74 , 203–208 (2020).
Marteau, T. M., Fletcher, P. C., Hollands, G. J. & Munafo, M. R. in Handbook of Behavior Change (eds. Hagger, M. S. et al.) 193–207 (Cambridge University Press, 2020).
Rutter, H. et al. The need for a complex systems model of evidence for public health. Lancet 390 , 2602–2604 (2017).
Sutherland, W. J., Fleishman, E., Mascia, M. B., Pretty, J. & Rudd, M. A. Methods for collaboratively identifying research priorities and emerging issues in science and policy. Methods Ecol. Evol. 2 , 238–247 (2011).
Hollands, G. J. et al. The TIPPME intervention typology for changing environments to change behaviour. Nat. Hum. Behav. 1 , 0140 (2017).
Michie, S. et al. The behavior change technique taxonomy (v1) of 93 hierarchically clustered techniques: Building an international consensus for the reporting of behavior change interventions. Ann. Behav. Med. 46 , 81–95 (2013).
Liu, J. et al. Framing sustainability in a telecoupled world. Ecol. Soc. 18 , 26 (2013).
Article CAS Google Scholar
Marteau, T. M., Hollands, G. J. & Fletcher, P. C. Changing human behavior to prevent disease: the importance of targeting automatic processes. Science 337 , 1492–1495 (2012).
Sumaila, U. R. et al. Updated estimates and analysis of global fisheries subsidies. Mar. Policy 109 , 103695 (2019).
Bernedo, M., Ferraro, P. J. & Price, M. The persistent impacts of norm-based messaging and their implications for water conservation. J. Consum. Policy 37 , 437–452 (2014).
Ferraro, P. J. & Price, M. K. Using nonpecuniary strategies to influence behavior: evidence from a large-scale field experiment. Rev. Econ. Stat. 95 , 64–73 (2013).
Dayer, A. A., Lutter, S. H., Sesser, K. A., Hickey, C. M. & Gardali, T. Private landowner conservation behavior following participation in voluntary incentive programs: recommendations to facilitate behavioral persistence. Conserv. Lett. 11 , e12394 (2018).
Kelly, R. et al. Ten tips for developing interdisciplinary socio-ecological researchers. SEPR 1 , 149–161 (2019).
Campbell, L. M. Overcoming obstacles to interdisciplinary research. Conserv. Biol. 19 , 574–577 (2005).
Download references
Acknowledgements
We are grateful for funding from the Cambridge Conservation Initiative Collaborative, Fund and Arcadia, RSPB and the Gund Institute for Environment, University of Vermont. A.B. is supported by a Royal Society Wolfson Research Merit award. E.E.G. was supported by a NERC studentship (grant number NE/L002507/1). We thank P. C. Stern for helpful discussion and feedback.
Author information
Authors and affiliations.
Department of Zoology, University of Cambridge, Cambridge, UK
Kristian Steensen Nielsen, Richard B. Bradbury, Emma E. Garnett & Andrew Balmford
Behaviour and Health Research Unit, University of Cambridge, Cambridge, UK
Theresa M. Marteau
Department of Management, Society and Communication, Copenhagen Business School, Frederiksberg, Denmark
Jan M. Bauer
Centre for Conservation Science, RSPB, Sandy, UK
Richard B. Bradbury
TRAFFIC, Cambridge, UK
Steven Broad & Gayle Burgess
Centre for Environmental Policy, Imperial College London, London, UK
Mark Burgman
Institute of Behavioral Science, University of Colorado, Boulder, CO, USA
Hilary Byerly
Psychology Department, The College of Wooster, Wooster, OH, USA
Susan Clayton
Center for Behavior and the Environment, Rare, Querétaro, México
Dulce Espelosin
Carey Business School and the Department of Environmental Health and Engineering, a joint department of the Bloomberg School of Public Health and the Whiting School of Engineering, Johns Hopkins University, Baltimore, MD, USA
Paul J. Ferraro
Gund Institute for Environment, University of Vermont, Burlington, VT, USA
Brendan Fisher & Taylor H. Ricketts
Environmental Program, Rubinstein School of Environment and Natural Resources, University of Vermont, Burlington, VT, USA
Cambridge Institute for Sustainability Leadership, University of Cambridge, Cambridge, UK
Emma E. Garnett
College of Environmental Sciences and Engineering, Bangor University, Bangor, UK
Julia P. G. Jones
Department of Animal Ecology and Tropical Biology, University of Würzburg, Würzburg, Germany
Mark Otieno
Department of Agricultural Resource Management, University of Embu, Embu, Kenya
Department of Applied Economics, University of Minnesota, St Paul, MN, USA
Stephen Polasky
Natural Capital Project, University of Minnesota, St Paul, MN, USA
Tropical Biology Association, Cambridge, UK
Rosie Trevelyan
Department of Psychology, University of Cambridge, Cambridge, UK
Sander van der Linden
Department of Zoology, University of Oxford, Oxford, UK
Diogo Veríssimo
You can also search for this author in PubMed Google Scholar
Contributions
All authors contributed to the conceptualization of the research. K.S.N., T.M.M. and A.B. wrote the manuscript. The other contributing authors (J.M.B., R.B.B., S.B., G.B., M.B., H.B., S.C., D.E., P.J.F., B.F., E.E.G., J.P.G.J., M.O., S.P., T.H.R., R.T., S.v.d.L. and D.V.) provided critical comments and revisions. All authors approved the final manuscript.
Corresponding author
Correspondence to Kristian Steensen Nielsen .
Ethics declarations
Competing interests.
The authors declare no competing interests.
Additional information
Peer review information Nature Human Behaviour thanks Rebecca Niemiec, Philip Seddon and Matthew Selinske for their contribution to the peer review of this work.
Publisher’s note Springer Nature remains neutral with regard to jurisdictional claims in published maps and institutional affiliations.
Rights and permissions
Springer Nature or its licensor (e.g. a society or other partner) holds exclusive rights to this article under a publishing agreement with the author(s) or other rightsholder(s); author self-archiving of the accepted manuscript version of this article is solely governed by the terms of such publishing agreement and applicable law.
Reprints and permissions
About this article
Cite this article.
Nielsen, K.S., Marteau, T.M., Bauer, J.M. et al. Biodiversity conservation as a promising frontier for behavioural science. Nat Hum Behav 5 , 550–556 (2021). https://doi.org/10.1038/s41562-021-01109-5
Download citation
Received : 30 November 2020
Accepted : 31 March 2021
Published : 13 May 2021
Issue Date : May 2021
DOI : https://doi.org/10.1038/s41562-021-01109-5
Share this article
Anyone you share the following link with will be able to read this content:
Sorry, a shareable link is not currently available for this article.
Provided by the Springer Nature SharedIt content-sharing initiative
This article is cited by
Realizing the full potential of behavioural science for climate change mitigation.
- Kristian S. Nielsen
- Viktoria Cologna
- Kimberly S. Wolske
Nature Climate Change (2024)
Achieving transformational change through the consilience of behavioral science and radical alternatives
- Daniel J. Read
- Matthew J. Selinske
Sustainability Science (2024)
A global analysis of coral reef conservation preferences
- Peter John Robinson
- Pieter van Beukering
- Luke Brander
Nature Sustainability (2023)
Pesticides drive patterns of insect visitors and pollination-related attributes of four crops in Buea, Southwest Cameroon
- Everdine Che Nchang
- Daniel Brice Kenko Nkontcheu
- Eric Bertrand Fokam
International Journal of Tropical Insect Science (2023)
Understanding the social impacts of enforcement activities on illegal wildlife trade in China
- Tien Ming Lee
Ambio (2022)
Quick links
- Explore articles by subject
- Guide to authors
- Editorial policies
Sign up for the Nature Briefing newsletter — what matters in science, free to your inbox daily.

Ecological Dynamics and Biodiversity in the Epipelagic Zone: a Detailed Analysis
This essay about the ecological dynamics and biodiversity of the epipelagic zone, or sunlight zone, explores its role as a critical marine layer from the surface to about 200 meters deep. It highlights how sunlight drives biological processes and nutrient cycles that support diverse life forms, from phytoplankton to whales. The text also discusses the impact of human activities like pollution, overfishing, and climate change on this zone, stressing the need for conservation efforts to maintain its ecological integrity and overall health.
How it works
The epipelagic zone, also known as the sunlight zone, is the uppermost layer of the world’s oceans, extending from the surface down to about 200 meters. This zone is a dynamic region characterized by its exposure to sunlight, making it one of the most biologically productive and diverse areas of the ocean. The ecological dynamics of this zone are crucial for understanding marine biodiversity, ecosystem health, and the overall functioning of oceanic systems.
Sunlight is a fundamental driver in the epipelagic zone, influencing both physical and biological processes.
It heats the surface water, creating temperature gradients that drive global ocean currents. These currents are essential for the transport of nutrients and organisms, linking disparate marine environments and facilitating the wide distribution of life forms. Additionally, sunlight supports photosynthesis, the primary process behind primary production in the ocean. Phytoplankton, microscopic plants that inhabit this upper layer, utilize sunlight to convert carbon dioxide into organic material, releasing oxygen in the process. This not only supports a vast array of marine life but also plays a significant role in the global carbon cycle, helping to regulate the Earth’s climate.
The biodiversity in the epipelagic zone is vast and includes everything from tiny planktonic organisms to the largest marine animals such as whales and sharks. Phytoplankton form the base of the oceanic food web, serving as the primary food source for zooplankton, which in turn are preyed upon by a variety of larger animals including fish, squids, and marine mammals. The diversity of life in this zone is not uniform and varies widely depending on geographical location, water temperature, salinity, and the availability of nutrients and light.
Nutrient availability is another crucial factor influencing the ecological dynamics of the epipelagic zone. Nutrients such as nitrogen, phosphorus, and iron are essential for phytoplankton growth and are typically brought to the surface waters by upwelling currents. These currents are generated by the wind-driven movement of water and the Earth’s rotation, which pushes water away from coastlines and pulls nutrient-rich deep water to the surface. Regions of strong upwelling, such as those found along the western coasts of continents, are often areas of high productivity and biodiversity. Conversely, regions with little to no upwelling tend to have lower productivity and biodiversity.
The epipelagic zone is also the region most affected by human activities. Pollution, overfishing, and climate change are having significant impacts on its ecological dynamics. Pollutants such as plastics and chemicals can accumulate in this zone, affecting the health of marine organisms and the overall ecosystem. Overfishing has led to the depletion of key species, which disrupts food webs and can lead to the collapse of local fisheries. Climate change, meanwhile, is altering temperature and nutrient distribution patterns in the ocean, affecting the distribution and productivity of phytoplankton and the species that depend on them.
The interactions between these various physical and biological factors create a complex and dynamic ecological framework in the epipelagic zone. This complexity is what makes the sunlight zone both a fascinating subject for scientific study and a critical area for conservation efforts. Protecting the biodiversity and ecological integrity of the epipelagic zone is essential not only for the health of the ocean but for the well-being of the entire planet. As such, it is crucial that efforts to mitigate human impacts are pursued vigorously. This includes enforcing sustainable fishing practices, reducing pollution, and addressing the global challenge of climate change. Only through such efforts can the ecological dynamics and biodiversity of the epipelagic zone be maintained for future generations.
In conclusion, the epipelagic zone is a vibrant, dynamic, and critically important part of the ocean. Its study not only enhances our understanding of marine biodiversity and ecological processes but also informs conservation strategies crucial for maintaining the health of the global ocean ecosystem. As research continues and our understanding deepens, the imperative to protect this invaluable marine layer becomes ever more apparent and urgent.

Cite this page
Ecological Dynamics and Biodiversity in the Epipelagic Zone: A Detailed Analysis. (2024, May 21). Retrieved from https://papersowl.com/examples/ecological-dynamics-and-biodiversity-in-the-epipelagic-zone-a-detailed-analysis/
"Ecological Dynamics and Biodiversity in the Epipelagic Zone: A Detailed Analysis." PapersOwl.com , 21 May 2024, https://papersowl.com/examples/ecological-dynamics-and-biodiversity-in-the-epipelagic-zone-a-detailed-analysis/
PapersOwl.com. (2024). Ecological Dynamics and Biodiversity in the Epipelagic Zone: A Detailed Analysis . [Online]. Available at: https://papersowl.com/examples/ecological-dynamics-and-biodiversity-in-the-epipelagic-zone-a-detailed-analysis/ [Accessed: 27 May. 2024]
"Ecological Dynamics and Biodiversity in the Epipelagic Zone: A Detailed Analysis." PapersOwl.com, May 21, 2024. Accessed May 27, 2024. https://papersowl.com/examples/ecological-dynamics-and-biodiversity-in-the-epipelagic-zone-a-detailed-analysis/
"Ecological Dynamics and Biodiversity in the Epipelagic Zone: A Detailed Analysis," PapersOwl.com , 21-May-2024. [Online]. Available: https://papersowl.com/examples/ecological-dynamics-and-biodiversity-in-the-epipelagic-zone-a-detailed-analysis/. [Accessed: 27-May-2024]
PapersOwl.com. (2024). Ecological Dynamics and Biodiversity in the Epipelagic Zone: A Detailed Analysis . [Online]. Available at: https://papersowl.com/examples/ecological-dynamics-and-biodiversity-in-the-epipelagic-zone-a-detailed-analysis/ [Accessed: 27-May-2024]
Don't let plagiarism ruin your grade
Hire a writer to get a unique paper crafted to your needs.
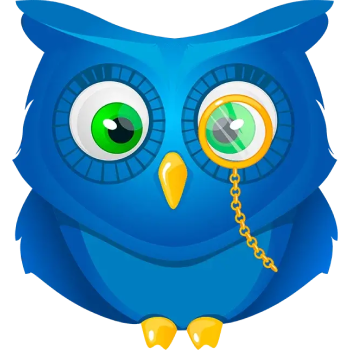
Our writers will help you fix any mistakes and get an A+!
Please check your inbox.
You can order an original essay written according to your instructions.
Trusted by over 1 million students worldwide
1. Tell Us Your Requirements
2. Pick your perfect writer
3. Get Your Paper and Pay
Hi! I'm Amy, your personal assistant!
Don't know where to start? Give me your paper requirements and I connect you to an academic expert.
short deadlines
100% Plagiarism-Free
Certified writers

- Search Search Search …
- Search Search …
Unlocking UNCLOS: How the ITLOS Advisory Opinion Delivers a Holistic Vision of Climate-relevant International Law
A long-standing conundrum of international environmental law is that the territorially-based, sectoral legal structures we have created to address environmental issues do not match the interconnected, interdependent nature of ecosystems. For many, this problem is writ large in the climate context. Whereas the science—synthesized in recent assessments and special reports by the Intergovernmental Panel on Climate Change (‘IPCC’)—emphasizes the deep interlinkages between issues of climate change, marine and terrestrial biodiversity loss, and ocean degradation, the international legal response is fragmented and uncoordinated. This gives rise to concerns of conflict and inconsistency between different international legal regimes, as well as the potential for ‘forum shopping’ in dispute settlement where different aspects of an environmental issue are presented to different judicial forums, which may reach differing conclusions on the interpretation of relevant international rules.
After many years during which international courts and tribunals have been largely silent on climate questions, 2024 has witnessed convergent advisory opinion proceedings taking place in three different bodies: the International Tribunal on the Law of the Sea (‘ITLOS’ or ‘Tribunal’), the International Court of Justice (‘ICJ’) and the Inter-American Court of Human Rights (‘IACHR’) . On one view, this might seem to set the stage for the exacerbation of a fragmented international legal response, ‘undermining the foundations of future cooperation combating climate change’ .
But the first international advisory opinion on climate change—delivered by ITLOS in its decision of 21 May 2024 —suggests a more hopeful outcome. Although primarily tasked with elucidating the ‘specific obligations of State Parties to the United Nations Convention on the Law of the Sea (‘UNCLOS’), including under Part XII’ , the Tribunal’s decision interweaves law of the sea requirements with those of other international laws relevant to climate change. In the process, the Tribunal illustrates how the fragmentation of international legal responses to climate change need not be seen as a weakness but could rather be a strength . The ITLOS advisory opinion crafts a series of interlocking and mutually reinforcing obligations across international climate law and international law of the sea that may ultimately serve to strengthen states’ duties to reduce greenhouse gas (‘GHG’) emissions and minimize the serious environmental harms resulting from climate change.
Unlocking UNCLOS as an Instrument of Climate Protection
When it comes to addressing the problem of climate change through international law, the global climate treaties—the 1992 United Nations Framework Convention on Climate Change (‘UNFCCC’) and the 2015 Paris Agreement —are undoubtedly the main game in town (see ITLOS advisory opinion, para. 222). But even from early on, they were not the sole locus of international climate law. The UNFCCC is applicable only to GHGs not controlled by the Montreal Protocol on Substances that Deplete the Ozone Layer and specific responsibilities for international aviation and maritime transport emissions are conferred on the International Civil Aviation Organization (‘ICAO’) and the International Maritime Organization (‘IMO’) . As the significance of climate threats has increased and the science has firmed, climate change is increasingly addressed in other areas of international law , from human rights, to international humanitarian law and trade law.
In this regard, international law of the sea is not an obvious candidate as a major site for action on climate change. UNCLOS was concluded in 1982 before climate change became a prominent issue of international concern, and the treaty lacks express references to GHG emissions or climate impacts. As argued in some of the state submissions before ITLOS, this might have led to a conclusion that law of sea obligations should give way to those of the international climate regime in dealing with the question of states’ obligations to address the impacts of GHG emissions and resultant climate change on the marine environment (see ITLOS advisory opinion, para. 220).
Instead, the Tribunal, in its advisory opinion, uses a series of interpretive ‘keys’ to unlock the potential of UNCLOS as a climate protection instrument. These include:
- A ‘broad’ approach to interpreting treaty language and the scope of relevant provisions relating to protection and preservation of the marine environment; and
- Seeking to ensure coordination and harmonization between UNCLOS and ‘external rules’ to ‘clarify and inform the meaning of the provisions of the Convention and to ensure that the Convention serves as a living instrument’ (see ITLOS advisory opinion, para. 130 [and also Christina Voigt’s contribution to this series]). In particular, ITLOS’ reference to rules of international climate law allowed the Tribunal to draw conceptual links between the animating concepts of climate law and the law of the sea.
Broad Treaty Interpretation Approach
An illustration of the Tribunal’s ‘broad’ approach to interpretation of UNCLOS treaty language is found in its analysis of whether anthropogenic GHG emissions to the atmosphere can be considered ‘pollution of the marine environment’ for the purposes of UNCLOS. (The Tribunal adopted a similar approach in its interpretation of UNCLOS article 192 dealing with the obligation to protect and preserve the marine environment (see, e.g. ITLOS advisory opinion, paras 385, 388)). Although UNCLOS does not provide a list of pollutants or forms of pollution of the marine environment, the Tribunal found it provides (cumulative) criteria to determine what constitutes such pollution (see UNCLOS, Art. 1(1)(4)), namely:
‘(1) there must be a substance or energy; (2) this substance or energy must be introduced by humans, directly or indirectly, into the marine environment; and (3) such introduction must result or be likely to result in deleterious effects.’
ITLOS noted that this ‘definition is general,’ meaning that ‘it encompasses whatever satisfies these criteria’ (see ITLOS advisory opinion, para. 161). Taking each of these components to have a ‘broad’ or ‘ordinary’ meaning (see, e.g., ITLOS advisory opinion, paras 163, 171, 172) the Tribunal reached a conclusion that ‘anthropogenic GHG emissions into the atmosphere constitute pollution of the marine environment within the meaning of article 1, paragraph 1, subparagraph 4, of the Convention’ (see ITLOS advisory opinion, para. 179).
Coordination with the Rules of International Climate Treaties
The Tribunal’s use of rules under international climate laws—particularly the Paris Agreement—to craft a coherent framework of climate protection across those rules and requirements of the international law of the sea is perhaps most evident in its analysis of the obligation under Article 194(1) of UNCLOS to take all measures ‘necessary to prevent, reduce and control pollution of the marine environment from any source’.
Referring to Article 4(1) of the Paris Agreement, which sets the aim of reaching net zero GHG emissions in the second half of this century, ITLOS sees consistency between this aim and the objective of Article 194(1) of UNCLOS (ITLOS advisory opinion, para. 200). Moreover, while acknowledging that UNCLOS does not provide specific criteria as to what constitute necessary measures to address pollution of the marine environment, the Tribunal found coherence between the kinds of measures articulated as examples under UNCLOS provisions (e.g. Article 194(3)) and the ‘mitigation measures’ to reduce GHG emissions specified by the international climate regime (ITLOS advisory opinion, para. 205).
Finally, the Tribunal included ‘[i]nternational rules and standards relating to climate change’ among the ‘various factors States should consider in their objective assessment of necessary measures to prevent, reduce and control marine pollution from anthropogenic GHG emissions’ (ITLOS advisory opinion, paras. 207, 214 and 222). It identified the Paris Agreement’s temperature goal (Art. 2(1)(a)) and its ‘timeline for emission pathways’ (Art. 4(1)) as ‘particularly relevant’ for informing the content of ‘necessary measures’ addressing marine pollution (see ITLOS advisory opinion, paras 215 and 222).
Separate Obligations but Mutually Reinforcing International Laws
While ITLOS in its advisory opinion sought harmony between obligations under UNCLOS and the requirements of international climate law, it nevertheless staked out a clear space of operation for law of the sea provisions in addressing GHG emissions and marine-related climate impacts. In so doing, the Tribunal showed how the law of the sea can play a complementary role to reinforce global goals to reduce GHG emissions and minimize the impacts of climate change.
This approach came to the fore in the Tribunal’s consideration of arguments from some participants that compliance with the UNFCCC and Paris Agreement would satisfy the specific obligation under UNCLOS to take measures regarding pollution of the marine environment arising from anthropogenic GHG emissions, and that Part XII of UNCLOS should not be interpreted as imposing emissions reduction obligations that are inconsistent with, or go beyond, those agreed under international climate treaties (ITLOS advisory opinion, paras 219-220). As the Paris Agreement leaves to each party decisions about domestic targets and timelines for reducing GHG emissions, this reasoning would have left the adoption of ‘necessary measures’ for addressing GHG-related ocean pollution entirely within the discretion of individual states, which might ‘frustrate the very goal of [UNCLOS]’ to protect and preserve the marine environment (ITLOS advisory opinion, para. 224).
The ITLOS advisory opinion charts a different course. It finds that the obligation under Article 194(1) of UNCLOS cannot be satisfied ‘simply by complying with the obligations and commitments under the Paris Agreement’ (ITLOS advisory opinion, para. 223; see also paras 201-2). According to the Tribunal, the Paris Agreement and UNCLOS ‘are separate agreements, with separate sets of obligations’ (ITLOS advisory opinion, para. 223). Hence the relationship between the two is complementary as regards the obligation to regulate marine pollution from anthropogenic GHG emissions; ‘the former does not supersede the latter’ (ibid). Indeed, the requirements of Article 194(1) of UNCLOS impose a distinct legal obligation on states parties ‘to take all necessary measures to prevent, reduce and control marine pollution from anthropogenic GHG emissions, including measures to reduce such emissions’ (ibid). Accordingly, if a state fails to comply with this specific obligation, its international responsibility would be engaged (ibid).
Conclusion: A Holistic Vision of Climate-relevant International Law
In this ‘critical decade’ for climate action, it can be easy to see the multiplicity of international laws with potential relevance for addressing climate change as more of a hindrance than a help. Some states and commentators have expressed anxiety that seeking multiple advisory opinions on climate obligations from different international courts and tribunals will only exacerbate the complexity of the international legal landscape for climate protection. But by advancing a more holistic vision of climate-relevant international law—one that seeks to harmonise but also allow for complementary interaction amongst the obligations set under different regimes—the ITLOS advisory opinion offers hope. It holds out the promise of a synergistic international legal response to climate change that better maps to the integrated and interconnected nature the ecosystems at stake and to the multi-pronged regulatory effort that will be needed to safeguard our climate system.
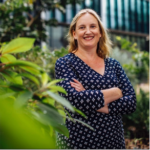
Jacqueline Peel
Jacqueline Peel is a Professor of Law at the Melbourne Law School and Director of the multidisciplinary climate initiative, Melbourne Climate Futures, at The University of Melbourne, Australia. She served as a Lead Author on the IPCC’s 6 th Assessment Report, Working Group III (Mitigation) and led the Hague Academy of International Law’s first-ever Centre for Studies and Research on Climate Change Law in 2022.
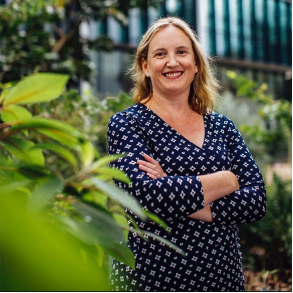
You may also like
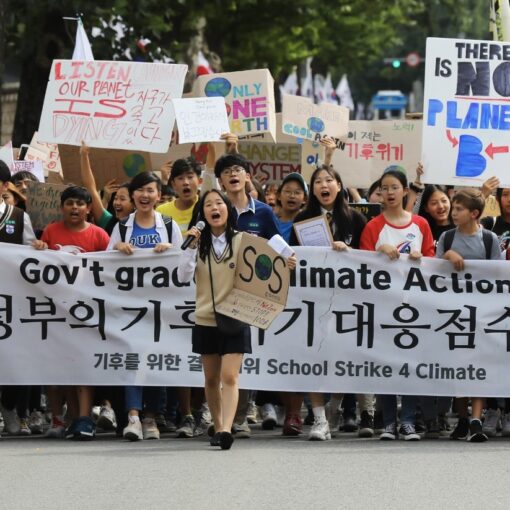
Future Trends in Climate Litigation Against Governments
We are in a critical decade for action on climate change. The world is on track to experience 3°C of warming and […]
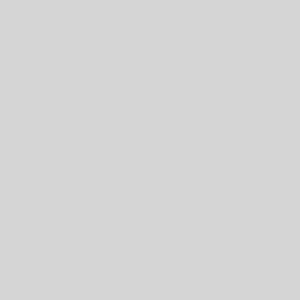
Mexico’s Congress Passes Climate Change Bill
By Julia Ciardullo Fellow On April 19, 2012, Mexico’s Senate unanimously passed the Ley General de Cambio Climático, or the General Law […]
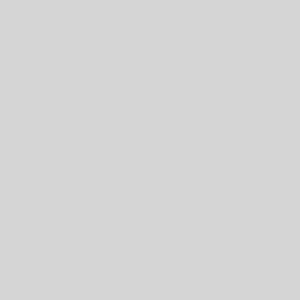
MAY 2015 UPDATE TO CLIMATE LITIGATION CHARTS
Each month, Arnold & Porter and the Sabin Center for Climate Change Law collect and summarize developments in climate-related litigation, which we […]
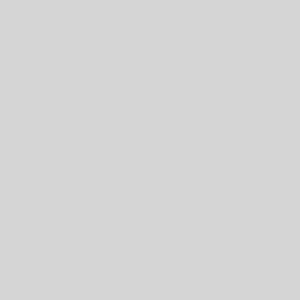
January Updates to the Climate Ligitation Charts
Update #58 January 2014 Each month, Arnold & Porter and the Center for Climate Change Law collect and summarize developments in […]

IMAGES
VIDEO
COMMENTS
Biodiversity is the variety of different forms of life on earth, including the different plants, animals, micro-organisms, the. genes they contain and the ecosystem they form. It refers to genetic ...
Conclusions are based on an analysis of more than 17,000 research articles published in ... Despite the continued increase in research papers, ... Our study shows the persistence of long-standing biases in research to conserve biodiversity. 9, 23, 26, 34, 41 These biases remain and will ultimately lead to uneven loss of biodiversity as ...
Categorization of papers to address questions 2 and 3 was determined primarily from our expert judgement, as few papers were explicit about the scales at which biodiversity benefits are delivered.
Towards a Biodiversity Conservation Research Strategy. Over 40 research topics are identified in the chapters of this volume. Such compilations of research opportunities need to be embraced within national strategies, matching needs with resources and priorities within a research agenda, while understanding the country's challenges.
Conclusion: As biodiversity underlies all goods and services provided by ecosystems that are crucial for human survival and well-being, this paper synthesizes and discusses observed and anticipated impacts of climate change on ... C., C. Folke, and K. G. Mäler. 1992. "The Ecology and Economics of Biodiversity Loss: The Research Agenda." ...
Abstract. Human beings are not only a part of our planet's ecosystems, but also, they are massively overusing them. This makes ecosystem protection, including biodiversity preservation, vital for humanity's future. The speed and scale of the threat are unprecedented in human history. The long arch of evolution has been confronted with such a ...
Concerns over the consequences of biodiversity loss for human well-being triggered the growth of biodiversity-ecosystem functioning (BEF) research, an important field of ecology over the past 25 ...
Conclusion: biodiversity requires diversity. ... where a first draft of this paper was presented and for editing the special issue of which this paper is part. ... 'Satellite Remote Sensing, Biodiversity Research and Conservation of the Future' 369 Philosophical Transactions of the Royal Society B: Biological Sciences 20130190.
The present study investigates the impact of climate change on biodiversity loss using global data consisting of 115 countries. In this study, we measure biodiversity loss using data on the total number of threatened species of amphibians, birds, fishes, mammals, mollusks, plants, and reptiles. The data were compiled from the Red List published by the International Union for Conservation of ...
Oecologia 110, 449-460 (1997)This paper raised several criticisms against early BEF research, which forced the reconsideration of conclusions with better experiments and more rigorous data analyses.
The term "biodiversity" is a contraction of "biotic diversity" or "biological diversity" and refers to the variety of life on Earth; from the diversity in genes and traits to species- and ecosystem-level diversity. The term was popularised in the mid-1980s by the late evolutionary biologist E.O. Wilson's 1988 book Biodiversity.
INTRODUCTION. One of the greatest attributes of the Earth is the biodiversity of her ecosystem. The Convention on Biological Diversity (Article 2) defined biological diversity or biodiversity" as the variability among living organisms from all sources including, inter alia, terrestrial, marine and other aquatic ecosystems and the ecological complexes of which they are part; this includes ...
The IPCC 4 Assessment Report (AR4) concluded that climate change will have significant impacts on many aspects of biological diversity. These impacts will include effects on ecosystems, on their component species and genetic diversity within species, and on ecological interactions. The implications of these impacts are significant for the long-term stability of the natural world and for the ...
In our introduction we outline the themes, introduce the papers in the thematic issue, and conclude with a synthesis of the main findings of the Forum. In doing so, we emphasize the research needed to better understand threats, opportunities and solutions regarding climate change and ecosystems.
Context Biodiversity loss is predicted to have significant impacts on ecosystem services based on previous ecological work at small spatial and temporal scales. However, scaling up understanding of biodiversity-ecosystem service (BES) relationships to broader scales is difficult since ecosystem services emerge from complex interactions between ecosystems, people, and technology. Objectives In ...
Abstract. As society strives to transition towards more sustainable development pathways, it is important to properly conceptualize the link between biodiversity (i.e. genes, traits, species and other dimensions) and human well-being (HWB; i.e. health, wealth, security and other dimensions). Here, we explore how published conceptual frameworks ...
Analysis of Warren et al. ( 2018) on a global scale on the effects of climate change on the distribution of insects, vertebrates and plants indicated that even with 2 °C temperature increase, approximately 18% of insects, 16% of plants and 8% of vertebrates species are projected to loose > 50% geographic range; this falls to 6% for insects, 8% ...
Abstract. Biodiversity faces unprecedented threats from rapid global change 1. Signals of biodiversity change come from time-series abundance datasets for thousands of species over large ...
Biodiversity loss and climate change are both globally significant issues that must be addressed through collaboration across countries and disciplines. With the December 2015 COP21 climate conference in Paris and the recent creation of the Intergovernmental Platform on Biodiversity and Ecosystem Services (IPBES), it has become critical to evaluate the capacity for global research networks to ...
This essay about cultural eutrophication explores the detrimental effects of nutrient pollution on aquatic biodiversity and water quality. It highlights how excessive nitrogen and phosphorus from human activities lead to algal blooms, depleting oxygen in water and creating dead zones.
The Cosmonaut Sea is one of the least accessed regions in the Southern Ocean, and our knowledge about the fish biodiversity in the region is sparse. In this study, we provided a description of demersal fish diversity in the Cosmonaut Sea by analysing cytochrome oxidase I (COI) barcodes of 98 fish samples that were hauled by trawling during the 37th and 38th Chinese National Antarctic Research ...
It is beyond the scope of this paper to describe such arguments, but philosophers have discussed the ethics of biodiversity conservation 7,8,9 and social scientists have identified public support ...
Biodiversity is a theoretical metaconcept based on the relationship between natural and functional concepts, which was proposed to raise awareness about nature conservation (Cordero-Rivera, 2017) and with the specific goal of highlighting the relevance of biological diversity in social and political discussions (Takacs, 1996).In short, biodiversity refers to the variety of living beings at all ...
This essay about the ecological dynamics and biodiversity of the epipelagic zone, or sunlight zone, explores its role as a critical marine layer from the surface to about 200 meters deep. ... In conclusion, the epipelagic zone is a vibrant, dynamic, and critically important part of the ocean. Its study not only enhances our understanding of ...
Conclusion: A Holistic Vision of Climate-relevant International Law. In this 'critical decade' for climate action, it can be easy to see the multiplicity of international laws with potential relevance for addressing climate change as more of a hindrance than a help. Some states and commentators have expressed anxiety that seeking multiple ...