
An official website of the United States government
The .gov means it’s official. Federal government websites often end in .gov or .mil. Before sharing sensitive information, make sure you’re on a federal government site.
The site is secure. The https:// ensures that you are connecting to the official website and that any information you provide is encrypted and transmitted securely.
- Publications
- Account settings
- My Bibliography
- Collections
- Citation manager

Save citation to file
Email citation, add to collections.
- Create a new collection
- Add to an existing collection
Add to My Bibliography
Your saved search, create a file for external citation management software, your rss feed.
- Search in PubMed
- Search in NLM Catalog
- Add to Search
The Applicability of Mouse Models to the Study of Human Disease
Affiliations.
- 1 Lung Biology Group, Lund, Sweden.
- 2 Department of Experimental Medical Science, Lund University, Lund, Sweden.
- 3 School of Life and Health Sciences, Aston University, Birmingham, UK. [email protected].
- PMID: 30788814
- PMCID: PMC7121329
- DOI: 10.1007/978-1-4939-9086-3_1
The laboratory mouse Mus musculus has long been used as a model organism to test hypotheses and treatments related to understanding the mechanisms of disease in humans; however, for these experiments to be relevant, it is important to know the complex ways in which mice are similar to humans and, crucially, the ways in which they differ. In this chapter, an in-depth analysis of these similarities and differences is provided to allow researchers to use mouse models of human disease and primary cells derived from these animal models under the most appropriate and meaningful conditions.Although there are considerable differences between mice and humans, particularly regarding genetics, physiology, and immunology, a more thorough understanding of these differences and their effects on the function of the whole organism will provide deeper insights into relevant disease mechanisms and potential drug targets for further clinical investigation. Using specific examples of mouse models of human lung disease, i.e., asthma, chronic obstructive pulmonary disease, and pulmonary fibrosis, this chapter explores the most salient features of mouse models of human disease and provides a full assessment of the advantages and limitations of these models, focusing on the relevance of disease induction and their ability to replicate critical features of human disease pathophysiology and response to treatment. The chapter concludes with a discussion on the future of using mice in medical research with regard to ethical and technological considerations.
Keywords: Disease; Ethics; Genetics; Immunology; Model; Mouse; Physiology.
PubMed Disclaimer
Similar articles
- Fibrocytes in Asthma and Chronic Obstructive Pulmonary Disease: Variations on the Same Theme. Dupin I, Contin-Bordes C, Berger P. Dupin I, et al. Am J Respir Cell Mol Biol. 2018 Mar;58(3):288-298. doi: 10.1165/rcmb.2017-0301PS. Am J Respir Cell Mol Biol. 2018. PMID: 29087726 Review.
- [Morphofunctional characteristics of pulmonary and bronchial arteries in bronchial asthma, chronic obstructive lung disease, idiopathic fibrosing alveolitis]. Mikhaleva LM, Cherniaev AL, Bykanova AV, Samsonova MV, Nekliudova GV. Mikhaleva LM, et al. Arkh Patol. 2008 Jan-Feb;70(1):35-7. Arkh Patol. 2008. PMID: 18368806 Russian.
- Investigating the role of relaxin in the regulation of airway fibrosis in animal models of acute and chronic allergic airway disease. Mookerjee I, Tang ML, Solly N, Tregear GW, Samuel CS. Mookerjee I, et al. Ann N Y Acad Sci. 2005 May;1041:194-6. doi: 10.1196/annals.1282.029. Ann N Y Acad Sci. 2005. PMID: 15956707
- Adenosine signaling in asthma and chronic obstructive pulmonary disease. Mohsenin A, Blackburn MR. Mohsenin A, et al. Curr Opin Pulm Med. 2006 Jan;12(1):54-9. doi: 10.1097/01.mcp.0000199002.46038.cb. Curr Opin Pulm Med. 2006. PMID: 16357580 Review.
- Do mouse models of allergic asthma mimic clinical disease? Epstein MM. Epstein MM. Int Arch Allergy Immunol. 2004 Jan;133(1):84-100. doi: 10.1159/000076131. Epub 2004 Jan 12. Int Arch Allergy Immunol. 2004. PMID: 14726635 Review.
- Improvement of a mouse infection model to capture Pseudomonas aeruginosa chronic physiology in cystic fibrosis. Duncan RP, Moustafa DA, Lewin GR, Diggle FL, Bomberger JM, Whiteley M, Goldberg JB. Duncan RP, et al. Proc Natl Acad Sci U S A. 2024 Aug 13;121(33):e2406234121. doi: 10.1073/pnas.2406234121. Epub 2024 Aug 5. Proc Natl Acad Sci U S A. 2024. PMID: 39102545 Free PMC article.
- Enhancing translation: A need to leverage complex preclinical models of addictive drugs to accelerate substance use treatment options. Corley C, Craig A, Sadek S, Marusich JA, Chehimi SN, White AM, Holdiness LJ, Reiner BC, Gipson CD. Corley C, et al. Pharmacol Biochem Behav. 2024 Oct;243:173836. doi: 10.1016/j.pbb.2024.173836. Epub 2024 Jul 26. Pharmacol Biochem Behav. 2024. PMID: 39067531 Review.
- Advances in an In Vitro Tuberculosis Infection Model Using Human Lung Organoids for Host-Directed Therapies. Kim SY, Choi JA, Choi S, Kim KK, Song CH, Kim EM. Kim SY, et al. PLoS Pathog. 2024 Jul 25;20(7):e1012295. doi: 10.1371/journal.ppat.1012295. eCollection 2024 Jul. PLoS Pathog. 2024. PMID: 39052544 Free PMC article.
- Hippocampal neuroimmune response in mice undergoing serial daily torpor induced by calorie restriction. Cogut V, Goris M, Jansma A, van der Staaij M, Henning RH. Cogut V, et al. Front Neuroanat. 2024 Apr 15;18:1334206. doi: 10.3389/fnana.2024.1334206. eCollection 2024. Front Neuroanat. 2024. PMID: 38686173 Free PMC article.
- Effects of increasing sensitizing doses of ovalbumin on airway hyperresponsiveness in asthmatic mice. Chen YJ, Yuan Y, Peng L, Dong XY, Xu YD, Wang Y, Yang YQ. Chen YJ, et al. Immun Inflamm Dis. 2024 Mar;12(3):e1225. doi: 10.1002/iid3.1225. Immun Inflamm Dis. 2024. PMID: 38533918 Free PMC article.
- Vandenbergh J. Use of house mice in biomedical research. ILAR J. 2000;41:133–135. doi: 10.1093/ilar.41.3.133. - DOI
- Hedrich H, editor. The laboratory mouse. Cambridge: Academic Press; 2012.
- Yue F, Cheng Y, Breschi A, Vierstra J, Wu W, et al. A comparative encyclopedia of DNA elements in the mouse genome. Nature. 2014;515:355–364. doi: 10.1038/nature13992. - DOI - PMC - PubMed
- Cheng Y, Ma Z, Kim BH, Wu W, Cayting P, et al. Principles of regulatory information conservation between mouse and human. Nature. 2014;515:371–375. doi: 10.1038/nature13985. - DOI - PMC - PubMed
- Bodenreider O, Hayamizu TF, Ringwald M, De Coronado S, Zhang S (2005) Of mice and men: aligning mouse and human anatomies. AMIA Annu Symp Proc 2005:61–65 - PMC - PubMed
- Search in MeSH
Related information
Linkout - more resources, full text sources.
- Europe PubMed Central
- PubMed Central
- MedlinePlus Consumer Health Information
- MedlinePlus Health Information
- Citation Manager
NCBI Literature Resources
MeSH PMC Bookshelf Disclaimer
The PubMed wordmark and PubMed logo are registered trademarks of the U.S. Department of Health and Human Services (HHS). Unauthorized use of these marks is strictly prohibited.
Thank you for visiting nature.com. You are using a browser version with limited support for CSS. To obtain the best experience, we recommend you use a more up to date browser (or turn off compatibility mode in Internet Explorer). In the meantime, to ensure continued support, we are displaying the site without styles and JavaScript.
- View all journals
- Explore content
- About the journal
- Publish with us
- Sign up for alerts
- Review Article
- Published: February 2007
Humanized mice in translational biomedical research
- Leonard D. Shultz 1 ,
- Fumihiko Ishikawa 2 &
- Dale L. Greiner 3
Nature Reviews Immunology volume 7 , pages 118–130 ( 2007 ) Cite this article
22k Accesses
995 Citations
17 Altmetric
Metrics details
There is a growing need for animal models to carry out in vivo studies of human biological systems without putting individuals at risk. Severely immunodeficient mice engrafted with human cells and tissues, known as 'humanized' mice, facilitate progress in studies of human haematopoiesis, immunity, gene therapy, infectious diseases, cancer and regenerative medicine.
Advances in the generation of humanized mice have depended on a systematic progression of genetic modifications in immunodeficient mouse hosts and on improvements in engraftment techniques.
Mice homozygous for the severe combined immunodeficiency ( scid ) gene mutation or for targeted mutations at the recombination-activating gene 1 ( Rag1 ) or Rag2 loci, accompanied by a targeted mutation at the interleukin-2 receptor γ-chain ( Il2rg ) locus, support greatly increased engraftment and function of human haematopoietic stem cells (HSCs) and peripheral-blood mononuclear cells (PBMCs) compared with previous immunodeficient mouse models.
The development of immunodeficient mice that are humanized by engraftment of human lymphoid tissues, HSCs and PBMCs provides an opportunity to carry out translational research on human immunity and autoimmune diseases. These models are also being used to study the biology of human pathogens responsible for AIDS and several other infectious diseases.
Humanized mice are being increasingly used as hosts for human malignant cells in studies of carcinogenesis, tumour metastasis and cancer therapy. The phenotypic and functional characterization of human tumour stem cells is also being advanced through the study of humanized mice.
The potential for new advances in our understanding of human immunology and other areas of human biology that is supported by studies in humanized mice remains promising. Additional genetic and technological modifications will accelerate progress towards the development of a functional human immune system in mice.
The culmination of decades of research on humanized mice is leading to advances in our understanding of human haematopoiesis, innate and adaptive immunity, autoimmunity, infectious diseases, cancer biology and regenerative medicine. In this Review, we discuss the development of these new generations of humanized mice, how they will facilitate translational research in several biomedical disciplines and approaches to overcome the remaining limitations of these models.
This is a preview of subscription content, access via your institution
Access options
Subscribe to this journal
Receive 12 print issues and online access
195,33 € per year
only 16,28 € per issue
Buy this article
- Purchase on SpringerLink
- Instant access to full article PDF
Prices may be subject to local taxes which are calculated during checkout
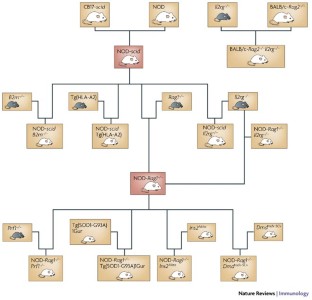
Similar content being viewed by others
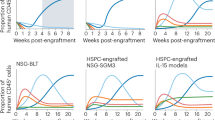
Humanized mouse models for immuno-oncology research
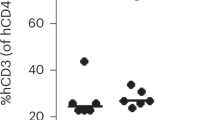
Generation of the NeoThy mouse model for human immune system studies
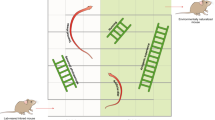
Naturalizing mouse models for immunology
Bosma, G. C., Custer, R. P. & Bosma, M. J. A severe combined immunodeficiency mutation in the mouse. Nature 301 , 527–530 (1983).
Article CAS PubMed Google Scholar
Mosier, D. E., Gulizia, R. J., Baird, S. M. & Wilson, D. B. Transfer of a functional human immune system to mice with severe combined immunodeficiency. Nature 335 , 256–259 (1988). The original description of the use of CB17- scid mice as hosts for human PBMCs, known as the Hu-PBL-SCID model.
McCune, J. M. et al. The SCID-hu mouse: murine model for the analysis of human hematolymphoid differentiation and function. Science 241 , 1632–1639 (1988). The original description of the use of CB17- scid mice as hosts for human haematopoietic fetal liver and thymus, resulting in the development of the SCID-hu model. This model is still used, in particular for testing HIV therapeutics.
Lapidot, T. et al. Cytokine stimulation of multi-lineage hematopoiesis from immature human cells engrafted in SCID mice. Science 255 , 1137–1141 (1992). The original description of the use of CB17- scid mice as hosts for human HSCs and the development of the Hu-SRC-SCID model.
Greiner, D. L., Hesselton, R. A. & Shultz, L. D. SCID mouse models of human stem cell engraftment. Stem Cells 16 , 166–177 (1998).
Fulop, G. M. & Phillips, R. A. The scid mutation in mice causes a general defect in DNA repair. Nature 347 , 479–482 (1990).
Mombaerts, P. et al. RAG-1-deficient mice have no mature B and T lymphocytes. Cell 68 , 869–877 (1992).
Shinkai, Y. et al. RAG-2-deficient mice lack mature lymphocytes owing to inability to initiate V(D)J rearrangement. Cell 68 , 855–867 (1992).
Shultz, L. D. et al. Multiple defects in innate and adaptive immunologic function in NOD/LtSz- scid mice. J. Immunol. 154 , 180–191 (1995).
CAS PubMed Google Scholar
Hesselton, R. M. et al. High levels of human peripheral blood mononuclear cell engraftment and enhanced susceptibility to HIV-1 infection in NOD/LtSz- scid/scid mice. J. Infect. Dis. 172 , 974–982 (1995).
Christianson, S. W. et al. Role of natural killer cells on engraftment of human lymphoid cells and on metastasis of human T-lymphoblastoid leukemia cells in C57BL/6J- scid mice and in C57BL/6J- scid bg mice. Cell. Immunol. 171 , 186–199 (1996).
Lowry, P. A. et al. Improved engraftment of human cord blood stem cells in NOD/LtSz- scid/scid mice after irradiation or multiple-day injections into unirradiated recipients. Biol. Blood Marrow Transplant. 2 , 15–23 (1996).
Pflumio, F. et al. Phenotype and function of human hematopoietic cells engrafting immune-deficient CB17-severe combined immunodeficiency mice and nonobese diabetic-severe combined immunodeficiency mice after transplantation of human cord blood mononuclear cells. Blood 88 , 3731–3740 (1996).
Ito, M. et al. NOD/SCID/γc null mouse: an excellent recipient mouse model for engraftment of human cells. Blood 100 , 3175–3182 (2002). This paper describes the development of a human immune system in NOD/Shi- scid Il2rg −/− mice engrafted as adults with human CD34 + cord-blood HSCs.
Traggiai, E. et al. Development of a human adaptive immune system in cord blood cell-transplanted mice. Science 304 , 104–107 (2004). This paper shows the development of a complete human immune system in BALB/c- Rag2 −/− Il2rg −/− mice engrafted intrahepatically as newborns with human cord-blood CD34 + HSCs.
Shultz, L. et al. Human lymphoid and myeloid cell development in NOD/LtSz- scid IL2rg null mice engrafted with mobilized human hematopoietic stem cells. J. Immunol. 174 , 6477–6489 (2005). One of the first studies to show the development of a complete human immune system in NOD- scid Il2rg −/− mice engrafted with human mobilized peripheral-blood CD34 + stem cells.
Ishikawa, F. et al. Development of functional human blood and immune systems in NOD/SCID/IL2 receptor γ-chain null mice. Blood 106 , 1565–1573 (2005). The first paper to show that engraftment of newborn NOD/LtSz- scid Il2rg −/− mice with human CD34 + HSCs leads to the generation of a complete haematopoietic system, including red blood cells and platelets.
Article CAS PubMed PubMed Central Google Scholar
Sugamura, K. et al. The interleukin-2 receptor γ-chain: its role in the multiple cytokine receptor complexes and T cell development in XSCID. Annu. Rev. Immunol. 14 , 179–205 (1996).
Cao, X. et al. Defective lymphoid development in mice lacking expression of the common cytokine receptor γ-chain. Immunity 2 , 223–238 (1995).
DiSanto, J. P., Muller, W., Guy-Grand, D., Fischer, A. & Rajewsky, K. Lymphoid development in mice with a targeted deletion of the interleukin 2 receptor γ-chain. Proc. Natl Acad. Sci. USA 92 , 377–381 (1995).
Ohbo, K. et al. Modulation of hematopoiesis in mice with a truncated mutant of the interleukin-2 receptor γ-chain. Blood 87 , 956–967 (1996).
Jacobs, H. et al. PIM1 reconstitutes thymus cellularity in interleukin-7- and common γ-chain-mutant mice and permits thymocyte maturation in Rag- but not CD3γ-deficient mice. J. Exp. Med. 190 , 1059–1068 (1999).
Yahata, T. et al. A highly sensitive strategy for SCID-repopulating cell assay by direct injection of primitive human hematopoietic cells into NOD/SCID mice bone marrow. Blood 101 , 2905–2913 (2003).
Gimeno, R. et al. Monitoring the effect of gene silencing by RNA interference in human CD34 + cells injected into newborn Rag2 −/− γ c −/− mice: functional inactivation of p53 in developing T cells. Blood 104 , 3886–3893 (2004).
Bryder, D., Rossi, D. J. & Weissman, I. L. Hematopoietic stem cells: the paradigmatic tissue-specific stem cell. Am. J. Pathol. 169 , 338–346 (2006).
Zanjani, E. D., Almeida-Porada, G. & Flake, A. W. Retention and multilineage expression of human hematopoietic stem cells in human–sheep chimeras. Stem Cells 13 , 101–111 (1995).
Clutterbuck, R. D. et al. Studies on the development of human acute myeloid leukaemia xenografts in immune-deprived mice: comparison with cells in short-term culture. Leuk. Res. 9 , 1511–1518 (1985).
Ganick, D. J., Sarnwick, R. D., Shahidi, N. T. & Manning, D. D. Inability of intravenously injected monocellular suspensions of human bone marrow to establish in the nude mouse. Int. Arch. Allergy Appl. Immunol. 62 , 330–333 (1980).
Shultz, L. D. et al. NOD/LtSz-Rag1 null mice: an immunodeficient and radioresistant model for engraftment of human hematolymphoid cells, HIV infection, and adoptive transfer of NOD mouse diabetogenic T cells. J. Immunol. 164 , 2496–2507 (2000).
Mazurier, F., Doedens, M., Gan, O. I. & Dick, J. E. Rapid myeloerythroid repopulation after intrafemoral transplantation of NOD-SCID mice reveals a new class of human stem cells. Nature Med. 9 , 959–963 (2003).
Schoeberlein, A. et al. Engraftment kinetics of human cord blood and murine fetal liver stem cells following in utero transplantation into immunodeficient mice. Stem Cells Dev. 13 , 677–684 (2004).
Article PubMed Google Scholar
Glimm, H. et al. Previously undetected human hematopoietic cell populations with short- term repopulating activity selectively engraft NOD/SCID-β2 microglobulin-null mice. J. Clin. Invest. 107 , 199–206 (2001).
Miyoshi, H., Smith, K. A., Mosier, D. E., Verma, I. M. & Torbett, B. E. Transduction of human CD34 + cells that mediate long-term engraftment of NOD/SCID mice by HIV vectors. Science 283 , 682–686 (1999).
Cohen-Haguenauer, O. et al. In vivo repopulation ability of genetically corrected bone marrow cells from Fanconi anemia patients. Proc. Natl Acad. Sci. USA 103 , 2340–2345 (2006).
Bleeker, W. K. et al. Dual mode of action of a human anti-epidermal growth factor receptor monoclonal antibody for cancer therapy. J. Immunol. 173 , 4699–4707 (2004).
Kolar, G. R., Yokota, T., Rossi, M. I., Nath, S. K. & Capra, J. D. Human fetal, cord blood, and adult lymphocyte progenitors have similar potential for generating B cells with a diverse immunoglobulin repertoire. Blood 104 , 2981–2987 (2004).
Yahata, T. et al. Functional human T lymphocyte development from cord blood CD34 + cells in nonobese diabetic/Shi- scid , IL-2 receptor-γ-null mice. J. Immunol. 169 , 204–209 (2002).
Legrand, N., Weijer, K. & Spits, H. Experimental models to study development and function of the human immune system in vivo . J. Immunol. 176 , 2053–2058 (2006).
Matsumura, T. et al. Functional CD5 + B cells develop predominantly in the spleen of NOD/SCID/γc null (NOG) mice transplanted either with human umbilical cord blood, bone marrow, or mobilized peripheral blood CD34 + cells. Exp. Hematol. 31 , 789–797 (2003).
Legrand, N. et al. Transient accumulation of human mature thymocytes and regulatory T cells with CD28 superagonist in 'human immune system' Rag2 −/− γ c −/− mice. Blood 108 , 238–245 (2006).
Banuelos, S. J. et al. Rejection of human islets and human HLA-A2.1-transgenic mouse islets by alloreactive human lymphocytes in immunodeficient NOD- scid and NOD- Rag1 null Prf1 null mice. Clin. Immunol. 112 , 273–283 (2004).
Camacho, R. E. et al. Intra-thymic/splenic engraftment of human T cells in HLA-DR1 transgenic NOD/scid mice. Cell. Immunol. 232 , 86–95 (2004).
Bajenoff, M. et al. Stromal cell networks regulate lymphocyte entry, migration, and territoriality in lymph nodes. Immunity 25 , 989–1001 (2006).
Suematsu, S. & Watanabe, T. Generation of a synthetic lymphoid tissue-like organoid in mice. Nature Biotechnol. 22 , 1539–1545 (2004).
Article CAS Google Scholar
Melkus, M. W. et al. Humanized mice mount specific adaptive and innate immune responses to EBV and TSST-1. Nature Med. 12 , 1316–1322 (2006).
Ifversen, P. & Borrebaeck, C. A. SCID-hu-PBL: a model for making human antibodies? Semin. Immunol. 8 , 243–248 (1996).
Murphy, W. J., Taub, D. D. & Longo, D. L. The huPBL-SCID mouse as a means to examine human immune function in vivo . Semin. Immunol. 8 , 233–241 (1996).
Shultz, L. D. et al. NOD/LtSz- Rag1 null Pfp null mice: a new model system to increase levels of human peripheral leukocyte and hematopoietic stem cell engraftment. Transplantation 76 , 1036–1042 (2003).
Christianson, S. W. et al. Enhanced human CD4 + T cell engraftment in β2-microglobulin-deficient NOD- scid mice. J. Immunol. 158 , 3578–3586 (1997).
Tary-Lehmann, M., Lehmann, P. V., Schols, D., Roncarolo, M. G. & Saxon, A. Anti-SCID mouse reactivity shapes the human CD4 + T cell repertoire in hu-PBL-SCID chimeras. J. Exp. Med. 180 , 1817–1827 (1994).
Greiner, D. L. & Shultz, L. D. in NOD Mice and Related Strains: Research Applications in Diabetes, AIDS, Cancer and Other Diseases (eds Leiter, E. & Atkinson, M.) 173–203 (Landes Bioscience, Austin, 1998).
Google Scholar
May, K. F. Jr et al. Anti-human CTLA-4 monoclonal antibody promotes T-cell expansion and immunity in a hu-PBL-SCID model: a new method for preclinical screening of costimulatory monoclonal antibodies. Blood 105 , 1114–1120 (2005).
Murray, A. G. et al. Human T-cell-mediated destruction of allogeneic dermal microvessels in a severe combined immunodeficient mouse. Proc. Natl Acad. Sci. USA 91 , 9146–9150 (1994).
Chang, S. K., Arendt, B. K., Darce, J. R., Wu, X. & Jelinek, D. F. A role for BLyS in the activation of innate immune cells. Blood 108 , 2687–2694 (2006).
Woodland, R. T. & Schmidt, M. R. Homeostatic proliferation of B cells. Semin. Immunol. 17 , 209–217 (2005).
Mosier, D. E. et al. EBV-induced human B cell lymphomas in hu-PBL-SCID mice. AIDS Res. Hum. Retroviruses 8 , 735–740 (1992).
Wagar, E. J. et al. Regulation of human cell engraftment and development of EBV-related lymphoproliferative disorders in Hu-PBL-scid mice. J. Immunol. 165 , 518–527 (2000).
van Rijn, R. S. et al. A new xenograft model for graft-versus-host disease by intravenous transfer of human peripheral blood mononuclear cells in Rag2 −/− γ c −/− double-mutant mice. Blood 102 , 2522–2531 (2003).
Jung, S. et al. In vivo depletion of CD11c + dendritic cells abrogates priming of CD8 + T cells by exogenous cell-associated antigens. Immunity 17 , 211–220 (2002).
Mosier, D. E. Human immunodeficiency virus infection of human cells transplanted to severe combined immunodeficient mice. Adv. Immunol. 63 , 79–125 (1996).
McCune, J. et al. The SCID-hu mouse: a small animal model for HIV infection and pathogenesis. Annu. Rev. Immunol. 9 , 399–429 (1991).
Davis, P. H. & Stanley, S. L. Jr Breaking the species barrier: use of SCID mouse–human chimeras for the study of human infectious diseases. Cel. Microbiol. 5 , 849–860 (2003).
Bente, D. A., Melkus, M. W., Garcia, J. V. & Rico-Hesse, R. Dengue fever in humanized NOD/SCID mice. J. Virol. 79 , 13797–13799 (2005).
Islas-Ohlmayer, M. et al. Experimental infection of NOD/SCID mice reconstituted with human CD34 + cells with Epstein–Barr virus. J. Virol. 78 , 13891–13900 (2004).
Kneteman, N. M. et al. Anti-HCV therapies in chimeric scid-Alb/uPA mice parallel outcomes in human clinical application. Hepatology 43 , 1346–1353 (2006).
Nelson, F. K., Greiner, D. L., Shultz, L. D. & Rajan, T. V. The immunodeficient scid mouse as a model for human lymphatic filariasis. J. Exp. Med. 173 , 659–663 (1991).
Guirado, E. et al. Passive serum therapy with polyclonal antibodies against Mycobacterium tuberculosis protects against post-chemotherapy relapse of tuberculosis infection in SCID mice. Microbes Infect. 8 , 1252–1259 (2006).
Morosan, S. et al. Liver-stage development of Plasmodium falciparum , in a humanized mouse model. J. Infect. Dis. 193 , 996–1004 (2006).
Moreno, A. et al. The course of infections and pathology in immunomodulated NOD/LtSz-SCID mice inoculated with Plasmodium falciparum laboratory lines and clinical isolates. Int. J. Parasitol. 36 , 361–369 (2006).
Macchiarini, F., Manz, M. G., Palucka, A. K. & Shultz, L. D. Humanized mice: are we there yet? J. Exp. Med. 202 , 1307–1311 (2005).
Lapenta, C. et al. Pertussis toxin B-oligomer inhibits HIV infection and replication in hu-PBL-SCID mice. Int. Immunol. 17 , 469–475 (2005).
Mosier, D. E. Viral pathogenesis in hu-PBL-SCID mice. Semin. Immunol. 8 , 255–262 (1996).
Ichiyama, K. et al. A duodenally absorbable CXC-chemokine receptor 4 antagonist, KRH-1636, exhibits a potent and selective anti-HIV-1 activity. Proc. Natl Acad. Sci. USA 100 , 4185–4190 (2003).
Baenziger, S. et al. Disseminated and sustained HIV infection in CD34 + cord blood cell-transplanted Rag2 −/− γc −/− mice. Proc. Natl Acad. Sci. USA 103 , 15951–15956 (2006).
Berges, B. K., Wheat, W. H., Palmer, B. E., Connick, E. & Akkina, R. HIV-1 infection and CD4 T cell depletion in the humanized Rag2 −/− γ c −/− (RAG-hu) mouse model. Retrovirology 3 , 76 (2006).
Article PubMed PubMed Central Google Scholar
Watanabe, S. et al. Hematopoietic stem cell-engrafted NOD/SCID/ Il2r γ null mice develop human lymphoid system and induce long-lasting HIV-1 infection with specific humoral immune responses. Blood 109 , 212–218 (2007).
Petersen, J. S. et al. Transfer of type 1 (insulin-dependent) diabetes mellitus associated autoimmunity to mice with severe combined immunodeficiency (SCID). Diabetologia 36 , 510–515 (1993).
Martin, A. et al. Characteristics of long-term human thyroid peroxidase autoantibody secretion in scid mice transplanted with lymphocytes from patients with autoimmune thyroiditis. Int. Arch. Allergy Immunol. 98 , 317–323 (1992).
Davis, L. S. et al. Inflammation, immune reactivity, and angiogenesis in a severe combined immunodeficiency model of rheumatoid arthritis. Am. J. Pathol. 160 , 357–367 (2002).
Tighe, H. et al. Autoantibody production by severe combined immunodeficient mice reconstituted with synovial cells from rheumatoid arthritis patients. Eur. J. Immunol. 20 , 1843–1848 (1990).
Gregersen, J. W., Holmes, S. & Fugger, L. Humanized animal models for autoimmune diseases. Tissue Antigens 63 , 383–394 (2004).
Friese, M. A., Jensen, L. T., Willcox, N. & Fugger, L. Humanized mouse models for organ-specific autoimmune diseases. Curr. Opin. Immunol. 18 , 704–709 (2006).
Takaki, T. et al. HLA-A * 0201-restricted T cells from humanized NOD mice recognize autoantigens of potential clinical relevance to type 1 diabetes. J. Immunol. 176 , 3257–3265 (2006).
Wen, L., Chen, N. Y., Tang, J., Sherwin, R. & Wong, F. S. The regulatory role of DR4 in a spontaneous diabetes DQ8 transgenic model. J. Clin. Invest. 107 , 871–880 (2001).
Marron, M. P., Graser, R. T., Chapman, H. D. & Serreze, D. V. Functional evidence for the mediation of diabetogenic T cell responses by HLA-A2.1 MHC class I molecules through transgenic expression in NOD mice. Proc. Natl Acad. Sci. USA 99 , 13753–13758 (2002).
Ellmerich, S. et al. High incidence of spontaneous disease in an HLA-DR15 and TCR transgenic multiple sclerosis model. J. Immunol. 174 , 1938–1946 (2005).
Mallone, R. et al. Differential recognition and activation thresholds in human autoreactive GAD-specific T cells. Diabetes 53 , 971–977 (2004).
Kent, S. C. et al. Expanded T cells from pancreatic lymph nodes of type 1 diabetic subjects recognize an insulin epitope. Nature 435 , 224–228 (2005).
Holst, J., Vignali, K. M., Burton, A. R. & Vignali, D. A. Rapid analysis of T-cell selection in vivo using T cell-receptor retrogenic mice. Nature Methods 3 , 191–197 (2006).
Fogh, J., Fogh, J. M. & Orfeo, T. One hundred and twenty-seven cultured human tumor cell lines producing tumors in nude mice. J. Natl Cancer Inst. 59 , 221–226 (1977).
Hudson, W. A., Li, Q., Le, C. & Kersey, J. H. Xenotransplantation of human lymphoid malignancies is optimized in mice with multiple immunologic defects. Leukemia 12 , 2029–2033 (1998).
Dick, J. E. & Lapidot, T. Biology of normal and acute myeloid leukemia stem cells. Int. J. Hematol. 82 , 389–396 (2005).
Kawano, N. et al. Efficient engraftment of primary adult T-cell leukemia cells in newborn NOD/SCID/β2-microglobulin null mice. Leukemia 19 , 1384–1390 (2005).
Nakamura, Y. et al. Engraftment of NOD/SCID/γc null mice with multilineage neoplastic cells from patients with juvenile myelomonocytic leukaemia. Br. J. Haematol. 130 , 51–57 (2005).
Pardal, R., Clarke, M. F. & Morrison, S. J. Applying the principles of stem-cell biology to cancer. Nature Rev. Cancer 3 , 895–902 (2003).
Reya, T., Morrison, S. J., Clarke, M. F. & Weissman, I. L. Stem cells, cancer, and cancer stem cells. Nature 414 , 105–111 (2001).
Bonnet, D. & Dick, J. E. Human acute myeloid leukemia is organized as a hierarchy that originates from a primitive hematopoietic cell. Nature Med. 3 , 730–737 (1997).
Pilarski, L. M. & Belch, A. R. Clonotypic myeloma cells able to xenograft myeloma to nonobese diabetic severe combined immunodeficient mice copurify with CD34 + hematopoietic progenitors. Clin. Cancer Res. 8 , 3198–3204 (2002).
PubMed Google Scholar
Al-Hajj, M., Wicha, M. S., Benito-Hernandez, A., Morrison, S. J. & Clarke, M. F. Prospective identification of tumorigenic breast cancer cells. Proc. Natl Acad. Sci. USA 100 , 3983–3988 (2003).
O'Brien, C. A., Pollett, A., Gallinger, S. & Dick, J. E. A human colon cancer cell capable of initiating tumour growth in immunodeficient mice. Nature 445 , 106–110 (2007).
Singh, S. K. et al. Identification of human brain tumour initiating cells. Nature 432 , 396–401 (2004).
Yoshida, S. et al. Long-term engraftment and self-renewal of AML stem cells in the newborn NOD-scid/ Il2rg null immunodeficient mouse model. Blood 106 , A1261 (2005).
Nomura, T. et al. Induction of cancer, actinic keratosis, and specific p53 mutations by UVB light in human skin maintained in severe combined immunodeficient mice. Cancer Res. 57 , 2081–2084 (1997).
Mitsiades, C. S. et al. Fluorescence imaging of multiple myeloma cells in a clinically relevant SCID/NOD in vivo model: biologic and clinical implications. Cancer Res. 63 , 6689–6696 (2003).
O'Reilly, M. S., Holmgren, L., Chen, C. & Folkman, J. Angiostatin induces and sustains dormancy of human primary tumors in mice. Nature Med. 2 , 689–692 (1996).
Siegler, U. et al. Activated natural killer cells from patients with acute myeloid leukemia are cytotoxic against autologous leukemic blasts in NOD/SCID mice. Leukemia 19 , 2215–2222 (2005).
Flavell, D. J. et al. The anti-CD20 antibody rituximab augments the immunospecific therapeutic effectiveness of an anti-CD19 immunotoxin directed against human B-cell lymphoma. Br. J. Haematol. 134 , 157–170 (2006).
Trieu, Y. et al. Soluble interleukin-13Rα2 decoy receptor inhibits Hodgkin's lymphoma growth in vitro and in vivo . Cancer Res. 64 , 3271–3275 (2004).
Dewan, M. Z. et al. Rapid tumor formation of human T-cell leukemia virus type 1-infected cell lines in novel NOD-SCID/γc null mice: suppression by an inhibitor against NF-κB. J. Virol. 77 , 5286–5294 (2003).
Watanabe, M. et al. A novel NF-κB inhibitor DHMEQ selectively targets constitutive NF-κB activity and induces apoptosis of multiple myeloma cells in vitro and in vivo . Int. J. Cancer 114 , 32–38 (2005).
Chalandon, Y. et al. BCR–ABL-transduced human cord blood cells produce abnormal populations in immunodeficient mice. Leukemia 19 , 442–448 (2005).
Serakinci, N. & Keith, W. N. Therapeutic potential of adult stem cells. Eur. J. Cancer 42 , 1243–1246 (2006).
Solter, D. From teratocarcinomas to embryonic stem cells and beyond: a history of embryonic stem cell research. Nature Rev. Genet. 7 , 319–327 (2006).
Kakinuma, S. et al. Human umbilical cord blood as a source of transplantable hepatic progenitor cells. Stem Cells 21 , 217–227 (2003).
Tateno, C. et al. Near completely humanized liver in mice shows human-type metabolic responses to drugs. Am. J. Pathol. 165 , 901–912 (2004).
Ishikawa, F. et al. Purified human hematopoietic stem cells contribute to the generation of cardiomyocytes through cell fusion. FASEB J. 20 , 950–952 (2006).
Ma, N. et al. Umbilical cord blood cell transplantation for myocardial regeneration. Transplant Proc. 38 , 771–773 (2006).
Torrente, Y. et al. Human circulating AC133 + stem cells restore dystrophin expression and ameliorate function in dystrophic skeletal muscle. J. Clin. Invest. 114 , 182–195 (2004).
Ishikawa, F. et al. Human cord blood- and bone marrow-derived CD34 + cells regenerate gastrointestinal epithelial cells. FASEB J. 18 , 1958–1960 (2004).
Droetto, S. et al. Vasculogenic potential of long term repopulating cord blood progenitors. FASEB J. 18 , 1273–1275 (2004).
Taguchi, A. et al. Administration of CD34 + cells after stroke enhances neurogenesis via angiogenesis in a mouse model. J. Clin. Invest. 114 , 330–338 (2004).
Pauwelyn, K. A. & Verfaillie, C. M. Transplantation of undifferentiated, bone marrow-derived stem cells. Curr. Top. Dev. Biol. 74 , 201–251 (2006).
Hess, D. et al. Bone marrow-derived stem cells initiate pancreatic regeneration. Nature Biotechnol. 21 , 763–770 (2003).
Yoshida, S. et al. Human cord blood-derived cells generate insulin-producing cells in vivo . Stem Cells 23 , 1409–1416 (2005).
Vassilopoulos, G., Wang, P. R. & Russell, D. W. Transplanted bone marrow regenerates liver by cell fusion. Nature 422 , 901–904 (2003).
Zhang, S. et al. Both cell fusion and transdifferentiation account for the transformation of human peripheral blood CD34 + cells into cardiomyocytes in vivo . Circulation 110 , 3803–3807 (2004).
Stamm, C., Liebold, A., Steinhoff, G. & Strunk, D. Stem cell therapy for ischemic heart disease: beginning or end of the road? Cell Transplant. 15 (Suppl. 1), 47–56 (2006).
Article Google Scholar
Brolen, G. K., Heins, N., Edsbagge, J. & Semb, H. Signals from the embryonic mouse pancreas induce differentiation of human embryonic stem cells into insulin-producing β-cell-like cells. Diabetes 54 , 2867–2874 (2005).
Cooke, M. J., Stojkovic, M. & Przyborski, S. A. Growth of teratomas derived from human pluripotent stem cells is influenced by the graft site. Stem Cells Dev. 15 , 254–259 (2006).
Przyborski, S. A. Differentiation of human embryonic stem cells after transplantation in immune-deficient mice. Stem Cells 23 , 1242–1250 (2005).
Wang, L. et al. Generation of hematopoietic repopulating cells from human embryonic stem cells independent of ectopic HOXB4 expression. J. Exp. Med. 201 , 1603–1614 (2005).
Tian, X., Woll, P. S., Morris, J. K., Linehan, J. L. & Kaufman, D. S. Hematopoietic engraftment of human embryonic stem cell-derived cells is regulated by recipient innate immunity. Stem Cells 24 , 1370–1380 (2006).
Galic, Z. et al. T-lineage differentiation from human embryonic stem cells. Proc. Natl Acad. Sci. USA 103 , 11742–11747 (2006).
Robert, J. S. The science and ethics of making part-human animals in stem cell biology. FASEB J. 20 , 838–845 (2006).
Sotiropoulou, P. A., Perez, S. A., Gritzapis, A. D., Baxevanis, C. N. & Papamichail, M. Interactions between human mesenchymal stem cells and natural killer cells. Stem Cells 24 , 74–85 (2006).
Flanagan, S. P. ' Nude ', a new hairless gene with pleiotropic effects in the mouse. Genet. Res. 8 , 295–309 (1966).
Mosier, D. E., Stell, K. L., Gulizia, R. J., Torbett, B. E. & Gilmore, G. L. Homozygous scid/scid ; beige/beige mice have low levels of spontaneous or neonatal T cell-induced B cell generation. J. Exp. Med. 177 , 191–194 (1993).
Lapidot, T. Mechanism of human stem cell migration and repopulation of NOD/SCID and B2m null NOD/SCID mice. The role of SDF-1/CXCR4 interactions. Ann. NY Acad. Sci. 938 , 83–95 (2001).
Nicolini, F. E., Cashman, J. D., Hogge, D. E., Humphries, R. K. & Eaves, C. J. NOD/SCID mice engineered to express human IL-3, GM-CSF and Steel factor constitutively mobilize engrafted human progenitors and compromise human stem cell regeneration. Leukemia 18 , 341–347 (2004).
Download references
Acknowledgements
We thank E. Leiter, D. Serreze, M. Berry and A. Rossini for valuable discussions. We are supported by the β-Cell Biology Consortium and Autoimmunity Prevention Centers of the National Institutes of Health (NIH; USA), the Juvenile Diabetes Research Foundation (International), the American Diabetes Association, and the Diabetes Endocrinology Research Center, the National Cancer Institute Center, the National Institute of Allergy and Infectious Diseases and the National Heart, Lung and Blood Institute of the NIH. The contents of this publication are solely the responsibility of the authors and do not necessarily represent the official views of the NIH.
Author information
Authors and affiliations.
The Jackson Laboratory, 600 Main Street, Bar Harbor, 04609, Maine, USA
Leonard D. Shultz
RIKEN, Research Center for Allergy and Immunology, Yokahama Institute, 1-7-22 Suehiro-cho, Tsurumi-Ku, Yokohama, 230-0045, Kanagawa, Japan
Fumihiko Ishikawa
University of Massachusetts Medical School, 373 Plantation Street, Biotech 2, Suite 218, Worcester, 01605, Massachusetts, USA
Dale L. Greiner
You can also search for this author in PubMed Google Scholar
Corresponding author
Correspondence to Leonard D. Shultz .
Ethics declarations
Competing interests.
Leonard D. Shultz and Dale L. Greiner are consultants for and receive financial support from Viacell, Inc. and Pfizer, Inc.
Related links
Further information.
The Jackson Laboratory
Leonard Shultz's homepage
( scid ). Mice homozygous for the scid mutation at the protein kinase, DNA activated, catalytic polypeptide ( Prkdc scid ) locus have a complete absence of mature T and B cells.
The spontaneous rearrangement of T- and B-cell receptors in scid mice, leading to the generation of mature T and B cells.
Two linked genes in which targeted mutations result in the complete inability to generate T and B cells expressing antigen-specific receptors.
Mice homozygous for a mutation in the forkhead box N1 ( Foxn1 ) gene, which causes both hairlessness and impaired development of the thymus, resulting in an inability to generate mature T cells.
Severe combined immunodeficiency ( scid ) mice engrafted with human fetal liver and thymus tissue under the renal capsule.
Severe combined immunodeficiency ( scid ) mice that have been sublethally irradiated and injected with human haematopoietic stem cells (HSCs). The HSCs are obtained from three main sources: bone marrow, umbilical cord blood or granulocyte colony-stimulating factor (G-CSF)-mobilized peripheral blood, which contains large numbers of human HSCs.
An immune reactivity of cells or antibody from one animal directed against cells or tissues of a different species.
(BLT mice). Non-obese diabetic (NOD)-severe combined immunodeficiency ( scid ) mice engrafted with human fetal liver (L) and thymus (T) under the renal capsule. Three weeks later, mice are irradiated and then injected with a suspension of CD34 + cells from the same human fetal liver sample. The injected fetal liver cells seed to the mouse bone marrow (B).
A type of autoimmune disease in which autoantibodies produced by the immune system overstimulate the thyroid gland, causing hyperthyroidism.
A term used for T-cell receptor (TCR)-transgenic mice generated by retrovirus-mediated transduction of haematopoietic stem cells (HSCs) with a vector carrying a TCR transgene. These transduced HSCs are then injected into conditioned mice to reconstitute the mice with T cells expressing the TCR transgene.
Refers to the ability of a non-stem cell to transform into a different type of cell lineage, or when an already partly differentiated stem cell creates cells of different lineages or cell types.
A tumour that derives from pluripotent germ cells, comprising disorganized tissues derived from all three embryonic germ layers (ectoderm, mesoderm and endoderm). It can arise spontaneously in the human gonads.
An episode of acute cardiac ischaemia that leads to death of heart-muscle cells. It is usually caused by a thrombotic atherosclerotic plaque.
Rights and permissions
Reprints and permissions
About this article
Cite this article.
Shultz, L., Ishikawa, F. & Greiner, D. Humanized mice in translational biomedical research. Nat Rev Immunol 7 , 118–130 (2007). https://doi.org/10.1038/nri2017
Download citation
Issue Date : February 2007
DOI : https://doi.org/10.1038/nri2017
Share this article
Anyone you share the following link with will be able to read this content:
Sorry, a shareable link is not currently available for this article.
Provided by the Springer Nature SharedIt content-sharing initiative
This article is cited by
Patient-derived xenograft models in cancer therapy: technologies and applications.
Signal Transduction and Targeted Therapy (2023)
CXCR1/2 dual-inhibitor ladarixin reduces tumour burden and promotes immunotherapy response in pancreatic cancer
- Carmine Carbone
- Giampaolo Tortora
British Journal of Cancer (2023)
- Jane Chuprin
- Hannah Buettner
- Michael A. Brehm
Nature Reviews Clinical Oncology (2023)
Application of a 3D hydrogel-based model to replace use of animals for passaging patient-derived xenografts
- Jennifer C. Ashworth
- Anna M. Grabowska
In vitro models (2023)
Quick links
- Explore articles by subject
- Guide to authors
- Editorial policies
Sign up for the Nature Briefing newsletter — what matters in science, free to your inbox daily.

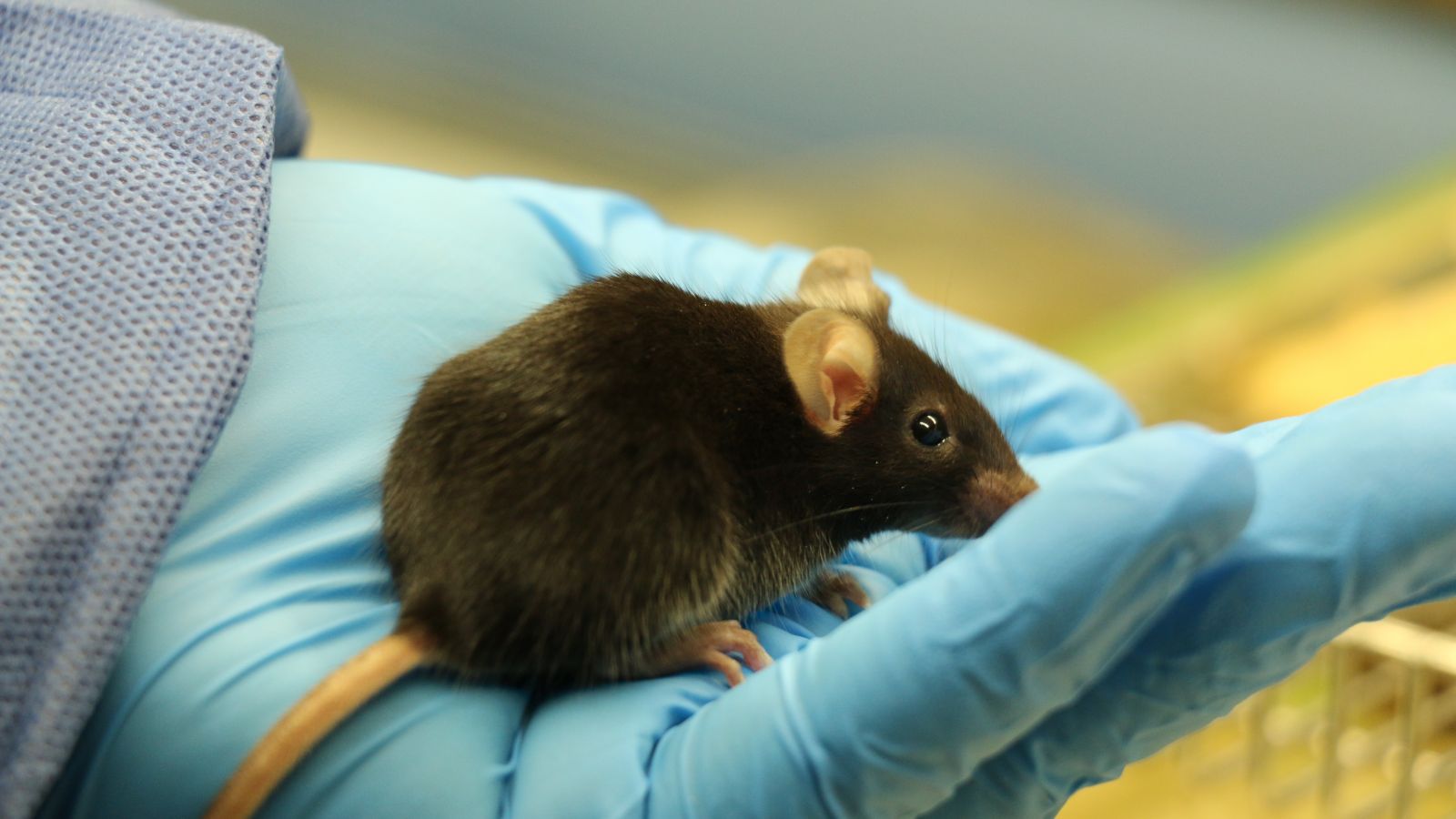
Mice are the most commonly used animals in medical research. This trend looks likely to continue now that both mouse and human genomes have been mapped ( 80% of human genes are exactly the same as those found in mice, and at least a further 10% are very similar) allowing human genetic disorders and diseases to be studied with greater accuracy.
Often, the only way of determining the function of a human gene is to insert it into, or remove it from, the mouse genome. Many thousands of mouse strains now exist, some frozen as embryos. Eventually, such techniques could lead to new methods of preventing, treating or even curing genetic diseases and other diseases with a genetic component.
Around 87% of all genetically modified animals used in research in the UK are mice [ UK 2020 figures ].
View this post on Instagram A post shared by Understanding Animal Research (@understandinganimalresearch)
Mice ( Mus musculus ) belong to the family rodentia (rodents) and are one of the most common mammals on Earth. They are small animals that grow to the size of 12cm long. Mice are omnivores, their diet consists of a mixture of both plant and animal matter, essentially mice can eat anything they like! Mice have been used in research for more than a century with the first use of mice in genetics dating back to 1902. They are the most commonly used animal in Great Britain.
Why are mice used in research?
Mice have many characteristics that make them ideal laboratory animals. Firstly, some diseases are modelled well in mice as human and mice share some anatomical, physiological, and genetic features. Conveniently, due to the successful sequencing of the mice genome, scientists can produce genetically modified mice and introduce or remove particular genetic features to make the mice better disease models. Mice have a relatively short gestation period and have multiple births, allowing researchers access to a lot of mice in a short amount of time. Their relatively fast ageing process also makes them great models for studying the effects and process of ageing. Finally, b ecause of their size, they are convenient for researchers and animal technicians to house and care for.
What types of research are mice used in?
Mice are versatile, they are used in a range of research from genetics to virology, oncology and many more. Notably, mice and other animals have been very important in the development of Herceptin, a monoclonal antibody used in certain types of breast cancer. Herceptin was the first monoclonal antibody successfully used to treat cancer. The HER2 protein, which makes breast cancer cells grow and duplicate was discovered in rat tumours, however, years later the monoclonal antibodies were used in mice to target the HER2, which successfully reduced tumour growth. The protein was discovered in rat tumours and years later, the antibodies were used to target the HER2 in mice. Herceptin is a humanised mice antibody, being 95% human and 5% mice.
More recently, mice have been instrumental in the search for a coronavirus vaccine. Researcher’s ability to genetically modify mice has been especially useful in breeding mice that are susceptible to the SAR-COV-2 virus. The mice ACE2 receptor is different from the human ACE2 receptor, exempting mice from being infected by the virus. Scientists altered the mice ACE2 receptor to become more human and allow the mice to get the virus and display symptoms of the covid-19 disease. When research began for the vaccine for the virus, mice with humanised ACE2 receptors were the best animal model at researchers’ disposal.
How are the mice looked after?
The use of animals in research is highly reg ulated, an important part of that regulation is ensuring the animals are housed and cared for correctly. Laboratory mice are housed similarly to pet mice, in cages lined with soft, absorbent bedding. In the laboratory the cages are made of see-through plastic so that they can be seen without disturbing them. Mice are given fresh food and water each day and are usually fed a specially constructed diet that meets all their nutritional needs. It is also important that animals have enrichment (things to entertain them), so they will usually have areas where they can hide away and objects to climb and gnaw on.
Because mice are highly social animals, it is very important that they are housed in groups, or at a minimum pairs. There are only a few exceptional circumstances where mice would be kept alone, usually for their own safety.
Find out more about mice in research with our '10 facts' infographic .
See also our pages on GM mice and breeding , mice and stem cell research , our video of mice, and animal research.info on mice and GM mice .
Mice in research: https://www.yourgenome.org/facts/why-use-the- mouse -in-research
Herceptin: https://www.understandinganimalresearch.org.uk/news/herceptin-first-monoclonal-antibody-treatment-for-cancer
Coronavirus and mice: https://www.sciencemag.org/news/2020/04/mice-hamsters-ferrets-monkeys-which-lab-animals-can-help-defeat-new-coronavirus
Mice: https://www.britannica.com/animal/ mouse -rodent/Geographic-distribution-and-habitat
Featured news

New video from UAR: Growing cancer in chicken eggs

The shameful case of Nellie Burnham

Improving openness: a survey to understand the student perspective
Subscribe to our newsletter.
Get the latest articles and news from Understanding Animal Research in your email inbox every month. For more information, please see our privacy policy .
Ethical care for research animals
WHY ANIMAL RESEARCH?
The use of animals in some forms of biomedical research remains essential to the discovery of the causes, diagnoses, and treatment of disease and suffering in humans and in animals., stanford shares the public's concern for laboratory research animals..
Many people have questions about animal testing ethics and the animal testing debate. We take our responsibility for the ethical treatment of animals in medical research very seriously. At Stanford, we emphasize that the humane care of laboratory animals is essential, both ethically and scientifically. Poor animal care is not good science. If animals are not well-treated, the science and knowledge they produce is not trustworthy and cannot be replicated, an important hallmark of the scientific method .
There are several reasons why the use of animals is critical for biomedical research:
• Animals are biologically very similar to humans. In fact, mice share more than 98% DNA with us!
• Animals are susceptible to many of the same health problems as humans – cancer, diabetes, heart disease, etc.
• With a shorter life cycle than humans, animal models can be studied throughout their whole life span and across several generations, a critical element in understanding how a disease processes and how it interacts with a whole, living biological system.
The ethics of animal experimentation
Nothing so far has been discovered that can be a substitute for the complex functions of a living, breathing, whole-organ system with pulmonary and circulatory structures like those in humans. Until such a discovery, animals must continue to play a critical role in helping researchers test potential new drugs and medical treatments for effectiveness and safety, and in identifying any undesired or dangerous side effects, such as infertility, birth defects, liver damage, toxicity, or cancer-causing potential.
U.S. federal laws require that non-human animal research occur to show the safety and efficacy of new treatments before any human research will be allowed to be conducted. Not only do we humans benefit from this research and testing, but hundreds of drugs and treatments developed for human use are now routinely used in veterinary clinics as well, helping animals live longer, healthier lives.
It is important to stress that 95% of all animals necessary for biomedical research in the United States are rodents – rats and mice especially bred for laboratory use – and that animals are only one part of the larger process of biomedical research.
Our researchers are strong supporters of animal welfare and view their work with animals in biomedical research as a privilege.
Stanford researchers are obligated to ensure the well-being of all animals in their care..
Stanford researchers are obligated to ensure the well-being of animals in their care, in strict adherence to the highest standards, and in accordance with federal and state laws, regulatory guidelines, and humane principles. They are also obligated to continuously update their animal-care practices based on the newest information and findings in the fields of laboratory animal care and husbandry.
Researchers requesting use of animal models at Stanford must have their research proposals reviewed by a federally mandated committee that includes two independent community members. It is only with this committee’s approval that research can begin. We at Stanford are dedicated to refining, reducing, and replacing animals in research whenever possible, and to using alternative methods (cell and tissue cultures, computer simulations, etc.) instead of or before animal studies are ever conducted.

Organizations and Resources
There are many outreach and advocacy organizations in the field of biomedical research.
- Learn more about outreach and advocacy organizations

Stanford Discoveries
What are the benefits of using animals in research? Stanford researchers have made many important human and animal life-saving discoveries through their work.
- Learn more about research discoveries at Stanford

Featured Topics
Featured series.
A series of random questions answered by Harvard experts.
Explore the Gazette
Read the latest.
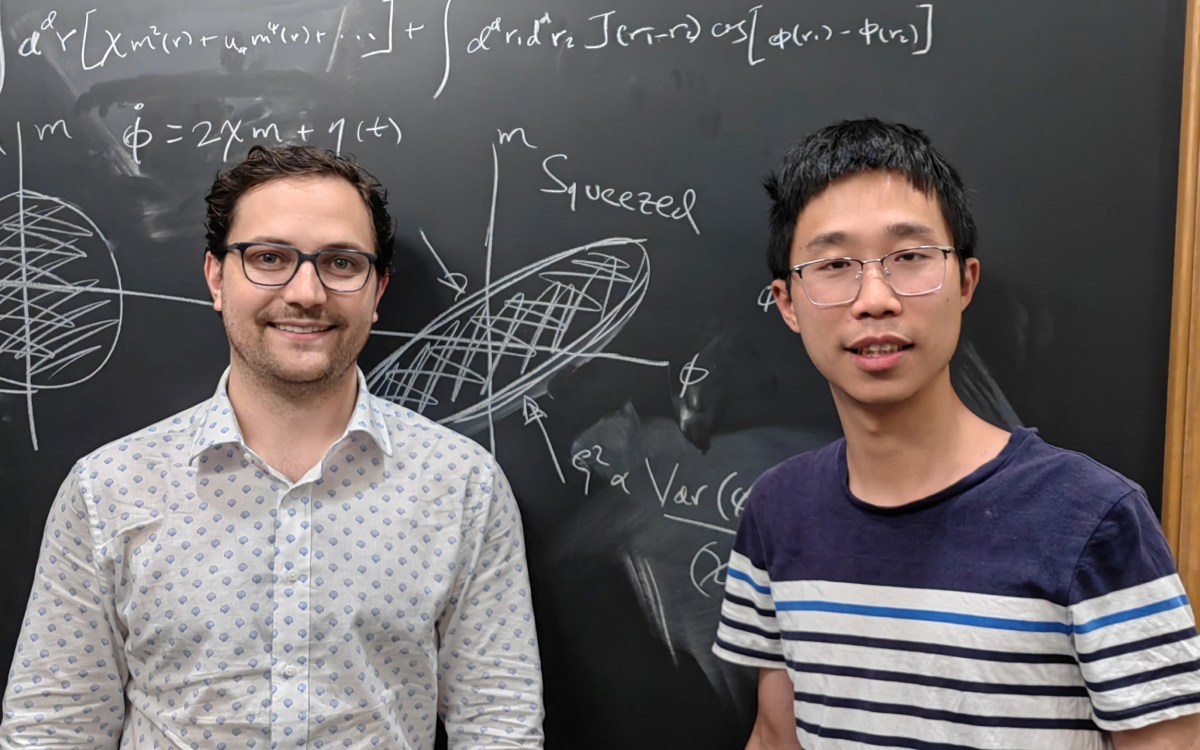
Spin squeezing for all
Should kids play wordle.
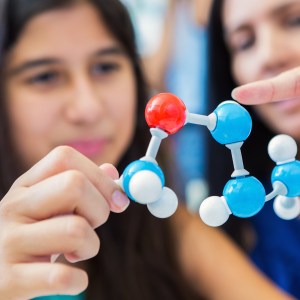
How moms may be affecting STEM gender gap
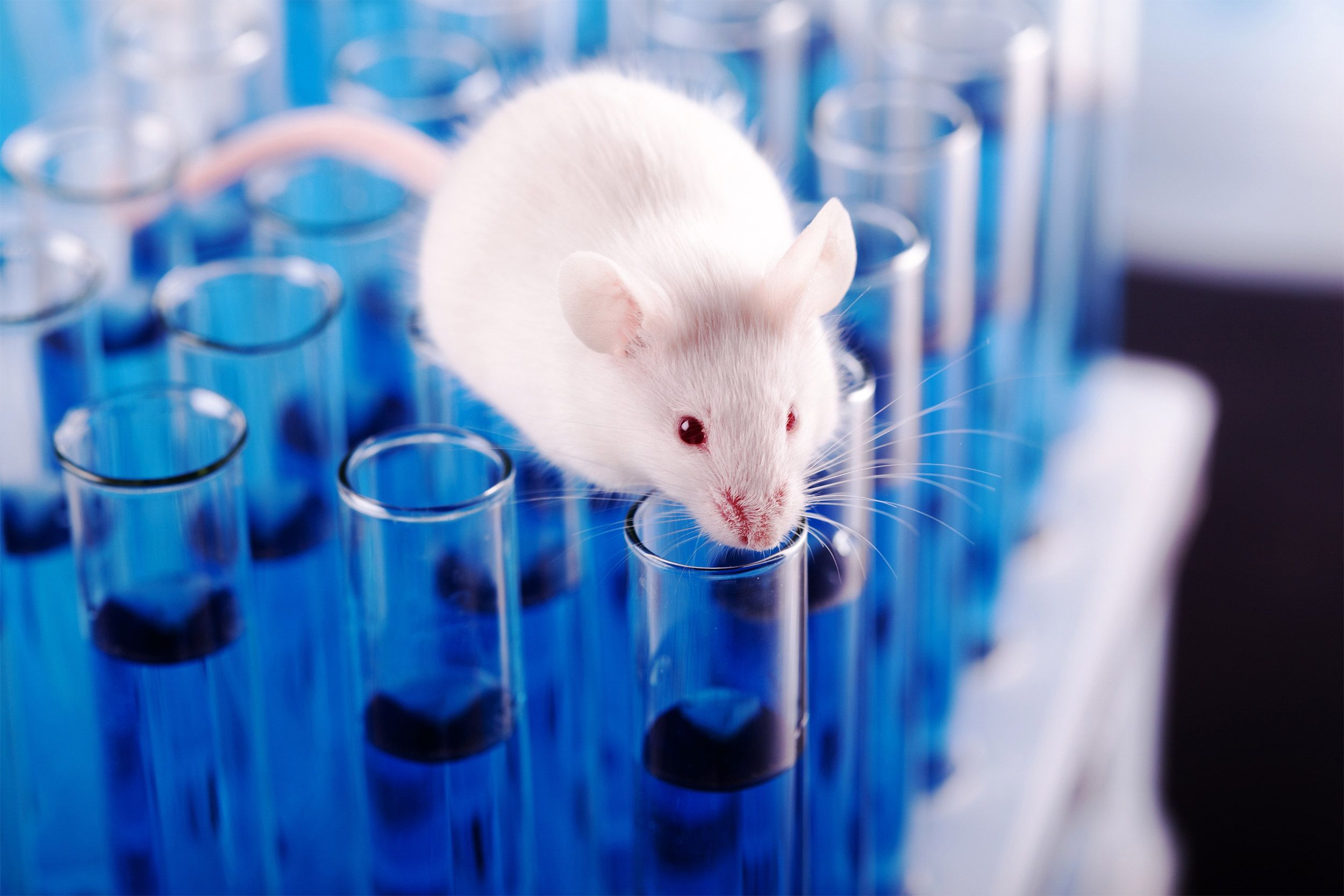
Why we need female mice in neuroscience research
Catherine Caruso
HMS Communications
Findings reveal that despite hormonal fluctuations, female mice exhibit more stable exploratory behavior than their male peers
Mice have long been a central part of neuroscience research, providing a flexible model that scientists can control and study to learn more about the intricate inner workings of the brain. Historically, researchers have favored male mice over female mice in experiments, in part due to concern that the hormone cycle in females causes behavioral variation that could throw off results.
But new research from Harvard Medical School challenges this notion and suggests that for many experiments, the concern may not be justified.
The study results, published March 7 in Current Biology , reveal that female mice, despite ongoing hormonal fluctuations, exhibit exploratory behavior that is more stable than that of their male peers.
Using a strain of mice commonly studied in lab settings, the researchers analyzed how the animals behaved as they freely explored an open space. They found that the hormone cycle had a negligible effect on behavior and that differences in behavior between individual female mice were much greater. Moreover, differences in behavior were even greater for males than for females, both within and between mice.
The results underscore the importance of incorporating both sexes into mouse studies, the research team said.
“I think this is really powerful evidence that if you’re studying naturalistic, spontaneous exploratory behavior, you should include both sexes in your experiments — and it leads to the argument that in this setting, if you can only pick one sex to work on, you should actually be working on females,” said Sandeep Robert Datta , professor of neurobiology in the Blavatnik Institute at HMS, who co-led the study with Rebecca Shansky of Northeastern University.
From rodents to humans: A history of bias
As neuroscientists strive to better understand the human brain, they routinely turn to the mouse, which Datta considers “the flagship vertebrate model for understanding how the brain works.”
This is because mouse and human brains share a considerable amount of structural organization and genetic information, so scientists can easily manipulate the mouse genome to address specific experimental questions and to build models of human diseases.
“Much of what we understand about the relationship between genes and neural circuits, and between neural activity and behavior, comes from basic research in the mouse, and mouse models are likely going to be really central tools in our fight against a broad array of neurological and psychological diseases,” Datta said.
“I think this is really powerful evidence that if you’re studying naturalistic, spontaneous exploratory behavior, you should include both sexes in your experiments — and it leads to the argument that in this setting, if you can only pick one sex to work on, you should actually be working on females.” Sandeep Robert Datta, professor of neurobiology in the Blavatnik Institute
For more than 50 years, researchers have preferentially used male mice in experiments, and nowhere has this practice been more prominent than in neuroscience. In fact, a 2011 analysis found that there were over five times as many single-sex neuroscience studies of male mice than of female mice. Over time, this practice has resulted in a poorer understanding of the female brain, likely contributing to the misdiagnosis of mental and neurological conditions in women, as well as the development of drugs that have more side effects for women — issues outlined by Shansky in a 2021 perspective in Nature Neuroscience .
The disparity in sex representation common in animal research has also been historically mirrored in research involving human subjects.
“This bias starts in basic science, but the repercussions are rolled into drug development, and lead to bias in drugs being produced, and how drugs are suited for the different sexes,” said lead author Dana Levy , a research fellow in neurobiology at HMS. For example, Levy noted that conditions such as anxiety, depression, and pain are known to manifest differently in female mice and women than in the male mice that are more often used in early-stage drug testing.
To address the problem of sex bias in scientific research, the National Institutes of Health published a policy in 2016 requiring researchers to include male and female subjects and samples in experiments. However, follow-up studies that look across scientific disciplines and examine neuroscience specifically indicate that progress has been slow.
The reasons for such a long-standing bias in neuroscience are complicated, Datta said: “Part of it is just plain old sexism, and part of it is conservatism in the sense that people have studied male mice for so long that they don’t want to make a change.”
Yet perhaps the biggest reason for excluding female mice, Datta said, stems from a widespread assumption that their behavior is broadly affected by cyclic variations in hormones such as estrogen and progesterone — the rodent version of a menstrual cycle, known as the estrous cycle. According to Datta and Levy, estrous status is known to have a strong effect on certain social and sexual behaviors in mice. However, data on the influence of estrous status in other behavioral contexts have been mixed, resulting in what Datta calls “a genuine disagreement in the literature.”
“We wanted to measure how much the estrous cycle seemed to influence basic patterns of exploration,” Datta said. “Our question was whether these ongoing changes in the hormonal state of the mouse affect other neural circuits in a way that’s confusing for researchers.”
“We assumed, like everybody else, that adding females was just going to complicate our experiments,” Levy added, “And so we said, ‘why not test this.’”
Testing assumptions
The researchers studied genetically identical males and females from a common strain of lab mouse in a circular open field — a standard lab setup for behavioral neuroscience experiments. In practice, the test involved placing a mouse in a 5-gallon Home Depot bucket for 20 minutes and using a camera to record the mouse’s movements and behaviors in 3D as it freely explored the space. The researchers swabbed each female mouse to determine its estrous status and repeated the bucket test with the same individual multiple times.
“This is a very interesting example of how assumptions that affect the way that we conduct and design our science are sometimes just assumptions — and it is important to directly test them, because sometimes they’re not true.” Dana Levy, a research fellow at Harvard Medical School
The team analyzed the videos with MoSeq, an artificial intelligence technology previously developed by the lab. The technology uses machine learning algorithms to break down a mouse’s movements into around 50 different “syllables,” or components of body language: short, single motions such as rearing up, pausing, stepping, or turning. With MoSeq, the researchers gathered in-depth, high-resolution data about the structure and pattern of mouse behavior during each session.
The researchers found that estrous status had very little effect on exploratory behavior in female mice. Instead, patterns of behavior tended to vary much more across female mice than they did throughout the estrous cycle.
“If you give me any random video from our pile, I can tell you which mouse it is. That’s how individualized the pattern of behavior is,” Datta said, which suggests that in behavioral studies, “a dominant aspect of variation in the data is the fact that individuals have subtly different life histories.”
When the researchers compared female and male mice, they found something that surprised them: Males also exhibited individuality of behavior, but they had more behavioral variation within a single mouse and between mice than females.
“People have been making this assumption that we can use male mice to reliably make comparisons within and across experiments, but our data suggest that female mice are more stable in terms of behavior despite the fact that they have the estrous cycle,” Datta said.
A case for change
Scientists generally agree that including female mice is important from a fairness perspective, Datta noted, yet some have remained concerned that it could complicate their research. For him, the new findings make a strong scientific case for using female mice in experiments.
“The fact that female behavior is more reliable suggests that including females might actually decrease the overall variability in your data under many circumstances,” Datta said.
Based on their findings, researchers in the Datta lab have already switched from male mice to mixed groups or female mice in their other experiments that involve circular, open-field testing.
Datta cautioned that the study looks at only one mouse strain in one lab setup, and so the results cannot be generalized to other strains and setups without further testing. However, he noted that the strain and setup are commonly used in neuroscience research, including in early-stage drug development to test how a potential drug affects mouse locomotion.
Datta said that the findings “should encourage folks who are interested in drug development in this context to include both sexes in their analysis.”
Now, Datta and Levy are interested in exploring how internal states beyond hormonal status, such as hunger, thirst, pain, and illness, affect exploratory behavior in mice.
“The question is, who wins in this tug-of-war between your current internal state and your individual identity,” Levy explained.
They also want to delve deeper into the neural basis of the individuality of mouse behavior that they saw in the study.
“I was shocked by how much stable variation between individuals we were observing — it’s like these mice really are individuals,” Datta said. “We’re used to thinking of lab mice as interchangeable widgets, but they’re not at all. So, what is controlling these individualized patterns of behavior?”
“We want to understand the mechanisms of individuality: how variability between individuals comes about, how it affects behavior, what can alter it, and what brain regions support it,” Levy added.
To this end, the Datta lab is examining mouse behavior from birth until death to understand how individualized patterns of behavior emerge and crystallize during development, and how they change throughout life.
The researchers also hope that their work will open the door for more rigorous, quantitative research on whether and how the estrous cycle affects mouse behavior in other contexts, such as completing complex tasks.
“This is a very interesting example of how assumptions that affect the way that we conduct and design our science are sometimes just assumptions — and it is important to directly test them, because sometimes they’re not true,” Levy said.
Additional authors include Nigel Hunter, Sherry Lin, Emma Robinson, Winthrop Gillis, Eli Conlin, and Rockwell Anyoha of HMS.
Datta is on the scientific advisory boards of Neumora, Inc., and Gilgamesh Pharmaceuticals, which have licensed the MoSeq technology.
The research was supported by the NIH (U19NS113201; RF1AG073625; R01NS114020), the Brain Research Foundation, the Simons Collaboration on the Global Brain, the Simons Collaboration for Plasticity in the Aging Brain, the Human Frontier Science Program, and the Zuckerman STEM Leadership Program.
Share this article
You might like.
Physicists ease path to entanglement for quantum sensing
Early childhood development expert has news for parents who think the popular online game will turn their children into super readers
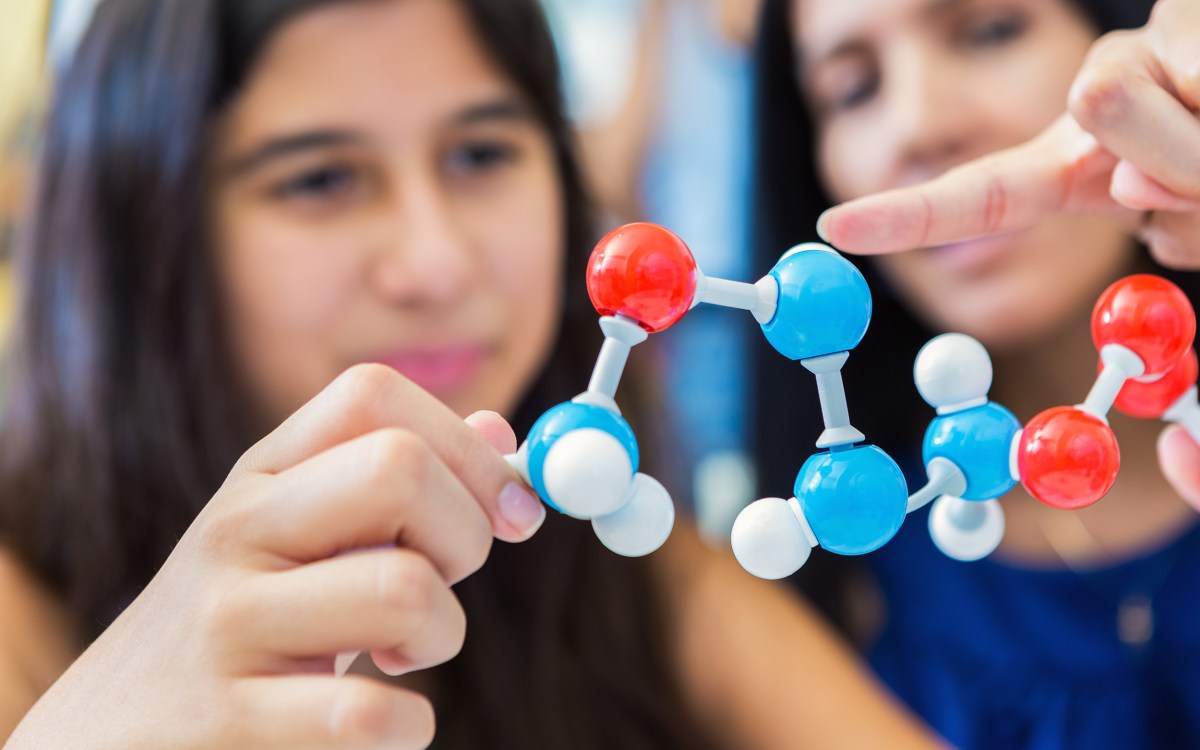
Research suggests encouragement toward humanities appears to be very influential for daughters
Good genes are nice, but joy is better
Harvard study, almost 80 years old, has proved that embracing community helps us live longer, and be happier
Examining new weight-loss drugs, pediatric bariatric patients
Researcher says study found variation in practices, discusses safety concerns overall for younger users
Shingles may increase risk of cognitive decline
Availability of vaccine offers opportunity to reduce burden of shingles and possible dementia

Why Do Medical Researchers Use Mice?
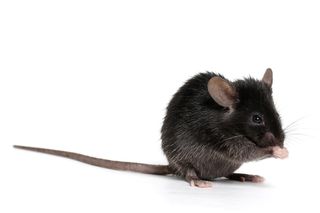
From formulating new cancer drugs to testing dietary supplements, mice and rats play a critical role in developing new medical wonders. In fact, 95 percent of all lab animals are mice and rats, according to the Foundation for Biomedical Research (FBR).
Scientists and researchers rely on mice and rats for several reasons. One is convenience: rodents are small, easily housed and maintained, and adapt well to new surroundings. They also reproduce quickly and have a short lifespan of two to three years, so several generations of mice can be observed in a relatively short period of time.
Mice and rats are also relatively inexpensive and can be bought in large quantities from commercial producers that breed rodents specifically for research. The rodents are also generally mild-tempered and docile, making them easy for researchers to handle, although some types of mice and rats can be more difficult to restrain than others . [ Why Do Mice Poop So Much? ]
Most of the mice and rats used in medical trials are inbred so that, other than sex differences, they are almost identical genetically. This helps make the results of medical trials more uniform, according to the National Human Genome Research Institute. As a minimum requirement, mice used in experiments must be of the same purebred species.
Another reason rodents are used as models in medical testing is that their genetic, biological and behavior characteristics closely resemble those of humans, and many symptoms of human conditions can be replicated in mice and rats. "Rats and mice are mammals that share many processes with humans and are appropriate for use to answer many research questions," said Jenny Haliski, a representative for the National Institutes of Health (NIH) Office of Laboratory Animal Welfare.
Over the last two decades, those similarities have become even stronger. Scientists can now breed genetically-altered mice called "transgenic mice" that carry genes that are similar to those that cause human diseases. Likewise, select genes can be turned off or made inactive, creating "knockout mice," which can be used to evaluate the effects of cancer-causing chemicals (carcinogens) and assess drug safety, according to the FBR.
Rodents also make efficient research animals because their anatomy, physiology and genetics are well-understood by researchers, making it easier to tell what changes in the mice's behaviors or characteristics are caused by.
Sign up for the Live Science daily newsletter now
Get the world’s most fascinating discoveries delivered straight to your inbox.
Some rodents, called SCID (severe combined immune deficiency) mice, are naturally born without immune systems and can therefore serve as models for normal and malignant human tissue research , according to the FBR.
Some examples of human disorders and diseases for which mice and rats are used as models include:
- Hypertension
- Respiratory problems
- Parkinson's disease
- Alzheimer's disease
- Cystic fibrosis
- HIV and AIDs
- Heart disease
- Muscular dystrophy
- Spinal cord injuries
— Why is the medical symbol a snake on a stick?
— Why Do Doctors Wear Green Or Blue Scrubs?
— Is the Placebo Effect Real?
Mice are also used in behavioral, sensory, aging, nutrition and genetic studies, as well as testing anti-craving medication that could potentially end drug addiction .
"Using animals in research is critical to scientific understanding of biomedical systems leading to useful drugs, therapies and cures," Haliski told Life's Little Mysteries.
Originally published on Live Science .
Why do cats hate closed doors?
Silky anteater: The tiny, boxing ball of fur
Antibiotic resistance makes once-lifesaving drugs useless. Could we reverse it?
Most Popular
- 2 Salps: The world's fastest-growing animals that look like buckets of snot
- 3 Supermoon Blue Moon 2024: Top photos from around the world
- 4 Why do cats hate closed doors?
- 5 'Doomsday glacier' won't collapse the way we thought, new study suggests

An official website of the United States government
Here's how you know
The .gov means it’s official. Federal government websites often end in .gov or .mil. Before sharing sensitive information, make sure you’re on a federal government site.
The site is secure. The https:// ensures that you are connecting to the official website and that any information you provide is encrypted and transmitted securely.
Cellular therapy targeting senescent cells may improve health in mice
Aging Biology Biomarkers Chronic Conditions Geroscience
A new cell therapy targeting senescent cells may improve metabolic and physical function in mice, according to an NIA-funded study. Senescent cells , which are cells that have stopped dividing but do not die off when they should, have been linked to many aspects of aging and disease. In the study, published in Nature Aging , senescent cells were safely and effectively removed from the tissues of mice, leading to better health.

Every day, damaged cells die and are replaced by new, healthy cells. As we age, the body may not remove damaged cells as efficiently as it used to, and although these cells stop dividing, they do not die. These damaged cells that linger — senescent cells — contribute to inflammation and age-related decline, such as metabolic dysfunction and decreased physical fitness. Drugs called senolytics eliminate senescent cells but require continuous treatment.
A new method of eliminating senescent cells involves modifying T cells, a type of immune cell, to express chimeric antigen receptors (CARs) on their surface. These CAR T cells are engineered to recognize and eliminate cells that express a specific protein found on many senescent cells. A team led by researchers from Cold Spring Harbor Laboratory, Memorial Sloan Kettering Cancer Center, and other institutions developed CAR T cells that recognize a protein found on many senescent cells.
The researchers tested the senolytic CAR T cell therapy in mice and found that it could eliminate senescent cells. The treatment improved metabolic function — for example, glucose tolerance — and exercise capacity in older mice. Next, they treated young mice given a high-fat diet to induce premature aging. These mice experienced similar improvements to their metabolic function. Additionally, the scientists gave young, healthy mice a single treatment of CAR T cells. The treatment prevented metabolic decline for more than a year; a substantial period, as their average lifespan is two years.
These findings suggest therapeutic potential for targeting senescent cells with CAR T cell therapy in humans. Future studies may explore the broader application of this targeted cellular therapy in addressing additional age-related diseases and potentially extending longevity, building on the outcomes observed in mouse studies.
This research was supported in part by NIA grants R01AG082800, R01AG065396, and U01AG077925.
Reference: Amor C, et al. Prophylactic and long-lasting efficacy of senolytic CAR T cells against age-related metabolic dysfunction . Nature Aging . 2024;4(3):336-349. doi: 10.1038/s43587-023-00560-5.
nia.nih.gov
An official website of the National Institutes of Health
- Skip to main content
- Keyboard shortcuts for audio player

Your Health
- Treatments & Tests
- Health Inc.
- Public Health
Drugs That Work In Mice Often Fail When Tried In People
Richard Harris

Most potential new drugs fail when they're tested in people. These failures are not only a major disappointment, they sharply drive up the cost of developing new drugs.
A major reason for these failures is that most new drugs are first tested out in mice, rats or other animals. Often those animal studies show great promise.
But mice aren't simply furry little people, so these studies often lead science astray. Some scientists are now rethinking animal studies to make them more effective for human health.
When scientists first started using animals in research over a century ago, the animals were not regarded as human stand-ins. Scientists studying rats were initially trying to understand rats, says Todd Preuss , an anthropologist at the Yerkes National Primate Research Center at Emory University.
"As this process went on, people stopped seeing them as specialized animals and started seeing them more and more as prototypical mammals," Preuss says.
But is a rat really a generic mammal? Preuss says emphatically no. But that's how rodents were pitched when they became products sold to scientists.
"It wasn't strictly a financial interest," he says. The sellers "really believed that you could do almost anything" with these animals. "You could learn about almost any feature of human organization, you could cure almost any disease by studying these animals."
That was a dangerous assumption. Rats and humans have been on their own evolutionary paths for tens of millions of years. We've developed our own unique features, and so have the rodents.
So it should come as no surprise that a drug that works in a mouse often doesn't work in a person. Even so, Preuss says there's tremendous momentum to keep using animals as human substitutes. Entire scientific communities are built up around rats, mice and other lab animals.
"Once these communities exist, then you have an infrastructure of knowledge: how to raise the animals, how to keep them healthy," Preuss says. "You have companies that spring up to provide you with specialized equipment to study these animals."

The rat holding facility at Hazelton Laboratories in Washington, D.C., in 1967. Fox Photos/Getty Images hide caption
The rat holding facility at Hazelton Laboratories in Washington, D.C., in 1967.
Chances are, people studying the same disease study the same tailor-made strain of animal. Journals and funding agencies actually expect it.
"So there's a whole institution that develops," Preuss says.
And it's hard to interrupt that culture. (Preuss spoke about this subject in a 2016 talk at the National Institutes of Health.)
You can get a glimpse of the scale of this enterprise by passing through one of hundreds of facilities nationwide devoted to the care and feeding of mice. On the Stanford University campus, attendants roll supply carts through fluorescent-lit hallways and past row after row of doors at an expansive mouse facility.
I'm guided through the labyrinth by Joseph Garner , a behavioral scientist at the Stanford University Medical Center. We go into a windowless room stacked floor to ceiling with seemingly identical plastic cages full of mice.
The philosophy behind mouse research has been to make everything as uniform as possible, so results from one facility would be the same as the identical experiment elsewhere.
But despite extensive efforts to be consistent, this setup hides a huge amount of variation. Bedding may differ from one facility to the next. So might the diet. Mice respond strongly to individual human handlers. Mice also react differently depending on whether their cage is up near the fluorescent lights or hidden down in the shadows.
Garner and colleagues tried to run identical experiments in six different mouse facilities, scattered throughout research centers in Europe. Even using genetically identical mice of the same age, the results varied all over the map.
Garner says scientists shouldn't even be trying to do experiments this way.
"Imagine you were doing a human drug trial and you said to the FDA, 'OK, I'm going to do this trial in 43-year-old white males in one small town in California,'" Garner says — a town where everyone lives in identical ranch homes, with the same monotonous diets and the same thermostat set to the same temperature.
"Which is too cold, and they can't change it," he goes on. "And oh, they all have the same grandfather!"
The FDA would laugh that off as an insane setup, Garner says.
"But that's exactly what we do in animals. We try to control everything we can possibly think of, and as a result we learn absolutely nothing."
Garner argues that research based on mice would be more reliable if it were set up more like experiments in humans — recognizing that variation is inevitable, and designing to embrace it rather than ignore it. He and his colleagues have recently published a manifesto , urging colleagues in the field to look at animals in this new light.
"Maybe we need to stop thinking of animals as these little furry test tubes that can be or even should be controlled," he says. "And maybe instead we should think of them as patients."
That could solve some of the problems with animal research, but by no means all.
Scientists make far too many assumptions about the underlying biology of disease when creating animal models of those illnesses, says Gregory Petsko , who studies Alzheimer's disease and other neurological disorders at the Weill Cornell Medical School.
"It's probably only when you get to try your treatments in people that you're really going to have any idea how right those assumptions were," Petsko says.
In his field, the assumptions are often poor, or downright misleading. And Petsko says this mindset has been counterproductive. Scientists in his field have "been led astray for many years by relying so heavily on animal models," he says.
For many years that was simply the best that science could do, Petsko says. So he doesn't fault his colleagues for trying.
"What I am saying is at some point you have to cut your losses. You have to say, 'OK, this took us as far as it could take us, quite some time ago.'"
For neurological diseases, Petsko says, scientists might learn more from studying human cells than whole animals. Animals are still useful for studying the safety of potential new treatments, but beyond that, he says, don't count on them.
Preuss at Emory agrees that using animals as models of disease is a big reason that many results in biomedical research aren't readily reproducible. "I think that we have means to resolve that, though."
How? "You have to think outside of the model box," he says. Mice and rats aren't simplified humans. Scientists should stop thinking they are.
But Preuss says scientists can still learn a lot about biology and disease by studying animals — for example, by comparing how humans and other animals differ, or where they share common traits. Those can reveal a lot about biology without assuming that what's true in a rat is likely true in a human.
"Scientists need to break out of a culture that is hampering progress," Preuss says. That's tough to do right now, in a world where science funding is on the chopping block. Many scientists are reluctant to take a risk that could backfire. But the upside could benefit us all, in the form of a better understanding of disease, and effective new drugs.
Richard Harris did some of the reporting for this story while researching his book Rigor Mortis: How Sloppy Science Creates Worthless Cures, Crushes Hope, and Wastes Billions. You can contact him at [email protected] .
- animal research
- Drug development
- medical research
- biomedical research
- U.S. Department of Health & Human Services

- Virtual Tour
- Staff Directory
- En Español
You are here
Nih research matters.
August 27, 2024
Keto diet enhances experimental cancer therapy in mice
At a glance.
- Researchers showed how a ketogenic diet can enhance the effects of an experimental anti-cancer drug and starve pancreatic tumors in mice.
- The findings suggest that diet might be paired with drugs to block the growth of certain types of cancerous tumors.

Cancer cells need fuel to survive and thrive. The energy they need usually comes from glucose in the blood. Some studies have shown that intermittent fasting or a ketogenic diet—high in fat and low in carbohydrates—can help to protect against cancer. These cause the body to break down fat to form molecules called ketones, which can serve as the body’s main energy source while glucose is scarce. Fasting and ketogenic diets likely work by limiting the amount of glucose available to feed cancer cells. But some cancers, such as pancreatic cancer, can also use ketones as an energy source.
A research team led by Dr. Davide Ruggero of the University of California, San Francisco, set out to better understand the underlying gene activities and metabolic pathways affected by diet and fasting. They hope to use this knowledge to enhance cancer therapies. The team focused on a protein called eIF4E (eukaryotic translation initiation factor), which is often hijacked by cancer cells. Results appeared in Nature on August 14, 2024.
The researchers found that chemical tags called phosphates are added to eIF4E as mice transition from fed to fasting. Further analyses showed that this phosphorylated eIF4E (P-eIF4E) plays an important role in coordinating the activity of genes involved in processing fats for energy during fasting. When mice were placed on a ketogenic diet instead of fasting, the P-eIF4E protein similarly triggered a shift to using fat for energy.
The scientists next asked how fasting activated eIF4E. They found that free fatty acids, the small molecules released by fat shortly after fasting begins, activated a chain of events leading to eIF4E phosphorylation. This suggests that free fatty acids have a dual role, serving both as an energy source and as signaling molecules that boost fat-based energy production during fasting.
To assess the relevance of these findings to cancers that can thrive on fat, the researchers combined a ketogenic diet with an experimental anti-cancer drug that blocks P-eIF4E. The drug is called eFT508 (or tomivosertib). They found that giving eFT508 alone did not slow the growth of pancreatic tumors in mice, likely because the tumors could survive with energy from carbohydrates. But when mice were given the drug while on a ketogenic diet, the cancer cells no longer had ready access to glucose or fat for energy. The cells then starved, and growth declined.
"Our findings open a point of vulnerability that we can treat with a clinical inhibitor that we already know is safe in humans,” Ruggero says. “We now have firm evidence of one way in which diet might be used alongside pre-existing cancer therapies to precisely eliminate a cancer.”
—by Vicki Contie
Related Links
- Research in Context: Obesity and Metabolic Health
- An mRNA Vaccine To Treat Pancreatic Cancer
- Keto Molecule Offers Clue for Preventing Colorectal Cancer
- Low-Fat Diet Compared to Low-Carb Diet
- Nutrition in Cancer Care
- Pancreatic Cancer
References: Remodelling of the translatome controls diet and its impact on tumorigenesis. Yang H, Zingaro VA, Lincoff J, Tom H, Oikawa S, Oses-Prieto JA, Edmondson Q, Seiple I, Shah H, Kajimura S, Burlingame AL, Grabe M, Ruggero D. Nature . 2024 Aug 14. doi: 10.1038/s41586-024-07781-7. Online ahead of print. PMID: 39143206.
Funding: NIH’s National Institute of Diabetes and Digestive and Kidney Diseases (NIDDK), National Cancer Institute (NCI), and National Institute of General Medical Sciences (NIGMS); American Heart Association; American Cancer Society; Howard Hughes Medical Institute.
Connect with Us
- More Social Media from NIH
Suggestions or feedback?
MIT News | Massachusetts Institute of Technology
- Machine learning
- Sustainability
- Black holes
- Classes and programs

Departments
- Aeronautics and Astronautics
- Brain and Cognitive Sciences
- Architecture
- Political Science
- Mechanical Engineering
Centers, Labs, & Programs
- Abdul Latif Jameel Poverty Action Lab (J-PAL)
- Picower Institute for Learning and Memory
- Lincoln Laboratory
- School of Architecture + Planning
- School of Engineering
- School of Humanities, Arts, and Social Sciences
- Sloan School of Management
- School of Science
- MIT Schwarzman College of Computing
Study reveals the benefits and downside of fasting
Press contact :, media download.
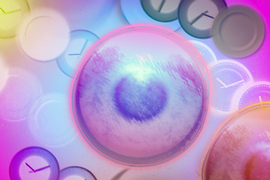
*Terms of Use:
Images for download on the MIT News office website are made available to non-commercial entities, press and the general public under a Creative Commons Attribution Non-Commercial No Derivatives license . You may not alter the images provided, other than to crop them to size. A credit line must be used when reproducing images; if one is not provided below, credit the images to "MIT."

Previous image Next image
Low-calorie diets and intermittent fasting have been shown to have numerous health benefits: They can delay the onset of some age-related diseases and lengthen lifespan, not only in humans but many other organisms.
Many complex mechanisms underlie this phenomenon. Previous work from MIT has shown that one way fasting exerts its beneficial effects is by boosting the regenerative abilities of intestinal stem cells, which helps the intestine recover from injuries or inflammation.
In a study of mice, MIT researchers have now identified the pathway that enables this enhanced regeneration, which is activated once the mice begin “refeeding” after the fast. They also found a downside to this regeneration: When cancerous mutations occurred during the regenerative period, the mice were more likely to develop early-stage intestinal tumors.
“Having more stem cell activity is good for regeneration, but too much of a good thing over time can have less favorable consequences,” says Omer Yilmaz, an MIT associate professor of biology, a member of MIT’s Koch Institute for Integrative Cancer Research, and the senior author of the new study.
Yilmaz adds that further studies are needed before forming any conclusion as to whether fasting has a similar effect in humans.
“We still have a lot to learn, but it is interesting that being in either the state of fasting or refeeding when exposure to mutagen occurs can have a profound impact on the likelihood of developing a cancer in these well-defined mouse models,” he says.
MIT postdocs Shinya Imada and Saleh Khawaled are the lead authors of the paper, which appears today in Nature .
Driving regeneration
For several years, Yilmaz’s lab has been investigating how fasting and low-calorie diets affect intestinal health. In a 2018 study , his team reported that during a fast, intestinal stem cells begin to use lipids as an energy source, instead of carbohydrates. They also showed that fasting led to a significant boost in stem cells’ regenerative ability.
However, unanswered questions remained: How does fasting trigger this boost in regenerative ability, and when does the regeneration begin?
“Since that paper, we’ve really been focused on understanding what is it about fasting that drives regeneration,” Yilmaz says. “Is it fasting itself that’s driving regeneration, or eating after the fast?”
In their new study, the researchers found that stem cell regeneration is suppressed during fasting but then surges during the refeeding period. The researchers followed three groups of mice — one that fasted for 24 hours, another one that fasted for 24 hours and then was allowed to eat whatever they wanted during a 24-hour refeeding period, and a control group that ate whatever they wanted throughout the experiment.
The researchers analyzed intestinal stem cells’ ability to proliferate at different time points and found that the stem cells showed the highest levels of proliferation at the end of the 24-hour refeeding period. These cells were also more proliferative than intestinal stem cells from mice that had not fasted at all.
“We think that fasting and refeeding represent two distinct states,” Imada says. “In the fasted state, the ability of cells to use lipids and fatty acids as an energy source enables them to survive when nutrients are low. And then it’s the postfast refeeding state that really drives the regeneration. When nutrients become available, these stem cells and progenitor cells activate programs that enable them to build cellular mass and repopulate the intestinal lining.”
Further studies revealed that these cells activate a cellular signaling pathway known as mTOR, which is involved in cell growth and metabolism. One of mTOR’s roles is to regulate the translation of messenger RNA into protein, so when it’s activated, cells produce more protein. This protein synthesis is essential for stem cells to proliferate.
The researchers showed that mTOR activation in these stem cells also led to production of large quantities of polyamines — small molecules that help cells to grow and divide.
“In the refed state, you’ve got more proliferation, and you need to build cellular mass. That requires more protein, to build new cells, and those stem cells go on to build more differentiated cells or specialized intestinal cell types that line the intestine,” Khawaled says.
Too much of a good thing
The researchers also found that when stem cells are in this highly regenerative state, they are more prone to become cancerous. Intestinal stem cells are among the most actively dividing cells in the body, as they help the lining of the intestine completely turn over every five to 10 days. Because they divide so frequently, these stem cells are the most common source of precancerous cells in the intestine.
In this study, the researchers discovered that if they turned on a cancer-causing gene in the mice during the refeeding stage, they were much more likely to develop precancerous polyps than if the gene was turned on during the fasting state. Cancer-linked mutations that occurred during the refeeding state were also much more likely to produce polyps than mutations that occurred in mice that did not undergo the cycle of fasting and refeeding.
“I want to emphasize that this was all done in mice, using very well-defined cancer mutations. In humans it’s going to be a much more complex state,” Yilmaz says. “But it does lead us to the following notion: Fasting is very healthy, but if you’re unlucky and you’re refeeding after a fasting, and you get exposed to a mutagen, like a charred steak or something, you might actually be increasing your chances of developing a lesion that can go on to give rise to cancer.”
Yilmaz also noted that the regenerative benefits of fasting could be significant for people who undergo radiation treatment, which can damage the intestinal lining, or other types of intestinal injury. His lab is now studying whether polyamine supplements could help to stimulate this kind of regeneration, without the need to fast.
“This fascinating study provides insights into the complex interplay between food consumption, stem cell biology, and cancer risk,” says Ophir Klein, a professor of medicine at the University of California at San Francisco and Cedars-Sinai Medical Center, who was not involved in the study. “Their work lays a foundation for testing polyamines as compounds that may augment intestinal repair after injuries, and it suggests that careful consideration is needed when planning diet-based strategies for regeneration to avoid increasing cancer risk.”
The research was funded, in part, by a Pew-Stewart Trust Scholar award, the Marble Center for Cancer Nanomedicine, the Koch Institute-Dana Farber/Harvard Cancer Center Bridge Project, and the MIT Stem Cell Initiative.
Share this news article on:
Press mentions, medical news today.
A new study led by researchers at MIT suggests that fasting and then refeeding stimulates cell regeneration in the intestines, reports Katharine Lang for Medical News Today . However, notes Lang, researchers also found that fasting “carries the risk of stimulating the formation of intestinal tumors.”
Prof. Ömer Yilmaz and his colleagues have discovered the potential health benefits and consequences of fasting, reports Max Kozlov for Nature . “There is so much emphasis on fasting and how long to be fasting that we’ve kind of overlooked this whole other side of the equation: what is going on in the refed state,” says Yilmaz.
MIT researchers have discovered how fasting impacts the regenerative abilities of intestinal stem cells, reports Ed Cara for Gizmodo . “The major finding of our current study is that refeeding after fasting is a distinct state from fasting itself,” explain Prof. Ömer Yilmaz and postdocs Shinya Imada and Saleh Khawaled. “Post-fasting refeeding augments the ability of intestinal stem cells to, for example, repair the intestine after injury.”
Previous item Next item
Related Links
- Omer Yilmaz
- Koch Institute
- Department of Biology
Related Topics
Related articles.
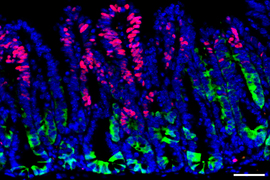
How early-stage cancer cells hide from the immune system
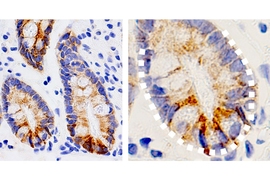
Study links certain metabolites to stem cell function in the intestine
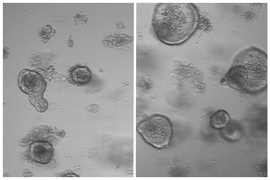
Fasting boosts stem cells’ regenerative capacity
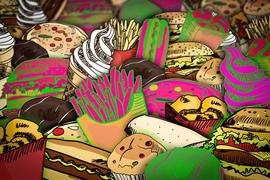
How diet influences colon cancer
More mit news.
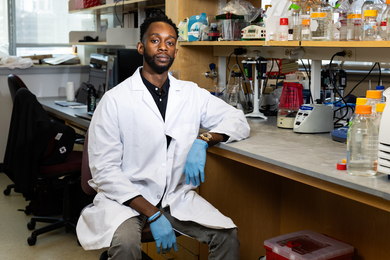
Designing better delivery for medical therapies
Read full story →
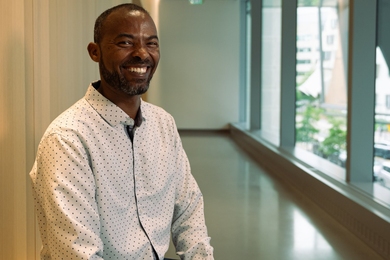
Making a measurable economic impact
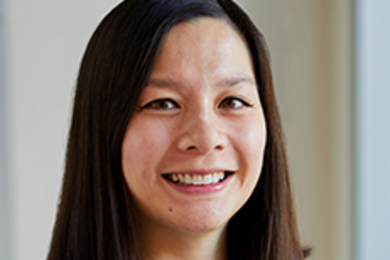
Faces of MIT: Jessica Tam
First AI + Education Summit is an international push for “AI fluency”

President Kornbluth welcomes the Class of 2028

Scientists find neurons that process language on different timescales
- More news on MIT News homepage →
Massachusetts Institute of Technology 77 Massachusetts Avenue, Cambridge, MA, USA
- Map (opens in new window)
- Events (opens in new window)
- People (opens in new window)
- Careers (opens in new window)
- Accessibility
- Social Media Hub
- MIT on Facebook
- MIT on YouTube
- MIT on Instagram
- Alzheimer's disease & dementia
- Arthritis & Rheumatism
- Attention deficit disorders
- Autism spectrum disorders
- Biomedical technology
- Diseases, Conditions, Syndromes
- Endocrinology & Metabolism
- Gastroenterology
- Gerontology & Geriatrics
- Health informatics
- Inflammatory disorders
- Medical economics
- Medical research
- Medications
- Neuroscience
- Obstetrics & gynaecology
- Oncology & Cancer
- Ophthalmology
- Overweight & Obesity
- Parkinson's & Movement disorders
- Psychology & Psychiatry
- Radiology & Imaging
- Sleep disorders
- Sports medicine & Kinesiology
- Vaccination
- Breast cancer
- Cardiovascular disease
- Chronic obstructive pulmonary disease
- Colon cancer
- Coronary artery disease
- Heart attack
- Heart disease
- High blood pressure
- Kidney disease
- Lung cancer
- Multiple sclerosis
- Myocardial infarction
- Ovarian cancer
- Post traumatic stress disorder
- Rheumatoid arthritis
- Schizophrenia
- Skin cancer
- Type 2 diabetes
- Full List »
share this!
August 27, 2024
This article has been reviewed according to Science X's editorial process and policies . Editors have highlighted the following attributes while ensuring the content's credibility:
fact-checked
Noncoding RNA Terc-53 and hyaluronan receptor Hmmr regulate aging in mice
by Higher Education Press

In a study appearing in Protein & Cell researchers investigated the physiological functions of Terc-53 by creating transgenic mice that overexpress this noncoding RNA. They observe that Terc-53 overexpression affects normal aging in mammals, contributing to cognitive decline and shortened lifespan.
The work is titled " Noncoding RNA Terc-53 and hyaluronan receptor Hmmr regulate aging in mice"
Mechanistically, they find that Terc-53 binds to and promotes the degradation of Hmmr, leading to enhanced inflammation in tissues and accelerated aging. They also note that Hmmr levels decrease with age in certain brain regions , similar to Terc-53's pattern, and that restoring Hmmr levels can improve cognitive abilities and reduce neuroinflammation markers.
Key findings from the study include:
- Terc-53's Role in Aging: Terc-53 overexpression in mice leads to age-related cognitive decline and a shorter lifespan, indicating its involvement in normal mammalian aging processes.
- Hmmr as Effector of Terc-53: Hmmr is identified as a target of Terc-53, with Terc-53 mediating its degradation. This degradation increases inflammation, contributing to accelerated aging.
- Restoration of Hmmr Improves Cognition: Supplementing Hmmr in the hippocampus of aging Terc-53 transgenic mice reverses cognitive decline, suggesting a potential therapeutic strategy for age-related cognitive issues.
- Tissue-Specific Aging Patterns of Hmmr: Hmmr's involvement in aging appears to be tissue-specific, with varying expression patterns across different organs.
The study highlights the complexity of aging in mammals and the significance of noncoding RNAs and proteins that emerged late in evolution. It demonstrates that Terc-53 regulates organismal aging through the stability of Hmmr and the modulation of neuroinflammation.
The findings open new avenues for understanding and potentially treating age-related physical disabilities and improving health span. By identifying Hmmr as a critical mediator of Terc-53's effects on aging, the research suggests that strategies aimed at stabilizing Hmmr could mitigate age-related cognitive decline and inflammation.
Explore further
Feedback to editors

Multipurpose vaccine shows new promise in the presence of pre-existing immunity
13 hours ago

Researchers develop affordable, rapid blood test for brain cancer

Discovery gives answers to parents of children with rare neurological gene mutation

Human-centered AI tool to improve sepsis management can identify missing information

AI-powered, big data research enhances understanding of systemic vasculitis

Team develops injectable heart stimulator for emergency situations
14 hours ago

Finding epilepsy hotspots before surgery: A faster, non-invasive approach
15 hours ago

Personalized brain stimulation significantly decreases depression symptoms in pilot study
16 hours ago

An ancient signaling pathway and 20 years of research offer hope for rare cancer

How mindset could affect the body's response to vaccination
Related stories.

Microtubule-associated protein governs chromosome segregation
Dec 13, 2021

Study identifies potential drug treatments for telomere diseases
Apr 22, 2020

Impact of exercise on aging: Highlighting muscle biomarkers
Aug 21, 2024

Geraniol attenuates oxidative stress and cognitive impairment in mouse aging model
Apr 10, 2024

Study finds loss of Menin helps drive the aging process, and dietary supplement can reverse it in mice
Mar 16, 2023

Chronic musculoskeletal pain may accelerate brain aging
Mar 26, 2024
Recommended for you

Scientist discover rare genotype causing early menopause
23 hours ago

Non-cognitive skills: DNA-based analyses suggest a hidden key to academic success
Aug 26, 2024

New insight into the protein mutations that cause Rett syndrome

New prognostic biomarker identified in small cell lung cancer

Looking for clues about your real age? Your grandparents' education may offer some insight
Let us know if there is a problem with our content.
Use this form if you have come across a typo, inaccuracy or would like to send an edit request for the content on this page. For general inquiries, please use our contact form . For general feedback, use the public comments section below (please adhere to guidelines ).
Please select the most appropriate category to facilitate processing of your request
Thank you for taking time to provide your feedback to the editors.
Your feedback is important to us. However, we do not guarantee individual replies due to the high volume of messages.
E-mail the story
Your email address is used only to let the recipient know who sent the email. Neither your address nor the recipient's address will be used for any other purpose. The information you enter will appear in your e-mail message and is not retained by Medical Xpress in any form.
Newsletter sign up
Get weekly and/or daily updates delivered to your inbox. You can unsubscribe at any time and we'll never share your details to third parties.
More information Privacy policy
Donate and enjoy an ad-free experience
We keep our content available to everyone. Consider supporting Science X's mission by getting a premium account.
E-mail newsletter

An official website of the United States government
The .gov means it’s official. Federal government websites often end in .gov or .mil. Before sharing sensitive information, make sure you’re on a federal government site.
The site is secure. The https:// ensures that you are connecting to the official website and that any information you provide is encrypted and transmitted securely.
- Publications
- Account settings
Preview improvements coming to the PMC website in October 2024. Learn More or Try it out now .
- Advanced Search
- Journal List
- Wiley - PMC COVID-19 Collection

An Overview of Typical Infections of Research Mice: Health Monitoring and Prevention of Infection
James r. fahey.
1 The Jackson Laboratory, Bar Harbor Maine
Haiyan Olekszak
There are many reasons to keep research mice healthy and free from infections. The two most important of these are to protect the health and welfare of research mice and to prevent infections from negatively impacting research. Just as the genetic integrity of a mouse strain will influence the reproducibility and validity of research data, so too will the microbiologic integrity of the animals. This has been repeatedly demonstrated in the literature of laboratory animal sciences wherein the direct impact of infections on physiologic parameters under study have been described. Therefore, it is of great importance that scientists pay close attention to the health status of their research animal colonies and maintain good communications with the animal facility personnel at their institution about mouse health issues. This overview provides information about animal health monitoring (HM) in research mouse colonies including commonly monitored agents, diagnostic methods, HM program, risk assessment, and animal facility biosecurity. Lastly, matters of communication with laboratory animal professionals at research institutions are also addressed. © 2015 by John Wiley & Sons, Inc.
Introduction
The quality of animals used in research has a direct impact on the value of that research. In the context of research mice, this means using animals that are genetically appropriate for the specific investigation and free of the variability induced by infections. From a genetic perspective research scientists address animal quality issues by testing their mice for consistency of genetic background, and in the case of genetically engineered mutant mice (GEM), for direct evidence that specific genes of interest are present (or absent in the case of knockouts). It is prudent to do this testing prior to embarking on an expensive research project (Fahey et al., 2013 ). Similarly, it is prudent to verify the health status of research mouse colonies to exclude mice compromised by infections. Even in the absence of clinical signs of disease, infections in mice are well known as a source of variability that can have adverse effects on research or may present a health hazard to laboratory staff (i.e., zoonotic infections of mice) and thus should be avoided when doing research.
Prevalence surveys of murine infectious agents taken at research institutions in North America (Carty, 2008 ), Europe (Mähler and Köhl, 2009 ), Japan (Hayashimoto et al., 2013 ), Australasia (McInnes et al., 2011 ), and Taiwan (Liang et al., 2009 ) over the past decade have demonstrated that infectious agents are still quite prevalent in research mouse colonies. The species and prevalence of the agents (viruses, bacteria, parasites and fungi) established in these surveys were dependent on the geographic areas and institutions under study. Nonetheless, these data point out that despite efforts taken at many research institutions worldwide to eliminate infectious agents and elevate the health status of their animal facilities, infectious agents of mice still persist. Moreover, novel infectious agents of mice are being discovered more frequently now as genome sequencing methods become faster and less expensive. Murine astrovirus (MuAstV) is such an example. The virus was first identified in 1985 by electron microscopy following an outbreak of diarrhea in a colony of nude mice and not further characterized until 2013 by use of viral metagenomics (Kjeldsberg and Hem, 1985 ; Ng et al., 2013 ). The availability of sophisticated molecular diagnostic techniques, the increased use of immunodeficient, transgenic, and humanized mice, and the increasing exchange of research animals and animal products among laboratories nationally and internationally guarantee that this trend of emerging/re‐emerging infectious agents will continue.
To circumvent problems generated by infections in research mice, mouse colonies should be regularly tested for infectious agents and measures taken to prevent, control and eliminate infections. A survey of animal health professionals at research institutions across the U.S. (Carty, 2008 ) reported that routine HM programs afford the optimal means of detecting disease outbreaks in animal facilities. The Federation of European Laboratory Science Associations (FELASA) regularly emphasizes this fact by meticulously outlining rodent HM guidelines for research animal facilities (Mähler et al., 2014 ). HM programs are generally based on long‐established standardized lists of target organisms (Tables (Tables1 1 and and2) 2 ) as well as recently discovered microorganisms. Yet, it is common for institutional laboratory animal veterinarians to tailor HM programs to meet their facilities’ specific needs in a cost‐effective manner, since the prevalence of murine pathogens varies by institution. Understanding HM programs is essential to the well‐being of one's animals as well as the preservation of data integrity. For this reason, it is important, especially for graduate students, postdoctoral fellows, new investigators and others with limited experience in dealing with infections in mouse colonies that they become familiar with the HM programs at their own institutional animal facilities. In addition to discussions with animal health professionals at your research institution, further resources on this subject are available at a number of laboratory animal associations (see Internet Resources below) and at professional mouse vendors to assist scientists in understanding the vagaries of health surveillance.
Viral Infectious Agents of Mice
Viral agents | Virion | Risk level /prevalence | Recommended tissues for PCR testing |
---|---|---|---|
Minute virus of mice | Non‐enveloped | B/low | Mesenteric lymph node, spleen, intestine |
Mouse parvovirus | Non‐enveloped | B/high | Mesenteric lymph node, spleen, intestine |
Ectromelia virus | Enveloped | A/low | Spleen, skin lesion, feces |
Murine adenovirus‐1 | Non‐enveloped | A/low | Lung |
Murine adenovirus‐2 | Non‐enveloped | B/low | Feces, intestine |
Murine cytomegalovirus | Enveloped | B/low | Salivary gland, spleen |
Mouse thymic virus | Enveloped | B/low | Salivary gland |
Mouse polyomavirus | Non‐enveloped | C/low | Mammary gland, skin |
Mouse hepatitis virus (polytropic) | Enveloped | A/high | Mesenteric lymph node, feces, lung |
Mouse hepatitis virus (enterotropic) | Enveloped | B/high | Mesenteric lymph node, feces |
Murine norovirus | Non‐enveloped | D/high | Feces, intestine |
Murine astrovirus | Non‐enveloped | D/high | Feces, intestine |
Theiler's murine encephalomyelitis virus | Non‐enveloped | B/low | Feces, intestine |
Lactate dehydrogenase‐elevating virus | Enveloped | B/low | Spleen |
Lymphocytic choriomeningitis virus | Enveloped | A/low | Kidney, urine, blood |
Sendai virus | Enveloped | A/low | Trachea, lung |
Hantavirus (ambisense ss RNA ±) | Enveloped | A/low | Trachea, lung |
Pneumonia virus of mice | Enveloped | C/low | Trachea, lung |
Mouse rotavirus (EDIM) | Non‐enveloped | B/high | Feces, intestine |
Reovirus | Non‐enveloped | C/low | Feces, liver, lung |
Common Bacterial, Mycoplasmal, Fungal, and Parasitic Infectious Agents of Mice
Agent | Sample | Methods of diagnosis |
---|---|---|
. | Respiratory swab, wash fluid | Microbiologic culture |
Cilia‐associated respiratory bacillus (CAR bacillus) | Serum/respiratory swab/respiratory wash fluid | MFI , ELISA , IFA , PCR |
Colon or cecum/feces | Microbiologic culture | |
Serum | MFI , ELISA , IFA | |
Skin scrape/swab, oropharyngeal swab | Microbiologic culture; PCR | |
Oropharyngeal swab | Microbiologic culture; PCR | |
. | Colon or cecum/feces | PCR |
Oropharyngeal swab/colon or cecum/feces | Microbiologic culture; PCR | |
Serum/oropharyngeal or nasal swab/lung wash | MFI ; PCR ; microbiologic culture | |
Oropharyngeal swab/colon or cecum/feces | Microbiologic culture; PCR | |
Oropharyngeal swab, colon or cecum/feces | Microbiologic culture; PCR | |
. | Colon or cecum/feces | Microbiologic culture; PCR |
. | Colon or cecum/feces | Microbiologic culture |
Oropharyngeal swab | Microbiologic culture; PCR | |
Oropharyngeal swab | Microbiologic culture | |
. | Oropharyngeal swab | Microbiologic culture; PCR |
Serum/urine/kidney/brain | MFI ; PCR | |
Lung | PCR | |
Cecum/colon | Microscopy; PCR | |
./flagellates | Cecum/colon | Microscopy; PCR |
Cecum/colon | Microscopy; PCR | |
Cecum/colon/anal tape sample | Microscopy; PCR | |
Intestine | Microscopy; PCR | |
Fur | Direct microscopy | |
Fur | Direct microscopy | |
Fur | Direct microscopy |
General Review of Mouse HM Programs
When performed regularly, animal HM provides animal facility veterinarians a continuous flow of test data enabling them to assess and adjust preventative medicine programs designed to reduce and eliminate pathogenic organisms. For example, in the 1960's and 1970's, early in the development of HM programs in animal facilities, murine pathogens were very prevalent and the goal of HM was primarily to identify the disease agents causing illness and deaths in mouse facilities. Today, many of these disease agents have been completely eliminated or severely reduced in mouse colonies and monitoring of mice is aimed at exclusion of unwanted infectious agents.
A HM program should contain the selection of infectious agents, diagnostic methods, preventive measures and response plans for dealing with biosecurity breaches and disease outbreaks. There are basic guidelines for establishment of standard HM programs (Mähler et al., 2014 ). However, designing such programs requires a risk assessment of the potential exposure of mice to infectious agents relevant to each particular institution and therefore must be adaptable and somewhat flexible. A variety of factors must be taken into consideration: the types of studies being conducted, caging systems, numbers and types of mouse strains being housed, local prevalence of specific infectious agents, animal care practices, health history, facility infrastructure, presence of non‐murine animal models, and available funding. Typically, as part of the HM planning process, the facility laboratory animal veterinarians engage in dialog with research investigators to acquire detailed information regarding researchers’ individual needs. As such, productive collaborations between research scientists and veterinarians are essential for developing and implementing a cost‐effective HM program.
With the rise of worldwide exchange of genetically engineered mutant mice (GEM) and biological materials, international harmonization of HM standards has been proposed along with promoting the 3Rs (i.e., replacement, reduction, and refinement) in animal use (Nicklas, 2008 ; National Centre for the Replacement, Refinement & Reduction of Animals in Research, 2014 ). Pharmaceutical companies and commercial vendors have made efforts toward such standards based on recommendations by FELASA and other international institutions. However, HM programs in research institutions and universities are more complex than commercial vendors, given the many different factors to be considered as mentioned above. Universal standards will thus not be applicable or feasible.
Infectious Agents of Research Mice
Viral infections of mice.
Table Table1 1 summarizes viruses that are commonly tested for in‐mouse HM programs. Viral infections of mice are the greatest concern to laboratory animal veterinarians and animal facility personnel because of their potential for spread across a facility, the diseases they can cause and their impact on research. There are seventeen viral species in Table Table1 1 many of which have substrains or additional serotypes that infect mice. However, the prevalence of these viruses varies greatly, with some no longer found in research animal facilities. Some viral infections, such as murine cytomegalovirus (MCMV), mouse thymic virus (MTV), murine polyomavirus (MPyV), ectromelia virus (ECTA), reovirus (REO) have declined significantly since the 1990's and have become rare or nonexistent in mouse colonies. Based on serological or polymerase chain reaction (PCR) data from the past fifteen years (Weisbroth, 1999 ; Prichett‐Corning et al., 2009 ; Mähler et al., 2014 ), the most prevalent viral agents in contemporary mouse facilities are mouse norovirus (MNV), mouse hepatitis virus (MHV), mouse parvovirus (MPV), and mouse rotavirus (MRV, known as epizootic diarrhea of infant mice, EDIM). Agents such as MNV and MPV are shed in mouse feces for as long as 2 months, even by immunocompetent mice. Additionally, small non‐enveloped viruses (i.e., MPV, MNV, MRV) are very resistant to chemical or environmental inactivation. In contrast, although MHV does not remain active for long periods in the environment, it is highly contagious and thus continues to be a major infectious agent in mouse facilities, especially in conventionally housed mice. It is likely that some agents may be prevalent simply because they are newly recognized (i.e, MNV, MuAstV; Kjeldsberg and Hem, 1985 ; Karst et al., 2003 ; Ng et al., 2013 ). A recently discovered murine virus, murine astrovirus (MuAstV; Ng et al., 2013 ) has not yet been the subject of widespread surveillance so the prevalence of this virus in research animal facilities is unknown at this time.
The significance of these viral infections to scientists is their potential impact on research, which varies depending on route of infection (i.e., natural versus experimental infection), host factors (i.e., mouse strain, age, immune competency), and virus factors (i.e., virulent versus avirulent strain; Fox et al., 2006 ; Percy and Barthold, 2007 ; Besselsen et al., 2008 ; Mähler et al., 2014 ). Laboratory animal veterinarians often classify murine viruses into risk groups according to the significance of interference with research, infectivity and potential for spread and difficulties with detection and elimination. In Table Table1, 1 , a simple classification scheme of A to D is based on these criteria. Viruses in groups A and B are more likely to adversely affect research and for group A, present the risk of human infection. Viruses in group C have minimal impact on research and are not common whereas those in group D are recently discovered viruses whose impact on research is not well known. For example, lymphocytic choriomeningitis virus (LCMV) and hantavirus are at risk level A because of their zoonotic potential, that is, their ability to infect humans as well as mice. A few other viral agents, including murine adenovirus‐1 (MAdV‐1), ECTA, MHV polytropic strains, and Sendai virus (SV), are also classified as risk level A because they are capable of causing clinical illness in both immunocompetent and immunodeficient adult mice. Viruses at risk level B may interfere with specific experiments involving the host immune system (parvovirus, MCMV, MTV, lactate dehydrogenase‐elevating virus [LDEV], MHV enterotropic strains), central nervous system (Theiler's murine encephalomyelitis virus, TMEV), or gastrointestinal systems (MAdV‐2, MRV).
Natural transmission of murine viruses occurs most commonly through the fecal‐oral route or fomites (e.g., MAdV, ECTA, MNV, MuAstV, EDIM, TMEV, MHV, REO). Other transmission routes include direct contact (e.g., parvovirus, MTV, MCMV, LCMV), respiratory (e.g., MPyV, pneumonia virus of mice [PVM], SV, MHV), and vertical transmission (e.g., LCMV, LDEV; Fox et al., 2006 ; Percy and Barthold, 2007 ).
Contaminated biological materials are a common source for inadvertent viral infection during experimentation. For example murine tumors, hybridomas, ascites fluid, embryonic stem cells, in vitro fertilized embryos, gametes, cell lines, and even viral stocks introduced into mice can cause infections unless the materials are screened for infectious agents first (Nicklas et al., 2010 ). This is particularly important for banked biologicals that may have been frozen before an infection was detected in the mouse from which the materials were derived, or before sufficiently sensitive tests were available for detection of the specific infectious agent.
Bacterial, Fungal and Parasitic Infections of Mice
Table Table2 2 demonstrates species of bacteria, fungi and parasites for which research mice are commonly tested. Regarding bacterial infections, the actual species of bacteria isolated from infected mice vary to a great extent depending on the genetics and phenotype, age, and immune status of the mice, as well as environmental factors such as heating, ventilation, and air conditioning (HVAC), human traffic flow, husbandry practices (e.g., bedding type, caging system), preparation of animal supplies (sterile versus non‐sterile), and human contact with mice. For example, virtually all mouse strains are susceptible to infection with opportunistic bacteria such as Staphylococcus aureus, Klebsiella oxytoca , Pasteurella pneumotropica , Proteus mirabilis and Helicobacter spp . and these species are commonly found in many mouse facilities (Prichett‐Corning et al., 2009 ; Treuting et al., 2012 ). Yet, taking certain precautions in mouse‐housing facilities can substantially reduce the prevalence of opportunistic bacterial infections. This topic will be discussed below under disease prevention. The preponderance of bacterial infections of mice is non‐lethal, subclinical infections. Asymptomatic mice may only be identified at the time of health monitoring when microbial cultures or PCR yield positive results. In a number of animals, however, infections with bacterial opportunists can present as clinical cases, such as bite‐wound and retrobulbar abscesses, conjunctivitis, otitis, rectal prolapse and other overt signs of infection (Treuting et al., 2012 ).
Fungal infections of mice are less common than bacterial infections. The two principle fungal infections of mice are caused by Encephalitozoon cuniculi and Pneumocystis murina . These are two unusual organisms previously considered to be protozoan parasites, but recently reclassified as fungi based on genomic sequencing data (Katinka et al., 2001 ; Chabe et al., 2011 ). Generally, Encephalitozoon infections are more of a concern in rabbits; however, mice can be susceptible to infection. Pneumocystis infections are of greatest concern in immunodeficient mouse strains as described below in the section Monitoring Immunodeficient and GEM Strains.
Both endo‐ and ecto‐parasite infections of mice are still prevalent in research mouse facilities, but their rate of prevalence is far below the more common bacterial and viral infectious agents discussed above (Pritchett‐Corning, et al., 2009 ). The existence of parasitic infections in research mouse facilities may be the result of unidentified or untreated enzootic infections of existing mouse colonies; most mice infested with endo‐ and ecto‐parasites are asymptomatic and may harbor a low parasite burden that is difficult to detect via standard HM methods. Other factors that may contribute to the continued presence of parasites in some research colonies may include unregulated movement of mice between institutional animal facilities and a lack of proper quarantine procedures that allow for ample time for testing or treatment of imported mice. Additionally, pinworm infections may be particularly problematic due to treatment failure, persistence of eggs in the environment and diagnostic inefficiencies. Although parasitic infections do not typically cause clinical signs in either immunocompetent or immunodeficient mice, there is evidence that these infections do cause physiologic changes that may significantly impact research studies (Beattie et al., 1980 ; Bugarski et al., 2006 ; Michels et al., 2006 ).
Principal Diagnostic Methods for Infectious Agents of Mice
The standard practice at most research institutions that house mice is to submit HM samples, or mice, to commercial diagnostic laboratories (see Internet Resources for commercial diagnostic laboratories). Some research institutions have in‐house laboratory animal diagnostic laboratories, however, these are generally small laboratories that lack the scientific capabilities of the commercial labs. Nonetheless, in‐house laboratories can provide quick answers to pressing questions about infections in mice by providing microbiologic culture, or other diagnostic procedures that do not require an intensive investment in scientific instrumentation as is done at commercial laboratories. The information below provides insight into the choice of diagnostic methods used in commercial diagnostic laboratories and the rationale for their use in determining whether or not an infection is present in a mouse colony.
Serology is the primary laboratory method used for monitoring viral infections in mouse colonies. Several non‐viral infectious agents of mice can also be detected by serology because these infectious agents also reliably provoke an antibody response in immunocompetent mice (e.g., Mycoplasma pulmonis , Cilia‐associated respiratory [CAR] bacillus, Clostridium piliforme and E. cuniculi ; Table Table2). 2 ). The benefits of serologic testing are that it is relatively inexpensive, yet provides information about current and previous infections that have occurred in a mouse colony because serum antibodies generally persist in mice for months after resolution of an infection. Additionally, current serologic test platforms such as the enzyme‐linked immunosorbent assay (ELISA) and multiplexed fluorometric immunoassay (MFI, Luminex) enable the detection of multiple infectious agents from a single serum sample. Both assay platforms can also be used as high throughput systems and are the most cost‐effective diagnostic systems for large‐scale surveillance. Another serology test, the indirect immuno‐fluorescence antibody (IFA) assay is often used to confirm equivocal results of ELISA or MFI because of its sensitivity and specificity. However, performing IFAs is labor intensive and highly dependent on the technical expertise of the observer, so it is not used as frequently as ELISA or MFI. Commercial diagnostic laboratories will provide the user information on how to prepare blood samples for serology testing as well as providing shipping forms and packaging. Furthermore, commercial diagnostic laboratories will provide information on choosing the best serologic test for a given circumstance.
Molecular diagnostics
PCR is the most common molecular assay for detection of DNA agents (DNA viruses, bacteria, parasites) and reverse transcription (RT)‐PCR for RNA viruses used in laboratory animal diagnostic laboratories. These assays detect a specific region of genomic nucleic acid (DNA or RNA) of infectious agents and can be used at any time during active infection. Confirmatory tests for positive PCR results include direct sequencing of PCR‐amplified DNA fragments, an alternative PCR assay with the same DNA/RNA, or the same PCR assay with different specimens. PCR has been increasingly used as a part of laboratory animal HM programs because of its significant advantages over serology or microbiologic culture (Compton and Riley, 2001 ). PCR is also valuable as a noninvasive antemortem test that has proved both practical and useful for detection of certain infectious agents shed in fecal samples (Tables (Tables1 1 and and2 2 ).
The major limitation of PCR as a diagnostic tool is that for most agents, PCR can only detect active infections. Therefore, PCR is best used as part of routine HM to detect the presence of unknown infections in samples regularly submitted to a diagnostic laboratory. PCR is, however, especially effective in detecting infections in immunodeficient, GEM, and aged mice since infections often persist in these mice and the probability of detecting a pathogen is increased. Since PCR is used to detect the infectious agent itself, tissue selection for PCR testing requires that the individual making this selection has an understanding of the pathogenesis of the specific infectious agent so that tissue tropism and duration of infection are taken into account when deciding on the most appropriate sample to collect (Tables (Tables1 1 and and2). 2 ). Another limitation of PCR is its dependence on proper sample handling during collection and processing to avoid cross‐contamination or sample degradation, which may result in false‐positive or false‐negative results.
Microbiologic culture
Microbiologic culture is utilized for detection of bacteria and fungi in samples from mice. Samples are cultured in nutrient broth and then subcultured on nutrient agar plates or selective media in agar when there is increased growth (seen as turbidity) in broth cultures. The presence of bacteria or fungi is assessed by the development of colonies on agar plates. Bacteria of interest can be further identified to species level using instruments such as the VITEK identification card system (BioMérieux), biochemical tests, or simply by colony characteristics (e.g., morphology, color, smell). Sample source and properties of bacteria being monitored determine types of medium (selective or differential) and incubation conditions (aerobic or anaerobic; Fox et al., 2006 ; Percy and Barthold, 2007 ). Microbiologic culture and identification of bacteria and fungi is labor, equipment and expertise intensive.
Parasitology
Direct microscopic examination of fresh samples is frequently used as the primary detection method for identification of endo‐ and ecto‐parasites. Endoparasites such as Trichomonas can be identified in cecum and colon tissues minced in saline. The same is true for other protozoan parasites. Identification of pinworms and pinworm eggs can also be done microscopically. To enhance this process the eggs can be concentrated by pre‐treatment of gut content samples with zinc or sodium sulfate whose specific gravities enable the eggs to “float” on top of the medium after centrifugation. The eggs are then adhered to a glass cover slide, attached to a microscope slide and observed under a microscope. Ectoparasites such as fur mites are also identified by direct microscopic evaluation of mice or of plucked fur samples from their head and neck. In all cases, expertise in microscopic identification of parasites is required to reliably confirm the presence of parasites. Currently, the trend in parasite diagnostics is leaning towards the use of PCR as the preferred method of detection.
Monitoring Immunodeficient and GEM Strains
Immunodeficient and GEM strains have greater susceptibility to infectious agents than their immunocompetent counterparts and this must be taken into consideration when testing them for infectious agents. For example, P. murina is a ubiquitous opportunistic pathogen that in immunocompetent mice produces a clinically silent infection controlled by a T‐cell mediated immune response. However in many immunodeficient mouse strains, Pneumocystis produces lethal pulmonary infections (Weisbroth, 2006 ). Since many GEM strains are “immunovague” (Treuting et al., 2012 ), that is, have an unknown or variable capacity to mount an effective acquired immune response, these strains should be considered potentially susceptible to lethal Pneumocystis infections. Currently, the best method of identifying Pneumocystis in mice is via PCR on a sample of lung tissue from potentially affected animals. This requires that the mice be humanely euthanized and a piece of lung submitted to a diagnostic laboratory. Ideally, for routine health surveillance of immunodeficient mouse colonies, mice taken directly from immunodeficient stocks or immunodeficient sentinel mice should be tested regularly for the presence of Pneumocystis in a mouse room.
Immunodeficient mice are also generally more susceptible to infections with opportunistic bacteria than are their immunocompetent counterparts. Abscesses, bite‐wound infections, otitis media, and unthrifty appearance due to internal infections can result from exposure of immunodeficient mouse strains to opportunistic bacteria that might otherwise be non‐pathogenic in immunocompetent mice. Helicobacter spp., K. oxytoca, Klebsiella pneumoniae, S. aureus , some species of coagulase negative Staphylococcus, Pasteurella pneumotropica and Corynebacterium bovis are among the more commonly identified opportunistic bacteria in infected immunodeficient mice. Sources of these bacteria (see Table Table2) 2 ) include the local environment, human caretakers and research personnel, unsterile materials (e.g., feed, caging, bedding) that have not been sterilized.
Infectious viral agents usually cause acute infection in immunocompetent mice, followed by complete recovery without clinical signs. In contrast, infections in immunodeficient mice may be asymptomatic and persistent. The mice become chronic carriers of virus and serve as a source of infection for other colonies, especially if the virus is shed in feces.
Prevention of Infections
Measures designed to prevent infections of research mice ensure the health and welfare of these animals that are valuable investments both experimentally and financially. Biosecurity measures, that is, the sum of risk management practices, employ barrier systems to minimize the risk of introduction (bio‐exclusion) and spread (bio‐containment) of infectious agents within or between laboratory animal units and can be implemented at all levels of a research animal facility from building design to personnel activity in a mouse room. Examples of biosecurity applied at the building level are high‐efficiency particulate air (HEPA) filtration of room air, room access controls for personnel so that only approved individuals can enter a mouse room, detailed procedures for movement of personnel and mice from room to room, use of construction materials that can withstand repeated chemical disinfection, and physical separation of areas where clean, sterilized caging materials are prepared and handled away from mouse waste and dirty cages. Generally speaking, research investigators, postdoctoral fellows and graduate students are not involved in the oversight or use of biosecurity measures at this level. These are overseen by the facility management staff. Nonetheless, especially for the novice mouse user, you can discuss the building level biosecurity measures at your institution with the facility management staff to gain an understanding of the current measures in place.
Mouse room level biosecurity is very critical and presents an opportunity for mouse users to participate in the protection of their animals from exposure to infectious agents. Mice are generally housed in either conventional cages with or without a filter top and placed on racks in an open room, or in individually ventilated cages (IVC), or rack and cage systems in which the cage (with or without filter) is securely attached to a specially designed rack that provides HEPA‐filtered input and exhaust air with no exposure to room air. IVC caging systems are considered very secure with regard to exposure of mice to adventitious infectious agents. Though IVC caging offers greater protection of mice to inadvertent exposure to infectious agents, for both cage types, IVC and conventional, the real risks are associated with handling of mice during cage changes, experimental use, and other handling that present opportunities for exposure of mice to infectious agents. Thus, applying strict biosecurity steps at these critical junctures pays off by reducing the risk of an outbreak.
What steps can help? Direct human contact with mice is inadvisable as it increases the risk of transmission of human opportunistic bacteria to the mice by exposure to human skin, breath, nasal or oral secretions. Forceps that have been treated with a disinfectant, or disinfected gloved hands should be used to pick up and handle mice. The specific combination of personal protective equipment (PPE) varies with the institution and is in most cases chosen by the animal facility and veterinary staff; however, in general, personnel that routinely work with mice should always wear gloves and a clean lab coat or scrubs. Mask, bonnet, and shoe covers or dedicated mouse room shoes should be worn in higher health status rooms. Immunodeficient and GEM mice housed in high level barrier rooms generally require more stringent PPE, such as sterilized PPE that may also include face shields or powered air‐purifying respirators (PAPRs) to further prevent inadvertent exposure of the mice to infectious agents. Additionally, sterilization of all of the materials to which mice in high barrier rooms are exposed (cages, bedding, feed and water) is performed to eliminate infectious agents that may be present in these materials. The specific PPE you use in preparation for handling mice should be discussed with your animal facility staff.
Following institutional biosecurity practices is prudent, as each of the PPE items mentioned serves to reduce exposure of mice to human microflora and mouse pathogens, as humans may also serve as unintentional fomites for agents that were acquired in the environment outside of the mouse facility.
Further biosecurity steps should be taken during experimental manipulation of mice and movement of animals back and forth to a laboratory, or during movement between mouse rooms. Research procedures ideally should be done in a mouse‐room‐associated procedure area to avoid transporting the mice out of the housing room. The procedure room and equipment should be routinely disinfected by the users to prevent the transmission of infectious agents between mice from different projects or, in the case of shared procedure rooms, between mice from different mouse rooms. In those cases in which mice are taken from a mouse room to a research laboratory for experimental manipulation, the same precautions for handling the mice in a mouse room apply to handling the mice in a laboratory. Additionally, if possible, the mice should be returned to a mouse room dedicated for the purpose of housing mice that have been in a laboratory or otherwise out of the mouse room of origin. Check with the animal facility staff at your institution to determine if such facilities are available.
It is also important to be aware of institutional procedures pertaining to the movement of mice between different housing rooms. While it would be ideal for all research mouse colonies to be free of all infectious agents and to maintain them using stringent biosecurity measures, this is often not feasible in many institutions due to the high cost of maintaining such operations on a daily basis. Therefore, physical separation of mice based on health status and regulation of mouse movement and human traffic is another biosecurity measure that is employed at many institutions. For this reason, movement of mice between mouse rooms is inadvisable unless it is a controlled move sanctioned by the veterinary staff. Additionally, each mouse room may have its own environmental microflora as well as that present in the mice. For this reason, moving mice around to different rooms has the potential of altering that microflora, which is a change that could impact research results.
It is not in the purview of this article to provide an exhaustive list or discussion of biosecurity procedures. However, it is important to note that testing all biologicals used in mice (e.g., cells, antibodies, proteins, other biological molecules) for the presence of pathogens is critical for the prevention of inadvertent transmission of infectious agents. Furthermore, quarantine of newly imported mice and testing them for infections prior to release into the general population will prevent the transmission of infections potentially carried by these mice. We strongly advise scientific personnel to contact their animal care staff for information on the biosecurity procedures at their institution.
Communication between Researchers and Animal Care Staff
Although modern detection methods and HM programs have facilitated the eradication of many infectious agents that were common in research colonies decades ago, contemporary research colonies require collaborative efforts in order to prevent unexpected outbreaks.
Generally speaking, animal care personnel consists of veterinarians, facility managers, and animal caretakers, who all share the common goal of ensuring research integrity through the proper care of research mice. To achieve this goal, the animal care staff must be familiar with each investigators’ research animal models and objectives. Communication between research personnel and members of the animal care staff plays a critical role in maintaining appropriate HM programs, biosecurity practices, and quarantine procedures that serve to prevent infectious outbreaks. Effective communication between research and animal care personnel ensures that the HM program and biosecurity measures employed are suitable for the types of research mice within a given mouse room and that health testing is properly performed, test results are correctly interpreted, and unforeseen events within the animal facility are promptly dealt with. When unexpected events occur, such as discovery of ill or dead animals or issues with the mouse housing room (e.g., variations in temperature, light, humidity), it is recommended that research personnel discuss the issues with the facility staff immediately to get advice and assistance in dealing with the situation.
The animal care staff can also assist new members of the scientific community in becoming familiar with the use of mice in their research. For example, research institutions have required training programs for new animal users. These programs present an opportunity for scientists to find out pertinent information about the overall facility, the animal health programs and the legal and institutional requirements for using mice. We urge you to communicate regularly with the animal care staff at your institution to maintain an open dialog about your research needs and to get updated information about the health status of the facility.
Fahey, J.R. and Olekszak, H. 2015. An overview of typical infections of research mice: Health monitoring and prevention of infection . Curr. Protoc. Mouse Biol. 5 :235‐245. doi: 10.1002/9780470942390.mo150023 [ PMC free article ] [ PubMed ] [ Google Scholar ]
Literature Cited
- Beattie, G. , Baird, S. , Lannom, R. , Slimmer, S. , Jensen, F.C. , and Kaplan, N.O. 1980. Induction of lymphoma in athymic mice: A model for study of the human disease . Proc. Natl. Acad. Sci. U.S.A. 77 :4971‐4974. doi: 10.1073/pnas.77.8.4971 [ PMC free article ] [ PubMed ] [ CrossRef ] [ Google Scholar ]
- Besselsen, D.G. , Franklin, C.L. , Livingston, R.S. , and Riley, L.K. 2008. Lurking in the shadows: Emerging rodent infectious diseases . ILAR J. 49 :277‐290. doi: 10.1093/ilar.49.3.277 [ PMC free article ] [ PubMed ] [ CrossRef ] [ Google Scholar ]
- Bugarski, D. , Jovčić, G. , Katić‐Radivojević, S. , Petakov, M. , Krstić, A. , Stojanović, N. , and Milenković, P. 2006. Hematopoietic changes and altered reactivity to IL‐17 in Syphacia obvelata ‐infected mice . Parasitol. Int. 55 :91‐97. doi: 10.1016/j.parint.2005.10.005 [ PubMed ] [ CrossRef ] [ Google Scholar ]
- Carty, A.J. 2008. Opportunistic infections in mice and rats: Jacoby and Lindsey revisited . ILAR J . 49 :272‐276. doi: 10.1093/ilar.49.3.272 [ PubMed ] [ CrossRef ] [ Google Scholar ]
- Chabe, M. , Aliouat‐Denis, C.‐M. , Delhaes, L. , Aliouat, E.M. , Viscogliosi, E. , and Dei‐Cas, E. 2011. Pneumocystis: From a doubtful unique entity to a group of highly diversified fungal species . FEMS Yeast Res . 11 :2‐17. doi: 10.1111/j.1567-1364.2010.00698.x [ PubMed ] [ CrossRef ] [ Google Scholar ]
- Compton, S.R. and Riley, L.K. 2001. Detection of infectious agents in laboratory rodents: Traditional and molecular techniques . Comp. Med. 51 :113‐119. [ PubMed ] [ Google Scholar ]
- Fahey, J.R. , Katoh, H. , Malcolm, R. , and Perez, A.V. 2013. The case for genetic monitoring of mice and rats used in biomedical research . Mamm. Genome 24 :89‐94. doi: 10.1007/s00335-012-9444-9 [ PMC free article ] [ PubMed ] [ CrossRef ] [ Google Scholar ]
- Fox, J.G. , Barthold, S.W. , Newcomer, C.E. , Smith, A.L. , and Quimby, F.W. 2006. The Mouse in Biomedical Research: Diseases, 2nd edition, Vol. 2 . Academic Press, New York. [ Google Scholar ]
- Hayashimoto, N. , Morita, H. , Ishida, T. , Yasuda, M. , Kameda, S. , Uchida, R. , Tanaka, M. , Ozawa, M. , Sato, A. , Takakura, A. , Itoh, T. , and Kagiyama, N. 2013. Current microbiological status of mice and rats in experimental facilities in Japan . Exp. Anim. 62 :41‐48. doi: 10.1538/expanim.62.41 [ PubMed ] [ CrossRef ] [ Google Scholar ]
- Karst, S.M. , Wobus, C.E. , Lay, M. , Davidson, J. , and Virgin, H.W. 2003. Stat1‐dependent innate immunity to a Norwalk‐like virus . Science 299 :1575‐1578. doi: 10.1126/science.1077905 [ PubMed ] [ CrossRef ] [ Google Scholar ]
- Katinka, M.D. , Duprat, S. , Cornillot, E. , Metenier, G. , Thomarat, F. , Prensier, G. , Barbe, V. , Peyretaillade, E. , Brottier, P. , Wincker, P. , Delbac, F. , El Alaoui, H. , Peyret, P. , Saurin, W. , Gouy, M. , Weissenbach, J. , and Vivarès, CP. 2001. Genome sequence and gene compaction of the eukaryotic parasite Encephalitozoan cuniculi . Nature 414 :450‐453. doi: 10.1038/35106579 [ PubMed ] [ CrossRef ] [ Google Scholar ]
- Kjeldsberg, E. and Hem, A. 1985. Detection of astroviruses in gut contents of nude and normal mice . Arch. Virol. 84 :35‐140. [ PMC free article ] [ PubMed ] [ Google Scholar ]
- Liang, C.T. , Shih, A. , Chang, Y.H. , Liu, C.W. , Lee, Y.T. , Hsieh, W.C. , Huang, Y.L. , Huang, W.T. , Kuang, C.H. , Lee, K.H. , Zhuo, Y.X. , Ho, S.Y. , Liao, S.L. , Chiu, Y.Y. , Hsu, C.N. , Liang, S.C. , and Yu, C.K. 2009. Microbial contaminations of laboratory mice and rats in Taiwan from 2004 to 2007 . J. Am. Assoc. Lab. Anim. Sci. 48 :381‐386. [ PMC free article ] [ PubMed ] [ Google Scholar ]
- Mähler, M. and Köhl, W. 2009. A serologic survey to evaluate contemporary prevalence of viral agents and Mycoplasma pulmonis in laboratory mice and rats in western Europe . Lab. Anim . 38 :161‐165. doi: 10.1038/laban0509-161 [ PMC free article ] [ PubMed ] [ CrossRef ] [ Google Scholar ]
- Mähler, M. , Berard, M. , Feinstein, R. , Gallagher, A. , Illgen‐Wilcke, B. , Pritchett‐Corning, K. , and Raspaet, M. 2014. FELASA recommendations for the HM of mouse, rat, hamster, guinea pig and rabbit, colonies in breeding and experimental units . Lab. Anim. 48 :178‐192. doi: 10.1177/0023677213516312 [ PubMed ] [ CrossRef ] [ Google Scholar ]
- McInnes, E.F. , Rasmussen, L. , Fung, P. , Auld, A.M. , Alvarez, L. , Lawrence, D.A. , Quinn, M.E. , del Fierro, G.M. , Vassallo, B.A. , and Stevenson, R. 2011. Prevalence of viral, bacterial and parasitological diseases in rats and mice used in research environments in Australasia over a 5‐y period . Lab. Anim. 40 :341‐350. doi: 10.1038/laban1111-341 [ PMC free article ] [ PubMed ] [ CrossRef ] [ Google Scholar ]
- Michels, C. , Goyal, P. , Neiuwenhuizen, N. , and Brombacher, B. 2006. Infection with Syphacia obvelata (pinworm) induces protective Th2 immune responses and influences ovalbumin‐induced allergic reactions . Infect. Immun. 74 :5926‐5932. doi: 10.1128/IAI.00207-06 [ PMC free article ] [ PubMed ] [ CrossRef ] [ Google Scholar ]
- National Centre for the Replacement, Refinement & Reduction of Animals in Research (NC3Rs) . 2014. Responsibility in the use of animals in bioscience research: Expectations of the major research council and charitable funding bodies . https://www.nc3rs.org.uk/responsibility-useanimals-bioscience-research
- Ng, T.F. , Kondov, N.O. , Hayashimoto, N. , Uchida, R. , Cha, Y. , Beyer, A.I. , Wong, W. , Pesavento, P.A. , Suemizu, H. , Muench, M.O. , and Delwart, E. 2013. Identification of an astrovirus commonly infecting laboratory mice in the US and Japan . PLoS One 8 :12161‐12175. [ PMC free article ] [ PubMed ] [ Google Scholar ]
- Nicklas, W. 2008. International harmonization of HM . ILAR J . 49 :338‐346. doi: 10.1093/ilar.49.3.338 [ PubMed ] [ CrossRef ] [ Google Scholar ]
- Nicklas, W. , Deeny, A. , Diercks, P. , Gobbi, A. , Illgen‐Wilcke, B. , and Seidelin, M. 2010. FELASA guidelines for the accreditation of HM programs and testing laboratories involved in HM . Lab. Anim. 39 :43‐48. doi: 10.1038/laban0210-43 [ PubMed ] [ CrossRef ] [ Google Scholar ]
- Percy, D.H. and Barthold, S.W. 2007. Pathology of Laboratory Rodents and Rabbits, 3rd edition . Blackwell Publishing, Ames, Iowa. [ Google Scholar ]
- Prichett‐Corning K.R., Cosetino J., and Clifford, C.B. 2009. Contemporary prevalence of infectious agents in laboratory mice and rats . Lab. Anim. 43 :165‐173. doi: 10.1258/la.2008.008009 [ PubMed ] [ CrossRef ] [ Google Scholar ]
- Treuting, P.M. , Clifford, C.B. , Sellers, R.S. , and Brayton, C.F. 2012. Of mice and microflora: Considerations for genetically engineered mice . Vet. Pathol. 49 :44‐63. doi: 10.1177/0300985811431446 [ PubMed ] [ CrossRef ] [ Google Scholar ]
- Weisbroth, S.H. 1999. Evolution of disease patterns in laboratory rodents: The post indigenous condition In 50 Years of Laboratory Animal Science (McPherson C.W. and Mattingly S., eds.) pp. 141‐146. American Association of Laboratory Animal Science, Memphis, Tenn. [ Google Scholar ]
- Weisbroth, S.H. 2006. Pneumocystis : New knowledge about the biology of this group of organisms in laboratory rats and mice . Lab. Anim . 35 :55‐61. doi: 10.1038/laban1006-55 [ PubMed ] [ CrossRef ] [ Google Scholar ]
Internet Resources
Guidelines for HM Programs/Laboratory Animal Health.
Commercial Laboratory Animal Diagnostic Laboratories.
Mouse Vendor Health Reports.
- http://www.felasa.eu/recommendations/recommendation/recommendations-for-health-monitoring-of-rodent-and-rabbit-colonies/
- http://dels.nas.edu/ilar
- http://www.aclam.org/
- https//www.aalas.org/
- http://www.criver.com/products-services/basic-research/health-monitoring-diagnostic-services
- http://www.idexxbioresearch.com/radil/Health_Monitoring/Health_Monitoring_Overview/
- http://jaxmice.jax.org/genetichealth/index.html
- http://www.taconic.com/breed-your-model/health-testing
- http://www.criver.com/products-services/basic-research/health-reports
- http://www.harlan.com/about_harlan_laboratories/quality_programs
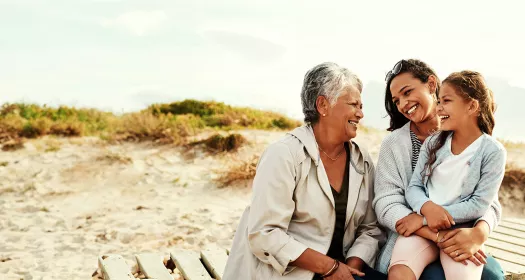
Research Scientist, Comparative Medicine- Research Collaborations
About the role.
Your Key Responsibilities:
- Perform state-of-the-art animal experimentation with the highest ethical and technical standards, setting up and animal models independently or through the team.
- Able to perform advanced, intermediate, and basic technical skills (compound administration by PO, IV, SC, IP, etc. routes, blood sample collection via different routes (ex: tail snip/vein, saphenous, abdominal aorta, vena cava, etc.). Tissue collection, clinical observation, necropsy etc.) on applicable in-house species (Mouse, Rat, Rabbit)
- Participate in scientific discussions, performing critical review of experimental protocols and identifying the best approach for in-vivo studies according to project needs.
- Continuously evaluate, and train on newest techniques and build scientific knowledge to ensure state-of-the-art in-vivo work.
- Closely interact with scientific customer base, providing optimal support, ability to build up a robust professional network and generate high quality data.
- Interest in data interpretation, statistics, and presentation, to propose next steps to customer.
- Be an excellent team player and show potential to operate within the Team to ensure appropriate performance, training, and professional development within the Team.
- Rota, weekend duties, early morning, or late evening dosing
- Routinely responsible for ensuring own compliance with all institutional and regulatory protocols, policies, and guidelines.
- Responsible for pursuing continuous learning/professional development opportunities, enhancing/expanding skill set, and reviewing CM techniques.
Role requirements:
- BS or Master’s in Life Sciences or equivalent experience
- Experience in a technical skills tract laboratory animal program or 3+ years preferred.
- Strong commitment to animal welfare, research support, and high quality in-vivo focused science
- Theoretical and practical expertise in animal experimentation with focus on small animals.
- Hands on expertise with multiple animal disease and mechanistic models
- High ethical approach, committed to high standards of animal welfare.
- Strong customer-orientation, used to communicate with a broad range of stakeholders, strong aptitudes as a team player.
- Fluency in English.
- Must be able to lift 50 lbs., be able to work from a standing position for prolonged periods of time and perform repetitive motion tasks.
Schedule: full time, shifted schedule
Includes either Saturday or Sunday
Some weekend on call, BR holiday coverage required.
Commitment to Diversity & Inclusion: The Novartis Group of Companies are Equal Opportunity Employers and take pride in maintaining a diverse environment. We do not discriminate in recruitment, hiring, training, promotion or other employment practices for reasons of race, color, religion, gender, national origin, age, sexual orientation, gender identity or expression, marital or veteran status, disability, or any other legally protected status. We are committed to building diverse teams, representative of the patients and communities we serve, and we strive to create an inclusive workplace that cultivates bold innovation through collaboration and empowers our people to unleash their full potential.
Novartis Compensation and Benefit Summary: The pay range for this position at commencement of employment is expected to be between $39.13- $58.72/year ; however, w hile salary ranges are effective from 1/1/24 through 12/31/24, fluctuations in the job market may necessitate adjustments to pay ranges during this period. Further, final pay determinations will depend on various factors, including, but not limited to geographical location, experience level, knowledge, skills, and abilities. The total compensation package for this position may also include other elements, including a sign-on bonus, restricted stock units, and discretionary awards in addition to a full range of medical, financial, and/or other benefits (including 401(k) eligibility and various paid time off benefits, such as vacation, sick time, and parental leave), dependent on the position offered. Details of participation in these benefit plans will be provided if an employee receives an offer of employment. If hired, employee will be in an “at-will position” and the Company reserves the right to modify base salary (as well as any other discretionary payment or compensation program) at any time, including for reasons related to individual performance, Company or individual department/team performance, and market factors.
Why Novartis: Helping people with disease and their families takes more than innovative science. It takes a community of smart, passionate people like you. Collaborating, supporting and inspiring each other. Combining to achieve breakthroughs that change patients’ lives. Ready to create a brighter future together? https://www.novartis.com/about/strategy/people-and-culture
Join our Novartis Network: Not the right Novartis role for you? Sign up to our talent community to stay connected and learn about suitable career opportunities as soon as they come up: https://talentnetwork.novartis.com/network
Benefits and Rewards: Read our handbook to learn about all the ways we’ll help you thrive personally and professionally: https://www.novartis.com/careers/benefits-rewards
EEO Statement:
The Novartis Group of Companies are Equal Opportunity Employers who are focused on building and advancing a culture of inclusion that values and celebrates individual differences, uniqueness, backgrounds and perspectives. We do not discriminate in recruitment, hiring, training, promotion or other employment practices for reasons of race, color, religion, sex, national origin, age, sexual orientation, gender identity or expression, marital or veteran status, disability, or any other legally protected status. We are committed to fostering a diverse and inclusive workplace that reflects the world around us and connects us to the patients, customers and communities we serve.
Accessibility & Reasonable Accommodations
The Novartis Group of Companies are committed to working with and providing reasonable accommodation to individuals with disabilities. If, because of a medical condition or disability, you need a reasonable accommodation for any part of the application process, or to perform the essential functions of a position, please send an e-mail to [email protected] or call +1(877)395-2339 and let us know the nature of your request and your contact information. Please include the job requisition number in your message.
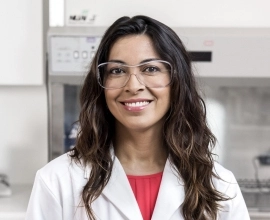

IMAGES
COMMENTS
Abstract. Mice and rats have long served as the preferred species for biomedical research animal models due to their anatomical, physiological, and genetic similarity to humans. Advantages of rodents include their small size, ease of maintenance, short life cycle, and abundant genetic resources. The Rat Resource and Research Center (RRRC) and ...
The Mouse: From Pest, to Pet, to Predominant Tool in Medical Research. Although the genetic lineages of mice and humans diverged around 75 million years ago, these two species have evolved to live together, particularly since the development of agriculture.
The impact of mouse-based research on biological discovery and medical progress over the past century has been profound. Read the background of most Nobel Prizes awarded in Physiology or Medicine and you'll find mice used for the research — in fact, 26 Nobel Prizes can be directly tied to JAX® Mice.
For practical and scientific reasons, mice have become the model organism of choice for a huge swath of biomedical research. Mice thrive and breed well in a laboratory environment, can be housed economically, and powerful experimental approaches are now widely available to alter gene expression and to specifically evaluate molecular, cellular ...
Mice are small and relatively economical to maintain, making them the ideal laboratory animal model. Thousands of laboratory mouse strains are now available, so scientists can therefore choose the ideal mouse model to study different diseases and disease processes. And the mouse genome is easily manipulated in order to create even more precise ...
Abstract. The laboratory mouse Mus musculus has long been used as a model organism to test hypotheses and treatments related to understanding the mechanisms of disease in humans; however, for these experiments to be relevant, it is important to know the complex ways in which mice are similar to humans and, crucially, the ways in which they differ.
Mice are essential in medical research because most diseases cannot be modeled in a test tube and require experimentation in a whole organism. The Jackson Laboratory pioneered the use of mice in disease research, and our mice and research programs have contributed to important medical breakthroughs ever since. Organ transplants, glaucoma ...
Humanized mice, or mouse-human chimaeras, have been developed to overcome these constraints and are now an important research tool for the in vivo study of human cells and tissues. Humanized ...
Mice are the most commonly used animals in medical research. This trend looks likely to continue now that both mouse and human genomes have been mapped (80% of human genes are exactly the same as those found in mice, and at least a further 10% are very similar) allowing human genetic disorders and diseases to be studied with greater accuracy.Often, the only way of determining the function of a ...
There are several reasons why the use of animals is critical for biomedical research: • Animals are biologically very similar to humans. In fact, mice share more than 98% DNA with us! • Animals are susceptible to many of the same health problems as humans - cancer, diabetes, heart disease, etc. • With a shorter life cycle than humans ...
Historically, researchers have favored male mice over female mice in experiments, in part due to concern that the hormone cycle in females causes behavioral variation that could throw off results. But new research from Harvard Medical School challenges this notion and suggests that for many experiments, the concern may not be justified. The ...
In fact, 95 percent of all lab animals are mice and rats, according to the Foundation for Biomedical Research (FBR). Scientists and researchers rely on mice and rats for several reasons. One is ...
INTRODUCTION. Both investigative and veterinary staffs monitor the health and well-being of mice that are used in research. Indeed, this level of responsibility and care is mandated by the Public Health Service based on the Guide for the Care and Use of Laboratory Animals (National Research Council. 2011).The Guide is "intended to assist investigators in fulfilling their obligation to plan ...
Mice whose endothelial cells lacked SIRT1 had poor exercise tolerance, managing to run only half the distance covered by their SIRT1-intact peers. ... The work was supported by the Glenn Foundation for Medical Research (grants RO1 AG028730 and RO1 DK100263), and by the National Institutes of Health/National Heart, Lung and Blood Institute ...
A new cell therapy targeting senescent cells may improve metabolic and physical function in mice, according to an NIA-funded study. Senescent cells, which are cells that have stopped dividing but do not die off when they should, have been linked to many aspects of aging and disease.In the study, published in Nature Aging, senescent cells were safely and effectively removed from the tissues of ...
It's important to note that the past seven decades of successful organ transplantations depended on research using mice. Jackson Laboratory scientist George Snell won the 1980 Nobel prize for his work in the 1940s establishing how the body's immune system recognizes the difference between its own tissues and foreign invaders.
Garner and colleagues tried to run identical experiments in six different mouse facilities, scattered throughout research centers in Europe. Even using genetically identical mice of the same age ...
In Boston labs, old, blind mice have regained their eyesight, developed smarter, younger brains and built healthier muscle and kidney tissue. On the flip side, young mice have prematurely aged ...
The drug is called eFT508 (or tomivosertib). They found that giving eFT508 alone did not slow the growth of pancreatic tumors in mice, likely because the tumors could survive with energy from carbohydrates. But when mice were given the drug while on a ketogenic diet, the cancer cells no longer had ready access to glucose or fat for energy.
Transgenic mice provide a basis for research of disease pathogenesis after infection with human-specific viruses. Today, humanized mice can be found at the very heart of this forefront of medical research allowing for recapitulation of disease pathogenesis and drug mechanisms in humans. This review discusses progress in the development and use ...
In a study of mice, MIT researchers have now identified the pathway that enables this enhanced regeneration, which is activated once the mice begin "refeeding" after the fast. They also found a downside to this regeneration: When cancerous mutations occurred during the regenerative period, the mice were more likely to develop early-stage ...
By identifying Hmmr as a critical mediator of Terc-53's effects on aging, the research suggests that strategies aimed at stabilizing Hmmr could mitigate age-related cognitive decline and inflammation.
Similarly, it is prudent to verify the health status of research mouse colonies to exclude mice compromised by infections. Even in the absence of clinical signs of disease, infections in mice are well known as a source of variability that can have adverse effects on research or may present a health hazard to laboratory staff (i.e., zoonotic ...
Research Collaborations (RC) is a centralized core unit within In vivo Science & Technology (IST), Comparative Medicine (CM). RC is responsible for performing best-in-class in-vivo studies together with developing key animal models and cutting-edge technologies needed for Biomedical Research (BR) drug discovery and development process.
US News is a recognized leader in college, grad school, hospital, mutual fund, and car rankings. Track elected officials, research health conditions, and find news you can use in politics ...